Structure–Function Relationships in Highly Modified Shoots of Cactaceae
结构功能主义英语
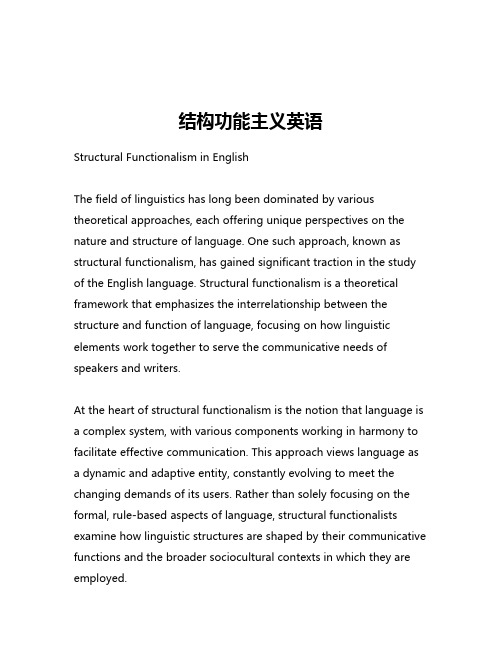
结构功能主义英语Structural Functionalism in EnglishThe field of linguistics has long been dominated by various theoretical approaches, each offering unique perspectives on the nature and structure of language. One such approach, known as structural functionalism, has gained significant traction in the study of the English language. Structural functionalism is a theoretical framework that emphasizes the interrelationship between the structure and function of language, focusing on how linguistic elements work together to serve the communicative needs of speakers and writers.At the heart of structural functionalism is the notion that language is a complex system, with various components working in harmony to facilitate effective communication. This approach views language as a dynamic and adaptive entity, constantly evolving to meet the changing demands of its users. Rather than solely focusing on the formal, rule-based aspects of language, structural functionalists examine how linguistic structures are shaped by their communicative functions and the broader sociocultural contexts in which they are employed.One of the key principles of structural functionalism is the idea that language is not merely a set of arbitrary rules and conventions, but rather a reflection of the cognitive and social processes that shape human interaction. Proponents of this approach argue that the structure of language is intimately tied to the ways in which it is used to convey meaning, express emotions, and negotiate social relationships. By understanding the functional motivations behind linguistic structures, scholars can gain deeper insights into the underlying mechanisms that govern language use and development.In the context of the English language, structural functionalism has made significant contributions to our understanding of various linguistic phenomena. For instance, the analysis of sentence structure and clause patterns has revealed how different grammatical constructions serve specific communicative purposes, such as expressing agency, foregrounding information, or establishing causal relationships. Similarly, the study of discourse-level features, such as cohesion and coherence, has shed light on the ways in which language users organize and structure their ideas to achieve particular rhetorical goals.Moreover, structural functionalism has also informed our understanding of language variation and change. By examining how linguistic structures are adapted and modified to suit the needs ofdifferent social, regional, or cultural contexts, scholars have gained valuable insights into the dynamic nature of language and the factors that drive its evolution over time. This perspective has challenged the traditional view of language as a static, monolithic entity, and has instead emphasized the importance of considering the diverse ways in which language is used and shaped by its speakers.One of the key strengths of structural functionalism in the study of English is its emphasis on the contextual and pragmatic aspects of language use. Rather than solely focusing on the formal properties of language, this approach encourages scholars to consider the ways in which linguistic structures are employed in real-world communicative situations, taking into account the social, cultural, and cognitive factors that influence language use. This holistic perspective has led to a deeper understanding of the complexities of language, and has opened up new avenues for research and pedagogical applications.For example, the structural functionalist approach has been instrumental in the development of discourse analysis, a field that examines the ways in which language is used to achieve specific communicative goals in various settings, such as academic writing, political discourse, or everyday conversation. By analyzing the linguistic choices made by speakers and writers, discourse analystscan shed light on the underlying social and cognitive processes that shape language use, and can offer valuable insights for improving language teaching and communication practices.Furthermore, structural functionalism has also informed the study of language acquisition and language learning. By emphasizing the role of communicative functions in shaping linguistic structures, this approach has challenged traditional, rule-based models of language learning, and has instead highlighted the importance of understanding the contextual and pragmatic aspects of language use. This perspective has led to the development of more communicative and task-based approaches to language instruction, which focus on helping learners develop the linguistic and pragmatic skills necessary for effective communication in a variety of real-world settings.Despite the significant contributions of structural functionalism to the study of the English language, it is important to acknowledge that this approach is not without its limitations. Like any theoretical framework, structural functionalism has been criticized for its potential to oversimplify the complexities of language, or to overlook important aspects of linguistic structure and variation. Moreover, some scholars have argued that the emphasis on communicative functions can sometimes lead to a neglect of the formal, rule-based aspects of language, which are also crucial to understanding its structure and development.Nevertheless, the structural functionalist perspective remains a valuable and influential approach to the study of the English language. By highlighting the intricate relationship between linguistic structures and their communicative functions, this approach has enriched our understanding of the ways in which language is used to navigate the social, cognitive, and cultural dimensions of human experience. As the field of linguistics continues to evolve, the insights and methodologies of structural functionalism are likely to remain an important part of the scholarly discourse, contributing to our ongoing efforts to unravel the complexities of this fundamental aspect of human communication.。
Structureandfunc...
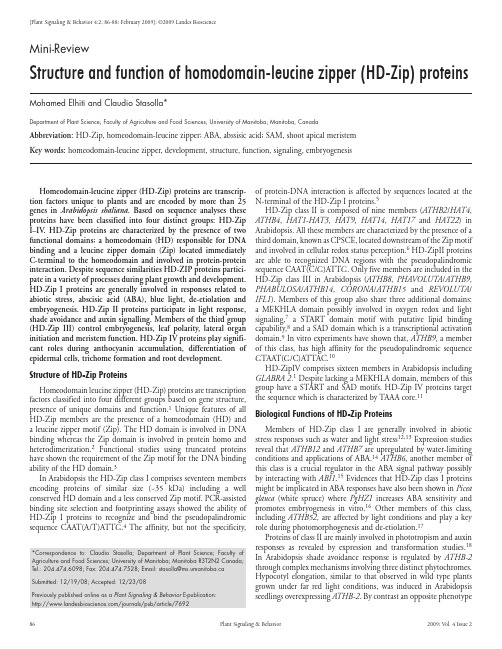
86Plant Signaling & Behavior2009; Vol. 4 Issue 2of protein-DNA interaction is affected by sequences located at the N-terminal of the HD-Zip I proteins.5HD-Zip class II is composed of nine members (ATHB2/HAT4, ATHB4, HAT1-HAT3, HAT9, HAT14, HAT17 and HAT22) in Arabidopsis. All these members are characterized by the presence of a third domain, known as CPSCE, located downstream of the Zip motif and involved in cellular redox status perception.6 HD-ZipII proteins are able to recognized DNA regions with the pseudopalindromic sequence CAAT(C/G)ATTG. Only five members are included in the HD-Zip class III in Arabidopsis (ATHB8, PHAVOLUTA /ATHB9, PHABULOSA /ATHB14, CORONA /ATHB15 and REVOLUTA /IFL1). Members of this group also share three additional domains: a MEKHLA domain possibly involved in oxygen redox and light signaling,7 a START domain motif with putative lipid binding capability,8 and a SAD domain which is a transcriptional activation domain.9 In vitro experiments have shown that, ATHB9, a member of this class, has high affinity for the pseudopalindromic sequence GTAAT(G/C)ATTAC.10HD-ZipIV comprises sixteen members in Arabidopsis includingGLABRA 2.1 Despite lacking a MEKHLA domain, members of this group have a START and SAD motifs. HD-Zip IV proteins targetthe sequence which is characterized by TAAA core.11Biological Functions of HD-Zip ProteinsMembers of HD-Zip class I are generally involved in abiotic stress responses such as water and light stress 12,13 Expression studies reveal that ATHB12 and ATHB7 are upregulated by water-limiting conditions and applications of ABA.14 ATHB6, another member of this class is a crucial regulator in the ABA signal pathway possibly by interacting with ABI1.15 Evidences that HD-Zip class I proteins might be implicated in ABA responses have also been shown in Picea glauca (white spruce) where PgHZ1 increases ABA sensitivity and promotes embryogenesis in vitro.16 Other members of this class, including ATHB52, are affected by light conditions and play a key role during photomorphogenesis and de-etiolation.17Proteins of class II are mainly involved in phototropism and auxin responses as revealed by expression and transformation studies.18In Arabidopsis shade avoidance response is regulated by ATHB-2 through complex mechanisms involving three distinct phytochromes. Hypocotyl elongation, similar to that observed in wild type plantsgrown under far red light conditions, was induced in Arabidopsisseedlings overexpressing ATHB-2. By contrast an opposite phenotype[Plant Signaling & Behavior 4:2, 86-88; February 2009]; ©2009 Landes BioscienceHomeodomain-leucine zipper (HD-Zip) proteins are transcrip-tion factors unique to plants and are encoded by more than 25 genes in Arabidopsis thaliana . Based on sequence analyses these proteins have been classified into four distinct groups: HD-Zip I–IV . HD-Zip proteins are characterized by the presence of two functional domains; a homeodomain (HD) responsible for DNA binding and a leucine zipper domain (Zip) located immediately C-terminal to the homeodomain and involved in protein-protein interaction. Despite sequence similarities HD-ZIP proteins partici-pate in a variety of processes during plant growth and development. HD-Zip I proteins are generally involved in responses related to abiotic stress, abscisic acid (ABA), blue light, de-etiolation and embryogenesis. HD-Zip II proteins participate in light response, shade avoidance and auxin signalling. Members of the third group (HD-Zip III) control embryogenesis, leaf polarity, lateral organ initiation and meristem function. HD-Zip IV proteins play signifi-cant roles during anthocyanin accumulation, differentiation of epidermal cells, trichome formation and root development.Structure of HD-Zip Proteins Homeodomain leucine zipper (HD-Zip) proteins are transcription factors classified into four different groups based on gene structure, presence of unique domains and function.1 Unique features of allHD-Zip members are the presence of a homeodomain (HD) and a leucine zipper motif (Zip). The HD domain is involved in DNA binding whereas the Zip domain is involved in protein homo and heterodimerization.2 Functional studies using truncated proteins have shown the requirement of the Zip motif for the DNA binding ability of the HD domain.3In Arabidopsis the HD-Zip class I comprises seventeen members encoding proteins of similar size (~35 kDa) including a well conserved HD domain and a less conserved Zip motif. PCR-assisted binding site selection and footprinting assays showed the ability of HD-Zip I proteins to recognize and bind the pseudopalindromic sequence CAAT(A/T)ATTG.4 The affinity, but not the specificity, Mini-ReviewStructure and function of homodomain-leucine zipper (HD-Zip) proteinsMohamed Elhiti and Claudio Stasolla*Department of Plant Science; Faculty of Agriculture and Food Sciences; University of Manitoba; Manitoba, CanadaAbbreviation: HD-Zip, homeodomain-leucine zipper; ABA, abssisic acid; SAM, shoot apical meristem Key words: homeodomain-leucine zipper, development, structure, function, signaling, embryogenesis*Correspondence to: Claudio Stasolla; Department of Plant Science; Faculty ofAgriculture and Food Sciences; University of Manitoba; Manitoba R3T2N2 Canada;Tel.:204.474.6098;Fax:204.474.7528;Email:*********************.caSubmitted: 12/19/08; Accepted: 12/23/08Previously published online as a Plant Signaling & Behavior E-publication: /journals/psb/article/7692 Plant Signaling & Behavior 87References1. Ariel FD, Manavella PA, Dezar CA, Chan RL. The true story of the HD-Zip family. T rendsPlant Sci 2007; 12:419-26.2. Lee YH, Chun JY. A new homeodomainleucine zipper gene from Arabidopsis thalianainduced by water stress and abscisic acid treatment. Plant Molec Biol 1998; 37:377-84. 3. T ron AE, Welchen E, Gonzalez DH. Engineering the loop region of a homeodomain-leucine zipper protein promotes efficient binding to a monomeric DNA binding site. Biochemistry 2004; 43:15845-51.4. Palena CM, Gonzalez DH, Chan RL. A monomer-dimer equilibrium modulates theinteraction of the sunflower homeodomain-leucine zipper protein HAHB-4 with DNA. Biochemistry 1999; 341:81-7.5. Palena CM, T ron AE, Bertoncini CW, Gonzalez DH, Chan RL. Positively charged residuesat the N-terminal arm of the homeodomain are required for efficient DNA binding by homeodomain-leucine zipper proteins. J Mol Biol 2001; 308:39-47.6. T ron AE, Bertoncini CW, Chan RL, Gonzalez DH. Redox regulation of plant homeodo-main transcription factors. J Biol Chem 2002; 277:34800-7.7. Mukherjee K, Burglin TR. MEKHLA, a novel domain with similarity to PAS domains, isfused to plant homeodomain-leucine zipper III proteins. Plant Physiol 2006; 140:1142-50.8. Schrick K, Nguyen D, Karlowski WM, Mayer KFX. START lipid/sterol-binding domainsare amplified in plants and are predominantly associated with homeodomain transcription factors. Genome Biol 2004; 41.9. De Caestecker MP , Yahata T, Wang D, Parks WT, Huang S, Hilli CS, Shioda T, Roberts AB,Lechleider RJ. The Smad 4 activation domain (SAD) is a proline-rich, p300-dependent transcriptional activation domain. J Biol Chem 2000; 3:2115-22.10. Sessa G, Steindler C, Morelli G, Ruberti I. The Arabidopsis ATHB-8, -9 and -14 genes aremembers of a small gene family coding for highly related HD-Zip proteins. Plant Mol Biol 1998; 38:609-22.11. Ohashi Y, Oka A, Pousada RR, Possenti M, Ruberti I, Morelli G, Aoyama T . Modulationof phospholipid signaling by GLABRA2 in root-hair pattern formation. Science 2003; 300:1427-30.12. Gago GM, Almoguera C, Jordano J, Gonzalez DH, Chan RL. Hahb-4, a homeobox-leucinezipper gene potentially involved in abscisic acid-dependent responses to water stress in sunflower. Plant Cell Environm 2002; 25:633-40.13. Wang Y, Henriksson E, Söderman E, Henriksson KN, Sundberg E, Engström P . TheArabidopsis homeobox gene, ATHB16, regulates leaf development and the sensitivity to photoperiod in Arabidopsis. Dev Biol 2003; 264:228-39.14. Olsson ASB, Peter Engstrom P , Soderman E. The homeobox genes ATHB12 and ATHB7encode potential regulators of growth in response to water deficit in Arabidopsis. Plant Mol Biol 2004; 55:663-77.15. Himmelbach A, Hoffmann T, Leube M, Hohener B, Grill E. Homeodoamin proteinATHB6 is a target of the protein phophatase ABI1 and regulates hormone responses in Arabidopsis. EMBO J 2002; 21:3029-38.16. Tahir M, Belmonte MF , Elhiti M, Flood H, Stasolla C. Identification and characterizationof PgHZ1, a novel homeodomain leucine-zipper gene isolated from white spruce (Picea glauca ) tissue. Plant Physiol Biochem 2008; 46:1031-9.17. Henriksson E, Olsson ASB, Johannesson H, Johansoon H, Hanson J, Engstrom P ,Soderman E. Homeodamin leucine zipper class I genes in Arabidopsis. Expression patterns and phylogentic relationships. Plant Physiol 2005; 139:509-18.18. Sessa G, Carabelli M, Sassi M, Ciolfi A, Possenti M, Mittempergher F , Becker J, Morelli G,Ruberti I. A dynamic balance between gene activation and repression regulates the shade avoidance response in Arabidopsis. Genes Dev 2005; 19:2811-5.19. Morelli G, Ruberti I. Light and shade in the photocontrol of Arabidopsis growth. T rendsPlant Sci 2002; 7:399-404.20. Sawa S, Ohgishi M, Goda H, Higuch K, Shimada Y, Yoshida S, Koshiba T . The HAT2 gene,a member of HD-Zip gene family, isolated as an auxin induciable gene by DNA microarray screening, effects auxin response in Arabidopsis. Plant J 2002; 32:1011-22.21. Rueda EC, Dezar CA, Gonzalez DH, Chan RL. Hahb , a sunflower homeodoamin-leucinezipper gene, is regulated by light quality and quantity, and promotes early flowering when expressed in Arabidopsis. Plant Cell Physiol 2005; 46:1954-63.22. Prigge MJ, Otsuga D, Alonso JM, Ecker JR, Drews GN, Clark SE. Class III homeodomain-leucine zipper gene family members have overlapping, antagonistic and distinct roles in Arabidopsis development. Plant Cell 2005; 17:61-76.23. Otsuga D, DeGuzman B, Prigge MJ, Drews GN, Clark SE. REVOLUTA regulates meristeminitiation at lateral position. Plant J 2001; 25:223-36.24. John L, Bowman JL, Floyd SK. Patterning and polarity in seed plant shoots. Annu RevPlant Biol 2008; 59:67-88.25. Emery JF , Floyd SK, Alvarez J, Eshed Y, Hawker NP , Izhaki A, Baum SF , Bowman JL.Radial patterning of Arabidopsis shoots by class III HD-Zip and KANADI genes. Curr Biol 2003; 13:1768-74.26. Baima S, Possenti M, Matteucci A, Wisman E, Altamura MM, Ruberti I, Morelli G. TheArabidopsis ATHB-8 HD-Zip protein acts as differentiation-promoting transcription factor of the vascular meristem. Plant Physiol 2001; 126:643-55.27. Abe M, Katsumata H, Komeda Y, Takahashi T. Regulation of shoot epidermal cell differen-tiation by a pair of homeodomain proteins in Arabidopsis. Development 2003; 130:635-43.was observed in plants with reduced ATHB-2 expression. A model explaining these phenotypes in relation to auxin distribution has been reviewed by Morelli and Ruberti.19 Tissue elongation was also induced by high expression of HAT-2, an auxin-inducible gene of the same class II.20 Response to dark and light conditions also involves HAHB-10, a sunflower gene with high similarity to ATHB-2. Ectopic expression of HAHB-10 in Arabidopsis produced a variety of phenotypic deviations, including dark cotyledons, planar leaves, reduced life cycle and accelerated flowering.21Members of HD-Zip class III play an important role during morphogenesis. Three proteins of this class, REV , PHB and PHV , control the pattern of apical formation during embryo develop-ment.22 Mutant analyses demonstrated the role of REV and PHB in regulating shoot apical meristem (SAM) maintenance and lateral organ initiation.23 Regulation of these morphogenic events might be caused by changes in auxin flow, since several members of HD-Zip class III have been implicated in events leading to changes of polar auxin transport.24 Several studies have elucidated the mode of action of REV , PHB and PHV , together with KANADI in control-ling abaxial-adaxial patterning of lateral organs.25 The abaxilation process and phloem differentiation are initiated by KANADI which also represses the expression of REV , PHB and PHV . This repres-sion is gradually released and expression of these three genes inhibits KANADI through feedback mechanisms and results in the adaxila-tion of the lateral organ and xylem formation.25 Another member of this class, ATHB-8 is also implicated in vascularization.26 Production of xylem is significantly increased in Arabidopsis plants overex-pressing this gene suggesting a possible role for ATHB-8 in inducing xylem element differentiation.26 This control however only occurs in the presence of specific cues since a reciprocal phenotype was not observed in lines with low ATHB-8 expression.The expression of several members of HD-Zip class IV is often restricted in the outer cells of plant organs where they regulate processes such as epidermal fate, trichome formation and antho-cyanin accumulation.23,27,28 The lack of epidermal cell identity in leaves of pdf2/atml1 double mutants argues for their involvement in epidermal identity acquisition. These genes might work in redundant fashion as denoted by the high degree of similarity. Proper trichome formation is also under the control of several members of this class. Genetic analyses revealed that hdg11/hdg12 double mutants produce highly branched trichomes. Further studies have revealed that HD-Zip IV members act redundantly with each other during devel-opmental events.29 Another gene of this class ANL2 is also involved in biosynthesis of anthocyanin in subepidermal tissues and cellular organization of primary root.28ConclusionsHD-Zip proteins are transcription factors unique to plants char-acterized by a homeodomain and a leucine zipper motif. Despite these structural similarities HD-Zip proteins participate in diverse and sometimes overlapping events ranging from stress responses to morphogenesis and development. Genetic analyses have revealed that their functions rely on the activation of downstream target genes the majority of which remain unknown. Elucidation of these downstream events will be key in understanding the role played by this important class of transcription factors.28. Kubo H, Peeters AJM, Aarts MGM, Pereira A, Koornneef M. ANTHOCYANINLESS2, ahomeobox gene affecting anthocyanin distribution and root development in Arabidopsis.Plant Cell 1999; 11:1217-26.29. Guan XY, Li QJ, Shan CM, Wang S, Mao YB, Wang LJ, Chen XY. The HD-Zip IV geneGaHOX1from cotton is a functional homologue of the Arabidopsis GLABRA2. PlantPhysiol 2008; 134:174-82.88Plant Signaling & Behavior2009; Vol. 4 Issue 2。
