Synthesis of micro and nanostructured MnO2
电化学沉积英语
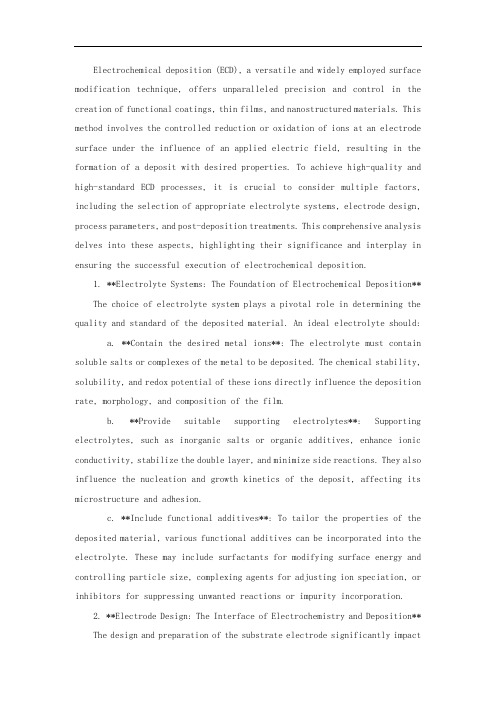
Electrochemical deposition (ECD), a versatile and widely employed surface modification technique, offers unparalleled precision and control in the creation of functional coatings, thin films, and nanostructured materials. This method involves the controlled reduction or oxidation of ions at an electrode surface under the influence of an applied electric field, resulting in the formation of a deposit with desired properties. To achieve high-quality and high-standard ECD processes, it is crucial to consider multiple factors, including the selection of appropriate electrolyte systems, electrode design, process parameters, and post-deposition treatments. This comprehensive analysis delves into these aspects, highlighting their significance and interplay in ensuring the successful execution of electrochemical deposition.1. **Electrolyte Systems: The Foundation of Electrochemical Deposition**The choice of electrolyte system plays a pivotal role in determining the quality and standard of the deposited material. An ideal electrolyte should:a. **Contain the desired metal ions**: The electrolyte must contain soluble salts or complexes of the metal to be deposited. The chemical stability, solubility, and redox potential of these ions directly influence the deposition rate, morphology, and composition of the film.b. **Provide suitable supporting electrolytes**: Supporting electrolytes, such as inorganic salts or organic additives, enhance ionic conductivity, stabilize the double layer, and minimize side reactions. They also influence the nucleation and growth kinetics of the deposit, affecting its microstructure and adhesion.c. **Include functional additives**: To tailor the properties of the deposited material, various functional additives can be incorporated into the electrolyte. These may include surfactants for modifying surface energy and controlling particle size, complexing agents for adjusting ion speciation, or inhibitors for suppressing unwanted reactions or impurity incorporation.2. **Electrode Design: The Interface of Electrochemistry and Deposition**The design and preparation of the substrate electrode significantly impactthe quality and standard of the deposited film. Key considerations include:a. **Substrate material**: The substrate material should possess adequate electrical conductivity, chemical compatibility with the electrolyte, and mechanical stability during the deposition process. Moreover, its surface chemistry and roughness can influence nucleation and adhesion of the deposited layer.b. **Surface pre-treatment**: Prior to deposition, the substrate surface may require cleaning, polishing, or activation treatments to remove contaminants, create a defined surface topography, or induce specific surface chemistries that promote nucleation and adhesion.c. **Electrode geometry**: The electrode geometry, including shape, size, and arrangement, can affect mass transport, current distribution, and the development of local electric fields, which in turn influence deposition uniformity, thickness control, and defect formation.3. **Process Parameters: The Fine-Tuning Elements**Optimizing process parameters is crucial for achieving high-quality and high-standard electrochemical deposition. Key parameters include:a. **Applied voltage or current**: The magnitude and mode (direct current, pulsed current, alternating current) of the applied electrical field govern the kinetics of ion reduction/oxidation, deposition rate, and energy input into the system. Careful control is essential for achieving desired film thickness, composition, and microstructure.b. **Temperature**: Temperature influences electrolyte conductivity, reaction kinetics, and mass transport. It can be used to modulate deposition rate, phase formation, and stress development in the deposited film.c. **Deposition time**: The duration of the deposition process determines the overall thickness of the film and can influence the evolution of microstructure and properties. Balancing deposition time with other parameters ensures efficient use of resources while maintaining desired film characteristics.4. **Post-Deposition Treatments: Enhancing and Preserving Deposit Quality**Post-deposition treatments are often employed to further refine the properties of the electrochemically deposited material or to ensure its long-term stability. Some common treatments include:a. **Annealing**: Thermal annealing can be used to homogenize the microstructure, relieve residual stresses, improve crystallinity, or induce phase transformations, thereby enhancing the mechanical, electrical, or optical properties of the deposited film.b. **Chemical or electrochemical passivation**: Surface passivation treatments can be applied to increase corrosion resistance, reduce surface roughness, or modify surface chemistry for improved adhesion or functionality in subsequent processing steps.c. **Mechanical or chemical polishing**: Polishing techniques can be employed to achieve a desired surface finish, remove defects, or control the thickness of the deposited layer with high precision.In conclusion, achieving high-quality and high-standard electrochemical deposition requires a holistic approach that encompasses careful selection of electrolyte systems, meticulous design of electrode substrates, optimization of process parameters, and strategic implementation of post-deposition treatments. Each of these aspects is interconnected and contributes uniquely to the overall performance and reliability of the deposited material. By systematically considering and fine-tuning these factors, researchers and engineers can harness the full potential of electrochemical deposition for the synthesis of advanced functional coatings, thin films, and nanostructured materials with tailored properties and exceptional performance.。
共沉淀法 英语
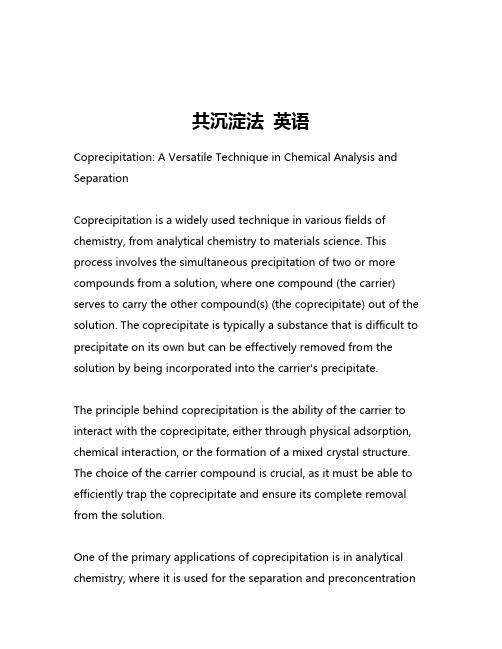
共沉淀法英语Coprecipitation: A Versatile Technique in Chemical Analysis and SeparationCoprecipitation is a widely used technique in various fields of chemistry, from analytical chemistry to materials science. This process involves the simultaneous precipitation of two or more compounds from a solution, where one compound (the carrier) serves to carry the other compound(s) (the coprecipitate) out of the solution. The coprecipitate is typically a substance that is difficult to precipitate on its own but can be effectively removed from the solution by being incorporated into the carrier's precipitate.The principle behind coprecipitation is the ability of the carrier to interact with the coprecipitate, either through physical adsorption, chemical interaction, or the formation of a mixed crystal structure. The choice of the carrier compound is crucial, as it must be able to efficiently trap the coprecipitate and ensure its complete removal from the solution.One of the primary applications of coprecipitation is in analytical chemistry, where it is used for the separation and preconcentrationof trace elements. In this context, the coprecipitate is often a metalor a metal complex that needs to be separated from the matrix for accurate analysis. The carrier compound, such as a hydroxide or a phosphate, can effectively remove the trace element from the solution, allowing for its subsequent determination by techniques like atomic absorption spectroscopy or inductively coupled plasma mass spectrometry.Another important application of coprecipitation is in the synthesisof materials, particularly in the field of nanomaterials. By carefully selecting the carrier and coprecipitate compounds, researchers can produce a wide range of nanostructured materials with unique properties. For example, the coprecipitation of metal ions with organic ligands can lead to the formation of metal-organic frameworks (MOFs), which have applications in areas like gas storage, catalysis, and drug delivery.Coprecipitation is also used in water treatment processes, where it is employed to remove heavy metals, radionuclides, and other contaminants from wastewater. In this case, the carrier compound, such as a metal hydroxide or a ferric hydroxide, can effectively trap the target pollutants and facilitate their removal from the water.The success of coprecipitation in various applications relies on several factors, including the solubility of the carrier andcoprecipitate compounds, the pH of the solution, the presence of competing ions, and the kinetics of the precipitation process. Careful optimization of these parameters is crucial to ensure the efficient and selective removal of the target species.In addition to the practical applications, coprecipitation also plays a significant role in the study of chemical equilibria and the thermodynamics of precipitation reactions. By understanding the factors that govern the coprecipitation process, researchers can gain insights into the underlying mechanisms and develop more accurate models for predicting the behavior of complex chemical systems.In conclusion, coprecipitation is a versatile and powerful technique that finds numerous applications in various fields of chemistry. Its ability to selectively remove and concentrate target compounds from complex matrices makes it an indispensable tool in analytical chemistry, materials science, and environmental remediation. As research in this area continues to evolve, we can expect to see further advancements in the understanding and utilization of coprecipitation for the development of innovative solutions to complex chemical problems.。
读书报告
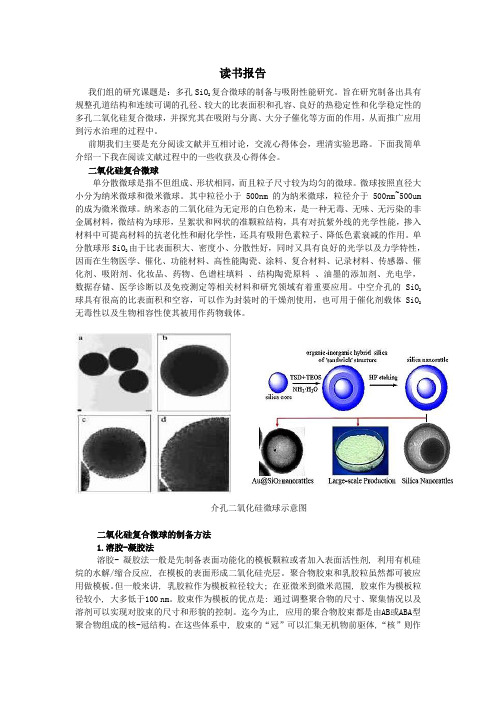
读书报告我们组的研究课题是:多孔SiO2复合微球的制备与吸附性能研究。
旨在研究制备出具有规整孔道结构和连续可调的孔径、较大的比表面积和孔容、良好的热稳定性和化学稳定性的多孔二氧化硅复合微球,并探究其在吸附与分离、大分子催化等方面的作用,从而推广应用到污水治理的过程中。
前期我们主要是充分阅读文献并互相讨论,交流心得体会,理清实验思路。
下面我简单介绍一下我在阅读文献过程中的一些收获及心得体会。
二氧化硅复合微球单分散微球是指不但组成、形状相同,而且粒子尺寸较为均匀的微球。
微球按照直径大小分为纳米微球和微米微球。
其中粒径小于500nm的为纳米微球,粒径介于500nm~500um 的成为微米微球。
纳米态的二氧化硅为无定形的白色粉末,是一种无毒、无味、无污染的非金属材料,微结构为球形,呈絮状和网状的准颗粒结构,具有对抗紫外线的光学性能,掺入材料中可提高材料的抗老化性和耐化学性,还具有吸附色素粒子、降低色素衰减的作用。
单分散球形SiO2由于比表面积大、密度小、分散性好,同时又具有良好的光学以及力学特性,因而在生物医学、催化、功能材料、高性能陶瓷、涂料、复合材料、记录材料、传感器、催化剂、吸附剂、化妆品、药物、色谱柱填料、结构陶瓷原料、油墨的添加剂、光电学,数据存储、医学诊断以及免疫测定等相关材料和研究领域有着重要应用。
中空介孔的 SiO2球具有很高的比表面积和空容,可以作为封装时的干燥剂使用,也可用于催化剂载体SiO2无毒性以及生物相容性使其被用作药物载体。
介孔二氧化硅微球示意图二氧化硅复合微球的制备方法1.溶胶-凝胶法溶胶- 凝胶法一般是先制备表面功能化的模板颗粒或者加入表面活性剂, 利用有机硅烷的水解/缩合反应, 在模板的表面形成二氧化硅壳层。
聚合物胶束和乳胶粒虽然都可被应用做模板。
但一般来讲, 乳胶粒作为模板粒径较大; 在亚微米到微米范围, 胶束作为模板粒径较小, 大多低于100 nm。
胶束作为模板的优点是: 通过调整聚合物的尺寸、聚集情况以及溶剂可以实现对胶束的尺寸和形貌的控制。
化学专业英语词汇
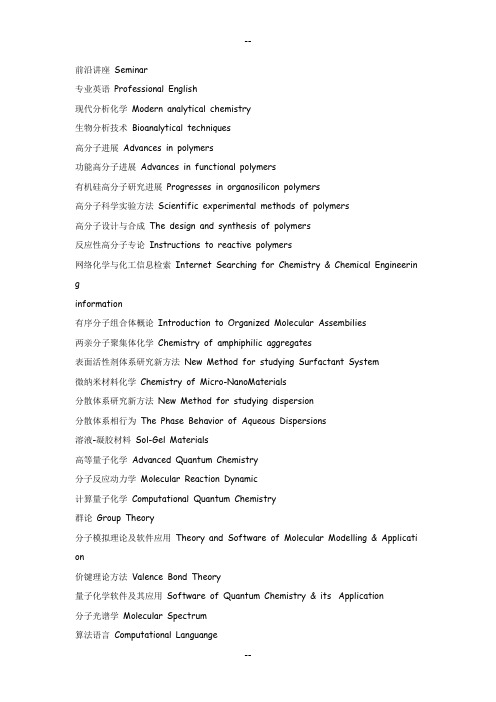
前沿讲座Seminar专业英语Professional English现代分析化学Modern analytical chemistry生物分析技术Bioanalytical techniques高分子进展Advances in polymers功能高分子进展Advances in functional polymers有机硅高分子研究进展Progresses in organosilicon polymers高分子科学实验方法Scientific experimental methods of polymers高分子设计与合成The design and synthesis of polymers反应性高分子专论Instructions to reactive polymers网络化学与化工信息检索Internet Searching for Chemistry & Chemical Engineerin ginformation有序分子组合体概论Introduction to Organized Molecular Assembilies两亲分子聚集体化学Chemistry of amphiphilic aggregates表面活性剂体系研究新方法New Method for studying Surfactant System微纳米材料化学Chemistry of Micro-NanoMaterials分散体系研究新方法New Method for studying dispersion分散体系相行为The Phase Behavior of Aqueous Dispersions溶液-凝胶材料Sol-Gel Materials高等量子化学Advanced Quantum Chemistry分子反应动力学Molecular Reaction Dynamic计算量子化学Computational Quantum Chemistry群论Group Theory分子模拟理论及软件应用Theory and Software of Molecular Modelling & Applicati on价键理论方法Valence Bond Theory量子化学软件及其应用Software of Quantum Chemistry & its Application分子光谱学Molecular Spectrum算法语言Computational Languange高分子化学Polymer Chemistry高分子物理Polymer Physics药物化学Medicinal Chemistry统计热力学Statistic Thermodynamics液-液体系专论Discussion on Liquid-Liquid System配位化学进展Progress in Coordination Chemistry无机材料及物理性质Inorganic Materials and Their Physical Properties物理无机化学Physical Inorganic Chemistry相平衡Phase Equilibrium现代无机化学Today's Inorganic Chemistry无机化学前沿领域导论Introduction to Forward Field in Inorganic Chemistry量子化学Quantum Chemistry分子材料Molecular Material固体酸碱理论Solid Acid-Base Theory萃取过程物理化学Physical Chemistry in Extraction表面电化学Surface Electrochemistry电化学进展Advances on Electrochemistry现代电化学实验技术Modern Experimental Techniques of Electrochemistry金属-碳多重键化合物及其应用Compounds with Metal-Carbon multiple bonds and T heir Applications叶立德化学:理论和应用Ylides Chemistry: Theory and Application立体化学与手性合成Stereochemistry and Chiral Synthesis杂环化学Heterocyclic Chemistry有机硅化学Organosilicon Chemistry药物设计及合成Pharmaceutical Design and Synthesis超分子化学Supramolecular Chemistry分子设计与组合化学Molecular Design and Combinatorial Chemistry纳米材料化学前沿领域导论Introduction to Nano-materials Chemistry纳米材料控制合成与自组装Controlled-synthesis and Self-assembly of Nano-materials前沿讲座Leading Front Forum专业英语Professional English超分子化学基础Basics of Supramolecular Chemistry液晶材料基础Basics of Liquid Crystal Materials现代实验技术Modern analytical testing techniques色谱及联用技术Chromatography and Technology of tandem发光分析及其研究法Luminescence analysis and Research methods胶束酶学Micellar Enzymology分析化学中的配位化合物Complex in Analytical Chemistry电分析化学Electroanalytical chemistry生物分析化学Bioanalytical chemistry分析化学Analytical chemistry仪器分析Instrument analysis高分子合成化学Polymers synthetic chemistry高聚物结构与性能Structures and properties of polymers有机硅化学Organosilicon chemistry功能高分子Functional polymers有机硅高分子Organosilicon polymers高分子现代实验技术Advanced experimental technology of polymers高分子合成新方法New synthetic methods of polymers液晶与液晶高分子Liquid crystals and liquid crystal polymers大分子反应Macromolecules reaction水溶性高分子Water-soluble polymers聚合物加工基础The basic process of polymers聚合物复合材料Composite materials高等化工与热力学Advanced Chemical Engineering and Thermodynamics 高等反应工程学Advanced Reaction Engineering高等有机化学Advanced Organic Chemistry高等有机合成Advanced Organic synthesis有机化学中光谱分析Spectrum Analysis in Organic Chemistry催化作用原理Principle of Catalysis染料化学Dye Chemistry中间体化学与工艺学Intermediate Chemistry and Technology化学动力学Chemical Kinetics表面活性剂合成与工艺Synthesis and Technology of Surfactants环境化学Environmental Chemistry化工企业清洁生产Chemical Enterprise Clean Production化工污染及防治Chemical Pollution and Control动量热量质量传递Momentum, Heat and Mass Transmission化工分离工程专题Separation Engineering耐蚀材料Corrosion Resisting Material网络化学与化工信息检索Internet Searching for Chemistry & Chemical Engineerin g information新型功能材料的模板组装Templated Assembly of Novel Advanced Materials胶体与界面Colloid and Interface纳米材料的胶体化学制备方法Colloid Chemical Methods for Preparing Nano-materia ls脂质体化学Chemistry of liposome表面活性剂物理化学Physico-chemistry of surfactants高分子溶液与微乳液Polymer Solutions and Microemulsions两亲分子的溶液化学Chemistry of Amphiphilic Molecules in solution介孔材料化学Mesoporous Chemistry超细颗粒化学Chemistry of ultrafine powder分散体系流变学The Rheolgy of Aqueous Dispersions量子化学Quantum Chemistry统计热力学Statistic Thermodynamics群论Group Theory分子模拟Molecular Modelling高等量子化学Advanced Quantum Chemistry价键理论方法Valence Bond Theory量子化学软件及其应用Software of Quantum Chemistry & its Application计算量子化学Computational Quantum Chemistry分子模拟软件及其应用Software of Molecular Modelling & its Application分子反应动力学Molecular Reaction Dynamic分子光谱学Molecular Spectrum算法语言Computational Languange高分子化学Polymer Chemistry高分子物理Polymer Physics腐蚀电化学Corrosion Electrochemistry物理化学Physical Chemistry结构化学structural Chemistry现代分析与测试技术(试验为主)Modern Analysis and Testing Technology(experimetally)高等无机化学Advanced Inorganic Chemistry近代无机物研究方法Modern Research Methods for Inorganic Compounds萃取化学研究方法Research Methods for Extraction Chemistry单晶培养Crystal Culture固态化学Chemistry of Solid Substance液-液体系专论Discussion on Liquid-Liquid System配位化学进展Progress in Coordination Chemistry卟啉酞箐化学Chemistry of Porphyrine and Phthalocyanine无机材料及物理性质Inorganic Materials and Their Physical Properties物理无机化学Physical Inorganic Chemistry相平衡Phase Equilibrium生物化学的应用Application of Biologic Chemistry生物无机化学Bio-Inorganic Chemistry绿色化学Green Chemistry金属有机化合物在均相催化中的应用Applied Homogeneous Catalysis with Organometallic Compounds功能性食品化学Functionalized Food Chemistry无机药物化学Inorganic Pharmaceutical Chemistry电极过程动力学Kinetics on Electrode Process电化学研究方法Electrochemical Research Methods生物物理化学Biological Physical Chemistry波谱与现代检测技术Spectroscopy and Modern Testing Technology理论有机化学theoretical Organic Chemistry合成化学Synthesis Chemistry有机合成新方法New Methods for Organic Synthesis生物有机化学Bio-organic Chemistry药物化学Pharmaceutical Chemistry金属有机化学Organometallic Chemistry金属-碳多重键化合物及其应用Compounds with Metal-Carbon multiple bonds and T heir Applications分子构效与模拟Molecular Structure-Activity and Simulation过程装置数值计算Data Calculation of Process Devices石油化工典型设备Common Equipment of Petrochemical Industry化工流态化工程Fluidization in Chemical Industry化工装置模拟与优化Analogue and Optimization of Chemical Devices化工分离工程Separation Engineering化工系统与优化Chemical System and Optimization高等化工热力学Advanced Chemical Engineering and Thermodynamics超临界流体技术及应用Super Critical Liguid Technegues and Applications膜分离技术Membrane Separation Technegues溶剂萃取原理和应用Theory and Application of Solvent Extraction树脂吸附理论Theory of Resin Adsorption中药材化学Chemistry of Chinese Medicine生物资源有效成分分析与鉴定Analysis and Detection of Bio-materials相平衡理论与应用Theory and Application of Phase Equilibrium计算机在化学工程中的应用Application of Computer in Chemical Engineering微乳液和高分子溶液Micro-emulsion