Mechanical Properties of Biomimetic Tissue Adhesive Based on
仿生学与天然蜘蛛丝仿生材料

1068高等学校化学学报V01.3l层层组装复合材料膜的固化过程,所制备的复合材料具有单一组分3倍的强度和韧性.通过这种多层次结构仿生层层组装法,制备高强度高分子复合体系材料,打破了传统物理复合增强方法局限于特殊纳米材料/高分子体系的格局,从一个完全不同的视野给人们展示了一种全新的仿生设计方法.高分子材料通常具有较低的密度,以高分子复合体系制备的天然蜘蛛丝仿生材料具有轻质特点.有关采用多层次结构仿生层层组装法制备天然蜘蛛丝仿生材料的研究报道很少,该方法还未发现用于天然蜘蛛丝仿生材料的制备.2.5金属元素仿生渗透注入法自然界某些生物体,如昆虫角质层、下颌骨、螫针、钳螯、产卵器等,由于含有极为少量的金属元素(如Zn,Mn,Ca,Cu等)而大大改善了这些部位的力学性能,特别是其刚度和硬度啪。
引.人们模仿生物体的这种特性,对天然蜘蛛丝自身进行了仿生修饰.Lee等Ⅲ。
通过多重脉冲气相渗透技术Fig.2SchematicofconsolidationofPU/PAAiayer.by.(MPI),将金属zn,Ti和Al引入到天然蜘蛛丝中,蛔era跚mblycompositem脚…他们认为在水蒸气和副产物气体(如甲烷或者异丙(A)ExperimentalprocedumforconsolidationofPU/PAAfilms:醇)破坏蜘蛛丝分子间氢键的同时,一方面,Zn2+,(1)thefilmsallowedswelinwator,(2)anynumberofA13+和Ti4+金属离子在氢键位点形成了金属.蛋白丘hm锄8眦ke8toge山”into““批“栅咖陀岫achl叭”‘络合物或更强的共价键,另外使卢一折叠片晶相尺寸:::‰芝,血e慧==譬乏u伽:减小,非晶相组分则相对增加,从而使天然蜘蛛丝eonsolidated岫kisl'emovedfromthepress;(B)photographof的强度、模量、伸长率及坚韧性大大提高.图3为100.bilay。
钛合金中碳化物的组成及形态的演变规律模板
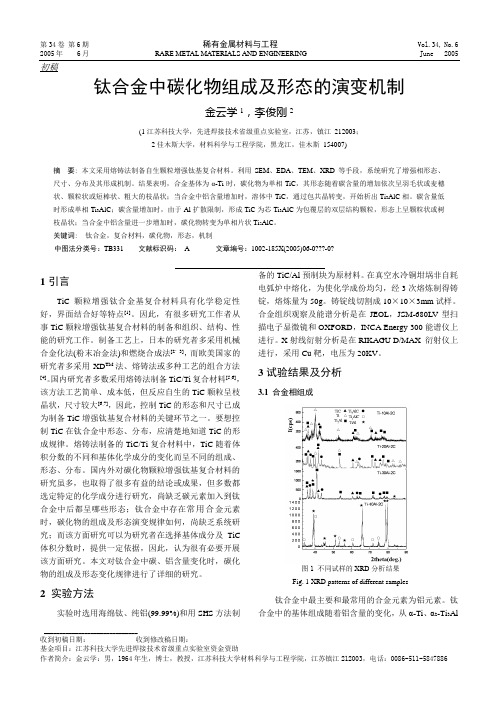
第34卷 第6期 稀有金属材料与工程 Vol.34, No.6 2005年 6月 RARE METAL MA TERIALS AND ENGINEERING June 2005 _______________________________ 收到初稿日期: 收到修改稿日期:基金项目:江苏科技大学先进焊接技术省级重点实验室资金资助作者简介:金云学:男,1964年生,博士,教授,江苏科技大学材料科学与工程学院,江苏镇江212003,电话:0086-511-5847886 初稿钛合金中碳化物组成及形态的演变机制金云学1,李俊刚2(1江苏科技大学,先进焊接技术省级重点实验室,江苏,镇江 212003;2佳木斯大学,材料科学与工程学院,黑龙江,佳木斯 154007)摘 要: 本文采用熔铸法制备自生颗粒增强钛基复合材料。
利用SEM 、EDA 、TEM 、XRD 等手段,系统研究了增强相形态、尺寸、分布及其形成机制。
结果表明,合金基体为α-Ti 时,碳化物为单相TiC ,其形态随着碳含量的增加依次呈羽毛状或麦穗状、颗粒状或短棒状、粗大的枝晶状;当合金中铝含量增加时,溶体中TiC ,通过包共晶转变,开始析出Ti 3AlC 相。
碳含量低时形成单相Ti 3AlC ;碳含量增加时,由于Al 扩散限制,形成TiC 为芯Ti 3AlC 为包覆层的双层结构颗粒,形态上呈颗粒状或树枝晶状;当合金中铝含量进一步增加时,碳化物转变为单相片状Ti 2AlC 。
关键词: 钛合金,复合材料,碳化物,形态,机制中图法分类号:TB331 文献标识码: A 文章编号:1002-185X(2005)06-0???-0?1引言TiC 颗粒增强钛合金基复合材料具有化学稳定性好,界面结合好等特点[1]。
因此,有很多研究工作者从事TiC 颗粒增强钛基复合材料的制备和组织、结构、性能的研究工作。
制备工艺上,日本的研究者多采用机械合金化法(粉末冶金法)和燃烧合成法[2,3],而欧美国家的研究者多采用XD TM 法、熔铸法或多种工艺的组合方法[4]。
力学生物的英文
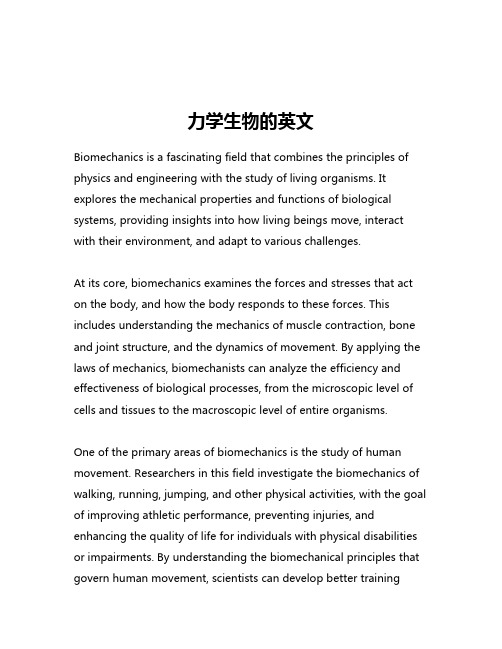
力学生物的英文Biomechanics is a fascinating field that combines the principles of physics and engineering with the study of living organisms. It explores the mechanical properties and functions of biological systems, providing insights into how living beings move, interact with their environment, and adapt to various challenges.At its core, biomechanics examines the forces and stresses that act on the body, and how the body responds to these forces. This includes understanding the mechanics of muscle contraction, bone and joint structure, and the dynamics of movement. By applying the laws of mechanics, biomechanists can analyze the efficiency and effectiveness of biological processes, from the microscopic level of cells and tissues to the macroscopic level of entire organisms.One of the primary areas of biomechanics is the study of human movement. Researchers in this field investigate the biomechanics of walking, running, jumping, and other physical activities, with the goal of improving athletic performance, preventing injuries, and enhancing the quality of life for individuals with physical disabilities or impairments. By understanding the biomechanical principles that govern human movement, scientists can develop better trainingmethods, design more effective prosthetic devices, and optimize the design of sports equipment.Beyond human movement, biomechanics also plays a crucial role in understanding the structure and function of other living organisms. For example, biomechanists study the locomotion of animals, such as the swimming of fish or the flight of birds, to gain insights into the evolutionary adaptations that have enabled these creatures to thrive in their respective environments. Similarly, biomechanics is essential for understanding the mechanical properties of plant tissues, which are essential for their growth, survival, and reproduction.In the field of medicine, biomechanics has numerous applications. Orthopedic surgeons use biomechanical principles to design and develop more effective prosthetic limbs, joint replacements, and other medical devices. Biomechanics also contributes to the understanding of injury mechanisms, allowing for the development of better protective equipment and rehabilitation strategies. Additionally, biomechanics plays a role in the design of medical imaging techniques, such as magnetic resonance imaging (MRI) and computed tomography (CT) scans, which provide valuable information about the structure and function of the human body.The applications of biomechanics extend beyond the realm of human health and performance. In the field of engineering, biomechanics isincreasingly being used to inspire the design of innovative technologies. Researchers are studying the remarkable abilities of various organisms, such as the adhesive properties of gecko feet or the efficient flight patterns of birds, to develop biomimetic solutions for a wide range of engineering challenges. These bioinspired designs have the potential to revolutionize fields like robotics, materials science, and energy production.As the field of biomechanics continues to evolve, it is becoming increasingly interdisciplinary, drawing on expertise from fields such as biology, physics, computer science, and mathematics. This collaborative approach allows for the development of more comprehensive and sophisticated models of biological systems, leading to groundbreaking discoveries and advancements in our understanding of the natural world.In conclusion, biomechanics is a dynamic and rapidly advancing field that has far-reaching implications for our understanding of living organisms and our ability to engineer innovative solutions to complex problems. By unraveling the mechanical principles that govern biological systems, biomechanists are paving the way for new breakthroughs in fields as diverse as medicine, sports science, and engineering. As we continue to explore the fascinating world of biomechanics, we can expect to witness even more remarkablediscoveries and applications that will shape the future of our understanding of the natural world.。
仿生鱼鳍单元设计及运动仿真分析
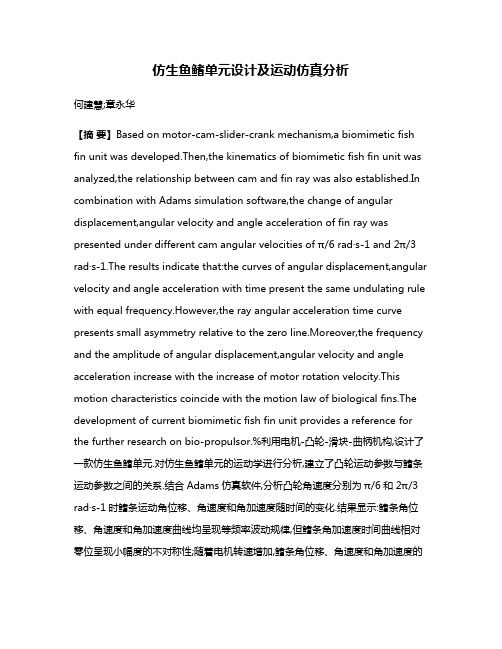
仿生鱼鳍单元设计及运动仿真分析何建慧;章永华【摘要】Based on motor-cam-slider-crank mechanism,a biomimetic fish fin unit was developed.Then,the kinematics of biomimetic fish fin unit was analyzed,the relationship between cam and fin ray was also established.In combination with Adams simulation software,the change of angular displacement,angular velocity and angle acceleration of fin ray was presented under different cam angular velocities of π/6 rad·s-1 and 2π/3 rad·s-1.The results indicate that:the curves of angular displacement,angular velocity and angle acceleration with time present the same undulating rule with equal frequency.However,the ray angular acceleration time curve presents small asymmetry relative to the zero line.Moreover,the frequency and the amplitude of angular displacement,angular velocity and angle acceleration increase with the increase of motor rotation velocity.This motion characteristics coincide with the motion law of biological fins.The development of current biomimetic fish fin unit provides a reference for the further research on bio-propulsor.%利用电机-凸轮-滑块-曲柄机构,设计了一款仿生鱼鳍单元.对仿生鱼鳍单元的运动学进行分析,建立了凸轮运动参数与鳍条运动参数之间的关系.结合Adams仿真软件,分析凸轮角速度分别为π/6和2π/3 rad·s-1时鳍条运动角位移、角速度和角加速度随时间的变化.结果显示:鳍条角位移、角速度和角加速度曲线均呈现等频率波动规律,但鳍条角加速度时间曲线相对零位呈现小幅度的不对称性;随着电机转速增加,鳍条角位移、角速度和角加速度的变化频率增大,幅值也随之增大.上述运动特征与生物鱼鳍的运动规律相符,该仿生鱼鳍单元结构设计为进一步研制仿生鳍推进器提供参考.【期刊名称】《系统仿真技术》【年(卷),期】2017(013)002【总页数】5页(P170-174)【关键词】仿生鱼鳍;鳍条;凸轮;曲柄-滑块机构【作者】何建慧;章永华【作者单位】台州职业技术学院机电工程学院,浙江台州 318000;台州职业技术学院机电工程学院,浙江台州 318000【正文语种】中文【中图分类】TP242.6鱼类因非凡的运动能力使其能够在复杂的水下环境中生存[1],这一点引起了研究人员广泛的关注,并于近二十年内开发了大量的仿生机器鱼推进器。
细胞膜制作
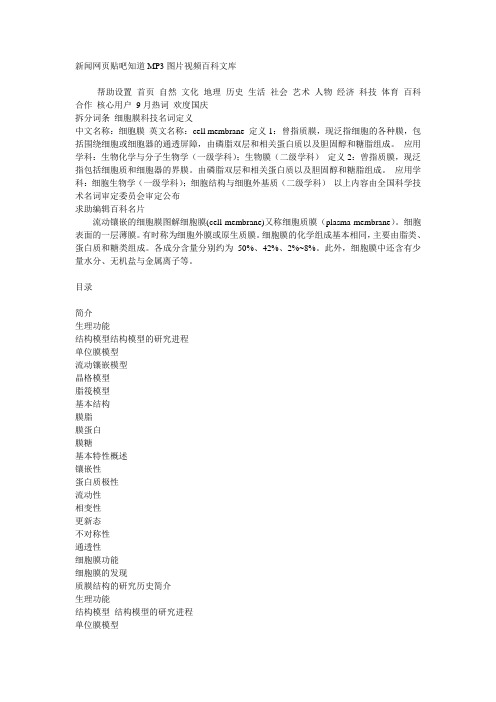
新闻网页贴吧知道MP3图片视频百科文库帮助设置首页自然文化地理历史生活社会艺术人物经济科技体育百科合作核心用户9月热词欢度国庆拆分词条细胞膜科技名词定义中文名称:细胞膜英文名称:cell membrane 定义1:曾指质膜,现泛指细胞的各种膜,包括围绕细胞或细胞器的通透屏障,由磷脂双层和相关蛋白质以及胆固醇和糖脂组成。