组织工程TissueEngineering
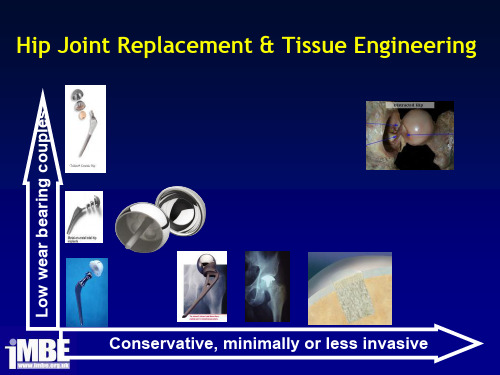
Cells, Tissues and Organs
• Cell anatomy
– Cell membrane A double layer of
phospholipids Proteins:
“Floating” proteins Integrins Specific receptor sites
• Cell anatomy
– Small membrane (cell membrane) bound compБайду номын сангаасrtment
– Nucleus (genetic inf.) – Mitochondria (energy) – Lysosomes (proteolytic enzymes) – Endoplasmic reticulum (protein
Inner layer (mucosa)
Connective tissue Epithelium
Outer layer (serosa) Epithelium Connective tissue
Lumen
Middle layer
muscle connective muscle
Organ Failure and Treatments
Cells, Tissues and Organs
• Basic tissues:
– Connective tissue
Skin dermis Pericardium, tendon Bone marrow, blood cells Cartilage, bone
– Muscle tissue
Organ Failure and Treatments
• Xeno-graft:
学术英语(管理)课文翻译
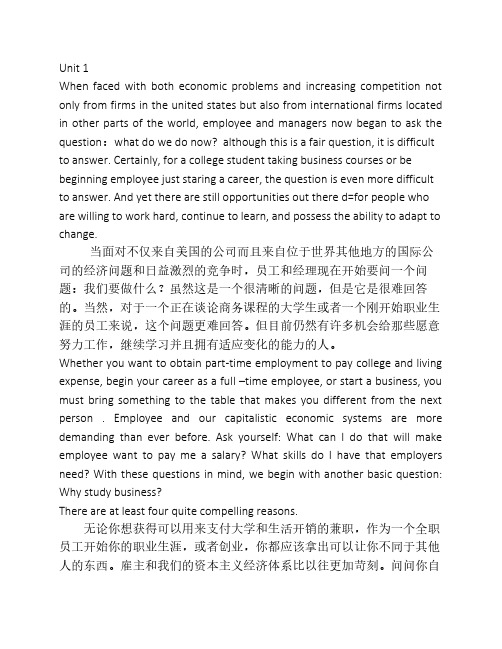
Unit 1When faced with both economic problems and increasing competition not only from firms in the united states but also from international firms located in other parts of the world, employee and managers now began to ask the question:what do we do now? although this is a fair question, it is difficult to answer. Certainly, for a college student taking business courses or be beginning employee just staring a career, the question is even more difficult to answer. And yet there are still opportunities out there d=for people who are willing to work hard, continue to learn, and possess the ability to adapt to change.当面对不仅来自美国的公司而且来自位于世界其他地方的国际公司的经济问题和日益激烈的竞争时,员工和经理现在开始要问一个问题:我们要做什么?虽然这是一个很清晰的问题,但是它是很难回答的。
当然,对于一个正在谈论商务课程的大学生或者一个刚开始职业生涯的员工来说,这个问题更难回答。
但目前仍然有许多机会给那些愿意努力工作,继续学习并且拥有适应变化的能力的人。
Whether you want to obtain part-time employment to pay college and living expense, begin your career as a full –time employee, or start a business, you must bring something to the table that makes you different from the next person . Employee and our capitalistic economic systems are more demanding than ever before. Ask yourself: What can I do that will make employee want to pay me a salary? What skills do I have that employers need? With these questions in mind, we begin with another basic question: Why study business?There are at least four quite compelling reasons.无论你想获得可以用来支付大学和生活开销的兼职,作为一个全职员工开始你的职业生涯,或者创业,你都应该拿出可以让你不同于其他人的东西。
药物设计的基本原理和方法

所选择参数之间不能有相关性,要有比较大的差异,并且生物活性数据的变化幅度应大于一个对数单位(即大于10倍),否则得不到足够的信息;
2 所设计化合物的物理化学性质差异要大
Hansch方法的一般操作过程
*
Hansch方程除了研究定量构效关系外, 还能用来解释药物作用机理,推测和描述可能的受体模型,研究除活性以外的其他药代动力学定量关系
分子对接法(Docking)
通过生长、旋转等得到基本骨架,按照受体的腔穴,定出靶标边界,这是一级结构的生成。从有关数据库搜索与受体受点结合的原子或原子团,设计新的化合物
*
二、间接药物设计(Indirect Drug Design)
间接药物设计法 受体的三维结构并不清楚
以小分子的构效关系为基础,从一组小分子化合物的结构和生物活性数据出发,研究结构与活性关系的规律
是一种新药设计的研究方法,可以作为先导化合物优化的一种手段。也是计算机辅助药物设计的一个重要内容
A=f(C)
生物活性
化合物的结构特征
由于学科的限制,并没有成功地将此关系用于药物设计
发展建立了三种定量构效关系的研究方法
*
20世纪 60年代 定量构效关系: 并根据信息进一步 结构参数 找出结构与活性间的
分子疏水性参数IogP,即分子的脂水分配系数(partition coefficient),表示分子的疏水性
化合物在有机相和水相中分配平衡时的量(摩尔)浓度Co和CW之比值,P=CO/CW
P值一般较大,常用IogP表示
当分子中有该取代基时I为1,当分子中没有该取代基时I为0。
logP的测定:
*
结构主义语言观 功能主义语言观 交互语言观 英文
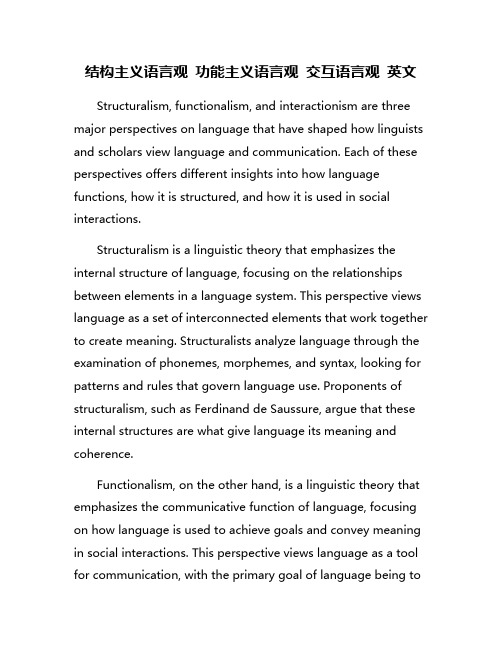
结构主义语言观功能主义语言观交互语言观英文Structuralism, functionalism, and interactionism are three major perspectives on language that have shaped how linguists and scholars view language and communication. Each of these perspectives offers different insights into how language functions, how it is structured, and how it is used in social interactions.Structuralism is a linguistic theory that emphasizes the internal structure of language, focusing on the relationships between elements in a language system. This perspective views language as a set of interconnected elements that work together to create meaning. Structuralists analyze language through the examination of phonemes, morphemes, and syntax, looking for patterns and rules that govern language use. Proponents of structuralism, such as Ferdinand de Saussure, argue that these internal structures are what give language its meaning and coherence.Functionalism, on the other hand, is a linguistic theory that emphasizes the communicative function of language, focusing on how language is used to achieve goals and convey meaning in social interactions. This perspective views language as a tool for communication, with the primary goal of language being toconvey meaning and achieve social cohesion. Functionalists analyze language through the study of speech acts, discourse analysis, and pragmatics, looking at how language is used in specific contexts to accomplish specific goals. Proponents of functionalism, such as Michael Halliday, argue that language is shaped by its communicative functions and its social purposes.Interactionism is a linguistic theory that emphasizes the dynamic and interactive nature of language use, focusing on how language is used in social interactions to create and maintain relationships. This perspective views language as a tool for social interaction, with the primary goal of language being to establish connections between individuals and negotiate meaning in shared contexts. Interactionists analyze language through the study of conversation analysis, discourse markers, and politeness strategies, looking at how language is used to navigate social interactions and establish rapport. Proponents of interactionism, such as Erving Goffman, argue that language is shaped by its use in social interactions and its role in creating and maintaining social relationships.Overall, these three perspectives offer unique insights into how language functions, how it is structured, and how it is used in social interactions. While structuralism focuses on the internalstructure of language, functionalism emphasizes the communicative function of language, and interactionism highlights the interactive nature of language use in social contexts. By considering these different perspectives, linguists and scholars can gain a more comprehensive understanding of the complexity of language and its role in human communication.。
语言学教程英文版
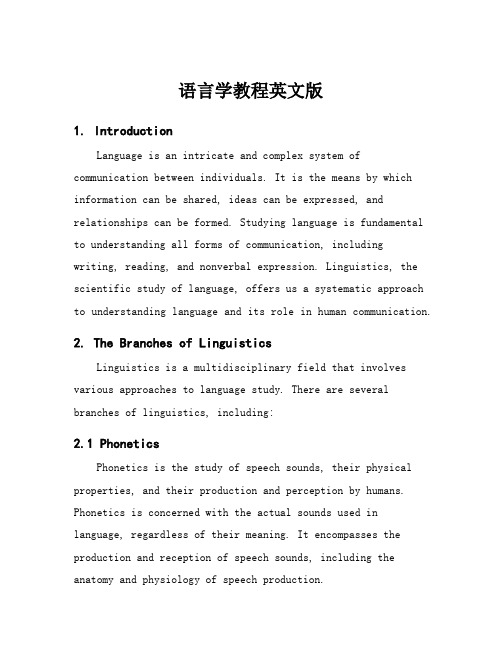
语言学教程英文版1. IntroductionLanguage is an intricate and complex system of communication between individuals. It is the means by which information can be shared, ideas can be expressed, and relationships can be formed. Studying language is fundamental to understanding all forms of communication, including writing, reading, and nonverbal expression. Linguistics, the scientific study of language, offers us a systematic approach to understanding language and its role in human communication.2. The Branches of LinguisticsLinguistics is a multidisciplinary field that involves various approaches to language study. There are several branches of linguistics, including:2.1 PhoneticsPhonetics is the study of speech sounds, their physical properties, and their production and perception by humans. Phonetics is concerned with the actual sounds used in language, regardless of their meaning. It encompasses the production and reception of speech sounds, including the anatomy and physiology of speech production.2.2 PhonologyPhonology is the study of the sound system of language, including the rules and patterns that govern the use and organization of speech sounds in a particular language. Phonology investigates the systematic relationships between sounds and how they are interpreted to convey meaning.2.3 MorphologyMorphology is the study of the structure of words and how they are formed from smaller units (morphemes) that carry meaning. Morphology is concerned with the internal structure of words, including morpheme identification, inflection, and derivation.2.4 SyntaxSyntax is the study of how words are combined to form meaningful phrases, clauses, and sentences. Syntax is concerned with the rules governing word order, grammatical agreement, and the use of function words (such as conjunctions and prepositions) to establish relationships between words.2.5 SemanticsSemantics is the study of meaning in language, including the meanings of words, phrases, and sentences. Semanticsanalyzes how meaning is conveyed through language and how different words and phrases can have multiple meanings.2.6 PragmaticsPragmatics is the study of language use in context and the ways in which speakers convey meaning beyond the literal meaning of words. Pragmatics investigates the social and situational factors that influence language use, including the speaker's intentions, the listener's expectations, and the shared cultural background of both.3. Key Concepts in LinguisticsLinguistics is concerned with understanding how language works and how it is used in everyday communication. There are several key concepts that are central to linguistic analysis: 3.1 Language UniversalsLanguage universals are patterns or tendencies that are found across all languages. These are features of language that are common to all human languages, such as the presence of consonants and vowels or the use of subject-verb word order.3.2 Language RelativityLanguage relativity is the idea that language and culture have a reciprocal relationship, with each influencing andshaping the other. This concept suggests that the structure and vocabulary of a language can shape the way its speakers perceive and understand the world around them.3.3 Language AcquisitionLanguage acquisition is the process by which humans learn a language. The study of language acquisition investigates how children learn to speak and understand their native language and how adults learn a second language.3.4 Language ChangeLanguage change is the process by which language evolves over time. This concept includes changes in the sound, structure, and meaning of language and can be influenced by social, cultural, and historical factors.4. ConclusionLinguistics is a fascinating field that helps us understand the intricate and complex nature of human communication. The study of linguistics provides us with a systematic approach to understanding language and its role in human society. With its focus on language universals, language relativity, language acquisition, and language change, linguistics offers us insights into how wecommunicate, how we learn, and how language shapes our understanding of the world.。
单分子综述-NATURE NANOTECHNOLOGY-Single-molecule junctions beyond electronic transport-2013
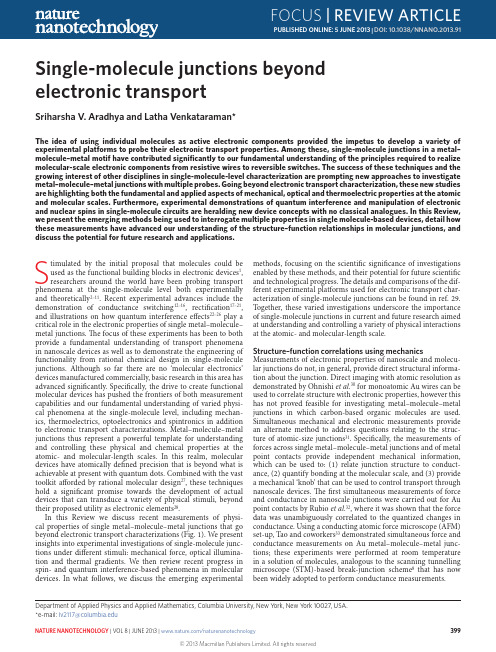
Stimulated by the initial proposal that molecules could be used as the functional building blocks in electronic devices 1, researchers around the world have been probing transport phenomena at the single-molecule level both experimentally and theoretically 2–11. Recent experimental advances include the demonstration of conductance switching 12–16, rectification 17–21, and illustrations on how quantum interference effects 22–26 play a critical role in the electronic properties of single metal–molecule–metal junctions. The focus of these experiments has been to both provide a fundamental understanding of transport phenomena in nanoscale devices as well as to demonstrate the engineering of functionality from rational chemical design in single-molecule junctions. Although so far there are no ‘molecular electronics’ devices manufactured commercially, basic research in this area has advanced significantly. Specifically, the drive to create functional molecular devices has pushed the frontiers of both measurement capabilities and our fundamental understanding of varied physi-cal phenomena at the single-molecule level, including mechan-ics, thermoelectrics, optoelectronics and spintronics in addition to electronic transport characterizations. Metal–molecule–metal junctions thus represent a powerful template for understanding and controlling these physical and chemical properties at the atomic- and molecular-length scales. I n this realm, molecular devices have atomically defined precision that is beyond what is achievable at present with quantum dots. Combined with the vast toolkit afforded by rational molecular design 27, these techniques hold a significant promise towards the development of actual devices that can transduce a variety of physical stimuli, beyond their proposed utility as electronic elements 28.n this Review we discuss recent measurements of physi-cal properties of single metal–molecule–metal junctions that go beyond electronic transport characterizations (Fig. 1). We present insights into experimental investigations of single-molecule junc-tions under different stimuli: mechanical force, optical illumina-tion and thermal gradients. We then review recent progress in spin- and quantum interference-based phenomena in molecular devices. I n what follows, we discuss the emerging experimentalSingle-molecule junctions beyond electronic transportSriharsha V. Aradhya and Latha Venkataraman*The id ea of using ind ivid ual molecules as active electronic components provid ed the impetus to d evelop a variety of experimental platforms to probe their electronic transport properties. Among these, single-molecule junctions in a metal–molecule–metal motif have contributed significantly to our fundamental understanding of the principles required to realize molecular-scale electronic components from resistive wires to reversible switches. The success of these techniques and the growing interest of other disciplines in single-molecule-level characterization are prompting new approaches to investigate metal–molecule–metal junctions with multiple probes. Going beyond electronic transport characterization, these new studies are highlighting both the fundamental and applied aspects of mechanical, optical and thermoelectric properties at the atomic and molecular scales. Furthermore, experimental demonstrations of quantum interference and manipulation of electronic and nuclear spins in single-molecule circuits are heralding new device concepts with no classical analogues. In this Review, we present the emerging methods being used to interrogate multiple properties in single molecule-based devices, detail how these measurements have advanced our understanding of the structure–function relationships in molecular junctions, and discuss the potential for future research and applications.methods, focusing on the scientific significance of investigations enabled by these methods, and their potential for future scientific and technological progress. The details and comparisons of the dif-ferent experimental platforms used for electronic transport char-acterization of single-molecule junctions can be found in ref. 29. Together, these varied investigations underscore the importance of single-molecule junctions in current and future research aimed at understanding and controlling a variety of physical interactions at the atomic- and molecular-length scale.Structure–function correlations using mechanicsMeasurements of electronic properties of nanoscale and molecu-lar junctions do not, in general, provide direct structural informa-tion about the junction. Direct imaging with atomic resolution as demonstrated by Ohnishi et al.30 for monoatomic Au wires can be used to correlate structure with electronic properties, however this has not proved feasible for investigating metal–molecule–metal junctions in which carbon-based organic molecules are used. Simultaneous mechanical and electronic measurements provide an alternate method to address questions relating to the struc-ture of atomic-size junctions 31. Specifically, the measurements of forces across single metal–molecule–metal junctions and of metal point contacts provide independent mechanical information, which can be used to: (1) relate junction structure to conduct-ance, (2) quantify bonding at the molecular scale, and (3) provide a mechanical ‘knob’ that can be used to control transport through nanoscale devices. The first simultaneous measurements of force and conductance in nanoscale junctions were carried out for Au point contacts by Rubio et al.32, where it was shown that the force data was unambiguously correlated to the quantized changes in conductance. Using a conducting atomic force microscope (AFM) set-up, Tao and coworkers 33 demonstrated simultaneous force and conductance measurements on Au metal–molecule–metal junc-tions; these experiments were performed at room temperature in a solution of molecules, analogous to the scanning tunnelling microscope (STM)-based break-junction scheme 8 that has now been widely adopted to perform conductance measurements.Department of Applied Physics and Applied Mathematics, Columbia University, New York, New York 10027, USA. *e-mail: lv2117@DOI: 10.1038/NNANO.2013.91These initial experiments relied on the so-called static mode of AFM-based force spectroscopy, where the force on the canti-lever is monitored as a function of junction elongation. I n this method the deflection of the AFM cantilever is directly related to the force on the junction by Hooke’s law (force = cantilever stiff-ness × cantilever deflection). Concurrently, advances in dynamic force spectroscopy — particularly the introduction of the ‘q-Plus’ configuration 34 that utilizes a very stiff tuning fork as a force sen-sor — are enabling high-resolution measurements of atomic-size junctions. In this technique, the frequency shift of an AFM cantilever under forced near-resonance oscillation is measuredas a function of junction elongation. This frequency shift can be related to the gradient of the tip–sample force. The underlying advantage of this approach is that frequency-domain measure-ments of high-Q resonators is significantly easier to carry out with high precision. However, in contrast to the static mode, recover-ing the junction force from frequency shifts — especially in the presence of dissipation and dynamic structural changes during junction elongation experiments — is non-trivial and a detailed understanding remains to be developed 35.The most basic information that can be determined throughsimultaneous measurement of force and conductance in metalThermoelectricsSpintronics andMechanicsOptoelectronicsHotColdFigure 1 | Probing multiple properties of single-molecule junctions. phenomena in addition to demonstrations of quantum mechanical spin- and interference-dependent transport concepts for which there are no analogues