直流电弧故障检测
光伏系统直流故障电弧特性与检测装
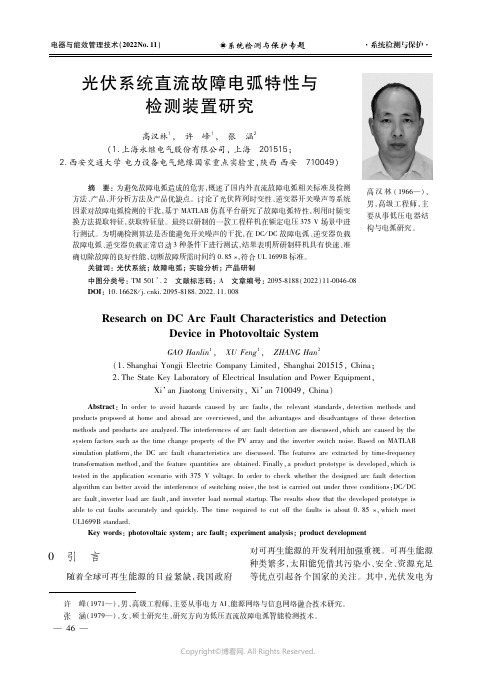
高汉林(1966—),男,高级工程师,主要从事低压电器结构与电弧研究。
许 峰(1971—),男,高级工程师,主要从事电力AI、能源网络与信息网络融合技术研究。
张 涵(1979—),女,硕士研究生,研究方向为低压直流故障电弧智能检测技术。
光伏系统直流故障电弧特性与检测装置研究高汉林1, 许 峰1, 张 涵2(1.上海永继电气股份有限公司,上海 201515;2.西安交通大学电力设备电气绝缘国家重点实验室,陕西西安 710049)摘 要:为避免故障电弧造成的危害,概述了国内外直流故障电弧相关标准及检测方法、产品,并分析方法及产品优缺点。
讨论了光伏阵列时变性、逆变器开关噪声等系统因素对故障电弧检测的干扰,基于MATLAB仿真平台研究了故障电弧特性,利用时频变换方法提取特征,获取特征量。
最终以研制的一款工程样机在额定电压375V场景中进行测试。
为明确检测算法是否能避免开关噪声的干扰,在DC/DC故障电弧、逆变器负载故障电弧、逆变器负载正常启动3种条件下进行测试,结果表明所研制样机具有快速、准确切除故障的良好性能,切断故障所需时间约0.85s,符合UL1699B标准。
关键词:光伏系统;故障电弧;实验分析;产品研制中图分类号:TM501+.2 文献标志码:A 文章编号:2095 8188(2022)11 0046 08DOI:10.16628/j.cnki.2095 8188.2022.11.008ResearchonDCArcFaultCharacteristicsandDetectionDeviceinPhotovoltaicSystemGAOHanlin1, XUFeng1, ZHANGHan2(1.ShanghaiYongjiElectricCompanyLimited,Shanghai201515,China;2.TheStateKeyLaboratoryofElectricalInsulationandPowerEquipment,Xi’anJiaotongUniversity,Xi’an710049,China)Abstract:Inordertoavoidhazardscausedbyarcfaults,therelevantstandards,detectionmethodsandproductsproposedathomeandabroadareoverviewed,andtheadvantagesanddisadvantagesofthesedetectionmethodsandproductsareanalyzed.Theinterferencesofarcfaultdetectionarediscussed,whicharecausedbythesystemfactorssuchasthetimechangepropertyofthePVarrayandtheinverterswitchnoise.BasedonMATLABsimulationplatform,theDCarcfaultcharacteristicsarediscussed.Thefeaturesareextractedbytime frequencytransformationmethod,andthefeaturequantitiesareobtained.Finally,aproductprototypeisdeveloped,whichistestedintheapplicationscenariowith375Vvoltage.Inordertocheckwhetherthedesignedarcfaultdetectionalgorithmcanbetteravoidtheinterferenceofswitchingnoise,thetestiscarriedoutunderthreeconditions:DC/DCarcfault,inverterloadarcfault,andinverterloadnormalstartup.Theresultsshowthatthedevelopedprototypeisabletocutfaultsaccuratelyandquickly.Thetimerequiredtocutoffthefaultsisabout0.85s,whichmeetUL1699Bstandard.Keywords:photovoltaicsystem;arcfault;experimentanalysis;productdevelopment0 引 言随着全球可再生能源的日益紧缺,我国政府对可再生能源的开发利用加强重视。
直流电弧故障预测方法
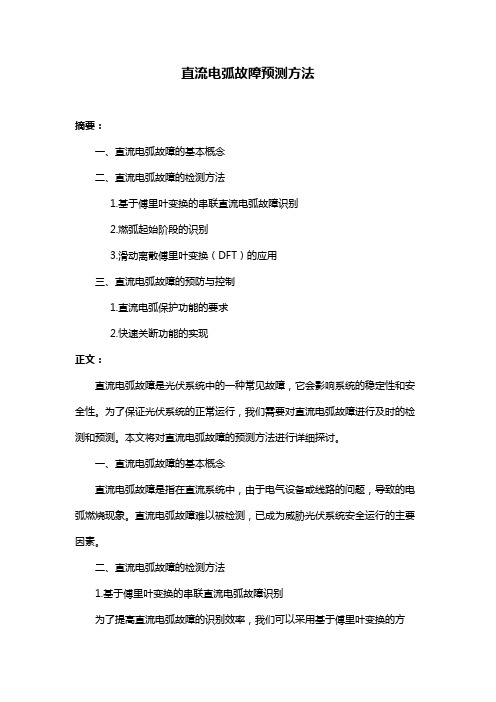
直流电弧故障预测方法摘要:一、直流电弧故障的基本概念二、直流电弧故障的检测方法1.基于傅里叶变换的串联直流电弧故障识别2.燃弧起始阶段的识别3.滑动离散傅里叶变换(DFT)的应用三、直流电弧故障的预防与控制1.直流电弧保护功能的要求2.快速关断功能的实现正文:直流电弧故障是光伏系统中的一种常见故障,它会影响系统的稳定性和安全性。
为了保证光伏系统的正常运行,我们需要对直流电弧故障进行及时的检测和预测。
本文将对直流电弧故障的预测方法进行详细探讨。
一、直流电弧故障的基本概念直流电弧故障是指在直流系统中,由于电气设备或线路的问题,导致的电弧燃烧现象。
直流电弧故障难以被检测,已成为威胁光伏系统安全运行的主要因素。
二、直流电弧故障的检测方法1.基于傅里叶变换的串联直流电弧故障识别为了提高直流电弧故障的识别效率,我们可以采用基于傅里叶变换的方法。
通过对电弧电流进行时域和频域分析,可以发现燃弧起始阶段是直流电弧故障识别的最佳时期。
根据该阶段电弧电流的频谱特征,确定滑动离散傅里叶变换(DFT)的分析频率点。
2.燃弧起始阶段的识别在燃弧起始阶段,电弧电流的波动较大,可通过观察电流波形的突变来识别直流电弧故障。
此外,还可以通过检测电弧电压、电弧电阻等参数的变化来判断故障的发生。
3.滑动离散傅里叶变换(DFT)的应用为了提高直流电弧故障识别的实时性,我们可以采用滑动离散傅里叶变换(DFT)方法。
根据燃弧起始阶段电弧电流的频谱特征,确定分析频率点为40kHz、80kHz和100kHz。
综合考虑算法分辨率和实时性要求,采用200us 的时间窗口对电弧电流进行分析。
三、直流电弧故障的预防与控制1.直流电弧保护功能的要求对于直流侧最大系统电压大于或等于120V的系统,应具备直流串联电弧保护功能。
保护功能应能够及时检测并切断故障电弧,以减小故障对系统的影响。
2.快速关断功能的实现为了提高直流电弧故障的应对能力,系统应具备快速关断功能。
Arc Detector直流故障电弧检测
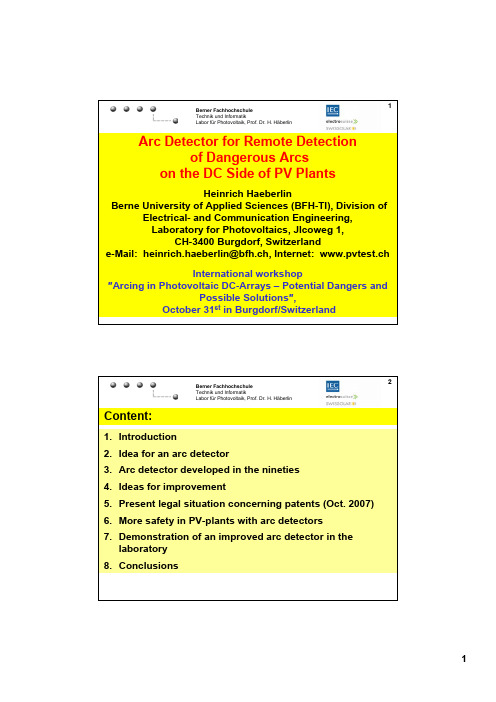
15
Berner Fachhochschule Technik und Informatik Labor für Photovoltaik, Prof. Dr. H. Häberlin
14
Berner Fachhochschule Technik und Informatik Labor für Photovoltaik, Prof. Dr. H. Häberlin
Increasing string voltages and currents rapidly increasing number of plants, increasing age of many plants, ⇒ Increasing risk of DC arcs ⇒ additional safety device required, which eliminates
e-Mail: heinrich.haeberlin@bfh.ch, Internet: www.pvtest.ch International workshop
″Arcing in Photovoltaic DC-Arrays – Potential Dangers and Possible Solutions″,
Heinrich Haeberlin Berne University of Applied Sciences (BFH-TI), Division of
Electrical- and Communication Engineering, Laboratory for Photovoltaics, Jlcoweg 1, CH-3400 Burgdorf, Switzerland
2
5
Berner Fachhochschule Technik und Informatik Labor für Photovoltaik, Prof. Dr. H. Häberlin
光伏系统直流串联故障电弧检测方法

第37卷第3期2021年3月电网与清洁能源Power System and Clean EnergyVol.37No.3Mar.2021——————————基金项目:中国博士后科学基金项目(2020M681928)。
