燃料电池研发部工作手册
燃料电池手册7_部分7
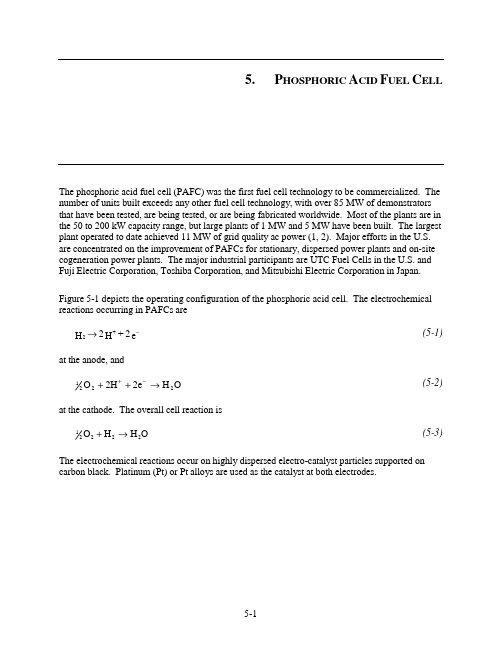
5. P HOSPHORIC A CID F UEL C ELLThe phosphoric acid fuel cell (PAFC) was the first fuel cell technology to be commercialized. The number of units built exceeds any other fuel cell technology, with over 85 MW of demonstrators that have been tested, are being tested, or are being fabricated worldwide. Most of the plants are in the 50 to 200 kW capacity range, but large plants of 1 MW and 5 MW have been built. The largest plant operated to date achieved 11 MW of grid quality ac power (1, 2). Major efforts in the U.S. are concentrated on the improvement of PAFCs for stationary, dispersed power plants and on-site cogeneration power plants. The major industrial participants are UTC Fuel Cells in the U.S. and Fuji Electric Corporation, Toshiba Corporation, and Mitsubishi Electric Corporation in Japan.Figure 5-1 depicts the operating configuration of the phosphoric acid cell. The electrochemical reactions occurring in PAFCs aree 2 + H 2 H +2−→(5-1)at the anode, andO H 2e 2H O 2221→++−+ (5-2)at the cathode. The overall cell reaction isO H H O 22221→+ (5-3)The electrochemical reactions occur on highly dispersed electro-catalyst particles supported on carbon black. Platinum (Pt) or Pt alloys are used as the catalyst at both electrodes.HydrogenFlowField Air (oxygen)Flow Field Waterand AirCathodeCurrentCollectorAnodeCurrentCollector AnodeBacking MEAHydrogenOutlet Cathode Backing e -e -ElectronsProtonsFigure 5-1 Principles of Operation of Phosphoric Acid Fuel Cell (Courtesy of UTC Fuel Cells)5.1Cell Components5.1.1 State-of-the-Art ComponentsThere have been only minor changes in cell design in recent years. The major U.S. manufacturer, UTC Fuel Cells, has concentrated on improving cell stability and life, and in improving the reliability of system components at reduced cost.The evolution of cell components from 1965 to the present day for PAFCs is summarized in Table 5-1. In the mid-1960s, the conventional porous electrodes were polytetrafluoroethylene (PTFE) - bonded Pt black, and the loadings were about 9 mg Pt/cm 2. During the past two decades, Pt supported on carbon black has replaced Pt black in porous PTFE-bonded electrode structures as the electro-catalyst. A dramatic reduction in Pt loading has also occurred; the loadings 13 are currently about 0.10 mg Pt/cm 2 in the anode and about 0.50 mg Pt/cm 2 in the cathode.The operating temperatures and acid concentrations of PAFCs have increased to achieve higher cell performance; temperatures of about 200 °C (392 °F) and acid concentrations of 100 percent H 3PO 4 are commonly used today. Although the present practice is to operate at atmospheric pressure, the operating pressure of PAFCs surpassed 8 atm in the 11 MW electric utilitydemonstration plant, confirming an increase in power plant efficiency. However, a number of 13. Assuming a cell voltage of 750 mV at 205 mA/cm 2 (approximate 11 MW design, 8 atmospheres) and the current Pt loadings at the anode and cathode, ~54 g Pt is required per kilowatt of power generated.issues remain whether to design and operate PAFC units at atmospheric vs. pressurized conditions.Primarily, small, multi-kW PAFC power units that were the focus of initial commercial applications led to atmospheric pressure operation. Although pressurization increased efficiency (lower fuel cost), it complicated the power unit - resulting in higher capital cost. The economic trade-off favored simpler, atmospheric operation for early commercial units.Another important issue, independent of power unit size, is that pressure promotes corrosion. Phosphoric acid electrolyte (H3PO4) produces a vapor. This vapor, which forms over the electrolyte, is corrosive to cell locations other than the active cell area. These cell locations are at a mixed voltage (open circuit and cell voltage), that can be over ~0.8V/cell. That is the limit above which corrosion occurs (active area limited to operation under ~0.8 V/cell). An increase in cell total pressure causes the partial pressure of the H3PO4 vapor to increase, causing increased corrosion in the cell. Cell temperature must also be increased with pressurized conditions to produce steam for the steam reformer (3).A major breakthrough in PAFC technology that occurred in the late 1960s was the development of carbon black and graphite for cell construction materials; this and other developments are reviewed by Appleby (4) and Kordesch (5). Carbon black and graphite were sufficiently stable to replace the more expensive gold-plated tantalum cell hardware used at the time. The use of high-surface-area graphite to support Pt permitted a dramatic reduction in Pt loading without sacrificing electrode performance. It was reported (4) that "without graphite, a reasonably inexpensive acid fuel cell would be impossible, since no other material combines the necessary properties of electronic conductivity, good corrosion resistance, low density, surface properties (especially in high area form) and, above all, low cost." However, carbon corrosion and Pt dissolution become an issue at cell voltages above ~0.8 V. Consequently, low current densities at cell voltage above 0.8 V and hot idle at open circuit potential should be avoided.The porous electrodes used in PAFCs have been described extensively in patent literature (6); see also the review by Kordesch (5). These electrodes contain a mixture of electro-catalyst supported on carbon black and a polymeric binder, usually PTFE (30 to 50 wt percent). The PTFE binds the carbon black particles together to form an integral, but porous, structure that is supported on a porous graphite substrate. The graphite structure serves as a support for the electro-catalyst layer, as well as the current collector. A typical graphite structure used in PAFCs has an initial porosity of about 90 percent, which is reduced to about 60 percent by impregnation with 40 wt percent PTFE. This wet-proof graphite structure contains macropores of 3 to 50 µm diameter (median pore diameter of about 12.5 µm) and micropores with a median pore diameter of about 34 Å for gas permeability. The composite structure, consisting of a carbon black/PTFE layer on the graphite substrate, forms a stable, three-phase interface in the fuel cell, with H3PO4 electrolyte on one side (electro-catalyst side) and the reactant gas environment on the other.Table 5-1 Evolution of Cell Component Technology for Phosphoric Acid Fuel CellsComponent ca. 1965 ca. 1975 Current Status a Anode PTFE-bonded Pt black PTFE-bonded Pt/C PTFE-bonded Pt/CXC-72aVulcan9 mg/cm20.25 mg Pt/cm20.25 mg Pt/cm2 Cathode PTFE-bonded Pt black PTFE-bonded Pt/C PTFE-bonded Pt/CXC-72aVulcan9 mg/cm20.5 mg Pt/cm20.5 mg Pt/cm2Ta mesh screen Graphite Structure Graphite Structure ElectrodeSupportGlass fiber paper PTFE-bonded SiC PTFE-bonded SiC ElectrolyteSupportElectrolyte 85 percent H3PO495 percent H3PO4100 percent H3PO4Porous graphite plate. Porous graphite plate. ElectrolyteReservoirCooler 1 per ~7 cells;imbedded (SS) tubesin graphite platea. - Over 40,000 hour component life demonstrated in commercial power plants.A bipolar plate separates the individual cells and electrically connects them in series in a fuel cell stack. In some designs, the bipolar plate also contains gas channels that feed the reactant gases to the porous electrodes and remove the reaction products and inerts. Bipolar plates made from graphite resin mixtures that are carbonized at low temperature (~900 °C/1,652 °F) are not suitable because of their rapid degradation in PAFC operating environments (7, 8). However, corrosion stability is improved by heat treatment to 2,700 °C (4,892 °F) (8), i.e., the corrosion current is reduced by two orders of magnitude at 0.8 V in 97 percent H3PO4 at 190°C (374 °F) and 4.8 atm (70.5 psi). The all-graphite bipolar plates are sufficiently corrosion-resistant for a projected life of 40,000 hours in PAFCs, but they are still relatively costly to produce.Several designs for the bipolar plate and ancillary stack components are used by fuel cell developers, and these are described in detail (9, 10, 11, 12). A typical PAFC stack contains cells connected in series to obtain the practical voltage level desired for the load. In such an arrangement, individual cells are stacked