净水
净水器中的纯水是什么原理
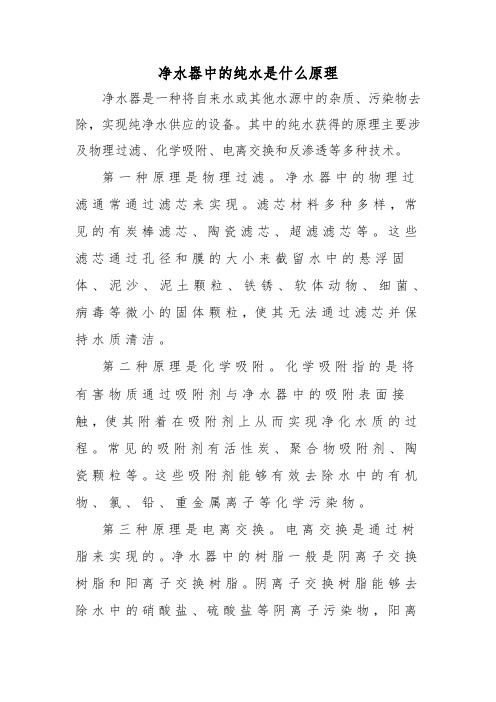
净水器中的纯水是什么原理净水器是一种将自来水或其他水源中的杂质、污染物去除,实现纯净水供应的设备。
其中的纯水获得的原理主要涉及物理过滤、化学吸附、电离交换和反渗透等多种技术。
第一种原理是物理过滤。
净水器中的物理过滤通常通过滤芯来实现。
滤芯材料多种多样,常见的有炭棒滤芯、陶瓷滤芯、超滤滤芯等。
这些滤芯通过孔径和膜的大小来截留水中的悬浮固体、泥沙、泥土颗粒、铁锈、软体动物、细菌、病毒等微小的固体颗粒,使其无法通过滤芯并保持水质清洁。
第二种原理是化学吸附。
化学吸附指的是将有害物质通过吸附剂与净水器中的吸附表面接触,使其附着在吸附剂上从而实现净化水质的过程。
常见的吸附剂有活性炭、聚合物吸附剂、陶瓷颗粒等。
这些吸附剂能够有效去除水中的有机物、氯、铅、重金属离子等化学污染物。
第三种原理是电离交换。
电离交换是通过树脂来实现的。
净水器中的树脂一般是阴离子交换树脂和阳离子交换树脂。
阴离子交换树脂能够去除水中的硝酸盐、硫酸盐等阴离子污染物,阳离子交换树脂能够去除水中的钠离子、钙离子、镁离子等阳离子污染物。
最后一种原理是反渗透。
反渗透是利用半透膜的特性将溶液通过膜表面的一个或多个孔的过程。
净水器中的半透膜可以有效地去除水中的无机盐、重金属、细菌、病毒等。
在反渗透过程中,水分子可以穿过微孔,但大多数溶质、溶剂不能通过,从而实现水的纯化。
综上所述,净水器中的纯水是通过多种原理来实现的。
不同类型的净水器可能采用不同的过滤方式和技术,但都旨在去除水中的微小固体颗粒、化学污染物、离子污染物等,提供纯净、安全的水源。
消费者可以选择适合自己需求的净水器,确保家庭健康饮水的需求。
家用净水器原理图解

家用净水器原理图解
家用净水器原理图解:
1. 水源:家用净水器的基本原理是将自来水或井水作为水源。
2. 预过滤:水进入净水器后,首先通过预过滤器。
预过滤器通常由一层或多层过滤网组成,它能够去除水中较大的悬浮颗粒物,如沙子、泥土和锈迹等。
3. 活性炭滤芯:经过预过滤后的水进入活性炭滤芯。
活性炭滤芯内填充着颗粒状的活性炭,它能够去除水中的异味、余氯和有机物等。
4. RO膜滤芯:经过活性炭滤芯处理后的水进入RO膜滤芯。
RO膜滤芯是家用净水器的核心部件,其内部包含成百上千个微孔,这些微孔能够只允许水分子通过,而拦截大多数溶解物质和微生物。
5. 后置活性炭滤芯:经过RO膜滤芯处理后的水进入后置活性炭滤芯。
后置活性炭滤芯主要是为了进一步去除残留的异味和有机化合物,提高水的口感。
6. 出水口:最后,经过多重过滤的净水通过出水口流出,供人们使用。
家用净水器通过预过滤、活性炭滤芯和RO膜滤芯等多级过滤方式,能够有效去除自来水中的悬浮颗粒、异味、余氯、有机
物和微生物等。
它不仅可以提供安全、干净的饮用水,还可以减少水垢对家电设备的损害,提高生活质量。
净水常用的几种方法
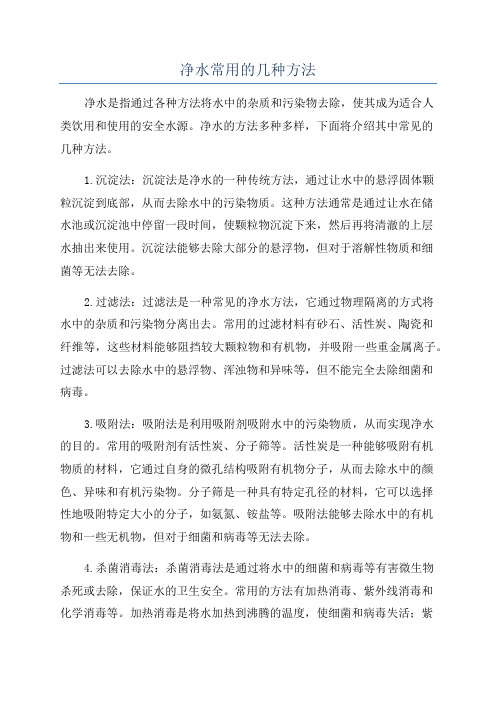
净水常用的几种方法净水是指通过各种方法将水中的杂质和污染物去除,使其成为适合人类饮用和使用的安全水源。
净水的方法多种多样,下面将介绍其中常见的几种方法。
1.沉淀法:沉淀法是净水的一种传统方法,通过让水中的悬浮固体颗粒沉淀到底部,从而去除水中的污染物质。
这种方法通常是通过让水在储水池或沉淀池中停留一段时间,使颗粒物沉淀下来,然后再将清澈的上层水抽出来使用。
沉淀法能够去除大部分的悬浮物,但对于溶解性物质和细菌等无法去除。
2.过滤法:过滤法是一种常见的净水方法,它通过物理隔离的方式将水中的杂质和污染物分离出去。
常用的过滤材料有砂石、活性炭、陶瓷和纤维等,这些材料能够阻挡较大颗粒物和有机物,并吸附一些重金属离子。
过滤法可以去除水中的悬浮物、浑浊物和异味等,但不能完全去除细菌和病毒。
3.吸附法:吸附法是利用吸附剂吸附水中的污染物质,从而实现净水的目的。
常用的吸附剂有活性炭、分子筛等。
活性炭是一种能够吸附有机物质的材料,它通过自身的微孔结构吸附有机物分子,从而去除水中的颜色、异味和有机污染物。
分子筛是一种具有特定孔径的材料,它可以选择性地吸附特定大小的分子,如氨氮、铵盐等。
吸附法能够去除水中的有机物和一些无机物,但对于细菌和病毒等无法去除。
4.杀菌消毒法:杀菌消毒法是通过将水中的细菌和病毒等有害微生物杀死或去除,保证水的卫生安全。
常用的方法有加热消毒、紫外线消毒和化学消毒等。
加热消毒是将水加热到沸腾的温度,使细菌和病毒失活;紫外线消毒是利用紫外线照射水中的微生物,破坏其遗传物质,从而达到杀菌的目的;化学消毒是通过加入氯、臭氧等化学物质,使其与细菌和病毒反应,杀灭其活性。
杀菌消毒法能够有效地去除水中的微生物,但对于有机物和重金属等无法去除。
5.反渗透法:反渗透法是一种高效的净水方法,通过半透膜将水中的溶解性物质和微小颗粒逆向渗透,从而实现水的净化。
这种方法通常是将水加压通过具有微孔的半透膜,膜孔大小约为0.1微米,可以阻挡细菌、病毒和溶解性物质等。
净水常用的几种方法
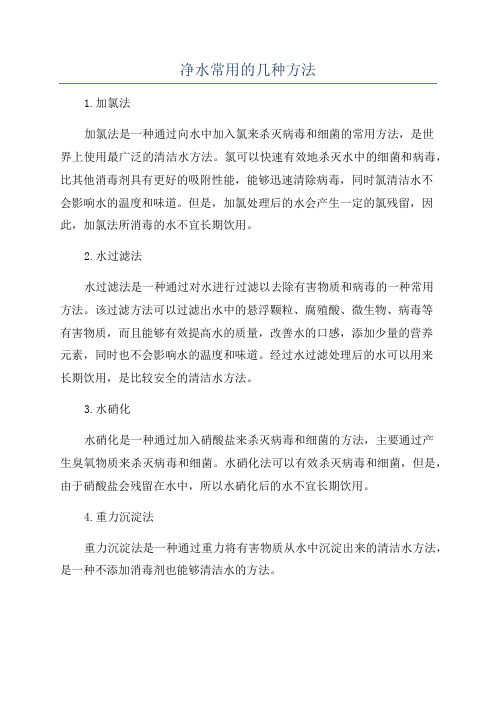
净水常用的几种方法
1.加氯法
加氯法是一种通过向水中加入氯来杀灭病毒和细菌的常用方法,是世
界上使用最广泛的清洁水方法。
氯可以快速有效地杀灭水中的细菌和病毒,比其他消毒剂具有更好的吸附性能,能够迅速清除病毒,同时氯清洁水不
会影响水的温度和味道。
但是,加氯处理后的水会产生一定的氯残留,因此,加氯法所消毒的水不宜长期饮用。
2.水过滤法
水过滤法是一种通过对水进行过滤以去除有害物质和病毒的一种常用
方法。
该过滤方法可以过滤出水中的悬浮颗粒、腐殖酸、微生物、病毒等
有害物质,而且能够有效提高水的质量,改善水的口感,添加少量的营养
元素,同时也不会影响水的温度和味道。
经过水过滤处理后的水可以用来
长期饮用,是比较安全的清洁水方法。
3.水硝化
水硝化是一种通过加入硝酸盐来杀灭病毒和细菌的方法,主要通过产
生臭氧物质来杀灭病毒和细菌。
水硝化法可以有效杀灭病毒和细菌,但是,由于硝酸盐会残留在水中,所以水硝化后的水不宜长期饮用。
4.重力沉淀法
重力沉淀法是一种通过重力将有害物质从水中沉淀出来的清洁水方法,是一种不添加消毒剂也能够清洁水的方法。
家庭用净水方案
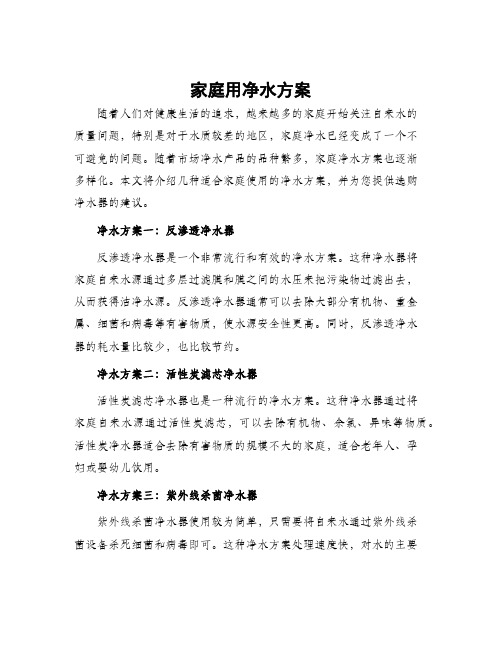
家庭用净水方案随着人们对健康生活的追求,越来越多的家庭开始关注自来水的质量问题,特别是对于水质较差的地区,家庭净水已经变成了一个不可避免的问题。
随着市场净水产品的品种繁多,家庭净水方案也逐渐多样化。
本文将介绍几种适合家庭使用的净水方案,并为您提供选购净水器的建议。
净水方案一:反渗透净水器反渗透净水器是一个非常流行和有效的净水方案。
这种净水器将家庭自来水源通过多层过滤膜和膜之间的水压来把污染物过滤出去,从而获得洁净水源。
反渗透净水器通常可以去除大部分有机物、重金属、细菌和病毒等有害物质,使水源安全性更高。
同时,反渗透净水器的耗水量比较少,也比较节约。
净水方案二:活性炭滤芯净水器活性炭滤芯净水器也是一种流行的净水方案。
这种净水器通过将家庭自来水源通过活性炭滤芯,可以去除有机物、余氯、异味等物质。
活性炭净水器适合去除有害物质的规模不大的家庭,适合老年人、孕妇或婴幼儿饮用。
净水方案三:紫外线杀菌净水器紫外线杀菌净水器使用较为简单,只需要将自来水通过紫外线杀菌设备杀死细菌和病毒即可。
这种净水方案处理速度快,对水的主要成分没有影响,而且基本不用更换材料。
但需要注意的是,紫外线杀菌净水器不能去除一些常见的化学污染物和重金属。
选购净水器选购净水器时,除了考虑用户的实际需求和安装环境外,对于净水器的品牌、过滤技术和净水效果也是需要考虑的关键因素。
首先,净水器的品牌和信誉度应该是首要考虑因素;其次,净水技术越先进的净水器,净水能力也越强,更安全、更健康;最后,要选择符合自己需求的净水器。
举例来说,如果居住在水质状况较好的地区,使用活性炭滤芯净水器已经可以满足需求,而当地自来水质量比较差,则应该选择更先进的反渗透净水器。
结论净水器可视为家庭健康饮水的一种选择,基于本文对家庭净水方案的介绍,我们可以看出不同类型的净水器都有适用的场景,如需购买净水器,可以根据自己家庭的实际情况、水质情况、使用范围等要素进行正确选择。
此外,购买净水器后,也需要遵循正确的维护方法,保障净水器的正常使用。
家用净水器原理

家用净水器原理
家用净水器是一种通过物理、化学或生物方法去除水中杂质和污染物的设备。
它的原理是利用滤芯、膜组合或其他净水技术,将自来水中的水质问题进行处理,提供更干净、更安全的饮用水。
家用净水器的工作原理根据不同的技术有所不同。
下面将介绍几种常见的家用净水器工作原理:
1. 活性炭滤芯:活性炭是一种能够吸附水中有机化学物质的材料。
家用净水器中的活性炭滤芯通过物理吸附和化学吸附的作用,去除自来水中的异味、颜色和余氯等物质。
2. 纤维滤芯:纤维滤芯可以过滤细小的悬浮物颗粒和杂质,如沙子、泥土、铁锈等。
通过纤维滤芯的层层过滤,可以有效去除自来水中的悬浮物质。
3. RO膜:RO膜是一种反渗透膜,能够过滤水中的无机盐和
微生物等。
当自来水通过RO膜时,较大颗粒和溶解物质被阻隔,纯净水则通过RO膜进入储水箱。
4. UV杀菌灯:UV杀菌灯利用紫外线照射,能够有效杀灭水
中的细菌和病毒。
通过UV杀菌灯的作用,可以提高饮用水的卫生安全性。
5. 陶瓷滤芯:陶瓷滤芯由微孔陶瓷组成,可以过滤掉水中的细菌、泥土和铁锈等杂质。
通过陶瓷滤芯的过滤,可以提供清澈
透明的饮用水。
总的来说,家用净水器通过不同的净水技术和材料,去除自来水中的杂质和污染物,从而提供更干净、更安全的饮用水。
不同的家用净水器可能采用单一的净水技术,也可能结合多种净水技术,以达到更好的过滤效果。
净水小知识
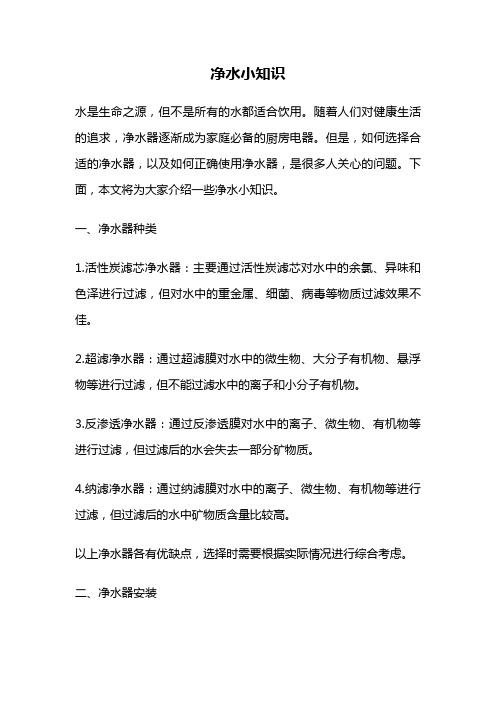
净水小知识水是生命之源,但不是所有的水都适合饮用。
随着人们对健康生活的追求,净水器逐渐成为家庭必备的厨房电器。
但是,如何选择合适的净水器,以及如何正确使用净水器,是很多人关心的问题。
下面,本文将为大家介绍一些净水小知识。
一、净水器种类1.活性炭滤芯净水器:主要通过活性炭滤芯对水中的余氯、异味和色泽进行过滤,但对水中的重金属、细菌、病毒等物质过滤效果不佳。
2.超滤净水器:通过超滤膜对水中的微生物、大分子有机物、悬浮物等进行过滤,但不能过滤水中的离子和小分子有机物。
3.反渗透净水器:通过反渗透膜对水中的离子、微生物、有机物等进行过滤,但过滤后的水会失去一部分矿物质。
4.纳滤净水器:通过纳滤膜对水中的离子、微生物、有机物等进行过滤,但过滤后的水中矿物质含量比较高。
以上净水器各有优缺点,选择时需要根据实际情况进行综合考虑。
二、净水器安装1.净水器安装尽量选择通风、干燥的地方,避免阳光直射和高温环境。
2.安装净水器时,要按照说明书的要求进行,不得私自改动或拆卸净水器。
3.净水器安装后,首次使用前应先放掉里面的水,再进行正常使用。
三、净水器日常保养1.定期更换滤芯:不同种类的净水器滤芯寿命不同,日常使用中要按照说明书的要求定期更换滤芯,以保证过滤效果。
2.清洗净水器:净水器长期使用后,容易出现污垢、沉淀等情况,应定期对净水器进行清洗,以保证净水器的工作效率。
3.避免长时间不用:长时间不使用净水器,应将净水器内的水放掉,并将净水器拆开晾干,避免滤芯变质,影响净水效果。
四、净水器选购1.品牌:选择知名品牌的净水器,可以更好地保证产品质量和售后服务。
2.过滤效果:根据实际水质情况选择过滤效果更好的净水器。
3.滤芯寿命:不同品牌、不同型号的净水器滤芯寿命不同,选择时要根据实际使用情况考虑。
4.价格:价格不是唯一标准,应根据产品质量、品牌信誉等综合考虑。
净水器是保障家庭健康的必备品。
选择合适的净水器、正确使用和维护净水器,能够更好地保障家庭饮水安全。
家用净水机的工作原理
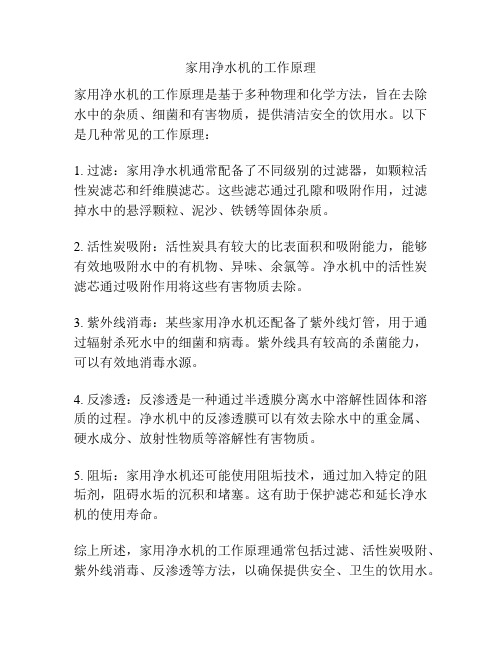
家用净水机的工作原理
家用净水机的工作原理是基于多种物理和化学方法,旨在去除水中的杂质、细菌和有害物质,提供清洁安全的饮用水。
