渗氮条件对马氏体时效钢疲劳强度的影响
2Cr13钢的表面气体渗氮处理

2Cr13钢系高铬马氏体不锈钢 ,具有较高的疲劳 强度和良好的综合性能 ,主要用于塑性要求较高及承 受冲击载荷的零件 ,如汽轮机叶片 、大型轴流式压缩机 叶片 、阀体 、紧固件 、轴泵套 、轴承 、耐蚀刃具等 。但由 于 2Cr13钢含碳量较低 ,耐磨和耐蚀性较差 ,在一些耐 磨和耐蚀性要求较高的场合 ,必须经表面强化后才能使 用。目前 ,已出现了一些利用表面强化技术改善 2Cr13 不锈钢摩擦磨损性能的报导 [123 ] ,采用气体渗氮处理不 锈钢主要集中奥氏体不锈钢和双相不锈钢 [4 ] ,而对马氏 体不锈钢进行气体渗氮的相关研究及报导尚不多见 。
Surface n itr id ing for 2Cr13 steel
YANG J ian2qun, L IU Yong, YE Zhu2yu, YANG De2zhuang ( School of M aterials Science and Engineering, Harbin Institute of Technology, Harbin Heilongjiang 150001, China) Abstract: The m icrostructure of 2Cr13 steel samp les after gas nitriding was analyzed w ith op tical m icroscopy, SEM , XRD and m icrohardness tester1W ear behavior of the gas nitrided 2Cr13 steel disk against unitrided 2Cr13 p ins was investigated using a p in2on2disk type tribometer1The results show that gas nitriding at 520 ℃ for 20 h can obtain the nitrided dep th of 2Cr13 steel up to 165 μm1The m icrohardness of samp les after nitriding increased by 215 times compared w ith unitrided samp les1The wear resistance of samp les are increased significantly after nitriding1 It is observed that there are no cracks appearing on the worn surfaces of nitrided 2Cr13 disks while severe cracks are appearing on the worn surfaces of unitrided 2Cr13 p ins1 Key words: 2Cr13 steel; gas nitriding; nitrided layer; wear resistance
新型钢材渗氮及其腐蚀性能研究

新型钢材渗氮及其腐蚀性能研究随着科技的进步和人们生活水平的提高,对各类金属材料的性能要求也越来越高。
钢材作为一种重要的结构材料,它的性能对于工程质量和安全性起着至关重要的作用。
为了提高钢材的综合性能,科研人员一直在不断努力,其中,渗氮技术被广泛应用于改性钢材的研究和应用。
渗氮是将氮气注入到钢材表面,并通过一定的热处理过程使氮原子渗入到钢的晶界和晶内,从而改变钢材的组织结构和性能。
渗氮钢通过渗氮处理能够显著提高钢材的硬度、强度、耐磨性和耐腐蚀性能等,具有很好的应用前景。
渗氮钢材的腐蚀性能是其性能的一个重要方面。
腐蚀是材料在外界介质作用下发生的破坏性变化。
钢材腐蚀主要包括氧化腐蚀、电化学腐蚀和应力腐蚀等。
渗氮钢材的腐蚀性能主要取决于渗氮过程中晶界处形成的化合物,这些化合物能够改善钢材的耐腐蚀性能,并提高其抗腐蚀能力。
对于渗氮钢材的腐蚀性能研究,首先需要对渗氮工艺进行优化。
通过不同的渗氮工艺参数的调整,可以得到不同性能的渗氮层。
针对不同的应用情况,可以选择合适的工艺参数来获得最佳的渗氮效果。
其次,需要对渗氮后的钢材进行腐蚀性能测试。
常见的腐蚀性能测试方法包括电化学测试和重量损失测试。
电化学测试主要包括极化曲线测试、交流阻抗谱测试等,它们可以通过测量钢材在腐蚀介质中的电化学行为来评估钢材的腐蚀性能。
重量损失测试是将钢材放置在腐蚀介质中一段时间后,测量其质量损失来评估钢材的腐蚀性能。
最后,需要综合分析和比较不同渗氮钢材的腐蚀性能数据,评估钢材的抗腐蚀能力。
在实际应用中,常常会选择具有良好腐蚀性能的渗氮钢材来替代常规钢材,以提高工程的耐久性和安全性。
总之,对渗氮钢材的腐蚀性能研究有助于深入了解渗氮工艺对钢材性能的影响,并为工程应用提供科学的依据,从而推动渗氮钢材的进一步发展和应用。
以上是关于新型钢材渗氮及其腐蚀性能研究的1200字以上的基本介绍。
这个题目在细节方面还可以再展开更多的内容,例如具体的渗氮工艺参数及其对腐蚀性能的影响、渗氮钢材在不同介质中的腐蚀行为等。
新型钢材渗氮及其腐蚀性能研究
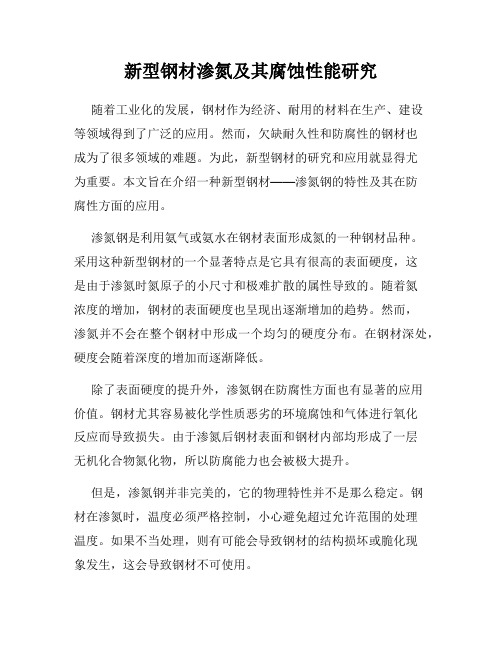
新型钢材渗氮及其腐蚀性能研究随着工业化的发展,钢材作为经济、耐用的材料在生产、建设等领域得到了广泛的应用。
然而,欠缺耐久性和防腐性的钢材也成为了很多领域的难题。
为此,新型钢材的研究和应用就显得尤为重要。
本文旨在介绍一种新型钢材——渗氮钢的特性及其在防腐性方面的应用。
渗氮钢是利用氨气或氨水在钢材表面形成氮的一种钢材品种。
采用这种新型钢材的一个显著特点是它具有很高的表面硬度,这是由于渗氮时氮原子的小尺寸和极难扩散的属性导致的。
随着氮浓度的增加,钢材的表面硬度也呈现出逐渐增加的趋势。
然而,渗氮并不会在整个钢材中形成一个均匀的硬度分布。
在钢材深处,硬度会随着深度的增加而逐渐降低。
除了表面硬度的提升外,渗氮钢在防腐性方面也有显著的应用价值。
钢材尤其容易被化学性质恶劣的环境腐蚀和气体进行氧化反应而导致损失。
由于渗氮后钢材表面和钢材内部均形成了一层无机化合物氮化物,所以防腐能力也会被极大提升。
但是,渗氮钢并非完美的,它的物理特性并不是那么稳定。
钢材在渗氮时,温度必须严格控制,小心避免超过允许范围的处理温度。
如果不当处理,则有可能会导致钢材的结构损坏或脆化现象发生,这会导致钢材不可使用。
此外,渗氮也有其炽热的过程。
必须使用一种称为渗氮的特殊设备来控制温度并保证钢材品质。
这就使得钢材的制造成本大大增加。
而渗氮后,钢材输入到模具制造、壳体制造等环节,可能出现裂口或者锈蚀等问题,这意味着维修成本也会更加昂贵。
在一些领域中,渗氮钢已经被广泛使用。
渗氮钢可以用于生产一些制造钢结构的设备,如各种模具、金属结构件等。
因为渗氮钢具有良好的耐腐蚀性能,所以在这些设备中有更长的使用寿命。
同时,渗氮钢也被应用于汽车制造领域,它的轮辋可以有较长的使用时间,同时进行高负荷、高速度条件下的运动时,可以有效延长车轮使用寿命。
总之,钢材渗氮已经被证明是一种极具应用价值的技术。
钢材渗氮不仅仅提高了表面硬度并提升了钢材的防腐能力。
在保障制造的质量以及使用寿命和安全方面这种特殊的钢材也具有它独特的价值。
合金钢表面渗氮处理
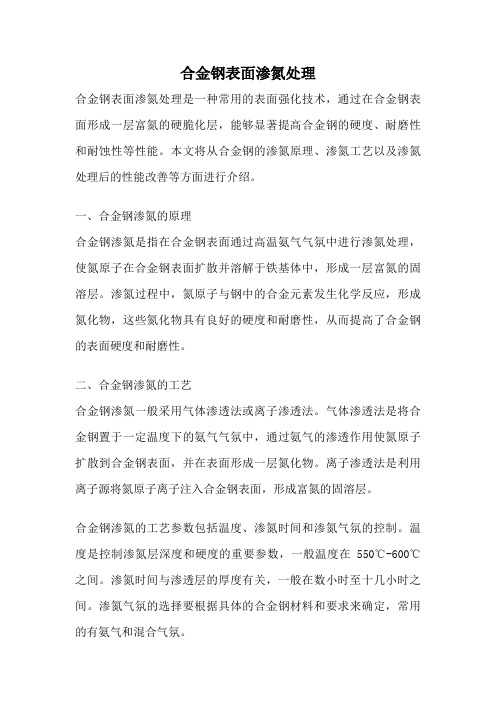
合金钢表面渗氮处理合金钢表面渗氮处理是一种常用的表面强化技术,通过在合金钢表面形成一层富氮的硬脆化层,能够显著提高合金钢的硬度、耐磨性和耐蚀性等性能。
本文将从合金钢的渗氮原理、渗氮工艺以及渗氮处理后的性能改善等方面进行介绍。
一、合金钢渗氮的原理合金钢渗氮是指在合金钢表面通过高温氨气气氛中进行渗氮处理,使氮原子在合金钢表面扩散并溶解于铁基体中,形成一层富氮的固溶层。
渗氮过程中,氮原子与钢中的合金元素发生化学反应,形成氮化物,这些氮化物具有良好的硬度和耐磨性,从而提高了合金钢的表面硬度和耐磨性。
二、合金钢渗氮的工艺合金钢渗氮一般采用气体渗透法或离子渗透法。
气体渗透法是将合金钢置于一定温度下的氨气气氛中,通过氨气的渗透作用使氮原子扩散到合金钢表面,并在表面形成一层氮化物。
离子渗透法是利用离子源将氮原子离子注入合金钢表面,形成富氮的固溶层。
合金钢渗氮的工艺参数包括温度、渗氮时间和渗氮气氛的控制。
温度是控制渗氮层深度和硬度的重要参数,一般温度在550℃-600℃之间。
渗氮时间与渗透层的厚度有关,一般在数小时至十几小时之间。
渗氮气氛的选择要根据具体的合金钢材料和要求来确定,常用的有氨气和混合气氛。
三、合金钢渗氮处理后的性能改善合金钢经过渗氮处理后,表面形成了一层氮化物,这些氮化物具有很高的硬度和耐磨性,能够显著提高合金钢的表面硬度和耐磨性。
同时,渗氮处理还能够提高合金钢的抗疲劳性能和抗腐蚀性能,延长合金钢的使用寿命。
渗氮处理后的合金钢在摩擦、磨损和腐蚀等方面表现出优异的性能。
渗氮层硬度高,能够有效抵抗磨损和划伤,提高合金钢的抗磨损性能。
渗氮层对于腐蚀介质的侵蚀能力较强,能够有效抵抗酸碱腐蚀和氧化腐蚀,提高合金钢的耐蚀性能。
此外,渗氮处理还能够提高合金钢的表面光洁度和装饰性能,使其更具美观性。
合金钢表面渗氮处理是一种有效的表面强化技术,能够显著提高合金钢的硬度、耐磨性和耐蚀性等性能。
通过合理控制渗氮工艺参数,可以获得理想的渗氮效果。
表面纳米化与离子渗氮对304不锈钢的影响

表面纳米化与离子渗氮对304不锈钢的影响王引真;冯雅;孙永兴;冯涛【摘要】为解决304不锈钢硬度低、耐磨性差的问题,本文采用预先表面纳米化,温度400、450℃,保温时间4、6 h,氮氢比1∶3的离子渗氮工艺对试样进行处理,研究纳米化以及渗氮工艺对304不锈钢渗氮层形貌和深度、硬度以及摩擦磨损性能的影响.利用金相显微镜、电子探针显微分析仪(EPMA)、能谱仪(EDS)、显微硬度计和磨损试验机对样品的显微组织、微观形貌、硬度及耐磨性进行了表征.结果表明:304不锈钢经表面纳米化与离子渗氮工艺处理后,渗氮层为0.1~0.2 mm,表面硬度约为1 200 HV0.1,比基体硬度提高了6~7倍,耐磨性也大大增强;但渗氮温度越高,保温时间越长,材料表面耐磨性越差.综合各种影响因素得出在本实验条件下最佳处理工艺为:预先表面纳米化,渗氮温度400℃、保温时间6 h.%To solve the problem of low hardness and poor wear resistance of 304 stainless steel, this research applied the surface nanocrystallization mechanism and treated the sample by plasma nitriding at 400 ℃and 450 ℃ with hol ding time 4 h and 6 h and nitrogen and hydrogen ratio of 1∶3. Influence of the nanocrystallization and nitriding process on the morphology, depth, hardness, and friction, and wear properties of nitrided layer of 304 stainless steel was studied. The microstructure, micro morphology, hardness, and wear resistance of the sample were measured and characterized by using metallographic microscope, EPMA, EDS, micro-hardness tester, and wear testing machine. Results show that after being treated by surface nanocrystallization and plasma nitriding composite process, the nitrided layer of 304 stainless steel was about 0.1~0.2 mm,the microhardness values reached 1 200 HV0.1, which is 6~7 times higher than that of the matrix, and the wear resistance was greatly improved. However, the higher the nitriding temperature was, the longer the holding time was, and the worse the wear resistance of the material surface became. Considering multiple factors, the optimum treatment process was obtained under the experimental condition when the nitriding temperature is 400 ℃ and the holding time is 6 h after surface nanocrystallization.【期刊名称】《材料科学与工艺》【年(卷),期】2019(027)001【总页数】6页(P59-64)【关键词】304不锈钢;表面纳米化;离子渗氮;硬度;耐磨性【作者】王引真;冯雅;孙永兴;冯涛【作者单位】中国石油大学(华东) 材料科学与工程学院, 山东青岛 266580;中国石油大学(华东) 材料科学与工程学院, 山东青岛 266580;中国石油大学(华东) 材料科学与工程学院, 山东青岛 266580;中国石油大学(华东) 材料科学与工程学院, 山东青岛 266580【正文语种】中文【中图分类】TG156.8奥氏体不锈钢具有很强的防锈、耐腐蚀性[1]和良好的可塑性、韧性,被广泛应用于工业、家具装饰行业和食品医疗行业等领域,但其较低的硬度和极差的耐摩擦磨损性能会导致设备因磨损而失效[2-3].奥氏体不锈钢不能同时兼顾耐磨和耐腐蚀特性,使其使用范围受到不小的影响[4].因此,迫切需要改善304不锈钢的耐磨损性能.优化材料表面的组织结构和性能可有效地提高零件的可靠性[5].近年来,常用的表面强化手段有压力扭转、等通道用挤压和表面机械研磨(SMAT)等[6].王少杰等[7]对304不锈钢采用SMAT与离子渗碳复合处理,发现处理后的材料组织内部发生了马氏体转变,渗层晶粒细化,材料的力学性能大为改善,复合处理也显著提高了材料的耐磨性.卢柯课题组[8]利用SMAT和气体渗氮复合技术对纯铁进行表面处理,结果显示,渗氮9 h后采用SMAT处理的材料渗氮层达10 μm,而未采用SMAT 处理的相同材料渗氮效果不佳.该组成员也探究了SMAT与渗氮复合处理对304不锈钢的影响,结果表明,材料的综合机械性能得到提升[9],得到了良好的效果.目前,对304不锈钢进行单一的纳米化处理或者渗氮处理的工艺研究已经比较纯熟,但关于304不锈钢表面纳米化与离子渗氮复合工艺的探索相对较少.本文首先采用超声冲击表面处理技术对304不锈钢进行表面纳米化处理,随后对纳米化工件和未纳米化工件同时进行渗氮处理,研究纳米化及渗氮工艺对304不锈钢渗氮层的形貌、深度、硬度以及摩擦磨损性能的影响.1 实验1.1 试样材料试样材料为304 奥氏体不锈钢,化学成分见表1.选用外径102 mm、管厚6 mm、长度260 mm的奥氏体不锈钢圆管,将其线切割成30 mm×10 mm×4 mm的长方体试样,然后用水砂纸由粗至细将试样打磨平整,随后用酒精冲洗去污.表1 304奥氏体不锈钢化学成分(质量分数/%)Table 1 Chemical composition of 304 austenitic stainless steel (wt.%) CSiMnCrNiMoTiFe0.050.81.517.59.50.0130.011Bal.1.2 试样制备1.2.1 表面超声处理材料表面超声处理采用HK30G型豪克能镜面加工设备,辅助设备为30M70数控加工中心,加工过程中豪克能加工设备的冲击装置代替加工中心原普通刀具加工试样.超声冲击频率为27.2 kHz,电流0.8 A,预压力0.3 MPa,进给速度1 700 mm/min,步距 0.2 mm.1.2.2 低温离子渗氮处理将未纳米化的试样和纳米化的试样同时放入LDMC-15A脉冲离子渗氮设备进行渗氮.氮气与氢气的气流量分别为40、120 mL/min,电压800 V,占空比0.7,温度450、400 ℃,保温时间4、6 h,随炉冷却.为方便分析说明,将不同工艺参数处理的试样标号,如表2所示.表2 304奥氏体不锈钢不同处理工艺的试样编号Table 2 Sample numbers of different processes of AISI 304 austenitic stainless steel编号工艺1#表面纳米化2#渗氮(400 ℃、4 h)3#渗氮(400 ℃、6 h)4#渗氮(450 ℃、4 h)5#渗氮(450 ℃、6 h)6#表面纳米化+渗氮(400 ℃、4 h)7#表面纳米化+渗氮(400 ℃、6 h)8#表面纳米化+渗氮(450 ℃、4 h)9#表面纳米化+渗氮(450 ℃、6 h)1.3 试样表征采用MDJ200 金相显微镜观察组织形貌,选用Marble溶液(CuSO4 4 g,HCl 20 mL,H2O 20 mL)为腐蚀液;采用JXA-8230型电子探针EPMA观察纳米化对试样表面的影响;通过EDS和WDS对渗氮层截面进行元素分布及含量分析;使用MH-3 型显微维氏硬度计测量硬度以及渗氮层深度,条件为试验力0.98 N,保荷15 s;在干摩擦条件下,采用M-2000A型磨损试验机考察基体与渗氮层的摩擦磨损性能,法向载荷50 N,转速360 r/min,时间60 min,摩擦副为GCr15滚动轴承钢,磨损实验前后使用精度为0.0001 g的FA2204B型电子天平测量样品质量,计算磨损量.2 结果与讨论2.1 组织分析2.1.1 表面纳米化对试样组织的影响图1为纳米化前后304不锈钢的表面显微组织形貌,其中图1(a)、(b)分别为母材和1#试样的金相组织形貌,图1(c)、(d)分别为母材和1#试样经电子探针扫描的组织形貌.由图1(a)、(b)可见,表面纳米化处理后晶粒尺寸明显减小,晶粒数量明显增多.这是因为表面纳米化使材料表面产生塑性变形,形成孪晶组织,同时塑性变形也使得晶粒破碎,细化了晶粒,增加了晶粒数量.由图1(c)、(d)可见,母材表面呈分散分布的犁沟,且朝向混乱.表面纳米化处理使得材料表面比母材更平整,且犁沟分散均匀,朝同一方向分布.图1 纳米化前后试样表面显微组织形貌Fig.1 Cross sectional microstructures of the sample before and after surface nanocrystallization: (a) Metallographic structure of the base metal;(b) Metallographic structure of sample 1#; (c) Micro morphology of base metal; (d) Micro morphology of sample 1#2.1.2 离子渗氮工艺参数对渗层组织的影响图2为不同渗氮工艺处理的渗层金相组织形貌.图2(a)、(b)分别为 2#和4#试样的渗层金相组织,可以明显看出,当渗氮时间相同时,450 ℃的白亮层比400 ℃时的厚,但存在较多的灰黑色物质.这是由于当渗氮温度升高时铬元素的扩散速率增加,并与氮原子形成CrN化合物.Cr是使不锈钢拥有耐蚀性的主要元素,铬含量的下降会使不锈钢耐蚀性下降,当被腐蚀剂腐蚀时,耐蚀性弱的区域就呈现出比基体更深的灰黑色.图2 不同渗氮工艺处理后的渗层金相组织Fig.2 Metallographic structure of nitrided layer treated by different nitriding processes: (a) sample 2#; (b) sample 4#图3为4#试样的表面经电子探针扫描的组织形貌.试样表面出现大量的点状凸起.氮原子的渗入和扩散致使周围的Cr向表面扩散,并与氮结合形成CrN;铬是体心立方结构,原子间隙较大,同时由于氮原子较小,所以,氮会固溶于铬形成固溶体.这些点状凸起是离子渗氮后形成的氮化物或者是氮的固溶体.图3 4#试样表面的微观形貌Fig.3 Micro morphology of the surface of sample 4#2.1.3 纳米化与离子渗氮复合工艺对试样组织的影响图4为6#试样的渗层金相组织形貌.可见预先进行表面纳米化处理后的渗层组织出现了缺陷.这是由于在离子渗氮过程中,由于工件是阴极,会经受氮氢阳离子的冲击,从而形成刻蚀痕[10].图4 6#试样渗层金相组织Fig.4 Metallographic structure of the nitrided layer of sample 6#图5为9#试样的表面经电子探针扫描的组织形貌.对比图3和图5可见,复合处理的试样表面比仅渗氮试样有更明显的点状凸起,且呈直线间断分布.这是因为纳米化促进氮扩散,使材料表面的氮固溶物增加;纳米化使材料表层形成朝同一方向分布的孪晶组织,氮在孪晶界的扩散速率高,形成了近似呈直线分布的点状凸起.图5 9#试样表面的微观形貌Fig.5 Micro morphology of the surface of sample 9#2.2 硬度及渗氮深度分析2.2.1 硬度分析图6为不同工艺处理后试样的硬度梯度分布.母材硬度171.8 HV0.1,纳米化后试样表面硬度340.4 HV0.1.渗氮温度对硬度的影响很大,450 ℃渗氮的表面硬度约是400 ℃的2倍,是基体硬度的5~6倍;保温时间对硬度的影响较小,随着保温时间的延长,硬度增加缓慢;渗氮后的表面硬度随距离的增加迅速下降,0.1 mm往后硬度变化曲线趋于与基体硬度持平;纳米化后硬度在0.05~0.5 mm时高于未纳米化50 HV0.1左右,纳米化产生的加工硬化提高了基体硬度.图6 不同工艺处理后试样的硬度梯度分布Fig.6 Microhardness gradient distribution of the sample after different treatments2.2.2 渗氮深度分析图7为不同渗氮工艺处理后的渗层深度分布.由图7可知:温度越高、保温时间越长,渗层越厚;当渗氮工艺参数相同时,纳米化使渗氮深度增加.温度影响氮原子的扩散速率,温度越高,氮原子扩散速率越大,渗氮层深度也就越高.氮扩散需要时间,随着时间的延长,氮扩散更充分,因此渗层越厚.纳米化使试样表面产生塑性变形,晶粒得到细化,形成了很多孪晶、位错等缺陷[11],为氮的扩散增加了额外的通道.此外,纳米化导致材料进行马氏体转变[12].因此,纳米化试样比未纳米化试样渗氮层更深.图7 不同渗氮工艺处理后的渗层深度分布Fig.7 Depth distribution of the nitrided layer after different nitriding processes2.3 摩擦磨损分析图8为不同工艺处理后的试样磨损失重.由图8可知:表面纳米化后摩擦失重增加,试样磨损量多于纳米化,在此渗氮工艺下,未纳米化试样耐磨性低于纳米化试样;随着渗氮温度的升高,保温时间的延长,磨损量越大.渗氮处理的试样磨损量明显小于未渗氮试样,且400 ℃时比450 ℃耐磨性更好,这是因为当渗氮温度较低时,材料表面会形成一层氮过饱和膨胀奥氏体,称为S相,与未渗氮的母材相比,耐磨性显著提高[13-14].图8 不同工艺处理后的试样磨损失重Fig.8 Weight lose of arburized sample after different process treatments 2.4 成分分析图9为4#渗氮试样的渗层经电子探针扫描的组织形貌,图10为其选点的元素的能谱图.图9 4#试样渗层微观形貌Fig.9 Micro morphology of the nitrided layer of sample 4#图10 选点元素能谱图Fig.10 Energy spectrum of the selection of elements表3为该点的元素含量,近似反映了渗氮层元素含量.渗氮层Cr的质量分数达到69.88%,N质量分数为13.60%,而原始304不锈钢的Cr质量分数仅为18%左右.表层富Cr严重将会导致材料表面的脆性增加[15].表3 渗氮层元素含量Table 3 Element composition in nitrided layer元素质量分数/%原子数分数/%N K13.6037.11Si K1.562.12Cr K69.8851.34MnK3.332.32Fe K8.695.95Mo L2.941.173 结论1)304不锈钢经表面纳米化与离子渗氮复合处理后,温度400 ℃时白亮层薄,且渗氮层较薄;温度450 ℃时白亮层厚但呈灰黑色,且渗层较厚.保温时间能增加渗氮层深度,但不明显.表面纳米化能够使试样在同种渗氮工艺下获得更深的渗氮层. 2)渗氮温度对304不锈钢硬度的影响很大,温度越高,获得的硬度越高.保温时间对硬度的影响较小,随着保温时间的延长,硬度有较小的提高;表面纳米化能够有效提高原始表面硬度以及渗后硬度.3)渗氮温度400 ℃、保温4 h,304不锈钢耐磨性最好.随着温度升高、保温时间延长,其硬度增加,耐磨性下降.渗氮处理可能导致材料表面脆化.在本实验条件下,304不锈钢最优处理工艺为:预先表面纳米化,渗氮温度400 ℃,保温时间4 h. 参考文献:【相关文献】[1] LO K H, SHEK C H, LAI J K L. Recent developments in stainless steels[J]. Materials Science & Engineering R, 2009, 65(4): 39-104.[2] 刘静,钱林茂,董汉山,等. 碳化、氮化与碳氮化对316LVM不锈钢微动腐蚀磨损性能的影响[J]. 摩擦学学报, 2009, 29(5): 399-404.LIU Jing, QIAN Linmao, DONG Hanshan, et al. Effect of surface treatment on the fretting wear behavior of medical grade austenitic stainless steels[J]. Tribology, 2009, 29(5): 399-404.[3] 王威,陈淑梅,严伟,等. 氮对冷变形高氮奥氏体不锈钢微观结构的作用[J]. 材料热处理学报, 2010, 31(7): 59-65.WANG Wei, CHEN Shumei, YAN Wei, et al. Effects of nitrogen on microstructure of cold deformed high nitrogen austenitic stainless steels[J]. Transactions of Materials and Heat Treatment, 2010, 31(7): 59-65.DOI:10.13289/j.issn.1009-6264.2010.07.029[4] 童幸生,张婷. 低温离子渗碳对304不锈钢耐磨性影响的研究[J]. 