Limiting SUSY-QCD spectrum and its application for decays of superheavy particles
稀土离子掺杂钙钛矿纳米晶的光学性质和应用
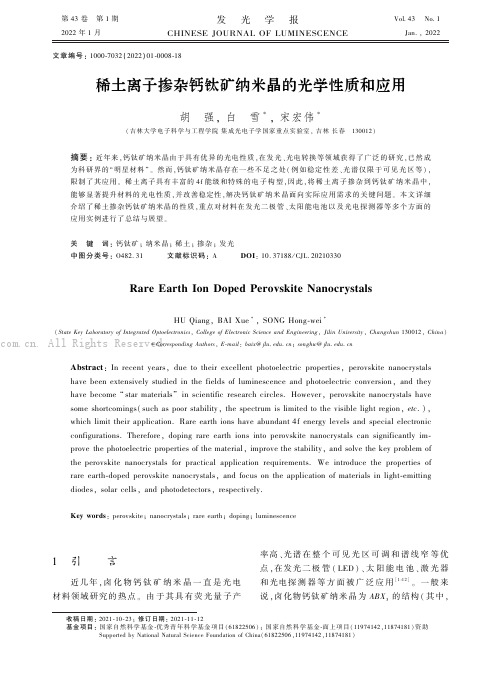
第43卷㊀第1期2022年1月发㊀光㊀学㊀报CHINESE JOURNAL OF LUMINESCENCEVol.43No.1Jan.,2022文章编号:1000-7032(2022)01-0008-18稀土离子掺杂钙钛矿纳米晶的光学性质和应用胡㊀强,白㊀雪∗,宋宏伟∗(吉林大学电子科学与工程学院集成光电子学国家重点实验室,吉林长春㊀130012)摘要:近年来,钙钛矿纳米晶由于具有优异的光电性质,在发光㊁光电转换等领域获得了广泛的研究,已然成为科研界的 明星材料 ㊂然而,钙钛矿纳米晶存在一些不足之处(例如稳定性差㊁光谱仅限于可见光区等),限制了其应用㊂稀土离子具有丰富的4f 能级和特殊的电子构型,因此,将稀土离子掺杂到钙钛矿纳米晶中,能够显著提升材料的光电性质,并改善稳定性,解决钙钛矿纳米晶面向实际应用需求的关键问题㊂本文详细介绍了稀土掺杂钙钛矿纳米晶的性质,重点对材料在发光二极管㊁太阳能电池以及光电探测器等多个方面的应用实例进行了总结与展望㊂关㊀键㊀词:钙钛矿;纳米晶;稀土;掺杂;发光中图分类号:O482.31㊀㊀㊀文献标识码:A㊀㊀㊀DOI :10.37188/CJL.20210330Rare Earth Ion Doped Perovskite NanocrystalsHU Qiang,BAI Xue ∗,SONG Hong-wei ∗(State Key Laboratory of Integrated Optoelectronics ,College of Electronic Science and Engineering ,Jilin University ,Changchun 130012,China )∗Corresponding Authors ,E-mail :baix @ ;songhw @ Abstract :In recent years,due to their excellent photoelectric properties,perovskite nanocrystals have been extensively studied in the fields of luminescence and photoelectric conversion,and they have become star materials in scientific research circles.However,perovskite nanocrystals have some shortcomings(such as poor stability,the spectrum is limited to the visible light region,etc .),which limit their application.Rare earth ions have abundant 4f energy levels and special electronic configurations.Therefore,doping rare earth ions into perovskite nanocrystals can significantly im-prove the photoelectric properties of the material,improve the stability,and solve the key problem of the perovskite nanocrystals for practical application requirements.We introduce the properties of rare earth-doped perovskite nanocrystals,and focus on the application of materials in light-emitting diodes,solar cells,and photodetectors,respectively.Key words :perovskite;nanocrystals;rare earth;doping;luminescence㊀㊀收稿日期:2021-10-23;修订日期:2021-11-12㊀㊀基金项目:国家自然科学基金-优秀青年科学基金项目(61822506);国家自然科学基金-面上项目(11974142,11874181)资助Supported by National Natural Science Foundation of China(61822506,11974142,11874181)1㊀引㊀㊀言近几年,卤化物钙钛矿纳米晶一直是光电材料领域研究的热点㊂由于其具有荧光量子产率高㊁光谱在整个可见光区可调和谱线窄等优点,在发光二极管(LED )㊁太阳能电池㊁激光器和光电探测器等方面被广泛应用[1-12]㊂一般来说,卤化物钙钛矿纳米晶为ABX 3的结构(其中,. All Rights Reserved.㊀第1期胡㊀强,等:稀土离子掺杂钙钛矿纳米晶的光学性质和应用9㊀A位为Cs+等一价金属离子,B位为Pb2+等二价金属离子,X位为F-㊁Cl-㊁Br-和I-)㊂但是,其本身具有一些不足,例如,稳定性差㊁含有毒元素等[13-15]㊂因而,为了克服这些缺点,研究者们提出了一些解决办法:调整X位元素的比例和成分[16-20]㊁控制钙钛矿纳米晶的尺寸[21-23]㊁在B 位置引入稀土(RE)离子或过渡金属离子[24-28]㊂其中,过渡金属和稀土离子掺杂被认为是最有效调节卤化物钙钛矿纳米晶的电子结构和光学性能的方法[29]㊂稀土元素共包括17种元素,由元素周期表中ⅢB族的15种镧系元素以及同属ⅢB族的钪(Sc)和钇(Y)组成㊂它们具有各种多价离子:Ln3+ (n=1~15)和RE3+离子具有[Xe]4f n-1的电子构型,Sc3+和Y3+分别具有[Ar]和[Kr]的电子构型,RE2+(Sm2+㊁Eu2+㊁Yb2+)和RE4+(Ce4+㊁Pr4+㊁Nd4+㊁Tb4+和Dy4+)离子分别具有[Xe]4f n和[Xe]4f n-2的电子结构[30-31]㊂稀土元素具有4f电子组态,并且处于未充满的状态,使其电子跃迁能级非常多,可以发射紫外㊁可见到红外区多个波段的光,激发寿命也很长,可以达到毫秒量级[32-34]㊂由于其电子结构以及可变价态,稀土离子具有独特的发光㊁电磁特性以及氧化还原性质㊂由于稀土离子丰富的4f能级和独特的电子排列,稀土离子掺杂已成为改善钙钛矿纳米晶光电性能的有效方法[35-40]㊂稀土离子内部存在的fңf和fңd电子跃迁过程能够增加钙钛矿纳米晶的发光强度和发光色纯度[41-42]㊂此外,稀土离子可以调节钙钛矿纳米晶的电学㊁光学和化学性质,从而增加其在光电领域的可应用性㊂稀土离子掺杂后的钙钛矿纳米晶展现出了固有的㊁高效的4f-4f窄带发射,同时也提高了钙钛矿纳米晶的光电性质以及稳定性㊂目前,研究者们已经制备出稀土掺杂的钙钛矿纳米晶,表现出优良的性能[35,43-45]㊂本文结合国内外最新研究进展,对稀土离子掺杂钙钛矿纳米晶的基本性质以及应用进行了系统总结㊂2㊀稀土离子掺杂钙钛矿纳米晶的性质金属卤化物钙钛矿纳米晶稳定性差(对水㊁氧㊁热等),其光学特性的可调性有限[46-48]㊂掺杂稀土离子能够更为有效地调控钙钛矿纳米晶的光学和电学特性㊂这些独特的性质主要是由于4f 子壳层中的电子被外部5s和5p子壳层中的电子有效地屏蔽[49]㊂在已经报道的稀土离子掺杂钙钛矿纳米晶的研究工作中,掺杂稀土离子不仅拓宽了钙钛矿材料的光谱范围[35,50],还极大地改善了金属卤化物钙钛矿纳米晶的发光效率和稳定性[51-53]㊂然而,为了保证稀土离子能够有效掺入卤化物钙钛矿纳米晶晶格中,获得结构稳定㊁性能优良的材料,不仅需要选择合适的掺杂元素,而且需要考虑合理的元素组成㊂元素周期表中很多元素都可以作为卤化物钙钛矿纳米晶的组成成分,但是能够形成稳定结构的元素却不多㊂尤其是,卤化物钙钛矿纳米晶的稳定性取决于材料本身的容忍因子t和八面体因子μ[54-55],这也决定了向卤化物钙钛矿体系中进行掺杂的难易程度㊂2.1㊀卤化物钙钛矿纳米晶的晶体结构卤化物钙钛矿满足ABX3的结构(A位为Cs+㊁Rb+㊁FA+等,B位为Pb2+等二价金属离子, X位为F-㊁Cl-㊁Br-和I-),通常呈现被A位包围的八面体结构,并且显示出6个B位与X配位[56]㊂根据A位原子与八面体的排列方式,把钙钛矿材料分为四种类型(如图1所示):零维(0D)㊁一维(1D)㊁二维(2D)和三维(3D)钙钛矿[57]㊂对于3D钙钛矿结构,BX6八面体在三维方向上与相邻的离子共享X位阴离子,八面体之间相互连接[58]㊂相比于3D钙钛矿结构,2D钙钛矿晶体中,BX6八面体仅在二维平面中与共享的X位阴离子连接[59]㊂特别地,当较大的基团占据A位时,例如长链烷基胺阳离子,典型的卤化铅钙钛矿结构将变成2D Ruddlesden-popper(RP)层状钙钛矿结构,此时需要按照层数进行分类[60]㊂同样,在1D钙钛矿晶体结构中,BX6八面体之间呈现一维连接[61]㊂0D钙钛矿晶体比较特别,其中过量的A位原子隔开了BX6八面体,八面体之间没有任何连接[43]㊂卤化物钙钛矿纳米晶具有特定的卤素元素以及不同的配位模式㊂大多数卤化物钙钛矿纳米晶为3D结构,而2D㊁1D和0D结构相对较少[58,61-62]㊂得益于多种类型和各种晶体结构,钙钛矿的结构具有更高的可调节性㊂因此,将稀土离子掺杂到钙钛矿结构中变得更加灵活[43,63-64]㊂㊀. All Rights Reserved.10㊀发㊀㊀光㊀㊀学㊀㊀报第43卷图1㊀钙钛矿的各种晶体结构㊂(a)3D双钙钛矿;(b)3D单钙钛矿;(c)2D钙钛矿;(d)1D钙钛矿;(e)~(h)0D钙钛矿㊂Fig.1㊀Various crystal structures of perovskites.(a)3D double perovskites.(b)3D single perovskites.(c)2D perovskites.(d)1D perovskites.(e)-(h)0D perovskites.2.2㊀容忍因子t和八面体因子μ理想钙钛矿体系中(满足ABX3结构),阳离子(A位㊁B位离子)和阴离子(X位离子)的半径应满足容忍因子t=(R A+R X) 2(R B+R A)()和八面体因子μ=R B R X(),其中,R A㊁R B和R X分别指A位㊁B位和X位离子的半径[55,65]㊂t值在0.81~1.11之间变化[66],如果超出该范围,立方相的晶体结构将发生扭曲,甚至被破坏㊂若t值较小,将会产生对称性较低的四方结构或正交结构㊂理想立方相结构中,t值在0.89~1.0之间变化[65]㊂μ值在0.44~ 0.90之间,μ值不仅决定了钙钛矿八面体结构的稳定性,而且进一步影响了钙钛矿结构的稳定性[67]㊂对于卤化物钙钛矿纳米晶,可以根据容忍因子t和八面体因子μ来选择合适的稀土离子取代其中B位二价金属离子,从而获取不同结构和性能的钙钛矿纳米晶㊂2.3㊀发光增强和光谱调控卤化物钙钛矿纳米晶具有优良的发光特性,却存在一些不足㊂它们的发光波长仅能在可见光区域内调节,很难达到近红外区,并且发射峰半峰宽(FWHM)通常比较宽[68-69]㊂而稀土离子具有特殊的发光特性:发射峰非常窄㊁FWHM仅有几纳米㊁发光衰减时间比较长(可以达到几微秒)[70-71]㊂最重要的是,稀土离子的发射光谱涵盖了从紫外区到近红外区的较宽范围[72-73]㊂通过掺杂稀土离子,可以实现卤化物钙钛矿纳米晶的发射光谱从可见光区到近红外区的调控㊂同时,稀土离子引入卤化物钙钛矿纳米晶后,不仅拓宽了钙钛矿材料的光谱范围(从可见光区拓宽到近红外区)[35,50],而且显著地改善了钙钛矿纳米材料本身的发光效率[53,74]㊂Pan等首先制备出了一系列稀土离子掺杂的CsPbCl3纳米晶,他们采用稀土卤化盐作为前驱体,在高温(200~240ħ)下通过热注入将稀土离子引入钙钛矿纳米晶[35]㊂荧光光谱表明,未掺杂样品仅有激子发射,而所有稀土掺杂样品均展现出掺杂稀土离子的发射和本身的激子发射,呈现多个发射峰㊂在各种稀土离子掺杂的CsPbCl3纳米晶中,Yb3+掺杂的样品呈现出最高的光致发光量子产率(PLQY,142.7%)㊂根据最早关于Yb3+掺杂CsPbCl3或CsPb(Cl/Br)3纳米晶的工作报道[35,44,75],掺杂后样品均呈现出超过100%的PLQY㊂后续也有报道,Yb3+掺杂的CsPbCl3薄膜和纳米晶均实现了~190%的PLQY[76]㊂这样的高荧光量子产率源自于Yb3+引入钙钛矿纳米晶后,产生的量子剪裁效应,这将在下一部分详细说明㊂另外,还有一些将稀土离子引入钙钛矿纳米晶中,实现了发光性能改善或是发光光谱调控,并未展现出量子剪裁效应㊂例如,Li等通过对CsPbCl3-x Br x(x=0,1,1.5,2,3)进行Eu3+掺杂实现了覆盖整个可见光谱的宽色域发射㊂如图2(a)所示,CsPb X3纳米晶的可调激子光致发光. All Rights Reserved.㊀第1期胡㊀强,等:稀土离子掺杂钙钛矿纳米晶的光学性质和应用11㊀覆盖了蓝光到绿光范围(400~520nm )的发射[77]㊂Yao 等通过简单的热注入将Ce 3+掺入CsPbBr 3纳米晶中,通过引入Ce 3+显著调控了PL动力学,提高了CsPbBr 3纳米晶的PLQY (89%)(图2(b))[53]㊂大部分稀土离子引入钙钛矿纳米晶中,都会对发光性能或发光光谱产生影响,可能是单一的光学特性增强,也可能是改变发光光谱的同时敏化了钙钛矿纳米晶的发光㊂图2㊀(a)CsPbCl 3-x Br x ʒEu 3+(x =0,1,1.5,2,3)纳米晶的光致发光光谱[77];(b)左图:未掺杂的和具有不同Ce /Pb 掺杂的CsPbBr 3纳米晶的PL 光谱(365nm 激发),右图:不同CeBr 3(0~50%)浓度下的PLQY [53]㊂Fig.2㊀(a)PL spectra of CsPbCl 3-x Br x ʒEu 3+(x =0,1,1.5,2,3)NCs [77].(b)Left:the PL spectra(excitation at 365nm)of undoped and doped CsPbBr 3NCs with different Ce /Pb ratios.Right:the PLQY versus dopant concentration ofCeBr 3(0-50%)[53].2.4㊀量子剪裁效应量子剪裁是一种下转换发光过程,其概念是Dexter 在1957年提出的,是指吸收一个高能量的光子(紫外或者蓝紫)转换为两个或者多个低能量的光子(可见或者红外)并发射出来的过程,其PLQY 一般会超过100%㊂2017年,Pan 等首次发现Yb 3+离子掺杂CsPbCl 3纳米晶可以实现PLQY 为142.7%的红外发光[35](如图3(a))㊂随后,Milstein 等提出了Yb 3+离子掺杂CsPbCl 3纳米晶的量子剪裁机制(如图3(b))[75]㊂他们指出,电荷补偿形成的Yb 3+-V Pb -Yb 3+(V Pb ʒPb 2+离子空位)电荷中性对在量子剪裁过程中扮演至关重要的角色㊂在导带边缘以下,该Yb 3+-V Pb -Yb 3+对感应出较浅的缺陷能级,这些缺陷能级与宿主材料的固有缺陷竞争来自于导带的皮秒量级非辐射能量转移,随后在单个量子剪裁过程中,几乎共振的能量转移至其中的两个Yb 3+离子㊂2017年,Zhou 等报道了Ce 3+和Yb 3+掺杂的CsPbCl 1.5Br 1.5体系中产生的量子剪裁效应(如图3(c))[44]㊂在该体系中,Ce 3+充当能量供体,Yb 3+充当能量受主㊂2019年,Li 等通过密度泛函理论(DFT )计算,结合之前提出的量子剪裁机制,提出了一种Yb 3+掺杂CsPbCl 3纳米晶的量子剪裁过程[33]㊂他们发现稀土离子掺杂会导致原始CsPbCl 3纳米晶的价带发生变化,而不是产生导带以下的浅缺陷能级,并且独特的Pb(RA)原子具有捕获激发图3㊀逐步能量转移机制(a)㊁掺杂Yb 3+的CsPbCl 3的量子剪裁机制(b)㊁将Ce 3+-Yb 3+掺入无机CsPb X 3中的量子剪裁机制(c)示意图[33]㊂Fig.3㊀Scheme of stepwise energy transfer mechanism(a),quantum cutting mechanism for Yb 3+-doped CsPbCl 3(b),the con-ventional quantum cutting mechanism with Ce 3+-Yb 3+incorporated into inorganic CsPb X 3(c)[33]. All Rights Reserved.12㊀发㊀㊀光㊀㊀学㊀㊀报第43卷态,与RA Yb 3+-V Pb -Yb 3+对相关联,定位光生电子,进而实现量子剪裁过程(如图4所示)㊂在该种量子剪裁过程中,Yb 3+-V Pb -Yb 3+的电荷中性对最可能存在于与Pb (RA )原子相关的晶体基质中;具有捕获激发态的Pb(RA)原子作为能量供体,在一个量子剪裁过程中激发两个Yb 3+离子㊂重要的是,由于Yb 3+-V Pb -Yb 3+电荷中性对与Pb (RA)原子结合产生的自然接近,有利于从Pb (RA)原子到两个相邻Yb 3+离子的能量转移,从图4㊀Yb 3+掺杂立方相CsPbCl 3量子剪裁机理示意图[33]Fig.4㊀Scheme of proposed quantumcutting mechanism forYb3+-doped CsPbCl 3of cubic phase[33]而同时激发两者,有助于实现量子剪裁㊂然而,需要进一步深入的实验和理论研究来印证量子剪裁机制㊂上述研究都是在带隙大于2.88eV (对应发光波长为430nm)的CsPbCl 3或CsPb(Cl /Br)3纳米晶中掺杂Yb 3+离子㊂而研究者们发现,将Yb 3+掺杂到带隙更窄的钙钛矿纳米晶中,例如CsPbBr 3和CsPbI 3纳米晶,具有一定的挑战性㊂因此,调节主体钙钛矿纳米晶的带隙对于了解带隙在量子剪裁效应中的角色十分重要㊂研究者们提出了以下两种策略来解决将Yb 3+离子掺杂到更窄带隙纳米晶中的挑战:(1)在合成后把Yb 3+掺杂到纳米晶中[38];(2)对于掺杂Yb 3+的CsPbCl 3纳米晶进行阴离子交换[75]㊂将Yb 3+离子掺杂进入合成后的CsPb X 3纳米晶(也掺入CsPbBr 3纳米片)中,实现了近红外区的Yb 3+发射[38](如图5(a))㊂然而,在CsPbBr 3和CsPbI 3纳米晶中,Yb 3+在近红外区的发射显著降低㊂在离子交换方法中,如果纳米晶带隙小于2.5eV,Yb 3+的近红外光发射急剧下降[75](图5(b ))㊂因此,将2.5eV 称为将Yb 3+掺杂进CsPbCl x Br 1-x 纳米晶实现量子剪裁效应的阈值,在带隙大于2.5eV 的钙钛矿纳米晶中才会实现量子剪裁效应㊂图5㊀(a)通过合成后Yb 3+掺杂获得的未掺杂和x %Yb 3+掺杂的CsPb X 3(X =Cl,Br,I)纳米晶以及CsPbBr 3钙钛矿纳米片(蓝色光谱)的光致发光光谱[38];(b)992nm 处Yb 3+的PLQY 随CsPb(Cl x Br 1-x )3纳米晶光学带隙的变化[75]㊂Fig.5㊀(a)PL spectra of undoped and x %Yb 3+-doped CsPb X 3(X =Cl,Br,I)perovskite nanocrystals along with CsPbBr 3per-ovskite nanoplatelets(NPLs,blue spectra)obtained through post synthesis Yb 3+doping [38].(b)PLQY of Yb 3+emissionat 992nm as a function of the optical band gap of CsPb(Cl x Br 1-x )3nanocrystals [75].3㊀应㊀㊀用卤化物型稀土离子掺杂及稀土基钙钛矿纳米晶的应用主要集中在发光领域,利用稀土离子调节卤化物钙钛矿纳米晶的发光光谱是该领域的研究热点㊂近几年,已经有系列工作报道了稀土离子掺杂卤化物钙钛矿纳米晶㊂对于发光二极管,掺杂稀土离子不仅提高了材料和器件的效率,拓宽了发光光谱范围,而且显著改善了材料和器件的稳定性[52-53,74,78-79]㊂在太阳能. All Rights Reserved.㊀第1期胡㊀强,等:稀土离子掺杂钙钛矿纳米晶的光学性质和应用13㊀电池中,基于稀土离子掺杂钙钛矿薄膜制备出的器件具有较高的光电转换效率和持久的稳定性[40,80]㊂3.1㊀稀土离子掺杂卤化物钙钛矿纳米晶在发光领域的应用钙钛矿纳米晶具有发光谱线窄和光谱范围可调等优异的光学性质,在发光领域一直备受关注㊂对卤化物钙钛矿纳米晶进行稀土离子掺杂后,能够将二者的发光特性结合,改善卤化物钙钛矿纳米晶发光特性,促进了卤化物钙钛矿纳米晶在LED领域的应用㊂由于稀土离子本身独特的电子结构,引入钙钛矿纳米晶晶格后,会对发光性能产生不同的影响,主要包括:(1)引入稀土离子仅敏化了钙钛矿纳米晶的发光,提升了材料本身的发光性能,而并未改变钙钛矿纳米晶本身的发光光谱,进而使电致发光LED的性能得以显著提升㊂例如,Yao等通过热注入方法将Ce3+离子掺杂到CsPbBr3纳米晶中,增强了PLQY并实现了高效的LED器件(图6(a))[53]㊂他们发现,当Ce3+掺杂量增加到2.88%(Ce与Pb的原子百分比),CsPbBr3纳米晶的PLQY达到89%;并表明掺杂Ce3+诱导的近带边缘态调节了CsPbBr3主体的PL动力学,进而大幅度提升了光学性质㊂采用Ce3+掺杂的CsPb-Br3纳米晶作为发光层制备LED,其外量子效率(EQE)从1.6%提高到4.4%㊂最近,Chiba等报道了三价镧系元素卤化物氯化钕(NdCl3)掺杂的钙钛矿纳米晶,并制备了蓝色电致发光LED[79]㊂掺杂NdCl3后,钙钛矿纳米晶在478nm展现蓝光发射,溶液PLQY高达97%㊂基于NdCl3掺杂钙钛矿纳米晶研制的蓝光LED的外量子效率为2.7%(图6(b)),器件性能显著提升㊂他们将这种性能提升归因于Nd3+对非辐射复合的有效抑制㊂同样地,Xie等通过向CsPbBr3纳米晶中掺杂Nd3+离子[81],实现了中心波长为459nm㊁光致发光量子产率高达90%的蓝光纳米晶㊂对于光学性能的提升,他们认为是由于Nd3+掺杂时价带和导带变平导致的激子结合能增加以及掺杂引起的晶格收缩导致的激子振荡强度增强的结果㊂(2)引入稀土离子不仅敏化了材料本身发光,还改变了材料发光光谱,使发光光谱覆盖整个可见光区,进而产生白光发射㊂对于稀土离子掺杂钙钛矿纳米晶产生白光发射的研究,一直备受关注㊂2018年,Pan等成功制备了Ce3+/Mn2+共掺杂的CsPbCl3钙钛矿纳米晶,获得了单一成分的稳定白光发射㊂Ce3+离子的引入不仅补充了蓝光和绿光成分,而且还敏化了Mn2+离子的红光发射㊂最终,采用2.7%Ce3+和9.1%Mn2+共掺杂的CsPbCl1.8Br1.2纳米晶实现了白光发射,PLQY 达到75%[74]㊂随后,他们采用365nm GaN LED 芯片和共掺杂钙钛矿纳米晶制备了光致发光白光LED,器件发光效率为51lm/W,显色指数为89 (图6(c))㊂通常,采用近紫外芯片激发稀土离子掺杂氧化物制备的白光LED能够产生高达94的显色指数,但是仅能实现23lm/W的低流明效率[82]㊂而对于近紫外光激发稀土离子掺杂的钙钛矿纳米晶却能在实现高显色指数的同时保持较高的器件流明效率㊂类似地,Cheng等将Eu3+和Tb3+引入CsPbBr3纳米晶玻璃中,并采用蓝光芯片激发,在20mA的电流下实现了85.7的显色指数和63.21lm/W的发光效率(图6(d))[52]㊂以上两个报道证明了稀土离子掺杂钙钛矿纳米晶在白光发光二极管上的巨大应用潜力㊂不同于Pan等采用近紫外芯片激发方式制备的光致发光LED,Sun等制备了电致发光白光LED,发光层材料采用Sm3+掺杂的CsPbCl3纳米晶㊂首先,她们通过一种改进的热注入方法制备了高效的Sm3+离子掺杂CsPbCl3纳米晶,Sm3+掺杂CsPbCl3纳米晶的PLQY达到85%㊂基于Sm3+掺杂的CsPbCl3纳米晶制备出的电致LED (如图6(e)~(f)所示)展现了出色的白光电致发光特性[78],得益于从CsPbCl3纳米晶主体到Sm3+的有效能量转移,实现了色坐标为(0.32,0.31)㊁最大亮度为938cd/m2㊁外量子效率为1.2%㊁显色指数为93的单组分白光钙钛矿电致LED㊂这是首次实现单组分白光钙钛矿LED,消除了与多组分钙钛矿进行离子交换的麻烦和串联钙钛矿LED器件结构设计的困难,具有重要意义㊂将稀土离子引入钙钛矿纳米晶后,无论是增强单色发光,还是实现白光发射,都能够有效地将钙钛矿纳米晶与稀土离子的优点充分地结合,实现更佳的光学性能㊂将稀土离子与钙钛矿纳米晶结合,促进了钙钛矿纳米晶今后在发光与显示领域的应用,同时也展现了二者结合后的巨大发展前景㊂. All Rights Reserved.14㊀发㊀㊀光㊀㊀学㊀㊀报第43卷图6㊀(a)分散在甲苯溶液中未掺杂的CsPbBr 3和掺杂Ce 3+的CsPbBr 3光致发光光谱及其在5V 电压下的电致发光光谱,插图显示了在5V 电压下相应器件的照片[53];(b)基于Nd 3+掺杂的钙钛矿纳米晶发光二极管的外量子效率-电流密度特性[79];(c)基于2.7%Ce 3+/9.1%Mn 2+掺杂的CsPbCl x Br 3-x 纳米晶LED 的CIE 色坐标(A (0.42,0.33)㊁B(0.39,0.32)㊁C(0.37,0.30)和D(0.33,0.29)),插图是在365nm 紫外灯下的2.7%Ce 3+/9.1%Mn 2+掺杂的CsPbCl x Br 3-x 纳米晶的光致发光图[74];(d)基于Eu 3+和Tb 3+共掺的CsPbBr 3纳米晶玻璃LED 的CIE 色坐标(0.3335,0.3413),插图为Tb3+/Eu 3+共掺杂CsPbBr 3纳米晶玻璃照片㊁制备出的LED 发射光谱和发光照片[52];(e)基于Sm 3+离子掺杂CsPbCl 3LED 器件结构示意图[78];(f)基于具有不同掺杂浓度的Sm 3+离子掺杂CsPbCl 3纳米晶LED 的CIE 色坐标,插图为具有不同Sm 3+离子掺杂浓度的钙钛矿LED 照片[78]㊂Fig.6㊀(a)EL spectra at an applied voltage of 5V and their corresponding PL emission spectra for undoped CsPbBr 3and Ce 3+-doped CsPbBr 3when dispersed in toluene solution [53].(b)EQE-current density characteristics of perovskite LEDs [79].(c)CIE chromaticity coordinate of the LED from 2.7%Ce 3+/9.1%Mn 2+-codoped CsPbCl x Br 3-x nanocrystals(A(0.42,0.33),B(0.39,0.32),C(0.37,0.30),and D(0.33,0.29)).The inset is PL images of 2.7%Ce 3+/9.1%Mn 2+-codoped CsPbCl x Br 3-x nanocrystals under a 365nm UV lamp [74].(d)CIE color coordinates based on Eu 3+and Tb 3+co-doped CsPbBr 3nanocrystalline glass LED(0.3335,0.3413).The illustration shows the photo of Tb 3+/Eu 3+co-doped CsPbBr 3nanocrystalline glass,the emission spectrum of the prepared LED and the luminescence photo of the working LED [52].(e)Sm 3+ion-doped CsPbCl 3LED device structure diagram [78].(f)CIE coordinates for the perovskite LED based on Sm 3+ion-doped CsPbCl 3nanocrystals with different doping concentrations.Inserts:photographs of perovskiteLEDs with different Sm 3+ion doping concentrations [78].3.2㊀稀土离子掺杂卤化物钙钛矿纳米晶在太阳能电池领域的应用稀土离子掺杂钙钛矿纳米晶在太阳能电池方面的应用主要有以下三种:(1)采用稀土离子掺杂钙钛矿作为功能层的钙钛矿太阳能电池;(2)采用稀土离子掺杂钙钛矿纳米晶作为量子剪裁层的硅太阳能电池;(3)利用稀土掺杂钙钛矿纳米晶量子剪裁效应制备的太阳能发光集中器㊂3.2.1㊀稀土掺杂钙钛矿太阳能电池(PSC )在卤化物钙钛矿材料中,铅基钙钛矿材料具有优秀的光伏性质,已经有较多的文章报道了基于卤化铅钙钛矿材料的太阳能电池,一直是研究热点㊂但是,铅基的卤化物钙钛矿材料在潮湿㊁高温和氧化还原环境下容易分解,导致器件性能显著下降,同时基于卤化铅钙钛矿材料的太阳能电池的功率转换效率(PCE )远未达到其理论极限[83-86]㊂掺入稀土离子是提高卤化铅钙钛矿材. All Rights Reserved.㊀第1期胡㊀强,等:稀土离子掺杂钙钛矿纳米晶的光学性质和应用15㊀料稳定性的重要手段[40,87-88],也可以进一步提升钙钛矿太阳能电池的PCE以及器件稳定性㊂例如,Duan等将一系列的稀土离子掺入CsPbBr3薄膜中,并制备了太阳能电池[40]㊂他们发现掺杂稀土离子后,延长了载流子迁移时间,显著抑制了钙钛矿膜表面的电子和空穴的复合㊂在不使用金属电极和空穴传输层的情况下,器件获得了10.14%的PCE,开路电压也达到1.59V㊂与此同时,电池表现出高稳定性,在80%相对湿度下存放110d,器件的PCE基本没有变化㊂此外,电池在80ħ下工作60d后仍保持高效率(如图7所示)㊂图7㊀(a)无空穴传输层㊁全无机PSC的横截面扫面电镜图像;(b)基于几种稀土离子掺杂的无机PSC特征电流-电压曲线;未封装的原始器件和Sm3+掺杂器件在25ħ和80%RH(c)㊁80ħ和0%RH(d)下的长期稳定性[40]㊂Fig.7㊀(a)The cross-sectional SEM image of a HTM-free,all inorganic PSC.(b)Based on inorganic PSC doped with several rare earth ions J-V curves.Long-term stability of the pristine and Sm3+doped devices without encapsulation under25ħand80%RH(c),80ħand0%RH(d)[40].在另外两个报道中,分别在卤化铅钙钛矿中进行了Nd3+和Yb3+掺杂㊂首先,Wang等制备了Nd3+掺杂MAPbI3薄膜的PSC[89]㊂与原始杂化钙钛矿材料相比,掺Nd3+杂化钙钛矿材料具有优异的薄膜质量,陷阱态密度大大降低,电荷载流子寿命明显延长,载流子迁移率提高,载流子传输更平衡㊂结果,由Nd3+掺杂的混合钙钛矿材料制成的平面异质结PSC表现出21.15%的高可重复PCE,并显著抑制了光电流滞后㊂随后,Shi等通过在合成过程中进行原位(Yb3+)掺杂,合成了CsPbI3纳米晶,并显示出优良的光电性能[90]㊂实验结果表明,Yb3+可有效减少材料表面和晶格空位引起的缺陷数量和陷阱态密度,有助于改善钙钛矿纳米晶的结晶度㊁热稳定性和载流子传输速率㊂采用Yb3+掺杂CsPbI3纳米晶制备的太阳能电池实现了13.12%的PCE(如图8(a)),器件稳定性明显得到改善(如图8(b))㊂. All Rights Reserved.16㊀发㊀㊀光㊀㊀学㊀㊀报第43卷图8㊀(a)基于Yb 3+掺杂的CsPbI 3太阳能电池电流-电压特性曲线,插图为掺杂后样品的透射电镜图[90];(b)无封装基于20%和50%Yb 3+掺杂CsPbI 3纳米晶器件的环境存储稳定性[90]㊂Fig.8㊀(a)The current-voltage characteristic curves of a solar cell based on Yb 3+doped CsPbI 3.The inset shows the TEM imageof the sample after doping [90].(b)The ambient storage stability of the devices based on CsPbI 3,20%Yb-doped and 50%Yb-doped CsPbI 3nanocrystals without encapsulation [90].图9㊀(a)基于掺杂0.15%不同M (acac)3(M =Eu 3+,Y 3+,Fe 3+)的(FA,MA,Cs)Pb(I,Br)3(Cl)PSC 的原始性能演变;(b)电流密度-电压曲线,稳定的输出(在0.97V 下测得)和掺有0.15%Eu 3+器件的参数;(c )掺有0.15%[M (acac)3(M =Eu 3+,Y 3+,Fe 3+)]MAPbI 3(Cl)制备PSC 的长期稳定性(在惰性条件下保存),在1太阳光照射或85ħ老化条件下,掺入Eu 3+-Eu 2+的器件和参比器件的PCE 演变;半个PSC(原始PCE:掺杂0.15%Eu 3+的PSC,(19.21ʃ0.54)%;参比PSC,(18.05ʃ0.38)%)(d)和完整的PSC(原始PCE:掺杂0.15%Eu 3+的PSC,(19.17ʃ0.42)%;参考PSC,(17.82ʃ0.30)%)(e),扫描速度为20mV /s;(f)在0.97V 和1太阳光照下测得的掺有0.15%Eu 3+器件归一化PCE 随时间的变化[87]㊂Fig.9㊀(a)Original performance evolution based on (FA,MA,Cs)Pb(I,Br)3(Cl)perovskite with the incorporation of 0.15%differ-ent M (acac)3(M =Eu 3+,Y 3+,Fe 3+).(b)The J-V curve,stable output(measured at 0.97V),and parameters of 0.15%Eu 3+-incorporated champion devices.(c)Long-term stability of PSCs based on MAPbI 3(Cl)perovskite absorber with the in-corporation of 0.15%different [M (acac)3(M =Eu 3+,Y 3+,Fe 3+)],stored in inert condition.The PCE evolution of Eu 3+-Eu 2+-incorporated and reference devices under 1sun illumination or 85ħaging condition.Half PSCs(original PCE:0.15%Eu 3+incorporated PSCs,(19.21ʃ0.54)%;reference PSCs,(18.05ʃ0.38)%)(d)and full PSCs(original PCE:0.15%Eu 3+incorporated PSCs,(19.17ʃ0.42)%;reference PSCs,(17.82ʃ0.30)%)(e).Scanning speed is 20mV /s.(f)Normalized PCE of of 0.15%Eu 3+-incorporated device as a function of time,measured at 0.97V and 1-sun illumination [87]. All Rights Reserved.。
分析仪器相关英文简称
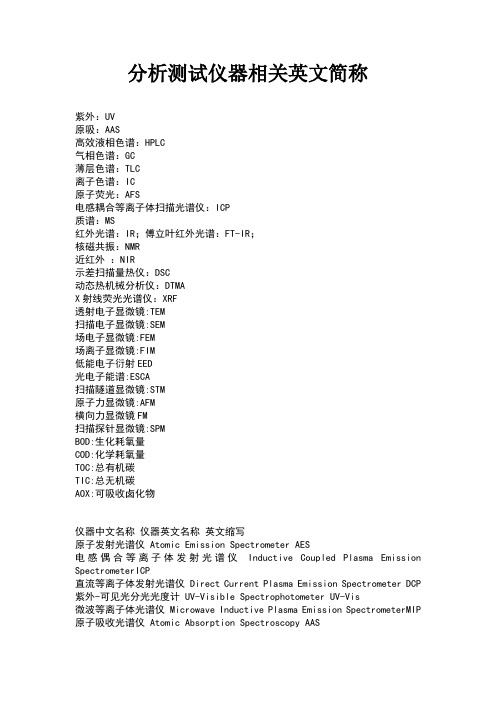
分析测试仪器相关英文简称紫外:UV原吸:AAS高效液相色谱:HPLC气相色谱:GC薄层色谱:TLC离子色谱:IC原子荧光:AFS电感耦合等离子体扫描光谱仪:ICP质谱:MS红外光谱:IR;傅立叶红外光谱:FT-IR;核磁共振:NMR近红外:NIR示差扫描量热仪:DSC动态热机械分析仪:DTMAX射线荧光光谱仪:XRF透射电子显微镜:TEM扫描电子显微镜:SEM场电子显微镜:FEM场离子显微镜:FIM低能电子衍射EED光电子能谱:ESCA扫描隧道显微镜:STM原子力显微镜:AFM横向力显微镜FM扫描探针显微镜:SPMBOD:生化耗氧量COD:化学耗氧量TOC:总有机碳TIC:总无机碳AOX:可吸收卤化物仪器中文名称仪器英文名称英文缩写原子发射光谱仪 Atomic Emission Spectrometer AES电感偶合等离子体发射光谱仪Inductive Coupled Plasma Emission SpectrometerICP直流等离子体发射光谱仪 Direct Current Plasma Emission Spectrometer DCP 紫外-可见光分光光度计 UV-Visible Spectrophotometer UV-Vis微波等离子体光谱仪 Microwave Inductive Plasma Emission SpectrometerMIP 原子吸收光谱仪 Atomic Absorption Spectroscopy AAS原子荧光光谱仪 Atomic Fluorescence Spectroscopy AFS傅里叶变换红外光谱仪 FT-IR Spectrometer FTIR傅里叶变换拉曼光谱仪 FT-Raman Spectrometer FTIR-Raman气相色谱仪 Gas Chromatograph GC高压/效液相色谱仪 High Pressure/Performance Liquid ChromatographyHPLC 离子色谱仪 Ion Chromatograph IC凝胶渗透色谱仪 Gel Permeation Chromatograph GPC体积排阻色谱 Size Exclusion Chromatograph SECX射线荧光光谱仪 X-Ray Fluorescence Spectrometer XRFX射线衍射仪 X-Ray Diffractomer XRD同位素X荧光光谱仪 Isotope X-Ray Fluorescence Spectrometer电子能谱仪 Electron Energy Disperse Spectroscopy能谱仪 Energy Disperse Spectroscopy EDS质谱仪 Mass Spectrometer MS核磁共振波谱仪 Nuclear Magnetic Resonance Spectrometer NMR电子顺磁共振波谱仪 Electron Paramagnetic Resonance Spectrometer ESR 极谱仪 Polarograph伏安仪 Voltammerter自动滴定仪 Automatic Titrator电导仪 Conductivity MeterpH计 pH Meter水质分析仪 Water Test Kits电泳仪 Electrophoresis System表面科学 Surface Science电子显微镜 Electro Microscopy光学显微镜 Optical Microscopy金相显微镜 Metallurgical Microscopy扫描探针显微镜 Scanning Probe Microscopy表面分析仪 Surface Analyzer无损检测仪 Instrument for Nondestructive Testing物性分析 Physical Property Analysis热分析仪 Thermal Analyzer粘度计 Viscometer流变仪 Rheometer粒度分析仪 Particle Size Analyzer热物理性能测定仪 Thermal Physical Property Tester电性能测定仪 Electrical Property Tester光学性能测定仪 Optical Property Tester机械性能测定仪 Mechanical Property Tester燃烧性能测定仪 Combustion Property Tester老化性能测定仪 Aging Property Tester生物技术分析 Biochemical analysisPCR仪 Instrument for Polymerase Chain Reaction PCRDNA及蛋白质的测序和合成仪 Sequencers and Synthesizers for DNA and Protein传感器 Sensors其他 Other/Miscellaneous流动分析与过程分析 Flow Analytical and Process Analytical Chemistry气体分析 Gas Analysis基本物理量测定 Basic Physics样品处理 Sample Handling金属/材料元素分析仪 Metal/material elemental analysis环境成分分析仪 CHN Analysis发酵罐 Fermenter生物反应器 Bio-reactor摇床 Shaker离心机 Centrifuge超声破碎仪 Ultrasonic Cell Disruptor超低温冰箱 Ultra-low Temperature Freezer恒温循环泵 Constant Temperature Circulator超滤器 Ultrahigh Purity Filter冻干机 Freeze Drying Equipment部分收集器 Fraction Collector氨基酸测序仪 Protein Sequencer氨基酸组成分析仪 Amino Acid Analyzer多肽合成仪 Peptide synthesizerDNA测序仪 DNA SequencersDNA合成仪 DNA synthesizer紫外观察灯 Ultraviolet Lamp分子杂交仪 Hybridization OvenPCR仪 PCR Amplifier化学发光仪 Chemiluminescence Apparatus紫外检测仪 Ultraviolet Detector电泳 Electrophoresis酶标仪 ELIASACO2培养箱 CO2 Incubators倒置显微镜 Inverted Microscope超净工作台 Bechtop(资料素材和资料部分来自网络,供参考。
太阳能电池阻抗谱测量方法及其应用进展

引用格式:李傲, 肖文波, 张濬哲, 等. 太阳能电池阻抗谱测量方法及其应用进展[J]. 中国测试,2024, 50(1): 1-8. LI Ao, XIAO Wenbo, ZHANG Junzhe, et al. Research progress of solar cell impedance spectroscopy measurement method and its application[J].China Measurement & Test, 2024, 50(1): 1-8. DOI: 10.11857/j.issn.1674-5124.2022080063太阳能电池阻抗谱测量方法及其应用进展李 傲1, 肖文波1, 张濬哲2, 吴华明1, 王树鹏3(1. 南昌航空大学 无损检测技术教育部重点实验室,江西 南昌 330063; 2. 南昌航空大学材料科学与工程学院,江西 南昌330063; 3. 中国航发沈阳黎明航空发动机有限责任公司,辽宁 沈阳 110043)摘 要: 阻抗谱测量技术是研究太阳能电池的重要手段。
该文首先对近几年提出的阻抗谱测量方法进行评述,分析各类方法的优缺点。
通过对阻抗谱测量方法的研究,发现不同测量方法之间的差异主要体现在其效率、精度以及成本等方面。
其次,分析阻抗谱在太阳电池故障检测、电子输运、界面研究等方面的应用情况,指出它们评价电池动态行为时存在的不足之处。
最后,总结阻抗谱测量方法未来发展方向及应用需求。
关键词: 太阳能电池; 阻抗谱; 故障评估; 电子输运; 界面研究中图分类号: TM930.12;TB9文献标志码: A文章编号: 1674–5124(2024)01–0001–08Research progress of solar cell impedance spectroscopy measurementmethod and its applicationLI Ao 1, XIAO Wenbo 1, ZHANG Junzhe 2, WU Huaming 1, WANG Shupeng 3(1. Key Laboratory of Nondestructive Testing, Ministry of Education, Nanchang Hangkong University, Nanchang 330063, China; 2. Material Science and Engineering Institute, Nanchang Hangkong University, Nanchang 330063,China; 3. AECC Shenyang Liming Aero-Engine Co., Ltd., Shenyang 110043, China)Abstract : Impedance spectroscopy is an important means of studying solar cells. Firstly, this paper reviews the impedance spectroscopy measurement methods proposed in recent years, and analyzes the advantages and disadvantages of each method. Through the study of impedance spectroscopy measurement methods, it is found that the differences between different measurement methods are mainly reflected in their efficiency,accuracy and cost. Secondly, the application of impedance spectroscopy in fault detection, electron transport,and interface research are analyzed, and their shortcomings in evaluating the dynamic behavior of cells are pointed out. Finally, the future development direction and application requirements of impedance spectroscopy measurement methods are summarized and analyzed.Keywords : solar cells; impedance spectrum; failure assessment; electron transport; interface research收稿日期: 2022-08-11;收到修改稿日期: 2022-10-05基金项目: 国家自然科学基金(12064027,62065014);研究生创新专项资金(YC2022-118,YC2022-113)作者简介: 李 傲(1999-),男,河北保定市人,硕士研究生,专业方向为光伏检测技术。