以下是几种常见的工作原理:
1. 过滤:家用净水机通常配备了不同级别的过滤器,如颗粒活性炭滤芯和纤维膜滤芯。
这些滤芯通过孔隙和吸附作用,过滤掉水中的悬浮颗粒、泥沙、铁锈等固体杂质。
2. 活性炭吸附:活性炭具有较大的比表面积和吸附能力,能够有效地吸附水中的有机物、异味、余氯等。
净水机中的活性炭滤芯通过吸附作用将这些有害物质去除。
3. 紫外线消毒:某些家用净水机还配备了紫外线灯管,用于通过辐射杀死水中的细菌和病毒。
紫外线具有较高的杀菌能力,可以有效地消毒水源。
4. 反渗透:反渗透是一种通过半透膜分离水中溶解性固体和溶质的过程。
净水机中的反渗透膜可以有效去除水中的重金属、硬水成分、放射性物质等溶解性有害物质。
5. 阻垢:家用净水机还可能使用阻垢技术,通过加入特定的阻垢剂,阻碍水垢的沉积和堵塞。
这有助于保护滤芯和延长净水机的使用寿命。
综上所述,家用净水机的工作原理通常包括过滤、活性炭吸附、紫外线消毒、反渗透等方法,以确保提供安全、卫生的饮用水。
净化程度最高的净水方法
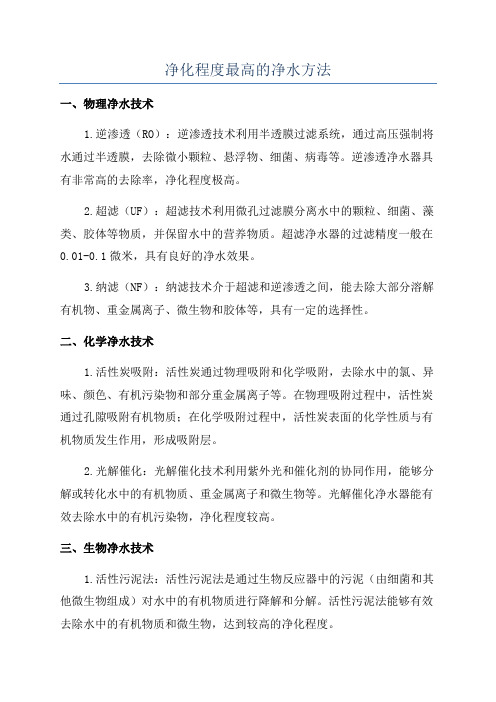
净化程度最高的净水方法一、物理净水技术1.逆渗透(RO):逆渗透技术利用半透膜过滤系统,通过高压强制将水通过半透膜,去除微小颗粒、悬浮物、细菌、病毒等。
逆渗透净水器具有非常高的去除率,净化程度极高。
2.超滤(UF):超滤技术利用微孔过滤膜分离水中的颗粒、细菌、藻类、胶体等物质,并保留水中的营养物质。
超滤净水器的过滤精度一般在0.01-0.1微米,具有良好的净水效果。
3.纳滤(NF):纳滤技术介于超滤和逆渗透之间,能去除大部分溶解有机物、重金属离子、微生物和胶体等,具有一定的选择性。
二、化学净水技术1.活性炭吸附:活性炭通过物理吸附和化学吸附,去除水中的氯、异味、颜色、有机污染物和部分重金属离子等。
在物理吸附过程中,活性炭通过孔隙吸附有机物质;在化学吸附过程中,活性炭表面的化学性质与有机物质发生作用,形成吸附层。
2.光解催化:光解催化技术利用紫外光和催化剂的协同作用,能够分解或转化水中的有机物质、重金属离子和微生物等。
光解催化净水器能有效去除水中的有机污染物,净化程度较高。
三、生物净水技术1.活性污泥法:活性污泥法是通过生物反应器中的污泥(由细菌和其他微生物组成)对水中的有机物质进行降解和分解。
活性污泥法能够有效去除水中的有机物质和微生物,达到较高的净化程度。
2.植物净水法:植物净水法是通过植物的吸收、蓄积和转化作用,去除水中的重金属离子、有机物质和微生物等。
植物净水法具有环保、经济的特点,能够将水污染物转化为植物生长的有机物。
3.生物滤池:生物滤池通过生物降解和吸附作用,去除水中的有机物质、氨氮和微生物等。
该技术具有较高的净化能力,对水中的有机物质和微生物有较好的去除效果。
综上所述,净化程度最高的净水方法要综合运用上述物理、化学和生物净水技术,如采用逆渗透、超滤等物理净水技术与活性炭吸附、光解催化等化学净水技术的组合,同时结合生物净水技术,才能够最大程度地去除水中的有害物质和污染物,实现最佳的净水效果。
净水常用方法
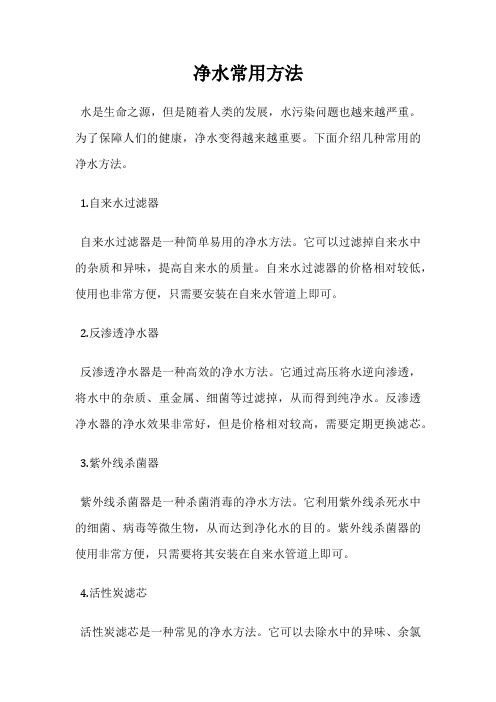
净水常用方法
水是生命之源,但是随着人类的发展,水污染问题也越来越严重。
为了保障人们的健康,净水变得越来越重要。
下面介绍几种常用的净水方法。
1.自来水过滤器
自来水过滤器是一种简单易用的净水方法。
它可以过滤掉自来水中的杂质和异味,提高自来水的质量。
自来水过滤器的价格相对较低,使用也非常方便,只需要安装在自来水管道上即可。
2.反渗透净水器
反渗透净水器是一种高效的净水方法。
它通过高压将水逆向渗透,将水中的杂质、重金属、细菌等过滤掉,从而得到纯净水。
反渗透净水器的净水效果非常好,但是价格相对较高,需要定期更换滤芯。
3.紫外线杀菌器
紫外线杀菌器是一种杀菌消毒的净水方法。
它利用紫外线杀死水中的细菌、病毒等微生物,从而达到净化水的目的。
紫外线杀菌器的使用非常方便,只需要将其安装在自来水管道上即可。
4.活性炭滤芯
活性炭滤芯是一种常见的净水方法。
它可以去除水中的异味、余氯
等有害物质,提高水的口感和质量。
活性炭滤芯的价格相对较低,使用也非常方便,只需要将其安装在自来水管道上即可。
净水是非常重要的,选择适合自己的净水方法可以保障家人的健康。
不同的净水方法有不同的优缺点,需要根据自己的需求和实际情况选择。
同时,定期更换滤芯也是保证净水效果的关键。
家用净水器原理是什么

家用净水器原理是什么
家用净水器的原理是通过一系列的物理、化学或生物方法去除水中的杂质和污染物,以提供干净、安全的饮用水。
一种常见的家用净水器原理是通过滤网去除水中的悬浮物、沉淀物和大颗粒污染物。
这种滤网通常由纤维材料或多孔陶瓷制成,具有能够阻挡杂质的微小孔隙。
当水通过滤网时,大颗粒物质会被滤网截留,而纯净的水则通过滤网流出。
其他一些家用净水器会利用活性炭或其他吸附材料来去除水中的化学污染物。
活性炭是一种多孔的材料,具有很大的表面积以吸附有机物和一些重金属离子。
当水流经过活性炭时,污染物会被吸附在其表面上,从而净化水质。
一些高级家用净水器可能会采用反渗透技术。
反渗透是通过半透膜筛选出溶解在水中的溶质和离子的方法。
当水通过反渗透膜时,其中的溶质和离子会被膜截留,而纯净的水则通过膜流出。
这种技术可以有效去除水中的溶解性无机盐、有机物和微生物。
有些家用净水器还会结合多种净化技术,如紫外线灭菌、臭氧氧化等,以提供更全面的净水效果。
总的来说,家用净水器的原理是通过物理、化学和生物作用来去除水中的杂质和污染物,提供安全、干净的饮用水。
净水常用方法
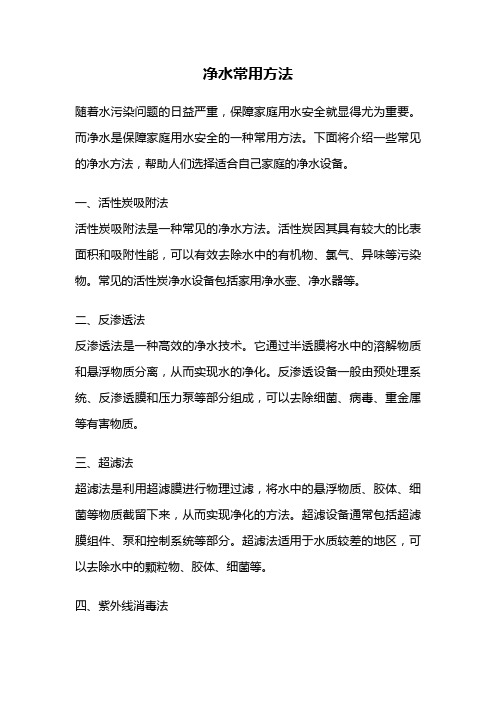
净水常用方法随着水污染问题的日益严重,保障家庭用水安全就显得尤为重要。
而净水是保障家庭用水安全的一种常用方法。
下面将介绍一些常见的净水方法,帮助人们选择适合自己家庭的净水设备。
一、活性炭吸附法活性炭吸附法是一种常见的净水方法。
活性炭因其具有较大的比表面积和吸附性能,可以有效去除水中的有机物、氯气、异味等污染物。
常见的活性炭净水设备包括家用净水壶、净水器等。
二、反渗透法反渗透法是一种高效的净水技术。
它通过半透膜将水中的溶解物质和悬浮物质分离,从而实现水的净化。
反渗透设备一般由预处理系统、反渗透膜和压力泵等部分组成,可以去除细菌、病毒、重金属等有害物质。
三、超滤法超滤法是利用超滤膜进行物理过滤,将水中的悬浮物质、胶体、细菌等物质截留下来,从而实现净化的方法。
超滤设备通常包括超滤膜组件、泵和控制系统等部分。
超滤法适用于水质较差的地区,可以去除水中的颗粒物、胶体、细菌等。
四、紫外线消毒法紫外线消毒法是一种物理杀菌方法。
利用紫外线的照射,可以破坏微生物的DNA结构,进而杀死细菌、病毒等。
紫外线消毒设备一般由紫外灯管、反应池和控制系统等组成。
紫外线消毒法适用于去除水中的细菌、病毒等微生物。
五、电解水法电解水法是一种利用电解原理进行净水的方法。
通过电解水设备,将水分解为酸性水和碱性水,可以去除水中的重金属离子、细菌、病毒等有害物质。
电解水法适用于改善水质和提高水的口感。
六、陶瓷过滤法陶瓷过滤法是一种常见的净水技术。
陶瓷过滤器由多孔陶瓷材料制成,具有较好的过滤效果。
它可以去除水中的悬浮物质、细菌等,同时保留水中的矿物质。
陶瓷过滤器使用方便,适用于户外活动和家庭生活等场景。
七、活性氧氧化法活性氧氧化法是一种利用活性氧氧化剂进行净水的方法。
活性氧能够快速氧化水中的有机物质,从而去除水中的有机污染物。
活性氧氧化法适用于去除水中的有机物质、异味等。
八、离子交换法离子交换法是一种通过离子交换树脂去除水中离子的方法。
通过选择性吸附和释放,离子交换树脂可以去除水中的硬度离子、重金属离子等。
如何净化水
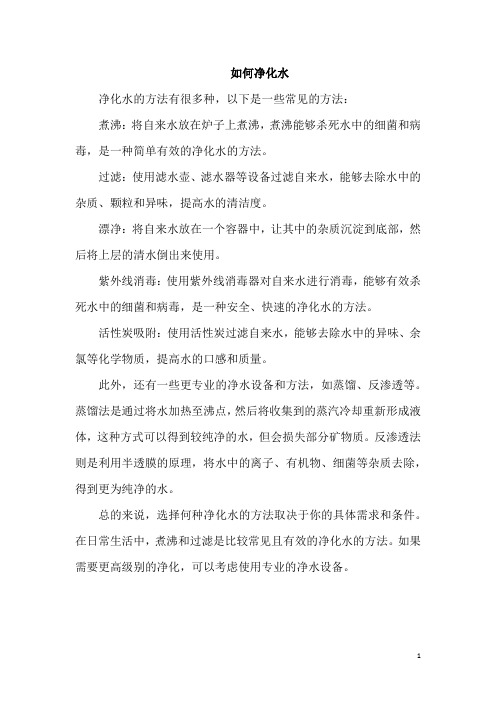
如何净化水
净化水的方法有很多种,以下是一些常见的方法:
煮沸:将自来水放在炉子上煮沸,煮沸能够杀死水中的细菌和病毒,是一种简单有效的净化水的方法。
过滤:使用滤水壶、滤水器等设备过滤自来水,能够去除水中的杂质、颗粒和异味,提高水的清洁度。
漂净:将自来水放在一个容器中,让其中的杂质沉淀到底部,然后将上层的清水倒出来使用。
紫外线消毒:使用紫外线消毒器对自来水进行消毒,能够有效杀死水中的细菌和病毒,是一种安全、快速的净化水的方法。
活性炭吸附:使用活性炭过滤自来水,能够去除水中的异味、余氯等化学物质,提高水的口感和质量。
此外,还有一些更专业的净水设备和方法,如蒸馏、反渗透等。
蒸馏法是通过将水加热至沸点,然后将收集到的蒸汽冷却重新形成液体,这种方式可以得到较纯净的水,但会损失部分矿物质。
反渗透法则是利用半透膜的原理,将水中的离子、有机物、细菌等杂质去除,得到更为纯净的水。
总的来说,选择何种净化水的方法取决于你的具体需求和条件。
在日常生活中,煮沸和过滤是比较常见且有效的净化水的方法。
如果需要更高级别的净化,可以考虑使用专业的净水设备。
1。
净水的基本原理是什么

净水的基本原理是什么净水是指通过一些特定的技术和方法,将水中的杂质、污染物质或者病原微生物等有害物质去除或减少至可接受标准以提供安全、干净、卫生的饮用水或者工业用水。
净水的基本原理涉及物理学、化学学和生物学等多个领域,常使用的净水技术包括过滤、沉淀、吸附、电离、反渗透等。
首先,净水的基本原理之一是过滤。
过滤是使用一定的过滤介质,例如砂、石英砂、活性炭等,通过筛选、截留和吸附等作用将悬浮物、大颗粒物质、泥沙等去除,使水体变得更加清澈与透明。
过滤是物理过程,能够有效去除可见的固体杂质,并减少浊度和颜色。
常见的过滤方式有常压过滤、压力过滤、砂滤等。
过滤的选择依赖于水源的特性和净水目的。
其次,沉淀是净水的另一个基本原理。
沉淀是指通过重力或电聚积等作用,使水中的固体杂质(如悬浮物、胶状物、胶体颗粒、铁锈等)与水分离,从而达到净水的目的。
常用的方法有沉淀池、沉淀池组合系统等。
在沉淀过程中,杂质的粒径较大,所以需要一个相对较长的停留时间才能完成沉淀。
此外,吸附也是净水的重要原理之一。
吸附是指通过活性炭、树脂、陶瓷等吸附材料的作用,吸附水中的有机物、异味、化学污染物等有害物质,使水质改善。
活性炭是一种经特殊处理的炭,具有较大的比表面积,因此能够吸附许多有害物质。
具体吸附原理是利用吸附材料的微孔和微生物体通过物理吸附和化学吸附将有机物质和其他化学污染物吸附在吸附材料上。
净水的基本原理还包括电离。
电离是利用电场力量,将水中的离子和溶解物质分离开来,并去除其中的有害物质。
常见的电离方法是电吸附、电渗析等。
电离净水可以移除水中的草酸根离子、硫酸根离子、氯离子等,使水的溶解固体含量达到可接受水平。
最后,净水的基本原理还包括反渗透。
反渗透是将水通过半透膜过滤,以排除其中的有害物质,获得高纯度水的一种技术。
通过施加高压,使水分子穿越半透膜,而溶质和悬浮物留在膜的一侧,从而实现物质的分离。
反渗透能够有效去除溶解在水中的无机盐、细菌、病毒和有机物等,得到高质量的纯净水。
净水器的工作原理
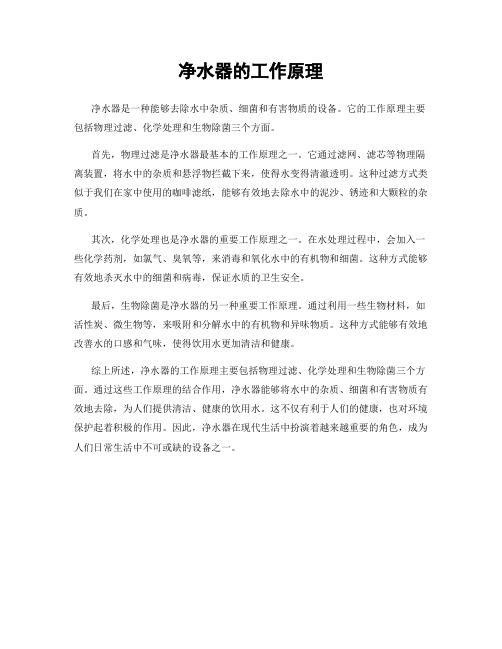
净水器的工作原理
净水器是一种能够去除水中杂质、细菌和有害物质的设备。
它的工作原理主要包括物理过滤、化学处理和生物除菌三个方面。
首先,物理过滤是净水器最基本的工作原理之一。
它通过滤网、滤芯等物理隔离装置,将水中的杂质和悬浮物拦截下来,使得水变得清澈透明。
这种过滤方式类似于我们在家中使用的咖啡滤纸,能够有效地去除水中的泥沙、锈迹和大颗粒的杂质。
其次,化学处理也是净水器的重要工作原理之一。
在水处理过程中,会加入一些化学药剂,如氯气、臭氧等,来消毒和氧化水中的有机物和细菌。
这种方式能够有效地杀灭水中的细菌和病毒,保证水质的卫生安全。
最后,生物除菌是净水器的另一种重要工作原理。
通过利用一些生物材料,如活性炭、微生物等,来吸附和分解水中的有机物和异味物质。
这种方式能够有效地改善水的口感和气味,使得饮用水更加清洁和健康。
综上所述,净水器的工作原理主要包括物理过滤、化学处理和生物除菌三个方面。
通过这些工作原理的结合作用,净水器能够将水中的杂质、细菌和有害物质有效地去除,为人们提供清洁、健康的饮用水。
