第一章智能电网1Smart Power Grids
智能电网知识读本
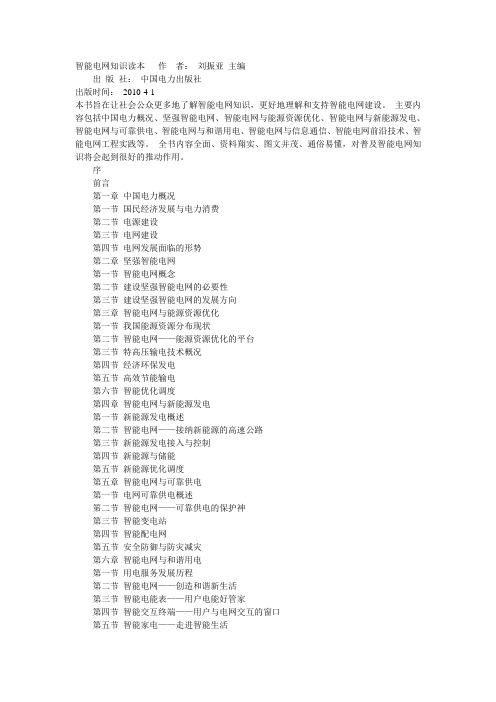
智能电网知识读本作者:刘振亚主编出版社:中国电力出版社出版时间:2010-4-1本书旨在让社会公众更多地了解智能电网知识,更好地理解和支持智能电网建设。
主要内容包括中国电力概况、坚强智能电网、智能电网与能源资源优化、智能电网与新能源发电、智能电网与可靠供电、智能电网与和谐用电、智能电网与信息通信、智能电网前沿技术、智能电网工程实践等。
全书内容全面、资料翔实、图文并茂、通俗易懂,对普及智能电网知识将会起到很好的推动作用。
序前言第一章中国电力概况第一节国民经济发展与电力消费第二节电源建设第三节电网建设第四节电网发展面临的形势第二章坚强智能电网第一节智能电网概念第二节建设坚强智能电网的必要性第三节建设坚强智能电网的发展方向第三章智能电网与能源资源优化第一节我国能源资源分布现状第二节智能电网——能源资源优化的平台第三节特高压输电技术概况第四节经济环保发电第五节高效节能输电第六节智能优化调度第四章智能电网与新能源发电第一节新能源发电概述第二节智能电网——接纳新能源的高速公路第三节新能源发电接入与控制第四节新能源与储能第五节新能源优化调度第五章智能电网与可靠供电第一节电网可靠供电概述笫二节智能电网——可靠供电的保护神第三节智能变电站第四节智能配电网第五节安全防御与防灾减灾第六章智能电网与和谐用电第一节用电服务发展历程第二节智能电网——创造和谐新生活第三节智能电能表——用户电能好管家第四节智能交互终端——用户与电网交互的窗口第五节智能家电——走进智能生活第六节智能用电楼宇——建筑也智能第七节家用太阳能、风能发电系统——用户也能发电第八节电动汽车与充电设施一汽车驶入“无油”时代第七章智能电网与信息通信第一节电力信息通信发展历程第二节信息通信——智能电网的神经系统界人士了解智能电网知识的参考用书第三节电力特种光缆第四节多媒体信息通信第五节物联网在智能电风中的应用第六节智能电网信息安全第八章智能电网前沿技术第一节新型发电技术第二节新型输电技术第三节新型变电技术第四节新型配电技术第五节新型用电技术第六节新材料与超导电力技术第九章智能电网工程实践第一节国内坚强智能电网工程实践第二节国外智能电网工程实践附录A 智能电网标准化简介附录B 智能电网相关的政府机构与研究组织附录C 名词术语中英文对照参考文献。
智能电网的运行与控制技术分析
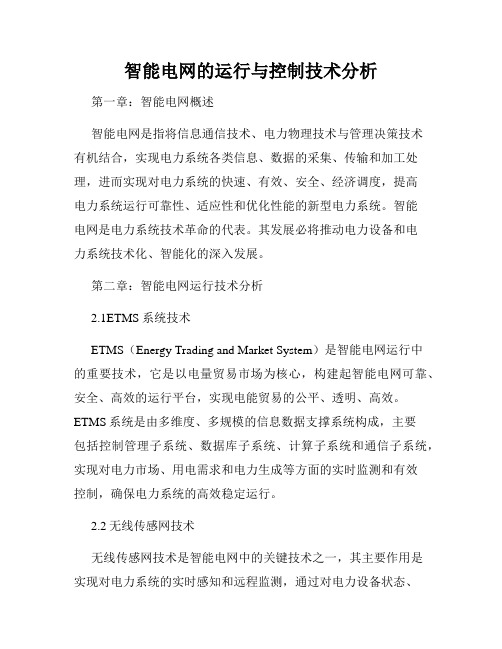
智能电网的运行与控制技术分析第一章:智能电网概述智能电网是指将信息通信技术、电力物理技术与管理决策技术有机结合,实现电力系统各类信息、数据的采集、传输和加工处理,进而实现对电力系统的快速、有效、安全、经济调度,提高电力系统运行可靠性、适应性和优化性能的新型电力系统。
智能电网是电力系统技术革命的代表。
其发展必将推动电力设备和电力系统技术化、智能化的深入发展。
第二章:智能电网运行技术分析2.1ETMS系统技术ETMS(Energy Trading and Market System)是智能电网运行中的重要技术,它是以电量贸易市场为核心,构建起智能电网可靠、安全、高效的运行平台,实现电能贸易的公平、透明、高效。
ETMS系统是由多维度、多规模的信息数据支撑系统构成,主要包括控制管理子系统、数据库子系统、计算子系统和通信子系统,实现对电力市场、用电需求和电力生成等方面的实时监测和有效控制,确保电力系统的高效稳定运行。
2.2无线传感网技术无线传感网技术是智能电网中的关键技术之一,其主要作用是实现对电力系统的实时感知和远程监测,通过对电力设备状态、供应负荷、电压等信息的采集和处理,对电力系统运行状态进行实时监测和分析,进而实现对电力系统的优化控制和快速响应。
无线传感网技术还可以实现对待处理数据的去重、压缩和加密,确保数据的透明度和安全性。
第三章:智能电网控制技术分析3.1 基于模型预测控制技术基于模型预测控制技术是一种先进的控制技术,它是通过对电力系统的运行数据进行建模和模拟,对未来的电力系统运行状态进行预测和优化,进而做出相应的控制策略。
该技术不仅能够对电力系统的稳态和暂态运行状态进行优化,还能够做出相应的应急响应策略,确保电力系统的稳定可靠运行。
3.2自适应控制技术自适应控制技术是指在电力系统运行过程中,对电网系统的控制和调节进行的智能化控制技术。
该技术利用电力系统运行的实时数据反馈信息,对电网系统进行分析和判断,通过计算机模拟和数据分析,对电力系统的运行状态进行精细控制和调整,确保电力系统的稳定运行。
智能电网的概念及实现
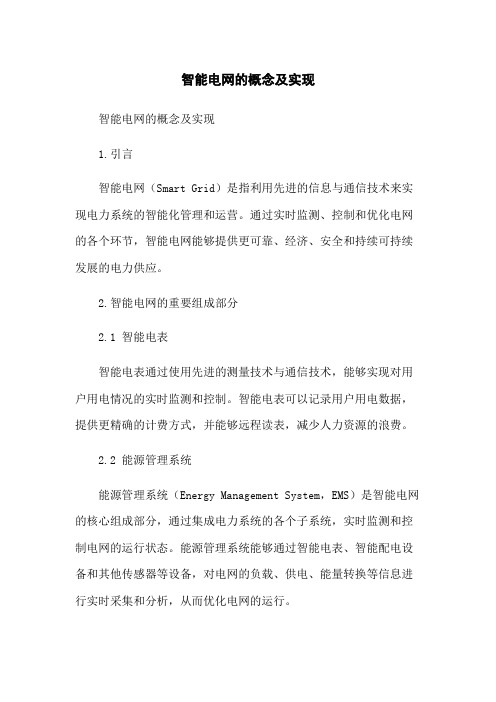
智能电网的概念及实现智能电网的概念及实现1.引言智能电网(Smart Grid)是指利用先进的信息与通信技术来实现电力系统的智能化管理和运营。
通过实时监测、控制和优化电网的各个环节,智能电网能够提供更可靠、经济、安全和持续可持续发展的电力供应。
2.智能电网的重要组成部分2.1 智能电表智能电表通过使用先进的测量技术与通信技术,能够实现对用户用电情况的实时监测和控制。
智能电表可以记录用户用电数据,提供更精确的计费方式,并能够远程读表,减少人力资源的浪费。
2.2 能源管理系统能源管理系统(Energy Management System,EMS)是智能电网的核心组成部分,通过集成电力系统的各个子系统,实时监测和控制电网的运行状态。
能源管理系统能够通过智能电表、智能配电设备和其他传感器等设备,对电网的负载、供电、能量转换等信息进行实时采集和分析,从而优化电网的运行。
2.3 功率电子设备功率电子设备在智能电网中起到关键作用。
它们能够对电能进行高效的转换、控制和调节,实现电网的优化运行。
例如,智能变压器、柔性直流输电技术和电力电子变流器等设备,能够有效地降低电网的损耗和发电成本。
2.4 通信网络智能电网利用现代通信技术构建了强大的通信网络,实现电力系统各个组成部分之间的信息传输与交互。
通信网络能够实现对电网的远程监控和控制,使得电力系统的管理更加智能化和高效。
3.智能电网的实现3.1 智能电网的数据管理智能电网需要对大量的电力数据进行采集、存储、管理和分析,以实现对电网的实时监测和优化控制。
数据管理涉及到数据采集设备、数据库管理系统和数据处理与分析算法等方面的技术。
3.2 智能电网的安全保障智能电网的安全保障是实现智能电网的重要环节。
智能电网涉及到大量的数据交互和信息传输,需要保障电网的数据安全、通信安全和系统安全,防止黑客攻击和信息泄露。
3.3 智能电网的监控与控制智能电网通过实时监测和控制电力系统的各个环节,提高电力系统的可靠性、稳定性和经济性。
智能电网文档
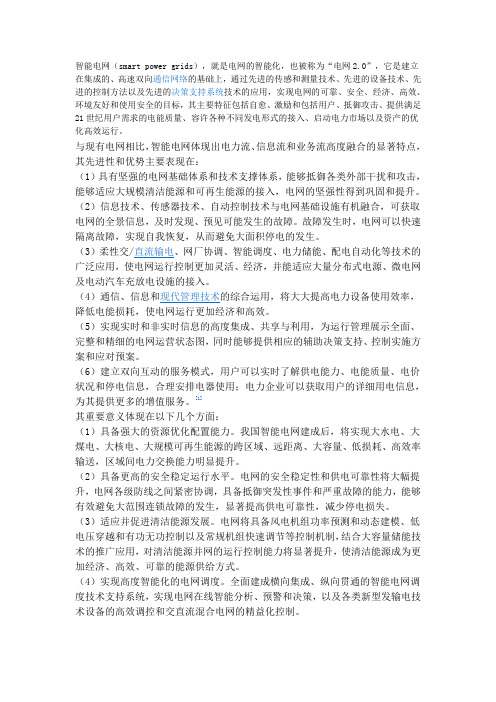
智能电网(smart power grids),就是电网的智能化,也被称为“电网2.0”,它是建立在集成的、高速双向通信网络的基础上,通过先进的传感和测量技术、先进的设备技术、先进的控制方法以及先进的决策支持系统技术的应用,实现电网的可靠、安全、经济、高效、环境友好和使用安全的目标,其主要特征包括自愈、激励和包括用户、抵御攻击、提供满足21世纪用户需求的电能质量、容许各种不同发电形式的接入、启动电力市场以及资产的优化高效运行。
与现有电网相比,智能电网体现出电力流、信息流和业务流高度融合的显著特点,其先进性和优势主要表现在:(1)具有坚强的电网基础体系和技术支撑体系,能够抵御各类外部干扰和攻击,能够适应大规模清洁能源和可再生能源的接入,电网的坚强性得到巩固和提升。
(2)信息技术、传感器技术、自动控制技术与电网基础设施有机融合,可获取电网的全景信息,及时发现、预见可能发生的故障。
故障发生时,电网可以快速隔离故障,实现自我恢复,从而避免大面积停电的发生。
(3)柔性交/直流输电、网厂协调、智能调度、电力储能、配电自动化等技术的广泛应用,使电网运行控制更加灵活、经济,并能适应大量分布式电源、微电网及电动汽车充放电设施的接入。
(4)通信、信息和现代管理技术的综合运用,将大大提高电力设备使用效率,降低电能损耗,使电网运行更加经济和高效。
(5)实现实时和非实时信息的高度集成、共享与利用,为运行管理展示全面、完整和精细的电网运营状态图,同时能够提供相应的辅助决策支持、控制实施方案和应对预案。
(6)建立双向互动的服务模式,用户可以实时了解供电能力、电能质量、电价状况和停电信息,合理安排电器使用;电力企业可以获取用户的详细用电信息,为其提供更多的增值服务。
[1]其重要意义体现在以下几个方面:(1)具备强大的资源优化配置能力。
我国智能电网建成后,将实现大水电、大煤电、大核电、大规模可再生能源的跨区域、远距离、大容量、低损耗、高效率输送,区域间电力交换能力明显提升。
(完整PPT)第一章 智能电网概述(第1讲)
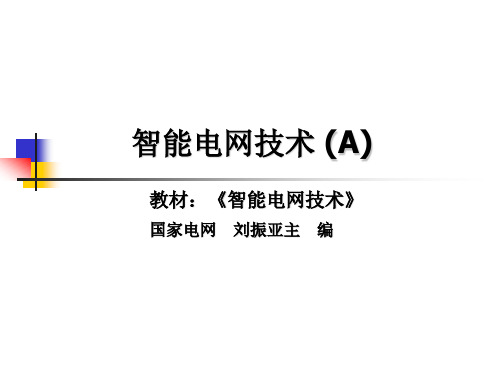
一、电网的发展历程
到2017年 底全国发电 装机容量已 超过17.8亿 kW
已建成六大 区域电网 建设北、中、 南三大西电东 送通道 推进全国联 网、建设坚强 国家电网
一、电网的发展历程
一、电网的发展历程
大规模西电东送
P16
新疆
华北
东北
西北
西藏水电
西藏 四川水 川渝
电 金沙江水
电
南方
华中
了解大规模新能源发电与并网技术、智能输电技术、智能 配电技术、智能用电技术和智能电网实践实例;
本课程主要以智能电网技术为主线,在学习中应加强基理 论的理解和工程设计基本技能的训练,同时尽量做到理论 联系实际,注意创新能力的培养和提高。
课程内容
1. 智能电网概述概述
(第一章)
2. 大规模新能源发电及并网技术 (第三章)
20世纪50年代后,电力工业发展迅速。1952年, 世界上第一条380kV交流输电线路在瑞典投运; 1964年,第一条500kV交流输电线路在苏联投 运;1965-1969年,加拿大、苏联和美国先后 建成了735kV、750kV和765kV交流线路; 1985年,苏联1150kV特高压输电线路投入试 验运行;2009年世界上第一条商业运行的 1000kV特高压输电线路在中国投运。
1888年,俄国的多布罗沃斯基提出三相交流 制,效率高的三相异步电机随之问世,交流电 的优越性逐渐显现。1891年,由劳芬至明兴河 畔的世界上第一条高压输电线路-法兰克福线 路在德国投入运行,总长175km,电压为
一、电网的发展历程
20世纪50~70年代,电力电子与微电子技术发 展迅速,促进了直流输电技术的发展。
3. 智能输电电网技术
(第四章)
智能电网ppt课件
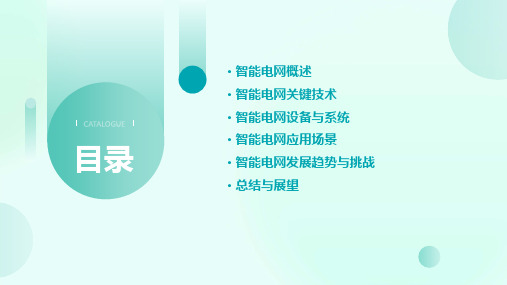
技术挑战
安全挑战
智能电网涉及多学科交叉,技术复杂度高, 需加强技术研发和人才培养。
随着智能化程度的提高,网络安全、数据安 全等问题日益突出,需建立完善的安全防护 体系。
市场挑战
政策挑战
智能电网建设投资大、周期长,需探索多元 化的投融资模式和市场化运营机制。
智能电网发展需要政策支持和引导,需加强 政策研究和制定,营造良好的发展环境。
发展历程
从传统的电力系统到智能电网的演进, 经历了自动化、信息化、互动化等阶段, 当前正处于向全面智能化发展的关键时 期。
智能电网特点与优势
特点
自愈能力、高安全性、优质电能质 量、高效资产利用、友好互动等。
优势
提高能源利用效率、减少环境污染、 促进可再生能源发展、提升电网运 行管理水平等。
国内外发展现状对比
智能电网在提高能源利用效率、减少环境污染、 促进经济发展等方面的作用。
未来智能电网发展趋势预测
能源互联网与智能电网的融合
随着能源互联网的快速发展,智能电网将与之深度融合,实现能源的 高效、安全、清洁利用。
人工智能与大数据技术的应用
人工智能和大数据技术的不断进步将为智能电网的发展提供有力支持, 提高电网的智能化水平。
数据安全与隐私保护 区块链技术提供去中心化、不可篡改的数据存储方式,保 障智能电网数据的安全性和隐私性。
电力交易与结算 区块链技术可实现去中心化的电力交易和结算,降低交易 成本和提高效率。
多方协同与信任机制 区块链技术构建多方协同的信任机制,促进智能电网中各 参与方的合作与共赢。
面临的主要挑战及应对策略
05 智能电网发展趋 势与挑战
人工智能在智能电网中的应用前景
负荷预测与调度优化
智能电网技术论文

智能电网技术论文智能电网是当前全球电力工业关注的热点,引领了电网的未来发展方向,涉及从发电到用户的整个能源转换和输送链。
店铺为大家整理的智能电网技术论文,希望你们喜欢。
智能电网技术论文篇一浅析智能电网摘要:智能配电网是一个庞大而有实际意义的工程,对国家、人民甚至对整个世界的经济发展和环境保护都有重大的贡献。
智能电网是当前全球电力工业关注的热点,引领了电网的未来发展方向,涉及从发电到用户的整个能源转换和输送链。
智能电网中应用到技术很多,是将现代先进的传感测量技术、信息技术、通讯技术、计算机技术和控制技术与物理电网高度集成,由此而形成的新型电网。
它的主要目的是:充分满足用户对电力的需求和优化资源配置、确保电力供应的安全性、可靠性和经济性、保证电能质量、适应电力市场化发展等,从而来实现可靠、经济、清洁、互动的电力供应和增值服务。
关键词:电力能源智能电网网络系统技术高效发展1 智能电网的概念及现状首先,我们来了解下智能电网的概念,从技术发展和应用的角度看,世界各国、各领域的专家普遍认同以下观点:智能电网就是将先进的传感测量技术、信息通信技术、分析决策技术、自动控制技术和能源电力技术相结合,并与电网基础设施高度集成而形成的新型现代化电网。
中国在电网的规模和投资方面取得的成绩有目共睹。
中国的电网企业在发展智能电网方面也具有自身优势。
中国目前在特高压与高压输配电领域的许多技术都是引领全球的。
另外,由于中国在可再生能源发电领域的迅速发展,可再生能源电力接入电网方面也具有一定的优势。
不过,智能电网是一个庞大而完整的体系,在电力消费端、需求响应等方面,欧美国家暂时处于领先地位,特别是在消费端的投入和重视程度上,欧美市场也相对比较成熟。
2 智能电网的特点将计算机应用于电网之中,将数字传感器、遥控装置等加入现有的电力传输及分配系统中,实现数据连接、采集、整合,再进行科学的分析,使电网变得更加智能、绿色、高效。
而且这样的“智能电网”能更加及时、透明地反应电网状况。
智能电网技术
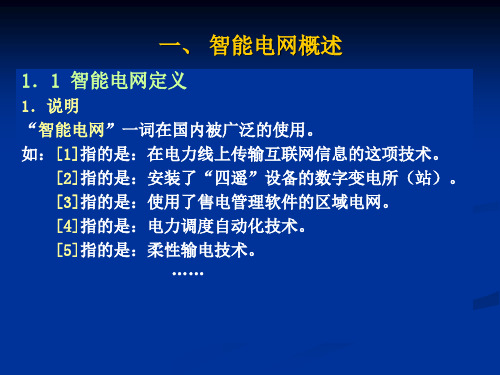
对远程的设备进行远程调试,如调节发电机输出功率
一、 智能电网概述
1.1 智能电网定义
2.智能电网定义: 智能电网的英文名字:IntelliGrid
Smart Grid 美国电力科学研究院对智能电网的定义被广泛引用: 智能电网: 利用传感器对发电、输电、配电、供电等关键设备的运行状况进行 全面的实时监控,然后把获得的数据通过网络系统进行收集、整 合,最后通过对数据的分析、挖掘,实现对整个电力系统运行的优 化管理。
一、 智能电网概述
1.2 智能电网的关键特征 3.互动性
系统运行与批发、零售电力市场实现无缝衔接, 支持电力交易的有效开展,实现资源优化配置;同 时通过市场交易更好地激励电力市场主体(用电客 户)参与电网安全管理,从而提升电力系统的安全 运行水平。
一、 智能电网概述
1.2 智能电网的关键特征 4.优化性
一、 智能电网概述
1.2 智能电网的关键特征
6.集成性
通过不断的流程优化,信息整合,实现企业管理、 生产管理、调度自动化与电力市场管理业务的集成,形 成全面的辅助决策支持体系,支撑企业管理的规范化和 精细化,不断提升电力企业的管理效率。
一、 智能电网概述
1.3 智能电网的特点 1.安全
更好地对人为或自然发生的扰动作出辨识与反应。 在遭遇自然灾害、人为破坏等不同情况下保证人身、设 备和电网的安全。
一、 智能电网概述
1.1 智能电网定义
1.说明 “智能电网”一词在国内被广泛的使用。 如:[1]指的是:在电力线上传输互联网信息的这项技术。
[2]指的是:安装了“四遥”设备的数字变电所(站)。 [3]指的是:使用了售电管理软件的区域电网。 [4]指的是:电力调度自动化技术。 [5]指的是:柔性输电技术。
智能电网论文
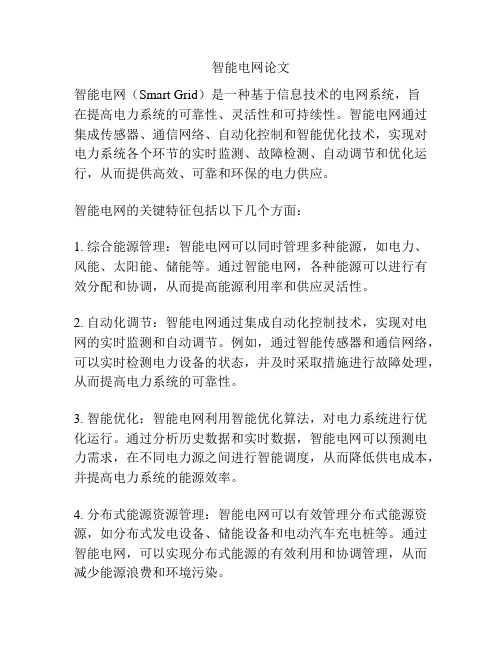
智能电网论文智能电网(Smart Grid)是一种基于信息技术的电网系统,旨在提高电力系统的可靠性、灵活性和可持续性。
智能电网通过集成传感器、通信网络、自动化控制和智能优化技术,实现对电力系统各个环节的实时监测、故障检测、自动调节和优化运行,从而提供高效、可靠和环保的电力供应。
智能电网的关键特征包括以下几个方面:1. 综合能源管理:智能电网可以同时管理多种能源,如电力、风能、太阳能、储能等。
通过智能电网,各种能源可以进行有效分配和协调,从而提高能源利用率和供应灵活性。
2. 自动化调节:智能电网通过集成自动化控制技术,实现对电网的实时监测和自动调节。
例如,通过智能传感器和通信网络,可以实时检测电力设备的状态,并及时采取措施进行故障处理,从而提高电力系统的可靠性。
3. 智能优化:智能电网利用智能优化算法,对电力系统进行优化运行。
通过分析历史数据和实时数据,智能电网可以预测电力需求,在不同电力源之间进行智能调度,从而降低供电成本,并提高电力系统的能源效率。
4. 分布式能源资源管理:智能电网可以有效管理分布式能源资源,如分布式发电设备、储能设备和电动汽车充电桩等。
通过智能电网,可以实现分布式能源的有效利用和协调管理,从而减少能源浪费和环境污染。
智能电网的应用可以从多个层面进行延展,如能源生产、传输、分配和消费等。
智能电网技术的应用可以提高能源利用效率,减少碳排放,降低能源成本,提升电力系统的可靠性和安全性。
然而,智能电网的建设和应用也面临一些挑战和难题,如安全和隐私问题、技术成本、法规和标准等。
因此,在智能电网的建设和应用过程中,需要综合考虑技术、经济、环境、社会和政策等多个因素,制定合理的发展策略和措施。