Project Supported by the China Postdoctoral Science Foundation(2020M681928).ABSTRACT :As arc faults have become a common cause of electrical fire accidents in photovoltaic (PV )systems ,it is of great significance to investigate a reliable DC arc fault detectionmethod to ensure the PV system operation and personal safety.In this paper ,an experimental platform of DC arc faults is builtin the PV system ,and typical arc fault current signals are collected.Next ,the stability analysis ,time-varying analysis ,correlation analysis and randomness analysis are carried out.Considering the global effective separation in whole arc faultburning stage ,less calculation loads and stronger anti-interference requirements ,Euler features are selected as theoptimal arc fault characteristic.Then an arc fault detection algorithm is constructed based on the above optimal Euler feature.The algorithm takes the multi-threshold judgment system as the core rule to discover the arc fault characteristic mode differentfrom the system transient processes.Finally ,the accuracy of the proposed arc fault detection algorithm is verified under conditions of different arc generation modes and system operating points ,and the result shows that the arc faults can be detected successfully in 0.5s under the conditions of different working points and arc generation modes of the photovoltaic system.KEY WORDS :photovoltaic system ;DC arc fault ;feature extraction ;detection method ;hardware implementation 摘要:故障电弧是光伏系统电气火灾事故的常见原因,研究可靠的光伏系统直流故障电弧检测方法对保障系统运行和人身安全有重要意义。
故障电弧检测概述
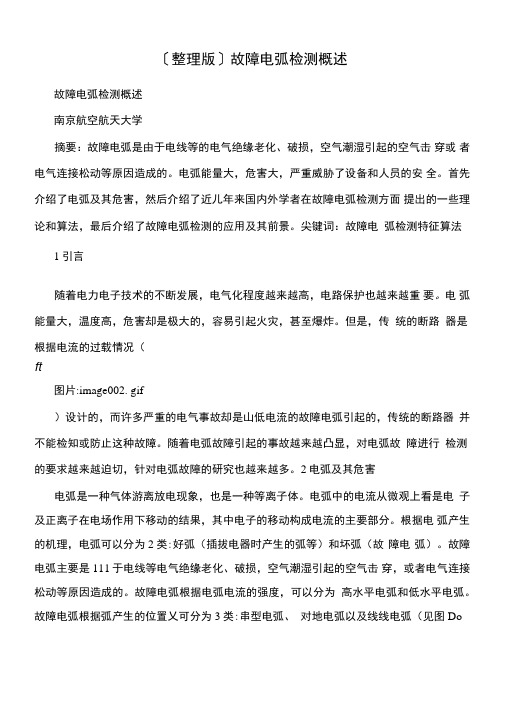
〔整理版〕故障电弧检测概述故障电弧检测概述南京航空航天大学摘要:故障电弧是由于电线等的电气绝缘老化、破损,空气潮湿引起的空气击穿或者电气连接松动等原因造成的。
电弧能量大,危害大,严重威胁了设备和人员的安全。
首先介绍了电弧及其危害,然后介绍了近儿年来国内外学者在故障电弧检测方面提出的一些理论和算法,最后介绍了故障电弧检测的应用及其前景。
尖键词:故障电弧检测特征算法1引言随着电力电子技术的不断发展,电气化程度越来越高,电路保护也越来越重要。
电弧能量大,温度高,危害却是极大的,容易引起火灾,甚至爆炸。
但是,传统的断路器是根据电流的过载情况(ft图片:image002. gif)设计的,而许多严重的电气事故却是山低电流的故障电弧引起的,传统的断路器并不能检知或防止这种故障。
随着电弧故障引起的事故越来越凸显,对电弧故障进行检测的要求越来越迫切,针对电弧故障的研究也越来越多。
2电弧及其危害电弧是一种气体游离放电现象,也是一种等离子体。
电弧中的电流从微观上看是电子及正离子在电场作用下移动的结果,其中电子的移动构成电流的主要部分。
根据电弧产生的机理,电弧可以分为2类:好弧(插拔电器时产生的弧等)和坏弧(故障电弧)。
故障电弧主要是111于电线等电气绝缘老化、破损,空气潮湿引起的空气击穿,或者电气连接松动等原因造成的。
故障电弧根据电弧电流的强度,可以分为高水平电弧和低水平电弧。
故障电弧根据弧产生的位置乂可分为3类:串型电弧、对地电弧以及线线电弧(见图Do图片:imageOOd・ jpg @)串型电弧(b)对地电弧(c)线线电弧图1故障电弧分类电弧的特点是温度很高,电流很小,持续时间短,一旦出现击穿点则会频繁出现。
电弧放电时,会产生大量的热,能引燃周围的易燃易爆品,造成火灾其至爆炸。
比如,《中国火灾统计年鉴》显示,因电气原因引发的火灾在各类火灾中高居榜首,约有30%的火灾是111住宅电气线路老化或配置不合理造成的,并正以平均每年1%的速度持续上升,6. 6%的人在使用插座板时曾有被电击的经历;美国联邦航空管理局(FAA)指出电气故障是无数商业飞机事故的主要问题;军方也认为电气故障是造成安全问题和飞机不能准时起飞的重要原因。
光伏直流电弧故障检测方法研究综述
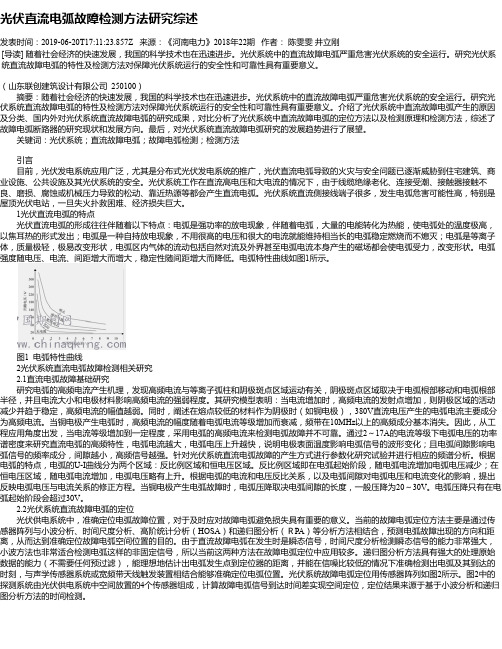
光伏直流电弧故障检测方法研究综述发表时间:2019-06-20T17:11:23.857Z 来源:《河南电力》2018年22期作者:陈雯雯井立刚[导读] 随着社会经济的快速发展,我国的科学技术也在迅速进步。
光伏系统中的直流故障电弧严重危害光伏系统的安全运行。
研究光伏系统直流故障电弧的特性及检测方法对保障光伏系统运行的安全性和可靠性具有重要意义。
(山东联创建筑设计有限公司 250100)摘要:随着社会经济的快速发展,我国的科学技术也在迅速进步。
光伏系统中的直流故障电弧严重危害光伏系统的安全运行。
研究光伏系统直流故障电弧的特性及检测方法对保障光伏系统运行的安全性和可靠性具有重要意义。
介绍了光伏系统中直流故障电弧产生的原因及分类、国内外对光伏系统直流故障电弧的研究成果,对比分析了光伏系统中直流故障电弧的定位方法以及检测原理和检测方法,综述了故障电弧断路器的研究现状和发展方向。
最后,对光伏系统直流故障电弧研究的发展趋势进行了展望。
关键词:光伏系统;直流故障电弧;故障电弧检测;检测方法引言目前,光伏发电系统应用广泛,尤其是分布式光伏发电系统的推广,光伏直流电弧导致的火灾与安全问题已逐渐威胁到住宅建筑、商业设施、公共设施及其光伏系统的安全。
光伏系统工作在直流高电压和大电流的情况下,由于线缆绝缘老化、连接受潮、接触器接触不良、磨损、腐蚀或机械压力导致的松动、靠近热源等都会产生直流电弧。
光伏系统直流侧接线端子很多,发生电弧危害可能性高,特别是屋顶光伏电站,一旦失火扑救困难、经济损失巨大。
1光伏直流电弧的特点光伏直流电弧的形成往往伴随着以下特点:电弧是强功率的放电现象,伴随着电弧,大量的电能转化为热能,使电弧处的温度极高,以焦耳热的形式发出;电弧是一种自持放电现象,不用很高的电压和很大的电流就能维持相当长的电弧稳定燃烧而不熄灭;电弧是等离子体,质量极轻,极易改变形状,电弧区内气体的流动包括自然对流及外界甚至电弧电流本身产生的磁场都会使电弧受力,改变形状。
直流故障电弧特性及检测方法
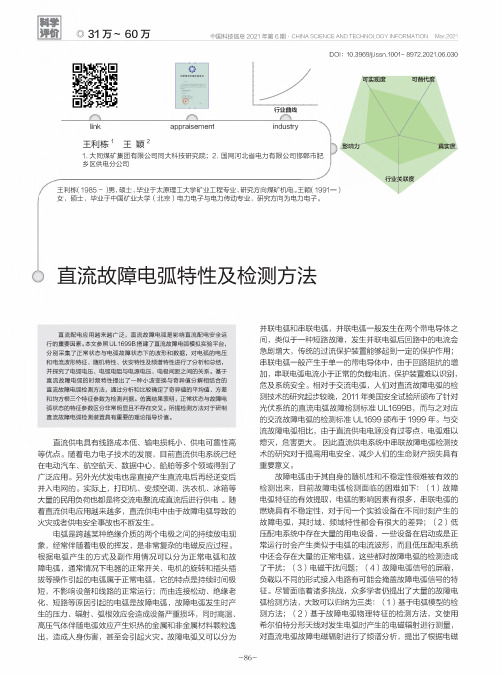
DOI:10.3969/j.issn.1001-8972.2021.06.030可实现度可替代行业曲线appraisement industry王利栋1王颖21•大同煤矿集团有限公司同大科技研究院;2.国网河北省电力有限公司邯郸市肥乡区供电分公司影响力行业关联度王利栋(1985-)男,硕士,毕业于太原理工大学矿业工程专业,研究方向煤矿机电。
王颖(1991-)女,硕士,毕业于中国矿业大学(北京)电力电子与电力传动专业,研究方向为电力电子。
6直流故障电弧特性及检测方法直流配电应用越来越广泛,直流故障电弧是影响直流配电安全运行的重要因素。
本文参照UL1699B搭建了直流故障电弧模拟实验平台,分别采集了正常状态与电弧故障状态下的波形和数据,对电弧的电压和电流波形特征、随机特性、伏安特性及频谱特性进行了分析和总结,并探究了电弧电压、电弧电阻与电源电压、电极间距之间的关系。
基于直流故障电弧的时频特性提出了一种小波变换与奇异值分解相结合的直流故障电弧检测方法,通过分析和比较确定了奇异值的平均值、方差和均方根三个特征参数为检测判据。
仿真结果表明,正常状态与故障电弧状态的特征参数区分非常明显且不存在交叉。
所提检测方法对于研制直流故障电弧检测装置具有重要的理论指导价值。
直流供电具有线路成本低、输电损耗小、供电可靠性高等优点。
随着电力电子技术的发展,目前直流供电系统已经在电动汽车、航空航天、数据中心、船舶等多个领域得到了广泛应用。
另外光伏发电也是直接产生直流电后再经逆变后并入电网的。
实际上,打印机、变频空调、洗衣机、冰箱等大量的民用负荷也都是将交流电整流成直流后进行供电。
随着直流供电应用越来越多,直流供电中由于故障电弧导致的火灾或者供电安全事故也不断发生。
电弧是跨越某种绝缘介质的两个电极之间的持续放电现象,经常伴随着电极的挥发,是非常复杂的电磁反应过程。
根据电弧产生的方式及副作用情况可以分为正常电弧和故障电弧,通常情况下电器的正常开关、电机的旋转和插头插拔等操作引起的电弧属于正常电弧,它的特点是持续时间极短,不影响设备和线路的正常运行;而由连接松动、绝缘老化、短路等原因引起的电弧是故障电弧,故障电弧发生时产生的压力、辐射、弧根效应会造成设备严重损坏,同时高温、高压气体伴随电弧效应产生炽热的金属和非金属材料颗粒逸出,造成人身伤害,甚至会引起火灾。
基于直流系统的故障电弧检测技术的研究
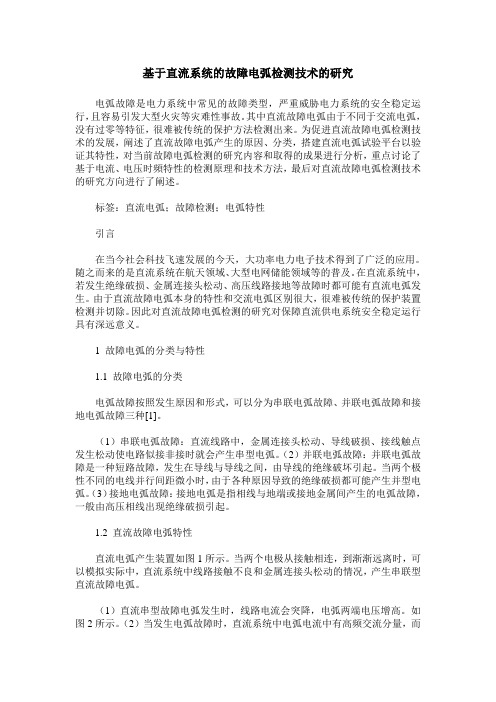
基于直流系统的故障电弧检测技术的研究电弧故障是电力系统中常见的故障类型,严重威胁电力系统的安全稳定运行,且容易引发大型火灾等灾难性事故。
其中直流故障电弧由于不同于交流电弧,没有过零等特征,很难被传统的保护方法检测出来。
为促进直流故障电弧检测技术的发展,阐述了直流故障电弧产生的原因、分类,搭建直流电弧试验平台以验证其特性,对当前故障电弧检测的研究内容和取得的成果进行分析,重点讨论了基于电流、电压时頻特性的检测原理和技术方法,最后对直流故障电弧检测技术的研究方向进行了阐述。
标签:直流电弧;故障检测;电弧特性引言在当今社会科技飞速发展的今天,大功率电力电子技术得到了广泛的应用。
