岩土工程专业翻译英文原文和译文
土木地质岩土工程专业毕业英文翻译原文和译文
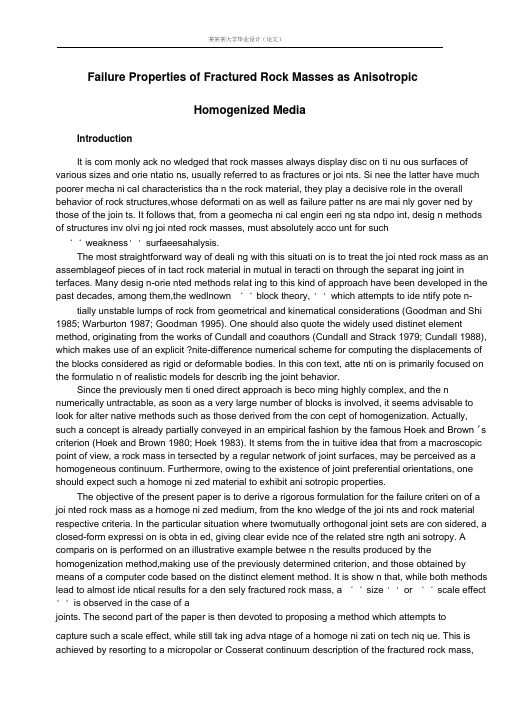
Failure Properties of Fractured Rock Masses as AnisotropicHomogenized MediaIntroductionIt is com monly ack no wledged that rock masses always display disc on ti nu ous surfaces of various sizes and orie ntatio ns, usually referred to as fractures or joi nts. Si nee the latter have much poorer mecha ni cal characteristics tha n the rock material, they play a decisive role in the overall behavior of rock structures,whose deformati on as well as failure patter ns are mai nly gover ned by those of the join ts. It follows that, from a geomecha ni cal engin eeri ng sta ndpo int, desig n methods of structures inv olvi ng joi nted rock masses, must absolutely acco unt for such‘‘ weakness' ' surfaeesahalysis.The most straightforward way of deali ng with this situati on is to treat the joi nted rock mass as an assemblageof pieces of in tact rock material in mutual in teracti on through the separat ing joint in terfaces. Many desig n-orie nted methods relat ing to this kind of approach have been developed in the past decades, among them,the wedlnown ‘‘ block theory, ' ' which attempts to ide ntify pote n- tially unstable lumps of rock from geometrical and kinematical considerations (Goodman and Shi 1985; Warburton 1987; Goodman 1995). One should also quote the widely used distinet element method, originating from the works of Cundall and coauthors (Cundall and Strack 1979; Cundall 1988), which makes use of an explicit ?nite-difference numerical scheme for computing the displacements of the blocks considered as rigid or deformable bodies. In this con text, atte nti on is primarily focused on the formulatio n of realistic models for describ ing the joint behavior.Since the previously men ti oned direct approach is beco ming highly complex, and the n numerically untractable, as soon as a very large number of blocks is involved, it seems advisable to look for alter native methods such as those derived from the con cept of homogenization. Actually, such a concept is already partially conveyed in an empirical fashion by the famous Hoek and Brown ' s criterion (Hoek and Brown 1980; Hoek 1983). It stems from the in tuitive idea that from a macroscopic point of view, a rock mass in tersected by a regular network of joint surfaces, may be perceived as a homogeneous continuum. Furthermore, owing to the existence of joint preferential orientations, one should expect such a homoge ni zed material to exhibit ani sotropic properties.The objective of the present paper is to derive a rigorous formulation for the failure criteri on of a joi nted rock mass as a homoge ni zed medium, from the kno wledge of the joi nts and rock material respective criteria. In the particular situation where twomutually orthogonal joint sets are con sidered, a closed-form expressi on is obta in ed, giving clear evide nce of the related stre ngth ani sotropy. A comparis on is performed on an illustrative example betwee n the results produced by the homogenization method,making use of the previously determined criterion, and those obtained by means of a computer code based on the distinct element method. It is show n that, while both methods lead to almost ide ntical results for a den sely fractured rock mass, a ‘‘ size ' ' or ‘‘ scale effect ' ' is observed in the case of ajoints. The second part of the paper is then devoted to proposing a method which attempts tocapture such a scale effect, while still tak ing adva ntage of a homoge ni zati on tech niq ue. This is achieved by resorting to a micropolar or Cosserat continuum description of the fractured rock mass,through the derivation of a generalized macroscopic failure condition expressed in terms of stresses and couple stresses. The implementation of this model is ?nally illustrated on a simple example, show ing how it may actually acco unt for such a scale effect.Problem Statement and Principle of Homogenization ApproachThe problem under consideration is that of a foundation (bridge pier or abutment) resting upon a fractured bedrock (Fig. 1), whose beari ngFig, 1, Bearing capacit>p of foundation on fractured rockmasscapacity needs to be evaluated from the knowledge of the strength capacities of the rock matrix and the joint in terfaces. The failure con diti on of the former will be expressed through the classical Mohr-Coulomb condition expressedby means of the cohesion C m and the friction angle m . Note that tensile stresses will be counted positive throughout the paper.Likewise, the joi nts will be modeled as pla ne in terfaces (represe nted by lines in the ?gure ' s plane). Their strength propertieiseadescribed by means of a condition involving the stress vector of comp onents ( c , T ) act ing at any point of those in terfacesF7(O-,T)=|T|+<J tan <f f—C r^0 (l)According to the yield design (or limit analysis) reasoning, the above structure will remai n safe un der a give n vertical load Q(force per un it le ngth along the Oz axis), if one can exhibit throughout the rock mass a stress distribution which satis?es the equilibrium equations along with the stress boundary conditions,while complying with the strength requirement expressed at any point of the structure.+This problem amounts to evaluating the ultimate load Q beyond which failure will occur, or equivalently within which its stability is ensured. Due to the strong heterogeneity of the joi nted rock mass, in surm oun table dif?culties are ilkely to arise whe n tryi ng to impleme nt the above reasoning directly. As regards, for instanee, the case where the strength properties of the joints are considerably lower than those of the rock matrix, the implementation of akinematic approach would require the use of failure mechanisms involving velocity jumps across the joi nts, since the latter would con stitute prefere ntial zones for the occurre nee offailure. In deed, such a direct approach which is applied in most classical desig n methods, is beco ming rapidly complex as the den sity of joi nts in creases, that is as the typical joi nt spaci ng l is beco ming small in comparis on with a characteristic len gth of the structure such as the foundation width B.In such a situati on, the use of an alter native approach based on the idea of homoge ni zati on and related con cept of macroscopic equivale nt continuum for the join ted rock mass, may be appropriate for dealing with such a problem. More details about this theory, applied in the con text of rein forced soil and rock mecha ni cs, will be found in (de Buha n et al. 1989; de Buhan and Sale nc ,on 1990; Bernaud et al. 1995).Macroscopic Failure Condition for Jointed Rock MassThe formulati on of the macroscopic failure con diti on of a join ted rock mass may be obtained from the solution of an auxiliary yield design boundary-value problem attached to a unit represe ntative cell of joi nted rock (Bekaert and Maghous 1996; Maghous et al.1998). It will now be explicitly formulated in the particular situation of two mutually orthogonal sets of joi nts un der pla ne strain con diti ons. Referri ng to an orth ono rmal frame O 1 2 whose axes are placed along the joints direct ions, and in troduci ng the follow ing cha nge of stress variables:p = ((T]|+(T22)/\2, I?=((T22-O,I1)/ \2, 1= \2(T|2 (2) such a macroscopic failure con diti on simply becomes(如mwh ere it will be assumed that15 = 5tan<p;.A convenient represe ntati on of the macroscopic criteri on is to draw the stre ngth env elope relating to an oriented facet of the homogenized material, whose unit normal n I is inclined by an an gle a with respect to the joint direct ion. Deno ti ng by - n and n the no rmal and shear comp onents of the stress vector act ing upon such a facet, it is possible to determ ine for any value of a the set of admissible stresses , n) deduced from conditions (3) expressed in terms of ( J,、, -12). The corresponding domain has been drawn in Fig. 2 in the particular case where 乞mTwo comme nts are worth being made:1. The decrease in stre ngth of a rock material due to the prese nceof joints is clearly illustrated by Fig.2. The usual strength envelope corresponding to the rock matrix failure condition is ‘‘ truncatedy two orthogonal semilines as soon as condition H j : H m is ful?lled.2. The macroscopic anisotropy is also quite apparent, since for instance the strength envelopedrawn in Fig. 2 is dependent on the facet orientation a. The usual notion of intrinsic curve should therefore be discarded, but also the con cepts of ani sotropic cohesi on and frictio n an gle as ten tatively in troduced by Jaeger (1960), or Mc Lamore and Gray (1967).Nor can such an anisotropy be properly described by means of criteria based on an extension of the classical Mohr-Coulomb condition using the concept of anisotropy tensor(Boehler and Sawczuk 1977; Nova 1980; Allirot and Bochler1981).Application to Stability of Jointed Rock ExcavationThe closed-form expressi on (3) obta ined for the macroscopic failure con diti on, makes it thenpossible to perform the failure design of any structure built in such a material, such as the excavati on show n in Fig. 3,where h and Bdenote the excavation height and the slope angle, respectively. Since noFig. 2. Strength envelope attached co facei of homogenized materialFig. 3. Stability analysis of jointed rock excavationsurcharge is applied to the structure, the speci?c weight y of the constituent material will obviously constitute the sole loading parameter of the system.Assessing the stability of this structure will amount to evaluati ng the maximum possible height F bey ond which failure will occur. A standard dimensional analysis of this problem shows that this critical height may be put in the formwhere 0 =jointorientation and K+=nondimensional factor governing the stability of the excavation. Upper-bound estimates of this factor will now be determined by means of the yield desig n kin ematic approach, using two kinds of failure mecha ni sms show n in Fig. 4.Rotational Failure Mechanism [Fig. 4(a)]The ?rst class of failure mecha ni sms con sidered in the an alysis is a direct tran spositi on of those usually employed for homogeneous and isotropic soil or rock slopes. In such a mecha nism a volume of homoge ni zed joi nted rock mass is rotati ng about a point Q angular velocity 3 . The curve separating thjisrvofrom the rest of the structure which iskept motionless is a velocity jump line. Since it is an arc of the log spiral of angle m andfocus Q the velocity disc on tin uity at any point of this line is in cli ned at an gle wm with respect to the tangent at the same point.The work done by the external forces and the maximum resisting work developed in such a mecha nism may be writte n as (see Chen and Liu 1990; Maghous et al. 1998)二丁3片片%(B;»i .42)_'' mr| P,「*申/A t,42 ) (')J mwhere W e and W me =dimensionless functions, and ® and %=angles specifying theposition of the center of rotation Q .Sinee the kinematic approach of yield design states tha n ecessary con diti on for the structure to be stable writes化w (6)it follows from Eqs. (5) and (6) that the best upper-bound estimatederived from this ?rstclass of mechanism is obtained by minimization with respect to 1 and 2 卩which may be determ ined nu merically.Piecewise Rigid-Block Failure Mechanism [Fig. 4(b)]The sec ond class of failure mecha ni sms invo Ives two tran slat ing blocks of homoge ni zed material. It is de?ned by ?ve an gular parameters .In order to avoid any misi nterpretati on, it should be speci?ed that the terminology of block does not refer here to the lumps of rock matrix in the initial structure but merely means that, in the framework of the yield design kin ematic approach, a wedge of homoge ni zed join ted rock mass is give n a (virtual) rigid-body moti on.The implementation of the upper-bound kinematic approach,making use of of this second class of failure mechanism, leads to the following results.叫=匕号沪叫(…;"gj 炉(…;山g)(8)where U representsthe norm of the velocity of the lower block. Hence, the following+upper-bo und estimate for K: Results and Comparison with Direct CalculationThe optimal bound has bee n computed nu merically for the follow ing set of parameters:0 = 75。
岩土基本术语英汉对照
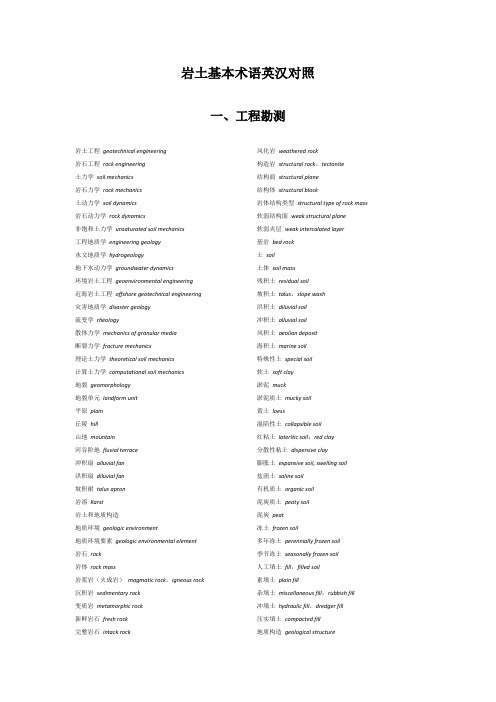
岩土基本术语英汉对照一、工程勘测岩土工程geotechnical engineering岩石工程rock engineering土力学soil mechanics岩石力学rock mechanics土动力学soil dynamics岩石动力学rock dynamics非饱和土力学unsaturated soil mechanics工程地质学engineering geology水文地质学hydrogeology地下水动力学groundwater dynamics环境岩土工程geoenvironmental engineering近海岩土工程offshore geotechnical engineering 灾害地质学disaster geology流变学rheology散体力学mechanics of granular media断裂力学fracture mechanics理论土力学theoretical soil mechanics计算土力学computational soil mechanics地貌geomorphology地貌单元landform unit平原plain丘陵hill山地mountain河谷阶地fluvial terrace冲积扇alluvial fan洪积扇diluvial fan坡积裙talus apron岩溶Karst岩土和地质构造地质环境geologic environment地质环境要素geologic environmental element岩石rock岩体rock mass岩浆岩(火成岩)magmatic rock,igneous rock 沉积岩sedimentary rock变质岩metamorphic rock新鲜岩石fresh rock完整岩石intack rock 风化岩weathered rock构造岩structural rock,tectonite结构面structural plane结构体structural block岩体结构类型structural type of rock mass 软弱结构面weak structural plane软弱夹层weak intercalated layer基岩bed rock土soil土体soil mass残积土residual soil坡积土talus,slope wash洪积土diluvial soil冲积土alluvial soil风积土aeolian deposit海积土marine soil特殊性土special soil软土soft clay淤泥muck淤泥质土mucky soil黄土loess湿陷性土collapsible soil红粘土lateritic soil;red clay分散性粘土dispersive clay膨胀土expansive soil, swelling soil盐渍土saline soil有机质土organic soil泥炭质土peaty soil泥炭peat冻土frozen soil多年冻土perennially frozen soil季节冻土seasonally frozen soil人工填土fill,filled soil素填土plain fill杂填土miscellaneous fill,rubbish fill冲填土hydraulic fill,dredger fill压实填土compacted fill地质构造geological structure褶曲fold背斜anticline向斜syncline断裂rupture,fracture裂隙fissure节理joint断层fault活断层active fault破碎带fracture zone产状attitude风化作用weathering风化壳weathered crust风化带weathered zone风化程度degree of weathering,intensity of weathering地表水surface water地下水groundwater包气带水aeration zone water上层滞水perched water潜水phreatic water承压水confined water层间水interlayer water裂隙水fissure water不透水层impervious layer径流区runoff area地下径流subsurface runoff水头water head储水系数storage coefficient导水系数transmissivity给水度specific yield持水度water retaining capacity容水量water bearing capacity影响半径radius of influence弥散系数dispersion coefficient地下水化学类型chemical type of groundwater地下水总矿化度total mineralization of groundwater地下水硬度groundwater hardness地下水腐蚀性groundwater corrosivity地下水污染roundwater pollution地下水等水位线图contour map of groundwater水文地质勘察hydrogeological investigation水文地质测绘hydrogeological survey,hydrogeological surveying and mapping水文地质钻探hydrogeological drilling岩土工程勘察geotechnical investigations 工程地质测绘engineering geological mapping工程地质勘探engineering geological prospecting岩芯采取率core recovery取土器soil sampler薄壁取土器thin wall sampler厚壁取土器thick wall sampler不扰动土样undisturbed soil sample扰动土样disturbed soil sample土试样质量等级quality grade of soil samples坑探pit exploration地球物理勘探geophysical exploration电法勘探electrical exploration磁法勘探magnetic exploration电磁法electromagnetic geophysical exploration探地雷达法ground penetrating radar method(GPR)地震勘探seismic exploration声波探测acoustic exploration红外探测infrared detection遥感勘测remote sensing prospecting原位测试in-situ testing平板载荷试验plate loading test螺旋板载荷试验screw plate loading test旁压试验pressuremeter test(PMT)扁铲侧胀试验flat dilatometer test(DMT)静力触探试验cone penetration test(CPT)孔压静力触探试验piezocone test,piezocone penetration test (CPTU)圆锥动力触探试验dynamic penetration test(DPT)标准贯入试验standard penetration test(SPT)十字板剪切试验vane test,vane shear test(VST)原位直接剪切试验in-situ shear test应力解除法stress relief method应力恢复法stress recovery method水力劈裂法hydraulic fracturing technique波速测试wave velocity test抽水试验pumping test注水试验water injection test渗水试验infiltration test;pit permeability test压水试验pump-in test连通试验connecting test弥散试验dispersion test水质分析chemical and physical analysis of water勘察成果与评价岩土工程勘察报告geotechnical investigation report勘察阶段investigation stage工程地质单元engineering geological unit工程地质图engineering geologic map综合工程地质图comprehensive engineering geologic map综合柱状图composite columnar section工程地质剖面图engineering geological profile探坑展示图extension chart of pit & shaft赤平极射投影polar-sterometric projection岩土工程分级classification of geotechnical projects岩土工程评价geotechnical evaluation工程测量engineering survey精密工程测量precise engineering survey高斯平面直角坐标系Gauss-Krueger plane rectangular coordinate system独立坐标系independent coordinate system高程elevation; height国家高程基准National Height Datum 1985水准测量leveling精密水准测量precise leveling测量标志surveying mark基准点datum point工作基点operating control point监测网monitoring control network限差tolerance点位误差position error 点位中误差mean square error of points相对中误差relative mean square error现场监测与检测现场监测in-situ monitoring原型监测prototype monitoring现场检测in-situ inspection地下水监测groundwater monitoring地下水动态观测groundwater dynamic observation孔隙水压力监测pore water pressure monitoring土压力监测earth pressure test岩土体位移监测displacement monitoring for soil and rock地面沉降监测ground subsidence monitoring地基变形监测ground deformation monitoring岩土体测斜inclination monitoring for soil and rock分层沉降观测layered settlement observation围岩收敛观测ambient rock convergence monitoring岩土环境污染监测monitoring for geotechnical environment pollution基槽检验foundation trench inspection桩底沉渣检测sludge measurement for bored pile锚杆基本试验basic tests of anchor锚杆验收试验anchor acceptance test土钉抗拔检测pull-out test of soil nail地下连续墙质量检测test of diaphragm wall复合地基载荷试验loading test of composite foundation二、岩土基本特性与室内试验土的组构soil fabric土的结构soil structure土骨架soil skeleton比表面积specific surface孔隙水pore water自由水free water重力水gravitational water毛细管水capillary water吸着水absorbed water粒径grain size粒径分布曲线grain size distribution curve 限制粒径constrained grain size有效粒径effective grain size粒组fraction巨粒类土over coarse-grained soil粗粒类土coarse-grained soil 细粒类土fine-grained soil漂石(块石)boulder(stone block)卵石(碎石)cobble砾类土gravelly soil砂类土sandy soil粉粒土silt soil黏粒土clay soil细粒土fines含粗粒的细粒土fines with coarse粉土silt粘性土cohesive soil无粘性土cohesionless soil不均匀系数coefficient of uniformity 曲率系数coefficient of curvature级配gradation良好级配土well-grade soil不良级配土poorly-grade soil不连续级配土gap-grade soil土试样soil specimen室内试验laboratory test含水率water content密度density重度unit weight土粒比重specific gravity of soil particle三相图three phase diagram孔隙率porosity孔隙比void ratio临界孔隙比critical void ratio饱和度degree of saturation饱和土saturated soil非饱和土unsaturated soil颗粒分析试验particle size analysis稠度界限consistency limit液限liquid limit塑限plastic limit缩限shrinkage limit塑性指数plasticity index液性指数liquidity index缩性指数shrinkage index活动性指数activity index塑性图plasticity chart膨胀力swelling force膨胀率swelling ratio自由膨胀率free swelling ratio线缩率linear shrinkage ratio体缩率volume shrinkage ratio冻胀frost heave冻胀力frost-heaving pressure冻胀量frost-heave capacity融陷性thaw collapsibility相对密度relative density压实性compactibility击实试验compaction test最大干密度maximum dry density最优含水率optimum moisture content压实度degree of compaction加州承载比California Bearing Ratio(CBR)土体渗透性permeability of soil渗透系数coefficient of permeability渗透试验permeability test 达西定律Darcy’s law水力梯度hydraulic gradient临界水力梯度critical hydraulic gradient渗流seepage渗透力seepage force压缩性compressibility固结consolidation单向固结unidirectional consolidation主固结primary consolidation次固结secondary consolidationK0 固结K0-consolidation固结试验consolidation test压缩系数coefficient of compressibility体积压缩系数coefficient of volume compressibility压缩指数compression index压缩模量constrained modulus回弹指数swelling index回弹模量rebound modulus固结度degree of consolidation固结系数coefficient of consolidation次固结系数coefficient of secondary consolidation固结压力consolidation pressure先期固结压力preconsolidation pressure超固结比overconsolidation ratio(OCR)正常固结土normally consolidated soil超固结土over consolidated soil欠固结土underconsolidated soil湿陷性collapsibility湿陷变形collapse deformation黄土湿陷试验collapsibility test of loess湿陷系数coefficient of collapsibility湿陷起始压力initial collapse pressure抗剪强度shear strength灵敏度sensitivity剪切试验shear test直剪试验direct shear test快剪试验quick shear test固结快剪试验consolidated quick shear test慢剪试验slow shear test无侧限抗压强度unconfined compressive strength无侧限抗压强度试验unconfined compressive strength test三轴压缩试验triaxial compression test不固结不排水三轴试验unconsolidated-undrained triaxial test 固结不排水三轴试验consolidated-undrained triaxial test固结排水三轴试验consolidated-drained triaxial test三轴伸长试验triaxial extension test强度包线strength envelope粘聚力cohesion内摩擦角internal friction angle天然休止角natural angle of repose触变性thixotropy剪胀性dilatancy应变软化strain softening应变硬化strain hardening破坏强度failure strength塑性破坏plastic failure脆性破坏brittle failure峰值强度peak strength残余强度residual strength孔隙水压力系数pore pressure parameter应力路径stress path应力路径试验stress path test真三轴试验true triaxial test平面应变试验plane strain test单剪试验simple shear test扭剪试验torsional shear test动三轴试验dynamic triaxial test动单剪试验dynamic simple shear test共振柱试验resonant column test土工离心模型试验geotechnical centrifugal model test岩体结构structure of rock mass岩块block岩石分类rock classification工程岩体engineering rock mass岩体结构面(不连续面)structural plane(discontinuity)结构面迹长trace of structural plane结构面密度density of structural plane原生结构面primary structure plane构造结构面constructive structure plane次生结构面secondary structure plane结构面分级classification of structure plane不连续岩体discontinuous rock mass延续性continuity粗糙度roughness张开度(开度)aperture充填物filling substance岩石物理性质physical properties of rock岩石力学性质mechanical properties of rock 岩石颗粒密度particle density of rock岩石块体密度block density of rock岩石含水率water content of rock岩石吸水率water-absorption of rock岩石饱和吸水率saturated water-absorption of rock节理连通率joint persistence ratio岩体体积节理数volumetric joint count of rock mass抗压强度compressive strength单轴抗压强度single axis compressive strength单轴抗压强度试验single axis compressive strength test单轴压缩变形试验single axis compression deformation test三轴抗压强度triaxial compressive strength三轴压缩强度试验triaxial compressive strength test压剪试验compressive shear test岩体直剪试验direct shear test of rock mass岩体结构面直剪试验direct shear test of structural plane抗剪断强度shearing strength抗剪试验anti-shear test抗切强度anti-cut strength抗切试验anti-cut test摩擦强度friction strength结构面的面摩擦强度surface friction strength of structure plane抗拉强度tensile strength岩石抗拉强度试验tensile strength test of rock抗弯试验bending test劈裂试验(巴西试验)split test点荷载强度试验point load strength test岩石膨胀压力swelling pressure of rock岩石自由膨胀率free swelling ratio of rock岩石侧向约束膨胀率lateral restraint swelling ratio of rock岩石膨胀性试验swelling test of rock岩石耐崩解性试验disintegration-resistance test of rock长期模量long-term modulus长期强度long-term strength抗冻性系数软化系数softening coefficient疲劳强度fatigue strength弹性后效delayed elasticity应力松弛stress relaxation松弛时间relaxation time流动特性flow behavior蠕变creep剪胀shear dilatation风化weathering泥化mudding泥化夹层siltized intercalation/muddy intercalation卸荷变形unloading deformation粘滞系数coefficient of viscosity微裂纹micro crack岩爆rock burst岩体初始应力(原岩应力)initial rock stress(geostress)初始应力场initial stress field尺度效应scale effect岩石扩容dilatancy of rock岩石声发射acoustic