PET 正电子发射型CT
医生要求做petct意味着什么
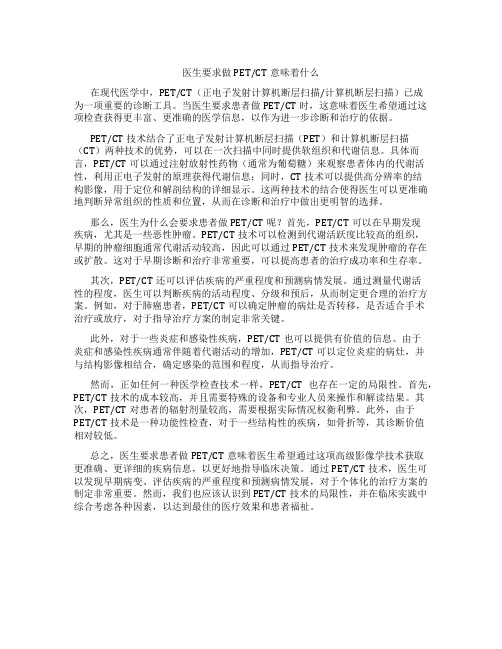
医生要求做PET/CT意味着什么在现代医学中,PET/CT(正电子发射计算机断层扫描/计算机断层扫描)已成为一项重要的诊断工具。
当医生要求患者做PET/CT时,这意味着医生希望通过这项检查获得更丰富、更准确的医学信息,以作为进一步诊断和治疗的依据。
PET/CT技术结合了正电子发射计算机断层扫描(PET)和计算机断层扫描(CT)两种技术的优势,可以在一次扫描中同时提供软组织和代谢信息。
具体而言,PET/CT可以通过注射放射性药物(通常为葡萄糖)来观察患者体内的代谢活性,利用正电子发射的原理获得代谢信息;同时,CT技术可以提供高分辨率的结构影像,用于定位和解剖结构的详细显示。
这两种技术的结合使得医生可以更准确地判断异常组织的性质和位置,从而在诊断和治疗中做出更明智的选择。
那么,医生为什么会要求患者做PET/CT呢?首先,PET/CT可以在早期发现疾病,尤其是一些恶性肿瘤。
PET/CT技术可以检测到代谢活跃度比较高的组织,早期的肿瘤细胞通常代谢活动较高,因此可以通过PET/CT技术来发现肿瘤的存在或扩散。
这对于早期诊断和治疗非常重要,可以提高患者的治疗成功率和生存率。
其次,PET/CT还可以评估疾病的严重程度和预测病情发展。
通过测量代谢活性的程度,医生可以判断疾病的活动程度、分级和预后,从而制定更合理的治疗方案。
例如,对于肺癌患者,PET/CT可以确定肿瘤的病灶是否转移,是否适合手术治疗或放疗,对于指导治疗方案的制定非常关键。
此外,对于一些炎症和感染性疾病,PET/CT也可以提供有价值的信息。
由于炎症和感染性疾病通常伴随着代谢活动的增加,PET/CT可以定位炎症的病灶,并与结构影像相结合,确定感染的范围和程度,从而指导治疗。
然而,正如任何一种医学检查技术一样,PET/CT也存在一定的局限性。
首先,PET/CT技术的成本较高,并且需要特殊的设备和专业人员来操作和解读结果。
其次,PET/CT对患者的辐射剂量较高,需要根据实际情况权衡利弊。
正电子发射型计算机断层扫描仪PETCT性能参数
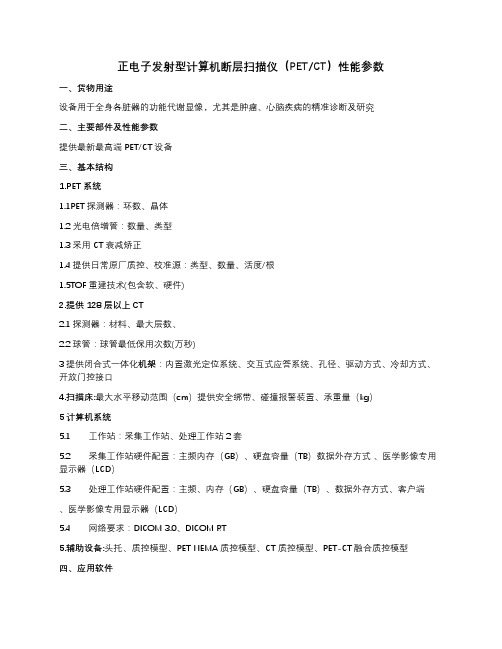
正电子发射型计算机断层扫描仪(PET/CT)性能参数一、货物用途设备用于全身各脏器的功能代谢显像,尤其是肿瘤、心脑疾病的精准诊断及研究二、主要部件及性能参数提供最新最高端PET/CT设备三、基本结构1.PET系统1.1PET探测器:环数、晶体1.2 光电倍增管:数量、类型1.3采用CT衰减矫正1.4提供日常原厂质控、校准源:类型、数量、活度/根1.5TOF重建技术(包含软、硬件)2.提供128层以上CT2.1探测器:材料、最大层数、2.2球管:球管最低保用次数(万秒)3提供闭合式一体化机架:内置激光定位系统、交互式应答系统、孔径、驱动方式、冷却方式、开放门控接口4.扫描床:最大水平移动范围(cm)提供安全绑带、碰撞报警装置、承重量(kg)5计算机系统5.1 工作站:采集工作站、处理工作站2套5.2 采集工作站硬件配置:主频内存(GB)、硬盘容量(TB)数据外存方式、医学影像专用显示器(LCD)5.3 处理工作站硬件配置:主频、内存(GB)、硬盘容量(TB)、数据外存方式、客户端、医学影像专用显示器(LCD)5.4 网络要求:DICOM 3.0、DICOM RT5.辅助设备:头托、质控模型、PET NEMA质控模型、CT质控模型、PET-CT融合质控模型四、应用软件1 PET应用软件1.1 图像采集软件(包含静态, 动态, 门控, 3D,List mode, 脑,心脏专用等)1.2 图像处理(重建)软件1.3 图像显示软件1.4 定量分析软件(SUV,VOI)1.5 校正软件1.6 质量控制软件1.7 NEMA测试软件1.8 3D迭代重建软件1.9 肿瘤疗效评估软件1.10 其他软件2 CT应用软件2.1 图像采集软件2.2 图像处理(重建)软件2.3 图像显示软件2.4 图像分析软件2.5 校正软件2.6 质量控制软件2.7 辐射剂量计算软件2.8 低剂量软件2.9 放疗模拟定位和放疗计划2.10 自动剂量调节软件2.11 其他软件3 PET/CT应用软件3.1 同机图像融合软件3.2 异机图像融合软件3.3 图像处理软件3.4 图像显示软件3.5 图像分析软件3.6 校正软件3.7 质量控制软件3.8 图像传输软件3.9 其他软件五、辅助配件1.高压注射器2台2.中文报告系统1套3.激光打印机1套4.放疗定位灯1台5.PET入墙注射防护台6.自动注射系统7.辐射防护用品7.1 钨合金药物分装翻转防护罐1个(30ml)7.2 注射器钨合金防护套(3cc和5cc规格)各1套7.3药物转运防护罐7.4正电子药物使用废物桶7.5个人防护:铅眼镜(平光、近视300、近视500度)、柔软性铅背心、铅帽、铅围脖四件为1套、共3套(0.5mmPb)7.6 数字化辐射剂量监测系统(需涵盖整个PET/CT楼放射工作场所及周边环境)1套8.阅片电脑+竖屏6套。
petct术语
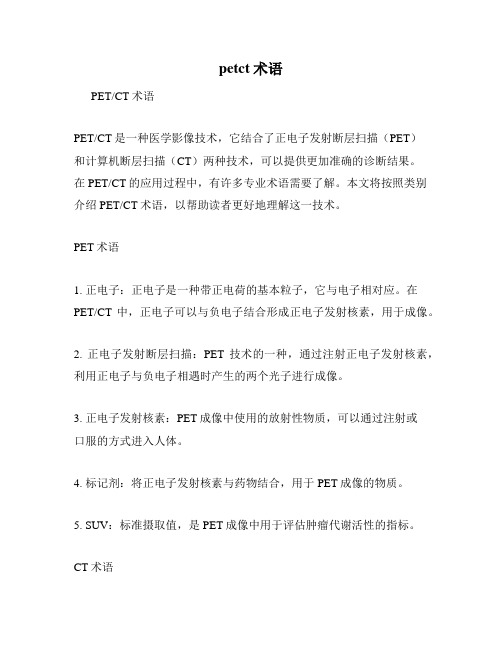
petct术语PET/CT术语PET/CT是一种医学影像技术,它结合了正电子发射断层扫描(PET)和计算机断层扫描(CT)两种技术,可以提供更加准确的诊断结果。
在PET/CT的应用过程中,有许多专业术语需要了解。
本文将按照类别介绍PET/CT术语,以帮助读者更好地理解这一技术。
PET术语1. 正电子:正电子是一种带正电荷的基本粒子,它与电子相对应。
在PET/CT中,正电子可以与负电子结合形成正电子发射核素,用于成像。
2. 正电子发射断层扫描:PET技术的一种,通过注射正电子发射核素,利用正电子与负电子相遇时产生的两个光子进行成像。
3. 正电子发射核素:PET成像中使用的放射性物质,可以通过注射或口服的方式进入人体。
4. 标记剂:将正电子发射核素与药物结合,用于PET成像的物质。
5. SUV:标准摄取值,是PET成像中用于评估肿瘤代谢活性的指标。
CT术语1. 计算机断层扫描:CT技术的一种,通过旋转X射线管和探测器,获取人体内部的断层图像。
2. 螺旋CT:一种高速计算机断层扫描技术,可以在短时间内获取更多的图像信息。
3. 造影剂:用于CT成像的物质,可以通过静脉注射或口服的方式进入人体。
4. CT值:计算机断层扫描中用于评估组织密度的指标。
PET/CT术语1. PET/CT融合图像:将PET和CT图像进行融合,可以提供更加准确的诊断结果。
2. PET/CT检查:一种结合了PET和CT技术的医学影像检查,可以用于诊断肿瘤、心血管疾病等疾病。
3. PET/CT报告:PET/CT检查结果的书面报告,包括图像和诊断结论。
4. PET/CT诊断:通过PET/CT检查得出的诊断结果,可以帮助医生制定治疗方案。
总结PET/CT技术是一种结合了PET和CT技术的医学影像技术,可以提供更加准确的诊断结果。
在PET/CT的应用过程中,有许多专业术语需要了解。
本文按照类别介绍了PET/CT术语,希望能够帮助读者更好地理解这一技术。
正电子发射断层扫描
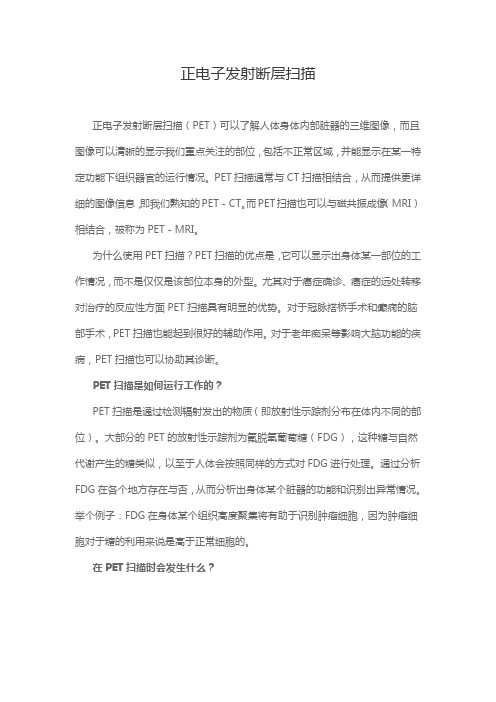
正电子发射断层扫描正电子发射断层扫描(PET)可以了解人体身体内部脏器的三维图像,而且图像可以清晰的显示我们重点关注的部位,包括不正常区域,并能显示在某一特定功能下组织器官的运行情况。
PET扫描通常与CT扫描相结合,从而提供更详细的图像信息,即我们熟知的PET-CT。
而PET扫描也可以与磁共振成像(MRI)相结合,被称为PET-MRI。
为什么使用PET扫描?PET扫描的优点是,它可以显示出身体某一部位的工作情况,而不是仅仅是该部位本身的外型。
尤其对于癌症确诊、癌症的远处转移对治疗的反应性方面PET扫描具有明显的优势。
对于冠脉搭桥手术和癫痫的脑部手术,PET扫描也能起到很好的辅助作用。
对于老年痴呆等影响大脑功能的疾病,PET扫描也可以协助其诊断。
PET扫描是如何运行工作的?PET扫描是通过检测辐射发出的物质(即放射性示踪剂分布在体内不同的部位)。
大部分的PET的放射性示踪剂为氟脱氧葡萄糖(FDG),这种糖与自然代谢产生的糖类似,以至于人体会按照同样的方式对FDG进行处理。
通过分析FDG在各个地方存在与否,从而分析出身体某个脏器的功能和识别出异常情况。
举个例子:FDG在身体某个组织高度聚集将有助于识别肿瘤细胞,因为肿瘤细胞对于糖的利用来说是高于正常细胞的。
在PET扫描时会发生什么?PET扫描一般在门诊进行,这就意味着你不用在医院过夜。
很重要的是,你必须按时到达你检查的地方,因为放射性示踪剂的有效期很短,如果迟到,你就只能放弃检查了。
准备工作你的预约信里会提到在你准备扫描前的一切注意事项。
六小时前禁食,可食用流质,但最好只是饮水。
预约后24小时尽量避免剧烈运动。
扫描的时候最好穿着宽松舒适的衣服,有的时候医院会要求你更换成病员服再进行扫描。
尽量避免穿戴首饰和含有金属的衣物(比如:拉链),如果穿戴上诉衣物在PET扫描的时候应先脱去。
放射性示踪剂的注射在扫描前,放射性示踪剂会从你手臂或手的静脉内注入,大约一个小时后,它将会被你身体内的细胞所吸收。
petct
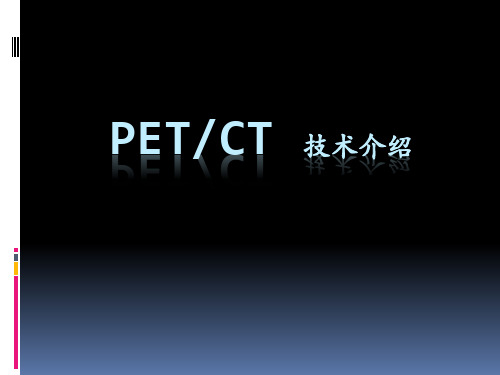
PET-CT(正电子发射断层显像 /X 线计算机体层成像仪)
将 PET(功能代谢显像)和CT(解剖结 构显像)两种先进的影像技术有机地结合在 一起的新型的影像设备. 它是将微量的正电子 核素示踪剂注射到人体内, 然后采用特殊的 体外探测 仪(PET)探测这些正电子核素人 体各脏器的分布情况,通过计算机断层显像 的方法显示人体的主要器官的生理代谢功能, 同时应用 CT 技术为这些核素分布情况进行精 确定位.
PET/CT
技术介绍
目录
PET/CT简介 发展历程 当前应用
PET -正电子发射型断层显像
Positron Emission Tomography
正电子发射成像(PET) 应用放射性示踪原理,以断面解剖形态 进行功能、代谢和受体显像的医学影像技术。 在分子水平上显示生物物质相应的生物活动 的空间分布、数量及其随时间的变化,故又 称为生化显像或分子显探测器接收到γ光子后产生一个定时脉冲,
这些定时脉冲分别输入符线路进行符合甄别, 挑选真符合事件。
符合线路设置了一个时间常数很小的时间窗
(通常≤15ns),同时落入时间窗的定时脉冲 被认为是同一个正电子湮灭事件中产生的γ光 子对,从而被符合电路记录。
时间窗排除了很多散射光子的进入。
PET/CT设备
性能: • 全身扫描20分钟完成 • 图像分辨率:4mm • 断层层厚:4.25mm • 各种图像定量分析软件
目前全球范围内有五家PET/CT制造商:西 门子医疗、GE医疗、日立医疗、飞利浦和东 芝医疗公司。下表总结的是由西门子分子影 像、GE医疗和飞利浦提供的PET/CT机型的 特点。
缺点: 放射量大
价格昂贵(仪器:
3000万+,做一次检 查7500-12000) 检查困难(机器少)
petct参数论证报告
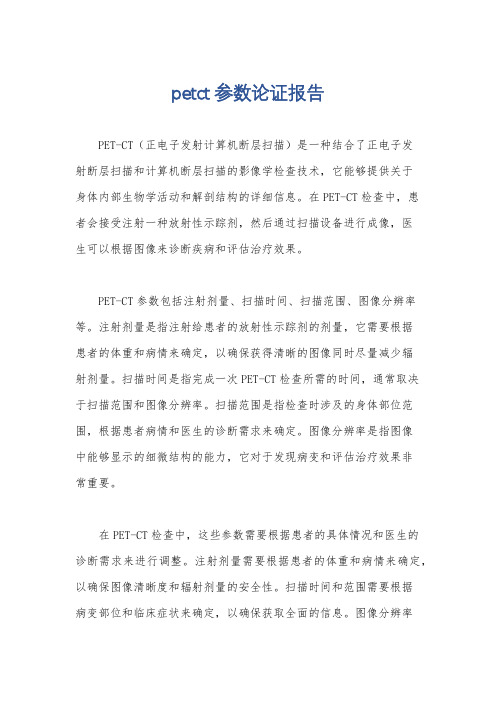
petct参数论证报告
PET-CT(正电子发射计算机断层扫描)是一种结合了正电子发
射断层扫描和计算机断层扫描的影像学检查技术,它能够提供关于
身体内部生物学活动和解剖结构的详细信息。
在PET-CT检查中,患
者会接受注射一种放射性示踪剂,然后通过扫描设备进行成像,医
生可以根据图像来诊断疾病和评估治疗效果。
PET-CT参数包括注射剂量、扫描时间、扫描范围、图像分辨率等。
注射剂量是指注射给患者的放射性示踪剂的剂量,它需要根据
患者的体重和病情来确定,以确保获得清晰的图像同时尽量减少辐
射剂量。
扫描时间是指完成一次PET-CT检查所需的时间,通常取决
于扫描范围和图像分辨率。
扫描范围是指检查时涉及的身体部位范围,根据患者病情和医生的诊断需求来确定。
图像分辨率是指图像
中能够显示的细微结构的能力,它对于发现病变和评估治疗效果非
常重要。
在PET-CT检查中,这些参数需要根据患者的具体情况和医生的
诊断需求来进行调整。
注射剂量需要根据患者的体重和病情来确定,以确保图像清晰度和辐射剂量的安全性。