这不仅有利于人们的健康,也对环境保护起着积极的作用。
因此,净水器在现代生活中扮演着越来越重要的角色,成为人们日常生活中不可或缺的设备之一。
净水机工作原理
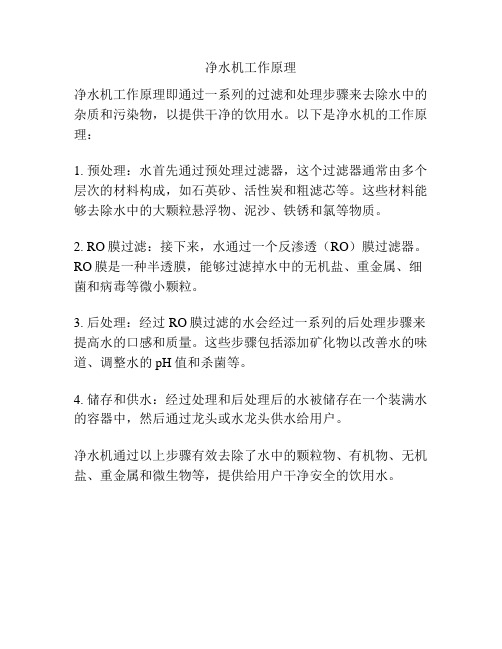
净水机工作原理
净水机工作原理即通过一系列的过滤和处理步骤来去除水中的杂质和污染物,以提供干净的饮用水。
以下是净水机的工作原理:
1. 预处理:水首先通过预处理过滤器,这个过滤器通常由多个层次的材料构成,如石英砂、活性炭和粗滤芯等。
这些材料能够去除水中的大颗粒悬浮物、泥沙、铁锈和氯等物质。
2. RO膜过滤:接下来,水通过一个反渗透(RO)膜过滤器。
RO膜是一种半透膜,能够过滤掉水中的无机盐、重金属、细菌和病毒等微小颗粒。
3. 后处理:经过RO膜过滤的水会经过一系列的后处理步骤来提高水的口感和质量。
这些步骤包括添加矿化物以改善水的味道、调整水的pH值和杀菌等。
4. 储存和供水:经过处理和后处理后的水被储存在一个装满水的容器中,然后通过龙头或水龙头供水给用户。
净水机通过以上步骤有效去除了水中的颗粒物、有机物、无机盐、重金属和微生物等,提供给用户干净安全的饮用水。
净水工作原理

净水工作原理
净水工作原理是通过物理、化学或生物等方法,将水中的杂质、污染物或有害物质过滤、分离、去除,从而使水变得干净、安全、适合人们使用或消费。
以下是一些常见的净水工作原理:
1. 滤网过滤:利用微孔过滤网将水中的固体颗粒、泥沙、锈迹等杂质拦截下来,从而净化水质。
2. 活性炭吸附:利用活性炭对水中的有机物质、异味物质、余氯等进行吸附,从而改善水的口感和气味。
3. 离子交换:利用离子交换树脂,将水中的硬度离子(如钙、镁离子)与树脂中的钠离子进行交换,从而减少水的硬度,防止水垢产生。
4. 膜分离:利用微孔膜或反渗透膜等进行分离过滤,将水中的溶解性固体、悬浮物、微生物、重金属等物质从水中分离出来,以获得纯净水。
5. 紫外线杀菌:利用紫外线照射水体,破坏细菌、病毒等微生物的遗传物质,达到杀菌消毒的目的。
以上只是一些常见的净水工作原理,不同的净水设备可能采用不同的工作原理或多种工作原理相结合,以达到净化水质的目的。
自来水厂净水方法
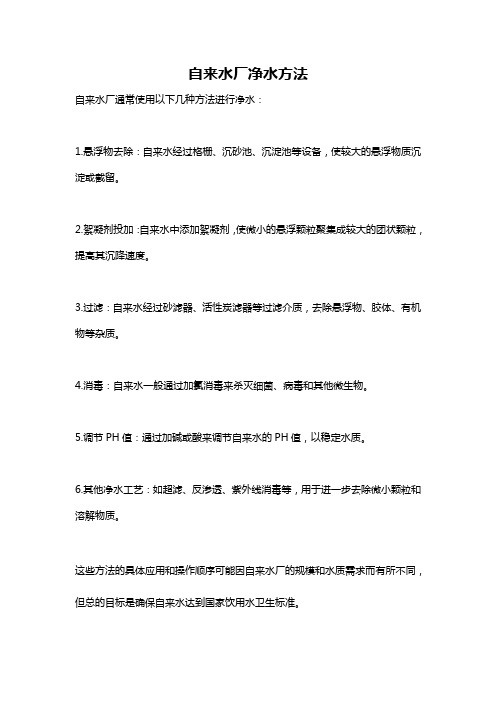
自来水厂净水方法
自来水厂通常使用以下几种方法进行净水:
1.悬浮物去除:自来水经过格栅、沉砂池、沉淀池等设备,使较大的悬浮物质沉淀或截留。
2.絮凝剂投加:自来水中添加絮凝剂,使微小的悬浮颗粒聚集成较大的团状颗粒,提高其沉降速度。
3.过滤:自来水经过砂滤器、活性炭滤器等过滤介质,去除悬浮物、胶体、有机物等杂质。
4.消毒:自来水一般通过加氯消毒来杀灭细菌、病毒和其他微生物。
5.调节PH值:通过加碱或酸来调节自来水的PH值,以稳定水质。
6.其他净水工艺:如超滤、反渗透、紫外线消毒等,用于进一步去除微小颗粒和溶解物质。
这些方法的具体应用和操作顺序可能因自来水厂的规模和水质需求而有所不同,但总的目标是确保自来水达到国家饮用水卫生标准。
净化水的原理

净化水的原理净化水的原理可以通过多种方法实现,主要包括物理净化、化学净化和生物净化。
下面我将详细解释这些原理。
一、物理净化物理净化是通过物理方式去除水中的杂质和污染物。
这种净化方法不改变水的化学性质和成分,只是通过一系列物理操作来分离和去除杂质。
1. 滤网过滤:水通过一定精度的滤网,可以拦截杂质、悬浮物和微生物。
常见的滤网材料有石英砂、石英石、陶土、活性炭等。
2. 沉淀:通过让水停留在一个容器中,很多重的杂质会沉积到容器的底部,形成沉淀物。
这需要依靠水的自然沉降速度,通常需要较长时间。
3. 蒸馏:将水加热到沸点,水蒸气升入冷凝器并冷却成液体状态,去除水中的溶解性杂质。
这是一种比较彻底的净化方法,但能耗较高。
4. 逆渗透:通过半透膜的作用,将水中的溶解性物质和杂质隔离出去。
逆渗透是一种高效的净水方法,广泛应用于户外旅行、海水淡化等领域。
二、化学净化化学净化是通过添加化学药剂,改变水的化学性质,使其中的污染物发生化学反应,并沉淀或形成可被过滤的固体物质。
1. 氧化还原反应:通过添加活性氧化剂(如过氧化氢、臭氧等)或还原剂(如亚硫酸氢钠、亚硝酸钠等),使有机物质氧化或还原,并转化成易于去除的物质。
2. 沉淀剂:通过添加沉淀剂(如硫酸铝、聚合氯化铝等),使溶解性无机物质形成沉淀,然后通过过滤等方法去除。
这种方法主要针对水中的悬浮物和重金属离子。
3. 吸附剂:通过添加吸附剂(如活性炭、硅胶等),使水中的有机物质吸附在表面,从而去除水中的污染物。
三、生物净化生物净化是利用微生物的生长代谢活动去除水中的有机物质和污染物。
1. 活性池:在水中加入适当的微生物培养基,引入一定适应环境的微生物种群,利用微生物对污染物的降解作用,来净化水。
2. 水生植物净化:选用适应水环境的水生植物,通过它们的根系、茎叶等部位与水体接触,吸收水中的营养物质和污染物,起到净化水质的作用。
3. 鱼类净化:通过鱼类的呼吸和摄食活动,吸收水中的氧气和有机物质,促进菌类的繁殖和代谢,从而净化水体。
净水的工艺

净水的工艺
净水的工艺是指将水中的污染物去除,使水达到安全、健康的标准。
下面是一些常见的净水工艺:
1. 滤网:使用不同孔径的滤网过滤水中的悬浮物和大颗粒物。
2. 活性炭吸附:使用活性炭吸附水中的有机物、余氯、重金属等物质。
3. 反渗透:利用半透膜的特性,通过压力差将水中的多种污染物分离。
4. 离子交换:使用离子交换树脂将水中的离子污染物如硬度离子(钙、镁等)去除。
5. 紫外线灭菌:利用紫外线的杀菌作用,灭活水中的细菌、病毒和其他微生物。
6. 臭氧氧化:通过臭氧氧化反应来去除水中的有机污染物和杀灭细菌。
7. 混凝絮凝:使用混凝剂和絮凝剂使水中的悬浮物和胶体物质聚集成大颗粒,方便后续过滤。
8. 砂滤:通过砂滤层将水中的颗粒物、悬浮物等去除。
这些净水工艺可以单独应用,也可以组合使用,根据水质状况和需要进行合理选择和设计,以确保净水效果。
- 1、下载文档前请自行甄别文档内容的完整性,平台不提供额外的编辑、内容补充、找答案等附加服务。
- 2、"仅部分预览"的文档,不可在线预览部分如存在完整性等问题,可反馈申请退款(可完整预览的文档不适用该条件!)。
- 3、如文档侵犯您的权益,请联系客服反馈,我们会尽快为您处理(人工客服工作时间:9:00-18:30)。
Water chemistry analysis of an industrial selective flocculation dispersion hematite ore concentrator plantHoward J.Haselhuhn,Joshua J.Carlson,S.Komar Kawatra ⁎Department of Chemical Engineering,Michigan Technological University,1400Townsend Drive,Houghton,MI 49931,USAa b s t r a c ta r t i c l e i n f o Article history:Received 29March 2011Received in revised form 26September 2011Accepted 2October 2011Available online 8October 2011Keywords:HematiteSelective flocculation dispersion Water chemistryHematite ore selective flocculation-dispersion process water is a complex system of ions and reagents work-ing together to produce a concentrated iron oxide product.The purpose of this study was to determine the process water concentrations of the important ionic species in a selective flocculation –dispersion hematite ore concentrating plant while process conditions were stable.This information was used to provide a detailed water chemistry analysis and to further understand how water quality affects the process.An analysis of the water chemistry of an operating selective flocculation-dispersion iron ore concentration plant has never be-fore been published.The pH of the process water was the most important factor in the selective flocculation and dispersion process because,according to prior studies,it has a direct relationship with the surface chem-istry of the particles (Carlson,2010).The pH also had a direct relationship with the solubilities of both calci-um and magnesium in the process.Water hardness was signi ficantly increased with the addition of calcite/dolomite flux which may cause issues in pelletization and in the reuse water.Soluble iron was removed in the flotation circuit suggesting that it may have been oxidized during flotation or it may have been absorbed by the collector.