新技术新工艺, 2013(5): 89-91.TONG Xingsheng, ZHANG Ting. Research on wear resistance of 304 stainless steel by low temperature plasma carbuirizing[J]. Journal of New Technology & New Process, 2013(5): 89-91.[5] LOU S, LI Y, ZHOU L, et al. Surface nanocrystallization of metallic alloys with different stacking fault energy induced by laser shock processing[J]. Materials & Design, 2016, 104: 320-326.DOI:10.1016/j.matdes.2016.05.028[6] JAYALAKSHMI M, HUILGOL P, BHAT B R, et al. Microstructural characterization of low temperature plasma-nitrided 316L stainless steel surface with prior severe shot peening[J]. Materials & Design, 2016, 108: 448-454.DOI:10.1016/j.matdes.2016.07.005[7] 王少杰,韩靖,韩月娇,等. 表面纳米化对304不锈钢渗碳层组织和性能的影响[J]. 中国表面工程, 2017, 30(3): 25-30.WANG Shaojie, HAN Jing, HAN Xuejiao, et al. Effect of surface nanocrystallization on the microstructure and properties of carburized layer of 304 stainless stee[J]. China Surface Engineering,2017, 30(3): 25-30.[8] TONG W P, TAO N R, WANG Z B, et al. Nitriding iron at lower temperatures[J]. Science, 2003, 299(5607): 686.[9] ZHANG H W, WANG L, HEI Z K, et al. Low temperature plasma nitriding of AISI 304 stainless steel with nano-structured surface layer[J]. Zeitschrift Fur Metallkunde, 2013,94(10): 1143-1147.[10] 沈烈. 奥氏体不锈钢形变/渗氮复合处理工艺及渗氮层结构与性能研究[D]. 大连:大连海事大学, 2013: 61-63.[11] 韩月娇,韩靖,张雪梅,等. 304不锈钢表面纳米化层的组织和性能[J]. 功能材料, 2016,47(8):8084-8088.HAN Yuejiao, HAN Jing, ZHANG Xue Mei, et al. Microstructure and properties of surface nanostructured layer of 304 stainless steel[J]. Journal of Functional Materials, 2016, 47(8): 8084-8088.DOI: 10.3969/j.issn.1001-9731.2016.08.014[12] 王晓军,侯瑞雪,赵文军,等. 304H不锈钢碳化物析出动力学研究[J]. 腐蚀科学与防护技术, 2014, 26(1): 45-49.WANG Xiaojun, HOU Ruixue, ZHAO Wenjun, et al. Precipitation kinetics of carbides of 304H austenitic stainless steel[J]. Corrosion Science and Protection Technology, 2014,26(1): 45-49.[13] LI C X, BELL T, DONG H. A study of active screen plasma nitriding[J]. Surface Engineering, 2002, 18(3): 174-181.[14] GALLO S C, DONG H. Study of active screen plasma processing conditions for carburising and nitriding austenitic stainless steel[J]. Surface & Coatings Technology, 2009, 203(24): 3669-3675.DOI:10.1016/j.surfcoat.2009.05.045[15] 王亮,许晓磊,许彬,等. 奥氏体不锈钢低温渗氮层的组织与耐磨性[J]. 摩擦学学报,2000, 20(1):67-69.WANG Liang, XU Xiaolei, XU Bin, et al. Microstructure and wear resistance of austenitic stainless steel nitrided layer at low temperature[J]. Tribology, 2000, 20(1): 67-69.DOI:10.16078/j.tribology.2000.01.017。
氮化处理对金属材料疲劳寿命的影响及优化研究
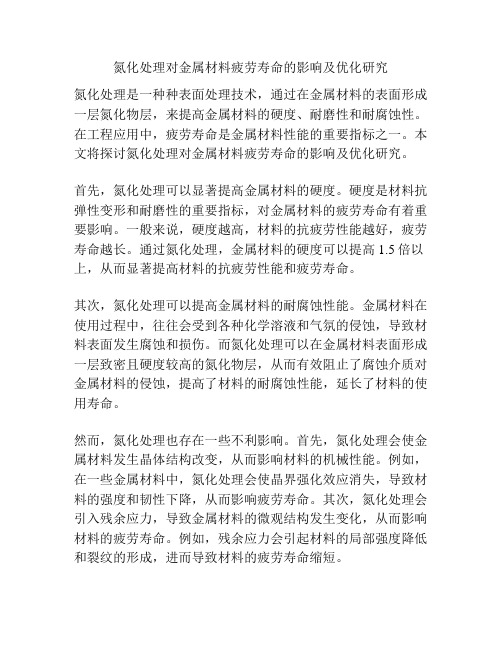
氮化处理对金属材料疲劳寿命的影响及优化研究氮化处理是一种种表面处理技术,通过在金属材料的表面形成一层氮化物层,来提高金属材料的硬度、耐磨性和耐腐蚀性。
在工程应用中,疲劳寿命是金属材料性能的重要指标之一。
本文将探讨氮化处理对金属材料疲劳寿命的影响及优化研究。
首先,氮化处理可以显著提高金属材料的硬度。
硬度是材料抗弹性变形和耐磨性的重要指标,对金属材料的疲劳寿命有着重要影响。
一般来说,硬度越高,材料的抗疲劳性能越好,疲劳寿命越长。
通过氮化处理,金属材料的硬度可以提高1.5倍以上,从而显著提高材料的抗疲劳性能和疲劳寿命。
其次,氮化处理可以提高金属材料的耐腐蚀性能。
金属材料在使用过程中,往往会受到各种化学溶液和气氛的侵蚀,导致材料表面发生腐蚀和损伤。
而氮化处理可以在金属材料表面形成一层致密且硬度较高的氮化物层,从而有效阻止了腐蚀介质对金属材料的侵蚀,提高了材料的耐腐蚀性能,延长了材料的使用寿命。
然而,氮化处理也存在一些不利影响。
首先,氮化处理会使金属材料发生晶体结构改变,从而影响材料的机械性能。
例如,在一些金属材料中,氮化处理会使晶界强化效应消失,导致材料的强度和韧性下降,从而影响疲劳寿命。
其次,氮化处理会引入残余应力,导致金属材料的微观结构发生变化,从而影响材料的疲劳寿命。
例如,残余应力会引起材料的局部强度降低和裂纹的形成,进而导致材料的疲劳寿命缩短。
为了优化氮化处理对金属材料疲劳寿命的影响,研究者们提出了一系列策略。
首先,研究者们通过调节氮化处理的工艺参数,如处理温度、处理时间和处理介质成分,来优化氮化层的性能。
例如,在一些研究中发现,通过降低氮化处理的温度和延长处理的时间,可以减小氮化层和基体之间的残余应力,从而提高材料的疲劳寿命。
其次,研究者们通过改变氮化处理的顺序和组合,如先氮化后退火处理,来优化材料的微观结构和性能。
例如,一些研究表明,通过先进行氮化处理,然后进行退火处理,可以减小氮化层和基体之间的残余应力,提高材料的疲劳寿命。
合金钢表面渗氮处理
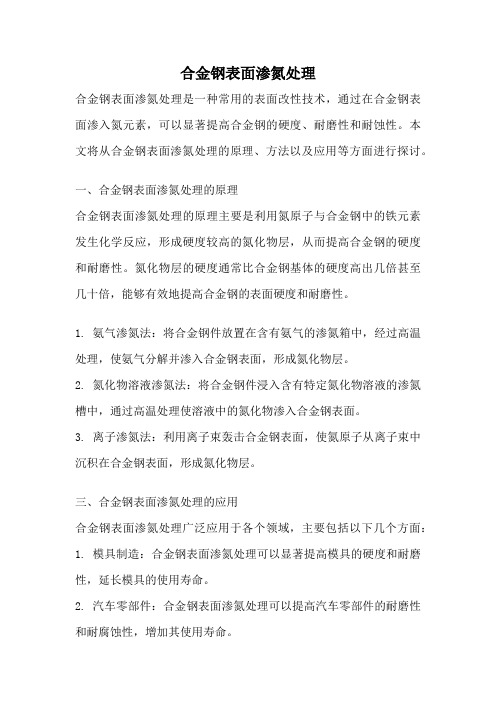
合金钢表面渗氮处理合金钢表面渗氮处理是一种常用的表面改性技术,通过在合金钢表面渗入氮元素,可以显著提高合金钢的硬度、耐磨性和耐蚀性。
本文将从合金钢表面渗氮处理的原理、方法以及应用等方面进行探讨。
一、合金钢表面渗氮处理的原理合金钢表面渗氮处理的原理主要是利用氮原子与合金钢中的铁元素发生化学反应,形成硬度较高的氮化物层,从而提高合金钢的硬度和耐磨性。
氮化物层的硬度通常比合金钢基体的硬度高出几倍甚至几十倍,能够有效地提高合金钢的表面硬度和耐磨性。
1. 氨气渗氮法:将合金钢件放置在含有氨气的渗氮箱中,经过高温处理,使氨气分解并渗入合金钢表面,形成氮化物层。
2. 氮化物溶液渗氮法:将合金钢件浸入含有特定氮化物溶液的渗氮槽中,通过高温处理使溶液中的氮化物渗入合金钢表面。
3. 离子渗氮法:利用离子束轰击合金钢表面,使氮原子从离子束中沉积在合金钢表面,形成氮化物层。
三、合金钢表面渗氮处理的应用合金钢表面渗氮处理广泛应用于各个领域,主要包括以下几个方面:1. 模具制造:合金钢表面渗氮处理可以显著提高模具的硬度和耐磨性,延长模具的使用寿命。
2. 汽车零部件:合金钢表面渗氮处理可以提高汽车零部件的耐磨性和耐腐蚀性,增加其使用寿命。
3. 工具制造:合金钢表面渗氮处理可以提高工具的硬度和耐磨性,使其更加适合高强度切削加工。
4. 轴承制造:合金钢表面渗氮处理可以提高轴承的硬度和耐磨性,降低摩擦系数,减少能量损失。
5. 航空航天领域:合金钢表面渗氮处理可以提高航空航天零部件的耐磨性和耐蚀性,提高其在恶劣环境下的使用性能。
四、合金钢表面渗氮处理的优点和局限性合金钢表面渗氮处理具有以下优点:1. 可以显著提高合金钢材料的硬度和耐磨性,延长其使用寿命。
2. 处理过程简单,操作方便,适用于大批量生产。
3. 处理后的合金钢表面光洁度高,具有较好的装饰性。
然而,合金钢表面渗氮处理也存在一定的局限性:1. 渗氮层深度有限,一般在几十微米到几百微米之间,无法满足某些特殊应用的需求。
外文翻译---离子渗氮对马氏体时效钢微观结构和性能的影响
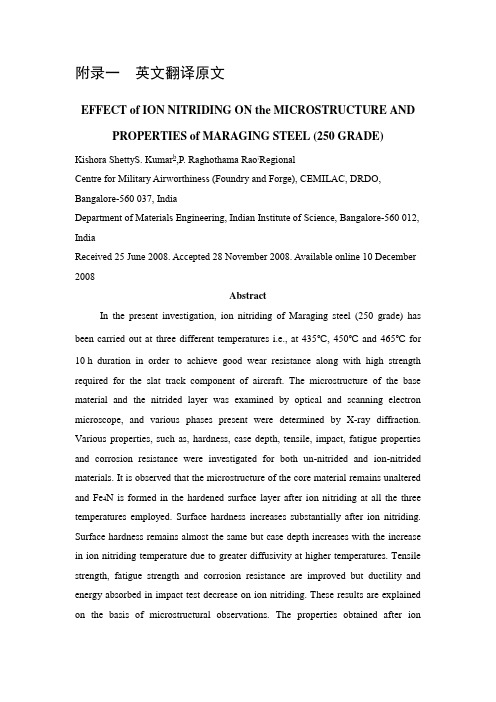
附录一英文翻译原文EFFECT of ION NITRIDING ON the MICROSTRUCTURE AND PROPERTIES of MARAGING STEEL (250 GRADE) Kishora ShettyS. Kumar b,P. Raghothama Rao;RegionalCentre for Military Airworthiness (Foundry and Forge), CEMILAC, DRDO, Bangalore-560 037, IndiaDepartment of Materials Engineering, Indian Institute of Science, Bangalore-560 012, IndiaReceived 25 June 2008. Accepted 28 November 2008. Available online 10 December 2008AbstractIn the present investigation, ion nitriding of Maraging steel (250 grade) has been carried out at three different temperatures i.e., at 435℃, 450℃ and 465℃ for 10 h duration in order to achieve good wear resistance along with high strength required for the slat track component of aircraft. The microstructure of the base material and the nitrided layer was examined by optical and scanning electron microscope, and various phases present were determined by X-ray diffraction. Various properties, such as, hardness, case depth, tensile, impact, fatigue properties and corrosion resistance were investigated for both un-nitrided and ion-nitrided materials. It is observed that the microstructure of the core material remains unaltered and Fe4N is formed in the hardened surface layer after ion nitriding at all the three temperatures employed. Surface hardness increases substantially after ion nitriding. Surface hardness remains almost the same but case depth increases with the increase in ion nitriding temperature due to greater diffusivity at higher temperatures. Tensile strength, fatigue strength and corrosion resistance are improved but ductility and energy absorbed in impact test decrease on ion nitriding. These results are explained on the basis of microstructural observations. The properties obtained after ionnitriding at 450 °C for 10 h are found to be optimum when compared to the other two ion nitriding temperatures.Keywords:Ionnitriding;Maragingsteel;Microstructure;Casedepth;Tensile properties;Fatigue properties1. IntroductionSurface engineering means ‘engineering the surface’ of a material or component to confer surface properties, which are different from the bulk properties of the base material [1]. The purpose may be to reduce wear, minimize corrosion, increase fatigue resistance, reduce frictional energy losses, act as a diffusion barrier, provide thermal insulation, exclude certain wave lengths of radiation, promote radiation, electronic interactions, electrically insulate or simply improve the aesthetic appearance of the surface. Surface engineering processes may broadly be grouped in three categories as (a) modifying surfaces without altering the substrate's chemical composition; these types of processes include transformation hardening, cold deformation, machining and peening, (b) changing the chemistry of the surface region; these types of processes include carburizing, nitriding, anodizing and ion implanting, and (c) adding a layer of material to the surface; these types of processes include weld overlay, painting, metal spraying, plasma spraying, electroplating, bonding, physical vapor deposition and chemical vapor deposition. Nitriding is a surface-hardening process by the introduction of nitrogen into the surface of steel [2]. Process methods for nitriding include gas nitriding, liquid or salt bath nitriding and plasma or ion nitriding. In gas nitriding this is done using a mixture of ammonia gas and dissociated ammonia in suitable proportions. In plasma or ion nitriding a glow discharge technology is used to introduce nascent (elemental) nitrogen to the surface of a metal part for subsequent diffusion into the material[3],[4]and[5]. The plasma assisted surface modification techniques offer a great flexibility and are capable of tailoring desirable chemical and structural surface properties independent of the bulk properties[3]. It has other advantages, such as, no or very thin white layer forms after nitriding and there is no machining or grinding involved after the process, which isparticularly beneficial for complex parts. The hardened surface layer becomes an integral part of the base material and there is no significant reduction in properties of the base material. It is also known to provide the modified surface without dimensional change and distortion of the component. Ion nitriding provides better control of case chemistry and uniformity [3],[6]and[7].Maraging steels belong to a new class of high strength steels with the combination of strength and toughness that are among the highest attainable in general engineering alloys[8]. The term maraging is derived from martensite age hardening and denotes the age hardening of a low carbon, iron–nickel lath martensite matrix [9] and [10]. These steels typically have very high nickel, cobalt and molybdenum and very low carbon content. They are strengthened by the precipitation of intermetallic compounds at a temperature of about 480℃ [11] and [12]. Carbon is treated as an impurity and is kept as low as commercially feasible (<0.03wt.%) in order to minimize the formation of titanium carbide (TiC), which can adversely affect strength, ductility and toughness [9] and [11]. Different maraging steels are designed to provide specific levels of yield strength from 1030 to 2420MPa (150 to 350ksi). Maraging steel (250 grade) possesses a yield strength of 250ksi.For aeronautical components requiring high strength and good wear resistance, such as, slat track, Maraging steel possesses high strength, and good wear resistance can be achieved by ion nitriding process of case nitriding. In conventional gas nitriding process the nitriding temperature is 500℃–550℃[2], which is above the ageing temperature of maraging steel and would result in the over-aging of the core. However, ion nitriding can be carried out at a temperature lower than the aging temperature. Ozbaysal and Inal have demonstrated that the surface hardening of maraging steels without a reduction in core hardness is possible using the ion nitriding process[13]. In the present investigation, ion nitriding of Maraging steel (250 grade) has been carried out at three different temperatures, i.e., 435℃, 450℃ and 465℃ for 10h duration. Microstructural changes are examined with the help of optical microscope, scanning electron microscope (SEM) and X-ray diffraction (XRD).C 0.03 max. 0.01Mn 0.10 max. 0.04Si 0.10 max. 0.04S 0.01 max. 0.005P 0.01 max. 0.006Ni 17.0–19.0 17.29Mo 4.60–5.20 4.89Co 7.0–8.50 7.90Ti 0.30–0.50 0.41Al 0.05–0.15 0.14Zr 0.02 max. 0.005B 0.003 max. 0.001Fe Balance Balance Fig. 1shows a simplified schematic of the ion nitriding installation used for ion nitriding of the material. The work load is supported on a hearth plate inside a double walled, water cooled vacuum chamber, connected to vacuum pumps and gas supply. The chamber is evacuated to a pressure of about 2.5⁎10−2mbar, a pressure low enough for the background level of oxygen to be within the acceptable limits (less than 50ppm) and is then filled with a low pressure mixture of hydrogen and nitrogen. The use of auxiliary AC heaters to heat the cathode to 250⁎ is desirable to minimize cycle time. It can also help to provide better temperature uniformity of the part in ionnitriding treatment. The discharge is ignited using a DC power supply, and pressure and temperature are raised to the desired operating values by controlling gas flow and pressure, applied voltage and current. The discharge can be monitored by control panel and viewed through inspection windows. The work is cathode and the vessel is anode. The furnace is electrically grounded, cool to touch, and quiet in operation. Maraging steel (250 grade) specimens are prepared from the material solutionized and aged at 480⁎ for 3h. These aged specimens are ion-nitrided in the nitriding furnace under vacuum. Plasma is obtained by passing the gas mixture of H2and N2gases in the ratio 3:1 into the chamber and maintaining the pressure of 5mbar. Ion nitriding is carried out at 435⁎., 450⁎ and 465⁎ for10h..Photograph of the specimens before ion nitriding and after ion nitriding at 465°C are shown inFig. 