TiO2_Nanoparticles_as_Functional_Building_Blocks
TIO2 nanobelts
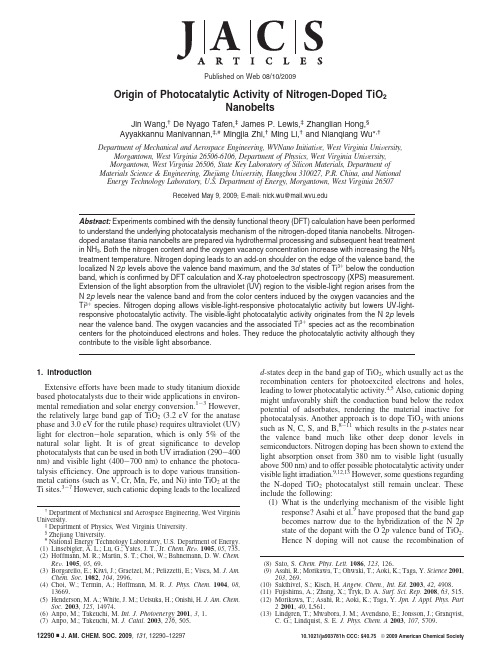
Origin of Photocatalytic Activity of Nitrogen-Doped TiO2NanobeltsJin Wang,†De Nyago Tafen,‡James P.Lewis,‡Zhanglian Hong,§Ayyakkannu Manivannan,‡,#Mingjia Zhi,†Ming Li,†and Nianqiang Wu*,†Department of Mechanical and Aerospace Engineering,WVNano Initiati V e,West Virginia Uni V ersity, Morgantown,West Virginia26506-6106,Department of Physics,West Virginia Uni V ersity,Morgantown,West Virginia26506,State Key Laboratory of Silicon Materials,Department of Materials Science&Engineering,Zhejiang Uni V ersity,Hangzhou310027,P.R.China,and NationalEnergy Technology Laboratory,U.S.Department of Energy,Morgantown,West Virginia26507Received May9,2009;E-mail:nick.wu@Abstract:Experiments combined with the density functional theory(DFT)calculation have been performed to understand the underlying photocatalysis mechanism of the nitrogen-doped titania nanobelts.Nitrogen-doped anatase titania nanobelts are prepared via hydrothermal processing and subsequent heat treatment in NH3.Both the nitrogen content and the oxygen vacancy concentration increase with increasing the NH3 treatment temperature.Nitrogen doping leads to an add-on shoulder on the edge of the valence band,the localized N2p levels above the valence band maximum,and the3d states of Ti3+below the conduction band,which is confirmed by DFT calculation and X-ray photoelectron spectroscopy(XPS)measurement. Extension of the light absorption from the ultraviolet(UV)region to the visible-light region arises from the N2p levels near the valence band and from the color centers induced by the oxygen vacancies and the Ti3+species.Nitrogen doping allows visible-light-responsive photocatalytic activity but lowers UV-light-responsive photocatalytic activity.The visible-light photocatalytic activity originates from the N2p levels near the valence band.The oxygen vacancies and the associated Ti3+species act as the recombination centers for the photoinduced electrons and holes.They reduce the photocatalytic activity although they contribute to the visible light absorbance.1.IntroductionExtensive efforts have been made to study titanium dioxide based photocatalysts due to their wide applications in environ-mental remediation and solar energy conversion.1-3However, the relatively large band gap of TiO2(3.2eV for the anatase phase and3.0eV for the rutile phase)requires ultraviolet(UV) light for electron-hole separation,which is only5%of the natural solar light.It is of great significance to develop photocatalysts that can be used in both UV irradiation(290-400 nm)and visible light(400-700nm)to enhance the photoca-talysis efficiency.One approach is to dope various transition-metal cations(such as V,Cr,Mn,Fe,and Ni)into TiO2at the Ti sites.3-7However,such cationic doping leads to the localized d-states deep in the band gap of TiO2,which usually act as the recombination centers for photoexcited electrons and holes, leading to lower photocatalytic activity.4,5Also,cationic doping might unfavorably shift the conduction band below the redox potential of adsorbates,rendering the material inactive for photocatalysis.Another approach is to dope TiO2with anions such as N,C,S,and B,8-11which results in the p-states near the valence band much like other deep donor levels in semiconductors.Nitrogen doping has been shown to extend the light absorption onset from380nm to visible light(usually above500nm)and to offer possible photocatalytic activity under visible light irradiation.9,12,13However,some questions regarding the N-doped TiO2photocatalyst still remain unclear.These include the following:(1)What is the underlying mechanism of the visible lightresponse?Asahi et al.9have proposed that the band gapbecomes narrow due to the hybridization of the N2pstate of the dopant with the O2p valence band of TiO2.Hence N doping will not cause the recombination of†Department of Mechanical and Aerospace Engineering,West Virginia University.‡Department of Physics,West Virginia University.§Zhejiang University.#National Energy Technology Laboratory,U.S.Department of Energy.(1)Linsebigler,A.L.;Lu,G.;Yates,J.T.,Jr.Chem.Re V.1995,95,735.(2)Hoffmann,M.R.;Martin,S.T.;Choi,W.;Bahnemann,D.W.Chem.Re V.1995,95,69.(3)Borgarello,E.;Kiwi,J.;Graetzel,M.;Pelizzetti,E.;Visca,M.J.Am.Chem.Soc.1982,104,2996.(4)Choi,W.;Termin,A.;Hoffmann,M.R.J.Phys.Chem.1994,98,13669.(5)Henderson,M.A.;White,J.M.;Uetsuka,H.;Onishi,H.J.Am.Chem.Soc.2003,125,14974.(6)Anpo,M.;Takeuchi,M.Int.J.Photoenergy2001,3,1.(7)Anpo,M.;Takeuchi,M.J.Catal.2003,216,505.(8)Sato,S.Chem.Phys.Lett.1986,123,126.(9)Asahi,R.;Morikawa,T.;Ohwaki,T.;Aoki,K.;Taga,Y.Science2001,293,269.(10)Sakthivel,S.;Kisch,H.Angew.Chem.,Int.Ed.2003,42,4908.(11)Fujishima,A.;Zhang,X.;Tryk,D.A.Surf.Sci.Rep.2008,63,515.(12)Morikawa,T.;Asahi,R.;Aoki,K.;Taga,Y.Jpn.J.Appl.Phys.Part22001,40,L561.(13)Lindgren,T.;Mwabora,J.M.;Avendano,E.;Jonsson,J.;Granqvist,C.G.;Lindquist,S.E.J.Phys.Chem.A2003,107,5709.Published on Web08/10/200910.1021/ja903781h CCC:$40.75 2009American Chemical Society 122909J.AM.CHEM.SOC.2009,131,12290–12297photoexcited charge carriers.In contrast,other calcula-tions have shown that no band gap narrowing occurs inthe N-doped TiO2.Instead N-doping induces localizedN2p states within the band gap just above the valenceband maximum of TiO2.14-16(2)Does nitrogen doping induce other defect states like Ti3d in the band gap that is usually associated with oxygenvacancies?(3)How does nitrogen doping affect the UV-excited pho-tocatalytic activity although it extends the light absorptionto the visible region?In a desirable case,nitrogen dopingshould enhance the UV-responsive photocatalytic activityinstead of diminishing it.Few studies have been per-formed on the UV-responsive photocatalytic activity ofN-doped TiO2.17(4)Is the photocatalytic activity of the highly N-doped TiO2different from that of the slightly N-doped TiO2?Basedon the calculation by Asahi et al.,9band gap narrowingwill occur when a high concentration of oxygen sites(6-12.5at%)are substituted by nitrogen.In contrast,Okato et al.18have claimed that band gap narrowing doesnot occur in the TiO2doped with a high concentrationof nitrogen atoms.To clarify the above-mentioned questions,pristine anatase TiO2nanobelts are synthesized by hydrothermal processing and subsequently heat treated in NH3to allow nitrogen atom doping into the TiO2lattice in the present work.The nitrogen concentration in the TiO2nanobelts is controlled by modulating the heat-treatment temperature.The photocatalytic activities of the N-doped TiO2nanobelts under the ultraviolet A(UVA) and the visible-light irradiations are evaluated by monitoring the degradation of methyl orange in an aqueous solution.The chemical status of the nitrogen dopant and the electronic structure of the N-doped TiO2nanobelts have been studied using the optical/photoemission spectroscopy combined with the density functional theory(DFT)simulation.The origin of the visible-light response and the dependence of the photocatalytic activity on the N-dopant concentration are discussed.In the present work,single-crystalline TiO2nanobelts are chosen as the test material,because one-dimensional(1D) nanostructures such as nanobelts,nanowires,and nanotubes have advantages over commonly used nanospheres or nanoparticles: (i)visible light scattering and absorption are highly enhanced in nanowires due to their high length-to-diameter ratio;19(ii) the1D geometry facilitates rapid,diffusion-free electron transport along the longest direction;20and(iii)the high degree of crystallinity of the nanowires/nanobelts and the low number of grain boundaries may exhibit lower recombination rates.It is well-known that grain boundaries often act as the hole-electron recombination sites.2.Experimental Section2.1.Synthesis of the N-Doped TiO2Nanobelts.The synthesis of pristine anatase TiO2nanobelts are described in detail in our previous paper.21Briefly,1.2g of anatase titanium dioxide particles (Alfa Aesar)were added to80mL of10M NaOH aqueous solution. The mixture was vigorously stirred for1h and then transferred to a100mL Teflon-lined stainless steel autoclave.The autoclave was sealed and put into a preheated oven to perform hydrothermal treatment at200°C for24h.After hydrothermal processing,a white fluffy powder was collected and washed with copious amounts of deionized water and0.1M hydrochloric acid until the pH of the washing solution was less than7.This resulted in the H2Ti3O7 nanobelts.21This product was then dried in an oven at80°C overnight.The samples were then heated in a quartz tube furnace at700°C for30min at a ramp rate of1°C/min.As a result,the pristine single-crystalline anatase TiO2nanobelts were obtained.21 Subsequently,anatase TiO2nanobelts were heat treated in an NH3flow in a tubular furnace equipped with a gasflow controller.Four N-doped samples were obtained by NH3treatment at different temperatures ranging from525to600°C for3h at a ramp rate of 10°C/min.The pristine sample was also annealed at550°C in ambient air for comparison.2.2.Characterization of the Nanobelts.The crystal structure of samples was characterized by powder X-ray diffraction(XRD) (X’Pert Pro PW3040-Pro,Panalytical Inc.)using Cu K R radiation. The morphology was observed with a Hitachi S4700field-emission scanning electron microscope(FE-SEM).For typical SEM sam-pling,1mg of nanobelts was added into5mL of absolute ethanol and then sonicated for10min to achieve a homogeneous whitish suspension.The suspension can stay stable for several days without obvious settling at the bottom of the container.The suspension was then dropped onto a smooth silicon wafer substrate(SPI Inc.)using a pipet and dried naturally in air.The dry powder specimen on the Si wafer was observed under SEM.The UV-vis absorption spectra were measured under the diffuse reflection mode using the integrating sphere(UV2401/2,Shimadzu) attached to a Shimadzu2550UV-vis spectrometer.The powders were pressed to form a pellet and placed on a BaSO4plate.The use of the diffuse reflection mode for solid pellets effectively excluded the artifacts and inferences from the variation due to the inhomogenity and dispersion of nanoparticles in liquid.The XPS experiments were performed on the titania nanobelts with PHI5000Versa Probe system(Physical Electronics,MN). The TiO2nanobelts were pressed to form a pellet prior to XPS measurement.The binding energy of the XPS spectra was calibrated with the reference to the C1s peak at284.8eV.2.3.Testing of the Photocatalysts.A commercial photoreactor (LUZ-4V,Luzchem)equipped with fourteen8W lamps was used. The lamps centered at350nm(LZC-UVA,Luzchem)were employed for UVA irradiation,and the Vis-420lamp centered at 420nm(LZC-420,Luzchem)was for visible-light irradiation.The photocatalytic activity was evaluated by the decomposition of methyl orange in a deionized water solution with an initial concentration of20mg/L.A total of0.01g of catalysts was added to10mL of methyl orange solution in a10mL polyethylene tube. Before irradiation,the suspensions were sonicated in the dark for 5min.During the radiation,the tubes were placed onto a carousel to ensure even exposure of every tube to the light.After desired time intervals of irradiation,the tubes were unloaded and centrifuged at10000rpm for1.5h to separate the supernatant liquid from the catalysts.The supernatant liquid was collected and analyzed by recording the characteristic absorption of methyl orange(464nm)(14)DiValentin,C.;Pacchioni,G.;Selloni,A.Phys.Re V.B2004,70,085116.(15)Lee,J.Y.;Park,J.;Cho,J.H.Appl.Phys.Lett.2005,87,011904.(16)Batzil,M.;Morales,E.H.;Diebold,U.Phys.Re V.Lett.2006,96,026103.(17)Irie,H.;Watanabe,Y.;Hashimoto,K.J.Phys.Chem.B2003,107,5483.(18)Okato,T.;Sakano,T.;Obara,M.Phys.Re V.B2005,72,115124.(19)(a)Tan,B.;Wu,Y.J.Phys.Chem.B2006,110,15932.(b)Zhu,K.;Neale,N.R.;Miedaner,A.;Frank,A.J.Nano Lett.2007,7,69.(20)(a)Adachi,M.;Murata,Y.;Takao,J.;Jiu,J.;Sakamoto,N.;Wang,F.J.Am.Chem.Soc.2004,126,14943.(b)Baxter,J.B.;Aydil,E.S.Appl.Phys.Lett.2005,86,053114.(c)Varghese,O.K.;Gong,D.;Paulose,M.;Ong,K.G.;Dickey,E.C.;Grimes,C.A.Ad V.Mater. 2003,15,624.(d)Wu,N.Q.;Zhao,M.;Zheng,J.-G.;Jiang,C.;Myers, B.;Chyu,M.;Li,S.;Mao,S.X.Nanotechnology2005,16,2878.(21)Wang,J.;Wu,N.Q.In Nanotechnology Research Ad V ances;Huang,X.,Ed.;Nova Science Publishers:New York,2008.J.AM.CHEM.SOC.9VOL.131,NO.34,200912291N-Doped TiO2Nanobelts A R T I C L E Susing the UV -vis spectrometer.The value of decomposition efficiency was calculated,based on the standard absorption curve of concentration and absorption.For the photocatalysis experiments including methanol into the system,0.2mL of methanol were added into 9.8mL of TiO 2aqueous suspension containing methyl orange to perform the same procedure.The experiments including oxygen and nitrogen into the system using the as-prepared 10mL aqueous suspension containing methyl orange and TiO 2was bubbled with the gas for 20min using a syringe needle tip connected to a gas flow controller.putational Methods and Models.FIREBALL,an ab initio tight binding code based on density functional theory (DFT)with nonlocal pseudopotentials within the local density approxima-tion (LDA)22was used to simulate the density of state (DOS)in TiO 2.In this method,the confined atomic-like orbitals were employed as a basis set for the determination of the occupied eigenvalues and eigenvectors of the one-electron Hamiltonian.For the calculations,a minimal basis set was chosen for N,O,and Ti,adding an excited p -state for polarization to the Ti ground-state configuration.This method has been successfully used for obtaining geometries and electronic properties of bulk TiO 2material 23as well as TiO 2nanobelts.24The atomic structure of the nanobelt was carved out from the anatase structure,which was found to be active in light absorption and photon-to-current conversion and was the prevalent one in experiments.25For each nanobelt the stoichiometry was preserved and no saturation of dangling bonds or charge embedding was applied.However,due to a long optimization procedure,surface atoms reconstruct and consequently are electronically passivated giving rise to a band gap free of surface states.The initial geometries were then fully optimized to the nearest local minimum structure.Based on the optimized geometries,nitrogen-doped TiO 2nanobelts were constructed by embedding a few nitrogen atoms in the interspaces of the supercell.Oxygen vacancies were formed by removing a few oxygen atoms from the supercell.The resulting structures,TiO 2-x N y (0.0e x ,y e 0.013),were then fully relaxed and analyzed through electronic properties,a fundamental ingredient in photocatalysis.Figure S1shows a segment of a TiO 2nanobelt comprising 96TiO 2units in the anatase phase.The dominant-area facet of the nanobelt is (101)which is stable and very reactive.The unit cells were repeated along the [010]direction to assemble the nanobelts.3.Results and Discussions3.1.Characterization of Morphology and Crystal Structure of the Nanobelts.Figure 1shows the SEM image of the pristineTiO 2nanobelts after hydrothermal processing and calcination.The nanobelts were 50-400nm wide and up to tens of micrometers long.XRD analysis revealed that the pristine TiO 2nanobelts have a monolithic anatase structure (Figure 2).The nanobelts were anatase single crystals with the growth direction along [010]and the two dominant surface facets as the (101)crystalline plane,as confirmed by transmission electron micro-scope (TEM)analysis (Figure S2in Supporting Information).The nanobelts treated in NH 3flow are denoted as N525,N550,N575,and N600,respectively.For example,N525was the sample after NH 3treatment at 525°C.The pristine anatase TiO 2nanobelts were pure white.N525,N550,and N575were yellow,and the color became dark yellow with an increase in the heat treatment temperature.N600showed a grayish green color.All the NH 3-treated samples retained a monolithic anatase phase.No other nitrogen-containing compounds or other titania pol-ymorphs were detected by XRD.Moreover,there was no evident change in the XRD peak position of anatase after treatment in NH 3.A major difference between the pristine and the doped samples was that the peak intensity decreased and the peaks were broadened after nitrogen doping,which could be clearly seen by comparing the patterns of N600and the pristine nanobelts.The size and shape of the nanobelts did not show any evident change after treatment in NH 3.Thus it is inferred that the orderliness of the crystal lattice of the anatase decreased upon nitrogen doping.3.2.Measurement of Chemical Status and Electronic Struc-ture by XPS.XPS measurement was performed on the N-dopedTiO 2nanobelts.The XPS spectrum of the Ti 2P core level obtained from the pristine TiO 2nanobelts exhibited a Ti 2P 3/2peak at 458.7eV and an O 1s peak at 530.3eV (Figures S3and S4in the Supporting Information).After treatment in NH 3at 600°C,the Ti 2P 3/2and the O 1s peaks shifted to 458.1and 529.5eV,respectively (as listed in Table 1).The shifts of Ti 2P 3/2and the O 1s peaks are mainly due to the introduction of oxygen vacancies into the TiO 2lattice.At a temperature higher(22)Lewis,J.P.;Glaesemann,K.R.;Voth,G.A.;Fritsch,J.;Demkov,A.A.;Ortega,J.;Sankey,O.F.Phys.Re V .B 2001,64,195103.(23)(a)Wang,H.;Lewis,J.P.J.Phys.:Condens.Matter 2005,17,L209.(b)Wang,H.;Lewis,J.P.J.Phys.:Condens.Matter 2006,18,421.(24)Tafen,D.N.;Wang,J.;Wu,N.Q.;Lewis,J.P.Appl.Phys.Lett.2009,94,093101.