and High Molecular Solution传递过程Transmision Process反应工程分析Reaction Engineering Analysis腐蚀电化学原理与应用Principle and Application of Corrosion Electrochemistry腐蚀电化学测试方法与应用Measurement Method and Application of Corrosion Ele ctrochemistry耐蚀表面工程Surface Techniques of Anti-corrosion缓蚀剂技术Inhabitor Techniques腐蚀失效分析Analysis of Corrosion Destroy材料表面研究方法Method of Studying Material Surfacc分离与纯化技术Separation and Purification Technology现代精细有机合成Modern Fine Organic Synthesis化学工艺与设备Chemical Technology and Apparatuas功能材料概论Functional Materials Conspectus油田化学Oilfield Chemistry精细化学品研究Study of Fine Chemicals催化剂合成与应用Synthesis and Application of Catalyzer低维材料制备Preparation of Low-Dimension Materials手性药物化学Symmetrical Pharmaceutical Chemistry光敏高分子材料化学Photosensitive Polymer Materials Chemistry纳米材料制备与表征Preparation and Characterization of Nanostructured material s溶胶凝胶化学Sol-gel Chemistry纳米材料化学进展Proceeding of Nano-materials Chemistry●化学常用词汇汉英对照表1●氨ammonia氨基酸amino acid铵盐ammonium salt饱和链烃saturated aliphatic hydrocarbon苯benzene变性denaturation不饱和烃unsaturated hydrocarbon超导材料superconductive material臭氧ozone醇alcohol次氯酸钾potassium hypochlorite醋酸钠sodium acetate蛋白质protein氮族元素nitrogen group element碘化钾potassium iodide碘化钠sodium iodide电化学腐蚀electrochemical corrosion电解质electrolyte电离平衡ionization equilibrium电子云electron cloud淀粉starch淀粉碘化钾试纸starch potassium iodide paper 二氧化氮nitrogen dioxide二氧化硅silicon dioxide二氧化硫sulphur dioxide二氧化锰manganese dioxide芳香烃arene放热反应exothermic reaction非极性分子non-polar molecule非极性键non-polar bond肥皂soap分馏fractional distillation酚phenol复合材料composite干电池dry cell干馏dry distillation甘油glycerol高分子化合物polymer共价键covalent bond官能团functional group光化学烟雾photochemical fog过氧化氢hydrogen peroxide合成材料synthetic material合成纤维synthetic fiber合成橡胶synthetic rubber核电荷数nuclear charge number核素nuclide化学电源chemical power source化学反应速率chemical reaction rate 化学键chemical bond化学平衡chemical equilibrium还原剂reducing agent磺化反应sulfonation reaction霍尔槽Hull Cell极性分子polar molecule极性键polar bond加成反应addition reaction加聚反应addition polymerization甲烷methane碱金属alkali metal碱石灰soda lime结构式structural formula聚合反应po1ymerization可逆反应reversible reaction空气污染指数air pollution index勒夏特列原理Le Chatelier's principle 离子反应ionic reaction离子方程式ionic equation离子键ionic bond锂电池lithium cell两性氢氧化物amphoteric hydroxide 两性氧化物amphoteric oxide裂化cracking裂解pyrolysis硫氰化钾potassium thiocyanate硫酸钠sodium sulphide氯化铵ammonium chloride氯化钡barium chloride氯化钾potassium chloride氯化铝aluminium chloride氯化镁magnesium chloride氯化氢hydrogen chloride氯化铁iron (III) chloride氯水chlorine water麦芽糖maltose煤coal酶enzyme摩尔mole摩尔质量molar mass品红magenta或fuchsine葡萄糖glucose气体摩尔体积molar volume of gas铅蓄电池lead storage battery强电解质strong electrolyte氢氟酸hydrogen chloride氢氧化铝aluminium hydroxide取代反应substitution reaction醛aldehyde炔烃alkyne燃料电池fuel cell弱电解质weak electrolyte石油Petroleum水解反应hydrolysis reaction四氯化碳carbon tetrachloride塑料plastic塑料的降解plastic degradation塑料的老化plastic ageing酸碱中和滴定acid-base neutralization titration 酸雨acid rain羧酸carboxylic acid碳酸钠sodium carbonate碳酸氢铵ammonium bicarbonate碳酸氢钠sodium bicarbonate糖类carbohydrate烃hydrocarbon烃的衍生物derivative of hydrocarbon烃基hydrocarbonyl同分异构体isomer同素异形体allotrope同位素isotope同系物homo1og涂料coating烷烃alkane物质的量amount of substance物质的量浓度amount-of-substance concentration of B 烯烃alkene洗涤剂detergent纤维素cellulose相对分子质量relative molecular mass相对原子质量relative atomic mass消去反应elimination reaction硝化反应nitratlon reaction硝酸钡barium nitrate硝酸银silver nitrate溴的四氯化碳溶液solution of bromine in carbon tetrachloride 溴化钠sodium bromide溴水bromine water溴水bromine water盐类的水解hydrolysis of salts盐析salting-out焰色反应flame test氧化剂oxidizing agent氧化铝aluminium oxide氧化铁iron (III) oxide乙醇ethanol乙醛ethana1乙炔ethyne乙酸ethanoic acid乙酸乙酯ethyl acetate乙烯ethene银镜反应silver mirror reaction硬脂酸stearic acid油脂oils and fats有机化合物organic compound元素周期表periodic table of elements元素周期律periodic law of elements原电池primary battery原子序数atomic number皂化反应saponification粘合剂adhesive蔗糖sucrose指示剂Indicator酯ester酯化反应esterification周期period族group(主族:main group)Bunsen burner 本生灯product 化学反应产物flask 烧瓶apparatus 设备PH indicator PH值指示剂,氢离子(浓度的)负指数指示剂matrass 卵形瓶litmus 石蕊litmus paper 石蕊试纸graduate, graduated flask 量筒,量杯reagent 试剂test tube 试管burette 滴定管retort 曲颈甑still 蒸馏釜cupel 烤钵crucible pot, melting pot 坩埚pipette 吸液管filter 滤管stirring rod 搅拌棒element 元素body 物体compound 化合物atom 原子gram atom 克原子atomic weight 原子量atomic number 原子数atomic mass 原子质量molecule 分子electrolyte 电解质ion 离子anion 阴离子cation 阳离子electron 电子isotope 同位素isomer 同分异物现象polymer 聚合物symbol 复合radical 基structural formula 分子式valence, valency 价monovalent 单价bivalent 二价halogen 成盐元素bond 原子的聚合mixture 混合combination 合成作用compound 合成物alloy 合金organic chemistry 有机化学inorganic chemistry 无机化学derivative 衍生物series 系列acid 酸hydrochloric acid 盐酸sulphuric acid 硫酸nitric acid 硝酸aqua fortis 王水fatty acid 脂肪酸organic acid 有机酸hydrosulphuric acid 氢硫酸hydrogen sulfide 氢化硫alkali 碱,强碱ammonia 氨base 碱hydrate 水合物hydroxide 氢氧化物,羟化物hydracid 氢酸hydrocarbon 碳氢化合物,羟anhydride 酐alkaloid 生物碱aldehyde 醛oxide 氧化物phosphate 磷酸盐acetate 醋酸盐methane 甲烷,沼气butane 丁烷salt 盐potassium carbonate 碳酸钾soda 苏打sodium carbonate 碳酸钠caustic potash 苛性钾caustic soda 苛性钠ester 酯gel 凝胶体analysis 分解fractionation 分馏endothermic reaction 吸热反应exothermic reaction 放热反应precipitation 沉淀to precipitate 沉淀to distil, to distill 蒸馏distillation 蒸馏to calcine 煅烧to oxidize 氧化alkalinization 碱化to oxygenate, to oxidize 脱氧,氧化to neutralize 中和to hydrogenate 氢化to hydrate 水合,水化to dehydrate 脱水fermentation 发酵solution 溶解combustion 燃烧fusion, melting 熔解alkalinity 碱性isomerism, isomery 同分异物现象hydrolysis 水解electrolysis 电解electrode 电极anode 阳极,正极cathode 阴极,负极catalyst 催化剂catalysis 催化作用oxidization, oxidation 氧化reducer 还原剂dissolution 分解synthesis 合成reversible 可逆的1. The Ideal-Gas Equation 理想气体状态方程2. Partial Pressures 分压3. Real Gases: Deviation from Ideal Behavior 真实气体:对理想气体行为的偏离4. The van der Waals Equation 范德华方程5. System and Surroundings 系统与环境6. State and State Functions 状态与状态函数7. Process 过程8. Phase 相9. The First Law of Thermodynamics 热力学第一定律10. Heat and Work 热与功11. Endothermic and Exothermic Processes 吸热与发热过程12. Enthalpies of Reactions 反应热13. Hess’s Law 盖斯定律14. Enthalpies of Formation 生成焓15. Reaction Rates 反应速率16. Reaction Order 反应级数17. Rate Constants 速率常数18. Activation Energy 活化能19. The Arrhenius Equation 阿累尼乌斯方程20. Reaction Mechanisms 反应机理21. Homogeneous Catalysis 均相催化剂22. Heterogeneous Catalysis 非均相催化剂23. Enzymes 酶24. The Equilibrium Constant 平衡常数25. the Direction of Reaction 反应方向26. Le Chatelier’s Principle 列·沙特列原理27. Effects of Volume, Pressure, Temperature Changes and Catalystsi. 体积,压力,温度变化以及催化剂的影响28. Spontaneous Processes 自发过程29. Entropy (Standard Entropy) 熵(标准熵)30. The Second Law of Thermodynamics 热力学第二定律31. Entropy Changes 熵变32. Standard Free-Energy Changes 标准自由能变33. Acid-Bases 酸碱34. The Dissociation of Water 水离解35. The Proton in Water 水合质子36. The pH Scales pH值37. Bronsted-Lowry Acids and Bases Bronsted-Lowry 酸和碱38. Proton-Transfer Reactions 质子转移反应39. Conjugate Acid-Base Pairs 共轭酸碱对40. Relative Strength of Acids and Bases 酸碱的相对强度41. Lewis Acids and Bases 路易斯酸碱42. Hydrolysis of Metal Ions 金属离子的水解43. Buffer Solutions 缓冲溶液44. The Common-Ion Effects 同离子效应45. Buffer Capacity 缓冲容量46. Formation of Complex Ions 配离子的形成47. Solubility 溶解度48. The Solubility-Product Constant Ksp 溶度积常数49. Precipitation and separation of Ions 离子的沉淀与分离50. Selective Precipitation of Ions 离子的选择沉淀51. Oxidation-Reduction Reactions 氧化还原反应52. Oxidation Number 氧化数53. Balancing Oxidation-Reduction Equations 氧化还原反应方程的配平54. Half-Reaction 半反应55. Galvani Cell 原电池56. Voltaic Cell 伏特电池57. Cell EMF 电池电动势58. Standard Electrode Potentials 标准电极电势59. Oxidizing and Reducing Agents 氧化剂和还原剂60. The Nernst Equation 能斯特方程61. Electrolysis 电解62. The Wave Behavior of Electrons 电子的波动性63. Bohr’s Model of The Hydrogen Atom 氢原子的波尔模型64. Line Spectra 线光谱65. Quantum Numbers 量子数66. Electron Spin 电子自旋67. Atomic Orbital 原子轨道68. The s (p, d, f) Orbital s(p,d,f)轨道69. Many-Electron Atoms 多电子原子70. Energies of Orbital 轨道能量71. The Pauli Exclusion Principle 泡林不相容原理72. Electron Configurations 电子构型73. The Periodic Table 周期表74. Row 行75. Group 族76. Isotopes, Atomic Numbers, and Mass Numbers 同位素,原子数,质量数77. Periodic Properties of the Elements 元素的周期律78. Radius of Atoms 原子半径79. Ionization Energy 电离能80. Electronegativity 电负性81. Effective Nuclear Charge 有效核电荷82. Electron Affinities 亲电性83. Metals 金属84. Nonmetals 非金属85. Valence Bond Theory 价键理论86. Covalence Bond 共价键87. Orbital Overlap 轨道重叠88. Multiple Bonds 重键89. Hybrid Orbital 杂化轨道90. The VSEPR Model 价层电子对互斥理论91. Molecular Geometries 分子空间构型92. Molecular Orbital 分子轨道93. Diatomic Molecules 双原子分子94. Bond Length 键长95. Bond Order 键级96. Bond Angles 键角97. Bond Enthalpies 键能98. Bond Polarity 键矩99. Dipole Moments 偶极矩100. Polarity Molecules 极性分子101. Polyatomic Molecules 多原子分子102. Crystal Structure 晶体结构103. Non-Crystal 非晶体104. Close Packing of Spheres 球密堆积105. Metallic Solids 金属晶体106. Metallic Bond 金属键107. Alloys 合金108. Ionic Solids 离子晶体109. Ion-Dipole Forces 离子偶极力110. Molecular Forces 分子间力111. Intermolecular Forces 分子间作用力112. Hydrogen Bonding 氢键113. Covalent-Network Solids 原子晶体114. Compounds 化合物115. The Nomenclature, Composition and Structure of Complexes 配合物的命名,组成和结构116. Charges, Coordination Numbers, and Geometries 电荷数、配位数、及几何构型117. Chelates 螯合物118. Isomerism 异构现象119. Structural Isomerism 结构异构120. Stereoisomerism 立体异构121. Magnetism 磁性122. Electron Configurations in Octahedral Complexes 八面体构型配合物的电子分布123. Tetrahedral and Square-planar Complexes 四面体和平面四边形配合物124. General Characteristics 共性125. s-Block Elements s区元素126. Alkali Metals 碱金属127. Alkaline Earth Metals 碱土金属128. Hydrides 氢化物129. Oxides 氧化物130. Peroxides and Superoxides 过氧化物和超氧化物131. Hydroxides 氢氧化物132. Salts 盐133. p-Block Elements p区元素134. Boron Group (Boron, Aluminium, Gallium, Indium, Thallium) 硼族(硼,铝,镓,铟,铊)135. Borane 硼烷136. Carbon Group (Carbon, Silicon, Germanium, Tin, Lead) 碳族(碳,硅,锗,锡,铅)137. Graphite, Carbon Monoxide, Carbon Dioxide 石墨,一氧化碳,二氧化碳138. Carbonic Acid, Carbonates and Carbides 碳酸,碳酸盐,碳化物139. Occurrence and Preparation of Silicon 硅的存在和制备140. Silicic Acid,Silicates 硅酸,硅酸盐141. Nitrogen Group (Phosphorus, Arsenic, Antimony, and Bismuth) 氮族(磷,砷,锑,铋)142. Ammonia, Nitric Acid, Phosphoric Acid 氨,硝酸,磷酸143. Phosphorates, phosphorus Halides 磷酸盐,卤化磷144. Oxygen Group (Oxygen, Sulfur, Selenium, and Tellurium) 氧族元素(氧,硫,硒,碲)145. Ozone, Hydrogen Peroxide 臭氧,过氧化氢146. Sulfides 硫化物147. Halogens (Fluorine, Chlorine, Bromine, Iodine) 卤素(氟,氯,溴,碘)148. Halides, Chloride 卤化物,氯化物149. The Noble Gases 稀有气体150. Noble-Gas Compounds 稀有气体化合物151. d-Block elements d区元素152. Transition Metals 过渡金属153. Potassium Dichromate 重铬酸钾154. Potassium Permanganate 高锰酸钾155. Iron Copper Zinc Mercury 铁,铜,锌,汞156. f-Block Elements f区元素157. Lanthanides 镧系元素158. Radioactivity 放射性159. Nuclear Chemistry 核化学160. Nuclear Fission 核裂变161. Nuclear Fusion 核聚变162. analytical chemistry 分析化学163. qualitative analysis 定性分析164. quantitative analysis 定量分析165. chemical analysis 化学分析166. instrumental analysis 仪器分析167. titrimetry 滴定分析168. gravimetric analysis 重量分析法169. regent 试剂170. chromatographic analysis 色谱分析171. product 产物172. electrochemical analysis 电化学分析173. on-line analysis 在线分析174. macro analysis 常量分析175. characteristic 表征176. micro analysis 微量分析177. deformation analysis 形态分析178. semimicro analysis 半微量分析179. systematical error 系统误差180. routine analysis 常规分析181. random error 偶然误差182. arbitration analysis 仲裁分析183. gross error 过失误差184. normal distribution 正态分布185. accuracy 准确度186. deviation偏差187. precision 精密度188. relative standard deviation 相对标准偏差(RSD)189. coefficient variation 变异系数(CV)190. confidence level 置信水平191. confidence interval 置信区间192. significant test 显著性检验193. significant figure 有效数字194. standard solution 标准溶液195. titration 滴定196. stoichiometric point 化学计量点197. end point滴定终点198. titration error 滴定误差199. primary standard 基准物质200. amount of substance 物质的量201. standardization 标定202. chemical reaction 化学反应203. concentration浓度204. chemical equilibrium 化学平衡205. titer 滴定度206. general equation for a chemical reaction化学反应的通式207. proton theory of acid-base 酸碱质子理论208. acid-base titration 酸碱滴定法209. dissociation constant 解离常数210. conjugate acid-base pair 共轭酸碱对211. acetic acid 乙酸212. hydronium ion水合氢离子213. electrolyte 电解质214. ion-product constant of water 水的离子积215. ionization 电离216. proton condition 质子平衡217. zero level零水准218. buffer solution缓冲溶液219. methyl orange 甲基橙220. acid-base indicator 酸碱指示剂221. phenolphthalein 酚酞222. coordination compound 配位化合物223. center ion 中心离子224. cumulative stability constant 累积稳定常数225. alpha coefficient 酸效应系数226. overall stability constant 总稳定常数227. ligand 配位体228. ethylenediamine tetraacetic acid 乙二胺四乙酸229. side reaction coefficient 副反应系数230. coordination atom 配位原子231. coordination number 配位数232. lone pair electron 孤对电子233. chelate compound 螯合物234. metal indicator 金属指示剂235. chelating agent 螯合剂236. masking 掩蔽237. demasking 解蔽238. electron 电子239. catalysis 催化240. oxidation氧化241. catalyst 催化剂242. reduction 还原243. catalytic reaction 催化反应244. reaction rate 反应速率245. electrode potential 电极电势246. activation energy 反应的活化能247. redox couple 氧化还原电对248. potassium permanganate 高锰酸钾249. iodimetry碘量法250. potassium dichromate 重铬酸钾251. cerimetry 铈量法252. redox indicator 氧化还原指示253. oxygen consuming 耗氧量(OC)254. chemical oxygen demanded 化学需氧量(COD) 255. dissolved oxygen 溶解氧(DO) 256. precipitation 沉淀反应257. argentimetry 银量法258. heterogeneous equilibrium of ions 多相离子平衡259. aging 陈化260. postprecipitation 继沉淀261. coprecipitation 共沉淀262. ignition 灼烧263. fitration 过滤264. decantation 倾泻法265. chemical factor 化学因数266. spectrophotometry 分光光度法267. colorimetry 比色分析268. transmittance 透光率269. absorptivity 吸光率270. calibration curve 校正曲线271. standard curve 标准曲线272. monochromator 单色器273. source 光源274. wavelength dispersion 色散275. absorption cell吸收池276. detector 检测系统277. bathochromic shift 红移278. Molar absorptivity 摩尔吸光系数279. hypochromic shift 紫移280. acetylene 乙炔281. ethylene 乙烯282. acetylating agent 乙酰化剂283. acetic acid 乙酸284. adiethyl ether 乙醚285. ethyl alcohol 乙醇286. acetaldehtde 乙醛287. β-dicarbontl compound β–二羰基化合物288. bimolecular elimination 双分子消除反应289. bimolecular nucleophilic substitution 双分子亲核取代反应290. open chain compound 开链族化合物291. molecular orbital theory 分子轨道理论292. chiral molecule 手性分子293. tautomerism 互变异构现象294. reaction mechanism 反应历程295. chemical shift 化学位移296. Walden inversio 瓦尔登反转n297. Enantiomorph 对映体298. addition rea ction 加成反应299. dextro- 右旋300. levo- 左旋301. stereochemistry 立体化学302. stereo isomer 立体异构体303. Lucas reagent 卢卡斯试剂304. covalent bond 共价键305. conjugated diene 共轭二烯烃306. conjugated double bond 共轭双键307. conjugated system 共轭体系308. conjugated effect 共轭效应309. isomer 同分异构体310. isomerism 同分异构现象311. organic chemistry 有机化学312. hybridization 杂化313. hybrid orbital 杂化轨道314. heterocyclic compound 杂环化合物315. peroxide effect 过氧化物效应t316. valence bond theory 价键理论317. sequence rule 次序规则318. electron-attracting grou p 吸电子基319. Huckel rule 休克尔规则320. Hinsberg test 兴斯堡试验321. infrared spectrum 红外光谱322. Michael reacton 麦克尔反应323. halogenated hydrocarbon 卤代烃324. haloform reaction 卤仿反应325. systematic nomenclatur 系统命名法e326. Newman projection 纽曼投影式327. aromatic compound 芳香族化合物328. aromatic character 芳香性r329. Claisen condensation reaction克莱森酯缩合反应330. Claisen rearrangement 克莱森重排331. Diels-Alder reation 狄尔斯-阿尔得反应332. Clemmensen reduction 克莱门森还原333. Cannizzaro reaction 坎尼扎罗反应334. positional isomers 位置异构体335. unimolecular elimination reaction 单分子消除反应336. unimolecular nucleophilic substitution 单分子亲核取代反应337. benzene 苯338. functional grou 官能团p339. configuration 构型340. conformation 构象341. confomational isome 构象异构体342. electrophilic addition 亲电加成343. electrophilic reagent 亲电试剂344. nucleophilic addition 亲核加成345. nucleophilic reagent 亲核试剂346. nucleophilic substitution reaction亲核取代反应347. active intermediate 活性中间体348. Saytzeff rule 查依采夫规则349. cis-trans isomerism 顺反异构350. inductive effect 诱导效应t351. Fehling’s reagent 费林试剂352. phase transfer catalysis 相转移催化作用353. aliphatic compound 脂肪族化合物354. elimination reaction 消除反应355. Grignard reagent 格利雅试剂356. nuclear magnetic resonance 核磁共振357. alkene 烯烃358. allyl cation 烯丙基正离子359. leaving group 离去基团360. optical activity 旋光性361. boat confomation 船型构象362. silver mirror reaction 银镜反应363. Fischer projection 菲舍尔投影式364. Kekule structure 凯库勒结构式365. Friedel-Crafts reaction 傅列德尔-克拉夫茨反应366. Ketone 酮367. carboxylic acid 羧酸368. carboxylic acid derivative 羧酸衍生物369. hydroboration 硼氢化反应370. bond oength 键长371. bond energy 键能372. bond angle 键角373. carbohydrate 碳水化合物374. carbocation 碳正离子375. carbanion 碳负离子376. alcohol 醇377. Gofmann rule 霍夫曼规则378. Aldehyde 醛379. Ether 醚380. Polymer 聚合物。
Synthesis
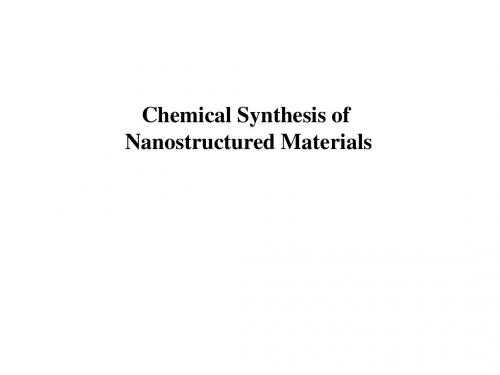
DLVO Theory
DVLO theory suggests that the stability of a colloidal system is determined by the sum of these van der Waals attractive (VA) and electrical double layer repulsive (VR) forces that exist between particles as they approach each other due to the Brownian motion they are undergoing. This theory proposes that an energy barrier resulting from the repulsive force prevents two particles approaching one another and adhering together. Therefore if the particles have a sufficiently high repulsion, the dispersion will resist flocculation and the colloidal system will be stable. However if a repulsion mechanism does not exist then flocculation or coagulation will eventually take place.