in conventional electronics.contacts is the relation between the measured current and force. An experimental study by Ternes et al.36 attempted to resolve a long-standing theoretical prediction 37 that indicated that both the tunnelling current and force between two atomic-scale metal contacts scale similarly with distance (recently revisited by Jelinek et al.38). Using the dynamic force microscopy technique, Ternes et al. effectively probed the interplay between short-range forces and conductance under ultrahigh-vacuum conditions at liquid helium temperatures. As illustrated in Fig. 2a, the tunnel-ling current through the gap between the metallic AFM probe and the substrate, and the force on the cantilever were recorded, and both were found to decay exponentially with increasing distance with nearly the same decay constant. Although an exponential decay in current with distance is easily explained by considering an orbital overlap of the tip and sample wavefunctions through a tunnel barrier using Simmons’ model 39, the exponential decay in the short-range forces indicated that perhaps the same orbital controlled the interatomic short-range forces (Fig. 2b).Using such dynamic force microscopy techniques, research-ers have also studied, under ultrahigh-vacuum conditions, forces and conductance across junctions with diatomic adsorbates such as CO (refs 40,41) and more recently with fullerenes 42, address-ing the interplay between electronic transport, binding ener-getics and structural evolution. I n one such experiment, Tautz and coworkers 43 have demonstrated simultaneous conduct-ance and stiffness measurements during the lifting of a PTCDA (3,4,9,10-perylene-tetracarboxylicacid-dianhydride) molecule from a Ag(111) substrate using the dynamic mode method with an Ag-covered tungsten AFM tip. The authors were able to follow the lifting process (Fig. 2c,d) monitoring the junction stiffness as the molecule was peeled off the surface to yield a vertically bound molecule, which could also be characterized electronically to determine the conductance through the vertical metal–molecule–metal junction with an idealized geometry. These measurements were supported by force field-based model calculations (Fig. 2c and dashed black line in Fig. 2d), presenting a way to correlate local geometry to the electronic transport.Extending the work from metal point contacts, ambient meas-urements of force and conductance across single-molecule junc-tions have been carried out using the static AFM mode 33. These measurements allow correlation of the bond rupture forces with the chemistry of the linker group and molecular backbone. Single-molecule junctions are formed between a Au-metal sub-strate and a Au-coated cantilever in an environment of molecules. Measurements of current through the junction under an applied bias determine conductance, while simultaneous measurements of cantilever deflection relate to the force applied across the junction as shown in Fig. 2e. Although measurements of current throughzF zyxCantileverIVabConductance G (G 0)1 2 3Tip–sample distance d (Å)S h o r t -r a n g e f o r c e F z (n N )10−310−210−11110−110−210−3e10−410−210C o n d u c t a n c e (G 0)Displacement86420Force (nN)0.5 nm420−2F o r c e (n N )−0.4−0.200.20.4Displacement (nm)SSfIncreasing rupture forcegc(iv)(i)(iii)(ii)Low HighCounts d9630−3d F /d z (n N n m −1)(i)(iv)(iii)(ii)A p p r o a chL i ft i n g110−210−4G (2e 2/h )2051510z (Å)H 2NNH 2H 2NNH 2NNFigure 2 | Simultaneous measurements of electronic transport and mechanics. a , A conducting AFM set-up with a stiff probe (shown schematically) enabled the atomic-resolution imaging of a Pt adsorbate on a Pt(111) surface (tan colour topography), before the simultaneous measurement of interatomic forces and currents. F z , short-range force. b , Semilogarithmic plot of tunnelling conductance and F z measured over the Pt atom. A similar decay constant for current and force as a function of interatomic distance is seen. The blue dashed lines are exponential fits to the data. c , Structural snapshots showing a molecular mechanics simulation of a PTCDA molecule held between a Ag substrate and tip (read right to left). It shows the evolution of the Ag–PTCDA–Ag molecular junction as a function of tip–surface distance. d , Upper panel shows experimental stiffness (d F /d z ) measurements during the lifting process performed with a conducting AFM. The calculated values from the simulation are overlaid (dashed black line). Lower panel shows simultaneously measured conductance (G ). e , Simultaneously measured conductance (red) and force (blue) measurements showing evolution of a molecular junction as a function of junction elongation. A Au point contact is first formed, followed by the formation of a single-molecule junction, which then ruptures on further elongation. f , A two-dimensional histogram of thousands of single-molecule junctionrupture events (for 1,4-bis(methyl sulphide) butane; inset), constructed by redefining the rupture location as the zero displacement point. The most frequently measured rupture force is the drop in force (shown by the double-headed arrow) at the rupture location in the statistically averaged force trace (overlaid black curve). g , Beyond the expected dependence on the terminal group, the rupture force is also sensitive to the molecular backbone, highlighting the interplay between chemical structure and mechanics. In the case of nitrogen-terminated molecules, rupture force increases fromaromatic amines to aliphatic amines and the highest rupture force is for molecules with pyridyl moieties. Figure reproduced with permission from: a ,b , ref. 36, © 2011 APS; c ,d , ref. 43, © 2011 APS.DOI: 10.1038/NNANO.2013.91such junctions are easily accomplished using standard instru-mentation, measurements of forces with high resolution are not straightforward. This is because a rather stiff cantilever (with a typical spring constant of ~50 N m−1) is typically required to break the Au point contact that is first formed between the tip and sub-strate, before the molecular junctions are created. The force reso-lution is then limited by the smallest deflection of the cantilever that can be measured. With a custom-designed system24 our group has achieved a cantilever displacement resolution of ~2 pm (com-pare with Au atomic diameter of ~280 pm) using an optical detec-tion scheme, allowing the force noise floor of the AFM set-up to be as low as 0.1 nN even with these stiff cantilevers (Fig. 2e). With this system, and a novel analysis technique using two-dimensional force–displacement histograms as illustrated in Fig. 2f, we have been able to systematically probe the influence of the chemical linker group44,45 and the molecular backbone46 on single-molecule junction rupture force as illustrated in Fig. 2g.Significant future opportunities with force measurements exist for investigations that go beyond characterizations of the junc-tion rupture force. In two independent reports, one by our group47 and another by Wagner et al.48, force measurements were used to quantitatively measure the contribution of van der Waals interac-tions at the single-molecule level. Wagner et al. used the stiffness data from the lifting of PTCDA molecules on a Au(111) surface, and fitted it to the stiffness calculated from model potentials to estimate the contribution of the various interactions between the molecule and the surface48. By measuring force and conductance across single 4,4’-bipyridine molecules attached to Au electrodes, we were able to directly quantify the contribution of van der Waals interactions to single-molecule-junction stiffness and rupture force47. These experimental measurements can help benchmark the several theoretical frameworks currently under development aiming to reliably capture van der Waals interactions at metal/ organic interfaces due to their importance in diverse areas includ-ing catalysis, electronic devices and self-assembly.In most of the experiments mentioned thus far, the measured forces were typically used as a secondary probe of junction prop-erties, instead relying on the junction conductance as the primary signature for the formation of the junction. However, as is the case in large biological molecules49, forces measured across single-mol-ecule junctions can also provide the primary signature, thereby making it possible to characterize non-conducting molecules that nonetheless do form junctions. Furthermore, molecules pos-sess many internal degrees of motion (including vibrations and rotations) that can directly influence the electronic transport50, and the measurement of forces with such molecules can open up new avenues for mechanochemistry51. This potential of using force measurements to elucidate the fundamentals of electronic transport and binding interactions at the single-molecule level is prompting new activity in this area of research52–54. Optoelectronics and optical spectroscopyAddressing optical properties and understanding their influence on electronic transport in individual molecular-scale devices, col-lectively referred to as ‘molecular optoelectronics’, is an area with potentially important applications55. However, the fundamental mismatch between the optical (typically, approximately at the micrometre scale) and molecular-length scales has historically presented a barrier to experimental investigations. The motiva-tions for single-molecule optoelectronic studies are twofold: first, optical spectroscopies (especially Raman spectroscopy) could lead to a significantly better characterization of the local junction structure. The nanostructured metallic electrodes used to real-ize single-molecule junctions are coincidentally some of the best candidates for local field enhancement due to plasmons (coupled excitations of surface electrons and incident photons). This there-fore provides an excellent opportunity for understanding the interaction of plasmons with molecules at the nanoscale. Second, controlling the electronic transport properties using light as an external stimulus has long been sought as an attractive alternative to a molecular-scale field-effect transistor.Two independent groups have recently demonstrated simulta-neous optical and electrical measurements on molecular junctions with the aim of providing structural information using an optical probe. First, Ward et al.56 used Au nanogaps formed by electromi-gration57 to create molecular junctions with a few molecules. They then irradiated these junctions with a laser operating at a wavelength that is close to the plasmon resonance of these Au nanogaps to observe a Raman signal attributable to the molecules58 (Fig. 3a). As shown in Fig. 3b, they observed correlations between the intensity of the Raman features and magnitude of the junction conductance, providing direct evidence that Raman signatures could be used to identify junction structures. They later extended this experimental approach to estimate vibrational and electronic heating in molecu-lar junctions59. For this work, they measured the ratio of the Raman Stokes and anti-Stokes intensities, which were then related to the junction temperature as a function of the applied bias voltage. They found that the anti-Stokes intensity changed with bias voltage while the Stokes intensity remained constant, indicating that the effective temperature of the Raman-active mode was affected by passing cur-rent through the junction60. Interestingly, Ward et al. found that the vibrational mode temperatures exceeded several hundred kelvin, whereas earlier work by Tao and co-workers, who used models for junction rupture derived from biomolecule research, had indicated a much smaller value (~10 K) for electronic heating61. Whether this high temperature determined from the ratio of the anti-Stokes to Stokes intensities indicates that the electronic temperature is also similarly elevated is still being debated55, however, one can definitely conclude that such measurements under a high bias (few hundred millivolts) are clearly in a non-equilibrium transport regime, and much more research needs to be performed to understand the details of electronic heating.Concurrently, Liu et al.62 used the STM-based break-junction technique8 and combined this with Raman spectroscopy to per-form simultaneous conductance and Raman measurements on single-molecule junctions formed between a Au STM tip and a Au(111) substrate. They coupled a laser to a molecular junction as shown in Fig. 3c with a 4,4’-bipyridine molecule bridging the STM tip (top) and the substrate (bottom). Pyridines show clear surface-enhanced Raman signatures on metal58, and 4,4’-bipy-ridine is known to form single-molecule junctions in the STM break-junction set-up8,15. Similar to the study of Ward et al.56, Liu et al.62 found that conducting molecular junctions had a Raman signature that was distinct from the broken molecu-lar junctions. Furthermore, the authors studied the spectra of 4,4’-bipyridine at different bias voltages, ranging from 10 to 800 mV, and reported a reversible splitting of the 1,609 cm–1 peak (Fig. 3d). Because this Raman signature is due to a ring-stretching mode, they interpreted this splitting as arising from the break-ing of the degeneracy between the rings connected to the source and drain electrodes at high biases (Fig. 3c). Innovative experi-ments such as these have demonstrated that there is new physics to be learned through optical probing of molecular junctions, and are initiating further interest in understanding the effect of local structure and vibrational effects on electronic transport63. Experiments that probe electroluminescence — photon emis-sion induced by a tunnelling current — in these types of molec-ular junction can also offer insight into structure–conductance correlations. Ho and co-workers have demonstrated simultaneous measurement of differential conductance and photon emissionDOI: 10.1038/NNANO.2013.91from individual molecules at a submolecular-length scale using an STM 64,65. Instead of depositing molecules directly on a metal sur-face, they used an insulating layer to decouple the molecule from the metal 64,65 (Fig. 3e). This critical factor, combined with the vac-uum gap with the STM tip, ensures that the metal electrodes do not quench the radiated photons, and therefore the emitted photons carry molecular fingerprints. Indeed, the experimental observation of molecular electroluminescence of C 60 monolayers on Au(110) by Berndt et al.66 was later attributed to plasmon-mediated emission of the metallic electrodes, indirectly modulated by the molecule 67. The challenge of finding the correct insulator–molecule combination and performing the experiments at low temperature makes electro-luminescence relatively uncommon compared with the numerous Raman studies; however, progress is being made on both theoretical and experimental fronts to understand and exploit emission pro-cesses in single-molecule junctions 68.Beyond measurements of the Raman spectra of molecular junctions, light could be used to control transport in junctions formed with photochromic molecular backbones that occur in two (or more) stable and optically accessible states. Some common examples include azobenzene derivatives, which occur in a cis or trans form, as well as diarylene compounds that can be switched between a conducting conjugated form and a non-conducting cross-conjugated form 69. Experiments probing the conductance changes in molecular devices formed with such compounds have been reviewed in depth elsewhere 70,71. However, in the single-mol-ecule context, there are relatively few examples of optical modula-tion of conductance. To a large extent, this is due to the fact that although many molecular systems are known to switch reliably in solution, contact to metallic electrodes can dramatically alter switching properties, presenting a significant challenge to experi-ments at the single-molecule level.Two recent experiments have attempted to overcome this chal-lenge and have probed conductance changes in single-molecule junctions while simultaneously illuminating the junctions with visible light 72,73. Battacharyya et al.72 used a porphyrin-C 60 ‘dyad’ molecule deposited on an indium tin oxide (I TO) substrate to demonstrate the light-induced creation of an excited-state mol-ecule with a different conductance. The unconventional transpar-ent ITO electrode was chosen to provide optical access while also acting as a conducting electrode. The porphyrin segment of the molecule was the chromophore, whereas the C 60 segment served as the electron acceptor. The authors found, surprisingly, that the charge-separated molecule had a much longer lifetime on ITO than in solution. I n the break-junction experiments, the illuminated junctions showed a conductance feature that was absent without1 μm Raman shift (cm –1)1,609 cm –1(–)Source 1,609 cm–1Drain (+)Low voltage High voltageMgPNiAl(110)STM tip (Ag)VacuumThin alumina 1.4 1.5 1.6 1.701020 3040200400Photon energy (eV)3.00 V 2.90 V 2.80 V 2.70 V 2.60 V2.55 V 2.50 VP h o t o n c o u n t s (a .u .)888 829 777731Wavelength (nm)Oxideacebd f Raman intensity (CCD counts)1,5001,00050000.40.30.20.10.01,590 cm −11,498 cm −1d I /d V (μA V –1)1,609 cm –11,631 cm–11 μm1 μmTime (s)Figure 3 | Simultaneous studies of optical effects and transport. a , A scanning electron micrograph (left) of an electromigrated Au junction (light contrast) lithographically defined on a Si substrate (darker contrast). The nanoscale gap results in a ‘hot spot’ where Raman signals are enhanced, as seen in the optical image (right). b , Simultaneously measured differential conductance (black, bottom) and amplitudes of two molecular Raman features (blue traces, middle and top) as a function of time in a p-mercaptoaniline junction. c , Schematic representation of a bipyridine junction formed between a Au STM tip and a Au(111) substrate, where the tip enhancement from the atomically sharp STM tip results in a large enhancement of the Raman signal. d , The measured Raman spectra as a function of applied bias indicate breaking of symmetry in the bound molecule. e , Schematic representation of a Mg-porphyrin (MgP) molecule sandwiched between a Ag STM tip and a NiAl(110) substrate. A subnanometre alumina insulating layer is a key factor in measuring the molecular electroluminescence, which would otherwise be overshadowed by the metallic substrate. f , Emission spectra of a single Mg-porphyrin molecule as a function of bias voltage (data is vertically offset for clarity). At high biases, individual vibronic peaks become apparent. The spectra from a bare oxide layer (grey) is shown for reference. Figure reproduced with permission from: a ,b , ref. 56, © 2008 ACS; c ,d , ref. 62, © 2011 NPG; e ,f , ref. 65, © 2008 APS.DOI: 10.1038/NNANO.2013.91light, which the authors assigned to the charge-separated state. In another approach, Lara-Avila et al.73 have reported investigations of a dihydroazulene (DHA)/vinylheptafulvene (VHF) molecule switch, utilizing nanofabricated gaps to perform measurements of Au–DHA–Au single-molecule junctions. Based on the early work by Daub et al.74, DHA was known to switch to VHF under illumina-tion by 353-nm light and switch back to DHA thermally. In three of four devices, the authors observed a conductance increase after irradiating for a period of 10–20 min. In one of those three devices, they also reported reversible switching after a few hours. Although