emission of rock 应力解除法stress-relief method应力恢复法stress restoration method高压压水试验high pressure water test岩体原位应力测试in-situ rock stress test承压板法试验bearing plate test钻孔变形试验borehole deformation test狭缝法试验slit method test岩体声波速度测试acoustic speed test of rock mass岩石坚硬程度hardness degree of rock岩石质量指标rock quality designation (RQD)岩体基本质量rock mass basic quality(BQ)岩体基本质量分级classification of rock mass basic quality三基本理论与计算分析半无限弹性体semi-infinite elastic body中心荷载(轴心荷载)central load偏心荷载eccentric load集中荷载(点荷载)concentrated load均布荷载uniformly distributed load条形荷载strip load线荷载line load交变荷载alternating load周期荷载cyclic load瞬时荷载transient load动荷载dynamic load体积力body force表面力surface force覆盖压力overburden pressure超载surcharge应力分布stress distribution应力集中stress concentration自重应力geostatic stress,self-weight stress基底压力(接触压力)contact pressure附加应力additional stress,superimposed stress 基底附加压力additional stress on the base剪应变shear strain体应变volumetric strain弹性应变elastic strain塑性应变plastic strain弹性模量(杨氏模量)modulus of elasticity变形模量modulus of deformation剪切模量shear modulus泊松比Poisson’s ratio 体积模量bulk modulus文克勒假定Winkler’s assumption沉降settlement沉降计算深度settlement calculation depth最终沉降final settlement初始沉降(瞬时沉降)immediate settlement主固结沉降primary consolidation settlement次固结沉降secondary consolidation settlement 固结沉降consolidation settlement不均匀沉降non-uniform settlement容许沉降allowable settlement工后沉降settlement after construction沉降速率rate of settlement太沙基固结理论Terzaghi’s consolidation theory 比奥固结理论Biot’s consolidation theory地基回弹rebound of foundation基坑底隆胀heaving of the bottom塑流plastic flow屈服yield屈服面yield surface应力空间stress space应变空间strain space应力历史stress history临塑荷载critical edge pressure塑性平衡状态state of plastic equilibrium塑性区plastic zone整体剪切破坏general shear failure局部剪切破坏local shear failure冲剪破坏punching shear failure容许承载力allowable bearing capacity承载力因数bearing capacity factors极限承载力ultimate bearing capacity块体理论block theory岩石的强度理论strength theory of rock摩尔-库仑定律Mohr-Coulomb Law极限平衡状态limit equilibrium极限平衡条件limit equilibrium condition有效应力原理principle of effective stress总应力total stress有效应力effective stress孔隙压力pore pressure孔隙水压力pore water pressure孔隙气压力pore air pressure孔隙压力比pore pressure ratio静水压力hydrostatic pressure超静水压力excess pore water pressure扬压力uplift pressure浮托力buoyancy稳定渗流steady seepage流网flow net流线flow line等势线equipotential line浸润线phreatic line渗透稳定性seepage stability长期稳定性long-term stability临界高度critical height(of slope)土的液化soil liquefaction液化势liquefaction potential液化破坏理论liquefaction failure theory渐近破坏progressive failure渐进破坏理论progressive failure theory凯塞效应Kaiser effect布辛涅斯克理论Boussinesq theory明德林解Mindlin’s solution角点法corner-point method分层总和法layerwise summation method沉降曲线settlement curve固结曲线consolidation curve摩尔-库仑破坏准则Mohr-Coulomb failure criterion 屈服准则yield criteria屈列斯卡屈服准则Tresca yield criteria米塞斯屈服准则Mises yield criteria格里菲斯强度准则Griffith’s strength criterion 应力水平stress level极限平衡法limit equilibrium method库尔曼图解法Kuhlman Graphic安全系数factor of safety稳定分析stability analysis总应力法total stress analysis有效应力法effective stress analysis主动土压力active earth pressure静止土压力earth pressure at rest被动土压力passive earth pressure库仑土压力理论Coulomb’s earth pressure theory朗肯土压力理论Rankine’s earth pressure theory条分法method of slice瑞典圆弧法Swedish circle method毕肖普简化条分法Bishop’s simplified method of slice稳定数stability number邓肯-张模型Duncan-Chang Model剑桥模型(临界状态模型)Cam-Clay model有限元法Finite Element Method(FEM)有限差分法Finite Difference Method(FDM)边界元法Boundary Element Method(BEM)离散元法Distinct Element Method(DEM)地基处理ground treatment,soil improvement置换法replacement振密、挤密法vibro-densification,compacting排水固结法consolidation,preloading灌入固化物法grouting curing material method加筋法reinforced method托换法underpinning复合地基composite ground,composite subgrade,composite foundation土工合成材料geosynthetics岩土锚固ground anchors, anchorage垫层cushion强夯置换法dynamic replacement褥垫法pillow抛石挤淤法rock filling replacement爆破挤淤法blasting replacement爆夯法blasting compaction轻量填土法light weight filling碾压法compaction by rolling强夯法dynamic consolidation,dynamic compaction振冲法vibro-flotation挤密砂(碎石)桩法densification by sand pile爆炸加密法densification by explosion振动压实法vibro-compaction排水砂井sand drain袋装砂井packed drain,fabric-enclosed drain塑料排水板(带)prefabricated strip drain,geodrain堆载预压法preloading真空预压法vacuum preloading真空堆载联合预压法vacuum combined surcharge preloading 电渗法electro-osmosis method砂垫层sand cushion水泥(稳定)土cement stabilization灰土lime treated soil高压喷射注浆法jet groutin挤密注浆法compaction grouting method深层搅拌法deep mixing method灌浆grouting固结灌浆consolidation grouting帷幕灌浆curtain grouting化学灌浆chemical grouting劈裂灌浆hydrofracture grouting土钉soil nailing铺网法fabric sheet reinforced earth加筋土reinforced earth纤维土textsoil,fiber soil纠倾托换rectification underpinning坑式托换pit underpinning桩基托换piles underpinning灌浆托换grouting underpinning锚杆静压桩托换pressed pile underpinning顶升纠倾jack-up leaning rectification堆载加压纠倾surcharge learning rectification掏土纠倾digging-out soil leaning rectification柔性桩复合地基flexible piles composite foundation刚性桩复合地基rigid piles composite foundationgranular material piles composite foundation长短桩复合地基long-short piles composite foundation大直径现浇混凝土管桩复合地基composite foundation of cast-in-place concrete large-diameter pipe pile桩网地基pile supported subgrade灰土挤密桩法compacted lime-soil pile石灰桩法lime pile砂石桩法sand-gravel pile水泥土桩法cement soil pile水泥粉煤灰碎石桩法cement-flyash-gravel pile (CFG pile) 桩土应力比stress ratio荷载分担比bearing ratio复合地基置换率replacement ratio土工合成材料土工织物geotextile织造土工织物woven geotextile非织造土工织物nonwoven geotextile土工膜geomenbrane土工格栅geogrid土工格室geocell土工模袋geofabriform土工复合材料geocomposite聚苯乙烯发泡材料expanded polystyrene (EPS)反滤filtration隔离separation防护protection等效孔径equivalent opening size (EOS)老化aging锚杆(索)anchor,anchorag tendon土层锚杆anchored bar in soil岩石锚杆rock anchor系统锚杆system of anchor bars预应力锚杆prestressed anchor非预应力锚杆non-prestressed anchor拉力型锚杆tensioned anchor压力型锚杆pressured anchor拉力分散型锚杆tensioned multiple-head anchor压力分散型锚杆pressured multiple-head anchor自由段unbonded tendon锚固段bonded tendon四、基础工程柔性基础flexible foundation弹性基础elastic foundation无筋扩展基础non-reinforced spread foundation 刚性基础(rigidity foundation)。
最新地质岩土英文文献翻译_冶金矿山地质_工程科技_专业资料
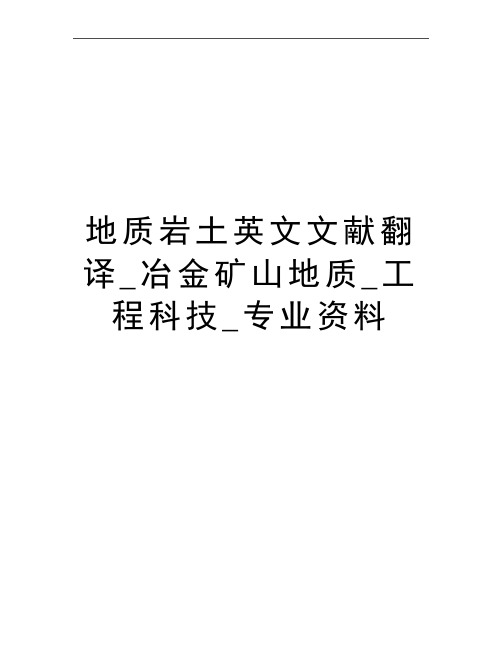
地质岩土英文文献翻译_冶金矿山地质_工程科技_专业资料International Journal of Rock Mechanics and Mining SciencesAnalysis of geo-structural defects in flexural topplingfailureAbbas Majdi and Mehdi Amini AbstractThe in-situ rock structural weaknesses, referred to herein asgeo-structural defects, such as naturally induced micro-cracks, are extremely responsive to tensile stresses. Flexural toppling failure occurs by tensile stress caused by the moment due to the weight ofthe inclined superimposed cantilever-like rock columns. Hence, geo-structural defects that may naturally exist in rock columns are modeled by a series of cracks in maximum tensile stress plane. The magnitude and location of the maximum tensile stress in rock columns with potential flexural toppling failure are determined. Then, the minimum factor of safety for rock columns are computed by means of principles of solid and fracture mechanics, independently. Next, a new equation is proposed to determine the length of critical crack in such rock columns. It has been shown that if the length of natural crack is smaller than the length of critical crack, then the result based on solid mechanics approach is more appropriate; otherwise, the result obtained based on the principles of fracture mechanics is more acceptable. Subsequently, for stabilization of the prescribed rock slopes, some new analytical relationships are suggested for determination the length and diameter of the required fully grouted rock bolts. Finally, for quick design of rock slopes against flexural toppling failure, a graphical approach along with some design curves are presented by which an admissible inclination of such rock slopes and or length of all required fully grouted rock bolts are determined.In addition, a case study has been used for practical verification of the proposed approaches.Keywords Geo-structural defects, In-situ rock structural weaknesses, Critical crack length1.IntroductionRock masses are natural materials formed in the course ofmillions of years. Since during their formation and afterwards, they have been subjected to high variable pressures both vertically and horizontally, usually, they are not continuous, and contain numerous cracks and fractures. The exerted pressures, sometimes, produce joint sets. Since these pressures sometimes may not be sufficiently high to create separate joint sets in rock masses, they can produce micro joints and micro-cracks. However, the results cannot be considered as independent joint sets. Although the effects of these micro-cracksare not that pronounced compared with large size joint sets, yet they may cause a drastic change of in-situ geomechanical properties ofrock masses. Also, in many instances, due to dissolution of in-situ rock masses, minute bubble-like cavities, etc., are produced, which cause a severe reduction of in-situ tensile strength. Therefore, one should not replace this in-situ strength by that obtained in the laboratory. On the other hand, measuring the in-situ rock tensile strength due to the interaction of complex parameters is impractical. Hence, an appropriate approach for estimation of the tensile strength should be sought. In this paper, by means of principles of solid and fracture mechanics, a new approach for determination of the effect of geo-structural defects on flexural toppling failure is proposed.2. Effect of geo-structural defects on flexural toppling failure2.1. Critical section of the flexural toppling failureAs mentioned earlier, Majdi and Amini [10] and Amini et al. [11] have proved that the accurate factor of safety is equal to that calculated for a series of inclined rock columns, which, by analogy, is equivalent to the superimposed inclined cantilever beams as shown in Fig. 3. According to the equations of limit equilibrium, the moment M and the shearing force V existing in various cross-sectional areas in the beams can be calculated as follows:(5)( 6)Since the superimposed inclined rock columns are subjected to uniformly distributed loads caused by their own weight, hence, the maximum shearing force and moment exist at the v ery fixed end, that is, at x=Ψ:(7)(8)If the magnitude of Ψ from Eq. (1) is substituted into Eqs. (7) and (8), then the magnitudes of shearing force and the maximum moment of equivalent beam for rock slopes are computed as follows:(9)(10)where C is a dimensionless geometrical parameter that is related to the inclinations of the rock slope, the total failure plane and the dip of the rock discontinuities that existin rock masses, and can be determined by means of curves shown in Fig.Mmax and Vmax will produce the normal (tensile and compressive) and the shear stresses in critical cross-sectional area, respectively. However, the combined effect of them will cause rock columns to fail. It is well understood that the rocks are very susceptible to tensile stresses, and the effect of maximum shearing force is also negligible compared with the effect of tensile stress. Thus, for the purpose of the ultimate stability, structural defects reduce the cross-sectional area of load bearing capacity of the rock columns and, consequently, increase the stress concentration in neighboring solid areas. Thus, the in-situ tensile strength of the rock columns, the shearing effect might be neglected and only the tensile stress caused due to maximum bending stress could be used.2.2. Analysis of geo-structural defectsDetermination of the quantitative effect of geo-structural defects in rock masses can be investigated on the basis of the following two approaches.2.2.1. Solid mechanics approachIn this method, which is, indeed, an old approach, the loads from the weak areas are removed and likewise will be transferred to the neighboring solid areas. Therefore, the solid areas of the rock columns, due to overloading and high stress concentration, will eventually encounter with the premature failure. In this paper, for analysis of the geo-structural defects in flexural toppling failure, a set of cracks in critical cross-sectional area has been modeled as shown in Fig. 5. By employing Eq. (9) and assuming that the loads from weak areas are transferred to the solid areas with higher load bearing capacity (Fig. 6), the maximum stresses could be computed by the following equation (see Appendix A for more details):(11)Hence, with regard to Eq. (11), for determination of the factor of safety against flexural toppling failure in open excavations and underground openings including geo-structural defects the following equation is suggested:(12)From Eq. (12) it can be inferred that the factor of safety against flexural toppling failure obtained on the basis of principles of solid mechanics is irrelevant to the length of geo-structuraldefects or the crack length, directly. However, it is related to the dimensionless parameter “joint persistence”, k, as it was defined earlier in this paper. Fig. 2 represents the effect of parameter k on the critical height of the rock slope. This figure also shows the=1) with a potential of limiting equilibrium of the rock mass (Fsflexural toppling failure.Fig. 2. Determination of the critical height of rock slopes with a potential of flexural toppling failure on the basis of principles of solid mechanics.2.2.2. Fracture mechanics approachGriffith in 1924 [13], by performing comprehensive laboratory tests on the glasses, concluded that fracture of brittle materials is due to high stress concentrations produced on the crack tips which causes the cracks to extend (Fig. 3). Williams in 1952 and 1957 and Irwin in 1957 had proposed some relations by which the stress around the single ended crack tips subjected to tensile loading at infinite is determined [14], [15] and [16]. They introduced a new factor in their equations called the “stress intensity factor” whichindicates the stress condition at the crack tips. Therefore if this factor could be determined quantitatively in laboratorial, then, the factor of safety corresponding to the failure criterion based on principles of fracture mechanics might be computed.Fig. 3. Stress concentration at the tip of a single ended crack under tensile loading Similarly, the geo-structural defects exist in rock columns with a potential of flexural toppling failure could be modeled. As it was mentioned earlier in this paper, cracks could be modeled in a conservative approach such that the location of maximum tensile stress at presumed failure plane to be considered as the cracks locations (Fig. 3). If the existing geo-structural defects in a rock mass, are modeled with a series cracks in the total failure plane, then by means of principles of fracture mechanics, an equation for determination of the factor of safety against flexural toppling failure could be proposed as follows:(13)where KIC is the critical stress intensity factor. Eq. (13) clarifies that the factor of safety against flexural toppling failure derived based on the method of fracture mechanics is directly related to both the “joint persistence” and the “length of cracks”. As such the length of cracks existing in the rock columns plays important roles in stress analysis. Fig. 10 shows the influence of the crack length on the critical height of rock slopes. This figure represents the limiting equilibrium of the rock mass with the potential of flexural toppling failure. As it can be seen, an increase of the crack length causes a decrease in the critical height of the rock slopes. In contrast to the principles of solid mechanics, Eq. (13) or Fig. 4 indicates either the onset of failure of the rock columns or the inception of fracture development.Fig. 4. Determination of the critical height of rock slopes with a potential of flexural toppling failure on the basis of principle of fracture mechanics.3. Comparison of the results of the two approachesThe curves shown in Fig. represent Eqs. (12) and (13), respectively. The figures reflect the quantitative effect of the geo-structural defects on flexural toppling failure on the basis of principles of solid mechanics and fracture mechanics accordingly. For the sake of comparison, these equations are applied to one kind of rock mass (limestone) with the following physical and mechanical properties [16]: , , γ=20kN/m3, k=0.75.In any case studies, a safe and stable slope height can be determined by using Eqs. (12) and (13), independently. The two equations yield two different slope heights out of which the minimum height must be taken as the most acceptable one. By equating Eqs. (12) and (13), the following relation has been derived by which a crack length, in this paper called critical length of crack, can be computed:(14a)where ac is the half of the average critical length of the cracks. Since ac appears on both sides of Eq. (14a), the critical length of the crack could be computed by trial and error method. If the lengthof the crack is too small with respect to rock column thickness, then the ratio t/(t−2ac) is slightly greater than one. Therefore one may ignore the length of crack in denominator, and then this ratiobecomes 1. In this case Eq. (14a) reduces to the following equation, by which the critical length of the crack can be computed directly:(14b)It must be born in mind that Eq. (14b) leads to underestimatethe critical length of the crack compared with Eq. (14a). Therefore, for an appropriate determination of the quantitative effect of geo-structural defects in rock mass against flexural toppling failure,the following 3 conditions must be considered: (1) a=0; (2) a<ac; (3) a>ac.In case 1, there are no geo-structural defects in rock columns and so Eq. (3) will be used for flexural toppling analysis. In case 2, the lengths of geo-structural defects are smaller than the critical length of the crack. In this case failure of rock column occurs dueto tensile stresses for which Eq. (12), based on the principles of solid mechanics, should be used. In case 3, the lengths of existing geo-structural defects are greater than the critical length. In this case failure will occur due to growing cracks for which Eq. (13), based on the principles of fracture mechanics, should be used for the analysis.The results of Eqs. (12) and (13) for the limiting equilibrium both are shown in Fig. 11. For the sake of more accurate comparative studies the results of Eq. (3), which represents the rock columnswith no geo-structural defects are also shown in the same figure. Asit was mentioned earlier in this paper, an increase of the crack length has no direct effect on Eq. (12), which was derived based on principles of solid mechanics, whereas according to the principles of fracture mechanics, it causes to reduce the value of factor of safety. Therefore, for more in-depth comparison, the results of Eq. (13), for different values of the crack length, are also shown in Fig. As canbe seen from the figure, if the length of crack is less than the critical length (dotted curve shown in Fig. 11), failure is considered to follow the principles of solid mechanics which results the least slope height. However, if the length of crack increases beyond the critical length, the rock column fails due to high stress concentration at the crack tips according to the principles of fracture mechanics, which provides the least slope height. Hence, calculation of critical length of crack is of paramount importance.4. Estimation of stable rock slopes with a potential of flexural toppling failureIn rock slopes and trenches, except for the soil and rock fills, the heights are dictated by the natural topography. Hence, the desired slopes must be designed safely. In rock masses with the potential of flexural toppling failure, with regard to the length of the cracks extant in rock columns the slopes can be computed by Eqs.