High-rate properties of LiFePO4-carbon composites as cathode materials for lithium-ion batteries
碳包覆LiFePO_4的结构与性能研究
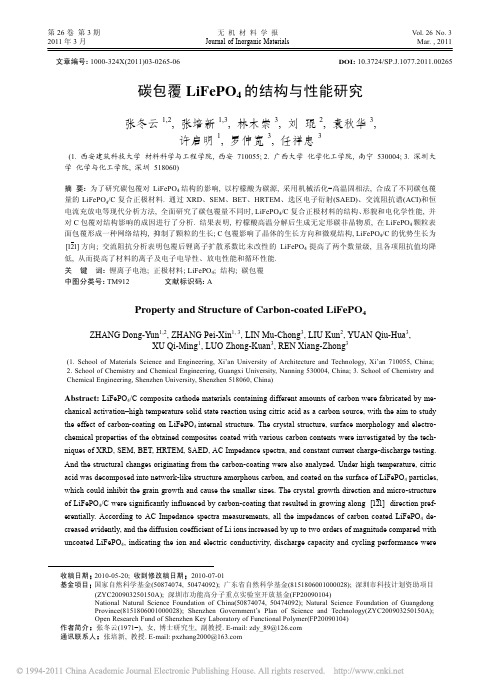
第26卷第3期无机材料学报Vol. 26No. 3 2011年3月Journal of Inorganic Materials Mar. , 2011文章编号: 1000-324X(2011)03-0265-06 DOI: 10.3724/SP.J.1077.2011.00265碳包覆LiFePO4的结构与性能研究张冬云1,2, 张培新1,3, 林木崇3, 刘琨2, 袁秋华3,许启明1, 罗仲宽3, 任祥忠3(1. 西安建筑科技大学材料科学与工程学院, 西安 710055; 2. 广西大学化学化工学院, 南宁 530004; 3. 深圳大学化学与化工学院, 深圳 518060)摘要: 为了研究碳包覆对LiFePO4结构的影响, 以柠檬酸为碳源, 采用机械活化−高温固相法, 合成了不同碳包覆量的LiFePO4/C复合正极材料. 通过XRD、SEM、BET、HRTEM、选区电子衍射(SAED)、交流阻抗谱(ACI)和恒电流充放电等现代分析方法, 全面研究了碳包覆量不同时, LiFePO4/C复合正极材料的结构、形貌和电化学性能, 并对C包覆对结构影响的成因进行了分析. 结果表明, 柠檬酸高温分解后生成无定形碳非晶物质, 在LiFePO4颗粒表面包覆形成一种网络结构, 抑制了颗粒的生长; C包覆影响了晶体的生长方向和微观结构, LiFePO4/C的优势生长为方向; 交流阻抗分析表明包覆后锂离子扩散系数比未改性的LiFePO4提高了两个数量级, 且各项阻抗值均降低, 从而提高了材料的离子及电子电导性、放电性能和循环性能.关键词: 锂离子电池; 正极材料; LiFePO4; 结构; 碳包覆中图分类号: TM912文献标识码: AProperty and Structure of Carbon-coated LiFePO4ZHANG Dong-Yun1,2, ZHANG Pei-Xin1, 3, LIN Mu-Chong3, LIU Kun2, YUAN Qiu-Hua3,XU Qi-Ming1, LUO Zhong-Kuan3, REN Xiang-Zhong3(1. School of Materials Science and Engineering, Xi’an University of Architecture and Technology, Xi’an 710055, China;2. School of Chemistry and Chemical Engineering, Guangxi University, Nanning 530004, China;3. School of Chemistry andChemical Engineering, Shenzhen University, Shenzhen 518060, China)Abstract: LiFePO4/C composite cathode materials containing different amounts of carbon were fabricated by me-chanical activation–high temperature solid state reaction using citric acid as a carbon source, with the aim to study the effect of carbon-coating on LiFePO4 internal structure. The crystal structure, surface morphology and electro-chemical properties of the obtained composites coated with various carbon contents were investigated by the tech-niques of XRD, SEM, BET, HRTEM, SAED, AC Impedance spectra, and constant current charge-discharge testing.And the structural changes originating from the carbon-coating were also analyzed. Under high temperature, citric acid was decomposed into network-like structure amorphous carbon, and coated on the surface of LiFePO4 particles, which could inhibit the grain growth and cause the smaller sizes. The crystal growth direction and micro-structure of LiFePO4/C were significantly influenced by carbon-coating that resulted in growing along [121] direction pref-erentially. According to AC Impedance spectra measurements, all the impedances of carbon coated LiFePO4 de-creased evidently, and the diffusion coefficient of Li ions increased by up to two orders of magnitude compared with uncoated LiFePO4, indicating the ion and electric conductivity, discharge capacity and cycling performance were收稿日期: 2010-05-20; 收到修改稿日期:2010-07-01基金项目:国家自然科学基金(50874074, 50474092); 广东省自然科学基金(8151806001000028); 深圳市科技计划资助项目(ZYC200903250150A); 深圳市功能高分子重点实验室开放基金(FP20090104)National Natural Science Foundation of China(50874074, 50474092); Natural Science Foundation of GuangdongProvince(8151806001000028); Shenzhen Government’s Plan of Science and Technology(ZYC200903250150A);Open Research Fund of Shenzhen Key Laboratory of Functional Polymer(FP20090104)作者简介: 张冬云(1971−), 女, 博士研究生, 副教授. E-mail: zdy_89@通讯联系人: 张培新, 教授. E-mail: pxzhang2000@266 无机材料学报第25卷greatly improved.Key words: lithium-ion batteries; cathode materials; LiFePO4; structure; carbon-coating近年来, 锂离子电池正极材料的研究与开发受到世界许多先进国家的高度重视[1-2], 然而电子电导率较低及锂离子扩散系数小限制了其应用. 目前国内外对LiFePO4的研究工作主要集中在材料的制备方法、工艺[3-4]、相关机理及性能改善的实验[5]研究上. 研究表明通过表面碳包覆[6-7]和金属离子掺杂[8-9]等技术手段可减小材料的颗粒尺寸, 增大材料的电导率, 从而提高LiFePO4的电子、离子传导率及其电化学性能.根据锂电池的工作原理, 晶格颗粒之间的导电性对电性能也非常重要, 提高颗粒间的导电性有利于Li+在颗粒间的穿梭能力, 从而改善材料的高倍率性能. 通过对LiFePO4进行碳包覆改性, 可以提高颗粒间的表观电导, 减少极化, 同时可以为LiFePO4提供电子隧道, 补偿Li+脱嵌过程中的电荷平衡, 提高其充放电的可逆性. Huang等[10]在原料中加入碳粉或其它添加剂后, 可以改善该材料的充放电性能,并且认为材料和碳是否紧密接触以及小的磷酸亚铁锂颗粒是提高容量的关键. 因此, 有必要对LiFePO4碳包覆后的结构进行研究, 以加深认识.基于上述考虑, 本工作对碳包覆前后的LiFePO4进行结构分析和充放电性能、交流阻抗谱研究, 以研究碳包覆改性的LiFePO4的结构和锂离子扩散的关系, 从而探讨使复合材料电化学性能提高的微观结构成因.1实验采用机械活化−高温固相法合成LiFePO4/C复合材料. 以Li2CO3(AR), FeC2O4·H2O(AR), NH4H2PO4(AR)为原料, 柠檬酸(AR)为碳源, 丙酮为介质, 球磨后的前驱体烘干, 转移至管式炉中, 在氩气(99.99%)保护下于350℃预分解6h, 冷却后取出, 充分研磨, 于氩气气氛中650℃下恒温20h, 得到LiFePO4/C.物相分析采用X射线粉末衍射仪(Bruker D8, 德国), 扫描范围为15°~60°. 表面形貌采用扫描电镜SEM(S-3400N, 日本). 比表面积测试在Nova1200e型比表面仪(美国康塔公司)上进行, 以N2为吸附质, 在液氮温度77K下进行吸/脱附, 样品于110℃真空下预处理3h. TEM测试在JEM2100型透射电镜(日本JEOL公司)上进行.25℃下采用恒电流充放电法测定材料的比容量和循环性能. 实验电池以金属锂片为负极, 隔膜采用Celgard2400, 电解液为l mol/L LiPF6/EC+DMC (1:2 vol%), 在无水厌氧手套箱中(Unilab2000, 德国)内组装成扣式电池后, 用Land电池测试系统(CT2001A, 中国)测试材料的充放电比容量. 充放电方式为先恒流充电, 静置, 再恒流放电. 充电电流均为0.1C, 充放电电压范围为2.6~4.2V.2结果与讨论2.1 LiFePO4/C复合材料的结构与形貌图1为不同碳包覆量的LiFePO4/C复合正极材料的XRD图谱. 各样品均有尖锐的衍射峰, 说明结晶很好. 与LiFePO4的标准XRD图谱(JCPDS 83-2092)完全一致, 没有观察到明显的杂质峰, 说明所合成的LiFePO4/C为单一的橄榄石结构. 图中也没有观察到晶态碳的衍射峰, 说明柠檬酸经过高温烧结后生成的碳最终以无定形的形式存在材料中. 由LiFePO4/C复合材料的晶胞参数(表1)可以看出, 包覆碳后, 晶胞体积随着碳包覆量的增加逐渐下降; 相对于未包覆的LiFePO4, LiFePO4/C复合材料各主要衍射峰的半峰宽基本上呈增加趋势, 且各衍射峰的强度逐渐减弱. 根据谢乐公式, 峰的宽化说明晶粒尺寸的减小, 因此碳包覆可以减小晶粒尺寸, 达到细化颗粒的目的.由SEM照片(图2)可见, 所有样品的表面形貌无规则: 未包覆碳的LiFePO4颗粒较大, 平均粒径图1 不同碳包覆量的LiFePO4/C复合材料的XRD图谱Fig. 1 XRD patterns of LiFePO4/C coated with different car-bon contents第3期张冬云, 等: 碳包覆LiFePO 4的结构与性能研究 267表1 不同碳包覆量的LiFePO 4/C 复合材料的晶胞参数及XRD 半峰宽Table 1 Crystal parameters of LiFePO 4/C coated with different carbon contentsLattice parametersHalf-width of main diffraction peaks (FWHM/rad)carbon content/wt%a /nmb /nmc /nmV /nm 3 (101)(111) (211) (311) 0 1.03375 0.60079 0.469050.29131 0.148 0.156 0.1360.1454 1.03237 0.60007 0.469180.29065 0.128 0.179 0.1800.2066 1.03189 0.60028 0.468870.29043 0.150 0.270 0.2100.1738 1.02947 0.59970 0.469500.28985 0.168 0.207 0.2520.20510 1.02819 0.59787 0.469490.28861 0.225 0.312 0.2060.244图2 碳包覆LiFePO 4的SEM 照片Fig. 2 SEM images for LiFePO 4 coated with different carbon contents ((a)-(e) corresponding to carbon content: 0, 4wt%, 6wt%, 8wt% and 10wt%)为1μm 左右, 存在一定的团聚; 包覆碳后, 粒径变小, 颗粒大小分布趋向均匀, 二次粒子的大小在200~500nm 之间. 由图中还可以看出, 包覆碳后样品的表面形貌变得更加蓬松和粗糙, 这是由于柠檬酸高温分解生成的无定形碳均匀地包覆在颗粒表面, 而这种无定形碳颗粒本身就具有多孔粗糙的表面.对LiFePO 4/C 复合正极材料进行BET 研究, 测得比表面积分别为: 5.56、12.43、20.26、32.81、41.68 m 2/g. 样品的比表面积随着碳包覆量的增加而增大, 表明晶体尺寸的减小. BET 和XRD 、SEM 的研究结果相一致: 碳包覆可以有效地抑制晶粒团聚, 使粒径分布趋向均匀.2.2 LiFePO 4/C 复合材料的电化学性能研究图3为LiFePO 4/C 复合材料在0.1C 倍率下的第二次充放电曲线. 与LiFePO 4相比, LiFePO 4/C 的放电比容量均提高, 充放电平台延长, 且充放电平台间的电压差减小, 说明碳包覆样品在充放电过程中极化小, 电化学性能优良. 含碳量为8wt%样品的放电比容量最大为150.5mAh/g, 达到理论容量的89%, 比未包覆的LiFePO 4提高了将近40%的比容量, 且具有最低的充电平台电位(3.45V)和最高放电平台电位(3.41V), 两平台间的电位差最小, 说明极化最小, 具有优良的电化学性能. 但含碳量为10%的样品放电比容量有所下降, 仅为135.6 mAh/g, 说明碳含量并不是越大越好.图4为LiFePO 4/C 复合材料0.1C 放电时的循环性能. 可以看出, 包覆碳后材料的循环性能得到有效的改善. 随着碳包覆量的增加, LiFePO 4/C 的循环性能逐步提高, 当碳含量为8wt%时, LiFePO 4/C 放电比容量最高, 容量最稳定, 具有最好的循环性能,268无 机 材 料 学 报 第26卷图3 0.1C 倍率下, LiFePO 4/C 的第二次充放电曲线Fig. 3 Second charge and discharge curves of LiFePO 4/C at rate of 0.1C图4 0.1C 倍率下, LiFePO 4/C 的循环性能Fig. 4 Cycling performance of LiFePO 4/C at rate of 0.1C基本无衰减.由于碳含量为8wt%的LiFePO 4/C 复合材料在0.1C 下表现出优良的电化学性能, 对其进一步进行高倍率的电性能研究, 充电倍率仍为0.1C , 放电倍率依次为0.1C 、0.5C 、1.0C 、2.0C , 第二次充放电曲线及前50次循环性能图如图5、图6所示.由图5可以看出, 样品在0.1C 、0.5C 、1.0C 、2.0C 的放电平台电位分别为3.41、3.38、3.35、3.28V , 逐图5 LiFePO 4/C 在不同的放电倍率下的第二次充放电曲线 Fig. 5 Second charge and discharge curves of LiFePO 4/C at different discharge rates图6 LiFePO 4/C 在不同的放电倍率下的循环性能Fig. 6 Cycling performance of LiFePO 4/C at different dis-charge rates (8wt%C)渐降低, 说明随着放电倍率的提高, 电池极化增大,造成可逆容量的损失; 第二次放电比容量分别为150.5、136.3、129.9和111.2 mAh/g, 容量依次下降. 循环性能图表明, 充放电循环50次后, 容量保持率分别为100%、95.4%、94.4%和89.3%, 特别是在2.0C 的放电倍率下循环50次后容量仍有101.0 mAh/g, 表现出优良的循环性能.2.3 碳包覆膜的形态研究碳包覆能提高LiFePO 4的电化学性能, 理论上说, 进行C 包覆改性时, 应该使C 均匀分布在正极材料表面, 以便整个表面能同时进行Li 的嵌入和脱出, 从而提高电流密度. 为了进一步了解无定形碳在颗粒表面的存在状态, 对LiFePO 4/C 复合材料进行HR-TEM 和SAED 研究.图7为包覆前后的TEM 照片. 由图7(a)可以看出纯相的LiFePO 4的一次颗粒近似球形, 存在团聚现象, 一次颗粒的大小分布范围较宽, 粒径在130~ 400nm 之间. 由图7(b)可以看出, 碳在颗粒的表面和颗粒间形成网络结构, 因此可以认为是包覆在颗粒表面的具有良好导电性的碳膜使材料性能提高. 包覆在颗粒表面的碳使得LiFePO 4/C 的二次颗粒晶形不是很明显, 大小在50nm 左右, 说明在合成LiFePO 4/C 复合材料的过程中, 原位形成的碳包覆有效抑制了颗粒的长大, 同前面的XRD 、SEM 、BET 的研究结果一致.图8为LiFePO 4/C 的高分辨透射电镜(HRTEM)及选区电子衍射(SAED)测试结果. 图8(a)显示出晶格中的原子以点阵的方式有序的排列, 没有观察到位错、孪晶等一维和二维缺陷, 最外层包覆一层厚度在2nm 左右的非晶物质, 此非晶层与LiFePO 4晶体结合完好, 故其可能是包覆在LiFePO 4晶体表面的无定形碳膜层. HR-TEM 和SAED 数据表明晶格第3期张冬云, 等: 碳包覆LiFePO 4的结构与性能研究 269图7 LiFePO 4包覆C 前后的TEM 照片Fig. 7 TEM images of LiFePO 4 before and after C-coating(a) LiFePO 4; (b) LiFePO 4/C图8 LiFePO 4/C 的HRTEM 照片(a)及选区电子衍射图(b) Fig. 8 HRTEM images of LiFePO 4/C (a) and the correspond-ing SAED pattern (b)中晶体生长方向为晶面间距0.3918nm 和0.4245nm 的两个晶面, 分别对应于LiFePO 4的(210)和(101)面. 由(210)和(101)面求得的晶带轴指数为[121], 因此LiFePO 4/C 的优势生长为[121]方向. 张淑萍等[11]也采用TEM 和SAED 研究了溶剂热法合成的LiFePO 4与导电添加剂的关系, 合成了沿[201]方向生长的棒状的LiFePO 4晶体, 并认为取向机理可能在于添加剂对晶体生长的吸附阻止作用. 而本课题组前期研究表明掺Nb 磷酸铁锂晶体生长方向为[112][12], 说明合成工艺、C 包覆和掺杂后由于缺陷的不同而使晶体生长方向不同, 从而影响了微观结构和其电化学性能.2.4 交流阻抗谱分析Li 离子的扩散性能是涉及正极材料性能优劣的关键因素, 锂离子在LiFePO 4电池正极材料中的扩散过程包括正极材料内部的扩散迁移、正极材料和膜界面的传递以及表面膜中扩散. 可通过交流阻抗(ACI)分析研究碳包覆如何降低材料的极性, 从而提高Li 离子的扩散性能.图9中点为样品的交流阻抗图谱实验值, 曲线是根据充放电时电极中锂离子运动过程由图中所示等效电路用Zview 软件拟合的结果. 可以看到, 拟图9 交流阻抗谱拟合等效电路及图谱Fig. 9 AC Impedance spectra and equivalent circuit of ACI modeling合曲线能较好地与实验值吻合, 说明本文所选用的等效电路能较好地描述锂离子在LiFePO 4电极中的扩散过程, 拟合的误差较小. 该图反映了锂离子在LiFePO 4电池正极材料中的三个扩散过程: 高频区(略为压缩的半圆)为锂离子在表面膜中扩散, 中高频区反映活性物质和膜界面电荷传递, 低频区(线性部分)反映锂离子在正极材料内部的扩散. 可以看到包覆后的样品较纯LiFePO 4的明显变小, 表明扩散阻力的减小.表2是阻抗的拟合结果, 包括溶液电阻(R s )、电极表面膜的阻抗(C 1和R 1)、LiFePO 4颗粒与表面膜之间以及LiFePO 4颗粒与颗粒间的阻抗(用电极双层电容C dl 与电化学反应电阻R ct 描述)、锂离子在LiFePO 4颗粒内部扩散引起的电阻(用Warburg 电阻Z w 描述). R ct 在整个阻抗中所占比例最大, 纯LiFePO 4的提高一个数量级; 从钝化膜阻抗R 1来看, 改性不利于钝化膜的形成, 从而减少锂离子在电极表面迁移阻抗. 对包覆改性后的材料而言, 各种阻抗值均降低, 从而提高了材料的电子电导性、放电性能和循环性能.此外, 由于Warburg 阻抗代表的是电极反应过程中的锂离子的扩散过程, 此阻抗表现为低频区的一条与坐标成45°的直线, 因此可计算出包覆前后锂离子的扩散系数D [13]分别为 1.19×10−14和1.54×10−12, 包覆后的样品比纯LiFePO 4的提高了两个数量级, 这是因为碳包覆使正极材料粒径减小, 从而促进锂离子的内部扩散.表2 阻抗拟合结果Table 2 Fitting results of impedance parameters Sample R s / ΩC 1/μFR 1/ ΩC dl /μFR ct / ΩLiFePO 4/C 8.17 2.6015 10.57 5.262 49.70LiFePO 417.991.0915 93.162.857 669.9270 无机材料学报第26卷以上研究表明碳包覆提高了LiFePO4的电化学性能, 主要有两方面原因: 首先碳包覆可以在LiFePO4颗粒表面形成一种具有良好导电性的碳网络结构, 不仅加强颗粒间的接触, 而且提高了颗粒间的电导率, 为充放电过程中电子的传输提高良好的通道, 减少了极化, 从而提高充放电的可逆性. 其次, 均匀包覆在颗粒表面的碳膜层可以在晶体生长过程中抑制晶粒的长大, 减小晶粒尺寸, 细化颗粒, 增加材料的比表面积, 晶粒的减小可以缩短Li+的脱嵌路径, 提高Li+的扩散系数, 而且比表面的增大使材料与电解液接触面积增加, 从而扩大了Li+的相间扩散面积. 但碳含量过多时, 反而会引起材料性能的下降, 这是因为碳含量过大, 造成单位面积的正极片中活性物质LiFePO4的含量减少, 容量下降; 如果包覆在颗粒表面的碳膜过厚, 可能造成Li+在表面的扩散困难; 碳含量过大, 材料的比表面积过大会导致极片涂膜过程和充放电循环过程出现掉粉的现象, 导致循环性能变差; 同时碳含量的增加将使材料的振实密度降低, 也影响其实际应用. 因此碳的包覆量应控制在一定的程度.3结论以柠檬酸为碳源, 通过机械活化−高温固相法制备了不同碳包覆量的LiFePO4/C复合正极材料, 通过对不同碳包覆量的LiFePO4的结构、形貌和电性能的研究, 得到如下结论:1) 柠檬酸高温分解后生成无定形碳非晶物质, 在LiFePO4颗粒表面包覆形成一种网络结构, 抑制了晶体颗粒的长大, 比表面积随着碳含量的增加而增大. 碳的加入没有改变LiFePO4的橄榄石结构.2) 电化学性能研究表明, 碳包覆提高了材料的充放电性能和循环性能. 以碳理论含量8wt%为最佳, 太大反而降低电化学性能.3) 碳包覆影响了晶体的生长方向和微观结构, 从而影响其电化学性能, LiFePO4/C的优势生长为[121]方向.4) 交流阻抗分析表明包覆后锂离子扩散系数比纯的LiFePO4提高了两个数量级, 且各项阻抗值均降低, 从而提高了材料的离子及电子电导性、放电性能和循环性能. 参考文献:[1] Padhi A K, Nanjundaswamy K S, Goodenough J B. Phospho-olivines as positive-electrode materials for rechargeable lithium batteries. Journal of the Electrochemical Society, 1997, 144(4):1188−1194.[2] Chen G Y, Song X Y, Richardson T J. Electron microscopy studyof the LiFePO4 to FePO4 phase Transition. Electrochemical and Solid-State Letters, 2006, 9(6): A295−A298.[3] Kim D H, Kim T R, Im J S, et al. A new method to synthesize oli-vine phosphate nanoparticles. Physica Scripta, 2007, T129: 31−34.[4] 张培新, 文衍宣, 刘剑洪, 等(ZHANG Pei-Xin, et al). 化学沉淀法制备掺杂磷酸铁锂的结构和性能研究. 稀有金属材料与工程(Rare Metal Mat. Eng.), 2007, 36(6): 954−958.[5] 卢俊彪, 唐子龙, 张中太, 等(LU Jun-Biao, et al). LiFePO4材料的制备与电池性能的研究. 无机材料学报(Journal of Inorganic Materials), 2005, 20(3): 666−670.[6] Kim J K, Choi J W, Cheruvally G, et al. A modified mechanicalactivation synthesis for carbon-coated LiFePO4 cathode in lithiumbatteries. Materials Letters, 2007, 61(18): 3822−3825.[7] Dominko R, Bele M, Gaberscek M, et al. Impact of the carboncoating thickness on the electrochemical performance of LiFePO4/C compostites. Journal of the Electrochemical Society, 2005, 152(3): A607−A610.[8] Chung S Y, Blocking J T, Chiang Y M. Electronically conductivephospho-olivines as lithium storage electrodes. Nature Materials,2002, 1: 123−128.[9] 陈宇, 王忠丽, 于春洋, 等(CHEN Yu, et al). 掺杂Mo的LiFePO4正极材料的电化学性能. 物理化学学报(ActaPhys-Chem. Sin.), 2008, 24(8): 1498−1502.[10] Huang H, Yin S C, Nazar L F. Approaching theoretical capacity ofLiFePO4 at room temperature at high rates. Electrochem. Solid State Lett., 2001, 4(10): A170−A172.[11] 张淑萍, 倪江锋, 周恒辉, 等(ZHANG Shu-Ping, et al). 溶剂热法控制合成规则的LiFePO4颗粒. 物理化学学报(ActaPhys-Chem. Sin.), 2007, 23(6): 830−834.[12] 林木崇. 锂离子电池正极材料LiFePO4的掺杂缺陷及电化学性能的研究. 深圳: 深圳大学硕士论文, 2010.[13] Liu H, Cao Q, Fu L J, et al. Doping effects of zinc on LiFePO4cathode material for lithium ion batteries. Electrochemistry Com-munications, 2006, 8(10): 1553−1557.。
正极材料综述

Recent progress in cathode materials research for advanced lithium ion batteries Bo Xu,Danna Qian,Ziying Wang,Ying Shirley Meng*Department of NanoEngineering,University of California San Diego,La Jolla,CA92093,USAContents1.Introduction (51)yered compounds LiMO2 (52)3.Spinel compounds LiM2O4 (56)4.Olivine compounds LiMPO4 (57)5.Silicate compounds Li2MSiO4 (59)6.Tavorite compounds LiMPO4F (62)7.Borate compounds LiMBO3 (63)8.Conclusion (64)Acknowledgements (64)References (64)1.IntroductionWith the worldwide energy shortage being one of the mountingproblems in21st century,efforts have been made to replace thenon-renewable fossil fuels by other green energy sources,such assolar,wind,and hydroelectric power.Different from the conven-tional fossil fuels,most of these green energy sources suffer fromtheir uncontrollable and intermittent nature,therefore thedifficulty in energy storage and regulation results in larger cost.This brings in enormous amount of research interests in materialdevelopments for energy storage.The LIB system is regarded as oneof the near-term solutions because of its high energy density andrelatively simple reaction mechanism.Current LIB technology iswell developed for the portable electronic devices and has beenwidely used in the past twenty years.However,to be implementedin the large-scale high-power system such as the plug-in hybridelectric vehicle(PHEV)or plug-in electric vehicle(PEV),perfor-mance requirements are raised especially from the aspects ofenergy/power density,cycling life and safety issues,thereforefurther LIB material and system developments are necessary.The basic working principles of LIB are shown in Fig.1.A lithiumion battery can work as the energy storage device by convertingelectric energy into electrochemical energy.There are three keycomponents in a LIB system:cathode,anode and electrolyte.Fortoday’s commercialized LIB system,both cathode and anodematerials are intercalation materials.The transition metal oxidesin cathode(graphite in anode)consist of a largely unchangeablehost with specific sites for Li ions to be intercalated in.All Li ionsMaterials Science and Engineering R73(2012)51–65A R T I C L E I N F OArticle history:Available online5June2012A B S T R A C TNew and improved materials for energy storage are urgently required to make more efficient use of ourfinite supply of fossil fuels,and to enable the effective use of renewable energy sources.Lithium ionbatteries(LIB)are a key resource for mobile energy,and one of the most promising solutions forenvironment-friendly transportation such as plug-in hybrid electric vehicles(PHEVs).Among the threekey components(cathode,anode and electrolyte)of LIB,cathode material is usually the most expensiveone with highest weight in the battery,which justifies the intense research focus on this electrode.In thisreview,we present an overview of the breakthroughs in the past decade in developing high energy highpower cathode materials for lithium ion batteries.Materials from six structural groups(layered oxides,spinel oxides,olivine compounds,silicate compounds,tavorite compounds,and borate compounds)arecovered.We focus on their electrochemical performances and the related fundamental crystalstructures,solid-state physics and chemistry are covered.The effect of modifications on both chemistryand morphology are discussed as well.ß2012Elsevier B.V.All rights reserved.*Corresponding author.E-mail address:shirleymeng@(Y.S.Meng).Contents lists available at SciVerse ScienceDirectMaterials Science and Engineering Rj ou r n a l h o m e p a g e:w w w.e l s e v i e r.co m/l oc a t e/m s e r0927-796X/$–see front matterß2012Elsevier B.V.All rights reserved./10.1016/j.mser.2012.05.003are in the cathode sides initially and the battery system is assembled in ‘‘discharged’’status.While charging,Li ions are extracted from the cathode host,solvate into and move through the non-aqueous electrolyte,and intercalate into the anode host.Meanwhile,electrons also move from cathode to anode through the outside current collectors forming an electric circuit.The chemical potential of Li is much higher in the anode than in the cathode,thus the electric energy is stored in the form of (electro)chemical energy.Such process is reversed when the battery is discharging where the electrochemical energy is released in the form of electric energy.The cathode region and anode region are separated by the separator,a micro-porous membrane that allows the electrolyte to penetrate and prevent shorting between the two electrodes.The electrolyte should be ionically conducting and electronically insulating in principle,however the actual properties of the electrolyte is much more complicated.During the first cycle,a so-called solid–electrolyte-interphase (SEI)layer will be formed on the surface of electrodes due to the decomposition of organic electrolyte at extreme voltage range (typically <1.2V or >4.6V).In current LIB technology,the cell voltage and capacities are mainly determined by the cathode material that is also the limiting factor for Li transportation rate.The developments of cathode materials therefore become ex-tremely crucial and receive much attention in recent decade.Since 1980when the LiCoO 2was demonstrated firstly as a possible cathode material for rechargeable lithium battery [1],the transition metal intercalation oxides have caught the major research interests as the LIB cathodes [2–8].Categorized by structure,the conventional cathode materials include layered compounds LiMO 2(M =Co,Ni,Mn,etc.),spinel compounds LiM 2O 4(M =Mn,etc.),and olivine compounds LiMPO 4(M =Fe,Mn,Ni,Co,etc.).Most of the researches are performed on these materials and their derivatives.New structure intercalation materials such as silicates,borates and tavorites are also gaining increasing attentions in recent years.During the materials optimization and development,following designing criterions are often considered:(1)energy density;(2)rate capability;(3)cycling performance;(4)safety;(5)cost.The energy density is determined by the material’s reversible capacity and operating voltage,which are mostly determined by the material intrinsic chemistry such as the effective redox couples and maximum lithium concentration in active materials.For rate capability and cycling performances,electronic and ionic mobilities are key determining factors,though particle morphologies are also important factors due to the anisotropic nature of the structures and are even playing a crucial role in some cases.Therefore materials optimizations are usually made from two important aspects,to change the intrinsic chemistry and to modify the morphology (surface property,particle size,etc.)of the materials.Fig.2compares the gravimetricenergy densities of different cathode materials that are currently under investigations.While some materials such as LiFeBO 3and LiFeSO 4F are already approaching their theoretical energy densi-ties,for other materials including conventional layered and spinel compounds,significant gaps are still present between their theoretical and practical energy densities.The materials with promising theoretical properties have high potentials as the candidates of future generation LIB cathode,therefore are under intensive studies.For certain materials such as the LiFePO 4olivine,significant property improvements have been achieved during the past decade with assistance of newly developed technologies.To review and summarize those researches could provide inspiring perspectives for further material optimizations.In this review,we will discuss the recent research progress in the past decade of different cathode materials following the structural category,and modifications on both chemistry and morphology will be discussed.yered compounds LiMO 2The ideal structure of layered compound LiMO 2is demonstrat-ed in Fig.3.The oxygen anions (omitted for clarity in the figures)form a close-packed fcc lattice with cations located in the 6-coordinated octahedral crystal site.The MO 2slabs and Li layers are stacked alternatively.Although the conventional layered oxide LiCoO 2has been commercialized as the LIB cathode for twenty years,it can only deliver about 140mAh/g capacity which is half of its theoretical capacity.Such limitation can be attributed to the intrinsic structural instability of the material when more thanhalfFig.1.Working principles of LIB(charging).Fig.2.Theoretical and practical gravimetric energy densities of different cathodematerials.Fig.3.Crystal structure of layered LiMO 2(blue:transition metal ions;red:Li ions).