温敏性的多孔硅纳米颗粒
温度响应性纳米介孔硅的制备、表征及其载药性能
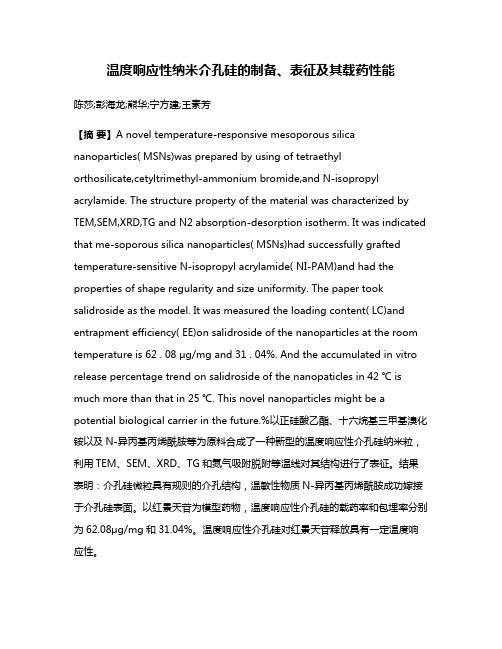
温度响应性纳米介孔硅的制备、表征及其载药性能陈莎;彭海龙;熊华;宁方建;王素芳【摘要】A novel temperature-responsive mesoporous silica nanoparticles( MSNs)was prepared by using of tetraethyl orthosilicate,cetyltrimethyl-ammonium bromide,and N-isopropyl acrylamide. The structure property of the material was characterized by TEM,SEM,XRD,TG and N2 absorption-desorption isotherm. It was indicated that me-soporous silica nanoparticles( MSNs)had successfully grafted temperature-sensitive N-isopropyl acrylamide( NI-PAM)and had the properties of shape regularity and size uniformity. The paper took salidroside as the model. It was measured the loading content( LC)and entrapment efficiency( EE)on salidroside of the nanoparticles at the room temperature is 62 . 08 μg/mg and 31 . 04%. And the accumulated in vitro release percentage trend on salidroside of the nanopaticles in 42 ℃ is much more than that in 25 ℃. This novel nanoparticles might be a potential biological carrier in the future.%以正硅酸乙酯、十六烷基三甲基溴化铵以及N-异丙基丙烯酰胺等为原料合成了一种新型的温度响应性介孔硅纳米粒,利用TEM、SEM、XRD、TG和氮气吸附脱附等温线对其结构进行了表征。
多级孔纳米硅
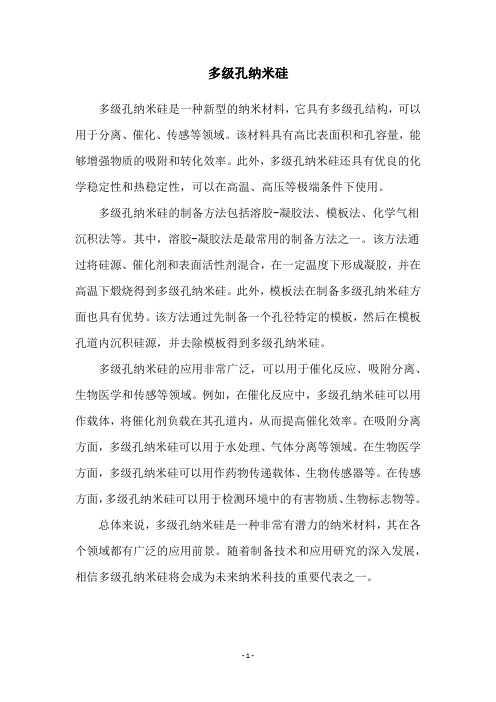
多级孔纳米硅
多级孔纳米硅是一种新型的纳米材料,它具有多级孔结构,可以用于分离、催化、传感等领域。
该材料具有高比表面积和孔容量,能够增强物质的吸附和转化效率。
此外,多级孔纳米硅还具有优良的化学稳定性和热稳定性,可以在高温、高压等极端条件下使用。
多级孔纳米硅的制备方法包括溶胶-凝胶法、模板法、化学气相沉积法等。
其中,溶胶-凝胶法是最常用的制备方法之一。
该方法通过将硅源、催化剂和表面活性剂混合,在一定温度下形成凝胶,并在高温下煅烧得到多级孔纳米硅。
此外,模板法在制备多级孔纳米硅方面也具有优势。
该方法通过先制备一个孔径特定的模板,然后在模板孔道内沉积硅源,并去除模板得到多级孔纳米硅。
多级孔纳米硅的应用非常广泛,可以用于催化反应、吸附分离、生物医学和传感等领域。
例如,在催化反应中,多级孔纳米硅可以用作载体,将催化剂负载在其孔道内,从而提高催化效率。
在吸附分离方面,多级孔纳米硅可以用于水处理、气体分离等领域。
在生物医学方面,多级孔纳米硅可以用作药物传递载体、生物传感器等。
在传感方面,多级孔纳米硅可以用于检测环境中的有害物质、生物标志物等。
总体来说,多级孔纳米硅是一种非常有潜力的纳米材料,其在各个领域都有广泛的应用前景。
随着制备技术和应用研究的深入发展,相信多级孔纳米硅将会成为未来纳米科技的重要代表之一。
- 1 -。
微纳米多孔Si-Ag颗粒掺杂石墨负极材料及其电性能

图像,对比图2(D)和2(c)可以看出,球磨2 h 后,Si粉粒径细化至0. 72 "Z,且球料比为
2。:1时分散性最好。Si粉在高能球磨机中,由
于受
氧化错球
,使得球与粉
之间
,外部的作用力使Si
,粉末受
的比容量,
为1100mAh/g,第
20
为 850 mAh/g,这个
372 mAh/g
,
有
储 的新
型特性。LI等卩10* 纳米管中添加其他活跃的负
极材料,利用其优异的电子导电性、机械和热稳定性
来改善电化学性能。KIM等g用石墨烯作为锂离
子电池负极材料,通过掺杂C&O』可进一步提高负
极材料的导电性能。LIN等)12*通过在人工石墨中
将上述方法获得的多孔Si-Ag复合粉体与人造
石墨 能球磨机中进行均匀复合,形成P-Si/Ag/C
复合材料结构。具体操作如下:取上述多孔Si-Ag复
合粉体100 g, 为80 [的干燥箱中干燥24 h(
随后,称量质量分数为30%的多孔Si-Ag复合粉末
与质量分数为70%人造石墨简单研磨。最后将混
合好的物料放 能球 磨 机 球 磨, 球 磨 线 速 为
SUN Bin,CAI Xiaolan,YAO Yaochun,ZHOU Lei,WNG Lei,WU Shaopeng,ZHU Xiaomeng,LI Wenhao
(Faculty of Metallurgical and Energy Engineering,Kunming University of Science and Technology,
多孔硅纳米材料的制备及在高能锂电池中的应用
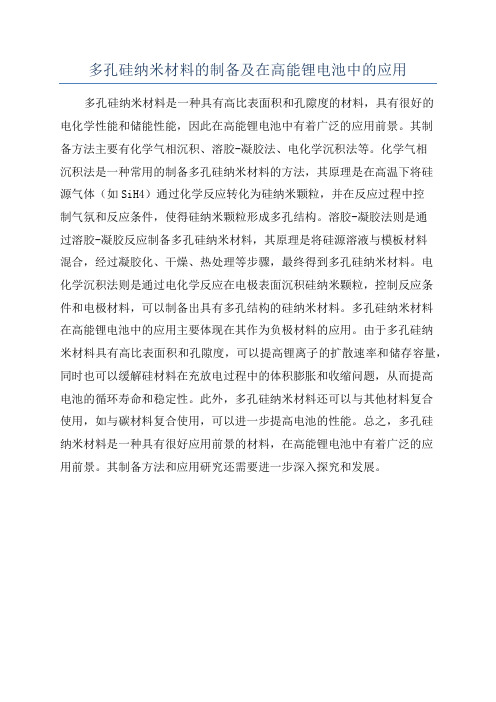
多孔硅纳米材料的制备及在高能锂电池中的应用
多孔硅纳米材料是一种具有高比表面积和孔隙度的材料,具有很好的
电化学性能和储能性能,因此在高能锂电池中有着广泛的应用前景。
其制
备方法主要有化学气相沉积、溶胶-凝胶法、电化学沉积法等。
化学气相
沉积法是一种常用的制备多孔硅纳米材料的方法,其原理是在高温下将硅
源气体(如SiH4)通过化学反应转化为硅纳米颗粒,并在反应过程中控
制气氛和反应条件,使得硅纳米颗粒形成多孔结构。
溶胶-凝胶法则是通
过溶胶-凝胶反应制备多孔硅纳米材料,其原理是将硅源溶液与模板材料
混合,经过凝胶化、干燥、热处理等步骤,最终得到多孔硅纳米材料。
电
化学沉积法则是通过电化学反应在电极表面沉积硅纳米颗粒,控制反应条
件和电极材料,可以制备出具有多孔结构的硅纳米材料。
多孔硅纳米材料
在高能锂电池中的应用主要体现在其作为负极材料的应用。
由于多孔硅纳
米材料具有高比表面积和孔隙度,可以提高锂离子的扩散速率和储存容量,同时也可以缓解硅材料在充放电过程中的体积膨胀和收缩问题,从而提高
电池的循环寿命和稳定性。
此外,多孔硅纳米材料还可以与其他材料复合
使用,如与碳材料复合使用,可以进一步提高电池的性能。
总之,多孔硅
纳米材料是一种具有很好应用前景的材料,在高能锂电池中有着广泛的应
用前景。
其制备方法和应用研究还需要进一步深入探究和发展。
多孔硅基VO纳米颗粒复合结构气敏传感元件的制备方法[发明专利]
![多孔硅基VO纳米颗粒复合结构气敏传感元件的制备方法[发明专利]](https://img.taocdn.com/s3/m/f16fb7a48662caaedd3383c4bb4cf7ec4bfeb65b.png)
专利名称:多孔硅基VO纳米颗粒复合结构气敏传感元件的制备方法
专利类型:发明专利
发明人:梁继然,张颖,吴文豪
申请号:CN202011185310.7
申请日:20201030
公开号:CN114441599A
公开日:
20220506
专利内容由知识产权出版社提供
摘要:本发明公开了一种多孔硅基VO2纳米颗粒复合结构气敏传感元件的制备方法,涉及气敏传感器技术领域,用于解决多孔硅对NO2气体响应灵敏度低的问题,所述制备方法包括以下步骤:清洗单晶硅片;利用电化学腐蚀法制备多孔硅;利用化学气相输运沉积在多孔硅基底沉积VO2纳米颗粒,形成多孔硅基VO2纳米颗粒复合结构气敏传感元件。
通过巨大的比表面积和异质结结构,多孔硅基VO2纳米颗粒复合结构气敏传感元件在室温下对NO2气体的灵敏度有较大的提高,同时有着良好的选择性。
申请人:天津大学
地址:300072 天津市南开区卫津路92号
国籍:CN
更多信息请下载全文后查看。
简述多孔纳米硅在生物医药中的应用

简述多孔纳米硅在生物医药中的应用
刘治鑫
【期刊名称】《当代化工研究》
【年(卷),期】2018(000)012
【摘要】目前,化学高纯硅已作为半导体微电子材料与太阳能电池的首要选择,同时,硅元素在生物医学应用中也极具潜力。
多孔纳米硅(Porous silicon,PSi)可利用外界能量活化体内生化反应和物理化学过程,外界刺激包括光源辐照、超声刺激(Ultrasound,US)、电磁射频(radio frequency,RF)等。
PSi纳米粒子的光敏性质已成功用于多种体外研究和实验中,而介孔级别PSi纳米粒子的光热效应可实现小动物肿瘤热消融和肿瘤细胞的生长抑制。
本文介绍了PSi纳米粒子的制备和在肿瘤治疗中的应用。
【总页数】2页(P188-189)
【作者】刘治鑫
【作者单位】[1]成都七中万达学校,四川610036
【正文语种】中文
【中图分类】R
【相关文献】
1.多孔硅发光—纳米硅量子海绵中的量子约束 [J], 翁渝民;宗祥福
2.氧化钒/多孔硅/硅结构的微观形貌、纳米力学及温敏特性 [J], 杨海波;胡明;梁继然;张绪瑞;刘志刚
3.简述多孔纳米硅在生物医药中的应用 [J], 刘治鑫
4.多孔硅纳米材料的制备及在高能锂电池中的应用 [J], 孙林; 谢杰; 刘涛; 黄松超; 张磊; 陈智栋; 姜瑞雨; 金钟
5.氧化多孔硅和纳米硅粒镶嵌氧化硅光致发光机制模型 [J], 秦国刚;李宇杰
因版权原因,仅展示原文概要,查看原文内容请购买。
MEMS微热敏器件中多孔硅绝热性能研究及模拟的开题报告
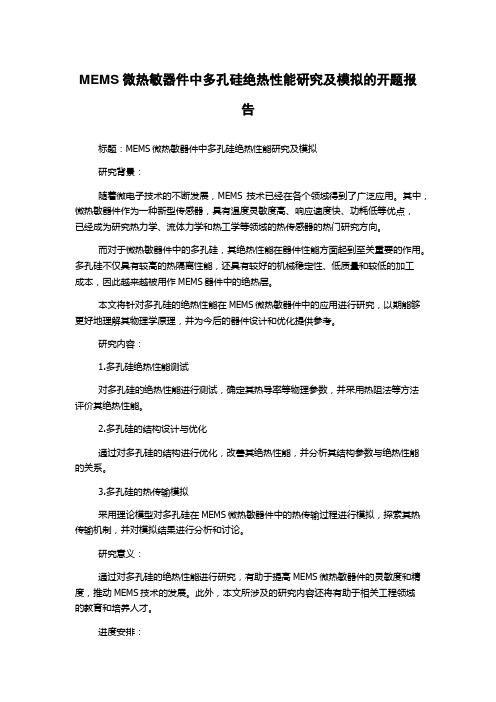
MEMS微热敏器件中多孔硅绝热性能研究及模拟的开题报告标题:MEMS微热敏器件中多孔硅绝热性能研究及模拟研究背景:随着微电子技术的不断发展,MEMS技术已经在各个领域得到了广泛应用。
其中,微热敏器件作为一种新型传感器,具有温度灵敏度高、响应速度快、功耗低等优点,已经成为研究热力学、流体力学和热工学等领域的热传感器的热门研究方向。
而对于微热敏器件中的多孔硅,其绝热性能在器件性能方面起到至关重要的作用。