bed
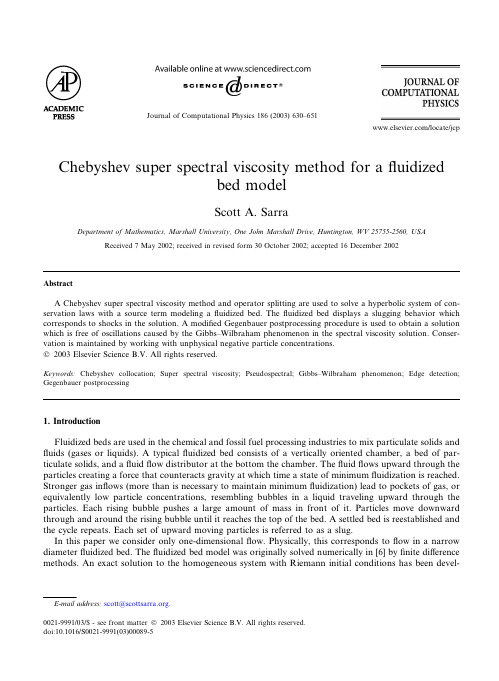
Chebyshev super spectral viscosity method for a fluidizedbed modelScott A.SarraDepartment of Mathematics,Marshall University,One John Marshall Drive,Huntington,WV 25755-2560,USAReceived 7May 2002;received in revised form 30October 2002;accepted 16December 2002AbstractA Chebyshev super spectral viscosity method and operator splitting are used to solve a hyperbolic system of con-servation laws with a source term modeling a fluidized bed.The fluidized bed displays a slugging behavior which corresponds to shocks in the solution.A modified Gegenbauer postprocessing procedure is used to obtain a solution which is free of oscillations caused by the Gibbs–Wilbraham phenomenon in the spectral viscosity solution.Conser-vation is maintained by working with unphysical negative particle concentrations.Ó2003Elsevier Science B.V.All rights reserved.Keywords:Chebyshev collocation;Super spectral viscosity;Pseudospectral;Gibbs–Wilbraham phenomenon;Edge detection;Gegenbauer postprocessing1.IntroductionFluidized beds are used in the chemical and fossil fuel processing industries to mix particulate solids and fluids (gases or liquids).A typical fluidized bed consists of a vertically oriented chamber,a bed of par-ticulate solids,and a fluid flow distributor at the bottom the chamber.The fluid flows upward through the particles creating a force that counteracts gravity at which time a state of minimum fluidization is reached.Stronger gas inflows (more than is necessary to maintain minimum fluidization)lead to pockets of gas,or equivalently low particle concentrations,resembling bubbles in a liquid traveling upward through the particles.Each rising bubble pushes a large amount of mass in front of it.Particles move downward through and around the rising bubble until it reaches the top of the bed.A settled bed is reestablished and the cycle repeats.Each set of upward moving particles is referred to as a slug.In this paper we consider only one-dimensional flow.Physically,this corresponds to flow in a narrow diameter fluidized bed.The fluidized bed model was originally solved numerically in [6]by finite difference methods.An exact solution to the homogeneous system with Riemann initial conditions has beendevel-Journal of Computational Physics 186(2003)630–/locate/jcpE-mail address:scott@.0021-9991/03/$-see front matter Ó2003Elsevier Science B.V.All rights reserved.doi:10.1016/S0021-9991(03)00089-5oped in[7].Thefluidized bed model can be put in the form of system of conservation laws with a source term asu tþfðuÞx¼bðuÞ:ð1ÞSpectral viscosity methods have been successfully applied to homogeneous systems of conservation laws. We use operator splitting to extend the methods to nonhomogeneous systems of conservation laws.If discontinuities are present in the solutions,the spectral viscosity approximations will be contaminated by the Gibbs–Wilbraham phenomenon,but the spectral viscosity solution may be postprocessed to obtain a better approximation.While the spectral viscosity is applied to the solution at every time level,postpro-cessing is only done at times for which a‘‘clean’’solution is desired.Several methods exist for postpro-cessing spectral approximations.They include spectral mollification[12,25,26,36]methods which involve using a two-parameterfilter,the Gegenbauer Reconstruction Procedure(GRP),and a recently developed Fourier–Pad e-based algorithm[9].Spectral mollification is a fairly robust method which may be used with or without the knowledge of edge locations.However,it will only recover spectral accuracy up to within a neighborhood of discontinuity locations.The GRP is capable of recovering spectral accuracy at every point,even at the locations of the discontinuities.In this paper,all numerical examples have been postprocessed using the GRP.One of our goals was to examine if the GRP,which has shown great promise on some simple examples,could be used to successfully postprocess PDE solutions which were either more detailed than piecewise linear or if the method could be used to postprocess solutions containing varying subintervals of detail.The solutions in the previous ap-plications[15,32]consisted of homogeneous features throughout the computational domain which allowed the parameters of the postprocessing method to be chosen globally.Thefluidized bed solutions contain features of varying detail throughout the computational domain and a different strategy must be used to choose the postprocessing parameters.Additionally,we examine what remains to be done if the GRP is to be used as a‘‘black box’’postprocessing method for spectral approximations.This paper is organized as follows:In Section2,the Chebyshev collocation method and super spectral viscosity methods are reviewed.Section3summarizes a method to locate edges in the spectral viscosity approximations.Edge locations will be necessary to apply the postprocessing procedure.Section4describes the GRP for non-periodic functions.Section5describes thefluidized bed model.Numerical results are presented in Section6.2.Chebyshev super spectral viscosity methodThe standard collocation points for a Chebyshev collocation(pseudospectral)method are usually de-fined byx j¼Àcosp jN;j¼0;1;...;N:ð2ÞThese points are extrema of the N th order Chebyshev polynomial,T kðxÞ¼cosðk arccosðxÞÞ:ð3ÞThe points are often labeled the Chebyshev–Gauss–Lobatto(CGL)points,a name which alludes to the points role in certain quadrature formulas.The CGL points cluster quadratically around the endpoints and are less densely distributed in the interior of the domain.The Chebyshev collocation method is based on assuming that an unknown PDE solution,u,can be represented by a global,interpolating,Chebyshev partial sum,S.A.Sarra/Journal of Computational Physics186(2003)630–651631u NðxÞ¼X Nn¼0a n T nðxÞ:ð4ÞThe discrete Chebyshev coefficients,a n,are defined bya n¼2N1c nX Nn¼0uðx jÞT nðx jÞc j;where c j¼2when j¼0;N;1otherwise:ð5ÞDerivatives of u at the collocation points are approximated by the derivative of the interpolating polynomial evaluated at the collocation points.Thefirst derivative,for example,is defined by,d u d x ¼X Nn¼0að1ÞnT nðxÞ:ð6ÞSince að1ÞNþ1¼0and að1ÞN¼0,the non-zero derivative coefficients can be computed in decreasing order by therecurrence relation:c n að1Þn ¼að1Þnþ2þ2ðnþ1Þa nþ1;n¼NÀ1;...;1;0:ð7ÞThe transform pair given by Eqs.(4)or(6)and(5)can be efficiently computed by a fast cosine transform. Equivalently,the interpolating polynomial and its derivatives can be computed in physical space using matrix multiplication[4].Special properties of the Chebyshev basis allow for differentiation via parity matrix multiplication[3](even–odd decomposition[33]),which can be performed by using slightly more than half as manyfloating point operations as standard matrix multiplication.More detailed information may be found in the standard references[4,10,11,18,19,37].After the spectral evaluation of spatial derivatives,the system of ordinary differential equationsd ud t¼Fðu;tÞresults,where u is the vector containing the unknown PDE solution at the collocation points.The system is typically integrated by a second,third,or fourth-order explicit Runge–Kutta method to advance the so-lution in time.A coordinate transformation may be necessary either to map a computational interval to½a;b from the interval½À1;1 ,or to redistribute the collocation points within an interval for the purpose of giving high resolution to regions of very rapid change.Popular maps used to redistribute the CGL points(2)are the Kosloff/Tal-Ezer map[27]x¼gðn;cÞ¼arcsinðcnÞarcsinðcÞ;ð8Þthe center map[1]x¼gðn;cÞ¼ð1:0ÀcÞn3þcn;ð9Þand the two parameter tangent map[2]x¼gðn;c;lÞ¼x0þtanðdnþxÞc;ð10Þwhere j¼arctanðcð1ÀlÞÞ,c¼arctanðcð1þlÞÞ,d¼0:5ðjþcÞ,x¼0:5ðjÀcÞ,and x0¼À1þ2ðlÀaÞ=ðbÀaÞ.632S.A.Sarra/Journal of Computational Physics186(2003)630–651If n denotes the original variable and x¼gðnÞthe new variable,then after a change of variable is performed Eq.(1)becomesu tþ1g0ðnÞfðuÞx¼bðuÞ:ð11ÞIf the PDE solution contains shocks,the spectral collocation method will not converge to the correct entropy solution[35].In this case,a spectrally small viscosity term must be added in order to stabilize the approximation and ensure convergence to the entropy solution.This can be done without sacrificing spectral accuracy and can be accomplished in several different ways,with each way being labeled a par-ticular type of spectral viscosity method.We have used the super spectral viscosity(SSV)method of[28], which for a conservation law in one space dimension,can be stated aso o t u Nþoo xfðu NÞ¼eðÀ1Þsþ1Q2s u N;ð12Þwhere the viscosity operator is given byQ¼ffiffiffiffiffiffiffiffiffiffiffiffiffi1Àx2p oo x:ð13ÞIt was shown in[28]that if e¼CN1À2s,with the parameter C chosen large enough to ensure stability and such that06C6N1=2,and with the parameter s chosen such that s6lnðNÞand allowing s to grow with N, that bounded solutions of(12)will converge to the correct entropy solution in the case bðuÞ¼0.Except for the ranges mentioned in order to ensure convergence to the entropy solution,the parameters s and C are problem dependent,depending mainly on the strength of the shocks involved.A direct implementation of(12)amounts to adding2s spatial derivatives to the equation.This would introduce additional stiffness which would severely limit the stable time step and increase the computational work involved by requiring the computation of higher order derivatives.Hence,the practical implementation of the SSV method is an important issue.The efficient implementation offthe SSV method wasfirst addressed in[8],where the authors recognized that the SSV method could be implemented as a spectralfilter.This fact is based on the examination of the viscosity operator Q2applied to the Chebyshev polynomial(3),T kðxÞ.Q2T kðxÞ¼ffiffiffiffiffiffiffiffiffiffiffiffiffi1Àx2p oo xffiffiffiffiffiffiffiffiffiffiffiffiffi1Àx2p oo xT kðxÞ¼Àk2T kðxÞ:ð14ÞAs a result of applying the viscosity operator to the Chebyshev polynomials,it can be noticed that the Chebyshev polynomials are the eigenfunctions of the operator Q2with eigenvalues k2.Expanding the viscosity term,which is the right-hand side of(12),we notice thateðÀ1Þsþ1Q2s u N¼ÀCNX Nk¼0kN2sa kðtÞT kðxÞ:ð15ÞIf we implement the SSV method via time splitting where in thefirst step we solveo o t u Nþoo xfðu NÞ¼0ð16Þand in the second step we solveo o t u N¼eðÀ1Þsþ1Q2s u N;ð17ÞS.A.Sarra/Journal of Computational Physics186(2003)630–651633the second equation,(17),in the split step can be written aso o tX Nk¼0a kðtÞT kðxÞ"#¼ÀCNX Nk¼0kN2sa kðtÞT kðxÞ;which can be solved analytically.Over one time step,the analytical solution modifies the Chebyshev co-efficients asa kðtþD tÞ¼a kðtÞexpðÀCN D tðk=NÞ2sÞ:Thus,the exact solution of the SSV split step can be written as thefiltered partial sumu NðxÞ¼X Nk¼0rkNa kðtÞT kðxÞ;ð18Þwherer k N¼expÀakNis an exponentialfilter of strength a and order b as described in[38].The Chebyshev SSV method is seen to be equivalent to applying the exponentialfilter with b¼2s and a¼CN D t.The method can be implemented with little additional cost.It should be stressed that while the SSV method is being implemented via the exponentialfiltering framework,that it is not a b th orderfilter as it does not meet the requirements set forth in[38].The amount of damping of the high modes is significantly less with the SSV method than with the application of a b th order exponentialfilter.An application of a b th order exponentialfilter typically takes a¼Àln e where e is machine zero(on a32-bit machine using double precisionfloating point operations, e¼2À52and lnðeÞ’À36:0437).Fig.1compares two exponentialfilters of different orders with an appli-cation of thefilter with the parameters set as a¼0:032and b¼4,which are possible settings that may be used if thefiltering framework is used to implement the SSV method.634S.A.Sarra/Journal of Computational Physics186(2003)630–6513.Edge detectionThe GRP recovers spectral accuracy up to the discontinuity points in each smooth subinterval of a piecewise analytic function.Thus,the GRP needs the exact location of discontinuities,or edges,in the function.If a PDE solution is being postprocessed and the solution contains rarefaction waves,disconti-nuities in the first derivative of the function will exist and need to be located as well.The method used to find the edges originated in [14]for periodic and non-periodic functions.The method is specialized to approximations of functions by Chebyshev methods and is summarized below.Denote the location of discontinuities as a j .Let½f ðx Þ:¼f ðx þÞÀf ðx ÀÞdenote a local jump in the function and defineue ðx Þ¼p ffiffiffiffiffiffiffiffiffiffiffiffiffi1Àx 2p N X N k ¼0a k d d x T k ðx Þ;ð19Þwhered d x T k ðx Þ¼k sin ðk arccos ðx ÞÞffiffiffiffiffiffiffiffiffiffiffiffiffi1Àx 2p :Essentially,we are looking at the derivative of the spectral projection of the numerical solution to de-termine the location of the discontinuities.The series ue ðx Þhas the convergence propertiesue ðx Þ!O ð1=N Þwhen x ¼a j ;½f ða j Þwhen x ¼a j :The series converges to both the height and direction of the jump at the location of a discontinuity.However,for the GRP,we only need the locations and magnitudes of the jumps,not the directions.While a graphical examination of the series ue ðx Þverifies that the series does have the desired convergence prop-erties,an additional step is needed to numerically pinpoint the location of the discontinuities.For that purpose,make a non-linear enhancement to the edge series asun ðx Þ¼N Q =2½ue ðx Þ Q :The values,un ðx Þ,will serve to amplify the separation of scales which has taken place in (19).The series has the convergence propertiesun ðx Þ!O ðN ÀQ =2Þwhen x ¼a j ;N Q =2½½f ða j Þ Q when x ¼a j :By choosing Q >1we enhance the separation between the O ð½1=N Q =2Þpoints of smoothness and the O ðN Q =2Þpoints of discontinuity.The parameter J ,whose value will be problem dependent,is a critical threshold value.Finally,redefine ue ðx Þasue ðx Þ¼j ue ðx Þj if un ðx Þ>J ;0otherwise :With Q large enough,one ends up with an edge detector ue ðx Þ¼0at all x except at the discontinuities x ¼a j .Only those edges with amplitude larger than J 1=Q ffiffiffiffiffiffiffiffiffi1=N p will be detected.S.A.Sarra /Journal of Computational Physics 186(2003)630–651635Often the series ue is slow to converge in the area of a discontinuity and the nonlinear enhancement has difficulty pinpointing the exact location of the edge.If an additional parameter,g,is added to the procedure this problem can be overcome in a simple manner.The parameter specifies that only one edge may be located in the intervalðx½iÀg ;x½iþg Þ,i¼0;...;N,with appropriate one sided intervals being considered near boundaries.The correct edge will be the maximum of ue in this subinterval.The value of g is problem-dependent and is best chosen after the edge detection procedure has been applied once.The edge detection parameters J,Q,and g,are all problem-dependent.Various combinations of the parameters may be used to successfully locate edges represented by jumps of a magnitude in a certain range.4.Gegenbauer reconstructionThe truncation error decays exponentially as N increases when spectral methods are used to approximate smooth functions.However,the situation changes when the function is discontinuous as the spectral ap-proximation no longer converges in the maximum norm.This is known as the Gibbs–Wilbraham phe-nomenon.Several methods exist for removing or reducing the effects of the Gibbs–Wilbraham phenomenon from spectral approximations.Most however,such as spectral mollification[17,36],only recover spectral accuracy up to within a neighborhood of each discontinuity.To date,the most powerful postprocessing method seems to be the GRP which is capable of recovering spectral accuracy up to and including at the location of discontinuities.Although the GRP has been shown to produce remarkable results on some simple problem,the method lacks robustness due to the fact that two parameters,for which an optimal choice for is currently not known,must be specified.The GRP was developed in[20–24]for the purpose of recovering exponential accuracy at all points, including at the discontinuities themselves,from the knowledge of a spectral partial sum of a discontinuous, but piecewise analytic function.While the SSV solution serves as a highly accurate approximation to the exact spectral partial sum,only partial theoretical justification can be found concerning using the GRP as a postprocessing method for the SSV solution.However,numerical results indicate that spectral accuracy can be achieved by applying the GRP to the SSV solution of homogeneous systems of conservation laws[12,15]. The same can be said about the edge detection method,as the theoretical results are limited to locating the jump discontinuities of a piecewise smooth function uðxÞ.However,numerical evidence also advocates applying the edge detection method to the SSV solution.The GRP works by expanding the function in another basis,the Gibbs complementary basis,via knowledge of the known Chebyshev coefficients and the location of discontinuities.The Chebyshev partial sums are projected onto a space spanned by the Gegenbauer polynomials.The associated weight functions increasingly emphasize information away from the discontinuities as the number of included modes grow. The approximation converges exponentially in the new basis even though it only converged very slowly in the original basis due to the Gibbs–Wilbraham phenomenon.The choice of a Gibbs complementary basis isthe Ultraspherical or Gegenbauer polynomials,C kn .The Gegenbauer polynomials are orthogonal polyno-mials of order n which satisfyZ1À1ð1Àx2ÞkÀ1=2C kkðxÞC knðxÞd x¼h kn;k¼n;0;k¼n;where(for k P0)h k n ¼p1=2C knð1ÞCðkþð1=2ÞÞCðkÞðnþkÞ636S.A.Sarra/Journal of Computational Physics186(2003)630–651withC k n ð1Þ¼Cðnþ2kÞn!Cð2kÞ:Whether the Gegenbauer basis is the optimal choice as the Gibbs complementary basis for the Chebyshev basis remains an open question.In other words,it may be possible to construct another basis in which the slowing converging Chebyshev approximation could be expanded in to obtain an approximation with better convergence properties than those of the Gegenbauer approximation.However,it is shown in[24] that the Gegenbauer basis is a Gibbs complementary basis for the Chebyshev basis.The Gegenbauer expansion of a function uðxÞ;x2½À1;1 isuðxÞ¼X1l¼0b f klC klðxÞ;where the continuous Gegenbauer coefficients,b f k l,of uðxÞareb f k l ¼1h klZ1À1ð1Àx2ÞkÀ1=2C klðxÞuðxÞd x:ð20ÞSince we do not know the function uðxÞ,implementing the GRP requires obtaining an exponentiallyaccurate approximation,b g kl ,to thefirst m coefficients b f k l in the Gegenbauer expansion from thefirst Nþ1Chebyshev coefficients of uðxÞ.The approximate Gegenbauer coefficients are defined as the integralb g k l¼1hl Z1À1ð1Àx2ÞkÀ1=2C klðxÞu NðxÞd x;ð21Þwhere u N is the Chebyshev partial sum(4).The integral should be evaluated by Gauss–Lobatto quadraturein order to insure sufficient accuracy.The coefficients b g kl are now used in the partial Gegenbauer sum toapproximate the original function asuðxÞ%u km ðxÞ¼X ml¼0b g k l C k lðxÞ:In practice,there will be discontinuities in the interval½À1;1 and the reconstruction must be done on each subinterval½a;b in which the solution remains smooth.To accomplish the reconstruction on each subinterval,define a local variable for each subinterval as xðnÞ¼ nþd where ¼ðbÀaÞ=2,d¼ðbþaÞ=2 and n j¼cosðp j=NÞ:The reconstruction in each subinterval is then accomplished byu k; m ð nþdÞ¼X ml¼0b g k ðlÞC k lðnÞ;whereb g k ðlÞ¼1hl ZÀ11ð1Àn2ÞkÀ1=2C klðnÞu Nð nþdÞd n:Notice that we have used collocation points on the entire interval½À1;1 to build the approximation in ½a;b .This is referred to as a global–local approach[22].The global–local approach seems to be best when postprocessing PDE solutions where u N is obtained from the time evolution of the PDE solution.The point values uðx iÞmay not be accurate,but the global interpolating polynomial u NðxÞis accurate.S.A.Sarra/Journal of Computational Physics186(2003)630–651637In order to show that the GRP yields uniform exponential accuracy for the approximation,it is nec-essary to select k and m such that k¼m¼b N,where b<2e=ð27ð1þ1=2pÞÞ,and p is the distance from ½À1;1 to the nearest singularity in the complex plane,in each subinterval where the function being re-constructed is assumed to be analytic[24].It is not necessary,and usually not advisable,to choose k¼m.In practice,we are often more concerned with obtaining results for afixed N,rather than achieving an ex-ponential convergence rate.If the function to be postprocessed consists homogeneous features,the reconstruction parameters can be successfully chosen as k¼k k N and m¼k m N for each subinterval where k k and k m are user chosen, globally applied parameters.We refer to this strategy as the global approach.In all previous applications of the GRP in the literature,the method was applied to such functions and it was possible to chose the parameters in this way[12,13].However,in problems with solutions with varying detail throughout the computational domain,the reconstruction parameters may need to be chosen independently in each subinterval[30].We refer to this strategy as the local approach.To date there is no known method to choose optimal values of the reconstruction parameters m and k.The parameters remain very problem dependent.Work is under way on choosing optimal parameters and results will be reported in a future paper.5.Fluidized bed equationsThe variable x denotes the vertical height in the bed.Let aðx;tÞdenote the concentration of particles by volume,vðx;tÞthe particle velocity,and mðx;tÞ¼aðx;tÞvðx;tÞthe particle momentum.The parameter a0is the concentration of particles at equilibrium(when v¼0)and a p is the packing concentration which sets an upper limit for a where a2ð0;1Þ.The parameter a0u denotes the particle concentration corresponding to the critical state dividing linearly stable and unstable states(the particle concentration at minimumflu-idization).The constant s¼3:5ð1Àa0uÞ2:5ða pÀa0uÞis related to the linear stability of the equilibrium solutions which correspond to states of uniformfluidization.The model can be put in the form of a system of conservation laws with a source term asa tþm x¼0;ð22Þm tþðm2=aþFðaÞÞx¼bða;mÞ;ð23ÞwhereFðaÞ¼s2aþs2a2paÀa pþ2s2a p lnðj aÀa p jÞ:The function bða;mÞin the source term is given bybða;mÞ¼Àaþa JÀm ð1ÀaÞ;where J¼ð1Àa0Þ3:5represents the total volumetricflux through the bed.Increasing J(or decreasing a0) corresponds to turning up the inflowing gas.Values a0<a0u correspond to large gasfluxes and have been shown to produce unstable states corresponding to slug-like solutions.Values a0>a0u give rise to stable states.From a mathematical point of view,the non-homogeneous system of conservation laws coincides with the Euler equations for an isentropic gasflow,subject to volumetric forces.The variables a,v,and FðaÞplay the role of density,velocity,and pressure respectively,in the Euler equations.638S.A.Sarra/Journal of Computational Physics186(2003)630–651S.A.Sarra/Journal of Computational Physics186(2003)630–6516395.1.Vacuums and unphysical particle concentrationsA vacuum is said to exist at a collocation point if the particle concentration is zero.Numerically,we will assume that a vacuum exists at a grid point if the concentration is either zero or it is very small (j a j<thres).The system becomes meaningless at vacuum points as m2=a is either undefinedða¼0Þor produces unrealistic valuesðj a j<thresÞ.At each vacuum point encountered in the numerical method,the corresponding values of v,and therefore m,are set equal to zero at that collocation point rather than using the spurious valueðj a j<thresÞor NaN valueða¼0Þ.Values of a such that j a j<thres are retained and not set to zero.Stable approximations by the spectral method always produced a<a p.In the spectral method,a must be allowed to take negative values even though a negative concentration in not physically meaningful,as this information is used in the GRP to postprocess the result.When it was attempted to artificially force the spectral method to work only with a>0,the quality of the postpro-cessed solution was adversely affected.More importantly,even though the spectral collocation method is conservative,if for a<0,a was redefined as a¼0,the conservative properties of the method were destroyed and the method started producing mass.If the values of a were allowed to be negative,the method was conservative and mass was preserved to as many as six decimal places.In all reported re-sults,the parameter thres was taken to be thres¼0:001.After postprocessing the solution,all concen-trations are such that a P0.6.Numerical resultsAll examples were postprocessed using the spectral signal processing[31]suite.In the reported results we have used a0u¼0:55and a p¼0:6.6.1.Homogeneous systemThefirst two problems solve the homogeneous system with Riemann initial data so that the Chebyshev SSV method with GRP postprocessing may be validated against an exact solution.Ourfirst example consists of a left-moving shock wave and a right moving rarefaction wave.The initial conditions are vðx;0Þ¼0for all x in a domain of½À0:2;0:2 and aðx;0Þ¼0:3if x<0and aðx;0Þ¼0:55if x P0.Fig.2shows the solution advanced to time t¼0:5with a fourth-order Runge–Kutta method.The grid consists of64points distributed by map(9)with c¼0:25.The use of the coordinate map has the effect of placing more points in the center of the domain.The SSV parameters used were C¼1and s¼4which produced a viscosity parameter of e¼CN1À2s¼2:27EÀ13(or a¼CN D t¼0:16and b¼8in the expo-nentialfilter).The rarefaction wave is characterized by the solution having a discontinuousfirst derivative,thus edge detection must be applied to thefirst derivative of the solution in addition to the solution itself.The edge detection procedure with Q¼1and J¼1locates jumps of magnitude greater than0.125.With these settings,the edge detection procedure locates edges in the function and thefirst derivative of the function at x¼À0:0331,x¼0:0331,and x¼0:1374.We were unable to get good postprocessed results by specifying the reconstruction parameters globally through the parameters k k and k m.Global parameter specification failed due to the solution containing three intervals of piecewise constant values and a fourth intervalð0:033;0:1374Þconsisting of a function requiring different reconstruction parameters.Good results were obtained by specifying the GRP parameters locally in each smooth subinterval as listed in Table1.The postprocessed solution in Fig.3.。
透射光栅和反射光栅对比-英文

Comparison of a transmission grating spectrometer to a reflective grating spectrometer for standoff laser-induced breakdown spectroscopymeasurementsArel Weisberg,1,*Joseph Craparo,1Robert De Saro,1and Romuald Pawluczyk2 1Energy Research Company,2571-A Arthur Kill Road,Staten Island,New York10309,USA 2P&P Optica,330Gage Avenue,Suite11,Kitchener,Ontario N2M5C6,Canada*Corresponding author:aweisberg@er‑Received7October2009;revised3March2010;accepted12March2010;posted15March2010(Doc.ID118281);published30March2010We evaluate a new transmission grating spectrometer for standoff laser-induced breakdown spectroscopy(LIBS)measurements.LIBS spectra collected from standoff distances are often weak,with smaller peaksblending into the background and noise.Scattered light inside the spectrometer can also contribute topoor signal-to-background and signal-to-noise ratios for smaller emission peaks.Further,collectingstandoff spectra can be difficult because most spectrometers are designed for laboratory environmentsand not for measurements in the field.To address these issues,a custom-designed small,lightweighttransmission grating spectrometer with no moving parts was built that is well suited for standoff LIBSfield measurements.The performance of the spectrometer was quantified through10m standoff LIBSmeasurements collected from aluminum alloy samples and measurements from spectra of a Hg–Ar lamp.The measurements were compared to those collected using a Czerny–Turner reflective grating spec-trometer that covered a similar spectral range and used the same ICCD camera.Measurements usingthe transmission grating spectrometer had a363%improved signal-to-noise ratio when measuredusing the669nm aluminum emission peak.©2010Optical Society of AmericaOCIS codes:050.0050,120.6200,230.1950,280.3420,300.6365.1.IntroductionLaser-induced breakdown spectroscopy(LIBS)has a set of unique strengths that make it well suited for standoff measurement applications,where the sens-ing device is some distance removed from the target material.This is because LIBS measurements re-quire no sample preparation,and the laser pulses and plasma emissions are readily delivered and col-lected via telescopic optics.A number of standoff LIBS instruments have been built,as described in a recent review[1].Applications in the scientific literature for standoff LIBS include material inspection in the metals industries[2],measurements of radioactive materials in the nuclear power industry[3],detection of residues of explosive compounds[4],and cleaning of sculpture and other cultural objects[5].Many of the described applications would benefit from improved throughput and sensitivity of the in-strument.Improved throughput and sensitivity can result in detection of an increased number of ele-ments,lower limits of detection,greater standoff range,and improved precision.For example,in the area of explosive residue detection,the value of a standoff LIBS instrument is directly tied to the smal-lest trace residues it can detect at a given standoff distance,as this will afford the user the greatest mar-gin of safety in the field.Operating LIBS instruments in the field also pre-sents its own unique challenges.Many of the lasers,0003-6935/10/13C200-11$15.00/0spectrometers,optics,and optomechanical compo-nents used in laboratory LIBS instruments are ill suited to field operation due to several factors.First, environmental conditions of temperature,humidity, vibrations,and dust can swing to extremes in the field.Second,robustness is of primary importance because making adjustments and repairs to equip-ment in the field is impractical.Also,the instrument will likely need to be shipped to the location where it will operate,so it must be rugged enough to survive the trip intact.Third,packaging constraints,such as the size and weight of individual components,may dictate limitations on the instrument design.This would be especially true for a standoff LIBS instru-ment that must be transported manually for some distance.In particular,a great deal of potential exists for im-proving the spectrometer systems used in standoff LIBS instruments.This is because most of the spec-trometers described in the applications in[1]are simply laboratory models taken out to the field. Generally,these spectrometers do not adequately meet the operational challenges listed above,which limits the LIBS systems’overall usability and perfor-mance envelope.Furthermore,inherent limitations in optical de-signs of these spectrometers limit their performance. LIBS spectrometer systems are generally of either the Czerny–Turner or echelle configuration[6],as well as some reported Paschen–Runge designs[7].All these configurations generate diffraction patterns by re-flecting light off ruled or etched holographic gratings. These gratings have certain general characteristics that limit the overall system performance.First,their efficiency is usually a strong function of wavelength, diminishing with wavelengths departing from the blaze wavelength.This directly impacts the through-put of the spectrometer,reducing the overall sensitiv-ity and precision and raising the limits of detection for some elements.Second,light throughput generally varies inversely with resolution.Therefore,achieving high-resolution LIBS spectra generally involves the trade-off of diminished throughput.Third,because the reflecting gratings diffract light back along the op-tical path,the potential for stray light to reach the de-tector is increased.Higher levels of stray light reduce signal-to-background levels in LIBS spectra,which results in lower sensitivity and higher limits of detection.We describe here a new LIBS spectrometer that has significant performance advantages over typical spectrometers used for standoff LIBS,both in terms of analytical performance and performance in the field.This spectrometer is based on a transmission grating rather than the more typical reflective grat-ing.Further,rather than a ruled grating,the grating is a volume phase holographic design that further re-duces stray light because it has no ruled ing this type of grating allows for a spectrometer that achieves greater light throughput,higher resolution,package with no moving parts.While other compar-isons between spectrometers[8]and detectors[9–11] for LIBS applications have been reported,there have not been,to our knowledge,any studies on the merits of spectrometer designs based on different grating technologies while using the same detector with both spectrometer systems.This important equalizing factor allows for a more straightforward comparison between the systems.2.Volume Phase Holographic GratingsThe heart of the P&P Optica spectrometers is a vo-lume phase holographic(VPH)diffraction gel grating. Gel gratings work on a different principle from tradi-tional relief reflecting gratings.The manufacturing process of a gel grating begins when a layer of gel or polymer that is able to change refractive index un-der the influence of light is exposed to an optical beam whose intensity periodically changes.After proces-sing,the exposed layer is sandwiched between two transparent plates.The entire assembly then acts as a diffraction grating.For comparison,traditional gratings are usually produced as a result of a highly disruptive mechanical or chemical process,which produces periodic grooves with microscopic cracks on the surface.Efficiency of the grating depends on the profile of the grooves, while microcracks are responsible for severe light scattering.Transmission gel gratings do not have pro-blems with light scattering microcracks.Further-more,the diffracted light propagates at an angle in relation to the transmitted beam;hence,they can be used in optimal working conditions.The result is a more efficient use of available light and dramati-cally lowered scattered light levels.Figure1provides a comparison of efficiency values for a typicalspectral Fig.1.Absolute and average diffraction efficiency(AE)of P&P Optica’s600l=mm gel VPH grating at9°of incidence in compar-ison with holographic relief reflection grating(HRG)and diamond ruled reflective grating(RRG).The AE of the gel grating is mea-sured for a dispersive nonscanning configuration used in all P&P Optica Inc.spectrometers,while the efficiency of the other two gratings is measured under impractical Littrow conditions,as re-ported in the manufacturer’s catalog,corrected to representrange covering a full octave for VPH and ruled gratings.There is a great misunderstanding regarding the measurement of grating performance,including effi-ciency.First,the grating efficiencies of traditional re-flecting gratings provided in catalogs are measured in relation to the intensity of the beam reflected from a mirror coated with the same material used for coat-ing the grating(typically an aluminum layer with a reflectivity of about90%);hence,the absolute diffrac-tion efficiency is about8%–12%lower than that provided in catalogs.Second,for a given grating fre-quency,the efficiency depends on both the angle of incidence of the incoming beam and the groove pro-file of the grating.It is well known that the highest efficiency can be achieved for grating grooves is with a sawtooth profile with a90°angle at the top of the tooth.The efficiency of such gratings is optimized at a particular“blaze”wavelength when the incident beam intersects the grating at a particular“blaze an-gle”such that the diffracted beam propagates in a direction opposite to the incident beam.The efficien-cies at wavelengths other than the blaze wavelength are lower,but can be slightly increased by rotating the grating such that the diffracted beam is back in the direction of the incident beam[12–14].The reported efficiencies for reflective gratings are measured at this optimum incoming beam angle for each individual wavelength(i.e.,the grating is physically rotated between each measurement to op-timize performance).This is commonly referred to as the“Littrow”configuration.While this configuration is optimal from an efficiency point of view,it is im-practical because it can lead to interferences between the delivery and the collection optics.For this reason, typical reflective grating spectrometers(such as the Czerny–Turner system)normally use a single detec-tor and a rotating grating to register one spectral component under the best possible conditions for the chosen geometry.In most LIBS applications,it is impractical to scan every wavelength across a fixed detector.Instead,for a single LIBS measurement,the grating is held in a fixed position and the light is dispersed over an array of detectors(typically the pixels of an ICCD camera). In this case,the efficiency of a reflective grating sys-tem is significantly reduced at those wavelengths that are oriented away from their optimal angle with respect to the incoming light.In some cases,the aver-age absolute efficiency of gratings in spectrometers operating under real conditions can be as low as 10%–20%even though the reported efficiencies are high as measured in the Littrow configuration. Many of the deficiencies of reflective grating spec-trometers can be eliminated by replacing the reflec-tive grating with a transmission VPH diffraction grating and using lenses,rather than focusing mir-rors,to form both the incident and the diffracted beams.In contrast to reflective gratings,VPH grat-ings are most efficient when the diffracted beam pro-in line with,the nondiffracted part of the beam trans-mitted through the grating.This eliminates interfer-ence between the optical systems forming the incident and diffracted beams,allowing VPH grat-ings to be practically implemented in the orientation of maximum efficiency.An additional advantage is that there is less nondiffracted light,which normally contributes to the scattered light background.Appli-cation of lenses in place of mirrors,while potentially reducing the usable spectral range,allows for the construction of more compact spectrometers with better optical performance over larger detector areas,better f-numbers,and higher-quality imaging for longer slits.The increase in grating efficiency has a twofold ef-fect on the performance of a spectrometer system. First,a larger percentage of the incoming light reaches the detector,resulting in an increase in re-corded signal.Second,the number of photons enter-ing the system that do not directly impinge on the detector is reduced.This is important because these stray photons can reach the detector indirectly through multiple reflections resulting in scattered light noise.Typically,the ratio of signal to stray light is of the order of15times greater for a VPH grating than for a similar reflective grating,as shown in Fig.2. The result is a significant increase in photometric dy-namic range.3.ApparatusA.SpectrometersThe spectrometer system used to compare to the P&P Optica Inc.transmission grating spectrometer is an Acton300i Czerny–Turner system(Princeton Instru-ments,Trenton,New Jersey)with a150grooves=mm Fig.2.