Intersite elastic coupling and invar effect
风力发电常用英语词汇

风电项目常用英语词汇tubular tower 塔筒式塔架lattice tower 桁架式塔架nacelle 机舱gearbox 齿轮箱rotor shaft 转轴generator 发电机yaw base 偏航基座、偏航盘yaw gear 偏航齿轮asynchronous generator 异步发电机turbine 涡轮hub 轮毂grid 电网blade 叶片diameter 直径area swept 扫掠面积speed of revolution 旋转速度operational interval 转速变化范围power regulation 功率调节air brake 空气制动cut-in speed 切入风速nominal wind speed 额定风速cut-out speed 切出风速stop wind speed 停止风速hub controller 轮毂控制器pitch cylinder 桨距调节气缸blade hub 叶片轮毂blade bearing 叶片轴承main shaft 主轴oil cooler 油冷却器parking brake 制动装置service crane 维护吊车transformer 变压器yaw 偏航revolution 旋转anemometer 测风仪vane 风向标planet/parallel axles 行星/平行轴microprocessor 微处理器pitch regulation 桨距调节synchronous 同步的asynchronous 异步的foundation 基础wind turbine generating 风力发电机组power performance 功率特性off-grid 离网grid connected 并网acoustic noise 噪声diameter 直径epoxy. 环氧的beam. 梁pitch angle. 桨距角perpendicular 正交的humidity 湿度operational interval 转速变化范围corrosion 腐蚀cable 电缆grid dropout 掉网earthing 接地terrain 地形tower shadow effect 塔影效应wind turbine wake 风轮机尾波vortexattack angle 攻角tip speed ratio 叶尖速比lift force 升力drag force (迎面)阻力thrust coefficient 推力系数stream tube theory 流管理论Rotor Disc Theory 转子盘理论blade element theory 叶片元理论(也有人称之为“叶片素理论”,或“叶素理论”) momentum theory 动量理论angular momentum theory 角动量理论abrasive研磨料abrasive disc磨料盘accumulator 储压罐acetone丙酮activation活动,赋活,激活,活化,激励,启用acute angle锐角adhesive 带粘性的, 胶粘, 粘合剂adjustable spanner 活动扳手admixture 混合, 混合物adversely逆地, 反对地adze扁斧aerial航空的, 生活在空气中的, 空气的, 高耸的,天线aerosol 浮质,气溶胶, 气雾剂, 烟雾剂aggregate 合计, 总计, 集合体aggressively 侵略地, 攻势地air inlet通风口air-cushion vehicle气垫船air gap气隙align对准,校直,定位;调,排列, 使结盟, 使成一行alkali-sensitive碱性感测allen key 六方allen wrench 六方扳手alloy 合金alteration变更, 改造alternator 交替符;交流发电机ammeter安培计,电流表anaerobic没有空气而能生活的, 厌氧性的anchor 锚, 抛锚, 锚定aneroid barometer无液气压表, 无液晴雨angle grinder角锉angle plate角盘annealing 退火annulus环面anode阳极, 正极anodization阳极氧化antenna 天线;触角antifriction减低或防止磨擦之物, 润滑剂anvil铁砧approximate近似, 接近, 约计adjarbor 树阴;凉亭;藤架〈机〉柄轴;心轴arc 弧, 弓形, 拱,电弧arc welding 电弧焊archimedean screw阿基米德螺线arrmature 电枢articulated接合,链接,有关节的assemble集合, 聚集, 装配assorted files分类排列;相匹配(文件)assume假定, 设想, 采取, 呈现attenuation变薄, 稀薄化, 变细, 衰减auger打孔钻,螺丝钻averagen. 平均, 平均水平, 平均数, 海损, 一般的, 通常的awln锥子axe斧, (经费的)大削减axle. 轮轴, 车轴Bback saw背锯backlash反冲,无效行程;间隙,偏移;退ball bearing滚珠轴ball saddle滚珠支撑ball-peen hammerband区;带,波段带子, 镶边, 波段, 队, 乐队. 联合, 结合bandsaw用带锯锯bar magnet磁条barometer气压计;晴雨表battery power drill电池钻beach海滨;湖滨;河滩bead 珠子, 水珠beam compass长臂圆规beam trammel骨架bearer 支架,托架,支座,载体bell crank曲柄belting制带的材料, 带类, 调带装置bessemer酸性转炉钢bessemer converter . 酸性转炉,贝塞麦转炉bevel gear斜角;斜齿轮bevel-edge shisel斜缘薄钢板biconcave lens两面凹镜biconvex lens两面凸镜bifurcated rivetbimetallic双金属的bin二进制;双态bit 位, 比特blade for iron saw剧刃blast 强风,过载blast furnace鼓风炉blast cleaning皮老虎bleed 放出(液体,气体等);漏出,漏入,泄漏,色料扩散bleeder valve 溢流阀block and tackle滑轮组blowlamp喷灯blowpipe吹风管blowtorch 吹管, 喷灯board 底板,板boiler锅炉;煮器;烧水器bolt螺栓,螺钉,支持,维持bone 骨剔除bonnet软帽,汽车发动机罩bore钻孔,钻bottom dead-centrebow-spring compassbox spanner管钳子box spanner insetbrac支柱, 带子, 振作精神bracket托架, 括弧, 支架brad曲头钉bradawl小锥Brakebrake lining闸, 刹车的衬里, 内层, 衬套brake shoe 闸轨branch of joint连接分支brass黄铜, 黄铜制品,brazing铜焊breast drillbreather 呼吸者, 喘息者, 剧烈的运动bricklayer's hammerbubble 磁泡,水泡,气泡bucket桶, 一桶的量, 铲斗Buffing wheelbulk大小, 体积, 大批, 大多数, 散装bulkhead隔壁, 防水壁bulldozer 推土机bunsen burner 本生灯burr芒刺;刺果植物;针球burstn. 突然破裂, 爆发, 脉冲bursting discbush 矮树丛, (机械)衬套bushing轴衬, 套管Ccabinet screw drivercable bundle 束,光纤束;捆,卷cable reel电缆盘cable shear电缆剪cable shoes电缆靴cable tie电缆带calibration标度, 刻度, 校准caliper测径器, 卡钳, 弯脚器cam凸轮camber 拱形camshaft凸轮轴cantilever伸臂,悬臂;悬臂梁cap帽,罩cap nut螺冒capaciron电容汞弧管capacitance电容;电容量capstan lathe绞盘车床carburettor 汽化器cardan shaft万向轴cartridge额盒式磁盘[带](机);夹头cast投;掷;抛casting. 铸件, 铸造castle nut城堡螺母catalyst 催化剂,触媒cathode 阴极cathode-ray tube阴极射线管catwalk桥上人行道, 狭小通道caulking 填...以防漏caution小心, 谨慎, 警告cement 水泥, 接合剂, 接合, 用水泥涂, 巩固, 粘牢center puncher中心冲centre bit中心位centrifugal离心centrifugal unit离心单元ceramics 陶瓷;陶瓷技术chain vice链式钳chain wheel 滑轮chain-grate stoker链条炉排加煤机change over 改变成,对调位chaser猎人, 驱逐舰cheese 干酪,垫砖cheese-head screw 有槽凸圆柱头螺钉chisel 凿子砍凿chloride氯化物,漂白粉choke窒息, 阻气门choke valve阻气阀chord弦, 和音, 情绪chrome 铬;铬矿石;氧化铬chuck. 轻拍, 抛掷, 驱逐, 丢弃, 用卡盘夹住circlip环形circular圆形,环;循环circular saw圆锯circulate 循环;流通circulating循环, 流通circumference周线;外围;周围clamp夹子, 夹具, 夹钳clamp ammeter钳形表claw hammer 拔钉锤clearancen清理,清除;出空,间空,间隔;距离cleat夹板clog填塞;塞满close grainedclout nail大帽钉club hammer锤子,榔头clutch离合器,联轴器coarse粗(糙,略),近似cobalt钴(符号为Co), 钴类颜料, 由钴制的深蓝色coil线圈,绕组coil spring弹圈collar 凸缘;圈,环,套环,轴;卡圈;安装环collar bolt凸缘螺栓combination结合, 联合, 合并, 化合, 化合物combination pliers台钳combustion chamber燃烧室compound 混合物, [化]化合物复合的, 混合, 配合compresso压缩物, 压缩机, 收缩肌comutator 换向器, 转接器concave len凹面镜concave-convex len凸凹镜concrete混凝土;具体concrete drill混凝土钻condenser冷凝器;凝结器,电容器conduit管道, 导管, 沟渠, 泉水, 喷泉cone数、物]锥形物, 圆锥体, (松树的)球果, 使成锥形cone and cup unionconical 圆锥的, 圆锥形的conjunction联合, 关联, 连接词connecting rod连接杆considerably 相当地consistency连结, 结合, 坚固性, 浓度, 密度, 一致性, 连贯性console 安慰, 藉慰,控制台container箱;罐;容器集装箱,货柜contaminate污染,弄污contrast使与...对比, 使与...对照, 和...形成对照, 对比, 对照, (对照中的) Controlconvection传送;运流;对流converter换流器;变换器;变流器convex lens凸透镜cooling冷却;冷却技术core sandcorrespond符合, 协调, 通信, 相当, 相应corrosion腐蚀,浸蚀cotter pincountersink bit装定位countersunk埋头孔, 暗钉眼countersunk rivetcountersunk-head screwcounting计算coupling bolt 联结, 接合, 耦合,耦合性,耦合技术coverage 覆盖;敷层;有效区域crack 裂纹,裂缝cramp 钳位(电路);压[夹板];卡子,夹(子);压[夹]紧crane起重机crank不稳定的,摇晃的,曲柄crankcase曲柄轴箱crankshaft曲轴;机轴criterion标准,判据,准则cross mark十字标记cross slotted screw十字长孔crosshead 小标题, 子题, [机]十字头, 丁字头cross-peen hammer 横头锤cross-section横断面;横切面;截面crosswise斜地, 成十字状地, 交叉地crowbar撬棍;铁棍;起货钩crown wheel 顶圈crucible坩锅, 严酷的考验cupola furnace园顶熔炉current ration电流定值customs 进口税, 海关cutter刀具, 切割机cutting disk切割盘cylinder block缸体cylinder head缸头cylinder-head gasket缸头垫片,垫圈;接合垫cylindrical圆柱形,圆柱体;柱面Ddampen使潮湿, 使沮丧damper风门;节气阀darwing board画图板deactivate释放;去激励;停用;退出工作;使无效debris碎片, 残骸decimal 十进的, 小数的,小数defective有缺陷的,欠缺的deflect (使)偏斜, (使)偏转deflection偏向;偏斜;转向deformation 变形,形变;畸变,失真degrease脱脂, 除油污degree celsius摄氏度deploy展开, 配置deposit 堆积物, 沉淀物, 存款, 押金, 保证金, 存放物depress使沮丧, 使消沉, 压下, 压低depression 沮丧, 消沉, 低气压, 低压depressurizes 使减压, 使降压depth gauge深度计detergent 清洁剂, 去垢剂deviation 偏差,偏移dial gauge量规dial micrometer千分尺dielectric 电介质, 绝缘体diestock螺丝攻differential gear差速齿轮differential protection差动保护diffuser 漫射体;(扬声器)纸盆;扩散器digger挖掘者挖掘机digital clock数字钟digitizing tablet数字面板dilute 冲淡, 变淡, 变弱, 稀释discharge 卸下, 放出, 清偿(债务), 履行(义务), 解雇, 开(炮), 放(枪), 射(箭). 卸货, 流注, 放电dismantle拆除,拆卸dismount 拆卸,卸下disposal 处理, 处置, 布置, 安排, 配置, 支配dissipation 分散, 浪费, 损耗,耗散,消耗distance ring间隔环distributor发行人,分电盘,配电器dividers圆规double phase两相dog clutchdolly洋娃娃,移动车,台车,,移动摄影车domed nut 圆顶螺母doubt不确定;疑惑dowel 木钉, 销子, 用暗销接合drain排水沟, 消耗, 排水drain tap排气阀draw bar绘图刀drawing pin图钉drawing point绘图点drift 漂移,偏差drill 训练, 钻孔, 条播, 钻头;锥子;钻孔机;钻床;钻drill gauge钻规driller 钻孔者, 钻孔机drilling machine 钻床drip pan油滴盘drop hammer落锤drum鼓, 鼓声drum brake鼓状刹车dryness干, 干燥duplex双(向,重),双工,二重durability 耐久性,耐用性duration宽度,持续时间dust 灰尘, 尘土, 尘埃dynamo 发电机dynamometer 测力计, 功率计Eearthmover 重型推土机eccentric古怪的;偏执的不同圆心的,离心的;不正圆的edge刀口, 利刃, 锋, 优势, 边缘, 优势, 尖锐elbow机械肘, 肘electric furnace电熔炉electric plier电气钳electric screw driver电钻electric welding电焊electricalelectrode 电极electrolysis 电解,电蚀electrolyte电解, 电解液electroplating 电镀, 电镀术eliminate消除,删去,排除;切断emery 金刚砂, 刚玉砂emery cloth砂布,金刚砂布emery wheel. 金刚砂旋转磨石, 砂轮endplate终板endwise末端朝前或向上的,向前的energizing使活跃, 给予精力, 加强, 给与...电压激励,赋能;接通epicyclic gear计数齿epoxy 环氧树脂epoxy-glued环氧胶equilateral triangle 等边三角形erection直立, 竖起, 建筑物exectric arc电弧exhaust pipe 排气管exhaust valve排气阀expanded metal膨胀金属expander扩展器,扩展电路,扩大器expansion bolt自攻螺丝explanatory quad填充铅块extension tube伸缩管extern外(面)的, 外来的external callipers外卡钳extrusion 挤压,挤压成形eye bolt吊耳eye screw螺丝眼Ffabrication 制造;生产;结构faceplate面板, 花盘facilitate使容易, 使便利, 推动, 帮助, 使容易, 促进fag bolt, 疲劳螺栓fake假货, 欺骗, 伪造, 赝造, 捏造, 假造, 仿造fan belt风扇皮带fan heate风扇加热器feeler gauge触规felling axe外轮轴fetch 接来, 取来, 带来, 售得, 引出, 吸引, 到达, 演绎出filament灯丝;细丝fire extinguisher灭火器firebrick 耐火砖fireman's axe消防斧firmer chisel . 凿子flange 边缘, 轮缘, 凸缘, 法兰flange coupling凸缘联轴器flanged nut凸缘螺母flanged union凸缘连接flank侧面, 军队侧翼, 侧腹, 胁flash welding 闪光焊flashlight 手电筒, 闪光灯flashpoint闪点flat 平坦的, 扁平的, 单调的, 倒下的, 浅的flat nut平螺母flat-head rivet平头铆钉flaw裂缝,缺陷,疵瑕flex弯曲(四肢), 伸缩, 折曲flexure 弯曲,挠曲float chamber浮子floating. 漂浮的, 浮动的, 移动的, 流动的, 不固定的flue烟洞, 烟道, 暖气管, 蓬松的东西flush刷新flux 磁通,通量;焊剂;流动, 熔化, 流出footpump脚泵forge炼炉;熔炉fork 派生(指令),分叉(指令),分支fork-lift truck叉架式运货车,铲车formation 构造,结构;形成,建立;形式forming印版foundry铸造, 翻砂, 铸工厂, 玻璃厂, 铸造厂foundation 基础, 根本, 建立, 创立, 地基,four-jaw chuck四爪卡盘four-stroke四冲程frame帧,画面;框架机架,架,机柜fret-saw线剧friction 摩擦, 摩擦力friction grip摩擦盘frictional摩擦的, 摩擦力的frost霜, 霜冻, 严寒, 结霜funnel 漏斗, 烟窗furnace炉子, 熔炉furnish 供应, 提供, 装备, 布置Ggale强风,大风galvanizing. 通电流于, 电镀gang saw 直锯gas burner. 煤气灶, 煤气火焰gas main. 煤气总管gas mask防毒面具gas pedal气体, 煤气, 毒气, 汽油, 瓦斯gas turbine 燃气涡轮gas well 天然气井gas works煤气厂gasket垫片,垫圈;接合垫gaskets垫片,垫圈;接合垫gasometer煤气厂, 气量计gate pole门极gate valve门阀gauge标准尺, 规格, 量规, 量表, 测量gavel 槌gear lever 变速杆gear train齿轮系gear wheel齿轮geiger counter盖格计数器gel-coated胶衣gimbals 平衡环,平衡架gimlet手钻;螺丝锥girder 梁, 钢桁的支架girder bridgegland腺,密封管glass cutter玻璃刀glaze釉料, 釉面, 光滑面, 上釉, 上光globe valve球形阀gloss注释;注解;评注, 光泽的表面, 光彩, 欺人的表面, 假象, gloss paint光滑涂料glossy 平滑的, 有光泽的glove手套glue胶, 胶水, 胶合, 粘贴, 粘合goggle 眼睛睁视, (复数)风镜, 护目镜gold leaf金叶goons细打包麻布gouge弧口凿,半圆凿governor 调节器;控制器grab 抢夺, 攫取, 夺取grader 分类机,分级机grain 颗粒,晶粒,粒度;纹理graph 图表, 曲线图grease油膏;润滑油grease gun注油枪;滑脂枪grease nipple油管grinding磨的, 磨擦的, 碾的grinding disk 磨擦盘grinding machine磨床grinding wheel 砂轮groove凹槽, 惯例, 最佳状态grout. 薄泥浆, 水泥浆grouting给…灌灰浆,给…涂薄胶泥grub screw自攻螺丝guide bars导向棍guide block导向块guide ring导向绳guiding shaft导向轴guillotine闸刀,处斩刑, 切(纸)gumming树胶分泌gyroscope 陀螺仪, 回旋装置, 回转仪, 纵舵调整器Hhacksaw可锯金属的弓形锯, 钢锯hairspring细弹簧, 游丝half shaft半轴half-round file半圆锉halfway中途的, 部分的, 不彻底的,半路地,在中途, 在半途handling 处理(技术,方法)hanging顶端对齐,悬挂hardener 固化剂,硬化剂harder硬的, 坚固的, (问题, 工作等)困难的, 艰苦的, 猛烈的, 确实的harness导线,装备;利用harrow耙hatch, 舱口, 舱口盖,开口,. 孵, 孵出,策划, 图谋hatchet. 短柄斧hazard 冒险(性);相关危险;事故,故障headscrew主轴螺杆headstock主轴箱helical gearing螺旋齿helix. 螺旋, 螺旋状物hemp 大麻, 纤维hereafter. 今后, 从此以后herring-bone gearhexagon screw diehexagon spanner六方hexagonal nut六角螺母hightensile 高强度high-tension cable高压电缆hissing发嘶嘶声, 蔑视hob滚刀, 铁架hoe 锄头,用锄耕地, 锄hoist升起;吊起;推起, 起重机, (台、架等)支持物, 固定器hollow空的,中空的hook bolt吊耳hook spanner 钩,弯脚扳手hook's jointhopper. 单足跳者horizontal地平线的, 水平的horseshoe magnet马蹄形磁铁hose 软管,胶皮管,蛇管hose clip管夹hot-dip热沾hottest. 热的,热烈的housing住房,房屋,护盖,框架hovercraft 水翼船hub rigidity轮毂刚度hubcap. 轮毂罩hull外壳, 船体humidity湿气, 潮湿, 湿度hydraulic水压的;液压的hydraulic block液压块hydraulic ram液压活塞hygrographs 自动湿度记录计hygrometer湿度计hysteresis. 滞后作用, [物]磁滞现象Ii-beamidler gear惰轮齿idler pulley惰轮,空转轮,导轮idling 空载,无载impeller 推进者, 叶轮impinge 撞击,冲击imprint 印记imprison监禁, 关押impulse推动, 刺激, 冲动, 推动力, 脉冲impurity杂质, 混杂物, 不洁, 不纯inductance 感应系数, 自感应,电感induction coil电感线圈inductive 诱导的, 感应的inflammable易燃的, 易怒的ingot条,块,锭initial 最初的, 词首的, 初始的initialize初始化initials缩写injector. 注射器injure 损害, 伤害inlet 进口, 入口inlet valve入口阀inner 内部的, 里面的, 内心的,内部inoperative不起作用的;无效的inspection 审查,检查instance 例图;事[实,范]例,样品,实例, 建议, 要求, 情况, 场合instantaneous瞬间的, 即刻的, 即时的,瞬时,立即insufficient不充足的,不适合的,不能胜任的insulation绝热;绝缘;隔离,绝缘体;绝缘材料intensive强烈的, 精深的, . 加强器interchange 互换,交换;交换机interlock连锁装置, 连锁internal callipers内卡钳internal-combustion engine内燃机inertia switch惯性开关interval 间隔,时间间隔,区间intervene干涉, 干预, 插入, 介入inverter反用换流器, 变极器, 反相器;"非"门irregularity 不规则, 无规律不规则性,不正则性irritation激怒;被激怒疼痛处isolating 孤立的,绝缘的isolation 隔绝, 孤立, 隔离, 绝缘, 离析isometric projection等边体isosceles triangle等边三角形Jjack 插孔,插口,插座;插头,接头;千斤顶jet 喷嘴;射流jib crane挺杆臂,转臂起重机jig 钻模;夹具;定位;模具jointing compound复合填料joist托梁,搁栅jubilee clipjumper 跨接线,跨接,跨[短]接片,跳接器,∏型短路线Kkeyhole sawkey-operate键盘操作keyway 键槽king dick spannerking pin大头钉kit成套工具, 用具包, 工具箱, 成套用具knob 按钮;调节器knuckle joint万向接头knurled nut凸螺母knurling多瘤的, 多节的Llabel 标签, 签条, 商标, 标志labyrinth迷路, 迷宫, 难解的事物ladder. 梯子, 阶梯ladle杓子, 长柄杓lager贮藏啤酒lagging绝缘层材料laminate 层压(制品),迭片,迭层板,绝缘板; 碾压lamp holder 灯座lapjoint搭接lap 盖板;搭接,重迭;研磨,抛光lapping compound研磨,抛光化合物large power drill大型钻latch 锁存器;门闩线路;凸轮;闩锁lathe 车床lathe tool车床工具layout 规划, 设计, 编排, 版面, 配线, 企划, 设计图案,布局图, 版面设计leaf spring叠簧leaflet 小叶, 传单leak漏洞, 漏出, 漏出物, 泄漏, 泄漏leakage 漏, 泄漏, 渗漏left-hand thread左旋螺纹lengthwise纵长的,纵向长的level水平, 水平面, 水准, 标准, 级别leverage杠杆作用liberal自由,丰富,充足lid. 盖子lifting提升light光, 日光, 发光体, 灯轻的, 发光的light metal spirit level光金属水平仪lighting conductor光导体limit gaugelining内衬,衬里link链环, 连结物, 火把, 链接, 连结, 联合lintel楣, 过梁lip. 嘴唇, 唇缘lithium saponify锂基脂litre 升local地方的, 当地的, 局部的, 乡土的,当地居民, 本地新闻, 慢车, 局部locate定位,位置location单元;位置,定位lock 加锁,锁(紧,定),封闭;自动跟踪locking nut自锁螺母locknut防松螺母,对开螺母locomotive 机车, 火车头log(运行)记录,(系统)日志logging saw伐木锯loom. 织布机, 织机, 隐现, 迫近lorry卡车, 铁路货车lost-head nail断头钉loudspeaker 扩音器, 喇叭lubricant润滑剂lubricate 润滑lug 接线柱;柄,把手;突起Mmachine tools 工作母机magnet 磁体, 磁铁magnetic 磁的, 有磁性的, 有吸引力的magnifier 放大器magnifying glass放大镜main主要部分, 体力, 力量, 大陆, 要点, 干线main spar主梁mallet木槌,球棍mandrel 半导体阴极金属心;心轴manhole人孔, 检修孔manner礼貌, 风格, 方式, 样式, 习惯manometer 流体压力计manometric. 压力计的, 用压力计测量的manual 手册,指南,人[手]工,手控,手动marine engine海用引擎masonry 石工术, 石匠职业masonry drill石钻masonry nail 水泥钉master征服,控制,精通master cylinder主液压缸mat 字模,垫块material 物质,材料;资料measureing scale测量刻度measuring 测量measuring tape卷尺mechanical机械的, 机械制的, 机械似的mechanical drawing机械图mechanism 机制;机械学,结构方式;机械装置;技巧;机械论medium媒体;介质;平均值;手段,方法;中(等);粗略,一般[M] membrane 薄膜;隔板;表层memory记忆, 记忆力, 回忆, 存储(器), 存储器,内存mention 提及, 说起mercury barometer 汞,水银气压计meshing接合;相合;啮合message 消息报文,电文;信息micrometer screw gauge螺旋测位器microprocessing unit 微处理器microscope显微镜microswitch 微动开关middle中间,中型;mill 碾磨, 磨细milling轧齿边milling machine 铣床mineral. 矿物, 矿石minimise 成极小,求最小值minor次要,局部,小minus 减(号);负missing 故障,损失,遗漏mitre block 斜接,斜面接合块mitre joint 斜接mitring machinemix 混合mixture混合, 混合物, 混合剂Monitoring监控mode方式, 模式, 样式, 时尚modem 调制解调器modification修改,改造,改变,变型,变体module 模数, 模块, 登月舱, 指令舱modulus模量,系数,模数moisture 湿气,湿度,潮湿molding塑造;铸型;造型塑造物;铸造物mole wrenchmomentary刹那间的;顷刻的;短暂的mortar 臼, 研钵, 灰泥mortise. 榫眼mortise chisel榫.Motor start马达启动moulding sand沙模multimeter 万用表,多用途计量器multi-meter万用表multiplication乘法, 增加, (动, 植的)繁殖, 增殖multiplier 增加者, 繁殖者,乘数, 增效器, 乘法器multiply 繁殖, 乘, 增加Nnacelle发动机舱,机舱nail指甲, 钉, 钉子needle file 针锉needle valve 针形阀, 针状活门negative 否定, 负数,阴性的;负极的network. 网络, 网状物, 广播网neutralization中立化, 中立状态, [化]中和noise噪声,噪音non-shrinking非收缩性normal正常,普通,标准nose cone. 火箭或飞弹之鼻锥体, 头锥体nozzle管口, 喷嘴nut 坚果, 螺母, 螺帽, 难解的问题nut tap自攻螺母nylon 尼龙Oobstruct 阻隔, 阻塞, 遮断(道路、通道等), 阻碍物, 障碍物obtuse angle 钝角obtuse-angled triangle钝角三角形occur 发生, 出现off-shore oil rig海上平台oil ringoil switch油开关oil well 油井oilstone油石oldham coupling欧氏联轴节on-site在场open ring wrench开口扳手open-hearth furnace平炉optimum最佳,最优orifice 孔, 口oscillation摆动, 振动outlet 输出;出口;引出线,电源插座output 产量, 输出, 输出量out yawing外偏航oval nail椭圆钉over speed过速overaging超龄的;老朽的;旧式的over current protection过电流保护overhead-valve engine 顶阀发动机,预置气门发动机overheat加热过度使发展过快,使过热overlap重叠,覆盖overload 超载,过载,过负荷overpressure过压overproduction生产过剩overspeed 超速over voltage过电压oxidation氧化[作用];氧化层oxyacetylene welding氧乙炔焊Ppackaging. 包装packaging 封装,组装packing 压[紧]缩;组装;包装,打包pad 焊点焊盘;填充,插入,拼凑篇幅;衰减器paint 上色,着色;涂料,油漆;paint brush漆刷paint roller. 漆滚筒, 辊子pallet扁平工具, 棘爪, 货盘panel pinpanel sawparallel 并行,并联,平行parallel connectionparallelogram平行四边形parallels平行线parameter 参数[量],变量parity奇偶particle粒子, 点, 极小量, 微粒, 质点pawl掣子;棘爪;制转杆peak 峰值,波峰pedal踏板;踏蹬pedestal 基架, 底座, 基础peg 钉, 栓, 桩, 销子pendulum 摆,振动体;钟摆perfect, 正确的, 熟练的, 精通的,完美的perform. 履行, 执行, 表演, 演出performance 性能,特性;实绩;【动】完成,实现,执行permanent永久的, 持久的permeate 弥漫, 渗透, 透过, 充满permissible 可允许的, 可容许程度的persistent持久性,不变personnel 人员, 职员perspective drawing透视图pestle and mortar 研杵petrol engine汽油机petroleum石油phase 阶段, 状态, 相, 相位phenomena 现象pick 抓[拾]取,挑,检,挑选,挑拣pile 堆积,堆,桩pile-driver打桩机pillar crane支柱起重机pilot 驾驶(飞机等), 领航, 引水pin 插针引脚,插头;引线;管脚pin puncherpin spannerpincers铁钳;钳子pinion 小齿轮pipe wrenchpiston活塞, 瓣piston ring. 活塞环pitch桨矩pitch angle桨矩角pivot枢;枢轴;支点plane平面, 飞机, 水平, 程度, 刨planet gear 行星齿轮planetary gear 行星planetary wheel行星架planimeter. 测面器, 求积计planing machine 刨床plano-concave lens 平凹镜plano-convex lens平凸镜plaster 石膏, 灰泥, 膏药, 橡皮膏plastic塑胶, 可塑体, 塑料制品, 整形plastic hammer塑胶榔头plate clutch 盘式离合器,联轴器platform)月台, 讲台, 讲坛平台platinum 白金, 铂plenty. 丰富, 大量pliers. 钳子, 老虎钳plug 插头;插塞, 插栓plumb line铅垂线pneumatic drill气[风]动钻polarization 偏振,极化polish 抛光,磨光polishing machine抛光机polyester 聚酯polymer聚合体;聚合物polymidwasherpoppet valve 提升阀pore 气孔,微孔port 端口,通信口,进出口position 位置,定位,部位;状态positive正(确;片,数,极),阳性,无条件,肯定potential-freepotentiometerrpour灌注, 倾泻, 涌入, 流power factor功率因数power hammerprecaution 预防, 警惕, 防范, 预防措施;预防方法precise 精确的, 准确的, 精确predetermine. 预定, 预先确定preserve 保护, 保持, 保存, 保藏press 压, 按, 印刷, 压力, 拥挤, 紧握, 新闻press plier压钳pressure 压, 压力, 电压, 压迫, 强制, 紧迫pressure gauge 压力计pressure switch压力开关pressurize增压, 密封, 使...加压prestressed concrete 钢筋混凝土pretension 借口, 要求, 主张, 自负, 骄傲prevailing流行,占优势prevent防止;预防priority 优先级,优先(权)prism 棱镜,棱柱(体);棱形procedure. 程序, 手续produce 提出, 出示, 生产, 制造, 结(果实),引起, 招致, 创作profile 剖面, 侧面, 外形, 轮廓prolong延长;伸展propeller螺旋桨;推进器propeller shaft桨轴property 财产, 所有物, 所有权, 性质,特性, (小)道具proportional dividers比列规protection保护protection glasses保护镜protocol 草案, 协议protractor量角器;分度规pulley 滑车, 滑轮pump泵,抽水机pump ability泵性能punch冲孔, 打孔punching machine冲孔, 打孔机punner夯,夯具purging清除,清理pyramid角锥、棱椎, 金字塔Qquality质量, 品质, 性质quantity 量,数量;大量quenching 淬火, 熄query 询问,查询;询问程序Rrace 竞争,追赶raceway水沟, (赛车用的)跑道rack and pinion机架,(框)架和小齿轮radiation 发散, 发光, 发热, 辐射, 放射,放射线, 放射物radius 半径, 范围, 辐射光线, 有效航程,范围, 界限rag. 抹布raised-head screwram 随机存储器rammer撞锤range 范围,(值,区)域;量[射]程rash鲁莽的;轻率的rasp. 粗锉, 锉磨ratch screw dieratchet 棘轮;棘爪ratchet handleratchet screwdriverratio of transformation 变换比rawhide hammerread out读出readjust重新调整, 再调整ready就绪;【修】准备好,已备ready-misedreamer 钻孔器, 刀, 铰床rear bearing后轴承rear lights 尾灯rebuild重构,重建receiver 接受者, 接收器, 收信机reciprocal互逆[反],可逆;倒数reciprocating engine往复式发动机recognition 承认, 重视, 公认, 赏识识别recognize认可, 承认, 公认, 赏识record 记录;录制recover 恢复,再现rectifier纠正者, 整顿者, 校正者, 整流器rectify 矫正, 调整, 整流,检波;(图形的)纠正;提纯,蒸馏,精炼reducer 缩减者, 减压器, 还原剂reduction gear 变形齿refill v. 再装满, 补充, 再充填n.新补充物, 替换物refining 精炼,提纯,细化refractory brickregion 区域,区,范围register记录, 登记簿, 登记, 注册, 寄存器reinforced concrete 钢筋混凝土reinitialisationreinstall 重新安装relation 关系;关系式relay 继电器,中继,(无线电)接力,转播relay circuit board继电器板release 释放;核发,发布;解除;(程序,软件)版(本)relevant相关,关联reliability 可靠性relief valve 安全阀remain. 保持, 逗留, 剩余, 残存remote control 遥控, 遥控装置, 遥控操作rendering 绘制;翻译;初涂;透视图repair 修理,修复replugreport 报表;报告representative典型;表示;代表rerticalreserve. 储备, 保存, 保留, 预定, 预约reservoir 容器;蓄水池,储藏处;贮存器reset 复位,(又称)重置;置"0",清除resettle重新定居residue 剩余;残数,留数;余式,余项, 残余物;残基;残渣resin树脂resist抗蚀剂;保护层restart重新启动result结果, 成效, 计算结果retain 保持, 保留retaining screwretraction收回reverberatory furnace反射炉revolution革命, 旋转revolution counter旋转计数器rheostat变阻器ribbed有罗纹的;有棱条纹的riffler 曲锉(用来雕模)rigging 索具, 绳索, 传动装置, 装备right angle直角right-angled screwdriver平口起子rigid硬,刚性;固定;严格ring环, 环形物, 环状, 铃声, 声调, 打电话ring circuit回路ring mainring renchring spanner环形扳子ripsaw粗齿锯rise上升, 增加, 上涨rivet铆钉,固定road rollerrocker arm摇臂rod杆, 棒rolled-steel joistroller滚轮(轴,筒),墨辊,印辊roller bearing 滚柱轴承rolling mill 轧钢厂rope 绳, 索, 绳索rotary旋转;循环rotary converterrotary engine旋转机rotating union旋转体rotor[route]①转子,电枢②转动体,旋转部(分),回(旋)转器③转[动]片,叶轮,round-head rivet铆钉round-head screw园头螺丝[钉];螺旋体routine 例行公事, 常规, 日常事务, 程序例程,例行程序;rubber 橡皮;橡胶rule 规则,法则;尺runway 飞机跑道, 斜坡跑道, 河道rust锈;衰退,迟钝rustling沙沙声rusty生锈的, 迟钝的, 腐蚀了的, 铁锈色的生了锈的saddle. 鞍, 鞍状物safety 安全, 保险, 安全设备, 保险装置sample. 标本, 样品, 例子sand blasting沙暴sander 沙, 沙子, [pl. ] 沙滩, 沙地sandingsandpaper砂纸sandwich夹层,层状[夹心]结构satisfactory符合要求的;合适的saturate. 使饱和, 浸透, 使充满saw 锯sawing machinescaffolding 脚手架scale 刻度,标度;比例尺,标尺;尺度,规模;【动】定标;换算;【WIN,NT】数值,缩放(比例scalene trangle不等边的(三角形)scarringscraper刮器,擦具;鞋刮子;刮刀,刮漆刀scratch 划痕,刻痕;【动】擦除;【修】临时screen 屏(幕,面),荧光屏;筛选;屏蔽;网screw螺丝钉, 螺旋, 螺杆, 螺孔, 螺旋桨,screw callipersscrew clampscrew driverscrew extractor拉马screw jack 螺旋千斤顶screw pitch gaugescrew tap 螺丝攻screw thread. 螺纹screwdriver 螺丝刀,起子scriber划线器, 描绘标记的用具scribing block划线笔sealing密封seam welding接合焊seat座, 座位, 所在地, 场所, 席位secger plierrsecure安全,可靠,保密securedsecurity 安全(性),保密(性),安全措施segregation分离,分凝法self-starter自动点火装置;自动启动器self-tapping screw自攻螺丝sensitivity 敏感, 灵敏(度), 灵敏性sensor 传感器,检测器;读出器,感测器;敏感元件sentsequence 顺序,时序;数列;定序,排序,序列series connectionservice 服务,服务程序;业务;维修,维护set screwset squaresetting设置[定]值;设置settle稳定,固定;调整,调度shackle 手铐, 脚镣, 桎梏, 束缚物shaft 轴, 杆状物shank 胫, 腿骨shaping machineshaping toolsharpness锐利shears 剪, 修剪, 剪切sheartubeshicknessshield屏蔽,罩;防护,庇护shim 垫片;填隙片shimming 摇动,震颤,晃动shock 冲击;震动shock absorber 减震器,震动吸收器short circuit. 短路shovel 铲, 铁铲shrink缩小,收缩shutdown 停止系统运行,停工[机],关机shutter 遮挡板,光闸,光阀;快门shuttle 梭;航天飞机,宇宙飞船,空间渡船;往返空间sidelight侧面射进来的光线, 侧灯,舷灯, 拾零, 杂闻, 间接说明side-valve enginesight glasssimultaneous同时的, 同时发生的sine wave正弦波single phase单相singnalsink 散热器,接收器,转接器;吸收(电流等);沟(半导体耗尽层的);汇点,收点;宿,汇集sintering烧结siphon 虹吸管site-mixedskew wind 歪斜,偏斜,扭斜[曲,动];相位偏移;变形,时滞(同一脉冲通过不同电路的时差);不齐,不齐量,偏离;【修】歪,斜,扭skin-halveskip 跳跃(进位);跳行[越,过];空指令slag. 矿渣, 炉渣, 火山岩渣sledge hammer大锤, 有压服力的东西sleeve 套筒,套管slide 滑动触头;【动】使滑动slide caliperrslide rule计算尺slide valve滑阀sliding滑动,活动;可调整slight 轻微的, 微小的slipping clutchslope 斜坡, 斜面, 倾斜slot 插槽,存储槽,槽口;开缝,存取窗口;裂缝[口],切口,沟;时间段片slow-running screwsmelting熔炼smooth平稳,平滑snips 剪断socket 插座[槽,孔,口];管座;套接字(报文包的);socket spanner套筒扳手soft cut in软切入software软件,软设备soldering 焊料,焊锡;焊接,结合soldering iron 烙铁solenoid螺线管solenoid valve电磁阀solitary单独,唯一solvent溶媒, 溶剂, 解决方法spacer. 取间隔的装置, 逆电流器,衬垫,衬套,衬片spade 铲, 铁锹spanner扳手;活络扳手;扳子sparking plug 火花塞spigot 插口;插头,塞子;(水)龙头spindle 主轴,心轴spinner 微调控制项spiral gear螺旋齿spirit level 水平仪spirit varnishsplash. 溅, 飞溅, 斑点spline方栓, 齿条, 止转楔, 花键splined shaftsplint夹板,托板split bearing分离轴承split pinspokeshave辐刀spot 斑点,点;地点;点焊spot welding点焊sprayer 喷出水沫者, 喷雾, 喷雾器sprig图钉spring 弹簧;【动】弹出spring balance弹簧秤spring washer弹垫spring-bow compasssprocket 链轮齿spur gear正齿轮spur wheel gear正齿轮square 平方;方块;【修】矩形[SQ],正方形的, 四方的,直角的, 正直的, 公平的, 结清的, 平方的, 彻底的square nut 四方螺母, 螺帽square thread矩形螺纹square-head bolt方头螺栓stabilizer稳定器;平衡器;安定剂stable稳定,稳态stage 级;阶段,程度;【动】登台,升级stain 着色,染色;着色剂;污点stainless 纯洁的, 无瑕疵的, 不锈的stall失速,使失速standard标准(型),规范,准则;【印】常身;正常体standing 直立的, 停滞的, 固定的, 常备的, 标准的, 常设的standstill静止状态;停顿star drillstart up 发动, 开动starter motor马达启动startup 启动steam engine 蒸汽机steam roller蒸汽压路机, 高压手段steam turbine 蒸汽轮机steel sheet scissorsteel wool钢丝线steering box舵盘steering gear方向齿steering wheel舵轮,方向盘step-down(up) transformer降压器stiffen 变粘, 变硬, 变猛烈stillson wrenchstipulate 规定, 保证stock 库存, 股票, 股份, 托盘, 祖先, 血统, 原料stoker 司炉, 烧炉工人, 加煤机stopcock管闩, 活塞, 活栓, 旋塞阀stoppage 中断, 填塞store 存储,存储器straight edge直刃strange陌生的, 生疏的, 前所未知的, 奇怪的, 奇异的, 不惯的strap 短接,跨接strap wrench绑扎带streak 条纹,拖尾strike 打, 打击, 罢工, 抓, 敲, 搏动, 打动, 穿透。
材料科学与基础英文词汇
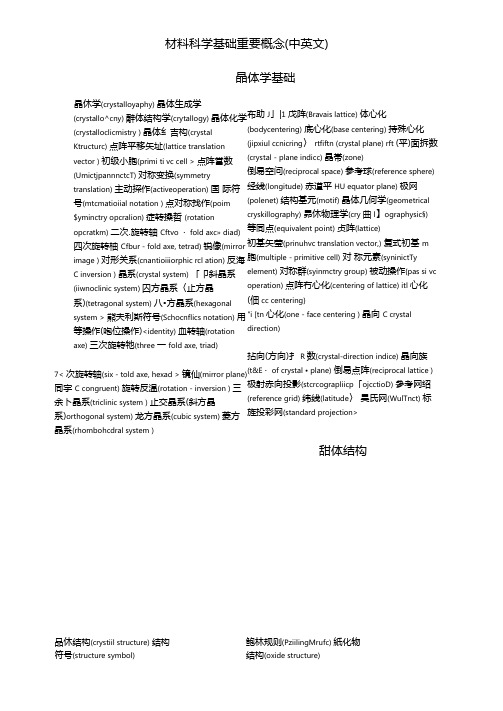
材料科学基础重要概念(中英文)晶体学基础布助J 」|1 戊阵(Bravais lattice) 体心化(bodycentering) 底心化(base centering) 持殊心化(jipxiul ccnicring 〉 rtfiftn (crystal plane) rft (平)面拆数(crystal - plane indicc) 晶帯(zone)倒易空问(reciprocal space) 参考球(reference sphere) 经线(longitude) 赤道平HU equator plane) 极网(polenet) 结构基元(motif) 晶体几何学(geometrical cryskillography) 昴休物理学(cry 曲l 】ographysic§)等同点(equivalent point) 贞阵(lattice)初基矢莹(prinuhvc translation vector,) 复式初基 m 胞(multiple - primitive cell) 对 称元素(syninictTyelement) 对称群(syinmctry group) 被动操作{pas si vcoperation) 点阵冇心化(centering of lattice) itl 心化(佃cc centering}"i [tn 心化(one - face centering ) 晶向 C crystaldirection)拈向(方向)扌R 数(crystal-direction indice) 晶向族(t&E ・ of crystal • plane) 倒易点阵(reciprocal lattice )极射赤向投影(stcrcograpliicp 「ojcctioD) 參考网绍(reference grid) 纬线(latitude 〉 昊氏网(WulTnct) 标旌投彩网(standard projection>甜体结构晶休学(crystalloyaphy) 晶体生成学(crystallo^cny) 辭体结构学(crytallogy) 晶体化学(crystalloclicmistry ) 晶体纟吉构(crystal Ktructurc) 点阵平移矢址(lattice translation vector ) 初级小胞(primi ti vc cell > 点阵當数(UmictjpannnctcT) 对称变换(symmetry translation) 主动探作(activeoperation) 国 际符号(mtcmatioiial notation ) 点对称找作(poim$yminctry opcralion) 症转操哲 (rotation opcratkm) 二次.旋转轴 Cftvo ・ fold axc» diad)四次旋转柚 Cfbur - fold axe, tetrad) 锐像(mirror image ) 对形关系(cnantioiiiorphic rcl ation) 反海 C inversion ) 晶系(crystal system) 「卩斜晶系(iiwnoclinic system) 囚方晶系〈止方晶系)(tetragonal system) 八•方晶系(hexagonalsystem > 熊夫利斯符号(Schocnflics notation) 用等操作(咆位操作)<identity) 血转轴(rotationaxe) 三次旋转牠(three 一 fold axe, triad)7< 次旋转轴(six - told axe, hexad > 镜仙(mirror plane)同宇 C congruent) 旋转反温(rotation - inversion ) 三余卜晶系(triclinic system ) 止交晶系(斜方晶系)orthogonal system) 龙方晶系(cubic system) 菱方晶系(rhombohcdral system )品休结构(crystiil structure) 结构符号(structure symbol)鲍林规则(PziilingMrufc) 紙化物结构(oxide structure)有序向溶休(超结构)[ordered solid solution 那晶(quadcrystal)(si^x:r lattice)]彭罗斯拼物(Penrose tiling)长程有宇参数(long-range order parameter) 短程有丹卷数(shot-Tangc order parameter)品体缺陷向 4ft (disclination)沃轻拉过程(M)lterraS process) 刃型位错(edge dislocation ) 嫖型位4ft (screwdislocation) 浪合型位箱(mixed dislocation) 柏氏冋路(Burgers circuit) 柏氏矢直:( Burgers vector) 位钳耳(dislocation loop) 位错密投(dislocation density) 位钳的孙性能(clastic energy of dislocation) 位错线张力(tension of dislocation)致密度(空问玻充效率)(efficiency of space filling) 配位数(coordination number} 配位多面体〈coordination polvhedra> 拓扑密堆相(ropologioally close - packed phase)金属晶休(meta 】 crystal) 离干晶体(ionic crystal) 共价晶休(cowulent crysUd) 分千晶体(molecularcrystal) 原干半径和离予半径(atomic radius and ionic rad: us)原干结构休积(volume odrurturc per storn) 体密疲(volumetric density,p v) 血巒度(plunw density, p P ) 綾密度(linear density; p L ) 金刚石结构(diamoiKlstructure) 纳米碳管(carbon nano tube) 轻换固溶休(substitutional solid solution ) 填陳向溶休(intcTStitiiil solid solution) 尺寸快I 素(sizedketor) 价电子浓度 C valanct electron concentration) 电子化合物(electron compound) 间隙化合物(interKtitial compound) 尺、JI 犬I 素化合物(Bizc~fhctwcQinfXHind 〉 Laves 相(Laws phase) 。
ELASTIC INTERFACES
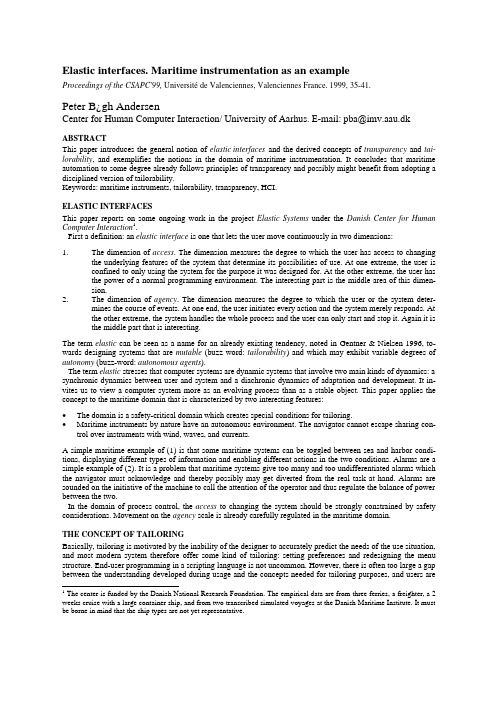
Elastic interfaces. Maritime instrumentation as an exampleProceedings of the CSAPC'99, Université de Valenciennes, Valenciennes France. 1999, 35-41.Peter Bøgh AndersenCenter for Human Computer Interaction/ University of Aarhus. E-mail: pba@imv.aau.dkABSTRACTThis paper introduces the general notion of elastic interfaces and the derived concepts of transparency and tai-lorability, and exemplifies the notions in the domain of maritime instrumentation. It concludes that maritime automation to some degree already follows principles of transparency and possibly might benefit from adopting a disciplined version of tailorability.Keywords: maritime instruments, tailorability, transparency, HCI.ELASTIC INTERFACESThis paper reports on some ongoing work in the project Elastic Systems under the Danish Center for Human Computer Interaction1.First a definition: an elastic interface is one that lets the user move continuously in two dimensions:1.The dimension of access. The dimension measures the degree to which the user has access to changingthe underlying features of the system that determine its possibilities of use. At one extreme, the user is confined to only using the system for the purpose it was designed for. At the other extreme, the user has the power of a normal programming environment. The interesting part is the middle area of this dimen-sion.2.The dimension of agency. The dimension measures the degree to which the user or the system deter-mines the course of events. At one end, the user initiates every action and the system merely responds. At the other extreme, the system handles the whole process and the user can only start and stop it. Again it is the middle part that is interesting.The term elastic can be seen as a name for an already existing tendency, noted in Gentner & Nielsen 1996, to-wards designing systems that are mutable (buzz word: tailorability) and which may exhibit variable degrees of autonomy (buzz-word: autonomous agents).The term elastic stresses that computer systems are dynamic systems that involve two main kinds of dynamics: a synchronic dynamics between user and system and a diachronic dynamics of adaptation and development. It in-vites us to view a computer system more as an evolving process than as a stable object. This paper applies the concept to the maritime domain that is characterized by two interesting features:•The domain is a safety-critical domain which creates special conditions for tailoring.•Maritime instruments by nature have an autonomous environment. The navigator cannot escape sharing con-trol over instruments with wind, waves, and currents.A simple maritime example of (1) is that some maritime systems can be toggled between sea and harbor condi-tions, displaying different types of information and enabling different actions in the two conditions. Alarms are a simple example of (2). It is a problem that maritime systems give too many and too undifferentiated alarms which the navigator must acknowledge and thereby possibly may get diverted from the real task at hand. Alarms are sounded on the initiative of the machine to call the attention of the operator and thus regulate the balance of power between the two.In the domain of process control, the access to changing the system should be strongly constrained by safety considerations. Movement on the agency scale is already carefully regulated in the maritime domain.THE CONCEPT OF TAILORINGBasically, tailoring is motivated by the inability of the designer to accurately predict the needs of the use situation, and most modern system therefore offer some kind of tailoring: setting preferences and redesigning the menu structure. End-user programming in a scripting language is not uncommon. However, there is often too large a gap between the understanding developed during usage and the concepts needed for tailoring purposes, and users are1 The center is funded by the Danish National Research Foundation. The empirical data are from three ferries, a freighter, a2 weeks cruise with a large container ship, and from two transcribed simulated voyages at the Danish Maritime Institute. It must be borne in mind that the ship types are not yet representative.often reluctant to accept this additional burden (Wasserschaff & Bentley 1997: 307). In Andersen 1997[1990]: 180 I suggested the following principle for tailorable systems:1.Experiences gained from using the system should be applicable for modifying and changing it.and later research in tailorability has emphasized the same point (Malone, T. W. K-Y. Lai & C. Fry. 1995: 178). In this paper I shall explore the following very simple and general principle:2.The geometry of tailorable artifacts should be self-similar.By self-similarity I mean the mathematical property that parts of the object are a scaled down version of the whole object (see Peitgen, Jürgens & Saupe 1992 for a good treatment of the concept and many examples). Self-similar objects have the property that if a part is enlarged it displays the same morphology as the whole object. Applied to computer systems it means that the internal structure of a module of the system is similar to the structure of the whole system. This enables experiences suitable for handling the whole system to be applicable for understanding the details of the parts too. Two specific criteria can be deduced from this. One pertains to understanding and one to acting:3.The Principle of Transparency: What goes on between two internal objects of the system is analogous towhat goes on between the user and the interface-objects.4.The Principle of Tailorability: Changing internal objects of the system is like changing the interface-objects of the system.WHY TRANSPARENCY AND TAILORABILITY?In this section I shall present two arguments for transparency and tailorability in systems for maritime navigation. The transparency argument concerns situations of malfunction of machinery, whereas the tailorability argument takes its point of departure in the fact that navigation is divided into phases and therefore causes the information needs to change.Transparency: Handling and Preventing MalfunctionsWhen I visited a modern ferry with integrated bridge and many useful systems for aiding the captain, the two navigators pulled me aside when I was leaving: they were quite satisfied with their equipment but they wanted to voice one worry: how do we know what is left when a component falls out?With the old equipment it was easy to figure out what was left if, for example, the GPS (Global Positioning System) stopped functioning. The GPS was an isolated instrument that showed the ship’s position, but had no interconnections with the other instruments. So the captain knew “what was left” when the GPS malfunctioned. This is much more difficult to assess in integrated bridges, where components deliver data to many other com-ponents. Thus, it is important that the user has a chance to understand the nature of malfunctions and figure out how the modules interact with each other.Even more important is the ability of acting in the case of malfunction. If the automatic steering system breaks down, the navigator should be able to easily revert to manual steering, because the crew cannot stop work because of an instrument failure, as the user of a safe PC can do.Prevention of malfunctions is another good reason for transparency. For example, in Maersk 1999 it is required that instruments and steering gear are regularly checked for correctness and functionality. If such control is to be possible at all, the workings of sensors and actuators must be understandable.But creating such understanding is no trivial problem: if the automatic systems are “strong, silent, clumsy, and difficult to direct” (Woods 1996: 6) they generate confusion and errors.Tailorability: PhasesA main argument for tailorability is the fact that navigation falls into distinct phases, each with their own infor-mation needs.There are three main phases: navigation in open waters, coastal waters and harbor2. The phases are clearly marked by different types of cooperation and information needs. For example, in open waters one officer can han-dle the navigation, whereas two to four may be needed in the two other cases.In one ship I visited there was the following sequence that reflects a decreasing curve of tension: (1) When things are difficult inside the harbor, the captain handles the steering advised by the pilot and helped by the chief officer. (2) Then the helmsman is called in and receives commands from the captain. (3) Outside the trafficked 2 In production plants, phases can be found e.g. in the start-up procedure.area, the pilot leaves, and the watch officer replaces the chief officer. (4) The helmsman leaves, and (5) in open waters (in the daytime and in clear weather) only the watch officer is on the bridge. The sequence 1⇒ 5 is used when leaving the harbor, whereas the reverse 5 ⇒ 1 is used during entry.Not only the work organization, butalso the place of work changes with thephases. During berthing, navigation canbe moved to the bridge wing so that thenavigator can see the ship’s side. Eachbridge wing must therefore contain acopy of the relevant displays and con-trols.Information needs change as well. Out-side the harbor, the radar is used to plotbearings and distances to other ships, butinside there may be too many vessels tooclose for this to be useful. In open wa-ters, the GPS information of latitude andcoastal waters. Inside a harbor, the importance of visual assessments ofdistances increases; some instruments increase their relevance as well,while others change their function. For example, drift and set become im-portant during berthing. The radar is now used for measuring the distanceto the quay, and the V(oyage) M(anagement) S(ystem) for assessing theposition of the ship in the basin. Other instruments become obsolete: forexample engine information about revolutions per minute (rpm)3, fins, andautopilot have nothing to do in the bridge wing that is specialized forberthing maneuvers. This is illustrated in Fig. 1. that displays the occur-rences of conversations dealing with distance and positions from a simu-lated voyage at the Danish Maritime Institute (see Andersen 1998b).With a metaphor, one may say that while navigating a ship is like drivinga train in open sea, it is more like riding a horse inside the harbor.These shifts of information needs are not reflected in instrumentation. Forexample, for some strange reason, many instruments give latitude and lon-gitude a prominent place in the display. In the conning display in Fig. 2, itoccupies the “best” place in the top half of the display. However, the vesselwas a ferry that always sailed in restricted waters and the numbers were never used (May 1999).In cases like this, one might suspect that some kind of tailoring of the interface would be appropriate, allowing the captain to select the information relevant for the phase he is in and give it a prominent place.This would not only benefit the person using the instruments, but also the other officers he is cooperating with since good bridge practice requires all to verbalize their actions so that everybody knows what goes on. This abil-ity is taught on courses, is encouraged in ship owner's guidelines (Maersk 1999) and can in fact be observed in good captains:(H, verbalizing)I am down on ninety nine, a hundred now, ninety nine, or ninety eight (E, volunteering information)We are to start the turn when we have the buoy to the northFor more examples, see Andersen 1998a, Section 2. This kind of discrete monitoring and helping out is not unique to maritime navigation but is probably common in all kinds of process control, cf. Heath & Luff 1992 that con-clude that in order for such mutual monitoring to occur, the size and location of the display is crucial.There is therefore reason to believe that giving the phase-relevant information a prominent position on the bridge may enhance the mutual monitoring and help.AGENCY AND ACCESS IN EXISTING TOOLSIn this section we shall see how the needs for transparency and tailorability are realized in existing instrumenta-tion.3 Although the exact rpm number is irrelevant, there is still a need for a more coarse engine feed back telling the captain whether his command is being executed: does the ship move in the right direction and do the revolutions go towards what he has ordered (e.g. full ahead).AgencyAgency is regulated in two ways, namely•Paradigmatic (either-or): Either the system or the user is doing the task, but not both at the same time. The system take over tasks which the navigator will perform under other circumstances.