RIE深刻蚀SiC工艺
反应离子刻蚀氮化硅与二氧化硅工艺的研究
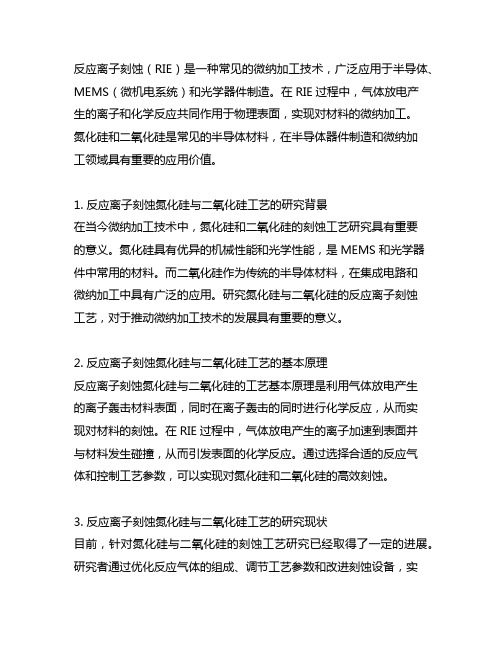
反应离子刻蚀(RIE)是一种常见的微纳加工技术,广泛应用于半导体、MEMS(微机电系统)和光学器件制造。
在RIE过程中,气体放电产生的离子和化学反应共同作用于物理表面,实现对材料的微纳加工。
氮化硅和二氧化硅是常见的半导体材料,在半导体器件制造和微纳加工领域具有重要的应用价值。
1. 反应离子刻蚀氮化硅与二氧化硅工艺的研究背景在当今微纳加工技术中,氮化硅和二氧化硅的刻蚀工艺研究具有重要的意义。
氮化硅具有优异的机械性能和光学性能,是MEMS和光学器件中常用的材料。
而二氧化硅作为传统的半导体材料,在集成电路和微纳加工中具有广泛的应用。
研究氮化硅与二氧化硅的反应离子刻蚀工艺,对于推动微纳加工技术的发展具有重要的意义。
2. 反应离子刻蚀氮化硅与二氧化硅工艺的基本原理反应离子刻蚀氮化硅与二氧化硅的工艺基本原理是利用气体放电产生的离子轰击材料表面,同时在离子轰击的同时进行化学反应,从而实现对材料的刻蚀。
在RIE过程中,气体放电产生的离子加速到表面并与材料发生碰撞,从而引发表面的化学反应。
通过选择合适的反应气体和控制工艺参数,可以实现对氮化硅和二氧化硅的高效刻蚀。
3. 反应离子刻蚀氮化硅与二氧化硅工艺的研究现状目前,针对氮化硅与二氧化硅的刻蚀工艺研究已经取得了一定的进展。
研究者通过优化反应气体的组成、调节工艺参数和改进刻蚀设备,实现了对氮化硅和二氧化硅的高质量刻蚀。
还有一些研究致力于在RIE 过程中减小残留应力、改善表面粗糙度和控制刻蚀剩余物等问题,以满足不同领域对于氮化硅和二氧化硅材料加工的要求。
4. 反应离子刻蚀氮化硅与二氧化硅工艺的发展趋势随着微纳加工技术的不断发展,反应离子刻蚀氮化硅与二氧化硅工艺将会朝着更加高效、精密和可控的方向发展。
未来的研究重点可能集中在以下几个方面:一是针对特定器件和应用领域的工艺优化,提高刻蚀质量和加工效率;二是开发新型的反应气体和刻蚀设备,拓展氮化硅与二氧化硅的刻蚀工艺窗口;三是结合表面修饰技术,实现对氮化硅和二氧化硅表面特性的精细调控。
台式反应离子刻蚀(RIE)系统
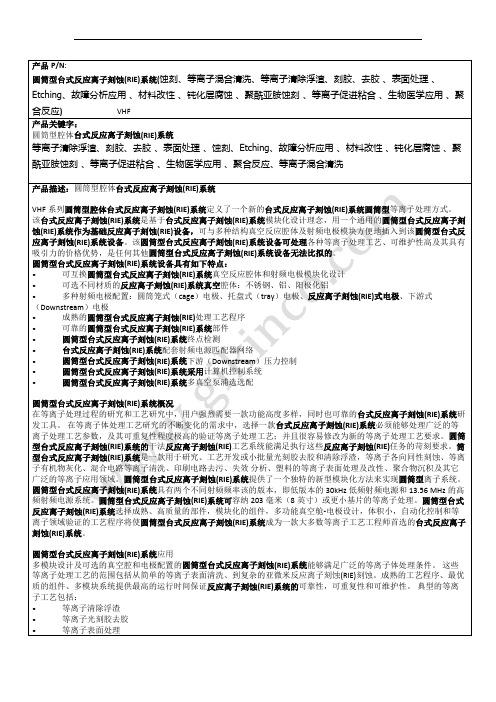
通用的圆筒型台式反应离子刻蚀(RIE)系统的基础系统平台包含所有必要的阀门、真空管路,射频电源、射频电源匹配器、工艺气体控制和系统逻辑提供一个完全自动化的圆筒型台式反应离子刻蚀(RIE)系统。圆筒型台式反应离子刻蚀(RIE)系统的基础平台设计可容纳各种模块化的真空腔及射频电极插入到基础系统单元之中。圆筒型台式反应离子刻蚀(RIE)系统可以在几分钟内从一般的圆筒型台式反应离子清洗系统转换成圆筒型台式反应离子刻蚀(RIE)系统或圆筒型台式平板电极系统。
产品P/N:
圆筒型台式反应离子刻蚀(RIE)系统(蚀刻、等离子混合清洗、等离子清除浮渣、刻胶、去胶、表面处理、Etching、故障分析应用、材料改性、钝化层腐蚀、聚酰亚胺蚀刻、等离子促进粘合、生物医学应用、聚合反应)VHF
产品关键字:
圆筒型腔体台式反应离子刻蚀(RIE)系统
等离子清除浮渣、刻胶、去胶、表面处理、蚀刻、Etching、故障分析应用、材料改性、钝化层腐蚀、聚酰亚胺蚀刻、等离子促进粘合、生物医学应用、聚合反应、等离子混合清洗
•成熟的圆筒型台式反应离子刻蚀(RIE)处理工艺程序
•可靠的圆筒型台式反应离子刻蚀(RIE)系统部件
•圆筒型台式反应离子刻蚀(RIE)系统终点检测
•台式反应离子刻蚀(RIE)系统配套射频电源匹配器网络
•圆筒型台式反应离子刻蚀(RIE)系统下游(Downstream)压力控制
•圆筒型台式反应离子刻蚀(RIE)系统采用计算机控制系统
圆筒型台式反应离子刻蚀(RIE)系统的模块化真空舱及射频电极配置
模块化的真空舱和射频电极组件是圆筒型台式反应离子刻蚀(RIE)系统的最独特的设计特点。钱真空舱材质可以是铝、阳极氧化铝和不锈钢。圆筒型台式反应离子刻蚀(RIE)系统的真空舱可以很容易互换从一种转换成另外一种。真空处理舱的组成包括几种不同的射频电极设计,其中包括水冷[温度控制]和用于反应离子刻蚀(RIE)系统的平行射频电极板,交变托盘式射频电极用于等离子表面清洁或处理,用于普通的为最大限度地减少离子损伤的下游电极和圆筒笼式(cage)电极。
sic蚀刻工艺

sic蚀刻工艺
一、概述
SIC蚀刻工艺是一种用于将硅碳化物材料制成器件的工艺。
该工艺主要利用气相反应将SiC材料表面进行蚀刻,以达到加工和制备器件的目的。
二、材料准备
1. SiC衬底:选择高质量的SiC衬底,表面应平整无瑕疵。
2. 掩模:根据器件设计要求选择合适的掩模,掩模应与SiC衬底紧密贴合。
3. 光刻胶:选择合适的光刻胶,并按照厂家说明书进行处理。
三、光刻
1. 清洗SiC衬底表面,去除污垢和有机物。
2. 在SiC衬底表面涂上光刻胶,并利用旋涂机将其均匀涂布在整个表面上。
3. 将掩模放置在光刻胶上,并利用紫外线曝光机进行曝光。
曝光时间和强度应根据厂家说明书和器件设计要求进行调整。
4. 去除未曝光部分的光刻胶,形成图案。
四、蚀刻
1. 在蚀刻室中,将SiC衬底放置在蚀刻夹具上,并将其与蚀刻室密封。
2. 开始气相反应,向蚀刻室中注入气体混合物(如Cl2、BCl3、H2等),并控制温度和压力。
3. 蚀刻时间应根据器件设计要求和材料特性进行调整。
4. 蚀刻后,将SiC衬底取出并清洗干净。
五、后续处理
1. 在SiC衬底表面进行电镀或其他加工,形成器件结构。
2. 清洗器件表面,并进行测试。
六、注意事项
1. 操作时应注意安全,避免气体泄漏和爆炸等危险。
2. 气相反应条件应根据材料特性和器件设计要求进行优化。
3. 光刻胶的选择和处理应严格按照厂家说明书进行操作。
硅片减薄蚀刻技术:RIE技术将硅片减薄到小于20微米
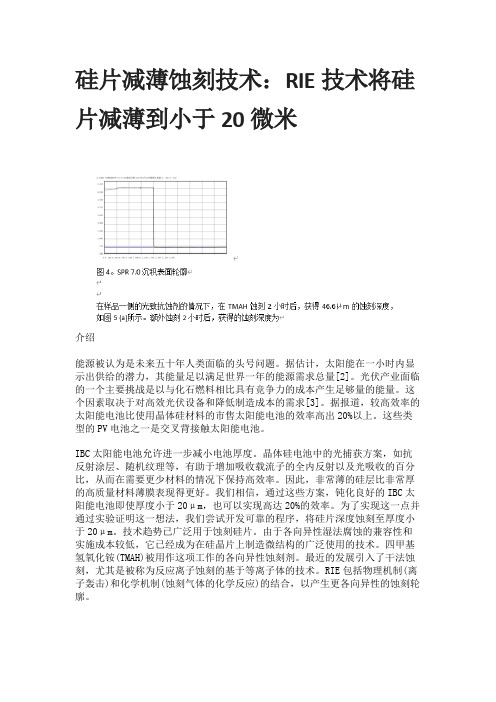
硅片减薄蚀刻技术:RIE技术将硅片减薄到小于20微米介绍能源被认为是未来五十年人类面临的头号问题。
据估计,太阳能在一小时内显示出供给的潜力,其能量足以满足世界一年的能源需求总量[2]。
光伏产业面临的一个主要挑战是以与化石燃料相比具有竞争力的成本产生足够量的能量。
这个因素取决于对高效光伏设备和降低制造成本的需求[3]。
据报道,较高效率的太阳能电池比使用晶体硅材料的市售太阳能电池的效率高出20%以上。
这些类型的PV电池之一是交叉背接触太阳能电池。
IBC太阳能电池允许进一步减小电池厚度。
晶体硅电池中的光捕获方案,如抗反射涂层、随机纹理等,有助于增加吸收载流子的全内反射以及光吸收的百分比,从而在需要更少材料的情况下保持高效率。
因此,非常薄的硅层比非常厚的高质量材料薄膜表现得更好。
我们相信,通过这些方案,钝化良好的IBC太阳能电池即使厚度小于20μm,也可以实现高达20%的效率。
为了实现这一点并通过实验证明这一想法,我们尝试开发可靠的程序,将硅片深度蚀刻至厚度小于20μm。
技术趋势已广泛用于蚀刻硅片。
由于各向异性湿法腐蚀的兼容性和实施成本较低,它已经成为在硅晶片上制造微结构的广泛使用的技术。
四甲基氢氧化铵(TMAH)被用作这项工作的各向异性蚀刻剂。
最近的发展引入了干法蚀刻,尤其是被称为反应离子蚀刻的基于等离子体的技术。
RIE包括物理机制(离子轰击)和化学机制(蚀刻气体的化学反应)的结合,以产生更各向异性的蚀刻轮廓。
本文介绍了使用RIE技术将硅片减薄到小于20微米的最终厚度。
这是通过使用SU-8光致抗蚀剂实现的,SU-8光致抗蚀剂是一种高对比度的负环氧基光致抗蚀剂,作为掩模层。
对这种类型的光致抗蚀剂在40微米和120微米的膜沉积厚度下的行为进行了研究。
使用SU-8光致抗蚀剂开发了基线光刻工艺。
使用SU-8和RIE方法的结果与流行的各向异性湿法化学蚀刻在蚀刻轮廓和最特别的蚀刻速率方面进行了比较。
对这一概念的理解有助于未来超薄IBC太阳能电池制造中的应用。
sic蚀刻工艺
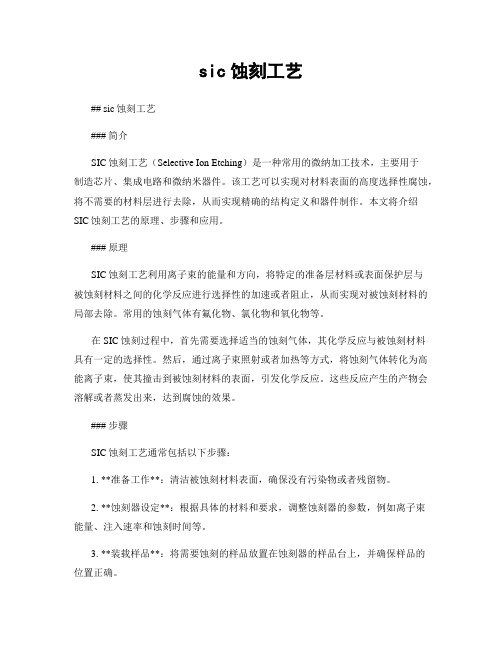
sic蚀刻工艺## sic蚀刻工艺### 简介SIC蚀刻工艺(Selective Ion Etching)是一种常用的微纳加工技术,主要用于制造芯片、集成电路和微纳米器件。
该工艺可以实现对材料表面的高度选择性腐蚀,将不需要的材料层进行去除,从而实现精确的结构定义和器件制作。
本文将介绍SIC蚀刻工艺的原理、步骤和应用。
### 原理SIC蚀刻工艺利用离子束的能量和方向,将特定的准备层材料或表面保护层与被蚀刻材料之间的化学反应进行选择性的加速或者阻止,从而实现对被蚀刻材料的局部去除。
常用的蚀刻气体有氟化物、氯化物和氧化物等。
在SIC蚀刻过程中,首先需要选择适当的蚀刻气体,其化学反应与被蚀刻材料具有一定的选择性。
然后,通过离子束照射或者加热等方式,将蚀刻气体转化为高能离子束,使其撞击到被蚀刻材料的表面,引发化学反应。
这些反应产生的产物会溶解或者蒸发出来,达到腐蚀的效果。
### 步骤SIC蚀刻工艺通常包括以下步骤:1. **准备工作**:清洁被蚀刻材料表面,确保没有污染物或者残留物。
2. **蚀刻器设定**:根据具体的材料和要求,调整蚀刻器的参数,例如离子束能量、注入速率和蚀刻时间等。
3. **装载样品**:将需要蚀刻的样品放置在蚀刻器的样品台上,并确保样品的位置正确。
4. **真空处理**:将蚀刻器的内部抽成真空状态,以防止气体泄漏和干扰蚀刻过程。
5. **气体处理**:将蚀刻气体注入蚀刻器,使其达到所需浓度。
6. **蚀刻处理**:开始蚀刻过程,控制离子束的能量和方向,使其仅蚀刻指定的区域。
7. **清洗和干燥**:蚀刻结束后,将样品从蚀刻器中取出,并进行清洗和干燥,以去除残留的蚀刻产物和污染物。
### 应用SIC蚀刻工艺在微纳加工领域具有广泛的应用。
首先,SIC蚀刻工艺可以用于集成电路的制造。
通过控制蚀刻气体的选择和加工参数,可以精确地定义集成电路的结构和形状,制造出高精度的电子器件。
其次,SIC蚀刻工艺还可以用于MEMS(微机电系统)器件的制造。
压电型微悬臂梁制备中RIE刻蚀硅工艺的研究
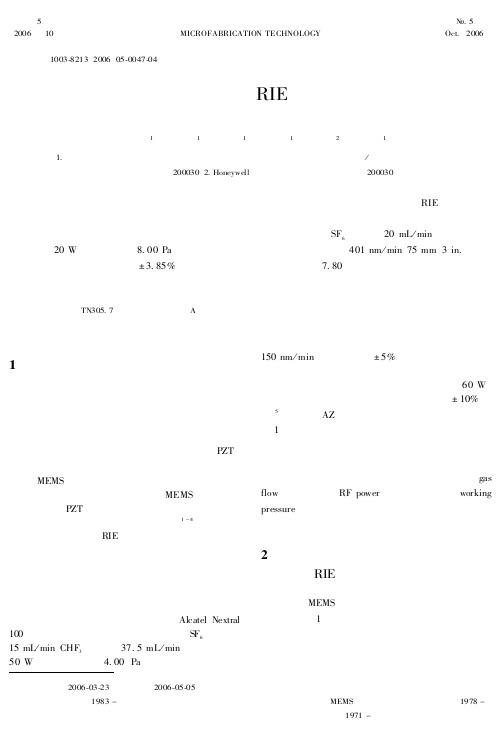
微细加工技术 &’()*+,-)’(,.’*/ .0(1/*2*34
56 ! *786 , "##$
压 电 型 微 悬 臂 梁 制 备 中
% % %
)’0
%
刻 蚀 硅 工艺 的 研 究
" %
