(1969)3456134_PIEZOELECTRIC_ENERGY_CONVERTER_F
Piezoelectric Energy Harvesting using Single Crystal PMN-PT Device 2009
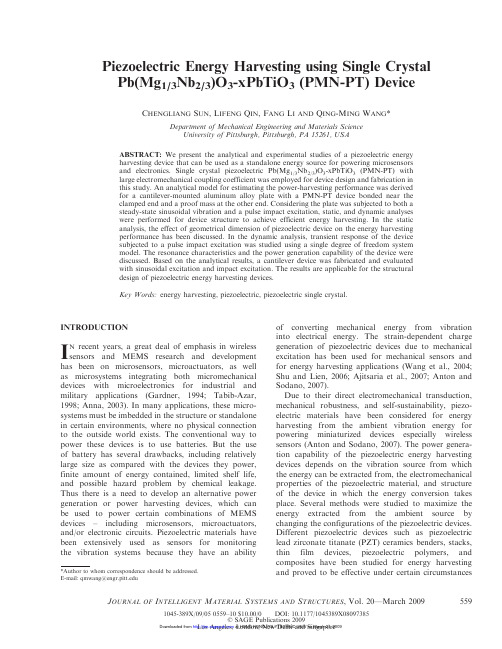
559
560
C. SUN ET AL.
(Churchill et al., 2003; Mohammadi et al., 2003; Sodano et al., 2004; Lee et al., 2005; Platt et al., 2005; Sodano et al., 2005). Improvement of materials property, and proper design of device configuration have been investigated to achieve a more efficient electromechanical coupling between the device and the vibration sources (Baker et al., 2005; Cho et al., 2005; Yang et al., 2005). The most commonly used piezoelectric devices for energy harvesting are cantilever beams or plates (Gurav et al., 2004; Ng and Iiao, 2005) because they can be easily implemented and are quite effective for harvesting energy from ambient vibrations using the mechanical amplification mechanism (bending mode). A similar way of improving the efficiency of piezoelectric energy harvesting is to use piezoelectric composite devices called ‘cymbal’ and ‘drum’ (Kim et al., 2004; Wang et al., 2007). Again mechanical amplification is achieved by converting normal force or stress into transverse stress in the piezoelectric devices. To get the maximum energy conversion, a method of tuning the resonant frequency of the harvesting devices to match the frequency of the ambient vibrations was also studied (Cornwell et al., 2005).
英文版原子物理课件

1.1 Introduction
The origins of atomic physics :quantum mechanics Bohr model of the H This introductory chapter surveys some of the early ideas: Spectrum of atomic H and Bohr Theory Einstein's treatment of interaction of atom with light the Zeeman effect Rutherford scattering And so on
Shanxi University Atomic Physics
1.2 Spectrum of atomic hydrogen_3
Wavenumbers may seem rather old-fashioned but they are very useful in atomic physics
the characteristic spectrum for atoms is composed of discrete lines that are the ‘fingerprint' of the element.
In 1888, the Swedish professor J. Rydberg found that the spectral lines in hydrogen obey the following mathematical formula:
Shanxi University Atomic Physics
Lyman series: n’ = 2; 3; 4; … n = 1. Balmer (n = 2), Paschen series: (n = 3), Brackett (n = 4) and Pfund (n = 5)
Mechanical and Aerospace Engineering and
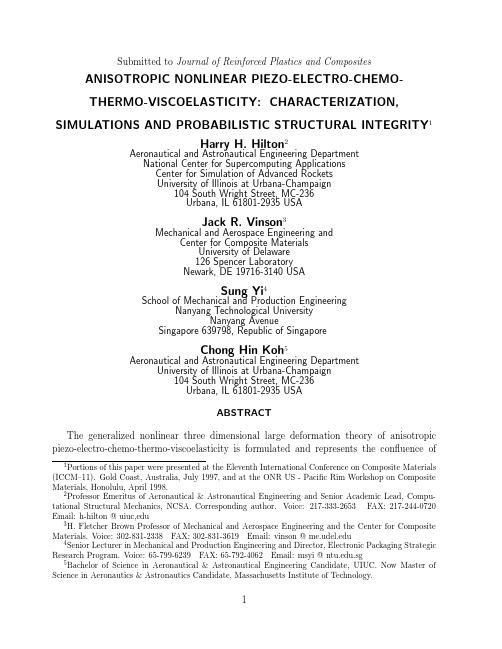
ANISOTROPIC NONLINEAR PIEZO-ELECTRO-CHEMOTHERMO-VISCOELASTICITY: CHARACTERIZATION, SIMULATIONS AND PROBABILISTIC STRUCTURAL INTEGRITY1 Harry H. Hilton2
Aeronautical and Astronautical Engineering Department National Center for Supercomputing Applications Center for Simulation of Aபைடு நூலகம்vanced Rockets University of Illinois at Urbana-Champaign 104 South Wright Street, MC-236 Urbana, IL 61801-2935 USA
Portions of this paper were presented at the Eleventh International Conference on Composite Materials (ICCM–11). Gold Coast, Australia, July 1997, and at the ONR US - Pacific Rim Workshop on Composite Materials, Honolulu, April 1998. 2 Professor Emeritus of Aeronautical & Astronautical Engineering and Senior Academic Lead, Computational Structural Mechanics, NCSA. Corresponding author. Voice: 217-333-2653 FAX: 217-244-0720 Email: h-hilton @ uiuc,edu 3 H. Fletcher Brown Professor of Mechanical and Aerospace Engineering and the Center for Composite Materials. Voice: 302-831-2338 FAX: 302-831-3619 Email: vinson @ 4 Senior Lecturer in Mechanical and Production Engineering and Director, Electronic Packaging Strategic Research Program. Voice: 65-799-6239 FAX: 65-792-4062 Email: msyi @ .sg 5 Bachelor of Science in Aeronautical & Astronautical Engineering Candidate, UIUC. Now Master of Science in Aeronautics & Astronautics Candidate, Massachusetts Institute of Technology.
基尔斯特 603C 微型压电压力传感器-测量和测试的应用说明书

© 2017 ... 2022 Kistler Group, Eulachstraße 22, 8408 Winterthur, Switzerland Tel.+41522241111,****************,. Kistler Group This information corresponds to the current state of knowledge. Kistler reserves the right to make technical changes. Liability for consequential damage resulting Page 1/8PressurePiezoelectric pressure sensorfor Test & Measurement applications603C _003-288e -11.22Type 603C...• • Acceleration compensated • Small sensor size• • • DescriptionDue to their high natural frequencies, piezoelectric pressure sensors can be used for a variety of applications where dynam-ic pressures need to be measured. Another unique character-istic of piezoelectric pressure sensors is their ability to measure small pressure fluctuations that are superimposed on top of high static pressures with exceptional resolution. By contrast, piezoresistive pressure sensors are the right choice when mea-suring static pressure curves.At the core of the all-welded, hermetically sealed 603C series there is a Quartz crystal. The pressure to be measured acts on the sensor’s diaphragm and compresses the Quartz crystal. The compressed crystal produces a charge which is proportional to the pressure. Finally, the charge signal needs to be converted, by a charge amplifier, into a voltage which can then be read.Typical applications• High pressure measurements on hydraulic and pneumatic systems, etc.• Highly dynamic pressure measurements on shock tubes, blast tests, etc.Charge (PE) vs. Voltage (IEPE) outputOne of the most important selection criteria for piezoelectric pressure sensors is the output signal. Two variants of the sen-sor are available, charge output (PE) and voltage output (IEPE resp. Piezotron).Piezoelectric pressure sensors are connected to an electronic circuit which converts the charge generated by the sensor into a proportional voltage. If the electronic circuit is integrated into the sensor housing, it is referred to as an IEPE sensor. If the electronics is an external device (charge amplifier), it is referred to as a PE sensor. Depending on the application, PE or IEPE sensors may be better suitable.IEPE sensors are the ideal solution for the long-term measure-ment of small pressure pulsations (without any static pressure content) or the measurement of dynamic pressure profiles (up to a few tenths of a millisecond only). IEPE sensors will how-ever, due to the built-in electronics, only work at moderate temperatures and come with a fixed measuring range.PE sensors on the other hand are the right solution for the long-term measurement of small pressure pulsations (without any static pressure content), quasi-static or dynamic pressure profiles (up to a few minutes). PE sensors will, due to the ex-ternal charge amplifier, operate at extreme temperatures too and allow for adjustable measuring ranges.The instruction manual and pressure catalogue provides fur-ther details on PE and IEPE sensors, measuring chains as well as applications each technology is best suited for.Page 2/8603C _003-288e -11.22© 2017 ... 2022 Kistler Group, Eulachstraße 22, 8408 Winterthur, Switzerland Tel.+41522241111,****************,. Kistler Group This information corresponds to the current state of knowledge. Kistler reserves the right to make technical changes. Liability for consequential damage resulting Technical data – IEPE sensors 1)Type 603CBA... 00014.0 00035.0 00070.0 00350.0 00690.0 01000.0Output signal V Voltage (IEPE)Pressure range bar 14 35 70 350 690 1 000psi200 500 1 000 5 000 10 000 15 000Maximum pressure bar / psi 1 000 / 15 000Overloadbar / psi 1 100 / 15 950Sensitivity (nom.) mV/bar 357 143 71 14 7 5 mV/psi 25 10 5 1 0.5 0.3Linearity%FSO ≤±1.0Operating temperature range °C / °F -55 ... +120 / -67 ... +248Rise time (10 ... 90%) μs<0.4Natural frequency 2)kHz >500Time constant (nom.) s 2 3Low frequency response -3 dB Hz 0.080 0.053 -5% Hz 0.242 0.161Noise (1 Hz ... 10 kHz) (typ.) μbar rms 32 79 160 810 1 620 2 268Acceleration sensitivity (axial) (typ.) bar/g 0.00014psi/g 0.00200Acceleration sensitivity (radial) (typ.) bar/g 0.0001psi/g 0.0015Supply voltage (by IEPE-Coupler) VDC 22 … 30Supply current (by IEPE-Coupler) mA 2 … 20Output bias voltage (nom.) VDC 11Output voltage FSO V±5Weightgrams 4.0Housing and diaphragm material– 17-4 S.S.1) Indications are valid for 23°C / 73°F (if not specified otherwise)2)Calculated from rise timeRise time (10 ... 90%)μs <0.4Natural frequency2)kHz>500Noise (1 Hz ... 10 kHz)(typ.)μbar rms 126Acceleration sensitivity (axial) (typ.)bar/g psi/g 0.000140.00200Acceleration sensitivity (radial) (typ.)bar/g psi/g 0.00010.0015Insulation resistance Ω≥1013WeightType 603CAA / 603CABgram 4.8 / 2.2Housing and diaphragm material17-4 S.S.Technical data – PE sensors 1)Type 603CA...Output signal pC Charge (PE)Pressure range bar 0 ... 1 000psi 0 ... 15 000Calibrated partial range % 7; 100Overloadbar psi 1 10015 950Sensitivity (nom.)pC/bar pC/psi -5.0-0.35Linearity (typ.) (max.)%FSO %FSO ≤±0.4≤±1.0Operating temperature range°C °F-196 ... +200-321 ... +392Page 3/8603C _003-288e -11.22© 2017 ... 2022 Kistler Group, Eulachstraße 22, 8408 Winterthur, Switzerland Tel.+41522241111,****************,. Kistler Group This information corresponds to the current state of knowledge. Kistler reserves the right to make technical changes. Liability for consequential damage resulting Mounting (sensors with standard housing)Sensors with charge output (PE) and voltage output (IEPE) are available with standard housing. Sensors with a standard hou-sing can either be installed directly or with an adapter.Direct MountingWhen the mounting location space is restricted, the sensor can be located in an appropriately dimensioned mounting bore, and then held in place with a floating clamp nut. Please note that for a reliable and accurate pressure measurement a precise machining of the mounting hole with special reamers and taps is required. F or details on mounting hole fabrication please check the manual.Adapter MountingFitting sensors into a mounting adapter greatly simplifies the installation process (when space and wall thickness are not a premium). Use of a Kistler mounting adapter will eliminate the need to provide a precise mounting configuration and allow the installer to provide only a threaded hole of the size and depth required for the adapter selected. Special reamers or taps are not required when using an adapter. For details on mounting thread fabrication please check the manual. All sen-sors and adapters are available for download as 3D CAD files from Kistler’s webpage.Cable with 10-32 connector(e.g. 1631C)Sensor with standard housing(e.g. 603CAA, 603CBAxxxxx.x)(e.g. 1131Bxx.xx)Adapter seal (e.g. 1113C0C)Direct Mounting Type 6503CxA Adapter Type 6503CxD Adapter Type 6507BxA Adapter Type 6503C3A Adapter Type 6509B Adapter • Flush mounting • C omplex drilling with special tool • M in. structuralinfluences on pressure measurement (mech-anical decoupling)• I deal for close matrix alignment of sensors• M10 and 3/8-24 UNF • S tainless steel (1.4542)• Flush mounting • M ax. Pressure1 000 bar (14 500 psi)• M10 and 3/8-24 UNF • Ground Isolating • P EEK GF30(glass fiber reinforced high performance plastic)• M ax. Pressure 100 bar (1 450 psi)• M ax. temperature 100°C (212°F)• M3 and 5-40 UNC • S tainless steel (1.4542)• Recessed mounting • M ax. Pressure 300 bar (4 350 psi)• 1/8-27 NPTF • S tainless steel (1.4542)• Almost flush mounting • M ax. Pressure1 000 bar (14 500 psi)• M14x1.25• Water Cooling • S tainless steel (1.4542)• M ax. Pressure 300 bar (4 350 psi)•Max. temperature400°C (752°F)Page 4/8603C _003-288e -11.22© 2017 ... 2022 Kistler Group, Eulachstraße 22, 8408 Winterthur, Switzerland Tel.+41522241111,****************,. Kistler Group This information corresponds to the current state of knowledge. Kistler reserves the right to make technical changes. Liability for consequential damage resulting Mounting (sensors with short housing)Sensors with charge output (PE) are also available with short housing. For the mounting of these sensors a connector ex-tender of Type 6482A is required. This mounting form is mostly only used where mechanical structures cannot be weakened.Connector extenders are made to order and are therefore not stock items (unlike floating clamp nuts of Type 6423B). Cus-tomers can choose between three different connectors, two different threads and a variable length (see ordering key).Please note that for a reliable and accurate pressure meas-urement a precise machining of the mounting hole with special reamers and taps is required (same as for direct mounting, see previous datasheet page). For details on mounting hole fabri-cation please check the manual. All connector extenders are available for download as 3D CAD files from Kistler’s webpage.10-32 connector BNC connectorTNC connectorSmall coaxial 10-32 connectors are widely used in the sensor in-dustry and therefore comes with a wide range of high impe-dance cable options. The small size further allows for sealing the sensor connector with thermo shrink slee-ves. Attention needs to be payed to clean-liness of these small connectors.The BNC connector (bayonet lock) is much bigger and therefor sturdier and less sensitive to con-taminations than the widely used 10-32 connector. It’s large dimensions and big mass as well the dif-ficulty to be sealed might however be a criterion for exclu-sion in some applica-tions.The TNC connector (thread lock) has about the same size, and offers the same advantages and disadvantages, as the BNC connector. Thanks to the thread lock a good sealing against exterior influ-ences is achieved and is therefore the pre-ferred choice when conducting tests outside a laboratoryenvironment.Ordering key (connector extender)Connector type BNC 0TNC 110-322Mounting thread M7x0.7505/16-24 UNF 1Length (in mm)10-1013 (200)-SP O rdering example Type6482A with BNC, M7 thread, 10 mm length 6482A00-106482A with TNC, M7 thread, 15 mm length 6482A10-SP156482A with 10-32, UNF thread, 125 mm length 6482A21-SP125Cable with 10-32, BNC orTNC connectorConnector Extender(6482Axxx)(603CAB)Sensor seal Max. operating temperaturePage 5/8603C _003-288e -11.22© 2017 ... 