(3), (12), and (13) proposed in this paper. These equations caneasily be converted into a series of design curves for selection of the slopes to replace the lengthy manual computations as well. [Fig. 12], [Fig. 13], [Fig. 14] and [Fig. 15] show several such design curves with the potential of flexural topping failures. If the lengths of existing cracks in the rock columns are smaller than the critical length of the crack, one can use the design curves, obtained on the basis of principles of solid mechanics, shown in [Fig. 12] and [Fig. 13], for the rock slope design purpose. If the lengths of the cracks existing in rock columns are greater than the critical length of the crack, then the design curves derived based on principles of fracture mechanics and shown in [Fig. 14] and [Fig. 15] must be used for the slope design intention. In all, these design curves, with knowing the height of the rock slopes and the thickness of the rockcolumns, parameter (H2/t) is computed, and then from the designcurves the stable slope is calculated. It must be born in mind thatall the aforementioned design curves are valid for the equilibrium condition only, that is, when FS=1. Hence, the calculated slopes from the above design curves, for the final safe design purpose must be reduced based on the desired factor of safety. For example, if the information regarding to one particular rock slope are given [17]:k=0.25, φ=10°, σt=10MPa, γ=20kN/m3, δ=45°, H=100m, t=1 m, ac>a=0.1 m, and then according to Fig. 12 the design slope will be 63°, which represents the condition of equ ilibrium only. Hence, the final and safe slope can be taken any values less than the above mentioned one, which is solely dependent on the desired factor of safety.Fig. 5. Selection of critical slopes for rock columns with the potential of flexural toppling failure on the basis of principles of solid mechanics when k=0.25.Fig. 6. Selection of critical slopes for rock columns with the potential of flexural toppling failure based on principles of solid mechanics when k=0.75..Fig. 7. Selection of critical slopes for rock columns with the potential of flexural toppling failure based on principles of fracture mechanics when k=0.25.Fig. 8. Selection of critical slopes for rock columns with the potential of flexural toppling failure based on principles of fracture mechanics when k=0.75.5. Stabilization of the rock mass with the potential of flexural toppling failureIn flexural toppling failure, rock columns slide over each other so that the tensile loading induced due to their self-weighting grounds causes the existing cracks to grow and thus failure occurs. Hence, if these slides, somehow, are prevented then the expected instability will be reduced significantly. Therefore, employing fully grouted rock bolts, as a useful tool, is great assistance in increasing the degree of stability of the rock columns as shown in Fig. 16 [5] and [6]. However, care must be taken into account that employing fully grouted rock bolts is not the only approach to stabilize the rock mass with potential of flexural toppling failure. Therefore, depending up on the case, combined methods such as decreasing the slope inclination, grouting, anchoring, retaining walls, etc., may even have more effective application than fullygrouted rock bolts alone. In this paper a method has been presentedto determine the specification of fully grouted rock bolts tostabilize such a rock mass. It is important to mention that Eqs. (15), (16), (17), (18), (19) and (20) proposed in this paper may also be used as guidelines to assist practitioners and engineers to definethe specifications of the desired fully grouted rock bolts to be used for stabilization of the rock mass with potential of flexuraltoppling failure. Hence, the finalized specifications must also be checked by engineering judgments then to be applied to rock masses. For determination of the required length of rock bolts for the stabilization of the rock columns against flexural toppling failure the equations given in previous sections can be used. In Eqs. (12)and (13), if the factor of safety is replaced by an allowable value, then the calculated parameter t will indicate the thickness of the combined rock columns which will be equal to the safe length of the rock bolts. Therefore, the required length of the fully grouted rock bolts can be determined via the following equations which have been proposed in this paper, based on the following cases.Fig. 9. Stabilization of rock columns with potential of flexural toppling failure withfully grouted rock bolts.Case 1: principles of solid mechanics for the condition when (a<a c):(15)Case 2: principles of fracture mechanics for the condition when(a>a c):(16)Where FSS is the allowable factor of safety, T is the length of the fully grouted rock bolts, and Ω is the angle between rock bolt longitudinal axis and the line of normal to the discontinuities of rock slope.Eqs. (15) and (16) can be converted into some design curves as shown in Fig. In some cases, one single bolt with a length T may not guarantee the stability of the rock columns against flexural toppling failure since it may pass through total failure plane. In such a case, the rock columns can be reinforced in a stepwise manner so that the thickness of the sewn rock columns becomes equal to T [11].Eq. (17) represents the shear force that exists at any cross-sectional area of the rock bolts. Therefore, both shear force and shear stress at any cross-sectional area can be calculated by the following proposed equations:(17)(18)where V is the longitudinal shear force function, τ is theshear stress function, and Q(y) is the first moment of inertia.According to the equations of equilibrium, in each element of a beam, at any cross-sectional area the shear stresses are equal tothat exist in the corresponding longitudinal section [18]. Hence, the total shear force S in the longitudinal section of the beam can be calculated as follows:The inserted shear force in the cross-sectional area of the rock bolt is equal to the total force exerted longitudinally as well. Therefore,the shear force exerted to the rock bolt's cross-section can be computed as follows:7. ConclusionsIn this paper, geo-structural defects existing in the in-situ rock columns with the potential of flexural toppling failure have been modeled with a series of central cracks. Thereafter on the basis of principles of both the solid and fracture mechanics some new equations have been proposed which can be used for stability analysis and the stabilization of such rock slopes. The final outcomes of this research are given as follows:1. Geo-structural defects play imperative roles in the stability of rock slopes, in particular, flexural toppling failure.2. The results obtained on the basis of principles of solid mechanics approach indicate that the length of cracks alone has no influence on the determination of factor of safety, whereas the value of joint persistence causes a considerable change in its value. On the other hand, the factor of safety obtained based on principles of fracture mechanics approach is strongly influenced by both the length of existing cracks in rock columns and joint persistence as well.3. The critical length of cracks represents the equality line of the results obtained from both approaches: solid mechanics and fracture mechanics.4. If the length of the crack is less than the critical length, failure is considered to follow the principles of solid mechanics. However, if the length of crack increases beyond the critical length, the rock column fails due to high stress concentration at the crack tips, according to the principles of fracture mechanics.5. The present proposed equations are also converted into some design graphs that can be used for ease of application and to reduce manual lengthy calculations for determining the critical height of rock slopes with the potential of flexural toppling failure.6. In this paper, on the basis of principles of both solid mechanics and fracture mechanics some equations are proposed to determine the safe length and the diameter of the fully grouted rock bolts for stabilization of rock slopes with the potential of flexural toppling failure.7. For simplicity of computations, some design graphs for determination of the length of the fully grouted rock bolts for stabilization of rock slopes with the potential of flexural toppling failure are also presented.8. Slope stability analysis of the Galandrood mine shows the new approach is well suited for the analysis of flexural toppling failure.国际岩石力学与工程学报地质结构缺陷对弯曲倾倒破坏的影响作者:Abbas Majdi and Mehdi Amini摘要原位岩石弱点,在此统称为地质结构缺陷,如自然诱发的微裂纹,对拉应力有很大影响。
岩土工程中英文对照外文翻译文献
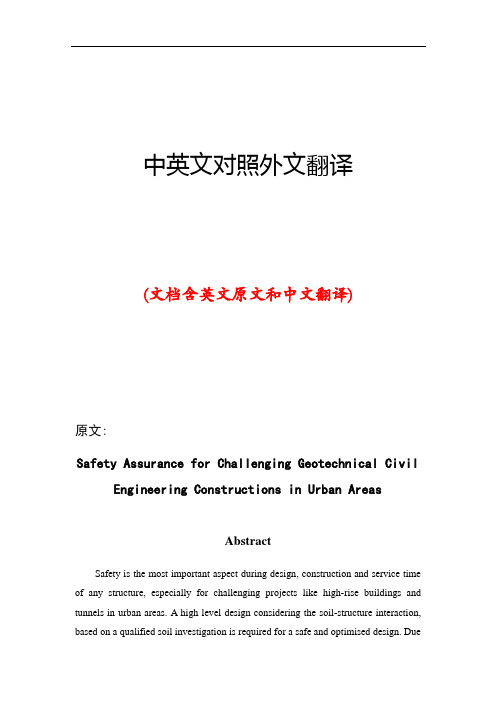
中英文对照外文翻译(文档含英文原文和中文翻译)原文:Safety Assurance for Challenging Geotechnical Civil Engineering Constructions in Urban AreasAbstractSafety is the most important aspect during design, construction and service time of any structure, especially for challenging projects like high-rise buildings and tunnels in urban areas. A high level design considering the soil-structure interaction, based on a qualified soil investigation is required for a safe and optimised design. Dueto the complexity of geotechnical constructions the safety assurance guaranteed by the 4-eye-principle is essential. The 4-eye-principle consists of an independent peer review by publicly certified experts combined with the observational method. The paper presents the fundamental aspects of safety assurance by the 4-eye-principle. The application is explained on several examples, as deep excavations, complex foundation systems for high-rise buildings and tunnel constructions in urban areas. The experiences made in the planning, design and construction phases are explained and for new inner urban projects recommendations are given.Key words: Natural Asset; Financial Value; Neural Network1.IntroductionA safety design and construction of challenging projects in urban areas is based on the following main aspects:Qualified experts for planning, design and construction;Interaction between architects, structural engineers and geotechnical engineers;Adequate soil investigation;Design of deep foundation systems using the FiniteElement-Method (FEM) in combination with enhanced in-situ load tests for calibrating the soil parameters used in the numerical simulations;Quality assurance by an independent peer review process and the observational method (4-eye-principle).These facts will be explained by large construction projects which are located in difficult soil and groundwater conditions.2.The 4-Eye-PrincipleThe basis for safety assurance is the 4-eye-principle. This 4-eye-principle is a process of an independent peer review as shown in Figure 1. It consists of 3 parts. The investor, the experts for planning and design and the construction company belong to the first division. Planning and design are done accordingto the requirements of the investor and all relevant documents to obtain the building permission are prepared. The building authorities are the second part and are responsible for the buildingpermission which is given to the investor. The thirddivision consists of the publicly certified experts.They are appointed by the building authorities but work as independent experts. They are responsible for the technical supervision of the planning, design and the construction.In order to achieve the license as a publicly certified expert for geotechnical engineering by the building authorities intensive studies of geotechnical engineering in university and large experiences in geotechnical engineering with special knowledge about the soil-structure interaction have to be proven.The independent peer review by publicly certified experts for geotechnical engineering makes sure that all information including the results of the soil investigation consisting of labor field tests and the boundary conditions defined for the geotechnical design are complete and correct.In the case of a defect or collapse the publicly certified expert for geotechnical engineering can be involved as an independent expert to find out the reasons for the defect or damage and to develop a concept for stabilization and reconstruction [1].For all difficult projects an independent peer review is essential for the successful realization of the project.3.Observational MethodThe observational method is practical to projects with difficult boundary conditions for verification of the design during the construction time and, if necessary, during service time. For example in the European Standard Eurocode 7 (EC 7) the effect and the boundary conditions of the observational method are defined.The application of the observational method is recommended for the following types of construction projects [2]:very complicated/complex projects;projects with a distinctive soil-structure-interaction,e.g. mixed shallow and deep foundations, retaining walls for deep excavations, Combined Pile-Raft Foundations (CPRFs);projects with a high and variable water pressure;complex interaction situations consisting of ground,excavation and neighbouring buildings and structures;projects with pore-water pressures reducing the stability;projects on slopes.The observational method is always a combination of the common geotechnical investigations before and during the construction phase together with the theoretical modeling and a plan of contingency actions(Figure 2). Only monitoring to ensure the stability and the service ability of the structure is not sufficient and,according to the standardization, not permitted for this purpose. Overall the observational method is an institutionalized controlling instrument to verify the soil and rock mechanical modeling [3,4].The identification of all potential failure mechanismsis essential for defining the measure concept. The concept has to be designed in that way that all these mechanisms can be observed. The measurements need to beof an adequate accuracy to allow the identification ocritical tendencies. The required accuracy as well as the boundary values need to be identified within the design phase of the observational method . Contingency actions needs to be planned in the design phase of the observational method and depend on the ductility of the systems.The observational method must not be seen as a potential alternative for a comprehensive soil investigation campaign. A comprehensive soil investigation campaignis in any way of essential importance. Additionally the observational method is a tool of quality assurance and allows the verification of the parameters and calculations applied in the design phase. The observational method helps to achieve an economic and save construction [5].4.In-Situ Load TestOn project and site related soil investigations with coredrillings and laboratory tests the soil parameters are determined. Laboratory tests are important and essential for the initial definition of soil mechanical properties of the soil layer, but usually not sufficient for an entire and realistic capture of the complex conditions, caused by theinteraction of subsoil and construction [6].In order to reliably determine the ultimate bearing capacity of piles, load tests need to be carried out [7]. Forpile load tests often very high counter weights or strong anchor systems are necessary. By using the Osterberg method high loads can be reached without install inganchors or counter weights. Hydraulic jacks induce the load in the pile using the pile itself partly as abutment.The results of the field tests allow a calibration of the numerical simulations.The principle scheme of pile load tests is shown in Figure 3.5.Examples for Engineering Practice5.1. Classic Pile Foundation for a High-Rise Building in Frankfurt Clay and LimestoneIn the downtown of Frankfurt am Main, Germany, on aconstruction site of 17,400 m2 the high-rise buildingproject “PalaisQuartier” has been realized (Figure 4). The construction was finished in 2010.The complex consists of several structures with a total of 180,000 m2 floor space, there of 60,000 m2 underground (Figure 5). The project includes the historic building “Thurn-und Taxis-Palais” whose facade has been preserved (Unit A). The office building (Unit B),which is the highest building of the project with a height of 136 m has 34 floors each with a floor space of 1340 m2. The hotel building (Unit C) has a height of 99 m with 24 upper floors. The retail area (Unit D)runs along the total length of the eastern part of the site and consists of eight upper floors with a total height of 43 m.The underground parking garage with five floors spans across the complete project area. With an 8 m high first sublevel, partially with mezzanine floor, and four more sub-levels the foundation depth results to 22 m below ground level. There by excavation bottom is at 80m above sea level (msl). A total of 302 foundation piles(diameter up to 1.86 m, length up to 27 m) reach down to depths of 53.2 m to 70.1 m. above sea level depending on the structural requirements.The pile head of the 543 retaining wall piles (diameter1.5 m, length up to 38 m)were located between 94.1 m and 99.6 m above sea level, the pile base was between 59.8 m and 73.4 m above sea level depending on the structural requirements. As shown in the sectional view(Figure 6), the upper part of the piles is in the Frankfurt Clay and the base of the piles is set in the rocky Frankfurt Limestone.Regarding the large number of piles and the high pile loads a pile load test has been carried out for optimization of the classic pile foundation. Osterberg-Cells(O-Cells) have been installed in two levels in order to assess the influence of pile shaft grouting on the limit skin friction of the piles in the Frankfurt Limestone(Figure 6). The test pile with a total length of 12.9 m and a diameter of 1.68 m consist of three segments and has been installed in the Frankfurt Limestone layer 31.7 m below ground level. The upper pile segment above the upper cell level and the middle pile segment between the two cell levels can be tested independently. In the first phase of the test the upper part was loaded by using the middle and the lower part as abutment. A limit of 24 MN could be reached (Figure 7). The upper segment was lifted about 1.5 cm, the settlement of the middle and lower part was 1.0 cm. The mobilized shaft friction was about 830 kN/m2.Subsequently the upper pile segment was uncoupled by discharging the upper cell level. In the second test phase the middle pile segment was loaded by using the lower segment as abutment. The limit load of the middle segment with shaft grouting was 27.5 MN (Figure 7).The skin friction was 1040 kN/m2, this means 24% higher than without shaft grouting. Based on the results of the pile load test using O-Cells the majority of the 290 foundation piles were made by applying shaft grouting. Due to pile load test the total length of was reduced significantly.5.2. CPRF for a High-Rise Building in Clay MarlIn the scope of the project Mirax Plaza in Kiev, Ukraine,2 high-rise buildings, each of them 192 m (46 storeys)high, a shopping and entertainment mall and an underground parking are under construction (Figure 8). The area of the project is about 294,000 m2 and cuts a 30 m high natural slope.The geotechnical investigations have been executed 70m deep. The soil conditions at the construction site are as follows: fill to a depth of 2 m to 3mquaternary silty sand and sandy silt with a thickness of 5 m to 10 m tertiary silt and sand (Charkow and Poltaw formation) with a thickness of 0 m to 24 m tertiary clayey silt and clay marl of the Kiev and But schak formation with a thickness of about 20 m tertiary fine sand of the But schak formation up to the investigation depthThe ground water level is in a depth of about 2 m below the ground surface. The soil conditions and a cross section of the project are shown in Figure 9.For verification of the shaft and base resistance of the deep foundation elements and for calibration of the numerical simulations pile load tests have been carried out on the construction yard. The piles had a diameter of 0.82 m and a length of about 10 m to 44 m. Using the results of the load tests the back analysis for verification of the FEM simulations was done. The soil properties in accordance with the results of the back analysis were partly 3 times higher than indicated in the geotechnical report. Figure 10 shows the results of the load test No. 2 and the numerical back analysis. Measurement and calculation show a good accordance.The obtained results of the pile load tests and of the executed back analysis were applied in 3-dimensionalFEM-simulations of the foundation for Tower A, taking advantage of the symmetry of the footprint of the building. The overall load of the Tower A is about 2200 MN and the area of the foundation about 2000 m2 (Figure11).The foundation design considers a CPRF with 64 barrettes with 33 m length and a cross section of 2.8 m × 0.8m. The raft of 3 m thickness is located in Kiev Clay Marl at about 10 m depth below the ground surface. The barrettes are penetrating the layer of Kiev Clay Marl reaching the Butschak Sands.The calculated loads on the barrettes were in the range of 22.1 MN to 44.5 MN. The load on the outer barrettes was about 41.2 MN to 44.5 MN which significantly exceeds the loads on the inner barrettes with the maximum value of 30.7 MN. This behavior is typical for a CPRF.The outer deep foundation elements take more loads because of their higher stiffness due to the higher volume of the activated soil. The CPRF coefficient is 0.88 =CPRF . Maximum settlements of about 12 cm werecalculated due to the settlement-relevant load of 85% of the total design load. The pressure under the foundation raft is calculated in the most areas not exceeding 200 kN/m2, at the raft edge the pressure reaches 400 kN/m2.The calculated base pressure of the outer barrettes has anaverage of 5100 kN/m2 and for inner barrettes an average of 4130 kN/m2. The mobilized shaft resistance increases with the depth reaching 180 kN/m2 for outer barrettes and 150 kN/m2 for inner barrettes.During the construction of Mirax Plaza the observational method according to EC 7 is applied. Especially the distribution of the loads between the barrettes and the raft is monitored. For this reason 3 earth pressure devices were installed under the raft and 2 barrettes (most loaded outer barrette and average loaded inner barrette) were instrumented over the length.In the scope of the project Mirax Plaza the new allowable shaft resistance and base resistance were defined for typical soil layers in Kiev. This unique experience will be used for the skyscrapers of new generation in Ukraine.The CPRF of the high-rise building project MiraxPlaza represents the first authorized CPRF in the Ukraine. Using the advanced optimization approaches and taking advantage of the positive effect of CPRF the number of barrettes could be reduced from 120 barrettes with 40 mlength to 64 barrettes with 33 m length. The foundation optimization leads to considerable decrease of the utilized resources (cement, aggregates, water, energy etc.)and cost savings of about 3.3 Million US$.译文:安全保证岩土公民发起挑战工程建设在城市地区摘要安全是最重要的方面在设计、施工和服务时间的任何结构,特别是对具有挑战性的项目,如高层建筑和隧道在城市地区。
岩土专业英汉词汇
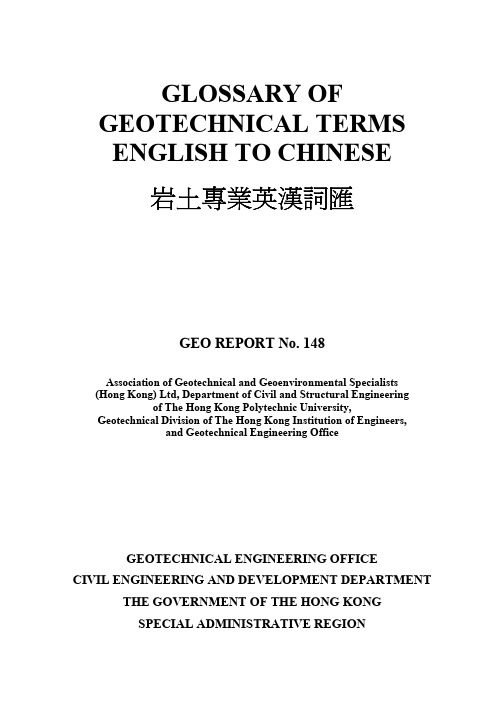
R.K.S. Chan Head, Geotechnical Engineering Office July 2004
f Chinese in oral and written communication on geotechnical and related subjects is getting common in Hong Kong. This Glossary contains a comprehensive collection of the Chinese translations of about 6,500 terms frequently met by geotechnical professionals in Hong Kong in their work. In some cases, a geotechnical term may be translated into Chinese in more than one way due to different local usage, language style, or personal preference. The different translations are listed in the Glossary for reference by readers. The Glossary was jointly produced by the Association of Geotechnical and Geoenvironmental Specialists (Hong Kong) Ltd., the Department of Civil and Structural Engineering of the Hong Kong Polytechnic University, the Geotechnical Division of the Hong Kong Institution of Engineers, and the Geotechnical Engineering Office of the Civil Engineering and Development Department. A Steering Group, comprising Dr C.K. Lau, Dr Victor K.S. Li, Dr David X.C. Li, Mr H.N. Wong and Professor J.H. Yin, oversaw the compilation of the Glossary. Professor John Wang prepared a glossary for terms used in Geotechnical Manual for Slopes; these terms have also been incorporated in this Glossary. Mr Philip W.K. Chung and Mr Jerry L. P. Ho assisted in the final phase of compilation of the Glossary. Many other geotechnical professionals in Hong Kong and elsewhere have provided invaluable advice, suggestions and assistance. We hope that the Glossary will facilitate the use of Chinese in the geotechnical profession and help standardize the Chinese translation of the commonly used geotechnical terms in Hong Kong. Comments and suggestions for improvement to this Glossary are most welcome. These should be addressed to Chief Geotechnical Engineer/Planning Division of Geotechnical Engineering Office, 11/F, Civil Engineering and Development Building, 101 Princess Margaret Road, Homantin, Kowloon. A proforma to facilitate provision of feedback is given at the end of this report.