扫描时间和范围需要根据
病变部位和临床症状来确定,以确保获取全面的信息。
图像分辨率
需要根据病变的大小和位置来确定,以确保能够清晰地显示病变部位。
总之,PET-CT参数的设定需要根据患者的具体情况和医生的诊
断需求来进行调整,以获得准确的诊断信息和评估治疗效果。
同时,医护人员在操作过程中需要严格遵守相关的操作规程和安全标准,
确保患者的安全和健康。
petct的原理和应用

PET/CT的原理和应用1. 基本介绍正电子发射断层扫描技术(Positron Emission Tomography,简称PET)结合计算机断层扫描技术(Computed Tomography,简称CT),即PET/CT,是一种医学成像技术。
它通过追踪和测量人体内放射性示踪剂在组织中的分布和代谢,提供精确的生物功能信息和解剖结构信息的结合,为医生提供病灶定位、细胞功能评估和治疗监测等方面的重要信息。
2. PET原理PET技术基于正电子放射性同位素的原理。
正电子是一种带正电荷的基本粒子,它和电子具有相同质量,但电荷相反。
在核子反应中,由于质子数目增加或中子数目减少,原子核内的质子与中子的比例变化,使原子核变得不稳定。
当核子组合得不稳定时,核子可以通过放射性衰变来恢复稳定状态。
正电子放射性同位素是由原子核产生的。
放射性示踪剂中的正电子放射性同位素会不稳定地衰变,释放出正电子。
这些正电子与体内的电子相撞,互相湮灭,产生能量释放,释放能量的过程称为正电子湮灭。
湮灭的能量以两个相对的方向以及同一方向的两个伽马光子的形式释放出来。
PET设备会检测这两个相对方向的伽马光子,进而计算出它们的发射位置。
通过多次记录在各个位置的伽马光子对的数据,可以恢复出体内放射性示踪剂的分布情况。
3. CT原理CT技术是一种断层扫描成像技术,通过旋转的X射线束在人体内部进行扫描。
CT技术的原理是不同组织对X射线的吸收程度不同,通过测量射线通过人体各部位的吸收程度,可以获得人体内部不同组织的密度分布信息。
CT扫描过程中,X射线管和探测器围绕人体旋转一圈,记录每个角度的X射线透过人体时的吸收情况。
计算机会根据这些数据重建出人体内部的横截面图像。
多个横截面图像组合在一起,可以得到人体的三维图像。
4. PET/CT的应用PET/CT技术在临床应用中具有广泛的应用价值。
•肿瘤诊断和分期:PET/CT技术可以提供非常高的灵敏度和特异性,可以检测癌症病灶及其转移,辅助肿瘤的分期和评估治疗效果。
PET-CT的临床应用
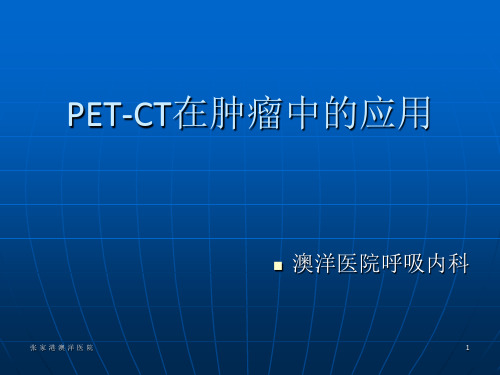
张家港澳洋医院
(六) Na18F Na18F是一种亲骨性代谢显像剂。18F通过与羟基磷灰石晶体中的羟基进行离子 交换沉积于骨质中。Na18F主要用于骨转移 癌的诊断及移植骨的监测。
张家港澳洋医院
14
(七)乏氧显像剂 18F-fluoromisonidazole (18F-MISO)是一种硝基咪 唑化合物,与乏氧细胞具有电子亲和力,可选择性地与肿 瘤乏氧细胞结合,是一种较好的乏氧显像剂。18F-MISO可 通过主动扩散通过细胞膜进入细胞,硝基(NO2)在硝基还 原酶的作用下被还原,在非乏氧细胞内,硝基还原产物可 立即被氧化;而在乏氧细胞内,硝基还原产物则不能发生 再氧化,还原产物与细胞内大分子物质发生不可逆结合, 滞留于乏氧细胞中,其浓聚程度与乏氧程度成正比。研究 结果证明,对于放射治疗,细胞在有氧状态下比在乏氧状 态下更敏感,因此,乏氧显像可用于预测放疗效果。18FMISO主要用于头颈部肿瘤如鼻咽癌的放疗效果预测。也可 用于估价心肌存活状态。
张家港澳洋医院 FDG主要用于恶性肿瘤的 诊断及良、恶性的鉴别诊断、临床分期、评价疗 效及监测复发等。 根据大脑的葡萄糖的代谢特点,18F-FDG主要用 于癫痫灶定位、早老性痴呆、脑血管疾病、抑郁 症诊断及研究;也用于研究大脑局部生理功能与 糖代谢关系,如视觉、听觉刺激、情感活动、记 忆活动等引起相应的大脑皮质区域的葡萄糖代谢 改变。 对于心肌主要用途是估测心肌存活。
12
张家港澳洋医院
(五) 11C-乙酸盐 11C-乙酸盐可被心肌细胞摄取,在线 粒体内转化为11C-乙酰辅酶A,并进入三羧 酸循环氧化为二氧化碳和水。能反映心肌 细胞的三羧酸循环流量,与心肌氧耗量成 正比。可用于估测心肌活力及肿瘤显像, 特别是对分化较高的原发性肝细胞癌具有 重要的诊断价值。
petct原理
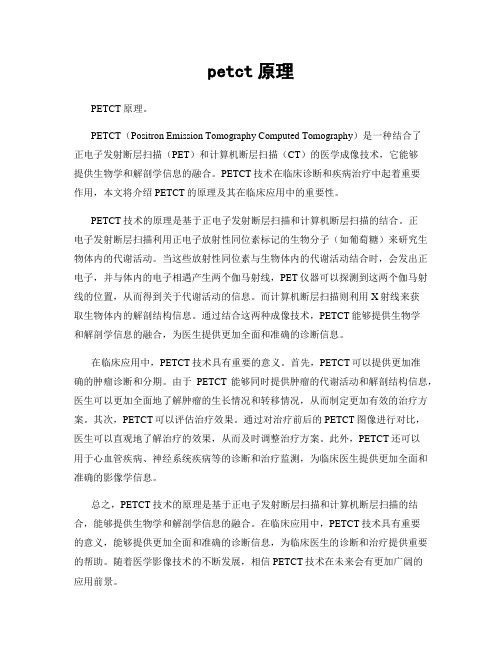
petct原理PETCT原理。
PETCT(Positron Emission Tomography Computed Tomography)是一种结合了正电子发射断层扫描(PET)和计算机断层扫描(CT)的医学成像技术,它能够提供生物学和解剖学信息的融合。
PETCT技术在临床诊断和疾病治疗中起着重要作用,本文将介绍PETCT的原理及其在临床应用中的重要性。
PETCT技术的原理是基于正电子发射断层扫描和计算机断层扫描的结合。
正电子发射断层扫描利用正电子放射性同位素标记的生物分子(如葡萄糖)来研究生物体内的代谢活动。
当这些放射性同位素与生物体内的代谢活动结合时,会发出正电子,并与体内的电子相遇产生两个伽马射线,PET仪器可以探测到这两个伽马射线的位置,从而得到关于代谢活动的信息。
而计算机断层扫描则利用X射线来获取生物体内的解剖结构信息。
通过结合这两种成像技术,PETCT能够提供生物学和解剖学信息的融合,为医生提供更加全面和准确的诊断信息。
在临床应用中,PETCT技术具有重要的意义。
首先,PETCT可以提供更加准确的肿瘤诊断和分期。
由于PETCT能够同时提供肿瘤的代谢活动和解剖结构信息,医生可以更加全面地了解肿瘤的生长情况和转移情况,从而制定更加有效的治疗方案。
其次,PETCT可以评估治疗效果。
通过对治疗前后的PETCT图像进行对比,医生可以直观地了解治疗的效果,从而及时调整治疗方案。
此外,PETCT还可以用于心血管疾病、神经系统疾病等的诊断和治疗监测,为临床医生提供更加全面和准确的影像学信息。
总之,PETCT技术的原理是基于正电子发射断层扫描和计算机断层扫描的结合,能够提供生物学和解剖学信息的融合。
在临床应用中,PETCT技术具有重要的意义,能够提供更加全面和准确的诊断信息,为临床医生的诊断和治疗提供重要的帮助。
随着医学影像技术的不断发展,相信PETCT技术在未来会有更加广阔的应用前景。
PET-CT 正电子发射计算机断层显像

PET-CTPET全称为正电子发射计算机断层显像(positron emission tomography PET),是反映病变的基因、分子、代谢及功能状态的显像设备。
它是利用正电子核素标记葡萄糖等人体代谢物作为显像剂,通过病灶对显像剂的摄取来反映其代谢变化,从而为临床提供疾病的生物代谢信息。
PET采用正电子核素作为示踪剂,通过病灶部位对示踪剂的摄取了解病灶功能代谢状态,可以宏观的显示全身各脏器功能,代谢等病理生理特征,更容易发现病灶。
CT可以精确定位病灶及显示病灶细微结构变化;PET/CT融合图像可以全面发现病灶,精确定位及判断病灶良恶性,故能早期,快速,准确,全面发现病灶。
作用PET的独特作用是以代谢显像和定量分析为基础,应用组成人体主要元素的短命核素如11C、13N、15O、18F等正电子核素为示踪剂,不仅可快速获得多层面断层影象、三维定量结果以及三维全身扫描,而且还可以从分子水平动态观察到代谢物或药物在人体内的生理生化变化,用以研究人体生理、生化、化学递质、受体乃至基因改变。
近年来,PET在诊断和指导治疗肿瘤、冠心病和脑部疾病等方面均已显示出独特的优越性。
原理一、PET显像的基本原理PET是英文Positron Emission Tomography的缩写。
其临床显像过程为:将发射正电子的放射性核素(如F-18等)标记到能够参与人体组织血流或代谢过程的化合物上,将标有带正电子化合物的放射性核素注射到受检者体内。
让受检者在PET的有效视野范围内进行PET显像。
放射核素发射出的正电子在体内移动大约1mm后与组织中的负电子结合发生湮灭辐射。
产生两个能量相等(511 KeV)、方向相反的γ光子。
由于两个光子在体内的路径不同,到达两个探测器的时间也有一定差别,如果在规定的时间窗内(一般为0-15 us),探头系统探测到两个互成180度(士0.25度)的光子时。
即为一个符合事件,探测器便分别送出一个时间脉冲,脉冲处理器将脉冲变为方波,符合电路对其进行数据分类后,送人工作站进行图像重建。
正电子发射型计算机断层显像-PET
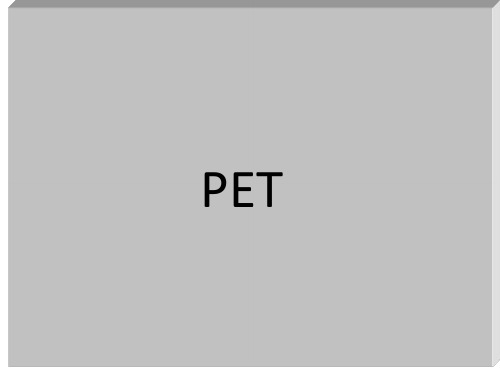
PET简介
PET ( 正 电 子 发 射 断 层 扫 描 技 术 ,Positron Emission Tomography)是继CT技术出现以来的又一种脑功能成像断 层技术,它首先向被试的体内注射一定的放射性物质,然 后再通过断层扫描技术测量脑的各个部位的放射量来测 量各个部位对葡萄糖的利用和局部的血流量,从而推测 不同脑区的活动情况。PET技术的发展和成熟使我们能 够安全准确地对正常人做某一心理活动的脑的部位进行 定位和监视。
PET用途
4.胰腺 胰腺疾病的诊断在临床上比较困难尤其是癌
病人常无症状PET 的解剖分辨率虽然不如X-CT 但 功能的改变总是早于形态的变化临床证明PET 对 胰腺病变诊断的准确率高于X-CT。
PET用途
5.肿瘤 有人用 1 3 N 1 5 O 观察肿瘤的血流和代谢变化,如
用C 1 5 O 2 测量局部血流发现肿瘤组织比正常组织代 谢快而坏死组织的血流量明显低还有人用18F-DG 研究脑胶质瘤的病理分级发现肿瘤分级高的代谢 率高而分级低的代谢低肿瘤周围组织的代谢则受 抑制这种方法可用于研究肿瘤生长的变化早期诊 断肿瘤有无复发。
✓ 60年代末出现了第一代商品化PET扫描仪,可进行断层面显像
✓ 1976年由Dr. Phelps和Dr. Hoffman设计,由ORTEC公司组装生产了第 一台用于临床的商品化的PET20世纪80年代更多公司投入了PET研制 ,岛津(Shimadzu,1980)、CTI公司(1983)、西门子公司(Siemens ,1986)通用电气公司(GE,1989)、日立公司(Hitachi,1989)和 ADAC公司(1989)POSITRON
PET影像的设备
正电子核素制备 正电子示踪剂制备 PET影像获取
petct医学名词解释
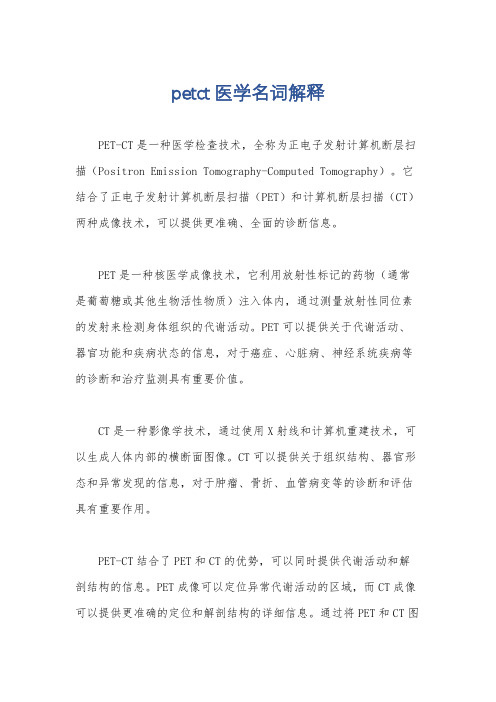
petct医学名词解释
PET-CT是一种医学检查技术,全称为正电子发射计算机断层扫描(Positron Emission Tomography-Computed Tomography)。
它结合了正电子发射计算机断层扫描(PET)和计算机断层扫描(CT)两种成像技术,可以提供更准确、全面的诊断信息。
PET是一种核医学成像技术,它利用放射性标记的药物(通常是葡萄糖或其他生物活性物质)注入体内,通过测量放射性同位素的发射来检测身体组织的代谢活动。
PET可以提供关于代谢活动、器官功能和疾病状态的信息,对于癌症、心脏病、神经系统疾病等的诊断和治疗监测具有重要价值。
CT是一种影像学技术,通过使用X射线和计算机重建技术,可以生成人体内部的横断面图像。
CT可以提供关于组织结构、器官形态和异常发现的信息,对于肿瘤、骨折、血管病变等的诊断和评估具有重要作用。
PET-CT结合了PET和CT的优势,可以同时提供代谢活动和解剖结构的信息。
PET成像可以定位异常代谢活动的区域,而CT成像可以提供更准确的定位和解剖结构的详细信息。
通过将PET和CT图
像进行叠加,医生可以更准确地评估病变的性质、范围和严重程度,有助于早期发现疾病、制定治疗方案和评估治疗效果。
总之,PET-CT是一种结合了正电子发射计算机断层扫描和计算
机断层扫描的医学成像技术,可以提供更准确、全面的诊断信息,
对于多种疾病的诊断和治疗监测具有重要价值。
公共基础知识PETCT基础知识概述
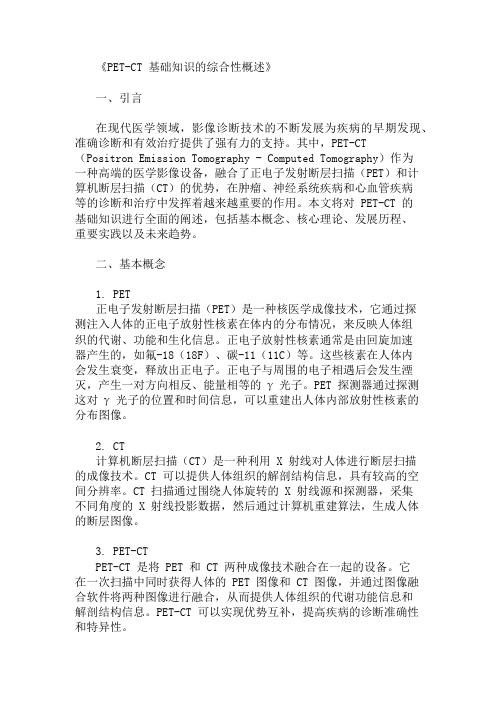
《PET-CT 基础知识的综合性概述》一、引言在现代医学领域,影像诊断技术的不断发展为疾病的早期发现、准确诊断和有效治疗提供了强有力的支持。