2022 Kistler Group, Eulachstraße 22, 8408 Winterthur, Switzerland Tel.+41522241111,****************,. Kistler Group This information corresponds to the current state of knowledge. Kistler reserves the right to make technical changes. Liability for consequential damage resulting Waterproof solution (IP68)F or pressure sensors subject to water splashes or even used under water the IP68 rated cable Type 1983A shall be used. The Type 1983A cable, made of FKM rubber, comes with a vulcanized 10-32 connector and is only compatible to pressure sensors with a standard housing.The Type 1983A cable can be screwed like any other standard cable, or optionally even be welded, to the pressure sensor. Welding, instead of screwing, the cable connector to the sen-sor offers protection against detachment of the cable in case of strong vibrations. Requirements to weld the connector to the sensor need to be stated at the time of order (see below ordering keys).Both, screwed and welded, solutions have successfully been qualified for IP68 rating in water with pressures ranging from vacuum to 16 bar.Ordering key (PE sensor with welded 1983A cable)Floating clamp nut Type 6423B00A 6423B11BCable Type1983AD (with BNC pos. connector)A 1983AC (with 10-32 pos. int. connector)BCable length (in m)1 m (for 1983AC cable only)1,02 m (for 1983AC and 1983AD cable)2,03 m (for 1983AC cable only)3,05 m (for 1983AD cable only)5,0Customized cable length (0.1 to 30.0 m)SPOrdering exampleType603CAA with 6423B00 and 2 m 1983AD cable 603CAA-WAA2,0603CAA with 6423B00 and 6 m 1983AD cable 603CAA-WAASP6,0603CAA with 6423B11 and 0.5 m 1983AC cable603CAA-WBBSP0,5Ordering key (IEPE sensor with welded 1983A cable)Ordering exampleType603CB00014.0 with 6423B00 and 2 m 603CBA-W00014.0-AA2,0 1983AD cable603CB00070.0 with 6423B00 and 6 m 603CBA-W00070.0-AASP6,0 1983AD cable603CB00350.0 with 6423B11 and 7 m 603CBA-W00350.0-BBSP7,01983AC cableCable with 10-32 connector (e.g. 1983AC, 1983AD)Floating clamp nut (e.g. 6423B00)Sensor with standard housing (e.g. 603CAA, 603CBAxxxxx.x)Sensor seal (e.g. 1131Bxx.xx)Sensor with welded cable and attached floating clamp nut (e.g. 603CAA-W, 603CAB-W)Page 6/8603C _003-288e -11.22© 2017 ... 2022 Kistler Group, Eulachstraße 22, 8408 Winterthur, Switzerland Tel.+41522241111,****************,. Kistler Group This information corresponds to the current state of knowledge. Kistler reserves the right to make technical changes. Liability for consequential damage resulting SensorsType 603CAA(dimensions in mm [inch])Type 603CAB(dimensions in mm [inch])Type 603CBAxxxxx.x (dimensions in mm [inch])Note: Mounting holes, tightening torques and more can be found in the instruction manual.3D CAD data can be downloaded free of charge from/cad-catalogSensor Typ 603CAAType 1131BB0.20(dimensions in mm [inch])Type 1131BC1.15(dimensions in mm [inch])Seals (Sensors)Floating clamp nutType 6423B00(dimensions in mm)HEX8HEX 7,1 /9/32"Type 6423B11(dimensions in inch)Page 7/8603C _003-288e -11.22© 2017 ... 2022 Kistler Group, Eulachstraße 22, 8408 Winterthur, Switzerland Tel.+41522241111,****************,. Kistler Group This information corresponds to the current state of knowledge. Kistler reserves the right to make technical changes. Liability for consequential damage resulting Note: Mounting holes, tightening torques and more can be found in the instruction manual.3D CAD data can be downloaded free of charge from/cad-catalogAdapterType 6503C0A(dimensions in mm)Type 6503C0D (dimensions in mm)Type 6507B0A(dimensions in mm)Type 6503C1A(dimensions in inch)Type 6503C1D(dimensions in inch)Type 6507B1A(dimensions in inch)Type 6503C3A(dimensions in inch)Type 6509B(dimensions in mm)Type 6501B0A(dimensions in mm)Page 8/8603C _003-288e -11.22© 2017 ... 2022 Kistler Group, Eulachstraße 22, 8408 Winterthur, Switzerland Tel.+41522241111,****************,. Kistler Group This information corresponds to the current state of knowledge. Kistler reserves the right to make technical changes. Liability for consequential damage resulting Accessories (included)Type/Art.-No.• Sensor seal copper (5 pcs.) 1131BA0.15Accessories (optional) Type/Art.-No.• Sensor seal copper (100 pcs) 1131BA0.15• Sensor seal nickel (100 pcs) 1131BB0.20• Floating clamp nut M7x0.75 6423B00• Floating clamp nut 5/16-24-UNF 6423B11• Adapter M10x11)6503C0A • Adapter seal (S.S. / 25 pcs) for 6503C0A 1113C0B • Adapter seal (Cu / 25 pcs) for 6503C0A 1113C0C • Adapter 3/8-24-UNF 1) 6503C1A • Adapter seal (S.S. / 25 pcs) for 6503C1A 1113C1B • Adapter seal (Cu / 25 pcs) for 6503C1A 1113C1C • Adapter M3x0.51)6507B0A • Adapter 5-40 UNC 1)6507B1A• Adapter seal for 6507BxA 1117B0C• Lubrication grease (Adapter) 1063• Adapter M10x1 (ground isolated) 6503C0D • Adapter 3/8-24 UNF (ground isolated) 6503C1D• Adapter 1/8-27 NPTF 6503C3A • Adapter M14x1.25 6501B0A• Adapter seal (S.S / 1pc) for 6501B0A 1100A11• Adapter M14x1.25 (water cooling) 6509B • Adapter seal (Cu / 1pc) for 6509B 1111• Temperature conditioning system 2621G • Connector extender (configurable product) 6482A • Extraction tool 1311• Dummy sensor (standard housing) 6487AA • Dummy sensor (short housing) 6487AB • Step reamer (SC H7 Ø6.35/Ø5.58) 1331C • Thread cutter M7x0.75 1351A0• Thread cutter 5/16-24 UNF 1351A1• RTV tool 1300A195• RTV seal (S.S. / 25 pcs) 1131BC1.15• RTV 1007A1)All of the adapters are delivered with 1 pc. of each adapter seal type and 1 pc. lubrication grease Type 1063 (except adapters Type 6503C0D, 6503C1D, 6503C3A).Ordering key (sensor)Output signal Charge (PE)A Voltage (IEPE)B HousingStandard housing (PE and IEPE)A Short housing (only PE)B Pressure range (only IEPE)14 bar / 200 psi 00014.035 bar / 500 psi 00035.070 bar / 1 000 psi 00070.0350 bar / 5 000 psi 00350.0690 bar / 10 000 psi 00690.01000 bar / 15 000 psi 01000.0O rderingexample Type PE sensor with standard housing 603CAAPE sensor with short housing 603CABIEPE sensor (350 bar / 5 000 psi) 603CBA00350.0。
python能量谱代码
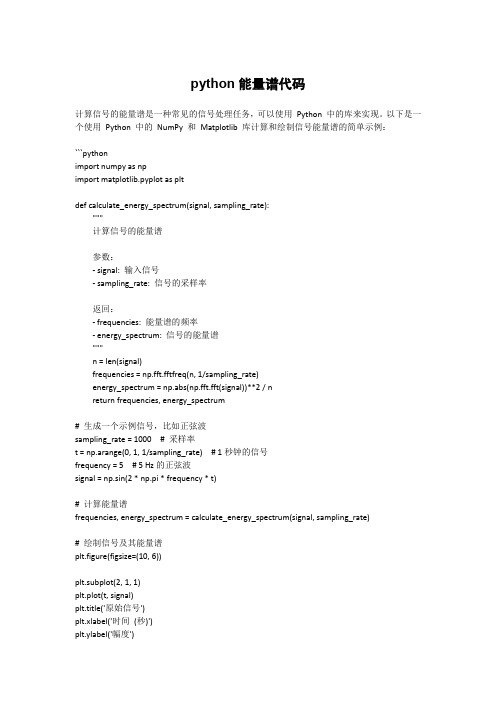
python能量谱代码计算信号的能量谱是一种常见的信号处理任务,可以使用Python 中的库来实现。
以下是一个使用Python 中的NumPy 和Matplotlib 库计算和绘制信号能量谱的简单示例:```pythonimport numpy as npimport matplotlib.pyplot as pltdef calculate_energy_spectrum(signal, sampling_rate):"""计算信号的能量谱参数:- signal: 输入信号- sampling_rate: 信号的采样率返回:- frequencies: 能量谱的频率- energy_spectrum: 信号的能量谱"""n = len(signal)frequencies = np.fft.fftfreq(n, 1/sampling_rate)energy_spectrum = np.abs(np.fft.fft(signal))**2 / nreturn frequencies, energy_spectrum# 生成一个示例信号,比如正弦波sampling_rate = 1000 # 采样率t = np.arange(0, 1, 1/sampling_rate) # 1秒钟的信号frequency = 5 # 5 Hz的正弦波signal = np.sin(2 * np.pi * frequency * t)# 计算能量谱frequencies, energy_spectrum = calculate_energy_spectrum(signal, sampling_rate)# 绘制信号及其能量谱plt.figure(figsize=(10, 6))plt.subplot(2, 1, 1)plt.plot(t, signal)plt.title('原始信号')plt.xlabel('时间(秒)')plt.ylabel('幅度')plt.subplot(2, 1, 2)plt.plot(frequencies, energy_spectrum)plt.title('能量谱')plt.xlabel('频率(Hz)')plt.ylabel('能量')plt.tight_layout()plt.show()```这段代码使用NumPy 中的FFT 函数来计算信号的频谱,然后计算能量谱。
piezoelectric effect 原理英文
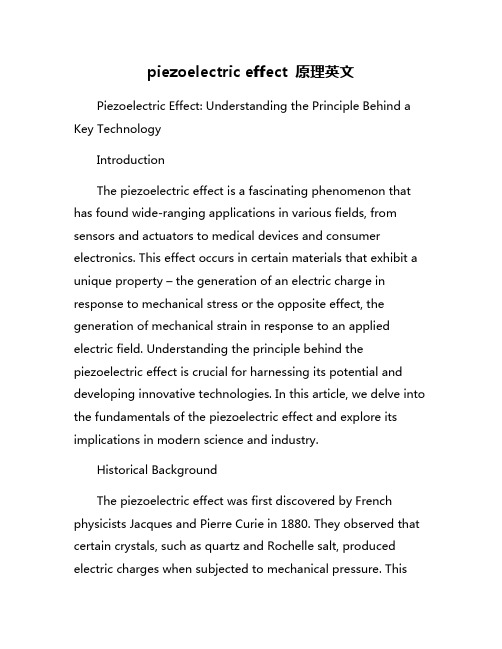
piezoelectric effect 原理英文Piezoelectric Effect: Understanding the Principle Behind a Key TechnologyIntroductionThe piezoelectric effect is a fascinating phenomenon that has found wide-ranging applications in various fields, from sensors and actuators to medical devices and consumer electronics. This effect occurs in certain materials that exhibit a unique property – the generation of an electric charge in response to mechanical stress or the opposite effect, the generation of mechanical strain in response to an applied electric field. Understanding the principle behind the piezoelectric effect is crucial for harnessing its potential and developing innovative technologies. In this article, we delve into the fundamentals of the piezoelectric effect and explore its implications in modern science and industry.Historical BackgroundThe piezoelectric effect was first discovered by French physicists Jacques and Pierre Curie in 1880. They observed that certain crystals, such as quartz and Rochelle salt, produced electric charges when subjected to mechanical pressure. Thisphenomenon sparked a wave of research into the properties of piezoelectric materials and laid the foundation for the development of piezoelectric technology. Over the years, scientists have identified a wide range of materials that exhibit piezoelectric behavior, including ceramics, polymers, and composites.Principle of the Piezoelectric EffectAt the heart of the piezoelectric effect lies the concept of polarization, where the positive and negative charges within a material become displaced in response to an external stimulus. In piezoelectric materials, such as quartz or lead zirconate titanate (PZT), the crystal structure is asymmetric, with positive and negative charges distributed unevenly along certain axes. When a mechanical force is applied to the material, the crystal lattice deforms, causing the charges to shift and creating an electric field. This leads to the generation of an electric charge on the surface of the material, known as the direct piezoelectric effect.Conversely, when an electric field is applied to a piezoelectric material, the charges within the crystal lattice rearrange, resulting in a change in shape or mechanical strain, known as the inverse piezoelectric effect. This bidirectionalrelationship between mechanical stress and electric field forms the basis of piezoelectric transduction, where energy is converted between mechanical and electrical forms.Applications of the Piezoelectric EffectThe piezoelectric effect has revolutionized a wide range of industries, enabling the development of innovative devices and technologies. Some of the key applications of piezoelectric materials include:1. Sensors and Actuators: Piezoelectric sensors are widely used in industrial applications, such as measuring pressure, force, and acceleration. Likewise, piezoelectric actuators are employed in precision positioning systems, ultrasonic motors, and vibration control devices.2. Energy Harvesting: Piezoelectric materials can convert mechanical vibrations or ambient vibrations into electrical energy, making them ideal for energy harvesting applications in wearable devices, wireless sensors, and self-powered systems.3. Medical Devices: Piezoelectric transducers are used in medical imaging technologies, such as ultrasound andnon-destructive testing. Piezoelectric bone conduction devicesand piezoelectric actuators are also used in hearing aids and implantable medical devices.4. Consumer Electronics: Piezoelectric materials are found ina variety of consumer electronics products, including piezoelectric buzzers, acoustic sensors, and haptic feedback devices in smartphones and tablets.Future Prospects and ChallengesAs the demand for miniaturized, energy-efficient devices continues to grow, the piezoelectric effect holds great promise for future technological advancements. Researchers are exploring new materials, such as flexible and bio-compatible polymers, for unique applications in wearable electronics, biomedical implants, and energy-efficient devices. However, there are still challenges to overcome, such as improving the performance and durability of piezoelectric materials, optimizing energy conversion efficiency, and reducing costs for large-scale production.ConclusionThe piezoelectric effect is a powerful phenomenon that has transformed the way we interact with technology and the world around us. By understanding the principles behind this effectand exploring its diverse applications, we can unlock new opportunities for innovation and create a sustainable future. As we continue to push the boundaries of material science and engineering, the piezoelectric effect will undoubtedly play a key role in shaping the technologies of tomorrow.。
Piezoelectric energy harvesting device optimization by synchronous electric charge extraction

of this, the design of vibration-powered electronic devices is made possible by the recent development of ultralow-power integrated circuits, able for instance, to feed sensors, to carry out fast signal processing, and to radio-transmit data (Elvin et al., 2001; Ayers et al., 2003; Roundy et al., 2003a, b; Amirtharajah and Chandrakasan, 2004).
Various techniques for the construction of such piezoelectric microgenerators have been investigated. Mechanical parts constitute an important aspect in design, but previous studies have shown that electrical energy conditioning system has also a determinant effect on the energy conversion effectiveness (Ottman et al., 2002, 2003).