Sonochemistry
Role of ultrasonic radiation: a) Dissociation of chalcogens and formation of E2b) Prevention of aggregation Application: a) Deposition of nanoparticles on ceramic and polymeric surfaces b) Insertion of nanomaterials into mesoporous materials c) Formation of protein-micro- and nanospheres
211262186_Cu-MOF_材料的合成及其对有机小分子的荧光识别
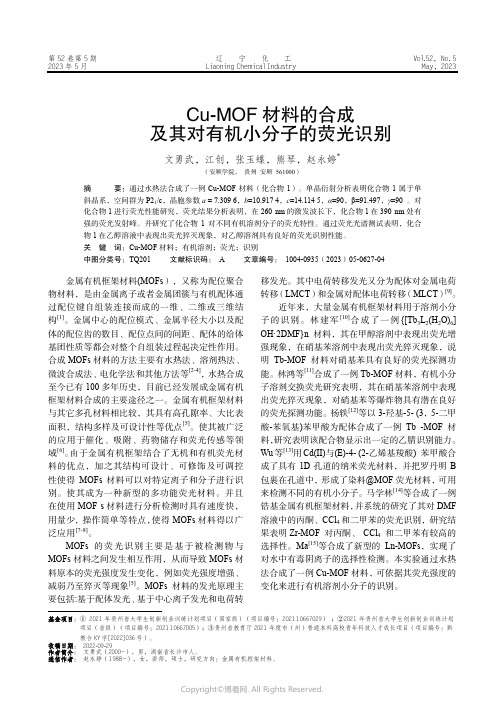
第52卷第5期 辽 宁 化 工 Vol.52,No. 5 2023年5月 Liaoning Chemical Industry May,2023基金项目:① 2021年贵州省大学生创新创业训练计划项目(国家级)(项目编号:202110667029) ;②2021年贵州省大学生创新创业训练计划 项目(省级)(项目编号:202110667005);③贵州省教育厅2021年度市(州)普通本科高校青年科技人才成长项目(项目编号:黔 教合KY 字[2022]036号)。
收稿日期: 2022-09-29Cu -MOF 材料的合成 及其对有机小分子的荧光识别文勇武,江创,张玉蝶,熊琴,赵永婷*(安顺学院, 贵州 安顺 561000)摘 要:通过水热法合成了一例Cu-MOF 材料(化合物1)。
单晶衍射分析表明化合物1属于单斜晶系,空间群为P21/c ,晶胞参数a = 7.309 6,b =10.917 4,c =14.114 5,α=90,β=91.497,γ=90 。
对化合物1进行荧光性能研究,荧光结果分析表明,在260 nm 的激发波长下,化合物1在390 nm 处有强的荧光发射峰。
并研究了化合物1对不同有机溶剂分子的荧光特性。
通过荧光光谱测试表明,化合物1在乙醇溶液中表现出荧光猝灭现象,对乙醇溶剂具有良好的荧光识别性能。
关 键 词:Cu-MOF 材料;有机溶剂;荧光;识别中图分类号:TQ201 文献标识码: A 文章编号: 1004-0935(2023)05-0627-04金属有机框架材料(MOFs ),又称为配位聚合物材料,是由金属离子或者金属团簇与有机配体通过配位键自组装连接而成的一维、二维或三维结 构[1]。
金属中心的配位模式、金属半径大小以及配体的配位齿的数目、配位点间的间距、配体的给体基团性质等都会对整个自组装过程起决定性作用。
合成MOFs 材料的方法主要有水热法、溶剂热法、微波合成法、电化学法和其他方法等[2-4],水热合成至今已有100多年历史,目前已经发展成金属有机框架材料合成的主要途径之一。
静电纺丝纳米纤维的制备工艺及其应用
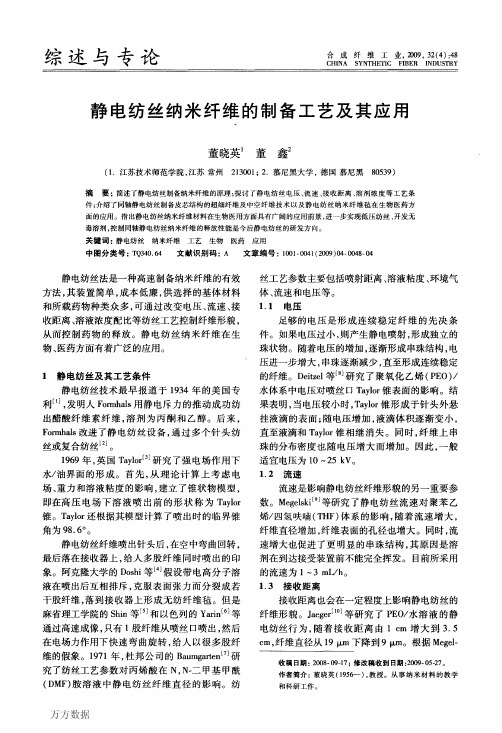
万方数据第4期董晓英等.静电纺丝纳米纤维的制备工艺及其应用49ski等∽o的实验,随着距离减小,聚苯乙烯纤维上串珠分布增多,其原因与流速增加相同,即溶剂在到达接受装置前不能完全挥发。
1.4溶液浓度静电纺丝需要适当的溶液浓度。
当溶液过稀时,溶液会从针头喷射,不能形成连续的纤维。
而当溶液浓度过大时,粘度过高,纺丝行为不稳定。
韩国的Lee等¨u研究了溶液浓度与串珠形貌的关系。
在电压为15kV,接收距离为12cnl的情况下,聚苯乙烯在1:1的THF/DMF溶液中进行静电纺丝,随着溶液质量分数从5%增加到15%,串珠逐渐变细,变长,直至消失。
康奈尔大学的Tan和Oberdorf¨21研究了不同浓度含5%氯化1,3.二氯-5,5.二甲基己内醯脲(DDMH)的尼龙6静电纺丝溶液粘度、电导率和纤维直径之间的关系。
随着溶液浓度增加,溶液粘度增大,电导率下降。
1.5溶剂挥发性静电纺丝溶液从针头喷出到达接收器的过程也是溶剂挥发的过程。
若溶剂挥发过快,则溶质易堵塞针头,影响纺丝的稳定性;若溶剂在到达接收器前不能完全挥发,则残留溶剂会溶蚀接收器上的纤维,进而破坏纤维形貌。
Megelski等一1研究了聚苯乙烯纤维在不同浓度的DMF和THF混合溶液中的静电纺丝行为。
两种极端情况下,在挥发性溶剂THF_中,纤维上小孔的分布密度最大,从而使纤维的比表面积增大20%~40%;而在低挥发性的DMF中,纤维表面趋于平滑。
2同轴静电纺丝单轴的静电纺丝既可以用一种材料的溶液纺出纤维¨3|,又可以对相容性体系的多种材料进行混纺¨4’15j。
但是,欲得到不互溶物间的理想静电纺丝材料,虽然人们通过乳液或悬浮液等分散的非均相体系也进行了一些尝试¨6’17o,但这些体系往往由于界面张力的不同而产生纤维内部分布的不均匀现象。
例如,美国纽约州立大学石溪分校的Kim等¨8J将亲水性药物头孢西丁钠负载于油性聚乙交酯.丙交酯(PLGA)基体中静电纺丝,结果药物在初期显示突释现象,引入亲水性链段PLGA/PLA/PEG.b.PLA后,突释现象只得到一定程度的抑制。
简单的溶剂热法制备分层的碲化铋微米结构
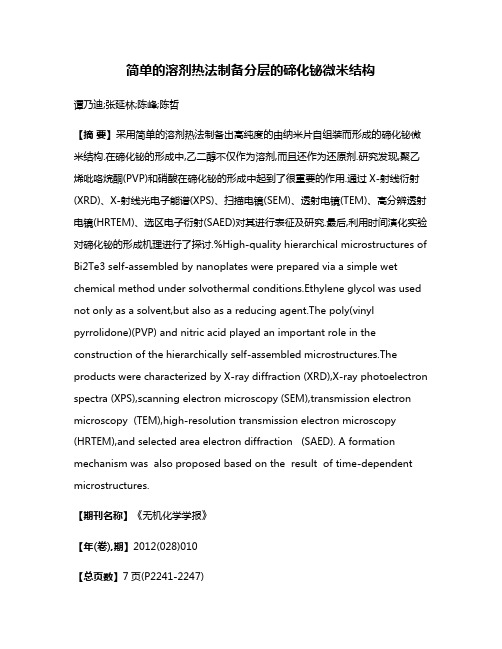
简单的溶剂热法制备分层的碲化铋微米结构谭乃迪;张延林;陈峰;陈哲【摘要】采用简单的溶剂热法制备出高纯度的由纳米片自组装而形成的碲化铋微米结构.在碲化铋的形成中,乙二醇不仅作为溶剂,而且还作为还原剂.研究发现,聚乙烯吡咯烷酮(PVP)和硝酸在碲化铋的形成中起到了很重要的作用.通过X-射线衍射(XRD)、X-射线光电子能谱(XPS)、扫描电镜(SEM)、透射电镜(TEM)、高分辨透射电镜(HRTEM)、选区电子衍射(SAED)对其进行表征及研究.最后,利用时间演化实验对碲化铋的形成机理进行了探讨.%High-quality hierarchical microstructures of Bi2Te3 self-assembled by nanoplates were prepared via a simple wet chemical method under solvothermal conditions.Ethylene glycol was used not only as a solvent,but also as a reducing agent.The poly(vinyl pyrrolidone)(PVP) and nitric acid played an important role in the construction of the hierarchically self-assembled microstructures.The products were characterized by X-ray diffraction (XRD),X-ray photoelectron spectra (XPS),scanning electron microscopy (SEM),transmission electron microscopy (TEM),high-resolution transmission electron microscopy (HRTEM),and selected area electron diffraction (SAED). A formation mechanism was also proposed based on the result of time-dependent microstructures.【期刊名称】《无机化学学报》【年(卷),期】2012(028)010【总页数】7页(P2241-2247)【关键词】碲化铋;分层微米结构;聚乙烯吡咯烷酮;硝酸【作者】谭乃迪;张延林;陈峰;陈哲【作者单位】吉林化工学院,吉林132022;东北电力大学,吉林 132012;吉林石化公司有机合成厂,吉林 132021;吉林化工学院,吉林132022【正文语种】中文【中图分类】O612.5In the past few decades,synthesis of micro-and nano-scale inorganic materials have attracted efforts to develop the materials because of their outstanding properties and potential applications correlated with the morphology ofthe nanomaterials[1-6].Specifically,hierarchical architecture via self-assembly has become an intensive and hot research topic because of their unique physical and chemical properties and potential applications in optics,electronics,medicine,ceramics,catalysis,and energy/chemical conversions,and their important roles in the systematic research of structureproperty relationships in recently years[7-11].Thus,three-dimensional(3D)hierarchical architectures assembled by nanostructured building blocks such as nanoplates,nanoparticles, nanoribbons, and nanorods, were extensively investigated[12-16].Up to now,a wide variety of inorganic materials,including metal oxides,sulfides,hydrates,and other minerals,have been prepared with hierarchical structures.Forinstance,Zhu,et al.[17]reported the synthesis of hierarchical AlOOH nanostructured microspheresbymicrowave-assisted solvothermalrge-scale CeO2hierarchical architectures composed of well-aligned nanorods were prepared through hydrothermal method controllably by Chen and co-workers[18].Up to the present,much advancehasbeen made,butthefabrication of hierarchical 3D architecture is still a big challenge.The binary chalcogenides of AV2BVI3(A=As,Sb,Bi;B=S,Se,Te)are a class of important semiconductor materials having applications in thermoelectric,optoelectronic devices,IRspectroscopy,paints,photoemitting diodes,and microwave switches,etc[19-21].Bismuth telluride is one of the best thermoelectric materials because they are available for thermoelectric application near room temperature.They are widely used in thermopiles, thermal sensors, and thermoelectric cooler for laser diodes[22-24].In this regard,much progress has been made in the synthesis of Bi2Te3multifarious morphology,such as Bi2Te3intermetallic compounds with hierarchical nanostructures synthesized via an electrochemical deposition route[25],Bi2Te3plate-like crystals with homogeneous hexagonal morphology synthesized using a microwave assisted wet chemical method[26],and Bi2Te3nanoparticles and nanowiresprepared bysolvothermalmethod with NaBH4as thereductant[27].Great progress has been achieved in the synthesis approach,however,most of the above investigations also use additional inorganic reducing agents,for example,NaBH4,N2H4,and soon.Thusdeveloping convenientand fastersynthetic process using inexpensive,environmentally benignant reagents at lower temperature arestill important themes.Herein,we report a solvothermal process to prepare well-definedBi2Te3nanostructures selfassembled through nanoplates using Bi(NO)3and Te powder as precursors,and poly(vinyl pyrrolidone)(PVP)as a soft template in ethylene glycol(EG).All chemicals were used as receieved without furtherpurification.Bi(NO)3·5H2O and PVP(K90)were purchased from Sinopharm Chemical Reagent Co.Ltd.;Te powder was from Jingchun Chemical Reagents Company;Ethylene glycol was from Tianjin Guangfu Chemical Reagents Company;HNO3(85wt%solution)was from Beijing Chemical Reagents Company.Bi2Te3with different nanostructures were prepared by a simple solvothermal method in the presence of PVP.In a typical synthesis,3 mmol·L-1Bi(NO)3·5H2O and 9 mmol·L-1PVP (K90)were dissolved in 60 mL ethyle ne glycol.Then,4.5 mmol·L-1Te powder,and an appropriate quantity of HNO3aqueous solution(5 mol·L-1)were added into the above solution and transferred into an 80 mL Teflon-lined stainless steel autoclave under stirring.The autoclave was maintained at 180 ℃f or 48 h and then cooled to room temperature in air.The resulting solid product was collected and washed with deionized water and ethanol,and finally dried at room temperature for 12 h.The resulting samples were characterized by Rigaku D/Max2500PC X-ray po wder diffraction(XRD)equipped with graphitemonochromatizedCu Kα radiation(λ=0.154 18 nm)under the following conditions:30 kV asaccelerating voltage;100 mA as emission current;and the 2θ range of 5°~90°at a scan rate of 4°·min-1.The morphology and dimension of samples were observed by field emission scanning electron microscopy (FE-SEM,JEOL 7500B)and transmission electron microscopy (TEM,H-800).The microstructure of samples was determined using highresolution transmission electron microscopy(HRTEM)on a JEM-2010 apparatus with an acceleration voltage of 200 kV.X-ray photoelectonspectroscopy(XPS,ESCALAB 250)was used to confirm the oxidation stateof iron.A typical powder X-ray diffraction (XRD)pattern of the as-synthesized powder formed via templateassisted solvothermal synthesis is shown in Fig.1.All of the diffraction peaks can be readily indexed to pure rhombohedral Bi2Te3crystal(PDF No.15-0863).No impurities such asBi2O3and others,which often appear in the Bi2Te3product synthesized by traditional routes,are observed.Furthermore,all diffraction peaks are strong and narrow,indicating the high crystallinity of the Bi2Te3sample.The X-ray photoelectron spectroscopy(XPS)of the as-prepared sample obtained in the presence of PVP at 180℃was measured to examine the composition of the surface.The wide XPS spectrum in Fig.2a shows that no obvious impurities are detected in the XPS survey spectrum of Bi2Te3.It should be noted that only ethylene glycol solvent was used and no other reducing agent was introduced in our system.To prove whether or not ethylene glycol could completely reduce the Te powder to Te2-,XPS was also consulted to unambiguously assign the crystal phase.Fig.2b presentsthe XPS spectrum of Bi and Te element on the surface of the sample.The two strong peaks at 572.0 eV and 582.3 eV can be attributed to the bonding energies of Te3d5/2.The peaks at 575.7 eV and 582.3 eV can be assigned to the bonding energy of Bi3d3/2transition,which perfectly match with the previously published spectra of Bi2Te3[28].The composition of the formed Bi2Te3is determined by EDX analysis(Fig.2c)attached to a SEM instrument.The EDS analysis microanalysis clearly demonstrates that only Bi and Te element in the sample with an atomic ratio of Bi/Te is1∶1.51,which is consistent with stoichiometric ratio of Bi2Te3.No other impurities are detected in the EDX analysis,such as C,H,and O,indicating the complete disappearance of the surfactants (PVP)during the washing with deionized water and ethanol later process.Si and Au in the EDS spectrum (Fig.2c)originate from the silicon wafer to support and a thin Au layer sputtered on the sample,respectively.Thus,the XRD,XPS,and EDX spectrum analysis further confirm the high purity forBi2Te3sample,respectively.The morphology and size of the as-prepared Bi2Te3sample were characterized by FE-SEM and TEM.A panoramic SEM (Fig.3a)image demonstrates that the product displays sawtooth-like pattern.No other morphologies are observed,indicating a high yield of this sample.Asshown bythehighermagnification SEM image in Fig.3b,these sawtoothlike samples are composed of uniform nanoplates with diameters of 30~40 nm.The representative TEM images of the Bi2Te3samples obtained at 180℃are shown in Fig.3c and Fig.3d.As indicated by the figures,the as-preparedBi2Te3samples are consisted of sawtooth rods with diameters of 30~40 nm.Closer observations are shown in Fig.3d,the individual sawtooth rod has an average diameter of 35±10 nm and length of 1 μm,in good accordance with the SEM images.Further structural characterizations for the Bi2Te3sampleswerecarried outbySAED and HRTEM (Fig.3e).A selected area electron diffraction(SAED)pattern (inset in Fig.3e)taken from the edge of sawtooth rods can be indexed as a pure crystalline feature.Fig.3e shows a high-resolution TEM(HRTEM)image taken on individualnanoplate,revealing that each nanoplate is ploy-crystalline and the clear crystal lattices with d-spacing of 0.345 nm,which is nearly consistent with the(003)reection plane spacing of the rhombohedral Bi2Te3phase.Thus,all the abovementioned results clearly demonstrate the successful fabrication of the title material.The current synthesis strategy provides powerful means to tailor the composition,purity,and assembly of Bi2Te3microstructures as a function ofreaction parameters such as concentration of reagents,molar ratio of nitric acid(HNO3),and addition of PVP.Firstly,to study the influences of nitric acid on the morphology of as-synthesized Bi2Te3,controllable experiments were performed by changing reaction parameters.The nitric acid is essential for the development of the sawtooth rod.Fig.4 shows the TEM image of the sample synthesized in the same experimental condition except the volume of nitric acid.Perfect rose-like microflowers with diameters of 1 ~1.5 μm are formed witho ut the addition of nitricacid(Fig.4a).When 2 mL of the above nitric acid aqueous solution is used,itcan be clearly seen that the products are comprised of a large number of irregular and nonuniform plates with different sizes and a little of sawtooth rod,as shown in Fig.4b.With the the nitric acid volume added (3 mL)is increased,the perfect 3D flowerlike nanostructures with well-arranged nanoplates are obtained (Fig.4c).Secondly,Fig.5 shows the influence of PVP on the assembly behavior of Bi2Te3.The irregular and nonuniform nanoplates with a small size are formed without the used of PVP as shown in Fig.5a.When the PVP usage is increased to 3 mmol·L-1(Fig.5b),both irregular nanoplates and sawtooth rods are obtained.Fig.5c further exhibits the morphologies o f the products when 9 mmol·L-1PVP is used,namely the optimum synthesized condition,and a great deal of well-defined sawtooth-like nanostructures self-assembled by nanoplates are observed.Further increase in the concentration of PVP (27 mmol·L-1)leads to the formation of sawtooth-like microstructures with large length and width.Thus,these observations clearly show that the concentration of PVP in the system also has a great effect on the morphology of the products(Fig.5d).Finally,the concentration of reactants is another important influence factor on the morphology of Bi2Te3sample.Fig.6 presents TEM imagesofBi2Te3nanostructures obtained with different concentrations of reactants.When 5 mmol·L-1of Bi(NO3)3is used,as shown in Fig.6a,a few of nanorods with different diametersare found in the product.When the concentration of initial reagents is increased from 0.5 mmol·L-1to 1 mmol·L-1and other reaction parameters are similar to the product shown in Fig.6b,lots of welldefined dispersed sawtooth-like microstructures areobtained.If only 3 mmol·L-1of Bi(NO3)3is used,the resulting sample has irregular morphology(Fig.6c).According to the above results,we can conclude that the sawtooth-like microstructures strongly depend on the concentrations of Bi(NO3)3and PVP,molar ratio of nitric acid,respectively. To further confirm the formation mechanism of these sawtooth-like microstructures,time-dependent experiments were performed.With a short reaction time (12 h,Fig.7a),the resulted sample are consisted of a great deal of irregular nanoplates and minor amount of nanorods.When the reaction time is up to 24 h,the amount of sawtooth-like morphology are enhanced and nanoplates are decreased comparing with Fig.7a (see Fig.7b).With increasing the reaction time to 36 h,sawtooth rods with different sizes are increased (Fig.7c).It is worth pointing out that nanoplates are far smaller than the one prepared through 24 h.After 36 h of reaction time,nanoplates disappeare, and 3D hierarchical sawtooth-like microstructures with a diameter of 35 nm are obtained(Fig.7d).Although the exactmechanism forthe formation of the sawtooth rods microstructures now is not clear,on the basis of the experimental results,a possible interpretation for the formation process of the sawtooth-like structure could be given as follows:at the initial stage of reaction,Te powder may be dissolved in nitric acid[29],and Te2-is produced from the reduction of Te powder by the EG.Then,tiny Bi2Te3crystal nuclei are formed between Bi3+and Te2-through homogeneous nucleation.Further growth of thesecrystalnucleiresultsin the formation of nanorodsand nanoplates because oftheirhigh activity.The initially formed nanoplatesbegin to assemble in face-to-face growth style and nanorods are located at the center,owning to the interaction with PVP.With time goingon,perfect 3D hierarchical sawtooth-like microstructures are formed.In summary,high purity Bi2Te3material with hierarchical microstructure self-assembled by nanoplates has been synthesized in mild ethylene glycol hydrothermal system. In the synthesis process,inorganic metal salts are used as raw materials,avoiding from the use of the complicated organic reactions and toxic reagents.Surfactant PVP is introduced as a template forthe formation ofBi2Te3hierarchical nanostructure.A formation mechanism is suggested on the basis of the time-dependent experimental results.【相关文献】[1]Yang H G,Zeng H C.Angew.Chem.,Int.Ed.,2004,43:5930-5933[2]Shen G Z,Chen D.J.Am.Chem.Soc.,2006,128:11762-11763[3]Gao P X,Wang Z L.J.Am.Chem.Soc.,2003,125:11299-11305[4]Hu J,Bando Y,Golberg D,et al.Adv.Mater.,2005,17:1964-1969[5]Shi H T,Qi L M,Ma J M.J.Am.Chem.Soc.,2003,125:3450-3451[6]Cao M H,Liu T F,Gao S.Angew.Chem.,Int.Ed.,2005,44:4197-4201[7]Yin Y D,Alivisatos A P.Nature,2005,437:664-670[8]Wang D L,Lieber C M.Nat.Mater.,2003,2:355-356[9]Wang Z L.J.Mater.Chem.,2005,15:1021-1024[10]Burda C,Chen X,El-Sayed M A,et al.Chem.Rev.,2001,105:1025-1102[11]Xia Y N,Yang P D,Sun Y G.Adv.Mater.,2003,15:353-389[12]Rhodes K H,Davis S A,Mann S,et al.Chem.Mater.,2000,12:2832-2834[13]Kim F,Kwan S,Yang P,et al.J.Am.Chem.Soc.,2001,123:4360-4361[14]Fan X,Meng X M,Lee S T,et al.Angew.Chem.,Int.Ed.,2006,45:2568-2571[15]Wu J,Duan F,Xie Y,et al.J.Phys.Chem.C,2007,111:12866-12871[16]Zhang X,Ai Z,Jia F,et al.J.Phys.Chem.C,2008,112:747-753[17]Zhang L,Zhu Y J.J.Phys.Chem.C,2008,112:16764-16768[18]Yu R B,Yan L,Zheng P,et al.J.Phys.Chem.C,2008,112:19896-19900[19]Arivouli D,Gnanam F D,Ramasamy P.J,Mater.Sci.Lett.,1988,7:711-713[20]Case T W.Phys.Rev.,1917,9:305-310[21]Tang Q,Zhou W I,Jiang K.Cryst.Growth Des.,2005,5:147-150[22]Harman T C,Taylor P J,Walsh M P,et al.Science,2002,297:2229-2232[23]Chen G,Dresselhaus M S,Dresselhauss G,et al.Int.Mater.Rev.,2003,48:45-66[24]Harman T C,Taylor P J,Spears D L,et al.J.Electron.Mater.,2000,29:L1-L4[25]Li G R,Zheng F L,Tong Y X.Gryst.Growth Des.,2008,8:1226-1232[26]Fan X A,Yang J Y,Xie Z.J.Phys.D:Appl.Phys.,2007,40:5975-5979[27]Zhao X B,Ji X H,Zhang Y H,et al.J.Alloys Comp.,2004,368:349-352[28]Kim S H,Kim J J,Suh S W.J.Ind.Eng.Chem.,2010,16:741-747[29]Marshall H.Tellurium(Ⅳ)Oxide:Tellurium Dioxide in Harold Simmons Booth eds.Inorganic Syntheses:Vol.1,2007:143-145,PublishedOnline.DOI:10.1002/9780470132326。
