布朗大学同位素地球化学3.Stableisotopefractionation
镧改性膨润土对底泥内源磷控制效果
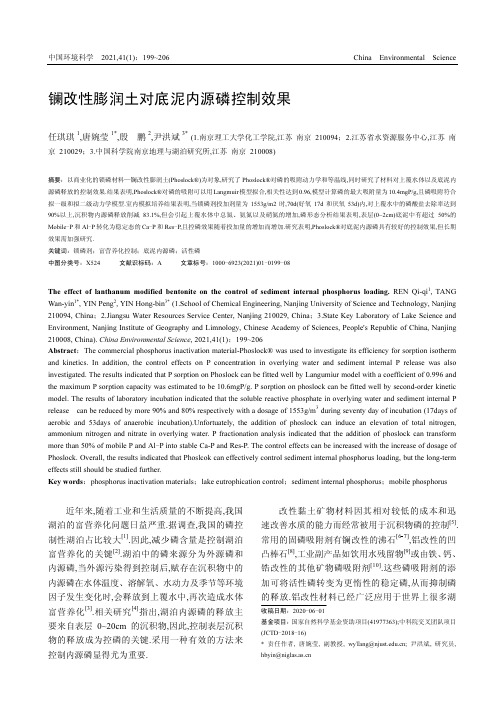
中国环境科学 2021,41(1):199~206 China Environmental Science 镧改性膨润土对底泥内源磷控制效果任琪琪1,唐婉莹1*,殷鹏2,尹洪斌3*(1.南京理工大学化工学院,江苏南京 210094;2.江苏省水资源服务中心,江苏南京 210029;3.中国科学院南京地理与湖泊研究所,江苏南京 210008)摘要:以商业化的锁磷材料—镧改性膨润土(Phoslock®)为对象,研究了Phoslock®对磷的吸附动力学和等温线,同时研究了材料对上覆水体以及底泥内源磷释放的控制效果.结果表明,Phoslock®对磷的吸附可以用Langmuir模型拟合,相关性达到0.96,模型计算磷的最大吸附量为10.4mgP/g,且磷吸附符合拟一级和拟二级动力学模型.室内模拟培养结果表明,当锁磷剂投加剂量为1553g/m2时,70d(好氧17d和厌氧53d)内,对上覆水中的磷酸盐去除率达到90%以上,沉积物内源磷释放削减83.1%,但会引起上覆水体中总氮、氨氮以及硝氮的增加,磷形态分析结果表明,表层(0~2cm)底泥中有超过50%的Mobile-P和Al-P转化为稳定态的Ca-P和Res-P,且控磷效果随着投加量的增加而增加.研究表明,Phoslock®对底泥内源磷具有较好的控制效果,但长期效果需加强研究.关键词:锁磷剂;富营养化控制;底泥内源磷;活性磷中图分类号:X524 文献识标码:A 文章标号:1000-6923(2021)01-0199-08The effect of lanthanum modified bentonite on the control of sediment internal phosphorus loading. R EN Qi-qi1, TANG Wan-yin1*, YIN Peng2, YIN Hong-bin3* (1.School of Chemical Engineering, Nanjing University of Science and Technology, Nanjing 210094, China;2.Jiangsu Water Resources Service Center, Nanjing 210029, China;3.State Key Laboratory of Lake Science and Environment, Nanjing Institute of Geography and Limnology, Chinese Academy of Sciences, People's Republic of China, Nanjing 210008, China). China Environmental Science, 2021,41(1):199~206Abstract:The commercial phosphorus inactivation material-Phoslock® was used to investigate its efficiency for sorption isotherm and kinetics. In addition, the control effects on P concentration in overlying water and sediment internal P release was also investigated. The results indicated that P sorption on Phoslock can be fitted well by Langumiur model with a coefficient of 0.996 and the maximum P sorption capacity was estimated to be 10.6mgP/g. P sorption on phoslock can be fitted well by second-order kinetic model. The results of laboratory incubation indicated that the soluble reactive phosphate in overlying water and sediment internal P release can be reduced by more 90% and 80% respectively with a dosage of 1553g/m3 during seventy day of incubation (17days of aerobic and 53days of anaerobic incubation).Unfortuately, the addition of phoslock can induce an elevation of total nitrogen, ammonium nitrogen and nitrate in overlying water. P fractionation analysis indicated that the addition of phoslock can transform more than 50% of mobile P and Al-P into stable Ca-P and Res-P. The control effects can be increased with the increase of dosage of Phoslock. Overall, the results indicated that Phoslcok can effectively control sediment internal phosphorus loading, but the long-term effects still should be studied further.Key words:phosphorus inactivation materials;lake eutrophication control;sediment internal phosphorus;mobile phosphorus近年来,随着工业和生活质量的不断提高,我国湖泊的富营养化问题日益严重.据调查,我国的磷控制性湖泊占比较大[1].因此,减少磷含量是控制湖泊富营养化的关键[2].湖泊中的磷来源分为外源磷和内源磷,当外源污染得到控制后,赋存在沉积物中的内源磷在水体温度、溶解氧、水动力及季节等环境因子发生变化时,会释放到上覆水中,再次造成水体富营养化[3].相关研究[4]指出,湖泊内源磷的释放主要来自表层0~20cm的沉积物,因此,控制表层沉积物的释放成为控磷的关键.采用一种有效的方法来控制内源磷显得尤为重要.改性黏土矿物材料因其相对较低的成本和迅速改善水质的能力而经常被用于沉积物磷的控制[5].常用的固磷吸附剂有镧改性的沸石[6-7],铝改性的凹凸棒石[8],工业副产品如饮用水残留物[9]或由铁、钙、锆改性的其他矿物磷吸附剂[10].这些磷吸附剂的添加可将活性磷转变为更惰性的稳定磷,从而抑制磷的释放.铝改性材料已经广泛应用于世界上很多湖收稿日期:2020-06-01基金项目:国家自然科学基金资助项目(41977363);中科院交叉团队项目(JCTD-2018-16)* 责任作者, 唐婉莹, 副教授,****************.cn;尹洪斌, 研究员, ***************.cn200 中国环境科学 41卷泊中,在控制内源磷方面取得了良好的效果.然而,由于藻华爆发期会极大地提高富营养化湖泊中上覆水的pH值,在高pH值(>9.50)条件下会增加沉积物中铝的氢氧化物释放出磷的风险[11].一种镧改性膨润土锁磷剂[12](商业名称为Phoslock®)是一种高效的吸附剂,由95%的膨润土和5%的稀土镧组成,在pH值为5~7时,H2PO4-对吸附剂表面具有最大的亲和力.与Al相比,Phoslock®施用时不会改变湖泊水的碱度和pH值,这可能是碱度较低湖泊的更好选择[12].另外,膨润土具有与沉积物相似的密度和颗粒大小,沉降后可以作为沉积物的组分从而限制物理再悬浮或生物扰动[13-14],是一种较为理想的磷钝化剂.为了研究Phoslock®的除磷效果,本研究进行了吸附实验探究其对磷的固定化性能,同时采用模拟室内培养测试Phoslock®在有氧和厌氧条件下控制沉积物内部磷的长期固定效率,以期为其实际应用提供参考.1 材料与方法1.1 水样、沉积物柱样采集与处理实验所用沉积物采集于安徽巢湖(31.700610°N, 117.368350°E),所用采样管为内径8.4cm、高度45cm的有机玻璃管,采集完沉积物样后两端用橡胶塞密封.在同一点位收集200L的湖水,这些沉积物柱状样和收集的水在低温中保存,并在4h内运回实验室.相关研究[4]指出,湖泊内源磷的释放主要来自表层0~20cm的沉积物,调整沉积物柱状样至20cm,上覆水至15cm[15],在进行培养实验之前样品一直保持黑暗状态.培养试验结束后用切板将底泥根据沉积物-水界面的距离分为5层:0~2cm、2~ 4cm、4~6cm、6~8cm、8~10cm,匀浆后自然风干研磨过100目筛备用.1.2吸附实验1.2.1 吸附等温线使用KH2PO4制备磷酸根溶液.称取若干份0.5g的Phoslock®于50mL离心管中,加入不同浓度(1,5,10,20,50,100,200,500,1000mg/L)的磷溶液25mL,磷溶液预先用0.1mol/LH2SO4或0.1mol/L的NaOH调整为pH=7,在180r/min,25℃的恒温摇床中震荡24h,离心后经0.45 μm水系滤膜过滤得上清液,并用于可溶性反应磷酸盐(SRP)分析.磷吸附结果拟合到Langmuir吸附模型,e L emL e1q K CqK C=+(1)Freundlich吸附模型由下式给出:m F enq K C= (2) 式中:q m代表磷吸附剂的最大吸附量,mg/g;K L和K F值表示吸附能力的强弱;C e为达到吸附平衡时溶液中的磷浓度,mg/L;q e表示达到吸附平衡时单位磷吸附剂的饱和吸附量,mg/g;n表示吸附的难易.1.2.2 吸附动力学称取若干份0.5g的Phoslock®于50mL离心管中,分别加入25mL初始磷浓度为0.5,10,50,200,500mg/L的磷溶液,pH值调至7,在180r/min,25℃的恒温摇床中振荡,设置不同时间取出(初始磷浓度为0.5,10,50mg/L分别在10,20,30,60,120,240,360min取出;初始磷浓度为200, 500mg/L分别在0.5,1,2,4,6,10,16,24,48h取出)后分析其上清液可溶性反应磷酸盐(SRP)浓度.用拟一级动力学和拟二级动力学模型来表示吸附过程中吸附量随时间的变化,拟合方程如下:拟一级动力学方程:()1e elog log2.303tkq q q t−=− (3) 拟二级动力学方程:22e e1tt tq k q q=+ (4) 式中:q e和q t分别为吸附平衡和t时刻磷吸附剂的吸附量,mg/g;k1、k2分别为一级、二级吸附速率常数,h-1.1.3 室内长期柱样培养实验1.3.1 样品处理与投加量为了研究好氧和厌氧条件Phoslock®对沉积物内部磷的长期控制效率,进行实验室模拟实验.根据表层沉积物中活性磷(0~5cm)含量和锁磷剂的最大磷吸附量来计算理论投加量,理论投加量的计算式如下:ma bVXSq+= (5)式中:X为理论投加量,g/m2;a是待处理的底泥中活性磷(mobile-P)总含量,g;b是待溶解的湖水中SRP的质量浓度,mg/L;V是待溶解的湖水体积,mL;S是柱状样采样管的底面积,m2;q m是由Langmuir等温吸附方程得到的Phoslock®对磷的理论最大吸附量,mg/g.柱状样表层5cm湿泥中的Mobile-P含量为86mg,已过滤的湖水中TP含量为0.19mg/L,Phoslock®的最大吸附量为10.4mg/g,因此,所需1期任琪琪等:镧改性膨润土对底泥内源磷控制效果 201Phoslock®的1、2倍理论投加量为1553g/m2和3105g/m2.1.3.2 实验搭建及过程室内柱状培养实验分别设置(1)空白对照组:不添加Phoslock®的柱状沉积物;(2) Phoslock®:Mobile-P=100:1(1X,1553g/m2);(3) Phoslock®:Mobile-P=200:1(2X,3106g/m2).每组实验均设置3组平行实验.先用虹吸法将所有柱状样中原先的上覆水缓慢抽出,空白对照组再缓慢注入1L 湖水,而第2,3材料处理组注入1L溶解有磷吸附剂的湖水,保持空白组和处理组的上覆水样一致.实验过程柱状样均放置在恒温培养箱(ILB-008-02型)中,保持温度为20°C,pH=7.实验分为好氧期和厌氧期2个阶段,其中第1~17d为好氧期阶段,第17~70d为厌氧期阶段.在好氧期阶段,所有柱子不加顶塞,以空气作为其氧气来源,并在2~3d内曝气,曝气强度以不扰动界面为宜;好氧实验结束后即刻向柱状样中通入氮气,盖紧顶塞,并且每24h向柱状样内通氮气以保持厌氧状态.每隔72h抽取50mL上覆水用于实验指标的测定,然后沿管壁再缓慢注入50mL的对应点位已过滤湖水;厌氧期阶段结束后,向柱内垂直插入Peeper装置[16-17],48h后取出测定.1.4 样品分析方法1.4.1 水体中氮、磷的测定将抽取的上覆水采用0.45µm的滤膜过滤,滤液中的SRP含量用钼蓝比色法进行测定,氨氮用纳氏试剂光度法[16]测定.用pH 计(雷磁,PHS-3C型)测定未过滤上覆水的pH值,将未过滤的上覆水消解后用钼蓝比色法测其TP含量.1.4.2 间隙水中氨氮、磷酸盐含量及其释放通量的测定本实验通过平衡式间隙水采样技术(Peeper)[17-18]获取沉积物间隙水,其技术属于原位被动采样范畴,基于内外膜的渗透压平衡原理,根据滤膜的特性使沉积物间隙水中一些可溶离子和分子通过滤膜与采样介质进行物质交换达到平衡[19].使用之前用N2曝气24h,然后垂直插入柱状沉积物中,48h后垂直取出装置并记录泥水界面的位置,用清水洗去表面的底泥,然后用移液枪刺穿一层渗透膜取出小室内的溶液置于酶标板中,加入显色剂之后于微孔板振荡器内(QB-9001型)震荡,间隙水中的磷酸盐和氨氮浓度采用分光光度计(Epoch BioTek 型)用微量比色法进行测定.对Peeper各小室中测出的数值即为相应位置沉积物间隙水中磷酸盐和氨氮的浓度,利用Fick第一扩散定律计算磷酸盐和氨氮的通量[19],通量由下式计算得到.()sJ cx xDδϕδ=⎛⎞=⎜⎟⎝⎠(6) 式中:φ指表层沉积物孔隙度;D S是沉积物扩散系数;cxδδ是整个沉积物界面上的孔隙水浓度梯度;J为沉积物-水界面的扩散通量.1.4.3 沉积物的磷形态的测定厌氧期实验结束后,用切板对沉积物进行分层,各层沉积物混合均匀后烘干、研磨过100目筛备用,通过分级提取法[20]测定其磷形态含量,简言之,在每一步提取步骤完成后测其过滤后的PO43-P浓度,即为每步提取液中不同磷形态的浓度,沉积物中的磷含量可以依次提取为(a)NH4Cl-P(Labile-P),(b)BD-P(Fe-P),(c)NaOH-rP(Al-P),(d)NaOH-nrP(Org-P)和(e)HCl-P(Ca-P).最后,残留物沉积物在马弗炉中于550℃灰化2h,然后用1mol/L HCl萃取,作为(f)残留P(Res-P).活性磷(mobile-P)为Labile-P,Fe-P和Org-P之和.2 结果与讨论2.1 吸附研究0200400 600 800246810Qe(mg/g)C e(mg/L)图1 Phosl ock®的等温吸附曲线Fig.1 Adsorption isotherms of P on Phoslock®2.1.1 等温吸附进行磷酸盐吸附等温线实验来研究Phoslock®的最大磷酸盐吸附能力,如表1所示,拟合结果如图1所示,计算出的模型参数列于表2.结果表明,用Langmuir等温线方程,Freundlich方程回归磷酸盐吸附等温线具有相对较高的相关参数. Langmuir方程计算得出的Phoslock®的最大吸附容202 中 国 环 境 科 学 41卷量为10.4mgP/g.镧和铝的组合对水和沉积物中的磷进行双重控制要优于单一金属负载吸附剂.这是因为单一的镧和单一的铝基磷吸附剂无法克服富营养化水中的高浓度有机碳和高pH 值的干扰[21],但是,磷吸附剂中两种元素的组合在高pH 值和高有机碳水中均表现良好.表1 Phosl ock ®等温吸附拟合参数Tabl e 1 Phosl ock ®isothermal adsorption fitting parametersLangmuir 吸附模型 Freundlich 吸附模型 磷吸附剂类型q m (mg/g)k L (L/mg)R 2k F (L/mg) 1/nR 2Phoslock® 10.4111 0.0098 0.9555 0.9972 0.34120.98642.1.2 吸附动力学 结果如图2和表2所示,Phoslock ®在初始磷浓度为0.5,10,200mgP/L 时,对磷的吸附均能很好地符合拟一级动力学和拟二级动力学模型,说明Phoslock ®对磷的吸附是物理吸附和化学吸附同时存在的.0 10 20 30 40 50时间(h)Q e(m g /g )图2 Phosl ock ®对磷的动力学吸附曲线Fig.2 Phosl ock ®kinetic adsorption curve of phosphorus表2 Phosl ock ®的动力学拟合参数 Tabl e 2 Phosl ock ® dynamics fitting parameters拟一级动力学 拟二级动力学初始磷浓度 (mg/L)q e(mg/g) k 1(1/min)R 2q e(mg/g) k 2(g/mg·minR 20.5 0.0242 7.497 0.98820.0248 16.7867 0.929610 0.4957 0.0924 0.98650.5267 2.0755 0.9681200 3.8955 0.13840.99684.6674 0.1505 0.99442.2 锁磷剂长期控磷效果2.2.1 上覆水pH 值变化特征 pH 值是影响沉积物磷循环和湖泊富营养化的重要因素之一,是评价湖泊水体质量的重要指标[22].上覆水pH 值随时间的变化如图3所示.投加锁磷剂后,总体上材料处理组pH 值和空白组相差不大.具体表现为好氧阶段的第1~10d 的pH 值基本无变化,第10~17d 时对照组和处理组pH 值均有所降低,随即进入厌氧阶段后,pH 值在第26d 时达到峰值后渐渐趋于平缓,pH 值均维持在7.2~7.7左右.说明锁磷剂的加入不会对上覆水pH 值造成影响.102030 40 50 6070678910p H 值时间(d)图3 上覆水pH 值随时间的变化Fig.3 Variation of pH value in overlying water with time2.2.2 上覆水水质变化特征 对于好氧期和厌氧期,锁磷剂处理柱状样后上覆水中TP 和SRP 的含量如图4所示,处理组的上覆水TP 和SRP 含量相对于未投加吸附剂的对照组明显降低,说明锁磷剂对水体有显著的控磷效果.在好氧条件下,对照组沉积物上覆水中的TP 和SRP 浓度保持相对恒定,而在无氧条件下,TP 和SRP 的浓度增加很多.相对而言,好氧条件下水柱中TP 浓度是对照沉积物中厌氧条件下水柱中TP 浓度的1.68倍.类似地,对照处理中厌氧条件下上覆水中的平均SRP 浓度是好氧条件下的5.0倍.在厌氧条件下,三价铁被还原为亚铁.因此,铁结合的磷被释放到水柱中.这与前期的研究结果一致[23-25].相反,与有氧和无氧条件相比,投加锁磷剂后的上覆水中SRP 和TP 浓度在有氧和无氧条件下都保持相对较低的水平(<0.3mg/L).这是因为沉积物中的La 结合磷对氧化还原电位变化不敏感,并且在缺氧条件下可以保持稳定.相对而言,与对照沉积物柱状样相比,1X 的Phoslock ®处理可以降低上覆水中82.6%的TP 和96.8%的SRP.并且与对照相比,高剂量的Phoslock ®1期 任琪琪等:镧改性膨润土对底泥内源磷控制效果 203对水柱中TP 和SRP 的控制效果更好.总体来看,锁磷剂对于柱状样水体中的磷有良好的去除效果,且能维持较长一段时间,因此具有较好的应用前景.0 10 20 3040 50 60 70T P 浓度(m g /L )时间(d)0 10 20 3040 50 60 70S R P 浓度(m g /L )时间(d)图4 上覆水TP 和SRP 随时间的变化Fig.4 Variation of TP and SRP in overlying water with time氮作为湖泊中一种重要的营养组分,当环境条件变化时也会改变底泥中氮的释放.上覆水中总氮、氨氮和硝态氮含量在好氧期和厌氧期随时间的变化如图5所示,在好氧期阶段,以总氮浓度为例,对比对照组发现,添加锁磷剂后,上覆水中总氮含量明显升高;随着培养时间越长,处理组上覆水总氮含量呈现出先增大后减小的趋势,在第14d 后和对照组中的总氮含量基本一致,且随着投加量的增大上覆水中总氮含量升高的越多.在厌氧期阶段,处理组中的总氮含量比对照组高,两个不同的处理组中上覆水的总氮含量随着时间均缓慢的增高,然后逐渐趋于稳定,在37d 后处理组始终比空白组中的总氮含量高.与对照沉积物柱状样相比,1X 的Phoslock ®处理在好氧期上覆水中总氮和氨氮相当于空白组增加了2倍和2.3倍,在厌氧期可以增加上覆水中49.0%的总氮和29.2%的氨氮.而在好氧期和厌氧期阶段材料处理组上覆水中的氨氮含量始终高于空白组,硝态氮的变化趋势和总氮的变化趋势有类似的规律.总的来说,添加锁磷剂后会造成柱状样上覆水中的总氮,氨氮和硝态氮含量的增加.有研究[26]指出连续萃取锁磷剂,膨润土基质的Phoslock ®含有氧化还原敏感的铁,当分散在水中时,Phoslock 可能会释放出铵.0102030 40 50 607012345678T N 质量浓度(m g /L )时间(d)102030 40 50 60700.51.01.52.02.53.03.54.04.5时间(d)N O 3-质量浓度(m g /L )0102030 40 50 60700.51.01.52.02.53.03.54.0时间(d)N H 4+质量浓度(m g /L )图5 上覆水TN 、NH 4+和NO 3-随时间的变化 Fig.5 Variation of TN, NH 4+ and NO 3- in overlying waterwith time2.2.3 底泥释放特征 沉积物中的大量可溶解性204 中国环境科学 41卷物质主要以间隙水作为介质通过表面扩散层向上覆水扩散迁移,因此沉积物间隙水中磷酸盐的多寡可以直接地反映出底质环境的优劣.