LIGO and the Detection of Gravitational Waves
科学启明星的英语作文

Science has always been a beacon of light,guiding humanity through the darkness of ignorance towards the enlightenment of knowledge.The journey of scientific discovery is akin to a constellation of stars,each one a milestone in our understanding of the universe and our place within it.The dawn of scientific thought can be traced back to the ancient Greeks,who laid the foundations for what would become modern science.Philosophers like Socrates,Plato, and Aristotle pondered the nature of the world,asking questions about the cosmos and the human condition.Their inquisitive minds sparked a flame that would burn brightly through the centuries.The Renaissance period saw a resurgence of scientific inquiry,with figures like Galileo Galilei and Nicolaus Copernicus challenging the established order and proposing new theories about the universe.Galileos observations through his telescope led to the heliocentric model of the solar system,which placed the sun,not the Earth,at the center.The Scientific Revolution of the17th century was a pivotal moment in history,with the likes of Isaac Newton and Robert Boyle making groundbreaking discoveries.Newtons laws of motion and universal gravitation revolutionized our understanding of physics, while Boyles work in chemistry laid the groundwork for the scientific method.The19th century brought about the Industrial Revolution,which was closely intertwined with scientific advancements.James Watts improvements to the steam engine and Michael Faradays experiments with electricity were instrumental in shaping the modern world.The20th century was marked by rapid scientific progress,with discoveries in fields such as quantum mechanics,relativity,and genetics.Albert Einsteins theory of relativity changed our perception of space and time,while Watson and Cricks discovery of the structure of DNA opened up new avenues in biology and medicine.Today,science continues to be a driving force for innovation and progress.From the exploration of space to the development of new technologies,the pursuit of knowledge is an unending quest.The Hubble Space Telescope has allowed us to gaze deeper into the cosmos,while advancements in artificial intelligence and biotechnology are reshaping our world.However,with great power comes great responsibility.The ethical implications of scientific advancements must be carefully considered.Issues such as climate change, genetic engineering,and artificial intelligence require a delicate balance betweenprogress and the wellbeing of society.In conclusion,science is the guiding star that lights our path to a brighter future.It is a testament to human curiosity and our unyielding desire to understand the world around us. As we continue to explore the unknown,we must do so with caution and wisdom, ensuring that our pursuit of knowledge benefits all of humanity.。
科学家与明星英语作文

