毫米波雷达
毫米波雷达工作原理
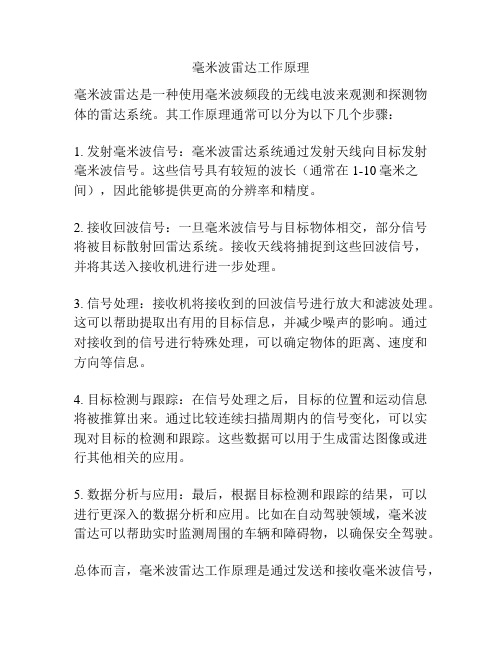
毫米波雷达工作原理
毫米波雷达是一种使用毫米波频段的无线电波来观测和探测物体的雷达系统。
其工作原理通常可以分为以下几个步骤:
1. 发射毫米波信号:毫米波雷达系统通过发射天线向目标发射毫米波信号。
这些信号具有较短的波长(通常在1-10毫米之间),因此能够提供更高的分辨率和精度。
2. 接收回波信号:一旦毫米波信号与目标物体相交,部分信号将被目标散射回雷达系统。
接收天线将捕捉到这些回波信号,并将其送入接收机进行进一步处理。
3. 信号处理:接收机将接收到的回波信号进行放大和滤波处理。
这可以帮助提取出有用的目标信息,并减少噪声的影响。
通过对接收到的信号进行特殊处理,可以确定物体的距离、速度和方向等信息。
4. 目标检测与跟踪:在信号处理之后,目标的位置和运动信息将被推算出来。
通过比较连续扫描周期内的信号变化,可以实现对目标的检测和跟踪。
这些数据可以用于生成雷达图像或进行其他相关的应用。
5. 数据分析与应用:最后,根据目标检测和跟踪的结果,可以进行更深入的数据分析和应用。
比如在自动驾驶领域,毫米波雷达可以帮助实时监测周围的车辆和障碍物,以确保安全驾驶。
总体而言,毫米波雷达工作原理是通过发送和接收毫米波信号,
以及对信号进行处理和分析,实现目标检测和跟踪的功能。
由于毫米波信号具有较短的波长和更高的分辨率,毫米波雷达在工业、军事和汽车等领域得到广泛应用。
2024年毫米波雷达市场发展现状
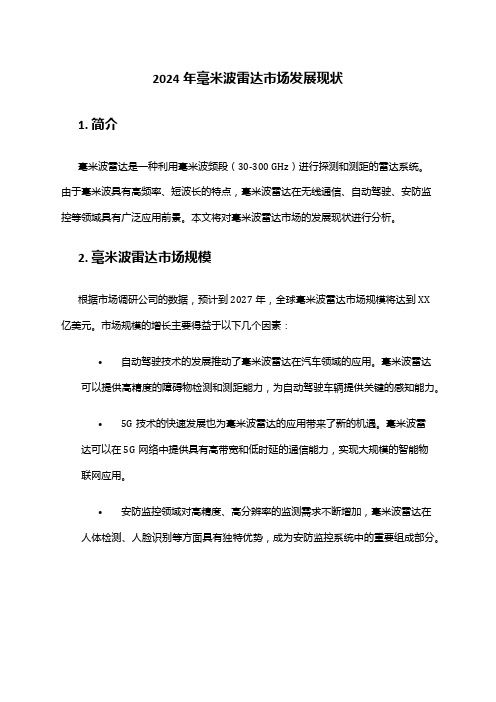
2024年毫米波雷达市场发展现状1. 简介毫米波雷达是一种利用毫米波频段(30-300 GHz)进行探测和测距的雷达系统。
由于毫米波具有高频率、短波长的特点,毫米波雷达在无线通信、自动驾驶、安防监控等领域具有广泛应用前景。
本文将对毫米波雷达市场的发展现状进行分析。
2. 毫米波雷达市场规模根据市场调研公司的数据,预计到2027年,全球毫米波雷达市场规模将达到XX亿美元。
市场规模的增长主要得益于以下几个因素:•自动驾驶技术的发展推动了毫米波雷达在汽车领域的应用。
毫米波雷达可以提供高精度的障碍物检测和测距能力,为自动驾驶车辆提供关键的感知能力。
•5G技术的快速发展也为毫米波雷达的应用带来了新的机遇。
毫米波雷达可以在5G网络中提供具有高带宽和低时延的通信能力,实现大规模的智能物联网应用。
•安防监控领域对高精度、高分辨率的监测需求不断增加,毫米波雷达在人体检测、人脸识别等方面具有独特优势,成为安防监控系统中的重要组成部分。
3. 毫米波雷达市场应用3.1 自动驾驶随着自动驾驶技术的快速发展,毫米波雷达成为自动驾驶系统中不可或缺的核心感知器。
毫米波雷达可以实现高精度的障碍物检测和测距,为自动驾驶车辆提供重要的环境感知信息。
3.2 5G通信毫米波雷达在5G通信中具有广泛的应用前景。
毫米波雷达可以提供高带宽、低时延的通信能力,支持大规模的智能物联网应用。
同时,毫米波雷达在5G通信中还可以实现多输入多输出(MIMO)技术,提升通信的可靠性和容量。
3.3 安防监控毫米波雷达在安防监控领域具有广泛应用。
由于毫米波雷达能够实现高分辨率的人体检测,可以在夜间或复杂环境下提供可靠的监测能力。
此外,毫米波雷达还可以进行人脸识别等高级监控功能,为安防系统提供更完善的功能。
4. 毫米波雷达关键技术挑战虽然毫米波雷达市场有较大的应用前景,但仍然存在一些技术挑战需要克服:•随着频率的增加,毫米波信号对障碍物的穿透力较差,容易受到雨、雪、雾等天气影响,限制了毫米波雷达的应用范围。
详细简介 什么是毫米波雷达 毫米波雷达分类及系统构成是怎么的
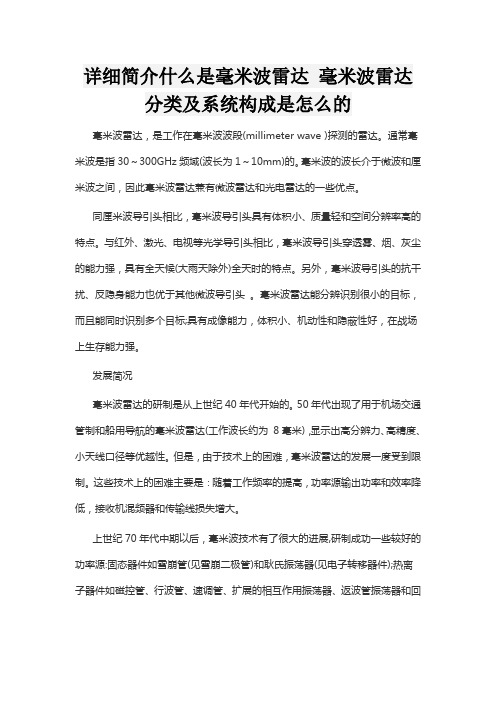
详细简介什么是毫米波雷达毫米波雷达分类及系统构成是怎么的毫米波雷达,是工作在毫米波波段(millimeter wave )探测的雷达。
通常毫米波是指30~300GHz频域(波长为1~10mm)的。
毫米波的波长介于微波和厘米波之间,因此毫米波雷达兼有微波雷达和光电雷达的一些优点。
同厘米波导引头相比,毫米波导引头具有体积小、质量轻和空间分辨率高的特点。
与红外、激光、电视等光学导引头相比,毫米波导引头穿透雾、烟、灰尘的能力强,具有全天候(大雨天除外)全天时的特点。
另外,毫米波导引头的抗干扰、反隐身能力也优于其他微波导引头。
毫米波雷达能分辨识别很小的目标,而且能同时识别多个目标;具有成像能力,体积小、机动性和隐蔽性好,在战场上生存能力强。
发展简况毫米波雷达的研制是从上世纪40年代开始的。
50年代出现了用于机场交通管制和船用导航的毫米波雷达(工作波长约为8毫米),显示出高分辨力、高精度、小天线口径等优越性。
但是,由于技术上的困难,毫米波雷达的发展一度受到限制。
这些技术上的困难主要是:随着工作频率的提高,功率源输出功率和效率降低,接收机混频器和传输线损失增大。
上世纪70年代中期以后,毫米波技术有了很大的进展,研制成功一些较好的功率源:固态器件如雪崩管(见雪崩二极管)和耿氏振荡器(见电子转移器件);热离子器件如磁控管、行波管、速调管、扩展的相互作用振荡器、返波管振荡器和回旋管等。
脉冲工作的固态功率源多采用雪崩管,其峰值功率可达5~15瓦(95吉赫)。
磁控管可用作高功率的脉冲功率源,峰值功率可达1~6千瓦(95吉赫)或1千瓦(140吉赫),效率约为10%。
回旋管是一种新型微波和毫米波振荡器或放大器,在毫米波波段可提供兆瓦级的峰值功率。
在低噪声混频器方面,肖特基二极管(见晶体二极管、肖特基结)混频器在毫米波段已得到应用,在100吉赫范围,低噪声混频器噪声温度可低至500K(未致冷)或100K(致冷)。
此外,在高增益天线、集成电路和鳍线波导等方面的技术也有所发展。
汽车毫米波雷达工作原理
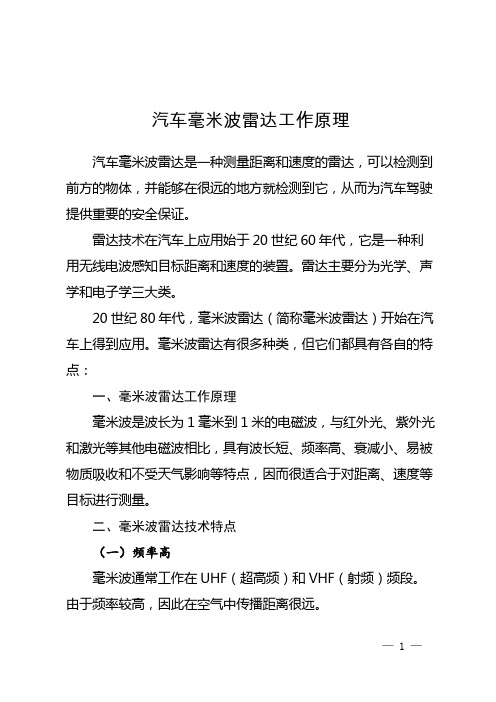
汽车毫米波雷达工作原理
汽车毫米波雷达是一种测量距离和速度的雷达,可以检测到前方的物体,并能够在很远的地方就检测到它,从而为汽车驾驶提供重要的安全保证。
雷达技术在汽车上应用始于20世纪60年代,它是一种利用无线电波感知目标距离和速度的装置。
雷达主要分为光学、声学和电子学三大类。
20世纪80年代,毫米波雷达(简称毫米波雷达)开始在汽车上得到应用。
毫米波雷达有很多种类,但它们都具有各自的特点:
一、毫米波雷达工作原理
毫米波是波长为1毫米到1米的电磁波,与红外光、紫外光和激光等其他电磁波相比,具有波长短、频率高、衰减小、易被物质吸收和不受天气影响等特点,因而很适合于对距离、速度等目标进行测量。
二、毫米波雷达技术特点
(一)频率高
毫米波通常工作在UHF(超高频)和VHF(射频)频段。
由于频率较高,因此在空气中传播距离很远。
— 1 —
(二)带宽宽
毫米波的带宽为1GHz至300GHz,是微波或红外的几倍甚至几十倍。
— 2 —。
毫米波雷达的工作原理
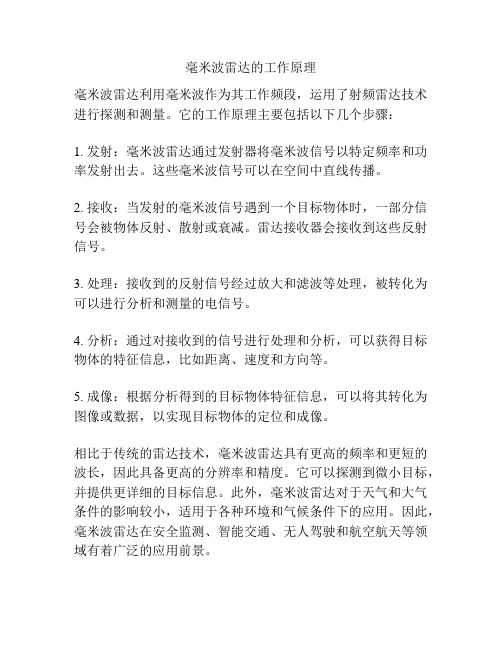
毫米波雷达的工作原理
毫米波雷达利用毫米波作为其工作频段,运用了射频雷达技术进行探测和测量。
它的工作原理主要包括以下几个步骤:
1. 发射:毫米波雷达通过发射器将毫米波信号以特定频率和功率发射出去。
这些毫米波信号可以在空间中直线传播。
2. 接收:当发射的毫米波信号遇到一个目标物体时,一部分信号会被物体反射、散射或衰减。
雷达接收器会接收到这些反射信号。
3. 处理:接收到的反射信号经过放大和滤波等处理,被转化为可以进行分析和测量的电信号。
4. 分析:通过对接收到的信号进行处理和分析,可以获得目标物体的特征信息,比如距离、速度和方向等。
5. 成像:根据分析得到的目标物体特征信息,可以将其转化为图像或数据,以实现目标物体的定位和成像。
相比于传统的雷达技术,毫米波雷达具有更高的频率和更短的波长,因此具备更高的分辨率和精度。
它可以探测到微小目标,并提供更详细的目标信息。
此外,毫米波雷达对于天气和大气条件的影响较小,适用于各种环境和气候条件下的应用。
因此,毫米波雷达在安全监测、智能交通、无人驾驶和航空航天等领域有着广泛的应用前景。
名词解释毫米波雷达

名词解释毫米波雷达
毫米波雷达是一种利用毫米波(mmWave)技术进行雷达探测和识别的电子设备,通常用于搜索、跟踪、识别和测距等应用。
它是雷达技术的一种重要分支,与传统的雷达相比,具有更高的穿透能力和更远的探测距离。
毫米波雷达的工作原理是利用毫米波在气体、固体和液体等材料中的传播特性,通过发送电磁波并测量其反射和回波的时间延迟和振幅等信息,实现对目标物体的探测和识别。
在毫米波雷达中,发送电磁波的波长通常在几十至几百毫米之间,而接收电磁波的波长则通常在几十至几百毫米之间。
这种新型雷达技术具有更高的频率,更远的探测距离和更高的探测精度,因此在军事、民用等领域得到了广泛的应用。
毫米波雷达的应用包括:搜索和跟踪飞机、导弹、坦克、车辆等目标;测距和定位;识别和跟踪隐形目标;以及进行环境感知和战争迷雾探测等。
此外,毫米波雷达还可以与其他技术结合,如计算机视觉和机器学习,以实现更高级别的应用,如自主飞行器的感知和自动驾驶等。
毫米波雷达的发展面临着一些挑战,如材料成本、信号处理算法的优化和系统设计的精度等。
未来,随着技术的不断发展和进步,毫米波雷达将会在更多领域得到应用,并推动雷达技术的发展。
毫米波雷达探测原理
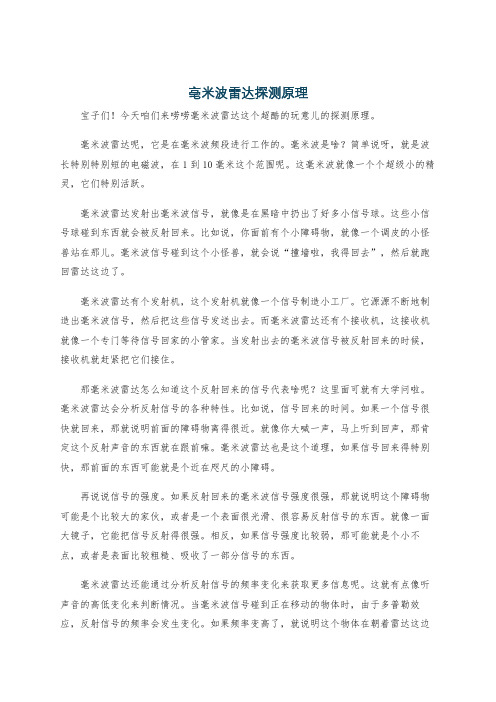
毫米波雷达探测原理宝子们!今天咱们来唠唠毫米波雷达这个超酷的玩意儿的探测原理。
毫米波雷达呢,它是在毫米波频段进行工作的。
毫米波是啥?简单说呀,就是波长特别特别短的电磁波,在1到10毫米这个范围呢。
这毫米波就像一个个超级小的精灵,它们特别活跃。
毫米波雷达发射出毫米波信号,就像是在黑暗中扔出了好多小信号球。
这些小信号球碰到东西就会被反射回来。
比如说,你面前有个小障碍物,就像一个调皮的小怪兽站在那儿。
毫米波信号碰到这个小怪兽,就会说“撞墙啦,我得回去”,然后就跑回雷达这边了。
毫米波雷达有个发射机,这个发射机就像一个信号制造小工厂。
它源源不断地制造出毫米波信号,然后把这些信号发送出去。
而毫米波雷达还有个接收机,这接收机就像一个专门等待信号回家的小管家。
当发射出去的毫米波信号被反射回来的时候,接收机就赶紧把它们接住。
那毫米波雷达怎么知道这个反射回来的信号代表啥呢?这里面可就有大学问啦。
毫米波雷达会分析反射信号的各种特性。
比如说,信号回来的时间。
如果一个信号很快就回来,那就说明前面的障碍物离得很近。
就像你大喊一声,马上听到回声,那肯定这个反射声音的东西就在跟前嘛。
毫米波雷达也是这个道理,如果信号回来得特别快,那前面的东西可能就是个近在咫尺的小障碍。
再说说信号的强度。
如果反射回来的毫米波信号强度很强,那就说明这个障碍物可能是个比较大的家伙,或者是一个表面很光滑、很容易反射信号的东西。
就像一面大镜子,它能把信号反射得很强。
相反,如果信号强度比较弱,那可能就是个小不点,或者是表面比较粗糙、吸收了一部分信号的东西。
毫米波雷达还能通过分析反射信号的频率变化来获取更多信息呢。
这就有点像听声音的高低变化来判断情况。
当毫米波信号碰到正在移动的物体时,由于多普勒效应,反射信号的频率会发生变化。
如果频率变高了,就说明这个物体在朝着雷达这边移动,就像一个小宠物欢快地向你跑来。
