磁控管工作原理
磁控管工作原理
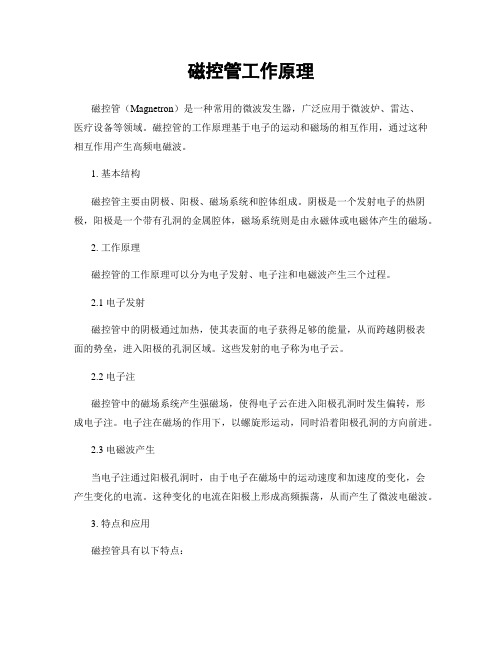
磁控管工作原理磁控管(Magnetron)是一种常用的微波发生器,广泛应用于微波炉、雷达、医疗设备等领域。
磁控管的工作原理基于电子的运动和磁场的相互作用,通过这种相互作用产生高频电磁波。
1. 基本结构磁控管主要由阴极、阳极、磁场系统和腔体组成。
阴极是一个发射电子的热阴极,阳极是一个带有孔洞的金属腔体,磁场系统则是由永磁体或电磁体产生的磁场。
2. 工作原理磁控管的工作原理可以分为电子发射、电子注和电磁波产生三个过程。
2.1 电子发射磁控管中的阴极通过加热,使其表面的电子获得足够的能量,从而跨越阴极表面的势垒,进入阳极的孔洞区域。
这些发射的电子称为电子云。
2.2 电子注磁控管中的磁场系统产生强磁场,使得电子云在进入阳极孔洞时发生偏转,形成电子注。
电子注在磁场的作用下,以螺旋形运动,同时沿着阳极孔洞的方向前进。
2.3 电磁波产生当电子注通过阳极孔洞时,由于电子在磁场中的运动速度和加速度的变化,会产生变化的电流。
这种变化的电流在阳极上形成高频振荡,从而产生了微波电磁波。
3. 特点和应用磁控管具有以下特点:- 高功率输出:磁控管可以产生高功率的微波电磁波,适用于需要大功率输出的应用,如微波炉。
- 高频稳定性:磁控管的频率稳定性较高,适用于需要精确频率控制的应用,如雷达。
- 长寿命:磁控管的寿命较长,可以连续工作数千小时。
- 调制性能好:磁控管可以通过调制电源的方式实现对微波信号的调制,适用于需要调制功能的应用,如通信设备。
磁控管的应用非常广泛,包括但不限于:- 微波炉:磁控管作为微波炉的核心部件,通过产生微波电磁波来加热食物。
- 雷达:磁控管作为雷达的发射源,产生高功率的微波信号,用于探测和跟踪目标。
- 医疗设备:磁控管在医疗设备中用于产生微波电磁波,如医用热疗设备。
总结:磁控管是一种基于电子运动和磁场相互作用的微波发生器。
通过加热阴极产生电子云,利用磁场使电子云形成电子注,最终产生高频电磁波。
磁控管具有高功率输出、高频稳定性、长寿命和调制性能好等特点,广泛应用于微波炉、雷达和医疗设备等领域。
磁控管工作原理
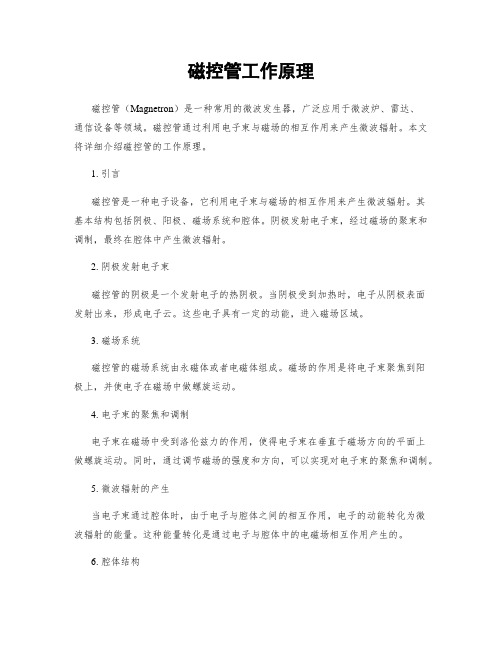
磁控管工作原理磁控管(Magnetron)是一种常用的微波发生器,广泛应用于微波炉、雷达、通信设备等领域。
磁控管通过利用电子束与磁场的相互作用来产生微波辐射。
本文将详细介绍磁控管的工作原理。
1. 引言磁控管是一种电子设备,它利用电子束与磁场的相互作用来产生微波辐射。
其基本结构包括阴极、阳极、磁场系统和腔体。
阴极发射电子束,经过磁场的聚束和调制,最终在腔体中产生微波辐射。
2. 阴极发射电子束磁控管的阴极是一个发射电子的热阴极。
当阴极受到加热时,电子从阴极表面发射出来,形成电子云。
这些电子具有一定的动能,进入磁场区域。
3. 磁场系统磁控管的磁场系统由永磁体或者电磁体组成。
磁场的作用是将电子束聚焦到阳极上,并使电子在磁场中做螺旋运动。
4. 电子束的聚焦和调制电子束在磁场中受到洛伦兹力的作用,使得电子束在垂直于磁场方向的平面上做螺旋运动。
同时,通过调节磁场的强度和方向,可以实现对电子束的聚焦和调制。
5. 微波辐射的产生当电子束通过腔体时,由于电子与腔体之间的相互作用,电子的动能转化为微波辐射的能量。
这种能量转化是通过电子与腔体中的电磁场相互作用产生的。
6. 腔体结构磁控管的腔体通常采用共振腔体结构,包括谐振腔和耦合装置。
谐振腔是一个封闭的空腔,其尺寸和形状决定了微波辐射的频率。
耦合装置用于将微波辐射从腔体中传出。
7. 工作频率调节通过调节磁场的强度和方向,可以改变电子束在腔体中的运动轨迹,从而改变微波辐射的频率。
这样就可以实现对磁控管的工作频率进行调节。
8. 总结磁控管是一种利用电子束与磁场相互作用产生微波辐射的设备。
其工作原理包括阴极发射电子束、磁场系统、电子束的聚焦和调制、微波辐射的产生等过程。
磁控管的工作频率可以通过调节磁场的强度和方向来实现。
磁控管在微波炉、雷达、通信设备等领域有着广泛的应用。
以上是关于磁控管工作原理的详细介绍,希翼对您有所匡助。
如果还有任何问题,请随时向我提问。
磁控管工作原理
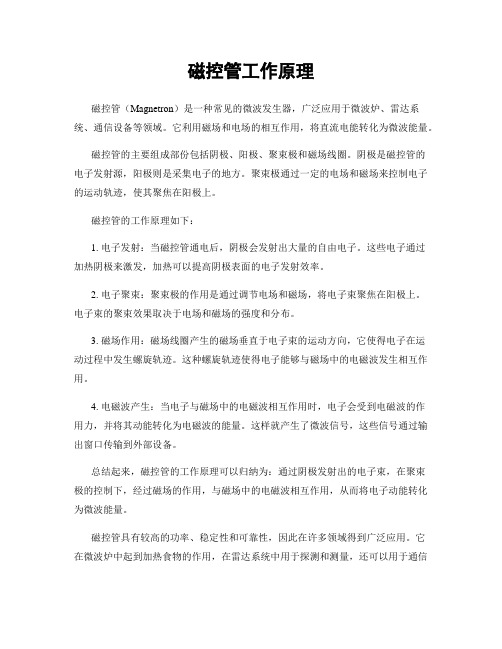
磁控管工作原理
磁控管(Magnetron)是一种常见的微波发生器,广泛应用于微波炉、雷达系统、通信设备等领域。
它利用磁场和电场的相互作用,将直流电能转化为微波能量。
磁控管的主要组成部份包括阴极、阳极、聚束极和磁场线圈。
阴极是磁控管的
电子发射源,阳极则是采集电子的地方。
聚束极通过一定的电场和磁场来控制电子的运动轨迹,使其聚焦在阳极上。
磁控管的工作原理如下:
1. 电子发射:当磁控管通电后,阴极会发射出大量的自由电子。
这些电子通过
加热阴极来激发,加热可以提高阴极表面的电子发射效率。
2. 电子聚束:聚束极的作用是通过调节电场和磁场,将电子束聚焦在阳极上。
电子束的聚束效果取决于电场和磁场的强度和分布。
3. 磁场作用:磁场线圈产生的磁场垂直于电子束的运动方向,它使得电子在运
动过程中发生螺旋轨迹。
这种螺旋轨迹使得电子能够与磁场中的电磁波发生相互作用。
4. 电磁波产生:当电子与磁场中的电磁波相互作用时,电子会受到电磁波的作
用力,并将其动能转化为电磁波的能量。
这样就产生了微波信号,这些信号通过输出窗口传输到外部设备。
总结起来,磁控管的工作原理可以归纳为:通过阴极发射出的电子束,在聚束
极的控制下,经过磁场的作用,与磁场中的电磁波相互作用,从而将电子动能转化为微波能量。
磁控管具有较高的功率、稳定性和可靠性,因此在许多领域得到广泛应用。
它
在微波炉中起到加热食物的作用,在雷达系统中用于探测和测量,还可以用于通信
设备中的信号发射和接收。
磁控管的工作原理的深入理解有助于我们更好地应用和改进这一技术。
磁控管工作原理

磁控管工作原理
磁控管(Magnetron)是一种常用于微波加热和雷达应用的真空电子设备。
它
利用磁场和电场的相互作用来产生和放大微波信号。
磁控管的工作原理涉及到电子束的产生、聚焦和调制。
1. 电子束的产生:
磁控管中的电子束是通过阴极和阳极之间的电场产生的。
当电子从阴极发射出
来后,受到阳极的吸引,形成一个电子束。
阴极通常是由钨丝制成,通过加热钨丝使其发射电子。
2. 电子束的聚焦:
为了将电子束聚焦到一个小的区域内,磁控管中使用了一个磁场。
这个磁场是
通过在阳极附近放置一个环形磁铁来产生的。
磁场的作用是使电子束发生螺旋运动,从而将其聚焦到阳极的小区域内。
3. 电子束的调制:
为了调制微波信号,磁控管中引入了一个调制极。
调制极是一个与阳极相连的
金属环,它的作用是改变电子束的轨迹,从而改变微波信号的强度。
当调制极的电压发生变化时,电子束的轨迹也会随之改变,从而调制微波信号。
总结:
磁控管的工作原理可以概括为:通过阴极发射电子,经过阳极的吸引形成电子束,通过磁场的作用将电子束聚焦到一个小的区域内,并通过调制极改变电子束的轨迹来调制微波信号。
磁控管在微波加热和雷达应用中具有广泛的应用,其工作原理的理解对于理解其工作性能和优化设计至关重要。
磁控管工作原理

磁控管工作原理引言概述:磁控管是一种重要的电子元件,广泛应用于电子设备和通信系统中。
它的工作原理基于磁场的控制,通过改变磁场来控制电子束的运动。
本文将详细介绍磁控管的工作原理,包括磁控管的基本结构、磁场的生成、电子束的控制以及应用案例等内容。
正文内容:1. 磁控管的基本结构1.1 真空管1.2 网格1.3 阴极1.4 高压电源1.5 引出电极2. 磁场的生成2.1 磁铁2.2 磁场的控制2.3 磁场的强度2.4 磁场的方向2.5 磁场的稳定性3. 电子束的控制3.1 网格电压3.2 阴极电流3.3 磁场与电子束的相互作用3.4 电子束的聚焦3.5 电子束的偏转4. 磁控管的工作原理4.1 电子发射4.2 电子束的加速4.3 电子束的聚焦4.4 电子束的偏转4.5 电子束的打印或显示5. 应用案例5.1 显示器5.2 激光打印机5.3 通信设备5.4 雷达系统5.5 医疗设备总结:通过本文的介绍,我们了解到磁控管的工作原理是基于磁场的控制,通过改变磁场来控制电子束的运动。
磁控管的基本结构包括真空管、网格、阴极、高压电源和引出电极。
磁场的生成依靠磁铁,通过控制磁场的强度、方向和稳定性来实现对电子束的控制。
电子束的控制主要通过调节网格电压、阴极电流和磁场与电子束的相互作用来实现。
磁控管的工作原理包括电子发射、电子束的加速、聚焦、偏转以及打印或显示。
最后,我们还介绍了磁控管在显示器、激光打印机、通信设备、雷达系统和医疗设备等领域的应用案例。
磁控管凭借其可靠性和高精度的性能,在现代科技领域发挥着重要的作用。
磁控管工作原理
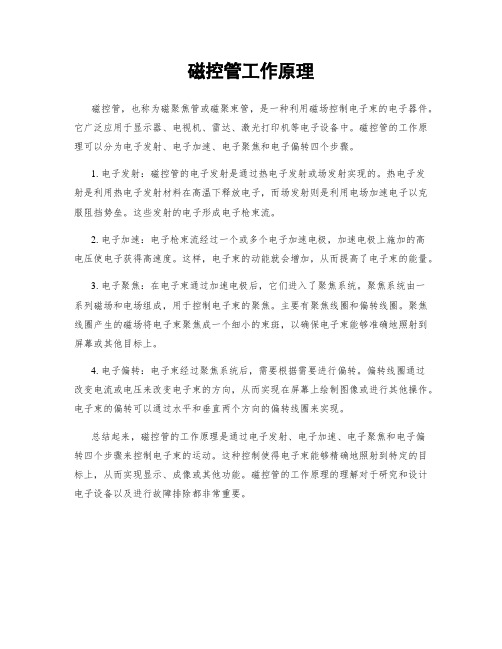
磁控管工作原理
磁控管,也称为磁聚焦管或磁聚束管,是一种利用磁场控制电子束的电子器件。
它广泛应用于显示器、电视机、雷达、激光打印机等电子设备中。
磁控管的工作原理可以分为电子发射、电子加速、电子聚焦和电子偏转四个步骤。
1. 电子发射:磁控管的电子发射是通过热电子发射或场发射实现的。
热电子发
射是利用热电子发射材料在高温下释放电子,而场发射则是利用电场加速电子以克服阻挡势垒。
这些发射的电子形成电子枪束流。
2. 电子加速:电子枪束流经过一个或多个电子加速电极,加速电极上施加的高
电压使电子获得高速度。
这样,电子束的动能就会增加,从而提高了电子束的能量。
3. 电子聚焦:在电子束通过加速电极后,它们进入了聚焦系统。
聚焦系统由一
系列磁场和电场组成,用于控制电子束的聚焦。
主要有聚焦线圈和偏转线圈。
聚焦线圈产生的磁场将电子束聚焦成一个细小的束斑,以确保电子束能够准确地照射到屏幕或其他目标上。
4. 电子偏转:电子束经过聚焦系统后,需要根据需要进行偏转。
偏转线圈通过
改变电流或电压来改变电子束的方向,从而实现在屏幕上绘制图像或进行其他操作。
电子束的偏转可以通过水平和垂直两个方向的偏转线圈来实现。
总结起来,磁控管的工作原理是通过电子发射、电子加速、电子聚焦和电子偏
转四个步骤来控制电子束的运动。
这种控制使得电子束能够精确地照射到特定的目标上,从而实现显示、成像或其他功能。
磁控管的工作原理的理解对于研究和设计电子设备以及进行故障排除都非常重要。
磁控管工作原理
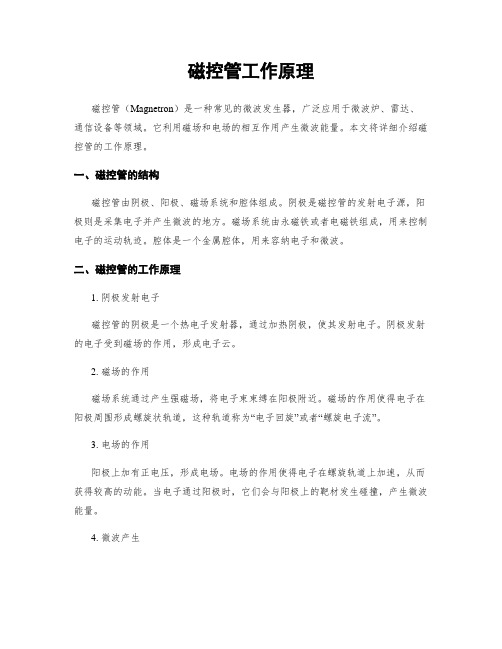
磁控管工作原理磁控管(Magnetron)是一种常见的微波发生器,广泛应用于微波炉、雷达、通信设备等领域。
它利用磁场和电场的相互作用产生微波能量。
本文将详细介绍磁控管的工作原理。
一、磁控管的结构磁控管由阴极、阳极、磁场系统和腔体组成。
阴极是磁控管的发射电子源,阳极则是采集电子并产生微波的地方。
磁场系统由永磁铁或者电磁铁组成,用来控制电子的运动轨迹。
腔体是一个金属腔体,用来容纳电子和微波。
二、磁控管的工作原理1. 阴极发射电子磁控管的阴极是一个热电子发射器,通过加热阴极,使其发射电子。
阴极发射的电子受到磁场的作用,形成电子云。
2. 磁场的作用磁场系统通过产生强磁场,将电子束束缚在阳极附近。
磁场的作用使得电子在阳极周围形成螺旋状轨道,这种轨道称为“电子回旋”或者“螺旋电子流”。
3. 电场的作用阳极上加有正电压,形成电场。
电场的作用使得电子在螺旋轨道上加速,从而获得较高的动能。
当电子通过阳极时,它们会与阳极上的靶材发生碰撞,产生微波能量。
4. 微波产生当电子与阳极碰撞时,它们会将动能转化为微波能量。
这是由于碰撞会导致靶材上的电子在电场的作用下振动,从而产生微波辐射。
微波能量通过腔体传播出来,用于实现各种应用。
三、磁控管的特点1. 高功率输出磁控管能够提供较高的功率输出,这是由于其独特的工作原理所决定的。
通过控制磁场和电场的强度,可以实现不同功率输出的需求。
2. 高效率磁控管的能量转换效率较高,能够将大部份电子动能转化为微波能量。
这使得磁控管成为微波领域中的重要组件。
3. 长寿命磁控管具有较长的寿命,这是由于其结构简单、稳定可靠所决定的。
它能够在恶劣的工作环境下长期稳定工作。
4. 频率稳定性好磁控管的频率稳定性较好,能够在一定范围内保持稳定的工作频率。
这使得磁控管在通信和雷达等领域中得到广泛应用。
总结:磁控管是一种利用磁场和电场相互作用产生微波能量的设备。
它通过控制磁场和电场的强度,实现电子的加速和微波的产生。
磁控管工作原理
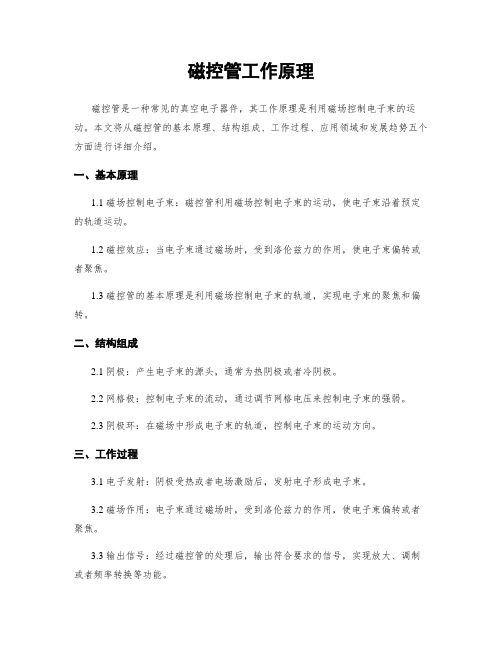
磁控管工作原理磁控管是一种常见的真空电子器件,其工作原理是利用磁场控制电子束的运动。
本文将从磁控管的基本原理、结构组成、工作过程、应用领域和发展趋势五个方面进行详细介绍。
一、基本原理1.1 磁场控制电子束:磁控管利用磁场控制电子束的运动,使电子束沿着预定的轨道运动。
1.2 磁控效应:当电子束通过磁场时,受到洛伦兹力的作用,使电子束偏转或者聚焦。
1.3 磁控管的基本原理是利用磁场控制电子束的轨道,实现电子束的聚焦和偏转。