随之而来的是直流系统在航天领域、大型电网储能领域等的普及。
在直流系统中,若发生绝缘破损、金属连接头松动、高压线路接地等故障时都可能有直流电弧发生。
由于直流故障电弧本身的特性和交流电弧区别很大,很难被传统的保护装置检测并切除。
因此对直流故障电弧检测的研究对保障直流供电系统安全稳定运行具有深远意义。
1 故障电弧的分类与特性1.1 故障电弧的分类电弧故障按照发生原因和形式,可以分为串联电弧故障、并联电弧故障和接地电弧故障三种[1]。
(1)串联电弧故障:直流线路中,金属连接头松动、导线破损、接线触点发生松动使电路似接非接时就会产生串型电弧。
(2)并联电弧故障:并联电弧故障是一种短路故障,发生在导线与导线之间,由导线的绝缘破坏引起。
当两个极性不同的电线并行间距微小时,由于各种原因导致的绝缘破损都可能产生并型电弧。
(3)接地电弧故障:接地电弧是指相线与地端或接地金属间产生的电弧故障,一般由高压相线出现绝缘破损引起。
1.2 直流故障电弧特性直流电弧产生装置如图1所示。
当两个电极从接触相连,到渐渐远离时,可以模拟实际中,直流系统中线路接触不良和金属连接头松动的情况,产生串联型直流故障电弧。
(1)直流串型故障电弧发生时,线路电流会突降,电弧两端电压增高。
基于机器学习方法的直流电弧故障检测

基于机器学习方法的直流电弧故障检测丁鑫;竺红卫;殷浩楠;王一闻【摘要】为了解决传统分析方法在直流供电系统中电弧故障检测的精确度不足及过程繁琐的问题,将直流电弧故障检测归为二分类问题,引入机器学习方法,通过直流电弧实验得到正常状态和电弧状态的数据,从时域中提取电流均值等4个特征,从频域中提取高频分量标准差等3个特征.利用提取到的特征对支持向量机(SVM)进行训练,利用求解得到的模型对测试数据集进行分类,分类准确率为94.483%.结果证明:所提方法能有效检测直流电弧故障,提高故障检测精度,且步骤精简,易于推广.%In order to solve the problems that in direct current(DC)power supply system,accuracy of arc fault detection is insufficient and the process is tedious with the traditional analysis method. The DC arc fault detection is classified into two classification problems while the machine learning method is used. The data of normal state and arc fault state are obtained by DC arc experiment. Extract four features from time domain,including the average current and so on. At the same time,extract three characteristics from frequency domain,such as standard deviation of high frequency component,etc. By training support vector machine(SVM)using the extracted features above,classification model is obtained. The accuracy of classification of the test data set by the model is 94. 483%,the result proves that this method can be used to detect DC arc fault effectively,improve detection precision,and can be popularized easily because of simple steps.【期刊名称】《传感器与微系统》【年(卷),期】2017(036)011【总页数】5页(P123-127)【关键词】直流电弧;故障检测;特征提取;机器学习;支持向量机【作者】丁鑫;竺红卫;殷浩楠;王一闻【作者单位】浙江大学电气工程学院,浙江杭州310000;浙江大学电气工程学院,浙江杭州310000;浙江大学电气工程学院,浙江杭州310000;浙江大学电气工程学院,浙江杭州310000【正文语种】中文【中图分类】TP391;TM501.2直流电源广泛应用于航天器的供电系统[1]、汽车的电气系统[2],这些电力电子系统也是发生直流电弧故障的重灾区[3],如果不能及时检测并排除故障,直流电弧将危害电源系统和控制系统,严重时还会引发火灾。
直流拉弧故障检测方法科华
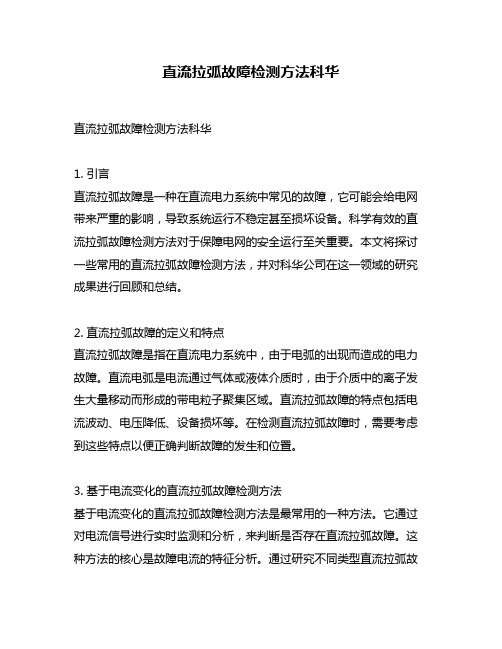
直流拉弧故障检测方法科华直流拉弧故障检测方法科华1. 引言直流拉弧故障是一种在直流电力系统中常见的故障,它可能会给电网带来严重的影响,导致系统运行不稳定甚至损坏设备。
科学有效的直流拉弧故障检测方法对于保障电网的安全运行至关重要。
本文将探讨一些常用的直流拉弧故障检测方法,并对科华公司在这一领域的研究成果进行回顾和总结。
2. 直流拉弧故障的定义和特点直流拉弧故障是指在直流电力系统中,由于电弧的出现而造成的电力故障。
直流电弧是电流通过气体或液体介质时,由于介质中的离子发生大量移动而形成的带电粒子聚集区域。
直流拉弧故障的特点包括电流波动、电压降低、设备损坏等。
在检测直流拉弧故障时,需要考虑到这些特点以便正确判断故障的发生和位置。
3. 基于电流变化的直流拉弧故障检测方法基于电流变化的直流拉弧故障检测方法是最常用的一种方法。
它通过对电流信号进行实时监测和分析,来判断是否存在直流拉弧故障。
这种方法的核心是故障电流的特征分析。
通过研究不同类型直流拉弧故障产生的电流信号特点,可以建立相应的故障诊断算法。
对于不同的电弧电流波形,可以采用小波分析方法进行特征提取和识别。
科华公司在这一领域做出了一些重要的研究工作,通过对实验数据的分析和建模,提出了一种高精度的电流波形识别算法,并应用于实际的电网故障监测中。
4. 基于电压变化的直流拉弧故障检测方法除了基于电流变化的方法外,还有一种基于电压变化的直流拉弧故障检测方法。
这种方法主要是通过对故障过程中电压信号的变化进行监测和分析来判断是否存在直流拉弧故障。
与基于电流变化的方法相比,基于电压变化的方法对于故障位置的判断更为准确,但同时也更加复杂。
科华公司在这一领域也进行了深入研究,提出了一种基于电压相位计算的故障诊断算法,通过对电压信号的相位变化进行分析,可以实现对直流拉弧故障的准确检测和定位。
5. 综合方法的应用和展望除了以上提到的单一方法外,还有一些综合方法被应用于直流拉弧故障的检测中。
直流拉弧检测步骤
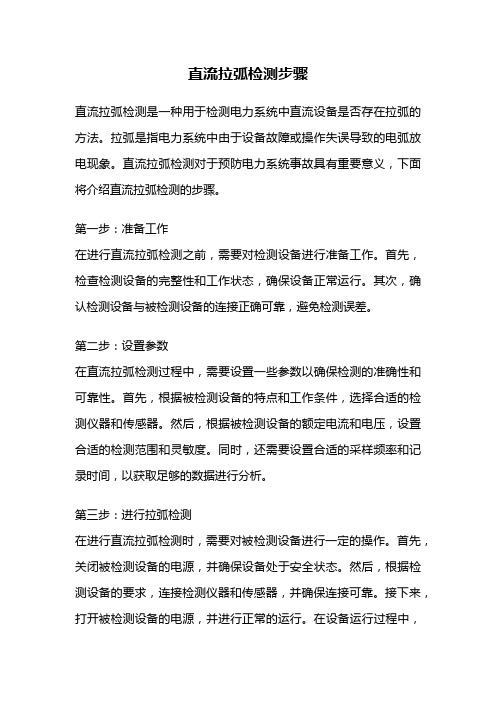
直流拉弧检测步骤直流拉弧检测是一种用于检测电力系统中直流设备是否存在拉弧的方法。
拉弧是指电力系统中由于设备故障或操作失误导致的电弧放电现象。
直流拉弧检测对于预防电力系统事故具有重要意义,下面将介绍直流拉弧检测的步骤。
第一步:准备工作在进行直流拉弧检测之前,需要对检测设备进行准备工作。
首先,检查检测设备的完整性和工作状态,确保设备正常运行。
其次,确认检测设备与被检测设备的连接正确可靠,避免检测误差。
第二步:设置参数在直流拉弧检测过程中,需要设置一些参数以确保检测的准确性和可靠性。
首先,根据被检测设备的特点和工作条件,选择合适的检测仪器和传感器。
然后,根据被检测设备的额定电流和电压,设置合适的检测范围和灵敏度。
同时,还需要设置合适的采样频率和记录时间,以获取足够的数据进行分析。
第三步:进行拉弧检测在进行直流拉弧检测时,需要对被检测设备进行一定的操作。
首先,关闭被检测设备的电源,并确保设备处于安全状态。
然后,根据检测设备的要求,连接检测仪器和传感器,并确保连接可靠。
接下来,打开被检测设备的电源,并进行正常的运行。
在设备运行过程中,检测仪器会实时采集数据,并记录下来。
第四步:数据分析在完成拉弧检测后,需要对采集到的数据进行分析。
首先,对数据进行清洗和整理,去除异常值和噪声干扰。
然后,根据拉弧的特征和规律,通过数据处理和算法分析,确定是否存在拉弧现象。
同时,还需对拉弧的强度和持续时间进行评估,以判断拉弧对设备和系统的影响。
第五步:结果判定根据数据分析的结果,可以对拉弧的情况进行判定。
如果存在拉弧现象,需要及时采取措施修复设备,以防止电力系统事故的发生。
如果未检测到拉弧,可以继续进行运行或定期进行检测,以确保设备的安全运行。
直流拉弧检测的步骤包括准备工作、设置参数、进行拉弧检测、数据分析和结果判定。
通过这些步骤,可以有效地检测直流设备中是否存在拉弧现象,并及时采取措施进行修复和预防,保障电力系统的安全运行。
一种基于多特征量的直流电弧故障检测方法
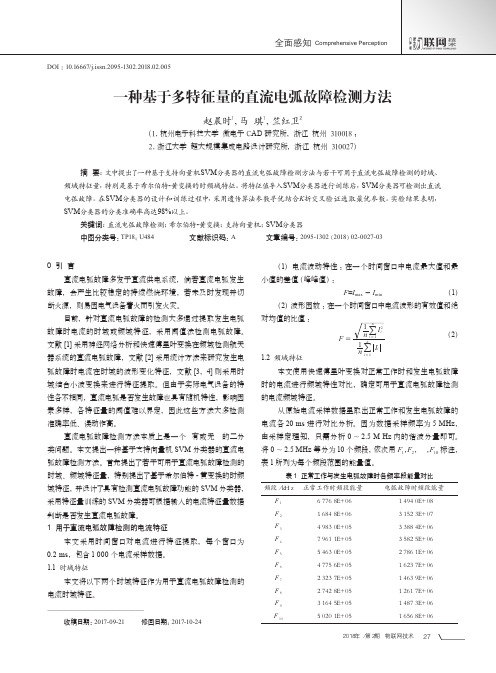
2018年 / 第2期 物联网技术270 引 言直流电弧故障多发于直流供电系统,倘若直流电弧发生故障,会产生比较稳定的持续燃烧环境,若未及时发现并切断火源,则易因电气设备着火而引发火灾。
目前,针对直流电弧故障的检测大多通过提取发生电弧故障时电流的时域或频域特征,采用阈值法检测电弧故障。
文献[1]采用神经网络分析和快速傅里叶变换在频域检测航天器系统的直流电弧故障,文献[2]采用统计方法来研究发生电弧故障时电流在时域的波形变化特征,文献[3,4]则采用时域结合小波变换来进行特征提取。
但由于实际电气设备的特性各不相同,直流电弧是否发生故障也具有随机特性,影响因素多样,各特征量的阈值难以界定,因此这些方法大多检测准确率低、误动作高。
直流电弧故障检测方法本质上是一个“有或无”的二分类问题。
本文提出一种基于支持向量机SVM 分类器的直流电弧故障检测方法。
首先提出了若干可用于直流电弧故障检测的时域、频域特征量,特别提出了基于希尔伯特-黄变换的时频域特征,并设计了具有检测直流电弧故障功能的SVM 分类器,采用特征量训练的SVM 分类器可根据输入的电流特征量数据判断是否发生直流电弧故障。
