High energy X-ray emission from recurrent novae in quiescence T CrB
染料敏化太阳能电池最高光电转换效率
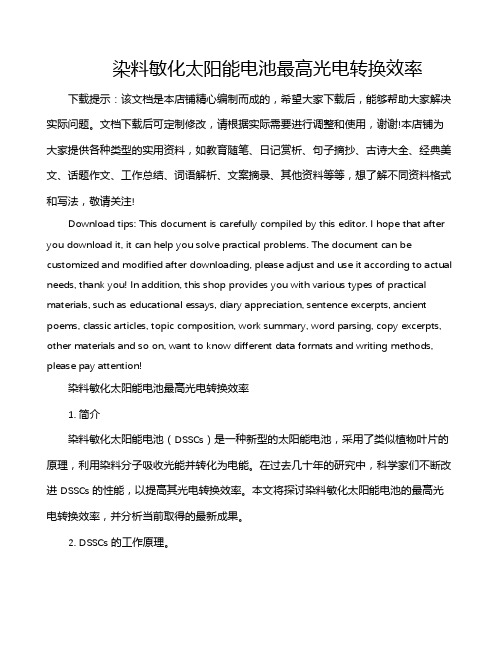
染料敏化太阳能电池最高光电转换效率下载提示:该文档是本店铺精心编制而成的,希望大家下载后,能够帮助大家解决实际问题。
文档下载后可定制修改,请根据实际需要进行调整和使用,谢谢!本店铺为大家提供各种类型的实用资料,如教育随笔、日记赏析、句子摘抄、古诗大全、经典美文、话题作文、工作总结、词语解析、文案摘录、其他资料等等,想了解不同资料格式和写法,敬请关注!Download tips: This document is carefully compiled by this editor. I hope that after you download it, it can help you solve practical problems. The document can be customized and modified after downloading, please adjust and use it according to actual needs, thank you! In addition, this shop provides you with various types of practical materials, such as educational essays, diary appreciation, sentence excerpts, ancient poems, classic articles, topic composition, work summary, word parsing, copy excerpts, other materials and so on, want to know different data formats and writing methods, please pay attention!染料敏化太阳能电池最高光电转换效率1. 简介染料敏化太阳能电池(DSSCs)是一种新型的太阳能电池,采用了类似植物叶片的原理,利用染料分子吸收光能并转化为电能。
X射线荧光光谱快速分析高碳铬铁中铬、硅、磷

总第267期2018年第3期HEBEI METALLURGYTotal No.2672018袁Number 3收稿日期:2017-10-25作者简介:郭利军(1974-),男,工程师,2011年毕业于河北联合大学院校冶金工程专业,现在河钢集团宣钢公司技术中心从事质量管理和化学分析,E -mail:G2375437@X 射线荧光光谱快速分析高碳铬铁中铬、硅、磷郭利军(河钢集团宣钢公司技术中心,河北宣化075100)摘要:介绍了采用X 射线荧光光谱法对高碳铬铁中铬、硅、磷进行含量测定的方法并对其准确度和精密度进行了验证。
通过对高碳铬铁合金样品进行压片制备,对X 射线荧光仪各参数的调整,建立了高碳铬铁工作曲线,满足了钢铁企业对高碳铬铁快速准确的分析要求。
关键词:X 射线荧光光谱法;高碳铬铁;铬、硅、磷测定中图分类号:TG 115.3 文献标识码:A文章编号:1006-5008(2018)03-0041-04.13630/ki.13-1172.2018.0311Guo Lijun(Technology Center of HBIS Group Xuansteel Company ,Xuanhua ,Hebei ,075100)The method of determining the content of chromium ,silicon and phosphorus in high carbon ferro⁃chrome by X ray fluorescence spectrometry is introduced ,and its accuracy and precision are verified.By the tablet compressing of high carbon ferrochrome alloy samples and adjustment of the parameters of X ray fluo⁃rescence spectrometer ,the working curve of high carbon ferrochrome has been established ,which meets the requirement of steelenterprises for fast and accurate analysis of high carbonferrochrome.X -ray fluorescence spectrometry ;high carbon ferrochromium ;determination of chromium ,and phosphorus0 引言高碳铬铁是炼钢生产中重要的铁合金原料,在轴承钢、不锈钢等工具钢的冶炼过程中被广泛应用。
最黑材料可吸收99.995%入射光
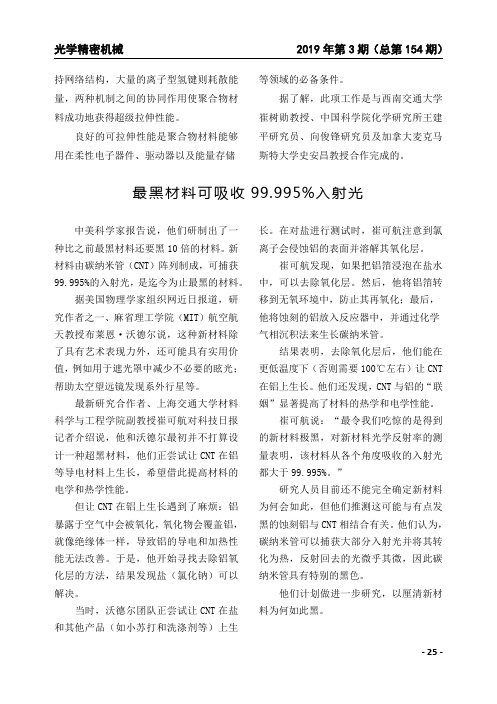
光学精密机械2019年第3期(总第154期)-25-持网络结构,大量的离子型氢键则耗散能量,两种机制之间的协同作用使聚合物材料成功地获得超级拉伸性能。
良好的可拉伸性能是聚合物材料能够用在柔性电子器件、驱动器以及能量存储等领域的必备条件。
据了解,此项工作是与西南交通大学崔树勋教授、中国科学院化学研究所王建平研究员、向俊锋研究员及加拿大麦克马斯特大学史安昌教授合作完成的。
最黑材料可吸收99.995%入射光中美科学家报告说,他们研制出了一种比之前最黑材料还要黑10倍的材料。
新材料由碳纳米管(CNT)阵列制成,可捕获99.995%的入射光,是迄今为止最黑的材料。
据美国物理学家组织网近日报道,研究作者之一、麻省理工学院(MIT)航空航天教授布莱恩·沃德尔说,这种新材料除了具有艺术表现力外,还可能具有实用价值,例如用于遮光罩中减少不必要的眩光;帮助太空望远镜发现系外行星等。
最新研究合作者、上海交通大学材料科学与工程学院副教授崔可航对科技日报记者介绍说,他和沃德尔最初并不打算设计一种超黑材料,他们正尝试让CNT 在铝等导电材料上生长,希望借此提高材料的电学和热学性能。
但让CNT 在铝上生长遇到了麻烦:铝暴露于空气中会被氧化,氧化物会覆盖铝,就像绝缘体一样,导致铝的导电和加热性能无法改善。
于是,他开始寻找去除铝氧化层的方法,结果发现盐(氯化钠)可以解决。
当时,沃德尔团队正尝试让CNT 在盐和其他产品(如小苏打和洗涤剂等)上生长。
在对盐进行测试时,崔可航注意到氯离子会侵蚀铝的表面并溶解其氧化层。
崔可航发现,如果把铝箔浸泡在盐水中,可以去除氧化层。
然后,他将铝箔转移到无氧环境中,防止其再氧化;最后,他将蚀刻的铝放入反应器中,并通过化学气相沉积法来生长碳纳米管。
结果表明,去除氧化层后,他们能在更低温度下(否则需要100℃左右)让CNT 在铝上生长。
他们还发现,CNT 与铝的“联姻”显著提高了材料的热学和电学性能。
211050371_正负压一体式无空气X_射线光电子能谱原位转移仓的开发及研制
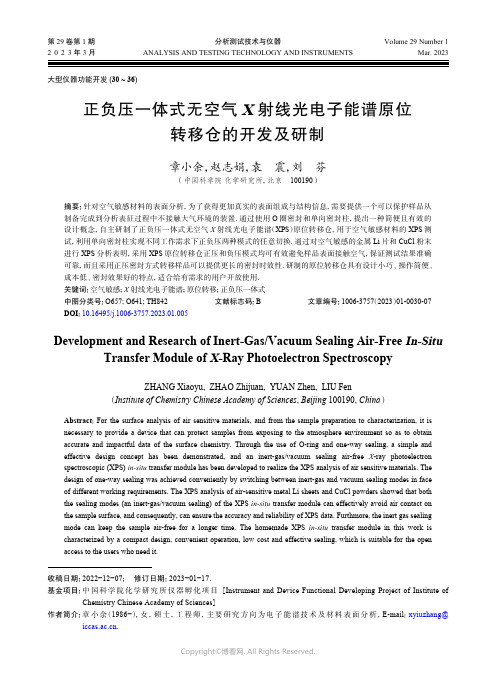
第 29 卷第 1 期分析测试技术与仪器Volume 29 Number 1 2023年3月ANALYSIS AND TESTING TECHNOLOGY AND INSTRUMENTS Mar. 2023大型仪器功能开发(30 ~ 36)正负压一体式无空气X射线光电子能谱原位转移仓的开发及研制章小余,赵志娟,袁 震,刘 芬(中国科学院化学研究所,北京 100190)摘要:针对空气敏感材料的表面分析,为了获得更加真实的表面组成与结构信息,需要提供一个可以保护样品从制备完成到分析表征过程中不接触大气环境的装置. 通过使用O圈密封和单向密封柱,提出一种简便且有效的设计概念,自主研制了正负压一体式无空气X射线光电子能谱(XPS)原位转移仓,用于空气敏感材料的XPS测试,利用单向密封柱实现不同工作需求下正负压两种模式的任意切换. 通过对空气敏感的金属Li片和CuCl粉末进行XPS分析表明,采用XPS原位转移仓正压和负压模式均可有效避免样品表面接触空气,保证测试结果准确可靠,而且采用正压密封方式转移样品可以提供更长的密封时效性. 研制的原位转移仓具有设计小巧、操作简便、成本低、密封效果好的特点,适合给有需求的用户开放使用.关键词:空气敏感;X射线光电子能谱;原位转移;正负压一体式中图分类号:O657; O641; TH842 文献标志码:B 文章编号:1006-3757(2023)01-0030-07 DOI:10.16495/j.1006-3757.2023.01.005Development and Research of Inert-Gas/Vacuum Sealing Air-Free In-Situ Transfer Module of X-Ray Photoelectron SpectroscopyZHANG Xiaoyu, ZHAO Zhijuan, YUAN Zhen, LIU Fen(Institute of Chemistry Chinese Academy of Sciences, Beijing 100190, China)Abstract:For the surface analysis of air sensitive materials, and from the sample preparation to characterization, it is necessary to provide a device that can protect samples from exposing to the atmosphere environment so as to obtain accurate and impactful data of the surface chemistry. Through the use of O-ring and one-way sealing, a simple and effective design concept has been demonstrated, and an inert-gas/vacuum sealing air-free X-ray photoelectron spectroscopic (XPS) in-situ transfer module has been developed to realize the XPS analysis of air sensitive materials. The design of one-way sealing was achieved conveniently by switching between inert-gas and vacuum sealing modes in face of different working requirements. The XPS analysis of air-sensitive metal Li sheets and CuCl powders showed that both the sealing modes (an inert-gas/vacuum sealing) of the XPS in-situ transfer module can effectively avoid air contact on the sample surface, and consequently, can ensure the accuracy and reliability of XPS data. Furthmore, the inert gas sealing mode can keep the sample air-free for a longer time. The homemade XPS in-situ transfer module in this work is characterized by a compact design, convenient operation, low cost and effective sealing, which is suitable for the open access to the users who need it.收稿日期:2022−12−07; 修订日期:2023−01−17.基金项目:中国科学院化学研究所仪器孵化项目[Instrument and Device Functional Developing Project of Institute of Chemistry Chinese Academy of Sciences]作者简介:章小余(1986−),女,硕士,工程师,主要研究方向为电子能谱技术及材料表面分析,E-mail:xyiuzhang@ .Key words:air-sensitive;X-ray photoelectron spectroscopy;in-situ transfer;inert-gas/vacuum sealingX射线光电子能谱(XPS)是一种表面灵敏的分析技术,通常用于固体材料表面元素组成和化学态分析[1]. 作为表面分析领域中最有效的方法之一,XPS广泛应用于纳米科学、微电子学、吸附与催化、环境科学、半导体、冶金和材料科学、能源电池及生物医学等诸多领域[2-3]. 其中在催化和能源电池材料分析中,有一些样品比较特殊,比如碱金属电池[4-6]、负载型纳米金属催化剂[7-8]和钙钛矿材料[9]对空气非常敏感,其表面形态和化学组成接触空气后会迅速发生改变,直接影响采集数据的准确性和有效性,因此这类样品的表面分析测试具有一定难度. 目前,常规的光电子能谱仪制样转移过程通常是在大气环境中,将样品固定在标准样品台上,随后放入仪器进样室内抽真空至1×10−6 Pa,再转入分析室内进行测试. 这种制备和进样方式无法避免样品接触大气环境,对于空气敏感材料,其表面很容易与水、氧发生化学反应,导致无法获得材料表面真实的结构信息.为了保证样品表面状态在转移至能谱仪内的过程中不受大气环境影响,研究人员采用了各种技术来保持样品转移过程中隔绝空气. 比如前处理及反应装置与电子能谱仪腔室间真空传输[10-12]、外接手套箱 [13-14]、商用转移仓[15-16]、真空蒸镀惰性金属比如Al层(1.5~6 nm)[17]等. 尽管上述技术手段有效,但也存在一些缺点,例如配套装置体积巨大、试验过程不易操作、投入成本高等,这都不利于在普通实验室内广泛应用. 而一些电子能谱仪器制造商根据自身仪器的特点也研发出了相应配套的商用真空传递仓,例如Thermofisher公司研发的一种XPS 真空转移仓,转移过程中样品处于微正压密封状态,但其价格昂贵,体积较大,转移过程必须通过手套箱大过渡舱辅助,导致传递效率低,单次需消耗至少10 L高纯氩气,因此购置使用者较少,利用率低.另外有一些国内公司也研发了类似的商品化气体保护原位传递仓,采用微正压方式密封转移样品,但需要在能谱仪器进样室舱门的法兰上外接磁耦合机械旋转推拉杆,其操作复杂且放置样品的有效区域小,单次仅可放置尺寸为3 mm×3 mm的样品3~4个,进样和测试效率较低. 因此,从2016年起本实验团队开始自主研制XPS原位样品转移装置[18],经过结构与性能的迭代优化[19],最终研制出一种正负压一体式无空气XPS原位转移仓[20](本文简称XPS原位转移仓),具有结构小巧、操作便捷、成本低、密封效果好、正压和负压密封两种模式转移样品的特点. 为验证装置的密封时效性能,本工作选取两种典型的空气敏感材料进行测试,一种是金属Li材料,其化学性质非常活泼,遇空气后表面迅速与空气中的O2、N2、S等反应导致表面化学状态改变. 另一种是无水CuCl粉末,其在空气中放置短时间内易发生水解和氧化. 试验结果表明,该XPS 原位转移仓对不同类型的空气敏感样品的无空气转移均可以提供更便捷有效的密封保护. 目前,XPS原位转移仓已在多个科研单位的实验室推广使用,支撑应用涉及吸附与催化、能源环境等研究领域.1 试验部分1.1 XPS原位转移仓的研制基于本实验室ESCALAB 250Xi型多功能光电子能谱仪器(Thermofisher 公司)的特点,研究人员设计了XPS原位转移仓. 为兼顾各个部件强度、精度与轻量化的要求,所有部件均采用钛合金材料.该装置从整体结构上分为样品台、密封罩和紧固挡板三个部件,如图1(a)~(c)所示. 在密封罩内部通过单向密封设计[图1(e)]使得XPS原位转移仓实现正负压一体,实际操作中可通过调节密封罩上的螺帽完成两种模式任意切换. 同时,从图1(e)中可以直观看到,密封罩与样品台之间通过O圈密封,利用带有螺钉的紧固挡板将二者紧密固定. 此外,为确保样品台与密封罩对接方位正确,本设计使用定向槽定位样品台与密封罩位置,保证XPS原位转移仓顺利传接到仪器进样室.XPS原位转移仓使用的具体流程:在手套箱中将空气敏感样品粘贴至样品台上,利用紧固挡板使样品台和密封罩固定在一起,通过调节密封罩上的螺帽将样品所在区域密封为正压惰性气氛(压强为300 Pa、环境气氛与手套箱内相同)或者负压真空状态,其整体装配实物图如图1(d)所示. 该转移仓结构小巧,整体尺寸仅52 mm×58 mm×60 mm,可直接放入手套箱小过渡舱传递. 由于转移仓尺寸小,其第 1 期章小余,等:正负压一体式无空气X射线光电子能谱原位转移仓的开发及研制31原料成本大大缩减,整体造价不高. 转移仓送至能谱仪进样室后,配合样品停放台与进样杆的同时双向对接,将转移仓整体固定在进样室内,如图1(f )所示. 此时关闭进样室舱门开始抽真空,当样品台与密封罩内外压强平衡后密封罩自动解除真空密封,但仍然处于O 圈密闭状态. 等待进样室真空抽至1×10−4Pa 后,使用能谱仪进样室的样品停放台摘除脱离的密封罩[如图1(g )所示],待真空抽至1×10−6Pa ,即可将样品送入分析室进行XPS 测试.整个试验过程操作便捷,实现了样品从手套箱转移至能谱仪内不接触大气环境.1.2 试验过程1.2.1 样品准备及转移试验所用手套箱是布劳恩惰性气体系统(上海)有限公司生产,型号为MB200MOD (1500/780)NAC ;金属Li 片购自中能锂业,纯度99.9%;CuCl 购自ALFA 公司,纯度99.999%.金属Li 片的制备及转移:将XPS 原位转移仓整体通过手套箱过渡舱送入手套箱中,剪取金属Li 片用双面胶带固定于样品台上,分别采用正压、负压两种密封模式将XPS 原位转移仓整体从手套箱中取出,分别在空气中放置0、2、4、8、18、24、48、72 h 后送入能谱仪内,进行XPS 测试.CuCl 粉末的制备及转移:在手套箱中将CuCl 粉末压片[21],使用上述同样的制备方法,将XPS 原位转移仓整体在空气中分别放置0、7、24、72 h 后送入能谱仪内,进行XPS 测试.1.2.2 样品转移方式介绍样品在手套箱中粘贴完成后,分别采用三种方式将其送入能谱仪. 第一种方式是在手套箱内使用标准样品台粘贴样品,将其装入自封袋密封,待能谱仪进样室舱门打开后,即刻打开封口袋送入仪器中开始抽真空等待测试,整个转移过程中样品暴露空气约15 s. 第二种方式是使用XPS 原位转移仓负压密封模式转移样品,具体操作步骤:利用紧固挡板将样品台和密封罩固定在一起,逆时针(OPEN )旋动螺帽至顶部,放入手套箱过渡舱并将其抽为真空,此过程中样品所在区域也抽至负压. 取出整体装置后再顺时针(CLOSE )旋动螺帽至底部,将样品所在区域进一步锁死密封. 样品在负压环境中转移至XPS 实验室,拆卸掉紧固挡板,随即送入能谱仪进样室内. 第三种方式是使用XPS 原位转移仓正压密封模式转移样品,具体操作步骤:利用紧固挡板将样品台和密封罩固定在一起,顺时针(CLOSE )旋螺帽抽气管限位板单向密封柱密封罩主体O 圈样品台紧固挡板(e) 密封罩对接停放台机械手样品台对接进样杆(a)(b)(c)(d)(g)图1 正负压一体式无空气XPS 原位转移仓系统装置(a )样品台,(b )密封罩,(c )紧固挡板,(d )整体装配实物图,(e )整体装置分解示意图,(f )样品台与密封罩在进样室内对接完成,(g )样品台与密封罩在进样室内分离Fig. 1 System device of inert-gas/vacuum sealing air-free XPS in-situ transfer module32分析测试技术与仪器第 29 卷动螺帽至底部,此时样品所在区域密封为正压惰性气氛. 直至样品转移至XPS 实验室,再使用配套真空抽气系统(如图2所示),通过抽气管将样品所在区域迅速抽为负压,拆卸掉紧固挡板,随即送入能谱仪进样室内.图2 能谱仪实验室内配套真空抽气系统Fig. 2 Vacuum pumping system in XPSlaboratory1.2.3 XPS 分析测试试验所用仪器为Thermo Fisher Scientific 公司的ESCALAB 250Xi 型多功能X 射线光电子能谱仪,仪器分析室基础真空为1×10−7Pa ,X 射线激发源为单色化Al 靶(Alk α,1 486.6 eV ),功率150 W ,高分辨谱图在30 eV 的通能及0.05 eV 的步长等测试条件下获得,并以烃类碳C 1s 为284.8 eV 的结合能为能量标准进行荷电校正.2 结果与讨论2.1 测试结果分析为了验证XPS 原位转移仓的密封性能,本文做了一系列的对照试验,选取空气敏感的金属Li 片和CuCl 粉末样品进行XPS 测试,分别采用上述三种方式转移样品,并考察了XPS 原位转移仓密封状态下在空气中放置不同时间后对样品测试结果的影响.2.1.1 负压密封模式下XPS 原位转移仓对金属Li片的密封时效性验证将金属Li 片通过两种(标准和负压密封)方式转移并在空气中放置不同时间,对这一系列样品进行XPS 测试,Li 1s 和C 1s 高分辨谱图结果如图3(a )(b )所示,试验所测得的Li 1s 半峰宽值如表1所列. 根据XPS 结果分析,金属Li 片采用标准样品台进样(封口袋密封),短暂暴露空气约15 s ,此时Li 1s 的半峰宽为1.62 eV. 而采用XPS 原位转移仓负压密封模式转移样品时,装置整体放置空气18 h 内,Li 1s 的半峰宽基本保持为(1.35±0.03) eV. 放置空气24 h 后,Li 1s 的半峰宽增加到与暴露空气15 s 的金属Li 片一样,说明此时原位转移仓的密封性能衰减,金属Li 片与渗入内部的空气发生反应生成新物质导致Li 1s 半峰宽变宽. 