为模板剂制备介孔碳材料
介孔碳材料的制备方法
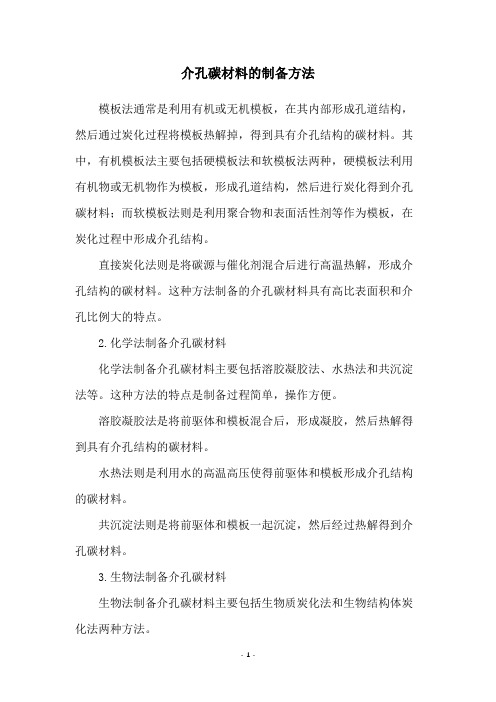
介孔碳材料的制备方法
模板法通常是利用有机或无机模板,在其内部形成孔道结构,然后通过炭化过程将模板热解掉,得到具有介孔结构的碳材料。
其中,有机模板法主要包括硬模板法和软模板法两种,硬模板法利用有机物或无机物作为模板,形成孔道结构,然后进行炭化得到介孔碳材料;而软模板法则是利用聚合物和表面活性剂等作为模板,在炭化过程中形成介孔结构。
直接炭化法则是将碳源与催化剂混合后进行高温热解,形成介孔结构的碳材料。
这种方法制备的介孔碳材料具有高比表面积和介孔比例大的特点。
2.化学法制备介孔碳材料
化学法制备介孔碳材料主要包括溶胶凝胶法、水热法和共沉淀法等。
这种方法的特点是制备过程简单,操作方便。
溶胶凝胶法是将前驱体和模板混合后,形成凝胶,然后热解得到具有介孔结构的碳材料。
水热法则是利用水的高温高压使得前驱体和模板形成介孔结构的碳材料。
共沉淀法则是将前驱体和模板一起沉淀,然后经过热解得到介孔碳材料。
3.生物法制备介孔碳材料
生物法制备介孔碳材料主要包括生物质炭化法和生物结构体炭化法两种方法。
生物质炭化法是利用生物质作为碳源,通过热解得到介孔碳材料。
生物结构体炭化法则是利用天然的生物结构体作为模板,形成介孔结构的碳材料。
总之,以上三种方法各有特点,可以根据具体需要选择不同的制备方法。
聚乙烯醇模板法制备介孔活性炭--1 (2)
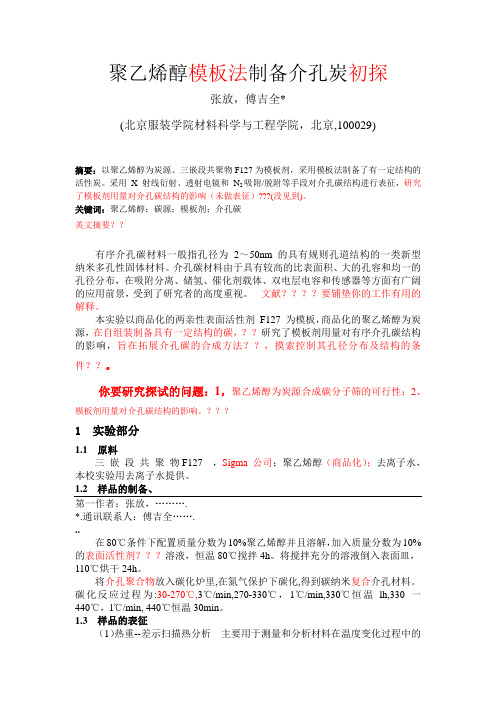
聚乙烯醇模板法制备介孔炭初探张放,傅吉全*(北京服装学院材料科学与工程学院,北京,100029)摘要:以聚乙烯醇为炭源、三嵌段共聚物F127为模板剂,采用模板法制备了有一定结构的活性炭。
采用X 射线衍射、透射电镜和N2吸附/脱附等手段对介孔碳结构进行表征,研究了模板剂用量对介孔碳结构的影响(未做表征)???(没见到)。
关键词:聚乙烯醇;碳源;模板剂;介孔碳英文摘要??有序介孔碳材料一般指孔径为2~50nm 的具有规则孔道结构的一类新型纳米多孔性固体材料。
介孔碳材料由于具有较高的比表面积、大的孔容和均一的孔径分布,在吸附分离、储氢、催化剂载体、双电层电容和传感器等方面有广阔的应用前景,受到了研究者的高度重视。
文献????要铺垫你的工作有用的解释。
本实验以商品化的两亲性表面活性剂F127 为模板,商品化的聚乙烯醇为炭源,在自组装制备具有一定结构的碳,??研究了模板剂用量对有序介孔碳结构的影响,旨在拓展介孔碳的合成方法??,摸索控制其孔径分布及结构的条件??。
你要研究探试的问题:1,聚乙烯醇为炭源合成碳分子筛的可行性;2、模板剂用量对介孔碳结构的影响。
???1实验部分1.1 原料三嵌段共聚物F127 ,Sigma 公司;聚乙烯醇(商品化);去离子水,本校实验用去离子水提供。
1.2 样品的制备、第一作者:张放,……….*.通讯联系人:傅吉全……...在80℃条件下配置质量分数为10%聚乙烯醇并且溶解,加入质量分数为10%的表面活性剂???溶液,恒温80℃搅拌4h。
将搅拌充分的溶液倒入表面皿,110℃烘干24h。
将介孔聚合物放入碳化炉里,在氮气保护下碳化,得到碳纳米复合介孔材料。
碳化反应过程为:30-270℃,3℃/min,270-330℃,1℃/min,330℃恒温lh,330一440℃,l℃/min, 440℃恒温30min。
1.3 样品的表征(1)热重--差示扫描热分析主要用于测量和分析材料在温度变化过程中的物理化学变化,研究样品的热失重行为和热量变化可为材料的研制提供有价值的热力学和动力学参数。
介孔碳cmk3
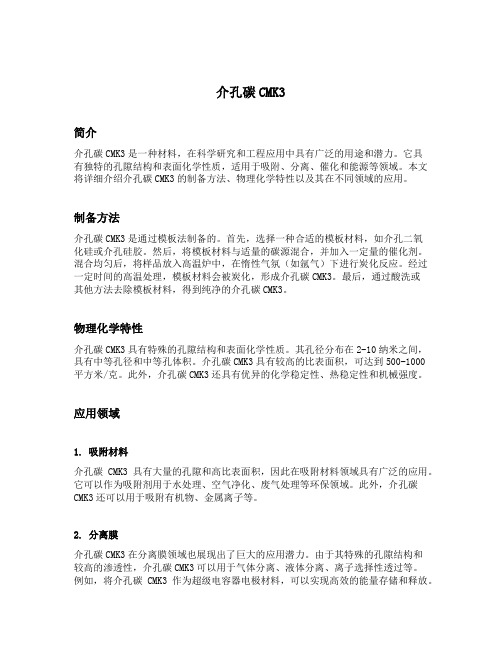
介孔碳CMK3简介介孔碳CMK3是一种材料,在科学研究和工程应用中具有广泛的用途和潜力。
它具有独特的孔隙结构和表面化学性质,适用于吸附、分离、催化和能源等领域。
本文将详细介绍介孔碳CMK3的制备方法、物理化学特性以及其在不同领域的应用。
制备方法介孔碳CMK3是通过模板法制备的。
首先,选择一种合适的模板材料,如介孔二氧化硅或介孔硅胶。
然后,将模板材料与适量的碳源混合,并加入一定量的催化剂。
混合均匀后,将样品放入高温炉中,在惰性气氛(如氩气)下进行炭化反应。
经过一定时间的高温处理,模板材料会被炭化,形成介孔碳CMK3。
最后,通过酸洗或其他方法去除模板材料,得到纯净的介孔碳CMK3。
物理化学特性介孔碳CMK3具有特殊的孔隙结构和表面化学性质。
其孔径分布在2-10纳米之间,具有中等孔径和中等孔体积。
介孔碳CMK3具有较高的比表面积,可达到500-1000平方米/克。
此外,介孔碳CMK3还具有优异的化学稳定性、热稳定性和机械强度。
应用领域1. 吸附材料介孔碳CMK3具有大量的孔隙和高比表面积,因此在吸附材料领域具有广泛的应用。
它可以作为吸附剂用于水处理、空气净化、废气处理等环保领域。
此外,介孔碳CMK3还可以用于吸附有机物、金属离子等。
2. 分离膜介孔碳CMK3在分离膜领域也展现出了巨大的应用潜力。
由于其特殊的孔隙结构和较高的渗透性,介孔碳CMK3可以用于气体分离、液体分离、离子选择性透过等。
例如,将介孔碳CMK3作为超级电容器电极材料,可以实现高效的能量存储和释放。
3. 催化剂载体介孔碳CMK3还可作为催化剂载体,用于催化反应。
其高比表面积和孔隙结构有利于催化剂的分散和反应物的扩散,提高催化反应的效率和选择性。
例如,将过渡金属纳米颗粒负载在介孔碳CMK3上,可用于催化氧化反应、催化还原反应等。
4. 能源存储介孔碳CMK3在能源存储领域也有广泛的应用。
其孔隙结构和电导性使其成为理想的电容器和电池材料。
介孔碳CMK3用作锂离子电池负极材料,具有高容量、长寿命和快速充放电性能。
一种介孔碳材料的合成方法与流程
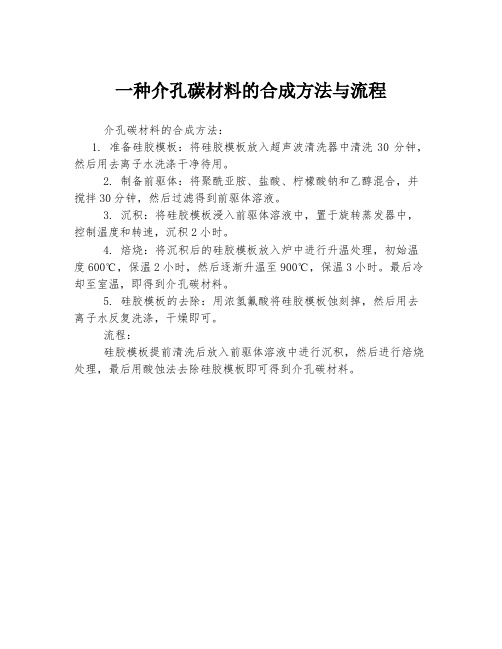
一种介孔碳材料的合成方法与流程
介孔碳材料的合成方法:
1. 准备硅胶模板:将硅胶模板放入超声波清洗器中清洗30分钟,然后用去离子水洗涤干净待用。
2. 制备前驱体:将聚酰亚胺、盐酸、柠檬酸钠和乙醇混合,并搅拌30分钟,然后过滤得到前驱体溶液。
3. 沉积:将硅胶模板浸入前驱体溶液中,置于旋转蒸发器中,控制温度和转速,沉积2小时。