间隙水中的SRP浓度随深度的变化如图6(a)所示,其中深度以“0”作为沉积物-水界面,沉积物-水界面以上用负值表示,沉积物-水界面以下用正值表示.对照组上覆水中的SRP浓度为0.33~0.55mg/L,间隙水中SRP浓度要远大于上覆水中的浓度,且随着深度的增加呈现出先增大后减小的趋势,在深度为2.8cm处达到最大值2.68mg/L,在5cm以后逐渐减小,这也验证了表层沉积物对内源磷的释放贡献较大,是控制内源磷的关键.在材料组中,不管是上覆水还是间隙水中的SRP浓度都要远远低于对照组,且材料的投加量不同SRP浓度的变化也不同,具体表现为随着投加量增大,SRP浓度降低.其中在沉积物-水界面以上,处理组的投加量不会引起上覆水SRP浓度的变化,处理后的SRP质量浓度为0.10~ 0.20mg/L,此处材料处理组中SRP的去除率为63.2%~82.8%;在沉积物-水界面以下,不同的材料组中的SRP浓度都表现出先增大后趋于稳定的趋势,且2X处理组中SRP的质量浓度低于1X处理组,1X 处理组在界面下1.2~2.8cm处的SRP去除率为71.9%~82.3%,2X处理组在界面下1.2~2.8cm处的SRP去除率为81.4%~90.1%.随着深度的增加去除效果略有降低,到5cm去除率仍有38%左右.综上所述,添加材料后可以大大降低上覆水和表层5cm左右间隙水中SRP的含量,且对沉积物-水界面下5~ 8cm仍有去除效果.SRP (mg/L)对照1X 2X1234P通量[mg/(m2⋅d)]深度(cm)图6 间隙水中SRP浓度的垂向变化和磷扩散通量变化Fig.6 Vertical change of SRP concentration and phosphorus diffusion flux in interstitial water 沉积物-水界面之间的磷交换量可以用扩散通量表示,扩散通量越大,则物质交换量越多.选取间隙水中的垂向浓度由菲克第一定律计算出的磷扩散通量如图6(b)所示,结果表明,锁磷剂的加入可以改变磷酸盐在沉积物间隙水中的分布趋势,使其浓度大大降低,穿过沉积物-水界面的磷通量也得到有效降低,同时随着锁磷剂的投加量增大,扩散通量减小.对照组,1X和2X沉积物中的磷释放通量分别为3.7;0.63和0.19mg/(m2⋅d),与对照组相比,分别从1X和2X处理组中减少了83.1%,94.9%的磷通量.综上所述,锁磷剂的添加会明显降低磷的扩散通量.2.2.4 底泥磷形态变化特征经过70d的室内培养,对照组和处理组沉积物的磷组分如图7所示,结果表明,在对照沉积物中的所有沉积物层中,NaOH-rP(Al-P)是TP的主要部分(51.4~58.9%),活性磷的含量(Labile-P,Fe-P和Org-P的总和)占总磷的20.0~28.9%.沉积物中的这3种磷被认为是磷的活泼形式,当环境条件发生改变时最容易从沉积物中释放出来[25-27].例如,Fe-P和Org-P的一部分最有可能在缺氧条件下和微生物活动期间从沉积物中释放出来,1期 任琪琪等:镧改性膨润土对底泥内源磷控制效果 205因此,Fe -P 和Org -P 可以增加上覆水中的TP 浓度[28-29].原位失活的目的是将磷的活泼形式(Labile -P,Fe -P 和Org -P)转移为惰性形式,与Fe -P 和Org -P 不同,Ca -P 和Res -P 等2个惰性磷形态通常稳定且生物利用度很低[30].投加锁磷剂可以对表层沉积物中的活性磷含量起到大大得削减作用,达到有效的控磷效果.对于表层0~2cm 的沉积物,1X 、2X 处理组沉积物中活性磷的含量分别降低了59.4%,62.7%.对于表层2~4cm 的沉积物,1X 、2X 处理组沉积物中活性磷的含量分别降低了29.6%,29.4%.随着深度的增加,Al -P 在部分条件下有可能会释放出来,有被生物利用的可能性.对比发现,添加材料的处理组沉积物中的Al -P 都有部分程度的削减,比对照组降低了超过30%的Al -P 含量.另外,由于La 3+和Al 3+与沉积物中可移动性P 的反应,观察发现添加Phoslock ®磷吸附剂可以增加Ca -P 和Res -P.这两种惰性P(Ca -P 和Res -P)的双重增加有利于富营养化湖泊的沉积物磷的长期控制[31].具体表现为:表层0~2cm 的沉积物,1X 处理组沉积物中Ca -P 的含量是对照组的4.02倍,Res -P 含量增加了36.5%.对于4~10cm 的沉积物,处理组对各种磷形态对比对照组并没有很明显的变化,可能是由于柱状样中底栖动物较少,不能通过生物的蠕动作用将磷吸附剂向深层沉积物渗透.综上所述,投加理论剂量的Phoslock ®对表层沉积物中的活性磷起到大大削减作用,能提高稳定结合态磷,对固定沉积物中活性磷起到积极作用.Res-PCa-PAl-PMobile-PX2磷含量(mg/kg) 深度(c m )深度(c m )深度(c m )图7 Phosl ock ®对沉积物中磷形态的影响Fig.7 Phosl ock ®'s effect on phosphorus forms in sediments3 结论3.1 根据Langmuir 模型计算得出,Phoslock ®的最大磷吸附量为10.4mgP/g,且其对磷的吸附均能较好符合拟一级动力学和拟二级动力学模型,说明其对磷的吸附是物理吸附和化学吸附同时存在的. 3.2 室内长周期模拟实验表明(17d 好氧,53d 厌氧),Phoslock ®显著降低上覆水总磷以及磷酸根的浓度,与对照沉积物柱状样相比,单倍理论投加量可以降低上覆水中82.6%的TP 和96.8%的SRP,但锁磷剂的添加会造成上覆水中的总氮、氨氮和硝态氮含量的增加.3.3 Phoslock ®的添加可以显著降低间隙水中磷酸根的浓度,与对照相比,单倍和双倍的理论投加量对沉积物-水界面磷的释放削减率分别为83.1%和94.9%.3.4 锁磷剂的添加可将底泥中活性磷和铝磷转化为稳定的钙磷和残渣态磷.单倍和双倍的投加量可对表层底泥中(0~2cm)活性磷削减比例分别达59.4%和62.7%,与对照相比,底泥中的钙磷可增加2倍和3倍.参考文献:[1] 金相灿,屠清瑛.湖泊富营养化调查规范(第二版) [M]. 北京:中国环境科学出版社, 1990,30-35.Jin X C, Tu Q Y. Specification for lake eutrophication investigation (Second edition) [M]. Beijing: China Environmental Science Press, 1990:30-35.[2] 陈镜伊.金属盐改性热处理凹土对水体及底泥磷钝化效果的影响[D]. 南京:南京理工大学, 2018.Chen J Y. Inactivation effects of phosphorus in water and sediment bymetal salt modified and thermal treated attapulgite [D]. NanJing: Nanjing University of Science and Technology, 2018.[3] Wang L Q, Liang T. Distribution patterns and dynamics of phosphorusforms in the overlying water and sediment of Dongting Lake [J]. Journal of Great Lakes Research, 2016,42(3):565-570.[4] Kong M, Yin H B, Wu Y, et al. Influence of thermal treatment on thephosphorus sorption capacity of natural calcium -rich clay minerals [J]. Journal of Environmental Sciences, 2013,33(12):3211-3218.[5] Spears B M, Maberly S C, Pan G, et al. Geo -engineering in lakes: Acrisis of confidence? [J]. Environmental Science & Technology, 2014, 48(17):9977-9979.[6] Lin J, He S, Zhang H, et al. Effect of zirconium -modified zeoliteaddition on phosphorus mobilization in sediments [J]. Science of the Total Environment, 2019,646(PT.1-1660):144-157.[7] Wang Z, Lu S, Wu D, et al. Control of internal phosphorus loading in206 中国环境科学 41卷eutrophic lakes using lanthanum-modified zeolite [J]. Chemical Engineering Journal, 2017,327:505-513.[8] Yin H B, Ren C, Li W. Introducing hydrate aluminum into porousthermally-treated calcium-rich attapulgite to enhance its phosphorus sorption capacity for sediment internal loading management [J].Chemical Engineering Journal, 2018,348:704-712.[9] Wang C, Wu Y, Wang Y, et al. Lanthanum-modified drinking watertreatment residue for initial rapid and long-term equilibrium phosphorus immobilization to control eutrophication [J]. Water Research, 2018:173-183.[10] Douglas G B, Hamilton D P, Robb M S, et al. Guiding principles forthe development and application of solid-phase phosphorus adsorbents for freshwater ecosystems [J]. Aquatic Ecology, 2016,50(3):385-405. [11] Rydin E, Huser B, Welch E B. Amount of phosphorus inactivated byalum treatments in Washington lakes [J]. Limnology & Oceanography, 2000,45(1):226-230.[12] Haghseresht F, Wang S, Do D D. A novel lanthanum-modifiedbentonite, Phoslock®, for phosphate removal from wastewaters [J].Applied Clay Science, 2009,46(4):0-375.[13] Diego C, K arin F, Marziali, et al. Eutrophication management insurface waters using lanthanum modified bentonite: A review [J].Water research, 2016:197-203.[14] 刘新,王秀,赵珍,等.风浪扰动对底泥内源磷钝化效果的影响[J]. 中国环境科学, 2017,37(8):3064-3071.Liu X, Wang X, Zhao Z, et al. Effect of wind and wave disturbance on passivation of internal phosphorus in sediment [J]. 2017,37(8):3064- 3071.[15] Reitzel K, Lotter S, Dubke M, et al. Effects of Phoslock treatment andchironomids on the exchange of nutrients between sediment and water [J]. Hydrobiologia, 2013,703(1):189-202.[16] 国家环保局本书编委会.水和废水监测分析方法 [M]. 中国环境科学出版社, 1989:35-40.National Environmental Protection Agency Book Editing Committee.Water and wastewater monitoring and analysis methods [M]. China Environmental Science Press, 1989:35-40.[17] Xu D, Wu W, Ding S, et al. A high-resolution dialysis technique forrapid determination of dissolved reactive phosphate and ferrous iron in pore water of sediments [J]. Science of the Total Environment, 2012,421-422:245-252.[18] Raymond, Hesslein H. An in situ sampler for close interval pore waterstudies1 [J]. Limnology and Oceanography, 1976,21(6).[19] Ding S , Sun Q, Xu D. Development of the DET technique forhigh-resolution determination of soluble reactive phosphate profiles in sediment pore waters [J]. International journal of Environmental Analytical Chemistry, 2010,90(14/15):1130-1138. [20] William J U, Robert C A. Diffusion coefficients in nearshore marinesediments1 [J]. Limnology and Oceanography, 1982,35(7).[21] Rydin E. Potentially mobile phosphorus in Lake Erken sediment [J].Water Research, 2000,34(7):2037-2042.[22] Yin H B, Du Y, K ong M, et al. Interactions of riverine suspendedparticulate matter with phosphorus inactivation agents across sediment-water interface and the implications for eutrophic lake restoration [J]. Chemical Engineering Journal, 2017,327:150-161. [23] 杨秀敏,任广萌,李立新,等.土壤pH值对重金属形态的影响及其相关性研究 [J]. 中国矿业, 2017,26(6):79-83.Yang X M, Ren G M, Li L X, et al. Effect of pH value on heavy metals form of soil and their relationship [J]. China Mining Magazine, 2017,26(6):79-83.[24] Fuchs E, Funes A, Saar K, et al. Evaluation of dried amorphous ferrichydroxide CFH-12® as agent for binding bioavailable phosphorus in lake sediments [J]. Science of the Total Environment, 2018,628-629: 990-996.[25] Nürnberg G K, Fischer R, Paterson A M. Reduced phosphorusretention by anoxic bottom sediments after the remediation of an industrial acidified lake area: Indications from P, Al, and Fe sediment fractions [J]. Science of the Total Environment, 2018,626:412-422. [26] Hupfer M, Jordan S, Herzog C, et al. Chironomid larvae enhancephosphorus burial in lake sediments: Insights from long-term and short-term experiments [J]. Science of the Total Environment, 2019, 663:254-264.[27] Reitzel K, Lotter S, Dubke M, et al. Effects of Phoslock treatment andchironomids on the exchange of nutrients between sediment and water [J]. Hydrobiologia, 2013,703(1):189-202.[28] Funes, A, Martinez, et al. Determining major factors controllingphosphorus removal by promising adsorbents used for lake restoration:A linear mixed model approach [J]. Water Research, 2018,141:377-386.[29] Upreti K, Maiti K, Victor H, et al. Microbial mediated sedimentaryphosphorus mobilization in emerging and eroding wetlands of coastal Louisiana [J]. Science of the Total Environment, 2019,651:122-133. [30] Huser, Brian J, Egemose, et al. Longevity and effectiveness ofaluminum addition to reduce sediment phosphorus release and restore lake water quality [J]. Water Research, 2016,97:122-132.[31] Xu R, Zhang M, Robert J G, et al. Enhanced phosphorus locking bynovel lanthanum/aluminum–hydroxide composite: Implications for eutrophication control [J]. Environmental Science and Technology, 2017,51(6):3418-3425.作者简介:任琪琪(1995-),女,安徽六安人,南京理工大学硕士研究生,主要从事水环境修复材料的研究.。
关于硫化物Re-Os同位素定年的一些思考
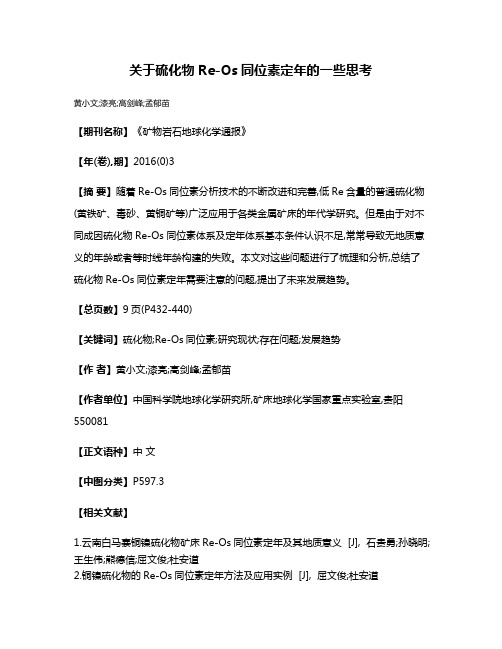
关于硫化物Re-Os同位素定年的一些思考
黄小文;漆亮;高剑峰;孟郁苗
【期刊名称】《矿物岩石地球化学通报》
【年(卷),期】2016(0)3
【摘要】随着Re-Os同位素分析技术的不断改进和完善,低Re含量的普通硫化物(黄铁矿、毒砂、黄铜矿等)广泛应用于各类金属矿床的年代学研究。
但是由于对不同成因硫化物Re-Os同位素体系及定年体系基本条件认识不足,常常导致无地质意义的年龄或者等时线年龄构建的失败。
本文对这些问题进行了梳理和分析,总结了硫化物Re-Os同位素定年需要注意的问题,提出了未来发展趋势。
【总页数】9页(P432-440)
【关键词】硫化物;Re-Os同位素;研究现状;存在问题;发展趋势
【作者】黄小文;漆亮;高剑峰;孟郁苗
【作者单位】中国科学院地球化学研究所,矿床地球化学国家重点实验室,贵阳550081
【正文语种】中文
【中图分类】P597.3
【相关文献】
1.云南白马寨铜镍硫化物矿床Re-Os同位素定年及其地质意义 [J], 石贵勇;孙晓明;王生伟;熊德信;屈文俊;杜安道
2.铜镍硫化物的Re-Os同位素定年方法及应用实例 [J], 屈文俊;杜安道
3.铜镍硫化物的Re-Os同位素定年方法及应用实例 [J], 屈文俊;杜安道
4.Re-Os同位素定年对岩浆型Cu-Ni硫化物矿床成矿时代的制约 [J], 屈文俊;陈江峰;杜安道;李超;王礼兵
5.河北木吉村斑岩铜矿辉钼矿Re-Os定年、成矿斑岩锆石U-Pb定年和Hf同位素组成研究 [J], 申志超;侯增谦;陈志宽;李秋耘;周玉谋;王志敏
因版权原因,仅展示原文概要,查看原文内容请购买。
江苏盘石山二辉橄榄岩包体的Nd、Sr、Pb同位素特征
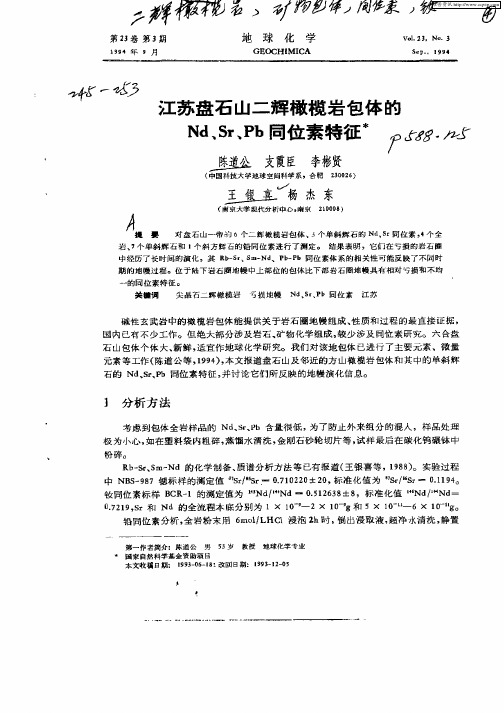
Fig. 2 T he diagram show ing the relation ̄hlp
between ”Sm/… Nd and 川 Nd,川 Nd (图 中 半 封 闭 曲 线 为 该 区 含 包 体 碱 性 玄 武
‘…Sr S1-
0.703045 O.703131 0.7037 l6
砖
r
— 2I.1 — 23.7 — 18.5 — 15.6
— 25.O 一 24.3 — 23.1 — 14.8
样 品 号
sm(x 10 ) Nd(x 10 )
P S2 P SS2 P SS3 P SS6 P SS7 P SS 卜 C PX P SS2-C PX P SS3一C PX L H FS