多孔硅不仅具有较高的热隔离性能,还具有较好的机械稳定性、低质量和较低的加工成本,因此越来越被用作MEMS器件中的绝热层。
本文将针对多孔硅的绝热性能在MEMS微热敏器件中的应用进行研究,以期能够更好地理解其物理学原理,并为今后的器件设计和优化提供参考。
研究内容:1.多孔硅绝热性能测试对多孔硅的绝热性能进行测试,确定其热导率等物理参数,并采用热阻法等方法评价其绝热性能。
2.多孔硅的结构设计与优化通过对多孔硅的结构进行优化,改善其绝热性能,并分析其结构参数与绝热性能的关系。
3.多孔硅的热传输模拟采用理论模型对多孔硅在MEMS微热敏器件中的热传输过程进行模拟,探索其热传输机制,并对模拟结果进行分析和讨论。
研究意义:通过对多孔硅的绝热性能进行研究,有助于提高MEMS微热敏器件的灵敏度和精度,推动MEMS技术的发展。
此外,本文所涉及的研究内容还将有助于相关工程领域的教育和培养人才。
进度安排:第一阶段:收集文献资料,了解目前多孔硅在MEMS微热敏器件中的应用情况。
第二阶段:对多孔硅的绝热性能进行测试,确定其热导率等物理参数,并采用热阻法等方法评价其绝热性能。
第三阶段:对多孔硅的结构进行优化,改善其绝热性能,并分析其结构参数与绝热性能的关系。
第四阶段:采用理论模型对多孔硅在MEMS微热敏器件中的热传输过程进行模拟,探索其热传输机制,并对模拟结果进行分析和讨论。
第五阶段:撰写论文并进行总结和讨论。
预期成果:通过本文的研究,可以在减小MEMS微热敏器件与外界环境的热交换过程中,提高器件的热阻值和灵敏度,同时也有助于更好地理解MEMS器件中的热传输机制。
多级孔纳米硅

多级孔纳米硅多级孔纳米硅是一种具有巨大应用潜力的纳米材料,其在能源存储、生物医药、传感器和催化等领域都具有重要的应用前景。
本文将从多个方面对多级孔纳米硅进行介绍,探讨其制备方法、特性以及应用前景。
多级孔纳米硅是一种具有多级孔结构的纳米材料,具有高比表面积和丰富的表面活性位点。
其制备方法主要包括溶胶-凝胶法、硅烷化学氧化法和电化学法等。
这些方法可以在纳米尺度上调控孔径大小、孔道结构和孔隙分布,从而实现对多级孔纳米硅的精密设计和调控。
多级孔纳米硅具有优异的物理化学性质,如高比表面积、优良的光学性能和独特的电化学性能。
其中,高比表面积为其在吸附分离、催化反应和传感检测等领域提供了良好的应用基础。
此外,多级孔结构还可以有效提高材料的稳定性和可控性,从而拓展了其在各种领域的应用。
在能源存储领域,多级孔纳米硅可以作为锂离子电池和超级电容器的电极材料,具有高能量密度、长循环寿命和快速充放电特性。
其高比表面积和多级孔结构为电荷传输和离子扩散提供了更多的通道,从而提高了能量转换效率和循环稳定性。
在生物医药领域,多级孔纳米硅可以用于药物载体、生物成像和肿瘤治疗等应用。
其多级孔结构可以有效载荷不同类型的药物分子,并实现药物的缓释和靶向释放。
同时,多级孔纳米硅还具有良好的生物相容性和生物可降解性,可以避免对人体的毒副作用。
在传感器和催化领域,多级孔纳米硅被广泛应用于气体传感、生物传感和催化反应等领域。
其高比表面积和丰富的表面活性位点为吸附分子和催化反应提供了更多的反应位点,从而提高了传感器的灵敏度和选择性,以及催化剂的活性和稳定性。
多级孔纳米硅作为一种新型纳米材料,具有广阔的应用前景和发展空间。
通过精密设计和调控其多级孔结构,可以实现对其物理化学性质的精确调控,从而拓展其在能源存储、生物医药、传感器和催化等领域的应用。
相信随着技术的不断进步和研究的深入,多级孔纳米硅将会在各个领域展现出更广阔的应用前景,为人类社会的发展和进步做出重要贡献。
一种多孔硅纳米颗粒粒径的调控方法
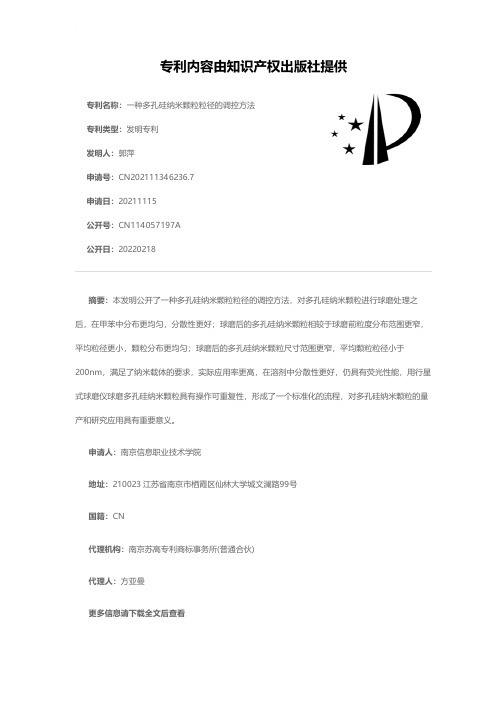
专利名称:一种多孔硅纳米颗粒粒径的调控方法专利类型:发明专利
发明人:郭萍
申请号:CN202111346236.7
申请日:20211115
公开号:CN114057197A
公开日:
20220218
专利内容由知识产权出版社提供
摘要:本发明公开了一种多孔硅纳米颗粒粒径的调控方法,对多孔硅纳米颗粒进行球磨处理之后,在甲苯中分布更均匀,分散性更好;球磨后的多孔硅纳米颗粒相较于球磨前粒度分布范围更窄,平均粒径更小,颗粒分布更均匀;球磨后的多孔硅纳米颗粒尺寸范围更窄,平均颗粒粒径小于
200nm,满足了纳米载体的要求,实际应用率更高,在溶剂中分散性更好,仍具有荧光性能,用行星式球磨仪球磨多孔硅纳米颗粒具有操作可重复性,形成了一个标准化的流程,对多孔硅纳米颗粒的量产和研究应用具有重要意义。
申请人:南京信息职业技术学院
地址:210023 江苏省南京市栖霞区仙林大学城文澜路99号
国籍:CN
代理机构:南京苏高专利商标事务所(普通合伙)
代理人:方亚曼
更多信息请下载全文后查看。
光控释药的可降解温敏介孔硅纳米粒子体系[发明专利]
![光控释药的可降解温敏介孔硅纳米粒子体系[发明专利]](https://img.taocdn.com/s3/m/2dcee1a3e43a580216fc700abb68a98271feaca7.png)
专利名称:光控释药的可降解温敏介孔硅纳米粒子体系
专利类型:发明专利
发明人:董文飞,彭佳惠,梅茜,葛明锋,从瑛哥,常智敏,李力,宁珊珊
申请号:CN202110168748.2
申请日:20210207
公开号:CN112957345B
公开日:
20220308
专利内容由知识产权出版社提供
摘要:本发明公开了一种光控释药的可降解温敏介孔硅纳米粒子体系,其包括:二硒桥连的介孔硅粒子、负载于所述二硒桥连的介孔硅粒子上的化学药物、包覆于所述二硒桥连的介孔硅粒子外部的温敏层以及负载于所述温敏层上的光敏剂。
本发明构建了一种包有温敏层的二硒桥连可降解介孔硅药物递送体系,其核心部位介孔硅粒子具有氧化还原双重响应性质,在肿瘤部位更易于降解从而减小载体材料所带来的蓄积毒性;另一方面,与常见两种药物共载入介孔硅粒子孔道之中不同的是,本体系将化药和光敏剂分级载入,从而可以实现通过光控开关孔道来控制其中化药释放的功能,最终实现光动力与化学药物联合的治疗模式。
申请人:济南国科医工科技发展有限公司,中国科学院苏州生物医学工程技术研究所
地址:250000 山东省济南市高新区综合保税区港兴三路北段1号济南药谷研发平台区3号楼401房间
国籍:CN
代理机构:北京远大卓悦知识产权代理有限公司
代理人:张川
更多信息请下载全文后查看。
- 1、下载文档前请自行甄别文档内容的完整性,平台不提供额外的编辑、内容补充、找答案等附加服务。
- 2、"仅部分预览"的文档,不可在线预览部分如存在完整性等问题,可反馈申请退款(可完整预览的文档不适用该条件!)。
- 3、如文档侵犯您的权益,请联系客服反馈,我们会尽快为您处理(人工客服工作时间:9:00-18:30)。
Temperature-Controlled Uptake and Release in PNIPAM-ModifiedPorous Silica NanoparticlesYe-Zi You,†Kennedy K.Kalebaila,‡Stephanie L.Brock,*,‡and David Oupicky´*,†Departments of Pharmaceutical Sciences and Chemistry,Wayne State Uni V ersity,Detroit,Michigan48202 Recei V ed No V ember27,2007.Re V ised Manuscript Recei V ed March10,2008Temperature-dependent uptake and release of small molecules within porous silica nanoparticles has been achieved by treatment of preformed,thiol-functionalized micro-to-mesoporous silica nanoparticles (MSN)with pyridyl disulfide-terminated poly(N-isopropylacrylamide)(PNIPAM-S-S-Py).The resulting nanoparticle-polymer composites show uptake and release offluorescein at room temperature(below the lower critical solution temperature,LCST,of the polymer)and a low level of leakage at38°C (above LCST,<2%after2h).The data are consistent with a mode of action in whichfluorescein diffusion occurs readily when the polymer is in the random coil conformation but is significantly retarded when the polymer adopts the globule conformation.This mode of action is opposite to that observed for systems in which the PNIPAM is grown from the porous silica surface or co-condensed with silica and is accompanied by a greater than10-fold improvement influorescein retention in the“pore-closed”conformation.IntroductionOf the M41S mesoporous silica materials,hexagonal MCM-41and cubic MCM-48have been of great interest because of their many attractive features,such as stable mesoporous structures,large surface areas,tunable pore sizes and volumes,good biocompatibility,and well-defined surface properties for subsequent organic functionalization.1–4M41S