4. 焙烧:将沉积后的硅胶模板放入炉中进行升温处理,初始温度600℃,保温2小时,然后逐渐升温至900℃,保温3小时。
最后冷却至室温,即得到介孔碳材料。
5. 硅胶模板的去除:用浓氢氟酸将硅胶模板蚀刻掉,然后用去离子水反复洗涤,干燥即可。
流程:
硅胶模板提前清洗后放入前驱体溶液中进行沉积,然后进行焙烧处理,最后用酸蚀法去除硅胶模板即可得到介孔碳材料。
介孔碳和介孔炭
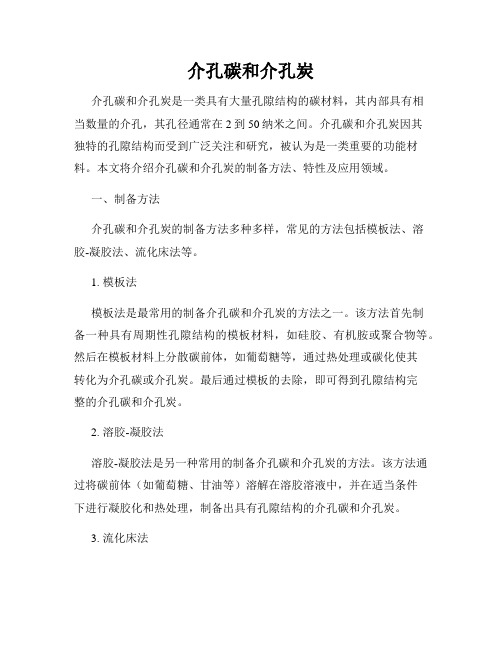
介孔碳和介孔炭介孔碳和介孔炭是一类具有大量孔隙结构的碳材料,其内部具有相当数量的介孔,其孔径通常在2到50纳米之间。
介孔碳和介孔炭因其独特的孔隙结构而受到广泛关注和研究,被认为是一类重要的功能材料。
本文将介绍介孔碳和介孔炭的制备方法、特性及应用领域。
一、制备方法介孔碳和介孔炭的制备方法多种多样,常见的方法包括模板法、溶胶-凝胶法、流化床法等。
1. 模板法模板法是最常用的制备介孔碳和介孔炭的方法之一。
该方法首先制备一种具有周期性孔隙结构的模板材料,如硅胶、有机胺或聚合物等。
然后在模板材料上分散碳前体,如葡萄糖等,通过热处理或碳化使其转化为介孔碳或介孔炭。
最后通过模板的去除,即可得到孔隙结构完整的介孔碳和介孔炭。
2. 溶胶-凝胶法溶胶-凝胶法是另一种常用的制备介孔碳和介孔炭的方法。
该方法通过将碳前体(如葡萄糖、甘油等)溶解在溶胶溶液中,并在适当条件下进行凝胶化和热处理,制备出具有孔隙结构的介孔碳和介孔炭。
3. 流化床法流化床法是一种高效的制备介孔碳和介孔炭的方法。
该方法首先将碳前体粉末放置在流化床反应器内,在适当条件下进行热解或碳化反应,生成介孔碳和介孔炭。
该方法制备的介孔碳和介孔炭孔隙结构较为均匀,具有较高的比表面积和孔容。
二、特性介孔碳和介孔炭具有许多独特的特性,主要包括以下几个方面:1. 高比表面积介孔碳和介孔炭由于其内部具有大量的介孔,因此具有较高的比表面积。
高比表面积使其有较强的吸附能力,可以吸附和储存大量的气体、液体和溶质,具有广泛的应用前景。
2. 调控孔径介孔碳和介孔炭的孔径可以通过制备方法的调控来实现。
不同孔径的介孔碳和介孔炭可以用于吸附、分离、催化等不同领域的应用。
因此,介孔碳和介孔炭的孔径调控对其应用性能具有重要影响。
3. 良好的化学稳定性介孔碳和介孔炭由于其具有较完整的碳骨架结构,因此具有良好的化学稳定性。
它们在酸碱环境、高温条件下都能保持稳定的结构和性能,具有较长的使用寿命。
介孔碳纳米结构
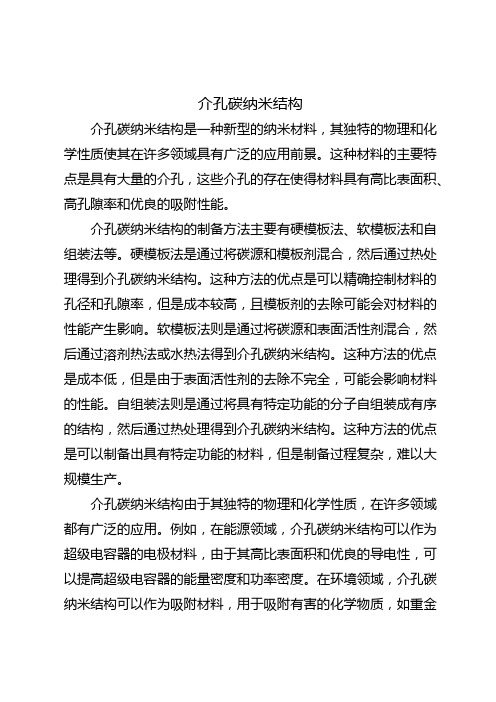
介孔碳纳米结构介孔碳纳米结构是一种新型的纳米材料,其独特的物理和化学性质使其在许多领域具有广泛的应用前景。
这种材料的主要特点是具有大量的介孔,这些介孔的存在使得材料具有高比表面积、高孔隙率和优良的吸附性能。
介孔碳纳米结构的制备方法主要有硬模板法、软模板法和自组装法等。
硬模板法是通过将碳源和模板剂混合,然后通过热处理得到介孔碳纳米结构。
这种方法的优点是可以精确控制材料的孔径和孔隙率,但是成本较高,且模板剂的去除可能会对材料的性能产生影响。
软模板法则是通过将碳源和表面活性剂混合,然后通过溶剂热法或水热法得到介孔碳纳米结构。
这种方法的优点是成本低,但是由于表面活性剂的去除不完全,可能会影响材料的性能。
自组装法则是通过将具有特定功能的分子自组装成有序的结构,然后通过热处理得到介孔碳纳米结构。
这种方法的优点是可以制备出具有特定功能的材料,但是制备过程复杂,难以大规模生产。
介孔碳纳米结构由于其独特的物理和化学性质,在许多领域都有广泛的应用。
例如,在能源领域,介孔碳纳米结构可以作为超级电容器的电极材料,由于其高比表面积和优良的导电性,可以提高超级电容器的能量密度和功率密度。
在环境领域,介孔碳纳米结构可以作为吸附材料,用于吸附有害的化学物质,如重金属离子和有机污染物。
在生物医学领域,介孔碳纳米结构可以作为药物载体,由于其大的孔隙率和良好的生物相容性,可以提高药物的载药量和释放效率。
尽管介孔碳纳米结构具有许多优点,但是其制备过程中仍然存在一些问题需要解决。
例如,如何精确控制材料的孔径和孔隙率,如何提高材料的电导率,如何提高材料的热稳定性等。
此外,介孔碳纳米结构的应用研究也需要进一步加强,以充分发挥其潜在的应用价值。
总的来说,介孔碳纳米结构是一种具有广泛应用前景的新型纳米材料。
随着科研技术的不断进步,我们有理由相信,介孔碳纳米结构将在未来的能源、环境和生物医学等领域发挥更大的作用。
简易模板法制备有序介孔碳

Q I U H u i - H u a L I U Y i n g - L i a n g Z E N G J i a n g - Hu a Z U O S h i — D i Z H E N G Mi n g — T a o
f D e p a r t m e n t o f C h e m i s t D ' , n M U n i v e r s i t y , G u a n  ̄h o u 5 1 0 6 3 2 )
Ab s t r a c t : Or d e r e d me s o p o r o u s c a r b o n ma t e r i a l s we r e s y n t h e s i z e d v i a a s i mpl e t e mpl a t e me t h o d b y a d d i n g s uc r o s e t o t he s ul f u r i c . - a c i d. . t r e a t e d s i l i c a / P1 2 3 t r i b l o c k c o p o l y me r c o mp o s i t e a nd f o l l o we d b y c a r b o ni z a t i o n a n d r e mo v a l o f t h e s i l i c a .T hi s t e c h n i q u e i s s i mp l e r a n d t h e c o s t i s l o we r t h a n t h e c o n v e n t i o n a l ha r d t e mp l a t e me t h o d.