科学家与明星英语作文In the contemporary world, the roles of scientists and celebrities are often seen as distinct, yet they share a common thread in shaping public perception and driving societal progress. This essay will explore the unique ways in which these two groups influence our lives and how their contributions intersect in the English-speaking world.Scientists, through their research and discoveries, have the power to alter the course of human history. Their work in fields such as medicine, technology, and environmental science not only propels innovation but also informs policy and education. The English language, being a global lingua franca, is instrumental in disseminating their findings to a broad audience. For instance, the works of scientists like Stephen Hawking and Jane Goodall have transcended academic circles to capture the imagination of the general public, largely due to their ability to communicate complex ideas in accessible English.On the other hand, celebrities, by virtue of their fame and influence, have the unique ability to bring attention to various causes and issues. Many celebrities use their platform to advocate for scientific initiatives, such as environmental conservation or medical research. For example, Leonardo DiCaprio's environmental activism has raised awareness about climate change, while Bill Gates has championed global health initiatives, often using the Englishlanguage to engage with international audiences.The intersection of scientists and celebrities can be particularly powerful in education and public outreach. When celebrities endorse scientific causes, they can inspire a new generation of students to pursue STEM fields. Similarly, scientists who can effectively communicate in English can reach a wider audience and spark interest in scientifictopics that might otherwise be overlooked.Moreover, the media plays a crucial role in bridging the gap between these two influential groups. English-language news outlets, documentaries, and social media platforms provide a platform for scientists to share their stories and for celebrities to amplify important messages. This symbiotic relationship can lead to increased funding for scientific research and greater public engagement with scientific issues.In conclusion, the English language serves as a common ground where the contributions of scientists and celebrities can converge to educate, inspire, and enact change. While their paths may differ, both groups have the potential to leave a lasting impact on society. It is through their combinedefforts that we can foster a more informed, engaged, and progressive global community.。
引力波观测原文PhysRevLett.116.061102
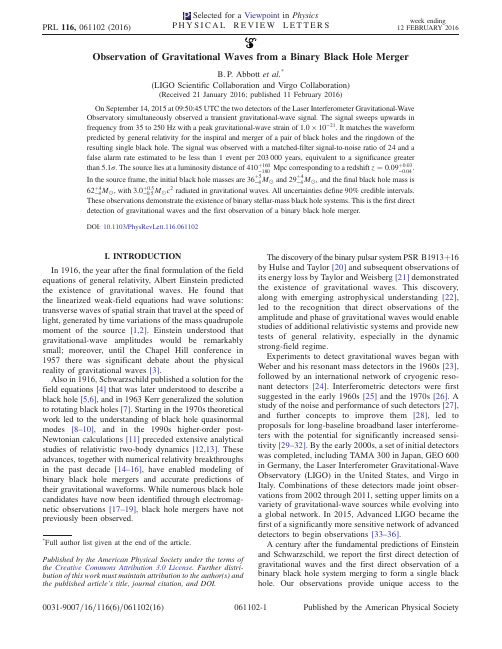
Observation of Gravitational Waves from a Binary Black Hole MergerB.P.Abbott et al.*(LIGO Scientific Collaboration and Virgo Collaboration)(Received21January2016;published11February2016)On September14,2015at09:50:45UTC the two detectors of the Laser Interferometer Gravitational-Wave Observatory simultaneously observed a transient gravitational-wave signal.The signal sweeps upwards in frequency from35to250Hz with a peak gravitational-wave strain of1.0×10−21.It matches the waveform predicted by general relativity for the inspiral and merger of a pair of black holes and the ringdown of the resulting single black hole.The signal was observed with a matched-filter signal-to-noise ratio of24and a false alarm rate estimated to be less than1event per203000years,equivalent to a significance greaterthan5.1σ.The source lies at a luminosity distance of410þ160−180Mpc corresponding to a redshift z¼0.09þ0.03−0.04.In the source frame,the initial black hole masses are36þ5−4M⊙and29þ4−4M⊙,and the final black hole mass is62þ4−4M⊙,with3.0þ0.5−0.5M⊙c2radiated in gravitational waves.All uncertainties define90%credible intervals.These observations demonstrate the existence of binary stellar-mass black hole systems.This is the first direct detection of gravitational waves and the first observation of a binary black hole merger.DOI:10.1103/PhysRevLett.116.061102I.INTRODUCTIONIn1916,the year after the final formulation of the field equations of general relativity,Albert Einstein predicted the existence of gravitational waves.He found that the linearized weak-field equations had wave solutions: transverse waves of spatial strain that travel at the speed of light,generated by time variations of the mass quadrupole moment of the source[1,2].Einstein understood that gravitational-wave amplitudes would be remarkably small;moreover,until the Chapel Hill conference in 1957there was significant debate about the physical reality of gravitational waves[3].Also in1916,Schwarzschild published a solution for the field equations[4]that was later understood to describe a black hole[5,6],and in1963Kerr generalized the solution to rotating black holes[7].Starting in the1970s theoretical work led to the understanding of black hole quasinormal modes[8–10],and in the1990s higher-order post-Newtonian calculations[11]preceded extensive analytical studies of relativistic two-body dynamics[12,13].These advances,together with numerical relativity breakthroughs in the past decade[14–16],have enabled modeling of binary black hole mergers and accurate predictions of their gravitational waveforms.While numerous black hole candidates have now been identified through electromag-netic observations[17–19],black hole mergers have not previously been observed.The discovery of the binary pulsar system PSR B1913þ16 by Hulse and Taylor[20]and subsequent observations of its energy loss by Taylor and Weisberg[21]demonstrated the existence of gravitational waves.This discovery, along with emerging astrophysical understanding[22], led to the recognition that direct observations of the amplitude and phase of gravitational waves would enable studies of additional relativistic systems and provide new tests of general relativity,especially in the dynamic strong-field regime.Experiments to detect gravitational waves began with Weber and his resonant mass detectors in the1960s[23], followed by an international network of cryogenic reso-nant detectors[24].Interferometric detectors were first suggested in the early1960s[25]and the1970s[26].A study of the noise and performance of such detectors[27], and further concepts to improve them[28],led to proposals for long-baseline broadband laser interferome-ters with the potential for significantly increased sensi-tivity[29–32].By the early2000s,a set of initial detectors was completed,including TAMA300in Japan,GEO600 in Germany,the Laser Interferometer Gravitational-Wave Observatory(LIGO)in the United States,and Virgo in binations of these detectors made joint obser-vations from2002through2011,setting upper limits on a variety of gravitational-wave sources while evolving into a global network.In2015,Advanced LIGO became the first of a significantly more sensitive network of advanced detectors to begin observations[33–36].A century after the fundamental predictions of Einstein and Schwarzschild,we report the first direct detection of gravitational waves and the first direct observation of a binary black hole system merging to form a single black hole.Our observations provide unique access to the*Full author list given at the end of the article.Published by the American Physical Society under the terms of the Creative Commons Attribution3.0License.Further distri-bution of this work must maintain attribution to the author(s)and the published article’s title,journal citation,and DOI.properties of space-time in the strong-field,high-velocity regime and confirm predictions of general relativity for the nonlinear dynamics of highly disturbed black holes.II.OBSERVATIONOn September14,2015at09:50:45UTC,the LIGO Hanford,W A,and Livingston,LA,observatories detected the coincident signal GW150914shown in Fig.1.The initial detection was made by low-latency searches for generic gravitational-wave transients[41]and was reported within three minutes of data acquisition[43].Subsequently, matched-filter analyses that use relativistic models of com-pact binary waveforms[44]recovered GW150914as the most significant event from each detector for the observa-tions reported here.Occurring within the10-msintersite FIG.1.The gravitational-wave event GW150914observed by the LIGO Hanford(H1,left column panels)and Livingston(L1,rightcolumn panels)detectors.Times are shown relative to September14,2015at09:50:45UTC.For visualization,all time series are filtered with a35–350Hz bandpass filter to suppress large fluctuations outside the detectors’most sensitive frequency band,and band-reject filters to remove the strong instrumental spectral lines seen in the Fig.3spectra.Top row,left:H1strain.Top row,right:L1strain.GW150914arrived first at L1and6.9þ0.5−0.4ms later at H1;for a visual comparison,the H1data are also shown,shifted in time by this amount and inverted(to account for the detectors’relative orientations).Second row:Gravitational-wave strain projected onto each detector in the35–350Hz band.Solid lines show a numerical relativity waveform for a system with parameters consistent with those recovered from GW150914[37,38]confirmed to99.9%by an independent calculation based on[15].Shaded areas show90%credible regions for two independent waveform reconstructions.One(dark gray)models the signal using binary black hole template waveforms [39].The other(light gray)does not use an astrophysical model,but instead calculates the strain signal as a linear combination of sine-Gaussian wavelets[40,41].These reconstructions have a94%overlap,as shown in[39].Third row:Residuals after subtracting the filtered numerical relativity waveform from the filtered detector time series.Bottom row:A time-frequency representation[42]of the strain data,showing the signal frequency increasing over time.propagation time,the events have a combined signal-to-noise ratio(SNR)of24[45].Only the LIGO detectors were observing at the time of GW150914.The Virgo detector was being upgraded, and GEO600,though not sufficiently sensitive to detect this event,was operating but not in observational mode.With only two detectors the source position is primarily determined by the relative arrival time and localized to an area of approximately600deg2(90% credible region)[39,46].The basic features of GW150914point to it being produced by the coalescence of two black holes—i.e., their orbital inspiral and merger,and subsequent final black hole ringdown.Over0.2s,the signal increases in frequency and amplitude in about8cycles from35to150Hz,where the amplitude reaches a maximum.The most plausible explanation for this evolution is the inspiral of two orbiting masses,m1and m2,due to gravitational-wave emission.At the lower frequencies,such evolution is characterized by the chirp mass[11]M¼ðm1m2Þ3=5121=5¼c3G596π−8=3f−11=3_f3=5;where f and_f are the observed frequency and its time derivative and G and c are the gravitational constant and speed of light.Estimating f and_f from the data in Fig.1, we obtain a chirp mass of M≃30M⊙,implying that the total mass M¼m1þm2is≳70M⊙in the detector frame. This bounds the sum of the Schwarzschild radii of thebinary components to2GM=c2≳210km.To reach an orbital frequency of75Hz(half the gravitational-wave frequency)the objects must have been very close and very compact;equal Newtonian point masses orbiting at this frequency would be only≃350km apart.A pair of neutron stars,while compact,would not have the required mass,while a black hole neutron star binary with the deduced chirp mass would have a very large total mass, and would thus merge at much lower frequency.This leaves black holes as the only known objects compact enough to reach an orbital frequency of75Hz without contact.Furthermore,the decay of the waveform after it peaks is consistent with the damped oscillations of a black hole relaxing to a final stationary Kerr configuration. Below,we present a general-relativistic analysis of GW150914;Fig.2shows the calculated waveform using the resulting source parameters.III.DETECTORSGravitational-wave astronomy exploits multiple,widely separated detectors to distinguish gravitational waves from local instrumental and environmental noise,to provide source sky localization,and to measure wave polarizations. The LIGO sites each operate a single Advanced LIGO detector[33],a modified Michelson interferometer(see Fig.3)that measures gravitational-wave strain as a differ-ence in length of its orthogonal arms.Each arm is formed by two mirrors,acting as test masses,separated by L x¼L y¼L¼4km.A passing gravitational wave effec-tively alters the arm lengths such that the measured difference isΔLðtÞ¼δL x−δL y¼hðtÞL,where h is the gravitational-wave strain amplitude projected onto the detector.This differential length variation alters the phase difference between the two light fields returning to the beam splitter,transmitting an optical signal proportional to the gravitational-wave strain to the output photodetector. To achieve sufficient sensitivity to measure gravitational waves,the detectors include several enhancements to the basic Michelson interferometer.First,each arm contains a resonant optical cavity,formed by its two test mass mirrors, that multiplies the effect of a gravitational wave on the light phase by a factor of300[48].Second,a partially trans-missive power-recycling mirror at the input provides addi-tional resonant buildup of the laser light in the interferometer as a whole[49,50]:20W of laser input is increased to700W incident on the beam splitter,which is further increased to 100kW circulating in each arm cavity.Third,a partially transmissive signal-recycling mirror at the outputoptimizes FIG. 2.Top:Estimated gravitational-wave strain amplitude from GW150914projected onto H1.This shows the full bandwidth of the waveforms,without the filtering used for Fig.1. The inset images show numerical relativity models of the black hole horizons as the black holes coalesce.Bottom:The Keplerian effective black hole separation in units of Schwarzschild radii (R S¼2GM=c2)and the effective relative velocity given by the post-Newtonian parameter v=c¼ðGMπf=c3Þ1=3,where f is the gravitational-wave frequency calculated with numerical relativity and M is the total mass(value from Table I).the gravitational-wave signal extraction by broadening the bandwidth of the arm cavities [51,52].The interferometer is illuminated with a 1064-nm wavelength Nd:Y AG laser,stabilized in amplitude,frequency,and beam geometry [53,54].The gravitational-wave signal is extracted at the output port using a homodyne readout [55].These interferometry techniques are designed to maxi-mize the conversion of strain to optical signal,thereby minimizing the impact of photon shot noise (the principal noise at high frequencies).High strain sensitivity also requires that the test masses have low displacement noise,which is achieved by isolating them from seismic noise (low frequencies)and designing them to have low thermal noise (intermediate frequencies).Each test mass is suspended as the final stage of a quadruple-pendulum system [56],supported by an active seismic isolation platform [57].These systems collectively provide more than 10orders of magnitude of isolation from ground motion for frequen-cies above 10Hz.Thermal noise is minimized by using low-mechanical-loss materials in the test masses and their suspensions:the test masses are 40-kg fused silica substrates with low-loss dielectric optical coatings [58,59],and are suspended with fused silica fibers from the stage above [60].To minimize additional noise sources,all components other than the laser source are mounted on vibration isolation stages in ultrahigh vacuum.To reduce optical phase fluctuations caused by Rayleigh scattering,the pressure in the 1.2-m diameter tubes containing the arm-cavity beams is maintained below 1μPa.Servo controls are used to hold the arm cavities on resonance [61]and maintain proper alignment of the optical components [62].The detector output is calibrated in strain by measuring its response to test mass motion induced by photon pressure from a modulated calibration laser beam [63].The calibration is established to an uncertainty (1σ)of less than 10%in amplitude and 10degrees in phase,and is continuously monitored with calibration laser excitations at selected frequencies.Two alternative methods are used to validate the absolute calibration,one referenced to the main laser wavelength and the other to a radio-frequencyoscillator(a)FIG.3.Simplified diagram of an Advanced LIGO detector (not to scale).A gravitational wave propagating orthogonally to the detector plane and linearly polarized parallel to the 4-km optical cavities will have the effect of lengthening one 4-km arm and shortening the other during one half-cycle of the wave;these length changes are reversed during the other half-cycle.The output photodetector records these differential cavity length variations.While a detector ’s directional response is maximal for this case,it is still significant for most other angles of incidence or polarizations (gravitational waves propagate freely through the Earth).Inset (a):Location and orientation of the LIGO detectors at Hanford,WA (H1)and Livingston,LA (L1).Inset (b):The instrument noise for each detector near the time of the signal detection;this is an amplitude spectral density,expressed in terms of equivalent gravitational-wave strain amplitude.The sensitivity is limited by photon shot noise at frequencies above 150Hz,and by a superposition of other noise sources at lower frequencies [47].Narrow-band features include calibration lines (33–38,330,and 1080Hz),vibrational modes of suspension fibers (500Hz and harmonics),and 60Hz electric power grid harmonics.[64].Additionally,the detector response to gravitational waves is tested by injecting simulated waveforms with the calibration laser.To monitor environmental disturbances and their influ-ence on the detectors,each observatory site is equipped with an array of sensors:seismometers,accelerometers, microphones,magnetometers,radio receivers,weather sensors,ac-power line monitors,and a cosmic-ray detector [65].Another∼105channels record the interferometer’s operating point and the state of the control systems.Data collection is synchronized to Global Positioning System (GPS)time to better than10μs[66].Timing accuracy is verified with an atomic clock and a secondary GPS receiver at each observatory site.In their most sensitive band,100–300Hz,the current LIGO detectors are3to5times more sensitive to strain than initial LIGO[67];at lower frequencies,the improvement is even greater,with more than ten times better sensitivity below60Hz.Because the detectors respond proportionally to gravitational-wave amplitude,at low redshift the volume of space to which they are sensitive increases as the cube of strain sensitivity.For binary black holes with masses similar to GW150914,the space-time volume surveyed by the observations reported here surpasses previous obser-vations by an order of magnitude[68].IV.DETECTOR VALIDATIONBoth detectors were in steady state operation for several hours around GW150914.All performance measures,in particular their average sensitivity and transient noise behavior,were typical of the full analysis period[69,70]. Exhaustive investigations of instrumental and environ-mental disturbances were performed,giving no evidence to suggest that GW150914could be an instrumental artifact [69].The detectors’susceptibility to environmental disturb-ances was quantified by measuring their response to spe-cially generated magnetic,radio-frequency,acoustic,and vibration excitations.These tests indicated that any external disturbance large enough to have caused the observed signal would have been clearly recorded by the array of environ-mental sensors.None of the environmental sensors recorded any disturbances that evolved in time and frequency like GW150914,and all environmental fluctuations during the second that contained GW150914were too small to account for more than6%of its strain amplitude.Special care was taken to search for long-range correlated disturbances that might produce nearly simultaneous signals at the two sites. No significant disturbances were found.The detector strain data exhibit non-Gaussian noise transients that arise from a variety of instrumental mecha-nisms.Many have distinct signatures,visible in auxiliary data channels that are not sensitive to gravitational waves; such instrumental transients are removed from our analyses [69].Any instrumental transients that remain in the data are accounted for in the estimated detector backgrounds described below.There is no evidence for instrumental transients that are temporally correlated between the two detectors.V.SEARCHESWe present the analysis of16days of coincident observations between the two LIGO detectors from September12to October20,2015.This is a subset of the data from Advanced LIGO’s first observational period that ended on January12,2016.GW150914is confidently detected by two different types of searches.One aims to recover signals from the coalescence of compact objects,using optimal matched filtering with waveforms predicted by general relativity. The other search targets a broad range of generic transient signals,with minimal assumptions about waveforms.These searches use independent methods,and their response to detector noise consists of different,uncorrelated,events. However,strong signals from binary black hole mergers are expected to be detected by both searches.Each search identifies candidate events that are detected at both observatories consistent with the intersite propa-gation time.Events are assigned a detection-statistic value that ranks their likelihood of being a gravitational-wave signal.The significance of a candidate event is determined by the search background—the rate at which detector noise produces events with a detection-statistic value equal to or higher than the candidate event.Estimating this back-ground is challenging for two reasons:the detector noise is nonstationary and non-Gaussian,so its properties must be empirically determined;and it is not possible to shield the detector from gravitational waves to directly measure a signal-free background.The specific procedure used to estimate the background is slightly different for the two searches,but both use a time-shift technique:the time stamps of one detector’s data are artificially shifted by an offset that is large compared to the intersite propagation time,and a new set of events is produced based on this time-shifted data set.For instrumental noise that is uncor-related between detectors this is an effective way to estimate the background.In this process a gravitational-wave signal in one detector may coincide with time-shifted noise transients in the other detector,thereby contributing to the background estimate.This leads to an overestimate of the noise background and therefore to a more conservative assessment of the significance of candidate events.The characteristics of non-Gaussian noise vary between different time-frequency regions.This means that the search backgrounds are not uniform across the space of signals being searched.To maximize sensitivity and provide a better estimate of event significance,the searches sort both their background estimates and their event candidates into differ-ent classes according to their time-frequency morphology. The significance of a candidate event is measured against the background of its class.To account for having searchedmultiple classes,this significance is decreased by a trials factor equal to the number of classes [71].A.Generic transient searchDesigned to operate without a specific waveform model,this search identifies coincident excess power in time-frequency representations of the detector strain data [43,72],for signal frequencies up to 1kHz and durations up to a few seconds.The search reconstructs signal waveforms consistent with a common gravitational-wave signal in both detectors using a multidetector maximum likelihood method.Each event is ranked according to the detection statistic ηc ¼ffiffiffiffiffiffiffiffiffiffiffiffiffiffiffiffiffiffiffiffiffiffiffiffiffiffiffiffiffiffiffiffiffiffiffi2E c =ð1þE n =E c Þp ,where E c is the dimensionless coherent signal energy obtained by cross-correlating the two reconstructed waveforms,and E n is the dimensionless residual noise energy after the reconstructed signal is subtracted from the data.The statistic ηc thus quantifies the SNR of the event and the consistency of the data between the two detectors.Based on their time-frequency morphology,the events are divided into three mutually exclusive search classes,as described in [41]:events with time-frequency morphology of known populations of noise transients (class C1),events with frequency that increases with time (class C3),and all remaining events (class C2).Detected with ηc ¼20.0,GW150914is the strongest event of the entire search.Consistent with its coalescence signal signature,it is found in the search class C3of events with increasing time-frequency evolution.Measured on a background equivalent to over 67400years of data and including a trials factor of 3to account for the search classes,its false alarm rate is lower than 1in 22500years.This corresponds to a probability <2×10−6of observing one or more noise events as strong as GW150914during the analysis time,equivalent to 4.6σ.The left panel of Fig.4shows the C3class results and background.The selection criteria that define the search class C3reduce the background by introducing a constraint on the signal morphology.In order to illustrate the significance of GW150914against a background of events with arbitrary shapes,we also show the results of a search that uses the same set of events as the one described above but without this constraint.Specifically,we use only two search classes:the C1class and the union of C2and C3classes (C 2þC 3).In this two-class search the GW150914event is found in the C 2þC 3class.The left panel of Fig.4shows the C 2þC 3class results and background.In the background of this class there are four events with ηc ≥32.1,yielding a false alarm rate for GW150914of 1in 8400years.This corresponds to a false alarm probability of 5×10−6equivalent to 4.4σ.FIG.4.Search results from the generic transient search (left)and the binary coalescence search (right).These histograms show the number of candidate events (orange markers)and the mean number of background events (black lines)in the search class where GW150914was found as a function of the search detection statistic and with a bin width of 0.2.The scales on the top give the significance of an event in Gaussian standard deviations based on the corresponding noise background.The significance of GW150914is greater than 5.1σand 4.6σfor the binary coalescence and the generic transient searches,respectively.Left:Along with the primary search (C3)we also show the results (blue markers)and background (green curve)for an alternative search that treats events independently of their frequency evolution (C 2þC 3).The classes C2and C3are defined in the text.Right:The tail in the black-line background of the binary coalescence search is due to random coincidences of GW150914in one detector with noise in the other detector.(This type of event is practically absent in the generic transient search background because they do not pass the time-frequency consistency requirements used in that search.)The purple curve is the background excluding those coincidences,which is used to assess the significance of the second strongest event.For robustness and validation,we also use other generic transient search algorithms[41].A different search[73]and a parameter estimation follow-up[74]detected GW150914 with consistent significance and signal parameters.B.Binary coalescence searchThis search targets gravitational-wave emission from binary systems with individual masses from1to99M⊙, total mass less than100M⊙,and dimensionless spins up to 0.99[44].To model systems with total mass larger than 4M⊙,we use the effective-one-body formalism[75],whichcombines results from the post-Newtonian approach [11,76]with results from black hole perturbation theory and numerical relativity.The waveform model[77,78] assumes that the spins of the merging objects are alignedwith the orbital angular momentum,but the resultingtemplates can,nonetheless,effectively recover systemswith misaligned spins in the parameter region ofGW150914[44].Approximately250000template wave-forms are used to cover this parameter space.The search calculates the matched-filter signal-to-noiseratioρðtÞfor each template in each detector and identifiesmaxima ofρðtÞwith respect to the time of arrival of the signal[79–81].For each maximum we calculate a chi-squared statisticχ2r to test whether the data in several differentfrequency bands are consistent with the matching template [82].Values ofχ2r near unity indicate that the signal is consistent with a coalescence.Ifχ2r is greater than unity,ρðtÞis reweighted asˆρ¼ρ=f½1þðχ2rÞ3 =2g1=6[83,84].The final step enforces coincidence between detectors by selectingevent pairs that occur within a15-ms window and come fromthe same template.The15-ms window is determined by the10-ms intersite propagation time plus5ms for uncertainty inarrival time of weak signals.We rank coincident events basedon the quadrature sumˆρc of theˆρfrom both detectors[45]. To produce background data for this search the SNR maxima of one detector are time shifted and a new set of coincident events is computed.Repeating this procedure ∼107times produces a noise background analysis time equivalent to608000years.To account for the search background noise varying acrossthe target signal space,candidate and background events aredivided into three search classes based on template length.The right panel of Fig.4shows the background for thesearch class of GW150914.The GW150914detection-statistic value ofˆρc¼23.6is larger than any background event,so only an upper bound can be placed on its false alarm rate.Across the three search classes this bound is1in 203000years.This translates to a false alarm probability <2×10−7,corresponding to5.1σ.A second,independent matched-filter analysis that uses adifferent method for estimating the significance of itsevents[85,86],also detected GW150914with identicalsignal parameters and consistent significance.When an event is confidently identified as a real gravitational-wave signal,as for GW150914,the back-ground used to determine the significance of other events is reestimated without the contribution of this event.This is the background distribution shown as a purple line in the right panel of Fig.4.Based on this,the second most significant event has a false alarm rate of1per2.3years and corresponding Poissonian false alarm probability of0.02. Waveform analysis of this event indicates that if it is astrophysical in origin it is also a binary black hole merger[44].VI.SOURCE DISCUSSIONThe matched-filter search is optimized for detecting signals,but it provides only approximate estimates of the source parameters.To refine them we use general relativity-based models[77,78,87,88],some of which include spin precession,and for each model perform a coherent Bayesian analysis to derive posterior distributions of the source parameters[89].The initial and final masses, final spin,distance,and redshift of the source are shown in Table I.The spin of the primary black hole is constrained to be<0.7(90%credible interval)indicating it is not maximally spinning,while the spin of the secondary is only weakly constrained.These source parameters are discussed in detail in[39].The parameter uncertainties include statistical errors and systematic errors from averaging the results of different waveform models.Using the fits to numerical simulations of binary black hole mergers in[92,93],we provide estimates of the mass and spin of the final black hole,the total energy radiated in gravitational waves,and the peak gravitational-wave luminosity[39].The estimated total energy radiated in gravitational waves is3.0þ0.5−0.5M⊙c2.The system reached apeak gravitational-wave luminosity of3.6þ0.5−0.4×1056erg=s,equivalent to200þ30−20M⊙c2=s.Several analyses have been performed to determine whether or not GW150914is consistent with a binary TABLE I.Source parameters for GW150914.We report median values with90%credible intervals that include statistical errors,and systematic errors from averaging the results of different waveform models.Masses are given in the source frame;to convert to the detector frame multiply by(1þz) [90].The source redshift assumes standard cosmology[91]. Primary black hole mass36þ5−4M⊙Secondary black hole mass29þ4−4M⊙Final black hole mass62þ4−4M⊙Final black hole spin0.67þ0.05−0.07 Luminosity distance410þ160−180MpcSource redshift z0.09þ0.03−0.04。
引力波探测原理札记:LIGO干涉相移的五个起源