应用学科:生物化学与分子生物学(一级学科);生物膜(二级学科)定义2:曾指质膜,现泛指包括细胞质和细胞器的界膜。
由磷脂双层和相关蛋白质以及胆固醇和糖脂组成。
应用学科:细胞生物学(一级学科);细胞结构与细胞外基质(二级学科)以上内容由全国科学技术名词审定委员会审定公布求助编辑百科名片流动镶嵌的细胞膜图解细胞膜(cell membrane)又称细胞质膜(plasma membrane)。
细胞表面的一层薄膜。
有时称为细胞外膜或原生质膜。
细胞膜的化学组成基本相同,主要由脂类、蛋白质和糖类组成。
各成分含量分别约为50%、42%、2%~8%。
此外,细胞膜中还含有少量水分、无机盐与金属离子等。
目录简介生理功能结构模型结构模型的研究进程单位膜模型流动镶嵌模型晶格模型脂筏模型基本结构膜脂膜蛋白膜糖基本特性概述镶嵌性蛋白质极性流动性相变性更新态不对称性通透性细胞膜功能细胞膜的发现质膜结构的研究历史简介生理功能结构模型结构模型的研究进程单位膜模型流动镶嵌模型晶格模型脂筏模型基本结构膜脂膜蛋白膜糖基本特性概述镶嵌性蛋白质极性流动性相变性更新态不对称性通透性细胞膜功能细胞膜的发现质膜结构的研究历史展开编辑本段简介细胞膜是防止细胞外物质自由进入细胞的屏障,它保证了细胞内环境的相对稳定,使各种生化反应能够有序运行。
但是细胞必须与周围环境发生信息、物质与能量的交换,才能完成特定的生理功能。
因此细胞必须具备一套物质转运体系,用来获得所需物质和排出代谢废物,据估计细胞膜上与物质转运有关的蛋白占核基因编码蛋白的15~30%,细胞用在物质转运方面的能量达细胞总消耗能量的三分之二。
羟基磷灰石相关复合材料的研究进展

第46卷第3期2021年6月广州化学Guangzhou ChemistryV ol. 46 No. 3Jun. 2021文章编号:1009-220X(2021)03-0022-08 DOI:10.16560/ki.gzhx.20210313羟基磷灰石相关复合材料的研究进展范云辉,王世革,黄明贤*(上海理工大学理学院,上海200093)摘要:羟基磷灰石(HAp)作为骨骼中的无机矿物成分,是天然的生物陶瓷材料。
由于特殊的结构组成,其具有良好的生物相容性和生物活性。
然而,纯的HAp纳米颗粒容易团聚且机械强度低,因此往往需要进一步改性和功能化用于合成HAp相关的复合材料。
本综述对元素或官能团掺杂、聚合物/HAp交联、HAp固定在载体材料表面等方式制备的HAp复合材料,及其在环境中分离纯化应用的研究进展进行讨论与分析。
最后对HAp相关复合材料的发展前景做出了总结与展望。
关键词:羟基磷灰石;复合材料;分离纯化中图分类号:O651 文献标识码:A对羟基磷灰石(HAp)材料的研究已经进行了半个多世纪,其作为动物骨骼的无机组分,是重要的生物材料。
近年来,随着纳米科学的不断发展,合成的纳米HAp的应用范围越来越广。
有关HAp的综述报道,主要是涉及不同的制备方法(包括固态法[1-2]、传统的化学沉淀法[3-4]、溶胶凝胶法[5-6]、水热法[7-8]、模板法[9-10]等)的总结[11],HAp纳米材料作为骨和牙齿替代物的应用,以及药物输送和癌症治疗方面的研究[12-15]。
可以发现,关于HAp复合材料及其在分离纯化中应用的报道较少。
因此,本文对HAp相关复合材料的结构设计和在环境中作为分离纯化材料的最新进展进行了简单的归纳总结。
1 概述HAp作为脊椎动物牙齿和骨骼中的主要无机成分,在生物陶瓷领域引起了广泛关注。
HAp属于磷酸钙,是磷灰石中的一种。
磷灰石的一般结构式可以表示为M10(ZO4)6X2,这里M,ZO4和X可以分别被替换成下列离子[16-17]:M=K,Mg,Mn,Pb,Co,Zn,Au,Ba,Cd,Al,Fe,La,Ce,Si,Ti等;ZO4=CO3,SiO4,HPO4,SeO2等;X=F,Cl等。
仿生材料研究与进展 王一安 刘志刚

齐齐哈尔大学综合实践课程论文题目仿生材料研究进展学院材料科学与工程学院专业班级无机非金属材料工程无机112班学生姓名王一安刘志刚指导教师李晓生成绩2014年 5月9 日仿生材料学研究进展摘要:仿生材料学以阐明生物体材料结构与形成过程为目标,用生物材料的观点来思考人工材料,从生物功能的角度来考虑材料的设计与制作。
仿生材料的当前研究热点包括贝壳仿生材料、蜘蛛丝仿生材料、骨骼仿生材料、纳米仿生材料等,它们具有各自特殊的微结构特征、组装方式及生物力学特性。
仿生材料正向着复合化、智能化、能动化、环境化的趋势发展,给材料的制备及应用带来革命性进步。
关键词:表面仿生超疏水材料、聚乙烯三元复合仿生材料、植物叶片仿生伪装材料、仿生层状结构壳聚糖医用材料Abstract:The“biomimeticmaterialsscience”formedbytheintersectionofmaterialscien ceandlifesciencehasgreattheoreticalandpracticalsignificance.Biomimeticmaterialsscie ncetakesmaterialstructureandformationastarget,considersartificialmaterialattheviewof bio2material,exploresthedesignandmanufactureofmaterialfromtheangleofbiologicalfu nction.Atpresent,thehotresearchesonbiomimeticmaterialsscienceincludeshellbiomime ticmaterial,spidersilkbiomimeticmaterial,bonebiomimeticmaterial,andnano2biomimet icmaterial,etc.whichhavetheirownspecialmicro2structuralcharacteristics,formationstyl e,andbio2mechanicalproperties.Biomimeticmaterialsaredevelopingtowardscompound ,intellectual,active,andenvironmentaltendency,willbringrevolutionaryimprovementfor manufactureandapplicationofmaterial,andwillchangegreatlythestatusofhumansociety.Keywords:Bionics,Materialsscience,Review1.前言仿生材料学以阐明生物体材料结构与形成过程为目标,用生物材料的观点来思考人工材料,从生物功能的角度来考虑材料的设计与制作。
超高真空下过渡金属Nb与Nb、Ta、Ti、W相互间粘附特性的试验研究
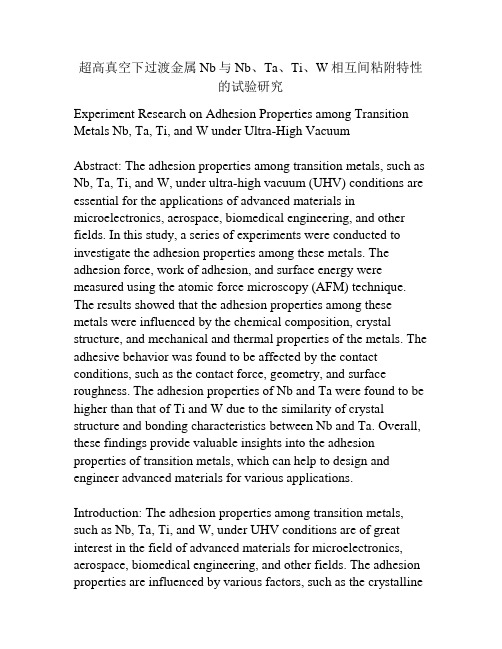
超高真空下过渡金属Nb与Nb、Ta、Ti、W相互间粘附特性的试验研究Experiment Research on Adhesion Properties among Transition Metals Nb, Ta, Ti, and W under Ultra-High VacuumAbstract: The adhesion properties among transition metals, such as Nb, Ta, Ti, and W, under ultra-high vacuum (UHV) conditions are essential for the applications of advanced materials in microelectronics, aerospace, biomedical engineering, and other fields. In this study, a series of experiments were conducted to investigate the adhesion properties among these metals. The adhesion force, work of adhesion, and surface energy were measured using the atomic force microscopy (AFM) technique. The results showed that the adhesion properties among these metals were influenced by the chemical composition, crystal structure, and mechanical and thermal properties of the metals. The adhesive behavior was found to be affected by the contact conditions, such as the contact force, geometry, and surface roughness. The adhesion properties of Nb and Ta were found to be higher than that of Ti and W due to the similarity of crystal structure and bonding characteristics between Nb and Ta. Overall, these findings provide valuable insights into the adhesion properties of transition metals, which can help to design and engineer advanced materials for various applications. Introduction: The adhesion properties among transition metals, such as Nb, Ta, Ti, and W, under UHV conditions are of great interest in the field of advanced materials for microelectronics, aerospace, biomedical engineering, and other fields. The adhesion properties are influenced by various factors, such as the crystallinestructure, chemical composition, roughness, and contact force. Among these factors, the chemical composition and crystal structure play a crucial role in determining the adhesive behavior of metals. For instance, the similarity of the crystal structure and bonding characteristics between Nb and Ta may promote the formation of strong adhesive bonds. In contrast, Ti and W have different crystal structures and bonding characteristics from Nb and Ta, which may lead to weaker adhesive behavior. Therefore, it is critical to investigate the adhesion properties among these metals and to identify their underlying mechanisms.Experimental methods: The adhesion properties among transition metals Nb, Ta, Ti, and W were investigated using AFM. The AFM was operated in force spectroscopy mode to measure the adhesion force, work of adhesion, and surface energy between the metals. The samples were prepared by polishing the metals to a mirror finish and were degassed under UHV conditions for at least 24 hours before the measurements. The measurements were conducted at room temperature and atmospheric pressure. Results and discussions: The adhesion force and work of adhesion between Nb and Ta were found to be higher than those between Ti and W. This may be attributed to the similarity of the crystal structure and bonding characteristics between Nb and Ta. Nb and Ta have a body-centered cubic (bcc) crystal structure with a lattice constant of approximately 3 Å, whereas Ti and W have a hexagonal close-packed (hcp) crystal structure with a lattice constant of approximately 2 Å. The bcc structure has a more open and less densely packed structure than the hcp structure, which may lead to a larger contact area and stronger adhesion.Furthermore, the surface energy of Nb and Ta was found to be higher than that of Ti and W. The surface energy is a measure of the energy required to create a unit area of surface, and it is related to the cohesive energy and bonding strength of the metals. The higher surface energy of Nb and Ta may promote the formation of stronger adhesive bonds between the metals. Additionally, the adhesive behavior was found to be influenced by the contact conditions, such as the contact force, geometry, and surface roughness. A higher contact force and a larger contact area may enhance the adhesive behavior, whereas a higher surface roughness may reduce the adhesive behavior.Conclusion: The adhesion properties among transition metals, such as Nb, Ta, Ti, and W, under UHV conditions were investigated using AFM. The adhesion force, work of adhesion, and surface energy were measured, and the results showed that the adhesion properties were influenced by the chemical composition, crystal structure, and mechanical and thermal properties of the metals. The adhesive behavior was found to be affected by the contact conditions, such as the contact force, geometry, and surface roughness. The adhesion properties of Nb and Ta were found to be higher than that of Ti and W due to the similarity of crystal structure and bonding characteristics between Nb and Ta. These findings provide valuable insights into the adhesion properties of transition metals and can help to design