机械工程-外文文献
机械工程科技论文 英文版

MICRO PLANETARY REDUCTION GEAR USINGSURFACE-MICROMACHININGAbstractA micro planetary gear mechanism featuring a high gear reduction ratio with compactness in size ispresented in this paper. SUMMiT V is employed for the fabrication method so that the redundancy of assembling parts is eliminated. The design rules of which has also been checked. To make full use of the benefits of the surface- micro - machining, the planetary reduction gear is designed toward using the on-chip micro- engine. The expected gearreduction ratio is calculated and compared with the conventional chain gear mechanism. The microplanetary gear mechanism presented in this paper is expected to have 162:1 reduction ratio utilizing less space consumption. This is an order of magnitude higher than the previously reported design in a single reduction gear train.Keywords:MEMS, planetary gear, reduction gear surface-micromachining, SUMMiT V processNomenclaturea sun gearb planet gearsc internal gear (fixed)d internal gear (rotary)n the number of units of gear trainD diameter of the pitch circleN number of teethP number of planetsa ngular velocityIntroductionThe gear mechanisms in microelectro mechanical systems(MEMS) are commonly expected to generate high torque in the confined micro-size systems. However, it is generally difficult for the micro-scale systems to have such a high torque without having multiple reduction systems.The design of the reduction gear drive based on a planetary paradox gear mechanism can increase the torque within a compact area, since the microplanetary gear system has an advantage of high reduction ratio per unit volume [1]. However its mechanism is so complicated that relatively few attempts have been made to miniaturize the gear systems [2-3]. Suzumori et al.[2] used the mechanical paradox planetary gear mechanism to drive a robot for 1-in pipes forward or backward. They employed a single motor to drive the gear mechanisms with high reduction ratio. Precise gear fabrication was enabled by micro wire electrical discharge machining (micro-EDM). These parts, however, should be assembled before the drive motor is attached to the gearbox. Takeuchi et. al. [3] also used micro-EDM to fabricate the microplanetary gears. They suggested special cermets or High Carbon Steel for possible materials. While the design can achieve a reduction ratio of 200, the gears should also be assembled and motor driven.To enable the driving of the planetary gear by onchip means, Sandia Ultra- planar Multi-level MEMS Technology (SUMMiT-V) process [4] for planetary gear fabrication is adopted in this study. The SUMMiT-V process is the only foundry process available which utilizes four layers of releasable polysilicon, for a total of five layers (including a ground plane) [5]. Due to this fact, it is frequently used in complicated gear mechanisms being driven by on-chip electrostatic actuators [5].However, in many cases, the microengines may not produce enough torque to drive the desired mechanical load, since their electrostatic comb drives typically only generate a few tens of micronewtons of force. Fortunately, these engines can easily be driven at tens of thousands of revolutions per minutes. This makes it very feasible to trade speed for torque [7].Rodgers et al. [7] proposed two dual level gears with an overall gear reduction ratio of 12:1. Thus six of these modular transmission assemblies can have a 2,985,984:1 reduction ratio at the cost of the huge space.With the desire for size compactness and at the same time, high reduction ratios, the planetary gear system is presented in this paper. It will be the first planetary gear mechanism using surface micromachining,to the authors knowledge. The principles of operations of the planetary gear mechanism, fabrication, and the expected performance of the planetary gear systems are described in this paper.Principles of operationAn alternative way of using gears to transmit torque is to make one or more gears, i.e., planetary gears, rotate outside of one gear, i.e. sun gear. Most planetary reduction gears, at conventional size, are used as well-known compact mechanical power transmission systems [1]. The schematic of the planetary gear system employed is shown in FigureSince SUMMiT V designs are laid out using AutoCAD 2000, the Figure 1 is generated automatically from the lay out masks (Appendix [1]). One unit of the planetary gear system is composed of six gears: one sun gear, a, three planetary gears, b, one fixed ring gear, c, one rotating ring gear, d, and one output gear. The number of teeth for each gear is different from one another except among the planetary gears. An input gear is the sun gear, a, driven by the arm connected to the micro-engine. The rotating ring gear, d, is served as an output gear. For example, if the arm drives the sun gear in the clockwise direction, the planetary gears, b, will rotate counter-clockwise at their own axis and at the same time, those will rotate about the sun gear in clockwise direction resulting in planetary motion. Due to the relative motion between the planetary gears, b, and the fixed ring gear, c, the rotating ring gear, d, will rotate counterclockwise direction. This is so called a 3K mechanical paradox planetary gear [1]. Fabrication procedure and test structuresThe features of the SUMMiT V process offer four levels of structural polysilicon layers and an electrical poly level, and also employ traditional integrated circuit processing techniques [4]. TheSUMMiT V technology is especially suitable for the gear mechanism. The planetary gear mechanism can be driven by the on-chip engine and thus is another reason of using the SUMMiT V process.Since the Sandia process is such a well-known procedure [5-7], only brief explanation is presented. Figure 2 represents the cross-sectional view of Figure 1, and also was generated from the AutoCAD layout masks (Appendix [1]). The discontinuity in the cross-section is for the etch holes. The poly1 (gray) is used for the hubs and also patterned to make the fixed ring gear, i.e., c, the sun gear, i.e., a, the rotating ring gear, i.e., c, and the output gear is patterned in the poly2. Since the planetary gear needs to contact both the fixed ring and rotating ring gear, poly2 is added to poly3, where the gear teeth are actually formed. The poly4 layer is used for the arm that drives the sun gear. After the release etch, the planetary gears will fall down so that those will engage both the ring gears.The figures for the test structures are presented in Appendix [2]. Since the aim of this paper is to suggest a gear reduction mechanism, the planetary gear system is decomposed to several gear units to verify its performance. The first test structure is about the arm, which rotates the sun gear, connected to the on-chip engine. The angular velocity of the arm depends on the engine output speed. The second test structure describes the point at which the sun gear and planetary gears are engaged to the fixed ring gear. Because of the fact that the ring gear is fixed, the planetary gear is just transmitting the torque from the sun gear to the fixed ring gear without planet motion, e.g., rotating its own axis not around the sun gear. When the rotating ring gear is mounted on top of the fixed ring gear, i.e., the third test structure, the planetary gears begin to rotate around the sun gear so that the planet motion are enabled. Therefore, once one output gear is attached to the rotating ring gear, i.e., the final test structure, the whole reduction unit is completed. Dismantling theplanetary gear into three test structures allows the pinpointing of possible errors in the gear system.Solutions procedure and expected performanceThe reduction ratio is defined as the ratio between the angular velocity of the driver gear and that of the driven gear. High reduction ratios indicate trading speed for torque. For example, a 10:1 gear reduction unit could increase torque an order of magnitude. Since the gears in the planetary system should be meshed to one another , the design of gear module should follow a restriction. For example, the number of teeth for the sun gear plus either that of the fixed ring gear or that of the rotating ring gear should be the multiple of the number of planets, P (equation 1). Equation 2, which represent the reduction ratio, should observe the equation 1 first. The N is the number of the teeth for corresponding gear.Gears, a, b, c, d in the planetary gear system have a tooth module of 4 m, which is a comparable size of the current gear reduction units[5], and the tooth numbers are 12, 29, 69, and 72 respectively. Therefore the overall reduction ratio is 162:1 from equation (2). Rodgers et al.[7] reported a 12:1 reduction unit using surface micromachining, which is less than order of magnitude for the gear reduction ratio of the planetary gear system. Although the reduction from Rodgers et al. [7] needs to be occupied in approximately 0.093 mm2, the planetary gear system only utilizes an area of approximately 0.076 mm2. Thus, this planetary reduction design can achieve an order of magnitude higher reduction ratio with less space. Since thereduction module is composed of several reduction units, the advantage of using a planetary gear system is self evident in Figure 3.Figure 3 shows the comparison of reduction ratios between the proposed planetary gear mechanism i.e. 162n, and the Sandia gear system [7], i.e. 12n, as a function of the number of units, i.e., n. The ordinate is drawn in log scale so that the orders of magnitude differences between two modules are evident. For example, in a module with five numbers of units, the reduction ratio difference between two is approximately six orders of magnitudes. Furthermore, the planetary gear system can save 8500 m2 in such a five unit reduction system.Conclusion and discussionsThe planetary gear reduction system using surface-micromachining, driven by an on-chip engine, first appears in this paper within the authors’ knowledge. The single reduction unit can achieve an order of magnitude higher reduction ratio than that of the previous design. However, due to the surface friction, and the backlash, which is inevitable for the gear manufacturing process, the overall reduction ratio may be less than 162:1 in the real situation. Even though some loss might be expected in the real application, the overall reduction ratio should be order of magnitude higher and the space consumption is less than the previous design [7].The authors learned a lot about the surfacemicromachining process during the project grant,and realized that a lot of the design needed to be revisited and corrected. This became prevalent when drawing the cross-sectional views of the design. Since the authors utilized the SUMMit V Advanced design Tools Software package and verified the design rules, the planetary gear layout is ready for fabrication. The authors hope that this planetary reduction unit will continue to be updated by successive researchers.AcknowledgementThe authors would acknowledge that discussions with Prof. Kris Pister, Prof. Arun Majumdar, Ms. Karen Cheung, and Mr. Elliot Hui contributed to this work tremendously.References1. Hori, K., and Sato, A., “Micro-planetary reduction gear” Proc. IEEE 2nd Int. Symp. Micro Machine and Human Sciences, pp. 53- 60 (1991).2. Suzumori, K., Miyagawa, T., Kimura, M., and Hasegawa, Y., “Micro Inspection Robot for 1-in Pipes”, IEEE/ASME Trans. On Mechatronics, Vol. 4., No. 3, pp. 286-292 (1999).3. Takeuchi, H., Nakamura, K., Shimizu, N., and Shibaike, N., “Optimization of Mechanical Interface for a Practical Micro-Reducer”, Proc. IEEE 13th Int. Symp. Micro Electro Mechanical Systems, pp. 170-175 (2000).4. Sandia National Laboratories, “Design Rules Design Rules”, Microelectronics Development Laboratory, Version 0.8, (2000)5. Krygowask, T. W., Sniegowask, J. J., Rodgers, M. S., Montague, S., and Allen, J. J., “Infrastructure, Technology and Applications of Micro-Electro-Mechanical Systems (MEMS)”, Sensor Expo 1999 (1999).6. Sniegowski, J. J., Miller, S. L., LaVigne, G. F., Rodgers, M. S., and McWhorter, P. J., “Monolithic G eared-Mechanisms Driven by aPolysilicon Surface-Micromachined On-Chip Electrostatic Microengine”, Solid-State Sensor and Actuator Workshop, pp. 178-182, (1996).7. Rogers, M. S., Sniegowski, S. S., Miller, S., and LaVigne, G. F., “Designing and OperatingE lectrostatically Driven Microengines”, Proceedings of the 44th International Instrumentation Symposium, Reno, NV, May 3-7, pp. 56-65 (1998).Figure 1. The schematic of the planetarygear mechanism generated from SUMMiT Vdesign layout softwareFigure 2. A schematic cross-section of the planetary gear system generated by SUMMiT-V technologyFigure 3. The comparison of reduction ratios as a function of the number of units采用表面微加工技术制造微型行星齿轮减速器摘要这篇文章论述了一种结构紧凑、传动比高的微型行星齿轮减速机构。
机械类论文参考文献(外文文献)2
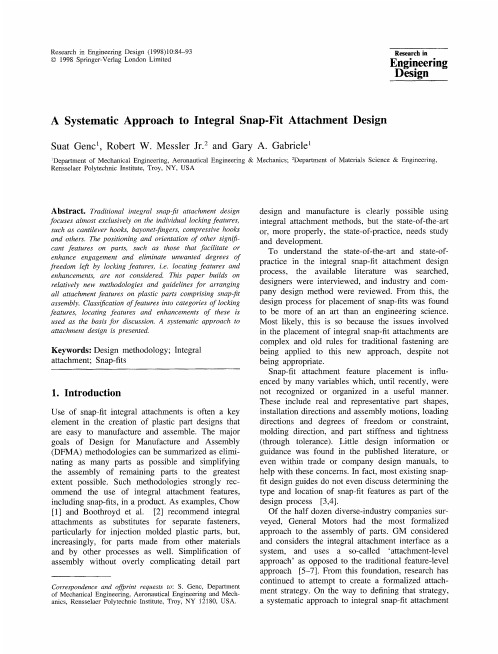
2. Integral Snap-Fit Attachment
Features
For the purpose of this paper, integral snap-fit attachments refer to the specific, detailed features molded into a plastic part to actually provide attachment functionality. These features can be further sub-classified based on sub-functions within attachment or assembly. This approach is important, since designers often do not know the full range of snapfit features available to them. Because of this, the best feature for a particular application is often not selected, with the consequence that problems are encountered during product assembly or during service. The following definitions and classifications were originally presented by Luscher et al. [8], and are summarized here in order to increase understanding of the features that can be found on plastic parts. Fundamental definitions can also be found in other papers [9,10].
机械类外文文献及翻译

机械类外文文献及翻译(文档含中英文对照即英文原文和中文翻译)原文:GEAR AND SHAFT INTRODUCTIONAbstract:The important position of the wheel gear and shaft can't falter in traditional machine and modern machines.The wheel gear and shafts mainly install the direction that delivers the dint at the principal axis box. The passing to process to make them can is divided into many model numbers, using for many situations respectively. So we must be the multilayers to the understanding of the wheel gear and shaft in many ways .Key words: Wheel gear; ShaftIn the force analysis of spur gears, the forces are assumed to act in a single plane. We shall study gears in which the forces have three dimensions. The reason for this, in the case of helical gears, is that the teeth are not parallel to the axis of rotation. And in the case ofbevel gears, the rotational axes are not parallel to each other. There are also other reasons, as we shall learn.Helical gears are used to transmit motion between parallel shafts. The helix angle is the same on each gear, but one gear must have a right-hand helix and the other a left-hand helix. The shape of the tooth is an involute helicoid. If a piece of paper cut in the shape of a parallelogram is wrapped around a cylinder, the angular edge of the paper becomes a helix. If we unwind this paper, each point on the angular edge generates an involute curve. The surface obtained when every point on the edge generates an involute is called an involute helicoid.The initial contact of spur-gear teeth is a line extending all the way across the face of the tooth. The initial contact of helical gear teeth is a point, which changes into a line as the teeth come into more engagement. In spur gears the line of contact is parallel to the axis of the rotation; in helical gears, the line is diagonal across the face of the tooth. It is this gradual of the teeth and the smooth transfer of load from one tooth to another, which give helical gears the ability to transmit heavy loads at high speeds. Helical gears subject the shaft bearings to both radial and thrust loads. When the thrust loads become high or are objectionable for other reasons, it may be desirable to use double helical gears. A double helical gear (herringbone) is equivalent to two helical gears of opposite hand, mounted side by side on the same shaft. They develop opposite thrust reactions and thus cancel out the thrust load. When two or more single helical gears are mounted on the same shaft, the hand of the gears should be selected so as to produce the minimum thrust load.Crossed-helical, or spiral, gears are those in which the shaft centerlines are neither parallel nor intersecting. The teeth of crossed-helical fears have point contact with each other, which changes to line contact as the gears wear in. For this reason they will carry out very small loads and are mainly for instrumental applications, and are definitely not recommended for use in the transmission of power. There is on difference between a crossed heli : cal gear and a helical gear until they are mounted in mesh with each other. They are manufactured in the same way. A pair of meshed crossed helical gears usually have the same hand; that is ,a right-hand driver goes with a right-hand driven. In the design of crossed-helical gears, the minimum sliding velocity is obtained when the helix angle areequal. However, when the helix angle are not equal, the gear with the larger helix angle should be used as the driver if both gears have the same hand.Worm gears are similar to crossed helical gears. The pinion or worm has a small number of teeth, usually one to four, and since they completely wrap around the pitch cylinder they are called threads. Its mating gear is called a worm gear, which is not a true helical gear. A worm and worm gear are used to provide a high angular-velocity reduction between nonintersecting shafts which are usually at right angle. The worm gear is not a helical gear because its face is made concave to fit the curvature of the worm in order to provide line contact instead of point contact. However, a disadvantage of worm gearing is the high sliding velocities across the teeth, the same as with crossed helical gears.Worm gearing are either single or double enveloping. A single-enveloping gearing is onein which the gear wraps around or partially encloses the worm.. A gearing in which each element partially encloses the other is, of course, a double-enveloping worm gearing. The important difference between the two is that area contact exists between the teeth of double-enveloping gears while only line contact between those of single-enveloping gears. The worm and worm gear of a set have the same hand of helix as for crossed helical gears, but the helix angles are usually quite different. The helix angle on the worm is generally quite large, and that on the gear very small. Because of this, it is usual to specify the lead angle on the worm, which is the complement of the worm helix angle, and the helix angle on the gear; the two angles are equal for a 0-deg. Shaft angle.When gears are to be used to transmit motion between intersecting shaft, some of bevel gear is required. Although bevel gear are usually made for a shaft angle of 0 deg. They may be produced for almost any shaft angle. The teeth may be cast, milled, or generated. Only the generated teeth may be classed as accurate. In a typical bevel gear mounting, one of the gear is often mounted outboard of the bearing. This means that shaft deflection can be more pronounced and have a greater effect on the contact of teeth. Another difficulty, which occurs in predicting the stress in bevel-gear teeth, is the fact the teeth are tapered.Straight bevel gears are easy to design and simple to manufacture and give very good results in service if they are mounted accurately and positively. As in the case of squr gears, however, they become noisy at higher values of the pitch-line velocity. In these cases it is often go : od design practice to go to the spiral bevel gear, which is the bevel counterpart of thehelical gear. As in the case of helical gears, spiral bevel gears give a much smoother tooth action than straight bevel gears, and hence are useful where high speed are encountered.It is frequently desirable, as in the case of automotive differential applications, to have gearing similar to bevel gears but with the shaft offset. Such gears are called hypoid gears because their pitch surfaces are hyperboloids of revolution. The tooth action between such gears is a combination of rolling and sliding along a straight line and has much in common with that of worm gears.A shaft is a rotating or stationary member, usually of circular cross section, having mounted upon it such elementsas gears, pulleys, flywheels, cranks, sprockets, and other power-transmission elements. Shaft may be subjected to bending, tension, compression, or torsional loads, acting singly or in combination with one another. When they are combined, one may expect to find both static and fatigue strength to be important design considerations, since a single shaft may be subjected to static stresses, completely reversed, and repeated stresses, all acting at the same time.The word “shaft” covers numerous v ariations, such as axles and spindles. Anaxle is a shaft, wither stationary or rotating, nor subjected to torsion load. A shirt rotating shaft is often called a spindle.When either the lateral or the torsional deflection of a shaft must be held to close limits, the shaft must be sized on the basis of deflection before analyzing the stresses. The reason for this is that, if the shaft is made stiff enough so that the deflection is not too large, it is probable that the resulting stresses will be safe. But by no means should the designer assume that they are safe; it is almost always necessary to calculate them so that he knows they are within acceptable limits. Whenever possible, the power-transmission elements, such as gears or pullets, should be located close to the supporting bearings, This reduces the bending moment, and hence the deflection and bending stress.Although the von Mises-Hencky-Goodman method is difficult to use in design of shaft, it probably comes closest to predicting actual failure. Thus it is a good way of checking a shaft that has already been designed or of discovering why a particular shaft has failed in service. Furthermore, there are a considerable number of shaft-design problems in which the dimension are pretty well limited by other considerations, such as rigidity, and it is only necessary for the designer to discover something about the fillet sizes, heat-treatment,and surface finish and whether or not shot peening is necessary in order to achieve the required life and reliability.Because of the similarity of their functions, clutches and brakes are treated together. In a simplified dynamic representation of a friction clutch, or brake, two in : ertias I and I traveling at the respective angular velocities W and W, one of which may be zero in the case of brake, are to be brought to the same speed by engaging the clutch or brake. Slippage occurs because the two elements are running at different speeds and energy is dissipated during actuation, resulting in a temperature rise. In analyzing the performance of these devices we shall be interested in the actuating force, the torque transmitted, the energy loss and the temperature rise. The torque transmitted is related to the actuating force, the coefficient of friction, and the geometry of the clutch or brake. This is problem in static, which will have to be studied separately for eath geometric configuration. However, temperature rise is related to energy loss and can be studied without regard to the type of brake or clutch because the geometry of interest is the heat-dissipating surfaces. The various types of clutches and brakes may be classified as fllows:. Rim type with internally expanding shoes. Rim type with externally contracting shoes. Band type. Disk or axial type. Cone type. Miscellaneous typeThe analysis of all type of friction clutches and brakes use the same general procedure. The following step are necessary:. Assume or determine the distribution of pressure on the frictional surfaces.. Find a relation between the maximum pressure and the pressure at any point. Apply the condition of statical equilibrium to find (a) the actuating force, (b) the torque, and (c) the support reactions.Miscellaneous clutches include several types, such as the positive-contact clutches, overload-release clutches, overrunning clutches, magnetic fluid clutches, and others.A positive-contact clutch consists of a shift lever and two jaws. The greatest differences between the various types of positive clutches are concerned with the design of the jaws. To provide a longer period of time for shift action during engagement, the jaws may be ratchet-shaped, or gear-tooth-shaped. Sometimes a great many teeth or jaws are used, and they may be cut either circumferentially, so that they engage by cylindrical mating, or on the faces of the mating elements.Although positive clutches are not used to the extent of the frictional-contact type, they do have important applications where synchronous operation is required.Devices such as linear drives or motor-operated screw drivers must run to definite limit and then come to a stop. An overload-release type of clutch is required for these applications. These clutches are usually spring-loaded so as to release at a predetermined toque. The clicking sound which is heard when the overload point is reached is considered to be a desirable signal.An overrunning clutch or coupling permits the driven member of a machine to “freewheel” or “overrun” bec ause the driver is stopped or because another source of power increase the speed of the driven. This : type of clutch usually uses rollers or balls mounted between an outer sleeve and an inner member having flats machined around the periphery. Driving action is obtained by wedging the rollers between the sleeve and the flats. The clutch is therefore equivalent to a pawl and ratchet with an infinite number of teeth.Magnetic fluid clutch or brake is a relatively new development which has two parallel magnetic plates. Between these plates is a lubricated magnetic powder mixture. An electromagnetic coil is inserted somewhere in the magnetic circuit. By varying the excitation to this coil, the shearing strength of the magnetic fluid mixture may be accurately controlled. Thus any condition from a full slip to a frozen lockup may be obtained.齿轮和轴的介绍摘要:在传统机械和现代机械中齿轮和轴的重要地位是不可动摇的。
机械工程专业外文文献及翻译
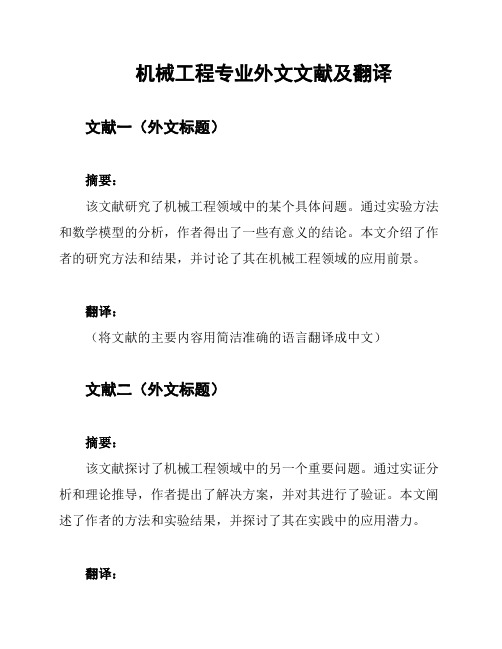
机械工程专业外文文献及翻译文献一(外文标题)
摘要:
该文献研究了机械工程领域中的某个具体问题。
通过实验方法和数学模型的分析,作者得出了一些有意义的结论。
本文介绍了作者的研究方法和结果,并讨论了其在机械工程领域的应用前景。
翻译:
(将文献的主要内容用简洁准确的语言翻译成中文)
文献二(外文标题)
摘要:
该文献探讨了机械工程领域中的另一个重要问题。
通过实证分析和理论推导,作者提出了解决方案,并对其进行了验证。