materials have been explored for many practical applications including catalysis,synthesis of optically active materials, polymerization,and separation technology.1Because the pore voids of this class of materials exhibit relatively narrow pore size distributions in the range of1-4nm,micro-to-mesoporous silicas can selectively host molecules of various sizes,shapes,and functionalities.1Accordingly,M41S-based materials are being investigated as passive molecular trans-porters.5–10Recently,significant effort has been devoted to the development of“smart”mesoporous silica-based delivery systems in which uptake and delivery of molecules in the pore voids can be controlled at will by a variety of external stimuli.Thus,coumarin modification of MCM-41allows photocontrolled release of guest molecules,11,12whereas chemically triggered release can be obtained with particles linked by reducible bonds to CdS or iron oxide nanoparticle “caps”.3,4Likewise,redox-or pH-controlled nanovalves based on switchable rotaxane molecules permit ready opening and closing of the nanovalves by chemical means,13–15and ionically controlled molecular-gate-based modified MCM-41enables the regulation of mass transport from the solution to the mesopores.16In most cases,effective use of these modified silica nanospheres is limited by the irreversibility of the pore opening(chemically bound caps)or the relative complexity of the synthesis of the molecular gates(reversible rotaxanes).A simple and reversible delivery system can be envisioned based on a combination of porous silica nanoparticles with stimuli-responsive or“smart”polymers in which access to the pores is controlled by application of a stimulus,such as a change in pH,ionic strength,or temperature.Poly(N-isopropylacrylamide)(PNIPAM)represents one of the most widely investigated temperature-responsive polymers and exhibits a lower critical solution temperature(LCST,the temperature at which the polymer undergoes a change from*To whom correspondence should be addressed.E-mail:oupicky@, sbrock@.†Department of Pharmaceutical Sciences.‡Department of Chemistry.(1)Stein,A.;Melde,B.J.;Schroden,R.C.Ad V.Mater.2000,12,1403–1419.(2)Sayari,A.;Hamoudi,S.Chem.Mater.2001,13,3151–3168.(3)Lai,C.-Y.;Trewyn,B.G.;Jeftinija,D.M.;Jeftinija,K.;Xu,S.-A.;Jeftinja,S.;Lin,V.S.-Y.J.Am.Chem.Soc.2003,125,4451–4459.(4)Giri,S.;Trewyn,B.G.;Stellmaker,M.P.;Lin,V.S.-Y.Angew.Chem.,Int.Ed.2005,44,5039–5044.(5)Azaı¨s,T.;Tourne´-Pe´teilh,C.;Aussenac,F.;Baccile,N.;Coelho,C.;Devoisselle,J.-M.;Babonneau,F.Chem.Mater.2006,18,6382–6390.(6)Horcajada,P.;Ra´mila,A.;Pe´rez-Pariente,J.;Vallet-Regı´,M.Mi-croporous Mesoporous Mater.2004,68,105–109.(7)Mun˜oz,B.;Ra´mila,A.;Pe´rez-Pariente,J.;Dı´az,I.;Vallet-Regı´,M.Chem.Mater.2003,15,500–503.(8)Arruebo,M.;Gala´n,M.;Navascue´s,N.;Te´llez,C.;Marquina,C.;Ibarra,M.R.;Santamarı´a,J.Chem.Mater.2006,18,1911–1919.(9)Izquierdo-Barba,I.;Martinez,A.;Doadrio,A.L.;Pe´rez-Pariente,J.;Vallet-Regı´,M.Eur.J.Pharm.Sci.2005,26,365–373.(10)Lu,J.;Liong,M.;Zink,J.I.;Tamanoi,F.Small2007,3,1341–1346.(11)Mal,N.K.;Fujiwara,M.;Tanaka,Y.Nature2003,421,350–353.(12)Mal,N.K.;Fujiwara,M.;Tanaka,Y.;Taguchi,T.;Matsukata,M.Chem.Mater.2003,15,3385–3394.(13)Nguyen,T.D.;Tseng,H.R.;Celestre,P.C.;Flood,A.H.;Liu,Y.;Stoddart,J.F.;Zink,J.I.PNAS2005,102,10029–10034.(14)Nguyen,T.D.;Liu,Y.;Saha,S.;Leung,K.C.-F.;Stoddart,J.F.;Zink,J.I.J.Am.Chem.Soc.2007,129,626–634.(15)Leung,K.C.-F.;Nguyen,T.D.;Stoddart,J.F.;Zink,J.I.Chem.Mater.2006,18,5919–5928.(16)Casasu´s,R.;Marcos,M.D.;Martinez,Ma´n˜ez.;Ros-Lis,J.V.;Soto,J.;Villaescusa,L.A.;Amoro´s,P.;Beltra´n,A.;Guillem,C.;Latorre, J.J.Am.Chem.Soc.2004,126,8612–8613.3354Chem.Mater.2008,20,3354–335910.1021/cm703363w CCC:$40.75 2008American Chemical SocietyPublished on Web04/25/2008a hydrated to a dehydrated state17,18)of∼32°C in water.19 Thus,at temperatures below the LCST,the polymer is in the coil(soluble)conformation,while above the LCST,it is in the globule or collapsed(insoluble)state.This property has been exploited in applications ranging widely from rheology modifications to drug delivery.20,21Studies on mesoporous silica-PNIPAM hybrids are few. Lo´pez and co-workers prepared hybrids by either conducting atom transfer radical polymerization(ATRP)of the NIPAM monomer from the surface of calcined MCM-41treated with an initiator or cogelation of an aminopropylsilyl-terminated PNIPAM with silica in the presence of a surfactant template.22,23Both methods produce materials capable of fluorescein uptake and release above the LCST of PNIPAM (40-50°C),and