二、结构组成2.1 阴极:产生电子束的源头,通常为热阴极或者冷阴极。
2.2 网格极:控制电子束的流动,通过调节网格电压来控制电子束的强弱。
2.3 阴极环:在磁场中形成电子束的轨道,控制电子束的运动方向。
三、工作过程3.1 电子发射:阴极受热或者电场激励后,发射电子形成电子束。
3.2 磁场作用:电子束通过磁场时,受到洛伦兹力的作用,使电子束偏转或者聚焦。
3.3 输出信号:经过磁控管的处理后,输出符合要求的信号,实现放大、调制或者频率转换等功能。
四、应用领域4.1 通信领域:磁控管广泛应用于射频放大器、频率变换器等通信设备中。
4.2 医疗领域:磁控管用于医疗成像设备中,如X射线机、CT机等。
4.3 工业领域:磁控管在激光器、等离子体喷涂设备等工业设备中也有应用。
五、发展趋势5.1 微型化:随着技术的不断发展,磁控管逐渐向微型化方向发展,体积更小、功耗更低。
5.2 集成化:磁控管与其他器件的集成化程度越来越高,功能更加多样化。
5.3 高性能:磁控管的性能不断提升,工作频率更广、输出功率更大、稳定性更高。
总结:磁控管作为一种重要的真空电子器件,其工作原理基于磁场控制电子束的运动。
通过对磁控管的基本原理、结构组成、工作过程、应用领域和发展趋势的介绍,我们可以更全面地了解磁控管在各个领域的应用及未来发展方向。
磁控管工作原理
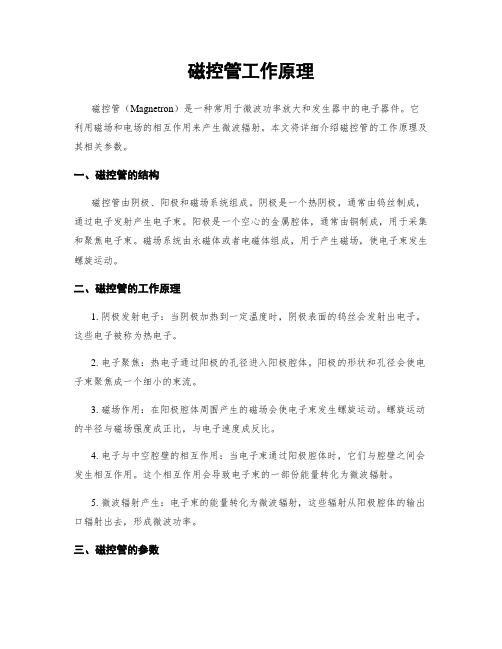
磁控管工作原理磁控管(Magnetron)是一种常用于微波功率放大和发生器中的电子器件。
它利用磁场和电场的相互作用来产生微波辐射。
本文将详细介绍磁控管的工作原理及其相关参数。
一、磁控管的结构磁控管由阴极、阳极和磁场系统组成。
阴极是一个热阴极,通常由钨丝制成,通过电子发射产生电子束。
阳极是一个空心的金属腔体,通常由铜制成,用于采集和聚焦电子束。
磁场系统由永磁体或者电磁体组成,用于产生磁场,使电子束发生螺旋运动。
二、磁控管的工作原理1. 阴极发射电子:当阴极加热到一定温度时,阴极表面的钨丝会发射出电子。
这些电子被称为热电子。
2. 电子聚焦:热电子通过阳极的孔径进入阳极腔体。
阳极的形状和孔径会使电子束聚焦成一个细小的束流。
3. 磁场作用:在阳极腔体周围产生的磁场会使电子束发生螺旋运动。
螺旋运动的半径与磁场强度成正比,与电子速度成反比。
4. 电子与中空腔壁的相互作用:当电子束通过阳极腔体时,它们与腔壁之间会发生相互作用。
这个相互作用会导致电子束的一部份能量转化为微波辐射。
5. 微波辐射产生:电子束的能量转化为微波辐射,这些辐射从阳极腔体的输出口辐射出去,形成微波功率。
三、磁控管的参数1. 阴极电流(Cathode Current):指通过阴极的电流。
阴极电流的大小决定了电子束的强度。
2. 阳极电压(Anode Voltage):指施加在阳极上的电压。
阳极电压的大小决定了电子束的速度。
3. 磁场强度(Magnetic Field Strength):指磁场的强度。
磁场强度的大小决定了电子束的螺旋半径。
4. 谐振频率(Resonant Frequency):指磁控管在特定工作模式下的输出频率。
谐振频率取决于磁场强度和电子束的速度。
5. 输出功率(Output Power):指磁控管输出的微波功率。
输出功率的大小与阴极电流和阳极电压有关。
四、磁控管的应用磁控管广泛应用于微波通信、雷达系统、微波炉等领域。
在微波通信中,磁控管用于放大微波信号,提高通信的传输距离和质量。
磁控管工作原理
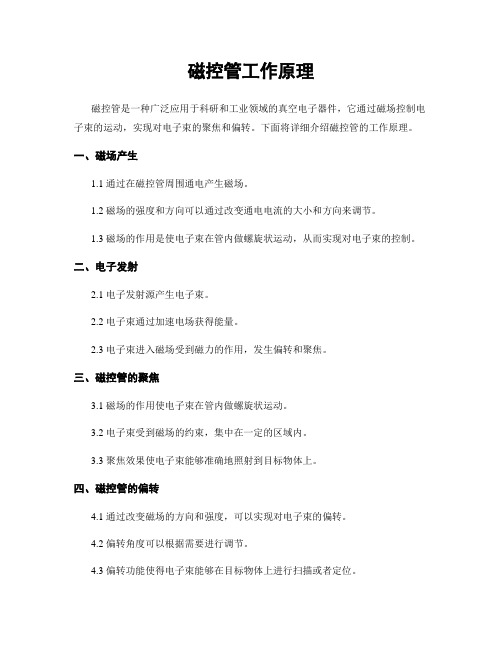
磁控管工作原理
磁控管是一种广泛应用于科研和工业领域的真空电子器件,它通过磁场控制电子束的运动,实现对电子束的聚焦和偏转。
下面将详细介绍磁控管的工作原理。
一、磁场产生
1.1 通过在磁控管周围通电产生磁场。
1.2 磁场的强度和方向可以通过改变通电电流的大小和方向来调节。
1.3 磁场的作用是使电子束在管内做螺旋状运动,从而实现对电子束的控制。
二、电子发射
2.1 电子发射源产生电子束。
2.2 电子束通过加速电场获得能量。
2.3 电子束进入磁场受到磁力的作用,发生偏转和聚焦。
三、磁控管的聚焦
3.1 磁场的作用使电子束在管内做螺旋状运动。
3.2 电子束受到磁场的约束,集中在一定的区域内。
3.3 聚焦效果使电子束能够准确地照射到目标物体上。
四、磁控管的偏转
4.1 通过改变磁场的方向和强度,可以实现对电子束的偏转。
4.2 偏转角度可以根据需要进行调节。
4.3 偏转功能使得电子束能够在目标物体上进行扫描或者定位。
五、应用领域
5.1 磁控管广泛应用于电子显微镜、电视机、激光打印机等领域。
5.2 在科研领域,磁控管也被用于粒子加速器和核物理实验中。
5.3 磁控管的工作原理为现代科技的发展提供了重要支持,具有广阔的应用前景。
总结:磁控管通过磁场控制电子束的运动,实现对电子束的聚焦和偏转,广泛应用于科研和工业领域。
深入了解磁控管的工作原理,有助于更好地理解其在现代科技中的重要作用。
磁控管的工作原理
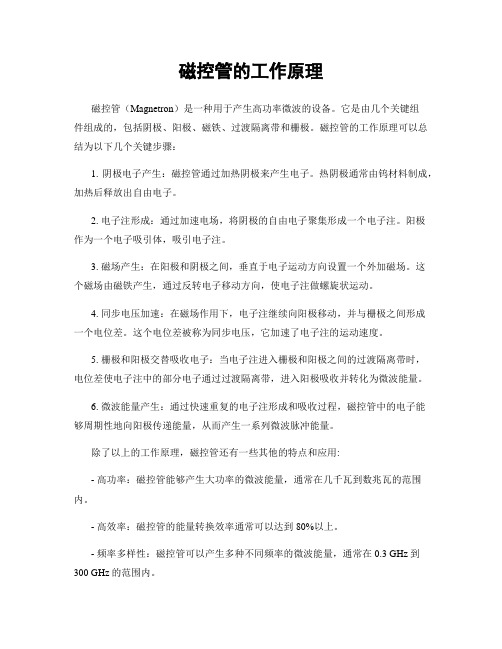
磁控管的工作原理磁控管(Magnetron)是一种用于产生高功率微波的设备。
它是由几个关键组件组成的,包括阴极、阳极、磁铁、过渡隔离带和栅极。
磁控管的工作原理可以总结为以下几个关键步骤:1. 阴极电子产生:磁控管通过加热阴极来产生电子。
热阴极通常由钨材料制成,加热后释放出自由电子。
2. 电子注形成:通过加速电场,将阴极的自由电子聚集形成一个电子注。
阳极作为一个电子吸引体,吸引电子注。
3. 磁场产生:在阳极和阴极之间,垂直于电子运动方向设置一个外加磁场。
这个磁场由磁铁产生,通过反转电子移动方向,使电子注做螺旋状运动。
4. 同步电压加速:在磁场作用下,电子注继续向阳极移动,并与栅极之间形成一个电位差。
这个电位差被称为同步电压,它加速了电子注的运动速度。
5. 栅极和阳极交替吸收电子:当电子注进入栅极和阳极之间的过渡隔离带时,电位差使电子注中的部分电子通过过渡隔离带,进入阳极吸收并转化为微波能量。
6. 微波能量产生:通过快速重复的电子注形成和吸收过程,磁控管中的电子能够周期性地向阳极传递能量,从而产生一系列微波脉冲能量。
除了以上的工作原理,磁控管还有一些其他的特点和应用:- 高功率:磁控管能够产生大功率的微波能量,通常在几千瓦到数兆瓦的范围内。
- 高效率:磁控管的能量转换效率通常可以达到80%以上。
- 频率多样性:磁控管可以产生多种不同频率的微波能量,通常在0.3 GHz到300 GHz的范围内。
- 广泛应用:磁控管在通信、雷达、微波烹饪、医学、科研等领域都有广泛的应用。
- 信号稳定性:磁控管能够产生稳定的微波信号,用于无线通信和雷达系统等。
总结起来,磁控管是一种用于产生高功率微波的关键设备。
它通过利用加热阴极产生电子,形成电子注,并在磁场和同步电压的作用下加速电子运动,并通过交替的吸收和释放电子能量来产生微波能量。
磁控管具有功率高、效率高、频率多样、应用广泛以及信号稳定等特点。
这使得磁控管在通信、雷达、微波烹饪和医学等领域都有重要的应用价值。
磁控管工作原理
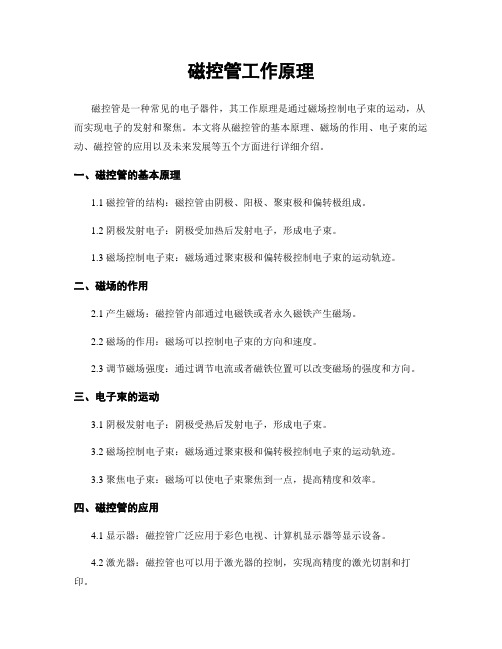
磁控管工作原理磁控管是一种常见的电子器件,其工作原理是通过磁场控制电子束的运动,从而实现电子的发射和聚焦。
本文将从磁控管的基本原理、磁场的作用、电子束的运动、磁控管的应用以及未来发展等五个方面进行详细介绍。
一、磁控管的基本原理1.1 磁控管的结构:磁控管由阴极、阳极、聚束极和偏转极组成。
1.2 阴极发射电子:阴极受加热后发射电子,形成电子束。
1.3 磁场控制电子束:磁场通过聚束极和偏转极控制电子束的运动轨迹。
二、磁场的作用2.1 产生磁场:磁控管内部通过电磁铁或者永久磁铁产生磁场。
2.2 磁场的作用:磁场可以控制电子束的方向和速度。
2.3 调节磁场强度:通过调节电流或者磁铁位置可以改变磁场的强度和方向。
三、电子束的运动3.1 阴极发射电子:阴极受热后发射电子,形成电子束。
3.2 磁场控制电子束:磁场通过聚束极和偏转极控制电子束的运动轨迹。
3.3 聚焦电子束:磁场可以使电子束聚焦到一点,提高精度和效率。
四、磁控管的应用4.1 显示器:磁控管广泛应用于彩色电视、计算机显示器等显示设备。
4.2 激光器:磁控管也可以用于激光器的控制,实现高精度的激光切割和打印。
4.3 医疗设备:磁控管在医疗成像设备中也有重要应用,如X射线机等。
五、未来发展5.1 磁控管的小型化:随着技术的发展,磁控管的尺寸将越来越小,应用范围也将更广泛。
5.2 高性能磁控管:未来的磁控管将具有更高的性能,如更快的响应速度和更高的分辨率。
5.3 新型应用领域:磁控管将在无人驾驶、虚拟现实等新兴领域得到更广泛的应用。
总结:磁控管作为一种重要的电子器件,通过磁场控制电子束的运动实现各种功能。
未来随着技术的发展,磁控管将在更多领域发挥重要作用,为人类生活带来更多便利和创新。
磁控管工作原理

磁控管工作原理
磁控管(Magnetron)是一种常用的微波发生器,广泛应用于微波炉、雷达、通信设备等领域。
它通过利用电子束与磁场的相互作用来产生微波辐射。
下面将详细介绍磁控管的工作原理。
1. 电子发射:磁控管中的发射极(阴极)通过加热或直接电子发射的方式产生自由电子。
这些自由电子被称为电子云。
2. 磁场引导:磁控管中的磁场由一个或多个永久磁铁或电磁铁产生。
这个磁场的作用是将电子束引导到特定的区域。
3. 电子束形成:磁场的作用下,电子云被束缚成一个密集的电子束。
磁控管中通常有一个中心的阳极,该阳极具有孔洞,只允许电子束通过。
4. 高频场形成:在磁控管的阳极周围,有一个腔体,该腔体被称为振荡腔。
振荡腔是一个封闭的空腔,内部有一定的介质,如空气或气体。
当电子束通过阳极孔洞时,它与振荡腔中的介质发生相互作用,产生高频电磁场。
5. 微波辐射:高频电磁场在振荡腔中反复振荡,形成微波辐射。
这些微波辐射通过振荡腔的出口传播出去,用于实现特定的应用。
磁控管的工作原理可以总结为:通过磁场引导电子束,使其通过阳极孔洞,与振荡腔中的介质相互作用,产生高频电磁场,最终形成微波辐射。
磁控管的优点包括:高功率输出、高效率、频率稳定、寿命长等。
然而,磁控管也存在一些缺点,如体积较大、重量较重、制造成本较高等。
总之,磁控管作为一种重要的微波发生器,通过电子束与磁场的相互作用来产生微波辐射。
它的工作原理简单明了,但在实际应用中起到了关键的作用。
磁控管工作原理
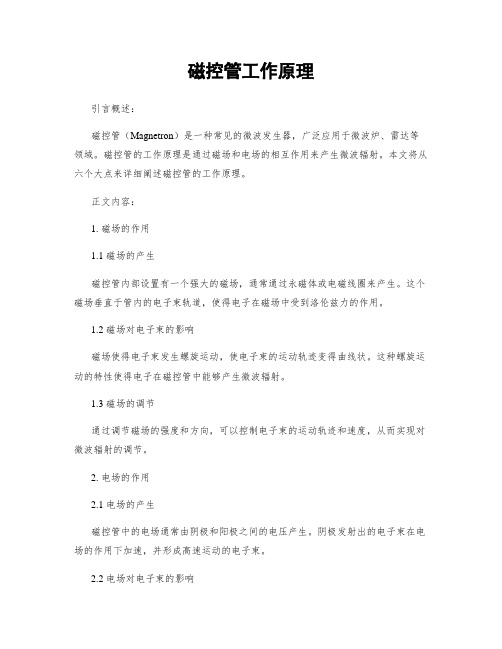
磁控管工作原理引言概述:磁控管(Magnetron)是一种常见的微波发生器,广泛应用于微波炉、雷达等领域。
磁控管的工作原理是通过磁场和电场的相互作用来产生微波辐射。
本文将从六个大点来详细阐述磁控管的工作原理。
正文内容:1. 磁场的作用1.1 磁场的产生磁控管内部设置有一个强大的磁场,通常通过永磁体或电磁线圈来产生。
这个磁场垂直于管内的电子束轨道,使得电子在磁场中受到洛伦兹力的作用。
1.2 磁场对电子束的影响磁场使得电子束发生螺旋运动,使电子束的运动轨迹变得曲线状。
这种螺旋运动的特性使得电子在磁控管中能够产生微波辐射。
1.3 磁场的调节通过调节磁场的强度和方向,可以控制电子束的运动轨迹和速度,从而实现对微波辐射的调节。
2. 电场的作用2.1 电场的产生磁控管中的电场通常由阴极和阳极之间的电压产生。
阴极发射出的电子束在电场的作用下加速,并形成高速运动的电子束。
2.2 电场对电子束的影响电场加速电子束的运动速度,使得电子束能够具有足够的能量来产生微波辐射。
同时,电场也控制着电子束的密度和形状。
2.3 电场的调节通过调节电场的电压和极性,可以控制电子束的速度和能量,进而调节微波辐射的频率和功率。
3. 电子束的形成3.1 阴极发射电子阴极通常由热阴极或冷阴极组成,通过热或电子轰击的方式发射出电子。
这些电子被称为电子束的前体。
3.2 电子束的聚束通过磁场和电场的作用,电子束的前体被聚束成为一个紧凑的束流,使得电子能够集中在一个小的空间范围内。
3.3 电子束的加速电子束在电场的作用下被加速,增加了电子的能量,为产生微波辐射提供了必要的条件。
4. 微波辐射的产生4.1 电子束的运动经过磁场和电场的作用,电子束以高速螺旋运动的方式在磁控管内运动。
4.2 电子束的辐射电子束的螺旋运动导致电子在磁场中不断加速和减速,产生了高频的电磁辐射,即微波辐射。
4.3 微波辐射的特性微波辐射具有较高的频率和能量,能够在空间中传播,并被接收器接收和利用。
磁控管工作原理
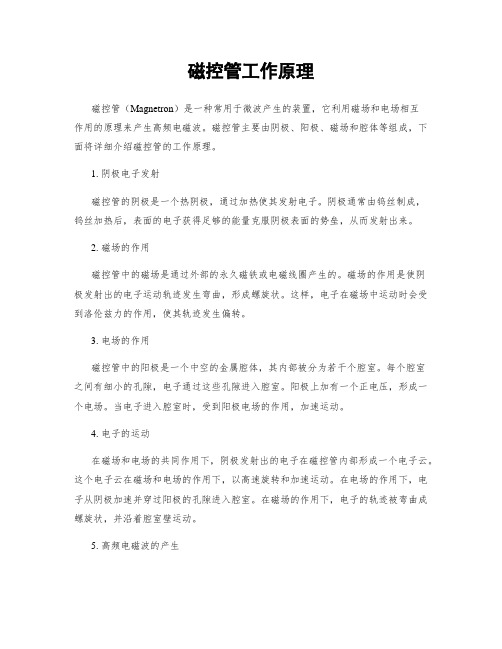
磁控管工作原理磁控管(Magnetron)是一种常用于微波产生的装置,它利用磁场和电场相互作用的原理来产生高频电磁波。
磁控管主要由阴极、阳极、磁场和腔体等组成,下面将详细介绍磁控管的工作原理。
1. 阴极电子发射磁控管的阴极是一个热阴极,通过加热使其发射电子。
