毕业论文外文翻译-负载银的掺氮石墨烯概论
氮掺杂石墨烯复合材料的制备及其电化学性能研究

氮掺杂石墨烯复合材料的制备及其电化学性能研究氮掺杂石墨烯(N-doped graphene)复合材料是一种具有重要应用潜力的新型材料,其制备方法和电化学性能研究一直是材料科学领域的热点研究方向之一。
本文将详细介绍氮掺杂石墨烯复合材料的制备方法及其电化学性能研究的进展。
首先,关于氮掺杂石墨烯的制备方法,目前常用的方法有热还原法、化学气相沉积法、溶胶-凝胶法和电化学法等。
其中,热还原法是较为常用的一种方法。
该方法通常是将石墨烯氧化物(GO)与含氮化合物(如尿素、三氯氰胺等)一起进行高温还原,通过热解的过程实现石墨烯的氮掺杂。
此外,化学气相沉积法是一种在高温下通过金属催化剂的作用将含氮化合物分解并封装在石墨烯层中的方法。
溶胶-凝胶法则是通过将石墨烯氧化物与含氮化合物溶解在溶液中,然后进行混合、干燥和热处理等步骤来实现氮掺杂。
最后,电化学法是通过电化学还原法将石墨烯氧化物在某种电解质溶液中还原为氮掺杂石墨烯。
利用以上方法制备的氮掺杂石墨烯复合材料具有较好的电化学性能。
例如,N-doped graphene具有较高的导电性和电化学活性,能够在电化学储能和传感器等领域发挥重要作用。
此外,氮掺杂还能够改变石墨烯的能带结构,增加其化学吸附活性,并提高其电催化性能。
此外,氮掺杂石墨烯还具有优异的光电性质,可应用于光电器件等领域。
在氮掺杂石墨烯复合材料的电化学性能研究方面,关键是研究其在储能和催化领域的应用。
例如,在储能方面,研究人员利用N-doped graphene构建超级电容器并取得了不错的电化学性能。
N-doped graphene具有较高的比表面积和良好的离子传输性能,可以增加储能电极的容量和循环寿命。
在催化方面,N-doped graphene在电催化还原CO2、氧化甲醇和氢氧化物等反应中表现出良好的活性和选择性。
研究人员还通过合成N-doped graphene/负载金属纳米材料复合体系来提高催化性能。
石墨烯英文版
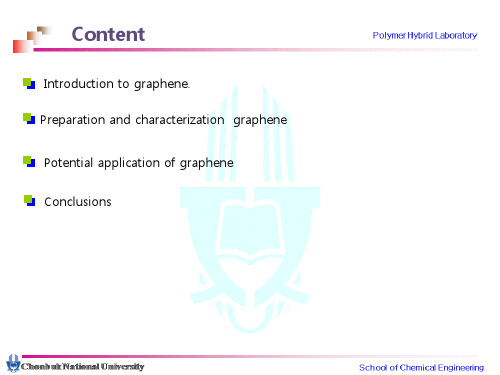
术语石墨烯首次出现在1987年,描述单石墨作为石墨层间化合物(GIC)的成分之一。 更大的石墨烯 分子或片(使得它们可以被认为是真正隔离的2D晶体)甚至在原理上也不能生长。 在20世纪30年代, Landau和Peierls(和Mermin,后来)显示热力学阻止2-d晶体在自由状态,物理今天的一篇文章
Introduction
Properties of graphene
Mechanical properties
- High Young’s modulus (~1,100 Gpa)高杨氏模量 High fracture strength (125 Gpa)高断裂强度 - Graphene is as the strongest material ever measured, some 200 times stronger than structural steel
• In 2004: Andre Geim and Kostya Novoselov at Manchester University managed to extract single-atom-thick crystallites (graphene) from bulk graphite: Pulled out graphene layers from graphite and transferred them onto thin silicon dioxide on a silicon wafer in a process sometimes called micromechanical cleavage or, simply, the Scotch tape technique. Since 2004, an explosion in the investigation of graphene in term of synthesis, characterization, properties as well as specifical potential application were reported. • 在2004年:曼彻斯特大学的Andre Geim和Kostya Novoselov设法从 块状石墨中提取单原子厚的微晶(石墨烯):从石墨中拉出石墨烯层 ,并将其转移到硅晶片上的薄二氧化硅上,有时称为微机械 切割, 或简单地,苏格兰带技术。 自2004年以来,报告了石墨烯在合成, 表征,性质以及特异性潜在应用方面的研究中的爆炸。
石墨烯外国文献翻译
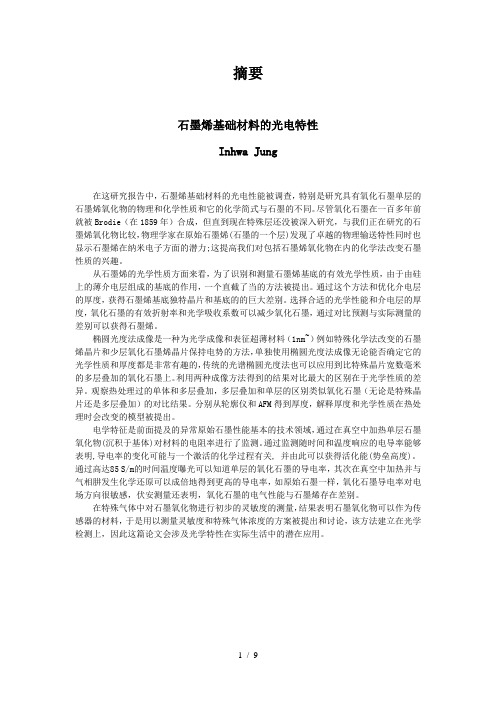
石墨烯基础材料的光电特性Inhwa Jung在这研究报告中,石墨烯基础材料的光电性能被调查,特别是研究具有氧化石墨单层的石墨烯氧化物的物理和化学性质和它的化学简式与石墨的不同。
尽管氧化石墨在一百多年前就被Brodie(在1859年)合成,但直到现在特殊层还没被深入研究,与我们正在研究的石墨烯氧化物比较,物理学家在原始石墨烯(石墨的一个层)发现了卓越的物理输送特性同时也显示石墨烯在纳米电子方面的潜力;这提高我们对包括石墨烯氧化物在内的化学法改变石墨性质的兴趣。
从石墨烯的光学性质方面来看,为了识别和测量石墨烯基底的有效光学性质,由于由硅上的薄介电层组成的基底的作用,一个直截了当的方法被提出。
通过这个方法和优化介电层的厚度,获得石墨烯基底独特晶片和基底的的巨大差别。
选择合适的光学性能和介电层的厚度,氧化石墨的有效折射率和光学吸收系数可以减少氧化石墨,通过对比预测与实际测量的差别可以获得石墨烯。
椭圆光度法成像是一种为光学成像和表征超薄材料(1nm~)例如特殊化学法改变的石墨烯晶片和少层氧化石墨烯晶片保持电势的方法,单独使用椭圆光度法成像无论能否确定它的光学性质和厚度都是非常有趣的,传统的光谱椭圆光度法也可以应用到比特殊晶片宽数毫米的多层叠加的氧化石墨上。
利用两种成像方法得到的结果对比最大的区别在于光学性质的差异。
观察热处理过的单体和多层叠加,多层叠加和单层的区别类似氧化石墨(无论是特殊晶片还是多层叠加)的对比结果。
分别从轮廓仪和AFM得到厚度,解释厚度和光学性质在热处理时会改变的模型被提出。
电学特征是前面提及的异常原始石墨性能基本的技术领域,通过在真空中加热单层石墨氧化物(沉积于基体)对材料的电阻率进行了监测。
通过监测随时间和温度响应的电导率能够表明,导电率的变化可能与一个激活的化学过程有关, 并由此可以获得活化能(势垒高度)。
通过高达85 S/m的时间温度曝光可以知道单层的氧化石墨的导电率,其次在真空中加热并与气相肼发生化学还原可以成倍地得到更高的导电率,如原始石墨一样,氧化石墨导电率对电场方向很敏感,伏安测量还表明,氧化石墨的电气性能与石墨烯存在差别。
“Graphene”研究及翻译

“Graphene”研究及翻译摘要:查阅近5年我国SCI、EI期源刊有关石墨烯研究873篇,石墨烯研究的有关翻译存在很大差异。
从石墨烯的发现史及简介,谈石墨烯内涵及研究的相关翻译。
指出“石墨烯”有关术语翻译、英文题目、摘要撰写应注意的问题。
关键词:石墨烯;石墨烯术语;翻译石墨烯是目前发现的唯一存在的二维自由态原子晶体,它是构筑零维富勒烯、一维碳纳米管、三维体相石墨等sp2杂化碳的基本结构单元,具有很多奇异的电子及机械性能。
因而吸引了化学、材料等其他领域科学家的高度关注。
近5年我国SCI、EI期源刊研究论文873篇,论文质量良莠不齐,发表的论文有35.97%尚未被引用过,占国际论文被引的4.84%左右。
石墨烯研究的有关翻译也存在很大差异。
为了更好的进行国际学术交流,规范化专业术语。
本文就“graphene”的内涵及翻译谈以下看法。
l “Graphene”的发现史及简介1962年,Boehm等人在电镜上观察到了数层甚至单层石墨(氧化物)的存在,1975年van Bom-mel等人报道少层石墨片的外延生长研究,1999年德克萨斯大学奥斯汀分校的R Ruoff等人对用透明胶带从块体石墨剥离薄层石墨片的尝试进行相关报道。
2004年曼彻斯特大学的Novoselov和Geim小组以石墨为原料,通过微机械力剥离法得到一系列叫作二维原子晶体的新材料——石墨烯,并于10月22日在Sclence期刊上发表有关少层乃至单层石墨片的独特电学性质的文章,2010年Gelm和No-voselov获得了诺贝尔物理学奖。
石墨烯有着巨大的比表面积(2630 m2/g)、极高的杨氏模量(1.06 TPa)和断裂应力(~130GPa)、超高电导率(~106 S/cm)和热导率(5000W/m·K)。
石墨烯中的载流子迁移率远高于传统的硅材料,室温下载流子的本征迁移率高达200000 cm2/V.s),而典型的硅场效应晶体管的电子迁移率仅约1000 cm2/V.s。
氮参杂石墨烯的制备及其在电化学生物传感中的应用
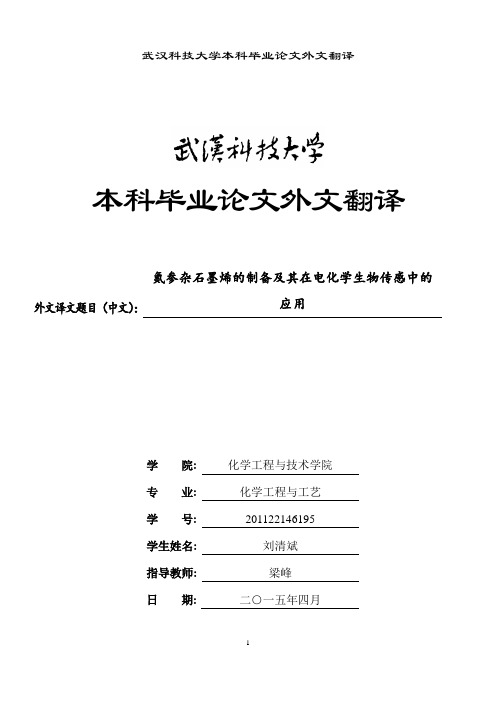
本科毕业论文外文翻译外文译文题目(中文):氮参杂石墨烯的制备及其在电化学生物传感中的应用学院: 化学工程与技术学院专业: 化学工程与工艺学号: 201122146195学生姓名: 刘清斌指导教师: 梁峰日期: 二○一五年四月Nitrogen-Doped Graphene and Its Application in ElectrochemicalBiosensingYing Wang, Yuyan Shao, Dean W. Matson, Jinghong Li,and Yuehe Lin American Chemical Society,2010,VOL. 4 ▪ NO. 4 ▪ 1790–1798氮参杂石墨烯的制备及其在电化学生物传感中的应用Ying Wang, Yuyan Shao, Dean W. Matson, Jinghong Li,and Yuehe Lin 美国化学学会,2010,第4卷,第4期,1790–1798。
生命有机磷化学及化学生物学重点实验室,清华大学,北京100084,中华人民共和国和太平洋西北国家实验室,Richland,华盛顿州99351摘要:化学掺杂外来原子是一种能本质上改变材料属性的有效方法。
其中,氮参杂在改善碳材料的电化学性能上有着关键的作用。
最近,石墨烯作为真正的二维碳材料,在生物电子学和生物传感器应用方面已经显示出引人入胜的前景。
在本文中,我们报告了一个浅显的策略,通过使用氮等离子体处理的石墨烯经化学方法合成制备N掺杂的石墨烯。
一种可能的示意图已被提出用来描述N掺杂的石墨烯的详细结构。
通过控制曝光时间,N 在石墨烯的比例可以调节,范围从0.11到1.35%。
最后所得到的N掺杂石墨烯对过氧化氢的还原、葡萄糖氧化酶快速直接电子转移动力学,显示出了高效的活性。
氮参杂石墨烯将进一步应用在伴有干扰存在且浓度低于0.01mM的葡萄糖的生物传感方面。
1.1关键词:石墨烯,氮参杂,直接电催化,电化学生物传感石墨烯作为一种真正的二维碳材料出现,已经展示出其在生物电催化和生物传感方面惊人的应用。
毕业论文外文翻译-负载银的掺氮石墨烯概论
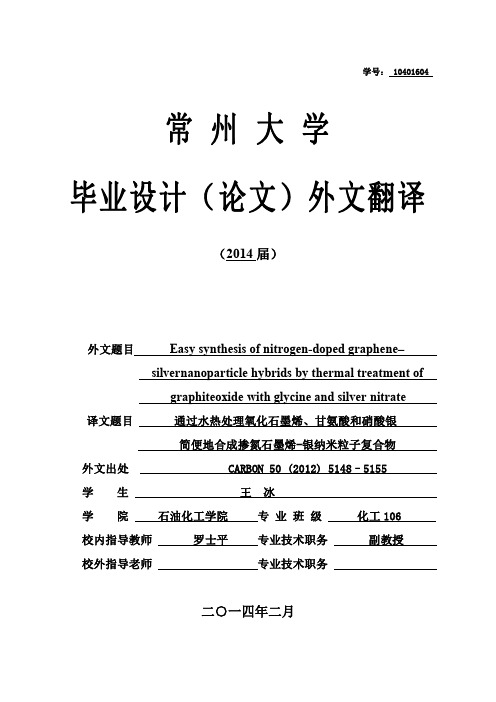
学号:10401604常州大学毕业设计(论文)外文翻译(2014届)外文题目Easy synthesis of nitrogen-doped graphene–silvernanoparticle hybrids by thermal treatment ofgraphiteoxide with glycine and silver nitrate 译文题目通过水热处理氧化石墨烯、甘氨酸和硝酸银简便地合成掺氮石墨烯-银纳米粒子复合物外文出处CARBON50(2012)5148–5155学生王冰学院石油化工学院专业班级化工106校内指导教师罗士平专业技术职务副教授校外指导老师专业技术职务二○一四年二月通过水热处理氧化石墨烯、甘氨酸和硝酸银简便地合成氮杂石墨烯-银纳米粒子杂合物Sundar Mayavan,Jun-Bo Sim,Sung-Min Choi摘要:氮杂石墨烯-银纳米粒子杂合物在500℃通过水热处理氧化石墨烯(GO)、甘氨酸和硝酸银制得。
甘氨酸用于还原硝酸根离子,甘氨酸和硝酸根混合物在大约200℃分解。
分解的产物可作为掺杂氮的来源。
水热处理GO、甘氨酸和硝酸银混合物在100℃可形成银纳米粒子,200℃时GO还原,300℃时产生吡咯型掺氮石墨烯,500℃时生成吡咯型掺氮石墨烯。
合成物质中氮原子所占百分比为13.5%.在合成各种纳米金属粒子修饰的氮杂石墨烯方面,该合成方法可能开辟了一个新的路径,其在能量储存和能量转换设备方面很有应用价值。
1.引言石墨烯是所有石墨材料的基本构件,其蜂窝状晶格由单层碳原子排列而成。
它表现出与结构有关的独特电子、机械和化学性质,具有较高的比表面积(2630-2965m2g-1)[1–3]。
化学掺杂杂原子石墨烯像掺杂氮原子,极大地引起了人们的兴趣,因其在传感器、燃料电池的催化剂和锂离子电池的电极等方面具有应用潜力[4–6]。
氮原子的掺杂改变了石墨烯的电子特性和结构特性,导致其电子移动性更强,产生更多的表面缺位。
氮掺杂石墨烯
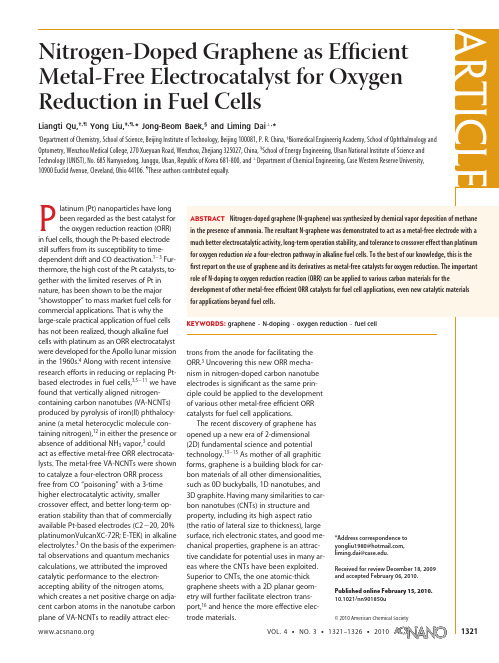
Although CNTs and their N-doped counterparts have been synthesized and studied for some years,the large-scale preparation of graphene sheets by chemical vapor deposition(CVD)is only the recent development.17,18More recently,attempts have been quartz purging min. furnaceFigure1.(a)A digital photo image of a transparent N-graphenefilmfloating on water after removal of the nickel layer by dissolving in an aqueous acid so-lution;(b,c)AFM images of the N-graphenefilm and the correpsonding height analyses along the lines marked in the AFM image(c1؊c3in panel c).Figure2.TEM and Raman analyses of the N-graphenefilms.(a)Low-magnification TEM image showing a few layers of the CVD-grown N-graphenefilm on a grid.Inset shows the correspondingelectron diffraction pattern.(b؊d)High magnification TEM imagesshowing edges of the N-graphenefilm regions consisting of(b)2,(c)4,and(d)ca.4؊8graphene layers.(e)The corresponding Ramanspectra of the N-graphenefilms of different graphene layers on aSiO2/Si substrate(Methods).XPS survey for the as-synthesized N-graphene shows the high-resolution N1s spectrum.the C-graphene suggests a stronger O2the former,an additional advantage asRRDE voltammograms for the ORR in air-saturated0.1M KOH at the C-graphene electrode(red line), line),and N-graphene electrode(blue line).Electrode rotating rate:1000rpm.Scan rate:0.01V/s.Mass Mass(N-grapene)؍7.5g.(b)Current density(j)؊time(t)chronoamperometric responses obtained at theN-graphene(square line)electrodes at؊0.4V in air saturated0.1M KOH.The arrow indicates the addition methanol into the air-saturated electrochemical cell.(c)Current(j)؊time(t)chronoamperometric response and N-graphene(square line)electrodes to CO.The arrow indicates the addition of10%(v/v)CO into KOH at؊0.4V;j o defines the initial current.(d)Cyclic voltammograms of N-graphene electrode inbefore(circle line)and after(square line)a continuous potentiodynamic swept for200000cycles at Scan rate:0.1V/s.。
