Derivation of ecotoxicity thresholds for uranium
生态学专业英语词汇

《生态学专业英语词汇》一、生态学基础词汇1. 生态系统(Ecosystem)2. 生物多样性(Biodiversity)3. 生态位(Niche)4. 食物链(Food Chain)5. 食物网(Food Web)6. 生物量(Biomass)7. 生产者(Producer)8. 消费者(Consumer)10. 环境因子(Environmental Factor)二、生态学过程与现象1. 竞争(Competition)2. 捕食(Predation)3. 共生(Symbiosis)4. 生物入侵(Biological Invasion)5. 生物放大(Biological Magnification)6. 生态演替(Ecological Succession)7. 生物地球化学循环(Biogeochemical Cycle)8. 碳循环(Carbon Cycle)9. 氮循环(Nitrogen Cycle)10. 水循环(Water Cycle)三、生态学分支与研究方向1. 景观生态学(Landscape Ecology)2. 生态系统生态学(Ecosystem Ecology)3. 行为生态学(Behavioral Ecology)4. 进化生态学(Evolutionary Ecology)5. 环境生态学(Environmental Ecology)6. 应用生态学(Applied Ecology)7. 恢复生态学(Restoration Ecology)8. 城市生态学(Urban Ecology)9. 乡村生态学(Rural Ecology)10. 海洋生态学(Marine Ecology)四、生态学实验与技术研究1. 生态调查(Ecological Survey)2. 样方调查(Quadrat Sampling)3. 实验设计(Experimental Design)4. 遥感技术(Remote Sensing)5. 地理信息系统(GIS)6. 生态模型(Ecological Model)7. 生态模拟(Ecological Simulation)8. 环境监测(Environmental Monitoring)9. 生态修复(Ecological Restoration)10. 生物指示物(Bioindicator)五、生态学政策与管理1. 生态保护(Ecological Conservation)2. 生态红线(Ecological Red Line)3. 生态补偿(Ecological Compensation)4. 生态规划(Ecological Planning)5. 生态风险评估(Ecological Risk Assessment)6. 可持续发展(Sustainable Development)7. 生态文明建设(Ecological Civilization Construction)8. 环境影响评价(Environmental Impact Assessment)9. 生态系统服务(Ecosystem Services)10. 自然保护区(Nature Reserve)六、生态学理论与概念深化1. 生态效率(Ecological Efficiency)描述能量或物质在生态系统中从一个营养级传递到下一个营养级的效率。
可持续性资本理论
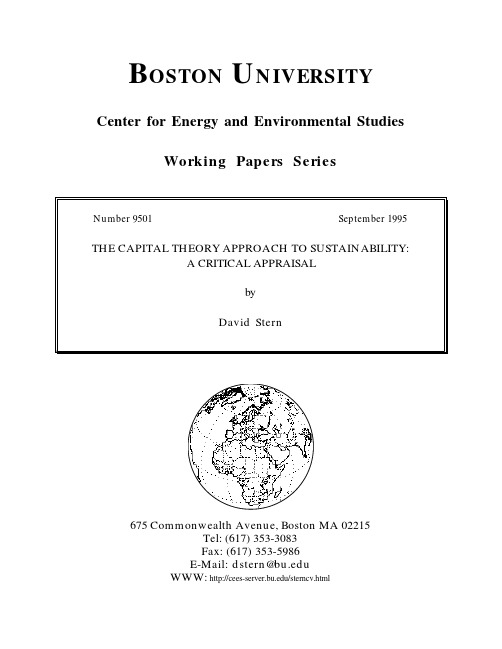
B OSTON U NIVERSITY Center for Energy and Environmental Studies Working Papers SeriesNumber 9501 September 1995 THE CAPITAL THEORY APPROACH TO SUSTAINABILITY:A CRITICAL APPRAISALbyDavid Stern675 Commonwealth Avenue, Boston MA 02215Tel: (617) 353-3083Fax: (617) 353-5986E-Mail: dstern@WWW: /sterncv.htmlThe Capital Theory Approach to Sustainability:A Critical AppraisalDavid I. SternBoston UniversityNovember 1995______________________________________________________________________________ Center for Energy and Environmental Studies, Boston University, 675 Commonwealth Avenue, Boston MA 02215, USA. Tel: (617) 353 3083 Fax: (617) 353 5986, E-Mail: dstern@The Capital Theory Approach to Sustainability:A Critical Appraisal______________________________________________________________________________ SummaryThis paper examines critically some recent developments in the sustainability debate. The large number of definitions of sustainability proposed in the 1980's have been refined into a smaller number of positions on the relevant questions in the 1990's. The most prominent of these are based on the idea of maintaining a capital stock. I call this the capital theory approach (CTA). Though these concepts are beginning to inform policies there are a number of difficulties in applying this approach in a theoretically valid manner and a number of critics of the use of the CTA as a guide to policy. First, I examine the internal difficulties with the CTA and continue to review criticisms from outside the neoclassical normative framework. The accounting approach obscures the underlying assumptions used and gives undue authoritativeness to the results. No account is taken of the uncertainty involved in sustainability analysis of any sort. In addition, by focusing on a representative consumer and using market (or contingent market) valuations of environmental resources, the approach (in common with most normative neoclassical economics) does not take into account distributional issues or accommodate alternative views on environmental values. Finally, I examine alternative approaches to sustainability analysis and policy making. These approaches accept the open-ended and multi-dimensional nature of sustainability and explicitly open up to political debate the questions that are at risk of being hidden inside the black-box of seemingly objective accounting.I.INTRODUCTIONThe Brundtland Report (WCED, 1987) proposed that sustainable development is "development that meets the needs of the present generation while letting future generations meet their own needs". Economists initially had some difficulty with this concept, some dismissing it1 and others proliferating a vast number of alternative definitions and policy prescriptions (see surveys by: Pezzey, 1989; Pearce et al., 