A fracture-resistant high-entropy alloy for cryogenic applications
TIG重熔对6005A-T6铝合金焊接接头组织及性能的影响
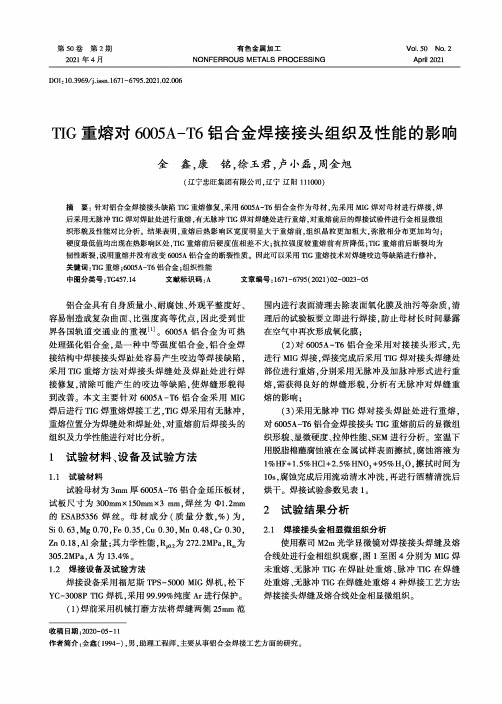
第50卷第2期2021年4月有色金属加工NONFERROUS METALS PROCESSINGVol.50No.2April2021DOI:10.3969/j.i$sn.1671-6795.2021.02.006TIG重熔对6005A-T6铝合金焊接接头组织及性能的影响金鑫,康铭,徐玉君,卢小磊,周金旭(辽宁忠旺集团有限公司,辽宁辽阳111000)摘要:针对铝合金焊接接头缺陷TIG重熔修复,采用6005A-T6铝合金作为母材,先采用MIG焊对母材进行焊接,焊后采用无脉冲TIG焊对焊趾处进行重熔,有无脉冲TIG焊对焊缝处进行重熔,对重熔前后的焊接试验件进行金相显微组织形貌及性能对比分析。
结果表明,重熔后热影响区宽度明显大于重熔前,组织晶粒更加粗大,弥散相分布更加均匀;硬度最低值均出现在热影响区处,TIG重熔前后硬度值相差不大;抗拉强度较重熔前有所降低;TIG重熔前后断裂均为韧性断裂,说明重熔并没有改变6005A铝合金的断裂性质。
因此可以采用TIG重熔技术对焊缝咬边等缺陷进行修补。
关键词:TIG重熔;6005A-T6铝合金;组织性能中图分类号:TG457.14文献标识码:A文章编号:1671-6795(2021)02-0023-05铝合金具有自身质量小、耐腐蚀、外观平整度好、容易制造成复杂曲面、比强度高等优点,因此受到世界各国轨道交通业的重视⑴。
6005A铝合金为可热处理强化铝合金,是一种中等强度铝合金,铝合金焊接结构中焊接接头焊趾处容易产生咬边等焊接缺陷,采用TIG重熔方法对焊接头焊缝处及焊趾处进行焊接修复,清除可能产生的咬边等缺陷,使焊缝形貌得到改善。
本文主要针对6005A-T6铝合金采用MIG 焊后进行TIG焊重熔焊接工艺,TIG焊采用有无脉冲,重熔位置分为焊缝处和焊趾处,对重熔前后焊接头的组织及力学性能进行对比分析。
1试验材料、设备及试验方法1.1试验材料试验母材为3mm厚6005A-T6铝合金延压板材,试板尺寸为300mmx150mmx3mm,焊丝为dl.2mm 的ESAB5356焊丝。
Microstructures and properties of high-entropy alloys
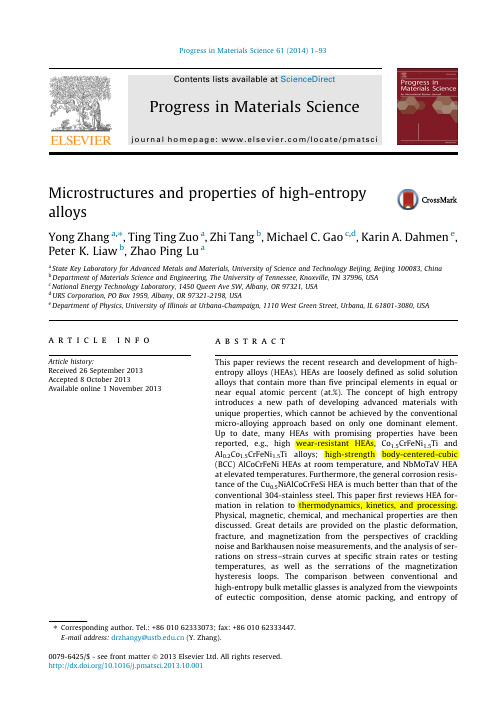
Microstructures and properties of high-entropyalloysYong Zhang a ,⇑,Ting Ting Zuo a ,Zhi Tang b ,Michael C.Gao c ,d ,Karin A.Dahmen e ,Peter K.Liaw b ,Zhao Ping Lu aa State Key Laboratory for Advanced Metals and Materials,University of Science and Technology Beijing,Beijing 100083,Chinab Department of Materials Science and Engineering,The University of Tennessee,Knoxville,TN 37996,USAc National Energy Technology Laboratory,1450Queen Ave SW,Albany,OR 97321,USAd URS Corporation,PO Box 1959,Albany,OR 97321-2198,USAe Department of Physics,University of Illinois at Urbana-Champaign,1110West Green Street,Urbana,IL 61801-3080,USA a r t i c l e i n f o Article history:Received 26September 2013Accepted 8October 2013Available online 1November 2013a b s t r a c tThis paper reviews the recent research and development of high-entropy alloys (HEAs).HEAs are loosely defined as solid solutionalloys that contain more than five principal elements in equal ornear equal atomic percent (at.%).The concept of high entropyintroduces a new path of developing advanced materials withunique properties,which cannot be achieved by the conventionalmicro-alloying approach based on only one dominant element.Up to date,many HEAs with promising properties have beenreported, e.g.,high wear-resistant HEAs,Co 1.5CrFeNi 1.5Ti andAl 0.2Co 1.5CrFeNi 1.5Ti alloys;high-strength body-centered-cubic(BCC)AlCoCrFeNi HEAs at room temperature,and NbMoTaV HEAat elevated temperatures.Furthermore,the general corrosion resis-tance of the Cu 0.5NiAlCoCrFeSi HEA is much better than that of theconventional 304-stainless steel.This paper first reviews HEA for-mation in relation to thermodynamics,kinetics,and processing.Physical,magnetic,chemical,and mechanical properties are thendiscussed.Great details are provided on the plastic deformation,fracture,and magnetization from the perspectives of cracklingnoise and Barkhausen noise measurements,and the analysis of ser-rations on stress–strain curves at specific strain rates or testingtemperatures,as well as the serrations of the magnetizationhysteresis loops.The comparison between conventional andhigh-entropy bulk metallic glasses is analyzed from the viewpointsof eutectic composition,dense atomic packing,and entropy of 0079-6425/$-see front matter Ó2013Elsevier Ltd.All rights reserved./10.1016/j.pmatsci.2013.10.001⇑Corresponding author.Tel.:+8601062333073;fax:+8601062333447.E-mail address:drzhangy@ (Y.Zhang).2Y.Zhang et al./Progress in Materials Science61(2014)1–93mixing.Glass forming ability and plastic properties of high-entropy bulk metallic glasses are also discussed.Modeling tech-niques applicable to HEAs are introduced and discussed,such asab initio molecular dynamics simulations and CALPHAD modeling.Finally,future developments and potential new research directionsfor HEAs are proposed.Ó2013Elsevier Ltd.All rights reserved. Contents1.Introduction (3)1.1.Four core effects (4)1.1.1.High-entropy effect (4)1.1.2.Sluggish diffusion effect (5)1.1.3.Severe lattice-distortion effect (6)1.1.4.Cocktail effect (7)1.2.Key research topics (9)1.2.1.Mechanical properties compared with other alloys (10)1.2.2.Underlying mechanisms for mechanical properties (11)1.2.3.Alloy design and preparation for HEAs (11)1.2.4.Theoretical simulations for HEAs (12)2.Thermodynamics (12)2.1.Entropy (13)2.2.Thermodynamic considerations of phase formation (15)2.3.Microstructures of HEAs (18)3.Kinetics and alloy preparation (23)3.1.Preparation from the liquid state (24)3.2.Preparation from the solid state (29)3.3.Preparation from the gas state (30)3.4.Electrochemical preparation (34)4.Properties (34)4.1.Mechanical behavior (34)4.1.1.Mechanical behavior at room temperature (35)4.1.2.Mechanical behavior at elevated temperatures (38)4.1.3.Mechanical behavior at cryogenic temperatures (45)4.1.4.Fatigue behavior (46)4.1.5.Wear behavior (48)4.1.6.Summary (49)4.2.Physical behavior (50)4.3.Biomedical,chemical and other behaviors (53)5.Serrations and deformation mechanisms (55)5.1.Serrations for HEAs (56)5.2.Barkhausen noise for HEAs (58)5.3.Modeling the Serrations of HEAs (61)5.4.Deformation mechanisms for HEAs (66)6.Glass formation in high-entropy alloys (67)6.1.High-entropy effects on glass formation (67)6.1.1.The best glass former is located at the eutectic compositions (67)6.1.2.The best glass former is the composition with dense atomic packing (67)6.1.3.The best glass former has high entropy of mixing (67)6.2.GFA for HEAs (68)6.3.Properties of high-entropy BMGs (70)7.Modeling and simulations (72)7.1.DFT calculations (73)7.2.AIMD simulations (75)7.3.CALPHAD modeling (80)8.Future development and research (81)Y.Zhang et al./Progress in Materials Science61(2014)1–9338.1.Fundamental understanding of HEAs (82)8.2.Processing and characterization of HEAs (83)8.3.Applications of HEAs (83)9.Summary (84)Disclaimer (85)Acknowledgements (85)References (85)1.IntroductionRecently,high-entropy alloys(HEAs)have attracted increasing attentions because of their unique compositions,microstructures,and adjustable properties[1–31].They are loosely defined as solid solution alloys that contain more thanfive principal elements in equal or near equal atomic percent (at.%)[32].Normally,the atomic fraction of each component is greater than5at.%.The multi-compo-nent equi-molar alloys should be located at the center of a multi-component phase diagram,and their configuration entropy of mixing reaches its maximum(R Ln N;R is the gas constant and N the number of component in the system)for a solution phase.These alloys are defined as HEAs by Yeh et al.[2], and named by Cantor et al.[1,33]as multi-component alloys.Both refer to the same concept.There are also some other names,such as multi-principal-elements alloys,equi-molar alloys,equi-atomic ratio alloys,substitutional alloys,and multi-component alloys.Cantor et al.[1,33]pointed out that a conventional alloy development strategy leads to an enor-mous amount of knowledge about alloys based on one or two components,but little or no knowledge about alloys containing several main components in near-equal proportions.Theoretical and experi-mental works on the occurrence,structure,and properties of crystalline phases have been restricted to alloys based on one or two main components.Thus,the information and understanding are highly developed on alloys close to the corners and edges of a multi-component phase diagram,with much less knowledge about alloys located at the center of the phase diagram,as shown schematically for ternary and quaternary alloy systems in Fig.1.1.This imbalance is significant for ternary alloys but becomes rapidly much more pronounced as the number of components increases.For most quater-nary and other higher-order systems,information about alloys at the center of the phase diagram is virtually nonexistent except those HEA systems that have been reported very recently.In the1990s,researchers began to explore for metallic alloys with super-high glass-forming ability (GFA).Greer[29]proposed a confusion principle,which states that the more elements involved,the lower the chance that the alloy can select viable crystal structures,and thus the greater the chanceand quaternary alloy systems,showing regions of the phase diagram thatand relatively less well known(white)near the center[33].4Y.Zhang et al./Progress in Materials Science61(2014)1–93solid-solutions even though the cooling rate is very high,e.g.,alloys of CuCoNiCrAlFeTiV,FeCrMnNiCo, CoCrFeNiCu,AlCoCrFeNi,NbMoTaWV,etc.[1,2,12–14].The yield strength of the body-centered cubic(BCC)HEAs can be rather high[12],usually compa-rable to BMGs[12].Moreover,the high strength can be kept up to800K or higher for some HEAs based on3d transition metals[14].In contrast,BMGs can only keep their high strength below their glass-transition temperature.1.1.Four core effectsBeing different from the conventional alloys,compositions in HEAs are complex due to the equi-molar concentration of each component.Yeh[37]summarized mainly four core effects for HEAs,that is:(1)Thermodynamics:high-entropy effects;(2)Kinetics:sluggish diffusion;(3)Structures:severe lattice distortion;and(4)Properties:cocktail effects.We will discuss these four core effects separately.1.1.1.High-entropy effectThe high-entropy effects,which tend to stabilize the high-entropy phases,e.g.,solid-solution phases,werefirstly proposed by Yeh[9].The effects were very counterintuitive because it was ex-pected that intermetallic compound phases may form for those equi-or near equi-atomic alloy com-positions which are located at the center of the phase diagrams(for example,a monoclinic compound AlCeCo forms in the center of Al–Ce–Co system[38]).According to the Gibbs phase rule,the number of phases(P)in a given alloy at constant pressure in equilibrium condition is:P¼Cþ1ÀFð1-1Þwhere C is the number of components and F is the maximum number of thermodynamic degrees of freedom in the system.In the case of a6-component system at given pressure,one might expect a maximum of7equilibrium phases at an invariant reaction.However,to our surprise,HEAs form so-lid-solution phases rather than intermetallic phases[1,2,4,17].This is not to say that all multi-compo-nents in equal molar ratio will form solid solution phases at the center of the phase diagram.In fact, only carefully chosen compositions that satisfy the HEA-formation criteria will form solid solutions instead of intermetallic compounds.The solid-solution phase,according to the classical physical-metallurgy theory,is also called a ter-minal solid solution.The solid-solution phase is based on one element,which is called the solvent,and contains other minor elements,which are called the solutes.In HEAs,it is very difficult to differentiate the solvent from the solute because of their equi-molar portions.Many researchers reported that the multi-principal-element alloys can only form simple phases of body-centered-cubic(BCC)or face-cen-tered-cubic(FCC)solid solutions,and the number of phases formed is much fewer than the maximum number of phases that the Gibbs phase rule allows[9,23].This feature also indicates that the high en-tropy of the alloys tends to expand the solution limits between the elements,which may further con-firm the high-entropy effects.The high-entropy effect is mainly used to explain the multi-principal-element solid solution. According to the maximum entropy production principle(MEPP)[39],high entropy tends to stabilize the high-entropy phases,i.e.,solid-solution phases,rather than intermetallic phases.Intermetallics are usually ordered phases with lower configurational entropy.For stoichiometric intermetallic com-pounds,their configurational entropy is zero.Whether a HEA of single solid solution phase is in its equilibrium has been questioned in the sci-entific community.There have been accumulated evidences to show that the high entropy of mixing truly extends the solubility limits of solid solution.For example,Lucas et al.[40]recently reported ab-sence of long-range chemical ordering in equi-molar FeCoCrNi alloy that forms a disordered FCC struc-ture.On the other hand,it was reported that some equi-atomic compositions such as AlCoCrCuFeNi contain several phases of different compositions when cooling slowly from the melt[15],and thus it is controversial whether they can be still classified as HEA.The empirical rules in guiding HEA for-mation are addressed in Section2,which includes atomic size difference and heat of mixing.Y.Zhang et al./Progress in Materials Science61(2014)1–935 1.1.2.Sluggish diffusion effectThe sluggish diffusion effect here is compared with that of the conventional alloys rather than the bulk-glass-forming alloys.Recently,Yeh[9]studied the vacancy formation and the composition par-tition in HEAs,and compared the diffusion coefficients for the elements in pure metals,stainless steels, and HEAs,and found that the order of diffusion rates in the three types of alloy systems is shown be-low:Microstructures of an as-cast CuCoNiCrAlFe alloy.(A)SEM micrograph of an etched alloy withBCC and ordered BCC phases)and interdendrite(an FCC phase)structures.(B)TEMplate,70-nm wide,a disordered BCC phase(A2),lattice constant,2.89A;(B-b)aphase(B2),lattice constant,2.89A;(B-c)nanoprecipitation in a spinodal plate,7nm(B-d)nanoprecipitation in an interspinodal plate,3nm in diameter,a disorderedarea diffraction(SAD)patterns of B,Ba,and Bb with zone axes of BCC[01[011],respectively[2].illustration of intrinsic lattice distortion effects on Bragg diffraction:(a)perfect latticewith solid solutions of different-sized atoms,which are expected to randomly distribute statistical average probability of occupancy;(c)temperature and distortion effectsY.Zhang et al./Progress in Materials Science61(2014)1–937 the intensities further drop beyond the thermal effect with increasing the number of constituent prin-cipal elements.An intrinsic lattice distortion effect caused by the addition of multi-principal elements with different atomic sizes is expected for the anomalous decrease in the XRD intensities.The math-ematical treatment of this distortion effect for the modification of the XRD structure factor is formu-lated to be similar to that of the thermal effect,as shown in Fig.1.3[41].The larger roughness of the atomic planes makes the intensity of the XRD for HEAs much lower than that for the single-element solid.The severe lattice distortion is also used to explain the high strength of HEAs,especially the BCC-structured HEAs[4,12,23].The severe lattice-distortion effect is also related to the tensileFCC-structured HEAs have very low strength[7],which certainly cannot be explained by thelattice distortion argument.Fundamental studies in quantification of lattice distortion of HEAs are needed.1.1.4.Cocktail effectThe cocktail-party effect was usually used as a term in the acousticsfield,which have been used to describe the ability to focus one’s listening attention on a single talker among a mixture of conversa-tions and background noises,ignoring other conversations.For metallic alloys,the effect indicates that the unexpected properties can be obtained after mixing many elements,which could not be obtained from any one independent element.The cocktail effect for metallic alloys wasfirst mentioned by Ranganathan[42],which has been subsequently confirmed in the mechanical and physical properties [12,13,15,18,35,43].The cocktail effect implies that the alloy properties can be greatly adjusted by the composition change and alloying,as shown in Fig.1.4,which indicates that the hardness of HEAs can be dramat-ically changed by adjusting the Al content in the CoCrCuNiAl x HEAs.With the increase of the Al con-lattice constants of a CuCoNiCrAl x Fe alloy system with different x values:(A)hardnessconstants of an FCC phase,(C)lattice constants of a BCC phase[2].CoNiCrAl x Fe alloy system with different x values,the Cu-free alloy has lower hardness.CoCrCuFeNiAl x[15,45].Cu forms isomorphous solid solution with Ni but it is insoluble in Co,Cr and Fe;it dissolves about20at.