the latter method produced membranescapable of rejecting crystal violet at low temperatures.The data are consistent with a mechanism whereby the polymers are encapsulated within the pores of MCM-41,acting to reduce small molecule diffusion when in the open coil configuration while facilitating diffusion in the globule conformation by effectively retreating to the pore walls. However,these systems are very leaky at room temperature, releasing one-half to one-third of the quantity offluorescein released at40-50°C after just2h,and the particles are very large(10-20µm)and thus poorly dispersible.22,23 Herein,we present PNIPAM-modified micro-to-meso-porous silica nanoparticles(MSN)with sizes on the order of100nm prepared by chemical attachment of preformed polymer to preprepared porous particles.Importantly,these new materials show minimal leakage in the“closed”conformation(<2%release over2h vs>25%release in the Lo´pez system22).These improvements appear to be related to a different mode of action than seen previously since the uptake and release in the presently reported system occurs above,rather than below,the LCST.Experimental SectionMaterials.Tetraethylorthosilicate(TEOS),3-mercaptopropyl-trimethoxysilane(MPTMS),N-cetyltrimethylammonium bromide (CTAB),5,5′-dithiobis-2-nitrobenzoic acid(Ellman’s reagent), N-isopropylacrylamide(NIPAM),2,2′-azobisisobutyronitrile(AIBN), and2,2′-dipyridyl disulfide(Aldrithiol-2)were all purchased from Aldrich and used as received.Nanopure water was dispensed from a Nanopure Diamond,Barnstead purification system. Synthesis of2-Dodecylsulfanylthiocarbonylsulfanyl-2-methyl Propionic Acid(DMP).1-Dodecanethiol(20.2g,0.10mol), acetone(58.0g,1.0mol),and tricaprylylmethylammonium chloride (1.0g,0.0025mol)were added into aflask and cooled to0°C under a nitrogen atmosphere.Sodium hydroxide solution(50%) (9.0g,0.11mol)was added over a period of10min.After the mixture was stirred for an additional20min,carbon disulfide(7.6 g,0.10mol)in acetone(10.0g)was added over30min,and the color turned gradually red.Chloroform(17.8g,0.15mol)was added,followed by dropwise addition of40g of50%sodium hydroxide solution over20min.The mixture was stirred overnight; then200mL of water was added,followed by80mL of concentrated HCl to acidify the aqueous solution.After removing organic solvents,the solid wasfiltered and then stirred in300mL of isopropanol.The insoluble solid wasfiltered off,leaving an isopropanol solution which was concentrated,and the resulting solid recrystallized from hexane to afford12.0g of product(yield33%). 1H NMR data:CDCl3(δ)0.89(t,3H,-C11CH23C H3),1.2-1.47 (m,18H,-CH2CH2C9H18CH3), 1.68(m,2H,-S-CH2C H2-C10H21),1.73(s,6H,-S-C(C H3)2COOH),3.3(t,2H,-S-C H2-C11H23),Supporting Information,Figure S1.RAFT Polymerization of NIPAM To Produce PNIPAM-S-C-S)-S-C12H25.The temperature-responsive polymer,PNI-PAM,containing a pyridyl disulfide end group was prepared via reversible addition fragmentation chain transfer(RAFT)polymer-ization in3steps(Scheme1).Polymerization of4.0g of NIPAM with120.0mg of DMP was conducted in the presence of4.0mg of AIBN in5.0mL of DMF in a20mL vial.The vial was sealed and immersed in a preheated water bath at60°C.Polymerization was halted by immediate exposure to air and cooling with dry ice. The product was precipitated in ether,filtered,and dried at room temperature.PNIPAM-S-C(S)-S-C12H25was characterized us-ing a combination of size exclusion chromatography(SEC)and gel permeation chromatography.Aminolysis of Trithiocarbonate-Terminated PNIPAM To Yield Thiol-Terminated PNIPAM.The trithiocarbonate-termi-nated PNIPAM(PNIPAM-S-C(S)-S-C12H25)was treated with hexylamine to yield thiol-terminated PNIPAM(PNIPAM-SH)as follows.A3.0g amount of trithiocarbonate-terminated PNIPAM in5mL of DMF was deoxygenated by a stream of nitrogen for 2h.To that solution,5drops of Na2S2O4solution was added to prevent oxidation of the thiols to disulfides.This was followed by addition of2.0mL of hexylamine.The mixture was stirred at room temperature for another12h.The thiol-terminated PNIPAM (PNIPAM-SH)was obtained by pouring the mixture into ether and filtering and drying in vacuum at room temperature.The trithio-carbonate absorbance at310nm was absent from the UV spectrum of PNIPAM-SH(Supporting Information,Figure S2),consistent with conversion of trithiocabonate groups to thiol groups. Preparation of Disulfide Pyridyl-Terminated PNIPAM.A solution containing2.5g of Aldrithiol-2,1.0mL of glacial acetic acid,and20mL of methanol was added to a100mL round-bottom flask with stir bar,followed by addition of PNIPAM-SH.After24h at room temperature,the reaction mixture was concentrated under(17)de las Heras Alarco´n,C.;Pennadam,S.;Alexander,C.Chem.Soc.Re V.2005,34,276–285.