新型碳气凝胶的制备及表征

新型碳气凝胶的制备及表征何蕊;刘振法【摘要】以氨水作为间苯二酚和甲醛反应的催化剂,经溶胶-凝胶制备有机气凝胶,再经过常温常压干燥、高温碳化形成碳气凝胶.采用X射线衍射、比表面仪、扫描电镜能谱分析仪对样品进行表征.结果表明:以氨水为催化剂所得碳气凝胶比表面积在900m2/g左右,呈现连续颗粒状.%Carbon aerogels are prepared by sol-gel process via reaction of resorcinol and formaldehyde with ammonia water as catalyst and afterward ambient drying followed by carbonization. The structure of products is characterized by X-ray diffraction, gas physisorption, scanning electron microscopy and energy spectrum analysis. Results indicte that the carbon aerogels with ammonia as catalyst show a coarser surface, and its specific surface area is about 900 m2/g, presenting continuous granular.【期刊名称】《河北科技大学学报》【年(卷),期】2013(034)001【总页数】4页(P26-29)【关键词】碳气凝胶;催化剂;氨水【作者】何蕊;刘振法【作者单位】河北省科学院能源研究所,河北石家庄050081;河北省科学院能源研究所,河北石家庄050081【正文语种】中文【中图分类】O648碳气凝胶是一种由高聚物分子构成的多空非晶凝聚态材料,可以用在力学、热学、光学及声学等方面,具有独特的性能和用途。
生物大分子功能化上转换纳米探针的制备及其成像研究
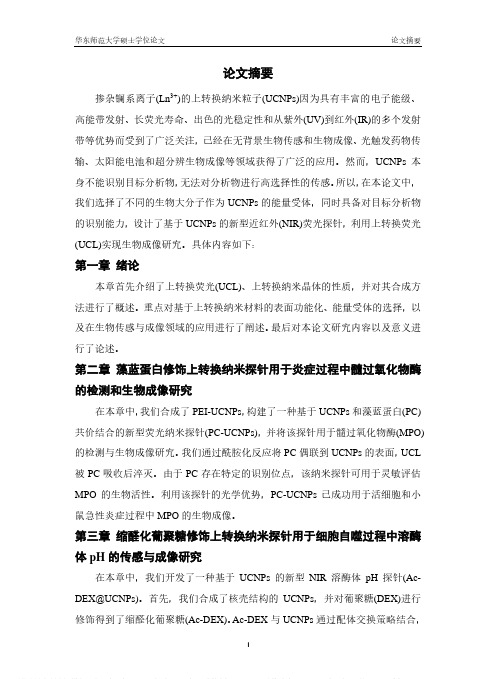
论文摘要掺杂镧系离子(Ln3+)的上转换纳米粒子(UCNPs)因为具有丰富的电子能级、高能带发射、长荧光寿命、出色的光稳定性和从紫外(UV)到红外(IR)的多个发射带等优势而受到了广泛关注,已经在无背景生物传感和生物成像、光触发药物传输、太阳能电池和超分辨生物成像等领域获得了广泛的应用。
然而,UCNPs本身不能识别目标分析物,无法对分析物进行高选择性的传感。
所以,在本论文中,我们选择了不同的生物大分子作为UCNPs的能量受体,同时具备对目标分析物的识别能力,设计了基于UCNPs的新型近红外(NIR)荧光探针,利用上转换荧光(UCL)实现生物成像研究。
具体内容如下:第一章绪论本章首先介绍了上转换荧光(UCL)、上转换纳米晶体的性质,并对其合成方法进行了概述。
重点对基于上转换纳米材料的表面功能化、能量受体的选择,以及在生物传感与成像领域的应用进行了阐述。
最后对本论文研究内容以及意义进行了论述。
第二章藻蓝蛋白修饰上转换纳米探针用于炎症过程中髓过氧化物酶的检测和生物成像研究在本章中,我们合成了PEI-UCNPs,构建了一种基于UCNPs和藻蓝蛋白(PC)共价结合的新型荧光纳米探针(PC-UCNPs),并将该探针用于髓过氧化物酶(MPO)的检测与生物成像研究。
我们通过酰胺化反应将PC偶联到UCNPs的表面,UCL 被PC吸收后淬灭。
由于PC存在特定的识别位点,该纳米探针可用于灵敏评估MPO的生物活性。
利用该探针的光学优势,PC-UCNPs已成功用于活细胞和小鼠急性炎症过程中MPO的生物成像。
第三章缩醛化葡聚糖修饰上转换纳米探针用于细胞自噬过程中溶酶体pH的传感与成像研究在本章中,我们开发了一种基于UCNPs的新型NIR溶酶体pH探针(Ac-DEX@UCNPs)。
首先,我们合成了核壳结构的UCNPs,并对葡聚糖(DEX)进行修饰得到了缩醛化葡聚糖(Ac-DEX)。
Ac-DEX与UCNPs通过配体交换策略结合,基于聚集诱导淬灭效应(ACQ),Ac-DEX@UCNPs的UCL淬灭。
硫化锑纳米材料的合成及其应用
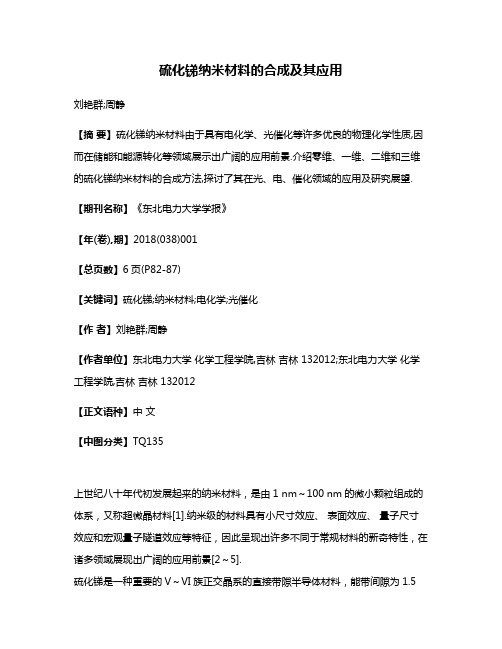
硫化锑纳米材料的合成及其应用刘艳群;周静【摘要】硫化锑纳米材料由于具有电化学、光催化等许多优良的物理化学性质,因而在储能和能源转化等领域展示出广阔的应用前景.介绍零维、一维、二维和三维的硫化锑纳米材料的合成方法,探讨了其在光、电、催化领域的应用及研究展望.【期刊名称】《东北电力大学学报》【年(卷),期】2018(038)001【总页数】6页(P82-87)【关键词】硫化锑;纳米材料;电化学;光催化【作者】刘艳群;周静【作者单位】东北电力大学化学工程学院,吉林吉林 132012;东北电力大学化学工程学院,吉林吉林 132012【正文语种】中文【中图分类】TQ135上世纪八十年代初发展起来的纳米材料,是由1 nm~100 nm的微小颗粒组成的体系,又称超微晶材料[1].纳米级的材料具有小尺寸效应、表面效应、量子尺寸效应和宏观量子隧道效应等特征,因此呈现出许多不同于常规材料的新奇特性,在诸多领域展现出广阔的应用前景[2~5].硫化锑是一种重要的V~VI族正交晶系的直接带隙半导体材料,能带间隙为1.5eV~2.2 eV[6~8],具有良好的光导性、光敏性和极高的热电性能[9].目前研究者们采用了不同方法制备出不同形貌的Sb2S3纳米材料,并探索其在不同领域的性能应用[10~12].本文论述了不同维度Sb2S3纳米材料的合成及其相关应用研究.1 不同形貌Sb2S3纳米材料根据纳米尺度的维数,可以将纳米材料划分为:零维(原子团簇和超微粒子等)、一维(纳米管、线等)、二维(纳米薄膜、多层膜等)以及三维材料(原子团簇及超微粒子的组成体等).目前国内外研究报道了零维、一维、二维以及三维纳米Sb2S3纳米材料的合成方法,典型形貌如图1所示.图1 不同维度Sb2S3纳米材料SEM图 (a)零维球状[13](b)一维管状[15](c)一维线状[16](d)二维带状[22](e)三维橄榄状[27](f)三维树枝状[28]1.1 零维Sb2S3纳米材料纳米颗粒是零维纳米材料的典型代表,一般为球形或类球形,其粒径分布窄、分散性良好.Senthil等[13]以C2H4N2S2为硫源,氯化锑为锑源,在柠檬酸以及PVP(聚乙烯吡咯烷酮)的共同作用下,在不同温度下采用水热和溶剂热法合成出Sb2S3纳米球,并通过控制温度,使产物形貌实现从零维到一维的转变.Kai等[14]以硫脲和氯化锑为原料,PVP为表面活性剂,通过溶剂热法制备出零维Sb2S3纳米颗粒,并负载于石墨烯上形成G-Sb2S3复合材料,将其用于降解罗丹明B,高的光催化降解活性及循环稳定性表明,复合材料具有优于单一材料的光催化性能. 1.2 一维Sb2S3纳米材料一维纳米材料在介观物理以及纳米器件制造领域独特的应用潜力,一直是材料界的研究热点.Xu等[15]以EDTA为表面活性剂,合成出表面光滑,壁厚为250 nm,内直径约为300 nm的纳米管,其禁带宽度约为1.55 eV,接近光电转化的最优值,在光电子和太阳能等领域有广泛的应用.实验结果表明,在不添加EDTA的条件下,无法合成出硫化锑管状形貌.Bao等[16]以酒石酸锑钾为原料,在添加3 g有机物(柠檬酸)条件下,成功合成出宽60 nm~200 nm,长约10 μm的Sb2S3纳米线,并且正交晶相的Sb2S3纳米线沿[001]方向生长.刘运等[17]以硫化钠和氯化锑为原料,添加尿素为矿化剂,水热法获得了直径约100 nm~250 nm,长约100 μm的Sb2S3纳米线,并通过调节反应时间,可控合成出不同长径比的Sb2S3纳米线.Jiang等[18]以离子液体[BMIM][BF4]作为反应介质,在微波辐射下合成出单晶Sb2S3纳米棒.随后,雍高兵等[19]利用回流法成功制备了直径约为70 nm~180 nm,长度为2 μm~5 μm的正交晶系Sb2S3单晶纳米棒.近几年内,研究者尝试以PVP作为表面活性剂,用来合成新型的自组装纳米材料,Kavinchen等[20]以氯化锑和硫代乙酰胺为原料,添加不同质量的PVP作为表面活性剂,通过微波法合成出沿[001]方向生长的哑铃状Sb2S3纳米棒. Zhou等[21]以PVP作为表面活性剂成功合成出Sb2S3纳米线束.同年吴松等[22]同样以PVP为表面活性剂,在乙二醇体系中以硫脲为硫源,在常压条件下回流合成出了的Sb2S3纳米棒束,单根纳米棒直径约300 nm~400 nm,长约5 μm~10 μm.1.3 二维Sb2S3纳米材料具有原子级别厚度的二维纳米材料,兼具良好电子传输能力和较大的比表面积,在许多领域内展示出优异的性能.2005年Yu等[23]以氯化锑为锑源,硫代硫酸钠为硫源,在不使用任何模板和表面活性剂的条件下,水热法合成出长几十微米到几毫米,厚65 nm,宽350 μm~400 μm的超长Sb2S3纳米带.2009年,Xiang等[24]更换新颖的硫源,在L-半胱氨酸协助下,氯化锑为锑源,在乙二醇和水的混合液中成功合成出截面宽约100 nm~300 nm的Sb2S3纳米带.随后陈广义等[25]以CTAB做表面活性剂,去离子水为溶剂,酒石酸锑钾和硫代乙酰胺为原料,成功制备了宽度约1 μm,厚约100 mm,并沿[001]晶面方向生长的高长径比Sb2S3纳米带.1.4 三维Sb2S3纳米材料三维形貌主要是由零维、一维和二维纳米结构通过自组装而成的有规则的纳米材料,由于具有大的比表面积、优异的电子传输能力、良好的力学性能、热传导性能以及稳定的多孔结构而受到广泛关注.2004年,Qian等[26]采用不同溶剂、不同硫源,在不使用任何表面活性剂的条件下,通过溶剂热法合成出羽毛状、放射性枝晶状、棱镜球体、圆盘形等新颖形貌的Sb2S3微晶.Han等[27]以氯化锑和硫化钠做原料,在酸性条件下水热合成出由800 nm×400 nm×100 nm 块状结构自组装而成的空心橄榄形Sb2S3微晶;随后该课题组[28]通过改变硫源,以柠檬酸和硫代乙酰胺为硫源,合成出由宽约300 nm~500 nm,长约5 μm~20 μm纳米棒组成的树枝状的Sb2S3.而叶明富等[29]以氯化锑和硫脲为原料,采用微波法,也成功制备出长约10 μm的树枝状Sb2S3纳米材料.由于表面活性剂具有固定的亲水亲油基团,在溶液的表面能定向排列,因此可有效地控制纳米颗粒的形态和结构.目前通过添加不同表面活性剂制备出不同形貌的Sb2S3纳米材料.Debao等[30]以酒石酸锑钾和硫代乙酰胺为原料,CTAB做表面活性剂,讨论了不同反应时间,不同反应温度对形貌的影响,并成功制备了长约30 μm~50 μm,直径约120 nm~500 nm的稻草束状Sb2S3.Chen等[31]酒石酸锑钾和硫代乙酰胺为原料,添加PVP作为表面活性剂,通过改变实验条件的参数,调节产物形貌,成功合成出片层分裂的Sb2S3纳米结构.Chen等[32]以酒石酸锑钾为锑源,硫粉为硫源,通过控制PVP的浓度,在双表面活性剂PVP和葡萄糖共同作用下,合成出长约10 μm~20 μm,大小均一的双束状Sb2S3纳米结构.结果表明,通过控制PVP的浓度,可以很容易地调节纳米棒的大小,并且加入的葡萄糖会对最终产品的形貌产生直接影响.Cheung等[33]以氯化锑和硫脲为原料,PEG-400为表面活性剂,溶剂热法制备出直径约(195±52) nm的纳米线自组装的蒲公英状Sb2S3,纳米线的禁带宽度约为1.67 eV,并初步推测了纳米线蒲公英的生长机理为晶体分裂所致.Lei等[34]以氯化锑和九水硫化钠为原料,酸性条件下加入DTAB成功合成出双花形的Sb2S3微晶.双花形的Sb2S3具有宽的广谱响应范围,在太阳能利用和光电转换等领域具有潜在的应用前景.随着人们对纳米材料的不断认识研究,发现纳米粒子的尺寸、形貌、维数等因素不同程度影响着其在光学、电学、磁学等领域的性能应用,因而不同形貌Sb2S3纳米材料的可控合成一直是研究者关注的重点问题之一.2 硫化锑纳米材料的应用目前,Sb2S3纳米材料在储能、传感器、光伏器件、光催化剂等领域有许多的应用.2.1 储能领域应用Sb2S3材料具有高的理论比容量以及优良的循环性能,可将其用作钠、锂离子电池的负极.Park等[35]在碳基质上合成出无定形Sb2S3复合材料,研究发现此材料具有较高的放电容量为757 mAh·g-1,100次循环后比容量仍大于600 mAh·g-1.随后Xiao等[36]通过化学方法成功制备Sb2S3纳米棒,并将其作为锂离子电池的电极材料,第一次放电容量高达850 mAh·g -1,但在嵌/放锂过程中因为巨大的体积变化导致材料在循环过程中容量很快衰减,而石墨烯的加入可以缓冲由充放电引起的体积变化.Prikhodchenko等[37]用热处理转换法,在辉锑矿上涂层还原石墨烯,首次成功制备硫化锑/石墨烯复合新材料,并将其作为锂电池负极.在电流密度为250 mA·g-1的条件下,50次循环后,充电容量高于720 mAh·g-1,具有较高的电化学吸放锂容量和良好的循环特性.2.2 传感器领域应用Sb2S3也可以应用于检测电化学活性物质的传感材料.Tao等[38]通过使用聚丙烯酸(PAA)作为形态控制试剂,溶剂热法合成出树状Sb2S3微晶,研究发现作为多巴胺(DA)电氧化的传感材料,Sb2S3微晶具有较低的检测限、宽的线性响应范围和良好的选择性,突显了Sb2S3微晶作为DA传感的潜在电化学性能.目前很少有报道涉及Sb2S3的电活性分子检测,对这一领域的研究将有利于进一步开发Sb2S3材料的应用范围.2.3 光伏器件领域应用由于Sb2S3具有较高的吸收系数(在450 nm处为1.8 × 105 cm -1),易捕获可见光光子,非常适合做光电器件敏化剂.Cardoso等[39]成功将Sb2S3沉积在TiO2纳米线阵列薄膜上,合成出低成本纳米结构薄膜光伏,然后将聚3-己基噻吩(P3HT)插入其中,为光吸收提供了大的表面积,可以进一步促进电荷分离和运输,增强光吸收.研究发现,AM 1.5 G光电转换效率可达到4.5%.2.4 光催化领域应用此外,锑硫化物因带隙较窄,在能量较低的可见光下即可被激发,能很好地利用太阳光来进行催化降解有机污染物,并且催化效率高,成本低,是一种具有广泛应用前景的可见光催化剂.Sun等[40]采用简单的湿化学法成功合成纳米棒,首次降解甲基橙(MO),可见光照射30分钟后,MO的光降解率可达97%.Zhang等[41]采用水热溶剂热法制备出线状Sb2S3纳米材料,并将其作为光催化剂用于降解MO染料,2.5 h后MO的降解率达75%.Kai Jiang等[14]将合成的Sb2S3/G复合物用于可见光下降解罗丹明B(RhB),实验结果表明,单一的Sb2S3催化降解率为35%,而Sb2S3/G 复合材料表现出较高的光催化降解活性,降解率达到93%.随后,Lu等[42]采用溶剂热法制备Sb2S3/G复合物,在H2O2存在条件下,将制得的Sb2S3/G复合物用以降解亚甲基蓝,测试结果表明,Sb2S3/G复合物催化亚甲基蓝的降解率高达98%.Sb2S3作为半导体功能材料,因其带隙能接近光伏转换的最佳值,具有独特的光学和光电子特性,可作为光催化剂、电池负极、光电导探测器、离子导体材料、太阳能转换等材料[43~46],因此开发性能优异的Sb2S3微纳米材料具有更迫切的研究意义.3 结语及展望综上所述,人们在Sb2S3纳米材料的合成及性能研究方面做了许多工作,可以在微观层面上对Sb2S3微纳米材料的结构、形貌、粒径和组成等方面进行调控.在未来的工作中,设计如何通过简易方法有效制备及控制微纳米材料的形貌,努力合成具有优良性能的Sb2S3纳米材料,探究结构和形貌等因素与材料性能之间的密切关系仍是研究的重点.参考文献[1] 孟弘.纳米材料制备研究进展[J].矿产保护与利用,2003 (4):14-18.[2] 张立德.纳米材料和纳米结构[J].中国科学院院刊,2001,16(6):444-445.[3] P.Rajeshwari,T.K.Dey.Novel HDPE nanocomposites containing aluminum nitride (nano) particles:micro-structural and nano-mechanical properties correlation[J].Materials Chemistry & Physics,2017,190:175-186.[4] D.An,X.Tong,J.Liu,et al.Template-free hydrothermal synthesis of ZnO micro/nano-materials and their application in acetone sensing properties[J].Superlattices & Microstructures,2015,77:1-11.[5] 周静,郑彩虹.微纳米Sb2O3的制备与生长机理研究进展[J].东北电力大学学报,2016,36(3):60-68.[6] Y.C.Choi,D.U.Lee,J.H.Noh,et al.Highly improved Sb2S3 sensitized-inorganic-organic heterojunction solar cells and quantification of traps by deep-level transient spectroscopy[J].Advanced Functional Materials,2014,24(23):3587-3592.[7] 刘峰,逯亚飞,叶明富,等.硫化锑纳米材料制备研究进展[J].上海化工,2014,39(11):27-30.[8] X.Zheng,Y.Xie,L.Zhu,et al.Growth of Sb(2)E(3) (E=S,Se) polygonal tubular crystals via a novel solvent-relief-self-seeding process[J].Inorganic Chemistry,2002,33(15):455-461.[9] P.Arun,A.G.Vedeshwar.Phase modification by instantaneous heat treatment of Sb2S3 films and their potential for photothermal optical recording[J].Journal of Applied Physics,1996,79(8):4029-4036.[10] M.Kriisa,M.Krunks,I.O.Acik,et al.The effect of tartaric acid in the deposition of Sb2S3,films by chemical spray pyrolysis[J].Materials Science in Semiconductor Processing,2015,40(1):867-872.[11] H.Zhang,L.Song,L.Luo,et al.TiO2/Sb2S3/P3HT based inorganic-organic hybrid heterojunction solar cells with enhanced photoelectric conversion performance[J].Journal of Electronic Materials,2017,46 (7):4670-4675.[12] J.C.Cardoso,C.A.Grimes,X.Feng,et al.Fabrication of coaxial TiO/Sb2S3 nanowire hybrids for efficient nanostructured organic-inorganicthin film photovoltaics[J].Chemical Communications,2012,48(22):2818-2820.[13] T.S.Senthil,X.Yu.Study of various Sb2S3 nanostructures synthesizedby simple solvothermaland hydrothermal methods[J].Materials Characterization,2014,95(3):164-170.[14] J.Kai.J.Chang,D.Wu,et al.Solvothermal synthesis of graphene-Sb2S3,composite and the degradation activity under visible light[J].Materials Research Bulletin,2013,48(2):538-543.[15] W.Xu,W.Chen,et al.Synthesis and characterization of single-crystal Sb2S3 nanotubes via an edta-assised hydrothermal route[J].Materials Chemistry & Physics.2010,123(1):236-240.[16] H.F.Bao,W.Zhang,et al.Synthesis and electrical transport properties of single-crystal antimony sulfide nanowires[J].Journal of Physical Chemistry C,2007,111(14):17131-17135.[17] 刘运,苗鸿雁,谈国强,等.Sb2S3纳米线的制备和表征[J].稀有金属材料与工程,2008,37(3):468-471.[18] Y.Jiang,Y.J.Zhu.Microwave-assisted synthesis of sulfide M2S3(M=Bi,Sb) nanorods using an ionic liquid[J].Journal of Physical Chemistry B,2005,109(10):4361-4364.[19] 雍高兵,朱启安,项尚,等.回流法合成硫化锑纳米棒及其光催化性能研究[J].化学学报,2010,68(21):2199-2205.[20] C.J.Kavin,T.Thongtema,S.Thongtem,et al.Cyclic microwave assistedsynthesis of Sb2S3 dumb-bells using polyvinylpyrrolidone as a template and splitting agent[J].Materials Letters,2010,64(21):2388-2391.[21] X.Z.Zhou.Synthesis and catalytic properties of Sb2S3 nanowire bundles as counter ele ctrodes for dye-sensitized solar cells[J].Journal of Physical Chemistry C,2013,117(20):10285-10290.[22] 吴松,杨保俊,袁新松,等.Sb2S3纳米棒束的回流法合成与表征[J].稀有金属与硬质合金,2013,41(3):20-23.[23] R.H.Yu.Q.Wang,et al.High-quality ultralong Sb2S3 nanoribbons on large scale[J].Journal of Physical Chemistry B.2005,109(9):23312-23315.[24] W.D.Xiang,Y.Liu,H.Wen,et al.L-cystine-assisted growth of Sb2S3 nanoribbons via solvothermal route[J].Materials Chemistry & Physics,2009,118(2/3):432-437.[25] 陈广义,张万喜,魏志勇,等.硫化锑单晶纳米带的水热制备表征及性能研究[J].功能材料,2010,41(11):1923-1926.[26] Y.T.Qian,Z.Wei,et al.Solvothermal growth of Sb2S3 microcrystallites with novel morphologies[J].Journal of Crystal Growth,2004,262(1/4):375-382.[27] Q.Han,J.Lu,X.Yang,et al.A template-free route to Sb2S3 crystals with hollow olivary architectures[J].Crystal Growth & Design,2008,8(2):395-398.[28] L.Chen,W.Zhu,Q.Han,et al.Preparation of rod-like Sb2S3 dendrites processed in conventional hydrothermal[J].Materials Letters,2009,63(6):1258-1261.[29] 叶明富,孔祥荣,潘正凯,等.微波法合成树枝状纳米硫化锑[J].安徽工业大学学报:自然科学版,2012,29(4):1-3.[30] D.B.Wang,X.F.Gao,Y.Hu.Growth of one-dimensional Sb2S3 andSb2Se3 crystals with straw-tied-like architectures[J].Journal of Crystal Growth,2005,281(2):611-615.[31] G.Y.Chen,L.Xu,J.H.Wang,et al.The fractal splitting growth of Sb2S3 and Sb2Se3 hierarchical nanostructures[J].Journal of Physical Chemistry C,2008,112(11):672-679.[32] C.Yan,G.Chen,D.Chen,et al.Double surfactant-directed controllable synthesis of Sb2S3 crystals with comparable electrochemicalperformances[J].CrystEngcomm,2014,16(33):7753-7760.[33] L.C.Cheung,G.Wang.Building crystalline Sb2S3 nanowire dandelions with multiple crystal splitting motif[J].Materials Letters,2012,67(1):222-225.[34] W.Lei,rge-scale synthesis of double cauliflower-like Sb2S3 microcrystallines by hydrothermal method[J].Journal of Alloys & Compounds,2013,572(36):56-61.[35] C.M.Park,Y.Hwa,N.E.Sung,et al.Stibnite (Sb2S3) and its amorphous composite as dual electrodes for rechargeable lithium batteries[J].Journal of Materials Chemistry,2010,20(6):1097-1102.[36] P.V.Prikhodchenko,J.Gun,S.Sladkevich,et al.Conversion of hydroperoxoantimonate coated graphenes to Sb2S3@ graphene for a superior lithium battery anode[J].Chemistry of Materials,2012,24(24):4750-4757.[37] K.Xiao,Q.Z.Xu,K.H.Ye,et al.Facile hydrothermal synthesis of Sb2S3 nanorods and their magnetic and electrochemical properties[J].Ecs Solid State Letters,2013,2(6):51-54.[38] W.Tao,J.Wang,D.Wu,et al.Solvothermal synthesis of antimony sulfide dendrites for electrochemical detection of dopamine[J].Dalton Trans,2013,42(32):11411-11417.[39] J.C.Cardoso,C.A.Grimes,X.Feng,et al.Fabrication of coaxialTiO2/Sb2S3 nanowire hybrids for efficient nanostructured organic-inorganic thin film photovoltaics[J].Chemical Communications,2012,48(22):2818-2820.[40] M.Sun,D.Li,W.Li,et al.New photocatalyst,Sb2S3,for degradation of methyl orange under visible-light irradiation[J].Journal of Physical Chemistry C,2010,112(46):18076-18081.[41] H.Zhang,C.Hu,Y.Ding et al.ChemInform abstract:synthesis of 1DSb2S3 nanostructures and its application in visible-light-driven photodegradation for mo[J].Journal of Alloys & Compounds,2015,625(12):90-94.[42] B.Lu,J.Tang.Facile,One-pot solvothermal method to synthesize ultrathin Sb2S3 nanosheets anchored on graphene[J].Dalton Transactions,2014,43(37):13948-13956.[43] J.George,M.K.Radhak.Electrical conduction in coevaporated antimony trisulphide films[J].Solid State Communications,1980,33(9):987-989. [44] B.Cheng,E.T.Samulski.One-step,ambient-temperature synthesis of antimony sulfide (Sb2S3) micron-size polycrystals with a spherical morphology[J].Materials Research Bulletin,2003,38(2):297-301.[45] K.Y.Rajpure,C.H.Bhosale.Effect of composition on the structural,optical and electrical properties of sprayed Sb2S3 thin films prepared from non-aqueous medium[J].Journal of Physics & Chemistry of Solids,2000,61(4):561-568.[46] K.C.Gödel,Y.C.Choi,B.Roose,et al.Efficient room temperature aqueous Sb2S3 synthesis for inorganic-organic sensitized solar cells with 5.1% efficiencies[J].Chemical Communications,2015,51(41):8640-8643.。
化学专业英语词汇
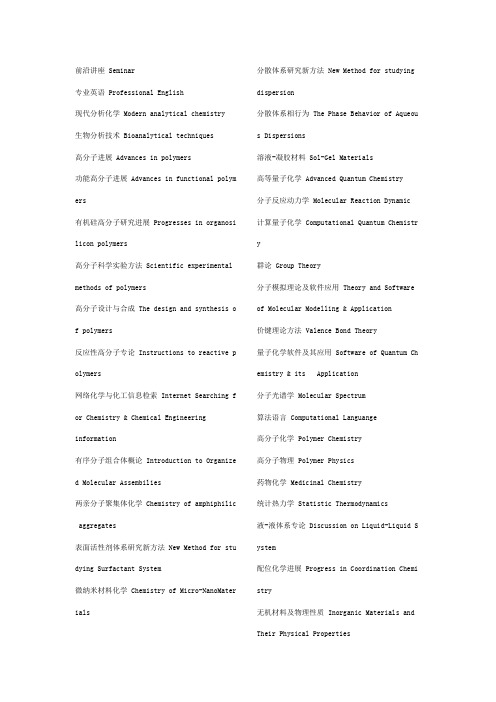