( X R D ) ,h i g h — r e s o l u t i o n t r a n s m i s s i o n e l e c t r o n m i c r o s c o p y ( H R T E M)a n d n i t r o g e n a d s o r p t i o n ・ d e s o pt r i o n .T h e
以模板法制备介孔碳及其性能研究

从近 于分子级别 的纳米尺度来设 计并控 制聚合物前驱 体结构的有效方法 , 通过 采用特殊 的炭化 过程 使这种 微观结 构得 以保存 并发生炭 化反应 , 从
而 得 到 与 传 统 意 义 上 完 全 不 同 的 多 孔 炭 材 料 。 而 以 纳 米 材 料 为 反 模 板 制 造 介 孔炭 的 方 法 是 比 较 新 的 制 备 方 法 , 研 究 得 较 少 ,其 基 本 原 理 是 利 用 纳 米 材 料 的 粒 径 分 布 ( -5 n )特 征 ,将 炭 前 驱 体 与 纳 米 材 料 以一 定 的 2 0m
王
清华大学 核能与新能 源技 术研究院 北京市精 细陶瓷重点实 验室 北京 1。 O。
的孑 径 分布较 宽 , L 按照 国际纯粹 与应用化学 联合会 (UP I AC) 的分 类“, 】 多
孔 炭 的 孔 大 小 分 为 :大 于 5 n 是 大 孔 ,2 0 m 为 中 孔 ,小 于 2 m 的 0m ~5 n n
确定 了最佳 的实验条件 为 :8 ℃ ,1 g蔗糖 ,2 g纳米碳 酸钙 ,3 ml 。 0 0 0 0 水
对原料 进行热 失重 ( G)分析 ,深入 地 了解 其在高 温炭化各 个温度段 的 T
中 国糟 伟 工 业 21年第1 0 0 期
The i ss
变化情 况 。采用 X射 线衍射 ( XRD)初
C
20
D
30
M C一 1
80
并用 于正 交实 验 的结果 分析 。
M C一2
M C一3 M C 一4
8 0
80 l 0
1 5
20 10
l 0
1 5 l 5
1 0
二氧化硅模板法制备介孔碳

二氧化硅模板法制备介孔碳介孔碳是一种具有特殊孔结构的碳材料,具有高比表面积、大孔径和可调控的孔分布特点,在吸附、催化、电化学等领域具有广泛的应用前景。
制备介孔碳材料的方法有很多种,其中二氧化硅模板法是一种常用且有效的方法。
二氧化硅模板法制备介孔碳的基本思路是:首先通过溶胶-凝胶法或搅拌法得到特定孔径、孔分布均匀的二氧化硅模板材料,然后利用模板材料作为模具,将其包覆一层碳源,如葡萄糖、蔗糖、麦芽糖等,通过热解或炭化反应,使碳源转化为碳材料,最后通过酸或碱等方法去除二氧化硅模板,得到具有介孔结构的碳材料。
二氧化硅模板法制备介孔碳的优点主要有以下几个方面:首先,该方法制备的介孔碳材料孔径可以在一定范围内调控。
通过合理选择二氧化硅模板的孔径和表面改性方法,可以得到不同孔径范围内的介孔碳材料。
这对于特定应用场景的需求非常重要,例如在吸附材料中,不同孔径的介孔碳对不同分子大小的吸附有着不同的选择性。
其次,制备过程简单、操作容易。
二氧化硅模板材料易于得到,制备过程相对简单,不需要复杂的设备和条件,因此该方法具有很高的可操作性和可实施性。
再次,制备的介孔碳材料具有较高的比表面积。
二氧化硅模板法制备的介孔碳材料具有大量的介孔结构,这种孔结构能够提供更多的活性表面积,从而提高材料的吸附、催化等性能。
此外,制备的介孔碳材料具有良好的化学和热稳定性。
由于碳材料的稳定性较好,制备的介孔碳材料在高温、酸碱等条件下表现出较高的稳定性和抗腐蚀性,具有较长的使用寿命。
需要注意的是,二氧化硅模板法制备介孔碳材料也存在一些挑战和难点。
首先,模板的选择和表面改性对最终碳材料的孔径和孔分布有着重要影响,因此需要针对具体需求进行合理选择。
其次,模板去除的方法和条件需要谨慎选择,以避免对碳材料结构和性质的不良影响。
另外,制备过程中还可能存在模板残留和碳材料内部缺陷的问题,对于一些特定应用场景需要特别注意。
总结而言,二氧化硅模板法是一种有效制备介孔碳材料的方法,具有孔径可调、制备简单、比表面积高和化学热稳定性好等优点。
介孔碳材料的合成及应用
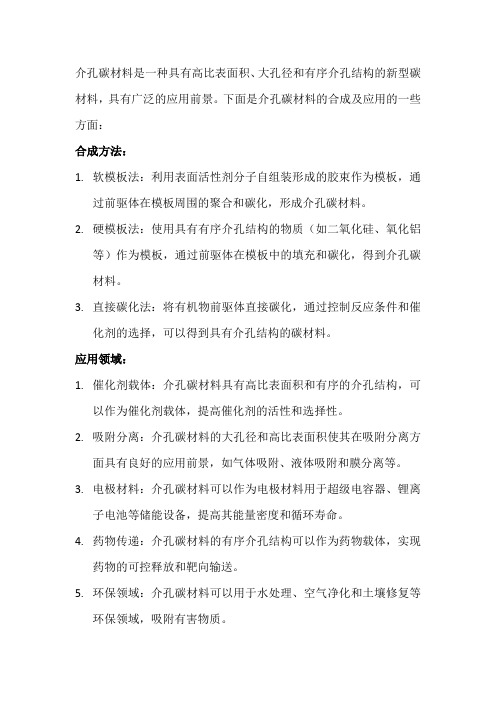
介孔碳材料是一种具有高比表面积、大孔径和有序介孔结构的新型碳材料,具有广泛的应用前景。
下面是介孔碳材料的合成及应用的一些方面:
合成方法:
1.软模板法:利用表面活性剂分子自组装形成的胶束作为模板,通
过前驱体在模板周围的聚合和碳化,形成介孔碳材料。
2.硬模板法:使用具有有序介孔结构的物质(如二氧化硅、氧化铝
等)作为模板,通过前驱体在模板中的填充和碳化,得到介孔碳材料。
3.直接碳化法:将有机物前驱体直接碳化,通过控制反应条件和催
化剂的选择,可以得到具有介孔结构的碳材料。
应用领域:
1.催化剂载体:介孔碳材料具有高比表面积和有序的介孔结构,可
以作为催化剂载体,提高催化剂的活性和选择性。
2.吸附分离:介孔碳材料的大孔径和高比表面积使其在吸附分离方
面具有良好的应用前景,如气体吸附、液体吸附和膜分离等。
3.电极材料:介孔碳材料可以作为电极材料用于超级电容器、锂离
子电池等储能设备,提高其能量密度和循环寿命。
4.药物传递:介孔碳材料的有序介孔结构可以作为药物载体,实现
药物的可控释放和靶向输送。
5.环保领域:介孔碳材料可以用于水处理、空气净化和土壤修复等
环保领域,吸附有害物质。
超分子聚集体为模板剂合成有序介孔碳材料

孔大小为22.6nm
反相两亲嵌段共聚物PPO-PEO-PPO作为模板剂
两种孔径分布:3.2–4.0 nm、5.4–6.9 nm
Y. H. Deng, T. Yu, Wan, et al. J. Am. Chem. Soc. 2007, 129, 1690-1697 Y. Huang, H. Q. Cai, et al. Angew. Chem. Int. Ed. 2007, 46, 1089 –1093
PS-PEO
氮系列
氧系列
最早报道有机模板法合成OMCs
P4VP-PS
n N H O m
氢键
HO
间苯二酚
OH + H OH
Resorcinol formaldehyde
O H
OH
OH
RF resin
孔径:33.7±2.5nm
C. D. Liang, K. L. Hong, G. A. Guiochon, J. W. Mays, S. Dai. Angew. Chem. Int. Ed. 2004, 43, 5785–5789
3.结论与展望
利用超分子聚集体为模板剂合成OMCs开辟了新的路线,并 可应用于大规模生产上。 采用超分子聚集体为模板剂合成OMCs,关键因素:
1. 嵌段共聚物中要有互不相容的两类嵌段,还要有与热固性树脂相
容的嵌段 2.模板剂与有机前驱体之间必须具有强的相互作用;前驱体必须足 够小才能在聚集体周围自组装; 3.碳前驱体是热固性的,才能在高温下保持住介孔结构
水溶液中合成OMCs
Resol 十六烷 P123
水相自组装方法,易调整孔尺寸和控制表面形态;重复性好,反应容器尺寸上限 制较少,有利于工业上生产。
以F127为模板制备介孔碳
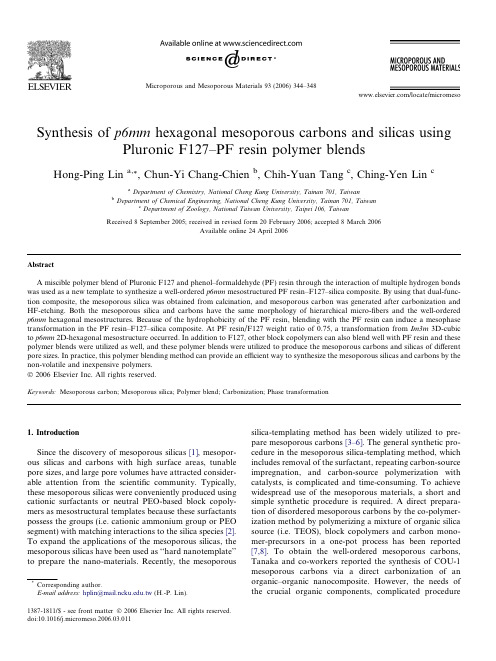
Synthesis of p6mm hexagonal mesoporous carbons and silicas usingPluronic F127–PF resin polymer blendsHong-Ping Lina,*,Chun-Yi Chang-Chien b ,Chih-Yuan Tang c ,Ching-Yen LincaDepartment of Chemistry,National Cheng Kung University,Tainan 701,TaiwanbDepartment of Chemical Engineering,National Cheng Kung University,Tainan 701,TaiwancDepartment of Zoology,National Taiwan University,Taipei 106,TaiwanReceived 8September 2005;received in revised form 20February 2006;accepted 8March 2006Available online 24April 2006AbstractA miscible polymer blend of Pluronic F127and phenol–formaldehyde (PF)resin through the interaction of multiple hydrogen bonds was used as a new template to synthesize a well-ordered p6mm mesostructured PF resin–F127–silica composite.By using that dual-func-tion composite,the mesoporous silica was obtained from calcination,and mesoporous carbon was generated after carbonization and HF-etching.Both the mesoporous silica and carbons have the same morphology of hierarchical micro-fibers and the well-ordered p6mm hexagonal mesostructures.Because of the hydrophobicity of the PF resin,blending with the PF resin can induce a mesophase transformation in the PF resin–F127–silica composite.At PF resin/F127weight ratio of 0.75,a transformation from Im3m 3D-cubic to p6mm 2D-hexagonal mesostructure occurred.In addition to F127,other block copolymers can also blend well with PF resin and these polymer blends were utilized as well,and these polymer blends were utilized to produce the mesoporous carbons and silicas of different pore sizes.In practice,this polymer blending method can provide an efficient way to synthesize the mesoporous silicas and carbons by the non-volatile and inexpensive polymers.