0.253 0.235 0.233 0.059 0.239 -.323 1.4 71 I.294 0.218
0.66 0 7 77 0.594 0 184 0.653 4 i69 4.081 3 969 0.947
注: 尾缀 CPX 指单 斜 辑石
_vS【D,… Nd l·’Nd‘ _ Nd
具 有 年 龄 意 义 ,在 下 节 讨 论。
u—Pb 7个 单 斜 辉 石 、 1个 斜 方 辉 石 和 4个 全 岩 及 浸 取 液 的 u、 Pb 含 量 及 铅 同位 素 组 成 列 于 表 2。 全 岩 和 斜 方 辉 石 铅 含 量 均 低 于 01× 1 0一,单 斜 辉 石
一 的同 位素 特 征 。
关 键调 尖 晶石 二辉 橄 榄岩 亏 损 地幔 Nd、sr、Pb 同位 素 江 苏
碱 性 玄 武 岩 中的 橄 榄 岩 包 体 能 提供 关 于 岩 石 圈 地 幔 组 成 、性 质和 过 程 的 最 直 接 证 据 , 国 内 已有 不 少 工 作 。 但 绝 大部 分 涉 及 岩 石 、矿 物 化学 组 成 ,较 少 涉 及 同位 素 研 究 。 六 合 盘 石 山 包体 个 体 大 、新 鲜 ,适 宜 作地 球 化 学 研 究 。 我 们 对 该地 包体 已进 行 了 主要 元 素 、微 量 元素 等工 作 (陈 道 公 等 ,1994),本 文 报 道 盘 石 山及 邻近 的 方 山橄 榄 岩 包体 和 其 中 的 单 斜 辉 石的 Nd、Sr、Pb 同 位 素 特 征 ,并 讨 论 它们 所 反 映 的地 幔演 化 信 息 。
美国布朗大学留学申请 布朗大学化学专业本科介绍
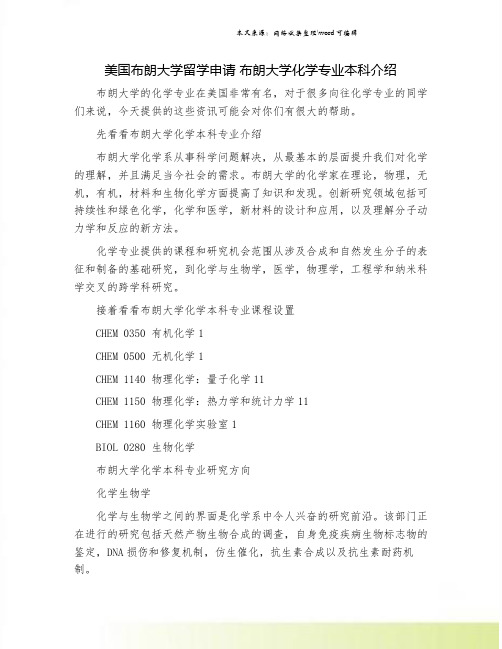
美国布朗大学留学申请布朗大学化学专业本科介绍布朗大学的化学专业在美国非常有名,对于很多向往化学专业的同学们来说,今天提供的这些资讯可能会对你们有很大的帮助。
先看看布朗大学化学本科专业介绍布朗大学化学系从事科学问题解决,从最基本的层面提升我们对化学的理解,并且满足当今社会的需求。
布朗大学的化学家在理论,物理,无机,有机,材料和生物化学方面提高了知识和发现。
创新研究领域包括可持续性和绿色化学,化学和医学,新材料的设计和应用,以及理解分子动力学和反应的新方法。
化学专业提供的课程和研究机会范围从涉及合成和自然发生分子的表征和制备的基础研究,到化学与生物学,医学,物理学,工程学和纳米科学交叉的跨学科研究。
接着看看布朗大学化学本科专业课程设置CHEM 0350 有机化学1CHEM 0500 无机化学1CHEM 1140 物理化学:量子化学11CHEM 1150 物理化学:热力学和统计力学11CHEM 1160 物理化学实验室1BIOL 0280 生物化学布朗大学化学本科专业研究方向化学生物学化学与生物学之间的界面是化学系中令人兴奋的研究前沿。
该部门正在进行的研究包括天然产物生物合成的调查,自身免疫疾病生物标志物的鉴定,DNA损伤和修复机制,仿生催化,抗生素合成以及抗生素耐药机制。
有机化学有机化学研究包括合成新型抗生素的分子结构单元,绿色化学,核酸和酶的化学,活性中间体的光谱表征和自组装。
无机化学布朗的无机化学专注于有机金属化合物,包括稀土金属,催化,二氧化碳还原,纳米材料的合成以及无机化合物在生物系统中的作用。
无机化学家与纳米/材料化学家和工程师合作,从事跨领域的尖端研究。
物理和理论化学理论和实验物理化学研究包括反应动力学的超快研究,计算化学,液体中的分子相互作用,光声学以及化学物理学在医学成像中的应用。
布朗物理化学实验研究的核心是使用多个超短脉冲激光系统,允许电子衍射,光谱和X射线吸收的超快时间分辨方法。
西双版纳绞杀植物斜叶榕的水分利用策略

西双版纳绞杀植物斜叶榕的水分利用策略*王平元1刘文杰1李金涛1,2(1中国科学院西双版纳热带植物园,云南勐仑666303;2中国科学院研究生院,北京100049)摘要以斜叶榕为研究对象,通过测定其不同生长阶段木质部与各潜在水源的稳定氢、氧同位素组成,以及土壤水分含量、土壤水势、叶片水势等参数,揭示西双版纳地区不同生长阶段的绞杀榕(斜叶榕)在不同季节的水分利用策略.结果表明:浅层土壤(10 50cm )的水势在干热季与雾凉季变化较大,较深土壤(51 120cm )的水势在各季节无明显变化;雾凉季与干热季的土壤含水量之间无显著差异(P =0.64);植物黎明前叶片水势与中午叶片水势随不同生长阶段而异;根据木质部水与各潜在水源的稳定氧同位素以及植物水势等其他参数判定,浅层土壤水是斜叶榕全年最主要的水分来源,不同生长阶段的斜叶榕在不同季节采取了不同的水分利用策略来应对环境的变化.关键词稳定同位素水分利用策略土壤水势叶片水势斜叶榕西双版纳文章编号1001-9332(2010)04-0836-07中图分类号Q948文献标识码AWater use strategy of Ficus tinctoria in tropical rainforest region of Xishuangbanna ,South-western China.WANG Ping-yuan 1,LIU Wen-jie 1,LI Jin-tao 1,2(1Xishuangbanna Tropical Bo-tanical Garden ,Chinese Academy of Sciences ,Menglun 666303,Yunnan ,China ;2Graduate Univer-sity of Chinese Academy of Sciences ,Beijing 100049,China ).-Chin.J.Appl.Ecol .,2010,21(4):836-842.Abstract :Based on the measurement of the stable isotope ratios of hydrogen and oxygen in soil ,fog ,rain ,and plant non-photosynthetic tissues ,as well as the gravimetric soil water content ,soilwater potential ,and leaf water potential ,this paper studied the water use strategy of F.tinctoria at its different life stages in Xishuangbanna of Southwestern China.The water potential in shallow soil layer (10-50cm )had a greater change between hot-dry season and foggy season ,whereas that indeeper soil layer (51-120cm )had less change during the seasons.No significant difference was observed in the soil water content between foggy season and hot-dry season.The leaf water potentialat predawn and midday varied with life stage.From the measurement of the stable isotope ratios and other parameters ,it was found that shallow soil water was the main water source for F.tinctoria ,and F.tinctoria had different water use strategy at its different life stages.Key words :stable isotope ;water use strategy ;soil water potential ;leaf water potential ;Ficus tinc-toria ;Xishuangbanna.*中国科学院“西部之光”人才计划项目和国家自然科学基金项目(30770368)资助.通讯作者.E-mail :pingyuan0920@ 2009-06-05收稿,2010-02-04接受.稳定同位素技术作为生态学研究的一种重要手段,近年来在生态学的诸多领域得到了广泛的应用.由于同位素分馏过程的存在,自然界中的不同水源具有不同的同位素组成[1].而在植物体内,除了一些排盐植物外[2],在植物根系对土壤水分的吸收过程中,稳定氢氧同位素一般不发生分馏;水分在被植物根系吸收后沿木质部向上运输是通过液流方式进行的,不存在汽化现象,一般在植物体内不存在稳定氢氧同位素分馏现象[3-5].因此,植物木质部水分的同位素组成能反映出植物利用不同水源的稳定同位素信息.如果不同来源的同位素组成差异显著,就可以通过对比植物木质部水分与各种水源的同位素组成确定植物究竟利用哪些来源的水分[6].日趋成熟的稳定同位素示踪技术为植物在不同季节从不同深度土壤获取水分的研究提供了捷径,国内外有许多学者利用稳定同位素技术对不同生态系统的植物水分利用策略进行了研究,如Valentini 等[7]对不同生应用生态学报2010年4月第21卷第4期Chinese Journal of Applied Ecology ,Apr.2010,21(4):836-842活型的植物研究发现,常绿地中海树种趋于利用雨水(浅层土壤中的水),而落叶树种则几乎毫无例外地依赖于地下水;Field等[8]对半附生植物Didymo-panax pittieri进行研究发现,该植物在其不同生长阶段采取了不同的水分获取策略,即:完全附生阶段从雾水和附生苔藓层中获取水分,乔木阶段从土壤中获取水分,而半附生阶段则同时采取以上两种方式.榕树是桑科(Moraceae)榕属(Ficus)植物的总称,主要分布在热带地区,尤以热带雨林最为集中[9],在维持热带雨林的生物多样性甚至整个生态系统的平衡中都起着十分重要的作用,是国内外公认的热带雨林中的一类关键类群[10-12].榕树物种的减少或灭绝将直接影响或改变整个热带雨林的物种多样性,没有榕树就形成不了热带雨林生态系统[9].榕树的一些种类是热带雨林中的绞杀植物,如高山榕(F.altissima)、垂叶榕(F.benjamina)、丛毛垂叶榕(F.benjamina var.nuda)、钝叶榕(F.cur-tipes)和斜叶榕(F.tinctoria)等.它们的种子通过鸟类的传播在热带雨林中的多种树木上发芽、生长,成为绞杀植物,有的种类还绞杀同种其他树木或另一种榕树.绞杀现象是热带雨林的一个重要特征,也是热带雨林中物种间复杂关系的体现,具有重要的生态学意义[13].被绞杀掉的树木死后,绞杀榕由于缺少支柱,很容易倒掉死亡,在它生长的地方就形成了一个林窗,有利于种子的萌发,使群落树种组成得以更新.同时,异质性环境也有利于森林中物种多样性的维持.另外,被榕树绞杀的多为多病的老树,所以,绞杀榕的存在有利于森林中树种的更新和森林生态系统的健康发展[14].榕树中的对叶榕、斜叶榕等是热带雨林中的先锋树种,多出现在受到一定破坏的林段、林窗与路旁.它们的种子主要靠动物传播,能够传很远的距离,且萌发力强,在光照充足的环境中生长迅速,很快就能长满林窗或被破坏的林段,在热带雨林的恢复中起到重要作用[9].绞杀榕的绞杀过程可分为3个明显的阶段:附生阶段、半附生阶段、乔木阶段.其中,我们根据缠绕在宿主树上与扎入土壤中树根的相对多少,将半附生阶段分为前期与后期.西双版纳地区具有特殊的气候条件,一年可以分为雨季、雾凉季与干热季,其中雾凉季降雨较少,而林下有大量滴落雾水补充土壤水,因此雾凉季的雾水有可能是绞杀榕的重要水分来源.本研究将以斜叶榕为研究对象,通过测定其木质部与各种潜在水源的氢氧同位素组成,以及不同生长阶段的叶片碳同位素组成,揭示西双版纳地区不同生长阶段的绞杀榕在不同季节的水分利用策略和生存机理,从而对热带雨林的保护提供参考.1研究地区与研究方法1.1自然概况西双版纳(21ʎ09ᶄ—22ʎ33ᶄN,99ʎ58ᶄ—101ʎ50ᶄE),受西南季风的影响,一年中有明显的雾凉季(11月—次年2月)、干热季(3—4月)和雨季(5—10月)之分,干热季与雾凉季又合称为干季.该地区年平均降雨量约1400mm,从图1可以看出,在雾凉季与干热季降水稀少,不足全年的13%,月均气温都在16ħ以上,温度较高,白天植物蒸散强烈,植物需水量增大,易受水分胁迫;雨季降雨占全年降雨量的87%以上.但干季几乎每日早晚都有浓雾出现(出现率>90%),且其总持续时间占干季时间的40%以上[15].本文以西双版纳勐仑地区中国科学院西双版纳热带植物园内不同生长阶段的斜叶榕为研究对象,定期测定不同深度土壤水势、叶片黎明水势与中午水势、土壤含水量,并定期收集雨水、林下滴落雾水、不同深度土壤水、木质部水分(用于测定其稳定性同位素比率δD、δ18O)以及不同生长阶段斜叶榕的叶片(用于测定其稳定性同位素比率δ13C).1.2研究方法1.2.1土壤水势的测定将土壤水势张力计(UIT,Germany)探头分不同层次(30、50、70cm)插入林下土壤中,测量土壤水势,3次重复,并在2007年8月、2007年12月与2008年2月、2008年4月分别测定雨季、雾凉季与干热季的土壤水势.土壤含水量图1西双版纳地区月均降水量(Ⅰ)与月均气温(Ⅱ)Fig.1Monthly precipitation(Ⅰ)and monthly average air temperature(Ⅱ)in Xishuangbanna(2007-05—2008-08).7384期王平元等:西双版纳绞杀植物斜叶榕的水分利用策略的测定、土壤水分与木质部水分以及叶片的取样时间均同土壤水势的测定时间.1.2.2叶片水势的测定在黎明前(5:00—6:00)和中午(12:00—14:00)用枝剪剪取不同生长阶段样树的叶片,用Pump-Up无气瓶植物压力室测定叶片水势[16].测定3片叶子,取平均值.于2007年10月与2008年3月分别测定雨季与干季的叶片水势. 1.2.3土壤质量含水量的测定用钻孔法钻取不同深度土壤样品(5、15、20、30、40、50、60、80、100、120 cm),实验室105ħ烘干,求取土壤的质量含水量.1.2.4大气降水的收集定期采集雾水样品,采集时间是干季9:00—10:00雾滴消失时,10d左右收集一次当日雾水水样并收集每次降雨水样.降雨水样采用中国科学院西双版纳热带雨林生态系统研究站采集的降水样品.1.2.5土壤水的取样用钻孔法钻取不同深度的土壤(5、15、20、30、40、50、60、80、100、120cm),同时取附生阶段与半附生阶段样树树干腐殖土.实验室内采用低温真空蒸馏法[17-18]提取土壤水.在数据处理时,我们认定表层土至50cm处为浅层土壤,50 cm以下为深层土壤.1.2.6植物木质部水分的取样8:00—9:00(干季为雾较浓重时),用枝剪剪取不同生长阶段样树的小枝样品(3 5个),实验室内采用低温真空蒸馏法[17-18]提取木质部水分.每个季节取样1 2次. 1.2.7叶片采集及δ13C的测定12:00—14:00,采摘不同生长阶段绞杀榕以及其宿主油棕(Elaeis gunieensis)林冠上的当年向阳叶片,分别在烘箱内60ħ烘干并研磨,过40目筛,用于叶片δ13C的测定.1.2.8雾水贡献比例的确定采用Brunel等[19]建立的同位素质量平衡模型计算植物利用不同来源水(雾水、雨水、地下水)的比例(P).模型假设植物获得的水分来自两部分:雾水或土壤水(雨水)以及地下水,对植物利用来说,雾水和土壤水具有同等可利用性.例如:模型计算的雾水利用比例(Pf)值不是雾水或雨水对地下水的简单比值,而是一个加权的比值.模型如下:当存在两个水分来源时:δD1x1+δD2x2=δD(1)δ18O1x1+δ18O1x2=δ18O(2)x 1+x2=1(3)如果存在3个水分来源,则:δ18O1x1+δ18O2x2+δ18O3x3=δ18O(4)δD1x1+δD2x2+δD3x3=δD(5)x1+x2+x3=1(6)式中:δ18O与δD分别指植物木质部水分的氧与氢的稳定同位素值;δD1(δ18O1)、δD2(δ18O2)、δD3(δ18O3)分别指可能利用的水源1、2、3的稳定氢(氧)同位素组成;x1、x2、x3指植物利用水源1、2、3的比例.所有水样与树叶样品寄送中国科学院兰州分院测试中心地球化学部采用稳定性同位素气体质谱仪测定其稳定同位素值.2007年8月—2008年5月期间,共收集雨水样品11个,雾水样品5个,土壤水样品113个,木质部水样品27个,树叶样品31个.水样品的稳定性氢氧同位素比率采用同位素质谱仪(氢的测定用Finnigan MAT-251,氧的测定用Finni-gan MAT-252,USA)测定.氢氧稳定性同位素比率的值是以相对于V-SMOW(Vienna Standard Mean Ocean Water)的千分率(ɢ)给出,分别以δD和δ18O 表示,精度分别为ʃ2.5ɢ和ʃ0.5ɢ.树叶样品采用同位素质谱仪(Finnigan MAT-252,USA)测定,碳稳定性同位素比率的值是以相对于PDB(Pee Dee Bel-emnite,一种出自美国南卡罗来那州的碳酸盐陨石)的千分率(ɢ)给出,以δ13C表示,精度为ʃ0.5ɢ.质谱分析的方程表达式为:δɢ=[(R sample/R standard)-1]ˑ1000(7)其中,Rsample与Rstandard分别表示样品与标准物的D与H、18O与16O、13C与12C的丰度之比.对不同季节的土壤水势、土壤含水量、叶片水势进行方差分析,数据的处理分析采用统计软件SPSS 13.0.采用绘图软件SigmaPlot10.0对文中各图进行绘制.2结果与分析2.1土壤水势的季节变化从图2可以看出,干热季的30cm土壤处水势最低值达到-0.0305MPa,此时土壤含水状况最差,随着深度的增加,土壤水势逐渐增大;最大水势出现在雾凉季时30cm深度土壤处,为-0.0145MPa.对数据进行差异显著性比较可知,雨季与干热季之间不同深度土壤的水势差异不显著,与雾凉季之间差异显著,雾凉季与干热季之间差异也显著.在雨季,随着土壤深度的增加,土壤水势逐渐变大.在30cm 处,不同季节之间土壤水势变化较大,而随着土壤深度的增加,不同季节的土壤水势逐渐趋向一致,到70cm处,在各个季节的土壤水势几乎没有差异.838应用生态学报21卷图2不同土壤深度的土壤水势季节变化Fig.2Comparisons of soil water potential at different depthsamong different seasons(meanʃSD).A:雨季Rainy season;B:雾凉季Foggy season;C:干热季Dry hotseason.下同The same below.2.2叶片水势的季节变化研究期间叶片黎明前、中午水势的季节变化如图3所示,在不同的生长阶段,雨季的叶片水势(包括黎明前与中午叶片水势)要明显大于干季的叶片水势,而干季时不同生长阶段叶片水势差异也较大.黎明前最低水势出现在干季时乔木阶段,为-0.35MPa,最高水势出现在雨季时半附生阶段前期,为-0.02MPa;中午最低水势出现在干季时附生阶段,为-0.4MPa;最高水势出现在雨季时半附生阶段后期,为-0.08MPa.图3雨季(A)与干季(B)叶片水势的季节变化Fig.3Comparisons of leaf water potential between rainy season(A)and dry season(B)(meanʃSD).Ⅰ:附生阶段Epiphytic;Ⅱ:半附生阶段前期Early stage of hemiepi-phytic;Ⅲ:半附生阶段后期Late stage of hemiepiphytic;Ⅳ:乔木阶段Arborescent.下同The same below.2.3土壤含水量的季节变化雨季的土壤水分含量为(19.05ʃ2.14)%,雾凉季为(14.93ʃ4.96)%,干热季为(14.53ʃ2.16)%(图4).雨季的土壤含水量极显著高于雾凉季与干热季(P<0.001),而雾凉季与干热季的土壤含水量则比较接近,差异不显著(P=0.64).2.4不同生长阶段斜叶榕的水分利用来源2.4.1雨季不同生长阶段斜叶榕的水分利用在雨季,雨水的稳定氧同位素值为(-9.37ʃ3.09)ɢ,浅层土壤水为(-7.76ʃ3.07)ɢ,深层土壤水为(-8.47ʃ1.7)ɢ,树干腐殖土水为(-8.9ʃ0.76)ɢ.根据Brunel等[19]建立的同位素质量平衡模型,我们可以用图中不同水源与木质部水分δ18O值之间的距离比较来表示各水源与木质部水分δ18O值的接近程度,从而揭示利用各种水分的相对比例,与木质部水分δ18O值距离越小,则该水源被利用的比例越大.只要木质部水分与某种潜在可利用水源的稳定同位素值大致处于同一区域或者有部分交叉,我们就可以认为植物利用该水源.但从图5A可以看出,不同生长阶段木质部水分的δ18O值普遍低于各潜在水源,主要是因为在蒸馏时未能将木质部中的水分提取充分,从而导致同位素发生分馏,使所得数值偏低.但根据斜叶榕的生态与生理习性可知,附生阶段由于整个植株都在宿主树上,因此只能利用雨水与树干腐殖土水;半附生阶段(包括前期与后期)则可以利用雨水、树干腐殖土水以及浅层土壤水(根系较浅不能扎入深层土壤);而乔木阶段则主要利用雨水与土壤水,由于斜叶榕的根系主要分布在土壤上层,加之浅层土壤水分含量较高,因此主要利用浅层土壤的水分.2.4.2雾凉季不同生长阶段斜叶榕的水分利用雨图4不同深度土壤含水量的季节变化Fig.4Soil water content at different depths among foggy,dryhot and rainy seasons(meanʃSD).9384期王平元等:西双版纳绞杀植物斜叶榕的水分利用策略图5不同生长阶段植物木质部水分与各潜在水源δ18O关系Fig.5Relationship ofδ18O between stem xylem water and theavailable water sources in(meanʃSD).a)可利用水源,指斜叶榕在不同生长阶段可以利用的水分来源,即图中的雨水(1)、树干腐殖土水(2)、深层土壤水(3)、浅层土壤水(4)、雾水(5)The available water sources,meant the water sources usedby F.tinctoria at different life stages,such as rain water(1),stem hu-mus water(2),deep soil water(3),shallow soil water(4)and fog drip(5);b)木质部水Stem xylem water.水的稳定氧同位素值为(-1.1ʃ0.14)ɢ,雾水为(-1.24ʃ0.15)ɢ,浅层土壤水为(-7.44ʃ2.18)ɢ,深层土壤水为(-8.02ʃ1.35)ɢ,树干腐殖土水为(-2.78ʃ3.34)ɢ.