1989; Rees, 1990; Lélé, 1991).In recent years, economists have made some progress in articulating their conception of sustainability. The large number of definitions of sustainability proposed in the 1980's have been refined into a smaller number of positions on the relevant questions in the 1990's. There is agreement that sustainability implies that certain indicators of welfare or development are non-declining over the very long term, that is development is sustained (Pezzey, 1989). Sustainable development is a process of change in an economy that does not violate such a sustainability criterion. Beyond this, the dominant views are based on the idea of maintaining a capital stock as a prerequisite for sustainable development. Within this school of thought there are opposing camps which disagree on the empirical question of the degree to which various capital stocks can be substituted for each other, though there has been little actual empirical research on this question.There is a consensus among a large number of economists that the CTA is a useful means of addressing sustainability issues.2 Capital theory concepts are beginning to inform policy, as in the case of the UN recommendations on environmental accounting and the US response to them (Beardsley, 1994; Carson et al., 1994; Steer and Lutz, 1993). There are, however, a growing number of critics who question whether this is a useful way to address sustainability (eg. Norgaard, 1991; Amir, 1992; Common and Perrings, 1992; Karshenas, 1994; Pezzey, 1994; Common and Norton, 1994; Faucheux et al., 1994; Common, 1995). The literature on sustainable development and sustainability is vast and continually expanding. There are also a large number ofsurveys of that literature (eg. Tisdell, 1988; Pearce et al., 1989; Rees, 1990; Simonis, 1990; Lélé, 1991; Costanza and Daly, 1992; Pezzey, 1992; Toman et al., 1994). I do not intend to survey this literature.The aim of this paper is to present a critique of the capital theory approach to sustainability (CTA henceforth) as a basis for policy. This critique both outlines the difficulties in using and applying the CTA from a viewpoint internal to neoclassical economics and problems with this approach from a viewpoint external to neoclassical economics. I also suggest some alternative approaches to sustainability relevant analysis and policy. The neoclasscial sustainability literature generally ignores the international dimensions of the sustainability problem. I also ignore this dimension in this paper.The paper is structured as follows. In the second section, I discuss the background to the emergence of the capital theory approach, while the third section briefly outlines the basic features of the approach. The fourth section examines the limitations of the CTA from within the viewpoint of neoclassical economics and the debate between proponents of "weak sustainability" and "strong sustainability". The following sections examine the drawbacks of this paradigm from a viewpoint external to neoclassical economics and discuss alternative methods of analysis and decision-making for sustainability. The concluding section summarizes the principal points.SHIFTING DEBATE: EMERGENCE OF THE CAPITAL THEORY II. THEAPPROACHMuch of the literature on sustainable development published in the 1980's was vague (see Lélé, 1991; Rees, 1990; Simonis, 1990). There was a general lack of precision and agreement in defining sustainability, and outlining appropriate sustainability policies. This confusion stemmed in part from an imprecise demarcation between ends and means. By "ends" I mean the definition ofsustainability ie. what is to be sustained, while "means" are the methods to achieve sustainability or necessary and/or sufficient conditions that must be met in order to do the same. As the goal of policy must be a subjective choice, considerable debate surrounded and continues to surround the definition of sustainability (eg. Tisdell, 1988). As there is considerable scientific uncertainty regarding sustainability possibilities, considerable debate continues to surround policies to achieve any given goal.Sharachchandra Lélé (1991) stated that "sustainable development is in real danger of becoming a cliché like appropriate technology - a fashionable phrase that