•Syntagmatic (both-and): Both system and user are active in performing the task, the system allowing the navigator to manipulate work objects of different granularity.Voyage Management Systems exemplify the paradigmatic type: they allow the navigator to draw a track the ship should follow, and when a plan is executing, the system replaces the navigator as the controller of the rudder. Integrated joysticks are examples of syntagmatic automation. On one ship, a joystick controlled the water-jets of the ship in unison, so that the navigator did not have to think about manipulating the individual jet, but could imagine that he manipulated the whole ship. The automation did not replace the navigator, but allowed him to work on a higher level of granularity.Because of its safety critical nature, maritime instrumentation is based on the principle that if higher level auto-mation falls out, there must always be a lower level that can be used. Steering is a good example. In one ship there were at least six following levels: VMS, Autopilot, Helm, N(on)F(ollow)U(p), Manual steering of rudder ma-chine, Physical movement of rudder by means of wires (Figs. 3-5). Similarly, instead of the integrated joystick the navigator could use individual sticks controlling the individual jets, and he could even manipulate their hydraulics directly.Fig. 3. VMS system on bridge Fig. 4. Autopilot on bridge Fig. 5. Manual steering of ruddermachine in engine room.Shifts of agency is carefully regulated in both instrumentation and operating guidelines.AccessWhereas shifts of agency play a major role in ship design, shifts of access are not so pronounced. However, elec-tronic charts can be tailored, so that only a selection of the available buoys, beacons, lights, depth contours, cau-tionary areas, and currents are displayed. The purpose is to avoid cluttering the chart. Another area is configuring instruments.USING TRANSPARENCY AND TAILORABILITY IN DESIGN OF MARITIME INSTRUMENTSIn this section I shall present some ideas for exploiting transparency and tailorability more systematically in the maritime domain.TransparencySome existing systems tend to conform partially to the principle of transparency. The higher level automation handles the lower level in the same way as the user does. For example, both VMS and user can input course or-ders to the autopilot, both autopilot and user can input rudder orders to the rudder system, and both rudder system and user can control the hydraulics of the rudder machine. Thus, the system is self-similar with respect to prag-matics, i.e. the user-system relation. I can understand the workings of the VMS in analogy to the way I myself interact with the autopilot.One could consider extending the pragmatic self-similarity to semantic self-similarity. This would mean that the internal system structure is based on the concepts the navigator uses when manipulating the whole system. The purpose is to make it easier for the user to move along the dimension of agency. Using action-based intentional concepts for process control has already been suggested in Lind 1990, 1994. In order to achieve semantic self-similarity, the action schemata must be extracted from the language pertaining to the usage of the instruments, andin the maritime domain this means maneuvers . The following schema is extracted from an analysis of typical ma-neuvers (Andersen 1998a).1.Goal: what we want to achieve, e.g. avoid collision with foreign ship.2.Means: actions that lead to the goal, e.g. using the rudder.3.Help: forces that enhance the means, e.g. exploiting the wind.4.Obstacles: forces that hinder the goal, e.g. low speed.5.Negative side-effects: negative state caused by the means, e.g. the turning moment produced during a crash stop achieved by putting the engine in reverse.6. Countermeasures: actions that aim at eliminating obstacles and negative side-effects, e.g. compensatingwith rudder in (5) or increasing speed in (4).For a system to be semantically self-similar its internal architecture must be based on concepts like these. If this is the case, the navigator can use his skills from maneuvering for understanding and repairing the system.TailorabilityWe cannot tailor collective, safety critical systems like a ship bridge in the same way as we do with our personal PC. Tailoring must be controlled, since it will not do that an officer has to look for the echo sounder in a new place each time he takes over the watch. Still, fixed and hardwired instruments do present problems for a work domain that is divided into phases. What is useful in one phase, is irrelevant for another. Furthermore, a panel that gives longitude and latitude a prominent position is well suited if the vessel is ocean-going, but becomes irrelevant if it is turned into a ferry.Here is a possible scenario: sensor signals are dissociated from individual displays, and the hardwired displays are replaced by a panel of screens that can be treated logically as one screen so that is possible to move interface objects from one screen to another. Some interface objects, such as the VMS and the radar, are produced by manufacturers, whereas others objects can be designed by ship-owner, captain, or officers according to a well-defined hierarchy of constraints. For example, maritime law will rule that some instruments are obligatory and must have a certain size.Judging from observations, the envisioned tool should offer facilities such as: moving and scaling objects, com-bining objects; changing mode of display. I conclude by two authentic ex-amples that illustrate this.1. An officer of the watch had forgotten that the rudder limit and R(ate)O(f) T(urn) limit were set and could not figure out why the wheel did notproduce the desired turning. The reason was that the ROT display and thelimits were represented in different places. In some autopilots limits areknobs whose position is difficult to see in the dark (see Fig. 4).After having heard the story, another officer produced on the spot Fig. 7 asa solution to the problem. In his redesign he combined four old displays into one: the rudder angle and ROT indicators, and the two knobs from theautopilot. The curved bars indicate the angles of the rudder and rate of turn,and the bullets the limits of both. By looking at the new display the officer ofthe watch can immediately see the correct cause of the failure.To realize this design, the officer needs facilities for changing the repre-sentation from the original digits to bars, and for combining four stand-aloneinstruments into one.2. A captain wants to use fuel most economically, i.e. to achieve most speed for the lowest fuel consumption. On the Maihak display in front of him (Fig. 8) he sees 3 numbers on a display:revolutions per minute (rpm), torque, and horse power.These numbers express the power produced by the engine. The captainwants to hit the most economical way of producing speed, so he wants tocompare the three numbers with the speed indicator placed some distanceaway.This is difficult two reasons: he must remember combinations of the fourfigures to hit upon the most economical one and his eye must move fromone place in the panel to another one. Therefore, instead of looking at thenumbers in front of him, he turns and follows the development of the rpm and speed on trend curves available on a screen behind him.The curves are better in two respects: the rpm and speed curves are drawnin the same diagram and therefore it is easy to compare them and see whenFig. 8. The Maihak display with rpm, torque and horse power.they increase in parallel and when speed starts to increase less than rpm. In addition, he does not need to remem-ber past values, because this is what trend curves do.To do this in reality the captain needs facilities for changing numbers into trend curves and combining two dis-plays (rpm and speed) into one.The examples are simple in the sense that they can be realized by changing representations and combining dis-plays. However, a tailorable bridge could also stimulate imagination of the officers and give rise to new displays that the landlubbers of the instrument manufacturers would never think of.REFERENCESANDERSEN, P. BØGH (1997 [1990]). A theory of computer semiotics. Semiotic approaches to construction and assessment of computer systems. Doctoral dissertation. Cambridge University Press.ANDERSEN, P. BØGH (1998a). Analysis of Maritime Operations II. Center for Human-Machine Interaction. Report CHMI-2-1998. http://www.chmi.dk/ANDERSEN, P. BØGH (1998b). Analysis of Maritime Operations III. Center for Human-Machine Interaction. Report CHMI-3-1998. http://www.chmi.dk/CARROLL, J. & M. ROSSON (1987). Paradox of the Active User. In J. Carroll (ed.): Interfacing Thought. Cambridge, MA: MIT Press, 80-111.DMI (1997). Manoeuver Theory.GENTNER, D. & J. NIELSEN (1996). The Anti-Mac Interface. CACM 8: 70 - 82.HEATH, C. & P. LUFF (1992). Collaboration and control. Crisis management and multimedia technology in London under-ground line control rooms. Computer Supported Work 1: 69-94.HUTCHINS, E. (1996). Cognition in the wild. Cambridge, Mass.: The MIT Press)LIND, M.(1990). Representing goals and functions of complex systems. Institute of Automatic Control Systems. DTU. 90-D-381.LIND, M. (1994). Modeling goals and functions of complex industrial plants. Applied Artificial Intelligence 8: 259-283. MACKAY, W. (1991). Triggers and Barriers to Customizing Software. In Proc. of CHI’91, New Orleans, Louisiana, 1991.New Work: The ACM Press, 153-160.MALONE, T. W. K-Y. LAI & C. FRY. (1995) Experiments with Oval: A Radically Tailorable Tool for Cooperative Work.ACM Transactions on Information Systems, 13/2: 177-205.MAY, M. (1999). Cognitive aspects of interface design and human-centered automation on the ship bridge: the example of ARPA/ECDIS integration. Presentation for PIC’99, Bath, June 21.23.MAERSK (1999). Guidelines for navigators. Maersk Vessels. Technical organisation, level 2 document. NAU610. Version April, 1999PEITGEN, H.O, H. JÜRGENS & D. SAUPE (1992). Chaos and fractals. Berlin: Springer-Verlag.WASSERSCHAFF, M. & R. BENTLEY (1997). Supporting Cooperation through Customisation: The Tviews Approach.Computer Supported Cooperative Work 6: 305-325.WOODS, D. D. (1996). Decomposing automation: apparent simplicity, real complexity. In: R. Paraduramen & M. Mouloua (eds.): Automation and human performance: theory and applications., 1-17. Mahwah, New Jersey: Erlbaum.。
名词索引——精选推荐
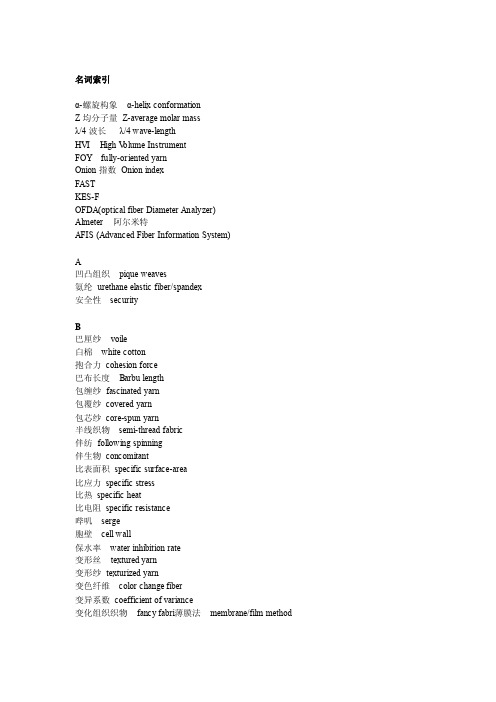
名词索引α-螺旋构象α-helix conformationZ均分子量Z-average molar massλ/4波长λ/4 wave-lengthHVI High V olume InstrumentFOY fully-oriented yarnOnion指数Onion indexFASTKES-FOFDA(optical fiber Diameter Analyzer)Almeter 阿尔米特AFIS (Advanced Fiber Information System)A凹凸组织pique weaves氨纶urethane elastic fiber/spandex安全性securityB巴厘纱voile白棉white cotton抱合力cohesion force巴布长度Barbu length包缠纱fascinated yarn包覆纱covered yarn包芯纱core-spun yarn半线织物semi-thread fabric伴纺following spinning伴生物concomitant比表面积specific surface-area比应力specific stress比热specific heat比电阻specific resistance哔叽serge胞壁cell wall保水率water inhibition rate变形丝textured yarn变形纱texturized yarn变色纤维color change fiber变异系数coefficient of variance变化组织织物fancy fabri薄膜法membrane/film method波长谱图wavelength spectrum波松分布poission distribution波松定律Poisson law波峰wave peak波谷valley波径指数wave-diameter index波动数petal number比表面积specific surface area表观厚度surface/apparent thickness表面结构surface structure表皮细胞cuticlar cell表面能surface energy表面积surface-area表面surface表面干爽性surface dry touch表面结构suface structure表面轮廓surface profile表面分析surface analysis表面性质surface character表面组份surface component表面比电阻surface apecific resistance表面散射光surface scattered light表层surface layer表面膜surface membrane表面张力surface tension表面能surface energy丙纶polypropylene fiber本色坯布greige cloth本构方程intrinsic equation编织物braided fabric编织加工braiding process编链组织chain stitch structure标准线性固体力学模型standard linear-solid model 并条drawing玻璃纤维glass fiber玻璃化温度glass-transition temperature玻璃态glassy state玻璃化转变glass transition倍克线Beckeline倍长纤维multiple length staple fiber不匀率irregularityC彩色棉color cotton草棉cotton长度length长绒棉long-staple cotton长丝纱filament yarn长丝织物filament yarn fabric长度分布length distribution长度界限length limit长度整齐度length evenness缠贴性knob & sticking property超细纤维ultra-fine fiber超细纤维superfine fiber超分子结构supermolecular structure超长纤维overlength staple fiber次表皮层exo-cuticle差别化纤维differential fiber差微摩擦效应differential friction effect 初生层primary layer初始模量initial modulus醋酯纤维acetate fiber成熟度maturity成熟程度maturity成型性formability/moldability成形性formability成熟度系数maturity coefficient成形性formation沉降弧sinker loop扯边纱条样法raveled- strip method产业用纺织品industrial textiles尺寸稳定性dimensional stability重组织backed weaves绸chou silk抽拔法pull-out method纯纺织物pure raw fabric纯纺纱线pure yarn刺激stimulate刺痒scratchiness刺扎stinging/prickle粗纺毛织物woolen cloth粗花呢fancy woolens粗糙度roughness/asperity/harshness触觉评价touch evaluation除杂cleaning除臭deodorization充满系数filling coefficient串套series loopD大衣呢overcoating大众呢popular cloth大麻hemp打成麻swingled hemp打结knot strength动物纤维animal fiber动态损耗模量dynamic loss modulus动态弹性模量dynamic elastic modulus动摩擦kinetic friction动态磨dynamic abrasion动压法dynamic pressure method涤纶polyester fiber导电纤维conductive fiber;electrically conductive fiber 导水wicking导热系数thermal conductivity导热系数thermal conductivity单缝撕裂(舌形撕裂)single-rip or single-tongue tear 低弹丝low- elastic yarn滴定法titration电阻electrical resistance电阻测湿moisture measurement of electrical resistance 电磁屏蔽纤维electromagnetism screen fiber电容式测湿electrical capacity-type moisture measure电磁屏蔽electromagnetism shielding电阻率resistivity电容式条干均匀度仪capacitance evenness tester电子织物electronic fabrics电磁辐射electromagnetism radiation点燃点ignite point点状spot adherence多孔结构geometric porosity多层多轴向针织物multiplayer multiaxial knitted fabric 多轴向经编针织物multi-axial warp knitted fabric带织物narrow fabric定点摩擦fixed-point friction定伸长弹性elasticity of constant elongation定负荷弹性elasticity of constant load定负荷constant load定应变constant strain灯心绒corduroy顶破性质bursting properties顶破强力bursting strength缎satin silks缎纹组织satin or sateen weaves缎纹变化组织satin derivative weaves等加负荷constant rate of loading等速伸长constant rate of elongation等长化纤cut-stable fiber断裂比功specific work of rupture断裂长度breaking length断裂功work of rupture断裂强度tenacity;breaking tenacity断裂强力breaking strength断裂伸长率extension at break ;tensility断裂应力breaking stress端毛羽end hair对称摩擦symmetric friction短纤维纱staple fibre yarn;staple yarns短纤维率short fiber contentE二醋酯纤维diacetate fiber二轴向斜交机织物biaxial bias-interlaced woven fabricF富强纤维polynosic rayon翻动磨tumble abrasion复合磨compound abrasion复合纱complex yarn;composite yarn复合纤维composite fiber复合纱composite yarn复杂组织织物compound-weave fabric复合织物compound fabric复模量complex modulus分束纺纱solospun缝纫线sewing thread副皮质para-cortex防紫外线纤维ultraviolet resistant fiber芳纶aramid fiber纺plain habutai纺丝spinning纺织纤维textile fiber纺丝成网法非织造布spun-laid nonwoven fabric仿麂皮织物suede fabric仿旧整理织物second-hand effect fabric防皱防缩整理crease防辐射纤维anti-radiation fiber resistant finish防霉整理mildew resistant finish防蛀整理insect-proof finish防污整理stain resistant finish防辐射整理radiation resistant finish防水性waterproof performance防火fire prevention防火织物fire-proof fabric防火服fire-fighting suit防护服protective clothing/suit防污anti-soil防霉mould proofing防蛀moth proofing防霉剂anti-mildew agent防血液感染blood-infectious protective防晒因子(SPF) Sun Protection Factor防紫外因子(UPF) UV Protection Factor放湿moisture liberation放湿过程moisture desorption process非洲棉African cotton非对称摩擦asymmetric friction非平衡态浸润nonequilibrium wetting非周期性不匀aperiodic unevenness非织造布nonwoven fabric非纤维类物质non-fiber substance飞数step number幅宽breadth/width浮线welt/float辅结构auxiliary/secondary structure附加不匀additional irregularity法兰绒flannel凡立丁valitin分等assortment, grading, ranking分子间结构the inter-molecule structure分子内结构the inner molecule structure分子结构molecular structure分解点温度decomposition temperature缝编法非织造布stitch-bonded nonwoven fabric 3 蜂巢组织brighton/honeycomb weave浮松组织huckback weaves负荷-伸长load - elongation curve府绸poplin辐射radiationG公制支数metric count干法成网非织造布dry-laid nonwoven fabric干法纺丝dry spinning干燥率drying rate干洗dry cleaning干洗剂dry-clean lotion干燥drying概率probability钢花呢homespun刚柔性stiffness and softness感官评定organolrptic evaluation各向异性anisotropic果实纤维fruit fiber构成constitution构型configuration构象conformation各向异性anisotropy高湿模量粘胶high wet modulus viscose高温高湿high temperature and humidity高频加热high-frequency heating高湿模量粘胶纤维high wet modulus rayon高收缩纤维high-shrinkage fiber高性能纤维high performance fiber高弹态high-elastic state高弹丝high-elastic yarn高能辐射high energy radiation股线plied yarn;ply yarn功能纤维functional fiber根数加权长度number weight length功能整理织物functional-finished fabric功系数work factor贡呢venetian公定回潮率official regain公制支数metric count光泽luster光电子条干均匀度仪photoelectric evenness tester 光纤optical fiber光敏变色纤维phototropic fiber光辐射light irradiation光致发光photoluminescence光程差opertical path difference工艺纤维technical fiber工艺要求technological requirements工业长丝industrial filament各向同性isotropy各向异性anisotropy勾丝snag/pulled thread勾接强度loop strength规格specification隔热性thermal insulation隔音sound insulation隔距gauge length葛poplin grosgrain刺割pierce耕犁作用plough古香缎suzhou brocadeH海军呢navy cloth含水率moisture content;percentage of water hold;moisture retention 含油率value of oilness汉密尔顿指数Hamilton index横向膨胀transverse swelling横向观察transverse observation横列course横向transverse厚度thickness后整理finishing后退角back angle化学老化chemical aging化学纤维chemical fiber化学短纤chemical short fiber化学溶解法chemical dissolution化学粘合法chemical bonding华达呢gabercord花呢fancy suiting花式线fancy or novel yarn滑脱长度slip length合成纤维synthetic fiber环锭纱ring spinning yarn灰棉gray cotton混纤丝combined filament yarn混纺纱线blended yarn混纺纱blended yarn混纺织物blended-spun-yarn fabric黄棉yellow cotton黄麻jute红麻red jute红外光谱法infrared spectroscopy /IR ;infrared absorption specturm红外光谱infrared absorption spectrum烘箱法oven drying method海岛棉Sea Island cotton横贡sateen横向形态transverse morphology虎木棉Toramomen缓弹性变形primary creep缓弹变形slow-elastic deformation / delayed deformation回复角recovery angle回弹性elastic resilience回潮率regain; moisture regain活泼率lively rateJ极化polarization极限氧指数limiting oxygon index/(LOI)极光效应polar lights effect极限不匀limit irregularity基原纤proto-fibril;elementary fibril急弹性变形immediate elastic deformatio基本风格basic style剪切变形shear deformation剪切刚度shearing rigidity家用纺织品household textiles降解degradation结构纱construction yarn结构系数structural factor接触角法contact angle method截面加权长度area-weight length聚集态state of aggregation聚合度degree of polymerization聚酯纤维polyester fibre聚酰胺纤维polyamide fibre/nylon聚合物挤出成网法非织造布polymer extruded nonwoven fabric 聚乳酸纤维polylactic fiber拒水water repellent拒水整理water repellent finish拒油整理oil-repellent finish间接吸收indirect absorption间接测试indirect measure巨原纤macrofibril集聚纺纱compact yarn加捻twisting卷曲crimp卷绕winding卷曲数crimp number卷曲比crimp ratio卷曲率crimp ratio结晶区crystalline zone结晶度degree of crystallinity近程short range静摩擦static friction静电电位序列static potential sequence静电纺electrostatic spinning静电electrostatic静压法hydrostatic pressure test锦brocades锦纶polyamide腈纶acrylic fiber ,polyacrylonitrile fiber精纺毛织物worsted cloth槿麻Ambari hemp经编织物warp knitted fabric金属纤维metallic fiber浸液法tincture/immersion test浸渍法非织造布impregnating bonded nonwoven fabric 浸润immersional wetting浸润滞后性wetting hysteresis浸渍法impregnation均匀系数wave evenness交织织物mixed fabric绞盘法capstan method机织物woven fabric机织纱weaving yarn加压厚度thickness under pressure交叉intersecting交织规律intersecting pattern交织角weaving angle结构厚度structure thickness结构相structural phase紧度tightness经编针织物warp-knitting fabric经缎组织lined satin经密end spacing/ warp sett经平组织locknit stitch / charmeuse纠缠kink/ entanglement卷边性curling热机械变形thermo-mechanical deformation机械加固法mechanical reinforcing机械成网非织造布mechanical-laid nonwoven fabric 机械波不匀mechanical wave unevenness锯齿棉saw ginned cotton绢纺silk spinning茧cocoon茧丝bave茧丝率bave yield茧丝长bave length接触角contact angle剑麻agave蕉麻Manila hemp剑杆引纬rapier wefting交联crosslinking镜面反射mirro reflection介电常数dielectric constant;relative permittivity 介电损耗dielectric loss介电损耗因子dielectric loss factor介电损耗正切dielectric loss tangent绢taffeta几何转移geometric migration解捻法untwist method解捻detwisting径向分布radial distribution绝热率heat insulation rate激光细度仪Sirolan-Laser scanK卡其khaki drill开松opening抗熔孔性melting-resistant抗静电整理antistatic finish抗静电纤维antistatic fiber抗起毛起球整理anti-pilling tendency finish抗静电性antistatic property抗菌防臭纤维anti-bacterial fiber抗皱性wrinkle resistance抗弯长度bending length抗刺割性pierce resistance抗切割度degree of pierce resistance抗菌antibacterial抗微生物antimicrobial防病毒anti-virus抗螨anti-acarid宽度width宽幅织物broad fabric矿物纤维mineral fiber克罗值CLO value客观评价objiective evaluation孔隙porosity空隙voidage/porosity空隙率porosity可燃性ignitability; combustibility可靠性reliability可加工性machinability可纺性spinnability可缝性sewability可变形性texturing property客观检验objective testing客观评价objective evaluation空气变形air texture空间厚度geometric thickness跨距长度space-length扩展extend/spread扩展速度spreading rate扩展比expansion ratio扩展取向度orientation in expansion抗静电处理antistatic treatment抗皱anti creaseL拉伸曲线tensile curve拉伸试验tensile test拉伸性能tensile property拉伸疲劳tensile fatique罗leno silk罗布麻apocynum落锤法撕裂falling pendulum tear骆驼绒camel down氯纶polyvinyl chloride fiber两型毛heterotypical fiber陆地棉uplands cotton力学模型mechanical model力学性质mechanical property立体织物cubic fabric立体机织物cubic woven fabric立体针织物cubic knitted fabric立体编织物cubic braided fabric立体非织造布cubic nonwoven fabric利萨如曲线Lissajou curve联合组织织物combined-weave fabric绫twills棱脊strip edge临界捻系数critical twist factor;critical twist coefficient帘子线cord, cord yarn龙卷风形cyclone-like罗纹组织ribbed stitch螺旋helix罗拉法roller measurement链节chain unit链段chain segment ;segment链节chain unit流动温度flow temperature磷光phosphorescenceM马海毛mohair马克隆值macronaire value马丁代尔Martindale马丁代尔公式Martindale formula麻纱织物dimity麦尔登melton美感系数aesthetic perception coefficient美感指数aesthetic index毛织物wool fabric毛巾组织terry weaves毛型纱线wool type yarn毛羽hairiness;hair毛细扩展capillary expanding毛丛wool staple漫反射diffusion reflection棉织物cotton fabric膜裂法非织造布film-splitting nonwoven fabric 膜结构用film-structure摩擦friction豪特长度Hauteur length摩擦性能friction property摩擦系数frictional coefficient磨损abrasion磨损性wear-resistance磨毛整理织物sanded fabric)密度density/ specific mass膜状membrane/film adherence棉哔叽cotton serge绵绸noil cloth毛纤维wool fiber毛细现象capillary phenomenon毛羽hair毛羽指数hair index绵羊绒sheep cashmere牦牛绒yak cashmere木棉KapokN纳米尺度nanoscale呢crepons粘胶viscose,rayon粘均分子量viscosity-average molar mass粘弹性viscoelastic property粘附adhere/adhesion粘结cohere/caking粘着功adhesion work粘滑现象stick slip phenomenon粘流态viscous-flow state耐热性heat endurance;heat-resistant耐久性durability耐刺割性stab-resistant and cut-resistant property 牛津纺oxford女式呢ladies cloth捻度twist捻幅twisting length捻回角twist angle捻缩twist shrinkage捻缩率percentage of twist shrinkage捻系数twist factor ;twist multiplier捻向direction of twist内不匀inner unevenness内表皮层endo-cuticle内旋转internal rotation内部反射光inner reflected light扭转性质torisonal property暖体假人thermal manikinP派力司palace泡泡纱crimp fabric排列密度packing density喷淋法spraying method膨胀dilatation/expansion /swelling匹长piece length破斜纹broken twill片梭引纬projectile wefting片晶lamellar crystal片段长度segment length泡沫法非织造布foam-bonded nonwoven fabric喷水引纬water-jet wefting喷气引纬air-jet wefting喷洒法非织造布spray-bonded nonwoven fabric 疲劳性质fatique property疲劳寿命fatique lifetime疲劳fatigue疲劳极限fatigue endurance limit漂白织物bleached fabric平磨flat abrasion平方米重weight per square meter平纹组织plain weave平行纤网parallel fiber web平布plain cloth平纹组plain weave平纹变化组织plain derivative weaves平衡回潮率equilibrium regain平衡态浸润equilibrium wetting平面偏振plane polarization平均长度mean length品质quality品质评定quality evaluation品质支数quality number品质要素quality factor普通粘胶纤维Rayon皮棍棉roller ginned cotton皮质细胞cortex cell皮芯Skin-core铺展spreading铺展系数spreading factor频数frequencyQ气流成网非织造布air-laid nonwoven fabric气相色谱法chromatography of gasses/GC气流仪air-flow meter起毛fuzzing起球pilling起毛组织pile weaves起毛起球fuzzing and pilling起拱变形bulging deformation乔其纱crepe georgette枪毛over hair前进角forward angle强化点strengthening point强力利用率strength efficiency强伸性strength and elongation properties切片skiving, sectioning切割条样法cut-strip method切割cutting切割系数cutting factor亲水基团hydrophilic group清花scotching, picking, blowing屈服点yield point屈曲波幅crimp amplitude屈曲波高crimp height曲磨flex abrasion取向orientation取向度orientation degree取向因子orientation factor去污soil removal牵伸drafting, drawing牵伸波不匀draft wave irregularity圈毛羽loop hair圈捻loop twist圈干needle loop圈高course spacing圈距lay/ knit stitchR燃烧温度combustion/burn temperature燃烧时间combustion/inflammation time燃烧性inflammability燃烧特征burning characters热传导thermal transmittance热定形thermosetting热变形thermo texturing热塑性thermo-plasticity热收缩thermo shrinkage热稳定性thermal-stability热风(烘)粘合法非织造布hot-air bonded nonwoven fabric 热粘合法thermo-bonded nonwoven热流变形纱thermal flow textured yarns热轧法非织造布calender-bonded nonwoven fabric热敏变色纤维thermochromatic fiber热粘合heat bonded热塑性thermo-plasticity热定形heat setting热湿舒适thermalhydro comfort热舒适性thermal comfortability热阻thermal resistance热稳定性thermal stability绒面组织suede/face/velours stitch柔软性softness柔软softness柔软剂softening agnet染色织物dyed fabric染色牢度color fastness绒velvet熔喷法非织造布melt-blown nonwoven fabric人造纤维man-made fiber人体组织工程human histology engineering韧皮纤维bast fiber熔融温度melt temperature熔融纺丝法(熔体纺丝)melt spinning溶液纺丝法solution spinning蠕变creep润湿wetting弱环定律weak-link theoryS啥味呢worsted flannel三轴向机织物triaxial woven fabric三轴织机triaxial loom三维立体结构three-dimensional cubic d structure 三维编织结构three-dimensional braid structure三维空间three dimensional space山形斜纹angled/reverse twill伸缩性dilation/ expand and contract生理学physiology生物防护biology protection生丝raw silk生物相容性biocompatibility生物可降解biodegradability声强acoustics intensity实体厚度substance/entity thickness实际回潮率actual regain;measured moisture regain 时间依赖性time dependence使用寿命life time湿冷clammy湿膨胀hygral expansion湿法纺丝wet spinning湿法非织造布wet-laid nonwoven fabric石棉asbestos视觉评价visual evaluation手感handle手扯长度hand staple松弛收缩relaxation shrinkage缩水shrinkage塑性变形plastic deformation双反面组织purl stitch双罗纹组织interlock stitch双轴拉伸double direction tensile tester水刺固结water needle binding水解hydrolysis随机排列random arrangement梳片法comb sorter双幅织物double-width fabric双层组织double-layer weaves双宫绸doupioni pongee双边分布bilateral distrubiton双折射birefringence双折射率specific birefringence萨那蒙补偿senamönt compensation闪光效应glitter effect散射scattering色织物yarn-dyed fabric纱gauze silk纱线yarn, thread纱织物single fabric纱罗组织gauze and leno weaves纱疵yarn defects/faults纱线断裂机理breakage mechanism of yarn 纱线捻度yarn multiplier纱线识别yarn identification纱线品质yarn quality纱线强力利用率strength efficiency for yarn 纱线交织阻力yarn interwave resistance伸直长度straight length收缩因素shrinking facto收缩不匀shrinkage unevenness随机不匀random unevenness山羊毛Cashmere hair山羊绒cashmere桑蚕丝mulberry silk死毛kempy wool撕裂性能tearing property撕裂强力tearing strength撕裂三角区delta region of tearing三醋酯纤维triacetate fiber丝纤维silk fiber丝织物silk fabric丝光棉mercerized cotton缫丝率silk reeling yield射流喷网法非织造布spun-laced nonwoven fabric手感目测handle and eyeballing;handle and visual measurement 舒适感comfortability数均分子量number-average molar mass髓质层medulla束纤维fiber bundle束纤维强力strength of fiber bundle塑性变形plastic deformation锁结lockingT太阳能服装solar energy textile天然纤维natural fiber陶瓷纤维ceramic fiber兔毛cony hair天丝tencel弹性纤维elastic fiber弹性结构elastic structure弹性回复elastic recovery弹性模量Y oung's modulus弹性回复率elastic recovery弹性elastic performance碳纤维fibrous carbon特种动物纤维Special animal wool特征鉴别characteristic identification特克斯tex退捻加捻法twist and untwist method炭化毛carbonized wool体积比电阻specific volume resistance体积分数volume fraction梯形撕裂trapezoid tear土工织物geo-textile贴服系数fitting factor团块状agglomerate脱散性ladderation/raveling property绨bengaline条格组织stripe and check weaves调湿conditioning透孔组织openwork weaves透光性transmission property透气率permeating rate透气性permeability透湿汽性moisture vapor transimi7透湿指数moisture permeability index透水性water permeability透通性transparent涂层coating涂层织物coating fabric凸条组织Bedford cord weavesW维纶vinylon,polyvinyl alcohol fiber纬编织物weft-knitted fabric纬编针织物weft-knitting fabric纬密pick/weft/filling density纬平组织weft plain stitch纹面组织face weave伪浸润pseudo-wetting微波microwave微波加热microwave heating微原纤microfibril微尺度micro-scale微环境microenviroment微气候microclimate外表皮层epi-cuticle外观指标index of aspect外观疵点outer defect外不匀external irregularity弯曲bending弯曲刚度flexural rigidity ;bending rigidity 弯曲疲劳bending fatique弯曲长度bending length弯曲模量bending modulus无机纤维inorganic fiber无序区amorphous region无定形disorder无规线团random coil网目组织distorted thread effect weaves纹织物jacquard-weave fabric温度temperature物理指标index of physics熨烫ironingX细绒棉medium cotton细布fine plain细胞间质CMC/cell membrane complex细度不匀fineness unevenness吸水吸湿纤维absorbency fiber吸附absorption吸收水absorption water吸湿性hydroscopicity吸湿指标adsorption index吸湿等湿等压线adsorption iso-humidity and isobar line 吸湿等温线adsorption isotherm吸湿剂moisture adsorbent吸湿微分热differential heat of sorption吸湿滞后hydroscopic hysteresis吸湿平衡equilibrium absorption of water吸湿膨胀hygroscopic expasion吸湿积分热integral heat of sorption吸湿法moisture adsorption吸湿absorbing moisture吸湿机理moisture sorption mechanism吸湿过程moisture sorption process吸声sound absorption系统鉴别systematical identification洗涤washing洗涤标志washing symbols纤维fiber纤维塞fiber plug纤维疵点defect of fiber纤维材料fibrous material纤维表面fiber surface纤维鉴别fiber identification纤维网fiber web/sheet纤维强力利用率strength efficiency for fiber纤维集合体fiber assembly纤维细度fineness纤维形态fiber morphology纤度size/titer线圈stitch/ coil/loop线圈长度stitch/loop length线圈歪斜loop spirality相系数phase coefficient斜纹组织twill weave显微镜观察法microscopic observation显微观察microscopic examination香味纤维perfumed fiber相变纤维phase-change fiber相位差phase difference相对湿度relative humidity相变材料phase change materials蓄热纤维Thermal storage fiber洗净毛clean wool洗涤剂washing agent蓄热纤维thermal storage fiber须丛曲线tuft curve小幅织物narrow-width fabric小提花组织Dobby design weaves绡sheer silks线织物ply-yarn fabric线密度linear density斜纹组twill weave斜纹变化组织twill derivative weaves 芯吸wicking芯吸高度wicking height心理学psychology性状behaviour and profile;property and morphology形态morphology/contour形貌morphology形状记忆shape memory形态结构morphological structure形状系数shape factor悬垂系数drape coefficient悬垂度drape factor学生呢student cloth循环次数cycle indexY亚麻flax亚麻织物flax亚洲棉Asiatic cotton压缩性质compressional property压缩性能compression property轧工质量quality of roll颜色color药品着色法staining test应力松弛stress relaxation应力-应变stress – stain隐身织物stealthy fabric荧光fluorescence叶纤维leaf fiber羊驼绒alpaca异形prifiled异形纤维profile fiber异纤维foreign fiber易护理easy care易去污soil release一端整齐alignment end远红外纤维far-infrared fiber远程long range衣分ginning outturn衣用纺织品apparel fabric翼形撕裂wing tear印花织物printed fabric印花粘合法非织造布print-bonded nonwoven fabric 延展线underlap/link英制支数English count液态水liquid water原纤fibril原组织织物elementary-weave fabric缨状微胞fringed micelle缨状片晶fringed lamellar crystal缨状原纤fringed fibril预调湿pre-conditioningZ主体长度major length杂质impurity造型系数multiwave折皱回复角crease recovery angle折边磨fold abrasion植物纤维plant fiber滞后常数delayed constant种子纤维seed fiber再生纤维regenerated fiber再生纤维素纤推regenerated cellulose fiber再生蛋白质纤维regenerated protein fiber再生淀粉纤维regenerated starch fiber再生角蛋白regenerated keratin再生丝蛋白regenerated silk fibroin胀破break in distention抓样法grasp method针编弧needle loop/ top arc针刺固结needling binding针织物knitting fabric纵行wale纵向longitudinal毡缩性felting shrinfage毡化felting中空纤维hollow fibre纵向形态longitudinal morphology纵向观察longitudinal observation苎麻ramie竹纤维bamboo fiber阻燃纤维flame retardant fiber转基因棉transgenic cotton转曲convolution/twist柞蚕丝tussah silk蜘蛛丝spider silk转曲convolution窄幅织物narrow fabric折皱整理织物crease-resist finished fabric折射率refraction index质量比电阻mass specific resistance质量要素element of quality质谱法mass spectrometry针刺法非织造布needle-punched nonwoven fabric 针织成型产品shaped knitwear针织坯布gray knits针织物knitted fabric针织物的歪斜spirality property of knitted fabric针织物的卷边lapel of knitted fabric蒸发法evaporation test智能防护intelligent protection智能纺织品材料intelligent/smart textiles制服呢uniform cloth织物fabric织锦缎satin brocade织缩率crimp contraction/percentage织物组织fabric weave织物的纰裂unwoven of fabric织物的耐疲劳性fabric fatigue-proof织物的耐老化性fabric resistance to aging织物的钩丝性fabric snagging property织物的耐磨损性fabric abrasion resistance织物的耐久性fabric durability织物的抗皱性fabric wrinkle resistance织物的褶裥保持性pleat retention of fabric织物的静态悬垂性fabric static drape织物的起球性fabric pilling property织物风格fabric style织物指纹图fabric fingerprint织物控制图fabric contral chart织物手感fabric handle织物保养fabric care织物要素fabric elements织物纹理fabric grain织物的尺寸稳定性fabric dimensional stability 织物的缩水性fabric water shrinkage property 织物结构fabric structure织物鉴别fabric identification张力转移tension distribution直接吸收direct absorption直贡twilled satin直接测量direct measurement直径diameter重量偏差weight deviation重均分子量weight-average molar mass直方图histogram直径不匀diameter unevenness中段切断称重法cut-weight measurement重量加权长度mass-weight length周期性不匀periodic irregularity转移migration绉crepes绉组织crepe weaves苎麻织物ramie正皮质ortho- cortex正切损耗loss tangent正反射光main reflected light自然长度natural length转曲conversion真空干燥vacuum drying主观评价subjiective evaluation噪音noise自适应self adapted紫外线ultraviolet radiation组织点interlacing/crossing point组织循环repetition of weave组合原位测量composite-in situ measurement 阻燃整理flame retardant finish阻燃flame retardant阻燃整理flame-retardant finish阻燃剂flame-retardant agent阻燃涂层flame-retardant coating阻燃纤维flame-retardant fiber。