由图3(b )中C 1s 高分辨谱图分析,结合能位于284.82 eV 的峰归属为C-C/污染C ,位于286.23 eV 的峰归属为C-OH/C-O-CBinding energy/eVI n t e n s i t y /a .u .Li 1s半峰宽增大暴露 15 s密封放置 24 h 密封放置 18 h 密封放置 8 h 密封放置 4 h 密封放置 0 h6058565452Binding energy/eVI n t e n s i t y /a .u .C 1s(a)(b)暴露 1 min 暴露 15 s 密封放置 24 h 密封放置 18 h 密封放置 0 h292290288284282286280图3 金属Li 片通过两种(标准和负压密封)方式转移并在空气中放置不同时间的(a )Li 1s 和(b )C 1s 高分辨谱图Fig. 3 High-resolution spectra of (a) Li 1s and (b) C 1s of Li sheet samples transferred by two methods (standard andvacuum sealings) and placed in air for different times第 1 期章小余,等:正负压一体式无空气X 射线光电子能谱原位转移仓的开发及研制33键,位于288.61~289.72 eV的峰归属为HCO3−/CO32−中的C[22]. 我们从C 1s的XPS谱图可以直观的看到,与空气短暂接触后,样品表面瞬间生成新的结构,随着暴露时间增加到1 min,副反应产物大量增加(HCO3−/CO32−). 而XPS原位转移仓负压密封模式下在空气中放置18 h内,C结构基本不变,在空气中放置24 h后,C结构只有微小变化. 因此根据试验结果分析,对于空气极其敏感的材料,在负压密封模式下,建议XPS原位转移仓在空气中放置时间不要超过18 h. 这种模式适合对空气极其敏感样品的短距离转移.表 1 通过两种(标准和负压密封)方式转移并在空气中放置不同时间的Li 1s的半峰宽Table 1 Full width at half maxima (FWHM) of Li 1stransferred by two methods (standard and vacuum sealings) and placed in air for different times样品说明进样方式半峰宽/eV密封放置0 h XPS原位转移仓负压密封模式转移1.38密封放置2 h同上 1.39密封放置4 h同上 1.36密封放置8 h同上 1.32密封放置18 h同上 1.32密封放置24 h同上 1.62暴露15 s标准样品台进样(封口袋密封)1.622.1.2 正压密封模式下原位转移仓对金属Li片的密封时效性验证将金属Li片通过两种(标准和正压密封)方式转移并在空气中放置不同时间,对这一系列样品进行XPS测试,Li 1s高分辨谱图结果如图4所示,所测得的Li 1s半峰宽值如表2所列. 根据XPS结果分析,XPS原位转移仓正压密封后,在空气中放置72 h内,Li 1s半峰宽基本保持为(1.38±0.04) eV,说明有明显的密封效果,金属Li片仍然保持原有化学状态. 所以对于空气极其敏感的材料,在正压密封模式下,可至少在72 h内保持样品表面不发生化学态变化. 这种模式适合长时间远距离(可全国范围内)转移空气敏感样品.2.1.3 负压密封模式下XPS原位转移仓对空气敏感样品CuCl的密封时效性验证除了金属Li片样品,本文还继续考察XPS原位转移仓对空气敏感样品CuCl的密封时效性. 图5为CuCl粉末通过两种(标准和负压密封)方式转移并在空气中放置不同时间的Cu 2p高分辨谱图. XPS谱图中结合能[22]位于932.32 eV的峰归属为Cu+的Cu 2p3/2,位于935.25 eV的峰归属为Cu2+的Cu 2p3/2,此外,XPS谱图中位于940.00~947.50 eV 处的峰为Cu2+的震激伴峰,这些震激伴峰被认为是表 2 通过两种(标准和正压密封)方式转移并在空气中放置不同时间的Li 1s的半峰宽Table 2 FWHM of Li 1s transferred by two methods(standard and inert gas sealings) and placed in air fordifferent times样品说明进样方式半峰宽/eV 密封放置0 h XPS原位转移仓正压密封模式转移1.42密封放置2 h同上 1.35密封放置4 h同上 1.35密封放置8 h同上 1.34密封放置18 h同上 1.38密封放置24 h同上 1.39密封放置48 h同上 1.42密封放置72 h同上 1.38暴露15 s标准样品台进样(封口袋密封)1.62Binding energy/eVIntensity/a.u.Li 1s半峰宽比正压密封的宽半峰宽=1.62 eV半峰宽=1.38 eV暴露 15 s密封放置 72 h密封放置 48 h密封放置 24 h密封放置 18 h密封放置 0 h605856545250图4 金属Li片通过两种(标准和正压密封)方式转移并在空气中放置不同时间的Li 1s高分辨谱图Fig. 4 High-resolution spectra of Li 1s on Li sheet samples transferred by two methods (standard and inert gas sealings) and placed in air for different times34分析测试技术与仪器第 29 卷价壳层电子向激发态跃迁的终态效应所产生[23],而在Cu +和Cu 0中则观察不到.根据XPS 结果分析,CuCl 在XPS 原位转移仓保护(负压密封)下,即使放置空气中72 h ,测得的Cu 2p 高分辨能谱图显示只有Cu +存在,说明CuCl 并未被氧化. 若无XPS 原位转移仓保护,CuCl 粉末放置空气中3 min 就发生了比较明显的氧化,从测得的Cu 2p 高分辨能谱图能够直观的看到Cu 2+及其震激伴峰的存在,并且随着放置时间增加到40 min ,其氧化程度也大大增加. 因此,对于空气敏感的无机材料、纳米催化剂和钙钛矿材料等,采用负压密封模式转移就可至少在72 h 内保持样品表面不发生化学态变化.3 结论本工作中自主研制的正负压一体式无空气XPS原位转移仓在空气敏感样品转移过程中可以有效隔绝空气,从而获得样品最真实的表面化学结构.试验者可根据样品情况和实验室条件选择转移模式,并在密封有效时间内将样品从实验室转移至能谱仪中完成测试. 综上所述,该XPS 原位转移仓是一种设计小巧、操作简便、密封性能优异、成本较低的样品无水无氧转移装置,因此非常适合广泛开放给有需求的试验者使用. 在原位和准原位表征技术被广泛用于助力新材料发展的现阶段,希望该设计理念能对仪器功能的开发和更多准原位表征测试的扩展提供一些启示.参考文献:黄惠忠. 论表面分析及其在材料研究中的应用[M ].北京: 科学技术文献出版社, 2002: 16-18.[ 1 ]杨文超, 刘殿方, 高欣, 等. X 射线光电子能谱应用综述[J ]. 中国口岸科学技术,2022,4(2):30-37.[YANG Wenchao, LIU Dianfang, GAO Xin, et al.TheapplicationofX -rayphotoelectronspectroscopy [J ]. China Port Science and Technology ,2022,4 (2):30-37.][ 2 ]郭沁林. X 射线光电子能谱[J ]. 物理,2007,36(5):405-410. [GUO Qinlin. X -ray photoelectron spectro-scopy [J ]. Physics ,2007,36 (5):405-410.][ 3 ]Malmgren S, Ciosek K, Lindblad R, et al. Con-sequences of air exposure on the lithiated graphite SEI [J ]. Electrochimica Acta ,2013,105 :83-91.[ 4 ]Zhang Y H, Chen S M, Chen Y, et al. Functional poly-ethylene glycol-based solid electrolytes with enhanced interfacial compatibility for room-temperature lithium metal batteries [J ]. Materials Chemistry Frontiers ,2021,5 (9):3681-3691.[ 5 ]周逸凡, 杨慕紫, 佘峰权, 等. X 射线光电子能谱在固态锂离子电池界面研究中的应用[J ]. 物理学报,2021,70(17):178801. [ZHOU Yifan, YANG Muzi,SHE Fengquan, et al. Application of X -ray photoelec-tron spectroscopy to study interfaces for solid-state lithium ion battery [J ]. Acta Physica Sinica ,2021,70(17):178801.][ 6 ]Huang J J, Song Y Y, Ma D D, et al. The effect of thesupport on the surface composition of PtCu alloy nanocatalysts: in situ XPS and HS-LEIS studies [J ].Chinese Journal of Catalysis ,2017,38 (7):1229-1236.[ 7 ]Koley P, Shit S C, Sabri Y M, et al. Looking into moreeyes combining in situ spectroscopy in catalytic bio-fuel upgradation with composition-graded Ag-Co core-shell nanoalloys [J ]. ACS Sustainable Chemistry &Engineering ,2021,9 (10):3750-3767.[ 8 ]Opitz A K, Nenning A, Rameshan C, et al. Enhancingelectrochemical water-splitting kinetics by polarization-driven formation of near-surface iron(0): an in situ XPS study on perovskite-type electrodes [J ]. Ange-wandte Chemie (International Ed in English),2015,54(9):2628-2632.[ 9 ]Czekaj I, Loviat F, Raimondi F, et al. Characterization[ 10 ]Binding energy/eVI n t e n s i t y /a .u .Cu 2pCu +Cu 2+暴露 3 min暴露 40 min 密封放置 7 h 密封放置 72 h 密封放置 24 h密封放置 0 h960950945935925955940930920图5 CuCl 粉末通过两种(标准和负压密封)方式转移并在空气中放置不同时间的Cu 2p 高分辨谱图Fig. 5 High-resolution spectra of Cu 2p on CuCl powder samples transferred by two methods (standard and vacuumsealings) and placed in air for different times第 1 期章小余,等:正负压一体式无空气X 射线光电子能谱原位转移仓的开发及研制35of surface processes at the Ni-based catalyst during the methanation of biomass-derived synthesis gas: X -ray photoelectron spectroscopy (XPS)[J ]. Applied Cata-lysis A:General ,2007,329 :68-78.Rutkowski M M, McNicholas K M, Zeng Z Q, et al.Design of an ultrahigh vacuum transfer mechanism to interconnect an oxide molecular beam epitaxy growth chamber and an X -ray photoemission spectroscopy analysis system [J ]. Review of Scientific Instruments ,2013,84 (6):065105.[ 11 ]伊晓东, 郭建平, 孙海珍, 等. X 射线光电子能谱仪样品前处理装置的设计及应用[J ]. 分析仪器,2008(5):8-11. [YI Xiaodong, GUO Jianping, SUN Haizhen, et al. Design of a sample pretreatment device for X -ray photoelectron spectrometer [J ]. Analytical Instrumentation ,2008 (5):8-11.][ 12 ]Aurbach D, Weissman I, Schechter A, et al. X -ray pho-toelectron spectroscopy studies of lithium surfaces pre-pared in several important electrolyte solutions. A comparison with previous studies by Fourier trans-form infrared spectroscopy [J ]. Langmuir ,1996,12(16):3991-4007.[ 13 ]Światowska-Mrowiecka J, Maurice V, Zanna S, et al.XPS study of Li ion intercalation in V 2O 5 thin films prepared by thermal oxidation of vanadium metal [J ].Electrochimica Acta ,2007,52 (18):5644-5653.[ 14 ]Weingarth D, Foelske-Schmitz A, Wokaun A, et al. Insitu electrochemical XPS study of the Pt/[BF 4]system [J ]. Electrochemistry Communications ,2011,13 (6):619-622.[ 15 ]Schneider J D, Agocs D B, Prieto A L. Design of asample transfer holder to enable air-free X -ray photo-electron spectroscopy [J ]. Chemistry of Materials ,2020,32 (19):8091-8096.[ 16 ]Karamurzov B S, Kochur A G, Misakova L B, et al.Calculation of the pure surface composition of the bin-ary alloy according to XPS data obtained after the al-loy surface contact with air [J ]. Journal of Structural Chemistry ,2015,56 (3):576-581.[ 17 ]章小余, 赵志娟. 一种半原位XPS 样品转移装置: 中国, 201620925237.5[P ]. 2017-02-15.[ 18 ]章小余, 袁震, 赵志娟. 一种半原位X 射线光电子能谱分析仪的样品转移装置: 中国, 201720056623.X [P ]. 2017-12-08.[ 19 ]袁震, 章小余, 赵志娟. 一种样品转移装置及转移方法: 中国, 2011203822.1[P ]. 2022-03-01.[ 20 ]刘芬, 赵志娟, 邱丽美, 等. XPS 分析固体粉末时的样品制备法研究[J ]. 分析测试技术与仪器,2007,13(2):107-109. [LIU Fen, ZHAO Zhijuan, QIU Limei, et al. Study of sample preparation method for XPS analysis of powdered samples [J ]. Analysis and Testing Technology and Instruments ,2007,13 (2):107-109.][ 21 ]Wagner C D, Riggs W M, Davis L E, et al. Handbookof X -ray photoelectron spectroscopy [M ]. Eden Prair-ie, Minnesota, 1978.[ 22 ]Watts J F, Wolstenholme J. 表面分析(XPS 和AES)引论[M ]. 吴正龙, 译. 上海: 华东理工大学出版社,2008.[ 23 ]36分析测试技术与仪器第 29 卷。
高光谱英文缩写

高光谱英文缩写Hyperspectral imaging, often referred to as HSI, is a powerful and versatile technology that has revolutionized the way we perceive and analyze the world around us. This advanced imaging technique goes beyond the capabilities of traditional digital cameras by capturing a vast array of spectral information from the electromagnetic spectrum, providing a wealth of data that can be used in a wide range of applications.At its core, hyperspectral imaging involves the acquisition of high-dimensional data cubes, where each pixel in the image contains a detailed spectral signature. This signature represents the unique reflectance or emission characteristics of the target material, allowing for the identification and classification of a wide variety of substances and materials. Unlike conventional RGB (red, green, blue) imaging, which captures only three color channels, hyperspectral sensors can record hundreds or even thousands of narrow spectral bands, creating a rich and detailed spectral profile.The power of hyperspectral imaging lies in its ability to revealinformation that is invisible to the human eye or traditional imaging techniques. By capturing the subtle nuances of the electromagnetic spectrum, HSI can detect and analyze a diverse range of materials, from minerals and vegetation to man-made objects and even chemical compounds. This capability has made it an indispensable tool in a variety of fields, including remote sensing, environmental monitoring, agriculture, and even medical diagnostics.In the realm of remote sensing, hyperspectral imaging has revolutionized the way we study and manage our natural resources. By analyzing the spectral signatures of different materials, researchers can map and monitor the distribution of minerals, identify areas of vegetation stress, and detect the presence of pollutants or contaminants in the environment. This information is invaluable for a wide range of applications, from mineral exploration and forestry management to environmental impact assessments and disaster response.In the agricultural sector, hyperspectral imaging has become a crucial tool for precision farming and crop monitoring. By analyzing the spectral signatures of plants, farmers can detect early signs of disease, nutrient deficiencies, or