%Al but also forms various stable intermetallic compounds with Al.Fig.1.6exhibits the hardness of some reported HEAs in the descending order with stainless steels as benchmark.The MoTiVFeNiZrCoCr alloy has a very high value of hardness of over800HV while CoCrFeNiCu is very soft with a value of less than200HV.Fig.1.7compares the specific strength,which yield strength over the density of the materials,and the density amongalloys,polymers and foam materials[5].We can see that HEAs have densitieshigh values of specific strength(yield strength/density).This is partiallyHEAs usually contain mainly the late transitional elements whoselightweight HEAs have much more potential because lightweightdensity of the resultant alloys will be lowered significantly.Fig.1.8strength of HEAs vs.Young’s modulus compared with conventional alloys.highest specific strength and their Young’s modulus can be variedrange of hardness for HEAs,compared with17–4PH stainless steel,Hastelloy,andYield strength,r y,vs.density,q.HEAs(dark dashed circle)compared with other materials,particularly structural Grey dashed contours(arrow indication)label the specific strength,r y/q,from low(right bottom)to high(left top).among the materials with highest strength and specific strength[5].Specific-yield strength vs.Young’s modulus:HEAs compared with other materials,particularly structural alloys.among the materials with highest specific strength and with a wide range of Young’s modulus[5].range.This observation may indicate that the modulus of HEAs can be more easily adjusted than con-ventional alloys.In addition to the high specific strength,other properties such as high hydrogen stor-age property are also reported[46].1.2.Key research topicsTo understand the fundamentals of HEAs is a challenge to the scientists in materials science and relatedfields because of lack of thermodynamic and kinetic data for multi-component systems in the center of phase diagrams.The phase diagrams are usually available only for the binary and ternary alloys.For HEAs,no complete phase diagrams are currently available to directly assist designing thealloy with desirable micro-and nanostructures.Recently,Yang and Zhang [28]proposed the Xparam-eter to design the solid-solution phase HEAs,which should be used combing with the parameter of atomic-size difference.This strategy may provide a starting point prior to actual experiments.The plastic deformation and fracture mechanisms of HEAs are also new because the high-entropy solid solutions contain high contents of multi-principal elements.In single principal-element alloys,dislo-cations dominate the plastic behavior.However,how dislocations interact with highly-disordered crystal lattices and/or chemical disordering/ordering will be an important factor responsible for plastic properties of HEAs.Interactions between the other crystal defects,such as twinning and stacking faults,with chemical/crystal disordering/ordering in HEAs will be important as well.1.2.1.Mechanical properties compared with other alloysFor conventional alloys that contain a single principal element,the main mechanical behavior is dictated by the dominant element.The other minor alloying elements are used to enhance some spe-cial properties.For example,in the low-carbon ferritic steels [47–59],the main mechanical properties are from the BCC Fe.Carbon,which is an interstitial solute element,is used for solid-solution strength-ened steels,and also to enhance the martensite-quenching ability which is the phase-transformation strengthening.The main properties of steels are still from Fe.For aluminum alloys [60]and titanium alloys [61],their properties are mainly related to the dominance of the elemental aluminum and tita-nium,respectively.Intermetallic compounds are usually based on two elements,e.g.,Ti–Al,Fe 3Al,and Fe 3Si.Interme-tallic compounds are typically ordered phases and some may have strict compositional range.The Burgers vectors of the ordered phases are too large for the dislocations to move,which is the main reason why intermetallic phases are usually brittle.However,there are many successful case studies to improve the ductility of intermetallic compound by micro-alloying,e.g.,micro-alloying of B in Ni 3Al[62],and micro-alloying of Cr in Fe 3Al [63,64].Amorphous metals usually contain at least three elements although binary metallic glasses are also reported,and higher GFA can be obtained with addition of more elements,e.g.,ZrTiCuNiBe (Vit-1),PdNiCuP,LaAlNiCu,and CuZrAlY alloys [65–69].Amorphous metals usually exhibit ultrahigh yield strength,because they do not contain conventional any weakening factors,such as dislocations and grain boundaries,and their yield strengths are usually three to five times of their corresponding crys-talline counterpart alloys.There are several models that are proposed to explain the plastic deforma-tion of the amorphous metal,including the free volume [70],a shear-transformation-zone (STZ)[71],more recently a tension-transition zone (TTZ)[72],and the atomic-level stress [73,74].The micro-mechanisms of the plastic deformation of amorphous metals are usually by forming shear bands,which is still an active research area till today.However,the high strength of amorphous alloys can be sustained only below the glass-transition temperature (T g ).At temperatures immediately above T g ,the amorphous metals will transit to be viscous liquids [68]and will crystallize at temperatures above the first crystallization onset temperature.This trend may limit the high-temperature applica-tions of amorphous metals.The glass forming alloys often are chemically located close to the eutectic composition,which further facilitates the formation of the amorphous metal–matrix composite.The development of the amorphous metal–matrix composite can enhance the room-temperature plastic-ity of amorphous metals,and extend application temperatures [75–78].For HEAs,their properties can be different from any of the constituent elements.The structure types are the dominant factor for controlling the strength or hardness of HEAs [5,12,13].The BCC-structured HEAs usually have very high yield strengths and limited plasticity,while the FCC-structured HEAs have low yield strength and high plasticity.The mixture of BCC +FCC is expected to possess balanced mechanical properties,e.g.,both high strength and good ductility.Recent studies show that the microstructures of certain ‘‘HEAs’’can be very complicated since they often undergo the spinodal decomposition,and ordered,and disordered phase precipitates at lower temperatures.Solution-strengthening mechanisms for HEAs would be much different from conventional alloys.HEAs usually have high melting points,and the high yield strength can usually be sustained to ultrahigh temperatures,which is shown in Fig.1.9for refractory metal HEAs.The strength of HEAs are sometimes better than those of conventional superalloys [14].10Y.Zhang et al./Progress in Materials Science 61(2014)1–931.2.2.Underlying mechanisms for mechanical propertiesMechanical properties include the Young’s modulus,yield strength,plastic elongation,fracture toughness,and fatigue properties.For the conventional one-element principal alloys,the Young’s modulus is mainly controlled by the dominant element,e.g.,the Young’s modulus of Fe-based alloys is about 200GPa,that of Ti-based alloys is approximately 110GPa,and that of Al-based alloys is about 75GPa,as shown in Fig.1.8.In contrast,for HEAs,the modulus can be very different from any of the constituent elements in the alloys [79],and the moduli of HEAs are scattered in a wide range,as shown in Fig.1.8.Wang et al.[79]reported that the Young’s modulus of the CoCrFeNiCuAl 0.5HEA is about 24.5GPa,which is much lower than the modulus of any of the constituent elements in the alloy.It is even lower than the Young’s modulus of pure Al,about 69GPa [80].On the other hand,this value needs to be verified using other methods including impulse excitation of vibration.It has been reported that the FCC-structured HEAs exhibit low strength and high plasticity [13],while the BCC-structured HEAs show high strength and low plasticity at room temperature [12].Thus,the structure types are the dominant factor for controlling the strength or hardness of HEAs.For the fracture toughness of the HEAs,there is no report up to date.1.2.3.Alloy design and preparation for HEAsIt has been verified that not all the alloys with five-principal elements and with equi-atomic ratio compositions can form HEA solid solutions.Only carefully chosen compositions can form FCC and BCC solid solutions.Till today there is no report on hexagonal close-packed (HCP)-structured HEAs.One reason is probably due to the fact that a HCP structure is often the stable structure at low tempera-tures for pure elements (applicable)in the periodic table,and that it may transform to either BCC or FCC at high temperatures.Most of the HEA solid solutions are identified by trial-and-error exper-iments because there is no phase diagram on quaternary and higher systems.Hence,the trial-and er-ror approach is the main way to develop high-performance HEAs.However,some parameters have been proposed to predict the phase formation of HEAs [17,22,28]in analogy to the Hume-Rothery rule for conventional solid solution.The fundamental thermodynamic equation states:G ¼H ÀTS ð1-2Þwhere H is the enthalpy,S is the entropy,G is the Gibbs free energy,and T is the absolute temperature.From Eq.(1-2),the TS term will become significant at high temperatures.Hence,preparing HEAs from the liquid and gas would provide different kinds of information.These techniques may include sput-Temperature dependence of NbMoTaW,VNbMoTaW,Inconel 718,and Haynes 230tering,laser cladding,plasma coating,and arc melting,which will be discussed in detail in the next chapter.For the atomic-level structures of HEAs,the neutron and synchrotron diffraction methods are useful to detect ordering parameters,long-range order,and short-range ordering[81].1.2.4.Theoretical simulations for HEAsFor HEAs,entropy effects are the core to their formation and properties.Some immediate questions are:(1)How can we accurately predict the total entropy of HEA phase?(2)How can we predict the phasefield of a HEA phase as a function of compositions and temperatures?(3)What are the proper modeling and experimental methods to study HEAs?To address the phase-stability issue,thermody-namic modeling is necessary as thefirst step to understand the fundamental of HEAs.The typical mod-eling techniques to address thermodynamics include the calculation of phase diagram(CALPHAD) modeling,first-principle calculations,molecular-dynamics(MD)simulations,and Monte Carlo simulations.Kao et al.[82]using MD to study the structure of HEAs,and their modeling efforts can well explain the liquid-like structure of HEAs,as shown in Fig.1.10.Grosso et al.[83]studied refractory HEAs using atomistic modeling,clarified the role of each element and their interactions,and concluded that4-and 5-elements alloys are possible to quantify the transition to a high-entropy regime characterized by the formation of a continuous solid solution.2.Thermodynamicsof a liquid-like atomic-packing structure using multiple elementsthird,fourth,andfifth shells,respectively,but the second and third shellsdifference and thus the largefluctuation in occupation of different atoms.2.1.EntropyEntropy is a thermodynamic property that can be used to determine the energy available for the useful work in a thermodynamic process,such as in energy-conversion devices,engines,or machines. The following equation is the definition of entropy:dS¼D QTð2-1Þwhere S is the entropy,Q is the heatflow,and T is the absolute temperature.Thermodynamic entropy has the dimension of energy divided by temperature,and a unit of Joules per Kelvin(J/K)in the Inter-national System of Units.The statistical-mechanics definition of entropy was developed by Ludwig Boltzmann in the1870s [85]and by analyzing the statistical behavior of the microscopic components of the system[86].Boltz-mann’s hypothesis states that the entropy of a system is linearly related to the logarithm of the fre-quency of occurrence of a macro-state or,more precisely,the number,W,of possible micro-states corresponding to the macroscopic state of a system:Fig.2.1.Illustration of the D S mix for ternary alloy system with the composition change[17].。
Fracture Toughness and Fatigue Crack Growth Behavior of As-Cast High-Entropy Alloys
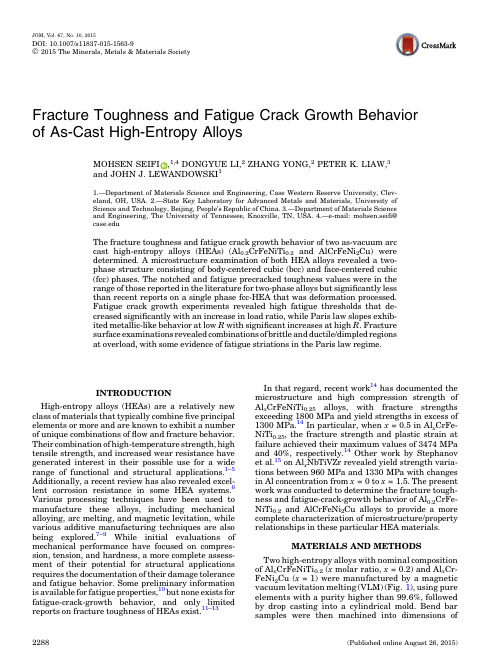
Fracture Toughness and Fatigue Crack Growth Behavior of As-Cast High-Entropy AlloysMOHSEN SEIFI ,1,4DONGYUE LI,2ZHANG YONG,2PETER K.LIAW,3and JOHN J.LEWANDOWSKI 11.—Department of Materials Science and Engineering,Case Western Reserve University,Clev-eland,OH,USA.2.—State Key Laboratory for Advanced Metals and Materials,University of Science and Technology,Beijing,People’s Republic of China.3.—Department of Materials Science and Engineering,The University of Tennessee,Knoxville,TN,USA.4.—e-mail:mohsen.seifi@The fracture toughness and fatigue crack growth behavior of two as-vacuum arc cast high-entropy alloys (HEAs)(Al 0.2CrFeNiTi 0.2and AlCrFeNi 2Cu)were determined.A microstructure examination of both HEA alloys revealed a two-phase structure consisting of body-centered cubic (bcc)and face-centered cubic (fcc)phases.The notched and fatigue precracked toughness values were in the range of those reported in the literature for two-phase alloys but significantly less than recent reports on a single phase fcc-HEA that was deformation processed.Fatigue crack growth experiments revealed high fatigue thresholds that de-creased significantly with an increase in load ratio,while Paris law slopes exhib-ited metallic-like behavior at low R with significant increases at high R .Fracture surface examinations revealed combinations of brittle and ductile/dimpled regions at overload,with some evidence of fatigue striations in the Paris law regime.INTRODUCTIONHigh-entropy alloys (HEAs)are a relatively new class of materials that typically combine five principal elements or more and are known to exhibit a number of unique combinations of flow and fracture behavior.Their combination of high-temperature strength,high tensile strength,and increased wear resistance have generated interest in their possible use for a wide range of functional and structural applications.1–5Additionally,a recent review has also revealed excel-lent corrosion resistance in some HEA systems.6Various processing techniques have been used to manufacture these alloys,including mechanical alloying,arc melting,and magnetic levitation,while various additive manufacturing techniques are also being explored.7–9While initial evaluations of mechanical performance have focused on compres-sion,tension,and hardness,a more complete assess-ment of their potential for structural applications requires the documentation of their damage tolerance and fatigue behavior.Some preliminary information is available for fatigue properties,10but none exists for fatigue-crack-growth behavior,and only limited reports on fracture toughness of HEAs exist.11–13In that regard,recent work 14has documented the microstructure and high compression strength of Al x CrFeNiTi 0.25alloys,with fracture strengths exceeding 1800MPa and yield strengths in excess of 1300MPa.14In particular,when x =0.5in Al x CrFe-NiTi 0.25,the fracture strength and plastic strain at failure achieved their maximum values of 3474MPa and 40%,respectively.14Other work by Stephanov et al.15on Al x NbTiVZr revealed yield strength varia-tions between 960MPa and 1330MPa with changes in Al concentration from x =0to x =1.5.The present work was conducted to determine the fracture tough-ness and fatigue-crack-growth behavior of Al 0.2CrFe-NiTi 0.2and AlCrFeNi 2Cu alloys to provide a more complete characterization of microstructure/property relationships in these particular HEA materials.MATERIALS AND METHODSTwo high-entropy alloys with nominal composition of Al x CrFeNiTi 0.2(x molar ratio,x =0.2)and Al x Cr-FeNi 2Cu (x =1)were manufactured by a magnetic vacuum levitation melting (VLM)(Fig.1),using pure elements with a purity higher than 99.6%,followed by drop casting into a cylindrical mold.Bend bar samples were then machined into dimensions ofJOM,Vol.67,No.10,2015DOI:10.1007/s11837-015-1563-9Ó2015The Minerals,Metals &Materials Society2288(Published online August 26,2015)3mm 95mm 947mm.The as-cast microstruc-tures were characterized using x-ray diffraction (XRD)as well as scanning electron microscopy (SEM)equipped with an energy-dispersive (EDX)x-ray spectrometer.The hardness was measured by aHXD-1000TM Vickers microhardness tester with a load of 500g and a dwell time of 15s.Fracture toughness was determined in three-point bending using single-edge notch (SEN)samples tested on a Model 810MTS servohydraulic machine in general accordance with ASTM E399standard.16The speci-mens were first notched using a slow-speed diamond wire saw to introduce notch root radius of about 75–100l m and then fatigue precracked to a crack depth/sample width (i.e.,a /W )of 0.45–0.55in accordance with the standard.The notched toughness tests using this same notch radius placed to a /W =0.5were also tested.All toughness tests were conducted to failure at a displacement rate of 0.25mm/min.Fatigue crack growth tests were performed at a cyclic frequency of 20Hz in room temperature air with relative humidity of 40%in accordance with ASTM E647standard (Ref.17)in the LT orientation.A direct current potential drop technique was used to monitor and control crack growth.Fracture Tech-nology Associates (FTA TM )software was used to monitor crack growth continuously in both the fati-gue and fracture toughness tests in order to also comply with existing ASTM requirements.In all cases,1A current input was used with voltage drop amplified by 10K gain.Fatigue crack growth tests at load ratios R =0.1,0.3,and 0.7were started at a low stress intensity range D K ,using constant cyclic loading to produce rising D K conditions until catas-trophic fracture.The Paris law slope and fatigue overload K c was calculated for multiple tests con-ducted in this manner.SEM of fractured samples was conducted on a FEI Quanta 200three-dimensional microscope (FEI Company,Hillsboro,OR)utilizing secondary elec-tron imaging (SE)operated at 20keV.RESULTSMicrostructuresThe XRD patterns obtained for Al 0.2CrFeNiTi 0.2and AlCrFeNi 2Cu alloys in the as-cast condition shown in Fig.2revealed a multiphase material,inMelting metalWater-cooling crucibleInduction coilFig.1.Schematicof VLM process.Fig.2.X-ray diffraction patterns of Al 0.2CrFeNiTi 0.2and AlCrFeNi 2Cu HEAs.Fig.3.SEM images of (a)Al 0.2CrFeNiTi 0.2and (b)AlCrFeNi 2Cu showing dendritic and interdendritic structures.Fracture Toughness and Fatigue Crack Growth Behavior of As-Cast High-Entropy Alloys 2289contrast to some HEAs.2,3,10–14The two main phases identified possess face-centered-cubic(fcc)and body-centered-cubic(bcc)structures.There are minor unidentified peaks at small angles and the structure corresponding to those peaks are being determined by a TEM study as part of future work.The SEM micrographs of the as-cast Al0.2CrFe-NiTi0.2,and AlCrFeNi2Cu alloys shown in Fig.3a and b,respectively,reveal a cast dendritic structure with interdendritic regions that also possess some microstructural features.The chemical composi-tions of both regions were analyzed by EDX and shown in Table I.The matrix in Al0.2CrFeNiTi0.2is enriched in Cr and Fe,whereas the particles and small,elongated precipitates are enriched in Al,Ni,and Ti.In contrast,the matrix in AlCrFeNi2Cu is enriched in Al,Ni,and Cu,whereas particles are enriched in Fe and Cr.Vickers microhardness results for both alloys are shown in Fig.4with Al0.2CrFeNiTi0.2exhibiting a hardness of510±7.7and AlCrFeNi2Cu with 320.9±7.8.Mechanical PropertiesTable II provides the fracture toughness and Rockwell C hardness for the present alloys,whereas Fig.5summarizes the effects of changing test temperature and notch root radius on the tough-ness.Both alloys exhibited load versus displace-ment traces at room temperature in the toughness tests and the AlCrFeNi2Cu exhibited a higher toughness but lower strength than Al0.2CrFeNiTi0.2. Toughness testing at200°C induced more plasticity (i.e.,nonlinear load versus displacement trace)and slightly improved the toughness(Fig.5).Table II provides a comparison of the present data with the data obtained on other HEAs11,13and a high toughness bulk metallic glass(BMG).18Fig.4.Vickers microhardness results.Table I.Chemical compositions(in wt.