学生石墨烯文献翻译
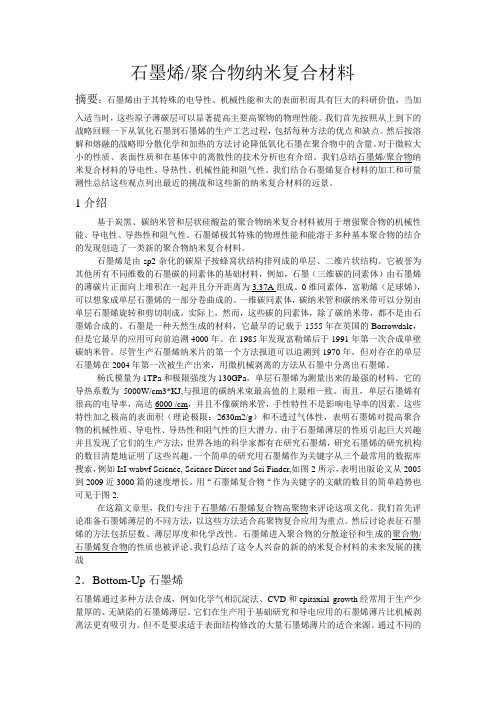
石墨烯/聚合物纳米复合材料摘要:石墨烯由于其特殊的电导性、机械性能和大的表面积而具有巨大的科研价值,当加入适当时,这些原子薄碳层可以显著提高主要高聚物的物理性能。
我们首先按照从上到下的战略回顾一下从氧化石墨到石墨烯的生产工艺过程,包括每种方法的优点和缺点。
然后按溶解和熔融的战略即分散化学和加热的方法讨论降低氧化石墨在聚合物中的含量。
对于微粒大小的性质、表面性质和在基体中的离散性的技术分析也有介绍。
我们总结石墨烯/聚合物纳米复合材料的导电性、导热性、机械性能和阻气性。
我们结合石墨烯复合材料的加工和可量测性总结这些观点列出最近的挑战和这些新的纳米复合材料的远景。
1介绍基于炭黑、碳纳米管和层状硅酸盐的聚合物纳米复合材料被用于增强聚合物的机械性能、导电性、导热性和阻气性。
石墨烯极其特殊的物理性能和能溶于多种基本聚合物的结合的发现创造了一类新的聚合物纳米复合材料。
石墨烯是由sp2杂化的碳原子按蜂窝状结构排列成的单层、二维片状结构。
它被誉为其他所有不同维数的石墨碳的同素体的基础材料,例如,石墨(三维碳的同素体)由石墨烯的薄碳片正面向上堆积在一起并且分开距离为3.37A组成。
0维同素体,富勒烯(足球烯),可以想象成单层石墨烯的一部分卷曲成的。
一维碳同素体,碳纳米管和碳纳米带可以分别由单层石墨烯旋转和剪切制成。
实际上,然而,这些碳的同素体,除了碳纳米带,都不是由石墨烯合成的。
石墨是一种天然生成的材料,它最早的记载于1555年在英国的Borrowdale,但是它最早的应用可向前追溯4000年。
在1985年发现富勒烯后于1991年第一次合成单壁碳纳米管。
尽管生产石墨烯纳米片的第一个方法报道可以追溯到1970年,但对存在的单层石墨烯在2004年第一次被生产出来,用微机械剥离的方法从石墨中分离出石墨烯。
杨氏模量为1TPa和极限强度为130GPa,单层石墨烯为测量出来的最强的材料。
它的导热系数为5000W/cm3*KJ,与报道的碳纳米束最高值的上限相一致。
石墨烯 英文综述 论文
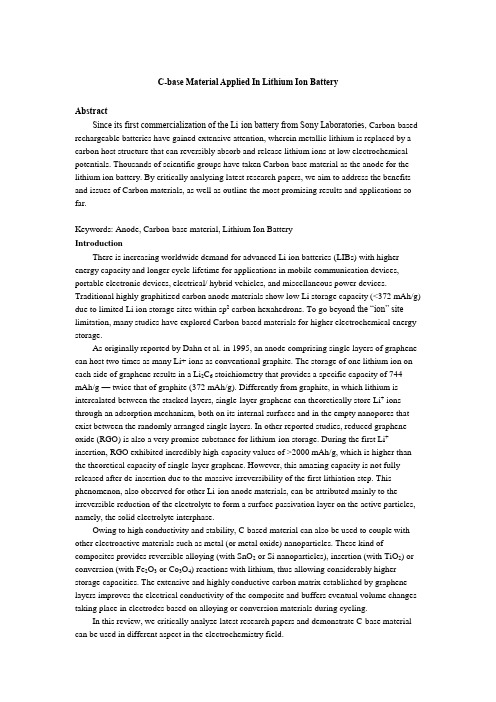
C-base Material Applied In Lithium Ion BatteryAbstractSince its first commercialization of the Li-ion battery from Sony Laboratories, Carbon-based rechargeable batteries have gained extensive attention, wherein metallic lithium is replaced by a carbon host structure that can reversibly absorb and release lithium ions at low electrochemical potentials. Thousands of scientific groups have taken Carbon-base material as the anode for the lithium ion battery. By critically analysing latest research papers, we aim to address the benefits and issues of Carbon materials, as well as outline the most promising results and applications so far.Keywords: Anode, Carbon-base material, Lithium Ion BatteryIntroductionThere is increasing worldwide demand for advanced Li ion batteries (LIBs) with higher energy capacity and longer cycle lifetime for applications in mobile communication devices, portable electronic devices, electrical/ hybrid vehicles, and miscellaneous power devices. Traditional highly graphitized carbon anode materials show low Li storage capacity (<372 mAh/g) due to limited Li ion storage sites within sp2 carbon hexahedrons. To go beyon d the “ion” site limitation, many studies have explored Carbon-based materials for higher electrochemical energy storage.As originally reported by Dahn et al. in 1995, an anode comprising single layers of graphene can host two times as many Li+ ions as conventional graphite. The storage of one lithium ion on each side of graphene results in a Li2C6 stoichiometry that provides a specific capacity of 744 mAh/g — twice that of graphite (372 mAh/g). Differently from graphite, in which lithium is intercalated between the stacked layers, single-layer graphene can theoretically store Li+ ions through an adsorption mechanism, both on its internal surfaces and in the empty nanopores that exist between the randomly arranged single layers. In other reported studies, reduced graphene oxide (RGO) is also a very promise substance for lithium-ion storage. During the first Li+ insertion, RGO exhibited incredibly high-capacity values of >2000 mAh/g, which is higher than the theoretical capacity of single-layer graphene. However, this amazing capacity is not fully released after de-insertion due to the massive irreversibility of the first lithiation step. This phenomenon, also observed for other Li-ion anode materials, can be attributed mainly to the irreversible reduction of the electrolyte to form a surface passivation layer on the active particles, namely, the solid electrolyte interphase.Owing to high conductivity and stability, C-based material can also be used to couple with other electroactive materials such as metal (or metal oxide) nanoparticles. These kind of composites provides reversible alloying (with SnO2 or Si nanoparticles), insertion (with TiO2) or conversion (with Fe2O3 or Co3O4) reactions with lithium, thus allowing considerably higher storage capacities. The extensive and highly conductive carbon matrix established by graphene layers improves the electrical conductivity of the composite and buffers eventual volume changes taking place in electrodes based on alloying or conversion materials during cycling.In this review, we critically analyze latest research papers and demonstrate C-base material can be used in different aspect in the electrochemistry field.Graphene as an active materialIn lithium-ion batteries (LIBs), Li+ ions continuously shuttle between a lithium-releasing cathode (commonly a layered lithium metal oxide) and a lithium-accepting anode (commonly graphite). The amount of ions hosted per gram of material determines the capacity — and thus the energy — of the battery. Similar to graphite, graphene can be used as an anode for hosting Li+, both as such and as a carbonaceous matrix in composites with other materials also capable of storing lithium.Graphene-prepared by different methodsGraphene nanosheets have significant disorder/defects, large specific surface area, and remaining surface functional groups. All these factors have ever been proposed to be related to additional Li storage capacity in nongraphite carbonaceous materials with high specific capacities. Dengyu Pan et al synthesize a highly disordered graphene nanosheet with an outstanding capacity of 794-1054 mAh/g. They prepare graphene nanosheets with different disorder level by changing reduction methods and conditions. A series of graphene nanosheet samples were prepared from graphene oxide (GO) sheets via three different routes including hydrazine reduction, pyrolytic deoxidation and electron beam irradiation.Figure 1 Raman spectra of four different reductionsRaman spectra with characteristic G and D bands sensitive to defects, disorder, and carbon grain size have extensively been used to characterize carbon materials. The G band arises from the zone center E2g mode, corresponding to ordered sp2 bonded carbon, whereas the D band is ascribed to edges, other defects, and disordered carbon. The I D/I G intensity ratio is a measure of disorder degree and average size of the sp2 domains. Figure 1 displays the Raman spectra of four different reduction samples. Figure 1b shows Electron Beam reduced GO has a I D/I G of 1.51 and Hydrazine Reduced GO has a smaller I D/I G of 0.74. Figure 1c displays the Raman spectra of the pristine and pyrolytic GO. For the 300℃pyrolytic GO, however, the G band disappears. The disappearance of the G band arises from the destruction of the E2g symmetry and structural distortion of sp2 domains induced by the low-temperature pyrolysis.Figure 2 shows reversible capability verse cycle Figure 2 Cycle performance number of above materials. (i) natural graphite (ii)pristine GO (iii) hydrazine-reduced GO (iv) 300℃pyrolytic GO (v) 600 ℃pyrolytic GO (vi) andelectron-beam-reduced GO. It can be easily to drawthe conclusion that the material with higher I D/I Gdisplays a better electrochemistry performance.They can correlate the reversible capacity with theRaman intensity ratio. That is, Raman intensityratio (I D/I G) may be the key structural parameter for evaluation of the reversible capacity. What is more, they propose a mechanism to explain how Raman intensity ratio is correlated with the reversible capacity. The I D/I G ratio describes disorder