本文阐述了作者的方法和实验结果,并探讨了其在实践中的应用潜力。
翻译:
(将文献的主要内容用简洁准确的语言翻译成中文)
文献三(外文标题)
摘要:
该文献研究了机械工程领域中的另一个新颖课题。
作者通过数
值模拟和实验验证,得出了一些有趣的发现。
本文介绍了作者的研
究过程和结果,并讨论了其对机械工程领域的影响。
翻译:
(将文献的主要内容用简洁准确的语言翻译成中文)
总结
本文档介绍了三篇机械工程专业的外文文献,包括摘要和翻译。
这些文献都对机械工程领域中的不同问题进行了研究,并提出了相
关的解决方案和发现。
希望这些文献能为机械工程专业的学生和研
究人员提供有价值的参考和启发。
机械专业英语英文专业文献

1. Introduction Turning instead of grinding hardened steel is an economical method to generate a high quality machined surface. During the past few years, there has been significant industrial interest in using dry machining rather than grinding of hardened steel and other difficult-to-machine materials. As an example, dry hard turning of automotive differential side gears is a successful industrial application of this technology. This technology reduces both the machining time and the specific cutting energy, and eliminates the health and environmental hazards associated with coolant usage in conventional machining operations. Although a large volume of literature exists on hard turning [1–10], the control of the tool wear and its effect on the machined surface’s physical properties represent a major technical challenge. Understanding the chip formation mechanism is essential to achieve a better insight of the machining process fundamentals. Saw toothed chip formation is observed during hard turning
机械类英文文献+翻译)

机械工业出版社2004年3月第1版20.9 MACHINABILITYThe machinability of a material usually defined in terms of four factors:1、Surface finish and integrity of the machined part;2、Tool life obtained;3、Force and power requirements;4、Chip control.Thus, good machinability good surface finish and integrity, long tool life, and low force And power requirements. As for chip control, long and thin (stringy) cured chips, if not broken up, can severely interfere with the cutting operation by becoming entangled in the cutting zone.Because of the complex nature of cutting operations, it is difficult to establish relationships that quantitatively define the machinability of a material. In manufacturing plants, tool life and surface roughness are generally considered to be the most important factors in machinability. Although not used much any more, approximate machinability ratings are available in the example below.20.9.1 Machinability Of SteelsBecause steels are among the most important engineering materials (as noted in Chapter 5), their machinability has been studied extensively. The machinability of steels has been mainly improved by adding lead and sulfur to obtain so-called free-machining steels.Resulfurized and Rephosphorized steels. Sulfur in steels forms manganese sulfide inclusions (second-phase particles), which act as stress raisers in the primary shear zone. As a result, the chips produced break up easily and are small; this improves machinability. The size, shape, distribution, and concentration of these inclusions significantly influence machinability. Elements such as tellurium and selenium, which are both chemically similar to sulfur, act as inclusion modifiers in resulfurized steels.Phosphorus in steels has two major effects. It strengthens the ferrite, causingincreased hardness. Harder steels result in better chip formation and surface finish. Note that soft steels can be difficult to machine, with built-up edge formation and poor surface finish. The second effect is that increased hardness causes the formation of short chips instead of continuous stringy ones, thereby improving machinability.Leaded Steels. A high percentage of lead in steels solidifies at the tip of manganese sulfide inclusions. In non-resulfurized grades of steel, lead takes the form of dispersed fine particles. Lead is insoluble in iron, copper, and alumin um and their alloys. Because of its low shear strength, therefore, lead acts as a solid lubricant (Section 32.11) and is smeared over the tool-chip interface du ring cutting. This behavior has been verified by the presence of high concentra tions of lead on the tool-side face of chips when machining leaded steels.When the temperature is sufficiently high-for instance, at high cutting spee ds and feeds (Section 20.6)—the lead melts directly in front of the tool, acting as a liquid lubricant. In addition to this effect, lead lowers the shear stress in the primary shear zone, reducing cutting forces and power consumption. Lead can be used in every grade of steel, such as 10xx, 11xx, 12xx, 41xx, etc. Le aded steels are identified by the letter L between the second and third numeral s (for example, 10L45). (Note that in stainless steels, similar use of the letter L means “low carbon,”a condition that improves their corrosion resistance.)However, because lead is a well-known toxin and a pollutant, there are se rious environmental concerns about its use in steels (estimated at 4500 tons of lead consumption every year in the production of steels). Consequently, there is a continuing trend toward eliminating the use of lead in steels (lead-free ste els). Bismuth and tin are now being investigated as possible substitutes for lea d in steels.Calcium-Deoxidized Steels. An important development is calcium-deoxidize d steels, in which oxide flakes of calcium silicates (CaSo) are formed. These f lakes, in turn, reduce the strength of the secondary shear zone, decreasing tool-chip interface and wear. Temperature is correspondingly reduced. Consequently, these steels produce less crater wear, especially at high cutting speeds.Stainless Steels. Austenitic (300 series) steels are generally difficult to mac hine. Chatter can be s problem, necessitating machine tools with high stiffness. However, ferritic stainless steels (also 300 series) have good machinability. Martensitic (400 series) steels are abrasive, tend to form a built-up edge, and req uire tool materials with high hot hardness and crater-wear resistance. Precipitati on-hardening stainless steels are strong and abrasive, requiring hard and abrasio n-resistant tool materials.The Effects of Other Elements in Steels on Machinability. The presence of aluminum and silicon in steels is always harmful because these elements com bine with oxygen to form aluminum oxide and silicates, which are hard and a brasive. These compounds increase tool wear and reduce machinability. It is es sential to produce and use clean steels.Carbon and manganese have various effects on the machinability of steels, depending on their composition. Plain low-carbon steels (less than 0.15% C) c an produce poor surface finish by forming a built-up edge. Cast steels are mor e abrasive, although their machinability is similar to that of wrought steels. To ol and die steels are very difficult to machine and usually require annealing pr ior to machining. Machinability of most steels is improved by cold working, w hich hardens the material and reduces the tendency for built-up edge formation.Other alloying elements, such as nickel, chromium, molybdenum, and vana dium, which improve the properties of steels, generally reduce machinability. T he effect of boron is negligible. Gaseous elements such as hydrogen and nitrog en can have particularly detrimental effects on the properties of steel. Oxygen has been shown to have a strong effect on the aspect ratio of the manganese sulfide inclusions; the higher the oxygen content, the lower the aspect ratio an d the higher the machinability.In selecting various elements to improve machinability, we should consider the possible detrimental effects of these elements on the properties and strengt h of the machined part in service. At elevated temperatures, for example, lead causes embrittlement of steels (liquid-metal embrittlement, hot shortness; see Se ction 1.4.3), although at room temperature it has no effect on mechanical prop erties.Sulfur can severely reduce the hot workability of steels, because of the fo rmation of iron sulfide, unless sufficient manganese is present to prevent such formation. At room temperature, the mechanical properties of resulfurized steelsdepend on the orientation of the deformed manganese sulfide inclusions (aniso tropy). Rephosphorized steels are significantly less ductile, and are produced so lely to improve machinability.20.9.2 Machinability of Various Other MetalsAluminum is generally very easy to machine, although the softer grades te nd to form a built-up edge, resulting in poor surface finish. High cutting speed s, high rake angles, and high relief angles are recommended. Wrought aluminu m alloys with high silicon content and cast aluminum alloys may be abrasive; they require harder tool materials. Dimensional tolerance control may be a pro blem in machining aluminum, since it has a high thermal coefficient of expans ion and a relatively low elastic modulus.Beryllium is similar to cast irons. Because it is more abrasive and toxic, t hough, it requires machining in a controlled environment.Cast gray irons are generally machinable but are. Free carbides in castings reduce their machinability and cause tool chipping or fracture, necessitating to ols with high toughness. Nodular and malleable irons are machinable with hard tool materials.Cobalt-based alloys are abrasive and highly work-hardening. They require sharp, abrasion-resistant tool materials and low feeds and speeds.Wrought copper can be difficult to machine because of built-up edge form ation, although cast copper alloys are easy to machine. Brasses are easy to ma chine, especially with the addition pf lead (leaded free-machining brass). Bronz es are more difficult to machine than brass.Magnesium is very easy to machine, with good surface finish and prolong ed tool life. However care should be exercised because of its high rate of oxi dation and the danger of fire (the element is pyrophoric).Molybdenum is ductile and work-hardening, so it can produce poor surfac e finish. Sharp tools are necessary.Nickel-based alloys are work-hardening, abrasive, and strong at high tempe ratures. Their machinability is similar to that of stainless steels.Tantalum is very work-hardening, ductile, and soft. It produces a poor surf ace finish; tool wear is high.Titanium and its alloys have poor thermal conductivity (indeed, the lowest of all metals), causing significant temperature rise and built-up edge; they can be difficult to machine.Tungsten is brittle, strong, and very abrasive, so its machinability is low, although it greatly improves at elevated temperatures.Zirconium has good machinability. It requires a coolant-type cutting fluid, however, because of the explosion and fire.20.9.3 Machinability of Various MaterialsGraphite is abrasive; it requires hard, abrasion-resistant, sharp tools.Thermoplastics generally have low thermal conductivity, low elastic modul us, and low softening temperature. Consequently, machining them requires tools with positive rake angles (to reduce cutting forces), large relief angles, small depths of cut and feed, relatively high speeds, and proper support of the work piece. Tools should be sharp.External cooling of the cutting zone may be necessary to keep the chips f rom becoming “gummy”and sticking to the tools. Cooling can usually be achi eved with a jet of air, vapor mist, or water-soluble oils. Residual stresses may develop during machining. To relieve these stresses, machined parts can be an nealed for a period of time at temperatures ranging from to ( to ), and then cooled slowly and uniformly to room temperature.Thermosetting plastics are brittle and sensitive to thermal gradients during cutting. Their machinability is generally similar to that of thermoplastics.Because of the fibers present, reinforced plastics are very abrasive and are difficult to machine. Fiber tearing, pulling, and edge delamination are significa nt problems; they can lead to severe reduction in the load-carrying capacity of the component. Furthermore, machining of these materials requires careful rem oval of machining debris to avoid contact with and inhaling of the fibers.The machinability of ceramics has improved steadily with the development of nanoceramics (Section 8.2.5) and with the selection of appropriate processi ng parameters, such as ductile-regime cutting (Section 22.4.2).Metal-matrix and ceramic-matrix composites can be difficult to machine, d epending on the properties of the individual components, i.e., reinforcing or wh iskers, as well as the matrix material.20.9.4 Thermally Assisted MachiningMetals and alloys that are difficult to machine at room temperature can be machined more easily at elevated temperatures. In thermally assisted machinin g (hot machining), the source of heat—a torch, induction coil, high-energy bea m (such as laser or electron beam), or plasma arc—is forces, (b) increased too l life, (c) use of inexpensive cutting-tool materials, (d) higher material-removal rates, and (e) reduced tendency for vibration and chatter.It may be difficult to heat and maintain a uniform temperature distribution within the workpiece. Also, the original microstructure of the workpiece may be adversely affected by elevated temperatures. Most applications of hot machi ning are in the turning of high-strength metals and alloys, although experiment s are in progress to machine ceramics such as silicon nitride.SUMMARYMachinability is usually defined in terms of surface finish, tool life, force and power requirements, and chip control. Machinability of materials depends n ot only on their intrinsic properties and microstructure, but also on proper sele ction and control of process variables.20.9 可机加工性一种材料的可机加工性通常以四种因素的方式定义:1、分的表面光洁性和表面完整性。
机械专业英语-英文专业文献1

Int J Adv Manuf TechnolDOI10.1007/s00170-005-0266-8ORIGINAL ARTICLERichard Y.Chiou.Lin Lu.Jim S.J.Chen.Mark T.NorthInvestigation of dry machining with embedded heat pipe cooling by finite element analysis and experimentsReceived:8March2005/Accepted:18May2005 / Published online: 30 March 2006#Springer-Verlag London Limited2006Abstract This paper investigates the performance of a cutting tool embedded with a heat pipe on reducing cutting temperature and wear in machining.The temperature of a tool plays an important role in thermal distortion and the machined part’s dimensional accuracy,as well as the tool life in machining.A new embedded heat pipe technology has been developed to effectively remove the heat gen-erated at the tool–chip interface in machining,thereby, reducing tool wear and prolonging tool life.In particular, the technique can effectively minimize pollution and con-tamination of the environment caused by cutting fluids,and the health problems of skin exposure and particulate inha-lation in manufacturing.The ANSYS finite element analysis simulations show that the temperature near the cutting edge drops significantly with an embedded heat pipe during machining.Temperature measurements at sev-eral locations on the cutting tool insert agree with the simulation results both with and without the heat pipe. Experiments were carried out to characterize the temper-ature distributions when performing turning experiments using a cutting tool installed with an embedded heat pipe. The performance of the heat pipe on reducing the cutting tool temperature was further supported by the observations of cutting tool material color,chip color,and the chip radius of curvature.Keywords Dry machining.Tool design.Heat pipe. Heat transfer.Wear.Temperature Nomenclaturea Insert lengthαT Tool thermal diffusivityb Insert widthc Insert heightC eff Heat pipe specific heatC S Specific heat of tool holder and shim seatC T Specific heat of tool insertd Depth of cutf Feed rate(mm/rev)h Convective heat transfer coefficientK eff Heat pipe effective thermal conductivityK G Thermal conductivity of thermal greaseK H Thermal conductivity of tool holderK S Thermal conductivity of shim seatK T Thermal conductivity of tool insertρeff Heat pipe densityρS Density of tool holder and shim seatρT Density of tool insertQ Heat generation rate(W)q c Heat flux flowing into the tool insert(W/m2)θAngle of heat zone on tool insert rake faceR Radius of heating zoneT TemperatureT0Initial temperaturet TimeV Cutting speed(m/min)X Coordinatex Position in the X directionY Coordinatey Position in the Y directionZ Coordinatez Position in the Z direction1IntroductionMachining is an important manufacturing operation in industry.The purpose of a machining process is to generate a surface having a specified shape and acceptable surface finish,and to prevent tool wear and thermal damage thatR.Y.Chiou(*).L.LuApplied Engineering Technology,Drexel University, Philadelphia,PA19104,USAe-mail:ryc23@J.S.J.ChenMechanical Engineering Department,Temple University, Philadelphia,PA19122,USAM.T.NorthThermacore,Inc.,Lancaster,PA17601,USA(2007)31:905–914leads to geometric inaccuracy of the finished part.The thermodynamic approach to the activity at the cutting edge attempts to account for the energy consumed[25].Re-search has shown that at least99%of the input energy is converted into heat by deformation of the chip and by friction of the chip and workpiece on the tool[2,23].The interface at which the chip slides over the tool is normally the hottest region during cutting.The actual temperature is strongly affected by workpiece material,cutting speed, feed,depth of cut,tool geometry,coolant,and many other variables[7,12,34,37].Due to the interaction of the chip and tool,which takes place at high pressures and high temperatures,the tool will always wear.The use of fluids inmachining is well known[3,4,13].Among the functions of a cutting fluid,cooling and lubrication are generally regarded as the most important,since they directly relate to heat generation and tool wear.However,significant negative consequences for environmental health and safety are associated with the use of cutting fluids[1,5,10,11, 15,17,28,32].Numerous methods have also been applied to predict temperatures in machining processes[8,18,19,26].Tay et al.[36]used a finite element method and Usui et al.[37] used a finite difference method to determine the proportion in which the cutting energy is distributed among the tool, chip,and workpiece.Radulescu and Kapoor[30]devel-oped an analytical model for the prediction of tool tem-perature fields in continuous or interrupted3-D cutting processes.The analysis predicts time-dependent heat fluxes in the cutting tool,and it only requires the cutting forces as inputs.Stephenson et al.[35]described a method for calculating cutting tool temperatures in contour turning based on Radulescu and Kapoor’s model for temperatures in interrupted cutting.Their model is adapted to account for variations in the dimensions of the heat source with time.In addition,insulated conditions are used on the boundaries exposed to the environment,in order to sim-plify input requirements and reduce computing time.A heat pipe is a passive heat transfer device with a very high thermal conductance.It is used to transport heat from one location to another by means of evaporation and sub-sequent condensation of an appropriate fluid,in which circulation of the fluid is maintained by capillary forces[6, 16,20,21,29,33].Heat pipes are used for cooling pur-poses in a wide range of applications,including electron-ics,die-casting and injection molding,heat recovery, aircraft de-icing,the cooling of batteries,and the control of manufacturing temperatures.The objective of this re-search is to contribute to the fundamental understanding of the new embedded heat pipe technology in metal cutting that can effectively carry away the heat generated at the tool–chip interface in machining,and,thus,reduce tool wear and prolong tool life.In this paper,a finite element model is presented for dry machining.This work departs from previous work in that a more realistic tool design has been adapted for the cutting process with an embedded heat pipe[9].Because the use of the heat pipe cooling system may reduce or eliminate the need for cutting fluid use,the pollution and contamination of the environment by cutting fluids and the health problems of skin exposure and particulate inhalation in manufacturing can be effectively minimized.2Heat generation in machiningIn machining operations,mechanical work is converted to heat through the plastic deformation involved in chip formation and through friction between the tool and the workpiece.Figure1shows the subsequent dissipation of that heat in the chip,tool,and the workpiece.Most of the analytical methods for steady-state temperature prediction in machining were developed based on Merchant’s model [27]for orthogonal cutting,which gives the shear and friction energy in terms of the measured cutting forces, tool–chip contact length,and chip thickness ratio.From Radulescu and Kapoor’s model[30]and Stephenson and Ali’s approach[34],the force model predicts the depen-dence of the forces on the cutting parameters and the workpiece’s geometry.It can predict the actual force magnitudes or their dependence on material types and cutting conditions.The heat generated during the metal cutting process where the work being done on the tool insert transforms itself in the form of heat.Consequently, the heat generation rate,Q(W),is given by Sata and Takeuchi[31]as:Q¼1:68df0:15V0:85(1) where d is the depth of cut(mm),f is the feed rate(mm/ rev),and V is the cutting speed(m/min).Fig.1Zones of heat generation and heat dissipation during the metal cutting processYFig.2Heating of a tool carbide insert9063Finite element simulation of cutting temperatureA finite element model (FEM)3-D model (ANSYS 6.1)was developed for the simulation of temperature based on the estimated machining heat flux over the tool –chip contact area on a tool insert of a cutting tool.Heat is generated at the tool –chip interface in the quarter-circular area (heat source area)of the tool insert rake surface,and a portion of it flows into the tool,as shown in Fig.2.The temperature distribution is obtained from the balance of the heat generated and transient thermal analysis for two cases:without and with heat pipe cooling.The real geometry of the cutting tool is chosen as the computational domain.Figures 3and 4show schematic views of the finite element analysis (FEA)models of the cutting tool for the two cases of without and with heat pipe cooling.To achieve more realistic and accurate simulation results,the model takes into account the real geometry of the cutting tool and the thermal resistance.By using con-stant thermophysical properties,the 3-D transient heat conduction equation becomes:@2T @x 2þ@2T @y 2þ@2T @z 2¼1αT @T@t(2)where αT is the tool thermal diffusivity.The initial condition is:T x ;y ;z ;t ¼0ðÞ¼T 0(3)Fig.3Schematic view of the finite element analysis (FEA)model without a heatpipeFig.4a Schematic illustration of an embedded heat pipe in a cutting tool in machining.b Schematic view of the FEA model with heat pipe cooling907The boundary condition for the present model in the heat source area is as follows:Àk T@T ðx ;y ;z ;t Þ@z j¼q c ;0 ffiffiffiffiffiffiffiffiffiffiffiffiffiffiffiffiffiffix 2þy 2p R ;z ¼0;0 θ 90h ðT ÀT 0Þ;otherwise()(4)where K T is the tool thermal conductivity,q c is the heat fluxflowing into the tool insert,R is the radius of heating zone,h is the convective heat transfer coefficient,and θis the angle of the heat zone on the tool insert rake face.The typical FEM used in this work for cutting sim-ulations consists of 4,228eight-node triangle elements for modeling the tool without a heat pipe and 6,915eight-node triangle elements for modeling with an installed heat pipe.The heat converted from the work of plastic deformation and friction is assumed to be the same for the two cases in the simulations.A typical simulation is based on a constant time step of 40s and a substep of 1s for a cutting time of 400s.To simulate the thermal resistance of the thermal grease,a layer of 0.1mm in height is placed between the heat pipe and the tool insert.The effective thermal conductivity of the heat pipe is obtained from experiments by Chiou et al.