沈建其
浙江大学紫金港校区光电学院,光及电磁波研究中心,浙江 杭州
收稿日期:2017年5月13日;录用日期:2017年5月28日;发布日期:2017年5月31日
摘
要
巨型迈克尔逊激光干涉仪是探测引力波的有效手段。本文研究了激光干涉引力波观察仪(Laser Interferometer Gravitational-Wave Observatory, LIGO)中的干涉臂长、干涉激光波长和频率等物理量在引 力波振幅与引力波潮汐力作用下的变化量。本文总结出引力波引起LIGO干涉相位移动的五个物理因素: ①干涉臂标准长度的纯几何改变(非潮汐力贡献); ②激光标准波长的纯几何改变(非潮汐力贡献); ③干涉 臂长度受潮汐力影响导致的改变(属引力波潮汐力贡献);④激光波长受潮汐力影响导致的胀缩效应(属引 力波潮汐力贡献);⑤激光频率的弱相干效应。在引力波作用下,在互相垂直的两条干涉臂中的激光会有 不同频移,这一频率弱相干条件也会影响干涉相位差。本文说明引力波潮汐力(引力落差)所导致的干涉 激光波长屈伸效应能在 LISA (Laser Interferometer Space Antenna) 中有测量意义。本文还讨论了 GW150914引力波信号频率变化率与描述螺旋合并双黑洞的引力波Blanchet频率方程之间的符合程度, 发现GW150914引力波信号观察与理论在±30%差距上相符。
Abstract
Giant Michelson interferometer is one of the essential means of gravitational wave detection. The influence of gravitational-tidal-force assisted laser wavelength stretch and laser-frequency soft coherence on the interference phase in LIGO (Laser Interferometer Gravitational-Wave Observatory) is investigated. It can be found that there are five physical origins of LIGO interference phase shift in LIGO gravitational wave detection: i) purely geometric change in standard length of interferometer arms (non-tidal contribution), ii) purely geometric change in standard length of laser wavelength (non-tidal contribution), iii) gravitational-wave tidal-force assisted change in interferometer arms (tidal contribution), iv) gravitational-wave tidal-force assisted change in laser wavelength (tidal contribution), and v) feeble coherence of laser frequency caused by gravitational wave in LIGO interferometer. The laser frequency shift in two vertical interference arms can occur due to gravitational wave, and hence such a faint coherence condition would also lead to interference pattern change when the gravitational wave propagates through the LIGO. The effect of flexion and extension in laser wavelength resulting from gravitational tidal force caused by a passing gravitational wave would have significance of measurement in LISA (Laser Interferometer Space Antenna). The frequency change rate of GW150914 gravitational wave signal resulting from in spiral, merger and ring-down of black-hole binaries has been addressed, and it can be found that the logarithm of the detected gravitational-wave frequency change rate agrees with the first-order Blanchet frequency equation within accuracy of ±30%.
我心目中的科学家英语作文 范文

