跳跃机器人的研究
机器人跳跃运动控制与优化

机器人跳跃运动控制与优化引言:机器人技术的发展正在改变着我们的生活方式和工作方式。
在许多领域,机器人的应用正在变得越来越重要,其中之一便是机器人的跳跃运动控制。
机器人跳跃是一项复杂而具有挑战性的任务,它需要机器人准确地调整姿势、力量和运动速度,以克服地心引力和其他外部因素,从而实现高效且稳定的跳跃。
一、机器人跳跃的挑战在机器人跳跃的控制过程中,有许多复杂因素需要考虑。
首先,机器人需要准确定位和判别所在环境的性质,以便做出相应的决策。
其次,机器人必须具备较强的计算能力和反应速度,以便在瞬息万变的环境中做出适时的调整。
最后,机器人还需要具备高度稳定性和精确性,以克服重力以及其他各种外部因素的干扰。
二、机器人跳跃的运动控制方法为了实现机器人跳跃的运动控制,目前主要采用以下几种方法:1. 传感器数据反馈控制机器人可以通过使用各种类型的传感器来感知其周围环境,从而根据反馈的数据做出相应调整。
例如,通过使用激光传感器来感知前方障碍物的位置和形状,机器人可以调整着陆点和跳跃角度,以避免碰撞并实现平稳的跳跃。
2. 机器学习控制机器学习技术可以在训练阶段对机器人进行大量的数据输入和模拟试验,从而使其能够学习到适应不同环境和任务的跳跃策略。
通过不断地反馈和调整,机器人可以逐渐提高跳跃的精确性和效率。
3. 优化算法控制优化算法是一种通过迭代优化的方式,寻找最佳控制策略的方法。
通过定义适应度函数和约束条件,机器人可以通过优化算法来找到最优的跳跃力量、角度和速度。
这种方法可以在较短的时间内找到接近最优解,并在跳跃过程中实时调整姿势和控制参数。
三、机器人跳跃的应用领域机器人跳跃技术在许多领域都有广泛的应用。
例如,在救援任务中,机器人可以通过跳跃来越过障碍物或深沟,以便更快地到达被困人员的位置,从而提高救援效率。
此外,机器人跳跃技术还可以应用于工业生产线上的物料运输和分拣过程中,能够提高运输效率和减少人力成本。
四、机器人跳跃的未来展望随着技术的不断进步,机器人跳跃的控制和优化算法将会越来越成熟和高效。
机器人跳跃运动控制与优化
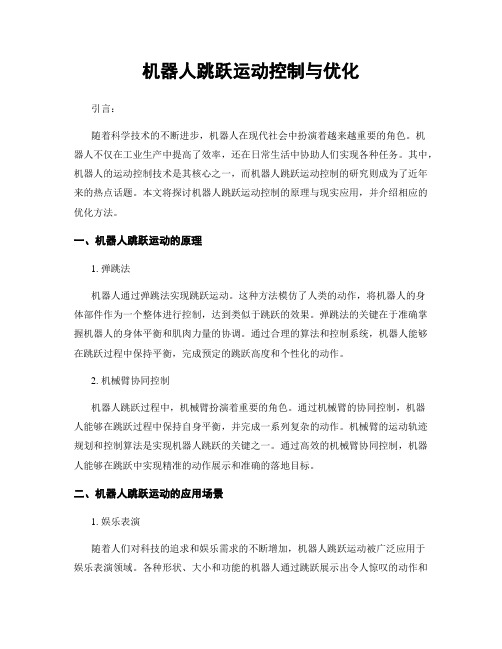
机器人跳跃运动控制与优化引言:随着科学技术的不断进步,机器人在现代社会中扮演着越来越重要的角色。
机器人不仅在工业生产中提高了效率,还在日常生活中协助人们实现各种任务。
其中,机器人的运动控制技术是其核心之一,而机器人跳跃运动控制的研究则成为了近年来的热点话题。
本文将探讨机器人跳跃运动控制的原理与现实应用,并介绍相应的优化方法。
一、机器人跳跃运动的原理1. 弹跳法机器人通过弹跳法实现跳跃运动。
这种方法模仿了人类的动作,将机器人的身体部件作为一个整体进行控制,达到类似于跳跃的效果。
弹跳法的关键在于准确掌握机器人的身体平衡和肌肉力量的协调。
通过合理的算法和控制系统,机器人能够在跳跃过程中保持平衡,完成预定的跳跃高度和个性化的动作。
2. 机械臂协同控制机器人跳跃过程中,机械臂扮演着重要的角色。
通过机械臂的协同控制,机器人能够在跳跃过程中保持自身平衡,并完成一系列复杂的动作。
机械臂的运动轨迹规划和控制算法是实现机器人跳跃的关键之一。
通过高效的机械臂协同控制,机器人能够在跳跃中实现精准的动作展示和准确的落地目标。
二、机器人跳跃运动的应用场景1. 娱乐表演随着人们对科技的追求和娱乐需求的不断增加,机器人跳跃运动被广泛应用于娱乐表演领域。
各种形状、大小和功能的机器人通过跳跃展示出令人惊叹的动作和技巧。
机器人跳跃运动的精准性和高度可控性使得演出更加精彩,吸引了大量观众的目光。
2. 救援和探索机器人跳跃运动在救援和探索领域也具有广阔的应用前景。
通过弹跳法,机器人能够跳跃过各种高低不平的地形,实现自主探索和救援任务。
例如,机器人可以通过跳跃越过障碍物,进入狭小空间进行救援任务,或者在恶劣环境中探索,收集数据。
机器人跳跃的自由度和适应性使其成为救援和探索领域中的有力工具。
三、机器人跳跃运动的优化方法1. 运动轨迹规划机器人跳跃运动的最关键问题之一是准确的轨迹规划。
通过优化路径规划算法,机器人可以根据目标高度和跳跃动作的需求,计算出最佳的跳跃路径。
对跳跃机器人研发的分析
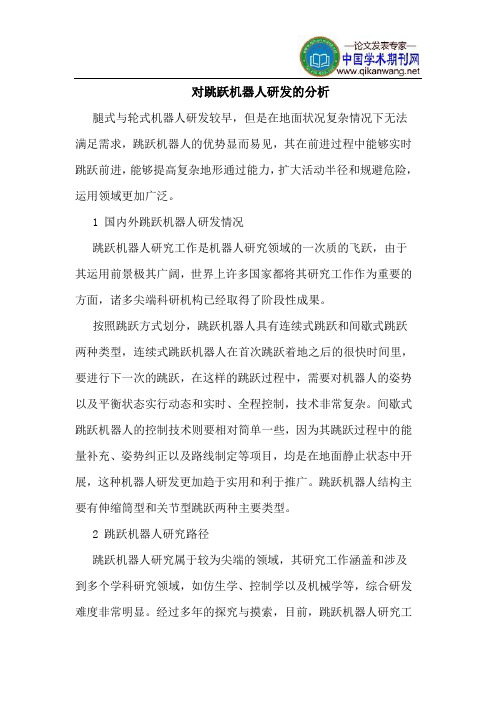
对跳跃机器人研发的分析腿式与轮式机器人研发较早,但是在地面状况复杂情况下无法满足需求,跳跃机器人的优势显而易见,其在前进过程中能够实时跳跃前进,能够提高复杂地形通过能力,扩大活动半径和规避危险,运用领域更加广泛。
1 国内外跳跃机器人研发情况跳跃机器人研究工作是机器人研究领域的一次质的飞跃,由于其运用前景极其广阔,世界上许多国家都将其研究工作作为重要的方面,诸多尖端科研机构已经取得了阶段性成果。
按照跳跃方式划分,跳跃机器人具有连续式跳跃和间歇式跳跃两种类型,连续式跳跃机器人在首次跳跃着地之后的很快时间里,要进行下一次的跳跃,在这样的跳跃过程中,需要对机器人的姿势以及平衡状态实行动态和实时、全程控制,技术非常复杂。
间歇式跳跃机器人的控制技术则要相对简单一些,因为其跳跃过程中的能量补充、姿势纠正以及路线制定等项目,均是在地面静止状态中开展,这种机器人研发更加趋于实用和利于推广。
跳跃机器人结构主要有伸缩筒型和关节型跳跃两种主要类型。
2 跳跃机器人研究路径跳跃机器人研究属于较为尖端的领域,其研究工作涵盖和涉及到多个学科研究领域,如仿生学、控制学以及机械学等,综合研发难度非常明显。
经过多年的探究与摸索,目前,跳跃机器人研究工作取得了长足的进展,为跳跃机器人的研究推广奠定了坚实的基础。
2.1 跳跃机器人驱动形式目前,在跳跃机器人驱动形式方面,主要有两种形式,一种为弹力机构驱动,另一种为内能以及化学能驱动。
相对来讲,弹力机构驱动属于复杂性稍低的驱动方式,主要借助于机械装置迸发的弹力完成跳跃,主要借助于弹簧和弹性杆这些构件来实现,这样的构建方式主要运用于较为简单的跳跃机器人,瑞士科学家研制出来的蚱蜢跳跃机器人就属于这样的类型。
内能与化学能驱动,主要借助于液压、气动产生的内能或者化学反应产生的化学能实施的跳跃机构,显著特点就是体积小但驱动力强劲等,运用此类驱动能够减轻跳跃机器人自身体积重量的同时,提高弹跳能力和扩大活动半径。
仿袋鼠跳跃机器人机构的动力学综合
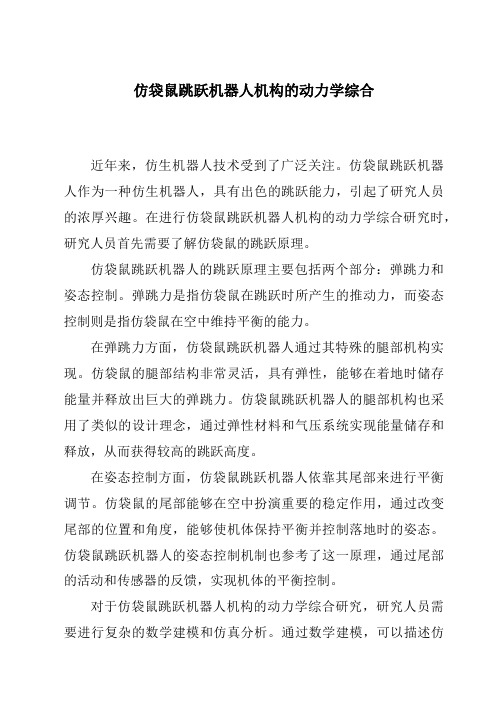
仿袋鼠跳跃机器人机构的动力学综合近年来,仿生机器人技术受到了广泛关注。
仿袋鼠跳跃机器人作为一种仿生机器人,具有出色的跳跃能力,引起了研究人员的浓厚兴趣。
在进行仿袋鼠跳跃机器人机构的动力学综合研究时,研究人员首先需要了解仿袋鼠的跳跃原理。
仿袋鼠跳跃机器人的跳跃原理主要包括两个部分:弹跳力和姿态控制。
弹跳力是指仿袋鼠在跳跃时所产生的推动力,而姿态控制则是指仿袋鼠在空中维持平衡的能力。
在弹跳力方面,仿袋鼠跳跃机器人通过其特殊的腿部机构实现。
仿袋鼠的腿部结构非常灵活,具有弹性,能够在着地时储存能量并释放出巨大的弹跳力。
仿袋鼠跳跃机器人的腿部机构也采用了类似的设计理念,通过弹性材料和气压系统实现能量储存和释放,从而获得较高的跳跃高度。
在姿态控制方面,仿袋鼠跳跃机器人依靠其尾部来进行平衡调节。
仿袋鼠的尾部能够在空中扮演重要的稳定作用,通过改变尾部的位置和角度,能够使机体保持平衡并控制落地时的姿态。
仿袋鼠跳跃机器人的姿态控制机制也参考了这一原理,通过尾部的活动和传感器的反馈,实现机体的平衡控制。
对于仿袋鼠跳跃机器人机构的动力学综合研究,研究人员需要进行复杂的数学建模和仿真分析。
通过数学建模,可以描述仿袋鼠跳跃机器人的运动学和动力学特性,进而分析机器人的跳跃性能并优化设计。
同时,仿真分析可以模拟仿袋鼠跳跃机器人的运动过程,验证数学模型的准确性,并为后续实验提供指导。
综上所述,仿袋鼠跳跃机器人机构的动力学综合研究对于提高机器人的跳跃性能和稳定性具有重要意义。
通过深入研究仿袋鼠的跳跃原理,并结合数学建模和仿真分析的方法,可以为仿袋鼠跳跃机器人的设计和控制提供理论依据和技术支持,推动仿生机器人技术的发展。
一种新型跳跃机器人的运动特性研究

2024年第48卷第2期Journal of Mechanical Transmission一种新型跳跃机器人的运动特性研究王建张文祥缪龙王树坤(南京工程学院机械工程学院,江苏南京211167)摘要针对一种新型的跳跃机器人,提出了其单腿跳跃机构的分析模型。
采用D-H方法,建立了跳跃机器人着地和腾空两个阶段的运动学方程;开展正、逆运动学研究,给出了机器人在跳跃过程中的运动位姿;利用Adams软件,对跳跃机器人的弹跳过程进行了动力学特性仿真。
研究结果表明,仿真得到的跳跃机器人水平/竖直方向的位移、水平/竖直方向的速度曲线的变化趋势均与试验结果相吻合,从而证明了所建立的新型跳跃机器人结构模型的可行性和机器人运动特性研究方法的有效性。
关键词跳跃机器人单腿模型运动学步态分析动力特征Research on Locomotion Characteristics of a Novel Hopping RobotWang Jian Zhang Wenxiang Miao Long Wang Shukun(School of Mechanical Engineering, Nanjing Institute of Technology, Nanjing 211167, China)Abstract One legged mechanism model of a novel hopping robot is presented. The kinematics equations during stance and flight phases are established and its direct and inverse kinematics characteristics are studied by the D-H method. The expression about the hopped position is given. Dynamic characteristics of the hopping robot are analyzed by Adams software. The simulation results confirm that the horizontal/vertical displacement curves and horizontal/vertical velocity curves are consistent with the experimental ones. The research shows the feasibility of this hopping model and validity of the research method.Key words Hopping robot One legged model Kinematics Gait analysis Dynamic characteristics0 引言能量转换效率是决定跳跃机器人弹跳性能优劣的最根本因素。