弹性力学专业英语词汇-elasticity
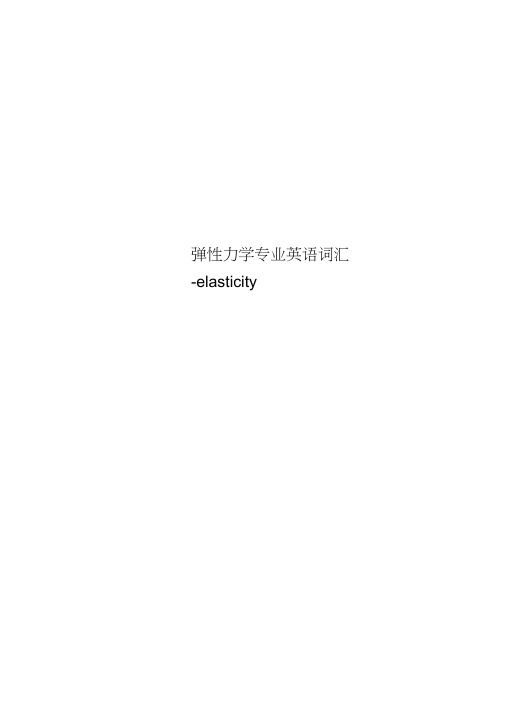
弹性力学专业英语词汇-elasticity弹性力学elasticity弹性理论theory of elasticity均匀应力状态homogeneous state of stress应力不变量stress invariant应变不变量strain invariant应变椭球strain ellipsoid均匀应变状态homogeneous state of strain应变协调方程equation of strain compatibility拉梅常量Lame constants各向同性弹性isotropic elasticity旋转圆盘rotating circular disk楔wedge开尔文问题Kelvin problem布西内斯克问题Boussinesq problem 艾里应力函数Airy stress function克罗索夫■穆斯赫利什维利法Kolosoff-Muskhelishvili method基尔霍夫假设Kirchhoff hypothesis 板Plate矩形板Rectangular plate板Circular plate环板Annular plate波纹板Corrugated plate 加劲板Stiffened plate,reinforced Plate 中厚板Plate of moderate thickness 弯[曲]应力函数Stress fun ctio n of ben di ng 壳Shell 扁壳Shallow shell 旋转壳Revolutionary shell 球壳Spherical shell[圆]柱壳Cylindrical shell 锥壳Coni cal shell 环壳Toroidal shell 封闭壳Closed shell 波纹壳Corrugated shell 扭[转]应力函数Stress function of torsion 翘曲函数Warping function 半逆解法semi-in verse method 瑞利里茨法Rayleigh-Ritz method 松弛法Relaxation method 莱维法Levy method 松弛Relaxation 量纲分析Dimensional analysis自相似[性]self-similarity 影响面In flue nee surface 接触应力Con tact stress 赫兹理论Hertz theory 协调接触Con formi ng con tact 滑动接触Slidi ng con tact 滚动接触Rolli ng con tact 压入Indentation 各向异性弹性An isotropic elasticity 颗粒材料Granular material 散体力学Mechanics of granular media 热弹性Thermoelasticity 超弹性Hyperelasticity 粘弹性Viscoelasticity 对应原理Correspondence principle 褶皱Wrinkle塑性全量理论Total theory of plasticity 滑动Sliding 微滑Microslip粗糙度Roughness 非线性弹性Nonlinear elasticity 大挠度Large deflection突弹跳变snap-through 有限变形Finite deformation 格林应变Green strain 阿尔曼西应变Almansi strain 弹性动力学Dynamic elasticity 运动方程Equation of motion 准静态的Quasi-static 气动弹性Aeroelasticity 水弹性Hydroelasticity 颤振Flutter 弹性波Elastic wave 简单波Simple wave 柱面波Cylindrical wave 水平剪切波Horizontal shear wave 竖直剪切波Vertical shear wave 体波body wave 无旋波Irrotational wave 畸变波Distortion wave 膨胀波Dilatation wave 壬瑞利波Rayleigh wave 等容波Equivoluminal wave 勒夫波Love wave界面波In terfacial wave 边缘效应edge effect 塑性力学Plasticity 可成形性Formability 金属成形Metal forming 耐撞性Crashworth in ess 结构抗撞毁性Structural crashworthi ness 拉拔Drawing破坏机构Collapse mechanism回弹Springback挤压Extrusion冲压Stamping穿透Perforation层裂Spalling塑性理论Theory of plasticity 安定[性]理论Shake-down theory 运动安定定理kinematic shake-down theorem静力安定定理Static shake-dow n theorem 率相关理论rate dependent theorem 载荷因子load factor加载准贝V Loading criterion加载函数Loading function 力口载面Loadingsurface 塑性加载Plastic loading 塑性加载波Plastic loading wave 简单加载Simple loading 比例加载Proportional loading 卸载Unloading 卸载波Unloading wave 冲击载荷Impulsive load 阶跃载荷step load 脉冲载荷pulse load 极限载荷limit load 中性变载nentral loading 拉抻失稳in stability in tension 力口速度波acceleration wave 本构方程constitutive equation 完全解completesolution 名义应力nominal stress 过应力over-stress 真应力true stress 等效应力equivale nt stress 流动应力flow stress应力间断stress disc on ti nuity 应力空间stress space 主应力空间principal stressspace 静水应力状态hydrostatic state ofstress 对数应变logarithmic strain 工程应变engineering strain 等效应变equivale nt strain 应变局部化strain localization 应变率strainrate 应变率敏感性strain rate sen sitivity 应变空间strain space 有限应变finite strain 塑性应变增量plastic strain in creme nt 累积塑性应变accumulated plastic strain 永久变形permanent deformation 内变量internalvariable 应变软化strain-softening理想刚塑性材料rigid-perfectly plastic Material刚塑性材料rigid-plastic material 理想塑性材料perfectl plastic material 材料稳定性stability of material应变偏张量deviatoric tensor of strain 应力偏张量deviatori tensor of stress 应变球张量spherical tensor of strain 应力球张量spherical tensor of stress 路径相关性path-dependency 线性强化linear strain-hardening 应变强化strain-hardening 随动强化kinematic hardening 各向同性强化isotropic harde ning 强化模量strain-hardening modulus 幂强化power hardening 塑性极限弯矩plastic limit bending Mome nt 塑性极限扭矩plastic limit torque 弹塑性弯曲elastic-plastic bending 弹塑性交界面elastic-plastic in terface 弹塑性扭转elastic-plastictorsion 粘塑性Viscoplasticity 非弹性Inelasticity理想弹塑性材料elastic-perfectly plastic Material极限分析limit analysis极限设计limit design 极限面limit surface上限定理upper bound theorem上屈服点upper yield point下限定理lower bound theorem 下屈服点lower yield point 界限定理bound theorem 初始屈服面initial yield surface 后继屈服面subsequent yield surface 屈服面[的]外凸性convexity of yield surface截面形状因子shape factor of cross-secti on沙堆比拟sand heap analogy屈服Yield屈服条件yield condition 屈服准则yieldcriterion 屈服函数yield function 屈服面yield surface 塑性势plastic potential 能量吸收装置energy absorb ing device 能量耗散率energy absorbing device 塑性动力学dynamicplasticity塑性动力屈曲dyn amic plastic buckli ng塑性动力响应dyn amic plastic resp onse 塑性波plastic wave运动容许场kinematically admissible Field 静力容许场statically admissible Field 流动法则flow rule速度间断velocity disc on ti nu ity滑移线slip-lines滑移线场slip-lines field移行塑性铰travelling plastic hinge塑性增量理论in creme ntal theory of Plasticity米泽斯屈服准则Mises yield criterio n 普朗特---------- 罗伊斯关系prandtl- Reussrelati on特雷斯卡屈服准则Tresca yield criteri on 洛德应力参数Lode stress parameter 莱维米泽斯关系Levy-Mises relation亨基应力方程Hencky stress equati on 赫艾一一韦斯特加德应力空间Haigh-Westergaard stress space洛德应变参数Lode strain parameter 德鲁克公设Drucker postulate_____ 盖林格速度方程Geiringer velocity Equati on 结构力学structural mechanics 结构分析structural analysis 结构动力学structuraldynamics 拱Arch三铰拱three-hinged arch 抛物线拱parabolicarch 圆拱circular arch 穹顶Dome 空间结构space structure 空间桁架space truss 雪载[荷]snow load 风载[荷]wind load 土压力earth pressure 地震载荷earthquake loading弹簧支座spring support 支座位移supportdisplacement 支座沉降support settlement 超静定次数degree of in determ inacy 机动分析kinematic analysis结点法method of joints 截面法method of sections 结点力joint forces 共轭位移conjugate displacement 影响线in flue nee line 三弯矩方程three-moment equation 单位虚力unit virtual force 刚度系数stiffness coefficient 柔度系数flexibility coefficient 力矩分配moment distribution 力矩分配法moment distribution method 力矩再分配moment redistribution 分配系数distribution factor 矩阵位移法matri displacement method 单元刚度矩阵eleme nt stiff ness matrix 单元应变矩阵eleme nt strain matrix 总体坐标global coord inates 贝蒂定理Betti theorem高斯---- 若尔当消去法Gauss-Jordan elim in ati on Method屈曲模态buckling mode 复合材料力学mecha nics of composites 复合材料composite material 纤维复合材料fibrouscomposite 单向复合材料uni directio nalcomposite 泡沫复合材料foamed composite颗粒复合材料particulate composite 层板Lam in ate 夹层板sandwich panel 正交层板cross-ply laminate 斜交层板an gle-ply lam inate 层片Ply多胞固体cellular solid膨胀Expansion 压实Debulk 劣化Degradation 脱层Delam in ati on 脱粘Debond 纤维应力fiber stress 层应力plystress 层应变ply strain 层间应力interlaminar stress 比强度specific strength 强度折减系数stre ngth reductio n factor强度应力比strength -stress ratio 横向剪切模量tran sverse shear modulus 横观各向同性tran sverse isotropy 正交各向异Orthotropy 剪滞分析shear lag analysis 短纤维chopped fiber 长纤维continuous fiber 纤维方向fiber directi on 纤维断裂fiber break 纤维拔脱fiber pull-out 纤维增强fiber reinforcement 致密化Densification 最小重量设计optimum weight desig n 网格分析法netting analysis 混合律rule of mixture 失效准贝V failure criterion蔡 --- 吴失效准则Tsai-W u failurecriteri on达格代尔模型Dugdale model 断裂力学fracture mechanics概率断裂力学probabilistic fracture Mecha nics格里菲思理论Griffith theory线弹性断裂力学linear elastic fracturemecha ni cs, LEFM弹塑性断裂力学 elastic-plastic fracture mecha-nics, EPFM断裂 Fracture脆性断裂 解理断裂 蠕变断裂 延性断裂 晶间断裂 微裂纹 Microcrack 折裂Kink 椭圆裂纹 elliptical crack 深埋裂纹 embedded crack [钱]币状裂纹 penny-shape crack预制裂纹 Precrack 短裂纹 short crackbrittle fracturecleavage fracturecreep fractureductile fracturein ter-gra nu lar fracture准解理断裂 quasi-cleavage fracture穿晶断裂 trans-granular fracture 裂纹 裂缝 缺陷 割缝 CrackFlawDefectSlit表面裂纹surface crack裂纹钝化crack blunting裂纹分叉crack branching裂纹闭合crack closure裂纹前缘crack front裂纹嘴crack mouth裂纹张开角crack ope ning an gle,COA 裂纹张开位移crack ope ning displaceme nt, COD 裂纹阻力crack resista nee裂纹面crack surface裂纹尖端crack tip裂尖张角crack tip opening angle, CTOA 裂尖张开位移crack tip opening displaceme nt, CTOD裂尖奇异场crack tip singularity Field 裂纹扩展速率crack growth rate 稳定裂纹扩展stable crack growth 定常裂纹扩展steadycrack growth 亚临界裂纹扩展subcriticalcrack growth裂纹[扩展]减速crack retardation止裂crack arrest止裂韧度arrest toughness 断裂类型fracturemode 滑开型sliding mode 张开型openingmode 撕开型tearing mode 复合型mixedmode 撕裂Tearing 撕裂模量tearingmodulus 断裂准贝V fracture criterion J 积分J-integralJ 阻力曲线J-resistanee curve 断裂韧度fracture toughness 应力强度因子stress inten sity factor HRR 场Hutchinson-Rice-Rosengren Field 守恒积分conservationintegral 有效应力张量effective stress ten sor应变能密度strain energy density 能量释放率energy release rate 内聚区cohesive zone 塑性区plastic zone张拉区stretched zone热影响区heat affected zone, HAZ延脆转变温度brittle-ductile tran siti on temperature剪切带shear band剪切唇shear lip无损检测non-destructive inspection双边缺口试件double edge no tched specime n, DEN specimen单边缺口试件sin gle edge no tched specime n, SEN specimen二点弯曲试件three poi nt bending specime n, TPB specime n中心裂纹拉伸试件center cracked tension specime n, CCT specime n中心裂纹板试件center cracked pane specime n, CCP specime n紧凑拉伸试件compact tension specimen, CT specime n大范围屈服large scale yielding 小范围攻屈服small scale yielding 韦布尔分布Weibulldistribution帕里斯公式paris formula 空穴化Cavitation 应力腐蚀stress corrosion 概率风险判定probabilistic risk assessme nt, PRA损伤力学damage mechanics损伤Damage连续介质损伤力学continuum damage mecha nics细观损伤力学microscopic damagemecha nics累积损伤accumulated damage脆性损伤brittle damage延性损伤ductile damage宏观损伤macroscopic damage细观损伤microscopic damage 微观损伤microscopic damage 损伤准贝V damagecriterion 损伤演化方程damage evoluti onequati on 损伤软化damage softening 损伤强化damage strengthening 损伤张量damagetensor损伤阈值damage threshold 损伤变量damage variable 损伤矢量damage vector 损伤区damage zone 疲劳Fatigue 低周疲劳low cycle fatigue 应力疲劳stress fatigue 随机疲劳random fatigue 蠕变疲劳creep fatigue腐蚀疲劳corrosion fatigue 疲劳损伤fatiguedamage 疲劳失效fatigue failure 疲劳断裂fatigue fracture 疲劳裂纹fatigue crack 疲劳寿命fatigue life 疲劳破坏fatigue rupture 疲劳强度fatigue strength 疲劳辉纹fatiguestriations 疲劳阈值fatigue threshold 交变载荷alternating load 交变应力alternatingstress 应力幅值stress amplitude 应变疲劳strain fatigue 应力循环stress cycle 应力比stress ratio 安全寿命safe life 过载效应overloading effect 循环硬化cyclic hardening循环软化cyclic softening 环境效应environmental effect 裂纹片crack gage 裂纹扩展crack growth, crack Propagation 裂纹萌生crack initiation 循环比cycle ratio 实验应力分析experime ntal stress An alysis 工作[应变]片active[strain] gage 基底材料backing material 应力计stress gage零[点]飘移zero shift, zero drift 应变测量strain measurement 应变计strain gage 应变指示器strain indicator 应变花strain rosette应变灵敏度strain sensitivity机械式应变仪mecha ni cal strain gage 直角应变花rectangular rosette弓丨伸仪Extensometer 应变遥测telemetering of strain 横向灵敏系数transverse gage factor 横向灵敏度transverse sensitivity 焊接式应变计weldable strain gage 平衡电桥balaneedbridge 粘贴式应变计bon ded stra in gage 粘贴箔式应变计bonded foiled gage 粘贴丝式应变计bon ded wire gage 桥路平衡bridge balancing 电容应变计capacitanee strain gage 补偿片compensation technique 补偿技术compensation technique 基准电桥referenee bridge 电阻应变计resista nee strain gage 温度自补偿应变计self-temperature compe nsati ng gage半导体应变计semic on ductor strain Gage 集流器slip ring应变放大镜strain amplifier疲劳寿命计fatigue life gage 电感应变计inductanee [strain] gage 光[测]力学Photomechanics 光弹性Photoelasticity 光塑性Photoplasticity 杨氏条纹Young fringe 双折射效应birefrigent effect 等位移线con tourof equal Displaceme nt 暗条纹dark fringe 条纹倍增fringe multiplication 干涉条纹interferenee fringe 等差线Isochromatic 等倾线Isoclinic 等和线isopachic 应力光学定律stress- optic law 主应力迹线Isostatic 亮条纹light fringe 光程差optical path differenee 热光弹性photo-thermo -elasticity 光弹性贴片法photoelastic coat ing Method 光弹性夹片法photoelastic sandwichMethod动态光弹性dynamic photo-elasticity 空间滤波spatial filtering空间频率spatial frequency起偏镜Polarizer反射式光弹性仪reflection polariscope残余双折射效应residual birefringent Effect应变条纹值strain fringe value应变光学灵敏度stra in-optic sen sitivity 应力冻结效应stress freez ing effect 应力条纹值stress fringe value 应力光图stress-opticpattern暂时双折射效应temporary birefri ngent Effect脉冲全息法pulsed holography透射式光弹性仪tran smissi on polariscope 实时全息干涉法real-time holographic in terferometry网格法grid method全息光弹性法holo-photoelasticity全息图Hologram全息照相Holograph全息干涉法holographic interferometry 全息云纹法holographic moire technique 全息术Holography全场分析法whole-field analysis 散斑干涉法speckle interferometry 散斑Speckle错位散斑干涉法speckle-shearing in terferometry, shearography散斑图Specklegram白光散斑法white-light speckle method 云纹干涉法moire interferometry[叠栅]云纹moire fringe[叠栅]云纹法moire method云纹图moire pattern离面云纹法off-plane moire method参考栅referenee grating 试件栅specimengrating 分析栅analyzer grating 面内云纹法in-plane moire method 脆性涂层法brittle-coating method 条带法strip coating method坐标变换transformation of Coordinates计算结构力学computational structural mecha nics力口权残量法weighted residual method 有限差分法finite differenee method 有限[单]元法finite element method 配点法pointcollocation 里茨法Ritz method广义变分原理generalized variational Prin ciple最小二乘法least square method胡[海昌]一鹫津原理Hu-Washizu prin ciple 赫林格-赖斯纳原理Helli nger-Reiss ner Prin ciple 修正变分原理modified variationalPrin ciple约束变分原理constrained variational Prin ciple混合法mixed method杂交法hybrid method边界解法boundary solution method有限条法finite strip method半解析法semi-analytical method协调元conforming element 非协调元non-conforming element 混合元mixed element 杂交元hybrid element 边界元boundary element 强迫边界条件forced boundary con diti on 自然边界条件n atural boundary con diti on 离散化Discretization 离散系统discrete system 连续问题continuous problem 广义位移generalized displacement 广义载荷generalized load 广义应变generalized strain 广义应力generalized stress 界面变量in terface variable 节点no de, no dal point [单]元Element 角节点corner node 边节点mid-side node 内节点in ter nal node 无节点变量nodeless variable 杆元bar element桁架杆元truss element 梁元beam element 二维元two-dimensional element 一维元one-dimensional element 三维元three-dimensional element 车由对称元axisymmetric element 板元plate element 壳元shell element 厚板元thick plate element 三角形元triangular element 四边形元quadrilateral element 四面体元tetrahedralelement 曲线元curved element 二次元quadratic element 线性元linear element 三次元cubic element 四次元quartic element 等参[数]元isoparametric element 超参数元super-parametric element 亚参数元sub-parametric element 节点数可变元variable-number-nodeeleme nt拉格朗日元Lagra nge eleme nt 拉格朗日族Lagrange family巧凑边点元serendipity element 巧凑边点族serendipity family 无限元infinite element 单元分析element analysis 单元特性elementcharacteristics 刚度矩阵stiffness matrix 几何矩阵geometric matrix 等效节点力equivalent no dal force 节点位移no dal displaceme nt 节点载荷no dal load 位移矢量displacementvector 载荷矢量load vector 质量矩阵massmatrix 集总质量矩阵lumped mass matrix 相容质量矩阵con siste nt mass matrix 阻尼矩阵damping matrix 壬瑞利阻尼Rayleighdamping刚度矩阵的组集assembly of stiffness Matrices载荷矢量的组集con siste nt mass matrix质量矩阵的组集assembly of mass matrices单元的组集assembly of elements 局部坐标系local coord in ate system 局部坐标localcoordinate 面积坐标area coordinates 体积坐标volume coordinates 曲线坐标curvilinearcoord inates 静凝聚static condensation 合同变换contragradient transformation 形状函数shape function 试探函数trial function 检验函数test function 权函数weight function 样条函数spline function 代用函数substitutefunction 降阶积分reduced integration 零能模式zero-energy mode P 收敛p-c on vergenee H 收敛h-c on verge nee 掺混插值blended interpolation 等参数映射isoparametric mapping双线性插值bilinear interpolation 小块检验patch test非协调模式in compatible mode 节点号node number 单元号element number 带宽band width 带状矩阵banded matrix 变带状矩阵profile matrix 带宽最小化mini mizatio n of band width 波前法frontal method 子空间迭代法subspace iterati on method 行列式搜索法determ inant search method 逐步法step-by-step method 纽马克法Newmark 威尔逊法Wilson 拟牛顿法quasi-Newton method 牛顿-拉弗森法Newton-Raphson method 增量法in creme ntal method 初应变initial strain 初应力initial stress 切线刚度矩阵tangent stiffness matrix 割线刚度矩阵seca nt stiffness matrix 模态叠加法mode superposition method 平衡迭代equilibrium iteration 子结构Substructure子结构法substructure technique 超单元super-element 网格生成mesh generation 结构分析程序structural an alysis program 前处理pre-processing 后处理post-processing 网格细化mesh refinement 应力光顺stress smoothing组合结构composite structure 流体动力学fluid dynamics 连续介质力学mechanics of continuousmedia 介质medium 流体质点fluid particle 无粘性流体non viscous fluid, i nviscid fluid 连续介质假设continuous medium hypothesis 流体运动学fluid kinematics 水静力学hydrostatics液体静力学hydrostatics 支配方程governing equation 伯努利方程Bernoulli equation 伯努利定理Bernonlli theorem 毕奥-萨伐尔定律Biot-Savart law 欧拉方程Euler equation 亥姆霍兹定理Helmholtz theorem 开尔文定理Kelvin theorem 涡片vortex sheet 库塔-茹可夫斯基条件Kutta-Zhoukowski con diti on布拉休斯解Blasius solution 达朗贝尔佯廖d&;am #39;Alembert paradox 雷诺数Reyno Ids n umber 施特鲁哈尔数Strouhal number 随体导数material derivative 不可压缩流体in compre ible fluid 质量守恒co ervation of ma 动量守恒co ervation of momentum 能量守恒co ervation of energy 动量方程momentum equation 能量方程energy equation控制体积control volume 液体静压hydrostatic pre ure 涡量拟能e trophy 压差differential pre ure 流[动]flow 流线stream line 流面stream surface 流管stream tube 迹线path, path line 流场flow field 流态flow regime 流动参量flow parameter 流量flow rate, flow discharge 涡旋vortex 涡量vorticity 涡丝vortex filament 涡线vortex line 涡面vortex surface 涡层vortex layer 涡环vortex ring 涡对vortex pair 涡管vortex tube涡街vortex street卡门涡街Karman vortex street 马蹄涡horseshoe vortex 对流涡胞convective cell 卷筒涡胞roll cell 涡eddy 涡粘性eddy viscosity 环流circulation 环量circulation速度环量velocity circulation 偶极子doublet, dipole 驻点stagnation point 总压[力]total pre ure 总压头total head 静压头static head 总焓total enthalpy 能量输运energy tra ort 速度剖面velocity profile 库埃特流Couette flow 单相流single phase flow 单组份流single-component flow 均匀流uniform flow非均匀流nonuniform flow 二维流two-dime ionalflow 三维流three-dime ional flow 准定常流quasi-steady flow 非定常流u teady flow, non-steady flow 暂态流tra ient flow 周期流periodic flow 振荡流oscillatory flow 分层流stratified flow 无旋流irrotational flow 有旋流rotational flow 车由对称流axisymmetric flow 不可压缩性incompre ibility 不可压缩流[动]incompre ible flow 浮体floating body 定倾中心metacenter 阻力drag, resista nee 减阻drag reduction 表面力surface force 表面张力surface te ion 毛细[管]作用capillarity 来流in com ing flow自由流free stream 自由流线free stream line 夕卜流external flow 进口entrance, inlet 出口exit, outlet 扰动disturbanee, perturbation 分布distribution 传播propagation 色散di ersion 弥散di ersion 附加质量added ma ,a oeiated ma 收缩eontraetion 镜象法image method 无量纲参数dime ionle parameter 几何相似geometric similarity 运动相似kinematic similarity 动力相似[性]dynamic similarity 平面流plane flow 势potential 势流potential flow 速度势velocity potential 复势complex potential复速度complex velocity 流函数stream function 源source 汇sink 速度[水]头velocity head 拐角流corner flow 空泡流cavity flow 超空泡supercavity 超空泡流supercavity flow 空气动力学aerodynamics 低速空气动力学low- eed aerod yn amics 高速空气动力学high- eed aerod yn amics 气动热力学aerothermodynamics 亚声速流[动]su onic flow 跨声速流[动]tra onic flow 超声速流[动]supersonic flow 锥形流coni cal flow 楔流wedge flow 叶栅流cascade flow非平衡流[动]non-equilibrium flow 细长体slender body 细长度slenderne钝头体bluff body 钝体blunt body 翼型airfoil 翼弦chord 薄翼理论thin-airfoil theory 构型configuration 后缘trailing edge 迎角angle of attack 失速stall 脱体激波detached shock wave 波阻wave drag 诱导阻力induced drag 诱导速度induced velocity 临界雷诺数critical Reyno Ids nu mber 前缘涡leading edge vortex 附着涡bound vortex 约束涡confined vortex 气动中心aerodynamic center 气动力aerodynamic force 气动噪声aerodynamic noise 气动加热aerodynamicheating 离解di ociation地面效应ground effect 气体动力学gas dynamics 稀疏波rarefaction wave 热状态方程thermal equation of state 喷管Nozzle普朗特-迈耶流Prandtl-Meyer flow 瑞利流Rayleigh flow 可压缩流[动]compre ible flow 可压缩流体compre ible fluid 绝热流adiabatic flow 非绝热流diabatic flow 未扰动流undisturbed flow 等熵流isentropic flow 匀熵流homoentropic flow 兰金-于戈尼奥条件Ran ki ne-Hugo niot con diti on 状态方程equation of state量热状态方程caloric equati on of state 完全气体perfect gas 拉瓦尔喷管Laval nozzle 马赫角Mach angle 马赫锥Mach cone马赫线Mach line 马赫数Mach number 马赫波Mach wave 当地马赫数local Mach number 冲击波shock wave 激波shock wave正激波normal shock wave 斜激波oblique shock wave 头波bow wave附体激波attached shock wave 激波阵面shock front 激波层shock layer 压缩波compre ion wave 反射reflection 折射refraction 散射scattering 衍射diffraction 绕射diffraction 出口压力exit pre ure 超压[强]over pre ure 反压back pre ure 爆炸explosion爆轰det on ati on 缓燃deflagration 水动力学hydrodynamics 液体动力学hydrodynamics 泰勒不稳定性Taylor i tability 盖斯特纳波Gerstner wave 斯托克斯波Stokes wave 壬瑞利数Rayleigh number 自由面free surface 波速wave eed, wave velocity 波高wave height 波列wave train 波群wave group 波能wave energy 表面波surface wave 表面张力波capillary wave 规则波regular wave 不规则波irregular wave 浅水波shallow water wave 深水波deep water wave 重力波gravity wave 椭圆余弦波enoidal wave 潮波tidal wave 涌波surge wave 破碎波breaking wave 船波ship wave 非线性波nonlinear wave 孤立子soliton 水动[力]噪声hydrodynamic noise 水击water hammer 空化cavitation 空化数cavitation number 空蚀cavitation damage 超空化流supercavitating flow 水翼hydrofoil 水力学hydraulics 洪水波flood wave 涟漪ri le 消能energy di ipation 海洋水动力学marine hydrod ynamics 谢齐公式Chezy formula 欧拉数Euler number 弗劳德数Froude number 水力半径hydraulic radius水力坡度hvdraulic slope 高度水头elevating head 水头损失head lo 水位water level 水跃hydraulic jump 含水层aquifer 排水drain age 排放量discharge 壅水曲线back water curve 压[强水]头pre ure head 过水断面flow cro -section 明槽流open cha el flow 孑L流orifice flow 无压流free surface flow 有压流pre ure flow 缓流subcritical flow 急流supercritical flow 渐变流gradually varied flow 急变流rapidly varied flow 临界流critical flow 异重流de ity current, gravity flow 堰流weir flow掺气流aerated flow含沙流sediment-laden stream 降水曲线dropdown curve 沉积物sediment, deposit 沉[降堆]积sedimentation, deposition 沉降速度settling velocity 流动稳定性flow stability 不稳定性i tability 奥尔-索末菲方程Orr-Sommerfeld equation 涡量方程vorticity equation 泊肃叶流Poiseuille flow 奥辛流Oseen flow 剪切流shear flow 粘性流[动]viscous flow 层流laminar flow分离流separated flow 二次流sec on dary flow 近场流near field flow 远场流far field flow 滞止流stagnation flow 尾流wake [flow]回流back flow反流reverse flow 射流jet 自由射流free jet 管流pipe flow, tube flow 内流internal flow 拟序结构cohere nt structure 猝发过程bursting proce 表观粘度 a arent viscosity 运动粘性kinematic viscosity 动力粘性dynamic viscosity 泊poise 厘泊centipoise 厘沱centistoke 剪切层shear layer 次层sublayer 流动分离flow separation 层流分离laminar separation 湍流分离turbulent separation 分离点separation point 附着点attachment point 再附reattachment 再层流化relam in arizati on 起动涡starting vortex 驻涡standing vortex 涡旋破碎vortex breakdown 涡旋脱落vortex shedding 压[力]降pre ure drop 压差阻力pre ure drag 压力能pre ure energy 型阻profile drag 滑移速度slip velocity 无滑移条件non-slip condition 壁剪应力skin friction, frictional drag 壁剪切速度friction velocity 磨擦损失friction lo 磨擦因子friction factor耗散di ipation 滞后lag 相似性解similar solution 局域相似local similarity 气体润滑gas lubrication 液体动力润滑hydrod yn amic lubricati on 浆体slurry泰勒数Taylor number纳维-斯托克斯方程Navier-Stokes equation 牛顿流体Newtonian fluid边界层理论boundary later theory 边界层方程boundary layer equation 边界层boundary layer 附面层boundary layer层流边界层laminar boundary layer 湍流边界层turbulent boundary layer 温度边界层thermal boundary layer 边界层转捩boundary layer tra ition 边界层分离boundary layer separation 边界层厚度boundary layer thickne 位移厚度di lacement thickne 动量厚度momentum thickne 能量厚度energy thickne 焓厚度enthalpy thickne 注入injection 吸出suction 泰勒涡Taylor vortex 速度亏损律velocity defect law 形状因子shape factor 测速法anemometry粘度测定法visco[si] metry 流动显示flow visualization 油烟显示oil smoke visualization 孑L板流量计orifice meter 频率响应frequency re o e 油膜显示oil film visualization 阴影法shadow method 纹影法schlieren method 烟丝法smoke wire method 丝线法tuft method 氢泡法nydrogen bu le method 相似理论similarity theory 相似律similarity law 咅B分相似partial similarity 定理pi theorem, Buck in gham theorem 静[态]校准static calibration 动态校准dynamic calibration 风洞wind tu el 激波管shock tube激波管风洞shock tube wind tu el 水洞water tu el 拖曳水池towing tank旋臂水池rotating arm basin 扩散段diffuser 测压孑L pre ure tap 皮托管pitot tube 普雷斯顿管preston tube 斯坦顿管Stanton tube 文丘里管Venturi tube U 形管U-tube 压强计manometer 微压计microma nometer 多管压强计multiple manometer 静压管static [pre ure]tube 流速计an emometer 风速管Pitot- static tube 激光多普勒测速计laser Do ler an emometer, laser Do ler velocimeter 热线流速计hot-wire an emometer 热膜流速计hot- film an emometer 流量计flow meter 粘度计visco[si] meter 涡量计vorticitymeter 传感器tra ducer, se or压强传感器pre ure tra ducer 热敏电阻thermistor 示踪物tracer 时间线time line 脉线streak line 尺度效应scale effect 壁效应wall effect 堵塞blockage 堵寒效应blockage effect 动态响应dynamic re o e 响应频率re o e frequency 底压base pre ure 菲克定律Fick law 巴塞特力Ba et force 埃克特数Eckert number 格拉斯霍夫数Grashof number 努塞特数Nu elt number 普朗特数prandtl number 雷诺比拟Reyno Ids an alogy 施密特数schmidt number 斯坦顿数Stanton number 对流convection自由对流n atural con vecti on,free con vec-ti on 强迫对流forced convection 热对流heat convection 质量传递ma tra fer 传质系数ma tra fer coefficient 热量传递heat tra fer 传热系数heat tra fer coefficient 对流传热convective heat tra fer 辐射传热radiative heat tra fer 动量交换momentum tra fer 能量传递energy tra fer 传导conduction 热传导conductive heat tra fer 热交换heat exchange 临界热通量critical heat flux 浓度concentration 扩散diffusion 扩散性diffusivity 扩散率diffusivity 扩散速度diffusion velocity 分子扩散molecular diffusion沸腾boiling蒸发evaporation 气化gasification 凝结conde ation 成核nucleation 计算流体力学computational fluid mecha nics多重尺度问题multiple scale problem 伯格斯方程Burgers equation对流扩散方程con vecti on diffusi on equati on KDU 方程KDV equation 修正微分方程modified differe ntial equati on 拉克斯等价定理Lax equivale nee theorem 数值模拟numerical simulation 大涡模拟large eddy simulation 数值粘性numerical viscosity 非线性不稳定性nonlinear i tability 希尔特稳定性分析Hirt stability an alysis 相容条件co istency conditionCFL 条件Courant- Friedrichs- Lewycon diti on ,CFL con diti on 狄里克雷边界条件Dirichlet bou ndarycon diti on熵条件entropy condition远场边界条件far field boundary con diti on 流入边界条件inflow boundary con diti on 无反射边界条件non reflect ing boundary con diti on数值边界条件numerical boundary con diti on流出边界条件outflow boundary con diti on 冯■诺伊曼条件von Neuma condition 近似因子分解法a roximate factorization method人工压缩artificial compre ion人工粘性artificial viscosity边界元法boundary element method 配置方法collocation method 能量法energy method 有限体积法finite volume method 流体网格法fluid in cell method, FLIC method通量校正传输法flux-corrected tra ort method通量矢量分解法flux vector litt ing method 伽辽金法Galerkin method 积分方法integral method标记网格法marker and cell method, MAC method 特征线法method of characteristics 直线法method of lines 矩量法moment method 多重网格法multi- grid method 板块法panel method 质点网格法particle in cell method, PIC method质点法particle method 预估校正法predictor-corrector method 投影法projection method 准谱法eudo- ectral method 随机选取法random choice method 激波捕捉法shock-capturing method 激波拟合法shock-fitting method 谱方法ectral method 稀疏矩阵分解法lit coefficient matrix method不定常法time-dependent method 时间分步法time litting method 变分法variational method 涡方法vortex method 隐格式implicit scheme 显格式explicit scheme交替方向隐格式alter nati ng directi on implicit scheme, ADI scheme反扩散差分格式anti-diffusion differenee scheme紧差分格式compact differenee scheme 守恒差分格式co ervation differenee scheme克兰克-尼科尔森格式Cran k-Nicolson scheme杜福特-弗兰克尔格式Dufort-Fra nkel scheme 指数格式exponential scheme 戈本诺夫格式Godu nov scheme 高分辨率格式high resoluti on scheme 拉克斯-温德罗夫格式Lax-We ndroff scheme 蛙跳格式leap-frog scheme单调差分格式monotone differe nee scheme保单调差分格式monotonicity preserving differe nee scheme穆曼-科尔格式Murman-Cole scheme 半隐格式semi-implicit scheme 斜迎风格式skew-u tream scheme 全变差下降格式total variation decreasing scheme TVD scheme迎风格式u tream scheme , upwind scheme计算区域computational domain物理区域physical domain 影响域domai n of in flue nee 依赖域domain of dependence 区域分解domain decomposition 维数分解dime ion al lit 物理解physical solution 弱解weak solution 黎曼解算子Riema solver 守恒型co ervation form 弱守恒型weak co ervation form 强守恒型strong coervation form 散度型diverge nee form 贴体曲线坐标body- fitted curvilinear coordi-nates[自]适应网格[self-] adaptive mesh 适应网格生成adaptive grid gen erati on 自动网格生成automatic grid gen erati on 数值网格生成n umerical grid gen erati on 交错网格staggered mesh 网格雷诺数cell Reynolds number 数植扩散numerical diffusion 数值耗散numerical di ipation 数值色散numerical di ersion 数值通量numerical flux 放大因子amplification factor 放大矩阵amplification matrix 阻尼误差damping error 离散涡discrete vortex 熵通量entropy flux 熵函数entropy function 分步法fractional step method 广义连续统力学generalized continuum mecha nics 简单物质simple material 纯力学物质purely mechanical material微分型物质material of differential type 积分型物质material of integral type 混合物组份co tituents of a mixture 非协调理论in compatibility theory 微极理论micropolar theory 决定性原理principle of determinism 等存在原理principle of equipresenee 局部作用原理prin ciple of objectivity 客观性原理。
The Delta(1232) at RHIC

a r X i v :n u c l -t h /0409026v 3 26 J a n 2005The ∆(1232)at RHICHendrik van Hees †and Ralf RappCyclotron Institute and Physics Department,Texas A&M University,CollegeStation,Texas 77843-3366E-mail:hees@,rapp@Abstract.We investigate properties of the ∆(1232)and nucleon spectral functions at finite temperature and baryon density within a hadronic model.The medium modifications of the ∆consist of a renormalization of its pion-nucleon cloud and resonant π∆scattering.Underlying coupling constants and form factors are determined by the elastic πN scattering phase shift in the isobar channel,as well as empirical partial decay widths of excited baryon resonances.For cold nuclear matter the model provides reasonable agreement with photoabsorption data on nuclei in the ∆-resonance region.In hot hadronic matter typical for late stages of central Au -Au collisions at RHIC we find the ∆-spectral function to be broadened by ∼65MeV together with a slight upward mass shift of 5-10MeV,in qualitative agreement with preliminary data from the STAR collaboration.PACS numbers:25.75.-q,21.65.+f,12.40.-y 1.Introduction At low energies,the main features of Quantum Chromodynamics (QCD)are confinement and the spontaneous breaking of chiral symmetry.The former implies that we onlyobserve hadrons (rather than quarks and gluons),while the latter is believed to govern the (low-lying)hadron-mass ttice-QCD calculations predict a phase transition from nuclear/hadronic matter to a deconfined,chirally symmetric state [1]at temperatures T ≃150-200MeV,dictating a major reshaping of the hadronic spectrum in terms of degenerate chiral partners.The observation of such medium modifications is therefore an important objective in relativistic heavy-ion collision rge theoretical efforts have been devoted to evaluate in-medium properties of vector mesons which are accessible experimentally through dilepton invariant-mass spectra [2].In most of these studies,baryon-driven effects are essential to account for the dilepton enhancement observed in P b -Au collisions at the SPS below the free ρmass [3,4].Thus,changes in the baryon properties themselves deserve further investigation.In addition,recent measurements of πN invariant-mass spectra in nuclear collisions [5,6,7]may open a more direct window on modifications of the ∆(1232).†presenting authorTo date,in-medium properties of the∆have mostly been assessed in cold nuclear matter[8,9,10,11,12,13],with few exceptions[14,15].In this article we will discuss properties of the nucleon and the∆(1232)in a hot and dense medium[16],employing a finite-temperaturefield theory framework based on hadronic interactions.Both direct interactions of the∆with thermal pions as well as modifications of its freeπN self-energy(incuding vertex corrections)will be accounted for.The article is organised as follows.In Sec.2we introduce the hadronic Lagrangian and outline how its parameters are determined using scattering and decay data in vacuum.In Sec.3we compute in-medium self-energies for nucleon and∆.In Sec.4we first check our model against photoabsorption cross sections on the nucleon and nuclei, followed by a discussion of the spectral functions under conditions expected to occur in high-energy heavy-ion collisions.We close with a summary and outlook.2.Hadronic Interaction Lagrangians in VacuumThe basic element of our analysis are3-point interaction vertices involving a pion and two baryons,πB1B2.Baryonfields are treated using relativistic kinematics, E2B(p)=m2B+p2,but neglecting anti-particle contributions and restricting Rarita-Schwinger spinors to their non-relativistic spin-3/2components.Pions are treated fully relativisticly(ω2π(k)=m2π+k2).The resulting interaction Lagrangians are thus of the usual nonrelativistic form involving(iso-)spin-1/2Pauli matrices,1/2to3/2transition operators,as well as spin-3/2matrices[9,17,18,19,20],see Ref.[16]for explicit expressions.To simulatefinite-size effects we employed hadronic form factors with auniform cutoffparameterΛπB1B2=500MeV(except forπNN andπN∆vertices).The imaginary part of the vacuum self-energy for the∆→Nπdecay takes the formImΣ(Nπ)∆(M)=−f2πN∆MF2(k cm)Θ(M−m N−mπ)(1)with k cm the center-of-mass decay momentum(an extra factor m N/E N(k cm)has been introduced in Eq.(1)to restore Lorentz-invariance),and the real part is determined via a dispersion relation.With a bare mass of m(0)∆=1302MeV,a form-factor cutoffΛπN∆=290MeV and a coupling constant fπN∆=3.2we obtain a satisfactoryfit to the experimentalδ33-phase shift[21,15,22].To account for resonant interactions of the∆with pions we identified the relevant excited baryons via their decay branchings B→π∆.The pertinent coupling constants have been determined assuming the lowest partial wave to be dominant(unless otherwise specified)[23],using pole masses and(total)widths of the resonances.The same procedure has been adopted for resonantπN interactions(which are used to evaluate finite-temperature effects on the nucleon).The resonances included are N(1440), N(1520),N(1535),∆(1600),∆(1620)and∆(1700).The total widthsfiguring into the resonance propagators have been obtained by scaling up the partialπN andπ∆channels,and vacuum renormalizations of the masses have been neglected.Figure 1.Diagrammatic representation ofπN∆vertex corrections(dashed lines:pion,solid lines:nucleon,double solid lines:∆(1232));a bubble with labelαcorresponds to a Lindhard functionΠα(α∈{1,2})attached to baryon lines withpertinent Migdal parameters,i.e.,g′12forα=1and g′22forα=2.Finally,the evaluation of the photoabsorption cross section requires aγN∆vertex for which we employ the magnetic coupling[10]LγN∆=−fγN∆3m2π d4lE N(l)k2F2π(|k|)(3)×{[Θ(k0)+σ(k0)fπ(|k0|)]Aπ(k)G N(l)−f N(l0)A N(l)Gπ(k)}, where k=p−l is the pion4-momentum.The thermal distributions are defined by f N(l0)=f fermi(l0−µN,T)and fπ(|k0|)=f bose(|k0|,T)exp(µπ/T),with f fermi and f bose the Fermi and Bose functions,respectively.For simplicity,finite pion-chemical potentials,µπ>0,are treated in the Boltzmann limit to avoid Bose singularities in the presence of broad pion spectral functions(a more detailed discussion of this point will be given elsewhere).In Eq.(3)positive energies k0>0correspond to outgoing pions,i.e.,∆→πN decays,while k0<0accounts for scattering with(incoming)pions from the heat bath.The key quantities in Eq.(3)are the in-medium pion and nucleon propagators, Gπand G N,and pertinent spectral functions A N=−2Im G N and Aπ=−2Im Gπ.The modifications of the pion propagator are implemetend via a self-energy,arising from two parts:(i)interactions with thermal pions modeled by a four-point interaction in second order(“sunset diagram”)[24],with a coupling constant adjusted to qualitatively reproduce the results of more elaborateππinteractions in s,p,and d-wave[25]; (ii)interactions with baryons via p-wave nucleon-and∆-hole excitations atfinite temperature,described by standard Lindhard functions,supplemented by short-range correlations encoded in Migdal parameters[26](our default values are g′NN=0.8, g′N∆=g′∆∆=0.33).These excitations induce a softening of the pion-dispersion relation which can even lead to a(near)vanishing of the pion group velocity atfinite momentum, inducing an artificial threshold enhancement in the∆self-energy[14].This feature isk γ[GeV]σγ/Ak γ[GeV]σγ/A [µb ]Figure 2.Photoabsorption cross sections on nucleons (left panel,data from [29])andnuclei (right panel;data from [30,31,32,33,34,35]).remedied by accounting for appropriate vertex corrections,which in the case of ρ→ππdecays are required to maintain a conserved vector current in the medium [27,28].Here we apply the same technique to the πN ∆vertex,cf.Fig.1.The nucleon self-energy is calculated in terms of resonant interactions with thermal pions,at the same level of approximation as the pion Lindhard functions (i.e.,neglecting offenergy-shell dependencies in the spectral functions of the excited baryons).The second contribution to the in-medium ∆self-energy consists of resonant π∆→B interactions,corresponding to the finite-temperature part of πB loops.The resulting self-energy expressions are similar to Eq.