董9 璐 , 方华斌 , 刘景全 , 徐9 东 , 徐峥 谊 , 蔡炳初
% % 在图 $ 所示的结构中, -./ 压电层关系着压电 微悬臂梁器件的工作性能, 是整个工艺过程中需要 重视的关键部分。 -./ 膜的制备采用新型 456 7896 技 [# ] 术, 通过多层涂覆 -./ 薄膜形成厚膜 , 其工艺过 程为: 以 !7甲氧 基乙 醇为溶 剂, 醋 酸铅、 四丁 氧基 钛、 异丙醇锆为溶质制备 -./ 先驱体溶液, 并在溶 液中另外添加摩尔百分含量为 !": 的富铅物, 然后 以0 """ ; < =’> 的转速涂覆在基底上, 时间为!" 4; 再 在 0"" ? 下进行 ! =’> 高温分解。重复以上步骤, 逐层进行, 经过 3 次涂覆, 得到 $@ # != 厚的膜。最 后在 #)" ? 下进行 0" =’> 快速热退火处理, 得到纯 钙钛矿相结 构的 -./ 膜。其刻蚀工艺采 用配比为 ! ( ABC) D ! ( BE6) D ! ( B ! ()F $D !)D 12 的刻蚀 液, 刻蚀速度约 "@ 0 != < =’>。 另外, -./ 膜的存在对后续的工艺也有着一定 影响。制备中发现暴露在硅的湿法刻蚀溶液 ( G(B 溶液) 中的电极会很快脱落, 其下露 出的 -./ 膜也 极易随电极脱落。原因是在湿法刻蚀硅中所使用的 G(B 溶液对 -./ 和电极有很强的破坏作用, 因此在 进行正面硅槽刻蚀 时, 考虑采 用反应离子刻 蚀法。 另外从背部深刻蚀硅形成悬臂梁结构时, 如果使用 湿法刻蚀直接刻 穿基片会造成刻蚀液渗入 硅片正 面, 对电极和 -./ 造成破坏; 而使用反应离子刻蚀 法直接刻穿基片将耗费大量的时间, 并且刻蚀不均 匀; 如采用 H*+, 则工艺成本昂贵。因此在研究中 采取如下 法: 先 将 基 片用 夹 具 单面 保 护, 再用 G(B 溶液刻蚀背面硅窗, 预留较薄一层硅 即停止, 最后用反应离子刻蚀将剩余的硅刻穿。
第三代半导体材料及制造工艺
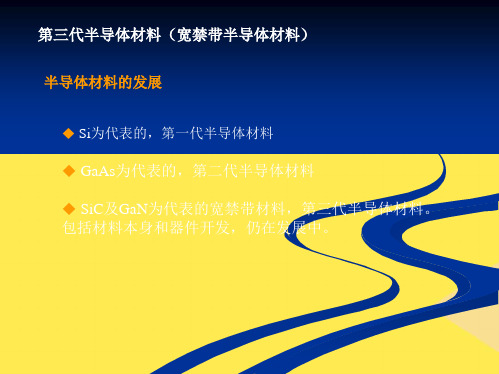
SiC 的结构
SiC结构示意图a) 3C-SiC;b) 2H-SiC; c) 4H-SiC;d) 6H-SiC。
a) ABCABC…, 3C-SiCb) ABAB…, 2H-SiC;c) ABCBABCB…, 4H-SiCd) ABCACB…, 6H-SiC
SiC 优良的物理和化学性能
力学性质: 高硬度(克氏硬度为3000 kg/mm2),可以切割红宝石;高耐磨性,仅次于金刚石。 热学性质: 热导率超过金属铜,是 Si 的3倍,是 GaAs 的 8-10 倍,散热性能好,对于大功率器件非常重要。SiC 的热稳定性较高,在常压下不可能熔化 SiC。 化学性质: 耐腐蚀性非常强,室温下几乎可以抵抗任何已知的腐蚀剂。SiC 表面易氧化生成 SiO2 薄层,能防止其进一步氧化,在高于1700 oC 时,这层 SiO2 熔化并迅速发生氧化反应。SiC能溶解于熔融的氧化剂物质。 电学性质: 4H-SiC 和 6H-SiC 的带隙约是 Si 的三倍,是 GaAs 的两倍;其击穿电场强度高于 Si 一个数量级,饱和电子漂移速度是 Si 的倍。4H-SiC 的带隙比 6H-SiC 更宽。
1824 年,瑞典科学家J. Jacob Berzelius 在试图制备金刚石时意外发现了这种新的化合物。1885 年,Acheson 用电弧熔炼法生长出 SiC, 但用这种方法形成的SiC质量较差,达不到大规模生产SiC器件所需的SiC单晶的质量要求。1955 年菲力浦研究室的 Lely 首先在实验室用升华法制备了杂质数量和种类可控的、具有足够尺寸的 SiC 单晶。具体过程:设计一个空腹的圆筒,将具有工业级的 SiC 块放入碳坩埚中,加热到 2500 oC, SiC 发生明显的分解与升华,产生 Si 和 SiC 的蒸汽,在高温炉内形成的温度梯度作用下向低温方向并凝聚在较低温度处,形成 SiC 晶体。此过程是一个“升华-凝聚”的过程,生长的驱动力是温度梯度。
sic反应离子刻蚀加工
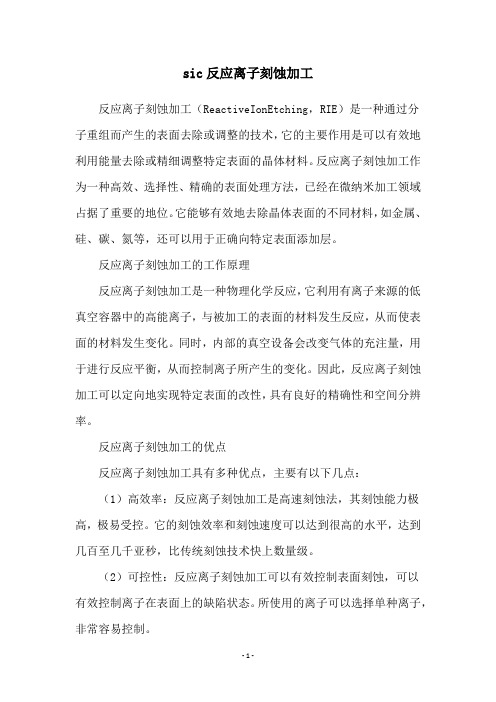
sic反应离子刻蚀加工反应离子刻蚀加工(ReactiveIonEtching,RIE)是一种通过分子重组而产生的表面去除或调整的技术,它的主要作用是可以有效地利用能量去除或精细调整特定表面的晶体材料。
反应离子刻蚀加工作为一种高效、选择性、精确的表面处理方法,已经在微纳米加工领域占据了重要的地位。
它能够有效地去除晶体表面的不同材料,如金属、硅、碳、氮等,还可以用于正确向特定表面添加层。
反应离子刻蚀加工的工作原理反应离子刻蚀加工是一种物理化学反应,它利用有离子来源的低真空容器中的高能离子,与被加工的表面的材料发生反应,从而使表面的材料发生变化。
同时,内部的真空设备会改变气体的充注量,用于进行反应平衡,从而控制离子所产生的变化。
因此,反应离子刻蚀加工可以定向地实现特定表面的改性,具有良好的精确性和空间分辨率。
反应离子刻蚀加工的优点反应离子刻蚀加工具有多种优点,主要有以下几点:(1)高效率:反应离子刻蚀加工是高速刻蚀法,其刻蚀能力极高,极易受控。
它的刻蚀效率和刻蚀速度可以达到很高的水平,达到几百至几千亚秒,比传统刻蚀技术快上数量级。
(2)可控性:反应离子刻蚀加工可以有效控制表面刻蚀,可以有效控制离子在表面上的缺陷状态。
所使用的离子可以选择单种离子,非常容易控制。
(3)有效性:反应离子刻蚀加工有很强的选择性,能够根据个性化的要求进行刻蚀,而不会损坏其他部位。
(4)精确性:反应离子刻蚀加工的精确度比其他表面处理方法高上许多,它可以实现精确的刻蚀,这对于集成电路表面处理有着重要的意义。
由于反应离子刻蚀加工的优势,它得以广泛应用在半导体加工、MEMS制造、MEMC制造、激光刻蚀加工以及制造等领域,为微纳米加工技术的发展贡献了巨大的力量。
反应离子刻蚀加工在微纳米加工中的重要性反应离子刻蚀加工作为高能量离子聚焦在表面上进行刻蚀,使得它在微纳米加工中有着至关重要的地位。
它的刻蚀效率和刻蚀速度极高,而且非常可控,在刻蚀特定表面材料时能够实现极高的精确度,是当今微纳米加工中最重要的表面加工技术之一。
4H-SiC材料干法刻蚀工艺的研究

4H-SiC材料干法刻蚀工艺的研究许龙来;钟志亲【摘要】鉴于SiC材料具有很强的稳定性以及湿法刻蚀的种种缺点,目前主要使用干法刻蚀来刻蚀SiC材料.但是干法刻蚀后样品表面的粗糙度对器件的性能有一定的影响.针对这一问题,采用电感耦合等离子体-反应离子刻蚀技术,对SiC材料进行SF6/02混合气体和SF6/CF4/O2混合气体的刻蚀,并且探究了压强、ICP功率和混合气体比例对样品表面粗糙度的影响.实验结果表明使用SF6/O2混合气体刻蚀后,样品的表面平整度较好.在一定RIE功率条件下,当ICP功率为700 W、压强为20 mT和SF6/O2为50/40 sccm时,样品表面的粗糙度最小.【期刊名称】《电子科技》【年(卷),期】2019(032)002【总页数】4页(P1-3,8)【关键词】碳化硅;电感耦合等离子体-反应离子刻蚀;粗糙度;压强;功率;SF6/O2【作者】许龙来;钟志亲【作者单位】电子科技大学电子薄膜与集成器件国家重点实验室,四川成都610054;电子科技大学电子薄膜与集成器件国家重点实验室,四川成都610054【正文语种】中文【中图分类】TN305SiC材料作为第三代宽禁带半导体材料,可用于制造出与传统硅基器件相比,能在更高温度、更高功率和更恶劣的环境下工作的器件[1-2]。
此外,SiC材料的高硬度及其化学稳定性使它在微机电系统中也有很好的应用前景[3-6]。
由于Si-C共价键很强,使SiC化学性质非常稳定。
常温下湿法腐蚀很难使SiC图形化,需要在高温辅助下进行强酸碱湿法化学腐蚀或者激光辅助光电化学刻蚀 [7]。
但是,这种腐蚀的缺点在于只有很少的金属可以用作掩模。
而且湿法腐蚀具有各向同性,致使侧壁出现严重的钻蚀现象,因此普遍使用干法刻蚀图案化SiC材料。
常用的干法刻蚀技术包括:反应离子刻蚀(RIE)[8]、电子回旋共振等离子体(ECR)以及电感耦合等离子体刻蚀(ICP)等[9-10]。
一种用于刻蚀硅的反应离子刻蚀方法[发明专利]
![一种用于刻蚀硅的反应离子刻蚀方法[发明专利]](https://img.taocdn.com/s3/m/77baa729fe00bed5b9f3f90f76c66137ee064fc5.png)
(10)申请公布号 CN 101928941 A(43)申请公布日 2010.12.29C N 101928941 A*CN101928941A*(21)申请号 200910053598.X(22)申请日 2009.06.23C23F 1/12(2006.01)(71)申请人中微半导体设备(上海)有限公司地址201201 上海市浦东华东路5001号金桥出口加工区(南区)中央大道188号(72)发明人崔在雄 安东炫 李柯奋 吴万俊(74)专利代理机构上海智信专利代理有限公司31002代理人王洁(54)发明名称一种用于刻蚀硅的反应离子刻蚀方法(57)摘要本发明提供一种用于刻蚀硅的反应离子刻蚀方法,属于半导体制造技术领域。
在该反应离子刻蚀方法中,采用的刻蚀气体包括含氟元素的气体和与硅反应形成钝化层的气体,刻蚀过程结束时,先停止通入氟元素的气体再停止通入与硅反应形成钝化层的气体。
通过该方法刻蚀硅形成的孔洞,具有相对较小的底切效应和碗形效应。
(51)Int.Cl.(19)中华人民共和国国家知识产权局(12)发明专利申请权利要求书 1 页 说明书 5 页 附图 2 页CN 101928941 A1/1页1.一种用于刻蚀硅的反应离子刻蚀方法,采用的刻蚀气体包括第一气体和第二气体,所述第一气体为含氟元素的气体,所述第二气体为用于与硅反应形成钝化层的气体,其特征在于,刻蚀过程结束时,先停止通入第一气体再停止通入第二气体。
2.根据权利要求1所述的反应离子刻蚀方法,其特征在于,刻蚀过程开始时,先通入第二气体再通入第一气体。
3.根据权利要求1或2所述的反应离子刻蚀方法,其特征在于,所述第一气体包括SF6、CF4、CHF4、NF3之一。
4.根据权利要求1或2所述的反应离子刻蚀方法,其特征在于,所述第二气体为CO2、CO、O2、N2之一或者CO2、CO、O2、N2任意组合的混合气体。
5.根据权利要求4所述的反应离子刻蚀方法,其特征在于,所述第二气体为CO2、CO、或者CO2与CO的混合气体时,所述与硅反应形成的钝化层为硅氧化合物或者碳硅化合物。