前沿讲座 Seminar专业英语 Professional English现代分析化学 Modern analytical chemistry 生物分析技术 Bioanalytical techniques高分子进展 Advances in polymers功能高分子进展 Advances in functional polym ers有机硅高分子研究进展 Progresses in organosi licon polymers高分子科学实验方法 Scientific experimental methods of polymers高分子设计与合成 The design and synthesis o f polymers反应性高分子专论 Instructions to reactive p olymers网络化学与化工信息检索 Internet Searching f or Chemistry & Chemical Engineering information有序分子组合体概论 Introduction to Organize d Molecular Assembilies两亲分子聚集体化学 Chemistry of amphiphilic aggregates表面活性剂体系研究新方法 New Method for stu dying Surfactant System微纳米材料化学 Chemistry of Micro-NanoMater ials 分散体系研究新方法 New Method for studying dispersion分散体系相行为 The Phase Behavior of Aqueou s Dispersions溶液-凝胶材料 Sol-Gel Materials高等量子化学 Advanced Quantum Chemistry 分子反应动力学 Molecular Reaction Dynamic 计算量子化学 Computational Quantum Chemistr y群论 Group Theory分子模拟理论及软件应用 Theory and Software of Molecular Modelling & Application价键理论方法 Valence Bond Theory量子化学软件及其应用 Software of Quantum Ch emistry & its Application分子光谱学 Molecular Spectrum算法语言 Computational Languange高分子化学 Polymer Chemistry高分子物理 Polymer Physics药物化学 Medicinal Chemistry统计热力学 Statistic Thermodynamics液-液体系专论 Discussion on Liquid-Liquid S ystem配位化学进展 Progress in Coordination Chemi stry无机材料及物理性质 Inorganic Materials and Their Physical Properties物理无机化学 Physical Inorganic Chemistry 相平衡 Phase Equilibrium现代无机化学 Today's Inorganic Chemistry 无机化学前沿领域导论 Introduction to Forwar d Field in Inorganic Chemistry量子化学 Quantum Chemistry分子材料 Molecular Material固体酸碱理论 Solid Acid-Base Theory萃取过程物理化学 Physical Chemistry in Extr action表面电化学 Surface Electrochemistry电化学进展 Advances on Electrochemistry 现代电化学实验技术 Modern Experimental Tech niques of Electrochemistry金属-碳多重键化合物及其应用 Compounds with Metal-Carbon multiple bonds and Their Applications叶立德化学:理论和应用 Ylides Chemistry: The ory and Application立体化学与手性合成 Stereochemistry and Chir al Synthesis杂环化学 Heterocyclic Chemistry有机硅化学 Organosilicon Chemistry药物设计及合成 Pharmaceutical Design and Sy nthesis超分子化学 Supramolecular Chemistry 分子设计与组合化学 Molecular Design and Com binatorial Chemistry纳米材料化学前沿领域导论 Introduction to Na no-materials Chemistry纳米材料控制合成与自组装 Controlled-synthes is and Self-assembly of Nano-materials前沿讲座 Leading Front Forum专业英语 Professional English超分子化学基础 Basics of Supramolecular Che mistry液晶材料基础 Basics of Liquid Crystal Mater ials现代实验技术 Modern analytical testing tech niques色谱及联用技术 Chromatography and Technolog y of tandem发光分析及其研究法 Luminescence analysis an d Research methods胶束酶学 Micellar Enzymology分析化学中的配位化合物 Complex in Analytica l Chemistry电分析化学 Electroanalytical chemistry生物分析化学 Bioanalytical chemistry分析化学 Analytical chemistry仪器分析 Instrument analysis高分子合成化学 Polymers synthetic chemistry高聚物结构与性能 Structures and properties of polymers有机硅化学 Organosilicon chemistry功能高分子 Functional polymers有机硅高分子 Organosilicon polymers高分子现代实验技术 Advanced experimental te chnology of polymers高分子合成新方法 New synthetic methods of p olymers液晶与液晶高分子 Liquid crystals and liquid crystal polymers大分子反应 Macromolecules reaction水溶性高分子 Water-soluble polymers聚合物加工基础 The basic process of polymer s聚合物复合材料 Composite materials高等化工与热力学 Advanced Chemical Engineer ing and Thermodynamics高等反应工程学 Advanced Reaction Engineerin g高等有机化学 Advanced Organic Chemistry 高等有机合成 Advanced Organic synthesis 有机化学中光谱分析 Spectrum Analysis in Org anic Chemistry催化作用原理 Principle of Catalysis染料化学 Dye Chemistry 中间体化学与工艺学 Intermediate Chemistry a nd Technology化学动力学 Chemical Kinetics表面活性剂合成与工艺 Synthesis and Technolo gy of Surfactants环境化学 Environmental Chemistry化工企业清洁生产 Chemical Enterprise Clean Production化工污染及防治 Chemical Pollution and Contr ol动量热量质量传递 Momentum, Heat and Mass Tr ansmission化工分离工程专题 Separation Engineering 耐蚀材料 Corrosion Resisting Material网络化学与化工信息检索 Internet Searching f or Chemistry & Chemical Engineering informa tion新型功能材料的模板组装 Templated Assembly o f Novel Advanced Materials胶体与界面 Colloid and Interface纳米材料的胶体化学制备方法 Colloid Chemical Methods for Preparing Nano-materials脂质体化学 Chemistry of liposome表面活性剂物理化学 Physico-chemistry of sur factants高分子溶液与微乳液 Polymer Solutions and Mi croemulsions两亲分子的溶液化学 Chemistry of Amphiphilic Molecules in solution介孔材料化学 Mesoporous Chemistry超细颗粒化学 Chemistry of ultrafine powder 分散体系流变学 The Rheolgy of Aqueous Dispe rsions量子化学 Quantum Chemistry统计热力学 Statistic Thermodynamics群论 Group Theory分子模拟 Molecular Modelling高等量子化学 Advanced Quantum Chemistry价键理论方法 Valence Bond Theory量子化学软件及其应用 Software of Quantum Ch emistry & its Application计算量子化学 Computational Quantum Chemistr y分子模拟软件及其应用 Software of Molecular Modelling & its Application分子反应动力学 Molecular Reaction Dynamic 分子光谱学 Molecular Spectrum算法语言 Computational Languange高分子化学 Polymer Chemistry高分子物理 Polymer Physics腐蚀电化学 Corrosion Electrochemistry物理化学 Physical Chemistry结构化学 structural Chemistry 现代分析与测试技术(试验为主) Modern Analysi s and Testing Technology(experimetally)高等无机化学 Advanced Inorganic Chemistry 近代无机物研究方法 Modern Research Methods for Inorganic Compounds萃取化学研究方法 Research Methods for Extra ction Chemistry单晶培养 Crystal Culture固态化学 Chemistry of Solid Substance液-液体系专论 Discussion on Liquid-Liquid S ystem配位化学进展 Progress in Coordination Chemi stry卟啉酞箐化学 Chemistry of Porphyrine and Ph thalocyanine无机材料及物理性质 Inorganic Materials and Their Physical Properties物理无机化学 Physical Inorganic Chemistry 相平衡 Phase Equilibrium生物化学的应用 Application of Biologic Chem istry生物无机化学 Bio-Inorganic Chemistry绿色化学 Green Chemistry金属有机化合物在均相催化中的应用 Applied Ho mogeneous Catalysis with Organometallic Compounds功能性食品化学 Functionalized Food Chemistr y无机药物化学 Inorganic Pharmaceutical Chemi stry电极过程动力学 Kinetics on Electrode Proces s电化学研究方法 Electrochemical Research Met hods生物物理化学 Biological Physical Chemistry 波谱与现代检测技术 Spectroscopy and Modern Testing Technology理论有机化学 theoretical Organic Chemistry 合成化学 Synthesis Chemistry有机合成新方法 New Methods for Organic Synt hesis生物有机化学 Bio-organic Chemistry药物化学 Pharmaceutical Chemistry金属有机化学 Organometallic Chemistry金属-碳多重键化合物及其应用 Compounds with Metal-Carbon multiple bonds and Their Applications分子构效与模拟 Molecular Structure-Activity and Simulation过程装置数值计算 Data Calculation of Proces s Devices石油化工典型设备 Common Equipment of Petroc hemical Industry 化工流态化工程 Fluidization in Chemical Ind ustry化工装置模拟与优化 Analogue and Optimizatio n of Chemical Devices化工分离工程 Separation Engineering化工系统与优化 Chemical System and Optimiza tion高等化工热力学 Advanced Chemical Engineerin g and Thermodynamics超临界流体技术及应用 Super Cratical Liguid Technegues and Applications膜分离技术 Membrane Separation Technegues溶剂萃取原理和应用 Theory and Application o f Solvent Extraction树脂吸附理论 Theory of Resin Adsorption 中药材化学 Chemistry of Chinese Medicine 生物资源有效成分分析与鉴定 Analysis and Det ection of Bio-materials相平衡理论与应用 Theory and Application o f Phase Equilibrium计算机在化学工程中的应用 Application of Com puter in Chemical Engineering微乳液和高分子溶液 Micro-emulsion and High Molecular Solution传递过程 Transmision Process反应工程分析 Reaction Engineering Analysis腐蚀电化学原理与应用 Principle and Applicat ion of Corrosion Electrochemistry腐蚀电化学测试方法与应用 Measurement Method and Application of Corrosion Elect rochemistry耐蚀表面工程 Surface Techniques of Anti-cor rosion缓蚀剂技术 Inhabitor Techniques腐蚀失效分析 Analysis of Corrosion Destroy 材料表面研究方法 Method of Studying Materia l Surfacc分离与纯化技术 Separation and Purification Technology现代精细有机合成 Modern Fine Organic Synthe sis化学工艺与设备 Chemical Technology and Appa ratuas功能材料概论 Functional Materials Conspectu s油田化学 Oilfield Chemistry精细化学品研究 Study of Fine Chemicals催化剂合成与应用 Synthesis and Application of Catalyzer低维材料制备 Preparation of Low-Dimension M aterials手性药物化学 Symmetrical Pharmaceutical Che mistry 光敏高分子材料化学 Photosensitive Polymer M aterials Chemistry纳米材料制备与表征 Preparation and Characte rization of Nanostructured materials溶胶凝胶化学 Sol-gel Chemistry纳米材料化学进展 Proceeding of Nano-materia ls Chemistry●化学常用词汇汉英对照表1●氨 ammonia氨基酸 amino acid铵盐 ammonium salt饱和链烃saturated aliphatic hydrocarbon苯 benzene变性 denaturation不饱和烃unsaturated hydrocarbon超导材料superconductive material臭氧 ozone醇 alcohol次氯酸钾potassium hypochlorite醋酸钠sodium acetate蛋白质 protein氮族元素nitrogen group element碘化钾potassium iodide碘化钠sodium iodide电化学腐蚀 electrochemical corrosion电解质 electrolyte电离平衡ionization equilibrium电子云electron cloud淀粉 starch淀粉碘化钾试纸starch potassium iodide paper二氧化氮nitrogen dioxide二氧化硅silicon dioxide二氧化硫sulphur dioxide二氧化锰manganese dioxide芳香烃 arene放热反应exothermic reaction非极性分子non-polar molecule非极性键non-polar bond肥皂 soap分馏fractional distillation酚 phenol复合材料 composite干电池 dry cell干馏dry distillation甘油 glycerol高分子化合物 polymer共价键covalent bond官能团functional group光化学烟雾photochemical fog过氧化氢hydrogen peroxide合成材料synthetic material合成纤维synthetic fiber合成橡胶synthetic rubber核电荷数nuclear charge number核素 nuclide化学电源chemical power source化学反应速率chemical reaction rate化学键chemical bond化学平衡chemical equilibrium还原剂reducing agent磺化反应sulfonation reaction霍尔槽 Hull Cell极性分子polar molecule极性键 polar bond加成反应addition reaction加聚反应addition polymerization甲烷 methane碱金属alkali metal碱石灰 soda lime结构式structural formula聚合反应 po1ymerization可逆反应reversible reaction空气污染指数 air pollution index勒夏特列原理Le Chatelier's principle离子反应ionic reaction离子方程式ionic equation离子键 ionic bond锂电池lithium cell两性氢氧化物amphoteric hydroxide两性氧化物amphoteric oxide裂化 cracking裂解 pyrolysis硫氰化钾potassium thiocyanate硫酸钠sodium sulphide氯化铵ammonium chloride氯化钡barium chloride氯化钾potassium chloride氯化铝aluminium chloride氯化镁magnesium chloride氯化氢hydrogen chloride氯化铁iron (III) chloride氯水chlorine water麦芽糖 maltose煤 coal酶 enzyme摩尔 mole摩尔质量molar mass品红magenta或fuchsine葡萄糖 glucose气体摩尔体积 molar volume of gas铅蓄电池lead storage battery强电解质strong electrolyte氢氟酸hydrogen chloride氢氧化铝aluminium hydroxide取代反应substitution reaction醛 aldehyde炔烃 alkyne燃料电池 fuel cell弱电解质weak electrolyte石油 Petroleum水解反应hydrolysis reaction四氯化碳carbon tetrachloride塑料 plastic塑料的降解plastic degradation塑料的老化plastic ageing酸碱中和滴定acid-base neutralization titration酸雨 acid rain羧酸carboxylic acid碳酸钠 sodium carbonate碳酸氢铵 ammonium bicarbonate碳酸氢钠 sodium bicarbonate糖类 carbohydrate烃 hydrocarbon烃的衍生物derivative of hydrocarbon烃基 hydrocarbonyl同分异构体 isomer同素异形体 allotrope同位素 isotope同系物 homo1og涂料 coating烷烃 alkane物质的量 amount of substance物质的量浓度 amount-of-substance concentration of B烯烃 alkene洗涤剂 detergent纤维素 cellulose相对分子质量relative molecular mass相对原子质量 relative atomic mass消去反应 elimination reaction硝化反应 nitratlon reaction硝酸钡 barium nitrate硝酸银 silver nitrate溴的四氯化碳溶液solution of bromine in carbon tetrachloride溴化钠 sodium bromide溴水 bromine water溴水 bromine water盐类的水解 hydrolysis of salts盐析 salting-out焰色反应 flame test氧化剂 oxidizing agent氧化铝 aluminium oxide氧化铁 iron (III) oxide乙醇 ethanol乙醛 ethana1乙炔 ethyne乙酸 ethanoic acid乙酸乙酯 ethyl acetate乙烯 ethene银镜反应silver mirror reaction硬脂酸 stearic acid油脂 oils and fats有机化合物 organic compound元素周期表periodic table of elements元素周期律 periodic law of elements原电池 primary battery原子序数 atomic number皂化反应 saponification粘合剂 adhesive蔗糖 sucrose指示剂 Indicator酯 ester酯化反应 esterification周期 period族 group(主族:main group)Bunsen burner 本生灯product 化学反应产物flask 烧瓶apparatus 设备PH indicator PH值指示剂,氢离子(浓度的)负指数指示剂matrass 卵形瓶litmus 石蕊litmus paper 石蕊试纸graduate, graduated flask 量筒,量杯reagent 试剂 test tube 试管burette 滴定管retort 曲颈甑still 蒸馏釜cupel 烤钵crucible pot, melting pot 坩埚 pipette 吸液管filter 滤管stirring rod 搅拌棒element 元素body 物体compound 化合物atom 原子gram atom 克原子atomic weight 原子量atomic number 原子数atomic mass 原子质量molecule 分子electrolyte 电解质ion 离子anion 阴离子cation 阳离子electron 电子isotope 同位素isomer 同分异物现象polymer 聚合物symbol 复合radical 基structural formula 分子式valence, valency 价monovalent 单价bivalent 二价halogen 成盐元素bond 原子的聚合mixture 混合combination 合成作用compound 合成物alloy 合金organic chemistry 有机化学inorganic chemistry 无机化学derivative 衍生物series 系列acid 酸hydrochloric acid 盐酸sulphuric acid 硫酸nitric acid 硝酸aqua fortis 王水fatty acid 脂肪酸organic acid 有机酸 hydrosulphuric acid 氢硫酸hydrogen sulfide 氢化硫alkali 碱,强碱ammonia 氨base 碱 hydrate 水合物hydroxide 氢氧化物,羟化物hydracid 氢酸hydrocarbon 碳氢化合物,羟anhydride 酐alkaloid 生物碱aldehyde 醛oxide 氧化物phosphate 磷酸盐acetate 醋酸盐methane 甲烷,沼气butane 丁烷salt 盐potassium carbonate 碳酸钾soda 苏打sodium carbonate 碳酸钠caustic potash 苛性钾caustic soda 苛性钠ester 酯gel 凝胶体analysis 分解fractionation 分馏endothermic reaction 吸热反应 exothermic reaction 放热反应 precipitation 沉淀to precipitate 沉淀to distil, to distill 蒸馏distillation 蒸馏to calcine 煅烧to oxidize 氧化alkalinization 碱化to oxygenate, to oxidize 脱氧,氧化 to neutralize 中和to hydrogenate 氢化to hydrate 水合,水化to dehydrate 脱水fermentation 发酵solution 溶解combustion 燃烧fusion, melting 熔解alkalinity 碱性isomerism, isomery 同分异物现象hydrolysis 水解electrolysis 电解electrode 电极anode 阳极,正极cathode 阴极,负极catalyst 催化剂catalysis 催化作用oxidization, oxidation 氧化reducer 还原剂dissolution 分解synthesis 合成reversible 可逆的1. The Ideal-Gas Equation 理想气体状态方程2. Partial Pressures 分压3. Real Gases: Deviation from Ideal Behavior 真实气体:对理想气体行为的偏离4. The van der Waals Equation 范德华方程5. System and Surroundings 系统与环境6. State and State Functions 状态与状态函数7. Process 过程8. Phase 相9. The First Law of Thermodynamics 热力学第一定律10. Heat and Work 热与功11. Endothermic and Exothermic Processes 吸热与发热过程12. Enthalpies of Reactions 反应热13. Hess’s Law 盖斯定律14. Enthalpies of Formation 生成焓15. Reaction Rates 反应速率16. Reaction Order 反应级数17. Rate Constants 速率常数18. Activation Energy 活化能19. The Arrhenius Equation 阿累尼乌斯方程20. Reaction Mechanisms 反应机理21. Homogeneous Catalysis 均相催化剂22. Heterogeneous Catalysis 非均相催化剂23. Enzymes 酶24. The Equilibrium Constant 平衡常数25. the Direction of Reaction 反应方向26. Le Chatelier’s Principle 列·沙特列原理27. Effects of Volume, Pressure, Temperature Changes and Catalystsi. 体积,压力,温度变化以及催化剂的影响28. Spontaneous Processes 自发过程29. Entropy (Standard Entropy) 熵(标准熵)30. The Second Law of Thermodynamics 热力学第二定律31. Entropy Changes 熵变32. Standard Free-Energy Changes 标准自由能变33. Acid-Bases 酸碱34. The Dissociation of Water 水离解35. The Proton in Water 水合质子36. The pH Scales pH值37. Bronsted-Lowry Acids and Bases Bronsted-Lowry 酸和碱38. Proton-Transfer Reactions 质子转移反应39. Conjugate Acid-Base Pairs 共轭酸碱对40. Relative Strength of Acids and Bases 酸碱的相对强度41. Lewis Acids and Bases 路易斯酸碱42. Hydrolysis of Metal Ions 金属离子的水解43. Buffer Solutions 缓冲溶液44. The Common-Ion Effects 同离子效应45. Buffer Capacity 缓冲容量46. Formation of Complex Ions 配离子的形成47. Solubility 溶解度48. The Solubility-Product Constant Ksp 溶度积常数49. Precipitation and separation of Ions 离子的沉淀与分离50. Selective Precipitation of Ions 离子的选择沉淀51. Oxidation-Reduction Reactions 氧化还原反应52. Oxidation Number 氧化数53. Balancing Oxidation-Reduction Equations 氧化还原反应方程的配平54. Half-Reaction 半反应55. Galvani Cell 原电池56. Voltaic Cell 伏特电池57. Cell EMF 电池电动势58. Standard Electrode Potentials 标准电极电势59. Oxidizing and Reducing Agents 氧化剂和还原剂60. The Nernst Equation 能斯特方程61. Electrolysis 电解62. The Wave Behavior of Electrons 电子的波动性63. Bohr’s Model of The Hydrogen Atom 氢原子的波尔模型64. Line Spectra 线光谱65. Quantum Numbers 量子数66. Electron Spin 电子自旋67. Atomic Orbital 原子轨道68. The s (p, d, f) Orbital s(p,d,f)轨道69. Many-Electron Atoms 多电子原子70. Energies of Orbital 轨道能量71. The Pauli Exclusion Principle 泡林不相容原理72. Electron Configurations 电子构型73. The Periodic Table 周期表74. Row 行75. Group 族76. Isotopes, Atomic Numbers, and Mass Numbers 同位素,原子数,质量数77. Periodic Properties of the Elements 元素的周期律78. Radius of Atoms 原子半径79. Ionization Energy 电离能80. Electronegativity 电负性81. Effective Nuclear Charge 有效核电荷82. Electron Affinities 亲电性83. Metals 金属84. Nonmetals 非金属85. Valence Bond Theory 价键理论86. Covalence Bond 共价键87. Orbital Overlap 轨道重叠88. Multiple Bonds 重键89. Hybrid Orbital 杂化轨道90. The VSEPR Model 价层电子对互斥理论91. Molecular Geometries 分子空间构型92. Molecular Orbital 分子轨道93. Diatomic Molecules 双原子分子94. Bond Length 键长95. Bond Order 键级96. Bond Angles 键角97. Bond Enthalpies 键能98. Bond Polarity 键矩99. Dipole Moments 偶极矩100. Polarity Molecules 极性分子101. Polyatomic Molecules 多原子分子102. Crystal Structure 晶体结构103. Non-Crystal 非晶体104. Close Packing of Spheres 球密堆积105. Metallic Solids 金属晶体106. Metallic Bond 金属键107. Alloys 合金108. Ionic Solids 离子晶体109. Ion-Dipole Forces 离子偶极力110. Molecular Forces 分子间力111. Intermolecular Forces 分子间作用力112. Hydrogen Bonding 氢键113. Covalent-Network Solids 原子晶体114. Compounds 化合物115. The Nomenclature, Composition and Structure of Complexes 配合物的命名,组成和结构116. Charges, Coordination Numbers, and Geometries 电荷数、配位数、及几何构型117. Chelates 螯合物118. Isomerism 异构现象119. Structural Isomerism 结构异构120. Stereoisomerism 立体异构121. Magnetism 磁性122. Electron Configurations in Octahedral Complexes 八面体构型配合物的电子分布123. Tetrahedral and Square-planar Complexes 四面体和平面四边形配合物124. General Characteristics 共性125. s-Block Elements s区元素126. Alkali Metals 碱金属127. Alkaline Earth Metals 碱土金属128. Hydrides 氢化物129. Oxides 氧化物130. Peroxides and Superoxides 过氧化物和超氧化物131. Hydroxides 氢氧化物132. Salts 盐133. p-Block Elements p区元素134. Boron Group (Boron, Aluminium, Gallium, Indium, Thallium) 硼族(硼,铝,镓,铟,铊)135. Borane 硼烷136. Carbon Group (Carbon, Silicon, Germanium, Tin, Lead) 碳族(碳,硅,锗,锡,铅)137. Graphite, Carbon Monoxide, Carbon Dioxide 石墨,一氧化碳,二氧化碳138. Carbonic Acid, Carbonates and Carbides 碳酸,碳酸盐,碳化物139. Occurrence and Preparation of Silicon 硅的存在和制备140. Silicic Acid,Silicates 硅酸,硅酸盐141. Nitrogen Group (Phosphorus, Arsenic, Antimony, and Bismuth) 氮族(磷,砷,锑,铋)142. Ammonia, Nitric Acid, Phosphoric Acid 氨,硝酸,磷酸143. Phosphorates, phosphorus Halides 磷酸盐,卤化磷144. Oxygen Group (Oxygen, Sulfur, Selenium, and Tellurium) 氧族元素(氧,硫,硒,碲)145. Ozone, Hydrogen Peroxide 臭氧,过氧化氢146. Sulfides 硫化物147. Halogens (Fluorine, Chlorine, Bromine, Iodine) 卤素(氟,氯,溴,碘)148. Halides, Chloride 卤化物,氯化物149. The Noble Gases 稀有气体150. Noble-Gas Compounds 稀有气体化合物151. d-Block elements d区元素152. Transition Metals 过渡金属153. Potassium Dichromate 重铬酸钾154. Potassium Permanganate 高锰酸钾155. Iron Copper Zinc Mercury 铁,铜,锌,汞156. f-Block Elements f区元素157. Lanthanides 镧系元素158. Radioactivity 放射性159. Nuclear Chemistry 核化学160. Nuclear Fission 核裂变161. Nuclear Fusion 核聚变162. analytical chemistry 分析化学163. qualitative analysis 定性分析164. quantitative analysis 定量分析165. chemical analysis 化学分析166. instrumental analysis 仪器分析167. titrimetry 滴定分析168. gravimetric analysis 重量分析法169. regent 试剂170. chromatographic analysis 色谱分析171. product 产物172. electrochemical analysis 电化学分析173. on-line analysis 在线分析174. macro analysis 常量分析175. characteristic 表征176. micro analysis 微量分析177. deformation analysis 形态分析178. semimicro analysis 半微量分析179. systematical error 系统误差180. routine analysis 常规分析181. random error 偶然误差182. arbitration analysis 仲裁分析183. gross error 过失误差184. normal distribution 正态分布185. accuracy 准确度186. deviation 偏差187. precision 精密度188. relative standard deviation 相对标准偏差(RSD)189. coefficient variation 变异系数(CV)190. confidence level 置信水平191. confidence interval置信区间192. significant test显著性检验193. significant figure 有效数字194. standard solution 标准溶液195. titration 滴定196. stoichiometric point 化学计量点197. end point 滴定终点198. titration error滴定误差199. primary standard 基准物质200. amount of substance 物质的量201. standardization 标定202. chemical reaction 化学反应203. concentration 