(3)but with only the scattering part (k 0<0)retained (note that this is consistent with our description of the ∆(1232)in vacuum where πB loops are not included).4.In-medium Spectral Properties of the ∆4.1.Photoabsorption on Nucleons and NucleiValuable constraints on the ∆spectral function in cold nuclear matter can be obtained from photoabsorption cross sections on nuclei.To leading order in αem ,the latter can be related to the photon self-energy (electromagnetic current correlator),Πγ,by [18]σabs γA k 12ImΠγ(k 0=k ),Πγ=1M N [GeV]-I m G N [G e V -1]k 0 [GeV]-I m G π [G e V -2]Figure 3.Left panel:nucleon spectral function at RHIC (solid line T =180MeV,̺N =0.68̺0;dashed line:T =100MeV,̺N =0.12̺0).Right panel:pion spectralfunction for cold nuclear matter (dashed line:T =0,̺N =0.68̺0)and at RHIC (solidline:T =180MeV,̺N =0.68̺0);the dash-dotted line corresponds to switching offbaryonic effects leaving only the 4-point interactions with thermal pions.Migdal parameters or the nuclear density is very moderate.Given our rather simple approach for the cross section,the agreement with data is fair.The discrepancies at low energy (which seem to be present already for the nucleon)could be due to interference with the background,collective effects involving direct NN −1-excitations,or transverse contributions with in-medium ρmesons in the vertex corrections of the ∆decay.At higher energies,further resonances in the photon self-energy need to be included.4.2.Hot Hadronic MatterLet us finally turn to the results for hot hadronic matter.In heavy-ion collisions one expects a hierarchy of chemical freeze-out (determining the ratios of stable hadrons)and thermal freeze-out (where elastic rescattering ceases).The former is characterized by a temperature T chem and a common baryon chemical potential µB .Thermal freezeout occurs at lower T fo ≃100MeV,which requires the build-up of additional chemical potentials for pions,kaons,etc.[36],to conserve the observed hadron ratios,including relative chemical equilibrium for elastic processes,e.g.πN ↔∆implying µ∆=µN +µπ.Under RHIC conditions the nucleon spectral function exhibits an appreciable broadening and a moderate downward mass shift (left panel of Fig.3)due to resonant scattering offthermal pions.The pion spectral function (right panel of Fig.3)is strongly broadened mostly due to scattering offbaryons,with little mass shift.Thermal motion completely washes out the multi-level structure visible at zero temperature (dashed line).Also for the ∆spectral function (left panel in Fig.4)the main effect is a broadening with a slight repulsive mass shift.Half of the increase of the in-medium width is due to baryon-resonance excitations (slightly enhanced due to in-medium pion propagators),adding to the contribution of the πN loop.In the real part,however,the predominantly repulsive contributions from baryon resonances are counterbalanced by net attraction in the πN loop (mostly due to the pion-Bose factor).At thermal freeze-out we find1 1.1 1.2 1.3 1.4 1.5 1.6M ∆ [GeV]0510152025-I m G ∆ [G e V -1]vacuum T=100 MeV T=180 MeV 1 1.1 1.2 1.3 1.4 1.5 1.6M ∆ [GeV]0510152025-I m G ∆ [G e V -1]vacuum T=70 MeV T=160 MeV Figure 4.In-medium ∆(1232)spectral functions in heavy-ion collisions compared tofree space (dash-dotted lines);left panel:RHIC;dashed line:T =100MeV,̺N =0.12̺0(µN =531MeV),µπ=96MeV;solid line:T =180MeV,̺N =0.68̺0(µN =333MeV),µπ=0.Right:future GSI facility;dashed line:T =70MeV,̺N =0.19̺0(µN =727MeV),µπ=105MeV;solid line:T =160MeV,̺N =1.80̺0(µN =593MeV),µπ=0.a peak position at about M ≃1.226GeV and a width Γ≃177MeV,to be compared to the corresponding vacuum values of M ≃1.219GeV and Γ≃110MeV,in qualitative agreement with preliminary data from STAR [7].For more conclusive comparison a detailed treatment of the freeze-out dynamics is mandatory.In the vicinity of T c ,the ∆width increases substantially.We expect this trend to be further magnified when including transverse parts in the vertex corrections,especially in combination with in-medium ρ-mesons [2].In the right panel of Fig.4we show the ∆-spectral function in a net-baryon rich medium,representative for the future GSI facility.Whereas in dilute matter the line shape is only little affected,the resonance structure has essentially melted close to T c ,mostly due to a strong renormalization of the pion propagator at high density.5.Conclusions and outlookBased on hadronic interaction Lagrangians employed within a finite-temperature many-body approach we have evaluated medium effects on pions,nucleons and deltas.The resulting ∆-spectral functions in cold nuclear matter provide fair agreement with photoabsorption data on nuclei.In hot hadronic matter,we found a significant broadening and a slight upward peak shift of the ∆resonance,qualitatively in line with preliminary measurements of πN invariant-mass spectra at RHIC.Future improvements of the πN ∆system in vacuum include u -channel exchange diagrams as well as spin-3/2-∆∗excitations which we expect to increase the rather low form-factor cut-offused so far.We further plan to implement in-medium baryon propagators into the description of axial-/vector mesons within a chiral framework to arrive at a more consistent picture of the equation of state of hadronic matter under extreme conditions [37]and the chiralphase transition.Another interesting ramification[38]concerns the role of the medium-modified∆spectral functions in the soft photon enhancement as recently observed at the SPS[39].AcknowledgmentsOne of us(HvH)acknowledges support from the Alexander-von-Humboldt Foundation as a Feodor-Lynen Fellow.References[1]Karsch F2002Lect.Notes Phys.583209[2]Rapp R and Wambach J2000Adv.Nucl.Phys.251[3]Agakishiev G et al.(CERES/NA45)1998Phys.Lett.B422405[4]Adamova D et al.(CERES/NA45)2003Phys.Rev.Lett.91042301[5]Hjort E L et al.1997Phys.Rev.Lett.794345[6]Pelte D et al.(FOPI)1997Z.Phys.A35955[7]Fachini P2004J.Phys.G30S735[8]Horikawa Y,Thies M and Lenz F1980Nucl.Phys.A345386[9]Oset E and Salcedo L L1987Nucl.Phys.A468631[10]Ericson T and Weise W1988Pions and Nuclei(Clarendon Press,Oxford)[11]Migdal A B,Saperstein E E,Troitsky M A et al.1990Phys.Rept.192179[12]Xia L H,Siemens P J and Soyeur M1994Nucl.Phys.A578493[13]Korpa C L and Lutz M F M2004Nucl.Phys.A742305[14]Ko C M,Xia L H and Siemens P J1989Phys.Lett.B23116[15]Korpa C L and Malfliet R1995Phys.Rev.C522756[16]van Hees H and Rapp R2004Preprint nucl-th/0407050[17]Cubero M1990Ph.D.thesis TH Darmstadt[18]Rapp R,Urban M,Buballa M et al.1998Phys.Lett.B4171[19]Urban M,Buballa M,Rapp R et al.2000Nucl.Phys.A673357[20]Nacher J C,Oset E,Vicente M J et al.2001Nucl.Phys.A695295[21]Moniz E J1981Nucl.Phys.A354535c[22]Weinhold W,Friman B and N¨o renberg W1998Phys.Lett.B433236[23]Hagiwara K et al.2002Phys.Rev.D6601001[24]van Hees H and Knoll J2002Phys.Rev.D6*******[25]Rapp R and Wambach J1995Phys.Lett.B35150[26]Migdal A B1978Rev.Mod.Phys.50107[27]Chanfray G and Schuck P1993Nucl.Phys.A555329[28]Herrmann M,Friman B L and N¨o renberg W1993Nucl.Phys.A560411[29]Lepretre A et al.1978Phys.Lett.B7943[30]Ahrens J et al.1984Phys.Lett.B146303[31]Ahrens J1985Nucl.Phys.A446229c[32]Frommhold T et al.1992Phys.Lett.B29528[33]Frommhold T et al.1994Z.Phys.A350249[34]Bianchi N et al.1993Phys.Lett.B299219[35]Bianchi N et al.1996Phys.Rev.C541688[36]Rapp R2002Phys.Rev.C66017901[37]Voskresensky D N2004Preprint hep-ph/0402020[38]Rapp R2004Mod.Phys.Lett.A191717[39]Aggarwal M M et al.(WA98)2004Phys.Rev.Lett.93022301。
PP木塑中界面设计和木纤维的增强特征:纳米氧化锌经过离子组装改性木纤维
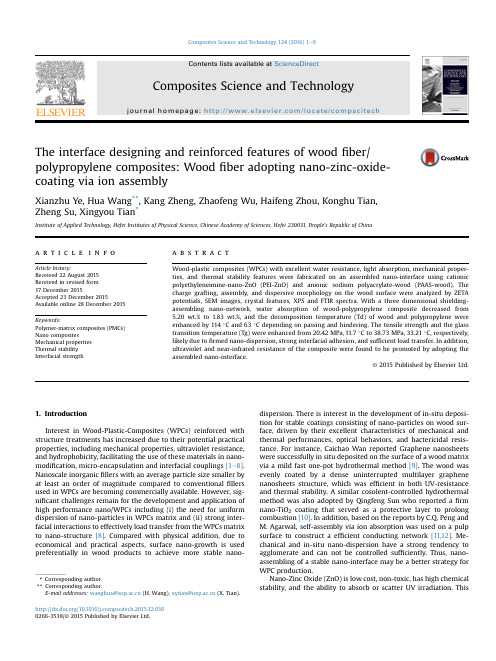
The interface designing and reinforced features of wood fiber/polypropylene composites:Wood fiber adopting nano-zinc-oxide-coating via ion assemblyXianzhu Ye,Hua Wang **,Kang Zheng,Zhaofeng Wu,Haifeng Zhou,Konghu Tian,Zheng Su,Xingyou Tian *Institute of Applied Technology,Hefei Institutes of Physical Science,Chinese Academy of Sciences,Hefei 230031,People's Republic of Chinaa r t i c l e i n f oArticle history:Received 22August 2015Received in revised form 17December 2015Accepted 23December 2015Available online 28December 2015Keywords:Polymer-matrix composites (PMCs)Nano compositesMechanical properties Thermal stability Interfacial strengtha b s t r a c tWood-plastic composites (WPCs)with excellent water resistance,light absorption,mechanical proper-ties,and thermal stability features were fabricated on an assembled nano-interface using cationic polyethyleneimine-nano-ZnO (PEI-ZnO)and anionic sodium polyacrylate-wood (PAAS-wood).The charge grafting,assembly,and dispersive morphology on the wood surface were analyzed by ZETA potentials,SEM images,crystal features,XPS and FTIR spectra.With a three dimensional shielding-assembling nano-network,water absorption of wood-polypropylene composite decreased from 5.20wt.%to 1.83wt.%,and the decomposition temperature (Td)of wood and polypropylene were enhanced by 114 C and 63 C depending on passing and hindering.The tensile strength and the glass transition temperature (Tg)were enhanced from 20.42MPa,11.7 C to 38.73MPa,33.21 C,respectively,likely due to firmed nano-dispersion,strong interfacial adhesion,and suf ficient load transfer.In addition,ultraviolet and near-infrared resistance of the composite were found to be promoted by adopting the assembled nano-interface.©2015Published by Elsevier Ltd.1.IntroductionInterest in Wood-Plastic-Composites (WPCs)reinforced with structure treatments has increased due to their potential practical properties,including mechanical properties,ultraviolet resistance,and hydrophobicity,facilitating the use of these materials in nano-modi fication,micro-encapsulation and interfacial couplings [1e 8].Nanoscale inorganic fillers with an average particle size smaller by at least an order of magnitude compared to conventional fillers used in WPCs are becoming commercially available.However,sig-ni ficant challenges remain for the development and application of high performance nano/WPCs including (i)the need for uniform dispersion of nano-particles in WPCs matrix and (ii)strong inter-facial interactions to effectively load transfer from the WPCs matrix to nano-structure [8].Compared with physical addition,due to economical and practical aspects,surface nano-growth is used preferentially in wood products to achieve more stable nano-dispersion.There is interest in the development of in-situ deposi-tion for stable coatings consisting of nano-particles on wood sur-face,driven by their excellent characteristics of mechanical and thermal performances,optical behaviors,and bactericidal resis-tance.For instance,Caichao Wan reported Graphene nanosheets were successfully in situ deposited on the surface of a wood matrix via a mild fast one-pot hydrothermal method [9].The wood was evenly coated by a dense uninterrupted multilayer graphene nanosheets structure,which was ef ficient in both UV-resistance and thermal stability.A similar cosolent-controlled hydrothermal method was also adopted by Qingfeng Sun who reported a firm nano-TiO 2coating that served as a protective layer to prolong combustion [10].In addition,based on the reports by C.Q.Peng and M.Agarwal,self-assembly via ion absorption was used on a pulp surface to construct a ef ficient conducting network [11,12].Me-chanical and in-situ nano-dispersion have a strong tendency to agglomerate and can not be controlled suf ficiently.Thus,nano-assembling of a stable nano-interface may be a better strategy for WPC production.Nano-Zinc Oxide (ZnO)is low cost,non-toxic,has high chemical stability,and the ability to absorb or scatter UV irradiation.This*Corresponding author.**Corresponding author.E-mail addresses:wanghua@ (H.Wang),xytian@ (X.Tian).Contents lists available at ScienceDirectComposites Science and Technologyjournal homepage:http://www.e lse /locate/compscitech/10.1016/pscitech.2015.12.0160266-3538/©2015Published by Elsevier Ltd.Composites Science and Technology 124(2016)1e 9material has potential use as afiller for composite,as demonstrated by some previous reports[13e16].The cell wall of wood is mainly composed of biopolymers,including cellulose,lignin and hemi-cellulose,and there are a large number of hydroxyl groups on cel-lulose.Chemical assembly can advantage of this structure making use of the hydroxyl groups on the surface of woodfiber with active nano-ZnO,to construct an efficient nano-coating on the wood surface,allowing construction of a dispersive three-dimensional nano-interface in WPCs.In terms of ionic self-assembly,this work used cationic polyethyleneimine-ZnO(PEI-ZnO)and anionic so-dium polyacrylate-woodfiber(PAAS-wood).The reasons for these selections are as follows:branched PEI with high electric density and activity can allow reaction of inorganic particles with the hy-droxyl groups on surface,efficiently making nano-ZnO cationic. Most importantly,in the process of ion assembly,the grafted PEI-coating structure enhances macroscopic smelting between wood fiber and polypropylene for its viscosity and liquidity[17,18].PAAS has excellent adhesive properties,and is endowed with excellent flocculent ability for its active anions(e COOÀ)[19].By grafting on wood surface,followed by ultrasonic treatment,cationic PEI-ZnO particles would be absorbed by PAAS-wood.In the present work,the assembling process adopted ultrasonic technology.By testing different assembling ratios,ion assembly was evaluated by ZETA potentials,nano-size,crystal features and absorptive structure.Additionally,particle emphasis was also placed on examining the internal mechanism of the dispersive nano-network in WPCs as well as the relative functions of wood-polypropylene composite,including dimensional stability,light absorption,mechanical reinforcements,and thermal shielding. 2.Materials and methods2.1.MaterialsPolypropylene(PP)particles were obtained from Dushanzi petrochemical company of china petroleum(Q/XJ1100-1998T30S, diameter:3mm).Poplarfiber was obtained from Shanghai Jia Feng, Ltd(diameter:5e20um).Sodium polyacrylate(PAAS)with40wt.% solid content was obtained from Sinopharm Chemical Reagent Co., Ltd(SCRC).Branched ethylene imine polymer(PEI,relative mo-lecular mass of about7000)and nano Zinc Oxide(ZnO,diameter: 50±10nm)were purchased from Aladdin Industrial Corporation (China).2.2.SynthesisWoodfibers and polypropylene particles were dried at110 C for 10h to eliminate moisture.Next,woodfibers were dispersed in PAAS solution(pH value¼10,16g woodfibers in500ml of 0.2wt.%,0.4wt.%,0.6wt.%,0.8wt.%,or1.0wt.%PAAS solution)and nano-ZnO particles were dispersed in PEI solution(0.4g,0.8g,1.2g, 1.6g,or1.8g in250ml-8wt.%-PEI solution),the ultrasonic time (50 C)was1,3,or5h,respectively.The resulting woodfibers and nano-ZnO particles were washed and isolated byfiltrationfive times,and assembled with each other for3h at50 C.The assembly adopted woodfibers grafted in0.4wt.%PAAS solution(3h)and exhibited different ratios of cationic nano-ZnO.After washing and isolation,the assembled wood(16g)was melted with dried poly-propylene(24g)at175 C in a torque rheometer.For avoiding wood decomposition,the success of smelting occurred when the shearing interaction and the torque-curve value became stable.Thefinal mixture was formed into a sheet shape using a plate rheometer at 180 C under pressure of5MPa,10MPa,or15MPa per3min.2.3.Functional characterizationThe morphology was observed under a sirion-200scanning electron microscope(SEM,FEI,America)with an accelerating voltage of10kV.Structure analysis based on chemical assembly was examined by a Nicolet Nexus Fourier transform infrared spectrometer(FTIR).Crystallization features were studied by X ray diffraction(XRD).For the wood-polypropylene composite,the weight ratio of the nano-ZnO mentionaed above was1wt.%,2wt.%, 3wt.%,4wt.%,or5wt.%.At room temperature(25 C,101.3kPa atmospheric condition),the contact angle was tested by a contact angle meter SL200A(Solon shanghai Co.,Ltd.)with2uL water droplet,and after drying at110 C,the water absorption was evaluatedfive according to formula(1) C for24h:A%¼W sÀW pW p*100%(1)where W s,W p are the weight of the primary composite and swelling composite respectively.Tensile properties(GB/T1040-92: 50mm*20(10)mm*3mm)andflexural properties(GB1042-79: 50mm*15mm*3mm)were determined by a CMT4204universal material testing system(Shenzhen SANS Test Machine Co.,Ltd, China)at room temperature with crosshead speed of20mm/min. The values reported here represent an average of the results of ten tests.Viscoelasticity was measured by the dynamic mechanical tester(DMA,tensile size:20mm*6mm*1mm) withÀ50 C e160 C range,the protecting gas was N2and heating rate was1 C/min at5Hz.The thermal decomposition of com-posites was analyzed by the TG with50 C e800 C range using N2 as the protecting gas.The light separation was measured by an ultraviolet-infrared spectrophotometer using1mm plate sample. Relative analysis referred to the Formula(2)and Formula(3):T¼II O¼expÀ"3V p xr34l4n pn mÀ1#!(2)where T is transmittance of nano-composite,V p is nano-volume,x was optical path,l is wavelength of incident light,n p and n m are refractive index of matrix and nano-particles,r is diameter of nano-particles.d¼4E13Nfd2À1(3)Kubo theory:d is gap;E1is Feimi energy,N is total number of conductive electron;d is supermicro-particle size.3.Results and discussion3.1.Nano-ZnO-coating based on ion absorption(chemical-assembly)The experimental design is shown in Fig.1.The grafting effect allows anionic PAAS-wood to assemble the cationic PEI-ZnO par-ticles,resulting in afirmed and dispersed nano-surface[20,21]. With high crystallinity,long polymer chain structure,and high mass fraction(~50%),the wood strength is mainly dependent on cellulose,which was observed mainly in an I crystal form((001) and(112)).Nano-ZnO is a six-party zinc crystal system,in which four Oxygen atoms surround a Zinc atom.The n-semiconductor and relative X-ray diffraction peaks focused on(100),(002),(101),(102), (110),(103),(112).Grafted by polyelectrolyte,in Fig.2,it is worth noting that the crystal structure of wood and nano-ZnO did not change obviously,suggesting that the relative performance ofX.Ye et al./Composites Science and Technology124(2016)1e9 2assembled wood would be maintained (Fig 2(3)).As shown in Fig.3,PAAS-wood and PEI-ZnO have stable anionic and cationic poten-tials,respectively.For suf ficient dispersion and ef ficient grafting,the highest zeta-potential of PAAS-wood and PEI-ZnO reached 55.2and À45mv,respectively.However,the value decreases if the so-lution concentration (PAAS)or addition ratio (ZnO)increases,which can be ascribed to bad dispersion and weak grafting ef fi-ciency.Further,compared with pure aggregates,the nano-particle size was decreased signi ficantly,showing that charge repulsion improved the nano-dispersion,mainly concentrated on 42e 133nm.Importantly,the results suggest that charge grafting based on polyeletrolyte is ef ficient for wood and nano-ZnO parti-cles,and the uniform interactions and firmed charges will promote ion assembly to construct an adhesive nano-interface in WPCs.The assembling morphology and structure of wood fiber are shown in Fig.4and Fig.5.In Fig.4(a)and (b),it is clear that for charge repulsion,PEI grafting (about 3nm thickness)promotes nano-dispersion to a certain extent and high-resolution phase of PEI-ZnO is maintained.Due to intense charge activity and the ad-hesive properties of PEI and PAAS,Fig.4(c)and (d)show that ion assembly allowed construction of an adhered and dispersed nano-coating on the wood surface.In addition,XPS (Table 1and Fig.5(1))showed the atomic composition before and after assembly;the N1s,O1s,and Zn2p peaks re flect the assembled components ef fi-ciently.Fig.5(2)shows the FTIR of PAAS-wood-PEI-ZnO in more detail.The disappearance of 1245cm À1is ascribed to the interac-tion of alcoholic (ether)hydroxyl on the wood surface with PEI.1500cm À1and 1725cm À1,characteristic peaks of primary amine (e NH 2)and C ]O,also weakened with assembly.The peak around 3300cm À1e 3600cm À1is attributed to e OH and e NH e ,which sharpens with PEI interaction [19].3.2.Water absorption of wood-polypropylene composite with nano-interfaceIn general,the water absorption of WPCs should be due to the mechanical interlock and the secondary forces (dispersion,induced dipole,dipole e dipole,H-bonds,etc.)between the polymer and the hydrophilic wood fillers [1,22].The coating on the wood surface consisted of three layers:PAAS-PEI-nano-ZnO.By reference to wetting theory,as seen in Fig.6(1),the contact angle increases with assembling WPCs,indicating the weakness of surface activity with ef ficient shielding.Similar results are seen in Fig 6(2),in which the assembling nano-interface firms the composite well in water compared with the pure composite.By soaking for 6h,the absorptive value decreases from 5.20wt.%to 1.83wt.%,indicating the shielding effect of assembly.The shielding can result from:(i)charge-grafting (coupling effect,H-bond,Van der Waals'force)weakens the wood activity and synchronously promotes the suf fi-ciency of the mechanical interlock for liquid and adhesive molec-ular structure on the wood surface.(ii)a firmed three-dimensional segregated nano-network separates the wetting ef ficiently.Fig.1.Designing route via ion assembly (anionic PEI-wood fiber and cationic PAAS-ZnO).X.Ye et al./Composites Science and Technology 124(2016)1e 933.3.Ultraviolet resistance with assembling nano-interfaceNano-ZnO shows absorption at 300e 400nm as its active bond with about 300e 400kJ/mol energy can produce obvious electronic migration in this range [13,16].According to Formula (2),ultraviolet transmittance is related with nano-size and nano-concentration if other parameters are constant,and small size can promote the transmittance to a certain extent [9].As seen in Fig.7,ultraviolet absorption is improved by the application of nano-assembly.Importantly,the absorption peak moves to 350e 370nm gradu-ally,suggesting the nano-ZnO is active and small as band gap is associated with particle size according to Kubo theory (Formula (3)).Due to suf ficient dispersion and firmed adhesion,the highest band gap is 3.52eV in the composite with the 2wt.%nano-interface,meaning that the transition energy is higher.Moreover,the near-infrared region produces more apparent absorption in the assembled composite in Fig.7.At 1600nm in the near-infrared region,the absorption value increased from 0.5to 1.33,meaning the separation protected the WPCs ef ficiently from near-infrared degradation effects including discoloration,fracturing and cloud-ing,and prolonged service lives of WPCs.In summary,results indicate that the assembled-network,interlaced well in WPCs,is feasible in light separation for its stable structure and ef ficient dispersion.3.4.Mechanical enhancements with assembling nano-interface There are four main requirements for effective reinforcement of nano-WPCs,including a large aspect ratio,good dispersion,align-ment,and interfacial stress transfer.Dispersion is probably the most fundamental obstacle to production of more uniform stressdistribution and minimum stress concentration [23,24].As can be seen in Table 2,after addition of 1,2,or 3wt.%nano-ZnO,the tensile strength was improved by about 43%,57%,and 90%.The elongation at break was improved by about 22%,40%,49%(from 1.63%of the pure composite to 1.99%,2.29%,2.43%respectively).The compre-hensive reinforced properties should result from:(i)assembly of nano-ZnO-particles were uniformly dispersed as the level of iso-lated nano-particles grafted by polyelectrolyte,required to achieve ef ficient load transfer to the nano-network.This also results in a more uniform stress distribution and minimizes the presence of stress concentration centers.(ii)assembly interaction of poly-electrolyte gives suf ficient micro-encapsulation on wood surface [11],and the coated molecular chains allow the construction of mechanical interlock between wood and polypropylene ef ficiently.The uniform dispersion of nano-ZnO and the strong interfacial adhesion between nano-ZnO and WPCs were con firmed by the SEM images of microcracks produced during fracture in Fig.8.Consistent with mechanical reinforcements,images of microcracks show that the nano-ZnO particles are dispersed well and uniformly embedded in the WPCs matrix (Fig.8b,c,d,e).However,apparent in Fig.8f,there are some apparent unevenness,likely due to higher nano-size and a weak grafting interaction.In Fig.8,the vicoelastic properties of composites are shown.The storage modulus based on self-assembly first increases and then reduces with the increase of the nano-ratio,consistent with the enhanced properties in Table 2.The signi ficant enhancement of the elastic modulus should be ascribed to the strong interfacial adhesion and suf ficient load transfer from WPCs to the nano-network.In addition,peaks (tan d )at about 11e 35 C,representing the glass transition temperature (Tg)of polypropylene increased in the assembled composite,the largest reaches 33.21 C while pure composite is only 11.7 C.ThisisFig.2.XRD spectra of pure wood,PAAS-wood,pure-ZnO,PEI-ZnO,PAAS-wood-PEI-ZnO.X.Ye et al./Composites Science and Technology 124(2016)1e 94Fig.3.Zeta potentials of nano-ZnO grafted by PEI and wood fibers grafted by PAAS treating,and particle size of nano-ZnO withPEI-treating.Fig.4.Images of transmission electron microscopy (TEM,a is PEI-ZnO,b is high-resolution phase of PEI-ZnO),and images of scanning electron microscopy (SEM,c and d are wood fiber (16g)with nano-ZnO (2.0g)assembling).X.Ye et al./Composites Science and Technology 124(2016)1e 95Fig.5.(1)The XPS spectra and (2)the infrared spectroscopy of pure wood and PEI-wood(16g)-PAAS-ZnO(1.2g)with chemical assembly.Table 1Atomic composition of pure wood and PEI-wood-PAAS-ZnO by X-ray photoelectron spectroscopy.SampleC (atomic %)O (atomic %)N (atomic %)Zn (atomic %)Na (atomic %)Pure wood66.9028.84 4.04e 0.23PEI-wood-PAAS-ZnO64.3624.028.902.390.32Fig.6.Contact angle (1)and water absorption (2)of composites with different nano-assembling ratios.X.Ye et al./Composites Science and Technology 124(2016)1e 96likely due to the surface activity of nano-particles and intense in-teractions between the active nano-particles and chain segments.With about 1wt.%e 4wt.%nano-ZnO network on interface of WPCs,the assembly by uniform nano-dispersion and the strong interfacial adhesion are controlled as predicted (see Fig.9).3.5.Thermal decomposition with assembling nano-interface In this work,wood polypropylene composites show two decomposition (Td)stages in the heating process.The first is wood decomposition,which occurs at about 207 C,and the second is mainly the decomposition process of polypropylene which occurs at about 342 C [3,25].In Fig.10,TG curves show that the first weight loss of pure composite (mass loss ~28wt.%)is greater than the assembled composites,owing to redundant nano-componentsof the assembled composites.Most importantly,composites with assembling nano-interface have higher temperature during these two decomposition stages.For example,the composite-3wt.%shows more compact shielding in Fig.10,and Td is up to 321 C and 438 C,respectively.These results can be attributed to:(i)disper-sive nano-ZnO interface transfers heat effectively,and hinders the diffusion of degradation components from the matrix to gaseous phase.(ii)Suf ficient melting,milling,and active interactions be-tween wood,polyelectrolyte-nano-ZnO and polypropylene inhibit decomposition to a certain extent.Results con firm that poly-electrolyte ion assembling to construct a three-dimensional nano-interface in WPCs enhances thermal shielding and stability ofWPCs.Fig.7.The absorption spectra (1)and re flection spectra (2)(ultraviolet e visible e near infrared light)of composites with different nano-assembling,and the band gap of nano-ZnO (3).Table 2Mechanical properties of composites with different wt.%of assembling nano-ZnO interface.SampleTensile propertiesFlexural propertiesTensile strength (MPa)Elastic modulus (MPa)Elongation at break (%)Flexural strength (MPa)Flexural modulus (MPa)Largest flexural force (N)Pure composite 20.42±1.392308.22±89.2 1.63±0.1852.21±2.89226.40±9.230.56±2.17composite-1wt.%29.20±2.142712.32±107.3 1.99±0.2356.88±2.31298.60±11.139.45±2.31composite-2wt.%32.12±2.682889.29±123.7 2.29±0.3857.12±2.19304.20±7.242.12±1.60composite-3wt.%38.73±2.123121.6±108.2 2.43±0.2766.29±2.34336.70±11.245.849±2.42composite-4wt.%31.39±3.213022.1±156.1 2.12±0.2861.18±2.67312.20±8.9943.12±1.21composite-5wt.%27.33±1.292631.2±112.62.04±0.1955.38±2.16287.16±4.2336.29±2.15X.Ye et al./Composites Science and Technology 124(2016)1e 974.ConclusionsIon assembly was used to construct a firm nano-ZnO interface into wood-polypropylene composite using PAAS-wood fibers and cationic PEI-ZnO particles.The dispersion,interactions,and assembling features were examined by ZETA potential,SEM im-ages,XRD spectra and FTIR.Importantly,mechanical properties,viscoelasticity,and the thermal stability of composite with assembling nano-interface were improved for the adhesive and dispersive nano-network.The flexural properties and the tensile strength of composite with 3wt.%nano-interface was enhanced about 90%.With a three-dimensional nano-shielding effect,sepa-ration in ultraviolet e visible e near-infrared light was also rein-forced as well as the water resistance,which decreasedfromFig.8.SEM images of wood-polypropylene composites with different wt.%of assembling nano-ZnO interface (a is pure composite;b,c,d,e,f are composites with 1wt.%,2wt.%,3wt.%,4wt.%,5wt.%nano-ZnO,respectively).Fig.9.(1)Storage modulus,(2)loss factor of composites with different wt.%of assembling nano-ZnO interface,and (3)Tg of polypropylene.X.Ye et al./Composites Science and Technology 124(2016)1e 985.20wt.%to 1.83wt.%after soaking for 6h.AcknowledgmentThe authors are grateful to the supports of National Natural Science Foundation of China (No.51103160).References[1]Yanjun Xie,Zefang Xiao,Timo Grüneberg,Holger Militz,Callum A.S.Hill,Leif Steuernagel,Carsten Mai,Effects of chemical modi fication of wood par-ticles with glutaraldehyde and 1,3-dimethylol-4,5-dihydroxyethyleneurea on properties of the resulting polypropylene composites,Compos Sci.Technol.70(13)(2010)2003e 2011.[2]Mohammad Reza M.Farahani,Fatemeh Banikarim,Effect of nano-zinc oxideon decay resistance of wood-plastic composites,Bioresources 8(4)(2013)5715e 5720.[3]Z.Farhadinejad,M.Ehsani, B.Khosravian,G.Ebrahimi,Study of thermalproperties of wood plastic composite reinforced with cellulose micro fibril and nano inorganic fiber filler,Eur.J.Wood Wood Prod.70(6)(2012)823e 828.[4]Jianli Li,Ping Xue,Wenying Ding,Jinmin Han,Guolin Sun,Micro-encapsulatedparaf fin/high-density polyethylene/wood flour composite as form-stable phase change material for thermal energy storage,Sol.Energ Mat.Sol.C 93(10)(2009)1761e 1767.[5]Omar Faruk,Andrzej K.Bledzki,Laurent M.Matuana,Microcellular foamedwood-plastic composites by different processes:a review,Macromol.Mater Eng.292(2)(2007)113e 127.[6]J.George,M.S.Sreekala,S.Thomas,A review on interface modi fication andcharacterization of natural fiber reinforced plastic composites,Polym.Eng.Sci.41(9)(2001)1471e 1485.[7]T.Gurunathan,Smita Mohanty,Sanjay K.Nayak,A review of the recent de-velopments in biocomposites based on natural fibres and their application perspectives,Compos Part A-Appl S 77(2015)1e 25.[8]Majid Chaharmahali,Yahya Hamzeh,Ghanbar Ebrahimi,Alireza Ashori,Ismail Ghasemi,Effects of nano-graphene on the physico-mechanical prop-erties of bagasse/polypropylene composites,Polym.Bull.71(2)(2014)337e 349.[9]Caichao Wan,Yue Jiao,Jian Li,In situ deposition of graphene nanosheets onwood surface by one-pot hydrothermal method for enhanced UV-resistant ability,Appl.Surf.Sci.347(2015)891e 897.[10]Qingfeng Sun,Haipeng Yu,Yixing Liu,Jian Li,Yongzhi Cui,Yun Lu,Prolongingthe combustion duration of wood by TiO2coating synthesized using cosolvent-controlled hydrothermal method,J.Mater Sci.45(24)(2010)6661e 6667.[11] C.Q.Peng,Y.S.Thio,R.A.Gerhardt,Conductive paper fabricated by layer-by-layer assembly of polyelectrolytes and ITO nanoparticles,NANOTECH-NOLOGY 19(50)(2008),505603.[12]M.Agarwal,Q.Xing,B.S.Shim,N.Kotov,K.Varahramyan,Y.Lvov,Conductivepaper from lignocellulose wood micro fibers coated with a nanocomposite of carbon nanotubes and conductive polymers,Nanotechnology 20(2009)215602.[13]Erjun Tang,Guoxiang Cheng,Xingshou Pang,Xiaolu Ma,Fubao Xing,Synthesisof nano-ZnO/poly(methyl methacrylate)composite microsphere through emulsion polymerization and its UV-shielding property,Colloid Polym.Sci.284(4)(2006)422e 428.[14]Dangge Gao,Chen Chen,Jianzhong Ma,Xiying Duan,Jing Zhang,Preparation,characterization and antibacterial functionalization of cotton fabric using dimethyl diallyl ammonium chloride-allyl glycidyl ether-methacrylic/nano-ZnO composite,Chem.Eng.J.258(2014)85e 92.[15]M.Ashraf,El Saeed,M.Abd El-Fattah,Ahmed M.Azzam,Synthesis of ZnOnanoparticles and studying its in fluence on the antimicrobial,anticorrosion andmechanical behavior of polyurethane composite for surface coating,Dyes Pigments 121(2015)282e 289.[16]Ying Huang,Tongwen Wang,Xiaolei Zhao,Xinlong Wang,Lu Zhou,Yuanyuan Yang,Fenghui Liao,Yaqing Ju,Poly(lactic acid)/graphene oxide-ZnO nanocomposite films with good mechanical,dynamicmechanical,anti-UV and antibacterial properties,J.Chem.Technol.Biot.90(9)(2015)1677e 1684.[17]Scott Renneckar,Yu Zhou,Nanoscale coatings on wood:polyelectrolyteadsorption and layer-by-layer assembled film formation,ACS Appl.Mater Inter.1(3)(2009)559e 566.[18]Christian Aulin,Imre Varga,Per M.Claesson,Lars Wågberg,Tom Lindstr €om,Buildup of polyelectrolyte multilayers of polyethyleneimine and micro-fibrillated cellulose studied by in situ dual-polarization interferometry and quartz crystal microbalance with dissipation,Langmuir 24(6)(2008)2509e 2518.[19]Bing-Qiang Lu,Ying-Jie Zhu,Xin-Yu Zhao,Guo-Feng Cheng,Yin-Jie Ruan,Sodium polyacrylate modi fied Fe 3O4magnetic microspheres formed by self-assembly of nanocrystals and their applications,Mater.Res.Bull.48(2)(2013)895e 900.[20]Wen Li,Lixin Wu,Nano-hybrid liquid crystals from the self-assembly ofsurfactant-encapsulated polyoxometalate complexes,Chin.J.Chem.33(1)(2015)15e 23.[21]Olga A.Guskova,Christian Seidel,Assembly of nano-particles on diblockcopolymer brushes:toward laterally nano-structured composites,Soft Matter 8(10)(2012)2833e 2843.[22]Irina Turku,Timo Karki,Reinforcing wood-plastic composites with macro-and micro-sized cellulosic fillers:comparative analysis,p.32(22)(2013)1746e 1756.[23]H.Nolte,C.Schilde,A.Kwade,Determination of particle size distributions andthe degree of dispersion in nanocomposites,Compos Sci.Technol.72(9)(2012)948e 958.[24]Songmin Shang,Lu Gan,Marcus Chun-wah Yuen,Improvement of carbonnanotubes dispersion by chitosan salt and its application in silicone rubber,Compos Sci.Technol.86(2013)129e 134.[25]Justo Lisperguer,Ximena Bustos,Yanina Saravia,Thermal and mechanicalproperties of wood flour-polystyrene blends from postconsumer plastic waste,J.Appl.Polym.Sci.119(1)(2011)443e 451.Fig.10.TG curves of composites with different wt.%of assembling nano-ZnO interface.X.Ye et al./Composites Science and Technology 124(2016)1e 99。