要是频率变低了,那这个物体就是在远离雷达,就像一个小坏蛋偷偷溜走啦。
毫米波雷达简介演示
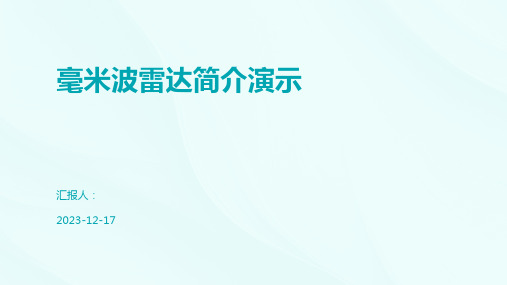
汇报人: 2023-12-17
目录
• 毫米波雷达概述 • 毫米波雷达技术原理 • 毫米波雷达性能指标及测试方
法 • 毫米波雷达在自动驾驶领域应
用案例分析
目录
• 毫米波雷达市场现状及发展趋 势预测
• 总结与展望:毫米波雷达未来 发展前景展望
01
毫米波雷达概述
定义与特点
定义
毫米波雷达是利用毫米波段电磁 波进行探测的雷达技术。
频段划分
毫米波频段通常指30-300GHz之间的电磁波,根据不同的应用场景,可划分为 多个子频段。
特点
毫米波具有波长短、频率高、衍射能力差但传播损耗小的特点,使其在雷达、 通信、医疗等领域具有广泛的应用前景。
发射与接收系统组成
发射系统
毫米波雷达的发射系统主要由发射天线、功率放大器和发射 信号源组成。通过调制发射信号的频率和幅度,实现不同距 离和角度的目标探测。
发展历程与趋势
发展历程
毫米波雷达技术经历了从模拟到数字 的发展过程,目前已经进入了太赫兹 频段,具有更高的分辨率和更强的抗 干扰能力。
发展趋势
未来毫米波雷达技术将朝着更高频率 、更低功耗、更小体积的方向发展, 同时将与其他传感器融合,实现更精 准的探测和识别。
02
毫米波雷达技术原理
毫米波频段划分及特点
特点
毫米波雷达具有频率高、波长短 、衍射能力弱等特点,适用于短 距离高分辨率的探测。
工作原理及应用领域
工作原理
毫米波雷达通过发射毫米波信号并接 收反射回来的信号,根据信号的往返 时间和强度等信息,计算出目标物体 的距离、速度和方位等参数。
应用领域
毫米波雷达广泛应用于无人驾驶、智 能交通、安防监控、航空航天等领域 。
毫米波雷达 范围

毫米波雷达范围
毫米波雷达是一种运用毫米波频段的雷达技术,其工作频率范围在30 GHz至300 GHz之间。
毫米波雷达具有高精度、高分辨率、抗干扰能力强等优点,因此在军事、安防、交通、医疗等领域都有广泛应用。
在军事领域,毫米波雷达被广泛应用于雷达侦察、预警、导航、反制等方面。
毫米波雷达可以通过穿透云雾、雨雪等天气干扰,实现对目标的高精度探测和跟踪,提高军事作战的效率和精度。
在安防领域,毫米波雷达也被广泛应用于空港、车站、商场等公共场所的人员检测和行李筛查等方面。
毫米波雷达可以检测到隐蔽的物品和藏匿的人员,有效提高安全防范能力。
在交通领域,毫米波雷达可以应用于智能交通系统中,实现车辆的自动识别、行驶轨迹跟踪、实时监控等功能。
毫米波雷达可以穿透雨雪等天气干扰,实现对车辆的高精度探测和跟踪,提高交通管理和安全保障水平。
在医疗领域,毫米波雷达可以应用于乳腺癌筛查、皮肤病诊断等方面。
毫米波雷达可以通过穿透皮肤、乳房等组织,实现对异常细胞的检测和诊断,提高疾病的早期发现率和治疗效果。
总的来说,毫米波雷达具有广泛的应用前景和市场空间,其范围涵盖了军事、安防、交通、医疗等多个领域,有望成为未来雷达技术的主流之一。
- 1 -。
毫米波雷达国标
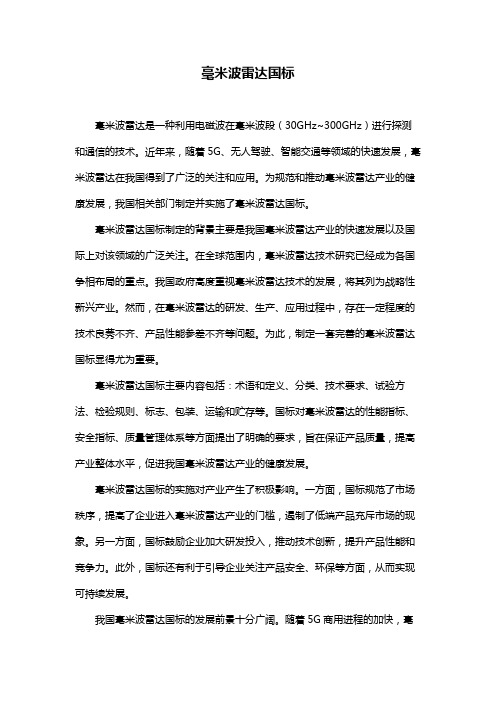
毫米波雷达国标毫米波雷达是一种利用电磁波在毫米波段(30GHz~300GHz)进行探测和通信的技术。
近年来,随着5G、无人驾驶、智能交通等领域的快速发展,毫米波雷达在我国得到了广泛的关注和应用。
为规范和推动毫米波雷达产业的健康发展,我国相关部门制定并实施了毫米波雷达国标。
毫米波雷达国标制定的背景主要是我国毫米波雷达产业的快速发展以及国际上对该领域的广泛关注。
在全球范围内,毫米波雷达技术研究已经成为各国争相布局的重点。
我国政府高度重视毫米波雷达技术的发展,将其列为战略性新兴产业。
然而,在毫米波雷达的研发、生产、应用过程中,存在一定程度的技术良莠不齐、产品性能参差不齐等问题。
为此,制定一套完善的毫米波雷达国标显得尤为重要。
毫米波雷达国标主要内容包括:术语和定义、分类、技术要求、试验方法、检验规则、标志、包装、运输和贮存等。
国标对毫米波雷达的性能指标、安全指标、质量管理体系等方面提出了明确的要求,旨在保证产品质量,提高产业整体水平,促进我国毫米波雷达产业的健康发展。
毫米波雷达国标的实施对产业产生了积极影响。
一方面,国标规范了市场秩序,提高了企业进入毫米波雷达产业的门槛,遏制了低端产品充斥市场的现象。
另一方面,国标鼓励企业加大研发投入,推动技术创新,提升产品性能和竞争力。
此外,国标还有利于引导企业关注产品安全、环保等方面,从而实现可持续发展。
我国毫米波雷达国标的发展前景十分广阔。
随着5G商用进程的加快,毫米波雷达在通信、物联网、无人驾驶等领域将迎来广泛应用。
此外,我国政府对科技创新的大力支持以及企业对国标的遵循和落实,将有助于我国毫米波雷达产业在国际竞争中脱颖而出,为实现产业升级和转型发展奠定坚实基础。
总之,毫米波雷达国标对于规范和推动我国毫米波雷达产业的健康发展具有重要意义。
毫米波雷达原理及器件-概述说明以及解释
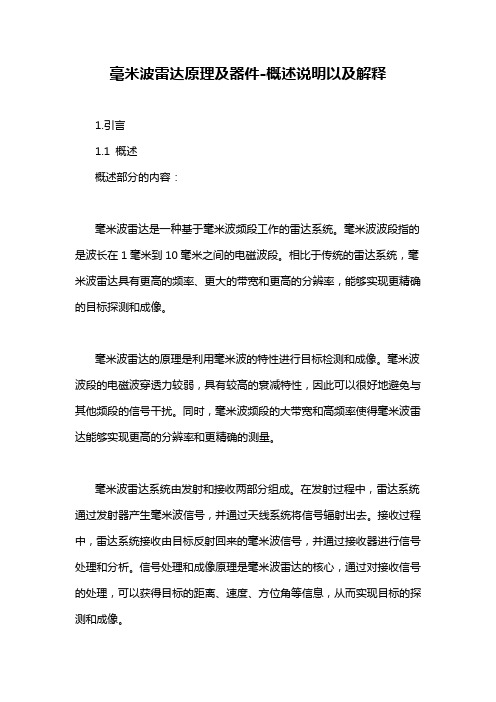
毫米波雷达原理及器件-概述说明以及解释1.引言1.1 概述概述部分的内容:毫米波雷达是一种基于毫米波频段工作的雷达系统。
毫米波波段指的是波长在1毫米到10毫米之间的电磁波段。
相比于传统的雷达系统,毫米波雷达具有更高的频率、更大的带宽和更高的分辨率,能够实现更精确的目标探测和成像。
毫米波雷达的原理是利用毫米波的特性进行目标检测和成像。
毫米波波段的电磁波穿透力较弱,具有较高的衰减特性,因此可以很好地避免与其他频段的信号干扰。
同时,毫米波频段的大带宽和高频率使得毫米波雷达能够实现更高的分辨率和更精确的测量。
毫米波雷达系统由发射和接收两部分组成。
在发射过程中,雷达系统通过发射器产生毫米波信号,并通过天线系统将信号辐射出去。
接收过程中,雷达系统接收由目标反射回来的毫米波信号,并通过接收器进行信号处理和分析。
信号处理和成像原理是毫米波雷达的核心,通过对接收信号的处理,可以获得目标的距离、速度、方位角等信息,从而实现目标的探测和成像。
毫米波雷达器件主要包括天线系统、频率合成器和发射机等。
天线系统负责发射和接收毫米波信号,其设计和性能直接影响了雷达系统的探测和成像能力。
频率合成器和发射机则负责产生稳定的毫米波信号,并将信号传输到天线系统进行辐射。
总之,毫米波雷达是一种利用毫米波频段工作的雷达系统,具有更高的分辨率和更精确的测量能力。
通过发射和接收毫米波信号,并经过信号处理和成像原理,毫米波雷达能够实现目标的探测和成像。
天线系统、频率合成器和发射机等是毫米波雷达的关键器件,其设计和性能对系统的性能具有重要影响。
未来,随着技术的不断进步和创新,毫米波雷达有望在多个领域得到广泛应用。
1.2 文章结构文章结构部分的内容应该包括对整篇文章的大致内容进行概述和介绍。
下面是文章结构部分的内容:文章结构:本文将介绍毫米波雷达的原理及器件。
文章主要分为以下几个部分:引言、正文和结论。
引言部分将对毫米波雷达进行一个概述,介绍其在科研和工业领域的应用以及当前的研究现状。
毫米波雷达的技术原理
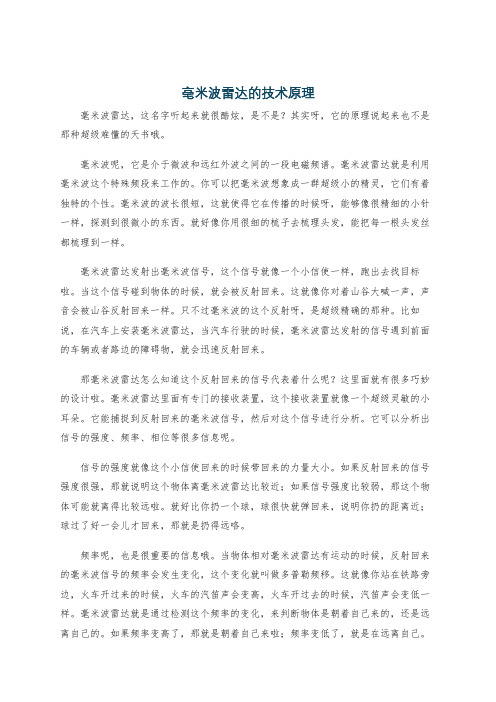
毫米波雷达的技术原理毫米波雷达,这名字听起来就很酷炫,是不是?其实呀,它的原理说起来也不是那种超级难懂的天书哦。
毫米波呢,它是介于微波和远红外波之间的一段电磁频谱。
毫米波雷达就是利用毫米波这个特殊频段来工作的。
你可以把毫米波想象成一群超级小的精灵,它们有着独特的个性。
毫米波的波长很短,这就使得它在传播的时候呀,能够像很精细的小针一样,探测到很微小的东西。
就好像你用很细的梳子去梳理头发,能把每一根头发丝都梳理到一样。
毫米波雷达发射出毫米波信号,这个信号就像一个小信使一样,跑出去找目标啦。
当这个信号碰到物体的时候,就会被反射回来。
这就像你对着山谷大喊一声,声音会被山谷反射回来一样。
只不过毫米波的这个反射呀,是超级精确的那种。
比如说,在汽车上安装毫米波雷达,当汽车行驶的时候,毫米波雷达发射的信号遇到前面的车辆或者路边的障碍物,就会迅速反射回来。
那毫米波雷达怎么知道这个反射回来的信号代表着什么呢?这里面就有很多巧妙的设计啦。
毫米波雷达里面有专门的接收装置,这个接收装置就像一个超级灵敏的小耳朵。
它能捕捉到反射回来的毫米波信号,然后对这个信号进行分析。
它可以分析出信号的强度、频率、相位等很多信息呢。
信号的强度就像这个小信使回来的时候带回来的力量大小。
如果反射回来的信号强度很强,那就说明这个物体离毫米波雷达比较近;如果信号强度比较弱,那这个物体可能就离得比较远啦。
就好比你扔一个球,球很快就弹回来,说明你扔的距离近;球过了好一会儿才回来,那就是扔得远咯。
频率呢,也是很重要的信息哦。
当物体相对毫米波雷达有运动的时候,反射回来的毫米波信号的频率会发生变化,这个变化就叫做多普勒频移。
这就像你站在铁路旁边,火车开过来的时候,火车的汽笛声会变高,火车开过去的时候,汽笛声会变低一样。
毫米波雷达就是通过检测这个频率的变化,来判断物体是朝着自己来的,还是远离自己的。
如果频率变高了,那就是朝着自己来啦;频率变低了,就是在远离自己。
毫米波雷达工作频率
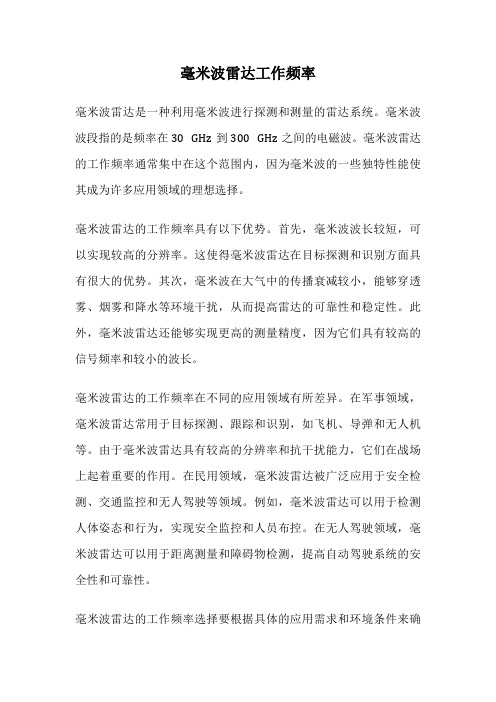
毫米波雷达工作频率毫米波雷达是一种利用毫米波进行探测和测量的雷达系统。
毫米波波段指的是频率在30 GHz到300 GHz之间的电磁波。
毫米波雷达的工作频率通常集中在这个范围内,因为毫米波的一些独特性能使其成为许多应用领域的理想选择。
毫米波雷达的工作频率具有以下优势。
首先,毫米波波长较短,可以实现较高的分辨率。
这使得毫米波雷达在目标探测和识别方面具有很大的优势。
其次,毫米波在大气中的传播衰减较小,能够穿透雾、烟雾和降水等环境干扰,从而提高雷达的可靠性和稳定性。
此外,毫米波雷达还能够实现更高的测量精度,因为它们具有较高的信号频率和较小的波长。
毫米波雷达的工作频率在不同的应用领域有所差异。
在军事领域,毫米波雷达常用于目标探测、跟踪和识别,如飞机、导弹和无人机等。
由于毫米波雷达具有较高的分辨率和抗干扰能力,它们在战场上起着重要的作用。
在民用领域,毫米波雷达被广泛应用于安全检测、交通监控和无人驾驶等领域。
例如,毫米波雷达可以用于检测人体姿态和行为,实现安全监控和人员布控。
在无人驾驶领域,毫米波雷达可以用于距离测量和障碍物检测,提高自动驾驶系统的安全性和可靠性。
毫米波雷达的工作频率选择要根据具体的应用需求和环境条件来确定。
在选择工作频率时,需要考虑目标距离、目标大小、环境干扰等因素。
通常情况下,较高的工作频率可以实现更高的分辨率和测量精度,但对于大范围目标的探测可能不够理想。
相反,较低的工作频率可以实现更大的探测范围,但分辨率和测量精度可能较低。
因此,需要根据具体的应用需求来选择适当的工作频率。
毫米波雷达的工作频率在30 GHz到300 GHz之间,具有较高的分辨率、较小的传播衰减和较高的测量精度等优势。
它们在军事、民用和无人驾驶等领域有着广泛的应用前景。
在选择工作频率时,需要根据具体的应用需求和环境条件来确定,以实现最佳的探测和测量效果。
毫米波雷达的发展将为各个领域带来更多的创新和应用机会。
毫米波雷达原理
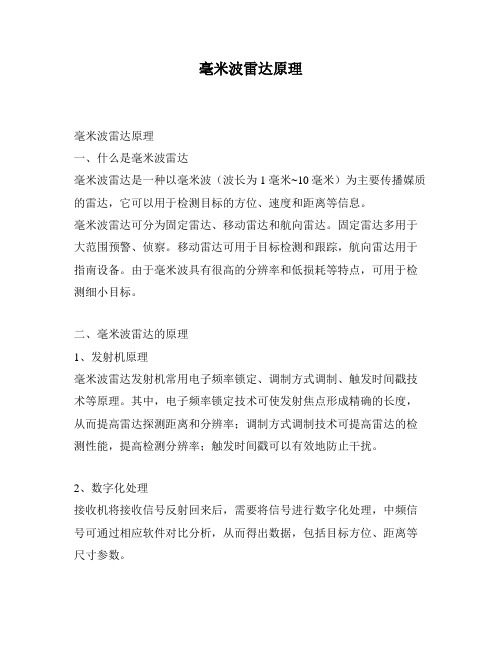