从图5B可以看出,附生阶段木质部δ18O值与雨水、雾水以及树干腐殖土水最为接近;半附生阶段木质部δ18O值与树干腐殖土水、浅层土壤水、深层土壤水最为接近;而乔木阶段木质部δ18O值与浅层土壤水以及深层土壤水最接近.2.4.3干热季不同生长阶段斜叶榕的水分利用雨水的稳定氧同位素值为(-4.87ʃ1.81)ɢ,浅层土壤水为(-6.01ʃ2.37)ɢ,深层土壤水为(-7.55ʃ0.图5C图6Fig.6gler fig可以看出,附生阶段木质部δ18O值与雨水最为接近;半附生阶段木质部δ18O值与雨水、树干腐殖土水以及浅层土壤水最为接近;而乔木阶段木质部δ18O值与浅层土壤水最接近.2.5油棕以及不同生长阶段的绞杀榕叶片碳同位素δ13C从图6可以看出,在不同季节,油棕的δ13C值比较稳定,各季节变化不大;叶片δ13C的最大值出现在干热季时附生阶段,最小值则出现在雾凉季时半附生阶段;半附生阶段后期与乔木阶段斜叶榕叶片的δ13C值普遍较大,且变化不大,而在附生与半附生阶段前期,雾凉季叶片的δ13C值明显比另外两个季节要小;在雨季,各个生长阶段的斜叶榕的δ13C值之间差异不显著.2.6雾凉季雾水的贡献比例在雾凉季时常有滴落雾水存在,雾水的存在对附生阶段斜叶榕的生长起着极其重要的作用.而对于半附生阶段的斜叶榕,雾水仍可作为其水分来源的一部分,经三元混合模型计算可知,在雾凉季,半附生阶段前期的斜叶榕利用的水分大约有7%来源于雾水,其他的绝大部分依赖于浅层土壤水(大约90%);而半附生阶段后期的斜叶榕几乎不利用雾水.3讨论西双版纳地区具有特殊的气候条件,降雨主要集中在雨季,干季时降雨极其稀少,但干季常有雾水出现,因此干季植物怎样获取水分以及利用哪种水分从而获得生存显得尤为重要.同时,由于斜叶榕具048应用生态学报21卷有特殊的生态习性,其生活史可以分为3个明显的生长阶段,各阶段潜在可利用水源不同,因此斜叶榕在不同季节不同生长阶段所利用的水源也可能存在不同.斜叶榕土壤最低水势出现在干热季较浅土壤30cm深度处,表明在干热季,30cm深度处的土壤水分含量极低,植物受水分胁迫严重.这可能是因为干热季降雨极其稀少,没有或很少有雾水补给,且气温较高,土壤蒸发与植物蒸腾非常大,导致浅层土壤水势较低.最高水势出现在雾凉季土壤30cm深度处,表明此时该处的土壤水分状况良好.这可能是因为雾凉季气温较低,植物蒸腾与土壤蒸发都较小,且浅层土壤有滴落雾水补给.在70cm深度处,各个季节的水势较高且趋向一致,说明在较深的土壤层水分含量较高并且变化较小,即斜叶榕很少利用深层土壤水.与土壤水势相比,叶片黎明前水势与中午水势明显偏低,显然这有利于植物从土壤中吸收水分.在各个生长阶段,雨季比干季的黎明前叶片水势要高,这可能是由于雨季多为阴雨天气,土壤含水量与空气相对湿度较高,叶片蒸腾较低,因而叶片水势较高.不同生长阶段的植物雨季与干季黎明前叶片水势的差异较大,说明不同生长阶段的绞杀榕对不同的水分状况表现不同的反应.黎明前叶片水势实际反映植物本身的吸水能力[20],在不同季节,植物的黎明前叶片水势随不同生长阶段而异,说明不同生长阶段的绞杀榕在不同季节具有不同的吸水能力,最低水势出现在干季乔木阶段,说明此阶段其吸水能力最强.中午时的叶片水势反映叶片水分的亏缺情况[20],最低水势出现在干季附生阶段,说明此时的叶片受水分胁迫最严重,这可能与干季时降水量较少以及气温较高有关.雨季中午叶片水势较高且差异不大,说明雨季时不同生长阶段的绞杀榕可利用水分状况良好且差异不大.由于在植物根系对土壤水分的吸收过程以及吸收后沿木质部向上运输过程中不存在稳定氢氧同位素分馏现象,各生长阶段斜叶榕木质部的稳定氧同位素值要普遍小于其各潜在水源,这可能是在取样过程或处理样品过程中出现同位素分馏现象造成的.在雨季,对于不同生长阶段的斜叶榕,雨水均为其主要水源;树干腐殖土水分也是附生阶段与半附生阶段的补充水源(树干腐殖土水分来源于雨水,同位素值与雨水接近);浅层土壤水是半附生阶段与乔木阶段的主要水源之一,这可能是因为雨季降雨充足,植物易于直接从雨水或浅层土壤中获取所需水分.在雾凉季,斜叶榕附生阶段的主要水源是雨水、雾水、树干腐殖土水;在半附生阶段前期,由于斜叶榕的根未能延伸足够长从而吸收深层土壤的水分,因此,半附生阶段前期的斜叶榕只能吸收树干腐殖土水、雾水以及浅层土壤水,而浅层土壤水与树干腐殖土水是其主要水源;而在半附生阶段后期与乔木阶段,土壤水(包括浅层与深层土壤水)是其主要水源;由于雾凉季降水很少,树干腐殖土水分含量低,因此树干腐殖土水分是半附生阶段的补充水源而非主要水源;雾水也是半附生阶段的补充水源.对于半附生阶段前期,大约有7%的水分来源于雾水,这是因为半附生阶段前期时裸露在地表上的根较多,而在半附生阶段后期,由于大部分根扎入地下,因此几乎不利用雾水.根据刘文杰等[21]对西双版纳地区的雾水与土壤水的稳定同位素研究发现,浅层土壤水主要来自于雨水与雾水的补给,但干季浅层土壤水中包含更多的雾水[21].同样,在本研究中,由于雾凉季降雨极其稀少,雾水成为浅层土壤水的主要补给水源,而浅层土壤水又是雾凉季斜叶榕半附生阶段以及乔木阶段的主要水分来源,因此,雾水虽然不会作为雾凉季斜叶榕最主要的直接水分来源,但由于其对浅层土壤水的补给作用,对雾凉季斜叶榕的生长也起着非常重要的作用.在干热季,由于附生阶段斜叶榕只有雨水与树干腐殖土水两个潜在可利用水源,且雨水与附生阶段木质部水的稳定同位素值接近,因此雨水是该阶段斜叶榕的主要水源;浅层土壤水是半附生阶段与乔木阶段的主要水源;由于干热季时降雨极少,树干腐殖质水分含量也极低,因此,雨水与树干腐殖土水是半附生阶段的补充水源;由于各生长阶段木质部同位素值与深层土壤水的同位素值相差较远,因此,各生长阶段几乎不利用深层土壤水,这是因为即使是乔木阶段斜叶榕的根也很浅,延伸不到深层土壤层.δ13C值与植物水分利用效率(WUE)呈正相关,与土壤水分含量呈负相关,油棕的δ13C比较稳定,说明油棕的水分来源也是稳定的水源,如深层土壤水,即使是在干季,受水分胁迫程度也较轻;半附生阶段后期与乔木阶段斜叶榕叶片的δ13C值普遍较大,且变化不大,说明这两个阶段植物需水量较大,且主要利用较稳定的水源,如土壤水;最大值出现在干热季的附生阶段,说明此时水分状况极差,WUE1484期王平元等:西双版纳绞杀植物斜叶榕的水分利用策略最大;雨季的各个生长阶段斜叶榕的δ13C变化不大,说明雨季时各生长阶段受水分胁迫较轻,WUE 较低.由于半附生阶段斜叶榕开始利用土壤水,乔木阶段的斜叶榕与油棕都主要利用土壤水,二者形成竞争,因此,斜叶榕生长到乔木阶段以后,根系逐渐发育完全,在与油棕的水分竞争中占据优势,油棕将逐渐死亡.综上所述,由于斜叶榕在不同季节内各生长阶段都具有不同的潜在水源,且各水源稳定同位素值存在差异,因此,根据稳定氧同位素以及植物水势等参数判定,斜叶榕不同生长阶段的植株在不同季节具有不同的水分利用来源,即在不同生长阶段采取不同的水分利用策略来应对环境的变化.参考文献[1]Hobson KA,Wasenaar LI.Stable isotope ecology:An introduction.Oecologia,1999,120:312-313[2]Lin GH,Sternberg L.Hydrogen isotopic fractionation by plant roots during water uptake in coastal wetlandplants//Ehleringer J,Hall A,Farqubar G,eds.StableIsotopes and Plant Carbon-Water Relations.San Diego,California:Academic Press,1993:497-510[3]Wershaw RL,Friedman I,Heller SJ.Hydrogen isotope fractionation of water passing through trees//Hobson F,Speers M,eds.Advances in Organic Geochemistry.New York:Pergamon Press,1966:55-67[4]Zimmermann V,Ehhalt D,Munnich KO.Soil-water movement and evapotranspiration:Changes in the iso-topic composition of water.Proceedings of the Symposi-um of Isotopes in Hydrology.Vienna,InternationalAtomic Energy Agency,1966:567-585[5]White JW,Cook ER,Lawrence JR.The D/H ratios of sap in trees:Implications of water sources and tree ringD/H ratios.Geochimica et Cosmochimica Acta,1985,49:237-246[6]Du D-Y(段德玉),Ouyang H(欧阳华).Application of hydrogen and oxygen isotope in analyzing plant wateruse sources.Ecology and Environment(生态环境),2007,16(2):655-660(in Chinese)[7]Valentini R,Scarascia Mugnozza GE,Ehleringer JR.Hydrogen and carbon isotope ratios of selected species ofa Mediterranean macchia ecosystem.Functional Ecolo-gy,1992,6:627-631[8]Field TS,Dawson TE.Water sources used by Didmo-panax pittieri at different life stages in a tropical cloudforest.Ecology,1998,79:1448-1452[9]XU Z-F(许再富),Zhu H(朱华),Yang D-R(杨大荣),et al.Species diversity and ecological signifi-cance of fig trees in tropical rainforests of southern Yun-nan//Xishuangbanna Tropical Botanical Garden,Chi-nese Academy Sciences,ed.Collected Research Paperson the Tropical Botany,Kunming:Yunnan UniversityPress,1996,4:1-15(in Chinese)[10]O’Brien TG,Kinnaird MF,Dierenfeld NL,et al.What’s so special about figs?Nature,1998,392:668[11]Zhang G-M(张光明),Gu H-Y(谷海燕),Song Q-S (宋启示),et parison of habitats and seasonallydifferentiated distribution patterns of fig wasp populationsassociated with Ficus racemosa in Xishuangbanna.Chi-nese Journal of Applied Ecology(应用生态学报),2004,15(4):627-633(in Chinese)[12]Yang D-R(杨大荣),Peng Y-Q(彭艳琼),Zhang G-M(张光明),et al.Structure and biodiversity of insectcommunity on syconia fruits of Ficus racemosa in tropicalrainforest of Xishuangbanna,China.Chinese Journal ofApplied Ecology(应用生态学报),2003,14(10):1710-1714(in Chinese)[13]Xu Z-F(许再富).Fig trees:A key species in tropical rainforests of southern Yunnan.Biodiversity Science(生物多样性),1994,2(1):21-23(in Chinese)[14]Wei Z-D(魏作东),Yang D-R(杨大荣),Peng Y-Q (彭艳琼),et al.Function of Ficus in the tropical rain-forest ecosystem in Xishuangbanna.Chinese Journal ofEcology(生态学杂志),2005,24(3):233-237(inChinese)[15]Liu W-J(刘文杰),Zhang Y-P(张一平),Liu Y-H (刘玉洪),et al.Fog throughfall at a seasonal rain for-est in Xishuangbanna,southwest China.Acta Phytoeco-logia Sinica(植物生态学报),2003,27(6):749-755(in Chinese)[16]Dawson TE,Ehleringer JR.Isotopic enrichment of water in‘woody’tissues of plants:Implications for plant-wa-ter source,water uptake and other studies which use sta-ble isotopic composition of cellulose.Geochimica et Cos-mochimica Acta,1993,57:3487-3492[17]Dawson TE.Fog in the California redwood forest:Eco-system inputs and use by plants.Oecologia,1998,117:476-485[18]Stratton LC,Goldstein G,Meinzer FC.Temporal and spatial partitioning of water resources among eight woodyspecies in a Hawaiian dry forest.Oecologia,2000,124:309-317[19]Brunel JP,Walker GR,Kennett-Smith AK.Field vali-dation of isotopic procedures for determining source wa-ter used by plants in a semi-arid environment.Journalof Hydrology,1995,167:351-368[20]Lin Z-F(林植芳),Sun G-C(孙谷畴),Lin G-Z(林桂珠),et al.Changes of leaf water potential in plantsfrom different sites at Dinghushan Biosphere Reserve//Sun H-L(孙鸿烈),ed.Papers on Tropical and Sub-tropical Forest Ecosystem.Beijing:China Meteorologi-cal Press,1998:110-118(in Chinese)[21]Liu W-J(刘文杰),Li P-J(李鹏菊),Li H-M(李红梅),et al.Fog interception and its relation with to soilwater in the tropical seasonal rain forest of Xishuangban-na,Southwest China.Acta Ecologica Sinica(生态学报),2006,26(1):9-15(in Chinese)作者简介王平元,男,1985年生,硕士.主要从事植物水分利用研究与植物专类园区管理工作.E-mail:pingyuan0920@ 责任编辑肖红248应用生态学报21卷。
氧同位素地球化学研究的新进展

氧同位素地球化学研究的新进展
穆治国
【期刊名称】《地球科学进展》
【年(卷),期】1991(6)4
【摘要】1989年在美国卡内基研究所,著名同位素地球化学家Zachary Sharp和James Oneil研制出了用于做硅酸盐和氧化物氧同位素分析的激光碳还原技术。
稳定同位素分析是能够获得制约各类岩石形成和变化条件信息的最有用的地球化学技术之一。
然而,从硅酸盐和氧化物中萃取氧的方法过去25年一直保持不变(我国是在13年前研制成的)。
由于这种分析必须有较大的样品量。
【总页数】2页(P43-44)
【关键词】氧;同位素;地球化学;碳;还原
【作者】穆治国
【作者单位】北京大学地质学系
【正文语种】中文
【中图分类】P597
【相关文献】
1.镁同位素地球化学研究新进展及其应用 [J], 柯珊;刘盛遨;李王晔;杨蔚;滕方振
2.铜同位素地球化学及研究新进展 [J], 王泽洲;刘盛遨;李丹丹;吕逸文;吴松;赵云
3.内蒙古阿扎哈达铜铋矿床流体包裹体和碳氧硫铅同位素地球化学研究 [J], 王银宏; 刘家军; 张梅; 张方方; 王康; 咸雪辰; 郭灵俊
4.钙同位素地球化学研究新进展及其在碳酸岩-共生硅酸盐研究中的应用 [J], 向蜜;龚迎莉;刘涛;田世洪
5.新疆北部同位素地球化学研究新进展 [J], 胡霭琴;张国新
因版权原因,仅展示原文概要,查看原文内容请购买。
环境同位素硫同位素的应用

中国地质大学研究生课程论文封面硫稳定同位素的应用课程名称:环境同位素教师姓名:研究生姓名:研究生学号:研究生专业:所在院系:类别: B.硕士日期:2014年12月24日评语注:1、无评阅人签名成绩无效;2、必须用钢笔或圆珠笔批阅,用铅笔阅卷无效;3、如有平时成绩,必须在上面评分表中标出,并计算入总成绩。
摘要硫酸盐存在于大多数天然环境中,例如地下岩层、土壤、湖泊、河水、海水、地下水以及大气圈中。
在海水中(SO42-)是第二个最丰富的阴离子,并且在全球生物地球化学循环中发挥着关键的作用。
大气圈中的硫化合物,特别是硫酸盐气溶胶是全球气候变化中的基本成分。
硫酸盐的硫同位素组成可以提供不同的自然环境中有关它的起源、迁移过程和转化的线索。
应用硫酸盐的硫同位素(34S)并结合环境同位素中的其他同位素(如氧同位素17O和18O等等)可探讨地表环境和水环境中(尤其是干旱地区)硫酸盐的起源(大气来源、基岩和风化来源、人类活动起源、风尘搬运、古火山活动或海盐起源等)。
目前,硫同位素作示踪剂在化学、地球化学、地质学、农业科学和环境科学研究中都有广泛的应用,硫同位素越来越多地被应用到人类生产生活的各个方面。
在自然界中,硫分布广泛,硫可以呈气态、液态和固态等多种形式存在于地球的各圈层中。
自然界的硫元素有5种同位素,即32S、33S、34S、35S、36S,除35S是放射性成因同位素外,其余四种都是稳定同位素其中,35S是大气40Ar受宇宙射线轰击而蜕变产生的。
硫元素的四种稳定同位素(32S、33S、34S、36S)的相对丰度为%、%、%、%。
由于33S和36S的丰度低,因此硫同位素研究中主要考虑分布最广泛、丰度较大的34S和32S这两种同位素的组成变化。
本文主要介绍硫同位素在地球化学,地质学,环境科学中的应用。
关键词:硫同位素应用;硫同位素分馏;稳定同位素应用;目录摘要第一章绪论 (1)§研究目的及意义 (1)研究目的及意义 (1)§研究现状 (1)国外研究现状 (1)国内研究现状 (1)第二章硫同位素概况 (3)§基本概况 (3)硫同位素组成 (3)硫同位素分布特征 (3)§硫同位素分馏 (5)硫同位素分馏 (5)硫同位素分馏分类 (5)第三章硫同位素的应用 (7)§硫同位素在地球化学中的应用 (7)硫同位素在地球化学中的应用 (7)§硫同位素在地质学中的应用 (7)硫同位素在水文地质中的应用 (7)硫同位素在沉积学中的应用 (8)硫同位素在矿床学中的应用 (8)硫同位素地质温度计 (9)§硫同位素在环境科学中的应用 (10)大气自然过程的硫来源分析 (10)硫稳定同位素在森林生态系统中的应用 (10)硫稳定同位素在水域生态系统中的应用 (11)硫稳定同位素在我国酸雨研究中的应用 (11)第四章结论与建议 (12)§ 结论与建议 (12)结论 (12)建议 (12)参考文献 (13)第一章绪论§研究目的及意义研究目的及意义硫酸盐存在于大多数天然环境中,例如地下岩层、土壤、湖泊、河水、海水、地下水以及大气圈中。
布朗大学同位素地球化学Carbonisotope

Ribulose-1,5-biphosphate carboxylase/oxygenase (Rubisco) for C3 plants (trees, aquatic plants and algae): “Rubisco” for short Phospoenol pyrurate carboxylase (PEP Carboxylase) for C4 plants (tropical grasses, sedges etc)
Key isotopic effects of steps leading to CO2 fixation in C3 plants
Process Isotope Discrimination Symbol effect (‰) (Δ) 1.0044 4.4 a
Diffusion of CO2 in air through the stomatal/pore
Dissolved inorganic carbon (DIC)
In equilibrium with atmospheric CO2, d13C values of DIC vary with pH, as carbonate speciation changes, because different carbonate species (CO2(g), CO2(aq), HCO3-, CO32-) have different d13C values
The Global Carbon Cycle
Carbonate system
CO2(g) ↔ CO2(aq)↔H2CO3*
KCO2
aH 2CO3* pCO2
3
101.46
铅同位素在示踪土壤重金属污染研究中的应用
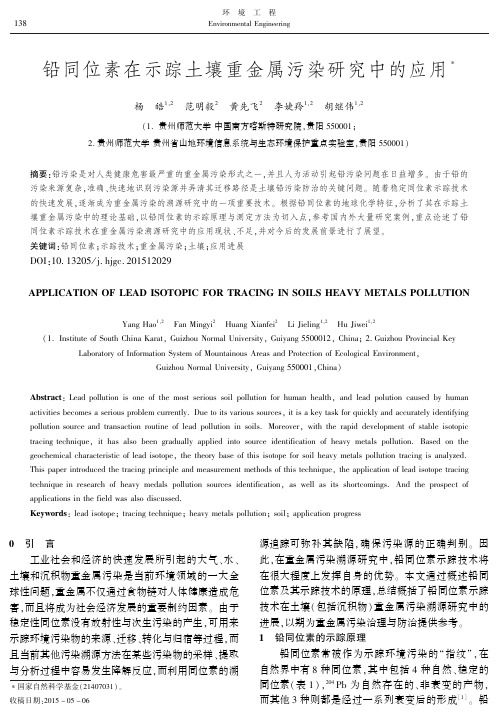
207
Pb
力较弱,但人为源的铅易溶解与易迁移,对环境的危