Ó2006Elsevier Inc.All rights reserved.Keywords:Mesoporous carbon;Mesoporous silica;Polymer blend;Carbonization;Phase transformation1.IntroductionSince the discovery of mesoporous silicas [1],mesopor-ous silicas and carbons with high surface areas,tunable pore sizes,and large pore volumes have attracted consider-able attention from the scientific community.Typically,these mesoporous silicas were conveniently produced using cationic surfactants or neutral PEO-based block copoly-mers as mesostructural templates because these surfactants possess the groups (i.e.cationic ammonium group or PEO segment)with matching interactions to the silica species [2].To expand the applications of the mesoporous silicas,the mesoporous silicas have been used as ‘‘hard nanotemplate’’to prepare the nano-materials.Recently,the mesoporoussilica-templating method has been widely utilized to pre-pare mesoporous carbons [3–6].The general synthetic pro-cedure in the mesoporous silica-templating method,which includes removal of the surfactant,repeating carbon-source impregnation,and carbon-source polymerization with catalysts,is complicated and time-consuming.To achieve widespread use of the mesoporous materials,a short and simple synthetic procedure is required.A direct prepara-tion of disordered mesoporous carbons by the co-polymer-ization method by polymerizing a mixture of organic silica source (i.e.TEOS),block copolymers and carbon mono-mer-precursors in a one-pot process has been reported [7,8].To obtain the well-ordered mesoporous carbons,Tanaka and co-workers reported the synthesis of COU-1mesoporous carbons via a direct carbonization of an organic–organic nanocomposite.However,the needs of the crucial organic components,complicated procedure1387-1811/$-see front matter Ó2006Elsevier Inc.All rights reserved.doi:10.1016/j.micromeso.2006.03.011*Corresponding author.E-mail address:hplin@.tw (H.-P.Lin)./locate/micromesoMicroporous and Mesoporous Materials 93(2006)344–348and silicon–substrate hampers the broad applications of the mesoporous carbons[8].In these previous reports,only one mesoporous material was produced from each synthe-sized composite.It is still very desirable tofind a simple synthetic procedure using cheap sources to create a dual-function composite,which can be converted to mesoporous silica and carbon at will.Based on the concept of polymer-blending[9],neutral PEO-based block copolymers can readily blend with other polymers via non-covalent bonding interaction.In this paper we describe the preparation of a miscible F127–PF polymer blend of Pluronic F127block-copolymer (EO106PO70EO106)and commercially available phenol–formaldehyde(PF)resin through multiple hydrogen bond-ing between hydroxyl groups(–OH)or hydroxylmethylene groups(–CH2OH)of the PF resin and ether groups(–O–) in the F127copolymer[10].In the F127–PF polymer blend, the PEO parts have a large affinity to aggregate with silica species via hydrogen-bonding interaction at near neutral pH[2],and the hydrophobic PF resin can raise the hydrophobicity of F127–PF resin blending micelles. Consequently,with effective control on the F127/PF resin weight ratio,the F127–PF resin blending micelles can assemble with the silica species at pH value of about5.0, and hence a F127–PF–silica composite with a well-ordered p6mm mesostructure was obtained.Because the F127–PF–silica composite is composed of the inorganic silica and the thermosetting PF resin,it is readily converted to carbon via a high-temperature pyrolysis[11].Using the dual-function F127–PF–silica composite,calcination in air produced well-ordered mesoporous silicafibers;on the other hand, carbonization and silica-removal obtained ordered meso-porous carbonfibers.When using other Pluronic copoly-mers,the mesoporous silicas and carbons of different pore sizes can be synthesized as well.2.ExperimentalIn a typical preparation of F127–PF resin–silica com-posite, 2.0g of Pluronic F127block copolymer (EO106PO70EO106,Aldrich)and0.5–3.0g of phenol–form-aldehyde resin(PF2180,phenol/aldehyde=0.8–0.9,M W ca.96,000,Chung-Chun Plastic,Taiwan)was dissolved in 20.0g ethanol to form a clear solution.After stirring for 2–6h,the F127–PF resin blend ethanol solution was poured into a neutralized sodium silicate aqueous solution at40°C.The neutralized silica solution was prepared by a mixture of7.5g of sodium silicate(Aldrich,SiO2% 27wt.%)and22.5g of water to300.0g of0.50M H2SO4 aqueous solution andfine-tuning the pH value of the sili-cate solution to4.0–5.0.Because silicate species condense quickly at near-neutral pH,light-yellow precipitates were formed in a few minutes.Filtration,washing and drying at100°C produced a F127–PF resin–silica composite. The F127–PF resin–silica composite was calcined in air at 580°C for6h to obtain the mesoporous silica.Alterna-tively,the mesoporous carbons were prepared via a pyroly-sis at1000°C under nitrogen atmosphere for2h with silica-etching using6wt%HF of water solution.3.Results and discussionFig.1A shows the XRD patterns of calcined mesopor-ous silica and silica-free mesoporous carbon prepared with PF resin/F127weight ratio of1.25.The mesoporous silica reveals four well-resolved peaks in the region of2h=0.5–2.0°,which are indexed to the100,110,200and210dif-fraction peaks of a space group p6mm hexagonal structure. Compared to the mesoporous silica,the XRD peaks of the mesoporous carbon are relatively broader and located at higher angles.This is because a structural distortion and lattice shrinkage rationally occurring in the2D-hexago-nal-packing carbon nanorods with little interconnection after silica removal[3].The unit cell sizes a0(2/p3d100) of mesoporous silica and carbon in p6mm symmetry are 13.2and11.6nm,respectively.Microtome transmission electron microscope(TEM)images further confirmed the well-ordered hexagonal structure of the mesoporous silica, and the