butyl(丁基橡胶)-石墨烯纳米复合材料
石墨烯橡胶基复合材料概论
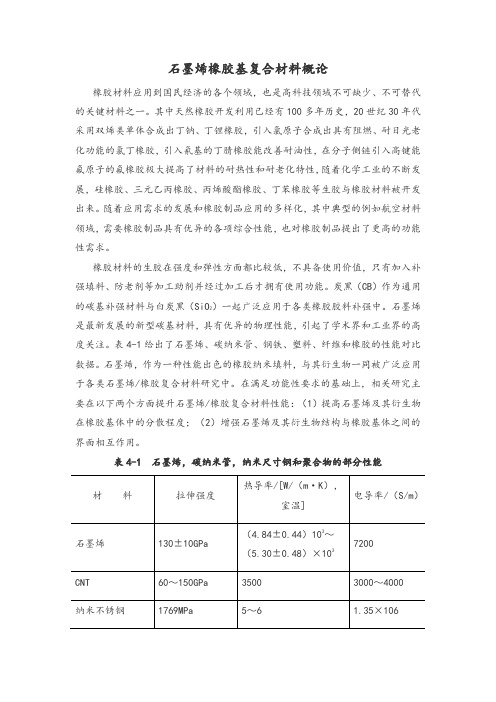
石墨烯橡胶基复合材料概论橡胶材料应用到国民经济的各个领域,也是高科技领域不可缺少、不可替代的关键材料之一。
其中天然橡胶开发利用已经有100多年历史,20世纪30年代采用双烯类单体合成出丁钠、丁锂橡胶,引入氯原子合成出具有阻燃、耐日光老化功能的氯丁橡胶,引入氰基的丁腈橡胶能改善耐油性,在分子侧链引入高键能氟原子的氟橡胶极大提高了材料的耐热性和耐老化特性,随着化学工业的不断发展,硅橡胶、三元乙丙橡胶、丙烯酸酯橡胶、丁苯橡胶等生胶与橡胶材料被开发出来。
随着应用需求的发展和橡胶制品应用的多样化,其中典型的例如航空材料领域,需要橡胶制品具有优异的各项综合性能,也对橡胶制品提出了更高的功能性需求。
橡胶材料的生胶在强度和弹性方面都比较低,不具备使用价值,只有加入补强填料、防老剂等加工助剂并经过加工后才拥有使用功能。
炭黑(CB)作为通用的碳基补强材料与白炭黑(SiO)一起广泛应用于各类橡胶胶料补强中。
石墨烯2是最新发展的新型碳基材料,具有优异的物理性能,引起了学术界和工业界的高度关注。
表4-1给出了石墨烯、碳纳米管、钢铁、塑料、纤维和橡胶的性能对比数据。
石墨烯,作为一种性能出色的橡胶纳米填料,与其衍生物一同被广泛应用于各类石墨烯/橡胶复合材料研究中。
在满足功能性要求的基础上,相关研究主要在以下两个方面提升石墨烯/橡胶复合材料性能:(1)提高石墨烯及其衍生物在橡胶基体中的分散程度;(2)增强石墨烯及其衍生物结构与橡胶基体之间的界面相互作用。
表4-1 石墨烯,碳纳米管,纳米尺寸钢和聚合物的部分性能了大量的研究成果,在材料、工艺、检测手段等方面也开辟了很多新的研究方向。
其发展历程、历史定位与发展基础已被为数众多的综述所记录。
本章将从石墨烯/橡胶复合材料应用的橡胶基体及典型应用出发,综述其制备及功能改性、结构、性能、相关测试方法及其应用方面的研究进展。
石墨烯橡胶基复合材料的制备方法
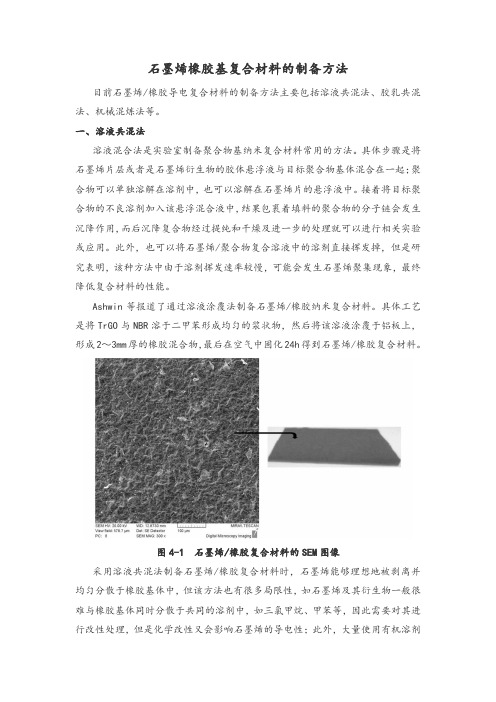
石墨烯橡胶基复合材料的制备方法目前石墨烯/橡胶导电复合材料的制备方法主要包括溶液共混法、胶乳共混法、机械混炼法等。
一、溶液共混法溶液混合法是实验室制备聚合物基纳米复合材料常用的方法。
具体步骤是将石墨烯片层或者是石墨烯衍生物的胶体悬浮液与目标聚合物基体混合在一起;聚合物可以单独溶解在溶剂中,也可以溶解在石墨烯片的悬浮液中。
接着将目标聚合物的不良溶剂加入该悬浮混合液中,结果包裹着填料的聚合物的分子链会发生沉降作用,而后沉降复合物经过提纯和干燥及进一步的处理就可以进行相关实验或应用。
此外,也可以将石墨烯/聚合物复合溶液中的溶剂直接挥发掉,但是研究表明,该种方法中由于溶剂挥发速率较慢,可能会发生石墨烯聚集现象,最终降低复合材料的性能。
Ashwin等报道了通过溶液涂覆法制备石墨烯/橡胶纳米复合材料。
具体工艺是将TrGO与NBR溶于二甲苯形成均匀的浆状物,然后将该溶液涂覆于铝板上,形成2~3mm厚的橡胶混合物,最后在空气中固化24h得到石墨烯/橡胶复合材料。
图4-1 石墨烯/橡胶复合材料的SEM图像采用溶液共混法制备石墨烯/橡胶复合材料时,石墨烯能够理想地被剥离并均匀分散于橡胶基体中,但该方法也有很多局限性,如石墨烯及其衍生物一般很难与橡胶基体同时分散于共同的溶剂中,如三氯甲烷、甲苯等,因此需要对其进行改性处理,但是化学改性又会影响石墨烯的导电性;此外,大量使用有机溶剂造成环境污染且成本大,与目前的环保趋势不符;橡胶硫化配合剂也很难通过溶液共混加入;另外,有研究表明,溶剂小分子极易进入并紧密吸附到石墨烯片层间,很难将其完全脱除,这为通过溶液共混法制备高性能复合材料带来了困难。
胶乳共混法可以避免这些缺点。
二、胶乳共混法胶乳共混法是首先将石墨烯或者GO分散在水相中,接着再与橡胶胶乳混合,搅拌均匀后进行破乳、干燥、硫化得到石墨烯/橡胶复合材料。
该方法无溶剂引入、污染小,工艺相对简单。
Li等通过在天然橡胶乳液中原位还原氧化石墨烯制备了石墨烯(GR)填充改性天然橡胶(NR),工艺路线见图4-2。
丁基橡胶纳米复合材料
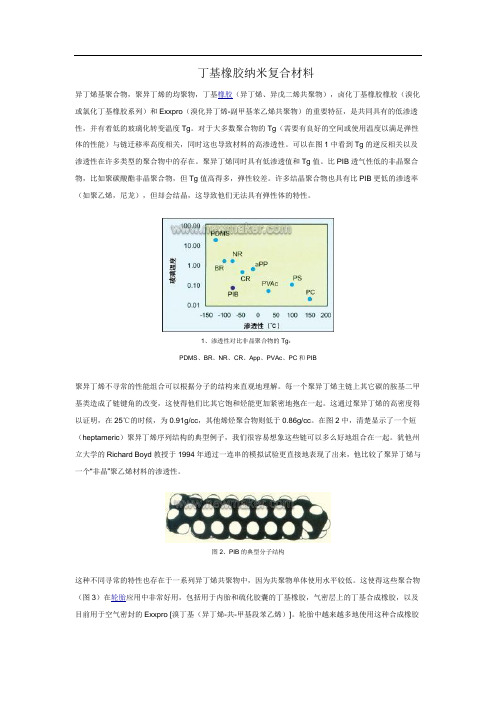
丁基橡胶纳米复合材料异丁烯基聚合物,聚异丁烯的均聚物,丁基橡胶(异丁烯、异戊二烯共聚物),卤化丁基橡胶橡胶(溴化或氯化丁基橡胶系列)和Exxpro(溴化异丁烯-副甲基苯乙烯共聚物)的重要特征,是共同具有的低渗透性,并有着低的玻璃化转变温度Tg。
对于大多数聚合物的Tg(需要有良好的空间或使用温度以满足弹性体的性能)与链迁移率高度相关,同时这也导致材料的高渗透性。
可以在图1中看到Tg的逆反相关以及渗透性在许多类型的聚合物中的存在。
聚异丁烯同时具有低渗透值和Tg值。
比PIB透气性低的非晶聚合物,比如聚碳酸酯非晶聚合物,但Tg值高得多,弹性较差。
许多结晶聚合物也具有比PIB更低的渗透率(如聚乙烯,尼龙),但却会结晶,这导致他们无法具有弹性体的特性。
1、渗透性对比非晶聚合物的Tg:PDMS、BR、NR、CR、App、PVAc、PC和PIB聚异丁烯不寻常的性能组合可以根据分子的结构来直观地理解。
每一个聚异丁烯主链上其它碳的胺基二甲基类造成了链键角的改变,这使得他们比其它饱和烃能更加紧密地抱在一起。
这通过聚异丁烯的高密度得以证明,在25℃的时候,为0.91g/cc,其他烯烃聚合物则低于0.86g/cc。
在图2中,清楚显示了一个短(heptameric)聚异丁烯序列结构的典型例子,我们很容易想象这些链可以多么好地组合在一起。
犹他州立大学的Richard Boyd教授于1994年通过一连串的模拟试验更直接地表现了出来,他比较了聚异丁烯与一个“非晶”聚乙烯材料的渗透性。
图2、PIB的典型分子结构这种不同寻常的特性也存在于一系列异丁烯共聚物中,因为共聚物单体使用水平较低。
这使得这些聚合物(图3)在轮胎应用中非常好用,包括用于内胎和硫化胶囊的丁基橡胶,气密层上的丁基合成橡胶,以及目前用于空气密封的Exxpro [溴丁基(异丁烯-共-甲基段苯乙烯)]。
轮胎中越来越多地使用这种合成橡胶使得轮胎气密性增加,从而提高安全性,以及更好的燃油经济性,这些都源于优秀的内胎。
低透气性丁基橡胶纳米复合材料

( C 和聚异丁烯( I )的渗透性与 T P) PB ] |
E x r[ 化 聚 ( 丁烯 一对 甲基 苯 乙烯 ) 用 于 x po 溴 异 ]
诸 多保压 领域 。这些 弹性体 在轮 胎 中越 来越 多的 应 用提 高 了轮 胎 的保压能力 , 而改 善了安全 性 , 从 并 且 由于降低 了气 密 层 厚度 , 因而 提 高 了燃 油 经 济 性 。这 些益 处在各 种车辆 轮胎 中随处 可见 。
明 。图 2清楚 地给 出了标准 的 P B的短序 ( 聚 ) I 七 结构 , 不难想 象 这些链 堆 积 得 的有 多 紧密 。Uth a 大学 的 Rc adB y i r o d教授 在 1 9 h 9 4年通 过 一系 列 P B和“ 晶形 ” 乙烯 的透 气性模 拟 比较 实验 给 I 非 聚
要进 一步 提高 轮胎 性 能 , 需 要新 技 术 和材 就
聚异 丁烯这 种反 常的性 能组合 与其分 子结构
有直 接关 系 。P B主链 上每 隔一 个碳 原 子上 成 双 I 的二 甲基导 致这 些链 的键 角 改变 , 因而 要 比其 他
饱 和烃 紧密 得多 。P B密度 ( 5 I 2 ℃下为 0 9 g c ) . 1 / c 比其 他烯 烃 聚合 物 密 度 ( . . 6 / c 高 就 是 证 0 0 8 gc)
~ cH
料 同时透气 性也 高 。如 图 1 示 , 所 Tg和渗 透性这 种反 向关 系对 于许 多类 型聚合 物都是 如此 。聚异
丁烯 与众不 同 , 透 气性 小 , 其 Tg值 低 。也 有一 些
车 一
CH3
H3
聚异 丁烯 ( I ) PB
CH3
,
透气 性低 于 P B 的非 晶 态 聚合 物 , 聚 碳 酸 酯 , I 如
可降低气体渗透性的丁基橡胶纳米复合材料
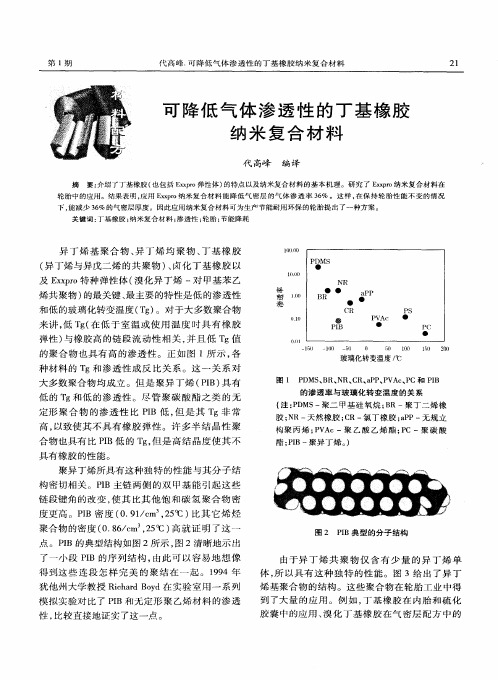
聚合 物 的密 度 ( . 6 c 2 高 就 证 明 了 这 一 0 8 / m ,5C) o
点 。PB的典 型结 构如 图 2所 示 , 2清晰地 示 出 I 图
图 2 P B典 型 的 分 子 结 构 I
了一 小 段 PB的 序 列结 构 , I 由此 可 以容 易 地 想 像
异 丁烯 基 聚 合 物 、 丁烯 均 聚 物 、 基 橡 胶 异 丁
( 丁烯 与异 戊 二烯 的共 聚 物 ) 卤化 丁 基 橡 胶 以 异 、
lm 0 ( 0
l { O 0)
及 E x r 种 弹性 体 ( 化 异 丁烯 一对 甲基 苯 乙 xpo特 溴 烯共聚物) 的最关 键 、 主 要 的特性 是 低 的 渗透 性 最
轮 胎 中 的 应 用 。结 果 表 明 , 用 E x r 米 复 合 材 料 能 降 低 气 密 层 的 气 体 渗 透 率 3 % 。这 样 , 保 持 轮 胎 性 能 不 变 的 情 况 应 xpo纳 6 在 下 . 减 少 3 % 的 气 密层 厚度 。 因 此 应 用 纳 米 复 合 材 料 可 为生 产 节 能 耐 用 环 保 的轮 胎 提 出 了 一 种 方 案 。 能 6 关 键 词 : 基 橡 胶 ; 米 复 合 材 料 ; 透 性 ; 胎 ; 能 降 耗 丁 纳 渗 轮 节