岩土专业部分术语中英文对照2

岩土专业部分术语中英文对照2来源:高凯强的日志水工建筑物抗震设计规范Specifications for seismic design of hydraulic structures DL 5073-97序号分类名词(中)名词(英)1 1. 综合类大地工程(岩土工程) geotechnical engineering2 1. 综合类反分析法back analysis method3 1. 综合类基础工程foundation engineering4 1. 综合类临界状态土力学critical state soil mechanics5 1. 综合类数值岩土力学numerical geomechanics6 1. 综合类土soil, earth7 1. 综合类土动力学soil dynamics8 1. 综合类土力学soil mechanics9 1. 综合类岩土工程geotechnical engineering10 1. 综合类应力路径stress path11 1. 综合类应力路径法stress path method12 2. 工程地质及勘察变质岩metamorphic rock13 2. 工程地质及勘察标准冻深standard frost penetration14 2. 工程地质及勘察冰川沉积glacial deposit15 2. 工程地质及勘察冰积层(台)glacial deposit16 2. 工程地质及勘察残积土eluvial soil, residual soil17 2. 工程地质及勘察层理beding18 2. 工程地质及勘察长石feldspar19 2. 工程地质及勘察沉积岩sedimentary rock20 2. 工程地质及勘察承压水confined water21 2. 工程地质及勘察次生矿物secondary mineral22 2. 工程地质及勘察地质年代geological age23 2. 工程地质及勘察地质图geological map24 2. 工程地质及勘察地下水groundwater25 2. 工程地质及勘察断层fault26 2. 工程地质及勘察断裂构造fracture structure27 2. 工程地质及勘察工程地质勘察engineering geological exploration28 2. 工程地质及勘察海积层(台)marine deposit29 2. 工程地质及勘察海相沉积marine deposit30 2. 工程地质及勘察花岗岩granite31 2. 工程地质及勘察滑坡landslide32 2. 工程地质及勘察化石fossil33 2. 工程地质及勘察化学沉积岩chemical sedimentary rock34 2. 工程地质及勘察阶地terrace35 2. 工程地质及勘察节理joint36 2. 工程地质及勘察解理cleavage37 2. 工程地质及勘察喀斯特karst38 2. 工程地质及勘察矿物硬度hardness of minerals39 2. 工程地质及勘察砾岩conglomerate40 2. 工程地质及勘察流滑flow slide41 2. 工程地质及勘察陆相沉积continental sedimentation42 2. 工程地质及勘察泥石流mud flow, debris flow43 2. 工程地质及勘察年粘土矿物clay minerals44 2. 工程地质及勘察凝灰岩tuff45 2. 工程地质及勘察牛轭湖ox-bow lake46 2. 工程地质及勘察浅成岩hypabyssal rock47 2. 工程地质及勘察潜水ground water48 2. 工程地质及勘察侵入岩intrusive rock49 2. 工程地质及勘察取土器geotome50 2. 工程地质及勘察砂岩sandstone51 2. 工程地质及勘察砂嘴spit, sand spit52 2. 工程地质及勘察山岩压力rock pressure53 2. 工程地质及勘察深成岩plutionic rock54 2. 工程地质及勘察石灰岩limestone55 2. 工程地质及勘察石英quartz56 2. 工程地质及勘察松散堆积物rickle57 2. 工程地质及勘察围限地下水(台)confined ground water58 2. 工程地质及勘察泻湖lagoon59 2. 工程地质及勘察岩爆rock burst60 2. 工程地质及勘察岩层产状attitude of rock61 2. 工程地质及勘察岩浆岩magmatic rock, igneous rock62 2. 工程地质及勘察岩脉dike, dgke63 2. 工程地质及勘察岩石风化程度degree of rock weathering64 2. 工程地质及勘察岩石构造structure of rock65 2. 工程地质及勘察岩石结构texture of rock66 2. 工程地质及勘察岩体rock mass67 2. 工程地质及勘察页岩shale68 2. 工程地质及勘察原生矿物primary mineral69 2. 工程地质及勘察云母mica70 2. 工程地质及勘察造岩矿物rock-forming mineral71 2. 工程地质及勘察褶皱fold, folding72 2. 工程地质及勘察钻孔柱状图bore hole columnar section73 3. 土的分类饱和土saturated soil74 3. 土的分类超固结土overconsolidated soil75 3. 土的分类冲填土dredger fill76 3. 土的分类充重塑土77 3. 土的分类冻土frozen soil, tjaele78 3. 土的分类非饱和土unsaturated soil79 3. 土的分类分散性土dispersive soil80 3. 土的分类粉土silt, mo81 3. 土的分类粉质粘土silty clay82 3. 土的分类高岭石kaolinite83 3. 土的分类过压密土(台)overconsolidated soil84 3. 土的分类红粘土red clay, adamic earth85 3. 土的分类黄土loess86 3. 土的分类蒙脱石montmorillonite87 3. 土的分类泥炭peat, bog muck88 3. 土的分类年粘土clay89 3. 土的分类年粘性土cohesive soil, clayey soil90 3. 土的分类膨胀土expansive soil, swelling soil91 3. 土的分类欠固结粘土underconsolidated soil92 3. 土的分类区域性土zonal soil93 3. 土的分类人工填土fill, artificial soil94 3. 土的分类软粘土soft clay, mildclay, mickle95 3. 土的分类砂土sand96 3. 土的分类湿陷性黄土collapsible loess, slumping loess97 3. 土的分类素填土plain fill98 3. 土的分类塑性图plasticity chart99 3. 土的分类碎石土stone, break stone, broken stone, channery, chat, cru shed stone, deritus100 3. 土的分类未压密土(台)underconsolidated clay101 3. 土的分类无粘性土cohesionless soil, frictional soil, non-cohesive soil 102 3. 土的分类岩石rock103 3. 土的分类伊利土illite104 3. 土的分类有机质土organic soil105 3. 土的分类淤泥muck, gyttja, mire, slush106 3. 土的分类淤泥质土mucky soil107 3. 土的分类原状土undisturbed soil108 3. 土的分类杂填土miscellaneous fill109 3. 土的分类正常固结土normally consolidated soil110 3. 土的分类正常压密土(台)normally consolidated soil111 3. 土的分类自重湿陷性黄土self weight collapse loess112 4. 土的物理性质阿太堡界限Atterberg limits113 4. 土的物理性质饱和度degree of saturation114 4. 土的物理性质饱和密度saturated density115 4. 土的物理性质饱和重度saturated unit weight116 4. 土的物理性质比重specific gravity117 4. 土的物理性质稠度consistency118 4. 土的物理性质不均匀系数coefficient of uniformity, uniformity coefficie nt119 4. 土的物理性质触变thixotropy120 4. 土的物理性质单粒结构single-grained structure121 4. 土的物理性质蜂窝结构honeycomb structure122 4. 土的物理性质干重度dry unit weight123 4. 土的物理性质干密度dry density124 4. 土的物理性质塑性指数plasticity index125 4. 土的物理性质含水量water content, moisture content126 4. 土的物理性质活性指数127 4. 土的物理性质级配gradation, grading128 4. 土的物理性质结合水bound water, combined water, held water129 4. 土的物理性质界限含水量Atterberg limits130 4. 土的物理性质颗粒级配particle size distribution of soils, mechanical c omposition of soil131 4. 土的物理性质可塑性plasticity132 4. 土的物理性质孔隙比void ratio133 4. 土的物理性质孔隙率porosity134 4. 土的物理性质粒度granularity, grainness, grainage135 4. 土的物理性质粒组fraction, size fraction136 4. 土的物理性质毛细管水capillary water137 4. 土的物理性质密度density138 4. 土的物理性质密实度compactionness139 4. 土的物理性质年粘性土的灵敏度sensitivity of cohesive soil140 4. 土的物理性质平均粒径mean diameter, average grain diameter141 4. 土的物理性质曲率系数coefficient of curvature142 4. 土的物理性质三相图block diagram, skeletal diagram, three phase dia gram143 4. 土的物理性质三相土tri-phase soil144 4. 土的物理性质湿陷起始应力initial collapse pressure145 4. 土的物理性质湿陷系数coefficient of collapsibility146 4. 土的物理性质缩限shrinkage limit147 4. 土的物理性质土的构造soil texture148 4. 土的物理性质土的结构soil structure149 4. 土的物理性质土粒相对密度specific density of solid particles150 4. 土的物理性质土中气air in soil151 4. 土的物理性质土中水water in soil152 4. 土的物理性质团粒aggregate, cumularpharolith153 4. 土的物理性质限定粒径constrained diameter154 4. 土的物理性质相对密度relative density, density index155 4. 土的物理性质相对压密度relative compaction, compacting factor, perc ent compaction, coefficient of compaction156 4. 土的物理性质絮状结构flocculent structure157 4. 土的物理性质压密系数coefficient of consolidation158 4. 土的物理性质压缩性compressibility159 4. 土的物理性质液限liquid limit160 4. 土的物理性质液性指数liquidity index161 4. 土的物理性质游离水(台)free water162 4. 土的物理性质有效粒径effective diameter, effective grain size, effectiv e size163 4. 土的物理性质有效密度effective density164 4. 土的物理性质有效重度effective unit weight165 4. 土的物理性质重力密度unit weight166 4. 土的物理性质自由水free water, gravitational water, groundwater, phr eatic water167 4. 土的物理性质组构fabric168 4. 土的物理性质最大干密度maximum dry density169 4. 土的物理性质最优含水量optimum water content170 5. 渗透性和渗流达西定律Darcy's law171 5. 渗透性和渗流管涌piping172 5. 渗透性和渗流浸润线phreatic line173 5. 渗透性和渗流临界水力梯度critical hydraulic gradient174 5. 渗透性和渗流流函数flow function175 5. 渗透性和渗流流土flowing soil176 5. 渗透性和渗流流网flow net177 5. 渗透性和渗流砂沸sand boiling178 5. 渗透性和渗流渗流seepage179 5. 渗透性和渗流渗流量seepage discharge180 5. 渗透性和渗流渗流速度seepage velocity181 5. 渗透性和渗流渗透力seepage force182 5. 渗透性和渗流渗透破坏seepage failure183 5. 渗透性和渗流渗透系数coefficient of permeability184 5. 渗透性和渗流渗透性permeability185 5. 渗透性和渗流势函数potential function186 5. 渗透性和渗流水力梯度hydraulic gradient187 6. 地基应力和变形变形deformation188 6. 地基应力和变形变形模量modulus of deformation189 6. 地基应力和变形泊松比Poisson's ratio190 6. 地基应力和变形布西涅斯克解Boussinnesq's solution191 6. 地基应力和变形残余变形residual deformation192 6. 地基应力和变形残余孔隙水压力residual pore water pressure193 6. 地基应力和变形超静孔隙水压力excess pore water pressure194 6. 地基应力和变形沉降settlement195 6. 地基应力和变形沉降比settlement ratio196 6. 地基应力和变形次固结沉降secondary consolidation settlement197 6. 地基应力和变形次固结系数coefficient of secondary consolidation 198 6. 地基应力和变形地基沉降的弹性力学公式elastic formula for settlement calculation199 6. 地基应力和变形分层总和法layerwise summation method200 6. 地基应力和变形负孔隙水压力negative pore water pressure201 6. 地基应力和变形附加应力superimposed stress202 6. 地基应力和变形割线模量secant modulus203 6. 地基应力和变形固结沉降consolidation settlement204 6. 地基应力和变形规范沉降计算法settlement calculation by specification 205 6. 地基应力和变形回弹变形rebound deformation206 6. 地基应力和变形回弹模量modulus of resilience207 6. 地基应力和变形回弹系数coefficient of resilience208 6. 地基应力和变形回弹指数swelling index209 6. 地基应力和变形建筑物的地基变形允许值allowable settlement of buildi ng210 6. 地基应力和变形剪胀dilatation211 6. 地基应力和变形角点法corner-points method212 6. 地基应力和变形孔隙气压力pore air pressure213 6. 地基应力和变形孔隙水压力pore water pressure214 6. 地基应力和变形孔隙压力系数A pore pressure parameter A215 6. 地基应力和变形孔隙压力系数B pore pressure parameter B216 6. 地基应力和变形明德林解Mindlin's solution217 6. 地基应力和变形纽马克感应图Newmark chart218 6. 地基应力和变形切线模量tangent modulus219 6. 地基应力和变形蠕变creep220 6. 地基应力和变形三向变形条件下的固结沉降three-dimensional consolida tion settlement221 6. 地基应力和变形瞬时沉降immediate settlement222 6. 地基应力和变形塑性变形plastic deformation223 6. 地基应力和变形谈弹性变形elastic deformation224 6. 地基应力和变形谈弹性模量elastic modulus225 6. 地基应力和变形谈弹性平衡状态state of elastic equilibrium226 6. 地基应力和变形体积变形模量volumetric deformation modulus227 6. 地基应力和变形先期固结压力preconsolidation pressure228 6. 地基应力和变形压缩层229 6. 地基应力和变形压缩模量modulus of compressibility230 6. 地基应力和变形压缩系数coefficient of compressibility231 6. 地基应力和变形压缩性compressibility232 6. 地基应力和变形压缩指数compression index233 6. 地基应力和变形有效应力effective stress234 6. 地基应力和变形自重应力self-weight stress235 6. 地基应力和变形总应力total stress approach of shear strength236 6. 地基应力和变形最终沉降final settlement237 7. 固结巴隆固结理论Barron's consolidation theory238 7. 固结比奥固结理论Biot's consolidation theory239 7. 固结超固结比over-consolidation ratio240 7. 固结超静孔隙水压力excess pore water pressure241 7. 固结次固结secondary consolidation242 7. 固结次压缩(台)secondary consolidation243 7. 固结单向度压密(台)one-dimensional consolidation244 7. 固结多维固结multi-dimensional consolidation245 7. 固结固结consolidation246 7. 固结固结度degree of consolidation247 7. 固结固结理论theory of consolidation248 7. 固结固结曲线consolidation curve249 7. 固结固结速率rate of consolidation250 7. 固结固结系数coefficient of consolidation251 7. 固结固结压力consolidation pressure252 7. 固结回弹曲线rebound curve253 7. 固结井径比drain spacing ratio254 7. 固结井阻well resistance255 7. 固结曼代尔-克雷尔效应Mandel-Cryer effect256 7. 固结潜变(台)creep257 7. 固结砂井sand drain258 7. 固结砂井地基平均固结度average degree of consolidation of sand-drai ned ground259 7. 固结时间对数拟合法logarithm of time fitting method260 7. 固结时间因子time factor261 7. 固结太沙基固结理论Terzaghi's consolidation theory262 7. 固结太沙基-伦杜列克扩散方程Terzaghi-Rendulic diffusion equation 263 7. 固结先期固结压力preconsolidation pressure264 7. 固结压密(台)consolidation265 7. 固结压密度(台)degree of consolidation266 7. 固结压缩曲线compression curve267 7. 固结一维固结one dimensional consolidation268 7. 固结有效应力原理principle of effective stress269 7. 固结预压密压力(台)preconsolidation pressure270 7. 固结原始压缩曲线virgin compression curve271 7. 固结再压缩曲线recompression curve272 7. 固结主固结primary consolidation273 7. 固结主压密(台)primary consolidation274 7. 固结准固结压力pseudo-consolidation pressure275 7. 固结K0固结consolidation under K0 condition276 8. 抗剪强度安息角(台)angle of repose277 8. 抗剪强度不排水抗剪强度undrained shear strength278 8. 抗剪强度残余内摩擦角residual angle of internal friction279 8. 抗剪强度残余强度residual strength280 8. 抗剪强度长期强度long-term strength281 8. 抗剪强度单轴抗拉强度uniaxial tension test282 8. 抗剪强度动强度dynamic strength of soils283 8. 抗剪强度峰值强度peak strength284 8. 抗剪强度伏斯列夫参数Hvorslev parameter285 8. 抗剪强度剪切应变速率shear strain rate286 8. 抗剪强度抗剪强度shear strength287 8. 抗剪强度抗剪强度参数shear strength parameter288 8. 抗剪强度抗剪强度有效应力法effective stress approach of shear stren gth289 8. 抗剪强度抗剪强度总应力法total stress approach of shear strength 290 8. 抗剪强度库仑方程Coulomb's equation291 8. 抗剪强度摩尔包线Mohr's envelope292 8. 抗剪强度摩尔-库仑理论Mohr-Coulomb theory293 8. 抗剪强度内摩擦角angle of internal friction294 8. 抗剪强度年粘聚力cohesion295 8. 抗剪强度破裂角angle of rupture296 8. 抗剪强度破坏准则failure criterion297 8. 抗剪强度十字板抗剪强度vane strength298 8. 抗剪强度无侧限抗压强度unconfined compression strength299 8. 抗剪强度有效内摩擦角effective angle of internal friction300 8. 抗剪强度有效粘聚力effective cohesion intercept301 8. 抗剪强度有效应力破坏包线effective stress failure envelope302 8. 抗剪强度有效应力强度参数effective stress strength parameter 303 8. 抗剪强度有效应力原理principle of effective stress304 8. 抗剪强度真内摩擦角true angle internal friction305 8. 抗剪强度真粘聚力true cohesion306 8. 抗剪强度总应力破坏包线total stress failure envelope307 8. 抗剪强度总应力强度参数total stress strength parameter308 9. 本构模型本构模型constitutive model309 9. 本构模型边界面模型boundary surface model310 9. 本构模型层向各向同性体模型cross anisotropic model311 9. 本构模型超弹性模型hyperelastic model312 9. 本构模型德鲁克-普拉格准则Drucker-Prager criterion313 9. 本构模型邓肯-张模型Duncan-Chang model314 9. 本构模型动剪切强度315 9. 本构模型非线性弹性模量nonlinear elastic model316 9. 本构模型盖帽模型cap model317 9. 本构模型刚塑性模型rigid plastic model318 9. 本构模型割线模量secant modulus319 9. 本构模型广义冯•米赛斯屈服准则extended von Mises yield criterion 320 9. 本构模型广义特雷斯卡屈服准则extended Tresca yield criterion 321 9. 本构模型加工软化work softening322 9. 本构模型加工硬化work hardening323 9. 本构模型加工硬化定律strain hardening law324 9. 本构模型剑桥模型Cambridge model325 9. 本构模型柯西弹性模型Cauchy elastic model326 9. 本构模型拉特-邓肯模型Lade-Duncan model327 9. 本构模型拉特屈服准则Lade yield criterion328 9. 本构模型理想弹塑性模型ideal elastoplastic model329 9. 本构模型临界状态弹塑性模型critical state elastoplastic model330 9. 本构模型流变学模型rheological model331 9. 本构模型流动规则flow rule332 9. 本构模型摩尔-库仑屈服准则Mohr-Coulomb yield criterion333 9. 本构模型内蕴时间塑性模型endochronic plastic model334 9. 本构模型内蕴时间塑性理论endochronic theory335 9. 本构模型年粘弹性模型viscoelastic model336 9. 本构模型切线模量tangent modulus337 9. 本构模型清华弹塑性模型Tsinghua elastoplastic model338 9. 本构模型屈服面yield surface339 9. 本构模型沈珠江三重屈服面模型Shen Zhujiang three yield surface me thod340 9. 本构模型双参数地基模型341 9. 本构模型双剪应力屈服模型twin shear stress yield criterion342 9. 本构模型双曲线模型hyperbolic model343 9. 本构模型松岗元-中井屈服准则Matsuoka-Nakai yield criterion344 9. 本构模型塑性形变理论345 9. 本构模型谈弹塑性模量矩阵elastoplastic modulus matrix346 9. 本构模型谈弹塑性模型elastoplastic modulus347 9. 本构模型谈弹塑性增量理论incremental elastoplastic theory348 9. 本构模型谈弹性半空间地基模型elastic half-space foundation model 349 9. 本构模型谈弹性变形elastic deformation350 9. 本构模型谈弹性模量elastic modulus351 9. 本构模型谈弹性模型elastic model352 9. 本构模型魏汝龙-Khosla-Wu模型Wei Rulong-Khosla-Wu model 353 9. 本构模型文克尔地基模型Winkler foundation model354 9. 本构模型修正剑桥模型modified Cambridge model355 9. 本构模型准弹性模型hypoelastic model356 10. 地基承载力冲剪破坏punching shear failure357 10. 地基承载力次层(台)substratum358 10. 地基承载力地基subgrade, ground, foundation soil359 10. 地基承载力地基承载力bearing capacity of foundation soil360 10. 地基承载力地基极限承载力ultimate bearing capacity of foundation s oil361 10. 地基承载力地基允许承载力allowable bearing capacity of foundation soil362 10. 地基承载力地基稳定性stability of foundation soil363 10. 地基承载力汉森地基承载力公式Hansen's ultimate bearing capacity f ormula364 10. 地基承载力极限平衡状态state of limit equilibrium365 10. 地基承载力加州承载比(美国)California Bearing Ratio366 10. 地基承载力局部剪切破坏local shear failure367 10. 地基承载力临塑荷载critical edge pressure368 10. 地基承载力梅耶霍夫极限承载力公式Meyerhof's ultimate bearing cap acity formula369 10. 地基承载力普朗特承载力理论Prandel bearing capacity theory370 10. 地基承载力斯肯普顿极限承载力公式Skempton's ultimate bearing cap acity formula371 10. 地基承载力太沙基承载力理论Terzaghi bearing capacity theory372 10. 地基承载力魏锡克极限承载力公式Vesic's ultimate bearing capacity f ormula373 10. 地基承载力整体剪切破坏general shear failure374 11. 土压力被动土压力passive earth pressure375 11. 土压力被动土压力系数coefficient of passive earth pressure376 11. 土压力极限平衡状态state of limit equilibrium377 11. 土压力静止土压力earth pressue at rest378 11. 土压力静止土压力系数coefficient of earth pressur at rest379 11. 土压力库仑土压力理论Coulomb's earth pressure theory380 11. 土压力库尔曼图解法Culmannn construction381 11. 土压力朗肯土压力理论Rankine's earth pressure theory382 11. 土压力朗肯状态Rankine state383 11. 土压力谈弹性平衡状态state of elastic equilibrium384 11. 土压力土压力earth pressure385 11. 土压力主动土压力active earth pressure386 11. 土压力主动土压力系数coefficient of active earth pressure387 12. 土坡稳定分析安息角(台)angle of repose388 12. 土坡稳定分析毕肖普法Bishop method389 12. 土坡稳定分析边坡稳定安全系数safety factor of slope390 12. 土坡稳定分析不平衡推理传递法unbalanced thrust transmission meth od391 12. 土坡稳定分析费伦纽斯条分法Fellenius method of slices392 12. 土坡稳定分析库尔曼法Culmann method393 12. 土坡稳定分析摩擦圆法friction circle method394 12. 土坡稳定分析摩根斯坦-普拉斯法Morgenstern-Price method395 12. 土坡稳定分析铅直边坡的临界高度critical height of vertical slope 396 12. 土坡稳定分析瑞典圆弧滑动法Swedish circle method397 12. 土坡稳定分析斯宾赛法Spencer method398 12. 土坡稳定分析泰勒法Taylor method399 12. 土坡稳定分析条分法slice method400 12. 土坡稳定分析土坡slope401 12. 土坡稳定分析土坡稳定分析slope stability analysis402 12. 土坡稳定分析土坡稳定极限分析法limit analysis method of slope sta bility403 12. 土坡稳定分析土坡稳定极限平衡法limit equilibrium method of slope stability404 12. 土坡稳定分析休止角angle of repose405 12. 土坡稳定分析扬布普遍条分法Janbu general slice method406 12. 土坡稳定分析圆弧分析法circular arc analysis407 13. 土的动力性质比阻尼容量specific gravity capacity408 13. 土的动力性质波的弥散特性dispersion of waves409 13. 土的动力性质波速法wave velocity method410 13. 土的动力性质材料阻尼material damping411 13. 土的动力性质初始液化initial liquefaction412 13. 土的动力性质地基固有周期natural period of soil site413 13. 土的动力性质动剪切模量dynamic shear modulus of soils414 13. 土的动力性质动力布西涅斯克解dynamic solution of Boussinesq 415 13. 土的动力性质动力放大因素dynamic magnification factor416 13. 土的动力性质动力性质dynamic properties of soils417 13. 土的动力性质动强度dynamic strength of soils418 13. 土的动力性质骨架波akeleton waves in soils419 13. 土的动力性质几何阻尼geometric damping420 13. 土的动力性质抗液化强度liquefaction stress421 13. 土的动力性质孔隙流体波fluid wave in soil422 13. 土的动力性质损耗角loss angle423 13. 土的动力性质往返活动性reciprocating activity424 13. 土的动力性质无量纲频率dimensionless frequency425 13. 土的动力性质液化liquefaction426 13. 土的动力性质液化势评价evaluation of liquefaction potential 427 13. 土的动力性质液化应力比stress ratio of liquefaction428 13. 土的动力性质应力波stress waves in soils429 13. 土的动力性质振陷dynamic settlement430 13. 