4H-SiC MESFET的反应离子刻蚀和牺牲氧化工艺
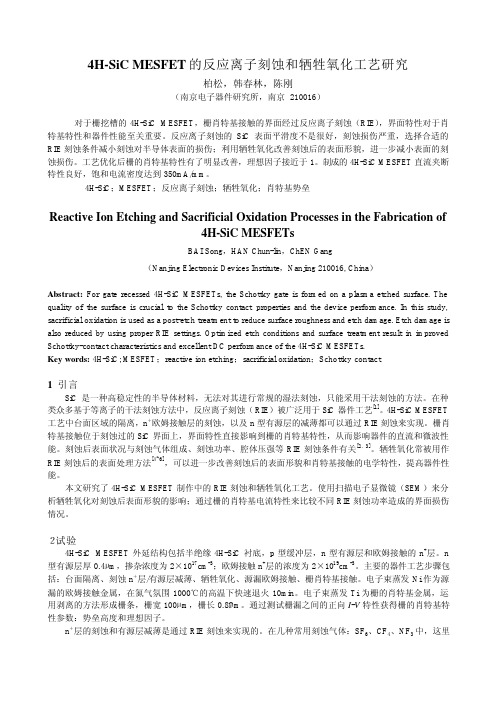
4H-SiC MESFET的反应离子刻蚀和牺牲氧化工艺研究柏松,韩春林,陈刚(南京电子器件研究所,南京 210016)摘要:对于栅挖槽的4H-SiC MESFET,栅肖特基接触的界面经过反应离子刻蚀(RIE),界面特性对于肖特基特性和器件性能至关重要。
反应离子刻蚀的SiC表面平滑度不是很好,刻蚀损伤严重,选择合适的RIE刻蚀条件减小刻蚀对半导体表面的损伤;利用牺牲氧化改善刻蚀后的表面形貌,进一步减小表面的刻蚀损伤。
工艺优化后栅的肖特基特性有了明显改善,理想因子接近于1。
制成的4H-SiC MESFET直流夹断特性良好,饱和电流密度达到350mA/mm。
关键词:4H-SiC;MESFET;反应离子刻蚀;牺牲氧化;肖特基势垒Reactive Ion Etching and Sacrificial Oxidation Processes in the Fabrication of4H-SiC MESFETsBAI Song,HAN Chun-lin,ChEN Gang(Nanjing Electronic Devices Institute,Nanjing 210016, China)Abstract: For gate recessed 4H-SiC MESFETs, the Schottky gate is formed on a plasma etched surface. The quality of the surface is crucial to the Schottky contact properties and the device performance. In this study, sacrificial oxidation is used as a post-etch treatment to reduce surface roughness and etch damage. Etch damage is also reduced by using proper RIE settings. Optimized etch conditions and surface treatment result in improved Schottky-contact characteristics and excellent DC performance of the 4H-SiC MESFETs.Key words: 4H-SiC; MESFET;reactive ion etching;sacrificial oxidation;Schottky contact1 引言SiC是一种高稳定性的半导体材料,无法对其进行常规的湿法刻蚀,只能采用干法刻蚀的方法。
氮化镓中使用光电化学刻蚀技术实现高纵横比深沟槽的进展

氮化镓中使用光电化学刻蚀技术实现高纵横比深沟槽
的进展
据麦姆斯咨询报道,近日,日本SCIOCS公司与法政大学报道了其在氮化镓(GaN)中使用光电化学(photo-electro-chemical,简称PEC)刻蚀技术实现高纵横比深沟槽的进展。
该小组希望这项技术能够利用GaN在高电场中的高击穿电场和高电子漂移速度等优良特性,为功率电子创造新的器件结构。
深刻蚀用来创建具有p型和n型材料柱的“超结”结构,当结合侧向场效应晶体管时,就会产生超过10kV的击穿电压。
同时,垂直器件也可以从超结漂移区域和其他深刻蚀结构中获益。
因此,激光二极管的脊形加工、晶圆切割应用和MEMS(微机电系统)等领域也需要高质量的快速刻蚀工艺。
如今,PEC技术已经应用于台面结构(mesa)、凹入式栅极(gate-recess)和垂直腔面发射激光器(VCSEL)制造工艺上。
据这项技术的研究者Horikiri 称,这项技术得到了日本环境部的大力支持。
该团队说道:“我们承诺将与GaN产业链分享此项PEC刻蚀技术,这是我们作为GaN衬底供应商的职责之一。
”通常,深刻蚀通过干法等离子体刻蚀实现,例如电感耦合等离子体反应离子刻蚀(ICP-RIE),但是该技术会造成较严重的表面损伤。
再加上由于GaN和掩模材料之间的干法刻蚀选择性低。
DRIE深反应离子刻蚀技术 PPT
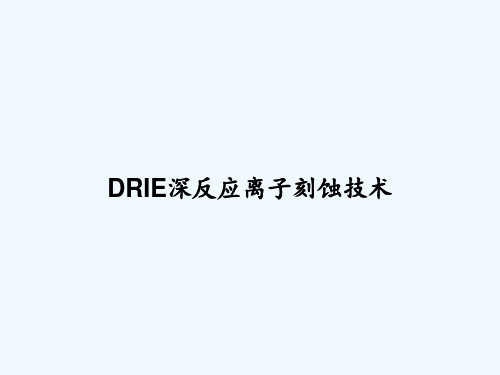
SiF4
Dry
腐蚀速率
• 腐蚀剂的产生
– 若没有腐蚀剂的产生,就没有腐蚀
• 扩散到表面
1 Generation of Etchant Species 6 Diffusion to Bulk Gas
– 腐蚀剂必须到表面才能和膜反应或腐蚀
2 Diffusion to Surface
– 运动到表面的方式将影响到选择比,
F+e-
F+eCF4 F+e-
F+e-
Cathode
-Vbias
e - + CF4 → CF3+ + F + 2e-
• RF: 频率 (典型 13.56 MHz)
– 电子在辉光区的震荡需要足够的能量以引起离化 – 气体离子太重,不能响应高频电场的变化
干法刻蚀工艺的类型
• 断面特征取决于:
– 能量 – 压力 (平均自由程) – 偏压 – 方向性 – 晶向
• 气体可以控制
• 尽管 CF4 只腐蚀 Si, 当更多的 Si 表面暴露时, 更多的 F 被消耗掉,导致 F/C 减小,腐蚀减慢
• 加入 H2 消耗 F – 易导致 polymerization • 加入 O2 消耗 C – 导致腐蚀
时间复用深腐蚀
开始
各向同性 SF6 腐蚀 加各向异性轰击
各向同性Polymer
过刻, 和均匀性
3 Adsorption
• 吸附和表面扩散
4 Reaction
– 能产生方向选择 (isotropic or anisotro• 反应
– 与温度关系密切 (Arrhenius relationship)
– 明显影响腐蚀速率
RIE深刻蚀SiC工艺

Published in Materials Research Society Symposium Proceedings Vol. 622 © 2000 Materials Research Society Deep RIE Process for Silicon Carbide Power Electronics and MEMSGlenn Beheim and Carl S. Salupo1NASA Glenn Research CenterCleveland, OH 441351Akima CorporationCleveland, OH 44135ABSTRACTReactive ion etching (RIE) of silicon carbide (SiC) to depths ranging from 10 µm to more than 100 µm is required for the fabrication of SiC power electronics and SiC MEMS. A deep RIE process using an inductively coupled plasma (ICP) etch system has been developed which provides anisotropic etch profiles and smooth etched surfaces, a high rate (3000 Å/min), and a high selectivity (80:1) to the etch mask. An etch depth of 100 µm is demonstrated.INTRODUCTIONDeep RIE processes for SiC are needed to realize the intrinsic advantages of SiC for power electronics and harsh environment MEMS. Etch depths from 10 µm to more than 100 µm are required for trench isolation of SiC power devices, through-wafer vias for advanced packaging schemes, and bulk micromachined SiC structures. The ideal deep RIE process would provide a high rate (at least several thousand Å/min), a highly anisotropic etch profile (e.g. vertical sidewalls with minimal bowing), and smooth etched surfaces. In addition, a high selectivity with respect to an easily deposited and patterned etch mask is required. Deep RIE of SiC has previously been demonstrated using conventional capacitive-type RIE systems [1]. Previously, inductively coupled plasma (ICP) etching has been shown to provide high rates for SiC [2-4]. The effectiveness of ICP for deep etching of SiC is demonstrated here.Key advantages of ICP relative to conventional RIE include: (1) a considerably higher plasma density, which provides a greater flux of energetic ions and reactive species (e.g. atomic fluorine) to the sample; (2) capability for operation at lower pressures, which helps minimize bowing of the etch