(Color online)Discharge from a He–Ne laser measured using Czerny–Turner and VPH spectrometers under identical con-ditions with dark signal subtracted.Background levels are propor-tional to stray light,seen here to be approximately150times lower for the VPH system.The graphs are not offset from their actualgrating blazed at 500nm and coupled to a Princeton Instruments PI-Max intensified CCD camera (1024×256pixel CCD array,25mm Gen II intensifier).The grating was mounted to a rotating turret that enables scanning across multiple wavelength ranges.The po-sition of the grating was fixed in this work such that the measured wavelength range was centered at 550nm.A photograph of the system is seen in Fig.3.This system is typical of many described in the LIBS literature,being a fairly compact and flexible design with a fiber optic cable attached to the input slit.The P&P Optica VPH transmission grating spec-trometer system coupled to the same PI-Max ICCD camera is shown in Fig.4.The compact form factor of the spectrometer is evident.A comparison of the spe-cifications of the two systems is seen in Table 1.The laser used for the LIBS experiments was a Quantel USA (Bozeman,Montana,formerly Big Sky Laser)CFR-400Nd:YAG Q -switched laser oper-ating at 1064nm.The laser outputs 330mJ =pulse in ∼7ns pulses,and it was operated in single-shot mode.The laser pulses were delivered to the aluminum alloy targets via a straightforward beam expansion and collimating optical arrangement followed by a Galilean telescope,with a ϕ25mm plano –concave lens and a ϕ50mm plano –convex lens,which,when combined,resulted in a 10m focal length for the telescope.The lenses were antireflection coated for 1064nm.The light from the LIBS plasma was collected with a ϕ200mm,f =4:9,f ¼1000mm,Newtonian tele-scope (SkyView Pro 8)from Orion Telescopes (Watsonville,California)that was modified by repla-cing the viewing eyepiece with a fiber optic cable SMA-905connector.A photograph of the modified telescope is seen in Fig.5,and a view down the barrel of the telescope toward the target samples placed 10m away is shown in Fig.6.A 2m long,600μm core,fiber optic cable was attached to the telescope.The mercury lamp used in some of the experiments was an Ocean Optics (Dunedin,Florida)CAL-2000mercury –argon wavelength calibration source with an SMA-905fiber optic cable connector.The input ends of the fiber optic cables attached to the spectro-meter systems are also fitted with SMA-905connectors.4.SamplesSix different Standard Reference Material (SRM)aluminum alloy samples were used as targets in this study.These SRMs were obtained from Brammer Standard (Houston,Texas).They are in theshapeFig.3.Acton Research 300i Czerny –Turner spectrometer system with PI-Max ICCD Camera and f -number matching fiber optic cable adapter attached.A 30cm ruler is also shown forscale.Fig. 4.P&P Optica transmission grating spectrometer and PI-Max ICCD camera.A 30cm ruler is also shown for scale.Table 1.Comparison of Specifications of Transmission Grating and Czerny –Turner Spectrometer Systems when Coupled to the PI-Max ICCDCamera Used in the ExperimentsP&P Optica Transmission GratingSpectrometerActon 300i Czerny –Turner Spectrometer Spectral range 366–796nm (Δλtotal ¼430nm)266–831nm (Δλtotal ¼565nm)f =#34Entrance slitNone —uses fiber optic bundle for 37μm ×6:6mm effective slit52μm ×1mmOptical fiber cross-section specifications68×50μm fibers arranged in a ϕ600μm circular bundle at the input end and in a vertical line at the spectrometer end 19×200μm fibers arranged in a ϕ1mm circular bundle at the input side and in a vertical line at the spectrometer end Optical fiber length1:5m 3:0m Fiber optic cable f -number matching moduleNoYesof approximately ϕ25–50mm disks.The concentra-tions of the elements examined in the tests reported below are given in Table 2.These alloys were selected to achieve a range of concentration values for each of these elements.5.Experimental Procedure A.Mercury Lamp TestsA mercury –argon line source,described in Section 3,was attached to each spectrometer via a simple col-limating and focusing optical design shown schema-tically in Fig.7.The positions of the fibers relative to the lenses were optimized for maximum throughput.The neutral density filter was needed to avoid satur-ating the ICCD detector.A procedure was also devel-oped that minimized the effects of attaching and removing the fiber optic cables from the optical assembly .Fifty mercury lamp spectra were collected with each spectrometer system using a temporal window of 5ms and an intensifier gain setting of zero.Dark spectra were also collected and automatically subtracted from the mercury lamp spectra by the software supplied by Princeton Instruments (Win-Spec).The resulting corrected spectra were averaged together.Repeatability was checked by collecting 25accu-mulations of 25spectra each,detaching the fibers from the optical assembly between each ing the 435and 579nm Hg emission lines,we measured a relative standard deviation (RSD)of 0.61%in the amplitudes of these lines in the set of spectral accumulations using the transmission grating spectrometer.B.Laser-Induced Breakdown Spectroscopy TestsThe SRM alloy samples were cleaned with acetone,followed by methyl alcohol,followed by isopropanol,prior to testing.The samples were then placed 10m from the telescope (Fig.6)and oriented vertically so that their surface was normal to the laser pulses.Fifty LIBS spectra were collected from each sam-ple by first applying 50laser shots (i.e.,“cleaning shots ”)to the same spot on the sample,followed by an analysis shot when the LIBS spectrum was re-corded.The sample was then rotated slightly for collecting the next spectrum from a new spot.The temporal settings for the ICCD camera relative to the laser shot were a delay of 3μs and a width of 1μs.The gain setting on the camera was 120(out of 255)for the transmission spectrometer and 160for the reflective grating spectrometer so that the peaks would be of roughly the same amplitude.The different gain setting amounts to an increase in absolute intensities (i.e.,counts)by a factor of 3.2and an increase in the RSD of the intensities by a factor of 1.3.These factors were taken into ac-count in the reported data.A PrincetonInstrumentsFig.5.Telescope used to collect the LIBS plasmalight.Fig.6.View from the Newtonian telescope used to collect the LIBS plasma light looking toward the target samples.Table 2.Concentrations of Elements in the Aluminum Alloy Standards Used in TestingStandard Cu (wt.%)Fe (wt.%)Mg (wt.%)Mn (wt.%)HG-40.0950.120.310.39HP-10.480.7220.11HP-2 1.50.5 1.250.25HP-33.520.220.790.42NCSHS53703-40.0330.350.040.055NCSHS53703-50.0791.310.1030.112programmable delay generator,Model ST133A,was used to control the laser –camera timing via the system ’s software package.Dark spectra were also collected with the same camera settings and automa-tically subtracted from the LIBS spectra.The fiber optic cable attached to the LIBS tele-scope (Fig.5)was connected to the fiber optic cables on the spectrometers via a simple SMA-905mating sleeve.This sleeve positions the ends of the two fiber optic cables up against each other to maximize the transmission of light from one fiber to the other.C.Data ComparisonThe procedure for the comparison between the two spectrometer systems was designed to compensate for the different system specifications that are unre-lated to the fundamental strengths and weaknesses of each system.The foremost example of this was the use of the same intensified CCD camera with both spectrometer systems.We also compensated for the difference in wavelength range between the grat-ings (Table 1).This is obviously a function of the par-ticular gratings and is unrelated to any benefits or drawbacks of the respective system optical config-urations.Therefore,the analysis presented below accounts for this difference.For the purpose of comparing the two systems,we did not attempt to compensate for the differences in how the optical fibers couple to their respective spec-trometer units.Each system ’s design has advantages and disadvantages in their fiber coupling method.The advantages of the P&P unit are the lack of an entrance slit and the low f -number of the unit (f =3).The advantages of the Acton unit are its fiber optic f -number matcher and the higher fill factor of its fiber optic cable bundle (the ratio of the total cross-sectional area of all the optical fibers in the bundle to the area of the front face of the fiber tip)as compared to the P&P unit (76%versus 47%).Given the difficulty in modeling and accounting for these differences accurately ,and the fact that the re-spectrometer units during the tests,the comparison presented here will consider the fiber optic cable as an integral part of the spectrometer unit.To compensate for the different spectral ranges of the spectrometers,the intensities of the spectra col-lected by the two units were normalized into counts/nanometer units.The spectral resolutions of the systems are reported in camera pixels rather than nanometers for this reason as well.6.ResultsA.Mercury Lamp TestsA comparison of the measured 435:8nm mercury emission peak for the reflective and transmissive grating spectrometers is presented in Fig.8and on a logarithmic scale in Fig.9.This clearly shows the throughput enhancement achieved by the transmis-sion grating spectrometer.The noise level for the re-flective spectrometer is approximately 13counts =nm ,while for the transmission spectrometer it is approxi-mately 20counts =nm .The peak areas by comparison are 5016and 11,091counts,resulting in signal-to-noise ratios of 394and 568for the reflective and transmission spectrometers,respectively .This is one example of the superior throughput and stray light minimization of the transmission grating spectrometer.Thesuperiorresolutionofthetransmissiondesignis demonstrated by the comparison of peak-normalized signals from the Hg 435:8nm emission line seen in Fig.10.The full width at half-maximum (FWHM)of the peak from the transmission spectrometer is mea-sured to be 2.37pixels,while the FWHM of the peak from the reflection spectrometer is 3.33pixels,or al-most 1pixel wider.The corresponding values for the 546:1nm Hg emission line are 2.67and 3.34pixels for the transmission and reflection grating spectro-meters,respectively .This indicates that theresolutionFig.7.(Color online)Schematic diagram of optical coupling arrangement for the mercurylamp.Fig.8.(Color online)Comparison of signals from the Hg 435:8nm emission line.Solid curve,transmission grating spectrometer;advantage of the transmission grating spectrometer persists across the wavelength range.The peak amplitudes,baseline,and noise levels of a number of Hg emission peaks were measured in all 50of the Hg spectra collected with each spectro-meter.The baseline was calculated as the average in-tensity of two 10nm wide regions on either side of the respective emission peak,and the noise level was calculated as the standard deviation of the intensity values in these two regions.The results are summar-ized in Table 3.In the table,throughput is measured as the peak area (baseline subtracted),the signal-to-background ratio is the peak area divided by the baseline value,and the signal-to-noise ratio is the peak area divided by the noise level.The repeatabil-ity was measured as the standard deviation of the peak heights over the 50Hg spectra divided by the mean amplitude value of the peak.The values in Table 3demonstrate how the perfor-mance advantage of the transmission grating spec-trometer as compared to the reflective grating spectrometer increases across the wavelength range.The signal-to-noise ratio improvement increasing from 6%to 130%highlights this trend especiallywell.This finding is in line with the general charac-teristic of reflection gratings that their efficiency is a strong function of wavelength.B.Laser-Induced Breakdown Spectroscopy TestsThe LIBS experiments were designed to highlight as-pects of spectrometer performance that are of most importance to standoff LIBS applications:through-put and limits of detection.Limits of detection are not only dependent upon how many of the incoming photons reach the detector (i.e.,efficiency),but are also dependent upon the level of scattered light.Since we used the same ICCD camera with both spec-trometers in these experiments,scattered light will be the dominant source of differences in the back-ground intensity levels.Spectral resolution was not evaluated using the LIBS spectra because,typically ,only a fraction of a LIBS spectral line ’s width is due to instrument broadening.LIBS spectral lines are often modeled as Voigt profiles,which are a convolution of a Lorent-zian profile primarily due to the Stark effect,and a Gaussian profile,which is due to a combination of instrument broadening and DopplerbroadeningFig.9.(Color online)Comparison of signals from the Hg 435:8nm emission line on a logarithmic scale.Solid curve,transmission grating spectrometer;dotted curve,reflective grating spectro-meter.Fig.10.(Color online)Comparison of normalized emissions from the Hg 435:8nm line.Solid circles,transmission spectrometer;open squares,reflective spectrometer.Table 3.Improvement of Transmission Spectrometer over Reflection Spectrometer as Measured by Three Hg Emission LinesHg 404:7nmHg 435:8nmHg 546:1nm Refl.Trans.Adv .a Refl.Trans.Adv .Refl.Trans.Adv .Throughput b1817307269%501611091121%350315377339%Signal/background c 647212%1781802%161374133%Signal/noise d 1351436%39456844%296679130%Repeatability e0.0730.04340%f0.0420.02639%0.0570.02066%a Adv .,transmission system advantage ¼ðtransmission value-reflection value)/reflection value.bIntegrated peak area in counts.cPeak area/mean background as described in text.dPeak area/mean noise as described in text.eRelative standard deviation (σ=μ)of peak amplitude over the 50collected spectra.[15].Therefore,quantitatively determining the in-strument broadening from a LIBS spectral line would require deconvolving the Voigt line shape and subtracting the Doppler broadening,which is de-pendent on the plasma temperature,from the total Gaussian width.Because of the potential inaccura-cies in this procedure,we chose to measure the reso-lution advantage of the transmission grating spectrometer using the Hg lamp spectra,as de-scribed in Subsection 6.A and shown in Fig.10,as they are not impacted by the LIBS plasma broaden-ing mechanisms [16].Sample 10m standoff LIBS spectra from the HP-3aluminum alloy is shown in Fig.11,taken at the same ICCD gain setting (120)with both spectro-meters.The figure demonstrates that the raw inten-sities vary from being 1order of magnitude greater in the transmission-grating spectrum to approaching 2orders of magnitude at the longer wavelengths.This is a clear indication that much more light is reaching the ICCD camera when the transmission grating spectrometer is used.While the increase in signal in-tensity is accompanied by an increase in background level,the signal levels of the LIBS peaks are enhanced by a greater amount,as evidenced by the improvements in the signal-to-background levels (see below).Consequently ,the increase in lightthroughput allows us to measure the smaller LIBS peaks in the spectrum without saturating the larger peaks.A comparison of the intensities from single laser shot LIBS spectra taken from the HG-4SRM is shown in Fig.12in the region of the Al I 669nm emis-sion line.This figure also shows the region over which baseline and noise statistics were gathered.As expected,the LIBS results verified the findings of the Hg lamp tests with regard to throughput,back-ground,and noise ing the Al I 669nm emis-sion line,the signal-to-noise ratio and repeatability of each spectrometer was quantified based upon all the single-shot LIBS spectra collected from the six alloys.This aluminum emission line is a nonreso-nance line that is also not self-absorbed.The results are summarized in Table 4.The relatively poor per-formance of the reflective grating system in this wavelength range illustrates one of the drawbacks of such systems for LIBS applications where the grating is fixed and one or more of the measured atomic emission lines is far from the angle at which the grating isoptimized.Fig.11.(Color online)Comparison of single laser shot LIBS spec-tra from the HP-3alloy .The plots are not offset from their actualvalues.Fig.12.(Color online)Comparison of Al 669nm peak from an HG-4alloy spectrum also showing the spectral region over which the baseline and noise statistics were computed.The curves are not artificially offset from each other.Top curve,transmission spec-trometer ICCD gain ¼120;middle curve,reflection spectrometer ICCD gain ¼160;bottom curve,reflection spectrometer ICCD gain ¼120.Table 4.Summary of Spectrometer System Performance as Measured by the Al I 669nm Emission Line StatisticsThroughputaAverage Signal/Background Ratio cAverage Signal/Noise Ratio dRepeatability eTransmission grating spectrometer 10953 3.1037.713%Reflection grating spectrometer 709b 2.888.15f 15%Transmission grating improvement1445%7.5%363%13%a Integrated area under the peak in counts.bCorrected for the 3.2times factor due to the increased gain setting (see text).cPeak area/mean background as described in text.dPeak area/mean noise as described in text.eRelative standard deviation (σ=μ)of peak amplitude over the 50collected spectra from each sample.。
QCD Description of Particle Spectra up to LEP-1.5 Energies and the Running of $alpha_s$
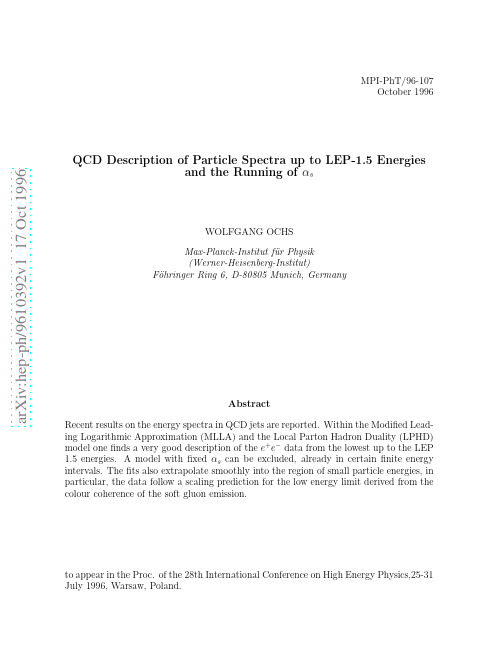