毫米波雷达原理
毫米波雷达原理
一、什么是毫米波雷达
毫米波雷达是一种以毫米波(波长为1毫米~10毫米)为主要传播媒质的雷达,它可以用于检测目标的方位、速度和距离等信息。
毫米波雷达可分为固定雷达、移动雷达和航向雷达。
固定雷达多用于大范围预警、侦察。
移动雷达可用于目标检测和跟踪,航向雷达用于指南设备。
由于毫米波具有很高的分辨率和低损耗等特点,可用于检测细小目标。
二、毫米波雷达的原理
1、发射机原理
毫米波雷达发射机常用电子频率锁定、调制方式调制、触发时间戳技术等原理。
其中,电子频率锁定技术可使发射焦点形成精确的长度,从而提高雷达探测距离和分辨率;调制方式调制技术可提高雷达的检测性能,提高检测分辨率;触发时间戳可以有效地防止干扰。
2、数字化处理
接收机将接收信号反射回来后,需要将信号进行数字化处理,中频信号可通过相应软件对比分析,从而得出数据,包括目标方位、距离等尺寸参数。
3、图像显示
使用图形化软件对接收的信号进行处理,可将信号转化为图形,然后
在图形中提取需要的数据,可用于语音、文本等报警,以便保证安全。
四、毫米波雷达的应用
1、运输方面
毫米波雷达可用于引导船只、汽车和飞机进行安全行驶。
2、武器瞄准
毫米波雷达还可以用于武器瞄准,比如火箭炮、飞弹等,可提高其准
确性和命中率。
3、安全
毫米波雷达可用于边界和海上等安全领域,可检测窃贼、潜水者、禁
足者以及非法进入的船只等。
4、运动传感
毫米波雷达可用于体育项目中的运动分析,可以准确测量单位时间内
运动者运动的距离、时间、力量等参数,为运动传感提供有效支持。
毫米波雷达国标
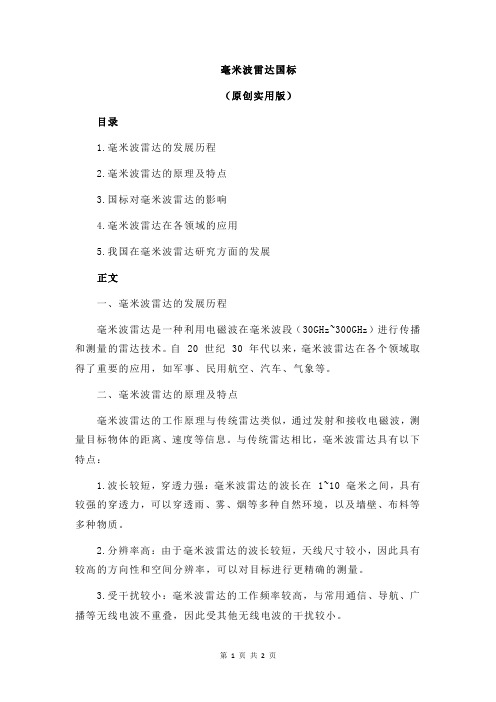
毫米波雷达国标(原创实用版)目录1.毫米波雷达的发展历程2.毫米波雷达的原理及特点3.国标对毫米波雷达的影响4.毫米波雷达在各领域的应用5.我国在毫米波雷达研究方面的发展正文一、毫米波雷达的发展历程毫米波雷达是一种利用电磁波在毫米波段(30GHz~300GHz)进行传播和测量的雷达技术。
自 20 世纪 30 年代以来,毫米波雷达在各个领域取得了重要的应用,如军事、民用航空、汽车、气象等。
二、毫米波雷达的原理及特点毫米波雷达的工作原理与传统雷达类似,通过发射和接收电磁波,测量目标物体的距离、速度等信息。
与传统雷达相比,毫米波雷达具有以下特点:1.波长较短,穿透力强:毫米波雷达的波长在 1~10 毫米之间,具有较强的穿透力,可以穿透雨、雾、烟等多种自然环境,以及墙壁、布料等多种物质。
2.分辨率高:由于毫米波雷达的波长较短,天线尺寸较小,因此具有较高的方向性和空间分辨率,可以对目标进行更精确的测量。
3.受干扰较小:毫米波雷达的工作频率较高,与常用通信、导航、广播等无线电波不重叠,因此受其他无线电波的干扰较小。
三、国标对毫米波雷达的影响我国在毫米波雷达领域制定了一系列国家标准,对毫米波雷达的技术要求、性能指标、测试方法等进行了详细的规定。
这些标准对毫米波雷达的研究、生产和应用起到了重要的指导作用,推动了我国毫米波雷达技术的发展和产业化。
四、毫米波雷达在各领域的应用毫米波雷达在多个领域具有广泛的应用前景,如:1.汽车:毫米波雷达在汽车领域的应用主要包括自动驾驶、自动泊车、碰撞预警等。
2.民用航空:毫米波雷达在民用航空领域的应用包括飞机着陆辅助、机场跑道监测等。
3.军事:毫米波雷达在军事领域的应用包括目标探测、跟踪、识别等。
4.气象:毫米波雷达在气象领域的应用包括降水测量、云层高度测量等。
五、我国在毫米波雷达研究方面的发展我国在毫米波雷达研究方面取得了显著的成果,已经在多个领域实现了毫米波雷达的应用。
毫米波雷达测量原理
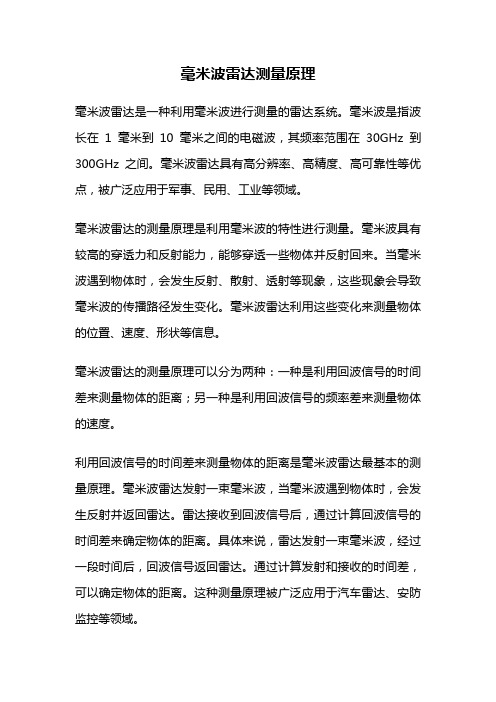
毫米波雷达测量原理毫米波雷达是一种利用毫米波进行测量的雷达系统。
毫米波是指波长在1毫米到10毫米之间的电磁波,其频率范围在30GHz到300GHz之间。
毫米波雷达具有高分辨率、高精度、高可靠性等优点,被广泛应用于军事、民用、工业等领域。
毫米波雷达的测量原理是利用毫米波的特性进行测量。
毫米波具有较高的穿透力和反射能力,能够穿透一些物体并反射回来。
当毫米波遇到物体时,会发生反射、散射、透射等现象,这些现象会导致毫米波的传播路径发生变化。
毫米波雷达利用这些变化来测量物体的位置、速度、形状等信息。
毫米波雷达的测量原理可以分为两种:一种是利用回波信号的时间差来测量物体的距离;另一种是利用回波信号的频率差来测量物体的速度。
利用回波信号的时间差来测量物体的距离是毫米波雷达最基本的测量原理。
毫米波雷达发射一束毫米波,当毫米波遇到物体时,会发生反射并返回雷达。
雷达接收到回波信号后,通过计算回波信号的时间差来确定物体的距离。
具体来说,雷达发射一束毫米波,经过一段时间后,回波信号返回雷达。
通过计算发射和接收的时间差,可以确定物体的距离。
这种测量原理被广泛应用于汽车雷达、安防监控等领域。
利用回波信号的频率差来测量物体的速度是毫米波雷达的另一种测量原理。
毫米波雷达发射一束毫米波,当毫米波遇到物体时,会发生反射并返回雷达。
由于物体的运动会导致回波信号的频率发生变化,因此可以通过计算回波信号的频率差来确定物体的速度。
具体来说,雷达发射一束毫米波,经过一段时间后,回波信号返回雷达。
通过计算发射和接收的频率差,可以确定物体的速度。
这种测量原理被广泛应用于交通监控、气象预报等领域。
毫米波雷达的测量原理还可以应用于测量物体的形状。
毫米波雷达发射一束毫米波,当毫米波遇到物体时,会发生反射并返回雷达。
由于物体的形状会导致回波信号的强度和相位发生变化,因此可以通过计算回波信号的强度和相位来确定物体的形状。
这种测量原理被广泛应用于三维成像、无人机避障等领域。
毫米波雷达 测角、测速、测距原理

毫米波雷达测角、测速、测距原理
毫米波雷达是一种利用毫米波频段的电磁波进行测量和探测的雷达系统。
它具有测角、测速和测距等多种功能,广泛应用于物流、交通、安防、军事等领域。
我们来看毫米波雷达的测角原理。
毫米波雷达通过发射一束狭窄的毫米波束,然后接收由目标物体反射回来的毫米波。
通过分析接收到的波形,可以确定目标物体的方向。
毫米波雷达的天线通常采用相控阵技术,通过改变天线阵列的相位和幅度,实现波束的方向控制。
通过扫描整个空间,毫米波雷达可以实现对目标物体的测角。
毫米波雷达还可以实现对目标物体的测速。
测速原理是基于多普勒效应,即当目标物体相对于雷达系统运动时,由于频率的变化,接收到的波形也会发生变化。
通过分析波形的频率变化,可以确定目标物体的速度。
毫米波雷达通常采用连续波或调频连续波的方式进行测速,具有高精度和高灵敏度的特点。
毫米波雷达还可以实现对目标物体的测距。
测距原理是基于雷达的工作原理,即通过测量发射和接收之间的时间差,从而计算出目标物体与雷达系统之间的距离。
毫米波雷达通常采用脉冲波的方式进行测距,具有较高的分辨率和精度。
毫米波雷达的测距能力通常可以达到几十米到几百米,适用于不同的应用场景。
毫米波雷达通过利用毫米波频段的电磁波进行测量和探测,具有测
角、测速和测距等多种功能。
它在物流、交通、安防、军事等领域发挥着重要作用。
通过合理的设计和优化,毫米波雷达可以实现高精度、高分辨率的目标检测和跟踪,为各行业的应用提供了可靠的技术支持。
未来随着技术的进一步发展,毫米波雷达有望在更多领域展现其潜力和优势。
- 1、下载文档前请自行甄别文档内容的完整性,平台不提供额外的编辑、内容补充、找答案等附加服务。
- 2、"仅部分预览"的文档,不可在线预览部分如存在完整性等问题,可反馈申请退款(可完整预览的文档不适用该条件!)。
- 3、如文档侵犯您的权益,请联系客服反馈,我们会尽快为您处理(人工客服工作时间:9:00-18:30)。
New Chirp Sequence Radar WaveformMATTHIAS KRONAUGEHERMANN ROHLING,Fellow,IEEETU Hamburg-HarburgGermanyThe general requirement in the automotive radar application is to measure the target range R and radial velocity v r simultaneously and unambiguously with high accuracy and resolution even in multitarget situations,which is a matter of the appropriate waveform design.Based on a single continuous wave chirp transmit signal,target range R and radial velocity v r cannot be measured in an unambiguous way.Therefore a so-called multiple frequency shift keying(MFSK)transmit signal was developed,which is applied to measure target range and radial velocity separately and simultaneously.In this case the radar measurement is based on a frequency and additionally on a phase measurement,which suffers from a lower estimation accuracy compared with a pure frequency measurement.This MFSK waveform can therefore be improved and outperformed by a chirp sequences waveform.Each chirp signal has in this case very short time duration T chirp.Therefore the measured beat frequency f B is dominated by target range R and is lessinfluenced by the radial velocity v r.The range and radial velocity estimation is based on two separate frequency measurements with high accuracy in both cases.Classical chirp sequence waveforms suffer from possible ambiguities in the velocity measurement.It is the objective of this paper to modify the classical chirp sequence to get an unambiguous velocity measurement even in multitarget situations.Manuscript