阴极通常由钨丝制成,钨丝加热后,表面的电子获得足够的能量克服阴极表面的势垒,从而发射出来。
2. 磁场的作用磁控管中的磁场是通过外部的永久磁铁或电磁线圈产生的。
磁场的作用是使阴极发射出的电子运动轨迹发生弯曲,形成螺旋状。
这样,电子在磁场中运动时会受到洛伦兹力的作用,使其轨迹发生偏转。
3. 电场的作用磁控管中的阳极是一个中空的金属腔体,其内部被分为若干个腔室。
每个腔室之间有细小的孔隙,电子通过这些孔隙进入腔室。
阳极上加有一个正电压,形成一个电场。
当电子进入腔室时,受到阳极电场的作用,加速运动。
4. 电子的运动在磁场和电场的共同作用下,阴极发射出的电子在磁控管内部形成一个电子云。
这个电子云在磁场和电场的作用下,以高速旋转和加速运动。
在电场的作用下,电子从阴极加速并穿过阳极的孔隙进入腔室。
在磁场的作用下,电子的轨迹被弯曲成螺旋状,并沿着腔室壁运动。
5. 高频电磁波的产生当电子在磁场和电场的作用下运动时,它们会与腔室壁产生相互作用。
这个相互作用会导致电子云中的电子释放出能量,产生高频电磁波。
这些电磁波通过腔室的孔隙传播出来,形成微波。
总结:磁控管通过利用磁场和电场的相互作用来产生高频电磁波。
阴极发射出的电子在磁场的作用下形成螺旋状轨迹,并在电场的作用下加速运动。
电子与腔室壁的相互作用导致电子释放能量,产生高频电磁波。
磁控管的工作原理是基于这些物理原理的。
通过合理设计磁场和电场的参数,可以实现对微波的产生和控制,使磁控管在通信、雷达、微波炉等领域得到广泛应用。
磁控管工作原理
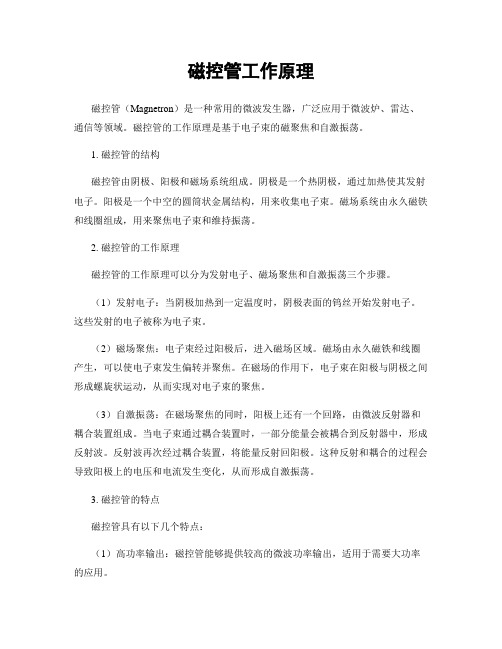
磁控管工作原理磁控管(Magnetron)是一种常用的微波发生器,广泛应用于微波炉、雷达、通信等领域。
磁控管的工作原理是基于电子束的磁聚焦和自激振荡。
1. 磁控管的结构磁控管由阴极、阳极和磁场系统组成。
阴极是一个热阴极,通过加热使其发射电子。
阳极是一个中空的圆筒状金属结构,用来收集电子束。
磁场系统由永久磁铁和线圈组成,用来聚焦电子束和维持振荡。
2. 磁控管的工作原理磁控管的工作原理可以分为发射电子、磁场聚焦和自激振荡三个步骤。
(1)发射电子:当阴极加热到一定温度时,阴极表面的钨丝开始发射电子。
这些发射的电子被称为电子束。
(2)磁场聚焦:电子束经过阳极后,进入磁场区域。
磁场由永久磁铁和线圈产生,可以使电子束发生偏转并聚焦。
在磁场的作用下,电子束在阳极与阴极之间形成螺旋状运动,从而实现对电子束的聚焦。
(3)自激振荡:在磁场聚焦的同时,阳极上还有一个回路,由微波反射器和耦合装置组成。
当电子束通过耦合装置时,一部分能量会被耦合到反射器中,形成反射波。
反射波再次经过耦合装置,将能量反射回阳极。
这种反射和耦合的过程会导致阳极上的电压和电流发生变化,从而形成自激振荡。
3. 磁控管的特点磁控管具有以下几个特点:(1)高功率输出:磁控管能够提供较高的微波功率输出,适用于需要大功率的应用。
(2)频率稳定性:磁控管的频率稳定性较好,适用于需要高精度的频率要求。
(3)可调性:通过调节磁场的强度和电源电压,可以实现对磁控管的频率和功率的调节。
(4)寿命长:磁控管的寿命较长,能够稳定工作数千小时。
4. 应用领域磁控管广泛应用于以下领域:(1)微波炉:磁控管是微波炉的核心部件,通过产生微波来加热食物。
(2)雷达:磁控管用于雷达系统中的发射和接收微波信号。
(3)通信:磁控管用于通信系统中的信号发射和接收。
(4)科学研究:磁控管在科学实验中也有广泛的应用,如等离子体研究、材料表面处理等。
总结:磁控管是一种常用的微波发生器,通过电子束的磁聚焦和自激振荡实现微波的产生。
磁控管工作原理
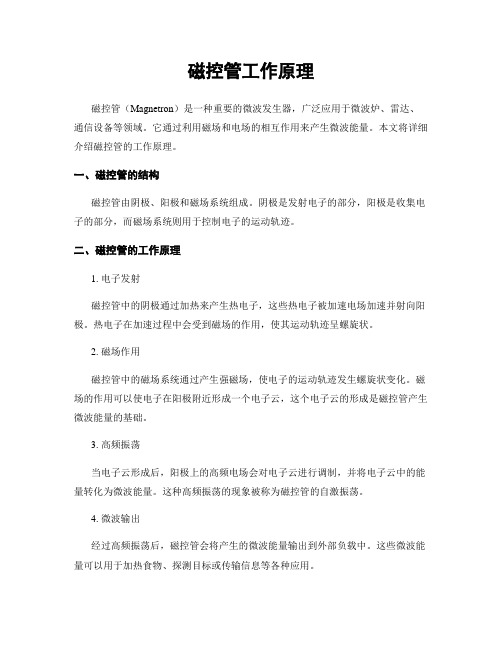
磁控管工作原理磁控管(Magnetron)是一种重要的微波发生器,广泛应用于微波炉、雷达、通信设备等领域。
它通过利用磁场和电场的相互作用来产生微波能量。
本文将详细介绍磁控管的工作原理。
一、磁控管的结构磁控管由阴极、阳极和磁场系统组成。
阴极是发射电子的部分,阳极是收集电子的部分,而磁场系统则用于控制电子的运动轨迹。
二、磁控管的工作原理1. 电子发射磁控管中的阴极通过加热来产生热电子,这些热电子被加速电场加速并射向阳极。
热电子在加速过程中会受到磁场的作用,使其运动轨迹呈螺旋状。
2. 磁场作用磁控管中的磁场系统通过产生强磁场,使电子的运动轨迹发生螺旋状变化。
磁场的作用可以使电子在阳极附近形成一个电子云,这个电子云的形成是磁控管产生微波能量的基础。
3. 高频振荡当电子云形成后,阳极上的高频电场会对电子云进行调制,并将电子云中的能量转化为微波能量。
这种高频振荡的现象被称为磁控管的自激振荡。
4. 微波输出经过高频振荡后,磁控管会将产生的微波能量输出到外部负载中。
这些微波能量可以用于加热食物、探测目标或传输信息等各种应用。
三、磁控管的特点1. 高功率输出:磁控管能够输出较高功率的微波能量,使其在各种应用中具有广泛的用途。
2. 高效率:磁控管的工作效率较高,能够将电能转化为微波能量。
3. 长寿命:磁控管的结构简单,使用寿命较长。
4. 可靠性:磁控管在工作过程中稳定可靠,能够长时间保持高频振荡。
四、磁控管的应用1. 微波炉:磁控管作为微波炉的核心部件,能够产生高功率的微波能量,用于食物的加热和烹饪。
2. 雷达系统:磁控管在雷达系统中被用作发射器,产生微波能量用于目标探测和跟踪。
3. 通信设备:磁控管在通信设备中被用作信号发生器,产生微波信号用于信息传输。
4. 医疗设备:磁控管在医疗设备中被用于产生微波能量,如医疗热疗设备等。
总结:磁控管是一种利用磁场和电场相互作用产生微波能量的重要设备。
它通过电子发射、磁场作用、高频振荡和微波输出等过程来实现工作。
磁控管工作原理
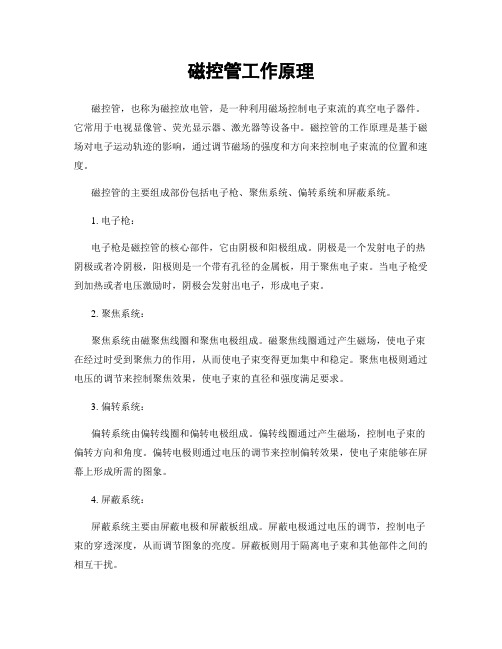
磁控管工作原理磁控管,也称为磁控放电管,是一种利用磁场控制电子束流的真空电子器件。
它常用于电视显像管、荧光显示器、激光器等设备中。
磁控管的工作原理是基于磁场对电子运动轨迹的影响,通过调节磁场的强度和方向来控制电子束流的位置和速度。
磁控管的主要组成部份包括电子枪、聚焦系统、偏转系统和屏蔽系统。
1. 电子枪:电子枪是磁控管的核心部件,它由阴极和阳极组成。
阴极是一个发射电子的热阴极或者冷阴极,阳极则是一个带有孔径的金属板,用于聚焦电子束。
当电子枪受到加热或者电压激励时,阴极会发射出电子,形成电子束。
2. 聚焦系统:聚焦系统由磁聚焦线圈和聚焦电极组成。
磁聚焦线圈通过产生磁场,使电子束在经过时受到聚焦力的作用,从而使电子束变得更加集中和稳定。
聚焦电极则通过电压的调节来控制聚焦效果,使电子束的直径和强度满足要求。
3. 偏转系统:偏转系统由偏转线圈和偏转电极组成。
偏转线圈通过产生磁场,控制电子束的偏转方向和角度。
偏转电极则通过电压的调节来控制偏转效果,使电子束能够在屏幕上形成所需的图象。
4. 屏蔽系统:屏蔽系统主要由屏蔽电极和屏蔽板组成。
屏蔽电极通过电压的调节,控制电子束的穿透深度,从而调节图象的亮度。
屏蔽板则用于隔离电子束和其他部件之间的相互干扰。
磁控管的工作过程如下:1. 电子发射:当磁控管通电后,阴极开始发射电子。
这些电子经过电子枪的聚焦系统,形成一个集中的电子束。
2. 磁场控制:磁聚焦线圈和偏转线圈产生的磁场分别对电子束进行聚焦和偏转。
聚焦磁场使电子束变得更加集中,而偏转磁场则控制电子束的偏转方向和角度。
3. 屏蔽控制:屏蔽电极和屏蔽板通过电压的调节,控制电子束的穿透深度和图象亮度。
4. 显示效果:经过聚焦、偏转和屏蔽的控制,电子束最终在屏幕上形成所需的图象。
总结:磁控管是一种利用磁场控制电子束流的真空电子器件。
它通过电子枪、聚焦系统、偏转系统和屏蔽系统的协同工作,实现对电子束的位置、速度和亮度的精确控制。
磁控管工作原理
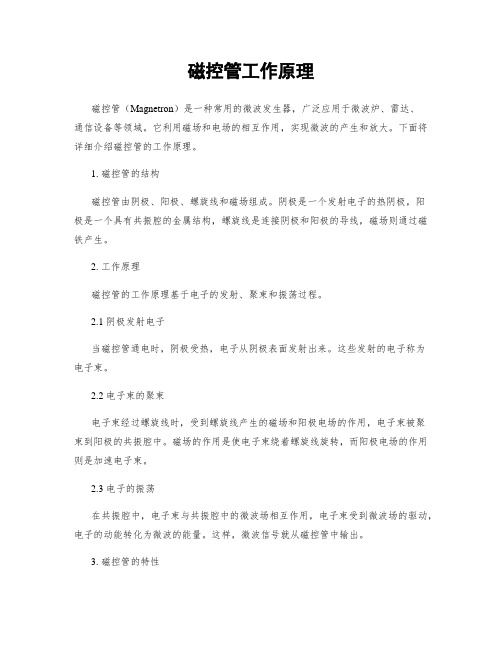
磁控管工作原理磁控管(Magnetron)是一种常用的微波发生器,广泛应用于微波炉、雷达、通信设备等领域。
它利用磁场和电场的相互作用,实现微波的产生和放大。
下面将详细介绍磁控管的工作原理。
1. 磁控管的结构磁控管由阴极、阳极、螺旋线和磁场组成。
阴极是一个发射电子的热阴极,阳极是一个具有共振腔的金属结构,螺旋线是连接阴极和阳极的导线,磁场则通过磁铁产生。
2. 工作原理磁控管的工作原理基于电子的发射、聚束和振荡过程。
2.1 阴极发射电子当磁控管通电时,阴极受热,电子从阴极表面发射出来。
这些发射的电子称为电子束。
2.2 电子束的聚束电子束经过螺旋线时,受到螺旋线产生的磁场和阳极电场的作用,电子束被聚束到阳极的共振腔中。
磁场的作用是使电子束绕着螺旋线旋转,而阳极电场的作用则是加速电子束。
2.3 电子的振荡在共振腔中,电子束与共振腔中的微波场相互作用,电子束受到微波场的驱动,电子的动能转化为微波的能量。
这样,微波信号就从磁控管中输出。
3. 磁控管的特性磁控管具有以下几个特性:3.1 高功率输出磁控管能够输出高功率的微波信号,这使得它在雷达和通信设备等领域得到广泛应用。
3.2 高效率磁控管具有高效率的特点,能够将电子束转化为微波能量。
3.3 频率稳定性磁控管具有较好的频率稳定性,能够在一定范围内保持稳定的工作频率。
3.4 宽带性能磁控管具有较宽的工作频率范围,能够满足不同应用场景的需求。
4. 应用领域磁控管广泛应用于以下领域:4.1 微波炉磁控管是微波炉的核心部件,通过产生微波能量,使食物加热。
4.2 雷达磁控管在雷达系统中用于产生和放大微波信号,实现目标探测和跟踪。
4.3 通信设备磁控管在通信设备中用于产生和放大微波信号,实现信号传输和接收。
4.4 医学设备磁控管在医学设备中用于产生和放大微波信号,如医学成像设备等。
总结:磁控管是一种利用磁场和电场相互作用产生和放大微波信号的器件。
它通过阴极发射电子,经过螺旋线的聚束和共振腔中的振荡,将电子的动能转化为微波的能量。
- 1、下载文档前请自行甄别文档内容的完整性,平台不提供额外的编辑、内容补充、找答案等附加服务。
- 2、"仅部分预览"的文档,不可在线预览部分如存在完整性等问题,可反馈申请退款(可完整预览的文档不适用该条件!)。
- 3、如文档侵犯您的权益,请联系客服反馈,我们会尽快为您处理(人工客服工作时间:9:00-18:30)。
Theory of OperationA magnetron is a high power microwave oscillator in which thepotential energy of an electron cloud near the cathode is convertedinto r.f. energy in a series of cavity resonators similar to the oneshown in Figure 1. As depicted by the low frequency analog, therear wall of the structure may be considered the inductive portion,and the vane tip region the capacitor portion of the equivalentresonant circuit. The resonant frequency of a microwave cavity isthereby determined by the physical dimension of the resonatortogether with the reactive effect of any perturbations to theinductive or capacitive portion of the equivalent circuit. This is animportant point and will be recalled later.In order to sustain oscillations in a resonant circuit, it is necessaryto continuously input energy in the correct phase. Referring to Figure 2, if the instantaneous r.f. field, due to steady state oscillations in the resonator, is in the direction shown, and, an electron with velocity was to travel through the r.f. field such that the r.f. field retarded the electron velocity by an amount, the decrease in electron energy will be exactly offset by an increase in the