单足跳跃式仿袋鼠机器人的动力学优化设计

单足跳跃式仿袋鼠机器人的动力学优化设计近年来,仿生机器人技术在机器人领域取得了显著的进展。
袋鼠作为一种优秀的跳跃动物,其独特的跳跃方式吸引了众多研究者的关注。
借鉴袋鼠的跳跃机理,研发出单足跳跃式仿袋鼠机器人具有广阔的应用前景。
本文旨在对单足跳跃式仿袋鼠机器人的动力学进行优化设计。
在设计过程中,首先需要对袋鼠的跳跃方式进行深入研究。
袋鼠通过将身体重心移到腿部,利用腿部的弹性能量储存和释放实现高效的跳跃。
因此,在仿袋鼠机器人的设计中,需要考虑到腿部的弹性特性,并通过合理的机械结构设计实现能量的储存和释放。
同时,还需要考虑到机器人的稳定性和控制策略。
通过对袋鼠跳跃过程的分析,可以发现其身体在跳跃过程中保持平衡的关键在于尾部的作用。
因此,在仿袋鼠机器人的设计中应添加类似尾部的结构,以提高机器人的稳定性。
其次,需要对仿袋鼠机器人的动力学进行优化。
通过对机器人跳跃过程的深入研究,可以发现机器人的跳跃高度和距离与多个因素相关。
其中最重要的因素之一是腿部的弹性特性。
通过调整腿部的弹性系数和刚度,可以实现不同高度和距离的跳跃。
另外,机器人的质量和能量的储存和释放方式也对跳跃性能有着重要影响。
因此,在动力学优化设计中,需要综合考虑这些因素,并通过合理的参数选择和控制策略来实现机器人的最优跳跃性能。
最后,需要进行仿真和实验验证。
通过建立仿真模型,可以对优化设计进行评估和验证。
通过仿真实验,可以优化机器人的设计参数和控制策略,以实现更好的跳跃性能。
同时,还需要进行实际的物理实验,验证仿袋鼠机器人的跳跃性能和稳定性。
通过与实际袋鼠的跳跃数据进行对比,可以评估仿袋鼠机器人的仿真模型的准确性和优化设计的有效性。
综上所述,单足跳跃式仿袋鼠机器人的动力学优化设计具有重要的研究意义和应用价值。
通过对袋鼠跳跃方式的研究,可以设计出具有高效跳跃性能的仿袋鼠机器人。
通过动力学优化设计和仿真实验验证,可以不断改进机器人的跳跃性能和稳定性,为机器人在特定环境中的应用提供有力支撑。
跳跃机器人的研究现状与发展趋势
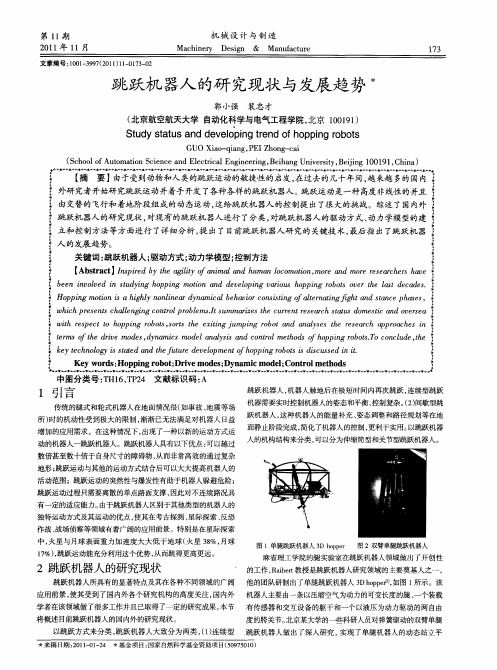
要】 由于受到动物和人类的跳跃运动的敏捷性的启发 , 在过去的几十年间, 越来越 多的国内
外研究者开始研 究跳跃运动并着手开发 了各种各样的跳跃机器人 。 跳跃运动是一种高度非线性的并且 由交替的飞行和着地阶段组成的动态运动 , 这给跳跃机器人 的控制提 出了 很大的挑战。综述了国内外 跳跃机器人的研 究现状, 对现有的跳跃机 器人进行 了分类, 对跳跃机器人的驱动方式、 动力学模型的建 立和控制方法等方面进行 了详细分析 , 出了目前跳跃机器人研 究的关键技术 , 提 最后指出了跳跃机器
H p i t nia i l nn na dnm c eai nii a e a n f h ad t c p ae, opn m i g y ol e a i bh o cs sn o lr tg gt a e h s g o o s hh ir y l a v ro tg f tn i i s s n n
第 1 期 1 21 年 l 01 1月
文章编号 :0 1 3 9 ( 0 1 1- 13 0 1 0 — 9 7 2 1 ) 10 7 — 2
机 械 设 计 与 制 造
Ma hi e y De in c n r sg & Ma u a t e n f cur 13 7
跳跃机 器人 的研 究现状与发展 趋势
人 的发展 趋势 。 关 键词 : 跃机器人 ; 跳 驱动方 式 ; 力学模型 ; 动 控制方 法
【 bt c】 n i db e g i n a adh m cm tnm r adm r r er e ae A s at I pr t i y fai l n u a l o o o , o o e a hr hv r s e yh al o m t n o i e n e s c s
仿青蛙跳跃机器人的结构优化与运动综合

仿青蛙跳跃机器人的结构优化与运动综合一、本文概述随着科技的快速发展,机器人技术已经成为当今研究的热点之一。
其中,仿青蛙跳跃机器人作为一种具有高效能量利用和出色越障能力的机器人类型,受到了广泛关注。
本文旨在探讨仿青蛙跳跃机器人的结构优化和运动综合问题,以期为未来机器人的设计和性能提升提供理论支持和实际应用指导。
本文将对仿青蛙跳跃机器人的研究背景和意义进行阐述,明确其在机器人技术领域的重要地位。
接着,我们将回顾和分析国内外在该领域的研究现状和发展趋势,指出当前存在的问题和挑战。
然后,本文将重点研究仿青蛙跳跃机器人的结构优化问题。
通过深入剖析青蛙的生物结构和运动机理,我们将探索如何将这些自然特性融入机器人设计中,以提高其跳跃性能和稳定性。
我们将关注机器人的结构参数优化、材料选择以及动力传递机制等方面,提出创新性的优化方案。
本文还将对仿青蛙跳跃机器人的运动综合进行研究。
我们将通过理论分析和实验验证,探索机器人在不同环境下的跳跃策略和运动规划,以实现高效、稳定的跳跃运动。
我们将关注机器人的感知、决策和执行等环节,以实现运动综合的最优化。
本文将对所提出的结构优化和运动综合方案进行总结和展望,评估其在实际应用中的潜力和价值。
我们希望通过本文的研究,能够为仿青蛙跳跃机器人的进一步发展和应用提供有益的参考和启示。
二、仿青蛙跳跃机器人结构设计在仿青蛙跳跃机器人的设计中,结构设计是至关重要的一环。
考虑到青蛙的跳跃机制,我们设计了一种具有弹性驱动和自适应落地机制的机器人结构。
主体结构设计:机器人的主体结构参考了青蛙的身体结构,包括头部、躯干和四肢。
头部集成了传感器和控制系统,用于感知环境和控制机器人的运动。
躯干部分则负责连接头部和四肢,以及存储能量。
弹性驱动机构:为了模拟青蛙的跳跃动作,我们在机器人的四肢上设计了弹性驱动机构。
这些机构由弹簧和驱动器组成,当驱动器收缩时,弹簧被压缩并储存能量,当驱动器放松时,弹簧释放能量,推动机器人跳跃。
可跳跃移动机器人机构设计与跳跃过程控制研究综述
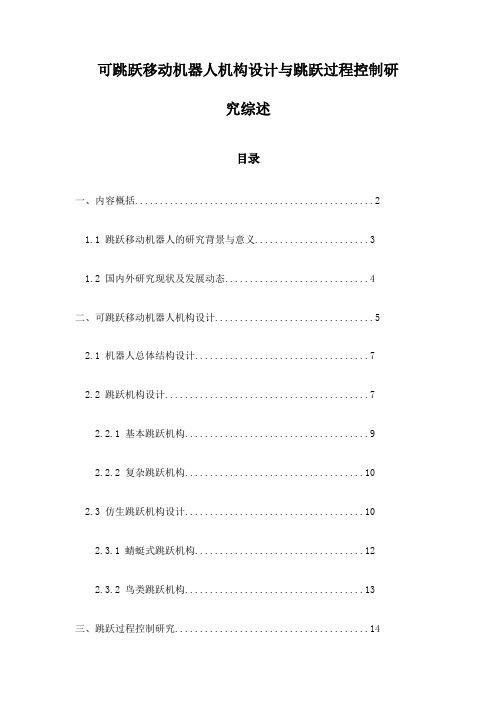
可跳跃移动机器人机构设计与跳跃过程控制研究综述目录一、内容概括 (2)1.1 跳跃移动机器人的研究背景与意义 (3)1.2 国内外研究现状及发展动态 (4)二、可跳跃移动机器人机构设计 (5)2.1 机器人总体结构设计 (7)2.2 跳跃机构设计 (7)2.2.1 基本跳跃机构 (9)2.2.2 复杂跳跃机构 (10)2.3 仿生跳跃机构设计 (10)2.3.1 蜻蜓式跳跃机构 (12)2.3.2 鸟类跳跃机构 (13)三、跳跃过程控制研究 (14)3.1 跳跃运动规划与控制策略 (15)3.1.1 基于预设轨迹的跳跃控制 (16)3.1.2 基于最优控制的跳跃控制 (18)3.1.3 基于模型预测控制的跳跃控制 (20)3.2 跳跃过程中的动力学分析与建模 (21)3.2.1 跳跃机器人的动力学建模 (22)3.2.2 跳跃过程中的力学分析 (24)3.3 跳跃机器人的感知与交互技术 (25)3.3.1 激光雷达感知技术 (26)3.3.2 触觉传感器感知技术 (28)3.3.3 人机交互技术 (30)四、实验与仿真分析 (31)4.1 实验环境搭建与实验方法 (33)4.2 实验结果与分析 (34)4.3 仿真结果与分析 (35)五、结论与展望 (36)5.1 研究成果总结 (37)5.2 存在问题与不足 (39)5.3 未来发展方向与展望 (40)一、内容概括随着科技的不断进步,可跳跃移动机器人作为一种具有高度自主性和灵活性的机器人形式,受到了广泛关注。
本文旨在对近年来可跳跃移动机器人机构设计与跳跃过程控制的研究进行综述,以期为该领域的发展提供参考和启示。
在可跳跃移动机器人机构设计方面,研究者们主要关注机器人的结构、驱动和跳跃性能等方面。
结构设计方面,为提高机器人的稳定性和机动性,往往采用多关节、柔性杆等复杂结构。
驱动方式上,除了传统的电机驱动外,还有采用生物启发式驱动(如仿生肌肉、形状记忆合金等)的机器人。
单足跳跃机器人运动控制系统研究
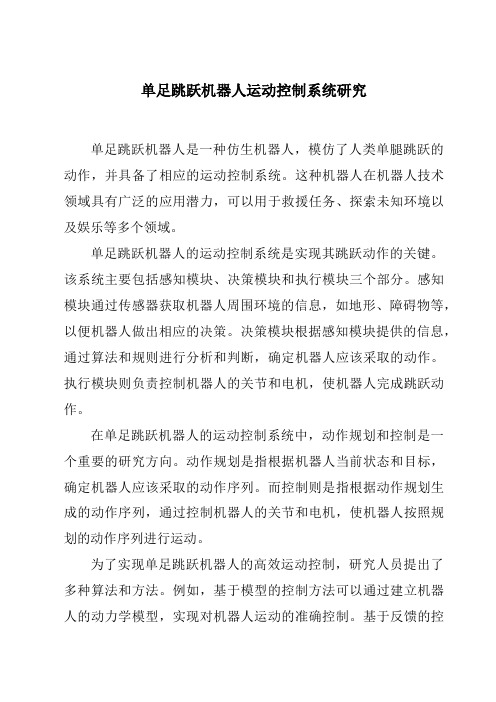
单足跳跃机器人运动控制系统研究单足跳跃机器人是一种仿生机器人,模仿了人类单腿跳跃的动作,并具备了相应的运动控制系统。
这种机器人在机器人技术领域具有广泛的应用潜力,可以用于救援任务、探索未知环境以及娱乐等多个领域。
单足跳跃机器人的运动控制系统是实现其跳跃动作的关键。
该系统主要包括感知模块、决策模块和执行模块三个部分。
感知模块通过传感器获取机器人周围环境的信息,如地形、障碍物等,以便机器人做出相应的决策。
决策模块根据感知模块提供的信息,通过算法和规则进行分析和判断,确定机器人应该采取的动作。
执行模块则负责控制机器人的关节和电机,使机器人完成跳跃动作。
在单足跳跃机器人的运动控制系统中,动作规划和控制是一个重要的研究方向。
动作规划是指根据机器人当前状态和目标,确定机器人应该采取的动作序列。
而控制则是指根据动作规划生成的动作序列,通过控制机器人的关节和电机,使机器人按照规划的动作序列进行运动。
为了实现单足跳跃机器人的高效运动控制,研究人员提出了多种算法和方法。
例如,基于模型的控制方法可以通过建立机器人的动力学模型,实现对机器人运动的准确控制。
基于反馈的控制方法则可以通过传感器获取的实时信息,对机器人的运动进行实时调整和修正。
此外,还有一些基于优化算法和机器学习的控制方法,可以通过不断学习和优化机器人的运动模式,提高机器人的运动性能和适应性。
然而,单足跳跃机器人的运动控制系统仍然面临一些挑战和难题。
例如,如何在复杂的环境中实现稳定的跳跃动作,如何提高机器人的运动速度和精度,以及如何实现机器人与环境的协调与交互等等。
这些问题需要进一步的研究和探索。
总的来说,单足跳跃机器人的运动控制系统是实现机器人跳跃动作的核心技术。
通过不断研究和创新,相信在不久的将来,单足跳跃机器人将会在各个领域展现出更加广阔的应用前景。