土的动力性质阻尼damping of soil431 13. 土的动力性质阻尼比damping ratio432 14. 挡土墙挡土墙retaining wall433 14. 挡土墙挡土墙排水设施434 14. 挡土墙挡土墙稳定性stability of retaining wall435 14. 挡土墙垛式挡土墙436 14. 挡土墙扶垛式挡土墙counterfort retaining wall437 14. 挡土墙后垛墙(台)counterfort retaining wall438 14. 挡土墙基础墙foundation wall439 14. 挡土墙加筋土挡墙reinforced earth bulkhead440 14. 挡土墙锚定板挡土墙anchored plate retaining wall441 14. 挡土墙锚定式板桩墙anchored sheet pile wall442 14. 挡土墙锚杆式挡土墙anchor rod retaining wall443 14. 挡土墙悬壁式板桩墙cantilever sheet pile wall444 14. 挡土墙悬壁式挡土墙cantilever sheet pile wall445 14. 挡土墙重力式挡土墙gravity retaining wall446 15. 板桩结构物板桩sheet pile447 15. 板桩结构物板桩结构sheet pile structure448 15. 板桩结构物钢板桩steel sheet pile449 15. 板桩结构物钢筋混凝土板桩reinforced concrete sheet pile450 15. 板桩结构物钢桩steel pile451 15. 板桩结构物灌注桩cast-in-place pile452 15. 板桩结构物拉杆tie rod453 15. 板桩结构物锚定式板桩墙anchored sheet pile wall454 15. 板桩结构物锚固技术anchoring455 15. 板桩结构物锚座Anchorage456 15. 板桩结构物木板桩wooden sheet pile457 15. 板桩结构物木桩timber piles458 15. 板桩结构物悬壁式板桩墙cantilever sheet pile wall459 16. 基坑开挖与降水板桩围护sheet pile-braced cuts460 16. 基坑开挖与降水电渗法electro-osmotic drainage461 16. 基坑开挖与降水管涌piping462 16. 基坑开挖与降水基底隆起heave of base463 16. 基坑开挖与降水基坑降水dewatering464 16. 基坑开挖与降水基坑失稳instability (failure) of foundation pit465 16. 基坑开挖与降水基坑围护bracing of foundation pit466 16. 基坑开挖与降水减压井relief well467 16. 基坑开挖与降水降低地下水位法dewatering method468 16. 基坑开挖与降水井点系统well point system469 16. 基坑开挖与降水喷射井点eductor well point470 16. 基坑开挖与降水铅直边坡的临界高度critical height of vertical slope 471 16. 基坑开挖与降水砂沸sand boiling472 16. 基坑开挖与降水深井点deep well point473 16. 基坑开挖与降水真空井点vacuum well point474 16. 基坑开挖与降水支撑围护braced cuts475 17. 浅基础杯形基础476 17. 浅基础补偿性基础compensated foundation477 17. 浅基础持力层bearing stratum478 17. 浅基础次层(台)substratum479 17. 浅基础单独基础individual footing480 17. 浅基础倒梁法inverted beam method481 17. 浅基础刚性角pressure distribution angle of masonary foundation 482 17. 浅基础刚性基础rigid foundation483 17. 浅基础高杯口基础484 17. 浅基础基础埋置深度embeded depth of foundation485 17. 浅基础基床系数coefficient of subgrade reaction486 17. 浅基础基底附加应力net foundation pressure487 17. 浅基础交叉条形基础cross strip footing488 17. 浅基础接触压力contact pressure489 17. 浅基础静定分析法(浅基础)static analysis (shallow foundation) 490 17. 浅基础壳体基础shell foundation491 17. 浅基础扩展基础spread footing492 17. 浅基础片筏基础mat foundation493 17. 浅基础浅基础shallow foundation494 17. 浅基础墙下条形基础495 17. 浅基础热摩奇金法Zemochkin's method496 17. 浅基础柔性基础flexible foundation497 17. 浅基础上部结构-基础-土共同作用分析structure- foundation-soil int eraction analysis498 17. 浅基础谈弹性地基梁(板)分析analysis of beams and slabs on elast ic foundation499 17. 浅基础条形基础strip footing500 17. 浅基础下卧层substratum501 17. 浅基础箱形基础box foundation502 17. 浅基础柱下条形基础503 18. 深基础贝诺托灌注桩Benoto cast-in-place pile504 18. 深基础波动方程分析Wave equation analysis505 18. 深基础场铸桩(台) cast-in-place pile506 18. 深基础沉管灌注桩diving casting cast-in-place pile507 18. 深基础沉井基础open-end caisson foundation508 18. 深基础沉箱基础box caisson foundation509 18. 深基础成孔灌注同步桩synchronous pile510 18. 深基础承台pile caps511 18. 深基础充盈系数fullness coefficient512 18. 深基础单桩承载力bearing capacity of single pile513 18. 深基础单桩横向极限承载力ultimate lateral resistance of single pile 514 18. 深基础单桩竖向抗拔极限承载力vertical ultimate uplift resistance of single pile515 18. 深基础单桩竖向抗压容许承载力vertical ultimate carrying capacity of single pile516 18. 深基础单桩竖向抗压极限承载力vertical allowable load capacity of si ngle pile517 18. 深基础低桩承台low pile cap518 18. 深基础地下连续墙diaphgram wall519 18. 深基础点承桩(台)end-bearing pile520 18. 深基础动力打桩公式dynamic pile driving formula521 18. 深基础端承桩end-bearing pile522 18. 深基础法兰基灌注桩Franki pile523 18. 深基础负摩擦力negative skin friction of pile524 18. 深基础钢筋混凝土预制桩precast reinforced concrete piles525 18. 深基础钢桩steel pile526 18. 深基础高桩承台high-rise pile cap527 18. 深基础灌注桩cast-in-place pile528 18. 深基础横向载荷桩laterally loaded vertical piles529 18. 深基础护壁泥浆slurry coat method530 18. 深基础回转钻孔灌注桩rotatory boring cast-in-place pile531 18. 深基础机挖异形灌注桩532 18. 深基础静力压桩silent piling533 18. 深基础抗拔桩uplift pile534 18. 深基础抗滑桩anti-slide pile535 18. 深基础摩擦桩friction pile536 18. 深基础木桩timber piles537 18. 深基础嵌岩灌注桩piles set into rock538 18. 深基础群桩pile groups539 18. 深基础群桩效率系数efficiency factor of pile groups540 18. 深基础群桩效应efficiency of pile groups541 18. 深基础群桩竖向极限承载力vertical ultimate load capacity of pile gro ups542 18. 深基础深基础deep foundation543 18. 深基础竖直群桩横向极限承载力544 18. 深基础无桩靴夯扩灌注桩rammed bulb ile545 18. 深基础旋转挤压灌注桩546 18. 深基础桩piles547 18. 深基础桩基动测技术dynamic pile test548 18. 深基础钻孔墩基础drilled-pier foundation549 18. 深基础钻孔扩底灌注桩under-reamed bored pile550 18. 深基础钻孔压注桩starsol enbesol pile551 18. 深基础最后贯入度final set552 19. 地基处理表层压密法surface compaction553 19. 地基处理超载预压surcharge preloading554 19. 地基处理袋装砂井sand wick555 19. 地基处理地工织物geofabric, geotextile556 19. 地基处理地基处理ground treatment, foundation treatment557 19. 地基处理电动化学灌浆electrochemical grouting558 19. 地基处理电渗法electro-osmotic drainage559 19. 地基处理顶升纠偏法560 19. 地基处理定喷directional jet grouting561 19. 地基处理冻土地基处理frozen foundation improvement562 19. 地基处理短桩处理treatment with short pile563 19. 地基处理堆载预压法preloading564 19. 地基处理粉体喷射深层搅拌法powder deep mixing method565 19. 地基处理复合地基composite foundation566 19. 地基处理干振成孔灌注桩vibratory bored pile567 19. 地基处理高压喷射注浆法jet grounting568 19. 地基处理灌浆材料injection material569 19. 地基处理灌浆法grouting570 19. 地基处理硅化法silicification571 19. 地基处理夯实桩compacting pile572 19. 地基处理化学灌浆chemical grouting573 19. 地基处理换填法cushion574 19. 地基处理灰土桩lime soil pile575 19. 地基处理基础加压纠偏法576 19. 地基处理挤密灌浆compaction grouting577 19. 地基处理挤密桩compaction pile, compacted column578 19. 地基处理挤淤法displacement method579 19. 地基处理加筋法reinforcement method580 19. 地基处理加筋土reinforced earth581 19. 地基处理碱液法soda solution grouting582 19. 地基处理浆液深层搅拌法grout deep mixing method583 19. 地基处理降低地下水位法dewatering method584 19. 地基处理纠偏技术585 19. 地基处理坑式托换pit underpinning586 19. 地基处理冷热处理法freezing and heating587 19. 地基处理锚固技术anchoring588 19. 地基处理锚杆静压桩托换anchor pile underpinning589 19. 地基处理排水固结法consolidation590 19. 地基处理膨胀土地基处理expansive foundation treatment591 19. 地基处理劈裂灌浆fracture grouting592 19. 地基处理浅层处理shallow treatment593 19. 地基处理强夯法dynamic compaction594 19. 地基处理人工地基artificial foundation595 19. 地基处理容许灌浆压力allowable grouting pressure596 19. 地基处理褥垫pillow597 19. 地基处理软土地基soft clay ground598 19. 地基处理砂井sand drain599 19. 地基处理砂井地基平均固结度average degree of consolidation of san d-drained ground600 19. 地基处理砂桩sand column601 19. 地基处理山区地基处理foundation treatment in mountain area602 19. 地基处理深层搅拌法deep mixing method603 19. 地基处理渗入性灌浆seep-in grouting604 19. 地基处理湿陷性黄土地基处理collapsible loess treatment605 19. 地基处理石灰系深层搅拌法lime deep mixing method606 19. 地基处理石灰桩lime column, limepile607 19. 地基处理树根桩root pile608 19. 地基处理水泥土水泥掺合比cement mixing ratio609 19. 地基处理水泥系深层搅拌法cement deep mixing method610 19. 地基处理水平旋喷horizontal jet grouting611 19. 地基处理塑料排水带plastic drain612 19. 地基处理碎石桩gravel pile, stone pillar613 19. 地基处理掏土纠偏法614 19. 地基处理天然地基natural foundation615 19. 地基处理土工聚合物Geopolymer616 19. 地基处理土工织物geofabric, geotextile617 19. 地基处理土桩earth pile618 19. 地基处理托换技术underpinning techniq。
土力学中英翻译
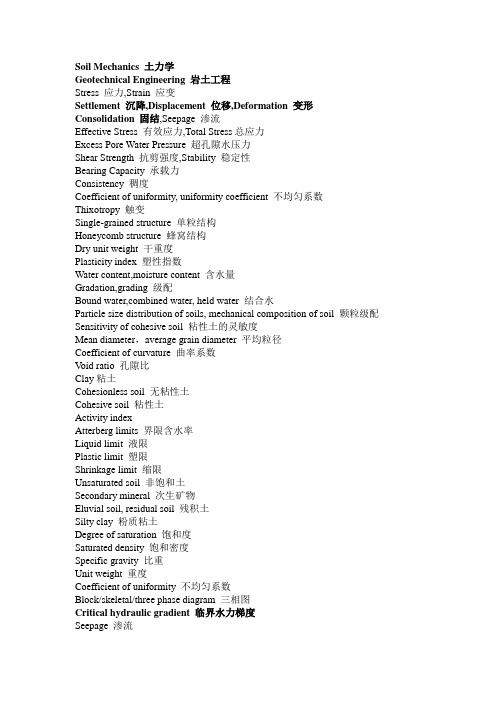
Soil Mechanics 土力学Geotechnical Engineering 岩土工程Stress 应力,Strain 应变Settlement 沉降,Displacement 位移,Deformation 变形Consolidation 固结,Seepage 渗流Effective Stress 有效应力,Total Stress总应力Excess Pore Water Pressure 超孔隙水压力Shear Strength 抗剪强度,Stability 稳定性Bearing Capacity 承载力Consistency 稠度Coefficient of uniformity, uniformity coefficient 不均匀系数Thixotropy 触变Single-grained structure 单粒结构Honeycomb structure 蜂窝结构Dry unit weight 干重度Plasticity index 塑性指数Water content,moisture content 含水量Gradation,grading 级配Bound water,combined water, held water 结合水Particle size distribution of soils, mechanical composition of soil 颗粒级配Sensitivity of cohesive soil 粘性土的灵敏度Mean diameter,average grain diameter 平均粒径Coefficient of curvature 曲率系数V oid ratio 孔隙比Clay粘土Cohesionless soil 无粘性土Cohesive soil 粘性土Activity indexAtterberg limits 界限含水率Liquid limit 液限Plastic limit 塑限Shrinkage limit 缩限Unsaturated soil 非饱和土Secondary mineral 次生矿物Eluvial soil, residual soil 残积土Silty clay 粉质粘土Degree of saturation 饱和度Saturated density 饱和密度Specific gravity 比重Unit weight 重度Coefficient of uniformity 不均匀系数Block/skeletal/three phase diagram 三相图Critical hydraulic gradient 临界水力梯度Seepage 渗流Seepage discharge 渗流量Seepage velocity 渗流速度Seepage force 渗透力Darcy’s law 达西定律Piping 管涌Permeability 渗透性Coefficient of permeability 渗透系数Seepage failure 渗透破坏Phreatic 浸润线Flowing soil 流土Hydraulic gradient 水力梯度Critical hydraulic gradient 临界水力梯度Flow function 流函数Flow net 流网Sand boiling 砂沸Potential function 势函数Capillary water 毛细水Constant/falling head test 常/变水头试验Modulus of deformation 变形模量Poisson’s ratio 泊松比Residual deformation 残余变形Excess pore water pressure 超静孔隙水压力Settlement 沉降Coefficient of secondary consolidation 次固结系数Elastic formula for settlement calculation 地基沉降的弹性力学公式Layerwise summation method 分层总和法Superimposed stress 附加应力Secant modulus 割线模量Consolidation settlement 固结沉降Settlement calculation by specification 规范沉降计算法Rebound deformation 回弹变形Modulus of resilience 回弹模量Coefficient of resilience 回弹系数Swelling index 回弹指数Allowable settlement of building 建筑物的地基变形允许值Corner-points method 角点法Tangent modulus 切线模量。
吉大岩土工程专业英语翻译第10课
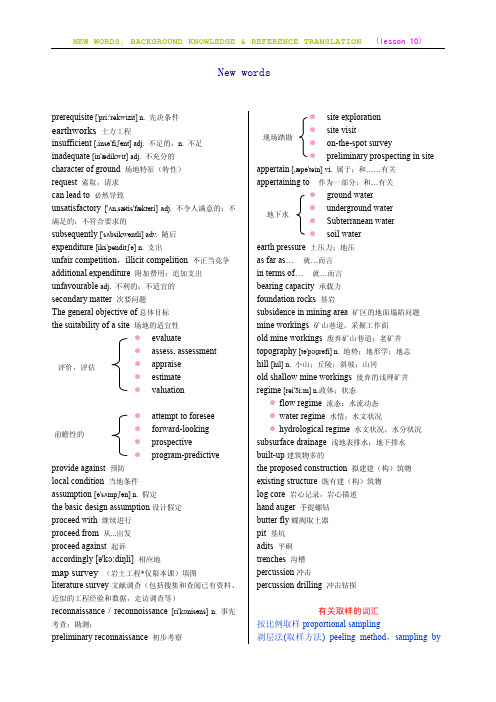
New wordsprerequisite ['pri:'rekwizit] n. 先决条件earthworks 土方工程insufficient [,insə'fiʃənt] adj. 不足的,n. 不足inadequate [in'ædikwit] adj. 不充分的character of ground 场地特征(特性)request 索取,请求can lead to 必然导致unsatisfactory ['ʌn,sætis'fæktəri] adj. 不令人满意的;不满足的;不符合要求的subsequently ['sʌbsikwəntli] adv. 随后expenditure [iks'penditʃə] n. 支出unfair competition,illicit compelition 不正当竞争additional expenditure 附加费用;追加支出unfavourable adj. 不利的;不适宜的secondary matter 次要问题The general objective of总体目标the suitability of a site 场地的适宜性z evaluatez assess, assessmentz appraisez estimatez valuationz attempt to foreseez forward-lookingz prospectivez program-predictive provide against 预防local condition 当地条件assumption [ə'sʌmpʃən] n. 假定the basic design assumption设计假定proceed with 继续进行proceed from 从...出发proceed against 起诉accordingly [ə'kɔ:diŋli] 相应地map survey (岩土工程*仅限本课)填图literature survey文献调查(包括搜集和查阅已有资料、近似的工程经验和数据,走访调查等)reconnaissance / reconnoissance [ri'kɔnisəns] n. 事先考查;勘测;preliminary reconnaissance 初步考察z site explorationz site visitz on-the-spot surveyz preliminary prospecting in site appertain [,æpə'tein] vi. 属于;和……有关appertaining to 作为一部分;和…有关z ground waterz underground waterz Subterranean waterz soil waterearth pressure 土压力;地压as far as… 就…而言in terms of… 就…而言bearing capacity 承载力foundation rocks 基岩subsidence in mining area 矿区的地面塌陷问题mine workings 矿山巷道,采掘工作面old mine workings 废弃矿山巷道;老矿井topography [tə'pɔɡrəfi] n. 地势;地形学;地志hill [hil] n. 小山;丘陵;斜坡;山冈old shallow mine workings 废弃的浅埋矿井regime [rei'ʒi:m] n.政体;状态z flow regime 流态;水流动态z water regime 水情;水文状况z hydrological regime 水文状况,水分状况subsurface drainage 浅地表排水;地下排水built-up建筑物多的the proposed construction 拟建建(构)筑物existing structure 既有建(构)筑物log core 岩心记录,岩心描述hand auger 手提螺钻butter fly蝶阀取土器pit 基坑adits 平硐trenches 沟槽percussion冲击percussion drilling 冲击钻探有关取样的词汇按比例取样proportional sampling剥层法(取样方法) peeling method,sampling by评价,评估前瞻性的现场踏勘地下水stripping沉落取样器drop sampler衬片取样器foil sampler重复取样repeated sampling, resampling地下取样subsurface sample地下水取样groundwater sampling冻结取样器cryogenic sampler对开式取样器split tube sampler多次取样multisampling二次取样subsample方格法(取样) quadrangle method分层取样stratified sampling固定活塞式取样器fixed piston sampler管式取样器tube sampler海底取样submarine sampling海底取样器kullenberg sampler盒式取样器(开斯顿取样器) kasten corer回转取样器rotary sampler井壁取样lateral coring井壁取样器side sampler; wall sampler井底取样器bottom-hole sample taker; bottom- hole sampler刻糟取样channel sampling刻槽取样法chip- channel method孔底取样器bottom sampler连续取样continuous sampling手动螺旋钻孔取样法auger sampling method泥泵取样器sample thief取岩心running coring取样sample collection; taking of sample; thief取样层位 sample horizon取样位置sample site取样法method of sampling取样格式 sampling dsign取样管bleeder / probe tube; sampling pepe取样厚度sampled thickness取样技术sampling technique取样|间隔sample interval; sample period取样间距interval of sampling取样流程 smpling flowsheet取样瓶 sample botlle取样器(深部) cheese tester取样枪sampling gun取样扰动sampling disturbance取样勺 sampling spoon 取样试验pick-test取样筒sampler barrel. sampling barrel. sampling tube取样系统sampling line取样钻进sample drilling取淤泥样sludge sampling双层取样double tube sampler双重岩心管取样器double tube core; barrelsampler 四分取样铲quartering shovel四分取样法quartering探槽取样pit sampling桶式取样方法barrel sampling外间隙比(取样器) outer clearance ratio无吊索取样管free-draining-fall corer系列取样serial sampling谢尔贝薄壁取样器Shelby tube sampler压力取样器pressure thief压入式取样器 jacker in sampler压人式取样简pressure-type core barrel液压活塞取样[土] hydraulic piston sampler移动式取样器moving machine sampler原地水取样器in-situ liquid sampler原状土取样器samplers for undisturbed samples真空取样器vacuum sampling tube真空岩心取样管vacuum corer重锤岩心取样gravity core sampling自返式取样管free-draining-fall core自由下落取样器free-draining-fall corer钻孔取样器messenger钻探(取样) drilling; bore; probe drilling; prospection drilling; exploralion drilling。
专业英语翻译(岩土系适用)

Abstract:The karst mud limestone of Triassic Badong formation (T2 b) is the serious engineering geological problem newly discovered in the population resettlement project in the Three Gorges Reservoir region. There are very complex structures in mud limestone, involving old structures, new structures and surface deformation structures, which coordinately control the karstification. In the old structures, the local structures such as folds and fault zones control the important segments and layers of karstification; and the mini structures such as joint and layer face popularize the karstification. The surface uplift and river cutting in new tectonic period put forward the unload and loose of rock mass, widening of karstification paths. The surface deformation structures densify the karstification paths and intensify the karstification. The mechanism of karst hazards yields to the regulation of structure controlling over karstification in mud limestone terrain, Three Gorges Reservoir region , which brings about hazards with features of broad range , huge scale and complex structure. The types of karst hazards involve uneven subsidence, fissure, landslide, collapse,mudflow and cave in.三峡库区三叠系巴东组(T2b)泥灰质岩石岩溶是移民迁建中发现的重大工程地质问题。
英文文献及翻译
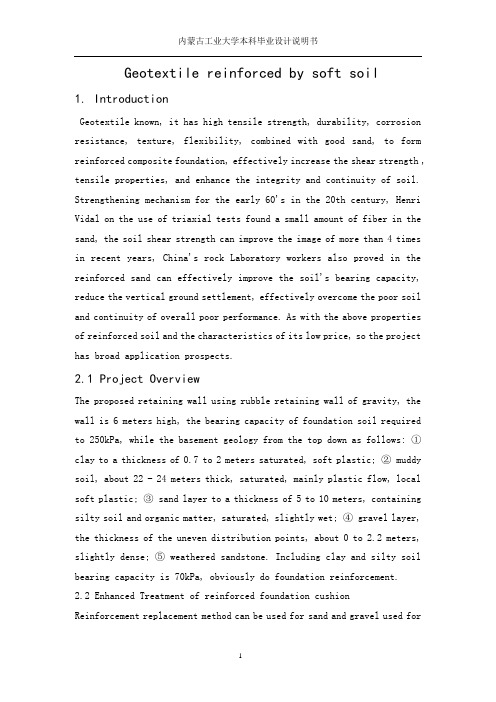