r.f. field strength.In a magnetron, the source of electrons is a heated cathode located on theaxis of an anode structure containing a number of microwave resonators.See Figure 3.Electrons leave the cathode and are accelerated toward the anode, due tothe dc field established by the voltage source E. The presence of a strongmagnetic field B in the region between cathode and anode produces aforce on each electron which is mutually perpendicular to the dc fieldand the electron velocity vectors, thereby causing the electrons to spiralaway from the cathode in paths of varying curvature, depending uponthe initial electron velocity a the time it leaves the cathode.As this cloud of electrons approaches the anode, it falls under theinfluence of the r.f. fields at the vane tips, and electrons will either be retarded in velocity, if they happen to face an opposing r.f.field, or accelerated if they are in the vicinity of an aiding r.f. field. Since the force on an electron due to the magnetic field B is proportional to the electron velocity through the field, the retarded velocity electrons will experience less "curling force" and will therefore drift toward the anode, while the accelerated velocity electrons willcurl back away from the anode.The result is an automatic collection of electron "spokes" as the cloud nears theanode (see Figure 4), with each spoke located at a resonator having an opposingr.f. field.On the next half cycle of r.f. oscillation, the r.f. field pattern will havereversed polarity and the spoke pattern will rotate to maintain its presence in anopposing field.The "automatic" synchronism between the electron spoke pattern and the r.f.field polarity in a crossed field device allows a magnetron to maintain relativelystable operation over a wide range of applied input parameters. For example, amagnetron designed for an output power of 200 kw peak will operate quite wellat 100 kw peak output by simply reducing the modulator drive level.Magnetron Theory of Operation150 Sohier RoadBeverly, Massachusetts 01915Phone: 978-922-6000 Fax: 978-922-2736E-Mail: marketing@Internet: /bmdYou will note that the instantaneous r.f. field pattern, shown in Figure 4, has exactly 180o of phase change (radians) between every adjacent pair of resonator vanes and is therefore called the mode. Other oscillation patterns (modes) could be supported by the anode structure; however, the mode pattern will product the maximum number of electron spokes, and therefore the maximum transfer of energy to the r.f. field, i.e., highest efficiency mode. Assuring that the magnetron maintains mode oscillation, to the exclusion of all other modes, is one of the prime concerns of the magnetron designer.The mode controlling techniques in a conventional magnetron, e.g.,electrically connecting alternate vane tips together to assure identicalpotential, employing geometrical similarities between alternateresonators to favor mode oscillation, will adequately maintain modecontrol in conventional magnetron anodes. Due to mode separationparameters, the number of resonators in conventional magnetron anodesis limited and rarely exceeds 20 resonator vanes. Since the physical sizeof each resonator is fixed by the desired output frequency, the overallsize of the anode is limited, thereby restricting cathode dimensions andheat dissipation capacity. The result is that at higher frequencies theconventional magnetron has reduced power output capability, lowerreliability and a shorter operating lifetime than can be realized at thelower microwave frequencies.The distinguishing feature of the coaxial magnetron is the presence of a high Q stabilizing cavity between the anode and the output waveguide.The theory of operation presented for a conventional magnetron applies equally to the anode-cathode region of the coaxial structure. However, the coaxial stabilizing cavity affords very significant improvements in overall magnetron performance.Superior mode control: Operating the cavity in the TE011 mode, and slot coupling alternate anode resonators to the cavity, produces anode control of such intensity as to permit the construction of coaxial magnetrons with many times the number of resonators that can be employed in a conventional type magnetron. This means lower cathode emission density, lower life and higher reliability.Reduced RF fields in the anode: Whereas all stored energy in a conventional is confined to the vane resonators, in a coaxial magnetron approximately 85% of the total stored energy is contained in the stabilizing cavity. This means reduced r.f. field intensity at the vane tips, and less tendency to arcing.Improved frequency stability: Theredistribution of stored energy in the coaxialmagnetron makes the high Q stabilizing cavitythe prime determiner of magnetron outputfrequency. This means a lower pushing figure, alower pulling figure, improved spectrum andreduced spurious emissions.Improved tuning: In the conventionalmagnetron, tuning is accomplished by insertinginductive pins in the rear portion of eachresonator, or by capacitive loading in the vane tipregion.Both techniques represent an adverse perturbationto the natural geometry of the resonators whichoften results in power output variation withtuning, starting instabilities, increasedsusceptibility to arcing and a generally reducedoperating lifetime for the magnetron. In contrastthe coaxial magnetron is tuned by moving anoncontacting plunger in the stabilizing cavity(see Figure 5). The result is a tuning characteristic with no discontinuities, broad tunable bandwidth, and none