得 到 这 些 连 段 怎 样 完 美 的 聚 结 在 一 起 。 19 9 4年
由于异 丁 烯 共 聚 物 仅 含 有 少 量 的 异 丁烯 单
体 , 以具 有 这 种 独 特 的性 能 。图 3给 出 了 异 丁 所 烯 基 聚合 物 的结 构 。这 些 聚合 物在 轮 胎工 业 中得
到 了大量 的应 用 。例 如 , 基橡 胶 在 内胎 和硫 化 丁 胶 囊 中的应 用 、 化 丁基 橡 胶 在气 密层 配 方 中 的 溴
石墨烯_橡胶纳米复合材料_唐征海

石墨烯是由单层碳原子通过 sp2 杂化紧密堆 积成二维蜂窝状晶格结构的一种新炭材料,它也 是构建其它维度碳质材料( 如零维富勒烯、一维 碳纳米管、三维石墨) 的基本单元. 石墨烯具有超 高的力学性能( 模量约 1100 GPa,断裂强度约 130 GPa) 、低密度( 约 2. 2 g / cm3 ) 、高导热性能且高度 各向 异 性 ( 面 内 5000 W / ( m K ) ,面 外 2 W / ( m K) ) 、高 电 子 迁 移 率 ( 高 达 2 × 104 cm2 ( V S) ) 、高比表面积( 极限值可达 2630 m2 / g) 和高阻 隔性能等[6 ~ 8]. 石墨烯表现出来的独特物理和电 子特性,使其在纳米器件、复合材料、传感器、锂电 池、储氢材料等领域有着巨大的应用前景[9 ~ 12]. 与其他填料如 CB、SiO2 、CNTs、黏土等相比,石墨 烯作 为 橡 胶 纳 米 填 料,具 有 更 高 的 比 表 面 积、强 度、弹性、热导率和电导率等. 目前,多种石墨烯 / 橡胶 复 合 材 料 被 广 泛 研 究,包 括 天 然 橡 胶 ( NR ) [13,14]、丁 腈 橡 胶 ( NBR ) [15]、乙 丙 橡 胶 ( EPDM) [16]、SBR[17]、IIR[18]、热塑性弹性体苯乙 烯-丁二烯-苯乙烯共聚物( SBS) [19]等. 石墨烯不 仅能明显提高复合材料的物理机械性能,同时赋 予其功能性[8]. 本文将综述石墨烯 / 橡胶复合材 料的制备与设计、结构和性能的研究进展.
橡胶在室温下具有独特的高弹性,其作为一种 重要的战略性物资,广泛应用于国民经济、高新技 术和国防军工等领域. 然而,未补强的橡胶强度 低、模量低、耐磨差、抗疲劳差,没有实用性,因此对 于绝 大 多 数 橡 胶 都 需 要 填 充 补 强. 目 前,炭 黑 ( CB) 和白炭黑( SiO2 ) 作为橡胶主要的补强剂,广 泛使用于各种橡胶制品中. 在橡胶补强的同时,由 于橡胶材料固有的黏弹滞后损耗和橡胶内部的填 料-填料、填 料-大 分 子 链 以 及 大 分 子 链 之 间 的 摩 擦,动态环境下使用的橡胶制品会产生大量的热 量,产生的热量不能及时地传导出去将导致橡胶内 部急剧升温,使其性能劣化,因此需要提高橡胶制 品的导热性来提高其动态使用下的使用性能和使 用寿命. 同时,随着橡胶制品的多样化,很多应用 领域如防过电流 / 过热元件、开关、传感器、密封器 件等需要橡胶制品具有抗静电、导电性或气体阻隔 性等. 面对橡胶制品的功能性需求,发展低填料含 量和高增强效率( 降低生热) ,同时具有功能性的 纳米填料( 黏土、碳纳米管( CNTs) 、石墨烯等) / 橡 胶复合材料的制备与研究显得尤为重要[1 ~3]. 如 Zhang 等通过溶液共混法制备有机改性黏土 / 丁基 橡胶( IIR) 复合材料,加入 8 vol% 有机改性黏土时, 复合材料的 300% 定伸强度和拉伸强度分别提高 约 1. 2 和 2. 3 倍,氮气渗透性下降 22%[4]. 对比炭 黑,CNTs 填充的丁苯橡胶( SBR) 具有更高的强度, 更高的导热率和电导率[5].
石墨烯特种橡胶复合材料
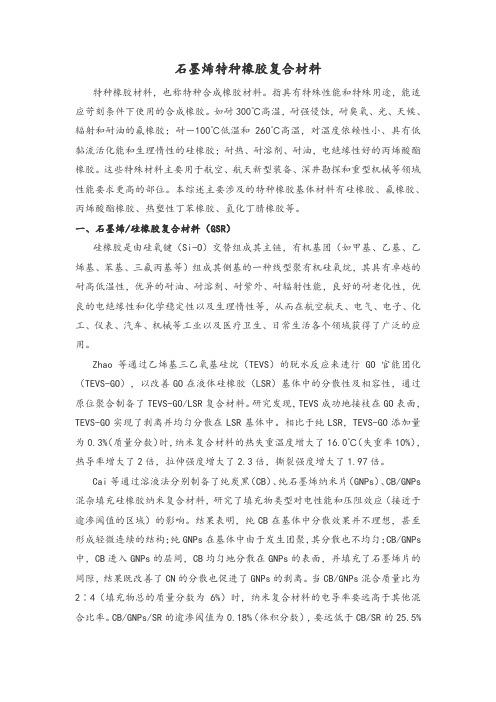
石墨烯特种橡胶复合材料特种橡胶材料,也称特种合成橡胶材料。
指具有特殊性能和特殊用途,能适应苛刻条件下使用的合成橡胶。
如耐300℃高温,耐强侵蚀,耐臭氧、光、天候、辐射和耐油的氟橡胶;耐-100℃低温和260℃高温,对温度依赖性小、具有低黏流活化能和生理惰性的硅橡胶;耐热、耐溶剂、耐油,电绝缘性好的丙烯酸酯橡胶。
这些特殊材料主要用于航空、航天新型装备、深井勘探和重型机械等领域性能要求更高的部位。
本综述主要涉及的特种橡胶基体材料有硅橡胶、氟橡胶、丙烯酸酯橡胶、热塑性丁苯橡胶、氢化丁腈橡胶等。
一、石墨烯/硅橡胶复合材料(GSR)硅橡胶是由硅氧键(Si-O)交替组成其主链,有机基团(如甲基、乙基、乙烯基、苯基、三氟丙基等)组成其侧基的一种线型聚有机硅氧烷,其具有卓越的耐高低温性,优异的耐油、耐溶剂、耐紫外、耐辐射性能,良好的耐老化性,优良的电绝缘性和化学稳定性以及生理惰性等,从而在航空航天、电气、电子、化工、仪表、汽车、机械等工业以及医疗卫生、日常生活各个领域获得了广泛的应用。
Zhao等通过乙烯基三乙氧基硅烷(TEVS)的脱水反应来进行GO官能团化(TEVS-GO),以改善GO在液体硅橡胶(LSR)基体中的分散性及相容性,通过原位聚合制备了TEVS-GO/LSR复合材料。
研究发现,TEVS成功地接枝在GO表面,TEVS-GO实现了剥离并均匀分散在LSR基体中。
相比于纯LSR,TEVS-GO添加量为0.3%(质量分数)时,纳米复合材料的热失重温度增大了16.0℃(失重率10%),热导率增大了2倍,拉伸强度增大了2.3倍,撕裂强度增大了1.97倍。
Cai等通过溶液法分别制备了纯炭黑(CB)、纯石墨烯纳米片(GNPs)、CB/GNPs 混杂填充硅橡胶纳米复合材料,研究了填充物类型对电性能和压阻效应(接近于逾渗阈值的区域)的影响。
结果表明,纯CB在基体中分散效果并不理想,甚至形成轻微连续的结构;纯GNPs在基体中由于发生团聚,其分散也不均匀;CB/GNPs 中,CB进入GNPs的层间,CB均匀地分散在GNPs的表面,并填充了石墨烯片的间隙,结果既改善了CN的分散也促进了GNPs的剥离。
石墨烯通用橡胶复合材料
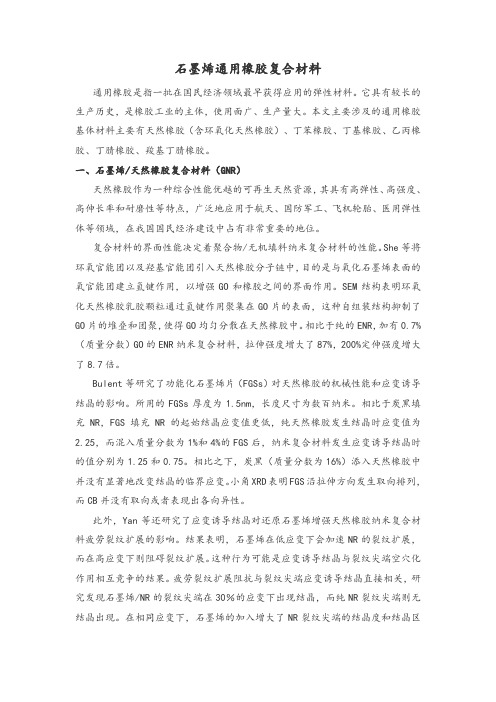
石墨烯通用橡胶复合材料通用橡胶是指一批在国民经济领域最早获得应用的弹性材料。
它具有较长的生产历史,是橡胶工业的主体,使用面广、生产量大。
本文主要涉及的通用橡胶基体材料主要有天然橡胶(含环氧化天然橡胶)、丁苯橡胶、丁基橡胶、乙丙橡胶、丁腈橡胶、羧基丁腈橡胶。
一、石墨烯/天然橡胶复合材料(GNR)天然橡胶作为一种综合性能优越的可再生天然资源,其具有高弹性、高强度、高伸长率和耐磨性等特点,广泛地应用于航天、国防军工、飞机轮胎、医用弹性体等领域,在我国国民经济建设中占有非常重要的地位。
复合材料的界面性能决定着聚合物/无机填料纳米复合材料的性能。
She等将环氧官能团以及羟基官能团引入天然橡胶分子链中,目的是与氧化石墨烯表面的氧官能团建立氢键作用,以增强GO和橡胶之间的界面作用。
SEM结构表明环氧化天然橡胶乳胶颗粒通过氢键作用聚集在GO片的表面,这种自组装结构抑制了GO片的堆叠和团聚,使得GO均匀分散在天然橡胶中。
相比于纯的ENR,加有0.7%(质量分数)GO的ENR纳米复合材料,拉伸强度增大了87%,200%定伸强度增大了8.7倍。
Bulent等研究了功能化石墨烯片(FGSs)对天然橡胶的机械性能和应变诱导结晶的影响。
所用的FGSs厚度为1.5nm,长度尺寸为数百纳米。
相比于炭黑填充NR,FGS填充NR的起始结晶应变值更低,纯天然橡胶发生结晶时应变值为2.25,而混入质量分数为1%和4%的FGS后,纳米复合材料发生应变诱导结晶时的值分别为1.25和0.75。
相比之下,炭黑(质量分数为16%)添入天然橡胶中并没有显著地改变结晶的临界应变。
小角XRD表明FGS沿拉伸方向发生取向排列,而CB并没有取向或者表现出各向异性。
此外,Yan等还研究了应变诱导结晶对还原石墨烯增强天然橡胶纳米复合材料疲劳裂纹扩展的影响。
结果表明,石墨烯在低应变下会加速NR的裂纹扩展,而在高应变下则阻碍裂纹扩展。
这种行为可能是应变诱导结晶与裂纹尖端空穴化作用相互竞争的结果。
一种高疏水性功能化二氧化硅石墨烯纳米复合材料的制备方法及其应用[发明专利]
![一种高疏水性功能化二氧化硅石墨烯纳米复合材料的制备方法及其应用[发明专利]](https://img.taocdn.com/s3/m/637541e509a1284ac850ad02de80d4d8d15a0195.png)
专利名称:一种高疏水性功能化二氧化硅/石墨烯纳米复合材料的制备方法及其应用
专利类型:发明专利
发明人:张丽园,朱林林,金效齐,李镇委
申请号:CN202010272047.9
申请日:20200409
公开号:CN111450801B
公开日:
20220422
专利内容由知识产权出版社提供
摘要:本发明公开了一种高疏水性功能化二氧化硅/石墨烯纳米复合材料的制备方法及其在油水分离中的应用。
