线性光耦hcnr200中文资料
模拟光耦HCNR200、HCNR201应用笔记

HCNR200和HCNR201模拟光电耦合器SPICE电路仿真应用笔记AN5545Jamshed Namdar Khan,安华高科技(Avago Technologies)隔离应用产品事业部光电耦合器应用工程师介绍本应用笔记的目的是展示PSpice软件如何通过使用安华高科技(Avago Technologies)提供的PSpice宏模型精确预测和仿真Avago公司HCNR200和HCNR201模拟光电耦合器的行为,聚焦集成电路仿真程序(SPICE, Sim-ulation Program with Integrated Circuit Emphasis)目前被认为是模拟电路设计工程师不可或缺的工具。
相对模拟光电耦合器数据表参数或规格,良好的宏模型应该精确预测电路性能,PSpice或SPICE仿真是任何设计工程师成功完成设计项目一个必备并且不可或缺的工具,电路仿真有助于原始设计概念的发想,从而允许工程师调整并优化原型电路取得最佳可能电路性能。
电路仿真的最大优势在于建构实体硬件或进行性能测试前可以先行验证并改善设计,极小化花费在原型测试的时间和相关费用成本。
为何需要仿真?不管电路仿真可以如何进行或带来什么,有一点它绝不可能做到的是为你提供实际的电路设计,因此我们首先列举几个吸引设计工程师进行电路仿真的原因。
进行电路仿真的主要动力是极小化预测目标电路设计性能的时间,相较于实际建立和进行原型测试等效电路的评估,使用SPICE电路进行评估所使用的时间相对上非常微小,另外,这些电路仿真也可以在各种不同温度、偏置条件以及零组件数值和误差条件下多次进行,但耗费时间仅为进行电路试验板设计并于工作台上进行评估的数分之一。
在进行光电耦合器SPICE仿真时,首先应该了解的是软件并无法仿真光电耦合器的两个基本特性,设计工程师使用光电耦合器主要有两个理由,分别是绝缘和隔离,SPICE软件并无法对这两个主要关键光电耦合器功能建立模型。
线性光耦隔离检测电压电路

线性光耦隔离检测电压电路耦合器以光为媒介传输电信号。
它对输入、输出电信号有良好的隔离作用,所以,它在各种电路中得到广泛的应用。
目前它已成为种类最多、用途最广的光电器件之一。
光耦合器一般由三部分组成:光的发射、光的接收及信号放大。
输入的电信号驱动发光二极管(LED),使之发出一定波长的光,被光探测器接收而产生光电流,再经过进一步放大后输出。
这就完成了电—光—电的转换,从而起到输入、输出、隔离的作用。
由于光耦合器输入输出间互相隔离,电信号传输具有单向性等特点,因而具有良好的电绝缘能力和抗干扰能力。
又由于光耦合器的输入端属于电流型工作的低阻元件,因而具有很强的共模抑制能力。
所以,它在长线传输信息中作为终端隔离元件可以大大提高信噪比。
在计算机数字通信及实时控制中作为信号隔离的接口器件,可以大大增加计算机工作的可靠性。
线性光耦的电流传输特性曲线接近直线,并且小信号时性能较好,能以线性特性进行隔离控制。
线性光耦TIL300 :主要技术指标如下:1.带宽>200Kz;2.传输增益稳定度为±0.05%/℃3.峰值隔离电压为3500VTIL300是一个由红外光LED照射分叉配置的隔离反馈光二极管和一个输出光二极管组成。
该器件采用特殊制造技术来补偿LED时间和温度特性的非线性,使输出信号与发出的伺服光通量成线性比例。
图1.TIL300电容C 防止电路产生震荡。
TIL300内部D0是发光二极管,其工作电流电If 可选为10mA 。
D1,D2为光敏二极管,他们受D0的激发分别产生电流Ip1和Ip2,其大小与If 有关:其中 、 表明 , 随 的变化,可称为光耦合函数。
由于D1,D2用相同的工艺做成并与D0封装在一起,因此,它们的光耦合函数变化规律一致,故可设:(1)实际上可以把K 看做常数,K 的值是TIL300的电器参数,典型值为1。
参数取值范围为0.75~1.25.。
U1构成一个负反馈放大器,其同相输入端和反相输入端的电压应近似相等,满足:(2) U2是一个电压跟随器,输出电压V0等于输入段电压:22i p V I R » (3) 于是电路的增益可由 (4)由于i V 的电压是由R3、R4、R5分压后输入U1同向端,所以: 0222111P i P V I R R K V I R R ==2p I 1p I f I 2f K I 11p fI K I = 1f K I 22p fI K I = 2211p f p f I K I K I K I == 11i p V I R »(5)于是: (6)可以看出输出0V 与E 是线性关系。
线性光耦hcnr201资料

1-418HHigh-Linearity Analog Optocouplers Technical DataHCNR200HCNR201CAUTION: It is advised that normal static precautions be taken in handling and assembly of this component to prevent damage and /or degradation which may be induced by ESD.I FIPD1I PD2NCNC PD2 CATHODEPD2 ANODELED CATHODELED ANODEPD1 CATHODEPD1 ANODEFeatures• Low Nonlinearity: 0.01%• K 3 (I PD2/I PD1) Transfer Gain HCNR200: ±15%HCNR201: ±5%• Low Gain Temperature Coefficient: -65ppm/°C • Wide Bandwidth – DC to >1 MHz• Worldwide Safety Approval-UL 1577 Recognized (5 kV rms/1 min Rating)-CSA Approved -BSI Certified-VDE 0884 Approved V IORM = 1414 V peak (Option #050)• Surface Mount Option Available(Option #300)• 8-Pin DIP Package - 0.400"Spacing• Allows Flexible Circuit Design• Special Selection forHCNR201: Tighter K 1, K 3and Lower Nonlinearity AvailableApplications• Low Cost Analog Isolation • Telecom: Modem, PBX• Industrial Process Control:Transducer IsolatorIsolator for Thermocouples 4mA to 20 mA Loop Isolation • SMPS Feedback Loop, SMPS Feedforward• Monitor Motor Supply Voltage • MedicalDescriptionThe HCNR200/201 high-linearity analog optocoupler consists of a high-performance AlGaAs LED that illuminates two closelymatched photodiodes. The input photodiode can be used tomonitor, and therefore stabilize,the light output of the LED. As a result, the nonlinearity and driftcharacteristics of the LED can be virtually eliminated. The output photodiode produces a photocur-rent that is linearly related to the light output of the LED. The close matching of the photodiodes and advanced design of the package ensure the high linearity and stable gain characteristics of the optocoupler.The HCNR200/201 can be used to isolate analog signals in a wide variety of applications that require good stability, linearity,bandwidth and low cost. The HCNR200/201 is very flexible and, by appropriate design of the application circuit, is capable of operating in many different modes, including: unipolar/bipolar, ac/dc and inverting/non-inverting. The HCNR200/201 is an excellent solution for many analog isolation problems.Schematic5965-3577E1-419Ordering Information:HCNR20x0 = ±15% Transfer Gain, 0.25% Maximum Nonlinearity 1 = ±5% Transfer Gain, 0.05% Maximum NonlinearityOption yyy050 = VDE 0884 V IORM = 1414 V peak Option 300 = Gull Wing Surface Mount Lead Option 500 = Tape/Reel Package Option (1 k min.)Option data sheets available. Contact your Hewlett-Packard sales representative or authorized distributor for information.Package Outline DrawingsFigure 1.MAX."V" = OPTION 050OPTION NUMBERS 300 AND 500 NOT MARKED.1-420Gull Wing Surface Mount Option #300240TIME – MINUTEST E M P E R A T U R E – °C220200180160140120100806040200260(NOTE: USE OF NON-CHLORINE ACTIVATED FLUXES IS RECOMMENDED.)Maximum Solder Reflow Thermal ProfileRegulatory InformationThe HCNR200/201 optocoupler features a 0.400" wide, eight pin DIP package. This package was specifically designed to meet worldwide regulatory require-ments. The HCNR200/201 has been approved by the following organizations:ULRecognized under UL 1577, Component Recognition Program,FILE E55361CSAApproved under CSA Component Acceptance Notice #5, File CA 88324BSICertification according to BS415:1994;(BS EN60065:1994);BS EN60950:1992(BS7002:1992) andEN41003:1993 for Class II applicationsVDEApproved according to VDE 0884/06.92(Available Option #050only)1.78 ± 0.15 MAX.BSCDIMENSIONS IN MILLIMETERS (INCHES).LEAD COPLANARITY = 0.10 mm (0.004 INCHES).Insulation and Safety Related SpecificationsParameter Symbol Value Units ConditionsMin. External Clearance L(IO1)9.6mm Measured from input terminals to output (External Air Gap)terminals, shortest distance through air Min. External Creepage L(IO2)10.0mm Measured from input terminals to output (External Tracking Path)terminals, shortest distance path along body Min. Internal Clearance 1.0mm Through insulation distance conductor to (Internal Plastic Gap)conductor, usually the direct distancebetween the photoemitter and photodetectorinside the optocoupler cavityMin. Internal Creepage 4.0mm The shortest distance around the border (Internal Tracking Path)between two different insulating materialsmeasured between the emitter and detector Comparative Tracking Index CTI200V DIN IEC 112/VDE 0303 PART 1Isolation Group IIIa Material group (DIN VDE 0110)Option 300 – surface mount classification is Class A in accordance with CECC 00802.VDE 0884 (06.92) Insulation Characteristics (Option #050 Only)Description Symbol Characteristic Unit Installation classification per DIN VDE 0110/1.89, Table 1For rated mains voltage ≤600 V rms I-IVFor rated mains voltage ≤1000 V rms I-IIIClimatic Classification (DIN IEC 68 part 1)55/100/21Pollution Degree (DIN VDE 0110 Part 1/1.89)2Maximum Working Insulation Voltage V IORM1414V peak Input to Output Test Voltage, Method b*V PR2651V peak V PR = 1.875 x V IORM, 100% Production Test witht m = 1 sec, Partial Discharge < 5 pCInput to Output Test Voltage, Method a*V PR2121V peak V PR = 1.5 x V IORM, Type and sample test, t m = 60 sec,Partial Discharge < 5 pCHighest Allowable Overvoltage*V IOTM8000V peak (Transient Overvoltage, t ini = 10 sec)Safety-Limiting Values(Maximum values allowed in the event of a failure,also see Figure 11)Case Temperature T S150°C Current (Input Current I F, P S = 0)I S400mA Output Power P S,OUTPUT700mW Insulation Resistance at T S, V IO = 500 V R S>109Ω*Refer to the front of the Optocoupler section of the current catalog for a more detailed description of VDE 0884 and other product safety regulations.Note: Optocouplers providing safe electrical separation per VDE 0884 do so only within the safety-limiting values to which they are qualified. Protective cut-out switches must be used to ensure that the safety limits are not exceeded.1-421Absolute Maximum RatingsStorage Temperature..................................................-55°C to +125°C Operating Temperature (T A)........................................-55°C to +100°C Junction Temperature (T J)............................................................125°C Reflow Temperature Profile...See Package Outline Drawings Section Lead Solder Temperature..................................................260°C for 10s (up to seating plane)Average Input Current - I F............................................................25 mA Peak Input Current - I F.................................................................40 mA (50 ns maximum pulse width)Reverse Input Voltage - V R..............................................................2.5 V (I R = 100 µA, Pin 1-2)Input Power Dissipation.........................................60 mW @ T A = 85°C (Derate at 2.2 mW/°C for operating temperatures above 85°C) Reverse Output Photodiode Voltage................................................30 V (Pin 6-5)Reverse Input Photodiode Voltage...................................................30 V (Pin 3-4)Recommended Operating ConditionsStorage Temperature....................................................-40°C to +85°C Operating Temperature.................................................-40°C to +85°C Average Input Current - I F.......................................................1 - 20 mA Peak Input Current - I F.................................................................35 mA (50% duty cycle, 1 ms pulse width)Reverse Output Photodiode Voltage...........................................0 - 15 V (Pin 6-5)Reverse Input Photodiode Voltage..............................................0 - 15 V (Pin 3-4)1-422Electrical Specifications1-423AC Electrical SpecificationsT A = 25°C unless otherwise specified.TestParameter Symbol Device Min.Typ.Max.Units Conditions Fig.Note LED Bandwidth f -3dB9MHz I F = 10 mAApplication Circuit Bandwidth:High Speed 1.5MHz167 High Precision10kHz177 Application Circuit: IMRRHigh Speed95dB freq = 60 Hz167, 8 Package CharacteristicsT= 25°C unless otherwise specified.Notes:1. K3 is calculated from the slope of thebest fit line of I PD2 vs. I PD1 with elevenequally distributed data points from5nA to 50 µA. This is approximatelyequal to I PD2/I PD1 at I F = 10 mA.2. Special selection for tighter K1, K3 andlower Nonlinearity available.3. BEST FIT DC NONLINEARITY (NL BF) isthe maximum deviation expressed as a percentage of the full scale output of a “best fit” straight line from a graph ofI PD2 vs. I PD1 with eleven equally distrib-uted data points from 5 nA to 50µA.I PD2 error to best fit line is the deviationbelow and above the best fit line,expressed as a percentage of the fullscale output.4. ENDS FIT DC NONLINEARITY (NL EF)is the maximum deviation expressed asa percentage of full scale output of astraight line from the 5 nA to the 50 µAdata point on the graph of I PD2 vs. I PD1.5. Device considered a two-terminaldevice: Pins 1, 2, 3, and 4 shortedtogether and pins 5, 6, 7, and 8 shortedtogether.6. In accordance with UL 1577, eachoptocoupler is proof tested by applyingan insulation test voltage of ≥6000 Vrms for ≥1 second (leakage detectioncurrent limit, I I-O of 5 µA max.). Thistest is performed before the 100%production test for partial discharge(method b) shown in the VDE 0884Insulation Characteristics Table (forOption #050 only).7. Specific performance will depend oncircuit topology and components.8. IMRR is defined as the ratio of thesignal gain (with signal applied to V IN ofFigure 16) to the isolation mode gain(with V IN connected to input commonand the signal applied between theinput and output commons) at 60 Hz,expressed in dB.