浓度204. chemical equilibrium 化学平衡205. titer 滴定度206. general equation for a chemical reaction 化学反应的通式207. proton theory of acid-base酸碱质子理论208. acid-base titration酸碱滴定法209. dissociation constant解离常数210. conjugate acid-base pair共轭酸碱对211. acetic acid 乙酸212. hydronium ion 水合氢离子213. electrolyte 电解质214. ion-product constant of water水的离子积215. ionization电离216. proton condition 质子平衡217. zero level 零水准218. buffer solution缓冲溶液219. methyl orange甲基橙220. acid-base indicator酸碱指示剂221. phenolphthalein 酚酞222. coordination compound配位化合物223. center ion中心离子224. cumulative stability constant累积稳定常数225. alpha coefficient酸效应系数226. overall stability constant总稳定常数227. ligand 配位体228. ethylenediamine tetraacetic acid乙二胺四乙酸229. side reaction coefficient副反应系数230. coordination atom配位原子231. coordination number 配位数232. lone pair electron孤对电子233. chelate compound 螯合物234. metal indicator 金属指示剂235. chelating agent 螯合剂236. masking 掩蔽237. demasking 解蔽238. electron 电子239. catalysis催化240. oxidation 氧化241. catalyst 催化剂242. reduction还原243. catalytic reaction催化反应244. reaction rate反应速率245. electrode potential电极电势246. activation energy 反应的活化能247. redox couple 氧化还原电对248. potassium permanganate 高锰酸钾249. iodimetry 碘量法250. potassium dichromate 重铬酸钾251. cerimetry 铈量法252. redox indicator 氧化还原指示253. oxygen consuming 耗氧量(OC)254. chemical oxygen demanded 化学需氧量(COD) 255. dissolved oxygen 溶解氧(DO) 256. precipitation 沉淀反应257. argentimetry 银量法258. heterogeneous equilibrium of ions 多相离子平衡259. aging 陈化260. postprecipitation 继沉淀261. coprecipitation 共沉淀262. ignition 灼烧263. fitration 过滤264. decantation 倾泻法265. chemical factor 化学因数266. spectrophotometry 分光光度法267. colorimetry 比色分析268. transmittance 透光率269. absorptivity 吸光率270. calibration curve 校正曲线271. standard curve 标准曲线272. monochromator 单色器273. source 光源274. wavelength dispersion 色散275. absorption cell 吸收池276. detector 检测系统277. bathochromic shift 红移278. Molar absorptivity 摩尔吸光系数279. hypochromic shift 紫移280. acetylene 乙炔281. ethylene 乙烯282. acetylating agent 乙酰化剂283. acetic acid 乙酸284. adiethyl ether 乙醚285. ethyl alcohol 乙醇286. acetaldehtde 乙醛287. β-dicarbontl compound β–二羰基化合物288. bimolecular elimination 双分子消除反应289. bimolecular nucleophilic substitution 双分子亲核取代反应290. open chain compound 开链族化合物291. molecular orbital theory 分子轨道理论292. chiral molecule 手性分子293. tautomerism 互变异构现象294. reaction mechanism 反应历程295. chemical shift 化学位移296. Walden inversio 瓦尔登反转n297. Enantiomorph 对映体298. addition rea ction 加成反应299. dextro- 右旋300. levo- 左旋301. stereochemistry 立体化学302. stereo isomer 立体异构体303. Lucas reagent 卢卡斯试剂304. covalent bond 共价键305. conjugated diene 共轭二烯烃306. conjugated double bond 共轭双键307. conjugated system 共轭体系308. conjugated effect 共轭效应309. isomer 同分异构体310. isomerism 同分异构现象311. organic chemistry 有机化学312. hybridization 杂化313. hybrid orbital 杂化轨道314. heterocyclic compound 杂环化合物315. peroxide effect 过氧化物效应t 316. valence bond theory 价键理论317. sequence rule 次序规则318. electron-attracting grou p 吸电子基319. Huckel rule 休克尔规则320. Hinsberg test 兴斯堡试验321. infrared spectrum 红外光谱322. Michael reacton 麦克尔反应323. halogenated hydrocarbon 卤代烃324. haloform reaction 卤仿反应325. systematic nomenclatur 系统命名法e 326. Newman projection 纽曼投影式327. aromatic compound 芳香族化合物328. aromatic character 芳香性r329. Claisen condensation reaction克莱森酯缩合反应330. Claisen rearrangement 克莱森重排331. Diels-Alder reation 狄尔斯-阿尔得反应332. Clemmensen reduction 克莱门森还原333. Cannizzaro reaction 坎尼扎罗反应334. positional isomers 位置异构体335. unimolecular elimination reaction 单分子消除反应336. unimolecular nucleophilic substitution 单分子亲核取代反应337. benzene 苯338. functional grou 官能团p339. configuration 构型340. conformation 构象341. confomational isome 构象异构体342. electrophilic addition 亲电加成343. electrophilic reagent 亲电试剂344. nucleophilic addition 亲核加成345. nucleophilic reagent 亲核试剂346. nucleophilic substitution reaction亲核取代反应347. active intermediate 活性中间体348. Saytzeff rule 查依采夫规则349. cis-trans isomerism 顺反异构350. inductive effect 诱导效应 t351. Fehling’s reagent 费林试剂352. phase transfer catalysis 相转移催化作用353. aliphatic compound 脂肪族化合物354. elimination reaction 消除反应355. Grignard reagent 格利雅试剂356. nuclear magnetic resonance 核磁共振357. alkene 烯烃358. allyl cation 烯丙基正离子359. leaving group 离去基团360. optical activity 旋光性361. boat confomation 船型构象362. silver mirror reaction 银镜反应363. Fischer projection 菲舍尔投影式364. Kekule structure 凯库勒结构式365. Friedel-Crafts reaction 傅列德尔-克拉夫茨反应366. Ketone 酮367. carboxylic acid 羧酸368. carboxylic acid derivative 羧酸衍生物369. hydroboration 硼氢化反应370. bond oength 键长371. bond energy 键能372. bond angle 键角373. carbohydrate 碳水化合物374. carbocation 碳正离子375. carbanion 碳负离子376. alcohol 醇377. Gofmann rule 霍夫曼规则378. Aldehyde 醛379. Ether 醚380. Polymer 聚合物。
高熵氧化物陶瓷的研究进展与展望

郭鑫,解勤兴(天津工业大学材料科学与工程学院,天津300160)高熵氧化物陶瓷是具有独特结构和物理化学性能的新型材料,成为了国内外研究的热点之一。
本文主要介绍了高熵氧化物陶瓷的分类;归纳了制备高熵氧化物陶瓷的方法;总结了高熵氧化物陶瓷在催化材料、锂电池电极材料、磁性材料以及介电材料等领域的应用。
最后简述高熵氧化物陶瓷的发展现状,展望了高熵氧化物陶瓷未来的发展趋势。
;制备方法;应用;发展趋势随着现代科技迅猛的发展,传统材料性能已无法适应现如今技术发展的需求,研发具有优异性能的新型材料显得尤为重要。
回顾材料发展的历史进程,界面工程[1]、基因工程[2]以及高通量计算[3]等技术方法为新型材料的研发提供了强有力的指导作用。
近些年来,“高熵材料”的问世为新型材料的研发提供了新颖的设计理念和思路。
高熵材料一般由多个不同元素(5种或5种以上)以等摩尔或近等摩尔比制备而成的固溶体结构材料[4]。
2004年,Yeh[5]教授指出形成单一相的固溶体与混合物的构型熵有关,从而提出“高熵合金”概念。
高熵合金材料因其独特的结构和优异的性能,如巨介电性能[6]、高效的电化学性能[7]以及独特的催化性能[8],很快便引起各国研究学者对高熵材料的关注与研发。
随着高熵材料体系的不断增加,人们对它的认识日渐加深。
之后,越来越多的学者将“高熵合金”的概念延伸到了其他领域。
2015年,Rost[9]团队采用高温固相反应法首次研发出了等摩尔比的五主元岩盐型高熵氧化物陶瓷。
等摩尔比的岩盐型(Co0.2Cu0.2Mg0.2Ni0.2Zn0.2)O高熵陶瓷的成功问世,证实了制备高熵陶瓷的可能性。
自此之后,国内外研究人员相继开发出种类繁多的高熵陶瓷体系。
依据化学成分的不同,主要划分为氧化物高熵陶瓷和非氧化物高熵陶瓷两大类。
其中氧化物高熵陶瓷可依晶体结构的不同进一步细分为:岩盐型高熵陶瓷[10]、钙钛矿型高熵陶瓷[11]、萤石型高熵陶瓷[12]等。
钨酸钠水热法合成晶态WO_3纳米棒及其表征
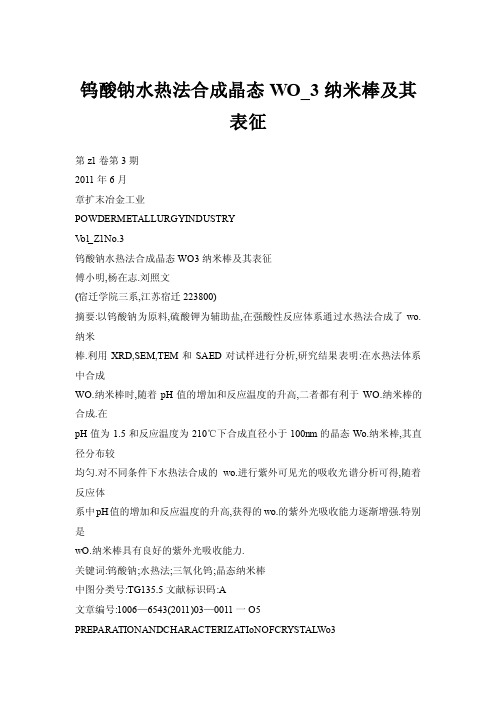
钨酸钠水热法合成晶态WO_3纳米棒及其表征第z1卷第3期2011年6月章扩末冶金工业POWDERMETALLURGYINDUSTRYV ol_Z1No.3钨酸钠水热法合成晶态WO3纳米棒及其表征傅小明,杨在志.刘照文(宿迁学院三系,江苏宿迁223800)摘要:以钨酸钠为原料,硫酸钾为辅助盐,在强酸性反应体系通过水热法合成了wo.纳米棒.利用XRD,SEM,TEM和SAED对试样进行分析,研究结果表明:在水热法体系中合成WO.纳米棒时,随着pH值的增加和反应温度的升高,二者都有利于WO.纳米棒的合成.在pH值为1.5和反应温度为210℃下合成直径小于100nm的晶态Wo.纳米棒,其直径分布较均匀.对不同条件下水热法合成的wo.进行紫外可见光的吸收光谱分析可得,随着反应体系中pH值的增加和反应温度的升高,获得的wo.的紫外光吸收能力逐渐增强.特别是wO.纳米棒具有良好的紫外光吸收能力.关键词:钨酸钠;水热法;三氧化钨;晶态纳米棒中图分类号:TG135.5文献标识码:A文章编号:1006—6543(2011)03—0011一O5 PREPARATIONANDCHARACTERIZA TIoNOFCRYSTALWo3NANoRODSSYNTHESIZEDWITHSODIUMTUNGSTATEBYTHEHYDR0THERMALMETHODFUXiao-ming,Y ANGZai—zhi,LIUZhao-wen(The3DepartmentofSuqianCollege,SuqianJiangsu223800,China)Abstract:WO3nanorodsaresynthesizedwithsodiumtungstateandpotassiumsulphateinaci dbythehydrotherma1method.ThesamplesarecharacterizedbyXRD,SEM,TEMand SAED.WiththeincrementofthepHvalueandthereactiontemperature,Wo3nanorodsare obtainedeasilybythehydrothermalmethod.CrystalWO3nanorodsoflessthan100nminthe diameterat210~CwithpH一1.5bythehydrothermalmethod.ThecrystalWO3nanorodsare homogeneousinthediameter.WhenitislessthanthesamplesarecharacterizedbyUV—VIS, withtheincrementofthepHvalueandthereactiontemperature,theabsorbentpoweroful—travioletlightofWo3synthesizedbythehydrothermalmethodenhancegradually.Especially,SinglecrystalWO3nanorodstakeonthegoodabsorbentpoweroful- travioletlight.Keywords:Sodiumtungstate;Hydrothermalmethod;Wo3;Crystalnanorod三氧化钨(WO.)是一种具有六方,立方等多种对称型结构的n型半导体材料,由于具有优异的电致变色,气致变色和光致变色等性能而备受关注Ⅲ.特别是自1973年s.K.Deb发现WO.具有光致变色现象以来,wo.在信息显示器件,高敏度光存储材料及变色玻璃等方面显示出巨大的应用前景,收稿日期:2Ol1一O1~O4基金项目:宿迁学院高级人才启动基金(SQCGJ2010002),江苏省大学生实践创新训练计划项目(2010SSJ02).作者简介:傅小明(1974一),男(汉),四川广元人,博士,讲师,主要从事粉末冶金的研究.12粉末冶金工业第21卷使其制备,结构及光致变色性能等成为国内外的研究热点之一l3].特别是纳米WO.因具有巨大的比表面积,其体积效应,表面效应,量子尺寸效应和宏观量子隧道效应显着,使得它的应用领域继续扩大, 可作为太阳能吸收材料,隐形材料,催化剂材料以及No,HS,NH.,H等的气敏感材料等【].目前,制备纳米WO.的方法主要有物理和化学方法,如活性溅射法_7],脉冲激光沉积法_8j,气体沉积法[,阳极电镀法l_j,高真空热蒸镀法,溶胶一凝胶法口和水热法口等.虽然通过这些制备方法对纳米Wo.的研究取得了不少进展,但是都还处于实验室研究阶段.具有应用前景的制备纳米wo.的方法是溶胶一凝胶法和水热法.对于溶胶一凝胶法,由于反应原料所用的金属醇盐比较昂贵, 且影响因素也较多,工艺稳定,在烘干过程巾容易出现团聚现象,因此,溶胶一凝胶法的应用受到_r一定的限制.而水热法具有简便,经济和参数易控制等优点,但是利用此方法合成WO.纳米棒的研究甚少.本文以钨酸钠为原料,硫酸钾为辅助盐,对利用水热法合成WO.纳米棒进行了研究.然后通过XRD,SEM,TEM和UV—VIS对试样分别进行了物相,形貌,晶态和光敏性分析.1实验方法1.1实验材料(1)分析纯钨酸钠;(2)分析纯硫酸钾;(3)分析纯草酸;(4)分析纯浓盐酸;(5)蒸馏水(自制).1.2实验设备(1)PHS一3C型精密酸度计(精度为0.1);(2)DF一101S型磁力搅拌器;(3)80—1型电动离心机;(4)DHGT一9101—1S型电热干燥箱(精度为1.0oC);(5)2(72-S型电热恒温箱(精度为1.0~C);(6)BS-224一S型电子天平(精度为0.1rag);(7)25mL移液管;(8>水热反应釜(内胆容量为20mL).1.3实验步骤(1)用电子天平称取适量的钨酸钠,硫酸钾和草酸同时溶解于一定量的蒸馏水中.(2)在磁力搅拌下,将浓盐酸按照一定速率滴加到(1)的混合溶液中,通过精密酸度计控制混合溶液的pH值,当混合溶液的pH值达到所需要的值时,停止浓盐酸的滴加,继续在磁力搅拌作用下的搅拌. 当混合溶液均匀时停止搅拌.(3)用移液管取一定量(2)的混合溶液滴加到水热反应釜中,使水热反应釜的填充量达到80,盖好水热反应釜的内盖和外盖.(4)将(3)的水热反应釜置于电热恒温箱中,加热到所需的温度,然后保温48.0h后停止加热,冷却. (5)将(4)制备出的混合溶液倒入离心管中,将此离心管放置到电动离心机中脱水,脱水后倒掉离心管的水,再往离心管中加人蒸馏水,然后再进行离心,反复进行3~4次.(6)将(5)中的离心管的试样放入电热恒温箱中,在80℃下干燥96.0h.然后重复(1)~(6)的步骤配置其他条件下的试样.(7)利用全自动粉末x射线衍射仪,热场发射扫描电子显微镜,紫外可见分光光度计分别对于燥后所得的样品进行物相,形貌,晶态和光敏性分析.1.4检测设备(1)D/max2500PC型全自动粉末x射线衍射仪;(2)JSM一7001F型热场发射扫描电子显微镜;(3)JEM一2100型高分辨透射电子显微镜;(4)UV一2450型紫外可见分光光度计.2实验结果与讨论2.1XRD分析不同反应温度和pH值下合成样品的XRD如图1.从图1可以看出,在反应体系pH值与反应温度不同的情况下所得到产物的XRD相同,与标准的X衍射粉末衍射卡片(JCPDS:33—1387)相对应,分别对应了wO.的(100),(001),(110),(1.0.)(1lo)i.,.1(202)(4o1)(a)j..4LL一一~..1㈣(d)10203040506070802o/(.)图1不同条件下48.0h水热法合成WO3的XRD(a)pH=10,150℃i(b)pH=1.5.150℃:(c)pH=15,180℃;(d)pH=15.210℃第3期傅小明等:钨酸钠水热法合成晶态WO.纳米棒及其表征?13? (200),(1l1),(201),(220),(202),(40O)和(401)晶面,是六方晶系的WO.,晶胞参数为口一7.298,c一3.899,所属空间群为P6/mm.2.2SEM分析在150~C和不同反应体系pH值条件下合成WO3的SEM如图2.图2(a)表明,当pH值为1.0时,通过水热法合成了短棒状的WO..图2(b)表明,随着pH值的增加,当pH值为1.5时,获得的棒状WO.变细.因此,在反应温度相同时,随着pH值的增加,有利于合成细棒状的WO..图2不同pH值150℃下水热法合成WO3的SEM(a)pH=10;(b)pH=1.5在反应体系pH值为1.5和不同反应温度条件下合成WO.的SEM如图3.图2(b)与图3(a)相比较可知,在反应体系pH值为1.5时,随着反应温度的升高,易合成较长的棒状WO..图3(a)与图3(b)相比较可得出,随着反应温度的进一步升高,更有利于合成较细长的棒状WO..所以,在反应体系pH值相同时,反应温度的升高,更有利于获得细长的棒状wO..图3pH值为15不同温度下水热法合成wo.的SEM(a)180℃;(b)210~C在反应体系pH值为1.5和反应温度为210~C下合成WO.的TEM和SAED如图4.图4的14?粉末冶金工业第21卷TEM表明,该条件下制备出了直径小于100Ylm的wO.纳米棒,此时的wo.纳米棒直径分布较均匀.图4的SAED显示该Wo.纳米棒为晶态纳米棒.其原因是:一方面,包酸钠在酸性环境中被酸化后,它形成了钨酸或者钨酸酐,然后在高温和高压的作用下钨酸或者钨酸酐被分解为w0..另一方面,w0.是一种各向异性的材料,更容易沿一维方向生长_1,因此,在高温和高压的作用下钨酸或者钨酸酐被分解为WO.更加容易生长成纳米棒.图4图3(bJ中WO的TEM和SAED2.3UV—VIS分析不同条件下合成WO.的紫外可见光的吸收光谱如图5.图5(a)与图5(b)相比较,在相同反应温度下,随着反应体系pH值的增大,合成WO.的吸收光向紫外光区域偏移,这表明在相同反应温度下, 随着反应体系pH值的增大,获得的WO.具有较好的紫外光吸收能力.图5(b)至图5(d)相比较,在反应体系的pH值相同的情况下,随着反应温度的升高,合成wO.的吸收光也向紫外光区域偏移,这说明在反应体系的pH值相同时,水热法合成的WO. 纳米棒具有良好的紫外光吸收能力.这是由于在紫外光的照射下,价带的电子被激发至导带,同时产生大量的空穴,新生成的电子与空穴在运动过程中若再次相遇便会以光或者热的形式释放能量,从而实现对紫外光的吸收所致¨1.因此,水热反应体系中合成纳米wo.棒时,不同pH值和温度影响了纳米w0.棒内部电子的排列,空穴和缺陷等的形成,从而使得不同条件下合成WO.的紫外吸收特性不同.图5不同条件下水热法48.0h合成wos的紫外可见光的吸收光谱图(a)pH=1.0,150℃;(b)pH=1.5.150℃;(C)pH=1.5,180℃;(d)pH=1.5,210℃3结论(1)在水热法体系中合成WO.纳米棒时,随着pH值的增加和反应温度的升高,pH值和反应温度都有利于WO.纳米棒的合成.在pH值为1.5和反应温度为210℃下合成直径小于]00nm的晶态wO.纳米棒,此WO.纳米棒直径分布较均匀.(2)对不同条件下水热法合成的WO.进行紫外可见光的吸收光谱分析可得,随着反应体系中pH值的增加和反应温度的升高,获得的WO.的紫外光吸收能力是逐渐增强的.特别是wO.纳米棒具有良好的紫外光吸收能力.参考文献[1]UlfTritthart,WolfgangGey,AlexanderGavrily—uk.NatureoftheopticalabsorptionhandinamorphousHWO3thinfilms[J].ElectrochimicaActa,1999,44(18):3039—3049.EelDebSK.Opticalandphotoelectricpropertiesandcol—ourcentresinthinfilmsoftungstenoxide[J].Phi一1OS.Mag.1973,27:801—822.[3]XuN,SunM,CaoYW,eta1.InfluenceofpHon structureandphotochromicbehaviorofnanocrystallinew()3films[J].AppliedSurfacesScience,2000,157(】一2):8184.E4]SunM,XuN,CaoYW,eta1.Preparation,microstruc—tureandphotochromismofanewnan0crystal1ineWO3film[J].Mater.Sci.Lett.,2000,19(16):1407—1409.[5]GrilliMI,BartolomeoEDi,TraversaE.NOsensors第3期傅小明等:钨酸钠水热法合成品态WO.纳米棒及其表征?15?行业动态?中国机械通用零部件协会粉末冶金分会发布2010年度创新优秀新产品奖和粉末冶金机械零件产量及应用领域统计中图分类号:TF12文献标识码:D根据"中国机械通用零部件行业创新优秀新产品的申报及评选办法"粉末冶金行业2010年创新优秀新产品经企业申报,专家评审并报中国机械通用零部件协会审批,现将获奖企业及项目公告如下:(1)东睦新材料集团股份有限公司获特等奖3项:VVT链轮F347729—0131,粉末冶金滚套,曲轴正时链轮.(2)扬州保来得科技实业有限公司获特等奖3项:粉末冶金汽车ABS速度检知盘,粉末冶金汽车转向器面凸轮,粉末冶金烧结焊偏心斜齿轮.(3)上海汽车粉末冶金有限公司获特等奖1项:BPSILON2转向管柱系列粉末冶金零件.(4)东睦(天津)粉末冶金有限公司获特等奖1项:变速箱换挡块.(5)杭州前进齿轮箱集团股份有限公司粉冶厂获优秀奖1项:某航空飞机制动器摩擦片研制.(6)黄石赛福摩擦材料有限公司获优秀奖1项:工程车辆湿式驱动桥用摩擦片.(7)海安县鹰球集团有限公司获优秀奖1项:粉末冶金高耐磨球铰.(8)湖南顶立科技有限公司获优秀1项:超细粉体材料专用无舟皿带式炉.(9)南通富士液压机床有限公司获优秀奖1项:FS79Z系列干粉自动成型液压机及模架.根据中国机械通用零部件协会粉末分会34家会员单位统计,2O10年粉末冶金机械零件生产应用情况为:产品产量铁基类:128185t,铜基类:8961t,总计:137146t..应用领域比例:运输机械(汽车,摩托车):62,工业机械(农机):1,电工机械(家电,电动工具):29,其他机械(工程机械等):8.中国机协粉末冶金分会秘书处basedoninterfacingyttriastabilizedzirconiawithPandn—typesemiconductingoxides[J].KeyEngineeringMa—terials,2002,206—213:12431246.[6]Xiaolanwei,PeikangShen.Electrochromicsofsinglecrys—tallinew.H2Onanorods[J]mun., 2006,8(2):293—298.[7]丁进月,钟良,张淑媛.溶胶一凝胶法制备纳米WO.气致变色材料EJ].化学进展,2009,21(6):1t71—1178. [8]BasergaA,RussoV,FonzoFDi,eta1.Nanostructured tungstenoxidewithcontrolledproperties:Synthesisand Ramancharacterization[J].ThinSolidFilms,2007,515 (16):6465—6469.[9]BlackmanS,ParkinIP.Atmosphericpressurechemical vapordepositionofcrystallinemonoclinicWO3andW()hthinfilmsfromreactionofWC16withO—contai—ningsolventsandtheirphotochromicandelectrochromic properties[J].Chem.Mater.,2005,17(6):1583—1590. DongJinKim,Su11Pyun.Hydrogentransport throughanodicw03films[J].ElectorchimActa,1998,43(16-17):2341-2347.CantaliniC,PelinoM,SunHT,eta1.Crosss'ensitivitv andstabilityofN02sensorsfromWO3thinfilm[J]. SensorsandActutorsB,1996,35(13):112-118.WuLZ,BharathiS,eta1.Sol—gelmaterialsinelectro—chemistry[J].Chem.Mater.,1997,11(9):2354—2375.陈亚琦.三氧化钨一维纳米结构气敏性能研究[D].长沙:湖南师范大学,2009.蔡万玲,宿新泰,王吉德.表面活性剂辅助超声合成纳米氧化钨粉体[J].中国钨业,2008,23(6):2628.王泽敏,刘勇,戢明,等.y-FeO.纳米粉末的光谱吸收特性研究[J].功能材料,2006,2(37):284286. 胡口l二I二J口。
化学专业英语前沿词汇-推荐下载

化学专业英语前沿讲座Seminar专业英语Professional English现代分析化学Modern analytical chemistry生物分析技术Bioanalytical techniques高分子进展Advances in polymers功能高分子进展Advances in functional polymers有机硅高分子研究进展Progresses in organosilicon polymers高分子科学实验方法Scientific experimental methods of polymers高分子设计与合成The design and synthesis of polymers反应性高分子专论Instructions to reactive polymers网络化学与化工信息检索Internet Searching for Chemistry & Chemical Engineering information 有序分子组合体概论Introduction to Organized Molecular Assembilies两亲分子聚集体化学Chemistry of amphiphilic aggregates 表面活性剂体系研究新方法New Method for studying Surfactant System微纳米材料化学Chemistry of Micro-NanoMaterials分散体系研究新方法New Method for studying dispersion分散体系相行为The Phase Behavior of Aqueous Dispersions溶液-凝胶材料Sol-Gel Materials高等量子化学Advanced Quantum Chemistry分子反应动力学Molecular Reaction Dynamic计算量子化学Computational Quantum Chemistry群论Group Theory分子模拟理论及软件应用Theory and Software of Molecular Modelling & Application 价键理论方法Valence Bond Theory量子化学软件及其应用Software of Quantum Chemistry & its Application分子光谱学Molecular Spectrum算法语言Computational Languange高分子化学Polymer Chemistry高分子物理Polymer Physics药物化学Medicinal Chemistry统计热力学Statistic Thermodynamics液-液体系专论Discussion on Liquid-Liquid System配位化学进展Progress in Coordination Chemistry无机材料及物理性质Inorganic Materials and Their Physical Properties物理无机化学Physical Inorganic Chemistry相平衡Phase Equilibrium现代无机化学Today's Inorganic Chemistry无机化学前沿领域导论Introduction to Forward Field in Inorganic Chemistry量子化学Quantum Chemistry分子材料Molecular Material固体酸碱理论Solid Acid-Base Theory萃取过程物理化学Physical Chemistry in Extraction表面电化学Surface Electrochemistry电化学进展Advances on Electrochemistry现代电化学实验技术Modern Experimental Techniques of Electrochemistry金属-碳多重键化合物及其应用Compounds with Metal-Carbon multiple bonds and The ir Applications叶立德化学:理论和应用Ylides Chemistry: Theory and Application立体化学与手性合成Stereochemistry and Chiral Synthesis杂环化学Heterocyclic Chemistry有机硅化学Organosilicon Chemistry药物设计及合成Pharmaceutical Design and Synthesis超分子化学Supramolecular Chemistry分子设计与组合化学Molecular Design and Combinatorial Chemistry纳米材料化学前沿领域导论Introduction to Nano-materials Chemistry纳米材料控制合成与自组装Controlled-synthesis and Self-assembly of Nano-materials 前沿讲座Leading Front Forum专业英语Professional English超分子化学基础Basics of Supramolecular Chemistry 液晶材料基础Basics of Liquid Crystal Materials 现代实验技术Modern analytical testing techniques色谱及联用技术Chromatography and Technology of tandem发光分析及其研究法Luminescence analysis and Research methods胶束酶学Micellar Enzymology分析化学中的配位化合物Complex in Analytical Chemistry电分析化学Electroanalytical chemistry生物分析化学Bioanalytical chemistry分析化学Analytical chemistry仪器分析Instrument analysis高分子合成化学Polymers synthetic chemistry高聚物结构与性能Structures and properties of polymers有机硅化学Organosilicon chemistry功能高分子Functional polymers有机硅高分子Organosilicon polymers高分子现代实验技术Advanced experimental technology of polymers高分子合成新方法New synthetic methods of polymers液晶与液晶高分子Liquid crystals and liquid crystal polymers大分子反应Macromolecules reaction水溶性高分子Water-soluble polymers聚合物加工基础The basic process of polymers聚合物复合材料Composite materials高等化工与热力学Advanced Chemical Engineering and Thermodynamics高等反应工程学Advanced Reaction Engineering高等有机化学Advanced Organic Chemistry高等有机合成Advanced Organic synthesis有机化学中光谱分析Spectrum Analysis in Organic Chemistry催化作用原理Principle of Catalysis染料化学Dye Chemistry中间体化学与工艺学Intermediate Chemistry and Technology化学动力学Chemical Kinetics表面活性剂合成与工艺Synthesis and Technology of Surfactants环境化学Environmental Chemistry化工企业清洁生产Chemical Enterprise Clean Production化工污染及防治Chemical Pollution and Control动量热量质量传递Momentum, Heat and Mass Transmission化工分离工程专题Separation Engineering耐蚀材料Corrosion Resisting Material网络化学与化工信息检索Internet Searching for Chemistry & Chemical Engineering information 新型功能材料的模板组装Templated Assembly of Novel Advanced Materials 胶体与界面Colloid and Interface纳米材料的胶体化学制备方法Colloid Chemical Methods for Preparing Nano-materials脂质体化学Chemistry of liposome 表面活性剂物理化学Physico-chemistry of surfactants高分子溶液与微乳液Polymer Solutions and Microemulsions两亲分子的溶液化学Chemistry of Amphiphilic Molecules in solution介孔材料化学Mesoporous Chemistry超细颗粒化学Chemistry of ultrafine powder分散体系流变学The Rheolgy of Aqueous Dispersions量子化学Quantum Chemistry统计热力学Statistic