采用一锅法制备乙烯基功能化二氧化硅/石墨烯纳米复合材料:以乙烯基三乙氧基硅烷(VETS)为原料,十六烷基三甲基溴化铵(CTAB)为模板剂,在碱性条件下合成乙烯基功能化的二氧化硅,然后加入石墨烯通过分子自组装制备得到高分散的乙烯基功能化二氧化硅/石墨烯纳米复合材料;该材料具有高疏水性、较大孔隙率和比表面积,可作为高效的吸附剂应用于油水分离(吸附率达99%以上,并且经再生后循环利用12次其吸附性能仍不发生改变)。
本发明涉及的乙烯基功能化二氧化硅/石墨烯纳米复合材料制备过程简单,作为吸附剂应用于油水分离时,可高选择性吸附芳香烃类化合物,具有吸附容量大、吸附效率高的特点;且仅通过简单的洗涤、过滤即可实现材料的再生和循环利用,具有优异的再生性能,经济环保,具有广阔的应用前景。
申请人:蚌埠学院
地址:233000 安徽省蚌埠市经济开发区曹山路1866号
国籍:CN
代理机构:安徽省蚌埠博源专利商标事务所(普通合伙)
代理人:朱恒兰
更多信息请下载全文后查看。
石墨烯填充丁腈橡胶纳米复合材料

烧和硫化时间。橡胶纳米 复合材料 的硬 度按照
N ̄ 8 5 , 0 5 测 试 。动态 机 械 性 能 分 析采 用 扭 转 模 式 由原始纳 米复 合 材 料 进行 , 获 取贮 能 模 量 与 填
是改善动态机械性能、 热及电性能的传统填料撰
具吸 引力 的替代 材料 。 这些纳 米填 料 的粒 子至少 有一维 尺寸是 纳米
含石墨的 橡胶复合材料的摩撩行为很复杂, 公 司产品 。氧化锌 和硬 脂酸作 为硫 黄交 联 的活化
强烈依赖于应变幅度和纳桊复合材料在制备过程
中的加 工条件 。X . C h e n等 发现 , C N T/ S B R改 善 了滞后 , 减少 了疲 劳 损 失 。弹性 体 的摩 擦行 为并 不符合 刚性 固体 的摩 擦 原理 , 因为 填 充纳 米 复合 材料具 有粘 弹特性 和大变形 能力 。 因此 弹性体 的
孔性 或表 面化学 性质 ( 极 性) 。
朗盛公 司 产 品 市 售 剥离 纳 米 石 墨 烯 粒 子 如
g C 7 5 O , x g M5 从 X G 科学 公 司 购得 , UF 1 C 9 8是 Kr o p f mu h l AMG公 司产 品 , 炭黑 N3 3 9是 卡博 特
剂, C B S为促进 剂 。所有 材料都 是 直接使 用 。
1 . 2 蜜验方法和胶料制备
1 . 2 . 1 用静 态气体吸 附等温缄测定 B E T 比表面积
摩擦力与表面结构和接触丽积有关 。
因为许 多现象 同时 发生 , 复杂 的测 试 条 件下
填料斡豢面特征, 如填料活性, B E T比表面 积或粗糙度都可通过静态气 体吸附等温线来获
1 实验
要影 响 , 如动 态模量 与应力 和频率 的依赖关 系 , 高
石墨烯基纳米材料在乳腺癌诊疗中的应用研究进展

石墨烯基纳米材料在乳腺癌诊疗中的应用研究进展
张博诚;王胜涛
【期刊名称】《现代肿瘤医学》
【年(卷),期】2024(32)7
【摘要】乳腺癌是女性最常见的恶性肿瘤,发病率逐年上升。
传统的诊治方法仍有不足,因此迫切需要探索新的诊治策略。
石墨烯基纳米材料(graphene-based nanomaterials,GBNs)具有靶标选择性、光致发光性、易功能化、化学增敏和高载药量等优点,是一种有前景的药物载体。
本文首先总结了GBNs在癌症治疗中的生物特性,然后重点介绍了GBNs在乳腺癌诊疗中的应用,包括化学治疗、光疗、靶向治疗、早期诊断和肿瘤显像、作为联合治疗的平台以及修饰策略。
强调了GBNs 在减毒增效、多药递送、生物传感、分子成像和联合治疗等方面的优势。
最后阐明了GBNs在乳腺癌诊疗领域亟待解决的问题与挑战,旨在为研究者们指明方向并提供新的思路。
【总页数】5页(P1370-1374)
【作者】张博诚;王胜涛
【作者单位】三亚中心医院(海南省第三人民医院)甲状腺乳腺外科;南方医科大学附属佛山妇幼保健院(佛山市妇幼保健院)
【正文语种】中文
【中图分类】R737.9
【相关文献】
1.石墨烯和石墨烯基纳米复合材料的制备及在生物医疗领域的应用
2.石墨烯基复合金属纳米材料在燃料电池应用中的研究进展
3.氧化石墨烯基纳米材料在重金属离子去除中的应用研究进展
4.石墨烯/铝基复合材料在纳米压痕过程中位错与石墨烯相互作用机制的模拟研究
5.功能化石墨烯及石墨烯基纳米复合材料润滑添加剂的研究进展
因版权原因,仅展示原文概要,查看原文内容请购买。
石墨烯丁基橡胶高导热复合材料
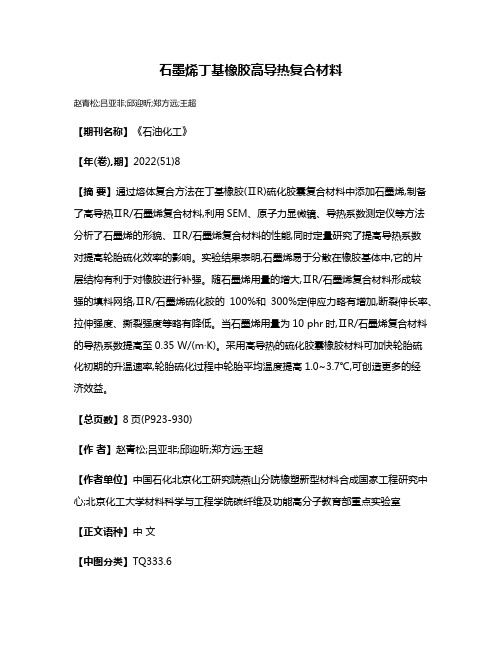
石墨烯丁基橡胶高导热复合材料赵青松;吕亚非;邱迎昕;郑方远;王超【期刊名称】《石油化工》【年(卷),期】2022(51)8【摘要】通过熔体复合方法在丁基橡胶(ⅡR)硫化胶囊复合材料中添加石墨烯,制备了高导热ⅡR/石墨烯复合材料,利用SEM、原子力显微镜、导热系数测定仪等方法分析了石墨烯的形貌、ⅡR/石墨烯复合材料的性能,同时定量研究了提高导热系数对提高轮胎硫化效率的影响。
实验结果表明,石墨烯易于分散在橡胶基体中,它的片层结构有利于对橡胶进行补强。
随石墨烯用量的增大,ⅡR/石墨烯复合材料形成较强的填料网络,ⅡR/石墨烯硫化胶的100%和300%定伸应力略有增加,断裂伸长率、拉伸强度、撕裂强度等略有降低。
当石墨烯用量为10 phr时,ⅡR/石墨烯复合材料的导热系数提高至0.35 W/(m·K)。
采用高导热的硫化胶囊橡胶材料可加快轮胎硫化初期的升温速率,轮胎硫化过程中轮胎平均温度提高1.0~3.7℃,可创造更多的经济效益。
【总页数】8页(P923-930)【作者】赵青松;吕亚非;邱迎昕;郑方远;王超【作者单位】中国石化北京化工研究院燕山分院橡塑新型材料合成国家工程研究中心;北京化工大学材料科学与工程学院碳纤维及功能高分子教育部重点实验室【正文语种】中文【中图分类】TQ333.6【相关文献】1.高导热定向石墨烯/环氧树脂复合材料的研究2.石墨烯含量对石墨烯/Cu复合材料组织及导热性能的影响3.改性氧化石墨烯/天然橡胶高导热复合材料4.一种纺丝级高导热石墨烯/尼龙复合材料原位聚合制备方法5.丁基橡胶/还原氧化石墨烯复合材料的制备及性能因版权原因,仅展示原文概要,查看原文内容请购买。
卤化丁基橡胶
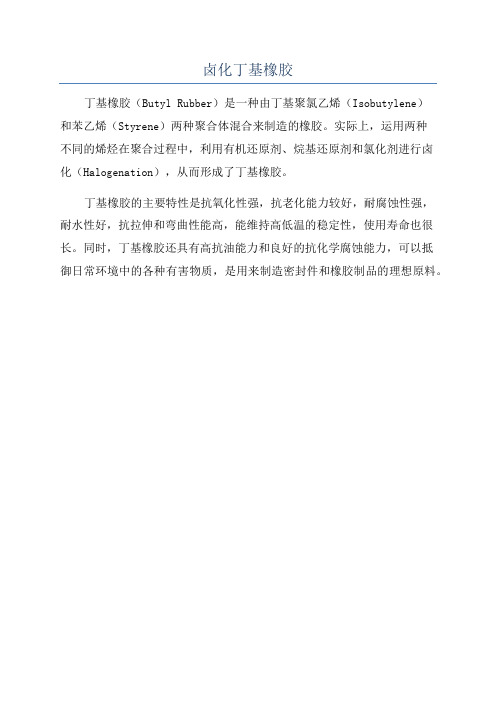
卤化丁基橡胶
丁基橡胶(Butyl Rubber)是一种由丁基聚氯乙烯(Isobutylene)
和苯乙烯(Styrene)两种聚合体混合来制造的橡胶。
实际上,运用两种
不同的烯烃在聚合过程中,利用有机还原剂、烷基还原剂和氯化剂进行卤
化(Halogenation),从而形成了丁基橡胶。
丁基橡胶的主要特性是抗氧化性强,抗老化能力较好,耐腐蚀性强,
耐水性好,抗拉伸和弯曲性能高,能维持高低温的稳定性,使用寿命也很长。
同时,丁基橡胶还具有高抗油能力和良好的抗化学腐蚀能力,可以抵
御日常环境中的各种有害物质,是用来制造密封件和橡胶制品的理想原料。
- 1、下载文档前请自行甄别文档内容的完整性,平台不提供额外的编辑、内容补充、找答案等附加服务。
- 2、"仅部分预览"的文档,不可在线预览部分如存在完整性等问题,可反馈申请退款(可完整预览的文档不适用该条件!)。
- 3、如文档侵犯您的权益,请联系客服反馈,我们会尽快为您处理(人工客服工作时间:9:00-18:30)。
Study on Modified Graphene/Butyl Rubber Nanocomposites.I.Preparation and CharacterizationHuiqin Lian,1,2,3Shuxin Li,1Kelong Liu,1Liangrui Xu,1Kuisheng Wang,2Wenli Guo11Department of Materials Science and Engineering,Beijing Institute of Petrochemical Technology,Beijing,China2Department of Materials Science and Engineering,Beijing University of Chemical Technology,Beijing,China 3Department of Chemical Engineering,Yanbian University,Beijing,ChinaButyl rubber,IIR nanocomposites based on modified graphene sheets,were fabricated by solution process-ing followed by compression molding.MG was pre-pared from natural graphite,NG through graphite oxide route.X-ray diffraction showed that the exfoliated MG was homogeneously dispersed in the IIR matrix with doping levels of1-10wt%as evidenced by the lack of the characteristic graphite reflection in the compo-sites.In contrast,the graphite retained its stacking order and showed the sharp characteristic peak in the NG-IIR composites.Scanning electron microscope images of the fracture surfaces of the IIR matrix showed that MG nanofillers exhibited better compati-bility than NG did.The mechanical properties of the MG-IIR nanocomposites were significantly improved due to the efficient distribution of the large surface area MG sheet.The tensile modulus of nanocomposite with doping level of MG10wt%was16times that of the pure IIR.POLYM.ENG.SCI.,00:000–000,2011.