with bipolar plates between the cells. The bipolar plates used in early PAFCs consisted of a single piece of graphite with gas channels machined on either side to direct the flow of fuel and oxidant. Currently, both bipolar plates of the previous design and new designs consisting of several components are being considered. In the multi-component bipolar plates, a thin impervious plate separates the reactant gases in adjacent cells in the stack, andseparate porous plates with ribbed channels are used to direct gas flow. In a cell stack, the impervious plate is subdivided into two parts, and each joins one of the porous plates. The electrolyte vaporizes so that a portion of H3PO4 escapes from the cell in the air stream over time. An electrolyte reservoir plate (ERP), made of porous graphite, provides enough electrolyte to achieve a 40,000-hour cell life goal (there is no electrolyte replacement). The ERP also accommodates increases in electrolyte volume due to an increase in H2O, so the porous graphite electrodes don’t flood. These fluctuations in electrolyte volume occur during start-up and during transient operation. The porous structure, which allows rapid gas transport, is also used to store additional acid to replenish the supply lost by evaporation during the cell operating life.In PAFC stacks, provisions must be included to remove heat generated during cell operation. In practice, heat has been removed by either liquid (two-phase water or a dielectric fluid) or gas (air) coolants that are routed through cooling channels located (usually about every fifth cell) in the cell stack. Liquid cooling requires complex manifolds and connections, but better heat removal is achieved than with air-cooling. The advantage of gas cooling is its simplicity, reliability, and relatively low cost. However, the size of the cell is limited, and the air-cooling passages must be much larger than the liquid- cooling passages.Improvements in state-of-the-art phosphoric acid cells are illustrated by Figure 5-2. Performance by the ~1 m2 (10 ft2) short stack, (f), results in a power density of nearly 0.31 W/cm2.Figure 5-2 Improvement in the Performance of H2-Rich Fuel/Air PAFCsa - 1977: 190 °C, 3 atm, Pt loading of 0.75 mg/cm2 on each electrode (13)b - 1981: 190 °C, 3.4 atm, cathode Pt loading of 0.5 mg/cm2 (14)c - 1981: 205 °C, 6.3 atm, cathode Pt loading of 0.5 mg/cm2 (14)d - 1984: 205 °C, 8 atm, electro-catalyst loading was not specified (15)e - 1992: 205 °C, 8 atm, 10 ft2 short stack, 200 hrs, electro-catalyst loading not specified (16)f - 1992: 205 °C, 8 atm, subscale cells, electro-catalyst loading not specified (16)5.1.2Development ComponentsPhosphoric acid electrode/electrolyte technology has reached a level of maturity at which developers commit resources for commercial capacity, multi-unit demonstrations and pre-prototype installations. UTC Fuel Cells has 25 (200 kW) atmospheric pressure power plants that have operated between 30,000 to 40,000 hours. Most cell parts are graphite, and there has been no electrolyte replacement over the cell life of 40,000 hours. Grid-independent units undergo extensive cycling. Cell components are manufactured at scale and in large quantities, demonstrating confidence that predicted performance will be met (3). However, further increases in power density and reduced cost are needed to achieve economic competitiveness with other energy technologies, as expressed in the early 1990s (17, 18). Fuel cell developers continue to address these issues.In 1992, UTC Fuel Cells' predecessor, International Fuel Cells, completed a government-sponsored, advanced water-cooled PAFC development project to improve the performance andreduce the cost of both its atmospheric and pressurized technology for both on-site and utilityapplications (16). The project focused on five major activities: 1) produce a conceptual design of a large stack with a goal of 175 W/ft2 (0.188 W/cm2), 40,000 hour useful life, and a stack cost of less than $400/kW; 2) test pressurized Configuration "B" single cells developed in a previous program, but improved with proprietary design advances in substrates, electrolyte reservoir plates, catalysts, seals, and electrolyte matrix to demonstrate the 175 W/ft2 (0.188 W/cm2) power density goal; 3) test a pressurized short stack with subscale size, improved component cells, and additional improvements in the integral separators and coolers to confirm the stack design; 4) test a pressurized short stack of improved full-size cell components, nominal 10 ft2 size (approximately 1 m2), to demonstrate the 175 W/ft2 (0.188 W/cm2) power density goal, and 5) test an advanced atmospheric "on-site" power unit stack with the improved components.A conceptual design of an improved technology stack, operating at 120 psi (8.2 atm) and 405 °F (207 °C), was produced based on cell and stack development tests. The stack was designed for 355 10 ft2 (approximately 1 m2) cells to produce over 1 MW dc power in the same physical envelope as the 670 kW stack used in the 11 MW PAFC plant built for Tokyo Electric Power. The improvements made to the design were tested in single cells and in subscale and full size short stacks.Table 5-2 summarizes the results. Single cells achieved an initial performance of 0.75 volts/cell at a current density of 400 A/ft2 (431 mA/cm2) at 8.2 atm and 207 °C. The power density,300 W/ft2 (0.323 W/cm2), was well above the project goal. Several cells were operated to600 A/ft2 (645 mA/cm2), achieving up to 0.66 volts/cell. The flat plate component designs were verified in a subscale stack prior to fabricating the full size short stack. The pressurized short stack of 10 ft2 cells achieved a performance of 285 W/ft2 (0.307 W/cm2). Although the average cell performance, 0.71 volts/cell at 400 A/ft2 (431 mA/cm2), was not as high as the single cell tests, the performance was 65 percent higher than the project goal. Figure 5-3 presents single cell and stack performance data for pressurized operation. The stack was tested for over3,000 hours. For reference purposes, Tokyo Electric Power Company's 11 MW power plant, operational in 1991, had an average cell performance of approximately 0.75 volts/cell at190 mA/cm2 or 0.142 W/cm2 (19).Table 5-2 Advanced PAFC PerformanceAverage Cell Voltage, VCurrent Density mA/cm 2 Power Density W/cm 2 IFC Pressurized:Project GoalSingle CellsFull Size Short Stack11 MW Reference0.75 to 0.66 0.71 0.75 431 to 645 431 190 0.188 0.323 0.307 0.142 IFC Atmospheric:Single CellsFull Size Short Stack0.75 0.65 242 215 0.182 0.139 Mitsubishi Electric AtmosphericSingle Cells 0.65 300 0.195Figure 5-3 Advanced Water-Cooled PAFC Performance (16)The atmospheric pressure short stack, consisting of 32 cells, obtained an initial performance of 0.65 volts/cell at 200 A/ft 2 (215 mA/cm 2) or 0.139 W/cm 2. The performance degradation rate was less than 4 mV/1,000 hours during the 4,500 hour test. Single cells, tested at atmospheric conditions, achieved a 500 hour performance of approximately 0.75 volts/cell at 225 A/ft 2 (242 mA/cm 2) or 0.182 W/cm 2.Mitsubishi Electric Corporation investigated alloyed catalysts, processes to produce thinnerelectrolytes, and increased utilization of the catalyst layer (20). These improvements resulted in an initial atmospheric performance of 0.65 mV at 300 mA/cm 2 or 0.195 W/cm 2, which was higher than the UTC Fuel Cells' performance mentioned above (presented in Table 5-2 for comparison). Note that this performance was obtained using small 100 cm 2 cells and may not yet have beendemonstrated with full-scale cells in stacks. Approaches to increase life are to use series fuel gasflow in the stack to alleviate corrosion, provide well-balanced micropore size reservoirs to avoidelectrolyte flooding, and use a high corrosion resistant carbon support for the cathode catalyst.These improvements resulted in the lowest PAFC degradation rate publicly acknowledged:2 mV/1,000 hours for 10,000 hours at 200 to 250 mA/cm2 in a short stack with 3,600 cm2 areacells. UTC Fuel Cells reported a similar degradation rate in 2002 for power units operating up to40,000 hours (3).Several important technology development efforts for which details have been published includecatalyst improvements, advanced gas diffusion electrode development, and