其中,PET-CT(Positron Emission Tomography - Computed Tomography)作为一种高端的医学影像设备,融合了正电子发射断层扫描(PET)和计算机断层扫描(CT)的优势,在肿瘤、神经系统疾病和心血管疾病等的诊断和治疗中发挥着越来越重要的作用。
本文将对 PET-CT 的基础知识进行全面的阐述,包括基本概念、核心理论、发展历程、重要实践以及未来趋势。
二、基本概念1. PET正电子发射断层扫描(PET)是一种核医学成像技术,它通过探测注入人体的正电子放射性核素在体内的分布情况,来反映人体组织的代谢、功能和生化信息。
正电子放射性核素通常是由回旋加速器产生的,如氟-18(18F)、碳-11(11C)等。
这些核素在人体内会发生衰变,释放出正电子。
正电子与周围的电子相遇后会发生湮灭,产生一对方向相反、能量相等的γ光子。
PET 探测器通过探测这对γ光子的位置和时间信息,可以重建出人体内部放射性核素的分布图像。
2. CT计算机断层扫描(CT)是一种利用 X 射线对人体进行断层扫描的成像技术。
CT 可以提供人体组织的解剖结构信息,具有较高的空间分辨率。
CT 扫描通过围绕人体旋转的 X 射线源和探测器,采集不同角度的 X 射线投影数据,然后通过计算机重建算法,生成人体的断层图像。
3. PET-CTPET-CT 是将 PET 和 CT 两种成像技术融合在一起的设备。
它在一次扫描中同时获得人体的 PET 图像和 CT 图像,并通过图像融合软件将两种图像进行融合,从而提供人体组织的代谢功能信息和解剖结构信息。
PET-CT 可以实现优势互补,提高疾病的诊断准确性和特异性。
三、核心理论1. 正电子放射性核素的代谢原理正电子放射性核素在人体内的分布取决于其参与的代谢过程。
什么是PETCT检查
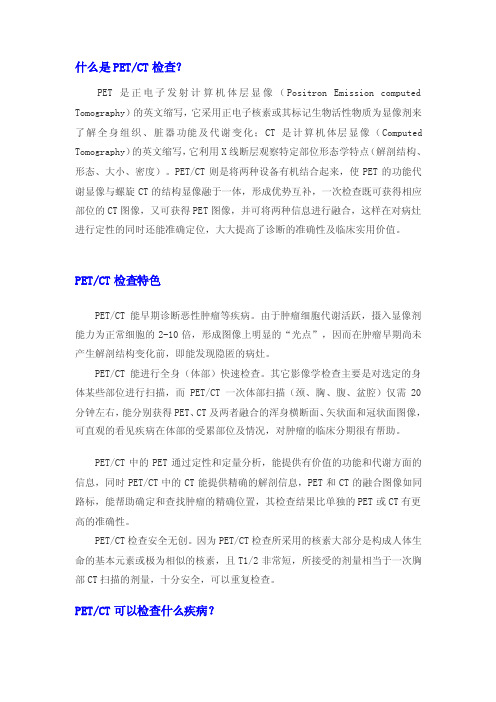
什么是PET/CT检查?PET是正电子发射计算机体层显像(Positron Emission computed Tomography)的英文缩写,它采用正电子核素或其标记生物活性物质为显像剂来了解全身组织、脏器功能及代谢变化;CT是计算机体层显像(Computed Tomography)的英文缩写,它利用X线断层观察特定部位形态学特点(解剖结构、形态、大小、密度)。
PET/CT则是将两种设备有机结合起来,使PET的功能代谢显像与螺旋CT的结构显像融于一体,形成优势互补,一次检查既可获得相应部位的CT图像,又可获得PET图像,并可将两种信息进行融合,这样在对病灶进行定性的同时还能准确定位,大大提高了诊断的准确性及临床实用价值。
PET/CT检查特色PET/CT能早期诊断恶性肿瘤等疾病。
由于肿瘤细胞代谢活跃,摄入显像剂能力为正常细胞的2-10倍,形成图像上明显的“光点”,因而在肿瘤早期尚未产生解剖结构变化前,即能发现隐匿的病灶。
PET/CT能进行全身(体部)快速检查。
其它影像学检查主要是对选定的身体某些部位进行扫描,而PET/CT一次体部扫描(颈、胸、腹、盆腔)仅需20分钟左右,能分别获得PET、CT及两者融合的浑身横断面、矢状面和冠状面图像,可直观的看见疾病在体部的受累部位及情况,对肿瘤的临床分期很有帮助。
PET/CT中的PET通过定性和定量分析,能提供有价值的功能和代谢方面的信息,同时PET/CT中的CT能提供精确的解剖信息,PET和CT的融合图像如同路标,能帮助确定和查找肿瘤的精确位置,其检查结果比单独的PET或CT有更高的准确性。
PET/CT检查安全无创。
因为PET/CT检查所采用的核素大部分是构成人体生命的基本元素或极为相似的核素,且T1/2非常短,所接受的剂量相当于一次胸部CT扫描的剂量,十分安全,可以重复检查。
PET/CT可以检查什么疾病?PET/CT则是将PET和CT有机结合一起起来,使用同一个检查床和同一个图像处理工作站,将PET图像和CT图像融合,可以同时放映病灶的病理生理变化和形态结构,明显提高诊断的准确性。
petct对人体的伤害有多大
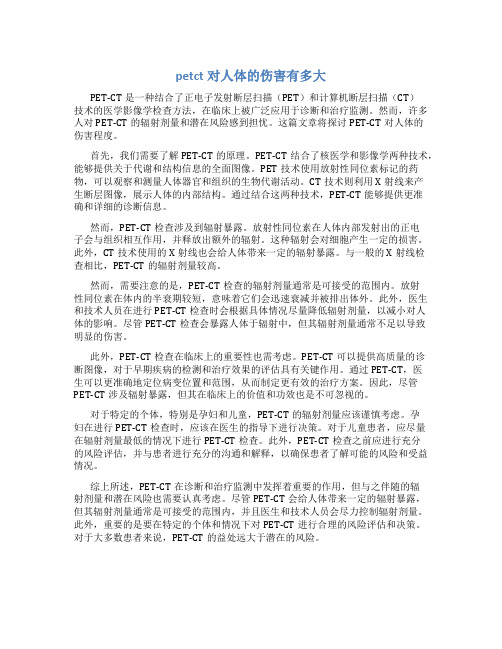
petct对人体的伤害有多大PET-CT是一种结合了正电子发射断层扫描(PET)和计算机断层扫描(CT)技术的医学影像学检查方法,在临床上被广泛应用于诊断和治疗监测。
然而,许多人对PET-CT的辐射剂量和潜在风险感到担忧。
这篇文章将探讨PET-CT对人体的伤害程度。
首先,我们需要了解PET-CT的原理。
PET-CT结合了核医学和影像学两种技术,能够提供关于代谢和结构信息的全面图像。
PET技术使用放射性同位素标记的药物,可以观察和测量人体器官和组织的生物代谢活动。
CT技术则利用X射线来产生断层图像,展示人体的内部结构。
通过结合这两种技术,PET-CT能够提供更准确和详细的诊断信息。
然而,PET-CT检查涉及到辐射暴露。
放射性同位素在人体内部发射出的正电子会与组织相互作用,并释放出额外的辐射。
这种辐射会对细胞产生一定的损害。
此外,CT技术使用的X射线也会给人体带来一定的辐射暴露。
与一般的X射线检查相比,PET-CT的辐射剂量较高。
然而,需要注意的是,PET-CT检查的辐射剂量通常是可接受的范围内。
放射性同位素在体内的半衰期较短,意味着它们会迅速衰减并被排出体外。
此外,医生和技术人员在进行PET-CT检查时会根据具体情况尽量降低辐射剂量,以减小对人体的影响。
尽管PET-CT检查会暴露人体于辐射中,但其辐射剂量通常不足以导致明显的伤害。
此外,PET-CT检查在临床上的重要性也需考虑。
PET-CT可以提供高质量的诊断图像,对于早期疾病的检测和治疗效果的评估具有关键作用。
通过PET-CT,医生可以更准确地定位病变位置和范围,从而制定更有效的治疗方案。
因此,尽管PET-CT涉及辐射暴露,但其在临床上的价值和功效也是不可忽视的。
对于特定的个体,特别是孕妇和儿童,PET-CT的辐射剂量应该谨慎考虑。
孕妇在进行PET-CT检查时,应该在医生的指导下进行决策。
对于儿童患者,应尽量在辐射剂量最低的情况下进行PET-CT检查。
pet一ct陪同人员辐射

pet一ct陪同人员辐射
PET-CT(正电子发射计算机断层扫描)是一种医学影像技术,它结合了正电子发射计算机断层扫描和CT(X射线计算机断
层扫描)技术,用于检测和评估肿瘤、心血管疾病和其他疾病。
PET-CT扫描需要受训的技术人员进行操作和监测。
这些人员
通常是医学影像技术师或核医学技术师,他们具有专业的知识和技能,能够准确进行扫描,并确保病人的安全。
辐射是PET-CT扫描过程中产生的一种副作用。
PET-CT扫描
使用放射性同位素进行标记,这些同位素发出的射线会被扫描设备检测和记录,从而生成图像。
尽管这些辐射剂量很小,但长期暴露可能会对人体健康产生影响。
为了减小辐射对人员的危害,PET-CT技术人员会采取一系列
的辐射防护措施。
他们通常会佩戴防护服和戴上辐射防护眼镜,以保护自己免受辐射。
此外,他们会与病人保持一定的距离,并尽量减少在扫描室内的停留时间,以降低辐射暴露的风险。
需要注意的是,PET-CT扫描对于病人来说通常是安全的,因
为辐射剂量被严格控制在可接受的范围内。
医疗机构也会根据相关指导和法规定期检测和监测辐射设备,以确保其符合安全标准。
总之,PET-CT扫描需要受训的技术人员进行操作和监测,他
们会采取一系列的辐射防护措施,以确保他们自身的安全,并减小辐射对人员的危害。
petct结构组成 -回复
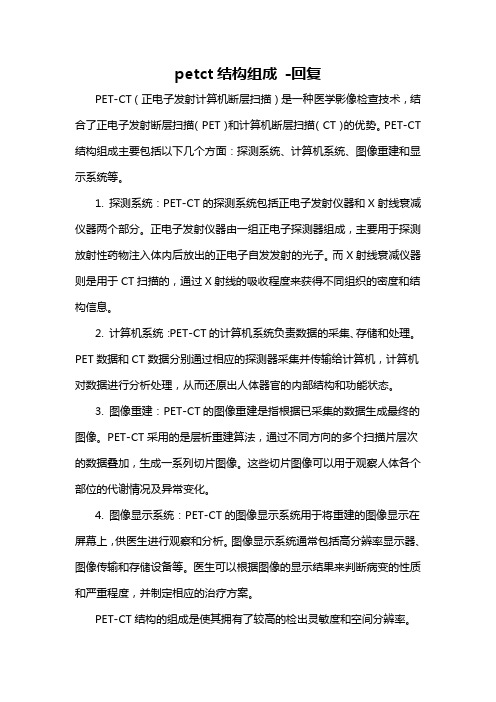
petct结构组成-回复PET-CT(正电子发射计算机断层扫描)是一种医学影像检查技术,结合了正电子发射断层扫描(PET)和计算机断层扫描(CT)的优势。
PET-CT 结构组成主要包括以下几个方面:探测系统、计算机系统、图像重建和显示系统等。
1. 探测系统:PET-CT的探测系统包括正电子发射仪器和X射线衰减仪器两个部分。
正电子发射仪器由一组正电子探测器组成,主要用于探测放射性药物注入体内后放出的正电子自发发射的光子。
而X射线衰减仪器则是用于CT扫描的,通过X射线的吸收程度来获得不同组织的密度和结构信息。
2. 计算机系统:PET-CT的计算机系统负责数据的采集、存储和处理。
PET数据和CT数据分别通过相应的探测器采集并传输给计算机,计算机对数据进行分析处理,从而还原出人体器官的内部结构和功能状态。
3. 图像重建:PET-CT的图像重建是指根据已采集的数据生成最终的图像。
PET-CT采用的是层析重建算法,通过不同方向的多个扫描片层次的数据叠加,生成一系列切片图像。
这些切片图像可以用于观察人体各个部位的代谢情况及异常变化。
4. 图像显示系统:PET-CT的图像显示系统用于将重建的图像显示在屏幕上,供医生进行观察和分析。
图像显示系统通常包括高分辨率显示器、图像传输和存储设备等。
医生可以根据图像的显示结果来判断病变的性质和严重程度,并制定相应的治疗方案。
PET-CT结构的组成是使其拥有了较高的检出灵敏度和空间分辨率。
PET可以提供组织的代谢信息,可以揭示肿瘤的生理和代谢状态;CT可以提供组织的解剖信息,可以展现不同组织的密度和结构。
通过PET和CT的结合,可以相辅相成,提供更全面和精确的诊断信息。
总之,PET-CT的结构组成主要包括PET探测系统、CT探测系统、计算机系统、图像重建和显示系统等几个方面。
这些组成部分的协同工作,使得PET-CT成为了一种非常重要的医学影像检查工具,广泛应用于肿瘤学、心脑血管疾病、神经科学等领域,为疾病的早期诊断和治疗提供了有效的支持和指导。
- 1、下载文档前请自行甄别文档内容的完整性,平台不提供额外的编辑、内容补充、找答案等附加服务。
- 2、"仅部分预览"的文档,不可在线预览部分如存在完整性等问题,可反馈申请退款(可完整预览的文档不适用该条件!)。
- 3、如文档侵犯您的权益,请联系客服反馈,我们会尽快为您处理(人工客服工作时间:9:00-18:30)。
Budinger, T. F.,VanBrocklin, H. F. “Positron-Emission Tomography (PET)”The Biomedical Engineering Handbook: Second Edition.Ed. Joseph D. BronzinoBoca Raton: CRC Press LLC, 200067Positron-EmissionTomography (PET)67.1 RadiopharmaceuticalsNuclear Reactor—Produced Radionuclides •Accelerator-Produced Radionuclides •Generator-ProducedRadionuclides •Radiopharmaceuticals •PET Radionuclides 67.2 InstrumentationBackground •PET Theory •PET Detectors •PhysicalFactors Affecting Resolution •Random Coincidences •Tomographic Reconstruction •Sensitivity •Statistical Properties of PET Thomas F. Budinger and Henry F. VanBrocklinSince the discovery of artificial radioactivity a half century ago, radiotracers, radionuclides, and radio-nuclide compounds have played a vital role in biology and medicine. Common to all is radionuclide (radioactive isotope) production. This section describes the basic ideas involved in radionuclide produc-tion and gives examples of the applications of radionuclides. The field of radiopharmaceutical chemistry has