*The Input-Output Momentary Withstand Voltage is a dielectric voltage rating that should not be interpreted as an input-output continuous voltage rating. For the continuous voltage rating refer to the VDE 0884 Insulation Characteristics Table (if applicable), your equipment level safety specification, or HP Application Note 1074, “Optocoupler Input-Output Endurance Voltage.”1-4241-425Figure 5. NL BF vs. Temperature.Figure 2. Normalized K3 vs. Input I PD .Figure 3. K3 Drift vs. Temperature.Figure 4. I PD2 Error vs. Input I PD (See Note 4).Figure 6. NL BF Drift vs. Temperature.Figure 7. Input Photodiode CTR vs.LED Input Current.Figure 8. Typical Photodiode Leakage vs. Temperature.Figure 9. LED Input Current vs.Forward Voltage.Figure 10. LED Forward Voltage vs.Temperature.I L K – P H O T O D I O D E L E A K A G E – n AT A – TEMPERATURE – °C D E L T A K 3 – D R I F T O F K 3 T R A N S F E R G A I NT A – TEMPERATURE – °C D E L T A N L B F – D R I F T O F B E S T -F I T N L – % P T ST A – TEMPERATURE – °C N O R M A L I Z E D K 1 – I N P U T P H O T O D I O D E C T RI F – LED INPUT CURRENT – mAV F – L E D F O R W A R D V O L T A G E – VT A – TEMPERATURE – °C-25-555356595125N O R M A L I Z E D K 3 – T R A N S F E R G AI NI PD1 – INPUT PHOTODIODE CURRENT – µAI PD1 – INPUT PHOTODIODE CURRENT – µAI P D 2 E R R O R F R O M B E S T -F I T L I N E (% O F F S )N L B F – B E S T -F I T N O N -L I N E A R I T Y – %T A – TEMPERATURE – °C 1.201000.10.0001V F – FORWARD VOLTAGE – VOLTS 1.30 1.501010.010.001 1.40 1.60I F – F O R W A R D C U R R E N T – m AT A = 25°C1-426Figure 12. Basic Isolation Amplifier.FV I OUTA) BASIC TOPOLOGYFigure 11. Thermal Derating Curve Dependence of Safety Limiting Value with Case Temperature per VDE 0884.V OUTV OUTA) POSITIVE INPUT B) POSITIVE OUTPUTD) NEGATIVE OUTPUTFigure 13. Unipolar Circuit Topologies.T S – CASE TEMPERATURE – °C1-427Figure 15. Loop-Powered 4-20 mA Current Loop Circuits.Figure 14. Bipolar Circuit Topologies.OUTV OUTA) SINGLE OPTOCOUPLERB) DUAL OPTOCOUPLERVOUT+I OUTA) RECEIVERB) TRANSMITTERV -I OUT1-428Figure 18. Bipolar Isolation Amplifier.Figure 16. High-Speed Low-Cost Analog Isolator.VOUTINPUT BNCV +15 V OUTPUT BNCFigure 17. Precision Analog Isolation Amplifier.MAGV IN1-429MAGV INSIGNFigure 20. SPICE Model Listing.Figure 19. Magnitude/Sign Isolation Amplifier..SUBCKT HCNR200Theory of Operation Figure 1 illustrates how the HCNR200/201 high-linearity optocoupler is configured. The basic optocoupler consists of an LED and two photodiodes. The LED and one of the photodiodes (PD1) is on the input leadframe and the other photodiode (PD2) is on the output leadframe. The package of the optocoupler is constructed so that each photo-diode receives approximately the same amount of light from the LED.An external feedback amplifier can be used with PD1 to monitor the light output of the LED and automatically adjust the LED current to compensate for any non-linearities or changes in light output of the LED. The feedback amplifier acts to stabilize and linearize the light output of the LED. The output photodiode then converts the stable, linear light output of the LED into a current, which can then be converted back into a voltage by another amplifier.Figure 12a illustrates the basic circuit topology for implementing a simple isolation amplifier using the HCNR200/201 optocoupler. Besides the optocoupler, two external op-amps and two resistors are required. This simple circuit is actually a bit too simple to function properly in an actual circuit, but it is quite useful for explaining how the basic isolation amplifier circuit works (a few more components and a circuit change are required to make a practical circuit, like the one shown in Figure 12b).The operation of the basic circuit may not be immediately obvious just from inspecting Figure 12a,particularly the input part of thecircuit. Stated briefly, amplifierA1 adjusts the LED current (I F),and therefore the current in PD1(I PD1), to maintain its “+” inputterminal at 0 V. For example,increasing the input voltage wouldtend to increase the voltage of the“+” input terminal of A1 above 0V. A1 amplifies that increase,causing I F to increase, as well asI PD1. Because of the way that PD1is connected, I PD1 will pull the “+”terminal of the op-amp backtoward ground. A1 will continueto increase I F until its “+”terminal is back at 0 V. Assumingthat A1 is a perfect op-amp, nocurrent flows into the inputs ofA1; therefore, all of the currentflowing through R1 will flowthrough PD1. Since the “+” inputof A1 is at 0 V, the currentthrough R1, and therefore I PD1 aswell, is equal to V IN/R1.Essentially, amplifier A1 adjusts I Fso thatI PD1 = V IN/R1.Notice that I PD1 depends ONLY onthe input voltage and the value ofR1 and is independent of the lightoutput characteristics of the LED.As the light output of the LEDchanges with temperature, ampli-fier A1 adjusts I F to compensateand maintain a constant currentin PD1. Also notice that I PD1 isexactly proportional to V IN, givinga very linear relationship betweenthe input voltage and thephotodiode current.The relationship between the inputoptical power and the outputcurrent of a photodiode is verylinear. Therefore, by stabilizingand linearizing I PD1, the lightoutput of the LED is alsostabilized and linearized. Andsince light from the LED falls onboth of the photodiodes, I PD2 willbe stabilized as well.The physical construction of thepackage determines the relativeamounts of light that fall on thetwo photodiodes and, therefore,the ratio of the photodiodecurrents. This results in verystable operation over time andtemperature. The photodiodecurrent ratio can be expressed asa constant, K, whereK = I PD2/I PD1.Amplifier A2 and resistor R2 forma trans-resistance amplifier thatconverts I PD2 back into a voltage,V OUT, whereV OUT = I PD2*R2.Combining the above threeequations yields an overallexpression relating the outputvoltage to the input voltage,V OUT/V IN = K*(R2/R1).Therefore the relationshipbetween V IN and V OUT is constant,linear, and independent of thelight output characteristics of theLED. The gain of the basic isola-tion amplifier circuit can beadjusted simply by adjusting theratio of R2 to R1. The parameterK (called K3 in the electricalspecifications) can be thought ofas the gain of the optocoupler andis specified in the data sheet.Remember, the circuit inFigure12a is simplified in orderto explain the basic circuit opera-tion. A practical circuit, more likeFigure12b, will require a fewadditional components to stabilizethe input part of the circuit, tolimit the LED current, or to1-4301-431second circuit requires two optocouplers, separate gain adjustments for the positive and negative portions of the signal,and can exhibit crossover distor-tion near zero volts. The correct circuit to choose for an applica-tion would depend on therequirements of that particular application. As with the basic isolation amplifier circuit inFigure 12a, the circuits in Figure 14 are simplified and would require a few additional compo-nents to function properly. Two example circuits that operate with bipolar input signals arediscussed in the next section.As a final example of circuit design flexibility, the simplified schematics in Figure 15 illustrate how to implement 4-20 mA analog current-loop transmitter and receiver circuits using the HCNR200/201 optocoupler. An important feature of these circuits is that the loop side of the circuit is powered entirely by the loop current, eliminating the need for an isolated power supply.The input and output circuits in Figure 15a are the same as the negative input and positive output circuits shown in Figures 13c and 13b, except for the addition of R3and zener diode D1 on the input side of the circuit. D1 regulates the supply voltage for the input amplifier, while R3 forms a current divider with R1 to scale the loop current down from 20mA to an appropriate level for the input circuit (<50 µA).As in the simpler circuits, the input amplifier adjusts the LED current so that both of its input terminals are at the same voltage.The loop current is then dividedoptimize circuit performance.Example application circuits will be discussed later in the data sheet.Circuit Design FlexibilityCircuit design with the HCNR200/201 is very flexible because the LED and both photodiodes are accessible to the designer. This allows the designer to make perf-ormance trade-offs that would otherwise be difficult to make with commercially available isolation amplifiers (e.g., bandwidth vs.accuracy vs. cost). Analog isola-tion circuits can be designed for applications that have either unipolar (e.g., 0-10 V) or bipolar (e.g., ±10 V) signals, with positive or negative input oroutput voltages. Several simplified circuit topologies illustrating the design flexibility of the HCNR200/201 are discussed below.The circuit in Figure 12a is configured to be non-inverting with positive input and output voltages. By simply changing the polarity of one or both of the photodiodes, the LED, or the op-amp inputs, it is possible toimplement other circuit configu-rations as well. Figure 13illustrates how to change the basic circuit to accommodate both positive and negative input and output voltages. The input and output circuits can bematched to achieve any combina-tion of positive and negative voltages, allowing for both inverting and non-inverting circuits.All of the configurations described above are unipolar (single polar-ity); the circuits cannot accommo-date a signal that might swing both positive and negative. It ispossible, however, to use the HCNR200/201 optocoupler to implement a bipolar isolation amplifier. Two topologies that allow for bipolar operation are shown in Figure 14.The circuit in Figure 14a uses two current sources to offset the signal so that it appears to be unipolar to the optocoupler.Current source I OS1 provides enough offset to ensure that I PD1is always positive. The second current source, I OS2, provides an offset of opposite polarity toobtain a net circuit offset of zero.Current sources I OS1 and I OS2 can be implemented simply as resistors connected to suitable voltage sources.The circuit in Figure 14b uses two optocouplers to obtain bipolar operation. The first optocoupler handles the positive voltage excursions, while the second optocoupler handles the negative ones. The output photodiodes are connected in an antiparallel configuration so that they produce output signals of opposite polarity.The first circuit has the obvious advantage of requiring only one optocoupler; however, the offset performance of the circuit isdependent on the matching of I OS1and I OS2 and is also dependent on the gain of the optocoupler.Changes in the gain of the opto-coupler will directly affect the offset of the circuit.The offset performance of the second circuit, on the other hand,is much more stable; it is inde-pendent of optocoupler gain and has no matched current sources to worry about. However, thebetween R1 and R3. I PD1 is equal to the current in R1 and is given by the following equation:I PD1 = I LOOP*R3/(R1+R3).Combining the above equation with the equations used for Figure 12a yields an overall expression relating the output voltage to the loop current,V OUT/I LOOP = K*(R2*R3)/(R1+R3).Again, you can see that the relationship is constant, linear, and independent of the charac-teristics of the LED.The 4-20 mA transmitter circuit in Figure15b is a little different from the previous circuits, partic-ularly the output circuit. The output circuit does not directly generate an output voltage which is sensed by R2, it instead usesQ1 to generate an output current which flows through R3. This output current generates a voltage across R3, which is then sensed by R2. An analysis similar to the one above yields the following expression relating output current to input voltage:I LOOP/V IN = K*(R2+R3)/(R1*R3).The preceding circuits were pre-sented to illustrate the flexibility in designing analog isolation circuits using the HCNR200/201. The next section presents several complete schematics to illustrate practical applications of the HCNR200/201.Example Application CircuitsThe circuit shown in Figure 16 is a high-speed low-cost circuit designed for use in the feedback path of switch-mode power supplies. This application requiresgood bandwidth, low cost andstable gain, but does not requirevery high accuracy. This circuit isa good example of how a designercan trade off accuracy to achieveimprovements in bandwidth andcost. The circuit has a bandwidthof about 1.5 MHz with stable gaincharacteristics and requires fewexternal components.Although it may not appear so atfirst glance, the circuit in Figure16 is essentially the same as thecircuit in Figure 12a. Amplifier A1is comprised of Q1, Q2, R3 andR4, while amplifier A2 iscomprised of Q3, Q4, R5, R6 andR7. The circuit operates in thesame manner as well; the onlydifference is the performance ofamplifiers A1 and A2. The lowergains, higher input currents andhigher offset voltages affect theaccuracy of the circuit, but notthe way it operates. Because thebasic circuit operation has notchanged, the circuit still has goodgain stability. The use of discretetransistors instead of op-ampsallowed the design to trade offaccuracy to achieve goodbandwidth and gain stability atlow cost.To get into a little more detailabout the circuit, R1 is selected toachieve an LED current of about7-10 mA at the nominal inputoperating voltage according to thefollowing equation:I F = (V IN/R1)/K1,where K1 (i.e., I PD1/I F) of theoptocoupler is typically about0.5%. R2 is then selected toachieve the desired output voltageaccording to the equation,V OUT/V IN = R2/R1.The purpose of R4 and R6 is toimprove the dynamic response(i.e., stability) of the input andoutput circuits by lowering thelocal loop gains. R3 and R5 areselected to provide enoughcurrent to drive the bases of Q2and Q4. And R7 is selected so thatQ4 operates at about the samecollector current as Q2.The next circuit, shown inFigure17, is designed to achievethe highest possible accuracy at areasonable cost. The highaccuracy and wide dynamic rangeof the circuit is achieved by usinglow-cost precision op-amps withvery low input bias currents andoffset voltages and is limited bythe performance of the opto-coupler. The circuit is designed tooperate with input and outputvoltages from 1 mV to 10 V.The circuit operates in the sameway as the others. The only majordifferences are the two compensa-tion capacitors and additionalLED drive circuitry. In the high-speed circuit discussed above, theinput and output circuits arestabilized by reducing the localloop gains of the input and outputcircuits. Because reducing theloop gains would decrease theaccuracy of the circuit, twocompensation capacitors, C1 andC2, are instead used to improvecircuit stability. These capacitorsalso limit the bandwidth of thecircuit to about 10 kHz and canbe used to reduce the outputnoise of the circuit by reducing itsbandwidth even further.The additional LED drive circuitry(Q1 and R3 through R6) helps