a r X i v :h e p -p h /9610392v 1 17 O c t 1996MPI-PhT/96-107October 1996QCD Description of Particle Spectra up to LEP-1.5Energiesand the Running of αsWOLFGANG OCHSMax-Planck-Institut f¨u r Physik (Werner-Heisenberg-Institut)F¨o hringer Ring 6,D-80805Munich,GermanyAbstractRecent results on the energy spectra in QCD jets are reported.Within the Modified Lead-ing Logarithmic Approximation (MLLA)and the Local Parton Hadron Duality (LPHD)model one finds a very good description of the e +e −data from the lowest up to the LEP 1.5energies.A model with fixed αs can be excluded,already in certain finite energy intervals.The fits also extrapolate smoothly into the region of small particle energies,in particular,the data follow a scaling prediction for the low energy limit derived from the colour coherence of the soft gluon emission.to appear in the Proc.of the 28th International Conference on High Energy Physics,25-31July 1996,Warsaw,Poland.QCD DESCRIPTION OF PARTICLE SPECTRA UP TO LEP-1.5ENERGIES ANDTHE RUNNING OF αsWOLFGANG OCHSMax-Planck-Institute f¨u r Physik (Werner-Heisenberg-Institut)F¨o hringer Ring 6,D-80805Munich,GermanyRecent results on the energy spectra in QCD jets are reported.Within the Modified Leading LogarithmicApproximation (MLLA)and the Local Parton Hadron Duality (LPHD)model one finds a very good description of the e +e −data from the lowest up to the LEP 1.5energies.A model with fixed αs can be excluded,already in certain finite energy intervals.The fits also extrapolate smoothly into the region of small particle energies,in particular,the data follow a scaling prediction for the low energy limit derived from the colour coherence of the soft gluon emission.1Introduction One of the important predictions of perturbative QCD on the intrinsic structure of jets concerns the energy spectra of particles.The particles of low energy E are not multiplied with increasing jet energy E jet because of colour coherence in the cascading process and this yields the bell shapedspectrum in the variable ξ=log Ejetαs .Termsof higher order are included as well,although not completely,which allow to fulfill the initial con-dition for the parton cascade at threshold.These predictions,at the parton level,involve only two parameters:the QCD scale Λwhich determines the running of the coupling αs and the transverse momentum cut-offQ 0of the gluon emission.Re-markably,the observed hadron spectra are rather well described by the spectrum of partons if a low value Q 0∼250MeV of the order of hadronic masses is used and this observation has led to the LPHD hypothesis 3.As a justification of this ap-proach is not yet available at a fundamental level it seems important to determine its range of ap-plicability and its limitations.Also one would like to know to what extent the predictions are sen-sitive to QCD as the underlying theory.In this contribution the sensitivity of the energy spectra to the running αs and the colour coherence -two properties specific to QCD as a field theory -are addressed.More details can be found in refs.4,5,6.2Moment Analysis of Particle SpectraTo analyse the effect of the running αs it is convenient to work with the moments of the ξ-spectrum of particles which evolve independently of each other with energy.They are defined by <ξq (Y,λ)>= dξξq D (ξ,Y,λ)/¯Nwhere we use the logarithmic variables Y =log(E jet /Q 0)and λ=log(Q 0/Λ);the normalization is by the mul-tiplicity ¯N.We consider finally the cumulant mo-ments K q which are obtained from the <ξq >mo-ments by K 1≡<ξ>=¯ξ,K 2≡σ2=<(ξ−¯ξ)2>,K 3=<(ξ−¯ξ)3>,K 4=<(ξ−¯ξ)4>−3σ4,...;also one introduces the reduced cumulants κq ≡K q /σq ,in particular the skewness s ≡κ3and the kurtosis k ≡κ4.The cumulant moments K q (Y,λ)behave at high energies like K q (Y,λ)=K q (Y 0,λ)++YY 0dy −∂¯N q k =0q k (N 1L (q )k +N 2R (q )k )(2)where N 1,N 2,L (q )k and R (q )k are known functionsof a =11N c /3+2n f /3N 2c ,b ≡(11N c -2n f )/3,and λwhere nf ,N C denote the numbers of flavours and paring these formulae to the ex-perimental data on moments,4best agreement is found for the “limiting spectrum”,where the two parameters coincide,i.e.,Q 0=Λ,or λ=0.In this case the formulae simplify and the moments can be expressed 7in terms of the parameter B ≡a/b and the variable z ≡Yq=P (q )0(B +1,B +2,z )++2I B +1(z )P (q )1(B +1,B +2,z )(3)where P (q )0and P (q )1are polynomials of order 2(q −1)in z .These expressions extrapolate smoothly to threshold,where they are determined by the ini-tial condition for a single parton.Similarly one can derive the complete MLLA results for fixed αs 4,which simplify for high energies to¯ξfix = 1+η2,σ2fix =γ20γ01¯γ0Y,k fix =3(4η2−γ20)Y(5)where η=aγ20/8N C ,¯γ0≡2N C αs /π.3Relating parton and hadron spectraStrictly speaking the QCD results are only reliablefor E ≫Q 0.In order to determine the moments one has to integrate the spectra over the full range and to extrapolate to the soft limit.The experi-mental particle densities are usually given as func-tion of momenta.The same kinematic limits of parton and hadron spectra is obtained if the same effective mass Q 0is assigned to the charged par-ticles which also limits the energy of the partons (E h =E p ≥Q 0).Furthermore,a common behaviour of the par-ton and hadron spectra near the boundary can be obtained if the spectra are related by 1,4E hdn (ξE )dp p≡K h D (ξE ,Y,λ)(6)at the same energy E or ξE ≡ξ=log(E jet /E ),where E h =d 3pap-proaching a constant value in the soft limit p →0,as suggested by the experimental data.The pre-scription (6)represents a minimal extension of the QCD prediction for E ≫Q 0towards the bound-ary at E =Q 0but is not unique;other prescrip-tions using a smaller value of Q 0∼m πhave also been applied (see discussion in 6).4Comparison with experimental dataThe moments <ξq >are determined 4from the spectra Edn/dp vs.ξE after appropriate trans-formation of the measured x p =2p/√p 2+Q 20andtherefore depend on Q 0.First a fit of the two parameters Q 0and Λis obtained by comparing the moments for a selected Q 0with the theoreti-cal predictions from Eq.(2)for different Λ.The best agreement with the data was obtained for Q 0≈Λ≈(270±20)MeV.It was not possible to obtain a satisfying description for the fixed αs case.Choosing γ0=0.64and the same Q 0=270MeV a good description of the multiplicity¯Nand the slope of ¯ξvs.Y could be obtained but not for the other quantities.In Fig.1we show the evolution of the first cumulant moments K q with enery for running αs and fixed αs .Note that these predictions depend only on the two parameters Q 0and Λwhich actually coincide in the fit.The ab-solute normalization of the moments is given at threshold Y 0=0by K q =0.As this is an application of perturbative QCD to an extreme limit one could make the weaker (more conventional)assumption and choose a higher starting energy,say Y 0=2(√0123456012345678-1-0.500.511.522.53012345678-0.200.20.40.60.811.2012345678-1.5-1-0.500.511.522.5312345678Figure 1:The first four cumulant moments K q of the charged particles’energy spectra Edn/dp vs.ξE ,shown as a function of Y for Q 0=270MeV.Predictions of the Limiting Spectrum of MLLA with running αs (solid lines),of MLLA with fixed αs (=0.214)(dashed lines)and of MLLA with fixed αs normalized by hand to the experimen-tal point at√s =44GeV).The trend at yet larger energies is again different,in particular for the higher moments.The dependence on the number of flavours n f has been studied as well 4,5.As the jet evolution is dominated at all energies by the gluon emission at the lower transverse momenta,the choice n f =3is a good approximation up to LEP energies.The recent LEP-1.5data are also well ac-counted for 5by the same theoretical scheme as is shown in Fig. 2.Here the experimental ξ-spectrum is shown in comparison with the lim-iting spectrum (setting ξp =ξE )which terminates at Y ∼5.3and after rescaling ξE →ξp with re-lation (6)which takes into account the boundary effects.Also the moments of the distribution and the position of the maximum are well reproduced by the theoretical predictions at this energy.Figure3:Invariant density Edn/d3p of charged particles in e+e−annihilation as a function of the particle energy E=s we now turn to the behaviour of thefits at low particle energy E.As the emis-sion of very soft gluons from all other partons in the jet is coherent the production rate of such par-ticles is nearly energy independent3.Indeed,the analytic results in the DLA and MLLA show the scaling behaviour of the spectrum in the soft limit and the production rate is essentially determined by the colour charge of the initial partons6.Re-markably,the observed hadrons follow the trend of this prediction as can be seen in Fig.3which shows the invariant particle density as a func-tion of particle energy E(assuming the effective charged particle mass Q0=270MeV).The data from all energies in e+e−annihilation approach a common limit for E→Q0(p→0).The curves which represent the MLLA calculation show again the scaling limit and reproduce the dependence√on energy E and cms-energy。
211050369_分散固相萃取-超高效液相色谱-串联质谱法测定海龙中11_种磺胺类抗菌药物残留
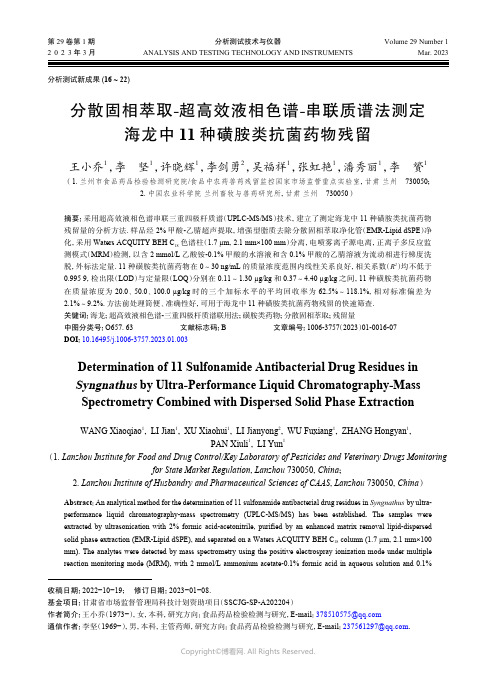
分析测试新成果 (16 ~ 22)分散固相萃取-超高效液相色谱-串联质谱法测定海龙中11种磺胺类抗菌药物残留王小乔1 ,李 坚1 ,许晓辉1 ,李剑勇2 ,吴福祥1 ,张虹艳1 ,潘秀丽1 ,李 赟1(1. 兰州市食品药品检验检测研究院/食品中农药兽药残留监控国家市场监管重点实验室,甘肃 兰州 730050;2. 中国农业科学院 兰州畜牧与兽药研究所,甘肃 兰州 730050)摘要:采用超高效液相色谱串联三重四极杆质谱(UPLC-MS/MS )技术,建立了测定海龙中11种磺胺类抗菌药物残留量的分析方法. 样品经2%甲酸-乙腈超声提取,增强型脂质去除分散固相萃取净化管(EMR-Lipid dSPE )净化,采用Waters ACQUITY BEH C 18色谱柱(1.7 µm ,2.1 mm×100 mm )分离,电喷雾离子源电离,正离子多反应监测模式(MRM )检测,以含2 mmol/L 乙酸铵-0.1%甲酸的水溶液和含0.1%甲酸的乙腈溶液为流动相进行梯度洗脱,外标法定量. 11种磺胺类抗菌药物在0~30 ng/mL 的质量浓度范围内线性关系良好,相关系数(R 2)均不低于0.995 9,检出限(LOD )与定量限(LOQ )分别在0.11~1.30 µg/kg 和0.37~4.40 µg/kg 之间,11种磺胺类抗菌药物在质量浓度为20.0、50.0、100.0 µg/kg 时的三个加标水平的平均回收率为62.5%~118.1%,相对标准偏差为2.1%~9.2%. 方法前处理简便、准确性好,可用于海龙中11种磺胺类抗菌药物残留的快速筛查.关键词:海龙;超高效液相色谱-三重四极杆质谱联用法;磺胺类药物;分散固相萃取;残留量中图分类号:O657. 63 文献标志码:B 文章编号:1006-3757(2023)01-0016-07DOI :10.16495/j.1006-3757.2023.01.003Determination of 11 Sulfonamide Antibacterial Drug Residues in Syngnathus by Ultra-Performance Liquid Chromatography-Mass Spectrometry Combined with Dispersed Solid Phase ExtractionWANG Xiaoqiao 1, LI Jian 1, XU Xiaohui 1, LI Jianyong 2, WU Fuxiang 1, ZHANG Hongyan 1,PAN Xiuli 1, LI Yun1(1. Lanzhou Institute for Food and Drug Control/Key Laboratory of Pesticides and Veterinary Drugs Monitoringfor State Market Regulation , Lanzhou 730050, China ;2. Lanzhou Institute of Husbandry and Pharmaceutical Sciences of CAAS , Lanzhou 730050, China )Abstract :An analytical method for the determination of 11 sulfonamide antibacterial drug residues in Syngnathus by ultra-performance liquid chromatography-mass spectrometry (UPLC-MS/MS) has been established. The samples were extracted by ultrasonication with 2% formic acid-acetonitrile, purified by an enhanced matrix removal lipid-dispersed solid phase extraction (EMR-Lipid dSPE), and separated on a Waters ACQUITY BEH C 18 column (1.7 µm, 2.1 mm×100mm). The analytes were detected by mass spectrometry using the positive electrospray ionization mode under multiple reaction monitoring mode (MRM), with 2 mmol/L ammonium acetate-0.1% formic acid in aqueous solution and 0.1%收稿日期:2022−10−19; 修订日期:2023−01−08.基金项目:甘肃省市场监督管理局科技计划资助项目(SSCJG-SP-A202204)作者简介:王小乔(1973−),女,本科,研究方向:食品药品检验检测与研究,E-mail :通信作者:李坚(1969−),男,本科,主管药师,研究方向:食品药品检验检测与研究,E-mail :.第 29 卷第 1 期分析测试技术与仪器Volume 29 Number 12023年3月ANALYSIS AND TESTING TECHNOLOGY AND INSTRUMENTS Mar. 2023formic acid in acetonitrile as mobile phases by a gradient elution program, and quantified by the external standard method. The results showed that the 11 antibacterial sulfonamides had good linearity in the range of 0~30 ng/mL, the correlation coefficient (R2) were all greater than 0.9959, the limit of detection (LOD) and quantitation (LOQ) were in the range of 0.11~1.30 µg/kg and 0.37~4.40 µg/kg, respectively. At three spiked levels of 20.0, 50.0 and 100.0 µg/kg, the spiked average recoveries of the analytes were in the range of 62.5%~118.1%, and the relative standard deviation was 2.1%~9.2%. The method is accurate, straightforward, and is suitable for the rapid screening of 11 sulfonamide antibacterial drug residues in Syngnathus.Key words:Syngnathus;UPLC-MS/MS;sulfonamides;solid phase extraction;residues海龙是一种药用价值很高的动物源性中药材,也是一种水产品,其为刁海龙Solenognathus hardwickii(Gray)、拟海龙Syngnathoidesbiaculeatus (Bloch)或尖海龙Syngnathus acus Linnaeus的干燥体[1-4],主要成分是甾体类、脂肪酸、蛋白质、氨基酸、微量元素等,具有温肾壮阳、散结消肿等功效,广泛应用于临床治疗中[5]. 因野生海龙资源稀缺,市场所见海龙主要来源于人工养殖. 在海龙养殖过程中,养殖户为了避免其受细菌感染而造成经济损失,常使用磺胺类药物进行预防治疗. 但有些不良养殖户违规超量使用磺胺类药物,导致药物残留在海龙体内,人类通过食用海龙进而转移到人体内[6-8]. 人类若食用磺胺类药物残留量较高的海龙,易产生药物蓄积,导致肾毒性与过敏反应,甚至会破坏胃肠道菌群平衡,引起白细胞减少等[9-10]. 当前,有关海龙中磺胺类抗菌药物残留量的常规监测未见文献报道,因此,从安全角度出发,很有必要建立简便、可靠的方法以满足海龙中磺胺类药物残留检测的需求.目前,测定动物源性食品中磺胺类药物应用最广泛的是超高效液相色谱-串联质谱法,其具有背景干扰少、灵敏度高、特异性强、选择性强等特点,适合复杂基质中微量成分的检测. 前处理方法应用最广泛的是分散固相萃取和固相萃取柱[11-14]. 传统的分散固相萃取难以去除动物源性食品中大部分脂质干扰物,固相萃取柱因需要活化、淋洗、洗脱和浓缩等,试验过程繁琐. 而增强型脂质去除分散固相萃取(enhanced matrix removal lipid-dispersed solid phase extraction,EMR-Lipid dSPE)可选择性去除样品中的主要脂类且不影响目标分析物的提取效率,相比传统的分散固相萃取和固相萃取柱,EMR-Lipid dSPE具有操作简单、杂质净化高效的优势.为了监测与评判海龙中磺胺类药物残留的暴露水平与风险,本研究采用2%甲酸-乙腈提取干燥样品中的目标待测物,使用EMR-Lipid dSPE净化技术去除脂类等非极性杂质,建立了一种基于超高效液相色谱质谱联用法同时测定海龙中11种磺胺类抗菌药物残留量的分析方法. 该方法快速简单、重复性好、定量准确,适用于海龙中磺胺类药物残留的风险监测.1 试验部分1.1 仪器与试剂Agilent 1290 Infinity II-6460C超高效液相色谱串联三重四极杆质谱联用仪(配置离子源为Agilent Jet Stream电喷雾离子源)、EMR-Lipid dSPE净化管(美国安捷伦科技有限公司);MS105DU型电子天平(瑞士梅特勒托利多有限公司);VXR涡旋混合器(德国艾卡仪器设备有限公司);H1850离心机(湖南湘仪实验室仪器开发有限公司);SB-800DT超声波清洗机(宁波新芝生物科技股份有限公司).乙腈(色谱纯,德国默克公司);乙酸铵、甲酸(色谱纯,日本东京化成工业株式会社);蒸馏水(广州屈臣氏食品饮料有限公司);其余试剂均为分析纯. 对照品:磺胺甲基嘧啶(批号:G982705,纯度:99.1%)、磺胺甲噁唑(批号:G150000,纯度:99.5%)、磺胺氯哒嗪(批号:G119228,纯度:99.1%)、磺胺邻二甲氧嘧啶(批号:G151421,纯度:99.1%)、磺胺噻唑(批号:G980077,纯度:99.2%)、磺胺间二甲氧嘧啶(批号:G122608,纯度:99.5%)、磺胺甲二唑(批号:G974370,纯度:99.3%)、磺胺甲氧哒嗪(批号:G150001,纯度:99.7%)均来源于德国Dr.Ehrenstorfer GmbH公司;磺胺嘧啶(批号:100026-201404,纯度:99.7%)、磺胺二甲嘧啶(批号:100411-200501,纯度:100%)来源于中国食品药品检定研究院;磺胺间甲氧嘧啶(批号:97864,纯度:99.1%)来源于曼哈格生物科技有限公司. 实际检测样品的信息如下:甘肃第 1 期王小乔,等:分散固相萃取-超高效液相色谱-串联质谱法测定海龙中11种磺胺类抗菌药物残留17中医药大学附属医院2批(批号:20141101);广西蓝正药业有限责任公司2批(批号:200901);甘肃陇脉药材有限公司1批(批号:190701).1.2 对照品溶液配制精密称取11种磺胺类药物对照品10.0 mg,分别置于10 mL棕色容量瓶中,加适量乙腈并超声使其溶解,取出放至室温,使用乙腈稀释至刻度,摇匀,得到质量浓度均为1 mg/mL的对照品储备溶液,于−18 ℃下保存备用. 分别准确吸取上述各对照品储备溶液0.025 mL,置于同一个25 mL的棕色容量瓶中,使用乙腈稀释至刻度,摇匀,得到质量浓度均为1 µg/mL的混合对照品中间溶液,于−18 ℃下保存备用. 再精密移取1.00 mL上述混合对照品中间溶液,置于10 mL棕色容量瓶,用乙腈稀释至刻度,摇匀,得到质量浓度均约为100 ng/mL的磺胺类药物混合对照品溶液,于4 ℃冷藏备用.1.3 供试品溶液配制取适量供试样品,粉碎,精密称取1 g,置于50 mL容量瓶中,加入2 mL水复原,静置20 min,精密加入10.00 mL 2%甲酸-乙腈,涡旋振荡2 min,超声处理20 min,再加入4 g硫酸钠和1 g氯化钠,涡旋振荡2 min,在3 900 r/min转速下离心15 min,精密移取上层清液2.00 mL置于EMR-Lipid dSPE 净化管,涡旋振荡1 min,在3 900 r/min转速下离心20 min,取上层清液,过0.22 µm有机滤膜,上机测定.1.4 色谱条件色谱柱:Waters ACQUITY BEH C18色谱柱(1.7µm,2.1 mm×100 mm). 流动相:A相为含2 mmol/L 乙酸铵和0.1%甲酸的水溶液,B相为含0.1%甲酸的乙腈溶液,梯度洗脱程序如下:0~1.0 min,15% B;1.0~2.0 min,30% B;2.0~3.0 min,40% B;3.0~4.5 min,90% B;4.5~6.5 min,90% B;6.5~7.0 min,15% B;7.0~8.0 min,15% B. 柱温:35 ℃;进样量:2 µL;流速:0.2 mL/min.1.5 质谱条件离子源:安捷伦喷射流电喷雾离子源(agilent jet stream electron spray ionization,AJS ESI);离子极性:正离子;采集模式:多反应监测模式(multiple reaction monitoring,MRM);气流温度:325 ℃;雾化气:N2;干燥气流速:6 L/min;雾化器压强:0.31 MPa;鞘气流速:10 L/min;鞘气温度:350 ℃;毛细管电压:4 000 V;各个磺胺类药物的质谱参数及保留时间如表1所列.2 结果与讨论2.1 前处理条件选择本试验检测的11种磺胺类药物在甲醇、乙腈中均能够很好的溶解,大多数被测目标化合物与乙腈极性接近,且乙腈能变性并沉淀蛋白,故选择乙腈作为海龙中磺胺类药物的提取溶剂. 由于磺胺类药物在酸性条件下容易发生质子化,酸化提取溶剂表 1 11种磺胺类药物的质谱参数及保留时间Table 1 Mass spectrum parameters and retention times of 11 sulfonamides序号名称保留时间/min母离子/(m/z)子离子/(m/z)碎裂电压/V碰撞能量/eV 1磺胺甲噁唑 4.665254.1156.0*/108.010010/25 2磺胺氯哒嗪 4.449285.0156.0*/108.010010/25 3磺胺间甲氧嘧啶 4.006281.1156.0*/108.010515/25 4磺胺甲基嘧啶 3.607265.1172.0/156.0*11012/15 5磺胺邻二甲氧嘧啶 4.585311.1156.0*/92.012015/30 6磺胺间二甲氧嘧啶 5.067311.0156.0*/108.013020/26 7磺胺二甲嘧啶 3.950279.1186.1*/156.112015/16 8磺胺嘧啶 2.902251.1156.0*/108.010010/22 9磺胺甲二唑 3.969271.0156.0*/108.09010/22 10磺胺噻唑 3.208256.0156.0*/108.010010/21 11磺胺甲氧哒嗪 4.287281.1156.0*/108.010515/25注:“*”为定量离子18分析测试技术与仪器第 29 卷有助于目标物的提取,本试验考察了在10 ng/mL 加标质量浓度下,乙腈、1%甲酸-乙腈、2%甲酸-乙腈、5%甲酸-乙腈的提取回收率和质谱响应. 结果发现,11种磺胺类药物在甲酸酸化乙腈中的质谱响应优于乙腈,当提取溶剂为2%甲酸-乙腈时,大部分磺胺类药物的质谱响应及提取回收率都优于其他甲酸酸化方案(如图1所示). 而EMR-Lipid dSPE 是一种新型分散固相萃取技术,常被用在QuEChERS 和蛋白质沉淀等净化流程中,可有效去除海龙中非极性的基质杂质,能够提高基质去除效率和分析重现性,与传统分散固相萃取相比,提高了净化效果.与固相萃取柱相比,净化流程简单快速,无繁琐的活化、上样、淋洗以及洗脱等步骤. 因此本试验最终采用2%甲酸-乙腈提取样品中目标待测物,EMR-Lipid dSPE 净化.2.2 色谱及质谱条件优化磺胺类药物含有相同的母体结构,在酸性条件下容易发生质子化,本试验选用了通用型超高效色谱柱Waters ACQUITY BEH C 18. 在正离子多反应监测采集模式下,流动相中加入适量甲酸能提高电喷雾源对目标检测物的离子化效率,加入适量乙酸铵能减少拖尾并改善峰形,因此选择在乙腈与水中加入不同浓度的甲酸、乙酸铵,考察峰形对称性、灵敏度与分离效果,寻找最佳流动相组合. 结果表明,在水相中加入2 mmol/L 乙酸铵和0.1%甲酸能显著改善峰形、提高灵敏度,在有机相中加入0.1%甲酸能提高离子化效果与重现性,稳定保留时间. 因此本试验采用含2 mmol/L 乙酸铵与0.1%甲酸的水溶液、含0.1%甲酸的乙腈作为流动相.分别将对照品溶液直接注入质谱仪,在正离子采集模式下,以全扫描(Scan )确定化合物准分子离子. 以选择离子扫描(Sim )确定碎裂电压,以子离子扫描(Product ion )确定丰度最高的2个子离子,分别作为定性离子与定量离子. 以多反应监测扫描(MRM )确定定性离子与定量离子的最佳碰撞能量,建立的仪器采集方法包含化合物名称、定性定量离子对、碰撞能量、碎裂电压以及驻留时间等.以多反应监测模式采集11种磺胺类抗菌药物的定性、定量离子对色谱图,其质量浓度为20ng/mL 的混合对照品溶液的提取离子流图如图2所示. 由图2可见,各个目标检测物监测通道互不干扰,所得采集峰形对称且响应良好.2.3 基质效应基质效应影响测定结果的准确性,因此,评价基质产生的基质效应十分有必要. 本试验通过考察同一浓度的空白样品基质溶液匹配混合对照品溶液,与2%甲酸-乙腈匹配混合对照品溶液质谱响应强度比值的百分比来评价基质效应(matrix effect ,ME ). ME 为(100±20)%,通常认为ME 弱. ME 大于120%或小于80%,分别为存在增强或抑制效应.如图3所示,磺胺间二甲氧嘧啶存在弱的增强效应,磺胺噻唑存在抑制效应,除此之外,其他目标检测物的基质效应较弱. 总体来说,基质效应不影响所有目标检测物的分析结果.2.4 方法学考察2.4.1 线性关系、检出限和定量限使用乙腈将混合对照品溶液依次稀释配制成质量浓度为0、1、2、5、10、20、30 ng/mL 的标准曲线磺胺间甲氧嘧啶磺胺甲噁唑回收率/%乙腈1% 甲酸乙腈2% 甲酸乙腈5% 甲酸乙腈140120100806040200磺胺氯哒嗪磺胺甲基嘧啶磺胺邻二甲氧嘧啶磺胺甲氧哒嗪磺胺间二甲氧嘧啶磺胺二甲嘧啶磺胺嘧啶磺胺甲二唑磺胺噻唑图1 不同提取溶剂的提取效率Fig. 1 Extraction efficiencies of different extraction solvents第 1 期王小乔,等:分散固相萃取-超高效液相色谱-串联质谱法测定海龙中11种磺胺类抗菌药物残留19溶液,外标法定量,以11种磺胺类药物的浓度(x )为横坐标,以定量离子的响应值(y )为纵坐标,绘制标准曲线. 取空白样品,逐级减少加入混合对照品溶液,按1.3项下方法处理样品,上机测定. 由提取的MRM 色谱图,以信噪比为3对应的质量浓度作为检出限(LOD ),以信噪比为10对应的质量浓度作为定量限(LOQ ),结果如表2所列. 由表2可见,11种目标检测物在0~30 ng/mL 的质量浓度范围内,线性关系良好,相关系数R 2不低于0.995 9,LOD 为0.11~1.30 µg/kg ,LOQ 为0.37~4.40 µg/kg.2.4.2 回收率与精密度选用海龙空白样品,分别添加20.0、50.0、100.0µg/kg 3个浓度水平的混合对照中间液,按照前处理方法处理后上机测定,每个浓度水平平行进行6次试验,每份待测样品溶液重复测定2次,计算回收率与精密度(RSD ),结果如表3所列. 由表3可见,11种目标待测物的平均回收率为62.5%~118.1%,RSD (n=6)为2.1%~9.2%,建立的分析方法完全能够满足实际样品的检测需求.2.5 样品测定采用本方法对5批海龙样品中11种磺胺类抗菌药物残留量进行测定,结果未检出目标检测物,如图4所示(其中1批阴性样品总离子流图),说明市售海龙中上述11种磺胺类抗菌药物残留在一定程度上处于低风险等级.3 结论本研究采用2%甲酸-乙腈作为提取溶剂,超声提取样品中目标待测物,EMR-Lipid dSPE 净化提取液,以正离子多反应监测模式检测,同时优化了液相色谱质谱检测条件,建立了一种超高效液相色谱质谱联用法同时测定海龙中11种磺胺类药物残留的分析方法,并进行了相关的方法学考察. 该方法具有线性关系良好、灵敏度高、准确度和精密度好的特点,且前处理简单、净化高效. 基质效应评价显示,虽然目标检测物存在不同程度的基质效应,但磺胺甲噁唑磺胺氯哒嗪磺胺间甲氧嘧啶磺胺甲基嘧啶磺胺邻二甲氧嘧啶磺胺甲氧哒嗪磺胺间二甲氧嘧啶磺胺二甲嘧啶磺胺嘧啶磺胺甲二唑磺胺噻唑2.0×103C o u n t s1.8×1031.6×1031.4×1031.2×1031.0×1030.8×1030.6×1030.4×1030.2×10300.81.2 1.62.0 2.4 2.83.23.64.44.0Acquisition time/min4.85.2 5.66.0 6.4 6.87.27.68.0图2 11种磺胺类药物对照品提取离子流图Fig. 2 Extract ion chromatogram of 11 standards of sulfonamides磺胺间甲氧嘧啶磺胺甲噁唑回收率/%140120100806040200磺胺氯哒嗪磺胺甲基嘧啶磺胺邻二甲氧嘧啶磺胺甲氧哒嗪磺胺间二甲氧嘧啶磺胺二甲嘧啶磺胺嘧啶磺胺甲二唑磺胺噻唑图3 11种磺胺类药物的基质效应Fig. 3 Matrix effects of 11 sulfonamides20分析测试技术与仪器第 29 卷不影响分析结果,符合准确定性定量要求. 该方法适用于海龙中磺胺类抗菌药物残留量的检测,可为监控海龙中磺胺类抗菌药物残留风险提供技术支撑.参考文献:国家药典委员会. 中华人民共和国药典(一部2020版)[M ]. 北京: 中国医药科技出版社, 2020:306.[ 1 ]吴伟建, 王燕, 王斌, 等. 基于聚类、主成分和判别分析的海龙红外指纹图谱研究[J ]. 中国药学杂志,2013,48(18):1540-1545. [WU Weijian, WANG Yan, WANG Bin, et al. Infrared fingerprint analysis of syngnathus' s ethanol extracts coupled with cluster[ 2 ]表 2 11种磺胺类药物的线性关系、检出限、定量限Table 2 Linear relationship, LOD, LOQ of 11 sulfonamides序号名称线性方程R2线性范围/(ng/mL )LOD/(µg/kg )LOQ/(µg/kg )1磺胺甲噁唑y =139.010x +28.158 70.995 90~300.48 1.602磺胺氯哒嗪y =107.763x +15.615 70.997 90~300.84 2.803磺胺间甲氧嘧啶y =171.804x +35.380 50.998 50~300.73 2.404磺胺甲基嘧啶y =141.980x +7.901 70.998 60~30 1.30 4.405磺胺邻二甲氧嘧啶y =1 038.220x +227.398 00.998 10~300.110.376磺胺间二甲氧嘧啶y =596.926x +90.787 30.998 60~300.38 1.307磺胺二甲嘧啶y =325.171x +42.142 30.998 80~300.170.548磺胺嘧啶y =146.238x +62.685 00.999 20~300.220.709磺胺甲二唑y =120.498x -3.966 50.999 20~300.95 3.1010磺胺噻唑y =117.540x +14.036 40.999 50~300.320.9711磺胺甲氧哒嗪y =165.395x+35.380 50.998 50~300.762.50表 3 11种磺胺类药物的回收率及RSD (n=6)Table 3 Recoveries and RSD of 11 sulfonamides 序号名称20.0 µg/kg50.0 µg/kg100.0 µg/kg加入质量/ng测得质量/ng 平均回收率/%RSD/%加入质量/ng 测得质量/ng 平均回收率/%RSD/%加入质量/ng测得质量/ng 平均回收率/%RSD/%1磺胺甲噁唑20.6814.0868.19.251.7038.8875.2 4.3103.4082.4179.7 3.92磺胺氯哒嗪21.7618.5485.27.754.4046.5185.5 3.7108.8088.4581.3 2.63磺胺间甲氧嘧啶21.6816.1974.7 4.854.2052.4696.8 3.2108.4083.3676.9 2.44磺胺甲基嘧啶22.0416.1873.4 3.855.1042.8777.8 6.9110.2085.0777.2 6.55磺胺邻二甲氧嘧啶21.7018.4485.0 2.754.2547.6887.9 3.0108.5098.0890.4 2.66磺胺间二甲氧嘧啶21.8219.7290.4 4.554.5555.80102.3 2.5109.10118.05108.22.17磺胺二甲嘧啶21.7621.3798.23.054.4053.9199.1 3.4108.80108.0499.3 2.88磺胺嘧啶22.7617.4176.5 4.656.9045.6380.2 5.8113.8084.8974.6 4.39磺胺甲二唑21.0024.42116.3 6.952.5062.00118.1 3.1105.00116.02110.54.710磺胺噻唑20.8013.0062.55.052.0032.9263.3 4.9104.0065.2162.76.011磺胺甲氧哒嗪22.5215.6769.64.356.3037.1065.92.9112.6074.6566.34.31.2×1031.0×1030.8×1030.6×1030.4×1030.2×1031.02.03.04.0Acquisition time/minC o u n t s5.06.07.08.0图4 阴性样品总离子流图Fig. 4 Total ion chromatogram of negative sample第 1 期王小乔,等:分散固相萃取-超高效液相色谱-串联质谱法测定海龙中11种磺胺类抗菌药物残留21analysis, principal component analysis and discrimin-ant analysis [J ]. Chinese Pharmaceutical Journal ,2013,48 (18):1540-1545.]王梦月, 韦静斐, 史海明, 等. 海龙药材及其伪品的HPLC 指纹图谱研究[J ]. 中国药学杂志,2009,44(24):1847-1851. [WANG Mengyue, WEI Jingfei,SHI Haiming, et al. Study on HPLC fingerprint of syn-gnathus and its adulterants [J ]. Chinese Pharmaceutic-al Journal ,2009,44 (24):1847-1851.][ 3 ]蒋超, 袁媛, 李军德, 等. 尖海龙商品调查与《中国药典》中海龙的基原考证[J ]. 中国实验方剂学杂志,2019,25(17):104-112. [JIANG Chao, YUAN Yuan,LI Junde, et al. Syngnathus acus in herbal markets and zoological origin of syngnathus in China pharmaco-poeia [J ]. Chinese Journal of Experimental Traditional Medical Formulae ,2019,25 (17):104-112.][ 4 ]颜洁. 海龙质量标准的定性研究[D ]. 青岛: 中国海洋大学, 2014. [YAN Jie. Qualitative studies on qual-ity control of sea dragon [D ]. Qingdao: Ocean Uni-versity of China, 2014.][ 5 ]丁晴, 仇雅静, 房克慧, 等. 动物来源中药材、饮片质量现状及原因分析[J ]. 中国中药杂志,2015,40(21):4309-4312. [DING Qing, QIU Yajing, FANG Kehui, et al. Animal drugs quality status and reason analysis [J ]. China Journal of Chinese Materia Medica ,2015,40 (21):4309-4312.][ 6 ]许晓辉, 李剑勇, 朱仁愿, 等. 动物源性中药材兽药残留检测技术研究进展[J ]. 中国兽药杂志,2021,55(6):45-52. [XU Xiaohui, LI Jianyong, ZHU Reny-uan, et al. Research progress of detecting technology of veterinary drug residues in animal-derived Chinese medicinal materials [J ]. Chinese Journal of Veterinary Drug ,2021,55 (6):45-52.][ 7 ]唐溱. 我国动物相关法律法规对动物类中药材利用与发展的影响[J ]. 中草药,2021,52(24):7718-7727. [TANG Zhen. Influence of legislations related to animal on utilization and development of animal tra-ditional Chinese medicine in China [J ]. Chinese Tradi-tional and Herbal Drugs ,2021,52 (24):7718-7727.][ 8 ]缪苗, 黄一心, 沈建, 等. 水产品安全风险危害因素来源的分析研究[J ]. 食品安全质量检测学报,2018,9(19):5195-5201. [MIAO Miao, HUANG Yixin,SHEN Jian, et al. Analysis and research on hazard sour-ces of aquatic products [J ]. Journal of Food Safety &Quality ,2018,9 (19):5195-5201.][ 9 ]陈胜军, 李来好, 杨贤庆, 等. 我国水产品安全风险来源与风险评估研究进展[J ]. 食品科学,2015,36(17):300-304. [CHEN Shengjun, LI Laihao,YANG Xianqing, et al. Progress in the risk sources and assessment of aquatic products in China [J ]. Food Science ,2015,36 (17):300-304.][ 10 ]周瑞铮, 陈锦杭, 张树权, 等. 分散固相萃取结合液相色谱-串联质谱法测定淡水鱼中9种磺胺类和3种喹诺酮类药物残留量[J ]. 食品安全质量检测学报,2021,12(17):6946-6952. [ZHOU Ruizheng, CHEN Jinhang, ZHANG Shuquan, et al. Determination of 9kinds of sulfonamides and 3 kinds of quinolones residues in freshwater fish by dispersive solid phase extraction coupled with liquid chromatography-tan-dem mass spectrometry [J ]. Journal of Food Safety &Quality ,2021,12 (17):6946-6952.][ 11 ]覃玲, 董亚蕾, 王钢力, 等. 分散固相萃取-液相色谱-串联质谱法测定常见动物源性食品中42种兽药残留[J ]. 色谱,2018,36(9):880-888. [QIN Ling,DONG Yalei, WANG Gangli, et al. Determination of 42 veterinary drug residues in common animal de-rived food by dispersive solid phase extraction coupled with liquid chromatography-tandem mass spectro-metry [J ]. Chinese Journal of Chromatography ,2018,36 (9):880-888.][ 12 ]余丽梅, 张聪, 宋超, 等. 分散固相萃取-超高液相色谱/质谱测定水产品中磺胺类抗生素的残留量[J ].中国农学通报,2017,33(26):128-135. [YU Limei,ZHANG Cong, SONG Chao, et al. Dispersive solid-phase extraction-ultra performance liquid chromato-graphy tandem mass spectrometry detecting sulfonam-ides residues in aquatic products [J ]. Chinese Agricul-tural Science Bulletin ,2017,33 (26):128-135.][ 13 ]张蓓蓓, 孙慧婧, 吉鑫. 混合型离子交换反相吸附固相萃取柱串联萃取-超高效液相色谱-串联质谱法测定地表水中32种抗生素的含量[J ]. 理化检验-化学分册,2022,58(8):893-901. [ZHANG Beibei, SUN Huijing, JI Xin. Determination of 32 antibiotics in sur-face water by ultra-high performance liquid chromato-graphy-tandem mass spectrometry after tandem extrac-tion with mixed ion exchange reversed phase adsorp-tion solid phase extraction columns [J ]. Physical Test-ing and Chemical Analysis (Part B:Chemical Analysis),2022,58 (8):893-901.][ 14 ]22分析测试技术与仪器第 29 卷。