总之,智能电网是电力系统发展的趋势,它不仅可以提高能源利用效率和供电质量,还可以促进能源的可持续发展。
因此,进一步研究和推广智能电网技术,对于实现清洁、安全、高效的能源供应具有重要意义。
Smart Power Grids
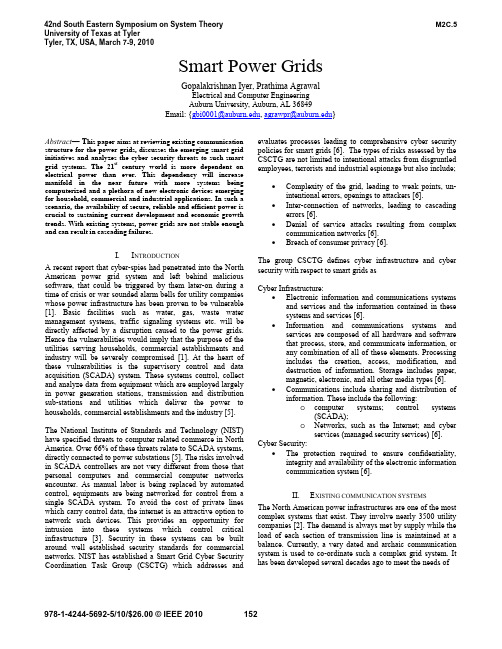
Smart Power GridsGopalakrishnan Iyer, Prathima Agrawal Electrical and Computer EngineeringAuburn University, Auburn, AL 36849 Email: {gbi0001@, agrawpr@}Abstract— T his paper aims at reviewing existing communication structure for the power grids, discusses the emerging smart grid initiatives and analyzes the cyber security threats to such smart grid systems. The 21st century world is more dependent on electrical power than ever. This dependency will increase manifold in the near future with more systems being computerized and a plethora of new electronic devices emerging for household, commercial and industrial applications. In such a scenario, the availability of secure, reliable and efficient power is crucial to sustaining current development and economic growth trends. With existing systems, power grids are not stable enough and can result in cascading failures.I.I NTRODUCTIONA recent report that cyber-spies had penetrated into the North American power grid system and left behind malicious software, that could be triggered by them later-on during a time of crisis or war sounded alarm bells for utility companies whose power infrastructure has been proven to be vulnerable [1]. Basic facilities such as water, gas, waste water management systems, traffic signaling systems etc. will be directly affected by a disruption caused to the power grids. Hence the vulnerabilities would imply that the purpose of the utilities serving households, commercial establishments and industry will be severely compromised [1]. At the heart of these vulnerabilities is the supervisory control and data acquisition (SCADA) system. These systems control, collect and analyze data from equipment which are employed largely in power generation stations, transmission and distribution sub-stations and utilities which deliver the power to households, commercial establishments and the industry [5]. The National Institute of Standards and Technology (NIST) have specified threats to computer related commerce in North America. Over 66% of these threats relate to SCADA systems, directly connected to power substations [5]. The risks involved in SCADA controllers are not very different from those that personal computers and commercial computer networks encounter. As manual labor is being replaced by automated control, equipments are being networked for control from a single SCADA system. To avoid the cost of private lines which carry control data, the internet is an attractive option to network such devices. This provides an opportunity for intrusion into these systems which control critical infrastructure [3]. Security in these systems can be built around well established security standards for commercial networks. NIST has established a Smart Grid Cyber Security Coordination Task Group (CSCTG) which addresses and evaluates processes leading to comprehensive cyber security policies for smart grids [6]. The types of risks assessed by the CSCTG are not limited to intentional attacks from disgruntled employees, terrorists and industrial espionage but also include;•Complexity of the grid, leading to weak points, un-intentional errors, openings to attackers [6].•Inter-connection of networks, leading to cascading errors [6].•Denial of service attacks resulting from complex communication networks [6].•Breach of consumer privacy [6].The group CSCTG defines cyber infrastructure and cyber security with respect to smart grids asCyber Infrastructure:•Electronic information and communications systems and services and the information contained in thesesystems and services [6].•Information and communications systems and services are composed of all hardware and softwarethat process, store, and communicate information, orany combination of all of these elements. Processingincludes the creation, access, modification, anddestruction of information. Storage includes paper,magnetic, electronic, and all other media types [6].•Communications include sharing and distribution of information. These include the following:o computer systems; control systems(SCADA);o Networks, such as the Internet; and cyberservices (managed security services) [6]. Cyber Security:•The protection required to ensure confidentiality, integrity and availability of the electronic informationcommunication system [6].II.E XISTING COMMUNICATION SYSTEMSThe North American power infrastructures are one of the most complex systems that exist. They involve nearly 3500 utility companies [2]. The demand is always met by supply while the load of each section of transmission line is maintained at a balance. Currently, a very dated and archaic communication system is used to co-ordinate such a complex grid system. It has been developed several decades ago to meet the needs of42nd South Eastern Symposium on System TheoryUniversity of Texas at TylerTyler, TX, USA, March 7-9, 2010M2C.5Fig. 1: Power grid communications today [2]a regulated power industry. Today, the de-regulation of the power sector has led to several thousand utility providers, pushing the existing transmission line infrastructure to its limits. Power generation stations, transmission and distribution centers and utility providers manage the delicate balance between demand and supply and ensure that power generated thousands of miles away reaches the target consumers just-in-time. This communication involves control centers, substations and Supervisory Control and Data Acquisition (SCADA) systems in a star topology [2]. Existing power grid communication system shown in fig. 1 consists of RTUs (Remote Terminal Units) at power plants and transmission and distribution centers which are linked to SCADA systems and a fault detection and correction scheme called Remedial Action Scheme (RAS) [2]. The link carries control and status information between each unit, which in this case can be different utilities with a sampling period of a few seconds. The control center at the utility consists of a central database connected to operation and commercial units. Many such control centers of different utilities are linked to each other by regional control centers. This type of a communication system will result in slow automatic control to balance load and generation. If a power outage occurs, the available systems with fast controls like the RAS will protect short-circuits and regulate voltages on lines, but it can neither detect nor correct rapidly occurring cascading failures in the power network[2]. It is evident that the communication system needs massive overhaul and upgrade to cater to the needs of the ever growing power grid.Devices like the IED (Intelligent electronic device) or Phasor measurement units (PMU’s) which are available today to monitor power quality and have ability to gather information several times in a single power cycle, are capable of allowing for planning and analysis of the power networks [2]. These devices are limited to deployment only at the substation level and not grid wide due to the dated communication infrastructure [2]. The SCADA systems were developed with performance in focus rather than security. These components used for automating the power infrastructures used proprietary communication standards and operating systems which were designed primarily for functionality and performance rather than security [1]. This is because security systems and automation systems developed at different rates and are isolated from each other. This meant that the vendor providing