ª2011 Society of Plastics EngineersINTRODUCTIONCommercial butyl rubber(IIR)is a copolymer of isobu-tylene and a small amount of isoprene.It is employed in the inner linings of automobile tires and in other specialty application due to its characteristics of chemical inertness, impermeability to gases and weatherability.However, unsaturated bonds in IIR,due to the presence of isoprene monomer units in the backbone,can be attacked by atmos-pheric ozone leading to oxidative degradation and chain cleavage.Also in somefields,such as aerospace,aircraft and high-vacuum systems,IIR does not meet the extremely high-gas barrier as well as the high mechanical properties requirements.Therefore,there exists a continuous interest in lowering gas permeability and improving the mechanical properties of IIR by various techniques[1,2].It is well known that polymeric nanocomposites are of great interest for both scientific challenges and industrial applications due to their enhanced mechanical properties and unique material properties[3–5].Compared with con-ventional systems,nanomaterials are more effective rein-forcements because the stress transfer from the matrix to the reinforcement is more efficient in nanocomposites due to the increased surface area,assuming good adhesion at the interface.Also,the crack propagation length at the interface becomes longer,improving the strength and toughness.In addition to improving the mechanical prop-erty,nanofillers,such as layered silicate or carbon nanotubes,can provide dramatic improvement in thermal stability,dimensional stability,heat-distortion tempera-ture,and barrier property[6–11].Recently,graphite oxide (GO)has attracted increasing interest as afiller for poly-mer nanocomposites due to its high dispersive capacity, long coherence length and the barrier property[12,13]. GO with a typical pseudo-two-dimensional structure gen-erally contains hydroxyl,carboxyl and ether groups, which causes GO to absorb polar molecules easily and thus GO/polymer composites can be formed.Such struc-tural nanocomposites can provide reinforcement to the base polymer matrix.Also,GO may also have other desirable properties,such as mass diffusion coefficients, coefficients of thermal expansion,dielectric constants, thermal/chemical stability,solvent resistance,selectivity, conductivity,and resistivity to membrane fouling and poisoning.However,as a reinforcement of IIR,the hydro-philic surface of GO makes it difficult to disperse in the hydrophobic IIR matrix.Therefore,it is an importantCorrespondence to:Wenli Guo;e-mail:wlguo2008@Contract grant sponsor:Natural Science Foundation of China(NSFC);contract grant number:51063009;contract grant sponsor:BeijingNatural Science Foundation of China;contract grant number:KZ200910017001.DOI10.1002/pen.21997Published online in Wiley Online Library().V C2011Society of Plastics EngineersPOLYMER ENGINEERING AND SCIENCE—-2011Administratorissue to improve the compatibility of the GO sheet with the IIR matrix.Moreover,methods used to prepare polymeric nano-composites include in-situ polymerization [14],solution mixing [15],melting compound [16],and cocoagulating of polymeric composite solution [17].Considering the manufacture process of the IIR,slurry and solution pro-cess,the solution mixing is promising to fabricate IIR hybrids in the IIR industry.In this study,we report for the first time the fabrication of well-dispersed modified graphene in IIR composites