2. After ion nitriding the specimens were subjected to buffing (cloth polishing) to remove the thin white layer formed..Fig. 2.Photograph of the specimens (a) before ion nitriding,Microstructural examination was carried out on NIKON optical microscope and Leo SEM. XRD was carried out on Philips Analytical X-ray Diffractometer. Hardness was measured using Vickers Hardness Tester (Buelher Micromet 2100) with a load of 100g. Tensile tests were carried out on cylindrical specimens having 4mm gauge diameter and 20mm gauge length using TIRA Test 2820S Universal Testing Machine.Impact tests were conducted on Charpy ‘U’ notch specimens having a dimension 10mm⁎10mm⁎55mm using FIE Charpy Impact Testing machine. Constant amplitude LCF tests were carried out on smooth round specimens with 4.5mm gauge diameter and 12mm gauge length using Zwick Roell Universal Testing Machine at an applied stress range of ±1172MPa having stress ratio of −1.Hardness values were measured at five places for each sample, for tensile and impact three samples were tested at each condition and for LCF five samples were tested at each condition and the average value along with standard deviation is reported. Corrosion tests were carried out on specimens having a dimension 10mm⁎10mm⁎18mm for un-nitrided samples and 10mm⁎1mm⁎20mm for ion-nitrided samples using 5% NaCl solution for 144h in a salt spray test chamber.3. Results and discussions3.1. Microstuctural examinationOptical and scanning electron micrographs of un-nitrided and ion-nitrided specimens are shown in Fig. 3 and Fig. 4. These micrographs show a case hardened nitrided layer and martensitic core structure. The thickness of the nitrided layer increases with the increase in ion nitriding temperature due to greater diffusivity at higher temperatures.Fig. 3.Optical micrographs of (a) un-nitrided specimen, (b) ion nitrided at 435℃, (c)Fig. 4.Scanning electron micrographs of (a) un-nitrided specimen, (b) ion nitrided atXRD patterns obtained from the surface of un-nitrided and ion-nitrided specimens are shown in Fig. 5. The un-nitrided specimen exhibits diffraction peaks only due to Fe, whereas the ion-nitrided specimens exhibit additional peaks due to Fe4N as well. The intensity of the Fe peaks progressively decreases and the intensity of the Fe4N peaks progressively increases with the increase in ion nitriding temperature. Thus, the iron nitride formed in the surface hardened layer on ion nitriding is Fe4N. The ratio of H2 and N2 in the gas mixture used for ion nitriding in our investigation is 3:1, which is termed as γ′ gas and forms mono phase (called as γ′ phase) crystal structure of Fe4N in the compound layer. Nitrogen atoms go in to the interstitial sites of iron lattice inanordered manner and form Fe4N [14].Fig. 5.X-ray diffraction pattern of (a) un-nitrided specimen, (b) ion nitrided at 43℃,3.2. Hardness and case depthThe hardness values obtained on un-nitrided and ion nitrided samples are given in Table 2. The average surface hardness of the un-nitrided samples is 616VHN. The average surface hardness of the samples ion nitrided at three different temperatures is more or less the same and is about 50% higher than the un-nitrided sample.Hardness values of the ion-nitrided samples obtained at various depths from surface for the three ion nitriding temperatures are listed in Table 3 and are plotted inFig. 6. The continuous decrease of hardness from surface to the core of the sample suggests the presence of a diffusion zone in which precipitates of nitrides of iron and other metals are formed at the grain boundaries as well as within the grains.These precipitates distort the lattice and pin crystal dislocations and thereby increase the hardness of the surface layer of the ion-nitrided samples[15]. Case depth is taken as the distance from the surface where hardness value is 100 units more than the core hardness. Accordingly, case depth is measured by drawing a line at a hardness value 716VHN and is obtained as 81, 87 and 99µm at 435℃, 450℃and 465℃ion-nitriding temperatures respectively from Fig. 6.Case depth increases with the increase in ion nitriding temperature due to greater diffusivity at higher temperatures. It should be noted that the core hardness at all the three ion nitriding temperatures remains the same as the hardness of the un-nitrided sample. This confirms that the core does not soften by over-aging at all the three ion nitriding temperatures.Table2.Surface hardness of un-nitrided and ion-nitrided specimens Before ion nitriding 616VHN±3After ion nitriding At 435°C 906 VHN±3At 450°C 901 VHN±2At 465°C 907 VHN±4Table3.Hardness values obtained at different depth from the surface afterion-nitridingDistance from Hardness (VHN)the edge(µm) Ion nitrided at435°C Ion nitrided at450°C Ion nitrided at465°C 50 749 ± 7 810 ± 8 822 ± 1075 725 ± 4 734 ± 4 737 ± 5100 689 ± 12 690 ± 5 714 ± 5125 633 ± 8 642 ± 5 659 ± 5150 626 ± 6 631 ± 2 636 ± 3175 618 ± 2 626 ± 4 628 ± 7200 618 ± 3 618 ± 2 620 ± 2225 618 ± 2 618 ± 2 619 ± 2Core 614 ± 3 618 ± 2 618 ± 2Fig. 6.Variation of hardness with depth from the surface for samples ion nitrided at 435℃, 450℃and 465℃.View high quality image (145K)3.3. Tensile propertiesTensile properties for the un-nitrided and ion-nitrided specimens at three different ion-nitriding temperatures are given inTable 4. It is observed that, as compared to the un-nitrided specimen, the nitrided specimens exhibit an increase in ultimate tensile strength (UTS) and 0.2% proof stress (0.2% PS) and a decrease in percentage elongation to fracture (%El) and percentage reduction in area (%RA). The higher UTS and 0.2% PS values and lower %El and %RA values exhibited by nitrided samples can be attributed to the hardened surface layer on ion nitriding.Table 4 Tensile, Impact and LCF properties of un-nitrided and ion-nitrided specimensProperty Un nitrided Ion-nitridedat435 °C Ion-nitrided at450 °C Ion-nitridedat465 °C UTS (MPa) 1697 ± 4 1740 ± 105 1863 ± 15 1699 ± 140.2% PS (MPa) 1616 ± 9 1711 ± 120 1793 ± 32 1628 ± 16% El 9.1 ± 0.3 5.8 ± 1.1 6.2 ± 0.5 7 ± 0% RA 57.0 ± 2.6 34.5 ± 0.7 27.7 ± 2.5 35 ± 0 Energy absorbedinimpact tes t 21.7 ± 0.6 18.7 ± 0.6 18.3 ± 1.2 14 ± 1 (Joules)No. of cycles to4700 ± 1113 11017 ± 5748 9366 ± 3557 9675 ± 6811 The nitrided specimen can be treated as a composite material consisting of martensitic core of about 3.7mm diameter surrounded by a thin nitrided layer of about 0.15mm thickness (as shown inFig.6), and a rough estimate of 0.2% PS can be obtained by applying the rule of mixture, as follows:(1)where,σC0.2% PS of the nitrided specimenσf0.2% PS of the nitrided layer (calculated below)σm0.2% PS of the un-nitrided core (assumed to be the same as that of the un-nitrided specimen, i.e., 1616MPa)A C cross section area of the nitrided specimen (12.57mm2)A f cross section area of the nitrided layer (1.82mm2)A m cross section area of the un-nitrided core (10.75mm2).It is observed fromFig. 6that the hardness of the nitrided specimens approaches the hardness of the core material at a depth of about 150 µm from the surface for all the three ion nitriding temperatures. The average hardness of the nitrided layer is obtained by calculating the area under the polynomial curve fitted up to a depth of 150 µm and dividing it by 150µm in Fig. 6for the samples ion nitrided at 435℃, 450℃and 465℃. These average hardness values are converted into 0.2% PS using Tabor's relation[16]and[17](2)σf=VHN/3.Thus,σf values at 435℃, 450℃and 465℃ion nitriding temperatures are obtained as 2390, 2449 and 2487MPa respectively. The σC values using Eq. (1) at 435℃, 450℃and 465℃ ion nitriding temperatures are obtained as 1728, 1737 and 1742 MPa respectively. Thus calculated 0.2% PS value matches closely with the experimentally obtained value for the specimens nitrided at 435℃, as shown inTable 4. The estimated value of 0.2% PS should further increase with the increase in ion nitriding temperature. However, the experimental results inTable 4show an increase in 0.2% PS value from 435℃to 450℃but a decrease from 450℃to 465℃. Perhaps, more number of tests is required to establish the experimental values without ambiguity. However, the important outcome from the application point of view is that the surface hardness has been substantially increased without sacrificing the strength of the bulk material.3.4. Impact propertiesEnergy absorbed by the Charpy ‘U’ notch specimens in the impact test for the un-nitrided and ion-nitrided samples are shown in Table 4. It is observed that the energy absorbed is reduced after ion nitriding and the reduction increases with the increase in ion nitriding temperature. Ion nitriding imparts a hardened surface layer all over the specimen including the notched area. This hardened surface layer will make the material more brittle and reduce the energy absorbed as compared to the un-nitrided specimen. The decrease in energy absorbed with the increase in ionnitriding temperature may be attributed to the increase in case depth with the increase in ion nitriding temperature.3.5. Low cycle fatigue propertiesNumber of cycles to failure in LCF tests for un-nitrided samples and ion-nitrided specimens are shown inTable 4. It is observed that there is a lot of scatter, as is usually encountered with fatigue data. It is also observed that the scatter is more for the ion-nitrided specimens as compared to the un-nitrided specimens. However, the ion-nitrided specimens undoubtedly exhibit an improvement in fatigue life. The formation of the precipitates of nitride in the diffusion zone results in the lattice expansion. The core material, in an attempt to maintain the original dimension, holds the nitrided case in compression. This compressive stress essentially lowers the magnitude of the applied tensile stress on the material and thus effectively increases the fatigue life of the specimen[15].3.6. Corrosion resistanceFig. 7shows the photograph of the samples after salt spray test for 144h exposure. It was observed that corrosion started in un-nitrided samples after 48h of exposure uniformly throughout the sample and there was no corrosion in ion-nitrided samples even after 144h of exposure. Thus, salt spray test results show an improvement in corrosion resistance after ion nitriding. It is reported that Fe4N formed after ion nitriding in the surface layer is relatively stable in corrosive medium[18]. The hardened surface layer is also uniform and continuous throughout the surface. These factors contribute to the improvement in the corrosion resistance of the material.Fig. 7.Photograph of the samples after the salt spray test.View high quality image (371K)4. ConclusionsIn the present investigation, Maraging steel (250 Grade) was ion nitrided at 435℃,450℃and 465℃. The following conclusions can be drawn from this investigation:1. Microstructure of core remains un-altered after ion nitriding.2. The iron nitride formed in the hardened surface layer is Fe4N for all the three ion nitriding temperatures.3. Ion nitriding improves the surface hardness of Maraging steel (250 grade) substantially. Surface hardness remains almost the same but case depth increases with the increase in ion nitriding temperature due to greater diffusivity at higher temperatures.4. Tensile strength, fatigue strength, and corrosion resistance also improve on ion nitriding. Tensile strength increases since the nitrided layer is much harder; fatigue strength improves due to residual compressive stresses at the surface arising from the formation of nitrided layer; and corrosion resistance improves because the nitrided layer is relatively stable in corrosive medium.5. Ductility in terms of %El and %RA and energy absorbed in impact test decrease on ion nitriding as a result of formation of hard and brittle nitrided layer.6. Properties obtained after ion nitriding at 450℃for 10h are optimum when compared with the other two ion nitriding temperatures for the same duration.AcknowledgementsThe authors wish to thank Mr. B. S. Vedaprakash, Group Director (Propulsion), and Mr. K. Tamilmani, Chief Executive (Airworthiness), CEMILAC, Bangalore, for their support.References[1]B. Kumar, N.C. Upadhyay,IIM Met. News, 8 (2005), p. 5[2] ASM Handbook, vol. 5, American Society for Metals, Metals Park, OH, 1999, 738.