(For interpretation of the references to color in this figure legend,the reader is referred to the web version of the article.)B.Xu et al./Materials Science and Engineering R 73(2012)51–6552of the Li ions are extracted.On the other hand,the presence of toxic and expensive Co ions in LiCoO 2has introduced the environmental problem as well as raised the cost of the LIB.The research focusing on layered compounds,therefore have moved from LiCoO 2to its derivatives in which Co ions are partially/fully substituted by more abundant and environmental friendly transition metal ions,such as Ni and Mn.The approaches include mixing the LiNiO 2and LiMnO 2with 1:1ratio,forming layered LiNi 0.5Mn 0.5O 2,and the formation of Li–Co–Ni–Mn–O layered compound (so-called NMC type materials).Good electrochemical data of LiNi 0.5Mn 0.5O 2was firstly reported in 2001by Ohzuku et al.[9].Fig.4shows the typical electrochemical performance of LiNi 0.5Mn 0.5O 2[10,11].The charge/discharge voltages of this material are around 3.6–4.3V where Ni 2+/Ni 4+act as the redox couple as confirmed from in situ X-ray absorption spectroscopy (XAS)study [12].Various methods including X-ray and neutron diffraction,nuclear magnetic reso-nance (NMR)spectroscopy,transmission electron microscope (TEM)and first-principles calculations [13–18]have been per-formed to investigate the structural change and local cation distribution of this material.The results showed that different from classic layered material composed of pure Li layer and pure MO 2slab,8–10%Ni ions are usually found in the Li layer of LiNi 0.5Mn 0.5O 2synthesized by solid state or sol–gel synthesis methods.It was suggested that such Li–Ni interlayer mixing might be partially reduced under high voltage (>4.6V)[19,20].For the transition metal layer,a flower-like in-plane cation ordering that Li in transition metal layer is surrounded sequentially by Mn rings and Ni rings was suggested by first-principles calculations [16]and confirmed by experiments [14,15].With MO 2slabs pined together by the Ni in Li layer,larger reversible capacity ($200mAh/g)can be obtained in LiNi 0.5Mn 0.5O 2at low rate (C /20)with little capacity fading even after 100cycles,therefore the energy density can be significantly improved.The material structure is thermally stable until $3008C,above which oxygen release and material decom-position would occur [21].Structural change including migration of transition metal ions to Li layer at high temperature was also reported both experimentally and computationally [21,22].However,with large amount of un-removable Ni in the Li layers blocking Li diffusion pathways,the Li mobility of the materials is negatively affected.The Li diffusion coefficient in LiNi 0.5Mn 0.5O 2is reported to be lower than that in LiCoO 2by one magnitude of order [23],resulting in the low rate capability of LiNi 0.5Mn 0.5O 2.It was also reported by Kang et al.[11]that the Li–Ni exchange is reduced to $4%in LiNi 0.5Mn 0.5O 2synthesized by ion-exchange method,therefore the rate capability can be significantly improved (shown in Fig.4(c)and (d)).Considering the Li–Ni disorder being major factor affecting the material rate capability,attempts to create new compounds of LiCo x Ni y Mn 1Àx Ày O 2are motivated.While good electrochemical performance of LiCo 1/3Ni 1/3Mn 1/3O 2was already reported in 2001by Ohzuku et al.[24],the importance of the series of Li–Co–Ni–Mn–O material is more recognized as the presence of Co ions can help to reduce the amount of defect Ni in Li layer.The LiCo 1/3Ni 1/3Mn 1/3O 2layer compound can be regarded as the solid solution of LiCoO 2,LiNiO 2and LiMnO 2.LiCo 1/3Ni 1/3Mn 1/3O 2deliver similar reversible capacity with LiNi 0.5Mn 0.5O 2.Their voltage profile are also similar in shape,but the operation voltage window of LiCo 1/3Ni 1/3Mn 1/3O 2can be extended to 3.6–4.7V.The material’s typical electrochemical performance is shown in Fig.5[25,26].With additional Co ions existing in the structure,the Li–Ni interlayer mixing can be much reduced to 1–6%[27–32].Though certain superlattice in the transition metal layer could be obtained from computations [33,34],diffraction [28]and NMR study [35],it is suggested that only short-range ordering can be found and Li in transition metal layer is surrounded primarily by Mn ions.The changes of transition metal valence state following cycling were investigated experimentally and computationally [29,30,32,34–36].In general,it is believed that,the transition metal ions are oxidized in sequence of Ni 2+to Ni 3+,Ni 3+to Ni 4+,Co 3+to Co4+Fig.4.Performance of layered LiNi 0.5Mn 0.5O 2:(a)compositional phase diagram,(b)cycling performance [10],(c)rate performance of LiNi 0.5Mn 0.5O 2synthesized by ion-exchange method [11],and (d)rate performance of LiNi 0.5Mn 0.5O 2synthesized by solid state method [11].B.Xu et al./Materials Science and Engineering R 73(2012)51–6553during charging.Mn 4+ions keep unchanged.However,due to the overlap of oxygen 2p band M 3+/4+band,at the end of charging,part of the electrons were also removed from the oxygen ions,causing possible oxygen release at high charging voltages (>4.5V).As shown in Fig.5(c)and (d),with certain morphology modifications,$90%of the capacity can be retained after 200cycles at room temperature and 84%of the capacity can be retained when at a discharge rate as high as 20C [26].The LiCo 1/3Ni 1/3Mn 1/3O 2compound also shows relatively good performance at elevated temperatures.It was reported [25]that more than 80%capacity can be retained at 558C and half of the capacity can still be achieved when the operation temperature is raised to 958C.We also want to point out that the reduced amount Co can be used to achieve the same benefits.It has been shown by Li et al.that as little as 20%Co (LiCo 0.2Ni 0.4Mn 0.4)can lead to excellent electrochemical perfor-mance [37].While the introduction of Co ions into LiNi 0.5Mn 0.5O 2could improve the material stability,the introduction of extra Li ions,on the other hand,could improve the material capacity.A series of Li-rich layered oxides Li[Li 1/3À2x /3Ni x Mn 2/3Àx /3]O 2therefore are created by making a compound between Li 2MnO 3and LiNi 0.5Mn 0.5O 2to achieve higher capacity beyond the limitation of one Li ion per MO 2formula.With excess Li ions introduced,the theoretical capacity of this series of materials can be increased to more than 300mAh/g.The compound Li[Li 1/9Ni 1/3Mn 5/9]O 2is firstly reported by Lu et al.in 2001[17].Different compositions (x =1/6,1/5,1/4,1/3,5/12,etc.)of this series of materials were studied by the same group later and similar electrochemical performances were shown [38].For this series of materials,the pristine samples are solid solutions phase following the layered structure in general,although it was reported that some of the spinel feature might be observed when x !1/3being heated to 6008C [38].The Li–Ni interlayer mixing is usually much smaller than that in LiNi 0.5Mn 0.5O 2.With x increasing,the Li–Ni interlayer mixing also increases while the c/a ratio decreases.The structuredetails and cation ordering were investigated by diffraction,TEM,NMR spectroscopy and first-principles calculations [39–44].The results show that the excess Li ions were located in the transition metal layer,surrounded mostly by 5or 6Mn 4+ions.The Ni/Mn zigzag ordering is regarded as another competent driving force for the in-plane cation ordering,and actual material may reflect a combination of these two orderings [39].A typical voltage profile of Li[Li 1/9Ni 1/3Mn 5/9]O 2for the first cycle charging is shown in Fig.6(c)[45].It is composed of a slopy region from the open circuit voltage to $4.5V followed by a plateau region between 4.5V and 4.6V.Such plateau,however,does not appear in following cycles causing a large first cycle irreversible capacity.While it is generally agreed that the slopy region originates from the oxidization of Ni 2+to Ni 4+,the mechanism of the anomalous high voltage capacity is still under debate.Several studies [46–48]proposed the mecha-nism of oxygen loss accompanied by Li removal,while other studies proposed the mechanisms involving surface reaction with electrode/electrolyte reduction [49]and/or hydrogen exchange [50].This series of materials deliver the highest reversible capacity (>250mAh/g)for current intercalation cathode materials,but only with low rate (C /50).It was reported that the excess Li ions in transition metal layer are electrochemically active and will migrate to Li layer becoming stable tetrahedral ions during cycling [44,51].Recent study [44]also suggested a possible layer to defective-spinel phase transformation happening near the material surface with significant migration of transition metal ions to lithium layer.These un-removable ions in lithium layer will block the lithium diffusion pathways,therefore may be one of the reasons that cause the low Li chemical diffusion coefficient in the plateau region [43]and the intrinsic poor rate capability of this series of materials.A recent study also suggested that the material rate and temperature performances may also be affected by the particle size as shown in Fig.6[45].For the Li-rich layered compounds,the surface characteristics can significantly affect the material electrochemicalperformanceFig.5.Performance of layered LiCo 1/3Ni 1/3Mn 1/3O 2:(a)compositional phase diagram,(b)cycling performance at elevated temperatures [25],(c)cycling performance at room temperature [26],and (d)rate performance at room temperature [26].B.Xu et al./Materials Science and Engineering R 73(2012)51–6554especially for thefirst-cycle irreversible capacity and rate capability.Different surface modifications,therefore,have been applied to this series of materials as further optimizations. Common coating materials applied include different types of metal oxides(Al2O3,Nb2O5,Ta2O5,ZrO2,and ZnO)[52–54],metal fluorides AlF3[55,56]and other polyanion compounds such as MPO4(M=Al,Co)[57,58].A systematic research on the metal oxides coating was performed by Myung et al.in2007[54].The area-specific impedance(ASI)results showed that during thefirst cycle,the ASI of un-coated samples dramatically increased,while the ASI of all the coated samples hardly changed.The cycling performance and rate capabilities of the materials were improved especially when coated with Al2O3.It was claimed that during the initial cycling,the oxide coating reacted with the electrolyte forming a solid stablefluoride layer which protected the active materials from further HF scavenger.Similar effect was also reported for AlF3coating[56].In addition,the coating can suppress the oxygen loss occurred in the active materials,therefore can also improve the material thermal stability[55,59].The coating morphology is also under optimization.Double layer coating combing two or more coating materials has been developed as well [58].Another approach involves the construction of composition gradient from surface to bulk and forming core–shell structured particles.The structure is shown in Fig.7[60].By introducing composition gradient,the performance of‘‘core’’active materials can be maintained,while the less active‘‘shell’’materials can actasFig.7.Demonstration of core–shell structured particles[60].Fig.6.Performance of layered Li[Li1/3À2x/3Ni x Mn2/3Àx/3]O2:(a)compositional phase diagram,(b)cycling performance(x=1/3)[45],and(c)rate and temperature performance (x=1/3)[45].B.Xu et al./Materials Science and Engineering R73(2012)51–6555a buffer layer and help improve the material performance in surface.Although there are general hypotheses of why the coating materials can improve the active materials’performance,the detailed mechanism is still unknown and under intensive investigations.In summary,the layered oxides LiMO2can deliver high capacities after activation at high voltages,therefore leading to promising energy densities.However,their practical reversible capacities are usually limited by the intrinsic structural instability at low lithium concentrations and high voltages,causing reduced efficiency of the active material utilization.Besides,for the cobalt-free lithium nickel manganese oxides,the intrinsic low rate capability has become the bottleneck problem impeding the commercialization of these materials.3.Spinel compounds LiM2O4The structure of LiM2O4spinel is shown in Fig.8.The oxygen framework of LiM2O4is the same as that of LiMO2layered structure.M cations still occupy the octahedral site but1/4of them are located in the Li layer,leaving1/4of the sites in transition metal layer vacant.Li ions occupy the tetrahedral sites in Li layer thatshare faces with the empty octahedral sites in the transition metal layer.The structure is based on a three-dimensional MO2host and the vacancies in transition metal layer ensure the three-dimensional Li diffusion pathways.The spinel LiMn2O4was proposed as the cathode of the lithium ion battery by Thackeray et al.in1983[61–63],but the material was found to encounter sever capacity fading problem.Two reasons have been considered as the main sources for the capacity fading:(1)dissolution of Mn2+ into the electrolyte generated by the disproportional reaction2 Mn3+!Mn4++Mn2+[64,65]and(2)generation of new phases during cycling and the related micro-strains[64,66].Substituting Mn with other metal ions has been used as an important approach to improve cycling performance of spinel materials.Multiple dopants including inactive ions such as Mg,Al,and Zn[67–69],first row transition metal ions such as Ti,Cr,Fe,Co,Ni,and Cu[70–74] and rare earth metal ions such as Nd and La[75–77]have been investigated and LiNi0.5Mn1.5O4shows the best overall electro-chemical performances among the above.LiNi0.5Mn1.5O4follows the spinel structure of LiMn2O4where Ni ions are located in the sites of Mn ions originally.With different synthesis conditions[78,79],LiNi0.5Mn1.5O4could possess two different structural symmetries,the ordered structure with space group P4332and the disordered structure with space group Fd¯3m. In ordered LiNi0.5Mn1.5O4,Ni ions occupy4b sites and Mn ions occupy12d sites forming an ordered pattern,while in disordered LiNi0.5Mn1.5O4,Ni and Mn ions are randomly distributed in16d sites.In stoichiometric LiNi0.5Mn1.5O4,the valence of Ni ions is2+ pushing all Mn ions to Mn4+.Comparing to LiMn2O4spinel,the redox couple of LiNi0.5Mn1.5O4is switched from Mn3+/Mn4+to Ni2+/ Ni4+and the voltage is lifted from 4.1V to 4.7V.Such high discharge voltage not only enlarges the energy density but also makes the material capable to be coupled with anode materials which have better safety but relatively higher voltage(Li4Ti5O12, etc.).However,phase-pure LiNi0.5Mn1.5O4is difficult to synthesize because impurities such as nickel oxides and/or lithium nickel oxides usually exist[78,80,81].As an alternative approach,the off-stoichiometric material LiNi0.5Mn1.5O4Àx which adopts the disor-dered structure is synthesized and the performances of these two materials are compared in Fig.9[71].InLiNi0.5Mn1.5O4Àx,there are small amount of Mn3+ions exist as the charge compensation of oxygen loss.The small voltage plateau$4V for LiNi0.5Mn1.5O4Àx therefore is attributed to the Mn3+/Mn4+couple.Different from other doped spinel materials the voltage profile of which is usually composed of two distinct plateaus,there is only oneflat plateauatFig.9.Performance of high voltage spinel LiNi0.5Mn1.5O4[71].Fig.8.Crystal structure of spinel LiM2O4(blue:transition metal ions;red:Li ions).(For interpretation of the references to color in thisfigure legend,the reader isreferred to the web version of the article.)B.Xu et al./Materials Science and Engineering R73(2012)51–6556$4.7V for LiNi0.5Mn1.5O4,although in LiNi0.5Mn1.5O4Àx,a small voltage step appears at half lithium concentration.The theoretical capacity of LiNi0.5Mn1.5O4is calculated as147mAh/g,and more than140mAh/g reversible capacity can be obtained experimen-tally.With most of the Mn ions keeping Mn4+unchanged during cycling,both ordered and disordered LiNi0.5Mn1.5O4exhibit good cycling performance for lower rate capability that there is little capacity fading after50cycles in room temperature(Fig.9(a)and (c))and elevated temperature(Fig.9(e)).However,their rate capabilities still need to be improved.The disordered LiNi0.5Mn1.5O4Àx shows better rate capability than ordered LiNi0.5Mn1.5O4for the material electronic conductivity is enhanced with the small amount of Mn3+ions.The ionic conductivity is regarded as another rate-limiting factor.The Li diffusion coefficient of LiNi0.5Mn1.5O4was reported to in a wide range between 10À10cm2/s and10À16cm2/s depending on different compositions and material morphologies[82–84].As a further optimization approach,doping small amount of metal ions into LiNi0.5Mn1.5O4forming‘‘bi-doped’’spinel has been widely used,and certain properties of LiNi0.5Mn1.5O4can be further improved.A recent review paper by Yi et al.[85]has summarized the effect of different doping ions including both cation substitu-tion and anion substitution.By doping other transition metal ions such as Fe,Cr,and Ti into ordered LiNi0.5Mn1.5O4,the impurity phases may be limited and the cation disorder could be enhanced [86–90].It was also reported that dopant such as Co and Cu may enhance the material electronic conductivity and/or lithium diffusion coefficient[91,92].These enhancements therefore could further improve the material cycling performance and rate capability(Fig.10[92]).The doped bivalence metal ions such as Cumay also shift the4.7V plateau to even higher voltage therefore could further improve the energy density.However,for most of the dopants,the high voltage capacity is shortened and the overall reversible capacity is reduced.It was reported[86,92,93]that some doped ions such as Fe,Cu,Al,and Mg tend to occupy the tetrahedral sites and become inactive ions,not only reducing the capacity but also blocking the lithium diffusion pathways,therefore may impose a negative effect to the material performances.Apart from chemistry modification,size minimization is also reported as an effective approach to improve the material rate capability.Highly crystalline LiNi0.5Mn1.5O4nano-sized particles can be successfully synthesized through different methods [79,94,95].It was shown that the bulk properties of nano-sized particles are generally the same compared to micro-sized particles, although their surface areas increase causing increasing surface reactions.However,the small size shortened the Li diffusion length inside the active materials thus largely enhanced the material ionic conductivity.The rate capability of the nano-sized LiNi0.5Mn1.5O4 therefore is highly improved as an overall effect[95,96].It is important to point out the volumetric energy density(Wh/L) suffers from the size minimization greatly as most of the nano-sized materials do not have the optimized packing scheme yet.In summary,the high voltage spinel material is promising due to its high energy density,perfect structural stability and good cycling performance under certain material modifications.The high voltage,however,is out of the voltage window of the current electrolyte,therefore causes the electrolyte decomposition and the formation of unstable SEI on composite cathode side during cycling.It is important to point out that the reversible capacity of this material is currently limited to0.5Li per MO2formula,which although is similar to the practical capacity of LiCoO2,still is significantly lower comparing to the lithium nickel manganese layered compounds.4.Olivine compounds LiMPO4Despite the early works back in1980s[97,98],intensive studies on polyanion materials have not been conducted until recent fifteen years.These materials are receiving growing attentions because of the inherent stability of the polyanion group,which can delay or minimize the oxygen loss happening in traditional layer and spinel oxides.Among all polyanion materials,olivine LiFePO4attracts the most interests due to its excellent electrochemical properties,as well as its low cost,non-toxicity,excellent thermal stability and environment friendliness.It wasfirst found by Goodenough and coworkers in1997[99,100].The structure of LiFePO4is shown in Fig.11.It contains slightly distorted hcp anion oxygen arrays with half of the octahedral sites occupied by Fe and one eighth by Li.The LiO6octahedra are edge-shared while the FeO6octahedra are corner-shared.Both of the LiO6and FeO6run parallel to the c axis and they alternate in the b direction.The a–c planes containing the Li atoms are bridged by PO4tetrahedral.Three different paths of Li diffusion were proposed[101,102]and computational studies suggested that the one along b axis is much more favored than other paths across the channels[101,102].In addition,in2008 Yamada et al.further confirmed from experiments that the Li ion diffusion path along the(010)is a curved one dimensionchainFig.11.Crystal structure of olivine LiMPO4(blue:transition metal ions;yellow:P ions;red Li ions).(For interpretation of the references to color in thisfigure legend, the reader is referred to the web version of thearticle.)Fig.10.Rate performance of rate capability of LiNi x Cu y Mn2ÀxÀy O4[92].B.Xu et al./Materials Science and Engineering R73(2012)51–6557。