[10].The weighted average method was used to evaluate the effective density and specific heat of the heat pipe in the simulations [24].The geometry and material properties of the tool and the heat pipe are listed in Tables 1and 2.To simulate the heat pipe ’s thermal be-havior,the convective heat transfer coefficients for the heat pipe ’s adiabatic section and condense section are set to 5W/m 2°C and 1,200W/m 2°C,respectively.The con-vective heat transfer coefficients for most surfaces on the tool holder are set to 10W/m 2°C,except the one for theTable 2Properties of the installed heat pipe Heat pipe length 152.4mm Heat pipe width 8.38mm Heat pipe thickness2.16mm Condenser length of heat pipe30mmHeat pipe effective thermal conductivity,K eff 11,960W/m·K Heat pipe density,ρeff3,395kg/m 3Heat pipe specific heat,C eff 1,985J/kg·K Initial temperature,T 025°CFig.5Cutting tool temperature distribution without heat pipe cooling (Q =15.7W)Table 1Input values of the FEA model for the cutting tool Insert,shim seat,and thermal grease width,a 9.525mm Insert,shim seat,and thermal grease length,b 9.525mm Insert thickness,c 1.5mm Shim seat thickness4.84mm Thermal grease thickness 0.1mm Tool holder length114.3mm Tool holder width and height19.05mm Thermal conductivity of insert,K T 112.5W/m·K Density of insert,ρT14,900kg/m 3Specific heat of insert,C T208J/kg·K Thermal conductivity of tool holder,K H 42.6W/m·K Thermal conductivity of shim seat,K S 42.6W/m·K Density of tool holder and shim seat,ρS7,850kg/m 3Specific heat of tool holder and shim seat,C S 473J/kg·K Thermal conductivity of thermal grease,K G 9.8W/m·K Initial temperature,T 025°C908back surface,which is set to 5W/m 2°C.Other convective heat transfer coefficients for the cutting tool top surface and the side surface are set to 20W/m 2°C and 15W/m 2°C,respectively,with the one for the shim seat set at 5W/m 2°C.All the case studies were conducted to simulate the heat transfer behavior by varying the input heat flux from 20×106W/m 2to 60×106W/m 2,applied to the top corner of the insert,where the heating zone on the corner was held constant:A=π×1mm 2/4=0.78×10−6m 2.A typical simula-tion for a cutting tool without a heat pipe in Fig.5for heat flow Q =15.7W shows that the maximum temperature near the heat source is 352°C.With the same heat flow as shown in Fig.6,the maximum tool tip temperature is reduced to 293°C with a heat pipe installed in the cutting tool,a reduction of 59°C compared to the no heat pipe case.It may be inferred that the heat-pipe system designed has lower thermal resistance than the non-heat-pipe system.Without a heat pipe,the heat transferred to the tool is resisted and accumulates on the tool –chip interface,and takes time to diffuse into the inner region of the cutting tool.4Experiments with an embedded heat pipe in a cutting toolAn experimental study was undertaken with the use of a heat pipe to remove heat from the tool –chip interface in a carbide insert.A series of cutting experiments were con-ducted on a conventional lathe using a commercially available SPU 422grade VC2with 11°rake angle un-coated carbide insert.The workpiece materials are AISI 1020and 1040carbon steels in the form of a 30-cm-long cylindrical rod with a diameter of 50mm.A standard tool holder manufactured by Valenite was modified for the purpose of the investigation.A VC2carbide insert was cut into the 1.5-mm-thick slot by wire electrical-discharge machining (EDM)to accommodate a flattened 6-mm copper water heat pipe.The heat pipe had a 135°bend to match the cutting rake insert geometry used.Arctic Silver thermal grease was used between the heat pipe,insert,and the tool holder to ensure good thermal contact.The proto-type insert fixture,carbide insert,and heat pipe were securely assembled and are shown in Fig.7a.Two system configurations (without and with heat pipe cooling)were studied and compared in order to determine the effect of the heat pipe on the removal of heat from themachiningFig.6Cutting tool temperature distribution with a heat pipe installed (Q =15.7W)Fig.7a Experimental setup with an embedded heat pipe in a cutting tool.b Locations of two thermocouples on the carbide insert.Temperature readings:(T c1,T c2)without heat pipe cooling;(T f1,T f2)with heat pipe cooling909processes.The flat-plate heat pipes with a 135°angle made by Thermacore Inc.to match the cutting rake insert geometry was studied in this project.Two 0.127-mm-diameter K-type (chromel-alumel)thermocouples (T c1and T c2,without heat pipe;T f1and T f2,with heat pipe)were affixed on the back side of the carbide insert,with a vertical distance (VD)of Z =1.5mm and horizontal distances (HD)of X =1.05mm and 4.22mm each from the cutting edge,as shown in Fig.7b.The depth of cut and the feed rate were fixed at 2mm and 0.1mm/rev,respectively,for all of thetests in machining AISI 1020steel.The thermocouples permitted the measurement of the transient temperature response at the side surface of the insert.Figure 8shows representative temperature variations for the two cases without and with heat pipe cooling at a cutting speed of 29.9m/min in machining AISI 1020steel.While the steady-state temperature T c1reaches 120°C for the cutting experiment without heat pipe cooling on the tool insert at the location of HD=1.05mm,the lower steady-state temperature T f1at the same locationreachesFig.8Temperature variation for the machining experiment without and with heat pipe cooling at a depth of cut of 2mm,a feed rate of 0.1mm/rev,and a cutting speed of 29.9m/minFig.9Temperature variations for the machining experiment without and with heat pipe cooling at a depth of cut of 2mm,a feed rate of 0.1mm/rev,and a cutting speed of 40.94m/min91096°C with heat pipe cooling.It can be seen that the temperature drop associated with the installed heat pipe is 24°C.Similarly,the steady-state temperature readings of T c2=110°C without heat pipe cooling and T f2=97°C with heat pipe cooling at the location of HD=4.22mm show a temperature drop of 23°C due to the embedded heat pipe.Figure 9shows the temperature variations for the same AISI 1020steel machining experiments at a higher cutting speed of 40.94m/min.For the case without heat pipe cooling,the steady-state temperature T c1at the location of HD=1.05mm is increased to 135°C.However,the steady-state temperature T f1with heat pipe cooling reaches about 106°C.The temperature drop in the case associated with the installed heat pipe is 29°C.The result at the location of HD=4.22mm is quite similar to that at the location of HD=1.05mm.The steady-state temperature readings of T c2=123°C without heat pipe cooling and T f2=95°C with heat pipe cooling at the same location show a temperature drop of 28°C.The experiments demonstrate the same results as the simulations indicating that the effect of the heat pipe is to reduce the steady-state temperature dependent upon the generated heat influx in machining.As predicted by the FEM analysis,the data shows that the thermal resistance of the heat pipe system is lower than the non-heat-pipe system.In order to estimate the temperature on the tool –chip interface and its effect on tool hardness and tool wear,a series of experiments in machining harder material AISI 1040steel with a diameter of 50mm were conducted.The same tendency appears in Fig.10for the effect of an installed heat pipe on steady-state temperature drops from the temperature readings at different locations of thermo-couples.In particular,the changes of the tool and chip material properties due to the temperature drop were also observed with the measurement of tool insert hardness and chip properties.It should be noted that similar methodshave been widely used to verify the cutting temperature for high-speed steel and carbide tool materials,since the material hardness is closely correlated to the history of heating [23].Figure 11shows the formation of the chips for the two cases without and with a heat pipe in machining a workpiece AISI 1040steel at a depth of cut of 1mm,a feed rate of 0.1mm/rev,and a cutting speed of 32.57m/min.It can be observed from the image that the formation of chips for the two cases are quite different.The chip radius of curvature for cutting without a heat pipe is longer than that in cutting with heat pipe cooling.Since chip generation is a major concern in machining ductile materials,it has drawn the interest of many researchers.It is well known from a number of papers [14,22]that the chip geometry is heavily dependent upon the temperature at which the material makes the transition from ductile to brittle.As observed from the research,the intense temper-ature rise at the tool –chip interface without a heat pipe tends to generate a higher heat flux in the secondary deformation zone,which makes up a metallurgicalstruc-Fig.10Temperature variation for the machining experiment without and with heat pipe cooling at a depth of cut of 1mm,a feed of 0.1mm/rev,and a cutting speed of 32.57m/minFig.11Formation of the chips.Top Cutting without heat pipe cooling.Bottom Cutting with heat pipe cooling911ture in the chip.Because the chip flow carries away a large portion of the heat generated,the temperature rise of the chip tends to make the chips more ductile,and,thus,increase the chip radius of curvature.It was also observed that the chip color is blue when the cutting is performed without a heat pipe;however,the chip color becomes dark brown when cutting is conducted with an installed heat pipe.The blue discoloration of the oxidized chip as a result of high temperature reveals that the tool –chip interface temperature is higher in cutting without heat pipe cooling.The corresponding wear rates of the tool insert for the cases without and with heat pipe cooling are also quite different.It is interesting to note that the location of maximum crater wear generally coincides with the location of the maximum temperature at some distance from the cutting edge of the rake face.One explanation of this behavior is that the tool –chip contact area combines the heat due to the frictional effect on the tool –chip interface and the heat from the primary deformation zone carried,largely convected with material flow along the interface,isconducted into the tool.Therefore,the temperature distri-bution at some distance away from the primary deforma-tion in the tool insert has a clear difference between the cases without and with a heat pipe.In addition,the shapes of both tool wear zones in the image confirm the assumed quarter-circular heating zone at the tool –chip interface at the tool insert rake surface in Fig.2.As shown in Fig.12,an examination of the test results reveals that there is a direct relation between the crater-wear rate and the tool –chip interface temperature.It can be seen in Fig.12a that there is sharp increase in crater wear after a higher temperature range has been reached for the tool insert without a heat pipe installed.Note the location of the crater-wear pattern and the discoloration of the tool insert (loss of temper)as a result of high temperatures.The discoloration profiles agree with the temperature shown by Kalpakjian and Schmid [23].From the photomicrographs in machining with and without an embedded heat pipe,it can be concluded that the installed embedded heat pipe reduces the temperature of the tool –chip interface.The temperature drop enhances the performance of the tool,reduces the tool wear,and increases the tool life,as shown in Fig.12b.The comparisons between the simulated and actual experimental data at different horizontal locations on the tool insert from the cutting edge are shown in Fig.13.While the two curves at the top of the figure represent the simulated data for the case without heat pipe cooling at the vertical distance (VD)of Z =1.5mm and the horizontal distances (HD)of X =1.5mm and 4.5mm from the tool –chip interface,the lower two temperature curves in the figure are at the locations of 2.1mm and 3.2mm from the cutting edge X =0for the case with heat pipe cooling.The overall trend of the FEA results shows reasonably good agreement with the measured results,except that the figure shows a lower measured value than thecalcu-Fig.12a Carbide insert without heat pipe cooling.b Carbide insert with heat pipe cooling at a depth of cut of 1mm,a feed of 0.1mm/rev,a cutting speed of 32.57m/min depth of cut,and a cutting time of 5min.(Photomicrographs,×50)Fig.13Comparison of simu-lated temperature profiles and actual experimental temperature measurements with the thermo-couples at different locations for two cases without and with heat pipe cooling912lated temperature predicted by the FEA model for the case without a heat pipe.This is because the time of150s for data acquisition of the steady-state temperature readings in the experiment was much lower than that which lasted for400s in the FEA simulation.In general, the installed heat pipe in the cutting tool has an effect of reducing the temperature on the tool–chip interface and other locations on the tool insert.Based on the experimental data at different locations,the extrapolated simulation results,the observed tool wear and chip properties,and the actual tool–chip interface tempera-tures are in good agreement with those predicted by the FEA simulation.5ConclusionsThe finite element model(FEM)analysis of heat transfer behavior and machining experimental results in the present study demonstrate that the heat generated in machining can be effectively removed by the use of a heat pipe installed on a cutting tool insert.The experimental results also agree with the analysis in the sense that the installed heat pipe in a cutting tool has a significant effect on the temperature drop at the tool–chip interface,tool wear reduction,and tool life prolongation in machining.The results based on the comparison between heat pipe and non-heat-pipe dry machining demonstrate the feasibility of the concept of using a heat pipe for reducing the generated heat and the use of cutting fluids in machining.Acknowledgement The authors would like to thank the National Science Foundation(grant nos.DMI-0115620and DMI-0342088) for its financial support of the project.References1.Ay H,Yang W-J(1998)Heat transfer and life of metal cuttingtools in turning.Int J Heat Mass Trans41(3):613–6232.Balaji AK,Sreeram G,Jawahir IS,Lenz E(1999)The effect ofcutting tool thermal conductivity on tool–chip contact length and cyclic chip formation in machining with grooved tools.Annals CIRP48(1):33–383.Beaubien SJ,Cattaneo AG(1964)A study of the role of cuttingfluid in machining operations.Lubr Eng10:74–794.Bishop KE,Lype EF,Raynor S(1950)The role of cutting fluidas a lubricant.Lubr Eng6:70–735.Byrne G,Scholta E(1993)Environmentally clean machiningprocesses—a strategic approach.Annals CIRP42(1):471–474 6.Cao Y,Faghri A(1994)Micro/miniature heat pipes andoperating limitations.J Enhanced Heat Trans1(3):265–274 7.Chao BT,Trigger KJ(1958)Temperature distribution at tool-chip and tool-work interface in metal cutting.Trans ASME 80:311–3208.Chandrasekaran H,Thuvander A(1998)Modeling tool stressesand temperature evaluation in turning using finite element method.Mach Sci Technol2(2):355–3679.Chiou RY,Chen JSJ,Cooper D,Ciuciu C(2000)Environmen-tally conscious investigation of cutting fluid mist behavior via particle image velocimetry in turning.In:Proceedings of the 2000ASME International Mechanical Engineering Congress and Exposition,Orlando,Florida,November2000,vol11,pp 829–83410.Chiou RY,Chen JSJ,Lu L,Cole I(2002)Prediction of heattransfer behavior of carbide inserts with embedded heat pipes for dry machining.In:Proceedings of the2002ASME International Mechanical Engineering Congress&Exposition, New Orleans,Louisiana,November2002,pp17–2211.Chen Z,Wong K,Li Wei,Stephenson DA,Liang SY(1999)Cutting fluid aerosol generation due to spin-off in turning operation:analysis for environmentally conscious machining.In:Proceedings of the ASME International Mechanical Engineering Conference and Exposition,Manufacturing Sci-ence and Engineering,Nashville,Tennessee,November1999, MED-vol10,pp285–29112.Chu TH,Wallbank J(1998)Determination of the temperatureof a machined surface.J Manuf Sci Eng120:259–26313.De Chiffre L(1988)Function of cutting fluids in machining.Lubr Eng44(6):514–51814.Ding Y,Hong SY(1998)Improvement of chip breaking inmachining low carbon steel by cryogenically precooling the workpiece.J Manuf Sci Eng—Trans ASME120:76–8315.Erdel BP(1999)Environmental issues in machining.SMEMachining Technology,vol10,no4,4th quarter16.Faghri A(1995)Heat pipe science and technology.Taylor andFrancis,Washington,DC17.Graham D(2000)Dry machining.Manuf Eng121(1):72–7818.Jen TC,Anagonye AU(2001)An improved transient model oftool temperatures in metal cutting.J Manuf Sci Eng—Trans ASME123(1):30–3719.Jen TC,Gutierrez G,Eapen S,Barber G,Zhao H,Szuba S,Manjunathaiah J,Labataille J(2002)Investigation of heat pipes cooling in drilling applications.Part I:preliminary numerical analysis and verifications.Int J Mach Tools Manuf42:643–652 20.Judd RL,Aftab K,Elbestawi MA(1994)An investigation ofthe use of heat pipes for machine tool spindle bearing cooling.Int J Mach Tools Manuf34(7):1031–104321.Judd RL,MacKenize HS,Elbestawi MA(1995)An investiga-tion of a heat pipe cooling system for use in turning on a lathe.Int J Mach Tools Manuf10(6):357–36622.Jawahir IS,van Luttervelt CA(1993)Recent developments inchip control research and applications.Annals CIRP42(2):659–69323.Kalpakjian S,Schmid SR(2001)Manufacturing engineeringand technology,4th edn.Prentice-Hall,Upper Saddle River, New Jersey24.Lu L(2003)Investigation of embedded heat pipe in cuttingtools for dry machining.Masters thesis,Temple University, Philadelphia,Pennsylvania25.Loewen EG,Shaw MC(1954)On the analysis of cutting-tooltemperatures.Trans ASME76:217–23126.Maekawa K,Nakano Y,Kitagawa T(1996)Finite elementanalysis of thermal behavior in metal machining.JSME Int J C 39(4):864–86927.Merchant ME(1945)Mechanics of the metal cutting process.I.Orthogonal cutting and a type2chip.J Appl Physi16(5):267–275 28.Olson WW,Sutherland JW(1994)Environmentally consciousmanufacturing.In:Proceedings of the Japan–USA Symposium on Flexible Automation,Kobe,Japan,July199429.Peterson GP(1999)Chapter12—heat pipes.In:RohnsenowWM,Hartnett JP,Cho YI(eds)McGraw-Hill handbook of heat transfer,3rd edn.McGraw-Hill,New York30.Radulescu R,Kapoor SG(1994)An analytical model forprediction of tool temperature fields during continuous and interrupted cutting.J Ind Eng—Trans ASME116:135–143913。
机械外文文献及翻译
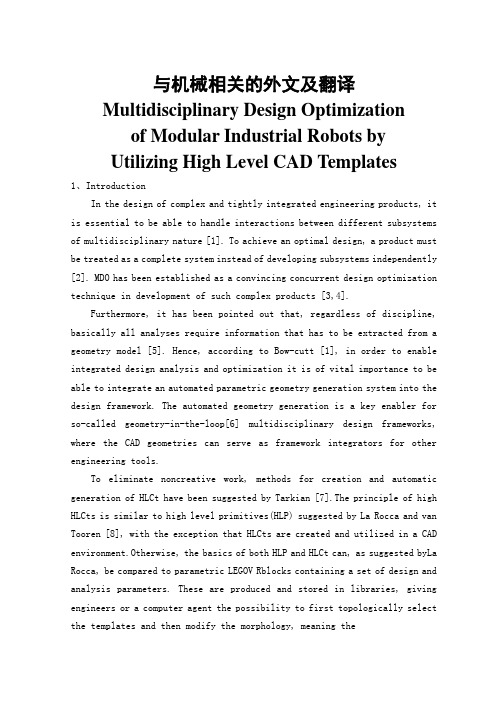
与机械相关的外文及翻译Multidisciplinary Design Optimization of Modular Industrial Robots by Utilizing High Level CAD Templates1、IntroductionIn the design of complex and tightly integrated engineering products, it is essential to be able to handle interactions between different subsystems of multidisciplinary nature [1]. To achieve an optimal design, a product must be treated as a complete system instead of developing subsystems independently [2]. MDO has been established as a convincing concurrent design optimization technique in development of such complex products [3,4].Furthermore, it has been pointed out that, regardless of discipline, basically all analyses require information that has to be extracted from a geometry model [5]. Hence, according to Bow-cutt [1], in order to enable integrated design analysis and optimization it is of vital importance to be able to integrate an automated parametric geometry generation system into the design framework. The automated geometry generation is a key enabler for so-called geometry-in-the-loop[6] multidisciplinary design frameworks, where the CAD geometries can serve as framework integrators for other engineering tools.To eliminate noncreative work, methods for creation and automatic generation of HLCt have been suggested by Tarkian [7].The principle of high HLCts is similar to high level primitives(HLP) suggested by La Rocca and van Tooren [8], with the exception that HLCts are created and utilized in a CAD environment.Otherwise, the basics of both HLP and HLCt can, as suggested byLa Rocca, be compared to parametric LEGOV Rblocks containing a set of design and analysis parameters. These are produced and stored in libraries, giving engineers or a computer agent the possibility to first topologically select the templates and then modify the morphology, meaning theshape,of each template parametrically.2、Multidisciplinary Design FrameworkMDO is a “systematic approach to design space exploration”[17], the implementation of which allows the designer to map the interdisciplinary relations that exist in a system. In this work, the MDO framework consists of a geometry model, a finite element(FE) model, a dynamic model and a basic cost model. The geometry model provides the analysis tools with geometric input. The dynamic model requires mass properties such as mass, center of gravity, and inertia. The FE model needs the meshed geometry of the robot as well as the force and torque interactions based on results of dynamic simulations.High fidelity models require an extensive evaluation time which has be taken into account. This shortcoming is addressed by applying surrogate models for the FE and the CAD models. The models are briefly presented below. 2.1 High Level CAD Template—Geometry ModelTraditionally, parametric CAD is mainly focused on morphological modifications of the geometry. However, there is a limit to morphological parameterization as follows:•The geometries cannot be radically modified.•Increased geometric complexity greatly increases parameterization complexity.The geometry model of the robot is generated with presaved HLCts, created in CATIA V5. These are topologically instantiated with unique internal design variables. Topological parameterization allows deletion, modification, and addition of geometricelements which leads to a much greater design space captured.Three types of HLCts are used to define the industrial robot topologically; Datum HLCt which includes wireframe references required for placement for the Actuator HLCTs and Structure HLCts, as seen Fig.2.Fig. 2 An industrial robot (left) and a modular industrial robot(right) The names of the references that must be provided for each HLCt instantiation are stored in the knowledge base (see Appen-dix A.4), which is searched through by the inference engine. In Appendix A, pseudocode examples describes how the references are retrieved and how they are stored in the knowledge base.The process starts by the user defining the number of degrees of freedom (DOF) of the robot (see Fig. 3) and is repeated until the number of axis (i) is equal to the user defined DOF.In order to instantiate the first Structure HLCt, two Datum and two actuator instances are needed. References from the two Datum instances help orienting the structure in space, while the geometries of the actuator instances, at both ends of the link, are used to construct the actuator attachments, as seen in Figs. 2 and 3. For the remaining links, only one new instance of both datum and actuator HLCts are required, since the datum and actuator instances from adjacent links are already available.Appendix A.2 shows a pseudocode example of an instantiation function. The first instantiated datum HLCt is defined with reference to the absolute coordinate system. The remaining datum HLCt instances are placed in a sequential order, where the coordinate system of previous instances is used as reference for defining the position in space according to user inputs (see also AppendixA.3). Furthermore, the type of each actuator and structure instance is user defined.Fig. 3 The high level CAD template instantiation process Since it is possible to create new HLCts in the utilized CAD tool, the users are not forced to merely choose from the templates available. New HLCts can be created, placed in the database and parametrically inserted into the models.2.2 Dynamic ModelThe objective of performing dynamic simulation of a robot is to evaluate system performance, such as predicting acceleration and time performance, but it also yields loads on each actuated axis, needed for actuator lifetime calculations and subsequent stress analysis based on FE calculations. Thedynamic model in the outlined framework is developed in Modelica using Dymola, and it constitutes a seven-axis robot arm based on the Modelica Standard library [18].The dynamic model receives input from the geometry model,as well as providing output to the FE model, which is further described in Sec. 2.3. However, to better understand the couplings between the models, the Newton –Euler formulation will be briefly discussed. In this formulation, the link velocities and acceleration are iteratively computed, forward recursivelyWhen the kinematic properties are computed, the force and torque interactions between the links are computed backward recursively from the last to the first link2.3 FE Surrogate ModelTo compute the structural strength of the robot, FE models for each robot link is created utilizing CATIA V5, see Fig. 4. For each HLCt, mesh and boundary conditions are manually preprocessed in order to allow for subsequent automation for FE-model creation. The time spent on preprocessing each FE-model is thus extensive. Nonetheless, the obtained parametric FE-model paves way for automated evaluation of a wide span of concepts. Each robot link is evaluated separately with the load conditions extracted from the dynamicmodel. The force (fi-11and fi) and torque (ţi-1and ti) are applied on the surfaceswhere the actuators are attached.2.4 Geometric Surrogate Models.Surrogate models are numerically efficient models to determine the relation between inputs and o utputs of a model [19]. The input variables for the proposed application are the morphological variables thickness and link height as well as a topological variable actuator type. The outputs of the surrogate models are mass m, Inertia I, and center of gravity ri,ci.To identify the most suitable type of surrogate model for the outlined problem, a range of surrogate models types are created and evaluated using 50 samples. The precision of each surrogate model is compared with the values of the original model with 20 new samples. The comparison is made using the relative average absolute error (RAAE) and relative maximum absolute error (RMAE) as specified by Shan et al. [20], as well as the normalized root mean square error (NRMSE), calculated as seen in Eq. (3). All precision metrics are desired to be as low as possible, since low values mean that the surrogate model is accurateThe resulting precision metrics can be seen in Appendix B and the general conclusion is that anisotropic kriging [21], neural networks [22], and radialbasis functions [23] are the most promising surrogate models. To investigate the impact of increasing number of samples, additional surrogate models of those three are fitted using 100 samples, and the results compiled in Appendix B. The resulting NRMSEs for 50 and 100 samples for anistotropic kriging, neural networks, and radial basis functions can be seen in Fig.5. The figures inside the parentheses indicate the number of samples used to fit the surrogate models.Fig. 5 Graph of the NRMSEs for different surrogate models,fitted using 50 and 100 samplesAccording to Fig. 5, anisotropic kriging outperforms the other surrogate models and the doubling of the number of samples usedfor fitting the surrogate model increases the precision dramatically.2.5 FE Surrogate ModelsFor generating FE surrogate models, the anisotropic kriging was also proven to be the most accurate compared to the methods evaluated in Sec. 2.4. Here, one surrogate model is created for each link. Inputs are thickness,actuators, force (fi-11and fi) and torque (ţi-1and ti). The output for eachsurrogate model is maximum stress (MS).A mean error of approximately 9% is reached when running 1400 samples for each link. The reason for the vast number of samples, compared to geometry surrogate models, has to do with a much larger design space.利用高水平CAD模板进行模块化工业机器人的多学科设计优化1 介绍指出,除了规则,基本上所有的分析都需要信息,而这些信息需要从一个几何模型中提取。
机械专业外文文献
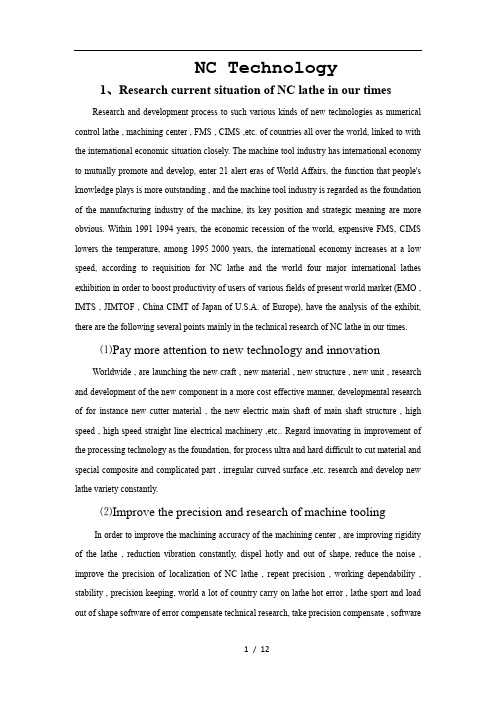