received December14,2012;revised July9and December 20,2013,and March7,2014;released for publication March26,2014. DOI.No.10.1109/TAES.2014.120813.Refereeing of this contribution was handled by N.Goodman. Authors’address:Department of Telecommunications,TUHamburg-Harburg,Eißendorfer Straße40,Hamburg,21073,Germany. E-mail:(matthias.kronauge@tu-harburg.de).0018-9251/14/$26.00C 2014IEEE I.INTRODUCTIONRadar systems are based on a suitable technique to meet the high performance requirements for automotive radar sensors.Target range R and radial velocity v r are estimated unambiguously and simultaneously with high accuracy and resolution.However these features are subject to the radar waveform design.There are several interesting continuous waveform(CW)proposals which have been developed in the last years for new radar systems to measure range and radial velocity simultaneously[1–10].Automotive radar sensors apply frequency modulated (FM)CW signals with a signal bandwidth f sw of150MHz, which guaranties a range resolution of1m[8].There are several FMCW transmit signals proposed for automotive applications with totally different performance and resulting computation complexity.The block diagram of an FMCW radar system with a single transmit and two receive inphase and quadrature channels is shown in Fig.1.In case of any CW radar it is a characteristicfirst step in signal processing that the receive echo signal is down-converted into baseband by the instantaneous transmit frequency.Therefore the bandwidth of the baseband signal is much smaller than the bandwidth of the transmit signal.The resulting difference frequency between transmit and receive signal is called beat frequency f B,which is measured directly by a fast Fourier transform(FFT) procedure with high accuracy and resolution.The system parameters affecting the beat frequency are the chirp duration T chirp,the sweep bandwidth f sw,and the carrier frequency f0.The carrier frequency f0and the sweep bandwidth f sw are assumed to be unchanged in the following.For a long chirp duration the beat frequency f B is small and vice versa.In general the beat frequency f B can be positive or negative.Therefore a two channel receiver with I-and Q-channel is necessary.However it is important to notice that the beat frequency f B is influenced simultaneously by the target range R and radial velocity v r in an unresolvable way.In this paper a new class of FMCW signals is described,which consists of FMCW modulated chirp sequences where each individual chirp signal has a very short duration T chirp,which increases the baseband signal bandwidth.In this case the target range is measured by an FFT procedure,which is applied to each single and individual chirp signal.The Doppler frequency is also measured by a second FFT applied to the chirp sequence. The estimation accuracy will be improved in this case. II.SINGLE CHIRP SIGNALFor the simultaneous measurement of target range R and radial velocity v r a linear FMCW transmit signal is considered as depicted in Fig.2.The receive signal(blue line)is directly down-converted by the instantaneous transmit frequency(red line).Therefore the resulting baseband beat frequency f B describes the differenceFig.1.Transmitter and dual channel(I/Q)receiver. between the instantaneous transmit and receive frequency. In a measurement situation without any moving targets and due to the linear frequency modulation,the propagation delayτof the signal from the transmitter to the reflecting object and back to the radar receiver causes a frequency shift f R.In this static case the beat frequency f B is influenced by the target range R only.However in a moving target situation the beat frequency f B consists additionally of the Doppler frequency f D in the following quantitative way:f B=f R−f D=−f swT chirp2cR+2λv r.(1)The ideal time continuous baseband receive signal (without any noise components)is described by the beat frequency f B and the phaseφin the following equation:s(t)=exp(j2π(f B·t+φ)).(2) For a single chirp signal,(1)shows that the measured beat frequency f B contains the information about the target range f R and radial velocity v r in an unresolvable way.In order to measure target range R and radial velocity v r separately several extended waveforms have been developed for automotive applications,e.g.an up-and down-chirp scheme[2].III.MULTIPLE FREQUENCY SHIFT KEYINGWAVEFORMThe limitations of a single chirp signal can be totally overcome by a so-called multiple frequency shift keying (MFSK)transmit signal.In this case two linear FM signals with the same frequency modulation and an additional frequency shift are combined in the transmit signal.The MFSK transmit scheme is depicted in Fig.3.The two FM signals are developed in an intertwinedway.Fig.2.FMCW transmit and receivesignal.Fig.3.MFSK transmit and receive signals.The echo signals are down-converted by the corresponding instantaneous transmit frequency.This results in the two intertwined baseband signals s1(t)ands2(t):s1(t)=exp(j2π(f B·t+φ1)),(3a)s2(t)=exp(j2π(f B·t+φ2)).