and engineer advanced materials for various applications.The findings of this study have important implications for the design and engineering of advanced materials with improved adhesion properties. For instance, the use of Nb and Ta may be preferred over Ti and W in applicationswhere strong adhesive bonds are required due to their higher adhesion properties. Furthermore, optimizing the contact conditions, such as the contact force, geometry, and surface roughness, may enhance the adhesive behavior of transition metals and improve their performance in various applications.In addition, the AFM technique used in this study provides a powerful tool for characterizing the adhesion properties of transition metals and other materials. AFM can be used to visualize and manipulate materials at the nanoscale level, providing valuable insights into their mechanical, electrical, and thermal properties. AFM can also be used to investigate the effects of various factors on the adhesive behavior of materials, such as temperature, humidity, and surface treatment.Future research can build on this study by investigating the adhesion properties of transition metals under more extreme conditions, such as high temperatures and pressures, and in different environments, such as under water or in acidic environments. Furthermore, future research can investigate the adhesion properties of other materials, such as polymers, ceramics, and composites, and their interactions with transition metals. Overall, the findings of this study provide a foundation for further research on the adhesion properties of materials and their applications in various fields.The adhesion properties of materials play a crucial role in a wide range of applications, from manufacturing processes to biomedical devices. Understanding the fundamental principles that govern adhesion at the nanoscale level is therefore essential for the development of new technologies and materials with improved functionality and performance.One promising area of research is the development of biomimetic adhesives that can mimic the adhesive properties of natural adhesives, such as the sticky feet of geckos and the adhesive proteins found in underwater organisms. By studying the adhesion mechanisms of natural systems, researchers can gain insights into how to design materials with superior adhesive properties.Another area of research is the development of self-healing materials, which can automatically repair themselves when damaged. Self-healing materials can provide significant advantages in applications where durability and longevity are important, such as in aerospace, automotive, and construction industries.Moreover, the adhesion properties of materials are critical in the development of novel electronic and optical devices, such as touch screens, solar cells, and sensors. The ability to control and manipulate the adhesion of materials at the nanoscale level can enable the development of new materials and devices that are more efficient, durable, and cost-effective.In conclusion, the study of adhesion properties of materials is a critical area of research with applications in a wide variety of fields, from advanced materials engineering to biotechnology and electronics. By gaining a better understanding of the complex mechanisms that govern adhesion at the nanoscale level, researchers can develop more effective and innovative materials and technologies that can enhance our lives and our environment.Another important area of research related toadhesion properties of materials is in the field of medical engineering. Researchers are constantly exploring new ways to develop materials with enhanced biocompatibility, such as coatings for medical implants, tissue engineering scaffolds, and drug delivery systems. Effective adhesion between the implants and the surrounding tissue, as well as between various component materials, can play a crucial role in the success of medical procedures.Furthermore, the study of adhesion properties of materials is also crucial in the development of energy storage devices, such as batteries and supercapacitors. The adhesion of various components within these devices can impact their efficiency, durability, and safety.Nanoengineering of surfaces has the potential for creating materials with tunable adhesion properties, which can have a significant impact in many applications. For example, surfaces that can switch from being very sticky to completely non-sticky by applying a small electrical stimulus can be very useful in creating gripping or releasing devices.Moreover, understanding the adhesion properties of materials is also important in the development of new adhesives and coatings for industrial and household applications. Innovations in adhesives can lead to the development of new products that can help to reduce waste and energy usage, for instance in the creation of more efficient packaging materials.In conclusion, the study of adhesion properties of materials has awide range of applications which include biomedical, industrial, and energy fields. The research in this area is driving innovation and development of new materials, devices and coatings with improved functionality and performance, which can have a significant impact on the way we live and work.As a language model AI, I do not have personal opinions but I can tell you that the importance of research on adhesion properties of materials cannot be overstated. It is an essential aspect of many industries, from biomedical to aerospace and electronics, and has the potential to lead to significant advancements in areas such as sustainability, efficiency, and safety. It is a constantly evolving field, with new discoveries and innovations being made regularly, and as such, it is important to continue supporting research in this area.。
羟基磷灰石及其复合涂层的研究现状

羟基磷灰石及其复合涂层的研究现状段希夕 高钦钦(新余学院机电工程学院 江西新余 338004)摘要:钛及其合金金属有良好的机械性能,羟基磷灰石是有优异生物性能的活性陶瓷,因而羟基磷灰石/钛(HA/Ti)复合涂层结合二者优势性能被广泛应用于人体骨组织和牙齿的修复中,以提高材料的医用价值。
该文采用冷喷涂、等离子喷涂、磁控溅射、激光熔覆、溶胶-凝胶、电化学沉积等其他技术制备HA/Ti复合粒子,并适当掺入其他金属合金,完善性能,探究其实验后的涂层特征、表面形态对力学性能和生物性能的影响。
关键词:羟基磷灰石 钛 喷涂 复合涂层中图分类号:TG146文献标识码:A 文章编号:1672-3791(2023)17-0087-07 Research Status of Hydroxyapatite and Its Composite CoatingsDUAN Xixi GAO Qinqin(School of Electrical and Mechanical Engineering, Xinyu University, Xinyu, Jiangxi Province, 338004 China) Abstract: Titanium and its alloy metals have good mechanical properties. Hydroxyapatite is an active ceramic with excellent biological properties, so hydroxyapatite/titanium ( HA/Ti ) composite coatings are widely used in the re‐pair of human bone tissue and teeth in combination with the advantages of the two to improve the medical value of materials. HA/Ti composite particles are prepared by cold spraying, plasma spraying, magnetron sputtering, laser cladding, sol-gel, electrochemical deposition and other technologies, other metal alloys are added to improve prop‐erties, and the effects of their coating characteristics and surface morphology after the experiment on mechanical properties and biological properties are explored.Key Words: Hydroxyapatite; Titanium; Spraying; Composite coatings21世纪以来,需要人工骨治疗患者的数量在全球约有3 000万人次[1-2]。
生物材料学-第9章 仿生材料
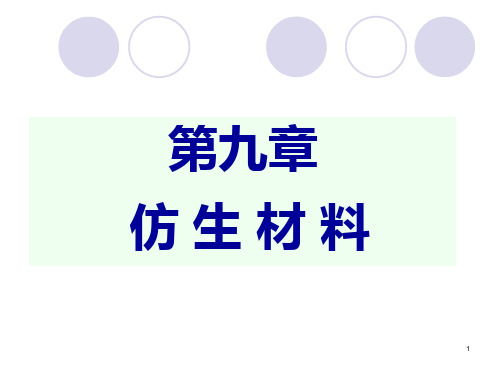
9.4 仿生材料研究的方法
仿生材料的研究期望通过结构仿生和功能仿生及其理论 计算与模拟,获得高效、低能耗、环境和谐与快速智能 应变的新材料及其新性质。
通过制备与生物相似结构或者形态,得到人造材料新的 性能和与自然界不同的特异性能,如人工类珐琅质、高 强韧陶瓷、仿生人工骨材料、仿蜘蛛人造纤维;
模仿的形态包括:体表形态结构、 翅的色彩花纹、翅的鳞片结构、足的 形状、昆虫巢穴形态及结构等。
6
仿蝴蝶色彩花纹形态构建军事伪装设施
7
蝴蝶翅膀鳞片仿生
然置功度阳 模 变不实而光 仿 化断现调照 蝴 的变对节射 蝶 控化人体方 翅 制而造温向 面 。引卫的自 上
起星原动的 温由理变鳞 度于,换片 骤位成角随
第九章 仿生材料
1
仿生学 ?
从自然到技术的思想或概念,对应的英文单词:
Bionics (仿生) Biomimetics (仿生)
Bionics取自拉丁文“bios” (生命方式)和词尾“nic”(具 有……性质的).
Biomimesis (生物拟态)
Biognosis (生命论/生物论)
bio-inspiration (生物灵感)
3
仿生学:生命科学、物质科学、信息科 学、工程技术、数学与力学、系统科 学等多学科的交叉.
仿生学可应用于所有技术领域和大多数 应用领域.