%)in theAlCrFeNi2Cu and Al0.2CrFeNiTi0.2Alloy Al Cr Fe Ni Ti CuAlCrFeNi2CuGray19.67 1.828.1252.04–18.34White7.8715.6622.4638.63–15.38Al0.2CrFeNiTi0.2Gray0.6838.6445.2214.43 1.03–White 2.9320.2533.8937.47 5.47–Table II.Summary of fracture toughness results of current study and other recent worksAlloyThickness,B(mm)Span,S(mm)Fracturetoughness,K q(MPaffiffiffiffiffimp)Rockwell C AlCrFeNi2Cu(HEA)32040,41,42,4536±1 AlCrFeNi2Cu(HEA)a32046,47NAAl0.2CrFeNiTi0.2(HEA)32032,3546±1 FeCoNiCrCuTiMoAlSiB0.5(HEA)NA NA51(Ref.13)NAAl23Cr23Co15Cu8Fe15Ni15(HEA)3245(Ref.11)NAZr61Ti2Cu25Al12(BMG)324106(Ref.18)NAa Test was performed at200°C.Fig.5.Effect of changes in notch root radius and test temperatureon the fracture toughness of HEA alloys.Seifi,Li,Yong,Liaw,and Lewandowski 2290Table III summarizes the fatigue-crack-growth results and includes D K th ,Paris law slope and K max at failure at various load ratios (i.e.,R =0.1,0.3and 0.7).Both alloys showed a load ratio effect on D K th ,whereby increases in R shifted the whole fatigue crack growth curve to the left as shown in Fig.6.These increases in R produced significant reduc-tions in D K th and increases in Paris law slope,somewhat similar to a recent report on an as-cast c -TiAl,19but much greater than what was reported in as-deposited Ti-6Al-4V (Ref.20)prepared by elec-tron beam processing.Both visual and SEM analyses of fracture sur-faces revealed macroscopically (and microscopically)rough surfaces for both HEAs.The high magnifica-tion image in Fig.7a illustrates fatigue striations on the fracture surface of the tougher AlCrFeNi 2Cu alloy in the Paris law regime,while isolated ductile/dimple-like features are evident in the overload region,as shown in Fig.7b,interspersed with more brittle regions as shown in Fig.7c and d.Figure 7c and d illustrate the large-scale roughness of the fracture surface in addition to revealing the combi-nation of ductile features as well as faceted brittle regions that appear in the overload region of this tougher HEA,in comparison to the stronger and more brittle Al 0.2CrFeNiTi 0.2shown in Fig.6.The less tough Al 0.2CrFeNiTi 0.2exhibited fewer ductile regions in the overload region and larger-scale brittle features in both the fatigue and over-load region as shown in Figs.8and 9.Some ductile/dimple-like features are evident in Fig.8d,but these were relatively few in extent and small in size,with a typical size of only 3–5l m.Fracture tough-ness experiments on this more brittle material similarly revealed a fracture surface dominated by large-scale brittle features (Fig.9),with isolated ductile features.DISCUSSIONWhile many of the HEA systems recently reviewed 2and experimentally evaluated 12have a single phase,there are a number of examples of multiphase HEA systems.2These may possess very different combinations of properties,depending on the crystal structure of the phases as well as their size,volume fraction,and distribution.The present work illustrates that the as-cast microstructures inAl 0.2CrFeNiTi 0.2and AlCrFeNi 2Cu presently tested exhibit a combination of bcc and fcc regions that produce levels of strength/toughness that approach/exceed the HEA systems listed in Table II but are far below that of a deformation-processed single fcc phase HEA that exhibits very high toughness 12along with fracture surface features that are 100%Table III.Summary of fatigue crack growth results at different load ratios for data shown in Fig.6AlloyR (load ratio)Fatigue overload K c (MPa ffiffiffiffiffim p )Paris slope (m)Threshold (MPa ffiffiffiffiffim p )AlCrFeNi 2Cu 0.156 3.417AlCrFeNi 2Cu 0.336 6.55AlCrFeNi 2Cu 0.73214.57Al 0.2CrFeNiTi 0.20.130 4.916Al 0.2CrFeNiTi 0.20.323 5.37Al 0.2CrFeNiTi 0.20.72125.85Fig.6.Fatigue crack growth behavior of (a)AlCrFeNi 2Cu and (b)Al 0.2CrFeNiTi 0.2.Fracture Toughness and Fatigue Crack Growth Behavior of As-Cast High-Entropy Alloys 2291ductile/dimpled fracture.An examination of the present fracture surfaces reveals combinations of brittle and ductile/dimpled features that roughly correspond to the size and volume fraction of the bcc and fcc microstructural constituents,respec-tively.At room temperature,both materials exhibit linear load versus displacement traces in the toughness tests in addition to the brittle fracture of the bcc constituent.Increasing the test tempera-ture to 200°C increases the toughness,while pro-ducing a nonlinear load versus displacement trace although the fracture surface features appeared similar to those of the room-temperature tests in that there appeared to be similar combinations of ductile/dimpled and brittle fracture.This is not uncommon in either single-phase bcc metals (e.g.,Nb),21,22ferritic steels,23or ductile-phase tough-ened composites containing a combination of bccand non-bcc phases.24In those cases,changes to the load versus displacement traces and toughness were obtained along with significant changes to the fracture surface details due to the additional plasticity required to reach stresses sufficient to trigger brittle (i.e.cleavage)fracture in the bcc metal/alloy 21–23or composite 24containing a bcc phase.Future work will examine the effects of testing at higher temperatures in order to induce a brittle-to-ductile transition in the bcc constituent in order to determine its effect on toughness of these two-phase HEA alloys.The effects of chang-ing the notch root radius on toughness are similar to that exhibited by many crystalline metallic systems,unlike that exhibited in some bulk metallic glasses 25where relatively minor changes in the notch root radius produce extreme increases in the load-carryingcapability.Fig.7.SEM images of AlCrFeNi 2Cu HEA alloy tested in fatigue:(a)fatigue striation features,(b)ductile/dimple-like features,and (c and d)brittle and ductile/dimple features.Seifi,Li,Yong,Liaw,and Lewandowski2292The preliminary fatigue-crack growth rate data obtained on the Al 0.2CrFeNiTi 0.2and AlCrFeNi 2Cu presently tested exhibit characteristics that are similar to that exhibited by some metallic alloys,26,27intermetallics,19and metal matrix composite sys-tems,28,29where metallic-like Paris slopes at low R (e.g.,3–5)significantly increase (e.g.,to >10)with an increase in R .In those systems,19,26–29the increase in Paris slope with increasing R coincided with an increase in static modes of brittle failure (e.g.,inter-granular or cleavage failure)in the Paris regime,thereby increasing the rate of crack growth in that regime and elevating the Paris law slope.The com-binations of brittle and ductile/dimpled features present on the fracture surfaces of the presently tested materials suggest that a similar mechanism may contribute to the significantly higher fatigue-crack growth rates and higher Paris law slopes obtained at higher R .Future work will examine the influence of changing the test temperature in order to induce more ductile behavior of the bcc constituent,as test temperature should have a significant effect on the fatigue-crack growth rate (and Paris law slope).Despite the predominance of brittle fracture in the fatigue regime,isolated areas of fatigue striations (cf.Fig.7a)were observed,indicating that some regions of the fatigue-fracture surface did fail in a locally ductile (i.e.,striation-controlled)manner.The fatigue threshold similarly revealed a rela-tively large effect of changes in the load ratio R with relatively high fatigue thresholds at low R and sig-nificant reductions in thresholds with increasing R .This trend likely results from the large surface roughness producing significant levels of fatigue-crack closure at low values of R ,as shown in many otherworks.Fig.8.SEM images of Al 0.2CrFeNiTi 0.2tested in fatigue:(a)brittle features,(b and c)higher magnification of faceted regions,and (d)isolated ductile/dimple-like features.Fracture Toughness and Fatigue Crack Growth Behavior of As-Cast High-Entropy Alloys 2293Whereas the present work was conducted on as-cast materials,future work should also focus on deformation-processed versions of these (and other)HEA materials.Deformation processing is known to produce significant effects on the mechanical behavior of cast composite systems,whether the matrix is metallic 30or intermetallic 31due to various beneficial microstructural changes that are pro-duced in the system under investigation.CONCLUSION1.Al 0.2CrFeNiTi 0.2and AlCrFeNi 2Cu HEA alloys produced via arc casting exhibited a combina-tion of bcc and fcc phases.2.The notched and fatigue precracked toughness values of these two-phase as-cast HEA alloys were in the range of those reported in the literature,although significantly less than those reported for a deformation-processed single fcc phase HEA.3.Fracture-surface examinations of the toughness tests revealed a combination of ductile/dimpled and brittle features that roughly corresponded to the fcc and bcc phases,respectively,in both HEA alloys presently tested.While both systems exhibited linear load versus displacement traces at room temperature,the lower hardness/strength AlCrFeNi 2Cu exhibited a higher tough-ness than the Al 0.2CrFeNiTi 0.2.Increasing the test temperature to 200°C produced nonlinear load versus displacement traces and a moderate increase in toughness,while increases in notch radius similarly produced modest increases to the load carrying capability.4.Fatigue-crack growth experiments conducted on as-cast Al 0.2CrFeNiTi 0.2and AlCrFeNi 2Cuexhibited high fatigue thresholds that decreased significantly with an increase in R ,while Paris law slopes exhibited metallic-like values (e.g.,3–5)at low R with significant increases at high R .Fracture-surface examinations revealed com-binations of brittle and ductile/dimpled regions in the overload region,with limited observations of fatigue striations in the Paris law regime.ACKNOWLEDGEMENTSThis work was partially supported by the Arthur P.Armington Professorship (J.J.L.),while mechan-ical characterization was performed in the Ad-vanced Manufacturing and Mechanical Reliability Center (AMMRC)at CWRU.ZY would like to thank the financial support of the National Science Foun-dation of China (Nos.51471025and 51210105006),111Project (B07003),and the Program for Chang-jiang Scholars and the Innovative Research Team of the University.PKL would like to acknowledge the support of the Department of Energy (DOE),Office of Fossil Energy,National Energy Technology Lab-oratory (DE-FE-0008855,DE-FE-0024054,and DE-FE-0011194),the Army Research Office (W911NF-13-1-0438),and the National Science Foundation (CMMI-1100080)with the program managers,Mr.V.Cedro,Mr.R.Dunst,Dr.J.Mullen,Dr.D.M.Stepp,and Dr.C.Cooper.REFERENCES1.J.W.Yeh,Ann.Chim.Sci.Des Mate´raux 31,633(2006).2.Y.Zhang,T.T.Zuo,Z.Tang,M.C.Gao,K.A.Dahmen,P.K.Liaw,and Z.P.Lu,Prog.Mater.Sci.61,1(2014).3. D.B.Miracle,ler,O.N.Senkov,C.Woodward,M.D.Uchic,and J.Tiley,Entropy 16,494(2014).4. C.J.Tong,Y.L.Chen,J.W.Yeh,S.J.Lin,S.K.Chen,T.T.Shun,C.H.Tsau,and S.Y.Chang,Metall.Mater.Trans.A 36A,881(2005).5. D.B.Miracle,Mater.Sci.Technol.31,1142(2015).Fig.9.SEM images of AlCrFeNi 2Cu after toughness test (a and b)reveals brittle features and some isolated ductile regions.Seifi,Li,Yong,Liaw,and Lewandowski22946.Y.Qiu,M.A.Gibson,H.L.Fraser,and N.Birbilis,Mater.Sci.Technol.10,1235(2015).7.Y.Brif,M.Thomas,and I.Todd,Scripta Mater.99,93(2015).8.J.Joseph,T.Jarvis,X.Wu,N.Stanford,P.Hodgson,andD.M.Fabijanic,Mater.Sci.Eng.A633,184(2015).9.T.Fujieda,H.Shiratori,K.Kuwabara,T.Kato,K.Yama-naka,Y.Koizumi,and A.Chiba,Mater.Lett.159,12 (2015).10.M.A.Hemphill,T.Yuan,G.Y.Wang,J.W.Yeh,C.W.Tsai,A.Chuang,and P.K.Liaw,Acta Mater.60,5723(2012).11.U.Roy,H.Roy,H.Daoud,U.Glatzel,and K.K.Ray,Mater.Lett.132,186(2014).12. B.Gludovatz,A.Hohenwarter,D.Catoor,E.H.Chang,E.P.George,and R.O.Ritchie,Science345,1153(2014).13.H.Zhang,Y.He,and Y.Pan,Scripta Mater.69,342(2013).14.S.Liu,M.C.Gao,P.K.Liaw,and Y.Zhang,J.Alloys Compd.619,610(2015).15.N.D.Stepanov,N.Y.Yurchenko,D.G.Shaysultanov,G.A.Salishchev,and M.A.Tikhonovsky,Mater.Sci.Technol.31, 1184(2015).16.ASTM E399,Standard Test Method for Linear-ElasticPlane-Strain Fracture Toughness KIc of Metallic Materials (West Conshohocken,PA:ASTM International,2012). 17.ASTM E647,Standard Test Method for Measurement ofFatigue Crack Growth Rates(West Conshohocken,PA: ASTM International,2013).18.J.Yi,S.M.Seifi,W.H.Wang,and J.J.Lewandowski,J.Mater.Sci.Technol.30,627(2014).19.M.S.Dahar,S.M.Seifi,B.P.Bewlay,and J.J.Lewandowski,Intermetallics57,73(2015).20.M.Seifi,M.Dahar,R.Aman,O.Harrysson,J.Beuth,andJ.J.Lewandowski,JOM67,597(2015).21. A.V.Samant and J.J.Lewandowski,Metall.Mater.Trans.A28,2297(1997).22. A.V.Samant and J.J.Lewandowski,Metall.Mater.Trans.A28,389(1997).23.R.O.Ritchie,J.F.Knott,and J.R.Rice,J.Mech.Phys.Solids21,395(1973).24.J.D.Rigney and J.J.Lewandowski,Metall.Mater.Trans.A27,3292–3306(1996).25.J.J.Lewandowski,M.Shazly,and A.S.Nouri,Scripta Ma-ter.54,337(2006).26.R.O.Ritchie and J.F.Knott,Acta Metall.21,639(1973).27. A.B.El-Shabasy and J.J.Lewandowski,Int.J.Fatigue26,305(2004).28.J.K.Shang,W.Yu,and R.O.Ritchie,Mater.Sci.Eng.A102,181(1988).29.W.A.Zinsser and J.J.Lewandowski,Metall.Mater.Trans.A29,1749(1998).30.G.A.Rozak,J.J.Lewandowski,J.F.Wallace,and A.Alt-misoglu,pos.Mater.26,2076(1992).31.M.G.Mendiratta,J.J.Lewandowski,and D.M.Dimiduk,Metall.Trans.A22,1573(1991).Fracture Toughness and Fatigue Crack Growth Behavior of As-Cast High-Entropy Alloys2295。
Indentation fracture toughness of neutron irradiated silicon carbide

Indentation fracture toughness of neutron irradiatedsilicon carbideS.Nogami a ,A.Hasegawaa,*,L.L.SneadbaDepartment of Quantum Science and Energy Engineering,Tohoku University,Aramaki-aza-Aoba 01,Aoba-ku,Sendai,Miyagi 980-8579,JapanbOa k Ridge Na tiona l La bora tory,Oa k Ridge,TN 378316138,USAAbstractThe indentation fracture toughness and other mechanical properties (elastic modulus and hardness)of high-puritystoichiometric chemical vapor deposition processed-SiC under neutron irradiation in a dose range of 1024–1025n/m 2(E n >0:1MeV)at the temperature range of 80–1150°C were investigated.Indentation fracture toughness decreased slightly at the temperatures below 400°C,and increased above 400°C,for example,from 5.08MPa m À1=2(non-irra-diated)to 6.52MPa m À1=2(800°C neutron irradiated).Mechanical properties investigated in this study were signifi-cantly dependent on the irradiation temperature up to 1150°C,while generally independent on the irradiation dose up to 7.7dpa signifying low-dose saturation.Ó2002Elsevier Science B.V.All rights reserved.1.IntroductionSilicon carbide (SiC)has been considered as a structural material for fusion applications because of its low neutron induced radioactivation and small degra-dation of mechanical properties at elevated temperatures [1–4].One of the significant issues for SiC as a structural material is its low fracture toughness.For this reason,SiC fiber reinforced SiC matrix (SiC/SiC)composites have been developed [1–3].The change of mechanical properties due to neutron irradiation is a central issue for fusion structural materials [1–3],and has received little study.For example,the behavior of fracture toughness under irradiation has not been well defined and may be of great importance.Considering this property is indicative of crack generation and propa-gation in SiC/SiC composites.The purpose of this study is to clarify the fracture toughness and other mechanical properties (elasticmodulus and hardness)of high-purity stoichiometric chemical vapor deposition (CVD)processed-SiC under neutron irradiation in a dose range of 1024–1025n/m 2(E n >0:1MeV)at various temperatures.The fracture toughness was evaluated by the tech-nique of indentation fracture toughness,which involves measurement of crack length introduced by an inden-tation using diamond tip defined by Evans [5].This is one of the best techniques available for measuring the toughness of a small and brittle material like an irradi-ated ceramic because it can be evaluated by a crack growth behavior in a very small region.2.Experimental and analysis procedure 2.1.Material and neutron irradiation testsMaterial used in this study was polycrystalline b -SiC,that was fabricated by CVD process by Rohm and Haas.This material has a stoichiometric composition with high purity.Bulk SiC samples were machined into 25mm Â1mm Â1mm bars.At least one side of the sample was mechanicallypolished.*Corresponding author.Tel.:+81-222177923;fax:+81-222177925.E-mail address:akira.hasegawa@qse.tohoku.ac.jp (A.Ha-segawa).0022-3115/02/$-see front matter Ó2002Elsevier Science B.V.All rights reserved.PI I :S 0022-3115(02)01055-3Neutron irradiation tests were performed in the High Flux Isotope Reactor(HFIR)at Oak Ridge National Laboratory and the High Flux Beam Reactor(HFBR) at Brookhaven National Laboratory.Samples were ir-radiated in HFIR up to$6and7:7Â1025n/m2 (E n>0:1MeV)at300and800°C and in HFBR up to $1:1Â1025n/m2and$0:15Â1025n/m2(E n>0:1MeV) at80–90,250–270,385,890–980and960–1150°C.In all cases thermocouples embedded in sample holders were used to monitor irradiation temperature.Irradiation conditions in this study are summarized in Table1, where doses were calculated using an assumption that 1Â1025n/m2(E n>0:1MeV)corresponds to1dis-placement per atom(dpa).2.2.Microindentation testMicroindentation test was performed using a Vickers diamond(Model Micromet TM3,Buehler,Ltd.),and doing so,Vickers hardness H v,indentation diagonal2a, and crack length c were measured.Each indentation was made at a load of2kg with a dwell time of15s.Cracks from the indentation sides and subsurface cracks were not included in the analysis.Indentations were spaced more than0.1mm apart to prevent an interaction be-tween indentations and free edge.The number of the indentation test was4–9in each sample in this study depending on goodness of data.2.3.Nanoindentation testNanoindentation test was performed using a NANO INDENTERâII(Nano-instruments,Oak Ridge,TN), which is an ultra-low load,ultra-low depth(nanometer scale)sensing machine.This machine is equipped with a Berkovich diamond tip.In this study,one indentation included three separate loading/unloading segments.Indentation depths and loading(unloading)rates were constant in each division;50nm and50l m/s,100nm and125l m/s,and200nm and300l m/s.The indenter was unloaded until90%of the load has been removed in each unloading segment and thermal drift corrected.The number of the indentation tests was12–15for each sample.Hardness H nano and elastic modulus E nano were eval-uated using an unloading curve by a method proposed by Oliver and Pharr[6].H nano is calculated from the following formula:H nano¼P max=Aðh cÞ;ð1Þwhere P max is the maximum indentation load and A, which is a function of constant indentation depth h c,is a contact area of the indenter with a sample surface.E nano is defined as follow:E nano¼ð1Àm2sÞ=ð1=E rþð1Àm2iÞ=E iÞ;ð2Þwhere m s,m i,and E i are a PoissonÕs ratio of the sample, that of the indenter(0.07for the diamond tip),and an elastic modulus of the indenter(1141GPa for the dia-mond tip),respectively.Reduced modulus E r in Eq.