Vibrations take place in most of the structures, but their potential energy often remains very low. In spite
Piezoelectric energy harvesting from transverse galloping of bluff bodies

Search
Collections
Journals
About
Contact us
My IOPscience
Piezoelectric energy harvesting from transverse galloping of bluff bodies
This content has been downloaded from IOPscience. Please scroll down to see the full text. 2013 Smart Mater. Struct. 22 015014 (/0964-1726/22/1/015014) View the table of contents for this issue, or go to the journal homepage for more
0964-1726/13/015014+11$33.00 1
several studies [18–24] have focused on the conversion of aeroelastic vibrations of wings to electrical power. Sirohi and Mahadik [25] proposed harvesting energy from transverse galloping of a prismatic structure that has an equilateral triangle section. Surface-bonded piezoelectric sheets attached to two beams connected to the structure were used to generate power. Their device generated more than 50 mW at a wind speed of 11.6 miles per hour; a power level that is sufficient to supply most of the commercially available wireless sensors. In this work, we consider the exploitation of transverse galloping of a bluff body with different cross-section geometries to harvest energy using a piezoelectric transducer. Transverse galloping of an elastic bluff body occurs when the wind speed exceeds a critical value and consequently the body starts to oscillate [26]. Many studies [27–36] have investigated the effects of various parameters on the galloping of different structures. These studies have also investigated the influence of the geometry of the cross-section
小学上册第十三次英语自测题(有答案)
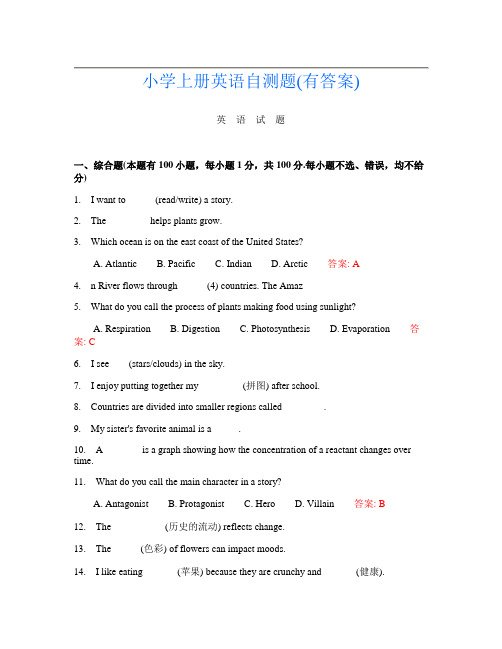
小学上册英语自测题(有答案)英语试题一、综合题(本题有100小题,每小题1分,共100分.每小题不选、错误,均不给分)1.I want to _____ (read/write) a story.2.The ________ helps plants grow.3.Which ocean is on the east coast of the United States?A. AtlanticB. PacificC. IndianD. Arctic答案: A4.n River flows through _____ (4) countries. The Amaz5.What do you call the process of plants making food using sunlight?A. RespirationB. DigestionC. PhotosynthesisD. Evaporation答案: C6.I see ___ (stars/clouds) in the sky.7.I enjoy putting together my ________ (拼图) after school.8.Countries are divided into smaller regions called ________.9.My sister's favorite animal is a _____.10. A _______ is a graph showing how the concentration of a reactant changes over time.11.What do you call the main character in a story?A. AntagonistB. ProtagonistC. HeroD. Villain答案: B12.The __________ (历史的流动) reflects change.13.The _____ (色彩) of flowers can impact moods.14.I like eating ______ (苹果) because they are crunchy and ______ (健康).15.What is the term for a group of wolves?A. PackB. PrideC. FlockD. School答案: A16.My dad is a ______. He enjoys fishing on weekends.17.My sister enjoys learning about ____ (geography).18.What do you call a group of lions?A. PackB. PrideC. FlockD. Herd答案: B19.What do we call a person who studies the effects of climate change?A. ClimatologistB. MeteorologistC. Environmental ScientistD. Ecologist答案: A20.She likes to ________ pictures.21.What do you call the science of classifying living things?A. TaxonomyB. BiologyC. EcologyD. Zoology答案:A22.I can ________ my shoes.23.My aunt works as a __________ (护士) in the clinic.24.Oxidation reactions involve the ________ of electrons.25.The chemical formula for lead(II) oxide is _______.26.We love to ______ (参加) community events.27.The ________ (地图测量) helps in navigation.28.I can ___ (skip) rope.29.The cat stretches after a long _______.30.I like to _____ (参加) parties.31.The snapping turtle can bite very _________ (痛).32.My grandma makes the best ________ (饼干).33.I see a _____ (fox) in the distance.34.He is reading a ___. (magazine)35.Turtles have a hard ______ for protection.36. A ______ is a method for visually representing data.37.The puma is also called a _________ (美洲狮).38.What is the capital of Finland?A. HelsinkiB. EspooC. TampereD. Oulu答案: A39.What is the term for a baby horse?A. CalfB. FoalC. KidD. Lamb答案: B40.Certain plants can ______ (适应) to new environments.41.I like to make ______ for special occasions.42.The lizard can lose its _______ (尾巴) to escape.43.My dad is a __________ (律师).44.I can ______ (克服) my fears.45.My brother is a ______. He enjoys acting in plays.46.I see a ___ (cloud/rainbow) above.47.The _______ (Trail of Tears) was a forced relocation of Native Americans.48.She is wearing a beautiful ___. (hat)49.Electrons have a ______ charge.50.The Great Wall is located in __________.51.The ice cream is _____ (cold/hot) and delicious.52.The chemical formula for potassium bromide is __________.53.They _____ (like/likes) to play outside.54.The ______ of a leaf can indicate how much sunlight it receives. (叶片的形状可以表明它接受多少阳光。
Two-dimensional Quantum Field Theory, examples and applications
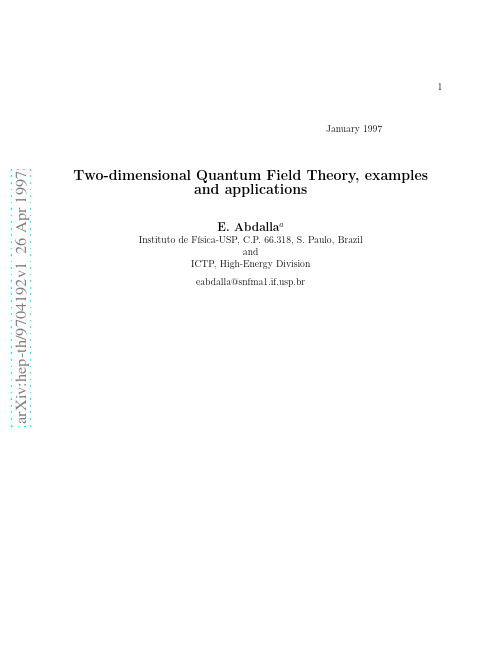
Abstract The main principles of two-dimensional quantum field theories, in particular two-dimensional QCD and gravity are reviewed. We study non-perturbative aspects of these theories which make them particularly valuable for testing ideas of four-dimensional quantum field theory. The dynamics of confinement and theta vacuum are explained by using the non-perturbative methods developed in two dimensions. We describe in detail how the effective action of string theory in non-critical dimensions can be represented by Liouville gravity. By comparing the helicity amplitudes in four-dimensional QCD to those of integrable self-dual Yang-Mills theory, we extract a four dimensional version of two dimensional integrability.
2 48 49 52 54 56
5 Four-dimensional analogies and consequences 6 Conclusions and Final Remarks
A comparative study on MEMS piezoelectric microgenerators
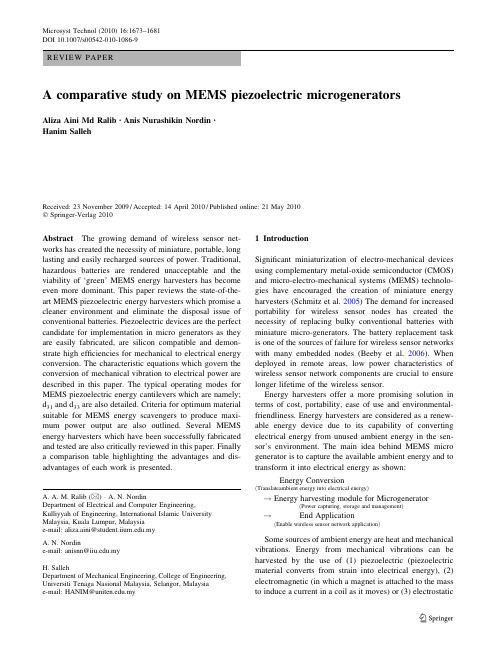
REVIEW PAPERA comparative study on MEMS piezoelectric microgeneratorsAliza Aini Md Ralib •Anis Nurashikin Nordin •Hanim SallehReceived:23November 2009/Accepted:14April 2010/Published online:21May 2010ÓSpringer-Verlag 2010Abstract The growing demand of wireless sensor net-works has created the necessity of miniature,portable,long lasting and easily recharged sources of power.Traditional,hazardous batteries are rendered unacceptable and the viability of ‘green’MEMS energy harvesters has become even more dominant.This paper reviews the state-of-the-art MEMS piezoelectric energy harvesters which promise a cleaner environment and eliminate the disposal issue of conventional batteries.Piezoelectric devices are the perfect candidate for implementation in micro generators as they are easily fabricated,are silicon compatible and demon-strate high efficiencies for mechanical to electrical energy conversion.The characteristic equations which govern the conversion of mechanical vibration to electrical power are described in this paper.The typical operating modes for MEMS piezoelectric energy cantilevers which are namely;d 31and d 33are also detailed.Criteria for optimum material suitable for MEMS energy scavengers to produce maxi-mum power output are also outlined.Several MEMS energy harvesters which have been successfully fabricated and tested are also critically reviewed in this paper.Finally a comparison table highlighting the advantages and dis-advantages of each work is presented.1IntroductionSignificant miniaturization of electro-mechanical devices using complementary metal-oxide semiconductor (CMOS)and micro-electro-mechanical systems (MEMS)technolo-gies have encouraged the creation of miniature energy harvesters (Schmitz et al.2005)The demand for increased portability for wireless sensor nodes has created the necessity of replacing bulky conventional batteries with miniature micro-generators.The battery replacement task is one of the sources of failure for wireless sensor networks with many embedded nodes (Beeby et al.2006).When deployed in remote areas,low power characteristics of wireless sensor network components are crucial to ensure longer lifetime of the wireless sensor.Energy harvesters offer a more promising solution in terms of cost,portability,ease of use and environmental-friendliness.Energy harvesters are considered as a renew-able energy device due to its capability of converting electrical energy from unused ambient energy in the sen-sor’s environment.The main idea behind MEMS micro generator is to capture the available ambient energy and to transform it into electrical energy as shown:Energy ConversionðTranslateambient energy into electrical energy Þ!Energy harvesting module for MicrogeneratorPower capturing ;storage and management ðÞ!End ApplicationEnable wireless sensor network application ðÞSome sources of ambient energy are heat and mechanical vibrations.Energy from mechanical vibrations can be harvested by the use of (1)piezoelectric (piezoelectric material converts from strain into electrical energy),(2)electromagnetic (in which a magnet is attached to the mass to induce a current in a coil as it moves)or (3)electrostaticA.A.M.Ralib (&)ÁA.N.NordinDepartment of Electrical and Computer Engineering,Kulliyyah of Engineering,International Islamic University Malaysia,Kuala Lumpur,Malaysia e-mail:aliza.aini@.my A.N.Nordine-mail:anisnn@.myH.SallehDepartment of Mechanical Engineering,College of Engineering,Universiti Tenaga Nasional Malaysia,Selangor,Malaysia e-mail:HANIM@.my123Microsyst Technol (2010)16:1673–1681DOI 10.1007/s00542-010-1086-9(in which a charge on the mass induces a voltage on a capacitor as it moves).Some of the applications of energy harvesting are long term medical monitoring and embedded sensors in buildings.The trend is now to develop micro power generators(Paradiso and Starner2005)that can harvest energy for condition monitoring applications.This device can be positioned on a gas turbine in power plants where at a critical vibration pattern it will generate power toactivate a wireless sensor to caution for maintenance. Wireless systems offer a huge advantage in condition monitoring applications because of theflexibility and ease of implementation at typically inaccessible locations.This paper emphasizes energy harvesters which utilize the piezoelectric conversion mechanism.Piezoelectric devices are the perfect candidate for implementation in micro generators as they can efficiently convert mechanical strain to electrical charge without any additional power. There has been a significant interest in vibration based energy harvesting for low power applications such as pagers,self powered emergency receivers,radio frequency identification(RFID)tags or locators(Shenck and Paradiso 2001).Choi et al.(2006)reported that one such MEMS device is a thinfilm piezoelectric power generator which employs the d33mode with measured performance of1l W. Another prototype of piezoelectric cantilever is capable of generating270nW when operating at the resonance fre-quency of229Hz(Kok et al.2008).To improve power output and to provide frequencyflexibility,Liu et al. (2008)proposed an array of MEMS piezoelectric power generators.This device array has a measured performance of3.98l W effective electrical power and3.93DC output voltages with bandwidth of226–234Hz.This paper explains the principle of piezoelectric energy harvesting and provides a comparative study on existing MEMS piezoelectric micro generators.The rest of the paper is organized as follows.Section2explains on the piezoelectric principle and design.Section3presents the state of the art MEMS devices and its emerging applica-tions.A comparative study of this recent work is tabulated and discussed in Sect.4.Finally,the conclusion is given in Sect.5.2Piezoelectric principle and design2.1Conversion designThe cantilever structure with proof mass at the end has proven to be the most popular structure for the energy conversion design from mechanical vibration to electrical power.Researchers have extensively applied this configu-ration for piezoelectric energy harvesting device(Kok et al.2008;Liu et al.2008;Choi et al.2006;Hyunuk et al.2008, 2009;Lee et al.2007).The direction of vibration is shown with an arrow in Fig.1.The piezoelectric energy conversion can be described using the equivalent linear spring mass system as shown in Fig.2.M€zþC_zþKz¼ÀM€yð1Þwhere z=x-y is the net displacement of mass,M is the lumped mass,K is the spring constant and C is the damping coefficient(Hyunuk et al.2009).The natural frequency of spring mass system,x n can be written asx n¼ffiffiffiffikmrð2ÞUsing the model of the power output of the system at resonance isP max¼mY2x3n4fð3ÞWhere m is the seismic mass,Y is the amplitude vibration x is the system resonance frequency and f is the relative damping ratio.The output power is directly proportional to mass which means that the converter size directly affects the power output produced as shown in(3).Power is inversely proportional to the damping ratio,which is dependent on the selection of materials and the design of the device.Output power of the system is optimized ifthe Fig.1Cantilever beam with tipmassFig.2Schematic of generic vibration converter1674Microsyst Technol(2010)16:1673–1681 123piezoelectric system is operating at its resonance frequency (Hyunuk et al.2009).In terms of excitation acceleration levels of the input vibration(a),the power output can be displayed in(4) where a=x n2Y(Beeby et al.2006).P max¼ma24x n fð4ÞAs shown in(4),the output power decreases as frequency increases because the input vibrations amplitude decreases with frequency.The output power also proportional to the square of acceleration(Hyunuk et al.2008).Furthermore, Liu et al.(2008)reported that most sources of ambient vibration are in low frequency(\1,000Hz).As such,to optimize output power,an energy harvesting device should operate at lower frequencies(Liu et al.2008).2.2Operating mode of piezoelectric transducerWith reference to the Fig.3,two frequent modes that are applied for energy harvesting devices are the d33and d31 modes.In the d31mode,stress is applied in the axial direction but voltage is obtained in the perpendicular direction.In contrast,for the d33mode,the applied stress has the same direction as the generated voltage.Choi et al. (2006)reported that the d31mode has separate top and bottom electrodes while the d33mode employs only top interdigital electrodes.The d33mode design gives a much higher open circuit voltage which is needed to overcome the forward bias of the rectifying diodes(Choi et al.2006).2.3Piezoelectric material selectionSuitable piezoelectric material is characterized by the product of piezoelectric energy constant(g)and piezo-electric strain coefficient(d)given as|d.g|(Hyunuk et al. 2009).The types of material can be categorized into four namely:piezoelectric polycrystalline ceramics,piezoelec-tric single crystal materials,piezoelectric and electrostric-tive polymers and piezoelectric thinfilms.PZT(lead zicronate titanate)is a polycrystalline ceramic which shows good piezoelectric strain and coupling coefficients as shown in Table1(Roundy et al.2003).PZT thinfilms can be constructed from materials such as Pb(Zr, Ti)O3(PZT),AlN and BaTiO3.They are commonly used for sensor applications.PZT polycrystalline thickfilms are widely used to fabricate MEMS scale energy harvesting devices(Hyunuk et al.2008).PZN-PT(lead zinc niobate–lead titanate)is a single crystal material which has excel-lent properties compared to PZT.However,PZN-PT is not typically used for microgenerators since they are difficult to fabricate in MEMS,are very expensive and only very small crystals can be produced(Roundy et al.2003).PVDF (polyvinylidenefluoride)is a semi crystalline polymer which is approximately half crystalline and half amor-phous.PZT is reported to be the optimum piezoelectric material for micro generators based on the comparison done;due to the ease of fabrication and high power output produced(Roundy et al.2003).3Recent techniques in MEMS micro generators There has been significant interest in the vibration based energy harvesting for low power applications research (Shenck and Paradiso2001).In this section,nine recent previous works will be discussed comprehensively.A complete comparison table is also attached at the end of the discussion.3.1Work1:MEMS based piezoelectric powergenerator array for vibration energy harvestingFang et al.(2006)demonstrated a generator formed of composite cantilever with nickel as its metal mass.The prototype generator was fabricated using MEMS process-ing techniques such as sol-gel,dry reactive-ion-etching (RIE)and wet chemical etching.The measured output performance was0.89AC peak–peak voltage output and 2.16l W power output at608Hz resonant frequency.Though the micro-generator is functioning satisfactorily, Liu et al.