LiFePO4-C_composite_with_network_connections_of_nano-carbon_wires
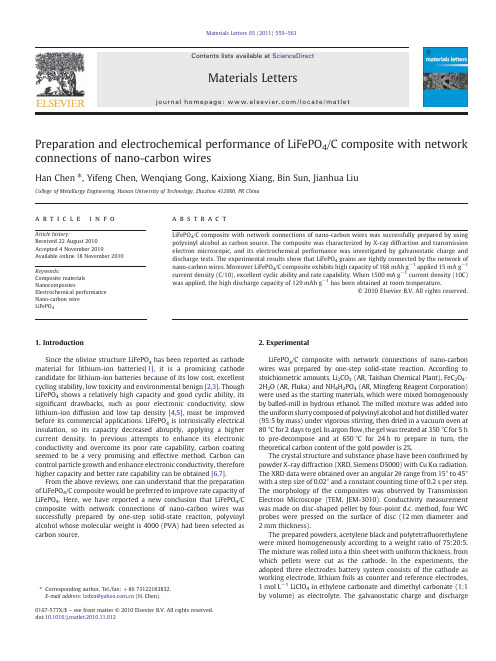
Preparation and electrochemical performance of LiFePO 4/C composite with network connections of nano-carbon wiresHan Chen ⁎,Yifeng Chen,Wenqiang Gong,Kaixiong Xiang,Bin Sun,Jianhua LiuCollege of Metallurgy Engineering,Hunan University of Technology,Zhuzhou 412000,PR Chinaa b s t r a c ta r t i c l e i n f o Article history:Received 22August 2010Accepted 4November 2010Available online 18November 2010Keywords:Composite materials NanocompositesElectrochemical performance Nano-carbon wire LiFePO 4LiFePO 4/C composite with network connections of nano-carbon wires was successfully prepared by using polyvinyl alcohol as carbon source.The composite was characterized by X-ray diffraction and transmission electron microscopic,and its electrochemical performance was investigated by galvanostatic charge and discharge tests.The experimental results show that LiFePO 4grains are tightly connected by the network of nano-carbon wires.Moreover LiFePO 4/C composite exhibits high capacity of 168mAh g −1applied 15mA g −1current density (C/10),excellent cyclic ability and rate capability.When 1500mA g −1current density (10C)was applied,the high discharge capacity of 129mAh g −1has been obtained at room temperature.©2010Elsevier B.V.All rights reserved.1.IntroductionSince the olivine structure LiFePO 4has been reported as cathode material for lithium-ion batteries [1],it is a promising cathode candidate for lithium-ion batteries because of its low cost,excellent cycling stability,low toxicity and environmental benign [2,3].Though LiFePO 4shows a relatively high capacity and good cyclic ability,its signi ficant drawbacks,such as poor electronic conductivity,slow lithium-ion diffusion and low tap density [4,5],must be improved before its commercial applications.LiFePO 4is intrinsically electrical insulation,so its capacity decreased abruptly,applying a higher current density.In previous attempts to enhance its electronic conductivity and overcome its poor rate capability,carbon coating seemed to be a very promising and effective method.Carbon can control particle growth and enhance electronic conductivity,therefore higher capacity and better rate capability can be obtained [6,7].From the above reviews,one can understand that the preparation of LiFePO 4/C composite would be preferred to improve rate capacity of LiFePO 4.Here,we have reported a new conclusion that LiFePO 4/C composite with network connections of nano-carbon wires was successfully prepared by one-step solid-state reaction,polyvinyl alcohol whose molecular weight is 4000(PVA)had been selected as carbon source.2.ExperimentalLiFePO 4/C composite with network connections of nano-carbon wires was prepared by one-step solid-state reaction.According to stoichiometric amounts,Li 2CO 3(AR,Taishan Chemical Plant),FeC 2O 4·2H 2O (AR,Fluka)and NH 4H 2PO 4(AR,Mingfeng Reagent Corporation)were used as the starting materials,which were mixed homogenously by balled-mill in hydrous ethanol.The milled mixture was added into the uniform slurry composed of polyvinyl alcohol and hot distilled water (95:5by mass)under vigorous stirring,then dried in a vacuum oven at 80°C for 2days to gel.In argon flow,the gel was treated at 350°C for 5h to pre-decompose and at 650°C for 24h to prepare in turn,the theoretical carbon content of the gold powder is 2%.The crystal structure and substance phase have been con firmed by powder X-ray diffraction (XRD,Siemens D5000)with Cu K αradiation.The XRD data were obtained over an angular 2θrange from 15°to 45°with a step size of 0.02°and a constant counting time of 0.2s per step.The morphology of the composites was observed by Transmission Electron Microscope (TEM,JEM-3010).Conductivity measurement was made on disc-shaped pellet by four-point d.c.method,four WC probes were pressed on the surface of disc (12mm diameter and 2mm thickness).The prepared powders,acetylene black and polytetra fluorethylene were mixed homogeneously according to a weight ratio of 75:20:5.The mixture was rolled into a thin sheet with uniform thickness,from which pellets were cut as the cathode.In the experiments,the adopted three electrodes battery system consists of the cathode as working electrode,lithium foils as counter and reference electrodes,1mol L −1LiClO 4in ethylene carbonate and dimethyl carbonate (1:1by volume)as electrolyte.The galvanostatic charge and dischargeMaterials Letters 65(2011)559–561⁎Corresponding author.Tel./fax:+8673122183832.E-mail address:lzdxn@ (H.Chen).0167-577X/$–see front matter ©2010Elsevier B.V.All rights reserved.doi:10.1016/j.matlet.2010.11.012Contents lists available at ScienceDirectMaterials Lettersj o u r na l ho m e p a g e :w w w.e l s ev i e r.c o m /l o c a t e /m a t l e ttests were carried out by BT-2000,Arbin instrument between2.5Vand4.1V at various current densities at room temperature.3.Results and discussionFig.1shows the XRD pattern of the prepared powders.All diffraction peaks can be indexed as the ordered orthorhombic crystal structure,No carbon diffraction peak is detected,indicating that the residual carbon is amorphous.The XRD result demonstrates that the polyvinyl alcohol can be used as carbon source to prepare single-phased LiFePO4materials. The carbon content in LiFePO4/C composite is only1.93%in weight tested by C/S analyzer.The electronic conductivity of the LiFePO4/C composite is about9×10−3S cm−1at room temperature,of which the value is much higher than that of bare LiFePO4(10−8S cm−1).Carbon plays a significantly important role to enhance the electronic conduc-tivity and control the particle size of LiFePO4,but residual carbon will reduce the energy density of composite.Therefore the above carbon content is just compromise between electronic conductivity and energy density.Fig.2illustrates representative TEM and HRTEM images of LiFePO4/C composite.LiFePO4grains and nano-carbon wires can be clearly distinguished in Fig.2.There are some interesting network interconnects,formed by nano-carbon wires,between LiFePO4grains. During the synthesis process,the continuous carbon chains of polyvinyl alcohol were carbonized into nano-carbon wires,which interlace excellent network interconnects.The nano-carbon network interconnects between LiFePO4grains can prevent overgrowth and adhesive aggregation of LiFePO4particles.Moreover they can lead to the electronic inter-particle connection,so the electronic conductivity of LiFePO4is improved significantly.The uniform andfine LiFePO4 grains connected by a network of nano-carbon wires increase the diffusivity of lithium-ion and contract the distance of lithium-ion diffusion during the process of charge and discharge.The discharge curves of LiFePO4/C composite at various rates are shown in Fig.3.Applying15mA g−1current(C/10),the initial discharge specific capacity of the composite are168mAh g−1,much higher than that of bare LiFePO4(121mAh g−1,A1),and there is no capacity loss through20cycles.In our experiment,various rates were applied at room temperature.With the increasing of current density,the discharge flats shorten and its potentials decrease gradually because of the rise of electrochemical polarization.When1500mA g−1current density(10C) was applied,the discharge capacity of LiFePO4/C composite with excellent network connections of nano-carbon wires is129mAh g−1,muchhigherFig.2.TEM(a)and HRTEM(b)images of LiFePO4/C composite.(a)LiFePO4grains can be clearly distinguished,the arrows shows the network of nano-carbon wires.(b)The nano-carbon wires can be distinguished more clearly at the arrowed-point.1520253035404530060090012001500321112221121421113131211111122Intensity(a.u.)2θ(°)11Fig.1.XRD pattern of olivine type LiFePO4/C composite.Voltage(V)Discharge capacity (mAhg-1)Fig.3.Discharge curves of LiFePO4/C composite at various rates,the discharge curves ofbare LiFePO4(A)and LiFePO4/C composite without network connections of nano-carbon wires(B)are inserted,(A1:sample discharges at C/10rate,A2and B:sample Aand B discharge at10C rate).560H.Chen et al./Materials Letters65(2011)559–561than those of bare LiFePO4(13mAh g−1,A2)and LiFePO4/C composite without network connections(81mAh g−1,B).Its capacity retention was over90%through400cycles under10C rate.The poor rate capability of LiFePO4can be overcome by preparation of LiFePO4/C composite with excellent network connections of nano-carbon wires.The network of nano-carbon wires provides the multi-dimension channels of electron diffusion for their rapid transportation and absorbed more electrolyte to speed the diffusion of lithium-ion.Moreover the carbon network is tightly integrated with LiFePO4grains so that they cannot be separated at high rate.Therefore the superior cyclic ability and rate capability can be attributed to the excellent network of nano-carbon wires.4.ConclusionsLiFePO4/C composite with the network connections of nano-carbon wires was successfully prepared by using polyvinyl alcohol as carbon source.It exhibits ordered olivine structure,perfect morphology and excellent electrochemical performance.Above all,the construction of the excellent network connections of nano-carbon wires between LiFePO4grains is an effective method of improving the electrochemical performance of LiFePO4.AcknowledgementsThis work was supported by the Project of Science and Technology of Hunan Province(2010GK3152)and the Education Department of Hunan Province(09B024).References[1]Padhi AK,Nanjundaswamy KS,Goodenough JB.J Electrochem Soc1997;144:1188–94.[2]Kwon SJ,Kim CW,Jeong WT,Lee KS.J Power Sources2004;137:93–9.[3]Gao F,Tang ZY.Electrochim Acta2008;53:5071–5.[4]Jin EM,Jin B,Jun DK,Park KH,Gua HB,Kim KW.J Power Sources2008;178:801–6.[5]Ni JF,Zhou HH,Chen JT,Zhang XX.Mater Lett2007;61:1260–4.[6]Cho YD,Feya GTK,Kao HM.J Power Sources2009;189:256–62.[7]Zhang WK,Zhou XZ,Tao XY,Huang H,Gan YP,Wang CT.Electrochim Acta2010;55:2592–6.561H.Chen et al./Materials Letters65(2011)559–561。
磷酸亚铁锂

湖南农业大学全日制普通本科生毕业论文FePO4制备工艺对流变相法合成LiFePO4/C性能的影响EFFECTS OF FEPO4 REACTION CONDITIONS ONELECTROCHEMICAL PROPERTIES OF LIFEPO4/C BYRHEOLOGICAL PHASE METHOD学生姓名:李季年级专业及班级:2010级材料化学(2)班指导老师及职称:钟美娥讲师学院:理学院湖南·长沙提交日期:20年月湖南农业大学全日制普通本科生毕业论文(设计)诚信声明本人郑重声明:所呈交的本科毕业论文(设计)是本人在指导老师的指导下,进行研究工作所取得的成果,成果不存在知识产权争议。
除文中已经注明引用的内容外,本论文不含任何其他个人或集体已经发表或撰写过的作品成果。
对本文的研究做出重要贡献的个人和集体在文中均作了明确的说明并表示了谢意。
本人完全意识到本声明的法律结果由本人承担。
毕业论文(设计)作者签名:20 年月日目录摘要 (1)关键词 (1)1 前言 (2)1.1 LiFePO4的研究现状 (2)1.2 LiFePO4与FePO4.2H2O的结构及特点 (3)1.3 锂离子电池的工作原理 (4)1.4 课题设计思路 (5)2实验部分 (6)2.2 试验方法 (7)2.2.1 样品的的制备与实验方案设计 (7)2.2.2 LiFePO4材料的结构表征 (8)2.2.3 电极的制备及模拟电池的装配 (8)2.2.4 模拟电池的电性能测试 (8)3 结果与讨论 (8)3.1 不同碳锂比 (8)3.2 不同反应温度 (9)3.3 不同反应pH (10)3.4 不同搅拌速度 (11)3.5 不同碳源 (11)3.6 掺杂 (12)4 实验结果的总结............................................................................ 错误!未定义书签。
电池电极纳米材料
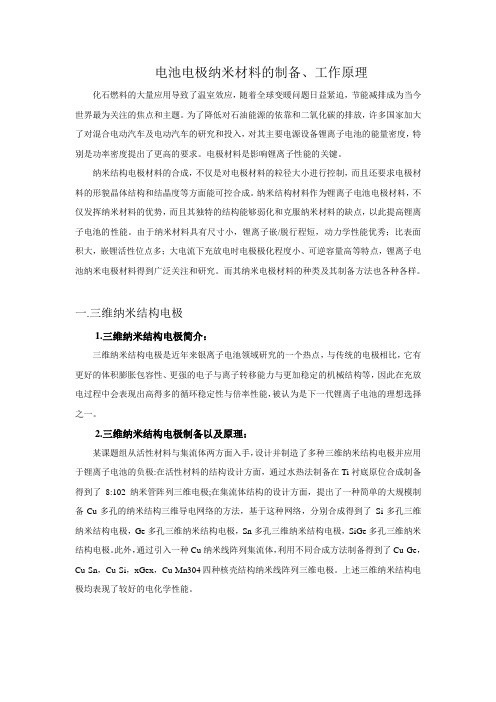
电池电极纳米材料的制备、工作原理化石燃料的大量应用导致了温室效应,随着全球变暖问题日益紧迫,节能减排成为当今世界最为关注的焦点和主题。
为了降低对石油能源的依靠和二氧化碳的排放,许多国家加大了对混合电动汽车及电动汽车的研究和投入,对其主要电源设备锂离子电池的能量密度,特别是功率密度提出了更高的要求。
电极材料是影响锂离子性能的关键。
纳米结构电极材料的合成,不仅是对电极材料的粒径大小进行控制,而且还要求电极材料的形貌晶体结构和结晶度等方面能可控合成。
纳米结构材料作为锂离子电池电极材料,不仅发挥纳米材料的优势,而且其独特的结构能够弱化和克服纳米材料的缺点,以此提高锂离子电池的性能。
由于纳米材料具有尺寸小,锂离子嵌/脱行程短,动力学性能优秀;比表面积大,嵌锂活性位点多;大电流下充放电时电极极化程度小、可逆容量高等特点,锂离子电池纳米电极材料得到广泛关注和研究。
而其纳米电极材料的种类及其制备方法也各种各样。
一.三维纳米结构电极1.三维纳米结构电极简介:三维纳米结构电极是近年来银离子电池领域研究的一个热点,与传统的电极相比,它有更好的体积膨胀包容性、更强的电子与离子转移能力与更加稳定的机械结构等,因此在充放电过程中会表现出高得多的循环稳定性与倍率性能,被认为是下一代锂离子电池的理想选择之一。
2.三维纳米结构电极制备以及原理:某课题组从活性材料与集流体两方面入手,设计并制造了多种三维纳米结构电极并应用于锂离子电池的负极:在活性材料的结构设计方面,通过水热法制备在Ti衬底原位合成制备得到了8:102纳米管阵列三维电极;在集流体结构的设计方面,提出了一种简单的大规模制备Cu多孔的纳米结构三维导电网络的方法,基于这种网络,分别合成得到了Si多孔三维纳米结构电极,Ge多孔三维纳米结构电极,Sn多孔三维纳米结构电极,SiGe多孔三维纳米结构电极。
此外,通过引入一种Cu纳米线阵列集流体,利用不同合成方法制备得到了Cu-Ge,Cu-Sn,Cu-Si,xGex,Cu-Mn304四种核壳结构纳米线阵列三维电极。
储能用LiFePO_(4)锂离子电池的热安全特性
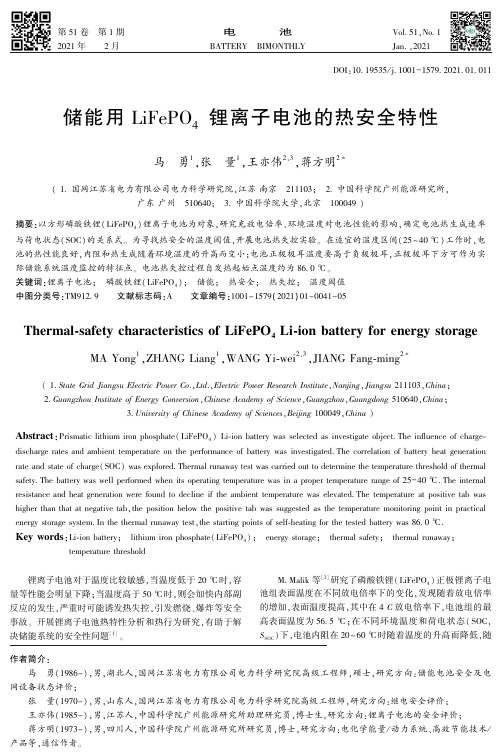
第51卷㊀第1期2021年㊀㊀2月电㊀㊀㊀池BATTERY㊀BIMONTHLYVol.51,No.1Jan.,2021作者简介:马㊀勇(1986-),男,湖北人,国网江苏省电力有限公司电力科学研究院高级工程师,硕士,研究方向:储能电池安全及电网设备状态评价;张㊀量(1970-),男,山东人,国网江苏省电力有限公司电力科学研究院高级工程师,研究方向:继电安全评价;王亦伟(1985-),男,江苏人,中国科学院广州能源研究所助理研究员,博士生,研究方向:锂离子电池的安全评价;蒋方明(1973-),男,四川人,中国科学院广州能源研究所研究员,博士,研究方向:电化学能量/动力系统㊁高效节能技术/产品等,通信作者㊂㊀㊀DOI:10.19535/j.1001-1579.2021.01.011储能用LiFePO 4锂离子电池的热安全特性马㊀勇1,张㊀量1,王亦伟2,3,蒋方明2∗(1.国网江苏省电力有限公司电力科学研究院,江苏南京㊀211103;㊀ 2.中国科学院广州能源研究所,广东广州㊀510640;㊀ 3.中国科学院大学,北京㊀100049)摘要:以方形磷酸铁锂(LiFePO 4)锂离子电池为对象,研究充放电倍率㊁环境温度对电池性能的影响,确定电池热生成速率与荷电状态(SOC )的关系式㊂为寻找热安全的温度阈值,开展电池热失控实验㊂在适宜的温度区间(25~40ħ)工作时,电池的热性能良好,内阻和热生成随着环境温度的升高而变小;电池正极极耳温度要高于负极极耳,正极极耳下方可作为实际储能系统温度监控的特征点㊂电池热失控过程自发热起始点温度约为86.0ħ㊂关键词:锂离子电池;㊀磷酸铁锂(LiFePO 4);㊀储能;㊀热安全;㊀热失控;㊀温度阈值中图分类号:TM912.9㊀㊀文献标志码:A㊀㊀文章编号:1001-1579(2021)01-0041-05Thermal-safety characteristics of LiFePO 4Li-ion battery for energy storageMA Yong 1,ZHANG Liang 1,WANG Yi-wei 2,3,JIANG Fang-ming 2∗(1.State Grid Jiangsu Electric Power Co .,Ltd .,Electric Power Research Institute ,Nanjing ,Jiangsu 211103,China ;㊀2.Guangzhou Institute of Energy Conversion ,Chinese Academy of Science ,Guangzhou ,Guangdong 510640,China ;㊀3.University of Chinese Academy of Sciences ,Beijing 100049,China )Abstract :Prismatic lithium iron phosphate(LiFePO 4)Li-ion battery was selected as investigate object.The influence of charge-discharge rates and ambient temperature on the performance of battery was investigated.The correlation of battery heat generationrate and state of charge(SOC)was explored.Thermal runaway test was carried out to determine the temperature threshold of thermalsafety.The battery was well performed when its operating temperature was in a proper temperature range of 25-40ħ.The internalresistance and heat generation were found to decline if the ambient temperature was elevated.The temperature at positive tab was higher than that at negative tab,the position below the positive tab was suggested as the temperature monitoring point in practicalenergy storage system.In the thermal runaway test,the starting points of self-heating for the tested battery was 86.0ħ.Key words :Li-ion battery;㊀lithium iron phosphate(LiFePO 4);㊀energy storage;㊀thermal safety;㊀thermal runaway;㊀temperature threshold㊀㊀锂离子电池对于温度比较敏感,当温度低于20ħ时,容量等性能会明显下降;当温度高于50ħ时,则会加快内部副反应的发生,严重时可能诱发热失控,引发燃烧㊁爆炸等安全事故㊂开展锂离子电池热特性分析和热行为研究,有助于解决储能系统的安全性问题[1]㊂M.Malik 等[2]研究了磷酸铁锂(LiFePO 4)正极锂离子电池组表面温度在不同放电倍率下的变化,发现随着放电倍率的增加,表面温度提高,其中在4C 放电倍率下,电池组的最高表面温度为56.5ħ;在不同环境温度和荷电状态(SOC,S SOC )下,电池内阻在20~60ħ时随着温度的升高而降低,随电㊀㊀㊀㊀池BATTERY㊀BIMONTHLY㊀第51卷着SOC的降低(90%~10%)而升高;当环境温度为20ħ㊁SOC 为10%时,最大内阻为9mΩ㊂X.N.Feng等[3]探究了25Ah 三元正极材料锂离子电池在极端高温但未引发热失控情况下的性能㊂电池在某一特定温度下突然中止热失控,并进行降温,在经过一次测试(120ħ)后,容量可能部分恢复,但容量损失可能高达20%;如果电池加热到140ħ,欧姆电阻呈指数级上升,即使经过冷却也会完全失效㊂沈嘉丽等[4]研究了不同环境温度(-5~45ħ)下18650型三元正极材料锂离子电池在不同倍率(1/5~3C)下放电的温升特性㊂在相同的环境温度下,放电倍率越大,电池表面温升增长速率也越大,且最终的表面温升也越大㊂在相同的放电倍率下,环境温度越低,表面温升越大;放电时间越短,放电性能越差㊂K.K.Wang等[5]研究了循环前后钛酸锂负极锂离子电池在不同充放电倍率下的内阻和产热量㊂电池无论新旧,放电产生的热量始终大于充电产生的㊂老化电池(在55ħ下循环2100次)在循环中,所有充放电倍率的发热量都比新电池要高;在较低倍率充电时,老化电池产生的不可逆热更多,主要是因为老化后的电池欧姆电阻更高㊂不同类型㊁不同形状的锂离子电池,电热特性不同,增加了储能系统安全管控的复杂性和难度㊂本文作者以国家电网江苏公司储能电站采用的某方形LiFePO4电池为研究对象,分析热安全特性㊂研究不同充放电倍率㊁SOC和环境温度下,电池充放电过程中电(容量㊁开路电压和内阻)㊁热(温度㊁产热)性能的变化㊂1㊀实验1.1㊀方形LiFePO4电池模块以储能用3.2V/40Ah LiFePO4锂离子电池单体(镇江产)为研究对象㊂电池的正极活性材料为LiFePO4㊁负极活性材料为石墨,外形尺寸为27mmˑ130mmˑ148mm,质量为1035g,充放电电压为2.5~3.6V,工作温度为0~45ħ㊂1.2㊀测试装置及方法电池的电热性能参数测试使用BTS-5V300A型充放电仪(深圳产,电压精度为ʃ1mV,电流精度为3mA)㊂整个试验过程电池都处于PU-400恒温箱(东莞产)内㊂用ARC-EV+绝热加速量热仪(英国产)进行电池的热安全温度边界测定,测试最小温升速率为0.02ħ/min㊂在实验过程中,同时对电池表面的温度分布进行测量㊂将K型热电偶(美国产,精度为ʃ0.3ħ)与34970A数据采集仪(美国产)连接,每隔1s采集一次数据㊂单体电池温度测试点布置见图1所示,测试点位置考虑了电池的对称性,其中,1㊁2分别为正㊁负极耳处,3~11位于外表面绝缘层上㊂除非特殊设置/目的,电池测试温度均保持在(25ʃ1)ħ㊂1.3㊀实验方法1.3.1㊀电池初始容量测试单体电池实际容量测试方法为[6]:将电池置于环境温度为25ħ左右的恒温箱中,以8A(即1/5C)恒流放完(放电至截止电压2.5V)剩余电量,再以1/5C倍率在2.5~3.6V图1㊀电池表面温度测试点Fig.1㊀The testing points on the battery surface进行小电流充放电,充㊁放电之间间隔1h,以减轻极化的影响㊂为保证测试结果的一致性和可靠性,重复此步骤3次㊂当容量误差在3%以内时,取3次测试的平均值作为电池实际容量㊂1.3.2㊀电池充放电倍率特性以及温度测试在25ħ环境温度下对电池进行恒流-恒压充电㊂以不同倍率(1/5C和1C)恒流充电至3.6V,转恒压充电至1/30C,搁置1h,以不同倍率(1/5C和1C)放电至2.5V㊂1.3.3㊀开路电压及内阻测试在不同环境温度(0ħ㊁25ħ㊁40ħ)下,通过混合脉冲(HPPC)方法[7]测量电池的开路电压和内阻(包括欧姆内阻和极化内阻)㊂HPPC测试的具体步骤是:以1/5C恒流充电至3.6V,转恒压充电至1/30C,搁置1h后,每恒流(1/5C)放电10%进行一次脉冲实验㊂脉冲测试步骤:以1C放电10s;搁置40s;以3/4C充电10s㊂80%SOC时的HPPC测试步骤见图2㊂图2㊀电池在80%SOC时的HPPC测试步骤Fig.2㊀HPPC test procedure for battery at80%SOC欧姆内阻R0和极化内阻R c分别按式(1)㊁(2)计算㊂R0=(U1-U2)/I p(1) R c=(U2-U3)/I p(2)式(1)㊁(2)中:U1㊁U2和U3为电池瞬态电压;I p为脉冲电流㊂1.3.4㊀熵变系数测试实验前,将电池在恒温25ħ下以1/5C充电至3.6V,转恒压充电至1/30C,然后静置6h㊂电池每20%SOC间隔24㊀第1期㊀马㊀勇,等:储能用LiFePO 4锂离子电池的热安全特性进行1次温度扫描,从40ħ降温至0ħ,每10ħ为1个台阶,每个温度保持6h,每次调整SOC 状态后,电池静置6h㊂例如,电池在SOC 为100%时,经过1次温度扫描后,以1/5C 放电至SOC 为80%,静置6h,再进行1次温度扫描㊂通过记录电池电压随温度的变化,可测得电池的熵变系数㊂1.3.5㊀电池热失控测试加速量热仪可提供一个近似绝热的环境,并通过 加热-等待-搜寻 模式对电池进行台阶加热,模拟电池在与环境没有热交换的状态下的热失控过程㊂将电池放置在绝热加速量热仪的量热腔内,在绝热环境中记录温度的变化㊂设置N 型热电偶(美国产),ARC 控温热电偶需要贴于电池大面正极极耳处(测温点3)㊂测试时,每个温度台阶为5ħ,自放热升温速率判断依据为温升速率>0.02ħ/min㊂1.4㊀实验误差测试的主要误差来自于热电偶和电池电压测试的误差㊂K 型热电偶测得的温度为θi ,测温绝对误差Δθi 为ʃ0.3ħ,在测试过程中的最小温度θmin 为环境温度25ħ,则相对误差Δθi /θi 为1.2%㊂电压测试误差为ʃ1mV,电流测试误差为ʃ3mA,电压(U )最小为2.5V,电流(I )最小为1.2A,因此,电压测试的相对误差ΔU /U 为0.04%,电流测试的相对误差ΔI /I 为0.25%㊂熵变系数(d U /d T )误差主要来自充放电设备的电压测量误差及恒温箱温度测量误差,熵变系数的相对误差Δ(d U /d T )/(d U /d T )为1.2%,其中T 为热力学温度㊂2㊀结果与讨论实验电池3次1/5C 放电容量分别是38.67Ah㊁38.65Ah和38.66Ah,3次测量值之间的偏差在ʃ5%以内㊂取3次测量值的平均值为该退役电池模组的初始容量38.66Ah,略低于40Ah 的标称容量㊂2.1㊀不同充放电倍率下电池端电压和容量的变化在25ħ的环境温度下,电池以不同倍率充放电的端电压变化见图3㊂图3㊀不同倍率充放电时电池端电压的变化Fig.3㊀Battery voltage change at different charge-discharge rates从图3可知,单体电池的端电压在充放电中期基本保持稳定,没有太大的波动,平台端电压在3.2V 左右;端电压在充电初期上升和放电末期下降都较快,主要是受正极材料性质的影响㊂电池在充电末期,恒压阶段时间也随倍率的增加而增加,主要是由于大电流充电,产生的极化较严重,恒压段时间相应延长㊂随着电池放电倍率的增加,电池能放出的容量有一定的减少(1/2C ㊁1C 放电容量分别约为1/5C 的99.1%㊁98.0%),主要是由于高放电倍率时,电池内部的电㊁热和物质不均匀分布加剧,容易更快地达到截止电压,使电池放出的容量减少㊂2.2㊀不同环境温度下电池端电压和容量的变化在不同环境温度下,电池以1C 充放电时的端电压变化见图4㊂图4㊀不同环境温度充放电时电池端电压的变化Fig.4㊀Battery voltage change at different ambient temperatures从图4可知,温度对电池的充放电电压和容量有很大的影响㊂环境温度越低,电池充电时的端电压越高㊁放电时的端电压越低㊂这主要是因为随着环境温度降低,电解液的离子扩散率及导电率降低,电化学极化㊁浓差极化和欧姆极化增强,电池的总内阻变大㊂环境温度越低,电池容量越小,主要是因为低温导致电池内部材料的电导率和扩散能力降低,同时,Li +迁移受阻,正负极间电解液中的Li +浓度差变大,放电截止电压提前㊂2.3㊀不同环境温度下开路电压(OCV )和内阻的变化在不同温度(0ħ㊁25ħ和40ħ)下,电池以1/5C 充放电时的OCV(U OCV )和内阻随温度的变化见图5㊂从图5可知,电池的OCV 和内阻随温度变化明显,在低温环境下尤为显著,主要是由于电池内部的活性物质㊁电化学反应速度与温度有密切的关系㊂温度下降,电池内部的活性离子迁移速度下降,欧姆内阻增加,Li +迁移速度下降导致电化学活性降低,正负极之间的离子浓差极化增强,极化内阻增加㊂25ħ和40ħ下的OCV 基本上没有差异,只在电池充放电起始和结束阶段有一点差别;不同温度条件下,中间区域SOC 下的OCV 基本相同,主要是由电池的平台电压所决定的㊂2.4㊀在不同充放电倍率下电池外表面温度分布及演化在25ħ环境温度下,电池以不同倍率充放电的温度变化见图6㊂从图6可知,电池在不同充放电倍率下的温升有所不同㊂在1/5C 充㊁放电结束时刻,电池的表面温升分别为1.8ħ和1.5ħ,各测点间的温差约为0.5ħ;在1C 充㊁放电结束时刻,电池的表面温升为4.9ħ㊁7.0ħ,各测点的最大温差34电㊀㊀㊀㊀池BATTERY㊀BIMONTHLY㊀第51卷图5㊀不同环境温度下电池OCV 和内阻变化㊀Fig.5㊀Battery OCV and internal resistance change at different ambienttemperatures图6㊀不同充放电倍率下电池表面温度分布和演化Fig.6㊀Temperature distribution and evolution on battery surfaceat different charge-discharge rates为1.0ħ左右㊂随着充放电倍率的增大,电池的表面温升增大,各测点的温差也相应的增加㊂这主要因为充放电电流增大使电池产热量增加,而外部环境温度基本不变,导致电池内热量积累㊁温度上升㊂电池温度变化曲线在充电过程中的某些时段出现下降的情况,主要与电池在低倍率放热过程中的可逆热有关㊂在充电末期,电池出现温度下降的情况,主要是由于电池进入恒压充电阶段,电池的电流变小,产热减少㊂总体来看,电池在各个倍率下,温升都有一定的增加,尤其在充放电末期,都处于快速升温的状态㊂从各测点的情况来看,电池正极下方(测温点3)始终为温度最高点,主要是由于正极极耳会产生大量的热,而正极下方外侧包裹了一层绝缘材料,产生了一定的热阻,导致外表面温度高于极耳处的温度㊂有鉴于此,建议在正极下方布置温度测点,作为电池热安全温度的监控点㊂2.5㊀不同SOC 下电池的熵变系数电池熵变系数随SOC 变化的情况见图7㊂图7㊀电池熵变系数随SOC 的变化Fig.7㊀Change of battery entropy coefficient with SOC从图7可知,整个曲线呈现倒 S 形,电池在30%SOC 以下时,熵变系数为负值,而在30%~100%SOC 时,熵变系数为正值㊂熵变系数关于SOC 的拟合公式为:d U d T=0.0279ˑS 5SOC -0.0654ˑS 4SOC +0.0568ˑS 3SOC -0.0247ˑS 2SOC +0.0063ˑS SOC -0.0007(3)由电池熵热[IT (d U /d T )]可知,当电流方向与熵变系数符号相同时,表现为放热;当电流方向与熵变系数符号相反时,表现为吸热㊂这说明,电池放电时可逆产热表现为吸热㊂2.6㊀电池产热评估锂离子电池在正常充放电状态下,内部产热主要有两部分:电池内阻带来的不可逆热;发生电化学反应产生的可逆热㊂D.Bernardi 等[8]基于锂离子电池内部材料均匀产热的假设,分析电池产热机理,提出了电池生成热的理论公式:q =ITd Ud T+I (U OCV -U )(4)44㊀第1期㊀马㊀勇,等:储能用LiFePO 4锂离子电池的热安全特性式(4)中:I (U OCV -U )表示因欧姆内阻和极化内阻等产生的不可逆热㊂由电流㊁温度㊁OCV㊁电压和熵变曲线,可得到电池在1/5C ㊁1/2C 和1C 放电时的生热曲线,如图8所示㊂图8㊀电池在不同放电倍率的生热曲线Fig.8㊀Battery heat generation curves at different discharge rates从图8可知,电池在放电初始阶段,即SOC 为100%时,生热量为负值,可逆热占主导;而在放电末期,不可逆热为主导,内部产热迅速增加,温度升高㊂在放电中间阶段,电池经历了一段缓慢生热的过程,可以从内阻看出,即电池内阻在中间区间SOC 时基本不变,产生的不可逆热相对稳定㊂2.7㊀热失控测试热失控测试对电池温度监控阈值有重要的参考作用,是进行热安全温度边界测量的重要方法[9]㊂电池热失控过程中温度随时间的变化见图9㊂图9㊀电池热失控温度变化Fig.9㊀Temperature changes of battery thermal runway从图9可知,电池自发热的起始温度(θs )为86.0ħ,在80~120ħ的自产热主要来源于固体电解质相界面(SEI)膜的分解放热㊂自发热发生后,电池温度进一步上升,内部热量进一步积累,温度(θvalve )到达173.4ħ后瞬时下降,是由于电池泄压阀打开,高温气液混合物从电芯内部喷出所致㊂随后,电池正负极发生短路,温度迅速上升,到达热失控起始温度(θb )193.7ħ左右时,温升速率达到1.0ħ/min,发生热失控,此时温度上升近乎呈指数变化,一直到达最高温度(θmax )325.2ħ,内部放热反应基本结束㊂3㊀结论本文作者以储能用方形LiFePO 4电池为研究对象,研究了不同环境温度和SOC 下,电池开路电压㊁内阻和容量的变化㊂锂离子电池的开路电压㊁内阻和容量受环境温度影响较大,在合适的环境温度下,整体性能有所增强㊂温度过低时,电池内阻增大,容量减小约8%,极化内阻相应变大㊂电池热特性的研究主要集中于电池表面温度变化和电池产热特性㊂实验研究了充放电倍率和环境对电池表面温度的影响㊂随着充放电倍率的增加,电池表面温度相应增加㊂正极下方温度为整个电池的温度最高点,一方面与电池正极极耳为铝材有关;另一方面,由于电池表面包裹绝缘膜,使热阻增加㊂建议在电池正极下方布置温度点,作为储能系统模组单体温度监控点㊂环境温度下降,电池内部Li +的移动速度减缓㊁内阻变大,电池产热增加㊁表面温度升高㊂对电池的产热特性进行分析,发现在小倍率电流放电时,电池初始阶段以可逆熵热为主,而随着SOC 的减小,电池的不可逆热占主导地位㊂电池自86.0ħ开始自放热,主要是由于电池内的SEI膜在高温下自放热引起的;到173.4ħ时,电池泄气阀打开,温度出现短暂的下降;而到193.7ħ时,电池热失控彻底触发,导致温度呈指数增加,仅17min 后,电池到达最高温度325.2ħ,热失控过程结束㊂参考文献:[1]㊀WANG S Q,LU L G ,REN D S,et al .Experimental investigationon the feasibility of heat pipe-based thermal management system to prevent thermal runaway propagation [J].J Electrochem Energy Convers Storage,2019,16(3):031006.[2]㊀MALIK M,MATHEW M,DINCER I,et al .Experimental investiga-tion and thermal modelling of a series connected LiFePO 4battery pack[J].Int J Therm Sci,2018,132:466-477.[3]㊀FENG X N,SUN J,OUYANG M G,et al .Characterization of largeformat lithium ion battery exposed to extremely high temperature[J].J Power Sources,2014,272:457-467.[4]㊀沈嘉丽,方奕栋,苏林,等.18650型锂动力电池热特性实验研究[J].农业装备与车辆工程,2019,57(12):67-71.[5]㊀WANG K K,GAO F,ZHU Y L,et al .Internal resistance and heatgeneration of soft package Li 4Ti 5O 12battery during charge and dis-charge[J].Energy,2018,149:364-374.[6]㊀CASTILLO E C.Standards for electric vehicle batteries and asso-ciated testing procedures [J].Adv Battery Technol Electric Vehi-cles,2015:469-494.[7]㊀US DOE .FreedomCAR battery test manual for power-assist hybridelectric vehicles,DOE /ID-11069[S].[8]㊀BERNARDI D,PAWLIKOWSKI E,NEWMAN J.A general energybalance for battery systems[J].J Electrochem Soc,1985,132(1):5-12.[9]㊀黄文才,胡广地,邓宇翔,等.锂离子电池的高温热失控模拟[J].电池,2019,49(3):204-207.收稿日期:2020-06-2954。