everyone pays homage to but nobody cares to define" (607). Lélé pointed out that different authors and speakers meant very different things by sustainability, and that even UNEP's and WCED's definitions of sustainable development were vague, and confused ends with means. Neither provided any scientific examination of whether their proposed policies would lead to increased sustainability. "Where the sustainable development movement has faltered is in its inability to develop a set of concepts, criteria and policies that are coherent or consistent - both externally (with physical and social reality) and internally (with each other)." (613). Judith Rees (1990) expressed extreme skepticism concerning both sustainable development and its proponents. “It is easy to see why the notion of sustainable development has become so popular ... No longer does environmental protection mean sacrifice and confrontation with dominant materialist values” (435). She also argued that sustainable development was just so much political rhetoric. A UNEP report stated: "The ratio of words to action is weighted too heavily towards the former" (quoted in Simonis, 1990, 35). In the early days of the sustainability debate, vagueness about the meaning of sustainability was advantageous in attracting the largest constituency possible, but in the longer run, greater clarity is essential for sustaining concern.In the 1990's many people have put forward much more precisely articulated definitions of sustainable development, conditions and policies required to achieve sustainability, and criteria toassess whether development is sustainable. This has coincided with a shift from a largely politically-driven dialogue to a more theory-driven dialogue. With this has come a clearer understanding of what kinds of policies would be required to move towards alternative sustainability goals, and what the limits of our knowledge are. There is a stronger awareness of the distinction between ends and means. Most, but not all (eg. Amir, 1992), analysts agree that sustainable development is a meaningful concept but that the claims of the Brundtland Report (WCED, 1987) that growth just had to change direction were far too simplistic.There is a general consensus, especially among economists, on the principal definition of sustainable development used by David Pearce et al. (1989, 1991): Non-declining average human welfare over time (Mäler, 1991; Pezzey, 1992; Toman et al., 1994).3 This definition of sustainability implies a departure from the strict principle of maximizing net present value in traditional cost benefit analysis (Pezzey, 1989), but otherwise it does not imply a large departure from conventional economics. John Pezzey (1989, 1994) suggests a rule of maximizing net present value subject to the sustainability constraint of non-declining mean welfare. It encompasses many but not all definitions of sustainability. For example, it excludes a definition of sustainability based on maintaining a set of ecosystem functions, which seems to be implied by the Holling-sustainability criterion (Common and Perrings, 1992; Holling, 1973, 1986) or on maintaining given stocks of natural assets irrespective of any contribution to human welfare. A sustainable ecosystem might not be an undesirable goal but it could be too strict a criterion for the goal of maintaining human welfare (Karshenas, 1994) and could in some circumstances lead to declining human welfare. Not all ecosystem functions and certainly not all natural assets may be necessary for human welfare. Some aspects of the natural world such as smallpox bacteria may be absolutely detrimental to people. In the context of the primary Pearce et al. definition, the Holling-sustainability criterion is a means not an end.The advantage of formalizing the concept of sustainability is that this renders it amenable to analysis by economic theory (eg. Barbier and Markandya, 1991; Victor, 1991; Common and Perrings, 1992; Pezzey, 1989, 1994; Asheim, 1994) and to quantitative investigations (eg. Repetto et al., 1989; Pearce and Atkinson, 1993; Proops and Atkinson, 1993; Stern, 1995). Given the above formal definition of sustainability, many economists have examined what the necessary or sufficient conditions for the achievement of sustainability