tests on materials thatoffer better carbon corrosion protection. Transition metal (e.g., iron, cobalt) organic macrocycles14from the families of tetramethoxyphenylporphyrins (TMPP), phthalocyanines (PC),tetraazaannulenes (TAA) and tetraphenylporphyrins (TPP) have been evaluated as O2-reductionelectro-catalysts in PAFCs. One major problem with these organic macrocycles is their limitedchemical stability in hot concentrated phosphoric acid. However, after heat treatment of theorganic macrocycle (i.e., CoTAA, CoPC, CoTMPP, FePC, FeTMPP) on carbon at about 500 to 800 °C (932 to1,472 °F), the pyrolyzed residue exhibits electro-catalytic activity that, in some instances, is comparable to that of Pt and has promising stability, at least up to about 100 °C/212 °F (21). Another successful approach for enhancing the electro-catalysis of O2 reduction is to alloy Pt with transition metals such as Ti (22), Cr (23), V (24), Zr, and Ta (24). The enhancement inelectro-catalytic activity has been explained by a correlation between the optimumnearest-neighbor distance of the elements in the alloy and the bond length in O2 (25). Conventional cathode catalysts comprise either platinum or platinum alloys supported on conducting carbon black at 10 wt percent platinum. Present platinum loadings on the anode and cathode are 0.1 mg/cm2 and 0.5 mg/cm2, respectively (12, 16). It has been suggested by Ito, et al., that the amount of platinum may have been reduced to the extent that it might be cost effective to increase the amount of platinum loading on the cathode (26). However, a problem exists in that fuel cell stack developers have not experienced satisfactory performance improvements when increasing the platinum loading. Johnson Matthey Technology Centre (J-M) presented data that resulted in improved performance nearly in direct proportion to that expected based on the increase in platinum (27). Initial tests by J-M confirmed previous results - that using platinum alloy catalyst with a 10 wt percent net platinum loading improves performance. Platinum/nickel alloy catalysts yielded a 49 wt percent increase in specific activity over pure platinum. This translated into a39 mV improvement in the air electrode performance at 200 mA/cm2.Johnson Matthey then determined that the platinum loading in the alloyed catalyst could beincreased up to 30 wt percent while retaining the same amount of platinum without any decrease inspecific activity or performance; the amount of nickel, hence the total amount of alloyed catalyst,decreased. Next, J-M researchers increased the amount of platinum from 10 to 30 wt percent whilekeeping the same nickel catalyst loading. The total amount of alloyed catalyst increased in thiscase. Results showed an additional 36 wt percent increase in specific activity, which providedanother 41 mV increase at 200 mA/cm2. The ideal voltage increase would have been 46 mV forthis increase in platinum. Thus, the performance increase obtained experimentally was nearly in 14. See Reference 21 for literature survey.direct proportion to the theoretical amount expected. The type of carbon support did not seem tobe a major factor, based on using several typical supports during the tests.The anode of a phosphoric acid fuel cell is subject to a reduction in performance when even lowamounts of contaminants are preferentially absorbed on the noble catalysts. Yet, hydrogen-richfuel gases, other than pure hydrogen, are produced with contaminant levels well in excess of theanode's tolerance limit. Of particular concern are CO, COS, and H2S in the fuel gas. The fuel stream in a state-of-the-art PAFC anode, operating at approximately 200 °C (392 °F), must contain 1 vol percent or less of CO (12), less than 50 ppmv of COS plus H2S, and less than 20 ppmv ofH2S (28). Current practice is to place COS and H2S cleanup systems and CO shift converters priorto the cell (normally in the fuel processor before reforming) to reduce the fuel stream contaminantlevels to the required amounts. Giner, Inc. performed experiments to develop a contaminant-tolerant anode catalyst in order to reduce or eliminate the cleanup equipment (29). An anodecatalyst, G87A-17-2, was identified that resulted in only a 24 mV loss from reference when exposed to a 75 percent H2, 1 percent CO, 24 percent CO2, 80 ppm H2S gas mixture at 190 °C (374 °F), 85 percent fuel utilization, and 200 mA/cm2. A baseline anode experienced a 36 mV loss from the reference at the same conditions. At 9.2 atm (120 psi) pressure, the anode loss was only 19 mV at 190 °C (374 °F) and 17 mV at 210 °C (410 °F) (compared with pure H2) with a gas of 71percent H2, 5 percent CO, 24 percent CO2, and 200 ppm H2S. Economic studies comparing thetradeoff between decreased cell performance with increased savings in plant cost showed noadvantage when the new anode catalyst was used with gas containing 1 percent CO/200 ppm H2S.A $7/kW increase resulted with the 5 percent CO gas (compared to a 1 percent CO gas) at a50 MW size. Some savings would result by eliminating the low temperature shift converter. Thereal value of the catalyst may be its ability to tolerate excessive CO and H2S concentrations duringfuel processor upsets, and to simplify the system by eliminating equipment.As previously mentioned, state-of-the-art gas diffusion electrodes are configured to provide anelectrolyte network and a gas network formed with the mixture of carbon black and PTFE. In the electrodes, carbon black agglomerates, consisting of small primary particles 0.02 to 0.04 µm, are mixed with much larger PTFE particles of ~0.3 µm. The carbon black surface may not be covered completely by the PTFE because of the large size of conventional PTFE particles. The space in the agglomerates or the space between the agglomerates and PTFE may act as gas networks at the initial stage of operation, but fill with electrolyte eventually because of the small contact angle of carbon black, uncovered with PTFE, to electrolyte (<90°), resulting in the degradation of cell performance. Attempts to solve this flooding problem by increasing the PTFE content have not been successful because of the offset in performance resulting from the reduction of catalyst utilization. Higher performance and longer lifetime of electrodes are intrinsically at odds, and there is a limit to the improvement in performance over life by optimizing PTFE content in the state-of-the-art electrode structures. Watanabe, et al. (30) proposed preparing an electrode utilizing 100 percent of catalyst clusters, where the functions of gas diffusion electrodes were allotted completely to a hydrophilic, catalyzed carbon black and a wet-proofed carbon black. The former worked as a fine electrolyte network, and the latter worked as a gas-supplying network in a reaction layer. Higher utilization of catalyst clusters and longer life at the reaction layer were expected, compared to state-of-the-art electrodes consisting of the uniform mixture of catalyzed carbon black and PTFE particles. The iR-free electrode potentials for the reduction of oxygen andair at 200 mA/cm2 on the advanced electrode were 10 mV higher than those of the conventional electrode.There is a trade-off between high power density and cell life performance. One of the major causes of declining cell performance over its life is that electrode flooding and drying, caused by migration of phosphoric acid between the matrix and the electrodes, occurs during cell load cycling. Researchers at Fuji Electric addressed two approaches to improve cell life performance while keeping power density high (31). In one, the wettability of the cathode and anode were optimized, and in the other a heat treatment was applied to the carbon support for the cathode catalyst. During tests, it was observed that a cell with low cathode wettability and high anode wettability was more than 50 mV higher than a cell with the reverse wetting conditions after 40 start/stop cycles.The use of carbon black with large surface area to improve platinum dispersion on supports was investigated as a method to increase the power density of a cell (32). However, some large surface area carbon blacks are fairly corrosive in hot potassium acid, resulting in a loss of catalytic activity. The corrosivity of the carbon support affects