NC Technology1、Research current situation of NC lathe in our timesResearch and development process to such various kinds of new technologies as numerical control lathe , machining center , FMS , CIMS ,etc. of countries all over the world, linked to with the international economic situation closely. The machine tool industry has international economy to mutually promote and develop, enter 21 alert eras of World Affairs, the function that people's knowledge plays is more outstanding , and the machine tool industry is regarded as the foundation of the manufacturing industry of the machine, its key position and strategic meaning are more obvious. Within 1991-1994 years, the economic recession of the world, expensive FMS, CIMS lowers the temperature, among 1995-2000 years, the international economy increases at a low speed, according to requisition for NC lathe and the world four major international lathes exhibition in order to boost productivity of users of various fields of present world market (EMO , IMTS , JIMTOF , China CIMT of Japan of U.S.A. of Europe), have the analysis of the exhibit, there are the following several points mainly in the technical research of NC lathe in our times.⑴Pay more attention to new technology and innovationWorldwide , are launching the new craft , new material , new structure , new unit , research and development of the new component in a more cost-effective manner, developmental research of for instance new cutter material , the new electric main shaft of main shaft structure , high speed , high-speed straight line electrical machinery ,etc.. Regard innovating in improvement of the processing technology as the foundation, for process ultra and hard difficult to cut material and special composite and complicated part , irregular curved surface ,etc. research and develop new lathe variety constantly.⑵Improve the precision and research of machine toolingIn order to improve the machining accuracy of the machining center , are improving rigidity of the lathe , reduction vibration constantly, dispel hotly and out of shape, reduce the noise , improve the precision of localization of NC lathe , repeat precision , working dependability , stability , precision keeping, world a lot of country carry on lathe hot error , lathe sport and load out of shape software of error compensate technical research, take precision compensate , softwarecompensate measure improve , some may make this kind of error dispel 60% already. And is developing retrofit constantly, nanometer is being processed.⑶Improve the research of the machine tooling productivityWorld NC lathe, machining center and corresponding some development of main shaft , electrical machinery of straight line , measuring system , NC system of high speed, under the prerequisite of boosting productivity.⑷What a lot of countries have already begun to the numerical control system melt intelligently, openly, study networkedlya、elligent research of the numerical control systemMainly showing in the following aspects: It is intelligent in order to pursue the efficiency of processing and process quality, the self-adaptation to the processing course is controlled, the craft parameter produces research automatically; Join the convenient one in order to improve the performance of urging and use intelligently, to the feedback control , adaptive operation , discerning automatically load selects models automatically , since carries on research whole definitely ,etc. of the electrical machinery parameter; There are such research of the respect as intelligent automatic programming , intelligent man-machine interface , intelligence diagnosing , intelligent monitoring ,etc..b、The numerical control system melts and studies openMainly showing in the following aspects: The development of the numerical control system is on unified operation platform, face the lathe producer and support finally, through changing, increasing or cutting out the structure target(numerical control target ), form the seriation, and can use users specially conveniently and the technical know-how is integrated in the control system, realize the open numerical control system of different variety , different grade fast, form leading brand products with distinct distinction. System structure norm of the open numerical control system at present, norm , disposing the norm , operation platform , numerical control systematic function storehouse and numerical control systematic function software developing instrument ,etc. are the core of present research to pass through.c、Meeting the manufacture system of the production line , demand for the information integration of the manufacturing company networkedly greatly of numerical control equipment, it is a basic unit of realizing the new manufacture mode too.2、Classification of the machining center⑴Process according to main shaft space position when it classifies to be as follows, horizontal and vertical machining center.Horizontal machining center, refer to the machining center that the axis level of the main shaft is set up . Horizontal machining center for 3-5 sport coordinate axis, a common one three rectilinear motion coordinate axis and one turn the coordinate axis of sports round (turn the workingbench round), it can one is it is it finish other 4 Taxi processing besides installing surfaces and top surfaces to insert to install in work piece, most suitable for processing the case body work piece . Compared with strength type machining center it, the structure is complicated, the floor space is large, quality is large, the price is high.Vertical machining center, the axis of the main shaft of the vertical machining center, in order to set up vertically, its structure is mostly the regular post type, the workingbench is suitable for processing parts for the slippery one of cross, have 3 rectilinear motion coordinate axis generally , can find a room for one horizontal numerical control revolving stage (the 4th axle ) of axle process the spiral part at workingbench. The vertical machining center is of simple structure, the floor space is small, the price is low, after allocating various kinds of enclosures , can carry on the processing of most work pieces .Large-scale gantry machining center, the main shafts are mostly set up vertically, is especially used in the large-scale or with complicated form work piece , is it spend the many coordinate gantry machining center to need like aviation , aerospace industry , some processing of part of large-scale steam turbine.Five machining centers, this kind of machining center has function of the vertical and horizontal machining center, one is it after inserting , can finish all five Taxi processing besides installing the surface to install in work piece, the processing way can make form of work pieceerror lowest , save 2 times install and insert working, thus improve production efficiency , reduce the process cost.⑵Classify by craft useIs it mill machining center to bore, is it mill for vertical door frame machining center , horizontal door frame mill the machining center and Longmen door frame mill the machining center to divide into. Processing technology its rely mainly on the fact that the door frame is milled, used in case body, shell and various kinds of complicated part special curve and large processes , curved surface of outline process, suitable for many varieties to produce in batches small.Complex machining center, point five times and compound and process mainly, the main shaft head can be turned round automatically, stand, lie and process, after the main shaft is turned round automatically, realize knowing that varies in the horizontal and vertical direction.⑶Classify by special functionSingle workingbench , a pair of workingbench machining center;Single axle , dual axle , three axle can change machining center , main shaft of case;Transfer vertically to the tower machining center and transfer ;One hundred sheets of storehouses adds the main shaft and changes one one hundred sheets of machining centers;One hundred sheets of storehouses connects and writes hands to add the main shaft and change one hundred sheets of machining centers;One hundred sheets of storehouses adds the manipulator and adds one pair of main shafts to transfer to the tower machining center.3、Development trend of the current numerical control latheAt present, the advanced manufacturing technology in the world is rising constantly, such application of technology as ultrafast cutting , ultraprecision processing ,etc., the rapid development of the flexible manufacturing system and integrated system of the computer one isconstant and ripe, have put forward higher demand to the process technology of numerical control. Nowadays the numerical control lathe is being developed in several following directions.The speed and precision at a high speed , high accuracy are two important indexes of the numerical control lathe , it concerns directly that processes efficiency and product quality. At present, numerical control system adopt-figure number, frequency high processor, in order to raise basic operation speed of system. Meanwhile , adopt the super large-scale integrated circuit and many microprocessors structure, in order to improve systematic data processing ability , namely improve and insert the speed and precision of mending operation. Adopt the straight line motor and urge the straight line of the lathe workingbench to be servo to enter to the way directly, it is quite superior that its responds the characteristic at a high speed and dynamically. Adopt feedforward control technology , make it lag behind error reduce greatly , thus improve the machining accuracy cut in corner not to track.For meet ultrafast demand that process, numerical control lathe adopt main shaft motor and lathe structure form that main shaft unite two into one, realize frequency conversion motor and lathe main shaft integrate , bearing , main shaft of electrical machinery adopt magnetism float the bearing , liquid sound pigeonhole such forms as the bearing or the ceramic rolling bearing ,etc.. At present, ceramic cutter and diamond coating cutter have already begun to get application.Multi-functional to is it change all kinds of machining centers of organization (a of capacity of storehouse can up to 100 of the above ) automatically to furnished with, can realize milling paring , boring and pares , bores such many kinds of processes as paring , turning , reaming , reaming , attacking whorl ,etc. to process at the same time on the same lathe , modern numerical control lathe adopt many main shaft , polyhedron cut also , carry on different cutting of way process to one different position of part at the same time. The numerical control system has because adopted many CPU structure and cuts off the control method in grades, can work out part processing and procedure at the same time on a lathe , realize so-called " the front desk processes , the backstage supporter is an editor ". In order to meet the needs of integrating the systematic one in flexible manufacturing system and computer, numerical control system have remote serial interface , can network , realize data communication , numerical control of lathe, can control many numerical control lathes directly too.Intelligent modern numerical control lathe introduce the adaptive control technology, according to cutting the change of the condition, automatic working parameter, make the processing course can keep the best working state , thus get the higher machining accuracy and roughness of smaller surface , can improve the service life of the cutter and production efficiency of the equipment at the same time . Diagnose by oneself , repair the function by oneself, among the whole working state, the system is diagnosed, checked by oneself to CNC system and various kinds of equipment linking to each other with it at any time. While breaking down , adopt the measure of shutting down etc. immediately , carry on the fault alarm, brief on position , reason to break down ,etc.. Can also make trouble module person who take off automatically , put through reserve module , so as to ensure nobody demand of working environment. For realize high trouble diagnose that requires , its development trend adopts the artificial intelligence expert to diagnose the system.Numerical control programming automation with the development of application technology of the computer, CAD/CAM figure interactive automatic programming has already get more application at present, it is a new trend of the technical development of numerical control. It utilize part that CAD draw process pattern , is it calculate the trailing punishing to go on by cutter orbit data of computer and then, thus produce NC part and process the procedure automatically, in order to realize the integration of CAD and CAM. With the development of CIMS technology , the full-automatic programming way in which CAD/CAPP/CAM integrates has appeared again at present, it, and CAD/CAM systematic programming great difference their programming necessary processing technology parameter needn't by artificial to participate in most, get from CAPP database in system directly.The dependability of the dependability maximization numerical control lathe has been the major indicator that users cared about most all the time . The numerical control system will adopt the circuit chip of higher integrated level , will utilize the extensive or super large-scale special-purpose and composite integrated circuit, in order to reduce the quantity of the components and parts , to improve dependability . Through the function software of the hardware, in order to meet various kinds of demands for controlling the function, adopt the module , standardization , universalization and seriation of the structure lathe noumenonn of the hardware at the same time , make not only improve the production lot of the hardware but also easy to is itproduce to organize and quality check on.. Still through operating and starting many kinds of diagnostic programs of diagnosing , diagnosing , diagnosing off-line online etc. automatically, realize that diagnoses and reports to the police the trouble to hardware , software and various kinds of outside equipment in the system. Utilize the warning suggestion, fix a breakdown in time; Utilize fault-tolerant technology, adopt and design the important part " redundantly ", in order to realize the trouble resumes by oneself; Utilize various kinds of test , control technology , exceed Cheng , one one hundred sheets of damages , interfering , cutting out ,etc. at the time of various kinds of accidents as production, carry on corresponding protection automatically.Control system miniaturization systematic miniaturization of numerical control benefit and combine the machine 、electric device for an organic whole. Adopt the super large-scale integrated component , multi-layer printed circuit board mainly at present, adopt the three-dimensional installation method , make the electronic devices and components must use the high density to install, narrow systematic occupying the space on a larger scale. And utilize the new-type slim display of colored liquid crystal to substitute the traditional cathode ray tube, will make the operating system of numerical control miniaturize further. So can install it on the machine tool conveniently, benefit the operation of the numerical control lathe correctly even more.数控技术1、当前世界NC机床的研究现状世界各国对数控机床、加工中心以至FMS、CIMS等各种新技术的研究与发展进程,是与世界经济形势紧密相连的。
机械英文参考文献及翻译
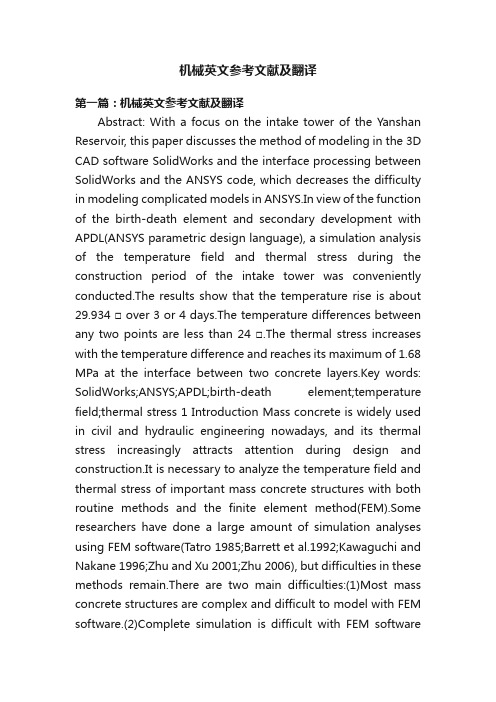
机械英文参考文献及翻译第一篇:机械英文参考文献及翻译Abstract: With a focus on the intake tower of the Yanshan Reservoir, this paper discusses the method of modeling in the 3D CAD software SolidWorks and the interface processing between SolidWorks and the ANSYS code, which decreases the difficulty in modeling complicated models in ANSYS.In view of the function of the birth-death element and secondary development with APDL(ANSYS parametric design language), a simulation analysis of the temperature field and thermal stress during the construction period of the intake tower was conveniently conducted.The results show that the temperature rise is about29.934 □ over 3 or 4 days.The temperature differences betweena ny two points are less than 24 □.The thermal stress increases with the temperature difference and reaches its maximum of 1.68 MPa at the interface between two concrete layers.Key words: SolidWorks;ANSYS;APDL;birth-death element;temperature field;thermal stress 1 Introduction Mass concrete is widely used in civil and hydraulic engineering nowadays, and its thermal stress increasingly attracts attention during design and construction.It is necessary to analyze the temperature field and thermal stress of important mass concrete structures with both routine methods and the finite element method(FEM).Some researchers have done a large amount of simulation analyses using FEM software(Tatro 1985;Barrett et al.1992;Kawaguchi and Nakane 1996;Zhu and Xu 2001;Zhu 2006), but difficulties in these methods remain.There are two main difficulties:(1)Most mass concrete structures are complex and difficult to model with FEM software.(2)Complete simulation is difficult with FEM softwarebecause of the complex construction processes and boundary conditions of concrete.The structure of the intake tower of the Yanshan Reservoir is complex.It is 34.5 m high and there is a square pressure tunnel at the bottom, the side length of which is 6 m.The intake tower was modeled in the 3D CAD software SolidWorks and imported into ANSYS with an interface tool.Then, using the APDL program, analysis of the temperature field and thermal stress during construction was conducted.2 Modeling in SolidWorks and interface processing between SolidWorks and ANSYS 2.1 Modeling in SolidWorks SolidWorks is a CAD/CAE/CAM/PDM desktop system, and the first 3D mechanical CAD software in Windows developed by the SolidWorks company.It provides product-level automated design tools(Liu and Ren 2005).The outside structure of the intake tower is simple but the internal structure is relatively complex.Therefore, the process of modeling is undertaken from the inside to the outside.The integrated and internal models of the intake tower are shown in Fig.1 and Fig.2.图片Fig.1 Integrated model Fig.2 Cross section 2.2 Interface processing between SolidWorks and ANSYS ANSYS is a type of large universal finite element software that has a powerful ability to calculate and analyze aspects of structure, thermal properties, fluid, electromagnetics, acoustics and so on.In addition, the interface of ANSYS can be used to import the CAD model conveniently(Zhang 2005), which greatly reduces the difficulties of dealing with complex models.The interface tools are given in Table 1.Table 1 CAD software packages and preferred interface tools 图表1After modeling in SolidWorks, it is necessary to save the model as a type of Parasolid(*.x_t)so as to import it into ANSYS correctly.Then, in ANSYS, the importing of the model iscompleted with the command “PARAIN, Name, Extension, Path, Entity, FMT, Scale” or the choice of “FileDImportDPARA...” in the GUI interface.There are two means of importing: selecting or not selecting “Allow Defeaturing”,the differences of which are shown in Fig.3 and Fig.4.图片Fig.3 Importing with defeaturing Fig.4 Importing without defeaturing 3 Analysis of temperature field of intake tower The temperature analysis of the intake tower during the construction period involves aspects of the temperature field and thermal stress.The calculation must deal with the problems of simulation of layered construction, dynamic boundary conditions, hydration heat, dynamic elasticity modulus, autogenous volume deformation of concrete and thermal creep stress, which are difficult to simulate directly in ANSYS.APDL is a scripting language based on the style of parametric variables.It is used to reduce a large amount of repetitive work in analysis(Gong and Xie 2004).This study carried out a simulation analysis of the temperature field considering nearly all conditions of construction, using the birth-death element and programming with APDL.3.1 Solving temperature field principle 3.1.1 Unsteady temperature field analysis The temperature of concrete changes during the construction period due to the effect of hydration heat of cement.This problem can be expressed as a heat conduction problem with internal heat sources in the area.The unsteady temperature field T(x, y, z,D)is written as(Zhu 1999): 公式1where □ is the thermal conductivity of concrete, c is the specific heat of concrete, □ is the density of concrete, □ is the adiabatic temperature rise of concrete, and □ is the age of concrete.In the 3D unsteady temperature field analysis, the functional form I e(T)is 公式2 where □R is a subfield of unit e;□0is the area on surface D , which is only in boundary units;c □ □ □□;□ is the exothermic coefficient;the thermal diffusivity c □ □ □ □;and isthe air temperature.a T3.1.2 Initial conditions and boundary conditions of concrete The initial conditions are the distribution laws of the initial transient temperature of internal concrete.The calculated initial temperature of concrete is 10 □.The index formula of hydration heat of cement is 公式 3 where t is the pouring time.The conversion between Q and □ is 公式4 The boundary conditions involve the laws of interaction between concrete and the surrounding medium.When concrete is exposed to the air, the boundary condition is 公式5 where n is the normal direction.Both and a T □ are constants or variables(Ashida and Tauchert 1998;Lin and Cheng 1997).During the maintenance period, the insulation materials of concrete are steel formworks and straws, and the exothermic coefficient of the outer surface is reduced as equivalent processing.The exothermic coefficients of the steel formwork and the straw are 45 kJ/(m2h+0)and 10 kJ/(m2h+0),respectively.Based on the local temperature during construction, the following formula can be fitted according to the temperature variation curve: 公式63.2 Analysis of temperature field in ANSYS The simulation scheme of layered construction, which is based on the real construction scheme, is shown in T able 2.The pouring days in Table 2 are all the total days of construction for each layer.A layer is not poured until the former layer is poured.图表2The feature points are selected in every layer above the base plate.The maximum temperatures and the temperature curvesare given in Table 3 and Fig.5, respectively.Table 3 Coordinates and maximum temperature of feature points 图表3 图片5 Fig.5 Maximum temperature curves Fig.5 shows that the maximum temperature of each layer occurs on the 3rd or 4th day after pouring, and then the temperature decreases with time, which is consistent with related literature(Lin and Cheng 1997;Luna and Wu 2000;Wu and Luna 2001).In Fig.5, the numbers of feature points from 2 to 8 are corresponding to their maximum temperature curves from Nodetemp 2 to Nodetemp 8, and the curve of Nodetemp 9 is the air temperature curve.Feature point 8, the maximum temperature of which is 29.934 □ , occurrin g on the 206th day of the total construction period, shows the maximum temperature rise during the construction period.Feature point 4, the coordinates of which are(16.4, 16.0, 5.0), shows the maximum temperature difference of 23.5340.4 Analysis of thermal stress of intake tower Expansion or contraction of the structure occurs during heating and cooling.If the expansion or contraction of different parts is inconsistent, then thermal stress occurs.The indirect method was adopted in this study: the temperature of nodes was first obtained in analysis of the temperature field, and then applied to the structure as a body load.4.1 Selection of calculating parameters The parameters of concrete are given in Table 4.The elasticity modulus is 公式7 T able 4 Parameters of concrete 图表4 The creep effect must be considered in analysis of temperature stress.The creep degree of concrete is influenced by the cement type, water-cement ratio and admixture.The formula of the creep degree is 公式8 Considering the creep degree, the formula of the elasticity modulus is adjusted to be 公式94.2 Analysis of thermal stress in ANSYS As in analysis of thetemperature field, feature points were selected in each layer above the base plate, and their coordinates were the same as those in the temperature field analysis.The maximum thermal stress of each point is shown in Table 5.Feature point 9, the coordinates of which are(17.4, 10.8, 8.0), is the point with the maximum thermal stress.Table 5 Maximum thermal stress of feature points 图表5The thermal stress curves of feature points are shown in Fig.6.图片6Fig.6 Maximum stress curves In Fig.6, the numbers of feature points from 2 to 9 are corresponding to their maximum stress curves from S1_2 to S1_9, and the S1_10 curve is the ultimate tensile stress o f concrete.The formula of concrete’s ultimate tensile stress is 公式10 The figures and table show that the maximum thermal stress of the intake tower is 1.68 MPa, occurring on the 90th day of the construction period, which is the end of the third layer maintenance period and the beginning of the pouring of the fourth layer.It is known that the thermal stress increases with the temperature difference.Feature point 9 is located at the interface between the third layer and the fourth layer.Thus, it is postulated that the maximum thermal stress is caused by the instantaneous temperature difference between two layers in the pouring period.In Fig.6, the S1_10 curve shows the ultimate tensile stress curve of concrete.It is known that the maximum thermal stress of each point in the intake tower during the construction period is less than the ultimate tensile stress of concrete.5 Conclusions ⑴ The problem of the interface between SolidWorks and ANSYS is resolved in this study, realizing an effective combination of the advantages of both SolidWorks and ANSYS and providing a basis for analysis in ANSYS.(2)Using abirth-death element and considering layered construction, dynamic boundary conditions, hydration heat, the dynamic elasticity modulus, autogenous volume deformation and creep of concrete, the temperature field and thermal stress during the construction period are conveniently obtained due to the virtues of secondary development with APDL.(3)The analysis of temperature shows that the temperature of concrete rises rapidly in the early stage of construction, reaches a maximum value of 29.934 □ on the 3rd or 4th day after pouring, drops thereafter, and is consistent with air temperature after about 30 days.The thermal stress increases with the temperature difference, and the occurrence time of the maximum thermal stress is consistent with that of the maximum temperature difference.The maximum thermal stress occurs at the interface of new and old layers and is caused by the instantaneous temperature difference, the value of which is 1.68 MPa.(4)The maximum thermal stress is less than the ultimate tensile stress of concrete, which illustrates that the curing measures in construction are effective.Meanwhile, in view of the fact that the maximum thermal stress occurs at the interface of new and old layers, more attention should be paid to it, especially when there is a long interval of time between the pouring of different layers.References Ashida, F., and Tauchert, T.R.1998.An inverse problem for determination of transient surface temperature from piezoelectric sensor measurement.Journal of Applied Mechanics, 65(2), 367-373.[doi:10.1115/1.2789064] Barrett, P.R., Foadian, H., James, R.J., and Rashid, Y.R.1992.Thermal-structural analysis methods for RCC dams.Proceedings of the Conference of Roller Concrete III, 407-422.San Diego: ASCE.Gong, S.G., and Xie, mands and Parametric Programming inANSYS.Beijing: China Machine Press.(in Chinese)Kawaguchi, T., and Nakane, S.1996.Investigations on determining thermal stress in massive concrete structures.ACI Materials Journal, 93(1), 96-101.Lin, J.Y., and Cheng, T.F.1997.Numerical estimation of thermal conductivity from boundary temperature measurements.Numerical Heat Transfer, 32(2), 187-203.[doi:10.1080/***87] Liu, L.J., and Ren, J.P.2005.Application of the secondary development in SolidWorks.Mechanical Management and Development,(1), 74-75.(in Chinese)Luna, R., and Wu, Y.2000.Simulation of temperature and stress fields during RCC dam construction.Journal of Construction Engineering and Management, ASCE, 126(5), 381-388.[doi: 10.1061/(ASCE)0733-9364(2000)126:5(381)] Tatro, S.B.and Schrader, E.K.1985.Thermal consideration for roller compacted concrete.ACI Structural Journal, 82(2), 119-128.Wu, Y., and Luna, R.2001.Numerical implementation of temperature and creep in mass concrete.Finite Elements in Analysis and Design, 37(2), 97-106.[doi:10.1016/S0168-874X(00)00022-6] Zhang, J.2005.Interface design between AutoCAD and ANSYS.Chinese Quarterly of Mechanics, 26(2), 257-262.(in Chinese)Zhu, B.F.1999.Thermal Stresses and Temperature Control of Mass Concrete.Beijing: China Electric Power Press.(in Chinese)Zhu, B.F., and Xu, P.2001.Methods for stress analysis simulating the construction process of high concrete dams.Dam Engineering, 6(4), 243-260.Zhu, B.F.2006.Current situation and prospect of temperature control and cracking prevention technology for concrete dam.Journal of Hydraulic Engineering, 37(12), 1424-1432.(in Chinese)第二篇:英文文献翻译(模版)在回顾D和H的文章时,我愿意第一个去单独地讨论每一篇,然后发表一些总体的观点。