through solution process.The MG nanosheets are homo-geneously dispersed in the IIR matrix with doping level of 1–10wt%.Compared with the pure IIR,the resulting nanocomposite membranes exhibit dramatic enhancement of mechanical properties.To the best of our knowledge,this is the first report of totally exfoliated graphite to reinforce IIR with outstanding mechanical property.The properties of vulcanization plateau,gas barrier,cure capa-bility,and rubber damping are under study and the results will be published in the near future.EXPERIMENTAL PROCEDURES MaterialsNatural graphite flakes with a partical size of 30l m were purchased from Aladdin Reagent Company (China).Cetyltrimethylammonium bromide (CTAB)was bought from Fuchen Chemical Reagents(Tianjin,China).Butyl rubber (IIR 1751)was obtained from YanShan Petrochem-ical Company of China.The other reagents (NaOH,NaNO 3,and KMnO 4)of analytical grade and 98%H 2SO 4,30%H 2O 2were purchased from Sinopharm Chemical Reagent Co.Ltd.(China)and were used as received with-out further purification.Ultrapure water with resistivity of 18M O was produced by a Milli-Q(Millipore,USA)and was used for solution preparation.Preparation of MG/NG-IIR Composite SheetsThe procedure used to prepare the MG-IIR nano-composite sheets was shown in Fig. 1.First,based on Hummers’method [18],the graphite was oxidized by con-centrated sulfuric acid to create polar hydrophilic groups (ÀÀCOOH,C ¼¼O,ÀÀOH)on the surface.The GO was dispersed in cetyltrimethylammonium bromide solution (20wt%)and ultrasonicated for 0.5h,followed by me-chanical stirring at 258C for 24h.During this process,the tertiary amine reacted with the carboxylic groups on the oxidized surface via an acid-base reaction or via hydrogen bonding between the surface ÀÀOH or C ¼¼O group and the amine groups.The suspension was filtrated and washed three times with water,dried at 408C in a vacuum for 24h.The resulting MG was added into the 15wt%solution of IIR in hexane by sonication for 0.5h to form a colloidal suspension.Then the mixture was stirred for 6h at 258C.The amounts of MG/NG added were 0,1,3,5,10wt%ofthe mass of rubber.The composite solution was then coa-gulated by adding methanol and the precipitated nanocom-posite was dried in a vacuum.Finally,sheet samples were prepared by vacuum compression molding using a 2mm thick spacer at 1008C under 10MPa for ing this procedure,the NG-IIR composite sheets were prepared.CHARACTERIZATION AND MEASUREMENTS The as-made membrane was characterized by X-ray diffraction (XRD,Scintag PAD X diffractometer,Cu K a source,operated at 45kV and 40mA).The samples were scanned with 58/min between 2y of 28–308.SEM observation was performed using Tecnai T12,at an acceleration voltage of 15kV with gold -posite samples were imaged by first fracturing in liquid nitrogen.TGA was performed using a TA Instrument Q500attached to an automatic programmer from ambient tem-perature to 5008C at a heating rate of 108C/min in a nitro-gen atmosphere.A TA instrument Q1000was used to record the DSC traces at a heating rate of 108C/min.Measurements of mechanical properties were con-ducted at 25628C according to relevant ISO standard (ISO 37).Tensile tests were measured on an Autograph AGS-J SHIMADZU universal testing machine at a cross-head speed of 500mm/min.The reported values were the average of five measurements.RESULTS AND DISCUSSIONThe FTIR spectra of NG,GO,and MG were shown in Fig.2.The FT-IR spectrum of NG showed no significant features.While that of GO showed quite differentFIG.1.Schematic representation for the fabrication of MG-IIR nano-composite membrane.2POLYMER ENGINEERING AND SCIENCE—-2011DOI 10.1002/pencharacter by the presence of new bands.The broad bandat3405cm21could be assigned to stretching of the ÀÀOH groups on the GO surface.The bands at1720and 1070cm21were associated with stretching of the C¼¼Oand CÀÀO stretching vibrations of carboxylic groupsrespectively.The FTIR spectrum of MG confirmed