(2008)concluded that with specific dimensions, such cantilevers havefixed and narrow frequencies and are capable of producing only l W level power output.To improve power output and to offer frequencyflexibility,an overlapping effect of resonance frequencies was introduced by designing a power generator array based on multiple cantilevers.The available bandwidth covers the range of minimum and maximum resonance frequencies of the cantilevers in an array.This generator array has a measured performance of3.98l W electrical power and3.93V DC output voltage to a resistive load and resonance frequencies ranging from226to234Hz(Liu et al.2008).As shown in Fig.4,the piezoelectric material used is thickfilm PZT with Pt/Ti as the top and bottom electrodes.Nimaterial Fig.3Operating mode of piezoelectric transducerMicrosyst Technol(2010)16:1673–16811675123was chosen for the proof mass.This design shows that the array of devices is a promising method to improve power output and bandwidth of the micro generator.3.2Work 2:Energy harvesting MEMS device based onthin film piezoelectric cantilevers A thin film piezoelectric MEMS cantilever energy harvester was developed by Choi et al.(2006).The dimensions of thecantilever were 1709260l m.The chosen structure of the design is bimorph with a proof mass at the end as shown in Fig.5.A thin film of lead zirconate titanate,Pb (Zr,Ti)O 3(PZT)was chosen as the piezoelectric material.The top Pt/Ti electrodes were patterned as interdigital electrodes on top of the PZT thin film to operate in the d 33mode.The generator had a measured performance of 1l W electrical power and 2.4V DC output voltage when measured across a 5.2M X resistance load.The device has three resonance modes namely;13.9,21.9and 48.5kHz (Choi et al.2006;Jeon et al.2005).A highlight of the work is a discussion on the relationship between the proof mass and the power output produced.The work reports that for a specific reso-nant frequency,the output power is proportional to the weight of the proof mass.P out ð€x B Þ¼m eff 1x N rk 2e R eq .R l X 2½1Àð1þ21m r ÞX 2 þ½ð1þk 2e Þr X þ21mX Àr X 3 ð5ÞThe generated power for a given input vibrationamplitude is derived (Choi et al.2006)as shown in (5)where P out is the output power,x B is the input vibration amplitude,m eff is the effective mass of the structure and x N is the natural frequency of the structure.The equivalent resistance (r )is equal to R l R p /(R l ?R p );where R l is theTable 1Comparison of piezoelectric materialsSource:(Roundy et al.2003)and (Hyunuk et al.2009)Material TypeStrain coefficient (10-12m/v)PZT 701Polycrystalline ceramics d 31320d 33650PZT-4Piezoelectric thin filmsd 33289PVDF Piezoelectric and electrostrictive polymers d 3120d 3330PZN-PTSingle crystal materials d 31950d 332,000BaTiO 3Piezoelectric thin filmsd 33191Fig.4Schematic configuration of single cantileverbeam Fig.5Side and top view of d33mode of piezoelectric devices1676Microsyst Technol (2010)16:1673–1681123load resistance,R p is the leak resistance of microgenerator,X is the relative frequency (x input /x N )and 1m is the mechanical damping coefficient (Choi et al.2006).Equation (5)indicated that the heavier the mass,the stiffer the beam and therefore,more power can be stored into the structure.3.3Work 3:A free standing thick film piezoelectricenergy harvester A free standing thick film cantilever was fabricated using conventional thick film and sacrificial layer fabrication techniques (Kok et al.2008).The dimensions of the can-tilever were 13.5mm long by 9mm wide with total thickness of 192l m.Again,thick film lead zirconate titanate (PZT)was chosen.The device produced an output voltage up to 130mV when driving a 60k X resistor and resonating at 229Hz.Ag/Pd was chosen as the top and lower electrodes and the device operates in the d 31mode.Figure 6shows the fabrication steps of a free standing thick film piezoelectric energy harvester.The resonant frequency of the cantilever decreases with added mass as shown in (6)where j the spring is constant is the effective proof mass V n 2is the eigenvalue of the n th mode of vibration and f n is the resonant frequency.m eff ¼j 12:71V 2n2P f n 2ð6ÞKok et al.(2008)also proved that additional proof mass improves the output electrical power.Typically,powers generated from the vibration devices are relatively small (*270nW).However,excessive proof mass leads to energy dissipation due to damping effects in the cantilever beam (Kok et al.2008).3.4Work 4:Two layered piezoelectric bender devicefor micro power generator A two layered piezoelectric bender device for micro gen-erator was designed as shown in Fig.7(Jeong et al.2008).The piezoelectric ceramic used was 0.2Pb (Mg 1/3Nb 2/3)O 3–0.8Pb (Zr 0.475Ti 0.525)O 3(PMNZT).Bender type pie-zoelectric devices show significant change in power with small deviations of frequency.As such,when the thick-nesses of the two layered device were varied in four samples,each layer shows a different resonant frequency as shown in Fig.8.The samples were placed under different frequencies of vibration (88,100,110and 120Hz)and the output voltage of each sample was measured with respect to time.Each sample showed maximum output voltage when excited at its resonant vibration frequency (Jeong et al.2008).The generator has a measured performance of peak to peak voltage of 2.0V and power of 0.5mW of acceleration 0.1G (G =0.981m/s 2)at 120Hz in reso-nance mode (Jeong et al.2008).Fig.6Fabrication steps for free standing piezoelectric energyharvesterFig.7Two layer structure of piezoelectric bender devices (Jeong et al.2008)Microsyst Technol (2010)16:1673–168116771233.5Work 5:Piezoelectric scavengers in MEMStechnology:fabrication and simulationSchmitz et al.(2005)designed and fabricated several pie-zoelectric devices differing in geometry and electrical connections on one wafer.Figure 9shows the piezoelectric generator located on top of a beam.The micro-generator consists of a piezoelectric layer sandwiched between top (Al)and bottom (Pt)electrodes.Three different mass dimensions (393mm 2,595mm 2,797mm 2)were designed.The change in length and width of the beam results in variation of the devices’resonance frequencies (300,700,and 1,000Hz).The devices are designed as single piezoelectric generators or as a series connection of four piezoelectric generators.Schmitz et al.(2005)pro-vided a comparison of fabrication using two different pie-zoelectric materials namely PZT and AlN.PZT has a higher piezoelectric constant compared to AlN.However,fabri-cation wise,the deposition of PZT layer is more time consuming and involves multiple steps compared to AlN (Schmitz et al.2005).AlN provides more favorable material parameters and has better composition control e.g.up to three times faster deposition and better composition due to AlN stoichiometric structure (Small Times 2009).The simplicity of AlN fabrication,which is merely sputtered on,makes it low-cost and easily compatible to standard CMOS and MEMS processes.Finally,the piezoelectric device is wafer bonded and encapsulated between two wafers.Suchprotection indicates that the microgenerator operates in vacuum and thus reducing air-damping of the beam.The finished piezoelectric energy harvester weighs only 34mg and is capable of generating 60l W electrical power at 500Hz resonance frequency.3.6Work 6:Piezoelectric harvesters and MEMStechnology This work illustrates a piezoelectric energy harvester with measured maximum output power of 40l W for input vibrations of 1.8kHz frequency and 80nm amplitude (Renaud et al.2007).As illustrated in Fig.10,the device is a piezoelectric capacitor formed using PZT layer with an Al electrode on the top and Pt electrode at the bottom.A small proof mass was attached to the tip of the cantilever.It was wafer-bonded between two capping wafers with electrical contacts openings and appropriate cavities allowing the motion of mass.Renaud et al.also presented a theoretical electromechanical model which estimates the output power of the encapsulated piezoelectric energy scavenger when connected to a resistive load.Some assumptions have been made during the development of the model such as;the frequency of the input vibration is close to one of the natural frequencies of the bender,the mass of the bending system is negligible with respect to the one attached at its end and the different modes of resonance of the structure do not interfere with each other.The model provided a method of approximating the outputpower.Fig.8Geometry of bender devices (t1=250l m,t1=220l m,t1=180l m,t1=160lm)Fig.9Piezoelectric scavengers in MEMS technology (Schmitz et al.2005)Fig.10Schematic drawing of piezoelectric scavenger (Renaud et al.2007)1678Microsyst Technol (2010)16:1673–1681123Minor discrepancies exist between the model and experi-mental results due to neglected nonlinear effects and losses (Renaud et al.2007).3.7Work 7:Laser machined piezoelectric cantileversfor mechanical energy harvesting A piezoelectric material based mechanical energy harvester fabricated using a combination of laser machining tech-nique and microelectronics packaging technology was reported (Hyunuk et al.2008).The device was tested at low frequency range of 50–1,000Hz at constant force of 8G (G =9.81m/s 2).Pb (Zr 0.52Ti 0.48)O 3–Pb(Zn 1/3Nb 2/3)O 3was chosen as the piezoelectric material.It was reported that laser machining process did not give significant effect on the electrical properties of piezoelectric material (Hyunuk et al.2008).Figure 11shows ten cantilevers on both sides of the bridge which were electrically connected in parallel.The tip masses were deposited on alternate cantilevers to ensure that each cantilever vibrates at a different resonance frequency,thus providing a wider fre-quency range (Hyunuk et al.2008).The whole package was mounted on the top of a shaker.The variation of the output voltage versus frequency were measured.Interest-ingly enough,the measured resonance peaks were at 870and 270Hz,which were far from the simulated value of 47.1and 14.8kHz.This deviation in frequency was attributed to the rocking and bouncing mode of the chip package which was mounted on an aluminum bar.Under such experimental conditions,the device has a measured performance of 1.13l W electrical power when resonating at 870Hz across 288.5k X load and 0.06l W electrical power when resonating at 270Hz across 949k X .The calculated power density is 301.3l W/cm 3.It can be con-cluded that a high energy density device can be fabricated using the combination of laser machining and IC fabrica-tion techniques.Optimum energy density is achieved when using the appropriate cantilever dimensions,number of cantilevers on a wafer,weight of the tip mass and PZT material characteristics (Hyunuk et al.2008).3.8Work 8:Power harvesting using piezoelectricMEMS generator with interdigital electrodes Lee et al.(2007)designed a piezoelectric MEMS generator consisting of a silicon beam structure laminated with PZT (lead zirconate titanate).Interdigital electrodes were placed on top of the PZT layer to transform mechanical strain energy into electrical charge when operating using the d 33mode.The gaps of the interdigital electrodes were varied and the power performance was evaluated.A proof mass was attached at the tip of the beam as shown in Fig.12.The beam structure was designed to operate at its resonant frequency of 570Hz to maximize stress,strain and output power.Varying the distance between interdigital electrodes in turn varies the output power since the output voltage is a function of the output charge and the capacitance between interdigital electrodes.The dimensions of the cantilever generator were 3,000l m 91,500l m with 22l m beam thickness.The thickness of the SOI wafer and PZT layer were and 10and 12l m respectively.A 50nm Ti and 200nm Pt metal layer was then deposited on top of the wafer using the E-beam evaporator.Experimental mea-surements of the device were conducted by mounting the piezoelectric MEMS generator on a shaker and measuring its output voltage via a buffer circuit.Devices of varying interdigital electrodes gaps of 20,30and 40l m were measured.Experimental results indicate that the higher the gap distances between electrodes,the larger the output power (Lee et al.2007).The maximum power output of 0.0471l W was obtained from the MEMS generator with 40l m gap.4Performance evaluation for piezoelectric energy harvesters Piezoelectric micro-generators based on the cantilever beam structure were chosen for discussion due to its sim-plicity and compatibility with MEMS technologies.MEMS energy harvesters have the challenge of havinglowFig.11Assembled PZT wafer with tip mass (Hyunuk et al.2008)Fig.12Schematic graph of the piezoelectric MEMS generator (Lee et al.2007)Microsyst Technol (2010)16:1673–16811679123T a b l e 2C o m p a r i s o n t a b l e f o r p i e z o e l e c t r i c e n e r g y h a r v e s t i n gP r e v i o u s w o r k P i e z o e l e c t r i c m a t e r i a l sD e s i g nR e s o n a n t f r e q u e n c y O u t p u t p o w e r o r v o l t a g e F a b r i c a t i o nW o r k 1.M E M S b a s e d p i e z o e l e c t r i c p o w e r g e n e r a t o r a r r a y f o r v i b r a t i o n e n e r g y h a r v e s t i n g (L i u e t a l .2008)T h i n fil m l e a d z i r c o n a t e t i t a n a t e P b (Z r ,T i )O 3(P Z T )C a n t i l e v e r s i z e :L e n g t h =2,000–3,500l m W i d t h =750–1,000l m 226–234H z d 31m o d eO u t p u t v o l t a g e o f 3.93V D C O u t p u t p o w e r o f 3.98l W F u n c t i o n a l fil m p r e p a r a t i o n a n d p a t t e r n ,b u l k s i l i c o n m i c r o m a c h i n i n g ,s t r u c t u r e r e l e a s e a n d m a s s a s s e m b l a g e W o r k 2.E n e r g y H a r v e s t i n g M E M S d e v i c e b a s e d o n t h i n fil m p i e z o e l e c t r i c c a n t i l e v e r s (C h o i e t a l .2006)T h i n fil m l e a d z i r c o n a t e t i t a n a t e P b (Z r ,T i )O 3(P Z T )C a n t i l e v e r s i z e :L e n g t h =170l m W i d t h =260l m 3m o d e :13.9,21.948.5k H z O u t p u t v o l t a g e o f 2.4V a t 5.2M X l o a d O u t p u t p o w e r o f 1.01l W T h r e e p h o t o m a s k s t e p s D e p o s i t i o n v i a s o l g e l m e t h o d ,p a t t e r n e d v i a R I EW o r k 3.A f r e e s t a n d i n g t h i c k fil m p i e z o e l e c t r i c e n e r g y h a r v e s t e r (K o k e t a l .2008)T h i c k fil m l e a d z i c r o n a t e t i t a n a t e (P Z T )C a n t i l e v e r s i z e :L e n g t h =13.5m m W i d t h =9m m T h i c k n e s s =192l m229H z d 31m o d e O u t p u t v o l t a g e o f 130m V O u t p u t P o w e r o f 270n W a t 9.81m /s 2S a c r i fic i a l l a y e r W o r k 4.T w o l a y e r e d p i e z o e l e c t r i c b e n d e r d e v i c e s f o r m i c r o p o w e r g e n e r a t o r (J e o n g e t a l .2008)0.2P b (M g 1/3N b 2/3)O 3–0.8P b (Z r 0.475T i 0.525)O 3(P M N Z T )C a n t i l e v e r s i z e :L e n g t h =10m m W i d t h =10m m120H zP e a k -t o -p e a k v o l t a g e o f 2.0V a n d a p o w e r o f 0.5m W i n r e s o n a n c e m o d e T h e s t r u c t u r e c o n s i s t o f f o u r l a y e r s w a s d e s i g n e d w i t h v a r i o u s t h i c k n e s sW o r k 5.P i e z o e l e c t r i c s c a v e n g e r s i n M E M S t e c h n o l o g y :f a b r i c a t i o n a n d s i m u l a t i o n (S c h m i t z e t a l .2005)1.A l N2.P Z T L e a d z i c r o n a t e t i t a n a t e P b Z r 0.53T i 0.47O 3T h e p i e z o e l e c t r i c g e n e r a t o r i s l o c a t e d o n t o p o f t h e b e a m a n d c o n s i s t s p i e z o e l e c t r i c l a y e r s a n d w i c h e d b e t w e e n t o p a n d b o t t o m e l e c t r o d e300–1,000H z V a r i a t i o n o f r e s o n a n c e f r e q u e n c i e s :300,700,1,000H z 1–100l W A l N l a y e r i s d e p o s i t e d a n d p a t t e r n e d b y u s i n g a c o m b i n a t i o n o f s p u t t e r i n g a n d l i t h o g r a p h yW o r k 6.P i e z o e l e c t r i c h a r v e s t e r s a n d M E M S t e c h n o l o g y (R e n a u d e t a l .2007)P Z TT h e d e v i c e i s p a c k a g e s u s i n g t w o w a f e r s 1.8k H z 40l WA p i e z o e l e c t r i c c a p a c i t o rf o r m e d b y a b o t t o m o f P t e l e c t r o d e ,a P Z T l a y e r a n d a t o p o f A l e l e c t r o d e i s f a b r i c a t e d o n a c a n t i l e v e r w h i c h s u p p o r t m a s s a t t a c h e d t o i t s t i pW o r k 7.L a s e r m a c h i n e d p i e z o e l e c t r i c c a n t i l e v e r s f o r m e c h a n i c a l e n e r g y h a r v e s t i n g (H y u n u k e t a l .2008)P b (Z r 0.52T i 0.48)O 3–P b (Z n 1/3N b 2/3)O 310c a n t i l e v e r s o n b o t h s i d e o f t h e b r i d g e ,5o f t h e m a r e p l a c e d w i t h t i p m a s s a l t e r n a t e l y .W i d t h =4m m L e n g t h =5.75m m870H z O u t p u t p o w e r o f 1.13l W a t 870H z t h r o u g h 288.5k X l o a d .P o w e r d e n s i t y o f 301.3l W /c m 3L a s e r m a c h i n i n gW o r k 8.P o w e r H a r v e s t i n g U s i n g P i e z o e l e c t r i c M E M S G e n e r a t o r w i t h I n t e r d i g i t a l E l e c t r o d e s (L e e e t a l .2007)L e a d Z i c r o n a t e T i t a n a t e (P Z T )C a n t i l e v e r s i z e :L e n g t h =3,000l m W i d t h =1,500l m T h i c k n e s s =22l m570–575H z O u t p u t v o l t a g e o f 1.127V P -P ,O u t p u t p o w e r o f 0.123l W D e p o s i t i o n ,b e a m s h a p e p a t t e r n a n d R I E a n d D R I E e t c h i n g1680Microsyst Technol (2010)16:1673–1681123。