(2) has the form:E r¼p1=2S=2b Aðh cÞ1=2;ð3Þwhere S and b is a slope of the initial unloading curve and a geometrical correlation factor(1.034),respec-tively.The theoretical geometrical shape function for Berkovich tip Aðh cÞrepresents the same area-to-depth ratio as the Vickers diamond tip.In practice,however, the shape of the indenter is not an ideal shape,therefore, Aðh cÞwas calibrated by procedure given by Oliver and Pharr[6].2.4.Indentation fracture toughnessEvans–Davis model[5]was used for the evaluation of indentation fracture toughness K c in this study.This model describes K c as follows:K c¼0:4636ðP v=a3=2ÞðE nano=H vÞ2=510F;ð4ÞF¼À1:59À0:34BÀ2:02B2þ11:23B3À24:97B4þ16:23B5;ð5ÞB¼log10ðc=aÞ;ð6Þwhere P v is a peak load in Vickers hardness test.Mod-ulus from the third division of the indentation curve in the nanoindentation test was used as E nano in calculating K c because the scattering of E nano among12–15inden-tations was the smallest.Behavior of H v change was different from that of H nano as described later,possiblyTable1Summary of the irradiation conditions in this studyReactor(cycle,capsule, position,etc.)Irradiationtemperature/°CDose/dpa_SiCHFIR14J30068007.7HFBR SiC-1Pos.1960–1150 1.1Pos.2(À2)960–1150 1.1Pos.2960–1150 1.1Pos.3890–980 1.1Pos.4250–270 1.1Pos.5385 1.1Pos.680–90 1.1V4Pos.11050.15Pos.21600.15Pos.32560.151164S.Nogami et al./Journal of Nuclear Materials307–311(2002)1163–1167due to the difference of the shape of indenter-tip and the calculation method between two tests.The relation between an ideal shaped tip are described as H v¼0:927H nano in the open literature[7].3.Results and discussionThe dependencies on the irradiation temperature of Vickers hardness H v and indentation hardness H nano of CVD-SiC irradiated up to various doses are shown in Figs.1and2,respectively.The error bars denote plus or minus of one standard deviation calculated from4to9 data points for H v and12to15data points for H nano at each irradiation conditions.From the results,it is clear that the hardness increased at all neutron doses and temperatures studied.The value H nano of the non-irra-diated sample($37.8GPa)was almost the same as the open literature values($36GPa)[8].Furthermore,re-sults suggest that the hardness of CVD-SiC is indepen-dent on the irradiation dose above a saturation value, while significantly dependent on the irradiation tem-perature.Such low-dose saturation in hardness was shown previously on identical materials to those used in this study irradiated in HFIR[8].The hardness is shown to rapidly increase with temperature below100°C and with almost no change observed above100°C.It isnoted that,for temperatures below$180°C,amorphi-zation of SiC is possible leading to a decreased hardness of$55%[9].However,the material of this study did not undergo amorphization.The ratio of the irradiated to the non-irradiated hardness was about119%for H v and 110%for H nano.The elastic modulus also exhibits a significant de-pendence on the irradiation temperature and indepen-dence on the irradiation dose shown in Fig.3.The error bars denote the same meaning as in H nano.The result show the rapid reduction of E nano with the irradiation temperature below100°C from$490GPa for the non-irradiated one to$400GPa for the irradiated one. Elastic modulus E nano of the irradiated samples,how-ever,gradually recovered and showed almost the same value as the non-irradiated value when irradiated above 1000°C.The observed trends in the elastic modulus can be related with the swelling summarized in the open liter-ature[10].The physical expansion for this decrease in as-irradiated elastic modulus is based on the accumula-tion of point defects(vacancies)in the SiC crystal.It is well known[11]that neutron-irradiation-induced defects are more stable during low-temperature irradiation and tend to self-anneal with increased irradiation tempera-ture until the vacanciesÕdensity responsible for lattice strain is so low at1000°C that essentially no swelling occur.The elastic modulus is considered to be physically dependent on the interatomic bond strength,atomic spacing and bond density,therefore,the modulus con-sidered to decrease as irradiation-induced strain is re-duced at high irradiationtemperatures.Fig.2.Dependence of the indentation hardness H nano on the irradiation temperature and dose,which are calculated using the method proposed by Oliver and Pharr[6].Fig.1.Dependence of the Vickers hardness H v on the irradi-ation temperature and dose.S.Nogami et al./Journal of Nuclear Materials307–311(2002)1163–11671165Fig.4shows the dependence of the indentation fracture toughness K c of CVD-SiC irradiated in various doses on the irradiation temperature.K c was calculated using Evans–Davis model [5]as described before,in which the average data of E nano in each irradiation conditions was used as the elastic modulus,and thus,the number of the data points of K c in each irradiation conditions corresponds to that of Vickers hardness test (4–9points).The error bars,therefore,only include the scatter of Vickers hardness H v ,indentation diagonal 2a ,and crack length c .The value K c of the non-irradiated CVD-SiC in this study (5.08MPa m À1=2)was about twice larger than that in the open literature [8].It is noted that,while this technique is quantitative,the measurement of crack length is somewhat subjective,hence data should be regarded as qualitative in nature.A small reduction of K c below $400°C,and an increase in it with increased irradiation temperature to $1000°C was observed.The value K c for CVD-SiC irradiated to 7.7dpa at 800°C in HFIR increased by about 30%compared with the non-irradiated value.Indentation fracture toughness of SiC is considered to be strongly affected by the plastic deformation,e.g.,dislocation motion,under compression introduced by indentation tests and crack growth behavior.The previous work suggested that carbon interstitials in SiC are considered to be mobile below about 800°C ac-cording to the molecular dynamics simulations [12],and they are considered to form a dislocation-loop.The previous work by Clinard et al.suggested that theincrease of the fracture toughness of Al 2O 3and MgO is attributed to crack pinning and deflection due to the defect clusters [13].The increase of K c for CVD-SiC with temperature in this study might be due to the increase of the mobility of a dislocation and crack pinning and deflection.Mechanical properties of CVD-SiC are significantly changed by neutron irradiation as described here,how-ever,in order to clarify the mechanism of the mechanical properties change,the detail of the microstructural de-velopment due to neutron irradiation must be investi-gated,for example by transmission electron microscopy,in future work.Additionally,data of the mechanical properties at temperature range of 400–800°C and above 1100°C are also required to complete the data systems of the neutron irradiated SiC.4.SummaryThe indentation fracture toughness of CVD-SiC ir-radiated up to 1024–1025n/m 2(E n >0:1MeV)at the temperature of 80–1150°C in HFIR and HFBR was investigated and was compared with other mechanical properties (hardness and elastic modulus).The following results were obtained:(1)The hardness (Vickers hardness H v and indentationhardness H nano )showed a similar irradiation-induced behavior.They rapidly increased with theirradiationFig.4.Dependence of the indentation fracture toughness K c on the irradiation temperature and dose,which are calculated by Evans–Davis model[5].Fig.3.Dependence of the elastic modulus E nano on the irradi-ation temperature and dose,which are calculated using the method proposed by Oliver and Pharr [6].1166S.Nogami et al./Journal of Nuclear Materials 307–311(2002)1163–1167temperature below100°C and showed almost no change above100°C.(2)The result showed the rapid reduction of the elasticmodulus E nano with the irradiation temperature be-low100°C.Elastic modulus of the irradiated sam-ples,however,gradually recovered and showed almost the same value as the non-irradiated value when irradiated above1000°C.(3)A small reduction in fracture toughness K c belowabout400°C was seen,with a significant increase as temperature is increased up to about1000°C. (4)Mechanical properties investigated in this studywere significantly dependent on the irradiation tem-perature,while generally independent on the irradi-ation dose.AcknowledgementsThe authors are grateful to all the staffs in Oak Ridge National Laboratory and Brookhaven National Labo-ratory relating to irradiation test,indentation test and sample preparation,etc.This work was supposed by the JUPITER(Japan–USA Program of Irradiation Testing for Fusion Research)program.References[1]A.Hasegawa,A.Kohyama,R.H.Jones,L.L.Snead,B.Riccardi,J.Nucl.Mater.283–287(2000)128.[2]P.Fenici,A.J.Frias Rebelo,R.H.Jones,A.Kohyama,L.L.Snead,J.Nucl.Mater.258–263(1998)215.[3]L.L.Snead,R.H.Jones,A.Kohyama,P.Fenici,J.Nucl.Mater.233–237(1996)26.[4]L.H.Rovner,G.R.Hopkins,Nucl.Tech.29(1976)274.[5]A.G.Evans,in:Proceedings of the11th National Sympo-sium on Fracture Mechanics,Part II,ASTM,Philadelphia, PA,1979,p.112.[6]W.C.Oliver,G.M.Pharr,J.Mater.Res.7(6)(1992)1564.[7]D.Tabor,in:Hardness of Metals,Oxford University,London,UK,1951,p.98.[8]M.C.Osborne,J.C.Hay,L.L.Snead,D.Steiner,J.Am.Ceram.Soc.82(9)(1999)2490.[9]L.L.Snead,J.C.Hay,J.Nucl.Mater.273(1999)213.[10]L.L.Snead,M.C.Osborne,R.A.Lowden,J.Strizak,R.J.Shinavaski,K.L.More,W.S.Eatherly,J.Bailey,A.M.Williams,J.Nucl.Mater.253(1998)20.[11]R.Blackstone,E.H.Voice,J.Nucl.Mater.39(1971)319.[12]H.Huang,N.Ghoniem,J.Nucl.Mater.212–215(1994)148.[13]F.W.Clinard,G.F.Hurley,R.A.Youngman,L.W.Hobbs,J.Nucl.Mater.133–134(1985)701.S.Nogami et al./Journal of Nuclear Materials307–311(2002)1163–11671167。
武汉“PEP”2024年11版小学4年级下册第8次英语第四单元综合卷

武汉“PEP”2024年11版小学4年级下册英语第四单元综合卷考试时间:100分钟(总分:110)A卷考试人:_________题号一二三四五总分得分一、综合题(共计100题)1、听力题:The gas released during fermentation is primarily _______.2、听力题:In a chemical equilibrium, the rate of the forward reaction equals the rate of the _____.3、听力题:A mixture that has a uniform appearance is called a ______ mixture.4、What is the primary function of leaves on a plant?a. To provide supportb. To absorb waterc. To make foodd. To store nutrients答案:c5、 Age marks the beginning of human ________ (文明). 填空题:The Suez6、填空题:The sun is _______ (在升起).7、填空题:The __________ (历史的探索精神) promotes inquiry.8、What is the name of the famous wizard in J.K. Rowling's series?A. GandalfB. DumbledoreC. Harry PotterD. Frodo答案:C9、听力题:A horse gallops across the _____.10、听力题:He rides his ___ (skateboard) to school.11、填空题:I saw a _______ (美丽的) butterfly.12、听力题:We eat ___ (breakfast/lunch) together.13、填空题:The ancient Greeks created ________ to tell stories about their gods.14、听力题:The chemical symbol for chromium is ____.15、填空题:The ________ was a major event in the history of Europe.16、What sound does a dog make?A. MeowB. MooC. BarkD. Quack17、填空题:The _______ (The American Civil War) had lasting impacts on US history.18、What is 10 3?A. 6B. 7C. 8D. 9答案: B. 719、听力题:We are going to _____ a movie tonight. (watch)20、填空题:A ________ (泥土) test can improve growth.21、填空题:The _____ (袋鼠) has a pouch to carry its baby. 袋鼠有一个育儿袋来携带它的宝宝。
骨科英语试题及答案

骨科英语试题及答案一、选择题(每题2分,共20分)1. Which of the following is not a common fracture type?A. Simple fractureB. Compound fractureC. Greenstick fractureD. Sprain2. The term "osteoporosis" refers to:A. A bone infectionB. A bone tumorC. A decrease in bone densityD. A bone fracture3. Which of the following is a symptom of osteoarthritis?A. RednessB. SwellingC. Joint pain and stiffnessD. Fever4. The primary function of the spine is to:A. Support the body weightB. Facilitate blood circulationC. Aid in respirationD. Assist in digestion5. The correct English term for "骨折" is:A. Bone breakB. Bone injuryC. FractureD. Bone disease6. The "ACL" in orthopedics stands for:A. Anterior cruciate ligamentB. Anterior cervical ligamentC. Anterior central ligamentD. Anterior cervical ligament7. What does "MRI" stand for in medical terms?A. Magnetic resonance imagingB. Medical radiology imagingC. Medical research imagingD. Medical radio imaging8. The most common site for a bone tumor is:A. SkullB. FemurC. HumerusD. Tibia9. Which of the following is a non-surgical treatment for fractures?A. CastingB. SurgeryC. AmputationD. Transfusion10. The term "osteotomy" refers to the surgical cutting of:A. MuscleB. LigamentC. BoneD. Tendon二、填空题(每空1分,共10分)1. The most common type of bone fracture is the __________ fracture.2. The medical term for a dislocation of the shoulder is__________.3. In orthopedics, "scoliosis" refers to a __________ of the spine.4. A __________ is a device used to immobilize a fractured limb.5. The process of bone healing is known as __________.6. The English term for "骨关节炎" is __________.7. The abbreviation "CT" stands for __________.8. A __________ is a type of joint replacement surgery.9. The English term for "骨密度" is __________.10. The most common cause of osteoporosis is __________.三、简答题(每题5分,共20分)1. What are the three main types of bone fractures?2. Describe the function of the knee joint.3. Explain the difference between osteoporosis and osteoarthritis.4. What are the common symptoms of a sprain?四、翻译题(每题5分,共10分)1. 翻译以下句子:"The patient has a history of osteoporosis and is at high risk for fractures."2. 翻译以下句子:"After a thorough examination, theorthopedic surgeon recommended an osteotomy to correct the deformity."五、论述题(共40分)1. Discuss the importance of physical therapy in the treatment and rehabilitation of orthopedic patients. (15分)2. Explain the role of orthopedic surgery in the management of traumatic injuries. (15分)3. Describe the process of bone healing and the factors that can affect it. (10分)答案:一、选择题1-5 D C C A C6-10 A A B A C二、填空题1. Simple2. Dislocation3. Lateral curvature4. Cast5. Ossification6. Osteoarthritis7. Computed Tomography8. Arthroplasty9. Bone density10. Aging三、简答题1. The three main types of bone fractures are simple, compound, and comminuted fractures.2. The knee joint is a complex hinge joint that allows for flexion and extension of the leg and contributes to stability and support during movement.3. Osteoporosis is a condition characterized by a decrease in bone density, while osteoarthritis is a degenerative joint disease that involves the breakdown of cartilage and bone at the joint's surface.4. Common symptoms of a sprain include pain, swelling, bruising, and limited range of motion.四、翻译题1. The patient has a history of osteoporosis and is at high risk for fractures.翻译:患者有骨质疏松症的病史,骨折风险较高。
高分子材料双语往年考题(2)
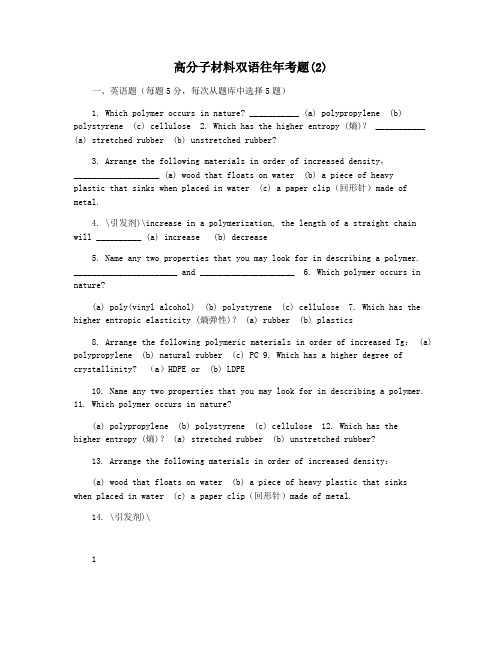
高分子材料双语往年考题(2)一、英语题(每题5分,每次从题库中选择5题)1. Which polymer occurs in nature? ___________ (a) polypropylene (b) polystyrene (c) cellulose2. Which has the higher entropy (熵)? ___________ (a) stretched rubber (b) unstretched rubber?3. Arrange the following materials in order of increased density,___________________ (a) wood that floats on water (b) a piece of heavyplastic that sinks when placed in water (c) a paper clip(回形针)made of metal.4. \引发剂)\increase in a polymerization, the length of a straight chainwill __________ (a) increase (b) decrease5. Name any two properties that you may look for in describing a polymer. _______________________ and _____________________6. Which polymer occurs in nature?(a) poly(vinyl alcohol) (b) polystyrene (c) cellulose 7. Which has the higher entropic elasticity (熵弹性)? (a) rubber (b) plastics8. Arrange the following polymeric materials in order of increased Tg: (a) polypropylene (b) natural rubber (c) PC 9. Which has a higher degree of crystallinity? (a)HDPE or (b) LDPE10. Name any two properties that you may look for in describing a polymer.11. Which polymer occurs in nature?(a) polypropylene (b) polystyrene (c) cellulose 12. Which has thehigher entropy (熵)? (a) stretched rubber (b) unstretched rubber?13. Arrange the following materials in order of increased density:(a) wood that floats on water (b) a piece of heavy plastic that sinkswhen placed in water (c) a paper clip(回形针)made of metal.14. \引发剂)\1increase in a polymerization, the length of a straight chain will (a) increase (b) decrease (c) keep constant15. Name any two properties that you may look for in describing a polymer.16. Which polymer occurs in nature?(a) polyethylene (b) polystyrene (c) proteins17. When we stretch a rubber, the conformational entropy (熵) of rubber chains will? (a) increase (b) decrease (c) keep constant18. Arrange the following polymeric materials in order of increased Tg:(a) polyisoprene (b) PE (c) PC19. Which has the higher cohesive energy density (内聚能密度)? (a) elastomer (b) fiber (c) thermoplastics 20. Which has a higher degree of crystallinity? (a) HDPE (b) LDPE21 Which of the following can not be used as elastomer? ( )A. EPDMB. polyethyleneC. SBSD. natural rubber 22 Which one can most easily crystallize in following polymers? ( ) A. PET B. POM C. PVC D. PMMA23 Which of the polymers is generally used to make agricultural films ?( A. PE B. PP C. PVC D. PS 24. Which will have the higher crystallinity? A. atactic PP B. isotactic PP?25 Which has the highest heat resistance in following polymers ( ) A. polycarbonate B. polyethylene C. polystyrene D. polyimide 26. Which ofthe following are polymers?( )A. ethyleneB. proteinC. celluloseD. polyethylene 27. Whichof the following are thermoplastics? ( )A. hard rubberB. BakeliteC. PVCD. polystyrene 28. Which hasthe higher cohesive energy density (CED) ( ) A. an elastomer B. a fiber?29. Which of the following are engineering resins? ( )) 2A. polystyreneB. polypropyleneC. polyimide30. Which of the following fibers can not manufactured by melt spinning ? ( ) A. PET B. nylon C. polypropylene D. polyacrylonitrile二、论述题1. 合成纤维有那几种主要纺丝方法?为什么不可以采用熔体纺丝的方法加工聚丙烯腈纤维?如果你想采用熔体纺丝方法加工聚丙烯腈纤维,你需要从原料上作那些改进?请说明原因。
稀土元素对AZ91D压铸镁合金高周疲劳性能的影响
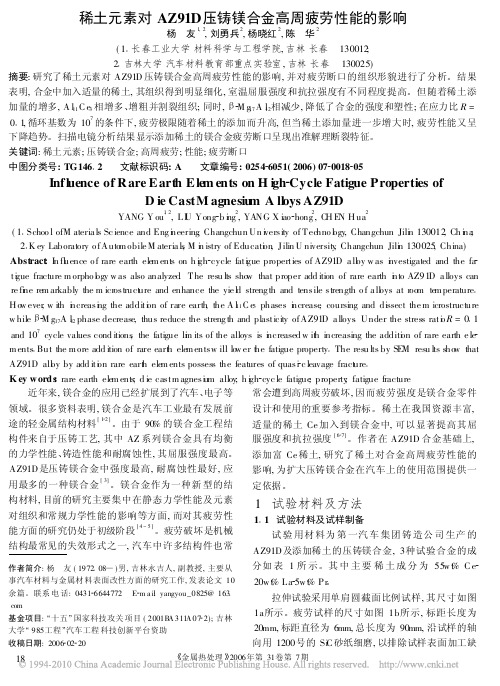
1 试验材料及方法
1 1 试验材料及试样制备 试 验 用材 料为 第 一汽 车 集团 铸 造公 司 生产 的 AZ91D 及添加稀土的压铸镁合金 , 3 种试验合金的成 分如 表 1 所 示。其 中 主 要 稀 土 成 分 为 55w % t Ce 20w % t L a 5w % t Pr 。 拉伸试验采用单肩圆截面比例试样 , 其尺寸如图 1a 所示。疲劳试样的尺寸如图 1b 所示 , 标距长度为 20mm, 标距直径为 6mm, 总长度为 90mm, 沿试样的轴 向用 1200 号的 SiC 砂纸细磨 , 以排除试样表面加工缺
7
2 试验结果与分析
2 1 显微组织观察与分析 图 2 为不同成分压铸镁合金的显微组织和相应的 X 射线衍射分析结果。由图 2a可见, 合金的组织是由 尺寸较大的块状白色基体相和不连续网状分布的灰色
图 1 拉伸 试样尺寸 ( a) 和高周疲劳试 样尺寸 ( b) F ig . 1 T he size o f tens ile sa m ple ( a) and h igh cyc le fatigue sa mp le( b)
。疲劳破坏是机械
结构最常见的失效形式之一, 汽车中许多结构件也常
作者简介 : 杨 友 ( 1972 08 ) 男 , 吉林永吉人 , 副教授 , 主要从 事汽车材料与金属材 料表面改性方面的研究工作 , 发表论文 10 余篇。联系 电 话 : 0431 6644772 com E m a i: l yangyou_0825@ 163.