(18)Yerushalmi,R.;Scherz,A.;van der Boom,M.E.;Kraatz,H.-B.J.Mater.Chem.2005,15,4480–4487.(19)You,Y.-Z.;Hong,C.-Y.;Pan,C.-Y.;Wang,P.-H.Ad V.Mater.2004,16,1953–1957.(20)Qiu,Y.;Park,K.Ad V.Drug Deli V ery Re V.2001,53,321–339.(21)Kopecek,J.Eur.J.Pharm.Sci.2003,20,1–16.(22)Fu,Q.;Rao,G.V.R.;Ista,L.K.;Wu,Y.;Andrzejewski,B.P.;Sklar,L.A.;Ward,T.L.;Lo´pez,G.P.Ad V.Mater.2003,15,1262–1266.(23)Fu,Q.;Rao,G.V.R.;Ward,T.L.;Lu,Y.;Lo´pez,ngmuir2007,23,170–174.Scheme1.Synthesis of Pyridyl Disulfide-TerminatedPNIPAM3355Chem.Mater.,Vol.20,No.10,2008PNIPAM-Modified Porous Silica Nanoparticlesvacuum and the concentrate added to5.0mL of THF.The disulfide pyridyl-terminated PNIPAM(PNIPAM-S-S-Py)was obtained by precipitating in ether,filtering,and drying in vacuum at room temperature.The presence of the pyridyl disulfide functionality was confirmed by1H NMR and UV-vis spectroscopy(Supporting Information,Figure S2).The molecular weight and PDI were determined by SEC(Supporting Information,Figure S3). Synthesis of MSN-SH.Micro-to-mesoporous silica nanoparticles (MSN)functionalized with a thiol linker were synthesized by reaction of TEOS with MPTMS according to the procedure reported by Lin and co-workers.3The ratio[MPTMS]/[TEOS]in the reaction was0.20.While Lin and co-workers appear to prepare MCM-41, Walcarius and co-workers note that ratios greater than0.1often lead to wormhole structures(MCM-48).24The chemically accessible thiol group surface coverage of the MSN-SH was estimated by treatment with Ellman’s reagent.Ellman’s reagent was prepared by dissolving12mg of reagent in5mL of buffer and kept in a freezer prior to use.In a typical analysis,2.5mg of the thiol linker was dissolved in10mL of Na2HPO4buffer(pH8.0),of which1 mL of this solution was mixed with1mL of Ellman’s reagent. Absorbance readings were taken at412nm( )14150cm-1for Ellman’s reagent)after20min of standing for the mixture,the blank (buffer solution),and the MSN-SH solution.The amount of free thiol in the10mL sample was calculated as described by Reiner and co-workers.25Surface Modification of MSN with Temperature-Responsive Polymer.Thiol-functionalized MSN was reacted with PNIPAM-S-S-Py to prepare the desired MSN-PNIPAM.The reaction mixture contained a suspension of0.10g of MSN-SH in20mL of methanol,0.5mL of glacial acetic acid,and2.0g of PNIPAM-S-S-Py.The mixture was stirred for24h at room temperature followed by centrifugation at4000rpm to obtain the product,which was dried at room temperature under vacuum. Characterization of MSN and MSN-PNIPAM Hybrids.Pow-der X-ray diffraction(PXRD)was used to study the crystallinity of the products using a diffractometer equipped with a rotating copper anode source.The data were collected over a range of1.5°e2θg10°at the Max Planck Institute of Colloids and Interfaces in Germany.Particle sizes and the morphology of the materials were investigated by transmission electron microscopy(TEM)on a JEOL2010F Analytical Electron Microscope at200kV.TEM samples were prepared by placing a drop of a sonicated acetone solution of MSN-SH or MSN-PNIPAM on a carbon-coated copper grid and then drying in a vacuum desiccator.The surface area,average pore size,cumulative pore volume, and pore size distributions were determined from nitrogen adsorp-tion/desorption isotherms acquired at77K using a30s equilibrium interval on an ASAP2010Micromeritics porosimeter.MSN-SH samples were degassed at100°C for24h and MSN-PNIPAM samples at50°C for12h,in order to remove any adsorbed molecules prior to the analysis.The surface area was computed using the BET(Brunauer-Emmett-Teller)model.The average pore size and cumulative pore volumes were obtained from the BJH(Barret-Joyner-Halenda)model.26The pore size distribution was obtained from density functional theory(DFT)modeling using the DFT package of the Micromeritics V2.00software over the entire range of the adsorption isotherm.5Thermal gravimetric analyses(TGA)were collected on a Perkin-Elmer Pyris1TGA instrument,under a nitrogen atmosphere,at a heating rate of5°C/ min.Fluorescein Loading and Release Studies.The temperature-responsive loading offluorescein in MSN-PNIPAM was achieved by vigorously stirring110mg of MSN-PNIPAM in a solution of fluorescein(4.0×10-6M)in10mL of pH7.4phosphate-buffer solution(PBS)at room temperature for24h.Then,MSN-PNIPAM loaded withfluorescein