1 用于直流电弧故障检测的电流特征本文采用时间窗口对电流进行特征提取,每个窗口为0.2ms ,包含1 000个电流采样数据。
1.1 时域特征本文将以下两个时域特征作为用于直流电弧故障检测的电流时域特征。
(1)电流波动特性:在一个时间窗口中电流最大值和最小值的差值(峰峰值):F =I max -I min(1)(2) 波形因数:在一个时间窗口中电流波形的有效值和绝对均值的比值:F n I 1i i 1=(2)1.2 频域特征本文使用快速傅里叶变换对正常工作时和发生电弧故障时的电流进行频域特性对比,确定可用于直流电弧故障检测的电流频域特征。
从原始电流采样数据里取出正常工作和发生电弧故障的电流各20 ms 进行对比分析。
因为数据采样频率为5 MHz ,由采样定理知,只需分析0~2.5 M Hz 内的谐波分量即可。
直流拉弧检测原理
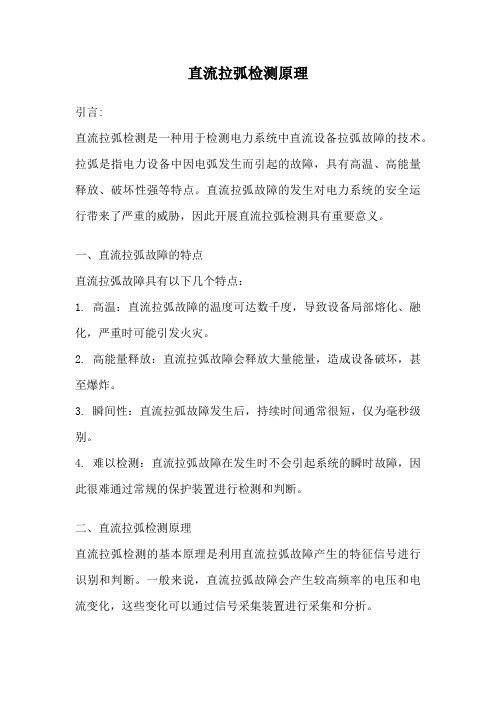
直流拉弧检测原理引言:直流拉弧检测是一种用于检测电力系统中直流设备拉弧故障的技术。
拉弧是指电力设备中因电弧发生而引起的故障,具有高温、高能量释放、破坏性强等特点。
直流拉弧故障的发生对电力系统的安全运行带来了严重的威胁,因此开展直流拉弧检测具有重要意义。
一、直流拉弧故障的特点直流拉弧故障具有以下几个特点:1. 高温:直流拉弧故障的温度可达数千度,导致设备局部熔化、融化,严重时可能引发火灾。
2. 高能量释放:直流拉弧故障会释放大量能量,造成设备破坏,甚至爆炸。
3. 瞬间性:直流拉弧故障发生后,持续时间通常很短,仅为毫秒级别。
4. 难以检测:直流拉弧故障在发生时不会引起系统的瞬时故障,因此很难通过常规的保护装置进行检测和判断。
二、直流拉弧检测原理直流拉弧检测的基本原理是利用直流拉弧故障产生的特征信号进行识别和判断。
一般来说,直流拉弧故障会产生较高频率的电压和电流变化,这些变化可以通过信号采集装置进行采集和分析。
直流拉弧检测系统的主要组成部分包括:1. 信号采集装置:负责采集直流设备上的电压和电流信号,并将其传输给后续的处理单元。
2. 信号处理单元:对采集到的信号进行滤波、放大、数字化等处理,提取出直流拉弧故障的特征信号。
3. 特征提取算法:根据直流拉弧故障的特点,设计相应的特征提取算法,用于判断是否发生拉弧故障。
4. 判断单元:根据特征提取算法的结果,判断当前是否发生直流拉弧故障,并进行相应的保护措施。
三、直流拉弧检测方法根据直流拉弧检测原理,可以采用以下几种方法进行检测:1. 电流比值法:通过对电流信号进行采样和比较,判断是否存在拉弧故障。
当电流比值超过设定的阈值时,即可判定为直流拉弧故障。
2. 频率分析法:对采集到的电压和电流信号进行频谱分析,提取出故障频率的成分,从而判断是否发生拉弧故障。
3. 波形分析法:对采集到的电压和电流波形进行分析,观察是否存在拉弧故障特征,如高频振荡、脉冲等。
4. 特征提取法:通过对采集到的信号进行数学处理和特征提取,提取出拉弧故障的特征参数,如能量、幅值、频率等,从而判断是否存在拉弧故障。
- 1、下载文档前请自行甄别文档内容的完整性,平台不提供额外的编辑、内容补充、找答案等附加服务。
- 2、"仅部分预览"的文档,不可在线预览部分如存在完整性等问题,可反馈申请退款(可完整预览的文档不适用该条件!)。
- 3、如文档侵犯您的权益,请联系客服反馈,我们会尽快为您处理(人工客服工作时间:9:00-18:30)。
PHOTOVOLTAIC DC ARC FAULT DETECTOR TESTING AT SANDIA NATIONALLABORATORIESJay Johnson1, Birger Pahl2, Charles Luebke2, Tom Pier2, Theodore Miller3,Jason Strauch1, Scott Kuszmaul1, and Ward Bower11Sandia National Laboratories, Albuquerque, NM, USA2Eaton Corporation, Milwaukee, WI, USA3Eaton Corporation, Pittsburgh, PA, USAABSTRACTThe 2011 National Electrical Code®(NEC®) added Article 690.11 that requires photovoltaic (PV) systems on or penetrating a building to include a listed DC arc fault protection device. To fill this new market, manufacturers are developing new Arc Fault Circuit Interrupters (AFCIs). Comprehensive and challenging testing has been conducted using a wide range of PV technologies, system topologies, loads and noise sources. The Distributed Energy Technologies Laboratory (DETL) at Sandia National Laboratories (SNL) has used multiple reconfigurable arrays with a variety of module technologies, inverters, and balance of system (BOS) components to characterize new Photovoltaic (PV) DC AFCIs and Arc Fault Detectors (AFDs). The device‘s detection capabilities, characteristics and nuisance tripping avoidance were the primary purpose of the testing. SNL and Eaton Corporation collaborated to test an Eaton AFD prototype and quantify arc noise for a wide range of PV array configurations and the system responses. The tests were conducted by generating controlled, series PV arc faults between PV modules. Arc fault detection studies were performed on systems using aged modules, positive- and negative-grounded arrays, DC/DC converters, 3-phase inverters, and on strings with branch connectors. The tests were conducted to determine if nuisance trips would occur in systems using electrically noisy inverters, with series arc faults on parallel strings, and in systems with inverters performing anti-islanding and maximum power point tracking (MPPT) algorithms. The tests reported herein used the arc fault detection device to indicate when the trip signal was sent to the circuit interrupter. Results show significant noise is injected into the array from the inverter but AFCI functionality of the device was generally stable. The relative locations of the arc fault and detector had little influence on arc fault detection. Lastly, detection of certain frequency bands successfully differentiated normal operational noise from an arc fault signal.INTRODUCTIONArc faults in photovoltaic systems have ignited a number of fires in residential, commercial, and utility installations [1-3]. Article 690.11 in the 2011 National Electrical Code requires new photovoltaic (PV) systems on or penetratinga building to include a listed DC arc fault protection device[4]. To enter this new market, PV DC Arc Fault Circuit Interrupter (AFCI) designs must be compatible with a wide range of PV technologies, inverters, and other BOS components.The Distributed