the automation systems, did not bundle security and the consumer had to implement different systems to ensure security. Protection systems are applied locally to safeguard specific components and by central control through the supervisory control and data acquisition (SCADA) system. The central control system is too slow, and the protection systems are limited to protection of specific components only [2].III.MOVING TOWARD THE SMART POWER GRIDIn order to make the electric power transmission system intelligent, processors should be incorporated into each component at substation level as well as at the power plant level. They must have a good operating system and should be able to act independently to communicate with other processors, creating a distributed computing environment [4]. Each component can be connected to sensors so that it can analyze its own operating conditions and report them to neighboring processors through the communication links [4]. For such a system to exist there should be a well defined information network. The NIST framework for Smart Grid Interoperability focuses on information networks having the following:•Status monitoring, fault detection, isolation and recovery for networks and network devices [7].•Routing capabilities to all network end-points [7].•Quality of service for wide range of bandwidth requirements, latency and loss requirements [7].Newly emerging standards for the power grid, call for a ‘smart grid’ which employs state-of-the-art communication technology with greater importance on security in such systems. There should be inter-operable standards which can be quickly adopted and merged with legacy systems in order to keep the interruption in power services to a minimum. An IP based standard for the smart grid has emerged as a favorite among the industry. Use of IP is a reference to the networking protocol for communications in the smart grid as opposed to the use of public internet [7]. The use of IP is favored over any other protocol because of the flexibilities offered.IP has the following favoring factors:•Large number of, widely-deployed IP standards exist [6]. •Plenty of tools and applications that can be applied to the Smart Grid Environment.Fig. 2: Advanced Metering Infrastructure based system[8]•Google’s Smart Meter Application is one such example[6].•Allows applications to be developed independent of underlying communication technology at the physical layer [6].•Protocols such as Multi Protocol Label Switching (MPLS) can be used for providing dedicated resources [6].Since the devices connected to the smart grid are largely heterogeneous, multiple IP protocol suites may be required to suite the requirements of the network.The revolutionary concept being employed in the smart grid is the two-way communication between the consumer and the utility at the appliance/meter level. This will enable the consumer to continuously monitor power usage and make instantaneous decisions related to power saving using applications developed based on IP protocols. Also, embedded controllers running these applications in appliances can communicate with Advanced Metering Infrastructure (AMI) to automatically control power consumption during peak and off-peak load times. This will ensure that power is not utilized by the appliance when the consumption of power is costliest. One such AMI system employs a wireless communications network that collects data from consumers and feeds it to collections points that sent it to the enterprise. This data is used for billing, load management, outage control and other functions [8].Fig. 2 shows one such AMI scheme in which the wide area communication network can be either wired or wireless based on the availability in a particular area. The scheme shown in fig. 2 is a business solution provided by Motorola [8] using the WiMAX technology to create a Home Area Network which connects AMI systems attached to homes to the power grid communications system. Although this system is flexible and allows consumers access to consumption data, it also involves security issues at the consumer level. The networks for such a system should isolate the consumer from the utility data and the data that is available to the consumer for monitoring. The security solutions must be extensible to handle any future state of the grid including incorporation of new technologies and advancing communication protocols.IV.SMART GRID CONCERNSOne of the main purposes of upgrading from the current electric power grid to the smart grid is power management. Through two-way communication between the utility and the consumer, there can be dynamic management of power consumption across several domains. Also, the concept of distributed power generation can become a reality. Consumers can offset their power bills by feeding into the grid using self-generated power by solar or other renewable energy sources. This is help in reduction of the global carbon footprint of each household. Although this two-way communication is at the core of the smart grid technology, it is also simultaneously the single largest security concern. This is because of the presence of both smart grid networks and public internet at the consumer end. It has to be ensured that the utility information accessed by applications from the consumer end cannot access grid information, as this will result in a serious exposure of grid components to the consumers.Security in communication networks built for the smart grid will be a great challenge. Organizations like NIST and the CSCTG are emphasizing on security in these systems and have a framework for the same [7]. The following areas of electric system fall under the ‘smart grid’ category:•Transmission and distribution lines [5].•End-consumer systems [5].•System co-ordination centers (control centers) [5].•Information networks (inter and intra-enterprise communications) [5].•Commercial systems [5].The following are the risks involved in smart grids:•Hacking: This form of intrusion is not new to information networks. Since the smart grid will bebased on an information network, hacking into AMIor utility databases is a huge threat. Intrusionvulnerability points include SCADA systems,modems used by components and other networkingcomponents [5].•Malware: Malwares or malicious software can be found across the public internet in large numbers. Theinter-connection of the smart grid network with thepublic internet will cause a potential spread of thesemalwares into grid software, causing disruption inpower services or false data being exchanged in thelink [5].•Espionage and information warfare from foreign sources [5].