[3] T. Spalvins,Ion Nitriding, Conference proceedings, ASM International (1986), p. 1[4] W. Moller, S. Parascandola, T. Telbizova, R. Gunzel, E. RichterSurf. Coat. Technol., 136 (2001), p. 73[5] ASM Handbook, vol. 5, American Society for Metals, Metals Park, OH, 1999, 759.[6] B. Edenhofer,Production ion nitriding,Met. Prog. (1976), p. 181[7]EPRI Centre for Materials Fabrication;Ion nitriding, 2 (5) (1994), p. 1[8] B. Gupta, V. Gopalakrishna, J.S. Yadav, A. Kumar, B. SahaAerospace Materials, S. Chand and Company, New Delhi (1996), p. 695[9] ASM Handbook, V ol. 1, American Society for Metals, Metals Park, OH, 1990, 793.[10] S. Morito, X. Huang, T. Furuhara, T. Maki, N. Hansen;Acta Mater., 54 (2006), p.5323[11] ASM Handbook, V ol. 4, American Society for Metals, Metals Park, OH, 1991, 219.[12] INCO Databook (1976) 351.[13] K. Ozbaysal, O.T. Inal;‘Ion Nitriding’, American Society for Metals, Metals Park,OH (1986), p. 97[14] W. Kovacs, W. Russel, An Introduction to Ion nitriding, What is it? Why is itused? Where is it used? Ion Nitriding Conference Proceeding, Cleveland, Ohio (1986) 9.[15] J. O Brien M, D. Goodman, Plasma (Ion) Nitriding, ASM Handbook, vol. 4,American Society for Metals, Metals Park, OH, 1991, 420.[16] A.E. Tekkaya;Steel Res., 71 (2001), p. 304[17] G.E. Dieter;Mechanical Metallurgy, Mc Graw-Hill Book Company, London(1988), p. 407[18] K.Y. Fu Ricky, D.L. Tang, G.J. Wan, K. Chu Paul;Surf. Coat. Technol., 201(2007), p. 4879附录二英文翻译离子渗氮对马氏体时效钢微观结构和性能的影响摘要目前调查表明,渗氮后的马氏体时效钢(250级)分别在435⁎、450⁎、465⁎下持续10小时,目的是为了使飞机机翼获得良好的耐磨性,伴随高强度。
渗氮工艺基本原理,特点及优缺点
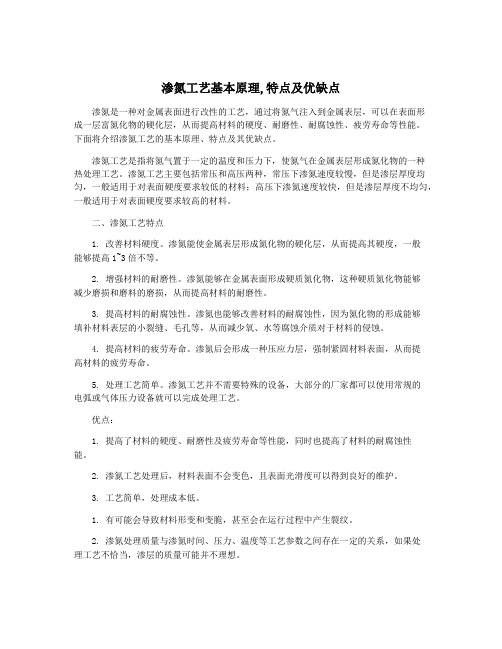
渗氮工艺基本原理,特点及优缺点渗氮是一种对金属表面进行改性的工艺,通过将氮气注入到金属表层,可以在表面形成一层富氮化物的硬化层,从而提高材料的硬度、耐磨性、耐腐蚀性、疲劳寿命等性能。
下面将介绍渗氮工艺的基本原理、特点及其优缺点。
渗氮工艺是指将氮气置于一定的温度和压力下,使氮气在金属表层形成氮化物的一种热处理工艺。
渗氮工艺主要包括常压和高压两种,常压下渗氮速度较慢,但是渗层厚度均匀,一般适用于对表面硬度要求较低的材料;高压下渗氮速度较快,但是渗层厚度不均匀,一般适用于对表面硬度要求较高的材料。
二、渗氮工艺特点1. 改善材料硬度。
渗氮能使金属表层形成氮化物的硬化层,从而提高其硬度,一般能够提高1~3倍不等。
2. 增强材料的耐磨性。
渗氮能够在金属表面形成硬质氮化物,这种硬质氮化物能够减少磨损和磨料的磨损,从而提高材料的耐磨性。
3. 提高材料的耐腐蚀性。
渗氮也能够改善材料的耐腐蚀性,因为氮化物的形成能够填补材料表层的小裂缝、毛孔等,从而减少氧、水等腐蚀介质对于材料的侵蚀。
4. 提高材料的疲劳寿命。
渗氮后会形成一种压应力层,强制紧固材料表面,从而提高材料的疲劳寿命。
5. 处理工艺简单。
渗氮工艺并不需要特殊的设备,大部分的厂家都可以使用常规的电弧或气体压力设备就可以完成处理工艺。
优点:1. 提高了材料的硬度、耐磨性及疲劳寿命等性能,同时也提高了材料的耐腐蚀性能。
2. 渗氮工艺处理后,材料表面不会变色,且表面光滑度可以得到良好的维护。
3. 工艺简单,处理成本低。
1. 有可能会导致材料形变和变脆,甚至会在运行过程中产生裂纹。
2. 渗氮处理质量与渗氮时间、压力、温度等工艺参数之间存在一定的关系,如果处理工艺不恰当,渗层的质量可能并不理想。
3. 渗氮处理的深度较浅,一般只能够处理金属的表层,如果需要提高整个材料的硬度,则需要采用其他处理工艺。
总之,渗氮工艺是一种较为常见的表面处理工艺,主要用于提高金属材料的硬度、耐磨性、耐腐蚀性等性能,工艺简单,处理成本低,但也存在一定的缺点。
钢的渗氮--及热处理不良分析
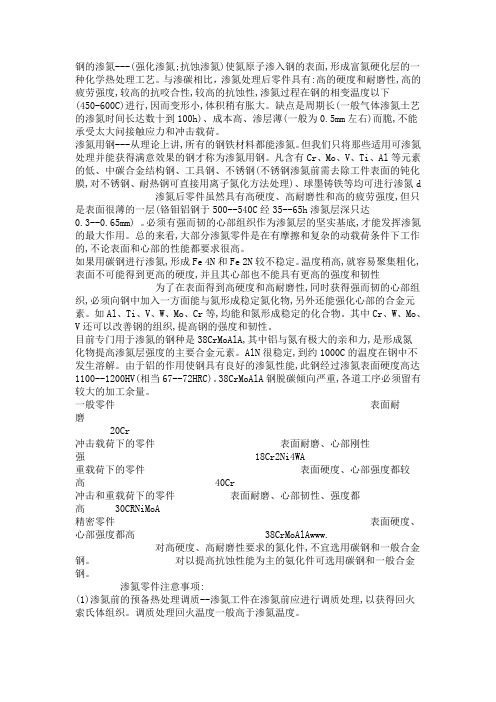
钢的渗氮---(强化渗氮;抗蚀渗氮)使氮原子渗入钢的表面,形成富氮硬化层的一种化学热处理工艺。
与渗碳相比,渗氮处理后零件具有:高的硬度和耐磨性,高的疲劳强度,较高的抗咬合性,较高的抗蚀性,渗氮过程在钢的相变温度以下(450-600C)进行,因而变形小,体积稍有胀大。
缺点是周期长(一般气体渗氮土艺的渗氮时间长达数十到100h)、成本高、渗层薄(一般为0.5mm左右)而脆,不能承受太大问接触应力和冲击载荷。
渗氮用钢---从理论上讲,所有的钢铁材料都能渗氮。
但我们只将那些适用可渗氮处理并能获得满意效果的钢才称为渗氮用钢。
凡含有Cr、Mo、V、Ti、Al等元素的低、中碳合金结构钢、工具钢、不锈钢(不锈钢渗氮前需去除工件表面的钝化膜,对不锈钢、耐热钢可直接用离子氮化方法处理)、球墨铸铁等均可进行渗氮d 渗氮后零件虽然具有高硬度、高耐磨性和高的疲劳强度,但只是表面很薄的一层(铬钼铝钢于500--540C经35--65h渗氮层深只达0.3--0.65mm) 。
必须有强而韧的心部组织作为渗氮层的坚实基底,才能发挥渗氮的最大作用。
总的来看,大部分渗氮零件是在有摩擦和复杂的动载荷条件下工作的,不论表面和心部的性能都要求很高。
如果用碳钢进行渗氮,形成Fe 4N和Fe 2N较不稳定。
温度稍高,就容易聚集粗化,表面不可能得到更高的硬度,并且其心部也不能具有更高的强度和韧性为了在表面得到高硬度和高耐磨性,同时获得强而韧的心部组织,必须向钢中加入一方面能与氮形成稳定氮化物,另外还能强化心部的合金元素。
如Al、Ti、V、W、Mo、Cr等,均能和氮形成稳定的化合物。
其中Cr、W、Mo、V还可以改善钢的组织,提高钢的强度和韧性。
目前专门用于渗氮的钢种是38CrMoAlA,其中铝与氮有极大的亲和力,是形成氮化物提高渗氮层强度的主要合金元素。
AlN很稳定,到约1000C的温度在钢中不发生溶解。
由于铝的作用使钢具有良好的渗氮性能,此钢经过渗氮表面硬度高达1100--1200HV(相当67--72HRC)。
氮对低碳马氏体不锈钢组织和性能的影响
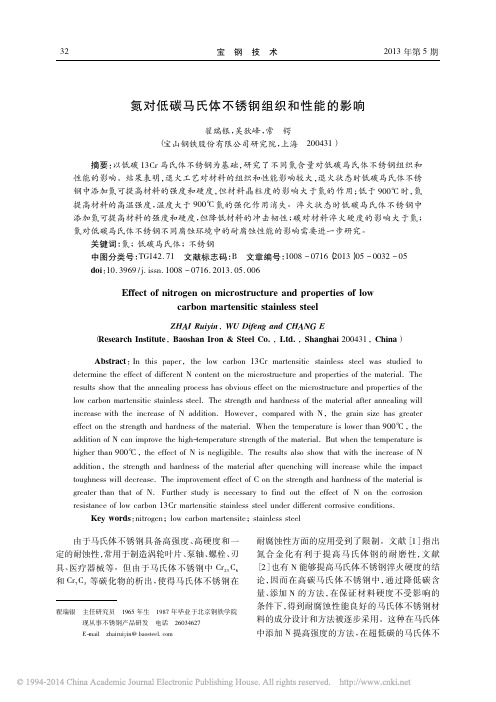
3# 0. 040 0. 44 1. 52 0. 012 0. 011 12. 29 0. 026 0
4# 0. 060 0. 31 1. 40 0. 007 0. 010 12. 47 0. 038 0
5# 0. 061 0. 31 1. 48 0. 006 0. 013 12. 75 0. 068 0
Fig. 2 Mechanical properties of annealed samples from 13Cr stainless steel with different N content
2. 2 高温强度 图 3 给出了 2#、4#、5#试样的高温强度。可见
而材料的冲击韧性呈现降低的趋势。
1 试验材料和方法
试验材料的化学成分见表 1。试验材料在 120kg 真空感应炉冶炼,然后浇注成小钢锭,钢锭 经 1 200℃ 锻造开坯,再由四辊热轧试验机轧制成 4. 5 mm 厚钢板。1#、3# 试样经 760℃ 保温 20 min 退火,4# ~ 6# 试样经 760℃ 保温 120 min 退火,检 测性能; 退火试样经 950℃ 保温 20 min 空冷淬火, 实验室检测性能。2 # 试样取自大生产热轧材料。
翟瑞银 主任研究员 1965 年生 1987 年毕业于北京钢铁学院 现从事不锈钢产品研发 电话 26034627 E-mail zhairuiyin@ baosteel. com
耐腐蚀性方面的应用受到了限制。文献[1]指出 氮合 金 化 有 利 于 提 高 马 氏 体 钢 的 耐 磨 性,文 献 [2]也有 N 能够提高马氏体不锈钢淬火硬度的结 论,因 而 在 高 碳 马 氏 体 不 锈 钢 中,通 过 降 低 碳 含 量、添加 N 的方法,在保证材料硬度不受影响的 条件下,得到耐腐蚀性能良好的马氏体不锈钢材 料的成分设计和方法被逐步采用。这种在马氏体 中添加 N 提高强度的方法,在超低碳的马氏体不
热处理知识——渗碳和渗氮
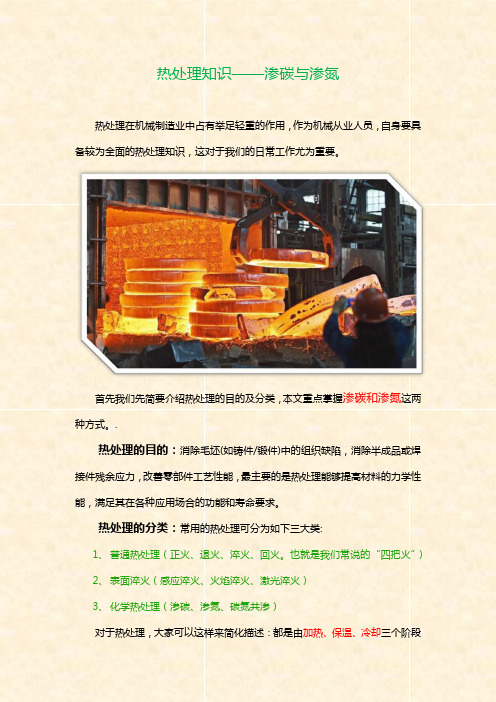
热处理知识——渗碳与渗氮热处理在机械制造业中占有举足轻重的作用,作为机械从业人员,自身要具备较为全面的热处理知识,这对于我们的日常工作尤为重要。
首先我们先简要介绍热处理的目的及分类,本文重点掌握渗碳和渗氮这两种方式。
.热处理的目的:消除毛坯(如铸件/锻件)中的组织缺陷,消除半成品或焊接件残余应力,改善零部件工艺性能,最主要的是热处理能够提高材料的力学性能,满足其在各种应用场合的功能和寿命要求。
热处理的分类:常用的热处理可分为如下三大类:1、普通热处理(正火、退火、淬火、回火。
也就是我们常说的“四把火”)2、表面淬火(感应淬火、火焰淬火、激光淬火)3、化学热处理(渗碳、渗氮、碳氮共渗)对于热处理,大家可以这样来简化描述:都是由加热、保温、冷却三个阶段组成。
不同热处理方法区别就在于这三个阶段,加热方式,加热温度或者加热的速度不同;保温方式,保温环境或者保温时间不同等;冷却的速度及冷却的环境不同等。
进入正文,主要介绍渗碳和渗氮渗碳定义:把零部件置于渗碳介质中,加热到单相奥氏体区,保温一定时间,使碳原子渗入钢表层的化学热处理工艺。
目的:使零件表面具有高硬度、耐磨及疲劳极限,心部具有较高的强度和韧性。
常用钢种:含碳量0.1%-0.25%的碳钢或合金(即低碳钢和低碳合金钢)渗碳方法:常用的气体渗碳法:工件置于密闭的加热炉中,滴入煤油、丙酮、甲苯等在高温(900-950°C)下分解出CO、CO2、CH4等,气体再分解出活性碳原子,随后活性碳原子溶于高温奥氏体中,随着时间进行,碳原子逐渐向内扩散,深度主要取决于保温时间,可大约按0.2mm/h的速度近似计算。
关键技术点:1)表面含碳量最好在0.85%-1.05%内,若含碳量过低,淬火、低温回火后得到的回火马氏体,含碳量较低,硬度低,耐磨性差。
2)在一定的渗碳层深度范围内,随渗碳层深度的增加,疲劳极限、抗弯强度及耐磨性都将增加,但渗碳层深度超过一定限度后,疲劳极限反随着渗碳层深度的增加而降低。
离子渗氮对2Cr13不锈钢磨损及冲蚀行为的影响(1)
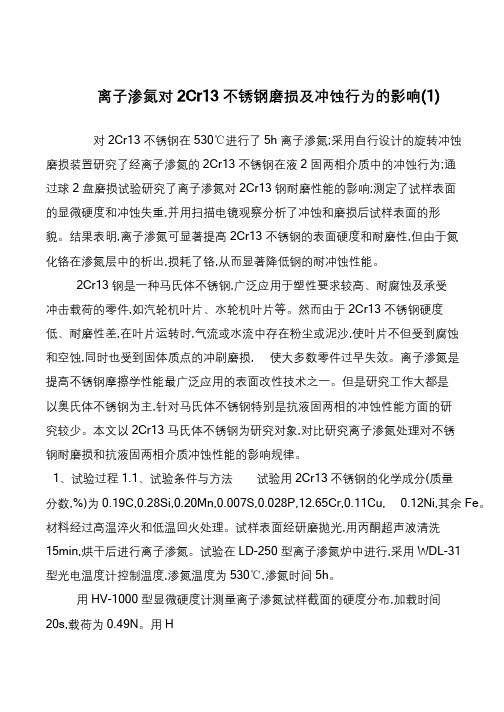
书山有路勤为径,学海无涯苦作舟离子渗氮对2Cr13 不锈钢磨损及冲蚀行为的影响(1)对2Cr13 不锈钢在530℃进行了5h 离子渗氮;采用自行设计的旋转冲蚀磨损装置研究了经离子渗氮的2Cr13 不锈钢在液2 固两相介质中的冲蚀行为;通过球2 盘磨损试验研究了离子渗氮对2Cr13 钢耐磨性能的影响;测定了试样表面的显微硬度和冲蚀失重,并用扫描电镜观察分析了冲蚀和磨损后试样表面的形貌。
结果表明,离子渗氮可显著提高2Cr13 不锈钢的表面硬度和耐磨性,但由于氮化铬在渗氮层中的析出,损耗了铬,从而显著降低钢的耐冲蚀性能。
2Cr13 钢是一种马氏体不锈钢,广泛应用于塑性要求较高、耐腐蚀及承受冲击载荷的零件,如汽轮机叶片、水轮机叶片等。
然而由于2Cr13 不锈钢硬度低、耐磨性差,在叶片运转时,气流或水流中存在粉尘或泥沙,使叶片不但受到腐蚀和空蚀,同时也受到固体质点的冲刷磨损, 使大多数零件过早失效。
离子渗氮是提高不锈钢摩擦学性能最广泛应用的表面改性技术之一。
但是研究工作大都是以奥氏体不锈钢为主,针对马氏体不锈钢特别是抗液固两相的冲蚀性能方面的研究较少。
本文以2Cr13 马氏体不锈钢为研究对象,对比研究离子渗氮处理对不锈钢耐磨损和抗液固两相介质冲蚀性能的影响规律。
1、试验过程1.1、试验条件与方法试验用2Cr13 不锈钢的化学成分(质量分数,%)为0.19C,0.28Si,0.20Mn,0.007S,0.028P,12.65Cr,0.11Cu, 0.12Ni,其余Fe。
材料经过高温淬火和低温回火处理。
试样表面经研磨抛光,用丙酮超声波清洗15min,烘干后进行离子渗氮。
试验在LD-250 型离子渗氮炉中进行,采用WDL-31型光电温度计控制温度,渗氮温度为530℃,渗氮时间5h。
用HV-1000 型显微硬度计测量离子渗氮试样截面的硬度分布,加载时间20s,载荷为0.49N。
用H。
渗氮钢实验报告

一、实验目的1. 了解渗氮钢的基本性质和渗氮工艺。
2. 掌握渗氮实验的操作步骤和注意事项。
3. 分析渗氮处理后渗氮钢的表面硬度、耐磨性、疲劳强度等性能变化。
二、实验原理渗氮钢是一种通过渗氮工艺处理,在钢表面形成一层富氮硬化层的材料。
渗氮工艺主要包括气体渗氮、离子渗氮和等离子体渗氮等。
本实验采用气体渗氮工艺,通过在钢表面引入氮原子,形成氮化层,从而提高钢的表面硬度、耐磨性、疲劳强度等性能。
三、实验材料及设备1. 实验材料:42CrMo渗氮钢棒材,直径30mm,长度100mm。
2. 实验设备:气体渗氮炉、温度控制器、炉内气氛分析仪、金相显微镜、显微硬度计、万能试验机。
四、实验步骤1. 预处理:将渗氮钢棒材进行表面清洗,去除油污和氧化物。
2. 氮气预处理:将渗氮钢棒材放入渗氮炉中,通入氮气进行预处理,温度控制在300℃左右,时间为2小时。
3. 渗氮:将预处理后的渗氮钢棒材放入渗氮炉中,通入氮气进行渗氮处理。
渗氮温度、时间、气氛等参数如下表所示:| 渗氮温度(℃) | 渗氮时间 (h) | 氮气流量 (L/h) | 氮气纯度 (%) ||--------------|--------------|----------------|--------------|| 530 | 35 | 100 | 99.999 |4. 冷却:渗氮结束后,将渗氮钢棒材取出,自然冷却至室温。
5. 性能测试:对渗氮后的渗氮钢棒材进行表面硬度、耐磨性、疲劳强度等性能测试。
五、实验结果与分析1. 表面硬度:渗氮处理后,渗氮钢棒材的表面硬度显著提高,达到HV 1000以上,比未渗氮钢的表面硬度提高了约50%。
2. 耐磨性:渗氮处理后,渗氮钢棒材的耐磨性得到显著提高,耐磨性提高了约40%。
3. 疲劳强度:渗氮处理后,渗氮钢棒材的疲劳强度得到显著提高,疲劳强度提高了约30%。
4. 金相组织:渗氮处理后,渗氮钢棒材的表面形成了一层富氮硬化层,主要由氮化物、碳化物和奥氏体组成。
QPQ盐浴复合热处理中渗氮温度对4145钢疲劳寿命的影响
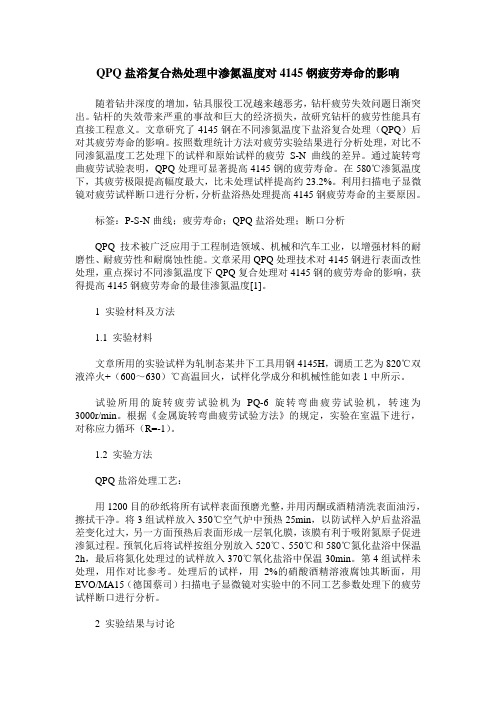
QPQ盐浴复合热处理中渗氮温度对4145钢疲劳寿命的影响随着钻井深度的增加,钻具服役工况越来越恶劣,钻杆疲劳失效问题日渐突出。
钻杆的失效带来严重的事故和巨大的经济损失,故研究钻杆的疲劳性能具有直接工程意义。
文章研究了4145钢在不同渗氮温度下盐浴复合处理(QPQ)后对其疲劳寿命的影响。
按照数理统计方法对疲劳实验结果进行分析处理,对比不同渗氮温度工艺处理下的试样和原始试样的疲劳S-N曲线的差异。
通过旋转弯曲疲劳试验表明,QPQ处理可显著提高4145钢的疲劳寿命。
在580℃渗氮温度下,其疲劳极限提高幅度最大,比未处理试样提高约23.2%。
利用扫描电子显微镜对疲劳试样断口进行分析,分析盐浴热处理提高4145钢疲劳寿命的主要原因。
标签:P-S-N曲线;疲劳寿命;QPQ盐浴处理;断口分析QPQ技术被广泛应用于工程制造领域、机械和汽车工业,以增强材料的耐磨性、耐疲劳性和耐腐蚀性能。
文章采用QPQ处理技术对4145钢进行表面改性处理,重点探讨不同渗氮温度下QPQ复合处理对4145钢的疲劳寿命的影响,获得提高4145钢疲劳寿命的最佳渗氮温度[1]。
1 实验材料及方法1.1 实验材料文章所用的实验试样为轧制态某井下工具用钢4145H,调质工艺为820℃双液淬火+(600~630)℃高温回火,试样化学成分和机械性能如表1中所示。
试验所用的旋转疲劳试验机为PQ-6旋转弯曲疲劳试验机,转速为3000r/min。
根据《金属旋转弯曲疲劳试验方法》的规定,实验在室温下进行,对称应力循环(R=-1)。
1.2 实验方法QPQ盐浴处理工艺:用1200目的砂纸将所有试样表面预磨光整,并用丙酮或酒精清洗表面油污,擦拭干净。
将3组试样放入350℃空气炉中预热25min,以防试样入炉后盐浴温差变化过大,另一方面预热后表面形成一层氧化膜,该膜有利于吸附氮原子促进渗氮过程。
预氧化后将试样按组分别放入520℃、550℃和580℃氮化盐浴中保温2h,最后将氮化处理过的试样放入370℃氧化盐浴中保温30min。
渗氮工艺基本原理,特点及优缺点
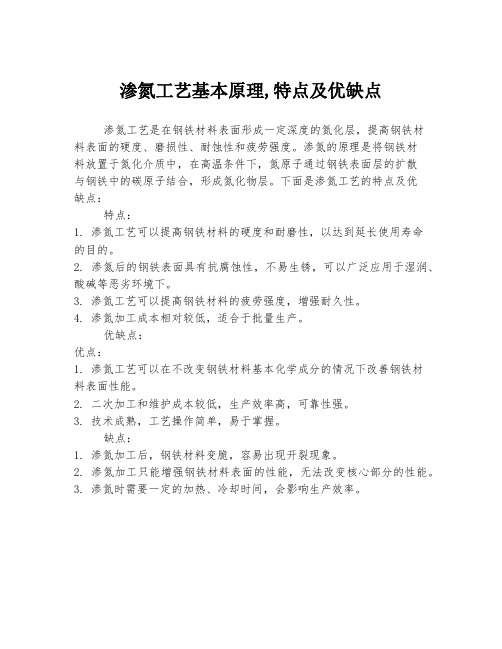
渗氮工艺基本原理,特点及优缺点
渗氮工艺是在钢铁材料表面形成一定深度的氮化层,提高钢铁材
料表面的硬度、磨损性、耐蚀性和疲劳强度。
渗氮的原理是将钢铁材
料放置于氮化介质中,在高温条件下,氮原子通过钢铁表面层的扩散
与钢铁中的碳原子结合,形成氮化物层。
下面是渗氮工艺的特点及优
缺点:
特点:
1. 渗氮工艺可以提高钢铁材料的硬度和耐磨性,以达到延长使用寿命
的目的。
2. 渗氮后的钢铁表面具有抗腐蚀性,不易生锈,可以广泛应用于湿润、酸碱等恶劣环境下。
3. 渗氮工艺可以提高钢铁材料的疲劳强度,增强耐久性。
4. 渗氮加工成本相对较低,适合于批量生产。
优缺点:
优点:
1. 渗氮工艺可以在不改变钢铁材料基本化学成分的情况下改善钢铁材
料表面性能。
2. 二次加工和维护成本较低,生产效率高,可靠性强。
3. 技术成熟,工艺操作简单,易于掌握。
缺点:
1. 渗氮加工后,钢铁材料变脆,容易出现开裂现象。
2. 渗氮加工只能增强钢铁材料表面的性能,无法改变核心部分的性能。
3. 渗氮时需要一定的加热、冷却时间,会影响生产效率。
氮化处理对金属材料疲劳裂纹扩展的影响研究
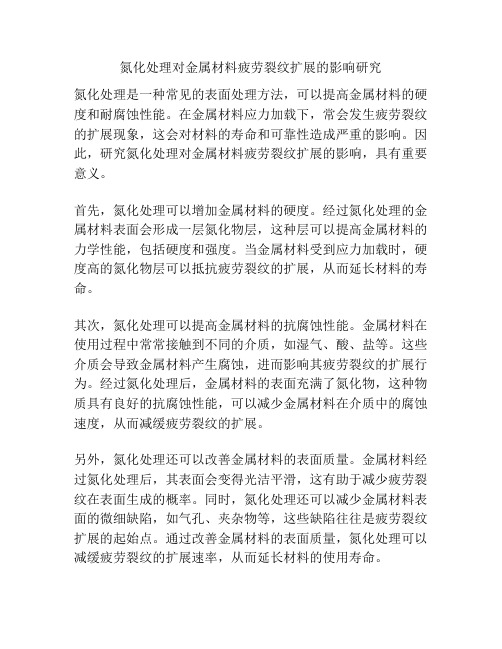
氮化处理对金属材料疲劳裂纹扩展的影响研究氮化处理是一种常见的表面处理方法,可以提高金属材料的硬度和耐腐蚀性能。
在金属材料应力加载下,常会发生疲劳裂纹的扩展现象,这会对材料的寿命和可靠性造成严重的影响。
因此,研究氮化处理对金属材料疲劳裂纹扩展的影响,具有重要意义。
首先,氮化处理可以增加金属材料的硬度。
经过氮化处理的金属材料表面会形成一层氮化物层,这种层可以提高金属材料的力学性能,包括硬度和强度。
当金属材料受到应力加载时,硬度高的氮化物层可以抵抗疲劳裂纹的扩展,从而延长材料的寿命。
其次,氮化处理可以提高金属材料的抗腐蚀性能。
金属材料在使用过程中常常接触到不同的介质,如湿气、酸、盐等。
这些介质会导致金属材料产生腐蚀,进而影响其疲劳裂纹的扩展行为。
经过氮化处理后,金属材料的表面充满了氮化物,这种物质具有良好的抗腐蚀性能,可以减少金属材料在介质中的腐蚀速度,从而减缓疲劳裂纹的扩展。
另外,氮化处理还可以改善金属材料的表面质量。
金属材料经过氮化处理后,其表面会变得光洁平滑,这有助于减少疲劳裂纹在表面生成的概率。
同时,氮化处理还可以减少金属材料表面的微细缺陷,如气孔、夹杂物等,这些缺陷往往是疲劳裂纹扩展的起始点。
通过改善金属材料的表面质量,氮化处理可以减缓疲劳裂纹的扩展速率,从而延长材料的使用寿命。
综上所述,氮化处理对金属材料疲劳裂纹扩展具有明显的影响。
它可以增加金属材料的硬度和抗腐蚀性能,改善材料的表面质量,从而减缓疲劳裂纹的扩展速率,延长金属材料的使用寿命。
然而,具体的影响因素还需要进一步的研究,以便更好地应用氮化处理技术来提高金属材料的性能和可靠性。
此外,氮化处理还可以提高金属材料的耐磨性。
在实际工程应用中,金属材料常常会因为受到摩擦、磨损等作用,导致疲劳裂纹的产生和扩展。
通过氮化处理,金属材料的表面形成了氮化物层,该层具有良好的耐磨性能,能够有效减少因摩擦引起的材料疲劳裂纹的扩展。
因此,氮化处理对金属材料的耐磨性具有显著的提高效果。
钢的渗氮技术及检验
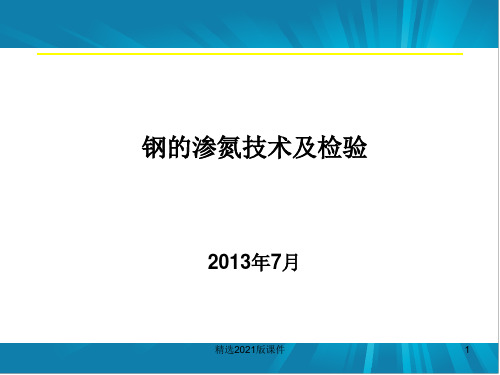
➢ 氮化物的稳定性按下列次序降低,即Ti、Al、V、Nb、 W、Mo、Cr、Mn、Fe的氮化物。
精选2021版课件
6
氮化方法
• 氮化工艺方法
– 硬氮化:学名‘渗氮’,也有人称为常规氮化。渗入钢表 面的是单一的‘氮’元素,在方法上有气体法和离子法等。
精选2021版课件
11
液体氮化
• 液含物,体Fe液软2N体氮ξ相软化氮氮主化化要物的不,方同ξ法是相是在化将氮合被化物处层硬理里脆工之在件有氮F,化e先处3N除理ε相锈上,,是F脱不e4脂良N,r于相预韧存热性在后的而再氮不置化 于氮化坩埚内,坩埚内是以TF – 1为主盐剂,被加温到560~600℃处 理数分至数小时,依工件所受外力负荷大小,而决定氮化层深度,在 处理中,必须在坩埚底部通入一支空气管以一定量之空气氮化盐剂分 解为CN或CNO,渗透扩散至工作表面,使工件表面最外层化合物8~ 9%wt的N及少量的C及扩散层,氮原子扩散入α – Fe基地中使钢件更 具耐疲劳性,氮化期间由于CNO之分解消耗,所以不断要在6~8小 时处理中化验盐剂成份,以便调整空气量或加入新的盐剂。
• 气 化之体钢氮种化,因分如含解有NHA3l进,行Cr渗,氮Mo效等率氮低化,元故素一,般否均则固氮定化选几用无适法用进于行氮, 一般使用强韧化处理又称调质,因Al,Cr,Mo等皆为提高变态点温 度之元素,故淬火温度高,回火温度亦较普通之构造用合金钢高, 此乃在氮化温度长时间加热之间,发生回火脆性,故预先施以调质 强 研磨韧,化而处且理时。间NH长3气不体经氮济化,,用因于为塑时胶间射长出表形面机粗的糙送,料硬管而及较螺脆旋不杆易的 氮化。
➢ γ′相是有序面心立点阵的间隙相,存在于680℃以 下。γ′相有较高的硬度(HV550)和韧性。
外文翻译---离子渗氮对马氏体时效钢微观结构和性能的影响