sidewalls and can also help to eliminate residues caused by the redeposition of nonvolatile etch products (e.g. sputtered mask materials) onto the etched surfaces; (3) capa-bility for independent control of the plasma density and the energy with which ions bombard the sample, through the use of separate RF generators for the coil and substrate bias electrode.EXPERIMENT A: ETCH RATE AND SELECTIVITY MEASUREMENTSFor this study deep ICP etching was performed on the silicon face of n-type 6H-SiC using an STS Multiplex ICP [5]. The 10-mm square SiC samples were attached to 100-mm diameter silicon carrier wafers using a drop of photoresist. Typically, the silicon carrier wafer etches at a fairly rapid rate (about 2 µm/min) because Si readily reacts to form a volatile product with atomic fluorine. The sacrificial carrier wafer helps to minimize roughness caused by the sputtering of nonvolatile materials onto the etched SiC surface, which leads to micromasking. The loading effect caused by the silicon carrier wafer varies with different process parameters. For the baseline process (described below), the same etch rate was obtained whether the SiCsample was mounted on a bare silicon wafer or a silicon wafer coated with nonreactive indium-tin-oxide (ITO). Nickel was used for thinner (≤2500 Å) and ITO for thicker etch masks (up to 3.5 µm). For thicknesses greater than about 2500 Å, Ni was too highly stressed, while the grain size of the ITO made it unsuitable for thinner masks. Liftoff was found to be a convenient means to pattern these evaporated films.A series of experiments was performed to determine the effects on SiC etch rate and selectivity that would be produced when the pressure, platen power (power to the substrate bias electrode) and oxygen flow were varied from the baseline process parameters. The baseline parameters, which were found in a preliminary investigation to provide a clean deep etch, are shown in table 1. A Ni etch mask was used for these measurements.Table 1. Baseline process parameters:Pressure SF 6 flowO 2 flow Coil power Platen power 5 mTorr 55 sccm 0 800 W 75 WFigure 1 shows the SiC etch rate as a function of pressure. Etch rate was found todecrease with increasing pressure. For these measurements the flow rate was maximized subject to the constraints imposed by the pumping system and flow controller. The SF 6 flow was 27 sccm at 3 mTorr, 55 sccm at 5 mTorr, and 110 sccm for 10 mTorr and higher.Figure 1. SiC etch rate as a function of pressure (27-110 sccm SF 6, 800 W coil, 75 W platen).Figure 2 shows the SiC etch rate and the selectivity to the Ni mask (ratio of the SiC and Ni etch rates) as functions of the platen power. The application of RF power to the platen causes ions from the plasma to be accelerated towards the substrate. Since the etch mask forms no volatile products with fluorine, its etch rate is strongly influenced by the platen power. For a platen power of 75 W or less, the selectivity is greater than 80:1.Figure 3 shows the effect of oxygen on the etch rates of SiC and Si. Here, the total flow rate was held fixed at 55 to 60 sccm, while the proportions of SF 6 and O 2 were varied. In general, the SiC etch rate decreased with increasing O 2 fraction. The slight increase in etch rate observed at 64% O 2 coincided with a rapidly decreasing Si etch rate; slower etching of the silicon carrier would make more atomic fluorine available for SiC etch.050010001500200025003000350051015202530354045Pressure (mTorr)S i C E t c h R a t e (Å/m i n )Figure 2. SiC etch rate and selectivity to Ni mask as functions of platen power (55 sccm SF 6, 5 mTorr pressure, 800 W coil power).Figure 3. SiC and Si etch rates as functions of the ratio of the O 2 flow to the total of the SF 6 and O 2 flows (55-60 sccm total flow, 5 mTorr pressure, 800 W coil and 75 W platen power).5001000150020002500300035000.00.10.20.30.40.50.60.70.80.91.0Fraction 02S i C R a t e (Å/m i n )5000100001500020000250003000035000S i R a t e (Å/m i n )0100020003000400050006000050100150200250Platen Power (Watts)S i C E t c h R a t e (Å/m i n )020406080100120S e l e c t i v i t yEXPERIMENT B: DEEP ETCHINGAn investigation of the various etch parameters for etches deeper than 10 µm found that the baseline process parameters provided a deep etch that was satisfactory for many applications.Relative to the baseline parameters, higher platen powers produced a lower selectivity to the mask, higher pressures and the addition of oxygen