仿青蛙跳跃机器人机械系统运动学研究
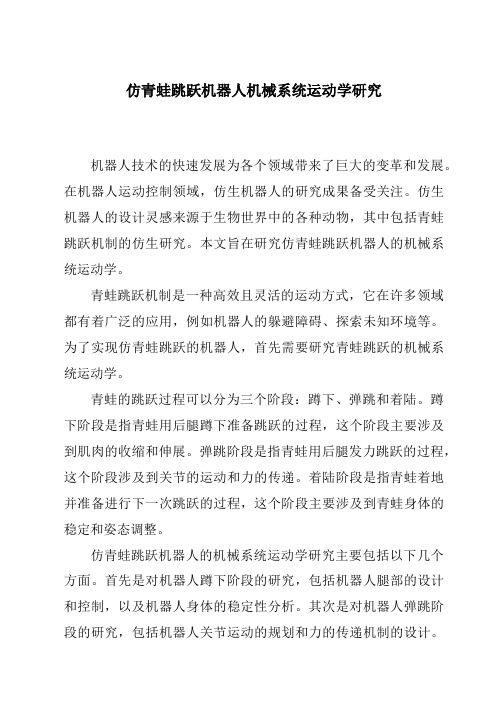
仿青蛙跳跃机器人机械系统运动学研究机器人技术的快速发展为各个领域带来了巨大的变革和发展。
在机器人运动控制领域,仿生机器人的研究成果备受关注。
仿生机器人的设计灵感来源于生物世界中的各种动物,其中包括青蛙跳跃机制的仿生研究。
本文旨在研究仿青蛙跳跃机器人的机械系统运动学。
青蛙跳跃机制是一种高效且灵活的运动方式,它在许多领域都有着广泛的应用,例如机器人的躲避障碍、探索未知环境等。
为了实现仿青蛙跳跃的机器人,首先需要研究青蛙跳跃的机械系统运动学。
青蛙的跳跃过程可以分为三个阶段:蹲下、弹跳和着陆。
蹲下阶段是指青蛙用后腿蹲下准备跳跃的过程,这个阶段主要涉及到肌肉的收缩和伸展。
弹跳阶段是指青蛙用后腿发力跳跃的过程,这个阶段涉及到关节的运动和力的传递。
着陆阶段是指青蛙着地并准备进行下一次跳跃的过程,这个阶段主要涉及到青蛙身体的稳定和姿态调整。
仿青蛙跳跃机器人的机械系统运动学研究主要包括以下几个方面。
首先是对机器人蹲下阶段的研究,包括机器人腿部的设计和控制,以及机器人身体的稳定性分析。
其次是对机器人弹跳阶段的研究,包括机器人关节运动的规划和力的传递机制的设计。
最后是对机器人着陆阶段的研究,包括机器人姿态调整和身体稳定性的控制。
在仿青蛙跳跃机器人的研究中,机械系统运动学是一个关键的研究方向。
通过对机器人蹲下、弹跳和着陆等运动过程的深入研究,可以更好地理解仿生机器人的运动原理,并为机器人的设计和控制提供参考。
此外,机械系统运动学的研究还可以为其他类型的仿生机器人的研究提供借鉴和启示。
总之,仿青蛙跳跃机器人机械系统运动学的研究是一个重要的课题。
通过对青蛙跳跃机制的仿生研究,可以为机器人技术的发展带来新的突破和创新。
希望本研究能够为仿生机器人的设计和控制提供有益的参考,推动机器人技术的发展。
气动肌肉驱动的仿青蛙跳跃机器人及其关键技术的
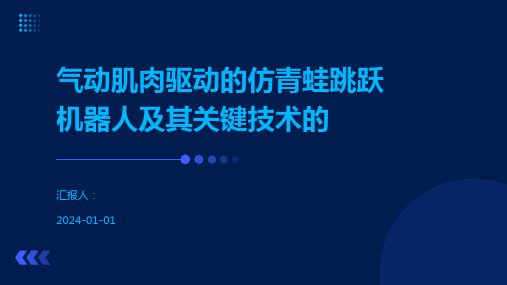
减震设计
优化机器人的着陆结构,采用减震材 料和设计,减小机器人跳跃时产生的 冲击力。
04
关键技术解析
气动肌肉驱动技术
气动肌肉是一种利用气压变化进行伸缩的装置,具有重量 轻、体积小、力矩大等优点。在仿青蛙跳跃机器人中,气 动肌肉被用作驱动器,通过向其内部充气或放气来控制机 器人的运动。
气动肌肉驱动技术能够实现快速响应和精确控制,使得仿 青蛙跳跃机器人在跳跃过程中能够快速启动和停止,并实 现连续跳跃。
目前,仿青蛙跳跃机器人的关键技术主要包括气动肌肉驱动 技术、姿态调整技术、起跳控制技术和落地缓冲技术等。这 些技术的发展和完善,对于提高仿青蛙跳跃机器人的性能和 实用性具有重要意义。
02
气动肌肉的工作原理与特性
气动肌肉的工作原理
气动肌肉通过充气和放气实现伸 缩,从而产生驱动力。
当向气动肌肉内充入压缩气体时 ,肌肉发生膨胀并产生推力;当 气体排出时,肌肉收缩并产生拉
VS
随着机器人技术的不断发展,仿生跳 跃机器人的研究逐渐成为机器人领域 的一个热点。这类机器人能够适应复 杂多变的环境,执行搜救、侦查等任 务,具有重要的应用前景。
仿生跳跃机器人的发展现状
近年来,仿生跳跃机器人的研究取得了显著的进展。研究者 们通过模仿自然界中动物的跳跃行为,设计出了多种具有跳 跃能力的机器人。其中,仿青蛙跳跃机器人在设计理念和实 现方式上具有代表性。
通过传感器技术,机器人能够感知自 身的姿态、速度、加速度等信息,同 时也能感知周围环境中的障碍物、地 形等信息,从而指导机器人进行合理 的跳跃和避障。
05
实验与验证
实验环境与设备
实验场地
选择一个平坦、无障碍物的场地 ,模拟自然环境中的地面条件。
跳跃机器人的知识点总结
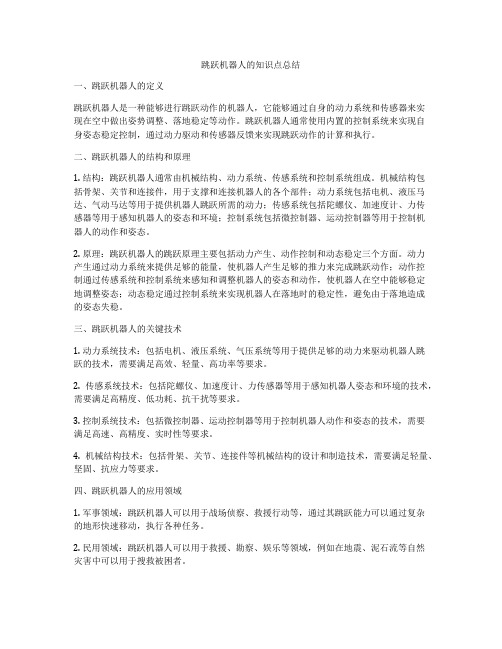
跳跃机器人的知识点总结一、跳跃机器人的定义跳跃机器人是一种能够进行跳跃动作的机器人,它能够通过自身的动力系统和传感器来实现在空中做出姿势调整、落地稳定等动作。
跳跃机器人通常使用内置的控制系统来实现自身姿态稳定控制,通过动力驱动和传感器反馈来实现跳跃动作的计算和执行。
二、跳跃机器人的结构和原理1. 结构:跳跃机器人通常由机械结构、动力系统、传感系统和控制系统组成。
机械结构包括骨架、关节和连接件,用于支撑和连接机器人的各个部件;动力系统包括电机、液压马达、气动马达等用于提供机器人跳跃所需的动力;传感系统包括陀螺仪、加速度计、力传感器等用于感知机器人的姿态和环境;控制系统包括微控制器、运动控制器等用于控制机器人的动作和姿态。
2. 原理:跳跃机器人的跳跃原理主要包括动力产生、动作控制和动态稳定三个方面。
动力产生通过动力系统来提供足够的能量,使机器人产生足够的推力来完成跳跃动作;动作控制通过传感系统和控制系统来感知和调整机器人的姿态和动作,使机器人在空中能够稳定地调整姿态;动态稳定通过控制系统来实现机器人在落地时的稳定性,避免由于落地造成的姿态失稳。
三、跳跃机器人的关键技术1. 动力系统技术:包括电机、液压系统、气压系统等用于提供足够的动力来驱动机器人跳跃的技术,需要满足高效、轻量、高功率等要求。
2. 传感系统技术:包括陀螺仪、加速度计、力传感器等用于感知机器人姿态和环境的技术,需要满足高精度、低功耗、抗干扰等要求。
3. 控制系统技术:包括微控制器、运动控制器等用于控制机器人动作和姿态的技术,需要满足高速、高精度、实时性等要求。
4. 机械结构技术:包括骨架、关节、连接件等机械结构的设计和制造技术,需要满足轻量、坚固、抗应力等要求。
四、跳跃机器人的应用领域1. 军事领域:跳跃机器人可以用于战场侦察、救援行动等,通过其跳跃能力可以通过复杂的地形快速移动,执行各种任务。
2. 民用领域:跳跃机器人可以用于救援、勘察、娱乐等领域,例如在地震、泥石流等自然灾害中可以用于搜救被困者。
仿青蛙跳跃机器人的结构优化与运动综合
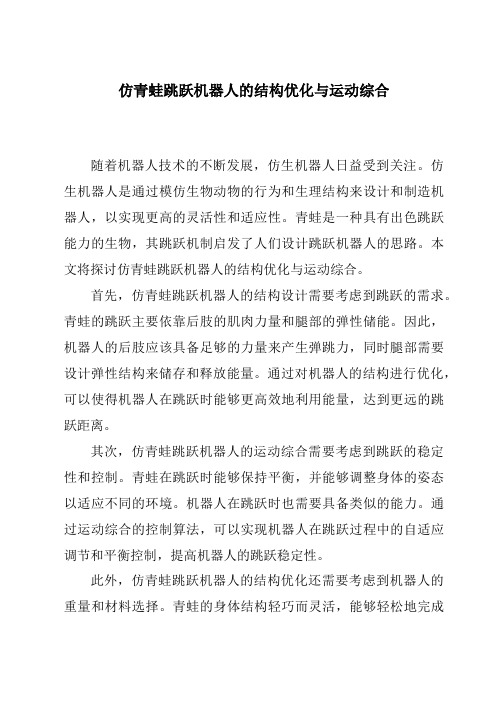
仿青蛙跳跃机器人的结构优化与运动综合随着机器人技术的不断发展,仿生机器人日益受到关注。
仿生机器人是通过模仿生物动物的行为和生理结构来设计和制造机器人,以实现更高的灵活性和适应性。
青蛙是一种具有出色跳跃能力的生物,其跳跃机制启发了人们设计跳跃机器人的思路。
本文将探讨仿青蛙跳跃机器人的结构优化与运动综合。
首先,仿青蛙跳跃机器人的结构设计需要考虑到跳跃的需求。
青蛙的跳跃主要依靠后肢的肌肉力量和腿部的弹性储能。
因此,机器人的后肢应该具备足够的力量来产生弹跳力,同时腿部需要设计弹性结构来储存和释放能量。
通过对机器人的结构进行优化,可以使得机器人在跳跃时能够更高效地利用能量,达到更远的跳跃距离。
其次,仿青蛙跳跃机器人的运动综合需要考虑到跳跃的稳定性和控制。
青蛙在跳跃时能够保持平衡,并能够调整身体的姿态以适应不同的环境。
机器人在跳跃时也需要具备类似的能力。
通过运动综合的控制算法,可以实现机器人在跳跃过程中的自适应调节和平衡控制,提高机器人的跳跃稳定性。
此外,仿青蛙跳跃机器人的结构优化还需要考虑到机器人的重量和材料选择。
青蛙的身体结构轻巧而灵活,能够轻松地完成高强度的跳跃动作。
机器人的结构优化可以通过减少材料使用和改进结构设计来降低机器人的重量,提高其跳跃效率和灵活性。
在仿青蛙跳跃机器人的结构优化与运动综合中,还可以借鉴其他生物的特点和运动机制。
比如,蚱蜢的跳跃能力和蛇的爬行能力都可以为机器人的设计提供思路和灵感。
通过将不同生物的特点和机制结合起来,可以设计出更加优化和高效的跳跃机器人。
总之,仿青蛙跳跃机器人的结构优化与运动综合是一个复杂而有挑战性的任务。
通过合理的结构设计和运动控制算法,可以实现机器人具备更高效的跳跃能力和稳定性。
未来的研究可以进一步探索跳跃机器人的应用领域,如救援、探险等,为人类创造更多的可能性。
仿青蛙跳跃机器人的研制
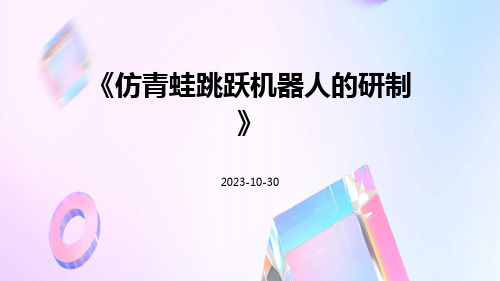
国外研究团队在仿生机器人领域具有 丰富的经验和技术积累,尤其在材料 选择和运动模拟方面取得了较多突破 。一些先进的技术已经被应用到跳跃 机器人的研制中,如柔性材料、传感 器融合、人工智能等。
发展趋势
随着机器人技术的不断发展,仿青蛙 跳跃机器人的研制将更加注重智能化 、自主化、高效化。未来,该领域的 研究将更加注重跨学科合作,融合多 学科前沿技术,以实现机器人性能的 全面提升
研究方法
本研究将采用理论建模、实验测试和 数值模拟等方法进行研究。首先,将 建立机器人的数学模型和动力学方程 ,以描述机器人的运动过程和控制策 略;其次,将进行实验测试,包括机 器人的跳跃高度、距离、稳定性等方 面的测试;最后,将通过数值模拟方 法对实验结果进行分析和优化。
02
青蛙跳跃机理分析
青蛙跳跃的生物力学原理
度的机器人控制系统。
03
跳跃机器人的设计
跳跃机器人的总体设计
1
跳跃机器人是一种能够模仿青蛙跳跃行为的机 器人,由机械结构、控械结构是跳跃机器人的基础,包括脚掌、膝 盖、大腿、腰部等部位,能够实现类似于青蛙 的跳跃动作。
3
控制系统是跳跃机器人的核心,通过传感器和 控制算法实现对机器人跳跃行为的精确控制。
《仿青蛙跳跃机器人的研制 》
2023-10-30
目 录
• 绪论 • 青蛙跳跃机理分析 • 跳跃机器人的设计 • 跳跃机器人的实现与优化 • 结论与展望
01
绪论
研究背景与意义
背景
青蛙是一种具有高度灵活性和适应性的动物,其跳跃能力引 起了科研人员的广泛关注。随着机器人技术的不断发展,研 制一种能够模拟青蛙跳跃行为的机器人成为了一个热门研究 方向。
跳跃机器人的机械结构
跳跃机器人研究现状和趋势
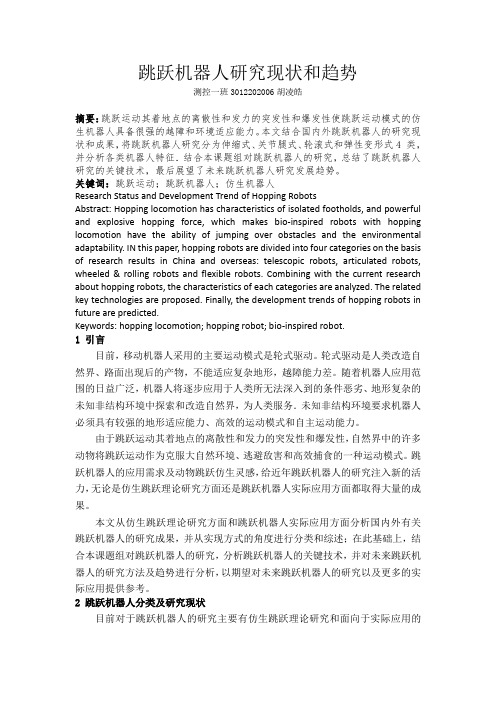
跳跃机器人研究现状和趋势测控一班3012202006胡凌皓摘要:跳跃运动其着地点的离散性和发力的突发性和爆发性使跳跃运动模式的仿生机器人具备很强的越障和环境适应能力。
本文结合国内外跳跃机器人的研究现状和成果,将跳跃机器人研究分为伸缩式、关节腿式、轮滚式和弹性变形式4 类,并分析各类机器人特征.结合本课题组对跳跃机器人的研究,总结了跳跃机器人研究的关键技术,最后展望了未来跳跃机器人研究发展趋势。