稀土元素对 AZ91D 压铸镁合金高周疲劳性能的影响
杨 友 , 刘勇兵 , 杨晓红 , 陈
1, 2 2 2
华
2
( 1 长春工业大学 材料科学与工程学院, 吉林 长春
12)2012机械合金化制备高熵合金研究进展_陈哲
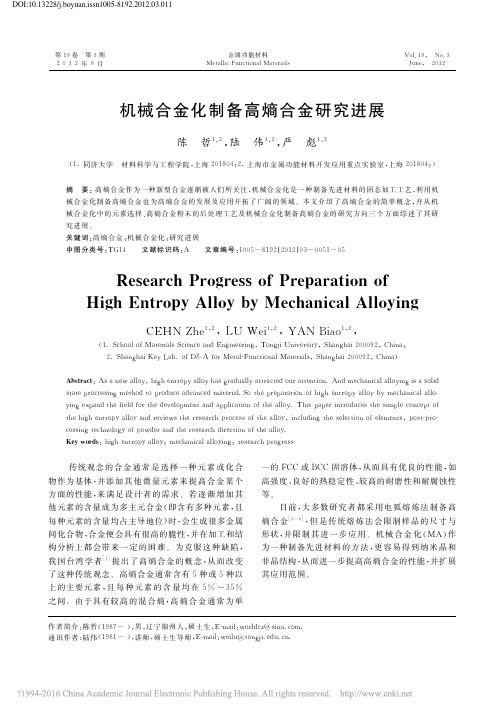
力 的 作 用 ,同 时 发 生 扩 散 和 固 相 反 应 ,最 后 可 以 获 得 组织和组分分布均匀的纳米晶或非晶颗粒。相比于 其他制备方法 ,机 [14~16] 械合金化制备的 高 熵 合 金 粉 末具有稳定的微观 结 构、良 好 的 化 学 均 质 性 和 优 异 的室温加工性能。
传统观念的合金通常是选择一种元素或化合 物作为基体,并添加 其 他 微 量 元 素 来 提 高 合 金 某 个 方面的性能,来 满 足 设 计 者 的 需 求。 若 逐 渐 增 加 其 他 元 素 的 含 量 成 为 多 主 元 合 金 (即 含 有 多 种 元 素 ,且 每 种 元 素 的 含 量 均 占 主 导 地 位 )时 ,会 生 成 很 多 金 属 间 化 合 物 ,合 金 便 会 具 有 很 高 的 脆 性 ,并 在 加 工 和 结 构分析上都会带来一定的困难。为克服这种缺陷, 我国 台 湾 学 者 提 [1] 出 了 高 熵 合 金 的 概 念,从 而 改 变 了这种传统观念。高熵合金通常含有5种或5种以 上的主要元 素,且 每 种 元 素 的 含 量 均 在 5% ~35% 之间。由于具有较 高 的 混 合 熵,高 熵 合 金 通 常 为 单
S.Varalakshmi等 人 在 [21] 制 备 高 熵 合 金 中 添 加了 Zn 元 素,成 功 制 备 了 CuNiCoZnAlTi高 熵 合 金,合金主要为 BCC 结构,晶粒尺寸在10nm 以下。 合金粉末经 过 真 空 热 压,其 相 对 密 度 为 99.5%,合 金的硬度 和 抗 压 强 度 分 别 达 到 7.55 GPa 和 2.36 GPa。Yu-Liang Chen等人 利 [22] 用机 械 合 金 化 制 备 了 Cu0.5NiAlCoCrFeTiMo,并 研 究 了 几 种 元 素 的 合 金化序列,合金体 系 的 合 金 化 顺 序 为:Al→Cu→Co →Ni→Fe→Ti→Cr→ Mo(按 合 金 化 率 递 减 的 顺 序 排列)。他们发现元 素 的 合 金 化 率 与 其 熔 点 的 相 关 性最大,这种关联的 机 制 通 过 熔 点 对 于 固 态 扩 散 的 影响和机械粉碎作 用,对 最 终 的 合 金 化 具 有 决 定 性 作用。
球差电镜难熔高熵合金
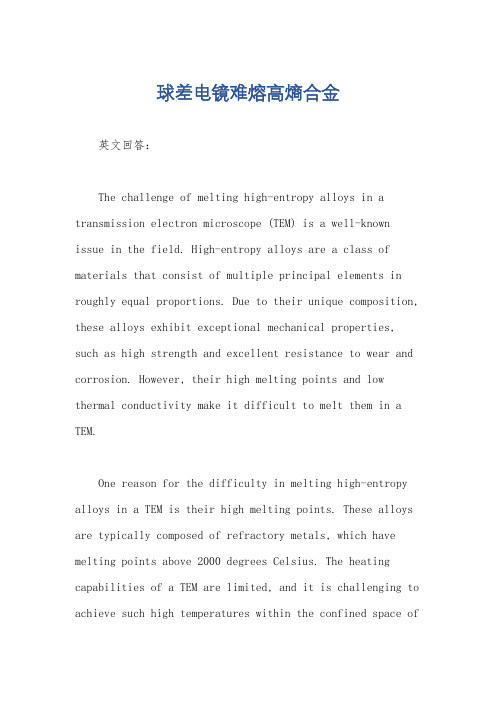
球差电镜难熔高熵合金英文回答:The challenge of melting high-entropy alloys in a transmission electron microscope (TEM) is a well-known issue in the field. High-entropy alloys are a class of materials that consist of multiple principal elements in roughly equal proportions. Due to their unique composition, these alloys exhibit exceptional mechanical properties, such as high strength and excellent resistance to wear and corrosion. However, their high melting points and low thermal conductivity make it difficult to melt them in a TEM.One reason for the difficulty in melting high-entropy alloys in a TEM is their high melting points. These alloys are typically composed of refractory metals, which have melting points above 2000 degrees Celsius. The heating capabilities of a TEM are limited, and it is challenging to achieve such high temperatures within the confined space ofthe microscope. As a result, the alloy may not reach its melting point, and the desired microstructural changes cannot be observed.Another factor that contributes to the challenge is the low thermal conductivity of high-entropy alloys. The low thermal conductivity prevents efficient heat transferwithin the material, making it difficult to achieve uniform heating. This can lead to localized melting or overheating, which may result in undesirable microstructural changes.To overcome these challenges, researchers have explored various techniques. One approach is to use a focused ion beam (FIB) to locally heat the alloy. By using a high-energy ion beam, it is possible to achieve localized heating and melt the alloy in specific regions of interest. This technique allows researchers to study the microstructural changes in the melted regions and gain insights into the behavior of high-entropy alloys under extreme conditions.Another technique involves the use of laser heating incombination with a TEM. By focusing a laser beam onto the alloy, it is possible to achieve higher temperatures and overcome the limitations of the TEM's heating capabilities. The laser heating technique has been successfully used to melt high-entropy alloys and study their microstructural changes in real-time.In conclusion, melting high-entropy alloys in a transmission electron microscope is a challenging task due to their high melting points and low thermal conductivity. However, researchers have developed techniques such as focused ion beam heating and laser heating to overcome these challenges and study the microstructural changes in these alloys. These advancements in TEM technology have provided valuable insights into the behavior of high-entropy alloys and have the potential to drive further advancements in materials science.中文回答:球差电镜难以熔化高熵合金是该领域一个众所周知的问题。
材料科技英语试题及答案
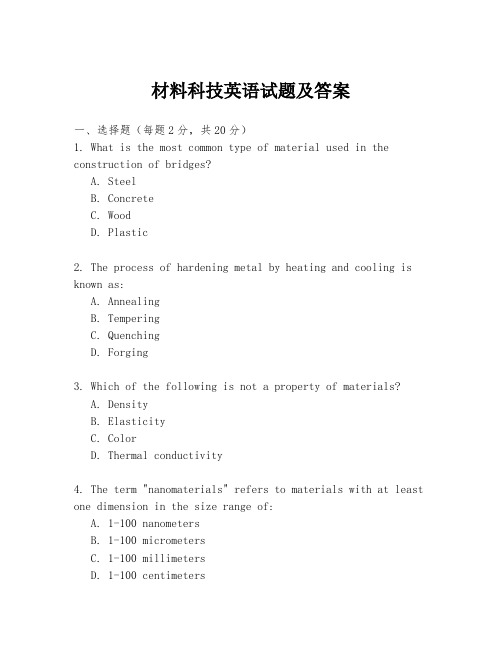
材料科技英语试题及答案一、选择题(每题2分,共20分)1. What is the most common type of material used in the construction of bridges?A. SteelB. ConcreteC. WoodD. Plastic2. The process of hardening metal by heating and cooling is known as:A. AnnealingB. TemperingC. QuenchingD. Forging3. Which of the following is not a property of materials?A. DensityB. ElasticityC. ColorD. Thermal conductivity4. The term "nanomaterials" refers to materials with at least one dimension in the size range of:A. 1-100 nanometersB. 1-100 micrometersC. 1-100 millimetersD. 1-100 centimeters5. What is the primary function of a catalyst in a chemical reaction?A. To increase the temperatureB. To provide energyC. To speed up the reaction without being consumedD. To slow down the reaction6. The strength of a material is often measured by its:A. DuctilityB. Tensile strengthC. MalleabilityD. Hardness7. Which of the following is a type of composite material?A. GlassB. CeramicC. AlloyD. Fiberglass8. The SI unit for measuring thermal expansion is:A. CelsiusB. KelvinC. JouleD. Degree Celsius per meter Kelvin9. What is the main difference between amorphous and crystalline materials?A. ColorB. ShapeC. Atomic arrangementD. Density10. The term "strain" in materials science refers to:A. The amount of deformation per unit lengthB. The force applied to a materialC. The change in shape of a materialD. The resistance to deformation二、填空题(每题2分,共20分)11. The process of changing the physical or chemical properties of a material is known as ________.12. The ________ of a material is its ability to resist deformation.13. The ________ is a material that can withstand high temperatures without significant loss of strength.14. The ________ of a material is the measure of its ability to conduct heat.15. A ________ is a material that can be easily deformed without breaking.16. The ________ of a material is its resistance to wear or abrasion.17. The ________ is the process of joining two pieces of metal by heating them to a molten state.18. The ________ is the process of removing excess material to create a desired shape.19. The ________ of a material is its ability to return to its original shape after deformation.20. The ________ is the study of the behavior of materials under various conditions.三、简答题(每题10分,共30分)21. Explain the difference between ductile and brittle materials.22. Describe the process of annealing and its effects on materials.23. Discuss the importance of material selection in the design of aeronautical components.四、论述题(每题15分,共30分)24. Discuss the role of materials science in the development of new technologies.25. Analyze the environmental impact of material production and disposal, and suggest ways to minimize these effects.答案:一、1-5: B C C A C6-10: B D D A C A二、11. Alteration12. Rigidity13. Refractory14. Thermal conductivity15. Ductile material16. Hardness17. Fusion18. Machining19. Elasticity20. Material science三、21. 略22. 略23. 略四、24. 略25. 略。
超低温力学性能测定
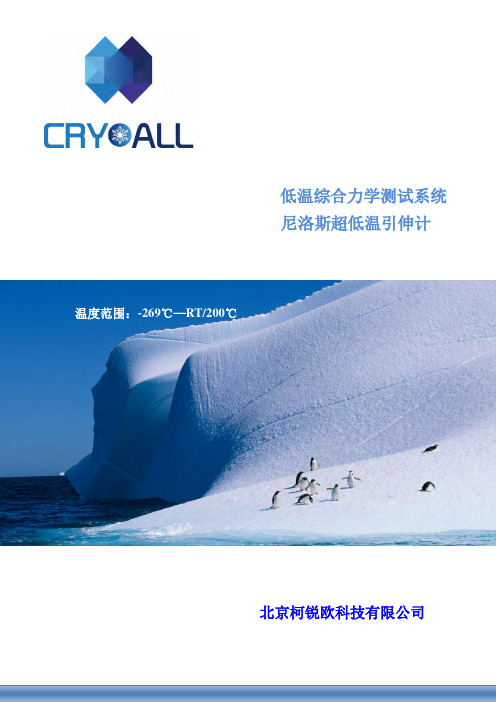
该系统一种降温方式是采用低温液体(液氮、液氦)降温,另一种降温方式是采用低温制冷机直接降温 (不消耗液氮和液氦)。两种方式可都可实现:4.2K-300K(-269℃—室温)连续控温;温度稳定性:±1K(±1℃).