water stress, allowing them to take targeted action to improve crop yields and reduce the environmental impact of their operations. Additionally, HSI can be used to map soil composition, monitor crop growth, and even detect the presence ofpests or weeds, enabling more efficient and sustainable farming practices.The medical field has also benefited greatly from the advances in hyperspectral imaging technology. In the area of diagnostics, HSI has shown promise in the early detection of various diseases, such as skin cancer, breast cancer, and cardiovascular conditions. By analyzing the unique spectral signatures of diseased tissues, healthcare professionals can identify subtle changes that may not be visible to the naked eye, enabling earlier intervention and improved patient outcomes.Beyond these applications, hyperspectral imaging has found its way into numerous other industries, including art conservation, forensics, and even aerospace engineering. In the field of art conservation, HSI can be used to identify pigments, detect forgeries, and monitor the condition of valuable artworks, while in forensics, it has been employed to analyze trace evidence and identify illicit substances.As the technology continues to evolve, the potential applications of hyperspectral imaging are virtually limitless. With advancements in sensor technology, data processing, and analytical algorithms, the future of HSI looks increasingly bright, promising new discoveries and innovations that will shape our understanding of the world around us.However, the widespread adoption of hyperspectral imaging technology is not without its challenges. The sheer volume of data generated by HSI systems, coupled with the complexity of the spectral analysis, can pose significant computational and storage challenges. Additionally, the cost of the specialized equipment and the expertise required to interpret the data can be barriers to entry for some organizations and individuals.Despite these challenges, the benefits of hyperspectral imaging are clear, and the technology continues to gain traction across a wide range of industries and disciplines. As researchers and engineers work to overcome the technical hurdles, the future of HSI looks increasingly promising, with the potential to unlock new insights and discoveries that will shape our understanding of the world around us.In conclusion, hyperspectral imaging is a transformative technology that has the power to revolutionize the way we perceive and interact with our environment. By capturing the rich spectral information that lies beyond the visible spectrum, HSI has opened up new frontiers of scientific exploration and practical applications, from remote sensing and precision agriculture to medical diagnostics and forensic analysis. As the technology continues to evolve and become more accessible, the potential of hyperspectral imaging to drive innovation and improve our understanding of the world around us is truly limitless.。
空间辐射环境模拟装置与空间辐射生物效应研究进展
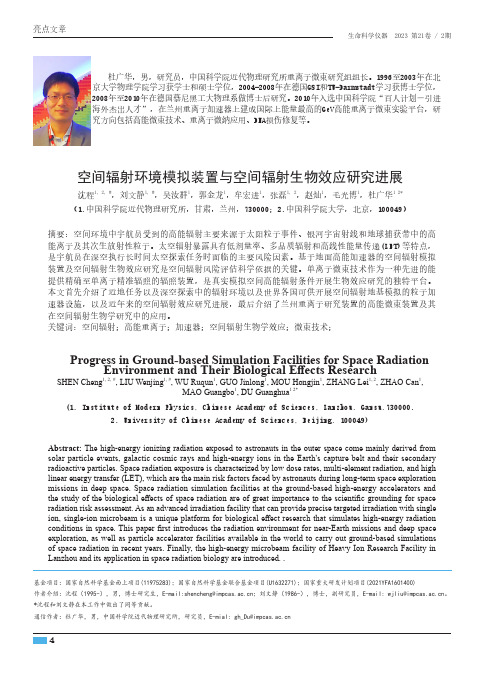
4基金项目:国家自然科学基金面上项目(11975283);国家自然科学基金联合基金项目(U1632271);国家重大研发计划项目(2021YFA1601400)作者介绍:沈程(1995-),男,博士研究生,E-mail:*******************.cn;刘文静(1986-),博士,副研究员,E-mail:***************.cn。
*沈程和刘文静在本工作中做出了同等贡献。
通信作者:杜广华,男,中国科学院近代物理研究所,研究员,E-mial:***************.cn空间辐射环境模拟装置与空间辐射生物效应研究进展沈程1, 2, #,刘文静1, #,吴汝群1,郭金龙1,牟宏进1,张磊1, 2,赵灿1,毛光博1,杜广华1 2*(1.中国科学院近代物理研究所,甘肃,兰州,730000;2.中国科学院大学,北京,100049)Abstract: The high-energy ionizing radiation exposed to astronauts in the outer space come mainly derived from solar particle events, galactic cosmic rays and high-energy ions in the Earth's capture belt and their secondary radioactive particles. Space radiation exposure is characterized by low dose rates, multi-element radiation, and high linear energy transfer (LET), which are the main risk factors faced by astronauts during long-term space exploration missions in deep space. Space radiation simulation facilities at the ground-based high-energy accelerators and the study of the biological effects of space radiation are of great importance to the scientific grounding for space radiation risk assessment. As an advanced irradiation facility that can provide precise targeted irradiation with single ion, single-ion microbeam is a unique platform for biological effect research that simulates high-energy radiation conditions in space. This paper first introduces the radiation environment for near-Earth missions and deep space exploration, as well as particle accelerator facilities available in the world to carry out ground-based simulations of space radiation in recent years. Finally, the high-energy microbeam facility of Heavy Ion Research Facility in Lanzhou and its application in space radiation biology are introduced. .Progress in Ground-based Simulation Facilities for Space RadiationEnvironment and Their Biological Effects ResearchSHEN Cheng 1, 2, #, LIU Wenjing 1, #, WU Ruqun 1, GUO Jinlong 1, MOU Hongjin 1, ZHANG Lei1, 2, ZHAO Can 1,MAO Guangbo 1, DU Guanghua 1 2*(1. Institute of Modern Physics, Chinese Academy of Sciences, Lanzhou, Gansu,730000.2. University of Chinese Academy of Sciences, Beijing, 100049)摘要:空间环境中宇航员受到的高能辐射主要来源于太阳粒子事件、银河宇宙射线和地球捕获带中的高能离子及其次生放射性粒子。
导师个人简介-许海波

导师个人简介-许海波光学教研室,博士生导师,许海波从事专业:光学互联网邮箱:xu_haibo@教育经历:1982.09-1986.07 北京师范大学物理系,获理学学士学位;1988.09-1991.07 华中科技大学物理系,获理学硕士学位;1998.09-2001.07 中国工程物理研究院研究生院,获理学博士学位工作经历1986.07-1998.09 河南师范大学物理系,历任助教、讲师、副教授;2001.07-至今北京应用物理与计算数学研究所,历任副研究员、研究员研究方向简介:主要从事高能光子、质子、电子、中子等粒子的辐射成像理论及图像处理方法研究。
结合国防、医学、工业等实际应用需求,发展粒子输运正算模拟和图像反演技术。
个人荣誉、所获奖项等国家科技进步二等奖1项;军队科技进步一等奖3项、二等奖1项、三等奖1项。
代表性研究成果列表(请按照参考文献引用格式提供详细信息)[1] Xu Haibo, Zhengna. Optimum angle-cut of collimator for dense objects in high-energyproton radiography, Chinese Physics C, 2016, 40(2): 028201Optimum angle-cut of collimator for dense objects in high-energy proton radiography[2] Xu Haibo, Zhengna. Monte Carlo simulation and parameterized treatment on the effect ofnuclear elastic scattering in high-energy proton radiography, Chinese Physics C, 2015, 39(7): 078201[3] 许海波,叶勇,郑娜. 高能X射线照相中降散射网栅失聚焦对图像的影响,强激光与粒子束,2015,27(11):115103[4] 许海波,郑娜,陈朝斌. 高能伽玛相机探测量子效率的蒙特卡罗模拟. 强激光与粒子束,2013,25(10):2734-2740[5] Xu Haibo, Hu Yuan, Wei Suhua. Quantitative simulation and density reconstruction inhigh-energy x-ray radiography. Chinese Journal of Computational Physics, 2011, 28(6):906-914[6]Xu Haibo, Peng Xianke, Chen Chaobin. The Monte Carlo simulation for bremsstrahlung and photoneutron yields in high-energy x-ray radiography. Chinese Physics B, 2010, 19(6): 062901[7] Zhengna,Xu Haibo. Transmission calculation by empirical numerical model and Monte Carlo simulation in high energy proton radiography of thick objects, Chinese Physics C, 2015, 39(10): 108201[8] 贾清刚,张发奎,许海波. 基于单脉冲康普顿电子高能伽玛能谱测量系统设计,物理学报,2017,66(1):010703[9] 杨波,应阳君,许海波等. 闪光照相X源光斑编码孔成像诊断可行性研究,原子能科学技术,2013,47(1):120-124[10] 胡渊,许海波. 利用单幅投影图像重建不对称客体密度的方法,强激光与粒子束,2011,23(9):2507-2511。
【美梦网】纽约大学理工学院拟研发生物材料
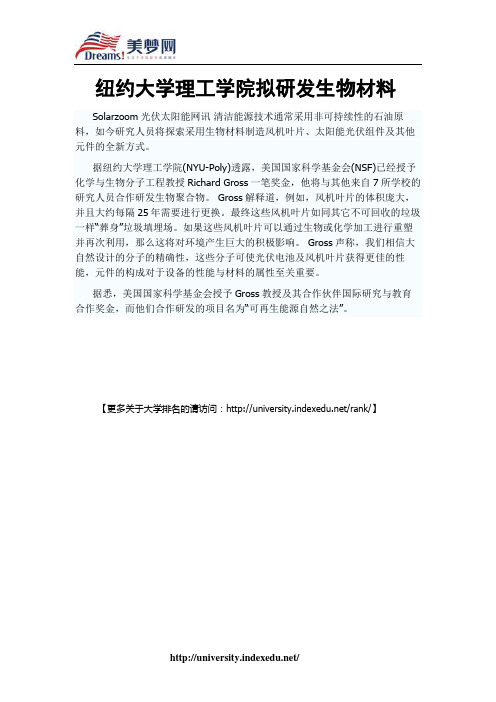
纽约大学理工学院拟研发生物材料
Solarzoom光伏太阳能网讯清洁能源技术通常采用非可持续性的石油原料,如今研究人员将探索采用生物材料制造风机叶片、太阳能光伏组件及其他元件的全新方式。
据纽约大学理工学院(NYU-Poly)透露,美国国家科学基金会(NSF)已经授予化学与生物分子工程教授Richard Gross一笔奖金,他将与其他来自7所学校的研究人员合作研发生物聚合物。
Gross解释道,例如,风机叶片的体积庞大,并且大约每隔25年需要进行更换。
最终这些风机叶片如同其它不可回收的垃圾一样“葬身”垃圾填埋场。
如果这些风机叶片可以通过生物或化学加工进行重塑并再次利用,那么这将对环境产生巨大的积极影响。
Gross声称,我们相信大自然设计的分子的精确性,这些分子可使光伏电池及风机叶片获得更佳的性能,元件的构成对于设备的性能与材料的属性至关重要。
据悉,美国国家科学基金会授予Gross教授及其合作伙伴国际研究与教育合作奖金,而他们合作研发的项目名为“可再生能源自然之法”。
【更多关于大学排名的请访问:/rank/】
/。
新型无机闪烁体的能量分辨率(中英文对照)
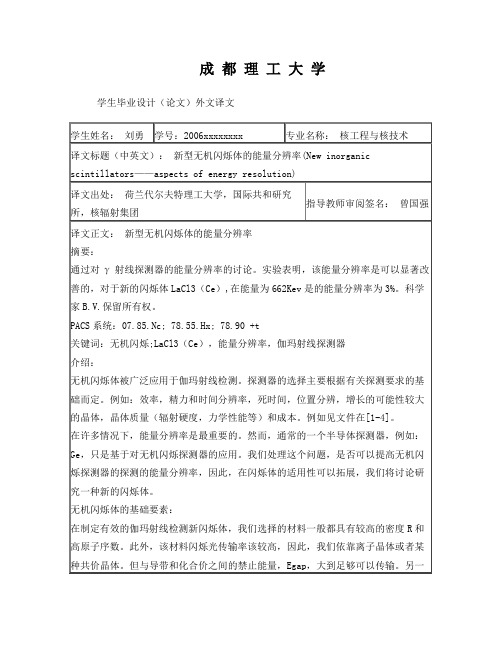
成都理工大学学生毕业设计(论文)外文译文极,(b)光电子是后来ηNph,(c)这些∝ηNph电子在第一倍增极和到达(d)倍增极的k(k = 1,2…)放大后为δk 并且我们假设δ1=δ2=δ3=δk=δ的,并且δ/δ1≈1的。
我们可以得出:R2=Rlid2=5.56δ/[∝ηNph(δ-1)] ≈5.56/Nel (3)Nel表示第一次到达光电倍增管的数目。
在试验中,δ1≈10>δ2=δ3=δk,因此,在实际情况下,我们可以通过(3)看出R2的值比实际测得大。
请注意,对于一个半导体二极管(不倍增极结构)(3)也适用。
那么Nel就是是在二极管产生电子空穴对的数目。
在物质不均匀,光收集不完整,不相称和偏差的影响从光电子生产过程中的二项式分布及电子收集在第一倍增极不理想的情况下,例如由于阴极不均匀性和不完善的重点,我们有:R2=Rsci2+Rlid2≈5.56[(νN-1/Nel)+1/Nel] (4)νN光子的产生包括所有非理想情况下的收集和1/Nel的理想情况。
为了说明,我们在图上显示,如图1所示。
ΔE/E的作为伽玛射线能量E的函数,为碘化钠:铊闪烁耦合到光电倍增管图。
1。
对ΔE/E的示意图(全曲线)作为伽玛射线能量E功能的碘化钠:铊晶体耦合到光电倍增管。
虚线/虚线代表了主要贡献。
例如见[9,10]。
对于Rsci除了1/(Nel)1/2的组成部分,我们看到有两个组成部分,代表在0-4%的不均匀性,不完整的光收集水平线,等等,并与在0-400代表非相称keV的最大曲线。
表1给出了E=662Kev时的数值(137Cs)在传统的闪烁体资料可见。
从图一我们可以清楚的看到在低能量E<100Kev,如果Nel,也就是Nph增大的话,是可以提高能量分辨率的。
这是很难达到的,因为光额产量已经很高了(见表1)在能量E>300Kev时,Rsci主要由能量支配其能量分辨率,这是没办法减小Rsci 的。
然而,在下一节我们将会讲到,可以用闪烁体在高能量一样有高的分辨率。
可吸收99%光线的“黑材料”
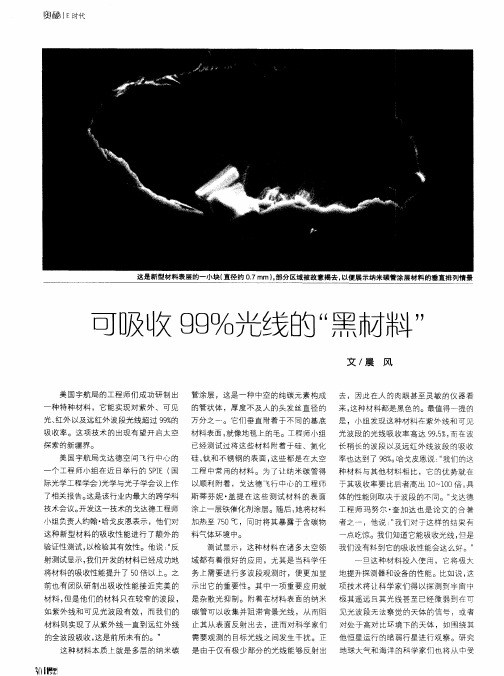
一
点 吃惊。我们知道 它能 吸收光线 , 但是
旦 这 种 材 料 投 入 使 用 ,它 将 极 大
测试显示 ,这种材料 在诸 多太空领 我们没有料到它的吸收性能会这么好。”
一
将材料的吸收性能提升了 5 倍 以上 。之 务上需要进行 多波段观测时 ,便更 加显 地提 升探测器和设备的性 能。比如说 , 0 这
负秘 ! 时代 E
这是 新型材料表层的一小块( 直径约 O7mm)部分区域被故意揭去 , _ , 以便展示纳米碳管涂层材料 的垂直排列情景
可 吸收 g %光线 “ 市料 " g 的 黑 / 丁
文 / 晨 风
美 国宇航局 的工程师们成功研 制出 管涂层 ,这是一种 中空 的纯 碳元素构成 去 ,因此 在人 的肉眼甚至灵敏 的仪器看
际光学工程学会 ) 光学与光子学会议上作 以顺利附着 ,戈达德 飞行中心的工程 师 于其吸收率要 比后 者高 出 1  ̄ 0 倍 , 0 i 0 具
技 术 会 议 。 发 这 一 技术 的戈 达德 工程 师 涂上一层铁催化剂涂层。随后 , 开 她将材料 工 程 师 玛 努 尔 ・ 加 达 也 是 论 文 的合 著 奎
们 会使 用涂 黑漆的办 法或者借 助其他 辅
助 设备来帮 助减少杂光 干扰 。然而黑漆 仅 能吸收大约 9 % 9 的入射 光 , 而纳米碳管 涂层产 生的 多层 反射则 能让材料 的总体 I A ' 更加优越 ,甚至能 将杂光减 少到原  ̄6
来 的 1以下。 %
, 立 5 f — ● 口
一
还 有一点很 重要 ,正 如戈达德 工程
音
这种材料 的超低 温性能进行 测试 , 目的 产生的红外辐射 , 将干扰和削弱设备 的灵 非常有前景的材料 。它非常有效 , 重量很 是检验其是 否适合 用在超高 灵敏度 的远 敏 度。 轻 , 且呈现极度 黑色。从长远来看 , 并 它 红 外线探测 设备上 。这种设备 工作于超
美研制出高性能超级电容材料
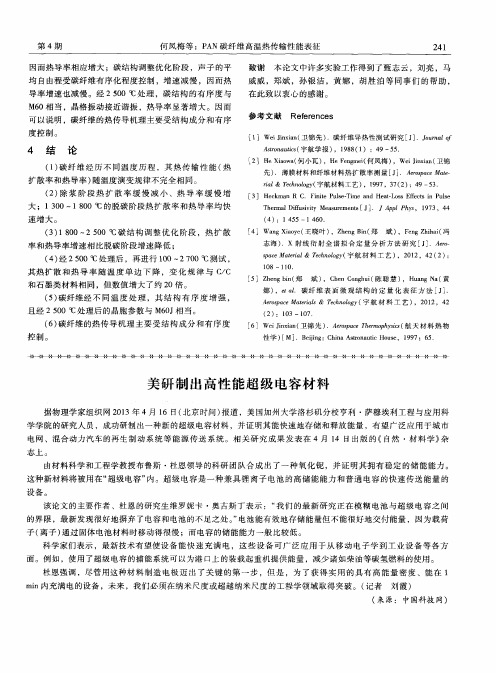
4 结 论
( 1 ) 碳 纤 维 经 历 不 同温 度 历 程 ,其 热 传 输 性 能 ( 热 扩 散 率 和热 导率 ) 随温 度演 变 规律 不 完全 相 同 。
第 4期
何 凤梅 等 :P AN碳 纤 维 高温 热传 输性 能 表征 致谢
2 41
因而 热导 率相 应 增大 ;碳 结 构调 整 优化 阶段 ,声 子 的平
本 论 文 中许 多实 验工 作得 到 了甄 志云 ,刘 亮 ,马
均 自由程 受碳 纤 维有 序化 程 度控 制 ,增 速 减慢 ,因而 热 导 率增 速 也减 慢 。经 2 5 0 0℃处 理 ,碳 结 构 的 有 序 度 与 M 6 0 相 当 ,晶格振 动 接近 谐 振 ,热导 率 显 著增 大 。因 而 可 以说 明 ,碳纤 维 的 热传 导机 理 主要 受 结构 成 分 和 有序
( 2 ) 除 浆 阶 段 热 扩 散 率 缓 慢 减 小 、热 导 率 缓 慢 增
[ 2 ]H e X i a o w a ( 何 小瓦),He F e n g m e i ( 何凤梅 ) ,We i J i n x i a n ( 卫锦 先) .薄膜材料和纤维材料 热扩散率测 量 [ J ] .A e r o s p a c e Ma t e —
A e r o s p a c e 抛 0 &T e c h n o l o g y ( 宇 航 材 料工 艺 ) ,2 0 1 2,4 2