theeffective functionalization of graphene.The double bandsat2849and2919cm21were antisymmetric and symmet-ric CÀÀH stretching vibrations of theÀÀCH2ÀÀgroups from surfactant molecules[19]respectively.The bands at 1463and1127cm21were corresponding to CÀÀH bend-ing and the C-N stretching vibration respectively.The spectrum also showed a C¼¼C peak at1574cm21corre-sponding to the skeletal vibration of graphene sheets[20]. These spectral features showed that MG was successfully synthesized.The XRD patterns of the NG,GO,MG were shown inFig.3.The sharp diffraction peak around26.5o for pris-tine graphite(Fig.3a)showed that the basal spacing was0.34nm.Because of the strong Van der Waals force andstatic electric force between the sheets of graphite,thesheet was difficult to disperse.Thus a relatively strongoxidative acid was used to oxidize the graphite creatingpolar groups on the surface of graphite sheet.The surfac-tant of cetyltrimethyl ammonium bromide was used to functionalize the oxidized graphite through acid-base reaction to obtain stable exfoliated graphene sheets.As shown in Fig.3a,the GO showed two diffraction peaks at 2y of9.7o and25.3o,corresponding to a d-spacing of0.91 and0.35nm,respectively,and indicated that the GO was not fully oxidized and the additional peak at25.3o was that of unoxidized graphite.From Fig.3b,MG showed no characteristic peak indicating that the modified graphene sheet had been exfoliated completely.The XRD patterns of MG/NG-IIR nanocomposites membranes with different doping levels were presented in Fig.4.As shown in Fig.4a,the broad peak of2y around 15o appeared in IIR and NG-IIR membranes,due to the amorphous phase of IIR.Toki et al.[21]reported that the amorphous peak of IIR changed during uniaxial deforma-tion.In this case,with NG loading increase,the broad peak shifted slightly,from2y of14.7o in IIR to14.4o in 10wt%NG-IIR composite.It was deduced that NG did not influence the crystallization behavior of IIR very much.From Fig.4a,the diffraction peak at2y around 26.5o appeared in NG and NG-IIR composites because the NG retained its stacking order in the composite.XRD diffraction curves of MG-IR nanocomposites were shown in Fig.4b.The diffraction ascribed to graphite or oxidized graphite did not appear in all of the XRD patterns of composites,indicating the complete exfoliation of the MG in the IIR matrix.SEM images of the fractured surfaces of the as-made MG-IIR and NG-IIR composites were shown in Fig.5. SEM images of NG-IIR composites showed a smooth to-pography with the NG remained its stacking order in the IIR matrix(Fig.5a and b).In contrast,the MG-IIR com-posites appeared a rough surface and the MG dispersed in IIR matrix homogenously(Fig.5c and d).This is likely due to the organic modifier on the surface of MG produc-ing good compatibility with IIR matrix.These results were coincident with the XRD test.TGA under a nitrogen atmosphere was performed on NG,MG,IIR and MG-IIR composites to obtain the struc-ture of MG and composites,as well as to determine the effects of the MG on the thermal stability of the compo-sites.The resulting curves were shown in Fig.6.From FIG.2.FT-IR spectra of NG,GO andMG.FIG.3.The XRD patterns of(a)NG,GO and(b)MG.The curves are shifted vertically for clarity.DOI10.1002/pen POLYMER ENGINEERING AND SCIENCE—-20113FIG.4.The XRD patterns of composites(a)NG-IIR and(b)MG-IIR.The curves are shifted vertically forclarity.FIG.5.SEM images of fracture surface of(a,b)NG-IIR nanocomposites and(c,d)MG-IIR nanocompo-sites.4POLYMER ENGINEERING AND SCIENCE—-2011DOI10.1002/penNG curve of Fig.6a,graphite maintained its weight under the test condition.The curve of MG indicated that MG was composed of 17wt%graphene and 83wt%organicmodifiers.The functional surface contributed to the dis-persion of MG in the IIR solution as well as to the com-patibility with the IIR matrix in the composite membrane.Figure 6b indicated that the IIR began to decompose at 2698C and degraded completely at 4158C.In the case of MG/IIR composites,the weight loss below 3008C was attributed to the decomposition of the small organic molecules on the surface of graphene.Table 1listed the temperature at 5wt%loss of the TGA curve of IIR and MG-IIR composites.The pure IIR lost 5wt%at 3248C and the value was the highest of all the curvesobtained.FIG.6.