carbon-nanorods bundles in a less ordered arrange-ment(Fig.1B and C).Comparing the scanning electron microscope(SEM)images of the mesoporous silica and carbon,we found they exist in the same morphology of micrometer-sizedfiber bundles,and thefiber lengths are distributed from a few to tens of micrometers(Fig.1D and Fig.S1).Morphology preservation of the mesoporous carbon indicates that the hierarchical structure(i.e.micro-sizedfiber is consisted of nano-sized nanorods or nanochannels)does not change during HF-etching[12]. Therefore,both mesoporous silica and carbon with hierar-chical structure can be produced with the PF resin–F127 polymer blend.N2adsorption–desorption isotherms of PF resin–F127-made mesoporous silica and carbon exhibit capillary con-densations at P/P0of about0.8and0.6,respectively (Fig.1E).As is typical of mesoporous materials,both the mesoporous silica and carbon have a high BET surface area (silica:495m2/g;carbon:1549m2/g)large pore size(silica: 10.2nm;carbon:5.0nm calculated by BJH method)and pore volume(silica:0.77cm3/g;carbon:1.16cm3/g).Due to the p6mm hexagonal structure,it is noteworthy that the PF resin–F127-templated mesoporous silica demon-strates a representative H1-type hysteresis loop of cylindri-cal pores rather than a cage-like pore of the F127-templated SBA-16silica.The thermal stability and phase purity of the mesopor-ous carbon can be examined on a TGA instrument.The TGA plot of the mesoporous carbon demonstrates a sharp weight-loss curve at a combustion temperature at around 550°C(Fig.1F),which indicates that the F127–PF resin blend can be converted to a phase-homogeneous and ther-mally stable carbon as well as pure PF resin[13].More-over,a low weight percentage of inorganic residue (<1.5wt.%)implies a nearly complete removal of the silica by HF-etching.H.-P.Lin et al./Microporous and Mesoporous Materials93(2006)344–348345In order to recognize the blending-homogeneity between the PF resin and F127embedded in the silica nanopores,TGA curves of the F127–PF–silica composites synthesized at different PF resin/F127weight ratios were examined (Fig.2A).One can clearly see that PF resin–F127blend-silica has a higher combustion temperature than that of F127–silica,and the combustion temperature increases with the PF resin content.This weighted average of elementary properties of each component occurs commonly in the mis-cible polymer blend [9].Moreover,the weight percentage of the organic parts in F127–PF resin–silica composites increases with the increase of the added PF resin content,suggesting a high compatibility between F127and the PF resin.To examine the effect of the PF resin blending on the mesostructure of the PF resin–F127–silica composite,we analyzed the N 2adsorption–desorption isotherms of the calcined mesoporous silica prepared at different PF resin/F127weight ratios (Fig.2B).At the PF resin/F127ratio (denoted as S value)less than 0.75,the mesoporous silicas demonstrate H2-type hysteresis characteristic of cage-like pores,and the pore size increases with the increase of the S value (Fig.2B,curves I and II).When S value was raised to 0.75,a shape-transformation in the isotherms from an H2-type hysteresis to an H1-type hysteresis of cylindrical pore takes bining with the XRD patterns (see the supplementary information,Fig.S2),we confirmed that a phase transformation from the Im3m 3D-cubic mesostructure to the p6mm 2D-hexagonal arrangement occurred at that component.Thus,blending with a proper amount of hydrophobic PF resin that enlarges the hydro-phobicity of the F127surfactants can induce the formation of the rod-like micelles.These PE resin–F127rod-like micelles self-assemble with the silicate species to generate the well-ordered p6mm mesostructure.According to our experimental results,we found the S value should be controlled within a range of 0.75–1.50to synthesize the mesoporous silicas with well-ordered p6mm hexagonal structure.However,the pore size of the p6mm hexagonal mesoporous silica was not apparently changed with the increase of the S value (Fig.2B,curves III and IV).Although the PF resin and F127can be arbitrarily blended by hydrogen-bonding interactions,the hydropho-bicity of PF–F127polymer blends depends on the fraction of PF resin.When the S value is larger than 2.0,the high PF resin causes the PF resin–F127blend to become too0.00.20.40.60.81.00200400600800V o l u m e a d s o r b e d /c m 3 g -1P/P 0100200300400500600700020406080100W e i g h t l o s s /%Temperature/oCEDFB200 nmIII0.5 1.0 1.5 2.0 2.5 3.0x3x4(200)(210)(200)(110)(100)(100)X -r a y i n t e n s i t y2θ/degreeAIII100 nmC346H.