( 2) :1 0 3—1 0 7
( 6 ) 碳纤 维 的热 传 导 机 理 主 要受 结构 成 分 和 有 序 度
MIT研制出薄如纸片的太阳能电池
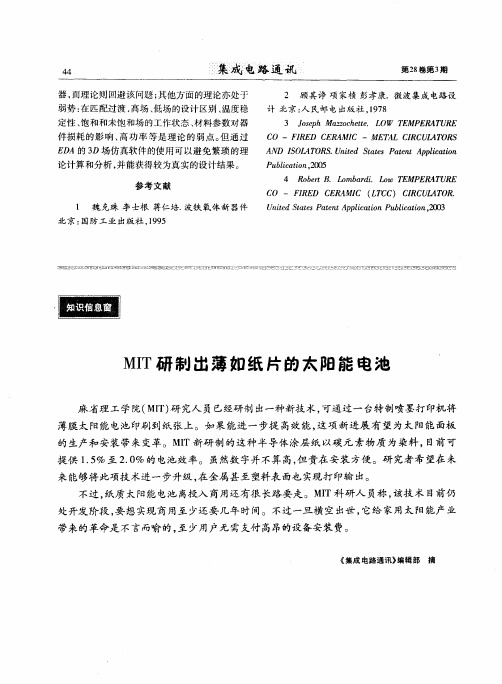
弱势 : 匹 配过渡 。 在 高场 、 低场 的设计 区别 、 温度稳
定 性 、 和 和未 饱 和场 的工 作状 态 、 料参数 对器 饱 材
件 损耗 的 影 响 、 功 率 等 是 理论 的 弱点 。 通 过 高 但 E A的 3 D D场仿 真 软件 的使 用 可 以避免 繁 琐 的题 ; 他 方面 的理论 亦处 于 其
第2 卷第3 8 期
2 顾 其诤 项 家桢 彭孝康.微 波集成 电路设
计 北京 : 民邮 电 出版社 ,9 8 人 17
3 J sp  ̄o ht . L oe h Ma c et e 0W E T MP R T RE EA U
处开发阶段 , 想 实现商用至少还要 几年 时间。不过一旦 横空 出世 , 要 它给 家用太阳能产 业
带来的革命是不言而喻 的, 至少用户无 需支付 高昂的设备安装 费。
《 集成 电路通讯》 编辑部 摘
论计 算 和分析 , 并能 获得较 为真 实的设 计结果 。 参考 文献 l 魏 克珠 李 士根 蒋仁培. 波铁 氧体 新 器件
北京: 国防 工业 出版 社 ,9 5 1 9
A ND S L 尺 . n td S a e a e t A p ia in I O A? S U i t ts e P tn p l t c o
田
巨曰虎
三 日
5 I ]卣 己 记
[ i’ 三
5目霸
_j ¨ 曩 二 1 _
明
咒
丘习夏
= 三 _
二 = 三 l_[日 巴芒[ 日髓 二 := 三
墨
日 l 芒罔 _ 广
为什么太阳那么热英语作文

为什么太阳那么热英语作文Why Is the Sun So Hot?The Sun is the center of our solar system and the primary source of energy for life on Earth. It is acolossal ball of hot plasma that emits vast amounts of electromagnetic radiation, including visible light, ultraviolet rays, and X-rays. The Sun's intense heat is essential for sustaining life on our planet, but whatfactors contribute to its extraordinarily high temperatures? Nuclear Fusion: The Sun's Energy Source.At the core of the Sun, the temperature reaches an astounding 27 million degrees Fahrenheit (15 milliondegrees Celsius). This extreme heat is generated by a process called nuclear fusion, where atomic nuclei combineto form heavier nuclei, releasing enormous amounts ofenergy in the process.Specifically, the Sun fuses hydrogen atoms into helium atoms through a series of nuclear reactions known as the proton-proton chain reaction. In this process, four hydrogen atoms are converted into one helium atom,releasing two positrons, two neutrinos, and a significant amount of energy in the form of gamma rays.Gravitational Pressure: Compressing the Sun's Core.The Sun's gravity plays a crucial role in maintaining the high temperatures necessary for nuclear fusion. The immense mass of the Sun exerts a gravitational force that compresses its core, increasing its density and pressure.This increased pressure forces the hydrogen atomscloser together, making it more likely that they willcollide and undergo nuclear fusion. The higher the pressure, the more frequent the fusion reactions become, and the greater the energy output.Radiative Transfer: Transporting Energy from the Core.The energy generated by nuclear fusion in the Sun's core must be transported to the surface in order to be radiated into space. This transfer of energy occurs through a combination of radiative and convective processes.In the core, energy is transferred outwards in the form of gamma rays. These high-energy photons travel through the Sun's interior, interacting with matter and gradually losing energy. As they move closer to the surface, the gamma rays are converted into lower-energy photons, such as visible light.Convection: Circulating the Sun's Outer Layers.In the outer layers of the Sun, energy is primarily transported by convection. Convection currents within the Sun's plasma cause hot material to rise from the interior and cooler material to sink. This circulation helps to distribute heat throughout the Sun's outer layers, ensuring that the surface temperature remains relatively stable.Sunspots and Solar Activity.The Sun's surface is not uniform in temperature. Darker regions called sunspots appear where the magnetic field lines emerge from the interior. These sunspots are cooler than the surrounding areas because the magnetic field lines inhibit the flow of hot plasma from the Sun's core.Solar activity, such as sunspots and solar flares, is caused by variations in the Sun's magnetic field. These disturbances can affect the amount of energy emitted by the Sun, and they can also cause disruptions to Earth's communication and power systems.Conclusion.The Sun's intense heat is the result of a complex interplay of factors, including nuclear fusion, gravitational pressure, radiative transfer, and convection. The Sun's gravity compresses its core, increasing the pressure and temperature to the point where nuclear fusion becomes possible. Energy generated by fusion is transported to the surface through a combination of radiative andconvective processes.The Sun's heat is essential for life on Earth, providing us with light, warmth, and the energy that drives our ecosystems. Understanding the factors that contribute to the Sun's high temperatures is crucial for comprehending the behavior of our star and its impact on our planet.。
高能量分辨率X射线光谱仪基于块状漂移检测器的设计说明书

A High Energy Resolution X-ray Spectrometer using SDDCHEN Er Lei 1, 2, a , FENG Chang Qing 1, 2, b *,YE Chun Feng 1, 2, and LIU Shu Bin 1, 21Modern Physics Department, University of Science and Technology of China2The State Key Laboratory of Particle Detection and Electronics, USTCa ****************,b ***************.cn*Correspondingauthor.Tel.**************.E-mailaddress:***************.cn.Keywords: Spectrometer, X-ray, Silicon Drift Detector, high energy resolution, FWHM.Abstract. A high energy resolution X-ray spectrometer based on Silicon Drift Detector is described in this paper. The spectrometer consists of the SDD detector module, the analog electronics for shaping and filtering and the digital electronics for peak detection and data transfer. The system can working at room temperature as a thermo electric cooler (Peltier Element) is integrated into the SDD chip. The dynamic range is about 1 keV to 10 keV. Test results indicated high energy resolution Full Width at Half Maximum (FWHM), which is better than 160 eV @ 5.9 keV with the incoming photon of radioisotopes (55Fe).IntroductionThe radiation of X-rays are widely used in many fields (such as airport security, X-ray astronomy, X-ray medical, X-ray crystallography, X-ray microscopic and so on) based on its properties [1]. X-rays with photon energies above 5 - 10 keV are called hard X-rays, while those with lower energy are called soft X-rays. A high energy resolution of Soft X-ray Detection (SXD) system is very necessary anduseful in scientific experiments and to explor the origin of the universe. Photon energy (keV)D e t e c t i o n e f f i c i e n c y (%)Fig. 1 SDD detector and its absolution efficiencySilicon Drift Detectors (SDD) are the state-of-the-art X-ray detectors based on silicon substrates. As shown in Fig. 1 is the used detector KETEK VITUS SDD H30 [2] and its absolution efficiency curve (about 70% at 1 keV and 98% at 10 keV), while the detector have 30 mm 2 active area with 8 µm Be window. An incoming photon will generate a number of electrons and holes dependent on its energy.A followed readout electronics based on the SDD will be discribed in the following sections. Joint International Mechanical, Electronic and Information Technology Conference (JIMET 2015)Structure of the readout electronicsA m p l i t u d e (V )A m p l i t u d e (m V )As shown in Fig. 2, is the structure of the readout electronics. The SDD detector is surpported bythe High Voltage Module and Temperature Controller to guarantee a good working environment, while the statues of the voltage and temperature were monitored by the Field Programmable Gate Array (FPGA) simultaneously. The output of the SDD is buffered by a low-noise & high-gain Pre-Amp, which is a voltage step well between ± 2 V because of the reset signal. The output of the Pre-Amp is followed by the CR-RC 2 [3] filter and shaper, after then the quasi-Gaussian output is fed to the Peak Hold module and then be digitalized by a 14-bit Analog to Digital Converter (ADC) (3 MHz sample rate). The digitized results is buffred and packaged in the FPGA, then be readout to remote PC. Detailes will be listed bellow.Silicon Drift DetectorU BACK(-65V)U OR (-130V)U IR(-20V)GND X-rayEntrance windowAnode FETC FBAs shown in Fig. 3 is the schematic of the SDD chip. The anode of the SDD is connected to a Field Effect Transistor (FET) and Feed Back Capacitance (C FB ) which forms the first part of a Charge Sensitive Amplifier (CSA). A certain rise time is dependent on the location of interaction of the X-ray with the SDD chip, while a certain amplitude is dependent on the energy of the incoming photon. SDDs require High Voltages Power Supply (low noise stabilized, U OR ‘-130V ’, U IR ‘-25V ’, U BACK ‘-65V ’,), Preamplifier Module (ultra-low-noise, charge-sensitive, ramped reset type, high-gain) andTemperature Controller Module (Peltier Element based thermo electric cooler) to guarantee a good working enviroment, meanwhile it is necessary to read out the temperature sensor of the SDD. Peak-Hold moduleOne kernel part of the readout electronics is the Peak Hold method, which combine with Analog to Digital Converter (ADC) for peak detection. The peak of the analog quasi-Gaussian output is detected and held by the Peak Hold module, then be injected to ADC for digitization. A high performance peak-hold chip (PH300) was chosen, which was designed for satellite instrumentation (equally useful in laboratory and commercial applications for its stability and characteristics).Detected peakFig. 