both the rate of catalyst loss and electrode flooding and, in turn, the life performance of a cell. Furnace black has been heat treated at high temperature by Fuji Electric to increase its resistance to corrosion. It was found that corrosion could be reduced and cell life performance improved by heat treating carbon supports at high temperature, at least to around 3,000 °C (5,432 °F).More recently, UTC Fuel Cells cites improvements to achieve 40,000 hour cell life through better cell temperature control, increasing H3PO4 inventory, and incorporating electrolyte reservoir plates in the cell stack (3).5.2PerformanceThere have been only minor changes in documented cell performance since the mid-1980s - mostly due to the operating conditions of the cell. The changes are reported in performance trends shown in this section that were primarily gained from contracts that UTC Fuel Cells had with the Department of Energy or outside institutions. New, proprietary PAFC performance data may likely have been observed by the manufacturer (3).Cell performance for any fuel cell is a function of pressure, temperature, reactant gas composition, and fuel utilization. In addition, performance can be adversely affected by impurities in both the fuel and oxidant gases.The sources of polarization in PAFCs (with cathode and anode Pt loadings of 0.5 mg Pt/cm2, 180 °C, 1 atm, 100 percent H3PO4) were discussed in Section 2 and were illustrated as half cell performance in Figure 2-4. From Figure 2-4 it is clear that the major polarization occurs at the cathode, and furthermore, the polarization is greater with air (560 mV at 300 mA/cm2) than with pure oxygen (480 mV at 300 mA/cm2) because of dilution of the reactant. The anode exhibits very low polarization (-4 mV/100 mA/cm2) on pure H2, and increases when CO is present in the fuel gas. The ohmic (iR) loss in PAFCs is also relatively small, amounting to about 12 mV at 100mA/cm2.Typical PAFCs will generally operate in the range of 100 to 400 mA/cm 2 at 600 to 800 mV/cell. Voltage and power constraints arise from increased corrosion of platinum and carbon components at cell potentials above approximately 800 mV.5.2.1 Effect of PressureEven though pressure operation is not being pursued, it is still of interest for possible future development. It is well known that an increase in the cell operating pressure enhances the performance of PAFCs (11, 33, 34). The theoretical change in voltage (∆V P ) as a function of pressure (P) is expressed as2P 1(3)(2.3RT)P (mV) = log V 2P ∆F (5-4)where 3(2.3)1382RT mV =F at 190°C (374 °F). Experimental data (35) reported that the effect of pressure on cell performance at 190°C (374 °F) and 323 mA/cm 2 is correlated by the equation:2P 1P (mV) = 146 log V P ∆ (5-5)where P 1 and P 2 are different cell pressures. The experimental data (35) also suggest that Equation (5-5) is a reasonable approximation for a temperature range of 177 °C < T < 218 °C (351 °F < T < 424 °F) and a pressure range of 1 atm < P < 10 atm (14.7 psi < P < 147.0 psi). Data from Appleby (14) in Figure 5-2 indicate that the voltage gain observed by increasing the pressure from3.4 atm (190 °C) to 6.3 atm (205 °C) is about 44 mV. According to Equation (5-5), the voltage gain calculated for this increase in pressure at 190 °C (374 °F) is 39 mV 15, which is in reasonable agreement with experimental data in Figure 5-2. Measurements (33) of ∆V P for an increase in pressure from4.7 to 9.2 atm (69.1 to 135.2 psia) in a cell at 190 °C (374 °F) show that ∆V P is a function of current density, increasing from 35 mV at 100 mA/cm 2 to 42 mV at 400 mA/cm 2 (50 percent O 2 utilization with air oxidant, 85 percent H 2 utilization with pure H 2 fuel). From Equation (5-4), ∆V p is 43 mV for an increase in pressure from 4.7 to 9.2 atm (69.1 to 135.2 psia) at 190 °C (374 °F), which is very close to the experimental value obtained at 400 mA/cm 2. Other measurements (36) for the same increase in pressure from 4.7 to 9.2 atm (69.1 to 135.2 psia), but at a temperature of 210 °C (410 °F) show less agreement between the experimental data and Equation (5-4).The improvement in cell performance at higher pressure and high current density can be attributed to a lower diffusion polarization at the cathode and an increase in the reversible cell potential. In addition, pressurization decreases activation polarization at the cathode because of the increased oxygen and water partial pressures. If the partial pressure of water is allowed to increase, a lower acid concentration will result. This will increase ionic conductivity and bring about a higher exchange current density. The net outcome is a reduction in ohmic losses. It was reported (33) that an increase in cell pressure (100 percent H 3PO 4, 169 °C (336 °F)) from 1 to 4.4 atm (14.7 to15. The difference in temperature between 190 and 205 °C is disregarded so Equation (5-5) is assumed to be valid at both temperatures.。
研发部工作手册-9.8
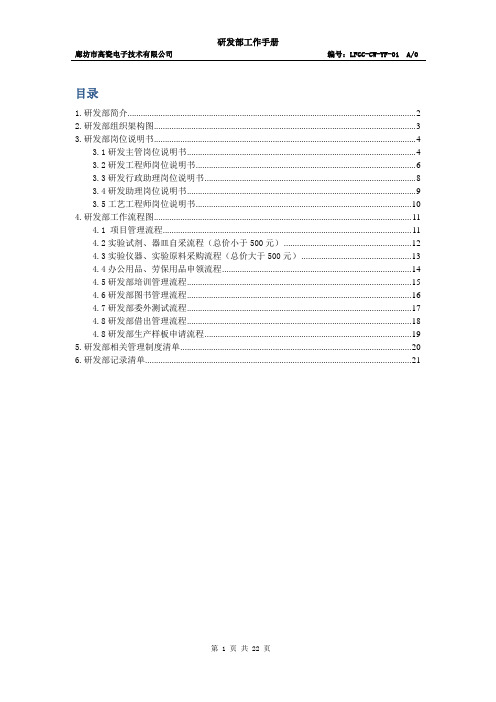
目录1.研发部简介 (2)2.研发部组织架构图 (3)3.研发部岗位说明书 (4)3.1研发主管岗位说明书 (4)3.2研发工程师岗位说明书 (6)3.3研发行政助理岗位说明书 (8)3.4研发助理岗位说明书 (9)3.5工艺工程师岗位说明书 (10)4.研发部工作流程图 (11)4.1 项目管理流程 (11)4.2实验试剂、器皿自采流程(总价小于500元) (12)4.3实验仪器、实验原料采购流程(总价大于500元) (13)4.4办公用品、劳保用品申领流程 (14)4.5研发部培训管理流程 (15)4.6研发部图书管理流程 (16)4.7研发部委外测试流程 (17)4.8研发部借出管理流程 (18)4.8研发部生产样板申请流程 (19)5.研发部相关管理制度清单 (20)6.研发部记录清单 (21)1.研发部简介本公司研发部致力于有机陶瓷板的研发,生产工艺的改进工作。
同时承担着其他电子材料的创新研发工作。
目前廊坊市高瓷电子有限公司研发中心已经形成12人的专业研发团队,总工为国家863计划带头人、中国著名高分子专家秦玉行,主要研发有机陶瓷覆铜板、线路印刷等项目,成员多为各大高校毕业研究生,研究生及以上学历占90%以上。
自研发中心成立以来,已成功申报获批实用新型专利技术一项,申报发明专利一项,另有4项发明专利,2项实用新型专利在筹备中。
产品研发已取得重大进展,成功制备出高导热有机陶瓷覆铜板,各项性能指标均已达到使用水平;另有低介电常数覆铜板、印制线路电路板等项目产品也已取得突破。
随着研发团队日臻成熟,研发体系趋于完善,研发中心将在公司的正确领导下,继续加强和完善自身建设,不断创新超越,为高瓷做大做强的宏伟蓝图,再添浓重的笔墨。
2.研发部组织架构图总经理研发主管研发工程师研发助理工艺工程师3.研发部岗位说明书3.1研发主管岗位说明书岗位名称研发主管所在部门研发部直接上级总经理直接下级研发工程师、工艺工程师、研发助理本职:依据企业研发计划,进行项目的研发及转产工作职责模块岗位职责责任角色分配履职指南工作标准具体工作事项牵头岗位配合岗位行政管理工作1、建立健全研发部管理制度2、做好研发部关于5S的工作3.关于实验室各类物品的的管控总经理研发行政助理1.制度经领导审批后实施2.督促各个岗位做好5S管理工作3.做好各类物品申领、采购的监督指导工作研发部5S工作良好执行;用品采购,领购,管理工作有条理,有计划实施新产品研发工作(实验室阶段)1. 负责项目的新产品技术立项总经理市场部主管对新项目立项做出项目可行性报告可行性评价可靠2. 负责项目的新产品技术开发总经理研发助理1. 详细制定研发计划,确保研发工作有序开展2. 产品开发过程中所有技术文件的收集、整理、存档3. 发表专利,技术文章研发工作开展有序;研发技术文件管理有序;按上级要求按时间与计划经费完成项目,并发表专利及技术文章3. 负责特殊原材料的采购和配置工作总经理研发助理对原材料的采购及配置做好保密工作采购与配置工作有条理产品中试1. 实现实验室阶段到生产阶段的转产总经理生产部与生产部合作,制定详细转产规划按上级要求转产计划在规定时间内完成2. 与质检部合作制定检验方法及流程总经理质检部与质检部合作,制定详细检验文件检验工作有序进行3. 根据市场部反应情况,对产品性能进行调试总经理市场部制定详细调试方法产品调试过后市场部认可技术支持客诉质量问题分析、解决总经理市场部根据客户反映情况解决产品问题问题解决80%以上职业行为禁区绝对禁止行为后果严重行为1、泄露公司技术文件及关键数据;任职资格权力教育水平最低:本科期望:硕士及以上学历1. 行政管理工作建议权专业最低:材料,化学相关专业期望:高分子复合材料相关专业2. 新项目开发一票否定权资格证书最低:不限期望:人力二级及以上3. 产品中试主导权工作经验最低:不限期望:2年及以上同岗位工作经验技能技巧熟悉复合材料的制备及各项性能的表征3.2研发工程师岗位说明书岗位名称研发工程师所在部门研发部直接上级研发主管直接下级研发助理本职:协助制定研发战略规划,完成研发任务,包括新产品的研发,小试、中试和量产中的配方调整等,实验技术文件等编写职责模块岗位职责责任角色分配履职指南工作标准具体工作事项牵头岗位配合岗位新品研发1、前期资料查阅研发主管营销部查阅相关文献、国内外相关信息,结合市场调研,确定产品技术指标。
中国氢能及燃料电池产业手册

中国氢能及燃料电池产业手册随着能源和环境问题的日益紧迫,新能源已经成为全球能源转型的重要方向之一,氢能作为可再生能源的代表之一,受到了各国政府的高度关注和重视。
近年来,中国氢能及燃料电池产业迅速发展,成为全球领先的市场之一。
本手册将介绍中国氢能及燃料电池产业的发展现状、政策体系、技术创新以及市场前景等内容,旨在为相关行业和机构提供全面而权威的参考资料。
一、产业概述自2003年中国启动“863”计划以来,氢能及燃料电池技术研发和产业化工作就启动了。
国家政策和产业基金的支持下,氢能及燃料电池产业逐渐萌芽、发展、壮大。
截至2021年,中国氢能及燃料电池产业已经形成了以汽车、供能、储能、备电为主要应用方向的完整产业链。
其中,燃料电池汽车逐渐成为整个产业的推动力。
当前,中国氢能及燃料电池产业已进入到了加速发展、形成产业体系的新阶段。
政策支持、市场需求、技术成熟度和供应链优势等多重因素,成为支撑中国氢能及燃料电池产业进一步发展的主要因素。
根据国家能源局预测,2025年中国氢能及燃料电池装机容量将达到200万千瓦,销售收入预计达到2000亿元。
二、政策环境目前,中国氢能及燃料电池产业的政策支持体系主要包括国家高层文件、地方政策、产业基金等。
其中,国家“十三五”规划中指出加快推进氢能利用的发展,以燃料电池汽车、储氢技术为重点,促进氢能产业的快速发展。
各地也陆续出台了支持氢能及燃料电池汽车产业发展的红利政策,包括财政补贴、税收优惠、用地政策等。
此外,产业基金也是目前支持中国氢能及燃料电池产业发展的重要途径之一。
国家和各地的氢能及燃料电池产业基金,已经资助了一大批相关技术研发和产业化水平提高的企业。
三、技术创新中国氢能及燃料电池产业的技术创新依托于国家“863”计划、国家新能源汽车产业创新工程等大型科技计划。
在燃料电池堆、电解水制氢、氢气储运等关键技术领域,国内企业已经获得了多项自主知识产权和专利技术,并在行业内处于领先地位。
动力电池研发――岗位说明书
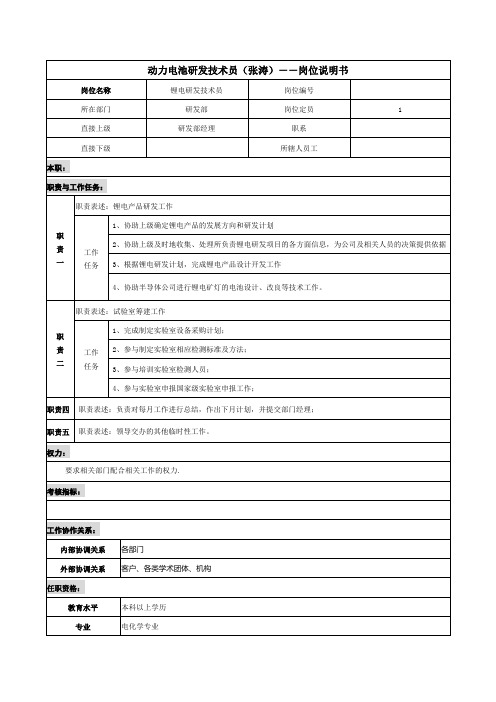
岗位名称
锂电研发技术员
岗位编号
所在部门
研发部
岗位定员
1
直接上级
研发部经理
职系
直接下级
所辖人员工
本职:
职责与工作任务:
职
责
一
职责表述:锂电产品研发工作
工作
任务Βιβλιοθήκη 1、协助上级确定锂电产品的发展方向和研发计划
2、协助上级及时地收集、处理所负责锂电研发项目的各方面信息,为公司及相关人员的决策提供依据
3、根据锂电研发计划,完成锂电产品设计开发工作
4、协助半导体公司进行锂电矿灯的电池设计、改良等技术工作。
职
责
二
职责表述:试验室筹建工作
工作
任务
1、完成制定实验室设备采购计划;
2、参与制定实验室相应检测标准及方法;
3、参与培训实验室检测人员;
4、参与实验室申报国家级实验室申报工作;
职责四
职责表述:负责对每月工作进行总结,作出下月计划,并提交部门经理;
时间:
计算机操作、管理技能
经验
具有行业工作二年以上的经验,了解熟悉本产品的制造工艺和质量标准
其它:
使用工具设备
计算机和相关设备
工作环境
办公室、实验室、车间
工作时间特征
在公司规定的时间内工作,有时需加班,偶尔出差;(建议规定加班时间和相应福利)
所需记录文档
述职人签字
任职人:
任职人上级:
人力资源部:
时间:
时间:
职责五
职责表述:领导交办的其他临时性工作。
权力:
要求相关部门配合相关工作的权力.