生活中的仿生学
凤蓬草 鱼尾 人眼晶状体 生物电 鸟
轮子 船橹 透镜 电池 飞机
4
昆虫是地球上分布 最广的动物
天地地水 上上下中 飞爬钻游
5
昆虫形态的仿生
模仿昆虫外部形态及其特征,构建 实用的技术系统或制造产品。
18
疏水——自然界的 启发
羟基磷灰石_壳聚糖生物复合材料的制备研究进展

.44.2008年02月第5卷第1期生物骨科材料与临床研究O PAEDIC B IOMECHANICS M ATERIALS A ND C LINICAL S TUDY羟基磷灰石/壳聚糖生物复合材料的制备研究进展徐挺何狄周银银汪涛[摘要]羟基磷灰石/壳聚糖复合材料因其生物相容性和合适的力学性能逐渐成为骨替代材料研究的热点。
本文综述了羟基磷灰石/壳聚糖复合材料的研究现状,探讨了其特点、制备和性能。
并在此基础上提出了此类材料今后的发展方向:三相复合材料和电、磁学性能的研究。
[关键词]羟基磷灰石;壳聚糖;生物材料;复合材料[中图分类号]R318.08[文献标识码]BProgress of hydroxyapatite/chitosan biomedical compositeXu Ting,He Di,zhou Yinyin,et al.Nanjing University of Aeronautics and Astronautics materials science and technologystudy,NaJing,211100[Abstract]Due to their biocompatibility and suitable mechanical properties,hydroxyapatite/chitosan composites havebecome the highlighted issue of the biomaterials for bone repairing.In the present paper,the recent applications and de-velopments of the composites are reviewed.Additionally,the characteristics,preparations and properties of the composi-tes are discussed as well.Finally,the developing trends of hydroxyapatite/chitosan biomedical composite materials are summarized and proposed.That is the study of three-phase composites and their electrical,magnetic properties.[Key words]Hydroxyapatite;Chitosan;Biomaterial;Composite1羟基磷灰石及壳聚糖的特点生物医学材料是指用于诊断、治疗、修复、替换人体组织、器官、或增进其功能的新型材料,有关此类材料的研究是近30年来发展起来的一门新兴交叉学科,而骨创伤事故的频繁发生使得骨修复与骨替代材料成为该领域中的研究重点。
仿生材料英语
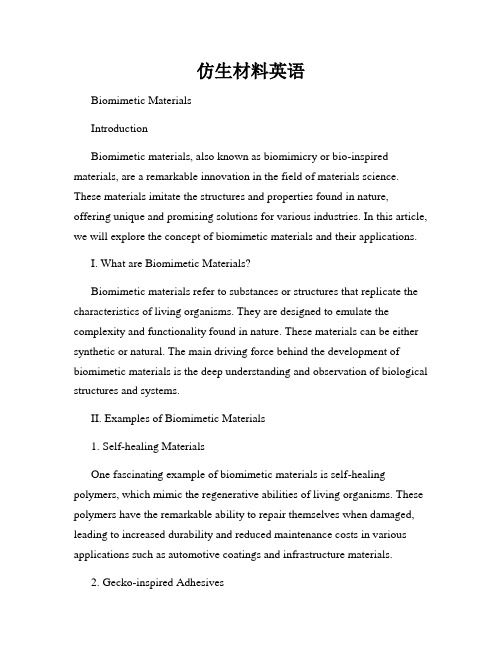
仿生材料英语Biomimetic MaterialsIntroductionBiomimetic materials, also known as biomimicry or bio-inspired materials, are a remarkable innovation in the field of materials science. These materials imitate the structures and properties found in nature, offering unique and promising solutions for various industries. In this article, we will explore the concept of biomimetic materials and their applications.I. What are Biomimetic Materials?Biomimetic materials refer to substances or structures that replicate the characteristics of living organisms. They are designed to emulate the complexity and functionality found in nature. These materials can be either synthetic or natural. The main driving force behind the development of biomimetic materials is the deep understanding and observation of biological structures and systems.II. Examples of Biomimetic Materials1. Self-healing MaterialsOne fascinating example of biomimetic materials is self-healing polymers, which mimic the regenerative abilities of living organisms. These polymers have the remarkable ability to repair themselves when damaged, leading to increased durability and reduced maintenance costs in various applications such as automotive coatings and infrastructure materials.2. Gecko-inspired AdhesivesGeckos are known for their exceptional ability to climb on smooth surfaces. Scientists have developed biomimetic adhesives that mimic the microscopic structure of gecko feet. These adhesives enable objects to stick to vertical surfaces without leaving residue or losing their adhesive properties. They have potential applications in robotics, aerospace, and medical devices.3. Lotus Leaf-inspired SurfacesThe lotus leaf has a unique ability to repel water. This natural phenomenon, known as the "lotus effect," has inspired the development of superhydrophobic surfaces. These biomimetic materials have water-repelling properties and can be used in various applications such as self-cleaning coatings, waterproof textiles, and anti-corrosion coatings.III. Advantages of Biomimetic Materials1. SustainabilityBiomimetic materials often offer sustainable solutions by reducing the use of toxic substances, minimizing waste, and promoting energy efficiency. By drawing inspiration from nature's design principles, these materials can contribute to a more environmentally friendly approach in various industries.2. Enhanced PerformanceBy imitating the intricate structures found in biological systems, biomimetic materials can achieve superior properties and performance. The implementation of such materials can lead to advancements in areas such as lightweight construction, energy storage, and drug delivery systems.3. VersatilityBiomimetic materials have a wide range of applications due to their diverse properties. They can be customized to meet specific requirements, making them suitable for various industries, including aerospace, electronics, healthcare, and architecture.IV. Challenges and Future PerspectivesWhile biomimetic materials offer immense potential, several challenges need to be addressed. The complexity of mimicking biological structures, the scalability of production, and the need for long-term durability are among the key issues that researchers face. However, continuous advancements in materials science and interdisciplinary collaboration hold promise for overcoming these challenges.In the future, biomimetic materials are expected to play an even more significant role in various fields. The integration of living organisms with synthetic materials, known as biohybrid materials, is a promising avenue for exploration. Additionally, the use of biomimetic materials in the development of autonomous systems and bio-inspired robots may revolutionize industries such as healthcare and manufacturing.ConclusionBiomimetic materials have emerged as a revolution in material science, offering innovative solutions inspired by nature. These materials imitate the ingenuity of biological systems and provide sustainable, high-performing alternatives in various industries. As research and technological advancements continue, the future holds great potential for the widespreadapplication of biomimetic materials, driving progress in a wide range of fields.。
汽车结构用590MPa级高屈服强度钢的研制_王科强

study on corrosion protection [D]. Hefei: Hefei University of [23]Palomino L M,Suegama P H,Aoki I V,et al. Electrochemical study
Technology,2009: 15-53.
alloys,and related materials for biomedical applications[J]. Materials
[20]张 林,谭帼馨,宁成云,等. 钛表面自组装硅烷化对仿生矿化
Science and Engineering R,2004,47( 3) : 49-121.
表 1 汽车结构用 590 MPa 级高屈服强度钢的 化学成分( 质量分数,%)
Table 1 Chemical composition of 590 MPa grade high yield strength steel for automobile structure ( mass fraction,%)
连铸坯在步进式加热炉中加热,为增加 Nb 和 Ti 的固溶量,提高析出强化效果,加热温度 ≥1250 ℃ 。 粗轧后高压除鳞在 1780 机组上精轧成 3 ~ 5 mm 厚板 卷。终轧温度≥890 ℃ ,轧后前段层流冷却,卷取温度 ≥650 ℃ 。热轧原料经酸洗后,在冷轧联合机组进行 轧制,此使钢组织中的珠光体团间距减小和珠光体被 破碎得较充分,为退火过程中的晶粒细化和再结晶提 供条件,冷轧压下率在 55% ~ 70% 之间。 2. 3 连续退火
and osteoblast proliferation [ J ]. Rare Metal Materials and
automotive steels[J]. Surface Engineering,2000,16( 4) : 315-320.
Effect of alloying elements on the microstructure and mechanical properties of nanostructured

LetterEffect of alloying elements on the microstructure and mechanical properties of nanostructured ferritic steels produced by spark plasmasinteringSomayeh Pasebani,Indrajit Charit ⇑Department of Chemical and Materials Engineering,University of Idaho,Moscow,ID 83844,USAa r t i c l e i n f o Article history:Received 23November 2013Received in revised form 23January 2014Accepted 29January 2014Available online 15February 2014Keywords:NanostructuresMechanical alloying Powder metallurgyTransmission electron microscopy High temperature alloya b s t r a c tSeveral Fe–14Cr based alloys with varying compositions were processed using a combined route of mechanical alloying and spark plasma sintering.Microstructural characteristics of the consolidated alloys were examined via transmission electron microscopy and atom probe tomography,and mechanical prop-erties evaluated using microhardness nthanum oxide (0.5wt.%)was added to Fe–14Cr leading to improvement in microstructural stability and mechanical properties mainly due to a high number den-sity of La–Cr–O-enriched nanoclusters.The combined addition of La,Ti (1wt.%)and Mo (0.3wt.%)to the Fe–14Cr base composition further enhanced the microstructural stability and mechanical properties.Nanoclusters enriched in Cr–Ti–La–O with a number density of 1.4Â1024m À3were found in this alloy with a bimodal grain size distribution.After adding Y 2O 3(0.3wt.%)along with Ti and Mo to the Fe–14Cr matrix,a high number density (1.5Â1024m À3)of Cr–Ti–Y–O-enriched NCs was also detected.For-mation mechanism of these nanoclusters can be explained through the concentrations and diffusion rates of the initial oxide species formed during the milling process and initial stages of sintering as well as the thermodynamic nucleation barrier and their enthalpy of formation.Ó2014Elsevier B.V.All rights reserved.1.IntroductionNanostructured ferritic steels (NFSs),a subcategory of oxide dis-persion strengthened (ODS)steels,have outstanding high temper-ature strength,creep strength [1,2]and excellent radiation damage resistance [3].These enhanced properties of NFSs have been attrib-uted to the high number density of Y–Ti–O-enriched nanoclusters (NCs)with diameter of 1–2nm [4].The Y–Ti–O-enriched NCs have been found to be stable under irradiation and effective in trapping helium [5].These NCs are formed due to the mechanical alloying (MA)of Fe–Cr–Ti powder with Y 2O 3during high energy ball milling followed by hot consolidation route such as hot isostatic pressing (HIP)or hot extrusion [6–8].Alinger et al.[4]have investigated the effect of alloying elements on the formation mechanism of NCs in NFSs processed by hot isostatic pressing (HIP)and reported both Ti and high milling energy were necessary for the formation of ler and Parish [9]suggested that the excellent creep properties in yttria-bearing NFSs result from the pinning of thegrain boundaries by a combined effect of solute segregation and precipitation.Although HIP and hot extrusion are commonly used to consoli-date the NFSs,anisotropic properties and processing costs are con-sidered challenging issues.Recently,spark plasma sintering (SPS)has been utilized to sinter the powder at a higher heating rate,low-er temperature and shorter dwell time.This can be done by apply-ing a uniaxial pressure and direct current pulses simultaneously to a powder sample contained in a graphite die [10].Except for a few studies on consolidation of simple systems such as Fe–9Cr–0.3/0.6Y 2O 3[11]and Fe–14Cr–0.3Y 2O 3[10],the SPS process has not been extensively utilized to consolidate the NFSs with complex compositions.Recently,the role of Ti and Y 2O 3in processing of Fe–16Cr–3Al–1Ti–0.5Y 2O 3(wt.%)via MA and SPS was investigated by Allahar et al.[12].A bimodal grain size distribution in conjunc-tion with Y–Ti–O-enriched NCs were obtained [12,13].In this study,Fe–14Cr (wt.%)was designed as the base or matrix alloy,and then Ti,La 2O 3and Mo were sequentially added to the ferritic matrix and ball milled.This approach allowed us to study the effect of individual and combined addition of solutes on the formation of NCs along with other microstructural evolutions.Furthermore,SPS instead of other traditional consolidation methods was used to consolidate the NFS powder.The mixture/10.1016/j.jallcom.2014.01.2430925-8388/Ó2014Elsevier B.V.All rights reserved.⇑Corresponding author.Tel.:+12088855964;fax:+12088857462.E-mail address:icharit@ (I.Charit).of Fe–Cr–Ti–Mo powder with Y2O3was also processed and characterized in a similar manner for comparison with the rest of the developed alloys.2.ExperimentalThe chemical compositions of all the developed alloys along with their identi-fying names in this study are given in Table1.High energy ball milling was per-formed in a SPEX8000M shaker mill for10h using Ar atmosphere with the milling media as steel balls of8mm in diameter and a ball to powder ratio(BPR) of10:1.A Dr.Sinter Lab SPS-515S was used to consolidate the as-milled powder at different temperatures(850,950and1050°C)for7min using the pulse pattern 12–2ms,a heating rate of100°C/min and a pressure of80MPa.The SPSed samples were in the form of disks with8mm in height and12mm in diameter.The density of the sintered specimens was measured by Archimedes’method. Vickers microhardness tests were performed using a Leco LM100microhardness tester operated at a load of1000g–f(9.8N).A Fischione Model110Twin-Jet Elec-tropolisher containing a mixture of CH3OH–HNO3(80:20by vol.