might be. Out of this activity has come the CTA described in the next section. The great attractiveness of this new approach is that it suggests relatively simple rules to ensure sustainability and relatively simple indicators of sustainability. This situation has seemingly cleared away the vagueness that previously attended discussions of sustainability and prompted relatively fast action by governments and international organizations to embrace specific goals and programs aimed at achieving this notion of the necessary conditions for sustainability.III. THE ESSENCE OF THE CAPITAL THEORY APPROACHThe origins of the CTA are in the literature on economic growth and exhaustible resources that flourished in the 1970s, exemplified by the special issue of the Review of Economic Studies published in 1974 (Heal, 1974). Robert Solow (1986) built on this earlier literature and the work of John Hartwick (1977, 1978a, 1978b) to formalize the constant capital rule. In these early models there was a single non-renewable resource and a stock of manufactured capital goods. A production function produced a single output, which could be used for either consumption or investment using the two inputs. The elasticity of substitution between the two inputs was one which implied that natural resources were essential but that the average product of resources could rise without bound given sufficient manufactured capital.The models relate to the notion of sustainability as non-declining welfare through the assumption that welfare is a monotonically increasing function of consumption (eg. Mäler, 1991). The path ofconsumption over time (and therefore of the capital stock) in these model economies depends on the intertemporal optimization rule. Under the Rawlsian maxi-min condition consumption must be constant. No net saving is permissible as this is regarded as an unjust burden on the present generation. Under the Ramsey utilitarian approach with zero discounting consumption can increase without bound (Solow, 1974). Here the present generation may be forced to accept a subsistence standard of living if this can benefit the future generations however richer they might be. Paths that maximize net present value with positive discount rates typically peak and then decline so that they are not sustainable (Pezzey, 1994). Pezzey (1989) suggested a hybrid version which maximizes net present value subject to an intertemporal constraint that utility be non-declining. In this case utility will first increase until it reaches a maximum sustainable level. This has attracted consensus as the general optimizing criterion for sustainable development. Geir Asheim (1991) derives this condition more formally.Under the assumption that the elasticity of substitution is one, non-declining consumption depends on the maintenance of the aggregate capital stock ie. conventional capital plus natural resources, used to produce consumption (and investment) goods (Solow, 1986). Aggregate capital, W t,and the change in aggregate capital are defined by:W t=p Kt K t + p Rt S t (1)∆W t=p Kt∆K t + p Rt R t (2)where S is the stock of non-renewable resources and R the use per period. K is the manufactured capital stock and the p i are the relevant prices. In the absence of depreciation of manufactured capital, maintenance of the capital stock implies investment of the rents from the depletion of the natural resource in manufactured capital - the Hartwick rule (Hartwick 1977, 1978a, 1978b). Income is defined using the Hicksian notion (Hicks, 1946) that income is the maximum consumption in a period consistent with the maintenance of wealth. Sustainable income is,therefore, the maximum consumption in a period consistent with the maintenance of aggregate capital intact (Weitzman, 1976; Mäler, 1991) and for a flow of income to be sustainable, the stock of capital needs to be constant or increasing over time (Solow, 1986).The initial work can be extended in various ways. The definition of capital that satisfies these conditions can be extended to include a number of categories of "capital": natural, manufactured, human, and institutional.4 Natural capital is a term used by many authors (it seems Smith (1977) was the first) for the aggregate of natural resource stocks that produce inputs of services or