PAM软模板法合成LiFePO_4_C纳米共生物

作者简介:杨书廷(1962-),男,河南人,河南师范大学化学与环境科学学院教授,研究方向:材料物理化学;赵娜红(1976-),女,河南人,河南师范大学化学与环境科学学院硕士生,研究方向:材料物理化学;尹艳红(1976-),女,河南人,河南师范大学化学与环境科学学院副教授,研究方向:无机材料;董红玉(1979-),女,河南人,河南师范大学化学与环境科学学院硕士生,研究方向:材料物理化学;岳红云(1981-),女,河南人,河南师范大学化学与环境科学学院硕士生,研究方向:材料物理化学;杨金鑫(1978-),男,河南人,河南师范大学化学与环境科学学院硕士生,研究方向:材料物理化学。
PAM 软模板法合成LiFePO 4/C 纳米共生物杨书廷,赵娜红,尹艳红,董红玉,岳红云,杨金鑫(河南师范大学化学与环境科学学院,河南新乡 453002)摘要:采用聚丙烯酰胺(PAM )软模板法合成了LiFePO 4/C 正极材料。
在011mA/cm 2电流密度下,PAM 软模板法合成的LiFePO 4/C 材料的放电比容量可达120mAh/g ,且可逆性增强。
材料的振实密度达到1119g/cm 3。
透射电镜和原子力显微镜图像研究表明:合成的活性材料和所掺杂的碳具有纳米共生结构,且颗粒具有较大的电化学活性比表面,颗粒间无团聚现象。
采用X 射线衍射实验,对PAM 软模板剂在合成过程中的改性作用进行了研究。
关键词:锂离子电池; 正极材料; LiFePO 4; 软模板剂中图分类号:TM91219 文献标识码:A 文章编号:1001-1579(2005)04-0263-03Synthesis of LiFePO 4/C nano 2structure intergrow th by PAM soft templating methodYAN G Shu 2ting ,ZHAO Na 2hong ,YIN Yan 2hong ,DON G Hong 2yu ,YU E Hong 2yun ,YAN G Jin 2xin(College of Chemist ry and Environmental Science ,Henan Norm al U niversity ,Xinxiang ,Henan 453002,China )Abstract :LiFePO 4/C cathode material was prepared by polyacrylamide (PAM )soft templating method 1Discharge specificcapacity of the LiFePO 4/C material prepared by PAM soft templating method reached 120mAh/g at 011mA/cm 2and its re 2versibility was increased 1The tap density of the material was 1119g/cm 31TEM and TP 2AFM pictures showed that nano 2struc 2tured intergrowth resided in the composite material and doped carbon with more electrochemical active specific surface and few ag 2gregates 1The composite effect of PAM soft template during preparing was studied by XRD test 1K ey w ords :Li 2ion battery ; cathode material ; LiFePO 4; soft template 锂离子电池正极材料橄榄石型LiFePO 4以低廉的成本、高安全性能赢得瞩目[1-2]。
改进固相法制备LiFePO_4_C正极材料及其性能
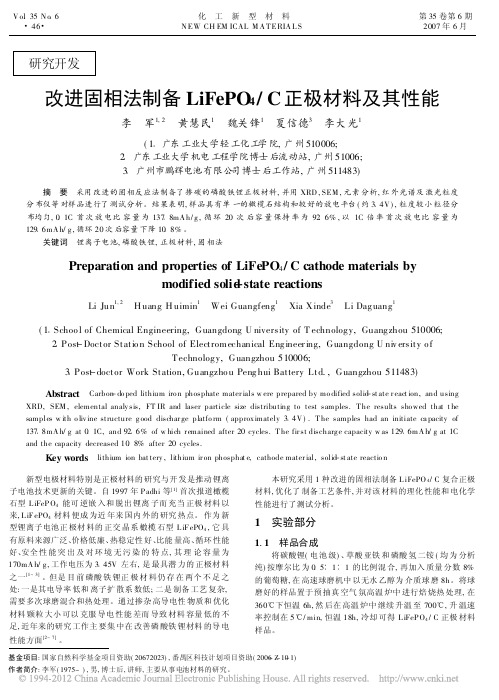
V ol 135N o 16#46#化 工 新 型 材 料N EW CH EM ICAL M A T ERIA L S 第35卷第6期2007年6月基金项目:国家自然科学基金项目资助(20672023),番禺区科技计划项目资助(2006-Z -10-1)作者简介:李军(1975-),男,博士后,讲师,主要从事电池材料的研究。
研究开发改进固相法制备LiFePO 4/C 正极材料及其性能李 军1,2 黄慧民1 魏关锋1 夏信德3 李大光1(11广东工业大学轻工化工学院,广州510006;21广东工业大学机电工程学院博士后流动站,广州51006;31广州市鹏辉电池有限公司博士后工作站,广州511483)摘 要 采用改进的固相反应法制备了掺碳的磷酸铁锂正极材料,并用XRD ,SEM ,元素分析,红外光谱及激光粒度分布仪等对样品进行了测试分析。
结果表明,样品具有单一的橄榄石结构和较好的放电平台(约314V ),粒度较小粒径分布均匀,011C 首次放电比容量为13718mA h/g ,循环20次后容量保持率为9216%,以1C 倍率首次放电比容量为12916mA h/g ,循环20次后容量下降1018%。
关键词 锂离子电池,磷酸铁锂,正极材料,固相法Preparation and properties of LiFePO 4/C cathode materials bymodified solid -state reactionsLi Jun1,2H uang H uimin 1 Wei Guangfeng 1 Xia Xinde 3 Li Dag uang1(11Schoo l of Chemical Engineering,Guangdong U niversity of T echnolog y,Guang zhou 510006;21Post -Doctor Statio n School of Electrom echanical Eng ineer ing,Guangdong U niv ersity o fTechnolog y,Guangzhou 510006;31Post -doctor Work Station,Guangzho u Peng hui Battery Ltd.,Guangzhou 511483)Abstract Carbon-do ped lithium iro n phosphate mater ials w ere prepared by mo dified solid -st ate r eact ion,and using XRD,SEM ,elemental analy sis,FT IR and laser particle size distributing to test samples.T he results sho wed that t hesamples w ith o liv ine structure g ood dischar ge platfo rm (approx imately 314V ).T he samples had an initiate ca pacity of 13718mA h/g at 011C,and 9216%of w hich r emained after 20cycles.T he fir st discharg e capacity w as 12916mA h/g at 1C and the capacity decreased 1018%after 20cycles.Key words lithium ion bat tery ,lithium ir on phosphat e,cathode mater ial,solid-st ate reactio n 新型电极材料特别是正极材料的研究与开发是推动锂离子电池技术更新的关键。
英文文献-溶胶-凝胶法制备LiFePO4改进其电化学性能

Electrochimica Acta 92 (2013) 248–256Contents lists available at SciVerse ScienceDirectElectrochimicaActaj o u r n a l h o m e p a g e :w w w.e l s e v i e r.c o m /l o c a t e /e l e c t a c taGel-combustion synthesis of LiFePO 4/C composite with improved capacity retention in aerated aqueous electrolyte solutionMilica Vujkovi´c a ,Ivana Stojkovi´c a ,Nikola Cvjeti´canin a ,Slavko Mentus a ,b ,∗,1a University of Belgrade,Faculty of Physical Chemistry,P.O.Box 137,Studentski trg 12-16,11158Belgrade,Serbia bThe Serbian Academy of Sciences and Arts,Kenz Mihajlova 35,11158Belgrade,Serbiaa r t i c l ei n f oArticle history:Received 2October 2012Received in revised form 3January 2013Accepted 5January 2013Available online 11 January 2013Keywords:Aqueous rechargeable Li-ion battery Galvanostatic cycling Gel-combustion Olivine LiFePO 4LiFePeO 4/C compositea b s t r a c tThe LiFePO 4/C composite containing 13.4wt.%of carbon was synthesized by combustion of a metal salt–(glycine +malonic acid)gel,followed by an isothermal heat-treatment of combustion product at 750◦C in reducing atmosphere.By a brief test in 1M LiClO 4–propylene carbonate solution at a rate of C/10,the discharge capacity was proven to be equal to the theoretical one.In aqueous LiNO 3solu-tion equilibrated with air,at a rate C/3,initial discharge capacity of 106mAh g −1was measured,being among the highest ones observed for various Li-ion intercalation materials in aqueous solutions.In addition,significant prolongation of cycle life was achieved,illustrated by the fact that upon 120charg-ing/discharging cycles at various rates,the capacity remained as high as 80%of initial value.The chemical diffusion coefficient of lithium in this composite was measured by cyclic voltammetry.The obtained val-ues were compared to the existing literature data,and the reasons of high scatter of reported values were considered.© 2013 Elsevier Ltd. All rights reserved.1.IntroductionThanks to its high theoretical Coulombic capacity (170mAh g −1)and environmental friendliness,LiFePO 4olivine became a desir-able cathodic material of Li-ion batteries [1,2],competitive to other commercially used cathodic materials (LiMnO 4,LiCoO 2).As evidenced in non-aqueous electrolyte solutions,a small vol-ume change (6.81%)that accompanies the phase transition LiFePO 4 FePO 4enables Li +ion insertion/deinsertion reactions to be quite reversible [1–3].The problem of low rate capability,caused by low electronic conductivity [4,5],was shown to be solv-able to some extent by reduction of mean particle size [6].Further improvements in both conductivity and electrochemical perform-ances were achieved by forming composite LiFePO 4/C,where in situ produced carbon served as an electronically conducting con-stituent [5,7–27].Ordinarily,both in situ formed carbon and carbon black additive,became unavoidable constituent of the LiFePO 4-based electrode materials [28–37].Zhao et al.[27]reported that Fe 2P may arise as an undesirable product during the synthesis of LiFePO 4/C composite under reducing conditions,however,other authors found later that this compound may contribute positively∗Corresponding author at:University of Belgrade,Faculty of Physical Chemistry,P.O.Box 137,Studentski trg 12-16,11158Belgrade,Serbia.Tel.:+381112187133;fax:+381112187133.E-mail address:slavko@ffh.bg.ac.rs (S.Mentus).1ISE member.to the electronic conductivity and improve the electrochemical per-formance of the composite [28–30].Severe improvement in rate capability and capacity retention was achieved by partial replace-ment of iron by metals supervalent relative to lithium [31–37].Thus one may conclude that the main aspects of practical applica-bility of LiFePO 4in Li-ion batteries with organic electrolytes were successively resolved.After the pioneering studies by Li and Dahn [38,39],recharge-able Li-ion batteries with aqueous electrolytes (ARLB)attracted considerable attention [40–50].The first versions of ARLB’s,suf-fered of very low Coulombic utilization and significantly more pronounced capacity fade relative to the batteries with organic electrolyte,regardless on the type of electrode materials [43].For the first time,LiFePO 4was considered as a cathode material in ARLB’s by Manickam et al.in 2006[44].He et al.[46],in an aqueous 0.5M Li 2SO 4solution,found that LiFePO 4displayed both a surprisingly high initial capacity of 140mAh g −1at a rate 1C and recognizable voltage plateau at a rate as high as 20C,which was superior relative to the other electrode materials in ARLB’s.Recently,the same authors reported a high capacity decay in aer-ated electrolyte solution,amounting to 37%after only 10cycles [48].In the same study,they demonstrated qualitatively by a brief cyclovoltammetric test,that a carbon layer deposited from a vapor phase over LiFePO 4particles,suppressed the capacity fade [48].Inspired by the recent discoveries about excellent rate capa-bility [46]but short cycle life [48]of LiFePO 4in aerated aqueous solution,we attempted to prolong the cycle life by means of protecting carbon layer over the LiFePO 4particles.Therefore we0013-4686/$–see front matter © 2013 Elsevier Ltd. All rights reserved./10.1016/j.electacta.2013.01.030M.Vujkovi´c et al./Electrochimica Acta92 (2013) 248–256249synthesized LiFePO4/C composite by a fast and simple glycine-nitrate gel-combustion technique.This method,although simpler than a classic solid state reaction method combined with ball milling[44,48],was rarely used for LiFePO4synthesis[19,27].It yielded a porous,foamy LiFePO4/C composite,easily accessible to the electrolyte.Upon the fair charging/discharging performance was confirmed by a brief test in organic electrolyte,we examined in detail the electrochemical behavior of this material in aqueous electrolyte,by cyclic voltammetry,complex impedance and cyclic galvanostatic charging/discharging methods.In comparison to pure LiFePO4studied in Ref.[48],this composite displayed markedly longer cycle life in aerated aqueous solutions.The chemical dif-fusion coefficient of lithium was also determined,and the reasons of its remarkable scatter in the existing literature were considered.2.ExperimentalThe LiFePO4/C composite was synthesized using lithium nitrate, ammonium dihydrogen phosphate(Merck)and iron(II)oxalate dihydrate(synthesized according to the procedure described else-where[51])as raw materials.Our group acquired the experience in this synthesis technique on the examples of spinels LiMn2O4 [52]and LiCr0.15Mn1.85O4[53],where glycine served as both fuel and complexing/gelling agent to the metal ions.A stoichiometric amount of each material was dissolved in deionized water and mixed at80◦C using a magnetic stirrer.Then,first glycine was added into the reaction mixture to provide the mole ratio of glycine: nitrate of2:1,and additionally,malonic acid(Merck)was added in an amount of60wt.%of the expected mass of LiFePO4.The role of malonic acid was to decelerate combustion and provide con-trollable excess of carbon[14].After removing majority of water by evaporation,the gelled precursor was heated to initiate the auto-combustion,resulting in aflocculent product.The combustion product was heated in a quartz tube furnacefirst at400◦C for3h in Ar stream,and then at750◦C for6h,under a stream of5vol.%H2in Ar.This treatment consolidated the olivine structure and enabled to complete the carbonization of residual organic matter.The VO2powder prepared by hydrothermal method was used as an active component of the counter electrode in the galvanostatic experiments in aqueous electrolyte solution.The details of the syn-thesis and electrochemical behavior of VO2are described elsewhere [54,55].The considerable stoichiometric excess of VO2was used,to provide that the LiFePO4/C composite only presents the main resis-tive element,i.e.,determines the behavior of the assembled cell on the whole.The XRD experiment was performed using Philips1050diffrac-tometer.The Cu K␣1,2radiation in15–70◦2Ârange,with0.05◦C step and2s exposition time was used.The carbon content in the composite was determined by its com-bustion in theflowing air atmosphere,by means of thermobalance TA SDT Model2090,at a heating rate of10◦C min−1.The morphology of the synthesized compounds was observed using the scanning electron microscope JSM-6610LV.For electrochemical investigations,the working electrode was made from LiFePO4/C composite(75%),carbon black-Vulcan XC72 (Cabot Corp.)(20%),poly(vinylidenefluoride)(PVDF)binder(5%) and a N-methyl-2-pyrrolidone solvent.The resulting suspension was homogenized in an ultrasonic bath and deposited on electron-ically conducting support.The electrode was dried at120◦C for 4h.Somewhat modified weight ratio,85:10:5,and the same drying procedure,were used to prepare VO2electrode.The non-aqueous electrolyte was1M LiClO4(Lithium Corpo-ration of America)dissolved in propylene carbonate(PC)(Fluka). Before than dissolved,LiClO4was dried over night at140◦C under vacuum.The aqueous electrolyte solution was saturated LiNO3solution.The cyclic voltammetry and complex impedance experiments were carried out only for aqueous electrolyte solutions,by means of the device Gamry PCI4/300Potentiostat/Galvanostat.The three electrode cell consisted of a working electrode,a wide platinum foil as a counter electrode,and a saturated calomel electrode(SCE) as a reference one.The experiments were carried out in air atmo-sphere.The impedance was measured in open-circuit conditions, at various stages of charging and discharging,within the frequency range10−2−105Hz,with7points per decade.Galvanostatic charging/discharging experiments were carried out in a two-electrode arrangement,by means of the battery testing device Arbin BT-2042,with two-terminal connectors only.In the galvanostatic tests in non-aqueous solution,working electrode was a2×2cm2platinum foil carrying2.3mg of compos-ite electrode material(1.5mg of olivine),while counter electrode was a2×2cm2lithium foil.The cell was assembled in an argon-filled glove box and cycled galvanostatically within a voltage range 2.1–4.2V.The galvanostatic tests in the aqueous electrolyte solution were carried out in a two-electrode arrangement,involving3mg of cathodic material,as a working electrode,and VO2in a multi-ple stoichiometric excess,as a counter electrode.According to its reversible potential of lithiation/delithiation reaction[55],VO2per-formed as an anode in this cell.The4cm2stainless steel plates were used as the current collectors for both positive and negative electrode.The cell was assembled in room atmosphere,and cycled within the voltage window between0.01and1.4V.3.Result and discussion3.1.The XRD,SEM and TG analysis of the LiFePO4/C compositeFig.1shows the XRD patterns of the composite LiFePO4/C pre-pared according to the procedure described in the Experimental Section.As visible,the diffractogram agrees completely with the one of pure LiFePO4olivine,found in the JCPDS card No.725-19. The narrow diffraction lines indicate complete crystallization and relatively large particle dimensions.On the basis of absence of diffraction lines of carbon,we may conclude that the carbonized product was amorphous one.Fig.2shows the SEM images of the LiFePO4/C composite at two different magnifications.Theflaky agglomerates,Fig.2left,with apparently smooth surface and low tap density,are due to a partial liquefaction and evolution of gas bubbles during gel-combustion procedure.These agglomerates consist of small LiFePO4/CFig.1.XRD patterns of LiFePO4/C composite in comparison to standard crystallo-graphic data.250M.Vujkovi´c et al./Electrochimica Acta 92 (2013) 248–256Fig.2.SEM images of LiFePO 4/C composite at two different magnification,20000×and 100000×.composite particles visible better at higher magnification,Fig.2,ly at the magnification of 100,000×,one may see that the size of majority of composite particles was in the range 50–100nm.The mean particle diameter,2r,as per SEM microphotograph amounted to 75nm.This analysis evidences that the gel-combustion method may provide nanodisprsed particles,desirable from the point of view of rate capability.For instance,Fey et al.[16]demonstrated that particle size reduction from 476to 205nm improved the rate capa-bility of LiFePO 4/C composite in organic electrolyte,illustrated by the increase of discharge capacity from 80mAh g −1to 140mAh g −1at discharging rate 1C.Also,carbon matrix prevented particles from agglomeration providing narrow size distribution,contrary to often used solid state reaction method of synthesis,when sintering of ini-tially nanometer sized particles caused the appearance of micron sized agglomerates [22].The SEM microphotograph (Fig.2)alone did not permit to rec-ognize carbon constituent of the LiFePO 4/C composite.However,carbonized product was evidenced,and its content measured,by means of thermogravimetry,as described elsewhere [9].The dia-gram of simultaneous thermogravimetry and differential thermal analysis (TG/DTA)of the LiFePO 4/C composite performed in air is presented in Fig.3.The process of moisture release,causing a slight mass loss of 1%,terminated at 150◦C.In the temperature range 350–500◦C carbon combustion took place,visible as a drop of the TG curve and an accompanying exothermic peak of the DTA curve.However,the early stage of olivine oxidation merged to some extent with the late stage of carbon combustion,and therefore,the minimum of the TG curve,appearing at nearly 500◦C,was not so low as to enable to read directly the carbon content.Fortunately,as proven by XRD analysis,the oxidation of LiFePO 4at tempera-ture exceeding 600◦C,yielded only Li 3Fe 2(PO 4)3and Fe 2O 3,whatFig.3.TGA/DTA curve of LiFePO 4/C under air flow at heating rate of 10C min−1.corresponded to the relative gain in mass of exactly 5.07%[9].Therefore,the weight percentage of carbonaceous fraction in the LiFePO 4/C composite was determined as equal to the difference between the TG plateaus at temperatures 300and 650◦C,aug-mented for 5.07%.According to this calculation the carbon fraction amounted to 13.4wt.