was isolated by centrifugation at4000rpm, washed extensively with50°C deionized water5times to remove physiosorbed matter,and dried at50°C.All the washings were collected.The solution temperature was increased from room temperature to38°C,resulting in a precipitate that wasfiltered and washed with a PBS solution at50°C.The amount offluorescein encapsulated was calculated from the difference in the concentration of the initial solution offluorescein and that of the reaction medium combined with the subsequent washings.Release studies were conducted at20and38°C in PBS.In a typical release experiment, two vials containing the MSN-PNIPAM loaded withfluorescein (∼10.0mg)and PBS solution(2.0mL,pH7.4)were kept at25or 38°C,respectively.Aliquots were removed at specified time intervals over several days,and the absorbance was measured at 490nm.Control Experiments.Nonporous silica nanoparticles function-alized with a thiol linker were synthesized by a modified Sto¨ber process using TEOS in ethanol as described by Rossi27and Rao.28 The number of accessible thiol groups on the MPTMS-function-alized SiO2-thiol was determined to be13.1mmol/g.PNIPAM-S-S-Py was grafted onto the nonporous silica particles as described for MSN.Loading and release experiments of the PNIPAM-modified dense silica spheres and thiol-functionalized mesoporous silica(MSN-SH)were conducted in a similar manner as for MSN-PNIPAM.Determination of Phase Transition Temperatures.Determi-nation of the phase transition temperature was performed using a ZetaPlus Particle Size analyzer(Brookhaven Instruments)equipped with35mW solid-state laser(658nm).To determine the transition temperature,the temperature dependence of the scattering intensity at90°from1mL of solution in a glass cuvette was measured.The temperature was increased by discrete temperature increments in the range25-50°C,and the readings were taken after3min equilibration at each temperature.Results and DiscussionMSN functionalized with thiol groups for linking to the modified PNIPAM polymer were synthesized by condensa-tion of tetraethylorthosilicate with mercaptopropyl trimethoxy-silane(MPTMS)in the presence of the micellar template, N-cetyltrimethylammonium bromide(CTAB).3Removal of CTAB by acid washing resulted in MSN with sizes of80-90 nm in diameter(Figure1a)and Braunaer-Emmett-Teller (BET)surface areas of1500m2/g.The pore diameters were computed by BJH and DFT methods to be1-4nm (Supporting Information,Figure S4),and the BJH cumulative pore volume was0.51cm3/g.X-ray powder diffraction (Figure2)shows only a single resolved peak at d)4nm, suggesting a low degree of order in the pore structure,making assignment of hexagonal(straight pores)or cubic(wormhole interconnected pores)polymorph ambiguous.Molecular transport can occur in either structure,although it is more facile in the interconnected pore structure.24The accessible(24)Walcarius,A.;Delacoˆte,C.Chem.Mater.2003,15,4181–4192.(25)Reiner,C.K.;Kada,G.;Gruber,H.J.Anal.Bioanal.Chem.2002,373,266–276.(26)Webb,P.A.;Orr,C.Analytical Methods in Fine Particle Technology;Micromeritics Instrument Corp.:Norcross,GA,1997.(27)Rossi,L.M.;Shi,L.;Quina,F.H.;Rosenzweig,ngmuir2005,21,4277–4280.(28)Rao,K.S.;El-Hami,K.;Kodaki,T.;Matsushige,K.;Makino,K.J.Colloid Interface Sci.2005,289,125–131.3356Chem.Mater.,Vol.20,No.10,2008You et al.thiol content of the MSN-SH material was computed to be 6.0×10-4mol/g by titration with Ellman’s reagent.The pyridyl disulfide-terminated PNIPAM polymer was synthesized in three steps (Scheme 1).First,a reversible addition-fragmentation chain transfer polymerization was employed to synthesize narrow polydispersity PNIPAM with a trithiocarbonate end group (PNIPAM -S -C(d S)-S -C 12H 25).The synthesized PNIPAM -S -C(d S)-S -C 12H 25(M n )11800,M w /M n )1.12)was then treated with hexylamine at room temperature to form PNIPAM with a terminal thiol group (PNIPAM-SH).Finally,PNIPAM-SH was treated with 2,2′-dipyridyl disulfide (Aldrithiol-2)to produce pyridyl disulfide-terminated PNIPAM (PNIPAM -S -S -Py,M n )12100,M w /M n )1.30).The thiol-functionalized MSN were reacted with PNIPAM -S -S -Py at room temperature to prepare the hybrids (MSN-PNIPAM)as illustrated in Scheme 2.The resultant hybrid particles show improved dispersion in water at room temperature relative to the native MSN,which we attribute to the solubilizing nature of PNIPAM.A comparison of thermal gravimetric analysis data for the hybrid,MSN-SH and PNIPAM -S -S -Py,suggests that ∼20wt %of the hybrid is due to the grafted polymer (Figure 