(完整版)机械类外文文献翻译
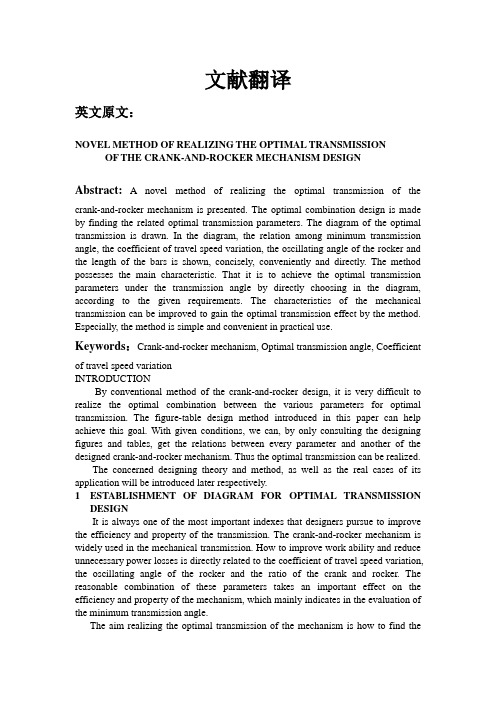
文献翻译英文原文:NOVEL METHOD OF REALIZING THE OPTIMAL TRANSMISSION OF THE CRANK-AND-ROCKER MECHANISM DESIGN Abstract: A novel method of realizing the optimal transmission of the crank-and-rocker mechanism is presented. The optimal combination design is made by finding the related optimal transmission parameters. The diagram of the optimal transmission is drawn. In the diagram, the relation among minimum transmission angle, the coefficient of travel speed variation, the oscillating angle of the rocker and the length of the bars is shown, concisely, conveniently and directly. The method possesses the main characteristic. That it is to achieve the optimal transmission parameters under the transmission angle by directly choosing in the diagram, according to the given requirements. The characteristics of the mechanical transmission can be improved to gain the optimal transmission effect by the method. Especially, the method is simple and convenient in practical use.Keywords:Crank-and-rocker mechanism, Optimal transmission angle, Coefficient of travel speed variationINTRODUCTIONBy conventional method of the crank-and-rocker design, it is very difficult to realize the optimal combination between the various parameters for optimal transmission. The figure-table design method introduced in this paper can help achieve this goal. With given conditions, we can, by only consulting the designing figures and tables, get the relations between every parameter and another of the designed crank-and-rocker mechanism. Thus the optimal transmission can be realized.The concerned designing theory and method, as well as the real cases of its application will be introduced later respectively.1ESTABLISHMENT OF DIAGRAM FOR OPTIMAL TRANSMISSION DESIGNIt is always one of the most important indexes that designers pursue to improve the efficiency and property of the transmission. The crank-and-rocker mechanism is widely used in the mechanical transmission. How to improve work ability and reduce unnecessary power losses is directly related to the coefficient of travel speed variation, the oscillating angle of the rocker and the ratio of the crank and rocker. The reasonable combination of these parameters takes an important effect on the efficiency and property of the mechanism, which mainly indicates in the evaluation of the minimum transmission angle.The aim realizing the optimal transmission of the mechanism is how to find themaximum of the minimum transmission angle. The design parameters are reasonably combined by the method of lessening constraints gradually and optimizing separately. Consequently, the complete constraint field realizing the optimal transmission is established.The following steps are taken in the usual design method. Firstly, the initial values of the length of rocker 3l and the oscillating angle of rocker ϕ are given. Then the value of the coefficient of travel speed variation K is chosen in the permitted range. Meanwhile, the coordinate of the fixed hinge of crank A possibly realized is calculated corresponding to value K .1.1 Length of bars of crank and rocker mechanismAs shown in Fig.1, left arc G C 2 is the permitted field of point A . Thecoordinates of point A are chosen by small step from point 2C to point G .The coordinates of point A are 02h y y c A -= (1)22A A y R x -= (2)where 0h , the step, is increased by small increment within range(0,H ). If the smaller the chosen step is, the higher the computational precision will be. R is the radius of the design circle. d is the distance from 2C to G .2cos )2cos(22cos 33ϕθϕϕ⎥⎦⎤⎢⎣⎡--+=l R l d (3) Calculating the length of arc 1AC and 2AC , the length of the bars of themechanism corresponding to point A is obtained [1,2].1.2 Minimum transmission angle min γMinimum transmission angle min γ(see Fig.2) is determined by the equations [3]322142322min 2)(cos l l l l l l --+=γ (4) 322142322max 2)(cos l l l l l l +-+=γ (5) max min180γγ-︒=' (6) where 1l ——Length of crank(mm)2l ——Length of connecting bar(mm)3l ——Length of rocker(mm)4l ——Length of machine frame(mm)Firstly, we choose minimum comparing min γ with minγ'. And then we record all values of min γ greater than or equal to ︒40 and choose the maximum of them.Secondly, we find the maximum of min γ corresponding to any oscillating angle ϕ which is chosen by small step in the permitted range (maximum of min γ is different oscillating angle ϕ and the coefficient of travel speed variation K ).Finally, we change the length of rockerl by small step similarly. Thus we3γcorresponding to the different length of bars, may obtain the maximum ofmindifferent oscillating angle ϕand the coefficient of travel speed variation K.Fig.3 is accomplished from Table for the purpose of diagram design.It is worth pointing out that whatever the length of rocker 3l is evaluated, the location that the maximum of min γ arises is only related to the ratio of the length of rocker and the length of machine frame 3l /4l , while independent of 3l .2 DESIGN METHOD2.1 Realizing the optimal transmission design given the coefficient of travelspeed variation and the maximum oscillating angle of the rockerThe design procedure is as follows.(1) According to given K and ϕ, taken account to the formula the extreme included angle θ is found. The corresponding ratio of the length of bars 3l /4l is obtained consulting Fig.3.︒⨯+-=18011K K θ (7) (2) Choose the length of rocker 3l according to the work requirement, the length of the machine frame is obtained from the ratio 3l /4l .(3) Choose the centre of fixed hinge D as the vertex arbitrarily, and plot an isosceles triangle, the side of which is equal to the length of rocker 3l (see Fig.4), andϕ=∠21DC C . Then plot 212C C M C ⊥, draw N C 1, and make angleθ-︒=∠9012N C C . Thus the point of intersection of M C 2 and N C 1 is gained. Finally, draw the circumcircle of triangle 21C PC ∆.(4) Plot an arc with point D as the centre of the circle, 4l as the radius. The arc intersections arc G C 2 at point A . Point A is just the centre of the fixed hinge of the crank.Therefore, from the length of the crank2/)(211AC AC l -= (8)and the length of the connecting bar112l AC l -= (9)we will obtain the crank and rocker mechanism consisted of 1l , 2l , 3l , and 4l .Thus the optimal transmission property is realized under given conditions.2.2 Realizing the optimal transmission design given the length of the rocker (or the length of the machine frame) and the coefficient of travel speed variationWe take the following steps.(1) The appropriate ratio of the bars 3l /4l can be chosen according to given K . Furthermore, we find the length of machine frame 4l (the length of rocker 3l ).(2) The corresponding oscillating angle of the rocker can be obtained consulting Fig.3. And we calculate the extreme included angle θ.Then repeat (3) and (4) in section 2.13 DESIGN EXAMPLEThe known conditions are that the coefficient of travel speed variation1818.1=K and maximum oscillating angle ︒=40ϕ. The crankandrockermechanism realizing the optimal transmission is designed by the diagram solution method presented above.First, with Eq.(7), we can calculate the extreme included angle ︒=15θ. Then, we find 93.0/43=l l consulting Fig.3 according to the values of θ and ϕ.If evaluate 503=l mm, then we will obtain 76.5393.0/504==l mm. Next, draw sketch(omitted).As result, the length of bars is 161=l mm,462=l mm,503=l mm,76.534=l mm.The minimum transmission angle is︒=--+=3698.462)(arccos 322142322min l l l l l l γ The results obtained by computer are 2227.161=l mm, 5093.442=l mm, 0000.503=l mm, 8986.534=l mm.Provided that the figure design is carried under the condition of the Auto CAD circumstances, very precise design results can be achieved.4 CONCLUSIONSA novel approach of diagram solution can realize the optimal transmission of the crank-and-rocker mechanism. The method is simple and convenient in the practical use. In conventional design of mechanism, taking 0.1 mm as the value of effective the precision of the component sizes will be enough.译文:认识曲柄摇臂机构设计的最优传动方法摘要:一种曲柄摇臂机构设计的最优传动的方法被提出。
机械类外文文献
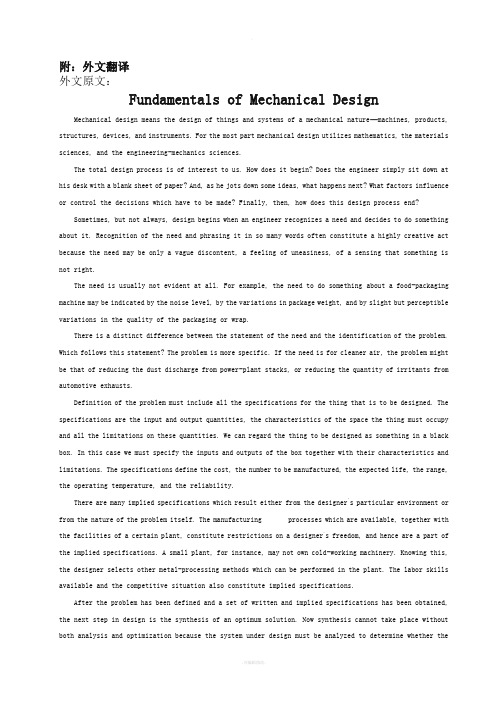
附:外文翻译外文原文:Fundamentals of Mechanical Design Mechanical design means the design of things and systems of a mechanical nature—machines, products, structures, devices, and instruments. For the most part mechanical design utilizes mathematics, the materials sciences, and the engineering-mechanics sciences.The total design process is of interest to us. How does it begin? Does the engineer simply sit down at his desk with a blank sheet of paper? And, as he jots down some ideas, what happens next? What factors influence or control the decisions which have to be made? Finally, then, how does this design process end?Sometimes, but not always, design begins when an engineer recognizes a need and decides to do something about it. Recognition of the need and phrasing it in so many words often constitute a highly creative act because the need may be only a vague discontent, a feeling of uneasiness, of a sensing that something is not right.The need is usually not evident at all. For example, the need to do something about a food-packaging machine may be indicated by the noise level, by the variations in package weight, and by slight but perceptible variations in the quality of the packaging or wrap.There is a distinct difference between the statement of the need and the identification of the problem. Which follows this statement? The problem is more specific. If the need is for cleaner air, the problem might be that of reducing the dust discharge from power-plant stacks, or reducing the quantity of irritants from automotive exhausts.Definition of the problem must include all the specifications for the thing that is to be designed. The specifications are the input and output quantities, the characteristics of the space the thing must occupy and all the limitations on these quantities. We can regard the thing to be designed as something in a black box. In this case we must specify the inputs and outputs of the box together with their characteristics and limitations. The specifications define the cost, the number to be manufactured, the expected life, the range, the operating temperature, and the reliability.There are many implied specifications which result either from the designer's particular environment or from the nature of the problem itself. The manufacturing processes which are available, together with the facilities of a certain plant, constitute restrictions on a designer's freedom, and hence are a part of the implied specifications. A small plant, for instance, may not own cold-working machinery. Knowing this, the designer selects other metal-processing methods which can be performed in the plant. The labor skills available and the competitive situation also constitute implied specifications.After the problem has been defined and a set of written and implied specifications has been obtained, the next step in design is the synthesis of an optimum solution. Now synthesis cannot take place without both analysis and optimization because the system under design must be analyzed to determine whether theperformance complies with the specifications.The design is an iterative process in which we proceed through several steps, evaluate the results, and then return to an earlier phase of the procedure. Thus we may synthesize several components of a system, analyze and optimize them, and return to synthesis to see what effect this has on the remaining parts of the system. Both analysis and optimization require that we construct or devise abstract models of the system which will admit some form of mathematical analysis. We call these models mathematical models. In creating them it is our hope that we can find one which will simulate the real physical system very well.Evaluation is a significant phase of the total design process. Evaluation is the final proof of a successful design, which usually involves the testing of a prototype in the laboratory. Here we wish to discover if the design really satisfies the need or needs. Is it reliable? Will it compete successfully with similar products? Is it economical to manufacture and to use? Is it easily maintained and adjusted? Can a profit be made from its sale or use?Communicating the design to others is the final, vital step in the design process. Undoubtedly many great designs, inventions, and creative works have been lost to mankind simply because the originators were unable or unwilling to explain their accomplishments to others. Presentation is a selling job. The engineer, when presenting a new solution to administrative, management, or supervisory persons, is attempting to sell or to prove to them that this solution is a better one. Unless this can be done successfully, the time and effort spent on obtaining the solution have been largely wasted.Basically, there are only three means of communication available to us. There are the written, the oral, and the graphical forms. Therefore the successful engineer will be technically competent and versatile in all three forms of communication. A technically competent person who lacks ability in any one of these forms is severely handicapped. If ability in all three forms is lacking, no one will ever know how competent that person is!The competent engineer should not be afraid of the possibility of not succeeding in a presentation. In fact, occasional failure should be expected because failure or criticism seems to accompany every really creative idea. There is a great to be learned from a failure, and the greatest gains are obtained by those willing to risk defeat. In the find analysis, the real failure would lie in deciding not to make the presentation at all.Introduction to Machine DesignMachine design is the application of science and technology to devise new or improved products for the purpose of satisfying human needs. It is a vast field of engineering technology which not only concerns itself with the original conception of the product in terms of its size, shape and construction details, but also considers the various factors involved in the manufacture, marketing and use of the product.People who perform the various functions of machine design are typically called designers, or design engineers. Machine design is basically a creative activity. However, in addition to being innovative, a designengineer must also have a solid background in the areas of mechanical drawing, kinematics, dynamics, materials engineering, strength of materials and manufacturing processes.As stated previously, the purpose of machine design is to produce a product which will serve a need for man. Inventions, discoveries and scientific knowledge by themselves do not necessarily benefit people; only if they are incorporated into a designed product will a benefit be derived. It should be recognized, therefore, that a human need must be identified before a particular product is designed.Machine design should be considered to be an opportunity to use innovative talents to envision a design of a product is to be manufactured. It is important to understand the fundamentals of engineering rather than memorize mere facts and equations. There are no facts or equations which alone can be used to provide all the correct decisions to produce a good design. On the other hand, any calculations made must be done with the utmost care and precision. For example, if a decimal point is misplaced, an otherwise acceptable design may not function.Good designs require trying new ideas and being willing to take a certain amount of risk, knowing that is the new idea does not work the existing method can be reinstated. Thus a designer must have patience, since there is no assurance of success for the time and effort expended. Creating a completely new design generally requires that many old and well-established methods be thrust aside. This is not easy since many people cling to familiar ideas, techniques and attitudes. A design engineer should constantly search for ways to improve an existing product and must decide what old, proven concepts should be used and what new, untried ideas should be incorporated.New designs generally have “bugs” or unforeseen problems which must be worked out before the superior characteristics of the new designs can be enjoyed. Thus there is a chance for a superior product, but only at higher risk. It should be emphasized that if a design does not warrant radical new methods, such methods should not be applied merely for the sake of change.During the beginning stages of design, creativity should be allowed to flourish without a great number of constraints. Even though many impractical ideas may arise, it is usually easy to eliminate them in the early stages of design before firm details are required by manufacturing. In this way, innovative ideas are not inhibited. Quite often, more than one design is developed, up to the point where they can be compared against each other. It is entirely possible that the design which ultimately accepted will use ideas existing in one of the rejected designs that did not show as much overall promise.Psychologists frequently talk about trying to fit people to the machines they operate. It is essentially the responsibility of the design engineer to strive to fit machines to people. This is not an easy task, since there is really no average person for which certain operating dimensions and procedures are optimum.Another important point which should be recognized is that a design engineer must be able to communicate ideas to other people if they are to be incorporated. Initially the designer must communicate a preliminary design to get management approval. This is usually done by verbal discussions in conjunction with drawinglayouts and written material. To communicate effectively, the following questions must be answered:(1)Does the design really serve a human need?(2)Will it be competitive with existing products of rival companies?(3)Is it economical to produce?(4)Can it be readily maintained?(5)Will it sell and make a profit?Only time will provide the true answers to the preceding questions, but the product should be designed, manufactured and marketed only with initial affirmative answers. The design engineer also must communicate the finalized design to manufacturing through the use of detail and assembly drawings.Quite often, a problem well occur during the manufacturing cycle. It may be that a change is required in the dimensioning or telegramming of a part so that it can be more readily produced. This falls in the category of engineering changes which must be approved by the design engineer so that the product function will not be adversely affected. In other cases, a deficiency in the design may appear during assembly or testing just prior to shipping. These realities simply bear out the fact that design is a living process. There is always a better way to do it and the designer should constantly strive towards finding that better way.MachiningTurning The engine lathe, one of the oldest metal removal machines, has a number of useful and highly desirable attributes. Today these lathes are used primarily in small shops where smaller quantities rather than large production runs are encountered.The engine lathe has been replaced in today's production shops by a wide variety of automatic lathes such as automatic of single-point tooling for maximum metal removal, and the use of form tools for finish and accuracy, are now at the designer's fingertips with production speeds on a par with the fastest processing equipment on the scene today.Tolerances for the engine lathe depend primarily on the skill of the operator. The design engineer must be careful in using tolerances of an experimental part that has been produced on the engine lathe by a skilled operator. In redesigning an experimental part for production, economical tolerances should be used.Turret Lathes Production machining equipment must be evaluated now, more than ever before, in terms of ability to repeat accurately and rapidly. Applying this criterion for establishing the production qualification of a specific method, the turret lathe merits a high rating.In designing for low quantities such as 100 or 200 parts, it is most economical to use the turret lathe. In achieving the optimum tolerances possible on the turret lathe, the designer should strive for a minimum of operations.Automatic Screw Machines Generally, automatic screw machines fall into several categories; single-spindle automatics, multiple-spindle automatics and automatic chucking machines. Originally designed for rapid, automatic production of screws and similar threaded parts, the automatic screw machine has long since exceeded the confines of this narrow field, and today plays a vital role in the mass production of a variety of precision parts. Quantities play an important part in the economy of the parts machined on the automatic to set up on the turret lathe than on the automatic screw machine. Quantities less than 1000 parts may be more economical to set up on the turret lathe than on the automatic screw machine. The cost of the parts machined can be reduced if the minimum economical lot size is calculated and the proper machine is selected for these quantities.Automatic Tracer Lathes Since surface roughness depends greatly upon material turned, tooling, and fees and speeds employed, minimum tolerances that can be held on automatic tracer lathes are not necessarily the most economical tolerances.Is some case, tolerances of ±0.05mm are held in continuous production using but one cut. Groove width can be held to ±0.125mm on some parts. Bores and single-point finishes can be held to ±0.0125mm. On high-production runs where maximum output is desirable, a minimum tolerance of ±0.125mm is economical on both diameter and length of turn.Milling With the exceptions of turning and drilling, milling is undoubtedly the most widely used method of removing metal. Well suited and readily adapted to the economical production of any quantity of parts, the almost unlimited versatility of the milling process merits the attention and consideration of designers seriously concerned with the manufacture of their product.As in any other process, parts that have to be milled should be designed with economical tolerances that can be achieved in production milling. If the part is designed with tolerances finer than necessary, additional operations will have to be added to achieve these tolerances——and this will increase the cost of the part.Grinding is one of the most widely used methods of finishing parts to extremely close tolerances and low surface roughness. Currently, there are grinders for almost for almost every type of grinding operation. Particular design features of a part dictate to a large degree the type of grinding machine required. Where processing costs are excessive, parts redesigned to utilize a less expensive, higher output grinding method may be well worthwhile. For example, wherever possible the production economy of center less grinding should be taken advantage of by proper design consideration.Although grinding is usually considered a finishing operation, it is often employed as a complete machining process on work which can be ground down from rough condition without being turned or otherwise machined. Thus many types of forgings and other parts are finished completely with the grinding wheel at appreciable savings of time and expense.Classes of grinding machines include the following: cylindrical grinders, center less grinders, internal grinders, surface grinders, and tool and cutter grinders.The cylindrical and center less grinders are for straight cylindrical or taper work; thus splices, shafts, and similar parts are ground on cylindrical machines either of the common-center type or the center less machine.Thread grinders are used for grinding precision threads for thread gages, and threads on precision parts where the concentricity between the diameter of the shaft and the pitch diameter of the thread must be held to close tolerances.The internal grinders are used for grinding of precision holes, cylinder bores, and similar operations where bores of all kinds are to be finished.The surface grinders are for finishing all kinds of flat work, or work with plain surfaces which may be operated upon either by the edge of a wheel or by the face of a grinding wheel. These machines may have reciprocating or rotating tables.译文:机械设计基础机械设计基础是指机械装置和机械系统——机器、产品、结构、设备和仪器的设计。
机械专业毕业设计外文翻译相关外文文献
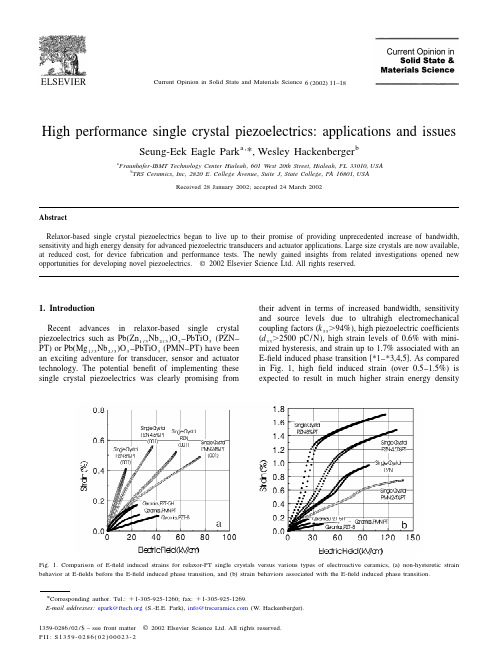
Fraunhofer-IBMT Technology Center Hialeah, 601 West 20 th Street, Hialeah, FL 33010, USA b TRS Ceramics, Inc, 2820 E. College Avenue, Suite J, State College, PA 16801, USA Received 28 January 2002; accepted 24 March 2002
* Corresponding author. Tel.: 1 1-305-925-1260; fax: 1 1-305-925-1269. E-mail addresses: epark@ (S.-E.E. Park), info@ (W. Hackenberger). 1359-0286 / 02 / $ – see front matter 2002 Elsevier Science Ltd. All rights reserved. PII: S1359-0286( 02 )00023-2
Fig. 1. Comparison of E-field induced strains for relaxor-PT single crystals versus various types of electroactive ceramics, (a) non-hysteretic strain behavior at E-fields before the E-field induced phase transition, and (b) strain behaviors associated with the E-field induced phase transition.