degree in carbonaceous materials because the D band is ascribed to disordered carbon, edge defects, and other defects.Scheme 1 The Li storage model of graphene nanosheetsThe Li storage model of graphene nanosheets is suggested in Scheme 1. The defects at the interface between the nanosheets and the electrolyte may accommodate irreversible Li present in SEI films, whereas the defects at edge sites and internal (basal-plane) defects (vacancies etc.) of the nanodomains embedded in graphene nanosheets may involve reversible Li storage because these defects do not come into contact with the electrolyte. Some Li ions may be stored reversibly between (002) planes, but the defect-based reversible storage may predominate. The notable capacity fading observed in the highly disordered samples may be ascribed to the disorder-induced structure instability.Graphene-doped by other elementsAnother method to improve defects and vacancies is to dope other elements to graphene. It is generally believe that lithium ion batteries (LIBs) using a bulk material such as layered graphite or metal oxide as an anode are capable of achieving a high energy density by storing charges in the bulk of the material, but suffer from a low power density compared to another important electrochemical storage device, electrochemical capacitors (ECs).In order to meet the requirements for LIB applications, Zhong-Shuai Wu et al synthesize the doped graphene electrodes with extremely high rate and large capacity made by heteroatom (N, B)- doped chemically derived graphene. At a low charge/discharge rate of 50 mA /g, the doped graphene electrodes exhibit a very high capacity of 1043 mAh/g for N-doped graphene and 1549 mAh/g for B-doped graphene with greatly improved Coulombic efficiency and cycle performance in comparison with undoped graphene. More importantly, the doped graphene can be quickly charged and discharged for a very short time of 1 h to several tens of seconds, showing high rate capability and excellent long-term cyclability at the same time.At a very high current rate of 25A /g (seen in Figure 3), corresponding to a charge time of28s (126C) for the N-doped graphene and 33s (106 C) for the B-doped graphene, the reversible capacity can still reach 199 mAh/g for the N-doped graphene and 235 mAh/g for the B-doped graphene.Figure 3 The rate performance of N-doped (c) and B-doped (d) graphene What’s more, these doped graphene sheets show a higher thermal stability of 531℃for the N-doped graphene and 588℃for the B-doped graphene than that of pristine graphene (502℃) due to the simultaneous doping and reduction during the doping process. The author suppose that improved electrical conductivity, and thermal stability of the doped graphene allow rapid surface Lit absorption and ultrafast Li+ diffusion and electron transport, thus making this material superior to conventional bulk electrode materials based on Li intercalation and conversion reactions. Graphene as an inactive materialLiCoO2, LiMn2O4 and LiFePO4 (hereafter referred to as LMO) are some of the most commonly used cathode materials in LIBs. The cycle life and rate capability of these materials are generally limited by their poor electrical conductivity. Introducing low-cost conductive additives (for example, carbon black) into the composite electrodes commonly solves this issue. Nevertheless, owing to their amorphous structure, carbon blacks possess a rather low electrical conductivity, with respect to more crystalline carbons such as graphene.Thousands of papers related to graphene are applied in LIB system, we demonstrate an interesting strategy that Shubin et al Yang synthesize a sandwich-like graphene-base titaniananosheets (seen scheme 2).Scheme 2 Illustration of sandwich compositeTo circumvent the drawbacks of titania, graphene with high electrical conductivity have beenutilized as matrices or conductive additives to improve its electrochemical performance. Thegraphene in the composite serve as a mini current collector, which can improve electronconductivity and leads to an dramatic improvement of electrochemical performance. As shown inthe Figure 4, it is striking that a high first discharge capacity of 269 mAh/g is achieved at a currentrate of 0.2 C (Figure 4), corresponding to a lithium insertion coefficient of 0.8 in Li0.8TiO2 . Thisvalue is much higher than the theoretical capacity of titania (167.5 mAh/g ) with the maximumcoefficient of 0.5 (Li0.5TiO2), suggesting the existence of additional lithium storage sites in ourG-TiO2 nanosheets.Figure 4 The cyclability of sandwich composite&TiO2ConclusionCarbon-base material, which can not only be served as an anode but also be used as an inactive material, plays an important role in lithium ion battery system. It arises from graphite, but it’s better than graphite for its breaking down the theoretical limitation of capacity( 372mAh/g ).This is particularly appropriate for the field of electrochemical energy storage, in which ‘graphene fever’ has reached rather high levels due to the continuous need for new materials that can meet the market’s performance requirements. We believe that Carbon-base material, especially graphene, will dominate the market of anode for a long time.Reference[1] Dengyu Pan et.a l. Li Storage Properties of Disordered Graphene Nanosheets. Chem. Mater. 2009, 21, 3136–3142[2] Zhong-Shuai Wu et.a l. Doped Graphene Sheets As Anode Materials with Superhigh Rate and Large Capacity for Lithium Ion Batteries. ACS Nano. 2011, Vol 5,No 7, 5463–5471[3]Shubin Yang et.al. Sandwich-Like, Graphene-Based Titania Nanosheets with High Surface Area for Fast Lithium Storage. Adv. Mate r. 2011, 23, 3575–3579[4] Arava Leela Mohana Reddy et.al. Synthesis Of Nitrogen-Doped Graphene Films For Lithium Battery Application. ACS Nano. 2010, Vol 4,No 11, 6337–6342[5] EunJoo Yoo et al. Large Reversible Li Storage of Graphene Nanosheet Families for Use in Rechargeable Lithium Ion Batteries. ACS2008 Vol.8 NO.8 2277-2282。
石墨烯的研究进展及应用的翻译讲稿
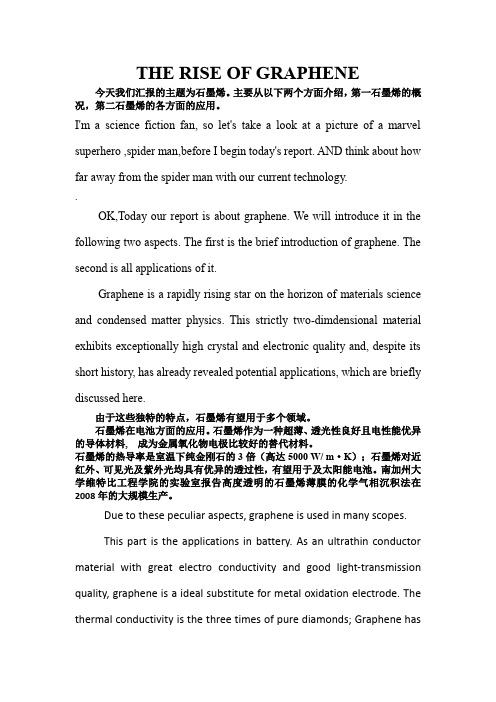
THE RISE OF GRAPHENE 今天我们汇报的主题为石墨烯。
主要从以下两个方面介绍,第一石墨烯的概况,第二石墨烯的各方面的应用。
I'm a science fiction fan, so let's take a look at a picture of a marvel superhero ,spider man,before I begin today's report. AND think about how far away from the spider man with our current technology..OK,Today our report is about graphene. We will introduce it in the following two aspects. The first is the brief introduction of graphene. The second is all applications of it.Graphene is a rapidly rising star on the horizon of materials science and condensed matter physics. Thisstrictly two-dimdensional material exhibits exceptionally high crystal and electronic quality and, despite itsshort history, has already revealed potential applications, which arebriefly discussed here.由于这些独特的特点,石墨烯有望用于多个领域。
石墨烯在电池方面的应用。
石墨烯作为一种超薄、透光性良好且电性能优异的导体材料, 成为金属氧化物电极比较好的替代材料。
氮掺杂褶皱石墨烯的自下而上制备及其氧气还原反应研究(英文)
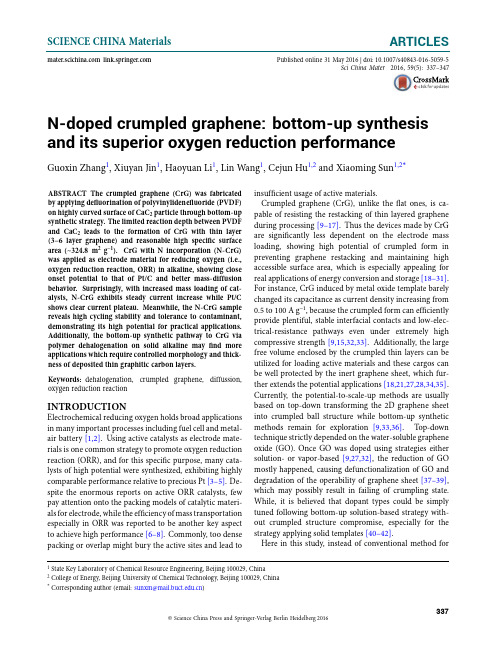