tomaintain the accuracy and band-width of the circuit over the entirerange of input voltages. Withoutthese components, the transcon-ductance of the LED driver would1-432decrease at low input voltages and LED currents. This would reduce the loop gain of the input circuit, reducing circuit accuracy and bandwidth. D1 prevents excessive reverse voltage from being applied to the LED when the LED turns off completely.No offset adjustment of the circuit is necessary; the gain can be adjusted to unity by simply adjusting the 50kohm poten-tiometer that is part of R2. Any OP-97 type of op-amp can be used in the circuit, such as theLT1097 from Linear Technology or the AD705 from Analog Devices, both of which offer pA bias currents, µV offset voltages and are low cost. The input terminals of the op-amps and the photodiodes are connected in the circuit using Kelvin connections to help ensure the accuracy of the circuit.The next two circuits illustrate how the HCNR200/201 can be used with bipolar input signals. The isolation amplifier inFigure18 is a practical implemen-tation of the circuit shown in Figure14b. It uses two opto-couplers, OC1 and OC2; OC1 handles the positive portions of the input signal and OC2 handles the negative portions.Diodes D1 and D2 help reduce crossover distortion by keeping both amplifiers active during both positive and negative portions of the input signal. For example, when the input signal positive, optocoupler OC1 is active while OC2 is turned off. However, the amplifier controlling OC2 is kept active by D2, allowing it to turn on OC2 more rapidly when the input signal goes negative, thereby reducing crossover distortion.Balance control R1 adjusts therelative gain for the positive andnegative portions of the inputsignal, gain control R7 adjusts theoverall gain of the isolationamplifier, and capacitors C1-C3provide compensation to stabilizethe amplifiers.The final circuit shown inFigure19 isolates a bipolaranalog signal using only oneoptocoupler and generates twooutput signals: an analog signalproportional to the magnitude ofthe input signal and a digitalsignal corresponding to the signof the input signal. This circuit isespecially useful for applicationswhere the output of the circuit isgoing to be applied to an analog-to-digital converter. The primaryadvantages of this circuit are verygood linearity and offset, withonly a single gain adjustment andno offset or balance adjustments.To achieve very high linearity forbipolar signals, the gain should beexactly the same for both positiveand negative input polarities. Thiscircuit achieves excellent linearityby using a single optocoupler anda single input resistor, whichguarantees identical gain for bothpositive and negative polarities ofthe input signal. This precisematching of gain for both polari-ties is much more difficult toobtain when separate componentsare used for the different inputpolarities, such as is the previouscircuit.The circuit in Figure19 is actuallyvery similar to the previouscircuit. As mentioned above, onlyone optocoupler is used. Becausea photodiode can conduct currentin only one direction, two diodes(D1 and D2) are used to steer theinput current to the appropriateterminal of input photodiode PD1to allow bipolar input currents.Normally the forward voltagedrops of the diodes would cause aserious linearity or accuracyproblem. However, an additionalamplifier is used to provide anappropriate offset voltage to theother amplifiers that exactlycancels the diode voltage drops tomaintain circuit accuracy.Diodes D3 and D4 perform twodifferent functions; the diodeskeep their respective amplifiersactive independent of the inputsignal polarity (as in the previouscircuit), and they also provide thefeedback signal to PD1 thatcancels the voltage drops ofdiodes D1 and D2.Either a comparator or an extraop-amp can be used to sense thepolarity of the input signal anddrive an inexpensive digitaloptocoupler, like a 6N139.It is also possible to convert thiscircuit into a fully bipolar circuit(with a bipolar output signal) byusing the output of the 6N139 todrive some CMOS switches toswitch the polarity of PD2depending on the polarity of theinput signal, obtaining a bipolaroutput voltage swing.HCNR200/201 SPICEModelFigure 20 is the net list of aSPICE macro-model for theHCNR200/201 high-linearityoptocoupler. The macro-modelaccurately reflects the primarycharacteristics of the HCNR200/201 and should facilitate thedesign and understanding ofcircuits using the HCNR200/201optocoupler.1-433。
HCNR200201
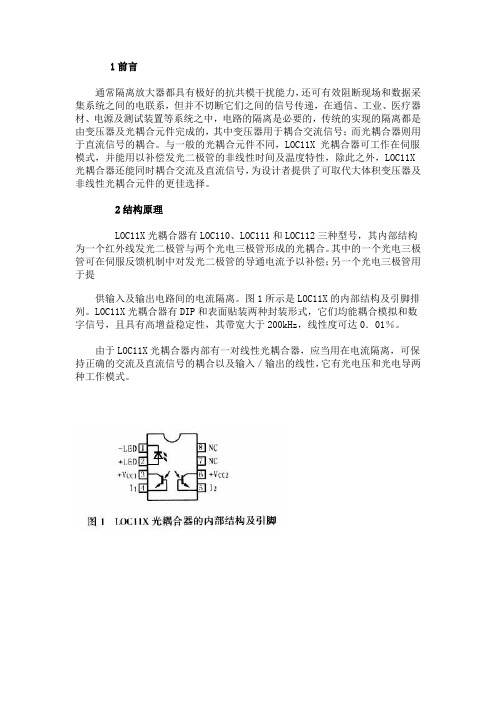
1前言通常隔离放大器都具有极好的抗共模干扰能力,还可有效阻断现场和数据采集系统之间的电联系,但并不切断它们之间的信号传递,在通信、工业、医疗器材、电源及测试装置等系统之中,电路的隔离是必要的,传统的实现的隔离都是由变压器及光耦合元件完成的,其中变压器用于耦合交流信号;而光耦合器则用于直流信号的耦合。
与一般的光耦合元件不同,LOC11X 光耦合器可工作在伺服模式,并能用以补偿发光二极管的非线性时间及温度特性,除此之外,LOC11X 光耦合器还能同时耦合交流及直流信号,为设计者提供了可取代大体积变压器及非线性光耦合元件的更佳选择。
2结构原理LOC11X光耦合器有LOC110、LOC111和LOC112三种型号,其内部结构为一个红外线发光二极管与两个光电三极管形成的光耦合。
其中的一个光电三极管可在伺服反馈机制中对发光二极管的导通电流予以补偿;另一个光电三极管用于提供输入及输出电路间的电流隔离。
图1所示是LOC11X的内部结构及引脚排列。
LOC11X光耦合器有DIP和表面贴装两种封装形式,它们均能耦合模拟和数字信号,且具有高增益稳定性,其带宽大于200kHz,线性度可达0.01%。
由于LOC11X光耦合器内部有一对线性光耦合器,应当用在电流隔离,可保持正确的交流及直流信号的耦合以及输入/输出的线性,它有光电压和光电导两种工作模式。
2.1光电导模式图2是LOC11X光耦合器在光电导模式下工作的典型电路,该电路被配置成光电三极管的集电极与基极反向偏压,这是LOC11X光耦合器在光电导模式下运作的典型接法。
当输入电压VIN在0V且IF为0mA时,U1有一个大的开环增益值,而随着VIN值的升高,U1的输出值开始进入VCC1的轨迹上,随着U1输出的增大,IF 开始有电流值,发光二极管也进入工作状态。
接着,光电三极管受到发光二极管所发出光的照射而导通并产生电流I1,当I1流经R1时,将在U1的反相端产生电压VA,从而使得放大器进入负反馈工作状态。
常用光耦简介及常见型号
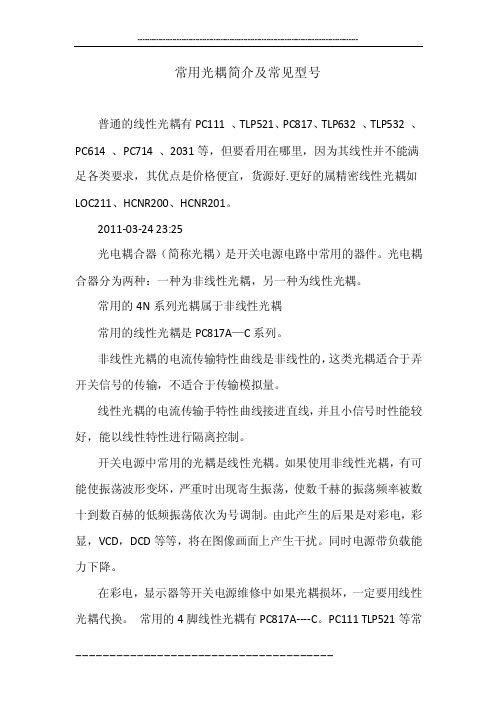
常用光耦简介及常见型号普通的线性光耦有PC111 、TLP521、PC817、TLP632 、TLP532 、PC614 、PC714 、2031等,但要看用在哪里,因为其线性并不能满足各类要求,其优点是价格便宜,货源好.更好的属精密线性光耦如LOC211、HCNR200、HCNR201。
2011-03-24 23:25光电耦合器(简称光耦)是开关电源电路中常用的器件。
光电耦合器分为两种:一种为非线性光耦,另一种为线性光耦。
常用的4N系列光耦属于非线性光耦常用的线性光耦是PC817A—C系列。
非线性光耦的电流传输特性曲线是非线性的,这类光耦适合于弄开关信号的传输,不适合于传输模拟量。
线性光耦的电流传输手特性曲线接进直线,并且小信号时性能较好,能以线性特性进行隔离控制。
开关电源中常用的光耦是线性光耦。
如果使用非线性光耦,有可能使振荡波形变坏,严重时出现寄生振荡,使数千赫的振荡频率被数十到数百赫的低频振荡依次为号调制。
由此产生的后果是对彩电,彩显,VCD,DCD等等,将在图像画面上产生干扰。
同时电源带负载能力下降。
在彩电,显示器等开关电源维修中如果光耦损坏,一定要用线性光耦代换。
常用的4脚线性光耦有PC817A----C。
PC111 TLP521等常用的六脚线性光耦有:TLP632 TLP532 PC614 PC714 PS2031等。
常用的4N25 4N26 4N35 4N36是不适合用于开关电源中的,因为这4种光耦均属于非线性光耦。
经查大量资料后,以下是目前市场上常见的高速光藕型号:100K bit/S:6N138、6N139、PS87031M bit/S:6N135、6N136、CNW135、CNW136、PS8601、PS8602、PS8701、PS9613、PS9713、CNW4502、HCPL-2503、HCPL-4502、HCPL-2530(双路)、HCPL-2531(双路)10M bit/S:6N137、PS9614、PS9714、PS9611、PS9715、HCPL-2601、HCPL-2611、HCPL-2630(双路)、HCPL-2631(双路)光耦合器的增益被称为晶体管输出器件的电流传输比(CTR),其定义是光电晶体管集电极电流与LED正向电流的比率(ICE/IF)。
针对HCNR200线性光耦隔离电路的带宽的脉冲测试法[发明专利]
![针对HCNR200线性光耦隔离电路的带宽的脉冲测试法[发明专利]](https://img.taocdn.com/s3/m/14fe69f0d4bbfd0a79563c1ec5da50e2524dd13d.png)
(19)中华人民共和国国家知识产权局(12)发明专利申请(10)申请公布号 (43)申请公布日 (21)申请号 201811273474.8(22)申请日 2018.10.30(71)申请人 北京航空航天大学地址 100191 北京市海淀区学院路37号(72)发明人 高成 寇震梦 黄姣英 王乐群 (74)专利代理机构 北京慧泉知识产权代理有限公司 11232代理人 王顺荣 唐爱华(51)Int.Cl.G01R 31/28(2006.01)G01R 31/00(2006.01)(54)发明名称针对HCNR200线性光耦隔离电路的带宽的脉冲测试法(57)摘要本发明提供一种针对HCNR200线性光耦隔离电路的带宽的脉冲测试法,其步骤如下:一:选择HCNR200线性光耦隔离电路并进行配置;二:搭建测试隔离电路带宽所用的测试系统;三:对HCNR200线性光耦隔离电路开展带宽测试;四:对HCNR200线性光耦隔离电路带宽测试数据进行处理和分析;通过以上步骤,针对HCNR200线性光耦隔离电路的带宽进行测试,利用被测隔离电路对脉冲信号的响应上升沿时间与被测电路的带宽之间的关系,发挥脉冲信号频率特性的优势,达到测试HCNR200线性光耦隔离电路的带宽的目的。
反映HCNR200线性光耦器件在实际应用中隔离传输高频信号的能力。
权利要求书2页 说明书4页 附图3页CN 109471013 A 2019.03.15C N 109471013A1.一种针对HCNR200线性光耦隔离电路的带宽的脉冲测试方法,其特征在于:其步骤如下:步骤一:选择HCNR200线性光耦隔离电路并进行配置;先查找HCNR200线性光耦数据手册中的隔离电路,选择高速低成本模拟型隔离电路;然后对选择的隔离电路进行配置;绘制电路结构图并制作高速低成本模拟型隔离电路的印制电路板即PCB电路板;将电阻、三极管和HCNR200线性光耦焊接到PCB电路板上;该HCNR200线性光耦为双列直插式封装即DIP8,管脚1连接三极管Q2N3904的集电极;管脚2连接5V DC电压源;管脚3连接R1电阻;管脚4、5连接地线;管脚6连接三极管Q2N3906的基极;管脚7、8空置;步骤二:搭建测试隔离电路带宽所用的测试系统;该测试系统由脉冲信号源、HCNR200线性光耦隔离电路、示波器、5V电压源和计算机组成;其中待测隔离电路的输入端与脉冲信号源的信号输出端连接,脉冲信号源的输出端连接示波器的一通道探头,待测隔离电路的输出端连接示波器的二通道探头,通用接口总线即GPIB连接脉冲源与计算机,GPIB线连接示波器与计算机;使用5V电压源对隔离电路供电使其能够正常工作;步骤三:对HCNR200线性光耦隔离电路开展带宽测试;首先调节脉冲信号源参数,然后将脉冲源调整至信号输出状态,使脉冲信号通过待测隔离电路,使用示波器采集到隔离电路的输入信号和隔离电路的响应信号波形;步骤四:对HCNR200线性光耦隔离电路带宽测试数据进行处理和分析;针对步骤三中示波器采集到的隔离电路的响应曲线,选取响应信号幅值上升至10%时所对应的时间记为t1,幅值上升至90%时所对应的时间记为t2;被测HCNR200线性光耦隔离电路对脉冲信号的响应上升沿时间为τr=t2-t1式中:τr为被测HCNR200线性光耦隔离电路对脉冲信号的响应上升沿时间;当脉冲信号通过HCNR200线性光耦隔离电路时,由于高频分量的丢失产生波形变化并体现在响应曲线上升沿时间上,经过时域和频域的转换分析,响应上升沿时间与电路的带宽具有如下的关系:式中:B是HCNR200线性光耦器件的隔离电路带宽;由此得HCNR200线性光耦隔离电路的带宽;通过以上步骤,利用HCNR200线性光耦隔离电路对脉冲信号的响应信号上升沿时间与电路的带宽之间的关系,发挥脉冲信号频率特性的优势,达到了测试HCNR200线性光耦隔离电路的带宽的目的;对于测试HCNR200线性光耦在实际应用时能够隔离传输的信号的频率范围具有重要的参考意义;本发明相对于扫频测试而言,不需要外加高频信号源来产生高频正弦波信号,而是采用脉冲信号源进行测试,能节约测试成本;取代多次扫频得出HCNR200线性光耦隔离电路的带宽的复杂步骤,经过一次脉冲信号的输入和一次响应信号的分析就能得到HCNR200线性光耦隔离电路的带宽,更加简单便捷。
HCNR201中文资料
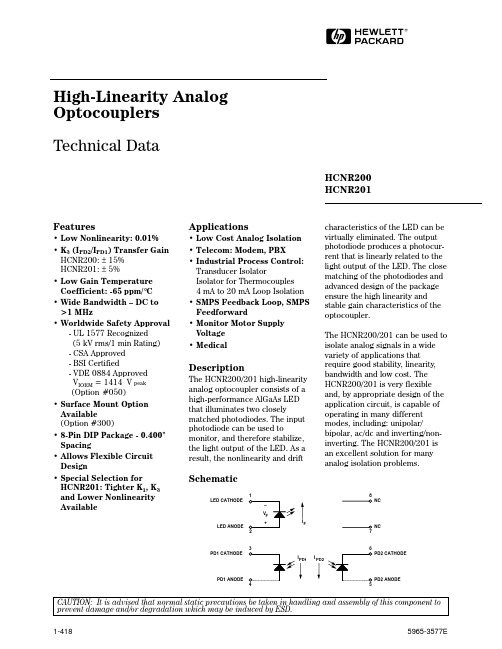
1-418HHigh-Linearity Analog Optocouplers Technical DataHCNR200HCNR201CAUTION: It is advised that normal static precautions be taken in handling and assembly of this component to prevent damage and /or degradation which may be induced by ESD.I FIPD1I PD2NCNC PD2 CATHODEPD2 ANODELED CATHODELED ANODEPD1 CATHODEPD1 ANODEFeatures• Low Nonlinearity: 0.01%• K 3 (I PD2/I PD1) Transfer Gain HCNR200: ±15%HCNR201: ±5%• Low Gain Temperature Coefficient: -65ppm/°C • Wide Bandwidth – DC to >1 MHz• Worldwide Safety Approval-UL 1577 Recognized (5 kV rms/1 min Rating)-CSA Approved -BSI Certified-VDE 0884 Approved V IORM = 1414 V peak (Option #050)• Surface Mount Option Available(Option #300)• 8-Pin DIP Package - 0.400"Spacing• Allows Flexible Circuit Design• Special Selection forHCNR201: Tighter K 1, K 3and Lower Nonlinearity AvailableApplications• Low Cost Analog Isolation • Telecom: Modem, PBX• Industrial Process Control:Transducer IsolatorIsolator for Thermocouples 4mA to 20 mA Loop Isolation • SMPS Feedback Loop, SMPS Feedforward• Monitor Motor Supply Voltage • MedicalDescriptionThe HCNR200/201 high-linearity analog optocoupler consists of a high-performance AlGaAs LED that illuminates two closelymatched photodiodes. The input photodiode can be used tomonitor, and therefore stabilize,the light output of the LED. As a result, the nonlinearity and driftcharacteristics of the LED can be virtually eliminated. The output photodiode produces a photocur-rent that is linearly related to the light output of the LED. The close matching of the photodiodes and advanced design of the package ensure the high linearity and stable gain characteristics of the optocoupler.The HCNR200/201 can be used to isolate analog signals in a wide variety of applications that require good stability, linearity,bandwidth and low cost. The HCNR200/201 is very flexible and, by appropriate design of the application circuit, is capable of operating in many different modes, including: unipolar/bipolar, ac/dc and inverting/non-inverting. The HCNR200/201 is an excellent solution for many analog isolation problems.Schematic5965-3577E1-419Ordering Information:HCNR20x0 = ±15% Transfer Gain, 0.25% Maximum Nonlinearity 1 = ±5% Transfer Gain, 0.05% Maximum NonlinearityOption yyy050 = VDE 0884 V IORM = 1414 V peak Option 300 = Gull Wing Surface Mount Lead Option 500 = Tape/Reel Package Option (1 k min.)Option data sheets available. Contact your Hewlett-Packard sales representative or authorized distributor for information.Package Outline DrawingsFigure 1.MAX."V" = OPTION 050OPTION NUMBERS 300 AND 500 NOT MARKED.1-420Gull Wing Surface Mount Option #300240TIME – MINUTEST E M P E R A T U R E – °C220200180160140120100806040200260(NOTE: USE OF NON-CHLORINE ACTIVATED FLUXES IS RECOMMENDED.)Maximum Solder Reflow Thermal ProfileRegulatory InformationThe HCNR200/201 optocoupler features a 0.400" wide, eight pin DIP package. This package was specifically designed to meet worldwide regulatory require-ments. The HCNR200/201 has been approved by the following organizations:ULRecognized under UL 1577, Component Recognition Program,FILE E55361CSAApproved under CSA Component Acceptance Notice #5, File CA 88324BSICertification according to BS415:1994;(BS EN60065:1994);BS EN60950:1992(BS7002:1992) andEN41003:1993 for Class II applicationsVDEApproved according to VDE 0884/06.92(Available Option #050only)1.78 ± 0.15 MAX.BSCDIMENSIONS IN MILLIMETERS (INCHES).LEAD COPLANARITY = 0.10 mm (0.004 INCHES).Insulation and Safety Related SpecificationsParameter Symbol Value Units ConditionsMin. External Clearance L(IO1)9.6mm Measured from input terminals to output (External Air Gap)terminals, shortest distance through air Min. External Creepage L(IO2)10.0mm Measured from input terminals to output (External Tracking Path)terminals, shortest distance path along body Min. Internal Clearance 1.0mm Through insulation distance conductor to (Internal Plastic Gap)conductor, usually the direct distancebetween the photoemitter and photodetectorinside the optocoupler cavityMin. Internal Creepage 4.0mm The shortest distance around the border (Internal Tracking Path)between two different insulating materialsmeasured between the emitter and detector Comparative Tracking Index CTI200V DIN IEC 112/VDE 0303 PART 1Isolation Group IIIa Material group (DIN VDE 0110)Option 300 – surface mount classification is Class A in accordance with CECC 00802.VDE 0884 (06.92) Insulation Characteristics (Option #050 Only)Description Symbol Characteristic Unit Installation classification per DIN VDE 0110/1.89, Table 1For rated mains voltage ≤600 V rms I-IVFor rated mains voltage ≤1000 V rms I-IIIClimatic Classification (DIN IEC 68 part 1)55/100/21Pollution Degree (DIN VDE 0110 Part 1/1.89)2Maximum Working Insulation Voltage V IORM1414V peak Input to Output Test Voltage, Method b*V PR2651V peak V PR = 1.875 x V IORM, 100% Production Test witht m = 1 sec, Partial Discharge < 5 pCInput to Output Test Voltage, Method a*V PR2121V peak V PR = 1.5 x V IORM, Type and sample test, t m = 60 sec,Partial Discharge < 5 pCHighest Allowable Overvoltage*V IOTM8000V peak (Transient Overvoltage, t ini = 10 sec)Safety-Limiting Values(Maximum values allowed in the event of a failure,also see Figure 11)Case Temperature T S150°C Current (Input Current I F, P S = 0)I S400mA Output Power P S,OUTPUT700mW Insulation Resistance at T S, V IO = 500 V R S>109Ω*Refer to the front of the Optocoupler section of the current catalog for a more detailed description of VDE 0884 and other product safety regulations.Note: Optocouplers providing safe electrical separation per VDE 0884 do so only within the safety-limiting values to which they are qualified. Protective cut-out switches must be used to ensure that the safety limits are not exceeded.1-421Absolute Maximum RatingsStorage Temperature..................................................