Current Opinion in Solid State and Materials Science 6 (2002) 11–18
英文文献翻译
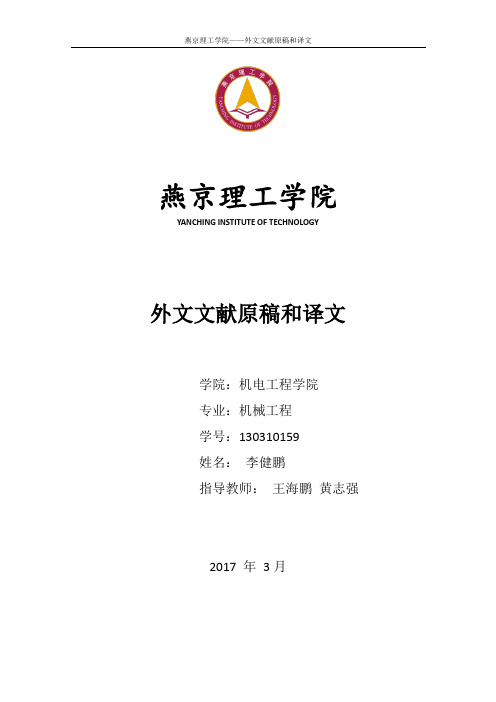
燕京理工学院YANCHING INSTITUTE OF TECHNOLOGY外文文献原稿和译文学院:机电工程学院专业:机械工程学号:130310159姓名:李健鹏指导教师:王海鹏黄志强2017 年3月外文文献原稿和译文原稿Style of materialsMaterials may be grouped in several ways. Scientists often classify materials by their state: solid, liquid, or gas. They also separate them into organic (once living) and inorganic (never living) materials.For industrial purposes, materials are divided into engineering materials or nonengineering materials. Engineering materials are those used in manufacture and become parts of products.Nonengineering materials are the chemicals, fuels, lubricants, and other materials used in the manufacturing process, which do not become part of the product.Engineering materials may be further subdivided into: ①Metal ②Ceramics③Composite ④Polymers, etc.Metals and Metal AlloysMetals are elements that generally have good electrical and thermal conductivity. Many metals have high strength, high stiffness, and have good ductility.Some metals, such as iron, cobalt and nickel, are magnetic. At low temperatures, some metals and intermetallic compounds become superconductors.What is the difference between an alloy and a pure metal? Pure metals are elements which come from a particular area of the periodic table. Examples of pure metals include copper in electrical wires and aluminum in cooking foil and beverage cans.Alloys contain more than one metallic element. Their properties can be changed by changing the elements present in the alloy. Examples of metal alloys include stainless steel which is an alloy of iron, nickel, and chromium; and gold jewelry which usually contains an alloy of gold and nickel.Why are metals and alloys used? Many metals and alloys have high densities andare used in applications which require a high mass-to-volume ratio.Some metal alloys, such as those based on aluminum, have low densities and are used in aerospace applications for fuel economy. Many alloys also have high fracture toughness, which means they can withstand impact and are durable.What are some important properties of metals?Density is defined as a material’s mass divided by its volume. Most metal s have relatively high densities, especially compared to polymers.Materials with high densities often contain atoms with high atomic numbers, such as gold or lead. However, some metals such as aluminum or magnesium have low densities, and are used in applications that require other metallic properties but also require low weight.Fracture toughness can be described as a material’s ability to avoid fracture, especially when a flaw is introduced. Metals can generally contain nicks and dents without weakening very much, and are impact resistant. A football player counts on this when he trusts that his facemask won’t shatter.Plastic deformation is the ability of bend or deform before breaking. As engineers, we usuallydesign materials so that they don’t def orm under normal conditions. You don’t want your car to lean to the east after a strong west wind.However, sometimes we can take advantage of plastic deformation. The crumple zones in a car absorb energy by undergoing plastic deformation before they break.The atomic bonding of metals also affects their properties. In metals, the outer valence electrons are shared among all atoms, and are free to travel everywhere. Since electrons conduct heat and electricity, metals make good cooking pans and electrical wires.It is impossible to see through metals, since these valence electrons absorb any photons of light which reach the metal. No photons pass through.Alloys are compounds consisting of more than one metal. Adding other metals can affect the density, strength, fracture toughness, plastic deformation, electrical conductivity and environmental degradation.For example, adding a small amount of iron to aluminum will make it stronger. Also, adding some chromium to steel will slow the rusting process, but will make itmore brittle.Ceramics and GlassesA ceramic is often broadly defined as any inorganic nonmetallic material.By this definition, ceramic materials would also include glasses; however, many materials scientists add the stipulation that “ceramic” must also be crystalline.A glass is an inorganic nonmetallic material that does not have a crystalline structure. Such materials are said to be amorphous.Properties of Ceramics and GlassesSome of the useful properties of ceramics and glasses include high melting temperature, low density, high strength, stiffness, hardness, wear resistance, and corrosion resistance.Many ceramics are good electrical and thermal insulators. Some ceramics have special properties: some ceramics are magnetic materials; some are piezoelectric materials; and a few special ceramics are superconductors at very low temperatures. Ceramics and glasses have one major drawback: they are brittle.Ceramics are not typically formed from the melt. This is because most ceramics will crack extensively (i.e. form a powder) upon cooling from the liquid state.Hence, all the simple and efficient manufacturing techniques used for glass production such as casting and blowing, which involve the molten state, cannot be used for th e production of crystalline ceramics. Instead, “sintering” or “firing” is the process typically used.In sintering, ceramic powders are processed into compacted shapes and then heated to temperatures just below the melting point. At such temperatures, the powders react internally to remove porosity and fully dense articles can be obtained.An optical fiber contains three layers: a core made of highly pure glass with a high refractive index for the light to travel, a middle layer of glass with a lower refractive index known as the cladding which protects the core glass from scratches and other surface imperfections, and an out polymer jacket to protect the fiber from damage.In order for the core glass to have a higher refractive index than the cladding, the core glass is doped with a small, controlled amount of an impurity, or dopant, whichcauses light to travel slower, but does not absorb the light.Because the refractive index of the core glass is greater than that of the cladding, light traveling in the core glass will remain in the core glass due to total internal reflection as long as the light strikes the core/cladding interface at an angle greater than the critical angle. The total internal reflection phenomenon, as well as the high purity of the core glass, enables light to travel long distances with little loss of intensity.CompositesComposites are formed from two or more types of materials. Examples include polymer/ceramic and metal/ceramic composites. Composites are used because overall propertiesof the composites are superior to those of the individual components.For example: polymer/ceramic composites have a greater modulus than the polymer component, but aren’t as brittle as ceramics.Two types of composites are: fiber-reinforced composites and particle-reinforced composites.Fiber-reinforced CompositesReinforcing fibers can be made of metals, ceramics, glasses, or polymers that have been turned into graphite and known as carbon fibers. Fibers increase the modulus of the matrix material.The strong covalent bonds along the fiber’s length give them a very high modulus in this direction because to break or extend the fiber the bonds must also be broken or moved.Fibers are difficult to process into composites, making fiber-reinforced composites relatively expensive.Fiber-reinforced composites are used in some of the most advanced, and therefore most expensive sports equipment, such as a time-trial racing bicycle frame which consists of carbon fibers in a thermoset polymer matrix.Body parts of race cars and some automobiles are composites made of glass fibers (or fiberglass) in a thermoset matrix.Fibers have a very high modulus along their axis, but have a low modulusperpendicular to their axis. Fiber composite manufacturers often rotate layers of fibers to avoid directional variations in the modulus.Particle-reinforced compositesParticles used for reinforcing include ceramics and glasses such as small mineral particles, metal particles such as aluminum, and amorphous materials, including polymers and carbon black.Particles are used to increase the modulus of the matrix, to decrease the permeability of the matrix, to decrease the ductility of the matrix. An example of particle-reinforced composites is an automobile tire which has carbon black particles in a matrix of polyisobutylene elastomeric polymer.Polymers A polymer has a repeating structure, usually based on a carbon backbone. The repeating structure results in large chainlike molecules. Polymers are useful because they are lightweight, corrosion resistant, easy to process at low temperatures and generally inexpensive.Some important characteristics of polymers include their size (or molecular weight), softening and melting points, crystallinity, and structure. The mechanical properties of polymers generally include low strength and high toughness. Their strength is often improved using reinforced composite structures.Important Characteristics of PolymersSize. Single polymer molecules typically have molecular weights between10,000 and 1,000,000g/mol—that can be more than 2,000 repeating units depending on the polymer structure!The mechanical properties of a polymer are significantly affected by the molecular weight, with better engineering properties at higher molecular weights.Thermal transitions. The softening point (glass transition temperature) and the melting point of a polymer will determine which it will be suitable for applications. These temperatures usually determine the upper limit for which a polymer can be used.For example, many industrially important polymers have glass transition temperatures near the boiling point of water (100℃, 212℉), and they are most useful for room temperature applications. Some specially engineered polymers can withstandtemperatures as high as 300℃(572℉).Crystallinity. Polymers can be crystalline or amorphous, but they usuallyhave a combination of crystalline and amorphous structures (semi-crystalline).Interchain interactions. The polymer chains can be free to slide past one another (thermo-plastic) or they can be connected to each other with crosslinks (thermoset or elastomer). Thermo-plastics can be reformed and recycled, while thermosets and elastomers are not reworkable.Intrachain structure. The chemical structure of the chains also has a tremendous effect on the properties. Depending on the structure the polymer may be hydrophilic or hydrophobic (likes or hates water), stiff or flexible, crystalline or amorphous, reactive or unreactive.The understanding of heat treatment is embraced by the broader study of metallurgy. Metallurgy is the physics, chemistry, and engineering related to metals from ore extraction to the final product.Heat treatment is the operation of heating and cooling a metal in its solid state to change its physical properties. According to the procedure used, steel can be hardened to resist cutting action and abrasion, or it can be softened to permit machining.With the proper heat treatment internal stresses may be removed, grain size reduced, toughness increased, or a hard surface produced on a ductile interior. The analysis of the steel must be known because small percentages of certain elements, notably carbon, greatly affect the physical properties.Alloy steel owe their properties to the presence of one or more elements other than carbon, namely nickel, chromium, manganese, molybdenum, tungsten, silicon, vanadium, and copper. Because of their improved physical properties they are used commercially in many ways not possible with carbon steels.The following discussion applies principally to the heat treatment of ordinary commercial steels known as plain carbon steels. With this process the rate ofcooling is the controlling factor, rapid cooling from above the critical range results in hard structure, whereas very slow cooling produces the opposite effect.A Simplified Iron-carbon DiagramIf we focus only on the materials normally known as steels, a simplifieddiagram is often used.Those portions of the iron-carbon diagram near the delta region and those above 2% carbon content are of little importance to the engineer and are deleted. A simplified diagram, such as the one in Fig.2.1, focuses on the eutectoid region and is quite useful in understanding the properties and processing of steel.The key transition described in this diagram is the decomposition of single-phase austenite(γ) to the two-phase ferrite plus carbide structure as temperature drops.Control of this reaction, which arises due to the drastically different carbon solubility of austenite and ferrite, enables a wide range of properties to be achieved through heat treatment.To begin to understand these processes, consider a steel of the eutectoid composition, 0.77% carbon, being slow cooled along line x-x’ in Fig.2.1. At the upper temperatures, only austenite is present, the 0.77% carbon being dissolved in solid solution with the iron. When the steel cools to 727℃(1341℉), several changes occur simultaneously.The iron wants to change from the FCC austenite structure to the BCC ferrite structure, but the ferrite can only contain 0.02% carbon in solid solution.The rejected carbon forms the carbon-rich cementite intermetallic with composition Fe3C. In essence, the net reaction at the eutectoid is austenite0.77%C→ferrite 0.02%C+cementite 6.67%C.Since this chemical separation of the carbon component occurs entirely in the solid state, the resulting structure is a fine mechanical mixture of ferrite and cementite. Specimens prepared by polishing and etching in a weak solution of nitric acid and alcohol reveal the lamellar structure of alternating plates that forms on slow cooling.This structure is composed of two distinct phases, but has its own set of characteristic properties and goes by the name pearlite, because oits resemblance to mother- of- pearl at low magnification.Steels having less than the eutectoid amount of carbon (less than 0.77%) are known as hypo-eutectoid steels. Consider now the transformation of such a material represented by cooling along line y-y’ in Fig.2.1.At high temperatures, the material is entirely austenite, but upon cooling enters aregion where the stable phases are ferrite and austenite. Tie-line and level-law calculations show that low-carbon ferrite nucleates and grows, leaving the remaining austenite richer in carbon.At 727℃(1341℉), the austenite is of eutectoid composition (0.77% carbon) and further cooling transforms the remaining austenite to pearlite. The resulting structure is a mixture of primary or pro-eutectoid ferrite (ferrite that formed above the eutectoid reaction) and regions of pearlite.Hypereutectoid steels are steels that contain greater than the eutectoid amount of carbon. When such steel cools, as shown in z-z’ of Fig.2.1 the process is similar to the hypo-eutectoid case, except that the primary or pro-eutectoid phase is now cementite instead of ferrite.As the carbon-rich phase forms, the remaining austenite decreases in carbon content, reaching the eutectoid composition at 727℃(1341℉). As before, any remaining austenite transforms to pearlite upon slow cooling through this temperature.It should be remembered that the transitions that have been described by the phase diagrams are for equilibrium conditions, which can be approximated by slow cooling. With slow heating, these transitions occur in the reverse manner.However, when alloys are cooled rapidly, entirely different results may be obtained, because sufficient time is not provided for the normal phase reactions to occur, in such cases, the phase diagram is no longer a useful tool for engineering analysis.HardeningHardening is the process of heating a piece of steel to a temperature within or above its critical range and then cooling it rapidly.If the carbon content of the steel is known, the proper temperature to which the steel should be heated may be obtained by reference to the iron-iron carbide phase diagram. However, if the composition of the steel is unknown, a little preliminary experimentation may be necessary to determine the range.译文材料的类型材料可以按多种方法分类。
机械类英文参考文献

Abrasive Wear of Fe-Mn-Si-Cr-Ni Shape MemoryStainless Steel:Preliminary ResultsChristian Egidio da Silva,Heide Heloise Bernardi,and Jorge Otubo (Submitted May 3,2010;in revised form February 10,2011)This study was developed to understand the influence of chemical composition and austenitic grain size on the wear resistance in stainless shape memory steel.A two-body abrasive wear device was used to understand the wear mechanism involved.They were tested pins with the following chemical composition:Fe-10.3Mn-5.3Si-9.9Cr-4.9Ni-0.006C and Fe-14.2Mn-5.3Si-8.8Cr-4.6Ni-0.008C after being austenitized at 900and 1050°C,followed by water quenching.The surface characterization was performed by optical microscopy and scanning electron microscopy,and the roughness profile evaluation was also conducted.The weight loss was measured after conducting the wear testing,and the wear rates were estimated.The results demonstrated that the alloy with less manganese and higher chromium content has the best wear resistance (between 17.5and 18.9%).With an increase of the austenitic grain size there was a small reduction on the wear resistance (between 3.0and 4.1%).The chemical composition demonstrated to have higher influence on the wear behavior than the austenitic grain size.Keywordsaerospace,mechanical testing,stainless steels,surface engineering,tribology1.IntroductionSince the 1990s,Fe-Mn-Si-based alloys such as Fe-Mn-Si-Cr-Ni and Fe-Mn-Si-Cr-Ni-Co have been studied as a candidate to substitute Ni-Ti.The shape memory effect on Fe-Mn-Si-Cr-Ni alloys is associated to a non thermoelastic martensitic transformation c (CFC)M e (HC).Due to its lower shape memory effect compared to Ni-Ti,the Fe-Mn-Si-based alloys are not employed in large-scale,however,they have many potential applications.A good shape memory effect would allow expanding their industrial application (Ref 1-3).The use of Fe-Mn-Si-based alloys is justified by their lower manufac-turing cost.In recent years,Fe-Mn-Si-based shape memory alloys have received much attention due to the possibility of using them in applications such as pipe joints,bolts,reinforce-ment of plasters,electrical actuators,thermal actuators,and vibration damping (Ref 4,5).Ferrous SMAs based on Fe-Mn alloy system may become a new class of SMAs of great technical importance.Works have been done by this group since 1994(Ref 6-13)to understand the physical metallurgy involved in this material,and to optimize its shape memory recovery capacity.These works have demonstrated that besides the chemical composi-tion,the grain size plays an important role on the shape recovery performance:the smaller the grain size the higher the shape recovery performance.Earlier works (Ref 10-13)have revealed that no grain growth is noticed up to 900°C of austenitizing temperature,which suggests that the energy to promote the grain growth was not enough.An abrupt grain size increase can be observed for samples austenitized in temperatures between 950and 1050°C.Alloys with less manganese and more chromium content are quite stable and there is no grain growth noticed up to 1000°C.It was also observed an increase of almost two times on the grain growth when compared to the ones from austenitizing temperatures of 900and 1050°C.According to Stachowiak and Batchelor (Ref 14),wear by abrasion is one form of wear caused by contact between particles and solid material.Considering that in several applications there is a mechanical contact among pieces,it is necessary to start studying wear resistance on shape memory alloy.There are no published articles studying wear mechanism on shape memory Fe-Mn-Si-Cr-Ni stainless steel.This article presents preliminary results about the influence of chemical composition and austenitic grain size on wear resistance.2.Experimental ProcedureIngots (65965mm 2)were prepared by vacuum induction melting and their chemical composition are shown in Table 1.The ingots were heated to 1180°C and hot forged down to 40940mm 2bars.These bars were solution treated at 1100°C for 3600s,then hot rolled to 20mm in diameter.This article is an invited paper selected from presentations at Shape Memory and Superelastic Technologies 2010,held May 16-20,2010,in Pacific Grove,California,and has been expanded from the original presentation.Christian Egidio da Silva,Heide Heloise Bernardi,and Jorge Otubo ,Mechanical Engineering Division,Technology Department,Aeronautical Technologic Institute,Sa ˜o Jose ´dos Campos,SP,Brazil;and Christian Egidio da Silva ,Product and Process Engineering,Gerdau Ac ¸os Especiais,Pindamonhangaba,SP,Brasil.Contact e-mails:christianegidio@ and christian.silva@.br.JMEPEG (2011)20:679–683ÓASM International DOI:10.1007/s11665-011-9888-11059-9495/$19.00The bars were submitted to a sequence of hot swaging followed by cold forging down to8mm offinal diameter, according to the following procedure:the20mm bars were initially heated to1050°C per2400s,and then hot swaged with intermediary annealing at1050°C,every swaging pass down to9.5mm in diameter;after that,these bars were cold forged down to afinal diameter of7.6mm.After reaching the final diameter,the bars were austenitized at900and1050°C for2400s.After concluding the heat treatment the bars were cut in [7.6mm912mm pins to perform wear testing.A two-body abrasive wear testing was used to assess the wear resistance, according to the following parameters:room temperature (24-28°C),static pin,#320grit(sandpaper)as abrasive surface,dry test(without coolingfluid),normal weight constant, 300rpm speed and different running times(120,240,360, and600s).Before conducting the wear testing the pins were mechanically grinded(#320to#600),and mechanically pol-ished with diamond paste(6,1,and¼l m).The same procedure was used on all the samples.The microstructures were analyzed by SEM(JEOL JSM-6490LV)and optical microscopy(LEICA LMDM).The surface was analyzed using optical microscopy tools.The wear rate was estimated using a balance METTLER H35AR (±0.0001g)after concluding each running time cycle on the two-body abrasive wear device.The abrasive surface(#320 grit)was changed after concluding each running cycle to avoid contamination.3.Results and Discussion3.1MicrostructureFigure1and2shows the microstructures of samples before the wear testing.It is possible to notice a heterogeneous microstructure for both alloys as well as the presence of twins inside the grain distributed along its area.The difference on the grain size is visible from900to1050°C of austenitizing temperature.Table1Chemical composition(wt.%)12 Cr9.98.8 Ni 4.9 4.6 Mn10.314.2 Si 5.3 5.3 C0.0060.008 Fe BalanceBalance Fig.1Microstructures of alloy1at900°C(a)and1050°C(b) before testing.SEM.Etching:marble Fig.2Microstructures of alloy2at900°C(a)and1050°C(b) before testing.SEM.Etching:marbleFig.3Roughness profile before testing:(a)Alloy1at900°C;(b)Alloy1at1050°C;(c)Alloy2at900°C;(d)Alloy2at1050°C.Optical microscopy.Etching:marbleFig.4Typical micrographs of alloy1(a)and2(b)after austenitizing at1050°C.SEM.Etching:marbleFig.5Overall wear rate for both materials at different austenitizing temperaturesFig.6Image of tracks on the surface after the contact with the abrasiveFig.7Roughness profile after testing:(a)Alloy 1at 900°C;(b)Alloy 1at 1050°C;(c)Alloy 2at 900°C;(d)Alloy 2at 1050°C.Optical microscopy3.2Surface Characterization Before Wear TestingFigure3shows the roughness profile of samples obtained by optical microscopy.It is possible to see that alloy1 presented a more continuous roughness profile along the scanning length(less dispersive)than alloy2.Figure3suggests that an increase of the austenitizing temperature leads to an increase of roughness profile.This effect on roughness can be explained by the difference of austenitic grain size:the larger the grain size,the bigger the difference on the surface because of its structure.Figure4shows typical micrographs for both materials after austenitizing at1050°C.Thisfigures help to understand the difference on roughness profile of samples‘‘1’’and‘‘2.’’It is noticed the existence of twins in both materials.Alloy1 (Fig.4a)is more homogeneously distributed than alloy2 (Fig.4b).3.3Wear ResistanceFigure5shows the overall wear rate for both materials after 600s of running time.Alloy1showed better wear resistance than alloy2:from17.5%(0.1038mg/m against0.1220mg/m, at1050°C)to18.9%(0.0997mg/m against0.1185mg/m,at 900°C).An increase of austenitizing temperature promoted a decrease of the wear resistance.The temperature of900C showed better wear resistance than of1050°C:from3.0% (0.1038mg/m against0.0997mg/m,for alloy1)to 4.1% (0.1220mg/m against0.1185mg/m,for alloy2).It is possible to infer that chemical composition has much more influence on the wear behavior than austenitic grain sizes (promoted by austenitizing heat treatment).Figure6shows typical tracks on the surface of samples after wear testing.Similar tracks were noticed on both materials,and at different austenitizing temperatures.It is possible to observe (in close detail)many particles displacement along the tracks.Figure7shows the roughness profile of the samples obtained by optical microscopy after wear testing.Alloy1 presented a more continuous roughness profile along the scanning length(less dispersive)than alloy2.This behavior can be explained by the difference in the microstructure(see Fig.3).Alloy2presented a larger austenitic grain size.This causes the appearance of many more crystallographic structures for alloy2than alloy1,which promotes the difference on roughness.4.ConclusionBoth Fe-Mn-Si-Cr-Ni shape memory stainless steel pre-sented a predominant abrasive wear micro-cutting mechanism. The material is removed as wear debris.The alloy with less manganese and higher chromium content showed better wear resistance.An increase of grain size resulted on a decrease of wear resistance.The larger the austenitic grain size,the rougher the profile.Chemical composition exhibits much more influence on wear resistance than austenitic grain sizes.References1.S.Kajiwara,Characteristic Features of Shape Memory Effect andRelated Transformation Behavior in Fe-Based Alloys,Mater.Sci.Eng., 1999,A273–275,p67–882.Y.H.Wen,M.Yan,and N.Li,Remarkable Improvement of ShapeMemory Effect in Fe-Mn-Si-Cr-Ni-C Alloy by Ageing with Deforma-tion,Scripta Mater.,2004,50,p835–8383.K.K.Jee,J.H.Han,and W.Y.Jang,Measurement of V olume Fractionof e Martensite in Fe-Mn Based Alloys,Mater.Sci.Eng.A,2004,378, p319–3224.A.Baruj and H.E.Troiani,The Effect of Pre-Rolling Fe-Mn-Si-BasedShape Memory Alloys:Mechanical Properties and Transmission Electron Microscopy Examination,Mater.Sci.Eng.A,2008,481–482,p574–5775.K.K.Jee,J.H.Han,and W.Y.Jang,A Method of Pipe Joining UsingShape Memory Alloys,Mater.Sci.Eng.A,2006,438–440,p1110–11126.J.Otubo,P.R.Mei,S.Koshimizu,A.H.Shinohara,and C.K.Suzuki,Relationship Between Thermomechanical Treatment,Microstructure and aÕMartensite in Stainless Fe-Based Shape Memory Alloys,Mater.Sci.Eng.A,1999,273–275,p533–5377.J.Otubo,F.C.Nascimento,P.R.Mei,L.P.Cardoso,and M.J.Kaufman,Influence of Austenite Grain Size on Mechanical Properties of Stainless SMA,Mater.Trans.,2002,43(5),p916–9198.J.Otubo,P.R.Mei,N.B.Lima,M.M.Serna,and E.Gallego,O Efeitodo Tamanho de gra˜o Austenı´tico no Nu´mero de Orientac¸o˜es das Variantes de Martensita em Ligas Inoxida´veis com Efeito de Memo´ria de Forma,Rev.Escola Minas,2007,p129–134(in Portuguese)9.F.C.Nascimento,J.Otubo,P.R.Mei,and L.P.Cardoso,Determinac¸a˜odas fases c,e e aÕpor difrac¸a˜o de raios-X em ligas inoxida´veis com efeito de memo´ria de forma,55ºCongresso Anual da ABM,2000(Rio de Janeiro,RJ,Brasil),ABM,CD-ROM(in Portuguese)10.C.E.Silva,A.R.Ju´nior,and J.Otubo,The Influence of AustenitizingTemperature and Chemical Composition on the Microstructure of Stainless Shape Memory Steel,18ºCongresso Brasileiro de Engenha-ria e Cieˆncia dos Materiais—CBECiMat,2008(Porto de Galinhas,PE, Brasil),CBECiMat,p5875–588211.C.E.Silva,J.Otubo,Influeˆncia da Temperatura de Solubilizac¸a˜o noTamanho de Gra˜o Austenı´tico de Ligas a`Base de Fe-Mn-Si com Efeito de Memo´ria de Forma:Resultados Preliminares,63ºCon-gresso Anual da ABM,2008(Santos,SP,Brasil),ABM,p2613–2621(in Portuguese)12.C.E.Silva,J.Otubo,and A.R.Ju´nior,A Influeˆncia do Tempo eTemperatura de Austenitizac¸a˜o e da Composic¸a˜o Quı´mica na Microe-strutura de Ligas Inoxida´veis com Efeito de Memo´ria de Forma,IX Semina´rio Brasileiro do Ac¸o Inoxida´vel,2008(Sa˜o Paulo,SP,Brasil), NU´CLEO INOX,p42–48(in Portuguese)13.C.E.Silva and J.Otubo,Influeˆncia da Temperatura de Austenitizac¸a˜ona Dureza,Resisteˆncia a`Compressa˜o e Capacidade de Recuperac¸a˜o de Forma de Ligas Inoxida´veis,64ºCongresso Anual da ABM,2009(Belo Horizonte,MG,Brasil),ABM,CD-ROM(in Portuguese)14.G.W.Stachowiak and A.W.Batchelor,Engineering tribology,3rded.,Elsevier:Butterworth-Heinemann,Burlington,2005,p501–551。