(3b) The frequency difference f shift of the two intertwined signals is very small compared with the carrier frequency, so that the baseband frequency f B will be the same for both intertwined receive signals.However the difference in the constant phase termsφ1andφ2is very important.In order to determine the beat frequency f B and the two phase termsφ1andφ2,the baseband signals s1(t)and s2(t)are sampled separately by the sampling interval T sa,one sample per frequency step,and transformed to the frequency domain by two separate FFT procedures:S1(m)=K−1k=0s1(k)·exp−j2πk·mK,(4a) S2(m)=K−1k=0s2(k)·exp−j2πk·mK,(4b)where k is the sample index of the time discrete baseband signal and m the beat frequency index.Both discrete spectra consist of K complex values indicating the amplitude and the phase of the signal at a given beat frequency.The beat frequencies caused by one target are identical in both spectra.However the phase terms at this specific beat frequency:φ1(m)=arg(S1(m)),(5a)φ2(m)=arg(S2(m))(5b) show a certain difference that depends on target range and Doppler frequency:φ(m)=φ2(m)−φ1(m)=2πf shift2c·R+f D·T sa.(6)Fig.4.MFSK waveform with signal processing scheme. The phase correction term f D·T sa is necessary due to the time shift T sa between the two signals s1(t)and s2(t).With the measured beat frequency f B[see(1)]and the measured phase difference φ(m)[see(6)]there are two equations that can be used to determine target range R and Doppler frequency f D simultaneously based on two measurement results.The complete signal processing procedure is illustrated in Fig.4.The MFSK waveform gives the opportunity to measure and to resolve targets in range and Doppler frequency simultaneously and unambiguously even in multitarget situations.However this technique suffers from low estimation accuracy due to the phase measurement.IV.CHIRP SEQUENCE WAVEFORMAnother important class of radar waveforms is described by chirp sequences[9].Fig.5shows such a chirp sequence with L coherently transmitted chirp signals with identical frequency modulation where each single chirp has a very small chirp duration T chirp.Due to the small chirp duration the received time continuous baseband signal of a single chirp has a large signal bandwidth.The beat frequency f B is calculated forFig.5.Chirp signal sequence.Fig.6.Two-dimensional beat and Doppler frequency matrix.each individual chirp signal by an FFT and contains again both the target range f R and Doppler frequency f D in an unsolvable way[see(1)].The received and down-converted echo signal of the chirp sequence contains the beat f B and the Doppler frequency f D as well as the phase informationφ.The time continuous baseband signal is analytically described by the following equation:s(t,l)=expj2πf B·t−f D·lT chirp+φ.(7) This baseband signal is sampled and FFT processed for each individual chirp signal,which splits the echo signal into K adjacent range gates:S(m,l)=K−1k=0s(k,l)·exp−j2πk·mK,(8)where k is the sample index of the time discrete baseband signal and m is the beat frequency index.The echo signal of each individual chirp signal contains the beat frequency f B.This procedure is repeated for each chirp signal and in total L FFTs are processed.The resulting spectra are stored column-wise in a two-dimensional matrix.In a second step the Doppler frequency f D is measured by applying a second FFT inside each single range gate. The FFT length in this case is L:Q(m,n)=L−1l=0S(m,l)·exp−j2πl·nL,(9)where n describes the discrete Doppler frequency index.In this case the FFT is applied to each row(range gate) of the above mentioned two-dimensional signal matrix. The described signal processing procedure is illustrated in Fig.6.For each detected target within the derived matrix the following linear system of equations is givenf B=f R−f D=−f swT chirp2c·R+2v rλ,(10a)Fig.7.Radar system with single receive channel.f D=−2v rλ.(10b)Due to these two different measurements of beat frequency f B and Doppler frequency f D the target range R and radial velocity v r can be calculated for each detected target as follows:R=−(f B+f D)·T chirpf swc2,(11a)v r=−f D λ2.(11b)Based on this chirp sequence waveform radar targets are resolved in range and in radial velocity separately.This feature is important for multiple target situations,which occur almost always in automotive applications.Two targets can be distinguished and resolved if they are separated in range byR=c2f sw.(12)Two moving targets can be resolved by their different radial velocities v r if they are separated at least byv r= f D·λ2=1L·T chirp ·λ2.