-55°C to +125°C Operating Temperature (T A)........................................-55°C to +100°C Junction Temperature (T J)............................................................125°C Reflow Temperature Profile...See Package Outline Drawings Section Lead Solder Temperature..................................................260°C for 10s (up to seating plane)Average Input Current - I F............................................................25 mA Peak Input Current - I F.................................................................40 mA (50 ns maximum pulse width)Reverse Input Voltage - V R..............................................................2.5 V (I R = 100 µA, Pin 1-2)Input Power Dissipation.........................................60 mW @ T A = 85°C (Derate at 2.2 mW/°C for operating temperatures above 85°C) Reverse Output Photodiode Voltage................................................30 V (Pin 6-5)Reverse Input Photodiode Voltage...................................................30 V (Pin 3-4)Recommended Operating ConditionsStorage Temperature....................................................-40°C to +85°C Operating Temperature.................................................-40°C to +85°C Average Input Current - I F.......................................................1 - 20 mA Peak Input Current - I F.................................................................35 mA (50% duty cycle, 1 ms pulse width)Reverse Output Photodiode Voltage...........................................0 - 15 V (Pin 6-5)Reverse Input Photodiode Voltage..............................................0 - 15 V (Pin 3-4)1-422Electrical Specifications1-423AC Electrical SpecificationsT A = 25°C unless otherwise specified.TestParameter Symbol Device Min.Typ.Max.Units Conditions Fig.Note LED Bandwidth f -3dB9MHz I F = 10 mAApplication Circuit Bandwidth:High Speed 1.5MHz167 High Precision10kHz177 Application Circuit: IMRRHigh Speed95dB freq = 60 Hz167, 8 Package CharacteristicsT= 25°C unless otherwise specified.Notes:1. K3 is calculated from the slope of thebest fit line of I PD2 vs. I PD1 with elevenequally distributed data points from5nA to 50 µA. This is approximatelyequal to I PD2/I PD1 at I F = 10 mA.2. Special selection for tighter K1, K3 andlower Nonlinearity available.3. BEST FIT DC NONLINEARITY (NL BF) isthe maximum deviation expressed as a percentage of the full scale output of a “best fit” straight line from a graph ofI PD2 vs. I PD1 with eleven equally distrib-uted data points from 5 nA to 50µA.I PD2 error to best fit line is the deviationbelow and above the best fit line,expressed as a percentage of the fullscale output.4. ENDS FIT DC NONLINEARITY (NL EF)is the maximum deviation expressed asa percentage of full scale output of astraight line from the 5 nA to the 50 µAdata point on the graph of I PD2 vs. I PD1.5. Device considered a two-terminaldevice: Pins 1, 2, 3, and 4 shortedtogether and pins 5, 6, 7, and 8 shortedtogether.6. In accordance with UL 1577, eachoptocoupler is proof tested by applyingan insulation test voltage of ≥6000 Vrms for ≥1 second (leakage detectioncurrent limit, I I-O of 5 µA max.). Thistest is performed before the 100%production test for partial discharge(method b) shown in the VDE 0884Insulation Characteristics Table (forOption #050 only).7. Specific performance will depend oncircuit topology and components.8. IMRR is defined as the ratio of thesignal gain (with signal applied to V IN ofFigure 16) to the isolation mode gain(with V IN connected to input commonand the signal applied between theinput and output commons) at 60 Hz,expressed in dB.*The Input-Output Momentary Withstand Voltage is a dielectric voltage rating that should not be interpreted as an input-output continuous voltage rating. For the continuous voltage rating refer to the VDE 0884 Insulation Characteristics Table (if applicable), your equipment level safety specification, or HP Application Note 1074, “Optocoupler Input-Output Endurance Voltage.”1-4241-425Figure 5. NL BF vs. Temperature.Figure 2. Normalized K3 vs. Input I PD .Figure 3. K3 Drift vs. Temperature.Figure 4. I PD2 Error vs. Input I PD (See Note 4).Figure 6. NL BF Drift vs. Temperature.Figure 7. Input Photodiode CTR vs.LED Input Current.Figure 8. Typical Photodiode Leakage vs. Temperature.Figure 9. LED Input Current vs.Forward Voltage.Figure 10. LED Forward Voltage vs.Temperature.I L K – P H O T O D I O D E L E A K A G E – n AT A – TEMPERATURE – °C D E L T A K 3 – D R I F T O F K 3 T R A N S F E R G A I NT A – TEMPERATURE – °C D E L T A N L B F – D R I F T O F B E S T -F I T N L – % P T ST A – TEMPERATURE – °C N O R M A L I Z E D K 1 – I N P U T P H O T O D I O D E C T RI F – LED INPUT CURRENT – mAV F – L E D F O R W A R D V O L T A G E – VT A – TEMPERATURE – °C-25-555356595125N O R M A L I Z E D K 3 – T R A N S F E R G AI NI PD1 – INPUT PHOTODIODE CURRENT – µAI PD1 – INPUT PHOTODIODE CURRENT – µAI P D 2 E R R O R F R O M B E S T -F I T L I N E (% O F F S )N L B F – B E S T -F I T N O N -L I N E A R I T Y – %T A – TEMPERATURE – °C 1.201000.10.0001V F – FORWARD VOLTAGE – VOLTS 1.30 1.501010.010.001 1.40 1.60I F – F O R W A R D C U R R E N T – m AT A = 25°C1-426Figure 12. Basic Isolation Amplifier.FV I OUTV PD2OUTA) BASIC TOPOLOGYB) PRACTICAL CIRCUITFigure 11. Thermal Derating Curve Dependence of Safety Limiting Valuewith Case Temperature per VDE 0884.VOUTV OUTA) POSITIVE INPUTB) POSITIVE OUTPUTD) NEGATIVE OUTPUTFigure 13. Unipolar Circuit Topologies.T S – CASE TEMPERATURE – °C1-427Figure 15. Loop-Powered 4-20 mA Current Loop Circuits.Figure 14. Bipolar Circuit Topologies.OUTV OUTA) SINGLE OPTOCOUPLERB) DUAL OPTOCOUPLERVOUT+I OUTA) RECEIVERB) TRANSMITTERV -I OUT1-428Figure 18. Bipolar Isolation Amplifier.Figure 16. High-Speed Low-Cost Analog Isolator.VOUTINPUT BNCV +15 V OUTPUT BNCFigure 17. Precision Analog Isolation Amplifier.MAGV IN1-429MAGV INSIGNFigure 20. SPICE Model Listing.Figure 19. Magnitude/Sign Isolation Amplifier..SUBCKT HCNR200Theory of Operation Figure 1 illustrates how the HCNR200/201 high-linearity optocoupler is configured. The basic optocoupler consists of an LED and two photodiodes. The LED and one of the photodiodes (PD1) is on the input leadframe and the other photodiode (PD2) is on the output leadframe. The package of the optocoupler is constructed so that each photo-diode receives approximately the same amount of light from the LED.An external feedback amplifier can be used with PD1 to monitor the light output of the LED and automatically adjust the LED current to compensate for any non-linearities or changes in light output of the LED. The feedback amplifier acts to stabilize and linearize the light output of the LED. The output photodiode then converts the stable, linear light output of the LED into a current, which can then be converted back into a voltage by another amplifier.Figure 12a illustrates the basic circuit topology for implementing a simple isolation amplifier using the HCNR200/201 optocoupler. Besides the optocoupler, two external op-amps and two resistors are required. This simple circuit is actually a bit too simple to function properly in an actual circuit, but it is quite useful for explaining how the basic isolation amplifier circuit works (a few more components and a circuit change are required to make a practical circuit, like the one shown in Figure 12b).The operation of the basic circuit may not be immediately obvious just from inspecting Figure 12a,particularly the input part of thecircuit. Stated briefly, amplifierA1 adjusts the LED current (I F),and therefore the current in PD1(I PD1), to maintain its “+” inputterminal at 0 V. For example,increasing the input voltage wouldtend to increase the voltage of the“+” input terminal of A1 above 0V. A1 amplifies that increase,causing I F to increase, as well asI PD1. Because of the way that PD1is connected, I PD1 will pull the “+”terminal of the op-amp backtoward ground. A1 will continueto increase I F until its “+”terminal is back at 0 V. Assumingthat A1 is a perfect op-amp, nocurrent flows into the inputs ofA1; therefore, all of the currentflowing through R1 will flowthrough PD1. Since the “+” inputof A1 is at 0 V, the currentthrough R1, and therefore I PD1 aswell, is equal to V IN/R1.Essentially, amplifier A1 adjusts I Fso thatI PD1 = V IN/R1.Notice that I PD1 depends ONLY onthe input voltage and the value ofR1 and is independent of the lightoutput characteristics of the LED.As the light output of the LEDchanges with temperature, ampli-fier A1 adjusts I F to compensateand maintain a constant currentin PD1. Also notice that I PD1 isexactly proportional to V IN, givinga very linear relationship betweenthe input voltage and thephotodiode current.The relationship between the inputoptical power and the outputcurrent of a photodiode is verylinear. Therefore, by stabilizingand linearizing I PD1, the lightoutput of the LED is alsostabilized and linearized. Andsince light from the LED falls onboth of the photodiodes, I PD2 willbe stabilized as well.The physical construction of thepackage determines the relativeamounts of light that fall on thetwo photodiodes and, therefore,the ratio of the photodiodecurrents. This results in verystable operation over time andtemperature. The photodiodecurrent ratio can be expressed asa constant, K, whereK = I PD2/I PD1.Amplifier A2 and resistor R2 forma trans-resistance amplifier thatconverts I PD2 back into a voltage,V OUT, whereV OUT = I PD2*R2.Combining the above threeequations yields an overallexpression relating the outputvoltage to the input voltage,V OUT/V IN = K*(R2/R1).Therefore the relationshipbetween V IN and V OUT is constant,linear, and independent of thelight output characteristics of theLED. The gain of the basic isola-tion amplifier circuit can beadjusted simply by adjusting theratio of R2 to R1. The parameterK (called K3 in the electricalspecifications) can be thought ofas the gain of the optocoupler andis specified in the data sheet.Remember, the circuit inFigure12a is simplified in orderto explain the basic circuit opera-tion. A practical circuit, more likeFigure12b, will require a fewadditional components to stabilizethe input part of the circuit, tolimit the LED current, or to1-4301-431second circuit requires two optocouplers, separate gain adjustments for the positive and negative portions of the signal,and can exhibit crossover distor-tion near zero volts. The correct circuit to choose for an applica-tion would depend on therequirements of that particular application. As with the basic isolation amplifier circuit inFigure 12a, the circuits in Figure 14 are simplified and would require a few additional compo-nents to function properly. Two example circuits that operate with bipolar input signals arediscussed in the next section.As a final example of circuit design flexibility, the simplified schematics in Figure 15 illustrate how to implement 4-20 mA analog current-loop transmitter and receiver circuits using the HCNR200/201 optocoupler. An important feature of these circuits is that the loop side of the circuit is powered entirely by the loop current, eliminating the need for an isolated power supply.The input and output circuits in Figure 15a are the same as the negative input and positive output circuits shown in Figures 13c and 13b, except for the addition of R3and zener diode D1 on the input side of the circuit. D1 regulates the supply voltage for the input amplifier, while R3 forms a current divider with R1 to scale the loop current down from 20mA to an appropriate level for the input circuit (<50 µA).As in the simpler circuits, the input amplifier adjusts the LED current so that both of its input terminals are at the same voltage.The loop current is then dividedoptimize circuit performance.Example application circuits will be discussed later in the data sheet.Circuit Design FlexibilityCircuit design with the HCNR200/201 is very flexible because the LED and both photodiodes are accessible to the designer. This allows the designer to make perf-ormance trade-offs that would otherwise be difficult to make with commercially available isolation amplifiers (e.g., bandwidth vs.accuracy vs. cost). Analog isola-tion circuits can be designed for applications that