fallen into subspecialties of positron-emission tomography (PET) chemistry and general radiophar-maceutical chemistry, including specialists in technetium chemistry, taking advantage of the imaging attributes of technetium-99m.The two general methods of radionuclide production are neutron addition (activation) from neutron reactors to make neutron-rich radionuclides which decay to give off electrons and gamma rays and charged-particle accelerators (linacs and cyclotrons) which usually produce neutron-deficient isotopes that decay by electron capture and emission of x-rays, gamma rays, and positrons. The production of artificial radionuclides is governed by the number of neutrons or charged particles hitting an appropriate target per time, the cross section for the particular reaction, the number of atoms in the target, and the half-life of the artificial radionuclide:(67.1)where A (t ) is the produced activity in number of atoms per second, N is the number of target nuclei, σis the cross section (probability that the neutron or charged particles will interact with the nucleus to form the artificial radioisotope) for the reaction, φ is the flux of charged particles, and T 1/2 is the half-life of the product. Note that Nis the target mass divided by the atomic weight and multiplied by Thomas F. BudingerUniversity of Californiaat Berkeley Henry F. VanBrocklin University of Californiaat BerkeleyAvogadro’s number (60.24 × 1023) and σ is measured in cm 2. The usual flux is about 1014 neutrons per second or, for charged particles, 10 to 100 µA, which is equivalent to 6.25 × 1013 to 6.25 × 1014 charged particles per second.Nuclear Reactor-Produced Radionuclides.Thermal neutrons of the order of 1014 neutrons/s/cm 2 are produced in a nuclear reactor usually during a controlled nuclear fission of uranium, though thorium or plutonium are also used. High specific activity neutron-rich radionuclides are produced usually through the (n, γ), (n, p ), or (n, α) reactions (Fig. 67.1a ).The product nuclides usually decay by β– followed by γ. Most of the reactor-produced radionuclides are produced by the (n, γ) reaction. The final step in the production of a radionuclide consists of the separation of the product nuclide from the target container by chemical or physical means.An alternative method for producing isotopes from a reactor is to separate the fission fragments from the spent fuel rods. This is the leading source of 99Mo for medical applications. The following two methods of 99Mo production are examples of carrier-added and no-carrier-added radionuclide synthesis, respec-tively. In Fig. 67.1a , the 99Mo is production from 98Mo. Only a small fraction of the 98Mo nuclei will be converted to 99Mo. Therefore, at the end of neutron bombardment, there is a mixture of both isotopes.These are inseparable by conventional chemical separation techniques, and both isotopes would partic-ipate equally well in chemical reactions. The 99Mo from fission of 238U would not contain any other isotopes of Mo and is considered carrier-free. Thus radioisotopes produced by any means having the same atomic number as the target material would be considered carrier-added. Medical tracer techniques obviate the need for carrier-free isotopes.Accelerator-Produced RadionuclidesCyclotrons and linear accelerators (linacs) are sources of beams of protons, deuterons, or helium ions that bombarded targets to produce neutron-deficient (proton-rich) radionuclides (Fig. 67.1b ). The neu-tron-deficient radionuclides produced through these reactions are shown in Table 67.1. These product nuclides (usually carrier-free) decay either by electron capture or by positron emission tomography or both, followed by γ emission. In Table 67.2, most of the useful charged-particle reactions are listed.The heat produced by the beam current on the target material can interfere with isotope production and requires efficient heat-removal strategies using extremely stable heat-conducting target materials such as metal foils, electroplates metals, metal powders, metal oxides, and salts melted on duralmin plate.All the modern targets use circulating cold deionized water and/or chilled helium gas to aid in cooling the target body and window foils. Cyclotrons used in medical studies have 11C, 13N, 15O, and 18F production capabilities that deliver the product nuclides on demand through computer-executed commands. The FIGURE 67.1(a ) High specific activity neutron-excess radionuclides are produced usually through the (n , γ), (n, p )or (n, α) reactions. The product nuclides usually decay β– followed by γ. Most of the reactor produced radionuclides are produced by the (n, γ) reaction. (b ) Cyclotrons and linear accelerators (linacs) are sources of beams of protons,deuterons, or helium ions which bombard targets to produce neutron-deficient radionuclides.Arsenic-74*17.9 d A positron emitting chemical analog of phosphorusBarium-128* 2.4 d Parent in the generator system for producing the positron emitting 128Cs, a potassium analog Berylium-7*53.37 d Berylliosis studiesBromine-7757 h RadioimmunotherapyBromin-8235.3 h Used in metabolic studies and studies of estrogen receptor contentCarbon-11*20.3 min Positron emitter for metabolism imagingCobalt-57*270 