Published online31May 2016 | doi: 10.1007/s40843-016-5059-5Sci China Mater 2016,59(5):337–347 N-doped crumpled graphene:bottom-up synthesis and its superior oxygen reduction performanceGuoxin Zhang1,Xiuyan Jin1,Haoyuan Li1,Lin Wang1,Cejun Hu1,2and Xiaoming Sun1,2*ABSTRACT The crumpled graphene(CrG)was fabricated by applying defluorination of polyvinylidenefluoride(PVDF) on highly curved surface of CaC2particle through bottom-up synthetic strategy.The limited reaction depth between PVDF and CaC2leads to the formation of CrG with thin layer (3−6layer graphene)and reasonable high specific surface area(~324.8m2g−1).CrG with N incorporation(N-CrG) was applied as electrode material for reducing oxygen(i.e., oxygen reduction reaction,ORR)in alkaline,showing close onset potential to that of Pt/C and better mass-diffusion behavior.Surprisingly,with increased mass loading of cat-alysts,N-CrG exhibits steady current increase while Pt/C shows clear current plateau.Meanwhile,the N-CrG sample reveals high cycling stability and tolerance to contaminant, demonstrating its high potential for practical applications. Additionally,the bottom-up synthetic pathway to CrG via polymer dehalogenation on solid alkaline may find more applications which require controlled morphology and thick-ness of deposited thin graphitic carbon layers. Keywords: dehalogenation,crumpled graphene,diffussion, oxygen reduction reactionINTRODUCTIONElectrochemical reducing oxygen holds broad applications in many important processes including fuel cell and metal-air battery[1,2].Using active catalysts as electrode mate-rials is one common strategy to promote oxygen reduction reaction(ORR),and for this specific purpose,many cata-lysts of high potential were synthesized,exhibiting highly comparable performance relative to precious Pt[3–5].De-spite the enormous reports on active ORR catalysts,few pay attention onto the packing models of catalytic materi-als for electrode,while the efficiency of mass transportation especially in ORR was reported to be another key aspect to achieve high performance[6–8].Commonly,too dense packing or overlap might bury the active sites and lead to insufficient usage of active materials.Crumpled graphene(CrG),unlike the flat ones,is ca-pable of resisting the restacking of thin layered graphene during processing[9–17].Thus the devices made by CrG are significantly less dependent on the electrode mass loading,showing high potential of crumpled form in preventing graphene restacking and maintaining high accessible surface area,which is especially appealing for real applications of energy conversion and storage[18–31]. For instance,CrG induced by metal oxide template barely changed its capacitance as current density increasing from 0.5to100A g−1,because the crumpled form can efficiently provide plentiful,stable interfacial contacts and low-elec-trical-resistance pathways even under extremely high compressive strength[9,15,32,33].Additionally,the large free volume enclosed by the crumpled thin layers can be utilized for loading active materials and these cargos can be well protected by the inert graphene sheet,which fur-ther extends the potential applications[18,21,27,28,34,35]. Currently,the potential-to-scale-up methods are usually based on top-down transforming the2D graphene sheet into crumpled ball structure while bottom-up synthetic methods remain for exploration[9,33,36].Top-down technique strictly depended on the water-soluble graphene oxide(GO).Once GO was doped using strategies either solution-or vapor-based[9,27,32],the reduction of GO mostly happened,causing defunctionalization of GO and degradation of the operability of graphene sheet[37–39], which may possibly result in failing of crumpling state. While,it is believed that dopant types could be simply tuned following bottom-up solution-based strategy with-out crumpled structure compromise,especially for the strategy applying solid templates[40–42].Here in this study,instead of conventional method for1State Key Laboratory of Chemical Resource Engineering,Beijing100029,China2College of Energy,Beijing University of Chemical Technology,Beijing100029,China *Corresponding author(email:sunxm@)CrG fabrication which is top-down from the structuring of GO[20,43],bottom-up strategy was applied to fabricate CrG via localized defluorination of polyvinylidenefluoride (PVDF)at the interfaces with particular CaC2,as shown in Scheme1.PVDF was used as the carbon source to wrap CaC2particles.Defluorination reaction was induced by the alkalinity of CaC2to form few-layered graphene.The final graphene can be bombed/cracked by as-formed C2H2due to the inner remaining CaC2reacting with water.Because of the limited reaction depth between PVDF and CaC2,and also the high melting point of CaC2,CrG with thin layer (3–6layers)and highly curved structure was formed.The bottom-up synthetic pathway of CrG applying polymer de-halogenation on solid alkaline has high potential to tailor the layer thickness or the morphology of the as-prepared CrG to meet desirable needs.The resulted CrG had spe-cific surface area(SSA)of324.8m2g−1,even after900°C an-nealing.Nitrogen was introduced in the carbon deposition stage using melamine to explore doped graphene of crum-pled form for the application of ORR.The annealed CrG samples,taking advantage of its crumpled form,high SSA, and N doping,show excellent ORR performance with high catalyst loading in alkaline electrolyte of0.1mol L−1KOH, comparable to20wt.%Pt/C.Its crumpled form further al-lows high catalyst loading for high output current density without triggering large overpotential,which demonstrates its high practical potential.EXPERIMENTALMaterialsPVDF was purchased from J&K Scientific Ltd.CaC2ofScheme1 Graphically depiction of the CrG fabrication through PVDF templated and defunctionalized by irregularly shaped alkaline CaC2 (blue).The interfacial CaC2will transfer to CaF2(yellow)but inner CaC2would probably stay their initial state of CaC2which can be used to generate C2H2(pink)to detonate the annealed product to crack the surficial graphene(black)into small sized CrG.grade1was obtained from Aladdin chemical Co.,Ltd. Melamine and N,N-dimethylactamide(DMAc)of A.R. grade were purchased from Beijing Chemical Factory and used as received.Bottom-up synthesis of N-doped CrGCrG was prepared via solvothermal treatment.Typically, 0.64g PVDF was dissolved in30mL DMAc by sonica-tion(~240kHz),followed by magnetically stirring until a homogeneous solution was formed.Afterwards,0.50g melamine was added into the suspension under thorough sonication.After a uniform suspension was formed,1.0 g fine CaC2powder freshly prepared was added into the uniform suspension,followed by a~10min magnetically stirring.Eventually,the suspension was transferred to a 40mL-packed Teflon-lined autoclave and heated at180°C for12h.Primary product was obtained after two cycles of wash with ethanol and70°C oven dry.Solid precursors were subscribed to inert annealing at700,800,and900°C. Calcined products were poured in dilute HCl solution and sonicated to remove the byproduct CaF2,followed by thor-ough washing and70°C oven dry.The sample annealed at 700,800,and900°C were labled as CrG-700/800/900,re-spectively.CharacterizationsThe morphologies and structures of the materials were examined using scanning electron microscopy(SEM) (Zeiss SUPRA55),transmission electron microscopy (TEM)(Hitachi800),high resolution TEM(HRTEM) (JEOL JEM-2100).Chemical compositions were tested using X-ray photoelectron spectroscopy(XPS)(Thermo Electron ESCALAB250).Powder X-ray diffraction (XRD)patterns of samples were recorded on a Shimadzu XRD-6000.Raman spectra were recorded on a LabRAM Aramis Raman spectrometer(HORIBA Jobin Yvon).Ni-trogen adsorption/desorption analyses were performed using Quantachrome Instruments(USA)Autosorb-1 at-196°C.Specific surface area was calculated from the ad-sorption branch according to the Brunauer-Emmett-Teller (BET)method.The pore size distribution plot was derived from the adsorption branch of the isotherm based on the Barrett-Joyner-Halenda(BJH)method. Electrochemical measurements5.0mg catalyst and10μL Nafion(DuPont,5wt.