关键词:跳跃运动;跳跃机器人;仿生机器人Research Status and Development Trend of Hopping RobotsAbstract: Hopping locomotion has characteristics of isolated footholds, and powerful and explosive hopping force, which makes bio-inspired robots with hopping locomotion have the ability of jumping over obstacles and the environmental adaptability. IN this paper, hopping robots are divided into four categories on the basis of research results in China and overseas: telescopic robots, articulated robots, wheeled & rolling robots and flexible robots. Combining with the current research about hopping robots, the characteristics of each categories are analyzed. The related key technologies are proposed. Finally, the development trends of hopping robots in future are predicted.Keywords: hopping locomotion; hopping robot; bio-inspired robot.1 引言目前,移动机器人采用的主要运动模式是轮式驱动。
轮式跳跃机器人设计及其弹跳性能研究开题报告

轮式跳跃机器人设计及其弹跳性能研究开题报告一、选题背景随着科技的发展和人类对机器人技术的需求日益增长,机器人的应用范围越来越广泛,已经成为现代科技的重要组成部分。
而跳跃机器人作为一种高度智能的机器人,已经被广泛应用于军事、医疗、救援等领域中。
传统的跳跃机器人多采用腿式结构,但腿部结构复杂、控制难度大,因此设计一种轮式跳跃机器人具有重要的意义。
二、选题意义本课题旨在设计一种轮式跳跃机器人,并研究其弹跳性能,为跳跃机器人技术的发展做出一定的贡献。
此外,本研究所设计的机器人还可以应用于以下领域:1.军事:可以用于侦查、侦察、排雷等任务。
2.医疗:可以用于患者康复训练,特别是针对下肢康复训练效果更佳。
3.救援:可以用于在地震、泥石流等灾难发生时进行救援等任务。
三、研究内容1.设计轮式跳跃机器人的结构和控制系统。
2.研究机器人在不同高度下的弹跳性能与稳定性。
3.优化机器人的设计,提高其弹跳高度和稳定性。
四、研究方法1.文献研究法:通过阅读相关文献,了解跳跃机器人的基础知识和发展现状,为设计轮式跳跃机器人提供理论指导。
2.实验研究法:利用物理实验和数字仿真方法,研究轮式跳跃机器人的弹跳性能,例如弹跳高度、弹跳时间和运动轨迹等。
3.优化设计法:根据实验结果,对机器人的结构和控制系统进行改进,提高机器人的弹跳高度和稳定性。
五、预期成果1.设计一种高效稳定的轮式跳跃机器人。
2.研究机器人在不同高度下的弹跳性能和稳定性。
3.优化机器人的设计,使其具有更好的弹跳高度和稳定性。
六、研究计划第1-2个月:文献研究,了解跳跃机器人的基础知识和现有技术。
第3-4个月:设计机器人的结构和控制系统,制作机器人样机。
第5-6个月:进行物理实验和数字仿真,研究机器人的弹跳性能。
第7-8个月:根据实验结果,对机器人进行优化设计。
第9-10个月:进行实验验证,测试机器人的弹跳高度和稳定性。
第11-12个月:撰写论文并进行口头答辩。
七、预算和资源1.硬件设备和材料费用:5000元。
- 1、下载文档前请自行甄别文档内容的完整性,平台不提供额外的编辑、内容补充、找答案等附加服务。
- 2、"仅部分预览"的文档,不可在线预览部分如存在完整性等问题,可反馈申请退款(可完整预览的文档不适用该条件!)。
- 3、如文档侵犯您的权益,请联系客服反馈,我们会尽快为您处理(人工客服工作时间:9:00-18:30)。
Biological Jumping Mechanism Analysis and Modeling for Frog RobotMeng Wang, Xizhe Zang, Jizhuang Fan, Jie ZhaoState Key Laboratory of Robotics and System, Harbin Institute of Technology, Harbin 150001, P. R. ChinaAbstractThis paper presents a mechanical model of jumping robot based on the biological mechanism analysis of frog. By biological observation and kinematic analysis the frog jump is divided into takeoffphase, aerial phase and landing phase. We find the similar trajectories of hindlimb joints during jump, the important effect of foot during takeoff and the role of forelimb in supporting the body. Based on the observation, the frog jump is simplified and a mechanical model is put forward. The robot leg is represented by a 4bar spring/linkage mechanism model, which has three Degrees of Freedom (DOF) at hip joint and one DOF (passive) at tarsometatarsal joint on the foot. The shoulder and elbow joints each has one DOF for the balancing function of arm. The ground reaction force of the model is analyzed and compared with that of frog during takeoff .The results show that the model has the same advantages of low likelihood of premature liftoff and high efficiency as the frog. Analysis results and the model can be employed to develop and control a robot capable of mimicking the jumping behavior of frog.Keywords: frog jump modality, kinematic analysis, mechanical model, jumping robot Copyright ) 2008, Jilin University. Published by Science Press and Elsevier Limited. All rights reserved.1 IntroductionBiological principles can improve the performance of robots. Raibert and colleagues developed one, two, and four leg hopping and running robots by incorporating biologically inspired dynamics into their designate.These robots have telescoping legs with an internal air spring for compliance, and hydraulic actuators. Researchers at Case Western Reserve University constructed a series of hexapod robots based on biological inspiration. Robots RI and RII were based upon stick insects that all the six legs are of the same construction. Robots RIII to RV modeled were on the Blaberus discoidalis that have a total of 24 DOF. Compared to the large R series of robots, a cricket microrobot can fit into a two inch cube, and could both walk and jump to navigate terrain with features much larger than itselft. Hyon and Mita developed a hopping robot "KenKen" inspired by dog. The robot has an articulated leg and uses two hydraulic actuators as muscles and a tensile spring as tendon. It could hop in a planer's. Yamakita et al. proposed a robotic system based on cat's behavior,which moves in a vertical direction as a cat kicks a wall to jump up to a roof.We select the frog as a model for the development