promoted residue formation, while lower pressures caused increased trenching. Figure 4 shows typical results for an off-baseline etchusing 20 sccm SF 6 and 35 sccm O 2, with all other parameters identical to the baseline. Figure 4 is an SEM image of a 1-mm diameter well which was etched to a depth of 40 µm. The application for this type of etch would be the fabrication of a diaphragm for a high temperature SiC pressure sensor.Figure 4. SEM image of a 1-mm diameter pit etched 40 µm using 20 sccm SF 6 and 35 sccm O 2, 5 mTorr pressure, 800 W coil and 75 W platen power.Figure 5. SEM image of a 1-mm diameter pit etched 46 µm using 55 sccm SF 6, 5 mTorr pressure, 800 W coil and 75 W platen power. The etch mask, which was not stripped prior to the SEM, was 3.5-µm thick ITO, and the duration of the etch was 150 min. The spike-like etch residue visible in Figure 4 was found to be almost entirely eliminated when the baseline parameters (55 sccm SF 6, no O 2) were used. The dimples in the etched surface, however, were a characteristic common to all the etch recipes which were tried. This texturing of the etched surface was found to be primarily determined by the condition of the SiC surface at the beginning of the etch. Organic residues and scratches, for example, were found to produce a high concentration of dimples. A number of cleaning procedures (solvents, hot sulfuric, oxidation followed by HF etch) were tried but none was found satisfactory. Several plasma cleaning processes, however, were found to be effective. Figure 5 shows an SEM of a 1-mm diameter pit etched to a depth of 46 µm using the baseline etch process (55 sccm SF 6, no O 2). Again the mask was 3.5 µm ITO, and the duration of the etch was 150 min. Prior toinitiation of the deep etch process, the sample was sputter etched in the ICP for 10 min using 50 sccm Ar, 2.5 mTorr pressure, 800 W coil and 75 W platen power.As can be seen in Figure 5, the ICP sputter etch pretreatment almost entirely eliminated the dimples from the etched surface. However, an entirely satisfactory cleaning procedure has not yet been developed. A disadvantage of the ICP sputter clean is the high rate at which iterodes the ITO mask. The 10 min sputter etch in the ICP was found to remove 1 µm of ITO, but only 500 Å of SiC. A higher pressure may provide a more favorable etch ratio. Preliminary results have shown that a sputter clean using conventional RIE can be effective at eliminating surface texture, with significantly less mask erosion.Figure 6. SEM image of a smaller feature etched in the sample shown in figure 5.Figure 7. SEM image of a via etched through a 100 µm thick SiC wafer.The baseline deep RIE process, which was used to etch the well shown in figure 5, also provides a satisfactory etch of structures with higher aspect ratios. Figure 6 shows a smaller feature, an alignment mark, from the same sample as is shown in figure 5. The residual ITO mask was not stripped. Enhanced etching (trenching) at the bottom of the sidewalls is caused by the deflection of ions that strike the sidewalls at grazing angles of incidence. The slope of the upper part of the sidewalls was a result of the excessive mask erosion during the sputter etch pretreatment. The baseline process is well suited for the etching of vias. Figure 7 shows a via etched through a 100-µm thick SiC wafer. The etch mask was 3.5-µm thick ITO which was readily electron-beam evaporated and patterned using by liftoff. The masked side of the wafer is shown here; the residual ITO was stripped prior to electron microscopy. Previously, the deepest SiC plasma etch reported was 80 µm, using conventional RIE [1]. In this case, a thick nickel mask, fabricated using selective electro-deposition, was used.A highly uniform etch is obtained using the baseline deep RIE process. Figure 8 shows the surface profile of a typical well in the sample shown in figure 5. The etch mask was not yet stripped from this sample. The depth of the well is uniform within ± 2000 Å or ± 0.2% (excluding the trench at the base of the wall, which is inaccessible to the stylus of the profilometer).Figure 8. Surface profile of the bottom of a well etched in the sample shown in figures 5 and 6. The inset shows the bottom of the well using an expanded vertical scale.CONCLUDING REMARKSAn ICP etch process for SiC has been developed which meets many of the deep etching requirements for SiC power devices and MEMS. This process provides a high rate (3000 Å/min), vertical sidewalls, smooth etched surfaces, and high selectivity to the etch mask (80:1). Further work is needed to optimize the surface cleaning procedure which precedes the deep etch process. In addition, work is planned to develop a process which produces smoother sidewalls, which are important in some applications, such as mesas for vertical structure high-voltage devices.ACKNOWLEDGEMENTS The technical assistance of Drago Androjna, Jeffrey Krotine, and David Spry is gratefully acknowledged.REFERENCES 1. D.C. Sheridan, et al., “Demonstration of Deep (80 µm) RIE Etching of SiC for MEMSand MMIC Applications,” in Proceedings of the International Conference on Silicon Carbide and Related Materials 1999, October 10-15, Research Triangle Park, NC. 2. F.A. Khan and I. Adesida, Appl. Phys. Letters 75, pp. 2268-2270 (1999). 3. P. Leerungawarat, et al., J. Vac. Sci. Technol. B 17 pp. 2050-2054 (1999). 4. Colin C. Welch, “Dry Etching of Materials Other than Silicon,” in Extremely HardMaterials for Micromechanics , IEE, London, UK (1997). 5. Surface Technology Systems, Newport, UK.-1.E+050.E+001.E+052.E+053.E+054.E+055.E+056.E+05Horizontal (µm)V e r t i c a l (Å) .。
- 1、下载文档前请自行甄别文档内容的完整性,平台不提供额外的编辑、内容补充、找答案等附加服务。
- 2、"仅部分预览"的文档,不可在线预览部分如存在完整性等问题,可反馈申请退款(可完整预览的文档不适用该条件!)。
- 3、如文档侵犯您的权益,请联系客服反馈,我们会尽快为您处理(人工客服工作时间:9:00-18:30)。
Published in Materials Research Society Symposium Proceedings Vol. 622 © 2000 Materials Research Society Deep RIE Process for Silicon Carbide Power Electronics and MEMSGlenn Beheim and Carl S. Salupo1NASA Glenn Research CenterCleveland, OH 441351Akima CorporationCleveland, OH 44135ABSTRACTReactive ion etching (RIE) of silicon carbide (SiC) to depths ranging from 10 µm to more than 100 µm is required for the fabrication of SiC power electronics and SiC MEMS. A deep RIE process using an inductively coupled plasma (ICP) etch system has been developed which provides anisotropic etch profiles and smooth etched surfaces, a high rate (3000 Å/min), and a high selectivity (80:1) to the etch mask. An etch depth of 100 µm is demonstrated.INTRODUCTIONDeep RIE processes for SiC are needed to realize the intrinsic advantages of SiC for power electronics and harsh environment MEMS. Etch depths from 10 µm to more than 100 µm are required for trench isolation of SiC power devices, through-wafer vias for advanced packaging schemes, and bulk micromachined SiC structures. The ideal deep RIE process would provide a high rate (at least several thousand Å/min), a highly anisotropic etch profile (e.g. vertical sidewalls with minimal bowing), and smooth etched surfaces. In addition, a high selectivity with respect to an easily deposited and patterned etch mask is required. Deep RIE of SiC has previously been demonstrated using conventional capacitive-type RIE systems [1]. Previously, inductively coupled plasma (ICP) etching has been shown to provide high rates for SiC [2-4]. The effectiveness of ICP for deep etching of SiC is demonstrated here.Key advantages of ICP relative to conventional RIE include: (1) a considerably higher plasma density, which provides a greater flux of energetic ions and reactive species (e.g. atomic fluorine) to the sample; (2) capability for operation at lower pressures, which helps minimize bowing of the etch sidewalls and can also help to eliminate residues caused by the redeposition of nonvolatile etch products (e.g. sputtered mask materials) onto the etched surfaces; (3) capa-bility for independent control of the plasma density and the energy with which ions bombard the sample, through the use of separate RF generators for the coil and substrate bias electrode.EXPERIMENT A: ETCH RATE AND SELECTIVITY MEASUREMENTSFor this study deep ICP etching was performed on the silicon face of n-type 6H-SiC using an STS Multiplex ICP [5]. The 10-mm square SiC samples were attached to 100-mm diameter silicon carrier wafers using a drop of photoresist. Typically, the silicon carrier wafer etches at a fairly rapid rate (about 2 µm/min) because Si readily reacts to form a volatile product with atomic fluorine. The sacrificial carrier wafer helps to minimize roughness caused by the sputtering of nonvolatile materials onto the etched SiC surface, which leads to micromasking. The loading effect caused by the silicon carrier wafer varies with different process parameters. For the baseline process (described below), the same etch rate was obtained whether the SiCsample was mounted on a bare