我心目中的科学家英语作文范文In my view, scientists are akin to modern-day alchemists, weaving intricate narratives of discovery within the fabric of the universe. They are the navigators of the unknown, wielding curiosity as their compass and reason as their sail. Let me take you on a journey through the corridors of my mind, where the portrait of a scientist unfolds in vibrant hues of intellect and ingenuity.Imagine a world where equations dance across chalkboards like cosmic ballets, where the language of atoms whispers secrets only the keenest ears can decipher. This is the realm of the scientist, where every question is a breadcrumb leading to the banquet of knowledge. They are the architects of understanding, building bridges between the tangible and the intangible.At the heart of scientific inquiry lies a relentlesspursuit of truth. It is a quest fueled not by ego, but by an insatiable hunger to unravel the mysteries of existence. From the microscopic dance of particles to the grandorchestration of galaxies, scientists peer through the veil of ignorance, seeking to illuminate the darkness with the torch of reason.Yet, amidst the chaos of experimentation and the labyrinth of data, there exists a quiet humility. For every answer uncovered reveals a dozen new questions, each more tantalizing than the last. The scientist is a humble pilgrim, journeying ever deeper into the unknown, guided by the twin beacons of curiosity and skepticism.But make no mistake, theirs is not a solitary endeavor. Science is a tapestry woven from the threads of collaboration and cooperation. Across continents and disciplines, scientists join hands in a symphony of discovery, harmonizing their efforts to conquer the frontiers of knowledge. In this global chorus, no voice is too small, no contribution too insignificant. For it is in diversity that the true power of science resides, drawing strength from the myriad perspectives that illuminate the path forward.And yet, for all their brilliance, scientists are not immune to the foibles of humanity. Egos clash like tectonic plates, and dogma can obscure the light of reason. But in the crucible of debate and discourse, truth emerges triumphant, tempered by the fire of scrutiny.So, what then defines the essence of a scientist? Is it the accolades adorning their walls or the equations etched in their minds? Perhaps it is neither, but rather the spark of curiosity that ignites their soul. For in the end, it isnot the destination that defines us, but the journey we undertake in pursuit of understanding.In my eyes, the scientist is more than a mere mortal; they are the custodians of curiosity, the stewards of skepticism, and the architects of enlightenment. They are the poets of the cosmos, crafting verses of truth in the language of the universe. And as long as there are questions left unanswered, their quest shall endure, a testament to the indomitable spirit of human intellect.。
Self-gravitating line sources of weak hypercharge