Thermodynamics群论Group Theory分子模拟Molecular Modelling高等量子化学Advanced Quantum Chemistry价键理论方法Valence Bond Theory量子化学软件及其应用Software of Quantum Chemistry & its Application计算量子化学Computational Quantum Chemistry分子模拟软件及其应用Software of Molecular Modelling & its Application分子反应动力学Molecular Reaction Dynamic分子光谱学Molecular Spectrum算法语言Computational Languange高分子化学Polymer Chemistry高分子物理Polymer Physics腐蚀电化学Corrosion Electrochemistry物理化学Physical Chemistry结构化学structural Chemistry现代分析与测试技术(试验为主)Modern Analysis and Testing Technology(experime tally)高等无机化学Advanced Inorganic Chemistry近代无机物研究方法Modern Research Methods for Inorganic Compounds萃取化学研究方法Research Methods for Extraction Chemistry单晶培养Crystal Culture固态化学Chemistry of Solid Substance液-液体系专论Discussion on Liquid-Liquid System配位化学进展Progress in Coordination Chemistry卟啉酞箐化学Chemistry of Porphyrine and Phthalocyanine无机材料及物理性质Inorganic Materials and Their Physical Properties物理无机化学Physical Inorganic Chemistry相平衡Phase Equilibrium生物化学的应用Application of Biologic Chemistry生物无机化学Bio-Inorganic Chemistry绿色化学Green Chemistry金属有机化合物在均相催化中的应用Applied Homogeneous Catalysis with Organometa llic Compounds功能性食品化学Functionalized Food Chemistry无机药物化学Inorganic Pharmaceutical Chemistry电极过程动力学Kinetics on Electrode Process电化学研究方法Electrochemical Research Methods生物物理化学Biological Physical Chemistry波谱与现代检测技术Spectroscopy and Modern Testing Technology理论有机化学theoretical Organic Chemistry合成化学Synthesis Chemistry有机合成新方法New Methods for Organic Synthesis生物有机化学Bio-organic Chemistry药物化学Pharmaceutical Chemistry金属有机化学Organometallic Chemistry金属-碳多重键化合物及其应用Compounds with Metal-Carbon multiple bonds and The ir Applications分子构效与模拟Molecular Structure-Activity and Simulation过程装置数值计算Data Calculation of Process Devices石油化工典型设备Common Equipment of Petrochemical Industry化工流态化工程Fluidization in Chemical Industry化工装置模拟与优化Analogue and Optimization of Chemical Devices化工分离工程Separation Engineering化工系统与优化Chemical System and Optimization高等化工热力学Advanced Chemical Engineering and Thermodynamics超临界流体技术及应用Super Cratical Liguid Technegues and Applications膜分离技术Membrane Separation Technegues溶剂萃取原理和应用Theory and Application of Solvent Extraction树脂吸附理论Theory of Resin Adsorption中药材化学Chemistry of Chinese Medicine生物资源有效成分分析与鉴定Analysis and Detection of Bio-materials相平衡理论与应用Theory and Application of Phase Equilibrium计算机在化学工程中的应用Application of Computer in Chemical Engineering微乳液和高分子溶液Micro-emulsion and High Molecular Solution传递过程Transmision Process反应工程分析Reaction Engineering Analysis腐蚀电化学原理与应用Principle and Application of Corrosion Electrochemistry腐蚀电化学测试方法与应用Measurement Method and Application of Corrosion Elect rochemistry耐蚀表面工程Surface Techniques of Anti-corrosion缓蚀剂技术Inhabitor Techniques腐蚀失效分析Analysis of Corrosion Destroy材料表面研究方法Method of Studying Material Surfacc分离与纯化技术Separation and Purification Technology现代精细有机合成Modern Fine Organic Synthesis化学工艺与设备Chemical Technology and Apparatuas功能材料概论Functional Materials Conspectus油田化学Oilfield Chemistry精细化学品研究Study of Fine Chemicals催化剂合成与应用Synthesis and Application of Catalyzer低维材料制备Preparation of Low-Dimension Materials手性药物化学Symmetrical Pharmaceutical Chemistry光敏高分子材料化学Photosensitive Polymer Materials Chemistry纳米材料制备与表征Preparation and Characterization of Nanostructured materials 溶胶凝胶化学Sol-gel Chemistry纳米材料化学进展Proceeding of Nano-materials Chemistry。
纳米材料在生活中的应用英语作文

纳米材料在生活中的应用英语作文Nanomaterials: A Revolutionary Leap in Technology.In the realm of science and technology, the advent of nanomaterials has ushered in a paradigm shift, unlocking unprecedented possibilities and transforming variousaspects of human life. These materials, characterized by their dimensions ranging from 1 to 100 nanometers, possess extraordinary properties that defy conventional materials.Unveiling the Unique Attributes of Nanomaterials.The defining feature of nanomaterials lies in their minuscule size, granting them an exceptionally high surface area-to-volume ratio. This attribute enables enhanced interactions with the surrounding environment, resulting in exceptional physical, chemical, and biological properties.Enhanced Reactivity: The increased surface area of nanomaterials facilitates efficient reactions and catalysis,accelerating processes that were previously sluggish or impossible. This property finds applications in fields such as energy conversion, pollution control, and drug delivery.Exceptional Strength and Durability: Nanomaterialsoften exhibit superior mechanical properties, including enhanced strength, hardness, and toughness. These characteristics make them ideal for use in lightweight materials, protective coatings, and high-performance electronics.Tunable Optical Properties: The optical properties of nanomaterials can be tailored by manipulating their size, shape, and composition. This allows for precise controlover light absorption, emission, and scattering, enabling applications in optics, photonics, and display technologies.Biocompatibility and Targeted Delivery: Certain nanomaterials exhibit biocompatibility, making themsuitable for biomedical applications. Their small size and ability to penetrate biological barriers facilitatetargeted drug delivery, enhancing treatment efficacy andminimizing side effects.Myriad Applications Transforming Industries.The remarkable properties of nanomaterials have spurred their adoption across a wide spectrum of industries, revolutionizing fields ranging from healthcare to energy to electronics.Healthcare: Nanomaterials play a pivotal role in drug delivery, diagnostics, and tissue engineering. Nanoparticles can encapsulate and deliver drugs directly to target sites, improving bioavailability and reducing toxicity. Nanosensors enable rapid and sensitive detection of diseases, while nanofibers facilitate the growth of new tissues for regenerative medicine.Energy: Nanomaterials offer promising solutions for sustainable energy production and storage. Nanostructured solar cells enhance light absorption and conversion efficiency, while nanobatteries provide high power density and extended cycle life. Fuel cells utilizing nanomaterialsexhibit improved performance and durability.Electronics: The miniaturization of electronic devices relies heavily on nanomaterials. Carbon nanotubes and graphene enable the development of flexible and transparent electronics, while nanoscale transistors enhance processing speed and power efficiency.Materials Science: Nanomaterials are incorporated into composite materials to improve mechanical, thermal, and electrical properties. Nanoclay additives enhance polymer strength, while nanocoatings provide corrosion resistance and self-cleaning abilities.Environmental Protection: Nanomaterials find applications in environmental remediation and pollution control. Nanoscale catalysts accelerate the breakdown of pollutants, while nanoporous membranes enable efficient water purification and desalination.Challenges and Future Prospects.Despite the transformative potential of nanomaterials, several challenges remain to be addressed. Concerns regarding potential health and environmental risks associated with their unique properties require careful assessment and risk management. Standardization of synthesis and characterization techniques is crucial to ensure quality and reproducibility.Looking ahead, the future of nanomaterials holds immense promise. Continued research and development efforts will lead to the discovery of new materials and applications, further expanding their impact on various sectors. The integration of nanomaterials with other emerging technologies, such as artificial intelligence and biotechnology, is expected to unlock even greater possibilities.Conclusion.Nanomaterials represent a groundbreaking advancement in technology, offering a plethora of novel opportunities and challenges. Their unique properties have revolutionizedindustries and hold immense potential for shaping the future of society. As research continues to unravel their full capabilities, the applications of nanomaterials will undoubtedly continue to expand, ushering in an era of unprecedented technological breakthroughs and societal transformations.。
球形硫化镉半导体纳米材料的合成

球形硫化镉半导体纳米材料的合成曹强;王赛赛;张倩【摘要】Objective:To synthesize CdS nanomaterials with different microstructure and morphologies by a new meth-od. Methods:CdS nanoparticles were synthesized using DMSO-solvothermal without any surfactant. Microstructure,mor-phologie and size control of the CdS nanomaterials were achieved by simple adjustments of the reactantsˊ concentration and the reaction time through adding potassium chloride as stabilizing agent. Resluts:Under different conditions,the XRD dif-fraction peaks of the synthesized CdS nanomaterials were all corresponded to the characteristic diffraction peaks of the Cad-mium sulfide(No. 41-1049 JCPDS),and no impurity peaks were obtained. Conclusion:By changing the reaction condi-tions,the structure and the micro size of the CdS can be controlled,and the synthesis is a low temperature,short time,low cost and simple operation method.%目的:用一种新的方法合成出球形的纳米硫化镉材料。
微乳法
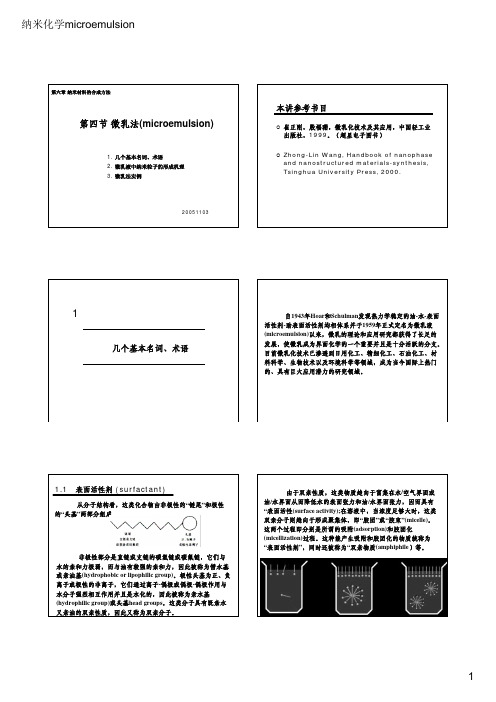
(5) Ostwald陈化(Ostwald Ripening), 根据Kelvin公式,小质点 (4)转相, 由于表面活性剂在油、水两相中的溶解度相对大小与 表面活性剂的亲水亲油平衡(Hydrophile-Lipophile Balance),即 HLB密切相关,因此可以说,表面括性剂的HLB是决定乳状液 类型的主要因素。非离子表面活性剂的HLB是温度的函数。在 低温下呈现水溶性的非离子表面活性剂在高温下则呈油溶性。 因此,用非离子表面活性剂作乳化剂时,若在低温下制得O/W 乳液,在高温下则会变为W/O型。发生变形时的温度称为相转 变温度(Phase Inversion Temperature),简称PIT。 式中Cr是微小晶体的溶解度,C是普通晶体的溶解度,γ(s) 及ρ(s)分别为固体的界面张力及密度。 将比大质点具有更大的溶解度。于是小质点将不断溶解,大质 点将不断长大。这一过程称为Ostwald陈化。在乳状液体系中, 它通过分散相经过连续相介质的分子扩散而进行。这一过程导 致体系的平均质点半径随时间增大,因此是一种不稳定过程。
4
纳米化溶剂中,随着双亲物质浓度的增大,也能形成 聚集体.这种聚集体通常以亲水基相互靠拢,而以亲油基朝向溶 剂,其构型与水相中的胶团正好相反,因此被称为反胶团或逆 胶团(Reversed or inserted Micelle)。例如,在水/油/非离子表面 活性剂体系中,低温时表面活性剂在水相形成胶团,但随着温 度的升高,表面活性剂逐步转移到油相,并形成反胶团。
2
纳米化学microemulsion
1.2.1 乳状液的形成 根据热力学理论,乳状液不能自发形成。因此,要使一 个油/水体系变成乳液,必须由外界提供能量。 制备乳状液的主要方法是分散法,即通过搅拌、超声波 作用或其它机械分散作用使两种流体充分混合,最终使得一相 分散在另一相中。 制备乳液时的个关键问题是制得的乳液是哪一种类型, 经验证明,影响乳液类型的因素有: ①两相的体积比。 ③表面活性剂的性质和浓度。 ②两相的粘度差异。 ④温度。
- 1、下载文档前请自行甄别文档内容的完整性,平台不提供额外的编辑、内容补充、找答案等附加服务。
- 2、"仅部分预览"的文档,不可在线预览部分如存在完整性等问题,可反馈申请退款(可完整预览的文档不适用该条件!)。
- 3、如文档侵犯您的权益,请联系客服反馈,我们会尽快为您处理(人工客服工作时间:9:00-18:30)。
ORIGINAL PAPERSynthesis of micro and nanostructured MnO 2and their comparative study in lithium batteryA.Pendashteh •M.F.Mousavi •M.A.Kiani •S.H.Kazemi •M.S.RahmanifarReceived:14March 2011/Accepted:23October 2011/Published online:5January 2012ÓIranian Chemical Society 2012Abstract Micro and nanostructured c -MnO 2are synthe-sized to investigate the size effect of cathode active materials in battery performance.MnSO 4and (NH 4)2S 2O 8were used as starting materials to prepare micro and nanostructured samples in the presence of stirring and ultrasonic irradiation,respectively.Structure optimization is done by changing values for temperature and manganese sulphate concentration.The MnO 2micro and nanoparticles are characterized by scanning electron microscopy and X-ray diffraction (XRD).The XRD results reveal that only c -MnO 2is formed under the reaction conditions.Under the optimized conditions,manganese dioxide nanoparticles,with an average particle size of 56nm,are obtained.Both micro and nanostructured MnO 2is used as the cathode active material in Li/MnO 2battery.Discharge profiles of stirrer-based cathode material (micro)and ultrasonic instrument-based one (nano)compared with each other in constant discharge currents of 50and 100mA g -1.The results demonstrated that nanosized materials show higher specific capacities and energies.Electrochemical imped-ance spectroscopy is used to investigate the size effect of cathode material on battery resistance and the results show a copious decrease in total resistance.Keywords Lithium battery ÁNanostructure ÁManganese dioxide ÁUltrasonicationIntroductionAmong different types of lithium batteries,the primary lithium/manganese dioxide cell was one of the first Li cells to be commercialized.The advantageous features of these cells include high nominal voltage (3V),high specific energy (230Wh kg -1),high energy density (535Wh L -1),and operation over a wide temperature range,superior shelf life,and low cost.Li/MnO 2primary battery as a high energy density,high drain power source uses heat-treated manga-nese dioxide (HTMD),a defect pyrolusite structure material as the cathode active material.Actually,c -MnO 2,which is the most promise candidate among the large variety of manganese oxides for use as positive electrode in non-aqueous batteries,requires a dehydration process above 300°C [1].Nanostructures have received steadily growing interest not only for their fundamental scientific significance but also for their many technological applications.These structures have been used extensively in various kinds of energy stor-age systems [2–4].Electrochemical properties of a material are extremely affected by the morphology and surface area of the electrode particles.Electrochemical performance of many materials has been improved significantly because of size effect of nanostructured active materials in the electrode [5–10].Nanostructured materials employed in electrodes decrease solid-state diffusion path length and thereby exhibit better electrochemical performance as expected.Among a variety of physicochemical methods,including hydrothermal [11],sol-gel [12],electrochemical methods [13]and others,the sonochemical methods have beenA.Pendashteh ÁM.F.Mousavi (&)ÁM.A.Kiani Department of Chemistry,Tarbiat Modares University,P.O.Box 14115-175,Tehran,Irane-mail:mfmousavi@;mousavim@modares.ac.ir S.H.KazemiDepartment of Chemistry,Institute for Advanced studies in Basic Sciences,Zanjan,Iran M.S.RahmanifarFaculty of Basic Sciences,Shahed University,Tehran,IranJ IRAN CHEM SOC (2012)9:389–395DOI 10.1007/s13738-011-0049-0shown to be very promising in the preparation of a variety of nanomaterials[14–18].The success of sonochemistry in creating nanostructured materials principally originates from acoustic cavitation;the formation,growth,and implosive collapse of bubbles in a liquid which causes intense local heating(5,000°C),pressure(1,800atm),and cooling rates greater than1010K s-1[19].Extensive interests have been focused on developing MnO2nanostructures and significant progress has been reported[20–31].Manganese dioxide can form different types of polymorphs,such as a,b,c,and d.Among them, especially c-MnO2has good electrochemical performances and is used in battery industries[32–34].This work extends our previous studies of various electrochemical energy storage systems,such as polyani-line battery[35–41],and lead–acid battery[42].Herein,we report the synthesis of micro and nanostructured manga-nese dioxide and some of reaction conditions including reactant concentration and temperature have been opti-mized.Synthesized samples were used in Li/MnO2bat-teries as cathode active material.The influence of particle size and structure of cathode active material on battery performance for lithium batteries will also be studied and compared.ExperimentalApparatusA multiwave ultrasonic generator(Sonicator-3000;Miso-nix,Inc.,Farmingdale,NY,USA),equipped with a con-verter/transducer and titanium oscillator(horn),12.5mm in diameter,operating at20kHz with a maximum power output of600W,was employed for the ultrasonic irradi-ation.The power level was adjusted automatically by the ultrasonic generator.Circulating water from a water bath (Optima,Tokyo,Japan)kept the solution temperature constant.An electrical furnace(Paragon E10,USA)was used to dehydrate the synthesized samples.Morphological studies of prepared samples were performed by SEM (Philips XL30).XRD(Philips X’pert diffractometer)and Cu K a radiation(k=0.15418nm)was used to study the phase composition of the prepared samples.Effective surface area of the samples was measured by Brunauer–Emmett–Teller(BET)(BEL Japan,Inc.).The EIS and discharge tests were carried out using Galvanostat/Poten-tiostat Autolab(PGSTAT30)connected to a PC. MaterialsMnSO4ÁH2O,(NH4)2S2O8and propylene carbonate(PC) were supplied from MERCK and used without any purification.LiClO4was purchased from ACROS for pre-paring the electrolyte and Lithium(from Fluka)was used as anode material.Doubly distilled water was used throughout.Synthesis of MnO2nanoparticlesA two-walled cylindrical glass cell with an interior volume of300ml was employed for the synthesis of MnO2.The redox reaction between manganese sulphate and ammonium peroxydisulphate was adopted for synthesis of c-MnO2. 