-P.Lin et al./Microporous and Mesoporous Materials 93(2006)344–348hydrophobic and hence a glue-like polymer-precipitation formed at the bottom of the silicate aqueous solution.At the S value less than0.5,carbon ash in low yield was obtained instead of mesoporous carbon.It is reasonable to suppose that excessively high content of the pyrolyzing F127leads to a dramatic decrease in carbon yield and the mesostructure cannot be maintained after silica removal [11].The PF resin/F127weight ratio must be controlled within0.75–1.5to prepare stable mesoporous carbons of well-ordered p6mm mesostructure.It is known that the pore volume of the mesoporous carbons is resulted from the silica removal.Therefore,the pore size of the p6mm mesoporous carbons is independent on the S value.To expand the pore size of the mesoporous carbon,the silica content in the PF resin–F127–silica composite should be increased,although to increase the silica content without disrupting the meso-structural orderness is still a challenge.The synthetic factors including pH value,temperature,and concentration of the silicate species will be further studied in the future.The basic concept of the polymer-blending template can be widely applied to other block-copolymer–PF resin composites.As with the F127copolymer,the PF resin can also blend well with other block copolymers such as P123(EO20PO70EO20),L64(EO13PO30EO13),and Brij76 (C18EO10).Table1demonstrates the physical properties of the prepared mesoporous silicas and porous carbons samples.Accordingly,all the mesoporous silica samples exhibit high surface area(>300m2/g),high pore volume (>0.40cm3/g)and tunable pore size.In addition,the por-ous carbon samples exhibits high surface area(>1200m2/ g)and high pore volume(>1.0cm3/g).In contrast to the F127copolymer,only disordered mesoporous silica and carbon were obtained with these copolymers.To improve mesostructural order of the silicas and carbons,other experimental factors(such as pH value,solvent properties, silicate concentration and temperature)that influence inter-action strength between the polymer blend and the tem-plate-silica self-assembling rate should be studied.4.ConclusionIn summary,polymer blends provide a new template to prepare both the mesoporous silica and carbons,and their morphologies and mesostructures could be changed by carefully controlling the compositions of the poly-mer blends.The unique advantage of using non-volatileTable1Physical properties of the mesoporous silicas and carbons prepared from calcination and carbonization of the block copolymer–PF resin–silica compositesPolymer blend template a Sample BET surfacearea(m2/g)Poresize(nm)Pore volume(cm3/g)P123–PF resin Silica3507.80.58Carbon1852 2.1 1.02Brij76–PF resin Silica638 3.00.48Carbon1442 2.3 1.38L64–PF resin Silica332 4.50.42Carbon1898 2.0 1.34a The block copolymer/PF resin weight ratio is1.0.The PF resin–blockcopolymer–silica composites were obtained from silicification of the PFresin–block copolymer blends in a sodium silicate solution of pH%5.0at40°C.H.-P.Lin et al./Microporous and Mesoporous Materials93(2006)344–348347polymer precursor can avoid the damages caused by using high volatility of the monomer-form precursors.In addi-tion,with proper interaction strength between com-mercially available polymers,development of a novel template of multi-component copolymer blends to produce the desired mesoporous silicas and carbons or introduce other elements(such as N,O,etc.)into carbon should be achieved[14].In practice,only one day was needed for the entire synthetic processes to prepare mesoporous car-bons of good electrical conductivity,and all the reaction agents used in this process were inexpensive.Although the HF-etching process is still required,our synthetic method should be highly efficient for mass production of mesoporous carbons.When physical and chemical proper-ties of mesoporous carbons and silicas can be easily manip-ulated,exceptional applications in adsorbents for large molecules,hard-templates of metal oxides,electrode mate-rials of methanol fuel cell and electrochemical double lay-ers capacitor will be further explored[15]. AcknowledgmentsWe thank Dr.Hwo-Shuenn Sheu for help in taking X-ray patterns(17A,NSRRC,Taiwan).Authors would also like to thank Chung-Chun Plastic,Taiwan for providing PEO and PF polymers.This work wasfinancially sup-ported by National Science Council,Taiwan(NSC93-2113-M-006-003and NSC93-2323-B-006-009).Appendix A.Supplementary dataSupplementary data associated with this article can be found,in the online version,at doi:10.1016/j.micromeso. 2006.03.011.References[1](a)T.Yanagisawa,T.Shimizu,K.Kuroda,C.Kato,Bull.Chem.Soc.Jpn.63(1990)988;(b)C.T.Kresge,M.E.Leonowicz,W.J.Roth,J.C.Vartuli,J.S.Beck,Nature359(1992)710.[2]D.Zhao,P.Yang,Q.Huo, B.F.Chmelka,G.D.Stucky,Curr.Opinion Solid State Mater.Sci.3(1998)111.[3](a)R.Ryoo,S.H.Joo,M.Kruk,M.Jaroniec,Adv.Mater.13(2001)677;(b)S.H.Joo,S.S.Chol,I.Oh,J.Kwak,Z.Liu,O.Terasaki,R.Ryoo,Nature412(2001)169.[4]Y.Xia,R.Mokaya,Adv.Mater.16(2004)1553.[5](a) C.-M.Yang, C.Weidenthaler, B.Spliethoff,M.Mayanna,F.Schuth,Chem.Mater.17(2005)355;(b)H.Yang,Y.Yan,Y.Liu,F.Zhang,R.Zhang,Y.Meng,M.Li,S.Xie,B.Tu,D.Zhao,J.Phys.Chem.B108(2004)17320.[6]T.Kyotani,T.Nagai,S.Inoue,A.Tomita,Chem.Mater.9(1997)609.[7](a)W.Wayne,G.D.Stucky,Chem.Mater.14(2002)1665;(b)J.Lee,J.Kim,Y.Lee,S.Yoon,S.M.Oh,T.Hyeon,Chem.Mater.16(2004)3323.[8]S.Tanaka,N.Nishiyama,Y.Egashira,K.Ueyama,mun.(2005)2125.[9]M.P.Steven,Polymer Chemistry,Oxford,New York,1999.[10]S.W.Kuo, C.L.Lin, F.C.Chang,Macromolecules35(2002)278.[11]M.Inagaki,K.Kaneko,T.Nishizawa,Carbon42(2004)1401.[12]B.Tian,S.Che,Z.Liu,X.Liu,W.Fan,T.Tatsumi,O.Terasaki,D.Zhao,mun.(2003)2726.[13]D.W.Chen,C.Y.Chang-Chien,Y.P.Lin,H.P.Lin,Chem.Lett.12(2004)1574.[14]M.C.Huang,H.Teng,Carbon41(2003)951.[15](a)J.Lee,S.Han,T.Hyeon,J.Mater.Chem.14(2004)478;(b)J.Roggenbuck,M.Timann,J.Am.Chem.Soc.127(2005)1096;(c)W.C.Li,A.H.Lu,C.Weidenthaler,F.Schu¨th,Chem.Mater.16(2004)5676.348H.-P.Lin et al./Microporous and Mesoporous Materials93(2006)344–348。
硬模板法制备介孔碳及其电化学性能研究