much more detailed studies are needed to establish the reliability of optical single-molecule switches, these experiments provide new platforms to perform in situ investigations of single-molecule con-ductance under illumination.We conclude this section by briefly pointing to the rapid pro-gress occurring in the development of optical probes at the single-molecule scale, which is also motivated by the tremendous interest in plasmonics and nano-optics. As mentioned previously, light can be coupled into nanoscale gaps, overcoming experimental chal-lenges such as local heating. Banerjee et al.75 have exploited these concepts to demonstrate plasmon-induced electrical conduction in a network of Au nanoparticles that form metal–molecule–metal junctions between them (Fig. 3f). Although not a single-molecule measurement, the control of molecular conductance through plas-monic coupling can benefit tremendously from the diverse set of new concepts under development in this area, such as nanofabri-cated transmission lines 76, adiabatic focusing of surface plasmons, electrical excitation of surface plasmons and nanoparticle optical antennas. The convergence of plasmonics and electronics at the fundamental atomic- and molecular-length scales can be expected to provide significant opportunities for new studies of light–mat-ter interaction 77–79.Thermoelectric characterization of single-molecule junctions Understanding the electronic response to heating in a single-mole-cule junction is not only of basic scientific interest; it can have a tech-nological impact by improving our ability to convert wasted heat into usable electricity through the thermoelectric effect, where a temper-ature difference between two sides of a device induces a voltage drop across it. The efficiency of such a device depends on its thermopower (S ; also known as the Seebeck coefficient), its electric and thermal conductivity 80. Strategies for increasing the efficiency of thermoelec-tric devices turned to nanoscale devices a decade ago 81, where one could, in principle, increase the electronic conductivity and ther-mopower while independently minimizing the thermal conductiv-ity 82. This has motivated the need for a fundamental understandingof thermoelectrics at the single-molecule level 83, and in particular, the measurement of the Seebeck coefficient in such junctions. The Seebeck coefficient, S = −(ΔV /ΔT )|I = 0, determines the magnitude of the voltage developed across the junction when a temperature dif-ference ΔT is applied, as illustrated in Fig. 4a; this definition holds both for bulk devices and for single-molecule junctions. If an addi-tional external voltage ΔV exists across the junction, then the cur-rent I through the junction is given by I = G ΔV + GS ΔT where G is the junction conductance 83. Transport through molecular junctions is typically in the coherent regime where conductance, which is pro-portional to the electronic transmission probability, is given by the Landauer formula 84. The Seebeck coefficient at zero applied voltage is then related to the derivative of the transmission probability at the metal Fermi energy (in the off-resonance limit), with, S = −∂E ∂ln( (E ))π2k 2B T E 3ewhere k B is the Boltzmann constant, e is the charge of the electron, T (E ) is the energy-dependent transmission function and E F is the Fermi energy. When the transmission function for the junction takes on a simple Lorentzian form 85, and transport is in the off-resonance limit, the sign of S can be used to deduce the nature of charge carriers in molecular junctions. In such cases, a positive S results from hole transport through the highest occupied molecu-lar orbital (HOMO) whereas a negative S indicates electron trans-port through the lowest unoccupied molecular orbital (LUMO). Much work has been performed on investigating the low-bias con-ductance of molecular junctions using a variety of chemical linker groups 86–89, which, in principle, can change the nature of charge carriers through the junction. Molecular junction thermopower measurements can thus be used to determine the nature of charge carriers, correlating the backbone and linker chemistry with elec-tronic aspects of conduction.Experimental measurements of S and conductance were first reported by Ludoph and Ruitenbeek 90 in Au point contacts at liquid helium temperatures. This work provided a method to carry out thermoelectric measurements on molecular junctions. Reddy et al.91 implemented a similar technique in the STM geome-try to measure S of molecular junctions, although due to electronic limitations, they could not simultaneously measure conductance. They used thiol-terminated oligophenyls with 1-3-benzene units and found a positive S that increased with increasing molecular length (Fig. 4b). These pioneering experiments allowed the iden-tification of hole transport through thiol-terminated molecular junctions, while also introducing a method to quantify S from statistically significant datasets. Following this work, our group measured the thermoelectric current through a molecular junction held under zero external bias voltage to determine S and the con-ductance through the same junction at a finite bias to determine G (ref. 92). Our measurements showed that amine-terminated mol-ecules conduct through the HOMO whereas pyridine-terminatedmolecules conduct through the LUMO (Fig. 4b) in good agree-ment with calculations.S has now been measured on a variety of molecular junctionsdemonstrating both hole and electron transport 91–95. Although the magnitude of S measured for molecular junctions is small, the fact that it can be tuned by changing the molecule makes these experiments interesting from a scientific perspective. Future work on the measurements of the thermal conductance at the molecu-lar level can be expected to establish a relation between chemical structure and the figure of merit, which defines the thermoelec-tric efficiencies of such devices and determines their viability for practical applications.SpintronicsWhereas most of the explorations of metal–molecule–metal junc-tions have been motivated by the quest for the ultimate minia-turization of electronic components, the quantum-mechanical aspects that are inherent to single-molecule junctions are inspir-ing entirely new device concepts with no classical analogues. In this section, we review recent experiments that demonstrate the capability of controlling spin (both electronic and nuclear) in single-molecule devices 96. The early experiments by the groups of McEuen and Ralph 97, and Park 98 in 2002 explored spin-depend-ent transport and the Kondo effect in single-molecule devices, and this topic has recently been reviewed in detail by Scott and Natelson 99. Here, we focus on new types of experiment that are attempting to control the spin state of a molecule or of the elec-trons flowing through the molecular junction. These studies aremotivated by the appeal of miniaturization and coherent trans-port afforded by molecular electronics, combined with the great potential of spintronics to create devices for data storage and quan-tum computation 100. The experimental platforms for conducting DOI: 10.1038/NNANO.2013.91。
Materials Characterization
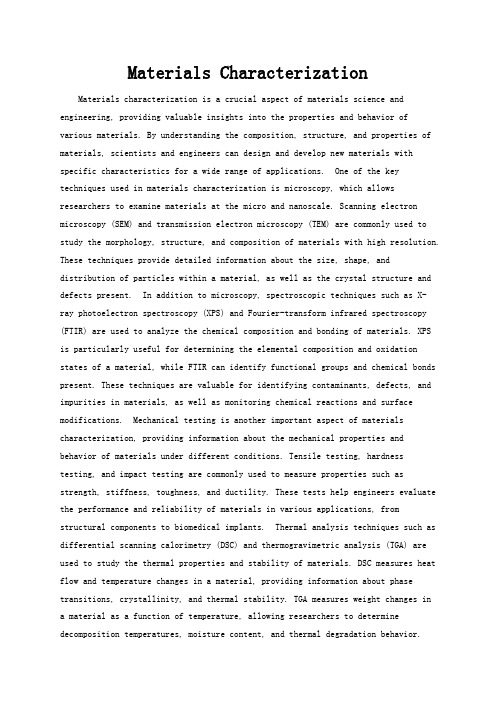
Materials Characterization Materials characterization is a crucial aspect of materials science and engineering, providing valuable insights into the properties and behavior of various materials. By understanding the composition, structure, and properties of materials, scientists and engineers can design and develop new materials with specific characteristics for a wide range of applications. One of the key techniques used in materials characterization is microscopy, which allows researchers to examine materials at the micro and nanoscale. Scanning electron microscopy (SEM) and transmission electron microscopy (TEM) are commonly used to study the morphology, structure, and composition of materials with high resolution. These techniques provide detailed information about the size, shape, anddistribution of particles within a material, as well as the crystal structure and defects present. In addition to microscopy, spectroscopic techniques such as X-ray photoelectron spectroscopy (XPS) and Fourier-transform infrared spectroscopy (FTIR) are used to analyze the chemical composition and bonding of materials. XPSis particularly useful for determining the elemental composition and oxidation states of a material, while FTIR can identify functional groups and chemical bonds present. These techniques are valuable for identifying contaminants, defects, and impurities in materials, as well as monitoring chemical reactions and surface modifications. Mechanical testing is another important aspect of materials characterization, providing information about the mechanical properties and behavior of materials under different conditions. Tensile testing, hardness testing, and impact testing are commonly used to measure properties such as strength, stiffness, toughness, and ductility. These tests help engineers evaluate the performance and reliability of materials in various applications, from structural components to biomedical implants. Thermal analysis techniques such as differential scanning calorimetry (DSC) and thermogravimetric analysis (TGA) are used to study the thermal properties and stability of materials. DSC measures heat flow and temperature changes in a material, providing information about phase transitions, crystallinity, and thermal stability. TGA measures weight changes ina material as a function of temperature, allowing researchers to determine decomposition temperatures, moisture content, and thermal degradation behavior.Electrical and magnetic characterization techniques are also important for studying the electronic and magnetic properties of materials. Electrical conductivity, dielectric constant, and magnetic susceptibility are key parameters that influence the performance of materials in electronic and magnetic devices. Techniques such as electrical impedance spectroscopy (EIS) and vibrating sample magnetometry (VSM) are used to measure these properties and understand the underlying mechanisms that govern them. Overall, materials characterization plays a crucial role in advancing materials science and engineering, enabling researchers to tailor materials for specific applications and optimize their performance. By combining multiple characterization techniques, scientists and engineers can gain a comprehensive understanding of the structure-property relationships in materials and develop new materials with enhanced properties and functionalities. This interdisciplinary approach is essential for driving innovation and addressing the complex challenges facing modern society.。
periodontal tissues

Structure of periodontal tissues in health and disease*A N T O NI O N A N C I &D I E T E R D.B O S S H A R D TThe periodontium,defined as those tissues support-ing and investing the tooth,comprises root cemen-tum,periodontal ligament,bone lining the tooth socket (alveolar bone),and that part of the gingiva facing the tooth (dentogingival junction).The wide-spread occurrence of periodontal diseases and the realization that lost tissues can be repaired and,perhaps,regenerated has generated considerable interest in the factors and cells regulating their for-mation and maintenance.It is important to under-stand that each of the periodontal components has its very specialized structure and that these structural characteristics directly define function.Indeed,proper functioning of the periodontium is only achieved through structural integrity and interaction between its components.In recent years,a number of detailed descriptions of the structural and compositional features of periodontal tissues have been published (3,5–7,9,15,17,46,50,56,58,61);we refer the reader to these for a comprehensive description of the develop-ment,formation,and structure of periodontal tissues.The present review will focus on structure–function relationships pertinent to understanding periodontal tissue breakdown and the repair ⁄regeneration of affected structures.Healthy periodontal tissuesDentogingival junctionThe dentogingival junction (gingiva facing the tooth)is an adaptation of the oral mucosa that comprises epithelial and connective tissue components.The epithelium is divided into three functional com-partments –gingival ,sulcular ,and junctionalepithelium –and the connective tissue into s uper-ficial and deep compartments.The junctional epi-thelium plays a crucial role since it essentially seals off periodontal tissues from the oral environment.Its integrity is thus essential for maintaining a healthy periodontium.Periodontal disease sets in when the structure of the junctional epithelium starts to fail,an excellent example of how structure determines function.The junctional epitheliumThe junctional epithelium arises from the reduced enamel epithelium as the tooth erupts into the oral cavity.It forms a collar around the cervical portion of the tooth that follows the cementoenamel junction (Fig.1).The free surface of this collar constitutes the floor of the gingival sulcus.Basically,the junctional epithelium is a nondifferentiated,stratified squa-mous epithelium with a very high rate of cell turn-over.It is thickest near the bottom of the gingival sulcus and tapers to a thickness of a few cells as it descends apically along the tooth surface.This epi-thelium is made up of flattened cells oriented paral-lel to the tooth that derive from a layer of cuboidal basal cells situated away from the tooth surface that rest on a basement membrane.Suprabasal cells have a similar ultrastructure and,quite remarkably,maintain the ability to undergo cell division.The cell layer facing the tooth provides the actual attachment of the gingiva to the tooth surface by means of a structural complex called the epithelial attachment .This complex consists of a basal lamina-like structure that is adherent to the tooth surface and to which the superficial cell layer is attached by hemidesmosomes.The basal lamina-like structure is a specialized extracellular matrix in which typical basement membrane constituents have not been immuno-detected in any significant quantity but which is*Parts of this article are adapted from Reference 50.11Periodontology 2000,Vol.40,2006,11–28Printed in the UK.All rights reservedCopyright ÓBlackwell Munksgaard 2006PERIODONTOLOGY 2000enriched in glycoconjugates and contains laminin 5.The latter matrix protein mediates cell adhesion and regulates the polarization and migration of kera-tinocytes (27).Junctional epithelial cells differ considerably from those of the gingival epithelium.They contain more cytoplasm,rough endoplasmic reticulum,and Golgi bodies.They exhibit fewer tonofilaments and des-mosomes,and wider intercellular spaces.The latter fluid-filled spaces normally contain polymorpho-nuclear leukocytes and monocytes that pass from the subepithelial connective tissue through the junc-tional epithelium and into the gingival sulcus.The mononuclear cells,together with molecules they secrete and others originating from junctional epi-thelial cells,blood and tissue fluid represent the first line of defense in the control of the perpetual microbial challenge.Among these molecules are a -and b -defensins,cathelicidin LL-37,interleukin (IL)-8,IL-1a and -1b ,tumor necrosis factor-a ,inter-cellular adhesion molecule-1,and lymphocyte func-tion antigen-3.Connective tissue compartmentThe connective tissue supporting the junctional epi-thelium is structurally different from that supporting the oral gingival epithelium.Even in clinically normal circumstances,it shows an inflammatory cell infil-trate.The gingival connective tissue adjacent to the junctional epithelium contains an extensive vascular plexus.Inflammatory cells such as polymorphonu-clear leukocytes and T-lymphocytes continually extravasate from this dense capillary and postcapil-lary venule network,and migrate across the junc-tional epithelium into the gingival sulcus and eventually the oral fluid.The vascular distribution in the gingival lamina propria is described in detail in Schroeder &Listgarten (58).One point of view considers the junctional epi-thelium as an incompletely developed stratified squamous epithelium.Alternatively,it may be viewed as a structure that evolves along a different pathway and produces the components of the epithelial attachment instead of progressing further into a keratinized epithelium.The special nature of the junctional epithelium is believed to reflect the fact that the connective tissue supporting it is function-ally different than that of the sulcular epithelium,a difference with important implications for under-standing the progression of periodontal disease and the regeneration of the dentogingival junction after periodontal surgery.The subepithelial connective tissue (lamina propria)is believed to provide instructive signals for the normal progression of stratified squamous epithelia (36,38).Such signaling presumably is absent from deeper connective tissues so that epithelium in contact with it does not attain the same degree of differentiation.Thus the sulcular epithelium,in marked distinc-tion to the gingival epithelium,is nonkeratinized,yet both are technically supported by a similar lamina propria.Indeed,this difference in epithelial expression may be attributed to inflammation.Even under normal clinical conditions,the connective tissue associated with the dentogingival junction is slightly inflamed.If the inflammatory process is removed by implementation of a strict regimen of oral hygiene combined with antibiotic coverage in experimental animals,the sulcular epithelium kera-tinizes (21,22).CementumCementum is the hard,avascular connective tissue that coats the roots of teeth and that servesprimarilyFig.1.Backscattered scanning electron micrograph of a decalcified tissue section showing the cervical region of a rat tooth with the junctional epithelium (JE),the enamel space (ES),and the cemento-enamel junction (CEJ).Numerous blood vessels (BV)are present in the connect-ive tissue (CT)of the lamina propria.Note how the enamel space extends between cementum and dentin (arrow-head),a situation which may give the impression that there is an intermediate layer between them.D,dentin.12Nanci &Bosshardtto invest and attach the principal periodontal liga-ment fibers.There are basically two varieties of ce-mentum distinguished on the basis of the presence or absence of cells within it and the origin of the colla-gen fibers of the matrix.Cementum varietiesAcellular extrinsic fiber cementum (primary cemen-tum or acellular cementum)is found on the cervical half to two thirds of the root (Fig.2–4).It develops very slowly and is considered to be acellular since the cells that form it remain on its surface.The very high number of principal periodontal ligament fibers inserting into the AEFC (where they are called Sharpey’s fibers)points to its important function in tooth attachment.The overall degree of mineraliza-tion of AEFC is about 45–60%,but soft X-ray examination reveals that the innermost layer is less mineralized and that the outer layers are character-ized by alternating bands of more and less mineral content that run parallel to the root surface.Cellular intrinsic fiber cementum (secondary cementum,cellular cementum)is distributed along the apical third or