Geotextile reinforced by soft soil1. IntroductionGeotextile known, it has high tensile strength, durability, corrosion resistance, texture, flexibility, combined with good sand, to form reinforced composite foundation, effectively increase the shear strength , tensile properties, and enhance the integrity and continuity of soil. Strengthening mechanism for the early 60's in the 20th century, Henri Vidal on the use of triaxial tests found a small amount of fiber in the sand, the soil shear strength can improve the image of more than 4 times in recent years, China's rock Laboratory workers also proved in the reinforced sand can effectively improve the soil's bearing capacity, reduce the vertical ground settlement, effectively overcome the poor soil and continuity of overall poor performance. As with the above properties of reinforced soil and the characteristics of its low price, so the project has broad application prospects.2.1 Project OverviewThe proposed retaining wall using rubble retaining wall of gravity, the wall is 6 meters high, the bearing capacity of foundation soil required to 250kPa, while the basement geology from the top down as follows: ①clay to a thickness of 0.7 to 2 meters saturated, soft plastic; ② muddy soil, about 22 - 24 meters thick, saturated, mainly plastic flow, local soft plastic; ③ sand layer to a thickness of 5 to 10 meters, containing silty soil and organic matter, saturated, slightly wet; ④ gravel layer, the thickness of the uneven distribution points, about 0 to 2.2 meters, slightly dense; ⑤ weathered sandstone. Including clay and silty soil bearing capacity is 70kPa, obviously do foundation reinforcement.2.2 Enhanced Treatment of reinforced foundation cushion Reinforcement replacement method can be used for sand and gravel used forsoil treatment, but due to loose bedding, based on past experience, witha gravel mat to treat a large settlement of the foundation always exist, even the characteristics of poor, often resulting in cracks in the superstructure, differential settlement of the image, this works for6-meter-high rubble retaining walls, height and large, and because the walls are 3 meters high wall, if there is differential settlement of retaining walls, cracks, will result in more serious consequences and thus should be used on the cushion reinforcement through economic and technical analysis, decide on the sand and gravel stratum were reinforced hardening. Reinforcement treatment method: first the design elevation and the basement excavation to 200mm thick layer of gravel bedding, and then capped with a layer of geotextile, and then in the thick sand and gravel on the 200, after leveling with the yellow sand using roller compaction; second with loaded bags of sand and gravel laying of geotextile, the gap filled with slag, geotextile bags capped 100 thick gravel, roller compaction. Its on repeat laying geotextile → → compacted gravel, until the design thickness of the cushion, the bridge is 1 m thick cushion, a total of 4 layers of geotextile, two bags of sand.This method works fast, simple machine, investment, after years of use, that reinforce good effect, building and construction units are satisfied.3 ExperienceTo achieve the reinforced soil reinforcement effect, must be reinforced earth construction technology, construction strict quality control: 1, geotextile should increase the initial pre-stress, and its end should be a reliable anchor to play the tensile strength of geotextile, anchoring more firmly, more capacity to improve, the foundation of the stress distribution more uniform, geotextile side Ministry of fixed length by laying end to ensure the fold, the folded end wrapped sand to increase its bond strength to ensure that the use will not be pulled out duringthe period.Second, the construction process have a significant effect on the reinforcement effect, the construction should be as soon as possible so that geotextile in tension, tensile strength geotextile can be played only when the deformation, so do not allow construction of geotextile crease occurs, the earth Fabric tension leveling as much as possible. Geotextile in order to have enough by the early Dutch strain, according to the following procedure works: ① laying geotextile; ② leveled the tension at both ends; both ends of the folded package gravel and sand filling at both ends; ③ center fill sand; ④ 2 higher end of sand; ⑤ Finally, the center of sand filling. Click here to enable the construction method of forming corrugated geotextile being stretched as soon as possible, to play a role in the early loaded.Third, the construction of geotextile-reinforced cushion should the level of shop using geotextile geotextile and laying of gravel bags cushion the turn to play bag cushion integrated turn out good, flexural rigidity, and dispersion of good and peace bedding layer of the overall continuity of good advantages.4 ConclusionGeotextile reinforced by soft soil is an effective, economical, safe, reliable, simple method, but the literature describes only qualitative, experience more components, yet the lack of rigorous The theoretical formula, reliable test data to be adequate, these are yet to be theoretical workers and the general engineering and technical personnel continue to explore.土工织物加筋垫层加固软土地基1. 引言土工织物又称土工聚合物,它具有高抗拉强度,耐久性、耐腐蚀性,质地柔韧,能与砂土很好地结合,组合成加筋土复合地基,有效地提高土的抗剪强度、抗拉性能,增强土体的整体性和连续性。
岩土工程英文介绍
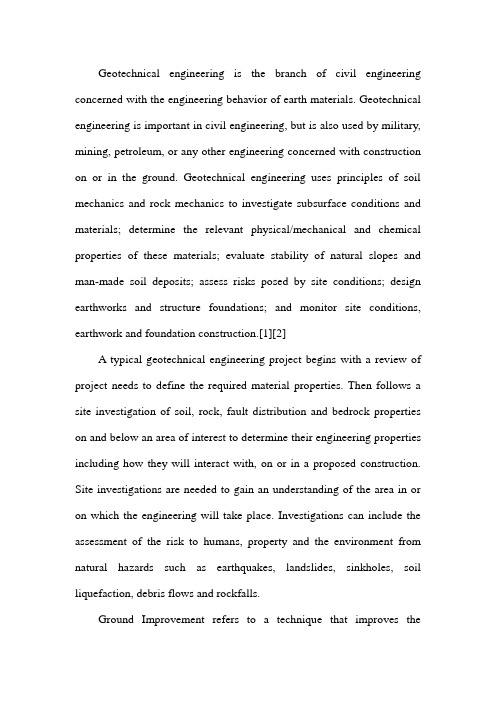
Geotechnical engineering is the branch of civil engineering concerned with the engineering behavior of earth materials. Geotechnical engineering is important in civil engineering, but is also used by military, mining, petroleum, or any other engineering concerned with construction on or in the ground. Geotechnical engineering uses principles of soil mechanics and rock mechanics to investigate subsurface conditions and materials; determine the relevant physical/mechanical and chemical properties of these materials; evaluate stability of natural slopes and man-made soil deposits; assess risks posed by site conditions; design earthworks and structure foundations; and monitor site conditions, earthwork and foundation construction.[1][2]A typical geotechnical engineering project begins with a review of project needs to define the required material properties. Then follows a site investigation of soil, rock, fault distribution and bedrock properties on and below an area of interest to determine their engineering properties including how they will interact with, on or in a proposed construction. Site investigations are needed to gain an understanding of the area in or on which the engineering will take place. Investigations can include the assessment of the risk to humans, property and the environment from natural hazards such as earthquakes, landslides, sinkholes, soil liquefaction, debris flows and rockfalls.Ground Improvement refers to a technique that improves theengineering properties of the soil mass treated. Usually, the properties that are modified are shear strength, stiffness and permeability. Ground improvement has developed into a sophisticated tool to support foundations for a wide variety of structures. Properly applied, i.e. after giving due to consideration to the nature of the ground being improved and the type and sensitivity of the structures being built, ground improvement often reduces directs costs and saves time.[3]A geotechnical engineer then determines and designs the type of foundations, earthworks, and/or pavement subgrades required for the intended man-made structures to be built. Foundations are designed and constructed for structures of various sizes such as high-rise buildings, bridges, medium to large commercial buildings, and smaller structures where the soil conditions do not allow code-based design.Foundations built for above-ground structures include shallow and deep foundations. Retaining structures include earth-filled dams and retaining walls. Earthworks include embankments, tunnels, dikes, levees, channels, reservoirs, deposition of hazardous waste and sanitary landfills.Geotechnical engineering is also related to coastal and ocean engineering. Coastal engineering can involve the design and construction of wharves, marinas, and jetties. Ocean engineering can involve foundation and anchor systems for offshore structures such as oil platforms.The fields of geotechnical engineering and engineering geology are closely related, and have large areas of overlap. However, the field of geotechnical engineering is a specialty of engineering, where the field of engineering geology is a specialty of geology.。
岩土工程专业英语 可删减

岩土工程专业外语词汇大全中英翻译1. 综合类大地工程geotechnical engineering临界状态土力学critical state soil mechanics数值岩土力学numerical geomechanics 岩土工程geotechnical engineering应力路径stress path2. 工程地质及勘察变质岩metamorphic rock标准冻深standard frost penetration残积土eluvial soil, residual soil层理beding长石feldspar沉积岩sedimentary rock次生矿物secondary mineral地质图geological map断裂构造fracture structure工程地质勘察engineering geological exploration花岗岩granite化学沉积岩chemical sedimentary rock 解理cleavage矿物硬度hardness of minerals流滑flow slide泥石流mud flow, debris flow粘土矿物clay minerals潜水ground water侵入岩intrusive rock砂岩sandstone深成岩plutionic rock石灰岩limestone 岩层产状attitude of rock岩浆岩magmatic rock, igneous rock岩石风化程度degree of rock weathering 岩石构造structure of rock岩石结构texture of rock页岩shale原生矿物primary mineral造岩矿物rock-forming mineral褶皱fold, folding3. 土的分类饱和土saturated soil超固结土overconsolidated soil冲填土dredger fill冻土frozen soil非饱和土unsaturated soil分散性土dispersive soil粉土silt粉质粘土silty clay红粘土red clay, adamic earth黄土loess粘性土cohesive soil, clayey soil膨胀土expansive soil, swelling soil欠固结粘土underconsolidated soil区域性土zonal soil人工填土fill, artificial soil软粘土soft clay, mildclay, mickle湿陷性黄土collapsible loess, slumping loess素填土plain fill塑性图plasticity chart碎石土stone, break stone, broken stone, channery, chat, crushed stone, deritus未压密土(台)underconsolidated clay 无粘性土cohesionless soil, frictional soil,non-cohesive soil淤泥muck, gyttja, mire, slush淤泥质土mucky soil原状土undisturbed soil正常固结土normally consolidated soil正常压密土(台)normally consolidated soil 自重湿陷性黄土self weight collapse loess4. 土的物理性质饱和度degree of saturation饱和密度saturated density饱和重度saturated unit weight比重specific gravity稠度consistency触变thixotropy干重度dry unit weight干密度dry density塑性指数plasticity index含水量water content, moisture content级配gradation, grading结合水bound water, combined water, held water界限含水量Atterberg limits颗粒级配particle size distribution of soils, mechanical composition of soil可塑性plasticity孔隙比void ratio孔隙率porosity毛细管水capillary water密实度compactionness年粘性土的灵敏度sensitivity of cohesive soil曲率系数coefficient of curvature三相土tri-phase soil湿陷起始应力initial collapse pressure湿陷系数coefficient of collapsibility缩限shrinkage limit 土粒相对密度specific density of solid particles相对密度relative density, density index 相对压密度relative compaction, compacting factor, percent compa ction, coefficient of compaction压密系数coefficient of consolidation压缩性compressibility液限liquid limit液性指数liquidity index游离水(台)free water重力密度unit weight自由水free water, gravitational water, groundwater, phreatic water组构fabric最大干密度maximum dry density最优含水量optimum water content5. 渗透性和渗流浸润线phreatic line临界水力梯度critical hydraulic gradient 流函数flow function渗流seepage渗流量seepage discharge渗透破坏seepage failure渗透系数coefficient of permeability渗透性permeability势函数potential function水力梯度hydraulic gradient6. 地基应力和变形变形deformation变形模量modulus of deformation残余变形residual deformation超静孔隙水压力excess pore water pressure沉降settlement次固结沉降secondary consolidation settlement次固结系数coefficient of secondary consolidation切线模量tangent modulus蠕变creep三向变形条件下的固结沉降three-dimensional consolidation settl ement弹性平衡状态state of elastic equilibrium 体积变形模量volumetric deformation modulus先期固结压力preconsolidation pressure压缩模量modulus of compressibility压缩系数coefficient of compressibility有效应力effective stress自重应力self-weight stress总应力total stress approach of shear strength7. 固结超固结比over-consolidation ratio超静孔隙水压力excess pore water pressure固结度degree of consolidation固结理论theory of consolidation固结曲线consolidation curve固结速率rate of consolidation固结系数coefficient of consolidation固结压力consolidation pressure回弹曲线rebound curve砂井sand drain砂井地基平均固结度average degree of consolidation of sand-drained ground时间对数拟合法logrithm of time fitting method太沙基固结理论Terzaghi s consolidation theory先期固结压力preconsolidation pressure压密度(台)degree of consolidation压缩曲线cpmpression curve一维固结one dimensional consolidation 有效应力原理principle of effective stress 预压密压力(台)preconsolidation pressure 原始压缩曲线virgin compression curve再压缩曲线recompression curve主固结primary consolidation主压密(台)primary consolidationK0固结consolidation under K0 condition8. 抗剪强度单轴抗拉强度uniaxial tension test动强度dynamic strength of soils峰值强度peak strength伏斯列夫参数Hvorslev parameter剪切应变速率shear strain rate抗剪强度shear strength抗剪强度参数shear strength parameter抗剪强度有效应力法effective stress approach of shear strength抗剪强度总应力法total stress approach of shear strength摩尔-库仑理论Mohr-Coulomb theory内摩擦角angle of internal friction粘聚力cohesion破裂角angle of rupture破坏准则failure criterion有效内摩擦角effective angle of internal friction有效粘聚力effective cohesion intercept有效应力破坏包线effective stress failure envelope有效应力强度参数effective stress strength parameter有效应力原理principle of effective stress 真内摩擦角true angle internal friction真粘聚力true cohesion总应力破坏包线total stress failure envelope总应力强度参数total stress strength parameter9. 本构模型本构模型constitutive model边界面模型boundary surface model层向各向同性体模型cross anisotropic model摩尔-库仑屈服准则Mohr-Coulomb yield criterion切线模量tangent modulus屈服面yield surface弹塑性模量矩阵elastoplastic modulus matrix弹塑性模型elastoplastic modulus10. 地基承载力冲剪破坏punching shear failure次层(台)substratum地基subgrade, ground, foundation soil地基承载力bearing capacity of foundation soil地基极限承载力ultimate bearing capacity of foundation soil地基允许承载力allowable bearing capacity of foundation soil地基稳定性stability of foundation soil整体剪切破坏general shear failure11. 土压力被动土压力passive earth pressure被动土压力系数coefficient of passive earth pressure极限平衡状态state of limit equilibrium静止土压力earth pressue at rest静止土压力系数coefficient of earth pressur at rest库仑土压力理论Coulomb s earth pressure theory库尔曼图解法Culmannn construction朗肯土压力理论Rankine s earth pressure theory朗肯状态Rankine state弹性平衡状态state of elastic equilibrium 土压力earth pressure 主动土压力active earth pressure主动土压力系数coefficient of active earth pressure12. 土坡稳定分析边坡稳定安全系数safety factor of slope土坡slope土坡稳定分析slope stability analysis分析土坡稳定极限分析法limit analysis method of slope stability分析土坡稳定极限平衡法limit equilibrium method of slope stability16. 基坑开挖与降水板桩围护sheet pile-braced cuts电渗法electro-osmotic drainage管涌piping基底隆起heave of base基坑降水dewatering基坑失稳instability (failure) of foundation pit基坑围护bracing of foundation pit减压井relief well降低地下水位法dewatering method井点系统well point system喷射井点eductor well point铅直边坡的临界高度critical height of vertical slope砂沸sand boiling深井点deep well point真空井点vacuum well point支撑围护braced cuts19. 地基处理表层压密法surface compaction超载预压surcharge preloading袋装砂井sand wick地工织物geofabric, geotextile地基处理ground treatment, foundation treatment电动化学灌浆electrochemical grouting 电渗法electro-osmotic drainage顶升纠偏法定喷directional jet grouting冻土地基处理frozen foundation improvement短桩处理treatment with short pile堆载预压法preloading粉体喷射深层搅拌法powder deep mixing method复合地基composite foundation干振成孔灌注桩vibratory bored pile高压喷射注浆法jet grounting灌浆材料injection material灌浆法grouting硅化法silicification夯实桩compacting pile化学灌浆chemical grouting换填法cushion灰土桩lime soil pile挤密灌浆compaction grouting锚固技术anchoring锚杆静压桩托换anchor pile underpinning 排水固结法consolidation膨胀土地基处理expansive foundation treatment劈裂灌浆fracture grouting浅层处理shallow treatment强夯法dynamic compaction人工地基artificial foundation软土地基soft clay ground砂井sand drain砂井地基平均固结度average degree of consolidation of sand-drained ground 22. 室内土工试验常水头渗透试验constant head permeability test单剪仪simple shear apparatus单轴拉伸试验uniaxial tensile test 固结排水试验consolidated drained triaxial test固结试验consolidation test含水量试验water content test黄土湿陷试验loess collapsibility test快剪试验quick direct shear test快速固结试验fast consolidation test离心模型试验centrifugal model test连续加荷固结试验continual loading test 慢剪试验consolidated drained direct shear test毛细管上升高度试验capillary rise test密度试验density test扭剪仪torsion shear apparatus膨胀率试验swelling rate test平面应变仪plane strain apparatus三轴伸长试验triaxial extension test三轴压缩试验triaxial compression test砂的相对密实度试验sand relative density test渗透试验permeability test湿化试验slaking test收缩试验shrinkage test塑限试验plastic limit test缩限试验shrinkage limit test土工模型试验geotechnical model test土工织物试验geotextile test无侧限抗压强度试验unconfined compression strength test真三轴仪true triaxial apparatus振动单剪试验dynamic simple shear test 直剪仪direct shear apparatus直接剪切试验direct shear test直接单剪试验direct simple shear test自振柱试验free vibration column testK0固结不排水试验K0 consolidated undrained testK0固结排水试验K0 consolidated drained test23. 原位测试标准贯入试验standard penetration test表面波试验surface wave test超声波试验ultrasonic wave test承载比试验Califonia Bearing Ratio Test 单桩横向载荷试验lateral load test of pile 单桩竖向静载荷试验static load test of pile 动力触探试验dynamic penetration test静力触探试验static cone penetration test 静力载荷试验plate loading test跨孔试验cross-hole test轻便触探试验light sounding test深层沉降观测deep settlement measurement十字板剪切试验vane shear test无损检测nondestructive testing下孔法试验down-hole test现场渗透试验field permeability test原位孔隙水压力量测in situ pore water pressure measurement原位试验in-situ soil test最后贯入度final setgabbro 辉长岩geographical mapping 地理勘察;地理测绘geological condition 地质状况geological map 地质图geological mapping 地质勘察;地质测绘geological profile 地质剖面geological survey 地质调查Geology Society of Hong Kong 香港地质学会geophysical exploration 地球物理勘探geostatic stress 地应力geotechnical 岩土;土力geotechnical appraisal 岩土评估;土力评估Geotechnical Area Study Programme 地区岩土研究计划geotechnical assessment 岩土评估;土力评估geotechnical assessment study 岩土评估研究;土力评估研究geotechnical consultant 岩土工程顾问;土力工程顾问geotechnical control 岩土工程管制geotechnical design assumption 岩土设计假定geotechnical investigation 岩土工程勘察;岩土工程勘探ground feature 地物;地貌ground investigation 土地勘测;土地勘探;探土ground profile 地形;地形剖面;地形切面ground settlement 土地沉降;地陷ground surface 地面ground survey 地面测量grounding electrode 接地电极groundwater catchment 地下水流域groundwater connectivity test 地下水连通实验groundwater discharge 地下水流量;地下水溢流groundwater drainage works 地下水排水工程groundwater flow direction 地下水流向groundwater level 地下水位groundwater monitoring 地下水监测groundwater pressure measurement 地下水水压测试groundwater regime 地下水体系groundwater table fluctuation 地下水位变动karst cave 岩溶洞karst topography 岩溶地形。
岩土工程外文翻译
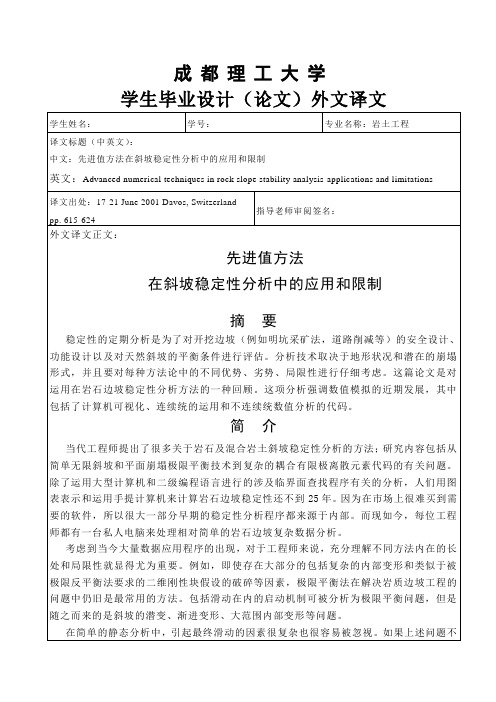
成都理工大学学生毕业设计(论文)外文译文在市场上可买到的极限平衡的计算机代码在近几年已经有了很大的进步。
这包括:有限元法和地下水应力分析(如GEO-SLOPE’s SIGMA/W, SEEP/W 和 SLOPE/W(6))的二维极限平衡法编码的集成。
三维极限平衡法的发展(例如 CLARA (7); 3D-SLOPE(8))。
概率极限平衡技术的发展。
允许多样的支持和加固的能力。
非饱和土抗剪强度标准的混合。
可视化的高度发展和前处理及后处理的制图学。
这些编码在土坡和高度蚀变岩斜坡的分析中起着至关重要的作用。
而在这些斜坡中,离散明确的表面容易发生滑动。
图2阐明了高岭土化的花岗岩斜坡崩塌的反分析法中的二维极限平衡程序的运用。
包括岩块内部的应力状态和复杂变形及脆性断裂影响是极其重要的。