4 The Function and Timing diagram of PH300 [4]PH300 is a peak-hold device, to track an analog input and keep the maximum amplitude as a peak voltage on a hold capacitor. As shown in Fig. 4, the input (marked as ‘IN’) is sensed by the error amplifier when the Gate is open (High), which is controlled by Transistor-Transistor Loigc (TTL) standard. The hold capacitor is charged though a charging diode and a hold resistor during the rise time of the signal (called charging mode marked as ‘C’). Then the device goes into the hold mode (marked as ‘H’) as soon as the input reaches V max and starts to decay. After completing the sample of the peak, the circuit was in fast discharge (was configured in the application) mode (marked as ‘D’), the hold capacitor is discharged through a large current for a short period of time. Finally, the circuit was ready for reciving a new peak, which is called the tracking mode (marked as ‘T’).The control signal is generated by the FPGA, which is converted from 3.3 V standard to 5V standard according to a schmitt CMOS 16-bit Bidirectional MultiPurpose Transceiver (UT54ACS164245S). The ‘GATE’ was set as HIGH in the application. The ‘PKDT’ was the output of th e PH300 when the peak is found and in the holding mode. The ‘RAMP’ is the input which starts from the rise time of the ‘IN’, and set as LOW when in the discharge mode. The ‘OUT’ from PH300 is sampled by a 14-bit ADC (AD9243) with 3 MHz samping rate. The results is buffered and packaged in FPGA, then transmitted to remote PC for further analyzing.Test resultsIn order to evaluate the X-ray spectrometer designed in this paper, a test platform was set up, as shown in Fig. 5. The test system is mainly consists of the signal source (AFG 3252), the radioisotope (55Fe), the SDD module and shaping board (marked as ‘A’), the digital board (marked as ‘B’) and power supply module.Firstly we measure the electronics linearity of the peak hold and ADC module, a series of quasi-Gaussian (generate by signal source AFG3252) were conducted with increasing amplitudemeasured by the readout electronics. The input amplitude ranges from 50 mV to 2500 mV with 300mV interval, which is equivalent to the dynamic range (1 keV to 10 keV).Fig. 5 Test platfrom of the soft X-ray spectrometerA linear curve is shown in Fig. 6, with an integral non-linearity less than 1%.After the X-ray spectrometer were assembled, including both the detector and electronics properties. We use the radioisotope (55Fe) as the input of the incoming photon [5].C ODE Input(mV) Fig. 6 The linear curve of the Peakhold and ADC circutC o u n t s Code Fig. 7 The test spectrum using the radioisotope 55FeAs shown in Fig. 7, the result of energy spectrum indicates that the energy resolution Full Width at HalfMaximum(FWHM)************************(usingX-rayastheincomingphotonfor SDD).SummaryA high energy resolution of soft X-ray spectrometer based on SDD is designed in this paper. Which achives a dynamic range of about 1 keV to 10 keV. The energy resolution (FWHM) is better than 160 *********.AcknowledgmentThis work was supported by the National Natural Science Funds of China (Grant No. 11205154).References[1] X-rays. NASA. Retrieved November 7, 2012. /ems/11_xrays.html.[2] KETEK GmbH. VITUS Silicon Drift Detectors. User’s Manual.[3] Wulleman J. Detector-noise suppression by appropriate CR-(RC)n shaping [J]. Electronics Letters, 1996, 32(21): 1953-1954.[4] AMP-TEK. PH300 Peak Hold Detector. PH300 Specifications.[5] Zhang F, Wang H Y, Peng W X, et al. High resolution solar soft X-ray spectrometer. Chinese Physics C. DOI: 10.1088/1674-1137/36/2/008.。
MPPC(硅光电倍增管)作为APD的替代者在PET中的应用
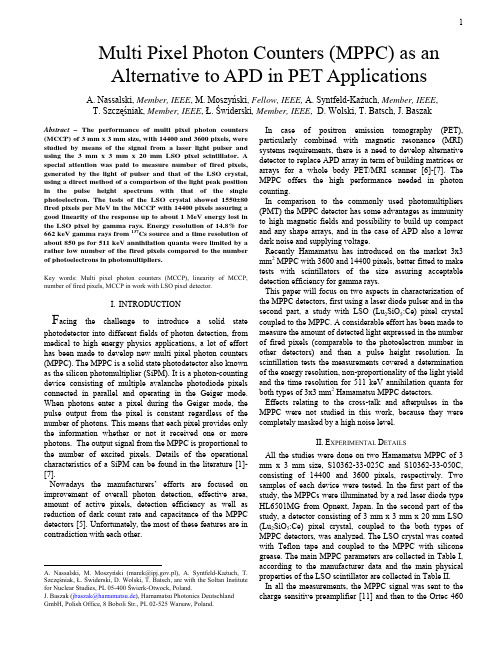
Multi Pixel Photon Counters (MPPC) as an Alternative to APD in PET Applications A. Nassalski, Member, IEEE, M. Moszyński, Fellow, IEEE, A. Syntfeld-Każuch, Member, IEEE, T. Szczęśniak, Member, IEEE, Ł. Świderski, Member, IEEE, D. Wolski, T. Batsch, J. BaszakAbstract – The performance of multi pixel photon counters (MCCP) of 3 mm x 3 mm size, with 14400 and 3600 pixels, were studied by means of the signal from a laser light pulser and using the 3 mm x 3 mm x 20 mm LSO pixel scintillator. A special attention was paid to measure number of fired pixels, generated by the light of pulser and that of the LSO crystal, using a direct method of a comparison of the light peak position in the pulse height spectrum with that of the single photoelectron. The tests of the LSO crystal showed 1550±80 fired pixels per MeV in the MCCP with 14400 pixels assuring a good linearity of the response up to about 1 MeV energy lost in the LSO pixel by gamma rays. Energy resolution of 14.8% for 662 keV gamma rays from 137Cs source and a time resolution of about 850 ps for 511 keV annihilation quanta were limited by a rather low number of the fired pixels compared to the number of photoelectrons in photomultipliers.Key words: Multi pixel photon counters (MCCP), linearity of MCCP, number of fired pixels, MCCP in work with LSO pixel detector.I.INTRODUCTIONF acing the challenge to introduce a solid state photodetector into different fields of photon detection, from medical to high energy physics applications, a lot of effort has been made to develop new multi pixel photon counters (MPPC). The MPPC is a solid state photodetector also known as the silicon photomultiplier (SiPM). It is a photon-counting device consisting of multiple avalanche photodiode pixels connected in parallel and operating in the Geiger mode. When photons enter a pixel during the Geiger mode, the pulse output from the pixel is constant regardless of the number of photons. This means that each pixel provides only the information whether or not it received one or more photons. The output signal from the MPPC is proportional to the number of excited pixels. Details of the operational characteristics of a SiPM can be found in the literature [1]-[7].Nowadays the manufacturers’ efforts are focused on improvement of overall photon detection, effective area, amount of active pixels, detection efficiency as well as reduction of dark count rate and capacitance of the MPPC detectors [5]. Unfortunately, the most of these features are in contradiction with each other.A. Nassalski, M. Moszyński (marek@.pl), A. Syntfeld-Każuch, T. Szczęśniak, Ł. Świderski, D. Wolski, T. Batsch, are with the Soltan Institute for Nuclear Studies, PL 05-400 Świerk-Otwock, Poland.J. Baszak (jbaszak@hamamatsu.de), Hamamatsu Photonics Deutschland GmbH, Polish Office, 8 Boboli Str., PL 02-525 Warsaw, Poland.In case of positron emission tomography (PET), particularly combined with magnetic resonance (MRI) systems requirements, there is a need to develop alternative detector to replace APD array in term of building matrices or arrays for a whole body PET/MRI scanner [6]-[7]. The MPPC offers the high performance needed in photon counting.In comparison to the commonly used photomultipliers (PMT) the MPPC detector has some advantages as immunity to high magnetic fields and possibility to build up compact and any shape arrays, and in the case of APD also a lower dark noise and supplying voltage.Recently Hamamatsu has introduced on the market 3x3 mm2 MPPC with 3600 and 14400 pixels, better fitted to make tests with scintillators of the size assuring acceptable detection efficiency for gamma rays.This paper will focus on two aspects in characterization of the MPPC detectors, first using a laser diode pulser and in the second part, a study with LSO (Lu2SiO5:Ce) pixel crystal coupled to the MPPC. A considerable effort has been made to measure the amount of detected light expressed in the number of fired pixels (comparable to the photoelectron number in other detectors) and then a pulse height resolution. In scintillation tests the measurements covered a determination of the energy resolution, non-proportionality of the light yield and the time resolution for 511 keV annihilation quanta for both types of 3x3 mm2 Hamamatsu MPPC detectors.Effects relating to the cross-talk and afterpulses in the MPPC were not studied in this work, because they were completely masked by a high noise level.II.E XPERIMENTAL D ETAILSAll the studies were done on two Hamamatsu MPPC of 3 mm x 3 mm size, S10362-33-025C and S10362-33-050C, consisting of 14400 and 3600 pixels, respectively. Two samples of each device were tested. In the first part of the study, the MPPCs were illuminated by a red laser diode type HL6501MG from Opnext, Japan. In the second part of the study, a detector consisting of 3 mm x 3 mm x 20 mm LSO (Lu2SiO5:Ce) pixel crystal, coupled to the both types of MPPC detectors, was analyzed. The LSO crystal was coated with Teflon tape and coupled to the MPPC with silicone grease. The main MPPC parameters are collected in Table I, according to the manufacturer data and the main physical properties of the LSO scintillator are collected in Table II.In all the measurements, the MPPC signal was sent to the charge sensitive preamplifier [11] and then to the Ortec 460Delay Line Amplifier, selected to reduce a shift of the baseline at the amplifier output caused by a high counting rate of noise pulses in the MPPC. A PC-based multichannel analyzer (MCA) Tukan 8K [12] recorded energy spectra. Peak positions and their full width at half maximum (FWHM) were obtained from Gaussian fit. In the analysis, the FWHM of the peaks was not corrected for the saturation effect in the MCCP.T ABLE IT HE MAIN PARAMETERS OF H AMAMATSU MPPCType S10362-33-025C S10362-33-050C Ser. No. 3 422 23Active area 3×3 mm 23×3 mm 2Number of pixels144003600Pixel size 25x25 µm 50x50 µm Fill factor 30.8 % 61.5 %Gain/bias voltage 2.75x105 /68.97 V 2.75x105/69.01 V 7.5x105 /68.66 V 7.53x105 /68.84 VSpectral resp. range270 -900 nmQ.E. 70% at 400 nmDark count0.45 Mc/s 0.51 MC/s 2.38 Mc/s3.65 Mc/sCapacitance 320 pFT ABLE IIT HE MAIN PROPERTIES OF THE STUDIED SCINTILLATORLSOLight output [ph/MeV] 31000 Peak emission [nm] 420 Decay time [ns] 40 – 47 Refr. Index1.82Density [g/cm 3] 7.4 ManufacturerSiemens (former CTI)Reference [9][10]III. R ESULTSA. The MPPC performanceThe performance of the MPPC S10362-33-025C is illustrated in Fig. 1. The MPPC dark noise spectrum was measured at 69 V bias and a temperature in the laboratory was kept below 20 °C. In the spectrum, the individual single, and double photon peaks are separated one from each other. A very high counting rate of dark noise pulses shifts up the base line at the output of the amplifier introducing a positive offset in the spectrum and destroys somewhat pulse height resolution. Thus, the position of the single