(25)Mor,G.K.;Varghese,O.K.;Paulose,M.;Shankar,K.;Grimes,C.A.Sol.Energy Mater.Sol.Cells 2006,90,2011.Figure 1.SEM image of the pristine TiO 2nanobelts.Figure 2.XRD patterns of the TiO 2nanobelts before and after heattreatment in ammonia flow at different temperatures.Table 1.XPS Peak Position and the N Content after Treatment of TiO 2Nanobelts in NH 3at Different Temperaturessampletreatment temperature (°C)N content (at%)N 1s peak position (eV)Ti 2p 3/2peak position (eV)O 1s peak position (eV)pristine ------458.7530.3N5255250.30399.6458.5529.8N550550 1.05399.5458.2529.5N575575 1.20399.7458.1529.5N600600 1.53399.6/395.6458.1529.512292J.AM.CHEM.SOC.9VOL.131,NO.34,2009A R T I C L E S Wang et al.than 550°C,NH 3is decomposed,releasing H 2.This reducing atmosphere can cause partial reduction of TiO 2.It has been reported that the reduction of stoichiometric TiO 2resulted in the shifts of both the Ti 2P 3/2and the O 1s peaks to lower binding energies.26In addition,the density functional theory (DFT)calculation has drawn a conclusion that nitrogen doping induces a substantial reduction of the formation energy of oxygen vacancies in bulk TiO 2.27This indicates that nitrogen doping is accompanied by oxygen vacancy formation.On the other hand,it has been reported that the incorporation of N atom into the TiO 2lattice can also lead to the shift of Ti 2P 3/2to lower binding energy.28,29Our XPS analysis determined that the nitrogen contents were 0.30at%for Sample N525,1.05at%for N550,1.20at%for N575,and 1.53at%for N600(as shown in Table 1).As the heat-treatment temperature increased,the nitrogen doping level increased.There is a leap in the concentration from 0.3%for N525to 1.05%for N550.This is due to the fact that the decomposition of NH 3into N 2and H 2started at 550°C.17The reducing atmosphere greatly facilitates the doping of nitrogen due to the formation of oxygen vacancies.Figure 3shows the N 1s XPS spectra of the pristine and the N-doped titania nanobelts.Two N 1s peaks are present in the spectra of N-doped samples.For all the N-doped samples,there is a peak at ∼399.6eV.For Sample N600,an additional peak appeared at 395.6eV.The N 1s peak at 395.6eV is characteristic of N 3-that corresponds to TiN.30,31The N 1s peak at 395-397eV obtained from N-doped TiO 2has also been assigned to the Ti -N bonding by other researchers.18,32-34TheN 1s peak at ∼399.6eV is ascribed to Ti -O -N or Ti -N -O oxynitride.One may argue that this peak could also be assigned to N -H or adsorbed NH 3,because its binding energy is close to the value (398.7-399.7eV)cited for NH 3.30,35In the present work,the N-doped samples were analyzed using Fourier Transform Infrared (FTIR)spectroscopy.No bands characteristic of N -H or NH 3were found in the FTIR spectra (Figure S5in the Supporting Information),which excludes the possibility that the peak at 399.6eV is derived from N -H or adsorbed NH 3.This peak cannot be assigned to the NO x molecules,because the N 1s binding energy for NO x is typically larger than 403eV.30Moreover,our FITR measurement did not detect any NO x molecules.Nitrogen can be located in the anatase lattice of TiO 2interstitially or/and substitutionally.As summarized by Fujish-ima et al.,11the N 1s peak at ∼400eV is typically assigned to the interstitial nitrogen dopant while the peak at ∼396eV is ascribed to the substitutional nitrogen dopant.The site of the N atoms in the TiO 2lattice depends on the synthetic route of N-doped TiO 2.Different synthetic routes make nitrogen species react with TiO 2in different fashions.Di Valentin et al.14have performed DFT calculations of nitrogen doping behaviors on the single-crystalline anatase TiO 2(101)surface.Their calcula-tions have showed that transition from the substitutional to the interstitial nitrogen doping undergoes an exothermic process by 0.8eV,which implies that substitutional nitrogen formation starting from the interstitial one will require more energy.Interstitial nitrogen doping is preferred under the oxygen-rich condition,whereas under oxygen-depleted conditions (at high reducing condition)after annealing at high temperature,sub-stitutional N in parallel with oxygen vacancy is favored.In addition,it has been reported that,at high temperature,oxygen vacancies in a single-crystalline TiO 2(110)surface occur at ∼580°C in a vacuum.Furthermore,the presence of nitrogen dopant facilitates the formation of oxygen vacancies.16,36,37In the present work,the pristine single-crystalline anatase TiO 2nanobelts with the (101)crystal plane as the dominant surface facets were obtained by calcinations in air of hydrogen titanate (H 2Ti 3O 7).During calcinations,water molecules are released,21leading to numerous dangling oxygen bonds exposed on the surface,which creates an oxygen-rich surface.Therefore,at relatively low temperature,the nitrogen atoms tend to sit in the interstitial sites (all the nitrogen atoms were located in the interstitial sites when the N content was below 1.2at%).At relatively high temperature (600°C)under reducing atmospheric conditions (more H 2are produced via decomposition of NH 3at higher temperature),some of the nitrogen atoms are incorporated into the TiO 2lattice substitutionally in addition to the presence of the interstitial nitrogen atoms.It is worth noting that the interstitial N atoms are limited in a very low range.Even at only 1.53at%N,excessive N atoms were present in the substititutional sites after the N atoms at the interstitial site become saturated.In summary,the present work reveals that interstitial nitrogen doping appears at a low nitrogen-dopant concentration at relatively low temperatures (525,550,and 575°C),while substitutional nitrogen atoms are also present in addition to the interstitial nitrogen dopant at relatively high temperature (in the(26)Rumaiz,A.K.;Ali,B.;Ceylan,A.;Boggs,M.;Beebe,T.;Shah,S.I.Solid State Commun.2007,144,334.(27)Valentin,C.D.;Pacchioni,G.;Selloni,A.;Livraghi,S.;Giamello,E.J.Phys.Chem.B 2005,109,11414.(28)Saha,N.C.;Tompkins,H.G.J.Appl.Phys.1992,72,3072.(29)Kitano,M.;Funatsu,K.;Matsuoka,M.;Ueshima,M.;Anpo,M.J.Phys.Chem.B 2006,110,25266.(30)Moulder,J.F.;Stickle,W.F.;Sobol,P.E.;Bomben,K.D.Handbookof X-ray photoelectron Spectroscopy ;Eden Prairie:1992.(31)Esaka,F.;Furuya,K.;Shimada,H.;Imamura,M.;Matsubayashi,N.;Sato,H.;Kawana,A.;Ichimura,H.;Kikuchi,T.J.Vac.Sci.Technol.,A 1997,15,2521.(32)Takahashi,I.;Payne,D.J.;Palgrave,R.G.;Egdell,R.G.Chem.Phys.Lett.2008,454,314.(33)Chen,H.;Nambu,A.;Wen,W.;Graciani,J.;Zhong,Z.;Hanson,J.C.;Fujita,E.;Rodriguez,J.A.J.Phys.Chem.B 2007,111,1366.(34)Nambu,A.;Graciani,A.N.;Rodriguez,J.A.;Wu,Q.;Fujita,E.;Fdez-Sanz,J.J.Chem.Phys.2006,125,094706.(35)Jansen,R.J.J.;Van Bekkum,H.Carbon 1995,33,1021.(36)Nakano,Y.;Morikawa,T.;Ohwaki,T.;Taga,Y.Appl.Phys.Lett.2005,86,132104.(37)Livraghi,S.;Paganini,M.C.;Giamello,E.;Selloni,A.;Valentin,C.D.;Pacchioni,G.J.Am.Chem.Soc.2006,128,15666.Figure 3.XPS spectra of the N 1s core level obtained from the anataseTiO 2nanobelts after heat treatment in ammonia flow at different temperatures.J.AM.CHEM.SOC.9VOL.131,NO.34,200912293N-Doped TiO 2Nanobelts A R T I C L E Ssample treated at 600°C).The result obtained in the present work is in disagreement with that reported by Okato et al.18Okato et al.reported that,at a low concentration,the N atoms were doped into the O sites substitutionally.The N dopant became saturated in the substitutional sites as the concentration of N increases in TiO 2.As a result,the excessive N atoms were interstitially doped into the TiO 2lattice.The controversy could be due to the difference in the synthesis route employed.They have employed a pulse-laser deposition method to make the N-doped TiO 2film by using the mixed TiO 2and TiN powders as the target.Figure 4shows the valence band spectra obtained from the pristine TiO 2nanobelt sample and the N-doped sample (N575,treated at 575°C).After nitrogen doping,the valence band edge has exhibited ∼0.3eV of red shift.Moreover,small bands occurred above the valence band maximum (VBM),and small bands existed close to the binding energy at zero.3.3.Calculation of the Density of States (DOS).The electronic structures of the anatase TiO 2nanobelts were further analyzed through calculated density of states.Figure 5a and 5b plot the density of states (DOS)and the projected DOS for the nitrogen-doped and the nitrogen -oxygen vacancy-containing nanobelts,respectively,and compared them with that of the pristine titanium oxide nanobelts.The calculated band gap of the pris-tine TiO 2nanobelt was 3.42eV compared to the experimental value 3.26eV derived from the UV -visible spectrum (Figure 6).The use of a local orbital basis set in our calculations compensates for the underestimating effect of the LDA on the optical gap.23The difference between the calculated and experimental band gaps is a quantum confinement effect due to the size of the calculated nanobelts compared to the experiments.For the pristine titanium oxide nanobelts (Figure 5a),the valence band (VB)and the conduction band (CB)consist of both the O 2p and Ti 3d states as in the case of the bulk.38As nitrogen atoms are doped into TiO 2,the N 2p states are delocalized (see Figure S6in the Supporting Information)and significantly contribute to the formation of impurity energy levels hybridized by O 2p states and Ti 3d states.For the interstitial nitrogen-doped nanobelts in the absence of oxygen vacancies (Figure 5a),two isolated impurity levels are located above the valence band maximum (VBM).The contribution of N 2p states to these impurity energy levels increase with an increase in the nitrogen concentration (Figure 5a).In addition,an add-on shoulder is present on the edge of the VBM.When N-doping is accompanied by the presence of the oxygen vacancies,an additional deep donor level is introduced below the conduction band minimum (CBM)(Figure 5b).The number of impurity energy levels depends on the concentration of oxygen vacancies in the nitrogen-doped nanobelts (Figure 5b).These energy levels mainly consist of the 3d states of Ti 3+.The deep donor level was located at a distance of 1.28and 1.20eV from the CBM for the oxygen vacancy concentrations of(38)Asahi,R.;Taga,Y.;Mannstadt,W.;Freeman,A.J.Phys.Re V .B 2000,61,7459.Figure 4.XPS spectra of TiO 2nanobelts,showing the valence bands forthe pristine and N575sample (treated in NH 3at 575°C).Figure 5.Calculated density of state (DOS)and the species-projected DOS (the dashed lines indicate the position of the Fermi level);(a)TiO 2with various nitrogen concentrations of dopant (0.33,0.69,and 1.37at%)in the absence of the oxygen vacancy;(b)N-doped TiO 2with a fixed nitrogen concentration (1.3at%)in the presence of different concentrations of oxygen vacancies (x )0.0034,0.0070).Black solid line,total DOS;green dash line,O 2p states;blue dot line,Ti 3d states;red dash line,N 2pstates.Figure 6.Diffuse reflectance spectra of the anatase TiO 2nanobelts beforeand after heat treatment in ammonia gas flow at different temperatures.12294J.AM.CHEM.SOC.9VOL.131,NO.34,2009A R T I C L E S Wang et al.。
氧气热处理的TiO_2纳米管阵列光电催化降解亚甲基蓝的研究
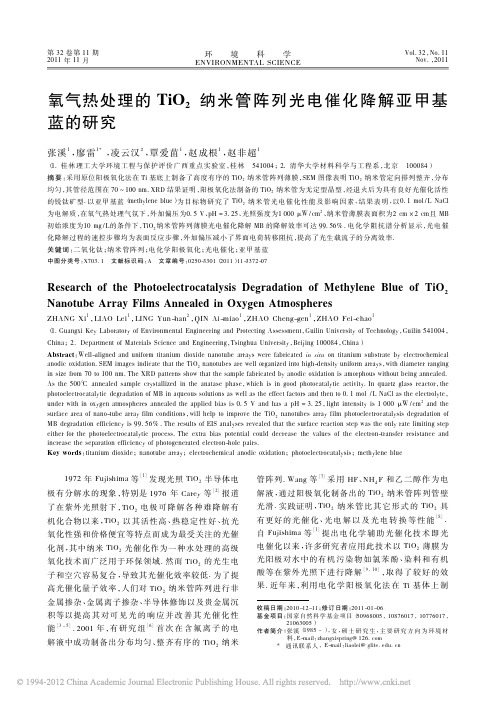
第32卷第11期2011年11月环境科学ENVIRONMENTAL SCIENCEVol.32,No.11Nov.,2011氧气热处理的TiO 2纳米管阵列光电催化降解亚甲基蓝的研究张溪1,廖雷1*,凌云汉2,覃爱苗1,赵成根1,赵非超1(1.桂林理工大学环境工程与保护评价广西重点实验室,桂林541004; 2.清华大学材料科学与工程系,北京100084)摘要:采用原位阳极氧化法在Ti 基底上制备了高度有序的TiO 2纳米管阵列薄膜,SEM 图像表明TiO 2纳米管定向排列整齐,分布均匀,其管径范围在70 100nm.XRD 结果证明,阳极氧化法制备的TiO 2纳米管为无定型晶型,经退火后为具有良好光催化活性的锐钛矿型.以亚甲基蓝(methylene blue )为目标物研究了TiO 2纳米管光电催化性能及影响因素,结果表明,以0.1mol /L NaCl为电解质,在氧气热处理气氛下,外加偏压为0.5V 、pH =3.25、光照强度为1000μW /cm 2、纳米管薄膜表面积为2cm ˑ2cm 且MB初始浓度为10mg /L 的条件下,TiO 2纳米管阵列薄膜光电催化降解MB 的降解效率可达99.56%.电化学阻抗谱分析显示,光电催化降解过程的速控步骤均为表面反应步骤,外加偏压减小了界面电荷转移阻抗,提高了光生载流子的分离效率.关键词:二氧化钛;纳米管阵列;电化学阳极氧化;光电催化;亚甲基蓝中图分类号:X703.1文献标识码:A文章编号:0250-3301(2011)11-3372-07收稿日期:2010-12-11;修订日期:2011-01-06基金项目:国家自然科学基金项目(50968005,10876017,10776017,21063005)作者简介:张溪(1985 ),女,硕士研究生,主要研究方向为环境材料,E-mail :zhangxispring@ *通讯联系人,E-mail :liaolei@glite.edu.cnResearch of the Photoelectrocatalysis Degradation of Methylene Blue of TiO 2Nanotube Array Films Annealed in Oxygen AtmospheresZHANG Xi 1,LIAO Lei 1,LING Yun-han 2,QIN Ai-miao 1,ZHAO Cheng-gen 1,ZHAO Fei-chao 1(1.Guangxi Key Laboratory of Environmental Engineering and Protecting Assessment ,Guilin University of Technology ,Guilin 541004,China ;2.Department of Materials Science and Engineering ,Tsinghua University ,Beijing 100084,China )Abstract :Well-aligned and uniform titanium dioxide nanotube arrays were fabricated in situ on titanium substrate by electrochemical anodic oxidation.SEM images indicate that the TiO 2nanotubes are well organized into high-density uniform arrays ,with diameter ranging in size from 70to 100nm.The XRD patterns show that the sample fabricated by anodic oxidation is amorphous without being annealed.As the 500ħannealed sample crystallized in the anatase phase ,which is in good photocatalytic activity.In quartz glass reactor ,the photoelectrocatalytic degradation of MB in aqueous solutions as well as the effect factors and then to 0.1mol /L NaCl as the electrolyte ,under with in oxygen atmospheres annealed the applied bias is 0.5V and has a pH =3.25,light intensity is 1000μW /cm 2and the surface area of nano-tube array film conditions ,will help to improve the TiO 2nanotubes array film photoelectrocatalysis degradation of MB degradation efficiency is 99.56%.The results of EIS analyses revealed that the surface reaction step was the only rate limiting step either for the photoelectrocatalytic process.The extra bias potential could decrease the values of the electron-transfer resistance and increase the separation efficiency of photogenerated electron-hole pairs.Key words :titanium dioxide ;nanotube array ;electrochemical anodic oxidation ;photoelectrocatalysis ;methylene blue1972年Fujishima 等[1]发现光照TiO 2半导体电极有分解水的现象,特别是1976年Carey 等[2]报道了在紫外光照射下,TiO 2电极可降解各种难降解有机化合物以来,TiO 2以其活性高、热稳定性好、抗光氧化性强和价格便宜等特点而成为最受关注的光催化剂,其中纳米TiO 2光催化作为一种水处理的高级氧化技术而广泛用于环保领域.然而TiO 2的光生电子和空穴容易复合,导致其光催化效率较低.为了提高光催化量子效率,人们对TiO 2纳米管阵列进行非金属掺杂、金属离子掺杂、半导体修饰以及贵金属沉积等以提高其对可见光的响应并改善其光催化性能[3 5].2001年,有研究组[6]首次在含氟离子的电解液中成功制备出分布均匀、整齐有序的TiO 2纳米管阵列.Wang 等[7]采用HF 、NH 4F 和乙二醇作为电解液,通过阳极氧化制备出的TiO 2纳米管阵列管壁光滑.实践证明,TiO 2纳米管比其它形式的TiO 2具有更好的光催化、光电解以及光电转换等性能[8].自Fujishima 等[1]提出电化学辅助光催化技术即光电催化以来,许多研究者应用此技术以TiO 2薄膜为光阳极对水中的有机污染物如氯苯酚、染料和有机酸等在紫外光照下进行降解[9,10],取得了较好的效果.近年来,利用电化学阳极氧化法在Ti 基体上制11期张溪等:氧气热处理的TiO2纳米管阵列光电催化降解亚甲基蓝的研究得的TiO2纳米管阵列电极已经引起了人们极大的关注,与纳米TiO2薄膜相比,TiO2纳米管阵列具有比表面积和量子阱效应高,纳米管尺寸、形貌和结构可控,阵列层与基体结合强度高等优点,并已成功地应用于光电催化降解有机污染物[11],获得了一些有价值的研究结果.曹长春等[12]以紫外光为光源,研究了活性艳红在不同条件下的降解行为.结果表明,只在单独的电场作用下溶液中活性艳红的浓度会有一定程度的降低,而且随着电压值的升高,降低速率就越快.刘惠玲等[13]研究表明在有外加电压下若丹明B的降解速率明显增加,在最初2h外加偏压使若丹明B的去除率较无外加偏压条件下增加约50%.张溪等[14]研究了不同热处理气氛对TiO2纳米管阵列薄膜光电催化性能的影响,结果表明在氧气热处理气氛下光电催化性能最好.孟耀斌等[15]研究指出,提高光强虽然可以增加光催化降解速率,但会降低其能量利用率.如何选择合适的光强既能顾及反应速度又能充分利用能量是一个有待解决的问题.目前,对TiO2纳米管阵列的光电性能和光电催化性能的研究及其机制的探讨也是该领域的研究重点,而用电化学阻抗技术对相关机制进行揭示尚在起步阶段.为此,本研究首先采用阳极氧化法制备TiO2纳米管薄膜光催化电极,考察在氧气热处理气氛下TiO2纳米管阵列电极的光电化学活性,在此基础上,以难生物降解的亚甲基蓝作为目标污染物,对TiO2纳米管阵列的光电性能、光电催化活性及影响降解效率的因素进行探讨,利用电化学阻抗技术对其光电催化性能进行研究,以期为光电催化技术的工业化应用提供理论依据.1材料与方法1.1TiO2纳米管的制备与表征金属钛箔纯度>99.6%,厚度为0.15mm;将钛箔剪切成2cmˑ2cm大小,然后分别在丙酮、乙醇和去离子水中超声10min,取出钛箔放在空气中风干.为了改善纳米管的表面质量,本文采用二次阳极氧化法,即在一次氧化反应完成后将制备好的TiO2纳米管放入HFʒHNO3ʒH2O=1ʒ3ʒ6的混合酸液中浸泡3s取出,迅速放到去离子水中超声直至薄膜脱落,然后将脱膜后光亮的钛片再进行第二次氧化,得到表面整齐均匀的纳米管.在阳极氧化过程中以钛箔为阳极,石墨为阴极.第一次阳极氧化电解液是0.5%(质量分数)NH4F+5%(体积分数)H2O的乙二醇溶液;反应条件为:电压50V,时间15min.第二次阳极氧化电解液是0.5%(质量分数)NH4F+10%(体积分数)H2O+0.2%HF的乙二醇溶液;反应条件为:电压50V,时间25min.第二次阳极氧化完成后,将样品放在马弗炉里以10ħ/min从室温升到500ħ,分别在O2中保温1h,然后冷却到室温,即得结晶的TiO2纳米管阵列薄膜,用在光电催化降解MB反应中.以下实验所用的TiO2纳米管均是在氧气气氛热处理后的样品.采用德国Bruker AXS公司的D8-Advance型X 射线衍射仪分析样品的晶型结构,测定条件为:室温Cu Kα射线(λ=0.15418nm),靶电压40kV,靶电流40mA,扫描范围10ʎ 80ʎ(2θ),步长0.02ʎ.1.2光电催化性能测试用Zahner IM6e电化学工作站三电极系统(如图1)进行TiO2纳米管光电催化降解MB的实验,其中工作电极为氧气中热处理的TiO2纳米管,大小为2cmˑ2cm,对电极为Pt,参比电极为饱和甘汞,光源用CMH-250金属卤素灯(主光谱有效范围在350 450nm之间,照度约为100mW/cm2),用10mg/L MB溶液为目标降解物.采用ST-86L弱光照度计(验光仪专用)对本实验弱光到强光进行准确的照度测量.利用紫外-可见吸收谱对纳米管薄膜的光吸收和MB溶液的降解效率进行分析.所用仪器型号为UV2100,波长范围190 850nm,分辨率0.1nm.电化学阻抗测试的交流扰动电位10mV,频率范围为1mHz 105Hz.A:工作电极B:参比电极(饱和甘汞电极)C:辅助电极(Pt)图1光电化学测试系统Fig.1Photoelectrochemical test system2结果与分析2.1TiO2纳米管的表征分析图2为未热处理和在氧气中热处理的TiO2纳米管阵列薄膜的XRD衍射图.从中可以看出,未经3733环境科学32卷热处理的TiO 2纳米管仅有Ti 基体的衍射峰(40.17ʎ、52.98ʎ、62.96ʎ、70.62ʎ和76.21ʎ),说明该薄膜为无定形.但经过氧气热处理的样品会有明显的TiO 2晶型衍射峰,主要物相为锐钛矿相,在25.35ʎ处出现特征峰,属于(101)晶面的衍射峰.(a )没有热处理;(b )在氧气气氛下500ħ热处理.图2TiO 2纳米管的XRD 衍射图Fig.2XRD patterns of TiO 2NT arrays图3为2次阳极氧化并经氧气热处理获得的TiO 2纳米管典型扫描电镜(SEM )照片,从中可以看出样品表明呈现出高度有序、规整的TiO 2纳米管阵列结构,管径约100nm ,管长约为2μm.图3TiO 2纳米管的SEM 照片Fig.3SEM image of TiO 2nanotubes2.2TiO 2纳米管光电性能的研究图4(a )为TiO 2纳米管阵列电极在光照和暗态下的Nyquist 图,从中可以看出,光照条件下TiO 2纳米管阵列电极的阻抗值相对暗态条件大幅度减小,这是由于在该条件下,TiO 2纳米管阵列电极表面产生大量的光生载流子,在相同的频率下,图上阻抗环半径小的曲线对应的电容常数大,产生的法拉第电流的阻抗值较小,因此在电极上面发生反应比较容易,需要克服的能垒比较小,电极反应速率加快.已有的研究表明,Nyquist 图上的圆弧半径对应着电荷转移电阻和光生电子-空穴对的分离效率,圆弧半径越小,光催化反应进行得越快.在相同的测试条件下,对TiO 2纳米管阵列电极施以0.5V 偏压,测定其在偏压下的阻抗.图4(b )为光照和光电协同作用下TiO 2纳米管阵列电极的Nyquist 图.从中可以看出,施加偏压后阻抗圆弧变小,阻抗值明显减小,说明对光阳极施加一定的阳极偏压更有利于光生电子-空穴对的分离.图4暗态及光照下和光照及光电协同作用下TiO 2纳米管阵列电极电化学阻抗谱的Nyquist 图Fig.4EIS Nyquist plots of TiO 2nanotube array electrode in dark andillumination as well as illumination and photoelectrochemical process2.3TiO 2纳米管光电催化性能的研究2.3.1电化学过程、直接光解、光催化、光电催化对MB 降解的比较图5所示是MB 的电化学降解、直接光解、以及473311期张溪等:氧气热处理的TiO2纳米管阵列光电催化降解亚甲基蓝的研究在TiO2纳米管的光催化降解、TiO2纳米管电极光电催化降解中MB降解效率的比较.亚甲基蓝的起始浓度均为10mg/L左右,pH=7.03,电解质NaCl,浓度0.01mol/L.电化学过程不加紫外光,外加偏压0.25V;光解实验无外加偏压,无TiO2纳米管电极;光催化实验无外加偏压,TiO2纳米管电极处的光强700μW/cm2;光电催化实验外加偏压0.2V,TiO2纳米管电极处的光强700μW/cm2.由图5(a)可知,在外加偏压0.2V的电化学过程中,MB几乎不发生降解,说明在接下来的光电催化反应中,施加的0.2V偏压不是使MB降解的主要原因.MB容易发生直接光解,在所给实验条件下,2h MB降解了19.58%.在随后的TiO2纳米管的光催化反应中,相同的时间内,MB降解了61.76%,TiO2纳米管显示出良好的光催化效果.