%)as the electrolyte and operated at aboutÀ40°C was used to prepare specimens for transmission elec-tron microscopy(TEM).A FEI Tecnai TF30–FEG STEM operating at300kV was used. The energy dispersive spectroscopy(EDS)attached with the STEM was used to roughly examine the chemical composition of the particles.A Quanta3D FEG instrument with a Ga-ion source focused ion beam(FIB)was used to prepare spec-imens for atom probe tomography(APT)studies on14L,14LMT and14YMT sam-ples.The APT analysis was carried out using a CAMECA LEAP4000X HR instrument operating in the voltage mode at50–60K and20%of the standing volt-age pulse fraction.The atom maps were reconstructed using CAMECA IVAS3.6soft-ware and the maximum separation algorithm to estimate the size and chemical composition of NCs.This was applied to APT datasets each containing20–30million ions for each specimen.Lower evaporationfield of the nanoparticles and trajectory aberrations caused estimation of higher Fe atoms in the nanoclusters.Although the contribution of Fe atoms from the matrix was examined here,the matrix-correction was not addressed in this study.3.Results and discussionThe TEM brightfield micrographs for the various alloys SPSed at 950°C for7min are illustrated in Fig.1a–d.The microstructure of 14Cr alloy shown in Fig.1a revealed a complex microstructure with submicron subgrain-like structures,relatively high density of dislocations and low number density of oxide nanoparticles. The nanoparticles were larger(25–65nm)than the other SPSed al-loys and found to have chemical compositions close to Cr2O3and FeCr2O4as analyzed by energy dispersive spectroscopy.The microstructure of the consolidated14L alloy is shown in Fig.1b.The microstructure consisted of more ultrafine grains (<1l m but>100nm),a few nanograins with sharp boundaries and a higher number of nanoparticles mainly in the grain interiors. The number density of nanoparticles was higher than that of14Cr alloy shown in Fig.1a but lower than14LMT(Fig.1c)and14YMT (Fig.1d).In14L alloy,the nanoparticles with2–11nm in diameter were found inside the grains(hard to be observed at magnification given in Fig.1b and micrographs taken at higher magnifications was used for this purpose)whereas the nanoparticles with 50–80nm in diameter were located at the grain boundary regions. The particles on the boundaries are likely to be mainly Cr2O3and LaCrO3,but the chemical analysis of those smallest particles could not be done precisely due to the significant influence of the ferritic matrix.Fig.1c shows the microstructure of the SPSed14LMT alloy, consisting of both ultrafine grains(as defined previously)and nanograins(6100nm).The nanoparticles present in the micro-structure were complex oxides of Fe,Cr and Ti.The nanoparticles with faceted morphology and smaller than10nm in diameter were enriched in La and Ti.No evidence of stoichiometric La2TiO5or La2Ti2O7particles was observed based on the EDS and diffraction data.A similar type of microstructure was revealed in the SPSed 14YMT alloy as shown in Fig.1d.The particle size distribution histograms of the14Cr,14L, 14LMT and14YMT alloys are plotted in Fig.2a–d,respectively. Approximately1000particles were sampled from each alloy to de-velop the histograms.The average particle size decreased in order of14Cr,14L,14LMT and14YMT.The highest fraction of the particle size as shown in the histograms of14Cr,14L,14LMT and14YMT was found to be associated with25±5nm(18±2.5%),10±5nm (28±3%),5±1nm(40±6%)and5±1nm(46±5%)in diameter, respectively.The number density of nanoparticles smaller than 5±1nm was higher in14YMT than14LMT alloy.The3-D APT maps for14L alloy revealed a number density (%3Â1022mÀ3)of CrO–La–O-enriched NCs.The average Guinier radius of these NCs was1.9±0.6nm.The average composition of the NCs in14L was estimated by using the maximum separation algorithm to be Fe–17.87±3.4Cr–32.61±3.2O–8.21±1.1La(at.%).A higher number density(%1.4Â1024mÀ3)and smaller NCs with average Guinier radius of 1.43±0.20nm were observed in the APT maps for14LMT alloy as shown in Fig.3a.The NCs were Cr–Ti–La–O-enriched with the average composition of Fe–10.9±2.8Cr–30.9±3.1O–17.3±2.5Ti–8.2±2.2La(at.%).According to the LEAP measurements,the chemical composition of NCs dif-fered considerably from stoichiometric oxides.A large amount of Fe and Cr was detected inside the NCs,and La/Ti and La/O ratios were not consistent with La2TiO5or La2Ti2O7as expected based on thermodynamic calculations,rather the ratios were sub-stoichi-ometric.The3-D APT maps for14YMT alloy were similar to14YMT alloy as shown in Fig.3b.The NCs with an average radius of 1.24±0.2nm and a number density of1.5Â1024mÀ3were Cr–Ti–Y–O-enriched.The chemical composition of NCs was estimated close to Fe–8.52±3.1Cr–37.39±4.5O–24.52±3.1Ti–10.95±3.1Y (at.%).The matrix-corrected compositions are currently being ana-lyzed and will be reported in a full-length publication in near future.The relative density of various alloys sintered at850–1050°C is shown in Fig.4a.Generally,a higher density was obtained in the specimens sintered at higher temperatures.At850and950°C, the density of unmilled14Cr specimen(97.2%and97.5%)was higher than the milled/SPSed14Cr(92.8%and95.5%)because the unmilled powder particles were less hard(due to absence of strain hardening)and plastically deformed to a higher degree than the milled powder leading to a higher density.Adding0.5and 0.7wt.%of La2O3and0.3wt.%Y2O3to the14Cr matrix significantly decreased the density of the specimen,especially at850and 950°C;however,adding Ti to14L and14Y improved the density to some extent.The microhardness data of various alloys processed at different temperatures are shown in Fig.4b.In general,microhardness in-creased with increasing SPS temperatures up to950°C and then decreased.Both Y and La increased the hardness due to the disper-sion hardening effect.The hardness increased at the higher content of La due to the greater effect of dispersion hardening.Adding Ti separately to the14Cr matrix improved the hardness due to theTable1The alloy compositions and processing conditions(milled for10h and SPSed at850-1050°C for7min).Alloy ID Elements(wt.%)Cr Ti La2O3Y2O3Mo Fe14Cr-unmilled140000Bal.14Cr140000Bal.14T141000Bal.14L1400.500Bal.14Y14000.30Bal.14LM1400.500.3Bal.14LT1410.500Bal.14LMT(0.3)1410.300.3Bal.14LMT1410.500.3Bal.14LMT(0.7)1410.700.3Bal.14YMT14100.30.3Bal.S.Pasebani,I.Charit/Journal of Alloys and Compounds599(2014)206–211207dispersion hardening but only at lower temperature(850°C).The coarsening of Ti-enriched particles at above850°C plausibly decreased the hardness.However,at950°C,higher hardness (457HV)was achieved by a combined addition of La and Ti toFig.2.Particle size frequency histogram for(a)14Cr,(b)14L,(c)14LMT and(d)14YMT alloys. Fig.1.TEM brightfield micrographs for various alloys(a)14Cr,(b)14L,(c)14LMT and(d)14YMT.the14Cr matrix to produce14LT.Further addition of Mo to14LT improved the hardness through solid solution strengthening in 14LMT(495HV).High dislocation density and no well-defined grain boundaries were characteristics of14Cr alloy as shown in Fig.1a.The presence of a low number density and larger oxide particles(FeCr2O4and Cr2O3)at the boundaries could not create an effective pinning effect during sintering.As a result,some of these particles became confined within the grain interiors.The coarse grains had the capacity to produce and store high density of dislocations that subsequently resulted in the strain hardening effect.The hardening mechanism in14Cr alloy can thus be attributed to greater disloca-tion activities and resulting strain hardening effect.The grain boundary or precipitation hardening cannot be the dominant mechanism because of larger particles,greater inter-particle spac-ing and weakened Zener drag effect at the temperature of sinter-ing.Such strain hardening capability in nanocrystalline Fe consolidated via SPS was reported by other researchers,too [14,15].Interestingly,the high hardness in Fe–14Cr alloy consoli-dated via SPS at1100°C for4min by Auger et al.[10]wasFig.3.Three-dimensional atom maps showing NCs for(a)14LMT–91Â34Â30nm3and(b)14YMT–93Â30Â30nm3.Fig.4.(a)The relative density and(b)microhardness values for different SPSed alloys processed at different SPS temperatures for a dwell time of7min.attributed to the formation of martensitic laths caused by higher carbon content diffusing from the die,possible Cr segregation and rapid cooling during SPS.It is noteworthy to mention that no martensite lath was observed in the consolidated14Cr alloy in the present study.The level of solutes in the bcc matrix could be much greater than the equilibrium level,associated with a large number of vacancies created during milling.Our recent study[16]has shown that high energy ball milling has a complex role in initiating nucle-ation of La–Ti–O-enriched NCs in14LMT alloy powder,with a mean radius of%1nm,a number density of3.7Â10À24mÀ3and a composition of Fe–12.11Cr–9.07Ti–4.05La(at.%).The initiation of NCs during ball milling of NFSs has also been investigated by other researchers[8,17,18].According to Williams et al.[8],due to a low equilibrium solubility of O in the matrix,the precipitation of nanoparticles is driven by an oxidation reaction,subsequently resulting in reduction of the free energy.As the SPS proceeds,the number density of NCs would decrease and larger grain boundary oxides would form with the grain structure developing simulta-neously during the sintering process[8].Formation of larger grain boundary oxides as shown in Fig.1a could have been preceded by segregation of O and Cr to grain boundaries leading to a decrease in the level of the solutes in the ferritic matrix.The initial oxides forming in a chromium-rich matrix can be Cr2O3as suggested by Williams et al.[8].However,formation of LaCrO3in14L alloy (shown in Fig.1b)was associated with a higher reduction in the free energy according to the enthalpy of formation of various oxi-des given in Table2.The presence of nanoparticles caused grain boundary pinning and subsequently stabilized the nanocrystalline grains.The high density of defects(dislocations and vacancies)in a supersaturated solid solution,such as14LMT and14YMT alloys, could dramatically increase the driving force for accelerated sub-grain formation during the initial stage of sintering.At the initial stage,the vacancies created during the milling are annihilated [8,17].Meanwhile,the temperature is not high enough to produce a significant number of thermal vacancies;subsequently,any nucleation of new NCs will be prevented.As the SPS proceeds with no nucleation of new NCs,the high concentrations of extra solutes in the matrix are thermodynamically and kinetically required to precipitate out to form larger oxide particles.The larger solute-enriched oxide particles can be formed more favorably on the grain boundaries due to the higher boundary diffusivity.On the other hand,it should be considered that there is a dynamic plastic deformation occurring within the powder particles during SPS. The interaction of larger particles and dislocations introduced by dynamic hot deformation can explain the coarsening in some grains;because larger particles could not effectively pin the dislo-cations and the grain boundary migration could be facilitated fol-lowing the orientation with lower efficiency of Zener drag mechanism[19].Once the extra solutes present in the matrix pre-cipitated out,the microstructure will remain very stable because of the grain boundary pinning by triple-junctions of the grain bound-aries themselves[20],along with the high density of NCs and other ultrafine oxide particles[8].Further coarsening of the grains will be prevented even for longer dwell times at950°C.Therefore,a bi-modal grain size distribution emerged.The hardening of14LMT and14YMT alloys were attributed to a combined effect of solid solution strengthening,Hall-Petch strengthening and precipitation hardening.Based on the APT studies of the as-milled powder[16]and for-mation mechanism of the oxide particles suggested by Williams et al.