考核指标:
研发部工作手册ok

目录1.研发部一体化治理体系职位图22. 研发部职能33. 研发部职位职责4部门领导职位职责4软件组组长职位职责5软件工程师职位职责5系统分析员职位职责6配置治理员职位职责6文档工程师职位职责7硬件组组长职位职责7研发工程师职位职责8研发技术员职位职责9结构工程师职位职责9测试组组长职位职责10应用软件测试工程师10系统软件测试工程师11硬件测试工程师11质检员124. 治理制度135 研发部质量/环境目标分解表146 研发部质量/环境目标考核表151. 研发部一体化治理体系组织结构图/职位图2. 研发部职能依照公司的质量/环境方针和质量/环境目标,组织开展方针、目标治理负责公司软件、硬件项目/产品的方案设计、详细设计负责对项目进行质量、环境活动的日常监督检查工作;负责组织项目的环境因素识别操纵工作,进行重要环境因素识别的报批。
负责软件、硬件项目/产品设计文件的修改、归档机治理。
负责软件、硬件项目/产品涉及的物资和设备的采购清单的编制。
负责公司软件、硬件项目/产品的安装调试和工程施工负责本部门一体化治理体系文件的编写、修改和相关外来文件资料的治理负责软件、硬件项目/产品交付和保护。
负责软件、硬件项目/产品涉及的物资和设备的治理。
负责相关备件的治理工作。
负责网络效劳产品的方案制作、销售拓展工作。
配合组织审定技术治理标准。
认真做好技术图张、技术资料的归档工作。
负责制定严格的技术资料交接、保管工作制度。
负责拟定本部门目标、工作打算。
组织实施检查监督及操纵。
负责产品实现的策划、设计和开发、软件、硬件项目/产品的进程操纵、设备设施的治理、工作环境的操纵,参与内审、治理评审,负责数据分析,参与纠正与预防方法的制定与实施。
负责软件、硬件项目/产品文档的治理。
3. 研发部职位职责领导职位职责3.1.1 负责本部门的业务治理工作;3.1.2 负责部门技术人员技术梯队的建设和培训;3.1.3 负责提高部门的技术专业水平和员工的软件、硬件项目/产品设计、开发及效劳能力;3.1.4 负责率领部门员工完成公司下达的考核指标;3.1.5 确保一体化治理体系在本部门正常运行;3.1.6 参加公司的治理评审;3.1.7 参加公司的内部审核;3.1.8 负责组织部门员工认真学习体系文件,将文件对本部门的要求分解到职位,并实施;3.1.9 负责组织将公司质量/环境目标分解,定制考核方式,并按时考核负责软件、硬件项目/产品销售的拓展工作;3.1.10负责组织编制作业指导文件,并监督执行;3.1.11负责软件、硬件项目/产品方案的审批;3.1.12编制施工作业打算并组织实施、检查和考核;3.1.13制定公司产品研发打算,撰写项目可行性研究报告,主持编写公司产品方案及标书;3.1.14科学制定本部门团队建设计划,不断提高本部门整体技术水平;3.1.15组织和分派研发部各项工作(项目、效劳)的开展和人员和所有资源的分派,并对整体的情形和每一个里程碑进行监督和操纵;3.1.16对产品质量/环境、治理、本钱工作负责;3.1.17与技术、销售、质检/采购等部门或人员做好和谐、沟通工作。
燃料电池应用技术手册
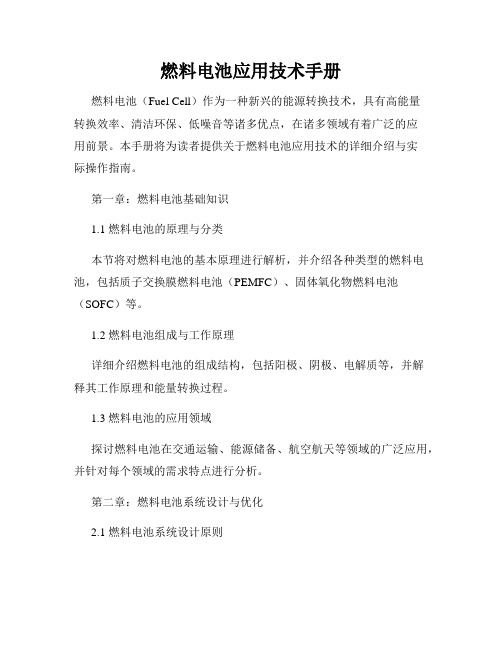
燃料电池应用技术手册燃料电池(Fuel Cell)作为一种新兴的能源转换技术,具有高能量转换效率、清洁环保、低噪音等诸多优点,在诸多领域有着广泛的应用前景。
本手册将为读者提供关于燃料电池应用技术的详细介绍与实际操作指南。
第一章:燃料电池基础知识1.1 燃料电池的原理与分类本节将对燃料电池的基本原理进行解析,并介绍各种类型的燃料电池,包括质子交换膜燃料电池(PEMFC)、固体氧化物燃料电池(SOFC)等。
1.2 燃料电池组成与工作原理详细介绍燃料电池的组成结构,包括阳极、阴极、电解质等,并解释其工作原理和能量转换过程。
1.3 燃料电池的应用领域探讨燃料电池在交通运输、能源储备、航空航天等领域的广泛应用,并针对每个领域的需求特点进行分析。
第二章:燃料电池系统设计与优化2.1 燃料电池系统设计原则介绍燃料电池系统设计的基本原则,包括能量匹配、负载管理、热管理等,并提供系统设计的实用方法和技巧。
2.2 燃料电池堆叠与集成技术讲解燃料电池堆叠设计的要点,包括堆叠结构、气体循环、热平衡等,并介绍堆叠与其他系统组件的集成技术。
第三章:燃料电池关键材料3.1 阳极材料详细介绍用于燃料电池阳极的常见材料,如铂、钯合金等,并探讨材料的制备方法和改性技术。
3.2 阴极材料介绍用于燃料电池阴极的材料,如钴酸锂、铁酸锂等,并分析材料的储氢性能和循环稳定性。
3.3 电解质材料讨论燃料电池中常用的电解质材料,如聚四氟乙烯(PTFE)、磺酸聚合物等,并研究其导电性能和稳定性。
第四章:燃料电池性能评价与测试技术4.1 燃料电池性能评价指标介绍评价燃料电池性能的关键指标,如电压、功率密度、效率等,并提供相应的测试方法和实验操作指南。
4.2 燃料电池测试技术讲解燃料电池性能测试的常用技术,包括极化曲线测试、循环伏安测试等,并介绍测试中的注意事项和数据处理方法。
第五章:燃料电池应用案例5.1 汽车领域应用案例介绍燃料电池在汽车领域的应用案例,包括燃料电池乘用车、货运车辆等,并分析其优势和挑战。
新能源开发与利用技术的员工手册
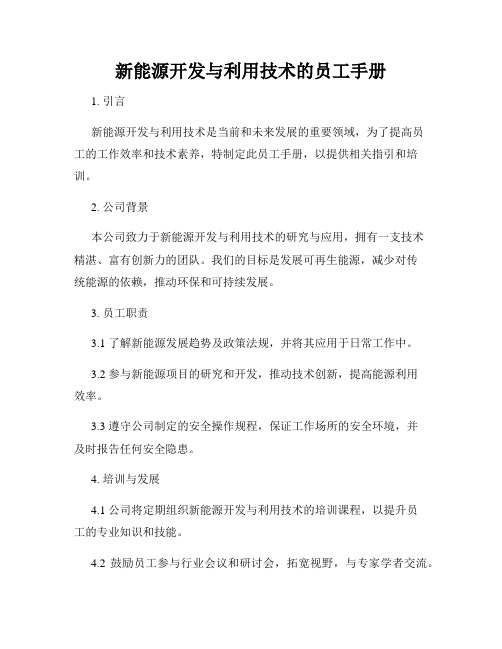
新能源开发与利用技术的员工手册1. 引言新能源开发与利用技术是当前和未来发展的重要领域,为了提高员工的工作效率和技术素养,特制定此员工手册,以提供相关指引和培训。
2. 公司背景本公司致力于新能源开发与利用技术的研究与应用,拥有一支技术精湛、富有创新力的团队。
我们的目标是发展可再生能源,减少对传统能源的依赖,推动环保和可持续发展。
3. 员工职责3.1 了解新能源发展趋势及政策法规,并将其应用于日常工作中。
3.2 参与新能源项目的研究和开发,推动技术创新,提高能源利用效率。
3.3 遵守公司制定的安全操作规程,保证工作场所的安全环境,并及时报告任何安全隐患。
4. 培训与发展4.1 公司将定期组织新能源开发与利用技术的培训课程,以提升员工的专业知识和技能。
4.2 鼓励员工参与行业会议和研讨会,拓宽视野,与专家学者交流。
5. 工作环境与设备5.1 公司将提供符合安全要求的工作环境,并配备必要的安全设备和防护用品。
5.2 员工应妥善使用和维护工作设备,定期检查设备的工作状况,确保正常运行。
6. 创新与合作6.1 鼓励员工提出新的创意和技术需求,促进技术创新和进步。
6.2 鼓励员工之间的合作与交流,共同攻克技术难题,提高工作效率。
7. 意识与责任7.1 强调员工对环境保护的责任意识,提倡节能减排,推动可持续发展。
7.2 公司将对出色工作的员工给予表彰和奖励,激励员工的积极性和创造力。
8. 问题与纠纷解决如遇到工作中的问题或纠纷,员工可以向相关主管或人力资源部门寻求帮助和解决方案。
9. 结语本手册为新能源开发与利用技术的员工提供了相关的指引和规范,希望员工能够遵守并将其应用于实际工作中,为公司的发展和可持续能源的进步做出贡献。
以上是新能源开发与利用技术的员工手册的内容。
希望员工能够了解手册的内容并按照其中的规定开展工作,使公司能够快速发展,并在新能源领域取得更大的成就。
感谢员工的支持与配合!。
新能源电池研发技术操作指南
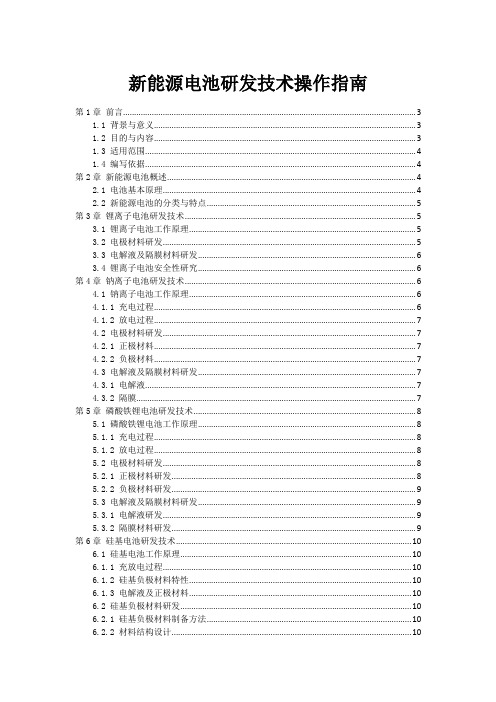
新能源电池研发技术操作指南第1章前言 (3)1.1 背景与意义 (3)1.2 目的与内容 (3)1.3 适用范围 (4)1.4 编写依据 (4)第2章新能源电池概述 (4)2.1 电池基本原理 (4)2.2 新能源电池的分类与特点 (5)第3章锂离子电池研发技术 (5)3.1 锂离子电池工作原理 (5)3.2 电极材料研发 (5)3.3 电解液及隔膜材料研发 (6)3.4 锂离子电池安全性研究 (6)第4章钠离子电池研发技术 (6)4.1 钠离子电池工作原理 (6)4.1.1 充电过程 (6)4.1.2 放电过程 (7)4.2 电极材料研发 (7)4.2.1 正极材料 (7)4.2.2 负极材料 (7)4.3 电解液及隔膜材料研发 (7)4.3.1 电解液 (7)4.3.2 隔膜 (7)第5章磷酸铁锂电池研发技术 (8)5.1 磷酸铁锂电池工作原理 (8)5.1.1 充电过程 (8)5.1.2 放电过程 (8)5.2 电极材料研发 (8)5.2.1 正极材料研发 (8)5.2.2 负极材料研发 (9)5.3 电解液及隔膜材料研发 (9)5.3.1 电解液研发 (9)5.3.2 隔膜材料研发 (9)第6章硅基电池研发技术 (10)6.1 硅基电池工作原理 (10)6.1.1 充放电过程 (10)6.1.2 硅基负极材料特性 (10)6.1.3 电解液及正极材料 (10)6.2 硅基负极材料研发 (10)6.2.1 硅基负极材料制备方法 (10)6.2.2 材料结构设计 (10)6.2.3 表面修饰 (10)6.3 电解液及正极材料研发 (11)6.3.1 电解液研发 (11)6.3.2 正极材料研发 (11)6.3.3 电解液与正极材料的匹配 (11)第7章固态电池研发技术 (11)7.1 固态电池工作原理 (11)7.1.1 电荷传输 (11)7.1.2 离子传输 (11)7.1.3 电极反应 (11)7.2 固态电解质材料研发 (12)7.2.1 硫化物固态电解质 (12)7.2.2 氧化物固态电解质 (12)7.2.3 铵盐固态电解质 (12)7.3 电极材料研发 (12)7.3.1 金属锂负极 (12)7.3.2 金属氧化物正极 (12)7.3.3 硫基正极材料 (13)7.3.4 复合电极材料 (13)第8章电池管理系统研发技术 (13)8.1 电池管理系统概述 (13)8.2 状态估计与安全管理 (13)8.2.1 状态估计 (13)8.2.2 安全管理 (13)8.3 热管理与均衡控制 (14)8.3.1 热管理 (14)8.3.2 均衡控制 (14)第9章电池制备与组装技术 (14)9.1 电极制备工艺 (14)9.1.1 活性物质的选择与处理 (14)9.1.2 导电剂与粘结剂的选择 (15)9.1.3 电极浆料的制备 (15)9.1.4 涂覆与干燥 (15)9.1.5 电极裁切与整形 (15)9.2 电解液及隔膜制备工艺 (15)9.2.1 电解液的选择与配制 (15)9.2.2 隔膜的选择与处理 (15)9.2.3 电解液与隔膜的匹配 (15)9.3 电池单体组装与封装 (15)9.3.1 电池单体的结构设计 (15)9.3.2 电池单体的装配 (15)9.3.3 电池单体的封装 (15)9.3.4 电池单体的化成与老化 (16)9.3.5 电池单体功能检测 (16)第10章电池测试与评价技术 (16)10.1 电池功能测试方法 (16)10.1.1 充放电功能测试 (16)10.1.2 阻抗测试 (16)10.1.3 自放电功能测试 (16)10.2 电池安全性评价 (16)10.2.1 安全性实验 (16)10.2.2 电池热管理系统评价 (16)10.2.3 内部短路风险评估 (16)10.3 电池循环寿命与可靠性评价 (16)10.3.1 循环寿命测试 (17)10.3.2 可靠性评估 (17)10.3.3 模块与系统级评价 (17)10.4 电池环境适应性评价 (17)10.4.1 高温适应性测试 (17)10.4.2 低温适应性测试 (17)10.4.3 湿度适应性测试 (17)10.4.4 振动与冲击测试 (17)第1章前言全球能源结构的转型与可持续发展战略的不断深入,新能源产业在我国得到了前所未有的关注与扶持。
燃料电池手册7_部分2
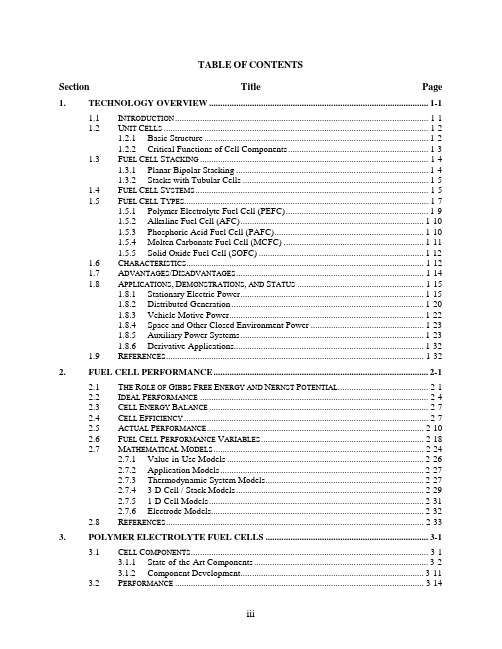
TABLE OF CONTENTSSection Title Page 1.TECHNOLOGY OVERVIEW.................................................................................................1-11.1I NTRODUCTION................................................................................................................1-11.2U NIT C ELLS.....................................................................................................................1-21.2.1Basic Structure...................................................................................................1-21.2.2Critical Functions of Cell Components..............................................................1-31.3F UEL C ELL S TACKING.....................................................................................................1-41.3.1Planar-Bipolar Stacking.....................................................................................1-41.3.2Stacks with Tubular Cells..................................................................................1-51.4F UEL C ELL S YSTEMS.......................................................................................................1-51.5F UEL C ELL T YPES............................................................................................................1-71.5.1Polymer Electrolyte Fuel Cell (PEFC)...............................................................1-91.5.2Alkaline Fuel Cell (AFC).................................................................................1-101.5.3Phosphoric Acid Fuel Cell (PAFC)..................................................................1-101.5.4Molten Carbonate Fuel Cell (MCFC)..............................................................1-111.5.5Solid Oxide Fuel Cell (SOFC).........................................................................1-121.6C HARACTERISTICS.........................................................................................................1-121.7A DVANTAGES/D ISADVANTAGES...................................................................................1-141.8A PPLICATIONS,D EMONSTRATIONS, AND S TATUS........................................................1-151.8.1Stationary Electric Power.................................................................................1-151.8.2Distributed Generation.....................................................................................1-201.8.3Vehicle Motive Power......................................................................................1-221.8.4Space and Other Closed Environment Power..................................................1-231.8.5Auxiliary Power Systems.................................................................................1-231.8.6Derivative Applications....................................................................................1-321.9R EFERENCES..................................................................................................................1-322.FUEL CELL PERFORMANCE...............................................................................................2-12.1T HE R OLE OF G IBBS F REE E NERGY AND N ERNST P OTENTIAL........................................2-12.2I DEAL P ERFORMANCE.....................................................................................................2-42.3C ELL E NERGY B ALANCE.................................................................................................2-72.4C ELL E FFICIENCY............................................................................................................2-72.5A CTUAL P ERFORMANCE................................................................................................2-102.6F UEL C ELL P ERFORMANCE V ARIABLES........................................................................2-182.7M ATHEMATICAL M ODELS.............................................................................................2-242.7.1Value-in-Use Models.......................................................................................2-262.7.2Application Models..........................................................................................2-272.7.3Thermodynamic System Models......................................................................2-272.7.43-D Cell / Stack Models...................................................................................2-292.7.51-D Cell Models...............................................................................................2-312.7.6Electrode Models..............................................................................................2-322.8R EFERENCES..................................................................................................................2-333.POLYMER