A review of power harvesting using piezoelectric materials (2003_2006)
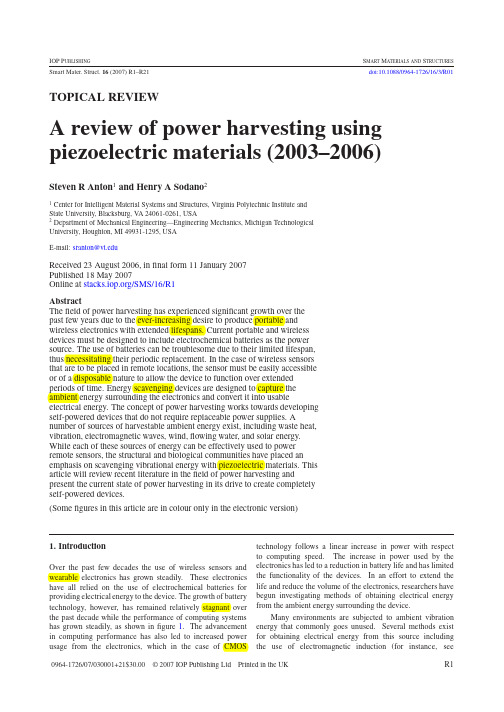
IOP P UBLISHING S MART M ATERIALS AND S TRUCTURES Smart Mater.Struct.16(2007)R1–R21doi:10.1088/0964-1726/16/3/R01TOPICAL REVIEWA review of power harvesting using piezoelectric materials(2003–2006)Steven R Anton1and Henry A Sodano21Center for Intelligent Material Systems and Structures,Virginia Polytechnic Institute andState University,Blacksburg,V A24061-0261,USA2Department of Mechanical Engineering—Engineering Mechanics,Michigan TechnologicalUniversity,Houghton,MI49931-1295,USAE-mail:sranton@Received23August2006,infinal form11January2007Published18May2007Online at /SMS/16/R1AbstractThefield of power harvesting has experienced significant growth over thepast few years due to the ever-increasing desire to produce portable andwireless electronics with extended lifespans.Current portable and wirelessdevices must be designed to include electrochemical batteries as the powersource.The use of batteries can be troublesome due to their limited lifespan,thus necessitating their periodic replacement.In the case of wireless sensorsthat are to be placed in remote locations,the sensor must be easily accessibleor of a disposable nature to allow the device to function over extendedperiods of time.Energy scavenging devices are designed to capture theambient energy surrounding the electronics and convert it into usableelectrical energy.The concept of power harvesting works towards developingself-powered devices that do not require replaceable power supplies.Anumber of sources of harvestable ambient energy exist,including waste heat,vibration,electromagnetic waves,wind,flowing water,and solar energy.While each of these sources of energy can be effectively used to powerremote sensors,the structural and biological communities have placed anemphasis on scavenging vibrational energy with piezoelectric materials.Thisarticle will review recent literature in thefield of power harvesting andpresent the current state of power harvesting in its drive to create completelyself-powered devices.(Somefigures in this article are in colour only in the electronic version)1.IntroductionOver the past few decades the use of wireless sensors and wearable electronics has grown steadily.These electronics have all relied on the use of electrochemical batteries for providing electrical energy to the device.The growth of battery technology,however,has remained relatively stagnant over the past decade while the performance of computing systems has grown steadily,as shown infigure1.The advancement in computing performance has also led to increased power usage from the electronics,which in the case of CMOS technology follows a linear increase in power with respect to computing speed.The increase in power used by the electronics has led to a reduction in battery life and has limited the functionality of the devices.In an effort to extend the life and reduce the volume of the electronics,researchers have begun investigating methods of obtaining electrical energy from the ambient energy surrounding the device.Many environments are subjected to ambient vibration energy that commonly goes unused.Several methods exist for obtaining electrical energy from this source including the use of electromagnetic induction(for instance,see0964-1726/07/030001+21$30.00©2007IOP Publishing Ltd Printed in the UK R1TopicalReviewFigure1.Advances in computer and battery technology since1990. (Derived from data in Paradiso and Starner2005.)Glynne-Jones et al2004),electrostatic generation(for instance,see Mitcheson et al2004),dielectric elastomers(for instance,see Kornbluh et al2002),and piezoelectric materials. While each of the aforementioned techniques can provide a useful amount of energy,piezoelectric materials have received the most attention due to their ability to directly convert applied strain energy into usable electric energy and the ease at which they can be integrated into a system.This energy conversion occurs because the piezoelectric molecular structure is oriented such that the material exhibits a local charge separation,known as an electric dipole.When strain energy is applied to the material it results in a deformation of the dipole and the formation of a charge that can be removed from the material and used to power various devices.The strain-dependent charge output of piezoelectric materials has typically been used for sensor applications and can be found in a variety of different devices including accelerometers,microphones,load cells,etc.More recently, the concept of shunt damping was developed,in which the electrical output of the piezoelectric material is used for damping purposes rather than sensing(Lesieutre1998). Because a portion of the vibration energy is converted to electrical energy by the piezoelectric material,when it is dissipated through Joule heating,energy is removed from the system resulting in a damping effect.The concept behind shunt damping is also used in power harvesting;however,rather than dissipating the energy it is used to power some other device.The rapid growth of research being performed in thefield of power harvesting has resulted in significant improvements to various energy scavenging techniques.This article will present a review of the recent advances in power harvesting using piezoelectric materials since Sodano et al(2004b)published a review of thefield in2004,which the reader is referred to as an introduction.This article will cover various topics in power harvesting using piezoelectric materials,including the design of efficient harvesting geometries,improving efficiency through circuitry,implantable and wearable power supplies,harvesting of ambientflows,microelectromechanical devices,self-powered sensors and comparisons of piezoelectric materials to other energy harvesting media.2.Improving efficiency and power generation through piezoelectric configurationsPiezoelectric materials can be configured in many different ways that prove useful in power harvesting applications.The configuration of the power harvesting device can be changed through modification of piezoelectric materials,altering the electrode pattern,changing the poling and stress direction, layering the material to maximize the active volume,adding prestress to maximize the coupling and applied strain of the material,and tuning the resonant frequency of the device.A large percentage of recent research in power harvesting with piezoelectric materials has focused on improving the efficiency of piezoelectric power harvesting systems.The following articles have all investigated ways to improve the efficiency of power harvesting by altering the configuration of the piezoelectric device in order to maximize the energy extracted from the ambient source.A review of these studies presents a wide variety of unique configurations,each of which proves to be advantageous under certain circumstances.The type of piezoelectric material selected for a power harvesting application can have a major influence on the harvester’s functionality and performance.To date,a number of different piezoelectric materials have been developed.The most common type of piezoelectric used in power harvesting applications is lead zirconate titanate,a piezoelectric ceramic, or piezoceramic,known as PZT.Although PZT is widely used as a power harvesting material,the piezoceramic’s extremely brittle nature causes limitations in the strain that it can safely absorb without being damaged.Lee et al(2005)note that piezoceramics are susceptible to fatigue crack growth when subjected to high frequency cyclic loading.In order to eliminate the disadvantages of piezoceramic materials and improve upon their efficiency,researchers have developed and tested other,moreflexible,piezoelectric materials that can be used in energy harvesting applications.Another common piezoelectric material is poly(vinylidene fluoride)(PVDF).PVDF is a piezoelectric polymer that exhibits considerableflexibility when compared to PZT. Lee et al(2004,2005)developed a PVDFfilm that was coated with poly(3,4-ethylenedioxy-thiophene)/poly(4-styrenesulfonate)[PEDOT/PSS]electrodes.They compared the PEDOT/PSS coatedfilms tofilms coated with the inorganic electrode materials,indium tin oxide(ITO)and platinum (Pt).When subjected to vibrations of the same magnitude over varying frequencies,it was found that thefilms with Pt electrodes began to show fatigue crack damage of the electrode surface at a frequency of33kHz.The ITO electrodes became damaged when operating at a frequency of213Hz.The PEDOT/PSSfilm,however,ran for10h at1MHz without electrode damage.One can conclude that,by utilizing a more durable electrode layer,a piezoelectric device can operate under more strenuous conditions.This may give the device the ability to harvest more power throughout its lifespan; however,the exact effect of a stronger electrode layer may vary depending on the specific application.Mohammadi et al(2003)developed afiber-based piezoelectric(piezofiber)material consisting of PZTfibers of various diameters(15,45,120,and250μm)that were aligned,laminated,and molded in an epoxy(Bent et al1995).R2TopicalReviewFigure2.Schematic of the cross section of an activefiber composite (AFC)actuator.(Figure from Wilkie et al2000.)This resulted inflexible composites with40%of the volume consisting of aligned piezoelectricfibers and the remaining 60%made up of epoxy.Several samples were made in which several34mm×11mm rectangular plates of various thicknesses(1.2–5.8mm)were diced from the composite such that thefibers were oriented in the plate thickness direction. The voltage output of the samples was tested by dropping a33.5g,20mm diameter stainless steel ball on them from a height of10cm.The peak power was also calculated considering a1M load resistance.A maximum voltage and power output of350V and120mW was obtained for the thickest transducer,5.85mm thick,with the smallestfiber diameter,15μm.Upon studying the relationship between voltage output of the harvester and its physical geometry,it was determined that thicker plates have the capability of larger fiber displacements,and that samples with smaller diameter fibers have the highest piezoelectric coefficient,d33and lowest dielectric constant defined in this study as K3,both of which contribute towards higher power outputs and more efficient systems.Piezofiber power harvesting materials have also been investigated by Churchill et al(2003)who tested a composite consisting of unidirectionally aligned PZTfibers of250μm diameter embedded in a resin matrix.It was found that when a 0.38mm thick sample of130mm length and13mm width was subjected to a180Hz vibration that caused a strain of300μεin the sample,the composite was able to harvest about7.5mW of power.The results of this study show that a relatively small fiber-based piezoelectric power harvester can supply useable amounts of power from cyclic strain vibration in the local environment.Sodano et al(2004a)presented a comparison of several piezoelectric composite devices for power harvesting that are normally used for sensing and actuation.The power harvesting ability of the macro-fiber composite(MFC),quick pack IDE (model QP10ni),and the quick pack model(QP10n)actuators was tested.The MFC contains piezofibers embedded in an epoxy matrix which affords it extremeflexibility,and it utilizes interdigitated electrodes,which allow the electricfield to be applied along the length of thefiber and act in the higher d33 coupling mode,as shown infigure2.A detailed explanation of the operation and of various applications of MFC devices is presented by Sch¨o necker et al(2006).The quick pack IDE contains interdigitated electrodes but conventional monolithic piezoceramic material,and the quick pack simply uses a traditional electrode pattern and a monolithic piezoceramic.To experimentally compare the efficiencies of these materials, all three were mounted to the same cantilever beam and thus subjected to the same vibration input.Tests were run at the first12vibration modes of the beam and the power output, which was normalized to volume because of the varying sizes of the specimens,was recorded for each device.It was found that at all vibration modes the quick pack proved to be the most efficient by harvesting the most energy,and that the MFC and quick pack IDE,while comparable,harvested considerably lower amounts of energy.The conclusion was made that the interdigitated electrode pattern of the MFC and the quick pack IDE results in low-capacitance devices which limit the amount of power that can be harvested.In a later study,Sodano et al(2005a)once again compared the efficiencies of three piezoelectric materials.The materials used in this study included a traditional PZT,a quick pack (QP)actuator,and the macro-fiber composite(MFC).Each specimen was excited at resonance,subjected to a0–500Hz chirp,and lastly exposed to random vibrations recorded from an air compressor of a passenger vehicle.The random vibrations recorded exhibited frequencies between0and 500Hz.Both the power into the system and the power harvested by the piezoelectrics were measured in order to directly compute the efficiencies of each specimen.It was found that the efficiency of the PZT for each vibration scheme was fairly consistent(4.5%at resonance,3.0%for a chirp,and 6.8%for random vibrations)and was higher than the other two devices.It was noted that the experimental configuration along with other factors varied between experiments so the efficiencies reported do not represent those of the actuators themselves,but simply present a comparison between the three actuators tested.The QP had efficiencies of0.6%at resonance, 1.4%for a chirp,and3%under random vibrations.The MFC had efficiencies of1.75%at resonance,0.3%for a chirp,and 1.3%for random vibrations.Again,these results suggest that the QP actuator is more efficient than the MFC,however,it is also concluded that the PZT is the most efficient of all three materials.A summary of the various piezoelectric materials discussed above can be found in table1.Flexible piezoelectric materials are attractive for power harvesting applications because of their ability to withstand large amounts of rger strains provide more mechanical energy available for conversion into electrical energy.A second method of increasing the amount of energy harvested from a piezoelectric is to utilize a more efficient coupling mode.Two practical coupling modes exist;the −31mode and the−33mode.In the−31mode,a force is applied in the direction perpendicular to the poling direction, an example of which is a bending beam that is poled on its top and bottom surfaces.In the−33mode,a force isapplied in the same direction as the poling direction,such as the compression of a piezoelectric block that is poled on its top and bottom surfaces.An illustration of each mode is presented infigure3.Conventionally,the−31mode hasbeen the most commonly used coupling mode:however,the −31mode yields a lower coupling coefficient,k,than the −33mode.Baker et al(2005)have shown that,for three different types of piezoelectric materials,the−31mode hasa lower coupling coefficient,k,than the−33mode.Uponcomparing a piezoelectric stack operating in the−33mode toR3Topical ReviewTable 1.Summary of several piezoelectric materials investigated.Author Type of material Advantages/disadvantages Power harvesting capabilities Lee et al (2005)Monolithic PZTMost common type of device.Not flexible.Susceptible to fatigue crack growth during cyclic loading N/ALee et al (2004,2005)PVDF film coated with PEDOT/PSS electrodesResistance to fatigue crack damage to electrodes N/AMohammadi et al (2003)Piezofiber composite Increased flexibility 120mW from 34×11mm plate of 5.85mm thickness Churchill et al (2003)Piezofiber composite Increased flexibility 7.5mW from 130×13mm patch of 0.38mm thickness Sodano et al (2004a )MFC composite,quick pack IDE,quick pack MFC–flexibilityMFC and quick pack IDE—low-capacitance devices quick pack—energy harvesting capability quick pack proved to harvest the most energySodano et al (2005a )Monolithic PZT,quick pack,MFCMFC—flexibilityquick pack and monolithic PZT—energy harvesting capabilityPZT proved to be most efficient (6.8%for random vibrationexcitation)Figure 3.Illustration of −33mode and −31mode operation for piezoelectric materials.