Energy Technologies Laboratory (DETL), at Sandia National Laboratories, shown in Figure 1, has been used as a test bed to integrate novel technologies into renewable energy systems for decades. SNL has partnered with inverter, module, and—most recently—AFD and AFCI manufacturers. With the adoption of the 2011 NEC, SNL is testing new AFDs and AFCIs on PV systems. SNL facilities have reconfigurable PV arrays with PV modules from different manufacturers and a diverse portfolio of PV technologies, ages, and I-V characteristics, along with a range of connectors, DC disconnects, combiner boxes, line lengths, and inverters. The variety of PV array technologies and configurations makes the facility ideal for testing the robustness of AFDs and AFCIs.Figure 1 A portion of the PV arrays at DETL.PV DC AFCI devices consist of an intelligent Arc Fault Detector (AFD) and an Arc Fault Interrupting Device (i.e., circuit interrupter) that physically disconnects the DC PV circuit. Some of the suggested AFCIs are installed on individual strings and detect the presence of a specific AC frequency or frequencies that are emitted by the arcing event. Based on this detection methodology, Sandia and Eaton engineers identified a set of tests—in addition to the UL 1699B testing [6]—to investigate the potential for two of the major AFD failure modes: (1) the AFD falsely indicates an arc (nuisance trips) due to antenna effects,crosstalk, or inverter noise or (2) the AFD fails to detect the arc fault because the frequency of the arcing signal was filtered, masked, or attenuated in the circuit components. Tests were conducted at DETL to measure baseline and arcing noise which the detector would encounter and be required to differentiate regardless of the arc fault location, module or inverter technology, and topology. This paper focuses on evaluating the types of noise that are encountered for various system configurations and conditions that can influence the ability of an AFD to detect arc faults. It will not report any proprietary pass/fail results or detection times of the AFD hardware under test.ARC FAULT DETECTION CHALLENGESThere are a number of challenges to remote arc fault detection. As shown in Fig. 2, the following behavior occurs during the arcing event:1. An arc fault initiates at some location in the stringand generates 1/f (―pink‖) AC noise on top of theDC current. This signal—illustrated on the left sideof Fig. 2—travels down the line through the system.2. As the signal passes through the PV modules andconnectors, a variable attenuation occurs in the PVmodules and connectors and changes thefrequency amplitudes (profile) of the electrical noiseas it propagates downstream.3. Antenna effects, crosstalk and other RFphenomena further modify the signal profile andcharacteristics.4. The modified arcing signal reaches the arc faultdetector in the AFCI. Depending on the PV celltechnology, system topology, metrologicalconditions, and health of the modules, the signalreaching the AFD is likely different than the originalarcing signal—possibly allowing an arcing conditionto go undetected.5. Inverter noise is injected onto the PV string andinverter switching generates switching frequencyand harmonics from 1kHz to >100 kHz [6].Figure 2 Arc fault signal propagation and detection block diagram.ARC FAULT DETECTION TESTSThe list in Table 1 outlines the tests performed at Sandia National Laboratories to verify the AFD was capable of arc fault detection without nuisance trips. The baseline measurements established system noise levels. Arcing tests monitored arc fault detection and nuisance events by inserting the arc fault generator (AFG) into different strings. With the diversity in PV systems, there was a need to test a number of special cases to satisfy different ―what if‖ scenarios. They include thin-film PV, 3-phase inverters, DC/DC converters, and noise from different inverter designs.Baseline MeasurementsPrior to inserting the arc fault detector or circuit interrupter into the PV system, the AC noise of the array was measured in three locations, (a) at the far end of the PV string, (b) after the combiner box, and (c) at the inverter. Repositioning the data acquisition (DAQ) system identified if there were any RF effects or filtering due to line lengths, connectors, disconnects, and switchgear between the string and the inverter. The