BA-TH05-521 CERN-PH-TH2005-216

BA-TH/05-521CERN-PH-TH/2005-216hep-th/0511039 Observable(?)cosmological signaturesof superstrings in pre-big bang models of inflationM.Gasperini1,2,3and S.Nicotri21CERN,Theory Unit,Physics Department,CH-1211Geneva23,Switzerland2Dipartimento di Fisica,Universit`a di Bari,Via G.Amendola173,70126Bari,Italy3Istituto Nazionale di Fisica Nucleare,Sezione di Bari,Bari,ItalyAbstractThe different couplings of the dilaton to the U(1)gaugefield of heterotic and Type Isuperstrings may leave an imprint on the relics of the very early cosmological evolu-tion.Working in the context of the pre-big bang scenario,we discuss the possibility ofdiscriminating between the two models through cross-correlated observations of cosmicmagneticfields and primordial gravitational-wave backgrounds.To appear in Phys.Lett.BCERN-PH-TH/2005-216November2005It is well known that thefive superstring models differ,at the level of the lowest-order bosonic effective action,for theirfield content and for the way in which the variousfields are coupled to the dilaton[1].Even in models with the same(boson)particle content,such as Type I and heterotic superstrings,there are differences due to the dilaton couplings,most notably for the Yang–Mills one form containing the U(1)gaugefield associated to standard electromagnetic(e.m.)interactions.The effective tree-level action for the photon–dilaton interactions,after appropriate dimensional reduction from ten to four dimensions,can be parametrized(in the string frame)as follows:S=−1−g f(b i)e−ǫφFµνFµν,Fµν=∂µAν−∂νAµ,(1)whereφis the ten-dimensional dilaton,(normalized in such a way that the string coupling parameter g corresponds to g2=expφ),and where the“form factor”f(b i)takes into account the possibe dynamical contribution of the internal modulifields b i,i=1, (6)The dilaton parameterǫhas values1,1/2,respectively,for heterotic and Type I superstrings, which are probably the best candidates for representing the low energy limit of standard model interactions.The value ofǫcould be different,however,were the e.m.field identified not as a component of the higher-dimensional gauge group,but as a one form present in the Ramond–Ramond or Neveu-Schwarz–Neveu-Schwarz sector of other string theory models. In that case also the coupling to the internal moduli would be different(see for instance [2]).The discussion of this paper will be concentrated on the case in which f(b i)simply represents the volume factor of the six-dimensional internal manifold,f(b i)=V6≡ 6i=1b i, and different string models are characterized by different values ofǫ.When the internal moduli are stabilized,indeed,the coupling function f(b i)becomes trivial,and the only relevant parameter isǫ.In any case,different values ofǫamount to different rescaling of the photon–dilaton coupling,and may have significant impact on cosmological processes where large variations of the dilatonfield,for long periods of time,may occur.The effect we shall consider here will concern the amplification of the quantumfluc-tuations of the e.m.field,and the possible production of“seeds”for the magneticfields currently observed on large scales[3].Working in the context of the so-called pre-big bang scenario of superstring cosmology[4,5]it will be shown in particular that,depending on the value ofǫ,the region of parameter space compatible with an efficient production of seeds may or may not overlap with the different regions of parameter space compatible with an observable production of cosmic gravitons.The cross-correlated analysis of cosmic magnetic fields and of the outputs of gravitational detectors may thus provide unique information, in principle,not only on the allowed region of parameter space of the considered class of string cosmology models,but also on the most appropriate superstring model–i.e.on the most appropriate M-theory limit–to be chosen for a realistic description of fundamental interactions during the primordial stages of our Universe.1We start by recalling that the e.m.fluctuations of the vacuum,according to the action (1),are represented by the canonical variableψµ,ψµ=zγAµ,zγ= 6 i=1b i 1/2e−ǫφ/2,(2)which diagonalizes the four-dimensional action.In a conformallyflat four-dimensional ge-ometry,gµν=a2ηµν,and in the radiation gauge A0=0=∂i A i,ψµsatisfies the canonical equation[6]:ψ′′i−∇2ψi−z−1γz′′γψi=0,(3) where the primes denote differentiation with respect to the conformal timeη.Notice that, because of the conformal invariance of the four-dimensional Maxwell action,thefluctuations ψi are decoupled from the four-dimensional geometry;the canonical equation only contains a coupling to thefirst and second derivatives of the dilaton background and of the internal moduli.A time-dependent dilaton(with the possible contribution of a time-dependent internal geometry)may thus act as an external“pumpfield”,and sustain the amplification of the e.m.fluctuations during the primordial phases of accelerated(inflationary)evolution.For the case of the heterotic couplingǫ=1the inflationary spectrum offluctuations produced by the dilaton has already been computed in[6,7].Here we shall derive the corresponding spectrum for a generic value ofǫ,using the same example of inflationary string-cosmology background as was adopted in[6],based on a“minimal”pre-big bang scenario consisting of two phases.During thefirst phase,ranging in conformal time from−∞toηs<0,the Universe is assumed to evolve from the string perturbative vacuum according to the exact(Kasner-like) solutions of the lowest order gravi-dilaton effective action[5]:a(η)∼(−η)β0(1−β0),φ(η)∼ iβi+3β0−13/2,ǫ′= iβi−ǫ( iβi+3β0−1)3(1−β0)(6)(in the special case of frozen internal dimensions,βi=0,β0=−1/√the higher-dimensional perturbative vacuum towards the four-dimensional strong coupling regime–that the internal dimensions are shrinking,and that the four-dimensional coupling, controlled byφ4=φ−ln V6=φ−6ln b i,is growing.The intersection with the Kasner condition then defines,forβ0andβi,the following allowed ranges of values:−1/√6.(7) During the second,high-curvature phase,ranging fromηs toη1<0,the(string-frame) curvature stays frozen at a constant value H1controlled by the string scale,H1∼M s;the internal moduli are also frozen,while the dilaton(and the string coupling g)keeps growing from the initial value g2s=expφ(ηs)≪1to afinal value g21=expφ(η1)∼1.The background evolution during this second“stringy”phase can be represented bya(η)∼(−η)−1,φ(η)∼−2βln(−η),zγ(η)∼(−η)ǫβ,β>0,ηs≤η≤η1<0,(8) whereβis a phenomenological parameter that possibly takes into account the effects of the higher orderα′corrections to the effective action.Eventually,forη>η1,the Universe is expected to enter the radiation-dominated,frozen-dilaton(decelerated)regime a∼η,φ= const,and to follow all subsequent steps of the standard cosmological evolution.For such a model of background,each polarization component of the canonical variable can be expanded in Fourier modesψk such that∇2ψk=−k2ψk,and Eq.(3)becomes a Bessel equation whose solution can be expressed in terms of thefirst-and second-kindHankel functions[5].By imposing the asymptotic normalization to a spectrum of quantum√vacuumfluctuations,ψk=exp(−ikη)/=Ωr(t0) H1ω1 3−|2ǫβ−1|,ωs<ω<ω1,d lnω=Ωr(t0) H1ω1 3−|2ǫβ−1| ω3−1|,ω<ωs,(9)where the parametersβ,βi,β0may vary in the ranges previously defined,and where we have assumedǫ>0(forǫ=0there is no amplification of e.m.fluctuations during the3string phase,and the above spectrum cannot be applied).Hereρc=3H0M2P is the criticalenergy density,M P=(8πG)−1is the Planck mass,Ωr(t0)≃4×10−5h−20is the present fraction of critical energy density in radiation,with h0=H0/(100km sec−1Mpc−1)the present(dimensionless)value of the Hubble parameter.Finally,ω1=(a|η1|)−1≃H1(a1/a) andωs=(a|ηs|)−1≃H s(a s/a),where H1∼M s is the value of the curvature scale at the inflation→radiation transition,whileωs is a free parameter of the given inflationaryscenario.Previous detailed computations of the above spectrum for the pure heterotic caseǫ=1can be found in[5,9](see[10]for a recent computation of the spectrum for a genericvalue ofǫ,but for the special case of frozen internal dimensions,i.e.withǫ′=ǫ).This primordial spectrum of e.m.fluctuations is distributed over a wide range of fre-quencies and can provide,in principle,the“seeds”required to trigger the galactic dynamoand to produce the cosmic magneticfields B G(of microgauss strength)currently observedon the galactic scale[3,6].The identification of the seedfields with the vacuumfluctua-tions,amplified by inflation,requires however that suchfluctuations be coherent and largeenough over a proper length scale that today roughly corresponds to the megaparsec scale,asfirst pointed out in[11].For a conservative estimate of the requiredfield strength[11]one can assume the exis-tence of the standard galactic dynamo mechanism,operating since the epoch of structureformation,characterized by an amplification factor∼1013,and can also take into accountthe additional amplification(∼104)due to magnetic-flux conservation in the collapse ofthe galactic structure from the Mpc to the10kpc scale.One obtains,in this way,the lowerbound B s>∼10−23gauss on the present amplitude of the magnetic seeds at the Mpc scale; the identification of the seeds with the inflationary spectrum of e.m.fluctuations leads then to the condition[11]:B2s(ωG,t0)ρr(t0)=Ωγ(ωG,t0)spanned by the variables{ω1,ωs,β,β0,H1},and the purpose of this paper is to compare such regions with analogous allowed regions associated to the amplification of tensor metric perturbations,and to the detectable production of cosmic gravitons,in the same given class of pre-big bang backgrounds.To this purpose we must consider the evolution equation of the canonical variable u ij, associated to the tensor perturbations h ij of the three-dimensional spatial sections of the metric background:u ij=z g h ij,z g=a 6 i=1b i 1/2e−φ/2.(12)In the transverse,traceless gauge∂i h i j=0=h i i,and in the string frame,such a canonical equation reads[12]u′′j i−∇2u i j−z−1g z′′g u i j=0,(13) for each spin-two polarization mode u i j.We will use the same model of background specified by Eqs.(4),(8),and we can notice that the graviton“pumpfield”z g,at low energies, is completely insensitive to the dynamics of the internal dimensions[13,14],unlike e.m.fluctuations:according to Eq.(4),indeed,z g∼(−η)1/2during the initial low-curvature phase,quite independently of the values ofβ0andβi(and even in the anisotropic case).In the second,high-curvature phase,the pumpfield is parametrized instead by z g∼(−η)β−1, withβ>0,according to Eq.(8).We can now follow the same procedure as before,expanding thefluctuations in Fourier modes,imposing the canonical normalization atη→−∞,and matching the solutions atηs andη1.The computation of the spectral energy density stored in the amplified gravitational radiation leads to thefinal(already known[14,15,5])result:Ωg(ω,t0)=Ωr(t0) H1ω1 3−|2β−3|,ωs<ω<ω1,=Ωr(t0) H1ω1 3−|2β−3| ωthe gravitational instability of the background[16].We shall thus restrict our discussion to the case0≤β≤3.We should warn the reader,however,that the considered graviton spectrum is typical of a stringy phase at constant curvature and linearly growing dilaton [17],which takes into account higher-derivative corrections but remains at the tree-level in the string coupling:the spectrum could be significantly different if higher-loop corrections were included.There are two main upper bounds on the amplitude of such a growing spectrum:one is obtained from the observations of millisecond pulsars(in particular,from the absence of a detectable distortion of pulsar timing),which imposes the bound[18]h20Ωg(ωp,t0)<∼10−8,ωp≃10−8Hz;(15) the other is obtained from the standard nucleosynthesis analysis,which imposes a bound on the total integrated energy density of the relic graviton spectrum at the nucleosynthesis epoch[19].Such a bound can also be expressed as an upper limit on the peak intensity of the spectrum[20]:Ωg(ω,t0)<∼10−1Ωr(t0),(16) which is valid at all frequency scales.These two upper bounds are in competition with the lower bounds to be imposed on the spectrum for its possible detection by a gravitational antenna of given sensitivity.Here we will use,as a typical reference value,the planned sensi-tivity of the Advanced LIGO interferometers,which are expected to detect a background of relic gravitons through cross-correlated observations around the frequency bandωL∼102 Hz,provided[21]:h20Ωg(ωL,t0)>∼10−11,ωL/2π≃102Hz.(17) See[22,5]for other sensitivities associated to different types of gravitational detectors.The set of constraints(15)–(17),imposed on the graviton spectrum(14),defines allowed regions in the parameter space of our class of background,which we shall now compare with the e.m.regions specified by the constraints(10),(11).For a clearer discussion we will first reduce the dimensions of the parameter space by exploiting the direct relationship existing between H1andω1,determined by the details of the post-inflationary evolution: considering the standard radiation-dominated→matter-dominated evolution wefind[5], in particular,ω1≃H1a1/a≃(H1/M P)1/21011Hz.Next,we willfix the transition scale H1 precisely at the present value of the string scale,H1=M s,assuming for M s the typical value M s=0.1M P,consistently with string models of unified gravitational and gauge interactions [23].This completely specifies the position and the“end point”of the graviton and photon spectrum in terms ofω1andΩ(ω1),and leaves us with four parameters,ωs,β,β0andǫ. The graviton spectrum,however,only depends onωs andβ:we will thus plot the allowed regions in the two-dimensional space spanned by the variables{ωs,β},at different,constant values of the parametersǫandβ0,chosen appropriately for the e.m.spectrum.6For the physical interpretation of thefinal result,however,it will be convenient to replaceωs andβwith an equivalent set of parameters,more directly related to the inflationarykinematics.This new set is obtained by noting that,according to Eq.(8),ωs is related tothe extension in time of the string phase through the e-folding factor z s=a1/a s=ηs/η1=k1/k s=ω1/ωs;the parameterβis related instead to the growth of the string coupling duringthe string phase,since g s/g1=(η1/ηs)β=z−βs.On the other hand,once we have imposed H1=M s=0.1M P,not onlyω1but also thefinal string coupling g1=exp(φ1/2)isfixed[20],at a value quite close to the currently expected value g1≃M s/M P=0.1(since the dilaton is assumed to be frozen during the subsequent standard evolution).The two variables{z s,g s} thus represent a complete and independent set of variables,equivalent to the set{ωs,β},for a convenient parametrization of the chosen class of string cosmology backgrounds[6].For practical purposes we shall work,in particular,with a decimal logarithmic scale,andwe shall plot thefigures in the plane parametrized by the coordinatesx=log z s=log(ω1/ωs)>0,y=log(g s/g1)=−βlog z s=−βx<0.(18) We are now in the position of discussing the possible overlap of the allowed regions for the photon and graviton spectra.We rewrite these in terms of the new variables{x,y} and we note,first of all,that the condition(16)is automatically satisfied because of the choice M s/M P=0.1and of the monotonic growth of the graviton spectrum,which imposes 0≤β≤3,and which translates into the stringent constraint−3x<y<0(19) on the allowed gravitational region.A similar argument can be applied to the e.m.spectrum(9)by noting that its dilaton(low-frequency)branch,controlled by the parameterǫ′,is always growing for all values ofβ0,βi included in the allowed range(7),and forǫvarying from0to1.The high-frequency branch of the spectrum might be decreasing,in principle,if y<−(2x/ǫ):a decreasing spectrum,however,has a peak atω=ωs,and is only marginally compatible with the homogeneity condition(11),which imposes,when applied to the peak value of the spectrum,y>−(2x/ǫ)−1/ǫ.In addition,a decreasing spectrum is ruled out if one would take into account the slightly more stringent(but model-dependent)boundΩγ<0.1Ωr, which follows from the presence of strong magneticfields at the nucleosynthesis epoch [24],which would impose y>−(2x/ǫ).As in the graviton case,we will thus restrict our discussion to a growing spectrum of e.m.fluctuations,which automatically satisfies Eq.(11)and the nucleosynthesis bound,and which imposes the constraint−2x/ǫ<y<0(20)on the allowed e.m.region.The allowed regions of the plane{x,y}determined by the set of conditions(10)(efficientseed production),(15)(pulsar bound),(17)(graviton detectability),(19)and(20)(growing7spectra)are illustrated in Fig.1,which presents in a compact form the main results of this paper.The regions marked by the thin border refer to the graviton spectrum(14),those marked by the bold border refer to the e.m.spectrum(9).We have plotted,for the e.m. spectrum,the two casesǫ=1andǫ=1/2,and we have explicitly indicated the application of the constraints to the high-frequency(string)or low-frequency(dilaton)branches of the spectrum,both for tensor and e.m.fluctuations.It should be stressed that the existence of two graviton regions is due to the absolute value appearing in the power of the graviton spectrum,and to the different kinematic behaviour of tensor perturbations outside the horizon[14,9],depending on the sign of 2β−3:the upper part of the allowed region corresponds to the class of backgrounds with pumpfield characterized by the powerβ−1<1/2,in which the amplitude of tensor perturbations stays constant outside the horizon;the lower part corresponds to backgrounds withβ−1>1/2,in which the amplitude grows outside the horizon,and the inflationary amplification is even more effective[5].No such distinction exists,instead,for the allowed regions of the photon spectrum,once the condition(20)has been imposed.Finally,the dashed lines present in the dilaton sector of the photon regions illustrate the possible effects of a non-trivial(primordial)dynamics of the internal dimensions,possibly producing different values of the effective parameterβ0according to Eqs.(4),(5).The bold line represents the seed condition imposed on the photon spectrum computed in the√case of frozen internal dimensions,and corresponding toβ0=−1/6),(1/3,1/3)for the caseǫ=1,and(−0.568,0.072),(−1/3,1/3), (0.063,0.406)for the caseǫ=1/2(the numerical values have been selected so as to include the maximal and minimal extension of the allowed region compatible with the range of variations of the parametersβ0andβi,given in Eq.(7)).Note that we have also included the possibility of a“bouncing”scenario with positiveβ0,in which the initial growth of the dilaton,at low energy,is associated with the shrinking of the four-dimensional geometry. The effects of the internal dynamics may enhance or reduce the photon allowed regions but, as clearly illustrated in the picture,it cannot lead to the overlap of the photon and graviton regions in the caseǫ=1.The main message of Fig.1is that an efficient production of seeds for the cosmic magneticfields(amplified by the dilaton in a string cosmology context)is in principle compatible with the associated production of a cosmic graviton background detectable by Advanced LIGO ifǫ=1/2(as in the case of Type I superstrings),and incompatible ifǫ=1 (as in the case of heterotic superstrings).This may give us direct experimental information on the possible primordial strength of the photon–dilaton coupling,and on the choice of the superstring model most appropriate to the early cosmological evolution.Let us suppose,indeed,that further studies of cosmic magneticfields will provide direct8x = log(ω1/ωs )y = l o g (g s /g 1) Figure 1:Allowed regions determined by the conditions (10),(15),(17),(19),(20),imposed on the spectra (9)and (14)with (H 1/M P )=0.1.The photon regions are plotted for the two cases ǫ=1and ǫ=1/2,and for appropriate values of β0and βi illustrating the maximal and minimal extension of the allowed region in the case of a non-trivial evolution of the moduli fields (dashed lines).independent confirmation of the expected seed production,exactly as predicted in the class of pre-big bang models considered here:for instance,by measuring the primordial spec-trum through its effects on the polarization and the anisotropy of the CMB radiation [3](primordial seeds can be produced,more or less naturally,with various mechanisms [10],but a monotonically growing spectrum seems to be peculiar only of the amplification of the vacuum fluctuations in pre-big bang cosmology).Then,a future detection of cosmic gravitons by the next generation of gravitational antennas will provide support in favour of Type I models,while the absence of detection (at the same sensitivity level)should be interpreted more in favour of the heterotic model of coupling (see [25]for a very recent,direct experimental bound on the energy density of a cosmic graviton background,and [26]for a discussion of the near-future sensitivities of LIGO in various regions of the parameter space of pre-big bang models).Such an argument cannot be directly applied to cosmological9models based on Type II superstrings,which are expected to describe the strong coupling limit of standard interactions.We may notice,as a conclusive remark,that the extension of the low-energy(dilaton) part of the allowed regions illustrated in Fig.1is rather strongly dependent on the values ofβ0andβi.A precise experimental determination of the spectrum of e.m.fluctuations may thus,in principle,open a direct window on the primordial dynamics of the extra dimensions.Work along these lines is currently in progress(see also[2,27]for previous studies on higher-dimensional modifications of the photon spectrum). AcknowledgementsIt is a pleasure to thank Carlo Angelantonj,Alessandra Buonanno,Massimo Giovannini, Augusto Sagnotti e Gabriele Veneziano for helfpful discussions and comments.10References[1]See for instance M.B.Green,J.Schwartz and E.Witten,Superstring theory(UniversityPress,Cambridge,1987);J.Polchinski,String theory(University Press,Cambridge, 1998);E.Kiritsis,Introduction to superstring theory(Leuwen Univ.Press,1998).[2]A.Buonanno,K.A.Meissner,C.Ungarelli and G.Veneziano,JHEP01,004(1998).[3]See for instance D.Grasso and H.R.Rubinstein,Phys.Rep.348,163(2001);M.Giovannini,Int.J.Mod.Phys.D13,391(2004).[4]M.Gasperini and G.Veneziano,Astropart.Phys.1,317(1993).[5]See for instance M.Gasperini and G.Veneziano,Phys.Rep.373,1(2003).[6]M.Gasperini,M.Giovannini and G.Veneziano,Phys.Rev.Lett.75,3796(1995).[7]D.Lemoine and M.Lemoine,Phys.Rev.D52,1955(1995).[8]See for instance N.D.Birrel and P.C.W.Davies,Quantumfields in curved spaces(University Press,Cambridge,1982);J.Garriga and E.Verdaguer,Phys.Rev.D39, 1072(1989).[9]M.Gasperini,in String gravity and physics at the Planck energy scale,eds.N.Sanchezand A.Zichichi(Kluwer Acad.Publ.,Dordrecht,1996),p.305.[10]M.Gasperini,in The origin and evolution of cosmic magnetism,eds.R.Beck,G.Brunetti,L.Feretti and B.Gaensler(Astronomische Nachrichten,Wiley,2005),in press.[11]M.S.Turner and L.M.Widrow,Phys.Rev.D37,2473(1988).[12]M.Gasperini and M.Giovannini,Phys.Rev.D47,1519(1993).[13]R.Brustein,M.Gasperini,M.Giovannini,V.Mukhanov and G.Veneziano,Phys.Rev.D51,6744(1995).[14]R.Brustein,M.Gasperini,M.Giovannini and G.Veneziano,Phys.Lett.B361,45(1995).[15]A.Buonanno,M.Maggiore and C.Ungarelli,Phys.Rev.D55,3330(1997).[16]S.Kawai,M.Sakagami and J.Soda,Phys.Lett.B347,284(1998).[17]M.Gasperini,M.Maggiore,and G.Veneziano,Nucl.Phys.B494,315(1997).[18]V.Kaspi,J.Taylor and M.Ryba,Astrophys.J.428,713(1994).[19]V.F.Schwarztmann,JETP Letters9,184(1969).11[20]R.Brustein,M.Gasperini and G.Veneziano,Phys.Rev D55,3882(1997).[21]B.Allen and J.D.Romano,Phys.Rev.D59,102001(1999).[22]M.Maggiore,Phys.Rep.331,283(2000).[23]V.Kaplunowski,Phys.Rev.Lett.55,11036(1985).[24]D.Grasso and H.R.Rubinstein,Astropart.Phys.3,95(1995).[25]B.Abbot et al.,astro-ph/0507254.[26]V.Mandic and A.Buonanno,astro-ph/0510341.[27]M.Giovannini,Phys.Rev.D62,123505(2000).12。
无线电力传输的电车辆应用-英文
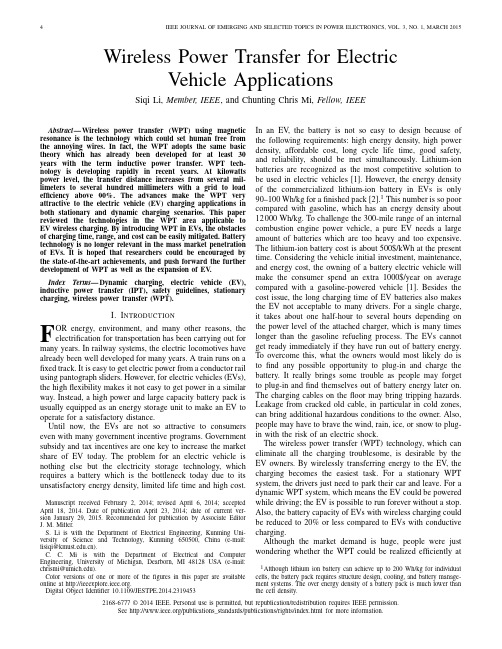
Wireless Power Transfer for Electric Vehicle ApplicationsSiqi Li,Member,IEEE,and Chunting Chris Mi,Fellow,IEEEAbstract—Wireless power transfer(WPT)using magnetic resonance is the technology which could set human free from the annoying wires.In fact,the WPT adopts the same basic theory which has already been developed for at least30 years with the term inductive power transfer.WPT tech-nology is developing rapidly in recent years.At kilowatts power level,the transfer distance increases from several mil-limeters to several hundred millimeters with a grid to load efficiency above90%.The advances make the WPT very attractive to the electric vehicle(EV)charging applications in both stationary and dynamic charging scenarios.This paper reviewed the technologies in the WPT area applicable to EV wireless charging.By introducing WPT in EVs,the obstacles of charging time,range,and cost can be easily mitigated.Battery technology is no longer relevant in the mass market penetration of EVs.It is hoped that researchers could be encouraged by the state-of-the-art achievements,and push forward the further development of WPT as well as the expansion of EV.Index Terms—Dynamic charging,electric vehicle(EV), inductive power transfer(IPT),safety guidelines,stationary charging,wireless power transfer(WPT).I.I NTRODUCTIONF OR energy,environment,and many other reasons,theelectrification for transportation has been carrying out for many years.In railway systems,the electric locomotives have already been well developed for many years.A train runs on a fixed track.It is easy to get electric power from a conductor rail using pantograph sliders.However,for electric vehicles(EVs), the highflexibility makes it not easy to get power in a similar way.Instead,a high power and large capacity battery pack is usually equipped as an energy storage unit to make an EV to operate for a satisfactory distance.Until now,the EVs are not so attractive to consumers even with many government incentive ernment subsidy and tax incentives are one key to increase the market share of EV today.The problem for an electric vehicle is nothing else but the electricity storage technology,which requires a battery which is the bottleneck today due to its unsatisfactory energy density,limited life time and high cost. Manuscript received February2,2014;revised April6,2014;accepted April18,2014.Date of publication April23,2014;date of current ver-sion January29,2015.Recommended for publication by Associate Editor ler.S.Li is with the Department of Electrical Engineering,Kunming Uni-versity of Science and Technology,Kunming650500,China(e-mail: lisiqi@).C. C.Mi is with the Department of Electrical and Computer Engineering,University of Michigan,Dearborn,MI48128USA(e-mail: chrismi@).Color versions of one or more of thefigures in this paper are available online at .Digital Object Identifier10.1109/JESTPE.2014.2319453In an EV,the battery is not so easy to design because of the following requirements:high energy density,high power density,affordable cost,long cycle life time,good safety, and reliability,should be met simultaneously.Lithium-ion batteries are recognized as the most competitive solution to be used in electric vehicles[1].However,the energy density of the commercialized lithium-ion battery in EVs is only 90–100Wh/kg for afinished pack[2].1This number is so poor compared with gasoline,which has an energy density about 12000Wh/kg.To challenge the300-mile range of an internal combustion engine power vehicle,a pure EV needs a large amount of batteries which are too heavy and too expensive. The lithium-ion battery cost is about500$/kWh at the present time.Considering the vehicle initial investment,maintenance, and energy cost,the owning of a battery electric vehicle will make the consumer spend an extra1000$/year on average compared with a gasoline-powered vehicle[1].Besides the cost issue,the long charging time of EV batteries also makes the EV not acceptable to many drivers.For a single charge, it takes about one half-hour to several hours depending on the power level of the attached charger,which is many times longer than the gasoline refueling process.The EVs cannot get ready immediately if they have run out of battery energy. To overcome this,what the owners would most likely do is tofind any possible opportunity to plug-in and charge the battery.It really brings some trouble as people may forget to plug-in andfind themselves out of battery energy later on. The charging cables on thefloor may bring tripping hazards. Leakage from cracked old cable,in particular in cold zones, can bring additional hazardous conditions to the owner.Also, people may have to brave the wind,rain,ice,or snow to plug-in with the risk of an electric shock.The wireless power transfer(WPT)technology,which can eliminate all the charging troublesome,is desirable by the EV owners.By wirelessly transferring energy to the EV,the charging becomes the easiest task.For a stationary WPT system,the drivers just need to park their car and leave.For a dynamic WPT system,which means the EV could be powered while driving;the EV is possible to run forever without a stop. Also,the battery capacity of EVs with wireless charging could be reduced to20%or less compared to EVs with conductive charging.Although the market demand is huge,people were just wondering whether the WPT could be realized efficiently at1Although lithium ion battery can achieve up to200Wh/kg for individual cells,the battery pack requires structure design,cooling,and battery manage-ment systems.The over energy density of a battery pack is much lower than the cell density.2168-6777©2014IEEE.Personal use is permitted,but republication/redistribution requires IEEE permission.See /publications_standards/publications/rights/index.html for more information.a reasonable cost.The research team from MIT published a paper in Science[3],in which60W power is transferred at a2-m distance with the so called strongly coupled magnetic resonance theory.The result surprised the academia and the WPT quickly became a hot research area.A lot of interesting works were accomplished with different kinds of innovative circuit,as well as the system analysis and control[4]–[9].The power transfer path can even be guided using the domino-form repeaters[10],[11].In order to transfer power more efficiently and further,the resonant frequency is usually selected at MHz level,and air-core coils are adopted.When the WPT is used in the EV charging,the MHz frequency operation is hard to meet the power and efficiency criteria.It is inefficient to convert a few to a few hundred kilowatts power at MHz frequency level using state-of-the-art power electronics devices.Moreover,air-core coils are too sensitive to the surrounding ferromagnetic objects.When an air-core coil is attached to a car,the magneticflux will go inside the chassis causing high eddy current loss as well as a significant change in the coil parameters.To make it more practical in the EV charging,ferrite as a magneticflux guide and aluminum plate as a shield are usually adopted in the coil design[12].With the lowered frequency to less than100kHz, and the use of ferrite,the WPT system is no different from the inductive power transfer(IPT)technology which has been developed for many years[13]–[39].In fact,since the WPT is based on the nonradiative and near-field electromagnetic,there is no difference with the traditional IPT which is based on magneticfield coupling between the transmitting and receiving coils.The IPT system has already been proposed and applied to various applications,such as underwater vehicles[32]–[34], mining systems[16],cordless robots in automation production lines[36]–[39],as well as the charging of electric vehicles [13],[14],[25]–[27].Recently,as the need of EV charging and also the progress in technology,the power transfer distance increases from several millimeters to a few hundred millimeters at kilowatts power level[12],[14],[40]–[60].As a proof-of-concept of a roadway inductively powered EV,the Partners for Advance Transit and Highways(PATH)program was conducted at the UC Berkeley in the late1970s[14],[54].A60kW, 35-passanger bus was tested along a213m long track with two powered sections.The bipolar primary track was supplied with1200A,400Hz ac current.The distance of the pickup from the primary track was7.6cm.The attained efficiency was around60%due to limited semiconductor technology.During the last15years,researchers at Auckland University have focused on the inductive power supply of movable objects. Their recent achievement in designing pads for the stationary charging of EV is worth noting.A766mm×578mm pad that delivers5kW of power with over90%efficiency for distances about200mm was reported[48],[55].The achieved lateral and longitudinal misalignment tolerance is 250and150mm,respectively.The knowledge gained from the on-line electric vehicle(OLEV)project conducted at the Korea Advanced Institute of Science and Technology(KAIST) also contributes to the WPT design.Three generations of OLEV systems have been built:a light golf cart as thefirst Fig.1.Typical wireless EV charging system.generation,a bus for the second,and an SUV for the third. The accomplishment of the second and the third is noteworthy: 60kW power transfer for the buses and20kW for the SUVs with efficiency of70%and83%,respectively;allowable vertical distance and lateral misalignment up to160mm and up to200mm,respectively[56],[57].In the United States, more and more public attention was drawn to the WPT since the publication of the2007Science paper[3].The WiTricity Corporation with technology from MIT released their WiT-3300development kit,which achieves90%efficiency over a 180mm gap at3.3kW output.Recently,a wireless charging system prototype for EV was developed at Oak Ridge National Laboratory(ORNL)in the United States.The tested efficiency is nearly90%for3kW power delivery[53].The research at the University of Michigan–Dearborn achieved a200mm distance,8kW WPT system with dc to dc efficiency as high as95.7%[61].From the functional aspects,it could be seen that the WPT for EV is ready in both stationary and dynamic applications.However,to make it available for large-scale commercialization,there is still abundant work to be done on the performance optimization,setup of the industrial standards, making it more cost effective,and so on.This paper starts with the basic WPT theory,and then gives a brief overview of the main parts in a WPT system, including the magnetic coupler,compensation network,power electronics converter,study methodology,and its control,and some other issues like the safety considerations.By introduc-ing the latest achievements in the WPT area,we hope the WPT in EV applications could gain a widespread acceptance in both theoretical and practical terms.Also,we hope more researchers could have an interest and make more brilliant contributions in the developing of WPT technology.II.F UNDAMENTAL T HEORYA typical wireless EV charging system is shown in Fig.1. It includes several stages to charge an EV wirelessly.First, the utility ac power is converted to a dc power source by an ac to dc converter with power factor correction.Fig.2.General two-coil WPT system.Then,the dc power is converted to a high-frequency ac todrive the transmitting coil through a compensation network.Considering the insulation failure of the primary side coil,a high-frequency isolated transformer may be inserted betweenthe dc-ac inverter and primary side coil for extra safety andprotection.The high-frequency current in the transmitting coilgenerates an alternating magneticfield,which induces an acvoltage on the receiving coil.By resonating with the secondarycompensation network,the transferred power and efficiencyare significantly improved.At last,the ac power is rectifiedto charge the battery.Fig.1shows that a wireless EV chargerconsists of the following main parts:1)the detached(or separated,loosely coupled)transmittingand receiving ually,the coils are built withferrite and shielding structure,in the later sections,theterm magnetic coupler is used to represent the entirety,including coil,ferrite,and shielding;2)the compensation network;3)the power electronics converters.The main difference between a wireless charger and aconventional conductive or wired charger is that a transformeris replaced by a set of loosely couple coils.To give a quickidea of the WPT principle,the coil and the compensationnetwork are pulled out separately,as shown in Fig.2,whereL1represents the self-inductance of the primary side transmit-ting coil and L2represents the self-inductance of the receivingcoil;˙I1and˙I2are the current in the two coils;˙U12is thevoltage in the secondary coil that is induced by the currentin the primary side coil.