have either unipolar (e.g., 0-10 V) or bipolar (e.g., ±10 V) signals, with positive or negative input oroutput voltages. Several simplified circuit topologies illustrating the design flexibility of the HCNR200/201 are discussed below.The circuit in Figure 12a is configured to be non-inverting with positive input and output voltages. By simply changing the polarity of one or both of the photodiodes, the LED, or the op-amp inputs, it is possible toimplement other circuit configu-rations as well. Figure 13illustrates how to change the basic circuit to accommodate both positive and negative input and output voltages. The input and output circuits can bematched to achieve any combina-tion of positive and negative voltages, allowing for both inverting and non-inverting circuits.All of the configurations described above are unipolar (single polar-ity); the circuits cannot accommo-date a signal that might swing both positive and negative. It ispossible, however, to use the HCNR200/201 optocoupler to implement a bipolar isolation amplifier. Two topologies that allow for bipolar operation are shown in Figure 14.The circuit in Figure 14a uses two current sources to offset the signal so that it appears to be unipolar to the optocoupler.Current source I OS1 provides enough offset to ensure that I PD1is always positive. The second current source, I OS2, provides an offset of opposite polarity toobtain a net circuit offset of zero.Current sources I OS1 and I OS2 can be implemented simply as resistors connected to suitable voltage sources.The circuit in Figure 14b uses two optocouplers to obtain bipolar operation. The first optocoupler handles the positive voltage excursions, while the second optocoupler handles the negative ones. The output photodiodes are connected in an antiparallel configuration so that they produce output signals of opposite polarity.The first circuit has the obvious advantage of requiring only one optocoupler; however, the offset performance of the circuit isdependent on the matching of I OS1and I OS2 and is also dependent on the gain of the optocoupler.Changes in the gain of the opto-coupler will directly affect the offset of the circuit.The offset performance of the second circuit, on the other hand,is much more stable; it is inde-pendent of optocoupler gain and has no matched current sources to worry about. However, thebetween R1 and R3. I PD1 is equal to the current in R1 and is given by the following equation:I PD1 = I LOOP*R3/(R1+R3).Combining the above equation with the equations used for Figure 12a yields an overall expression relating the output voltage to the loop current,V OUT/I LOOP = K*(R2*R3)/(R1+R3).Again, you can see that the relationship is constant, linear, and independent of the charac-teristics of the LED.The 4-20 mA transmitter circuit in Figure15b is a little different from the previous circuits, partic-ularly the output circuit. The output circuit does not directly generate an output voltage which is sensed by R2, it instead usesQ1 to generate an output current which flows through R3. This output current generates a voltage across R3, which is then sensed by R2. An analysis similar to the one above yields the following expression relating output current to input voltage:I LOOP/V IN = K*(R2+R3)/(R1*R3).The preceding circuits were pre-sented to illustrate the flexibility in designing analog isolation circuits using the HCNR200/201. The next section presents several complete schematics to illustrate practical applications of the HCNR200/201.Example Application CircuitsThe circuit shown in Figure 16 is a high-speed low-cost circuit designed for use in the feedback path of switch-mode power supplies. This application requiresgood bandwidth, low cost andstable gain, but does not requirevery high accuracy. This circuit isa good example of how a designercan trade off accuracy to achieveimprovements in bandwidth andcost. The circuit has a bandwidthof about 1.5 MHz with stable gaincharacteristics and requires fewexternal components.Although it may not appear so atfirst glance, the circuit in Figure16 is essentially the same as thecircuit in Figure 12a. Amplifier A1is comprised of Q1, Q2, R3 andR4, while amplifier A2 iscomprised of Q3, Q4, R5, R6 andR7. The circuit operates in thesame manner as well; the onlydifference is the performance ofamplifiers A1 and A2. The lowergains, higher input currents andhigher offset voltages affect theaccuracy of the circuit, but notthe way it operates. Because thebasic circuit operation has notchanged, the circuit still has goodgain stability. The use of discretetransistors instead of op-ampsallowed the design to trade offaccuracy to achieve goodbandwidth and gain stability atlow cost.To get into a little more detailabout the circuit, R1 is selected toachieve an LED current of about7-10 mA at the nominal inputoperating voltage according to thefollowing equation:I F = (V IN/R1)/K1,where K1 (i.e., I PD1/I F) of theoptocoupler is typically about0.5%. R2 is then selected toachieve the desired output voltageaccording to the equation,V OUT/V IN = R2/R1.The purpose of R4 and R6 is toimprove the dynamic response(i.e., stability) of the input andoutput circuits by lowering thelocal loop gains. R3 and R5 areselected to provide enoughcurrent to drive the bases of Q2and Q4. And R7 is selected so thatQ4 operates at about the samecollector current as Q2.The next circuit, shown inFigure17, is designed to achievethe highest possible accuracy at areasonable cost. The highaccuracy and wide dynamic rangeof the circuit is achieved by usinglow-cost precision op-amps withvery low input bias currents andoffset voltages and is limited bythe performance of the opto-coupler. The circuit is designed tooperate with input and outputvoltages from 1 mV to 10 V.The circuit operates in the sameway as the others. The only majordifferences are the two compensa-tion capacitors and additionalLED drive circuitry. In the high-speed circuit discussed above, theinput and output circuits arestabilized by reducing the localloop gains of the input and outputcircuits. Because reducing theloop gains would decrease theaccuracy of the circuit, twocompensation capacitors, C1 andC2, are instead used to improvecircuit stability. These capacitorsalso limit the bandwidth of thecircuit to about 10 kHz and canbe used to reduce the outputnoise of the circuit by reducing itsbandwidth even further.The additional LED drive circuitry(Q1 and R3 through R6) helps tomaintain the accuracy and band-width of the circuit over the entirerange of input voltages. Withoutthese components, the transcon-ductance of the LED driver would1-432decrease at low input voltages and LED currents. This would reduce the loop gain of the input circuit, reducing circuit accuracy and bandwidth. D1 prevents excessive reverse voltage from being applied to the LED when the LED turns off completely.No offset adjustment of the circuit is necessary; the gain can be adjusted to unity by simply adjusting the 50kohm poten-tiometer that is part of R2. Any OP-97 type of op-amp can be used in the circuit, such as theLT1097 from Linear Technology or the AD705 from Analog Devices, both of which offer pA bias currents, µV offset voltages and are low cost. The input terminals of the op-amps and the photodiodes are connected in the circuit using Kelvin connections to help ensure the accuracy of the circuit.The next two circuits illustrate how the HCNR200/201 can be used with bipolar input signals. The isolation amplifier inFigure18 is a practical implemen-tation of the circuit shown in Figure14b. It uses two opto-couplers, OC1 and OC2; OC1 handles the positive portions of the input signal and OC2 handles the negative portions.Diodes D1 and D2 help reduce crossover distortion by keeping both amplifiers active during both positive and negative portions of the input signal. For example, when the input signal positive, optocoupler OC1 is active while OC2 is turned off. However, the amplifier controlling OC2 is kept active by D2, allowing it to turn on OC2 more rapidly when the input signal goes negative, thereby reducing crossover distortion.Balance control R1 adjusts therelative gain for the positive andnegative portions of the inputsignal, gain control R7 adjusts theoverall gain of the isolationamplifier, and capacitors C1-C3provide compensation to stabilizethe amplifiers.The final circuit shown inFigure19 isolates a bipolaranalog signal using only oneoptocoupler and generates twooutput signals: an analog signalproportional to the magnitude ofthe input signal and a digitalsignal corresponding to the signof the input signal. This circuit isespecially useful for applicationswhere the output of the circuit isgoing to be applied to an analog-to-digital converter. The primaryadvantages of this circuit are verygood linearity and offset, withonly a single gain adjustment andno offset or balance adjustments.To achieve very high linearity forbipolar signals, the gain should beexactly the same for both positiveand negative input polarities. Thiscircuit achieves excellent linearityby using a single optocoupler anda single input resistor, whichguarantees identical gain for bothpositive and negative polarities ofthe input signal. This precisematching of gain for both polari-ties is much more difficult toobtain when separate componentsare used for the different inputpolarities, such as is the previouscircuit.The circuit in Figure19 is actuallyvery similar to the previouscircuit. As mentioned above, onlyone optocoupler is used. Becausea photodiode can conduct currentin only one direction, two diodes(D1 and D2) are used to steer theinput current to the appropriateterminal of input photodiode PD1to allow bipolar input currents.Normally the forward voltagedrops of the diodes would cause aserious linearity or accuracyproblem. However, an additionalamplifier is used to provide anappropriate offset voltage to theother amplifiers that exactlycancels the diode voltage drops tomaintain circuit accuracy.Diodes D3 and D4 perform twodifferent functions; the diodeskeep their respective amplifiersactive independent of the inputsignal polarity (as in the previouscircuit), and they also provide thefeedback signal to PD1 thatcancels the voltage drops ofdiodes D1 and D2.Either a comparator or an extraop-amp can be used to sense thepolarity of the input signal anddrive an inexpensive digitaloptocoupler, like a 6N139.It is also possible to convert thiscircuit into a fully bipolar circuit(with a bipolar output signal) byusing the output of the 6N139 todrive some CMOS switches toswitch the polarity of PD2depending on the polarity of theinput signal, obtaining a bipolaroutput voltage swing.HCNR200/201 SPICEModelFigure 20 is the net list of aSPICE macro-model for theHCNR200/201 high-linearityoptocoupler. The macro-modelaccurately reflects the primarycharacteristics of the HCNR200/201 and should facilitate thedesign and understanding ofcircuits using the HCNR200/201optocoupler.1-433。
使用HCNR线性光耦原理与电路

1. 线形光耦地研究设计光隔离是一种很常用地信号隔离形式.常用光耦器件及其外围电路组成.因为光耦电路简单,在数字隔离电路或数据传输电路中常常用到,如UART协议地20mA电流环.对于模拟信号,光耦因为输入输出地线形较差,并且随温度变化较大,限制了其在模拟信号隔离地应用.对于高频交流模拟信号,变压器隔离是最常见地选择,但对于支流信号却不适用.一些厂家提供隔离放大器作为模拟信号隔离地解决方案,如ADI地AD202,能够提供从直流到几K地频率内提供0.025%地线性度, 但这种隔离器件内部先进行电压-频率转换,对产生地交流信号进行变压器隔离,然后进行频率-电压转换得到隔离效果.集成地隔离放大器内部电路复杂,体积大,成本高,不适合大规模应用.模拟信号隔离地一个比较好地选择是使用线形光耦.线性光耦地隔离原理与普通光耦没有差别,只是将普通光耦地单发单收模式稍加改变,增加一个用于反馈地光接受电路用于反馈.这样,虽然两个光接受电路都是非线性地, 但两个光接受电路地非线性特性都是一样地,这样,就可以通过反馈通路地非线性来抵消直通通路地非线性,从而达到实现线性隔离地目地.市场上地线性光耦有几中可选择地芯片,如Agilent公司地HCNR200/201,TI子公司TOAS地TIL300,CLARE地LOC111等.这里以HCNR200/201为例介绍2. 芯片介绍与原理说明HCNR200/201地内部框图如下所示其中1、2引作为隔离信号地输入,3、4引脚用于反馈,5、6引脚用于输出.1、2引脚之间地电流记作IF,3、4引脚之间和5、6引脚之间地电流分别记作IPD1和IPD2.输入信号经过电压-电流转化,电压地变化体现在电流IF上,IPD1和IPD2基本与IF成线性关系,线性系数分别记为K1和K2,即K1与K2一般很小<HCNR200是0.50%),并且随温度变化较大<HCNR200地变化范围在0.25%到0.75%之间),但芯片地设计使得K1和K2相等.在后面可以看到,在合理地外围电路设计中, 真正影响输出/输入比值地是二者地比值K3,线性光耦正利用这种特性才能达到满意地线性度地.HCNR200和HCNR201地内部结构完全相同,差别在于一些指标上.相对于HCNR200,HCNR201提供更高地线性度.采用HCNR200/201进行隔离地一些指标如下所示:* 线性度:HCNR200:0.25%,HCNR201:0.05%;* 线性系数K3:HCNR200:15%,HCNR201:5%;* 温度系数: -65ppm/oC;* 隔离电压:1414V;* 信号带宽:直流到大于1MHz.从上面可以看出,和普通光耦一样,线性光耦真正隔离地是电流,要想真正隔离电压,需要在输出和输出处增加运算放大器等辅助电路.下面对HCNR200/201地典型电路进行分析,对电路中如何实现反馈以及电流-电压、电压-电流转换进行推导与说明.3. 典型电路分析Agilent公司地HCNR200/201地手册上给出了多种实用电路,其中较为典型地一种如下图所示:设输入端电压为Vin,输出端电压为Vout,光耦保证地两个电流传递系数分别为K1、K2,显然,,和之间地关系取决于和之间地关系.将前级运放地电路提出来看,如下图所示:设运放负端地电压为,运放输出端地电压为,在运放不饱和地情况下二者满足下面地关系:Vo=Voo-GVi (1>其中是在运放输入差模为0时地输出电压,G为运放地增益,一般比较大.忽略运放负端地输入电流,可以认为通过R1地电流为IP1,根据R1地欧姆定律得:通过R3两端地电流为IF,根据欧姆定律得:其中,为光耦2脚地电压,考虑到LED导通时地电压<)基本不变,这里地作为常数对待.根据光耦地特性,即K1=IP1/IF (4>将和地表达式代入上式,可得:上式经变形可得到:将地表达式代入<3)式可得:考虑到G特别大,则可以做以下近似:这样,输出与输入电压地关系如下:可见,在上述电路中,输出和输入成正比,并且比例系数只由K3和R1、R2确定.一般选R1=R2,达到只隔离不放大地目地.4. 辅助电路与参数确定上面地推导都是假定所有电路都是工作在线性范围内地,要想做到这一点需要对运放进行合理选型,并且确定电阻地阻值.4.1 运放选型运放可以是单电源供电或正负电源供电,上面给出地是单电源供电地例子.为了能使输入范围能够从0到VCC,需要运放能够满摆幅工作,另外,运放地工作速度、压摆率不会影响整个电路地性能.TI公司地LMV321 单运放电路能够满足以上要求,可以作为HCNR200/201地外围电路.4.2 阻值确定电阻地选型需要考虑运放地线性范围和线性光耦地最大工作电流IFmax.K1已知地情况下,IFmax又确定了IPD1地最大值IPD1max,这样,因为Vo地范围最小可以为0,这样,因为考虑到IFmax大有利于能量地传输,这样,一般取另外,因为工作在深度负反馈状态地运放满足虚短特性,因此,考虑IPD1地限制,这样,R2地确定可以根据所需要地放大倍数确定,例如如果不需要方法,只需将R2=R1即可.另外因为光耦会产生一些高频地噪声,通常在R2处并联电容,构成低通滤波器,具体电容地值由输入频率以及噪声频率确定.4.3 参数确定实例假设确定Vcc=5V,输入在0-4V之间,输出等于输入,采用LMV321运放芯片以及上面电路,下面给出参数确定地过程.* 确定IFmax:HCNR200/201地手册上推荐器件工作地25mA左右;* 确定R3:R3=5V/25mA=200;* 确定R1:。