浅析电感耦合等离子体原子发射光谱仪最新进展
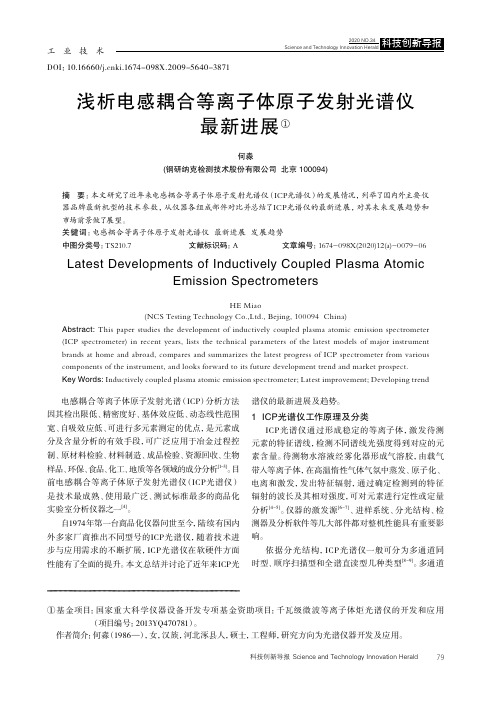
DOI:10.16660/ki.1674-098X.2009-5640-3871浅析电感耦合等离子体原子发射光谱仪最新进展①何淼(钢研纳克检测技术股份有限公司 北京 100094)摘 要:本文研究了近年来电感耦合等离子体原子发射光谱仪(ICP光谱仪)的发展情况,列举了国内外主要仪器品牌最新机型的技术参数,从仪器各组成部件对比并总结了ICP光谱仪的最新进展,对其未来发展趋势和市场前景做了展望。
关键词:电感耦合等离子体原子发射光谱仪 最新进展 发展趋势中图分类号:TS210.7 文献标识码:A 文章编号:1674-098X(2020)12(a)-0079-06Latest Developments of Inductively Coupled Plasma AtomicEmission SpectrometersHE Miao(NCS Testing Technology Co.,Ltd., Bejing, 100094 China)Abstract: This paper studies the development of inductively coupled plasma atomic emission spectrometer (ICP spectrometer) in recent years, lists the technical parameters of the latest models of major instrument brands at home and abroad, compares and summarizes the latest progress of ICP spectrometer from various components of the instrument, and looks forward to its future development trend and market prospect.Key Words: Inductively coupled plasma atomic emission spectrometer; Latest improvement; Developing trend①基金项目:国家重大科学仪器设备开发专项基金资助项目:千瓦级微波等离子体炬光谱仪的开发和应用(项目编号:2013YQ470781)。
放射性核素γ能谱相关标准分析
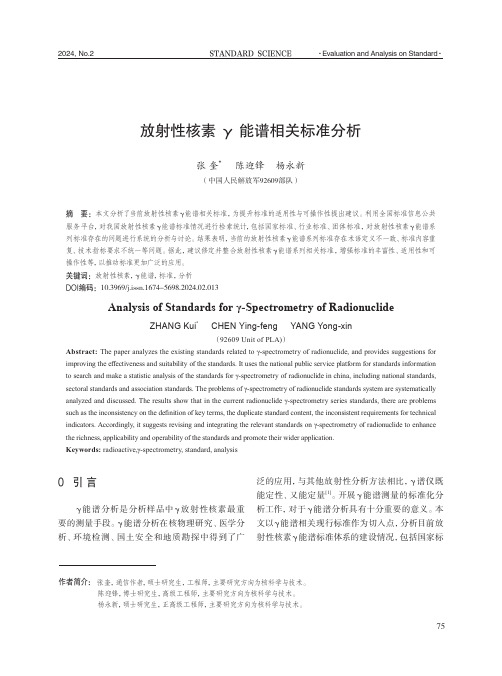
放射性核素γ能谱相关标准分析张 奎* 陈迎锋 杨永新(中国人民解放军92609部队)摘 要:本文分析了当前放射性核素γ能谱相关标准,为提升标准的适用性与可操作性提出建议。
利用全国标准信息公共服务平台,对我国放射性核素γ能谱标准情况进行检索统计,包括国家标准、行业标准、团体标准,对放射性核素γ能谱系列标准存在的问题进行系统的分析与讨论。
结果表明,当前的放射性核素γ能谱系列标准存在术语定义不一致、标准内容重复、技术指标要求不统一等问题。
据此,建议修定并整合放射性核素γ能谱系列相关标准,增强标准的丰富性、适用性和可操作性等,以推动标准更加广泛的应用。
关键词:放射性核素,γ能谱,标准,分析 DOI编码:10.3969/j.issn.1674-5698.2024.02.013Analysis of Standards for γ-Spectrometry of RadionuclideZHANG Kui * CHEN Ying-feng YANG Yong-xin(92609 Unit of PLA))Abstract: The paper analyzes the existing standards related to γ-spectrometry of radionuclide, and provides suggestions for improving the effectiveness and suitability of the standards. It uses the national public service platform for standards information to search and make a statistic analysis of the standards for γ-spectrometry of radionuclide in china, including national standards, sectoral standards and association standards. The problems of γ-spectrometry of radionuclide standards system are systematically analyzed and discussed. The results show that in the current radionuclide γ-spectrometry series standards, there are problems such as the inconsistency on the definition of key terms, the duplicate standard content, the inconsistent requirements for technical indicators. Accordingly, it suggests revising and integrating the relevant standards on γ-spectrometry of radionuclide to enhance the richness, applicability and operability of the standards and promote their wider application.Keywords: radioactive,γ-spectrometry, standard, analysis作者简介:张奎,通信作者,硕士研究生,工程师,主要研究方向为核科学与技术。
微波辅助提取-原子吸收光谱法测定食品中铅、镉、锰、锌
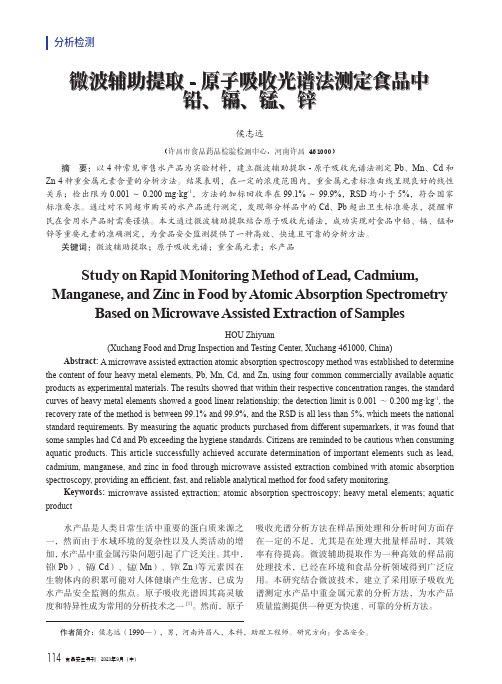
分析检测微波辅助提取-原子吸收光谱法测定食品中铅、镉、锰、锌侯志远(许昌市食品药品检验检测中心,河南许昌 461000)摘 要:以4种常见市售水产品为实验材料,建立微波辅助提取-原子吸收光谱法测定Pb、Mn、Cd和Zn 4种重金属元素含量的分析方法。
结果表明,在一定的浓度范围内,重金属元素标准曲线呈现良好的线性关系;检出限为0.001~0.200 mg·kg-1,方法的加标回收率在99.1%~99.9%,RSD均小于5%,符合国家标准要求。
通过对不同超市购买的水产品进行测定,发现部分样品中的Cd、Pb超出卫生标准要求,提醒市民在食用水产品时需要谨慎。
本文通过微波辅助提取结合原子吸收光谱法,成功实现对食品中铅、镉、锰和锌等重要元素的准确测定,为食品安全监测提供了一种高效、快速且可靠的分析方法。
关键词:微波辅助提取;原子吸收光谱;重金属元素;水产品Study on Rapid Monitoring Method of Lead, Cadmium, Manganese, and Zinc in Food by Atomic Absorption Spectrometry Based on Microwave Assisted Extraction of SamplesHOU Zhiyuan(Xuchang Food and Drug Inspection and Testing Center, Xuchang 461000, China) Abstract: A microwave assisted extraction atomic absorption spectroscopy method was established to determine the content of four heavy metal elements, Pb, Mn, Cd, and Zn, using four common commercially available aquatic products as experimental materials. The results showed that within their respective concentration ranges, the standard curves of heavy metal elements showed a good linear relationship; the detection limit is 0.001~0.200 mg·kg-1, the recovery rate of the method is between 99.1% and 99.9%, and the RSD is all less than 5%, which meets the national standard requirements. By measuring the aquatic products purchased from different supermarkets, it was found that some samples had Cd and Pb exceeding the hygiene standards. Citizens are reminded to be cautious when consuming aquatic products. This article successfully achieved accurate determination of important elements such as lead, cadmium, manganese, and zinc in food through microwave assisted extraction combined with atomic absorption spectroscopy, providing an efficient, fast, and reliable analytical method for food safety monitoring.Keywords: microwave assisted extraction; atomic absorption spectroscopy; heavy metal elements; aquatic product水产品是人类日常生活中重要的蛋白质来源之一,然而由于水域环境的复杂性以及人类活动的增加,水产品中重金属污染问题引起了广泛关注。
简版SpectRx近红外光谱系统课件

Incoming Inspection and Verifications 输入检验和确认
In-process Inspection
在线检测
Maintains Consistent Quality
保持品质一致性
Accuracy ( Concentration ): 0.01% 含量精确度:万分之一
Active Ingredients 活性成分 Impurities 杂质 Concentration 浓度 Homogeneity 同质性
简版SpectRx近红外光谱系统课件
6
SpectRx™ NIR
Tablets/Capsules
Diffused Reflectance 漫反射系数 Tablet Hardness 硬度 Tablet Disintegration 崩解 Tablet/Capsule Moisture Contents 湿度含量 Tablet Friability 脆性 Tablet Capsule Active Ingredients 活性组分 Tablet/Capsule Impurities 杂质 Tablet Cracks 裂纹 Capsule Deterioration 变质 Tablet/Capsule Homogeneity 药片-胶囊同质性
库), 95%的机会可以发现一个未知的成分,除非组成的信号信噪比太小。
简版SpectRx近红外光谱系统课件
10
On Line Production 在线生产 (Including raw materials release 包括原材料放行)
简版SpectRx近红外光谱系统课件
11
Rotary Inspection in Lab (实验室旋转式检测)
MJD350 300V PNP高电压传输器TO252(DPAK)商品说明书
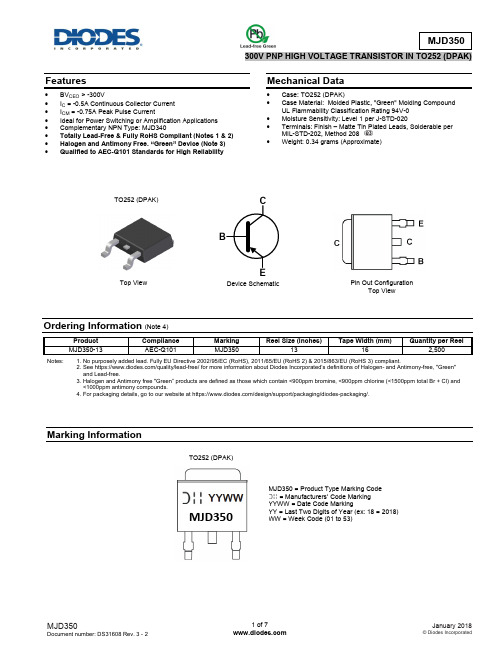
Features∙ BV CEO > -300V∙ I C = -0.5A Continuous Collector Current ∙ I CM = -0.75A Peak Pulse Current∙ Ideal for Power Switching or Amplification Applications ∙ Complementary NPN Type: MJD340∙ Totally Lead-Free & Fully RoHS Compliant (Notes 1 & 2) ∙ Halogen and Antimony Free. “Green” Device (Note 3) ∙ Qualified to AEC-Q101 Standards for High ReliabilityMechanical Data∙ Case: TO252 (DPAK)∙ Case Material: Molded Plastic, "Green" Molding Compound UL Flammability Classification Rating 94V-0 ∙ Moisture Sensitivity: Level 1 per J-STD-020∙ Terminals: Finish – Matte Tin Plated Leads, Solderable per MIL-STD-202, Method 208 ∙Weight: 0.34 grams (Approximate)Ordering Information (Note 4)Notes: 1. No purposely added lead. Fully EU Directive 2002/95/EC (RoHS), 2011/65/EU (RoHS 2) & 2015/863/EU (RoHS 3) compliant.2. See https:///quality/lead-free/ for more information about Diodes Incorporated’s definitions of Hal ogen- and Antimony-free, "Green" and Lead-free.3. Halogen and Antimony free "Green” products are defined as those which contain <900ppm bromine, <900ppm chlorine (<1500ppm tot al Br + Cl) and <1000ppm antimony compounds.4. For packaging details, go to our website at https:///design/support/packaging/diodes-packaging/.Marking InformationTop ViewDevice SchematicPin Out ConfigurationTop ViewMJD350 = Product Type Marking Code = Manufacturers’ Code Marking YYWW = Date Code MarkingYY = Last Two Digits of Year (ex: 18 = 2018) WW = Week Code (01 to 53)TO252 (DPAK)TO252 (DPAK)MJD350Thermal Characteristics(@T A = +25°C, unless otherwise specified.)Notes: 5. For a device mounted on FR-4 PCB with minimum recommended pad layout.6. Refer to JEDEC specification JESD22-A114 and JESD22-A115.Thermal Characteristics and Derating InformationElectrical Characteristics(@T A = +25°C, unless otherwise specified.)Note: 7. Measured under pulsed conditions. Pulse width ≤300μs. Duty cycle ≤2%.Typical Electrical Characteristics(@T A = +25°C, unless otherwise specified.)Package Outline DimensionsPlease see /package-outlines.html for the latest version.TO252 (DPAK)Suggested Pad LayoutPlease see /package-outlines.html for the latest version.TO252 (DPAK)Note:For high voltage applications, the appropriate industry sector guidelines should be considered with regards to creepage and clearance distances between device Terminals and PCB tracking.7°±1°Seating Plane。
!Emission spectroscopy and energy transfer in Tm3+, Tm3+-Ho3+ and Tm3+-Yb3+ doped tellurite fibers

Emission spectroscopy and energy transfer in Tm3+, Tm3+-Ho3+ andTm3+-Yb3+ doped tellurite fibersBilly Richards*a, Shaoxiong Shen a, Animesh Jha aa Institute for Materials Research, Houldsworth Building, University of Leeds, Clarendon Road,Leeds, LS2 9JTABSTRACTThis paper examines the steady state and time resolved emission spectroscopy of Tm3+ doped and Tm3+-Ho3+, Tm3+-Yb3+ co-doped tellurite fibers for mid-IR fiber laser design which find applications for lidar. These doped fibers show promising properties for compact and tunable laser sources in the visible and mid-IR when pumped at 800 nm, 980 nm and 1480 nm which can be used for remote chemical sensing and atmospheric monitoring. Tellurite glass has a lower cut-off phonon energy than silica glass and is more environmentally stable than fluoride glass, and coupling these properties with its high rare-earth ion solubility and high refractive index make this glass a very interesting material in which to study the fluorescence properties of these rare earth ions. We have measured the mid-IR fluorescence properties in varying lengths of multi-mode and single-mode fiber for the 3H4-3H6 (~1.85 µm), 3H4-3F4 (~1.46 µm) transitions in Tm3+ and the 5I7-5I8 (~2.05 µm) transition in Ho3+. We have also measured the visible emission from these fibers due to excited state absorption (ESA) as there is blue and green emission in Tm3+ and Tm3+-Ho3+ doped fibers respectively when pumped at 800 nm, and strong red and blue emission in the Tm3+-Yb3+ when pumped at 980 nm. These results in fiber are compared to bulk glass results and are used to describe the pumping schemes and energy transfer mechanisms of these rare earth ions in tellurite fiber.Keywords: Tellurite, fiber, fluorescence, thulium, holmium, ytterbium, laser1. INTRODUCTIONTellurite glass has several properties which make it an interesting candidate for mid-IR fiber laser design and use in other optical devices. The transmission range of tellurium based glasses extends into the mid infrared up to around 5 µm and has a low cut-off phonon energy of around 800cm-1.1 Both of these properties compare well against other oxide based glasses such as silicates. This feature, coupled with the high rare earth ion solubility, makes tellurium oxide based glass a good host within which to examine the longer wavelength transitions of thulium and holmium ions. Tellurite glass has a high refractive index which increases the absorption and emission cross-sections2, good optical and mechanical properties and is relatively easy to manufacture having a low melting point.Mid-IR fiber lasers in the range 1.8-2.9 µm and beyond have various potential applications including eye-safe laser lidar and remote chemical sensing, including the characterization of CO2 and OH which both have very strong absorption bands in the mid-IR. The strong absorption from water in this region makes these compact laser sources attractive for medical surgery applications. There are many reports of thulium doped silica fiber lasers operating at around 2 µm3-7, and of thulium and thulium/holmium co-doped fluoride based fiber lasers operating in the 1.8 to 2.9 µm region.8-12 There are however very few reports of tellurite fiber lasers operating in the longer wavelength end of this range. The development of these lasers in tellurite fiber is desirable due to the fact that the glass is much more stable and resistant to corrosion than fluoride glass leading to fewer design and operation problems.13The thulium ions can be pumped directly into the 3H4 level with an 800 nm source, and will show emission at around 1.46 µm (3H4-3F4) and 1.8 µm (3F4-3H6). Holmium cannot be pumped directly with an 800 nm source due to the lack of an appropriate absorption band. In a thulium-holmium co-doped glass, the 3H4 thulium level is populated by the 800 nm pump source and a quenching mechanism transfers energy to the 5I7 holmium level which then radiatively depopulates to the holmium ground state (5I8) giving rise to emission at around 2.0 µm.14*prebdor@; phone +44(0)113 3432352; fax +44(0)1133432384Lidar Technologies, Techniques, and Measurements for Atmospheric Remote Sensing II,edited by Upendra N. Singh, Proc. of SPIE Vol. 6367, 63670G, (2006)0277-786X/06/$15 · doi: 10.1117/12.689619Thulium and holmium can also be co-doped with ytterbium which acts as an effective sensitizer. The ytterbium ions can be pumped with a 980 nm source into the 2F5/2 level which can then through energy transfer mechanisms, populate the thulium 3H5 and holmium 5I6 levels. The absorption band of ytterbium at 980 nm makes it very convenient for use with readily available diode lasers, and because of the ytterbium ion energy level structure there is no possibility of excited state absorption making ytterbium co-doped devices more efficient. 15This paper details the results of the emission spectroscopy of Tm3+, Ho3+ and Yb3+ doped tellurite fiber and also Tm3+ doped silica fiber in the infrared and visible regions and uses these results to explain the pumping schemes and energy transfer processes within these rare-earth ion dopants.2. EXPERIMENTALThe tellurite fibers were manufactured from glass with the composition 78TeO2-12ZnO-10Na2O (mol%) for the core and 75TeO2-15ZnO-10Na2O (mol%) for the cladding. Tm2O3 (0.5 wt%) doped, Tm2O3-Ho2O3 (0.5-1.0 wt%) codoped and Tm2O3-Yb2O3 (0.5-1.0 wt%) codoped fibers were investigated. The starting chemicals for the core and cladding glasses were weighed, ground, mixed and then placed into gold crucibles and melted in separate electric tube furnaces in a dry O2 atmosphere. The powders are initially dried overnight and then melted at 820ºC. The mixtures were stirred after 1 hour with a gold rod and then homogenized at 720ºC for 2 hours. The fiber preform is cast using the suction method into a preheated brass mould and then annealed at 300ºC for 3 hours, after which it is allowed to cool slowly in the furnace to room temperature. The preforms are extruded into structured rods which can then be further extruded with cladding tubes made by the rotation method to reduce the core diameter. Fiber is drawn at each stage providing a range of fibers with varying core diameter. The silica fiber tested was bought from the National Optics Institute, (INO, Canada), and was a 10 m Tm3+ doped double-clad fiber with a D-shaped inner cladding and a 20 µm core diameter.The infrared fluorescence spectra of the samples were measured using an FLS920 Steady State and Time Resolved Fluorescence Spectrometer (Edinburgh Instruments). The spectrometer uses an indium gallium arsenide (InGaAs) detector for measurements in the wavelength range 1.3-2.2 µm. Visible measurements were made using a cooled photomultiplier tube in the range 400-850 nm. The samples were pumped with either a 5 W 808 nm, or a 250 mW 976 nm laser diode. The excitation radiation was launched into the fibers using a 40× microscope objective and the emitted fluorescence was collected and delivered to the spectrometer using a commercial silica fiber which was butt-coupled to the end of the test fiber. Steady-state spectra were measured using a mechanical chopper and lock-in amplifier. Lifetime measurements were made by modulating the laser diode current supply with an external function generator and capturing the fluorescence decay using a digital oscilloscope (Tektronics, TDS3012). All experiments were carried out at room temperature. The spectra were measured with an 800 nm filter placed between the sample and the detector. This was required to attenuate the high intensity pump light reaching the detector which had not been fully absorbed by the test fiber. This was necessary because without the filter, the spectra showed a large sharp peak at 1.6 µm which is the first harmonic of the pump laser. Spectra were taken with and without the filter and then normalized. Although the filter did slightly reduce the intensity of the entire spectrum, the shapes of the peaks were not altered, and the peak at 1.6 µm was removed.3. RESULTS AND DISCUSSIONS3.1. Infrared emission spectroscopy of Tm3+ doped tellurite fiberFigure 1 shows the emission spectra of a range of lengths of multi-mode Tm3+ doped tellurite fiber with a core diameter of approximately 12 µm when pumped with an 808 nm laser diode source. The doping concentration in this fiber is 0.5 wt%. The spectra have been normalized with respect to the peak at ~1.86 µm which is attributed to 3F4→3H6 transition of Tm3+. As the length of the fiber increases, the peak shifts to longer wavelengths due to ground-state absorption and re-emission of light within these two energy levels. The figure also compares the fiber emission spectra to that of the bulk glass sample with the same doping concentration. The 3F4→3H6 peaks measured in the fiber samples are narrower than the bulk glass sample and also shifted to longer wavelengths. In fiber the peak at 1.46 µm due to the 3H→3F4 transition is lower in intensity relative to the ~1.86 µm peak than in the bulk sample showing that the 4confinements of the fiber geometry are increasing the energy transfer efficiency between Tm 3+ ions. The emission spectra of different lengths of single-mode Tm 3+ doped tellurite fiber were also measured which showed the same trends as for the multi-mode fibers.The measured fluorescence lifetime from the 3F 4 Tm 3+ level was 1633 µs in bulk glass and increased from 1941 µs to 2290 µs in increasing lengths of singly doped multi-mode fiber. This increase in lifetime is due to the 3F 4 population increasing due to the enhanced energy transfer in longer fibers.140015001600170018001900200021000.00.20.40.60.81.065mm 100mm 137mm 172mm 210mm BulkN o r m a l i s e d i n t e n s i t y (a .u .)Wavelength (nm)Fig. 1. The emission spectra of 0.5 wt% Tm 3+ doped tellurite fiber (core diameter = 12µm) and bulk glass when excited withan 808 nm laser source. The spectra have been normalized with respect to the peak at ~1.86 µm.3.2. Infrared emission spectroscopy of Tm 3+-Ho 3+ codoped tellurite fiberFigure 2 shows the emission spectra of varying lengths of 0.5 wt% Tm 3+ and 1.0 wt% Ho 3+ codoped telluritefiber which are compared to the bulk glass sample of the same dopant concentration when excited with the same 808 nm laser source. The fibers are multi-mode with a core diameter of approximately 12 µm. The spectra have been normalized with respect to the peak at ~2.0 µm which is attributed to the 