%)were dispersed in1.0mL N,N-dimethylformamide(DMF)by at least10min sonication to form a homogeneous ink. 5.0μL ink(containing25.0μg catalyst)was loaded on a glassycarbon rotating disk electrode (RDE)of 5mm in diame-ter (Pine Instruments)giving a loading of 0.1274mg cm −2.Cyclic voltammetry (CV)(using PARSTAT 2273potentio-stat from Princeton Applied Research)was conducted in an electrochemical cell using the saturated calomel electrode (SCE)as the reference electrode,a 1.0cm 2platinum foil as the counter electrode and the sample modified glassy car-bon electrode as the working electrode.The electrolyte (0.1mol L −1KOH)was saturated with oxygen by bubbling O 2prior to the measurement.A flow of O 2was kept over the electrolyte during the data recording.The working elec-trode was cycled at least 5times before data were collected with a scanning rate of 50mV s −1.For the RDE mea-surements,the working electrode was scanned cathodically with a scanning rate of 5mV s −1and varied rotating speed from 400to 2025rpm.Koutecky-Levich plots (j −1vs .ω−1/2)were studied at various electrode potentials.The slopes of the linear fit lines were used to calculate the number of electrons transferred (n )according to the Koutecky-Levich equation [44]:J J J B J 11111,L K 1/2K =+=+(1)B nFC D v0.62(),o o 2/31/6=(2)J nFkC ,K o =(3)where J is the measured current density,J K and J L are the ki-netic-and diffusion-limiting current densities,ωis the an-gular velocity in rpm,n is the transferred electron number,F is the Faraday constant,C o is the bulk concentration of O 2,D o is the O 2diffusion coefficient,v is the kinematic vis-cosity of the electrolyte,and k is the electron-transfer rate constant.For the rotating ring-disk electrode (RRDE)measure-ments,the catalyst inks and electrodes were prepared by dissolving 5.0mg solid samples in 500μL DMF,the cata-lysts’loading were the same as that in RDE measurements,which was 0.1274mg cm −2.The ink was dried slowly under infrared light in air and the drying condition was adjusted by trial and error until a homogeneous catalyst distribution across the electrode surface was obtained.The disk elec-trode was scanned cathodically with a scanning rate of 5mV s −1and the ring potential was constant at 1.50V vs .re-versible hydrogen electrode (RHE).The H 2O 2percentage and the electron transferred number (n )were determined by the following equations:I NI I N %HO 200/(/),2r d r =×+(4)n I I I N 4(/),dd r =×+(5)where I d is disk current,I r is ring current,and N is the col-lection efficiency (0.37)of the Pt ring electrode.RESULTS AND DISCUSSIONEffective dehalogenation of PVDF was conducted by the high affinity between Ca on CaC 2and F on PVDF;mean-while,strong alkalinity of CaC 2and high polarity of solvent DMF can also facilitate the leaving of F-functionality.As demonstrated in Scheme 1,thin layered carbonization products and the in situ generated insoluble CaF 2will form at the interface and deposit on the highly curved CaC 2particle surface,which would prevent further reaction between CaC 2and PVDF,and form a solid replica of the initial CaC 2morphology with atomic thickness.The CrG precursor,the encapsulating CaF 2and the inner remaining CaC 2were purified and subscribed to inert annealing to graphitize the thin carbon layer.After performing anneal-ing,the inner enclosed CaF 2,CaC 2and a few Ca(OH)2generated in the drying process can be completely removed by sonication in diluted HCl.During this washing stage,the remaining CaC 2will react with water to form C 2H 2gas,which can effectively fragment the templated CrG,leading to the formation of micro-sized CrG.Heteroatom source,for instance,in this study,melamine,can be incorporated into the thin carbon shell formed on CaC 2,which allow us to further explore electrochemical applications of CrG products due to its highly N-doped trait.The irreplaceable role of CaC 2in the dehalogenation of PVDF was evidenced with controlled experiments using blank or different types of alkaline to defunctionalize PVDF.(Experiments No.1,2,3,4,and 5represent for the solvothermal treatments of melamine,PVDF,PVDF/melamine,PVDF/CaC 2,and PVDF/CaC 2/melamine,respectively.)As shown in Fig.S1a,obvious carbonization can be observed from Exper-iments No.3,4,and 5except the other two,in which organic (melamine)or inorganic (CaC 2)alkaline were involved to defluorinated PVDF.No visual carbonized products were found for solvothermal treatment of solo PVDF or solo melamine which clearly evidenced that no carbonization happened.Furthermore,Experiment No.3applying solo organic alkaline of melamine obtained very few solid product even after 10min centrifugation at 12,000rpm (Fig.S1c).This limited product was further verified to be dominated by melamine,not originated from carbonization of PVDF,as illustrated in Fig.S2.While for the product of Experiment No.4applying inorganic CaC 2,big-sized particles can be found,after dryness.Theseparticles were characterized presenting large amount of CaF2and a few Ca(OH)2(Ca(OH)2was generated by CaC2 reacting with H2O during washing and drying),matching our abovementioned statements(Fig.S3).After complete removal of soluble components in diluted HCl and further cracked by C2H2gas bubbles,the thin layered CrG was obtained,as revealed in Fig.S4.In order to demonstrate the feasibility of the bottom-up strategy in fabricating applicable CrG,organic alkaline melamine as well as one common N source was introduced in the solvothermal synthetic condition to co-dehalogenate PVDF and dope the carbon matrix with nitrogen.As shown in Fig.S5,the solvothermal treated suspension (Experiment No.5)of PVDF/CaC2/melamine results in particular products with highly curved surface,which was characterized to be dominated by CaF2.Alike to Experiment No.4,Ca(OH)2was formed during the dry-ing process(Fig.S5c).Annealed sample of Experiment No.5went through the explosive purification process to form fragmented CrG.The final CrG annealed at900°C (yield~20.8%)was subscribed to electron microscopy mea-surements,and revealed to be highly crumpled and even thinner than products from Experiment No.5,as shown in Fig.1a.Typical morphology of highly crumpled graphene of CrG-900(annealed at900°C),abundant wrinkles and inner voids can be observed.A few enclosed carbon cap-sules can be also observed in900°C-annealed CrG,which was formed with the assistance of the in situ generated CaF2and CaC2template,as displayed in Fig.1b.CrG was substantiated to be highly graphitized,clear crystallized graphene layers can be found and the layers’number falls in4–6graphene sheet(Fig.1b,CrG-700and CrG-800are shown in Fig.S6,whose yields are at~30%),which thanks to the limited reaction depth between PVDF and CaC2. Fig.1c shows abundant micropores presenting in CrG-900. Cyclic narrow strip of N2adsorption/desorption(A/D) isotherms in the inset of Fig.1c confirm the presence of mesopores which are indexed toⅣtype pore according to its A/D isothermal,corresponds to cone-and plate-shape mesopores[20].The other two CrG samples(i.e.,annealed at700and800°C)are also attributed to typeⅣpore(Fig. S7).Meanwhile,sharp peaks of(002)graphene lattice of XRD profiles(Fig.1d)and typical graphitization bands of Raman spectra are presenting in all CrG samples(Fig.S8). Considerable defect was revealed in the N-CrG by Ra-man spectra(Fig.S8,I D/I G~1.09–1.15).The I D/I G ratio is comparable to that of the liquid phase exfoliated graphene but much lower than that of the chemically converted graphene oxide even after reduction(I D/I G~1.5–2.5),demonstrating the reasonable graphitization degree [20,45,46].The defect on CrG may provide plentiful ac-tive catalytic sites for adsorbing reaction molecules and conveying efficient catalysis.Fig.S9a shows the XPS ele-mental survey for the CrG samples,revealing the presence of mainly three peaks located at~285,400,and533eV for C1s,N1s,and O1s,respectively.The N content was estimated to be up to7.2at.