使用线性光耦HCNR200制作交流电压测量模块

使用线性光耦HCNR200制作交流电压测量模块测量范围:幅值30V测量精度:0.5%误差电路自行设计,要求写好设计报告,制作出实物模块。
参考原理见/hiwxzh/blog/item/e145eff1965a43bba40f52a7.html1. 线性光耦介绍光隔离是一种很常用的信号隔离形式。
常用光耦器件及其外围电路组成。
由于光耦电路简单,在数字隔离电路或数据传输电路中常常用到,如UART协议的20mA电流环。
对于模拟信号,光耦因为输入输出的线形较差,并且随温度变化较大,限制了其在模拟信号隔离的应用。
对于高频交流模拟信号,变压器隔离是最常见的选择,但对于支流信号却不适用。
一些厂家提供隔离放大器作为模拟信号隔离的解决方案,如ADI的AD202,能够提供从直流到几K的频率内提供0.025%的线性度,但这种隔离器件内部先进行电压-频率转换,对产生的交流信号进行变压器隔离,然后进行频率-电压转换得到隔离效果。
集成的隔离放大器内部电路复杂,体积大,成本高,不适合大规模应用。
模拟信号隔离的一个比较好的选择是使用线形光耦。
线性光耦的隔离原理与普通光耦没有差别,只是将普通光耦的单发单收模式稍加改变,增加一个用于反馈的光接受电路用于反馈。
这样,虽然两个光接受电路都是非线性的,但两个光接受电路的非线性特性都是一样的,这样,就可以通过反馈通路的非线性来抵消直通通路的非线性,从而达到实现线性隔离的目的。
市场上的线性光耦有几中可选择的芯片,如Agilent公司的HCNR200/201,TI 子公司TOAS的TIL300,CLARE的LOC111等。
这里以HCNR200/201为例介绍2. 芯片介绍与原理说明HCNR200/201的内部框图如下所示其中1、2引作为隔离信号的输入,3、4引脚用于反馈,5、6引脚用于输出。
1、2引脚之间的电流记作IF,3、4引脚之间和5、6引脚之间的电流分别记作IPD1和IPD2。
输入信号经过电压-电流转化,电压的变化体现在电流IF上,IPD1和IPD2基本与IF成线性关系,线性系数分别记为K1和K2,即K1与K2一般很小(HCNR200是0.50%),并且随温度变化较大(HCNR200的变化范围在0.25%到0.75%之间),但芯片的设计使得K1和K2相等。
模拟光耦HCNR200、HCNR201应用笔记

HCNR200和HCNR201模拟光电耦合器SPICE电路仿真应用笔记AN5545Jamshed Namdar Khan,安华高科技(Avago Technologies)隔离应用产品事业部光电耦合器应用工程师介绍本应用笔记的目的是展示PSpice软件如何通过使用安华高科技(Avago Technologies)提供的PSpice宏模型精确预测和仿真Avago公司HCNR200和HCNR201模拟光电耦合器的行为,聚焦集成电路仿真程序(SPICE, Sim-ulation Program with Integrated Circuit Emphasis)目前被认为是模拟电路设计工程师不可或缺的工具。
相对模拟光电耦合器数据表参数或规格,良好的宏模型应该精确预测电路性能,PSpice或SPICE仿真是任何设计工程师成功完成设计项目一个必备并且不可或缺的工具,电路仿真有助于原始设计概念的发想,从而允许工程师调整并优化原型电路取得最佳可能电路性能。
电路仿真的最大优势在于建构实体硬件或进行性能测试前可以先行验证并改善设计,极小化花费在原型测试的时间和相关费用成本。
为何需要仿真?不管电路仿真可以如何进行或带来什么,有一点它绝不可能做到的是为你提供实际的电路设计,因此我们首先列举几个吸引设计工程师进行电路仿真的原因。
进行电路仿真的主要动力是极小化预测目标电路设计性能的时间,相较于实际建立和进行原型测试等效电路的评估,使用SPICE电路进行评估所使用的时间相对上非常微小,另外,这些电路仿真也可以在各种不同温度、偏置条件以及零组件数值和误差条件下多次进行,但耗费时间仅为进行电路试验板设计并于工作台上进行评估的数分之一。
在进行光电耦合器SPICE仿真时,首先应该了解的是软件并无法仿真光电耦合器的两个基本特性,设计工程师使用光电耦合器主要有两个理由,分别是绝缘和隔离,SPICE软件并无法对这两个主要关键光电耦合器功能建立模型。
4-20MA

4~20mA电流变送器的工业控制应用4~20mA电流环工作原理在工业现场,用一个仪表放大器来完成信号的调理并进行长线传输,会产生以下问题:第一,由于传输的信号是电压信号,传输信号就会受到噪声的干扰而不纯洁;第二,传输线的电阻会产生电压降,那么接收端的信号就会产生误差;第三,在现场如何提供仪表放大器的不同的工作电压也是个问题。
为了解决上述问题和避开相关噪声的影响,我们用电流来传输信号,因为电流对噪声并不敏感。
4~20mA的电流环便是用4mA表示零信号,用20mA表示信号的满刻度,而低于4mA 高于20mA的信号用于各种故障的报警。
4~20mA电流环有两种类型:二线制和三线制。
当监控系统需要通过长线驱动现场的驱动器件如阀门等时,一般采用三线制变送器,这里XTR位于监控的系统端,由系统直接向XTR供电,供电电源是二根电流传输线以外的第三根线。
二线系统是XTR和传感器位于现场端,由于现场供电问题的存在,一般是接收端利用4~20mA的电流环向远端的XTR供电,通过4~20mA来反映信号的大小。
4~20mA产品的典型应用是传感和测量应用,在工业现场有许多种类的传感器可以被转换成4~20mA的电流信号,TI拥有一些很方便的用于RTD和电桥的变送器芯片。
由于TI的变送器芯片含有通用的功能电路比如电压激励源、电流激励流、稳压电路、仪表放大器等,所以可以很方便地把许多传感器的信号转化为4~20mA的信号。
4~20mA的校正传统的4~20mA校正,要求特殊的夹具固定,需要特别的激光或手动电阻器调整,而调整是相互影响的,需要一个测试、调整,再测试、再调整的过程,调整次数和范围有限。
电子器件和传感器调整起来不够方便。
现代的数字化4~20mA校正,它允许电子器件和传感器在封装之后进行调整;可通过计算机计算出校正系数来简化数值调整;可以有无限的调整次数,并且有很好的分辨率和较宽的调整范围;调整过程中不存在相互影响;电子器件和传感器可以很方便地调整。
4-20ma信号
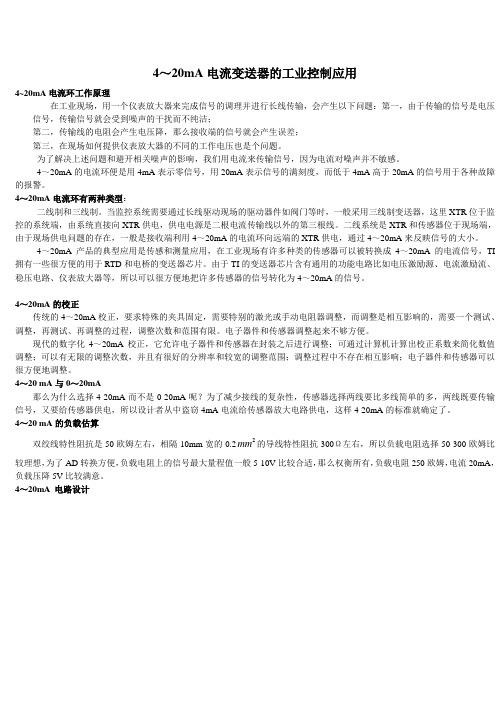
4~20mA电流变送器的工业控制应用4~20mA电流环工作原理在工业现场,用一个仪表放大器来完成信号的调理并进行长线传输,会产生以下问题:第一,由于传输的信号是电压信号,传输信号就会受到噪声的干扰而不纯洁;第二,传输线的电阻会产生电压降,那么接收端的信号就会产生误差;第三,在现场如何提供仪表放大器的不同的工作电压也是个问题。
为了解决上述问题和避开相关噪声的影响,我们用电流来传输信号,因为电流对噪声并不敏感。
4~20mA的电流环便是用4mA表示零信号,用20mA表示信号的满刻度,而低于4mA高于20mA的信号用于各种故障的报警。
4~20mA电流环有两种类型:二线制和三线制。
当监控系统需要通过长线驱动现场的驱动器件如阀门等时,一般采用三线制变送器,这里XTR位于监控的系统端,由系统直接向XTR供电,供电电源是二根电流传输线以外的第三根线。
二线系统是XTR和传感器位于现场端,由于现场供电问题的存在,一般是接收端利用4~20mA的电流环向远端的XTR供电,通过4~20mA来反映信号的大小。
4~20mA产品的典型应用是传感和测量应用,在工业现场有许多种类的传感器可以被转换成4~20mA的电流信号,TI 拥有一些很方便的用于RTD和电桥的变送器芯片。
由于TI的变送器芯片含有通用的功能电路比如电压激励源、电流激励流、稳压电路、仪表放大器等,所以可以很方便地把许多传感器的信号转化为4~20mA的信号。
4~20mA的校正传统的4~20mA校正,要求特殊的夹具固定,需要特别的激光或手动电阻器调整,而调整是相互影响的,需要一个测试、调整,再测试、再调整的过程,调整次数和范围有限。
电子器件和传感器调整起来不够方便。
现代的数字化4~20mA校正,它允许电子器件和传感器在封装之后进行调整;可通过计算机计算出校正系数来简化数值调整;可以有无限的调整次数,并且有很好的分辨率和较宽的调整范围;调整过程中不存在相互影响;电子器件和传感器可以很方便地调整。
HCNR201高线性度模拟光电耦合器评估电路板使用手册

HCNR201/200高线性度模拟光电耦合器 评估电路板使用手册硬件指南介绍HCNR200/201高线性度模拟光电耦合器包含一个用来照射两个紧密匹配光电二极管的高性能铝砷化镓(AlGaAs, Aluminium Gallium Arsenide)发光二极管(LED, Light Emitting Diode),输入光电二极管可以作为监测从而稳定LED 的光输出,因此基本上可以消除LED 的非线性和漂移特性,输出光电二极管则可产生线性相关于LED 光输出的光电流,光电二极管间的紧密匹配和先进的封装设计可以确保光电耦合器的高线性度和稳定增益特性。
HCNR200/201可以使用于需要良好稳定性、线性度、带宽和低成本等各种广泛的模拟信号隔离应用,HCNR200/201具备高灵活度,通过适当的应用电路设计,可在多个不同的模式下工作,包括单极/双极、交流/直流以及反向/非反向等,HCNR200/201是许多模拟隔离问题的良好解决方案。
HCNR201/200评估电路板可以帮助设计工程师快速评估这些高线性度模拟光电耦合器,图1为评估电路板的结构电路,图2则是电路板的照片。
除了HCNR201/200外,这个电路板还分别在输入侧和输出侧加入两个运算放大器,请参考电路操作说明了解电路的详细工作原理。
这个评估电路板可以立即应用于电机控制设计中的电流感应,虽然评估电路板面向特定应用设计,但功能多样的高线性度模拟光电耦合器HCNR200/201适合许多应用,如电压电流感应和模拟信号耦合等。
图1:评估电路板的电路结构。
輸入側輸出側D1VCC2 (+3 to +5V)GND22评估电路板的连接和操作1. 输入侧电源:连接+15V 电源到连接器J1的脚位1,-15V 到J1的脚位4,0V 电源到J1的脚位2或3,电机驱动电路板中±15V 电源非常普遍,也可由实验室工作环境取得这些电源。
2. 输出侧电源:连接VCC2电源到评估电路板输出侧J2的脚位1,GND2到J2的脚位2,VCC2可以接受3V 到5V 的灵活电源电压范围以满足电机控制电路板上3.3V 或5V 的微控制器系统,VCC2同样也可以连接到实验室电源。
4-20ma信号
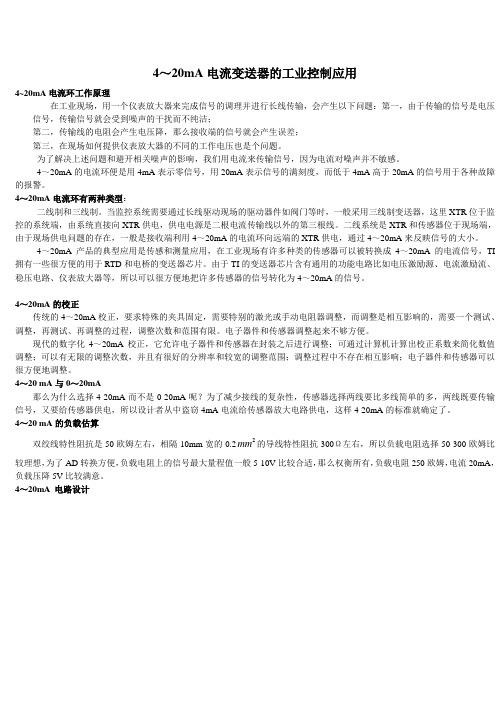
4~20mA电流变送器的工业控制应用4~20mA电流环工作原理在工业现场,用一个仪表放大器来完成信号的调理并进行长线传输,会产生以下问题:第一,由于传输的信号是电压信号,传输信号就会受到噪声的干扰而不纯洁;第二,传输线的电阻会产生电压降,那么接收端的信号就会产生误差;第三,在现场如何提供仪表放大器的不同的工作电压也是个问题。
为了解决上述问题和避开相关噪声的影响,我们用电流来传输信号,因为电流对噪声并不敏感。
4~20mA的电流环便是用4mA表示零信号,用20mA表示信号的满刻度,而低于4mA高于20mA的信号用于各种故障的报警。
4~20mA电流环有两种类型:二线制和三线制。
当监控系统需要通过长线驱动现场的驱动器件如阀门等时,一般采用三线制变送器,这里XTR位于监控的系统端,由系统直接向XTR供电,供电电源是二根电流传输线以外的第三根线。
二线系统是XTR和传感器位于现场端,由于现场供电问题的存在,一般是接收端利用4~20mA的电流环向远端的XTR供电,通过4~20mA来反映信号的大小。
4~20mA产品的典型应用是传感和测量应用,在工业现场有许多种类的传感器可以被转换成4~20mA的电流信号,TI 拥有一些很方便的用于RTD和电桥的变送器芯片。
由于TI的变送器芯片含有通用的功能电路比如电压激励源、电流激励流、稳压电路、仪表放大器等,所以可以很方便地把许多传感器的信号转化为4~20mA的信号。
4~20mA的校正传统的4~20mA校正,要求特殊的夹具固定,需要特别的激光或手动电阻器调整,而调整是相互影响的,需要一个测试、调整,再测试、再调整的过程,调整次数和范围有限。
电子器件和传感器调整起来不够方便。
现代的数字化4~20mA校正,它允许电子器件和传感器在封装之后进行调整;可通过计算机计算出校正系数来简化数值调整;可以有无限的调整次数,并且有很好的分辨率和较宽的调整范围;调整过程中不存在相互影响;电子器件和传感器可以很方便地调整。
线性光偶HCNR200的原理与电路设计
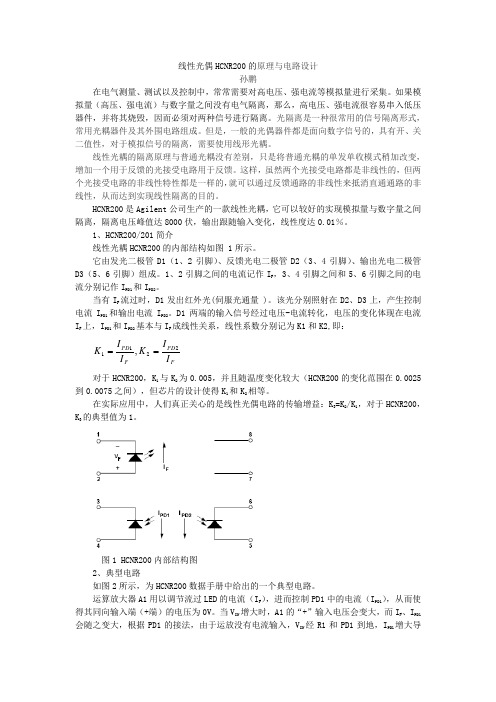
线性光偶HCNR200的原理与电路设计孙鹏在电气测量、测试以及控制中,常常需要对高电压、强电流等模拟量进行采集。
如果模拟量(高压、强电流)与数字量之间没有电气隔离,那么,高电压、强电流很容易串入低压器件,并将其烧毁,因而必须对两种信号进行隔离。
光隔离是一种很常用的信号隔离形式,常用光耦器件及其外围电路组成。
但是,一般的光偶器件都是面向数字信号的,具有开、关二值性,对于模拟信号的隔离,需要使用线形光耦。
线性光耦的隔离原理与普通光耦没有差别,只是将普通光耦的单发单收模式稍加改变,增加一个用于反馈的光接受电路用于反馈。
这样,虽然两个光接受电路都是非线性的,但两个光接受电路的非线性特性都是一样的,就可以通过反馈通路的非线性来抵消直通通路的非线性,从而达到实现线性隔离的目的。
HCNR200是Agilent 公司生产的一款线性光耦,它可以较好的实现模拟量与数字量之间隔离,隔离电压峰值达8000伏,输出跟随输入变化,线性度达0.01%。
1、HCNR200/201简介线性光耦HCNR200的内部结构如图 1所示。
它由发光二极管D1(1、2引脚)、反馈光电二极管D2(3、4引脚)、输出光电二极管D3(5、6引脚)组成。
1、2引脚之间的电流记作I F ,3、4引脚之间和5、6引脚之间的电流分别记作I PD1和I PD2。
当有I F 流过时,D1发出红外光(伺服光通量 )。
该光分别照射在D2、D3上,产生控制电流I PD1和输出电流I PD2。
D1两端的输入信号经过电压-电流转化,电压的变化体现在电流I F 上,I PD1和I PD2基本与I F 成线性关系,线性系数分别记为K1和K2,即:FPD F PD I I K I I K 2211,== 对于HCNR200,K 1与K 2为0.005,并且随温度变化较大(HCNR200的变化范围在0.0025到0.0075之间),但芯片的设计使得K 1和K 2相等。
在实际应用中,人们真正关心的是线性光偶电路的传输增益:K 3=K 2/K 1,对于HCNR200,K 3的典型值为1。
线性光耦hcnr 中文

1. 线形光耦介绍光隔离是一种很常用的信号隔离形式。
常用光耦器件及其外围电路组成。
由于光耦电路简单,在数字隔离电路或数据传输电路中常常用到,如UART协议的20mA 电流环。
对于模拟信号,光耦因为输入输出的线形较差,并且随温度变化较大,限制了其在模拟信号隔离的应用。
对于高频交流模拟信号,变压器隔离是最常见的选择,但对于支流信号却不适用。
一些厂家提供隔离放大器作为模拟信号隔离的解决方案,如ADI的AD202,能够提供从直流到几K的频率内提供0.025%的线性度,但这种隔离器件内部先进行电压-频率转换,对产生的交流信号进行变压器隔离,然后进行频率-电压转换得到隔离效果。
集成的隔离放大器内部电路复杂,体积大,成本高,不适合大规模应用。
模拟信号隔离的一个比较好的选择是使用线形光耦。
线性光耦的隔离原理与普通光耦没有差别,只是将普通光耦的单发单收模式稍加改变,增加一个用于反馈的光接受电路用于反馈。
这样,虽然两个光接受电路都是非线性的,但两个光接受电路的非线性特性都是一样的,这样,就可以通过反馈通路的非线性来抵消直通通路的非线性,从而达到实现线性隔离的目的。
市场上的线性光耦有几中可选择的芯片,如Agilent公司的HCNR200/201,TI子公司TOAS的TIL300,CLARE的LOC111等。
这里以HCNR200/201为例介绍2. 芯片介绍与原理说明HCNR200/201的内部框图如下所示其中1、2引作为隔离信号的输入,3、4引脚用于反馈,5、6引脚用于输出。
1、2引脚之间的电流记作IF,3、4引脚之间和5、6引脚之间的电流分别记作IPD1和IPD2。
输入信号经过电压-电流转化,电压的变化体现在电流IF上,IPD1和IPD2基本与IF成线性关系,线性系数分别记为K1和K2,即K1与K2一般很小(HCNR200是0.50%),并且随温度变化较大(HCNR200的变化范围在0.25%到0.75%之间),但芯片的设计使得K1和K2相等。
HCNR200中文资料

1-418HHigh-Linearity Analog Optocouplers Technical DataHCNR200HCNR201CAUTION: It is advised that normal static precautions be taken in handling and assembly of this component to prevent damage and /or degradation which may be induced by ESD.I FIPD1I PD2NCNC PD2 CATHODEPD2 ANODELED CATHODELED ANODEPD1 CATHODEPD1 ANODEFeatures• Low Nonlinearity: 0.01%• K 3 (I PD2/I PD1) Transfer Gain HCNR200: ±15%HCNR201: ±5%• Low Gain Temperature Coefficient: -65ppm/°C • Wide Bandwidth – DC to >1 MHz• Worldwide Safety Approval-UL 1577 Recognized (5 kV rms/1 min Rating)-CSA Approved -BSI Certified-VDE 0884 Approved V IORM = 1414 V peak (Option #050)• Surface Mount Option Available(Option #300)• 8-Pin DIP Package - 0.400"Spacing• Allows Flexible Circuit Design• Special Selection forHCNR201: Tighter K 1, K 3and Lower Nonlinearity AvailableApplications• Low Cost Analog Isolation • Telecom: Modem, PBX• Industrial Process Control:Transducer IsolatorIsolator for Thermocouples 4mA to 20 mA Loop Isolation • SMPS Feedback Loop, SMPS Feedforward• Monitor Motor Supply Voltage • MedicalDescriptionThe HCNR200/201 high-linearity analog optocoupler consists of a high-performance AlGaAs LED that illuminates two closelymatched photodiodes. The input photodiode can be used tomonitor, and therefore stabilize,the light output of the LED. As a result, the nonlinearity and driftcharacteristics of the LED can be virtually eliminated. The output photodiode produces a photocur-rent that is linearly related to the light output of the LED. The close matching of the photodiodes and advanced design of the package ensure the high linearity and stable gain characteristics of the optocoupler.The HCNR200/201 can be used to isolate analog signals in a wide variety of applications that require good stability, linearity,bandwidth and low cost. The HCNR200/201 is very flexible and, by appropriate design of the application circuit, is capable of operating in many different modes, including: unipolar/bipolar, ac/dc and inverting/non-inverting. The HCNR200/201 is an excellent solution for many analog isolation problems.Schematic5965-3577E1-419Ordering Information:HCNR20x0 = ±15% Transfer Gain, 0.25% Maximum Nonlinearity 1 = ±5% Transfer Gain, 0.05% Maximum NonlinearityOption yyy050 = VDE 0884 V IORM = 1414 V peak Option 300 = Gull Wing Surface Mount Lead Option 500 = Tape/Reel Package Option (1 k min.)Option data sheets available. Contact your Hewlett-Packard sales representative or authorized distributor for information.Package Outline DrawingsFigure 1.MAX."V" = OPTION 050OPTION NUMBERS 300 AND 500 NOT MARKED.1-420Gull Wing Surface Mount Option #300240TIME – MINUTEST E M P E R A T U R E – °C220200180160140120100806040200260(NOTE: USE OF NON-CHLORINE ACTIVATED FLUXES IS RECOMMENDED.)Maximum Solder Reflow Thermal ProfileRegulatory InformationThe HCNR200/201 optocoupler features a 0.400" wide, eight pin DIP package. This package was specifically designed to meet worldwide regulatory require-ments. The HCNR200/201 has been approved by the following organizations:ULRecognized under UL 1577, Component Recognition Program,FILE E55361CSAApproved under CSA Component Acceptance Notice #5, File CA 88324BSICertification according to BS415:1994;(BS EN60065:1994);BS EN60950:1992(BS7002:1992) andEN41003:1993 for Class II applicationsVDEApproved according to VDE 0884/06.92(Available Option #050only)1.78 ± 0.15 MAX.BSCDIMENSIONS IN MILLIMETERS (INCHES).LEAD COPLANARITY = 0.10 mm (0.004 INCHES).Insulation and Safety Related SpecificationsParameter Symbol Value Units ConditionsMin. External Clearance L(IO1)9.6mm Measured from input terminals to output (External Air Gap)terminals, shortest distance through air Min. External Creepage L(IO2)10.0mm Measured from input terminals to output (External Tracking Path)terminals, shortest distance path along body Min. Internal Clearance 1.0mm Through insulation distance conductor to (Internal Plastic Gap)conductor, usually the direct distancebetween the photoemitter and photodetectorinside the optocoupler cavityMin. Internal Creepage 4.0mm The shortest distance around the border (Internal Tracking Path)between two different insulating materialsmeasured between the emitter and detector Comparative Tracking Index CTI200V DIN IEC 112/VDE 0303 PART 1Isolation Group IIIa Material group (DIN VDE 0110)Option 300 – surface mount classification is Class A in accordance with CECC 00802.VDE 0884 (06.92) Insulation Characteristics (Option #050 Only)Description Symbol Characteristic Unit Installation classification per DIN VDE 0110/1.89, Table 1For rated mains voltage ≤600 V rms I-IVFor rated mains voltage ≤1000 V rms I-IIIClimatic Classification (DIN IEC 68 part 1)55/100/21Pollution Degree (DIN VDE 0110 Part 1/1.89)2Maximum Working Insulation Voltage V IORM1414V peak Input to Output Test Voltage, Method b*V PR2651V peak V PR = 1.875 x V IORM, 100% Production Test witht m = 1 sec, Partial Discharge < 5 pCInput to Output Test Voltage, Method a*V PR2121V peak V PR = 1.5 x V IORM, Type and sample test, t m = 60 sec,Partial Discharge < 5 pCHighest Allowable Overvoltage*V IOTM8000V peak (Transient Overvoltage, t ini = 10 sec)Safety-Limiting Values(Maximum values allowed in the event of a failure,also see Figure 11)Case Temperature T S150°C Current (Input Current I F, P S = 0)I S400mA Output Power P S,OUTPUT700mW Insulation Resistance at T S, V IO = 500 V R S>109Ω*Refer to the front of the Optocoupler section of the current catalog for a more detailed description of VDE 0884 and other product safety regulations.Note: Optocouplers providing safe electrical separation per VDE 0884 do so only within the safety-limiting values to which they are qualified. Protective cut-out switches must be used to ensure that the safety limits are not exceeded.1-421Absolute Maximum RatingsStorage Temperature..................................................-55°C to +125°C Operating Temperature (T A)........................................