Pb /
害更加严重 [11] 。 随着重金属污染问题的进一 步 加
。 铅同位素示踪技术最早应用
将铅同位素的比值与浓度变化引入混合模型之中,能
于大气颗粒物铅污染源的研究,此后逐步扩展到土壤
有效地区分出自然来源与人为来源,并能推算出自然
与沉积物中。 Jaime 等分析了美国佛罗里达州湖泊的
似的地球化学性质,采用铅同位素示踪工具研究了土
。 刘茜进一步对湘江入
壤中 Ti 的污染特征,发现污染土壤中 Ti 分布与 Pb
质的铅同位素数据,发现部分样品与矿石的铅同位素
组成相似,污染源可能为矿山,污染传播方式可能为
大气沉降 [9] 。 而长春市城市土壤的铅同位素示踪结
果显示:土壤铅污染主要来自于历史遗留的汽车尾气
释质谱法( IDMS) 和电感耦合等离子体质谱法( ICP⁃
污染、工业燃煤排放以及建筑尘,为多种污染源共同
异位素干扰。 而随着激光技术的兴起,激光共振电离
环 境 工 程
Environmental Engineering
138
铅同位素在示踪土壤重金属污染研究中的应用
∗
杨 皓1,2 范明毅2 黄先飞2 李婕羚1,2 胡继伟1,2
(1. 贵州师范大学 中国南方喀斯特研究院,贵阳 550001;
2. 贵州师范大学 贵州省山地环境信息系统与生态环境保护重点实验室,贵阳 550001)
applications in the field was also discussed.
Keywords: lead isotope; tracing technique; heavy metals pollution; soil; application progress
地球化学科学前沿:Stable isotopes
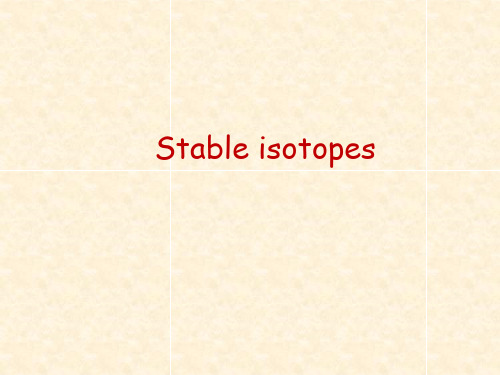
Carbon: the standard is abbreviated as PDB which stands for the Pee Dee Formation of South Carolina. It is a belemnite formation and the carbon isotope ratio of the CO2 liberated from the reaction with acid is considered a universal standard. 13C/12C = 0.01124.
– H2 – CO2 – SO2 – N2 – CO Therefore complex organic molecules must be
broken down to the simpler gas molecules.
HOW DO WE EXPRESS THE QUANTITY OF ISOTOPE IN A SAMPLE?
12C = light stable isotope of carbon (~98.9%) 13C=heavy stable isotope of carbon (~1.1%)
16O=light stable isotope of oxygen (~99.759%) 18O=heavy stable isotope of oxygen (~.204%) 17O=heavy stable isotope of oxygen (~.037%)
用天然_13_C丰度法评估贵州茂兰喀斯特森林区玉米地土壤中有机碳的来源

3 期 朴河春等: 用天然13C 丰度法评估贵州茂兰喀斯特森林区玉米地土壤中有机碳的来源 43 5
留物, 因此, 植物残留物的13C 12C 比值对土壤有机质的 ∆13C 值有直接的影响。 因此, 利用土壤有机质的稳
定碳同位素比值可以重建生态时间尺度和地质时间尺度上的植被动态过程 [4, 5]。当森林生态系统或草地生
can efficien tly supp ly nu trien ts to p lan t a re very sm a ll. T herefo re, it is necessa ry to op tim ize the m anage2 m en t of p lan t residues inco rpo ra ted in to the so ils. Key words: fo rest eco system ; agricu ltu ra l eco systm ; stab le C iso top e; p lan t residues; Ka rst 文章编号: 100020933 (2001) 0320434206 中图分类号: Q 143 文献标识码: A
∆13C 值。
2 结果与讨论
211 植物和土壤的 ∆13C 值
森林植被的 ∆13C 值, 其不同组织间的差异较大, 从树叶的- 30. 7‰±0. 7‰变化到树根的- 2714‰±
012‰, 树枝为- 29. 3‰±0. 3‰ (表 1) , 其平均值为- 2911‰±1. 3‰, 是属于 C3 植物。 而农田植被的 ∆13C 值各组织间的差异较小, 如玉米各组织间的差异仅为 0. 02‰, 其平均值为- 11. 4‰±0. 01‰, 属于 C4 植
态系统转变成农田生态系统时, 会把 C4 植物 (一般其 ∆13C = - 12‰~ - 14‰) 残留物导入到曾在 C3 植
Stable isotopes in plant ecology

Annu.Rev.Ecol.Syst.2002.33:507–59doi:10.1146/annurev.ecolsys.33.020602.095451Copyright c 2002by Annual Reviews.All rights reservedFirst published online as a Review in Advance on August 14,20021Center for Stable Isotope Biogeochemistry and the Department of Integrative Biology,University of California,Berkeley,California 94720;email:tdawson@,mambelli@,ktu@,agneta@ 2Ecosystem Sciences Division,Department of Environmental Science,Policy and Management,University of California,Berkeley,California 94720;email:ptempler@Key Words plant-environment interactions,resources,tracers,integrators,scaling,methodsAbstract The use of stable isotope techniques in plant ecological research has grown steadily during the past two decades.This trend will continue as investigators realize that stable isotopes can serve as valuable nonradioactive tracers and nondestruc-tive integrators of how plants today and in the past have interacted with and responded to their abiotic and biotic environments.At the center of nearly all plant ecological re-search which has made use of stable isotope methods are the notions of interactions and the resources that mediate or influence them.Our review,therefore,highlights recent advances in plant ecology that have embraced these notions,particularly at different spatial and temporal scales.Specifically,we review how isotope measurements asso-ciated with the critical plant resources carbon,water,and nitrogen have helped deepen our understanding of plant-resource acquisition,plant interactions with other organ-isms,and the role of plants in ecosystem studies.Where possible we also introduce how stable isotope information has provided insights into plant ecological research being done in a paleontological context.Progress in our understanding of plants in natural environments has shown that the future of plant ecological research will continue to see some of its greatest advances when stable isotope methods are applied.At the core of all plant ecological investigations is the notion of plant-environment interactions,be these with the physical environment or with other organisms.Investigations that seek to understand the nature of these interactions commonly focus on particular resources such as light,water,carbon dioxide,or nutrients and how the interaction is influenced or mediated by that resource.Understanding the importance of a particular resource in a plant ecological context requires the0066-4162/02/1215-0507$14.00507A n n u . R e v . E c o l . S y s t . 2002.33:507-559. D o w n l o a d e d f r o m a r j o u r n a l s .a n n u a l r e v i e w s .o r g b y I n s t i t u t e o fB o t a n y o n 06/10/07. F o r p e r s o n a l u s e o n l y .508DAWSON ET AL.acquisition of observational and experimental data;the collection of such data,in turn,requires suitable methods and measurements.As with most areas of science our ability to obtain appropriate measurements that aid us with addressing the unanswered questions in plant ecology have of-ten been limited or constrained by available tools.In this regard,stable isotope methods have recently emerged as one of the more powerful tools for advancing understanding of relationships between plants and their environment.Stable iso-tope techniques have permitted plant ecologists to address issues that have seemed intractable using other methods.They have therefore had a significant and positive impact on the science of plant ecology much like modern molecular techniques have for the fields of genetics,biochemistry,and evolutionary biology.Stable iso-tope information has provided insights across a range of spatial scales from the cell to the plant community,ecosystem,or region and over temporal scales from seconds to centuries.The elegance of stable isotope methods derives from the fact that it is generally easy to learn and the behavior of stable isotopes in ecological systems and biogeochemical cycles is reasonably well understood owing to the pioneering work of isotope chemists and geochemists.Areas in need of a deeper understanding seem well within our reach as stable isotope investigations and methods become more refined.Arguably,stable isotope methods are now among the most important empirical tools in modern plant ecological research,and the information they provide has yielded some of the newest and most important in-sights about plants in natural environments since the advent of the common garden experiment.Our review highlights recent advances in plant ecology that have used stable isotope data to address questions at a variety of scales.We focus on carbon (C),water (H 2O),and nitrogen (N)because they are three of the most important re-sources influencing plant function,growth,distribution,and the biogeochemical cycles in which plants participate.We begin by briefly reviewing stable isotope terminology.Some essential principles for understanding how and why isotopes vary in nature and how isotope values alone or in mixtures are calculated are found in special topic boxes within the text.The sections that follow review studies that illustrate particular issues and identify where emerging trends or patterns exist,where areas of controversy and/or disagreement remain,and where promising ar-eas of future research lie.For the newcomer,our hope is that this review enhances understanding of how stable isotopes might be used in plant ecological research.For the seasoned user,this review serves as a place to retrieve information on what we know,do not know,and need to know.Because space is limited,our review is not comprehensive but we hope that we have not been superficial and we apologize to scientists whose work we could not include.Wherever we feel it is helpful and possible we direct the reader toward other literature that provides more in-depth discussions of particularly important issues or areas of st,we restrict our review to H,C,N,and O isotopes and terrestrial systems and attempt to show how both natural abundance and enrichment studies can be used.A n n u . R e v . E c o l . S y s t . 2002.33:507-559. D o w n l o a d e d f r o m a r j o u r n a l s .a n n u a l r e v i e w s .o r g b y I n s t i t u t e o fB o t a n y o n 06/10/07. F o r p e r s o n a l u s e o n l y .STABLE ISOTOPES IN PLANT ECOLOGY509As noted previously (Peterson &Fry 1987),ecological studies have been informed by using stable isotopes at naturally occurring levels (called “natural abundance”;Table 1B)and at levels well outside the natural range of values (called “enriched”levels);enriched isotope studies therefore use “labeled”substances.Isotope abun-dance in any sample,enriched or not,is measured using a mass spectrometer.The details of how these measurements are made and how the mass spectrometer worksTABLE 1The (A )isotope abundance ratios measured and their internationally accepted reference standards,and (B )the elements,their isotopes,their percent abundance,common form,relative molecular mass difference,and range (in ‰)measured in terrestrial environments of the principle stable isotopes discussed in this review Ratio Abundance ratio Isotope measured Standard of reference standard A2H (D)a 2H/1H (D/H)V-SMOW b 1.557510413C 13C/12C V-PDB c 1.123710215N 15N/14N N 2-atm.d 3.676410318O18O/16OV-SMOW, 2.0052103V-PDB 2.0672103Form &relative Percent molecular mass Terrestrial e Element Isotopeabundance differencerange BHydrogen 1H 99.9841HD/1H 1H 700‰2H (D)0.0156(3/2),50%Carbon 12C 98.98213C 16O 16O/12C 16O 16O 100‰13C 1.108(45/44),2.3%Nitrogen 14N 99.6315N 14N/14N 14N 50‰15N 0.3663(29/28),3.6%Oxygen16O 99.75912C 16O 18O/12C 16O 16O 100‰17O 0.037(46/44),4.5%18O0.204a The hydrogen stable isotope with mass two is also called deuterium,D.b The original standard was standard mean ocean water (SMOW)which is no longer available;however,Vienna-SMOW is available from the IAEA.cThe original standard was a belemnite from the PeeDee formation (PDB)which is no longer available;however,“Vienna”-PDB is available from the IAEA.datm.atmospheric gas.e Approximaterange measured in all analyzed substances from Earth (gasses,solids,biological materials).A n n u . R e v . E c o l . S y s t . 