of a jumping robot because of its remarkable jumping capability (a bullfrog can jump more than 15 times its body length). In order to produce such a robot, we need to identify a model that can jump. Kinematic observation and analysis of the frog can serve as the raw data for simulation and provide insights into the robot Construction.2 Biological observation and analysis2.1 Frog morphologyFive frogs (Rananigromaculata) were obtained from a commercial supplier. They werekept at approximately 20 0C in plastic containers measuring 35 cm (width) X 50 cm (length) X 30 cm (height), and provided with clean water. The frogs were fed with crickets at least twice a week. Immediately after obtaining the frogs from the supplier, each animal's body's mass (Mb) in grams, snoutvent length (LS,,), hindlimb length (Lhl) and forelimb length (L}), as well as the distance from thesacral joint to the vent (Lsa}) in millimeters were measured (Table 1).Frogs have evolved remarkable disparity in the relative size of their hindlimbs and forelimbs. The forelimbs that serve in supporting and steering are small and weak; while the hindlimbs are relatively large and strong,enabling them to propel the frog in both jumping andswimming.The jumping patterns of frogs differ from those of humans and other mammals in several important ways.In frogs, the hindlimb bones do not lie in a single plane throughout the jump, and hindlimb joint rotations other than extension are prominent. Further, the tarsometatarsal (TM) joint at the foot, which is nearly fixed in humans, is flexible in frogs, and may contribute greatlyto jumping}lo'I}}.The forelimb and hindlimb each has five distinct segments (Fig. 1 a). Starting from the body attachment point, the forelimb segments are humerus, radioulna,metacarpal, wrist and phalanx (without web between digits). The hindlimb segments are femur, tibiofibula,tarsal, metatarsal and phalange (with web between digits)}12}. We regard metacarpal, wrist and phalanx of forelimb as a whole segment, also metatarsal and phalange of hindlimb as a whole segment for the convenfence of observation. To realize complex positioning of the entire hindlimb, the hip joint has 3 DOF. The knee,ankle and TM (displacement of the tarsal relative to the metatarsal) joints are simple 1 DOF joints and act in the same plane. For the same reason, the shoulder joint has 3 DOF. Elbow and wrist are simple 1 DOF joints.Although the forelimb and hindlimb are not arranged in completely orthogonal planar fashion, we defined a relative x yz axis orientation to assist the analysis. By definition, the animal's horizontal, longitudinal headtotail line is the xaxis; the lateral, horizontal sidetoside line is the zaxis; and the vertical line direcred upward is the yaxis.Fig. 1 Skeleton of frog (a) Side view of frog with digitizing points marked and segments identified;(b) Dorsal (top) view of frog with digitizing points and joints identified.2.2 Experimental setupThe jumping movements of frog are explosive and involve coordination of its extremity. In order to observe and measure these movements, we built a platform to collect the necessary data such as individual joint angles.The platform consists of a highspeed video (100 frames per second), a mirror and a 3D staff gauge (Fig. 2). The mirror was mounted above the arena at 450 to allow simultaneously filming the side and top views. A 3D staff gauge that provides x (longitudinal), y (vertical), and:(lateral) orientation coordinates, helps us get accurate data. 3D information of the red dots locating thejoints of limbs and body was obtained from video images by program (Fig. 1 and Fig. 2). After correction of digitized data from the effect of perspective, the program reconstructed the true angles of the joints in 3D as frog jumped on the arena}l3}. The temperature was kept at 20 0C一25 0C during the experiment.2.3 Jumping performanceOn the basis of the actions and positions of the body, forelimbs and hindlimbs, three phases in the jump were identified from the video recordings (Fig. 3): (i) the takeoff phase, which lasts from the ` jumpready" or crouching position to the point at which the frog leaves the ground; (ii) the aerial phase, which lasts from this point to the first touching point of the ground; (iii) the landing phase, measured as the time from the first contact with the ground to the resumption of the "jumpready" position.Fig. 3The jumping movement is divided into three phases: takeoff phase, from image 1 