3).FT-IR data are also consistent with a chemical linkage between MSN and PNIPAM.A comparison of FT-IR spectra of MSN-SH,MSN-PNIPAM,and PNIPAM(Figure 4),shows that the two characteristic peaks for PNIPAM at 1650(amide stretch)and 1553cm -1(N -H stretch)29appear in the MSN-PNIPAM but not in MSN-SH.In addition,the silica framework of MSN in MSN-PNIPAM remains unchanged upon PNIPAM grafting,as evidenced by preservation of peaks at 461,800,and 1090cm -1,associated with silica.30The overall morphology and particle size also remain unchanged in MSN-PNIPAM,as shown in Figure 1,but the surface area is decreased by 2orders of magnitude,suggesting that grafting the polymer onto MSN blocks the pores in the solid state (Supporting Information).Indeed,the extremely low surface area (23m 2/g)and the broad,featureless pore-size distribution plot (Supporting Information)suggest that only the outer surface and voids between precipitated particles are being probed.Loading and release studies were conducted with fluores-cein as a model drug molecule.First,to demonstrate temperature-controlled uptake of small organic molecules into MSN-PNIPAM,experiments were performed with(29)Xie,R.;Chu,L.-Y.;Chen,W.-M.;Xiao,W.;Wang,H.-D.;Qu,J.-B.J.Membr.Sci.2005,258,157–166.(30)Gu,G.;Ong,P.P.;Chu,C.J.Phys.Chem.Solids 1999,60,943–947.Figure 1.TEM image of (a)as-prepared mesoporous MSN-SH and (b)MSN-PNIPAMnanoparticles.Figure 2.Low-angle PXRD pattern of MSN-SH and MSN-PNIPAM.The reduction in peak intensity for MSN-PNIPAM is attributed to decreased contrast due to the polymer.1,32,33Scheme 2.Reaction of MSN-SH with PNIPAM -S -S -Py ToFormMSN-PNIPAMFigure 3.TGA curves for MSN-SH,MSN-PNIPAM,and PNIPAM.3357Chem.Mater.,Vol.20,No.10,2008PNIPAM-Modified Porous Silica Nanoparticlesfluorescein (4×10-6M)in phosphate-buffered saline (PBS).The MSN-PNIPAM was stirred vigorously in a solution of fluorescein at room temperature for 24h.Then,the temper-ature of the solution was increased to 38°C,and the precipitated MSN-PNIPAM was filtered,washed extensively with 50°C PBS solution to remove fluorescein molecules adsorbed on the surface,and dried at 50°C.The loading was performed for 24h at temperatures above and below the LCST of PNIPAM.The results showed that no detectable amount of fluorescein could be loaded into the MSN-PNIPAM above the LCST (38°C).Fluorescein,however,entered the MSN-PNIPAM below the LCST (25°C).The 25°C loaded samples contained 8×10-8mol fluorescein per gram of MSN-PNIPAM.On the basis of the BJH cumulative pore volume of 0.51cm 3/g for MSN-SH and taking into account a 20%increase in mass due to PNIPAM (computed from TGA analyses),this suggests the concentra-tion of fluorescein within the pores is on the order of 2×10-5M,similar to the fluorescein concentration in the original solution (4×10-6M).We then investigated whether,in addition to temperature-controlled loading,the MSN-PNIPAM also showed temper-ature-dependent release of fluorescein.The release data at 25(below LCST)and 38°C (above LCST)are shown in Figure 5.Similar to the loading experiments,the soluble PNIPAM chains rendered the entrances of pores open below the phase transition at 25°C.This was demonstrated by a gradual release of the fluorescein loaded in the voids of MSN.On the other hand,PNIPAM chains are insoluble in PBS at 38°C,the entrances of pores are blocked by the collapsed PNIPAM chains,and the release of fluorescein from the voids of MSN is significantly obstructed.To further investigate the link between the conformation of the polymer and its pore opening/closing capability,we determined the total amount of fluorescein released in 16h at different temperatures and compared this data to the light-scattering behavior of the polymer sample at that temperature.We expected that the transformation from the soluble,random coil conformation of PNIPAM to the insoluble,globule form would result in a blocking of the pores of the hybrid MSN-PNIPAM materials.As shown in Figure 6a,the amount of released fluorescein decreases dramatically around 30°C,concomitant with a clear increase in scattering intensity.Thus,release of small organic molecules loaded in MSN-PNIPAM can be controlled by the phase state of the modifying PNIPAM chains.However,it appears that the polymer alone cannot act as an effective system for stimuli-responsive uptake and release.Control experiments in which the uptake was attempted with PNIPAM grafted onto dense (nonporous)silica spheres showed no evidence for fluores-cein encapsulation,regardless of temperature,suggesting that