100mL of MnSO4ÁH2O and100mL of(NH4)2S2O8solu-tions with definite concentrations were mixed together in reaction vessel and the solution was sonicated for1h.Then the solution was centrifuged at7,500rpm for5min.A dark brown precipitate was obtained,washed several times with doubly distilled water and dried in an oven at80°C for6h. The obtained powder was annealed in350°C in electrical furnace for2.5h to dehydrate and form HTMD[1].The experiment was also repeated using stirring in the long enough time of24h instead of ultrasonication and the resulting precipitate was centrifuged,washed,and dried as described above.CharacterizationThe crystalline phases of the synthesized samples were characterized by X-ray diffraction(XRD)using Cu K a radiation(k=0.15418nm).Morphological studies of synthesized samples were performed by scanning electron microscopy,SEM.The BET method[43]was used to measure effective surface area by calculating the amount of absorption/desorption of N2gas in a sample.Electrode fabrication and electrochemical measurementsThe positive electrode was fabricated by mixing of the synthesized MnO2as the cathode active material,activated carbon(Merck),and polytetrafluoroethylene(PTFE)(60% water based solution from SUTECH,Iran)as binder with a weight ratio of85:10:5.The resultant slurry was coated onto a nickel plate(1cm in diameter),pressed under a pressure of100kg cm-2and then dried in the oven at 120°C for24h.Cells were composed by stacking cathode against a Li anode,separated by polypropylene separator (Celgard3401)soaked in electrolyte(1M LiClO4in PC). The schematic diagram of the Teflon cell used in the experiments is shown in Fig.1.Because of the high reac-tivity of metallic lithium toward atmospheric oxygen,cell assembly and all of the electrochemical tests were per-formed in an argon atmospheric glove box.EIS was carried out by applying an ac signal with amplitude of 5mV at the frequency varying from 100kHz to 100mHz at an open circuit voltage (OCV).Data anal-ysis was done based on CNLS method of Boukamp using Zview2software (Scribner Associates),using an appro-priate equivalent circuit described in the text.The discharge experiments were examined in constant current densities,50and 100mA g -1in the low cut-off voltage of 1.5V versus Li at 25°C.Results and discussion X-ray studiesSuccessful synthesis of manganese dioxide was demon-strated by XRD of the prepared samples in all designed conditions.Figure 2a shows the XRD spectrum of the sample prepared by using stirrer.As it can be demonstrated from this spectrum,product of the reaction is Mn(OOH)2(JCPS card no.17-0510),a mixture of different manganese oxides with the oxidation states of 2?and 4?.The exactformula of the product is Mn (1-x )4?Mn x 2?O (2-2x )(OH)2x when x =0.06–0.07.Manganese content with the oxida-tion state of 2?reveals that despite assigning the long enough time (24h)for reaction to be completed,the oxi-dant (ammonium peroxydisulphate)could not complete the oxidation of whole manganese content.But irradiating the solution by ultrasonic wave completed the oxidation in a short time of 1h and resulted the MnO 2as the only product.It has been demonstrated that ultrasonic irradiation can prompt and complete the oxidation process by hydro-xyl radical generation [15].The oxidation proceeds in the presence of ultrasonication as follows:MnSO 4ÁH 2O þðNH 4S 2O 8þH 2O ÞÀ!ÞÞÞMnO 2þðNH 4Þ2SO 4þ2H 2SO 4The X-ray powder diffraction spectrum of nanostructured c -MnO 2is shown in Fig.2b.As it can be seen from XRD spectra,micro-sized samples have clear and sharp peaks which indicate that c -MnO 2have big crystallite size unlikenanosized sample with broader peaks showing the small size of particles.SEM studiesFigure 3shows SEM images of micro and nanostructures of c -MnO 2,synthesized by using stirrer and sonicator,respectively.Figure 3a and b are concerned to the samples,prepared through a simple chemical oxidation using stirrer during the reaction in 50,and 60°C,respectively.It can be seen from both images that the synthesized particles are aggregated and do not have a definite morphology.We also investigated the effects of manganese sulphate concentra-tion (0.05,0.1,and 0.2M)and temperature (40,50,and 60°C)on the particle size,and morphology of the prepared manganese dioxide.In previous works,it has been found that the applied ultrasonic wave determines the particle size.Increasing the ultrasonic wave amplitude decreases the rate of particle growth [15].Therefore,in this work,we have used constant sonication wave amplitude of about 84l m.The synthesis efficiency at the temperature of 40°C was too low that the amount of prepared samples was not adequate to be characterized by SEM.Tempera-tures higher than 60°C was not investigated because of high vaporization which yields significant concentration changes.Figure 3c,d,and e shows the SEM images of samples synthesized at temperature of 50°C,but in dif-ferent concentrations of manganese sulphate of 0.05,0.1,and 0.2M,respectively.As it can be observed from these images,size of MnO 2particles has been reduced by increase in MnSO 4concentration.Furthermore,the increase in MnSO 4concentration has led to change in morphology of prepared samples.Low concentration (0.05M)leads to the formation of rice-shape particles (Fig.3c).The prepared nanoparticles in this situation did not grow a lot in length,probably due to the effect of ultrasonication.As the concentration of MnSO 4is raised,the morphology of the synthesized particles changed from rice-shape to globular nanoparticles (Fig.3d,e).As it can be seen in Fig.3e,increasing MnSO 4concentrationtoFig.1Schematic diagram of the Teflon cell used in electrochemicalexperimentsFig.2X-ray powder diffraction pattern for a sample prepared in the presence of stirrer (micro),b nanostructured c -MnO 2in the presence of sonicator0.2M led to formation of MnO 2nanoparticles with an average size of 65nm (57–90nm).This decrease in size is attributed to more nucleation centres in high concentration of MnSO 4,which prevents the nucleation growth process to proceed and therefore leads to smaller particles in size.Figure 3f shows the SEM image of the sample which was synthesized at 60°C and in MnSO 4concentration of 0.2M while ultrasonication was applied during the reaction.An increase in temperature reaction from 50to 60°C caused a change in solution color from light brown to dark brown,which clearly shows the increase in reaction rate in high temperatures.Figure 3e shows a more compact assemblies or aggregates than those in Fig.3f,which may be attributed to the partial expansion of nano-MnO 2aggregates caused by increasing the temperature from 50to 60°C.This leads to an increase in the specific surface area of these assem-blies.Therefore,the synthesis condition of the sample shown in Fig.3f was considered as optimized to preparation of the most uniform morphology and the largest specific surface area (20.80m 2g -1).Thus,all of the electrochem-ical tests done to investigate the use of nanoparticles as the cathode material of Li/MnO 2battery were performed by using the samples synthesized in condition of using soni-cator,0.2M MnSO 4,and 60°C.Fig.3SEM micrographs of micro and nanostructured c -MnO 2synthesized in the presence of stirrer and sonicator,respectively.a stirrer,0.1M MnSO 4,50°C,b stirrer,0.1M MnSO 4,60°C,c sonicator,0.05M MnSO 4,50°C,d sonicator,0.1M MnSO 4,50°C,e sonicator,0.2M MnSO 4,50°C,f sonicator,0.2M MnSO 4,60°CPorosity measurementsBET was used to measure and compare the effective sur-face area of prepared microstructured and nanostructured c-MnO2.As expected,BET measurements showed larger effective reactive surface area for nanostructured MnO2 against microstructured one.According to these measure-ments,specific surface area of micro-synthesized sample is 14.63m2g-1;while that for nanostructure sample syn-thesized in50°C is17.20and20.80m2g-1for the sample synthesized in60°C.Electrochemical studiesElectrochemical impedance measurement in a battery reflects a combined effect of the impedances of negative electrode,electrolyte,and positive electrode and is a powerful method for analysis of internal battery resistance. Typical Nyquist plot of synthesized c-MnO2with micro and nanostructure at OCV are shown in Fig.4.The spectra display two arcs,one in high-frequency region which is related to the Li-ion migration resistance through the solid electrolyte interface(SEI)film(R1coupled with SEIfilm capacitance),and the second arc in the medium-frequency, corresponds to charge transfer resistance coupled with double layer capacitance[44].The inset in Fig.4depicts the equivalent circuit used tofit experimental data.The parameters of impedance spectra in Fig.4werefitted by Zview software.The results are listed in Table1.In a two-electrode Li/cathode configuration,the contributions of the electrolyte and lithium electrode to the EIS are believed to be constant due to a result of the instant stripping of lith-ium,which remains the surface of lithium constantly fresh [44].Therefore,all of the variations in the EIS by changing the cathode material structure can be intrinsically attributed to the structure.The EIS results show that the total resis-tance is decreased from131.91to88.54X cm2as the MnO2structure changed from micro to nano.It is obvious that using nanosized material declined the charge transfer resistance,which can be attributed to the decreased diffu-sion path length due to the presence of small particles in the cathode layer.Discharge studiesFigure5a shows the discharge profiles of c-MnO2,micro and nanostructures in a constant discharge current density of50mA g-1.As it can be seen,the cell with micro-structured materials showed a discharge capacity of about 171.26mAh g-1and the one with nanostructures showed a capacity equal to213.48mAh g-1which shows about25% improvement.In Fig.5b,it has been shown that the dis-charge capacity of micro and nanostructurecathode Fig.4Comparison of Nyquist plots of micro and nanostructured c-MnO2(MnO2/Li system,1M LiClO4in PC,at OCV).The inset shows the corresponding equivalent circuit used tofit the experimen-tal dataTable1Impedance parameters of equivalent circuit used tofit the experimental dataMnO2Resistance(X cm2)R s R1R2Micro 3.3974.2054.32 Nano 2.3737.2248.95Fig.5Comparison of discharge profile of micro and nanostructured c-MnO2,at two different current discharge,a50mA g-1and b100mA g-1material is158.94and193.02mAh g-1at the constant discharge current of100mA g-1(about22%capacity improvement).As seen from discharge profiles in both currents,using nanostructured MnO2as cathode material rendered higher average voltage,which it yields higher specific energy.Specific energy(SE)of the battery systems calculated from the following equation:SE¼IVt2MÂ3;600!ð1Þwhere I is the discharge current density,V is the average voltage,t is the discharge time and M is the amount of active materials in the electrode.In discharge current of 50mA g-1,the value of specific energy for nanostructured material is254.41Wh kg-1;while for microstructured one is195.91Wh kg-1.As seen,there is58.5Wh kg-1 improvement in specific energy by using nanostructured active materiel.It can be seen from discharge profiles that the discharge capacity for nanostructure material showed higher values compared to that of bulk c-MnO2.The spe-cific capacity increase in the nanoparticles can be attributed to their small particle size which causes an increase in the active surface area.The increase in the specific surface area,increases the efficiency of the electrochemical inser-tion of the Li?ions into the structure of MnO2.The nanoparticles have enhanced reactivity due to the increase in surface area.Furthermore,going to the nanosized materials decrease the solid-state diffusion path lengths. All of these lead to an improvement in electrochemical behavior.The discharge capacity at high rate is directly related to the active surface area of the electrodes and thus to the current density[45].The effective current density (current density per reactive surface area)decreases as the surface area increases.Therefore,the higher surface area of nanostructure cathode material lowers the discharge cur-rent density and thereby increases its discharge capacity. According to a literature survey,some of the investigated systems by others listed in Table2.Capacity values of the current study demonstrate the size effect on battery per-formance and have a good accordance with reported data of nanostructured MnO2and are much higher than that of the related bulk materials.ConclusionNanostructured manganese dioxide was synthesized in the presence of ultrasonic radiation,which facilitated oxidation of manganese sulphate with ammonium peroxydisulphate as an oxidant.The structure of the micro and nanoparticles were characterized by XRD and SEM.The prepared MnO2 nanoparticles were used as cathode active material in Li/MnO2primary cell.Capacities compared to capacities of those cells with the micro-sized cathode material prepared by using stirrer.It is demonstrated that using nanoparticles showed higher capacities of213.48and193.02mAh g-1at discharge current of50and100mA g-1,respectively. Electrochemical impedance spectra demonstrated that electrochemical reaction activity of the electrode material with synthesized nanostructure is dramatically improved and the total resistance of the battery is decreased. Acknowledgments We gratefully acknowledge the Tarbiat Mod-ares University Research Council forfinancial support of this work.References1.W.Bowden,T.Bofinger, F.Zhang,N.Iltchev,R.Sirotina,Y.Paik,J.Power Sources165,609(2007)2.Kh.Ghanbari,M.F.Mousavi,M.Shamsipur,Electrochim.Acta.52,1514(2006)3.S.Ghasemi,M.F.Mousavi,H.Karami,M.Shamsipur,S.H.Kazemi,Electrochim.Acta.52,1596(2006)4.H.R.Ghenaatian,M.F.Mousavi,S.H.Kazemi,M.Shamsipur,Syn.Met.159,1717(2009)5.T.Doi,Y.Iriyama,T.Abe,Z.Ogumi,Chem.Mat.17,1580(2005)6.H.Li,L.Shi,Q.Wang,L.Chen,X.Huang,Solid State Ionics148,247(2002)7.S.S.Zhang,M.S.Ding,K.Xu,J.Allen,T.R.Jow,Electrochem.Solid-State Lett.4,A206(2001)8.X.Wu,H.Li,L.Chen,X.Huang,Solid State Ionics149,185(2002)9.H.Li,L.H.Shi,W.Lu,X.J.Huang,L.Q.Chen,J.Electrochem.Soc.148,A915(2001)10.Kh.Ghanbari,S.Z.Bathaie,M.F.Mousavi,Biosen.Bioelectron.23,1825(2008)11.Q.H.Zhang,S.Sun,S.Li,H.Jiang,J.G.Yu,Chem.Eng.Sci.62,4869(2007)12.M.Sugantha,P.A.Ramakrishnan,A.M.Hermann,C.P.Warm-singh,D.S.Ginley,Int.J.Hydrogen Energy28,597(2003)Table2Comparison of the current study with some of the investigated systems beforeSystem Particlesize(nm)Electrolyte Discharge current(mA g-1)Voltagerange(V)Capacity(mAh g-1)Referencec-MnO2(bulky)/Li–LiPF6-DMC/EC20 4.0–2.0181Lee et al.[46]a-MnO2nanorods/Li10LiPF6-DMC/EC50 3.8–1.5206.7Wang et al.[47] c-MnO2nanorods/Li20–100_50 3.8–1.5210Cheng et al.[48] MnO2nanowires/Li50–100LiPF6-DMC/EC150 3.9–2.0160Kim et al.[49] MnO2nanowires/Li50–100LiPF6-DMC/EC300 3.9–2.0150Kim et al.[49] Current study56(avg.)LiClO4-PC50(100) 3.4–1.5213.48(193.02)–13.M.Wu,L.Zhang,J.Gao,C.Xiao,D.Wang,A.Chen,S.Zhang,J.Electroanal.Chem.613,125(2008)14.M.A.Kiani,M.F.Mousavi,S.Ghasemi,J.Power Sources195,5794(2010)15.S.Ghasemi,M.F.Mousavi,M.Shamsipur,H.Karami,Ultrason.Sonochem.15,448(2008)16.A.Zolfaghari,F.Ataherian,M.Ghaemi,A.Gholami,Electro-chim.Acta.52,2806(2007)17.K.H.Kim,K.B.Kim,Ultrason.Sonochem.15,1019(2008)18.E.Ohayan,A.Gedanken,Ultrason.Sonochem.17,173(2010)19.K.S.Suslick,Sonochemistry,Kirk-Othmer Encyclopedia ofChemical Technology,4th edn.,vol.26(Wiley,New York,1998), pp.517–54120.L.Yuan,Z.Li,J.Sun,K.Zhang,Y.Zhou,Mater.Lett.57,1945(2003)21.M.S.Wu,Appl.Phys.Lett.87,153102(2005)22.M.S.Wu,J.T.Lee,Y.Y.Wang,C.C.Wan,J.Phys.Chem.B108,16331(2004)23.L.Li,Y.Pan,L.Chen,G.Li,J.Solid State Chem.180,2896(2007)24.F.Y.Cheng,J.Chen,X.L.Gou,P.W.Shen,Adv.Mater.17,2753(2005)25.F.Y.Cheng,J.Z.Zhao,W.E.Song,C.S.Li,H.Ma,J.Chen,P.W.Shen,Inorg.Chem.45,2038(2006)26.V.Subramanian,H.W.Zhu,R.Vajtai,P.M.Ajayan,B.Q.Wei,J.Phys.Chem.B109,20207(2005)27.Z.Y.Yuan,Z.Zhang,G.Du,T.Z.Ren,B.L.Su,Chem.Phys.Lett.378,349(2003)28.C.Z.Wu,Y.Xie,D.Wang,J.Yang,T.W.Li,J.Phys.Chem.B107,13583(2003)29.Y.Xiong,Y.Xie,Z.Li,C.Wu,Chem.Eur.J.9,1645(2003)30.X.Wang,Y.D.Li,mun.764(2002)31.X.Wang,Y.D.Li,J.Am.Chem.Soc.124,2880(2002)32.B.Ammundsen,J.Paulsen,Adv.Mater.13,943(2001)33.M.S.Whittingham,Chem.Rev.104,4271(2004)34.M.M.Thackeray,Prog.Solid State Chem.25,1(1997)35.M.S.Rahmanifar,M.F.Mousavi,M.Shamsipur,J.Power Sour-ces110,229(2002)36.H.Karami,M.F.Mousavi,M.Shamsipur,J.Power Sources117,255(2003)37.H.Karami,M.F.Mousavi,M.Shamsipur,J.Power Sources124,303(2003)38.M.S.Rahmanifar,M.F.Mousavi,M.Shamsipur,M.Ghaemi,J.Power Sources132,296(2004)39.M.S.Rahmanifar,M.F.Mousavi,M.Shamsipur,H.Heli,Syn.Met.155,480(2005)40.H.Karami,M.F.Mousavi,M.Shamsipur,S.Riahi,J.PowerSources154,298(2006)41.Kh.Ghanbari,M.F.Mousavi,M.Shamsipur,H.Karami,J.PowerSources170,513(2007)42.H.Karami,M.Shamsipur,S.Ghasemi,M.F.Mousavi,J.PowerSources164,896(2007)43.S.Brunauer,P.H.Emmett,E.Teller,J.Am.Chem.Soc.60,309(1938)44.S.S.Zhang,K.Xu,T.R.Jow,Electrochim.Acta.51,1636(2006)45.J.Drews,R.Wolf,G.Fehrmann,R.Staub,J.Power Sources65,129(1997)46.J.Lee,J.M.Lee,S.Yoon,S.O.Kim,J.S.Sohn,K.I.Rhee,H.J.Sohn,J.Power Sources183,325(2008)47.H.Wang,Z.Lu,D.Qian,S.Fang,J.Zhang,J.Alloys Compd.466,250(2008)48.F.Cheng,J.Zhao,W.Song,C.Li,H.Ma,J.Chen,P.Shen,Inorg.Chem.45,2038(2006)49.J.H.Kim,T.Ayalasomayajula,V.Gona, D.Choi,J.PowerSources183,366(2008)。