noise injected into the system from different inverters was measured and quantified to determine if this influenced arc fault detection time or nuisances trips. Last, the number of strings that were connected within the combiner box and with branched connections was varied to determine if system size and topology influenced the noise signatures.Arcing TestsThe arcing tests were designed to determine if the AFD functioned properly over a range of PV systems. First, the arc fault detector was placed on the PV array side of the combiner box, and then the arc fault was initiated at different locations within the array. The time required for detection was recorded for each location to determine if there was consistency. Other variations included:∙changing the inverter type∙changing the circuit layout with and without MC4 Y-connectors∙varying the AFD location∙testing on strings with aged modules with hot spotsThere are many possible testing permutations. Experiments focused on encompassing worst case situations: noisy inverters, long line lengths, parallel strings connected with Y-connectors, and aged modules containing hot spots from many years of degradation. The goal was to verify these variables would not affect the AFD performance. Table 2 shows a subset of the components and configurations used for the characterization tests.Special CasesAFDs and AFCIs must be robust enough to operate with various attenuation effects from the components while being exposed to external noise sources. The AFD under test was found to operate on a small—but representative—subset of PV technologies, BOS components and configurations. These special configurations included DC/DC converters, positive- and negative-grounded arrays, PV arrays with partial shading, single and 3-phase inverters, and thin-film PV systems. The susceptibility to nuisance tripping events due tomaximum power point tracking (MPPT) algorithms and anti-islanding fluctuations were also tested to verify they would not cause nuisance tripping. Also, there was concern that an arc fault in a parallel PV string would cause AFDs to trip due to crosstalk or other coupling. Tests were conducted to verify AFD selectivity.Data Acquisition SystemThe string current was measured with a Tektronix TCP0150A; the string voltage and arcing voltage were measured with Tektronix P5205 probes; and the AC current was measured with an Ion Physics CM-1-L current transformer. A Tektronix MSO4054 500-MHz mixed signal oscilloscope was used to visualize and record the data. The data was collected at a 10 MHz sample rate.RESULTSTesting was performed at the DETL facility at SNL to compare baseline noise and arcing noise on a range of systems. The most significant test results are presented in this paper. Baseline measurements were taken to quantify the noise in six different PV strings with different inverters, shown in Table 2. For the six configurations, the inverter places a unique noise signature on the line as shown in Figure 3.Figure 3 DC string current on different PV arrays with different inverters.The noise signatures are composed of harmonics from 60 Hz AC current being reflected onto the DC side of the inverter, 120 Hz signals (shown in Figure 4) resulting from inverter switching in the single-phase H-bridge topology, and other inverter switching frequencies and broadband noise. Inverters also update the maximum power point tracking set-point at regular intervals (often 1 second), which induces noise at that point, shown in Figure 4. Further, inverters that have active anti-islanding algorithms regularly produce a prognostic spike on the AC side that may couple to the DC side of the inverter. Many noisecomponents are identifiable by taking the Discrete Fourier Transform (DFT) of the inverter noise signatures. The resulting spectral content for the configurations in Table 2 is shown in Figure 5.Figure 4 120 Hz noise from the inverter compared to a resistive load bank noise (left) and the effect of MPPT on current (right).Figure 5 Noise in six PV arrays with different inverters.Depending on the PV system topology and components, arc faults in the PV system may result in different MPPT or anti-islanding operation. In single string tests, the operating dc current and voltage drops initially because the string impedance suddenly increased from the arc fault event. The