d Calibration of imaging instrumentsCopper-629.8 min Heart perfusionCopper-6412.8 h Used as a clinical diagnostic agent for cancer and metabolic disordersCopper-6758.5 h RadioimmunotherapyChromium-5127.8 d Used to assess red blood cell survivalFluorine-18109.7 min Positron emitter used in glucose analogs uptake and neuroreceptor imaging.Gallium-6868 min Required in calibrating PET tomographs. Potential antibody levelGermanium-68*287 d Parent in the generator system for producing the positron emitting 68GaIndium-111* 2.8 d RadioimmunotherapyIodine-122 3.76 min Positron emitter for blood flow studiesIodine-123*13.3 h SPECT brain imaging agentIodine-124* 4.2 d Radioimmunotherapy, positron emitterIodine-12560.2 d Used as a potential cancer therapeutic agentIodine-1318.1 d Used to diagnose and treat thyroid disorders including cancerIron-52*8.2 h Used as an iron tracer, positron emitter for bone-marrow imagingMagnesium-28*21.2 h Magnesium tracer which decays to 2.3 in aluminum-28Magnese-52m 5.6 d Flow tracer for heart muscleMercury-195m*40 h Parent in the generator system for producing 195m Au, which is used in cardiac blood poolstudiesMolybdenum-9967 h Used to produce technetium-99m, the most commonly used radioisotope in clinical nuclearmedicineNitrogen-13*9.9 min Positron emitter used as 13NH for heart perfusion studiesOsmium-19115 d Decays to iridium-191 used for cardiac studiesOxygen-15*123 s Positron emitter used for blood flow studies as H152OPalladium-10317 d Used in the treatment of prostate cancerPhosphorus-3214.3 d Used in cancer treatment, cell metabolism and kinetics, molecular biology, genetics research,biochemistry, microbiology, enzymology, and as a starter to make many basic chemicalsand research productsRhenium-18817 h Used for treatment of medullary thyroid carcinoma and alleviation of pain in bonemetastasesRubidium-82* 1.2 min Positron emitter used for heart perfusion studiesRuthemiun-97* 2.9 d Hepatobiliary function, tumor and inflammation localizationSamarium-145340 d Treatment of ocular cancerSamarium-15346.8 h Used to radiolabel various molecules as cancer therapeutic agents and to alleviate bonecancer painScandium-47 3.4 d RadioimmunotherapyScandium-47* 3.4 d Used in the therapy of cancerStrontium-82*64.0 d Parent in the generator system for producing the positron emitting 82Rb, a potassiumanalogueStrontium-8564 d Used to study bone formation metabolismStrontium-8952 d Used to alleviate metastatic bone painSulfur-3587.9 d Used in studies of cell metabolism and kinetics, molecular biology, genetics research,biochemistry, microbiology, enzymology, and as a start to make many basic chemicals andresearch productsTechnetium-99m 6 h The most widely used radiopharmaceutical in nuclear medicine and produced frommolybdenum-99Thalium-201*74 h Cardiac imaging agentTin-117m14.0 d Palliative treatment of bone cancer painTritium (hydrogen-3)12.3 yr Used to make tritiated water which is used as a starter for thousands of different researchproducts and basic chemicals; used for life science and drug metabolism studies to ensurethe safety of potential new drugsradionuclide is remotely transferred into a lead-shielded hot cell for processing. The resulting radionu-clides are manipulated using microscale radiochemical techniques: small-scale synthetic methodology,ion-exchange chromatography, solvent extraction, electrochemical synthesis, distillation, simple filtra-tion, paper chromatography, and isotopic carrier precipitation. Various relevant radiochemical techniques have been published in standard texts.Generator-Produced RadionuclidesIf the reactor, cyclotron, or natural product radionuclide of long half-life decays to a daughter with nuclear characteristics appropriate for medical application, the system is called a medical radionuclide generator. There are several advantages afforded by generator-produced isotopes. These generators rep-resent a convenient source of short-lived medical isotopes without the need for an on-site reactor or particle accelerator. Generators provide delivery of the radionuclide on demand at a site remote from the production facility. They are a source of both gamma- and positron-emitting isotopes.The most common medical radionuclide generator is the 99Mo → 99m Tc system, the source of 99m Tc,a gamma-emitting isotope currently used in 70% of the clinical nuclear medicine studies. The 99Mo has a 67-hour half-life, giving this generator a useful life of about a week. Another common generator is the 68Ge → 68Ga system. Germanium (half-life is 287 days) is accelerator-produced in high-energy accelerators (e.g., BLIP , LAMPF, TRIUMF) through the alpha-particle bombardment of 66Zn. The 68Ge decays to 68Ga,a positron emitter, which has a 68-minute half-life. Gallium generators can last for several months.The generator operation is fairly straightforward. In general, the parent isotope is bound to a solid chemical matrix (e.g., alumina column, anionic resin, Donux resin). As the parent decays, the daughter nuclide grows in. The column is then flushed (“milked”) with a suitable solution (e.g., saline, hydrochloric acid) that elutes the daughter and leaves the remaining parent absorbed on the column. The eluent may be injected directly or processed into a radiopharmaceutical.Radiopharmaceuticals99m Tc is removed from the generator in the form of TcO 4– (pertechnetate).This species can be injected directly for imaging or incorporated into avariety of useful radiopharmaceuticals. The labeling of 99m Tc usually involvesreduction complexation/chelation. 