photoelectron peak was corrected on the offset determined from the calibration based on the single and double photoelectron peaks. It was used further, as the reference, to measure the number of the fired pixels for the light flashes from the laser pulser or LSO crystal.The MPPC response to the light pulse from the laser diode is presented in Fig. 2, as observed at Tektronix TDS5034B digital scope. The laser was triggered by the short pulse of 3 ns at FWHM. The light pulse of laser of 6 ns at FWHM was monitored by the very fast R5320 PMT, characterized by a rise time of the single photoelectron pulse of 700 ps.1x1042x1043x1044x1045x104N u m b e r o f c o u n t sChannel number (ch)Fig. 1. Separated single photoelectrons obtained with the S10362-33-025C MPPC detector.Fig. 2. The S10362-33-025C MPPC output pulse, the light pulse from laser, as seen by R5320 fast PMT and the electrical pulse triggered laser. Upper panel presents output signal on 50 ohms loading resistor at the input of a scope, while at the lower panel the second 50 ohms resistor was placed at the anode of the MCCP. Note a faster fall time due to a shorter time constant at the MCCP output.A slow rise time of the MCCP is evidently due to the laser pulse, while its fall time is the effect of the large capacitance of 320 pF, which forms the time constant of 16 ns, together with the 50 ohms load resistor at the input of the scope. It is confirmed at the lower panel of Fig. 2 displaying the same signal but with the second 50 ohms resistor at the anode of the MCCP itself. The fall time is shorter by about factor of 2. To characterize the detector, tests of the photon-counting and a proportionality of the output signal to the number of the detected photons, were performed. Since each pixel detects only whether or not, one or more photons have entered the detector, the total number of the fired pixels does not directly correspond to the number of detected photons. If two or more photons are triggering one pixel then the photon detection linearity degrades because the number of incident photons becomes larger than the number of fired pixels. It is reflected in Eq. (1) presenting the relation of the fired pixels number versus a number of detected photons for a given number of pixels in the MPPC [2].⎥⎥⎦⎤⎢⎢⎣⎡⎟⎟⎠⎞⎜⎜⎝⎛×−−×=total photon totalfired N PDE N N N exp 1, (1) were:fired N : number of excited pixels,total N : total number of pixels,photon N : number of incident photons,PDE : photon detection efficiency.The photon detection efficiency, PDF, is the product of the quantum efficiency (QE), the fill factor or geometrical factor (GF) and the probability that an incoming photon trigger a breakdown (Pt) [2].PDE = QE x GF x Pt (2)The number of detected photons is described, in turn, by:N detect = N photon x PDE (3)Fig. 3 presents the number of measured fired pixels versus the number of detected photons, calculated, following the equation (1) and (3). It reflects the deviation from the linear dependence represented by the solid line, calculated for the infinite number of pixels in the MPPC. Error bars are within the point size. What is observed, that the nonlinearity of both 14400 and 3600 pixels MPPC are presenting the same behavior. However it is clear that the range of linearity is more extended in case of the MPPC with a higher number of active pixels.In the next experiment the MPPC was illuminated by light pulses from the laser of different intensities and then their pulse height spectra were stored at MCA. For each position of the peak, the corresponding number of the fired pixels wasdetermined in relation to the single photoelectron peak and its respective FWHM, expressed in the number of the fired pixels was obtained from the Gaussian fit. The results are presented in Fig. 4, where the plot of the measured FWHM versus the square root of the measured number of fired pixels is presented. The nonlinear response of MPPC is reflected by a deviation of the FWHM from the continuous line calculated from Poisson statistic, for a perfectly linear photodetector. In case if two or more photons are triggering one pixel then the photon detection linearity lowers because the number of incident photons becomes larger than the number of excited pixels. Fig. 4 reflects clearly an influence of nonlinear response of MPPC for larger light signals.50010001500200025003000N f i r e dN detectedFig 3. The number of excited pixels versus the number of detected photons, for both 14400 and 3600 pixels Hamamatsu MPPC, error bars are within the point size.10203040506020406080100120140F W H Msqrt NFig. 4. The FWHM of the light peaks versus the square root of fired pixels.The above discussion of the MPPC linearity and the corresponding pulse height resolution confirm also the numbers of the fired pixels measured in a direct way by a comparison of the light pulse peak position to that of the single photoelectrons.B. Gamma-ray SpectrometryIn all the study, the MPPC signal was sent to the charge sensitive preamplifier [11] and then to the Ortec 460 Delay Line Amplifier operated with 0.25 µs integrating time constant. The energy spectra of single and double photoelectrons and gamma rays of a 137Cs source are presented in Fig. 5. It allows to measure the number of the fired pixels by the method used in the scintillation detectors based on the PMT light readout [13].In this method the number of photoelectrons is measured directly by comparing the position of the full energy peak of γ-rays detected in the crystal to the position of the single photoelectron peak. The observed positions of the single and double photoelectrons was used to correct for the offset introduced into spectrum by a high counting rate of noise pulses.Table III summarizes the performance of the detectors in gamma spectrometry with LSO scintillator in comparison to the Photonis XP2020Q PMT and the Hamamatsu S8550 APD array [14]. Since the pixel size in the S8550 APD array is only 1.8 mm x 1.8 mm, the tests were done with a 2 mm x 2 mm x 15 mm LSO crystal.In the third column, the number of the fired pixels is collected for the tested 3x3x20 mm 3 LSO crystal. Note somewhat lower number of photoelectrons for the 14400 pixel detector in comparison to the 3600 pixels detector, also confirmed by a poorer energy resolution, collected in the forth column. It confirms a lower fill factor quoted for this MPPC by the manufacturer.010002000200400600800137Cs 1pheN u m b e r o f c o u n t sChannel number (ch)x 200FWHM 14.8%Fig. 5. The energy spectra of singe photoelectron and cesium source (137Cs), measured for LSO crystal with 14400 pixels Hamamatsu MPPC.To compare the MPPC performance two types of photodetector were selected: a commonly used Photonis photomultiplier XP2020Q, characterized by a photocathode blue sensitivity of 10.1 µA/lmF (where F stands for filtered blue sensitivity) [15] and a Hamamatsu S8550 APD array, previously tested by the author in [14]. Note the observed bya factor of two and four higher photoelectron and electron-hole pair numbers for PMT and APD, respectively, than that of the MPPC and significantly better energy resolution.T ABLE IIIR ESULTS OF LSO GAMMA -RAY SPECTROMETRY MEASURMENTSPhotodetector Number of pixels N phe a)∆E/E b)MPPC 025C 14400 1550±80 14.8±0.6 MPPC 050C 3600 1700±90 14.0±0.6 PMT XP2020Q -3950±20011.3±0.5APD S8550 c)- 7010±350 12.8±0.6a) Number of photoelectrons, phe/MeV, 137Cs (662keV),b)Energy resolution, %, 137Cs (662keV), c)2x2x15 mm 3 LSO crystal coupled to Hamamatsu S8550 APD array [14].The measured photoelectron number with the XP2020Q PMT allows for estimation of the photon detection efficiency of the tested MPPC devices for the LSO pixel crystal. First the light output of the LSO pixel was calculated assuming the integral quantum efficiency of the XP2020Q, following [13], see Table IV. For the MPPCs the procedure was reversed and the PDE was calculated based on the measured light output of the LSO crystal.The estimated PDEs of about 10% is comparable to that measured in [3] for SiPM of comparable size of pixels. It could be improved by a larger over-voltage, but the bias voltages of both MPPC was very close to those recommended by the manufacturer, see Table I. In the case of the MPPC 050C, the estimated PDE could be affected partly by a nonlinear response for 662 keV peak in LSO, see Fig. 7 below. A low number of the fired pixels in the MPPCs is also confirmed by a poor energy resolution, see Table III.T ABLE IVL IGHT OUTPUT OF THE LSO PIXEL CRYSTAL AND PHOTON DETECTIONEFFICIENCY OF MPPC PHOTOEDETECTORSPhotodetector N phe /MeV QE (PMT)or PDE(MCCP) Light output[ph/MeV]PMT XP2020Q 3950±200 21.9%a)18000±1800 b)MPPC 025C1550±80 8.6±1% 18000±1800 b)MPPC 050C1700±909.2±1%18000±1800 b)a)see Ref [13],b) for 3 mm x 3 mm x 20 mm LSO pixel.In the Fig. 6, the non-proportionality characteristics of the LSO pixel crystal, as measured with the MPPC, with 14400 and 3600 pixels, in comparison to the XP2020Q PMT, are presented. It confirms that the LSO light signal is within the linear response of the MPPC up to about 1 MeV gamma ray energy in case of the 14400 pixels detector. The bending down of the curve at about 1 MeV is common for the MCCP and XP2020 PMT, characterized by the excellent linearity of the current pulse up to 100 mA. This effect is characteristic for the pixel crystals and it is related to a reduced light outputof high energy gamma rays detected by a multiple Compton scattering, probably because of the escape of KX-rays in the final detection by the photoeffect. In case of the 3600 pixels MPPC detector, the lower number of active pixels limits the linearity of the detector below 500 keV gamma ray energy.1010010000.40.50.60.70.80.91.01.11.2L i g h t y i e l d (f r a c t i o n o f 662 k e V )Energy (keV)Fig. 6. The non-proportionality characteristics for LSO crystal measured