©2011Elsevier B.V.All rights reserved.1.IntroductionSelective flocculation and dispersion is a method of concentrating the valuable constituents of hematite ore bodies.This is accomplished by adding reagents that either flocculate or disperse the particles in a slurry of ground ore by altering the surface chemistry of the ore par-ticles.This methodology has been used sparingly in the mineral pro-cessing industry due to dif ficulties in controlling the surface chemistry characteristics that affect selectivity and high reagent costs (Department of Energy,2001).For these reasons,it is generally not believed that selective flocculation and dispersion is a cost effec-tive method for concentrating iron ore.A Lake Superior district hematite ore concentrating facility has been effectively using selective flocculation and dispersion for con-centrating hematite ore bodies since 1974.Selective flocculation is the process of choice because of the relatively non-magnetic nature and small liberation size (b 25μm)of the iron minerals (Paananen and Turcotte,1980).For selective flocculation and dispersion methods to work,precise control of the surface chemistry of the par-ticles is required (Carlson,2010).Water chemistry is one of the most important aspects of the process because of its direct relation to the surface properties of particles.Very small changes in water chemistry can have a disastrous effect on the grade and recovery of the process.Many years ago there were studies carried out on comminution in this hematite concentrator to determine the effectiveness of grinding aids.During this study,the flotation froth became ineffective for an unknown reason (Klimpel,1990).It was speculated that this plant failure was due to changes in viscosity or water chemistry.There have been many publications on how water chemistry im-pacts the ef ficacy of the hematite ore selective flocculation dispersion process on a laboratory scale.These publications demonstrate the abilities of common dispersants and flocculants in ideally prepared water samples of known chemistry.The detailed water chemistry of an operating selective flocculation-dispersion hematite ore concen-trating facility has never been reported.The purpose of this study was to determine the process water con-centration of a selective flocculation-dispersion hematite ore concen-trating plant while process conditions were stable to provide a detailed water chemistry analysis and further understand the effects of the flocculants and dispersants used throughout the process.2.Background 2.1.Process flow sheetThe selective flocculation dispersion process for hematite ore con-centration begins with crushing and grinding the hematite ore to the liberation size of the hematite particles.The process flow sheet for the hematite concentrator is shown in Fig.1.Twelve autogenous grinding mills with a cone crusher to crush intermediate products feed 24linesInternational Journal of Mineral Processing 102–103(2012)99–106⁎Corresponding author.Tel.:+19064873132;fax:+19064873213.E-mail address:skkawatra@ (S.K.Kawatra).0301-7516/$–see front matter ©2011Elsevier B.V.All rights reserved.doi:10.1016/j.minpro.2011.10.002Contents lists available at SciVerse ScienceDirectInternational Journal of Mineral Processingj o u rn a l ho m e p a g e :w w w.e l s e v i e r.c o m /l o c a t e /i j mi n p r oof pebble mills,cyclones,and deslime thickeners.The deslimed slurry enters twelve flotation lines followed by four concentrate thickeners and slurry tanks.This system feeds eight lines of six disc filters per line for concentrate dewatering.All tailings are sent to two tailings thickeners to recover process water (Keranen,1986).2.2.Grinding and deslimingThe most important step in hematite ore concentration is the deslime process in which fine particles are removed from the ground feed.In the best selective flocculation-dispersion conditions,15to 30%of the feed weight can be removed in the deslime thickeners with only a 5%loss in iron concentration (Colombo,1980).These conditions are obtained by adding the correct types and amounts of flocculants and dispersants.Selective desliming for hematite ore utilizes a combination of several dispersants to disperse siliceous gangue followed by a starch to flocculate hydrated hematite particles (Iwasaki,1989).Sodium hydroxide (Caustic soda)is added during grinding to in-crease the alkalinity (pH between 10.5and 11)(Green and Colombo,1984).This increase in alkalinity is necessary to disperse all fine parti-cles in the slurry.At high pH values,the surface charge of the slurry par-ticles become negative,causing dispersion.Minerals that dissolve at higher pH values add metallic cations,namely calcium and magnesium,to the slurry.These positively charged cations adsorb onto the negative-ly charged surfaces as CaOH +and MgOH +and disrupt the sign and magnitude of the surface charge,inhibiting dispersion (Iwasaki,1989).Glass-H®is added to the process after primary comminution to precipitate calcium metaphosphates and magnesium metaphos-phates.The (Ca,Mg)OH +are removed from the particle surfaces allowing retention of the dispersive qualities of the negatively charged surfaces (Iwasaki,1989).Glass-H®is a trade name for sodi-um hexametaphosphate (SHMP).Starch is added to the deslime thickener feed as a selective floccu-lant for hematite.The starch forms hydrogen bonds with the oxide surfaces of hematite particles and selectively flocculates the hematite particles (Balajee and Iwasaki,1969).The effectiveness of starchadsorption is relative to the mineralogy of the ore and the water qual-ity.An over-abundance of calcium or magnesium ions can cause the particle surfaces to become completely coated in metaphosphate pre-cipitates and hinder starch adsorption and therefore hematite floccu-lation (Iwasaki,1989).2.3.Flotation and dewateringThe deslime thickener over flow is sent for treatment in one of the tailings thickeners.The under flow,which has been increased to 35to 40%iron in the desliming process,is sent to a conditioner where it is once again mixed with starch that coats the hematite particles (Benner and Goetzman,1994).This creates a barrier to both surface charge and Van der Waals forces to eliminate any attraction of the silica to the he-matite.The conditioned slurry feed is fed into the reverse cationic flota-tion circuit consisting of a rougher followed by four stages of scavenger flotation.It is mixed with an 8–10chain primary ether amine that acts as both a cationic collector and frothing agent in the rougher feed box (Keranen,1986).Ether amine and starch are also added to the scaven-ger flotation cells to increase the recovery of the hematite.The concen-trate of each scavenger is fed back into the rougher.The flotation concentrate is pumped to the concentrate thickener where it is thickened and fed into a slurry tank.Pressurized carbon di-oxide is mixed with the slurry at one of 6injection points to lower the pH of the slurry and prepare the slurry for filtration (Keranen,1986).A limestone (CaCO 3)/dolomite (CaMg(CO 3)2)flux is added to the slurry tank to improve reduction properties of the iron in the blast furnace (Benner and Goetzman,1994).The fluxed slurry is filtered using vacuum disc filters.The fluxed hematite filter cake is then sent for pelletization.3.Materials and methodsSamples were taken at 11sampling locations throughout the he-matite concentrator.Fig.2shows the general layout of the hematite concentrating plant and the sampling locations.Each locationhasFig.1.Hematite ore concentrator circuit as described by Keranen (1986).100H.J.Haselhuhn et al./International Journal of Mineral Processing 102–103(2012)99–106been assigned a number for ease of data presentation.Table 1shows descriptions for each sampling location along with their assigned numbers for future reference.All water chemistry data presented will refer to these location numbers.The samples were taken in 1gal plastic buckets and brought back to Michigan Technological University immediately after being taken.The supernatant fluid from each sample was decanted into 1L glass sample bottles and tested for conductivity and pH.The 1L samples were kept in a refrigerator and sent in a cooler packed with ice packs to a certi fied laboratory for further testing.Tests were conducted on each sample to determine the concentra-tions of cations (calcium,magnesium,sodium,potassium,and iron),anions (chloride,sulfate,fluoride,nitrate,nitrite,carbonate and bicar-bonate),hardness,total organic carbon (TOC),Kjeldahl nitrogen,and grease/oil.pH was also re-tested at the certi fied laboratory to con firm the results obtained at Michigan Technological University and to de-termine if there is potential for pH drift over time.4.Results and discussionTest results were received from the laboratory detailing the water chemistry of the hematite ore concentrating facility.4.1.pHFig.3shows the results of the pH tests conducted at Michigan Technological University's Advanced Sustainable Iron and Steel Mak-ing Center (ASISC)and at the certi fied laboratory.pH played a signif-icant role in the removal of silica in the deslime thickener and in flotation.Fine silica and other gangue minerals were removed in the deslime thickener at a pH of around 11.This pH was necessary be-cause hematite particles exhibit a net negative surface charge allow-ing for a near complete dispersion of the fine silica particles from the hematite surfaces.Most of the remaining silica particles were removed in the flota-tion process.This was kept at a pH of 11to maintain the negative sur-face charge of silica and maximize the effectiveness of the cationic amine collector.After flotation,the concentrate entered the concen-trate thickener,where it was thickened prior to filtration.The concen-trate thickener under flow was sparged with CO 2.The addition of CO 2caused a drop in pH,yielding a decrease in surface charge of the he-matite particles.This caused silica fines to flocculate with hematiteparticles increasing the effective particle size and therefore filtration rate (Esumi et al.,1988).A pH drift was noticed for these samples over the course of several days.The pH of each sample tended to decrease slowly with time as shown by the higher pH results obtained while the samples were at Michigan Technological University prior to shipment to the certi fied laboratory.4.2.ConductivityFig.4shows the conductivity of the process water by location throughout the hematite ore concentrating process.The conductivity remained consistent between 1907and 2100μS/cm (μΩ−1cm −1)until the addition of flux where the conductivity increased to 2389μS/cm.This may have been due to the dissolution of the lime-stone and dolomite in the flux and the dissolved carbonate/bicarbon-ate ions present from the addition of carbon dioxide.4.3.CalciumCalcium concentration after primary comminution was found to be 4.47mg/L at location one prior to Glass-H®addition,see Fig.5.Glass-H®is typically added to a slurry as a dispersant,in