˙U21is the voltage in the primarycoil that is induced by the current in secondary side coil dueto coupling,or mutual inductance between the primary andsecondary coils.S1and S2are the apparent power goes intoL1and L2,respectively.S3and S4are the apparent powerprovided by the power converter.S12and S21represent theapparent power exchange between the two coils.The form ofthe compensation network is not specified.The characteristicsof the compensation network will be discussed later.As shown in Fig.2,neglecting the coil resistance andmagnetic losses,we can calculate the simplified form ofexchanged complex power from L1to L2˙S12=−˙U12˙I∗2=−jωM˙I1˙I∗2=ωM I1I2sinϕ12−jωM I1I2cosϕ12(1)˙S21=−˙U21˙I∗1=−jωM˙I2˙I∗1=−ωM I1I2sinϕ12−jωM I1I2cosϕ12(2)where I1and I2are the root mean square value andϕ12isthe phase difference between˙I1and˙I2.The active powertransfer from the primary side to the secondary side can beexpressed asP12=ωM I1I2sinϕ12.(3)The system shown in Fig.2can transfer active power inboth directions.In the analysis below,we assume the poweris transferred from L1to L2.Whenϕ12=π/2,which means˙I1leads˙I2by a quarter cycle,the maximum power can betransferred from L1to L2.The total complex power goes into the two-coil system is˙S=˙S1+˙S2=jωL1˙I1+ωM˙I2˙I∗1+jωL2˙I2+ωM˙I1˙I∗2=jωL1I21+L2I22+2M I1I2cosϕ12.(4)Therefore,the total reactive power goes into the two-coilsystem isQ=ωL1I21+L2I22+2M I1I2cosϕ12.(5)For a traditional transformer,the reactive power representsthe magnetizing power.Higher magnetizing power bringshigher copper and core loss.To increase the transformerefficiency,the ratio between the active power and reactivepower should be maximized.The ratio is defined byf(ϕ12)=|P12||Q|=ωM I1I2sinϕ12ωL1I21+ωL2I22+2ωM I1I2cosϕ12=k1−cos2ϕ12L1L2I1I2+L2L1I2I1+2k cosϕ21=k1−cos2ϕ12x+1x+2k cosϕ12(6)whereπ/2<ϕ12<πx=L1L2I1I2>0k is the coupling coefficient between L1and L2.To achieve the maximum value of f(ϕ12),we solve thefollowing equations:∂∂ϕ12f(ϕ12)=0,∂2∂2ϕ12f(ϕ12)<0(7)and the solutions arecosϕ12=−2kx+1x,sinϕ12=1−4k2x+1x2.(8)When k is close to1,it is a traditional transformer.In this case,if˙I2is an induced current by˙I1,x will be close to1. Thus,cosϕ12≈−1.The phase difference between˙I1and˙I2 is nearly180°.While for WPT,k is close to0.f(ϕ12)is maximized at sinϕ12=1,at which point the transferred power is also maximized.The phase between˙I1and˙I2is around90°instead of180°.Hence we can see the difference between the tightly and the loosely coupled coils.The degree of coupling affects the design of the compensa-tion network.Taking the series–series topology as an example, there are two ways to design the resonant capacitor.One way is design the capacitor to resonate with the leakage inductance [46],[62]which could achieve a higher f(ϕ12).Another way is to resonate with the coil self-inductance[27],[41],[63] which could maximum the transferred power at a certain coil current.When the coupling is tight with a ferrite,like k>0.5, it is important to increase f(ϕ12)to achieve better efficiency. In this case,resonate with the coil self inductance,which makesϕ12=π/2and lowers f(ϕ12),is not recommended. Otherwise the magnetizing loss may significantly increase. When the capacitor resonates with the leakage inductance,it is like the leakage inductance is compensated.This makes the transformer perform as a traditional one and increases f(ϕ12). However,the overall system does not work at a resonant mode.When the coupling is loose,like k<0.5,which is the case for the EV wireless charging,usually the capacitor is tuned with the self inductance to make the system working at a resonate mode to achieve maximum transferred power at a certain coil current.In this case,most of the magnetic field energy is stored in the large air gap between the two coils.The hysteresis loss in the ferrite is not so relative to the magnetizing power.However,the loss in the copper wire is proportional to the square of the conducting current. To efficiently transfer more power at a certain coil current,the induced current˙I2should lag˙I1by90°.Since the induced voltage˙U12on the receiving coil lags˙I1by90°,˙U12and˙I2 should be in phase.The secondary side should have a pure resistive characteristic seen from˙U12at the frequency of˙I1. At the meanwhile,the primary side input apparent power S3 should be minimized.At cosϕ12=0,the complex power˙S1is˙S1=jωL1I21+ωM I1I2.(9) Ideally,the primary side compensation network should cancel the reactive power and make S3=ω0M I1I2,where ω0is the resonant frequency.From the above analysis,we see for a certain transferred power,it is necessary to make the secondary side resonant to reduce the coil volt-ampere(V A) rating,which reduces the loss in the coils;and to make the primary side resonant to reduce the power electronics converter V A rating,which reduces the loss in the power converter. Therefore,we transfer power at the magnetic resonance. With the above analysis,we can calculate the power transfer efficiency between the two coils at the resonant frequency.We haveU12=I2(R2+R Le)=ωM I1=ωkL1L2I1(10) where R2is the secondary winding resistance and R Le is the equivalent load resistance.By defining the quality factor of the two coils, Q1=ωL1/R1,Q2=ωL2/R2,the transferred efficiency can be expressed asη=I22R LeI21R1+I22R2+I22R Le=R Le(R2+R Le)2k2Q1Q2R2+R2+R Le.(11)By defining a=R Le/R2,we obtain the expression of efficiency as a function of aη(a)=1a+1a+2k2Q1Q2+1a+1.(12)The maximum efficiency is obtained by solving the follow-ing equations:∂∂aη(a)=0,∂2∂2aη(a)<0.(13) The maximum efficiencyηmax=k2Q1Q21+1+k2Q1Q22is achieved at aηmax=1+k2Q1Q21/2.In[64],the maximum efficiency is also derived based on several different kinds of compensation network.The results are identical and accord with the above results.The analysis here does not specify a particular compensation form.It can be regarded as a general formula to evaluate the coil performance and estimate the highest possible power transfer efficiency. In EV wireless charging applications,the battery is usu-ally connected to the coil through a diode-bridge rectifier. Most of the time,there is some reactive power required. The reactive power can be provide by either the coil or the compensation network like a unit-power-factor pickup.The battery could be equivalent to a resistance R b=U b/I b,where U b and I b is the battery voltage and current,respectively. If the battery is connected to the rectifier directly in a series-series compensation form,the equivalent ac side resistance could be calculated by R ac=8/π2·R b.Thus,a battery load could be converted to a resistive load.The R ac equation is different for different battery connection style,like with or without dc/dc converter,parallel or series compensation. Most of the time,the equivalent R ac could be derived.Some typical equivalent impendence at the primary side is given in paper[42].By calculating the equivalent ac resistances,the above equations could also be applied to a battery load with rectifier.For stationary EV wireless charging,the coupling between the two coils is usually around0.2.If both the sending and receiving coils have a quality factor of300,the theoretical maximum power transfer efficiency is about96.7%.More efficiency calculations under different coupling and quality factors are shown in Fig.3.Fig.3.Theoretical maximum transfer efficiency between two coils.III.M AGNETIC C OUPLER D ESIGNTo transfer power wirelessly,there are at least two magnetic couplers in a WPT system.One is at the sending side,named primary coupler.The other is at the receiving side,named pickup coupler.Depending on the application scenarios,the magnetic coupler in a WPT for an EV could be either a pad or a track form.For higher efficiency,it is important to have high coupling coefficient k and quality factor Q. Generally,for a given structure,the larger the size to gap ratio of the coupler is,the higher the k is;the thicker the wire and the larger the ferrite section area is,the higher the Q is. By increasing the dimensions and materials,higher efficiency can be achieved.But this is not a good engineering approach. It is preferred to have higher k and Q with the minimum dimensions and cost.Since Q equalsωL/R,high frequency is usually adopted to increase the value of Q.The researchers at Massachusetts Institute of Technology(MIT)used a frequency at around10MHz and the coil Q value reached nearly 1000[3].In high power EV WPT applications,the frequency is also increased to have these benefits.In Bolger’s early design,the frequency is only180Hz[13].A few years later, a400Hz frequency EV WPT system was designed by System Control Technology[14].Neither180Hz nor400Hz is high enough for a loosely coupled system.Huge couplers were employed in the two designs.Modern WPT system uses at least10kHz frequency[15].As the technical progress of power electronics,100kHz could be achieved[65]at high power level.The WiTricity Company with the technology from MIT adopts145kHz in their design.In the recent researches and applications,the frequency adopted in an EV WPT system is between20and150kHz to balance the efficiency and cost.At this frequency,to reduce the ac loss of copper coils, Litz wire is usually adopted.Besides the frequency,the coupling coefficient k is sig-nificantly affected by the design of the magnetic couplers, which is considered one of the most important factors in a WPT system.With similar dimensions and materials,different coupler geometry and configuration will have a significant difference of coupling coefficient.A better coupler design may lead to a50%–100%improvement compared with some nonoptimal designs[48].Fig.4.Mainflux path of double-sided and single-sided coupler.(a)Double-sided type.(b)Single-sided type.A.Coupler in the Stationary ChargingIn a stationary charging,the coupler is usually designed in a pad form.The very early couplers are just like a simple split core transformer[19],[38],[56].Usually this kind of design could only transfer power through a very small gap. To meet the requirements for EV charging,the deformations from spilt core transformers and new magnetic coupler forms are presented for large gap power transfer[12],[31],[37],[42], [47]–[50],[66]–[71].According to the magneticflux dis-tribution area,the coupler could be classified as the double-sided and single-sided types.For the double-sided type,theflux goes to both sides of the coupler[12], [31],[67].Aflattened solenoid inductor form is pro-posed in[12]and[67].Because theflux goes through the ferrite like through a pipe,it is also called aflux-pipe coupler.To prevent the eddy current loss in the EV chassis,an aluminum shielding is usually added which bring a loss of1%–2%[12].When the shielding is added, the quality factor of aflux-pipe coupler reduces from 260to86[48].The high shielding loss makes the double-sided coupler not the optimal choice.For the single-sided coupler, most of theflux exists at only one side of the coupler.As shown in Fig.4,the mainflux pathflows through the ferrite in a single-sided coupler.Unlike the double-sided coupler having half of the mainflux at the back,the single-sided coupler only has a leakageflux in the back.This makes the shielding effort of a single-sided type much less.Two typical single-sidedflux type pads are shown in Fig.5. One is a circular unipolar pad[47].Another one is a rectan-gular bipolar pad proposed by University of Auckland,which is also named DD pad[48].Besides the mechanical support material,a single-sided pad is composed of three layers.The top layer is the coil.Below the coil,a ferrite layer is inserted for the purpose of enhancing and guiding theflux.At the bottom is a shielding layer.To transfer power,the two pads are put closed with coil to coil.With the shielding layer, most of the high-frequency alternating magneticflux can be confined in the space between the two pads.A fundamental flux path concept was proposed in theflux pipe paper[67].Fig. 5.Two typical single-sidedflux type pads.(a)Circular pad.(b)DD pad.Theflux path height of a circular pad is about one-fourth ofthe pad’s diameter.While for a DD pad,the height is abouthalf of the pad’s length.For a similar size,a DD pad has asignificant improvement in the coupling.The charge zone fora DD pad could be about two times larger than a circular padwith similar material cost.The DD pad has a good tolerant inthe y-direction.This makes the DD pad a potential solutionfor the dynamic charging when the driving direction is alongwith the y-axis.However,there is a null point for DD pad inthe x-direction at about34%misalignment[48].To increasethe tolerant in x-direction,an additional quadrature coil namedQ coil is proposed to work together with the DD pad,whichis called DDQ pad[48],[49],[68].With a DDQ receivingpad on a DD sending pad,the charge zone is increased tofivetimes larger than the circular configuration.As the additionalQ coil in the receiver side,the DDQ over DD configurationuses almost two times copper compared with the circularone[48].A variant of a DDQ pad,which is called a newbipolar pad,was also proposed by University of Auckland[49],[50].By increasing the size of each D pad and havingsome overlap between the two D coils,the new bipolar padcould have a similar performance of a DDQ pad with25%less copper.With all the efforts,at200mm gap,the cou-pling between the primary and secondary pads could achieve0.15–0.3with an acceptable size for an EV.Referred to Fig.3,at this coupling level,efficiency above90%could possibly beachieved.B.Coupler in the Dynamic ChargingThe dynamic charging,also called the OLEVs[56]orroadway powered electric vehicles[14],is a way to chargethe EV while driving.It is believed that the dynamic chargingcan solve the EVs’range anxiety,which is the main reasonlimits the market penetration of EVs.In a dynamic chargingsystem,the magnetic components are composed of a primaryside magnetic coupler,which is usually buried under the road,and a secondary side pickup coil,which is mounted under anEV chassis.There are mainly two kinds of primary magneticcoupler in the dynamic charging.Thefirst kind is a long trackcoupler[26],[31],[57],[70],[72]–[76].When an EV withaFig.6.Top view of W-shape and I-shape track configuration.pickup coil is running along with the track,continues powercan be transferred.The track can be as simple as just twowires[37],[77],or an adoption of ferrites with U-type orW-type[26],[56]to increase the coupling and power trans-fer distance.Further,a narrow-width track design with anI-type ferrite was proposed by KAIST[72],[73].The dif-ferences between the W-type and I-type are shown in Fig.6.For W-type configuration,the distribution area of the ferrite Wdetermines the power transfer distance,as well as the lateraldisplacement.The total width of W-type should be about fourtimes the gap between the track and the pickup coil.For I-typeconfiguration,the magnetic pole alternates along with the road.The pole distance W1is optimized to achieve better coupling atthe required distance.The width of pickup coil W2is designedto meet the lateral misalignment requirement.The relationbetween track width and transfer distance is decoupled andthe track can be built at a very narrow form.The width forU-type and W-type is140and80cm,respectively[73].ForI-type,it could be reduced to only10cm with a similar powertransfer distance and misalignment capacity.35kW power wastransferred at a200mm gap and240mm displacement usingthe I-type configuration[73].With the narrowed design,theconstruction cost could be reduced.Also,the track is far awayfrom the road side,the electromagneticfield strength exposedto pedestrians can also be reduced.The problem of the track design is that the pickup coil onlycovers a small portion of the track,which makes the couplingcoefficient very small.The poor coupling brings efficiencyand electromagnetic interference(EMI)issues.To reduce theEMI issue,the track is built by segments[52],[70],[75]with a single power converter and a set of switches to powerthe track.The excitation of each segment can be controlledby the switches’ON-OFF state.The electromagneticfieldabove the inactive segments is reduced significantly.However,there is always a high-frequency currentflowing through thecommon supply cables,which lowers the system efficiency.The published systems efficiency is about70%–80%,whichis much lower than the efficiency achieved in the stationarycharging.When each segment is short enough,the track becomes likea pad in the stationary charging,which is the other kind ofthe primary magnetic coupler.Each pad can be driven by anindependent power converter.Thus,the primary pads can beselectively excited without a high-frequency common current.Also,the energized primary pad is covered by the vehicle.Theelectromagneticfield is shielded to have a minimum impact。
双极化平面反射阵天线
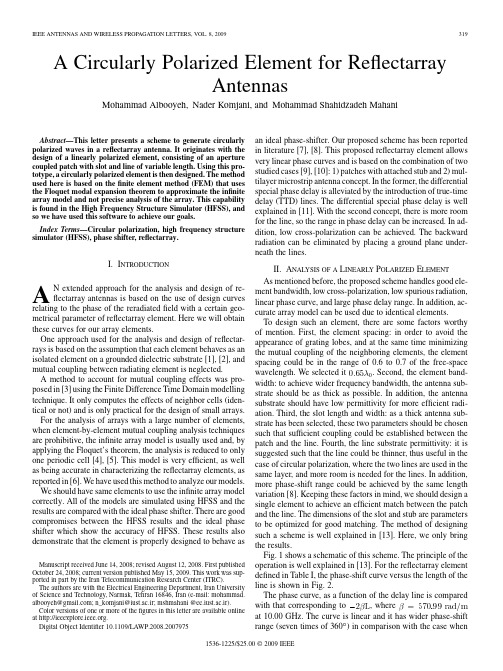
A Circularly Polarized Element for ReflectarrayAntennasMohammad Albooyeh,Nader Komjani,and Mohammad Shahidzadeh MahaniAbstract—This letter presents a scheme to generate circularly polarized waves in a reflectarray antenna.It originates with the design of a linearly polarized element,consisting of an aperture coupled patch with slot and line of variable ing this pro-totype,a circularly polarized element is then designed.The method used here is based on thefinite element method(FEM)that uses the Floquet modal expansion theorem to approximate the infinite array model and not precise analysis of the array.This capability is found in the High Frequency Structure Simulator(HFSS),and so we have used this software to achieve our goals.Index Terms—Circular polarization,high frequency structure simulator(HFSS),phase shifter,reflectarray.I.I NTRODUCTIONA N extended approach for the analysis and design of re-flectarray antennas is based on the use of design curves relating to the phase of the reradiatedfield with a certain geo-metrical parameter of reflectarray element.Here we will obtain these curves for our array elements.One approach used for the analysis and design of reflectar-rays is based on the assumption that each element behaves as an isolated element on a grounded dielectric substrate[1],[2],and mutual coupling between radiating element is neglected.A method to account for mutual coupling effects was pro-posed in[3]using the Finite Difference Time Domain modelling technique.It only computes the effects of neighbor cells(iden-tical or not)and is only practical for the design of small arrays. For the analysis of arrays with a large number of elements, when element-by-element mutual coupling analysis techniques are prohibitive,the infinite array model is usually used and,by applying the Floquet’s theorem,the analysis is reduced to only one periodic cell[4],[5].This model is very efficient,as well as being accurate in characterizing the reflectarray elements,as reported in[6].We have used this method to analyze our models. We should have same elements to use the infinite array model correctly.All of the models are simulated using HFSS and the results are compared with the ideal phase shifter.There are good compromises between the HFSS results and the ideal phase shifter which show the accuracy of HFSS.These results also demonstrate that the element is properly designed to behave asManuscript received June14,2008;revised August12,2008.First published October24,2008;current version published May15,2009.This work was sup-ported in part by the Iran Telecommunication Research Center(ITRC).The authors are with the Electrical Engineering Department,Iran University of Science and Technology,Narmak,Tehran16846,Iran(e-mail:mohammad. albooyeh@;n_komjani@iust.ac.ir;mshmahani@ee.iust.ac.ir). Color versions of one or more of thefigures in this letter are available online at .Digital Object Identifier10.1109/LAWP.2008.2007975an ideal phase-shifter.Our proposed scheme has been reported in literature[7],[8].This proposed reflectarray element allows very linear phase curves and is based on the combination of two studied cases[9],[10]:1)patches with attached stub and2)mul-tilayer microstrip antenna concept.In the former,the differential special phase delay is alleviated by the introduction of true-time delay(TTD)lines.The differential special phase delay is well explained in[11].With the second concept,there is more room for the line,so the range in phase delay can be increased.In ad-dition,low cross-polarization can be achieved.The backward radiation can be eliminated by placing a ground plane under-neath the lines.II.A NALYSIS OF A L INEARLY P OLARIZED E LEMENTAs mentioned before,the proposed scheme handles good ele-ment bandwidth,low cross-polarization,low spurious radiation, linear phase curve,and large phase delay range.In addition,ac-curate array model can be used due to identical elements.To design such an element,there are some factors worthy of mention.First,the element spacing:in order to avoid the appearance of grating lobes,and at the same time minimizing the mutual coupling of the neighboring elements,the element spacing could be in the range of0.6to0.7of the free-space wavelength.We selectedit.Second,the element band-width:to achieve wider frequency bandwidth,the antenna sub-strate should be as thick as possible.In addition,the antenna substrate should have low permittivity for more efficient radi-ation.Third,the slot length and width:as a thick antenna sub-strate has been selected,these two parameters should be chosen such that sufficient coupling could be established between the patch and the line.Fourth,the line substrate permittivity:it is suggested such that the line could be thinner,thus useful in the case of circular polarization,where the two lines are used in the same layer,and more room is needed for the lines.In addition, more phase-shift range could be achieved by the same length variation[8].Keeping these factors in mind,we should design a single element to achieve an efficient match between the patch and the line.The dimensions of the slot and stub are parameters to be optimized for good matching.The method of designing such a scheme is well explained in[13].Here,we only bring the results.Fig.1shows a schematic of this scheme.The principle of the operation is well explained in[13].For the reflectarray element defined in Table I,the phase-shift curve versus the length of the line is shown in Fig.2.The phase curve,as a function of the delay line is compared with that correspondingto,whereat10.00GHz.The curve is linear and it has wider phase-shift range(seven times of360)in comparison with the case when1536-1225/$25.00©2009IEEEFig.1.Geometry of a phase-shifter element based on aperture-coupled patches with slots and lines of variable length:(a)top view and (b)3D view.TABLE ID IMENSIONS AND D IELECTRIC L AYERS OF THE R EFLECTARRAY ELEMENTwe used a lower permittivity()for the line substrate (four times of 360).It should be noted that the best matchingtakes placewhenis set to 0.7mm.III.A NALYSIS OF A C IRCULARLY P OLARIZED E LEMENT We have used the element type of the previous case to achievea circularly polarized reflected wave.We have used two slots instead of one.The element is defined in Fig.3and Table II.We have excited the element by means of a linearly polarized wave.The electric field of the excitation wave is polarized in a way that the two slots could be excited,i.e.,along the patch diagonal.We have two reflected waves in this case,created by two modes;excited in two directions,each of them along one of the patch lengths.The two delay lines have an electrical lengthdifferenceof(a total differenceof ,when the waves move along the lines and reflected from their open ends)and therefore produce a 90phase shift between the tworeflectedFig.2.Phase-shift curve versus the line length compared with the ideal phase-shifter 02 L ,simulation frequency is set to 10.00GHz.Fig.3.Geometry of an element that is used for generating circular polarization.waves.A electric phase difference is equal to a 1.4-mmphysical lengthfor.We should notice another point here:the cell type used in HFSS.We know that the excitation direction of the electric field is along the patch diagonal,so the angle between the elements’alignment and the excitation direction is 45.As discussed be-fore,the infinite array model would also be used and the electric and magnetic-walls should be applied as the periodic boundary conditions.The cell shape is selected as Fig.4.By this selection,the cell could be extended in two directions in the array plane and the boundary conditions (periodic boundaryconditions,-and -walls)could be used correctly.The dimensions are defined in Table II.As explained before and from Figs.3and 4,the cell lengthis.The axial ratio curves are shown in Fig.5.As can beseen from these curves,a good circular polarization is achieved.ALBOOYEH et al.:CIRCULARLY POLARIZED ELEMENT FOR REFLECTARRAY ANTENNAS 321TABLE IID IMENSIONS AND D IELECTRIC L AYERS OF THE R EFLECTARRAY ELEMENTFig.4.The cell used in HFSS to analyze the model that is used to produce a circularly polarized wave,topview.Fig.5.Axial ratio of reflected wave (a)versus the length of the shorter line,the operation frequency is 10.00GHz (b)versus frequency,the length of the shorter line is set to 1.8mm.An axial ratio bandwidth()of approximately 3%isachieved.Fig.6.Phase-shift at normal incidence at three frequencies.IV .E LEMENT ’S B ANDWIDTHBefore starting section,it should be noted that more fre-quency bandwidth occurs when the phase curves are parallel.For more information about the element bandwidth explana-tions refer to [13].To investigate the bandwidth of the design,we have analyzed it in three frequencies.Fig.6shows the results.As can be seen from this figure,the two curves are completely parallel at 9.5and 10GHz;that shows the lower frequency band of the element is near to 9.5GHz,but the phase-shift curves are not completely parallel at 10and 10.5GHz.So we can prog-nosticate that the upper frequency band is near 10.3GHz that shows an 8%frequency bandwidth for this scheme.Finally,it should be noted that the CPU time for analyzing such a scheme with HFSS is approximately 15min,using a computer with 2-GHz CPU speed and 2GB RAM,that demonstrate reasoning and efficiency capabilities of HFSS in calculating the phase characteristics of such a scheme.V .T HE E FFECT OF I NCIDENCE A NGLESAs stated in Section I,the design curve approach is used for the analysis and design of reflectarry antennas.But this ap-proach assumes that the phase response is independent of the angle of incidence of the impinging wave.This assumption is valid for the central elements of the centered fed reflectarray,which is for angles not too far from the normal incidence.As the largest fraction of the incidence power is reflected by the central part of the reflectarray,the normal incidence assumption can provide good predictions for center-fed reflectarrays with reasonably large F/D.It was shown in [12]that for a 40angle of incidence on rectangular patches of variable size,the phase changes around 25with respect to the response of normal in-cidence.The change in phase goes to 50for an incidence angle of 60.Note that the offset configuration is normally used to avoid the feed shadow effect and the angles of incidence are in order of 40and larger near one reflectarray edge.Thus,this approach may not be accurate for those elements near the edge and the angle of incidence must be considered in the design.We have shown the effect of incidence angle in this section.The phase-shift curves are shown in Fig.7for different angles of incidence322IEEE ANTENNAS AND WIRELESS PROPAGATION LETTERS,VOL.8,2009Fig.7.Phase-shift curve versus the line length for different incidence angles,simulation frequency is set to 10.00GHz.and polarization.The differences in phase-shift for the two or-thogonal polarizations,with the electric fieldin -and -direc-tion,can be larger than 60for and incidence of 45(,),and the phase shift also differ from that correspondingto the normal incidence up to 63for -polarization and up to 130for -polarization.So,it has been proven that in the case of such a circularly polarized design,to achieve an accurate re-sponse,the effect of the incidence angle should be considered accurately for the edge elements.VI.C ONCLUSIONA circularly polarized phasing element for reflectarray an-tennas has been analyzed here.The design originates from a lin-early polarized element that is consisted of an aperture coupled patch with slot and line of variable length.A circularly polar-ized element is then designed.Its characteristics have been in-vestigated.The effect of incidence angle has been investigated and explained.We have achieved an 8%frequency bandwidth.A good circular polarization has been achieved too.The methodused here is based on the finite element method (FEM)that uses the Floquet modal expansion theorem to approximate the infi-nite array model and not precise analysis of the array.We have also shown the HFSS efficiency for the analysis of such a peri-odic problem.R EFERENCES[1]T.Metzler and D.Shaubert,“Scattering from a stub loaded microstripantenna,”in Antenna and Propagation Society Int.Symp.Digest ,Jun.1989,pp.446–449.[2]F.Venneri,G.Angiulli,and G.Di Massa,“Design of microstrip reflec-tarray using data from isolated patch,”Microw.Opt.Technol.Lett.,vol.34,pp.411–414,Sep.2002.[3]D.Cadoret,isne,on,R.Gillard,and H.Legay,“FDTDanalysis of reflectarray radiating cells,”in IEEE/ACES Int.Conf.on Wireless Commun.and putat.Electromagn.,Apr.2005,pp.853–856.[4]D.M.Pozar and D.H.Schaubert,“Analysis of an infinite array of rect-angular microstrip patches with idealized probe feeds,”IEEE Trans.Antennas Propag.,vol.32,pp.1101–1107,Oct.1984.[5]D.M.Pozar,“Analysis of an infinite phased array of aperture cou-pled microstrip patches,”IEEE Trans.Antennas Propag.,vol.37,pp.418–425,Apr.1989.[6]D.M.Pozar,“Microstrip reflectarrays myths and realities,”in Proc.Int.Symp.on Antennas JINA ,Nice,France,Nov.2004,pp.175–179.[7]E.Carrasco,M.Barba,and J.A.Encinar,“Aperture-coupled reflec-tarray element with wide range of phase delay,”Electron.Lett.,vol.42,no.12,pp.667–668,Jun.2006.[8]E.Carrasco,M.Barba,and J.A.Encinar,“Reflectarray element basedon aperture-coupled patches with slots and lines of variable length,”IEEE Trans.Antennas Propag.,vol.55,no.3,pp.820–825,Mar.2007.[9]R.E.Munson and H.Haddad,“Microstrip Reflectarray for SatelliteCommunication and RCS Enhancement and Reduction,”U.S.patent 4684952,Aug.4,1987.[10]J.A.Encinar,“Design of two-layer printed reflectarrays using patchesof variable size,”IEEE Trans.Antennas Propag.,vol.49,no.10,pp.1403–1410,Oct.2001.[11]J.Huang,Analysis of a microstrip reflectarray antenna for microspace-craft applications JPL TDA,1995,Progress Rep.42–120.[12]S.D.Targonski and D.M.Pozar,“Analysis and design of a microstripreflectarray using patchs of variable size,”in Antennas Propag.Society Int.Symp.Digest ,Jun.1994,pp.1820–1823.[13]J.Haung and J.A.Encinar ,Reflectarray Antennas ,1st ed.Hoboken,NJ:Wiley,2008.。
Elastic coupling

专利内容由知识产权出版社提供
专利名称:Elastic coupling 发明人:CORDTYER,
CH RIST IAN 申请号:EP 03014 828.2 申请日:20030630 公开号:EP1382870A3 公开日:2004 0901
摘要:A coupling for connecting a driving machine part and a driven machine part, such as shafts, wheel hubs or the like, includes an intermediate shaft and articulated lever couplings situated at the ends of the intermediate shaft. Each articulated lever coupling has at least three identical articulated levers, which engage via elastic joints situated at their ends with the intermediate shaft and with the adjacent machine parts to be connected. The axes of the elastic joints of each articulated lever are oriented perpendicularly to an axial plane, the axial plane being parallel to the articulated lever and intersecting the axis of the intermediate shaft and the axes of the machine parts to be connected.
chapter2-new

9
Two Physical Origins of Elasticity
Energetic and Entropic Stresses
σij
σij U σij = T εij T
T σij T
εij is constant.