(a)TG curves of NG and MG;(b)TG curves of MG-IIR nano-composites and (c)DTG curves of MG-IIR nanocomposites.TABLE 1.Thermal propertiesofpureIIRandMG-IIRnanocomposites.IIR 1%MG-IIR 3%MG-IIR 5%MG-IIR 10%MG-IIRT 5wt%(8C)a 324297321256236T mrv (8C)b 372368375378383a Temperature at 5wt%loss.bTemperature at the maximum reactivevelocity.FIG.7.DSC curves of MG-IIRnanocomposites.FIG.8.Tensile stress-strain curve of (a)MG-IIR nanocomposites and (b)NG-IIR nanocomposites.DOI 10.1002/pen POLYMER ENGINEERING AND SCIENCE—-20115The temperature at5wt%loss decreased with the increas-ing MG content when the MG contents were3,5,and10wt%in composites respectively.This might be due to theamount of small molecule of organic modifier increasedwith the increasing MG content.Thefirst derivative of the TGA curve(DTA curvesshown in Fig.6c showed the variation in weight withtime(dW/dT)as a function of temperature.The DTApeaks indicated the temperature of the maximum reactivevelocity.Table1listed the temperature at the maximumreactive velocity of the DTA curve of IIR and MG-IIRcomposites.Pure rubber reached the maximum reactivevelocity at3728C.The value of1wt%MG-IIR nanocom-posite was3688C and it was lower than that of IIR.Thismight be that the amount of graphene was too low toinfluence the thermal stability of the composite,whilesmall organic molecules on the surface of graphene withlow decomposition temperature induced the thermaldecomposition.For all other composites,the temperatureat the maximum reactive velocity increased with increas-ing graphene content,and for the10wt%composite itwas increased by118C compared with pure IIR.Thisindicated that the thermal stability of IIR was improvedby the addition of graphene.A significant number ofpapers had reported increased thermal stability of variouspolymers using graphene asfiller[15,22,23].T.Kuilaet al.reported that the better thermal stability of gra-phene/polyethylene composite system was due to the highaspect ratio of the monodispersed graphene layers whichacted as a barrier and inhibited the emission of small gas-eous molecules[23].DSC for the MG/IIR nanocomposites was shown inFig.7.The glass transition(T g)temperature of IIR wasobserved at270.58C,and the MG loading increased,theT g values of composites which were269.28C,268.68C, 268.98C,and269.18C with the loading levels of1,3,5, 10wt%,respectively.All the composites showed aslightly higher T g than that of pure IIR;among them thehighest one was268.68C of3wt%MG-IIR nanocompo-site.It indicated that the effective exfoliation of the MGincreased the T g due to the interaction between MG andpared with the results of graphene/polystyrenenanocomposite[24],the incorporation of PS nanoparticleson graphene sheets resulted in an increase in T g by178C,while the exfoliated MG did not influence the T g of MG-IIR nanocomposite very much.Therefore,the properties of nano-filler influenced the phase behavior of polymer matrix.The interaction between MG and IIR is under further study.The typical stress–strain curves for the MG-IIR and NG-IIR compositesfilms were presented in Fig.8.The mechanical performance of MG/IIR and NG/IIR compos-itefilms was significantly increased compared with pure IIRfilm(Fig.8a and b).The effect of MG content on mechanical properties of the nanocomposites was shown in Table 2.Stresses at 100%,200,and300%elongation of composites increased along with the MG loading increase.Therefore,in this study,MG-IIR nanocomposites had higher mechanical properties than that of pure IIR.On the basis of these stress–strain curves of tensile tests(Fig.8a and b),Young’s moduli were taken