[8]it could be speculated that in14LMT and14YMT alloys, Cr–O species formfirst and then absorb Ti and La/Y.This is associ-ated with a change in the interfacial energy of Cr–O species even though it is not thermodynamically the most favorable oxide.It has been established that the driving force for the oxide precipi-tates to form is the low solubility limit of oxygen in the ferritic ma-trix.The change in free energy due to oxidation reaction and nucleation of oxide nanoparticles is the leading mechanism[8].The majority of the oxygen required to generate the oxide nano-particles may be provided from the surface oxide during milling process.Furthermore,higher concentrations of Cr led to greater nucleation of Cr–O by influencing the kinetics of oxide formation. Concentrations and diffusivities of the oxide species along with the energy barrier for nucleation will control the nucleation of oxide nanoparticles.After the Cr–O formed during sintering,the Ti–O and Y/La-enriched clusters could form.The sub-stoichiome-tric NCs in14LMT and14YMT alloys were not due to insufficient level of O in the matrix[8].Formation of stoichiometric Y2Ti2O7 and Y2TiO5requires very high temperatures[8],which were outside the scope of this study.4.ConclusionThe SPSed Fe–14Cr alloy was found to have a higher hardness at room temperature due to the strain hardening effect.The stability of its microstructure at high temperatures was improved by addi-tion of La forming the Cr–La–O-enriched NCs.Adding La and Ti to Fe–14Cr matrix significantly improved the mechanical behavior and microstructural stability further due to the high number density of Cr–Ti–La–O-enriched NCs in14LMT alloy.It is demon-strated that the potential capability of La in developing new NFSs is promising but further investigations on their thermal and irradiation stability will still be required.AcknowledgementThis work was supported partly by the Laboratory Directed Research and Development Program of Idaho National Laboratory (INL),and by the Advanced Test Reactor National Scientific User Facility(ATR NSUF),Contract DE-AC07-05ID14517.The authors gratefully acknowledge the assistance of the staff members at the Microscopy and Characterization Suite(MaCS)facility at the Center for Advanced Energy Studies(CAES).References[1]M.J.Alinger,G.R.Odette,G.E.Lucas,J.Nucl.Mater.307–311(2002)484.[2]R.L.Klueh,J.P.Shingledecker,R.W.Swindeman,D.T.Hoelzer,J.Nucl.Mater.341(2005)103.[3]M.J.Alinger,G.R.Odette,D.T.Hoelzer,J.Nucl.Mater.329–333(2004)382.[4]M.J.Alinger,G.R.Odette,D.T.Hoelzer,Acta Mater.57(2009)392.Table2The standard enthalpies of formation of various oxide compounds at25°C[8,21,22].Element CompositionÀD H f(kJ molÀ1(oxide))Cr Cr2O31131CrO2583Fe Fe3O41118Fe2O3822Ti TiO543TiO2944Ti2O31522Ti3O52475Y Y2O31907YCrO31493Y2Ti2O73874La La2O31794La2Ti2O73855LaCrO31536210S.Pasebani,I.Charit/Journal of Alloys and Compounds599(2014)206–211[5]G.R.Odette,M.L.Alinger,B.D.Wirth,Annu.Rev.Mater.Res.38(2008)471.[6]ai,T.Okuda,M.Fujiwara,T.Kobayashi,S.Mizuta,H.Nakashima,J.Nucl.Sci.Technol.39(2002)872.[7]ai,M.Fujiwara,J.Nucl.Mater.307–311(2002)749.[8]C.A.Williams,P.Unifantowicz,N.Baluc,G.D.Smith,E.A.Marquis,Acta Mater.61(2013)2219.[9]ler,C.M.Parish,Mater.Sci.Technol.27(2011)729.[10]M.A.Auger,V.De Castro,T.Leguey,A.Muñoz,Pareja,R,J.Nucl.Mater.436(2013)68.[11]C.Heintze,M.Hernández-Mayoral, A.Ulbricht, F.Bergner, A.Shariq,T.Weissgärber,H.Frielinghaus,J.Nucl.Mater.428(2012)139.[12]K.N.Allahar,J.Burns,B.Jaques,Y.Q.Wu,I.Charit,J.I.Cole,D.P.Butt,J.Nucl.Mater.443(2013)256.[13]Y.Q.Wu,K.N.Allahar,J.Burns,B.Jaques,I.Charit,D.P.Butt,J.I.Cole,Cryst.Res.Technol.(2013)1,/10.1002/crat.201300173.[14]K.Oh-Ishi,H.W.Zhang Hw,T.Ohkubo,K.Hono,Mater.Sci.Eng.A456(2007)20.[15]B.Srinivasarao,K.Ohishi,T.Ohkubo,K.Hono,Acta Mater.57(2009)3277.[16]S.Pasebani,I.Charit,Y.Q.Wu, D.P.Butt,J.I.Cole,Acta Mater.61(2013)5605.[17]M.L.Brocq,F.Legendre,M.H.Mathon,A.Mascaro,S.Poissonnet,B.Radiguet,P.Pareige,M.Loyer,O.Leseigneur,Acta Mater.60(2012)7150.[18]M.Brocq,B.Radiguet,S.Poissonnet,F.Cuvilly,P.Pareige,F.Legendre,J.Nucl.Mater.409(2011)80.[19]H.K.D.H.Bhadeshia,Mater.Sci.Eng.A223(1997)64.[20]H.K.D.H.Bhadeshia,Mater.Sci.Technol.16(2000)1404.[21]W.Gale,T.Totemeier,Smithells Metals Reference Book,Amsterdam,Holland,2004.[22]T.J.Kallarackel,S.Gupta,P.Singh,J.Am.Ceram.Soc.(2013)1,http:///10.1111/jace.12435.S.Pasebani,I.Charit/Journal of Alloys and Compounds599(2014)206–211211。
蚕丝蛋白生物医学材料的研究进展
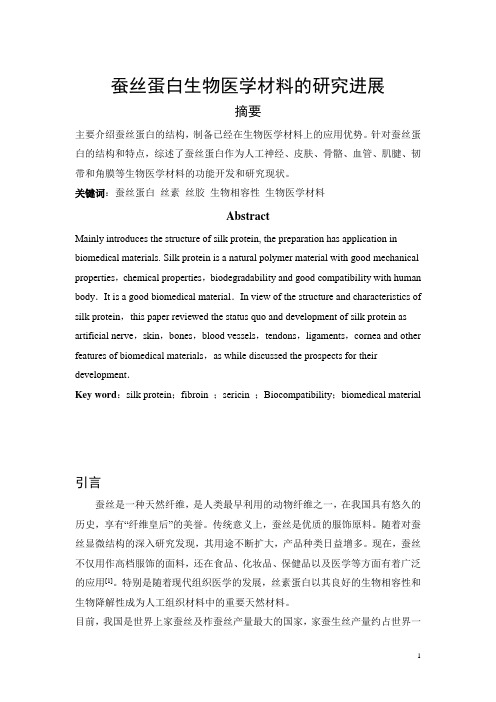
蚕丝蛋白生物医学材料的研究进展摘要主要介绍蚕丝蛋白的结构,制备已经在生物医学材料上的应用优势。
针对蚕丝蛋白的结构和特点,综述了蚕丝蛋白作为人工神经、皮肤、骨骼、血管、肌腱、韧带和角膜等生物医学材料的功能开发和研究现状。
关键词:蚕丝蛋白丝素丝胶生物相容性生物医学材料AbstractMainly introduces the structure of silk protein, the preparation has application in biomedical materials. Silk protein is a natural polymer material with good mechanical properties,chemical properties,biodegradability and good compatibility with human body.It is a good biomedical material.In view of the structure and characteristics of silk protein,this paper reviewed the status quo and development of silk protein as artificial nerve,skin,bones,blood vessels,tendons,ligaments,cornea and other features of biomedical materials,as while discussed the prospects for their development.Key word:silk protein;fibroin ;sericin ;Biocompatibility;biomedical material引言蚕丝是一种天然纤维,是人类最早利用的动物纤维之一,在我国具有悠久的历史,享有“纤维皇后”的美誉。
仿生材料研究进展

羟基磷灰石(Hydroxyapatite, HA)
• 羟基磷灰石(Hydroxyapatite, HA)其化学式为 Ca10(PO4)6(OH)2,是一种含有羟基的钙磷盐,Ca/P 为1.67。HA属六方晶系,空间群为P63/m,点阵常数 为a=0.9423nm,c=0.6875nm。磷灰石——“Apatite” 一词是1970年Werner从希腊文“apato”转用过来的。 它是对一类成分为M10(ZO)6X2的结晶矿物的通称。其 中M—Ca、Sr、Ba、Cr等;Z—P、V、As、S、Si、Ge、 CO3等;X—F、Cl、OH、Br、CO3、空位等。HA具有优 秀的骨、皮肤和肌肉生物相容性以及骨传导性,在骨 修复和替代材料领域包括从骨的填充、修补到全关节 的置换,都获得了日渐广泛的应用。
• 甲壳的纤维片条中存在许多“钉柱”以及由“钉 柱”支撑而形成的空隙,这样的结构形式使材料 既较轻而又具有较好的刚度和面内抗剪强度, 满足 了昆虫外甲壳自然复合材料对提高材料强度、刚 度、减轻材料重量以及释放或减轻材料内应力的 要求。在昆虫外甲壳中的传感器官和传输物质的 管道及孔洞附近的纤维具有较高的密度及保持连 续地绕过, 这与孔边的高应力场相适应, 当外甲壳 发生断裂时在这些地方遇到强烈的抵抗而消耗大 量的能量, 使材料在孔洞附近具有很好的强度和止 裂能力。据此结构制备的复合材料有更高的强度 和断裂韧性[17]。
• 为满足上述要求,生物医学复合材料是较佳选择。 医用金属、高分子材料、生物陶瓷等均可作为生 物医学复合材料的基体或增强体,经过适当的组 合、搭配,可得到大量性质各异、满足不同功能 要求的生物医学复合材料。此外,生物体中绝大 多数组织均可视为复合材料。 • 通过生物技术,把一些活体组织、细胞和诱导组 织再生的生长因子等引入生物医学材料,给无生 命的材料赋予生命的活力,并使其具有药物治疗 功能,成为一类新型生物医学复合材料——可吸 收生物医学复合材料,这些材料的发展为获得真 正仿生的复合材料开辟了途径。
最新基础生命科学名词解释汇总

干细胞:具有自我更新能力和高度增殖以及多向分化潜能的细胞。
细胞凋亡(apoptosis):细胞在一定的生理和病理条件下,受内在遗传机制的控制自动结束生 命的过程。
细胞程序性死亡(programmed cell death, PCD)生物在发育过程中一定生理刺激的反应性死 亡,它需要一定基因表达。
1)干细胞:具有分裂和分化潜能的细胞称为干细胞,干细胞在适合的条件下可分化形成不
同类型的细胞群•
2)单能干细胞:仅能分裂形成同类型的子代细胞•
2)多能干细胞:仅可分化为少数不同类型的细胞
3)全能干细胞:可以分化所有细胞类型的细胞
同化作用/合成代谢:生物体将简单小分子合成复杂大分子并消耗能量的过程
异化作用/分解代谢:生物体将复杂化合物分解成简单小分子并放出能量的反应
脱下的H+穿过内膜从线粒体的基质到内膜外的膜间腔中,造成跨膜的质子梯度。紧接着化
学渗透发生,质子顺浓度梯度从外腔经内膜通道返回到线粒体基质中,在ATP合成酶的作用
下,所释放的能量使ADP与磷酸结合生成了ATP
光系统:在类囊体膜上由叶绿素分子及其蛋白复合物、天线色素系统和电子受体等组成光系
统。
希尔反应:人们将叶绿体在光下所进行的水分解,并释放氧气的反应称为希尔反应。
基因工程 就是从生物体中把生物遗传物质分离出来,或人工合成一个DNA分子,用人工的
方法对遗传物质进行搭配、组合,然后转入某细胞内,通过改变其遗传物质的结构,来改变 它的遗传特性,使之定向地产生符合人类需要的新型生物物种、类型。
将重组的DNA分子引入到合适的宿主细胞
1转化:某NA,而使其基因型和表型
受体(receptor):存在于细胞表面或细胞内的特殊蛋白质,有三个必备条件:
- 1、下载文档前请自行甄别文档内容的完整性,平台不提供额外的编辑、内容补充、找答案等附加服务。
- 2、"仅部分预览"的文档,不可在线预览部分如存在完整性等问题,可反馈申请退款(可完整预览的文档不适用该条件!)。
- 3、如文档侵犯您的权益,请联系客服反馈,我们会尽快为您处理(人工客服工作时间:9:00-18:30)。
Mechanical Properties of Biomimetic Tissue Adhesive Based on the Microbial Transglutaminase-Catalyzed Crosslinking ofGelatinMartin K.McDermott,†Tianhong Chen,‡Christina M.Williams,§Kolleen M.Markley,|andGregory F.Payne*,‡,⊥Division of Mechanics and Materials Science,Office of Science and Technology, Food and Drug Administration,9200Corporate Blvd.,HFZ-150,Rockville,Maryland20850, Center for Biosystems Research,University of Maryland Biotechnology Institute,5115Plant Sciences Building,College Park,Maryland20742,Department of Biomedical Engineering,Texas A&M University, College Station,Texas77843-0100,Department of Biomedical Engineering,Marquette University, Milwaukee,Wisconsin53201-1881,and Department of Chemical and Biochemical Engineering, University of Maryland,Baltimore County,1000Hilltop Circle,Baltimore,Maryland21250 Received December15,2003;Revised Manuscript Received March11,2004Fibrin sealants are a type of soft tissue adhesive that employs biochemical reactions from the late stages of the blood coagulation cascade.Intrinsic to these adhesives are a structural protein and a transglutaminase crosslinking enzyme.We are investigating an alternative biomimetic adhesive based on gelatin and a calcium-independent microbial transglutaminase(mTG).Rheological measurements show that mTG catalyzes the conversion of gelatin solutions into hydrogels,and gel times are on the order of minutes depending on the gelatin type and concentration.Tensile static and dynamic loading of the adhesive hydrogels in bulk form demonstrated that the Young’s modulus ranged from15to120kPa,and these bulk properties were comparable to those reported for hydrogels obtained from fibrin-based p-shear adhesion tests of porcine tissue were performed using a newly published American Society for Testing and Materials(ASTM)standard for tissue adhesives.The gelatin-mTG adhesive bound the opposing tissues together with ultimate adhesive strengths of12-23kPa which were significantly higher than the strength observed for fibrin sealants.Even after failure,strands of the gelatin-mTG adhesive remained attached to both of the opposing tissues.These results suggest that gelatin-mTG adhesives may offer the benefits of fibrin sealants without the need for blood products.IntroductionSoft tissue adhesives are required to perform a variety of functions:stop bleeding,seal leaks,bind tissue,and/or facilitate healing.1,2Typically,these functions are met through the in situ generation of a three-dimensional polymeric network that is bonded to the tissue.There are two chemical approaches commonly used for generating such networks: the in situ polymerization of reactive monomers and the in situ crosslinking of preformed polymers.An example of the former is the cyanoacrylate-based adhesives that undergo rapid in situ polymerization upon contact with the tissue. An example of the latter is the gelatin-based adhesives that are in situ crosslinked by resorcinol and formaldehyde.A disadvantage of both of these adhesives is the toxicity of formaldehyde which is a degradation product of cyanoacry-late adhesives3,4and a reactant in gelatin-resorcinol-formaldehyde adhesives.There has been considerable recent effort to develop soft tissue adhesives that are less toxic and address other limitations of currently marketed adhesives. These efforts include the use of alternative materials(e.g., albumin,polysaccharides,poly(ethylene oxide)s),5-10better crosslinkingchemistries(e.g.,glutaraldehyde,carbodiimide),11-15 and more controllable polymerization reactions(e.g.,photo-initiated polymerization).16-18Biochemical crosslinking approaches could offer a more biocompatible alternative to synthetic crosslinking for the in situ generation of the three-dimensional polymeric network required for adhesion.One biochemical approach is to exploit the water-resistant adhesive that allows mussels to attach to wet and submerged surfaces(i.e.the mussel glue).19,20 Scheme1a shows that the mussel uses an enzyme to oxidize phenolic residues(i.e.,tyrosine or dihydroxyphenylalanine residues)of its adhesive protein.These oxidized residues undergo subsequent uncatalyzed crosslinking reactions to yield the three-dimensional polymeric network required for adhesion.21-24A second biochemical crosslinking approach relies on the reactions that naturally occur during blood coagulation.Scheme1b shows that during coagulation thrombin cleaves the blood protein fibrinogen into fibrin that undergoes subsequent crosslinking by factor XIIIa.As*To whom correspondence should be addressed.Phone:(301)405-8389.Fax:(301)314-9075.E-mail:payne@.