(Figure from Roundy et al 2003,©2003,with permission from Elsevier.)a cantilever beam operating in the 31mode of equal volumes,however,it was observed that,although the stack was more robust and had a higher coupling coefficient,the cantilever produced two orders of magnitude more power when subjected to the same force.This result is due to the high mechanical stiffness in the stack configuration which makes straining of the material difficult.It was concluded that in a small force,low vibration level environment,the −31configuration cantilever proved most efficient,but in a high force environment,such as a heavy manufacturing facility or in large operating machinery,a stack configuration would be more durable and generate useful energy.This result was also presented by Roundy et al (2003)who concluded that the resonant frequency of a system operating in the −31mode is much lower,making the system more likely to be driven at resonance in a natural environment,thus providing more power.Analytically,Yang et al (2005)have shown that,for a piezoelectric plate operating in the −33mode,the output power of the device is proportional to the coupling coefficient,k ,and the dielectric constant,ε.This confirms that devices with higher coupling coefficients will produce more power and behave more efficiently.Also,through their analytical calculations it was shown that,when the driving frequency is near a resonant frequency of the system,the output power is significantly increased.This is because when a system operates at resonance,much higher displacements and strains are observed than when operating slightly above or below resonance.Richards et al (2004)present a similar study in which a general approach to establishing the relationship between the coupling coefficient,quality factor,Q ,and the efficiency is presented.The quality factor,Q ,is inversely proportional to the damping in an oscillating system caused by energy loss via heat transfer.A system with a high Q value,therefore,does not lose much energy to heat,thus more energy is available for harvesting through a piezoelectric device.Richards et al found that generally,high efficiencies can be achieved with moderate coupling coefficients but large quality factors are necessary for the reasons described above.It should be noted,however,that higher coupling coefficients do lead to greater efficiencies.It can be concluded that the quality factor of systems deployed in field applications is an important design issue in order to optimize the power harvesting ability of the system.Cho et al (2005a )continued the work presented by Richards et al (2004)by analytically optimizing the coupling coefficient in a piezoelectric power harvesting system and then testing the optimization scheme experimentally.First,an analytical model was created for a rectangular thin-film PZT membrane consisting of two layers,a passive elastic material and a piezoelectric material with a variable sized electrode on either side.Their model predicted that the coupling coefficient increases with electrode size and reaches a maximum when the electrode covers 42%of the membrane area.It was also found that the coupling coefficient can be increased by increasingR4TopicalReviewFigure 4.(a)A series triple layer type piezoelectric sensor.(b)A parallel triple layer type piezoelectric sensor.(c)A unimorph piezoelectric sensor.the stiffness of the passive elastic layer and that an optimal piezoelectric layer thickness exists for each substrate layer stly,of all the process and design parameters,the residual stress was found to have the greatest effect on the coupling coefficient.Decreasing the residual stress in the device leads to significant gains in the coupling coefficient.Experimentally,Cho et al (2005b )show that an electrode coverage of about 60%proved to give the best coupling.Also,for a substrate thickness of 2μm,increasing the PZT thickness from 1to 3μm increases the coupling coefficient by a factor of about four.Finally,for a membrane with an initial residual stress of 80MPa,the coupling coefficient increased by 150%through reductions in the stress.Another method of changing the configuration of a system in order to improve its power harvesting capabilities is to add multiple pieces of piezoelectric material to the system.Many conventional systems consist of a single piezoceramic in bending mode,referred to as a unimorph.The design of such a unimorph cantilever beam is described by Johnson et al (2006).Another common configuration is a bimorph,which consists of two bonded piezoelectrics in bending.Sodano et al (2004c )developed a mathematical model to predict the energy generated from a piezoelectric bimorph cantilever beam.Upon experimentally validating the model,a maximum error of 4.61%was found.Ng and Liao (2004,2005)presented two types of bimorphs along with a unimorph piezoelectric harvester.The unimorph consisted of a single piezoelectric patch mounted to a metallic cantilever beam.The first bimorph,referred to as the series triple layer,consisted of two piezoelectrics with a metallic layer sandwiched between them.The piezoelectric patches were connected electrically in series.The second bimorph,called the parallel triple layer,was the same as the series triple layer except that the piezoelectrics were connected electrically in parallel.The configuration of each device can be seen in figure 4.Findings showed that under low load resistances and excitation frequencies the unimorph generated the highest power,under medium load resistances and frequencies the parallel triple layer had the highest power output,and under high load resistances and frequencies the series triple layer produced the greatest power.This result is due to the concept that maximum power transfer from the piezoelectric device occurs when the load resistance is matched to the impedance of the piezoelectric device.A series connection increases the device impedance,leading to more efficient operation at higher loads,as was found.In a similar study,Mateu and Moll (2005)analyzed a homogeneous bimorph which contained two pieces of piezoelectric material bonded to each other,a heterogeneousbimorph that contained two pieces of piezoelectric material bonded on either side of a non-piezoelectric material that simply provided elastic function,and a heterogeneous unimorph that consisted of a single piezoelectric patch bonded to a non-piezoelectric beam.It was determined that,for harvesters with the same piezoelectric material volume,the heterogeneous unimorph generated the most power because the piezoelectric material was furthest away from the neutral bending axis,thus causing higher strains in the active material and greater energy generation.Jiang et al (2005)also investigated methods of increasing the efficiency of a piezoelectric bimorph.Their study involved modeling a cantilever bimorph with a proof mass attached to its end and using the model to determine the relationship between performance and physical and geometrical parameters.Results showed that,by both reducing the thickness of the bimorph’s elastic layer and by increasing the proof mass attached to the end of the cantilever,the resonant frequency of the system was substantially decreased.The maximum power harvested was shown to be greater for lower resonant frequencies.Anderson and Sexton (2006)arrived at a similar conclusion when optimizing the physical and geometrical parameters of a similar bimorph.By varying the proof mass,length,and width,they discovered that changes to the proof mass had the largest effect on the power harvested by the system.The work of Gurav et al (2004)focused on optimizing the power output of micro-scale piezoelectric cantilever harvesters.Taking into consideration the high tolerances on shapes and the large variation in material properties involved with micro-scale machining operations,an uncertainty-based design optimization technique was utilized to determine the best possible design parameters for a micro-scale cantilever power harvester.In order to avoid impossible designs,limits were set on each of the geometrical parameters to be optimized.The resulting geometry obtained from the optimization routine was analytically compared to the geometry of a baseline cantilever and a 30%increase in power output over the baseline design was found for the optimized geometry.Another effective way to improve the energy output of a power harvesting device is to stack a large number of thin piezoceramic wafers together,called the stack configuration,with the electric field applied along the length of the stack.Platt et al (2005a )investigated power harvesting using approximately 145PZT wafers stacked mechanically in series,but electrically in parallel,to form a 1.0cm square stack with a height of 1.8cm.A solid monolithic cylinder of PZT with a diameter of 1.0cm and a height of 2.0cm was tested for comparison.The monolithic cylinder had a low capacitance of about 47pF and a very high open circuit voltage of around 10000V .The PZT stack,however,had an increased capacitance in the range of 1–10μF and a decreased open circuit voltage of around 30V .Through experimentation it was found that stacked and monolithic PZTs of the same geometry produce the same power if the load resistance is matched to the physical system,but that the matching load is in the k range for stacked configurations and in the G range for monolithic elements.It was concluded that both the voltage output and the matching resistive load are much more manageable in a PZT stack than in a monolithic configuration,thus making the stack a more useful option.Table 2presents a summary ofR5Topical ReviewTable2.Summary of various devices using multiple piezoelectric patches.Author Piezoelectric configuration Advantages/disadvantageNg and Liao(2004,2005)Unimorph,Series triplelayer bimorph,Parallel triple layerbimorph Unimorph—good under low excitations and loadsSeries triple layer—good under high excitations and loadsParallel triple layer—goodunder mediumexcitations and loadsMateu and Moll(2005)Homogeneous bimorph,heterogeneous bimorph,heterogeneous unimorph Heterogeneous unimorph generated most powerPlatt et al(2005a)145stacked PZT wafers,solid monolithic PZTcylinder Matching resistance is in k range for stacked and in the Grange for monolithicthe various devices discussed that use multiple piezoelectric patches.Similar to some of the stacked configurations using multiple pieces of piezoelectric material described above, Bayrashev et al(2004)have developed a method of piezoelectric power harvesting in which a piezoelectric patch is sandwiched between two magnetostrictive materials.When subjected to a magneticfield,the magnetostrictive material changes shape and causes the piezoelectric patch to strain and harvest energy.An experimental device containing a0.5mm thick,7mm diameter piezoelectric patch surrounded by two 1.5mm thick,7mm diameter Terfenol-D discs was created and tested.The device was subjected to a low frequency magnetic field and was found to generate high voltages up to285V,and power in the range of10–80μW,depending on how far away the device was located from the magneticfield source.The overall efficiency of the device was found to be3.1%.The most commonly used geometrical configuration in piezoelectric power harvesting is the rectangular cantilever beam.The cantilever beam harvester has been well developed and has proven to be easy to implement and effective for harvesting energy from ambient vibrations.Various other geometries,however,have been studied in order to improve upon the conventional cantilever design and to better suit other power harvesting applications.Mateu and Moll(2005) presented a brief analytical comparison between a rectangular cantilever and a triangular shaped cantilever with the large end clamped and the small end free.It was proven mathematically that a triangular cantilever with base and height dimensions equal to the base and length dimensions of a rectangular beam will have a higher strain and maximum deflection for a given load.Higher strains and deflections in piezoelectric materials translate to higher power outputs;therefore,a triangular cantilever beam will produce more power per unit area than a rectangular beam.Additionally,Roundy et al(2005)suggested that,with an increasingly trapezoidal shaped cantilever,the strain can be more evenly distributed throughout the structure as opposed to a rectangular beam that contains a non-uniform strain distribution.Also stated was that,for the same volume of PZT,a trapezoidal cantilever can generate more than twice the energy than a rectangular beam.Continuing the work of Roundy et al(2005),Baker et al(2005)experimentally tested a nearly triangular trapezoidal cantilever against a rectangular cantilever of the same volume and determined that the trapezoidal beam produced30%more power than the rectangular beam.It was concluded that,by using a trapezoidal configuration,a smaller and less expensive harvester could be used to satisfy a given power requirement.Rather than altering the profile of the conventional rectangular cantilever,Mossi et al(2005)changed the end constraints on the beam and created a so-called‘unimorph prestressed bender’.This is an initially curved,arc shaped, rectangular piezoelectric device that elongates when a force is applied to the top of the arc.The elongation causes strain in the active material which produces a voltage.The device is simply supported and allows for movement only in the lateral direction.Typically,these devices are used as actuators; however,research(Kymissis et al1998,Yoon et al2005)has shown that they are capable of producing useable energy as power harvesters.The effects of varying different physical parameters of the prestressed bender mentioned in this study are presented.The conductivity of the adhesive layer between the piezoelectric material and the passive metal layer,the thickness of the PZT layer,the thickness and type of the metal layer,and the width of the device were investigated.Varying the metal thickness and type had a significant effect on the amount of curvature of the beam,also known as dome height. Larger dome heights correspond to larger strains and energy generation when the harvester is compressed,thus altering the metal thickness and type can affect the power output of the bimorph.The most notable increase in power generation occurred,however,when the conductivity of the adhesive layer was increased by adding nickel particles.This resulted in a 15.2%increase in the energy produced.Danak et al(2003)also researched ways to optimize the design of an initially curved PZT unimorph power harvester.A mathematical model was created that predicts the power output of the device.From this model,relationships between generated charge and initial dome height,substrate thickness, PZT thickness,and substrate stiffness were established.It was found that increasing the dome height gave the greatest increase in charge output.Increasing the substrate and PZT thickness both gave higher charge outputs;however,increasing the substrate thickness had a greater effect than increasing the PZT stly,it was found that,by increasing the stiffness of the substrate,more charge could be generated.In a subsequent study,Yoon et al(2005)not only investigated the effects of altering the initial dome height,R6。
高三英语生物英语阅读理解30题
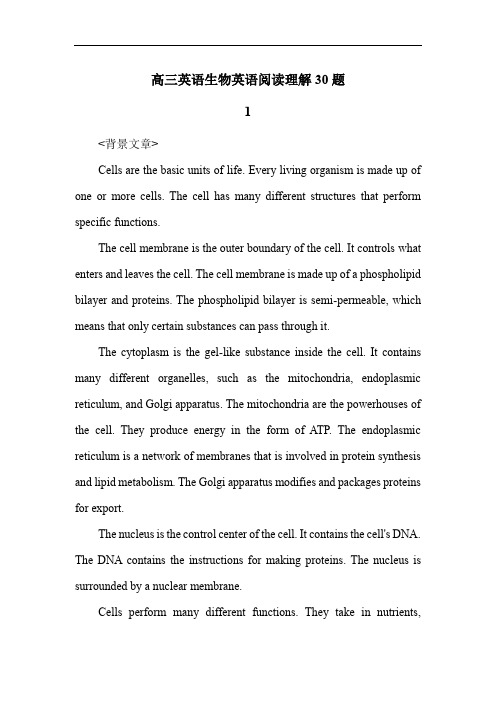
高三英语生物英语阅读理解30题1<背景文章>Cells are the basic units of life. Every living organism is made up of one or more cells. The cell has many different structures that perform specific functions.The cell membrane is the outer boundary of the cell. It controls what enters and leaves the cell. The cell membrane is made up of a phospholipid bilayer and proteins. The phospholipid bilayer is semi-permeable, which means that only certain substances can pass through it.The cytoplasm is the gel-like substance inside the cell. It contains many different organelles, such as the mitochondria, endoplasmic reticulum, and Golgi apparatus. The mitochondria are the powerhouses of the cell. They produce energy in the form of ATP. The endoplasmic reticulum is a network of membranes that is involved in protein synthesis and lipid metabolism. The Golgi apparatus modifies and packages proteins for export.The nucleus is the control center of the cell. It contains the cell's DNA. The DNA contains the instructions for making proteins. The nucleus is surrounded by a nuclear membrane.Cells perform many different functions. They take in nutrients,produce energy, and get rid of waste. They also grow, divide, and respond to their environment.1. What is the outer boundary of the cell?A. The cytoplasmB. The nucleusC. The cell membraneD. The mitochondria答案:C。
材料物理性能3
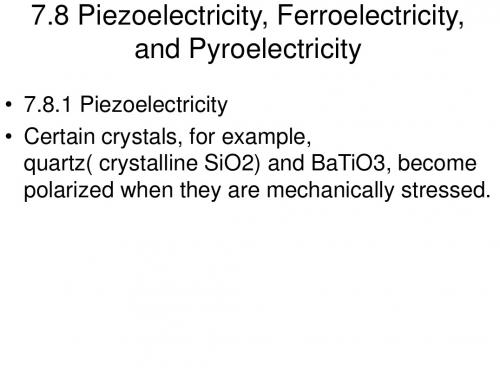
k 2 Mechanical energy converted to electrical energy Input of mechanical energy
k = electromechanical coupling factor
Converse Piezoelectric Effect
Sj = dij Ei