entropy stress
energetic stress
Chapter 2.3
26
Tensor Description of Elasticity
Voigt Symmetry
Voigt relation of the elasticity tensor
Cijkl = C jikl = Cijlk = C klij
27
Chapter 2.3
Tensor Description of Elasticity
Long Chain Polymers Characteristic:
highly elastic in terms of large extensibility low elastic modulus nearly incompressible Gough-Joule effect
17
Chapter 2.2
0
Index
Elasticity of Solids
Definition of Elasticity Two Physical Origins of Elasticity Tensor Description of Elasticity Physical Foundation of Elastic Symmetry
14
Chapter 2.2
Two Physical Origins of Elasticity
CDN-UTP8 ED3耦合和去耦合网络说明书

LARGEST RANGE OF IMPULSE TESTERS UP TO 100KV/100KA Technical SpecificationE-CDN-UTP8 Ed3.docRevised: 29. August 20131 CDN Network Type CDN-UTP8 ED3(Unshielded Twisted Pairs)1 CDN Network Type CDN-UTP8 ED3 11.1 Introduction 1 2 General 22.1 Application range2 3Function of the Network 3 3.1 De-coupling part 3 3.2 Coupling part 4 3.3 Mechanical dimensions, climatic conditions4 4Accessories and use with EMC PARTNER generators 5 4.1 Accessories to CDN-UTP8 ED3 5 4.2 CDN-UTP8 ED3 network can be used with following EMC-PARTNER generators 5 4.3 Other EMC-PARTNER coupling, de-coupling networks61.1 IntroductionThe CDN-UTP8 ED3 is a sophisticated coupling and de-coupling network for superimposing surge impulses on balanced communication lines in accordance with IEC61000-4-5 ED3 Figure: 9,unshielded unsymmetrical interconnection lines; Figure 10 unshielded symmetrical interconnection lines, ITU-K20, K21 and FCC part 68. Figure A.4It is designed for 1.2/50us and 10/700us pulses up to 6.6kVProvided by:(800)404-ATECAdvanced Test Equipment Corp .® Rentals • Sales • Calibration • Service2 General2.1 Application rangeThe CDN-UTP8 ED3 is specially designed to fulfil the requirements of modern high speedcommunication lines. For the following communication ports the CDN-UTP8 ED3 is recommended:Application Typical data Remarks CDN-UTP8 ED3 Gigabit Ethernet 10Mbits/s, 100Mbits/s, 1Gbit/s 8 wires, 4 pairs IEEE 802.RJ45 connectorscoupling and decoupling with GDTNeeded PN 105839 ADAPTER BOX RJ45Ethernet : 10Base-T 100ohm, 4wire 10Mbit/s and 100Mbit/s IEEE 802.3RJ45 connectorscoupling and decoupling with GDTNeeded PN 105839 ADAPTER BOX RJ45USB 90ohm, 2-wire + 2-wire supply 5V, 200mA1.5Mbit/s, 12Mbit/s, 480Mbit/s USB = Universal Serial BusRJ45 connectors coupling and decoupling with GDTNeeded PN 105839 ADAPTER BOX RJ45CDN-UTP ED3 and CDN-UTP8 ED3 Analogue subscriber lines (a/b-line) 600ohm, 2-wire 24V..60V, 20..100mA,100Hz..3.4kHz ( 50Hz..16kHz)Including modems up to 56kBit/s ISDN 100ohm, 4-wire0.75V ( supply 40V )25kHz..80kHz(120kHz) 192Kbit/sIntegrated Services Digital Network (ISDN) S0-Bus (CCITT 1.430) ADSL resp. xDSL 100ohm, 4-wire, 1 V25kHz..1104kHzup to 8MBit/sADSL = Asymmetric Digital Subscriber Line. Different protocols for 2- and 4-wire at different data-rates Interbus4-wire + 1wire ground5V, 800mA up to 500kBit/sRS-485 Profibus 2-wire up to 500kBit/s RS-485EN50170ASI 2-wire 2V, 100mAASI = Actor Sensor InterfaceCan-Bus 2-wire RS-485 RS-432 2-wire 20k, 115kbits/sIEC61000-4-5: 2013, Electromagnetic compatibility (EMC) - Part 4 Testing and measurement techniques – Section 2: SURGE immunity testsITU-T K.20 Resistibility of telecommunication switching equipment to overvoltages and overcurrents ITU-T K.21 Resistibility of subscriber’s terminals to overvoltages and overcurrents ITU-T K.22 Overvoltage resistibility of equipment connected to an ISDN T/S busITU-T K.44 Resistibility tests for telecommunication equipment exposed to overvoltages and overcurrents - Basic Recommendation3 Function of the NetworkThe third edition or IEC61000-4-5 cancels and replaces the second edition published in 2005, and constitutes a technical revision.This edition includes the following significant technical additions with respect to the previous edition:a) New Annex E on mathematical modelling of surge waveforms;b) New Annex F on measurement uncertainty;c) New Annex G on method of calibration of impulse measuring systems;d) New Annex H on coupling/Decoupling surges to lines rated above 200 A.Moreover while surge test for ports connected to outside telecommunication lines was addressed in sub- clause 6.2 of Edition 2, in this Edition 3 the normative Annex A is fully dedicated to this topic. In particular it gives the specifications of the 10/700 µs combined wave generator.3.1 De-coupling partCurrent compensated inductor :Common mode inductance 20mHStray inductance 12uHDC-Resistance 3.5ohm each lineMaximum unbalance < 0.003ohm line to lineLine capacitance 2nF line to lineStray capacitance 150pF line to groundSignal line characteristicsNumber of lines up to 8 (one up to 4 pairs balanced)Maximum signal current per line 1A ac or dc Sum of all lines max. 2A Signal voltage range 0V .. 300Vdc No switching contactsinside CDN-UTP8 ED3 Signal transmissionMaximum pulse voltage 6400V 1.2/50us or 10/700us Saturation No relevant saturation up to 6kV10/700usV x T > 1VsFrequency responseInsertion loss < 1dB at 1MHzNear end crosstalk (NEXT) > 40dB at 1MHzTransmission line impedance 100ohmBandwidth (50 ohm source/load) >5MHz - 3dBBandwidth (100 ohm source/load) >10MHz - 3dBBandwidth (600 ohm source/load) >300kHz - 3dBProtection of auxiliary equipment :Selectable1 ) 90V gas arrestor (GTD)PN 104409 ADAPTER BOX 90VG V signal < 90VV residual peak < 300VV clamping = 0VInsertion capacitance < 2pFV signal = maximumusable wanted signalvoltage2 ) adapter for other clamp elements PN 104412 EMPTY BOX Empty boxesFor customer designincluded3.2 Coupling partCoupling resistors :SelectableUnsymmetric lines4 x 40 Ohm IEC 1,2/50µs up to 4 wires Up to 6.6kV Symmetric lines (pairs)2 x 80 ohm IEC 1.2/50us on 2-wires up to 6.6kV 4 x 160 ohm IEC 1.2/50us on 4-wires up to 6.6kV 8 x 320 ohm IEC 1.2/50us on 8-wires up to 6.6kV IEC/ITU 10/700 µs8 x 25 ohm ITU-K20 up to 6.6kV Coupling elements :Selectable1 ) direct2 ) 90V gas arrestor (GTD)PN 104409 ADAPTER BOX 90VG V signal maximum < 90VV surge minimum > 1000V Insertion capacitance < 2pF3 ) 05µF capacitorPN 106526 ADAPTER BOX C05 V signal maximum < 300V V surge minimum > 250V4 adapter for other clamp elements PN 104412 EMPTY BOX Empty boxesFor customer designincludedCoupling elements : IEC/EN61000-4-5 ED3 recommends a gas discharge tube (GTD) of 90V for unsymmetric and symmetric operated lines. The 0.5µF isspecified in some product standard specified.3.3 Mechanical dimensions, climatic conditionsMIG type Dimensions [mm] Weight [kg] Versionswidth x depth x heightCDN-UTP8 ED3 450 x 600 x 370 77 19" Rack 8 UHEnvironment conditionsTemperature range °C 0 to 35 °CHumidity rh % 25 to 80%Pressure kPa 86 to 1064 Accessories and use with EMC PARTNER generators 4.1 Accessories to CDN-UTP8 ED34.2 CDN-UTP8 ED3 network can be used with following EMC-PARTNERgeneratorsMIG0603IN2 1.2/50µs and 10/700µs up to 6kVMIG0603IN3 1.2/50µs and 10/700µs up to 6kVTRA3000 1.2/50µs up to 4kV1.2/50µs and 10/700µs up to 6kVIMU3000 Versions with S6-T6circuits4.3 Other EMC-PARTNER coupling, de-coupling networksCDN-UTP Ed3 1.2/50us and 10/700us up to 6kV for 2 pairs (4 lines)CDN-KIT1000 Ed3 1.2/50 µs surge coupling-decoupling network for unbalanceddata lines in accordance with IEC 61000-4-5.。
Rexnord Omega Elastomer Couplings Installation Ins

Installation Instructions• Rexnord® Omega® ElastomerCouplings(Page 1 of 6) Type E and ES•Sizes 2-140 This is the Original Document in English LanguageT he designation ATEX (A tmosphere E xplosibles) has established itself for the new guidelines. ATEX 100a controls allregulations for the condition of explosion-proof equipment.Model No. _____________________Category ______________________Reference _____________________Mfg Year _______________________Max Temperature _______________1. General Information1.1. Omega Couplings are designed to provide a mechanical connection between the rotating shafts of mechanical equipment, using atorsionally soft flexible element to accommodate inherent misalignment while transmitting the power and torque between the shafts.1.2. These instructions are intended to help you to install and maintain your Omega coupling. Please read these instructions prior toinstalling the coupling, and prior to maintenance of the coupling and connected equipment. Keep these instructions near the coupling installation and available for review by maintenance personnel.1.3. Rexnord Industries, LLC owns the copyright of this material. These Installation and Maintenance instructions may not bereproduced in whole or in part for competitive purposes.1.4. Symbol descriptions:Danger of injury to persons.Damages on the machine possible.Pointing to important items.2. Safety and Advice HintsDANGER!2.1. Safety should be a primary concern in all aspects of coupling installation, operation, and maintenance.2.2. All rotating power transmission products are potentially dangerous and can cause serious injury. They must be guarded incompliance with OSHA, ANSI, ATEX and any other local standard for the applications they are used. It is the responsibility ofthe user to provide proper guarding.2.3. Failure to secure capscrews properly could cause coupling component(s) to dislodge during operation and result in personalinjury. See table 3 for proper tightening torques.2.4. Do not use on turbine drives if the coupling cannot be protected from steam leakage or overspeed situations beyond thecouplings published speed rating.2.5. Before installing this coupling on systems involving sleeve bearings, herringbone gearsets or other axially sensitive devices,consult Rexnord.2.6. Elastomeric couplings can hold a static electric charge that may discharge and ignite in an explosive environment Both shafts ofthe connected equipment must have a path to ground.Installation Instructions • Rexnord ® Omega ® Elastomer Couplings (Page 2 of 6) Type E and ES • Sizes 2-1403. Rexnord Omega Coupling Design and Part NumbersType EType ES4.Drive AlignmentDANGER!Stop the motor and lock it out to prevent start-up during installation of coupling.A TTENTION! Improper alignment of the equipment or hubs may result in hub contact and sparking.⑥⑤①④⑥⑦③②Table 1 - Omega part numbersSizeElastomer ElementHubsElementcapscrews METRIC ⑥High speedrings⑦SleeveextensionE①ES ②Rough bore③BSW UNFQD hub⑤Taper Bush hub ④Taper Bush hub ④27300005M 7300075M 7300215M ---7301410-37300010M 7300080M 7300240M 7300795M 7300730M-73014207369574M 47300015M 7300085M 7300270M 7300800M 7300740M 7300860M 73014207369575M 57300020M 7300090M 7300305M 7300805M 7300745M 7300865M 73014207369576M 107300025M 7300095M 7300340M 7300810M 7300750M 7300870M 73014507369577M207300030M 7300100M 7300650M 7300815M 7300755M 7300875M 7393101 7301100M 7369578M 307300035M 7300105M 7300660M 7300820M 7300760M 7300880M 7393101 7301105M 7369579M 407300040M 7300110M 7300670M 7300825M 7300765M 7300885M 7393105 7301110M 7369580M 507300045M 7300115M 7300680M 7300830M 7300770M 7300890M 7393105 7301115M 7369581M 607300050M 7300120M 7300690M 7300835M 7300775M 7300895M 7393109 7301120M 7369582M 707300055M 7300125M 7300700M 7300840M 7300780M 7300900M 7393109 7301125M 7369583M 807300060M 7300130M 7300710M 7300845M 7300785M 7300905M 7393109 7301130M 7369584M1007300065M -7300720M 7300850M 7300850M -7301530-7369834M 1207300070M -7300725M 7300855M 7300855M -7301540-7369835M1407300071M-7300727M7300858M7300857M-7301545--5. Rexnord Omega Coupling InstallationSTEp 15.1. Clean dirt and burrs from shafts and hub bores.5.2. Be sure the keys fit shafts properly .5.3. Position both hubs on the shaft without tighteningthe setscrews.5.4. Use a half element to set proper hub spacing. 5.5. When the hubs are properly spaced, tighten the setscrews.5.6.When using tapered bushings, follow bushing manufacturers instructions.STEp 25.7. Mount first half element to the hubs using cap screws provided.5.8. Rotate the shaft 180 degrees and secure second half element.5.9.If shaft cannot be rotated, mount half elements at 90 degrees.STEp 35.10. Tighten all cap screws to the torques specified in Table 3. 5.11. Align equipment.5.12. Install proper guarding prior to equipment start up.A TTENTION! When installing the element, first seat all the cap screws with a light torque, then tighten all cap screws to proper torque using a torque wrench.Type E Type ESType EType ESType EType ESTable 2 - Drive Alignment23451020304050607080100120140(b-a)mm 3456767911881091214Δ Kr mm222222222333555Installation Instructions • Rexnord ® Omega ® Elastomer Couplings (Page 4 of 6) Type E and ES • Sizes 2-1406. Rexnord Omega Hub Mounting Options6.1.Hubs can be installed:• flush with the shaft end (D)• extended beyond the end of the shaft (E) • recessed behind the shaft end (F)A TTENTION! Shaft engagement length should be >0,8 times shaft diameter , bushed hubs must engage 100%.7. Cap Screw Torque7.1.Do not lubricate cap screws threads.7.2.Cap screws must have a thread-locking adhesive applied.7.3. Tighten cap screws by using torque wrench.A TTENTION! Do not lubricate cap screw threads 8. Rexnord Omega “Type E” Mounting OptionsArrangementAArrangementBCE Table 3 - Cap Screw Torque Couplingsize Quantity Torque - DRYMetricIn. Lbs Nm Part number Steel Part number Stainless steel Thread size Wrench size28+82042373014107301417M61038+87301420730142748+87301420730142758+8730142073014271012+87301450730145720124685373931017393102M101630127393101739310240167393105739310650167393105739310660168169273931097393110M12187016739310973931108016739310973931101002032403707301530-M203012024730154073015471403270808007301545-M24Table 4 - Type E Mounting Options23451020304050607080100120140A 368888131281181817445776B 4127273434394142515364837091102C4646465959656975919710914995124127Installation Instructions • Rexnord® Omega® ElastomerCouplings(Page 5 of 6) Type E and ES•Sizes 2-140 9.Rexnord Omega “Type ES” Mounting Optionsoutward One hub mounted inwardinwardTable 5 - Spacer coupling (ES) Hub mounting options for industry shaft gaps* Hub mounted inboardInstallation Instructions • Rexnord ® Omega ® Elastomer Couplings (Page 6 of 6) Type E and ES • Sizes 2-14010. Preventative MaintenanceDANGER!Do not make contact with the coupling when it is rotating and/or in operation10.1. Periodic visual inspection is necessary to evaluate the condition of the flex element. Inspection can be done during the operationusing a strobe light.10.2. When inspecting the element look for:• Fatigue cracks at element splits • Discoloration• Surface cracking in body of element.A TTENTION! Replace Element if necessary.11. Element ReplacementDANGER!Stop the motor and lock it out to prevent start-up during installation of coupling.11.1.Always replace both half elements.11.2. Install both half elements from the same box.11.3. Follow installation instructions (see Section 5, Rexnord Omega Coupling Installation).11.4.Tighten element cap screws to proper torque (see Table 3).。
and Nuclear Engineering,
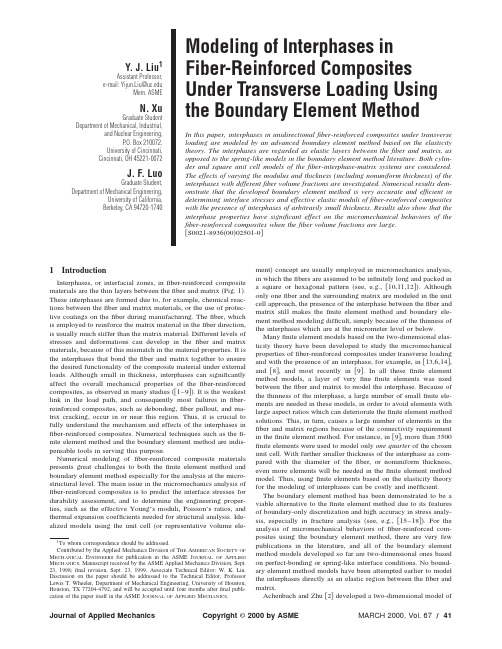
Y.J.Liu1Assistant Professor,e-mail:Yijun.Liu@Mem.ASMEN.XuGraduate Student Department of Mechanical,Industrial,and Nuclear Engineering,P.O.Box210072,University of Cincinnati,Cincinnati,OH45221-0072J.F.LuoGraduate Student, Department of Mechanical Engineering,University of California,Berkeley,CA94720-1740Modeling of Interphases inFiber-Reinforced Composites Under Transverse Loading Using the Boundary Element MethodIn this paper,interphases in unidirectionalfiber-reinforced composites under transverse loading are modeled by an advanced boundary element method based on the elasticity theory.The interphases are regarded as elastic layers between thefiber and matrix,as opposed to the spring-like models in the boundary element method literature.Both cylin-der and square unit cell models of thefiber-interphase-matrix systems are considered. The effects of varying the modulus and thickness(including nonuniform thickness)of the interphases with differentfiber volume fractions are investigated.Numerical results dem-onstrate that the developed boundary element method is very accurate and efficient in determining interface stresses and effective elastic moduli offiber-reinforced composites with the presence of interphases of arbitrarily small thickness.Results also show that the interphase properties have significant effect on the micromechanical behaviors of the fiber-reinforced composites when thefiber volume fractions are large.͓S0021-8936͑00͒02501-0͔1IntroductionInterphases,or interfacial zones,infiber-reinforced composite materials are the thin layers between thefiber and matrix͑Fig.1͒. These interphases are formed due to,for example,chemical reac-tions between thefiber and matrix materials,or the use of protec-tive coatings on thefiber during manufacturing.Thefiber,which is employed to reinforce the matrix material in thefiber direction, is usually much stiffer than the matrix material.Different levels of stresses and deformations can develop in thefiber and matrix materials,because of this mismatch in the material properties.It is the interphases that bond thefiber and matrix together to ensure the desired functionality of the composite material under external loads.Although small in thickness,interphases can significantly affect the overall mechanical properties of thefiber-reinforced composites,as observed in many studies͓͑1–9͔͒.It is the weakest link in the load path,and consequently most failures infiber-reinforced composites,such as debonding,fiber pullout,and ma-trix cracking,occur in or near this region.Thus,it is crucial to fully understand the mechanism and effects of the interphases in fiber-reinforced composites.Numerical techniques such as thefi-nite element method and the boundary element method are indis-pensable tools in serving this purpose.Numerical modeling offiber-reinforced composite materials presents great challenges to both thefinite element method and boundary element method especially for the analysis at the micro-structural level.The main issue in the micromechanics analysis of fiber-reinforced composites is to predict the interface stresses for durability assessment,and to determine the engineering proper-ties,such as the effective Young’s moduli,Poisson’s ratios,and thermal expansion coefficients needed for structural analysis.Ide-alized models using the unit cell͑or representative volume ele-ment͒concept are usually employed in micromechanics analysis, in which thefibers are assumed to be infinitely long and packed in a square or hexagonal pattern͑see,e.g.,͓10,11,12͔͒.Although only onefiber and the surrounding matrix are modeled in the unit cell approach,the presence of the interphase between thefiber and matrix still makes thefinite element method and boundary ele-ment method modeling difficult,simply because of the thinness of the interphases which are at the micrometer level or below. Manyfinite element models based on the two-dimensional elas-ticity theory have been developed to study the micromechanical properties offiber-reinforced composites under transverse loading and with the presence of an interphase,for example,in͓13,6,14͔, and͓8͔,and most recently in͓9͔.In all thesefinite element method models,a layer of veryfinefinite elements was used between thefiber and matrix to model the interphase.Because of the thinness of the interphase,a large number of smallfinite ele-ments are needed in these models,in order to avoid elements with large aspect ratios which can deteriorate thefinite element method solutions.This,in turn,causes a large number of elements in the fiber and matrix regions because of the connectivity requirement in thefinite element method.For instance,in͓9͔,more than3500finite elements were used to model only one quarter of the chosen unit cell.With further smaller thickness of the interphase as com-pared with the diameter of thefiber,or nonuniform thickness, even more elements will be needed in thefinite element method model.Thus,usingfinite elements based on the elasticity theory for the modeling of interphases can be costly and inefficient. The boundary element method has been demonstrated to be a viable alternative to thefinite element method due to its features of boundary-only discretization and high accuracy in stress analy-sis,especially in fracture analysis͑see,e.g.,͓15–18͔͒.For the analysis of micromechanical behaviors offiber-reinforced com-posites using the boundary element method,there are very few publications in the literature,and all of the boundary element method models developed so far are two-dimensional ones based on perfect-bonding or spring-like interface conditions.No bound-ary element method models have been attempted earlier to model the interphases directly as an elastic region between thefiber and matrix.Achenbach and Zhu͓2͔developed a two-dimensional model of1To whom correspondence should be addressed.Contributed by the Applied Mechanics Division of T HE A MERICAN S OCIETY OF M ECHANICAL E NGINEERS for publication in the ASME J OURNAL OF A PPLIED M ECHANICS.Manuscript received by the ASME Applied Mechanics Division,Sept. 23,1998;final revision,Sept.23,1999.Associate Technical Editor:W.K.Liu. Discussion on the paper should be addressed to the Technical Editor,Professor Lewis T.Wheeler,Department of Mechanical Engineering,University of Houston, Houston,TX77204-4792,and will be accepted until four months afterfinal publi-cation of the paper itself in the ASME J OURNAL OF A PPLIED M ECHANICS.a square unit cell using the boundary element method.To studythe effect of the interphase,the continuity of the tractions acrossthe interface offiber and matrix is maintained,while a linearrelation between the displacement differences and the tractionsacross the interface is introduced.This simple relation represents aspring-like model of the interphase.The proportionality constantsused in this model characterize the stiffness of the interphase.Based on this model,it was shown that the variations of the in-terphase parameters can cause pronounced changes in the stressdistributions in thefiber and matrix.The initiation,propagationand arrest of the interface cracks were also analyzed.The sameapproach to the interphase modeling was extended in͓3͔to studyhexagonal-arrayfiber composites,and in͓4͔to study the micro-mechanical behaviors of a cluster offibers.Oshima and Watari ͓19͔calculated the transverse effective Young’s modulus using a two-dimensional boundary element method for a square unit cellmodel.No interphase was modeled and perfect bonding betweenthefiber and the matrix was assumed.Nevertheless,the boundaryelement method results using constant elements were shown to bein very good agreement with the experimental data.Gulrajani andMukherjee͓20͔studied the sensitivities and optimal design ofcomposites with a hexagonal array offibers.A two-dimensionalboundary element method model with the same spring-like inter-phase model as in͓2͔was used.The sensitivities of stresses at theinterphase were calculated and employed to optimize the value ofthe stiffness of an interphase in order to minimize the possibilityof failure of a composite.Most recently,Pan,Adams,and Rizzo ͓21͔developed a similar two-dimensional boundary element method model using the same interphase relation as in͓2͔to study the perfectly bonded as well as imperfectly bondedfiber-reinforced composites.A main component in this research was the development of a library of Green’s functions͑or matrices of boundary element method equations͒for analyzingfiber-reinforced composite materials,which can be used by engineers in the design of such composites.Although successful to some ex-tent,all the above boundary element method models of the unit cells forfiber-reinforced composites with the spring-like inter-phase relations are incapable of providing other important infor-mation about the properties of composites,such as effects of changes of the thickness and nonuniform distribution of the inter-phases.Furthermore,in order to avoid overlapping of thefiber and matrix in the spring-like model,an iteration approach is needed.A trial calculation needs to be donefirst to check the sign of the normal traction at the interface.If the spring is in compression, continuity of the normal displacement is resumed and the bound-ary element method is applied again.This procedure is inefficient and can be costly.An improved boundary element method model of the interphases based on elasticity theory is desirable.Interphases,as infiber-reinforced composites,are thin shell-like structures.For this class of structures,there have been twomajor concerns in applying the boundary element method.Thefirst concern is whether or not the conventional boundary integralequation for elasticity can be applied successfully to thin struc-tures.It is well known in the boundary integral equation/boundaryelement method literature that the conventional boundary integralequation will degenerate when it is applied to cracks or thin voidsin structures because of the closeness of the two crack surfaces ͑see,e.g.,͓16͔and͓22͔͒.One of the remedies to such degeneracy in the conventional boundary integral equation for crack-likeproblems͑exterior-like problems͒,is to employ the hypersingularboundary integral equation͑see,e.g.,͓18,23–25͔͒.Does this de-generacy occur when the conventional boundary integral equationis applied to thin structures͑interior-like problems͒,such as thinshells?It was not clear in the boundary element method literatureand the boundary element method based on elasticity had beenavoided in analyzing thin shell-like structures for a long time dueto this concern.Recently,it was shown in͓26͔and͓27͔,bothanalytically and numerically,that the conventional boundary inte-gral equation will not degenerate,contrary to the case of crack-like problems,when it is applied to thin shell-like structures if thedisplacement boundary conditions are not imposed at all theboundaries.Further discussions on this nondegeneracy issue forthe boundary element method applied to shell-like structures canbe found in͓26͔and͓28͔.Based on these new results,the degen-eracy issue should no longer be a concern when the conventionalboundary integral equation is applied to thin structures,once thesecond concern,that is,the numerical difficulty is addressed.The numerical difficulty in the boundary integral equation is thenearly singular integrals which arise in thin structures when twoparts of the boundary become close to each other.Detailed studieson the behaviors of the nearly singular integrals and comprehen-sive reviews of the earlier work in this regard can be found in͓29͔and͓30͔.One of the most efficient and accurate approaches todeal with the nearly singular integrals in the boundary elementmethod for three-dimensional problems is to transform these͑sur-face͒integrals to line integrals analytically before the numericalintegration͓͑22,26,31͔͒.A similar approach can be established fortwo-dimensional elasticity problems͓͑27͔͒.It has been demon-strated in͓27͔that very accurate numerical solutions can be ob-tained for thin structures with the thickness-to-length ratio in themicro and even nanoscales,using the newly developed boundaryelement method approach,without seeking refinement of themeshes as the thickness decreases.Once the degeneracy issue for the conventional boundary inte-gral equation in thin structure problems has been clarified and thenearly singular integrals can be dealt with accurately and effi-ciently,it is believed that the boundary element method can nowbe applied to a wide range of engineering problems,includingsimulations of thin shell-like structures͓͑26͔͒thin-film,and coat-ings in the micro or nanoscales͓͑27͔͒and in particular,the inter-phases infiber-reinforced composite materials.In this paper,detailed two-dimensional models for the inter-phases infiber-reinforced composite materials have been devel-oped based on the elasticity theory to study their micromechanicalbehaviors under transverse loading.All the regions—thefiber,matrix,and interfacial zone contained in a unit cell,are modeled using the advanced two-dimensional boundary element method with thin-body capabilities͓͑27͔͒and extended to multidomain cases.The interphases can have uniform thickness of any arbi-trarily small values or nonuniform thickness.Interface stresses in the interphases and effective elastic moduli in the transverse di-rections are computed using this approach.This two-dimensional model of the interphases can provide more accurate interface stresses and therefore a more accurate account on the microme-chanical behaviors offiber-reinforced composites than the current spring-like models in the boundary element methodliterature. Fig.1The interphase in afiber-reinforced composite2The Boundary Element Method FormulationFor the unit cell models under transverse loading ͑Fig.2͒,the following boundary integral equation for two-dimensional elastic-ity problems can be applied in each material domain ͑index nota-tion is used in this section,where repeated subscripts imply sum-mation ͒:C i j ͑P 0͒u j ͑͒͑P 0͒ϭ͵S͓U i j ͑͒͑P ,P 0͒t j ͑͒͑P ͒ϪT i j ͑͒͑P ,P 0͒ϫu j ͑͒͑P ͔͒dS ͑P ͒,(1)in which u i ()and t i ()are the displacement and traction fields,respectively;U i j ()(P ,P 0)and T i j ()(P ,P 0)the displacement and traction kernels ͑Kelvin’s solution or the fundamental solution ͒,respectively;P the field point and P 0the source point;and S the boundary of the single material domain,͑Fig.2͒.C i j (P 0)is a constant coefficient matrix depending on the smoothness of the curve S at the source point P 0͑e.g.,C i j (P 0)ϭ1/2␦i j if S is smooth at point P 0,where ␦i j is the Kronecker delta ͒.The super-script on the variables in Eq.͑1͒signifies the dependence of these variables on the material domains,as specified below:ϭf :fiber domain ͑S ϭS 1͒;ϭi :interphase domain ͑S ϭS 1ഫS 2͒;ϭm :matrix domain ͑S ϭS 2ഫS 3͒.The two kernel functions U i j ()(P ,P 0)and T i j ()(P ,P 0)in boundary integral equation ͑1͒are given as follows for plane-strain problems:U i j ͑͒͑P ,P 0͒ϭ18͑͒͑1Ϫ͑͒͒ͫ͑3Ϫ4͒␦i jln ͩ1r ͪϩr ,i r ,j ͬ,T i j ͑͒͑P ,P 0͒ϭϪ14͑1Ϫ͑͒͒1r ͕r ,n͓͑1Ϫ2͑͒͒␦i j ϩ2r ,i r ,j ͔ϩ͑1Ϫ2͑͒͒͑r ,j n i Ϫr ,i n j ͖͒,(2)where ()is the shear modulus and ()the Poisson’s ratio forthe three different domains,respectively;r the distance from the source point P 0to the field point P ;n i the directional cosines of the outward normal n ;and (),i ϭץ()/ץx i with x i being the coor-dinates of the field point P .In Eq.͑1͒the integral containing the U i j ()(P ,P 0)kernel isweakly singular,while the one containing T i j ()(P ,P 0)is strongly singular and must be interpreted in the Cauchy principal value sense.There is a vast body of literature on how to deal with the Cauchy principle value integrals in the boundary element method formulations for bulky-shaped structures,either analytically for some special cases or numerically for other cases.An alternative approach is to transform the boundary integral equation in the form of Eq.͑1͒into a weakly singular form by using some simple solutions or integral identities for the fundamental solution͓͑32,33͔͒,before doing any numerical work.However,when the structure becomes thin in shape,such as the interphase shown in Fig.2,both integrals in Eq.͑1͒are difficult to deal with when the source point is on one side and the integration is carried out on the other side of the thin structure.These types of integrals are called nearly singular integrals since the distance r is very small in this case but is still not zero.Most techniques for dealing with the singular integrals do not work for nearly singular integrals and special attention is needed.Recently,several techniques,includ-ing singularity subtractions,analytical integration,and nonlinear coordinate transformations have been developed for the two-dimensional elasticity boundary integral equation to calculate the nearly singular integrals arising in thin structures ͓͑27͔͒.The com-bination of these techniques is found to be extremely effective and efficient in computing the nearly singular integrals in the two-dimensional boundary integral equation,no matter how close the source point is to the element of integration.Very accurate bound-ary element method results have been obtained using this ap-proach for thin structures,such as coatings on macroscale struc-tures,with the coating thickness-to-length ratios in the micro to nanoscales and with a small number of boundary elements.The same approach in ͓27͔is applied in this paper to compute the nearly singular integrals arising in the modeling of the inter-phases.Employing the boundary elements ͑line elements in two-dimensional ͒on the boundary and interfaces S 1,S 2,and S 3,the discretized equations of the three boundary integral equations as given in ͑1͒for the fiber,interphase,and matrix can be written as follows ͑cf.,e.g.,͓17͔͒:T 1͑f ͒u 1͑f ͒ϭU 1͑f ͒t 1͑f ͒,͑in fiber domain ͒(3)T 1͑i ͒u 1͑i ͒ϩT 2͑i ͒u 2͑i ͒ϭU 1͑i ͒t 1͑i ͒ϩU 2͑i ͒t 2͑i ͒,͑in interphase domain ͒(4)T 2͑m ͒u 2͑m ͒ϩT 3͑m ͒u 3͑m ͒ϭU 2͑m ͒t 2͑m ͒ϩU 3͑m ͒t 3͑m ͒,͑in matrix domain ͒(5)in which U and T are matrices generated from the U i j ()(P ,P 0)and T i j ()(P ,P 0)kernels,respectively;u and t the displacement and traction vectors,respectively.The superscripts indicate the material domain,while the subscripts indicate the interface or boundary (S 1,S 2,or S 3)on which the integration is performed.Assuming perfect bonding at the fiber/interphase (S 1)and interphase/matrix (S 2)interfaces,one can write the following in-terface conditions:On S 1:u 1͑f ͒ϭu 1͑i ͒ϵu 1,͑continuity ͒(6)t 1͑f ͒ϭϪt 1͑i ͒ϵt 1,͑equilibrium ͒(7)On S 2:u 2͑i ͒ϭu 2͑m ͒ϵu 2,͑continuity ͒(8)t 2͑i ͒ϭϪt 2͑m ͒ϵt 2,͑equilibrium ͒(9)where u 1,t 1,u 2,and t 2are defined as the interface displacementor traction vectors.Applying the interface conditions ͑6͒–͑9͒in Eqs.͑3͒–͑5͒,one obtains the following system:ͫT 1͑f ͒0T 1͑i ͒T 2͑i ͒T 2͑m ͒T 3͑m ͒ͬͭu 1u 2u 3ͮϭͫU 1͑f ͒0ϪU 1͑i ͒U 2͑i ͒0ϪU 2͑m ͒U 3͑m ͒ͬͭt 1t 2t 3ͮ,where u 3ϵu 3(m )and t 3ϵt 3(m )have been used for simplicity.Rear-ranging the columns and moving all the ͑unknown ͒interface vari-ables to the left-hand side,one finally arrivesatFig.2Two unit cell models of the fiber-interphase-matrix sys-temͫT1͑f͒ϪU1͑f͒000T1͑i͒U1͑i͒T2͑i͒ϪU2͑i͒0 00T2͑m͒U2͑m͒T3͑m͒ͬΆu1t1u2t2u3·ϭͫ00U3͑m͕͒ͬt3͖.(10)The last column in the matrix on the left-hand side and the matrix on the right-hand side may need to be rearranged again according to the boundary conditions specified on S3.Equation͑10͒is the global system of equations for thefiber-interphase-matrix model.The system has a banded matrix due to the multidomain nature of the problem.This system of equations satisfies both the continuity and equilibrium conditions at the in-terfaces explicitly,which is an advantage of the boundary element method approach over thefinite element method in which only the continuity of displacementfields can be satisfied explicitly.By solving Eq.͑10͒,one can obtain the displacements and tractions at the two interfaces and the boundary,and then calculate the inter-face stresses based on the traction and displacementfields.3Two Unit Cell Models With the InterphaseTwo unit cell models are used in this paper,namely,the con-centric cylinder model and the square model͑see,e.g.,͓12͔͒both of which include the interphase͑Fig.2͒.For the cylinder model, analytical solutions are obtained for the displacement and stress fields,which can be employed to validate the boundary element method results.For the square model,manyfinite element and boundary element solutions are available in the literature for the effective elastic moduli which will be compared with the data from the present boundary element method approach.3.1Concentric Cylinder Model.For the concentric cylin-der model,Fig.3,the response of the composite in the x-y plane is axisymmetric if the applied load or displacement on the bound-ary S3is also axisymmetric.Here it is assumed that a radial dis-placement␦is given on S3͑at rϭc,Fig.3͒.Applying the theory of elasticity for plane strain case in the polar coordinate system (r,),one can derive the following expressions for the radialdisplacement and stressfields in thefiber,interphase,and matrix, respectively͑see the Appendix for details͒:u͑f͒͑r͒ϭA͑f͒r,͑0рrрa͒u͑i͒͑r͒ϭA͑i͒rϩB͑i͒r,͑aрrрb͒u͑m͒͑r͒ϭA͑m͒rϩB͑m͒r,͑bрrрc͒(11)andr͑f͒ϭ͑f͒ϭk͑f͒A͑f͒,͑0рrрa͒r͑i͒ϭk͑i͒ͫA͑i͒Ϫ͑1Ϫ2͑i͒͒B͑i͒r2ͬ,͑i͒ϭk͑i͒ͫA͑i͒ϩ͑1Ϫ2͑i͒͒B͑i͒r2ͬ,͑aрrрb͒(12)r͑m͒ϭk͑m͒ͫA͑m͒Ϫ͑1Ϫ2͑m͒͒B͑m͒r2ͬ,͑m͒ϭk͑m͒ͫA͑m͒ϩ͑1Ϫ2͑m͒͒B͑m͒r2ͬ,͑bрrрc͒where the constants A(),B(),and k()(ϭf,i and m͒are givenin the Appendix.From the above expressions,one can compute the radial dis-placement and stress components at any point in the three do-mains within the cylinder model for any small values of the inter-phase thickness.3.2Square Model.As shown in Fig.4,the boundary con-ditions for the square model under tension arealong AB:u xϭ␦,t yϭ0;along BC:u yϭϪC0,t xϭ0;along CD:u xϭ0,t yϭ0,(13)except at yϭ0where u xϭu yϭ0;along DA:u yϭC0,t xϭ0;where u x,u y,t x,and t y are the displacement and traction com-ponents,respectively;␦the given displacement͑Fig.4͒;and C0an unknown constant.This unknown constant is meant to keep theedges BC and DA straight after the deformation.This representsthe constraint of the neighboring cells to the one under study.Inthe literature,there are several ways in dealing with these subtleboundary conditions along the top and bottom edges.For ex-ample,in͓9͔C0is chosen as zero in one case and nonzero inanother case.This is equivalent to another given displacementcondition besides the one imposed along the two vertical edges.In͓2͔and recently in͓21͔,C0is regarded as an unknown and thecondition͐ϪL Ly dxϭ0along the top or bottom edges is used toprovide the additional equation needed for solving this unknowntogether with other unknown boundary variables.Discretization ofthis simple equation using shape functions is needed.In͓19͔,however,this straight-line constraint is totally ignored,and t xϭt yϭ0͑traction-free conditions͒are assumed.It is found thatresults for the unit cell model is not very sensitive to all thedifferent techniques mentioned above.In this paper,C0isas-Fig.3Concentric cylindricalmodelFig.4Square model under tensionsumed to be unknown,but a different approach is employed toenforce the straight-line condition,instead of solving for this un-known constant with additional equations.Here the penalty method used in the finite element method for multipoint con-straints ͑see,e.g.,͓34͔͒is introduced in the boundary element method equations to enforce that all the nodes along edges BC and DA remain along straight lines after deformation.To imple-ment this penalty method in the boundary element method equa-tions a very large number ͑penalty ͒with a proper sign is placed in the locations in the matrix corresponding to the related displace-ment components.Then these displacement components will have the same value after the system of equations is solved.It is very easy to implement this penalty method in the boundary element method equations and no additional equation is needed.Once stresses on the boundary are determined,the average ten-sile stress along the edge AB is evaluated byx ϭ12L͵ϪLLx ͑L ,y ͒dy .(14)The effective Young’s modulus in the transverse direction and under the plane-strain condition is thus determined byE x Јϭx xϭ͐ϪL L x ͑L ,y ͒dy␦,(15)where ⑀x ϭ␦/2L is the average tensile strain.The effective Pois-son’s ratio under the plane-strain condition can be determined byv xyЈϭϪy x,(16)in which y is the average strain in the y -direction.For the square model under shear deformation,Fig.5,the boundary conditions arealong AB :u x ϭ0,u y ϭ;along BC :u x ϭ0,t y ϭ0;along CD :u x ϭ0,u y ϭ0;along DA :u x ϭ0,t y ϭ0.(17)The average shear stress along edge AB can be evaluated byxy ϭ12L͵ϪLLxy ͑L ,y ͒dy ,(18)and the effective shear modulus in the transverse plane and under the plane-strain condition isG xy Јϭxy ␥xyϭ͐ϪL Lxy ͑L ,y ͒dy ,(19)where ␥xy ϭ/2L is the average shear strain.Finally,one recognizes that the material constants E x Ј,xyЈ,and G xyЈgiven in Eqs.͑15͒,͑16͒,and ͑19͒,respectively,are deter-mined under the plane-strain condition which accounts for the constraint in the z -direction (z ϭ0).These constants are related to the intrinsic material properties by the following relations ͑cf.,e.g.,͓35͔and ͓21͔͒:E x ϭ1ϩ2xy Ј͑1ϩxyЈ͒2E x Ј,xy ϭxy Ј1ϩxyЈ,G xy ϭG xy Ј,(20)which are the effective Young’s modulus,Poisson’s ratio,andshear modulus,respectively,in the transverse direction for the composite.4Numerical Examples4.1Cylinder Model.The cylinder model ͑Fig.3͒is studiedfirst to validate the developed boundary element method formula-tion and the solution strategy,since for this idealized geometry the analytical solutions are available ͑see Eqs.͑11͒–͑12͒and the Ap-pendix ͒.The specified radial displacement on the boundary ͑at r ϭc )is ␦.The following material constants for a glass/epoxy com-posite are used:for fiber:E ͑f ͒ϭ72.4GPa ͑10.5ϫ106psi),͑f ͒ϭ0.22;for interphase:E ͑i ͒ϭ36.2GPa ͑5.25ϫ106psi),͑i ͒ϭ0.30;for matrix:E ͑m ͒ϭ3.45GPa ͑0.5ϫ106psi),͑m ͒ϭ0.35;where the Young’s modulus of the interphase has been taken ashalf of that of the fiber;and the dimensions used area ϭc /2,b ϭa ϩh ,with h being the thickness of the interphase,which is varying.Quadratic line elements are employed in the discretization and two meshes are tested,one with 24elements ͑eight on each circle ͒and another one with 48elements ͑16on each circle͒.DifferencesFig.5Square model under shear deformationTable 1Results of the radial displacement u …Ã10À2␦…for the cylinder modelin the results from the two meshes are less thanfive percent and the results from the refined mesh͑48elements͒are reported.The radial displacements and stresses at selected points͑Fig.3͒are given in Table1and Table2,respectively.It is observed that the maximum errors of the displacement and stress using the devel-oped boundary element method are less than0.05percent in all the cases with different thickness of the interphase.These results demonstrate that the developed boundary element method ap-proach is extremely accurate and effective in modeling the inter-phases with any small thickness,as has been confirmed in the context of single material problems͓͑27͔͒.4.2Square Model(a)Calculation of Effective Young’s Modulus With Varying Interphase Property.First,the square model under a stretch in the x-direction is considered͑Fig.4͒.The properties of the con-stituent materials considered areforfiber:E͑f͒ϭ84.0GPa,͑f͒ϭ0.22;for interphase:E͑i͒ϭ4.0ϳ12.0GPa,͑i͒ϭ0.34;for matrix:E͑m͒ϭ4.0GPa,͑m͒ϭ0.34;and,aϭ8.5m,bϭaϩh,2Lϭ21.31m͑fiber volume fraction V fϭ50percent).Young’s modulus for the interphase is changing in the range between4.0and12.0GPa.The effect of the varia-tions in the interphase material on the effective Young’s modulus of the composite is of the primary interest here.A total of64 quadratic boundary elements are used,with16elements on each of the two circular interfaces and32elements on the outer bound-ary.Table3shows the effective Young’s moduli obtained from the boundary element method stress data using Eq.͑15͒and then Eq.͑20͒,and compared with those from thefinite element method quarter model with3518linear triangular elements in͓9͔for the thickness hϭ1.0m.The boundary element method results are slightly lower than those from thefinite element method data. This may be caused by the use of linear triangular element in the finite element method which tends to overestimate the stiffness of the structure.It is noticed that the different boundary conditions along the top and bottom edges of the square model͑free-traction or straight-line conditions͒have very little influences on thefinal effective Young’s modulus.It should also be pointed out that for thefinite element results in͓9͔,the only thickness considered is hϭ1.0m which is relatively large compared with thefiber ra-dius(aϭ8.5m).If a smaller thickness were used in thefinite element model,a much larger number of elements would have been needed in order to avoid large aspect ratios in thefinite element mesh,as demonstrated in a similar study͑see͓27͔͒.How-ever,for the boundary element method employed here,the same number of elements can be used no matter how small the thick-ness of the interphase is.(b)Effect of the Interphase Thickness.Figure6shows the effect of different interphase thicknesses to the effective Young’s modulus.In order to compare with the data in͓21͔and͓19͔,the same material constants as listed in Section4.1͑for the cylinder model͒are used.It is found that the effect of the thickness is not significant on the effective Young’s moduli when thefiber volume fraction V f is small͑50percent and less͒,while significant effect is observed when V f is large͑70percent͒.This may be due to the fact that the effective elastic moduli are obtained by evaluating the average stress on the outer boundary of the matrix͑edge AB,Fig. 4͒.When thefiber volume fraction is small,the interphase is away from the matrix outer boundary and thus changing the interphase thickness does not considerably affect the stresses on the edge AB.This will change if thefiber volume fraction is large͑e.g.,70 percent͒when the interphase becomes closer to the outer bound-ary of the matrix.It should also be pointed out that when thefiber volume fraction is large,it will present additional difficulty in the modeling using thefinite element method and earlier boundary element method formulation,because of the thinness of the matrix region.However,for the current boundary element method for-mulation,this additional thinness of the matrix domain does not present any problem.(c)Effect of Nonuniform Thickness.Next,the effect of non-uniform thickness of the interphase on the interface stresses and effective elastic moduli is investigated.The starting model͑Fig. 4͒is the same as the one used for Table3with the material constants listed at the beginning of this subsection͑with E(i)ϭ42.0GPa).To form the nonuniform distribution of the inter-phase,the outer boundary of the interphase is shifted to the leftTable2Results of the radial stressr…ÃE…m…␦Õc…for the cylindermodel Table3Effective transverse elastic modulus…GPa…using the square unit cellmodel。