to image 4; aerial phase, from image 4 to image 6; landing phase, from image 6 to image 8.At the beginning of the takeoff phase, the hip and knee are greatly flexed; in fact, the thigh and shank that bend underneath the body are completely folded. The TM joint is flexed about 1200 to elevate the ankle so that it is not in contact with the ground. The forelimbs support the body in position for takeoff.As the muscles shorten, the generated power leads to a rapid extension of the hindlimbs which, in turn, propels the frog into the air. While the hip, knee, and ankle extend during initial takeoff phase, most of the foot (metatarsals and phalanges) remains in contact withthe ground, and the TM joint extends just slightly. But while the TM joint extends, there is a concurrent dor soflexion at metatarsophalangeal (MP) joint that causes the tarsals to rock forward until to the distal toe joint, as in a human foot shifting from the heel to the ball of the foot}la}.During the initial takeoff, the elbow extends and the humerus retracts and adducts as the forelimbs come off the ground. It seems unlikely that this extension of the small forearm segments contributes much, if any, power to the jump. Nevertheless, the forelimbs probably supply balancing and steering as the animal initiates a dump.During the aerial phase, the hip, knee, ankle, and TM joints of hindlimbs begin flexing, and the forelimbs are extended to prepare for landing.In the final landing phase, the hindlimb flexion is completed. The forelimbs support and decelerate the frog before its body is pivoted ventrally about the shoulder until the hindlimbs contact the ground. At this point, the bulk of the body weight is transferred to the hindlimbs, and the frog is ready to jump again.During a jump, the duration of the takeoff phase is quite variable, ranging from 90 ms to 220 ms (40 jumps were chosen for research). The duration decreases with increasing jumping distance (linear regression, r2一0.28, t二一1.711+204, P<0.0001, t is takeoff duration (ms), l is jump distance (cm); Fig. 4). Because of its ballistic nature, jump performance, as measured by the linear horizontal distance traveled by the animal, is largely a function of takeoff velocity and angle. Given similar amount of limb extension, a jump with shorter takeoff phase should have higher accelerations of the center of mass and, given similar takeoff angles, the jump should cover a greater distance.Fig. 4 Scatterplot of 40 separate jumps-the relationship between jumping distance and takeoff duration.The long, soft and mufti一jointed foot is a critical element for successful jump performance. Its movement, with the legs keep most of the plantar surface in contact with the ground as long as possible, causes the site of push off to shift forward from the back of the foot. For an animal with feet as long as a frog's, this allows for more controlled extension. Keeping the broadest part of the foot in contact with the ground throughout propul sion also permits continuous adjustment of balance so that rapid changes in direction and/or trajectory could be made. Additionally, these movements maximize the velocity of limb extension and the final takeoff velocity by maximizing the contact of the foot (to keep pushing for the possible longest time as the upper limb segments extend) and by combining velocities of as many joints as possible}}5}. Thus, the elongated foot of the frog funclions to produce very large takeoff velocitiesllb}, and the foot with the TM joint should be considered in robot design.2.4 Analysis of joint trajectoryIn order to find the characteristics of the jump, we compared and analyzed the joint trajectory based on the kinematic data from the experiment. Five similar jumps (the takeoff angle is about 300 and jumping distance is about 0.5 m, takeoff angle was measured from the frame at takeoff and corrected geometrically for the deviation of the jumping plane from the focal plane of camera) were selected. Fig. 5a and Sb show the mean joint angles of the forelimb and hindlimb respectively.From Fig. 5a, the shoulder and elbow joints change greatly from the time that the frog touches the ground. But the former has more extension, perhaps because it contributes more to support and decelerate the frog.Fig. 5 The mean joint angles of 5 jumps: (a) Elbow and shoulder joints of forelimb; (b) Hip, knee, ankle and TM joint angles of hindlimb; The time of take off from the ground is about 360ms.During the takeoff phase, joint