-55°C to +100°C Junction Temperature (T J)............................................................125°C Reflow Temperature Profile...See Package Outline Drawings Section Lead Solder Temperature..................................................260°C for 10s (up to seating plane)Average Input Current - I F............................................................25 mA Peak Input Current - I F.................................................................40 mA (50 ns maximum pulse width)Reverse Input Voltage - V R..............................................................2.5 V (I R = 100 µA, Pin 1-2)Input Power Dissipation.........................................60 mW @ T A = 85°C (Derate at 2.2 mW/°C for operating temperatures above 85°C) Reverse Output Photodiode Voltage................................................30 V (Pin 6-5)Reverse Input Photodiode Voltage...................................................30 V (Pin 3-4)Recommended Operating ConditionsStorage Temperature....................................................-40°C to +85°C Operating Temperature.................................................-40°C to +85°C Average Input Current - I F.......................................................1 - 20 mA Peak Input Current - I F.................................................................35 mA (50% duty cycle, 1 ms pulse width)Reverse Output Photodiode Voltage...........................................0 - 15 V (Pin 6-5)Reverse Input Photodiode Voltage..............................................0 - 15 V (Pin 3-4)1-422Electrical Specifications1-423AC Electrical SpecificationsT A = 25°C unless otherwise specified.TestParameter Symbol Device Min.Typ.Max.Units Conditions Fig.Note LED Bandwidth f -3dB9MHz I F = 10 mAApplication Circuit Bandwidth:High Speed 1.5MHz167 High Precision10kHz177 Application Circuit: IMRRHigh Speed95dB freq = 60 Hz167, 8 Package CharacteristicsT= 25°C unless otherwise specified.Notes:1. K3 is calculated from the slope of thebest fit line of I PD2 vs. I PD1 with elevenequally distributed data points from5nA to 50 µA. This is approximatelyequal to I PD2/I PD1 at I F = 10 mA.2. Special selection for tighter K1, K3 andlower Nonlinearity available.3. BEST FIT DC NONLINEARITY (NL BF) isthe maximum deviation expressed as a percentage of the full scale output of a “best fit” straight line from a graph ofI PD2 vs. I PD1 with eleven equally distrib-uted data points from 5 nA to 50µA.I PD2 error to best fit line is the deviationbelow and above the best fit line,expressed as a percentage of the fullscale output.4. ENDS FIT DC NONLINEARITY (NL EF)is the maximum deviation expressed asa percentage of full scale output of astraight line from the 5 nA to the 50 µAdata point on the graph of I PD2 vs. I PD1.5. Device considered a two-terminaldevice: Pins 1, 2, 3, and 4 shortedtogether and pins 5, 6, 7, and 8 shortedtogether.6. In accordance with UL 1577, eachoptocoupler is proof tested by applyingan insulation test voltage of ≥6000 Vrms for ≥1 second (leakage detectioncurrent limit, I I-O of 5 µA max.). Thistest is performed before the 100%production test for partial discharge(method b) shown in the VDE 0884Insulation Characteristics Table (forOption #050 only).7. Specific performance will depend oncircuit topology and components.8. IMRR is defined as the ratio of thesignal gain (with signal applied to V IN ofFigure 16) to the isolation mode gain(with V IN connected to input commonand the signal applied between theinput and output commons) at 60 Hz,expressed in dB.*The Input-Output Momentary Withstand Voltage is a dielectric voltage rating that should not be interpreted as an input-output continuous voltage rating. For the continuous voltage rating refer to the VDE 0884 Insulation Characteristics Table (if applicable), your equipment level safety specification, or HP Application Note 1074, “Optocoupler Input-Output Endurance Voltage.”1-4241-425Figure 5. NL BF vs. Temperature.Figure 2. Normalized K3 vs. Input I PD .Figure 3. K3 Drift vs. Temperature.Figure 4. I PD2 Error vs. Input I PD (See Note 4).Figure 6. NL BF Drift vs. Temperature.Figure 7. Input Photodiode CTR vs.LED Input Current.Figure 8. Typical Photodiode Leakage vs. Temperature.Figure 9. LED Input Current vs.Forward Voltage.Figure 10. LED Forward Voltage vs.Temperature.I L K – P H O T O D I O D E L E A K A G E – n AT A – TEMPERATURE – °C D E L T A K 3 – D R I F T O F K 3 T R A N S F E R G A I NT A – TEMPERATURE – °C D E L T A N L B F – D R I F T O F B E S T -F I T N L – % P T ST A – TEMPERATURE – °C N O R M A L I Z E D K 1 – I N P U T P H O T O D I O D E C T RI F – LED INPUT CURRENT – mAV F – L E D F O R W A R D V O L T A G E – VT A – TEMPERATURE – °C-25-555356595125N O R M A L I Z E D K 3 – T R A N S F E R G AI NI PD1 – INPUT PHOTODIODE CURRENT – µAI PD1 – INPUT PHOTODIODE CURRENT – µAI P D 2 E R R O R F R O M B E S T -F I T L I N E (% O F F S )N L B F – B E S T -F I T N O N -L I N E A R I T Y – %T A – TEMPERATURE – °C 1.201000.10.0001V F – FORWARD VOLTAGE – VOLTS 1.30 1.501010.010.001 1.40 1.60I F – F O R W A R D C U R R E N T – m AT A = 25°C1-426Figure 12. Basic Isolation Amplifier.FV I OUTV PD2OUTA) BASIC TOPOLOGYB) PRACTICAL CIRCUITFigure 11. Thermal Derating Curve Dependence of Safety Limiting Valuewith Case Temperature per VDE 0884.VOUTV OUTA) POSITIVE INPUTB) POSITIVE OUTPUTD) NEGATIVE OUTPUTFigure 13. Unipolar Circuit Topologies.T S – CASE TEMPERATURE – °C1-427Figure 15. Loop-Powered 4-20 mA Current Loop Circuits.Figure 14. Bipolar Circuit Topologies.OUTV OUTA) SINGLE OPTOCOUPLERB) DUAL OPTOCOUPLERVOUT+I OUTA) RECEIVERB) TRANSMITTERV -I OUT1-428Figure 18. Bipolar Isolation Amplifier.Figure 16. High-Speed Low-Cost Analog Isolator.VOUTINPUT BNCV +15 V OUTPUT BNCFigure 17. Precision Analog Isolation Amplifier.MAGV IN1-429MAGV INSIGNFigure 20. SPICE Model Listing.Figure 19. Magnitude/Sign Isolation Amplifier..SUBCKT HCNR200Theory of Operation Figure 1 illustrates how the HCNR200/201 high-linearity optocoupler is configured. The basic optocoupler consists of an LED and two photodiodes. The LED and one of the photodiodes (PD1) is on the input leadframe and the other photodiode (PD2) is on the output leadframe. The package of the optocoupler is constructed so that each photo-diode receives approximately the same amount of light from the LED.An external feedback amplifier can be used with PD1 to monitor the light output of the LED and automatically adjust the LED current to compensate for any non-linearities or changes in light output of the LED. The feedback amplifier acts to stabilize and linearize the light output of the LED. The output photodiode then converts the stable, linear light output of the LED into a current, which can then be converted back into a voltage by another amplifier.Figure 12a illustrates the basic circuit topology for implementing a simple isolation amplifier using the HCNR200/201 optocoupler. Besides the optocoupler, two external op-amps and two resistors are required. This simple circuit is actually a bit too simple to function properly in an actual circuit, but it is quite useful for explaining how the basic isolation amplifier circuit works (a few more components and a circuit change are required to make a practical circuit, like the one shown in Figure 12b).The operation of the basic circuit may not be immediately obvious just from inspecting Figure 12a,particularly the input part of thecircuit. Stated briefly, amplifierA1 adjusts the LED current (I F),and therefore the current in PD1(I PD1), to maintain its “+” inputterminal at 0 V. For example,increasing the input voltage wouldtend to increase the voltage of the“+” input terminal of A1 above 0V. A1 amplifies that increase,causing I F to increase, as well asI PD1. Because of the way that PD1is connected, I PD1 will pull the “+”terminal of the op-amp backtoward ground. A1 will continueto increase I F until its “+”terminal is back at 0 V. Assumingthat A1 is a perfect op-amp, nocurrent flows into the inputs ofA1; therefore, all of the currentflowing through R1 will flowthrough PD1. Since the “+” inputof A1 is at 0 V, the currentthrough R1, and therefore I PD1 aswell, is equal to V IN/R1.Essentially, amplifier A1 adjusts I Fso thatI PD1 = V IN/R1.Notice that I PD1 depends ONLY onthe input voltage and the value ofR1 and is independent of the lightoutput characteristics of the LED.As the light output of the LEDchanges with temperature, ampli-fier A1 adjusts I F to compensateand maintain a constant currentin PD1. Also notice that I PD1 isexactly proportional to V IN, givinga very linear relationship betweenthe input voltage and thephotodiode current.The relationship between the inputoptical power and the outputcurrent of a photodiode is verylinear. Therefore, by stabilizingand linearizing I PD1, the lightoutput of the LED is alsostabilized and linearized. Andsince light from the LED falls onboth of the photodiodes, I PD2 willbe stabilized as well.The physical construction of thepackage determines the relativeamounts of light that fall on thetwo photodiodes and, therefore,the ratio of the photodiodecurrents. This results in verystable operation over time andtemperature. The photodiodecurrent ratio can be expressed asa constant, K, whereK = I PD2/I PD1.Amplifier A2 and resistor R2 forma trans-resistance amplifier thatconverts I PD2 back into a voltage,V OUT, whereV OUT = I PD2*R2.Combining the above threeequations yields an overallexpression relating the outputvoltage to the input voltage,V OUT/V IN = K*(R2/R1).Therefore the relationshipbetween V IN and V OUT is constant,linear, and independent of thelight output characteristics of theLED. The gain of the basic isola-tion amplifier circuit can beadjusted simply by adjusting theratio of R2 to R1. The parameterK (called K3 in the electricalspecifications) can be thought ofas the gain of the optocoupler andis specified in the data sheet.Remember, the circuit inFigure12a is simplified in orderto explain the basic circuit opera-tion. A practical circuit, more likeFigure12b, will require a fewadditional components to stabilizethe input part of the circuit, tolimit the LED current, or to1-4301-431second circuit requires two optocouplers, separate gain adjustments for the positive and negative portions of the signal,and can exhibit crossover distor-tion near zero volts. The correct circuit to choose for an applica-tion would depend on therequirements of that particular application. As with the basic isolation amplifier circuit inFigure 12a, the circuits in Figure 14 are simplified and would require a few additional compo-nents to function properly. Two example circuits that operate with bipolar input signals arediscussed in the next section.As a final example of circuit design flexibility, the simplified schematics in Figure 15 illustrate how to implement 4-20 mA analog current-loop transmitter and receiver circuits using the HCNR200/201 optocoupler. An important feature of these circuits is that the loop side of the circuit is powered entirely by the loop current, eliminating the need for an isolated power supply.The input and output circuits in Figure 15a are the same as the negative input and positive output circuits shown in Figures 13c and 13b, except for the addition of R3and zener diode D1 on the input side of the circuit. D1 regulates the supply voltage for the input amplifier, while R3 forms a current divider with R1 to scale the loop current down from 20mA to an appropriate level for the input circuit (<50 µA).As in the simpler circuits, the input amplifier adjusts the LED current so that both of its input terminals are at the same voltage.The loop current is then dividedoptimize circuit performance.Example application circuits will be discussed later in the data sheet.Circuit Design FlexibilityCircuit design with the HCNR200/201 is very flexible because the LED and both photodiodes are accessible to the designer. This allows the designer to make perf-ormance trade-offs that would otherwise be difficult to make with commercially available isolation amplifiers (e.g., bandwidth vs.accuracy vs. cost). Analog isola-tion circuits can be designed for applications that have either unipolar (e.g., 0-10 V) or bipolar (e.g., ±10 V) signals, with positive or negative input oroutput voltages. Several simplified circuit topologies illustrating the design flexibility of the HCNR200/201 are discussed below.The circuit in Figure 12a is configured to be non-inverting with positive input and output voltages. By simply changing the polarity of one or both of the photodiodes, the LED, or the op-amp inputs, it is possible toimplement other circuit configu-rations as well. Figure 13illustrates how to change the basic circuit to accommodate both positive and negative input and output voltages. The input and output circuits can bematched to achieve any combina-tion of positive and negative voltages, allowing for both inverting and non-inverting circuits.All of the configurations described above are unipolar (single polar-ity); the circuits cannot accommo-date a signal that might swing both positive and negative. It ispossible, however, to use the HCNR200/201 optocoupler to implement a bipolar isolation amplifier. Two topologies that allow for bipolar operation are shown in Figure 14.The circuit in Figure 14a uses two current sources to offset the signal so that it appears to be unipolar to the