ELECTROLYTE FUEL CELLS........................................................................3-13.1C ELL C OMPONENTS.........................................................................................................3-13.1.1State-of-the-Art Components.............................................................................3-23.1.2Component Development.................................................................................3-113.2P ERFORMANCE..............................................................................................................3-143.3PEFC S YSTEMS..............................................................................................................3-163.3.1Direct Hydrogen PEFC Systems......................................................................3-163.3.2Reformer-Based PEFC Systems.......................................................................3-173.3.3Direct Methanol Fuel Cell Systems.................................................................3-193.4PEFC A PPLICATIONS.....................................................................................................3-213.4.1Transportation Applications.............................................................................3-213.4.2Stationary Applications....................................................................................3-223.5R EFERENCES..................................................................................................................3-224.ALKALINE FUEL CELL.........................................................................................................4-14.1C ELL C OMPONENTS.........................................................................................................4-54.1.1State-of-the-Art Components.............................................................................4-54.1.2Development Components.................................................................................4-64.2P ERFORMANCE................................................................................................................4-74.2.1Effect of Pressure...............................................................................................4-84.2.2Effect of Temperature........................................................................................4-94.2.3Effect of Impurities..........................................................................................4-114.2.4Effects of Current Density................................................................................4-124.2.5Effects of Cell Life...........................................................................................4-144.3S UMMARY OF E QUATIONS FOR AFC.............................................................................4-144.4R EFERENCES..................................................................................................................4-165.PHOSPHORIC ACID FUEL CELL........................................................................................5-15.1C ELL C OMPONENTS.........................................................................................................5-25.1.1State-of-the-Art Components.............................................................................5-25.1.2Development Components.................................................................................5-65.2P ERFORMANCE..............................................................................................................5-115.2.1Effect of Pressure.............................................................................................5-125.2.2Effect of Temperature......................................................................................5-135.2.3Effect of Reactant Gas Composition and Utilization.......................................5-145.2.4Effect of Impurities..........................................................................................5-165.2.5Effects of Current Density................................................................................5-195.2.6Effects of Cell Life...........................................................................................5-205.3S UMMARY OF E QUATIONS FOR PAFC...........................................................................5-215.4R EFERENCES..................................................................................................................5-226.MOLTEN CARBONATE FUEL CELL..................................................................................6-16.1C ELL C OMPONENTS.........................................................................................................6-46.1.1State-of-the-Art Componments..........................................................................6-46.1.2Development Components.................................................................................6-96.2P ERFORMANCE..............................................................................................................6-136.2.1Effect of Pressure.............................................................................................6-156.2.2Effect of Temperature......................................................................................6-196.2.3Effect of Reactant Gas Composition and Utilization.......................................6-216.2.4Effect of Impurities..........................................................................................6-256.2.5Effects of Current Density................................................................................6-306.2.6Effects of Cell Life...........................................................................................6-306.2.7Internal Reforming...........................................................................................6-306.3S UMMARY OF E QUATIONS FOR MCFC..........................................................................6-346.4R EFERENCES..................................................................................................................6-387.SOLID OXIDE FUEL CELLS..................................................................................................7-17.1C ELL C OMPONENTS.........................................................................................................7-27.1.1Electrolyte Materials..........................................................................................7-27.1.2Anode Materials.................................................................................................7-37.1.3Cathode Materials..............................................................................................7-57.1.4Interconnect Materials........................................................................................7-67.1.5Seal Materials.....................................................................................................7-97.2C ELL AND S TACK D ESIGNS...........................................................................................7-137.2.1Tubular SOFC..................................................................................................7-137.2.1.1 Performance........................................................................................7-207.2.2Planar SOFC.....................................................................................................7-317.2.2.1 Single Cell Performance......................................................................7-357.2.2.2 Stack Performance...............................................................................7-397.2.3Stack Scale-Up.................................................................................................7-417.3S YSTEM C ONSIDERATIONS............................................................................................7-457.4R EFERENCES..................................................................................................................7-458.FUEL CELL SYSTEMS............................................................................................................8-18.1S YSTEM P ROCESSES........................................................................................................8-28.1.1Fuel Processing..................................................................................................8-28.2P OWER C ONDITIONING..................................................................................................8-278.2.1 Introduction to Fuel Cell Power Conditioning Systems...................................8-288.2.2 Fuel Cell Power Conversion for Supplying a Dedicated Load [2,3,4].............8-298.2.3 Fuel Cell Power Conversion for Supplying Backup Power to a LoadConnected to a Local Utility............................................................................8-348.2.4 Fuel Cell Power Conversion for Supplying a Load Operating in ParallelWith the Local Utility (Utility Interactive)......................................................8-378.2.5 Fuel Cell Power Conversion for Connecting Directly to the Local Utility......8-378.2.6 Power Conditioners for Automotive Fuel Cells...............................................8-398.2.7 Power Conversion Architecture for a Fuel Cell Turbine Hybrid InterfacedWith a Local Utility..........................................................................................8-418.2.8 Fuel Cell Ripple Current..................................................................................8-438.2.9 System Issues: Power Conversion Cost and Size.............................................8-44R EFERENCES (Sections 8.1 and 