Sj = induced stain along the j direction, dij = piezoelectric coefficients, Ei = electric field along the i direction
If we graw a vector from O to any charge and draw the reverse vector, we will find the same type of charge.
A hexagonal unit cell has no center of symmetry.
below 130℃, the structure of barium titanate is tetragonal, in which the Ti4+ atom is not located at the center of mass of the negative charges. The crystal is therefore polarized by the separation of the centers of mass of the negative and positive charges. The crystal possesses a finite polarization vector P and is ferroelectric.
斐波拉切数列 能量 -回复
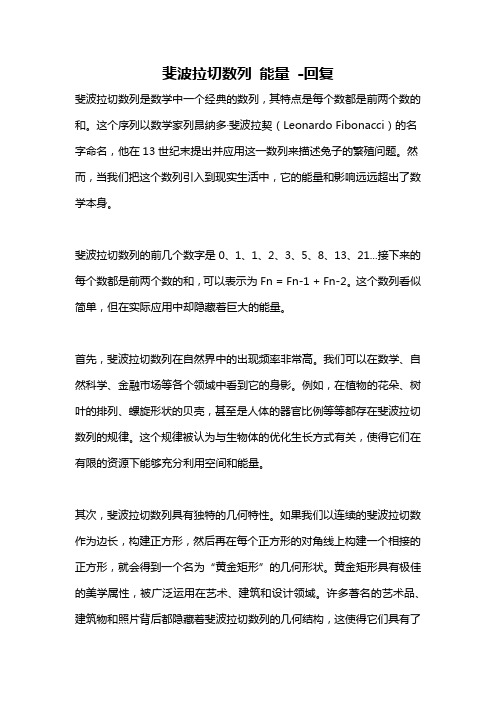
斐波拉切数列能量-回复斐波拉切数列是数学中一个经典的数列,其特点是每个数都是前两个数的和。
这个序列以数学家列昂纳多·斐波拉契(Leonardo Fibonacci)的名字命名,他在13世纪末提出并应用这一数列来描述兔子的繁殖问题。
然而,当我们把这个数列引入到现实生活中,它的能量和影响远远超出了数学本身。
斐波拉切数列的前几个数字是0、1、1、2、3、5、8、13、21...接下来的每个数都是前两个数的和,可以表示为Fn = Fn-1 + Fn-2。
这个数列看似简单,但在实际应用中却隐藏着巨大的能量。
首先,斐波拉切数列在自然界中的出现频率非常高。
我们可以在数学、自然科学、金融市场等各个领域中看到它的身影。
例如,在植物的花朵、树叶的排列、螺旋形状的贝壳,甚至是人体的器官比例等等都存在斐波拉切数列的规律。
这个规律被认为与生物体的优化生长方式有关,使得它们在有限的资源下能够充分利用空间和能量。
其次,斐波拉切数列具有独特的几何特性。
如果我们以连续的斐波拉切数作为边长,构建正方形,然后再在每个正方形的对角线上构建一个相接的正方形,就会得到一个名为“黄金矩形”的几何形状。
黄金矩形具有极佳的美学属性,被广泛运用在艺术、建筑和设计领域。
许多著名的艺术品、建筑物和照片背后都隐藏着斐波拉切数列的几何结构,这使得它们具有了独特的美感和吸引力。
此外,斐波拉切数列在金融市场中也扮演着重要的角色。
通过斐波拉切数列,分析师可以预测股票、商品、外汇和加密货币等市场的价格变动趋势。
这是因为斐波拉切数列在市场波动中展现出明显的“支撑位”和“阻力位”,即价格反弹或回落的位置。
许多投资者和交易者运用这个原理来进行交易决策,以获取更高的收益和降低风险。
此外,斐波拉切数列还在心理学中发挥作用。
研究表明,人们对斐波拉切数列的构图、比例和对称性有着强烈的好感和认同感。
这使得它成为广告、品牌设计和网页界面等领域中常用的设计元素。
斐波拉切数列的应用可以调动人们的情绪和共鸣,增强与群众的互动和连接。
Martin J. Osborne_An introduction to game theory_5_References
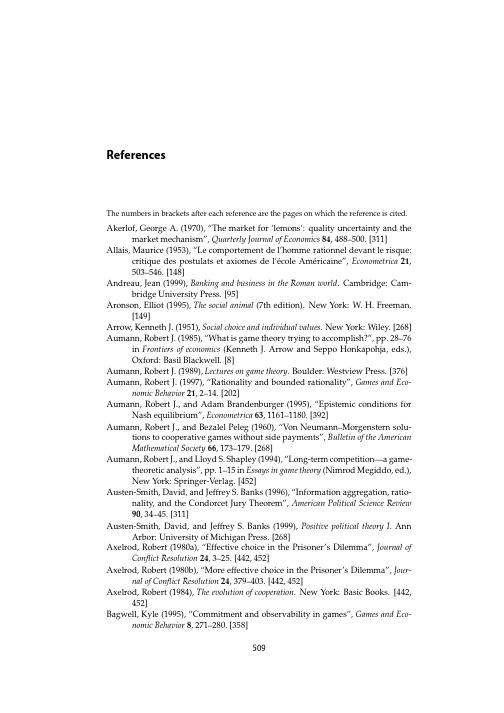
ReferencesThe numbers in brackets after each reference are the pages on which the reference is cited. Akerlof,George A.(1970),“The market for‘lemons’:quality uncertainty and the market mechanism”,Quarterly Journal of Economics84,488–500.[311] Allais,Maurice(1953),“Le comportement de l’homme rationnel devant le risque: critique des postulats et axiomes de l’école Américaine”,Econometrica21, 503–546.[148]Andreau,Jean(1999),Banking and business in the Roman world.Cambridge:Cam-bridge University Press.[95]Aronson,Elliot(1995),The social animal(7th edition).New York:W.H.Freeman.[149]Arrow,Kenneth J.(1951),Social choice and individual values.New York:Wiley.[268] Aumann,Robert J.(1985),“What is game theory trying to accomplish?”,pp.28–76 in Frontiers of economics(Kenneth J.Arrow and Seppo Honkapohja,eds.), Oxford:Basil Blackwell.[8]Aumann,Robert J.(1989),Lectures on game theory.Boulder:Westview Press.[376] Aumann,Robert J.(1997),“Rationality and bounded rationality”,Games and Eco-nomic Behavior21,2–14.[202]Aumann,Robert J.,and Adam Brandenburger(1995),“Epistemic conditions for Nash equilibrium”,Econometrica63,1161–1180.[392]Aumann,Robert J.,and Bezalel Peleg(1960),“Von Neumann–Morgenstern solu-tions to cooperative games without side payments”,Bulletin of the American Mathematical Society66,173–179.[268]Aumann,Robert J.,and Lloyd S.Shapley(1994),“Long-term competition—a game-theoretic analysis”,pp.1–15in Essays in game theory(Nimrod Megiddo,ed.), New York:Springer-Verlag.[452]Austen-Smith,David,and Jeffrey S.Banks(1996),“Information aggregation,ratio-nality,and the Condorcet Jury Theorem”,American Political Science Review 90,34–45.[311]Austen-Smith,David,and Jeffrey S.Banks(1999),Positive political theory I.Ann Arbor:University of Michigan Press.[268]Axelrod,Robert(1980a),“Effective choice in the Prisoner’s Dilemma”,Journal of Conflict Resolution24,3–25.[442,452]Axelrod,Robert(1980b),“More effective choice in the Prisoner’s Dilemma”,Jour-nal of Conflict Resolution24,379–403.[442,452]Axelrod,Robert(1984),The evolution of cooperation.New York:Basic Books.[442, 452]Bagwell,Kyle(1995),“Commitment and observability in games”,Games and Eco-nomic Behavior8,271–280.[358]509510References Banks,Jeffrey S.(1991),Signaling games in political science.Chur:Harwood Aca-demic Publishers.[358]Banks,Jeffrey S.(1995),“Singularity theory and core existence in the spatial model”, Journal of Mathematical Economics24,523–536.[268]Baye,Michael R.,Dan Kovenock,and Casper G.de Vries(1996),“The all-pay auction with complete information”,Economic Theory8,291–305.[149] Baye,Michael R.,and John Morgan(1999),“A folk theorem for one-shot Bertrand games”,Economic Letters65,59–65.[149]Becker,Gary S.(1974),“A theory of social interactions”,Journal of Political Economy 82,1063–1093.[178]Bellman,Richard(1957),Dynamic programming.Princeton:Princeton University Press.[177]Bellman,Richard,and David Blackwell(1949),“Some two-person games involving bluffing”,Proceedings of the National Academy of Sciences of the United States ofAmerica35,600–605.[358]Benoît,Jean-Pierre(1984),“Financially constrained entry in a game of incomplete information”,Rand Journal of Economics15,490–499.[178,235]Benoît,Jean-Pierre,and Lewis A.Kornhauser(2002),“Game theoretic analysis of legal rules and institutions”,pp.???–???in Handbook of Game Theory withEconomic Applications(Robert J.Aumann and Sergiu Hart,eds.),Amster-dam:North-Holland.[95]NOT DEFINITIVEBenoît,Jean-Pierre,and Vijay Krishna(1985),“Finitely repeated games”,Economet-rica53,905–922.[466]Benoît,Jean-Pierre,and Vijay Krishna(1987),“Nash equilibria offinitely repeated games”,International Journal of Game Theory16,197–204.[466] Bergstrom,Carl T.,and Michael Lachmann(1997),“Signalling among relatives.I.Is costly signalling too costly?”,Philosophical Transactions of the Royal Societyof London,Series B(Biological Sciences)352,609–617.[358]Bergstrom,Theodore C.(1989),“A fresh look at the rotten kid theorem—and other household mysteries”,Journal of Political Economy97,1138–1159.[178] Bergstrom,Theodore C.(1995),“On the evolution of altruistic ethical rules for siblings”,American Economic Review85,58–81.[414,416,420] Bergstrom,Theodore C.,Lawrence E.Blume,and Hal R.Varian(1986),“On the private provision of public goods”,Journal of Public Economics29,25–49.[52]Bergstrom,Theodore C.,and Hal R.Varian(1987),Workouts in intermediate microe-conomics,3rd edition.New York:Norton.[420]Bernheim,B.Douglas(1984),“Rationalizable strategic behavior”,Econometrica52, 1007–1028.[392]Bernoulli,Daniel(1738),“Specimen theoriae novae de mensura sortis”,translated by Louise Sommer as“Exposition of a new theory on the measurement ofrisk”,Econometrica22(1954),23-36.[148]Bertrand,Joseph(1883),“Review of‘Théorie mathématique de la richesse sociale’by Léon Walras and‘Recherches sur les principes mathématiques de la théorieReferences511 des richesses’by Augustin Cournot”,Journal des Savants,September,499-508.(Translated by Margaret Chevaillier as“Review by Joseph Bertrand oftwo books”,History of Political Economy24(1992),646–653.)[61,68,94] Binmore,Kenneth G.(1985),“Bargaining and coalitions”,pp.269–304in Game-theoretic models of bargaining(Alvin E.Roth,ed.),Cambridge:CambridgeUniversity Press.[493]Binmore,Kenneth G.(1987),“Nash bargaining theory II”,pp.61–76in The eco-nomics of bargaining(Kenneth G.Binmore and Partha Dasgupta,eds.),Ox-ford:Blackwell.[493]Binmore,Kenneth G.(1992),Fun and games.Lexington,Massachusetts:D.C.Heath.[358]Binmore,Kenneth G.,Ariel Rubinstein,and Asher Wolinsky(1986),“The Nash bargaining solution in economic modelling”,Rand Journal of Economics17,176–188.[493]Black,Duncan(1958),The theory of committees and elections.Cambridge:Cambridge University Press.[268]Black,Duncan,and R.A.Newing(1951),Committee decisions with complementary valuation.London:William Hodge.[268]Blackwell,David,and M.A.Girshick(1954),Theory of games and statistical decisions.New York:Wiley.[95]Bolton,Gary E,and Axel Ockenfels(2000),“ERC:a theory of equity,reciprocity, and competition”,American Economic Review90,166–193.[202,235]Bolton,Gary E,and Rami Zwick(1995),“Anonymity versus punishment in ulti-matum bargaining”,Games and Economic Behavior10,95–121.[182]Borel,Émile(1921),“La théorie du jeu et les equations intégralesànoyau symétrique”, Comptes Rendus Hebdomadaires des Séances de l’Académie des Sciences(Paris)173,1304–1308.(Translated by Leonard J.Savage as“The theory of play andintegral equations with skew symmetric kernels”,Econometrica21(1953),97–100.)[51,148]Borel,Émile(1924),Eléments de la théorie des probabilités(third edition).Paris:Li-brairie Scientifique,J.Hermann.(Pp.204–221translated by Leonard J.Sav-age as“On games that involve chance and the skill of the players”,Econo-metrica21(1953),101–115.)[148,149]Borel,Émile(1927),“Sur les systèmes de formes linéairesàdéterminant symétrique gauche et la théorie générale du jeu”,Comptes Rendus Hebdomadaires desSéances de l’Académie des Sciences(Paris)184,52–54.(Translated by LeonardJ.Savage as“On systems of linear forms of skew symmetric determinantand the general theory of play”,Econometrica21(1953),116–117.)[148]Borel,Émile(1938),Traitédu calcul des probabilités et de ses applications,Tome.IV, Fascicule II(Applications aux jeux de hasard)(Lectures byÉmile Borel,tran-scribed by Jean Ville).Paris:Gauthier-Villars.[358]Boylan,Richard T.(2000),“An optimal auction perspective on lobbying”,Social Choice and Welfare17,55–68.[95]Braess,Dietrich(1968),“Über ein Paradoxon der Verkehrsplanung”,Unternehmensforschung512References 12,258–268.[52]Brams,Steven J.,and Peter C.Fishburn(1978),“Approval voting”,American Polit-ical Science Review72,831–847.[52]Brams,Steven J.,and Peter C.Fishburn(1983),Approval voting.Boston:Birkhäuser.[52]Brams,Steven J.,D.Marc Kilgour,and Morton D.Davis(1993),“Unraveling in games of sharing and exchange”,pp.195–212in Frontiers of Game Theory(Kenneth G.Binmore,Alan Kirman,and Piero Tani,eds.),Cambridge,Mass.:MIT Press.[52,311]Brams,Steven J.,and Philip D.Straffin,Jr.(1979),“Prisoners’dilemma and pro-fessional sports drafts”,American Mathematical Monthly86,80–88.[172,178]Brams,Steven J.,and Alan D.Taylor(1996),Fair division.Cambridge University Press:Cambridge.[202]Brockmann,H.Jane,Alan Grafen,and Richard Dawkins(1979),“Evolutionarily stable nesting strategy in a digger wasp”,Journal of Theoretical Biology77,473–496.[403,419,420]Brown,George W.(1951),“Iterative solution of games byfictitious play”,pp.374–376in Activity analysis of production and allocation(Tjalling C.Koopmans,ed.),New York:Wiley.[149]Brown,James N.,and Robert W.Rosenthal(1990),“Testing the minimax hypothe-sis:a re-examination of O’Neill’s game experiment”,Econometrica58,1065–1081.[374,375]Brown,John P.(1973),“Toward an economic theory of liability”,Journal of Legal Studies2,323–349.[95]Brown,Roger(1986),Social psychology,the second edition.New York:Free Press.[149]Bryant,John(1983),“A simple rational expectations Keynes-type model”,Quar-terly Journal of Economics98,525–528.[28]Bryant,John(1994),“Coordination theory,the stag hunt and macroeconomics”, pp.207–225in Problems of coordination in economic activity(James W.Fried-man,ed.),Boston:Kluwer.[28]Bulmer,Michael G.(1994),Theoretical evolutionary ecology.Sunderland,Massachusetts: Sinauer Associates.[420]Burgess,J.Wesley(1976),“Social spiders”,Scientific American234(3),100–106.[412]Camerer,Colin(1995),“Individual decision making”,pp.587–703in The handbook of experimental economics(John H.Kagel and Alvin E.Roth,eds.),Princeton:Princeton University Press.[102]Capen,E.C.,R.V.Clapp,and W.M.Campbell(1971),“Competitive bidding in high-risk situations”,Journal of Petroleum Technology23,641–653.[311] Carcopino,Jérôme(1940),Daily life in ancient Rome(edited with bibliography and notes by Henry T.Rowell,translated by E.O.Lorimer).New Haven:YaleUniversity Press.[372]References513 Cassady,Ralph,Jr.(1967),Auctions and auctioneering.Berkeley:University of California Press.[79,95]Cho,In-Koo,and David M.Kreps(1987),“Signaling games and stable equilibria”, Quarterly Journal of Economics102,179–221.[358]Conlisk,John(1989),“Three variants on the Allais example”,American Economic Review79,392–407.[102,103,148]Cooper,Russell,Douglas V.DeJong,Robert Forsythe,and Thomas W.Ross(1996),“Cooperation without reputation:experimental evidence from Prisoner’s Dilemma games”,Games and Economic Behavior12,187–218.[26] Cournot,Antoine A.(1838),Recherches sur les principes mathématiques de la théorie des richesses.Paris:Hachette.(Translated by Nathaniel T.Bacon as“Re-searches into the mathematical principles of the theory of wealth”,New York:Macmillan,1897.)[51,53,94,149]Court,Gordon S.(1996),“The seal’s own skin game”,Natural History105.8,36–41.[112]Cramton,Peter C.(1995),“Money out of thin air:the nationwide narrowband PCS auction”,Journal of Economics and Management Strategy4,267–343.[311] Cramton,Peter C.(1997),“The FCC spectrum auctions:an early assessment”, Journal of Economics and Management Strategy6,431–495.[311] Cramton,Peter C.(1998),“The efficiency of the FCC spectrum auctions”,Journal of Law and Economics41,727–736.[311]Crawford,Vincent P.,and Joel Sobel(1982),“Strategic information transmission”, Econometrica50,1431–1451.[358]Darwin,Charles(1871),The descent of man,and selection in relation to sex.London: John Murray.[401]Darwin,Charles(1874),The descent of man,and selection in relation to sex(Second edition,revised and augmented).London:John Murray.[420] Denzau,Arthur T.,and Robert J.Mackay(1983),“Gatekeeping and monopoly power of committees:an analysis of sincere and sophisticated behavior”, American Journal of Political Science27,740–761.[201]Deutsch,Morton(1958),“Trust and suspicion”,Journal of Conflict Resolution2,265–279.[26]Dhillon,Amrita,and Ben Lockwood(1999),“When are plurality rule voting games dominance-solvable?”,unpublished paper.[393]NOT DEFINITIVE Diamond,Peter A.(1974),“Accident law and resource allocation”,Bell Journal of Economics and Management Science5,366–405.[95]Diermeier,Daniel,and Timothy J.Feddersen(1998),“Cohesion in legislatures and the vote of confidence procedure”,American Political Science Review92,611–621.[235]Dixit,Avinash,and Barry Nalebuff(1991),Thinking strategically.New York:Nor-ton.[178]Downs,Anthony(1957),An economic theory of democracy.New York:Harper and Row.[95]Dubey,Pradeep,and Mamoru Kaneko(1984),“Information patterns and Nash514References equilibria in extensive games:I”,Mathematical Social Sciences8,111–139.[234]Dubins,Lester E.,and David A.Freedman(1981),“Machiavelli and the Gale-Shapley algorithm”,American Mathematical Monthly88,485–494.[269] Dugatkin,Lee Alan(1991),“Dynamics of the tit for tat strategy during predator inspection in the guppy(Poecilia reticulata)”,Behavioral Ecology and Sociobi-ology29,127–132.[448]Edgeworth,Francis Y.(1881),Mathematical psychics.London:Kegan Paul.[268] Elster,Jon(1998),“Emotions and economic theory”,Journal of Economic Literature 36,47–74.[8]Ewerhart,Christian(2000),“Chess-like games are dominance solvable in at most two steps”,Games and Economic Behavior33,41–47.[393]Farquharson,Robin(1969),Theory of voting.New Haven:Yale University Press.[52,235]Feddersen,Timothy J.,and Wolfgang Pesendorfer(1996),“The swing voter’s curse”, American Economic Review86,408–424.[311]Feddersen,Timothy J.,and Wolfgang Pesendorfer(1998),“Convicting the inno-cent:the inferiority of unanimous jury verdicts under strategic voting”, American Political Science Review92,23–35.[307,311]Feddersen,Timothy J.,Itai Sened,and Stephen G.Wright(1990),“Rational vot-ing and candidate entry under plurality rule”,American Journal of Political Science34,1005–1016.[235]Fellner,William(1949),Competition among the few.New York:Alfred A.Knopf.[68] Fisher,Ronald A.(1930),The genetical theory of natural selection.Oxford:Clarendon Press.[420]Flood,Merrill M.(1958/59),“Some experimental games”,Management Science5, 5–26.[51,438,452]Forsythe,Robert,Joel L.Horowitz,N.E.Savin,and Martin Sefton(1994),“Fairness in simple bargaining experiments”,Games and Economic Behavior6,347–369.[182]Frank,Robert H.,Thomas Gilovich,and Dennis T.Regan(1993),“Does studying economics inhibit cooperation?”,Journal of Economic Perspectives7(2),159–171.[26]Friedman,James W.(1971),“A non-cooperative equilibrium for supergames”,Re-view of Economic Studies38,1–12.[452]Fudenberg,Drew,and David K.Levine(1998),The theory of learning in games.Cambridge,Massachusetts:MIT Press.[149]Fudenberg,Drew,and Eric S.Maskin(1986),“The folk theorem in repeated games with discounting or with incomplete information”,Econometrica54,533–554.[452,466]Fudenberg,Drew,and Eric S.Maskin(1991),“On the dispensability of public ran-domization in discounted repeated games”,Journal of Economic Theory53, 428–438.[452,466]References515 Fudenberg,Drew,and Jean Tirole(1991),“Perfect Bayesian equilibrium and se-quential equilibrium”,Journal of Economic Theory53,236–260.[358] Gabay,Daniel,and HervéMoulin(1980),“On the uniqueness and stability of Nash-equilibria in noncooperative games”,pp.271–293in Applied stochasticcontrol in econometrics and management science(Alain Bensoussan,Paul Klein-dorfer,and Charles S.Tapiero,eds.),Amsterdam:North-Holland.[393] Gale,David(1953),“A theory of N-person games with perfect information”,Pro-ceedings of the National Academy of Sciences of the United States of America39,496–501.