commodities for the economy. Some of the components of natural capital may be renewable resources. Manufactured capital refers to the standard neoclassical definition of "a factor of production produced by the economic system" (Pearce, 1992). Human capital also follows the standard definition. Institutional capital includes the institutions and knowledge necessary for the organization and reproduction of the economic system. It includes the ethical or moral capital referred to by Fred Hirsch (1976) and the cultural capital referred to by Fikret Berkes and Carl Folke (1992). For convenience I give the name 'artificial capital' to the latter three categories jointly. None of these concepts is unproblematic and natural capital is perhaps the most problematic. Technical change and population growth can also be accommodated (see Solow, 1986).Empirical implementation of the CTA tends to focus on measurement of sustainable income (eg. El Serafy, 1989; Repetto, 1989) or net capital accumulation (eg. Pearce and Atkinson, 1993; Proops and Atkinson, 1993) rather than on direct estimation of the capital stock.5 The theoretical models that underpin the CTA typically assume a Cobb-Douglas production function with constant returns to scale, no population growth, and no technological change. Any indices of net capital accumulation which attempt to make even a first approximation to reality must take these variables into account. None of the recent empirical studies does so. For example, David Pearce and Giles Atkinson (1993) present data from eighteen countries on savings and depreciation of natural andmanufactured capital as a proportion of GNP. They demonstrate that only eight countries had non-declining stocks of total capital, measured at market prices, and thus passed a weak sustainability criterion of a constant aggregate capital stock, but their methodology ignores population growth, returns to scale or technological change.IV.INTERNAL APPRAISAL OF THE CAPITAL THEORY APPROACHIn this section, I take as given the basic assumptions and rationale of neoclassical economics and highlight some of the technical problems that are encountered in using the CTA as an operational guide to policy. From a neoclassical standpoint these might be seen as difficulties in the positive theory that may lead to difficulties in the normative theory of sustainability policy. In the following section, I take as given solutions to these technical difficulties and examine some of the problems inherent in the normative neoclassical approach to sustainability.a.Limits to Substitution in Production and "Strong Sustainability"Capital theorists are divided among proponents of weak sustainability and strong sustainability. This terminology is confusing as it suggests that the various writers have differing ideas of what sustainability is.6 In fact they agree on that issue, but differ on what is the minimum set of necessary conditions for achieving sustainability. The criterion that distinguishes the categories is the degree of substitutability believed to be possible between natural and artificial capital.7The weak sustainability viewpoint follows from the early literature and holds that the relevant capital stock is an aggregate stock of artificial and natural capital. Weak sustainability assumes that the elasticity of substitution between natural capital and artificial capital is one and therefore that there are no natural resources that contribute to human welfare that cannot be asymptotically replaced by other forms of capital. Reductions in natural capital may be offset by increases inartificial capital. It is sometimes implied that this might be not only a necessary condition but also a sufficient condition for achieving sustainability (eg. Solow, 1986, 1993).Proponents of the strong sustainability viewpoint such as Robert Costanza and Herman Daly (1992) argue that though this is a necessary condition for sustainability it cannot possibly be a sufficient condition. Instead, a minimum necessary condition is that separate stocks of aggregate natural capital and aggregate artificial capital must be maintained. Costanza and Daly (1992) state: "It is important for operational purposes to define sustainable development in terms of constant or nondeclining total natural