8.2).................................................................8-458.2.108.3S YSTEM O PTIMIZATION.................................................................................................8-468.3.1Pressure............................................................................................................8-468.3.2Temperature.....................................................................................................8-488.3.3Utilization.........................................................................................................8-498.3.4Heat Recovery..................................................................................................8-508.3.5Miscellaneous...................................................................................................8-518.3.6Concluding Remarks on System Optimization................................................8-518.4F UEL C ELL S YSTEM D ESIGNS........................................................................................8-528.4.1Natural Gas Fueled PEFC System...................................................................8-528.4.2Natural Gas Fueled PAFC System...................................................................8-538.4.3Natural Gas Fueled Internally Reformed MCFC System.................................8-568.4.4Natural Gas Fueled Pressurized SOFC System................................................8-588.4.5Natural Gas Fueled Multi-Stage Solid State Power Plant System...................8-628.4.6Coal Fueled SOFC System...............................................................................8-668.4.7Power Generation by Combined Fuel Cell and Gas Turbine System..............8-708.4.8Heat and Fuel Recovery Cycles.......................................................................8-708.5F UEL C ELL N ETWORKS.................................................................................................8-828.5.1Molten Carbonate Fuel Cell Networks: Principles, Analysis andPerformance.....................................................................................................8-828.5.2MCFC Network................................................................................................8-868.5.3Recycle Scheme...............................................................................................8-868.5.4Reactant Conditioning Between Stacks in Series.............................................8-868.5.5Higher Total Reactant Utilization....................................................................8-878.5.6Disadvantages of MCFC Networks..................................................................8-888.5.7Comparison of Performance.............................................................................8-888.5.8Conclusions......................................................................................................8-898.6H YBRIDS........................................................................................................................8-898.6.1Technology.......................................................................................................8-898.6.2Projects.............................................................................................................8-928.6.3World’s First Hybrid Project............................................................................8-938.6.4Hybrid Electric Vehicles (HEV)......................................................................8-938.7F UEL C ELL A UXILIARY P OWER S YSTEMS.....................................................................8-968.7.1System Performance Requirements..................................................................8-978.7.2Technology Status............................................................................................8-988.7.3System Configuration and Technology Issues.................................................8-998.7.4System Cost Considerations...........................................................................8-1028.7.5SOFC System Cost Structure.........................................................................8-1038.7.6Outlook and Conclusions...............................................................................8-1048.8R EFERENCES................................................................................................................8-1049.SAMPLE CALCULATIONS....................................................................................................9-19.1U NIT O PERATIONS...........................................................................................................9-19.1.1Fuel Cell Calculations........................................................................................9-19.1.2Fuel Processing Calculations...........................................................................9-139.1.3Power Conditioners..........................................................................................9-169.1.4Others...............................................................................................................9-169.2S YSTEM I SSUES..............................................................................................................9-169.2.1Efficiency Calculations....................................................................................9-179.2.2Thermodynamic Considerations.......................................................................9-199.3S UPPORTING C ALCULATIONS........................................................................................9-229.4C OST C ALCULATIONS....................................................................................................9-259.4.1Cost of Electricity.............................................................................................9-259.4.2Capital Cost Development...............................................................................9-269.5C OMMON C ONVERSION F ACTORS.................................................................................9-279.6A UTOMOTIVE D ESIGN C ALCULATIONS.........................................................................9-289.7R EFERENCES..................................................................................................................9-2910.APPENDIX...............................................................................................................................10-110.1E QUILIBRIUM C ONSTANTS............................................................................................10-110.2C ONTAMINANTS FROM C OAL G ASIFICATION................................................................10-210.3S ELECTED M AJOR F UEL C ELL R EFERENCES,1993 TO P RESENT...................................10-410.4L IST OF S YMBOLS........................................................................................................10-1010.5F UEL C ELL R ELATED C ODES AND S TANDARDS..........................................................10-1410.5.1Introduction....................................................................................................10-1410.5.2Organizations.................................................................................................10-1510.5.3Codes & Standards.........................................................................................10-1610.5.4Codes and Standards for Fuel Cell Manufacturers.........................................10-1710.5.5Codes and Standards for the Installation of Fuel Cells..................................10-1910.5.6Codes and Standards for Fuel Cell Vehicles..................................................10-1910.5.7Application Permits........................................................................................10-1910.5.8References......................................................................................................10-2110.6F UEL C ELL F IELD S ITE D ATA......................................................................................10-2110.6.1Worldwide Sites.............................................................................................10-2110.6.2DoD Field Sites..............................................................................................10-2410.6.3IFC Field Units...............................................................................................10-2410.6.4FuelCell Energy..............................................................................................10-2410.6.5Siemens Westinghouse...................................................................................10-2410.7H YDROGEN..................................................................................................................10-3110.7.1Introduction....................................................................................................10-3110.7.2Hydrogen Production.....................................................................................10-3210.7.3DOE’s Hydrogen Research............................................................................10-3410.7.4Hydrogen Storage...........................................................................................10-3510.7.5Barriers...........................................................................................................10-3610.8T HE O FFICE OF E NERGY E FFICIENCY AND R ENEWABLE E NERGY W ORK IN F UELC ELLS..........................................................................................................................10-3610.9R ARE E ARTH M