the porosity of the MSN is integral to the storage of fluorescein.The mechanism of action in the presently reported materi-als is clearly different from that reported by Lo ´pez and co-workers,where release was observed abo V e LCST.22,23These materials were prepared by either preforming MSN and growing the PNIPAM polymer from the surface or preform-ing PNIPAM and co-condensing it with silica during formation of MSN.In either case,incorporation of the polymer within the pores can occur readily.Thus,contraction above LCST would be expected to effectively open the pores,while the random coil network of polymer present below LCST would be expected to act as a diffusion barrier.We surmise that the present method of synthesis,in whichbothFigure 4.FT-IR spectra of MSN-SH,MSN-PNIPAM,andPNIPAM.Figure 5.Relative amount of fluorescein released with time at 38(2)and 25°C (b).Figure 6.(a)Correlation between release of fluorescein from MSN-PNIPAM (b )and phase transition of PNIPAM (indicated by the increase of light scattering intensity)(2).(b)Schematic representation of the principle of action.3358Chem.Mater.,Vol.20,No.10,2008You et al.PNIPAM and MSN components are preformed,results in significant grafting at the pore surface.Thus,above LCST, the polymer forms“stoppers”that prevent uptake and release, whereas below this temperature,the random coil conforma-tion permits diffusion into and out of the pores(Figure6b). The presence of the polymer preferentially at the pore surface would be expected based on the significant molecular weight of the polymer(M n)12100),the relatively small size of the pores(1-4nm),and the rapid kinetics of the reaction of pyridyl disulfide with free thiol.The ability to exclude the access of molecules to mesoporous silica based on their size is a characteristic feature of these materials.31Although we cannot rule out the presence of polymer within the pores, the observed response strongly suggests that surface polymer dominates the temperature-dependent uptake and release. The presence of the polymer is clearly active in moderating diffusion,as can be seen when the release profile of the unmodified MSN-SH is compared(Supporting Information). Approximately twice as muchfluorescein is released after 24h for MSN-SH than for the MSN-PNIPAM hybrid in the “open”configuration(below LCST).Indeed,diffusion into and from the pores for the present system in the“pore-open”configuration appears to be slower than that for the co-condensed materials in the“pore-closed”configuration (∼10%vs∼30%release after2h);in the“pore-closed”configuration,<20%release occurs after16h in the new materials.Thus,application of preformed polymer to pre-formed porous particles appears to enable much greater control over the release.ConclusionThe present work demonstrates that the phase state of a temperature-responsive polymer can control opening and closing of the pores when grafted onto preformed micro-to-mesoporous silica nanoparticles.Further work is underway to apply this approach to the development of porous nanoparticles in which the molecular gates around the pores are reversibly switched on and off by other stimuli,such as pH,and develop new chemical attachment strategies that will result in polymer-particle tethers that are not subject to cleavage under physiological conditions.This general ap-proach can be used to expand the choice of stimulus-controlled drug and gene delivery systems in which a fully reversible loading and release mechanism is required. Acknowledgment.This work was supported by NIH Grants EB0043588and EB008164from the National Institute of Biomedical Imaging and Bioengineering and the Institute of Manufacturing Research of Wayne State University.We thank Ingrid Zenke and Hans Riegler of the Max Planck Institute of Colloids and Interfaces for X-ray diffraction data. Supporting Information Available:1H NMR of DMP;details on the characterization of PNIPAM-S-S-Py along with absorbance spectra and GPC trace;details on surface area analyses of MSN materials and isotherm/pore-size distribution plots;data forfluo-rescein release from MSN-SH(control reaction)(PDF).This material is available free of charge via the Internet at http://pubs. .CM703363W(31)Zapilko,C.;Anwander,R.Chem.Mater.2006,18,1479–1482.(32)Parala,H.;Winkler,W.;Kolbe,M.;Wohlfart,A.;Fischer,R.A.;Schmechel,R.;von Seggern,H.Ad V.Mater.2000,12,1050–1055.(33)Zhang,W.-H.;Shi,J.-L.;Chen,H.-R.;Hua,Z.-L.;Yan,D.-S.Chem.Mater.2001,13,648–654.3359 Chem.Mater.,Vol.20,No.10,2008PNIPAM-Modified Porous Silica Nanoparticles。