this case,to form calcium and magnesium precipitates and remove multivalent cations from the surface of the slurry particles (Iwasaki,1989).This allows for greater dispersion of the negatively charged particles.Calcium concentrations above 25mg/L in the flotation circuit are known to be detrimental to iron recovery (Benner and Goetzman,Fig.2.Hematite concentrator process flow sheet with water analysis sample locations.Each numbered circle represents a sample location and data is characterized based on these sample location numbers.Table 1Sampling location descriptions.Location Description1Screen under flow before glass –h addition 2Screen under flow after glass –h addition 3Cyclone over flow4Deslime thickener over flow 5Deslime thickener under flow 6Flotation feed 7Scavenger returns 8Scavenger tails9Rougher concentrate10Concentrate thickener under flow11Filter feed after CO 2and flux addition101H.J.Haselhuhn et al./International Journal of Mineral Processing 102–103(2012)99–1061994).This is caused by ionic competition of the cationic collector (Iwasaki,1989).These concentrations were averted by using Glass-H®in the desliming process.The calcium concentration throughout the flotation circuit was found to be between 4and 10mg/L.The calcium concentration remained low until the addition of the limestone/dolomite flux.The flux addition increased the calcium con-centration to 71mg/L showing that some of the limestone and dolomite dissolved into the solution.This could be detrimental to downstream processes in which bentonites are used to bind the concentrated hema-tite ore into pellets.Calcium displaces the sodium in bentonite as an ex-changeable cation which converts the bentonite from a highly active sodium bentonite into a relatively inactive calcium bentonite.It has been shown that the calcium concentration of water extracted from a filtered hematite ore concentrate can be as high as 550times that of the calcium concentration found in the filtrate (Ripke and Kawatra,2003).This increase in concentration just prior to filtration could be ex-tremely detrimental to the pelletization process.4.4.MagnesiumMagnesium,like calcium,is a multivalent cation that plays a sig-ni ficant role in the dispersion of hematite from silica.The entering concentration of magnesium into the concentrator was 2.67mg/L,see Fig.6.The deslime thickener was operated at a magnesium con-centration of about 5mg/L.The magnesium concentration dropped to nearly zero throughout the flotation process suggesting that the ether amine absorbs the magnesium.Iwasaki (1989)showed that flo-tation recovery dropped abruptly to nearly zero with magnesium concentrations above 10−3M (~24mg/L)which supports this hy-pothesis.If the amount of collector added is not enough to absorball of the magnesium,magnesium hydroxide precipitates on the quartz surfaces,hindering flotation (Iwasaki,1989).The magnesium concentration increased to 58mg/L after the addition of flux.This was most likely due to the dissociation of magnesium from dolomite in the flux.4.5.SodiumTable 2shows the sodium concentration by location throughout the hematite ore concentrating process.The entering sodium concen-tration was 455mg/L and increased with the addition of Glass-H®.This was to be expected because the sodium in Glass-H®(sodium hexametaphosphate)was exchanged with calcium and magnesium ions in solution.The deslime thickener seemed to remove some sodi-um along with silica at location 4;there was a 32mg/L concentration difference between the sodium concentration of the over flows and under flows.This suggests that sodium left the process adsorbed to the negatively charged silica surfaces.The concentrate thickener seemed to concentrate the sodium as well by increasing the concentration by 27mg/L.This also suggests that the sodium can be found near the negatively charged particle surfaces.The addition of CO 2and flux also caused an increase in sodi-um concentration by 35mg/L most likely due to impurities in the limestone/dolomite flux.4.6.PotassiumMonovalent ionic species such as potassium are less likely to cause harmful effects on flocculation-dispersion processes;howeverFig.3.pH of process water by location.pH remains stable throughout process until in-troduction of CO 2and flux at filter feed (Location 11in Fig.2).Fig.4.Conductivity of process water by location.Conductivity increases signi ficantly with the addition of flux at filter feed (Location 11in Fig.2).Fig.5.Calcium concentration of process water by location.Calcium concentration in-creases signi ficantly at filter feed suggesting that flux is dissolving (Location 11in Fig.2).Fig.6.Magnesium concentration of process water by location.Magnesium concentra-tion increases signi ficantly at filter feed suggesting that dolomite is dissolving (Loca-tion 11in Fig.2).102H.J.Haselhuhn et al./International Journal of Mineral Processing 102–103(2012)99–106potassium fluoride has been shown to inhibit the activation of quartz particles in selective flocculation –dispersion processes of hematite (Attia,1982).Fig.7shows the potassium concentration by location throughout the hematite ore concentrating process.The entering po-tassium concentration