在此基础上施加的0.2V偏压,更使MB的降解效率大大提高,2h内,MB浓度从10mg/L降到了0.1mg/L,去除率达到99.56%.图5(b)是TiO2纳米管在4种过程中降解MB的动力学曲线.TiO2纳米管对MB的降解均符合一级反应,其速率常数分别为0.153h-1(EC)、0.387h-1(DP)、0.872h-1(PC)和4.724h-1(PEC).图54种过程MB随时间变化降解效率曲线和动力学曲线Fig.5Degradation efficiency curve of MB using differentprocess and the kinetic curves 2.3.2外加偏压对MB降解的影响本研究考察了外加偏转电压对MB降解的影响,因为外加偏压能够有效地将光生电子转移到对电极,防止其与空穴的复合,因此,外加偏压是影响光电催化过程的重要因素.从图6(a)中可以看出,随着外加偏压的增加,MB的去除率在2h的反应时间里从96.73%提高的99.57%.而在不施加外加偏压的光催化过程中,MB的去除率为66.96%.还可以看出:增大外加电压可明显提高反应的氧化降解性能.当施加的外加电压为0V时,此时溶液中发生的反应仅为TiO2光催化反应.当外加电压增大时,体系对MB的降解效率迅速增大,这是因为当外加电压低于TiO2/Ti光电极的弯曲能带时,外加电压的增大会压迫弯曲能带,使禁带宽度变窄,从而加速了光生电子和空穴的分离,提高了TiO2/Ti电极的光催化效率.图6(b)是TiO2纳米管在不同偏压下降解MB的动力学曲线.TiO2纳米管对MB 的降解均符合一级反应,其速率常数分别为0.893h-1(0.0V)、2.878h-1(0.25V)和4.724h-1(0.5V).图6在不同偏压下MB在氧气中热处理TiO2纳米管光电催化降解MB的降解效率曲线和动力学曲线Fig.6Degradation efficiency curve of MB degradation for TiO2nanotube arrays annealed in oxygen atmospheres andthe kinetic curves under the bias in different5733环境科学32卷2.3.3溶液初始pH 值对MB 降解的影响在污水处理过程中,在给定的过程中pH 值通常是影响污染物去除的重要参数.本研究考察了3个具有代表性的pH 值,即3.25、6.29、8.00,分别代表酸性溶液、锐钛矿型TiO 2等电点附近、碱性溶液.如图7(a )所示,溶液pH 值越低,光催化剂对目标降解物亚甲基蓝的降解效率越高.这表明酸性条件有助于光电催化降解有毒有机物.pH 值可通过很多方面影响光电催化过程,比如改变TiO 2平带电位,影响目标物在TiO 2上的吸附能力等.目前的现象有可能是这些个别因素综合影响的结果.图7(b )是TiO 2纳米管在不同偏压下降解MB 的动力学曲线TiO 2其速率常数分别为1.008h -1(0.0V )、4.724h -1(0.25V )和8.08h -1(0.5V ).图7在不同溶液初始pH 下MB 在氧气中热处理TiO 2纳米管光电催化降解MB 的降解效率曲线和动力学曲线Fig.7Degradation efficiency curve of MB degradation for TiO 2nanotube arrays annealed in oxygen atmospheres and the kinetic curves at different initial solution pH2.3.4电解质浓度对MB 降解的影响与传统的电化学过程一样,电解质的种类和浓度能够影响光电催化过程.本研究选择了NaCl 作为电解质,并考察了3个不同水平的浓度,即0、0.05和0.1mol /L.结果表明[图8(a )],NaCl 的加入增加了MB 的降解,并且随着NaCl 浓度的增加,MB 的去除趋势也随之增加.由于电解质的存在提高了溶液的导电性,加速了电子的转移,因此增强了光电催化过程.图8(b )是TiO 2纳米管在不同偏压下降解MB 的动力学曲线TiO 2其速率常数分别为0.181h -1(0.0V )、0.804h -1(0.25V )和4.724h -1(0.5V ).图8在不同电解质浓度下MB 在氧气中热处理TiO 2纳米管光电催化降解MB 的降解效率曲线和动力学曲线Fig.8Degradation efficiency curve of MB degradation for TiO 2nanotube arrays annealed in oxygen atmospheres and the kinetic curves at different electrolyte concentrations2.3.5光强对MB 降解的影响在其他实验条件相同而光强不同对MB 光电催化降解影响,考察了3个不同的光强400、620及1000μW /cm 2.由图9(a )可以看出,TiO 2纳米管薄膜光电催化对MB 的降解效率随辐射光源强度的增大而增大.当体系无紫外光照射时,此时体系对有机物的降解主要是由体系中生成的H 2O 2引起的,在光强为400μW /cm 2,经120min ,TiO 2纳米管薄膜光电催化对MB 的降解效率仅为75.32%,当向体系中施加紫外光照射,TiO 2/Ti 光电极被激发产生光生电子-空穴对,电子-空穴对在外电路发生定向偏转迁移产生光电流.由光电效应可知,在入射光辐射频率一定的条件下,光电流强度随入射光源强度的增大673311期张溪等:氧气热处理的TiO 2纳米管阵列光电催化降解亚甲基蓝的研究而增大.所以,光源辐射强度的提高促进了光生电子-空穴对的生成和定向迁移,提高了光生电流的强度,增大了TiO 2/Ti 光电极表面空穴的浓度,光生空穴有很强的氧化氛围,产生了更多的·OH ,从而促进了TiO 2/Ti 光电极的光催化氧化效率,提高了TiO 2纳米管薄膜光电催化对MB 的降解效率.图9(b )是TiO 2纳米管在不同偏压下降解MB的动力学曲线TiO 2其速率常数分别为1.168h-1(0.0V )、1.362h -1(0.25V )和 4.724h -1(0.5V ).图9在不同光照强度下MB 在氧气中热处理TiO 2纳米管光电催化降解MB 的降解效率曲线和动力学曲线Fig.9Degradation efficiency curve of MB degradation for TiO 2nanotube arrays annealed in oxygen atmospheres and the kinetic curves in different illumination intensity2.3.6TiO 2纳米管薄膜电极面积对MB 降解的影响为了考察TiO 2纳米管薄膜电极面积对MB 光电催化的影响,本研究考察了其他条件完全相同,只有TiO 2纳米管薄膜电极面积不同对MB 去除的影响,电极面积分别为1cm ˑ1cm 、1cm ˑ2cm 及2cm ˑ2cm.结果如图10(a )所示,增大薄膜电极面积,MB 的去除率从93.79%提高到99.56%.图10在不同电极面积下MB 在氧气中热处理TiO 2纳米管光电催化降解MB 的降解效率曲线和动力学曲线Fig.10Degradation efficiency curve of MB degradation for TiO 2nanotube arrays annealed in oxygen atmospheres and the kinetic curves at different the electrode area2.4产物紫外-可见吸收光谱分析光照时间直接影响到亚甲基蓝的降解矿化程度和中间产物的种类以及量的多少.因而本研究考察了每隔20min 溶液中MB 的紫外吸收光谱图,其结果如图11所示,随着降解时间的增加,MB 在664nm 处的吸收峰强度逐渐减弱,这是因为降解物对光特征吸收强度的变化反应了目标物中某些基团的含量因破坏而减少,所以在本实验中纳米管薄膜对目标物的光电催化降解效率通过评估不同降解时刻UV-Vis 光谱的吸收峰(664nm 处)强度的变化来实现.随着光照时间的增加,溶液中的MB 的紫外吸收值减弱,说明MB 逐渐降解.光照射时间越长,产生的光致空穴就越多,MB 捕获的空穴与羟基自由基就越多.溶液中MB 浓度在20min 前随着时间的增加剧烈衰减,120min 时MB 降解率达到99.56%.吸光度与浓度的关系式如下:c =A /Kb(1)式中,c 表示溶液的浓度;A 表示物质对光的吸收程7733环境科学32卷度,即吸光度;K 表示物质的特性常数即吸光系数(K 值可以从手册或文献中查到);b 表示单位液层厚度(在本实验中表示比色皿的厚度:1cm );在式(1)中Kb 为常数,c 与A 成正比关系.在光电催化降解后,在任意时刻(t )亚甲基蓝的降解率为η,则:η=(c t -c 0)/c 0%(2)式中,c 0为亚甲基蓝溶液初始浓度,c t 为亚甲基蓝在某一降解时刻的浓度.将式(1)代入式(2)得:η=(A t /Kb -A 0/Kb )/(A 0/Kb )%,化简得:η=(A t -A 0)/A 0%,式中,A t 为t 时刻降解MB 的吸光度,A 0为初始时刻MB 的吸光度.从而计算出MB 的降解效率.图11光电催化降解MB 吸光值随时间变化的紫外光谱Fig.11UV spectrum of absorbance with time changes of MB solution using PEC degradation3结论采用2次阳极氧化法制备了高度有序的TiO 2纳米管阵列薄膜,探讨了氧气热处理的TiO 2纳米管阵列薄膜光电催化降解MB 反应的影响因素,结果表明,以0.1mol /L NaCl 为电解质时,外加偏压越大,较低的pH ,较强的光照强度和纳米管的表面积越大将有助于TiO 2纳米管阵列薄膜光电催化降解MB 反应的降解效率提高.电化学阻抗谱的测试揭示了TiO 2纳米管阵列电极光生电子-空穴对的分离及传输特性.外加偏压减小了界面电荷转移阻抗,提高了光生载流子的分离效率.氧气热处理气氛的TiO 2纳米管在可见光区域的光吸收与其半导体性质有关,但目前还没有系统研究,对于以上相关问题还有待于进一步研究.参考文献:[1]Fujishima A ,Honda K.Electrochemical photolysis of water at asemiconductor electrode [J ].Nature ,1972,238(53-58):37-38.[2]Carey J H ,Lawrence J ,Tosine H W.Photodechlorination of PCB'sin the presence of titanium dioxide in aqueous suspension [J ].Bulletin of Environmental Contamination and Toxicology ,1976,16(6):697-701.[3]Shankar K ,Tep K C ,Grimes C A.N-doped and N ,F-codopedTiO 2photoelectrodes :An electrochemical strategy to incorporate anionic dopants [J ].Journal of Physics D :Applied Physics ,2006,39(11):2361-2366.[4]赵慧敏,陈越,全燮.Zn 掺杂TiO 2纳米管电极制备及其对五氯酚的光电催化降解[J ].科学通报,2007,52(2):158-162.[5]Paramasivam I ,Macak J M ,Schmuki P.Photocatalytic activity ofTiO 2nanotube layers loaded with Ag and Au nanoparticles [J ].Electrochemistry Communications ,2008,10:71-75.[6]Gong D ,Grimes C A ,Varghese O K ,et al.Titanium oxidenanotube arrays prepared by anodic oxidation [J ].Journal of Materials Research ,2001,16(12):3331-3334.[7]Wang D A ,Yu B ,Wang C W ,et al.A novel protocol towardperfect alignment of anodized TiO 2nanotubes [J ].Advanced Materials ,2009,21(19):1964-1967.[8]Macak J M ,Tsuchiya H ,Ghicov A ,et al.TiO 2nanotubes :Self-organized electrochemical formation ,properties and applications [J ].Current Opinion in Solid State and Materials Science ,2007,11(1-2):3-18.[9]Vinodgopal K ,Hotechandani S ,Kamat P V.Electrochemicallyassisted photocatalysis :titania particulate film electrodes for photocatalytic degradation of 4-chlorophenol [J ].Physics and Chemistry of Minerals ,1993,97(35):9040-9044.[10]Li X Z ,Liu H L ,Yue P T ,et al.Photoelectrocatalytic oxidation ofrose Bengal in aqueous solution using a Ti /TiO 2mesh electrode [J ].Environmental Science and Technology ,2000,34(20):4401-4406.[11]Liu Z Y ,Zhang X T ,Nishimoto S ,et al.Highly ordered TiO 2nanotube arrays with controllable length for potoelectrocatalytic degradation of phenol [J ].Journal of Physical Chemistry C ,2008,112(1):253-259.[12]曹长春,蒋展鹏,余刚,等.TiO 2薄膜光电协同催化氧化降解活性艳红[J ].环境科学,2002,23(6):108-110.[13]刘惠玲,周定,李湘中,等.网状Ti /TiO 2电极光电催化氧化若丹明B [J ].环境科学,2002,23(4):47-51.[14]张溪,凌云汉,廖雷,等.热处理气氛对TiO 2纳米管阵列薄膜光电催化性能的影响[J ].催化学报,2010,31(10):1300-1304.[15]孟耀斌,黄霞,钱易.不同波段紫外光在TiO 2悬浊液中的消光特点[J ].环境科学,2001,22(2):46-50.8733。
TiO_2纳米管薄膜的裂纹起源、扩展与饱和(英文)
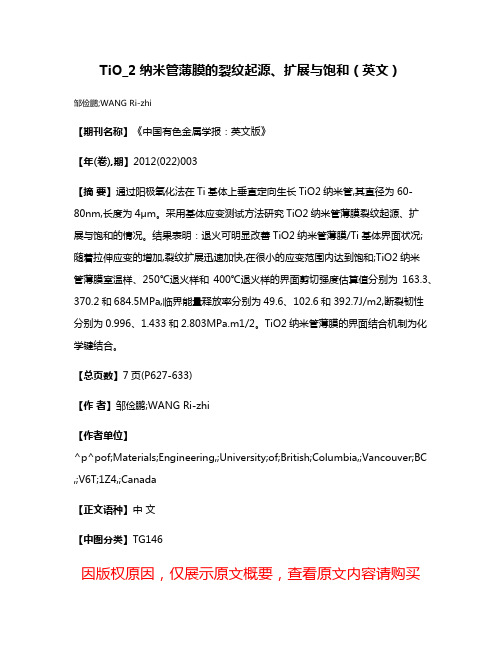
TiO_2纳米管薄膜的裂纹起源、扩展与饱和(英文)
邹俭鹏;WANG Ri-zhi
【期刊名称】《中国有色金属学报:英文版》
【年(卷),期】2012(022)003
【摘要】通过阳极氧化法在Ti基体上垂直定向生长TiO2纳米管,其直径为60-
80nm,长度为4μm。
采用基体应变测试方法研究TiO2纳米管薄膜裂纹起源、扩
展与饱和的情况。
结果表明:退火可明显改善TiO2纳米管薄膜/Ti基体界面状况;
随着拉伸应变的增加,裂纹扩展迅速加快,在很小的应变范围内达到饱和;TiO2纳米
管薄膜室温样、250℃退火样和400℃退火样的界面剪切强度估算值分别为163.3、370.2和684.5MPa,临界能量释放率分别为49.6、102.6和392.7J/m2,断裂韧性分别为0.996、1.433和2.803MPa.m1/2。
TiO2纳米管薄膜的界面结合机制为化学键结合。
【总页数】7页(P627-633)
【作者】邹俭鹏;WANG Ri-zhi
【作者单位】
^p^pof;Materials;Engineering,;University;of;British;Columbia,;Vancouver;BC ,;V6T;1Z4,;Canada
【正文语种】中文
【中图分类】TG146
因版权原因,仅展示原文概要,查看原文内容请购买。
自组织tio2纳米管阵列的制备及形成机理
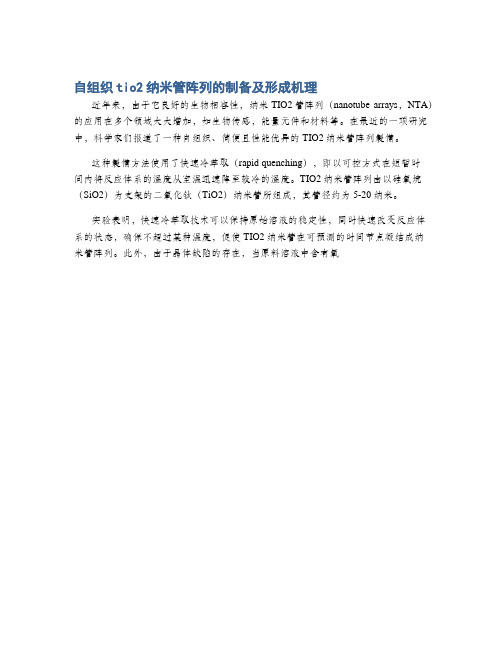
自组织tio2纳米管阵列的制备及形成机理
近年来,由于它良好的生物相容性,纳米TIO2管阵列(nanotube arrays,NTA)的应用在多个领域大大增加,如生物传感,能量元件和材料等。
在最近的一项研究中,科学家们报道了一种自组织、简便且性能优异的TIO2纳米管阵列製備。
这种製備方法使用了快速冷萃取(rapid quenching),即以可控方式在短暂时
间内将反应体系的温度从室温迅速降至较冷的温度。
TIO2纳米管阵列由以硅氧烷(SiO2)为支架的二氧化钛(TiO2)纳米管所组成,其管径约为5-20纳米。
实验表明,快速冷萃取技术可以保持原始溶液的稳定性,同时快速改变反应体系的状态,确保不超过某种温度,促使TIO2纳米管在可预测的时间节点凝结成纳
米管阵列。
此外,由于晶体缺陷的存在,当原料溶液中含有氧。
建立具有习晶特征的anatase_TiO2的MS第一性原理模型例子

MS 纳米颗粒/团簇建模一例:建立具有习晶特征的锐钛矿结构TiO2团簇
Emuch:gkd_0000
有时候我们需要计算团簇的电子结构,但是很多情况下制备的纳米颗粒都具有一定的晶体学构造特征。
如例1和例2。
那么在做计算的时候建立符合习晶特征的团簇模型显然更符合实际情况。
如果原子数较少的话,可以通过输入坐标来建模。
但是原子数很多的话就非常麻烦了。
实例1
实例2
因为最近需要计算不同大小的锐钛矿结构TiO2团簇的电子结构,所以我琢磨了半天,终于在MS中把建这个模型的办法搞出来了,给大家分享一下,高手勿笑,欢迎指正。
第一步,在MS中输入锐钛矿的结构,在structure里面有现成的。
第二步,build -> build nanostructure ->nanocluster , 选shape里面的square pyramid,这是个金子塔的形状,高度:n×c,base edge:2n×a,其中a(=b)和c分别为TiO2原胞的[1 0 0]方向基矢长度和[0 0 1]方向基矢长度。
Build。
这样就得到了一个金子塔形状的团簇。
第三步,体力活,放正模型(x轴对正自己,z轴竖直),在金字塔高度的一半左右的下方删不要的原子,可以一层一层的删,也挺快的,注意上下的对称性。
删完y方向,y轴对正自己删x方向的原子。
这样就差不多了。
上下两个尖尖根据需要可以删掉不同的原子层。
结果如下图。
纳米TiO2固相光催化降解固体废弃塑料
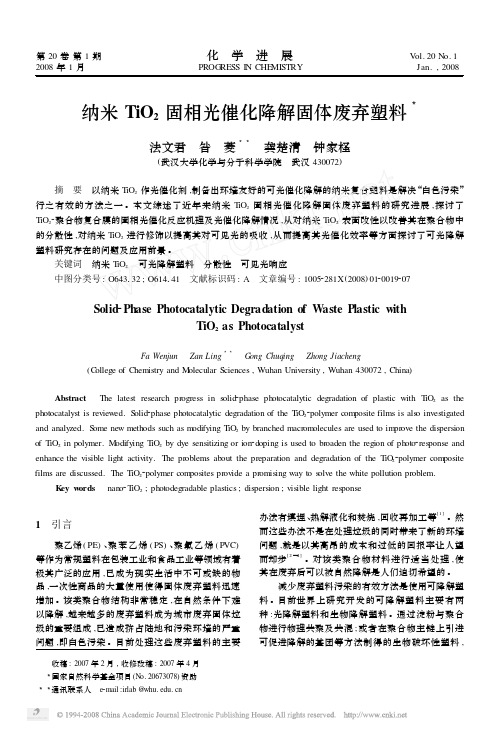
办法有填埋 、 热解液化和焚烧 ,回收再加工等 。然 而这些办法不是在处理垃圾的同时带来了新的环境 问题 ,就是以其高昂的成本和过低的回报率让人望 [2 — 4] 而却步 。对该类聚合物材料进行适当处理 , 使 其在废弃后可以被自然降解是人们迫切希望的 。 减少废弃塑料污染的有效方法是使用可降解塑 料 。目前世界上研究开发的可降解塑料主要有两 种 : 光降解塑料和生物降解塑料 。通过淀粉与聚合 物进行物理共聚及共混 ; 或者在聚合物主链上引进 可促进降解的基团等方法制得的生物破坏性塑料 ,
2. 2 纳米 TiO2 光催化降解聚苯乙烯 ( PS) 塑料
朱永法等
[22 ]
报道了用纳米 TiO2 作光催化剂 ,固
相光催化降解 PS 塑料 。紫外光照射前复合膜和纯 膜有几乎相同的分子量 ,红外光谱也显示 ,其特征吸 收峰基本一致 。实验证明二氧化钛粉末与聚苯乙烯 之间的相互作用为物理过程 , 而非化学过程 。照射 一段时间后 , 相对于纯膜 , PS2TiO2 复合膜的苯环特 征吸 收 峰 有 明 显 的 降 低 , 并 且 分 别 在 1 742 及 - 1 1 217cm 处出现新的 C O 和 C — O 伸缩振动峰 。 用气相色谱检测了复合膜光氧化过程中释放的二氧 化碳及挥发性有机气体产物 。在相同的条件下 , 复 合膜产生的中间有机气体 ( 例如乙烯 、 乙烷 、 丁烷 、 甲 醛、 乙醛和乙醇等) 总量少于纯膜 , 大部分更快地转 化为 CO2 气体释放出来 。这也说明 PS2TiO2 复合膜 不仅降解效果较好 ,而且是环境友好的 。 2. 3 纳米 TiO2 固相光催化降解聚乙烯 ( PE) 塑料 昝菱等
TiO2纳米单晶及其介晶的生长
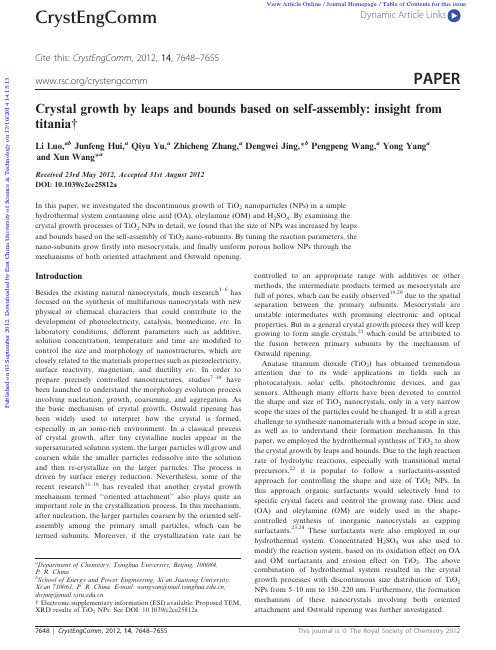
Crystal growth by leaps and bounds based on self-assembly:insight from titania {Li Luo,ab Junfeng Hui,a Qiyu Yu,a Zhicheng Zhang,a Dengwei Jing,*b Pengpeng Wang,a Yong Yang a and Xun Wang *aReceived 23rd May 2012,Accepted 31st August 2012DOI:10.1039/c2ce25812aIn this paper,we investigated the discontinuous growth of TiO 2nanoparticles (NPs)in a simple hydrothermal system containing oleic acid (OA),oleylamine (OM)and H 2SO 4.By examining the crystal growth processes of TiO 2NPs in detail,we found that the size of NPs was increased by leaps and bounds based on the self-assembly of TiO 2nano-subunits.By tuning the reaction parameters,the nano-subunits grow firstly into mesocrystals,and finally uniform porous hollow NPs through the mechanisms of both oriented attachment and Ostwald ripening.IntroductionBesides the existing natural nanocrystals,much research 1–6has focused on the synthesis of multifarious nanocrystals with new physical or chemical characters that could contribute to the development of photoelectricity,catalysis,biomedicine,etc.In laboratory conditions,different parameters such as additive,solution concentration,temperature and time are modified to control the size and morphology of nanostructures,which are closely related to the materials properties such as piezoelectricity,surface reactivity,magnetism,and ductility etc.In order to prepare precisely controlled nanostructures,studies 7–10have been launched to understand the morphology evolution process involving nucleation,growth,coarsening,and aggregation.As the basic mechanism of crystal growth,Ostwald ripening has been widely used to interpret how the crystal is formed,especially in an ionic-rich environment.In a classical process of crystal growth,after tiny crystalline nuclei appear in the supersaturated solution system,the larger particles will grow and coarsen while the smaller particles redissolve into the solution and then re-crystallize on the larger particles.The process is driven by surface energy reduction.Nevertheless,some of the recent research 11–18has revealed that another crystal growth mechanism termed ‘‘oriented attachment’’also plays quite an important role in the crystallization process.In this mechanism,after nucleation,the larger particles coarsen by the oriented self-assembly among the primary small particles,which can be termed subunits.Moreover,if the crystallization rate can becontrolled to an appropriate range with additives or other methods,the intermediate products termed as mesocrystals are full of pores,which can be easily observed 19,20due to the spatial separation between the primary subunits.Mesocrystals are unstable intermediates with promising electronic and optical properties.But in a general crystal growth process they will keep growing to form single crystals,21which could be attributed to the fusion between primary subunits by the mechanism of Ostwald ripening.Anatase titanium dioxide (TiO 2)has obtained tremendous attention due to its wide applications in fields such as photocatalysis,solar cells,photochromic devices,and gas sensors.Although many efforts have been devoted to control the shape and size of TiO 2nanocrystals,only in a very narrow scope the sizes of the particles could be changed.It is still a great challenge to synthesize nanomaterials with a broad scope in size,as well as to understand their formation mechanism.In this paper,we employed the hydrothermal synthesis of TiO 2to show the crystal growth by leaps and bounds.Due to the high reaction rate of hydrolytic reactions,especially with transitional metal precursors,22it is popular to follow a surfactants-assisted approach for controlling the shape and size of TiO 2NPs.In this approach organic surfactants would selectively bind to specific crystal facets and control the growing rate.Oleic acid (OA)and oleylamine (OM)are widely used in the shape-controlled synthesis of inorganic nanocrystals as capping surfactants.23,24These surfactants were also employed in our hydrothermal system.Concentrated H 2SO 4was also used to modify the reaction system,based on its oxidation effect on OA and OM surfactants and erosion effect on TiO 2.The above combination of hydrothermal system resulted in the crystal growth processes with discontinuous size distribution of TiO 2NPs from 5–10nm to 150–220nm.Furthermore,the formation mechanism of these nanocrystals involving both oriented attachment and Ostwald ripening was further investigated.aDepartment of Chemistry,Tsinghua University,Beijing,100084,P.R.China bSchool of Energy and Power Engineering,Xi’an Jiaotong University,Xi’an 710061,P.R.China.E-mail:wangxun@;dwjing@{Electronic supplementary information (ESI)available:Proposed TEM,XRD results of TiO 2NPs.See DOI:10.1039/c2ce25812aCrystEngCommDynamic Article LinksCite this:CrystEngComm ,2012,14,7648–7655/crystengcommPAPERP u b l i s h e d o n 03 S e p t e m b e r 2012. D o w n l o a d e d b y E a s t C h i n a U n i v e r s i t y o f S c i e n c e & T e c h n o l o g y o n 17/10/2014 14:15:13.View Article Online / Journal Homepage / Table of Contents for this issueExperimental sectionSynthesisAll the regents were purchased from the Sinopharm Chemical Reagent Co.,Ltd and used without any further purification.They include oleic acid (OA),oleylamine (OM),titanium sulfate (Ti(SO 4)2),Sulfuric acid (H 2SO 4,98%),nitric acid (HNO 3),hydrochloric acid (HCl),acetic acid (CH 3COOH),sodium sulfate (Na 2SO 4),hexamethylene (C 6H 12)and ethanol (C 2H 5OH).Tiny anatase TiO 2NPs:in a typical synthesis,3mL of OA and 1mL of OM were successively added into a 40mL Teflon-lined autoclave.5mL of Ti(SO 4)2solution (0.25mol L 21)were then slowly added into the system with continuous stirring at room temperature.With the increasing amount of Ti(SO 4)2solution,the mixture changed quickly from a turbid liquid to a viscous colloid.This was accompanied by a color change from yellowish to milky white,just like cream.Then,the reaction system was sealed and kept at 150u C for 8h.After it was cooled to room temperature,the product was washed with anhydrous ethanol and hexamethylene and then separated by centrifugation.Finally,the sample was dried at 60u C in air for 6h.Mesocrystal and hollow structure anatase TiO 2NPs:the synthesis procedure was the same as above except that 0.5mL of H 2SO 4were mixed with 5mL of Ti(SO 4)2solution before adding into the system.The mesocrystal TiO 2NPs were calcined at 300u C for 3h to remove the residual organics.For further investigation,we obtained products by changing the reaction temperature,time and the amount of H 2SO 4.A two-step process was employed to synthesize the uniform hollow spindle-shaped TiO 2NPs by adding 1mL of excess H 2SO 4into the system after TiO 2mesocrystal formation and arranging another 8h reaction at the same hydrothermal conditions.In order to investigate the effects of H 2SO 4addition on the process of crystal growth,control experiments were carried out using other acids (HNO 3,HCl,CH 3COOH)and Na 2SO 4with the equimolar quantity of hydrogen ion and sulfate ion as in H 2SO 4.CharacterizationThe size and morphology of the products were characterized by JEOL JEM-1200EX transmission electron microscope (TEM)with an accelerating voltage of 100kV,a Tecnai TF20S-Twin high-resolution transmission electron microscope (HRTEM)with an accelerating voltage of 200kV and a JEOL JSM-6700F scanning electron microscope (SEM).Samples were prepared by dropping a hexamethylene dispersion of the products onto the surface of a carbon coated copper grid for TEM,and spreading the powder of the products onto a conducting resin for SEM,respectively.The phase and structure of the products were examined by XRD on a Bruker D8Advance X-ray diffractometer using Cu K a radiation (l =1.5418A˚).The sizes of small NPs,mesocrystals and hollow structures are the statistical average values.The surface area of the products was calculated by using the nitrogen adsorption–desorption method,and pore size distribution was calculated from the desorption branch of sorption isotherms by using the Barrett–Joyner–Halenda (BJH)theory.Results and discussionHere,the mechanism of the TiO 2crystal growth with discontin-uous sizes from 5–10nm to 150–220nm was investigated.Tiny TiO 2NPs were formed in the system with the additives of OA and OM.Uniform TiO 2porous mesocrystals and hollow structures were obtained by introducing H 2SO 4into the system.Fig.1(a–c)are HRTEM images of tiny and uniform TiO 2NPs obtained at the hydrothermal conditions of 210u C after 8h.From the Fourier transform pattern shown in the insert of Fig.1(c),uniform lattice fringes representing the (101),(200)and (204)planes of the anatase TiO 2can be clearly observed.The lattice distance of the (101)plane is labelled as 0.352nm.In addition,more products with sizes between 5–10nm (y 7.6nm on average)were obtained if H 2SO 4was not added into the hydrothermal system,as shown in Fig.S1(ESI {).As shown in Fig.1(d),the typical X-ray diffraction (XRD)patterns of the tiny TiO 2NPs fit well with anatase phase (JCPDS no.21-1272).The diffraction peaks are considerable broadened due to the small scale of TiO 2NPs.It is normal that TiO 2NPs have better crystallinity when prepared at higher temperatures.As shown in Fig.2,it is interesting to note that the TiO 2NPs in the SEM images have grown into spindle-shapes and larger sizes of around 150–220nm in length (y 180nm on average)after adding an appropriate amount of H 2SO 4into the system.Fig.2(d)indicates that these larger NPs also belong to the anatase phase.In order to investigate the specific structure of these spindle-shaped NPs,we used the Scherrer Equation to calculate their average size.The calculated result is ca.38nm,which is significantly different from the actual size of y 180nm as observed by TEM images (Fig.2(a)and (b)).The reason for the discrepancy is further investigated in our following studies.Temperature and time played quite important roles in the formation of TiO 2porous mesocrystals and hollow structures.Under 90u C there were only amorphous precursors.However,Fig.1(a)–(c)TEM images of tiny TiO 2NPs obtained after 8h at 180u C with the OA and OM in the reaction system.