%in the CrG-700,which would decrease to2.9at.%for the CrG-900upon annealing at 900°C(Figs1e and S9a).More importantly,curve-fitting of the high-resolution XPS N1s peaks(Fig.S9c)indicates up to~68.9at.%N in the form of graphitic N,which was reported to be one of the most active catalytic N sites for ORR and could also improve the overall conductivity [16,47–49].The curve-fitted XPS C1s spectrum of the CrG-900is given in Fig.1f,which shows80.7%graphitic carbon,18.2%C−N/C−O,and8.1%C=O.The presence of O-containing groups could enhance the O2adsorption while the high content of graphitic N species could act as active sites for ORR.Interestingly,it was found that the control sample of CrG synthesized without melamine still possessed~1.85at.%N doping(Fig.S10).Considering the only N source in this case was the solvent of DMAc, we may safely conclude that the on-site defluorinated carbon atoms were extremely reactive and could even extract heteroatoms from stable organic molecules[39,50], which further demonstrated the effectiveness of our syn-thetic strategy for various of doped carbon materials.The morphology of control sample(the sample synthesized without melamine addition was annealed at900°C and denoted as CrG-9-no-ME)was still crumpled form with thin layers(Fig.S10a).XRD profile(Fig.S10b)reveals the high graphitization degree that CrG-9-no-ME possessed, similar to other CrG samples.To evaluate the ORR performance of the CrG samples, we fabricated them into electrodes and performed CV and polarization measurements using a RRDE unless otherwise stated.As shown in Fig.2a,the CrG-900exhibits a CV with the same onset potential and the maximum current peak relative to that of commercial20wt.%Pt/C.Fig.2b re-produces the linear scanning voltammetry(LSV)curve for the CrG-900,showing a highly comparable onset and even better half-wave potential to those of the Pt/C electrode, but left big gap of limiting current density being much less than that of20wt.%Pt/C.The CrG-900exhibited advanced onset and half-wave potentials than that of the previously reported CrG which made by the conventional top-down methods(Table S1)[51–55].Fig.2c reproduces the Tafel plots of the CrG-900and Pt catalyzing ORR, which wasFigure 1 Typical morphology of the CrG which was annealed at 900°C:(a)TEM image,inset shows the SEM image of the CrG-900,in which thin layered and crumpled form can be observed.(b)Manified TEM image of the CrG-900.(c)BET measurements:pore volume of the CrG-900,inset shows the N 2A/D curve.(d)XRD patterns of the CrG samples.XPS analysis:(e)C1s and (f)N1s spectra of the CrG-900.Graphitic N takes nearly 70%of overall N species.originated from LSV curves.As implied by the slightly small slope of the CrG-900(52.2mV dec −1),it was sug-gested that the ORR could be facilitated similarly fast on the CrG-900as it on precious Pt.As shown in Fig.2d ,a diffusion-controlled 4e-ORR process occurs over a wide potential window from 0to 0.85V ,as the case for the Pt/C electrode.Similar to the Pt/C electrode,the amount H 2O 2generated is also very low (<8%,Fig.2d ).Compared with the CrG-900,the CrG-700/800exhibits bigger cyclic areas (Fig.S11a),presumably due to their higher (O-containing)functionality contents but smaller limiting current densi-ties and lower onset potentials (Fig.S11b,measured on a RDE)associated with the lower contents of graphitic N (Fig.S9c).Figure2 The ORR performance of the CrG-900(red)relative to the commercial20wt.%Pt/C(black).(a)CV;(b)polarization curves(including disk current density and ring current(inset),1600rpm-line was selected);(c)Tafel plot of the ORR on CrG-900and Pt/C catalysts.(d)Electron transfer-ring number and generating H2O2percentage.Catalyst loading=0.3mg cm−2.All electrochemical measurements abovementioned were conducted in O2-saturated0.1mol L−1KOH electrolyte and copying the1600rpm polarization curve of disk(or plus ring)current.Electrochemical impedance spectroscopy(EIS)isfrequently used to investigate the electrode reactions in-volving mass transportation[56].The cases with heavyloading catalysts of0.45mg cm−2(three fold of catalystloading on the abovementioned case for activity compar-ison between Pt and CrG-900)were performed with theEIS measurements.The EIS of CrG-900collected at0.8V vs.RHE against that of Pt/C with the same catalystloading density was displayed in Fig.3a.At high frequencyregion,the Pt/C shows bigger system impedance thanCrG-900,implying inner resistance increase caused byunavoidably increased contacts between densely packedparticles.Due to the nature of high activity of Pt,verysmall electrochemical reaction semicircle was observed.Thereafter,the EIS comes into the medium frequencywhere the information of mass/charge transfer resistanceand short-region diffusion impedance could be obtained,the impedance could be also called as Warburg resistance[18].In our cases,Pt exhibits planar electrode behaviorand the CrG-900shows much slower increasing trendwhich indicates the highly rough surface of the CrG-900(Fig.3b)[56].In low-frequency region,the CrG-900,with mutual supporting crumpled GO sheets,shows muchbigger slope than Pt on particle-like carbon,indicatingthat smooth mass transportation occurs[9,21].At given rotation rate,it was conceivable that the trans-portation of the solvated O2forced from bulk solutionto border of catalyst layer(CL)would be close(schemat-ically shown in Fig.3c).However,those two cases ofCrG-900and Pt/C are different,for particle-like Pt/Ccatalyst,densely packed CL was formed,leaving few voidsas electrolyte reservoir.When the inner O2runs out,itneeds to migrate from CL border all the way to the deepCL.While in the CrG-900case,naturally formed voidsand void space between mutual supporting CrG-900couldreserve quite a lot of electrolyte as broad pathways for thesolvated O2channel and reservoir.When CL is thick anddensely loaded with catalyst materials,it is also very hardfor ions to penetrate the electrode materials with thin porestructuring,therefore,minor catalysts deep inside the CLwould stay inactive due to O2deficiency.Providing broaddiffusion pathways for the solvated O2could be of greatsignificance for the electrode materials free of impact ofdense catalyst packing,on one hand,taking full use of CL,Figure 3 (a)Enlarged part of high frequency region of (b).(b)EIS of electrodes made of Pt/C and CrG-900at potential=0.8V (vs .RHE).