5I 7→5I 8 Ho 3+ transition. The spectra show that the shape of the emission peak from this transition is different when measured in fiber to bulk glass. In fiber the peak is narrowed and shifted to longer wavelengths and also appears as a single peak instead of a double peak in bulk glass. Ground state absorption and re-emission in the Ho 3+ ions due to the increased path length and energy confinement in the fiber results in emission only from the longer wavelength levels in the 5I 7 manifold. The peak at 1.46 µm and shoulder at ~1.86 µm are due to the 3H 4→3F 4 and 3F 4→3H 6 transitions respectively in Tm 3+. The intensities of these peaks relative to the ~2.0 µm peak are much lower in fiber than bulk glass. Ho 3+ ions cannot be directly excited with an 808 nm source which means that energy must be transferred to Ho 3+ from the Tm 3+ ions. The reduction in intensity of the Tm 3+ peaks in figure 3 shows that the energy transfer processes from Tm 3+ to Ho 3+ are becoming more efficient in fiber over bulk glass. The intensity of the peak at 1.46 µm increases with fiber length suggesting a back energy transfer process from Ho 3+ to Tm 3+ ions due to the upconversion in Ho 3+ which increases in longer fibers. This also suggests that for operation at ~2.0 µm in this fiber, shorter lengths are preferable. In single-mode geometries of this fiber the spectra shape are similar but with smaller 1.46 µm peak intensities confirming that the increased energy confinement in the fiber geometry is increasing the energy transfer from the 3H 4→3F 4 Tm 3+ transition to the 5I 8→5I 7 Ho 3+ transition.The measured fluorescence lifetime of the 5I 7 Ho 3+ level is 3148 µs in bulk glass and 4200-4500 µs in multi-mode fiber but does not appear to vary with fiber length. The increase in lifetime in fiber over bulk glass further confirms the increased energy transfer to the 5I 7 level in the fiber geometry.1400150016001700180019002000210022000.00.20.40.60.81.061mm 120mm 176mm 226mm 279mm BulkN o r m a l i s e d i n t e n s i t y (a .u .)Wavelength (nm)Fig. 2. The emission spectra of 0.5 wt% Tm 3+-1.0 wt% Ho 3+ codoped tellurite fiber (core diameter = 12µm) and bulk glasswhen excited with an 808 nm laser source. The spectra have been normalized with respect to the peak at ~2.0 µm.N o r m a l i s e d i n t e n s i t y (a .u .)Wavelength (nm)Fig. 3. The emission spectra of 0.5 wt% Tm 3+-1.0 wt% Yb 3+ codoped tellurite fiber (core diameter = 12µm) when excitedwith an 978 nm laser source. The spectra have been normalized with respect to the peak at ~1.86 µm.3.3. Infrared emission of Tm 3+-Yb 3+ codoped tellurite fiberFigure 3 shows the emission spectra of 0.5 wt% Tm 3+ and 1.0 wt% Yb 3+ codoped fiber of varying length with acore diameter of approximately 12 µm. The use of Yb 3+ as a sensitizer in this fiber allowed the use of a 978 nm pump source to populate the 3H 5 level of Tm 3+ through energy transfer from Yb 3+. Yb 3+ has an absorption peak centered close to 978 nm due to the 2F 7/2→2F 5/2 transition which is more intense than the Tm 3+ absorption peaks making this an efficient pumping scheme.2 Coupling of this pump source into the fiber was much improved over the 808 nm source dueto the single-mode nature of the pump diode output. The emission spectra have been normalized with respect to the peak from the 3F 4→3H 6 Tm 3+ transition. The results again show a narrowing and shifting to longer wavelengths with increasing fiber length. The peak at ~1.5 µm is from the 3H 4→3F 4 transition of Tm 3+ which must be caused by upconversion in Tm 3+ due to the fact that energy transfer from Yb 3+ populates the lower lying 3H 5 level of Tm 3+. The intensity of this peak is lower in shorter lengths of fiber and in the single-mode geometry. The lifetime of the 3F 4 level in this fiber was generally slightly shorter than in the pure Tm 3+ doped fiber due to the increased upconversion, and did not seem to vary with length.3.4. Visible upconversion in Tm 3+, Tm 3+-Ho 3+ and Tm 3+-Yb 3+ dope tellurite fibersStrong visible emission due to upconversion was observed in all these fibers, so to further understand thefluorescence and energy transfer mechanisms, the visible emission spectra were also measured at room temperature using the same pump sources as for the infrared measurements. These results can be found in figure 4 and are all measured using single-mode fiber. Graphs (a) and (b) do not show the emission at 800 nm from the 3H 4 level of Tm 3+ because a filter had to be used to attenuate the unabsorbed pump light which was saturating the detector. This emission can be seen in graph (c) however as the pump (at 978 nm) was out of the detection range thus not requiring the filter. The Tm 3+ doped fiber shows weak blue emission at 480 nm due to the 1G 4→3H 6 transition arising from a two pump photon excited state absorption (ESA) process. In graph (a) there also appears to be emission at 525 and 550 nm but this is not due to the Tm 3+ ions. These peaks have been seen in other fibers, (including undoped ones), and is believed to be due to some possible contamination arising during the fiber fabrication process. The intensity of the blue emission in the Tm 3+ doped fiber is extremely low compared to the emission in the other two fibers which is why these contamination peaks are visible in this spectrum but not in the other two. The Tm 3+-Ho 3+ doped fiber shows additional emission at 550 nm which is due to the (5S 2, 5F 4)→5I 8 Ho 3+ transition. In this fiber the blue and green emission lines are significantly narrowed which suggests superfluorescence and promise for visible laser operation. The Tm 3+-Yb 3+ codoped fiber shows strong emission at 480 and 650 and 800 nm due to the 1G 4→3H 6, 1G 4→3F 4 and 3H 4→3H 6 Tm 3+ transitions respectively.N o r m a l i s e d i n t e n s i t y (a u )Wavelength (nm)N o r m a l i s e d i n t e n s i t y (a u )Wavelength (nm)N o r m a l i s e d i n t e n s i t y (a .u .)Wavelength (nm)Fig. 4. The visible upconversion emission spectra for (a) 0.5 wt% Tm 3+ doped tellurite fiber with 808 nm pump (b) 0.5 wt%Tm 3+ - 1.0 wt% Ho 3+ codoped tellurite fiber with 808 nm pump and (c) 0.5 wt% Tm 3+ - 1.0 wt% Yb 3+ codoped tellurite fiber with 978 nm pump.Figure 5 shows the energy level diagrams for the three systems studied showing the pumping schemes, energytransfer and upconversion mechanisms. The 808 nm pump excites the 3H 4 Tm 3+ level which decays to the 3F 4 level emitting a 1.46 µm photon and then to the 3H 6 level emitting the 1.86 µm photon. At high Tm 3+ concentration and in fiber, a quenching mechanism takes place between Tm 3+ ions where the 1.46 µm photon excites a neighboring ion to the 3F 4 level which decays emitting a further 1.86 µm photon. Stokes energy transfer also occurs from an ion decaying from the 3F 4 level exciting another ion to the 3F 4 level. ESA excites a Tm 3+ ion from the 3H 5 level to the 1G 4 level which decays to the ground state giving rise to the small 480 nm peak in fig 5 (a). This peak is very low in intensity because the upconversion to 1G 4 is originating from the 3H 5 level which will undergo fast non-radiative decay to 3F 4. Upconversion emission has been observed at ~376 nm in Tm 3+ doped fluoride glass due to the 1D 2→3H 6 transition 16 which is not seen in our tellurite glass because the corresponding energy of this transition falls in the UV absorption edge and is therefore quenched.In the Tm 3+-Ho 3+ fiber the Tm 3+ ions are pumped in the same way as above but the energy from the emission at1.86 µm is transferred to a Ho 3+ ion exciting it from the ground state to the 5I 7 level where it decays emitting a2.05 µm photon. Upconversion excites the Ho 3+ ion to the 5I 5 level which then decays non-radiatively to the 5I 6 level, is back transferred to the 3H 5 Tm 3+ level which decays non-radiatively to the 3F 4 level where energy is transferred back to the Ho 3+ 5I 7 level emitting a 2.05 µm photon.14 Upconversion also populates the 5S 2 and 5F 4 levels of Ho 3+ which de-excite to the ground state giving rise to the green emission at 550 nm. The addition of the Ho 3+ ions enhances the upconversion to the 1G 4 Tm 3+ level and consequently the blue emission intensity at 480 nm.In the Tm 3+-Yb 3+ codoped fiber the 976 nm pump excites the 2F 5/2 Yb 3+ level which exhibits fluorescence at1020 nm pumping the Tm 3+ ions into the 3H 5 level. This level decays non-radiatively to 3F 4 and then radiatively to the ground state emitting the 1.86 µm photon. Two photon upconversion populates the 3H 4 Tm 3+ level which decays to the ground state emitting an 800 nm photon and three photon upconversion populates the 1G 4 which decays to the 3F 4 and 3H 6 levels giving strong emission at 650 nm (red) and 480 nm (blue) respectively.17,185101520Yb 3+Tm3+Tm 3+Ho3+E n e r g y (103c m -1)Tm 3+ Tm3+Fig. 5. Energy level diagrams for the Tm 3+-Tm 3+, Tm 3+-Ho 3+ and Tm 3+-Yb 3+ systems showing the pumping schemes,multiple photon upconversion and energy transfer, (represented by the dashed arrows).3.5. Tm 3+ doped silica fiberFigure 6 shows the infrared emission spectrum of a 10 m length of Tm 3+ doped double clad silica fiber whenpumped with around 1.8 W of 808 nm laser source. The graph also shows the spectrum of the 210 mm length of Tm 3+ doped multi-mode tellurite fiber for comparison. The silica fiber has emission peaks at around 1.45 and 1.85 µm which are due to the 3H 4-3F 4 and 3F 4-3H 6 transitions respectively. Although these peaks are due to the same transitions as in the tellurite fiber, the peak shapes are different due to the change in host glass causing the ratio of transitions from the various Stark levels within the manifolds to change. The spectrum for the tellurite fiber is narrower than the silica fiber and the 1.85 µm peak is shifted to longer wavelengths. This suggests that the emission in the tellurite fiber is starting to superfluoresce. The silica fiber also showed weak emission at 480 nm due to upconversion and emission due to the 1G 4-3H 6 transition. Figure 7 shows the decay curve for emission at 1852 nm in the silica fiber when pumped with a modulating 808 nm laser source. The lifetime of the 3F 4 level in silica level was measured to be around 650 µs compared to around 2290 µs in the 210 mm length of tellurite fiber. A long upper laser level lifetime is desirable for non-terminating CW laser operation, and these results confirm the potential advantages of tellurite fiber for compact fiber laser sources.N o r m a l i s e d i n t e n s i t y (a .u .)Wavelength (nm)Fig. 6. The emission spectra of Tm 3+ doped silica and tellurite fiber when excited with an 808 nm laser source. The spectrahave been normalized with respect to the peak at ~1.86 µm.0.00.5 1.0 1.5 2.0 2.5e-2e-1eN o r m a l i s e d i n t e n s i t y (a .u .)Time (ms)Fig. 7. The emission decay curve at 1852 nm for the Tm 3+ doped silica fiber when excited with a modulated 808 nm lasersource.4. CONCLUSIONMid-IR emission in the 1.8 – 2.1 µm range has been measured in Tm 3+ doped and Tm 3+-Ho 3+ codoped telluritefibers when excited with an 808 nm pump. Tm 3+ doped fiber shows strong emission at ~1.86 µm while the Tm 3+-Ho 3+ codoped fiber shows emission at ~2.05 µm through energy transfer to the Ho 3+ ions. Emission has also been observed at ~1.86 µm in Tm 3+-Yb 3+ codoped fiber when pumped with a 976 nm source. These measurements show narrowing and red-shifting of emission peaks and increased energy transfer in the smaller geometries associated with these fibers. Thevisible upconversion emission has been measured and used to help explain the energy transfer processes involved. Emission has also been measured in a 10 m length of Tm3+ doped double-clad silica fiber which exhibits a broader peak and a shorter lifetime at ~1.86 µm than in the tellurite fiber. These results suggest that short lengths (<0.5 m) of doped tellurite fiber can be used for mid-IR fiber laser sources.REFERENCES1.L. D. da Vila, L. Gomes, C. R. Eyzaguirre, E. Rodriguez, C. L. Cesar, L. C. Barbosa, Opt. Mater. 27, 1333-1339(2005).2.L. Huang, S. Shen, A. Jha, J. Non-Cryst. Solids,345&346, 349-353 (2004).3.Y. H. Tsang, D. J. Coleman, T. A. King, Opt. Comm. 231, 357-364 (2004).4. D. C. Hanna, I. R. Perry, J. R. Lincoln, Opt. Comm. 80(1), 52-56 (1990).5. B. C. Dickinson, S. D. Jackson, T. A. King, Opt. Comm. 182, 199-203 (2000).6.W. A. Clarkson, N. P. Barnes, P. W. Turner, J. Nilsson, D. C. Hanna, Opt. Lett. 27(22), 1989-1991 (2002).7. A. F. El-Sherif, T. A. King, Opt. Lett. 28(1), 22-24 (2003).8.J. Y. Allain, M. Monerie, H. Poignant, Electron. Lett. 25(24), 1660-1662 (1989).9.R. G. Smart, J. N. Carter, A. C. Tropper, D. C. Hanna, Opt. Comm.82(5,6), 563-570 (1991).10.M. Doshida, M. Obara, Jpn. J. Appl. Phys. 34, 6079-6083 (1995).11.L. Esterowitz, R. Allen, I. Aggarwal, Electron. Lett. 24(17), 1104 (1988).12.S. D. Jackson, Electron. Lett. 37(13), 821-822 (2001).13.S. Shen, A. Jha, E. Zhang, S. J. Wilson, C. R. Chimie, 5, 921-938 (2002).14.X. Zou, H. Toratani, J. Non-Cryst Solids, 195, 113-124 (1996).15.L. C. Courrol, L. V. G. Tarelho, L. Gomes, N. D. Vieira Jr, F. C. Cassanjes, Y. Messaddeq, S. J. L. Ribeiro, J. Non-Cryst Solids, 284, 217-222 (2001).16.N. Rakov, G. Maciel, M. Sundheimer, L. Menezes, A. Gomes, Y. Messaddeq, F. Cassanjes, G. Poirier, S. Ribeiro,J. App. Phys. Comm. 92(10), 6337-6339 (2002).17.S. Shen, A. Jha, L. Huang, P. Joshi, Opt. Lett. 30(12), 1437-1439 (2005).18.G. Wang, S. Dai, J. Zhang, L. Wen, J. Yang, Z. Jiang, Spectrochim. Acta A, 64, 349-354 (2005).。
FREQUENCY SPECTRUM MANAGEMENT APPARATUS AND METHOD
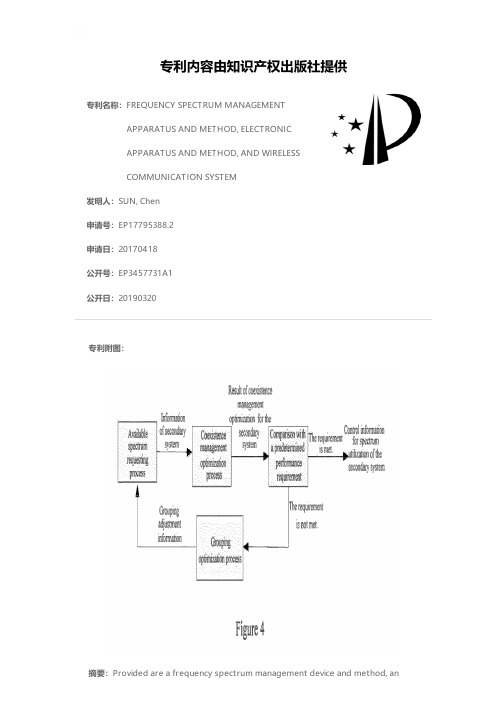
专利名称:FREQUENCY SPECTRUM MANAGEMENTAPPARATUS AND METHOD, ELECTRONICAPPARATUS AND METHOD, AND WIRELESSCOMMUNICATION SYSTEM发明人:SUN, Chen申请号:EP17795388.2申请日:20170418公开号:EP3457731A1公开日:20190320专利内容由知识产权出版社提供专利附图:摘要:Provided are a frequency spectrum management device and method, anelectronic device and method, and a wireless communication system. The frequency spectrum management device comprises a processor, and the processor is configured to: adjust, in response to a coexistence management request from one or more secondary system apparatuses, current grouping of the one or more secondary system apparatuses according to a predetermined performance requirement, wherein the coexistence management request comprises at least information about available spectrum resources requested from a geographic location database by the one or more secondary system apparatuses according to the current grouping; determine, according to a result of the adjustment, regrouping information of the one or more secondary system apparatuses, so that the one or more secondary system apparatuses re-request the geographic location database for available spectrum resources according to the regrouping information; and optimize, according to the available spectrum resources re-requested by the one or more secondary system apparatuses, spectrum utilization of the one or more secondary system apparatuses According to the embodiments of the disclosure, the frequency spectrum utilization efficiency can be optimized, and the system performance can be improved.申请人:Sony Corporation地址:1-7-1 Konan Minato-ku Tokyo 108-0075 JP国籍:JP代理机构:D Young & Co LLP更多信息请下载全文后查看。
GBT 38947-2020《磁选设备磁感应强度检测方法》国家标准解读
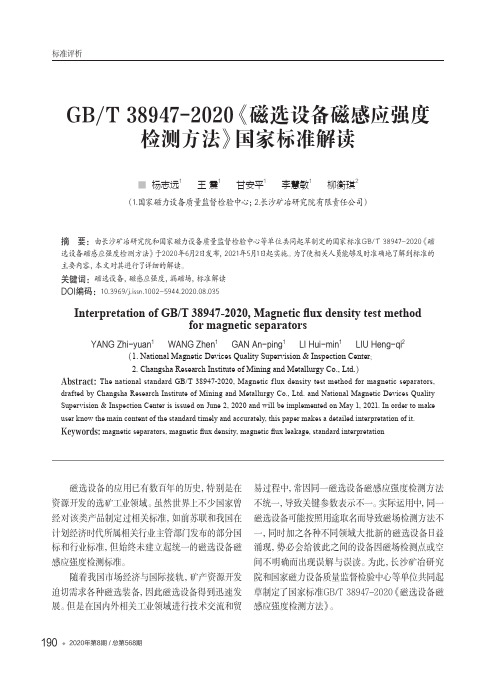
GB/T 38947-2020《磁选设备磁感应强度检测方法》国家标准解读■ 杨志远1 王 震1 甘安平1 李慧敏1 柳衡琪2(1.国家磁力设备质量监督检验中心;2.长沙矿冶研究院有限责任公司)摘 要:由长沙矿冶研究院和国家磁力设备质量监督检验中心等单位共同起草制定的国家标准GB/T 38947-2020《磁选设备磁感应强度检测方法》于2020年6月2日发布,2021年5月1日起实施。
为了使相关人员能够及时准确地了解到标准的主要内容,本文对其进行了详细的解读。
关键词:磁选设备,磁感应强度,漏磁场,标准解读DOI编码:10.3969/j.issn.1002-5944.2020.08.035Interpretation of GB/T 38947-2020, Magnetic flux density test methodfor magnetic separatorsYANG Zhi-yuan1 WANG Zhen1 GAN An-ping1 LI Hui-min1 LIU Heng-qi2(1. National Magnetic Devices Quality Supervision & Inspection Center;2. Changsha Research Institute of Mining and Metallurgy Co., Ltd.)Abstract: The national standard GB/T 38947-2020, Magnetic flux density test method for magnetic separators, drafted by Changsha Research Institute of Mining and Metallurgy Co., Ltd. and National Magnetic Devices Quality Supervision & Inspection Center is issued on June 2, 2020 and will be implemented on May 1, 2021. In order to make user know the main content of the standard timely and accurately, this paper makes a detailed interpretation of it. Keywords: magnetic separators, magnetic flux density, magnetic flux leakage, standard interpretation标准评析磁选设备的应用已有数百年的历史,特别是在资源开发的选矿工业领域。
GBT29639
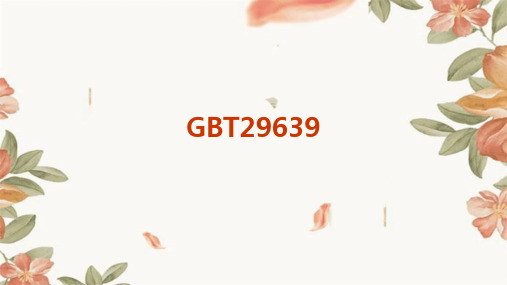
3
The development process involves extensive research, consultation, and collaboration among industry experts, government agencies, and other stakeholders
Security management system
Establish and improve the security management system, formulate security management regulations and operating procedures, and strictly implement them
03
Risk assessment and control
Risk assessment me risk assessment: This method involves the use of expert judgment and experience to identify and assess the potential risks associated with a specific situation or activity It typically depends on subjective analysis and may include techniques such as brainstorming, interviews, or surveys
• The management system requirements establish a framework for organizations to manage their energy and environmental performance, including the development of policies, targets, action plans, and monitoring and reporting mechanisms
370570_钽铌湿法冶炼配水洗涤监控系统设计
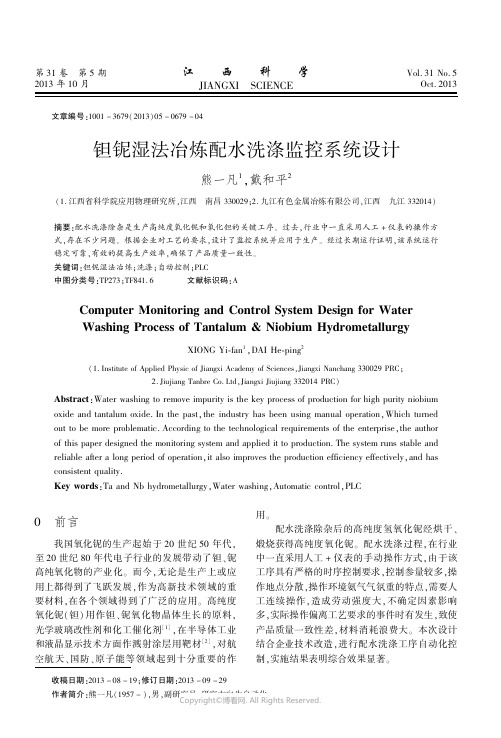
收稿日期:2013-08-19;修订日期:2013-09-29作者简介:熊一凡(1957-),男,副研究员,研究方向为自动化。
第31卷 第5期2013年10月江 西 科 学JIANGXI SCIENCEVol.31No.5Oct.2013 文章编号:1001-3679(2013)05-0679-04钽铌湿法冶炼配水洗涤监控系统设计熊一凡1袁戴和平2(1.江西省科学院应用物理研究所,江西 南昌330029;2.九江有色金属冶炼有限公司,江西 九江332014)摘要:配水洗涤除杂是生产高纯度氧化铌和氧化钽的关键工序。
过去,行业中一直采用人工+仪表的操作方式,存在不少问题。
根据企业对工艺的要求,设计了监控系统并应用于生产。
经过长期运行证明,该系统运行稳定可靠,有效的提高生产效率,确保了产品质量一致性。
关键词:钽铌湿法冶炼;洗涤;自动控制;PLC 中图分类号:TP273;TF841.6 文献标识码:AComputer Monitoring and Control System Design for Water Washing Process of Tantalum &Niobium HydrometallurgyXIONG Yi⁃fan 1,DAI He⁃ping 2(1.Institute of Applied Physic of Jiangxi Academy of Sciences,Jiangxi Nanchang 330029PRC;2.Jiujiang Tanbre Co.Ltd,Jiangxi Jiujiang 332014PRC)Abstract :Water washing to remove impurity is the key process of production for high purity niobium oxide and tantalum oxide.In the past,the industry has been using manual operation,Which turned out to be more problematic.According to the technological requirements of the enterprise,the author of this paper designed the monitoring system and applied it to production.The system runs stable and reliable after a long period of operation,it also improves the production efficiency effectively,and hasconsistent quality.Key words :Ta and Nb hydrometallurgy,Water washing,Automatic control,PLC0 前言我国氧化铌的生产起始于20世纪50年代,至20世纪80年代电子行业的发展带动了钽、铌高纯氧化物的产业化。
RCA Clean 清洗过程手册说明书
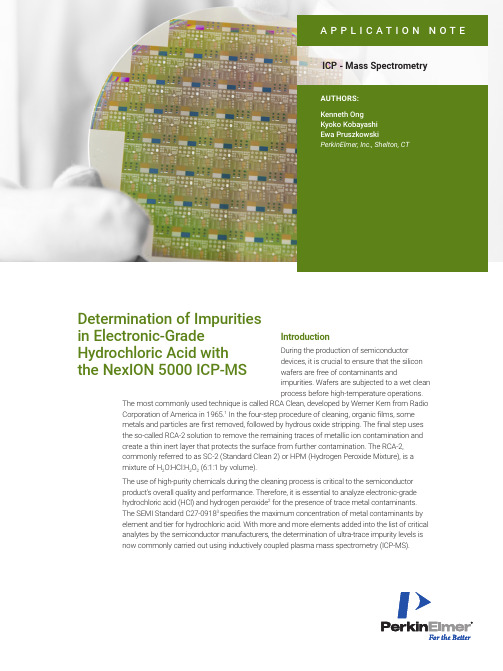