INERALS.............................................................................................10-3810.9.1Introduction....................................................................................................10-3810.9.2Outlook...........................................................................................................10-4010.10R EFERENCES................................................................................................................10-4111.INDEX.......................................................................................................................................11-1LIST OF FIGURESFigure Title Page Figure 1-1 Schematic of an Individual Fuel Cell...................................................................1-2Figure 1-2 Expanded View of a Basic Fuel Cell Unit in a Fuel Cell Stack (1).....................1-4Figure 1-3 Fuel Cell Power Plant Major Processes................................................................1-7Figure 1-4 Relative Emissions of PAFC Fuel Cell Power Plants Compared to StringentLos Angeles Basin Requirements......................................................................1-13Figure 1-5 PC-25 Fuel Cell..................................................................................................1-16Figure 1-6 Combining the SOFC with a Gas Turbine Engine to Improve Efficiency........1-19Figure 1-7 Overview of Fuel Cell Activities Aimed at APU Applications.........................1-24Figure 1-8 Overview of APU Applications.........................................................................1-24Figure 1-9 Overview of typical system requirements..........................................................1-25Figure 1-10 Stage of development for fuel cells for APU applications................................1-26Figure 1-11 Overview of subsystems and components for SOFC and PEFC systems.........1-28Figure 1-12 Simplified process flow diagram of pre-reformer/SOFC system......................1-29Figure 1-13 Multilevel system modeling approach...............................................................1-30Figure 1-14 Projected Cost Structure of a 5kWnet APU SOFC System. .............................1-32Figure 2-1 H2/O2 Fuel Cell Ideal Potential as a Function of Temperature............................2-5Figure 2-2 Effect of fuel utilization on voltage efficiency and overall cell efficiencyfor typical SOFC operating conditions (800 °C, 50% initial hydrogenconcentration)....................................................................................................2-10Figure 2-3 Ideal and Actual Fuel Cell Voltage/Current Characteristic...............................2-11Figure 2-4 Example of a Tafel Plot.....................................................................................2-13Figure 2-5 Example of impedance spectrum of anode-supported SOFC operated at°C................................................................................................................2-14850Figure 2-6 Contribution to Polarization of Anode and Cathode..........................................2-17Figure 2-7 Voltage/Power Relationship..............................................................................2-19Figure 2-8 The Variation in the Reversible Cell Voltage as a Function of ReactantUtilization..........................................................................................................2-23Figure 2-9 Overview of Levels of Fuel Cell Models...........................................................2-26Figure 2-10 Conours of Current Density on Electrolyte.......................................................2-31Figure 2-11 Typical Phenomena Considered in a 1-D Model (17).......................................2-32Figure 2-12 Overview of types of electrode models (9)........................................................2-33Figure 3-1 (a) Schematic of Representative PEFC (b) Single Cell Structure ofPEFC...........................................................................................3-2RepresentativeFigure 3-2 PEFC Schematic (4, 5).........................................................................................3-3Figure 3-3 Polarization Curves for 3M 7 Layer MEA (12)...................................................3-7Figure 3-4 Endurance Test Results for Gore Primea 56 MEA at Three CurrentDensities.............................................................................................................3-10Figure 3-5 Multi-Cell Stack Performance on Dow Membrane (9)......................................3-12Figure 3-6 Effect on PEFC Performance of Bleeding Oxygen into the Anode(1)................................................................................................3-13CompartmentFigure 3-7 Evolutionary Changes in PEFCs Performance [(a) H2/O2, (b) H2/Air,(c) Reformate Fuel/Air, (d) H2/unkown)] [24, 10, 12, , ]..................................3-14。
研发部工作手册范本

研发中心工作手册
1.研发中心定位
研发部依据公司的战略,制定新品研发计划和策略,对对外直接负责新品项目的开发、跟踪及市场开拓;以考核、激励等多种方式建立人才梯队;新品开发中的知识产权计划。
1.研发中心架构图
2.研发中心人员编制图(常设人员4人)
研发中心研发主任负责具体相关工作。
3.研发中心职责
5.岗位描述
5.1研发主任
5.2总工
5.3财务成本审核
5.4研发组长
5.5新品销售经理
6.研发中心工作流程
6.1新品研发流程
6.1.1控制目标
有效控制资金风险,提高工作效率,使加工业务工作有序有效的进行。
6.1.2流程跟踪者
总工程师
6.2新品设计流程
6.2.1控制目标
根据客户要求,按时完成设计任务要求。
6.2.2流程跟踪者
总工程师
6.2.3流程图说明
6.3新品成本核算及报价流程
6.3.1控制目标
准确核定项目成本,合理制作新品报价。
6.3.2流程跟踪者
财务成本审核
6.3.3流程图说明
7、研发中心相关表单
7.1项目立项与审批
项目科研计划
研发项目知识产权状况分析报告
项目立项申请审批单
项目科研计划
研发项目知识产权状况分析报告编号:
编制:项目组长审核:总工批准:研发主任
项目立项申请审批单
编号:。
(完整word版)燃料电池产品操作说明书

KAT-115型燃料电池实训系统产品说明书由于产品的不断更新、完善,本资料中的内容可能与实际产品不完全相符,敬请谅解。
如需查询产品的更新情况,请联系当地经销商。
操作手册如有改动,恕不另行通知。
目录1、燃料电池 (4)1)安全警告及担保 (4)2) EOS50系列产品说明 (5)2.1 EOS50系列产品结构 (5)2.2 EOS50系列主要特点 (6)2.3 EOS50系列测试参数 (7)2.4 EOS50性能曲线图 (7)2.5 EOS50装配说明 (8)3)燃料电池操作运行环境 (10)3.1 工作环境要求 (10)3.2 供氢设备使用注意事项 (12)4)燃料电池系统运行操作步骤 (15)5)操作注意事项 (16)6)燃料电池活化操作步骤及注意事项 (17)2、氢气减压器 (19)3、逆变器 (20)1、安全警告及担保操作人员在进行任何有关燃料电池设备的操作之前,需要仔细阅读所在地的安全规范和相关操作规程。
手册中提到的安全注意事项只作为当地安全规范的补充。
操作人员进行设备安装、操作和维护时,必须充分领会该用户手册,系统掌握正确的操作方法及各种安全注意事项后方可进行设备的各项操作。
不正确的操作可能会导致设备损坏或人身伤害。
出于安全性的考虑,不建议在狭小的密闭空间操作燃料电池。
因为一旦氢气泄露极有可能酿成危险。
同时禁止靠近热源、火源操作燃料电池系统。
必须保证提供充足的氢气燃料,如果氢气供应不足会造成燃料电池的严重不可恢复的损坏。
EOS50型电池在额定功率下瞬时需要氢气流量为(20.05)L/min(排气瞬间),稳定氢气消耗为0.85L/min。
操作时严禁佩戴手表、手链、手镯、戒指等易导电物体。
操作时必须使用绝缘工具。
在进行直流带电作业时必须严格检查线缆和接口端子的极性。
在连接电缆之前,必须先确认电缆、电缆标识与实际安装情况相符后再进行连接。
燃料电池设备仅能由专业的维修人员予以维修。
本公司不承担任何因违反安全操作要求或违反设计、生产和使用设备安全标准所造成的后果的责任。
燃料电池应用手册(横河)JAPAN解析
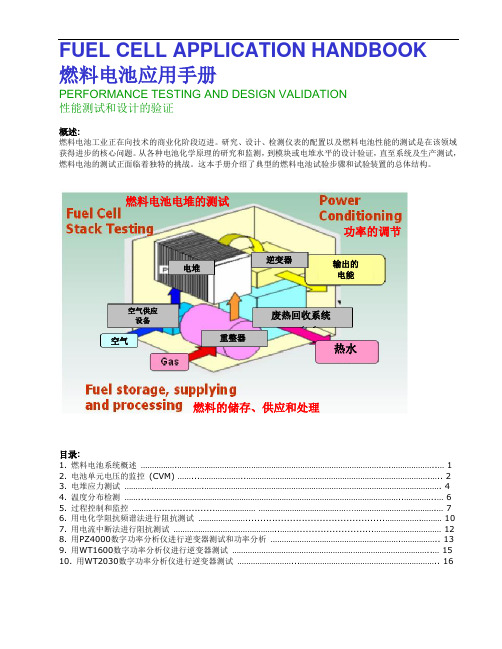
FUEL CELL APPLICATION HANDBOOK 燃料电池应用手册PERFORMANCE TESTING AND DESIGN VALIDATION 性能测试和设计的验证概述:燃料电池工业正在向技术的商业化阶段迈进。
研究、设计、检测仪表的配置以及燃料电池性能的测试是在该领域获得进步的核心问题。
从各种电池化学原理的研究和监测,到模块或电堆水平的设计验证,直至系统及生产测试,燃料电池的测试正面临着独特的挑战。
这本手册介绍了典型的燃料电池试验步骤和试验装置的总体结构。
目录:1. 燃料电池系统概述 ...................................................................................................................................... 1 2. 电池单元电压的监控 (CVM) .................................................................................................................... 2 3. 电堆应力测试 ............................................................................................................................................. 4 4. 温度分布检测 ............................................................................................................................................ 6 5. 过程控制和监控 ................................................ ................................................................................. 7 6. 用电化学阻抗频谱法进行阻抗测试 ............................................................................................. 10 7. 用电流中断法进行阻抗测试 .............................................................................................................. 12 8. 用PZ4000数字功率分析仪进行逆变器测试和功率分析 ........................................................................... 13 9. 用WT1600数字功率分析仪进行逆变器测试 ............................................................................................ 15 10. 用WT2030数字功率分析仪进行逆变器测试 .. (16)逆变器电堆重整器空气空气供应 设备废热回收系统输出的 电能热水燃料电池电堆的测试功率的调节燃料的储存、供应和处理应用:由于单一电池单元所产生的电压在0.8-1.5VDC 范围内, 必须将电池单元串联成电堆才能发出足够的电能。
燃料电池系统开发工程师工作说明书

燃料电池系统开发工程师工作说明书燃料电池电动汽车是指由电动机驱动,由燃料电池提供部分或全部电能的新能源汽车,主要由电机系统、燃料电池系统、储氢系统、整车控制器(VCU)、燃料电池控制器(FC-ECU)、蓄电池、电池管理系统(BMS)等组成,如图1所示。
与常规电动汽车类似,燃料电池电动汽车的动力源为电机,整车控制器根据驾驶员需求,控制电机转矩的大小,从而驱动整车运动;与常规电动汽车的不同之处在于,常规电动汽车的能量源为蓄电池,而燃料电池电动汽车为燃料电池或燃料电池和蓄电池的组合。
燃料电池以氢气和空气为燃料,在燃料电池堆中通过电化学反应产生电流,通过DC/DC逆变器实现与蓄电池的耦合,共同为电机供电,从而实现整车驱动。
燃料电池控制器根据VCU的控制指令,控制输入燃料电池的氢气和空气的流量,从而实现对燃料电池输出电流的控制。
1.对产品初步的结构进行报价和评估,检讨产品结构的可行性,为客户提供参考依据;
2.实施产品结构设计;
3.召集评审会议,对设计的产品进行全面评审,确认结构设计的可行性及可操作性;
4.按照项目进度进行产品结构设计输出,确保产品结构设计输出及时、准确;
5.负责结构物料的开发﹑打样,安排样品制作﹑测试和追踪;
6.跟进项目试产并解决试产中的出现的结构问题;。
固体氧化物燃料电池科研岗位的岗位职责
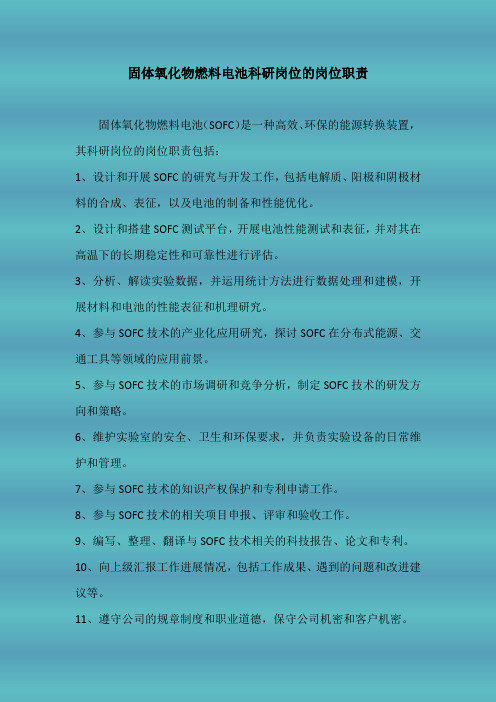
固体氧化物燃料电池科研岗位的岗位职责
固体氧化物燃料电池(SOFC)是一种高效、环保的能源转换装置,其科研岗位的岗位职责包括:
1、设计和开展SOFC的研究与开发工作,包括电解质、阳极和阴极材料的合成、表征,以及电池的制备和性能优化。
2、设计和搭建SOFC测试平台,开展电池性能测试和表征,并对其在高温下的长期稳定性和可靠性进行评估。
3、分析、解读实验数据,并运用统计方法进行数据处理和建模,开展材料和电池的性能表征和机理研究。
4、参与SOFC技术的产业化应用研究,探讨SOFC在分布式能源、交通工具等领域的应用前景。
5、参与SOFC技术的市场调研和竞争分析,制定SOFC技术的研发方向和策略。
6、维护实验室的安全、卫生和环保要求,并负责实验设备的日常维护和管理。
7、参与SOFC技术的知识产权保护和专利申请工作。
8、参与SOFC技术的相关项目申报、评审和验收工作。
9、编写、整理、翻译与SOFC技术相关的科技报告、论文和专利。
10、向上级汇报工作进展情况,包括工作成果、遇到的问题和改进建议等。
11、遵守公司的规章制度和职业道德,保守公司机密和客户机密。
12、积极参与学术交流与合作,提高专业技能和职业素养。
13、完成其他相关职责与任务。
- 1、下载文档前请自行甄别文档内容的完整性,平台不提供额外的编辑、内容补充、找答案等附加服务。
- 2、"仅部分预览"的文档,不可在线预览部分如存在完整性等问题,可反馈申请退款(可完整预览的文档不适用该条件!)。
- 3、如文档侵犯您的权益,请联系客服反馈,我们会尽快为您处理(人工客服工作时间:9:00-18:30)。
研发部工作手册
一、研发中心主要职能
1、产品研发与设计:策划、需求识别与确定、研发与设计(包括
软件、电子、动力、机械等)、
产品研发与设计过程的评审、验证、确认)
2、工艺工程:产品实现策划、制作物质清单、工艺规范、检验规
范、产品说明;
3、样品管理;
4、质量风险管理:产品研发过程质量风险的识别与控制。
董事长
总经理
研发部经
理
制氢主管燃料电池工
程师测试工程
师
装配工程
师
焊接工程
师
机械工程
师
二、各岗位职责和权限
三、研发作业流程与规范
四、管理目标
1、质量目标
1)按计划完成任务:95%;
定义:绘制出图纸装配无异常,间隙不能超过1mm
考核周期:一个月一次
计算公式:异常次数/总绘图次数X100%=错误率
奖惩:出现异常一处绩效考核扣1分
方案或措施:如果出现异常跟进装配位置是否为外观面进行考虑是否需要重新加工配件。
2)设计图纸准确率97%;
定义:绘制出图纸装配无异常,间隙不能超过0.3mm
考核周期:一个月一次
计算公式:异常次数/总绘图次数X100%=错误率
奖惩:出现异常一处绩效考核扣1分
方案或措施:如果出现异常跟进装配位置是否为外观面进行考虑是否需要重新加工配件。
3、记录清单:序号、文件名称、文件编号、对应工序、保存年限;。