(d)XRD patterns of the tiny TiO 2NPs prepared from different temperature:(1)210,(2)180,(3)150,(4)120u C.P u b l i s h e d o n 03 S e p t e m b e r 2012. D o w n l o a d e d b y E a s t C h i n a U n i v e r s i t y o f S c i e n c e & T e c h n o l o g y o n 17/10/2014 14:15:13.when the temperature was increased to 120u C homogeneously porous mesocrystals could also be obtained (Fig.S1(e,f)in ESI {).With an increase of the reaction temperature the corrosion of TiO 2happened and the mesocrystals evolved into hollow structures (Fig.2(b))and finally,a via hole was formed through the (001)direction of the NPs formed (Fig.2(c)).Samples obtained under 150u C with different reaction times were observed by TEM.As illustrated in Fig.3(a),we can find that there was no crystal formed when the hydrothermal time was only 0.5h.The amorphous properties of the precursors were further confirmed by XRD (Fig.S2in ESI {).With increasing reaction time and temperature,the precursors gradually dis-appeared and the porous mesocrystals appeared and maintained the size of y 180nm.The corrosion effect began and then led to a symmetrical hollow structure,which can be clearly observed in the insert in Fig.2(b).Fig.4indicates the discontinuous size distribution of the TiO 2NPs formed in the hydrothermal procedures at different reaction temperatures (Fig.4(a))and time (Fig.4(b)).In normal crystal growth processes,the size distribu-tion of the products always maintains at a certain range and often has a relatively continuous variation under the different reaction conditions.This process was often interpreted by the mechanism of Ostwald ripening.However,in our crystal growth processes,the size of the products was y 6.7in the non-H 2SO 4system and leaped to y 180nm after introducing H 2SO 4into the system,and we proposed that this phenomenon could be attributed to another crystal growth mechanism termed ‘‘oriented attachment’’result-ing from the effect of the H 2SO 4addition into the system.This view will be confirmed in the following parts.As is well known,in order to prepare small scale anatase TiO 2in high dispersion,the hydrolysis rate needs to be slowed down to avoid aggregation of TiO 2NPs into bulk.OA and OM played quite important parts as capping agents in the formation of tiny anatase TiO 2crystals.9,24With the carboxylic acid functional groups and amidogen functional groups,OA and OM have been demonstrated to tightly bind onto the (001)and (101)crystal faces of TiO 2,respectively.25,26In this procedure,Ti(SO 4)2was partly hydrolyzed and formed complexes with surfactants of OA and OM.Nucleation then began and the crystal nucleus coarsened in the mechanism of Ostwald ripening via a hydrolytic condensation or a nonhydrolytic condensation process.The shape and size of TiO 2NPs can be tuned by controlling the ratio of OA :OM due to the their binding effect on the surface of the TiO 2.9As shown in Fig.1,truncated rhombic TiO 2NPs were formed when the ratio of OA :OM is 3:1.HRTEM images were taken to study the details of the TiO 2mesocrystals and hollow structures,as shown in Fig.5.We can clearly see the homogeneous pores in Fig.5(a),which formed by an aggregation of tiny nano subunits through oriented attach-ment.It was demonstrated in the literature that it is normal to form porous structures by the growth mechanism of oriented attachment.19As shown in the magnified TEM image in Fig.5(d),there is no obvious boundary of the subunits.But uniform lattice fringes,which are similar to a single crystal-lographic orientation,representing the (112)atomic planes of the anatase TiO 2with a space of 0.232nm (Fig.5(d)),could be found.This further proves that the TiO 2mesocrystals were formed from the subunits by oriented attachment.As showninFig.2(a)–(c)SEM images of mesocrystals and hollow structured TiO 2NPs synthesized by introducing H 2SO 4into the OA and OM system at different temperatures:(a)150,(b)180,(c)210u C.The inserts in (a),(b)and (c)are the corresponding TEM images.(d)XRD pattern of as-prepared TiO 2NPs of (a).P u b l i s h e d o n 03 S e p t e m b e r 2012. D o w n l o a d e d b y E a s t C h i n a U n i v e r s i t y o f S c i e n c e & T e c h n o l o g y o n 17/10/2014 14:15:13.Fig.5(b),it is interesting to note that there are four petal-like structures at one end of the NPs,indicating that the intermediate TiO 2NPs are mesocrystals formed from the assembly of nano subunits instead of single crystals formed by the mechanism of Ostwald ripening.From the Fourier transform patterns in Fig.5(e)and (f),the uniform lattice fringes with a space of 0.225nm corresponding to (112)facets and 0.19nm correspond-ing to (101)facets also suggest a single crystal-like structure.However,with prolonged reaction time,the pores of the mesocrystals do not seem to be as homogeneous as the previous products and some bigger NPs appear in the mesocrystals with indistinct boundary,as highlighted in the insert of Fig.5(c).The phenomenon could be attributed to the fusion and recrystalliza-tion in the mechanism of Ostwald ripening between adjacent nano subunits.27Furthermore,with longer reaction time and at higher temperature,some small hollows were generated in the mesocrystals caused by the corrosion effects action of the concentrated H 2SO 4.A series of experiments were designed to find more details about the intermediates during the crystal growth process of TiO 2.As shown in Fig.6,the products are close in final size to the intermediates,including some smaller NPs and mesocrystals,under the condition of 150u C for 10h,with tripling the dosage of all the synthetic materials.In such cases,both the assembly and fusion of the NPs were stopped to some extent due to the inhomogenous reaction.From the marked areas in Fig.6(a),weFig.3TEM images of precipitation obtained at 150u C from different reaction times:(a)0.5,(b)1,(c)2,(d)8,(e)24,(f)48h.Fig.4TiO 2particle size distribution at (a)different reaction temperatures for 8h and (b)different reaction times at 150u C.P u b l i s h e d o n 03 S e p t e m b e r 2012. D o w n l o a d e d b y E a s t C h i n a U n i v e r s i t y o f S c i e n c e & T e c h n o l o g y o n 17/10/2014 14:15:13.can clearly see that the particles have grown into short rods along the (001)direction,suggesting that the assembly of small TiO 2NPs takes place in the way of oriented attachment.Moreover,an incomplete mesocrystal with lots of small particles around it,presented in Fig.6(b),reveals the process of crystal growth.Fig.6(c)is the enlarged view of the marked part of Fig.6(b).An ellipse-marked nanoparticle in the edge of the mesocrystal shows its distinct boundaries,indicating that the nanoparticles have not recrystallized completely into the mesocrystal.A small and complete nanoparticle attaching to the edge of the mesocrystal is marked,which has an obvious lattice.As shown by Fourier transform patterns corresponding to the areas in Fig.6(c),the edge part of the mesocrystal marked with 2has different lattice fringe orientations,which are similar to those of multicrystals.In contrast,the area in the middle of the mesocrystal marked with 1has a single crystallographic orientation.At this point,with all the discussion above,we can assume that after premier aggregation the NPs adjust themselves to attach with each other along the (001)direction and then fuse into a mesocrystal with the same lattice fringe orientation.At the beginning of the reaction in the system with H 2SO 4,H 2SO 4had no obvious effect on the system due to the low temperature (no more than 90u C,see Fig.S1(e),ESI {).As mentioned before,Ti(SO 4)2partly hydrolyzed and some com-plexes,as the precursor,were formed with the surfactants of OA and OM.After increasing the reaction time and temperature,functional groups or even carbon chains of OA and OM could be destroyed by H 2SO 4,which were mixed well with Ti(SO 4)2in the solution.While the adsorption and protection effect of OAandFig.5HRTEM images of TiO 2mesocrystals and hollow structures obtained under different reaction times (a),(d)1h,(b),(e)3h,(c),(f)12h on the same reaction temperature of 150uC.Fig.6HRTEM of products obtained under the condition of 150u C for 10h.(c)shows the detail (with enlarged scale)of the marked part of (b).The Fourier transform patterns 1and 2correspond to the areas 1and 2in (c).P u b l i s h e d o n 03 S e p t e m b e r 2012. D o w n l o a d e d b y E a s t C h i n a U n i v e r s i t y o f S c i e n c e & T e c h n o l o g y o n 17/10/2014 14:15:13.OM were weakened,the unstable nano-subunits of TiO 2could aggregate quickly together with each other in order to reduce the surface activation energy and become a relatively stable phase with low energy after their nucleation.As is well known,the (001)facets of anatase TiO 2with the biggest number of dangling bonds have higher surface free energy than the (101)facets.25,28Thus,it is reasonable for the TiO 2crystal to be assembling and fusing together along the (001)direction,exposing the (101)facets and being spindle-shaped NPs at last.As shown by the TEM images of Fig.S1(a)and (e),ESI,{we can figure out that tiny TiO 2NPs could crystallize at a low reaction temperature of 90u C,but there were no crystals formed in the system with H 2SO 4under the same hydrothermal condition.Therefore,it could be confirmed that the assembly of TiO 2nano-subunits only appeared after nucleation at a higher temperature.And in the presence of H 2SO 4,the low temperature of 90u C might not be able to provide enough energy for nucleation,not to mention the aggregation.With this in mind,it is easy to understand why there were still some precursors in the products obtained at lower temperature or with less time.Different amounts of H 2SO 4were introduced into the hydrothermal system to further reveal their effect on TiO 2crystalization.The results are shown in Fig.S3,ESI.{From the TEM images,with less H 2SO 4in the reaction system,the extent of nano subunits aggregation was restricted in a small scale by the protection effect of the capping agents of OA and OM.With increasing the amount of H 2SO 4,the size of the mesocrystal became uniform and the morphology of the NPs trended to change from spindle-shaped into spherical-shape due to the aggregation of the nano-subunits on the (101)facets.As comparison,a series of control experiments were carried out using other inorganic acids,organic acid and sodium sulfate instead of H 2SO 4,with the equimolar quantity to the hydrogen ions and sulfate ions,and the results are shown in Fig.S4and Fig.S5,ESI.{In Fig.S4(ESI {),the TiO 2NPs are monodisperse and have no obvious assembly,which means that the sulfate ion does not have a conspicuous effect on the formation of TiO 2porous mesocrystals and hollow structures.When different kinds of acids were added into the system with Na 2SO 4,we can find the assembly happened more easily in the system with strong acid:HNO 3and HCl.In the system with weak acid,CH 3COOH,the products are monodisperse NPs (see Fig.S5(a,c,e),ESI {).Additionally,the variation trend of the crystal growth is almost the same in the system without Na 2SO 4,which confirms that the hydrogen ion plays a more important role than the sulfate ion in the hydrothermal reaction system.Among these results,the products obtained from the system with HCl have similar spindle-shapes with those prepared in the system with H 2SO 4.However,the length of these is only around 83nm,smaller than the 150–220nm for the system with H 2SO 4.In general,the hydrogen ion plays an important role in the assembly of TiO 2NPs because of their interaction with OA and OM under the hydrothermal condition,but only H 2SO 4has a corrosion action on TiO 2so that the hollow structures can be obtained.With increasing reaction time and temperature,the nano-subunits with little protection of surfactants in the middle of the mesocrystals were firstly etched and then the mesocrystals turned to be a hollow structure inside (shown in Fig.2(b,c)).We wanted to know what we would get from a two-step process forintroducing more H 2SO 4into the system.That is,after the mesocrystals are formed in the first step process,then excess H 2SO 4is added in a second step process.Fig.7shows the HRTEM and HAADF-STEM images of the products from a two-step paring to OA and OM with long carbon chains,H 2SO 4(as small molecules)could easily go through the pores and into the inside of the mesocrystal.Meanwhile,the residual OA and OM were tightly bound to the surface of the mesocrystal acting as protective films,which could prevent the erosion caused by H 2SO 4,making the shell more firm.So it iseasyFig.7TEM and HAADF-STEM images of hollow spindle-shaped TiO 2obtained via a two-step process at a temperature of 150uC.Fig.8The crystal growth schematic diagram of tiny anatase TiO 2NPs and TiO 2mesocrystals and hollow structures.P u b l i s h e d o n 03 S e p t e m b e r 2012. D o w n l o a d e d b y E a s t C h i n a U n i v e r s i t y o f S c i e n c e & T e c h n o l o g y o n 17/10/2014 14:15:13.to understand why the erosion of TiO 2started in the middle of the mesocrystals.But with increasing duration of the reaction,the erosion happened from core to the shell in some hollow NPs,which are displayed in Fig.7.As illustrated in Fig.7(c,d),we can clearly find that on one half of the particle there is only a skeleton structure after the erosion.From Fig.7(e,f),it can be observed that there is only a half of the shell.In general,we have provided a new and simple way to synthesize hollow anatase TiO 2structures without any template.We drew a schematic diagram in Fig.8to show the process of crystal growth.Tiny anatase TiO 2NPs formed in the non-H 2SO 4system by the mechanism of Ostwald ripening,and TiO 2mesocrystals and hollow structures were then obtained,involving both oriented attachment and Ostwald ripening.After being calcined at 300u C for 3h,the mesocrystals obtained from the temperature of 150u C for 8h exhibit 62.3m 2g 21of surface area by a nitrogen Brunauer–Emmett–Teller (BET)measurement,comparing to 40.5m 2g 21of the products formed by prolonging the reaction time to 48h.The reduction of surface area can be attributed to the decrease of the amount of pores caused by the fusion in the adjacent nano-subunits and the erosion by H 2SO 4that resulted in hollow structures.Fig.9(b)indicates that the nanopores in the mesocrystals have a narrow distribution centered at 3.7nm.This result confirms that the products are mesocrystals with quite uniform nanopores formed in the self-assembly process by the mechanism of oriented attachment.ConclusionsIn conclusion,we developed a new route to synthesize anatase TiO 2NPs whose size changed based on the self-assembly of primary nanocrystals.By tuning the reaction conditions,the crystal growth processes were investigated in detail.Based on our results,porous mesocrystal TiO 2NPs and uniform hollow NPs could be obtained in the system involving the effect of adsorption and protection of OA and OM surfactants on the surface of TiO 2nano crystals,and of damage in functional groups or even in carbon chains of OA and OM and the corrosion in TiO 2NPs caused by H 2SO 4.In the case of theformation of larger TiO 2NPs,the process could be described as the co-effect of both oriented attachment and Ostwald ripening.Our work may afford an insight into the understanding of crystal growth mechanism and provide a simple method to synthesize anatase TiO 2mesocrystals and hollow structures,which have potential in several application areas,although more efforts are needed in future because there are still some more unknown details in the crystallization procedure.AcknowledgementsThis work was supported by NSFC (91127040,20921001),the State Key Project of Fundamental Research for Nanoscience and Nanotechnology (2011CB932402).References1X.Wang,J.Zhuang,Q.Peng and Y.Li,Nature ,2005,437,121–124.2H.G.Yang,C.H.Sun,S.Z.Qiao,J.Zou,G.Liu,S.C.Smith,H.M.Cheng and G.Q.Lu,Nature ,2008,453,638–641.3 B.Liu and H.C.Zeng,Small ,2005,1,566–571.4 D.W.Jing,R.Li,M.C.Liu and L.J.Guo,I &EC ,2011,8,446–457.5J.Su,L.Guo,N.Bao and C.A.Grimes,Nano Lett.,2011,11,1928–1933.6J.Hui and X.Wang,Chem.–Eur.J.,2011,17,6926–6930.7S.Ding,J.S.Chen,Z.Wang,Y.L.Cheah,S.Madhavi,X.Hu and X.W.Lou,J.Mater.Chem.,2011,21,1677.8G.Xiang,J.Zhuang and X.Wang,Inorg.Chem.,2009,48,10222–10230.9 C.-T.Dinh,T.-D.Nguyen,F.Kleitz and T.-O.Do,ACS Nano ,2009,3,3737–3743.10 C.Chen,R.Hu,K.Mai,Z.Ren,H.Wang,G.Qian and Z.Wang,Cryst.Growth Des.,2011,11,5221–5226.11V.M.Yuwono,N.D.Burrows,J.A.Soltis and R.L.Penn,J.Am.Chem.Soc.,2010,132,2163–2165.12R.L.Penn,J.Phys.Chem.B ,2004,108,12707–12712.13R.L.Penn and J.F.Banfield,Geochim.Cosmochim.Acta ,1999,63,1549–1557.14Y.Liu,D.Wang,Q.Peng,D.Chu,X.Liu and Y.Li,Inorg.Chem.,2011,50,5841–5847.15Z.Zhang,H.Sun,X.Shao,D.Li,H.Yu and M.Han,Adv.Mater.,2005,17,42–47.16H.Xu,W.Wang,W.Zhu,L.Zhou and M.Ruan,Cryst.GrowthDes.,2007,7,2720–2724.17 D.Zitoun,N.Pinna,N.Frolet and C.Belin,J.Am.Chem.Soc.,2005,127,15034–15035.18Q.Zhang,S.-J.Liu and S.-H.Yu,J.Mater.Chem.,2009,19,191.Fig.9(a)N 2adsorption–desorption isotherms and (b)the corresponding pore size distribution curves obtained from the desorption branch of porous anatase TiO 2mesocrystals synthesized at 150u C after 8h.P u b l i s h e d o n 03 S e p t e m b e r 2012. D o w n l o a d e d b y E a s t C h i n a U n i v e r s i t y o f S c i e n c e & T e c h n o l o g y o n 17/10/2014 14:15:13.。