half of the root and in furcation areas (Fig.5).As cellular intrinsic fiber cementum is also produced as a repair tissue that fills resorptive defects and root fractures,it may also be found further coro-nally.Collagen produced by cementoblasts (intrinsic collagen fibers)and the presence of cementoblasts entrapped in lacunae within the matrix they produce (cementocytes)are the characteristic features of cel-lular intrinsic fiber cementum.The heterogeneous collagen organization,its rapid speed of formation,and the presence of cells and lacunae may be the reason why this cementum variety is less well miner-alized than acellular extrinsic fiber cementum.Cellular intrinsic fiber cementum constitutes the intrinsic component of cellular mixedstratifiedFig.2.Backscattered scanning electron micrographs showing the development of acellular extrinsic fiber ce-mentum (AEFC)in a human premolar from apical (A)to cervical (B,C).A)Following disintegration of Hertwig’s epithelial root sheath (HERS),cells on the exposed root surface implant a collagenous fiber fringe (FF)into the not yet mineralized dentin matrix (PD ¼predentin).B)The fiber fringe is oriented perpendicular to the root surface and engulfs the adjacent cells.C)When the cementum layer has attained a thickness of approximately 10l m,most of the fringe fibers are still short,while others have elongated into the periodontal ligament (PL)space.D,dentin;MF,mineralization front;P,pulp;Od,odonto-blasts.13Structure of periodontal tissuescementum,which possesses a stratification that is derived from consecutively deposited,alternating layers of acellular extrinsic fiber cementum and cellular intrinsic fiber cementum.Cellular mixed stratified cementum is not found in rodent molars but is always present in human teeth.BecausetheFig.3.Transmission electron micro-graph illustrating the cervical root surface of a human tooth.acellular extrinsic fiber cementum (AEFC),which prevails in this root region,is characterized by densely packed periodontal ligament fibers (PLF)that enter the cementum layer at the miner-alization front (MF).Cb,cementoblast;N,nucleus.Fig.4.Electron micrographs of acellular extrinsic fiber cementum (AEFC)from tissue sections following im-munogold labeling for bone sialoprotein (BSP).A)Perio-dontal ligament fibers (PLF)enter the cementum layer at the mineralization front (MF).Labeling predominates over the interfibrillar cementum matrix.B)In the region of the dentino-cemental junction,cemental and dentinal collagen fibrils overlap and interdigitate.noncollagenous proteins like bone sialoprotein fill the wide interfibrillar spaces.D,dentin.14Nanci &Bosshardtintrinsic cementum variety can be formed very rap-idly and focally,it may serve as a means to adjust the tooth position to new requirements.Biochemical composition of cementumThe composition of cementum resembles that of bone.As a bulk,it contains about 50%mineral (substituted apatite)and 50%organic matrix.Type I collagen is the predominant organic component,constituting up to 90%of the organic matrix.Other collagens associated with cementum include type III,a less cross-linked collagen found in high concen-trations during development and repair ⁄regener-ation of mineralized tissues,and type XII,a fibril-associated collagen with interrupted triple helices (FACIT)that binds to type I collagen and also to noncollagenous matrix proteins.Trace amounts of other collagens,including type V,VI,and type XIV,are also found in extracts of mature cementum;however,these may be contaminants from the peri-odontal ligament region associated with fibers inserted into cementum.Almost all noncollagenous matrix proteins identified in cementum are also found in bone (7).These include bone sialoprotein (Fig.4),dentin matrix protein 1(DMP-1)(20,24,44),dentin sialoprotein (1),fibronectin,osteocalcin,osteonectin,osteopontin,tenascin (47,69),proteo-glycans,proteolipids,and several growth factors including cementum growth factor that appears to be an insulin-like growth factor (IGF)-like molecule.Enamel proteins have also been suggested to be present in cementum.It has been reported that Hertwig’s epithelial root sheath (HERS)cells may synthesize amelogenins that accumulate on the forming root surface to form a layer,referred to as intermediate cementum (40–42,60).To date,how-ever,there is no conclusive evidence that either amelogenins or nonamelogenins accumulate in nor-mal cementum matrix constituents or even form a distinct layer between dentin and cementum.Whenever enamel matrix proteins are found on the root,their presence is limited to a very short,cervical region which likely represents the cervical extremity of the crown onto which cementum is deposited (11,12).Sporadic expression of enamel proteins has also been reported along the root in porcineteethFig.5.Backscattered scanning electron micrographs showing the development of cellular intrinsic fiber cementum (CIFC)in a human premolar from apical (A)to a coronal direction (B,C).A)Following disintegration of Hertwig’s epithelial root sheath (HERS),cementoblasts (Cb)on the exposed root surface rapidly deposit the cementum matrix onto the not yet mineralized dentin matrix (PD =predentin).B)Some cementoblasts become embedded as cementocytes (Cc)in their own matrix.C)In a more mature,thicker cementum layer,the cementocytes lodge in lacunae.D,dentin;Od,odontoblasts;PGE 2,prostaglandin E 2.15Structure of periodontal tissues(Fig.6)(13),and in rodent molars in association with epithelial cells entrapped in cellular intrinsic fiber cementum (12,25,26,63).Finally,an apparently unique cementum attachment protein has also been identified in cementum (64).Cementum developmentCementum formation takes place along the entire root and during the entire life of the tooth.However,its initiation is limited to the advancing root edge during root formation.At this site,Hertwig’s epithe-lial root sheath,which derives from the apical extension of the inner and outer enamel epithelium,is believed to send an inductive message,possibly by secreting some enamel matrix proteins,to the facing ectomesenchymal pulp cells.These cells differentiate into odontoblasts and produce a layer of predentin.Soon after,HERS becomes fragmented and ectome-senchymal cells from the inner portion of the dental follicle can now come in contact with the predentin.Some cells from the fragmented root sheath form discrete masses surrounded by a basement mem-brane,known as epithelial rests of Malassez that persist in the mature periodontal ligament.Followingthese events,cementoblasts will differentiate and deposit cementum matrix onto the forming radicular dentin.The origin of cementoblasts and series of events that culminates in their differentiation is still unresolved and will be discussed below.Cementum formationAcellular extrinsic fiber cementum :during root development in human teeth,the first cells that align along the newly formed,but not yet mineralized,mantle dentin surface exhibit fibroblastic character-istics (Fig.2).These cells deposit collagen within the unmineralized dentin matrix so that fibrils from both matrices interdigitate.Mineralization of the mantle dentin starts internally and does not reach the sur-face until blending of collagen fibrils from both layers has occurred.It then spreads across into cementum matrix,thereby establishing the dentin–cementum junction.Initial acellular extrinsic fiber cementum thus consists of a thin mineralized layer with a short fringe of collagen fibers implanted perpendicular to the root surface.The cells on the root surface con-tinue to deposit collagen so that the fiber fringe lengthens and thickens.At the same time,theyalsoFig.6.Transmission electron micro-graph showing the cervical root surface at the beginning of root formation in a porcine tooth;the tissue section was processed for immunogold labeling with anti-amelogenin antibody.Matrix masses containing amelogenin (arrowheads)are sporadically observed along the dentin (D)surface.They co-localize with the collagenous pre-cementum (PC)matrix.AMEL;amelogenin;N,nucleus.16Nanci &Bosshardtsecrete noncollagenous matrix proteins thatfill in the spaces between the collagenfibers and regulate mineralization of the forming cementum layer (Fig.4).This activity continues until about15–20l m of cementum has been formed,at which time the intrinsicfibrous fringe becomes connected to the developing periodontal ligamentfiber bundles (Fig.3).Thereafter,acellular extrinsicfiber cemen-tum formative cells will be essentially engaged in synthesis of noncollagenous matrix proteins;collagen fibrils that embed in it will be formed by periodontal ligamentfibroblasts.No morphologically distinct layer of cementoid,akin to osteoid or predentin, exists on the surface of acellular extrinsicfiber cementum.Although this cementum variety is classi-fied as having extrinsicfibers,one may question whether its initial part should rather be classified as having intrinsicfibers.As described above,the col-lagenous matrix of thefirst-formed cementum is the result of cementum-associated cells and is elaborated before the periodontal ligament forms;therefore, the collagen is of local origin and thus of intrinsic derivation.Cellular intrinsicfiber cementum:after at least half of the root has been formed,cementoblasts start forming a less mineralized variety of cementum that is distinctive in that its constituent collagenfibrils are produced by the cementoblasts themselves(Fig.5). In all cases,thefirst collagen is deposited onto the unmineralized dentin surface such thatfibrils from both layers intermingle.As for acellular extrinsicfiber cementum,cellular intrinsicfiber cementum-form-ing cementoblasts also manufacture a number of noncollagenous matrix proteins thatfill in the spaces between the collagenfibrils,regulate mineral deposition and impart cohesion to the mineralized layer.A layer of unmineralized matrix,termed cementoid,is established at the surface of the min-eralized cementum matrix,with the mineralization front at the interface between the two layers.In contrast to osteoid,cementoid is not as regular and readily discernible.As the process proceeds,some cementoblasts become trapped in the matrix they form.These entrapped cells,with reduced secretory activity,are called cementocytes and sit in lacunae. The structural organization of the matrix and the presence of cells in it give cellular intrinsicfiber cementum a bone-like appearance.Collagenfibrils are produced rapidly and deposited haphazardly during the initial phase;however,subsequently the bulk offibrils organize as bundles oriented mostly parallel to the root surface.When the periodontal ligament becomes organized,cementum may form around some of the periodontal ligamentfiber bun-dles–they are thus incorporated into cementum and become partially mineralized.In human teeth, incorporation of periodontal ligamentfibers into cellular intrinsicfiber cementum occurs only rarely, essentially in the acellular extrinsicfiber cementum component of cellular mixed stratified cementum.How does cementum hold onto dentin?The attachment mechanism of cementum to dentin is both of biological interest and of clinical relevance, since pathological alterations and clinical interven-tions may influence the nature of the exposed root surface and hence the quality of the new attachment that forms when repair cementum is deposited.The mechanism by which these hard tissues bind together is essentially the same for acellular extrinsic fiber cementum and cellular intrinsicfiber cemen-tum.Mineralization of the mantle dentin starts internally and does not reach the surface until the collagenfibrils of dentin and cementum have had time to blend together.It then spreads through the surface layer of dentin,across the dentin–cementum junction and into cementum,essentially resulting in an amalgamated mass of mineral.Whereas dentin mineralization is initiated by matrix vesicles,the subsequent spread of mineral deposition is under the regulatory influence of noncollagenous matrix proteins.From a biomechanical perspective,this arrangement appears optimal for a strong union between dentin and cementum.In acellular extrinsic fiber cementum of rodent teeth,cementum is deposited onto mineralized dentin,making amalga-mation of dentin and cementum impossible and establishing a weakened interface.Indeed,histologi-cal sections of rodent teeth often show a separation between dentin and cementum in the cervical third of the root.Although tissue processing is commonly held responsible for tissue separation in histologic sections,arguments have been raised to question this generalized interpretation(16).Interestingly,repair cementum adheres very well to the root surface if a resorptive phase precedes new matrix deposition (14,8),implying that odontoclasts favorably precon-dition the root surface.Chemical preconditioning of the root surface with acids or chelators(Fig.7B)is an often-applied step in periodontal therapy(43,45). Following various regenerative procedures,tissue separation between repair cementum and the treated root surface is frequently observed,implying poor attachment quality(8,16)and suggesting that there17Structure of periodontal tissuesstill is room for improvements in chemical root sur-face conditioning of periodontitis-affected teeth.Origin of cementoblasts and periodontal ligament fibroblastsThere are still several fundamental issues that need to be resolved and whose clarification is not only essential to understand the process of cemento-genesis but,most importantly,to devise targeted therapeutic approaches for the prevention and treatment of periodontal diseases.These include determining the following:•the precursors of cementoblasts;•whether cementoblasts are a distinct cell popula-tion that expresses unique gene products;•whether acellular and cellular cementum are dis-tinct tissues;•what regulates formation and maintenance of periodontal ligament vs.cementum,thus pre-venting fusion of the root to the alveolar bone (ankylosis).The long-standing view is that precursors of cementoblasts and periodontal ligament fibroblasts reside in the dental follicle and that factors within the local environment regulate their ability to function as cementoblasts that form root cementum,fibroblasts of the periodontal ligament or osteoblasts forming bone tissue (32).It is widely held that infiltrating dental follicle cells receive a reciprocal inductive signal from the forming dentin and differentiate into cementoblasts.However,there is increasing evidence that HERS cells may undergo epithelial–mesenchy-mal transformation into cementoblasts during development (7).This is a fundamental process in developmental biology that occurs,among others,as ectodermal cells migrate away from the neural crest and during medial edge fusion of the palatal shelves.Structural and immunocytochemical data support the possibility that cementoblasts derive,at least in part,from transformed epithelial cells of HERS.In rodents,initial formation of acellular cementum takes place in the presence of epithelial cells and it has been shown that enamel organ cells are capable of producing typical mesenchymal products such as type I collagen,bone sialoprotein,and osteopontin (10,12,13,18,48).There is still debate as to whether acellular (pri-mary)and cellular (secondary)cementum are pro-duced by distinct populations of cells expressing spatio-temporal behaviors that result in the charac-teristic histological differences between these tissues.The possibility has been raised that acellularextrinsicFig.7.Transmission electron micrographs of human teeth affected by periodontitis and conservatively treated with root scaling and planing,and root surface deminer-alization using EDTA.A)A long junctional epithelium (LJE)may establish on the treated root.Note the presence of bacteria (arrowheads)among the epithelial cells.B)The EDTA treatment aims at exposing collagen fibrils (CF).AEFC,acellular extrinsic fiber cementum.18Nanci &Bosshardtfiber cementum is formed by HERS-derived cells, whereas cellular intrinsicfiber cementum is pro-duced by cells that derive from the dental follicle(68). Experimental evidence supports the concept that the periodontal ligament is a repository for cells involved in the formation of cementum,periodontal ligament itself,and alveolar bone(37);however,the nature and precise location of progenitor cells remain to be determined.It is also not known whether dis-tinct precursor cell lines exist for each of the three support tissues or whether periodontal ligament fibroblasts,cementoblasts,and osteoblasts arise from a common precursor.The complexity of the perio-dontal ligament is enhanced by the fact that it con-tains several cell types(fibroblastic subpopulations, osteoblasts,cementoblasts,endothelial cells,peri-vascular cells,blood-borne cells,and epithelial cells). In addition,recentfindings also suggest the presence of cells with stem cell characteristics(59).A com-prehensive review of the literature on cell origin and differentiation has recently been published by Bosshardt(7).This review highlights several lines of evidence that support the concept that cemento-blasts producing both acellular extrinsicfiber cementum and cellular intrinsicfiber cementum are unique phenotypes that differ from osteoblasts,and proposes a model that may explain how cell diversity evolves in the periodontal ligament.This new theory proposes that cells derived from HERS play an essential role in tissue development and mainten-ance,and that periodontal regeneration recapitulates tooth development.Cells descending from HERS may give direct rise to cells that form new cementum and periodontal ligament tissues,or play an indirect role by producing the necessary signaling molecules for cell recruitment and differentiation.Understanding cell origin and cell differentiation mechanisms within the periodontal ligament is mandatory for the development of more effective therapies aimed at achieving true and significant periodontal regener-ation.Molecular factors regulating cementogenesisBone morphogenetic proteins:Bone morphogenic proteins are members of the transforming growth factor b(TGF-b)superfamily that act through transmembrane serine⁄threonine protein kinase receptors.These signaling molecules have a variety of functions during morphogenesis and cell differ-entiation and,in teeth,they are considered to be part of the network of epithelial–mesenchymal signaling molecules regulating crown development. The roles for bone morphogenic proteins in root development,including whether they are involved in epithelial–mesenchymal signaling,and the sign-aling pathways and transcription factors involved in modulating their behavior remain to be defined. However,it is known that several of the bone morphogenic proteins,including BMP-2,-4,and-7, promote differentiation of preosteoblasts and putative cementoblast precursor cells.In this con-text,bone morphogenic proteins have been suc-cessfully used to induce periodontal regeneration in a number of experimental models but their clinical use is still lagging behind.Epithelial factors:The same two populations of cells involved in crown morphogenesis,i.e.enamel epithelium and ectomesenchymal cells,also take part in root formation.It would thus not be surprising if some of the same signaling molecules implicated in crown morphogenesis were also active during development of the root.Prospective candidates include enamel matrix proteins,parathyroid hor-mone-related protein and basement membrane constituents.In the case of enamel matrix proteins, the debate centers on the fact that they have not been consistently detected along the root,in every species and in all teeth.However,this inconsistency does not rule out their participation in root forma-tion.Some proteins may still be transiently secreted in limited amounts at early stages of root formation by HERS cells to influence odontoblast or cemento-blast differentiation;such a limited expression would be difficult to detect.Matrix proteins:as indicated above,bone sialoprotein and osteopontin are fundamental con-stituents of cementum matrix,during both its development and repair.Present data suggest that osteopontin is involved in regulating mineral growth, whereas bone sialoprotein promotes mineral forma-tion on the root surface(18,49).They may also be involved in cellular events through their RGD cell-binding motifs.Since no tooth root develop-mental anomalies have to date been reported in osteopontin knockout mice,it is likely that other noncollagenous matrix proteins compensate for the absence of osteopontin in these animals.BoneÔGlaÕprotein(osteocalcin)is a marker for maturation of osteoblasts,odontoblasts and cementoblasts that may regulate the extent of mineralization.No root development and forma-tion problems have so far been reported in knockout mice.Transcription factors:Runx-2(runt-related tran-scription factor2),also known as Cbfa1(core binding factor alpha1),and osterix,downstream from Cbfa1,19Structure of periodontal tissues。