%,and by means of this value,the electro-chemical parameters discussed in the next sections were correlated to pure LiFePO 4.Specific surface area of LiFePO 4,required for the measurement of diffusion constant,was determined from SEM image (Fig.2).Assuming a spherical particle shape and accepting mean particle radius r =37.5nm,the specific surface area was estimated on the basis of equation [17,22,45,46]:S =3rd(1)where the bulk density d =3.6g cm −3was used .This calculation resulted in the value S =22.2m 2g −1.In this calculation the contri-bution of carbon to the mean particle radius was ignored,however the error introduced in such way is more acceptable than the error which may arise if standard BET method were applied to the com-posite with significant carbon ly,due to a usually very developed surface area of carbon,the measured specific sur-face may exceed many times the actual surface area of LiFePO 4.3.2.Electrochemical measurements3.2.1.Non-aqueous electrolyte solutionIn order to compare the behavior of the synthesized LiFePO 4/C composite to the existing literature data,available predominantly for non-aqueous solutions,a brief test was performed in non-aqueous 1M LiClO 4+propylene carbonate solution by galvano-static experiments only.The results for the rates C/10,C/3and C,within the voltage limits 2.1–4.2V,were presented in Fig.4.The polarizability of the lithium electrode was estimated on the basis of the study by Churikov [56–67],who measured the current–voltage curves of pure lithium electrode in LiClO 4/propylene carbon-ate solutions at various temperatures.To the highest rate of 1C =170mA g −1in nonaqueous electrolyte,the corresponding cur-rent amounted to 0.25mA,which was equal to the current density of 0.064mA cm −2through the Li counter electrode.According to Fig.2in Ref.[67],for room temperature,the corresponding over-voltage amounted to only 6mV.Since lithium electrode is thus practically non-polarizable in this system,the voltages presented on the ordinate of the left diagram are the potentials of the olivine electrode expressed versus Li/Li +reference electrode.The clear charge and discharge plateaus at about 3.49V and 3.40V,respec-tively,correspond to the LiFePO 4 FePO 4phase equilibria [5].At discharging rate of C/10,the initial discharge capacity,within the limits of experimental error,was close to a full theoreticalM.Vujkovi´c et al./Electrochimica Acta 92 (2013) 248–256251Fig.4.The initial charge/discharge curves (a)and cyclic performance (b)of LiFePO 4/C composite in 1M LiClO 4+PC at different rates within a common cut-off voltage of2.1–4.2V.Fig.5.Charge/discharge profile and corresponding cyclic behavior of LiFePO 4/C in 1M LiClO 4+PC at the rate of 1C.capacity of LiFePO 4(170mAh g −1).This value is higher than that for LiFePO 4/C composite obtained by glycine [19],malonic acid [14]and adipic acid/ball milling [15]assisted methods.As usual,the discharge capacity decreased with increasing discharging rate (Fig.4b),and amounted to 127mAh g −1at C/3,and 109mAh g −1at 1C.For practical application of Li-ion batteries,a satisfactory rate capability and long cycle life are of primary importance.The charge/discharge profiles and dependence of capacity on the cycle number at the rate 1C are presented in Fig.5.The capacity was almost independent on the number of cycles,similarly to theearlier reports by Fey et al.[37–39].For comparison,Kalaiselvi et al.[19],by a glycine assisted gel-combustion procedure,with an additional amount (2wt.%)of carbon black,produced a similar nanoporous LiFePO 4/C composite displaying somewhat poorer per-formance,i.e.,smaller discharge capacity of 160mAh g −1at smaller discharging rate of C/20.On the other hand,better rate capability of LiFePO 4/C com-posite,containing only 1.1–1.8wt.%of carbon,in a non-aqueous solution,was reported by Liu et al.[21].For instance they mea-sured 160mAh g −1at the rate 1C,and 110at even 30C [21].This may be due to a thinner carbon layer around the LiFePO 4olivine particles.However the advantage of here applied thicker carbon layer exposed itself in aqueous electrolyte solutions,as described in the next section.3.2.2.Aqueous electrolyte solution3.2.2.1.Cyclic voltammetry.By the cyclic voltammetry method (CV)the electrochemical behavior of LiFePO 4/C composite in satu-rated aqueous LiNO 3solution was preliminary tested in the voltage range 0.4–1V versus SCE.The cyclic voltammograms are pre-sented in Fig.6.The highest scan rate of 100mV s −1,tolerated by this material,was much higher than the ones (0.01–5mV s −1)used in previous studies in both organic [13,24,25]and aqueous electrolyte solutions [47,48].Since one deals here with the thin layer solid redox electrode,limited in both charge consumption and diffusion length,the voltammogram is more complicated for interpretation comparing with the classic case of electroactive species in a liquid solution.A sharp,almost linear rise of current upon achieving reversible potential,with overlapped rising parts at various scan rates,similar to ones reported elsewhere [21,25],resembles closely the voltammogram of anodic dissolution ofaFig.6.Cyclic voltammograms of LiFePO 4/C in saturated LiNO 3aqueous electrolyte with a scan rate of 1mV s −1(left)and at various scan rates in the range 1–100mV s −1.252M.Vujkovi´c et al./Electrochimica Acta 92 (2013) 248–256Fig.7.Anodic and cathodic peak current versus square root of scan rate forLiFePO 4/C composite in aqueous LiNO 3electrolyte solution.thin metal layer [56],which proceeds under constant reactant activity.Since the solid/solid phase transitions LiFePO 4 FePO 4accompanies the redox processes in this system [5,8,57,58],the positive scan of the voltammograms depict the phase transition of LiFePO 4to FePO 4,while the negative scan depicts the phase transi-tion FePO 4to LiFePO 4.As shown by Srinivasan et al.[5],LiFePO 4may be exhausted by Li not more than 5mol.%before to trans-form into FePO 4,while FePO 4may consume no more than 5%Li before to transform into LiFePO 4,i.e.cyclic voltammetry exper-iments proceeds under condition of almost constant activity of the electroactive species.Although these aspects of the Li inser-tion/deinsertion process do not fit the processes at metal/liquid electrolyte boundary implied by Randles–Sevcik equation:i p =0.4463F RT1/2C v 1/2AD 1/2(2)this equation was frequently used to estimate apparent diffusion coefficient in Li insertion processes [5,17,21,46,59].To obtain peak current,i p ,in amperes,the concentration of lithium,C =C Li ,should be in mol cm −3,the real surface area exposed to the electrolyte in cm 2,chemical diffusion coefficient of lithium through the solid phase,D =D Li ,in cm 2s −1,and sweep rate,v ,in V s −1.The Eq.(2)pre-dicts the dependence of the peak height on the square root of sweep rate to be linear,as found often in Li-ion intercalation processes [17,21,25,59,60].This condition is fulfilled in this case too,as shown in Fig.7.The average value of C Li may be estimated as a reciprocal value of molar volume of LiFePO 4(V M =44.11cm 3mol −1),hence C Li =2.27×10−2mol cm −3.The determination of the actual surface area of olivine is a more difficult task,due to the presence of carbon in the LiFePO 4/C ly,classical BET method of sur-face area measurement may lead to a significantly overestimated value,since carbon surface may be very developed and participate predominantly in the measured value [15].Thus the authors in this field usually calculated specific surface area by means of Eq.(1),using mean particle radius determined by means of electron microscopy [17,22,45,46].Using S =22.2m 2g −1determined by means of Eq.(1),and an actual mass of the electroactive substance applied to the elec-trode surface (0.001305g),the actual electrode surface area was calculated to amount to A =290cm 2.This value introduced in Randles–Sevcik equation yielded D Li ∼0.8×10−14cm 2s −1.From the aspect of capacity retention,the insolubility of olivine in aqueous solutions is advantageous compared to the vanadia-based Li-ion intercalation materials,such as Li 1.2V 3O 8[61],LiV 3O 8[62]and V 2O 5[63],the solubility of which in LiNO 3solution was perceivable through the yellowish solutioncoloration.Fig.8.The Nyquist plots of LiFePO 4/C composite in aqueous LiNO 3solution at var-ious stages of delithiation;inset:enlarged high-frequency region.3.2.2.2.Impedance measurements.Figs.8and 9present the Nyquist plots of the LiFePO 4/C composite in aqueous LiNO 3solution at various open circuit potentials (OCV),during delithiation (anodic sweep,Fig.8)and during lithiation (cathodic sweep,Fig.9).The delithiated phase,observed at OCV =1V,as well as the lithi-ated phase,observed at OCV =0V,in the low-frequency region (f <100Hz)tend to behave like a capacitor,characteristic of a surface thin-layered redox material with reflective phase bound-ary conditions [64].At the OCV not too far from the reversible one (0.42V during delithiation,0.308V during lithiation),where both LiFePO 4and FePO 4phase may be present,within the whole 10−2–105Hz frequency range,the reaction behaves as a reversible one (i.e.shows the impedance of almost purely Warburg type).The insets in Figs.8and 9present the enlarged parts of the impedance diagram in the region of high frequencies,where one may observe a semicircle,the diameter of which corresponds theoretically to the charge transfer resistance.As visible,the change of open circuit potential between 0and 1V,in spite of the phase transition,does not cause significant change in charge transfer resistance.The small charge transfer resistance obtained with the carbon participation of 13.4%,being less than 1 ,is the smallest one reported thus far for olivine based materials.This finding agrees with the trend found by Zhao et al.[27],that the charge transfer resistance scaleddownFig.9.The Nyquist plots of LiFePO 4/C composite in aqueous LiNO 3solution at var-ious stages of lithiation;inset:enlarged high-frequency region.M.Vujkovi´c et al./Electrochimica Acta 92 (2013) 248–256253Fig.10.The dependence Z Re vs.ω−1/2during lithiation at 0.308V (top)and delithi-ation at 0.42V (down)in the frequency range 72–2.68Hz.to 1000,400and 150 when the amount of in situ formed carbon in the LiFePO 4/C composite increased in the range 1,2.8and 4.8%.For OCV corresponding to the cathodic (0.42V)and anodic (0.308V)peak maxima,the Warburg constant W was calculated from the dependence [21]:Z Re =R e +R ct + W ω−1/2(3)In the frequency range 2.7–72Hz,almost purely Warburg impedance was found to hold (i.e.the slope of the Nyquist plot very close to 45degrees was found).At the potential of cathodic current maximum (0.42V),from Fig.10, W was determined to amount to 7.96 s −1/2.At the potential of anodic maxima,0.308V, W was determined to amount to 9.07 s −1/2.In the published literature,for the determination of diffusion coefficient on the basis of impedance measurements,the following equation was often used [66,68,69]:D =0.5V M AF W ıE ıx2(4)where V M is molar volume of olivine,44.1cm 3, W is Warburg con-stant and ıE /ıx is the slope of the dependence of electrode potential on the molar fraction of Li (x )for given value of x .However,the potentials of CV maxima in the here studied case correspond to the x range of two-phase equilibrium,where for an accurate deter-mination of ıE /ıx a strong control of perturbed region of sample particles is required [69],and thus the determination of diffusion coefficients was omitted.3.2.2.3.Galvanostatic measurements.The galvanostatic measure-ments of LiFePO 4/C in saturated LiNO 3aqueous solution were performed in a two-electrode arrangement using hydrother-mally synthesized VO 2[55]as the active material of thecounterFig.11.Capacity versus cycle number and charge/discharge profiles (inset)for thecell consisting of LiFePO 4/C composite as cathode,and VO 2in large excess as anode,in saturated LiNO 3aqueous electrolyte observed at rate C/3.electrode.Preliminary cyclovoltammetric tests of VO 2in saturated LiNO 3solution at the sweep rate 10mV s −1,evidenced excellent cyclability and stable capacity of about 160mAh g −1during at least 50cycles.The voltage applied to the two-electrode cell was cycled within the limits 0and 1.4V.Due to a significant stoichiometric excess of VO 2over LiFePO 4/C composite (5:1)the actual voltage may be considered to be the potential versus reference VO 2/Li x VO 2electrode.Fig.11shows the dependence of the discharging Coulombic capacity of the LiFePO 4/C composite on the number of galvano-static cycles at discharging rate C/3,as well as (in the inset)the voltage vs.charging/discharging degree for 1st,2nd and 50th cycle.The charge/discharge curves do not change substantially in shape upon cycling,indicating stable capacity.For an aqueous solution,a surprisingly high initial discharge capacity of 106mAh g −1and low capacity fade of only 6%after 50charge/discharge cycles were evidenced.This behavior is admirable in comparison to other elec-trode materials in aqueous media reported in literature (LiTi 2(PO 4)3[42],LiV 3O 8[57]),and probably enabled by a higher thermody-namic stability of olivine structure [1].Fig.12presents the results of cyclic galvanostatic investigations of LiFePO 4/C composite in aqueous LiNO 3solution at various dis-charging rates.The charging/discharging rate was initially C/3for 80cycles and then was increased stepwise up to 3C.ThecapacityFig.12.Cyclic performance of LiFePO 4/C in saturated LiNO 3aqueous electrolyte at different charging/discharging rates.。
磷酸铁锂粉末电导率
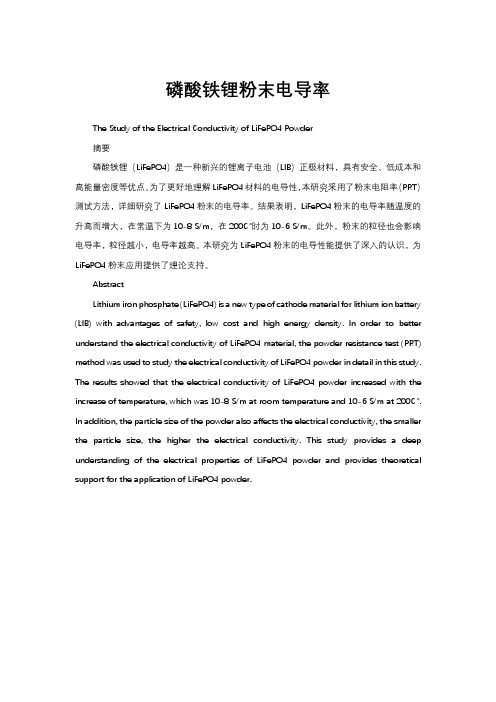
磷酸铁锂粉末电导率The Study of the Electrical Conductivity of LiFePO4 Powder摘要磷酸铁锂(LiFePO4)是一种新兴的锂离子电池(LIB)正极材料,具有安全、低成本和高能量密度等优点。
为了更好地理解LiFePO4材料的电导性,本研究采用了粉末电阻率(PRT)测试方法,详细研究了LiFePO4粉末的电导率。
结果表明,LiFePO4粉末的电导率随温度的升高而增大,在常温下为10-8 S/m,在200℃时为10-6 S/m。
此外,粉末的粒径也会影响电导率,粒径越小,电导率越高。
本研究为LiFePO4粉末的电导性能提供了深入的认识,为LiFePO4粉末应用提供了理论支持。
AbstractLithium iron phosphate (LiFePO4) is a new type of cathode material for lithium ion battery (LIB) with advantages of safety, low cost and high energy density. In order to better understand the electrical conductivity of LiFePO4 material, the powder resistance test (PRT) method was used to study the electrical conductivity of LiFePO4 powder in detail in this study. The results showed that the electrical conductivity of LiFePO4 powder increased with the increase of temperature, which was 10-8 S/m at room temperature and 10-6 S/m at 200℃. In addition, the particle size of the powder also affects the electrical conductivity, the smaller the particle size, the higher the electrical conductivity. This study provides a deep understanding of the electrical properties of LiFePO4 powder and provides theoretical support for the application of LiFePO4 powder.。
碳纳米管在锂电池中的应用研究进展
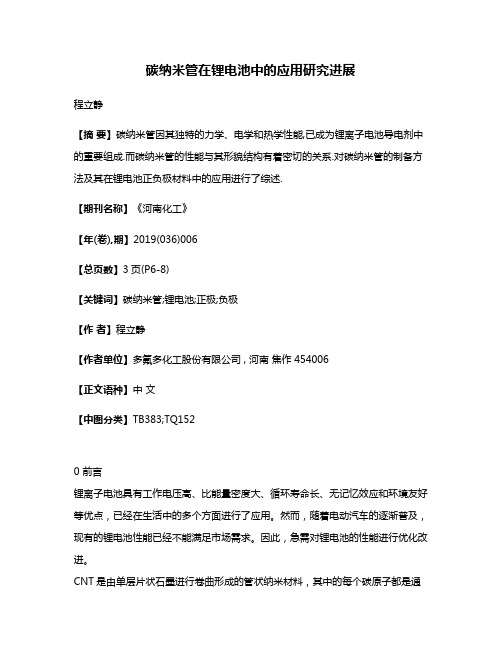
碳纳米管在锂电池中的应用研究进展程立静【摘要】碳纳米管因其独特的力学、电学和热学性能,已成为锂离子电池导电剂中的重要组成.而碳纳米管的性能与其形貌结构有着密切的关系.对碳纳米管的制备方法及其在锂电池正负极材料中的应用进行了综述.【期刊名称】《河南化工》【年(卷),期】2019(036)006【总页数】3页(P6-8)【关键词】碳纳米管;锂电池;正极;负极【作者】程立静【作者单位】多氟多化工股份有限公司 , 河南焦作 454006【正文语种】中文【中图分类】TB383;TQ1520 前言锂离子电池具有工作电压高、比能量密度大、循环寿命长、无记忆效应和环境友好等优点,已经在生活中的多个方面进行了应用。
然而,随着电动汽车的逐渐普及,现有的锂电池性能已经不能满足市场需求。
因此,急需对锂电池的性能进行优化改进。
CNT是由单层片状石墨进行卷曲形成的管状纳米材料,其中的每个碳原子都是通过SP2杂化方式,与周边的碳原子形成六边形。
按照石墨层数的不同进行划分,CNT可以分为单臂CNT(SWNT)和多臂CNT(MWNT),其结构如图1所示[1]。
图1 SWNT和MWNT结构示意图本文主要针对CNT的制备方法,在正极材料和负极材料中的应用研究进展进行综述。
1 CNT的制备方法CNT常用的制备方法主要有电弧法、激光蒸发法和化学气相沉积法(CVD)等多种工艺。
1.1 电弧法CNT是由Lijima采用电弧法于1991年在日本首次发现的。
该制备方法需要严格控制电弧的电压、载气、电流强度等多个实验参数。
JOURNET等[3]以氢气对载气,通过大量的实验探索,提出了一种半连续式电弧工艺,该工艺可以显著提升CNT的制备速率。
虽然,经过多个科学家对该工艺的不断优化,该工艺仍然存在产品杂质含量高、产品结晶度高等缺点。
1.2 激光蒸发法该工艺是通过将激光束的热量转移至石墨靶上,使其气化变成气态,同时,在催化剂的作用下,气态碳激发生成碳原子,该原子在载气的气氛中,发生碰撞形成CNT。
关于磷酸铁锂的英文文献(含中文翻译)
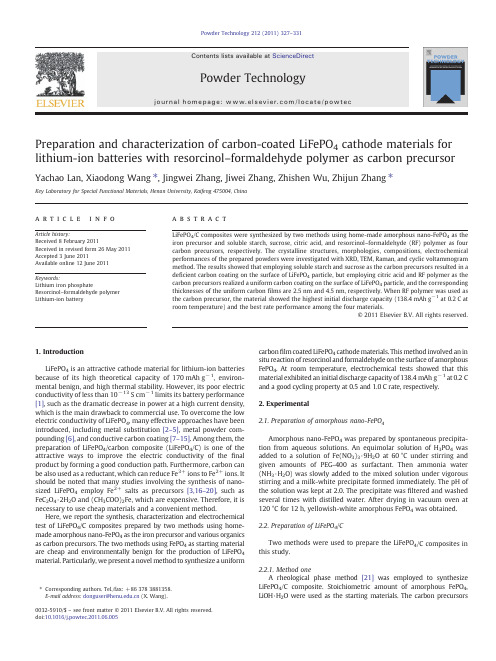
Preparation and characterization of carbon-coated LiFePO 4cathode materials for lithium-ion batteries with resorcinol –formaldehyde polymer as carbon precursorYachao Lan,Xiaodong Wang ⁎,Jingwei Zhang,Jiwei Zhang,Zhishen Wu,Zhijun Zhang ⁎Key Laboratory for Special Functional Materials,Henan University,Kaifeng 475004,Chinaa b s t r a c ta r t i c l e i n f o Article history:Received 8February 2011Received in revised form 26May 2011Accepted 3June 2011Available online 12June 2011Keywords:Lithium iron phosphateResorcinol –formaldehyde polymer Lithium-ion batteryLiFePO 4/C composites were synthesized by two methods using home-made amorphous nano-FePO 4as the iron precursor and soluble starch,sucrose,citric acid,and resorcinol –formaldehyde (RF)polymer as four carbon precursors,respectively.The crystalline structures,morphologies,compositions,electrochemical performances of the prepared powders were investigated with XRD,TEM,Raman,and cyclic voltammogram method.The results showed that employing soluble starch and sucrose as the carbon precursors resulted in a de ficient carbon coating on the surface of LiFePO 4particle,but employing citric acid and RF polymer as the carbon precursors realized a uniform carbon coating on the surface of LiFePO 4particle,and the corresponding thicknesses of the uniform carbon films are 2.5nm and 4.5nm,respectively.When RF polymer was used as the carbon precursor,the material showed the highest initial discharge capacity (138.4mAh g −1at 0.2C at room temperature)and the best rate performance among the four materials.©2011Elsevier B.V.All rights reserved.1.IntroductionLiFePO 4is an attractive cathode material for lithium-ion batteries because of its high theoretical capacity of 170mAh g −1,environ-mental benign,and high thermal stability.However,its poor electric conductivity of less than 10−13S cm −1limits its battery performance [1],such as the dramatic decrease in power at a high current density,which is the main drawback to commercial use.To overcome the low electric conductivity of LiFePO 4,many effective approaches have been introduced,including metal substitution [2–5],metal powder com-pounding [6],and conductive carbon coating [7–15].Among them,the preparation of LiFePO 4/carbon composite (LiFePO 4/C)is one of the attractive ways to improve the electric conductivity of the final product by forming a good conduction path.Furthermore,carbon can be also used as a reductant,which can reduce Fe 3+ions to Fe 2+ions.It should be noted that many studies involving the synthesis of nano-sized LiFePO 4employ Fe 2+salts as precursors [3,16–20],such as FeC 2O 4·2H 2O and (CH 3COO)2Fe,which are expensive.Therefore,it is necessary to use cheap materials and a convenient method.Here,we report the synthesis,characterization and electrochemical test of LiFePO 4/C composites prepared by two methods using home-made amorphous nano-FePO 4as the iron precursor and various organics as carbon precursors.The two methods using FePO 4as starting material are cheap and environmentally benign for the production of LiFePO 4material.Particularly,we present a novel method to synthesize a uniformcarbon film coated LiFePO 4cathode materials.This method involved an in situ reaction of resorcinol and formaldehyde on the surface of amorphous FePO 4.At room temperature,electrochemical tests showed that this material exhibited an initial discharge capacity of 138.4mAh g −1at 0.2C and a good cycling property at 0.5and 1.0C rate,respectively.2.Experimental2.1.Preparation of amorphous nano-FePO 4Amorphous nano-FePO 4was prepared by spontaneous precipita-tion from aqueous solutions.An equimolar solution of H 3PO 4was added to a solution of Fe(NO 3)3·9H 2O at 60°C under stirring and given amounts of PEG-400as surfactant.Then ammonia water (NH 3·H 2O)was slowly added to the mixed solution under vigorous stirring and a milk-white precipitate formed immediately.The pH of the solution was kept at 2.0.The precipitate was filtered and washed several times with distilled water.After drying in vacuum oven at 120°C for 12h,yellowish-white amorphous FePO 4was obtained.2.2.Preparation of LiFePO 4/CTwo methods were used to prepare the LiFePO 4/C composites in this study.2.2.1.Method oneA rheological phase method [21]was employed to synthesize LiFePO 4/C composite.Stoichiometric amount of amorphous FePO 4,LiOH·H 2O were used as the starting materials.The carbon precursorsPowder Technology 212(2011)327–331⁎Corresponding authors.Tel./fax:+863783881358.E-mail address:donguser@ (X.Wang).0032-5910/$–see front matter ©2011Elsevier B.V.All rights reserved.doi:10.1016/j.powtec.2011.06.005Contents lists available at ScienceDirectPowder Technologyj o u r n a l h o me p a g e :w w w.e l sev i e r.c o m /l oc a t e /pow t e care soluble starch(50.0g/1mol LiOH·H2O),sucrose(35.0g/1mol LiOH·H2O),citric acid monohydrate(21.0g/1mol LiOH·H2O),respec-tively.These carbon precursors were respectively solved in an appropri-ate amount of distilled water under stirring and heating.Then the amorphous FePO4and LiOH·H2O were added under vigorous stirring. Subsequently,the mixtures were respectively dried in an oven at120°C for6h,heated at350°C for1h in argonflow,treated at750°C for12h in argonflow,and ground.Finally,the LiFePO4/C composites were obtained and were denoted as sample A,sample B and sample C,respectively. 