99m Tc-Sestamibi (Fig. 67.2) is a radiop-harmaceutical used to evaluate myocardial perfusion or in the diagnosis ofcancer. There are several reduction methods employed, including Sn(II)reduction in NaHCO 3 at pH of 8 and other reduction and complexationreactions such as S 2O 3 + HCl, FeCl 3 + ascorbic acid, LiBH 4, Zn + HCl, HCl,Fe(II), Sn(II)F 2, Sn(II) citrate, and Sn(II) tartrate reduction and complex-ation, electrolytic reduction, and in vivo labeling of red cells following Sn(II)Tungsten-178*21.5 d Parent in generator system for producing 178Ta, short lived scanning agent Tungsten-18869 d Decays to rhenium-188 for treatment of cancer and rheumatoid arthritis Vanadium-48*16.0 d Nutrition and environmental studies Xenon-122*20 h Parent in the generator system for producing the positron emitting 122I Xenon-127*36.4 d Used in lung ventilation studies Xenon-1335.3 d Used in lung ventilation and perfusion studies Yttrium-88*106.6 d Radioimmunotherapy Yttrium-9064 h Used to radiolabel various molecules as cancer therapeutic agents Zinc-62*9.13 h Parent in the generator system for producing the positron emitting 62Cu Zirconium-89*78.4 h Radioimmunotherapy, positron emitter * Produced by accelerated charged particles. Others are produced by neutron reactors.TABLE 67.2Important Reactions for Cyclotron-2.p, 2n 8.p, 2p 3.d, n 9.d, p 4.d, 2n 10.d, 4He 5.p, 4He 11.p, d 6.p, 4He n 12.4He, n 13.3He, ppyrophosphate or Sn(II) DTPA administration. 131I,125I, and 123I labeling requires special reagents or con-ditions such as chloramine-T, widely used for proteinlabeling at 7.5 pH; peroxidase + H 2O 2 widely used forradioassay tracers; isotopic exchange for imaging trac-ers; excitation labeling as in 123Xe → 123I diazotizationplus iodination for primary amines; conjugation label-ing with Bolton Hunter agent (N -succinimidyl 3-[4-hydroxy 5-(131,125,123I)iodophenyl] propionate);hydroboration plus iodination; electrophilic destanny-lation; microdiffusion with fresh iodine vapor; andother methods. Radiopharmaceuticals in common usefor brain perfusion studies are N -isopropyl-p [123I]iodoamphetamine and 99m Tc-labeled hexamethyl-propyleneamine.PET RadionuclidesFor 11C, 13N, 15O, and 18F, the modes of production ofshort-lived positron emitters can dictate the chemicalform of the product, as shown in Table 67.3. Onlinechemistry is used to make various PET agents andprecursors. For example, 11C cyanide, an important precursor for synthesis of other labeled compounds,is produced in the cyclotron target by first bombarding N 2 + 5% H 2 gas target with 20-MeV protons.The product is carbon-labeled methane, 11CH 4, which when combined with ammonia and passed over a platinum wool catalyst at 1000°C becomes 11CN –, which is subsequently trapped in NaOH.Molecular oxygen is produced by bombarding a gas target of 14N 2 + 2% O 2 with deuterons (6 to 8 MeV). A number of products (e.g., 15O 2, C 15O 2, N 15O 2, 15O 3, and H 215O) are trapped by soda lime followed by charcoal to give 15O 2 as the product. However, if an activated charcoal trap at 900°C is used before the soda lime trap, the 15O 2 will be converted to C 15O. The specific strategies for other online PET agents is given in Rayudu [1990].Fluorine-18 (18F) is a very versatile positron emitting isotope. With a 2-hour half-life and two forms (F + and F –), one can develop several synthetic methods for incorporating 18F into medically useful compounds [Kilbourn, 1990]. Additionally, fluorine forms strong bonds with carbon and is roughly the same size as a hydrogen atom, imparting metabolic and chemical stability of the molecules without drastically altering biologic activity. The most com-monly produced 18F radiopharmaceutical is 2-deoxy-2-[18F]fluoroglucose (FDG). This radiotracer mimics part of the glucose metabolic pathway and has shown both hypo- and hypermetabolic abnormalities in cardiology,oncology, and neurology. The synthetic pathway for theproduction of FDG is shown in Fig. 67.3.The production of positron radiopharmaceuticals requires the rapid incorporation of the isotope into the desired molecule. Chemical techniques and synthetic strategies have been developed to facilitate these reac-tions. Many of these synthetic manipulations require hands-on operations by a highly trained chemist. Addi-tionally, since positrons give off 2- to 511-keV gamma rays upon annihilation, proximity to the source can increase one’s personal dose. The demand for the routineFIGURE 67.299m Tc-Sestamibi is a radiopharma-ceutical used to evaluate myocardial perfusion or in the diagnosis of cancer using both computed tomog-raphy and scintigraphy techniques.TABLE 67.3Major Positron-Emitting 121114N (p, α) 11C Nitrogen-1310 min 16O (p, α) 13N 13C (p, n ) 13NOxygen-15 2 min15N (p, n ) 15O 14N (d, n ) 15O Fluorine-18110 min 18O (p, n ) 18F 20Ne (d, α) 18F Note:A (x, y )B: A is target, x is the bombardingparticle, y is the radiation product, and