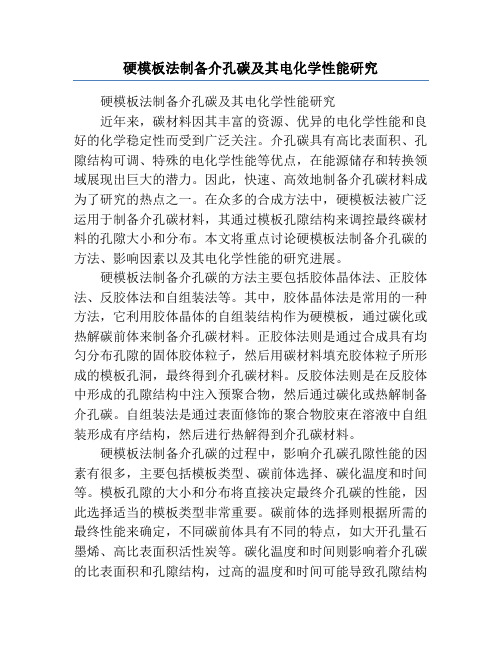
硬模板法制备介孔碳及其电化学性能研究硬模板法制备介孔碳及其电化学性能研究近年来,碳材料因其丰富的资源、优异的电化学性能和良好的化学稳定性而受到广泛关注。
介孔碳具有高比表面积、孔隙结构可调、特殊的电化学性能等优点,在能源储存和转换领域展现出巨大的潜力。
因此,快速、高效地制备介孔碳材料成为了研究的热点之一。
在众多的合成方法中,硬模板法被广泛运用于制备介孔碳材料,其通过模板孔隙结构来调控最终碳材料的孔隙大小和分布。
本文将重点讨论硬模板法制备介孔碳的方法、影响因素以及其电化学性能的研究进展。
硬模板法制备介孔碳的方法主要包括胶体晶体法、正胶体法、反胶体法和自组装法等。
其中,胶体晶体法是常用的一种方法,它利用胶体晶体的自组装结构作为硬模板,通过碳化或热解碳前体来制备介孔碳材料。
正胶体法则是通过合成具有均匀分布孔隙的固体胶体粒子,然后用碳材料填充胶体粒子所形成的模板孔洞,最终得到介孔碳材料。
反胶体法则是在反胶体中形成的孔隙结构中注入预聚合物,然后通过碳化或热解制备介孔碳。
自组装法是通过表面修饰的聚合物胶束在溶液中自组装形成有序结构,然后进行热解得到介孔碳材料。
硬模板法制备介孔碳的过程中,影响介孔碳孔隙性能的因素有很多,主要包括模板类型、碳前体选择、碳化温度和时间等。
模板孔隙的大小和分布将直接决定最终介孔碳的性能,因此选择适当的模板类型非常重要。
碳前体的选择则根据所需的最终性能来确定,不同碳前体具有不同的特点,如大开孔量石墨烯、高比表面积活性炭等。
碳化温度和时间则影响着介孔碳的比表面积和孔隙结构,过高的温度和时间可能导致孔隙结构发生变化或被破坏,影响电化学性能。
介孔碳的电化学性能研究主要包括对其在储能器件(如超级电容器和锂离子电池)中的应用和性能评价。
介孔碳作为电极材料的特殊结构和优异的导电性能使其在超级电容器领域表现出色。
研究发现,介孔碳具有较高的比容量和优异的循环稳定性,且可以快速进行电化学反应,这些特点使得介孔碳成为理想的超级电容器材料。
二氧化硅模板法制备介孔碳

二氧化硅模板法制备介孔碳
介孔碳是一种具有大孔径和高比表面积的碳材料,广泛用于能源存储、催化剂载体和吸附剂等领域。
二氧化硅模板法是一种将二氧化硅作为模板,通过炭化或热解过程制备介孔碳的方法。
首先,二氧化硅模板法的制备步骤如下:首先,选择一个适当的有机硅化合物作为二氧化硅的前体物质,如正硅酸乙酯。
然后,将有机硅化合物溶解在适当的有机溶剂中,并加入一定量的嵌段共聚物,如聚乙烯氧化物-聚丙烯酸酯。
接下来,通过适当的加热和搅拌使溶液均匀混合。
其次,将混合溶液倒入模具中,使溶液均匀涂布在模具内壁上。
然后,将模具放入高温炉中,在惰性气氛下进行炭化或热解过程。
在炭化或热解过程中,有机硅化合物会分解形成二氧化硅,而嵌段共聚物会产生微相分离,形成连续有序的孔道结构。
最后,经过脱硅和洗涤等步骤,就可以得到二氧化硅模板法制备的介孔碳材料。
二氧化硅模板法制备的介孔碳具有以下优点:首先,通过选择适当的二氧化硅前体物质和嵌段共聚物,可以调控介孔碳的孔径和孔道结构。
其次,该方法制备的介孔碳材料具有较大的比表面积和孔体积,有利于提高其吸附和传质性能。
此外,该方法操作简单、成本较低,并且可以实现批量生产。
总而言之,二氧化硅模板法是一种制备介孔碳的有效方法,通过调控二氧化硅和嵌段共聚物的选择和合适的炭化或热解条件,可以制备具有理想孔径和孔道结构的介孔碳材料。
这种介孔碳材料具有广泛的应用潜力,在能源和环境等领域有着重要的应用前景。
一纳米氧化镁为模板一步法制备多级孔炭材料解读

第一部分文献综述1.1 多孔炭的研究背景与意义伴随着全球经济的快速发展和科技水平的进步,煤、石油和天然气等化石燃料消耗逐年增加,日渐枯竭,并且化石燃料的利用造成严重的环境污染,如温室效应、酸雨、大气颗粒物污染、臭氧层破坏和生态环境破坏等。
人类正面临着资源短缺、环境污染、生态破坏等迫切需要解决的问题,全球经济和会的可持续发展也面临着严峻的考验。
人们迫切需要开发利用新能源和可再生清洁能源来解决日趋短缺的能源问题和日益严重的环境污染。
化学储能装置具有使用方便,性能可靠,便于携带,容量、电流和电压可在相当大的范围内任意组合和对环境无污染等许多优点,在新能源技术的开发和利用中占有重要地位。
储氢、储锂和超级电容器等储能装置的电极材料的研究成为材料研究中的热点。
在所有的储能材料中,多孔碳材料由于具有大的比表面积,均一的孔径分布,孔结构可调等优点,是迄今为止最理想的储能材料。
除此之外,多孔碳材料由于具有均匀的孔径分布,吸收储存气体和液体性能也非常优秀,常被应用于环境保护,制药和化工等领域,作为有毒气体和液体的净化吸收剂。
在近十几年间,有关多孔碳材料方面的报告和论文大批量在国际会议和国际学术刊物上发表,表明多孔碳材料已经成为当今科学界的研究热点。
经过科研人员多年不断的试验研究,大批量孔径尺寸分布均匀且可以调控、结构组成可以变化、排列样式和孔道形态多种多样的多孔碳材料可以通过各种各样的合成方法被制备出来。
尽管人们已经取得了许多成果,但是多孔碳材料仍然存在许多不足,需要我们去探索和解决,多孔碳材料的性能与实际应用有一定的差距,也有待进一步提高。
未来仍然需要我们不断努力去开发成本低,制备过程简单,性能优越的多孔碳材料,并不断推进在电化学领域的广泛应用。
1.2 多孔碳材料概述多孔碳材料是指一种具有多孔性结构的含碳物质,具有高度发达的孔隙结构,大的比表面积,且其孔径可以根据实际要求进行调控。
多孔碳材料主要包括普通活性碳、超级活性碳(高比表面积活性炭)、活性炭纤维、泡沫碳材料、碳分子筛等不同形态的碳材料。
一纳米氧化镁为模板一步法制备多级孔炭材料解读

第一部分文献综述1.1 多孔炭的研究背景与意义伴随着全球经济的快速发展和科技水平的进步,煤、石油和天然气等化石燃料消耗逐年增加,日渐枯竭,并且化石燃料的利用造成严重的环境污染,如温室效应、酸雨、大气颗粒物污染、臭氧层破坏和生态环境破坏等。
人类正面临着资源短缺、环境污染、生态破坏等迫切需要解决的问题,全球经济和会的可持续发展也面临着严峻的考验。
人们迫切需要开发利用新能源和可再生清洁能源来解决日趋短缺的能源问题和日益严重的环境污染。
化学储能装置具有使用方便,性能可靠,便于携带,容量、电流和电压可在相当大的范围内任意组合和对环境无污染等许多优点,在新能源技术的开发和利用中占有重要地位。
储氢、储锂和超级电容器等储能装置的电极材料的研究成为材料研究中的热点。
在所有的储能材料中,多孔碳材料由于具有大的比表面积,均一的孔径分布,孔结构可调等优点,是迄今为止最理想的储能材料。
除此之外,多孔碳材料由于具有均匀的孔径分布,吸收储存气体和液体性能也非常优秀,常被应用于环境保护,制药和化工等领域,作为有毒气体和液体的净化吸收剂。
在近十几年间,有关多孔碳材料方面的报告和论文大批量在国际会议和国际学术刊物上发表,表明多孔碳材料已经成为当今科学界的研究热点。
经过科研人员多年不断的试验研究,大批量孔径尺寸分布均匀且可以调控、结构组成可以变化、排列样式和孔道形态多种多样的多孔碳材料可以通过各种各样的合成方法被制备出来。
尽管人们已经取得了许多成果,但是多孔碳材料仍然存在许多不足,需要我们去探索和解决,多孔碳材料的性能与实际应用有一定的差距,也有待进一步提高。
未来仍然需要我们不断努力去开发成本低,制备过程简单,性能优越的多孔碳材料,并不断推进在电化学领域的广泛应用。
1.2 多孔碳材料概述多孔碳材料是指一种具有多孔性结构的含碳物质,具有高度发达的孔隙结构,大的比表面积,且其孔径可以根据实际要求进行调控。
多孔碳材料主要包括普通活性碳、超级活性碳(高比表面积活性炭)、活性炭纤维、泡沫碳材料、碳分子筛等不同形态的碳材料。
软模板法合成介孔碳材料的研究进展
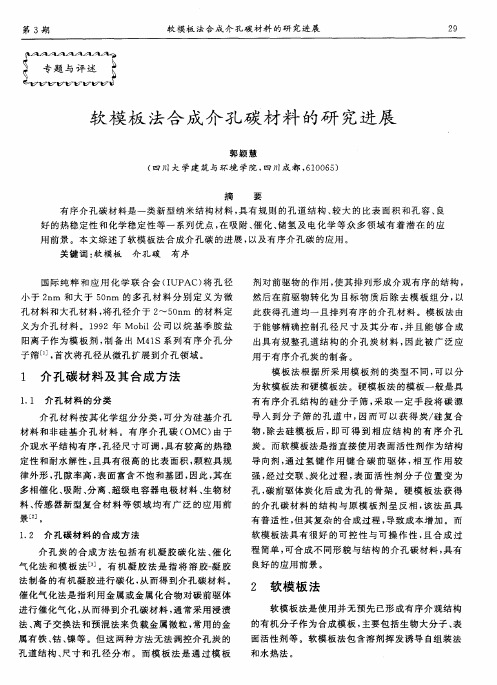
的有 机分 子作 为合 成模 板 , 主要 包括 生 物大分 子 、 表
面活 性剂 等 。软模 板法 包 含溶剂 挥 发诱导 自组 装法 和水 热法 。
孑 L 道结 构 、 尺寸 和孔 径 分 布 。而模 板 法 是 通 过 模 板
3 0 2 . 1 溶剂挥 发 诱导 自组 装法 (
软 模板 法合成 介孔 碳材 料 的研 究进展
2 9
《 专 题 与 评 述 2
t t t l t t l l t
软 模 板 法合 成 介 孔 碳 材 料 的研 究进 展
郭 颖 慧
( 四川 大学 建筑 与环境 学院 , 四川成 都 , 6 1 0 0 6 5 )
介孑 L 材 料按 其 化 学 组 分分 类 , 可分 为 硅 基 介 孔 材 料和 非硅 基 介 孔 材 料 。有 序介 孔 碳 ( 0ME) 由于
介 观水 平结 构有 序 , 孔径 尺 寸可 调 , 具 有较 高 的热稳
定性 和 耐水 解性 , 且 具有很 高的 比表 面积 , 颗粒 具规
关键 词 : 软模板 介孔 碳 有序
国际 纯 粹 和 应 用 化 学 联 合 会 ( I UP AC) 将 孔 径
剂 对前 驱物 的作用 , 使 其排 列形 成介 观 有序 的结 构 , 然后 在前 驱 物转化 为 目标 物 质 后 除 去 模 板组 分 , 以
此 获得孑 L 道 均一且 排列 有 序 的介 孑 L 材 料 。模板 法 由
进行 催化 气化 , 从 而得 到 介孑 L 碳材料 , 通常 采用 浸渍 法、 离 子交 换 法 和预混 法 来负 载金 属微 粒 , 常用 的金
属有铁、 钴、 镍 等 。但 这两 种方 法无 法调 控介 孔 炭 的
介孔碳 合成

介孔碳合成介孔碳是一种具有大孔径、高比表面积和良好化学稳定性的碳材料。
它具有介孔结构,表面积可达到几百到几千平方米每克,孔径分布均匀且可调控。
介孔碳的合成方法多种多样,下面将介绍几种常见的合成方法。
一种常用的合成方法是模板法。
该方法通过选择适当的模板剂,如有机聚合物或纳米颗粒,来控制介孔碳的孔径和结构。
首先,选择模板剂并与碳源混合,形成混合物。
然后,在高温条件下进行热处理,使模板剂分解或挥发,留下介孔碳。
最后,通过洗涤和热处理等步骤,去除残留物,得到纯净的介孔碳。
另一种合成方法是硬模板法。
该方法使用硬模板剂,如硅胶或氧化铝,作为模板,通过碳源的浸渍和热处理来制备介孔碳。
首先,选择合适的硬模板剂,并将其浸渍在碳源溶液中。
然后,将浸渍后的硬模板剂进行热处理,使其分解或挥发,留下介孔碳。
最后,通过酸洗或高温处理等方法,去除硬模板剂,得到纯净的介孔碳。
还有一种合成方法是软模板法。
该方法使用软模板剂,如表面活性剂或聚合物,来调控介孔碳的孔径和结构。
首先,选择合适的软模板剂,并将其与碳源混合。
然后,通过溶胶-凝胶法或水热法等方法,形成凝胶体系。
最后,通过热处理或碳化等步骤,将凝胶转化为介孔碳。
除了以上几种常见的合成方法,还有其他一些特殊的合成方法。
例如,气相法利用气相沉积技术,在适当的反应条件下,将气体或蒸汽中的碳源转化为介孔碳。
电化学法利用电化学沉积技术,在电解质溶液中通过电极反应,将碳源转化为介孔碳。
此外,还有一些新兴的合成方法,如微乳液法和热压法等,可以制备具有特殊结构和性能的介孔碳材料。
介孔碳具有许多优异的性能和广泛的应用。
由于其大孔径和高比表面积,介孔碳可以用作吸附剂、催化剂载体和电化学电极材料。
它还具有良好的化学稳定性和热稳定性,可以应用于储能、分离和环境治理等领域。
此外,通过调控介孔碳的孔径和结构,还可以实现对其性能的定制和优化。
介孔碳是一种具有重要应用潜力的碳材料。
通过选择合适的合成方法和调控条件,可以制备出具有不同孔径和结构的介孔碳材料。
一种利用盐模板制备高比表面积大孔-介孔碳的方法及应用[发明专利]
![一种利用盐模板制备高比表面积大孔-介孔碳的方法及应用[发明专利]](https://img.taocdn.com/s3/m/ad494080011ca300a7c39061.png)
专利名称:一种利用盐模板制备高比表面积大孔-介孔碳的方法及应用
专利类型:发明专利
发明人:储伟,李敬,骞伟中
申请号:CN201811021947.5
申请日:20180904
公开号:CN109553098A
公开日:
20190402
专利内容由知识产权出版社提供
摘要:本发明公开了一种利用盐模板制备高比表面积大孔‑介孔碳材料的方法,包括以下步骤:将生物质明胶溶热水形成溶胶,加入无机盐作为模板剂,冷却至室温后放置于4℃的恒温箱中形成水凝胶,然后在‑20℃下继续放置形成冻胶;将冻胶冷冻干燥,得气凝胶在氩气氛围下400‑1000℃焙烧得黑色碳化产物。