of the disadvantages resulting from perturbations in the anode-cathode region.Typical Magnetron ParametersThe following is a discussion and explanation of typical magnetron specification parameters.Thermal DriftAt the time high voltage is first applied to a magnetron,the thermal equilibrium of the device is suddenlyaltered. The anode vanes being to heat at the tips due toelectron bombardment and the entire anode/cathodestructure undergoes a transient change in thermalprofile. During the time required for each part of themagnetron to stabilize at its normal operatingtemperature, the output frequency of the magnetron will"drift." The curve of output frequency vs. time duringthe period following initial turn on is called the"Thermal Drift" curve. Generally speaking, themaximum drift occurs during the first few minutes afterturn on, and slowly approaches equilibrium over a period ranging from 10 to 30 minutes depending upon the structure mass, power output, type of cooling and basic magnetron design. Thermal drift curves across a variety of magnetron types operating at the same frequency and output power may differ radically from each other. Each type is usually designed for a particular purpose and subtle differences in the internal magnetron configuration can produce radical differences in the thermal drift curve.It should be noted that a thermal drift effect will occur not only at initial turn-on, but whenever the peak or average input power to the magnetron is changed, e.g., a change of pulse duration, PRF or duty. Figure 7 shows typical thermal drift curves for a particular magnetron plotted as a function of duty. The dotted line indicates the effect of a change in duty from .001 to .0005 after thermal equilibrium has been initially achieved.Temperature CoefficientAfter the thermal drift period has expired and a stable operating frequency has been achieved, changes to ambient conditions which cause a corresponding change in the magnetron temperature will produce a change in the output frequency. In this content ambient changes include cooling air temperature or pressure in air cooled magnetrons; mounting plate temperature in heat sink cooled magnetrons; and flow rate or temperature in liquid cooled magnetrons.The change in magnetron output frequency for each degree change in body temperature, as measured at a specified point on the outside surface of the magnetron body, is defined as the Temperature Coefficient for the magnetron and is usually expressed in MHz/o C. For most magnetrons the temperature coefficient is a negative (frequency decreases as temperature increases) and is essentially constant over the operating range of the magnetron.When estimating magnetron frequency change due to temperature coefficient, keep in mind that the temperature coefficient relates magnetron frequency to body temperature and there is not necessarily a 1:1 relation between body temperature and, for example, ambient air temperature. In addition, for airborne systems, the cooling effect of lower air temperature at altitude may offset by a corresponding reduction in air density.Pushing FigureThe pushing figure of a magnetron is defined as the change in magnetron frequency due to a change in the peak cathode current. Referring back to the earlier theory discussion, we noted that the resonant frequency of a vane resonator is determined by its mechanical dimensions plus the reactive effect of any perturbation. The presence of electrons in the vicinity of the vane tips affects the equivalent capacitance of the resonator by an amount proportional to the density of the electrons and,since electron density is similarly related to peak pulse current, changes in pulse current level will produce changes in output frequency. The pushing figure expressed in MHz/Amp is represented by the slope of a frequency vs. peak current curve plotted for a particular magnetron typeFrom the curve of Figure 8, it can be seen that the slope is not a constant over the full range of operating current. It is therefore meaningless to talk about a specific value for the pushing figure unless one also specifies the range of peak current over which it appliesIt should be noted that since power output is proportional to peak current in a magnetron, the pushing figure at peak current levels well below the normal operating point of the magnetron are usually unimportant because the power output at these current levels is low.The primary importance of a low pushing figure near the magnetronoperating point is that the pushing figure will determine intrapulse FM,and thereby will affect the spectral quality of the transmitting pulse.The Pulling Figure is defined as the maximum change in output frequencythat results when an external, fixed amplitude mismatch, located in theoutput waveguide, is moved through a distance of one half wavelengthrelative to the magnetron. Stated somewhat less formally, the pullingfigure is a measure of a magnetron's ability to maintain a constant outputfrequency against changes in load mismatch.During the design of a magnetron, the degree to which the outputwaveguide is electrically coupled to the internal resonator structure isselected to optimize certain performance parameters. Strong couplingincreases output power and efficiency but also increases time jitter andsensitivity to changes to load mismatch. Generally, the coupling is chosento obtain the best compromise between efficiency and stability.Depending upon the phase relation between incident and reflected power at the output port of a magnetron, reflected