optocoupler.Current source I OS1 provides enough offset to ensure that I PD1is always positive. The second current source, I OS2, provides an offset of opposite polarity toobtain a net circuit offset of zero.Current sources I OS1 and I OS2 can be implemented simply as resistors connected to suitable voltage sources.The circuit in Figure 14b uses two optocouplers to obtain bipolar operation. The first optocoupler handles the positive voltage excursions, while the second optocoupler handles the negative ones. The output photodiodes are connected in an antiparallel configuration so that they produce output signals of opposite polarity.The first circuit has the obvious advantage of requiring only one optocoupler; however, the offset performance of the circuit isdependent on the matching of I OS1and I OS2 and is also dependent on the gain of the optocoupler.Changes in the gain of the opto-coupler will directly affect the offset of the circuit.The offset performance of the second circuit, on the other hand,is much more stable; it is inde-pendent of optocoupler gain and has no matched current sources to worry about. However, thebetween R1 and R3. I PD1 is equal to the current in R1 and is given by the following equation:I PD1 = I LOOP*R3/(R1+R3).Combining the above equation with the equations used for Figure 12a yields an overall expression relating the output voltage to the loop current,V OUT/I LOOP = K*(R2*R3)/(R1+R3).Again, you can see that the relationship is constant, linear, and independent of the charac-teristics of the LED.The 4-20 mA transmitter circuit in Figure15b is a little different from the previous circuits, partic-ularly the output circuit. The output circuit does not directly generate an output voltage which is sensed by R2, it instead usesQ1 to generate an output current which flows through R3. This output current generates a voltage across R3, which is then sensed by R2. An analysis similar to the one above yields the following expression relating output current to input voltage:I LOOP/V IN = K*(R2+R3)/(R1*R3).The preceding circuits were pre-sented to illustrate the flexibility in designing analog isolation circuits using the HCNR200/201. The next section presents several complete schematics to illustrate practical applications of the HCNR200/201.Example Application CircuitsThe circuit shown in Figure 16 is a high-speed low-cost circuit designed for use in the feedback path of switch-mode power supplies. This application requiresgood bandwidth, low cost andstable gain, but does not requirevery high accuracy. This circuit isa good example of how a designercan trade off accuracy to achieveimprovements in bandwidth andcost. The circuit has a bandwidthof about 1.5 MHz with stable gaincharacteristics and requires fewexternal components.Although it may not appear so atfirst glance, the circuit in Figure16 is essentially the same as thecircuit in Figure 12a. Amplifier A1is comprised of Q1, Q2, R3 andR4, while amplifier A2 iscomprised of Q3, Q4, R5, R6 andR7. The circuit operates in thesame manner as well; the onlydifference is the performance ofamplifiers A1 and A2. The lowergains, higher input currents andhigher offset voltages affect theaccuracy of the circuit, but notthe way it operates. Because thebasic circuit operation has notchanged, the circuit still has goodgain stability. The use of discretetransistors instead of op-ampsallowed the design to trade offaccuracy to achieve goodbandwidth and gain stability atlow cost.To get into a little more detailabout the circuit, R1 is selected toachieve an LED current of about7-10 mA at the nominal inputoperating voltage according to thefollowing equation:I F = (V IN/R1)/K1,where K1 (i.e., I PD1/I F) of theoptocoupler is typically about0.5%. R2 is then selected toachieve the desired output voltageaccording to the equation,V OUT/V IN = R2/R1.The purpose of R4 and R6 is toimprove the dynamic response(i.e., stability) of the input andoutput circuits by lowering thelocal loop gains. R3 and R5 areselected to provide enoughcurrent to drive the bases of Q2and Q4. And R7 is selected so thatQ4 operates at about the samecollector current as Q2.The next circuit, shown inFigure17, is designed to achievethe highest possible accuracy at areasonable cost. The highaccuracy and wide dynamic rangeof the circuit is achieved by usinglow-cost precision op-amps withvery low input bias currents andoffset voltages and is limited bythe performance of the opto-coupler. The circuit is designed tooperate with input and outputvoltages from 1 mV to 10 V.The circuit operates in the sameway as the others. The only majordifferences are the two compensa-tion capacitors and additionalLED drive circuitry. In the high-speed circuit discussed above, theinput and output circuits arestabilized by reducing the localloop gains of the input and outputcircuits. Because reducing theloop gains would decrease theaccuracy of the circuit, twocompensation capacitors, C1 andC2, are instead used to improvecircuit stability. These capacitorsalso limit the bandwidth of thecircuit to about 10 kHz and canbe used to reduce the outputnoise of the circuit by reducing itsbandwidth even further.The additional LED drive circuitry(Q1 and R3 through R6) helps tomaintain the accuracy and band-width of the circuit over the entirerange of input voltages. Withoutthese components, the transcon-ductance of the LED driver would1-432decrease at low input voltages and LED currents. This would reduce the loop gain of the input circuit, reducing circuit accuracy and bandwidth. D1 prevents excessive reverse voltage from being applied to the LED when the LED turns off completely.No offset adjustment of the circuit is necessary; the gain can be adjusted to unity by simply adjusting the 50kohm poten-tiometer that is part of R2. Any OP-97 type of op-amp can be used in the circuit, such as theLT1097 from Linear Technology or the AD705 from Analog Devices, both of which offer pA bias currents, µV offset voltages and are low cost. The input terminals of the op-amps and the photodiodes are connected in the circuit using Kelvin connections to help ensure the accuracy of the circuit.The next two circuits illustrate how the HCNR200/201 can be used with bipolar input signals. The isolation amplifier inFigure18 is a practical implemen-tation of the circuit shown in Figure14b. It uses two opto-couplers, OC1 and OC2; OC1 handles the positive portions of the input signal and OC2 handles the negative portions.Diodes D1 and D2 help reduce crossover distortion by keeping both amplifiers active during both positive and negative portions of the input signal. For example, when the input signal positive, optocoupler OC1 is active while OC2 is turned off. However, the amplifier controlling OC2 is kept active by D2, allowing it to turn on OC2 more rapidly when the input signal goes negative, thereby reducing crossover distortion.Balance control R1 adjusts therelative gain for the positive andnegative portions of the inputsignal, gain control R7 adjusts theoverall gain of the isolationamplifier, and capacitors C1-C3provide compensation to stabilizethe amplifiers.The final circuit shown inFigure19 isolates a bipolaranalog signal using only oneoptocoupler and generates twooutput signals: an analog signalproportional to the magnitude ofthe input signal and a digitalsignal corresponding to the signof the input signal. This circuit isespecially useful for applicationswhere the output of the circuit isgoing to be applied to an analog-to-digital converter. The primaryadvantages of this circuit are verygood linearity and offset, withonly a single gain adjustment andno offset or balance adjustments.To achieve very high linearity forbipolar signals, the gain should beexactly the same for both positiveand negative input polarities. Thiscircuit achieves excellent linearityby using a single optocoupler anda single input resistor, whichguarantees identical gain for bothpositive and negative polarities ofthe input signal. This precisematching of gain for both polari-ties is much more difficult toobtain when separate componentsare used for the different inputpolarities, such as is the previouscircuit.The circuit in Figure19 is actuallyvery similar to the previouscircuit. As mentioned above, onlyone optocoupler is used. Becausea photodiode can conduct currentin only one direction, two diodes(D1 and D2) are used to steer theinput current to the appropriateterminal of input photodiode PD1to allow bipolar input currents.Normally the forward voltagedrops of the diodes would cause aserious linearity or accuracyproblem. However, an additionalamplifier is used to provide anappropriate offset voltage to theother amplifiers that exactlycancels the diode voltage drops tomaintain circuit accuracy.Diodes D3 and D4 perform twodifferent functions; the diodeskeep their respective amplifiersactive independent of the inputsignal polarity (as in the previouscircuit), and they also provide thefeedback signal to PD1 thatcancels the voltage drops ofdiodes D1 and D2.Either a comparator or an extraop-amp can be used to sense thepolarity of the input signal anddrive an inexpensive digitaloptocoupler, like a 6N139.It is also possible to convert thiscircuit into a fully bipolar circuit(with a bipolar output signal) byusing the output of the 6N139 todrive some CMOS switches toswitch the polarity of PD2depending on the polarity of theinput signal, obtaining a bipolaroutput voltage swing.HCNR200/201 SPICEModelFigure 20 is the net list of aSPICE macro-model for theHCNR200/201 high-linearityoptocoupler. The macro-modelaccurately reflects the primarycharacteristics of the HCNR200/201 and should facilitate thedesign and understanding ofcircuits using the HCNR200/201optocoupler.1-433。
线性光耦HCNR200中文数据手册
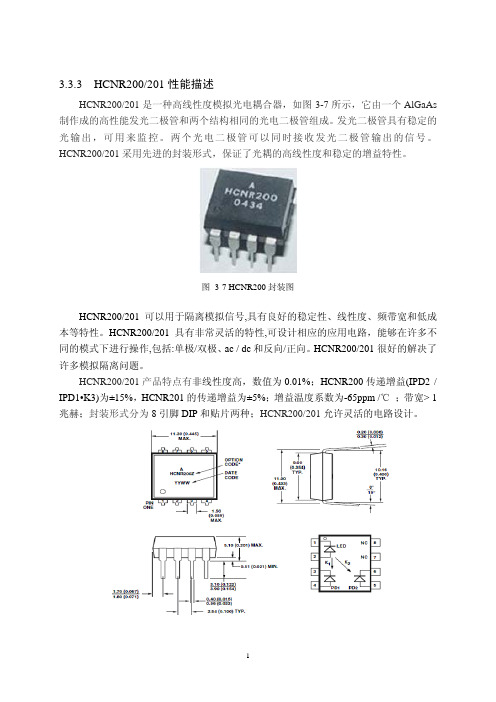
3.3.3 HCNR200/201性能描述HCNR200/201是一种高线性度模拟光电耦合器,如图3-7所示,它由一个AlGaAs 制作成的高性能发光二极管和两个结构相同的光电二极管组成。
发光二极管具有稳定的光输出,可用来监控。
两个光电二极管可以同时接收发光二极管输出的信号。
HCNR200/201采用先进的封装形式,保证了光耦的高线性度和稳定的增益特性。
图 3-7 HCNR200封装图HCNR200/201可以用于隔离模拟信号,具有良好的稳定性、线性度、频带宽和低成本等特性。
HCNR200/201具有非常灵活的特性,可设计相应的应用电路,能够在许多不同的模式下进行操作,包括:单极/双极、ac / dc和反向/正向。
HCNR200/201很好的解决了许多模拟隔离问题。
HCNR200/201产品特点有非线性度高,数值为0.01%;HCNR200传递增益(IPD2 / IPD1•K3)为±15%,HCNR201的传递增益为±5%;增益温度系数为-65ppm /℃;带宽> 1兆赫;封装形式分为8引脚DIP和贴片两种;HCNR200/201允许灵活的电路设计。
图 3-8 HCNR200内部管脚图HCNR200/201主要应用在低成本的模拟信号隔离、工业过程控制、电子反馈回路、监测电机电源电压、医疗等领域。
其内部结构图如图3-8所示。
图3-8说明了HCNR200/201是一种高线性度的光耦。
它由一个发光二极管(LED)和两个同种工艺的光电二极管组成。
其中一个光电二极管(PD1)在隔离电路的输入部分,另一个光电二极管(PD2)构成隔离电路的输出部分。
由于光耦的封装,使每一个光电二极管可从LED上接收大致相同数量的光线。
放大器与PD1组成的外部反馈电路可用来监控发光二极管(LED)发出的光,并且可以补充流过LED的电流起到对LED的调节作用。
使得LED输出的光信号更加稳定,当PD2接收到光信号后,可以再通过另一个运算放大器把接收到的电流信号转化为电压信号。
- 1、下载文档前请自行甄别文档内容的完整性,平台不提供额外的编辑、内容补充、找答案等附加服务。
- 2、"仅部分预览"的文档,不可在线预览部分如存在完整性等问题,可反馈申请退款(可完整预览的文档不适用该条件!)。
- 3、如文档侵犯您的权益,请联系客服反馈,我们会尽快为您处理(人工客服工作时间:9:00-18:30)。
1. 线形光耦介绍
光隔离是一种很常用的信号隔离形式。
常用光耦器件及其外围电路组成。
由于光耦电路简单,在数字隔离电路或数据传输电路中常常用到,如UART协议的20mA 电流环。
对于模拟信号,光耦因为输入输出的线形较差,并且随温度变化较大,限制了其在模拟信号隔离的应用。
对于高频交流模拟信号,变压器隔离是最常见的选择,但对于支流信号却不适用。
一些厂家提供隔离放大器作为模拟信号隔离的解决方案,如ADI的AD202,能够提供从直流到几K的频率内提供0.025%的线性度,但这种隔离器件内部先进行电压-频率转换,对产生的交流信号进行变压器隔离,然后进行频率-电压转换得到隔离效果。
集成的隔离放大器内部电路复杂,体积大,成本高,不适合大规模应用。
模拟信号隔离的一个比较好的选择是使用线形光耦。
线性光耦的隔离原理与普通光耦没有差别,只是将普通光耦的单发单收模式稍加改变,增加一个用于反馈的光接受电路用于反馈。
这样,虽然两个光接受电路都是非线性的,但两个光接受电路的非线性特性都是一样的,这样,就可以通过反馈通路的非线性来抵消直通通路的非线性,从而达到实现线性隔离的目的。
市场上的线性光耦有几中可选择的芯片,如Agilent公司的HCNR200/201,TI子公司TOAS的TIL300,CLARE的LOC111等。
这里以HCNR200/201为例介绍
2. 芯片介绍与原理说明
HCNR200/201的内部框图如下所示
其中1、2引作为隔离信号的输入,3、4引脚用于反馈,5、6引脚用于输出。
1、2引脚之间的电流记作IF,3、4引脚之间和5、6引脚之间的电流分别记作IPD1和IPD2。
输入信号经过电压-电流转化,电压的变化体现在电流IF上,IPD1和IPD2基本与IF成线性关系,线性系数分别记为K1和K2,即
K1与K2一般很小(HCNR200是0.50%),并且随温度变化较大(HCNR200的变化范围在0.25%到0.75%之间),但芯片的设计使得K1和K2相等。
在后面可以看到,在合理的外围电路设计中,真正影响输出/输入比值的是二者的比值K3,线性光耦正利用这种特性才能达到满意的线性度的。
HCNR200和HCNR201的内部结构完全相同,差别在于一些指标上。
相对于HCNR200,HCNR201提供更高的线性度。
采用HCNR200/201进行隔离的一些指标如下所示:
* 线性度:HCNR200:0.25%,HCNR201:0.05%;
* 线性系数K3:HCNR200:15%,HCNR201:5%;
* 温度系数: -65pp m/o C;
* 隔离电压:1414V;
* 信号带宽:直流到大于1M H z。
从上面可以看出,和普通光耦一样,线性光耦真正隔离的是电流,要想真正隔离电压,需要在输出和输出处增加运算放大器等辅助电路。
下面对HCNR200/201的典型电路进行分析,对电路中如何实现反馈以及电流-电压、电压-电流转换进行推导与说明。
3. 典型电路分析
Agilent公司的HCNR200/201的手册上给出了多种实用电路,其中较为典型的一种如下图所示:
图2
设输入端电压为V in,输出端电压为Vou t,光耦保证的两个电流传递系数分别为K1、K2,显然,,和之间的关系取决于和之间的关系。
将前级运放的电路提出来看,如下图所示:
设运放负端的电压为,运放输出端的电压为,在运放不饱和的情况下二者满足下面的关系:
Vo=Voo-GV i (1)
其中是在运放输入差模为0时的输出电压,G为运放的增益,一般比较大。
忽略运放负端的输入电流,可以认为通过R1的电流为IP1,根据R1的欧姆定律得:
通过R3两端的电流为IF,根据欧姆定律得:
其中,为光耦2脚的电压,考虑到LED导通时的电压()基本不变,这里的作为常数对待。
根据光耦的特性,即
K1=IP1/IF (4)
将和的表达式代入上式,可得:
上式经变形可得到:
将的表达式代入(3)式可得:
考虑到G特别大,则可以做以下近似:
这样,输出与输入电压的关系如下:
可见,在上述电路中,输出和输入成正比,并且比例系数只由K3和R1、R2确定。
一般选R1=R2,达到只隔离不放大的目的。
4. 辅助电路与参数确定
上面的推导都是假定所有电路都是工作在线性范围内的,要想做到这一点需要对运放进行合理选型,并且确定电阻的阻值。
4.1 运放选型
运放可以是单电源供电或正负电源供电,上面给出的是单电源供电的例子。
为了能使输入范围能够从0到V CC,需要运放能够满摆幅工作,另外,运放的工作速度、压摆率不会影响整个电路的性能。
TI公司的L MV321单运放电路能够满足以上要求,可以作为HCNR200/201的外围电路。
4.2 阻值确定
电阻的选型需要考虑运放的线性范围和线性光耦的最大工作电流IFm ax。
K1已知的情况下,IFm ax又确定了IPD1的最大值IPD1m ax,这样,由于Vo的范围最小可以为0,这样,由于
考虑到IFm ax大有利于能量的传输,这样,一般取
另外,由于工作在深度负反馈状态的运放满足虚短特性,因此,考虑IPD1的限制,
这样,
R2的确定可以根据所需要的放大倍数确定,例如如果不需要方法,只需将R2=R1即可。
另外由于光耦会产生一些高频的噪声,通常在R2处并联电容,构成低通滤波器,具体电容的值由输入频率以及噪声频率确定。
4.3 参数确定实例
假设确定Vcc=5V,输入在0-4V之间,输出等于输入,采用L MV321运放芯片以及上面电路,下面给出参数确定的过程。
*确定IFm ax:HCNR200/201的手册上推荐器件工作的25mA左右;
*确定R3:R3=5V/25mA=200;
*确定R1:;
*确定R2:R2=R1=32K。
5. 总结
本文给出了线性光耦的简单介绍以及电路设计、参数选择等使用中的注意事项与参考设计,并对电路的设计方法给出相应的推导与解释,供广大电子工程师参考。