with Hamamatsu MPPC 14400 and 3600 pixels detector in comparison to the XP2020Q PMT.Fig. 7 presents the energy resolution measured with the LSO crystal as a function of incident γ-rays energy. The curves are linear in a double logarithmic scale. The MPPC detectors present the same behavior of the energy resolution in the whole range of energies. The energy resolution measured with the PMT is superior in the whole measuredenergy range. It is evidently associated with a larger number of photoelectrons produced at the PMT photocathode than that of the MPPC. 10100100010100E n e r g y R e s o l u t i o n (%)Energy (keV)Fig. 7. The energy resolution (FWHM) characteristics for LSO crystal measured with Hamamatsu MPPC 14400 and 3600 pixels detector in comparison to the XP2020Q PMT.C. Time Resolution StudyTo check the usefulness of the MPPC detector for PET instrumentation, the coincidence time resolution of the tested detectors for 511 keV annihilation quanta was measured using the same LSO pixel crystal. As the reference detector, a truncated conical BaF 2 crystal 25 mm in diameter and 15 mm high was used. The crystal was coupled to the Photonis XP20Y0 QDA PMT equipped with a screening grid at the anode. Its time resolution was equal to 128±4 ps for 511 keV, as reported in [16]. The timing measurements wereperformed with a 22Na γ-source. The energy windows in the side channels of the detectors were set at the 511 keV full energy peaks.101102103104105N u m b e r o f c o u n t sChannel number (ch)Fig. 8. The time resolution spectra for LSO crystal measured with Hamamatsu MPPC 14400 and 3600 pixels detector in comparison to theXP2020Q PMT.In the time setup, the Ortec 935 four channels Constant Fraction Discriminator (CFD) was used. Note that the CFDwas used in the leading-edge mode with a short circuit ofshaping delay and its threshold was set just above the noise level. The output signals from the CFD channels provided start and stop input signals for the Ortec 567 Time-to-Amplitude Converter (TAC). The TAC output was recordedby a PC-based multichannel analyzer Tukan 8K [12]. The output signals were gated by the full energy peaks of 511 keV γ-rays. The time spectra are shown in Fig. 8 and the results of the time resolution measurements are given in Table V.In the third column the number of photoelectrons (fired pixels for MPPC) corresponding to the 511 keV peaks are listed. The time resolution corrected for the contribution of the reference detector (128 ps) is presented in the fourth column. The last column shows the time resolution of the tested detectors normalized to the number of photoelectrons. It is not done for the APD, since the measured time resolution is mainly determined by the signal-to-noise ratio at the input of a fast discriminator.T ABLE VT IME RESOLUTION OF THE LSO SCINTILLATORPhotodetector Number of pixelsN phe a)δt b)[ps] δt*N 1/2 x 103MPPC 025C 14400 790±40 840±40 23.6±1.2MPPC 050C 3600 870±40 770±40 22.7±1.2PMT XP2020Q - 2000±100 203±10 9.8±0.6APD S8550 c)- 3180±160 2150±110d)-a)Number of photoelectrons for 511 keV, b) Time resolution corrected for the contribution of the reference detector, ps,(FWHM), 22Na (511keV), c)2x2x15 mm 3 LSO crystal coupled to Hamamatsu S8550 APD array [14], d)The main contribution is form the dark noise.The best time resolution was measured with the XP2020Q PMT, three times better that those measured with the MPPCs. It follows, first of all, a factor two larger photoelectron number obtained with the PMT. However, the normalized time resolution to the photoelectron number, presented in the last column, reflects also a higher speed of the PMT in comparison to the MPPCs. This is the effect of a large time constant of 16 ns due to the 50 ohms load resistor and the MCCP capacitance of 320 pF, see Fig. 2. For the LSO light pulse, with the decay time of 40 ns, it leads to the rise time of the output pulse of the MPPC of about 20 ns [15]. A 1 mm x 1 mm SiPM is preferable in this respect, due to 9 times lower capacitance. In the tested MPPC, it could be largely improved by a drastic reduction of the load resistor, below 10 ohms [17]. Both tested MPPCs give comparable time resolution, which follows measured numbers of fired pixels. It is well reflected in the same values of normalized time resolution. The strength of SiMPs in the fast timing is their excellent time resolution for 1 photoelectron of about 100 ps [2]. However, for slow decaying scintillators, like LSO, the time resolution is more governed by the decay of the light pulse than the time jitter of photodetector [16]. Thus, the high number of photoelectrons is more important than a low time jitter [18]. A low time jitter of SiPMs will be reflected in timing with fast light pulses of plastic scintillators or Cherenkov radiation. However, the MPPCs are superior in comparison to the APD array. The measured time resolution is more than twice better than in case of APD arrays. It is the result of dark noise of the APD array which limits mainly the time resolution.The presented timing study of the tested detectors, confirmed a future possibility to introduce the proposed detectors in PET applications.IV. C ONCLUSIONSMulti pixel photon counters are novel type of solid state photodetectors with significant advantages such as a high gain, fast timing, compactness and insensitivity to magnetic field. All of them make MPPC very attractive in application to nuclear medicine and high energy physics.The present studies showed that the new Hamamatsu S10362-33-025C / 050C MPPC could be easily applied in nuclear medicine, in particular in detectors for positron emission tomography, as an alternative to APD arrays. Alarge number of pixels in the detector assure a good linearity of the response to the light from LSO pixel crystals and a good energy resolution. A slow output pulse of MPPC for theLSO crystal, associated with a high capacitance of thedevices, limits the time resolution; however, it is still superior in comparison to APDs. A considerable effort was done to determine the number of fired pixels and its relation to the pulse height and time resolutions. The estimated photon detection efficiency for the light from LSO crystal showed a value of about 10%, typical for silicon photomultipliers. R EFERENCES[1] P. Buzhan et al., “Silicon Photomultipliers and its possibleapplications”, Nucl. Instr. And Meth. A 504, pp. 48-52, 2003.[2] D. Renker, “Geiger-mode avalanche photodiodes, history, propertiesand problems”, Nucl. Instrum. Meth., A , vol. 567, no. 1, pp. 48-56, November 2006.[3] G. Llosá, et al. “Novel Silicon Photomultipliers for PET Applications”,IEEE Trans. Nucl. Sci., vol. 55, no. 3, pp. 877-881, June. 2008.[4] G. Llosá, et al. “Silicon Photomultiplier and SiPM matrices asphotodetectors in nuclear medicine”, 2007 Nucl. Sci. Symp. Conf. Rec., pp. 3220- 3223, N14-4.[5] C. Piemonte, M. Boscardin, G. F. Dalla Betta, M. Melchiorri, N. Zorzi,R. Battiston, A. Del Guerra, G. Llosa, “Recent developments on silicon photomultipliers produced at FBK-irst”, 2007 Nucl. Sci. Symp. Conf. Rec., pp. 2089-2092, N41-2.[6] D. P. McElroy, V. Saveliev, A. Reznik, and J. A. Rowlands,“Evaluation of silicon photomultipliers: A promising new detector for MR compatible PET”, Nucl. Instr. Meth. vol. 571A pp. 106-109, Feb 2007.[7] A.N. Otte, J. Barral, B. Dolgoshein, J. Hose, S. Klemin, E. Lorenz, R.Mirzoyan, E. Popova, M. Teshima, “A test of silicon photomultipliers as readout for PET”, Nucl. Instrum. Meth., A , vol. 545, no. 3, pp. 705-715, June 2005.[8] Q. Xie, R. G. Wagner, G. Drake, P. DeLurgio, Y. Dong, C. T. Chen,and C. M. Kao, “Performance evaluation of multi-pixel photon counters for PET imaging”, 2007 Nucl. Sci. Symp. Conf. Rec., pp. 969-974, N18-1.[9] C. L. Melcher and J.S. Schweitzer, …Cerium doped lutetiumoxyorthosilicate: A fast efficient new scintillator”, IEEE Trans. Nucl. Sci., vol. 39, 502-505, 1992.[10] M. Kapusta, P Szupryczy ński, C. Melcher, M. Moszy ński,M. Balcerzyk, A.A. Carey, W. Czarnacki, A. Spurrier, A. Syntfeld, …Non-proportionality and thermoluminescence of LSO:Ce”, IEEE Trans. Nucl. Sci., vol. 52, no. 4, pp. 1098-1104, Aug. 2005.[11] M. Kapusta, P. Crespo, D. Wolski, M. Moszy ński, W. Enghardt,…Hamamatsu S8550 APD arrays for high-resolution scintillator matrices readout”, Nucl. Instrum. Meth., A , vol. 504, pp. 139–142, 2003.[12] Z. Guzik, S. Borsuk, K. Traczyk, M. Plominski, …TUKAN – An 8Kpulse height analyzer and multi-channel scaler with PCI or USB interface”, IEEE Trans. Nucl. Sci., vol 55, no 1, pp. 231-235, Feb. 2006.[13] M. Moszy ński, M. Kapusta, M. Mayhugh, D. Wolski, S.O. Flyckt,"Absolute Light Output of Scintillators”, IEEE Trans. Nucl. Sci., vol. 44, no. 3, pp 1052-1061, June 1997.[14] A. Nassalski, M. Moszy ński, A. Syntfeld-Ka żuch, Ł. Świderski, T.Szcz ęśniak, D. Wolski, T. Batsch, “Application of Hamamatsu S8550 APD array to the Common PET/CT Detector”, IEEE Trans. Nucl. Sci., vol 55, no 5, pp., Oct. 2008.[15] Photonis, …Photomultiplier tubes principles and applications”,September 2002.[16] M. Moszy ński, M. Kapusta, A. Nassalski, T. Szcz ęśniak, D. Wolski,L. Eriksson, C. L. Melcher, “New prospects for Time-of-Flight PET with LSO scintillators”, IEEE Trans. Nucl. Sci., vol. 53, no. 5, pp. 2484-2488, Oct. 2006.[17] S. Seifert, D.R. Schaart, H.T. van Dam, J. Huizenga, R. Vinke, P.Dendooven, H. Lohner, F.J. Beekman, “A High BandwidthPreamplifier for SiPM-Based TOF PET Scintillation Detectors”, 2008 Nucl. Sci. Symp. Conf. Rec., pp. 1616-1619, NM1-2.[18]T. Szczęśniak, M. Moszyński, Ł. Świderski, A. Nassalski, P. Lavoute,M. Kapusta, …Fast Photomultipliers for TOF PET”, IEEE Trans. Nucl.Sci., vol. 56, no. 1, pp. 173-181, Febr. 2009.。
- 1、下载文档前请自行甄别文档内容的完整性,平台不提供额外的编辑、内容补充、找答案等附加服务。
- 2、"仅部分预览"的文档,不可在线预览部分如存在完整性等问题,可反馈申请退款(可完整预览的文档不适用该条件!)。
- 3、如文档侵犯您的权益,请联系客服反馈,我们会尽快为您处理(人工客服工作时间:9:00-18:30)。