2.2.2.Method twoIn a typical synthesis,0.10g of CTAB was solved in30ml of distilled water solution under continuous stirring.Subsequently,1.52g FePO4·3H2O,0.055g resorcinol(R)and0.10ml formaldehyde(F)were successively added.When the temperature of water bath was up to85°C,LiOH·H2O was added.The mixture was kept stirred up in the dark for2h,dried in an oven at120°C for6h,heated at 350°C for1h in argonflow,treated at750°C for12h in argonflow, andfinally ground to obtain the LiFePO4/C composites(denoted as sample D).These four samples and their corresponding parameters are listed in Table1.The carbon contents of the samples were calculated by the loss on ignition of the four LiFePO4/C composites in air.2.3.CharacterizationThermogravimetric(TG)and differential thermal analysis(DTA) analyses were conducted with an EXSTAR6000thermal analysis system at a heating rate of10°C min−1.The powder X-ray diffraction (XRD,X'Pert Pro MPD,Philips)using Cu Ka radiation was employed to identify the crystalline phase of the prepared materials.Raman spectrum was recorded on a Renishaw RM-1000Microscopic Raman spectrometer with457.5nm excitation requiring a10mW power at room temperature.Low-magnification and high-magnification TEM images were taken on a JEM-2010transmission electron microscope (using an accelerating voltage of200kV).Electrodes were fabricated from a mixture of prepared carbon-coated LiFePO4powders(80wt.%),carbon black(12wt.%),and polyvinylidenefluoride in N-methylpyrrolidinon(8wt.%).The slurry was spread onto Al foil and dried in vacuum at120°C for12h.The carbon-coated LiFePO4loading was2mg cm−2in the experimental cells.The cells were assembled in an argon-atmosphere-filled glove box.The electrolyte was1M LiPF6in a mixture of ethylene carbonate (EC)and dimethyl carbonate(DMC)(1:1volume).The cells were galvanostatically charged and discharged at a voltage range of2.5–4.2V with LAND battery testing system at room temperature.Cyclic voltammograms were run on an IM6impedance and electrochemicalmeasurement system(Zahner,Germany)at a scan rate of0.1mV s−1 between2.5and4.0V.3.Results and discussionThe TEM images of the amorphous nano-FePO4were shown in Fig.1.The morphology of the as-prepared FePO4is an irregular particle with an average diameter of30nm.Most of the particles connected to each other because of their high surface energy which results from their small sizes.Fig.2a shows the TG/DTA curves of the FePO4·3H2O powder with a heating rate of10°C/min from room temperature to850°C in air.On the DTA curve near150°C,there is a very strong endothermic peak, associating with the sharp weight loss on the TG curve,which is related to the quick dehydration of FePO4·3H2O.During150–550°C, 26.3%weight loss on the TG curve indicates the slow elimination of residual H2O in FePO4·3H2O,exactly corresponding to the loss of crystalline water of FePO4·3H2O.And one exothermic peak is displayed at a higher temperature of590°C,which is not accompa-nied by appreciable weight loss in the TG curve,indicating the transformation of the amorphous FePO4to hexagonal FePO4crystal. The XRD patterns of FePO4·3H2O before and after calcination have been investigated in Fig.2b.As illustrated in pattern A,it can be seen that there is no evidence of diffraction peaks before calcination, indicating the synthesized FePO4·3H2O is just amorphous.While for the calcinated FePO4·3H2O at600°C for6h in air,it exhibits strong and narrow peaks revealing a well-crystallized material in pattern B. All of the diffraction peaks of the prepared FePO4are indexed to a single-phase hexagonal structure with a P3121space group and without any impurities,which is in good agreement with the standard card(JCPDS card no:72–2124).Table1Carbon precursors and residual carbon content of samples A,B,C and D.Samples A B C DCarbon precursor Starch Sucrose Citric acid RF polymer Final carbon content(wt.%) 5.48.5 4.35.1Fig.1.TEM images of the prepared amorphous nano-FePO4.n et al./Powder Technology212(2011)327–331The XRD diffraction patterns of LiFePO 4/C powders prepared with different carbon precursors were shown in Fig.3.All peaks can be indexed as a single phase with an ordered olivine structure indexed to the orthorhombic space group,Pnmb (JCPDS card no.83–2092).The obtained lattice parameters are sample A:a=10.2956Å,b=6.0367Å,and c =4.7001Å,sample B:a =10.1992Å,b =6.0483Å,andc=4.6971Å,sample C:a=10.2472Å,b=6.0208Å,and c=4.6882Åand sample D:a=10.3372Å,b=5.9993Å,and c=4.6932Å,respec-tively.There is no evidence of diffraction peaks for carbon,though some amorphous masses and films attached to the LiFePO 4particles were observed from TEM images (see Fig.4).This indicates the carbon contents are very low.Morphologies of these LiFePO 4/C composites were shown in Fig.4.It is obvious that the samples show different carbon distribution on LiFePO 4particle surface.From Fig.4a,c,e and g,we observed that the samples were composed of agglomerated particles whose sizes range from 50to 300nm.From Fig.4b and d,there is not enough carbon coating to spread throughout the substrate particles.In contrast to sample A and sample B,there are uniform carbon thin films on the grain surfaces of sample C and sample D,and the thickness of the carbon films are about 2.5nm (Fig.4f)and 4.5nm (Fig.4h),respectively.The reason may lie in that different carbon precursors have different adsorbabilities on the surface of FePO 4·3H 2O particles,resulting in different carbon distribution on the surface of LiFePO 4particle after the post treatment.Soluble starch and sucrose possess plentiful hydroxyl groups,by which soluble starch and sucrose molecules could probably weakly adsorb on the surface of FePO 4·3-H 2O particles in the hydrogen bonding.In the post treatment process,part of soluble starch and sucrose molecules desorbed from the surface of FePO 4·3H 2O particles,resulting in the de ficient carbon coating.But citric acid possesses carboxyl groups,which may be partially esteri fied by hydroxyl groups on the FePO 4·3H 2O particles,forming a tight connection.This results in more complete carbon coating after the post treatment.For sample D,we suppose that,in the present synthetic system,the surfactant CTAB may con fine the resorcinol –formaldehyde (RF)polymer molecules and FePO 4·3H 2O particles in plenty of tiny spaces,so the RF polymer molecules were tightly attached to FePO 4·3H 2O particles.After the post treatment,the RF polymer was transformed into the carbon film which tightly stuck on the surface of LiFePO 4particle.In addition,from the HRTEM image of sample D (shown in Fig.4h),the d-spacing of 0.294nm corresponds to the (211)plane of LiFePO 4.As an important aid investigating the structure of the carbon,the Raman measurement was adopted,and the results were shown in Fig.5.Every Raman spectrum consists of a small band at 940cm −1,which corresponds to the symmetric PO 4stretching vibration in LiFePO 4.The intense broad bands at 1350and 1590cm −1can be attributed to the characteristic Raman spectra of carbon.The bands at 1590cm −1mainly correspond to graphitized structured carbon of G band,while that at 1350cm −1corresponds to disordered structured carbon of D band [22,23].The graphitized carbon contains sp 2hybrid bonding,which is positively correlated with the electronic conduc-tivity of carbon,and the disordered carbon mainly corresponds to sp 3hybrid bonding.As shown in Fig.5,the integrated intensity ratios of sp 2/sp 3of the LiFePO 4/C composites synthesized with different carbon precursors are 0.865(curve A),0.857(curve B),0.856(curve C)and 0.860(curve D),respectively.So the similar sp 2/sp 3ratios of the four samples give us few clues to explain the difference in their electrochemical performances.Fig.6shows the cycling performance curves of all the samples at different rates.As shown in Fig.6,the initial discharge capacities of sample A,sample B,sample C and sample D at room temperature at 0.2C rate are 110.4,118.8,137.7and 138.4mAh g −1,respectively.The capacity of sample D gradually increases in the initial cycles.This may be due to the incomplete dispersion of the electrolyte into the electrode material at the beginning.Moreover,the capacity of sample D is highest among the four samples at 0.5C and 1.0C,indicating that method two is better than method one.The lower capacities of sample A and sample B must be due to the incomplete carbon coating on the LiFePO 4particles.The higher capacity of sample D than that of sample C may be attributed to the thicker carbon film of sample D keeping the crystal structure of LiFePO 4morestable.Fig.2.(a)TG/DTA curves of the FePO 4·3H 2O.(b)XRD patterns of the FePO 4samples before (A)and after (B)calcination inair.Fig. 3.XRD patterns of LiFePO 4/C composites synthesized with different carbon precursors.329n et al./Powder Technology 212(2011)327–331In order to further understand the electrochemical properties of the four samples,the cyclic voltammogram (CV)curves were performed at a scan rate of 0.1mV s −1at room temperature (as shown in Fig.7).Each of the CV curves consists of an oxidation peak and a reduction peak,corresponding to the charge reaction and discharge reaction of the Fe 2+/Fe 3+redox couple.In the CV pro files of the LiFePO 4cathode material,the smaller voltage difference between the charge and discharge plateaus and the higher peak current means better electrode reaction kinetics,and consequently better rate performance.Sample A and sample B electrodes have broad peaks in CV curves.In contrast,sample C and sample D electrodes demonstrate sharp redox peaks,which indicate an improvement in the kinetics of the lithium intercalation/de-intercalation at the electrode/electrolyte interface.The voltage difference of sample D is smaller than that of sample C,so sample D demonstrates a better rate performance.4.ConclusionsLiFePO 4/C composites were synthesized by two methods using home-made amorphous nano-FePO 4as the iron precursor and various organics as carbon precursors.It was found that employing soluble starch and sucrose as the carbon precursors resulted in a de ficient carbon coating on the surface of LiFePO 4particle,but employing citric acid and RF polymer as the carbon precursors realized a uniform carbon coating on the surface of LiFePO 4particle.Particularly,when RF polymer was used as the carbon precursor,the carbon film is thicker,and the material showed the highest initial discharge capacity (138.4mAh g −1at 0.2C at room temperature)and the best rate performance among the four materials.The intensities of redox peak and the voltage differences in the CV curves of the four samples are consistent with their rateperformance.Fig.4.TEM images of synthesized LiFePO 4/C composite synthesized with different carbon precursors.(a)and (b)sample A,(c)and (d)sample B,(e)and (f)sample C,(g)and (h)sampleD.Fig. 5.Raman shift of LiFePO 4/C composites synthesized with different carbonprecursors.Fig.6.The cycling performance curves of the samples with different carbon precursors at various discharge rates.n et al./Powder Technology 212(2011)327–331References[1] A.K.Padhi,K.S.Nanjundaswamy,J.B.Goodenough,Phospho-olivines as positive-electrode materials for rechargeable lithium batteries,J.Electrochem.Soc.144(1997)1188–1194.[2]T.Nakamura,Y.Miwa,M.Tabuchi,Y.Yamada,Structural and surfacemodi fications of LiFePO 4olivine particles and their electrochemical properties,J.Electrochem.Soc.153(2006)A1108–A1114.[3]S.-Y.Chung,J.T.Bloking,Y.-M.Chiang,Electronically conductive phospho-olivinesas lithium storage electrodes,Nat.Mater.1(2002)123–128.[4]P.S.Herle,B.Ellis,N.Coombs,L.F.Nazar,Nano-network electronic conduction iniron and nickel olivine phosphates,Nat.Mater.3(2004)147–152.[5]G.X.Wang,S.Bewlay,S.A.Needham,H.K.Liu,R.S.Liu,V.A.Drozd,J.-F.Lee,J.M.Chend,Synthesis and characterization of LiFePO 4and LiTi 0.01Fe 0.99PO 4cathode materials,J.Electrochem.Soc.153(2006)A25–A31.[6] F.Croce,A.D'Epifanio,J.Hasson,A.Duptula,T.Olczac,B.Scrosati,A novel conceptfor the synthesis of an improved LiFePO 4lithium battery cathode,Electrochem.Solid-State Lett.5(2002)A47–A50.[7]H.Huang,S.C.Yin,L.F.Nazar,Approaching theoretical capacity of LiFePO 4at roomtemperature at high rates,Electrochem.Solid-State Lett.4(2001)A170–A172.[8]M.Herstedt,M.Stjerndahl,A.Nyten,T.Gustafsson,H.Rensmo,H.Siegbahn,N.Ravert,M.Armand,J.O.Thomas,K.Edstroem,Surface chemistry of carbon-treated LiFePO 4particles for Li-ion battery cathodes studied by PES,Electrochem.Solid-State Lett.6(2003)A202–A206.[9]M.M.Doeff,Y.Hu,F.McLarnon,R.Kostecki,Effect of surface carbon structure onthe electrochemical performance of LiFePO 4,Electrochem.Solid-State Lett.6(2003)A207–A209.[10]Y.Hu,M.M.Doeff,R.Kostecki,R.Finones,Electrochemical performance of sol –gelsynthesized LiFePO 4in lithium batteries,J.Electrochem.Soc.151(2004)A1279–A1285.[11]X.Z.Liao,Z.Ma,L.Wang,X.Zhang,Y.Jiang,Y.He,A novel synthesis route forLiFePO 4/C cathode materials for lithium-ion batteries,Electrochem.Solid-State Lett.7(2004)A522–A525.[12] C.H.Mi,X.B.Zhao,G.S.Cao,J.P.Tu,In situ synthesis and properties of carbon-coated LiFePO 4as Li-ion battery cathodes,J.Electrochem.Soc.152(2005)A483–A487.[13]R.Dominko,M.Bele,M.Gaberscek,M.Remskar,D.Hanzel,S.Pejovnik,J.Jamnik,Impact of the carbon coating thickness on the electrochemical performance of LiFePO 4/C composites,J.Electrochem.Soc.152(2005)A607-A607.[14]K.Zaghib,J.Shim,A.Guer fi,P.Charest,K.A.Striebel,Effect of carbon source asadditives in LiFePO 4as positive electrode for lithium-ion batteries,Electrochem.Solid-State Lett.8(2005)A207–A210.[15]K.S.Park,J.T.Son,H.T.Chung,S.J.Kim,C.H.Lee,K.T.Kang,H.G.Kim,Surfacemodi fication by silver coating for improving electrochemical properties of LiFePO 4,Solid State Commun.129(2004)311–314.[16] A.Yamada,S.C.Chung,K.Hinokuma,Optimized LiFePO 4for lithium batterycathodes,J.Electrochem.Soc.148(2001)A224–A229.[17]N.Meethong,H.-Y.Shadow Huang,W.C.Carter,Y.-M.Chiang,Size-dependentlithium miscibility gap in nanoscale Li 1−x FePO 4,Electrochem.Solid-State Lett.10(2007)A134–A138.[18] C.Delacourt,P.Poizot,S.Levasseur,C.Masquelier,Size effects on carbon-freeLiFePO 4powders,Electrochem.Solid-State Lett.9(2006)A352–A355.[19] D.Choi,P.N.Kumta,Surfactant based sol –gel approach to nanostructured LiFePO 4for high rate Li-ion batteries,J.Power Sources 163(2007)1064–1069.[20]K.Zaghib,A.Mauger,F.Gendron,C.M.Julien,Surface effects on the physical andelectrochemical properties of thin LiFePO 4particles,Chem.Mater.20(2008)462–469.[21]Y.H.Huang,H.B.Ren,S.Y.Yin,Y.H.Wang,Z.H.Peng,Y.H.Zhou,Synthesis ofLiFePO 4/C composite with high-rate performance by starch sol assisted rheolog-ical phase method,J.Power Sources 195(2010)610–613.[22]M.M.Doeff,J.D.Wilcox,R.Kostecki,u,Optimization of carbon coatings onLiFePO 4,J.Power Sources 163(2006)180–184.[23]G.L.Yang,A.F.Jalbout,Y.Xu,H.Y.Yu,X.G.He,H.M.Xie,R.S.Wang,Effect ofpolyacenic semiconductors on the performance of olivine LiFePO 4,Electrochem.Solid-State Lett.11(2008)A125–A128.Fig.7.The CV pro files of the different samples at the scan rate of 0.1mV s −1.331n et al./Powder Technology 212(2011)327–331。
北大考研-化学与分子工程学院研究生导师简介-周恒辉

爱考机构-北大考研-化学与分子工程学院研究生导师简介-周恒辉周恒辉分析化学博士、副教授、博士生导师兼北京市动力锂离子电池工程技术研究中心主任,先进电池材料与技术北京市重点实验室副主任电话/传真:86-010-********电子信箱:hhzhou@1963年生,1989年获湘潭大学理学硕士学位,1998年获北京大学理学博士学位。
研究领域和兴趣:能量储存及转换材料的设计、合成和表征化学电源中的电极/电解液界面研究可移动能源器件的设计及应用近年本课题方向承担的主要课题:北京市科技计划项目,新一代正极材料LiFePO4的中试研究,Z0004106040221,400万元,2004-2006信息产业部“电子信息产业发展基金”项目,高比能量聚合物锂离子电池开发与产业化,信部运[2004]479,200万元,2004-2006中关村科技园区重点支持产业领域专项,基于新型能源的LED道路照明示范工程重大产业化项目,350万元,2007-2008北京市科技计划项目,新一代正极材料LiFePO4的产业化技术研究,Z070901310701,180万元,2007-2008国家科技部863项目,改善磷酸铁锂高温性能的关键技术研究,2006AA03Z229,89万元,2007-2008工信部“电子信息产业发展基金”项目,磷酸铁锂正极材料的研发与产业化,工信部财[2008]192号,400万元,2008-2010。
工信部“电子信息产业发展基金”项目,磷酸铁锂动力电池及系统集成技术研发与产业化,300万元,2009-2011,副组长单位。
国家科技部863重点项目,铁锂正极材料规模化生产和应用关键技术研究,2009AA0352002,2000万元,2010-2012主要论文:ChengF.Q.,WanW.,TanZ.,HuangY.Y.,ZhouH.H.*,ChenJ.T.,ZhangX.X.,Highpowerperformanceofn ano-LiFePO4/Ccathodematerialsynthesizedvialauricacid-assistedsolid-statereaction,Electrochimic aActa,2011,56:2999-3005LiJ.M.,WaW.,ZhouH.H.*,LiJ.J.,XuD.S.*,HydrothermalsynthesisofTiO2(B)nanowireswithultrahig hsurfaceareaandtheirfastcharginganddischargingpropertiesinLi-ionbatteries,mun.,2011,47:3439-3441YeJ.F.,LiuW.,CaiJ.G.,ChenS.,ZhaoX.W.,ZhouH.H.,QiL.M.*,NanoporousAnataseTiO2Mesocrystal s:Additive-FreeSynthesis,RemarkableCrystalline-PhaseStability,andImprovedLithiumInsertionBeh avior,J.Am.Chem.Soc.,2011,133:933–940HuangY.Y.,ChenJ.T.,ChengF.Q.,WanW.,LiuW.,ZhouH.H.*,ZhangX.X.,Amodi?edAl2O3coatingpr ocesstoenhancetheelectrochemicalperformanceofLi(Ni1/3Co1/3Mn1/3)O2anditscomparisonwithtr aditionalAl2O3coatingprocess,J.PowerSources,2010,195:8267–8274HuangYY,NiJF,ZhouHH*,ChenJT,ZhangXX,AmodifiedZrO2-coatingprocesstoimproveelectrochemicalperformanceofLi(Ni1/3Co1/3Mn1/3)O2, J.PowerSources,2009,188(2):538-545NiJF,ZhouHH*,ChenJT,ZhangXX,ImprovedelectrochemicalperformanceoflayeredLiNi0.4Co0.2Mn0.4O2viaLi2ZrO3coating,ElectrochimicaActa,53(7):3075-3083,2008QiuJY,ZhaoL,ZhaiML?,NiJF,ZhouHH??,PengJ,LiJQ,WeiGS,Pre-irradiationgraftingofstyreneandmaleicanhydrideontoPVDFmembraneandsubsequentsulfonatio nforapplicationinvanadiumredoxbatteries,JournalofPowerSources,177:617–623,2008NiJF,ZhouHH*,ChenJT,ZhangXX,MoltensaltsynthesisandelectrochemicalpropertiesofsphericalLiFePO4particles,MaterialsLetters,61(3-4):1260~1264,2007QiuJY,LiMY,NiJF,ZhaiML*,PengJ,XuL,ZhouHH*,LiJQ,WeiGS,PreparationofETFE-basedanionex changemembranetoreducepermeabilityofvanadiumionsinvanadiumredoxbattery,JournalofMembraneScience,297(1-2):174-180,2007NiJF,ZhouHH*,ChenJT,ZhangXX,LiFePO4dopedwithionspreparedbyco-precipitationmethod,MaterialsLetters,59(18)2361-2365,2005主要专利:成富圈、周恒辉、黄友元、陈继涛、张新祥,一种制备聚阴离子型锂离子电池正极材料的方法,中国专利,公开号:CN101794878A,2010倪江锋、周恒辉、陈继涛、张新祥,一种可调控其颗粒形貌的磷酸铁锂制备方法,中国专利,专利号:ZL200710000683.0,2009倪江锋,周恒辉,陈继涛,张新祥,一种合成类球形磷酸金属锂盐的方法中国专利,专利号:ZL200610011378.7,2008周恒辉,陈继涛,锂离子电池正极材料磷酸亚铁锂的制备方法中国专利,专利号:ZL200410039176.4,2006周恒辉,陈继涛,孙杰,锂离子电池的电极及其制备方法和锂离子电池中国专利,专利号:ZL03153874.6,2005陈继涛,周恒辉,常文保,慈云祥,锂离子电池用复合石墨负极材料的制备方法、负极和电池,中国专利,专利号:ZL02159166.0,2005周恒辉,杨正,任献举,锂离子电池正极活性材料钴酸锂的制备方法,中国专利,专利号:ZL02155659.8,2005周恒辉,陈继涛,常文保,慈云祥,一种苯萘聚合物及其制备方法和应用,中国专利,专利号:ZL01123429.6,2004陈继涛,周恒辉,常文保,慈云祥,一种锂离子电池负极材料的制备方法、负极和电池,中国专利,专利号:ZL01123430.X,2004周恒辉,杨正,任献举,一种提高钴酸锂的中位径和振实密度的方法,中国专利,专利号:ZL01123431.8,2004欢迎校内外对可移动能源材料与器件有兴趣的本科生、研究生和博士后加入本研究方向,在校内奖学金基础上为学生提供优厚的北大先行奖学金。
磷酸锰铁锂文献

磷酸锰铁锂文献磷酸锰铁锂是一种重要的锂离子电池正极材料,具有高比容量、良好的循环稳定性和较高的工作电压。
以下是一些相关的文献:1. An Optimized Electrode Slurry for High-Energy Li-Ion Cells Based on LiNi0.5Mn1.5O4 and a Mn-RichLi1.2Ni0.22Mn0.54Co0.24O2 Cathode: Electrochemical, Electrical Characterization and Safety Aspects. Journal of The Electrochemical Society, 2020. 167 (11): 110559.2. Synthesis and properties of LiFe0.94Mn0.06PO4/C composite cathode materials for lithium-ion batteries. Journal of Power Sources, 2016. 301: 101-105.3. Electrochemical per formance of LiFe1−yMgyPO4/C for Li-ion batteries. Journal of Solid State Electrochemistry, 2012. 16: 2599-2604.4. Ionothermal Synthesis of LiFePO4 and LiFe0.97Mn0.03PO4 Electrodes. Journal of The Electrochemical Society, 2007. 154 (9): A849-A852.5. Synthesis and characterizations of LiMn2-xFex(PO4)3/C cathode materials for lithium ion batteries. Electrochimica Acta, 2011. 56: 193-199.这些文献提供了关于磷酸锰铁锂的合成方法、结构特性、电化学性能以及应用方面的研究成果。
不同碳源对LiFePO_4_C电化学性能的影响