capital, rather than in terms of nondeclining utility" (39).8 Other analysts such as members of the "London School" hold views between these two extremes (see Victor, 1991). They argue that though it is possible to substitute between natural and artificial capital there are certain stocks of "critical natural capital" for which no substitutes exist. A necessary condition for sustainability is that these individual stocks must be maintained in addition to the general aggregate capital stock.The weak sustainability condition violates the Second Law of Thermodynamics, as a minimum quantity of energy is required to transform matter into economically useful products (Hall et al., 1986) and energy cannot be produced inside the economic system.9 It also violates the First Law on the grounds of mass balance (Pezzey, 1994). Also ecological principles concerning the importance of diversity in system resilience (Common and Perrings, 1992) imply that minimum quantities of a large number of different capital stocks (eg. species) are required to maintain life support services. The London School view and strong sustainability accommodate these facts by assuming that there are lower bounds on the stocks of natural capital required to support the economy, in terms of the supply of materials and energy, and in terms of the assimilative capacity of the environment, and that certain categories of critical natural capital cannot be replaced by other forms of capital.Beyond this recognition it is an empirical question as to how far artificial capital can substitute for natural capital. There has been little work on this at scales relevant to sustainability. However, the econometric evidence from studies of manufacturing industry suggest on the whole that energy and capital are complements (Berndt and Wood, 1979).In some ways the concept of maintaining a constant stock of aggregate natural capital is even more bizarre than maintaining a non-declining stock of total capital. It seems more reasonable to suggest that artificial capital might replace some of the functions of natural capital than to suggest that in general various natural resources may be substitutes for each other. How can oil reserves substitute for clean air, or iron deposits for topsoil? Recognizing this, some of the strong sustainability proponents have dropped the idea of maintaining an aggregate natural capital stock as proposed by Costanza and Daly (1992) and instead argue that minimum stocks of all natural resources should be maintained (Faucheux and O'Connor, 1995). However, this can no longer really be considered an example of the CTA. Instead it is an approach that depends on the concept of safe minimum standards or the precautionary principle. The essence of the CTA is that some aggregation of resources using monetary valuations is proposed as an indicator for sustainability.The types of models which admit an index of aggregate capital, whether aggregate natural capital or aggregate total capital, is very limited. Construction of aggregate indices or subindices of inputs depend on the production function being weakly separable in those subgroups (Berndt and Christensen, 1973). For example it is only possible to construct an index of aggregate natural capital if the marginal rate of substitution between two forms of natural capital is independent of the quantities of labor or capital employed. This seems an unlikely proposition as the exploitation of many natural resources is impractical without large capital stocks. For example, in the production of caught fish, the marginal rate of substitution, and under perfect competition the price ratio, between stocks of fresh water fish and marine fish should be independent of the number of fishingboats available. This is clearly not the case. People are not likely to put a high value on the stock of deep sea fish when they do not have boats to catch them with.If substitution is limited, technological progress might reduce the quantity of natural resource inputs required per unit of output. However, there are arguments that indicate that technical progress itself is bounded (see Pezzey, 1994; Stern, 1994). One of these (Pezzey, 1994) is that, just as in the case of substitution, ultimately the laws of thermodynamics limit the minimization of