B is the isotope produced.production of positron radiopharmaceuticals such as FDG has led to the development of remote synthetic devices. These devices can be human-controlled (i.e., flipping switches to open air-actuated valves),computer-controlled, or robotic. A sophisticated computer-controlled chemistry synthesis unit has been assembled for the fully automated production of 18FDG [Padgett, 1989]. A computer-controlled robot has been programmed to produce 6α-[18F]fluoroestradiol for breast cancer imaging [Brodack, 1986;Mathias, 1987]. Both these types of units increase the availability and reduce the cost of short-lived radiopharmaceutical production through greater reliability and reduced need for a highly trained staff.Additionally, these automated devices reduce personnel radiation exposure. These and other devices are being designed with greater versatility in mind to allow a variety of radiopharmaceuticals to be produced just by changing the programming and the required reagents.These PET radionuclides have been incorporated into a wide variety of medically useful radiophar-maceuticals through a number of synthetic techniques. The development of PET scanner technology has added a new dimension to synthetic chemistry by challenging radiochemists to devise labeling and purification strategies that proceed on the order of minutes rather than hours or days as in conventional synthetic chemistry. To meet this challenge, radiochemists are developing new target systems to improve isotope production and sophisticated synthetic units to streamline routine production of commonly desired radiotracers as well as preparing short-lived radiopharmaceuticals for many applications.AcknowledgmentThis work was supported in part by the Director, Office of Energy Research, Office of Health and Environmental Research, Medical Applications and Biophysical Research Division of the U.S. Department of Energy, under contract No. DE-AC03-SF00098, and in part by NIH Grant HL25840.ReferencesBrodack JW, Dence CS, Kilbourn MR, Welch MJ. 1988. Robotic production of 2-deoxy-2-[18F]fluoro-D -glucose: A routine method of synthesis using tetrabutylammonium [18F]fluoride. Int J Radiat Appl Instrum Part A: Appl Radiat Isotopes 39(7):699.Brodack JW, Kilbourn MR, Welch MJ, Katzenellenbogen JA. 1986. Application of robotics to radiophar-maceutical preparation: Controlled synthesis of fluorine-18 16 alpha-fluoroestradiol-17 beta.J Nucl Med 27(5):714.Hupf HB. 1976. Production and purification of radionuclides. In Radiopharmacy. New Y ork, Wiley.FIGURE 67.3Schematic for the chemical production of deoxyglucose labelled with fluorine-18. Here K222 refersto Kryptofix and C18 denotes a reverse-phase high-pressure liquid chromatography column.Kilbourn MR. 1990. Fluorine-18 Labeling of Radiopharmaceuticals. Washington, National Academy Press.Lamb J, Kramer HH. 1983. Commercial production of radioisotopes for nuclear medicine. In Radiotrac-ers for Medical Applications, pp 17–62. Boca Raton, Fla, CRC Press.Mathias CJ, Welch MJ, Katzenellenbogen JA, et al. 1987. Characterization of the uptake of 16 alpha-([18F]fluoro)-17 beta-estradiol in DMBA-induced mammary tumors. Int J Radiat Appl Instrum Part B: Nucl Med Biol 14(1):15.Padgett HC, Schmidt DG, Luxen A, et al. 1989. Computed-controlled radiochemical synthesis: A chem-istry process control unit for the automated production of radiochemicals. Int J Radiat Appl Instrum Part A: Appl Radiat Isotopes 40(5):433.Rayudu GV. 1990. Production of radionuclides for medicine. Semin Nucl Med 20(2):100.Sorenson JA, Phelps ME. 1987. Physics in Nuclear Medicine. New Y ork, Grune & Stratton.Steigman J, Eckerman WC. 1992. The Chemistry of Technetium in Medicine. Washington, National Academy Press.Stocklin G. 1992. Tracers for metabolic imaging of brain and heart: Radiochemistry and radiopharma-cology. Eur J Nucl Med 19(7):527.Thomas F. BudingerBackgroundThe history of positron-emission tomography (PET) can be traced to the early 1950s, when workers in Boston first realized the medical imaging possibilities of a particular class of radioactive substances. It was recognized then that the high-energy photons produced by annihilation of the positron from positron-emitting isotopes could be used to describe, in three dimensions, the physiologic distribution of “tagged”chemical compounds. After two decades of moderate technological developments by a few research centers, widespread interest and broadly based research activity began in earnest following the development of sophisticated reconstruction algorithms and improvements in detector technology. By the mid-1980s, PET had become a tool for medical diagnosis and for dynamic studies of human metabolism.Today, because of its million-fold sensitivity advantage over magnetic resonance imaging (MRI) in tracer studies and its chemical specificity, PET is used to study neuroreceptors in the brain and other body tissues. In contrast, MRI has exquisite resolution for anatomic (Fig. 67.4) and flow studies as well as unique attributes of evaluating chemical composition of tissue but in the millimolar range rather than the nanomolar range of much of the receptor proteins in the body. Clinical studies include tumors of the brain, breast, lungs, lower