(13)In case of a very short chirp duration T chirp the measured beat frequency f B is in any case negative and will never be positive.In this case the radar receiver can be developed by a single receive channel(inphase component)only. This property of a single receive channel reduces the hardware complexity and improves the estimation accuracy.This is an important advantage for chirp signals with very short chirp duration T chirp.Any channel calibration procedures can be avoided in this case.Fig.7 shows the structure of the radar system by a block diagram and a single channel receiver.V.AMBIGUITIES IN DOPPLER FREQUENCYIn case of a chirp sequence waveform and the resulting signal processing based on FFT processing for each chirp signal and additionally by FFT within each range gate, some ambiguities can occur in the Doppler frequency f D domain.The Doppler frequency is determined byFig.8.Ambiguous Doppler frequency measurement.TABLE ISystem Design Example ISystem Parameter Symbol Value Carrier Frequency f024GHzChirp Duration T chirp1msMaximum Radial Velocity v r,max 6.25m/sTime on Target T oT=L×T chirp64msVelocity Resolution v r0.1m/sSweep Bandwidth f sw150MHzRange Resolution R1mevaluating the baseband signal described in(7)from one chirp to the next.Therefore the maximum unambiguous Doppler frequency is defined by the chirp duration T chirp and is denoted byf D,max=1T chirp.(14)The unambiguous Doppler frequency f D,unamb can be obtained by adding a multiple q of the chirp repetition frequency1T chirpto the ambiguously estimated Doppler frequency f D,amb:f D,unamb=f D,amb+q·f D,max,q∈Z.(15) The unknown factor of q represents the ambiguity of the Doppler frequency measurement,as depicted in Fig.8.If q can be determined,the measured ambiguities are resolved.The system parameters of such an automotive radar system based on a chirp sequence waveform with L=64 adjacent chirp signals are given in Table I.The described 24GHz radar sensor has a target range resolution of1m due to the sweep bandwidth of150MHz.The radial velocity resolution is0.1m/s due to the64ms time on target.The unambiguous radial velocity,which can be measured by this waveform depends on the single chirp duration T chirp and results in6.25m/s=22.5km/h,which is clearly too small for automotive applications.These Doppler frequency ambiguities show a strong disadvantage of chirp sequence waveforms compared with the MFSK waveform.VI.NEW CHIRP SEQUENCE WAVEFORMThe main idea of this section and this new waveform is to design a chirp sequence waveform,which can avoid the discussed ambiguities in the Doppler frequency domain. This waveform willfinally fulfill all requirements of an automotive application.It will be a combination of the classical chirp sequence and an additional frequency shift keying or frequency modulation component.TheFig.9.New chirp sequence waveform. waveform design principle can be compared with the MFSK waveform of Section III.The new chirp sequence waveform consists of two sequences,which are intertwined to each other.The length of the two intertwined waveforms is2L·T chirp.Each chirp signal has the same frequency modulation,the same bandwidth,and the same chirp duration.However there is a frequency shift between two adjacent chirp signals.The new waveform with two intertwined chirp sequences is down-converted to the baseband domain in the classical way.The two resulting baseband real valued signals are denoted by s1(t)and s2(t).The new waveform is shown in Fig.9.The signal s1(t)consists of all even chirp numbers(2l =0;2;4...(2L–2)),while the signal s2(t)consists of all odd chirp numbers((2l+1)=1;3;5...(2L–1)).The two resulting time continuous signals are described bys1(t,l)=cos(j2π(f B·t−f D,amb·2l·T chirp+φ1)),s2(t,l)=cos(j2π(f B·t−f D,amb·(2l+1)·T chirp+φ2)).(16)These two time continuous and intertwined real valued baseband signals with beat frequency f B and ambiguous Doppler frequency f D,amb are sampled individually.Since in this case every second chirp is included in the FFT procedure,the maximum unambiguous Doppler frequency corresponds to twice the chirp duration:f D,max=12·T chirp.(17)The two time discrete signals are processed by afirst FFT to calculate the beat frequency f B and by a second range gate specific FFT to measure the ambiguous Doppler frequency f D,amb.After these FFT processing steps two different two-dimensional signal matrices are obtained as shown in Fig.10.The frequency shift f shift is chosen so small that the beat frequency measurement and ambiguous Doppler frequency measurement are not influenced by this frequency shift waveform parameter.That means in case of this new chirp sequence waveform the beat frequency f B and the ambiguous Doppler frequency f D,amb are measured for each target twice.Targets will be resolved by these two frequency measurements in range R and in ambiguous Doppler frequency f D,amb separately.This is shown in the two-dimensional matrix of Fig.10.Fig.10.Two-dimensional signal matrices.Each point inside the matrix is a complex value consisting of amplitude and phase(Q1(m,n)and Q2(m, n)).The signal phase depends on the target range R information only and is described analytically as follows:φ1(m,n)=arg(Q1(m,n)),(18a)φ2(m,n)=arg(Q2(m,n)).