数值模拟技术也应用其中。
(如图2所示).图 1. SWEDGE 分析(右)建立在DIPS立体图输入的基础上(LEFT).图 2. 用极限平衡法对瓷土边坡进行分析来寻求滑动平面(左)和有线差分来模拟剪应变发展(右)石雨模拟器是另一分析法的传统模式,其中包括用来评估单个坠落方块的危害的工具。
像ROCFALL (2) 的程序用来分析从给定坡面几何上滚动或弹动的岩块在速率发生变水特征; 原位应力状态料、间断运行和液压机械及动态分析);能够评估不稳定性参数变化的影响样;需要注意缩放效果;需要模拟典型间断几何(间距, 持久性等等);节理特性可使用的的数据有限(例如jkn, jks).混合物/耦合模拟列出独立模型的输入参数的组合耦合有限元/离散单元模型能够模拟节理和层状媒介上的完整的裂缝延伸和断裂复杂问题需要高端内存容量;在实践中相对来说有较少的经验;需要持续校准和限制连续统建模连续统建模最适合应用于由大量完整岩石、软弱岩石、类土或严重断裂的岩块构成的斜坡。
大多数连续统代码有含离散断裂的设备,如断层和层面。
但是不适用于不均介质的分析。
石坡稳定性中的连续统方法包括有线差分法和有限元素法。
土力学专业英语
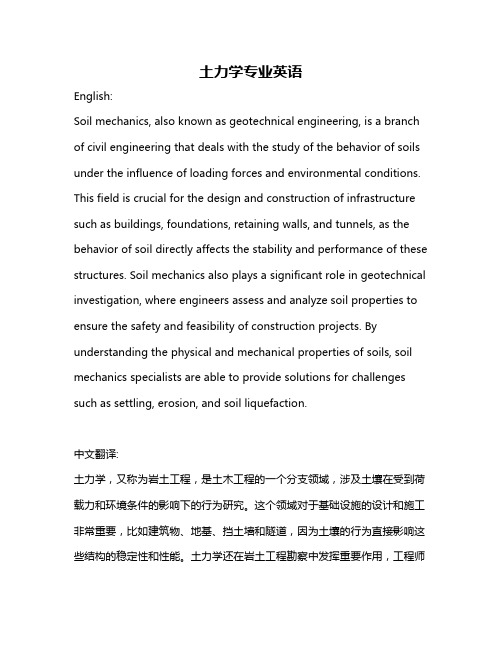
土力学专业英语English:Soil mechanics, also known as geotechnical engineering, is a branch of civil engineering that deals with the study of the behavior of soils under the influence of loading forces and environmental conditions. This field is crucial for the design and construction of infrastructure such as buildings, foundations, retaining walls, and tunnels, as the behavior of soil directly affects the stability and performance of these structures. Soil mechanics also plays a significant role in geotechnical investigation, where engineers assess and analyze soil properties to ensure the safety and feasibility of construction projects. By understanding the physical and mechanical properties of soils, soil mechanics specialists are able to provide solutions for challenges such as settling, erosion, and soil liquefaction.中文翻译:土力学,又称为岩土工程,是土木工程的一个分支领域,涉及土壤在受到荷载力和环境条件的影响下的行为研究。
岩土工程锚杆中英文对照外文翻译文献
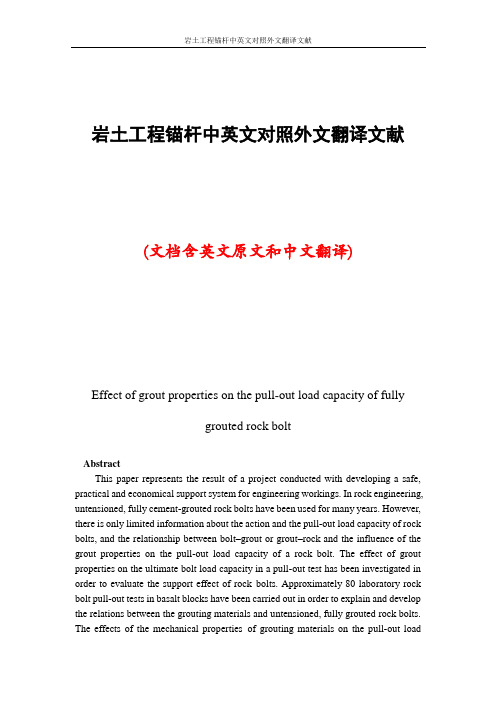
岩土工程锚杆中英文对照外文翻译文献(文档含英文原文和中文翻译)Effect of grout properties on the pull-out load capacity of fullygrouted rock boltAbstractThis paper represents the result of a project conducted with developing a safe, practical and economical support system for engineering workings. In rock engineering, untensioned, fully cement-grouted rock bolts have been used for many years. However, there is only limited information about the action and the pull-out load capacity of rock bolts, and the relationship between bolt–grout or grout–rock and the influence of the grout properties on the pull-out load capacity of a rock bolt. The effect of grout properties on the ultimate bolt load capacity in a pull-out test has been investigated in order to evaluate the support effect of rock bolts. Approximately 80 laboratory rock bolt pull-out tests in basalt blocks have been carried out in order to explain and develop the relations between the grouting materials and untensioned, fully grouted rock bolts. The effects of the mechanical properties of grouting materials on the pull-out loadcapacity of a fully grouted bolt have been qualified and a number of empirical formulae have been developed for the calculating of the pull-out load capacity of the fully cement-grouted bolts on the basis of the shear strength, the uniaxial compressive strength of the grouting material, the bolt length, the bolt diameter, the bonding area and the curing time of the grouting material.Keywords: Rock bolt; Grouting materials; Bolt pull-out load capacity; Bolt geometry; Mortar1. IntroductionIn rock engineering, rock bolts have been used to stabilise openings for many years. The rock bolting system may improve the competence of disturbed rock masses by preventing joint movements, forcing the rock mass to support itself (Kaiser et al., 1992). The support effect of rock bolt has been discussed by many researchers(e.g. Hyett et al., 1992; Ito et al., 2001; Reichert et al., 1991 and Stillborg, 1984). Rock bolt binds together a laminated, discontinued, fractured and jointed rock mass. Rock bolting not only strengthens or stabilizes a jointed rock mass, but also has a marked effect on the rock mass stiffness (Chappell, 1989). Rock bolts perform their task by one or a combination of several mechanisms. Bolts often act to increase the stress and the frictional strength across joints, encouraging loose blocks or thinly stratified beds to bind together and act as a composite beam (Franklin and Dusseault, 1989). Rock bolts reinforce rock through a friction effect, through a suspension effect, or a combination of two. For this reason, rock bolt technique is acceptable for strengthening of mine roadway and tunnelling in all type of rock ( Panek and McCormick, 1973).Generally rock bolts can be used to increase the support of low forces due to the diameter and the strength of the bolt materials. They enable high anchoring velocity to be used at closer spacing between bolts.Their design provides either mechanical clamping or cement grouting against the rock (Aldorfand Exner,1986).Anchorage system of rock bolt is normally made of solid or tube formed steel installed untensioned or tensioned in the rock mass (Stillborg, 1986). Rock bolts can be divided into three main groups according to their anchorage systems (Franklin and Dusseault, 1989;Aldorfand Exner, 1986; Hoek and Wood, 1989; Cybulski and Mazzoni, 1989). First group is the mechanically anchored rock bolts that can be divided into two groups: slit and wedge type rock bolt, expansion shell anchor. They can be fixed in the anchoring part either by a wedge-shaped clamping part or by a threaded clamping part. Second group is the friction-anchored rock bolts that can be simply divided into two groups: split-set and swellex. Friction-anchored rock bolts stabilise the rock mass by friction of the outer covering of bolt against the drill hole side. The last group is the fully grouted rock bolts that can also be divided into twogroups: cement-grouted rock bolts, resin grouted rock bolts.A grouted rock bolt (dowel) is a fully grouted rock bolt without mechanical anchor, usually consisting of a ribbed reinforcing bar, installed in a drill hole and bonded to the rock over its full length (Franklin and Dusseault, 1989). Special attention should be paid to cement-grouted bolts and bolts bonded (glued, resined) by synthetics resins for bolt adjustment. Grouted bolts fix the using of the coherence of the sealing cement with the bolt rod and the rock for fastening the bolts. Synthetic resin (resined bolt) and cement mortar (reinforced-concrete bolt) can be used for this type rock bolt. These bolts may be anchored in all type of rock. Anchoring rods may be manufactured of several materials such as ribbed steel rods, smooth steel bars, cable bolts and other special finish (Aldorfand Exner, 1986).Grouted bolts are widely used in mining for the stabilisation of tunnelling, mining roadway, drifts and shafts for the reinforcing of its peripheries. Simplicity of installation, versatility and relatively low cost of rebars are further benefits of grouted bolts is comparison to their alternative counterparts (Indraratna and Kaiser,1990).Bolts are self-tensioning when the rock starts to move and dilate. They should therefore be installed as soon as possible after excavation, before the rock has started to deform, and before it has lost its interlocking and shear strength.Although several grout types are available, in many applications where the rock has a measure of short term stability, simple Portland cement-grouted reinforcing dowels are sufficient. They can be installed by filling the drill hole with lean, quickly set mortar into which the bar is driven. The dowel is retained in up holes either by a cheap form of end anchor, or by packing the drill hole collar with cotton waste, steel wool, or wooden wedges (Franklin and Dusseault, 1989).Concrete grouted bolts use cement mortar as a bonding medium. In drill holes at minimum of15 8 below the horizontal plane, the mortar can simply poured in, whereas in raising drill holes various design of bolts or other equipment is used to prevent the pumped mortar from flowing out (Aldorfand Exner,1986).The load bearing capacity off ully cement-grouted rock bolts depends on the bolt shape, the bolt diameter, the bolt length, rock and grout strength. The bond strength off ully cement-grouted rock bolts is primarily frictional and depends on the shear strength at the bolt–grout or grout–rock interface. Thus any changes in this interfaces shear strength must affect the bolt bond strength and bolt load capacity.This laboratory testing program was executed to evaluate the shear strength effect on the bond strength of the bolt–grout interface of a threaded bar and the laboratory test results confirm the theory.2. Previous solutionsThe effectiveness of a grouted bolt depends on its length relative to the extent ofthe zone of overstressed rock or yield zone. The shear and axial stress distributions of a grouted bolt are also related to the bolt length because equilibrium must be achieved between the bolt and the surrounding ground (Indraratna and Kaiser,1990).Bearing capacities of cement-grouted rock bolts (P b) and their anchoring forces are a function of the cohesion of the bonding agent and surrounding rock, and the bolting bar. The ultimate bearing capacity of the bolt (P m) is expressed as follows (Aldorf and Exner, 1986):(1)where k b, safety coefficient (usually k b=1.5); C1, cohesion of the bonding material on bolting bar, l d, anchored length of the bolt, d s, bolt diameter.(2)where d v, drill hole diameter;C2,cohesion of the bonding material with surrounding rock(carboniferousrocks and polyester resins C2 =3 MPa).(3)where C3, shearing strength of the bonding material.The maximum (ultimate) bearing capacity of the bolt (P m) will be the lowest value from P1to P111.Bearing capacities of all type bolts must also be evaluated from the view point of the tensile strength of the bolt material (P ms), which must not be lower than the ultimate bearing capacity resulting from the anchoring forces of bolts in drill holes (P m). It holds that(4)where P ms, the ultimate bearing capacity of the bolt with respect of the tensile strength of the bolt material;P m, the ultimate bearing capacity of the bolt.3. Laboratory study3.1. ExperimentsThe pull-out tests were conducted on rebars, grouted into basalt blocks with cement mortar in laboratory. The relations between bolt diameter (d b) and pull-out load of bolt (P b) (Fig. 2), bolt area (A b) and pull-out load of bolt (P b) (Fig. 3), bolt length (L b) and pull-out load of bolt (P b) (Fig. 5), water to cement ratio (w/c) and bolt bond strength (τb) (Fig. 7), mechanical properties of grout material and bolt bond strength (τb) (Fig. 9,Figs. 10 and 11), and curing time (days) and bolt strength (Figs.12 and 13) were evaluated by simple pull-out test programme.The samples consisted of rebars (ranging 10–18 mm diameters two by two) bonded into the basalt blocks. These basalt blocks used have a Youn g’s modulus of27.6 GPa and a uniaxial compressive strength (UCS g) of 133 MPa. Drilling holes which were 10 mm larger than the bolt diameter, having a diameter of 20 –28 mm for installation of bolts, were drilled up to 15–32 cm in depth. The bolt was grouted with cement mortar. The grout was a mixture of Portland cement with a water to cement ratio of 0.34, 0.36, 0.38 and 0.40 cured for 28 days. In order to obtain different grout types that have different mechanical properties, siliceous sand <100μm; 500 μm> and fly ash <10μm; 200μm> were added in a proportion of 10% of cement weight and white cement with a water to cement ratio of 0.40. The sand should be well graded, with a maximum grain size of v2 mm (Schack et al., 1979). The Young’s modulus of the grouts was measured during unconfined compression tests and shear strength was calculated by means of ring shear tests.The test set-up is illustrated schematically in Fig. 1 and the procedure is explained below:1. After filling prepared grout mortar into the hole, bolt is inserted to the centre of drilling hole.2. After curing time, the rebars in the rock were axially loaded and the load was gradually increased until the bolt failed.3. The bond strength (τb)was then calculated by dividing the load (P b)by surface area (A b)of the bolt bar in contact with the grout.4. Pull-out tests were repeated for various grout types, bolt dimensions and curing times.The influence of the bolt diameter and the bond area on the bond strength of a rock bolt can be formulated as follows (Littlejohn and Bruce, 1975):(5)where τb, ultimate bolt bond strength (MPa); P b, maximum pull-out load of bolt (kN); d b, bolt diameter (mm); l b, bolt length (cm); πd b l b , bonded area (cm2).3.2. Analysis of laboratory test results3.2.1. Infl uence of the bolt materialBolt diameters of 10, 12, 14, 16 and 18 mm were used in pull-out tests. Typical results are represented in Table 1, Figs. 2 and 3. The most important observationswere:(1)The maximum pull-out load (P b) increases linearly with the section of the bolt while embedment length was constant.(2) Bolt section depends upon bolt diameter. The relation between bolt diameter and bolt bearing capacity can be explained as follow empiric formulae (Fig. 2).(6)(3) The values of bolt bond strength were calculated between 5.68 and 5.96 MPa(Table 1).Bolt lengths of 15.0, 24.7, 27.0, 30.0 and 32.0 cm were used in pull-out tests as seen in Fig. 4. Typical results are represented in Table 2, and Figs. 5 and 6.The most important observations were:(1) The pull-out force of a bolt increases linearly with the embedded length of the bolt.(7)(2) Maximum pull-out strength of a bolt is limited to the ultimate strength of the bolt shank.3.2.2. Influence of grouting materialThe water to cement ratio should be no greater than 0.40 by weight; too much water greatly reduces the long-term strength. Because, part of the mixing water is consumed by the hydration of cement used. Rest of the mixing water evaporates and then capillary porosities exist which results in unhomogenities internal structure of mortar. Thus, this structure reduces the long-term strength by irregular stress distribution (Neville, 1963;Atis, 1997). To obtain a plastic grout, bentonit clay can be added in a proportion of up to 2% of the cement weight. Other additives can accelerate the setting-time, improve the grout fluidity allowing injection at lower water to cement ratios, and make the grout expand and pressurize the drill hole. Additives, if used at all, should be used with caution and in the correct quantities to avoid harmful side effect such as weakening and corrosion (Franklin and Dusseault, 1989).The water to cement ratio (w/c) in grouting materials considerably affects pull-out strength of bolt. As seen in Table 3, UCS g and shear strength (t g) of grout in high w/c ratio show lower values whereas in low w/c ratio higher values. The ratio between 0.34 and 0.40 presents quite good results. Although the w/c ratio of 0.34 gives the best bond strength, groutibility (pumpability) decreases and a number of difficulties in application appear. In high w/c ratio, the pumpability of grouting materials into the drilling hole is easy but the bond strength of bolt decreases (Figs. 7 and 8).The bond strength off ully cement-grouted rock bolts is primarily frictional and depends on the shear strength at the bolt–grout or grout–rock interface. Thus any change in this shear strength of interfaces affects the bolt bond strength and load capacity. The influences of mechanical properties of grouting materials on the bearing capacity of bolt can be described as follows:(1) The uniaxial compressive and shear strength of the grouting materials has an important role on the behaviour of rock bolts. It was observed that increasing shear strength of the grouting material logarithmically increases bolt bond strength as shown in Table 4 and Fig. 9. The relation between grout shear strength and bolt bond strength was formulated as follows:(8)(2) Table 4 and Fig. 10 show that increasing grout compressive strength considerable increases the bond strength of the grouted bolts.(9)(3) In Fig. 11 and Table 4 show that there is another relationship between Young’s modulus of grout and bolt bond strength. Increasing the Young’s modulus increases bolt bond strength.