[392]Gale,David,and Lloyd S.Shapley(1962),“College admissions and the stability of marriage”,American Mathematical Monthly69,9–15.[269]Gardner,Martin(1959),The Scientific American book of mathematical puzzles and di-versions.New York:Simon and Schuster.[178]Ghemawat,Pankaj,and Barry Nalebuff(1985),“Exit”,Rand Journal of Economics16, 184–194.[235]Gillies,Donald B.(1959),“Solutions to general non-zero-sum games”,pp.47–85in Contributions to the theory of games,Volume IV(Annals of Mathematics Stud-ies,40)(A.W.Tucker and R.D.Luce,eds.),Princeton:Princeton UniversityPress.[268]Gilligan,Thomas W.,and Keith Krehbiel(1987),“Collective decisionmaking and standing committees:an informational rationale for restrictive amendmentprocedures”,Journal of Law,Economics,and Organization3,287–335.[358] Godfray,H.Charles J.,and Rufus A.Johnstone(2000),“Begging and bleating: the evolution of parent-offspring signalling”,Philosophical Transactions of theRoyal Society of London,Series B(Biological Sciences)355,1581–1591.[358] Gordon,H.Scott(1954),“The economic theory of a common-property resource: thefishery”,Journal of Political Economy62,124–142.[52]Green,Edward J.,and Robert H.Porter(1984),“Noncooperative collusion under imperfect price information”,Econometrica52,87–100.[466]Greville,Fulke(1986),The prose works of Fulke Greville,Lord Brooke(John Gouws, ed.).Oxford:Clarendon Press.[358]Groseclose,Tim,and James M.Snyder,Jr.(1996),“Buying supermajorities”,Amer-ican Political Science Review90,303–315.[201]Grout,Paul A.(1984),“Investment and wages in the absence of binding contracts:a Nash bargaining approach”,Econometrica52,449–460.[201]Guilbaud,Georges T.(1961),“Faut-il jouer au plusfin?(notes sur l’histoire de la théorie des jeux)”,pp.171–182in La décision,Paris:Éditions du CentreNational de la Recherce Scientifique.[148,375]Güth,Werner,and Steffen Huck(1997),“From ultimatum bargaining to dictatorship—an experimental study of four games varying in veto power”,Metroeconom-ica48,262–279.[182]Güth,Werner,Rolf Schmittberger,and Bernd Schwarze(1982),“An experimental analysis of ultimatum bargaining”,Journal of Economic Behavior and Organi-zation3,367–388.[182,201]516References Hald,Anders(1990),A history of probability and statistics and their applications before 1750.New York:Wiley.[371]Halmos,Paul R.(1973),“The legend of John von Neumann”,American Mathemati-cal Monthly80,382–394.[4,8]Hamilton,W.D.(1967),“Extraordinary sex ratios”,Science156,477–488.[401] Hammerstein,Peter,and Susan E.Riechert(1988),“Payoffs and strategies in ter-ritorial contests:ESS analyses of two ecotypes of the spider Agelenopsisaperta”,Evolutionary Ecology2,115–138.[413,420]Hammerstein,Peter,and Reinhard Selten(1994),“Game theory and evolution-ary biology”,pp.929–993in Handbook of Game Theory,Volume2(Robert J.Aumann and Sergiu Hart,eds.),Amsterdam:Elsevier.[405,420]Hardin,Garrett(1968),“The tragedy of the commons”,Science162,1243–1248.[52] Harris,Christopher,and John Vickers(1985),“Perfect equilibrium in a model of a race”,Review of Economic Studies52,193–209.[201]Harsanyi,John C.(1967/68),“Games with incomplete information played by‘Bayesian’players,Parts I,II,and III”,Management Science14,159–182,320–334,and486–502.[311]Herodotus(1998),The histories(translated by Robin Waterfield).Oxford:Oxford University Press.[95]Heuer,Gerald A.(1995),“Solution to problem1069”,Journal of Recreational Math-ematics27,146–158.[Author’s middle initial incorrectly given as“N”injournal.][149]Hey,John D.(1997),“Experiments and the economics of individual decision mak-ing under risk and uncertainty”,pp.173–205in Advances in economics andeconometrics:theory and applications(Seventh world congress),Volume I(DavidM.Kreps and Kenneth F.Wallis,eds.),Cambridge:Cambridge UniversityPress.[148]Hirshleifer,Jack,and Juan Carlos Martinez Coll(1988),“What strategies can sup-port the evolutionary emergence of cooperation?”,Journal of Conflict Resolu-tion32,367–398.[442,452]Hoffman,Elizabeth,Kevin A.McCabe,and Vernon L.Smith(1996),“On expecta-tions and the monetary stakes in ultimatum games”,International Journal ofGame Theory25,289–301.[182]Holt,Charles A.,Jr.,and Roger Sherman(1982),“Waiting-line auctions”,Journal of Economic Perspectives90,280–294.[95]Hotelling,Harold(1929),“Stability in competition”,Economic Journal39,41–57.[71,95]Jervis,Robert(1977/78),“Cooperation under the security dilemma”,World Politics 30,167–214.[52]Kalai,Ehud,Dov Samet,and William Stanford(1988),“A note on reactive equilib-ria in the discounted Prisoner’s Dilemma and associated games”,Interna-tional Journal of Game Theory17,177–186.[452]Karlin,Samuel(1959a),Mathematical methods and theory in games,programming,and economics,Volume I.Reading,Massachusetts:Addison-Wesley.[149]References517 Karlin,Samuel(1959b),Mathematical methods and theory in games,programming,and economics,Volume II.Reading,Massachusetts:Addison-Wesley.[95,358] Kim,Yong-Gwan(1994),“Evolutionarily stable strategies in the repeated pris-oner’s dilemma”,Mathematical Social Sciences28,167–197.[443,452] Kinnaird,Clark(1946),Encyclopedia of puzzles and pastimes.New York:Citadel Press.[235]Klemperer,Paul(1999),“Auction theory:a guide to the literature”,Journal of Eco-nomic Surveys13,227–286.[311]Kohlberg,Elon,and Jean-François Mertens(1986),“On the strategic stability of equilibria”,Econometrica54,1003–1037.[358]Kohler,David A.,and R.Chandrasekaran(1971),“A class of sequential games”, Operations Research19,270–277.[178]Kreps,David M.,and Robert B.Wilson(1982),“Sequential equilibria”,Econometrica 50,863–894.[358]Krishna,Vijay,and John Morgan(2001),“Asymmetric information and legisla-tive rules:some amendments”,American Political Science Review95,435–452.[358]Kuhn,Harold W.(1950a),“A simplified two-person poker”,pp.97–103in Con-tributions to the theory of games,Volume I(Annals of Mathematics Studies,24)(Harold W.Kuhn and Albert W.Tucker,eds.),Princeton,New Jersey:Princeton University Press.[358]Kuhn,Harold W.(1950b),“Extensive games”,Proceedings of the National Academy of Sciences of the United States of America36,570–576.[177,358]Kuhn,Harold W.(1953),“Extensive games and the problem of information”,pp.193–216in Contributions to the theory of games,Volume II(Annals of MathematicsStudies,28)(Harold W.Kuhn and A.W Tucker,eds.),Princeton:PrincetonUniversity Press.[177,358]Kuhn,Harold W.(1968),“Preface”and translation of excerpt from a letter from Pierre Rémond de Monmort to Nicholas Bernoulli”,pp.3–9in Precursors inmathematical economics:an anthology(Series of Reprints of Scarce Works onPolitical Economy,19)(William J.Baumol and Stephen M.Goldfeld,eds.),London:London School of Economics and Political Science.[148,371,375] Kuhn,Harold W.(1996),“Introduction”,Duke Mathematical Journal81,i–v.[21,52] Kuhn,Harold W.,John C.Harsanyi,Reinhard Selten,Jörgen W.Weibull,Eric van Damme,John F.Nash,Jr.,and Peter Hammerstein(1995),“The work ofJohn Nash in game theory”,pp.280–310in Les prix Nobel1994,Stockholm:Almqvist and Wiksell.(Reprinted in Journal of Economic Theory69(1996),153–185.)[21,52]Kuhn,Harold W.,and Sylvia Nasar(eds.)(2001),The essential John Nash.Princeton: Princeton University Press.[52]NOT DEFINITIVEKummer,H.,W.Götz,and W.Angst(1974),“Triadic differentiation:an inhibitory process protecting pair bonds in baboons”,Behaviour49,62–87.[413,420] Lagunoff,Roger,and Akihiko Matsui(1997),“Asynchronous choice in repeated coordination games”,Econometrica65,1467–1477.[466]518References Langdon,Merle(1994),“Public auctions in ancient Athens”,pp.253–265in Rit-ual,finance,politics(Robin Osborne and Simon Hornblower,eds.),Oxford: Clarendon Press.[79,95]Latané,Bibb,and Steve Nida(1981),“Ten years of research on group size and helping”,Psychological Bulletin89,308–324.[149]Ledyard,John O.(1981),“The paradox of voting and candidate competition:a gen-eral equilibrium analysis”,pp.54–80in Essays in contemporaryfields of eco-nomics(George Horwich and James P.Quirk,eds.),West Lafayette,Indiana: Purdue University Press.[235]Ledyard,John O.(1984),“The pure theory of large two-candidate elections”,Public Choice44,7–41.[235]Leininger,Wolfgang(1989),“Escalation and cooperation in conflict situations:the dollar auction revisited”,Journal of Conflict Resolution33,231–254.[178] Leonard,Janet L.(1990),“The hermaphrodite’s dilemma”,Journal of Theoretical Biology147,361–371.[52]Leonard,Robert J.(1994),“Reading Cournot,reading Nash:the creation and sta-bilisation of the Nash equilibrium”,Economic Journal104,492–511.[52,68, 95]Leonard,Robert J.(1995),“From parlor games to social science:von Neumann, Morgenstern,and the creation of game theory1928–1944”,Journal of Eco-nomic Literature33,730–761.[8]Lewis,David K.(1969),Convention.Cambridge:Harvard University Press.[52] Linster,Bruce G.(1992),“Evolutionary stability in the infinitely repeated Prisoners’Dilemma played by two-state Moore machines”,Southern Economic Journal 58,880–903.[442,452]Linster,Bruce G.(1994),“Stochastic evolutionary dynamics in the repeated Prison-ers’Dilemma”,Economic Inquiry32,342–357.[442,452]Lipman,Barton L.,and Ruqu Wang(2000),“Switching costs in frequently repeated games”,Journal of Economic Theory93,149–190.[452]Luce,R.Duncan,and Howard Raiffa(1957),Games and decisions.New York:John Wiley and Sons.[52,268,392,393,452]Machina,Mark J.(1987),“Choice under uncertainty:problems solved and un-solved”,Journal of Economic Perspectives1(1),121–154.[148]MacKie-Mason,Jeffrey K.,and Hal R.Varian(1995),“Pricing the internet”,pp.269–314in Public access to the internet(Brian Kahin and James Keller,eds.),Cam-bridge,Massachusetts:MIT Press.[95]Magnan de Bornier,Jean(1992),“The‘Cournot-Bertrand debate’:a historical per-spective”,History of Political Economy24,623–656.[95]Marx,Karl(1973),Economic and philosophic manuscripts of1844(edited with an intro-duction by Dirk J.Struik,translated by Martin Milligan).London:Lawrence and Wishart.[474]Maynard Smith,John(1972a),“Game theory and the evolution offighting”,pp.8–28in On evolution(John Maynard Smith),Edinburgh:Edinburgh University Press.[401,420]References519 Maynard Smith,John(1972b),On evolution.Edinburgh:Edinburgh University Press.[401]Maynard Smith,John(1974),“The theory of games and the evolution of animal conflicts”,Journal of Theoretical Biology47,209–221.[95,420]Maynard Smith,John(1982),Evolution and the theory of games.Cambridge:Cam-bridge University Press.[403,412,420]Maynard Smith,John(1991),“Honest signalling:the Philip Sydney game”,Animal Behaviour42,1034–1035.[358]Maynard Smith,John,and G.A.Parker(1976),“The logic of asymmetric contests”, Animal Behaviour24,159–175.[420]Maynard Smith,John,and G.R.Price(1973),“The logic of animal conflict”,Nature 246,15–18.[52,401,420]McAfee,R.Preston,and John McMillan(1996),“Analyzing the airwaves auction”, Journal of Economic Perspectives10(1),159–175.[311]McKelvey,Richard D.,and Richard G.Niemi(1978),“A multistage game represen-tation of sophisticated voting and binary procedures”,Journal of Economic Theory18,1–22.[235]McMillan,John(1994),“Selling spectrum rights”,Journal of Economic Perspectives8(3),145–162.[300,311]Milgrom,Paul R.(1981),“Rational expectations,information acquisition,and com-petitive bidding”,Econometrica49,921–943.[311]Milgrom,Paul R.,Douglass C.North,and Barry R.Weingast(1990),“The role of institutions in the revival of trade:the law merchant,private judges,and the Champagne fairs”,Economics and Politics2,1–23.[451,452]Milgrom,Paul R.,and D.John Roberts(1986),“Price and advertising signals of product quality”,Journal of Political Economy94,796–821.[358]Milinski,Manfred(1987),“Tit for tat in sticklebacks and the evolution of coopera-tion”,Nature325,433–435.[448,452]Milinski,Manfred(1993),“Cooperation wins and stays”,Nature364,12–13.[448, 452]Miller,Nicholas R.(1977),“Graph-theoretical approaches to the theory of voting”, American Journal of Political Science21,769–803.[235]Miller,Nicholas R.(1995),Committees,agendas,and voting.Chur,Switzerland: Harwood Academic Publishers.[235]Milnor,John W.(1995),“A Nobel prize for John Nash”,Mathematical Intelligencer 17(3),11–17.[21]Morris,Stephen,Rafael Rob,and Hyun Song Shin(1995),“p-dominance and belief potential”,Econometrica63,145–157.[311]Moulin,Hervé(1979),“Dominance solvable voting schemes”,Econometrica47,1337–1351.[392]Moulin,Hervé(1981),“Prudence versus sophistication in voting strategy”,Journal of Economic Theory24,398–412.[177]Moulin,Hervé(1984),“Dominance solvability and Cournot stability”,Mathemati-cal Social Sciences7,83–102.[393]。
- 1、下载文档前请自行甄别文档内容的完整性,平台不提供额外的编辑、内容补充、找答案等附加服务。
- 2、"仅部分预览"的文档,不可在线预览部分如存在完整性等问题,可反馈申请退款(可完整预览的文档不适用该条件!)。
- 3、如文档侵犯您的权益,请联系客服反馈,我们会尽快为您处理(人工客服工作时间:9:00-18:30)。
3,456,134
Patented July 15, 1969
2
small series capacity Co limits the conversion efficiency and hence, the output power eo to a very small value. An attempt to overcome these difficulties is made both by the construction shown in FIG. 2 and the circuit modification of FIG. 4. Referring to FIG. 2, the structure of the implant comprises a container 15 made of metal, glass, or plastic but of such metal, glass, or plastic which would not be affected by the surrounding body elements in which the implant takes place. Inside this sealed container 15 there is found a crystal 10, in the form of a cantilever beam, anchored at 17 to and supported by the container 15. Attached to the free end of the crystal cantilever beam 10 is a weight 18. The relative size of container 15, cantilever beam crystal /10, weight 18, and the placement of the cantilever beam 10 in container 15 should be such that the cantilever beam 10 is free to swing its fullest extent S' without contacting the inner sides of container 15. This amplitude of swing S' is determined by the length and material of cantilever beam 10, the size of weight 18, and in addition, by the amount of movement due to body motion through which container 15 is moved. The body motion which moves container 15 with the end loaded cantilever beam 10 causes the cantilever beam 10 to resonate at a suitable frequency corresponding to the mechanical driving source or body. As the container base is moved periodically, the crystal cantilever beam 10 is caused to vibrate at its natural frequency with varying amplitude. It, thus, generates trains of electrical voltage as shown in FIG. 3. In this figure, the amplitude S is shown in registration for comparison with the voltage Eo generated by the cantilever beam crystal !10. In order to utilize the crystal in the structure described and to fully utilize the capacitance of the crystal, a voltage doubler circuit, as illustrated in FIG. 4, was developed for combination !with the crystal. This circuit uses diodes for rectification, an external capacitor Cand the crystal capacitor Co for voltage doubler; the crystal 10 may be of a type known as a Clevite PZT-5H crystal. With such an energy converter, when the device is driven at a mechanical pulse rate of 80 pulses per minute and with a motion similar to the heart motion of a dog, upon which it was tested, the maximum output is 4.0 volts at 105 ohms load, or 160 microwatts. This power is sufficient to drive a pacemaker and some of the existing implant telemetry systems. In tests, the performance of this new operation mode has been shown to be more desirable than the conventional mode. In addition, this design has eliminated the package problem wherein the implant is placed in the body, while increasing the output of the converter over the original output of prior art converters. It will be obvious to those skilled in the art that various changes may be made without departing from the scope of the invention and the invention is not to be considered limited to what is shown in the drawings and described in the specification. What is claimed is: 1. A converter of body motion to electrical energy for use with electronic implants in the body comprising: a closed container of a material not affected by body fluids, a piezoelectric crystal in the form of a cantilevered beam within said container and extending inwardly from a wall of said container with one end anchored in said container wall and the opposite end free to move, a weight mounted on said free end of said crystal cantilevered beam, and means connecting said crystal to the electronic implants in the body.
10
BRIDGE
RECTIFIER
FIG.2.
'-----'-----r-~~: .---" 1 I s :
L --'
;?'lG. 4 .
,-------,
I I I I
Ro
Co
I I
IFLeabharlann G. 3.l~Eo
I I
I I I
~
I I
I
I
~_.J
L
•+
10
INVENTOR
WEN
H. Ko
BY~~
~ 1(.....
ATTORNEY
United States Patent 0 ce
1
3,456,134 PIEZOELECTRIC ENERGY CONVERTER FOR . ELECTRONIC IMPLANTS Wen H. Ko, Palo Alto, Calif., assignor to the United States of America as represented by the Secretary of 5 Health, Education, and Welfare . Filed Oct. 5, 1967, Ser. No. 673,115 Int. Cl. HOlv 7102 U.S. CI. 310-8.5 3 Claims 10 ABSTRACT OF THE DISCLOSURE A piezoelectric converter for electronic implants for convertin