•V.CONCLUSIONCyber security risks also involve wireless intrusion, weak network encryption and weak password protection. These threats can be eliminated by incorporating encryption in SCADA systems, which were not considered when developing these systems. In case of a compromise in security for such an automation infrastructure, the person analyzing data would be fairly efficiently be able to decipher the contents of communication and even be able to modify data or shut down the systems with ease.The conclusions that arise from the study of such systems is that the linking of the age-old communication infrastructure of the power grid to the latest communication systems as an attempt to upgrade these dated systems, only created new holes and openings for potential hacks which could compromise the functioning of not only the attack point but also create a cascading effect across all the interconnections that the system has. This scenario can be compared to a worm or a virus on the internet which can spread very quickly doing harm in its path. Malware in such wide networks can create much bigger losses because of their link to critical infrastructure that play a vital role in everyday lives. The future of power grids lies in making them intelligent, capable of real-time adaptation to disturbances, man-made or natural. In a way, the smart grid will resemble modern computer networks but will be free from the flaws and pit-falls that today’s computer networks face. In fact, the super-networked power grid, if implemented, simply cannot afford to allow the security flaws that exist in protocols which are widely employed in today’s communication networks. New networking standards need to be established for such a large network to work flawlessly in the face of growing cyber threats. According to the NIST and the CSCTG, the smart grid communication infrastructure is likely to be based on the following standards:•Wired communication links: Wavelength Division Multiplexing (WDM) techniques, SONET/SDH fiberlinks, Passive Optical Networks(PON), GigabitEthernet (GbE) [6].•Wireless communication links: IEEE 802.15(WPANs or Wireless Personal Area Networks), IEEE802.11(WLAN), IEEE 802.16, 3/4G cellularstandards [6].The future of smart grids will thus depend on the level of security that applications, devices and networking components incorporate rather than their efficiency, reliability or cost.R EFERENCES[1]Dong Wei; Yan Lu; Paul Skare; Mohsen Jafari; Kenneth Rohde;Michael Muller, “Power Infrastructure Security: Fundamental Insights ofPotential Cyber Attacks and Their Impacts on Power Grid,” Part of project “Protecting Intelligent Distributed Power Grids against CyberAttacks” for DOE.[2]Carl H. Hauser; David E. Bakken; Anjan Bose; “A Failure toCommunicate,” Power and Energy Magazine, IEEE, pp. 47-55, Mar-Apr, 2005.[3]David Watts, “Security and Vulnerability in Electric Power Systems,”NAPS 2003, 35th North American Power Symposium, University of Missouri-Rolla in Rolla Missouri, October 20-21,2003. Pp.559-566.[4]Massoud Amin S.; Wollenberg Bruce F., “Toward a Smart Grid,” Powerand Energy Magazine, IEEE, pp. 34-41, sep-oct, 2005.[5]Paul Oman and Edmund O. Schweitzer,III ; Deborah Frincke, “ConcernsAbout Intrusions Into Remotely Accessible Substation Controllers AndScada Systems.”[6]National Institute of Standards and Technology; “NIST Framework andRoadmap for Smart Grid Interoperability Standards Release 1.0,”; U.S.Department of Commerce, September 2009.[7]The Cyber Security Co-ordination Task Group;“Smart Grid CyberSecurity Strategy and Requirements”, September 2009.[8]Solution Brief, MOTOROLA INC.“AMI and Beyond: How WirelessBroadband enables the Smart Grid Today and Tomorrow,” 2009.。
智能电网(1).ppt
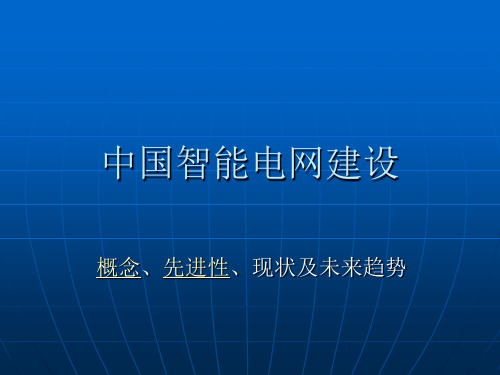
网必须依靠智能化手段不断提高其安全防御能力和自愈能力。为降低运营成本,促进
节能减排,电网运行必须更为经济高效,同时须对用电设备进行智能控制,尽可能减
少用电消耗。分布式发电、储能技术和电动汽车的快速发展,改变了传统的供用电模
式,促使电力流、信息流、业务流不断融合,以满足日益多样化的用户需求。
电力技术的发展,使电网逐渐呈现出诸多新特征,如自愈、兼容、集成、优化,
其比 缺恢等一 先, 乏复都个 进智 良能缺刚 性能 好力乏性 和电 的完较系 优网 信全好统 势体 息依的, 主现 共赖灵智 要出 享于活能 表电 机物性化 现力 制理,程 在流 。冗电度 :、 余网不
信 ;的高 息 对协。 流 用调电 和 户控源 业 的制的 务 服能接 流 务力入 高 形不与 度 式理退
网系统功能。
(1)智能电网是电网技术发展的必然趋势。近年来,通信、计算机、自动化等技
术在电网中得到广泛深入的应用,并与传统电力技术有机融合,极大地提升了电网的
智能化水平。传感器技术与信息技术在电网中的应用,为系统状态分析和辅助决策提
供了技术支持,使电网自愈成为可能。调度技术、自动化技术和柔性输电技术的成熟
可力
辅管
设 应自
积故机 网外 融 简想出现
以、
助理
备 大动
停障融 的部 合 单;、有
获电 ( 决展( 使( 量化( 电发合( 坚干( 的与 、系电电
取能 六 策示五 用四 分等三 的生,二 强扰一 显现 信统能网
用质 ) 支全) 效) 布技) 发时可) 性和) 著有 息自量总
户量 建 持面实 率通 式术柔 生,获信 得攻具 特电 开发利用提供了基本保障。通信网络的完善和用
户信息采集技术的推广应用,促进了电网与用户的双向互动。随着各种新技术的进一
诠释智能电网中的AMI

诠释智能电网中的AMI智能电网(smart power grids),就是电网的智能化,也被称为“电网2.0”,它是建立在集成的、高速双向通信网络的基础上,通过先进的传感和测量技术、先进的设备技术、先进的控制方法以及先进的决策支持系统技术的应用,实现电网的可靠、安全、经济、高效、环境友好和使用安全的目标,其主要特征包括自愈、激励和包括用户、抵御攻击、提供满足21世纪用户需求的电能质量、容许各种不同发电形式的接入、启动电力市场以及资产的优化高效运行。
智能电网主要由4 部分组成:1)高级量测体系(advanced metering infrastructure,AMI);2)高级配电运行(advanced distribution operation,ADO);3)高级输电运行(advanced transmission operation,ATO);4)高级资产管理(advanced asset management,AAM)。
高级量测体系(AMI)AMI 主要功能是授权给用户,使系统同负荷建立起联系,使用户能够支持电网的运行。
AMI 是许多技术和应用集成的解决方案,其技术组成和功能主要包括:1)智能电表。
可以定时或即时取得用户带有时标的分时段的(如15 min,1h 等)或实时(或准实时)的多种计量值,如用电量、用电功率、电压、电流和其他信息;事实上已成为电网的传感器。
2)通信网络。
采取固定的双向通信网络,能把表计信息(包括故障报警和装置干扰报警)接近于实时地从电表传到数据中心,是全部高级应用的基础。
3)计量数据管理系统(MDMS)。
这是一个带有分析工具的数据库,通过与AMI 自动数据收集系统的配合使用,处理和储存电表的计量值。
4)用户室内网(HAN)。
通过网关或用户入口把智能电表和用户户内可控的电器或装置(如可编程的温控器)连接起来,使得用户能根据电力公司的需要,积极参与需求响应或电力市场。
5)提供用户服务(如分时或实时电价等)。
智能电网建设与运维规范
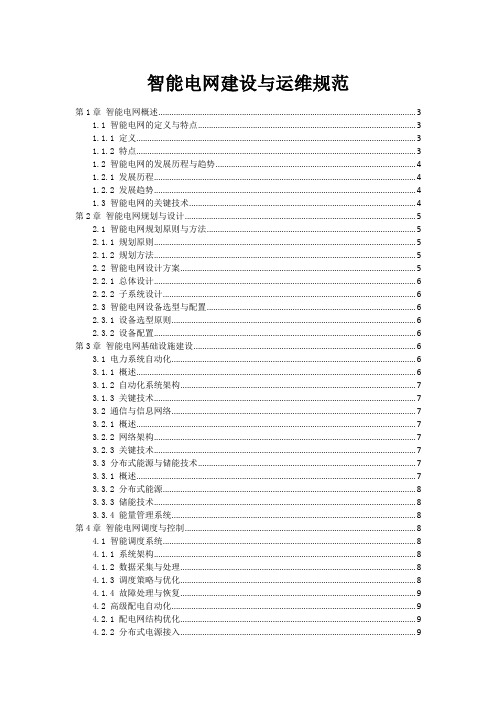
智能电网建设与运维规范第1章智能电网概述 (3)1.1 智能电网的定义与特点 (3)1.1.1 定义 (3)1.1.2 特点 (3)1.2 智能电网的发展历程与趋势 (4)1.2.1 发展历程 (4)1.2.2 发展趋势 (4)1.3 智能电网的关键技术 (4)第2章智能电网规划与设计 (5)2.1 智能电网规划原则与方法 (5)2.1.1 规划原则 (5)2.1.2 规划方法 (5)2.2 智能电网设计方案 (5)2.2.1 总体设计 (6)2.2.2 子系统设计 (6)2.3 智能电网设备选型与配置 (6)2.3.1 设备选型原则 (6)2.3.2 设备配置 (6)第3章智能电网基础设施建设 (6)3.1 电力系统自动化 (6)3.1.1 概述 (6)3.1.2 自动化系统架构 (7)3.1.3 关键技术 (7)3.2 通信与信息网络 (7)3.2.1 概述 (7)3.2.2 网络架构 (7)3.2.3 关键技术 (7)3.3 分布式能源与储能技术 (7)3.3.1 概述 (7)3.3.2 分布式能源 (8)3.3.3 储能技术 (8)3.3.4 能量管理系统 (8)第4章智能电网调度与控制 (8)4.1 智能调度系统 (8)4.1.1 系统架构 (8)4.1.2 数据采集与处理 (8)4.1.3 调度策略与优化 (8)4.1.4 故障处理与恢复 (9)4.2 高级配电自动化 (9)4.2.1 配电网结构优化 (9)4.2.2 分布式电源接入 (9)4.2.3 集成化保护与控制 (9)4.2.4 远程控制与维护 (9)4.3 需求侧管理 (9)4.3.1 负荷预测 (9)4.3.2 需求响应 (9)4.3.3 能效管理 (9)4.3.4 用户互动 (9)第5章智能电网安全防护 (10)5.1 智能电网安全策略 (10)5.1.1 安全目标 (10)5.1.2 安全体系 (10)5.1.3 风险评估与管理 (10)5.2 网络安全防护技术 (10)5.2.1 防火墙技术 (10)5.2.2 入侵检测与防御系统 (10)5.2.3 安全审计 (10)5.2.4 数据加密与传输 (10)5.3 设备与数据安全 (10)5.3.1 设备安全管理 (10)5.3.2 数据安全管理 (10)5.3.3 数据备份与恢复 (11)5.3.4 安全防护设备部署 (11)第6章智能电网运维管理 (11)6.1 运维组织与管理体系 (11)6.1.1 运维组织架构 (11)6.1.2 管理体系 (11)6.2 运维流程与规范 (11)6.2.1 运维流程 (11)6.2.2 运维规范 (11)6.3 智能巡检与维护 (12)6.3.1 智能巡检 (12)6.3.2 智能维护 (12)6.3.3 智能化技术应用 (12)第7章智能电网设备检测与评估 (12)7.1 设备检测技术 (13)7.1.1 检测方法 (13)7.1.2 检测技术 (13)7.2 设备状态评估 (13)7.2.1 评估方法 (13)7.2.2 评估模型 (13)7.3 预防性维护策略 (13)7.3.1 维护策略制定 (13)7.3.2 维护策略实施 (13)7.3.3 维护效果评估 (14)第8章智能电网与新能源接入 (14)8.1 新能源发展现状与趋势 (14)8.1.1 国际新能源发展概况 (14)8.1.2 我国新能源发展现状 (14)8.1.3 新能源发展趋势 (14)8.2 新能源并网技术 (14)8.2.1 风电并网技术 (14)8.2.2 太阳能并网技术 (14)8.2.3 储能技术 (14)8.3 智能电网与新能源互动 (15)8.3.1 智能电网对新能源的支撑作用 (15)8.3.2 新能源在智能电网中的应用 (15)8.3.3 智能电网与新能源协同发展 (15)第9章智能电网与能源互联网 (15)9.1 能源互联网概述 (15)9.2 智能电网与能源互联网的融合 (15)9.3 智能电网在能源互联网中的作用 (15)第10章智能电网案例分析与发展展望 (16)10.1 国内外智能电网案例分析 (16)10.1.1 国内智能电网案例 (16)10.1.2 国外智能电网案例 (16)10.2 智能电网发展面临的挑战与机遇 (17)10.2.1 挑战 (17)10.2.2 机遇 (17)10.3 智能电网未来发展趋势与展望 (17)10.3.1 技术发展趋势 (17)10.3.2 市场与产业展望 (18)10.3.3 政策与管理创新 (18)第1章智能电网概述1.1 智能电网的定义与特点1.1.1 定义智能电网,又称智能化电网,是基于现代信息技术、通信技术、自动控制技术、物联网技术等先进技术,实现电力系统的高效、安全、环保、可靠运行,为用户提供优质服务的现代化电网。
智能电网Smart Grid
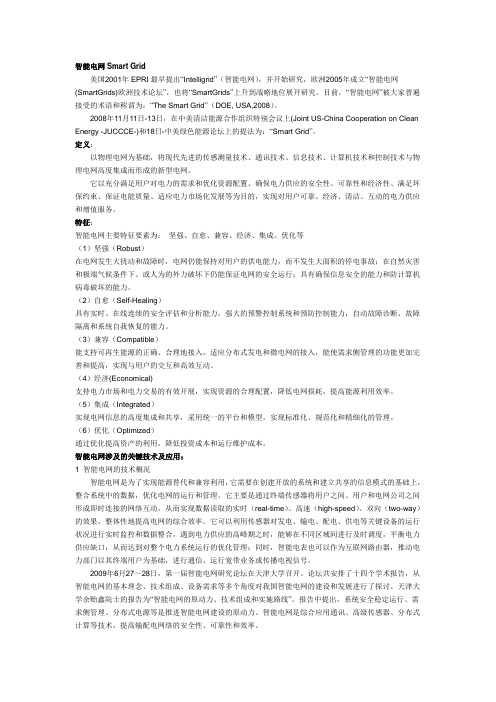
智能电网Smart Grid美国2001年EPRI最早提出“Intelligrid”(智能电网),并开始研究,欧洲2005年成立“智能电网(SmartGrids)欧洲技术论坛”,也将“SmartGrids”上升到战略地位展开研究。
目前,“智能电网”被大家普遍接受的术语和称谓为:“The Smart Grid”(DOE, USA,2008)。
2008年11月11日-13日,在中美清洁能源合作组织特别会议上(Joint US-China Cooperation on Clean Energy -JUCCCE-)和18日-中美绿色能源论坛上的提法为:“Smart Grid”。
定义:以物理电网为基础,将现代先进的传感测量技术、通讯技术、信息技术、计算机技术和控制技术与物理电网高度集成而形成的新型电网。
它以充分满足用户对电力的需求和优化资源配置、确保电力供应的安全性、可靠性和经济性、满足环保约束、保证电能质量、适应电力市场化发展等为目的,实现对用户可靠、经济、清洁、互动的电力供应和增值服务。
特征:智能电网主要特征要素为:坚强、自愈、兼容、经济、集成、优化等(1)坚强(Robust)在电网发生大扰动和故障时,电网仍能保持对用户的供电能力,而不发生大面积的停电事故;在自然灾害和极端气候条件下、或人为的外力破坏下仍能保证电网的安全运行;具有确保信息安全的能力和防计算机病毒破坏的能力。
(2)自愈(Self-Healing)具有实时、在线连续的安全评估和分析能力,强大的预警控制系统和预防控制能力,自动故障诊断、故障隔离和系统自我恢复的能力。
(3)兼容(Compatible)能支持可再生能源的正确、合理地接入,适应分布式发电和微电网的接入,能使需求侧管理的功能更加完善和提高,实现与用户的交互和高效互动。
(4)经济(Economical)支持电力市场和电力交易的有效开展,实现资源的合理配置,降低电网损耗,提高能源利用效率。
智能电网基础知识
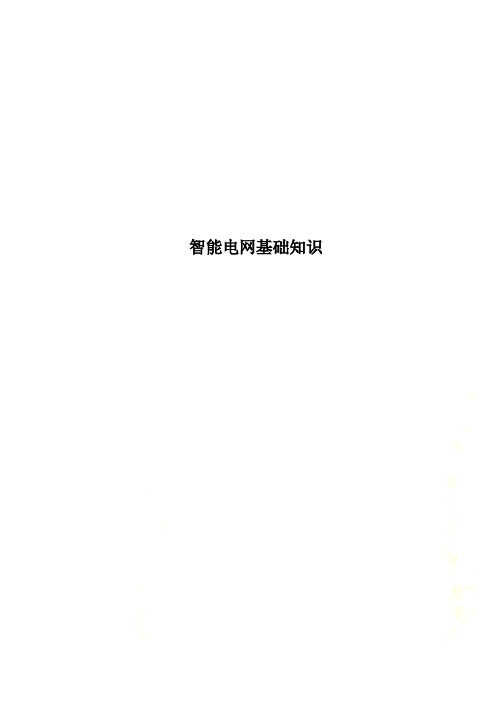
智能电网基础知识智能电网基础知识二0一一年二月一、智能电网概述二、“智能电网关键设备(系统)研制规划”摘录及解读三、配电自动化基础知识附录:物联网技术基础知识一、智能电网(smart power grids)概述智能电网就是电网的智能化,也被称为“电网2.0”,它是建立在集成的、高速双向通信网络的基础上,通过先进的传感和测量技术、先进的设备技术、先进的控制方法以及先进的决策支持系统技术的应用,实现电网的可靠、安全、经济、高效、环境友好和使用安全的目标,其主要特征包括自愈、激励和包括用户、抵御攻击、提供满足21世纪用户需求的电能质量、容许各种不同发电形式的接入、启动电力市场以及资产的优化高效运行。