a rXiv:g r-qc/99257v 119Feb1999Self-gravitating Line Sources of Weak Hypercharge T.Dereli Department of Physics,Middle East Technical University 06531Ankara,Turkey E.mail:tekin@.tr Robin W Tucker Department of Physics,University of Lancaster Bailrigg,14YB,UK E.mail:r.tucker@ (15August 1998)We explore the role of the Cremmer-Scherk mechanism in the context of low energy effective string theory by coupling the antisymmetric 3-form gauge field to an Abelian gauge potential carrying weak hypercharge.The theory admits a class of exact self-gravitating solutions in the spontaneously broken phase in which dual fields acquire massive perturbative modes.Despite the massive nature of these fields they admit non-perturbative progressive longitudinal modes that together with pp-type gravitational waves travel in a direction of a line source at the speed of light.Considerable effort has been devoted to the search for classical string-like solutions in relativistic field theories.Such solutions range from the pioneering work on vortices as models for dual strings [1]to more recent investigations on the properties of global and superconducting cosmic strings [2]-[4].A common feature in recent work has been the role played by singular sources as models for the strings themselves.Such source descriptions often lend themselves to a formulation in terms of de Rham periods.Thus in the Higgs vacuum of the global Abelian Higgs model [3],the phase θof a complex scalar field satisfies the massless field equations d ∗dθ=0in a regular space-time domain.In such a domain one may introduce a 2-form potential ˆB by d ˆB =∗dθ.Classical sources enter the theory as solutions with C dθ=2πfor some closed space-like curve C =∂Σ2bounding a space-like disc Σ2.For such solutions,ˆB can be promoted to a distribution on space-time satisfying d ∗d ˆB =2πδwith Σ2δ=1.One then identifies the solution as a line source threading C at each instant and such ”axionic”strings have interesting cosmological implications [5].In a recent note we have suggested that fields arising in low energy effective string actions may have consequences for the standard model of the electroweak interactions [7].By coupling the antisymmetric 3-form gauge field H to an Abelian gauge potential 1-form A carrying weak hypercharge via a gauge covariant derivative of the standard Higgs weak isospinor,we showed explicitly how the masses of the W ±,Z 0could depend on this coupling.A salient feature of this generalised Cremmer-Scherk mechanism [6]was the manner in which the H field became assimilated into the physical degrees of freedom of the vector bosons via a spontaneous breakdown of a local gauge symmetry.Since the H field along with the dilaton φis thought to have implications for cosmology,it is of interest to explore the gravitational sector of the low energy effective string action in the presence of the Cremmer-Scherk interaction.Although the model discussed in Ref.[7]involves the full non-Abelian SU (2)×U (1)gauge theory of the electroweak standard model,we shall here restrict attention to a single local Abelian internal symmetry gauge group for simplicitybut retain the hypercharge interpretation.The Cremmer-Scherk mechanism is controlled by a coupling constant λand we are interested in the phase with λ=0.Thus to lowest order in string fields we investigate the dynamics derived from the action density 4-formΛ[g ,φ,A,B ]=κR ∗1−(2α−3)2e −2φdB ∧∗dB +1(2α−3)e −2φ(dB ∧∗dB +dA ∧∗dA ),(4)κwhereτa[φ]=(2α−3)2e−2φ(ιa dA∧∗dA−dA∧ιa∗dA)τa[B]=1λd f0,˜B=B−1(2α−3)e2φ˜A∧∗˜A+22R bc∧∗(e a∧e b∧e c)=τa[φ]+τa[˜A]+λ2(2α−3)e2φ˜B∧∗˜B+22R bc∧∗(e a∧e b∧e c)=τa[φ]+τa[˜B]+λ2φ=φ0.(18) It follows from(7)that the corresponding solution for˜B will have the form˜B=−e−2φ0ρβ′−µ20β=0,(20)e2φ0κ(h′′+1µ02e2φ0h(ρ)= ρ1ρ′lnρ′(K1(µ0ρ′)2+K0(µ0ρ′)2)dρ′+1κe−2φ0lnρare displayed in Figure1forκ=µ0=1,φ0=0.The interpretation of these solutions depends on the form of f(u).When f is constant the solution is static.In terms of the coordinates(t,x,y,z)wheret=12(u+v),z=12(v−u),x=ρcosψ,y=ρsinψwe identifyρ=0as a line source for{g,˜A}along the z-axis at each instant.Writing thefield strength d˜A in terms of hyper-electric e and hyper-magnetic bfields with respect to dt,onefinds that a radial e emanates from this source and it is everywhere transverse to b.The fact that C1b for a closed space-like contour C1and theflux of e through afinite space-like cylinder depend on the extent of the integration regions is a reflection of the massive nature of the˜Afield.When f(u)is a non-constant bounded function,the solution describes a progressive gravitational wave with am-plitude h(ρ)that propagates together with˜A with amplitudeβ(ρ)in the z direction at the speed of light.In the other spatial directions the˜Afield falls offexponentially to zero at infinity while the behaviour of the gravitatonal field is determined by h(ρ).If one interprets the singular domainρ=0as a straight wire,then it acts as a kind of gravitational opticalfibre that guides a pp-type gravitational wave.Such an interpretation has potential astrophysical implications.Given the surprising properties of these exterior self-gravitating Einstein-Proca solutions it may be of interest to explore the generalised Cremmer-Scherk mechanism[7]on the gravitational sector of low energy effective string theory.It may be of further interest to note that any Einstein-Proca solution can be used to generate a solution to non-Riemannian theories of gravity[8].The authors are grateful to TUBITAK for the support of this research.RWT is also grateful to the Department of Physics,Middle East Technical University for hospitality.I.REFERENCES1.H.B.Nielsen,P.Olesen,Nucl.Phys.B61(1973)45D.F¨o rster,Nucl.Phys.B81(1975)842.E.Witten,Phys.Lett.153B(1985)243A.Vilenkin,T.Vachaspati,Phys.Rev.D35(1987)11383.R.L.Davis,E.P.S.Shellard,Phys.Lett.214B(1988)219R.L.Davis,E.P.S.Shellard,Phys.Rev.Lett.63(1989)20214.B.Carter,Phys.Lett.224B(1989)61B.Carter,Phys.Lett.228B(1989)466B.Carter,Nucl.Phys.B412(1994)3455.E.Copeland,M.Hindmarsh,N.Turok,Phys.Rev.Lett.58(1987)1910E.Copeland,D.Haws,M.Hindmarsh,N.Turok,Nucl.Phys.B306(1988)9086.E.Cremmer,J.Scherk,Nucl.Phys.B72(1974)117M.Kalb,P.Ramond,Phys.Rev.D9(1974)22737.T.Dereli,R.W.Tucker,Stringfields and the standard model,hep-th/98080598.T.Dereli,M.¨Onder,J.Schray,R.W.Tucker,C.Wang,Class.Q.Grav.13(1996)L103。
万有引力是自然科学中重大发现之一作文

Gravitational force is one of the major discoveriesin natural scienceGravitational force is one of the major discoveries in natural science. It is a fundamental concept that explains the interaction between objects in the universe. The understanding of gravitational force has had a profound impact on our understanding of the universe and various aspects of science.The discovery of gravitational force was made by physicist Isaac Newton, who formulated the laws of motion and universal gravitation. This discovery explained why objects fall to the ground, why planets orbit the sun, and how celestial bodies are held together in the universe.Gravitational force plays a crucial role in many fields of science. It is essential in astronomy for understanding the motion of planets, stars, and galaxies. In astrophysics, it helps us explain the formation and evolution of celestial objects. Gravitational waves, recently detected, provide new insights into the most extreme events in the universe.Moreover, gravitational force has practical applications in our daily lives. Satellite navigation systems rely on the precise measurement of gravitational forces to determine our location. The study of gravitational force also leads toadvancements in space exploration and the understanding of black holes.The significance of the discovery of gravitational force lies not only in its scientific importance but also in its profound impact on human civilization. It has expanded our knowledge of the universe, inspired technological innovations, and continues to drive scientific progress.In conclusion, the discovery of gravitational force is a remarkable achievement in the field of natural science. It has unlocked many secrets of the universe and continues to shape our understanding of the world we live in.。
the nature of scientific reasoning

本次翻译练习的难度比较大,文章出自北京师范大学研究生英语阅读与翻译课程所用的授课材料,作者布洛诺夫斯基是英国著名的数学家和散文家,剑桥大学数学博士。
这篇文章从科学发展史的角度出发,论述的问题主要是科学并不排斥想象力和创造力。
因此标题翻译成“科学理性的本质”或“科学推理的本质”是比较恰当的。
要翻译好这篇文章不仅应在在宏观的层面牢牢把握文章的主旨,也需要从微观的角度考虑作者使用的语言在语法和修辞上的特点,这样才能在理解的基础上恰当的表达。
当然,这篇文章相对于大家目前的英语水平,在理解和表达两个方面都具有不小的挑战性。
下面通过对这次翻译比较好的赵新平同学作业的点评,来分段落说一说这篇文章究竟有哪些细节部分需要注意,以及相应的翻译策略。
1What is the insight in which the scientist tries to see into nature? Can it indeed be called either imaginative or creative? To the literary man the question may seem merely silly. He has been taught that science is a large collection of facts; and if this is true, then the only seeing which scientists need to do is, he supposes, seeing the facts. He pictures them, the colorless professionals of science, going off to work in the morning into the universe in a neutral, unexposed state. They then expose themselves like a photographic plate. And then in the darkroom or laboratory they develop the image, so that suddenly and startlingly it appears, printed in capital letters, as a new formula for atomic energy.原译:什么是洞察力?科学家一直试图弄清它的本质。
最伟大的物理学家Top10(国外英文资料)