机械类外文文献及翻译大全
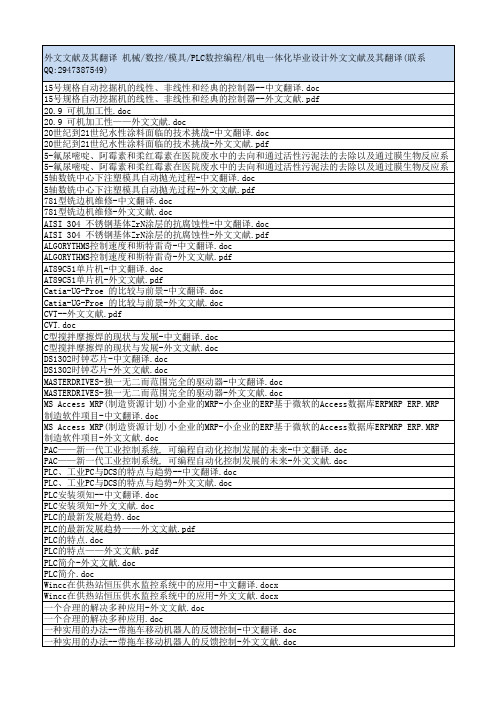
农业节水技术采用的决定因素:研究中国的10个省份--中文翻译.doc 农业节水技术采用的决定因素:研究中国的10个省份--外文文献.doc 冲压变形-中文翻译.docx 冲压变形-外文文献.doc 冲压模具设计中侧壁起皱的分析-中文翻译.doc 冲压模具设计中侧壁起皱的分析-外文文献.pdf 冷冲模具使用寿命的影响及对策-中文翻译.docx 冷冲模具使用寿命的影响及对策-外文文献.doc 凸轮设计的基本内容-中文翻译.docx 凸轮设计的基本内容-外文文献.doc 刀具成本的检测--外文文献.doc 刀具成本的检测文档.doc 切削加工新概念-中文翻译.docx 切削加工新概念-外文文献.docx 利用CAD - CAM - CAE软件及网络设施的产品开发-中文翻译.docx 利用CAD - CAM - CAE软件及网络设施的产品开发-外文文献.docx 利用三坐标测量仪确定聚苯乙烯材料表面形态--中文翻译.doc 利用三坐标测量仪确定聚苯乙烯材料表面形态--外文文献.doc 利用只装有车轮速度传感器的制动防抱死系统做路面情况鉴定-中文翻译.doc 利用只装有车轮速度传感器的制动防抱死系统做路面情况鉴定-外文文献.doc 利用曲臂3个自由度的相同并列式微型机械结构的实验设计-中文翻译.doc 利用曲臂3个自由度的相同并列式微型机械结构的实验设计-外文文献.doc 制造工程与技术(机加工)-中文翻译.doc 制造工程与技术(机加工)-外文文献.pdf 动态优化的一种新型高速,高精度的三自由度机械手-中文翻译.doc 动态优化的一种新型高速,高精度的三自由度机械手-外文文献.PDF 单刃刀具-中文翻译.doc 单刃刀具-外文文献.doc 单片机-中文翻译.doc 单片机-外文文献.pdf 单片机系统-中文翻译.doc 单片机系统-外文文献.doc 单片集成MEMS技术--中文翻译.doc 单片集成MEMS技术-外文文献.doc 压塑和压铸工艺-中文翻译.docx 压塑和压铸工艺-外文文献.docx 压电液压驱动器的设计和测试-中文翻译.docx 压电液压驱动器的设计和测试-外文文献.docx 原型基于颜色的图像检索与MATLAB -中文翻译.docx 原型基于颜色的图像检索与MATLAB -外文文献.docx 叠层陶瓷喷嘴的冲蚀磨损-中文翻译.doc 叠层陶瓷喷嘴的冲蚀磨损-外文文献.pdf 可伸缩悬臂式装煤机的研制--中文翻译.doc 可伸缩悬臂式装煤机的研制--外文文献.doc 可机加工性--中文翻译.doc 可机加工性--外文文献.doc 可编程控制器技术讨论与未来发展.doc 可编程控制器技术讨论与未来发展——外文文献.doc 可视化的PLC程序使用XML--中文翻译.doc 可视化的PLC程序使用XML——外文文献.doc 可靠性仿真与优化设计机械维修-中文翻译.docx 可靠性仿真与优化设计机械维修-外文文献.pdf 含有碳氮化合物的高压力钢应用于自动传动齿轮的发展-中文翻译.docx 含有碳氮化合物的高压力钢应用于自动传动齿轮的发展-外文文献.docx
机械专业外文论文
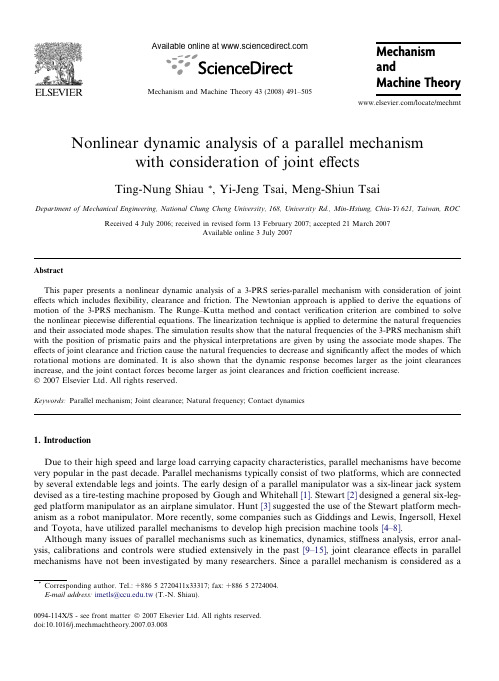
Nonlinear dynamic analysis of a parallel mechanismwith consideration of joint effectsTing-Nung Shiau *,Yi-Jeng Tsai,Meng-Shiun TsaiDepartment of Mechanical Engineering,National Chung Cheng University,168,University Rd.,Min-Hsiung,Chia-Yi 621,Taiwan,ROCReceived 4July 2006;received in revised form 13February 2007;accepted 21March 2007Available online 3July 2007AbstractThis paper presents a nonlinear dynamic analysis of a 3-PRS series-parallel mechanism with consideration of joint effects which includes flexibility,clearance and friction.The Newtonian approach is applied to derive the equations of motion of the 3-PRS mechanism.The Runge–Kutta method and contact verification criterion are combined to solve the nonlinear piecewise differential equations.The linearization technique is applied to determine the natural frequencies and their associated mode shapes.The simulation results show that the natural frequencies of the 3-PRS mechanism shift with the position of prismatic pairs and the physical interpretations are given by using the associate mode shapes.The effects of joint clearance and friction cause the natural frequencies to decrease and significantly affect the modes of which rotational motions are dominated.It is also shown that the dynamic response becomes larger as the joint clearances increase,and the joint contact forces become larger as joint clearances and friction coefficient increase.Ó2007Elsevier Ltd.All rights reserved.Keywords:Parallel mechanism;Joint clearance;Natural frequency;Contact dynamics1.IntroductionDue to their high speed and large load carrying capacity characteristics,parallel mechanisms have become very popular in the past decade.Parallel mechanisms typically consist of two platforms,which are connected by several extendable legs and joints.The early design of a parallel manipulator was a six-linear jack system devised as a tire-testing machine proposed by Gough and Whitehall [1].Stewart [2]designed a general six-leg-ged platform manipulator as an airplane simulator.Hunt [3]suggested the use of the Stewart platform mech-anism as a robot manipulator.More recently,some companies such as Giddings and Lewis,Ingersoll,Hexel and Toyota,have utilized parallel mechanisms to develop high precision machine tools [4–8].Although many issues of parallel mechanisms such as kinematics,dynamics,stiffness analysis,error anal-ysis,calibrations and controls were studied extensively in the past [9–15],joint clearance effects in parallel mechanisms have not been investigated by many researchers.Since a parallel mechanism is considered as a0094-114X/$-see front matter Ó2007Elsevier Ltd.All rights reserved.doi:10.1016/j.mechmachtheory.2007.03.008*Corresponding author.Tel.:+88652720411x33317;fax:+88652724004.E-mail address:imetls@.tw (T.-N.Shiau).Available online at Mechanism and Machine Theory 43(2008)491–505/locate/mechmtandMachine Theorymultibody system,joints are important components to connect an individual body together.The joint behav-iors such as clearance,friction,lubrication and impact forces can have a significant effect on dynamic response of the parallel system.For instance,the impact forces due to clearance might induce noise and vibration,and results in a loss of precision and a life shortage.Early researchers treat a body’s connections in parallel mechanism as ideal joints;that is,a joint is consid-ered as a spring with no clearance [16,17].In investigating non-ideal joints’behaviors,including joint clear-ances,damping and stiffness,most researches were only focused on planar mechanisms with rigid links,and have proposed some mathematical joint models to study joint effects on planar mechanisms [18–21].Experiments were performed to verify the proposed models [22–25].Liu and Lin [26]included the lubrication effect to establish a more complete joint model.For spatial mechanisms,contact dynamics of a spherical joint was presented by Tzou and Rong [27].Furthermore,stochastic simulation of dynamic characteristics of elas-tically jointed structures has been developed by Rong and Tzou [28].Bauchau and Rodriguez [29]proposed a spatial model including the effects of joint clearance and lubrication for three dimension mechanisms.How-ever,numerical simulation in their papers only demonstrated their results in planar mechanism.In this paper,a hybrid machine tool developed by Taiwan Industrial Technique Research Institute is con-sidered.The hybrid machine tool consists of a 3-PRS series-parallel mechanism and an X –Y table as shown in Fig.1.The nonlinear dynamic behavior analysis of the 3-PRS series-parallel mechanism is studied.Previous studies on the inverse kinematics [30]and forward kinematics [31]of the 3-PRS mechanism are stated and included.The Newtonian approach is applied to derive the dynamic equations of motion.The joint model is developed together with the derived equations of motion.Due to the highly nonlinear properties of the par-allel mechanism,the natural frequencies are first determined by using the impulse response function.Then the linearized dynamic equations of motion are developed.The natural frequencies distribution using the linear-ized dynamic equations is compared with those obtained by the impulse response approach.The joint clear-ance effects including joint flexibility,joint clearance and friction effect are discussed particularly for the spatial mechanism.2.The formulation of the equations of motionThe 3-PRS mechanism under investigation consists of three ball screws,three sliders,three links,and a moving platform as shown in Fig.2.The connection between the ball screw and slider is regarded as a pris-matic pair.The revolute joints are used to connect the sliders and the links,and the spherical joints are utilized to connect the links and the moving platform.A spindle is mounted on the moving platform when the 3-PRS mechanism is applied to the machine tool.The position and posture of the moving platform can becontrolled492T.-N.Shiau et al./Mechanism and Machine Theory 43(2008)491–505by the feed system of the sliders and the ball screws.For precision positioning of the moving platform,the dynamic analysis of the 3-PRS mechanism is very important.However,the dynamics of the 3-PRS mechanism with flexible linkages and joints is complicated.Moreover,the stiffness of the joints is usually less than 1/10of that of the link,and they are the weakest components in the parallel mechanism.Neglecting the stiffness of the joints is not sensible in a practical application.In order to achieve manageable equations for the 3-PRS mech-anism,the dynamic system is derived based on the following assumptions:(1)The feed system,links and the moving platform are assumed to be rigid.(2)The joint contact action is presented as an isotropic contact stiffness/damping set.Based on the assumptions,one can decide the degrees of freedom of the flexible 3-PRS mechanism.As shown in Fig.2,the links are restrained by the revolute joints to move on the three specific planes,which are X ¼ffiffiffi3p Y ,X =0and X ¼Àffiffiffi3p Y ;three generalized coordinates (y L i ,z L i ,h L i )are needed to describe the planar motion for each link.The moving platform connects with three spherical joints,and so six generalized coordinates x p ,y p ,z p ,a p ,b p ,c p are defined to describe the motion of moving platform.a p ,b p and c p are the rotational displacements of the moving platform which rotates about the Z -axis with c p first,and then rotates about the X -axis with a p and finally rotates about the Y -axis with b p .The total degrees of freedom of the 3-PRS mechanism with flexible joints equals to 15.Therefore,the coordinates of B i ,C i ,D i ,and T i can be expressed with these generalized coordinates.The expressions of B i are as follows:B 1¼R Z ;À60 B Ã1ð1ÞB 2¼R Z ;À180 B Ã2ð2ÞB 3¼R Z ;60 B Ã3ð3Þwhere R denotes the rotation matrix.B Ãi ¼f 0;ÀR B ;S i g Tare the position of the sliders in the three specific frames mentioned above.R B is the radius of the base platform and S i is the position of sliders.The expressions of C i and D i are as follows:C 1¼R Z ;À60 ðG ÃL1ÀR X ;Àh L1L ÃÞð4ÞFig.2.The coordinate system of the 3-PRS mechanism.T.-N.Shiau et al./Mechanism and Machine Theory 43(2008)491–505493C 2¼R Z ;À180 ðG ÃL2ÀR X ;Àh L2L ÃÞð5ÞC 3¼R Z ;60 ðG ÃL3ÀR X ;Àh L3L ÃÞð6ÞD 1¼R Z ;À60 ðG ÃL1þR X ;Àh L1L ÃÞð7ÞD 2¼R Z ;À180 ðG ÃL2þR X ;Àh L2L ÃÞð8ÞD 3¼R Z ;60 ðG ÃL3þR X ;Àh L3L ÃÞð9Þwhere G ÃL i ¼f 0;y L i ;z L i g Tare the mass centers of the links in the three specific frames.L üf 0;12L L ;0g Tis the length vector which is measured from the mass center of the link to the ends.L L is the length of the link.The expressions of T i are given as follows:T 1¼G p þR Y ;b R X ;a R Z ;c R Z ;À60 T Ãð10ÞT 2¼G p þR Y ;b R X ;a R Z ;c R Z ;À180 T Ãð11ÞT 3¼G p þR Y ;b R X ;a R Z ;c R Z ;60 T Ãð12Þwhere G p ¼f x p ;y p ;z p g Tis the mass center of the moving platform.T üf 0;ÀR S ;0g Tis the length vector which is measured from the mass center of the moving platform to the spherical joint.R S is the radius of the moving platform.After defining the coordinates,the dynamic equations of motion can be formulated.The free body diagram of link 1is illustrated in Fig.3.Applying Newton’s second law,the equations of motion of link 1can be for-mulated as follows:R Z ;60 ðf 1þf 4Þ¼m L1a L1ð13ÞR Z ;60 ðððC 1ÀG L1ÞÂf 1þðD 1ÀG L1ÞÂf 4ÞþM L1Þ¼I L1a L1ð14Þwhere a L1¼f 0;€y L1;€z L1g T is the acceleration of link 1.m L1is the mass of link 1.f 1¼k r ðB 1ÀC 1Þþc r ð_B1À_C 1Þand f 4¼k s ðT 1ÀD 1Þþc s ð_T1À_D 1Þare the contact force of the revolute joint and spherical joint,respectively.ðk r ,c r Þand ðk s ;c s Þare the contact stiffness and damping coefficients of the revolute and spherical joints,respec-tively.Because the motion of link 1is constrained to the specific plane X ¼ffiffiffi3p Y ,the force exerted on the revolute joint are only perpendicular to the direction of the joint axis.The component f 1x shown in Fig.3Fig.3.The free body diagrams of link 1and the moving platform (a)link 1;(b)moving platform.494T.-N.Shiau et al./Mechanism and Machine Theory 43(2008)491–505is the reacted force of f 4x .M L1is the reacted moments of the revolute joint.I L1is the mass moment of inertia ofthe link about G L1.G L1is the mass center of link 1.a L1¼f €h L1;0;0g Tis the angular acceleration of link 1.Similarly,the equations of motion of links 2and 3can be formulated as follows:R Z ;180 ðf 2þf 5Þ¼m L2a L2ð15ÞR Z ;180 ðððC 2ÀG L2ÞÂf 2þðD 2ÀG L2ÞÂf 5ÞþM L2Þ¼I L2a L2ð16ÞR Z ;À60 ðf 3þf 6Þ¼m L3a L2ð17ÞR Z ;À60 ðððC 3ÀG L3ÞÂf 3þðD 3ÀG L3ÞÂf 6ÞþM L3Þ¼I L3a L3ð18Þwhere the definition of symbols used in Eqs.(15)–(18)are similar to that in Eqs.(13)and (14).For the moving platform,the free body diagram is shown in Fig.3.The spindle mounted on the moving platform is assumed not to rotate and the equations of motion can be formulated as follows:Àf 4Àf 5Àf 6Àm p g þf C ¼m p a pð19ÞÀr 1Âf 4Àr 2Âf 5Àr 3Âf 6þl t Âf C ¼d d t ðI 0px p Þð20Þwhere m p is the mass of the moving platform.g is the gravity.f C is the cutting force acting at the tool-tip.a p ¼f €x p ;€y p ;€z p g Tis the acceleration of the moving platform.r i ¼T i ÀG p are the vectors measured from the center of the moving platform to the spherical joints.l t is the vector measured form the center of the mov-ing platform to the tool-tip.I 0p ¼R p I p R T p is the mass moment of inertia of the moving platform in the global coordinate system.R p ¼R Y ;b R X ;a R Z ;c is the rotation matrix of the moving platform from the local coordinate system to the global coordinate system.I p is the mass moment of inertia of the moving platform in local coor-dinate system mounted on the moving platform.Since the rotation matrix of the moving platform is defined,the angular velocity of the moving platform,x p ,can be obtained form the matrix _R p R T p.The components of the angular velocity,x PX ,x PY and x PZ ,are the elements (3,2),(1,3)and (2,1)of the matrix _R p R T p,respectively.The x p can be expressed as x p ¼f _a p cos b p þ_c p cos a p sin b p ;_b T p À_c p sin a p ;À_a p sin b p þ_c p cos a p cos b p g .3.Joint clearance modelIn this section,the joint clearance model will be developed and incorporated with the dynamic model in theprevious section.To investigate the joint effects,the mathematical model of a three-dimensional spherical joint is shown in Fig.4.The joint clearance d s is defined as the difference of the inner radius of the socket and the outer radius of the ball.It is assumed that the joint contact is decomposed into two components.The first is the normal contact and the second is the friction force acting in the tangential direction.It is also assumed that the clearance is large enough to allow relative motion of the socket and ball,so that the joint ‘‘sticktion’’is notconsidered.T.-N.Shiau et al./Mechanism and Machine Theory 43(2008)491–505495The normal contact force f4n,due to the joint stiffness and damping,is expressed asf 4n ¼k k sðj T1ÀD1jÀd sÞT1ÀD1j T1ÀD1jþc sð_T1À_D1Þð21Þwhere k is a step function and is defined ask¼0;if j T1ÀD1jÀd s<01;if j T1ÀD1jÀd s>0ð22ÞWhen k=0denotes the free-flight mode which the ball and the socket is not in contact.k=1means the impact mode and the friction effect should be counted.In this study,the joint clearance is only10À5–10À4m. Thus,the direction of the friction force can be approximated to the opposite of the tangential velocity and perpendicular to the relative displacement and angular velocity vectors at the contact point,and thus it can be given asf4f?ðT1ÀD1Þ;f4f?X4ð23Þwhere X4¼x pÀR Z;À60 x L1is the related angular velocity between the moving platform and the link1. x L1¼f_h L1;0;0g T is the angular velocity of link1.The friction force is defined asf 4f ¼l s f4nj jðT1ÀD1ÞÂX4ðT1ÀD1ÞÂX4j jð24Þwhere l s is the friction coefficient of the spherical joint.The total contact force is sum of f4n and f4ff4¼f4nþf4fð25ÞThe joint contact forces of the dynamic formulations in Eqs.(13),(14),(19)and(20)are replaced by Eq.(25)with consideration of joint stiffness,clearance and friction effects.Other joint forces can be formulated by the same approaches.The contact detection is the most important task for the numerical ually coming into contact is detected by a change of sign in the relative distance of B i C i and D i T i between the discrete moments in time t n and t nþ1ðB i C iðqðt nÞÞÞÁðB i C iðqðt nþ1ÞÞÞ<0ð26ÞðD i T iðqðt nÞÞÞÁðD i T iðqðt nþ1ÞÞÞ<0ð27ÞThis zero crossing can be found by a bisection or a Newton–Raphson procedure.The dynamic response can be simulated with the modified Runge–Kutta method.In this study,the time step is selected as10À4s for the excitation frequency of10Hz.4.Natural frequency and mode shapeThe natural frequencies of the3-PRS mechanism are very important when it is applied to a machine tool.A nonlinear system does not have natural frequencies.However,one canfind natural frequencies at the given positions under the assumption of small vibrations.Generally,there are two approaches tofind natural fre-quencies for a nonlinear system.One is the nonlinear simulation using an impulse response to excite the sys-tem,and the other is the linear analysis where the nonlinear dynamic equations are linearized into a set of ordinary differential equations.The impulse response approach is typically used in experiments and can be applied to obtain the system natural frequencies,and modal testing can be used tofind the mode shape. But it might not be suitable for symmetric mechanism because one particular natural frequency can corre-spond to several mode shapes.In our case,five mode shape couples correspond to the same natural frequency at the position of S1¼0,S2¼0and S3=0.The linearized equations of motion can be utilized to recognize the repeated natural frequencies,and the mode shapes can also be obtained.However,the friction and clear-496T.-N.Shiau et al./Mechanism and Machine Theory43(2008)491–505ance effects are not continuous functions,and the effects are neglected first in the linearization process.Their effects are analyzed by using the impulse response method.The linearized equations can be obtained by expanding the nonlinear Eqs.(13)–(20)in the Taylor series regarding the equilibrium position.By ignoring the high order terms,the linearized equations of motion regarding the equilibrium can be represented in matrix form asM €q þC _q þK ðq Àq e Þ¼U ðq e ;_q e ;€q e Þ¼0ð28Þwhere q is the set of the generalized coordinates,q e is the equilibrium position._qe and €q e are the velocity and acceleration at the equilibrium position which equals to zero.U is the functions to stand for the left hand sideof equations of motion.M is the system mass matrix.C ¼o U i ðq e ;_q e Þj is the system damping matrix.K ¼o U i ðq e ;_qe Þjis the system stiffness matrix,for i =1–15and j =1–15.To determine the equilibrium position,the Newton–Raphson method is employed to find the equilibrium position q e by iterating Eq.(29)with a set of initial estimates q 0while the D q n are convergedD q n ¼ÀK À1U ðq n ;0Þq n þ1¼q n þD q n (ð29ÞIn this study,the tolerance is selected as 10À8due to the complex triangle functions.For analyzing the nat-ural frequency of the 3-PRS mechanism,Eq.(28)can be rearranged as ½E 1 f _a g þ½E 2 f a g ¼0ð30Þwhere ½E 1 ¼M C 0M ;½E 2 ¼KÀM;f a g ¼f _qqg T Table 1The parameters of the 3-PRS mechanism Symbol ValueSymbol Value L L 1.1m m L60kg R B 0.349m m p 80kgR s0.2mI L6.0875kg m 2k r and k s 1·106N/mc r and c s600N s/mI pI p ¼1:03930001:03930000:81252435kg m 2T.-N.Shiau et al./Mechanism and Machine Theory 43(2008)491–505497T.-N.Shiau et al./Mechanism and Machine Theory43(2008)491–505499Assuming the eigenvalues to be the form ofL i¼r iþj x i;i¼1–30ð31ÞThe natural frequencies and the associated mode shapes can be obtained by solving Eq.(31).L i are the com-plex frequencies which the imaginary part x i are the damped natural frequencies and the real part r i are the corresponding damping exponents.5.Numerical results and discussionsWith the developed equations,the numerical results of system dynamic behaviors are analyzed in this sec-tion.The natural frequencies and mode shapes are obtained and the variations of the natural frequencies at different sliders’positions are discussed.The joint effects on the natural frequencies,dynamic responses and contact forces are also analyzed.The system parameters used in the simulations are listed in Table1unless stated otherwise.Fig.5shows the power spectrums of the tool-tip displacement with undamped and damped systems using an impulse response.A100N impulsive force acts at the edge of the moving platform in the direction of f cos30 ;sin30 ;cos45 g T,and it can excite all of the generalized coordinates.Fig.5shows that the damping effect reduces the amplitude of the tool-tip displacement and some modes are not well excited due to the damp-ing effect such as the modes at16.72Hz,44.49Hz,50.36Hz and53.59Hz.However,there are only nine res-onance frequencies excited in Fig.5.The linear analysis is used to help infinding the repeated natural frequencies.Table2presents the natural frequencies and their associated mode shapes calculated by using the linearized model.Fifteen modes are obtained by solving the characteristic equation of the linearized equa-tions of motion.Fig.6shows the variation of the natural frequencies versus the position of slider2.Table3presents the natural frequencies and their associated mode shapes at the configuration of S1¼0m,S2¼0:01m and S3=0m.Note that the modes are numbered by their order at S1¼0m,S2¼0:01m and S3=0m;the mode numbers are not rearranged for other paring Tables2,3and Fig.6,it can be found that some of the natural frequencies will change with the slider’s position.However,some of them,such as modes1,6,7, 8and9,almost remain the same as the slider changes its position.Close observation of the above mode shapes are shown in Tables2and3,and the corresponding weighting factors,a p and b p,in the above modes are close to zero.Thus,the variation of the natural frequencies may directly correlate to the posture of the moving plat-form.This is due to the posture of the moving platform will influence the distribution of the equivalent stiff-ness.Furthermore,rotating the mode shapes of the three links listed in Table2to the global frame,the mode shapes of the3-PRS mechanism can be illustrated as shown in Fig.7.The mode shapes can be categorized into five classes and are shown as follows:(1)Class1represents the motion which is dominated by vertical motion such as modes1and9.The linksand the moving platform vibrate in the same direction for mode1but in opposite direction for mode9.(2)Class2represents the motion which is dominated by horizontal motion of the links such as mode6.(3)Class3represents the motion which is dominated by the rotational motion such as modes12and15.(4)Class4represents the motion that link2does not vibrate,links1and3vibrate in opposite directionssuch as modes3,4,7,11and13and the moving platform only vibrates in the X-direction.