附录一英文翻译原文EFFECT of ION NITRIDING ON the MICROSTRUCTURE AND PROPERTIES of MARAGING STEEL (250 GRADE) Kishora ShettyS. Kumar b,P. Raghothama Rao;RegionalCentre for Military Airworthiness (Foundry and Forge), CEMILAC, DRDO, Bangalore-560 037, IndiaDepartment of Materials Engineering, Indian Institute of Science, Bangalore-560 012, IndiaReceived 25 June 2008. Accepted 28 November 2008. Available online 10 December 2008AbstractIn the present investigation, ion nitriding of Maraging steel (250 grade) has been carried out at three different temperatures i.e., at 435℃, 450℃ and 465℃ for 10 h duration in order to achieve good wear resistance along with high strength required for the slat track component of aircraft. The microstructure of the base material and the nitrided layer was examined by optical and scanning electron microscope, and various phases present were determined by X-ray diffraction. Various properties, such as, hardness, case depth, tensile, impact, fatigue properties and corrosion resistance were investigated for both un-nitrided and ion-nitrided materials. It is observed that the microstructure of the core material remains unaltered and Fe4N is formed in the hardened surface layer after ion nitriding at all the three temperatures employed. Surface hardness increases substantially after ion nitriding. Surface hardness remains almost the same but case depth increases with the increase in ion nitriding temperature due to greater diffusivity at higher temperatures. Tensile strength, fatigue strength and corrosion resistance are improved but ductility and energy absorbed in impact test decrease on ion nitriding. These results are explained on the basis of microstructural observations. The properties obtained after ionnitriding at 450 °C for 10 h are found to be optimum when compared to the other two ion nitriding temperatures.Keywords:Ionnitriding;Maragingsteel;Microstructure;Casedepth;Tensileproperties;Fatigue properties1. IntroductionSurface engineering means ‘engineering the surface’ of a material or component to confer surface properties, which are different from the bulk properties of the base material[1]. The purpose may be to reduce wear, minimize corrosion, increase fatigue resistance, reduce frictional energy losses, act as a diffusion barrier, provide thermal insulation, exclude certain wave lengths of radiation, promote radiation, electronic interactions, electrically insulate or simply improve the aesthetic appearance of the surface. Surface engineering processes may broadly be grouped in three categories as (a) modifying surfaces without altering the substrate's chemical composition; these types of processes include transformation hardening, cold deformation, machining and peening, (b) changing the chemistry of the surface region; these types of processes include carburizing, nitriding, anodizing and ion implanting, and (c) adding a layer of material to the surface; these types of processes include weld overlay, painting, metal spraying, plasma spraying, electroplating, bonding, physical vapor deposition and chemical vapor deposition. Nitriding is a surface-hardening process by the introduction of nitrogen into the surface of steel[2]. Process methods for nitriding include gas nitriding, liquid or salt bath nitriding and plasma or ion nitriding. In gas nitriding this is done using a mixture of ammonia gas and dissociated ammonia in suitable proportions. In plasma or ion nitriding a glow discharge technology is used to introduce nascent (elemental) nitrogen to the surface of a metal part for subsequent diffusion into the material[3],[4]and[5]. The plasma assisted surface modification techniques offer a great flexibility and are capable of tailoring desirable chemical and structural surface properties independent of the bulk properties[3]. It has other advantages, such as, no or very thin white layer forms after nitriding and there is no machining or grinding involved after the process, which isparticularly beneficial for complex parts. The hardened surface layer becomes an integral part of the base material and there is no significant reduction in properties of the base material. It is also known to provide the modified surface without dimensional change and distortion of the component. Ion nitriding provides better control of case chemistry and uniformity[3],[6]and[7].Maraging steels belong to a new class of high strength steels with the combination of strength and toughness that are among the highest attainable in general engineering alloys[8]. The term maraging is derived from martensite age hardening and denotes the age hardening of a low carbon, iron–nickel lath martensite matrix [9] and [10]. These steels typically have very high nickel, cobalt and molybdenum and very low carbon content. They are strengthened by the precipitation of intermetallic compounds at a temperature of about 480℃[11] and [12]. Carbon is treated as an impurity and is kept as low as commercially feasible (<0.03wt.%) in order to minimize the formation of titanium carbide (TiC), which can adversely affect strength, ductility and toughness [9] and [11]. Different maraging steels are designed to provide specific levels of yield strength from 1030 to 2420MPa (150 to 350ksi). Maraging steel (250 grade) possesses a yield strength of 250ksi.For aeronautical components requiring high strength and good wear resistance, such as, slat track, Maraging steel possesses high strength, and good wear resistance can be achieved by ion nitriding process of case nitriding. In conventional gas nitriding process the nitriding temperature is 500℃–550℃[2], which is above the ageing temperature of maraging steel and would result in the over-aging of the core. However, ion nitriding can be carried out at a temperature lower than the aging temperature. Ozbaysal and Inal have demonstrated that the surface hardening of maraging steels without a reduction in core hardness is possible using the ion nitriding process[13]. In the present investigation, ion nitriding of Maraging steel (250 grade) has been carried out at three different temperatures, i.e., 435℃, 450℃ and 465℃ for 10h duration. Microstructural changes are examined with the help of optical microscope, scanning electron microscope (SEM) and X-ray diffraction (XRD).C 0.03 max. 0.01Mn 0.10 max. 0.04Si 0.10 max. 0.04S 0.01 max. 0.005P 0.01 max. 0.006Ni 17.0–19.0 17.29Mo 4.60–5.20 4.89Co 7.0–8.50 7.90Ti 0.30–0.50 0.41Al 0.05–0.15 0.14Zr 0.02 max. 0.005B 0.003 max. 0.001Fe Balance Balance Fig. 1shows a simplified schematic of the ion nitriding installation used for ion nitriding of the material. The work load is supported on a hearth plate inside a double walled, water cooled vacuum chamber, connected to vacuum pumps and gas supply. The chamber is evacuated to a pressure of about 2.5⁎10− 2mbar, a pressure low enough for the background level of oxygen to be within the acceptable limits (less than 50ppm) and is then filled with a low pressure mixture of hydrogen and nitrogen. The use of auxiliary AC heaters to heat the cathode to 250℃ is desirable to minimize cycle time. It can also help to provide better temperature uniformity of the part in ionnitriding treatment. The discharge is ignited using a DC power supply, and pressure and temperature are raised to the desired operating values by controlling gas flow and pressure, applied voltage and current. The discharge can be monitored by control panel and viewed through inspection windows. The work is cathode and the vessel is anode. The furnace is electrically grounded, cool to touch, and quiet in operation. Maraging steel (250 grade) specimens are prepared from the material solutionized and aged at 480℃ for 3h. These aged specimens are ion-nitrided in the nitriding furnace under vacuum. Plasma is obtained by passing the gas mixture of H2 and N2 gases in the ratio 3:1 into the chamber and maintaining the pressure of 5mbar. Ion nitriding is carried out at 435℃., 450℃ and 465℃ for10h..Photograph of the specimens before ion nitriding and after ion nitriding at 465°C are shown inFig. 2. After ion nitriding the specimens were subjected to buffing (cloth polishing) to remove the thin white layer formed..Fig. 2.Photograph of the specimens (a) before ion nitriding,Microstructural examination was carried out on NIKON optical microscope and Leo SEM. XRD was carried out on Philips Analytical X-ray Diffractometer. Hardness was measured using Vickers Hardness Tester (Buelher Micromet 2100) with a load of 100 g. Tensile tests were carried out on cylindrical specimens having 4mm gauge diameter and 20mm gauge length using TIRA Test 2820S Universal Testing Machine.Impact tests were conducted on Charpy ‘U’ notch specimens having a dimension 10mm⁎10mm⁎55mm using FIE Charpy Impact Testing machine. Constant amplitude LCF tests were carried out on smooth round specimens with 4.5mm gauge diameter and 12mm gauge length using Zwick Roell Universal Testing Machine at an applied stress range of ±1172MPa having stress ratio of −1.Hardness values were measured at five places for each sample, for tensile and impact three samples were tested at each condition and for LCF five samples were tested at each condition and the average value along with standard deviation is reported. Corrosion tests were carried out on specimens having a dimension 10mm⁎10mm⁎18mm for un-nitrided samples and 10mm⁎1mm⁎20mm for ion-nitrided samples using 5% NaCl solution for 144h in a salt spray test chamber.3. Results and discussions3.1. Microstuctural examinationOptical and scanning electron micrographs of un-nitrided and ion-nitrided specimens are shown in Fig. 3 and Fig. 4. These micrographs show a case hardened nitrided layer and martensitic core structure. The thickness of the nitrided layer increases with the increase in ion nitriding temperature due to greater diffusivity at higher temperatures.Fig. 3.Optical micrographs of (a) un-nitrided specimen, (b) ion nitrided at 435℃, (c) ion nitrided at 450℃and (d) ion nitrided at 465℃.View high quality image (2381K)XRD patterns obtained from the surface of un-nitrided and ion-nitrided specimens are shown inFig. 5. The un-nitrided specimen exhibits diffraction peaks only due to Fe, whereas the ion-nitrided specimens exhibit additional peaks due to Fe4N as well. The intensity of the Fe peaks progressively decreases and the intensity of the Fe4N peaks progressively increases with the increase in ion nitriding temperature. Thus, the iron nitride formed in the surface hardened layer on ion nitriding is Fe4N. The ratio of H2and N2in the gas mixture used for ion nitriding in our investigation is 3:1, which is termed as γ′ gas and forms mono phase (called as γ′ phase) crystal structure of Fe4N in the compound layer. Nitrogen atoms go in to the interstitial sites of iron lattice inanorderedmannerandform Fe4N[14].3.2. Hardness and case depthThe hardness values obtained on un-nitrided and ion nitrided samples are given inTable 2. The average surface hardness of the un-nitrided samples is 616VHN. The average surface hardness of the samples ion nitrided at three different temperatures is more or less the same and is about 50% higher than the un-nitrided sample.Hardness values of the ion-nitrided samples obtained at various depths from surface for the three ion nitriding temperatures are listed in Table 3and are plotted inFig. 6. The continuous decrease of hardness from surface to the core of the sample suggests the presence of a diffusion zone in which precipitates of nitrides of iron and other metals are formed at the grain boundaries as well as within the grains.These precipitates distort the lattice and pin crystal dislocations and thereby increase the hardness of the surface layer of the ion-nitrided samples[15]. Case depth is taken as the distance from the surface where hardness value is 100 units more than the core hardness. Accordingly, case depth is measured by drawing a line at a hardness value 716VHN and is obtained as 81, 87 and 99µm at 435℃, 450℃and 465℃ion-nitriding temperatures respectively fromFig. 