2002.33:507-559. D o w n l o a d e d f r o m a r j o u r n a l s .a n n u a l r e v i e w s .o r g b y I n s t i t u t e o fB o t a n y o n 06/10/07. F o r p e r s o n a l u s e o n l y .510DAWSON ET AL.can be found in reviews by Criss (1999),Ehleringer et al.(2000b),and Dawson &Brooks (2001).Natural abundance stable isotopes are used as both natural integrators and tracers of ecological processes.As integrators,they permit ecologists to evalu-ate the net outcome of many processes that vary both spatially and temporally,while not disrupting the natural activity or behavior of the element in that sys-tem (Handley &Raven 1992,H¨o gberg 1997,Robinson 2001).As tracers,they allow ecologists to follow the fates and transformations of a ing nat-ural abundance isotopes as tracers requires that the different potential sources have repeatable and distinct values (Equation 1)that are broader than the natu-ral range of plant values measured.Furthermore,for tracers to be most useful there must not be significant fractionation (Text Box 1)or mixing of sources dur-ing the steps that move the resource from its source to the plant.Because it can be very difficult to fulfill all these requirements,many plant ecological investi-gations cannot use natural abundance isotope data to determine sources or pro-cess rates (Handley &Scrimgeour 1997);these studies rely on enriched isotope approaches.BOX 1Natural Abundance Stable Isotope FractionationChanges in the partitioning of heavy and light isotopes between a source substrate and the product(s)is termed isotope fractionation.Fractionation occurs because physical and chemical processes that influence the representation of each isotope in a particular phase (e.g.,liquid vs.vapor)are proportional to their mass.In plant ecological investigations,though these fractionations are typically quite small,they are nonetheless important and must be understood for proper data interpretation.Isotope fractionations are categorized as primarily of two types;equilibrium frac-tionation and kinetic fractionation.Equilibrium isotope fractionation occurs during isotope exchange reactions that convert one phase (e.g.,liquid)to another phase (e.g.,vapor).The forward and back reaction rates of the rare isotope that leads to isotope redistribution are identical to each other.These reactions are often incom-plete or take place in an open system that result in unequal (or nonequilibrium)representation of all of the isotope species in the mixture in all of the phases.If the system in which the isotope exchange reaction is taking place is closed and/or the reaction is allowed to go to completion (full exchange has taken place),there will be no net fractionation.This can occur under natural conditions,for example,when all of a particular substrate is consumed,but under many circumstances these reactions are incomplete and therefore net fractionation does exist.Kinetic isotope fractionation occurs when the reaction is unidirectional and the reaction rates are mass-dependent.In biological systems,kinetic fractionations are often catalyzed by an enzyme that discriminates among the isotopes in the mixture such that the substrate and product end up isotopically distinct from one another.Biologically mediated isotope fractionation is also called isotope discrimination.Fractionations exist because the lighter isotope (with a lower atomic mass)forms bonds that are more easily broken.Therefore,the lighter isotope is more reactive and likely to be concentrated in the product faster and more easily than the heavierA n n u . R e v . E c o l . S y s t . 2002.33:507-559. D o w n l o a d e d f r o m a r j o u r n a l s .a n n u a l r e v i e w s .o r g b y I n s t i t u t e o fB o t a n y o n 06/10/07. F o r p e r s o n a l u s e o n l y .STABLE ISOTOPES IN PLANT ECOLOGY511isotope (Kendall &McDonnell 1998,Dawson &Brooks 2001).Many biochemical and biogeochemical processes discriminate against the heavier isotopic species in a mixture (e.g.,against 13CO 2more than 12CO 2during C3photosynthetic C fixation).This discrimination leads to marked variation in the isotopic ratios of source and product pools at different stages of a chemical reaction or biogeochemical cycle and of the different resources used by the organisms from these pools.Fractionations involved in biogeochemical reactions can provide information about processes.The resulting isotope ratio of any substance that is part of the reaction can act also as a fingerprint for that resource or transitional form.It can therefore be used as a tracer to follow the reaction products through complex cycles or into diets (e.g.,you are what you eat 0.1to 1‰for 13C,and 3to 4‰for 15N;see Griffiths 1998,Robinson 2001)or along an isotope gradient of continuum such as when water moves from soils through plants and into the atmosphere (Gat 1996,Dawson et al.1998).Enriched isotope methods involve applying trace amounts of a labeled sub-stance.This procedure permits one to follow the flows and fates of an element without altering its natural behavior (Schimel 1993;go to Text Box 3).Because the substances are enriched,relative to the background,tracer studies remove or minimize problems of interpretation brought about by fractionation (Text Box 1)among pools that mix because the signal (the label)is amplified relative to the noise (variation caused by fractionation).Thus,the addition of an enriched sub-stance acts as a powerful tracer for following a specific element’s cohort through a system as well as for determining rates of biological process within the system (see Nadelhoffer &Fry 1994).For natural abundance work we express the stable isotope composition of a particular material or substance as a ratio relative to an internationally accepted reference standard (Table 1A)as,XXE 1000R sample R standard1‰ 1.where E is the element of interest (e.g.,2H or D,13C,15N or 18O),“XX”is the mass of the rarest (and heavier)isotope in the abundance ratio,and R is the abundance ratio of those isotopes (e.g.,18O/16O).Absolute abundance ratios are often very small (on the order of a few parts per thousand;Table 1B),so expressing isotope values relative to a standard and multiplying these by 1000simply expresses the very small fractional differences in convenient “per mil”(or ppt)notation shown as ‰.The final value is expressed as the amount of the rarest to commonest (heavy to light)isotope in the sample with higher values indicating greater amounts of the heavier isotope.By definition,standards have a value of 0‰.A positive value therefore indicates that the sample contains more of the heavy isotope than the standard whereas a negative value indicates that the sample contains less than the standard.A n n u . R e v . E c o l . S y s t . 2002.33:507-559. D o w n l o a d e d f r o m a r j o u r n a l s .a n n u a l r e v i e w s .o r g b y I n s t i t u t e o fB o t a n y o n 06/10/07. F o r p e r s o n a l u s e o n l y .512DAWSON ET AL.For studies using enriched materials,the labeled substance added (e.g.,15NO 3)has an isotopic composition that significantly differs (usually exceeds)from any natural occurring level.The expression of the isotopic composition of this type of material is referred to in “atom %”(A b )which is defined as,A bX heavyX heavy X light100R sample R sample1%2.where X heavy and X light are the numbers of heavy and light atoms present in the sample and R sample is the isotope ratio (as above).Equation 2is most commonly used when values of A b exceed 0.5atom %(or 500‰).Atom %is thus the percentage contribution of the heavy isotope to the total number of atoms of that element in the sample.The sections that follow are arranged hierarchically.We begin with the individual plant and how it interacts with the environment to acquire the resources it needs.We then review examples of how isotopes have informed us about interactions that occur between plants and other organisms;this section therefore focuses on the population and community scales.The final section reviews ecosystem studies where plants play a central role.We conclude with our views on future directions in plant ecology where we believe stable isotopes can have a positive impact.The acquisition of resources is the dominant theme that encapsulates stable isotope research at the individual plant level.Stable isotope information has been most informative in studies focused on water,carbon,and nitrogen—three resources that influence and limit plant growth,survival,and distribution.The sections that follow are organized around these resources.The utility of using C isotopes as an ecological index of plant functionstems from the correlation between habitat quality and the biochemical discrimina-tion (Text Box 1)against 13CO 2during gas exchange,noted here as .In C3plants,discrimination ()against 13C by the carboxylating enzyme,Rubisco (27‰),is linked to photosynthesis via c i /c a ,the ratio of intercellular to atmospheric CO 2concentrations (Farquhar et al.1982,Brugnoli et al.1988).This ratio reflects the relative magnitudes of net assimilation (A )and stomatal conductance (g )that relate to demand and supply of CO 2,respectively.Carbon-13data are thus a useful index for assessing intrinsic water use efficiency (A /g ;the ratio of carbon acquired to water vapor losses via stomatal conductance,g )and may even provide informa-tion on actual water use efficiency (the ratio of assimilation to transpiration)when the leaf-to-air vapor pressure difference is known (Farquhar et al.1989).In C4A n n u . R e v . E c o l . S y s t . 