阳极氧化法制备TiO_2纳米管阵列膜及其应用
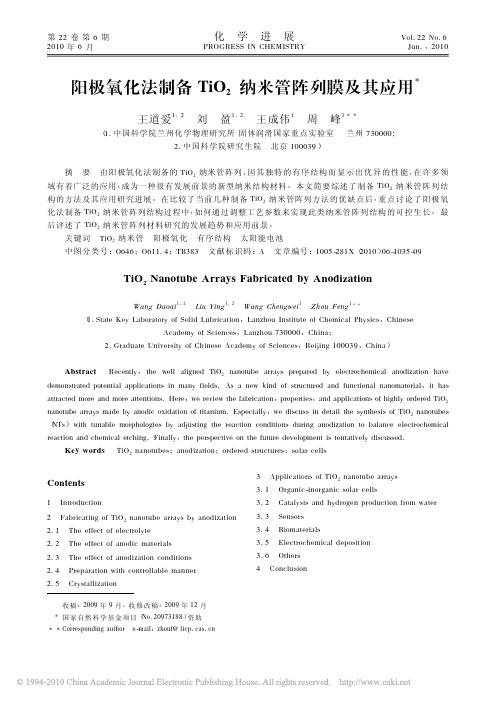
, 在国际上
。
阳极 氧 化 法 制 备 TiO 2 纳 米 管 阵 列 一 般 采 用 两 电极体系, 阳 极 为 钛 箔 板, 阴极为铂电极或石墨电 极, 电解液采用含氟 离 子 的 水 溶 液 或 其 他 有 机 溶 剂 ( 图 1 ) 。 当在 两 极 施 加 一 定 电 压 时, 钛箔板被氧化 形成管状无定形 TiO 2 阵列膜, 经不同温度热处理后 即可得到不 同 晶 型 的 TiO 2 纳 米 管 阵 列 膜 。 此 种 方 法制备的 TiO 2 纳米管与 基 底 垂 直 取 向,管 径 、 管长 度以及壁厚 均 匀 可 控, 与 钛 基 底 结 合 牢 固, 不易脱 落 。 由于该材料为高度有序的纳米管阵列结构,具
Contents
1 2 2. 1 2. 2 2. 3 2. 4 2. 5 Introduction Fabricating of TiO 2 nanotube arrays by anodization The effect of electrolyte The effect of anodic materials The effect of anodization conditions Preparation with controllable manner Crystallization
demonstrated potential applications in many fields. As a new kind of structured and functional nanomaterial ,it has attracted more and more attentions. Here ,we review the fabrication, properties,and applications of highly ordered TiO2 nanotube arrays made by anodic oxidation of titanium. Especially ,we discuss in detail the synthesis of TiO2 nanotubes ( NTs) with tunable morphologies by adjusting the reaction conditions during anodization to balance electrochemical reaction and chemical etching. Finally ,the perspective on the future development is tentatively discussed. Key words TiO 2 nanotubes ; anodization ; ordered structures ; solar cells 3 3. 1 3. 2 3. 3 3. 4 3. 5 3. 6 4 Applications of TiO 2 nanotube arrays Organic-inorganic solar cells Catalysis and hydrogen production from water Sensors Biomaterials Electrochemical deposition Others Conclusion
表面修饰二氧化钛纳米粒子的结构表征及形成机理
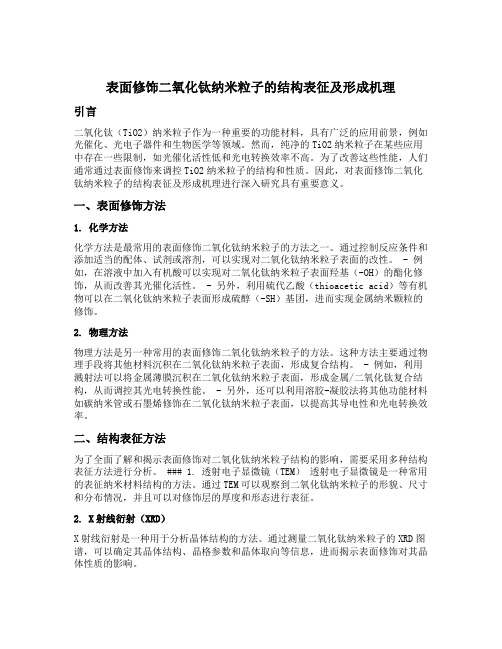
表面修饰二氧化钛纳米粒子的结构表征及形成机理引言二氧化钛(TiO2)纳米粒子作为一种重要的功能材料,具有广泛的应用前景,例如光催化、光电子器件和生物医学等领域。
然而,纯净的TiO2纳米粒子在某些应用中存在一些限制,如光催化活性低和光电转换效率不高。
为了改善这些性能,人们通常通过表面修饰来调控TiO2纳米粒子的结构和性质。
因此,对表面修饰二氧化钛纳米粒子的结构表征及形成机理进行深入研究具有重要意义。
一、表面修饰方法1. 化学方法化学方法是最常用的表面修饰二氧化钛纳米粒子的方法之一。
通过控制反应条件和添加适当的配体、试剂或溶剂,可以实现对二氧化钛纳米粒子表面的改性。
- 例如,在溶液中加入有机酸可以实现对二氧化钛纳米粒子表面羟基(-OH)的酯化修饰,从而改善其光催化活性。
- 另外,利用硫代乙酸(thioacetic acid)等有机物可以在二氧化钛纳米粒子表面形成硫醇(-SH)基团,进而实现金属纳米颗粒的修饰。
2. 物理方法物理方法是另一种常用的表面修饰二氧化钛纳米粒子的方法。
这种方法主要通过物理手段将其他材料沉积在二氧化钛纳米粒子表面,形成复合结构。
- 例如,利用溅射法可以将金属薄膜沉积在二氧化钛纳米粒子表面,形成金属/二氧化钛复合结构,从而调控其光电转换性能。
- 另外,还可以利用溶胶-凝胶法将其他功能材料如碳纳米管或石墨烯修饰在二氧化钛纳米粒子表面,以提高其导电性和光电转换效率。
二、结构表征方法为了全面了解和揭示表面修饰对二氧化钛纳米粒子结构的影响,需要采用多种结构表征方法进行分析。
### 1. 透射电子显微镜(TEM)透射电子显微镜是一种常用的表征纳米材料结构的方法。
通过TEM可以观察到二氧化钛纳米粒子的形貌、尺寸和分布情况,并且可以对修饰层的厚度和形态进行表征。
2. X射线衍射(XRD)X射线衍射是一种用于分析晶体结构的方法。
通过测量二氧化钛纳米粒子的XRD图谱,可以确定其晶体结构、晶格参数和晶体取向等信息,进而揭示表面修饰对其晶体性质的影响。
TiO_2基钙钛矿太阳能电池的界面修饰及其性能研究
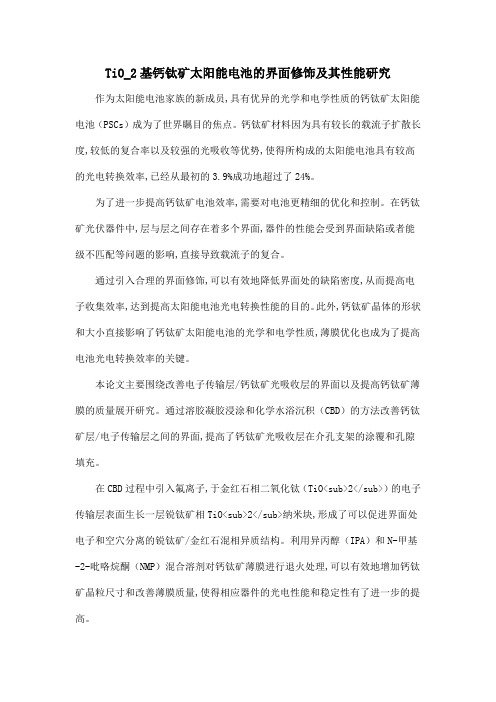
TiO_2基钙钛矿太阳能电池的界面修饰及其性能研究作为太阳能电池家族的新成员,具有优异的光学和电学性质的钙钛矿太阳能电池(PSCs)成为了世界瞩目的焦点。
钙钛矿材料因为具有较长的载流子扩散长度,较低的复合率以及较强的光吸收等优势,使得所构成的太阳能电池具有较高的光电转换效率,已经从最初的3.9%成功地超过了24%。
为了进一步提高钙钛矿电池效率,需要对电池更精细的优化和控制。
在钙钛矿光伏器件中,层与层之间存在着多个界面,器件的性能会受到界面缺陷或者能级不匹配等问题的影响,直接导致载流子的复合。
通过引入合理的界面修饰,可以有效地降低界面处的缺陷密度,从而提高电子收集效率,达到提高太阳能电池光电转换性能的目的。
此外,钙钛矿晶体的形状和大小直接影响了钙钛矿太阳能电池的光学和电学性质,薄膜优化也成为了提高电池光电转换效率的关键。
本论文主要围绕改善电子传输层/钙钛矿光吸收层的界面以及提高钙钛矿薄膜的质量展开研究。
通过溶胶凝胶浸涂和化学水浴沉积(CBD)的方法改善钙钛矿层/电子传输层之间的界面,提高了钙钛矿光吸收层在介孔支架的涂覆和孔隙填充。
在CBD过程中引入氟离子,于金红石相二氧化钛(TiO<sub>2</sub>)的电子传输层表面生长一层锐钛矿相TiO<sub>2</sub>纳米块,形成了可以促进界面处电子和空穴分离的锐钛矿/金红石混相异质结构。
利用异丙醇(IPA)和N-甲基-2-吡咯烷酮(NMP)混合溶剂对钙钛矿薄膜进行退火处理,可以有效地增加钙钛矿晶粒尺寸和改善薄膜质量,使得相应器件的光电性能和稳定性有了进一步的提高。
主要内容如下:(1)利用TiO<sub>2</sub>溶胶凝胶浸涂的方法对TiO<sub>2</sub>纳米棒表面进行修饰,通过分析形貌和结构信息,探究TiO<sub>2</sub>纳米颗粒对PSCs光电性能的影响。
TiO-2纳米半导体材料的制备及其光探测性能

TiO_2纳米半导体材料的制备及其光探测性能半导体光探测器在军事和国民经济的各个领域均有广泛应用,如光纤通讯系统、成相遥感、环境监测以及光度计量等。
已经商业化的光探测器主要基于Si、GaAs等半导体材料,但是这些材料因具有较窄的禁带宽度,紫外光选择性较差,并需要可见、红外光过滤器。
TiO2纳米半导体材料,因其较宽的禁带宽度,具有良好的紫外光选择性。
同时还具有稳定的化学性质、较强的耐候性、高的电子注入率和传导性能等其它半导体材料所不具备的优点,在光电探测领域发挥越来越重要的作用。
在TiO2紫外光探测器的研究中,一直存在高光响应度和高响应速率难以兼得的问题。
本文采用具有高紫外光吸收能力的聚合物以及纳米颗粒支化纳米棒的结构改性,提高了TiO2紫外光探测器光响应度的同时得到了较快的响应速率。
为了拓展TiO2对可见光的探测应用,又采用有机染料和导电聚合物聚吡咯对TiO2改性,制备出具有单波长可见光选择性的光谱探测器,并验证了这种可见光探测器具有普适性,可以扩展到其它半导体体系。
为提高TiO2紫外光探测器的光响应度,提出采用聚苯乙烯磺酸(PSS)改性TiO2纳米颗粒来增强紫外光吸收和增加光生电子-空穴对。
分别以TiO2纳米颗粒及其PSS改性复合材料构建了三明治结构的光导型紫外光探测器。
TiO2纳米颗粒光探测器的光响应度和光/暗电流比为1.2AW-1和40,响应时间为22.63s(上升时间)和15.93s(衰减时间),紫外/可见抑制比为86,表现出良好的紫外光选择性。
PSS改性后的复合材料紫外光探测器的光响应度随着PSS层数增多而增大,10层PSS/TiO2复合材料的光响应度比5层PSS复合材料高出1个数量级。
10层PSS/TiO2复合材料的光响应度和光/暗电流比分别为602AW-1和1932,与纯TiO2纳米颗粒相比,均提高了3个数量级。
这一高光响应度比大部分报道的TiO2纳米颗粒紫外光探测器高出1-2个数量级。
TiO2纳米结构、复合及其光催化性能研究共3篇
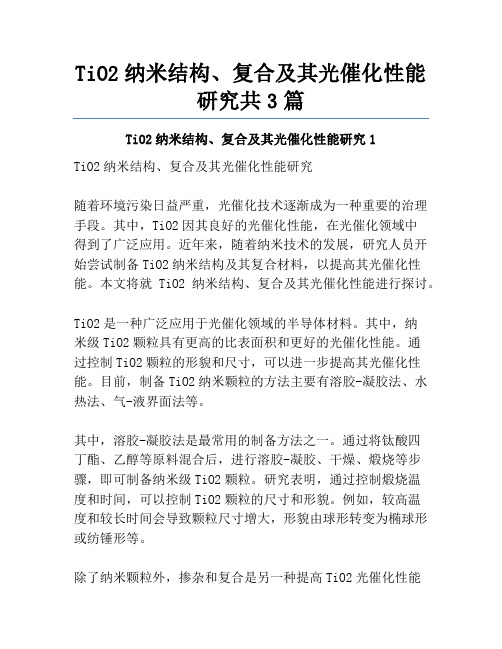
TiO2纳米结构、复合及其光催化性能研究共3篇TiO2纳米结构、复合及其光催化性能研究1TiO2纳米结构、复合及其光催化性能研究随着环境污染日益严重,光催化技术逐渐成为一种重要的治理手段。
其中,TiO2因其良好的光催化性能,在光催化领域中得到了广泛应用。
近年来,随着纳米技术的发展,研究人员开始尝试制备TiO2纳米结构及其复合材料,以提高其光催化性能。
本文将就TiO2纳米结构、复合及其光催化性能进行探讨。
TiO2是一种广泛应用于光催化领域的半导体材料。
其中,纳米级TiO2颗粒具有更高的比表面积和更好的光催化性能。
通过控制TiO2颗粒的形貌和尺寸,可以进一步提高其光催化性能。
目前,制备TiO2纳米颗粒的方法主要有溶胶-凝胶法、水热法、气-液界面法等。
其中,溶胶-凝胶法是最常用的制备方法之一。
通过将钛酸四丁酯、乙醇等原料混合后,进行溶胶-凝胶、干燥、煅烧等步骤,即可制备纳米级TiO2颗粒。
研究表明,通过控制煅烧温度和时间,可以控制TiO2颗粒的尺寸和形貌。
例如,较高温度和较长时间会导致颗粒尺寸增大,形貌由球形转变为椭球形或纺锤形等。
除了纳米颗粒外,掺杂和复合是另一种提高TiO2光催化性能的有效手段。
掺杂主要是通过将其他元素掺入TiO2晶格中,以改变其电子结构,提高光催化性能。
目前常用的掺杂元素包括银、氮、碳等。
复合则是将TiO2与其他材料复合,以提高其光催化稳定性和性能。
常用的复合材料包括金属氧化物、石墨烯、聚合物等。
对于掺杂TiO2,研究发现,掺杂银元素可以增加TiO2的光催化活性和稳定性。
由于银元素具有良好的表面等离子共振吸收效应,可促进TiO2的光吸收和电子传输。
同时,掺杂氮和碳元素可以缩小TiO2带隙,增强光吸收效果。
对于复合TiO2,研究发现,纳米级TiO2颗粒与金属氧化物复合,可以提高其光吸收和电子传输效果,从而提高光催化性能。
总体而言,制备TiO2纳米结构、掺杂和复合是提高TiO2光催化性能的有效手段。
(2011)Synthesis of TiO2 nanoparticles by premixed stagnation swirl flames

Synthesis of TiO 2nanoparticles by premixedstagnation swirl flamesJunjing Wang a ,Shuiqing Li a ,*,Wen Yan a ,Stephen D.Tse a ,b ,**,Qiang Yao aaKey Laboratory for Thermal Science and Power Engineering of Ministry of Education,Department of Thermal Engineering,Tsinghua University,Beijing 100084,ChinabDepartment of Mechanical and Aerospace Engineering,Rutgers,The State University of New Jersey,Piscataway,NJ 08854,USAAvailable online 6August 2010AbstractA new turbulent,premixed,stagnation swirl flame (SSF)is used to synthesize titanium dioxide (TiO 2)nanopowders.Synthesis conditions under two flame modes,i.e.,burner-and substrate-stabilized SSF,are investigated,for the same equivalence ratio,but different inert-dilution ratios.The particles (collected on the substrate)have high anatase purity,with mean diameters of 5–10nm,determined using BET and TEM,for all cases studied.For the same mean nanoparticle diameter synthesized,the SSF can accommodate higher precursor loading fluxes than that produced by others using laminar premixed stagnation flat flames.Particles in the flow field are determined to be non-agglomerated.For the particles deposited on the substrate,molecular dynamics simulations support the experimental results that sintering and growth of TiO 2nanoparticles do not occur on the substrate after the deposition,and the high anatase-phase purity is maintained.Ó2010The Combustion Institute.Published by Elsevier Inc.All rights reserved.Keywords:Flame synthesis;TiO 2nanoparticles;Stagnation flame;Swirl flame;MD simulation1.IntroductionUltrafine titanium oxides (e.g.,TiO 2)are expected to play important roles in addressing various environmental and energy challenges,due to their unique properties applicable for solar-energy conversion based on photocatalytic,photovoltaic,and water-splitting devices [1–3].TiO 2properties are strongly influenced by size,polymorph,and phase purity.For example,ana-tase titania,rather than rutile,possesses the requi-site properties for the above applications [4].The thermodynamic phase stability of nanocrystalline aggregates during growth is dependent on the ini-tial particle sizes [5].It has been reported that,for equally sized nanoparticles,anatase is thermody-namically stable for sizes <11nm,that brookite is stable for sizes between 11and 35nm,and that rutile is stable for sizes >35nm [6].Photocatalytic activity is enhanced with decreasing TiO 2particle size,with an increasing fraction of atoms located at the particle surface.On the other hand,while the increase in band-gap energy in relation to a decrease in nanoparticle1540-7489/$-see front matter Ó2010The Combustion Institute.Published by Elsevier Inc.All rights reserved.doi:10.1016/j.proci.2010.05.022*Corresponding author.Fax:+861062794068.**Corresponding author.Fax:+17324453124.E-mail addresses:lishuiqing@ (S.Li),sdytse@ (S.D.Tse).Proceedings of the Combustion Institute 33(2011)1925–1932/locate/prociProceedings of theCombustion Institutesize enhances the redox potential of the valence band holes and the conduction band electrons [1],the efficiency of TiO2nanoparticles as photo-catalysts becomes limited as only a small percent-age of the sunlight spectrum can be utilized.As such,studies have been conducted tofind optimal sizes for specific photocatalytic reactions.For example,in trying to achieve maximum photocat-alytic efficiency in the decomposition of chloro-form,Ref.[7]found an improvement in activity when the particle size is decreased from21to 11nm,while the activity decreases when the size is reduced further to6nm.In this work,the opti-mum mean particle size is found to be$10nm, where bulk recombination of charge carriers in lar-ger TiO2nanoparticles balances surface recombi-nation in smaller particles.In addition,it has been reported that7nm particles show1.6times better photocatalytic activity than those of TiO2 P25and other15-and30-nm particles in the decomposition of2-propanol[8].Therefore,pro-ducing anatase-dominated TiO2nanoparticles with sizes<10nm,which is highly dependent on the method and conditions of synthesis,is of con-siderable interest.Flame synthesis is an attractive technique, where particle size,crystallinity,and phase purity can be precisely controlled,along with the poten-tial scale up for high-throughput production,giv-ing it an advantage over other methods such as wet-chemical and chemical vapor deposition[3]. Moreover,flame-made catalysts(compared with wet-made catalysts),e.g.,TiO2composites,can be fabricated with an open-pore structure that should facilitate mass-transfer limited reactions [9].Reportedflame synthesis configurations include co-flow diffusionflame[9,10],counterflow diffusionflame[11],multi-element diffusionflame [12],burner-stabilized premixed stagnationflat flame[13,14],and divergence-stabilized premixed stagnationflatflame(SFF)[3,15].In the latter two SFF configurations[3,13–15],the cooled stag-nation substrate provides many advantages,such as:(i)steep temperature gradient for nanoparticle formation,(ii)controllable temperature histories based on burner–substrate distance,(iii)flame sta-bilization,(iv)collection source for TiO2nanopar-ticles deposition by thermophoresis,and(v) electricfield application.Thus,using the stagna-tion configuration,synthesis of ultrafine anatase TiO2nanocrystals ranging from3to10nm is readily achieved.Finally,by setting the substrate at an“elevated”temperature,nanoparticles can sinter upon arrival,allowing for one-step continu-ous synthesis of porous TiO2nanocrystalfilms, e.g.,for use in dye-sensitized solar cells[15,16].Although the SFF can produce ultrafine (<10nm)TiO2photocatalysts,operation in the laminar regime may limit further scale-up to com-mercial production.Moreover,burners based on shower-head design are subject to particle clog-ging by upstream thermophoretic transport,mak-ing them troublesome for long-term industrial operation[13,14].The turbulent low-swirlflame, with swirl number S<0.6,has received consider-able attention in combustion applications because of its stability,ultralow NO x emissions,and ultra-lean operation[17–19].In a previous study,using identical methaneflow rate and precursor loading rate as that used in this paper,we found that introducing swirl into a free-jetflame(without stagnation substrate)producedfiner,less-aggre-gated TiO2nanoparticles[20].In this work,a novel turbulentflame configuration,termed here as stagnation-point swirlflame(SSF),is utilized for nanomaterials synthesis,combining the advantages of both substrate-established stagna-tionflames and low-swirlflames.The SSF is always detached from the burner,moderating over-heating and particle deposition.Addition-ally,theflame can beflexibly stabilized at either the burner or the substrate.As previously men-tioned,depending on substrate temperature,as well as temperature gradient along the burner–substrate axis,the sintering or growth of TiO2 nanoparticles may occur not only in theflowfield, but also on the substrate itself.Several publica-tions on modeling have investigated particle growth dynamics in theflowfield using the popu-lation balance model(PBM)[13,14,21].Compara-tively,theoretical studies on the sintering and growth of TiO2particles on the substrate are rare.The current study aims to demonstrate that anatase-phase TiO2of a few nanometers,with a narrow size distribution,can be synthesized using a novel SSF configuration,with promising scale-up prospects for high-throughput production. Specifically,the influences of the substrate-to-bur-ner distance and the precursor loading rate are investigated.Properties such as specific surface area(SAA),morphology and size,and crystalline phase of the particles collected on the substrate are characterized by Brunauer–Emmet–Teller (BET),transmission electron microscopy(TEM), and X-ray diffraction(XRD),respectively. Finally,molecular dynamics(MD)simulation is conducted to compare particle sintering and growth on the substrate with that observed in the experiments.2.ExperimentalFig.1depicts the SSF setup,along with the reactant and precursor delivery system.The SSF setup is composed of three main parts:(i)a down-ward stainless steel tube of18mm i.d.,(ii)a swir-ler located within the tube that is73mm above the tube exit,and(iii)a substrate below the burner exit(with adjustable distance).The swirler com-ponent consists of circumferential annular vanes with a tubular central bypassfitted with a perfo-1926J.Wang et al./Proceedings of the Combustion Institute33(2011)1925–1932rated screen,as shown in Fig.2.The swirler is 14mm long and has eight blades with an angle of 45°.The swirl number is dependent on the ratio of the jet flow in the central bypass to the swirl flow through the annular vanes.The outer diame-ter of the vane is 18mm,while that of the central bypass is 8.0mm.It can be altered by adjusting the mesh density of the perforated screen.The swirl number (S )is measured as 0.248to ensure operation in low-swirl combustion mode [19,20].The substrate is a 90mm Â90mm plate,which is mounted on a three-axis translation stage.The upper surface of the substrate is an aluminum sheet attached to a copper plate embedded with cooling-water channels.The temperature of the substrate surface (monitored by a 1-mm type K thermocouple located 0.5mm below the substrate surface)is maintained at 110±5°C,avoiding serious particle sintering and water vapor condensation.Nitrogen-diluted oxidizer is preheated to 170°C,combined with methane,and passed through a pre-mixing chamber,prior to delivery to the burner.CH 4/O 2/N 2mixture into the SSF setup.The liquid TTIP is heated by an electrical mantle to 80°C,with the downstream line heated to 130°C to avoid con-densation.The reactant flow exiting the premixing chamber is maintained above 130°C.Three precursor loading rates of TTIP are investigated in this work by adjusting the carrier gas flow rates from 0.5to 1.5L/min (where 1.0L/min gas delivers 0.025g/min TTIP precur-sor).For all synthesis runs,the equivalence ratio is purposely fixed at U =0.68,with flow rates of the CH 4and O 2set at 2.25and 6.60L/min,respectively.We isolate the effects of characteristic temperature by adjusting the dilution ratio of the oxidizer,i.e.,a =O 2/(O 2+N 2),via N 2dilution with nitrogen flow rates ranging from 20.0to 27.15L/ing the operation map (Fig.3,to be discussed later),two N 2dilution rates are utilized for the synthesis runs:one at 20.0L/minFig.1.Schematic diagram of the SSF nanopowder synthesis setup.Schematic of the swirler component.where a=0.2481(oxygen-enriched air),and the other at24.9L/min where a=0.2095(standard air).Theflame temperatures are measured by a 0.25mm type B thermocouple coated with SiO2 to minimize surface catalytic effects,in which radi-ation corrections are made for measurement preci-sion.Specifically,a TEM grid is mounted on a holder,which rotates in and out of theflowfield, similar to Refs.