《英语语言学概论》-课程教学大纲
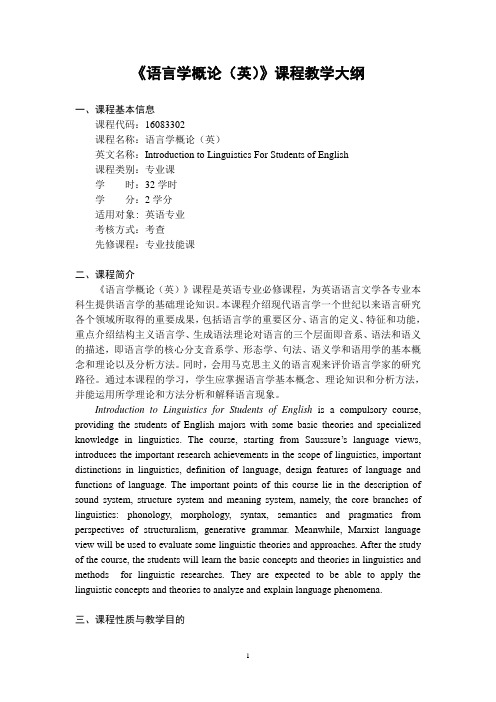
《语言学概论(英)》课程教学大纲一、课程基本信息课程代码:16083302课程名称:语言学概论(英)英文名称:Introduction to Linguistics For Students of English课程类别:专业课学时:32学时学分:2学分适用对象: 英语专业考核方式:考查先修课程:专业技能课二、课程简介《语言学概论(英)》课程是英语专业必修课程,为英语语言文学各专业本科生提供语言学的基础理论知识。
本课程介绍现代语言学一个世纪以来语言研究各个领域所取得的重要成果,包括语言学的重要区分、语言的定义、特征和功能,重点介绍结构主义语言学、生成语法理论对语言的三个层面即音系、语法和语义的描述,即语言学的核心分支音系学、形态学、句法、语义学和语用学的基本概念和理论以及分析方法。
同时,会用马克思主义的语言观来评价语言学家的研究路径。
通过本课程的学习,学生应掌握语言学基本概念、理论知识和分析方法,并能运用所学理论和方法分析和解释语言现象。
Introduction to Linguistics for Students of English is a compulsory course, providing the students of English majors with some basic theories and specialized knowledge in linguistics. The course, starting from Saussure’s language views, introduces the important research achievements in the scope of linguistics, important distinctions in linguistics, definition of language, design features of language and functions of language. The important points of this course lie in the description of sound system, structure system and meaning system, namely, the core branches of linguistics: phonology, morphology, syntax, semantics and pragmatics from perspectives of structuralism, generative grammar. Meanwhile, Marxist language view will be used to evaluate some linguistic theories and approaches. After the study of the course, the students will learn the basic concepts and theories in linguistics and methods for linguistic researches. They are expected to be able to apply the linguistic concepts and theories to analyze and explain language phenomena.三、课程性质与教学目的《语言学概论(英)》课程是为英语专业本科生开设的英语专业必修课之一。
如何处理好父母和孩子之间的关系英语作文

如何处理好父母和孩子之间的关系英语作文全文共3篇示例,供读者参考篇1How to Handle the Relationship Between Parents and ChildrenThe relationship between parents and children is one of the most important and complex relationships in our lives. It can be both incredibly rewarding and challenging at the same time. In order to ensure a healthy and thriving relationship between parents and children, it is important to consider a few key factors.First and foremost, communication is key. It is essential for parents and children to communicate openly and honestly with each other. This means listening to each other’s thoughts, feeli ngs, and concerns, and expressing one’s own thoughts and feelings in a respectful and compassionate manner. Effective communication helps build trust and understanding between parents and children, and can help prevent misunderstandings and conflicts.Secondly, it is important for parents to set boundaries and rules for their children, while also allowing them some independence and autonomy. Boundaries and rules provide structure and guidance for children, while autonomy allows them to develop their own identities and make their own decisions. Finding the right balance between structure and freedom is crucial for a healthy parent-child relationship.Thirdly, it is important for parents to show love and affection towards their children on a regular basis. Expressing love and affection through words, actions, and gestures helps strengthen the bond between parents and children, and fosters a sense of security and belonging. It is important for children to know that they are loved and valued by their parents, no matter what.Additionally, it is important for parents and children to spend quality time together. Quality time allows parents and children to bond, create memories, and build a deeper connection with each other. Whether it’s going for a walk in the park, cooking a meal together, or watching a movie, spending time together can strengthen the relationship between parents and children.Furthermore, it is important for parents to be good role models for their children. Children learn by observing theirparents’ behavior, attitudes, and values. Therefore, it is important for parents to demonstrate positive qualities such as honesty, kindness, and respect towards others. Being a good role model can help children develop into responsible, compassionate, and well-adjusted individuals.In conclusion, the relationship between parents and children is a complex and multifaceted one. By following the tips outlined above – communicating effectively, setting boundaries, showing love and affection, spending quality time together, and being good role models – parents can foster a healthy and thriving relationship with their children. Remember, a strong and loving relationship between parents and children is one of the most important gifts we can give each other.篇2How to Handle the Relationship between Parents and ChildrenThe relationship between parents and children is one of the most important relationships in our lives. It sets the foundation for our emotional and social development, and can have a lasting impact on our overall well-being. However, this relationship can be complicated and challenging at times, asboth parents and children have their own needs, desires, and emotions. In order to maintain a healthy and positive relationship, it is important for both parties to communicate effectively, show respect and empathy, and work together to build trust and understanding.Effective communication is key to building a strong relationship between parents and children. Parents should take the time to listen to their children's thoughts, feelings, and concerns, and validate their experiences. It is important for parents to create a safe and open environment where children feel comfortable sharing their thoughts and emotions without fear of judgment or criticism. Parents should also be open and honest with their children, and strive to communicate in a clear and respectful manner. By fostering open lines of communication, parents and children can better understand each other's perspectives and work together to address any issues or conflicts that may arise.Respect and empathy are also essential components of a positive parent-child relationship. Parents should show respect for their children's individuality, autonomy, and opinions, and avoid imposing their own beliefs and values onto them. It is important for parents to acknowledge and validate theirchildren's emotions, and show empathy and understanding in times of distress or conflict. By demonstrating respect and empathy, parents can help their children feel valued, heard, and appreciated, which can strengthen the bond between them and foster a sense of trust and connection.Building trust and understanding is another important aspect of maintaining a healthy relationship between parents and children. Trust is the foundation of any relationship, and is built through consistent and reliable communication, honesty, and respect. Parents should strive to be consistent in their actions and words, and follow through on their promises and commitments. By demonstrating trustworthiness, parents can help their children feel secure and confident in their relationship, and foster a sense of mutual understanding and support.In conclusion, the relationship between parents and children is a complex and dynamic one that requires effort, patience, and understanding from both parties. By fostering effective communication, showing respect and empathy, and building trust and understanding, parents and children can develop a strong and positive relationship that can withstand the challenges and obstacles of life. Through love, support, and cooperation, parents and children can navigate the ups anddowns of their relationship and create a bond that is strong, resilient, and enduring.篇3How to Handle Parent-Child RelationshipsParent-child relationships are among the most important and complex relationships we will ever have in our lives. These relationships can have a profound impact on our emotional well-being, self-esteem, and future success. However, conflicts and misunderstandings between parents and children are not uncommon, and it can sometimes be difficult to navigate these relationships in a healthy and constructive way. In this article, we will explore some tips on how to handle parent-child relationships in a positive manner.1. Communication: Effective communication is key to a healthy parent-child relationship. Both parents and children should strive to communicate openly and honestly with each other. Parents should create a safe and supportive environment where children feel comfortable sharing their thoughts and feelings. Likewise, children should be respectful and listen to their parents' perspectives. By actively listening to each other and expressing thoughts and emotions in a respectful manner,both parties can develop a deeper understanding and stronger bond.2. Respect: Mutual respect is essential in any relationship, including parent-child relationships. Parents should treat their children with respect and dignity, valuing their opinions and emotions. Children, in turn, should show respect towards their parents, acknowledging their wisdom and life experiences. By treating each other with respect, parents and children can establish a foundation of trust and support.3. Setting boundaries: Boundaries are important in any relationship, especially in parent-child relationships. Parents should set clear and firm boundaries for their children, outlining expectations, rules, and consequences. At the same time, parents should also respect their children's boundaries, giving them space to develop their independence and make their own decisions. By establishing healthy boundaries, parents can instill discipline and structure while still fostering a sense of autonomy and self-expression in their children.4. Quality time: Spending quality time together is essential for building a strong parent-child relationship. Parents should make an effort to engage with their children in meaningful activities, such as family dinners, game nights, or outings. Thesemoments of connection allow parents and children to bond, share experiences, and create lasting memories. Quality time also provides an opportunity for open communication and emotional support, strengthening the parent-child relationship.5. Support and encouragement: Parents play a crucial role in supporting and encouraging their children to pursue their passions and goals. Parents should provide emotional support, guidance, and motivation to help their children overcome challenges and achieve success. By showing interest in their children's interests and dreams, parents can empower them to reach their full potential and build confidence. Encouraging words, praise, and appreciation go a long way in nurturing a positive parent-child relationship.6. Resolving conflicts: Conflicts are normal in any relationship, including parent-child relationships. When conflicts arise, it is important to handle them in a calm and respectful manner. Both parents and children should strive to listen to each other's perspectives, express their feelings, and work towards finding a resolution. Compromise, understanding, and forgiveness are key in resolving conflicts and moving forward in a positive direction. Seeking professional help or counseling canalso be beneficial in addressing complex conflicts and improving communication within the family.In conclusion, parent-child relationships are dynamic and evolving, requiring effort, patience, and understanding from both parties. By practicing effective communication, mutual respect, setting boundaries, spending quality time together, providing support and encouragement, and resolving conflicts amicably, parents and children can cultivate a healthy and fulfilling relationship. Remember that every parent-child relationship is unique, and it is important to approach each relationship with love, empathy, and a willingness to learn and grow together.。
关于规则的英语拓展作文

关于规则的英语拓展作文Exploring the Significance of Rules。
Rules play an indispensable role in every aspect of our lives. From the moment we are born, we are surrounded by rules societal norms, regulations, laws, and even personal guidelines. These rules serve as the framework that shapes our behaviors, interactions, and the functioning of society as a whole. In this essay, we will delve into the significance of rules, exploring their importance in various spheres of life and reflecting on their impact on individuals and communities.The Foundation of Society。
First and foremost, rules are the foundation upon which societies are built. They provide structure, order, and stability, ensuring that members of a community can coexist harmoniously. Without rules, chaos would ensue, leading to conflict, confusion, and ultimately, the breakdown ofsocial cohesion. Imagine a world where there are no traffic rules – roads would be chaotic, and accidents would be rampant. Thus, rules establish the necessary boundaries and expectations that allow societies to function smoothly.Promoting Fairness and Justice。
中药有效成分及其类似物对乙酰胆碱酯酶
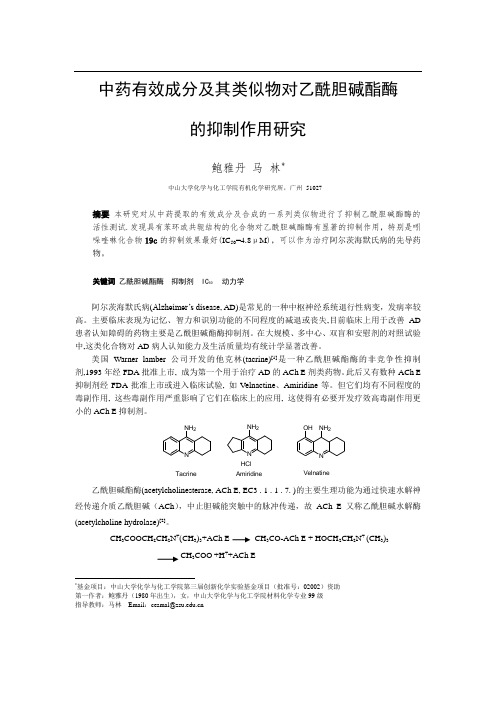
中药有效成分及其类似物对乙酰胆碱酯酶的抑制作用研究鲍雅丹马林*中山大学化学与化工学院有机化学研究所,广州51027摘要本研究对从中药提取的有效成分及合成的一系列类似物进行了抑制乙酰胆碱酯酶的活性测试.发现具有苯环或共轭结构的化合物对乙酰胆碱酯酶有显著的抑制作用,特别是吲哚喹啉化合物19c的抑制效果最好(IC50=4.8μM),可以作为治疗阿尔茨海默氏病的先导药物。
关键词乙酰胆碱酯酶抑制剂 IC50 动力学阿尔茨海默氏病(Alzheimer’s dis ease, AD)是常见的一种中枢神经系统退行性病变,发病率较高。
主要临床表现为记忆、智力和识别功能的不同程度的减退或丧失,目前临床上用于改善AD 患者认知障碍的药物主要是乙酰胆碱酯酶抑制剂。
在大规模、多中心、双盲和安慰剂的对照试验中,这类化合物对AD病人认知能力及生活质量均有统计学显著改善。
美国Warner lamber 公司开发的他克林(tacrine)[1]是一种乙酰胆碱酯酶的非竞争性抑制剂,1993年经FDA批准上市, 成为第一个用于治疗AD的ACh E剂类药物。
此后又有数种ACh E 抑制剂经FDA批准上市或进入临床试验, 如Velnactine、Amiridine等。
但它们均有不同程度的毒副作用, 这些毒副作用严重影响了它们在临床上的应用, 这使得有必要开发疗效高毒副作用更小的ACh E抑制剂。
NNH2 TacrineNNH2HClAmiridineNNH2OHVelnatine乙酰胆碱酯酶(acetylcholinesterase, ACh E, EC3 . 1 . 1 . 7. )的主要生理功能为通过快速水解神经传递介质乙酰胆碱(ACh),中止胆碱能突触中的脉冲传递,故ACh E又称乙酰胆碱水解酶(acetylcholine hydrolase)[2]。
CH3COOCH2CH2N+(CH3)3+ACh E CH3CO-ACh E + HOCH2CH2N+ (CH3)3CH3COO-+H++ACh E*基金项目:中山大学化学与化工学院第三届创新化学实验基金项目(批准号:02002)资助第一作者:鲍雅丹(1980年出生),女,中山大学化学与化工学院材料化学专业99级指导教师:马林Email:cesmal@为了筛选出高效、低毒的ACh E 抑制剂,我们参考各种ACh E 抑制剂的结构类型,研究了从中药山芝麻和筋骨草分离出的有效成分及其类似物对ACh E 的抑制作用。
Microbial Community Function
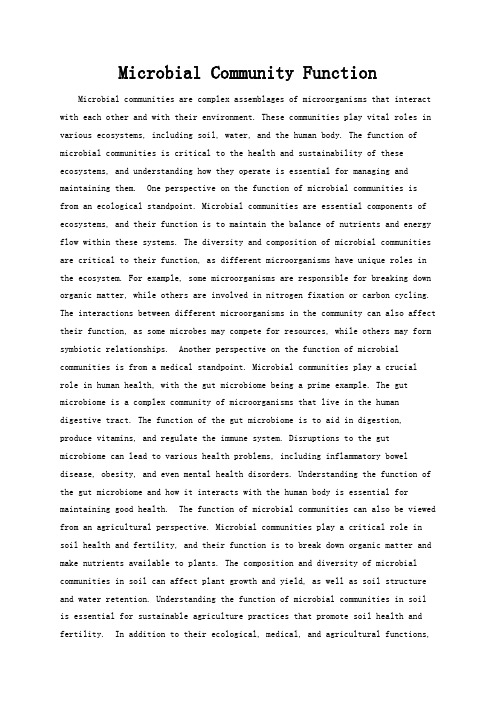
Microbial Community Function Microbial communities are complex assemblages of microorganisms that interact with each other and with their environment. These communities play vital roles in various ecosystems, including soil, water, and the human body. The function of microbial communities is critical to the health and sustainability of these ecosystems, and understanding how they operate is essential for managing and maintaining them. One perspective on the function of microbial communities is from an ecological standpoint. Microbial communities are essential components of ecosystems, and their function is to maintain the balance of nutrients and energy flow within these systems. The diversity and composition of microbial communities are critical to their function, as different microorganisms have unique roles in the ecosystem. For example, some microorganisms are responsible for breaking down organic matter, while others are involved in nitrogen fixation or carbon cycling. The interactions between different microorganisms in the community can also affect their function, as some microbes may compete for resources, while others may form symbiotic relationships. Another perspective on the function of microbial communities is from a medical standpoint. Microbial communities play a crucialrole in human health, with the gut microbiome being a prime example. The gut microbiome is a complex community of microorganisms that live in the human digestive tract. The function of the gut microbiome is to aid in digestion, produce vitamins, and regulate the immune system. Disruptions to the gut microbiome can lead to various health problems, including inflammatory bowel disease, obesity, and even mental health disorders. Understanding the function of the gut microbiome and how it interacts with the human body is essential for maintaining good health. The function of microbial communities can also be viewed from an agricultural perspective. Microbial communities play a critical role in soil health and fertility, and their function is to break down organic matter and make nutrients available to plants. The composition and diversity of microbial communities in soil can affect plant growth and yield, as well as soil structure and water retention. Understanding the function of microbial communities in soil is essential for sustainable agriculture practices that promote soil health and fertility. In addition to their ecological, medical, and agricultural functions,microbial communities also have industrial applications. Microorganisms are used in various industries, including food production, pharmaceuticals, and biotechnology. The function of microbial communities in these industries is to produce enzymes, antibiotics, and other compounds that are essential for these processes. Understanding the function of microbial communities in these industries is essential for developing new and innovative products and processes. In conclusion, the function of microbial communities is multifaceted and essential to various ecosystems, including the human body, soil, and industrial processes. Understanding the diversity, composition, and interactions of microbial communities is critical for managing and maintaining these ecosystems and developing new and innovative products and processes. As our understanding of microbial communities continues to grow, so does our ability to harness their power for the benefit of society.。
中英文科技论文写作
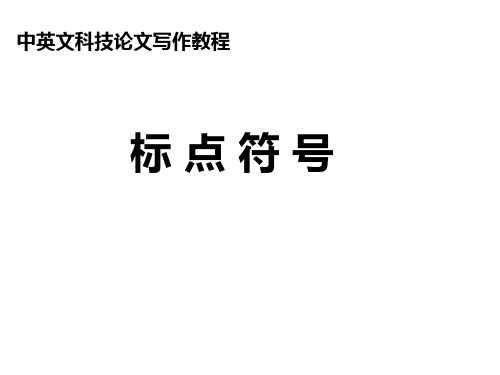
英文 em dash “–” 表示范围en 表示范围 dash“–” “
en dash“–”构成对等复合词 “ hyphen“-”构成不对等复合词 “
一.中文连接号
半字线: – 半字线: 一字线:- 一字线:- 波浪线:~ 波浪线:~ 二字线:——
二.句内括号、句外括号、引号末尾点号 句内括号、句外括号、
句内括号:注释句中某些词语
例:光学显微镜可以给出很有价值的结 构信息,比如结晶的高级结构( 构信息,比如结晶的高级结构(树枝状晶 体、球晶、单晶)、试样取向等。 球晶、单晶)、试样取向等。 )、试样取向等
句号 (period) 句号是用于陈述句的末尾, 句号是用于陈述句的末尾,但不与另外 的标点重复使用。诸如: 的标点重复使用。诸如: (1) 引语 She asked, “May I go?” ” (2)如句子以含有实圆点的某一缩略结尾, 如句子以含有实圆点的某一缩略结尾, 如句子以含有实圆点的某一缩略结尾 则不再另加句号。 则不再另加句号。 The abbreviation of for example is e.g.