inductive inverter components increase the dc-side time constant, so the response is significantly slower with the inverter. Baseline and arcing measurements on a string with an inverter and with a resistive load bank are shown in Figures 6 and 7.The inverter and arcing noise can be quantified by examining the DFT of the current from 0.05-0.8 s shown in Figure 6. Arcing frequencies are characterized by 1/f (pink) noise content [5], but, as shown in Figure 8, the noise is perturbed as the signal travels to the remotely located detector by filtering from the PV modules, antenna effects, or inverter noise. Shown in the binned result in Figure 9, this particular inverter puts more noise on the line for frequencies below 1 kHz, whereas the arc fault puts more uniform broadband noise on the line. Interestingly, the arcing noise is larger than the non-arcing noise between 1 Hz and 100 kHz regardless of the inverter noise. When performing the DFT on the voltage time series, as shown in Figure 10, the differentiation between arcing and non-faulted strings is more challenging using the decade binning technique.Figure 6 DC string current for arcing and non-arcing conditions with an inverter and a resistive load bank. The arc initiated at 0 seconds and continued for more than 0.8 seconds.Figure 7 DC string voltage for arcing and non-arcing conditions with an inverter and a resistive load bank.Figure 8 Frequency content of the string current for arcing and non-arcing conditions with an inverter and a resistive load bank.Switching Frequencyfor Inverter in Configuration 660 Hz Noise120 Hz NoiseArcs, inverterNo arc, inverterArcs, load bankNo arc, load bankArcs, inverterArcs, load bankNo arcs, inverterNo arcs, load bankArc fault begins at 0 sec.Arcs, inverterArcs, load bankNo arcs, inverterNo arcs, load bankArc fault begins at 0 sec.Figure 9 Current frequency content binned each decade for arcing and non-arcing conditions with an inverter and a resistive load bank.Figure 10 Voltage frequency content binned each decade for arcing and non-arcing conditions with an inverter and a resistive load bank.To create the ignition of an arc fault, the voltage exceeds the permittivity of free space, current begins flowing between the electrodes, and the gas ionizes to create a plasma. To determine if the initial period of the arc fault contained different frequency content than subsequent time periods, the arcing time domain was subdivided into 0.10 second blocks and the DFT of each time subsection was calculated. Shown in Figure 11, there was a slight variation in the frequency domain as the arcing time increased. Over most of the frequency domain, and especially between 10-100 kHz, the arcing noise amplitudes increased slightly over the duration of the arc fault. Since the arc was ―drawn out‖ by separating opposing electrodes, the increase in noise may be caused by the increased arc gap.It was believed that the location of the arc fault and the location of the detector could affect the measured frequency content. The arc fault generator was located before and after each module in a 6-module string and the arcing current was measured. While the amplitude of allarcing frequencies was significantly larger than the baseline measurements, the location of the arc fault had little effect on the frequency content at the AFD. Likewise, when the detector was placed at the end of the string and at a location ~30 m farther along the conductor, there was no significant effect on the detected frequencies.Figure 11 DFT data for 0.10 s arc fault subsections.DETL has three subarrays connected to the same 3-phase inverter. The subarrays were composed of four 21 module strings of 90 W c-Si (crystalline silicon) modules, four 22 module strings with 80 W c-Si, and four 22 module 80 W p-Si (polycrystalline silicon) strings —each from different module manufacturers —and labeled subarray 1, 2, and 3. Baseline measurements and arcing measurements at the centers of the strings were taken. The binned frequency content (Figure 12) illustrates that certain frequency ranges are superior for arc fault detection. From 1-100 Hz and 100 kHz to 5 MHz there is little or no difference in the arcing noise content when compared to the baseline current frequencies. However, for the frequencies between 100 Hz-100 kHz, there is a clear separation between the arcing noise