IntroductionDuring the production of semiconductor devices, it is crucial to ensure that the silicon wafers are free of contaminants andimpurities. Wafers are subjected to a wet clean process before high-temperature operations.The most commonly used technique is called RCA Clean, developed by Werner Kern from Radio Corporation of America in 1965.1 In the four-step procedure of cleaning, organic films, some metals and particles are first removed, followed by hydrous oxide stripping. The final step uses the so-called RCA-2 solution to remove the remaining traces of metallic ion contamination and create a thin inert layer that protects the surface from further contamination. The RCA-2, commonly referred to as SC-2 (Standard Clean 2) or HPM (Hydrogen Peroxide Mixture), is a mixture of H 2O:HCl:H 2O 2 (6:1:1 by volume).The use of high-purity chemicals during the cleaning process is critical to the semiconductor product’s overall quality and performance. Therefore, it is essential to analyze electronic-grade hydrochloric acid (HCl) and hydrogen peroxide 2 for the presence of trace metal contaminants. The SEMI Standard C27-09183 specifies the maximum concentration of metal contaminants by element and tier for hydrochloric acid. With more and more elements added into the list of critical analytes by the semiconductor manufacturers, the determination of ultra-trace impurity levels is now commonly carried out using inductively coupled plasma mass spectrometry (ICP-MS).Determination of Impurities in Electronic-Grade Hydrochloric Acid with the NexION 5000 ICP-MSA P P L I C A T I O N N O T EAUTHORS:Kenneth Ong Kyoko Kobayashi Ewa PruszkowskiPerkinElmer, Inc., Shelton, CTICP - Mass SpectrometryTable 1. Chloride Interferences Observed during HCl Analysis.In an ICP-MS analysis, in addition to spectral interferences from Ar, O 2, N 2 and H 2, the HCl matrix could generate some further polyatomic interferences. Examples of chloride-based interferences observed during the analysis of HCl are listed in Table 1.This application note demonstrates the extreme power of PerkinElmer’s NexION ® 5000 Multi-Quadrupole ICP-MS to remove interferences in order to achieve low BECs (background equivalent concentrations) in HCl for all analytes.ExperimentalSample and Standard PreparationA direct analysis was carried out on undiluted 20% ultra-pure HCl (Tamapure-AA 10, Tama Chemicals, Tokyo, Japan) using a method of standard addition (MSA). Standard solutions were prepared from 10 mg/L multi-element standards (PE Pure,PerkinElmer, Shelton, Connecticut USA) via serial dilutions, with the final standards being spiked directly into 20% HCl. InstrumentationThe instrument used for the described experiments was a NexION 5000 Multi-Quadrupole ICP-MS 4 (PerkinElmer). The NexION 5000’s Universal Cell Technology (UCT) function, while operating in Reaction (DRC) mode, is an extremely effective technique to eliminate atomic and polyatomic interferences even in hot plasma conditions. The UCT uses a quadrupole mass filter to create dynamic bandpass tuning, wherein only ions of a specific mass range pass through the cell, thus allowing only controlled reactions to take place, preventing undesirable by-product ions from forming within the cell even when very reactive gases, such as 100% NH 3 and O 2, are used. The NexION 5000 ICP-MS – running on a patented 34-MHzsolid-state RF generator (patent no. 9,420,679), especially created for ICP-MS operation, together with the LumiCoil™ RF coil and the second-generation Triple Cone Interface (TCI) with OmniRing™ technology – provides superior analytical performance with great sensitivity and stability.Fast power-switching between Cold and Hot Plasma modes is made possible by the balanced and free-running RF generator, allowing multi-mode methods to leverage these technologies in combination with a cell-based Reaction mode (in the Universal Cell) and multi-quadrupole technology. As a result, superior polyatomic interference removal is achieved that further improves detection limits (DLs) and background equivalent concentrations (BECs).Instrumental ConditionsAll preparation and analysis steps were performed under a Class 100 performance clean booth within a controlled laboratory room. In this work, three reaction gases (NH 3, H 2, and O 2) were used to remove all spectral interferences on measured analytes. To analyze P , Ti, As, and Se most effectively, O 2 is recommended as a reaction gas in Mass Shift mode to create oxide ions with analytes and measure them at higher masses away from interferences existing on their original masses. NH 3 isapplicable for 18 elements analyzed mostly in MS/MS mode. While this work demonstrates Cold Plasma conditions being applied for few elements to provide the lowest backgroundequivalent concentrations (BECs), the NexION 5000 ICP-MS can achieve ppt levels of BECs in this matrix even in Hot Plasma mode. The operating conditions were determined using a 200-ppt standard solution in 1% HNO 3. Instrumental parameters andsample introduction components are shown in Table 2.Table 2. NexION 5000 ICP-MS Instrumental Conditions.Results and Discussion20% HCl was quantitatively analyzed using the method of standard addition (MSA). The calibration curves for K, V , Cr, Ge, As, and Se are shown in Figure 1. Even though they were obtained using different modes (MS/MS and Mass Shift), reaction gases (NH 3 and O 2) and plasma conditions (Hot and Cold), they demonstrate good linearity and low BECs, which is only possible when all the polyatomic interferences are removed by reactions with pure, undiluted NH 3 and O 2 combined with the Universal Cell’s dynamic bandpass tuning.Figure 1. Calibration curves for K, V, and Cr in MS/MS mode with NH 3, Ge in Mass Shift mode with NH 3, and As and Se in Mass Shift mode with O 2.K 39/39V 51/51Cr 52/52Ge 74/90As 75/91Se 80/96One very interesting case is the analysis of Ge because its major isotope at mass 74 suffers serious interference from the dimer of 37Cl 2. While NH 3 can remove the dimer of 37Cl 2 via a charge transfer reaction, Ge itself is quite reactive to NH 3, resulting in lower intensity at mass 74. Taking advantage of Ge’s reactivity and the Mass Shift capability of NexION 5000 ICP-MS, Ge can be measured as a GeNH 2+ cluster at mass 90, or as Ge(NH 3)NH 2+ cluster at mass 107, moving it away from the 37Cl 2 interference at mass 74. The cluster formation can be demonstrated by a product ion scan for 74Ge with Q1 set at mass 74 and Q3scanning from mass 62 to 120 (Figure 2). Sensitivity of each cluster depends on the NH 3 flows.Another example of the power of the NexION 5000 system is the 35Cl 16O + and 35Cl 16OH + interference removal on 51V and 52Cr. V + and Cr + ions have a low reactivity rate with NH 3, while 35Cl 16O + and 35Cl 16OH + react spontaneously. This spontaneous reaction, nevertheless, will also create by-products such as 35ClNH 2+and (NH 3)2NH 4+ interfering with V + and Cr + in the absence of bandpass within the reaction cell, as is the case of an octupole cell or a quadrupole cell when the bandpass is open with a low RPq value setting.Figure 2. 74Ge Product ion scan showing two Ge clusters with NH 3 at mass 90 and 107.The importance of bandpass can be demonstrated through a spectral scan of a clean HCl sample with a minimal presence of V , when Q1 is set at mass 51 (51V and 35Cl 16O +), and Q3 is scanning masses from 34 to 53. With RPq set to a low value of 0.25, equivalent to the operation of an octupole, quite substantial counts can be observed at mass 35 and some at masses 51 and 52 (Figure 3). It was concluded that the ions observed at mass 35 are NH 3NH 4+, at mass 51 are 35ClNH 2, and at mass 52 are (NH 3)2NH 4+.When RPq is increased to a higher value, the side reactions that produce theby-product interferences at masses 51 and 52 are stopped, allowing very low BEC detection of V and Cr in HCl, as shown in Figure 4. More information on the advantage of Reaction Cell with dynamic bandpass tuning can be found in “Multi-Quadrupole ICP-MS: Pushing limits of detection to the next decimal”.5Figure 3. Product ion scan of a 20% HCl with Q2 bandpass open acting as ion guide(low RPq).Figure 4. Product ion scan of a 20% HCl with Q2 bandpass for mass-dependent cut off (high RPq).The detection limits (DLs) and background equivalentconcentrations (BECs) were determined in 20% HCl. DLs were calculated by multiplying the standard deviation of sevenmeasurements of the blank by three. These values are greatly affected by the impurity level in samples.A spike recovery test was carried out based on SEMI guidelines. According to SEMI, the spike level must be at least 50% of the proposed specification level, and the recovery should be between 75% and 125% of the expected value. The specification level for Tier C grade is 100 ppt in 37% HCl. However, 10 ppt spikes in 20% HCl were used in this experiment, much lower than required. Theresults are summarized in Table 3.Table 3. BECs, DLs and Spike Recoveries in 20% HCl.Table 3. BECs, DLs and Spike Recoveries in 20% HCl continued...Another important attribute of good analysis, besides low BECs and excellent DLs, is the long-term stability of an ICP-MS system to handle corrosive matrices, such as concentratedhydrochloric acid. Figure 5 shows excellent stability of 50-ppt multi-element spikes into 20% HCl with RSDs of < 3% and variation <15% over five hours. This stability is brought about through the novel design of the second-generation Triple ConeInterface with OmniRing, developed specifically for the NexION 5000 ICP-MS. More information on this can be found in the technical note “Advantages of a Novel Interface Design for NexION 5000 ICP-MS".6ConclusionThe NexION 5000 Multi-Quadrupole ICP-MS is shown to be robust and suitable for the routine quantification of ultra-trace impurities at the ng/L level in concentrated HCl. The data demonstratesthe effectiveness of Reaction mode in the UniversalFigure 5. Signal stability for a multi-mode analysis of 50 ppt spiked in undiluted (20%) HCl.Cell coupled with multi-quadrupole operation to eliminate chloride-derived interferences. By applying the most effective Reaction mode, 20% HCl can be analyzed directly for contaminants at SEMI Tier C levels, without the need for cumbersome sample pre-treatment or dilution. Employing computer-controlled switching between Hot and Cold Plasma as well as Standard and Reaction modes in the Universal Cell, interference-free analysis for all elements crucial to semiconductor manufacturing is possible in a single sample run.For a complete listing of our global offices, visit /ContactUsCopyright ©2021-2022, PerkinElmer, Inc. All rights reserved. PerkinElmer ® is a registered trademark of PerkinElmer, Inc. All other trademarks are the property of their respective owners.528626 (174514) PKIPerkinElmer, Inc. 940 Winter StreetWaltham, MA 02451 USA P: (800) 762-4000 or (+1) Consumables UsedReferences1. W olf S. et al., "Silicon Processing for the VLSI Era," Vol. 1, Lattice Press, Sunset Beach, CA, 2000.2. O ng K. et al., “Analysis of Semiconductor-Grade Hydrogen Peroxide Using the NexION 5000 ICP-MS”, PerkinElmer Application Note, 2020.3. S EMI Standard C27-0918, Specification and Guide for Hydrochloric Acid.4. “NexION 5000 Multi-Quadrupole ICP-MS”, PerkinElmer Product Note, 2020.5. K roukamp E. et al. “Multi-Quadrupole ICP-MS: Pushing limits of detection to the next decimal”, Spectroscopy 35 (9) 19, 2020.6. B adiei H. et al., “Advantages of a Novel InterfaceDesign for NexION 5000 ICP-MS”, PerkinElmer Technical Note, 2020.。
- 1、下载文档前请自行甄别文档内容的完整性,平台不提供额外的编辑、内容补充、找答案等附加服务。
- 2、"仅部分预览"的文档,不可在线预览部分如存在完整性等问题,可反馈申请退款(可完整预览的文档不适用该条件!)。
- 3、如文档侵犯您的权益,请联系客服反馈,我们会尽快为您处理(人工客服工作时间:9:00-18:30)。
a r X i v :h e p -p h /9803500v 3 22 J u n 1998Limiting SUSY-QCD spectrum and its application for decays of superheavy particlesV.Berezinsky 1,2and M.Kachelrieß11INFN,Laboratori Nazionali del Gran Sasso,I–67010Assergi (AQ),Italy2Institute for Nuclear Research,Moscow,RussiaThe supersymmetric generalization of the limiting and Gaussian QCD spectra is obtained.These spectra are valid for x ≪1,when the main contribution to the parton cascade is given by gluons and gluinos.The derived spectra are applied to decaying superheavy particles with masses up to the GUT scale.These particles can be relics from the Big Bang or produced by topological defects and could give rise to the observed ultrahigh energy cosmic rays.General formulae for the fluxes of protons,photons and neutrinos due to decays of superheavy particles are obtained.PACS numbers:11.30.Pb,12.38.Bx,96.40.-z.I.INTRODUCTIONThe spectra of hadrons produced in deep-inelastic scattering and e +e −annihilation are formed due to QCD cas-cading of the partons.In the Leading Logarithmic Approximation (LLA)which takes into account ln(Q 2)terms this cascade is described by the Gribov-Lipatov-Altarelli-Parisi-Dokshitzer (GLAPD)equation [1].The Modified LeadingLogarithmic Approximation (MLLA)takes into account additionally ln(x )terms,where x =k /k maxand k is the longitudinal momentum of the produced hadron.Color coherence effects are described in MLLA.Two approximate analytic solutions to the MLLA evolution equations have been obtained.These are the limiting spectrum [2]and the Gaussian spectrum [3,4],in which we include the distorted Gaussian spectrum [5–7].The limiting spectrum is the most accurate one among them.In fact,it describes well the experimental data at large x ,too,and this is natural,though accidental (for an explanation see Ref.[6]).The limiting spectrum has a free normalization constant,K lim ,which cannot be calculated theoretically and is found from comparison with experimental data.This constant has to be considered as a basic parameter of the theory,and it can be used at all energies,where the physical assumptions,under which the limiting spectrum is derived,are valid.For detailed calculations of hadron spectra in e +e −annihilation and comparison with experimental data see Ref.[8].Up to energies of existing e +e −colliders,√s >∼1TeV the production of supersymmetric particles might substantially change the QCD spectra.Apart from future experiments at LHC,supersymmetry (SUSY)might strongly reveal itself in the decays of superheavy particles.They can appear as relics of the Big Bang,or be produced by topological defects (TD),and can be the sources of the observed ultrahighenergy cosmic rays (UHECR)at E >∼1·1010GeV.The range of masses,m X ,of interest for UHECR goes from the GUT scale (m X ∼1016GeV)or less down to m X ∼1012−1014GeV.In this paper we obtain the generalization of the limiting and Gaussian QCD spectra for the SUSY case.Although the influence of SUSY on,e.g.,the evolution of parton distributions or the running coupling constant was considered in many works in the 80’s [9],this is,to the best of our knowledge,the first time that fragmentation spectra of hadrons are examined for large√k 2⊥αs (k 2⊥)4πlnQ 2∂zΦC A (z )D BC (x/z,ξ)−C1dz z ΦC A (z )D BA (x,ξ),(2)where ΦB A (z )is the splitting function characterizingthe decayA →B +C .The supersymmetrization of Eq.(2)is simple:each parton A should be substituted by the supermultiplet whichcontains A and its superpartner ˜A.We shall generalize here the limiting spectrum of QCD given e.g.in [6]to SUSY-QCD.This spectrum is valid for small x ,where gluons strongly dominate the other partons.Therefore,we restrict ourselves to calculations taking into account only two partons,namely gluons g and gluinos λ,in the tree diagrams.However,in the loop diagrams which govern the running of αs (k 2⊥)we take into account also quarks and squarks.Multiplying Eq.(2)by x j +1and integrating it over x ,we obtain an equation for the moments D BA (j,ξ),∂z+zz(7)Φλλ(z )=2N c1+z 2∂ξD (j,ξ)=H (j )D (j,ξ)(9)whereH (j )=νg (j )Φλg (j )Φgλ(j )νλ(j )(10)νg (j )=1dz z j −1−z Φg g (z )−Φλg(z ) (11)νλ(j )=10dz z j −1−1 Φλλ(z ).(12)The procedure of solving Eq.(9)is identical to that given in Ref.[6].After diagonalization of H (j )with the help of(D +,D −),the eigenvalues of H areν±=1ω−a +O (ω)(14)with a=11Γ(B) π/2−π/2dτ8N c sinhαY B I B(y).(15)b√s is the c.m.energy of an Here,Y now is Y=ln[e+e−pair or the mass m X of the superheavy decaying particle.The parameters depending on the structure of the theory are a=11αbc/Y−c/Y)with c≈0.39in QCD and c≈0.84in SUSY-QCD. Therefore,the SUSY spectra are shifted to the right,and since they are also narrower than the QCD spectra(see Eq.(19)below),the SUSY maxima are dramatically higher(by a factor of30)than the QCD ones.We remind the reader that the value of the maximum is given by the multiplicity.We compare these spectra also with the Gaussian approximation obtained for the MLLA solution[5,7].This√approximation can be easily generalized to the SUSY case[12]and has for x≪1as function of x=2E/exp −ln2x/x mx24 6ln3/2 s√III.APPLICATIONSWe shall apply now the limiting spectrum in SUSY-QCD to the calculation of the spectrum of ultrahigh energy cosmic rays (UHECR)generated by the decay of superheavy particles with masses 1012−1015GeV.Let us discuss first the problem of the normalization of spectrum.We remind the reader that the limiting spectrum has been derived for x≪1,though at least for small s it describes well the experimental data for x up to x ∼1(see [6]for discussion).In Ref.[10],the normalization constant was fixed by a comparison with experimental data on e +e −annihilation to K lim =2.6.This value is fixed by D lim (l,Y )at maximum (see Fig.1),i.e.by multiplicity.Since the shape of the spectrum and the position of its peak change dramatically,if one goes from QCD and √s ∼1012−1015GeV,we cannot use this value of K lim .Instead we use asnormalization condition Eq.(17)replacing the factor 2by 2f i ,where i runs through N (all nucleons)and π±and π0(charged and neutral pions),while f i is the fraction of energy carried by the hadron i .Note that the main contribution to the integral in Eq.(17)comes from large values x ∼1,where the limiting spectrum might have large uncertainties.However,this is,in our opinion,the most physical way of normalization.The numerical values of f i are unknown at large s .One can assume that f π≈1−f LSP ,where f LSP is the energy fraction taken away by the lightest supersymmetric particle (LSP).According to the simplified Monte-Carlo simulation of [14],f LSP ∼0.4.For the ratio f N /f πwe use ∼0.05inspired by Z 0decay.Let us assume that the decay rate of X particles ˙n X in the extragalactic space does not depend on distance and time.Then taking into account the energy losses of UHE protons and the absorption of UHE photons due to pair production (γ+γ→e ++e −)on the radio and microwave background,the diffuse flux of UHE protons and antiprotons isI p +¯p (E )=1m X ∞dt g D N (x g ,Y )dE g (E,t g )dE=(1+z g )expz g 0dz∂EE =E g (z ),(22)where H 0is the Hubble constant and z the redshift.In the case that the energy looses on microwave radiation are much larger than the adiabatic ones,Eq.(22)reduces todE g (E,z g )b (E,0).(23)The diffuse spectrum of UHE photons can be calculated asI γ(E )=˙n X m X 12E/m xdxt3+p.(25)The number of neutrinos with energy E produced per decay of one X particle can be approximately calculated asN ν(E )≈12xD π±(x,Y ).(26)Combining Eqs.(25)and (26),we obtain for the diffuse neutrino fluxI ν(E )=3˙n X (t 0)2p 14E (1+z )xD π±(x,Y ),(27)where 1+z max (E )≈m X /(4E ).In Fig.3,the spectra of UHE protons,photons,and neutrinos are shown together with experimental data[17]for the model of cosmic necklaces–topological defects which consists of monopoles connected by strings[18].We use the model of Ref.[13],wherer2µ˙n X=[1]V.N.Gribov and L.N.Lipatov,Sov.J.Nucl.Phys.15,438and675(1972);G.Altarelli and G.Parisi,Nucl.Phys.B126,298(1977);Yu.L.Dokshitzer,Sov.Phys.JETP46,641(1977).[2]Yu.L.Dokshitzer and S.I.Troyan,Leningrad preprint,LNPI-922(1984);Ya.I.Azimov,Yu.L.Dokshitzer,V.A.Khoze andS.I.Troyan,Z.Phys.C27,65(1985);ibid.C31,213(1986).[3]A.H.Mueller,Proc.1981Int.Symp.on Lepton and Photon Interactions,ed.W.Pfeil,Bonn,689(1981).[4]Yu.L.Dokshitzer,V.S.Fadin and V.A.Khoze,Phys.Lett.B115,242(1982).[5]C.P.Fong and B.R.Webber,Phys.Lett.B229,289(1989);Nucl.Phys.B355,54(1991).[6]Yu.L.Dokshitzer,V.A.Khoze,A.H.Mueller and S.I.Troyan,Basics of Perturbative QCD,Editions Fronti`e res1991.[7]R.K.Ellis,W.J.Stirling and B.R.Webber,QCD and Collider Physics,Cambridge Univ.Press1996.[8]Yu.L.Dokshitzer,V.A.Khoze and S.I.Troyan,Z.Phys.C55,107(1992);V.A.Khoze and W.Ochs,Int.J.Mod.Phys.A12,2949(1997).[9]S.K.Jones and C.H.Lewellyn-Smith,Nucl.Phys.B217,145(1983)and references therein.[10]Yu.L.Dokshitzer and S.I.Troyan,Leningrad preprint,LNPI-922(1984);Yu.L.Dokshitzer,V.A.Khoze and S.I.Troyan,Int.J.Mod.Phys.A7,1875(1992).[11]I.S.Gradshteyn and I.M.Ryzhik,Table of Integrals,Series and Products,Academic Press1994.[12]V.Berezinsky,X.Martin and A.Vilenkin,Phys.Rev.D56,2024(1997).[13]V.Berezinsky and A.Vilenkin,Phys.Rev.Lett.79,5202(1997).[14]V.Berezinsky and M.Kachelrieß,Phys.Lett.B422,163(1998).[15]V.S.Berezinsky and S.I.Grigorieva,Astron.Astrophys.199,1(1988).[16]R.J.Protheroe and P.L.Biermann,Astropart.Phys.6,45(1996),Erratum ibid.7,181(1997).[17]M.Nagano,private communication.[18]M.B.Hindmarsh and T.W.B.Kibble,Phys.Rev.Lett.55,2398(1985).4.0e48.0e41.2e51.6e52.0e52.4e52.8e53.2e5051015202530350.010.11101001e-061e-050.00010.0010.010.12222.52323.52424.52525.52626.51919.52020.52121.5。