power will appear as a reactance across the coupling transformer and effectively change the degree of coupling. Therefore, using a fixed mismatch and varying its distance from the magnetron output port will cause the magnetron frequency to shift and the output power to vary concurrently.To standardize the measurement values, pulling figure is normally measured using a fixed 1.5:1 VSWR; however, in very high power magnetrons a 1.3:1 VSWR is often used. When referring to the pulling figure of a magnetron one should always indicate the VSWR value used in the measurement.Frequency AgilityFrequency agility (FA) in regard to radar operations, is defined as the capability to tune the output frequency of the radar with sufficient speed to produce a pulse-to-pulse frequency change greater than the amount required to effectively obtain decorrelation of adjacent radar echoes.It has been firmly established that FA, together with appropriate receiver integration circuits, affords reduced target scintillation/glint, improved ability to detect targets in a clutter environment, elimination of 2nd time around echoes, and improved resistance to electronic countermeasures, over that possible with a fixed frequency or tunable radar system. It is important to note that, with the exception of ECM resistance, increasing the pulse-to-pulse frequency spacing will increase the amount of system performance improvement that can be realized to a maximum occurring at the point where full pulse echo decorrelation is obtained (nominally 1/tp). Pulse-to-pulse frequency spacings greater than this critical value produce no further increase in system performance, and, in fact, may result in a performance decrease due to the large "IF" inaccuracies arising from the need for the AFC to correct larger pulse to pulse frequency errors.On the other hand, as regards resistance to electronic jamming (ECCM), the greater the pulse-to-pulse frequency spacing, the more difficult it will be to center a jamming transmitter on the radarfrequency to effectively interfere with system operation.Each radar system application must be considered separately to determine which FA parameters will best satisfy the particular need. Just as the FA requirements of each radar differ, so also do the mechanisms differ for optimally producing the required agility parameters. No single tuning scheme has been found which will universally satisfy the requirements of every FA application. For this reason, CPI produces a broad range of FA tuning mechanisms for coaxial magnetrons; each mechanism offering the optimum combination of parameters for a particular application.Frequency Agile Magnetron ClassesFrequency agile magnetrons fall into four classes:·Dither Magnetrons (D) -- Output rf frequency varies periodically with a constant excursion, constant rate and a fixed-center frequency.·Tunable/Dither Magnetrons (T/D) -- Output rf frequency varies periodically with a constant excursion and constant rate.The center frequency may be slowly tuned by hand or by external servomotor drive to any point within the tunable band.·Accutune(tm) Magnetrons (A) -- Output rf frequency variations are determined by the waveshape of an externally generated, low level, voltage signal. With appropriate selection of a tuning waveshape, the Accutune magnetron combines the features of dither and tunable/dither magnetrons.·Accusweep(tm) Magnetrons (As) -- Our best and most versatile tuning system. The output rf tuning rate and waveshape are infinitely variable within the design limits of each device. Customer inputs are typically any waveform from random to square wave and a + 5 volt command.All CPI frequency-agile magnetrons provide a reference voltage output which is an accurate analog of the instantaneous rf output frequency. This signal greatly simplifies automatic frequency control of the system local oscillator frequency. The analog voltage is produced either by a self-generating, permanent magnet device requiring no external drive, or by a precision resolve or LVDT (Linear Voltage Displacement Transducer) acting in conjunction with one of CPI's solid-state frequency readout modules.The Accutune and Accusweep magnetrons operate with a servo loop, feedback control, tuner drive and thereby utilize CPI's solid-state servo amplifier together with the frequency readout module.Frequency Agile Magnetrons150 Sohier RoadBeverly, Massachusetts 01915Phone: 978-922-6000 Fax: 978-922-2736 E-Mail: marketing@ Internet: /bmdAgile Magnetron Design ConsiderationsAt first glance one might conclude that the largest frequency change at the highest rate will give the best radar performance. Unfortunately, this is not a true statement.There have been many separate theoretical studies and comprehensive experiments performed to establish the relationship between radar performance improvement and pulse-to-pulse frequency difference. An understanding of the theoretical basis for the conclusions reached in these efforts is important. In order to preserve the continuity of our discussion, we will show only the results of these studies in this section.Effective performance improvement is achieved when the frequency difference between radar pulses is large enough to eliminate any correlation between the return echoes. A plot of correlation coefficient versus pulse to pulse frequency difference is shown below.Using this relationship, one finds that a radar operating at a 0.5m s pulse duration will have efficient decorrelation betweentarget echoes if pulses differ in frequency by at least 2 MHz.Note that