†Food and Drug Administration.‡University of Maryland Biotechnology Institute.§Texas A&M University.|Marquette University.⊥University of Maryland,Baltimore County.1270Biomacromolecules2004,5,1270-127910.1021/bm034529a CCC:$27.50©2004American Chemical SocietyPublished on Web04/21/2004illustrated in Scheme 1b,factor XIIIa is a calcium-dependent transglutaminase enzyme.25-27Fibrin sealants are currently used in clinical practice 28,29and are prepared by mixing two solutions,typically one contains thrombin and calcium chloride and the second contains fibrinogen and factor XIII (factor XIII is a zymogen that is activated into factor XIIIa by thrombin and calcium 27).One concern with these bio-chemical approaches is the source of the protein;the mussel’s adhesive protein is difficult to obtain,and components of the fibrin sealant are derived from blood.We propose an alternative adhesive that is analogous to the fibrin sealant.Like the fibrin sealant,Scheme 1c shows that this proposed adhesive exploits the same biochemical crosslinking chemistry as the blood coagulation cascade.The differences are that the proposed adhesive uses gelatin as the “structural”protein (not fibrin)and a calcium-independent microbial transglutaminase as the crosslinking catalyst (not factor XIIIa).An adhesive based on gelatin and microbial transglutami-nase (mTG)should be nontoxic since gelatin is already used in various medical products while mTG is approved for food use.30(It should be noted that mTG’s approval for food use does not necessarily indicate that it will be biocompatible in medical applications.)A gelatin -mTG adhesive should also be simple to produce since both components are commercially available and derived from sources more convenient than blood.Finally,a gelatin -mTG adhesive may be simpler to use and subject to fewer sources of variability since there are fewer components and only one reaction step (as illustrated in Scheme 1b,the fibrin sealant requires twothrombin-catalyzed reactions and one transglutaminase-catalyzed reaction).Here we examined whether the mTG-crosslinked gelatin hydrogels offer appropriate mechanical properties for ap-plications as a soft tissue adhesive.There are two challenges to characterizing the mechanical properties of such adhesives.First,when such an adhesive “sets”,its bulk mechanical properties undergo dramatic changes and these changes are not readily observed using a single measurement method.Typically,“setting”is initiated by mixing the structural and crosslinking components.31As the three-dimensional poly-meric network is being formed,the sample undergoes a characteristic sol -gel transition that yields an elastic gel.Rheology is commonly used to examine the sol -gel transi-tion (i.e.,to measure gel time),monitor the initial evolution of mechanical properties,and evaluate the properties of weak gels.32-36Once the hydrogels become strong,however,rheology becomes less convenient;gels formed outside the rheometer cannot be easily loaded onto the instrument while gels formed within the instrument adhere to the parts (i.e.,to the cone and plate).To characterize the mechanical properties of strong gels,standard tensile tests and dynamic mechanical analysis can be used.37The second challenge in evaluating the mechanical proper-ties of a tissue adhesive is the testing method for establishing its adhesive strength.Numerous ASTM methods exist for industrial adhesives.However,these methods are not ap-propriate for evaluating tissue adhesives because tissues are mechanically compliant,have variable moisture levels,and present chemically complex surfaces.The lack of appropriate methods coupled with an increasing interest in medical adhesives has resulted in the development of a new ASTM method for testing tissue adhesives.This method is based on traditional lap-shear methods but employs porcine skin as the model tissue.38In the current study,we designed fixtures to create lap-shear adhesive joints that could be tested following this ASTM method.Materials and MethodsMaterials.Gelatin (type A from porcine)was obtained at two separate bloom values,175and 300(Sigma-Aldrich,St.Louis,MO).Bloom is a standard industrial measure used to rate gelatin “quality”and is related to the shear modulus of physical gelatin gels.We selected a gelatin prepared by acid treatment (type A)and not base treatment (type B),because base treatment hydrolyzes the amide groups of glutamine residues 39and suppresses enzymatic crosslinking.40The calcium-independent microbial transglutaminase (mTG;Activa TI)was obtained from Ajinomoto and was used without further purification.This enzyme is supplied as a proprietary formulation with a maltodextrin support and is reported by the manufacturer to have a specific activity of 100U/gm (lot number L-04207).The skin tissue was obtained from Brennen Medical (St.Paul,MN)and was nonperforated Porcine Mediskin Xenograft (S-106)that was supplied frozen in 7×9cm 2sections with a thickness of 0.032(0.005cm.The fibrin sealant,BaxTisseel Fibrin Sealant,was kindly supplied by Baxter Healthcare Corp.(Glendale,CA).Scheme1Biomimetic Tissue AdhesiveBiomacromolecules,Vol.5,No.4,20041271A concentrated enzyme solution was prepared for each experiment by mixing0.5gm of mTG per mL of deionized water.This enzyme solution was stored on ice until it was ready for use.A concentrated gelatin solution was prepared by adding50gm of gelatin to100mL of warm deionized water and mixing at50°C until the protein was dissolved. The pH of this concentrated gelatin solution was adjusted to6.0by adding small amounts of1M NaOH.Aliquots from this concentrated gelatin solution were then mixed with deionized water to prepare solutions with differing gelatin concentrations.The gelatin solutions were either used immediately,or stored at4°C for no more than4days. Gelatin solutions that were refrigerated were melted at50°C for no more than1h prior to use.Rheological Testing of Adhesive.Rheological tests were performed to examine the dynamics of gel formation.Gel formation was initiated by adding mTG solution to gelatin solutions to achieve a final enzyme activity of15U/gm-gelatin.41After mixing mTG,the solution was immediately loaded on top of the bottom plate of the rheometer,bubbles were removed from the solution using a pipet,and therheometer’s top plate(i.e.,the cone)was lowered onto the sample.Excess solution that exuded from the gap between the top cone and bottom plate was removed using a paper towel,silicon oil was placed along the edge of top plate to limit sample drying,and the rheometer test was initiated. Less than2min were required from the time mTG was mixed with the gelatin solution until the rheological test was initiated.Rheological tests were performed using a Thermo Haake RheoStress1rheometer(Thermo Electron Corpora-tion,Waltham,MA)at a temperature of37(1°C.A6.0 cm diameter cone-and-plate sensor was used with an angle of1°and a gap distance of0.052mm.The samples were subjected to an oscillatory stress of0.5Pa at a frequency of 0.1Hz.Bulk Mechanical Testing of Adhesive.The bulk me-chanical properties of the gelatin-mTG gels were character-ized by tensile static and dynamic testing.The samples were prepared by mixing mTG with gelatin solutions to a final enzyme activity of15U/gm-gelatin.After adding mTG,the solutions were poured into a Petri dish,sealed with a dampened lid and allowed to undergo crosslinking on a level surface at37°C.After incubating for90min,the crosslinked hydrogel was lifted from the Petri dish and cut into tensile specimens having cross-sectional dimensions of0.95(0.03 cm and0.32(0.01cm and lengths of2to2.5cm.After cutting the specimens and measuring their dimensions at room temperature(which required about20min),the tensile specimens were returned to the humid,37°C incubator.The first sample was tested150min after mixing the enzyme into the gelatin solution.The mechanical tests were performed using a dynamic mechanical thermal analyzer(DMTA)V Instrument(Rheo-metric Scientific,Inc.,Piscataway,NJ).The tensile samples were removed from the incubator and placed in the tensile fixture grips that were separated by a1cm gap.A0.03cm diameter wire thermocouple was inserted into a portion of the sample that protruded above the top grip.After closing the sample chamber,2-5min were required for the sample to reequilibrate to37(1°C.The sample was then loaded at a strain rate of1%/sec to a strain of20%and then unloaded back to0%strain.Initial studies showed that the stress-strain curves were different for the first and second loading/ unloading cycles.Such differences are commonly observed with soft,biological materials.42The stress-strain curves for subsequent loading/unloading cycles resulted in stress-strain curves that were similar to that for the second cycle(we examined4loading/unloading cycles in our initial studies). Thus,we used the stress-strain curve from the sample’s second loading/unloading cycle to determine the Young’s modulus(E).E was calculated as the slope of the linear regression line for data between0and4%strain.Immediately after completing the tensile static test,the sample was dynamically loaded at a peak strain of1.5%and frequency of0.5Hz.These dynamic tests allowed the determination of the complex modulus(E*),storage modulus(E′),loss modulus(E′′),and loss tangent(tanδ)E′′/E′).Lap-Shear Testing of Adhesive p-shear testing was performed following the procedures in ASTM F2255-03(“Test Method for Strength Properties of Tissue Adhesives in Lap-Shear by Tension Loading”).Figure1 shows that0.90(0.04cm2porcine skin tissue samples were attached to the aluminum backing using ethyl cyanoacrylate glue(Krazy Glue,Toagosel Co.,Ltd,Japan).As illustrated in Figure1,about0.15mL of gelatin-mTG adhesive was applied to one tissue surface,and then the two surfaces were lapped together.A47.5(0.3gm lead weight was placed on the adhesive joint directly over the tissues.The adhesive joint with the weight were incubated at37(1°C in enough water to cover the adhesive joint(but not the weight).After 120min,the adhesive joint was removed from the water and attached to the tension fixture of the DMTA.After the sample temperature was equilibrated to37(1°C in1-2 min,the upper and lower grips were separated at a speed of 5mm/min to measure the adhesive shearstrength.p-shear adhesive test.Schematics illustrate how the adhesive joints were formed and tested.Photographs of an adhesive joint alone and after mounting in the mechanical analyzer.1272Biomacromolecules,Vol.5,No.4,2004McDermott et al.Results and DiscussionFormation of Adhesive Hydrogel.Rheological methods were used to characterize the dynamics of gel formation.In these experiments,a gelatin solution was mixed with transglutaminase and immediately loaded onto the rheometer where the samples were subjected to oscillatory stresses.Figure 2shows typical results for samples prepared with gelatins of 175and 300bloom.Figure 2a shows that immediately after mixing mTG with the 175bloom gelatin,the sample behaved as a solution with the elastic modulus (G ′)being less than the viscous modulus (G ′′).Over time,Figure 2a shows that both moduli increased,but G ′increased more rapidly and became equal to G ′′after 28min.The point at which G ′becomes equal to G ′′is typically used as a rheological measure of the gel-point.32,36Figure 2b showsresults for a sample prepared with a 300bloom gelatin.As expected,the higher bloom gelatin had higher initial moduli and gelled more rapidly compared to the lower bloom gelatin of Figure 2a.A convenient comparison of the two samples is summarized in Figure 2c that shows the complex viscosity (η*)increases more rapidly for the higher bloom gelatin.The results in Figure 2show that transglutaminase catalyzed the conversion of gelatin solutions into gels (gelatin solutions lacking transglutaminase do not form gels at 37°C).These gels formed as a result of the covalent crosslinking of the gelatin chains and differ from the thermally reversible physical gelatin gels that are induced by cooling.40,41,43A series of rheological tests were performed with samples containing different gelatin concentrations.Figure 3a shows that the gel time depends on the gelatin bloom with lower bloom gelatin taking longer to gel.Depending on the gelatin concentration,we observed gel times to vary between 10and 29min for the 175bloom gelatin,and 2.7to 5.1min for the 300bloom gelatin.These time frames areconvenientFigure 2.Evolution of mechanical properties for gels formed by the mTG-catalyzed crosslinking of gelatin.Elastic (G ′)and viscous (G ′′)moduli for hydrogels formed from (a)175bloom gelatin and (b)300bloom gelatin.