(18b) This additional phase information will now be used for a resolution of ambiguities in the Doppler frequency domain.The calculated phase difference φ(m,n),which depends on the target range R and on the known frequency shift f shift,can be calculated as follows:φ(m,n)=φ2(m,n)−φ1(m,n)=2πf shift2c·R+f D,amb·T chirp.(19)The phase correction term f D,amb·T chirp[see(6)]is again necessary due to the time shift T chirp between the receive signals s1(t,l)and s2(t,l).The maximum unambiguous target range R max in terms of the phase measurement can be adjusted by the frequency shift:R max=c21f shift,(20)which results e.g.,into300m in case of a frequency shift of500kHz.The signal processing and parameter estimation procedure to calculate an unambiguous Doppler frequency starts with an unambiguous rangeˆR measurement basedFig.11.Resolution of ambiguities in Doppler frequency measurement.on the measured phase difference φ(m,n ):ˆR= φ(m,n )2π−f D ,amb ·T chirp 1f shift c 2.(21)Due to the phase measurement this first range estimationstep has a limited accuracy.The target range R contribution to the beat frequency is f R :f R =−f sw T chirp 2c·ˆR.(22)Based on the measured range information theunambiguous Doppler frequency ˆf D ,unamb can therefore be obtained from the measured beat frequency f B and the estimated target range dependent frequency f R :ˆf D ,unamb =f B −f R .(23)With this information the Doppler frequency measurement ˆf D ,unamb is now unambiguous but due to the phasemeasurement is of low estimation accuracy.At this point the two ambiguous Doppler frequency measurementsf D,amb come into play.The ambiguous Doppler frequencies f D,amb are measured by FFT processing and have therefore a high estimation accuracy.To resolve the ambiguities the unambiguous Doppler frequency f D,unamb can be represented by the ambiguous Doppler frequency f D,amb and a parameter q as shown in (15):f D ,unamb =f D ,amb +q ·f D ,max .(24)The parameter q is calculated by the comparison of (24)and the unambiguously but inaccurately measured Doppler frequency ˆf D ,unamb of (23).This procedure is illustrated in Fig.11.From a signal processing point of view the parameter q is the nearest integer value obtained from (24)with the unambiguous Doppler frequency ˆf D ,unamb obtained from the phase measurement:q =round ˆf D ,unamb −f D ,ambf D ,max .(25)Finally target range R can be determined by the unambiguous and high accuracy Doppler frequency f D,unamb measurement:R =−(f B −f D ,unamb )·T chirp f sw c2.(26)The first Doppler frequency measurement is very accurate,however ambiguous.The second Doppler frequency measurement is unambiguous,however not accurate dueto the phase measurement.The combination of the two independent Doppler frequency estimation procedures gives finally the desired result.VII.DISCUSSION OF THE PROPOSED WAVEFORMIn automotive radar as in many other applications a simultaneous,accurate,and unambiguous measurement of target range and radial velocity is required even in multitarget situations.The considered MFSK waveform and the related signal processing scheme of the received signal is based on a simultaneous frequency and phase measurement.In additive noise situations the signal phase measurement is limited in accuracy.Therefore the target range and radial velocity measurements result in some accuracy limitations as well.The classical chirp sequence waveform has a high target range and radial velocity measurement accuracy because it is based on two frequency measurements with high accuracy each.Table I shows a system designexample.Due to the chirp duration of T chirp =1ms and the carrier frequency of 24GHz,the maximum unambiguous radial velocity is v r,max =6.25m/s,corresponding to 22.5km/h,which is not sufficient in almost all radarapplications.Therefore,the new chirp sequence waveform has been designed to avoid the ambiguities of the radial velocity measurement.The target range and radial velocity measurement accuracy is unchanged compared with the classical chirp sequence.Therefore,the new chirp sequence fulfills all requirements of a simultaneous,highly accurate,and unambiguous measurement of target range and radial velocity even in multitarget situations.VIII.CONCLUSIONAutomotive radar sensors require accurate,simultaneous,and unambiguous measurements of target range R and radial velocity v r .Both target parameters contribute to the beat frequency f B of each single chirp