设备名称
电液伺服 低温万能试验机系统
电子低温万能试验机
主要参数
轴向载荷:10kN-10000 kN 扭 矩:1000 N•m 频率范围:0.01-30Hz 轴向载荷:5 N-600 kN
UK 杜伦大学 英国科学磁体公司
Switzerland 布鲁克拜厄斯宾有限公司(核磁共振) 日内瓦大学
Spain 融合能源,巴塞罗那(核研究)
USA 美国国家标准与技术研究院 NIST 休斯顿大学 美国 Affiliated Engineering 公司 塔夫斯大学
Japan 日本宇宙航空研究开发机构 JAXA 日本国立材料研究所 NIMS 京都大学 岩手大学
超轻重量:0.6-10 克;标距范围:10-50mm;测量范围:±2mm-±4mm; 适用于线状材料或薄带状材料的测试。如:低温超导线,高温超导带材
典型用户
Germany 德国 EAS 公司 卡尔斯鲁厄理工学院 KIT 德国布鲁克 HTS 公司 林德集团 西门子
France 法国原子能研究中心 CEA 液化空气集团
多年以来,中国科学院理化技术研究所材料低温力学性能测试平台一直为我国航天航空部门、大科学工 程、国内外高校和科研院所提供低温力学测试服务,获得广泛好评。
此低温力学性能测试平台承担了我国ITER采购包中重 力支撑、绝缘材料、导体铠甲及线圈盒等结构材料低温力学 性能表征任务。此平台测试的ITER大型超导磁体超导导体铠 甲材料低温力学性能数据均通过与ITER 计划低温力学测试 仲裁机构-德国卡尔斯鲁厄理工学院(KIT)测试比对。获得 了ITER 国际组织低温力学测试平台的资质认证。我国ITER 采购包中所有结构材料低温力学性能表征均在此低温力学性 能测试平台完成。
第一原理计算高熵合金CoCrFeNi的弹性性质
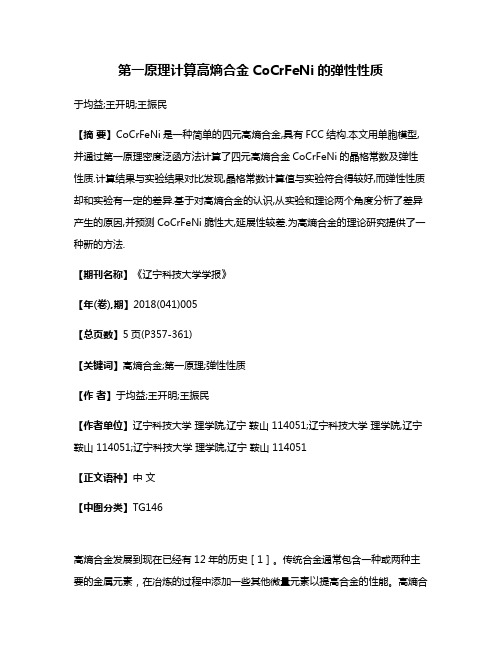
第一原理计算高熵合金CoCrFeNi的弹性性质于均益;王开明;王振民【摘要】CoCrFeNi是一种简单的四元高熵合金,具有FCC结构.本文用单胞模型,并通过第一原理密度泛函方法计算了四元高熵合金CoCrFeNi的晶格常数及弹性性质.计算结果与实验结果对比发现,晶格常数计算值与实验符合得较好,而弹性性质却和实验有一定的差异.基于对高熵合金的认识,从实验和理论两个角度分析了差异产生的原因,并预测CoCrFeNi脆性大,延展性较差.为高熵合金的理论研究提供了一种新的方法.【期刊名称】《辽宁科技大学学报》【年(卷),期】2018(041)005【总页数】5页(P357-361)【关键词】高熵合金;第一原理;弹性性质【作者】于均益;王开明;王振民【作者单位】辽宁科技大学理学院,辽宁鞍山 114051;辽宁科技大学理学院,辽宁鞍山 114051;辽宁科技大学理学院,辽宁鞍山 114051【正文语种】中文【中图分类】TG146高熵合金发展到现在已经有12年的历史[1]。
传统合金通常包含一种或两种主要的金属元素,在冶炼的过程中添加一些其他微量元素以提高合金的性能。
高熵合金是由多种等量或大约等量金属形成的合金,具有硬度高,强度大,耐磨耐腐蚀及热稳定性[2]良好等特点,引起了广泛关注和研究兴趣[3-5]。
弹性性质是衡量材料力学性质的重要参数,其对高熵合金的筛选以及应用都起着关键作用。
高熵合金含有多种元素,实验上很难合成一致性好且没有缺陷的合金块体样品。
这就导致大部分高熵合金的弹性性能无法测量。
第一原理计算晶体材料弹性性质的正确性已经被很多实验所证实,因此可以作为研究高熵合金弹性性质的重要补充手段。
高熵合金由于含有多种元素,按照传统的方式利用原子的占位进行理论计算筛选是无法实现的。
迄今为止,SQS(Special quasirandom structure),EMTO (Exact muffin-tin orbitals),MaxEnt(Maximum entropy)这3种方法被用于研究高熵合金。
医护英语试题及答案
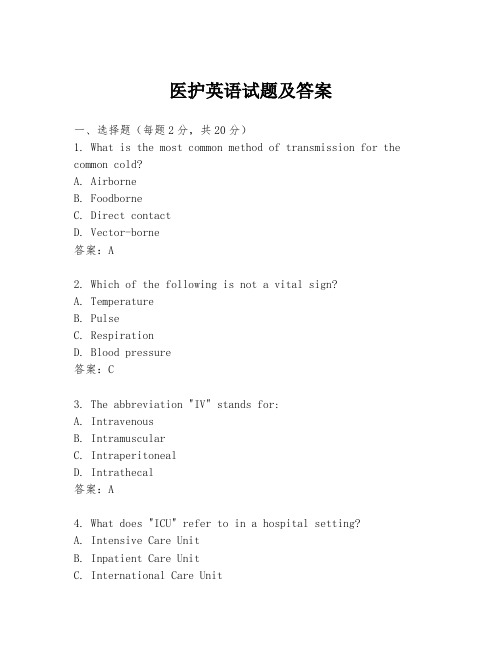
医护英语试题及答案一、选择题(每题2分,共20分)1. What is the most common method of transmission for the common cold?A. AirborneB. FoodborneC. Direct contactD. Vector-borne答案:A2. Which of the following is not a vital sign?A. TemperatureB. PulseC. RespirationD. Blood pressure答案:C3. The abbreviation "IV" stands for:A. IntravenousB. IntramuscularC. IntraperitonealD. Intrathecal答案:A4. What does "ICU" refer to in a hospital setting?A. Intensive Care UnitB. Inpatient Care UnitC. International Care UnitD. Intermittent Care Unit答案:A5. Which of the following is a symptom of anemia?A. FatigueB. High feverC. Excessive sweatingD. Rapid weight gain答案:A6. The term "auscultation" refers to listening to sounds within the body using a:A. StethoscopeB. OtoscopeC. OphthalmoscopeD. Thermometer答案:A7. A patient is said to be in "shock" when:A. They are experiencing severe painB. They are unconsciousC. Their blood pressure is significantly lowD. They have a high fever答案:C8. What is the purpose of a "suture" in medical terms?A. To provide anesthesiaB. To close a woundC. To remove a foreign objectD. To diagnose a condition答案:B9. The "HCG" test is used to detect:A. DiabetesB. PregnancyC. AnemiaD. Infection答案:B10. Which of the following is a type of imaging technique used in medical diagnostics?A. X-rayB. Blood testC. BiopsyD. Electrocardiogram答案:A二、填空题(每题2分,共20分)1. The medical term for a broken bone is ____________.答案:fracture2. A doctor's written instructions for the use of medication are called a ____________.答案:prescription3. The process of removing a damaged or diseased organ is called ____________.答案:surgery4. The study of the causes and control of diseases is known as ____________.答案:epidemiology5. A ____________ is a healthcare professional who specializes in the care of patients with mental disorders.答案:psychiatrist6. The medical term for a surgical incision is ____________. 答案:incision7. The ____________ is the part of the hospital where critically ill patients are treated.答案:intensive care unit8. A ____________ is a healthcare professional who provides care to patients in their homes.答案:nurse9. The ____________ is a medical device used to measure blood pressure.答案:sphygmomanometer10. The term ____________ refers to the process of removing a patient's blood for analysis.答案:blood draw三、简答题(每题10分,共40分)1. Explain the difference between a "nurse" and a "nurse practitioner".答案:A nurse is a healthcare professional who provides care to patients under the supervision of a physician. A nurse practitioner (NP) is an advanced practice registered nurse who has completed additional education and training, allowingthem to diagnose and treat patients, prescribe medications, and often act as a primary care provider.2. Describe the role of a "physician assistant" in a medical setting.答案:A physician assistant (PA) is a healthcare professional who practices medicine under the supervision of a physician. They can perform physical exams, diagnose and treat illnesses, order and interpret tests, assist in surgery, and prescribe medications.3. What is the purpose of a "dialysis" treatment?答案:Dialysis is a medical treatment that removes waste products and excess fluids from the blood when the kidneysare unable to do so. It is used to treat patients with kidney failure or severe kidney disease.4. Explain the concept of "informed consent" in medical procedures.答案:Informed consent is the process by which a patient is given information about a medical procedure or treatment, including its risks and benefits, and then voluntarily agrees to undergo the procedure. It is a fundamental ethicalprinciple in healthcare that ensures the patient's autonomy and right to make decisions about their own medical care.。
热处理对CoCrFeMnNi-5%Mo高熵合金组织及性能的影响

热处理对CoCrFeMnNi-5%Mo高熵合金组织及性能的影响李田野;姚小飞;吕煜坤;周学阳;高巍【摘要】The paper aims to study the distribution of the second phase in CoCrFeMnNi-5%Mo high entropy alloy and its effect on the alloy's mechanical properties.The microstructure and properties of the CoCrFeMnNi-5% Mo high entropy alloy heat treated by different cooling methods were studied.The results are as follows.The as-cast CoCrFeMnNi-5%Mo high entropy alloys are of FCC single-phase organization.After being heat treated at 1 000 ℃for 4 hours, the s econd phase sigma phase (MoCr) was formed and its microstructure was of the FCC+sigma phase two phase.With the cooling speed increasing, the relative amounts of sigma phase in the CoCrFeMnNiMo-5% Mo high entropy alloy decreases.The sigma phase changes gradually from intracrystalline precipitation into grain boundaryprecipitation, and the distribution pattern changes from the intracrystalline strip and continuous shape into grain boundary strip.The sigma phase formed in the heat-treated CoCrFeMnNi-5%Mo high entropy alloy has the second phase strengthening effect, and its yield strength and tensile strength were significantly improved, and its plasticity was not significantly reduced, The fracture is a dimple fracture formed by micropore aggregation.%为了研究CoCrFeMnNi-5%Mo高熵合金中第二相的分布及其对力学性能的影响,采用不同冷却方式对CoCrFeMnNi-5%Mo高熵合金进行热处理,研究了其在不同冷却方式下的微观组织及性能.结果表明:铸态CoCrFeMnNi-5%Mo高熵合金为FCC单相组织,1 000℃/4h热处理后形成第二相σ相(MoCr相),其显微结构为FCC+σ相两相组织,随着冷却速度的增大,CoCrFeMnNi-5%Mo高熵合金中σ相的相对量减少,σ相由晶内析出逐渐变为晶界析出,分布形态由晶内条状和晶界连续状转变为晶界处条状.热处理态CoCrFeMnNi-5%Mo高熵合金中形成的σ相具有第二相强化作用,其屈服强度和抗拉强度均显著提高,塑性没有明显的降低,断裂为微孔聚集形成的韧窝型韧性断裂.【期刊名称】《西安工业大学学报》【年(卷),期】2019(039)001【总页数】6页(P80-85)【关键词】CoCrFeMnNi-5%Mo高熵合金;热处理;微观结构;力学性能【作者】李田野;姚小飞;吕煜坤;周学阳;高巍【作者单位】西安工业大学材料与化工学院, 西安 710021;西安工业大学材料与化工学院, 西安 710021;西安工业大学材料与化工学院, 西安 710021;西安工业大学材料与化工学院, 西安 710021;西安工业大学材料与化工学院, 西安 710021【正文语种】中文【中图分类】TG156.1高熵合金采用新型材料设计理念,具有高强度、高硬度、耐磨、耐腐蚀及高温力学性能好等特征[1-3],加之高熵合金灵活的成分和结构设计,增加了材料种类的多样性,因此具有重大的学术意义与应用价值,备受国内外学者的关注[4-6]。
断裂强力的英语
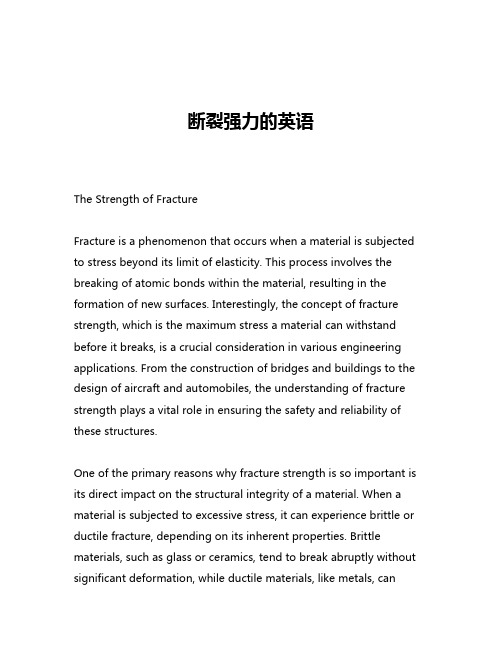
断裂强力的英语The Strength of FractureFracture is a phenomenon that occurs when a material is subjected to stress beyond its limit of elasticity. This process involves the breaking of atomic bonds within the material, resulting in the formation of new surfaces. Interestingly, the concept of fracture strength, which is the maximum stress a material can withstand before it breaks, is a crucial consideration in various engineering applications. From the construction of bridges and buildings to the design of aircraft and automobiles, the understanding of fracture strength plays a vital role in ensuring the safety and reliability of these structures.One of the primary reasons why fracture strength is so important is its direct impact on the structural integrity of a material. When a material is subjected to excessive stress, it can experience brittle or ductile fracture, depending on its inherent properties. Brittle materials, such as glass or ceramics, tend to break abruptly without significant deformation, while ductile materials, like metals, canundergo significant plastic deformation before reaching their fracture point. The ability to accurately predict and control the fracture behavior of a material is crucial in engineering design, as it allows for the development of structures that can withstand the expected loads and stresses they will encounter during their lifetime.Moreover, the study of fracture strength has led to the development of advanced materials and manufacturing techniques. For instance, the understanding of fracture mechanics has enabled the creation of high-strength alloys and composite materials that are widely used in aerospace and automotive applications. These materials are designed to exhibit specific fracture characteristics, such as high toughness or resistance to crack propagation, which can significantly enhance the safety and performance of the final product.In the field of civil engineering, the consideration of fracture strength is equally important. The construction of bridges, buildings, and other infrastructure requires a thorough understanding of the materials' fracture behavior to ensure that these structures can withstand the forces of nature, such as earthquakes, wind, and snow loads. Engineers must carefully analyze the fracture characteristics of the materials used in construction, taking into account factors like the size and geometry of the structural elements, the loading conditions, and the environmental factors that may influence the material's performance.The importance of fracture strength extends beyond the realm of structural engineering. In the medical field, the study of fracture mechanics has led to the development of advanced medical implants and prosthetic devices. These implants, such as hip and knee replacements, must be designed to withstand the stresses and loads imposed by the human body throughout the patient's lifetime. Fracture strength is a critical consideration in the design and manufacturing of these medical devices, as failure could lead to serious complications and the need for additional surgical interventions.Furthermore, the concept of fracture strength is not limited to the physical world. In the digital realm, the idea of fracture strength has been applied to the security of computer systems and networks. Cybersecurity experts often use the principles of fracture mechanics to analyze the resilience of digital systems against cyber attacks. By understanding how vulnerabilities and weaknesses can propagate through a network, these experts can develop strategies to strengthen the overall system and mitigate the impact of potential breaches.In conclusion, the strength of fracture is a fundamental concept in various fields of engineering and beyond. Its importance lies in its ability to predict and control the behavior of materials under stress,which is crucial for the design and construction of safe and reliable structures, devices, and systems. As technology continues to advance, the understanding of fracture strength will undoubtedly play an increasingly vital role in shaping the future of our built environment, medical advancements, and digital security. The continued research and application of fracture mechanics will undoubtedly lead to even more remarkable innovations and breakthroughs in the years to come.。
医学院英语试题及答案
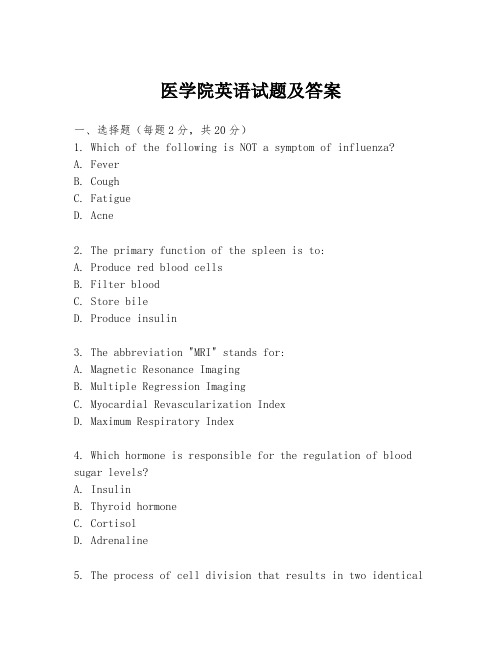
医学院英语试题及答案一、选择题(每题2分,共20分)1. Which of the following is NOT a symptom of influenza?A. FeverB. CoughC. FatigueD. Acne2. The primary function of the spleen is to:A. Produce red blood cellsB. Filter bloodC. Store bileD. Produce insulin3. The abbreviation "MRI" stands for:A. Magnetic Resonance ImagingB. Multiple Regression ImagingC. Myocardial Revascularization IndexD. Maximum Respiratory Index4. Which hormone is responsible for the regulation of blood sugar levels?A. InsulinB. Thyroid hormoneC. CortisolD. Adrenaline5. The process of cell division that results in two identicalcells is called:A. MitosisB. MeiosisC. ApoptosisD. Cytokinesis6. In medical terms, "icterus" refers to:A. JaundiceB. AnemiaC. EdemaD. Hemorrhage7. The "ABCs" of first aid are:A. Airway, Breathing, CirculationB. Ambulance, Bandage, CPRC. Alert, Breathe, CompressionD. Assess, Bleed, Clean8. The study of the structure of the body is called:A. PhysiologyB. AnatomyC. PathologyD. Pharmacology9. Which of the following is a type of cancer?A. MelanomaB. DiabetesC. InfluenzaD. Pneumonia10. The standard unit of measurement for blood pressure is:A. mmHgB. cmH2OC. kPaD. mmol/L二、填空题(每题2分,共20分)1. The largest organ in the human body is the __________.2. The medical term for a broken bone is __________.3. The __________ is the part of the brain responsible for voluntary movement.4. A __________ is a medical professional who specializes in the diagnosis and treatment of diseases of the heart and blood vessels.5. The process by which the body maintains a stable internal environment is called __________.6. The __________ is the largest gland in the human body and is responsible for metabolism.7. The __________ is a type of white blood cell that plays a critical role in the immune response.8. A __________ is a medical condition characterized by a persistently high level of glucose in the blood.9. The __________ is the study of the causes and effects of diseases.10. The __________ is a medical device used to measure blood pressure.三、阅读理解(每题2分,共20分)Read the following passage and answer the questions that follow.The human body is a complex system composed of various organsand systems that work together to maintain life. Thecirculatory system, for example, is responsible for transporting blood, oxygen, and nutrients throughout the body. The respiratory system facilitates the exchange of gases,while the digestive system processes food and absorbs nutrients. Each system plays a crucial role in the overall health and well-being of an individual.1. What is the primary function of the circulatory system?2. Which system is responsible for gas exchange?3. What does the digestive system do?4. How many systems are mentioned in the passage?5. What is the importance of these systems to an individual's health?四、翻译题(每题5分,共20分)1. 请将以下句子翻译成英文:"糖尿病是一种以高血糖为特征的慢性疾病。
气机英语
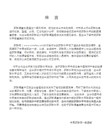
coefficient 系数
cogeneration 联合供热
cogeneration power plant 热电厂
cold start 冷态启动
combined thrust and journal bearing 联合轴承
come into operation 投入运行
built-up 组装
burst disc 破碎盘
bushing 衬管、套管
butterfly valve 蝶阀
bypass 旁路
bypass valve 旁路阀
C
cabinet 箱、柜
cam 凸轮
cam gear 凸轮装置
capability of overload 过负荷能力
convection (热)传导、对流
cool down 冷却
cooling water 冷却水
corrosion 腐蚀
counter clockwise 逆时针
countered 抵消
countersunk 埋头孔
coupling 联轴器
coupling bolt 联轴器螺栓
coupling face 耦合面
branch 支管
brass 铜
breadth 宽度
break-away torque 启动力矩
bridge gauge 桥规
brittle 易碎的
brittle failure 脆断、脆性破坏
brittle fracture 脆性断裂
buckstay 支柱
buffer 阻尼器、缓冲器
阅读理解B篇 (解析+词汇+变式+技巧+模拟) -2024年浙江1月卷深度解析及变式训练 (原卷版)
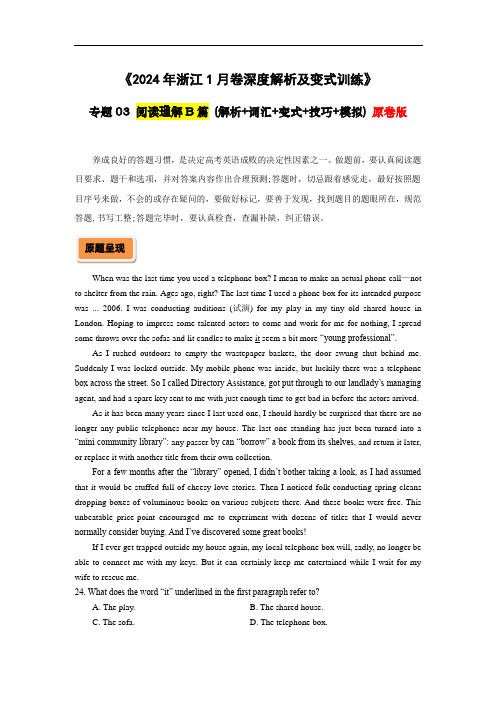
《2024年浙江1月卷深度解析及变式训练》专题03 阅读理解B 篇 (解析+词汇+变式+技巧+模拟) 原卷版养成良好的答题习惯,是决定高考英语成败的决定性因素之一。
做题前,要认真阅读题目要求、题干和选项,并对答案内容作出合理预测;答题时,切忌跟着感觉走,最好按照题目序号来做,不会的或存在疑问的,要做好标记,要善于发现,找到题目的题眼所在,规范答题,书写工整;答题完毕时,要认真检查,查漏补缺,纠正错误。
When was the last time you used a telephone box? I mean to make an actual phone call —not to shelter from the rain. Ages ago, right? The last time I used a phone box for its intended purpose was ... 2006. I was conducting auditions (试演) for my play in my tiny old shared house in London. Hoping to impress some talented actors to come and work for me for nothing, I spread some throws over the sofas and lit candles to make it seem a bit more “young professional”.As I rushed outdoors to empty the wastepaper baskets, the door swung shut behind me. Suddenly I was locked outside. My mobile phone was inside, but luckily there was a telephone box across the street. So I called Directory Assistance, got put through to our landlady’s managing agent, and had a spare key sent to me with just enough time to get bad in before the actors arrived.As it has been many years since I last used one, I should hardly be surprised that there are no longer any public telephones near my house. The last one standing has just been turned into a “mini community library”: any passer-by can “borrow” a book from its shelves, and return it later, or replace it with another title from their own collection.For a few months after the “library” opened, I didn’t bother taking a look, as I had assumed that it would be stuffed full of cheesy love stories. Then I noticed folk conducting spring cleans dropping boxes of voluminous books on various subjects there. And these books were free. This unbeatable price-point encouraged me to experiment with dozens of titles that I would never normally consider buying. And I’ve discovered some great books!If I ever get trapped outside my house again, my local telephone box will, sadly, no longer be able to connect me with my keys. But it can certainly keep me entertained while I wait for my wife to rescue me.24. What does the word “it” underlined in the first paragraph refer to?A. The play.B. The shared house.C. The sofa.D. The telephone box.25. Why did the author use the telephone box in 2006?A. To place an urgent call.B. To put up a notice.C. To shelter from the rain.D. To hold an audition.26. What do we know about the “mini community library”?A. It provides phone service for free.B. Anyone can contribute to its collection.C. It is popular among young readers.D. Books must be returned within a month.27. Why did the author start to use the “library”?A. He wanted to borrow some love stories.B. He was encouraged by a close neighbour.C. He found there were excellent free books.D. He thought it was an ideal place for reading.1. actual a.2. shelter vt.3. conduct vt4. talented a.5. sofa n.6. candle n.7.light(lit, lit) vt8. professional a.9. swing(swung, swung) v.10. directory n.11. assistance n.12. agent n.13. spare a.14. passer-by n.15. collection n.16. title n.17. assume vt.18. stuff vt.19. cheesy a. 20. voluminous a.21. unbeatable a.22. price-point n.23. entertained a.24. urgent a.25. ideal a.26. the last time…27. make a phone call28. light candles29. replace A with B 用B)30. don’t bother doing31. experiment with32. dozens of33. wait for sb to do34. refer to35. put up a notice36. contribute to37. be popular among38. for free1. The last time I used a phone box for its ____________(intend) purpose was ... 