(10)3.2.3. Influence of the curing timeAn important problem in the application of cementgrouted bolts is the setting time of the mortar, which strongly affects the stabilizing ability of bolt. Cementgrouted dowels cannot be used for immediate support because of the timeneeded for the cement to set and harden (Franklin and Dusseault, 1989).In the pull-out tests, eight group ofbolts having same length and mortar with a water to cement ratio of0.4 were used for determining the effects of curing time on the bolt bond strength. Each group ofr ock bolt testing was performed after different setting times (Table 5). As can be seen in Figs. 12 and 13, the strength of bolt bond increases rapidly in 7 days due to curing time. However, the bond strength of bolt continues to increase rather slowly after 7 days.Rock bolts may lose their supporting ability because of yielding of bolt material, failure at the bolt–grout or grout–rock interface, and unravelling of rock between bolts. However, laboratory tests and field observations suggest that the most dominant failure mode is shear at the bolt–grout interface (Hoek and Wood, 1989). So, this laboratory study focussed on the interface between rock bolt and rock and the mechanical properties of grouting materials.4. ConclusionsThe laboratory investigation showed that the bolt capacity depends basically on the mechanical properties of grouting materials which can be changed by water to cement ratio, mixing time, additives, and curing time.Increasing the bolt diameter and length increases the bolt bearing capacity. However, this increase is limited to the ultimate tensile strength of the bolt materials.Mechanical properties of grouting materials have an important role on the boltbearing capacity. It is offered that the optimum water to cement ratio must be 0.34~0.4 and the mortar have to be well mixed before poured into drill hole. Improving the mechanical properties of the grouting material increases the bolt bearing capacity logarithmically. The best relationship was observed between grout shear strength and bolt bond strength.Increasing the curing time increases the bolt bond strength. Bolt bond strength of 19 kg/cm2 in first day,77 kg/cm2in 7 days and 86 kg/cm2in 35 days was determined respectively. The results show that bolt bond strength increases quickly in first 7 days and then the increase goes up slowly.Bond failure in the pull-out test occurred between the bolt and cement grout, of which the mechanical behaviour is observed by shear spring.This explains the development of bolt bond strength and the failure at the bolt–grout interface considering that the bond strength is created as a result of shear strength between bolt and grout. This means that any change at the grout strength causes to the changing of bolt capacity. The failure mechanism in a pull-out test was studied in order to clarify the bond effect of rock bolt. Thus one main bond effect was explained from bond strength of rock bolts.中文翻译水泥浆性能对充分注浆锚杆拉拔承载力的影响A. Kılıc, E. Yasar*, A.G. Celik摘要:本文代表了一项在安全、实用、经济的支持系统指导下的工程结果。
- 1、下载文档前请自行甄别文档内容的完整性,平台不提供额外的编辑、内容补充、找答案等附加服务。
- 2、"仅部分预览"的文档,不可在线预览部分如存在完整性等问题,可反馈申请退款(可完整预览的文档不适用该条件!)。
- 3、如文档侵犯您的权益,请联系客服反馈,我们会尽快为您处理(人工客服工作时间:9:00-18:30)。
毕业设计---外文翻译原作题目:Failure Properties of Fractured Rock Masses asAnisotropic Homogenized Media译作题目:均质各向异性裂隙岩体的破坏特性专业:土木工程姓名:吴雄指导教师:吴雄志河北工程大学土木工程学院2012年5月21日Failure Properties of Fractured Rock Masses as AnisotropicHomogenized MediaIntroductionIt is commonly acknowledged that rock masses always display discontinuous surfaces of various sizes and orientations, usually referred to as fractures or joints. Since the latter have much poorer mechanical characteristics than the rock material, they play a decisive role in the overall behavior of rock structures,whose deformation as well as failure patterns are mainly governed by those of the joints. It follows that, from a geomechanical engineering standpoint, design methods of structures involving jointed rock masses, must absolutely account for such ‘‘weakness’’ surfaces in their analysis.The most straightforward way of dealing with this situation is to treat the jointed rock mass as an assemblage of pieces of intact rock material in mutual interaction through the separating joint interfaces. Many design-oriented methods relating to this kind of approach have been developed in the past decades, among them,the well-known ‘‘block theory,’’ which attempts to identify poten-tially unstable lumps of rock from geometrical and kinematical considerations (Goodman and Shi 1985; Warburton 1987; Goodman 1995). One should also quote the widely used distinct element method, originating from the works of Cundall and coauthors (Cundall and Strack 1979; Cundall 1988), which makes use of an explicit finite-difference numerical scheme for computing the displacements of the blocks considered as rigid or deformable bodies. In this context, attention is primarily focused on the formulation of realistic models for describing the joint behavior.Since the previously mentioned direct approach is becoming highly complex, and then numerically untractable, as soon as a very large number of blocks is involved, it seems advisable to look for alternative methods such as those derived from the conc ept of homogenization. Actually, such a concept is already partially conveyed in an empirical fashion by the famous Hoek and Brown’s criterion (Hoek and Brown 1980; Hoek 1983). It stems from the intuitive idea that from a macroscopic point of view, a rock mass intersected by a regular network of joint surfaces, may be perceived as a homogeneous continuum. Furthermore, owing to the existence of joint preferential orientations, one should expect such a homogenized material to exhibit anisotropic properties.The objective of the present paper is to derive a rigorous formulation for the failure criterion of a jointed rock mass as a homogenized medium, from the knowledge of the joints and rock material respective criteria. In the particular situation where twomutually orthogonal joint sets are considered, a closed-form expression is obtained, giving clear evidence of the related strength anisotropy. A comparison is performed on an illustrative example between the results produced by the homogenization method,making use of the previously determined criterion, and those obtained by means of a computer code based on the distinct element method. It is shown that, while both methods lead to almost identical results for a densely fractured rock mass, a ‘‘size’’ or ‘‘scale effect’’ is observed in the case of a limited number of joints. The second part of the paper is then devoted to proposing a method which attempts to capture such a scale effect, while still taking advantage of a homogenization technique. This isachieved by resorting to a micropolar or Cosserat continuum description of the fractured rock mass, through the derivation of a generalized macroscopic failure condition expressed in terms of stresses and couple stresses. The implementation of this model is finally illustrated on a simple example, showing how it may actually account for such a scale effect.Problem Statement and Principle of Homogenization ApproachThe problem under consideration is that of a foundation (bridge pier or abutme nt) resting upon a fractured bedrock (Fig. 1), whose bearingcapacity needs to be evaluated from the knowledge of the strength capacities of the rock matrix and the joint interfaces. The failure condition of the former will be expressed through the classical Mohr-Coulomb condition expressed by means of the cohesionC and themfriction angle. Note that tensile stresses will be counted positive throughout the paper.mLikewise, the joints will be modeled as plane interfaces (represented by lines in the figure’s plane). Their strength properties are described by means of a condition involving the stress vector of components (σ, τ) acting at any point of those interfacesAccording to the yield design (or limit analysis) reasoning, the above structure will remain safe under a given vertical load Q(force per unit length along the Oz axis), if one can exhibit throughout the rock mass a stress distribution which satisfies the equilibrium equations along with the stress boundary conditions,while complying with the strength requirement expressed at any point of the structure.This problem amounts to evaluating the ultimate load Q﹢beyond which failure will occur, or equivalently within which its stability is ensured. Due to the strong heterogeneity of the jointed rock mass, insurmountable difficulties are likely to arise when trying to implement the above reasoning directly. As regards, for instance, the case where the strength properties of the joints are considerably lower than those of the rock matrix, the implementation of a kinematic approach would require the use of failure mechanisms involving velocity jumpsacross the joints, since the latter would constitute preferential zones for the occurrence offailure. Indeed, such a direct approach which is applied in most classical design methods, is becoming rapidly complex as the density of joints increases, that is as the typical joint spacing l is becoming small in comparison with a characteristic length of the structure suc h as the foundation width B.In such a situation, the use of an alternative approach based on the idea of homogenization and related concept of macroscopic equivalent continuum for the jointed rock mass, may be appropriate for dealing with such a problem. More details about this theory , applied in the context of reinforced soil and rock mechanics, will be found in (de Buhan et al. 1989; de Buhan and Salenc ,on 1990; Bernaud et al. 1995).Macroscopic Failure Condition for Jointed Rock MassThe formulation of the macroscopic failure condition of a jointed rock mass may be obtained from the solution of an auxiliary yield design boundary-value problem attached to a unit representative cell of jointed rock (Bekaert and Maghous 1996; Maghous et al.1998). It will now be explicitly formulated in the particular situation of two mutually orthogonal sets of joints under plane strain conditions. Referring to an orthonormal frame O 21ξξwhose axes are placed along the joints directions, and introducing the following change of stress variables:such a macroscopic failure condition simply becomeswhere it will be assumed thatA convenient representation of the macroscopic criterion is to draw the strength envelope relating to an oriented facet of the homogenized material, whose unit normal n I is inclined by an angle a with respect to the joint direction. Denoting by n σ and n τthe normal and shearcomponents of the stress vector acting upon such a facet, it is possible to determine for any value of a the set of admissible stresses (n σ , n τ) deduced from conditions (3) expressed interms of (11σ,22σ , 12σ). The corresponding domain has been drawn in Fig. 2 in theparticular case where m ϕα≤ .Two comments are worth being made:1. The decrease in strength of a rock material due to the presence of joints is clearly illustrated by Fig.2. The usual strength envelope corresponding to the rock matrix failure condition is ‘‘truncated’’ by two orthogonal semilines as soon as condition m j H H is fulfilled.2. The macroscopic anisotropy is also quite apparent, since for instance the strength envelope drawn in Fig. 2 is dependent on the facet orientation a. The usual notion of intrinsic curve should therefore be discarded, but also the concepts of anisotropic cohesion and friction angle as tentatively introduced by Jaeger (1960), or Mc Lamore and Gray (1967).Nor can such an anisotropy be properly described by means of criteria based on an extension of the classical Mohr-Coulomb condition using the concept of anisotropy tensor(Boehler and Sawczuk 1977; Nova 1980; Allirot and Bochler1981).Application to Stability of Jointed Rock ExcavationThe closed-form expression (3) obtained for the macroscopic failure condition, makes it then possible to perform the failure design of any structure built in such a material, such as the excavation shown in Fig. 3,where h and β denote the excavation height and the slope angle, respectively . Since nosurcharge is applied to the structure, the specific weight γ of the constituent material will obviously constitute the sole loading parameter of the system.Assessing the stability of this structure will amount to evaluating the maximum possible height h + beyond which failure will occur. A standard dimensional analysis of this problem shows that this critical height may be put in the formwhere θ=joint orientation and K +=nondimensional factor governing the stability of the excavation. Upper-bound estimates of this factor will now be determined by means of the yield design kinematic approach, using two kinds of failure mechanisms shown in Fig. 4.Rotational Failure Mechanism [Fig. 4(a)]The first class of failure mechanisms considered in the analysis is a direct transposition of those usually employed for homogeneous and isotropic soil or rock slopes. In such a mechanism a volume of homogenized jointed rock mass is rotating about a point Ω with an angular velocity ω. The curve separating this volume from the rest of the structure which is kept motionless is a velocity jump line. Since it is an arc of the log spiral of angle m andfocus Ω the velocity discontinuity at an y point of this line is inclined at angle wm with respect to the tangent at the same point.The work done by the external forces and the maximum resisting work developed in such a mechanism may be written as (see Chen and Liu 1990; Maghous et al. 1998)where e w and me w =dimensionless functions, and μ1 and μ2=angles specifying theposition of the center of rotation Ω.Since the kinematic approach of yield design states that a necessary condition for the structure to be stable writesit follows from Eqs. (5) and (6) that the best upper-bound estimate derived from this first class of mechanism is obtained by minimization with respect to μ1 and μ2which may be determined numerically.Piecewise Rigid-Block Failure Mechanism [Fig. 4(b)]The second class of failure mechanisms involves two translating blocks of homogenized material. It is defined by five angular parameters. In order to avoid any misinterpretation, it should be specified that the terminology of block does not refer here to the lumps of rock matrix in the initial structure, but merely means that, in the framework of the yield design kinematic approach, a wedge of homogenized jointed rock mass is given a (virtual) rigid-body motion.The implementation of the upper-bound kinematic approach,making use of of this second class of failure mechanism, leads to the following results.where U represents the norm of the velocity of the lower block. Hence, the following upper-bound estimate for K+:Results and Comparison with Direct CalculationThe optimal bound has been computed numerically for the following set of parameters:The result obtained from the homogenization approach can then be compared with that derived from a direct calculation, using the UDEC computer software (Hart et al. 1988). Since the latter can handle situations where the position of each individual joint is specified, a series of calculations has been performed varying the number n of regularly spaced joints, inclined at the same angleθ=10° with the horizontal, and intersecting the facing of the excavation, as sketched in Fig. 5. Thecorresponding estimates of the stability factor have been plotted against n in the same figure. It can be observed that these numerical estimates decrease with the number of intersecting joints down to the estimate produced by the homogenization approach. The observed discrepancy between homogenization and direct approaches, could be regarded as a ‘‘size’’ or ‘‘scale effect’’ which is not included in the classicalhomogenization model. A possible way to overcome such a limitation of the latter, while still taking advantage of the homogenization concept as a computational time-saving alternative for design purposes, could be to resort to a description of the fractured rock medium as a Cosserat or micropolar continuum, as advocated for instance by Biot (1967); Besdo(1985); Adhikary a nd Dyskin (1997); and Sulem and Mulhaus (1997) for stratified or block structures. The second part of this paper is devoted to applying such a model to describing the failure properties of jointed rock media.均质各向异性裂隙岩体的破坏特性概述由于岩体表面的裂隙或节理大小与倾向不同,人们通常把岩体看做是非连续的。