silicon wafer or a silicon wafer coated with nonreactive indium-tin-oxide (ITO). Nickel was used for thinner (≤2500 Å) and ITO for thicker etch masks (up to 3.5 µm). For thicknesses greater than about 2500 Å, Ni was too highly stressed, while the grain size of the ITO made it unsuitable for thinner masks. Liftoff was found to be a convenient means to pattern these evaporated films.A series of experiments was performed to determine the effects on SiC etch rate and selectivity that would be produced when the pressure, platen power (power to the substrate bias electrode) and oxygen flow were varied from the baseline process parameters. The baseline parameters, which were found in a preliminary investigation to provide a clean deep etch, are shown in table 1. A Ni etch mask was used for these measurements.Table 1. Baseline process parameters:Pressure SF 6 flowO 2 flow Coil power Platen power 5 mTorr 55 sccm 0 800 W 75 WFigure 1 shows the SiC etch rate as a function of pressure. Etch rate was found todecrease with increasing pressure. For these measurements the flow rate was maximized subject to the constraints imposed by the pumping system and flow controller. The SF 6 flow was 27 sccm at 3 mTorr, 55 sccm at 5 mTorr, and 110 sccm for 10 mTorr and higher.Figure 1. SiC etch rate as a function of pressure (27-110 sccm SF 6, 800 W coil, 75 W platen).Figure 2 shows the SiC etch rate and the selectivity to the Ni mask (ratio of the SiC and Ni etch rates) as functions of the platen power. The application of RF power to the platen causes ions from the plasma to be accelerated towards the substrate. Since the etch mask forms no volatile products with fluorine, its etch rate is strongly influenced by the platen power. For a platen power of 75 W or less, the selectivity is greater than 80:1.Figure 3 shows the effect of oxygen on the etch rates of SiC and Si. Here, the total flow rate was held fixed at 55 to 60 sccm, while the proportions of SF 6 and O 2 were varied. In general, the SiC etch rate decreased with increasing O 2 fraction. The slight increase in etch rate observed at 64% O 2 coincided with a rapidly decreasing Si etch rate; slower etching of the silicon carrier would make more atomic fluorine available for SiC etch.050010001500200025003000350051015202530354045Pressure (mTorr)S i C E t c h R a t e (Å/m i n )Figure 2. SiC etch rate and selectivity to Ni mask as functions of platen power (55 sccm SF 6, 5 mTorr pressure, 800 W coil power).Figure 3. SiC and Si etch rates as functions of the ratio of the O 2 flow to the total of the SF 6 and O 2 flows (55-60 sccm total flow, 5 mTorr pressure, 800 W coil and 75 W platen power).5001000150020002500300035000.00.10.20.30.40.50.60.70.80.91.0Fraction 02S i C R a t e (Å/m i n )5000100001500020000250003000035000S i R a t e (Å/m i n )0100020003000400050006000050100150200250Platen Power (Watts)S i C E t c h R a t e (Å/m i n )020406080100120S e l e c t i v i t yEXPERIMENT B: DEEP ETCHINGAn investigation of the various etch parameters for etches deeper than 10 µm found that the baseline process parameters provided a deep etch that was satisfactory for many applications.Relative to the baseline parameters, higher platen powers produced a lower selectivity to the mask, higher pressures and the addition of oxygen promoted residue formation, while lower pressures caused increased trenching. Figure 4 shows typical results for an off-baseline etchusing 20 sccm SF 6 and 35 sccm O 2, with all other parameters identical to the baseline. Figure 4 is an SEM image of a 1-mm diameter well which was etched to a depth of 40 µm. The application for this type of etch would be the fabrication of a diaphragm for a high temperature SiC pressure sensor.Figure 4. SEM image of a 1-mm diameter pit etched 40 µm using 20 sccm SF 6 and 35 sccm O 2, 5 mTorr pressure, 800 W coil and 75 W platen power.Figure 5. SEM image of a 1-mm diameter pit etched 46 µm using 55 sccm SF 6, 5 mTorr pressure, 800 W coil and 75 W platen power. The etch mask, which was not stripped prior to the SEM, was 3.5-µm thick ITO, and the duration of the etch was 150 min. The spike-like etch residue visible in Figure 4 was found to be almost entirely eliminated when the baseline parameters (55 sccm SF 6, no O 2) were used. The dimples in the etched surface, however, were a characteristic common to all the etch recipes which were tried. This texturing of the etched surface was found to be primarily determined by the condition of