was 11.4mg/L after autogenous grinding.The addition CO 2and flux nearly doubled the concentration of potassium,likely due to impurities in the flux.4.7.Soluble ironFig.8shows the soluble iron concentration by location throughout the hematite ore concentrating process.The entering concentration of soluble iron was 16.4mg/L.The soluble iron concentrations slowly in-creased throughout the grinding and deslime process.It immediately dropped to near zero in the flotation circuit.This suggests that the ether amine cationic collector may have absorbed the soluble iron species in solution or the soluble iron was oxidizing in the flotation circuit.The soluble iron concentration remains less than 1mg/L at every location downstream of the flotation circuit.4.8.ChlorideTable 2shows the chloride concentration by location throughout the hematite ore concentrating process.As shown,the chloride con-centration did not change by more than 10mg/L throughout the en-tire process.4.9.FluorideFluorides present in the water can be attributed to the grinding of a fluorapatite.These fluorides are readily precipitated as calcium fluo-ride and were not prevalent in the concentrator water chemistry analysis.Table 2shows the fluoride concentration by location throughout the hematite ore concentrating process.It has been shown that fluoride concentration is limited by the presence of calci-um and is promoted by the presence of sodium (Engesser,2003).All three of these ions were present throughout the concentrator at con-sistent concentrations and no relationship could be adequately deter-mined based on this data.4.10.SulfateTable 2shows the sulfate concentration by location throughout the hematite ore concentrating process.The entering sulfate concentration was 90mg/L after autogenous grinding and progressively lowered throughout the plant to a minimum of 83mg/L at the concentrate thick-ener feed.The addition of a sulfosuccinate tailings treatment at the con-centrate thickener feed may have increased the concentration of sulfates in solution to 110mg/L (Keranen,1986).The concentration was then lowered to 100mg/L following the addition of CO 2and flux.4.11.NitrateFig.9shows the nitrate concentration by location throughout the hematite ore concentrating process.The entering nitrate concentrationTable 2Sodium,chloride,fluoride,and sulfate concentration of process water by location.Con-centrations remain relatively stable throughout process.Sulfate concentration has a slight increase upon flux addition.LocationSodium (mg/L)Chloride (mg/L)Sulfate (mg/L)Fluoride (mg/L)145511090 4.12465110904345411088 3.9447811088 3.75446110894644811088 3.8743710085 3.8845910085 3.8943310083 3.810460110110 5.511495100100 4.2Average457106.91 4.1Standard deviation17.75.07.80.5Fig.7.Potassium concentration of process water by location.Potassium concentration remains stable with a spike at filter feed (Location 11in Fig.2)possibly due to impuri-ties in flux.Fig.8.Soluble iron concentration of process water by location.Soluble iron steadily in-creases until flotation (Location 7in Fig.2),where it either binds with the collector or isoxidized.Fig.9.Nitrate concentration of process water by location.Nitrate concentration re-mains stable until filter feed (Location 11in Fig.2)where the nitrates may be affected by the change in pH.103H.J.Haselhuhn et al./International Journal of Mineral Processing 102–103(2012)99–106was 16mg/L and remained fairly constant throughout the process until CO 2and flux were added between locations 10and 11.4.12.NitriteFig.10shows the nitrite concentration by location throughout the hematite ore concentrating process.The nitrite concentration remained almost undetectably low (bb 1mg/L)throughout the process.4.13.Carbonate and bicarbonateFigs.11and 12show the carbonate and bicarbonate concentra-tion by location throughout the hematite ore concentrating process.The carbonate and bicarbonate concentration deviated opposite to each-other throughout the process suggesting that they are in equilibrium with each other as shown in Eq.(1).The equilibrium concentrations of these carbonate species are dependent on the pH of the solution.As Fig.13shows,lower pH values (~7–10)cor-respond to higher concentrations of the bicarbonate ion,whereas higher pH values (~10+)correspond to higher concentrations of the carbonate ion.CO 2−3þH 2O ↔HCO −3þOH−HCO −3þH 2O ↔H 2CO 3þOH−(1)Carbonate –Bicarbonate –Carbonic Acid EquilibriumAs seen in Fig.3,after the addition of CO 2the pH dropped consid-erably down to 7.67,explaining the loss of all carbonate ions and theappearance of 1295mg/L of bicarbonate ions in solution.This showed that the process water takes on approximately 550mg/L of carbonate ions as a result of the forced injection of CO 2and the addition of the limestone/dolomite flux.Literature states that the solubilities of both limestone and dolo-mite increase with a decrease in pH (Cowan and Weintritt,1976).The presence of carbonate species in solution slightly lowers the sol-ubility of calcium carbonate.The relationship between calcium car-bonate solubility,pH and carbonate concentration is shown on the surface plot in Fig.14.The decreased flux solubility