the required frequency separation is a function onlyof the pulse duration.According to the plot of the figure, as frequency separationincreases above the value 1/T, pulse decorrelation continues toimprove, however, the amount of improvement is negligiblysmall for large increases in pulse frequency separation. Inpractical situations, the improvement in decorrelation obtainedby increasing the frequency separation to values greater than 1/T is usually more than offset by other factors. For example, as pulse-to-pulse frequency difference increases, the receiver circuitry needed to assure stable LO (Local Oscillator) tracking also increases, in both complexity and physical size. The accuracy necessary for LO tracking relates directly to the IF bandwidth needed to pass the resultant video signal. Any increase in IF bandwidth, needed to offset inaccuracies in LO tracking, will reduce overall receiver sensitivity and tend to defeat the original purpose. Experience has shown that if one designs for pulse-to-pulse frequency separation as near as possible to, but not le ss than, 1 /T (where T is the shortest pulse duration used in the radar) optimum system performance will be achieved. Experimental studies have shown that performance improvement varies as N, where N is the number of independent (decorrelated) pulses integrated within the receiver circuitry, up to a maximum of 20 pulses.It should be noted that the number of pulses, which can beeffectively integrated, cannot be greater than the number ofpulses placed on the target during one scan of the antenna and,therefore, the antenna beamwidth and scan rate become factorswhich must also be considered in determining the integrationperiod of the radar.Using the above, a design value for Agile Excursion can nowbe expressed in terms of radar operating parameters.Agile Excursion = N/TWhere N is the number of pulses placed on the target duringone radar scan, or 20 whichever is smaller, and T is the shortestpulse duration used in the system.Determination of the required agile rate is now required. Theobject is to traverse the full agile excursion range in the timeneeded to transmit the number of pulses on the target duringone antenna scan.Example:Assume one desires to add agility to a radar having the following operating parameters:Pulse duration - 0.25, 0.5 & 1.0 m Sec.Duty Ratio - 0.001Pulses on target - 16 per scanUsing the formulas derived above one obtains:Agile excursion= N/T = 16/0.25 = 64 MHzPulse to pulse frequency separation = 1/T = 1/ 0.25 = 4 MHzPRR = Duty / T = 0.001/ (0.25x10-6) = 4000 HzTime for 16 pulses = 16/ 4000 = 0.004 SecAgile Rate* = 1/(2x0.004) = 125 Hz* The 2 in the denominator accounts for the fact that two excursions through the agile frequency range occur during each cycle of agile rate.The agile parameters used above were derived using clutter reduction as the prime objective. Elimination of target scintillation requires the satisfaction of one additional constraint, namely that the agile excursion in Mhz should be at least equal to 150/D, where D is the characteristic distance, in meters, between major reflecting points on the target cross section. For most practical situations, an excursion which satisfied the requirements of clutter reduction will usually be sufficient to satisfy the requirements of target scintillation also.Further information and data on agile coaxial magnetrons can be obtained by requesting the Frequency Agile Magnetron Story booklet.Beacon magnetrons are small conventional magnetrons with peak power output less than 4 kW and average power output of less than 5 watts. Typically, they weigh 8 ounces.The technical requirements for this class of magnetrons demand precise frequency control of the magnetron. The temperature stability factor is of great importance since it allows frequency control without additional electronics in the total radar transponder.The magnetron itself requires tunability but must have theproperties of a fixed frequency magnetron after adjustment andlocking. Thus, the techniques of temperature compensation mustwork over a band of frequencies. Also, frequency stability isessential over typical temperature ranges of -65o C to +100o C, andtypical shock of 100G, and vibration environments of 15G(generally those of missile and aircraft electronic systems).Construction of beacon magnetrons can be simplified to containfive basic building blocks. They are the anode, tuner, cathode,output, and magnet.These may be arranged in block diagram fashion as shown inFigure 1.The following sections will discuss each of the five parts of themagnetron.AnodeThe anode is the foundation of the magnetron circuit. It generally consists of an even number of microwave cavities arranged in radial fashion as shown. There are three possible anode configurations:· Hole and slot · Vane tip · Rising sunAdvantages and disadvantages of each type involveconsideration of operating characteristics andconstruction techniques. The hole-and-slot and vanetype normally have every other cavity strapped toeach other by a conducting metal strip. The hole-and-slot type and the rising sun type are usually machinedby hobbing methods out of solid copper stock. Thevane type is generally made up of individual vanesassembled and brazed into a support ring. Thisrequires assembly labor and brazing fixtures.The anode provides the basic magnetron with itsoperating frequency. The central area provides C (capacitance) and the outer perimeter contributes L (inductance) to fulfill the relationshipF = 1/(2p √LC)Each anode is cold checked for "Q" - value and frequency. This involves general microwave impedance and resonance measurements techniques.Beacon Magnetrons150 Sohier RoadBeverly, Massachusetts 01915Phone: 978-922-6000 Fax: 978-922-2736E-Mail: marketing@Internet: /bmdTunerThe tuner is the device which provides some magnetrons with the ability to vary from the basic frequency determined by the anode. Tuners fall into three basic categories:·Capacitive·Inductive·Combination of bothA fundamental description of each is shown in Figure 3. Thecapacitive type is so named because in it a tuning member isintroduced into the anode cavities affecting the E-field andhence the capacitance of the anode. This type can beconstructed of either metal (copper) fingers which are insertedbetween adjacent anode vanes in the central portion of theanode or a dielectric or metallic ring which is inserted into theanode between its central vane straps.The inductive type tuner is much the same as the capacitive butthe tuning member enters the cavities in the back wall regionwhere the H-field and Inductance are affected. The combinationof the two is a complicated affair which affects both L and C and is used whereextremely wide tunability is required. The attachment must necessarily involve abellows or diaphragm arrangement in order to allow for mechanical movement andstill contain the necessary vacuum envelope.Figure 4 shows a simplified capacitive tuner-anode assembly. The magnetron tuner isgenerally composed of two parts, internal and external. The internal portiondescribed above is that part which is enclosed by the vacuum envelope. The externalportion is attached to the internal portion by some mechanical means and providesthe drive mechanism to actually move the tuner the required distance to change Land C and therefore change frequency.CathodeThe cathode of a magnetron is the part which makes the magnetron an activedevice. This provides the electrons through which the mechanism of energytransfer is accomplished. The cathode is usually located in the center of the anodeand is made up of a hollow cylinder of emissive material surrounding a heater.A cross- section of a simple magnetron cathode is shown in Figure 5. Many typesof magnetron cathodes have been developed; each designed for a specificadvantage. The fabrication of magnetron cathodes is carried out in verymeticulous and precise environments. Each braze and weld must be inspected forcompleteness in order not to upset the designed heat flow characteristics.Magnetron cathodes are designed to operate at particular temperatures and owingto the phenomenon called “back bombardment” they cannot tolerate widevariations in construction and assembly techniques. As a further check onoperating temperature of cathodes used in high reliability magnetrons, thecathode-heater assembly alone is evacuated and operated at a predeterminedheater voltages and the cathode temperature checked with an optical pyrometer.This technique reveals any flaw or defect in construction prior to the time thecathode is actually assembled in a magnetron.The next step in the magnetron's construction is to attach the cathode to the tuner-anodeassembly. This procedure also requires extreme care in the axial line-up and orientationof the cathode and anode. Any eccentricity between anode and cathode will producevariations in magnetron operation and can cause serious internal arcing or malfunction.Figure 6 shows a simplified cathode-tuner-anode assembly.OutputThe output circuit in a magnetron is that portion of the device which provides thecoupling to the external load. The RF energy produced in the cavities may be coupledby either a coaxial or waveguide type of output. The figure here shows both types. Thecoaxial design involves either a probe, a loopor a tapped vane coupling to the anode andconcentric coaxial line through the vacuum envelope to the output connector. Suitablematching sections must be included along the line to provide for the correct impedancetransformations and coupled load which appears at the anode. The center conduction ofthe coaxial line is insulated and supported along its length by either glass or ceramicbeads.The waveguide type of output is made up of a coupling slot in the back wall of a cavity,a 1/4 transformer, a vacuum seal window (either glass or ceramic) and a section ofoutput waveguide. The sizes of the coupling slot and 1/4 transformer are determined byfrequency, bandwidth and load coupling considerations. The type of vacuum sealwindow used is determined by the power output and pressurization requirements.Placement of the output window is extremely critical as far as position along the line isconcerned, because any high VSWR which may be reflected back from the load thatwill cause a voltage maximum at the window will cause overheating and subsequentrupture of the vacuum seal.Magnetic CircuitThe magnetic circuit associated with the magnetron is necessary to provide thecrossed field type of operation which provides for the synchronization of theelectron trajectories. The magnetic circuit shown here is composed of anexternal permanent magnet and associated internal pole pieces. The type andcomposition of the permanent magnet vary with particular requirements offield strength and stability. Size and weight are also important considerations.The transmission and focusing of the magnetic field from the externalpermanent magnet to the interaction gap between the anode and cathode isaccomplished by the use of high permeability metal pole pieces shaped tofocus the field lines as sharply as possible.。