extension appears to be temporally staggered, with the hip and knee beginning to extend prior to or initially faster than the more distal ankle joint (Fig. 5b). The hip and knee joints finish extending near the point of takeoff (when the feet leave the ground), while the ankle joint still extends for some time (about 30 ms). However, the hip, knee and ankle joints of hindlimbs have very similar trajectory and range during the whole jump (Table 2).The TM joint has relatively small range and extends until the end of takeoff phase. This movement pushes the tarsus upward and forward throughout propulsion to realize the function of foot mentioned above.2.5 Biological summaryKinematic analysis of a frog jumping provides critical 3D space point data for modeling. Notably, the role of the forelimb (in supporting and positioning more than in taking off), the similar trajectories of hindlimb joints during the jump and the function of the foot during takeoff phase are issues that must be addressed in design.3 A dynamic model of frogWe developed a dynamic model of frog to help us understand the biological data and to aid the design of the robot (Fig. 6). There are 18 DOF in the model; five for each of the hindlimbs and four for each of forelimbs. Each hindlimb has 3 DOF at the hip joint, 1 DOF at the knee joint, and 1 DOF at the ankle joint. Each forelimb has 3 DOF at the shoulder joint, and 1 DOF at the elbow joint.The inputs to the simulation include the lengths and inertias of the hindlimb and forelimb segments and the body, the joint angle trajectories obtained from the observation of frog jump. We choose the parameters of frog No. O 1, shown in Table 1, as the inputs to the model. The outputs from the simulation are the overall body movement and ground reaction forces.Fig. 7 shows the ground forces during takeoff phase. The result displays a similar time course to those recorded by Calow and Alxander}l}}. In the early stage of takeoff, the forces exerted on the ground are low (the ground force in Fig. 7 at onset includes the gravity of frog) and thenincrease to a peak before falling rapidly as the frog approaches takeoff. The high peak force in the late stage of takeoff could be explained by the redis tribution of power output by elastic elements, for example tendons, in series with muscles}'g}. This characteristic is very important in the design of a mechanism.4 A mechanical model of frogAccording to the kinematic analysis of frog jumping, in which the hip, knee and ankle joints of the hindlimbs have similar trajectory, we put forward a kind of model to mimic the movement of hindlimb (Fig. 8). Like the elastic elements that are used as energy storage, we employed springs as a convenient and robust storage mechanism. Three joints could be simplified and com pressed by one motor via a cable.The main part of the model is a 4bar spring/linkage mechanism. We can obtain the relationship of the thrust force and linkage displacement for this mechanism as follows. From Fig.9 one can easily derive an expression for y as a function of x:This equation can be solved for x:The principle of virtual work states that for an infinitesimal displacement of the mechanism, FXdx=FYdy FX is the spring force along xaxis, andFYis the thrust force in the ydirection. From this we obtain:where k and so are the spring constant and undistorted length respectively. An expression for FY as a function of y can be obtained by substituting Eq. (2) into Eq. (3). For the particular case, where a=b (the length of thigh is equal to that of shank).Fig. 10 plotsFYvs y for the case where the spring constant is normalized, k=1, and so= a=1.5.One can find that the model has ground reaction force similar to the frog during takeoff phase by comparing Fig. 10 with Fig. 7. At the onset of takeoff, the thrust force is quite low and then increases to its maximum before falling as the mechanism approaches the end of takeoff. The jump with this characteristic possesses the advantages of low likelihood of premature liftoff and high efficiency}l9}.Besides the linkage mechanism as the main part of robot's leg, there should be 1 DOF (passive) at the TM joint on the foot for its important role in frog jump. It could also prevent slipping and enhance stability by keeping the plantar surface in contact with the ground as long as possible during takeoff. Moreover, the leg requires another 3 DOF (rotation about xaxis, yaxis and zaxis, Fig. 11) at hip joint for adjusting the direction