and the baseline for all strings. Thus, it is likely that the same arc detection algorithm could be used for these three module technologies.Figure 12 DFT of string current for three string topologies with arcing and non-arcing conditions.Arcs, load bankArcs, inverterNo arcs, inverter No arcs, load bankArcs, inverterNo arcs, inverterArcs, load bankNo arcs, load bankThe 2011 NEC requires AFCIs to have enunciators to indicate that an arcing fault was detected. Ideally this will help identify the possible locations where the arc fault occurred so repairs can be completed quickly. However, if an arc fault on a parallel string causes the AFCI to trip, it will be more difficult to locate the fault location. To determine if the Eaton AFD was susceptible to false trips due to crosstalk, an arc fault was generated on a parallel string. The test setup is shown in Figure 13 and the frequency results for the crosstalk test and an arc fault on the monitored string are shown in Figure 14. The results show that the arcing frequency content and crosstalk noise on parallel strings is very similar. This indicates that more than just the frequency content of the string may be required to differentiate arcing noise from crosstalk noise. Figure 13 Crosstalk test experimental configuration.Figure 14 Crosstalk tests on Array.CONCLUSIONSArticle 690.11 of the 2011 National Electrical Code requires DC arc fault circuit protection on PV systems greater than 80 V mounted on or penetrating a building. Underwriters Laboratories, Inc has created Subject 1699B, which prescribes a series of tests to list the AFCI, including temperature, humidity, mechanical and electrical loads, radiation, and a representative set of arc fault tests [6]. The arc fault tests however do not cover the full range of field situations in which arc fault detectors will be required to perform. AFDs and AFCIs must function on a wide range of PV, BOS, and inverter technologies, topologies, and array sizes, while also being resistant to nuisance trips from different forms of system noise. Additional AFD and AFCI tests using various PV technologies and system topologies were performed at the Distributed Energy Technologies Laboratory at Sandia National Laboratories.Eaton Corporation collaborated with Sandia to run a battery of tests with a prototype AFCI. These tests focused on verifying accurate arc fault detection without false tripping. By also capturing current and voltage time series data of the baseline and arcing cases for the range of configurations, detection strategies and variabilities were measured. Inverters injected significant signal profiles on the PV strings. From the binned DFT results, it was shown that a range of noise frequencies are emitted above the baseline level during an arc fault, indicating these may be most appropriate for arc fault detection. Arc fault and detector locations did not appreciably affect the probability of detection for tests conducted, but some abnormalities were observed and will be further investigated. Difficulties distinguishing between arcing and crosstalk noise for some PV system configurations will also be an area for future study.ACKNOWLEDGEMENTSandia National Laboratories is a multi-program laboratory managed and operated by Sandia Corporation, a wholly owned subsidiary of Lockheed Martin Corporation, for the U.S. Department of Energy's National Nuclear Security Administration under contract DE-AC04-94AL85000. This work was funded by the US Department of Energy Solar Energy Technologies Program.REFERENCES[1] Haeberlin, H. ―Arc Detector as an External Accessory Device for PV Inverters for Remote Detection of Dangerous Arcs on the DC Side of PV Plants‖ European Photovoltaic Solar Energy Conference Valencia, Spain 2010.[2] Ji, L. PV Fire: Experience and Studies, International Photovoltaic Reliability Workshop II, 31 July, 2009.[3] Jackson, P. ―Target Roof PV Fire of 4-5-09,‖ City of Bakersfield, CA Development Services/Building Department Memorandum, 4-29-2009.[4] National Electrical Code, 2011 Edition, NFPA70, National Fire Protection Association, Batterymarch, MA.[5] Strobl, C. and Meckler, P. ―Arc Faults in Photovoltaic Systems,‖ 25th ICEC, 4 Oct, 2010.[6] Outline of Investigation for Photovoltaic (PV) DC Arc-Fault Circuit Protection, Subject 1699B, UnderwritersLaboratories, Northbrook, IL, 29 April, 2011.。