美国电力科学研究院将智能电网定义为:一个由众多自动化的输电和配电系统构成的电力系统,以协调、有效和可靠的方式实现所有的电网运作,具有自愈功能;快速响应电力市场和企业业务需求;具有智能化的通信架构,实现实时、安全和灵活的信息流,为用户提供可靠、经济的电力服务。
中国的智能电网的基本特征是在技术上要实现信息化、自动化、互动化。
1、基本简介智能电网概念的发展有3个里程碑:第一个就是2006年,美国IBM公司提出的“智能电网”解决方案。
IBM的智能电网主要是解决电网安全运行、提高可靠性,从其在中国发布的《建设智能电网创新运营管理-中国电力发展的新思路》白皮书可以看出,解决方案主要包括以下几个方面:一是通过传感器连接资产和设备提高数字化程度;二是数据的整合体系和数据的收集体系;三是进行分析的能力,即依据已经掌握的数据进行相关分析,以优化运行和管理。
该方案提供了一个大的框架,通过对电力生产、输送、零售的各个环节的优化管理,为相关企业提高运行效率及可靠性、降低成本描绘了一个蓝图。
是IBM一个市场推广策略。
第二个是奥巴马上任后提出的能源计划,除了已公布的计划,美国还将着重集中对每年要耗费1200亿美元的电路损耗和故障维修的电网系统进行升级换代,建立美国横跨四个时区的统一电网;发展智能电网产业,最大限度发挥美国国家电网的价值和效率,将逐步实现美国太阳能、风能、地热能的统一入网管理;全面推进分布式能源管理,创造世界上最高的能源使用效率。
- 1、下载文档前请自行甄别文档内容的完整性,平台不提供额外的编辑、内容补充、找答案等附加服务。
- 2、"仅部分预览"的文档,不可在线预览部分如存在完整性等问题,可反馈申请退款(可完整预览的文档不适用该条件!)。
- 3、如文档侵犯您的权益,请联系客服反馈,我们会尽快为您处理(人工客服工作时间:9:00-18:30)。
Chapter 1Smart Power GridsAli KeyhaniGreen Energy laboratoryThe Ohio State UniversityKeyhani@1.1 IntroductionEnergy technology plays a central role in societal economic and social development. Fossil fuel-based technologies have advanced our quality of life, but at the same time, these advancements have come at a very high price. Fossil fuel sources of energy are the primary cause of environmental pollution and degradation; they have irreversibly destroyed aspects of our environment. Global warming [4, 75, 76] is a result of our fossil fuel consumption. Our relentless search for and need to control the valuable fossil fuel resources such as oil and natural gas have promoted political strife. We are now dependent on energy sources that are unsustainable as our energy needs grow and we deplete our limited resources. As oil supplies dwindle, it will become increasingly urgent to find energy alternatives that are sustainable as well as safe for the environment and humanity.The restructuring of the electric power industry was a critical step for individual stakeholders, facilitating their wide participation in the production, delivery, utilization of energy and efficient use of electric energy. The restructuring is facilitating integration of internet technology in protection, control and real-time pricing. Furthermore, the worldwide awaking of global warming has created a market for integration of renewable and green energy sources. Now, technology is rapidly moving in a direction where every energy user can become energy producer.Amin [43] coined the term “smart grid” in 2005. Shahidehpour integrated many definitions of smart grids in his forward section of Keyhani’s [1] book on smart power grids as“The smart grid has further offered alternatives to participants looking to enhance the reliability, sustainability, and capability for customer choices in energy systems. The smart grid has made it possible to set up microgrids that could be operated as stand-alone islands in critical operating conditions. Such small installations can enhance the reliability of regional electric power systems when the larger grid is faced with major contingencies. There are several practical examples of microgrid installations which have demonstrated that2 A.Keyhanithe use of smart switches in distributed power grids could reduce the number andthe duration of outages. In addition, the smart grid allows microgrids to optimizethe use of volatile and intermittent renewable energy resources and enhance thesustainability of regional power systems. The applications of solar photovoltaic,which mostly follows the daily load profile for power generation, on-site or localwind energy, along with storage devices for microgrid installations could providean inexpensive and sustainable means of supplying microgrid loads. Theprinciples of a widespread utilization of energy storage can also be found in theemerging market of plug-in electric vehicles, which would utilize wind energy atoff-peak hours. Such microgrid applications could also eliminate the need forextensive additions of high voltage lines for the transmission of renewable energyacross densely populated regions of the world. However, the evolutions in theelectric power industry that I believe will truly revolutionize the way we deliverelectricity to individual consumers are smart grid applications related to real-timepricing, hourly demand response, and the expansion of customer choices forpromoting energy efficiency. The use of new smart grid innovations would makeit possible for consumers to prioritize their energy use according to their dailyschedules, needs, and preferences, taking into account a variable cost of electricityto save money. Smart grid advancements would also enable automated controlsystems to optimize energy use at home or for businesses, identifying the mostappropriate times for device operation to reduce the cost of electricity. Customerparticipation will offer a number of incentives for the optimization of electricpower operations, including lower operation costs by eliminating the commitmentof costly generating units at peak hours, mitigating mandatory system upgradesthat are required for responding to a few hundred hours of annual peak loads, andreducing the chance of transmission congestion, which could otherwise operatethe power system at a state close to its critical point of collapse. Demand responsecould also offer a less fluctuating and flatter daily profile, which would make itpossible to forecast the daily load profile and schedule fuel and hydroconsumption more comprehensively and efficiently for power generation.” Thisdescription of a smart power grid ushers the design and development of electricenergy system for centuries to come.1.2 Solar EnergySolar energy is a readily available renewable energy; it reaches earth in the formof electromagnetic waves (radiation). Many factors affect the amount of radiationreceived at a given location on earth. These factors include location, season,humidity, temperature, air mass, and the hour of day. Insolation refers to exposureto the rays of the sun, i.e., the word “insolation” has been used to denote the solarradiation energy received at a given location at a given time. The phrase incidentsolar radiation is also used; it expresses the average irradiance in watts per squaremeter (W/m2) or kilowatt per square meter (kW/m2). The surface of the earth iscoordinated with imaginary lines of latitude and longitude. Latitudes on the1 Smart Power Grids 3 surface of the earth are imaginary parallel lines measured in degrees. The lines subtend to the plane of the equator are called latitudes. The latitudes vary from 90° south (S) to 90° north (N). The longitudes are imaginary lines that vary from 180° east (E) to 180° west (W). The longitudes converge at the poles (90° north and 90° south). The radiation of the sun on the earth varies with the location based on the latitudes. Approximately, the region between 30° S and 30° N has the highest irradiance as depicted in Fig.1.1. The latitude on which the sun shines directly overhead between these two latitudes depends on the time of the year. If the sun lies directly above the northern hemisphere, it is summer in the north and winter in the southern hemisphere. If it is above the southern hemisphere, it is summer in the southern hemisphere and winter in the north.Fig. 1.1 The Global Irradiation Values for the World (kWh/m2) [44]1.3 Wind EnergyWind energy, as one of our most abundant resources, is the fastest growingrenewable energy technology worldwide as shown in Fig. 1.2. Improved turbineKeyhani4 A.and power converter designs have promoted a significant drop in wind energygeneration cost making it the least-expensive source of electricity—from 37cents/kWh in 1980 down to 4 cents/kWh