低通滤波器
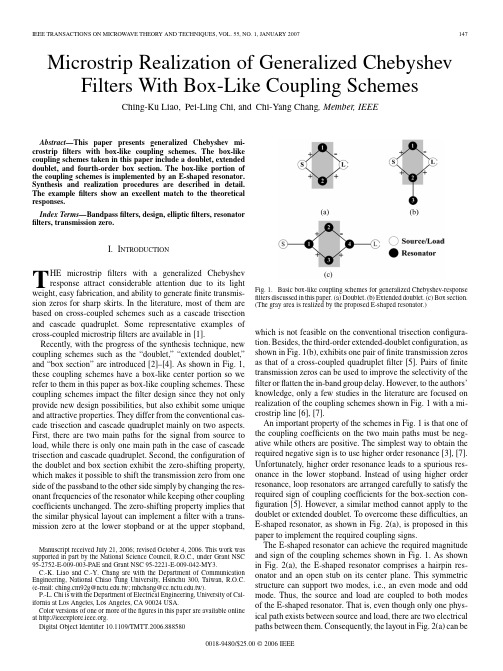
Microstrip Realization of Generalized Chebyshev Filters With Box-Like Coupling SchemesChing-Ku Liao,Pei-Ling Chi,and Chi-Yang Chang,Member,IEEEAbstract—This paper presents generalized Chebyshev mi-crostripfilters with box-like coupling schemes.The box-like coupling schemes taken in this paper include a doublet,extended doublet,and fourth-order box section.The box-like portion of the coupling schemes is implemented by an E-shaped resonator. Synthesis and realization procedures are described in detail. The examplefilters show an excellent match to the theoretical responses.Index Terms—Bandpassfilters,design,ellipticfilters,resonator filters,transmission zero.I.I NTRODUCTIONT HE microstripfilters with a generalized Chebyshev response attract considerable attention due to its light weight,easy fabrication,and ability to generatefinite transmis-sion zeros for sharp skirts.In the literature,most of them are based on cross-coupled schemes such as a cascade trisection and cascade quadruplet.Some representative examples of cross-coupled microstripfilters are available in[1]. Recently,with the progress of the synthesis technique,new coupling schemes such as the“doublet,”“extended doublet,”and“box section”are introduced[2]–[4].As shown in Fig.1, these coupling schemes have a box-like center portion so we refer to them in this paper as box-like coupling schemes.These coupling schemes impact thefilter design since they not only provide new design possibilities,but also exhibit some unique and attractive properties.They differ from the conventional cas-cade trisection and cascade quadruplet mainly on two aspects. First,there are two main paths for the signal from source to load,while there is only one main path in the case of cascade trisection and cascade quadruplet.Second,the configuration of the doublet and box section exhibit the zero-shifting property, which makes it possible to shift the transmission zero from one side of the passband to the other side simply by changing the res-onant frequencies of the resonator while keeping other coupling coefficients unchanged.The zero-shifting property implies that the similar physical layout can implement afilter with a trans-mission zero at the lower stopband or at the upper stopband,Manuscript received July21,2006;revised October4,2006.This work was supported in part by the National Science Council,R.O.C.,under Grant NSC 95-2752-E-009-003-PAE and Grant NSC95-2221-E-009-042-MY3.C.-K.Liao and C.-Y.Chang are with the Department of Communication Engineering,National Chiao Tung University,Hsinchu300,Taiwan,R.O.C. (e-mail:ching.cm92g@.tw;mhchang@.tw).P.-L.Chi is with the Department of Electrical Engineering,University of Cal-ifornia at Los Angeles,Los Angeles,CA90024USA.Color versions of one or more of thefigures in this paper are available online at .Digital Object Identifier10.1109/TMTT.2006.888580Fig.1.Basic box-like coupling schemes for generalized Chebyshev-responsefilters discussed in this paper.(a)Doublet.(b)Extended doublet.(c)Box section.(The gray area is realized by the proposed E-shaped resonator.)which is not feasible on the conventional trisection configura-tion.Besides,the third-order extended-doublet configuration,asshown in Fig.1(b),exhibits one pair offinite transmission zerosas that of a cross-coupled quadrupletfilter[5].Pairs offinitetransmission zeros can be used to improve the selectivity of thefilter orflatten the in-band group delay.However,to the authors’knowledge,only a few studies in the literature are focused onrealization of the coupling schemes shown in Fig.1with a mi-crostrip line[6],[7].An important property of the schemes in Fig.1is that one ofthe coupling coefficients on the two main paths must be neg-ative while others are positive.The simplest way to obtain therequired negative sign is to use higher order resonance[3],[7].Unfortunately,higher order resonance leads to a spurious res-onance in the lower stopband.Instead of using higher orderresonance,loop resonators are arranged carefully to satisfy therequired sign of coupling coefficients for the box-section con-figuration[5].However,a similar method cannot apply to thedoublet or extended doublet.To overcome these difficulties,anE-shaped resonator,as shown in Fig.2(a),is proposed in thispaper to implement the required coupling signs.The E-shaped resonator can achieve the required magnitudeand sign of the coupling schemes shown in Fig.1.As shownin Fig.2(a),the E-shaped resonator comprises a hairpin res-onator and an open stub on its center plane.This symmetricstructure can support two modes,i.e.,an even mode and oddmode.Thus,the source and load are coupled to both modesof the E-shaped resonator.That is,even though only one phys-ical path exists between source and load,there are two electricalpaths between them.Consequently,the layout in Fig.2(a)can be 0018-9480/$25.00©2006IEEEFig.2.Doublet filter.(a)Proposed layout (gray area indicate the E-shaped res-onator).(b)Corresponding coupling scheme.modeled by the coupling scheme,a doublet,shown in Fig.2(b).The doublet filter illustrates how a E-shaped resonator directly couples to external feeding network.Furthermore,based on the proposed E-shaped resonator,filters with an extended-doublet and a box-section con figuration can be realized as well.The E-shaped resonator can use either its even mode or odd mode to couple an extra resonator.Thus,the extended-doublet con figu-ration in Fig.1(b)is achievable.Besides,the E-shaped resonator can couple to external resonators with two of its modes simul-taneously and forms the box-section con figuration in Fig.1(c).The feasibility of realization of the basic coupling schemes in Fig.1with the proposed E-shaped resonator makes it possible to realize a class of coupled microstrip filters in a uni fied ap-proach.II.C IRCUIT M ODELINGA.Filters in the Doublet ConfigurationThe E-shaped resonator filter in Fig.2(a)was originally re-ported in [8].In [8],the E-shaped resonator was not modeled as a two-mode resonator.Instead,the circuit was modeled as two quarter-wavelength resonators with a tapped open stub in the center plane.The open stub is considered as a K-inverter between two quarter-wavelength resonators to control the cou-pling strength,and as a quarter-wave open stub to generate a transmission zero at the desired frequency.However,the filter cannot be designed with a prescribed quasi-elliptic response since there is no suitable prototype corresponding to the circuit model in [8].In this paper,a doublet,as shown in Fig.2(b),is used to model the circuit in Fig.2(a).In Fig.2(b),resonator 1rep-resents the odd-mode resonance,where the center plane of the E-shaped resonator is an electric wall (-plane).On the other hand,resonator 2represents even-mode resonance,where the center plane of the E-shaped resonator is a magnetic wall(-plane).With the notation shown in Fig.2(b),the corre-sponding couplingmatrixcan be written down as[3](1)There are some interesting properties of the doublet filter in Fig.2(a).First,since the E-shaped resonator exhibits sym-metry,therelationshipand holds.Second,is always true for this structure since the coupling strength between the odd mode and external feeding network is always larger than that of the even mode.To get more insight of how to control a transmission zero of a doublet filter in this con figuration,an explicit expression relating the coupling elements and the transmissionzero is provided in a low-pass prototype asfollows:(2)Note that the mapping between normalizedfrequency and actualfrequencyis ,whereand are center frequency and bandwidth of a filter,re-spectively.Based on the (2),observations are summarized as follows.1)The transmission zero is always located at finite frequencysince.In other words,the structure exhibits finite transmission zero inherently.2)The transmission zero can be moved from the upper stop-band to lower stopband,or vice versa,by changing the signofand simultaneously.This property makes it possible to generate upper stopband or lower stopband fi-nite transmission zero with similar structure.3)Ifand,would be greater than zero.In a more explicitexpression,and can be related to the resonant frequencies of oddmodeand evenmode ,respectively by the followingequations:(3)(4)whereand are the center frequency and bandwidthof a filter,respectively;i.e.,ifand ,the transmission zero would be on the upper stopband.4)Ifand,would be smaller than zero;i.e.,ifand ,the transmission zero would be on the lower stopband.To get the related electrical parameters indicated in Fig.2(a),one can take the following procedures.First,synthesize a cou-plingmatrixcorresponding to the prescribed response by methods provided in [7].Then consider parameters concerning the odd mode only by removing the open stub on the center plane.Once the open stub is removed,the circuit becomes a first-order hairpin filter.The first-order hairpin filter can be syn-thesized by the conventional method [8]with the center fre-quency set to be the resonant frequency of odd mode,whichcan be expressedas.At this step,one can specify the valuesof,,and and obtain the valuesofand by an analytical method [8].Second,put the open stub back.The two parameters of the open stub,i.e.,and ,can be adjusted to achieve the desired reso-nant frequency and the needed external coupling strength of theLIAO et al.:MICROSTRIP REALIZATION OF GENERALIZED CHEBYSHEV FILTERS WITH BOX-LIKE COUPLING SCHEMES149Fig.3.Responses generated from the coupling matrix and from electrical net-work shown in Fig.2(a)with synthesis parameters.even mode.Here,the resonant frequency of the even modeis.To illustrate the procedure,an example is taken of a second-order generalized Chebyshevfilter with a passband return loss of20dB and a single transmission zero at a normalizedfrequency.The corresponding coupling coefficientsare,,,and.Forfilter with centerfrequency GHz and fractionalbandwidth,the ideal response is depicted in Fig.3as solid lines.After getting the couplingmatrix,couldfirstbe specified.Here,weset,,andandobtainand fora uniform impedance resonator with characteristicimpedanceatfrequency GHz.Next,put the openstub back and adjust the valuesofand by the optimizationmethod to let the response of the circuit match with the idealresponse calculated fromthe matrix.The optimized valuesofand are62and86.8,respectively,atfrequencyGHz.According to the obtained electrical parametersin Fig.2(a),the corresponding response is shown in Fig.3ascircled lines.The frequency response contributed only by theodd mode is also depicted in Fig.3as dashed lines to let usunderstand the procedures clearer.B.Extended-Doublet FiltersBased on the doubletfilters developed in Section II-A,theemphasis here is put on how to extend the design to extended-doubletfilters[4].There are two possible arrangements suitableto form extended-doubletfilters.One possible arrangement isindicated in Fig.4,where the extended doubletfilter consists ofa doubletfilter plus a grown resonator.The grown resonator isa half-wavelength resonator with both ends open.In this case,the grown resonator would mainly couple to the odd mode ofthe E-shaped resonator.For the even mode of the E-shaped res-onator,it acts as a nonresonant element,which slightly perturbsthe resonant frequency of the even mode.Another possible de-sign is shown in Fig.5where both ends of the grown resonatorare shorted to ground.In this case,the grown resonator mainlycouples to the even mode of the E-shaped resonator and acts as anonresonant element to the odd mode of the E-shapedresonator.Fig. yout of extended-doubletfilter and its corresponding couplingscheme.The design is forflat group-delayresponse.yout of extended-doubletfilter and corresponding coupling scheme.The design is for skirt selectivity response.To clarify the coupling relationship between each resonator,thecoupling routes are accompanied with layouts in Figs.4and5.The extended-doubletfilter has a pair offinite transmissionzeros[4].For the design in Fig.4,the pair of transmission zerosis on the imaginary-frequency axis.On the other hand,to gen-erate a pair of real-frequency transmission zeros,the design inFig.5must be adopted.The difference between the two de-signs can be understood from the governing equation offinitetransmission zeros.Since the proposed extended doubletfiltersare symmetric structures,therelationsandalways hold.Thus,the governing equation offinite transmission zero can be expressedas(5)As discussed in the design of the doublet,the coupling co-efficient of source to odd mode is stronger than that of sourceto even mode.Thus,for the design in Fig.4,,which leadsto.On the contrary,for the design in Fig.5,,which resultsin.In conclusion,thedesign in Fig.4can be used to generate delay-flattening trans-mission zeros,while the design in Fig.5can be used to generatea pair of attenuation poles.To illustrate the procedure of design,a generalized Cheby-shevfilter with passband return loss of20dB and a pair of trans-mission zerosat is taken as an example.The design ofan extended doublet starts from the synthesis of the couplingmatrix,which can be done using the technique in[9].The syn-thesized coupling matrix is shown in Fig.6(a).Using the infor-mationofand,one can construct the doublet by themethod provided in Section II-A.Excludingand inthe coupling matrix,one can calculate the response contributed150IEEE TRANSACTIONS ON MICROWA VE THEORY AND TECHNIQUES,VOL.55,NO.1,JANUARY2007Fig.6.Extended-doubletfilter with in-band return loss RL=20dB,normal-ized transmission zeros at =62.(a)Its coupling matrix.(b)Responses of extended doubletfilter and responses contributed by doublet only.from the doublet only.For instance,if the center frequency and fractional bandwidth of the designedfilter are2.4GHz and5%, respectively,the responses of the doublet are shown as dotted lines in Fig.6(b).After getting the initial design of the doublet,add the grown resonator.Since in this case,the layoutin Fig.5must be adopted.Ideally,the response of the extended doublet would be the solid lines shown in Fig.6.The physical implementation of this design will be presented in Section III to confirm the validity.C.Box-Section FiltersThe fourth-orderfilter in the“box-section”configuration was first proposed in[2]and realized by coaxial resonators.With the zero-shifting property,it is possible to use the similarfilter struc-ture to realize thefinite transmission zero either on the upper or lower stopband.The box-sectionfilter is suitable for the com-plementaryfilters of a transmit/receive duplexer[7]since it has an asymmetric response with high selectivity on one side of the passband.The microstrip box-sectionfilter wasfirst reported in [6]with open square loop resonators.Since the box-section cou-pling diagram is symmetricwhere,,,and should be held in the couplingroute shown in Fig.7(a).Therefore,it is preferable to layoutthefilter symmetrically because a symmetrical-layoutfilter caninherently obtain symmetrical coupling coefficients.The asym-metrical layout causes thefilters in[6]to be difficult to keep thecoupling coefficients symmetric.Another microstrip box-sec-tionfilter was proposed in[7].Although the layout of thefiltersin[7]is symmetric,it suffers from a spurious response in thefilter’s lower stopband due to one of thefilter’s resonators beinga higher order mode resonator.In this paper,the layout depictedin Fig.7(b)solves the problems mentioned.The E-shaped res-onator is symmetric and is free from lower stopbandspuriousFig.7.Box-sectionfilter.(a)Filter’s coupling scheme.(b)Proposed layout.resonances.Due to the symmetry,only half of the electrical pa-rameters are shown in Fig.7(b).As explained in the doubletfilter,the circuit layout in Fig.7(b)satisfies the required sign ofcouplings.To illustrate how to obtain the corresponding electrical pa-rameters in Fig.7(b)from a prescribed response,examples aretaken as follows.Thefirst example is a fourth-order general-ized Chebyshevfilter with a passband return loss of20dB,and a single transmission zeroat,which gives alobe level of48dB on the lower side of the passband.Thecorresponding couplingmatrix is shown in Fig.8(a).Afterthe low-pass-to-bandpass transformation,the ideal bandpass re-sponse of thisfilter with a center frequency of2.4GHz and frac-tional bandwidth of5%is shown in Fig.8(b).The design procedures are described as follows.First,removethe open stub in the E-shaped resonator in Fig.7(b),which isequivalent to discarding the even mode[resonator3in Fig.7(a)]of the E-shaped resonator.After removing the open stub,thecircuit becomes a third-order hairpin-likefilter.The couplingmatrix of this hairpin-likefilter is identical to the couplingmatrix in Fig.8(a),exceptand are zero.The idealresponse of this hairpin-likefilter can be calculated fromthematrix,as denoted by circled lines in Fig.8(b).To get theelectrical parameters associated with the asynchronously tunedthird-order hairpin-likefilter,a synchronous tuned third-orderhairpinfilter provides the initial design and is synthesizedfirst.The synchronous tuned hairpinfilter has the couplingmatrix,which is identicalto,except.When synthe-sizing the synchronously tuned hairpinfilter,weset,,,andat,and thecharacteristic impedance of each resonator to50.With thesesettings,the electrical parameters of the synchronously tunedhairpinfilter are calculated and shown in Table I(a),which pro-vides the initial values for the asynchronous-tuned hairpin-likefilter.An optimization routine is then involved.The goal of theLIAO et al.:MICROSTRIP REALIZATION OF GENERALIZED CHEBYSHEV FILTERS WITH BOX-LIKE COUPLING SCHEMES151Fig.8.Fourth-order box-section filter.(a)Its coupling matrix.(b)Responses of the box-section filter and ideal responses of the asynchronous tuned third-order hairpin-like filter calculated by M matrix.TABLE IE LECTRICAL P ARAMETERS C ORRESPONDING TO B OX -S ECTIONF ILTERS S HOWNIN F IG .7(b).H ERE ,#=90,#=60,Z =50 ,AND Z =50 .A LL OF THE E LECTRICAL L ENGTHS C ORRESPOND TO THE C ENTER F REQUENCY OF THE F ILTER .D ESIGN 1:I N -B AND R ETURN L OSS RL =20dB, =02:57,AND FBW =5%D ESIGN 2:I N -B AND R ETURN L OSS RL =20dB,=2:57,AND FBW =5%optimization routine is to find a set of electrical parameters,which can make the response match with the response of the ideal asynchronously tuned hairpin-like filter calculated fromthematrix.The optimized parameters are shown in Table I for comparison.Note that the optimized values of associated parameters are nearly identical to the initial values;therefore,the optimization routine can converge within a few times.Fi-nally,put the open stub back and optimize theparametersand to make the response match with the response of the de-sired box-section filter ’s response,as denoted by solid lines inFig.8(b).The optimized valuesofand are given in Table I as well.Instead of a low-pass prototype filter with a transmission zeroatin the first example,the second example locates the transmission zero at a normalizedfrequencyFig.9.Responses of the box-section filters.(a)Responses obtained by elec-trical parameters of design #1in Table I and its coupling matrix,respectively.(b)Responses obtained by electrical parameters of design #2in Table I and its coupling matrix,respectively.and keeps all other parameters unchanged.According to the synthesis procedures in [2],the inter-resonator couplings are unchanged,but self-couplings [principal diagonal matrix ele-ments,etc.,of the coupling matrix in Fig.8(a)]must change sign.Following the same procedures in the pre-vious design,one can get the electrical parameters given in Table I.In Table I,the column of design #1corresponds to alow-pass prototype filter transmission zeroatand the column of design #2corresponds to a low-pass prototypefilter transmission zeroat.The responses obtained from the electrical parameters are listed in Table I and responsescalculated fromthematrix in Fig.8(a)are both plotted in Fig.9for comparison.III.D ESIGN E XAMPLES AND E XPERIMENTAL R ESULTS The extended-doublet filter discussed in Section II-B with its ideal response.shown in Fig.6,and the design #1of box-section filter discussed in Section II-C with its ideal response,shown in Fig.9(a),are fabricated to verify the designs.Although all of the electrical parameters obtained in Section II can be trans-formed to physical parameters,it does not include the junction effect.Therefore,a commercial electromagnetic (EM)simulator Sonnet [11]is adopted to take all the EM effects into consider-ation.To ef ficiently tune the physical dimensions of the filter to achieve the prescribed response,the diagnosis and tuning152IEEE TRANSACTIONS ON MICROWA VE THEORY AND TECHNIQUES,VOL.55,NO.1,JANUARY2007Fig.10.Fabricated extended-doubletfilter.(a)Layout(unit:mils).(b)Simu-lated and measured response.methods proposed in[12]are taken.Fig.10shows the phys-ical dimensions and the corresponding responses for the ex-tended-doubletfilter where an RO6010substrate with a dielec-tric constant of10.8and thickness of50mil is used.Fig.11 depicts the physical dimensions and corresponding responses for the box-sectionfilter where an RO4003substrate with a di-electric constant of3.63and a thickness of20mil is used.The measured in-band insertion loss of thefilters in Figs.10and11 are1.4and2.7dB,respectively.In Fig.10(b),the experimental results show a larger passband than the simulated ones.The de-viation mainly results from the fabrication error.In Fig.11(b), the measured response is shifted about30MHz.Further inves-tigation showed that the dielectric constant of the substrate is closer to3.4rather than3.63.The wideband measurement re-sults of the fabricated box-sectionfilter are shown in Fig.11(c).IV.D ISCUSSIONIn Section II,we have discussed how to get the electrical pa-rameters of afilter network in a doublet,an extended-doublet, and a box-section configuration from the corresponding cou-pling matrices.With the understanding of the correspondence between the coupling matrix and physical structure,the layout is not limited to those provided in this paper.Afilter canbe Fig.11.Fabricated box-sectionfilter.(a)Layout(unit:mil).(b)Simulated and measured response.(c)Measured widebandresponse.Fig.12.Possiblefilter layout that can be modeled as a doublet configuration. modeled by the box-like coupling scheme,as long as it contains a two-mode resonator that is physically symmetric and supports two resonant modes.For instance,thefilters in Fig.12can also be modeled as a doubletfilter since it is symmetric and has two resonant modes.However,for thefilter in Fig.12,it is not easy to get the initial physical dimensions.On the contrary,the ini-tial dimensions of the layouts proposed in this paper can easilyLIAO et al.:MICROSTRIP REALIZATION OF GENERALIZED CHEBYSHEV FILTERS WITH BOX-LIKE COUPLING SCHEMES153be obtained.Besides,using the E-shaped resonator and the de-sign procedures provided in this paper,all electrical parame-ters of afilter with box-like coupling schemes can be easily obtained.These parameters can be applied tofilters with the same low-pass prototype and fractional bandwidth,but a dif-ferent center frequency and a different substrate.Having clear initial dimensions of afilter can save quite a lot of time in the design when comparing to the conventional design procedures of cross-coupledfilters,e.g.,thefilters in[5].In the design of a conventional cross-coupledfilter,once the substrate,shape of the resonator,or center frequency of afilter is changed,one must redo the design from the very beginning of the procedures. The sensitivity analysis of the box-like coupling routes can be performed by the method proposed in[13].The most sensitive part of the proposed structures is the coupling section between the E-shaped resonator and the source/load or other resonators because the coupling section controls the coupling strengths of two modes of the E-shaped resonator to an external circuit si-multaneously.V.C ONCLUSIONThe three box-like coupling schemes,namely,the doublet, extended doublet,and box section,have been illustrated.How an E-shaped resonator constructs the box-like portion of the coupling schemes has been explained.The couplings between an E-shaped resonator,external feeding network,and other single-mode resonators have also been modeled.The corre-spondence between an electrical network and coupling matrix has been established,which make it possible to get the initial dimensions of afilter from the information of a coupling matrix. Besides,with the aid of a proper coupling matrix served as a surrogate model,a systematic way of tuning afilter has been applied,which saves a lot of time for optimizing the response of afilter.The proposedfilters have provided an effective way to design a class offilters exhibiting a generalized Chebyshev response to meet the stringent specification in modern commu-nication systems.R EFERENCES[1]J.S.Hong and ncaster,Microstrip Filters for RF/MicrowaveApplications.New York:Wiley,2001.[2]R.J.Cameron,A.R.Harish,and C.J.Radcliffe,“Synthesis ofadvanced microwavefilters without diagonal cross-couplings,”IEEETrans.Microw.Theory Tech.,vol.50,no.12,pp.2862–2872,Dec.2002.[3]U.Rosenberg and S.Amari,“Novel coupling schemes for microwaveresonatorfilters,”IEEE Trans.Microw.Theory Tech.,vol.50,no.12,pp.2896–2902,Dec.2002.[4]S.Amari and U.Rosenberg,“New building blocks for modular de-sign of elliptic and self-equalizedfilters,”IEEE Trans.Microw.TheoryTech.,vol.52,no.2,pp.721–736,Feb.2004.[5]J.S.Hong and ncaster,“Couplings of microstrip squareopen-loop resonators for cross-coupled planar microwavefilters,”IEEE Trans.Microw.Theory Tech.,vol.44,no.12,pp.2099–2108,Dec.1996.[6]S.Amari,G.Tadeson,J.Cihlar,R.Wu,and U.Rosenberg,“Pseudo-el-liptic microstrip linefilters with zero-shifting properties,”IEEE Mi-crow.Wireless Compon.Lett.,vol.14,no.7,pp.346–348,Jul.2004.[7]mperez and M.S.Palma,“High selectivity X-band planardiplexer with symmetrical box sectionfilters,”in Eur.Microw.Conf.,Paris,France,Oct.2005,vol.1,pp.105–108.[8]J.R.Lee,J.H.Cho,and S.W.Yun,“New compact bandpassfilterusing microstrip =4resonator with open stub inverter,”IEEE Microw.Guided Wave Lett.,vol.12,no.12,pp.526–527,Dec.2000.[9]R.J.Cameron,“Advanced coupling matrix synthesis techniques formicrowavefilters,”IEEE Trans.Microw.Theory Tech.,vol.51,no.1,pp.1–10,Jan.2003.[10]M.Makimoto and S.Yamashita,Microwave Resonators and Filters forWireless Communication.New York:Springer,2001.[11]Em User’s Manual.Liverpool,NY:Sonnet Software Inc.,2004.[12]mperez,S.L.Romano,M.S.Palma,and T.K.Sarka,“Fastdirect electromagnetic optimization of a microwavefilter without diag-onal cross-couplings through model extraction,”in Eur.Microw.Conf.,Munich,Germany,2003,vol.2,pp.1361–1364.[13]S.Amari and U.Rosenberg,“On the sensitivity of coupled resonatorfilters without some direct couplings,”IEEE Trans.Microw.TheoryTech.,vol.51,no.6,pp.1767–1773,Jun.2003.Ching-Ku Liao was born in Taiwan,R.O.C.,onOctober16,1978.He received the B.S.degree inelectrophysics and M.S.degree in communicationengineering from National Chiao-Tung University,Hsinchu,Taiwan,R.O.C.,in2001and2003,respec-tively,and is currently working toward the Ph.D.degree in communication engineering at NationalChiao-Tung University.Since March2006,he has been a Visiting Re-searcher with University of Florida,Gainesville,under a sponsorship of the National Science Council Graduate Student Study Abroad Program(GSSAP).His research interests include the analysis and design of microwave and millimeter-wavecircuits.Pei-Ling Chi was born in Taiwan,R.O.C.,on March25,1982.She received the B.S.and M.S.degreesin communication engineering from National ChiaoTung University,Hsinchu,Taiwan,R.O.C.,in2004and2006,respectively,and is currently workingtoward the Ph.D.degree in electrical engineering atthe University of California at Los Angeles.Her research interests include the analysis and de-sign of microwave and millimeter-wavecircuits.Chi-Yang Chang(S’88–M’95)was born in Taipei,Taiwan,R.O.C.,on December20,1954.He receivedthe B.S.degree in physics and M.S.degree in elec-trical engineering from National Taiwan University,Taipei,Taiwan,R.O.C.,in1977and1982,respec-tively,and the Ph.D.degree in electrical engineeringfrom the University of Texas at Austin,in1990.From1979to1980,he was with the Department ofPhysics,National Taiwan University,as a TeachingAssistant.From1982to1988,he was with theChung-Shan Institute of Science and Technology (CSIST),as an Assistant Researcher,where he was in charge of development of microwave integrated circuits(MICs),microwave subsystems,and mil-limeter-wave waveguide E-plane circuits.From1990to1995,he returned to CSIST as an Associate Researcher in charge of development of uniplanar circuits,ultra-broadband circuits,and millimeter-wave planar circuits.In1995, he joined the faculty of the Department of Communication,National Chiao Tung University,Hsinchu,Taiwan,R.O.C.,as an Associate Professor and became a Professor in2002.His research interests include microwave and millimeter-wave passive and active circuit design,planar miniaturizedfilter design,and monolithic microwave integrated circuit(MMIC)design.。
- 1、下载文档前请自行甄别文档内容的完整性,平台不提供额外的编辑、内容补充、找答案等附加服务。
- 2、"仅部分预览"的文档,不可在线预览部分如存在完整性等问题,可反馈申请退款(可完整预览的文档不适用该条件!)。
- 3、如文档侵犯您的权益,请联系客服反馈,我们会尽快为您处理(人工客服工作时间:9:00-18:30)。
a r X i v :c o n d -m a t /0402374v 1 [c o n d -m a t .s t r -e l ] 13 F eb 2004Intersite elastic coupling and invar effectD.I.Khomskii1and F.V.Kusmartsev21II Physikalisches Institut,Universit ¨a t zu K ¨o ln,Z ¨u lpicher Str.7750937K ¨o ln,Germany2Department of Physics,Loughborough University,LE113TU,UK(Dated:20.01.2004)The invar phenomenon (very small thermal expansion in some iron alloys or compounds)is usually explained by the thermally-induced transitions between different spin states of F e ,having different atomic volumes.We consider these processes taking into account elastic interaction between F e atoms in different spin states.Inclusion of these interactions explains why thermal expansion may be close to zero in a broad temperature interval and thus gives rise to the invar effect.Invar behaviour –the absence of the dependence of the lattice parameter on temperature in F e −Ni alloys in certain concentration range,was discovered in 1897[1],and similar behaviour was found later in certain other systems -e.g.in ordered and disordered F e 3P t and F e 3P d.[2].The most plausible explanation of this phenomenon was suggested by Weiss [3]who postulated the existence of two states of iron,close in energy:the ground state with the high spin,or the high-moment (HM )state with large specific volume,and the low-lying excited state with low spin or low moment (LM ),having smaller atomic radius or specific volume.According to this picture,thermal excitation of the LM low-volume states causes lattice contraction,which counteracts and may cancel the usual positive thermal expansion.Although there is yet no definite proof of the existence of such two states in invar alloys,many experimented facts are naturally explained in this picture [2,4,5].The existence of almost degenerate states with different moments and different specific volumes is also corroborated by the detailed band-structure calculations [6,7].Recent neutron scattering studies [8]have confirmed the importance of magnetoelastic coupling for the invar effect −apparently not the usual coupling present in magnetic materials with a given spin of the ions,but of the coupling with mutliplet excitations,e.g.HM −LM excitations iron.Although many particular details are still not clear,all these results confirm the general validity of the Weiss two-state model.This simple explanation of the invar effect is very appealing.However,one problem in this explanation becomes immediately apparent.The conventional thermal expansion is usually more or less linear in temperaturea (T )=a 0+α0T,(1)where a (T )is the lattice parameter at a temperature T and α0is the conventional thermal expan-sion coefficient.On the other hand the thermal population of the low-spin state with smaller radius in simplest case of two well-defined LM and HM states would be exponential in temperature:a (T )=a 0−c exp(−∆transform one site from a HM to a LM state with smaller volume,it would be favourable to have close to this small-volume LM ion the larger,i.e.HM ions.This interaction will modify the temperature dependence of the occupation of different spin-states,and,consequently,will change the extra contribution to thermal expansion,effectively stretching the exponential temperature dependence(2).This would help to explain the almost full compensation of two mechanisms of thermal expansion–the usual one(1)and the additional stretched contribution,givingfinally the invar effect in a rather broad temperature interval.One can describe this situation introducing the pseudospin operators,which describe two spinstates,so that the stateτz i=+12to a LMstate of it.The fact that these states have different ions radii(or atomic volumes)gives rise to a coupling of these states to the lattice,which classically can be written as:H=−gτz i v i−v0 +B2 v L+v H),where v L and v H are the corresponding atomic volumes of the respectively LM and HM states,and g=B v H−v L is the effective coupling constant.By minimizing the average energy E=<H>with respect to volume,we can indeed see thatv i=v0+g2 =v H,v i τ=−1ωkThe effective sign of an intersite interaction depends on the detailed k-dependence of the spin-phonon matrix element˜g k,on the phonon dispersionωk and on the type of the lattice.One can easily show that the coupling via short-wavelength phonons leads to a nearest-neighbor repulsion J>1,i.e.to an antiferromagnetic interaction between pseudospinsτ,in accordance with the qualitative considerations presented above(the large HM stateτz1=+12).Longer range interactions may in general havedifferent sign[12],but usually the nn interactions dominate,and this is what we will assume further on.With this assumption we can reduce our model to an antiferromagnetic Ising model with nn coupling J in a parallelfield.For invar systems,the parameters of the model should be chosensuch that the ground state corresponds to the HM state,i.e.allτz1=+12th∆−2J zτBτ(T)(8)It is convenient to rewrite Eq.(7)asτ=12−τ2−exp −˜∆2exp −˜∆2−exp −˜∆2−τ(T) wouldgradually increase with temperature,making the extra negative contribution to lattice parameter more smooth.If we now add to this term the usual positive thermal expansion(qualitatively shown in Fig.1by thin line),we indeed see that with J=0one can get a better cancellation of the normal and anomalous contributions to thermal expansion(although this cancellation is not exact).50100150200250Temperature ,K0.20.30.40.50.60.7T h e r m a l E x p a n s i o n ,ΤFig.1The extra negative thermal expansion(see Eq.(9))without intersite elastic interaction (dotted line)and with this interaction taken into account (solid line).Thin line is a conventional positive thermal expansion.This is the main conclusion of the present paper.We used the fact that the elastic interaction between different spin (and volume)states of F e in invar alloys is always present.We show that its inclusion helps to resolve some of the problems inherent to the two-state (Weiss)model traditionally used in this field.The essence of our results is that due to this interaction the effective energy separating low moment/small volume and high moment/large volume states in invar alloys becomes temperature-dependent.This modifies the temperature dependence of the thermal expansion and finally guarantees the invar behaviour in a broad temperature interval.Extra consequences of our treatment are,first,that due to this effect the energy separation of these two states becomes dependent on the local coordination (occupation of neighbouring sites);this can hinder the direct observation of these two-level-systems e.g.by the neutron scattering.On the other hand,there should appear certain correlation in the occupation of different magnetic states;this effect should be observable experimentally.This could even lead to the formation of some textures in the invar samples.Many of the problems in this field still remain open.One of them is the role of magnetic ordering for the invar phenomenon,which was not included in the present treatment.Another problem is the account of the metallic nature of most of the invar systems.Nevertheless,even in this simplified form the model considered above,with the inclusion of the intersite elastic interactions,can explain the main features of the invar systems,and these interactions definitely have to be taken into account in the full theory of the invar effect.We are grateful to M.Abd-Elmeguid,K.Neumann and K.R.A.Ziebeck for useful discussions.This work was supported by the German Physical Society via SFB 608and by the Leverhulme Trust.[1]C.E.Guillaume,Compt.Rend-Acad.Sci125(1987)[2]V.L.Sedov,Antiferromagnetism of gamma-iron.The invar problem.Nauka Publ.,Moscow1987(inRussian).[3]Y.Weiss,Proc.Roy.Soc.London82,281(1963)[4]Y.Nakamura,IEEE Trans.Magn MAG-12,278(1976)[5]M.M.Abd-Elmeguid and H.Micklitz,Phys.Rev.B40,7395(1989)[6]P.Entel,E.Hoffmann,P.Mohn,K.Schwarz and V.L.Moruzzi,Phys.Rev.B47,8706(1993)[7]M.van Schilfgaarde,I.A.Abrikosov and B.Johansson,Nature422,58(2003)[8]P.Y.Brown,T.Kanomata,M.Matsumoto,K.U.Neumann and K.R.A.Ziebeck,J.Magn.Magn.Mater242-245,781(2002)[9]M.E.Gr¨u ner,R.Meyer and P.Entel,Eur.Phys.J.B2,107(1998)[10]U.Low and D.Khomskii,cond-mat/0106135[11]G.Gerhing and K.A.Gehring,Rep.Progr.Phys.38,1(1975)[12]K.I.Kugel and D.I.Khomskii,Europhysics Letters55,208(2001);Phys.Rev B67,134401(2003)。