6.Case depth increases with the increase in ion nitriding temperature due to greater diffusivity at higher temperatures. It should be noted that the core hardness at all the three ion nitriding temperatures remains the same as the hardness of the un-nitrided sample. This confirms that the core does not soften by over-aging at all the three ion nitriding temperatures.Table2.Surface hardness of un-nitrided and ion-nitrided specimens Before ion nitriding 616VHN±3After ion nitriding At 435°C 906 VHN±3At 450°C 901 VHN±2At 465°C 907 VHN±4Table3.Hardness values obtained at different depth from the surface afterion-nitridingDistance from Hardness (VHN)the edge(µm) Ion nitrided at435°CIon nitrided at450°C Ion nitrided at465°C50 749 ± 7 810 ± 8 822 ± 1075 725 ± 4 734 ± 4 737 ± 5100 689 ± 12 690 ± 5 714 ± 5125 633 ± 8 642 ± 5 659 ± 5150 626 ± 6 631 ± 2 636 ± 3175 618 ± 2 626 ± 4 628 ± 7200 618 ± 3 618 ± 2 620 ± 2225 618 ± 2 618 ± 2 619 ± 2Core 614 ± 3 618 ± 2 618 ± 2Fig. 6.Variation of hardness with depth from the surface for samples ion nitrided at 435℃, 450℃and 465℃.View high quality image (145K)3.3. Tensile propertiesTensile properties for the un-nitrided and ion-nitrided specimens at three different ion-nitriding temperatures are given inTable 4. It is observed that, as compared to the un-nitrided specimen, the nitrided specimens exhibit an increase in ultimate tensile strength (UTS) and 0.2% proof stress (0.2% PS) and a decrease in percentage elongation to fracture (%El) and percentage reduction in area (%RA). The higher UTS and 0.2% PS values and lower %El and %RA values exhibited by nitrided samples can be attributed to the hardened surface layer on ion nitriding.Table 4 Tensile, Impact and LCF properties of un-nitrided and ion-nitrided specimensProperty Un nitrided Ion-nitridedat435 °CIon-nitrided at450 °CIon-nitridedat465 °C UTS (MPa) 1697 ± 4 1740 ± 1051863 ± 15 1699 ± 140.2% PS (MPa) 1616 ± 9 1711 ± 1201793 ± 321628 ± 16% El 9.1 ± 0.35.8 ± 1.16.2 ± 0.57 ± 0% RA 57.0 ± 2.6 34.5 ± 0.727.7 ± 2.5 35 ± 0Energy absorbedinimpact test 21.7 ± 0.618.7 ± 0.618.3 ± 1.2 14 ± 1(Joules)No. of cycles to4700 ± 1113 11017 ± 5748 9366 ± 35579675 ± 6811The nitrided specimen can be treated as a composite material consisting of martensitic core of about 3.7mm diameter surrounded by a thin nitrided layer of about 0.15mm thickness (as shown inFig.6), and a rough estimate of 0.2% PS can be obtained by applying the rule of mixture, as follows:(1)where,σC0.2% PS of the nitrided specimenσf0.2% PS of the nitrided layer (calculated below)σm0.2% PS of the un-nitrided core (assumed to be the same as that of the un-nitrided specimen, i.e., 1616MPa)A C cross section area of the nitrided specimen (12.57mm2)A f cross section area of the nitrided layer (1.82mm2)A m cross section area of the un-nitrided core (10.75mm2).It is observed fromFig. 6that the hardness of the nitrided specimens approaches the hardness of the core material at a depth of about 150 µm from the surface for all the three ion nitriding temperatures. The average hardness of the nitrided layer is obtained by calculating the area under the polynomial curve fitted up to a depth of 150 µm and dividing it by 150µm inFig. 6for the samples ion nitrided at 435℃, 450℃and 465℃. These average hardness values are converted into 0.2% PS using Tabor's relation[16]and[17](2)σf=VHN/3.Thus,σf values at 435℃, 450℃and 465℃ion nitriding temperatures are obtained as 2390, 2449 and 2487MPa respectively. The σC values using Eq. (1) at 435℃, 450℃and 465℃ ion nitriding temperatures are obtained as 1728, 1737 and 1742 MPa respectively. Thus calculated 0.2% PS value matches closely with the experimentally obtained value for the specimens nitrided at 435℃, as shown inTable 4. The estimated value of 0.2% PS should further increase with the increase in ion nitriding temperature. However, the experimental results inTable 4show an increase in 0.2% PS value from 435℃to 450℃but a decrease from 450℃to 465℃. Perhaps, more number of tests is required to establish the experimental values without ambiguity. However, the important outcome from the application point of view is that the surface hardness has been substantially increased without sacrificing the strength of the bulk material.3.4. Impact propertiesEnergy absorbed by the Charpy ‘U’ notch specimens in the impact test for the un-nitrided and ion-nitrided samples are shown inTable 4. It is observed that the energy absorbed is reduced after ion nitriding and the reduction increases with the increase in ion nitriding temperature. Ion nitriding imparts a hardened surface layer all over the specimen including the notched area. This hardened surface layer will make the material more brittle and reduce the energy absorbed as compared to the un-nitrided specimen. The decrease in energy absorbed with the increase in ionnitriding temperature may be attributed to the increase in case depth with the increase in ion nitriding temperature.3.5. Low cycle fatigue propertiesNumber of cycles to failure in LCF tests for un-nitrided samples and ion-nitrided specimens are shown inTable 4. It is observed that there is a lot of scatter, as is usually encountered with fatigue data. It is also observed that the scatter is more for the ion-nitrided specimens as compared to the un-nitrided specimens. However, the ion-nitrided specimens undoubtedly exhibit an improvement in fatigue life. The formation of the precipitates of nitride in the diffusion zone results in the lattice expansion. The core material, in an attempt to maintain the original dimension, holds the nitrided case in compression. This compressive stress essentially lowers the magnitude of the applied tensile stress on the material and thus effectively increases the fatigue life of the specimen[15].3.6. Corrosion resistanceFig. 7shows the photograph of the samples after salt spray test for 144h exposure. It was observed that corrosion started in un-nitrided samples after 48h of exposure uniformly throughout the sample and there was no corrosion in ion-nitrided samples even after 144h of exposure. Thus, salt spray test results show an improvement in corrosion resistance after ion nitriding. It is reported that Fe4N formed after ion nitriding in the surface layer is relatively stable in corrosive medium[18]. The hardened surface layer is also uniform and continuous throughout the surface. These factors contribute to the improvement in the corrosion resistance of the material.Fig. 7.Photograph of the samples after the salt spray test.View high quality image (371K)4. ConclusionsIn the present investigation, Maraging steel (250 Grade) was ion nitrided at 435℃,450℃and 465℃. The following conclusions can be drawn from this investigation:1. Microstructure of core remains un-altered after ion nitriding.2. The iron nitride formed in the hardened surface layer is Fe4N for all the three ion nitriding temperatures.3. Ion nitriding improves the surface hardness of Maraging steel (250 grade) substantially. Surface hardness remains almost the same but case depth increases with the increase in ion nitriding temperature due to greater diffusivity at higher temperatures.4. Tensile strength, fatigue strength, and corrosion resistance also improve on ion nitriding. Tensile strength increases since the nitrided layer is much harder; fatigue strength improves due to residual compressive stresses at the surface arising from the formation of nitrided layer; and corrosion resistance improves because the nitrided layer is relatively stable in corrosive medium.5. Ductility in terms of %El and %RA and energy absorbed in impact test decrease on ion nitriding as a result of formation of hard and brittle nitrided layer.6. Properties obtained after ion nitriding at 450℃for 10h are optimum when compared with the other two ion nitriding temperatures for the same duration.AcknowledgementsThe authors wish to thank Mr. B. S. Vedaprakash, Group Director (Propulsion), and Mr. K. Tamilmani, Chief Executive (Airworthiness), CEMILAC, Bangalore, for their support.References[1]B. Kumar, N.C. Upadhyay,IIM Met. News, 8 (2005), p. 5[2] ASM Handbook, vol. 5, American Society for Metals, Metals Park, OH, 1999, 738.[3]T. Spalvins,Ion Nitriding, Conference proceedings, ASM International (1986), p. 1[4]W. Moller, S. Parascandola, T. Telbizova, R. Gunzel, E. RichterSurf. Coat. Technol., 136 (2001), p. 73[5] ASM Handbook, vol. 5, American Society for Metals, Metals Park, OH, 1999, 759.[6]B. Edenhofer,Production ion nitriding,Met. Prog. (1976), p. 181[7]EPRI Centre for Materials Fabrication;Ion nitriding, 2 (5) (1994), p. 1[8]B. Gupta, V. Gopalakrishna, J.S. Yadav, A. Kumar, B. SahaAerospace Materials, S. Chand and Company, New Delhi (1996), p. 695[9] ASM Handbook, V ol. 1, American Society for Metals, Metals Park, OH, 1990, 793.[10]S. Morito, X. Huang, T. Furuhara, T. Maki, N. Hansen;Acta Mater., 54 (2006), p.5323[11] ASM Handbook, V ol. 4, American Society for Metals, Metals Park, OH, 1991, 219.[12] INCO Databook (1976) 351.[13]K. Ozbaysal, O.T. Inal;‘Ion Nitriding’, American Society for Metals, Metals Park,OH (1986), p. 97[14] W. Kovacs, W. Russel, An Introduction to Ion nitriding, What is it? Why is itused? Where is it used? Ion Nitriding Conference Proceeding, Cleveland, Ohio (1986) 9.[15] J. O Brien M, D. Goodman, Plasma (Ion) Nitriding, ASM Handbook, vol. 4,American Society for Metals, Metals Park, OH, 1991, 420.[16]A.E. Tekkaya;Steel Res., 71 (2001), p. 304[17]G.E. Dieter;Mechanical Metallurgy, Mc Graw-Hill Book Company, London(1988), p. 407[18]K.Y. Fu Ricky, D.L. Tang, G.J. Wan, K. Chu Paul;Surf. Coat. Technol., 201(2007), p. 4879附录二英文翻译离子渗氮对马氏体时效钢微观结构和性能的影响摘要目前调查表明,渗氮后的马氏体时效钢(250级)分别在435℃、450℃、465℃下持续10小时,目的是为了使飞机机翼获得良好的耐磨性,伴随高强度。
外文翻译---离子渗氮对马氏体时效钢微观结构和性能的影响

附录一英文翻译原文EFFECT of ION NITRIDING ON the MICROSTRUCTURE AND PROPERTIES of MARAGING STEEL (250 GRADE) Kishora ShettyS. Kumar b,P. Raghothama Rao;RegionalCentre for Military Airworthiness (Foundry and Forge), CEMILAC, DRDO, Bangalore-560 037, IndiaDepartment of Materials Engineering, Indian Institute of Science, Bangalore-560 012, IndiaReceived 25 June 2008. Accepted 28 November 2008. Available online 10 December 2008AbstractIn the present investigation, ion nitriding of Maraging steel (250 grade) has been carried out at three different temperatures i.e., at 435℃, 450℃ and 465℃ for 10 h duration in order to achieve good wear resistance along with high strength required for the slat track component of aircraft. The microstructure of the base material and the nitrided layer was examined by optical and scanning electron microscope, and various phases present were determined by X-ray diffraction. Various properties, such as, hardness, case depth, tensile, impact, fatigue properties and corrosion resistance were investigated for both un-nitrided and ion-nitrided materials. It is observed that the microstructure of the core material remains unaltered and Fe4N is formed in the hardened surface layer after ion nitriding at all the three temperatures employed. Surface hardness increases substantially after ion nitriding. Surface hardness remains almost the same but case depth increases with the increase in ion nitriding temperature due to greater diffusivity at higher temperatures. Tensile strength, fatigue strength and corrosion resistance are improved but ductility and energy absorbed in impact test decrease on ion nitriding. These results are explained on the basis of microstructural observations. The properties obtained after ionnitriding at 450 °C for 10 h are found to be optimum when compared to the other two ion nitriding temperatures.Keywords:Ionnitriding;Maragingsteel;Microstructure;Casedepth;Tensileproperties;Fatigue properties1. IntroductionSurface engineering means ‘engineering the surface’ of a material or component to confer surface properties, which are different from the bulk properties of the base material[1]. The purpose may be to reduce wear, minimize corrosion, increase fatigue resistance, reduce frictional energy losses, act as a diffusion barrier, provide thermal insulation, exclude certain wave lengths of radiation, promote radiation, electronic interactions, electrically insulate or simply improve the aesthetic appearance of the surface. Surface engineering processes may broadly be grouped in three categories as (a) modifying surfaces without altering the substrate's chemical composition; these types of processes include transformation hardening, cold