[3,22].The vertical distance of the TEM grid to the substrate surface is<1mm,and the sample collection time for each period is <21ms.The temperature of the TEM grid is <50°C.3.MD simulationTo assess the sintering behavior of nanoparti-cles on the substrate,atomic-level simulations of TiO2particle dynamics are performed using the molecular dynamics simulation open code, DL_POLY version2.18,from Daresbury labora-tories[23,24].The Matsui–Akaogi(MA)poten-tial,which has been validated by experimental data describing the structure and stability of TiO2particles[25–27],is employed to represent the detailed interactions between the constituent atoms of TiO2as given below:V ij¼qiqjr ijÀC i C jr6ijþfðB iþB jÞexpA iþA jÀr ijB iþB jð1Þwhere V ij is the interaction energy between atoms i and j.The three terms in the right-hand side of the equation represents Coulomb,dispersion,and repulsion interactions,respectively.The values of the parameters in Eq.(1)are given in Table1. The charges(q i)for titanium and oxygen are +2.196andÀ1.098,respectively,in atomic units.We extend the simulation procedure in Ref.[27]of the interaction of two TiO2nanoparticles from initial-stage sintering to complete sintering or coalescence.First,a perfect lattice is con-structed using parameters for anatase,given that anatase-phase dominates for TiO2nanocrystals with d p<10nm.Then,a2nm spherical nanopar-ticle is“excised”from the lattice structure.The excess titanium ions or oxygen ions are removed from the nanoparticle to obtain neutrality. Second,the neutral nanoparticle is simulated using the canonical ensemble(NVT)for15ns (3,000,000time steps with5fs steps),which is sufficient for approaching equilibrium at the given temperature.Third,the particle configuration is replicated and translated along the x-axis,to establish two particles with1nm separation dis-tance.Fourth,the sintering of two nanoparticles is investigated using the microcanonical ensemble (NVE).Simulations are run until the time-aver-aged mean temperature becomes constant,repre-senting system equilibrium.The initial particle temperatures examined are573,973,1273,1473, and1673K,respectively,with the simulation time ranging from2to10ns.4.Results and discussionFirst,we map the operating limits of the stag-nation-swirlflame to determine the appropriate conditions for TiO2synthesis.Withfixed U,the dilution ratio a can be varied to determine the dilution blowout(DBO)limit.Fig.3illustrates the dilution blowout limit(DBO)and synthesis conditions of the SSF burner with S=0.248, CH4=2.25L/min,and O2=6.6L/min(i.e., U=0.64).As the substrate–burner distance(d sÀb) increases,the DBO limit decreases,reaching a minimum value of a=0.1956at d sÀb=18mm, and then increases again.Note that d sÀb at the minimum DBO limit(a)nearly equals the inside diameter of the burner ing this operating map,we set eight runs for TiO2synthesis.Three runs are operated at a=0.2095with d sÀb of12, 18and24mm,respectively.We denote these cases as s-SSF since theflames are stabilized close to the substrate surface,whoseflame configurations, with and without the synthesis,are both shown in Fig.1.The otherfive synthesis runs are oper-ated at a=0.24812with d sÀb ranging from6to 36mm.We denote these cases as b-SSF because theflames are stabilized close to the burner tip, also shown in Fig.1.Nevertheless,the SSF is always detached from the burner,permitting long-time operation with negligible powder depo-sition on the tube tip.All experiments are run for 45min,ensuring sufficient nanopowder produc-tion for collection at the substrate.Fig.4illustrates the effect of substrate–burner distance d sÀb on the SSA of as-synthesized TiO2 nanopowders,as determined by BET for all cases. For the b-SSF cases,the SSA decreases from277 to184m2/g as d sÀb increases from6to36mm. Correspondingly,assuming spherical,monodis-perse primary particles with homogeneous density (q=3840kg/m3),the calculated average BET particle diameter,d BET,increases from 5.7to8.5nm.This trend is expected as the increase ofd sÀb from6to36mm results in both a decrease in the average temperature gradient between the burner and substrate(170–25K/mm)and an increase in mean particle residence time in theflow field.As shown in Fig.3,the SSF stabilizes closeTable1The interaction parameters for Matsui–Akaogipotential.q A B CTi 2.196 1.18230.07722.5OÀ1.098 1.63390.11754.01928J.Wang et al./Proceedings of the Combustion Institute33(2011)1925–1932to the burner tip,and the maximum flame temper-atures are $1850±50K for all five d s Àb studied.However,for the s-SSF cases,the SSA remains relatively constant in a range of 274–290m 2/g,insensitive to the variation in d s Àb .The corre-sponding d BET of the nanoparticles are about 5.4–5.7nm.In contrast to the b-SSF,the s-SSF is stabilized close to the substrate surface.Precur-sor oxidation and nanoparticle nucleation/coagu-lation are likely confined to the thin zone (see Fig.3)between the flame sheet (1680±40K)and the substrate (383±5K),in which the tem-perature gradient and temperature history of the particles are similar for different d s Àb .In addition,this difference between b-SSF and s-SSF may also be attributed to the difference in characteristic flame temperature.Since s-SSF has a relatively low flame temperature,particles may coagulate but not seriously coalesce,with longer burner-substrate distance resulting in growth in aggregate size,but not decrease in SSA.As can be seen,both b-SSF and s-SSF cases produce ultrafine TiO 2nanocrystals with sizes of 5–10nm,satisfying the characteristic size of ther-modynamically-stable anatase TiO 2for use as photocatalysts [1,6].Moreover,the SSFthe TiO 2nanopowders uniformly,laminar burner-stabilized SFF [14]and gence-stabilized SFF [15],but at higher The scale-up of the SSF will also ing the dependence of nanoparticle size sor loading.Fig.5illustrates the effect gas flow rate (and thus precursor on the SSA of TiO 2nanoparticles the s-SSF case where d s Àb =18mm.the heated temperature of the 1.0L/min carrier gas flow rate delivers min TTIP precursor to the burner.As gas flow rate increases from 0.5to 2.0SSA of the produced TiO 2nanopowders almost linearly from 313.08to 188.35m 2sponding to d BET from 5.0to 8.4nm.tively,TEM imaging gives from 5.9to 9.3nm,in the range of that calculated based on BET.A case with carrier flow rate of 1.0L/min that produces TiO 2nanoparticles of d BET =5.8nm is compared with similar 6nm TiO 2nanoparticles produced by burner-stabilized [14]and diver-gence-stabilized SFFs [15].For producing the sim-ilar $6nm TiO 2,the TTIP loading rate per burner nozzle flow-area (mass flux)of the s-SSF is about 98.4g/(min m 2),while those of burner-stabilized and divergence-stabilized SFFs are 53.7and 62.4g/(min m 2)[14,15],respectively.Again,the SSF in this work is not subject to burner clogging,which may be an issue for burner-stabilized SFFs,e.g.,those based on a Mckenna-type burner,thus hindering industrial use.Also,difficulties exist in scaling up burners for premixed laminar flames,since flame shape and stability are strongly influ-enced by the issuing velocity profile.As such,the SSF possesses advantages over the SFF mak-ing it promising for commercial production.The nanopowders collected from the substrate for all eight runs are analyzed by TEM,with the micrographs appearing very similar for all cases.Fig.6.TEM images of TiO 2particles collected on the substrate produced by b-SSF (a)d s Àb =12mm and (b)s Àb =36mm).J.Wang et al./Proceedings of the Combustion Institute 33(2011)1925–19321929and8.6nm,respectively.The collected nanopow-ders are in aggregate form,posing the question if significant sintering and growth of particles occurs after their deposition on the substrate.From XRD results,Zhao et al.[3]reported that partial phase transformation to rutile,coupled with sintering of anatase crystallites,occurred on the substrate after ing TEM,they also compared the sizes of particles collected from the substrate with those sampled from a point in theflowfield just above it,finding the particle growth factor to be2–3upon deposition.Fig.7shows TEM images of the particles obtained in theflowfield just above the substrate, for the b-SSF case with d sÀb=12mm,using a rapid-insertion sampler.As can be seen,the parti-cles mainly exist as individual particle,which can be attributed to the sintering time of ultrafine par-ticles(<10nm)being much smaller than the colli-sion time inflowfield[16,28].The particle size is 6.4±0.8nm,which is very close to the 6.8±1.1nm of the particles collected from the substrate shown in Fig.6(left).The results indicate that sin-tering and growth processes are weak after particle deposition,different from thefindings of Ref.[3]. XRD(Fig.8)shows that the particles collected on the substrate,for all eight runs,are anatase dominant,with almost negligible rutile content.TiO2nanoparticles are performed at573,973, 1273,1473,and1673K.In this work,the collected particles from the experiments are about5nm. Simulations of particles with2,3,and5nm were conducted,with the results for the2nm particles selected to be presented because smaller particles have a greater tendency to sinter than larger ones do,due to larger surface tension forces.Two clas-sic sintering modes are manifested.Fig.9a shows that with initial low particle temperature, e.g., 573K,the temperature increases sharply by $60K after20ps,due to decrease in surface energy.Subsequently,the two particles neck,but cannot completely sinter into one large particle,Fig.7.TEM images of TiO2particles sampled fromposition in theflowfield<1mm above the substrate byrapid-insertion technique(b-SSF with d sÀb=12mm).MD simulations on temporal evolution of particle temperature during the sintering of two2nm TiO2particles 573K initial particle temperature;(b)1473K initial particle temperature).1930J.Wang et al./Proceedings of the Combustion Institute33(2011)1925–1932573K used in the MD simulations),it is unreason-able for the4–5nm TiO2nanoparticles,as shown in Fig.7,to sinter and grow after their deposition on the substrate.Therefore the results of the MD simulations fully support our experimentalfind-ings(Figs.6and7).Of course,if cooling of the substrate is insufficient and the particles reach ele-vated temperatures,then considerable sintering and particle growth will result in particle aggre-gates on the substrate,as found by others and shown in the MD simulations(e.g.,Fig.9b).5.ConclusionsThe results of the work demonstrate that a novel premixed,stagnation swirlflame(SSF)can produce ultrafine,high-purity anatase TiO2nano-particles,with sizes of5–10nm,suitable for pho-tocatalytic applications.The main conclusions drawn from this work are given below:(1)Above the DBO limit using SSF,synthesisof anatase titania nanopowders can beaccomplished forflames stabilized near theburner(b-SSF)or near the substrate(s-SSF).(2)With increasing substrate–burner distance,d sÀb,the BET diameters of the nanoparti-cles increase from 5.7to8.5nm for theb-SSF cases,but remain nearly constantwithin the narrow ranges of 5.4–5.7nmfor the s-SSF cases.(3)By increasing TTIP precursor loading ratefrom0.0125to0.05g/min,the BET averagediameters of collected particles linearlyincreases from5.0to8.4nm under the con-ditions of s-SSF with d sÀb¼18mm.Forproducing the same6nm titania particles,our SSF can accommodate much higher pre-cursor loading rates than that for laminarpremixed SFFs reported in the literature.(4)TEM imaging shows that the particles intheflowfield,prior to deposition on thesubstrate,are non-agglomerated.The parti-cle sizes before and after deposition are asclose as6.4and6.8nm,respectively. (5)The nanopowders collected from the sub-strate are almost completely anatase,withnegligible rutile content.Molecular dynam-ics simulation of the sintering behavior oftwo TiO2nanoparticles strongly supportsthe experimentalfindings that sinteringand growth of TiO2nanoparticles do notoccur on the cold substrate. AcknowledgementsThis work was funded by the National Natural Science Fund of China(No.50776054).We also acknowledge the Joint project between the Natu-ral Science Fund of China and the Academy of Finland(NSFC-AF,No.50711130637),as well as partial support from the U.S.National Science Foundation(Grant No.CBET-0755615)for co-author,SDT.Thanks are due to Prof.Richard Axelbaum and Prof.Pratim Biswas from WUSTL and to Prof.Lutz Ma¨dler from the University of Bremen for useful communications.The helpful work of Mr.Yiyang Zhang and Ms.Sili Deng is appreciated.References[1]X.Chen,S.S.Mao,Chem.Rev.107(2007)2891–2959.[2]M.Gratzel,Nature414(2001)338–344.[3]B.Zhao,K.Uchikawa,J.R.McCormick,C.Y.Ni,J.G.Chen,H.Wang,bust.Inst.30 (2005)2569–2576.[4]H.K.Kammler,L.Ma¨dler,S.Pratsinis,Chem.Eng.Technol.24(2001)583–596.[5]A.S.Barnard,P.Zapol,Phys.Rev.B70(2004)235403/1.[6]H.Zhang,J.F.Banfield,J.Phys.Chem.B104(2000)3481.[7]C.C.Wang,Z.Zhang,J.Y.Ying,Nanostruct.Mater.9(1997)583.[8]S.Y.Chae,M.K.Park,S.K.Lee,T.Y.Kim,S.K.Kim,W.I.Lee,Chem.Mater.15(2003)3326. [9]B.Schimmoeller,H.Schulz,S.E.Pratsinis, A.Bareiss,A.Reitzmann,B.Kraushaar-Czarnetzki, J.Catal.243(2006)82–92.[10]W.J.Starkm,K.Wegner,S.E.Pratsinis,A.Baiker,J.Catal.197(2001)182–191.[11]Y.Xing,U.O.Koylu, D.E.Rosner,Combust.Flame107(1996)85–102.[12]M.S.Wooldridge,P.V.Torek,M.T.Donovan,D.L.Hall,ler,T.R.Palmer,C.R.Schrock,Combust.Flame131(2002)98–109.[13]H.Zhao,X.Liu,S.D.Tse,J.Nanopart.Res.10(2008)907–923.[14]H.Zhao,X.Liu,S.D.Tse,J.Aerosol Sci.40(2009)919–937.[15]E.D.Tolmachoff, A.D.Abid, D.J.Phares, C.S.Campbell,H.Wang,bust.Inst.32(2009) 1839–1845.[16]E.Thimsen,P.Biswas,AIChE J.53(2007)1727–1735.[17]R.K.Cheng,Combust.Flame101(1995)1–14.[18]D.Littlejohn,R.K.Cheng,bust.Inst.31(2007)3155–3162.[19]Y.Huang,A.Ratner,J.Propul.Power25(2009)365–373.[20]B.Ma,S.Q.Li,Z.J.Cai,Q.Yao,J.Eng.Thermo-phys.29(2008)1423–1426.[21]S.Tsantilis,H.K.Kammler,S.E.Pratsinis,Chem.Eng.Sci.57(2002)2139–2156.[22]J.Han,H.Chang,J.Lee,H.Chang,Aerosol Sci.Technol.37(2003)550–564.[23]W.Smith,T.R.Forester,J.Mol.Graphics14(1996)136–141.[24]W.Smith,C.W.Yong,P.M.Rodger,Mol.Simul.28(2002)385–471.J.Wang et al./Proceedings of the Combustion Institute33(2011)1925–19321931[25]M.Matsui,M.Akaogi,Mol.Simul.6(1991)239.[26]P.K.Naicker,P.T.Cummings,Z.H.Zhang,J.F.Banfield,J.Phys.Chem.B109(2005)15243–15249.[27]V.N.Koparde,P.T.Cummings,J.Phys.Chem.B109(2005)24280–24287.[28]T.Hawa,M.R.Zachariah,Phys.Rev.B69(2004)035417.[29]R.N.Grass,S.Tsantilis,S.E.Pratsinis,AIChE J.52(2006)1318–1324.1932J.Wang et al./Proceedings of the Combustion Institute33(2011)1925–1932。
阳极氧化TiO_2纳米管阵列的制备与掺杂
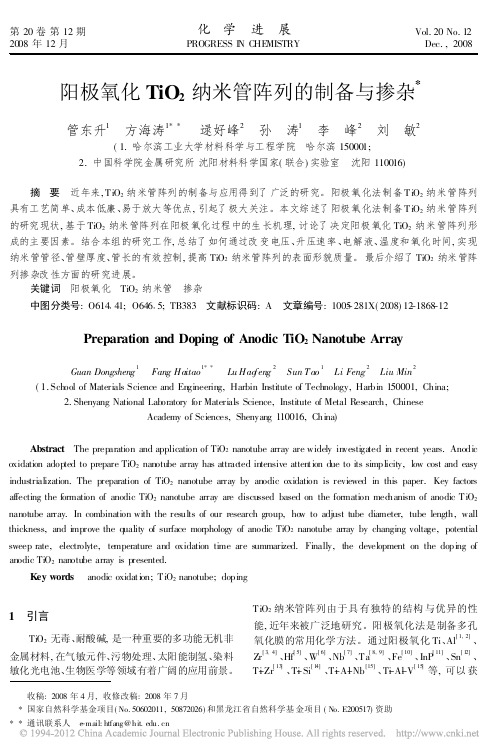
1
引言
TiO2 无毒、 耐酸碱, 是一种重要的多功能无机非
、
金属材料 , 在气敏元件、 污物处理、 太阳能制氢、 染料 敏化光电池、 生物医学等领域有着广阔的应用前景。
收稿 : 2008 年 4 月 , 收修改稿 : 2008 年 7 月
2
Liu Min
2
( 1. School of Materials Science and Engineering, Harbin Institute of Technology, Harbin 150001, China; 2. Shenyang National Laboratory for Materials Science, Institute of Metal Research, Chinese Academy of Sciences, Shenyang 110016, China) Abstract The preparation and application of TiO2 nanotube array are widely investigated in recent years. Anodic oxidation adopted to prepare TiO2 nanotube array has attracted intensive attent ion due to its simplicity, low cost and easy industrialization. The preparation of TiO2 nanotube array by anodic oxidation is reviewed in this paper. Key factors affecting the formation of anodic TiO2 nanotube array are discussed based on the formation mechanism of anodic T iO2 nanotube array. In combination with the results of our research group, how to adjust tube diameter, tube length, wall thickness, and improve the quality of surface morphology of anodic TiO2 nanotube array by changing voltage, potential sweep rate, electrolyte, temperature and oxidation time are summarized. Finally, the development on the doping of anodic TiO2 nanotube array is presented. Key words anodic oxidat ion; T iO2 nanotube; doping T iO2 纳米管阵列由于具 有独特的结构 与优异的性 能 , 近年来被广泛地研究。阳极氧化法是制备多孔 氧化膜的常用化学方法。通过阳极氧化 Ti、 Al
《二维有序大孔结构纳米二氧化钛光电子特性研究》

《二维有序大孔结构纳米二氧化钛光电子特性研究》篇一一、引言随着纳米科技的飞速发展,纳米材料因其独特的物理和化学性质在光电子领域展现出巨大的应用潜力。
其中,二维有序大孔结构的纳米二氧化钛(TiO2)因其高效的光吸收、光催化以及光电转换性能,成为了光电子领域的研究热点。
本文旨在探讨二维有序大孔结构纳米二氧化钛的光电子特性,通过实验研究和理论分析,揭示其优异性能背后的机理,并为进一步的应用研究提供理论依据。
二、文献综述TiO2作为一种重要的半导体材料,其纳米结构的研究已经取得了显著的进展。
特别是二维有序大孔结构的TiO2,因其具有高的比表面积、良好的电子传输性能以及优异的光吸收能力,被广泛应用于光催化、光电器件、太阳能电池等领域。
然而,其光电子特性的研究仍需深入。
目前,关于其光生电子-空穴对的分离、传输以及在界面处的反应机制等方面的研究尚不充分。
因此,对二维有序大孔结构纳米二氧化钛的光电子特性进行深入研究具有重要的科学意义和应用价值。
三、实验方法本实验采用溶胶-凝胶法结合模板法,制备了二维有序大孔结构的纳米二氧化钛。
通过X射线衍射(XRD)、扫描电子显微镜(SEM)、透射电子显微镜(TEM)等手段对样品的结构和形貌进行表征。
利用紫外-可见光谱(UV-Vis)和光电化学工作站对样品的光吸收性能和光电子特性进行测试和分析。
四、结果与讨论1. 结构与形貌分析通过XRD和SEM等手段对制备的二维有序大孔结构纳米二氧化钛进行表征,发现其具有明显的二维有序大孔结构,且晶型良好。
与传统的TiO2相比,其具有更高的比表面积和更优的孔道结构。
2. 光吸收性能分析紫外-可见光谱测试结果表明,二维有序大孔结构纳米二氧化钛具有优异的光吸收性能,特别是在可见光区域有明显的吸收增强。
这归因于其大的比表面积和特殊的孔道结构,能够有效地增加光程和光吸收效率。
3. 光电子特性分析通过光电化学工作站测试发现,二维有序大孔结构纳米二氧化钛具有较高的光电转换效率和较好的电子传输性能。
海报(poster)模板(英文)
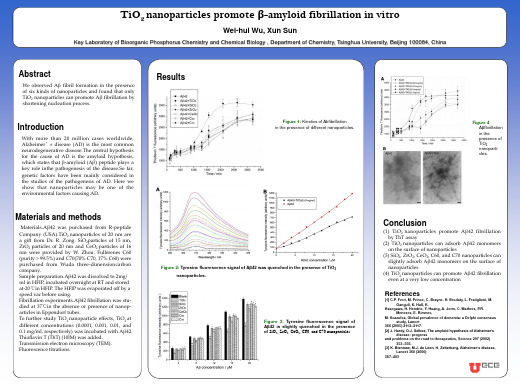
[1] C.P. Ferri, M. Prince, C. Brayne, H. Brodaty, L. Fratiglioni, M. Ganguli, K. Hall, K. Hasegawa, H. Hendrie, Y. Huang, A. Jorm, C. Mathers, P.R. Menezes, E. Rimmer, M. Scazufca, Global prevalence of dementia: a Delphi consensus study, Lancet 366 (2005) 2112–2117. [2] J. Hardy, D.J. Selkoe, The amyloid hypothesis of Alzheimer’s disease: progress and problems on the road to therapeutics, Science 297 (2002) 353 –356. [3] K. Blennow, M.J. de Leon, H. Zetterberg, Alzheimer’s disease, Lancet 368 (20ቤተ መጻሕፍቲ ባይዱ6) 387–403
Figure 2: Tyrosine fluorescence signal of Aβ42 was quenched in the presence of TiO2 nanoparticles.