2002.33:507-559. D o w n l o a d e d f r o m a r j o u r n a l s .a n n u a l r e v i e w s .o r g b y I n s t i t u t e o fB o t a n y o n 06/10/07. F o r p e r s o n a l u s e o n l y .STABLE ISOTOPES IN PLANT ECOLOGY513plants,variations in c i /c a and are relatively small despite variation in A and g (Farquhar 1983,Henderson et al.1992,Buchmann et al.1996a).In CAM plants,values generally lie between that for C3(15to 25‰or 20to 35‰using 13C notation)and C4( 2.5to 5‰or 11to 15‰using 13C notation)plants (Griffiths 1992).Variation in of nonvascular plants is similar to that in C3plants (Rundel et al.1979,Teeri 1981,Proctor et al.1992).In contrast to gas exchange techniques that provide measurements of photo-synthetic rates at a single time,13C integrates photosynthetic activity throughout the period the leaf tissue was synthesized.Moreover,leaf 13C values reflect the interplay among all aspects of plant carbon and water relations and are thereby more useful than plant gas exchange measurements as integrators of whole plant function (Figure 1).As such,the earliest observations of 13C values in plant tissues (Wickman 1952,Craig 1953,Baertschi 1953)quickly established that C-isotope analyses were an important tool for integrating photosynthetic performance across ecological gradients in both space and time.As reviewed by Ehleringer (1988,1993a,b),C isotopes have also been instrumental in revealing how species adjust their gas exchange metabolism,strategies of resource acquisition and use,and life-history patterns to ensure competitiveness and survival in a given habitat.Variation in is caused by genetic and environmental factors that combine to influence gas exchange through morphological and functional plant responses.Discrimination has been observed to vary in response to soil moisture (Ehleringer &Cooper 1988;Ehleringer 1993a,1993b;Stewart et al.1995;Korol et al.1999),low hu-midity (Madhavan et al.1991,Comstock &Ehleringer 1992,Panek &Waring 1997),irradiance (Ehleringer et al.1986,Zimmerman &Ehleringer 1990),tem-perature (Welker et al.1993,Panek &Waring 1995),nitrogen availability (Condon et al.1992,H¨o gberg et al.1993,Guehl et al.1995),salinity (Bowman et al.1989,Sandquist &Ehleringer 1995,Poss et al.2000),and atmospheric CO 2concen-tration (Bettarini et al.1995,Ehleringer &Cerling 1995,Williams et al.2001).Furthermore,morphological features also impose constraints on the physiologi-cal response to these various conditions through their influence on such factors as leaf boundary layer resistance,hydraulic conductivity through xylem,and leaf internal resistance to CO 2and H 2O.Accordingly,variation in has been found in relation to leaf size (Geber &Dawson 1990)and thickness (Vitousek et al.1990,Hanba et al.1999,Hultine &Marshall 2000),stomatal density (Hultine &Marshall 2000),branch length (Waring &Silvester 1994,Panek &Waring 1995,Panek 1996,Walcroft et al.1996,Warren &Adams 2000),and canopy height (Yoder et al.1994,Martinelli et al.1998).Finally,is,to a large extent,genetically determined,as relative rankings within and among genotypes are maintained irrespective of varia-tions in the environment or plant condition (Farquhar et al.1989,Ehleringer 1990,Ehleringer et al.1990,Geber &Dawson 1990,Johnson et al.1990,Schuster et al.1992a,Dawson &Ehleringer 1993,Zhang et al.1993,Donovan &Ehleringer 1994,Johnsen &Flanagan 1995,Zhang &Marshall 1995,Damesin et al.1998,Johnsen et al.1999).In contrast to vascular plants,genetic variation among non-vascular plants appears to have little effect on whereas environmental factorsA n n u . R e v . E c o l . S y s t . 2002.33:507-559. D o w n l o a d e d f r o m a r j o u r n a l s .a n n u a l r e v i e w s .o r g b y I n s t i t u t e o fB o t a n y o n 06/10/07. F o r p e r s o n a l u s e o n l y .514DAWSON ETAL.Figure 1Isotopic composition of C,O,and H pools in the carbon and water cycles.Boxes represent pools and arrows represent processes.The values are rough approximations and can vary greatly with geographical location and environmental conditions.For demonstration purposes,we include data based on an example from Israel;D values were estimated from 18O using the local meteoric water line for the same region following Gat &Carni (1970).The main advantages of the isotopic approach lie in the unique labeling of flux components;leaf transpiration and soil evaporation are isotopically very different;root and soil respiration can have distinct 13C labeling;photosynthesis (depleted uptake)tends to enrich the atmosphere,while respiration (depleted release)tends to deplete the atmosphere in 18O and 13C.OM refers to organic matter.indicates discrimination occurs during photosynthetic assimilation.Values are on the SMOW and PDB scales for 18O and 13C values,respectively.[Modified from Yakir &Sternberg (2000).]such as moisture availability and water content are most important (Rice &Giles 1996,Williams &Flanagan 1996,Rice 2000).But unlike in vascular plants,tends to increase with water limitation in nonvascular plant taxa (Williams &Flanagan 1996,1998).The factors cited above explain much of the variation in observed with respect to phenology (Lowden &Dyck 1974,Smedley et al.1991,Ehleringer et al.1992,Damesin et al.1998),development (Geber &Dawson 1990),age (YoderA n n u . R e v . E c o l . S y s t . 2002.33:507-559. D o w n l o a d e d f r o m a r j o u r n a l s .a n n u a l r e v i e w s .o r g b y I n s t i t u t e o fB o t a n y o n 06/10/07. F o r p e r s o n a l u s e o n l y .STABLE ISOTOPES IN PLANT ECOLOGY515et al.1994,Fessenden &Ehleringer 2002),and gender (Dawson &Ehleringer 1993,Kohorn et al.1994,Retuerto et al.2000,Ward et al.2002).In addition,the aforementioned factors also explain spatial gradients in found within canopies (Medina &Minchin 1980,Garten &Taylor 1992,Buchmann et al.1997a,Hanba et al.1997,Le Roux et al.2001),across landscapes (Williams &Ehleringer 1996,Moore et al.1999),and with altitude (K¨o rner et al.1991,Morecroft &Woodward 1990,Hultine &Marshall 2000).It should be noted that the causes of variation in are clearly complex and are,at times,not straightforward.This complexity can make correlations between and a single factor such as hydraulic conductivity (Cernusak &Marshall 2001),water availability (Warren et al.2001),or rainfall (Miller et al.2001)problematic.Further,variations in 13CO 2of source-air owing to recycling of respired CO 2within canopies (Figure 1)may confound the ecological interpretation of 13C or in leaf tissues (Schleser &Jayasekera 1985,Sternberg et al.1989,Broadmeadow et al.1992,Buchmann et al.1997b,Yakir &Sternberg 2000).Because of the integrative response of to multiple eco-physiological con-straints through time,C isotopes can be used to assess traits that co-vary with gas exchange,C gain,and water relations,including water use efficiency (WUE)(Farquhar &Richards 1984,Henderson et al.1998),photosynthetic capacity (Virgona &Farquhar 1996),stomatal conductance (Condon et al.1987,Ehleringer 1990,Ehleringer et al.1990,Virgona et al.1990,Meinzer et al.1992),leaf nitro-gen content (Sparks &Ehleringer 1997,Schulze et al.1998),leaf mass per area (Vitousek et al.1990,Hultine &Marshall 2000,Williams &Ehleringer 2000),longevity (DeLucia et al.1988,Schuster et al.1992b),and relative growth rate (Ehleringer 1993b,Poorter &Farquhar 1994).For example,working in boreal ecosystems,Brooks et al.(1997)used 13C as a surrogate for physiological char-acteristics and found that life form (deciduous or evergreen trees,shrubs,forbs,and mosses)can be a robust indicator of functional group membership related to carbon and water fluxes (see also Flanagan et al.1997a).Whereas these data are consistent with those gathered by Marshall &Zhang (1994),Kelly &Woodward (1995)found that life form had no effect on among three altitude catagories.In another example,Smedley et al.(1991)examined the seasonal time-course of 13C among grassland species and found lower WUE among the taxa active during the initial,less stressful months of the growing season.Further,WUE increased with evaporative demand as soil moisture declined.In a related fashion,Kloeppel et al.(1998)used 13C of leaf tissue to assess WUE and determined that,in general,larches (Larix spp.)use water less efficiently and maintain higher photosynthetic capacity (based on foliar N concentration)than co-occurring evergreen conifers from 20locations in the northern hemisphere.Their results suggest that water is not the most limiting resource at these high elevation (3000–4000m)sites.Finally,Flanagan et al.(1992)and Valentini et al.(1992)used 13C of leaves to assess WUE of species from different functional groups in a Pinyon-Juniper Woodland and the Mediterranean macchia,respectively.By concurrently measuring D in xylem water to distinguish surface versus groundwater sources (see next section),A n n u . R e v . E c o l . S y s t . 2002.33:507-559. D o w n l o a d e d f r o m a r j o u r n a l s .a n n u a l r e v i e w s .o r g b y I n s t i t u t e o fB o t a n y o n 06/10/07. F o r p e r s o n a l u s e o n l y .516DAWSON ET AL.they found that species with more negative 13C values and therefore lower WUE had deeper rooting depths and a more reliable water supply than species that relied on rain water in the upper soil layers (also see Lajtha &Marshall 1994).To separate the independent effects of photosynthetic capacity and stomatal conductance on c i /c a ,Scheidegger et al.