第30卷第3期福建师范大学学报(自然科学版)Vol.30,No.3(2014年5月)Journal of Fujian Normal University (Natural Science Edition )May 2014文章编号:1000-5277(2014)03-0047-06不同碳源对LiFePO 4/C 电化学性能的影响黄艳丹,余蕊冰,杨艳敏,林应斌,赖恒,黄志高(福建师范大学物理与能源学院,福建省量子调控与新能源材料重点实验室,福建福州350117)摘要:分别以蔗糖、柠檬酸、酒石酸为碳源,采用高温固相法制备了LiFePO 4/C 正极复合材料.利用X-射线衍射(XRD )、热重差热分析(TGA )、扫描电子显微镜(SEM )、拉曼光谱(Raman )、充放电测试、循环伏安及交流阻抗测试系统研究了不同碳源包覆对材料微结构及电化学性能的影响.XRD 结果表明合成的材料均具有单一的橄榄石型结构;热重差热分析表明3个样品的质量度分数均为7%.SEM 结果发现以蔗糖为碳源的LiFePO 4/C 团聚现象严重,以柠檬酸为碳源的LiFePO 4/C 颗粒最大,以酒石酸为碳源的LiFePO 4/C 颗粒最小.Raman 表明以蔗糖或酒石酸为碳源的LiFePO 4/C 具有很好的石墨化碳.电化学性能表明以酒石酸为碳源的LiFePO 4/C 具有最佳的倍率和循环性能.关键词:锂离子电池;LiFePO 4/C ;高温固相法;碳源中图分类号:O469文献标志码:A收稿日期:2013-10-26基金项目:国家973计划项目(2011CBA00200);国家自然科学基金资助项目(11344008);福建省自然科学基金资助项目(2013J01007)通信作者:黄志高(1964-),男,教授,博士,从事新能源材料研究.zghuang@Effect of Carbon Source on Electrochemical Properties of LiFePO 4/CHUANG Yan-dan ,YU Rui-bing ,YANG Yan-min ,LIN Ying-bin ,LAI Heng ,HUANG Zhi-gao(College of Physics and Energy ,Fujian Normal University ,Fujian Provincial KeyLaboratory of Quantum Manipulation and New Energy Materials ,Fuzhou 350117,China )Abstract :Olivine LiFePO 4/C cathode materials were successfully prepared by solid state reac-tion with different carbon source (sucrose ,citric acid and tartaric acid ),and the effect of carbon source on the structure and the electrochemical performances were systematically investigated by XRD ,TGA ,SEM ,Raman ,charge-discharge test ,cyclic voltammetry (CV ),and AC imped-ance.Analysis from XRD results show that all-prepared powers can be indexed to be a single-phase of an orthorhombic olivine-type structure.Thermogravimetric analysis (TGA )reveals that the carbon content in every sample is about 7%.More particle agglomeration is found in LiFePO 4/C using su-crose as carbon source ,LiFePO 4/C using citric acid as carbon source has largest particle-size while LiFePO 4/C using tartaric acid as carbon source has smallest particle-size.Raman spectra demon-strate LiFePO 4/C using sucrose or tartaric acid as carbon source has well-graphitized carbon.Analy-sis from the electrochemical measurements indicate that LiFePO 4/C prepared by tartaric acid shows best electrochemical performances.Key words :lithium-ion battery ;LiFePO 4/C ;solid state reaction ;carbon source锂离子电池作为新型二次电池的代表,以其消耗低、能量密度高、寿命长等优点,在逐步应用中已显示出巨大的优势,被广泛应用于便携式电子产品以及混合动力电动车等[1-3].与传统的LiCoO 2、LiMn 2O 4等正极材料相比,LiFePO 4材料的制备原料来源广泛、环境相容性好,用作正极材料时具有热稳定性好、循环性能优良、安全性高以及比容量高(170mAh ·g -1)等优点,是极具潜力的高能福建师范大学学报(自然科学版)2014年动力电池正极材料[4-5].然而LiFePO 4较低的电子传导率和离子扩散系数是阻碍其功率型应用的主要因素[6-7].许多研究结果表明,表面碳包覆是提高LiFePO 4电导率的主要途径之一[8-9].Ravet 等[10]首先对LiFePO 4颗粒进行碳包覆,合成了LiFePO 4/C 复合材料,极大提高了材料的动力学性能及循环性能,10次循环后,样品放电容量保持率为96%,提高了近一倍.Molenda [11]等采用高温固相法添加聚合物合成LiFePO 4/C 复合材料,其电导率实现了7个数量级的提高,在0.1C 下放电比容量约为130mAh ·g -1.Wu [12]等用多元醇法复合LiFePO 4、碳纳米管以及单层石墨烯,形成三维复合材料,在0.1C 下放电比容量稳定在160mAh ·g -1.虽然以葡萄糖、柠檬酸、蔗糖和酒石酸为碳源固相法制备LiFePO 4/C 的相关研究报道很多,但碳源在裂解过程中对材料微结构及电化学性能的影响研究不够深入.本文分别以蔗糖、柠檬酸和酒石酸为碳源,利用高温固相法合成LiFePO 4/C 正极材料,在碳包覆质量分数均为7%的前提下,研究不同碳源对材料微结构及其电化学性能的影响.1实验部分1.1样品制备按物质的量比n (Li )ʒn (Fe )ʒn (P )=1ʒ1ʒ1称取一定化学计量的分析纯LiC 2H 3O 2、C 2FeO 4·2H 2O 和NH 4H 2PO 4分散于装有无水乙醇的玛瑙罐中,分别加入碳质量分数为7%的蔗糖、柠檬酸、酒石酸作为还原剂和碳源,在160r ·min -1的转速下球磨48h.将所得前驱体置于鼓风干燥箱中,70ħ干燥10h ,直至无水乙醇完全挥发.将所得前驱体置于管式炉中,在Ar 气保护下350ħ恒温加热6h 后在700ħ下煅烧12h ,随炉冷却至室温后取出,得到不同碳源包覆的LiFePO 4/C 正极材料粉末.1.2电池组装将LiFePO 4/C 正极活性材料、导电炭黑(Super P )和聚偏氟乙烯(PVDF )按质量比8ʒ1ʒ1混合,分散于适量N-甲基吡咯烷酮(NMP )有机溶剂中搅拌成泥浆状,并均匀涂敷于铝箔后置于真空干燥箱中,110ħ真空干燥12h.使用专用模具切片,将其裁成直径为12.5mm 的圆片,在充满高纯Ar 的手套箱中,将所得电极作为正极,锂片作为参考负极,微孔聚丙烯(Celgard 2300)作为隔膜,以1mol ·L -1LiPF6/EC +DEC +EMC (体积比为1ʒ1ʒ1)为电解液,组装成CR2025扣式电池.组装后的电池静置12h 后进行电化学性能测试.1.3材料表征及电化学性能测试图1不同碳源合成的LiFePO 4/C 样品的XRD 图谱Fig.1XRD patterns of LiFePO 4/C composites with different carbon sources采用理学Rigaku MiniFlex ⅡX 射线衍射仪对材料进行物相结构分析,测试使用Cu-K α辐射源,扫描角度从15ʎ 65ʎ.采用耐驰STA 449F3同步热分析仪测定LiFePO 4/C 材料中的碳含量;采用JSM-7500LV 型扫描电子显微镜(SEM )观测样品的颗粒大小和表面形貌特征;采用Renishaw 光谱仪记录拉曼光谱,测试范围从100 2500cm -1.采用武汉蓝电电池测试系统(LAND CT-2001A ),用恒流-恒压的充电方式对电池进行充放电性能测试.测试电压范围为2.5 4.3V.采用上海华辰的电化学工作站(CHI660C )在2.5 4.3V 电压区间内对模拟电池进行循环伏安特性测试,扫描速率为0.05mV ·s -1,同时也进行了交流阻抗性能测试,频率范围为0.01 100kHz ,振幅为5mV.2结果与讨论图1给出了不同有机物作为碳源合成的LiFePO 4/C 样品的XRD 图谱,其中样品A 、B 、C 分别为以蔗糖、柠檬酸、酒石酸为碳源包覆的样品.从图1可以看到,样品的衍射峰均与标准XRD 图(JCPDS 83-2092)一致,具有单一的橄榄石结构,属于正交晶系(Pnma 空间群)[13].XRD 图中未84第3期黄艳丹等:不同碳源对LiFePO 4/C 电化学性能的影响发现其他杂相衍射峰且衍射峰形尖锐有序,表明晶型结构完整,结晶度高.图2为LiFePO 4/C 样品的SEM 图谱.从图2可以发现碳纳米颗粒分布在LiFePO 4颗粒表面,碳的存在可以有效抑制生长过程中LiFePO 4晶粒的长大,进而缩短锂离子在晶粒之间的扩散距离,有利于增强材料的离子扩散速率,提高材料的电导率[14].SEM 结果还发现以蔗糖为碳源的LiFePO 4/C 团聚现象严重(图2(a ));以酒石酸为碳源的LiFePO 4/C 颗粒最小(图2(c ));以柠檬酸酸为碳源的LiFePO 4/C 颗粒最大,且存在部分游离状态的碳纳米微粒(图2(b )).这是因为柠檬酸的分解温度较低[15],使其不能在LiFePO 4晶体生长阶段有效抑制晶体的生长,因此其颗粒最大.图2不同碳源合成的LiFePO 4/C 样品的SEM 图Fig.2The SEM images of LiFePO 4/C composites with different carbon sources图3是样品A 、B 、C 的热重曲线,升温速率为5ħ·min -1,气氛环境体积分数为80%氮气和20%氧气的混合气,测试范围是30 900ħ.从热重曲线可以发现3个样品的碳质量分数几乎一致,均为7.6%.图4给出了不同碳源包覆合成的LiFePO 4/C 样品的拉曼光谱,从图4明显发现在1335cm -1以及1590cm -1附近出现了两个宽度较宽的特征驼峰,D 峰代表的是无定形碳的振动模式,G 峰代表了石墨化碳的振动模式[16].通过计算,得到样品A 、B 、C 的特征峰的强度之比(I G /I D )分别为0.625,0.523,0.627,说明酒石酸制备的样品C 和A 的表面比B 具有更大的石墨化碳的比例,这对应于较佳的导电性和较低的接触电阻[17].图3不同碳源合成的LiFePO 4/C 样品的热重曲线Fig.3The TGA curves of LiFePO 4/Ccomposites 图4不同碳源合成的LiFePO 4/C 样品的拉曼光谱Fig.4Raman spectra of LiFePO 4/C samples 图5为不同碳源合成的LiFePO 4/C 样品在室温下以0.5C 充放电的首次充放电曲线.从图5可以看出,A 、B 、C 3个样品的充放电平台电压差依次为0.12,0.28,0.07V ,以样品C 有最小的极化,样品B 的放电比容量较低,且平台较倾斜.这主要是因为柠檬酸分解形成的无定型碳比例大,导致LiFePO 4颗粒的接触不够好、降低了颗粒之间、LiFePO 4与碳层之间导电效果.蔗糖和酒石酸分解形成的石墨化碳的比例较大,导致LiFePO 4颗粒的接触较好、电导率得到明显提高,从而改善了它们的电化学性能[18].图6给出不同有机物为碳源合成的LiFePO 4/C 样品的循环伏安特性曲线,扫描速率为0.05mV ·s -1、扫描范围为2.5 4.3V.由图6可见,样品均有一对明显且尖锐的氧化还原峰,对应LiFePO 494福建师范大学学报(自然科学版)2014年材料在相应电位下脱嵌锂离子时Fe2+/Fe3+之间的转化[19].样品A的峰形最尖锐,峰电流最强,表明以蔗糖为碳源合成的LiFePO4/C样品的充放电动力学性能较强[20].A、B、C三个样品的Fe2+/ Fe3+电对氧化还原电位差分别为0.251,0.296,0.245V.以样品C的电位差最小,说明其具有最小的极化,一定程度上也表明了该材料具有更好的Li+脱嵌可逆性,循环性能最为稳定[21].图5不同碳源合成的LiFePO4/C 样品在0.5C下的首次充放电曲线Fig.5First cycle charge-discharge curvesof LiFePO4/C samples at0.5C图6不同碳源合成的LiFePO4/C样品的循环伏安特性曲线Fig.6Cyclic voltammograms of LiFePO4/C samples图7为不同有机物为碳源合成的LiFePO4/C样品电极在不同倍率(0.5,1.0,2.0,3.0,0.5C)下的放电比容量.结果表明,以酒石酸为碳源制备的LiFePO4/C样品具有最好的倍率性能和循环性能,在0.5,3C下其放电比容量分别为145,102mAh·g-1;以柠檬酸为碳源制备的LiFePO4/C样品的倍率性能最差,在0.5,3C下其放电比容量分别为137,49mAh·g-1.样品倍率性能的改善主要归因于材料电导率的提高,这与循环伏安分析结果即极化减小结果相对应.图7样品的倍率(0.5,1,2,3C)性能Fig.7Rate performances of Samples at0.5,1,2,3C图8不同碳源合成的LiFePO4/C样品的交流阻抗图谱及等效电路图Fig.8Nyquist plots and equivalent circuit of LiFePO4/C samples after80cycles at charged state图8为不同有机物为碳源合成的LiFePO4/C样品电极在0.5C下循环50次后的电化学阻抗谱.图谱是由高频区的低压圆弧和低频区的斜线组成.圆弧反映了在电极和电解液接触面发生电荷转移时Li+的脱嵌过程,低频的斜线反映了锂离子扩散过程中所引起的Warburg阻抗.采用Zsimpwin软件对交流阻抗图谱进行模拟电路分析,等效电路如插图所示,在等效电路中,Rs为电解液的总电阻,即欧姆电阻;Rct表示电荷传递极化电阻,即电化学阻抗.双电层电容和SEI膜电容由恒相位原件(CPE)替代,Zw为Warburg阻抗[22],由等效电路模拟得到的样品A、B、C的电荷传递极化电阻Rct 分别为332,809,264Ω.因此,样品C最有利于Li+在电极界面的脱出与嵌入,具有最好的电化学性能.锂离子在样品A、B和C电极中的扩散系数可根据阻抗谱中低频率部分进行计算,计算公式05第3期黄艳丹等:不同碳源对LiFePO 4/C 电化学性能的影响为[23]D +Li =2RT 槡2n 2F 2σw ()AC 2=2R2T 2n 4F 4σ2w A 2C 2,(1)式中R为气体常数,A 为电极的表面积,n 为反应电荷数,F 为法拉第常数,C 为锂离子浓度,σw 为图9低频率区样品A 、B 和C 的Z' ω-1/2曲线Fig.9Z'vs.plots in the low frequency of Sample A ,B andC Warburg 系数.交流阻抗的实部Z'与Warburg 系数σw 的关系为[24]Z'=RS +Rct +σωω-1/2.(2)图9为低频率区的Z'和ω-1/2之间的线性关系曲线,可以计算出锂离子在样品A 、B 和C 电极中的扩散系数分别为 2.07746ˑ10-11,3.13351ˑ10-12,4.12187ˑ10-11cm 2·s -1.以柠檬酸作为碳源烧结的颗粒最大,其锂离子扩散路径最长.虽然以蔗糖作为碳源烧结的与酒石酸源的颗粒相当,但团聚现象严重,颗粒与颗粒之间的界限不甚分明,影响了其电锂离子扩散速率.以酒石酸作为碳源烧结的颗粒最小且团聚现象最小,活性表面积最大,因此其表现出最佳的电化学性能.3实验结论本文利用高温固相法,成功制备了不同碳源(蔗糖、柠檬酸、酒石酸)包覆合成碳含量均为7.6%的LiFePO 4/C 正极材料.包覆碳后得到的样品具有单一的橄榄石结构.电化学性能测试表明以酒石酸为碳源的LiFePO 4/C 正极材料具有最佳的倍率性能和循环性能,这主要归因于其表面包覆的碳具有最佳的石墨化程度,即具有最高的电子电导率.同时交流阻抗测试表明,以酒石酸为碳源的LiFePO 4/C 正极材料具有最小的电荷迁移电阻和最大的锂离子扩散系数,这是因为该材料具有最小的颗粒和最小的团聚现象.参考文献:[1]Maschler T G ,Friedrich B ,Weyhe R,et al.Development of a recycling process for Li-ion batteries [J ].Journal ofPower Sources ,2012,207:173-182.[2]Chen M ,Du C Y ,Song B ,et al.High-performance LiFePO 4cathode material from FePO 4microspheres with carbonnanotube networks embedded for lithium ion batteries [J ].Journal of Power Sources ,2013,223:100-106.[3]Hu X S ,Bi L S ,Peng H.A comparative study of equivalent circuit models for Li-ion batteries [J ].Journal of PowerSources ,2012,198:359-367.[4]Chen Z Y ,Du B L ,Xu M ,et al.Polyacene coated carbon /LiFePO 4cathode for Li ion batteries :understanding the sta-bilized double coating structure and enhanced lithium ion diffusion kinetics [J ].Electrocheimica Acta ,2013,109:262-268.[5]Zhou J ,Liu B H ,Li Z P.Nanostructure optimization of LiFePO 4/carbon aerogel composites for performance enhance-ment [J ].Solid State Ionics ,2013,244:23-29.[6]Uchida S ,Yamagata M ,Ishikawa M.Novel rapid sythesis method of LiFePO 4/C cathode material by high-frequency in-duction heating [J ].Journal of Power Sources ,2013,243:481-487.[7]Chang Q Q ,Yao G H ,Pan F F ,et al.Investigatin on interfacial interaction and origin of Fe 3+in LiFePO 4/C [J ].Electrocheimica Acta ,2013,108:211-218.[8]Luo S H ,Tang Z L ,Lu J B ,et al.Electrochmical properties of carbon-mixed LiFePO 4cathode materrial synthesized bythe ceramic granulation method [J ].Science Direct ,2008,34:1349-1351.15福建师范大学学报(自然科学版)2014年[9]熊学,戴永年,陈海清,等.包覆碳对LiFePO4正极材料性能的影响[J].湖南有色金属,2009,25(1):29-32.[10]Ravet N,Chouinard Y,Magnan J F,et al.Electroactivity of natural and synthetic triphylite[J].Journal of Power Sources,2001,97/98:503-507.[11]Molenda M,Swietoslawski M,Milewska A,et al.Carbon nanocoatings for C/LiFePO4composite cathode[J].SolidState Ionics,2013,251:47-50.[12]Wu G,Zhou Y K,Shao Z P.Carbon nanotube and graphene nanosheet Co-modified LiFePO4nanoplate composite cath-ode material by a facile polyol process[J].Applied Surface Science,2013,283:999-1005.[13]Uchida S,Yamagata M,Ishikawa M.Optimized condition of high-frequency induction heating for LiFePO4with idealcrystal structure[J].Journal of Power Sources,2013,243:617-621.[14]Jegal J P,Kim K B.Carbon nanotube-embedding LiFePO4as a cathode material for high rate lithium ion batteries[J].Journal of Power Sources,2013,243:859-864.[15]蔡卓,关瑾,文衍宣,等.不同碳源LiFePO4/C复合材料的表征与性能分析[J].广西大学学报:自然科学版,2010,35(3):432-436.[16]Zheng Z M,Wang Y,Zhang A,et al.Porous Li2FeSiO4/C nanocomposite as the cathode material of lithium-ion batter-ies[J].Journal of Power Sources,2012,18:229-235.[17]Yan Z P,Cai S,Miao L J,et al.Synthesis and characterization of in situ carbon-coated Li2FeSiO4cathode materials forlithium ion battery[J].Journal of Power Sources,2012,511:101-106.[18]Lv Y J,Su J,Long Y F,et al.Effects of ball-to-powder weight ratio on the performance of LiFePO4/C prepared by wet-milling assisted carbothermal reduction[J].Powder Technology,2014,253:467-473.[19]Konarova M,Taniguchi I.Synthesis of carbon-coated LiFePO4nanoparticles with high rate performance in lithium sec-ondary batteries[J].Journal of Power Sources,2010,195:3661-3667.[20]Lin Y B,Lin Y,Zhou T,et al.Enhanced electrochemical performances of LiFePO4/C by surface modification with Sn nanoparticles[J].Journal of Power Sources,2013,226:20-26.[21]Miao C,Bai P F,Jiang Q Q,et al.A novel synthesis and characterization of LiFePO4of LiFePO4/C as a cathode mate-rial for lithium-ion battery[J].Journal of Power Sources,2014,245:232-238.[22]叶一斌,李加新,李晓炜,等.LiFePO4-MWCNTs复合正极材料的制备与电化学性能研究[J].福建师范大学学报:自然科学版,2012,28(2):56-59.[23]Chen C,Liu G B,Wang Y,et al.Preparation and electrochemical properties of LiFePO4/C nanocomposite usingFePO4·2H2O nanoparticles by introduction of Fe3(PO4)2·8H2O at low cost[J].Electrochim Acta,2013,113:464-469.[24]Huang X B,Chen H H,Zhou S B,et al.Synthesis and characterization of nano-Li1.95FeSiO4/C composite as cathodematerial for lithium-ion batteries[J].Electrochim Acta,2012,60:239-243.(责任编辑:黄家瑜)25。
磷酸铁锂碳源研究进展
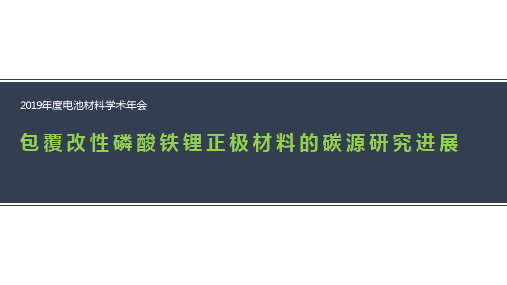
新能源汽车
电机
电池
电控
4
1 前言
锂离子电池
正极
成 本 隔膜
负极
5
1 前言
材料主成分
理论能量密度 (mAh/g) 实际能量密度 (mAh/g) 电压(V) 循环性(次)
过渡金属
磷酸铁锂
LiFePO4 170
130-140 3.2-3.7 >2000 非常丰富
环保性
安全性能 适用温度(℃)
无毒
好 -20~75
该材料的结晶度高,缺陷少,在10C、30C下的放电比容量分别达到132.2mAh/g和113.3mAh/g。
[9] Lv Y J,Long Y F,Su J,et al. Synthesis of bowl-like mesoporous LiFePO4/C composites as cathode materials for lithium ion batteries[J]. Electrochimica Acta, 2014, 119(0): 155-163. [10] Li J L,Wu J H,Wang Y,et al. Synthesis of LiFePO4/C composite with high rate capability using spheniscidite as a facile precursor[J]. Materials Letters, 2014, 136(0): 282-285. [11] 陈明鸣.一种高倍率型磷酸铁锂/炭及其制备方法[P].中国专利:103904325,2014-03-21.
composite from mechanical activation using sucrose as carbon source[J]. Electrochimica Acta, 2009, 54(10): 2861-2868.
163disk[1].com_24-231630
![163disk[1].com_24-231630](https://img.taocdn.com/s3/m/6cce652ae2bd960590c6772c.png)
Yuan, L., Wang, Z., Zhang, W., Hu, X., Chen,J., Huang, Y., Goodenough, J. B. Energy Environ. Sci.,2011, 4, 26-284. 12
Aleees’ Approach: Nanosize LFP M20
Press Density : 1.8 g/cm3 ~ 2.0 g/cm3
17
Aleees’ Two Grade LFP Comparison
Coin half cell M121
Primary particle size (nm) BET Specific surface 400-500 13±2
.tw/enews/epaper/10102/e01.htm
6
Emerging Application
E-Bus E-scooter PHEV/ HEV/ EV/ BEV
E-boat
UPS
Power tool
Military
Medical Application
立凱亞以士能源科技 (上海)有限公司
上海市徐汇区虹漕路421号65幢3楼301室 /cn Tel: +86-21-6420-1418 Fax: +86-21-6420-0419 20
14
Electrochemical Impedance Spectroscopy
Coin half cell (Li as a counter and reference electrode) M20 M121
M20 has superior impedance property.
15
Low Temperature Performance
富锂铁酸锂合成工艺流程
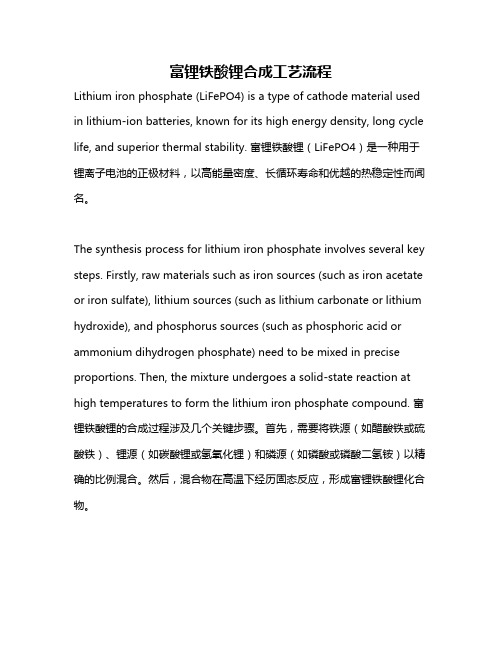
富锂铁酸锂合成工艺流程Lithium iron phosphate (LiFePO4) is a type of cathode material used in lithium-ion batteries, known for its high energy density, long cycle life, and superior thermal stability. 富锂铁酸锂(LiFePO4)是一种用于锂离子电池的正极材料,以高能量密度、长循环寿命和优越的热稳定性而闻名。
The synthesis process for lithium iron phosphate involves several key steps. Firstly, raw materials such as iron sources (such as iron acetate or iron sulfate), lithium sources (such as lithium carbonate or lithium hydroxide), and phosphorus sources (such as phosphoric acid or ammonium dihydrogen phosphate) need to be mixed in precise proportions. Then, the mixture undergoes a solid-state reaction at high temperatures to form the lithium iron phosphate compound. 富锂铁酸锂的合成过程涉及几个关键步骤。
首先,需要将铁源(如醋酸铁或硫酸铁)、锂源(如碳酸锂或氢氧化锂)和磷源(如磷酸或磷酸二氢铵)以精确的比例混合。
然后,混合物在高温下经历固态反应,形成富锂铁酸锂化合物。
Fullprof软件在不同温度下合成LiFePO4材料中的应用研究
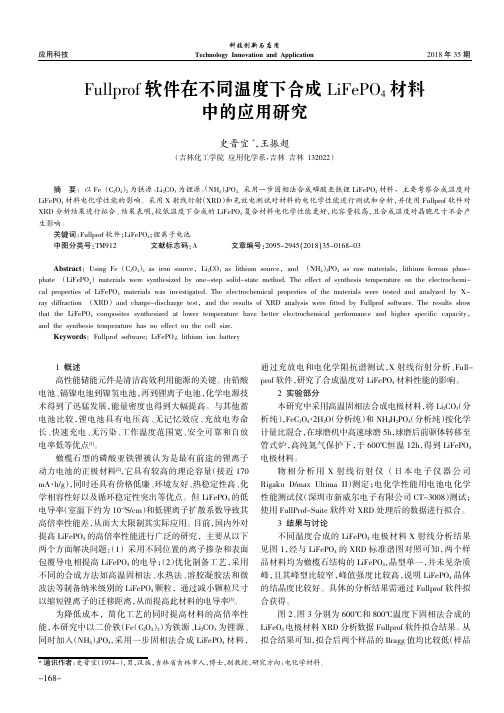
2018年35期应用科技科技创新与应用Technology Innovation and ApplicationFullprof 软件在不同温度下合成LiFePO 4材料中的应用研究史晋宜*,王振超(吉林化工学院应用化学系,吉林吉林132022)1概述高性能储能元件是清洁高效利用能源的关键。
由铅酸电池、镉镍电池到镍氢电池,再到锂离子电池,化学电源技术得到了迅猛发展,能量密度也得到大幅提高。
与其他蓄电池比较,锂电池具有电压高、无记忆效应、充放电寿命长、快速充电、无污染、工作温度范围宽、安全可靠和自放电率低等优点[1]。
橄榄石型的磷酸亚铁锂被认为是最有前途的锂离子动力电池的正极材料[2],它具有较高的理论容量(接近170mA ·h/g ),同时还具有价格低廉、环境友好、热稳定性高、化学相容性好以及循环稳定性突出等优点。
但LiFePO 4的低电导率(室温下约为10-9S/cm )和低锂离子扩散系数导致其高倍率性能差,从而大大限制其实际应用。
目前,国内外对提高LiFePO 4的高倍率性能进行广泛的研究,主要从以下两个方面解决问题:(1)采用不同位置的离子掺杂和表面包覆导电相提高LiFePO 4的电导;(2)优化制备工艺,采用不同的合成方法如高温固相法、水热法、溶胶凝胶法和微波法等制备纳米级别的LiFePO 4颗粒,通过减小颗粒尺寸以缩短锂离子的迁移距离,从而提高此材料的电导率[3]。
为降低成本,简化工艺的同时提高材料的高倍率性能,本研究中以二价铁(Fe (C 2O 4)2)为铁源、Li 2CO 3为锂源、同时加入(NH 4)3PO 4,采用一步固相法合成LiFePO 4材料,通过充放电和电化学阻抗谱测试,X 射线衍射分析、Full -prof 软件,研究了合成温度对LiFePO 4材料性能的影响。
2实验部分本研究中采用高温固相法合成电极材料,将Li 2CO 3(分析纯),FeC 2O 4·2H 2O (分析纯)和NH 4H 2PO 4(分析纯)按化学计量比混合,在球磨机中高速球磨5h ,球磨后前驱体转移至管式炉,高纯氮气保护下,于600℃恒温12h ,得到LiFePO 4电极材料。
形貌对磷酸铁锂电池内阻特性影响
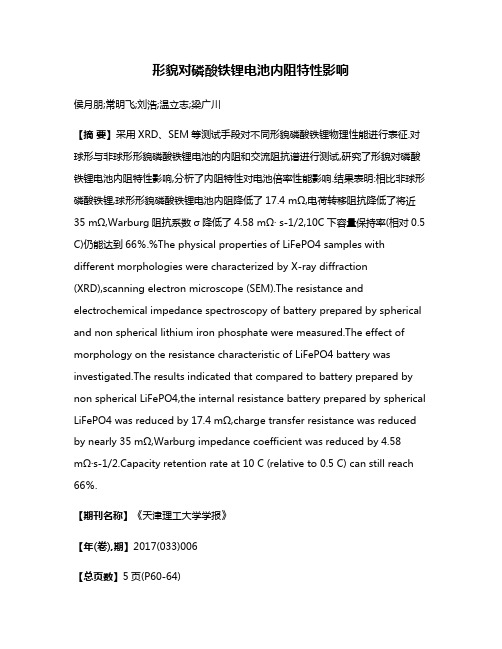
形貌对磷酸铁锂电池内阻特性影响侯月朋;常明飞;刘浩;温立志;梁广川【摘要】采用XRD、SEM等测试手段对不同形貌磷酸铁锂物理性能进行表征.对球形与非球形形貌磷酸铁锂电池的内阻和交流阻抗谱进行测试,研究了形貌对磷酸铁锂电池内阻特性影响,分析了内阻特性对电池倍率性能影响.结果表明:相比非球形磷酸铁锂,球形形貌磷酸铁锂电池内阻降低了17.4 mΩ,电荷转移阻抗降低了将近35 mΩ,Warburg阻抗系数σ降低了4.58 mΩ· s-1/2,10C下容量保持率(相对0.5C)仍能达到66%.%The physical properties of LiFePO4 samples with different morphologies were characterized by X-ray diffraction (XRD),scanning electron microscope (SEM).The resistance and electrochemical impedance spectroscopy of battery prepared by spherical and non spherical lithium iron phosphate were measured.The effect of morphology on the resistance characteristic of LiFePO4 battery was investigated.The results indicated that compared to battery prepared by non spherical LiFePO4,the internal resistance battery prepared by spherical LiFePO4 was reduced by 17.4 mΩ,charge transfer resistance was reduced by nearly 35 mΩ,Warburg impedance coefficient was reduced by 4.58mΩ·s-1/2.Capacity retention rate at 10 C (relative to 0.5 C) can still reach 66%.【期刊名称】《天津理工大学学报》【年(卷),期】2017(033)006【总页数】5页(P60-64)【关键词】形貌;磷酸铁锂电池;内阻;交流阻抗谱;倍率【作者】侯月朋;常明飞;刘浩;温立志;梁广川【作者单位】河北工业大学材料科学与工程学院河北省新型功能材料重点实验室,天津300130;河北工业大学材料科学与工程学院河北省新型功能材料重点实验室,天津300130;河北工业大学材料科学与工程学院河北省新型功能材料重点实验室,天津300130;河北工业大学材料科学与工程学院河北省新型功能材料重点实验室,天津300130;河北工业大学材料科学与工程学院河北省新型功能材料重点实验室,天津300130【正文语种】中文【中图分类】TQ152由于磷酸铁锂材料结构的特点,其电子导电性和离子扩散系数较低,导致磷酸铁锂电池内阻较高[1-4].内阻是衡量电池性能的重要参数,与电池的寿命、容量、倍率、安全等性能密切相关.高内阻问题一直是阻碍磷酸铁锂电池被广泛应用的一大难题.因此研究磷酸铁锂电池内阻特性具有重要的现实意义.近年,磷酸铁锂电池内阻特性逐渐成为研究热点.林春景等[5]研究了环境温度对磷酸铁锂电池内阻特性的影响.席安静等[6]研究了磷酸铁锂电池EIS参数随SOC变化的规律.孟仙雅等[7]研究了导电剂对磷酸铁锂电池性能的影响.结果表明,采用SP/KS-6/CNT新型组合导电剂制备的磷酸铁锂电池内阻降低70%.然而目前关于形貌对磷酸铁锂电池内阻特性影响的研究较少.本文针对市场上常见的球形形貌磷酸铁锂和非球形形貌磷酸铁锂来进行研究,分别测试了两种形貌磷酸铁锂电池的内阻和交流阻抗谱,深入研究了磷酸铁锂形貌对电池内阻特性的影响,讨论了内阻对电池倍率性能所产生的影响.1 实验1.1 材料的制备实验中球形和非球形磷酸铁锂都是以FePO4·2H2O为铁源,葡萄糖为碳源,LiCO3为锂源.不同的是球形磷酸铁锂的前驱体是通过喷雾干燥法制备,直接加入旋转式烧结炉中高温烧结而成.而非球形磷酸铁锂的前驱体是通过微波干燥法制备,经过辊压后,再加入烧结炉中高温烧结而成.为了便于分析,将球形和非球形磷酸铁锂材料分别记为LFPQ、LFPN.1.2 材料的表征采用D8 FOCUS型X射线多晶衍射仪,来分析材料的物相和结晶度.并通过Jade6.0软件对材料的晶胞结构进行计算.测试条件为CuKα靶,波长λ为0.154 06 nm,电压40 KV,步长 0.02,扫描范围2θ为10-80°.采用Nova Nano 450型扫描电子显微镜分析材料的微观形貌.采用LS-800型激光粒度分析仪对材料粒径进行测试.1.3 电池制作及性能测试分别采用球形和非球形磷酸铁锂作为活性物质,采用PVDF-5130作为粘结剂制备电池正极片.负极片采用石墨作为活性物质,CMC/SBR作为粘结剂制备.将制备好的正负极片经过辊压,裁片,焊极耳,卷绕等工序制备成电芯,然后将电芯用铝塑膜封装起来,在手套箱中进行注液,封口,制备成14500型全电池.采用NZY-200型电池内阻仪对电池进行内阻测试.采用深圳新威尔电子有限公司生产的电池性能测试仪测试电池的倍率性能.采用CHI660C型电化学工作站来测试电池交流阻抗谱,测试频率范围为10-2Hz~105Hz,振幅为5 mV.用ZView2软件对电池交流阻抗谱进行等效电路拟合.2 结果与讨论2.1 物相分析图1为不同形貌LiFePO4样品的XRD图谱.从图1中可以看出,两种不同形貌的衍射图谱都与LiFePO4的标准衍射图谱相吻合,并且没有杂质衍射峰的出现,从而说明两种不同方法合成的样品纯度都较高,都为具有橄榄石结构的纯LiFePO4相.衍射图谱中所有的衍射峰都比较尖锐,没有碳的衍射峰出现,说明两种样品的结晶度较高,并且碳以非晶态存在.通过Jade6.0软件对两种不同形貌样品的晶体结构进行分析,从而得出两种不同形貌样品的晶胞参数.表1为两种不同形貌LiFePO4样品的晶胞参数.从表中可以看出,两种不同形貌样品的晶胞参数变化非常小,几乎没有变化,说明不同方法制备的不同形貌LiFePO4样品晶格结构没有明显差异.图1 不同形貌LiFePO4样品的XRD图谱Fig.1 XRD patterns ofLiFePO4samples with different morphologies表1 不同形貌LiFePO4样品的晶胞参数Tab.1 Lattice parameters ofLiFePO4samples with different morphologies样品 a/nm b/nm c/nm V/nm3 LFPQ 1.0331 0.6002 0.4692 0.29094 LFPN 1.0324 0.6003 0.4689 0.29060 2.2 材料形貌分析图2为两种不同形貌LiFePO4样品的SEM图.由图可以看出两种样品的形貌相差较大.喷雾干燥法制成的样品LFPQ为规则球形形貌,这是由于喷雾干燥使样品保持了液滴的球形.而微波干燥法制成的样品LFPN为不规则非球形形貌,这是由于烧结完成后对样品进行了粉碎、筛分等处理,对样品颗粒形貌造成了严重影响.图2(a)和图 2(b)为样品LFPQ的SEM图.由图中可见,样品LFPQ的颗粒呈标准球形,微米级的大颗粒由纳米级的小颗粒组成,并且小颗粒形貌和粒径分布均匀.图2(c)和图2(d)为样品LFPN的SEM图,由图可知,样品LFPN的颗粒大小不一,形状不规则,且粒径较小.对比两种样品的SEM图,还可以发现,样品LFPQ颗粒被碳网包围,且在颗粒间碳网分布均匀,而在样品LFPN颗粒间未形成均匀的碳网,碳分布极不均匀,有些颗粒间碳含量过多,而有些颗粒间未见碳分布. 表2为不同形貌LiFePO4样品的物理性能.由表可见样品LFPQ颗粒粒径较样品LFPN大.这是由于样品LFPN后续经过粉碎处理,使用物理方法粉碎了粒径较大的颗粒,导致样品LFPN二次颗粒粒径变小.样品LFPQ具有球形形貌,使得颗粒间排列紧密,并且样品LFPQ的粒径分布较好,小颗粒完美填充了大颗粒间的空隙.因此相比样品LFPN,样品LFPQ的振实密度和压实密度都较高.另外由于样品LFPQ中颗粒间有均匀的碳网形成,从而极大改善了样品LFPQ的电导率.而样品LFPN颗粒间碳分布不均匀,对样品的电导率提升不明显.因此样品LFPN的电导率较低.图2 不同形貌LiFePO4样品的SEM图Fig.2 SEM images of LiFePO4samples with different morphologies表2 不同形貌LiFePO4样品的物理性能Tab.2 Physical properties ofLiFePO4samples with different morphologies样品粒径分析(μm) 振实密度(g/cm3)电导率(S/cm)D10 D50 D90压实密度(g/cm3)LFPQ 8.206 18.101 29.769 1.37 2.45 5.5×10-2 LFPN 0.535 1.549 2.320 1.02 2.32 3.5×10-22.3 形貌对电池内阻影响表3为不同形貌磷酸铁锂电池化成后内阻和电压.从表中可以看出样品LFPQ的电池内阻为50.6 mΩ,样品LFPN电池内阻为68.0 mΩ.相比于样品LFPN,样品LFPQ电池内阻降低了17.4 mΩ,将近样品LFPN全电池电阻的四分之一.由此可见磷酸铁锂形貌对电池内阻影响非常明显.表3 不同形貌LiFePO4样品电池化成后内阻和电压Tab.3 Internal resistance and voltage of LiFePO4battery after formationLFPQ 50.6 3.38 LFPN 68.03.38样品LFPQ由于颗粒为规则的球形颗粒,具有较高振实密度和压实密度,减小了充放电时电子和锂离子的传递距离.且样品LFPQ的电导率较高,降低了电子和离子的传递阻力,因此样品LFPQ电池内阻较低.而样品LFPN颗粒振实密度和压实密度都较低,电子和锂离子的传递距离较大.另外样品LFPN电导率低,电子和离子的传递阻力较大,从而导致样品LFPN电池内阻较高.2.4 形貌对电池交流阻抗影响图3为不同形貌样品全电池交流阻抗谱曲线.从图中可以看出两不同形貌样品所作电池交流阻抗都由低频区的一条直线和高频区一个半圆组成.其中高频区的半圆为电荷的界面转移阻抗Rct,低频区的直线是锂离子在活性物质内扩散的Warburg 阻抗.图中的电路图为样品电池的拟合电路.其中Rs为接触阻抗,包括集流体、电解液、隔膜等产生的欧姆内阻.Rct为电荷转移阻抗,Wo则代表锂离子扩散产生的Warburg阻抗[8].电池交流阻抗谱测试是在电池充放电循环一周后进行.由于循环初期磷酸铁锂电池SEI膜的阻抗较小,在阻抗谱中表现不明显.另外,两不同形貌样品电池所用负极相同,且SEI膜主要在负极产生.因此本实验阻抗分析时不考虑SEI产生的阻抗.从图3中还可以看出样品LFPQ的电荷转移阻抗Rct与样品LFPN 电池相比有明显降低.这是由于良好的球形形貌和合理的粒径分布增强了电解液的润湿性,降低了充放电过程中锂离子的脱嵌阻力.图4为不同形貌样品电池Z′与ω-1/2的关系图.ω代表低频角频率,Z′代表阻抗的实部.图中直线斜率则为Warburg系数σ.其可由下式求出:从图中可以看出样品LFPQ斜线的斜率小于LFPN样品,这表示LFPQ样品的Warburg系数σ比样品LFPN小.而Warburg系数σ越小,锂离子扩散阻抗越小.样品LFPQ电池的扩散阻抗较样品LFPN电池扩散阻抗低.用ZVeiw2软件对电池EIS曲线进行拟合可得到电池阻抗的各参数值.表4列出了不同形貌样品电池的EIS参数值.由表可见,两样品电池的接触阻抗Rs相差较小.但转移阻抗Rct相差明显.样品LFPQ的转移阻抗Rct较样品LFPN降低了将近35 mΩ,Warburg系数σ降低了4.58 mΩ·s-1/2.说明形貌能够影响磷酸铁锂电池的锂离子转移阻抗和扩散阻抗.球形磷酸铁锂电池具有较低的锂离子转移阻抗和扩散阻抗.图3 不同形貌LiFePO4样品电池交流阻抗谱曲线Fig.3 Electrochemical impedance spectra of LiFePO4battery图4 样品电池Z′与ω-1/2的关系图Fig.4 Relationship between Z′and ω-1/2of LiFePO4battery表4 不同形貌样品电池EIS参数值Tab.4 EIS parameters of LiFePO4battery样品Rs/mΩ Rct/mΩ σ/mΩ·s-1/2 LFPQ 43.62 55.99 16.09 LFPN 49.69 90.63 21.672.5 内阻对电池倍率性能影响图5为两样品电池在不同倍率下的放电曲线.由图可以看出,两样品电池随着放电倍率的增加,放电容量均有所降低.但由于样品LFPQ电池内阻较小,电池极化现象不明显,随放电倍率的增加,放电容量下降较慢.10 C倍率下样品LFPQ电池的放电容量仍能达到363.8 mAh,而样品LPFN电池的放电容量仅为223.6 mAh.表5为两样品电池不同倍率下的容量保持率.由表可见,相比0.5 C倍率,样品LFPQ 电池10 C倍率下,放电容量保持率能达到66.0%.由于电池内阻高,样品LFPN电池10 C的容量保持率仅为42.0%.由此可以看出磷酸铁锂电池内阻对电池倍率性能有直接影响.样品LFPQ电池具有良好的倍率性能.图5 LiFePO4样品电池在不同倍率下的放电曲线图Fig.5 Discharge curve of LiFePO4battery at different rates表5 LiFePO4样品电池不同倍率下的放电容量保持率Tab.5 Discharge capacity retention of LiFePO4battery at different rates样品 0.5C/% 1C/% 2C/% 5C/% 10C/%LFPQ 100 98.6 94.9 91.5 66.0 LFPN 100 93.5 84.2 78.9 42.03 结论本文以常见的球形和非球形形貌磷酸铁锂作为研究对象,探讨了磷酸铁锂形貌与电池内阻等特性之间的关系,对进一步提高锂离子电池性能具有实际意义.具体结论如下:1)形貌对磷酸铁锂电池内阻具有显著影响.相比非球形磷酸铁锂,球形形貌磷酸铁锂电池内阻降低了17.4 mΩ.2)磷酸铁锂形貌能够影响电池的交流阻抗.球形磷酸铁锂电池的电荷转移阻抗和锂离子扩散阻抗较低.3)内阻降低对磷酸铁锂电池倍率性能提升效果明显.低内阻的球形磷酸铁锂电池表现出优异的倍率性能.参考文献:[1]Chung S Y,Bloking J T,Chiang Y M.Electronically conductive phospho-olivines as lithium storage electrodes.[J].Nature Materials,2002,1(2):123-8.[2]Prosini P P,Lisi M,Zane D,et al.Determination of the chemical diffusion coefficient of lithium in LiFePO4[J].Solid State Ionics,2002,148(1-2):45-51.[3]常照荣,吕豪杰,付小宁.LiFePO4的研究进展、问题及解决方法[J].材料导报,2009,23(1):16-19.[4]Hsieh C T,Pai C T,Chen Y F,et al.Preparation of lithium iron phosphate cathode materials with different carbon contents using glucoseadditive for Li-ion batteries[J].Journal of the Taiwan Institute of Chemical Engineers,2014,45(4):1501-1508.[5]林春景,李斌,常国峰,等.不同温度下磷酸铁锂电池内阻特性实验研究[J].电源技术,2015,39(1):22-25.[6]席安静,田光宇,白鹏.磷酸铁锂锂离子电池EIS参数随 SOC 变化的规律[J].电池,2012,42(2):77-80.[7]孟仙雅,王睿,钟小华,等.导电剂对LiFePO4锂离子电池性能的影响[J].电池工业,2012,17(5):296-298.[8]Rodriguesa S,Munichandraiah N,Shukla A K.A review of state-of-charge indication of batteries by means of a.c.impedance measurements [J].Journal of Power Sources,2000,87(1-2):12-20.。