deformation, machining and peening, (b) changing the chemistry of the surface region; these types of processes include carburizing, nitriding, anodizing and ion implanting, and (c) adding a layer of material to the surface; these types of processes include weld overlay, painting, metal spraying, plasma spraying, electroplating, bonding, physical vapor deposition and chemical vapor deposition. Nitriding is a surface-hardening process by the introduction of nitrogen into the surface of steel[2]. Process methods for nitriding include gas nitriding, liquid or salt bath nitriding and plasma or ion nitriding. In gas nitriding this is done using a mixture of ammonia gas and dissociated ammonia in suitable proportions. In plasma or ion nitriding a glow discharge technology is used to introduce nascent (elemental) nitrogen to the surface of a metal part for subsequent diffusion into the material[3],[4]and[5]. The plasma assisted surface modification techniques offer a great flexibility and are capable of tailoring desirable chemical and structural surface properties independent of the bulk properties[3]. It has other advantages, such as, no or very thin white layer forms after nitriding and there is no machining or grinding involved after the process, which isparticularly beneficial for complex parts. The hardened surface layer becomes an integral part of the base material and there is no significant reduction in properties of the base material. It is also known to provide the modified surface without dimensional change and distortion of the component. Ion nitriding provides better control of case chemistry and uniformity[3],[6]and[7].Maraging steels belong to a new class of high strength steels with the combination of strength and toughness that are among the highest attainable in general engineering alloys[8]. The term maraging is derived from martensite age hardening and denotes the age hardening of a low carbon, iron–nickel lath martensite matrix [9] and [10]. These steels typically have very high nickel, cobalt and molybdenum and very low carbon content. They are strengthened by the precipitation of intermetallic compounds at a temperature of about 480℃[11] and [12]. Carbon is treated as an impurity and is kept as low as commercially feasible (<0.03wt.%) in order to minimize the formation of titanium carbide (TiC), which can adversely affect strength, ductility and toughness [9] and [11]. Different maraging steels are designed to provide specific levels of yield strength from 1030 to 2420MPa (150 to 350ksi). Maraging steel (250 grade) possesses a yield strength of 250ksi.For aeronautical components requiring high strength and good wear resistance, such as, slat track, Maraging steel possesses high strength, and good wear resistance can be achieved by ion nitriding process of case nitriding. In conventional gas nitriding process the nitriding temperature is 500℃–550℃[2], which is above the ageing temperature of maraging steel and would result in the over-aging of the core. However, ion nitriding can be carried out at a temperature lower than the aging temperature. Ozbaysal and Inal have demonstrated that the surface hardening of maraging steels without a reduction in core hardness is possible using the ion nitriding process[13]. In the present investigation, ion nitriding of Maraging steel (250 grade) has been carried out at three different temperatures, i.e., 435℃, 450℃ and 465℃ for 10h duration. Microstructural changes are examined with the help of optical microscope, scanning electron microscope (SEM) and X-ray diffraction (XRD).C 0.03 max. 0.01Mn 0.10 max. 0.04Si 0.10 max. 0.04S 0.01 max. 0.005P 0.01 max. 0.006Ni 17.0–19.0 17.29Mo 4.60–5.20 4.89Co 7.0–8.50 7.90Ti 0.30–0.50 0.41Al 0.05–0.15 0.14Zr 0.02 max. 0.005B 0.003 max. 0.001Fe Balance Balance Fig. 1shows a simplified schematic of the ion nitriding installation used for ion nitriding of the material. The work load is supported on a hearth plate inside a double walled, water cooled vacuum chamber, connected to vacuum pumps and gas supply. The chamber is evacuated to a pressure of about 2.5⁎10− 2mbar, a pressure low enough for the background level of oxygen to be within the acceptable limits (less than 50ppm) and is then filled with a low pressure mixture of hydrogen and nitrogen. The use of auxiliary AC heaters to heat the cathode to 250℃ is desirable to minimize cycle time. It can also help to provide better temperature uniformity of the part in ionnitriding treatment. The discharge is ignited using a DC power supply, and pressure and temperature are raised to the desired operating values by controlling gas flow and pressure, applied voltage and current. The discharge can be monitored by control panel and viewed through inspection windows. The work is cathode and the vessel is anode. The furnace is electrically grounded, cool to touch, and quiet in operation. Maraging steel (250 grade) specimens are prepared from the material solutionized and aged at 480℃ for 3h. These aged specimens are ion-nitrided in the nitriding furnace under vacuum. Plasma is obtained by passing the gas mixture of H2 and N2 gases in the ratio 3:1 into the chamber and maintaining the pressure of 5mbar. Ion nitriding is carried out at 435℃., 450℃ and 465℃ for10h..Photograph of the specimens before ion nitriding and after ion nitriding at 465°C are shown inFig. 2. After ion nitriding the specimens were subjected to buffing (cloth polishing) to remove the thin white layer formed..Fig. 2.Photograph of the specimens (a) before ion nitriding,Microstructural examination was carried out on NIKON optical microscope and Leo SEM. XRD was carried out on Philips Analytical X-ray Diffractometer. Hardness was measured using Vickers Hardness Tester (Buelher Micromet 2100) with a load of 100 g. Tensile tests were carried out on cylindrical specimens having 4mm gauge diameter and 20mm gauge length using TIRA Test 2820S Universal Testing Machine.Impact tests were conducted on Charpy ‘U’ notch specimens having a dimension 10mm⁎10mm⁎55mm using FIE Charpy Impact Testing machine. Constant amplitude LCF tests were carried out on smooth round specimens with 4.5mm gauge diameter and 12mm gauge length using Zwick Roell Universal Testing Machine at an applied stress range of ±1172MPa having stress ratio of −1.Hardness values were measured at five places for each sample, for tensile and impact three samples were tested at each condition and for LCF five samples were tested at each condition and the average value along with standard deviation is reported. Corrosion tests were carried out on specimens having a dimension 10mm⁎10mm⁎18mm for un-nitrided samples and 10mm⁎1mm⁎20mm for ion-nitrided samples using 5% NaCl solution for 144h in a salt spray test chamber.3. Results and discussions3.1. Microstuctural examinationOptical and scanning electron micrographs of un-nitrided and ion-nitrided specimens are shown in Fig. 3 and Fig. 4. These micrographs show a case hardened nitrided layer and martensitic core structure. The thickness of the nitrided layer increases with the increase in ion nitriding temperature due to greater diffusivity at higher temperatures.Fig. 3.Optical micrographs of (a) un-nitrided specimen, (b) ion nitrided at 435℃, (c) ion nitrided at 450℃and (d) ion nitrided at 465℃.View high quality image (2381K)XRD patterns obtained from the surface of un-nitrided and ion-nitrided specimens are shown inFig. 5. The un-nitrided specimen exhibits diffraction peaks only due to Fe, whereas the ion-nitrided specimens exhibit additional peaks due to Fe4N as well. The intensity of the Fe peaks progressively decreases and the intensity of the Fe4N peaks progressively increases with the increase in ion nitriding temperature. Thus, the iron nitride formed in the surface hardened layer on ion nitriding is Fe4N. The ratio of H2and N2in the gas mixture used for ion nitriding in our investigation is 3:1, which is termed as γ′ gas and forms mono phase (called as γ′ phase) crystal structure of Fe4N in the compound layer. Nitrogen atoms go in to the interstitial sites of iron lattice inanorderedmannerandform Fe4N[14].3.2. Hardness and case depthThe hardness values obtained on un-nitrided and ion nitrided samples are given inTable 2. The average surface hardness of the un-nitrided samples is 616VHN. The average surface hardness of the samples ion nitrided at three different temperatures is more or less the same and is about 50% higher than the un-nitrided sample.Hardness values of the ion-nitrided samples obtained at various depths from surface for the three ion nitriding temperatures are listed in Table 3and are plotted inFig. 6. The continuous decrease of hardness from surface to the core of the sample suggests the presence of a diffusion zone in which precipitates of nitrides of iron and other metals are formed at the grain boundaries as well as within the grains.These precipitates distort the lattice and pin crystal dislocations and thereby increase the hardness of the surface layer of the ion-nitrided samples[15]. Case depth is taken as the distance from the surface where hardness value is 100 units more than the core hardness. Accordingly, case depth is measured by drawing a line at a hardness value 716VHN and is obtained as 81, 87 and 99µm at 435℃, 450℃and 465℃ion-nitriding temperatures respectively fromFig. 6.Case depth increases with the increase in ion nitriding temperature due to greater diffusivity at higher temperatures. It should be noted that the core hardness at all the three ion nitriding temperatures remains the same as the hardness of the un-nitrided sample. This confirms that the core does not soften by over-aging at all the three ion nitriding temperatures.Table2.Surface hardness of un-nitrided and ion-nitrided specimens Before ion nitriding 616VHN±3After ion nitriding At 435°C 906 VHN±3At 450°C 901 VHN±2At 465°C 907 VHN±4Table3.Hardness values obtained at different depth from the surface afterion-nitridingDistance from Hardness (VHN)the edge(µm) Ion nitrided at435°CIon nitrided at450°C Ion nitrided at465°C50 749 ± 7 810 ± 8 822 ± 1075 725 ± 4 734 ± 4 737 ± 5100 689 ± 12 690 ± 5 714 ± 5125 633 ± 8 642 ± 5 659 ± 5150 626 ± 6 631 ± 2 636 ± 3175 618 ± 2 626 ± 4 628 ± 7200 618 ± 3 618 ± 2 620 ± 2225 618 ± 2 618 ± 2 619 ± 2Core 614 ± 3 618 ± 2 618 ± 2Fig. 6.Variation of hardness with depth from the surface for samples ion nitrided at 435℃, 450℃and 465℃.View high quality image (145K)3.3. Tensile propertiesTensile properties for the un-nitrided and ion-nitrided specimens at three different ion-nitriding temperatures are given inTable 4. It is observed that, as compared to the un-nitrided specimen, the nitrided specimens exhibit an increase in ultimate tensile strength (UTS) and 0.2% proof stress (0.2% PS) and a decrease in percentage elongation to fracture (%El) and percentage reduction in area (%RA). The higher UTS and 0.2% PS values and lower %El and %RA values exhibited by nitrided samples can be attributed to the hardened surface layer on ion nitriding.Table 4 Tensile, Impact and LCF properties of un-nitrided and ion-nitrided specimensProperty Un nitrided Ion-nitridedat435 °CIon-nitrided at450 °CIon-nitridedat465 °C UTS (MPa) 1697 ± 4 1740 ± 1051863 ± 15 1699 ± 140.2% PS (MPa) 1616 ± 9 1711 ± 1201793 ± 321628 ± 16% El 9.1 ± 0.35.8 ± 1.16.2 ± 0.57 ± 0% RA 57.0 ± 2.6 34.5 ± 0.727.7 ± 2.5 35 ± 0Energy absorbedinimpact test 21.7 ± 0.618.7 ± 0.618.3 ± 1.2 14 ± 1(Joules)No. of cycles to4700 ± 1113 11017 ± 5748 9366 ± 35579675 ± 6811The nitrided specimen can be treated as a composite material consisting of martensitic core of about 3.7mm diameter surrounded by a thin nitrided layer of about 0.15mm thickness (as shown inFig.6), and a rough estimate of 0.2% PS can be obtained by applying the rule of mixture, as follows:(1)where,σC0.2% PS of the nitrided specimenσf0.2% PS of the nitrided layer (calculated below)σm0.2% PS of the un-nitrided core (assumed to be the same as that of the un-nitrided specimen, i.e., 1616MPa)A C cross section area of the nitrided specimen (12.57mm2)A f cross section area of the nitrided layer (1.82mm2)A m cross section area of the un-nitrided core (10.75mm2).It is observed fromFig. 