(c)Graphical depiction of the difference of ORR occurring on (a)Pt/C and (b)CrG-900.on the other hand,achieving high energy of overall devices.The CrG-900sample holds crumpled morphology which is credited to be beneficial for mass transportation.In order to obtain high energy of fuel cells or metal-O 2battery for actual use,sufficiently high density of catalyst loading should be performed to counter the whole pack-age including current collector,electrolyte,separator,and wrappings.Fig.2a displays high ORR performance of Pt/C catalyst with low catalyst loading of 0.15mg cm −2;however,the considered aspects especially the limiting current den-sities show minor advances or even fading after the loading areal density slightly increased over 0.25mg cm −2(Fig.4a ).While on the contrary,the CrG-900sample with crumpled morphology,capable of reserving channels for ion pass-ing at very high catalyst loading density,as tested by EIS,suffers no such heavy adverseness,and shows unstopped increase of current density until areal density of catalyst loading reaching as high as 0.75mg cm −2,as shown in Fig.4b .Remarkably,the current density of CrG electrode with catalyst loading reaching 0.60mg cm −2,surpasses that of Pt/C with a 0.35mA cm −2(Fig.4c )at low overpotential of430mV (U =0.8V),which highlights the advantages of the structure of crumpled N-doped CrG.To investigate the stability of the CrG electrode toward ORR and MeOH tolerance,we carried out chronoamper-ometric measurements at a constant voltage of 0.6V (vs .RHE)within the diffusion-controlled region.As shown in Fig.5a ,the CrG-900electrode exhibits a significantly bet-ter long-term stability than the commercial Pt/C catalyst.In Fig.5b ,the CrG-900electrode is almost free from the methanol crossover effect whereas a significant variation of CV profile and a considerable activity variation in the ORR performance happened with the Pt/C electrode.Therefore,the CrG-900fabricated following our newly developed bot-tom-up synthetic strategy is of high practical importance.CONCLUSIONSIn summary,a bottom-up synthetic strategy of CrG was de-veloped applying defluorination of PVDF on highly curved surface of CaC 2particle.Following this strategy,the CrG with thin layer (3–6layer graphene)and large specific sur-face area (~324.8m 2g −1) was obtained. Layer thicknessFigure4 Investigations of the effect of catalyst’s loading amount on ORR performance:(a)20wt.%Pt/C and(b)CrG-900.The data point of current density at given potential of(c)0.8V and(d)0.6V.All the polarization curves were collected with1600rpm rotationspeed.Figure5 (a)The cycling stability and(b)test of tolerance to methanol.can be controlled due to the limited reaction depth between PVDF and CaC2.The CrG-900with N dopant and crum-pled form was applied as electrode material for reducing oxygen in alkaline.Thanks to the structure of crumpled morphology which forms lots of voids inside and between CrG,the solvated O2transportation can be greatly facil-itated,leading to the current density of the CrG-900in-creasing along with the increase of areal density of catalyst loading.Remarkably,at lower overpotential of0.43V and loading density of0.60mg cm−2,the current density of CrG surpasses that of Pt with0.35mA cm−2.Moreover,much higher cycling stability and tolerance to contaminant are observed for N-doped CrG-900,demonstrating high po-tentials of CrG for practical applications.Additionally,thebottom-up synthetic pathway to the CrG via polymer de-halogenation by solid alkaline may find more applications in controlling morphology and thickness of deposited thin graphitic carbon layers.Received28April2016;accepted24May2016;published online31May20161Wu G,Santandreu A,Kellogg W,et al.Carbon nanocomposite cat-alysts for oxygen reduction and evolution reactions:From nitro-gen doping to transition-metal addition.Nano Energy,2016,doi:10.1016/j.nanoen.2015.12.0322Zhang T,Tao Z,Chen J.Magnesium–air batteries:from principle to application.Mater Horiz,2014,1:196–2063Dai L,Xue Y,Qu L,et al.Metal-free catalysts for oxygen reduction reaction.Chem Rev,2015,115:4823–48924Zhang J,Dai L.Heteroatom-doped graphitic carbon catalysts for effi-cient electrocatalysis of oxygen reduction reaction.ACS Catal,2015, 5:7244–72535Elumeeva K,Fechler N,Fellinger TP,et al.Metal-free ionic liq-uid-derived electrocatalyst for high-performance oxygen reduction in acidic and alkaline electrolytes.Mater Horiz,2014,1:588–594 6Jang HD,Kim SK,Chang H,et al.Three-dimensional crumpled graphene-based platinum–gold alloy nanoparticle composites as su-perior electrocatalysts for direct methanol fuel cells.Carbon,2015, 93:869–8777Dai L.Functionalization of graphene for efficient energy conversion and storage.Acc Chem Res,2013,46:31–428Kim JY,Lim J,Jin HM,et al.3D tailored crumpling of block-copoly-mer lithography on chemically modified graphene.Adv Mater, 2016,28:1591–15969Luo J,Jang HD,Huang J.Effect of sheet morphology on the scal-ability of graphene-based ultracapacitors.ACS Nano,2013,7: 1464–147110Zang J,Ryu S,Pugno N,et al.Multifunctionality and control of the crumpling and unfolding of large-area graphene.Nat Mater,2013, 12:321–32511Cranford SW,Buehler MJ.Packing efficiency and accessible surface area of crumpled graphene.Phys Rev B,2011,84:12Baimova A,Korznikova EA,Dmitriev SV,et al.Review on crumpled graphene:unique mechnical properties.Rev Adv Mater Sci,2014, 39:6913Lin Z,Waller GH,Liu Y,et al.3D Nitrogen-doped graphene prepared by pyrolysis of graphene oxide with polypyrrole for elec-trocatalysis of oxygen reduction reaction.Nano Energy,2013,2: 241–24814Xiao L,Damien J,Luo J,et al.Crumpled graphene particles for mi-crobial fuel cell electrodes.J Power Sources,2012,208:187–192 15Guo F,Creighton M,Chen Y,et al.Porous structures in stacked, crumpled and pillared graphene-based3D materials.Carbon,2014, 66:476–48416Zhang C,Hao R,Liao H,et al.Synthesis of amino-functionalized graphene as metal-free catalyst and exploration of the roles of var-ious nitrogen states in oxygen reduction reaction.Nano Energy, 2013,2:88–9717Deng S,Berry V.Wrinkled,rippled and crumpled graphene:an overview of formation mechanism,electronic properties,and applications.Mater Today,2016,19:197–21218Smith AJ,Chang YH,Raidongia K,et al.Molybdenum sulfide supported on crumpled graphene balls for electrocatalytic hydrogen production.Adv Energy Mater,2014,4:59–6219Lee JY,Lee KH,Kim YJ,et al.Sea-urchin-inspired3D crumpled graphene balls using simultaneous etching and reduction process for high-density capacitive energy storage.Adv Funct Mater,2015,25: 3606–361420Wen Z,Wang X,Mao S,et al.Crumpled nitrogen-doped graphene nanosheets with ultrahigh pore volume for high-performance super-capacitor.Adv Mater,2012,24:5610–561621Du FH,Liu YS,Long J,et al.Incorporation of heterostructured Sn/SnO nanoparticles in crumpled nitrogen-doped graphene nanosheets for application as anodes in lithium-ion batteries.Chem Commun,2014,50:9961–996422An C,Wang Y,Li L,et al.Effects of highly crumpled graphene nanosheets on the electrochemical performances of pseudocapaci-tor electrode materials.Electrochim Acta,2014,133:180–18723Mao S,Wen Z,Huang T,et al.High-performance bi-functional electrocatalysts of3D crumpled graphene–cobalt oxide nanohybrids for oxygen reduction and evolution reactions.Energy Environ Sci, 2014,7:609–61624Liu YZ,Chen CM,Li YF,et al.Crumpled reduced graphene oxide by flame-induced reduction of graphite oxide for supercapacitive en-ergy storage.J Mater Chem A,2014,2:5730–573725Zou Y,Kinloch IA,Dryfe RAW.Nitrogen-doped and crumpled graphene sheets with improved supercapacitance.J Mater Chem A, 2014,2:19495–1949926Wang Y,Wu Y,Huang Y,et al.Preventing graphene sheets from