(5)Class5represents the motion in which link2vibrates in opposite direction of links1and3such as modes2,5,8,10and14and the moving platform vibrates only in Y-direction.In classes4and5,the links and the moving platform vibrate in the same direction for the lower modes such as modes2,3,4and5,and in the opposite direction for the higher modes such as modes10,11,13and14. This physical phenomenon is similar to the double pendulum system in which the two pendulums vibrate in the same direction for the lower resonance frequency and in the opposite direction for the higher resonance frequency.Class1also conforms to the same phenomenon in which the links and the moving platform vibrate in the same direction for mode1and in the opposite direction for mode9.Furthermore,the shifting of natural frequencies is related to the total equivalent stiffness of the revolute and spherical joints when the3-PRS mech-anism is treated as a double pendulum system.The total equivalent stiffness of the revolute and spherical joints changes with the inclined angle of the links as the sliders move.The natural frequencies of modes3,5,8,11, and14increase as slider2moves up,and the natural frequencies of modes2,4,7,10,and13decrease as slider 2moves down.Fig.8shows the response of the tool-tip with the joint clearance effect.The excitation force is chosen as f¼m p gþ100sinð20p tÞN acting at the tool-tip in the Z-direction.The constant part of the excitation force is selected as m p g N.If the excitation force is not large enough to overcome the weight of the moving platform, the free-flight mode of the joint clearance will not occur,and the joint clearance effect can be neglected. Fig.8(b)shows that the tool-tip displacement becomes larger as joint clearance increases since the free moving space is increased.Fig.9(a)shows the power spectrum of the tool-tip displacement in X-direction under impulsive excitation.It is shown that the natural frequencies of thefirst,third and fourth resonances decrease as the joint clearance becomes larger.It is because the joint clearance leads to the contact period being increased,which results in less contact between the ball and the socket within a given period.Moreover,by comparing the frequency responses due to the joint clearance and friction effects in Fig.9(a),it is found that the friction particularly increases the magnitude of the fourth resonance more significantly as compared to other mode excitation.The friction has more influence on the rotational mode due to the moment caused by the friction force,and the fourth resonance is just mainly formed by the rotational motion of links.Based on these results and observations of the mode shapes,it is found that the joint clearance and friction signif-icantly affect the modes by which the rotational motions are dominate.This observation can provide more insights in designing joints under friction effects,and no research has been performed to study the shifting of natural frequencies due to friction effect.A similar phenomenon is also shown in Fig.9(b).It is mentioned that the super harmonic of mode12is excited with the joint clearance and friction effects.Fig.10shows the power spectrum of the deformationof revolute and spherical joints,j B iÀC i j and j D iÀT i j.It is presented that mode12is also the most remark-able mode.The joints might break while working near mode12and its super harmonic modes.Fig.11shows the trend of the joint clearance effect on natural frequencies which decrease as the joint clearance increases. Based on these results and observations of the mode shapes,one also found that the joint clearance signifi-cantly affect the modes by which the rotational motions are dominated.Fig.12shows the effect of joint clearance on contact forces.The excitation force is also selected as f¼m p gþ100sinð20p tÞN acting at the tool-tip in the Z-direction.The contact forces of revolute andspherical joints increase as the joint clearance and friction coefficient increase.It is because the contact velocity becomes large since the duration of acceleration increases as the joint clearance increases.6.ConclusionsIn this study,the nonlinear dynamic behaviors of the3-PRS series-parallel mechanism with consideration of joint effects includingflexibility,clearance and friction is investigated.The Newtonian approach is utilized to derive the nonlinear dynamic equations of motion of the3-PRS series-parallel mechanism.The Runge–Kutta numerical method and the contact verification criterion are combined to evaluate the dynamic response. The linearization technique is used to determine the natural frequencies and their associated mode shapes.The impulse response is helped to deal with the joint effects on the natural frequencies.Based on the numerical results,ourfindings can be summarized as follows:(1)The natural frequencies are the function of the sliders’location,and the shifting trends can be explained by analyzing their associated mode shapes.(2)The joint clearance significantly affects the modes by which the rotational motions are dominated.Some of the natural frequencies decrease as the joint clearance increase.Especially,the mode12and its super harmonic frequencies are obviously induced due to the joint clearance and friction effect.(3)The dynamic response becomes larger as the joint clearances increase,and the contact force increases as the joint clearance and the friction coefficient increase.These observations can provide useful information towards the design of parallel mechanisms. References[1]V.E.Gough,S.G.Whitehall,Universal Tyre Test Machine,in:Proceedings of Ninth International Technical Congress,F.I.S.I.T.A.,1962,p.177.[2]D.Stewart,A platform with six degrees of freedom,Proceedings of the Institution of Mechanical Engineers180(5)(1965)371–386.[3]K.H.Hunt,Kinematic Geometry of Mechanism,Clarendon Press,Oxford,1978.[4]P.Bailey,The merits of hexapods for robotics applications,in:Conference on Next Steps for Industrial Robotics,London,1994,pp.11/8–16/8.[5]C.R.Boer,L.Molinari-Tosatti,K.S.Smith,Parallel Kinematic Machines,Springer,London,1999.[6]J.Hollingum,Features:hexapods to take over?Industrial Robot24(1997)428–431.[7]uffer,T.Hinnerichs,C.P.Kuo,B.Wada,D.Ewaldz,B.Winfough,N.Shankar,Milling machine for the21st century–goals,approach,characterization and modeling,in:Proceedings of SPIE–The International Society for Optical Engineering Smart Structures and Materials1996:Industrial and Commercial Applications of Smart Structures Technologies,San Diego,2721,1996,pp.326–340.[8]T.H.Chang,I.Inasaki,K.Morihara,J.J.Hsu,The development of a parallel mechanism of5D.O.F.hybrid machine tool,in:Y2000Parallel Kinematic Machines International Conference,Ann Arbor,MI,USA,2000.[9]E.F.Fichter,A Stewart platform-based manipulator:general theory and practical construction,International Journal of RoboticsResearch5(2)(1986)157–182.[10]B.Dasgupta,T.S.Mruthyunjaya,A canonical formulation of the direct position kinematics problem for a general6-6Stewartplatform,Mechanism and Machine Theory29(6)(1994)819–927.。
- 1、下载文档前请自行甄别文档内容的完整性,平台不提供额外的编辑、内容补充、找答案等附加服务。
- 2、"仅部分预览"的文档,不可在线预览部分如存在完整性等问题,可反馈申请退款(可完整预览的文档不适用该条件!)。
- 3、如文档侵犯您的权益,请联系客服反馈,我们会尽快为您处理(人工客服工作时间:9:00-18:30)。
The content of mechanical engineeringMechanical engineering services and multi-faceted, who use machinery, tools, and energy and material production department of mechanical engineering, need the service. The summary mentions, modern machinery engineering has five services: development and provide energy conversion, and provide for mechanical manufacturing all kinds of products and machinery, provide various services in the machinery, the development and provide family and personal life, the development and the application of the machines provide various mechanical weapons.Regardless of what a field service to the working content of mechanical engineering, basic and same, mainly include:The establishment and development of mechanical engineering construction and theoretical basis. For example, research force and motion and fluid mechanics, engineering mechanics, Metallic and non-metallic materials to study the performance, and its application in engineering materials, The heat conduction, research and conversion of thermodynamics, All kinds of mechanical components are independent of the function of the working principle, structure, design and calculation of mechanical principle and mechanical parts; Study of metallic and nonmetallic machining and forming technology of metal and technology, etc.Research, design and development of new products and continuously improving existing machine mechanical products and new generation of mechanical products, in order to adapt to the current and future needs.Mechanical products production, including: the planning and production facilities, Production plan and production scheduling, Prepare and implement manufacturing process, Design and manufacturing tools, moulds, Determine the quota and material quota, Organization processing, assembling and commissioning and package delivery, For product quality effectively control. Mechanical manufacturing enterprise operation and management. Mechanical general is composed of many each have unique shape, processing precision parts assembled into a complex products. Production batch have single piece and small batch, also have a large batch, until production. Throughout the entire industry and sales target individual, family. And sales in the social and economic situations, may appear under the influence of the big wave. Therefore, mechanical manufacturing enterprise management and operation of enterprises, especially complex production management, planning and management of research are also began in machinery industry.The application of mechanical products. This includes selection, order, acceptance, installation, adjustment and operation, maintenance, repair and transformation of the industry by use of machinery and complete sets of equipment, to ensure the long-term use of mechanical products in the reliability and efficiency.The application of mechanical products. This includes selection, order, acceptance, installation, adjustment and operation, maintenance, repair and transformation of the industry by use of machinery and complete sets of equipment, to ensure the long-term use of mechanical products in the reliability and efficiency.Research in the manufacturing process of mechanical products, especially in the use of the natural resources and environmental pollution, excessive consumption issues, andtreatment measures. This is a modern mechanical engineering is particularly important task, and its importance.Mechanical engineering classificationMechanical variety, can according to several different ways into various categories, such as: the function can be divided into mechanical power, material handling machinery, machine etc, According to the service industry could be divided into agricultural machinery, mining machinery, textile machinery, etc. According to the principle of work can be divided into the thermal fluid machinery, mechanical, bionic machine, etc. In addition, machinery in the research, development, design, manufacture, application process to work through several stages of nature. According to the different stage, mechanical engineering and can be divided into each other, with several branches system, such as mechanical research, mechanical design, mechanical manufacturing, mechanical using and maintenance, etc.According to the different aspects of the various branches of system is divided into each other, thus overlap, mechanical engineering may be differentiated into hundreds of branches. For example, according to the function of dynamic mechanical, it points with the working principle of mechanical, thermal fluid machinery, turbine machinery, reciprocating machinery, steam power machinery, nuclear power plant, internal combustion engines, gas turbine, according to industry and the center of power equipment, industrial power plant, locomotives, ship, automobile engineering Marine engineering are complex and overlapping relation. Marine steam turbine power machinery, also is the thermal fluid machinery, mechanical and turbine machinery, it belongs to the Marine power plant, steam power plant, may also belongs to the nuclear power plant etc.The development of mechanical engineeringHumans become "modern" sign is manufacturing tools. The Stone Age, all sorts of this stone hammers and wood, leather simple tools is rough pioneer of the machinery. From a simple tool to make manufacturing by multiple parts, components of modern machinery, experienced a long process.Thousands of years ago, humanity has created a shell and crushed grain used to carry mortar and grinding, the JieGao engineer-in-chief with wheels, with the car, the ship sailing in rivers, buildings, and the rudder. The power from the physical, one's own development, using animal, hydraulic and wind. Materials from the natural stone, wood, clay, leather, synthetic materials development. The earliest artificial material is made of pottery and porcelain, is already has the pottery, transmission and three parts of the complete machine work.Humans from the Stone Age to the Bronze Age, and then to the iron age, to flourish of drum wind blows of the development plays an important role. Have strong enough, the drum wind to get a high enough metallurgical furnace temperature, can have refined metal from ore in. In China, the former 900 years to use the drum for metallurgy, and gradually from the human drum wind to work and hydraulic drum wind.15-16 centuries ago, mechanical engineering development is slow. But in practice, the millennium project in machinery development and has accumulated much experience and technical knowledge, became the mechanical engineering development potential. Since the 17th century, capitalism in the English, French and European countries, commodity production began to become the center of social problems.In the 18th century, the application of steam from mining to textiles, flour, metallurgy, etc. The main material of production machines from wood to use more tough, but with manual processing of metal. Mechanical manufacturing industry, and began to form in the decades to become an important industry.Mechanical engineering through expanding from the practice, the dispersion, mainly depends on the needs of individual talents and craft a skill, gradually develop a theoretical guidance, system and independent engineering technology. Mechanical engineering is 18-19 century and the industrial revolution, the capitalist mechanical production main technological factors.Power is an important factor of the development of production. In the 17th century, along with the development and improvement of machinery, metal ore with coal and the requirements of increased year by year, people feel rely on human and animal will not produce to a new stage.In Britain, textile, grinding industries are increasingly using workshop in the river, will work to drive hydraulic machinery. But when the coal mine, the copper ore, etc, with lots of groundwater is only to ascend and excluded. Animal In this production needs, 18 century appeared newcomen atmospheric type steam, mine drainage pumps to drive. But the steam engine fuel consumption, high, basically applies only to mine.In 1765, watt invented the steam engine have separate condenser, reduce the fuel consumption. 1781 watt and create the steam engine, turning provide motivation of steam expanding the scope of application. The steam engine invented and development, mining and industrial production, railways and shipping to mechanical power. The steam engine was almost in the 19th century, but the only power and steam boiler, condenser, cooling water system huge volume, bulky, application is not easy.19 century, the power supply system, and the development and popularization of motor start. At the beginning of the 20th century, the motor in industrial production has replaced the steam, become the basic mechanical drive all kinds of work. Production mechanization, already from electrification of electrified by mechanization production function.Power for the initial application of the steam engine. In the early 20th century, high efficiency, high speed, high steam turbine, also appeared to water resources of the turbine power supply system, promote the vigorous development.The invention of the late 19th century, has improved combustion through light and small, high efficiency, easy manipulation, and can always start the engine. It is used to drive to work without power supply of land, and mechanical for automobile, mobile machinery and ships, until the middle of 20th century began for locomotives. Steam turbine engine in the crowd, and are no longer important power machinery. After the invention of internal combustion engine and gas turbine, jet engines, is the development of aircraft, such successful development foundation spacecraft technical factor.Before the industrial revolution, mostly of wood, mechanical hand made by the carpenter. Metal (mainly copper, iron) only to manufacture equipment, lock, clocks, pump and mechanical parts of the small wood. Metal processing of the machine mainly by seiko spy, achieves the need of precision. Steam power plant, and the promotion of the mining, metallurgical appears, ships, large machinery development of locomotive, forming and cutting processing of metal parts, growing more and more and more is also high accuracyrequirements. The application of metal materials from copper and iron development to steel.Mechanical processing including forging, forging, sheet metal work, welding, and heat treatment technology and equipment, and machining technology and machine tools, measuring tools, etc, is rapidly developing, ensuring each industry development for production equipment supply.The development of social economy, the demand of mechanical products explosion. Production batch of increase and precision machining technology development, promote the formation of large production methods, such as division of professional production and interchangeability parts and collaboration, water processing line and assembly line etc.Simple interchangeable parts and division of professional production, in ancient collaboration has appeared. In mechanical engineering, the interchangeability embodied in mo for 1797 Bates USES its created by threading lathe of bolts and nuts production. At the same time, the American engineers with interchangeable manufacturing production methods, Whitney, shows the feasibility and superiority of interchangeable. The production methods, in the United States has formed the so-called "American production methods".In the early 20th century, ford car manufacturing and created the assembly line. Mass production technology in the 19th century and Taylor was that the scientific management method, automobile and other mass production machinery production efficiency quickly reached the height of past cannot imagine.In the late 20th century, the main characteristics, mechanical processing, continuously improve the machine is processing speed and accuracy, reducing the dependence of craftsmanship, Improve machining and forming, cutting and assembly of mechanization and automation, Using CNC processing center, the group technology, etc, to develop flexible manufacturing systems, small volume and multiple varieties of production efficiency is to improve the level of production, Study hard and improve the processing of metallic and non-metallic materials, new forming and machining technology.18 centuries ago, mechanical artisan, intuition and experience in mechanical manufacture craft, and almost no scientific association. To 18-19 century, in the emerging of capitalist economy, promote the scientific knowledge of master production, and people start paying attention to direct production needs to start to learn scientific and cultural knowledge, communication between them and made great achievements in mutual inspiration. In this process, and gradually formed a set of basic theory on mechanical engineering.Mechanical power with the advanced scientific combination. The steam engine invented Avery, pizza, applied physicist watt pat Penn and black theory, Based on the practice in the steam engine, physicists cano, LanJinHe kelvin established a new science - thermodynamics. Engine is based on the theory of France in 1862 LuoSha founded, In 1876, the application of theory LuoSha otto thoroughly improve he had created the rough heavy, noise, low efficiency of internal combustion and laid the position. Other such as steam turbine, gas turbine, etc. In theory, and under the guidance of the theory in practice and improvement.In early BC, China has applied the car in guide in complex system of gear was applied to the burner and level of the place turn, etc. Ancient Greece had cylindrical gears, bevel gears and worm transmission. However, the instantaneous speed on gear tooth shape and the relationship of tooth profile curve and the 17th century, until after a theory.The agency's hand and pedal pioneers of crank rod system in the ancient civilization has a long history, but in the form of crank rod system, movement and dynamic analysis and synthesis, exactly is modern organization learning achievements. As a specialized agencies, until the early 19th century in higher engineering college for the technology institute (Paris). Through the theoretical study, people can accurately analysis of various institutions, including the complex space of movement, and then according to need a new comprehensive.Mechanical engineering work object is dynamic mechanical, it will happen. This change is sometimes randomly and unforeseeable, Actual application materials are incomplete, there may be various defects, Machining accuracy has certain deviation, etc.With the static structure for the civil engineering work, mechanical engineering in more difficult problems in theory to solve accurate. Therefore, the early application of mechanical engineering only simple theoretical concept, combined with practical experience. Experience in design and calculation formula for more on, To ensure safety, rather conservative, results of large and heavy machinery, high cost and low productivity, energy consumption.In the 18th century, a new theory of birth, and the development of mathematics method, design calculation precision of continuous improvement. In the 20th century, appear all sorts of experimental stress analysis method, the experimental method to detect with people already on the physical model and the stress. 20 century, and the finite element method and the wide application of electronic computers, which makes the complex machinery and zero. Component force, moment, stress analysis and calculation of such as possible. To master have sufficient practice or experimental dataMechanical or its components, can use statistical techniques, according to the requirements of reliability, mechanical design scientifically.The development of mechanical engineeringTo increase the production of mechanical engineering and improve labor productivity, improve the production efficiency of research and development for the goal to new mechanical products. In the future development of the new products, to reduce the consumption of resources to develop clean and renewable energy, management, reduce and eliminate environmental pollution as super economic goals and tasks.The persons involved with both hands and mechanical can eyes and ears, feet and cannot be directly directly, and finish the job finished faster and better. Modern mechanical engineering to create more and more exquisite and increasingly complex machinery and mechanical device, the past many fantasy to become a reality.Humans can now upstream sky and the universe, diving deep ocean, and nearly 100 million light-years out till the cellular and molecular. The emerging electronic computer hardware and software to strengthen human has begun to replace the brain, and part of the scientific and technological means, this is the artificial intelligence. This is a new development has shown great influence in the future, it will constantly create people cannot imagine the miracle.The growth of human wisdom does not reduce the hands, but instead as more and more delicate hands, more complex, so more promote the function of hand. The practice of hand, in turn, promote the wisdom of the human brain. In the process of evolution of humanity, and the growth of each individual in the process, the brain and the hand is mutual promoting peace line of evolution.Artificial intelligence and the relationship between mechanical engineering in the brain and the hand approximation, the relationship between the difference is only in artificial intelligence will need to use the hardware machinery manufacturing. In the past, all kinds of machinery without man's operating and controlling the reaction speed, accuracy and operating by the brain evolved slowly and nervous system restriction, artificial intelligence will eliminate this restriction. Computer science and mechanical engineering, the mutual promotion between parallel, will make the mechanical engineering at the higher level is the beginning of a new round of development,In the 19th century, mechanical engineering knowledge is limited in European universities and colleges, it is generally for a comprehensive and civil engineering disciplines, civil engineering, called the 19th century has become an independent discipline. In the 20th century, with the mechanical engineering technology development and growth of knowledge, mechanical engineering and began to decompose the specialization of branch appeared. The decomposition of the trend in the middle of the twentieth century, and that in the end of the second world war during peak reached before.Due to mechanical engineering knowledge has been expanded to be far from certain professional master, all is indispensable. But the excessive specialized knowledge, caused by excessive vision narrow orthodoxy and as a whole, not the whole of the project scale slightly, and shrink and technical exchanges, hampering the new techniques and technology progress on the whole, the ability to adapt to the change of external conditions is very poor. Closure of professional experts have acquired knowledge, consider problems in work, had only with difficulty, also coordinate when to self-study. Unfavorable Therefore, since the 20th century, and the comprehensive trend appears. People pay more attention the basic theory, widen the field of merger, division meticulous professional.Comprehensive - professional differentiation - again, is the comprehensive knowledge of the development process of the reasonable and necessary. Different professional experts have different exquisite specialized knowledge, and have enough knowledge to the comprehensive understanding, understanding other subject and overall appearance, can form each other powerful collective work.Comprehensive and professional is mutiple level. In mechanical engineering expertise and internal contradictions are integrated, In the comprehensive engineering technology also has a comprehensive and professional problem. In all human knowledge, including social sciences and natural sciences and the engineering technology, also have a higher layer, in more comprehensive and professional macro problem.。