(c)Complex viscosity (η*)of these two samples.Gels were prepared by incubating gelatin (16.5%)with mTG (15U/gm-gelatin)at 37°C while these samples were subjected to oscillatory stresses (0.5Pa at 0.1Hz).Figure 3.Effect of gelatin bloom and concentration on gel formation.(a)Gel time is the time where the elastic and viscous moduli cross (i.e.,G ′)G ′′).(b)Complex viscosity (η*)evaluated at the gel point.(c)Elastic moduli evaluated at the gel point.Tests were performed in triplicate.Biomimetic Tissue Adhesive Biomacromolecules,Vol.5,No.4,20041273in the sense that it allows the gelatin and mTG to be thoroughly mixed before being applied to the tissue.If necessary,it should be possible to adjust the gel time by adjusting the mTG activity or adding other ingredients(not investigated here).For instance,previous studies showed that the gel time is significantly reduced by the inclusion of a high molecular weight polysaccharide(i.e.chitosan)to the solution.41Figure3,parts b and c,shows the complex viscosity(η*) and elastic moduli at the gel point(G′)G′′)for samples prepared at differing gelatin concentrations.These parameters provide a measure of the flow properties and gel strength at a single time,the gel-point,and provide an indication of the ease of mixing and applying the adhesive to the tissue surface.31If an adhesive is too weak or is applied before it has begun to gel,then it will have low viscosities and elasticities.In this instance,the adhesive will be able to flow into and mechanically“interlock”with tissue to improve adhesive-tissue bonding.However,a lower viscosity ad-hesive may lack sufficient cohesive strength to be retained where it is applied and it may flow away.This problem can be particularly important if the adhesive must be applied to wet tissue.In contrast,stronger gels or gels that have been allowed to set longer have greater cohesive strength(i.e., larger G′),retain their shape,but may not effectively penetrate and interlock with tissue.Thus,the adhesive’s flow properties and gel strength are practically important and the desired values will depend on the specific situation(e.g., wet or dry tissue).The adhesive’s flow properties and gel strength can be controlled by the enzyme activity,the gelatin bloom,and the gelatin concentration,whereas the results in Figure2show that these properties are rapidly changing during crosslinking.Bulk Mechanical Properties of Adhesive Hydrogel.The bulk mechanical properties of the mTG-catalyzed gelatin gels were characterized by static and dynamic tensile loading. For these studies,mTG was mixed with a gelatin solution and incubated in a Petri dish to form hydrogels that were subsequently cut into tensile specimens.Approximately 2.5-3h after initiating the reaction,the specimens weremounted in tensile fixtures for analyses.In the first study, the specimen was loaded in tension at a rate of1%/s(0.01 cm/s)to20%strain and then unloaded back to0%strain. Loading and unloading were performed for two cycles,and results from the second cycle were analyzed.The typical stress-strain curve of Figure4a shows that little hysteresis was observed between loading and unloading which indicates that the sample underwent little plastic deformation.This behavior is expected for a crosslinked elastic network.The Young’s modulus(E)was calculated from Figure4a using the slope of the linear regression line for strains between0 and4%.The value of E determined for the sample of Figure 4a is61kPa.Tensile static tests were performed for samples prepared at differing gelatin concentrations.Figure4b shows that the magnitude of E was between15and120kPa.This range is similar to that reported for fibrin-based adhesives containing high fibrin content(15and30kPa for sealants prepared with 30and70mg/mL fibrinogen,respectively).44Figure4b also shows that E tended to increase with increasing gelatin concentration which is also consistent with results from fibrin-based adhesives.44Finally,Figure4b shows that bulk properties of the gelatin-mTG adhesive were significantly dependent on the gelatin bloom with higher bloom gelatin yielding higher values for E.After completing the tensile static tests,each sample was then dynamically loaded at a peak strain of1.5%and a frequency of0.5Hz.These measurements allow the follow-ing properties to be determined;complex modulus(E*), storage modulus(E′),loss modulus(E′′),and tanδ()E′′/ E′).Results from these studies are summarized in Table1. For each of the samples prepared with the300bloom gelatin, the values for E*and E′are nearly the same,whereas the values for tanδare substantially less than0.1.These results indicate that these materials behave as elastic solids.Con-sistent with the results in Figure4b,Table1shows that samples prepared from the175bloom gelatin had lower E* and E′values compared to samples prepared from300bloom Figure4.Tensile static testing of mTG-catalyzed gelatin-based gels. Samples were prepared by reacting gelatin with mTG(15U/gm-gelatin)in a Petri dish to form thin hydrogels.After reaction,the gels were cut into tensile specimens that were mounted in tensile fixtures, loaded to20%strain and then unloaded.(a)Typical stress-strain curve for a sample prepared with300bloom gelatin(16.5%)during its second loading/unloading cycle.(b)Young’s modulus(E)for gelatin-mTG gels prepared with differing gelatin blooms and con-centrations.Tests were performed with5-10replicates.Table1.Bulk Mechanical Properties of Hydrogels Formed by mTG-Catalyzed Crosslinking of Gelatingelatinbloom(%)E(kPa)E*(kPa)E′(kPa)E′′(kPa)tanδ17516.515(38.5(3.27.8(3.3 2.8(1.70.39(0.22 17526.540(732(732(7 2.5(0.90.08(0.04 30016.560(560(560(5 1.9(0.30.03(0.01 30019.977(1272(1472(14 1.9(0.20.03(0.01 30023.191(1399(2499(24 6.8(5.10.06(0.04 30026.5120(22130(20130(20 6.4(4.20.05(0.031274Biomacromolecules,Vol.5,No.4,2004McDermott et al.gelatin.Also,Table 1shows good agreement in results obtained from tensile static testing and dynamic loading of the samples (i.e.,E ≈E *).It is desirable to relate the observed mechanical properties for the mTG-crosslinked gelatin gel to the underlying network structure.A strict chemical approach to assess network structure (i.e.,the types and number of crosslinks)is complicated by the facts that gelatin is not a simple monodisperse starting material,and the crosslinks are difficult to detect/quantify because the linkage is not unique (i.e.,an amide crosslink replaces an amide side chain).An alternative (although approximate)approach to estimate network struc-ture is to employ simplified physical models and use averaged values for gelatin.As outlined in the Appendix,we employed an idealized model for rubber elasticity as suggested by Sperling.45Also,we used an average amino acid composition for type A gelatin obtained from Rose 39and an average molecular weight for our gelatin provided by the supplier.This method indicates that the crosslink density for our gels reaches 8mole crosslink/m 3and 2crosslinks per gelatin chain,but only about 20%of the glutamine and lysine residues undergo crosslinking.Strength of Gelatin -mTG Adhesive.The testing of tissue adhesives generally results in high variability for numerous reasons:the starting tissue can have varying properties (e.g.,moisture levels);alignment of the tissue surfaces prior to contact can be challenging;and the adhesive joints can be damaged in sample handling.To minimize these problems,we constructed the alignment fixture shown in Figure 5that allows seven adhesive joints to be formed rapidly and reproducibly.Initially,skin tissue was bonded to aluminum backings (using cyanoacrylate adhesive)and the tissue was kept moist by covering it with gauze that had been soaked in PBS buffer.Seven backings with tissues attached were placed onto the alignment fixture to create the lower tissue surfaces of the seven adhesive joints.As illustrated in Figure 5,the adhesive (≈0.15mL)was spread onto each upper tissue surface,and then the upper and lower surfaces were contacted to create an adhesive joint.A weight was attached to the upper backing to apply a consistent force to each adhesive joint while it was curing.The sliding spacer bars shown in Figure 5ensured that the upper and lower tissue surfaces were aligned when they were being contacted.These spacer bars were removed immediately after the tissue surfaces were contacted and the adhesive joint was formed.Removing the spacer bars avoids the possibility that any adhesive that is exuded from between the tissue surfaces can form a bond with the surface of the spacer.Any bonds formed between the adhesive joint and other surfaces can make it difficult to remove the adhesive joint from the alignment fixture and risks damage to the adhesive bond formed between the two tissue surfaces.Approximately 3.5min were required to complete the formation of all seven adhesive joints in the alignment fixture.After forming the seven adhesive joints,the alignment fixture was submerged in a water bath at 37°C for 2h while the adhesive was curing.When incubation was complete,the adhesive joints were removed from the alignment fixture,mounted on the DMTA and tested by applying loads to separate the surfaces at a rate of 5mm/min.Figure 6a shows a typical force versus deflection curve for this lap-shear test in which failure was defined as the maximum load achieved (the maximum load for the sample in Figure 6a was 1.6N).When the sample was strained past failure,the force resisting further deflection diminished slowly indicating that failure was not brittle.The adhesive’s toughness results because strands of gel remain connected to the two skin surfaces even after failure.In fact,Figure 6b shows that some strands remain even after the resisting force diminished to zero and the samples were removed from the DMTAgrips.Figure 5.Alignment fixture to assemble adhesive joints.Schematics and photographs show adhesive joints in various stages of formation in the alignment fixture.Seven aluminum backings with tissue were placed in the fixture separated by removable spacer bars.Adhesive (≈0.15mL)was applied to the upper tissue surfaces and the two tissue surfaces were contacted to create the adhesive joint.The spacer bar separating two finished adhesive joints was removed as shown,leaving the adhesive joints aligned and exposed to the environment.The completed adhesive joints in the alignment fixture were immersed in water and allowed to cure at 37°C.Biomimetic Tissue Adhesive Biomacromolecules,Vol.5,No.4,20041275。