resource inputs per unit output. Stern (1994) argues that unknown useful knowledge is itself a nonrenewable resource. Technological progress is the extraction of this knowledge from the environment and the investment of resources in this activity will eventually be subject to diminishing returns.Limits to substitution in production might be thought of in a much broader way to include nonlinearities and threshold effects. This view is sometimes described as the "ecological" viewpoint on sustainability (Common and Perrings, 1992; Common, 1995) or as the importance of maintaining the "resilience" of ecological systems rather than any specific stocks or species. This approach derives largely from the work of Holling (1973, 1986). In this view ecosystems are locally stable in the presence of small shocks or perturbations but may be irreversibly altered by large shocks. Structural changes in ecosystems such as those that come about through human interference and particularly simplification, may make these systems more susceptible to losing resilience and being permanently degraded. There is clearly some substitutability between species or inorganic elements in the role of maintaining ecosystem productivity, however, beyond a certain point this substitutability may suddenly fail to hold true. This approach also asks us to look at development paths as much less linear and predictable than is implied in the CTA literature.All things considered, what emerges is a quite different approach to sustainability policy. It is probable that substitution between natural and artificial capital is limited, as is ultimately technicalchange. Additionally the joint economy-ecosystem system may be subject to nonlinear dynamics. This implies that eventually the economy must approach a steady state where the volume of physical economic activity is dependent on the maximum economic and sustainable yield of renewable resources or face decline ie. profit (or utility) maximizing use of renewable resources subject to the sustainability constraint. As in Herman Daly's vision (Daly, 1977) qualitative change in the nature of economic output is still possible. Sustainability policy would require not just maintaining some stocks of renewable resources but also working to reduce "threats to sustainability" (Common, 1995) that might cause the system to pass over a threshold and reduce long-run productivity.The notion of Hicksian income originally applied to an individual price-taking firm (Faucheux and O'Connor, 1995). However, even here it is not apparent that the myopic policy of maintaining capital intact from year to year is the best or only way to ensure the sustainability of profits into the future. If a competing firm makes an innovation that renders the firm's capital stock obsolete, the latter's income may drop to zero. This is despite it previously following a policy of maintaining its capital intact. The firm's income measured up to this point is clearly seen to be unsustainable. In fact its policy has been shown to be irrelevant to long-run sustainability. In the real world firms will carry out activities that may not contribute to the year to year maintenance of capital and will reduce short-run profits such as research and development and attempts to gain market share.10 These activities make the firm more resilient against future shocks and hence enhance sustainability.b.Prices for AggregationSupposing that the necessary separability conditions are met so that aggregation of a capital stock is possible, analysts still have to obtain an appropriate set of prices so that the value of the capital stock is a sustainability relevant value. The CTA is more or less tautological if we use the "right" prices. However, these correct "sustainability prices" are unknown and unknowable. A number of。
关于森林的英语作文
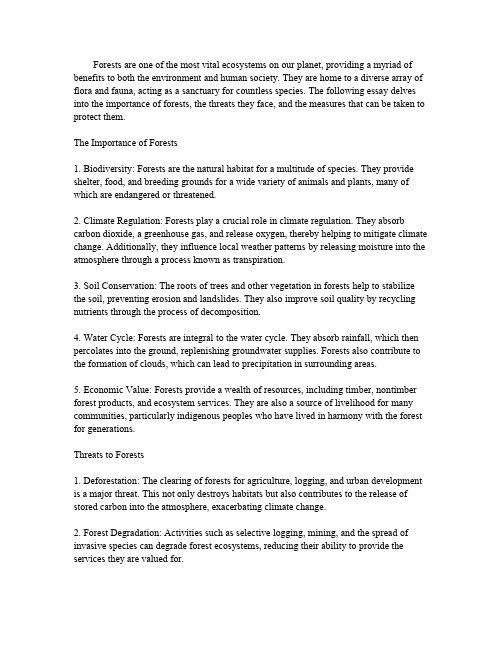