References
Figure 3: Tyrosine fluorescence signal of Aβ42 is slightly quenched in the presence of SiO 2 , ZrO 2 , CeO 2 , C60, and C70 nanoparticles.
Titanium Dioxide Nanomaterials Synthesis, Properties, Modifications, and
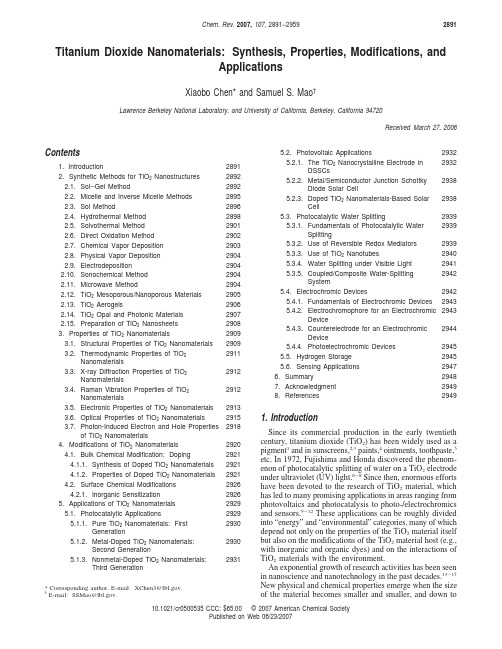
Titanium Dioxide Nanomaterials:Synthesis,Properties,Modifications,andApplicationsXiaobo Chen*and Samuel S.Mao†Lawrence Berkeley National Laboratory,and University of California,Berkeley,California94720Received March27,2006Contents1.Introduction28912.Synthetic Methods for TiO2Nanostructures28922.1.Sol−Gel Method28922.2.Micelle and Inverse Micelle Methods28952.3.Sol Method28962.4.Hydrothermal Method28982.5.Solvothermal Method29012.6.Direct Oxidation Method29022.7.Chemical Vapor Deposition29032.8.Physical Vapor Deposition29042.9.Electrodeposition29042.10.Sonochemical Method29042.11.Microwave Method29042.12.TiO2Mesoporous/Nanoporous Materials29052.13.TiO2Aerogels29062.14.TiO2Opal and Photonic Materials29072.15.Preparation of TiO2Nanosheets29083.Properties of TiO2Nanomaterials29093.1.Structural Properties of TiO2Nanomaterials29093.2.Thermodynamic Properties of TiO2Nanomaterials29113.3.X-ray Diffraction Properties of TiO2Nanomaterials29123.4.Raman Vibration Properties of TiO2Nanomaterials29123.5.Electronic Properties of TiO2Nanomaterials29133.6.Optical Properties of TiO2Nanomaterials29153.7.Photon-Induced Electron and Hole Propertiesof TiO2Nanomaterials29184.Modifications of TiO2Nanomaterials29204.1.Bulk Chemical Modification:Doping29214.1.1.Synthesis of Doped TiO2Nanomaterials29214.1.2.Properties of Doped TiO2Nanomaterials29214.2.Surface Chemical Modifications29264.2.1.Inorganic Sensitization29265.Applications of TiO2Nanomaterials29295.1.Photocatalytic Applications29295.1.1.Pure TiO2Nanomaterials:FirstGeneration29305.1.2.Metal-Doped TiO2Nanomaterials:Second Generation29305.1.3.Nonmetal-Doped TiO2Nanomaterials:Third Generation 29315.2.Photovoltaic Applications29325.2.1.The TiO2Nanocrystalline Electrode inDSSCs29325.2.2.Metal/Semiconductor Junction SchottkyDiode Solar Cell29385.2.3.Doped TiO2Nanomaterials-Based SolarCell29385.3.Photocatalytic Water Splitting29395.3.1.Fundamentals of Photocatalytic WaterSplitting2939e of Reversible Redox Mediators2939e of TiO2Nanotubes29405.3.4.Water Splitting under Visible Light29415.3.5.Coupled/Composite Water-SplittingSystem29425.4.Electrochromic Devices29425.4.1.Fundamentals of Electrochromic Devices29435.4.2.Electrochromophore for an ElectrochromicDevice29435.4.3.Counterelectrode for an ElectrochromicDevice29445.4.4.Photoelectrochromic Devices29455.5.Hydrogen Storage29455.6.Sensing Applications29476.Summary29487.Acknowledgment29498.References29491.IntroductionSince its commercial production in the early twentiethcentury,titanium dioxide(TiO2)has been widely used as apigment1and in sunscreens,2,3paints,4ointments,toothpaste,5etc.In1972,Fujishima and Honda discovered the phenom-enon of photocatalytic splitting of water on a TiO2electrodeunder ultraviolet(UV)light.6-8Since then,enormous effortshave been devoted to the research of TiO2material,whichhas led to many promising applications in areas ranging fromphotovoltaics and photocatalysis to photo-/electrochromicsand sensors.9-12These applications can be roughly dividedinto“energy”and“environmental”categories,many of whichdepend not only on the properties of the TiO2material itselfbut also on the modifications of the TiO2material host(e.g.,with inorganic and organic dyes)and on the interactions ofTiO2materials with the environment.An exponential growth of research activities has been seenin nanoscience and nanotechnology in the past decades.13-17New physical and chemical properties emerge when the sizeof the material becomes smaller and smaller,and down to*Corresponding author.E-mail:XChen3@.†E-mail:SSMao@.2891 Chem.Rev.2007,107,2891−295910.1021/cr0500535CCC:$65.00©2007American Chemical SocietyPublished on Web06/23/2007the nanometer scale.Properties also vary as the shapes of the shrinking nanomaterials change.Many excellent reviews and reports on the preparation and properties of nanomaterials have been published recently.6-44Among the unique proper-ties of nanomaterials,the movement of electrons and holes in semiconductor nanomaterials is primarily governed by the well-known quantum confinement,and the transport proper-ties related to phonons and photons are largely affected by the size and geometry of the materials.13-16The specific surface area and surface-to-volume ratio increase dramati-cally as the size of a material decreases.13,21The high surface area brought about by small particle size is beneficial to many TiO 2-based devices,as it facilitates reaction/interaction between the devices and the interacting media,which mainly occurs on the surface or at the interface and strongly depends on the surface area of the material.Thus,the performance of TiO 2-based devices is largely influenced by the sizes of the TiO 2building units,apparently at the nanometer scale.As the most promising photocatalyst,7,11,12,33TiO 2mate-rials are expected to play an important role in helping solvemany serious environmental and pollution challenges.TiO 2also bears tremendous hope in helping ease the energy crisis through effective utilization of solar energy based on photovoltaic and water-splitting devices.9,31,32As continued breakthroughs have been made in the preparation,modifica-tion,and applications of TiO 2nanomaterials in recent years,especially after a series of great reviews of the subject in the 1990s.7,8,10-12,33,45we believe that a new and compre-hensive review of TiO 2nanomaterials would further promote TiO 2-based research and development efforts to tackle the environmental and energy challenges we are currently facing.Here,we focus on recent progress in the synthesis,properties,modifications,and applications of TiO 2nanomaterials.The syntheses of TiO 2nanomaterials,including nanoparticles,nanorods,nanowires,and nanotubes are primarily categorized with the preparation method.The preparations of mesopo-rous/nanoporous TiO 2,TiO 2aerogels,opals,and photonic materials are summarized separately.In reviewing nanoma-terial synthesis,we present a typical procedure and repre-sentative transmission or scanning electron microscopy images to give a direct impression of how these nanomate-rials are obtained and how they normally appear.For detailed instructions on each synthesis,the readers are referred to the corresponding literature.The structural,thermal,electronic,and optical properties of TiO 2nanomaterials are reviewed in the second section.As the size,shape,and crystal structure of TiO 2nanomate-rials vary,not only does surface stability change but also the transitions between different phases of TiO 2under pressure or heat become size dependent.The dependence of X-ray diffraction patterns and Raman vibrational spectra on the size of TiO 2nanomaterials is also summarized,as they could help to determine the size to some extent,although correlation of the spectra with the size of TiO 2nanomaterials is not straightforward.The review of modifications of TiO 2nanomaterials is mainly limited to the research related to the modifications of the optical properties of TiO 2nanoma-terials,since many applications of TiO 2nanomaterials are closely related to their optical properties.TiO 2nanomaterials normally are transparent in the visible light region.By doping or sensitization,it is possible to improve the optical sensitiv-ity and activity of TiO 2nanomaterials in the visible light region.Environmental (photocatalysis and sensing)and energy (photovoltaics,water splitting,photo-/electrochromics,and hydrogen storage)applications are reviewed with an emphasis on clean and sustainable energy,since the increas-ing energy demand and environmental pollution create a pressing need for clean and sustainable energy solutions.The fundamentals and working principles of the TiO 2nanoma-terials-based devices are discussed to facilitate the under-standing and further improvement of current and practical TiO 2nanotechnology.2.Synthetic Methods for TiO 2Nanostructures2.1.Sol −Gel MethodThe sol -gel method is a versatile process used in making various ceramic materials.46-50In a typical sol -gel process,a colloidal suspension,or a sol,is formed from the hydrolysis and polymerization reactions of the precursors,which are usually inorganic metal salts or metal organic compounds such as metal plete polymerization and loss of solvent leads to the transition from the liquid sol into a solid gel phase.Thin films can be produced on a pieceofDr.Xiaobo Chen is a research engineer at The University of California at Berkeley and a Lawrence Berkeley National Laboratory scientist.He obtained his Ph.D.Degree in Chemistry from Case Western Reserve University.His research interests include photocatalysis,photovoltaics,hydrogen storage,fuel cells,environmental pollution control,and the related materials and devicesdevelopment.Dr.Samuel S.Mao is a career staff scientist at Lawrence Berkeley National Laboratory and an adjunct faculty at The University of California at Berkeley.He obtained his Ph.D.degree in Engineering from The University of California at Berkeley in 2000.His current research involves the development of nanostructured materials and devices,as well as ultrafast laser technologies.Dr.Mao is the team leader of a high throughput materials processing program supported by the U.S.Department of Ener-gy.2892Chemical Reviews,2007,Vol.107,No.7Chen and Maosubstrate by spin-coating or dip-coating.A wet gel will form when the sol is cast into a mold,and the wet gel is converted into a dense ceramic with further drying and heat treatment.A highly porous and extremely low-density material called an aerogel is obtained if the solvent in a wet gel is removed under a supercritical condition.Ceramic fibers can be drawn from the sol when the viscosity of a sol is adjusted into a proper viscosity range.Ultrafine and uniform ceramic powders are formed by precipitation,spray pyrolysis,or emulsion techniques.Under proper conditions,nanomaterials can be obtained.TiO2nanomaterials have been synthesized with the sol-gel method from hydrolysis of a titanium precusor.51-78This process normally proceeds via an acid-catalyzed hydrolysis step of titanium(IV)alkoxide followed by condensa-tion.51,63,66,79-91The development of Ti-O-Ti chains is favored with low content of water,low hydrolysis rates,and excess titanium alkoxide in the reaction mixture.Three-dimensional polymeric skeletons with close packing result from the development of Ti-O-Ti chains.The formation of Ti(OH)4is favored with high hydrolysis rates for a medium amount of water.The presence of a large quantity of Ti-OH and insufficient development of three-dimensional polymeric skeletons lead to loosely packed first-order particles.Polymeric Ti-O-Ti chains are developed in the presence of a large excess of water.Closely packed first-order particles are yielded via a three-dimensionally devel-oped gel skeleton.51,63,66,79-91From the study on the growth kinetics of TiO2nanoparticles in aqueous solution using titanium tetraisopropoxide(TTIP)as precursor,it is found that the rate constant for coarsening increases with temper-ature due to the temperature dependence of the viscosity of the solution and the equilibrium solubility of TiO2.63Second-ary particles are formed by epitaxial self-assembly of primary particles at longer times and higher temperatures,and the number of primary particles per secondary particle increases with time.The average TiO2nanoparticle radius increases linearly with time,in agreement with the Lifshitz-Slyozov-Wagner model for coarsening.63Highly crystalline anatase TiO2nanoparticles with different sizes and shapes could be obtained with the polycondensation of titanium alkoxide in the presence of tetramethylammonium hydroxide.52,62In a typical procedure,titanium alkoxide is added to the base at2°C in alcoholic solvents in a three-neck flask and is heated at50-60°C for13days or at90-100°C for6h.A secondary treatment involving autoclave heating at175and200°C is performed to improve the crystallinity of the TiO2nanoparticles.Representative TEM images are shown in Figure1from the study of Chemseddine et al.52A series of thorough studies have been conducted by Sugimoto et ing the sol-gel method on the formation of TiO2nanoparticles of different sizes and shapes by tuning the reaction parameters.67-71Typically,a stock solution of a0.50M Ti source is prepared by mixing TTIP with triethanolamine(TEOA)([TTIP]/[TEOA])1:2),followed by addition of water.The stock solution is diluted with a shape controller solution and then aged at100°C for1day and at140°C for3days.The pH of the solution can be tuned by adding HClO4or NaOH solution.Amines are used as the shape controllers of the TiO2nanomaterials and act as surfactants.These amines include TEOA,diethylenetri-amine,ethylenediamine,trimethylenediamine,and triethyl-enetetramine.The morphology of the TiO2nanoparticles changes from cuboidal to ellipsoidal at pH above11with TEOA.The TiO2nanoparticle shape evolves into ellipsoidal above pH9.5with diethylenetriamine with a higher aspect ratio than that with TEOA.Figure2shows representative TEM images of the TiO2nanoparticles under different initial pH conditions with the shape control of TEOA at[TEOA]/ [TIPO])2.0.Secondary amines,such as diethylamine,and tertiary amines,such as trimethylamine and triethylamine, act as complexing agents of Ti(IV)ions to promote the growth of ellipsoidal particles with lower aspect ratios.The shape of the TiO2nanoparticle can also be tuned from round-cornered cubes to sharp-edged cubes with sodium oleate and sodium stearate.70The shape control is attributed to the tuning of the growth rate of the different crystal planes of TiO2 nanoparticles by the specific adsorption of shape controllers to these planes under different pH conditions.70A prolonged heating time below100°C for the as-prepared gel can be used to avoid the agglomeration of the TiO2nano-particles during the crystallization process.58,72By heating amorphous TiO2in air,large quantities of single-phase ana-tase TiO2nanoparticles with average particle sizes between 7and50nm can be obtained,as reported by Zhang and Banfield.73-77Much effort has been exerted to achieve highly crystallized and narrowly dispersed TiO2nanoparticles using the sol-gel method with other modifications,such as a semicontinuous reaction method by Znaidi et al.78and a two-stage mixed method and a continuous reaction method by Kim et al.53,54By a combination of the sol-gel method and an anodic alumina membrane(AAM)template,TiO2nanorods have been successfully synthesized by dipping porous AAMs into a boiled TiO2sol followed by drying and heating processes.92,93In a typical experiment,a TiO2sol solution is prepared by mixing TTIP dissolved in ethanol with a solution containing water,acetyl acetone,and ethanol.An AAM is immersed into the sol solution for10min after being boiled in ethanol;then it is dried in air and calcined at400°C for 10h.The AAM template is removed in a10wt%H3PO4 aqueous solution.The calcination temperature can be used to control the crystal phase of the TiO2nanorods.At low temperature,anatase nanorods can be obtained,while at high temperature rutile nanorods can be obtained.The pore size of the AAM template can be used to control the size of these TiO2nanorods,which typically range from100to300 nm in diameter and several micrometers in length.Appar-ently,the size distribution of the final TiO2nanorods is largely controlled by the size distribution of the pores of the AAM template.In order to obtain smaller and mono-sized TiO2nanorods,it is necessary to fabricate high-quality AAM templates.Figure3shows a typical TEM for TiO2 nanorods fabricated with this method.Normally,the TiO2 nanorods are composed of small TiO2nanoparticles or nanograins.By electrophoretic deposition of TiO2colloidal suspensions into the pores of an AAM,ordered TiO2nanowire arrays can be obtained.94In a typical procedure,TTIP is dissolved in ethanol at room temperature,and glacial acetic acid mixed with deionized water and ethanol is added under pH)2-3 with nitric acid.Platinum is used as the anode,and an AAM with an Au substrate attached to Cu foil is used as the cathode.A TiO2sol is deposited into the pores of the AMM under a voltage of2-5V and annealed at500°C for24h. After dissolving the AAM template in a5wt%NaOH solution,isolated TiO2nanowires are obtained.In order toTitanium Dioxide Nanomaterials Chemical Reviews,2007,Vol.107,No.72893fabricate TiO 2nanowires instead of nanorods,an AAM with long pores is a must.TiO 2nanotubes can also be obtained using the sol -gel method by templating with an AAM 95-98and other organic compounds.99,100For example,when an AAM is used as the template,a thin layer of TiO 2sol on the wall of the pores of the AAM is first prepared by sucking TiO 2sol into the pores of the AAM and removing it under vacuum;TiO 2nanowires are obtained after the sol is fully developed and the AAM is removed.In the procedure by Lee and co-workers,96a TTIP solution was prepared by mixing TTIP with 2-propanol and 2,4-pentanedione.After the AAM was dipped intothisFigure 1.TEM images of TiO 2nanoparticles prepared by hydrolysis of Ti(OR)4in the presence of tetramethylammonium hydroxide.Reprinted with permission from Chemseddine,A.;Moritz,T.Eur.J.Inorg.Chem.1999,235.Copyright 1999Wiley-VCH.Figure 2.TEM images of uniform anatase TiO 2nanoparticles.Reprinted from Sugimoto,T.;Zhou,X.;Muramatsu,A.J.Colloid Interface Sci.2003,259,53,Copyright 2003,with permission from Elsevier.2894Chemical Reviews,2007,Vol.107,No.7Chen and Maosolution,it was removed from the solution and placed under vacuum until the entire volume of the solution was pulled through the AAM.The AAM was hydrolyzed by water vapor over a HCl solution for 24h,air-dried at room temperature,and then calcined in a furnace at 673K for 2h and cooled to room temperature with a temperature ramp of 2°C/h.Pure TiO 2nanotubes were obtained after the AAM was dissolved in a 6M NaOH solution for several minutes.96Alternatively,TiO 2nanotubes could be obtained by coating the AAM membranes at 60°C for a certain period of time (12-48h)with dilute TiF 4under pH )2.1and removing the AAM after TiO 2nanotubes were fully developed.97Figure 4shows a typical SEM image of the TiO 2nanotube array from the AAM template.97In another scheme,a ZnO nanorod array on a glass substrate can be used as a template to fabricate TiO 2nanotubes with the sol -gel method.101Briefly,TiO 2sol isdeposited on a ZnO nanorod template by dip-coating with a slow withdrawing speed,then dried at 100°C for 10min,and heated at 550°C for 1h in air to obtain ZnO/TiO 2nanorod arrays.The ZnO nanorod template is etched-up by immersing the ZnO/TiO 2nanorod arrays in a dilute hydro-chloric acid aqueous solution to obtain TiO 2nanotube arrays.Figure 5shows a typical SEM image of the TiO 2nanotube array with the ZnO nanorod array template.The TiO 2nanotubes inherit the uniform hexagonal cross-sectional shape and the length of 1.5µm and inner diameter of 100-120nm of the ZnO nanorod template.As the concentration of the TiO 2sol is constant,well-aligned TiO 2nanotube arrays can only be obtained from an optimal dip-coating cycle number in the range of 2-3cycles.A dense porous TiO 2thick film with holes is obtained instead if the dip-coating number further increases.The heating rate is critical to the formation of TiO 2nanotube arrays.When the heating rate is extra rapid,e.g.,above 6°C min -1,the TiO 2coat will easily crack and flake off from the ZnO nanorods