分号 (semicolon) ) 分隔不是以连词连接的独立分句。 (1) 分隔不是以连词连接的独立分句。 All solvents were distilled from an appropriate drying agent; tetrahydrofuran and diethyl ether were also pretreated with activity I alumina. 用于一系列单词、短语或数据组的各项之间, (2)用于一系列单词、短语或数据组的各项之间, 如 果一个或多个项已包含逗号。 果一个或多个项已包含逗号。 (3) 用 于 以 连 接 副 词 或 诸 如 “ that is” , “ however”,“ therefore”,“ hence”,“ indeed”, , , , , “accordingly”,“besides”,和“thus”等常用短语 , , ” 连接的独立分句之间。 连接的独立分句之间。
核酸检测报告定性

核酸检测报告定性1. 引言核酸检测是一种常用的生物学技术,用于检测特定的DNA或RNA序列。
根据不同的目的,核酸检测可以分为定性检测和定量检测两种类型。
本文将重点介绍核酸检测报告中的定性结果。
2. 定性检测的原理定性检测是通过比较待测样品中的核酸序列与已知的参考序列进行对比来判断样品中是否存在目标序列。
其中,常用的定性检测方法包括聚合酶链式反应(PCR)和核酸杂交等。
下面将对这两种方法进行详细介绍。
2.1 聚合酶链式反应(PCR)PCR是一种用于扩增DNA片段的技术,其原理是利用两个引物(前向引物和反向引物)将待扩增的目标序列特异性地扩增为大量的DNA片段。
在定性检测中,PCR可以被用于扩增待测样品中的目标序列,并通过凝胶电泳等方法进行可视化分析。
2.2 核酸杂交核酸杂交是将待测样品中的核酸序列与已知的参考序列进行杂交,通过检测杂交信号的强度来判断目标序列的存在与否。
核酸杂交的方法包括Southern blotting、Northern blotting和in situ杂交等。
3. 核酸检测报告定性内容在核酸检测报告中,定性结果是最基本的信息之一,其表明目标序列在待测样品中的存在与否。
通常,定性结果以阳性或阴性的形式呈现。
3.1 阳性结果阳性结果表示在待测样品中存在目标序列。
在核酸检测报告中,阳性结果通常会附带一些补充信息,例如扩增的片段大小、杂交信号的强度等。
此外,如果多个目标序列被检测到,可能会进一步说明每个目标序列的检测结果。
3.2 阴性结果阴性结果表示在待测样品中未检测到目标序列。
阴性结果并不排除目标序列的存在,可能是由于目标序列浓度低于检测的灵敏度或样品处理过程中的污染等原因导致的。
在核酸检测报告中,阴性结果通常会附带一些注意事项,例如重新检测或采取其他措施来进一步验证结果。
4. 定性检测的应用定性检测在许多领域中都有重要的应用。
以下是一些常见的应用场景:4.1 传染病检测定性检测可用于检测各种传染病病原体的存在与否,包括病毒、细菌和寄生虫等。
- 1、下载文档前请自行甄别文档内容的完整性,平台不提供额外的编辑、内容补充、找答案等附加服务。
- 2、"仅部分预览"的文档,不可在线预览部分如存在完整性等问题,可反馈申请退款(可完整预览的文档不适用该条件!)。
- 3、如文档侵犯您的权益,请联系客服反馈,我们会尽快为您处理(人工客服工作时间:9:00-18:30)。
INVITED REVIEWStructure–Function Relationships in Highly Modified Shoots of CactaceaeJAMES D.MAUSETH*Section of Integrative Biology,1University Station,A6700,University of Texas,Austin,TX 78712,USAReceived:22March 2006Returned for revision:28April 2006Accepted:4May 2006Published electronically:4July 2006Background and Aims Cacti are extremely diverse structurally and ecologically,and so modified as to be intimidating to many biologists.Yet all have the same organization as most dicots,none differs fundamentally from Arabidopsis or other model plants.This review explains cactus shoot structure,discusses relationships between structure,ecology,development and evolution,and indicates areas where research on cacti is necessary to test general theories of morphogenesis.Scope Cactus leaves are diverse;all cacti have foliage leaves;many intermediate stages in evolutionary reduction of leaves are still present;floral shoots often have large,complex leaves whereas vegetative shoots have microscopic leaves.Spines are modified bud scales,some secrete sugar as extra-floral nectaries.Many cacti have juvenile/adult phases in which the flowering adult phase (a cephalium)differs greatly from the juvenile;in some,one side of a shoot becomes adult,all other sides continue to grow as the juvenile phase.Flowers are inverted:the exterior of a cactus ‘flower’is a hollow vegetative shoot with internodes,nodes,leaves and spines,whereas floral organs occur inside,with petals physically above stamens.Many cacti have cortical bundles vascularizing the cortex,however broad it evolves to be,thus keeping surface tissues alive.Great width results in great weight of weak parenchymatous shoots,correlated with reduced branching.Reduced numbers of shoot apices is compensated by great increases in number of meristematic cells within individual SAMs.Ribs and tubercles allow shoots to swell without tearing during wet seasons.Shoot epidermis and cortex cells live and function for decades then convert to cork cambium.Many modifications permit water storage within cactus wood itself,adjacent to vessels.Key words:Cactus,epidermis,flower,leaf development,phase change,plant anatomy,shoot apical meristem,structure/function,wood evolution,xerophyte.INTRODUCTIONThe first two objectives of this review are to introduce readers to many of the exotic and extreme aspects of cactus biology,and also to show that even the most bizarre cacti are easy to understand because all have the fundamental tissues and organs of an ordinary dicot.People familiar with arabidopsis will find that cacti have the same basic body organization,just having a bit more cortex,smaller leaves,and axillary buds that develop as clusters of spines.A third objective is to emphasize the diversity of structure,ecology and reproduction in the family.Probably no other plant family exceeds Cactaceae in diversity of structure;its members include trees,vines,dwarfs,giants,epiphytes and geophytes.Many are dimorphic,producing different types of anatomy or morphology at different stages of their lives.The final and main objective is to point out that many research topics in many fields can be studied with this family;there is already a solid foundation of existing knowledge that can be a basis for further studies of morphogenesis,ecology,physiology,evolution and many more areas.Cactus evolution has been a process of diversification.Starting from some ancestral organization of stems,leaves and roots,cacti have diversified into a multiplicity of body forms.Members of subfamily Pereskioideae (Fig.1A and Table 1)are shrubs or large trees with thin,broad,ordinary-looking leaves and hard,woody,non-succulent trunks;they are not adapted to dry,hot conditions.Subfamilies Maihuenioideae and Opuntioideae containplants with small but still easily visible foliage leaves,and plants vary from being trees to dwarfs (Fig.1B and C).The largest subfamily,Cactoideae (Fig.1D),differs from the others by having foliage leaves that are always microscopic:all photosynthesis is carried out by shoot cortex cells covered by a persistent epidermis and stomata,all of which live and function for decades or centuries,as long as the shoot is green.Members of Cactoideae and Opuntioideae occupy almost every terrestrial habitat:hot deserts;cold deserts;grasslands;shady forests;rainforests;and cold,wet or snow-covered alpine zones above the treeline (Mauseth et al .,2002).Several genera of Cactoideae display what appears to be unparalleled in any other group:an absolutely amazing morphogenetic phase change in which the adult body (able to flower)looks nothing at all like the juvenile body (unable to flower).Almost every aspect of shoot morphogenesis changes,each plant produces two totally distinct types of body (Figs 1E and F and 2E).Various aspects of cactus biology have been reviewed recently,so I will emphasize either newer discoveries or fields that have not received sufficient attention.Two monographs are recommended for numerous excellent photographs and general details of plant form and distribution:Anderson (2001)and Hunt (2006).The older work of Backeberg (1958–1962)lacks many recently discovered species and modern ideas but has much more detail than any other source (4041pages in six volumes).Natural histories are provided by Rauh (1979)and Mauseth et al .(2002;A Cactus Odyssey being especially recommended for a less technical,more*E-mail j.mauseth@Annals of Botany 98:901–926,2006doi:10.1093/aob/mcl133,available online at ÓThe Author 2006.Published by Oxford University Press on behalf of the Annals of Botany Company.All rights reserved.For Permissions,please email:journals.permissions@902Mauseth—Structure and Biology of Cactus ShootsF I G.1.Cactus shoot structure.(A)Pereskia sacharosa with leaves.Stem about6mm across.(B)Maihuenia poeppigii,a geophyte;all green colour is due to small leaves.The yellow structure is a fruit.The entire plant is about1m across.(C)Growing cladode(long-shoot)of Opuntia violacea,with leaves still present(two indicated by arrows).Young cladode is emerging from an axillary bud of an older cladode.The spines of the axillary buds of the older cladode are bud scales.Slightly smaller than life size.(D).Shoot tip of Cereus forbesii.Three of four ribs are visible;spine clusters(axillary buds,areoles)are located along the rib apex.Spines are bud scales,dormant axillary bud shoot apical meristem is located just above each spine cluster,hidden by a mass of white trichomes.Spines are present even on very young axillary buds thus protecting the shoot apical meristem,which is not the highest point of the shoot(shoot apex is concave).Almost life size.(E)Old plant of Melocactus intortus with juvenile portion of shoot(green)produced during thefirst10–15years of the plant’s life,and the adult portion(red,the cephalium),which is probably at least10years old.This is a single shoot(not a graft of two unrelated plants), produced by a single shoot apical meristem.No new chlorenchyma has been produced for years.(F).Shoots of Espostoa with lateral cephalia;flowers are produced only by axillary buds within the adult(cephalium)portion,not from the juvenile(green)portions.Note disrupted phyllotaxy.Shoots are about5cm across.(G)Areole(axillary bud)of Ferocactus.Spines are modified bud scales.The location of the bud apical meristem is indicated by an arrow,below the mass of trichomes.Trichomes(yellow)are abundant in the areole but absent from the rest of the shoot.Spines and trichomes emerge from a depression about 3mm deep.About four times life size.(H)Leaves onfloral bud of Browningia candelaris;the largest scale is about1cm across.Vegetative shoots of the same plant have only microscopic foliage leaves.(I)Longitudinal section of fully developed foliage leaf(L)of Oreocereus trollii;present are epidermis,stomata (not visible here),vascular tissue,chlorenchyma,dorsiventral asymmetry.Leaf is450m m tall.Axillary bud SAM(out of view on left)has produced leaf primordia,one of which is developing as a spine(S).Cells in spine base are meristematic,those in upper portion are elongating intofibres.Scale bar=300m m.inclusive account of cactus biology).Cactus structure is reviewed by Buxbaum(1950),Gibson and Nobel (1986),Terrazas Salgado and Mauseth(2002)and Terrazas and Arias(2003).Ecophysiology is summarized by Nobel(1988).Techniques for extracting DNA from even mucilaginous cacti are now available(Griffith and Porter,2003),and DNA-based phylogenies have been proposed(Nyffeler,2002;Crozier,2005;Edwards et al., 2005;Griffith,2005).HABITIn every species,the cactus body organization is fundamentally the same as that of ordinary dicots.Most cactus leaves are microscopically small(Fig.1I)and the cortex of most species is gigantically enlarged,but still each cactus shoot has the basic dicot organization:all consist of internodes,nodes,leaves and axillary buds produced by shoot apical meristems(SAMs).Without exception their primary body has an epidermis(with stomata),cortex,eustele(single ring of collateral vascular bundles each with primary xylem and phloem)and pith. No cactus is an annual or an herb.All produce a secondary body consisting of secondary xylem(wood), secondary phloem and bark.All genetic programmes that guide basic dicot morphogenesis are probably still present and functional in cacti.ORGANS OF THE CACTUS SHOOT LeavesEvolutionary modification of leaf morphogenesis has been extensive in all cacti,and has resulted in great diversity of leaf types within each individual plant.All cacti produce foliage leaves(microscopically small in most)and spines (modified leaves);some also produce glands(modified spines),most have large,thin leaves on the surface of theirfloral shoots(Fig.1C,D and G–I;see Floral shoots below).Diversity of cactus leaves is associated with an extreme polymorphism present in all cactus shoots.The green, photosynthetic body of an unbranched cactus is a single shoot known as a‘long-shoot’;if branched,all the green,fleshy branches are also long-shoots(Fig.1A,C and D). Almost all familiar plants consist only of long-shoots so the term is usually unnecessary and rarely used.But in cacti,each axillary bud immediately produces leaf primordia,which in most other plants would become small,flat,waxy bud scales;in cacti,however,they develop into spines(Fig.1D,G and I;Boke,1944,1952, 1967;Buxbaum,1950).Many morphologists have considered a cluster of cactus spines to be just an axillary bud;others interpret it as a‘short-shoot’,a shoot with extremely short,narrow internodes and without the broad, succulent tissues of the long-shoot.Long-shoot/short-shoot dimorphism is not unusual in seed plants.For example, most of an apple tree(Malus)consists of long-shoots which have ordinary photosynthetic leaves with axillary buds enclosed by bud scales,but the axillary buds themselves perennially produce bothflowers and photo-synthetic leaves on shoots with extremely short inter-nodes:the axillary buds become short-shoots,often called spur shoots.In this case,long-shoots and short-shoots bear leaves that are virtually indistinguishable.In contrast, most of the body of a pine tree(Pinus)consists of long-shoots which bear small brown papery scale leaves(easily overlooked);the axillary buds of the scale leaves develop into short-shoots with needle-like leaves.The familiar pine needles are not the leaves of the familiar pine branches(long-shoots)but instead are the leaves of almost invisible short-shoots(Foster and Gifford,1974). Foliage leaves.In all cacti,SAMs(see below)of long-shoots produce leaf primordia(Boke,1951,1980;T A B L E1.Subfamilies of Cactaceae(see Leuenberger,1986,1997;Barthlott and Hunt,1993;Anderson,2001;Griffith,2005;Hunt,2006)1.Pereskioideae(Fig.1A)Similar to ordinary dicots:leaves broad,thin with reticulate venation;shoots are trees or shrubs(vines inP.aculeata)with slender stems with thin,relatively nonsucculent cortex;shoot epidermis is ephemeral,being replaced by bark while the stem is only1or2years old.Pereskia aculeata,P.diaz-romeroana,P.grandifolia and P.sacharosa are easy to cultivate;P.diaz-romeroana is self-fertile and produces seedswhen only2–4years old2.Maihuenioideae(Fig.1B)Two Patagonian species in one genus,Maihuenia(do not confuse with Maihueniopsis in Opuntioideae).Plants are xeric-adapted small shrubs(M.patagonica of hot lowlands)or geophytes(M.poeppigii,with most of body buried,and only the leafy shoot tips visible above ground;of cold highlands)3.Opuntioideae(Fig.1C)A large,diverse group but all members are more highly modified than those of Pereskioideae,all look lesslike ordinary dicots and are more easily recognized as cacti.Most have green,photosynthetic succulentstems.Although small,opuntioid leaves are green,photosynthetic and always easily visible on young shoots.Stems areflattened cladodes(‘pads’or‘ears’)in some,have radial symmetry in others,or have cylindricaltrunks with cladodes as lateral branches(Consolea,Brasiliopuntia).Only opuntioids have glochids.Shoots inmost are articulated;each stem has a determinate growth period after which the SAM disorganizes and furthergrowth is by several axillary buds.Many shoots easily break apart at these joints,form adventitious roots and establish extensive clones4.Cactoideae(Figs1D–I, 2A–I and3A–D)A large highly diverse group,none of which would be confused with an ordinary leafy non-succulent dicot.All foliage leaves are too tiny to be visible without aid(except Matucana aurantiaca and several Rhipsalis). Stems vary from long,slender and moderately succulent(Hylocereus,Leptocereus,Selenicereus)to moderately thick to extremely broad and tall(Carnegiea,Pachycereus,Trichocereus)or broad and globose(Ferocactus, Echinocactus,Echinopsis,Eriosyce),to tiny globose(Table2).Plants may be highly branched or with few or no branches.Many have extreme phase change between juvenile and adult phases(see Cephalia in text) Mauseth—Structure and Biology of Cactus Shoots903904Mauseth—Structure and Biology of Cactus ShootsF I G.2.Specialized features of cacti.(A)Elongated axillary buds of Oroya peruviana,with spines in two rows,not in spiral phyllotaxy around the bud SAM, which is located at the top of each areole.Each areole is about5mm long.(B)Tubercles of Coryphantha clavata,each with an elongate areole containing one or two secretory spines(arrow).Note ordinary non-secretory spines at the tips of each tubercle(out of focus in foreground).Tubercles are about12mm long.(C)Elongated areoles of Neoraimondia roseiflora.Whenfirst formed,these resembled ordinary areoles as in Fig.1D,but each hasflowered numerous times over many years,growing longer each time.Each has bark,cortex,stele and pith.These are about60mm long.(D)Dimorphic areoles of Mammillaria camptotricha.Each areole SAM has divided into two,one being carried outward with the tubercle apex where it makes only spines,the other remaining at the tubercle base where it produces aflower or a vegetative shoot.The openflower is about8mm across.(E)Terminal cephalia of a single,branched plant of Backebergia militaris.Green portions are juvenile,cephalia are the adult body.Each shoot tip will be abscised about4cm below the cephalium,then one or two axillary buds will grow as new juvenile bodies for several years,then convert to making cephalia.The plant is about6m tall.