in 2008. In 2008, wind energy systemsworldwide generated 331,600 million kWh, which is 1.6% of total electricitygeneration—making wind the second highest resource after hydroelectric power(16.6%), while the photovoltaic (PV) technology contribution was only 0.1%.The United States alone possesses more than 8,000 GW of land-based windresources suitable for harnessing, and an extra 2,000 GW of shallow offshoreresources. With U.S. total electricity generating capacity at 1,109 GW in 2008, theuntapped wind sources in United States is almost 8 times as large. Global windmovement is predicated on the earth’s rotation, and regional and seasonalvariations of sun irradiance and heating. Local effects on wind include thedifferential heating of the land and the sea, and topography such as mountainsvalleys. We always describe wind by its speed and direction. The speed of thewind is determined by an anemometer, which measures the angular speed ofrotation and translates it into its corresponding linear wind speed in meters persecond or miles per hour. The average wind speed determines the wind energypotential at a particular site. Wind speed measurements are recorded for a 1-yearperiod and then compared to a nearby site with available long-term data toforecast wind speed and the location’s potential to supply wind energy.Fig. 1.2 Worldwide Electricity Generations for Wind, Biomass, Geothermal, and SolarResources, Years 2000–2008 [69]1 Smart Power Grids 5A wind energy resource map for the contiguous United States from the NREL[80] is shown in Fig. 1.3. This map is based on a location’s annual average wind speed and wind power density (in W/m2) at 60-m (164 ft) tower height; it can be used for initial site assessment. The coastal areas have high wind energy potential; nevertheless, 90% of the U.S. usable wind resources lie in the wind belt spanning the 11 Great Plains states.Fig. 1.3 Wind Resource Map of the Contiguous United States with Wind Power Classifications Indicated by Region [69].Table 1.1. Wind Power Classification.Wind Power Class Resource Potential Wind PowerDensity at 50 m(W / m2)Wind Speed aat 50 m (m / s)Wind Speed aat 50 m (mph)3 Fair 300 – 400 6.4–7.0 14.3–15.74 Good 400 – 500 7.0–7.5 15.7–16.85 Excellent 500 – 600 7.5–8.0 16.8–17.96 Outstanding 600 – 800 8.0–8.8 17.9–19.77 Superb 800 – 1800 8.8–11.1 19.7–24.8a Wind speeds are based on a Weibull’s [8] value of 2.0.6 A.Keyhani1.4 Microgrid of Renewable and Green (MRG) Power GridsFigure 1.4 depicts a microgrid of renewable and green (MRG) energy system.The MRG systems consists of PV, wind power of renewable energy sources andgreen energy sources such as fuel cells and high speed micro turbine generatingstations.The microgrids of MRG are designed to supply power to local grid or importpower on prior contract agreements. The combined heat and power (CHP) andmicro turbine generating stations and some induction generator based windgenerating are directly connected to AC bus of MRG systems. However, PV,variable speed wind, and fuel cell generating stations produced DC power. The PVpower can be directly connected to DC-AC inverters to AC bus of MRG systems.Alternative design is based on using a DC-DC boost converter to step up the DCvoltage and use higher rating DC-AC inverters. When MRG systems aresynchronized to AC bus of the local power grids, the AC bus voltage andfrequency are controlled by the local power grid’s energy management. However,upon separation of MRG systems from the local power grid, the energymanagement of MRG must control the MRG AC bus voltage and frequency forstable operation. The load control is essential in proper balance of MRG’s loadsand generation when the MRG is separated from the local power grid.1.5 Control Operation of Interconnected Network Bulk PowerGridsInitially designed in the early 1900s, today’s power grid has evolved to become alarge interconnected network that connects thousands of generating stations andload centers through a system of power transmission lines. A power grid system isdesigned based on the long-term load forecast of the power grid load centers,which is developed according to the anticipated needs of the community it serves.Then, an analytical model of the system is developed to project the grid’s real-time operation. In a smart power grid system, a large number of microgridsoperate as part of an interconnected power grid. For example, a photovoltaic- (PV-) based residential system with its local storage system and load would be one ofthe smallest microgrids in the smart power grid system. To understand the newparadigm of tomorrow’s smart power grid design and operation, we need tounderstand today’s electric power grid operation and costs of design.1 Smart Power Grids 7Ethernet TCP / IP Local LAN/HTTPBrowserFig. 1.4 A Microgrid Renewable and Green (MRG) Power GridTo ensure security and reliability, power plant facilities and resources must first be planned then managed effectively. A large power grid is comprised of many elements including generating units, transmission lines, transformers, and circuit breakers. As new green energy sources are adopted into the power grid and a smart power grid is put in place, additional equipment such as DC/DC converters and DC/ AC converters must be integrated and scheduled for power grid operation. In addition, market structure and real-time pricing of energy need to be evaluated.For stable operation of power grid, we will need to schedule power generation to supply the system loads for every second of the system’s operation. The energy resources of a large power system consist of hydro and nuclear energy, fossil fuel, renewable energy sources such as wind and solar energy, as well as green energy sources such as fuel cells, combined heat and power (CHP; also known as cogeneration), and microturbines. These resources must be managed and synchronized to satisfy the load demand of the power grid. The load demand of a power grid is cyclic in nature and has a daily peak demand over a week, a weekly peak demand over a month, and a monthly peak demand over a year. Energy resources must be optimized to satisfy the peak demand of each load cycle, such that the total cost of production and distribution of electric energy is minimized.8 A. KeyhaniFigure 1.5 depicts a 24-hour load variation sampled every 5 minutes. From Fig. 1.5, it can be seen that peak demand is twice the minimum power demand. The power system operator must plan the power grid energy resources and facilities to satisfy the varying load conditions.E r r o r (M W * 1000) Y a n d Y ^(M W * 1000) P .u E r r o rFig. 1.5 A Twenty-Four Hour Load Variation Sampled Every 5 MinutesThe system load has a general pattern of increasing slowly during the day and then decreasing at night. The cost of generated power is not the same for all generating units. Therefore, more power generation are assigned to the least costly units. In addition, a few lines connect one power grid to another neighboring power grid. These lines are referred to as tie lines. Tie lines are controlled to import or export power according to set agreed contracts. When power is exported from a power system to a neighboring power system through the tie lines, the exported power is considered as load; conversely when imported, it is considered as power generation. The control of the power flow through these lines is prespecified on agreed schedules and they are based on secure operation and economic transactions. To control both the power flow through transmission tie lines and the system frequency, the concept of area control error (ACE) is defined asf P ACE TL