2006.2. ____________(hope) to impress some ____________(talent) actors to come and work for me for nothing, I spread some throws over the sofas and ____________(light) candles…3. As I rushed outdoors____________(empty) the wastepaper baskets, the door ____________ (swing) shut behind me.4. My mobile phone was inside, but ____________(lucky) there was a telephone box across the street.5. So I called Directory Assistance, ____________(get) put through to our landlady’s managing agent, and had a spare key ____________(send) sent to me.6. As it has been many years ____________ I last used one, I should hardly ____________(surprise) that there are no longer any public telephones near my house.7. For a few months after the “library” opened, I didn’t bother taking a look, as I ____________ (assume) that it would be stuffed full of cheesy love stories.8. Then I noticed folk ____________(conduct) spring cleans dropping boxes of voluminous books on various ____________(subject) there.9. This price-point encouraged me ____________(experiment) with dozens of titles ___________I would never normally consider ____________(buy).10. If I ever get ____________(trap) outside my house again, my local telephone box will, sadly, no longer be able to connect me with my keys.11. But it can certainly keep me ____________(entertain) while I wait for my wife ____________ (rescue) me.Live with roommates? Have friends and family around you? Chances are that if you’re looking to live a more sustainable lifestyle, not everyone around you will be ready to jump on that bandwagon.I experienced this when I started switching to a zero waste lifestyle five years ago, as I was living with my parents, and I continue to experience this with my husband, as he is not completely zero waste like me. I’ve learned a few things along the way though, which I hope you’ll find encouraging if you’re doing your best to figure out how you can make the change in a not-always-supportive household.Zero waste was a radical lifestyle movement a few years back. I remember showing my parents a video of Bea Johnson, sharing how cool I thought it would be to buy groceries with jars, and have so little trash! A few days later, I came back with my first jars of zero waste groceries, and my dad commented on how silly it was for me to carry jars everywhere. It came off as a bitdiscouraging.Yet as the months of reducing waste continued, I did what I could that was within my own reach. I had my own bedroom, so I worked on removing things I didn’t need. Since I had my own toiletries (洗漱用品), I was able to start personalising my routine to be more sustainable. I also offered to cook every so often, so I portioned out a bit of the cupboard for my own zero waste groceries. Perhaps your household won’t entirely make the switch, but you may have some control over your own personal spaces to make the changes you desire.As you make your lifestyle changes, you may find yourself wanting to speak up for yourself if others comment on what you’re doing, which can turn itself into a who le household debate. If you have individuals who are not on board, your words probably won’t do much and can often leave you feeling more discouraged.So here is my advice: Lead by action.24. What do the underlined words “jump on that bandwagon” mean in the first paragraph?A. Share an apartment with you.B. Join you in what you’re doing.C. Transform your way of living.D. Help you to make the decision.25. What was the attitude of the author’s father toward buying groceries with jars?A. He disapproved of it.B. He was favorable to it.C. He was tolerant of it.D. He didn’t care about it.26. What can we infer about the author?A. She is quite good at cooking.B. She respects others’ privacy.C. She enjoys being a housewife.D. She is a determined person.27. What is the text mainly about?A. How to get on well with other family members.B. How to have one’s own personal space at home.C. How to live a zero waste lifestyle in a household.D. How to control the budget when buying groceries.阅读理解记叙文解题指导一、“人物传记”类记叙文解题策略人物传记是高中记叙文考试中常遇到的体裁形式。
- 1、下载文档前请自行甄别文档内容的完整性,平台不提供额外的编辑、内容补充、找答案等附加服务。
- 2、"仅部分预览"的文档,不可在线预览部分如存在完整性等问题,可反馈申请退款(可完整预览的文档不适用该条件!)。
- 3、如文档侵犯您的权益,请联系客服反馈,我们会尽快为您处理(人工客服工作时间:9:00-18:30)。
DOI: 10.1126/science.1254581, 1153 (2014);345 Science et al.Bernd Gludovatz A fracture-resistant high-entropy alloy for cryogenic applicationsThis copy is for your personal, non-commercial use only.clicking here.colleagues, clients, or customers by , you can order high-quality copies for your If you wish to distribute this article to othershere.following the guidelines can be obtained by Permission to republish or repurpose articles or portions of articles): September 13, 2014 (this information is current as of The following resources related to this article are available online at/content/345/6201/1153.full.html version of this article at:including high-resolution figures, can be found in the online Updated information and services, /content/suppl/2014/09/03/345.6201.1153.DC1.html can be found at:Supporting Online Material /content/345/6201/1153.full.html#ref-list-1, 2 of which can be accessed free:cites 45 articles This article/cgi/collection/mat_sci Materials Sciencesubject collections:This article appears in the following registered trademark of AAAS.is a Science 2014 by the American Association for the Advancement of Science; all rights reserved. The title Copyright American Association for the Advancement of Science, 1200 New York Avenue NW, Washington, DC 20005. (print ISSN 0036-8075; online ISSN 1095-9203) is published weekly, except the last week in December, by the Science o n S e p t e m b e r 13, 2014w w w .s c i e n c e m a g .o r g D o w n l o a d e d f r o mdid not observe the formation of any well-defined structures in the absence of an applied magnetic field (see,e.g.,fig.S8J).24.A.Dong et al .,Nano Lett.11,841–846(2011).25.S.Brooks,A.Gelman,G.Jones,X.-L.Meng,Handbook of Markov Chain Monte Carlo (Chapman &Hall,London,2011).26.Z.Kakol,R.N.Pribble,J.M.Honig,Solid State Commun.69,793–796(1989).27.Ü.Özgür,Y.Alivov,H.Morkoç,J.Mater.Sci.Mater.Electron.20,789–834(2009).28.The formation of helices,and the self-assembly of NCs in our system in general,is likely facilitated by entropic forces;OA used in large excess during self-assembly may act as adepletion agent,inducing crystallization of NCs during hexane evaporation as reported previously (29).29.D.Baranov et al .,Nano Lett.10,743–749(2010).30.On the basis of measurements of electrophoretic mobility [see (34)]and the lack of literature reports on electric dipole moments of magnetite nanoparticles,we did not considerelectrostatic and electric dipole-dipole interactions in our analysis of interparticle interactions.At the same time,we cannot exclude 31.S.Srivastava et al .,Science 327,1355–1359(2010).32.S.Das et al .,Adv.Mater.25,422–426(2013).33.J.V.I.Timonen,tikka,L.Leibler,R.H.A.Ras,O.Ikkala,Science 341,253–257(2013).34.Previous self-assembly experiments performed in nonpolarsolvents excluded a significant role played by electrostatic interactions [e.g.,(35,36)].Although the degree ofdissociation of OA in hexane (dielectric constant =1.84)is negligible,the large excess of OA as well as the nature of our experimental setup (self-assembly at the liquid-air interface)might potentially promote dissociation of OA;to verify this possibility,we used a Malvern Zetasizer Nano ZS to perform electrophoretic mobility (m e )measurements of our nanocubes in hexane both in the absence and in the presence of additional OA (5%v/v).The results [0.00706(T 0.00104)×10−4cm 2V –1s –1and 0.0218(T 0.00710)×10−4cm 2V –1s –1,respectively]indicate that in both cases,the nanocubes are essentially neutral [compare with (37)].35.Z.Chen,J.Moore,G.Radtke,H.Sirringhaus,S.O ’Brien,J.Am.Chem.Soc.129,15702–15709(2007).37.S.A.Hasan,D.W.Kavich,J.H.Dickerson,mun.2009,3723–3725(2009).ACKNOWLEDGMENTSSupported by Israel Science Foundation grant 1463/11,theG.M.J.Schmidt-Minerva Center for Supramolecular Architectures,and the Minerva Foundation with funding from the Federal German Ministry for Education and Research (R.K.)and byNSF Division of Materials Research grant 1309765and American Chemical Society Petroleum Research Fund grant 53062-ND6(P.K.).SUPPLEMENTARY MATERIALS/content/345/6201/1149/suppl/DC1Materials and Methods Figs.S1to S28References (38–92)31March 2014;accepted 14July 2014Published online 24July 2014;METAL ALLOYSproperties required for structural applica-tions.Consequently,alloying elements are added to achieve a desired microstructure or combination of mechanical properties,such as strength and toughness,although the re-sulting alloys invariably still involve a single dom-inant constituent,such as iron in steels or nickel in superalloys.Additionally,many such alloys,such as precipitation-hardened aluminum alloys,rely on the presence of a second phase for me-chanical performance.High-entropy alloys (1–3)represent a radical departure from these notions.they contain high concentrations (20to 25atomic percent)of multiple elements with different crystal structures but can crystallize as a single phase (4–7).In many respects,these alloys rep-resent a new field of metallurgy that focuses attention away from the corners of alloy phase diagrams toward their centers;we believe that as this evolving field matures,a number of fas-cinating new materials may emerge.The CrMnFeCoNi alloy under study here is a case in point.Although first identified a decade ago (1),the alloy had never been investigated mechanically until recently (5,6,8),yet is clearly scientifically interesting from several perspec-tives.It is not obvious why an equiatomic five-element alloy —where two of the elements (Cr and Fe)crystallize with the body-centered cubic (bcc)structure,one (Ni)as face-centered cubic (fcc),one (Co)as hexagonal close-packed (hcp),and one (Mn)with the complex A 12structure —should form a single-phase fcc structure.Fur-thermore,several of its properties are quite unlike those of pure fcc metals.Recent studies indicatethat the alloy exhibits a strong temperature de-of the yield strength between ambient cryogenic temperatures,reminiscent of bcc and certain fcc solid-solution alloys (6).any temperature-dependent effect of rate on strength appears to be marginal (6).the marked temperature-dependent in strength is accompanied by a substan-increase in tensile ductility with decreasing between 293K and 77K (6),which counter to most other materials where an dependence of ductility and strength is seen (9).Preliminary indications sug-that this may be principally a result of the ’s high work-hardening capability,possi-associated with deformation-induced nano-which acts to delay the onset of any instability (i.e.,localized plastic deforma-that can lead to premature failure)to higher (5).We prepared the CrMnFeCoNi alloy with high-elemental starting materials by arc melting drop casting into rectangular-cross-section copper molds,followed by cold forging and cross rolling at room temperature into sheets roughly 10mm thick.After recrystallization,the alloy had an equiaxed grain structure.Uniaxial tensile spec-imens and compact-tension fracture toughness specimens in general accordance with ASTM standard E1820(10)were machined from these sheets by electrical discharge machining.[See (11)for details of the processing procedures,sam-ple sizes,and testing methods.]Figure 1A shows a backscattered electron (BSE)micrograph of the fully recrystallized micro-structure with ~6-m m grains containing numer-ous recrystallization twins.Energy-dispersive x-ray (EDX)spectroscopy and x-ray diffraction (XRD)indicate the equiatomic elemental dis-tribution and single-phase character of the al-loy,respectively.Measured uniaxial stress-strain curves at room temperature (293K),in a dry ice –alcohol mixture (200K),and in liquid nitrogen (77K)are plotted in Fig.1B.With a decrease in temperature from 293K to 77K,the yield strength s y and ultimate tensile strength s utsSCIENCE 5SEPTEMBER 2014•VOL 345ISSUE 62011153RESEARCH |REPORTS1Materials Sciences Division,Lawrence Berkeley National Laboratory,Berkeley,CA 94720,USA.2Department of Materials Physics,Montanuniversität Leoben and Erich Schmid Institute of Materials Science,Austrian Academy of Sciences,Leoben 8700,Austria.3Materials Sciences and Technology Division,Oak Ridge National Laboratory,Oak Ridge,TN 37831,USA.4Materials Sciences and Engineering Department,University of Tennessee,Knoxville,TN 37996,USA.5Department of Materials Science and Engineering,University of California,Berkeley,CA 94720,USA.*Corresponding author.E-mail:georgeep@ (E.P.G.);roritchie@ (R.O.R.)increased by ~85%and ~70%,to 759and 1280MPa,respectively.Similarly,the tensile ductility (strain to failure,e f )increased by ~25%to >0.7;the strain-hardening exponent n remained high at ~0.4,such that there was an enhancement in the frac-ture energy (12)by more than a factor of 2.Table S1provides a detailed summary of the stresses and strains at the three different temperatures,as well as the corresponding strain-hardening exponents.In light of the extensive plasticity involved in the deformation of this alloy,we evaluated the fracture toughness of CrMnFeCoNi with non-linear elastic fracture mechanics,specifically with crack-resistance curve (R curve)measurements in terms ofthe J integral.Analogous to the stress intensity K for linear elastic analysis,provided that specific validity criteria are met,J unique-ly characterizes the stress and displacement fields in the vicinity of the crack tip for a non-linear elastic solid;as such,it is able to capture both the elastic and plastic contributions to the fracture process.J is also equivalent to the strain energy release rate G under linear elastic conditions;consequently,K values can be back-calculated from J measurements assuming a mode I equivalence between K and J :specifically,J =K 2/E ´,with E ´=E (Young ’s modulus)in plane stress and E /(1–n 2)(where n is Poisson ’s ratio)in plane strain.E and n values were determined by resonance ultrasound spectroscopy at each tem-perature (13).Our toughness results for the CrMnFeCoNi alloy at 293K,200K,and 77K are plotted in Fig.1C,in terms of J R (D a )–based resistance curves showing crack extension D a in precracked and side-grooved compact-tension specimens as a function of the applied J .Using these R curves to evaluate the fracture toughness for both the initiation and growth of a crack,we measured a crack initiation fracture toughness J Ic ,deter-mined essentially at D a →0,of 250kJ/m 2at 293K,which in terms of a stress intensity gives K JIc =217MPa·m 1/2.Despite a markedly increased strength at lower temperature,K JIc values at 200K and 77K remained relatively constant at K JIc =221MPa·m 1/2(J Ic =260kJ/m 2)and K JIc =219MPa·m 1/2(J Ic =255kJ/m 2),respectively.After11545SEPTEMBER 2014•VOL 345ISSUE SCIENCEFig.1.Microstructure and mechanical properties of the CrMnFeCoNi high-entropy alloy.(A )Fully recrystallized microstructure with an equiaxed grain structure and grain size of ~6m m;the composition is approximately equiatomic,and the alloy is single-phase,as shown from the EDX spectroscopy and XRD insets.(B )Yield strength s y ,ultimate tensile strength s uts ,and ductility (strain to failure,e f )all increase with decreasing temperature.The curves are typical tests at the individual temperatures,whereas the data points are means T SD of multiple tests;see table S1for exact values.(C )Fracture toughness measure-ments show K JIc values of 217MPa·m 1/2,221MPa·m 1/2,and 219MPa·m 1/2at 293K,200K,and 77K,respectively,and an increasing fracture resistance in terms of the J integral as a function of crack extension D a [i.e.,resistance curve (R curve)behavior].(D )Similar to austenitic stainless steels (e.g.,304,316,or cryogenic Ni steels),the strength of the high-entropy alloy (solid lines)increases with decreasing temperature;although the toughness of the other materials decreases with decreasing temperature,the toughness of the high-entropy alloy remains unchanged,and by some measures it actually increases at lower temperatures.(The dashed lines in the plots mark the upper and lower limits of data found in the literature.)RESEARCH |REPORTSinitiation,the fracture resistance further increased with extensive subcritical crack growth;after just over 2mm of such crack extension,a crack growth toughness exceeding K =300MPa·m 1/2(J =500kJ/m 2)was recorded [representing,in terms of ASTM standards,the maximum (valid)crack extension capacity of our samples].Such toughness values compare favorably to those of highly alloyed,austenitic stainless steels such as 304L and 316L,which have reported tough-nesses in the range of K Q =175to 400MPa·m 1/2at room temperature (14–16),and the best cryogenic steels such as 5Ni or 9Ni steels,with K Q =100to 325MPa·m 1/2at 77K (17–19).Similar to the high-entropy alloy,these materials show an expected increase in strength with decreasing temper-ature to 77K;however,unlike the high-entropy alloy,their reported fracture toughness values are invariably reduced with decreasing temperature (20)(Fig.1D)and furthermore are rarely valid (i.e.,they are size-and geometry-dependent and thus not strictly material parameters).The high fracture toughness values of the CrMnFeCoNi alloy were associated with a 100%ductile fracture by microvoid coalescence,with the extent of deformation and necking behavior being progressively lessapparent at the lower temperatures (Fig.2,A and B).EDX analysis of the particles,which were found inside the voids of the fracture surface and acted as initiation sites for their formation,indicated either Cr-rich or Mn-rich compounds (Fig.2B,inset).These particles are likely oxides associated with the Mn additions;preliminary indications are that they are absent in the Mn-free (CoCrFeNi)alloy (6).Both microvoid size and particle size varied markedly;the microvoids ranged in size from ~1m m to tens of micrometers,with particle sizes ranging from <1m m to ~5m m (Fig.2B,inset)with an average size of 1.6m m and average spacing d p ≈49.6m m,respectively.To verify the high measured fracture tough-ness values,we used three-dimensional (3D)ster-eophotogrammetry of the morphology of these fracture surfaces to estimate local crack initia-tion toughness (K i )values for comparison with the global,ASTM-based K JIc measurements.This technique is an alternative means to characterize the onset of cracking,particularly under large-scale yielding conditions.Under mode I (tensile)loading,the crack surfaces completely separate from each other,with the regions of first sepa-ration moving the farthest apart and progres-sively less separation occurring in regions that crack later.Accordingly,the formation and coa-lescence of microvoids and their linkage with the crack tip allow for the precise reconstruction of the point of initial crack advance from the juxta-position of the stereo images of each fracture sur-face.This enables an evaluation of the crack tip opening displacement at crack initiation,CTOD i ,which then can be used to estimate the local stress intensity K i at the midsection of the sample at the onset of physical crack extension,where D a =0(21).Specifically,we used an automatic fracture surface analysis system that creates 3D digital surface models from stereo-image pairs of the corresponding fracture surfaces taken in the scan-ning electron microscope (Fig.2C);digitally re-constructing the crack profiles by superimposing the stereo-image pairs allows for a precise mea-surement of the CTOD i s of arbitrarily chosen crack paths (which must be identical on both fracture surfaces).Figure 2D indicates two examples of the approximately 10crack paths taken on both fracture surfaces of samples tested at 293K and 77K.The two corresponding profiles show the point at which the first void,formed ahead of the fatigue precrack,coalesced with this pre-crack to mark the initial crack extension,there-by locally defining the crack initiation event andSCIENCE 5SEPTEMBER 2014•VOL 345ISSUE 62011155Fig.2.Images of fractured CrMnFeCoNi samples.