due to great tensile stress between the TiO 2coat and the ZnO template,and a TiO 2film with loose,porous nanostructure is obtained.2.2.Micelle and Inverse Micelle MethodsAggregates of surfactant molecules dispersed in a liquid colloid are called micelles when the surfactant concentration exceeds the critical micelle concentration (CMC).The CMC is the concentration of surfactants in free solution in equilibrium with surfactants in aggregated form.In micelles,the hydrophobic hydrocarbon chains of the surfactants are oriented toward the interior of the micelle,and the hydro-philic groups of the surfactants are oriented toward the surrounding aqueous medium.The concentration of the lipid present in solution determines the self-organization of the molecules of surfactants and lipids.The lipids form a single layer on the liquid surface and are dispersed in solution below the CMC.The lipids organize in spherical micelles at the first CMC (CMC-I),into elongated pipes at the second CMC (CMC-II),and into stacked lamellae of pipes at the lamellar point (LM or CMC-III).The CMC depends on the chemical composition,mainly on the ratio of the head area and the tail length.Reverse micelles are formed in nonaqueous media,and the hydrophilic headgroups are directed toward the core of the micelles while the hydrophobic groupsareFigure 3.TEM image of anatase nanorods and a single nanorod composed of small TiO 2nanoparticles or nanograins (inset).Reprinted from Miao,L.;Tanemura,S.;Toh,S.;Kaneko,K.;Tanemura,M.J.Cryst.Growth 2004,264,246,Copyright 2004,with permission fromElsevier.Figure 4.SEM image of TiO 2nanotubes prepared from the AAO template.Reprinted with permission from Liu,S.M.;Gan,L.M.;Liu,L.H.;Zhang,W.D.;Zeng,H.C.Chem.Mater.2002,14,1391.Copyright 2002American ChemicalSociety.Figure 5.SEM of a TiO 2nanotube array;the inset shows the ZnO nanorod array template.Reprinted with permission from Qiu,J.J.;Yu,W.D.;Gao,X.D.;Li,X.M.Nanotechnology 2006,17,4695.Copyright 2006IOP Publishing Ltd.Titanium Dioxide Nanomaterials Chemical Reviews,2007,Vol.107,No.72895directed outward toward the nonaqueous media.There is no obvious CMC for reverse micelles,because the number of aggregates is usually small and they are not sensitive to the surfactant concentration.Micelles are often globular and roughly spherical in shape,but ellipsoids,cylinders,and bilayers are also possible.The shape of a micelle is a function of the molecular geometry of its surfactant molecules and solution conditions such as surfactant concentration,tem-perature,pH,and ionic strength.Micelles and inverse micelles are commonly employed to synthesize TiO2nanomaterials.102-110A statistical experi-mental design method was conducted by Kim et al.to optimize experimental conditions for the preparation of TiO2 nanoparticles.103The values of H2O/surfactant,H2O/titanium precursor,ammonia concentration,feed rate,and reaction temperature were significant parameters in controlling TiO2 nanoparticle size and size distribution.Amorphous TiO2 nanoparticles with diameters of10-20nm were synthesized and converted to the anatase phase at600°C and to the more thermodynamically stable rutile phase at900°C.Li et al. developed TiO2nanoparticles with the chemical reactions between TiCl4solution and ammonia in a reversed micro-emulsion system consisting of cyclohexane,poly(oxyethyl-ene)5nonyle phenol ether,and poly(oxyethylene)9nonyle phenol ether.104The produced amorphous TiO2nanoparticles transformed into anatase when heated at temperatures from 200to750°C and into rutile at temperatures higher than 750°C.Agglomeration and growth also occurred at elevated temperatures.Shuttle-like crystalline TiO2nanoparticles were synthesized by Zhang et al.with hydrolysis of titanium tetrabutoxide in the presence of acids(hydrochloric acid,nitric acid,sulfuric acid,and phosphoric acid)in NP-5(Igepal CO-520)-cyclohexane reverse micelles at room temperature.110The crystal structure,morphology,and particle size of the TiO2 nanoparticles were largely controlled by the reaction condi-tions,and the key factors affecting the formation of rutile at room temperature included the acidity,the type of acid used, and the microenvironment of the reverse micelles.Ag-glomeration of the particles occurred with prolonged reaction times and increasing the[H2O]/[NP-5]and[H2O]/[Ti-(OC4H9)4]ratios.When suitable acid was applied,round TiO2 nanoparticles could also be obtained.Representative TEM images of the shuttle-like and round-shaped TiO2nanopar-ticles are shown in Figure6.In the study carried out by Lim et al.,TiO2nanoparticles were prepared by the controlled hydrolysis of TTIP in reverse micelles formed in CO2with the surfactants ammonium carboxylate perfluoropolyether(PFPECOO-NH4+)(MW587)and poly(dimethyl amino ethyl methacrylate-block-1H,1H,2H,2H-perfluorooctyl meth-acrylate)(PDMAEMA-b-PFOMA).106It was found that the crystallite size prepared in the presence of reverse micelles increased as either the molar ratio of water to surfactant or the precursor to surfactant ratio increased.The TiO2nanomaterials prepared with the above micelle and reverse micelle methods normally have amorphous structure,and calcination is usually necessary in order to induce high crystallinity.However,this process usually leads to the growth and agglomeration of TiO2nanoparticles.The crystallinity of TiO2nanoparticles initially(synthesized by controlled hydrolysis of titanium alkoxide in reverse micelles in a hydrocarbon solvent)could be improved by annealing in the presence of the micelles at temperatures considerably lower than those required for the traditional calcination treatment in the solid state.108This procedure could produce crystalline TiO2nanoparticles with unchanged physical dimensions and minimal agglomeration and allows the preparation of highly crystalline TiO2nanoparticles,as shown in Figure7,from the study of Lin et al.1082.3.Sol MethodThe sol method here refers to the nonhydrolytic sol-gel processes and usually involves the reaction of titanium chloride with a variety of different oxygen donor molecules, e.g.,a metal alkoxide or an organic ether.111-119Figure6.TEM images of the shuttle-like and round-shaped(inset) TiO2nanoparticles.From:Zhang,D.,Qi,L.,Ma,J.,Cheng,H.J. Mater.Chem.2002,12,3677(/10.1039/b206996b). s Reproduced by permission of The Royal Society ofChemistry.Figure7.HRTEM images of a TiO2nanoparticle after annealing. Reprinted with permission from Lin,J.;Lin,Y.;Liu,P.;Meziani, M.J.;Allard,L.F.;Sun,Y.P.J.Am.Chem.Soc.2002,124,11514. Copyright2002American Chemical Society.TiX4+Ti(OR)4f2TiO2+4RX(1)TiX4+2ROR f TiO2+4RX(2)2896Chemical Reviews,2007,Vol.107,No.7Chen and MaoThe condensation between Ti -Cl and Ti-OR leads to the formation of Ti -O -Ti bridges.The alkoxide groups can be provided by titanium alkoxides or can be formed in situ by reaction of the titanium chloride with alcohols or ethers.In the method by Trentler and Colvin,119a metal alkoxide was rapidly injected into the hot solution of titanium halide mixed with trioctylphosphine oxide (TOPO)in heptadecane at 300°C under dry inert gas protection,and reactions were completed within 5min.For a series of alkyl substituents including methyl,ethyl,isopropyl,and tert -butyl,the reaction rate dramatically increased with greater branching of R,while average particle sizes were relatively unaffected.Variation of X yielded a clear trend in average particle size,but without a discernible trend in reaction rate.Increased nucleophilicity (or size)of the halide resulted in smaller anatase nanocrystals.Average sizes ranged from 9.2nm for TiF 4to 3.8nm for TiI 4.The amount of passivating agent (TOPO)influenced the chemistry.Reaction in pure TOPO was slower and resulted in smaller particles,while reactions without TOPO were much quicker and yielded mixtures of brookite,rutile,and anatase with average particle sizes greater than 10nm.Figure 8shows typical TEM images of TiO 2nanocrystals developed by Trentler et al.119In the method used by Niederberger and Stucky,111TiCl 4was slowly added to anhydrous benzyl alcohol under vigorous stirring at room temperature and was kept at 40-150°C for 1-21days in the reaction vessel.The precipitate was calcinated at 450°C for 5h after thoroughly washing.The reaction between TiCl 4and benzyl alcohol was found suitable for the synthesis of highly crystalline anatase phase TiO 2nanoparticles with nearly uniform size and shape at very low temperatures,such as 40°C.The particle size could be selectively adjusted in the range of 4-8nm with the appropriate thermal conditions and a proper choice of the relative amounts of benzyl alcohol and titanium tetrachloride.The particle growth depended strongly on temperature,and lowering the titanium tetrachloride concentration led to a considerable decrease of particle size.111Surfactants have been widely used in the preparation of a variety of nanoparticles with good size distribution and dispersity.15,16Adding different surfactants as capping agents,such as acetic acid and acetylacetone,into the reaction matrixcan help synthesize monodispersed TiO 2nanoparticles.120,121For example,Scolan and Sanchez found that monodisperse nonaggregated TiO 2nanoparticles in the 1-5nm range were obtained through hydrolysis of titanium butoxide in the presence of acetylacetone and p -toluenesulfonic acid at 60°C.120The resulting nanoparticle xerosols could be dispersed in water -alcohol or alcohol solutions at concentrations higher than 1M without aggregation,which is attributed to the complexation of the surface by acetylacetonato ligands and through an adsorbed hybrid organic -inorganic layer made with acetylacetone,p -toluenesulfonic acid,and wa-ter.120With the aid of surfactants,different sized and shaped TiO 2nanorods can be synthesized.122-130For example,the growth of high-aspect-ratio anatase TiO 2nanorods has been reported by Cozzoli and co-workers by controlling the hydrolysis process of TTIP in oleic acid (OA).122-126,130Typically,TTIP was added into dried OA at 80-100°C under inert gas protection (nitrogen flow)and stirred for 5min.A 0.1-2M aqueous base solution was then rapidly injected and kept at 80-100°C for 6-12h with stirring.The bases employed included organic amines,such as trimethylamino-N-oxide,trimethylamine,tetramethylammonium hydroxide,tetrabut-ylammonium hydroxyde,triethylamine,and tributylamine.In this reaction,by chemical modification of the titanium precursor with the carboxylic acid,the hydrolysis rate of titanium alkoxide was controlled.Fast (in 4-6h)crystal-lization in mild conditions was promoted with the use of suitable catalysts (tertiary amines or quaternary ammonium hydroxides).A kinetically overdriven growth mechanism led to the growth of TiO 2nanorods instead of nanoparticles.123Typical TEM images of the TiO 2nanorods are shown in Figure 9.123Recently,Joo et al.127and Zhang et al.129reported similar procedures in obtaining TiO 2nanorods without the use of catalyst.Briefly,a mixture of TTIP and OA was used to generate OA complexes of titanium at 80°C in1-octadecene.Figure 8.TEM image of TiO 2nanoparticles derived from reaction of TiCl 4and TTIP in TOPO/heptadecane at 300°C.The inset shows a HRTEM image of a single particle.Reprinted with permission from Trentler,T.J.;Denler,T.E.;Bertone,J.F.;Agrawal,A.;Colvin,V.L.J.Am.Chem.Soc.1999,121,1613.Copyright 1999American ChemicalSociety.Figure 9.TEM of TiO 2nanorods.The inset shows a HRTEM of a TiO 2nanorod.Reprinted with permission from Cozzoli,P.D.;Kornowski,A.;Weller,H.J.Am.Chem.Soc.2003,125,14539.Copyright 2003American Chemical Society.Titanium Dioxide Nanomaterials Chemical Reviews,2007,Vol.107,No.72897。
不同形貌TiO2纳米粒子制备及应用研究现状

第26卷 第4期中国陶瓷工业Vol.26,No.42019年8月CHINA CERAMIC INDUSTRYAug. 2019收稿日期:2018‒03‒20。
修订日期:2018‒03‒25。
Received date: 2018‒03‒20. Revised date: 2018‒03‒25. 基金项目:辽宁省自然科学基金(2015020182);Correspondent author: LIU Shimin, male, Associate Professor. 辽宁省教育厅计划项目(JDL2017008)。
E-mail : lsm@通信联系人:刘世民,男,副教授。
DOI: 10.13958/ki.ztcg.2019.04.005不同形貌TiO 2纳米粒子制备及应用研究现状王 竞,郭 玉,王祉诺,李孝通,刘世民,姜薇薇,刘超前,王华林,王 楠,丁万昱(大连交通大学材料科学与工程学院,辽宁 大连 116028)摘 要:二氧化钛(TiO 2)纳米粒子是一种禁带宽度较大的n 型半导体材料,其性能优良,用途广泛,具有稳定性好、无毒、低成本等特点,常应用于太阳能电池和光催化等领域。
但TiO 2 由于其带隙较宽的特点,使其仅能吸收波长小于380 nm 的紫外光,对太阳光的利用率不足5%,并且光生电子-空穴易于复合,降低了光量子效率,使得TiO 2纳米材料的实际应用受到了阻碍。
目前,越来越多的科学家通过控制TiO 2的形貌、晶型等手段来调控TiO 2禁带使其吸收光谱向可见光区域扩展并且降低光生电子-空穴对复合率。
研究人员对于制备性能优异的TiO 2纳米粒子投入了巨大的精力,使这一课题不断科学化、完善化。
本文主要综述了近年来不同形貌TiO 2光催化剂研究进程,同时展望了未来的研究和发展方向。
关键词:TiO 2;光催化;不同形貌;综述;纳米粒子;制备;应用;研究进展中图分类号:TQ174.75 文献标志码:A 文章编号:1006-2874(2019)04-0018-06Research Status of Preparation and Application of TiO 2Nanoparticles with Different MorphologyWANG Jing, GUO Yu, WANG Zhinuo, LI Xiaotong, LIU Shimin *, JIANG Weiwei,LIU Chaoqian, WANG Hualin, WANG Nan, DING Wanyu(School of Materials Science and Engineering, Dalian Jiaotong University, Dalian 116028, Liaoning, China)Abstract: Titanium dioxide (TiO 2) nanoparticles are n-type semiconductor materials with large bandgap width, which have ex-cellent performance, wide application, good stability, non-toxic, low cost and other characteristics. TiO 2 nanoparticles are often used in fields such as solar cells and photocatalysis. However, due to its wide band gap, TiO 2 can only absorb ultraviolet light whose wavelength is less than 380nm, and therefore its utilization rate of sunlight is less than 5%. At the same time, the photoge-nerated electrons and holes are easy to recombined, and the photo quantum efficiency is low, which hinders the practical applica-tion of TiO 2 photocatalytic materials. At present, more and more scientists regulate TiO 2 band gap by controlling the morphology and crystal shape of TiO 2 to expand the absorption spectrum to the visible region and reduce the photogenerated electron-hole pair recombination rate. How to prepare TiO 2 nanoparticles with excellent properties has become one of the active topics in material research, which has important scientific significance. In this paper, the research progress of TiO 2 photocatalyst with different morphology in recent years has been reviewed, and the future research and development strategy are predicted. Key words: TiO 2; photocatalytic; different shape; review; nanoparticles; preparation; application; research0 引 言近年来,随着资源短缺和环境污染问题的日益加重,人们对清洁能源太阳能的利用及环境污染控制的关注日趋紧密。
tio2量子点溶胶

tio2量子点溶胶《关于TiO₂量子点溶胶的那些事儿》TiO₂量子点溶胶,听起来就像是一个充满科技感的小玩意儿。
它就像是纳米世界里的一颗小星星,虽然小,但是本事可不小呢。
先来说说TiO₂,这可是个大名鼎鼎的物质。
在我们的日常生活中,TiO₂就像一个勤劳的小卫士。
它常常出现在防晒霜里,想象一下,当我们在阳光下欢快奔跑的时候,TiO₂就在那里默默地为我们阻挡紫外线的侵袭,就像一把小小的遮阳伞,只不过它是在微观世界里发挥作用。
而量子点这个概念呢,就更有趣了。
量子点就像是一群特别的小精灵,它们的大小在纳米级别,因为这个小小的尺寸,它们就有了一些非常奇特的性质。
那TiO₂量子点溶胶呢?这溶胶就像是量子点的一个小集体,大家和谐地待在一起。
制作这个溶胶可有点像做一道精致的小点心。
你得小心地选择原料,精准地控制各种条件。
这过程就像小心翼翼地调配一杯独特的饮品,差一点味道就不对了。
有时候可能会遇到各种小状况,就像你做饭的时候盐放多了或者少了一样。
不过一旦成功了,那可就是满满的成就感。
TiO₂量子点溶胶在很多领域都有它的用武之地。
在光催化领域,它就像是一个超级催化剂。
比如说在处理污水的时候,它就像一个小小的清洁机器人,在污水里跑来跑去,把那些脏东西分解掉。
它利用光能,就像太阳能小超人一样,把光能转化为分解污染物的能量。
在太阳能电池方面,它又像是一个能量小助手。
想象一下,它在电池里就像一个勤劳的搬运工,努力地提高电池的光电转换效率,让电池能够更好地收集和储存能量。
在研究TiO₂量子点溶胶的时候,也有很多有趣的事情。
有时候观察它就像在看一场微观世界的表演。
那些量子点在溶胶里的运动,就像一群小蚂蚁在搬家,每个小蚂蚁都有自己的路线和任务。
科学家们就像是观众,同时也是导演,他们想要弄清楚这些小蚂蚁的规律,然后让它们按照自己的想法去工作。
不过呢,TiO₂量子点溶胶也不是完美无缺的。
它就像一个有点小脾气的孩子。
比如说它的稳定性可能会是个问题,就像一个情绪容易波动的小朋友。
- 1、下载文档前请自行甄别文档内容的完整性,平台不提供额外的编辑、内容补充、找答案等附加服务。
- 2、"仅部分预览"的文档,不可在线预览部分如存在完整性等问题,可反馈申请退款(可完整预览的文档不适用该条件!)。
- 3、如文档侵犯您的权益,请联系客服反馈,我们会尽快为您处理(人工客服工作时间:9:00-18:30)。
TiO2 Nanoparticles as Functional Building Blocks
Lixia Sang,† Yixin Zhao,‡ and Clemens Burda*,§
†
Key Laboratory of Enhanced Heat Transfer and Energy Conservation, Ministry of Education and Key Laboratory of Heat Transfer and Energy Conversion, Beijing Municipality, College of Environmental andniversity of Technology, Beijing 100124, China ‡ School of Environmental Science and Engineering, Shanghai Jiao Tong University, Shanghai 200240, China § Center for Chemical Dynamics and Nanomaterials Research, Department of Chemistry, Case Western Reserve University, 10900 Euclid Avenue, Cleveland, Ohio 44106, United States
7.2.1. Improving Charge Separation with Metal NPs 7.2.2. Core−Shell Metal@TiO2 7.2.3. Increasing UV and Visible Light Absorption with Plasmonics NPs 7.3. TiO2−Semiconductor Nanoheterostructures 8. TiO2 NPs as Charge Separation Centers 8.1. Photoinduced Charge Separation in TiO2 NPs 8.2. Charge Extraction for Photocatalytic Redox Reactions 8.2.1. Charge Extraction for Photocatalytic Purification of Water and Air 8.2.2. Charge Extraction for Solar Water Splitting 8.2.3. Charge Extraction for Photocatalytic Reduction of CO2 8.3. Charge Separation and Injection in Grätzeltype Solar Cells 9. Outlook Author Information Corresponding Author Notes Biographies Acknowledgments References T U U V X X X X Y Z AA AB AB AB AB AB AC AC
CONTENTS
1. Introduction: The Development of the TiO2 Nanoparticle Research Field 2. Structural Evolution of Molecule−Cluster−Nanoparticles 3. Crystal Phases and Phase Transformation 4. Synthesis and Characterization of TiO2 NPs 4.1. Novel Aspects to the Synthesis Methods of TiO2 NPs 4.1.1. Chemical Synthesis of Sub-2-nm TiO2 NPs 4.1.2. Low-Temperature Synthesis of Sub-5nm TiO2 Nanoparticles 4.1.3. Nonaqueous Preparation of TiO2 NPs 4.1.4. Controlling Shapes and Crystal Facets of TiO2 4.2. Characterization Approaches 4.2.1. Structural Properties via XRD and Raman Spectroscopy 4.2.2. Optoelectronic Properties via UV-DRS, XPS, EPR, IR, and PL Spectroscopy 4.2.3. Laser Spectroscopic Characterization of Charge Carrier Dynamics 5. TiO2 NPs as Growth Centers: Evolution of TiO2Based Nanomaterials 5.1. One-Dimensional (1-D) Growth 5.2. TiO2 with Higher Organized Architectures 5.3. Breaking One-Dimensional TiO2 Arrays into Zero-Dimensional TiO2 NPs 6. Chemical and Physical Modifications of TiO2 NPs 6.1. TiO2 NPs as Hosts for Metal and Nonmetal Dopants 6.2. Codoping 6.3. Photonic Crystals Constructed from TiO2 NPs 6.4. Ti3+ Self-Doped TiO2 7. TiO2 NP Heteronanostructures 7.1. Mixed Phase TiO2 7.2. TiO2−Metal Nanostructures A B C E E E E F G H H H J L L N N O O P Q R S S T
Special Issue: 2014 Titanium Dioxide Nanomaterials Received: November 5, 2013
© XXXX American Chemical Society
A
/10.1021/cr400629p | Chem. Rev. XXXX, XXX, XXX−XXX
1. INTRODUCTION: THE DEVELOPMENT OF THE TIO2 NANOPARTICLE RESEARCH FIELD TiO2 has been commercially manufactured by the millions of tons to be widely utilized as pigment, paint additive, and sunscreen to name a few uses, due to its photostability and light dispersion, yet simultaneously strong UV light filtering. TiO2 has also been one of most investigated engineering materials during recent decades, especially in the arena of energy and environmental applications. The momentum of this research and its historical development has been significantly impacted by two milestone research reports in 1972. In 1972, Fujishima and Honda published the finding of photocatalytic splitting of water on a TiO2 electrode under ultraviolet (UV) light.1 The discovery of this phenomenon spurred a tremendous amount of
Chemical Reviews research related to TiO2 photocatalysis including solar fuels and environmental remediation. Second, and also in 1972, Tributsch demonstrated the idea of a dye-sensitized solar cell (DSSC), fabricating a chlorophyll-sensitized zinc oxide (ZnO) electrode to convert visible light radiation into an electric current by charge injection from the excited dye molecules into the wide band gap metal oxide.2 Although in this first DSSC concept report the author used ZnO, not TiO2, as the wide band gap semiconductor, TiO2 was soon to become the most popular wide band gap semiconductor material for later DSSCs, mainly due to its better photostability. These two reports mark the start of a remarkable development toward the promi