(2000)have recently proposed measuring both 13C and 18O in leaf organic matter (Figure 1).Whereas 13C reflects c i /c a ,18O generally varies with ambient humidity,which in turn reflects changes in water use [g ](Ball et al.1987,Grantz 1990;but see also Mott &Parkhurst 1991,Monteith 1995).The 18O of leaf and tree ring cellulose are largely determined by the integrated leaf-to-air vapor pressure gradient during photosynthetic gas ex-change (Farquhar et al.1998).This leaf-air vapor pressure gradient changes with environmental conditions (atmospheric humidity,soil moisture,air temperature)and plant response to these environmental changes (e.g.,g ,leaf temperature,A ).So measurement of the 18O composition of plant tissues aids with the interpretation of differences in 13C among individual plants growing in the same location and among species in different environments.Moreover,the determination of WUE is greatly improved by the simultaneous use of 13C and 18O in plant tissues (Saurer et al.1997).By considering concurrent variations 13C and 18O,one can distin-guish between biochemical and stomatal limitations to photosynthesis in response to a change in environmental conditions.Alternative methods to distinguish such effects rely on instantaneous gas exchange measurements (Farquhar &Sharkey 1982)that are more difficult to extend through time or to apply simultaneously on a large number of samples.Although further research is needed to develop a quan-titative dual (C and O)isotope model,this approach should improve our ability to relate gas exchange characteristics to 13C and 18O signals in plant leaves and tree rings.In fact,bulk wood or purified cellulose obtained from tree rings has provided some of the best samples for isotope analyses because the 13C,D,18O,and even 15N isotopes in the wood can record a great deal about the ecophysiology of the plants (Leavitt &Long 1986,1988,1989,1991;Livingston &Spittehouse 1993;Bert et al.1997;Saurer et al.1997;Borella &Saurer 1999;Roden &Ehleringer 1999a,b),the resources they use (Roden et al.2000,Ward et al.2002),and the environments they inhabit,both now (Barbour et al.2000,Roden et al.2000)and in the past (Edwards et al.1985;Leavitt 1993;Lipp et al.1996;Switsur et al.1996;Feng 1999;Hemming et al.1998,2000;Monserud &Marshall 2001).Recent modeling efforts that use 13C (Hemming et al.2000)and D and 18O (Roden &Ehleringer 2000;Barbour et al.2000,2001)have very much improved our interpretations of isotope variation in tree rings.In these models,fractionations are better understood and accounted for,allowing one to make more precise inferences about environmental conditions (temperature and humidity),plant resource status (water-use efficiency and/or sources of water and nitrogen),and environmental change (Epstein &Krishnamurthy 1990,Duquesnay et al.1998).In addition to the use of natural abundance 13C to deepen our understanding of plant gas exchange,stable isotope techniques based on 13-C labeling (see TextA n n u . R e v . E c o l . S y s t . 2002.33:507-559. D o w n l o a d e d f r o m a r j o u r n a l s .a n n u a l r e v i e w s .o r g b y I n s t i t u t e o fB o t a n y o n 06/10/07. F o r p e r s o n a l u s e o n l y .。
第六章稳定性同位素地球化学
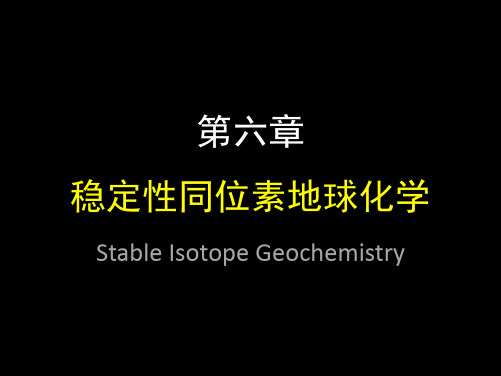
• 物理化学过程识别(Process identification)
如对动物化石进行C同位素分析,确定其是否属于 C4(木本?)或C3(草本?)类植物的食用动物
稳定同位素组成表达形式
• 采用同位素比值
• 通常情况下,稳定同位素的比值采用质量数大的同位素为分 子,而质量数小的同位素为分母。如18O/16O、2H/1H、13C/12C 和34S/32S等;
• 为直观反映样品稳定同位素组成,将同位素比值与标准样品 进行比较,并用差值的千分率()表示。如:
R R
A St
1 1000
• 与放射成因同位素表达方式不同的是,标样的同位素组成是 常数,不随时间改变,且标准化值放大系数为103
ZN 10 11 66 67 77 78 88 89 8 10 16 16 16 17 16 18 16 20
Atomic Mass 1.0078 2.0141 12 13.0034 14.0031 15.0001 15.9949 16.9991 17.9992 31.9721 32.9714 33.9676 35.9671
Standard
Hydrogen δD D/H (2H/1H)
SMOW
Lithium
δ7Li
7Li/6Li
NBS L-SVEC
Boron
δ11B
11B/10B
NBS 951
Carbon
δ13C 13C/12C
PDB
Nitrogen δ15N 15N/14N
atmosphere
Oxygen
δ18O
18O/16O
同位素基础获奖课件
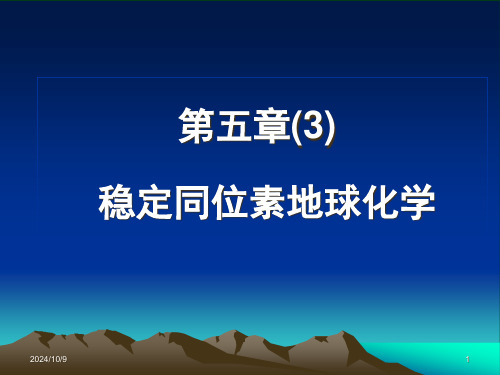
■从矿床底部到顶部,δS34具有增大趋势 ■在共生矿物中: δS34黄铁矿 >δS34闪锌矿 >δS34方铅矿
三、硫同位素旳地质应用
1、鉴别成岩物质起源
■在地质作用过程中,因为多种硫化物旳形 成条件不同,相应旳硫同位素构成也不同, 所以硫同位素构成也就能够用来鉴别成岩 物质起源。
2024/10/9
17
每个测定样品旳δ(‰)值可正可负,正值表达与原 则相比所测样品中重同位素有一定旳富集,而负值则 表达重同位素有一定旳贫化,亦即轻同位素有所富集。
不同相(不同矿物、液体、气体)中同位素构成不 同,即产生了同位素分馏,两相间同位素比值之商称 为同位素分馏系数
R / R, RA 、RB分别为A相及B相中重同位素
(
D H
)标准
1000
(
D H
)标准
2024/10/9
15
同位素分析资料要能够进行世界范围内旳比 较,就必须建立世界性旳原则样品。世界各国所 采用旳原则样品已基本统一。国际原则样品旳名 称及其同位素绝对比值见下:
氢、碳、氧、硫同位素原则样品
元
标
准
素
H 平均大洋水标准(Standard Mean Ocean Water)
24
2、花岗岩旳硫化物 ■因为花岗岩成因复杂、多样,故其硫化物旳
δS34值也不相同 ■一般由幔源衍生而来旳花岗岩,其硫化物中
旳δS34值在-3~+8‰之间,且单个岩体中δS34 值变化范围窄,阐明成岩物质比较均匀
■ S花岗岩δS34值为-9.4~+7.6 ‰ ■ I花岗岩δS34值为-3.6~+5.0 ‰
与轻同位素A旳比值B。
2024/10/9
大气N2O的稳定氮同位素质量平衡探讨

大气N2O的稳定氮同位素质量平衡探讨
徐文彬
【期刊名称】《地质地球化学》
【年(卷),期】1999(027)002
【摘要】地表释放源释放的N2O均比对流层大气N2O贫^15N,对流层N2O的氮同位素质量由平流层回流的富^15N的N2O来平衡,若全球N2O源汇质量估算结果是平衡的,则地表释放源对对流层N2O的氮同位素质量的贡献量应与平流层回流的贡献量相等。
【总页数】7页(P22-28)
【作者】徐文彬
【作者单位】中国科学院地球化学研究所环境地球化学国家重点实验室
【正文语种】中文
【中图分类】X511
【相关文献】
1.大气浓度下N2O中氮稳定同位素比值测定的GasBench-IRMS系统改造 [J], 崔杰华;孙辞;李国琛;李波;王颜红
2.大气浓度下N2O、CH4和CO2中氮、碳和氧稳定同位素比值的质谱测定 [J], 曹亚澄;孙国庆;韩勇;孙德玲;王曦
3.N2O的氮,氧稳定同位素真空制样系统探讨 [J], 徐文彬
4.大气气溶胶硝酸盐中稳定氮氧同位素比值测定 [J], 赵祝钰;曹芳;张雯淇;翟晓瑶;
方言;范美益;章炎麟
5.关于对流层大气N_2O氮、氧稳定同位素负荷平衡的初步探讨 [J], 徐文彬因版权原因,仅展示原文概要,查看原文内容请购买。
中国农科院稳定同位素实验室
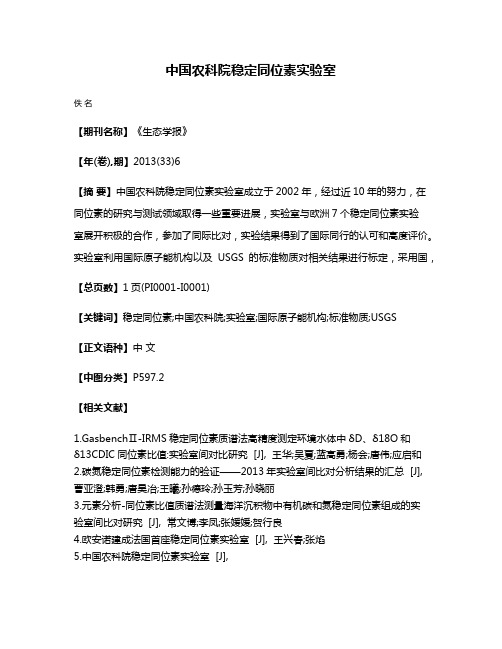
中国农科院稳定同位素实验室
佚名
【期刊名称】《生态学报》
【年(卷),期】2013(33)6
【摘要】中国农科院稳定同位素实验室成立于2002年,经过近10年的努力,在同位素的研究与测试领域取得一些重要进展,实验室与欧洲7个稳定同位素实验
室展开积极的合作,参加了同际比对,实验结果得到了国际同行的认可和高度评价。
实验室利用国际原子能机构以及USGS的标准物质对相关结果进行标定,采用国,【总页数】1页(PI0001-I0001)
【关键词】稳定同位素;中国农科院;实验室;国际原子能机构;标准物质;USGS
【正文语种】中文
【中图分类】P597.2
【相关文献】
1.GasbenchⅡ-IRMS稳定同位素质谱法高精度测定环境水体中δD、δ18O和
δ13CDIC同位素比值:实验室间对比研究 [J], 王华;吴夏;蓝高勇;杨会;唐伟;应启和2.碳氮稳定同位素检测能力的验证——2013年实验室间比对分析结果的汇总 [J], 曹亚澄;韩勇;唐昊冶;王曦;孙德玲;孙玉芳;孙晓丽
3.元素分析-同位素比值质谱法测量海洋沉积物中有机碳和氮稳定同位素组成的实
验室间比对研究 [J], 常文博;李凤;张媛媛;贺行良
4.欧安诺建成法国首座稳定同位素实验室 [J], 王兴春;张焰
5.中国农科院稳定同位素实验室 [J],
因版权原因,仅展示原文概要,查看原文内容请购买。
- 1、下载文档前请自行甄别文档内容的完整性,平台不提供额外的编辑、内容补充、找答案等附加服务。
- 2、"仅部分预览"的文档,不可在线预览部分如存在完整性等问题,可反馈申请退款(可完整预览的文档不适用该条件!)。
- 3、如文档侵犯您的权益,请联系客服反馈,我们会尽快为您处理(人工客服工作时间:9:00-18:30)。
E2 KT E4 KT
3 (20) e
/ 4 (18)3 / 2 e
1
combine combine
1
4618 ( ) e 44 20
3 2
( E1 E2 )( E3 E4 ) KT ECO2 EH 2O KT
1
4618 ( ) e 44 20
The changes in isotopic ratios in these processes are called “isotopic fractionation”
Expression of isotopic fractionation
For a process from reactant to product, the isotopic fractionation factor α is defined as:
The exchange reactions involve breaking of old bonds and formation of new bonds The strength of bonds formed by the light vs. heavier isotopes is different, resulting in difference in reaction rates
Thermodynamic equilibrium of isotope fractionation
Fractionation can be regarded as an exchange of isotopes between two molecular species or phases that are participating in a reaction H218O+C16O16O H216O+C16O18O
What is isotopic fractionation?
Isotopes of an element have slightly different chemical and physical properties due to the mass difference In chemical, physical or biological processes, the reactants and products may have different isotopic ratios
Friedman: found δ18O and δD change in coordinated fashion in precipitation Harmon Craig: studied δ18O and δD partition in precipitation, established meteoric water line (1961)
At lower temperatures, ∆E plays a greater role in changing the Q, hence larger isotopic fractionation
Requirement of isotopic equilibrium
Chemical equilibrium, forward and backward reaction rates are equal Forward and backward reactions have proceeded enough times to mix the isotopes between reactants and products
This process needs to occur many times to ensure full exchange, sometimes stirring is necessary, the exchange also proceeds faster when pH<4.5
Pቤተ መጻሕፍቲ ባይዱrtition functions (Q)
Dissociation energy of a molecule is related to its partition function (Q), at vibrational frequency
Q = -1m3/2∑e-E/kT
where, = a symmetry value m = mass E = the energy state, from zero point energy to the energy of the dissociated molecule (J*mole-1) K = Boltzmann constant (gas constant per molecule)=n1.380658 10-23JK-1 T = thermodynamic temperature K
Temperature impact
At higher temperature, the influence of difference in E (zero point energy difference) on Q is smaller, hence the isotopic fractionation between different isotopic species is smaller
Objectives
Understand the fundamental theory of stable isotope fractionation Familiar with the types of isotopic fractionation
Familiar with different ways to describe fractionation (sometimes confusing)
1. Equilibrium constant = α
X + Y* X* + Y H2 + HDO HD + H2O
e.g.,
K = [X*][Y]/[X][Y*] = {[X*]/[X]}÷{[Y*]/[Y]} = Rx/Ry = α
Concentration ratio = ratio of Q
For example, dissolution of CO2 in H2O CO2 + H2O H2CO3
The equilibrium involves exchange of O atoms between CO2 and H2O H218O+C16O16O H216O+C16O18O
Rx = [X*]/[X] = Qx* / Qx
C16O2/C16O18O ↔ H216O/H218O
QC16O18O / QC16O QH 18O / QH 16O
2 2 2
3 2
(46) e
3/ 2 1 3/ 2
1 1
E1 KT E3 KT
/ 2 (44) e
3/ 2
1
Later work demonstrates how isotopes are partitioned through other systems, such as 13C in the carbon cycle
Types of isotope fractionation
Equilibrium isotope fractionation
The heavy isotope has a stronger bond and require greater energy to dissociate than a light isotope
Lighter nuclei react more quickly In a equilibrium reaction, stronger bonds survive longer, so that usually the heavy isotopic species are partitioned into the more condensed phase, e.g., liquid phase in vapor-liquid reactions
Kinetic (non-equilibrium) isotope fractionation Diffusive isotope fractionation (a special case of kinetic isotope fractionation) Non-mass dependent isotope fractionation
Equilibrium isotopic fractionation
Redistribution of isotopes of an element among various reactants and products At equilibrium, isotope ratios in each compound are constant
Stable Isotope Fractionation
Topics in this class
Why do isotopic ratios change (fractionate) in nature?
Types of stable isotope fractionation How do we express the isotopic fractionation in a quantitative manner? How could isotopic fractionation be used for environmental and geological research?