and trajectory.Because the function of the forelimb is balancing and steering, we need only 1 DOF (rotation about zaxis, Fig. 11) at the shoulder joint to simplify the mechanism. There is also 1 DOF (passive) at the elbow joint to support and decelerate the robot during the landing phase.Hence, we get the whole model of frog for robot design (half of the symmetrical body showed in Fig. 11). The desired stance for takeoff can be achieved by controlling the shoulder and hip joints. When the cable is released, the leg could push the robot into the air. During the aerial phase, the leg will be retracted by cable and the shoulder joint will be adjusted to prepare for landing.5 ConclusionThe biological kinematic data of a jumping frog were obtained. These data and the function of the main joints at each phase were analyzed. A kind of mechanism model was put forward and studied. The forces exerted on the ground by a frog during the takeoff phase of jump were derived from the simulation, and compared with that of the model. The result shows that the mechanical model could be used as a part of robot leg. The model has five actuators which could all be fixed on the body. The relatively light leg could reduce the energy loss of jumplt9l, and the passive joint at elbow and TM could absorb the impact during landing phase.Continuing work will involve the design of jump robot, and corresponding control methods.AcknowledgementThis work was financially supported by the National High Technology Research and Development Program of China NO.0.2006AA04Z245), and partially supported by Program for ChangJiang fang Scholars and Innovative Research Team in University (PCSIRT)(IRT0423).References[1] Raibert M H. Legged Robots that Balanced, The MIT Press, Cambrige, MA, USA, 1986.[2] Nelson G M, Quinn R D, Bachmann R J, Flannigan W C, Ritzmann R E, Watson J T. Design and simulation of a cockroachlike hexapod robot. Proceedings of the IEEE In ternational Conference on Robotics and Automation, 1997, 2,11061111.[3] Kingsley D A. A Cockroach Inspired Robot with Artificial Muscles, PhD Dissertation, Dept. of Mechanical and Aero space Engineering, Case Western Reserve University, Cleveland, 2005[4] Quinn R D, Ritzmann R E. Construction of a hexapod robot with cockroach kinematics benefits both robotics and biology. Connection Science, 1998, 10, 239254.[5] Birch M C, Quinn R D, Hahm q Philips S M, Drennan B, Fife A, Verma H, Beer R Cx Design of a cricket microrobot.Proceedings of the IEEE International Conference on Robotics and Automation, 2000, 2, 1109一川4.[6] Laksanacharoen S, Pollack A J, Nelson G M, Quinn R D, Ritzmann R E. Biomechanics and simulation of cricket for microrobot design. Proceedings of the IEEE International Conference on Robotics and Automation, 2000, 2, 10881094.[7] Hyon S H, Mita T. Development of a biologically inspired hopping robotkenken. Proceedings of the IEEE International Conference on Robotics and Automation, 2002, 4, 39843991.[8] Yamakita M, Omagari Y, Taniguchi Y Jumping cat robot with kicking a wall. Journalof the Robotics Society oflapan, 1994, 9, 934938.[9] Young M C. Guinness Book of World Records, Guinness Press, Stamford CT, USA, 1997.[ 10] Lombard W P, Abbott F M. The mechanical effects produced by the contraction of individual muscles of the thigh of the frog. American Journal of Physiology, 1907, 20, 160.[11] Gans C, Parsons T S. On the origin of the jumping mechanism in frogs. Evolution, 1966, 20, 9299.[ 12] Liu L Y, Zheng G M. Ordinary Zoology, Higher Education Press, Beijing, China, 2005. (in Chinese)[13] Wang M, Zang X Z, Zhao J. An extraction method of frog jumping trajectory for biomimetic robot design. Journal of Beijing University of Posts and Telecommunications, 2008, 31, 41一5. (in Chinese)[14] Emerson S B, Jongh H J. Muscle activity at the iliosacral articulation of frogs. International Journal of Morphology, 2005, 166, 129144.[15] Hildebrand M. Walking and Running, Belknap Press, Cambridge MA, USA, 1985.[16] Emerson S B. Jumping and Leaping, Belknap Press, Cambridge MA, USA, 1985.[ 17] Calow L J, Alexander R M. A mechanical analysis of a hind leg of a frog (Rana temporaria). Journal of Zoology, 1973,171,293321.[ 18] Marsh R L, JohnAlder H B. Jumping performance of hylid frogs measured with highspeed cine film. Journal of Experimental Biology,1994,188,131141.[19] Burdick J, Fiorini P Minimalist jumping robots for celestial exploration. International Journal of Robotics Research, 2003, 22, 6536 74.。