due to the pres-ence of more carbonate ions was not enough to counteract the in-crease in solubility due to the drop in pH.This explains the increase in calcium and magnesium concentration seen upon flux addition.As stated in Sections 4.3and 4.4,an increase in the calcium or magne-sium concentration can be detrimental to downstream processes.4.14.HardnessFig.15shows the hardness of the process water by location throughout the hematite ore concentrating process.The entering hardness after comminution was 22mg/L and generally followed the trend of both calcium and magnesium.The water remained rela-tively soft throughout the process until the addition of CO 2and flux.A sample of the process water at the concentrate thickener under-flow was sparged with CO 2until the solution was saturated.The water analysis of this sample was compared to the samples at locations 9and 11to show how CO 2affects the water chemistry with regardstoFig.10.Nitrite concentration of process water by location.Nitrite concentration re-mains extremely low and possibly at the threshold ofdetectability.Fig.11.Carbonate concentration of process water by location.Carbonate concentration remains stable until filter feed (Location 11in Fig.2)when the change in pH causes all carbonates to hydrolyze tobicarbonates.Fig.12.Bicarbonate concentration of process water by location.Bicarbonates remain at near zero until the pH is adjusted with an addition of CO 2(Location 11in Fig.2).The bicarbonate concentration at location 11includes all hydrolyzed carbonates,dissolved CO 2,and dissolved flux.Fig.13.Concentration of H x CO 3ions with respect to the total CO 3concentration (C T )as a function of pH (Zasoski,2001).104H.J.Haselhuhn et al./International Journal of Mineral Processing 102–103(2012)99–106carbonate,bicarbonate and hardness concentrations.The results of this test are shown in Table 3.With no CO 2or flux added,the pH was highenough to retain all the carbonate concentration as CO 32-.With the addi-tion of only CO 2,the pH dropped and carbonate equilibrium shifted tothe bicarbonate (HCO 3-)species.Some change was noticed in the hard-ness suggesting that some calcium may be dissolved into the solution from particles surfaces at the lower pH.Addition of the limestone/dolomite flux increased both the bicarbonate concentration and the hardness of the process water.This is another positive indication that some of the flux was dissolving in the slurry.4.15.Total organic carbonFig.16shows the total organic carbon (TOC)concentration by lo-cation throughout the hematite ore concentrating process.The TOCremained fairly low throughout the process until the flux and CO 2are added at location 11.The hematite particle charge reversal seen at the lowered pH at location 11may have released adsorbed posi-tively charged organic carbons from hematite particle surfaces caus-ing the spike to 113mg/L of total organic carbon.4.16.Kjeldahl nitrogenKjeldahl nitrogen refers to a testing method used for determining the organic nitrogen,amine,and ammonium concentration of a solu-tion.Fig.17shows that there was no increase in nitrogen concentra-tion until the addition of the ether amine cationic collector in the flotation circuit.The rougher concentrate contained 5.12mg/L of ni-trogen possibly from a cationic exchange of soluble iron with the amine collector.The excess nitrogen was removed in the concentrate thickener over flow.The nitrogen concentration increased again with the addition of CO 2and flux indicating that the change in pH may have released cationic nitrogen based species from particle surfaces;similar to the effect seen with organic carbons.4.17.Oil/greaseFig.18shows the oil and grease concentration by location throughout the hematite ore concentrating process.This may give clues to areas where oil and grease may be being added unintention-ally from bearings or other mechanical devices.It appeared that the presence of oil and grease in the process water was sporadic and does not consistently correlate to reagentadditions.Fig.14.Calcium carbonate solubility as a function of both pH and dissolvedcarbonates.Fig.15.Hardness of process water by location.Hardness peaks at filter feed (Location 11in Fig.2)suggesting the dissolution of flux.Table 3Water analysis comparison of concentrate thickener under flow (Location 10in Fig.2)reagent additions.CO 2injection alone cannot create the difference in bicarbonate con-centration and water hardness;flux must be dissolving to some extent.Concentrate thickener under flow addition Carbonateconcentration (mg/L)Bicarbonateconcentration (mg/L)Hardness (mg/L)None 752021CO 2082652CO 2and flux1295416Fig.16.Total organic carbon (TOC)concentration of process water by location.Change in surface charge of hematite particles may be releasing organic carbons from surface at location 11in Fig.2.Fig.17.Kjeldahl nitrogen testing results for process water by location.Ether amine cat-ionic collector may be leaving in rougher concentrate (Location 9in Fig.2).Ether amine may also be desorbed from hematite surfaces at lower pH at filter feed (Location 11in Fig.2).105H.J.Haselhuhn et al./International Journal of Mineral Processing 102–103(2012)99–106。