Top 10 Greatest Physicists of All Time (Based on Foreign English Resources)1. Albert EinsteinWithout a doubt, Albert Einstein tops the list of the greatest physicists of all time. His theory of relativity revolutionized our understanding of space, time, and gravity. Einstein's famous equation, E=mc², demonstrated the relationship between energy and mass, opening up new possibilities in the field of physics.2. Isaac NewtonSir Isaac Newton is another physicist who has left an indelible mark on the scientific world. His laws of motion and universal gravitation laid the foundation for classical mechanics. Newton's work in optics and the development of the reflecting telescope also contributed significantly to the advancement of physics.3. Niels BohrNiels Bohr, a Danish physicist, played a crucial role in the development of quantum mechanics. His model of the atom, which incorporated the concept of quantized energy levels, helped to explain the behavior of electrons and the stability of atoms.4. James Clerk MaxwellScottish physicist James Clerk Maxwell is renowned forhis formulation of the classical theory of electromagnetic radiation. His set of equations, known as Maxwell's equations, unified the understanding of electricity, magnetism, andlight.5. Richard FeynmanRichard Feynman was an American theoretical physicist who made significant contributions to quantum mechanics andparticle physics. His development of the path integral formulation of quantum mechanics and his work on the theoryof quantum electrodynamics earned him a Nobel Prize in Physics.6. Max PlanckGerman physicist Max Planck is considered the father of quantum theory. His discovery of Planck's constant and his proposal that energy is radiated in discrete packets, or quanta, marked the beginning of quantum physics.7. Werner HeisenbergWerner Heisenberg, another prominent figure in quantum mechanics, formulated the uncertainty principle, which states that it is impossible to simultaneously know the exactposition and momentum of a particle.8. Galileo GalileiGalileo Galilei, often referred to as the "Father of Modern Science," made significant contributions to physics, astronomy, and the scientific method. His work on inertia, falling objects, and the laws of motion laid the groundwork for Newton's theories.9. Stephen Hawking10. Marie CurieMarie Curie, a Polishborn physicist and chemist, was the first woman to win a Nobel Prize and remains the only person to win Nobel Prizes in two different sciences (Physics and Chemistry). Her work on radioactivity opened up new avenues in medical research and laid the foundation for the field of atomic physics.Continuing the exploration of the most influential physicists in history, let's delve into the lives and achievements of these extraordinary individuals who have shaped our understanding of the universe.11. Paul DiracPaul Dirac, an English theoretical physicist, is often celebrated for his prediction of the existence of antimatter, a discovery that was later confirmed the experimental work of Carl Anderson. Dirac's formulation of quantum mechanics, particularly the Dirac equation, was pivotal in the development of quantum field theory.12. Michael FaradayMichael Faraday was a British scientist who contributed immensely to the study of electromagnetism. His experiments led to the discovery of electromagnetic induction, the laws of electrolysis, and the invention of the Faraday cage. Faraday's work laid the groundwork for the future development of electric motors and generators.13. Ludwig BoltzmannAustrian physicist Ludwig Boltzmann was a key figure in the development of statistical mechanics and thermodynamics. His insight into the behavior of molecules and his formulation of the Boltzmann equation helped to explain the concepts of entropy and the statistical nature of physical laws.14. Ernest RutherfordErnest Rutherford, a New Zealandborn British physicist, is known as the father of nuclear physics. His groundbreaking gold foil experiment led to the discovery of the atomic nucleus and the understanding that most of an atom's mass is concentrated in a tiny, central nucleus.15. Murray GellMann16. J.J. ThomsonSir Joseph John Thomson, an English physicist, discovered the electron in 1897, demonstrating that atoms are notindivisible and consist of smaller particles. His work on cathode rays and the discovery of the masstocharge ratio of electrons was fundamental in the development of atomic physics.17. Enrico FermiEnrico Fermi, an Italian physicist, was pivotal in the development of nuclear technology. His work on nuclear reactions led to the construction of the first nuclear reactor and the first controlled nuclear chain reaction. Fermi's contributions to quantum theory and particle physics are also noteworthy.18. Lise MeitnerLise Meitner, an AustrianSwedish physicist, was involved in the discovery of nuclear fission. Despite facing discrimination as a woman in science, Meitner's work was crucial in understanding the process which heavy nuclei can split into lighter nuclei, releasing a significant amount of energy.19. Roger PenroseBritish mathematician and physicist Roger Penrose has made significant contributions to the understanding of general relativity and cosmology. His work on black holes, particularly the Penrose process and the Penrose singularitytheorem, has deepened our knowledge of the most extreme phenomena in the universe.20. Kip ThorneKip Thorne, an American theoretical physicist, has been at the forefront of gravitational physics and astrophysics. His work on the detection of gravitational waves, as part of the LIGO collaboration, confirmed a key prediction of Einstein's theory of general relativity and opened a new window into the cosmos.Each of these physicists has left an indelible mark on the field, pushing the boundaries of human knowledge and challenging our understanding of the natural world. Their legacies continue to inspire and guide the next generation of scientists in their quest to uncover the secrets of the universe.As we further unravel the rich tapestry of scientific achievement, let's continue to honor the contributions of more extraordinary physicists whose insights have transformed our understanding of the cosmos and the fundamental forces that govern it.21. Andrei SakharovAndrei Sakharov, a Soviet nuclear physicist, played a crucial role in the development of the Soviet hydrogen bomb. However, he is perhaps best known for his advocacy of civilliberties and human rights in the Soviet Union. His work on the concept of "metric elasticity" also contributed to the field of general relativity.22. ChenNing YangChenNing Yang, a ChineseAmerican physicist, made significant contributions to theoretical physics,particularly in the area of parity nonconservation in weak interactions. His work with TsungDao Lee led to a Nobel Prize in Physics, demonstrating that certain processes are not mirrorsymmetric.23. TsungDao LeeTsungDao Lee, a ChineseAmerican physicist, collaborated with ChenNing Yang to challenge the symmetry principles in physics. Their proposal that weak interactions do not conserve parity was a groundbreaking discovery that revolutionized particle physics.24. Sheldon GlashowSheldon Glashow, an American theoretical physicist, is known for his work on the electroweak theory, which unified the weak nuclear force and electromagnetism. Hiscontributions to particle physics, including the prediction of the W and Z bosons, were recognized with a Nobel Prize in Physics.25. Abdus SalamAbdus Salam, a Pakistani theoretical physicist, sharedthe Nobel Prize in Physics with Sheldon Glashow and Steven Weinberg for their work on the electroweak unification. Salam was instrumental in developing the mathematical frameworkthat described the weak and electromagnetic forces asdifferent aspects of the same force.26. Edward WittenEdward Witten, an American theoretical physicist and mathematician, is often regarded as one of the leadingfigures in string theory. His work has deepened our understanding of the mathematical underpinnings of theuniverse and has earned him numerous accolades, including the Fields Medal.27. Julian Schwinger28. SinItiro Tomonaga29. George GamowGeorge Gamow, a RussianAmerican physicist and cosmologist, made significant contributions to the fields of nuclearphysics and cosmology. He was one of the first to propose the Big Bang theory as the origin of the universe and also made important contributions to the understanding of stellar nucleosynthesis.30. Freeman DysonFreeman Dyson, a BritishAmerican theoretical physicist and mathematician, has made numerous contributions to quantum electrodynamics and solidstate physics. His work on the unification of the electromagnetic and gravitational forces, as well as his speculations on Dyson spheres, have been influential in theoretical physics.These physicists, through their relentless pursuit of knowledge, have not only advanced the frontiers of science but have also inspired a sense of wonder and curiosity in generations of scholars and laypeople alike. Their work continues to be a testament to the power of human intellect and the boundless potential of scientific inquiry.。
著名的科学家英语作文高中