Δ−Δ=β(1.1) whereActual Sch TL P P P −=ΔActual S f f f −=Δ (1.2)1 Smart Power Grids 9Sch P : The scheduled power flow between two power networksActual P : The actual power flow between two power networkss f : The reference frequency, i.e., the rated frequencyactual f : The actual measured system frequencyβ: The frequency biasThe AGC software control is designed to accomplish the following objectives:1. Match area generation to area load, i.e., match the tie-line interchangeswith the schedules and control the system frequency.2. Distribute the changing loads among generators to minimize theoperating costs.The above condition is also subject to additional constraints that might be introduced by power grid security considerations such as loss of a line or a generating station.Fig. 1.6 The Automatic Generation Control (AGC).The first objective involves the supplementary controller and the concept of tie-line bias. The term βis defined as bias and it is a tuning factor that is set when AGC is implemented. A small change in the system load produces proportional changes in the system frequency. Hence, the area control error (f P ACE TL Δ−Δ=β) provides each area with approximate knowledge of the load change and directs the supplementary controller for the area to manipulate the turbine valves of the regulating units. To obtain a meaningful regulation (i.e., reducing the ACE to zero), the load demands of the system are sampled every few10 A.Keyhaniseconds. The second objective is met by sampling the load every few minutes (1–5minutes) and allocating the changing load among different units to minimize theoperating costs. This assumes the load demand remains constant during eachperiod of economic dispatch. Figure 1.7 depicts the AGC control block diagram.The AGC also controls the connected microgrids in a large interconnectedpower grid. The microgrid concept assumes a cluster of loads and itsmicrosources, such as photovoltaic, wind, and combined heat and power (CHP)are operating as a single controllable power grid. To the local power grid, thiscluster becomes a single dispatchable load. When a microgrid is connected to apower grid, the microgrid bus voltage is controlled by the local power grid.Furthermore, the power grid frequency is controlled by the power grid operator.The microgrid cannot change the power grid bus voltage and the power gridfrequency. Therefore, when a microgrid is connected to a local power grid, itbecomes part of the power grid network, and is subject to the power griddisturbances. Therefore, the AGC control system is designed to follow the systemload fluctuations.Fig. 1.7 The Automatic Generation Control (AGC) Block Diagram1.6 Smart Power GridIn a classical power grid, a fixed price is charged to energy users. However, thecost of energy is highest during the daily peak load operation. The classical powersystem operation has no control over the loads except in an emergency situationwhen a portion of the loads can be dropped as needed to balance the powergrid generation with its loads. Therefore, most elements of grid are used for ashort time during the peak power demand and they remain idle during the dailyoperation.For an efficient smart power grid system design and operation, substantialinfrastructure investment in the form of a communication system, cyber network,sensors, and smart meters must be installed to curtail the system peak loads whenthe cost of electric energy is highest. The smart power grid introduces a sensing,monitoring, and control system that provides end users with the cost of energy atany moment through real-time pricing. In addition, the advanced control systemsof smart metering provide the energy users with the ability to respond to real-time pricing. Furthermore, the smart power grid supplies the platform for the use of renewable and green energy sources and adequate emergency power for major metropolitan load centers. It safeguards against a complete blackout of the interconnected power grids due to man-made events or environmental calamity. It also allows for the break-up of the interconnected power grid into smaller, regional clusters. In addition, the smart power grid enables every energy user to become an energy producer by giving the user the choice of PV or wind energy, fuel cells, and combined heat and power (CHP) energy sources and to participate in the energy market by buying or selling energy through the smart meter connection.The bulk power grid of the United States and many other countries are already operating as a large interconnected network. The mission of the North American Electric Reliability Corporation(NERC) is to ensure the reliability and security of the America’s bulk power grid. Fig. 1.8 depicts the North American electric reliability centers [75].Fig. 1.8 North American Electric Reliability Centers (NERC). ERCOT, Electric Reliability Council of Texas; FRCC, Florida Reliability Coordinating Council; MRO, Midwest Reliability Organization; NPCC, Northeast Power Coordinating Council, Inc.; RFC, Reliability First Corporation; SERC, SERC Reliability Corporation; SPP, Southwest Power Pool, Inc.; WECC, Western Electricity Coordinating Council [25].The industry is experiencing a gradual transformation that will have a long-term effect on the development of the infrastructure for generating, transmitting, and distributing power. This fundamental change will incorporate renewable generation and green energy sources in a new distributed generation program based on increased levels of distributed monitoring, automation, and control as well as new sensors. The power grid control will rely on data and informationcollected on each microgrid for decentralized control. In return, the microgrids and interconnected power grid will be able to operate as a more reliable, efficient, and secure energy supplier.The technology of the power grid and microgrids has a number of key elements. Adaptive and autonomous decentralized controls respond to changing conditions. Predictive algorithms capture the power grid state (phasor measurements) for a wide area and are able to identify potential outages. The system also provides market structure for real-time pricing and interaction between customers, grid networks, and power markets. Furthermore, the smart grid provides a platform to maximize reliability, availability, efficiency, economic performance, and higher security from attack and naturally occurring power disruptions.The implementation of an advanced metering infrastructure provides real-time pricing to the energy end users. In parallel, the penetration of renewable energy sources is providing a platform for autonomous control or local control of connected microgrids to the local power grid. A distributed autonomous control will provide reliability through fault detection, isolation, and restoration. The autonomous control and real-time pricing also delivers efficiency in feeder voltage to minimize feeder losses and to reduce feeder peak demand of plug-in electric vehicles. The maturing storage technology will provide community energy storage, which becomes yet another important element for microgrid control and allows the energy user to become an energy producer. These interrelated technologies require a coordinated modeling, simulation, and analysis system to achieve the benefits of a smart power grid.Table 1.2 A Comparison of the Current Grid and the Smart Grid.Current Grid Smart GridSystem communicationsLimited to powercompaniesExpanded, real-timeInteraction with energy usersLimited to largeenergy usersExtensive two-waycommunicationsOperation & maintenance Manual anddispatchingDistributed