- 1、下载文档前请自行甄别文档内容的完整性,平台不提供额外的编辑、内容补充、找答案等附加服务。
- 2、"仅部分预览"的文档,不可在线预览部分如存在完整性等问题,可反馈申请退款(可完整预览的文档不适用该条件!)。
- 3、如文档侵犯您的权益,请联系客服反馈,我们会尽快为您处理(人工客服工作时间:9:00-18:30)。
High-rate properties of LiFePO 4/carbon composites as cathodematerials for lithium-ion batteriesAkira Kuwahara *,Shinya Suzuki 1,Masaru Miyayama 1Research Center for Advanced Science and Technology,The University of Tokyo,4-6-1Komaba,Meguro,Tokyo 153-8904,JapanAvailable online 29September 2007AbstractElectrochemical properties of LiFePO 4/carbon composites were investigated to achieve a high-rate lithium electrode performance.LiFePO 4/carbon composites were synthesized by a hydrothermal reaction of a solution of FeSO 4Á7H 2O,H 3PO 4,and LiOH ÁH 2O mixed with carbon powders under nitrogen atmosphere followed by annealing under 1%H 2–99%Ar atmosphere.Particle size of the obtained LiFePO 4/carbon composites observed by scanning electron microscopy was less than 100nm.At a high current density of 1000mA g À1,the LiFePO 4/carbon composites showed a high discharge capacity of 113mA h g À1,and a flat discharge potential plateau was observed around 3.4V .The discharge capacity at the high current density,85%of that at a low current density of 30mA g À1,is a quite high value for LiFePO 4cathodes.Homogeneous microstructure consisting of small particles contributed to the high-rate properties of the LiFePO 4/carbon composites.#2007Elsevier Ltd and Techna Group S.r.l.All rights reserved.Keywords:E.Batteries;High-rate properties;LiFePO 4;Cathode1.IntroductionThe development of energy storage devices with a high energy density and a high power density is devoted for use as power sources of electric and hybrid vehicles.Lithium-ion batteries [1]have been applied in many portable electronic devices because of a high energy density.Lithium-ion batteries with a high energy density are required for an improvement of high-rate performance in order to use high power devices as power sources of electric and hybrid vehicles.Besides long cycle life,environmental safety and low cost are required.Lithium iron phosphate (LiFePO 4)is a promising candidate as a cathode material for lithium-ion batteries [2].The olivine-based structure of LiFePO 4consists of polyoxyanionic frame-work containing LiO 6octahedra,FeO 6octahedra and PO 4tetrahedra [3].LiFePO 4has a high theoretical capacity of 170mA h g À1and a long cycle life [4].A flat potential plateau is observed when the insertion/extraction of lithium proceedsvia a two-phase of LiFePO 4/FePO 4.Moreover,LiFePO 4is nontoxic and inexpensive material.However,discharge capacities of LiFePO 4at high current densities decrease by a low lithium diffusion constant (approximately 10À14to 10À16cm 2s À1)[5]and a low electronic conductivity in the LiFePO 4(approximately 10À9to 10À10S cm À1)[6].A low lithium diffusion constant and a low electronic conductivity in electrodes lead to capacity losses due to lithium diffusion limitations and ohmic drops.Many studies have been conducted in order to eliminate lithium diffusion limitations in LiFePO 4by reducing particle size or enhance electronic conductivities by coating LiFePO 4with conductive carbon.Yang et al.reported a hydrothermal synthesis method of LiFePO 4,and the hydrothermally synthesized LiFePO 4showed a discharge capacity of 100mA h g À1at a low current density [7].The particle size of LiFePO 4synthesized by hydrothermal reactions was smaller than that of LiFePO 4synthesized by solid-state reactions.Yamada et al.reported that LiFePO 4with small particle size synthesized by a solid state reaction sintering at 5508C showed a large discharge capacity of 162mA h g À1at a low current density [8].Reducing particle size leads to a reduction of a lithium diffusion distance.Therefore,lithium diffusion limitations are eliminated by reducing particle size.They also analyzed the oxidation state of Fe with Mo¨ssbauer /locate/ceramintAvailable online at Ceramics International 34(2008)863–866*Corresponding author.Tel.:+81354525082;fax:+81354525083.E-mail addresses:fwd2akira@crm.rcast.u-tokyo.ac.jp (A.Kuwahara),sin@crm.rcast.u-tokyo.ac.jp (S.Suzuki),miyayama@rcast.u-tokyo.ac.jp (M.Miyayama).1Tel.:+81354525082;fax:+81354525083.0272-8842/$34.00#2007Elsevier Ltd and Techna Group S.r.l.All rights reserved.doi:10.1016/j.ceramint.2007.09.037spectroscopy and indicated that the oxidation state of Fe was Fe2+in the most part of the LiFePO4.Preventing the formation of Fe3+in LiFePO4contributes to achieve a high lithium intercalation charge/discharge capacity.Chen et al.reported that LiFePO4particle coated with a carbon synthesized by sintering with sugar showed a large capacity of125mA h gÀ1 at a high current density of850mA gÀ1[9].The electronic conductivity in electrodes was enhanced by coating LiFePO4 with conductive carbon.The enhanced electronic conductivity contributed to the high discharge capacity at the high current density.Kudo et al.reported sufficient high-rate charge/ discharge performances for V2O5/carbon composites,prepared by mixing V2O5sol and carbon powders followed by heat treatment[10].Thin V2O5layers on carbon particles can lead to high discharge capacities at sufficient high current densities for the following reason.The formation of such a homogeneous composite contributes to a reduction of lithium diffusion distance and an increase of a specific surface area as is the case with reducing particle size and an enhancement of an electronic conductivity in electrodes.Kimura et al.reported that a homogeneous LiFePO4/carbon composite based on the microstructure of the composite suggested by Kudo et al.[10]showed high-rate charge/discharge properties,synthesized from an aqueous solution mixed with carbon powders by hydrothermal reactions followed by annealing[11].Discharge capacities of the LiFePO4/carbon composite were90mA h gÀ1at a high current density of 1000mA gÀ1.However,the discharge capacity of the LiFePO4/ carbon composite was120mA h gÀ1at a low current density, and Fe3+contents as impurities produced through oxidation of Fe2+by oxygen dissolved in the aqueous solution would exist in LiFePO4.Preventing the oxidation to Fe3+is required in a synthesis of a homogeneous LiFePO4/carbon composite in order to achieve high capacities and high-rate properties.In this work,hydrothermal reactions of LiFePO4precursor mixed with carbon powders under nitrogen atmosphere were conducted in order to synthesize a homogeneous LiFePO4/ carbon composite without oxidation of Fe2+by oxygen dissolved in an aqueous solution and to achieve a high-rate lithium intercalation/deintercalation properties.The detailed structure and lithium intercalation properties of the LiFePO4/ carbon composite were evaluated.2.Experimental procedureLiFePO4/carbon composites were synthesized by hydrother-mal reactions[11]under nitrogen atmosphere.FeSO4Á7H2O (Wako Chemicals),H3PO4(Wako Chemicals),and LiOHÁH2O (High Purity Chemicals)with molar ratio1.0:1.0:3.0were dissolved in deionized water under nitrogen atmosphere. Polyethylene glycol(Wako Chemicals)was added to the solution by a half volume of the mixture solution in order to obtainfine particles[7].Acetylene black(Denki Kagaku Kogyo)was added to the solution and dioxane(Kanto Chemical)as a surfactant between the aqueous solution and carbon particles was added by a same volume of the mixture solution.The carbon was added by the same weight as that of LiFePO4to be yielded.A homogeneous dispersion obtained by stirring for24h and the nitrogen gas were put into Teflon crucible,and the sealed Teflon crucible was set in pressure-resistant container.Hydrothermal reactions were performed for3h at1508C under nitrogen atmosphere,and obtained LiFePO4/carbon composites were annealed under1%hydrogen–99%argon atmosphere for1h at 5008C.The crystal structure of the LiFePO4/carbon composites was evaluated by X-ray diffraction methods using Cu K a radiation(Bruker AXS,D8diffractometer).The X-ray diffrac-tion data were corrected in the angle interval from158to558(2u) at steps of0.028(2u).The local structure of the LiFePO4/carbon composites was characterized by diffuse reflectance Fourier transform infrared spectroscopy(Shimadzu,IR Prestage-21). The particle sizes of the LiFePO4/carbon composites were observed by scanning electron microscopy(Hitachi,S-4500). The specific surface areas of the LiFePO4/carbon composites were evaluated by nitrogen adsorption–desorption measure-ments(Micromeritics,TriStar3000).The weight ratio of carbon in the LiFePO4/carbon composites was evaluated by thermo-gravimetry(Rigaku,TG8120).The electrodes for electrochemical measurements were fabricated by pressing the mixture of the LiFePO4/carbon composites with10wt.%Teflon binder(Du Pont)onto Nickel mesh.The mass loading of LiFePO4in the electrodes was approximately1Â10À2g cmÀ2.The thickness of the electro-des was approximately0.3mm.Electrochemical measure-ments were performed using a three-electrode cell with a counter electrode and a reference electrode of lithium metal.An electrolyte was1M LiClO4in propylene carbonate(Kishida Chemical).The cells were assembled in an argon-filled glove box at room temperature.Cyclic voltammetry measurements were carried out at a sweep rate of0.1mV sÀ1,and galvanostatic charge and discharge measurements were carried out in a current density range of30–2000mA gÀ1.All measurements were performed in the voltage range of2.7–4.3V with potentiostat/galvanostat(Hokuto Denko,HAG-5001)at room temperature.3.Results and discussionFig.1shows the X-ray diffraction(XRD)patterns for the LiFePO4/carbon composites.Thefigure also shows theposition Fig.1.X-ray diffraction patterns for the LiFePO4/carbon composites.A.Kuwahara et al./Ceramics International34(2008)863–866 864and the relative intensity of peaks for LiFePO 4(JCPDS,No.40-1499).The XRD pattern of the LiFePO 4/carbon composites included broad peak originated from carbon around 268.All sharp peaks of the LiFePO 4/carbon composites were indexed to LiFePO 4.Therefore,no impurities in the LiFePO 4/carbon composites were detected by X-ray diffraction measurements.The impurity nano-sized phases can be detected by Fourier transform infrared spectra [12].Fig.2shows the Fourier transform infrared spectra for the LiFePO 4/carbon composites,acetylene black and LiFePO 4.The intramolecular vibrations of the (PO 4)3Àappear in the wavenumber range of 372–1139cm À1[13].Symmetric and antisymmetric O–P–O bending modes exist in the range of 372–647cm À1.Symmetric and antisymmetric P–O stretching modes exist in the range of 945–1139cm À1[13].In Fig.2,these bending and stretching modes in the LiFePO 4/carbon composites and LiFePO 4were observed.Additional peaks in the LiFePO 4/carbon composites compared with the peaks in LiFePO 4were not observed.Therefore,no inclusions as impurities were detected by Fourier transform infrared spectra in the LiFePO 4/carbon composites.Fig.3shows the scanning electron microscopic images for (a)the LiFePO 4/carbon composites and (b)acetylene black using as carbon sources.The particle size of the LiFePO 4/carbon composites was less than 100nm.The particle size of acetylene black was approximately 50nm,and this particle size of acetylene black was smaller than that of the LiFePO 4/carbon composites.Then,the LiFePO 4/carbon composites are expected to have a microstructure where each carbon particles with a size of approximately 50nm would be covered with thin LiFePO 4layer or buried in LiFePO 4aggregates,as reported previously [11].The Brunauer–Emmett–Teller (B.E.T.)surface area of the LiFePO 4/carbon composites was 39m 2g À1measured by nitrogen adsorption–desorption measurements.A specific surface area of the LiFePO 4/carbon composites estimated in the ideal state in which carbon with a diameter of 50nm was uniformly coated with LiFePO 4is 40m 2g À1.The estimated surface area was nearly equal to the measured B.E.T.surface area.These results of scanning electron microscopic images and B.E.T.surface area indicated that the LiFePO 4/carbon composites were constructed with carbon particles coveredwith a thin LiFePO 4layer.A thickness of the LiFePO 4layer denoted a lithium diffusion distance for the LiFePO 4/carbon composites,and the layer thickness could be estimated to be a few nanometer.The weight ratio of carbon in the LiFePO 4/carbon composites was evaluated by using thermogravimetry.Fig.4shows the thermogravimetric curves for the LiFePO 4/carbon composites and LiFePO 4.The amount of weight change in the LiFePO 4/carbon composites and LiFePO 4was evaluated by heating in air at 8008C.The difference of weight change in the LiFePO 4/carbon composites and LiFePO 4denoted the mass of carbon content in the composites as the carbon in the composites was burned off by heating in air at 8008C.The mass of LiFePO 4increased approximately 3%by an oxidation of Fe 2+in LiFePO 4.The carbon in the LiFePO 4/carbonFig.2.Fourier transform infrared spectra for the LiFePO 4/carbon composites.The spectrum in the middle represents the patterns of acetylene black.The spectrum on the bottom represents the patterns of LiFePO 4.Fig.3.SEM images for LiFePO 4/carbon composites and acetylene black using as carbon sources.(a)LiFePO 4/carbon composites and (b)acetyleneblack.Fig.4.Thermogravimetric curves for the LiFePO 4/carbon composites and LiFePO 4.Heating rate was 10K min À1.A.Kuwahara et al./Ceramics International 34(2008)863–866865composites was burned off at 6008C,and the total mass of the composites decreased by 52%.As a result,the carbon weight ratio in the LiFePO 4/carbon composites was 52wt.%as against a total mass of the composites.Fig.5shows the discharge curves for the LiFePO 4/carbon composites in a current density range of 30–2000mA g À1.Current densities and discharge capacities were normalized to the mass of LiFePO 4.A discharge capacity for the LiFePO 4/carbon composites was 133mA h g À1at a low current density of 30mA g À1and a flat discharge potential plateau was observed around 3.4V .Discharge capacities for the LiFePO 4/carbon composites decreased with increasing current density,however significant capacity losses were not observed.Discharge capacities for the LiFePO 4/carbon composites were 125mA h g À1at a current density of 100mA g À1,116mA h g À1at 300mA g À1,113mA h g À1at 1000mA g À11and 113mA h g À1at 2000mA g À1,respectively.In a current density range of 30–1000mA g À1,flat discharge potential plateaus were observed around 3.4V ,and the discharge capacity at the high current density was approximately 85%of that at the low current density in the LiFePO 4/carbon composites.The flat discharge potential plateaus around 3.4V in a current density range of 30–1000mA g À1indicates that the LiFePO 4/carbon composites has a high electronic conductivity and a low lithium diffusion distance in the electrodes.The homogeneous microstructure of the LiFePO 4/carbon compo-sites led to an increase in an electronic conductivity by close contact of LiFePO 4/carbon interface and a reduction of a lithium diffusion distance in the LiFePO 4layer with a few nanometer thickness as shown above.The discharge capacity at the highest current density of 2000mA g À1,85%of that at a low current density of 30mA g À1,is a quite high value for LiFePO 4cathodes.Then,high-rate lithium intercalation/deintercalation performances for the LiFePO 4/carbon compo-sites were achieved.On the other hand,a volumetric capacity for the LiFePO 4/carbon composites was approximately 50mA h cm À3.The small volumetric capacity is due to a high carbon content,which is necessary for preparing such homogeneous LiFePO 4/carbon composites.Improvement of composite microstructure such as use of smaller carbonparticles with a low content will enable an increase in volumetric capacity.4.ConclusionsLiFePO 4/carbon composites were synthesized by hydro-thermal reactions under nitrogen atmosphere and their electrochemical properties were investigated.The particle size of the LiFePO 4/carbon composites was less than 100nm.The LiFePO 4/carbon composites were constructed with carbon particles covered with a thin LiFePO 4layer.At a high current density of 1000mA g À1,the LiFePO 4/carbon composites showed a high discharge capacity of 113mA h g À1,and the flat discharge potential plateau was observed around 3.4V .The discharge capacity at the high current density was approxi-mately 85%of that at the low current density in the LiFePO 4/carbon composites.The homogeneous microstructure of the LiFePO 4/carbon composites led to an increase in an electronic conductivity by close contact of LiFePO 4/carbon interface and a reduction of a lithium diffusion distance in LiFePO 4layer with a few nanometer thickness.Therefore,high-rate lithium intercalation/deintercalation performances for the LiFePO 4/carbon composites were achieved.References[1]R.J.Brodd,K.R.Bullock,R.A.Leising,R.L.Middaugh,ler,E.Takeuchi,Batteries,1977to 2002,J.Electrochem.Soc.151(3)(2004)K1–K11.[2]M.S.Whittingham,Lithium batteries and cathode materials,Chem.Rev.104(2004)4271–4301.[3]V .A.Streltsov,E.L.Belokoneva,V .G.Tsirelson,N.Hansen,Multipoleanalysis of the electron density in triphylite,LiFePO 4,using X-ray diffraction data,Acta Crystallogr.Section B:Struct.Sci.B49(1993)147–153.[4]A.K.Padhi,K.S.Nanjundaswamy,C.Masquelier,S.Okada,J.B.Good-enough,Effect of structure on the Fe 3+/Fe 2+redox couple in iron phos-phates,J.Electrochem.Soc.144(5)(1997)1609–1613.[5]P.P.Prosini,M.Lisi,D.Zane,M.Pasquali,Determination of the chemicaldiffusion coefficient of lithium in LiFePO 4,Solid State Ionics 148(2002)45–51.[6]S.Y .Chung,J.T.Bloking,Y .M.Chiang,Electronically conductive phos-pho-olivines as lithium storage electrodes,Nat.Mater.1(2002)123–128.[7]S.Yang,P.Y .Zavalij,M.S.Whittingham,Hydrothermal synthesis of lithiumiron phosphate cathodes,mun.3(2001)505–508.[8]A.Yamada,S.C.Chung,K.Hinokuma,Optimized LiFePO 4for lithiumbattery cathodes,J.Electrochem.Soc.148(3)(2001)A224–A229.[9]Z.Chen,J.R.Dahn,Reducing carbon in LiFePO 4/C composite electrodesto maximize specific energy,volumetric energy,and tap density,J.Electrochem.Soc.149(9)(2002)A1184–A1189.[10]T.Kudo,Y .Ikeda,T.Watanabe,M.Hibino,M.Miyayama,H.Abe,K.Kajita,Amorphous V 2O 5/carbon composites as electrochemical super-capacitor electrodes,Solid State Ionics 152–153(2002)833–841.[11]K.Kimura,M.Miyayama,Li intercalation properties of LiFePO 4/carboncomposites prepared by hydrothermal method,Key Eng.Mater.269(2004)139–142.[12]A.A.Salah,A.Mauger,C.M.Julien,F.Gendron,Nano-sized impurityphases in relation to the mode of preparation of LiFePO 4,Mater.Sci.Eng.B 129(2006)232–244.[13]C.M.Burba,R.Frech,Raman,FTIR spectroscopic study of Li x FePO 4,J.Electrochem.Soc.151(7)(2004)A1032–A1038.Fig.5.Discharge curves for the LiFePO 4/carbon composites.Current density range was 30–2000mA g À1.A.Kuwahara et al./Ceramics International 34(2008)863–866866。