as the linear regression of the initial linear part of stress–strain curves.Figure9a showed the representative calculated Young’s moduli of IIR,NG-IIR,and MG-IIR.The corre-sponding Young’s modulus values were shown in Fig.9b. The addition of MG grapheneflakes significantly increased the Young’s modulus.Remarkably,the Young’s modulus of10wt%MG-IIR was16times that of pure IIR.In comparison,the10wt%NG-IIR compositesTABLE 2.Mechanical properties of pure IIR and MG-IIR nanocomposites.Stress at 100%(MPa)Stress at200%(MPa)Stress at300%(MPa)IIR0.170.190.181%MG-IIR0.250.230.183%MG-IIR0.440.380.295%MG-IIR0.540.430.3110%MG-IIR0.770.590.40FIG.9.(a)Representative calculated Young’s moduli of IIR,NG-IIR,and MG-IIR based on the slope of the elastic region.(b)Dependence ofYoung’s modulus on loading offillers in MG-IIR and NG-IIR nanocom-posites.6POLYMER ENGINEERING AND SCIENCE—-2011DOI10.1002/penimproved3.5times only,as shown in Fig.9b.It might be that the NG stacks did not exfoliate or intercalate in the IIR matrix.This result coincided with the results of the XRD and SEM analysis.Therefore,from the result of mechanical properties,it was deduced that the modifier on the surface of the gra-phene sheet not only caused the sheet to disperse in the IIR matrix,but also bonded to the large rubber chain in two dimensions,which gave the nanocomposite better tensile performance.According to the reference[25],gra-phene layers in poly(vinyl chloride)matrix enhanced the mechanical properties of PVC,because of the strong interfacial adhesion.Therefore,in our case,MG-IIR nano-composites had higher mechanical properties than the pure IIR,which may be due to the homogenous distribu-tion of exfoliated MG and the good compatibility with polymer matrix.CONCLUSIONMG-IIR nanocomposites were prepared by solution processing.SEM and XRD analysis indicated that the exfoliated MG was homogeneously disperse in the IIR matrix.The addition of MG greatly improved the mechan-ical property of the nanocomposites.The nanocomposites with10wt%MG showed the highest Young’s modulus, 3.4MPa,which was about16times higher than that of pure IIR,0.21MPa.REFERENCES1.S.Takahashi,H.A.Goldberg,C.A.Feeney,D.P.Karim,M.Farrell,K.O’Leary,and R.Paul,Polymer,47,3083 (2006).2.Y.Liang,W.Cao,Z.Li,Y.Wang,Y.Wu,and L.Zhang,Polym.Test.,27,270(2008).3.N.A.Kotov,Nature,442,254(2006).4.M.A.Pulickel and M.T.James,Nature,447,1066(2007).5.E.P.Giannelis,Adv.Mater.,8,29(1996).6.Camenzinda,W.R.Caserib,and S.E.Pratsinis,Nano Today,5,48(2010).7.S.C.Tjong,Mater.Sci.Eng.,R53,73(2006).8.Suresha,B.N.Ravi Kumar,M.Venkataramareddy,and T.Jayaraju,Mater.Des.,31,1993(2010).9.H.Kim and C.W.Macosko,Polymer,50,3797(2009).10.Y.Liang,Y.Wang,Y.Wu,Y.Lu,H.Zhang,and L.Zhang,Polym.Test.,24,12(2005).11.E.Burgaz,H.Lian,R.H.Alonso,L.Estevez,and E.P.Gian-nelis,Polymer,50,2348(2009).12.Y.Lian,Y.Liu,T.Jiang,J.Shu,H.Lian,and M.Cao,J.Phys.Chem.C,114,21(2010).13.K.Kalaitzidou,H.Fukushima,and L.T.Drzal,Compos.Sci.Technol.,67,2045(2007).14.J.M.Herrera-Alonso,Z.Sedlakova,and E.Marand,J.Membr.Sci.,349,251(2010).15.S.Ansari and E.P.Giannelis,J.Polym.Sci.Part B:Polym.Phys.,47,888(2009).uki, A.Tukigase,and M.Kato,Polymer,43,2185(2002).17.L.Q.Zhang,Y.Z.Wang,Y.Q.Wang,Y.Sui,and D.S.Yu,J.Appl.Polym.Sci.,78,1873(2000).18.W.S.Hummers and R.E.Offeman,J.Am.Chem.Soc.,80,1339(1958).19.P.J.Thistlethwaite and M.S.Hook,Langmuir,16,4993(2000).20.T.Szabo,O.Berkesi,and I.Dekany,Carbon,43,3186(2005).21.S.Toki,I.Sics,B.S.Hsiao,S.Murakami,M.Tosaka,S.Poompradub,S.Kohjiya,and Y.Ikeda,J.Polym.Sci.Part B:Polym.Phys.,42,956(2004).22.Q.L.Bao,H.Zhang,J.X.Yang,S.Wang,D.Y.Tong,R.Jose,S.Ramakrishna,C.T.Lim,and K.P.Loh,Adv.Funct.Mater.,20,1(2010).23.T.Kuila,S.Bose,C.E.Hong,M.E.Uddin,P.Khanra,N.H.Kim,and J.H.Lee,Carbon,49,1033(2011).24.A.S.Patole,S.P.Patole,H.Kang,J.Yoo,T.Kim,and J.Ahn,J.Colloid Interface Sci.,350,530(2010).25.S.Vadukumpully,J.Paul,N.Mahanta,and S.Valiyaveettil,Carbon,49,198(2011).DOI10.1002/pen POLYMER ENGINEERING AND SCIENCE—-20117。