the SiC surface at the beginning of the etch. Organic residues and scratches, for example, were found to produce a high concentration of dimples. A number of cleaning procedures (solvents, hot sulfuric, oxidation followed by HF etch) were tried but none was found satisfactory. Several plasma cleaning processes, however, were found to be effective. Figure 5 shows an SEM of a 1-mm diameter pit etched to a depth of 46 µm using the baseline etch process (55 sccm SF 6, no O 2). Again the mask was 3.5 µm ITO, and the duration of the etch was 150 min. Prior toinitiation of the deep etch process, the sample was sputter etched in the ICP for 10 min using 50 sccm Ar, 2.5 mTorr pressure, 800 W coil and 75 W platen power.As can be seen in Figure 5, the ICP sputter etch pretreatment almost entirely eliminated the dimples from the etched surface. However, an entirely satisfactory cleaning procedure has not yet been developed. A disadvantage of the ICP sputter clean is the high rate at which iterodes the ITO mask. The 10 min sputter etch in the ICP was found to remove 1 µm of ITO, but only 500 Å of SiC. A higher pressure may provide a more favorable etch ratio. Preliminary results have shown that a sputter clean using conventional RIE can be effective at eliminating surface texture, with significantly less mask erosion.Figure 6. SEM image of a smaller feature etched in the sample shown in figure 5.Figure 7. SEM image of a via etched through a 100 µm thick SiC wafer.The baseline deep RIE process, which was used to etch the well shown in figure 5, also provides a satisfactory etch of structures with higher aspect ratios. Figure 6 shows a smaller feature, an alignment mark, from the same sample as is shown in figure 5. The residual ITO mask was not stripped. Enhanced etching (trenching) at the bottom of the sidewalls is caused by the deflection of ions that strike the sidewalls at grazing angles of incidence. The slope of the upper part of the sidewalls was a result of the excessive mask erosion during the sputter etch pretreatment. The baseline process is well suited for the etching of vias. Figure 7 shows a via etched through a 100-µm thick SiC wafer. The etch mask was 3.5-µm thick ITO which was readily electron-beam evaporated and patterned using by liftoff. The masked side of the wafer is shown here; the residual ITO was stripped prior to electron microscopy. Previously, the deepest SiC plasma etch reported was 80 µm, using conventional RIE [1]. In this case, a thick nickel mask, fabricated using selective electro-deposition, was used.A highly uniform etch is obtained using the baseline deep RIE process. Figure 8 shows the surface profile of a typical well in the sample shown in figure 5. The etch mask was not yet stripped from this sample. The depth of the well is uniform within ± 2000 Å or ± 0.2% (excluding the trench at the base of the wall, which is inaccessible to the stylus of the profilometer).Figure 8. Surface profile of the bottom of a well etched in the sample shown in figures 5 and 6. The inset shows the bottom of the well using an expanded vertical scale.CONCLUDING REMARKSAn ICP etch process for SiC has been developed which meets many of the deep etching requirements for SiC power devices and MEMS. This process provides a high rate (3000 Å/min), vertical sidewalls, smooth etched surfaces, and high selectivity to the etch mask (80:1). Further work is needed to optimize the surface cleaning procedure which precedes the deep etch process. In addition, work is planned to develop a process which produces smoother sidewalls, which are important in some applications, such as mesas for vertical structure high-voltage devices.ACKNOWLEDGEMENTS The technical assistance of Drago Androjna, Jeffrey Krotine, and David Spry is gratefully acknowledged.REFERENCES 1. D.C. Sheridan, et al., “Demonstration of Deep (80 µm) RIE Etching of SiC for MEMSand MMIC Applications,” in Proceedings of the International Conference on Silicon Carbide and Related Materials 1999, October 10-15, Research Triangle Park, NC. 2. F.A. Khan and I. Adesida, Appl. Phys. Letters 75, pp. 2268-2270 (1999). 3. P. Leerungawarat, et al., J. Vac. Sci. Technol. B 17 pp. 2050-2054 (1999). 4. Colin C. Welch, “Dry Etching of Materials Other than Silicon,” in Extremely HardMaterials for Micromechanics , IEE, London, UK (1997). 5. Surface Technology Systems, Newport, UK.-1.E+050.E+001.E+052.E+053.E+054.E+055.E+056.E+05Horizontal (µm)V e r t i c a l (Å) .。