restacking for high-capacitance performance.J Phys Chem C,2011, 115:23192–2319727Luo J,Zhao X,Wu J,et al.Crumpled graphene-encapsulated si nanoparticles for lithium ion battery anodes.J Phys Chem Lett, 2012,3:1824–182928Choi SH,Lee JK,Kang YC.Three-dimensional porous graphene-metal oxide composite microspheres:preparation and application in Li-ion batteries.Nano Res,2015,8:1584–159429Yan J,Xiao Y,Ning G,et al.Facile and rapid synthesis of highly crumpled graphene sheets as high-performance electrodes for su-percapacitors.RSC Adv,2013,3:2566–257130Zang J,Cao C,Feng Y,et al.Stretchable and high-performance supercapacitors with crumpled graphene papers.Sci Rep,2014,4: 649231Wen G,Wu S,Li B,et al.Active sites and mechanisms for direct oxidation of benzene to phenol over carbon catalysts.Angew Chem Int Ed,2015,54:4105–410932Luo J,Jang HD,Sun T,et pression and aggregation-resistant particles of crumpled soft sheets.ACS Nano,2011,5:8943–8949 33Mao S,Wen Z,Kim H,et al.A general approach to one-pot fabri-cation of crumpled graphene-based nanohybrids for energy applica-tions.ACS Nano,2012,6:7505–751334Wang H,Yang Y,Liang Y,et al.Graphene-wrapped sulfur particles as a rechargeable lithium–sulfur battery cathode material with high capacity and cycling stability.Nano Lett,2011,11:2644–264735Jang HD,Kim SK,Chang H,et al.Three-dimensional crumpled graphene-based platinum–gold alloy nanoparticle composites as su-perior electrocatalysts for direct methanol fuel cells.Carbon,2015, 93:869–87736Wang X,Maeda K,Thomas A,et al.A metal-free polymeric photo-catalyst for hydrogen production from water under visible light.Nat Mater,2008,8:76–8037Luo D,Zhang G,Liu J,et al.Evaluation criteria for reduced graphene oxide.J Phys Chem C,2011,115:11327–1133538Zhang G,Xu Y,Wang L,et al.Rational design of graphene oxide and its hollow CoO composite for superior oxygen reduction reaction.Sci China Mater,2015,58:534–542。
WSe_2负载掺氮三维石墨烯的制备及储锂性能
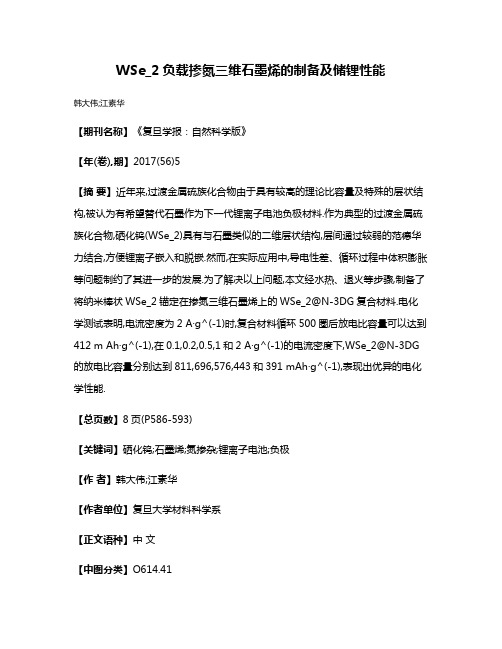
WSe_2负载掺氮三维石墨烯的制备及储锂性能韩大伟;江素华【期刊名称】《复旦学报:自然科学版》【年(卷),期】2017(56)5【摘要】近年来,过渡金属硫族化合物由于具有较高的理论比容量及特殊的层状结构,被认为有希望替代石墨作为下一代锂离子电池负极材料.作为典型的过渡金属硫族化合物,硒化钨(WSe_2)具有与石墨类似的二维层状结构,层间通过较弱的范德华力结合,方便锂离子嵌入和脱嵌.然而,在实际应用中,导电性差、循环过程中体积膨胀等问题制约了其进一步的发展.为了解决以上问题,本文经水热、退火等步骤,制备了将纳米棒状WSe_2锚定在掺氮三维石墨烯上的WSe_2@N-3DG复合材料.电化学测试表明,电流密度为2 A·g^(-1)时,复合材料循环500圈后放电比容量可以达到412 m Ah·g^(-1),在0.1,0.2,0.5,1和2 A·g^(-1)的电流密度下,WSe_2@N-3DG 的放电比容量分别达到811,696,576,443和391 mAh·g^(-1),表现出优异的电化学性能.【总页数】8页(P586-593)【关键词】硒化钨;石墨烯;氮掺杂;锂离子电池;负极【作者】韩大伟;江素华【作者单位】复旦大学材料科学系【正文语种】中文【中图分类】O614.41【相关文献】1.石墨烯负载NiO纳米盘的制备与储锂性能 [J], 武永鑫;田雷雷;刘佳奇;庄全超2.3D α-Fe2O3/掺氮石墨烯/碳纳米管复合材料及其储锂性能 [J], 刘晓峰;米常焕;张文庆3.三维石墨烯包覆的硫掺杂碳负载二硫化硒复合材料的制备及其储锂性能研究 [J], 胡金龙;任逸伦;张灵志4.超薄SnS2纳米片嵌入氮掺杂石墨烯复合材料的制备及其储锂/钠性能研究 [J], 陶石;吴大军;韩志达;钱斌;江学范5.高性能氮掺杂石墨烯的制备及其储锂性能 [J], 沈进冉; 郭翠静; 陈赫; 周淑琴; 徐斌; 官亦标因版权原因,仅展示原文概要,查看原文内容请购买。
- 1、下载文档前请自行甄别文档内容的完整性,平台不提供额外的编辑、内容补充、找答案等附加服务。
- 2、"仅部分预览"的文档,不可在线预览部分如存在完整性等问题,可反馈申请退款(可完整预览的文档不适用该条件!)。
- 3、如文档侵犯您的权益,请联系客服反馈,我们会尽快为您处理(人工客服工作时间:9:00-18:30)。
学号:10401604常州大学毕业设计(论文)外文翻译(2014届)外文题目Easy synthesis of nitrogen-doped graphene–silvernanoparticle hybrids by thermal treatment ofgraphiteoxide with glycine and silver nitrate 译文题目通过水热处理氧化石墨烯、甘氨酸和硝酸银简便地合成掺氮石墨烯-银纳米粒子复合物外文出处CARBON50(2012)5148–5155学生王冰学院石油化工学院专业班级化工106校内指导教师罗士平专业技术职务副教授校外指导老师专业技术职务二○一四年二月通过水热处理氧化石墨烯、甘氨酸和硝酸银简便地合成氮杂石墨烯-银纳米粒子杂合物Sundar Mayavan,Jun-Bo Sim,Sung-Min Choi摘要:氮杂石墨烯-银纳米粒子杂合物在500℃通过水热处理氧化石墨烯(GO)、甘氨酸和硝酸银制得。
甘氨酸用于还原硝酸根离子,甘氨酸和硝酸根混合物在大约200℃分解。
分解的产物可作为掺杂氮的来源。
水热处理GO、甘氨酸和硝酸银混合物在100℃可形成银纳米粒子,200℃时GO还原,300℃时产生吡咯型掺氮石墨烯,500℃时生成吡咯型掺氮石墨烯。
合成物质中氮原子所占百分比为13.5%.在合成各种纳米金属粒子修饰的氮杂石墨烯方面,该合成方法可能开辟了一个新的路径,其在能量储存和能量转换设备方面很有应用价值。
1.引言石墨烯是所有石墨材料的基本构件,其蜂窝状晶格由单层碳原子排列而成。
它表现出与结构有关的独特电子、机械和化学性质,具有较高的比表面积(2630-2965m2g-1)[1–3]。
化学掺杂杂原子石墨烯像掺杂氮原子,极大地引起了人们的兴趣,因其在传感器、燃料电池的催化剂和锂离子电池的电极等方面具有应用潜力[4–6]。
氮原子的掺杂改变了石墨烯的电子特性和结构特性,导致其电子移动性更强,产生更多的表面缺位。
氮原子上孤对电子的存在改进了石墨烯的活性和催化性能。
在碱性条件下,与已商业化的Pt催化剂相比,掺氮石墨烯(NG)在氧化还原反应(ORR)中活性更高,稳定性更强[5]。
因掺氮石墨烯中氮原子电子接受能力较高,其可以创造出碳正粒子促进氧的吸附,所以NG 在ORR反应中表现出较高的活性。
Pt纳米粒子负载在NG上比负载未掺杂石墨烯上催化电化学反应时活性和稳定更高。
Pt负载在NG上比负载在石墨烯上具有更高的能量密度,因为它增加了NG的导电性,提高了对Pt的吸附力[7]。
最近有报道,在NG上长出的CO3O4纳米晶体催化剂具有较高的氧化还原活性[8],这增加了NG催化剂的应用前景。
NG的合成方法主要有化学气相沉积法(CVD)、在氮前驱体存在的石墨烯弧光放电法、激光烧蚀、氮或氨等离子处理法[9-11]。
所有这些合成方法各有特点,但均能耗高、使用昂贵的设备、反应条件苛刻、处理特殊、反应步骤多。
本文以氧化石墨烯(GO)为原料,提出了一种简单的合成氮杂石墨烯-Pt纳米粒子杂合物的方法。
水热法处理GO、甘氨酸(GLY)和硝酸银制备上述杂合物。
甘氨酸作为辅助原料,以减少硝酸根离子的用量,使用甘氨酸-硝酸盐混合物在150~200℃发生分解反应。
分解产物作为掺杂氮的来源,同时还原表面氧官能团。
与Pt负载在未掺杂石墨烯上,NG-纳米粒子杂合物显示出了良好的活性和电催化稳定性。
2.实验部分2.1NG-Ag催化剂的合成先采用改进的Hummers方法以天然石墨粉为原料制备GO。
X射线衍射和热重分析法证实所合成物质为氧化石墨烯[12,13]。
GO与甘氨酸、硝酸盐(硝酸银或硝酸钯)按重量比1:2:2的比例溶解于水中。
混合物超声处理两个小时,然后倒入氧化铝坩埚。
在氩气保护下,逐渐将温度从室温升到500℃,500℃恒温2h,生成Ag或Pd修饰的氮杂石墨烯。
产品直接从坩埚中收集。
Pd纳米粒子石墨烯(G-Pd)以同样的方式制备,只是不需要加入氮源甘氨酸。
通过ICP分析,石墨烯上负载的Pd纳米粒子浓度为34.4wt%,NG上为55.1wt%。
2.2仪器使用高分辨率色散拉曼显微镜(1MwHe-Ne激光、波长633nm)记录了合成物质的拉曼光谱。
XRD衍射图由高分辨率XRD衍射仪获得。
XPS图在西格玛探针XPS上测定,Al Ka射线作为辐射源。
TGA分析氮气氛围下TA仪器上分析(升温速率10℃/min)。
NG-Ag的表面形态使用场发射扫描电子显微镜测定。
同时采用Philips-Tecnai F20观察NG-Ag的形貌(操作电压200KV)。
能量色散X射线分析(EDX)在EDAX探测器上执行,其安装在FE-SEM上。
TEM试样的制备方法是将NG-Ag的水分散体滴加到碳图层铜网格上,然后干燥得到试样。
2.3电化学测试伏安曲线在传统的三电极电化学工作站上进行,铂电极为对电极,氯化银电极为参比电极(SCE)(0.2V可逆氢电极校准)。
工作电极由薄膜法制备。
抛光玻璃碳电极(GC、5mm直径)被作为衬底。
10微升NG-Pd水性悬浮液滴加到衬底上,80℃干燥形成催化剂层。
3.结果与讨论将GO、硝酸银和甘氨酸混合,500℃热处理2h,制备得NG-Ag催化剂。
FE-SEM 图(见图1a)显示石墨烯上覆盖有纳米颗粒(白点)。
NG-Ag的EDX图像显示杂合物上有银的存在。
NG-Ag的FE-TEM图像表明修饰有Ag纳米粒子的石墨烯薄片随机地堆放在一起,显示出褶皱的丝绸面纱波浪形状,与原始的石墨烯类似[14,15]。
纳米粒子存在于少数石墨烯薄层之间的表面。
采用热重分析法测定了AgNO3–Gly–GO和AgNO3–Gly的热分解过程(见图2)。
在约200℃两个都有最大的重量损失,这种损失源于甘氨酸-硝酸盐的分解(通过自燃反应,甘氨酸被硝盐氧化)。
AgNO3–Gly–GO更早些的重量损失与GO表面的含氧官能团有关(像OH和COOH)[16,17]。
在150℃到200℃之间的重量损失表明甘氨酸-硝酸盐的燃烧和GO的还原同时发生,生成了CO2和NH3气[16,17]。
众所周知,甘氨酸-硝酸盐燃烧生成NH3。
事实上,基于甘氨酸-硝酸盐的燃烧制备了各种金属粉末和氧化物。
最近采用甘氨酸-硝酸盐的燃烧,制备了铜镍合金纳米金属粉末[17]。
至于AgNO3–Gly–GO 体系,氨和氮氧化物从甘氨酸-硝酸盐的分解中得到,并作为氮源,同时促进了GO的还原,最终掺氮杂到还原石墨烯的薄层中。
为了了解Ag纳米粒子的形成、GO的还原和石墨烯上氮物种的变换过程,AgNO3–Gly–GO在不同的温度下退火,然后采用XRD、XPS和拉曼光谱进行检测。
为进行这些检测,AgNO3–Gly–GO以2℃/min的速率加热到不同的温度(100℃、300℃和500℃),然后再氮气的氛围下恒温2h。
检测前氩气保护冷却至室温。
AgNO3–Gly–GO不同温度退火的XRD图见图3.图1—(a)Ag-掺氮石墨烯的FE-SEM图和FE-TEM图2—AgNO3–Gly–GO和AgNO3–Gly的热分解图谱图3—AgNO3–Gly–GO不同温度的X射线衍射图100℃的XRD图在(111)面、(200)面、(220)面、(311)面出现了银的明显峰值,同时还有相应的AgNO3–Gly的峰,这显然说明银纳米粒子的形成。
当硝酸银和甘氨酸混合物不含GO在100℃退火时,XRD图上未找到Ag晶体的峰,以此确定了在Ag 离子还原时,GO所起的作用。
银离子的还原只有在GO存在的条件下才会发生,因为还原时需要GO表面的羟基进行电子传递。
在100℃未观察到GO的峰,说明GO已经消失[18]。
在200℃,AgNO3–Gly相应的峰消失,说明AgNO3–Gly已经反应(这与TGA 的分析一致)[19]。
200℃在2θ大约为25.8°附近显示有一个小宽峰,这可能与石墨烯薄片的堆积有关。
在300℃500℃AgNO3–Gly相应的峰彻底地消失,只剩下晶体银和石墨烯相应的峰。
在不同温度退火的AgNO3–Gly–GO的XPS图见图4.。
所有试样的C、H、O、N和Ag光电吸收峰都进行了检测。
银的3d吸收峰在各个温度和预期的金属银吸收峰一样,集中在368.1ev和374.1ev,与XRD的结果一致[20]。
在100℃,高分辨率的C1s的XPS峰为两个分开的峰,这与含氧基团所占比例较高造成了石墨的氧化有关[21]。
当温度提高到300到500℃,C=C占据主导地位,284.5ev处显示出一个单峰,这说明热处理后大量的含氧基团被还原。
在高束缚能方向上C1s峰的不对称性加大,表明氮已经进入石墨薄层形成了C-N键[15]。
此外,O1s的峰随着温度的升高而下降,温度从100℃升高到500℃,XPS能谱测定的氧原子的组成从39.4%下降到7.38%。
说明热处理过程中GO被还原。
AgNO3–Gly–GO 在500℃的热重分析也显示500℃时GO发生了还原。
图4–XPS 全谱(a )高分辨率的C 1S 图谱;(b )N 1S;(C)AgNO 3–Gly–GO 在不同温度下的光谱;(d )不同含氮量的NG-Ag 示意图随着温度变化,石墨烯上氮的形态改变见图4c 。
在100℃,N1s 的XPS 图显示在吡咯吡啶406.8ev和401.6ev处有两个分开的峰,分别对应硝酸盐上的氮和甘氨酸上的氮。
当退火温度提高到300℃,这两峰消失,意味着硝酸盐和甘氨酸的分解(与TGA和XRD结果一致)。
400ev处出现一个新吸收峰,标志着吡啶型氮出现。
500℃时,吡咯型氮峰强度增加,同时398.8ev处又出现一个新峰,说明有吡啶型氮存在。
吡啶型氮的峰强度比吡咯型氮的峰强度更高,说明所合成NG-Ag试样中吡啶型氮含量较高(见图4d)。
从XPS 图谱计算得的NG-Ag上氮原子百分含量为13.5%(原子百分比)。
这表明,与最近出现的三聚氰胺和尿素合成方法一样,氮杂石墨烯(携带有Ag纳米颗粒)可以通过热处理甘氨酸和硝酸银来合成[15,25]。
在100℃退火的AgNO3–GlY和AgNO3–GLY–GO的拉曼光谱见图5a。
AgNO3–GLY 的拉曼光谱在1045cm-1处显示有一个吸收峰,对应银离子聚合物上的硝酸根离子的伸展带。
这清楚地表明AgNO3–Gly中硝酸银主要以离子聚合物的形式存在[26]。
AgNO3–GLY–GO在1045cm-1处的峰消失,说明GO表面的银离子大部分被还原,因此硝酸银离子聚合物的数量大大的减少。
200℃退火的AgNO3–GLY–GO在1300cm-1(D 带)和1600cm-1(G带)处显示出很强的峰带。
值得指出的是200℃的G带向高频迁移了15cm-1,这可能与银离子沉积在GO上有关[22,23]。