(A )Stereomicroscopic photographs of the fracture surfaces after testing indicate less lateral defor-mation and necking-like behavior with decreasing temperature.(B )SEM image of the fracture surface of a sample tested at room temperature shows ductile dimpled fracture where the void initiation sites are mainly Mn-rich or Cr-rich par-ticles,as shown by the EDX data (insets).(C )Three-dimensional digital fracturesurface models were derived from SEM stereo-image pairs,which indicate the transition from fatigue precrack to ductile dimpled fracture and the presence of the stretch zone.(D )Profiles of identical crack paths from both fracture halves of the fracture surface models were extracted to evaluate the crack tip opening displacement at the first physical crack extension,CTOD i ,which was then converted to J i using the relationship of the equivalence of J and CTOD (50).RESEARCH |REPORTSthe fracture toughness (22).Using these pro-cedures,the initial crack tip opening displace-ments at crack initiation were found to be CTOD i =57T 19m m at 293K and 49T 13m m at ing the standard J-CTOD equivalence relationship of J i ºs o CTOD i =K i 2/E ´gives es-timates of the crack initiation fracture toughness:K i =191MPa·m 1/2and 203MPa·m 1/2at 293K and 77K,respectively.These values are slightly con-servative with respect to the global R curve –based values in Fig.1C;however,this is to be expected,as theyare estimated at the initial point of physical contact of the first nucleated void with the precrack,whereas the ASTM-based measurements use an operational definition of crack initiation involving subcritical crack ex-tension of D a =200m m.To discern the micromechanisms underlying the excellent fracture toughness behavior,we fur-ther analyzed the fracture surfaces of samples tested at 293K and 77K by means of stereomi-croscopy and scanning electron microcopy (SEM).Some samples were additionally sliced in two halves,embedded,and metallographically pol-ished for BSE microscopy and electron back-scatter diffraction (EBSD)analysis of the region in the immediate vicinity of the crack tip and in the wake of the crack,close to the crack flanks,specifically “inside ”the sample where fully plane-strain conditions prevail.SEM images of the crack tip region of sam-ples tested at ambient and liquid nitrogen tem-peratures show the formation of voids and their coalescence characteristic of the microvoid co-alescence fracture process (Fig.3A).A large population of the particles that act as the void11565SEPTEMBER 2014•VOL 345ISSUE 6201 SCIENCEFig.3.Deformation mechanisms in the vicinity of the crack tip in the center (plane-strain)section of CrMnFeCoNi high-entropy alloy samples.(A )Low-magnification SEM images of samples tested at 293K and 77K show ductile fracture by microvoid coalescence,with a somewhat more distorted crack path at the lower temperature.(B )EBSD images show numerous annealing twins and pronounced grain misorientations due to dislocations —the primary defor-mation mechanism at 293K.(C )At 77K,BSE images taken in the wake of the propagated crack show the formation of pronounced cell structures resulting from dislocation activity.Both BSE and EBSD images show deformation-induced nanotwinning as an additional mechanism at 77K.[The EBSD image is an overlay to an image quality (IQ)map,which is a measure of the quality of the collected EBSD pattern used to visualize certain microstructural features.]RESEARCH |REPORTSinitiation sites can be seen on the fracture surfaces (Fig.2B);these particles have a substan-tial influence on material ductility and likely contribute to the measured scatter in the failure strains (Fig.1B).Macroscopically,fracture sur-faces at 77K appear significantly more deviated from a mode I (K II =0)crack path than at 293K (Fig.3A).Although such deflected crack paths act to reduce the local crack-driving force at the crack tip (23)and hence contribute to the rising R curve behavior (i.e.,crack growth toughness),this mech-anism cannot be responsible for the exceptional crack initiation toughness of this alloy.Such high K i values are conversely derived from the large CTOD s at crack initiation and are associated with the intrinsic process of microvoid coalescence;as such,they are highly dependent on the formation and size of voids,the prevailing deformation and flow conditions,and the presence of steady strain hardening to suppress local necking.Using simple micromechanical models for fracture (24),we can take advantage of a stress state –modified critical strain criterion for ductile fracture to derive estimates for these high tough-ness values (25–27).This yields expressions forthe fracture toughness in the form J Ic ≈s o e f l *o,where s o is the flow stress,e f is the fracture strain in the highly constrained stress state in the vicinity of the crack tip [which is roughly an order of magnitude smaller than the un-iaxial tensile ductility (28)],and l *ois the char-acteristic distanceahead of the tip over which this critical strain must be met for fracture (which can be equated to the particle spacing d p ).Assum-ing Hutchinson-Rice-Rosengren (HRR)stress-strain distributions ahead of a crack tip in plane strain for a nonlinear elastic,power-law hard-ening solid (strain-hardening coefficient of n )(29,30),and the measured properties,specifically E ,s o ,e f ,n ,n ,and d p ,for this alloy (11),estimates of the fracture toughness of K JIc =(J Ic E ´)1/2of ~150to 215MPa·m 1/2can be obtained for the measured particle spacing of d p ~50m m.Although approx-imate,these toughness predictions from the critical fracture strain model are completely consistent with a fracture toughness on the order of 200MPa·m 1/2,as measured for the CrMnFeCoNi alloy in this study (Fig.1C).In addition to crack initiation toughnesses of 200MPa·m 1/2or more,this alloy develops even higher crack growth toughness with stable crack growth at “valid ”stress intensities above 300MPa·m 1/2.These are astonishing toughness levels by any standard,particularly because they are retained at cryogenic temperatures.A primary factor here is the mode of plastic deformation,which induces a steady degree of strain hardening to suppress plastic instabilities;expressly,the mea-sured strain-hardening exponents of n ~0.4are very high relative to the vast majority of metals,particularly at this strength level.Recent studies have shown that,similar to mechanisms known for binary fcc solid solutions (31,32),plastic de-formation in the CrMnFeCoNi alloy at ambienttemperatures is associated with planar glide of 1/2〈110〉dislocations on {111}planes leading to the formation of pronounced cell structures at higher strains (5).However,at 77K,in addition to planar slip,deformation-induced nanoscale twinning has been observed both previously (5)and in the present study (Fig.3C)and contributes to the increased ductility and strain hardening at lower temperatures.Both the planar slip and nanotwinning mechanisms are highly active in the vicinity of the crack tip during fracture,as illustrated in Fig.3.EBSD images taken ahead of the crack tip inside the sample of a fracture toughness test performed at room temperature show grain misorientations resulting from dis-location activity as the only deformation mech-anism (Fig.3B).Aside from numerous annealing twins resulting from the recrystallization step during processing,twinning does not play a role at ambient temperatures,with only a few single nanotwins in evidence.With decrease in tem-perature,cell structure formation is more appar-ent,as shown by the BSE image in Fig.3C,taken in the wake of a crack propagating at 77K.Here,however,excessive deformation-induced nano-scale twinning occurs simultaneously with planar dislocation slip,leading to a highly distorted grain structure,which can be seen in both the BSE and IQ +EBSD images in the vicinity of the growing crack.[The EBSD image is shown as an overlay of an image quality (IQ)map to enhance visual-ization of structural deformations of the grains.]Note that several other classes of materials show good combinations of strength and ductility when twinning is the dominant deformation mecha-nism.These include copper thin films (33–36)and the recently developed twinning-induced plas-ticity (TWIP)steels (37–40),which are of great interest to the car industry as high-Mn steels (41–44).We believe that the additional plasticity mechanism of nanotwinning in CrMnFeCoNi is critical to sustaining a high level of strain hard-ening at decreasing temperatures;this in turn acts to enhance the tensile ductility,which,to-gether with the higher strength at low tem-peratures,preserves the exceptional fracture toughness of this alloy down to 77K.We conclude that the high-entropy CrMnFeCoNi alloy displays remarkable fracture toughness properties at tensile strengths of 730to 1280GPa,which exceed 200MPa·m 1/2at crack initiation and rise to >300MPa·m 1/2for stable crack growth at cryogenic temperatures down to 77K.The alloy has toughness levels that are comparable to the very best cryogenic steels,specifically cer-tain austenitic stainless steels (15,16)and high-Ni steels (17–19,45–48),which also have outstanding combinations of strength and ductility.With respect to the alloy ’s damage tolerance,a comparison with the other major material classes is shown on the Ashby plot of fracture toughness versus yield strength (49)in Fig.4.There are clearly stronger materials,which is understand-able given that CrMnFeCoNi is a single-phase material,but the toughness of this high-entropy alloy exceeds that of virtually all pure metals and metallic alloys (9,49).SCIENCE 5SEPTEMBER 2014•VOL 345ISSUE 62011157Fig.4.Ashby map showing fracture toughness as a function of yield strength for high-entropy alloys in relation to a wide range of material systems.The excellent damage tolerance (toughness combined with strength)of the CrMnFeCoNi alloy is evident in that the high-entropy alloy exceeds the toughness of most pure metals and most metallic alloys (9,49)and has a strength comparable to that of structural ceramics (49)and close to that of some bulk-metallic glasses (51–55).RESEARCH |REPORTSREFERENCES AND NOTES1. B.Cantor,I.T.H.Chang,P.Knight,A.J.B.Vincent,Mater.Sci.Eng.A 375–377,213–218(2004).2.J.-W.Yeh et al .,Adv.Eng.Mater.6,299–303(2004).3. C.-Y.Hsu,J.-W.Yeh,S.-K.Chen,T.-T.Shun,Metall.Mater.Trans.A 35,1465–1469(2004).4.O.N.Senkov,G.B.Wilks,J.M.Scott,D.B.Miracle,Intermetallics 19,698–706(2011).5. F.Otto et al .,Acta Mater.61,5743–5755(2013).6. A.Gali,E.P.George,Intermetallics 39,74–78(2013).7. F.Otto,Y.Yang,H.Bei,E.P.George,Acta Mater.61,2628–2638(2013).8.W.H.Liu,Y.Wu,J.Y.He,T.G.Nieh,Z.P.Lu,Scr.Mater.68,526–529(2013).9.R.O.Ritchie,Nat.Mater.10,817–822(2011).10.E08Committee,E1820-13Standard Test Method forMeasurement of Fracture Toughness (ASTM International,2013).11.See supplementary materials on Science Online.12.As a preliminary estimate of the fracture resistance,thearea under the load displacement curve of a tensile test was used to compute the fracture energy (sometimes termed the work to fracture),which was calculated from this area divided by twice the area of the crack surface.13.J.Maynard,Phys.Today 49,26–31(1996).14.K Q values refer to fracture toughnesses that are notnecessarily valid by ASTM standards (i.e.,they do not meet the J -validity and/or plane strain conditions).Consequently,these toughnesses are likely to be inflated relative to truly valid numbers and are size-and geometry-dependent;they are not strictly material parameters.When comparing these values to the toughnesses measured in this study forCoCrFeMnNi,it is important to note that all values determined here for the high-entropy alloy were strictly valid,meeting ASTM standards for both J validity and plane ls,Int.Mater.Rev.42,45–82(1997).16.M.Sokolov et al .,in Effects of Radiation on Materials:20thInternational Symposium ,S.Rosinski,M.Grossbeck,T.Allen,A.Kumar,Eds.(ASTM International,West Conshohocken,PA,2001),pp.125–147.17.J.R.Strife,D.E.Passoja,Metall.Trans.A 11,1341–1350(1980).18.C.K.Syn,J.W.Morris,S.Jin,Metall.Trans.A 7,1827–1832(1976).19.A.W.Pense,R.D.Stout,Weld.Res.Counc.Bull.205,1–43(1975).20.Note that despite the uncertainty in the (valid)toughnessvalues for the stainless and high Ni steels,their upper toughness range could possibly be higher than the current measurements for the CrMnFeCoNi alloy.It must beremembered,however,that these materials are microalloyed and highly tuned with respect to grain size/orientation,tempering,precipitation hardening,etc.,to achieve their mechanical properties,whereas the current CrMnFeCoNi alloy is a single-phase material that undoubtedly can be further improved through second-phase additions and grain control.21.J.Stampfl,S.Scherer,M.Gruber,O.Kolednik,Appl.Phys.A 63,341–346(1996).22.J.Stampfl,S.Scherer,M.Berchthaler,M.Gruber,O.Kolednik,Int.J.Fract.78,35–44(1996).23.B.Cotterell,J.Rice,Int.J.Fract.16,155–169(1980).24.R.O.Ritchie,A.W.Thompson,Metall.Trans.A 16,233–248(1985).25.A.C.Mackenzie,J.W.Hancock,D.K.Brown,Eng.Fract.Mech.9,167–188(1977).26.R.O.Ritchie,W.L.Server,R.A.Wullaert,Metall.Trans.A 10,1557–1570(1979).27.Details of the critical strain model for ductile fracture (25,26)and the method of estimating the fracture toughness are described in the supplementary materials.28.J.R.Rice,D.M.Tracey,J.Mech.Phys.Solids 17,201–217(1969).29.J.W.Hutchinson,J.Mech.Phys.Solids 16,13–31(1968).30.J.R.Rice,G.F.Rosengren,J.Mech.Phys.Solids 16,1–12(1968).31.H.Neuhäuser,Acta Metall.23,455–462(1975).32.V.Gerold,H.P.Karnthaler,Acta Metall.37,2177–2183(1989).33.M.Dao,L.Lu,Y.F.Shen,S.Suresh,Acta Mater.54,5421–5432(2006).34.L.Lu,X.Chen,X.Huang,K.Lu,Science 323,607–610(2009).35.K.Lu,L.Lu,S.Suresh,Science 324,349–352(2009).36.A.Singh,L.Tang,M.Dao,L.Lu,S.Suresh,Acta Mater.59,2437–2446(2011).37.R.A.Hadfield,Science 12,284–286(1888).38.V.H.Schumann,Neue Hütte 17,605–609(1972).39.L.Remy,A.Pineau,Mater.Sci.Eng.28,99–107(1977).40.T.W.Kim,Y.G.Kim,Mater.Sci.Eng.A 160,13–15(1993).41.O.Grässel,G.Frommeyer,C.Derder,H.Hofmann,J.Phys.IV07,C5-383–C5-388(1997).42.O.Grässel,L.Krüger,G.Frommeyer,L.W.Meyer,Int.J.Plast.16,1391–1409(2000).43.G.Frommeyer,U.Brüx,P.Neumann,ISIJ Int.43,438–446(2003).44.L.Chen,Y.Zhao,X.Qin,Acta Metall.Sin.Engl.Lett.26,1–15(2013).45.D.T.Read,R.P.Reed,Cryogenics 21,415–417(1981).46.R.D.Stout,S.J.Wiersma,in Advances in CryogenicEngineering Materials ,R.P.Reed,A.F.Clark,Eds.(Springer,New York,1986),pp.389–395.47.Y.Shindo,K.Horiguchi,Sci.Technol.Adv.Mater.4,319–326(2003).48.J.W.Sa et al .,in Twenty-First IEEE/NPS Symposium on FusionEngineering 2005(IEEE,Piscataway,NJ,2005),pp.1–4.49.M.F.Ashby,in Materials Selection in Mechanical Design ,M.F.Ashby,Ed.(Butterworth-Heinemann,Oxford,ed.4,2011),pp.31–56.50.C.F.Shih,J.Mech.Phys.Solids 29,305–326(1981).51.C.J.Gilbert,R.O.Ritchie,W.L.Johnson,Appl.Phys.Lett.71,476–478(1997).52.A.Kawashima,H.Kurishita,H.Kimura,T.Zhang,A.Inoue,53.A.Shamimi Nouri,X.J.Gu,S.J.Poon,G.J.Shiflet,J.J.Lewandowski,Philos.Mag.Lett.88,853–861(2008).54.M.D.Demetriou et al .,Appl.Phys.Lett.95,041907,041907–3(2009).55.M.D.Demetriou et al .,Nat.Mater.10,123–128(2011).ACKNOWLEDGMENTSSponsored by the U.S.Department of Energy,Office ofScience,Office of Basic Energy Sciences,Materials Sciences and Engineering Division.All data presented in this article can additionally be found in the supplementary materials.Author contributions:E.P.G.and R.O.R.had full access to theexperimental results in the study and take responsibility for the integrity of the data and the accuracy of the data analysis.The alloys were processed by D.C.and mechanically characterized by B.G.,A.H.,and D.C.Study design,interpretation and analysis of data,and preparation of the manuscript were performed jointly by B.G.,A.H.,D.C.,E.H.C.,E.P.G.,and R.O.R.The authors declare no conflict of interest.SUPPLEMENTARY MATERIALS/content/345/6201/1153/suppl/DC1Materials and Methods Supplementary Text Fig.S1Table S19April 2014;accepted 18July 2014process,representing the initial transfor-mation of a disordered phase into an or-dered one.It is also the most difficult part of the process to observe because it hap-pens on very short time and length scales.In thebate as to whether classical nucleation theory (CNT),as initially developed by Gibbs (1),is a suitable framework within which to describe the process,or whether nonclassical elements such as dense liquid phases (2–4)or (meta)stable clusters (5)play important roles.Furthermore,uncertainty exists as to whether a final,stable phase can nucleate directly from solution or whether it forms through a multistep,multi-phase evolution (6,7).In the case of multistep nucleation pathways,whether transformation from one phase to another occurs through nu-cleation of the more stable phase within the11585SEPTEMBER 2014•VOL 345ISSUE 6201 SCIENCE1Department of Materials Science and Engineering,University of California,Berkeley,CA 94720,USA.2Molecular Foundry,Lawrence Berkeley National Laboratory,Berkeley,CA 94720,USA.3Physical Sciences Division,Pacific Northwest National Laboratory,Richland,WA 99352,USA.4Department ofMaterials Science and Engineering,University of Washington,Seattle,WA 98195,USA.*Corresponding author.E-mail:james.deyoreo@RESEARCH |REPORTS。