(F)Section of afloral shoot of Neocardenasia.The outer portion is a long-shoot with leaves,nodes and internodes.Trueflower organs occur only along the inner surface(upper arrow indicates boundary between vegetative andfloral organs);petals and stamens,although located physically above the ovary and style base,are morphologically lower(proximal).After fertilization,all tissues above the lower arrow will abscise,removing style,stamens,petals and much vegetative tissue.The region below the lower arrow will develop into a true fruit surrounded by a false fruit.(G)External structure offloral shoot of Echinocereus.Although referred to as a ‘flower,’this is long-shoot tissue with tiny foliage leaves,axillary buds(bud scales are spines),nodes and internodes.Truefloral structures are present inside this shoot(some petal bases are visible at the top).Almost life size.(H)Cortical bundle in Lepismium,with xylem(x),phloem(ph)and a cap of phloemfibres (f).All conducting cells are extremely narrow.Scale bar=100m m.(I)Collapsible cortex in pletely turgid palisade cortex cells are<500m m away in the same region.Scale bar=100m m.Mauseth and Halperin,1975;Mauseth,1976,1977,1978d, 1980a,2004d).In Pereskia,these develop into large,thin, fully functional photosynthetic foliage leaves with a broad lamina(Fig.1A;lamina to23cm long,6cm wide;Bailey, 1960;Leuenberger,1986;Mauseth and Landrum,1997). These are the main sites of photosynthesis and persist for months but abscise when plants become dormant. Pereskia foliage leaves may be slightly thickened but not remarkably so,and palisade and spongy mesophyll are only weakly differentiated.An extensive reticulate venation of collateral vascular bundles is present.In Maihuenioideae and Opuntioideae,green photosynthetic leaves are present and all are large enough to be easily visible by the naked eye(Fig.1C;Mauseth,1999a,2005; Leuenberger,1997).They areflattened with a small thick, succulent lamina in Pereskiopsis and Quiabentia,but are radially symmetrical in all other Opuntioideae and Maihuenia,and usually are narrow(2–5mm),short (range3–12mm,but120mm long in Austrocylindropuntia subulata)and ephemeral(persistent in M.poeppigii, Pereskiopsis,Quiabentia and Austrocylindropuntia).Their photosynthesis is probably insignificant except when relatively large and long-lived.If an opuntioid long-shoot is more than1or two months old,there may be nothing other than a tiny leaf scar immediately below the cluster of spines.Long-shoot leaves in all Cactoideae have been greatly reduced evolutionarily but most have all the tissue types typical of an ordinary foliage leaf(Fig.1I;Boke,1951, 1952,1957b;J.D.Mauseth,unpubl.res.)and thus they probably still have leaf development genes similar to those of other plants(Fleming,2005).They range from very small(maximum2Á3mm long in Matucana aur-antiaca)to microscopic,<500m m long in most.All but the most miniscule have stomata,at least one vascular bundle,and dorsiventral asymmetry(the vascular bundle is located closer to the adaxial epidermis,and the abaxial mesophyll is slightly aerenchymatous).At least a few have a noticeable lamina(up to1776m m wide in Epi-phyllum)but none has a petiole or abscission zone.The most reduced long-shoot leaves in Cactoideae do not develop beyond the leaf primordium stage,but instead remain as just a tiny bump(50m m tall)of epidermis covering several mesophyll cells;their leaf trace typically runs only to the axillary bud SAM and spines,not to the leaf itself.Evolutionary restriction of foliage leaf development had consequences other than the obvious ones of reducing the shoot’s surface area and surface:volume(S:V)ratio, reducing transpirational water loss,and reducing photo-synthetic surface area.It also reduced leaf venation,which is the site of vascular loading and unloading.Ordinary foliage leaves have an extensive set of leaf veins consisting of primary xylem and phloem and having a tremendous length and surface area in contact with living mesophyll.Shoots of Cactoideae with their highly reduced foliage leaves have little or no leaf vascular tissue, so water must be unloaded from bundles in the cortex(see Cortical bundles below),from leaf/bud traces or from secondary xylem(wood).But in most non-cactus woody plants,water is almost never unloaded from or loaded into secondary xylem(roots load water into primary xylem); vessels of wood are surrounded by a matrix of woodfibres or a bit of paratracheal parenchyma,and they do not have extensive surface contact with a voluminous parenchyma capable of absorbing the water they transport.Wood vessels instead transfer water to primary xylem of leaves,flowers,and so on.Loss of leaf venation in cacti almost certainly resulted in selection pressure to alter secondary xylem such that it has increased amounts of paratracheal tissues able to unload,store and transfer water[Fig.3G; see Secondary xylem(wood)below].Loss of leaf venation also affected phloem loading; secondary phloem in the central vascular cylinder does not load sugars directly,it only receives them from primary phloem in leaf traces.Because cacti store water in a voluminous cortex,the outer photosynthetic cortex is too distant from the secondary phloem of the central cylinder to allow it to load sugars directly.All loading of sugars apparently must occur in cortical bundles or perhaps leaf/ bud traces.Spines.Cactus spines are the modified bud scales of an axillary bud;alternatively they can be considered the modified leaves of a short-shoot(Mauseth,1976;Boke, 1980).Differences between the two interpretations are not obvious.Being leaves of an axillary bud,cactus spines almost always occur in clusters,a character which distinguishes this family from all others.Several cacti have only one spine per cluster,and spines are completely absent in Blossfeldia(Mauseth,2006a)and some epiphytic rainforest cacti(some Epiphyllum,Lepismium, Rhipsalis;Fig.3D).Almost as soon as the axillary bud SAM becomes recognizable,it develops zonation typical of any angiosperm,having a uniseriate tunica over a corpus composed of central cells,peripheral zone and pith-rib meristem.It immediately produces leaf primordia; these resemble long-shoot leaf primordia in being small swellings of ground meristem covered by protoderm.As the axillary bud’s leaf primordia enlarge,their tip cells vacuolate and elongate,and quickly the young spine consists of three regions:a basal meristem;a zone of elongation/differentiation and an apical zone of mature; and dead lignifiedfibres(Fig.1I;Mauseth,1977).The spine basal meristem consists of only a unistratose protoderm surrounding a mass of ground meristem.No vascular tissue or procambium has been reported.Most cell division produces daughter cells aligned parallel to the spine’s long axis,but occasional divisions in other planes widen the basal meristem gradually,thus cactus spines taper from a narrow tip to a broader base(Fig.1G). Spines are frequently circular in transverse section but can beflattened on one side(usually the adaxial side; Ferocactus latispinus)or their basal meristem becomes so broad but thin that the spine isflat and papery, mimicking a dry blade of grass(Leuchtenbergia principis, Tephrocactus articulatus:spines4mm wide,0Á3mm thick,to15cm long).Factors that control morphogenesis in spine basal meristems are unknown,but in many cacti these meristems are accessible large masses of uniformMauseth—Structure and Biology of Cactus Shoots905906Mauseth—Structure and Biology of Cactus ShootsF I G.3.Cactus structures.(A)Prostrate shoot of Harrisia pomanensis.The shoot tip is elevated by reaction cortex near the phloem on the lower side of the shoot.The visible part of the shoot is about40cm long;the entire shoot is several metres long,branched and growing in various directions.The lower side has adventitious roots.(B)Three ribs of a columnar cactus,Coleocephalocereus.As the shoot loses water and volume,ribs become narrower but do not change surface area.Each spine cluster is an axillary bud;subtending foliage leaves(like those in Fig.1I)are microscopic.Each areole could potentially produce a vegetative long-shoot(a branch);because this species has lateral cephalia,these are juvenile phase areoles and cannot bloom.Each rib base is about10mm across.(C)Tubercles of Mammillaria magnimamma,produced in obvious phyllotactic spirals.Tuberculate shoots shrink vertically as well as radially as water is lost.Being a Mammillaria,this has divided,dimorphic areoles:areole SAMs at tubercle tips produce only spines;areole SAMs at tubercle bases(hidden by white trichomes)producefloral shoots or vegetative shoots(none are present in the photograph).Each tubercle is about10mm long.(D)Dimorphic shoots of Epiphyllum caudatum;the branch on the left was initially terete but immediately switched to distichous phyllotaxy with just two tall,thin ribs.The‘midvein’is the central vascular cylinder,the‘blade’is the two ribs and notches along the rib crests are the axillary buds(spines are microscopic).The vertical shoot on the right is terete here,but its tip had also switched to growing as a two-ribbed leaf-like structure.The cladode is about30mm across.(E)Wide-band tracheid wood of Thelocactus bicolor in transverse section.The double-headed arrow in the lower portion indicates the region with many vessels(dark red,narrower walls),perhaps earlywood.The upper portion of the micrograph is mostly WBTs(perhaps latewood),with two vessels(arrows).In many WBTs,the band-like secondary wall almost occludes the lumen.Scale bar=100m m.(F)Tangential section of WBT wood in Thelocactus;WBTs are short and imperforate,and in this species the secondary wall occurs as one or two helices per cell.Blue is theflexible primary wall;despite the thick secondary wall,these cells shorten and lengthen as the water content changes.Cells near the left,lacking wide-bands,are ray parenchyma cells.Scale bar=100m m.(G)Transverse view (macroscopic)of Consolea wood.Rays(white,arrowed)are very wide(1to3mm);vessels within the axial masses(tan)are close to water stored in the rays. These rays interconnect water stored in the pith and cortex.The image is about30mm across.(H)Dimorphic wood of Stenocereus.The double-headed arrow indicates primary xylem andfirst-formed secondary xylem,both lackingfibres and instead having WBTs,vessels and xylem parenchyma.After several months,the vascular cambium switched to makingfibrous wood(above upper arrowhead),consisting of vessels,xylem parenchyma and xylaryfibres but no WBTs.Scale bar=100m m.(I)Transverse section of secondary phloem of Corryocactus.The arrow indicates collapsed phloem,below which are abundant sieve tube members and companion cells.A phloemfibre cap is at the top of the image,secondary xylem at the bottom.Scale bar=100m m.meristematic cells which are active for weeks and thus could be an excellent experimental system.Genes that maintain SAM cells in a meristematic state,such as WUSCHEL and CLAVATA,may also act in spine basal meristems.As cells are pushed upward in the meristem,at some point they cross into the spine’s zone of elongation and maturation(Mauseth,1977).All cells in both the surface (protoderm)and centre(mesophyll)develop intofibres. Mesophyll cells elongate greatly and have only a few simple pits in their extremely thick,hard walls.In most spines,cell elongation is uniform throughout,producing a remarkably straight spine,but some have predictable, differential growth rates,elongating more on their adaxial side and thus becoming curved or hooked downward(especially Ferocactus,Mammillaria,Parodia). Once mature,the cells die,but it is not known if they undergo programmed cell death or merely starve as they are pushed far away from the nutrient supply in the basal meristem and separated from it by younger cells that are also sclerifying.Spine protoderm cells also elongate, deposit a sclerified secondary wall then die.Cactus spine epidermis lacks stomata;in a few species some spine epidermis cells elongate outward as trichomes(Mammil-laria plumosa,Turbinicarpus;Sotomayor and Arredondo, 2004).Spines of Cylindropuntia are covered by a loose sheath assumed to be deciduous epidermis.At maturity,cactus spines lack almost all characters of leaves,even of the reduced long-shoot foliage leaves of Cactoideae(Fig.1I;Mauseth,1977).They have no guard cells,no stomata,no hypodermis,no chlorenchyma (except in the basal meristem),no spongy mesophyll,no phloem and no xylem.Instead they consist of just two cell types that never occur in long-shoot foliage leaves of cacti:libriformfibres and sclerified epidermis.Axillary bud leaf primordia must repress virtually all foliage leaf morphogenesis genes and instead activate genes that normally are only expressed in xylem or phloemfibres. In tissue culture,cactus leaf morphogenesis is easily controlled by hormones.Cytokinins cause cultured axillary bud SAMs of Opuntia polyacantha to convert to long-shoot SAMs and produce primordia that develop as foliage leaves,whereas gibberellins cause cultured SAMs to continue as short-shoot SAMs,producing more spine primordia(Mauseth and Halperin,1975;Mauseth,1976, 1977).If cultured SAMs are transferred from one hormone to the other,or given both hormones simultaneously, lateral organs develop combinations of spine and foliage leaf characters(J.D.Mauseth,unpubl.res.).A single axillary bud usually produces several types of spines,each differing in size,shape,colour and texture, varying in a predictable sequence(Figs1G and3B; Buxbaum,1950).This is an extreme form of heteroblasty. The outermost spines(radial spines)are usually smaller, shorter,more delicate whereas those in the centre(central spines)are more robust and have different pigmentation. Radial and central spines are totally distinctive with no intermediates in many species,but intergrade in others. Spine colour may be important for camouflage(spines often have the colour of dry grass)or for recognition by pollinators and seed dispersers,but the basis of spine pigmentation is unknown.In a few species,all spines of an axillary bud are similar,differing only slightly from each other(Fig.2A).The phyllotactic arrangement of spine primordia around the axillary bud SAM in cacti is unusual and may challenge theories of phyllotaxy and leaf initiation.In most(all?)cacti,spines are produced only on the side of the axillary bud SAM adjacent to the subtending leaf, they are not produced in a radially symmetrical pattern centred on the SAM.An easily observed exotic phyllotaxy is that of Pelecyphora aselliformis,Turbinicarpus pseudopectinatus and Oroya peruviana(Fig.2A;Boke, 1959);spines occur in two parallel rows,which atfirst appears to be distichous phyllotaxy,but both rows are located on the same side of the SAM,and all other sides are free of primordia.In some species,spine primordia remain small and quiescent after they are formed and none develops until the full complement is present,but then the most recently initiated primordia develop into spinesfirst,and thefirst-initiated primordia are the last to enlarge(Boke1952,1955,1957a,b,1961a,b). Unusual spines called glochids occur in all members of Opuntioideae(except Puna clavarioides;Kiesling,1984) but no other subfamily.Glochids are short and narrow, occur in high numbers per axillary bud,have retrorsely barbed epidermis cells at their tip,and,unlike all other spines,glochids always abscise from their base(Robinson, 1974).After breaking away,they remain in place unless disturbed because they are so tightly crowded together.An incautious touch results in glochids in skin,clothing, equipment and laboratory.Glochids are modified spines and thus modified leaves;they are initiated by the axillary bud SAM as extremely slender leaf primordia in a phyllotactic pattern;these aspects of SAM function and leaf morphogenesis are unstudied.Spines provide more than protection from herbivores. When abundant,they shade photosynthetic cortex from intense insolation and UV.Spine epidermis and mesophyll of several cacti have deepfissures as a part of normal development;in Turbinicarpus klinkerianus,Discocactus horstii and Opuntia invicta,radioactive phosphate or safranin dye applied to the spines was absorbed into the cactus body(Schill and Barthlott,1973;Porembski,1994); water absorption through such spines may be significant in fog zones.Spines areflammable,increasing damage to cacti during wildfires(Emming,2005,2006).Spines as glands.Spines of several genera are secretory glands but this is almost completely unstudied.Ants are often seen at the glands of some species of Ancistrocactus, Coryphantha,Cylindropuntia,Ferocactus and Opuntia when they have droplets of clear liquid,presumably sugar and water(Fig.2B).Ants are attracted to the glands of Cylindropuntia acanthocarpa and protect it from insects (Pickett and Clark,1979),and ant visits to extra-floral nectaries of Opuntia stricta increase fruit set(Oliveira et al.,1999).Secretory spines on axillary buds offlowers of Neoraimondia arequipensis attract ants to theflower itself.Mauseth—Structure and Biology of Cactus Shoots907。