gastrointestinal tract, and other sites. Additional clinical uses include Alzheimer’s disease, Parkinson’s disease, epilepsy, and coronary artery disease affecting heart muscle metabolism and flow. Its use has added immeasurably to our current understanding of flow, oxygen utilization, and the metabolic changes that accompany disease and that change during brain stimulation and cognitive activation.PET TheoryPET imaging begins with the injection of a metabolically active tracer—a biologic molecule that carries with it a positron-emitting isotope (e.g., 11C, 13N, 15O, or 18F). Over a few minutes, the isotope accumulates in an area of the body for which the molecule has an affinity. As an example, glucose labeled with 11C, or a glucose analogue labeled with 18F, accumulates in the brain or tumors, where glucose is used as the primary source of energy. The radioactive nuclei then decay by positron emission. In positron (positive electron) emission, a nuclear proton changes into a positive electron and a neutron. The atom maintainsFIGURE 67.4The MRI image shows the arteriovenous malformation (AVM) as an area of signal loss due to blood flow. The PET image shows the AVM as a region devoid of glucose metabolism and also shows decreased metabolism in the adjacent frontal cortex. This is a metabolic effect of the AVM on the brain and may explain some of the patient’s symptoms.its atomic mass but decreases its atomic number by 1. The ejected positron combines with an electron almost instantaneously, and these two particles undergo the process of annihilation. The energy associated with the masses of the positron and electron particles is 1.022 MeV in accordance with the energy E to mass m equivalence E = mc2, where c is the velocity of light. This energy is divided equally between two photons that fly away from one another at a 180-degree angle. Each photon has an energy of 511 keV. These high-energy gamma rays emerge from the body in opposite directions, to be detected by an array of detectors that surround the patient (Fig. 67.5). When two photons are recorded simultaneously by a pair of detectors, the annihilation event that gave rise to them must have occurred somewhere along the line connecting the detectors. Of course, if one of the photons is scattered, then the line of coincidence will be incorrect. After 100,000 or more annihilation events are detected, the distribution of the positron-emitting tracer is calculated by tomographic reconstruction procedures. PET reconstructs a two-dimen-sional (2D) image from the one-dimensional projections seen at different angles. Three-dimensional (3D) reconstructions also can be done using 2D projections from multiple angles.PET DetectorsEfficient detection of the annihilation photons from positron emitters is usually provided by the com-bination of a crystal, which converts the high-energy photons to visible-light photons, and a photomul-tiplier tube that produces an amplified electric current pulse proportional to the amount of light photons interacting with the photocathode. The fact that imaging system sensitivity is proportional to the square of the detector efficiency leads to a very important requirement that the detector be nearly 100% efficient. Thus other detector systems such as plastic scintillators or gas-filled wire chambers, with typical individual efficiencies of 20% or less, would result in a coincident efficiency of only 4% or less.Most modern PET cameras are multilayered with 15 to 47 levels or transaxial layers to be reconstructed (Fig. 67.6). The lead shields prevent activity from the patient from causing spurious counts in the tomograph ring, while the tungsten septa reject some of the events in which one (or both) of the 511-keV photons suffer a Compton scatter in the patient. The sensitivity of this design is improved by collectionof data from cross-planes (Fig. 67.6). The arrangement of scintillators and phototubes is shown in Fig. 67.7.The “individually coupled” design is capable of very high resolution, and because the design is very parallel (all the photomultiplier tubes and scintillator crystals operate independently), it is capable of very high data throughput. The disadvantages of this type of design are the requirement for many expensive photomultiplier tubes and, additionally, that connecting round photomultiplier tubes to rectangular FIGURE 67.5The physical basis of positron-emission tomography. Positrons emitted by “tagged” metabolically active molecules annihilate nearby electrons and give rise to a pair of high-energy photons. The photons fly off in nearly opposite directions and thus serve to pinpoint their source. The biologic activity of the tagged molecule can be used to investigate a number of physiologic functions, both normal and pathologic.FIGURE 67.6Most modern PET cameras are multilayered with 15 to 47 levels or transaxial layers to be recon-structed. The lead shields prevent activity from the patient from causing spurious counts in the tomograph ring,while the tungsten septa reject some of the events in which one (or both) of the 511-keV photons suffer a Comptonscatter in the patient. The sensitivity of this design is improved by collection of data from cross-planes.。