a r X i v :0711.0725v 1 [a s t r o -p h ] 5 N o v 2007**FULL TITLE**ASP Conference Series,Vol.**VOLUME**,**YEAR OF PUBLICATION****NAMES OF EDITORS**High energy X-ray emission from recurrent novae in quiescence:T CrBGerardo J.M.Luna 1,J.L.Sokoloski 2&Koji Mukai 3Abstract.We present Suzaku X-ray observations of the recurrent nova T CrB in quiescence.T CrB is the first recurrent nova to be detected in the hard-X-ray band (E ∼40.0keV)during quiescence.The X-ray spectrum is consistent with cooling-flow emission emanating from an optically thin region in the boundary layer of an accretion disk around the white dwarf.The detection of strong stochastic flux variations in the light curve supports the interpretation of the hard X-ray emission as emanating from a boundary layer.1.IntroductionSymbiotic stars are binary systems in which a compact object accretes from red giant,the wind of which forms a dense nebula around the binary.The nebula is ionized by the hot compact source,which is usually a white dwarf (Kenyon 1986).Among the known symbiotic stars (Belczy´n ski et.al.2000),four objects have shown recurrent nova (RN)eruptions:T CrB,RS Oph,V3890Sgr and V745Sco.These eruptions are triggered by a thermonuclear runaway on the white dwarf (WD)surface after accretion of a critical amount of H rich material from the companion.Orio et al.(2001)suggested that during quiescence,the X-ray emission from RN could originate in the boundary layer of an accretion disc that was reconstructed around the WD after the outburst.Therefore,X-ray observa-tions should allow one to determine a lower limit for the accretion rate.The low X-ray luminosity measured in 350novae and recurrent novae in quiescence (Orio et al.2001)imply a low accretion rate,inconsistent with the observed recurrence times.For example,in the case of RS Oph (Orio 1993),the short recurrence time of ∼20years requires an accretion rate of ∼10−8M ⊙/yr,incon-sistent with measured X-ray fluxes.This inconsistency is known as the “missing boundary layer problem”.T CrB is a well known recurrent nova with recorded outbursts in 1866and 1946.Its quiescent-state hard X-ray emission (E max ∼100keV)was first revealed during a 9month Swift survey (Tueller et al 2005).Two ad-2Luna et al.ditional Swift/XRT observations were analyzed by Kennea et al.(2007),who reported highly absorbed emission that was wellfit with a coolingflow model with kT max=23.1keV.The absorber consisted of components that both fully covered and partially covered the hard X-ray source.Here we describe Suzaku X-ray Imaging Spectrometer(XIS)and Hard X-ray Detector(HXD)observa-tions of the recurrent nova T CrB performed on September6,2006.2.Observations and data reductionOn September6th2006,the Astro-E2/Suzaku X-ray observatory performed a 46ks(GO)observation of T CrB(ObsId401043010start time22:44:21UT). We reduced the data according to standard procedures1using the software pack-age HEASOFT6.22.After determining the XIS background count rate from a nearby blank region,we extracted spectra and light curves for the XIS and HXD detectors.We extracted two sets of light curves for each detector–one with a bin size of360s(used to search for stochastic bright variations)and the other grouping photons every8s.We used the light curve with the smaller time bins for power spectrum analysis.3.Analysis and Results3.1.Spectral analysisVisual inspection of the extracted spectrum showed prominent Fe lines around ∼6.5keV.As these lines are an indication of the presence of thermal emis-sion from a plasma with temperatures∼107−8K,wefirst attempted tofit the spectrum with a single-temperature plasma plus neutral absorption.But no satisfactoryfit was obtained.Even using more complex absorbers,such as an absorber that only partially covers the source,or absorption from an ionized plasma,did not produce acceptablefits.We did,however,find acceptablefits (χ2=1.07)for a multi-temperature,coolingflow plasma model(mkcflow).The model required a complex absorber consisting of one absorbing system that fullycovered the source[n H(full)=17.819.216.6×1022cm−2,which includes interstellarabsorption of0.047×1022cm−2]and and another that only partially covered thesource[n H(partial)=35.642.232.3×1022cm−2,partial covering fraction of0.680.720.66].The minimum temperature of the cooling-flow model is consistent with the small-est value allowed by the mkcflow model(kT=0.0808keV),and the maximumtemperature is kT=57.468.348.0keV.We also added a gaussian emission-line profileto the model to account for the Fe Kαfluorescence line.Figure1shows the spectra from the XIS and HXD detectors,with the cooling-flow model overplot-ted.To estimate the parameters for the Fe lines,we used a simple powerlaw fit to establish the continuum level,and thenfit the Fe Kα,Fe XXV and Fe XXVI emission lines with three Gaussian profiles(seefig.1,right panel).Theequivalent widths obtained were185187183,135137134and151153149eV respectively.tween 0.3and 40.0keV.The top spectrum on the low-energy side is the sum of spectra from the front-side illuminated XIS detectors (0,2,and 3).The bottom spectrum on the low-energy side is from the back-side illuminated XIS detector (1).The high-energy spectrum is from the HXD.The best-fit absorbed cooling flow model is overplotted.Right panel :Fe line region (∼6.5keV),with best-fit powerlaw continuum plus 3gaussian profiles overplotted.The botton panels show the residuals with respect to the models.3.2.Timing analysisWe examined the XIS time series binned at 360s (Figure 2)and found significant stochastic variability above the level expected from Poisson noise.The fractional amplitude of the stochastic variations,represented as the ratio between the measured rms variation,s ,and that expected from Poisson fluctuations alone,s exp ,is 3.75.In the case of HXD light curve,no stochastic variations were found above the Poisson level.Although by eye there appears to be a periodic (or quasi-periodic)variation in the light curve at a period of around 100minutes,we did not detect a statistically significant oscillation at this period in our preliminary Lomb-Scargle analysis (taking into account the underlying broadband continuum Figure 2.Mean normalized XIS light curve (all four detectors added)with a 360s bin size.The plot shows the flux as a function of time in the energy range 0.3-12.0keV.The X-ray emission is clearly variable on a time scale of minutes.4.DiscussionHard X-ray emission with energy up to 100keV is observed from the dwarf nova SS Cyg in quiescence (Barlow et al.2006).Dwarf novae observed with XMM-Newton in quiescence also display spectra with energies up to ∼12keV(Pandel et al.2005).For low accretion rates (<3×10−9M ⊙/yr),Narayan &Popham4Luna et al.(1993)showed that the most internal part of an accretion disc around a1M⊙WD,the boundary layer,radiates as an optically thin plasma at∼10keV.The hardness of the X-ray emission detected from T CrB strongly suggest that it comes from the accretion region closest to the WD.From the spectral model fitted,the accretion rate is˙M∼4.2×10−9M⊙yr−1(d/1kpc)2.The detection of rapidflickering,which typically emanates from regions close to the WD,sup-ports an accretion origin for the detected emission.Moreover,the cooling-flow spectral model,as with boundary layer emission from non-magnetic cataclysmic variables(CVs;Mukai et al.2003)and other accreting high-mass white dwarfs (Luna&Sokoloski2007;Smith et al.2007),provides a natural context for the flickering.The EW of the neutral Fe Kαline suggests that the X-ray source is sur-rounded by large amounts of neutral material(Inoue1985).Reflection of radia-tion offof the WD surface can also contribute to the formation of this line.From George&Fabian(1991),the EW of the Fe Kαline when a2πsource reflects bremsstrahlung photons with∼10keV is100-200eV.Therefore the intense Fe Kαline is consistent either with large amounts of neutral material,as with RT Cru and CD-573057(Luna&Sokoloski2007;Smith et al.2007;Kennea et al. 2007),or with reflection from the surface of the WD.5.ConclusionsUsing the Suzaku X-ray satellite,we observed hard-X-ray emission from the recurrent nova T CrB in quiescence.Assuming that a more detailed timing analysis confirms the lack of periodic variations,the presence offlickering on a timescale of minutes and the cooling-flow type spectrum suggest that the accretion onto the WD proceeds through a disc with an optically thin boundary layer.T CrB is thefirst recurrent nova to show hard-X-ray emission from a boundary layer during quiescence.Acknowledgments.G.J.M.Luna acknowledge CNPq and CAPES/PROEX support.J.L.Sokoloski acknowledge NASA(award number NNX06AI16G)in support of the Suzaku observation of T CrB.ReferencesBarlow E.J.,Knigge C.,Bird A.J.,Dean A.,Clark D.J.,Hill A.B.,Molina M.,Sguera V.,2006, MNRAS,372,224Belczy´n sky,K,Miko l ajewska,J.,Munari,U.,Ivison,R.J.and Friedjung,M.,2000,A&AS,146,407 George I.,Fabian A.,1991,MNRAS,249,352Hachisu I.&Kato M.,2001,ApJ,558,323Hachisu,I.,Kato,M.,Luna,G.J.M.,2007,ApJL,Inoue H.,1985,Sp.Sci.Rev.,40,317Kennea,J.&Mukai,K.,Sokoloski J.,2007in prep.Kenyon,S.J.,The symbiotic stars,1986,Cambridge University PressLuna,G.J.M.&Sokoloski J.L.,2007,ApJ,in pressMukai K.,Kinkhabwala A.,Peterson J.R.,Kahn S.M.,Paerels F.,2003,ApJ,586,77Narayan R.&Popham R.,1993,Nature,362,820Nelson T.,Orio M.,Cassinelli J.,Still M.,Leibowitz E.,Mucciarelli P.,2007,ApJ,in pressOrio M.,1993,A&A,274,L41Orio M.,Covington J.,¨Ogelman H.,2001,A&A,373,542Pandel D.,C´o rdova F.A.,Mason K.O.,Priedhorsky W.C.,2005,ApJ,626,396Smith R.,Mushotzki R.,Mukai K.,Kallman T.,Markwardt C.,Tueller J.,2007,PASJ,submetido Sokoloski,J.L.,Luna,G.J.M.,Mukai,K.,Kenyon,S.J.,2006,Nature,442,276High energy X-ray emission from recurrent novae5Tueller,J.,Barthelmy,S.,Burrows,D.,Falcone,A.,Gehrels,N.,Grupe,D.,Kennea,J.,Markwardt,C.B.,Mushotzky,R.F.,Skinner,G.K.,The Astronomer’s Telegram,669。