When discussing the topic of famous scientists,one cannot help but be drawn into a world of curiosity,discovery,and innovation.It is through the eyes of these great minds that we have been able to understand the universe,the earth,and the very essence of life itself.In this essay,we will delve into the lives and contributions of some of the most renowned scientists in history,exploring their impact on the world and the legacy they have left behind.Isaac Newton16421727Isaac Newton is often hailed as one of the most influential scientists of all time.His groundbreaking work in physics,particularly in the field of mechanics,laid the foundation for classical physics.Newtons laws of motion and his law of universal gravitation are still taught in schools today.His work on optics,where he discovered that white light is made up of a spectrum of colors,was also revolutionary.Newtons PhilosophiæNaturalis Principia Mathematica,published in1687,is considered one of the most important works in the history of science.Marie Curie18671934Marie Curie was a physicist and chemist who conducted pioneering research on radioactivity.She was the first woman to win a Nobel Prize and remains the only person to have won Nobel Prizes in two different scientific fields physics and chemistry.Curies discovery of the elements polonium and radium,along with her work on radioactivity, paved the way for medical treatments such as cancer therapy.Her dedication to her research,despite the health risks associated with radiation exposure,is a testament to her commitment to science.Albert Einstein18791955Albert Einsteins theories have fundamentally changed the way we understand the universe.His theory of relativity,which includes the famous equation Emc², revolutionized physics by introducing the concept that mass can be converted into energy and vice versa.Einsteins work has had profound implications for the development of nuclear energy and the understanding of the cosmos.His contributions to the field of quantum mechanics,while sometimes controversial,have been instrumental in shaping modern physics.Charles Darwin18091882Charles Darwins work on evolution has had a lasting impact on our understanding ofbiology.His book On the Origin of Species introduced the theory of natural selection, which posits that species evolve over time through a process of variation and environmental selection.Darwins work has been foundational in the development of modern evolutionary biology and has influenced fields as diverse as genetics,ecology, and anthropology.Stephen Hawking19422018Stephen Hawking was a theoretical physicist whose work on black holes and the nature of the universe captivated both the scientific community and the public at large.His book A Brief History of Time brought complex scientific concepts to a wider audience. Hawkings work on the singularity theorems of general relativity and his contributions to the field of cosmology have been pivotal in our understanding of the origins and fate of the universe.Rosalind Franklin19201958Rosalind Franklins work in molecular biology was crucial in understanding the structure of DNA.Her Xray diffraction images of DNA were instrumental in revealing the molecules double helix structure,a discovery for which James Watson and Francis Crick are often credited.Franklins meticulous research and scientific rigor were essential in advancing our understanding of genetics.ConclusionThe contributions of these scientists have not only expanded our knowledge of the natural world but have also shaped the way we approach scientific inquiry.Their dedication to understanding the unknown has led to countless innovations and has inspired generations of scientists to continue the pursuit of knowledge.As we look to the future,it is with the hope that the legacy of these great minds will continue to inspire curiosity,discovery,and the relentless pursuit of truth.。
高二英语科学家名称单选题20题

高二英语科学家名称单选题20题1.Who is known for inventing papermaking?A.ConfuciusB.Zhang HengC.Cai LunD.Sima Qian答案:C。
蔡伦发明了造纸术。
孔子是思想家教育家,张衡发明地动仪等,司马迁是史学家。
2.In ancient times, who made great contributions to astronomy?A.Li ShizhenB.Bian QueC.Guo ShoujingD.Zhang Heng答案:D。
张衡在天文学方面有很大贡献,发明了地动仪等观测仪器。
李时珍是医学家,扁鹊也是医学家,郭守敬在天文学和水利方面有贡献,但本题主要突出古代对天文学贡献最大的是张衡。
3.Who is famous for writing "Compendium of Materia Medica"?A.Sun SimiaoB.Li ShizhenC.Hua TuoD.Cheng Ying答案:B。
李时珍编写了《 本草纲目》。
孙思邈也是医学家但不是写《本草纲目》的,华佗也是著名医学家,程婴不是医学家也没有写过 本草纲目》。
4.Who is renowned for his contributions to mathematics in ancient China?A.Zu ChongzhiB.Xu GuangqiC.Kuai XiangD.Yang Zhenning答案:A。
祖冲之在古代中国对数学有很大贡献。
徐光启在农学等方面有贡献,蒯祥是建筑师,杨振宁是现代物理学家不是古代的。
5.Who is remembered for his invention of the seismograph?A.Cai LunB.Zhang HengC.Li BaiD.Du Fu答案:B。
张衡发明了地动仪。
蔡伦发明造纸术,李白和杜甫是诗人不是发明家。
广义相对论全文

广义相对论全文介绍广义相对论是由爱因斯坦于1915年提出的一种描述引力的理论。
它是与牛顿引力理论相对立的一种物理学理论,通过重新定义了引力的本质,提供了一种更加准确的描述自然界中引力现象的方式。
广义相对论在宇宙学、黑洞研究以及引力波探测等领域起着重要的作用。
本文将对广义相对论的基本原理、数学形式和相关实验验证进行全面的探讨。
基本原理广义相对论的基本原理可以总结为以下几点:1.等效原理:等效原理指出,在引力场中的质点自由下落的过程中,其运动状态与在没有引力场中匀速直线运动的状态是等效的。
也就是说,引力场中的物体运动状态是由空间的弯曲决定的。
2.弯曲时空:广义相对论认为,质量和能量会弯曲时空,形成引力场。
这种弯曲是由物质的分布和运动引起的,被称为时空的曲率。
3.弯曲路径:在弯曲时空中,物体沿着一条路径运动时,会呈现出弯曲的轨迹。
这条路径被称为测地线,描述了物体在引力场中的运动轨迹。
4.引力是几何效应:广义相对论认为,引力不是通过作用力进行传递的,而是通过时空的几何效应产生的。
物体在弯曲时空中自由运动,看起来就像是受到了引力的作用。
数学形式广义相对论使用了爱因斯坦场方程来描述引力场的性质。
爱因斯坦场方程的数学形式如下:R_{\mu\nu} - \frac{1}{2} g_{\mu\nu} R = \frac{8\pi G}{c^4} T_{\mu\nu}其中,R_{\mu\nu}是时空的曲率张量,g_{\mu\nu}是时空的度规张量,R是时空的标量曲率,G是引力常数,c是光速。
T_{\mu\nu}是物质能量动量张量,描述了物质对时空的影响。
爱因斯坦场方程可以通过求解时空的度规张量来得到。
求解爱因斯坦场方程是一个非线性的偏微分方程问题,需要借助于数值方法来进行求解。
目前的研究主要集中在通过数值模拟来研究引力场的性质和时空的演化过程。
实验验证广义相对论的预言已经得到了多个实验的验证。
下面列举一些重要的实验验证结果:1.光线偏转:1919年,爱因斯坦的广义相对论的一项重要预言,在太阳附近的背景星星上观测到了太阳光的弯曲。
我们应该追科学家英语作文牛顿

我们应该追科学家英语作文牛顿The Enduring Legacy of Isaac Newton: Why We Should Emulate the Scientist.Isaac Newton, a name synonymous with scientific revolution, revolutionized the way we understand the universe. His contributions to physics, mathematics, and astronomy are still felt today, more than three centuries after his death. Newton's legacy is not just about his groundbreaking discoveries but also about the qualitiesthat made him a great scientist: his curiosity, dedication, and perseverance. These qualities are as relevant today as they were in Newton's time, making him a timeless figure worthy of emulation.Curiosity Driven by Inquiry.Newton's scientific journey began with a simple question: why does an apple fall to the ground? This curiosity led him to delve into the mysteries of gravity,ultimately formulating the law of universal gravitation.His curiosity was not just about satisfying his own wonder but about understanding the fundamental principles that govern the universe. This kind of inquiry-driven curiosityis essential for scientific progress. It drives us to question the unknown, to push the boundaries of knowledge, and to seek answers to the deepest mysteries of existence.Dedication to Scientific Pursuits.Newton's dedication to his work was unparalleled. He spent years isolated in his study, meticulously calculating and observing, often neglecting his personal life. This dedication allowed him to make breakthroughs that changedthe course of science. His dedication reminds us that true success requires hard work, perseverance, and a relentless pursuit of knowledge. In today's world, where distractions are plentiful, Newton's dedication serves as a reminder to stay focused on our goals and to persist despite challenges.Perseverance in the Face of Difficulties.Newton's scientific career was not without its setbacks. He faced criticism, skepticism, and even ridicule for his theories. Yet, he never gave up. His perseverance in the face of difficulties is a powerful lesson for us all. It teaches us that success often comes through failure, that setbacks are merely temporary, and that only through perseverance can we overcome obstacles and achieve our goals. Newton's perseverance reminds us that no matter how tough the journey may be, it is always worth it in the end.The Relevance of Newton's Work Today.Newton's work is still relevant today because it forms the foundation of much of modern science. His laws ofmotion and gravity underlie our understanding of physics, engineering, and even astronomy. His mathematical contributions, such as the development of differential and integral calculus, have revolutionized the way we approach problems in various fields, from economics to biology. The fact that Newton's work is still relevant after centuries underscores the importance of fundamental research and the value of a deep understanding of the natural world.The Enduring Lessons of Newton.Isaac Newton's life and work teach us valuable lessons about curiosity, dedication, perseverance, and the importance of fundamental research. His legacy is not just about his scientific discoveries but also about the qualities that made him a great scientist. These qualities are as relevant today as they were in Newton's time, making him a timeless figure worthy of emulation. As we look to the future of science and technology, let us remember the enduring lessons of Isaac Newton and strive to emulate his qualities in our own pursuits of knowledge and understanding.。
gravitational wave - 英译汉
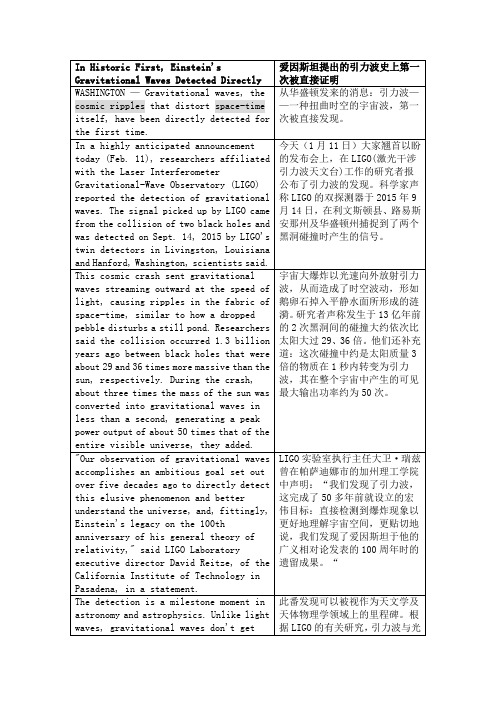
In Historic First, Einstein's Gravitational Waves Detected Directly
爱因斯坦提出的引力波史上第一次被直接证明
WASHINGTON — Gravitational waves, thecosmic ripplesthat distortspace-timeitself, have been directly detected for the first time.
此番发现可以被视作为天文学及天体物理学领域上的里程碑。根据LIGO的有关研究,引力波与光波不同,它们不会在穿越空间地过程中被介质扭曲或者改变;因此能传播有关造成其形成的物质及事件的“纯粹”信息。
好奇探星空的英语作文