monitoring and diagnostics, predictiveGeneration Centralized Centralized and distributed,substantial renewable resources,energy storagePower flowcontrolLimited More extensiveReliability Based on static, off-line models andsimulations Proactive, real-timepredictions, more actual systemdataRestoration Manual Decentralized control Topology Mainly radial NetworkThe optimum operation and control of power grid systems where every energy user can be energy user and energy producer may be designated as a smart power grid.1.7 Cyber Controlled Smart Power GridsIt is natural to expect that future cyber-controlled smart grid systems will be developed for the NERC-mandated reliability centers of the U.S. grid. A future cyber controlled system is depicted in Fig. 1.9.The cyber-fusion point (CFP) represents a node of the smart grid system where the renewable and green energy system is connected to large-scale interconnected systems. The U.S. interconnected system has eight regional reliability centers as shown in Fig. 1.8. It is expected that renewable microgrids will be connections of regional reliability centers such as Reliability First Corporation (RFC) transmission systems. The CFP is the node in the system that receives data from upstream, i.e., from the interconnected network, and downstream, i.e., from the microgrid renewable and green energy (MRG) system and its associated smart metering systems. The CFP node is the smart node of the system where the status of the network is evaluated and controlled, and where economic decisions are made as to how to operate the local MRG system. A CFP also evaluates whether its MRG system should be operated as an independent grid system or as a grid system separate from the large interconnected system. A cyber system is the backbone of the communication system for the collection of data on the status of the interconnected network system. The security of the cyber network is essential for the security of the grid. Fig. 1.9 illustrates such a future cyber-instrumented power grid.Two-way communication is a key characteristic of the smart power grid energy system. It enables end users to adjust the time of their energy usage for nonessential activities based on the expected real-time price of energy. The knowledge gained from smart meters permits the power grid operators to spot power outages more quickly and smooth demand in response to real-time pricing as the cost of power varies during the day.The cyber control of a smart grid is the subject of research by many disciplines in electrical and computer engineering. It requires a control system that analyzes the performance of the power grid using distributed, autonomous, and intelligent controllers. The cyber system will learn on-line from the sensors, the smart grid, and microgrid states. The control system analyzes the system for possible impeding failure. By sensor measurements and monitoring, the cyber control system governs grid behavior based on real-time data in the face of ever-changing operating conditions and new equipment. The system uses electronic switches that control multiple MRG systems with varying costs of generation and reliability.As a result, a cyber-controlled smart grid requires consumers to pay the real-time price of produced electric power. Table 1.3 presents the cost of electric energy as of 2009 from different sources.Fig. 1.9 A Cyber-Controlled Smart Grid.Table 1.3 The Cost of Electric Energy in 2009 [75]. Energy Source Cost perKilowatt Hour(Cents)Typical Uses Typical Installation SizeSolar energy (photovoltaics)20–40Base load power source 1–10,000 kW Microturbines 10–15 Can be used in base load, peaking or cogeneration applications 30–300 kW Fuel cells 10–15Rural (off-grid) power Transportation Appropriate for base load applications 1–200 kW Wind turbines5–10 5–10 MW Internalcombustionengines 1.5–3.5Well-established, long history as back up or in peaking applications 50 kW–5 MW Central power generation1.7–3.7Base load/peaking electricity generation500–3000 MWReal-time pricing was introduced by F. Schweppes in July 1978 during an energy crisis. A simple analysis of the cost to the supplier per unit of energy delivered explains the relationship between cost and plant utilization. The real-time price of electricity is a function of load factor, load demand, and unexpected events. The first cost is the utilization of a power plant and its operating costs. To build a large plant, many issues must be addressed. In a regulated market, large plants take years to build and are located far away from load centers; electric power is transferred by long transmission lines. Normally, coal-fired power plants are built close to a coal mine. From an operational viewpoint, the sudden loss of a large plant creates instant real-time price change in the power market because the allocated real-time reserve is limited due to cost. Small power plants are normally gas-fired; they are built over a short time and their construction costs can be accurately estimated. Gas-fired plants can be placed close to load centers because these plants need limited space. Furthermore, when plants are close to load centers and their power does not need to be shipped over long-distance transmission lines, these plants have lower system losses. These plants also tend to have good system security and are generally more reliable and less consequential when they are subjected to sudden outage. Combined cycle units are highly desirable because of their high efficiency. Cogeneration facilities are also attractive because they typically have lower ratings. Plants fueled by renewable energy sources are attractive as well because of their low operating cost. Due to many sources of power and their associated cost, the cost of real-time power is a variable and needs to be determined as sources of electric energy to supply the system load changes.There are a number of advantages to smart MRG systems. It empowers the individual to provide his or her own energy needs. This participation by energy end users has been called the "democratization of energy.” This allows matching the characteristics’ of loads to their generators. This makes these types of microgrids independent from the interconnected grid power failures. Smart MRG technologies have the ability to identify potential stability problem. Real-time information enables MRG systems to separate themselves from the interconnected grid and operate as an “island.” During normal synchronized operation, the interconnected smart grid with its smart monitoring of power grids can control and manage the interconnected power grid to control the system loads and avoid system-wide black out.When a smart microgrid DG is connected to a power grid, then the smart microgrid generating station should operate, using a master and slave control technology. The master is the EMS of the power grid system. The EMS controls the infinite bus voltage located at the connection node of one microgrid to its local power grid. The smart grid is connected to the power grid system by a transformer. The slave controller controls the AC bus voltage of the microgrid inverter (bus voltage magnitude and phase angle) and the inverter current. Therefore, the slave controller of the microgrid inverter also controls active and reactive power. The microgrid inverter is designed to operate as a unity power factor and to leave the voltage control, that is, the reactive power (Vars) control, to the EMS of the power grid system. Or, the inverter of microgrid operates with a leading power factor, a lagging power factor. If the smart grid is suddenly。