signal simultaneously but in an unresolvable way.The new chirp sequence waveform fulfills all these requirements of unambiguous measurements even in multitarget environments.The list of system parameters given in Table I can be updated for the new waveform.The new chirp sequence waveform measures the Doppler frequency f Dunambiguously.Therefore,no maximum unambiguous radial velocity parameter v r,max is given.However,the maximum radial velocity will be limited by the phase measurement accuracy or simply by the signal-to-noise ratio (SNR).Table II shows the system parameters and performance features for the new waveform.In contrast to the classic chirp sequence waveform,the new scheme makes use of a phase measurement,which is unambiguous within a certain maximum target range interval R max as defined in (20).For completeness this system parameter is added in Table II .With a frequency shift f shift =500kHz the maximum unambiguous targetTABLE IISystem Design Example IISystem Parameter Symbol ValueCarrier Frequency f024GHzChirp Duration T chirp1msTime on Target T oT=2L×T chirp128msVelocity Resolution v r0.05m/sSweep Bandwidth f sw150MHzRange Resolution R1mFrequency Shift f shift500kHzMaximum Range R max300mrange of R max=300m is achieved,which is sufficient for all automotive applications.The signal processing of the new waveform is based on two different ways to measure an unambiguous Doppler frequency f D,unamb.Thefirst measurement is unambiguous,however of low estimation accuracy due to the phase measurement.The second estimation of the unambiguous Doppler frequency f D,unamb is based on an accurate,however ambiguous,frequency measurement. The combination of these two measurements gives the desired result.The two-dimensional resolution of targets in range R and in Doppler frequency f D direction and the unambiguous measurements show strong advantages for the new chirp sequence waveform.REFERENCES[1]Fitzgerald,R.J.Effects of range-Doppler coupling on chirp radar trackingaccuracy.IEEE Transactions on Aerospace and Electronic Systems,AES-10,4(1974),528–532.[2]Rohling,H.,and Lissel,E.77GHz radar sensor for car application.Proceedings of the IEEE International Radar Conference,Alexandria,V A,May1995,pp.373–379.[3]Griffiths,H.D.New ideas in FM radar.Electronics&Communication Engineering Journal,2,5(Oct.1990),185–194.[4]Rihaczek,A.W.,and Mitchell,R.L.Design of zigzag FM signals.IEEE Transactions on Aerospace and Electronic Systems,AES-4,5(1968),680–692.[5]Barna,A.Pseudorandom frequency modulation in range-Doppler radar.IEEE Transactions on Aerospace and Electronic Systems,AES-5,2(1969),221–229.[6]Albanese,D.F.,and Klein,A.M.Pseudorandom code waveform design for CW radar.IEEE Transactions on Aerospace and Electronic Systems,AES-15,1(1979),67–75.[7]Artis,J.-P.,and Kemkemian,S.The radar in the automotive domain.Annals of Telecommunications,60,3–4(2005),326–356. [8]Rohling,H.,and Meinecke,M.-M.Waveform design principles for automotive radar systems.Proceedings of the CIE International Conference on Radar,Beijing,Oct.2001.[9]Stove,A.G.Linear FMCW radar techniques.IEE Proceedings on Radar and Signal Processing,139,5(Oct.1992),343–350.[10]Rohling,H.,and Kronauge,M.Continuous waveforms for automotive radar systems.In Gini,F.,De Maio,A.,and Patton,L.(Eds.),WaveformDesign and Diversity for Advanced Radar Systems(Radar,Sonar and Navigation Series22).London:Institution ofEngineering and Technology,2012,pp.173–205. Matthias Kronauge received the Dipl.-Ing.degree in electrical engineering from the Hamburg University of Technology(TUHH),Germany,in Oct.2009.Since Nov.2009he has been working with the Department of Telecommunications, TUHH.His main research interests include various aspects of radar signal processing, target detection,and waveform design for automotive radar and area surveillance applications.Hermann Rohling(F’05)is the head of the Institute of Telecommunications at Hamburg University of Technology,where he has developed an international reputationfor mobile communications and automotive radar systems.Prof.Rohling started his career at the AEG Research Institute,Ulm,Germany,as a researcher working in the area of digital signal processing for radar and communication applications.His research interests include signal theory,digital radar signal processing,detection,estimation, wideband mobile communications based on multicarrier transmission techniques (OFDM)for future broadband systems(4G),and differential GPS for high precision navigation.Prof.Rohling is the President of the German Institute of Navigation(DGON)and a member of Informationstechnische Gesellschaft(ITG).Every year he is the organizer of an International Radar Symposium(IRS)and an International OFDM Workshop.He was the Vice President of the Hamburg University of Technology,Germany for more than six years.。