Curiosity has always been a driving force for human exploration and discovery.The vast expanse of the cosmos has long captivated our imagination,prompting us to delve into the mysteries of the universe.In this essay,I will discuss the significance of exploring the stars and the impact it has had on our understanding of the world.The desire to explore the stars is rooted in our innate curiosity about the unknown.Since ancient times,humans have gazed at the night sky,marveling at the celestial bodies that adorn it.The stars have inspired countless myths,legends,and philosophical inquiries about our place in the universe.As our knowledge and technology have advanced,so too has our ability to explore the cosmos.One of the most significant contributions of star exploration is the advancement of science.Through the study of celestial bodies,we have gained a deeper understanding of the physical laws that govern the universe.For example,the observation of stars and planets has led to the development of the laws of motion and universal gravitation. Furthermore,the study of distant galaxies has provided insights into the origins and evolution of the universe.In addition to scientific discovery,exploring the stars has also had a profound impact on our culture and society.The romanticism of space travel has inspired countless works of literature,film,and art.The idea of venturing beyond our planet has become a symbol of human ambition and our quest for knowledge.It has also sparked discussions about the potential for life beyond Earth and the ethical implications of colonizing other planets. The practical applications of star exploration are also significant.Satellite technology, which has its roots in space exploration,has revolutionized communication,navigation, and meteorology.Additionally,the development of space travel has led to innovations in various fields,such as materials science,engineering,and computer technology. However,exploring the stars is not without its challenges.The vast distances and harsh conditions of space present numerous obstacles to overcome.The financial and human resources required for space exploration are substantial,and there are ongoing debates about the allocation of these resources.Despite these challenges,the potential benefits of star exploration make it a worthy endeavor.In conclusion,the exploration of the stars is a testament to human curiosity and our relentless pursuit of knowledge.It has expanded our understanding of the universe, enriched our culture,and led to numerous technological advancements.As we continue to push the boundaries of our exploration,we may one day unlock even greater mysteries of the cosmos.。
科学之光指迷津的英语作文

The light of science has always been a beacon of hope and progress for humanity.It guides us through the darkness of ignorance and uncertainty,illuminating the path to a brighter future.In this essay,we will explore the various ways in which the light of science has helped to dispel confusion and lead us towards a better understanding of the world around us.Firstly,the light of science has been instrumental in advancing our knowledge of the natural world.Through rigorous observation,experimentation,and analysis,scientists have uncovered the fundamental laws that govern the behavior of matter and energy.This has allowed us to develop a deeper understanding of the universe and its many wonders, from the smallest subatomic particles to the vast expanses of space.Secondly,the light of science has played a crucial role in improving our quality of life. Medical breakthroughs,technological innovations,and agricultural advancements are just a few examples of how scientific discoveries have transformed the way we live.By addressing pressing issues such as disease,hunger,and environmental degradation, science has the power to alleviate suffering and create a more sustainable future for all.Moreover,the light of science fosters critical thinking and problemsolving skills.By encouraging us to question our assumptions and seek evidencebased solutions,science helps to cultivate a mindset that values reason and logic.This is particularly important in todays world,where misinformation and superstition can easily spread and lead to misguided decisions.However,it is important to recognize that the light of science is not without its challenges. The pursuit of knowledge can sometimes lead to ethical dilemmas,as seen in cases of scientific research involving animals or human subjects.Additionally,the rapid pace of technological advancement can create a sense of unease and uncertainty about the future. In conclusion,the light of science is a powerful force that has the potential to guide us through the complexities of life and help us navigate the unknown.By embracing the spirit of inquiry and striving for a greater understanding of the world,we can harness the full potential of science to create a brighter,more enlightened future for all.。
插上科学的翅膀飞的英语作文缩,

插上科学的翅膀飞的英语作文缩,Science, the unceasing quest for knowledge and understanding, has propelled humanity to remarkable heights, empowering us to soar above limitations and grasp the intricacies of our universe. Like majestic birds taking flight, we have harnessed the power of scientific inquiryto explore uncharted realms, unravel mysteries, and forge a path towards a future filled with limitless possibilities.Science has bestowed upon us the gift of sight,enabling us to peer beyond the confines of our immediate surroundings. Through telescopes that pierce the celestial tapestry, we have glimpsed distant galaxies, witnessed the birth and death of stars, and marveled at the vastness of cosmic horizons. Microscopes, on the other hand, have revealed a hidden world teeming with microscopic organisms, unlocking the secrets of life at its most fundamental level.Moreover, science has granted us the power of prediction. By meticulously observing patterns andformulating mathematical models, scientists can forecast weather patterns, anticipate earthquakes, and even predict the behavior of distant celestial bodies. This ability to anticipate future events has proven invaluable in safeguarding lives, mitigating disasters, and guiding our decisions.The advancements in medicine stand as a testament to the transformative power of science. Vaccines have eradicated dreaded diseases that once ravaged humanity, while antibiotics have empowered us to combat infections that were once thought to be invincible. Surgical techniques have become increasingly sophisticated, giving hope to those suffering from debilitating conditions. Science has extended our lifespans, enhanced our quality of life, and given us the opportunity to live healthier, more fulfilling lives.Science has also revolutionized the way we communicate and connect with the world. The internet, a global network of interconnected computers, has shattered geographical barriers, allowing us to share information, ideas, andexperiences with unprecedented ease. Social media platforms have fostered virtual communities, bridging distances and fostering connections between individuals from all walks of life.In the realm of transportation, science has paved the way for high-speed trains that traverse vast distances in a matter of hours, airplanes that soar through the skies with remarkable speed and efficiency, and spacecraft that have ventured beyond the confines of our planet, carrying the hopes and dreams of humanity to distant destinations.Science has not only expanded our physical horizons but has also enriched our intellectual landscape. Through the study of history, we have gained a profound understanding of our past, enabling us to avoid the mistakes of our ancestors and build upon their achievements. Philosophy has challenged our fundamental beliefs, encouraging us to question the nature of reality, the meaning of existence, and the purpose of human life. Psychology has delved into the depths of the human psyche, shedding light on our emotions, motivations, and behaviors.The pursuit of scientific knowledge has spurred technological advancements that have transformed our daily lives. From the humble light bulb that illuminated our homes to the sleek smartphones that connect us to the world, science has provided us with countless conveniences that enhance our comfort, productivity, and overall well-being.However, the power of science is not without its responsibilities. As we continue to push the boundaries of human knowledge, we must proceed with both wisdom and humility. It is essential to consider the ethical implications of our scientific endeavors and to ensure that they are used for the betterment of humanity, not for its destruction.Science has granted us the wings of knowledge and understanding, empowering us to soar above the constraintsof ignorance and superstition. By embracing the scientific method, we have gained an unparalleled ability to explore, discover, and create. As we continue our journey throughthe vast expanse of scientific inquiry, let us harness thepower of science for the betterment of our planet and for the generations to come. May we always strive to fly with the wings of science, guided by the unwavering pursuit of knowledge and the boundless possibilities that lie ahead.。
天体物理学报LIGO引力波探测器释疑PPT课件

⟹ Electromagnetic radiation
The General Theory of Relativity and theory of Gravity (1916)
Gravity described as a warpage of spacetime, not a force acting at a distance
Joseph Taylor
Russell Hulse
17 / sec Very precise clock
~ 8 hr
forbitaltoday ~ 0.000035 Hz (35 microHertz)
forbital300,000,000 yrs ~ 100 Hz (in LIGO band)
LIGO-G1401149-v1
Outline
The LIGO mission Gravitational waves and their detection Astronomical sources for LIGO The Advanced LIGO Project Prospects for a global network
suspended (‘freely falling’) mirrors
Passing GWs stretch and compress the distance between the end test mass and the beamsplitter
On behalf of the LIGO Scientific Collaboration
13 October 2014 Eastern Forum of Science and Technology (EFST)