6that the hardness of the nitrided specimens approaches the hardness of the core material at a depth of about 150 µm from the surface for all the three ion nitriding temperatures. The average hardness of the nitrided layer is obtained by calculating the area under the polynomial curve fitted up to a depth of 150 µm and dividing it by 150µm inFig. 6for the samples ion nitrided at 435℃, 450℃and 465℃. These average hardness values are converted into 0.2% PS using Tabor's relation[16]and[17](2)σf=VHN/3.Thus,σf values at 435℃, 450℃and 465℃ion nitriding temperatures are obtained as 2390, 2449 and 2487MPa respectively. The σC values using Eq. (1) at 435℃, 450℃and 465℃ ion nitriding temperatures are obtained as 1728, 1737 and 1742 MPa respectively. Thus calculated 0.2% PS value matches closely with the experimentally obtained value for the specimens nitrided at 435℃, as shown inTable 4. The estimated value of 0.2% PS should further increase with the increase in ion nitriding temperature. However, the experimental results inTable 4show an increase in 0.2% PS value from 435℃to 450℃but a decrease from 450℃to 465℃. Perhaps, more number of tests is required to establish the experimental values without ambiguity. However, the important outcome from the application point of view is that the surface hardness has been substantially increased without sacrificing the strength of the bulk material.3.4. Impact propertiesEnergy absorbed by the Charpy ‘U’ notch specimens in the impact test for the un-nitrided and ion-nitrided samples are shown inTable 4. It is observed that the energy absorbed is reduced after ion nitriding and the reduction increases with the increase in ion nitriding temperature. Ion nitriding imparts a hardened surface layer all over the specimen including the notched area. This hardened surface layer will make the material more brittle and reduce the energy absorbed as compared to the un-nitrided specimen. The decrease in energy absorbed with the increase in ionnitriding temperature may be attributed to the increase in case depth with the increase in ion nitriding temperature.3.5. Low cycle fatigue propertiesNumber of cycles to failure in LCF tests for un-nitrided samples and ion-nitrided specimens are shown inTable 4. It is observed that there is a lot of scatter, as is usually encountered with fatigue data. It is also observed that the scatter is more for the ion-nitrided specimens as compared to the un-nitrided specimens. However, the ion-nitrided specimens undoubtedly exhibit an improvement in fatigue life. The formation of the precipitates of nitride in the diffusion zone results in the lattice expansion. The core material, in an attempt to maintain the original dimension, holds the nitrided case in compression. This compressive stress essentially lowers the magnitude of the applied tensile stress on the material and thus effectively increases the fatigue life of the specimen[15].3.6. Corrosion resistanceFig. 7shows the photograph of the samples after salt spray test for 144h exposure. It was observed that corrosion started in un-nitrided samples after 48h of exposure uniformly throughout the sample and there was no corrosion in ion-nitrided samples even after 144h of exposure. Thus, salt spray test results show an improvement in corrosion resistance after ion nitriding. It is reported that Fe4N formed after ion nitriding in the surface layer is relatively stable in corrosive medium[18]. The hardened surface layer is also uniform and continuous throughout the surface. These factors contribute to the improvement in the corrosion resistance of the material.Fig. 7.Photograph of the samples after the salt spray test.View high quality image (371K)4. ConclusionsIn the present investigation, Maraging steel (250 Grade) was ion nitrided at 435℃,450℃and 465℃. The following conclusions can be drawn from this investigation:1. Microstructure of core remains un-altered after ion nitriding.2. The iron nitride formed in the hardened surface layer is Fe4N for all the three ion nitriding temperatures.3. Ion nitriding improves the surface hardness of Maraging steel (250 grade) substantially. Surface hardness remains almost the same but case depth increases with the increase in ion nitriding temperature due to greater diffusivity at higher temperatures.4. Tensile strength, fatigue strength, and corrosion resistance also improve on ion nitriding. Tensile strength increases since the nitrided layer is much harder; fatigue strength improves due to residual compressive stresses at the surface arising from the formation of nitrided layer; and corrosion resistance improves because the nitrided layer is relatively stable in corrosive medium.5. Ductility in terms of %El and %RA and energy absorbed in impact test decrease on ion nitriding as a result of formation of hard and brittle nitrided layer.6. Properties obtained after ion nitriding at 450℃for 10h are optimum when compared with the other two ion nitriding temperatures for the same duration.AcknowledgementsThe authors wish to thank Mr. B. S. Vedaprakash, Group Director (Propulsion), and Mr. K. Tamilmani, Chief Executive (Airworthiness), CEMILAC, Bangalore, for their support.References[1]B. Kumar, N.C. Upadhyay,IIM Met. News, 8 (2005), p. 5[2] ASM Handbook, vol. 5, American Society for Metals, Metals Park, OH, 1999, 738.[3]T. Spalvins,Ion Nitriding, Conference proceedings, ASM International (1986), p. 1[4]W. Moller, S. Parascandola, T. Telbizova, R. Gunzel, E. RichterSurf. Coat. Technol., 136 (2001), p. 73[5] ASM Handbook, vol. 5, American Society for Metals, Metals Park, OH, 1999, 759.[6]B. Edenhofer,Production ion nitriding,Met. Prog. (1976), p. 181[7]EPRI Centre for Materials Fabrication;Ion nitriding, 2 (5) (1994), p. 1[8]B. Gupta, V. Gopalakrishna, J.S. Yadav, A. Kumar, B. SahaAerospace Materials, S. Chand and Company, New Delhi (1996), p. 695[9] ASM Handbook, V ol. 1, American Society for Metals, Metals Park, OH, 1990, 793.[10]S. Morito, X. Huang, T. Furuhara, T. Maki, N. Hansen;Acta Mater., 54 (2006), p.5323[11] ASM Handbook, V ol. 4, American Society for Metals, Metals Park, OH, 1991, 219.[12] INCO Databook (1976) 351.[13]K. Ozbaysal, O.T. Inal;‘Ion Nitriding’, American Society for Metals, Metals Park,OH (1986), p. 97[14] W. Kovacs, W. Russel, An Introduction to Ion nitriding, What is it? Why is itused? Where is it used? Ion Nitriding Conference Proceeding, Cleveland, Ohio (1986) 9.[15] J. O Brien M, D. Goodman, Plasma (Ion) Nitriding, ASM Handbook, vol. 4,American Society for Metals, Metals Park, OH, 1991, 420.[16]A.E. Tekkaya;Steel Res., 71 (2001), p. 304[17]G.E. Dieter;Mechanical Metallurgy, Mc Graw-Hill Book Company, London(1988), p. 407[18]K.Y. Fu Ricky, D.L. Tang, G.J. Wan, K. Chu Paul;Surf. Coat. Technol., 201(2007), p. 4879附录二英文翻译离子渗氮对马氏体时效钢微观结构和性能的影响摘要目前调查表明,渗氮后的马氏体时效钢(250级)分别在435℃、450℃、465℃下持续10小时,目的是为了使飞机机翼获得良好的耐磨性,伴随高强度。
- 1、下载文档前请自行甄别文档内容的完整性,平台不提供额外的编辑、内容补充、找答案等附加服务。
- 2、"仅部分预览"的文档,不可在线预览部分如存在完整性等问题,可反馈申请退款(可完整预览的文档不适用该条件!)。
- 3、如文档侵犯您的权益,请联系客服反馈,我们会尽快为您处理(人工客服工作时间:9:00-18:30)。
试验研究渗氮条件对马氏体时效钢疲劳强度的影响华东交通大学机电学院 (南昌 330014) 黄 勇 编译0 前言马氏体时效钢是实用钢中强度最高而且韧性也很高的材料。
但是,它存在着与高的静强度相比,疲劳强度低这一高强度钢所特有的问题。
为此,进行了旨在改善疲劳特性的多项研究。
森野数博等人弄清了若从组织学观点出发,通过改变时效条件,使之生成适当的逆相变奥氏体,就能改善疲劳强度。
此外,表面改性也是有效的方法。
为此,探讨了喷丸硬化和渗氮的影响,报道了利用这些方法得以大幅度提高疲劳强度的结果。
但是,这样一些疲劳强度的改善,其效果和机理均有所不同。
例如,逆相变奥氏体的影响在低应力条件下明显,此外渗氮的效果也随应力水平之不同而异。
在高应力条件下,由硬化导致的脆性是疲劳强度低下的主要原因。
因此,实际讨论这种改善疲劳强度的策略时,必须先弄清各不同方法的最适宜条件,由此,也就必须弄清渗氮的利弊。
以此观点探讨了渗氮马氏体时效钢的疲劳特性,但对于被列为讨论对象的寿命区域,在107以下的疲劳特性及破坏机理尚不一定十分清楚。
因此,在本研究中,森野数博等人根据迄今的研究结果,通过改变渗氮条件,使渗氮层组织和深度发生变化,探讨其对疲劳强度的影响,以弄清通过渗氮来改善疲劳强度时所存在的问题。
1 材料、试样及实验方法实验所用材料为市面出售的300级马氏体时效钢,在固熔化处理的状态下被采用。
其化学成分示于表1。
将材料机械加工成图1所示的形状后,渗氮材料在渗氮处理前,时效材料在时效处理后,将表面电解抛光约20 m 。
时效条件要进行预备实验,选择了不完全时效状态的480 、2h 和6h,以及生成与此相同硬度之逆相变奥氏体的过时效条件的570 、2h 。
选择480 是因为它是通常采用的时效温度,同时也接近渗氮温度,并考虑到了2h 和6h 乃是疲劳强度最高的范围。
此外,由于渗氮与时效同时进行,为使内部硬度达到相同,选择了与时效条件相对应的加热温度和时间,以图2所示的三种条件进行实验。
表1 化学成分(质量分数)%CSiMnPSNiMoCo Al Ti 0.0050.030.040.0020.00218.69 4.898.920.10.91本研究采用的渗氮方法是可以控制表面化合物层的基本的渗氮法。
以下用处理条件的温度、时间和处理法标记各种材料,将480 、570 的时效材料记为48A_2、48A_6和57A_2材料,将480 、570渗氮材料记为48N_1、48N_5及57N_1材料。
图3表示各种渗氮材料的组织照片。
无论哪种材料都能确第25卷第6期2004年12月国外金属热处理GUOWAI JINS HU REC HULI Vol.25,No.6Dec,2004认其渗氮层,但渗氮时间长或温度高者渗氮层深。
硬度及残留应力的测定分别采用显微硬度计及X 射线应力测定装置进行。
疲劳试验采用小野式旋转弯曲疲劳试验机(容量:15N m;旋转数:约50HZ)在室温大气中进行。
2 实验结果及考察图4表示各种渗氮材料的硬度分布。
所有材料的表面硬化深度均为50 m 到100 m 左右,而48N_5材料的硬化深度最深。
而且,所有的材料的内部硬度均大致相同,为维氏硬度620~650。
对于57N_1材料虽然表面相当硬,但在表面下方的硬度降低比其它材料大,因此,可以认为表层的高硬度是受化合物层影响所致。
此外,所有渗氮材料表面均存在压缩残留应力,其大小为500MPa左右。
图5表示各种材料的S_N 曲线。
图中!/∀标记表示有内部破坏。
如后面所述,对于48N_1材料和48N_5材料,在高应力条件下发生表面破坏,而在低应力条件下发生内部破坏。
因此,48N_1材料和48N_5材料的S_N 曲线形状成为二级弯折曲线。
此外,通过渗氮虽然提高了疲劳强度,但其提高程度随应力水平及渗氮条件的不同而不同。
而且,若渗氮时间延长,则在表面发生破坏的高应力条件下,其寿命缩短,但在内部发生破坏的低应力条件下则形成大致相等的疲劳寿命。
另一方面,对于57N_1材料即使超过107周次,也和时效材料48A_2、48A_6和57A_2材料一样均发生表面破坏,其S_N 曲线形状也是随着应力降低疲劳寿命增大的普通形状(关于达到更长寿命区域情况的破坏机理尚须研究)。
此外,57N_1材料的疲劳强度由硬度分布、残留应力差异所推断出的结果比48N_1、48N_5的低得多,仅高于时效材料的结果。
此外,关于57A_2材料及48A_6材料与57A_2材料疲劳强度的差异,正如目前所知道的,由于是在过时效条件下生成逆相变奥氏体,故在低应力条件下,57A_2材料的疲劳强度稍高。
图6~8表示各种材料的疲劳断裂面。
图6表示作为时效材料例子的48A_2材料的结果。
但在时效材料的情况下,所有应力均导致表面破坏,而对渗氮材料,在57N_1材料的全部应力区域和48N_1、48N_5材料的高应力区域,破坏均由表面发生(图7)。
另一方面,如图8所示,48N_1、48N_5材料在低应力区域发生以夹杂物为起点的内部破坏。
图8所示各种材料中鱼眼的宏观照片(a_1)、(b_1)和试样表面方向的鱼眼边界(a _2)、(b_2),鱼眼中央部份(a _3)、(b_3),以及试样内部方向的鱼眼边界(a _4)、(b_4)的显微照片。
由放大照片可知,在鱼眼边缘,能观察到凹凸不平的严重的原奥氏体晶界裂纹,而且在其周20 国外金属热处理第25卷围观察到在马氏体时效处理钢中经常能看到的马氏体板条裂纹。
根据这一事实,可以推测到达鱼眼边界后的裂纹传播迅速进展,以至于最终发生断裂。
渗氮材料中原奥氏体晶界裂纹不仅在鱼眼边界,而且在试样表面层也能被观察到,但其比率以渗氮时间长或渗氮温度高者为高。
此外,所有材料的内部裂纹在渗氮硬化层的传播都受到阻碍。
鱼眼的边界位置在试样表面下方的21 第6期黄 勇:渗氮条件对马氏体时效钢疲劳强度的影响内部,但48N_5材料与48N_1材料相比,前者在试样表面方向的边界不清楚,这也表明由渗氮引起的脆化使裂纹的传播加速。
有报告称,在高强度钢长寿命区域发生内部破坏时,在鱼眼起点部位观察到粒状断裂面,而且寿命愈长,其范围愈大。
图9是以48N_1材料为例表示鱼眼起点部位。
本研究材料的起点部位周围也能观察到粒状断裂面。
且长寿命者此范围较大。
图10表示48N_1材料和48N_5材料内部破坏的起点深度d inc 和疲劳寿命Nf 的关系。
所有材料,其破坏起点都是分布在硬化层边界附近的夹杂物。
图11表示48N_1材料和48N_5材料的鱼眼尺寸2b 和疲劳寿命Nf 的关系。
疲劳寿命愈长,鱼眼就愈大,未见渗氮时间对此关系之影响。
表2表示各种材料的机械性质。
表2 表示材料的机械性质 0.2/MPaB /MPa T /MPa (%)48A_248A_657A_248N_148N_557N_119412020206121002150194419702090207421172155200026852740288425882370214455.654.353.447.131.512.9图12表示各种材料拉伸断裂后的表面照片。
由于渗氮处理使塑性下降,且渗氮时间愈长或者温度愈高,则降低愈甚。
特别是57N_1材料,虽拉伸强度也降低,但塑性则是大幅度降低。
与此相对应,在57N_1材料的情况下,渗氮材料的表面也发生脆性裂纹,且试样的缩颈非常小,即脆化很大。
这种状况也与疲劳性能方面已被确认的渗氮层脆化(图7,8)相对应。
图13是为了观察渗氮处理对裂纹传播特性的影响,而将57N_1材料表面裂纹的传播曲线与48A_6材料的该曲线进行比较。
图14是表示两种材料裂纹形态复膜的照片。
图13中横座标上的空缺标记表示在此交变下未确认裂纹。
马氏体时效钢与其它高强度材料一样,裂纹一旦发生之后,就迅速传播。
48A_6材料也一样,但对57N_1材料而言,虽然裂纹发生受到抑制,但裂纹传播却更加迅速,从而全部寿命中几乎100%都是发生裂纹的寿命。
这点从裂纹的形态上,前者为塑性的而后者为脆性的这一事实,亦可明了。
22 国外金属热处理第25卷如上所述,进行渗氮处理,可延长疲劳寿命,但若延长渗氮时间或提高渗氮温度,则在发生表面破坏的区域,疲劳寿命缩短。
可以认为这是由于通过渗氮使氮在表面扩散的同时生成了氮化物,这些氮化物主要析出于原奥氏体晶界,结果随着塑性的大幅度降低,产生了晶界裂纹。
也就是说,表面脆化所产生的不良影响大于渗氮所引起的表面硬化的效果,从而在高应力下产生粒界裂纹,导致寿命缩短。
另一方面,可以认为在产生内部破坏的应力区域中,内部裂纹的传播主要发生在硬化层边界附近,其理由是,在那里两种材料的硬度几乎相同。
此外,在57N_1材料的情况下,如前所述,渗氮温度乃是逆相变奥氏体析出的温度条件,这些奥氏体析出于组织边界。
可以认为,这一现象与上述的晶界开裂行为有关,但逆相变奥氏体对渗氮的影响目前尚不清楚。
4 小结为了弄清有关马氏体时效钢渗氮时的疲劳强度的时效性及问题,通过改变渗氮温度和时间以改变渗氮层性质和深度,探讨了这些因素对疲劳强度的影响,获得的主要结论如下:1)通过渗氮虽提高了疲劳强度,但其提高程度受渗氮温度和渗氮时间的影响很大。
也就是说,在不完全时效条件的480进行1h和5h渗氮时,能大幅度提高疲劳强度,但在过时效状态的570的渗氮温度下,疲劳强度提高不大。
2)处于不完全时效状态的渗氮材料,在短寿命区域发生表面破坏,而在长寿命区域则发生以内部夹杂物为起点的鱼眼破坏。
与此相关,S_N曲线变成了二级弯折曲线。
另一方面,在过时效状态下渗氮时,在整个寿命区域均发生表面破坏。
3)通过渗氮可提高疲劳强度,其主要原因是表面硬化和产生了压缩残留应力,而渗氮引起的原奥氏体的晶界裂纹则是其上升程度减少的原因。
(上接第11页)[3] Kelton,K.F.Inter.Mater.Rev.1993,28(3):105_137[4] 木村久道等.轻金属.1997,47(10):539_544.[5] 伊藤尚等.粉体粉末冶金.1998,45(2):182_188.[6] Benjamin,J.S.M etall.Trans.1970,1(10):2943_2951.[7] 汤浅荣二,矶西和夫.塑性 加工.第42卷,第489号。
[8] 新家光雄等.轻金属.1997,47(10):521_526.[9] 小林庆三等.粉体 !粉末冶金,1998,45(11):1050_1054.[10] 长柄毅一等.粉体 !粉末冶金,2001,48(6):531_538.[11] 特集.放电烧结∀基础 应用.粉体#!粉未冶金,2001,48(6):530_570.23第6期黄 勇:渗氮条件对马氏体时效钢疲劳强度的影响。