经水洗,抽滤,烘干得到最终产品。
本发明方法采用可溶性生物质碳源和无机盐为原料,方法简便成本低,不涉及难溶金属模板,不需要酸洗,不残留金属杂质,绿色环保;所制备的碳材料具有高比表面积(2872cm g),三维连续,丰富的大孔(50‑150nm)和介孔(2‑4 nm),氮原子掺杂等特征。
将其应用于4V离子液体超级电容器中,能够提供92Wh kg的能量密度,以及优异的功率密度。
申请人:四川大学
地址:610065 四川省成都市武侯区一环路南一段24号
国籍:CN
更多信息请下载全文后查看。
- 1、下载文档前请自行甄别文档内容的完整性,平台不提供额外的编辑、内容补充、找答案等附加服务。
- 2、"仅部分预览"的文档,不可在线预览部分如存在完整性等问题,可反馈申请退款(可完整预览的文档不适用该条件!)。
- 3、如文档侵犯您的权益,请联系客服反馈,我们会尽快为您处理(人工客服工作时间:9:00-18:30)。
In this study, ordered mesoporous carbons (OMCs) with different pore structures, namely 2D hexagonal CMK-3 and 3D cubic CMK-8 prepared by the nanocasting method using mesoporous silicas SBA-15 and KIT-6 as hard templates, respectively, in their pure forms are used as anode materials in lithium ion batteries (LIBs) to evaluate the role of mesoporous structures in their electrochemical performances. The results demonstrate that the CMK-8 electrode exhibits a higher reversible capacity and better cycling stability and rate capability, as compared to the CMK-3 electrode, due to its unique 3D cubic mesostructure. The initial capacities of 1884 and 964 mA h gÀ1 are obtained for the CMK-8 and CMK-3 electrodes, respectively. The CMK-8 electrode exhibits a higher capacity value (around 37.4% higher) than
b
layers is still a concern for using these materials as anodes in LIBs. To overcome this shortcoming and to further improve the LIB anode performance, much of the recent research has been focused on the incorporation of metal oxide nanoparticles (e.g., Co3O4, Fe3O4, SnO2, MoO2, TiO2 and others) into carbonaceous materials to integrate the advantages of metal oxides and carbons.16–25 Although it is considered to be a promising strategy, the development of new carbonaceous materials with favorable structural properties, such as morphology, crystallinity, and porosity, as ideal carbon matrices for the high performance of LIBs is highly desirable. In recent years, ordered mesoporous carbons (OMCs), e.g., CMK-3, have gained more attention to be used as the anode materials in lithium-ion batteries due to their unique structural characteristics such as ordered mesoporous channels, high surface areas, large pore volumes and uniform pore size distribution.16,17,26 The ordered mesoporous channels and large surface areas of OMCs have the advantages to shorten the diffusion length of lithium ions. Moreover, their pore structures provide a continuous pathway for rapid transport of electrons, and thus exhibit high conductivities.27 Among various types of OMCs, the mesoporous carbon CMK-3, a reverse replica of mesoporous silica SBA-15 with a twodimensional (2D) hexagonal pore structure, and its composites have become the most widely investigated electrode materials for LIBs.18,19,27 For example, Zhou et al. have tested the performance of CMK-3 in a three-electrode cell and obtained a high reversible specic capacity.27 Zhang et al. have prepared
RSC Advances
Published on 05 May 2015. Downloaded by East China University of Science & Technology on 09/10/2015 16:39:18.
PAPER
View Article Online
View Journal | View Issue
Received 24th March 2015 Accepted 5th May 2015 DOI: 10.1039/c5ra05168a /advances
the CMK-3 electrode at the 100th cycle. The enhanced electrochemical performance of CMK-8 is mainly attributable to its unique 3D channel networks, which are beneficial for efficient Li storage and volume change. Although CMK-3 is the most investigated OMCs used in LIBs, herein we demonstrate that CMK8 is a better carbon matrix for the fabrication of the electrode materials composed of mesoporous carbons.
Cite this: RSC Adv., parative study of ordered mesoporous carbons with different pore structures as anode materials for lithium-ion batteries†
a
Department of Chemistry, National Central University, Chung-Li 32054, Taiwan, Republic of China. E-mail: hmkao@.tw; Fax: +886-3-4227664; Tel: +8863-4275054 Department of Fuel Cell Materials and Advanced Capacitors, Division of Energy Storage Materials and Technology, Material and Chemical Laboratories, Industrial Technology Research Institute, Hsin-Chu 300, Republic of China † Electronic supplementary information (ESI) available: Small angle XRD and nitrogen adsorption–desorption isotherms of SBA-15 and KIT-6, dynamic light scattering measurements of CMK-3 and CMK-8, and SEM images of CMK-3 and CMK-8 samples aer 100 cycles. See DOI: 10.1039/c5ra05168a
Introduction
Rechargeable lithium-ion batteries (LIBs) are promising power sources that are currently used in many portable electronic devices and electric vehicles due to their high energy density, high operating voltage, low self-discharge, long cycle life and low toxicity.1–5 To date, graphite is still the most commonly used anode material in LIBs because of its desirable characteristics such as low cost, easy processability and chemical stability. However, the Li storage capacity of graphite is limited to 372 mA h gÀ1 due to the formation of LiC6 in the lithium-ion intercalation process, which greatly restricts the development of LIBs with high energy density.6 Other carbonaceous materials, in their various forms such as amorphous carbons, carbon nanotubes, carbon nanobers, graphene and large porous carbon monoliths, have been extensively studied in order to achieve higher specic capacities.7–15 However, the high irreversible capacity due to the formation of solid electrolyte interface (SEI)