Forests are one of the most vital ecosystems on our planet,providing a myriad of benefits to both the environment and human society.They are home to a diverse array of flora and fauna,acting as a sanctuary for countless species.The following essay delves into the importance of forests,the threats they face,and the measures that can be taken to protect them.The Importance of Forests1.Biodiversity:Forests are the natural habitat for a multitude of species.They provide shelter,food,and breeding grounds for a wide variety of animals and plants,many of which are endangered or threatened.2.Climate Regulation:Forests play a crucial role in climate regulation.They absorb carbon dioxide,a greenhouse gas,and release oxygen,thereby helping to mitigate climate change.Additionally,they influence local weather patterns by releasing moisture into the atmosphere through a process known as transpiration.3.Soil Conservation:The roots of trees and other vegetation in forests help to stabilize the soil,preventing erosion and landslides.They also improve soil quality by recycling nutrients through the process of decomposition.4.Water Cycle:Forests are integral to the water cycle.They absorb rainfall,which then percolates into the ground,replenishing groundwater supplies.Forests also contribute to the formation of clouds,which can lead to precipitation in surrounding areas.5.Economic Value:Forests provide a wealth of resources,including timber,nontimber forest products,and ecosystem services.They are also a source of livelihood for many communities,particularly indigenous peoples who have lived in harmony with the forest for generations.Threats to Forests1.Deforestation:The clearing of forests for agriculture,logging,and urban development is a major threat.This not only destroys habitats but also contributes to the release of stored carbon into the atmosphere,exacerbating climate change.2.Forest Degradation:Activities such as selective logging,mining,and the spread of invasive species can degrade forest ecosystems,reducing their ability to provide the services they are valued for.3.Climate Change:Rising temperatures and changing precipitation patterns can lead to shifts in forest composition and the spread of pests and diseases,threatening the health and resilience of forests.4.Illegal Logging:Illegal logging undermines sustainable forest management and contributes to the loss of biodiversity and forest cover.Conservation Efforts1.Protected Areas:Establishing and effectively managing protected areas is a key strategy for conserving forests.These areas can serve as refuges for biodiversity and help maintain ecological processes.2.Sustainable Forest Management:Implementing sustainable practices in logging, agriculture,and other forest uses can help maintain the health and productivity of forests.munity Involvement:Engaging local communities in forest management can ensure that their needs and knowledge are taken into account,leading to more sustainable and equitable outcomes.cation and Awareness:Raising public awareness about the value of forests and the threats they face is essential for garnering support for conservation efforts.5.International Cooperation:Forests are a global resource,and their conservation requires international cooperation to address issues such as illegal logging and the global trade in forest products.In conclusion,forests are a treasure trove of ecological and economic value,and their protection is essential for the health of our planet and the wellbeing of future generations. It is incumbent upon all of us to recognize the importance of forests and to take action to ensure their conservation for the benefit of all life on Earth.。
2019年成考《生态学基础》知识点精解(2)

2019年成考《生态学基础》知识点精解(2)第二章生物与环境本章重点一、名词解释1.生态环境2.生境4.限制因子5.趋同适合和趋异适合3.生态幅(ecologicalamplitute):生物对每一种生态因子都有其耐受的上限和下限,上下限之间是生物对这种生态因子的耐受范围,称为生态幅或生态价。
6.生活型和生态型:(1)趋同适合的生物,具有类似的形态、生理和生态特性的物种类群称为生活型。
(2)趋异适合的生物,分化形成的形态、生理和生态特性不同的基因型类群称为生态型。
二、问答题1.简述谢尔福德耐性定律。
2.简述利比希最小因子定律及其补充。
9、当光强度不足时,C02浓度的适当提升,则使植物光合作用强度不致于降低,这种作用称为(C)A.综合作用B.阶段性作用C.补偿作用D.不可替代作用10、在诸多生态因子中,(BD)因子称为主导因子。
A.能替代少数其它因子B.对植物生长有明显影响C.把其它因子的直接作用变为间接作用D.对其他因子有影响作用的因子11、地形因子对生物的作用属于(B)A.直接作用B.间接作用C.替代作用D.补偿作用12、试述生态因子的补偿性和不可替代性当某个生态因子在数量上不足时,能够由其它因子来补偿,结果仍可获得相似的生态效应。
例如光强减弱所引起的光合作用下降能够依靠CO2浓度的增加得到补偿,这也是森林林冠下幼苗能够存活生长的一个因素。
但是,这种补偿作用不是没有限度的,它只能在一定的范围内作部分的补充,生态因子虽非等价,但都不可缺少,一个因子的缺失不能由另一个因子来替代。
13、生物与环境的相互作用包括(作用)(适合)和(反作用)。
14、简述李比希最小因子定律植物的生长取决于那些处于最少量状态的营养元素。
两点补充:(1)Liebig定律只能严格地适用于稳定状态。
(2)是要考虑因子间的替代作用。
15、对于某种作物,当土壤中的氮可维持250kg产量,钾可维持350kg产量,磷可维持500kg产量,则实际产量一般会在(A)A.250kg左右B.350kg左右C.500kg左右D.大于500kg16、用图形表示耐性定律,并简要说明各区域生物的特点。
2023年高中生物竞赛辅导资料:生态学第三版名词解释
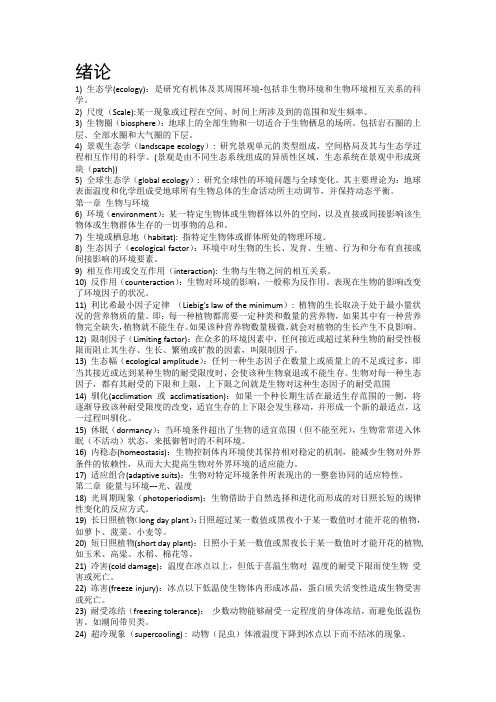
绪论1) 生态学(ecology):是研究有机体及其周围环境-包括非生物环境和生物环境相互关系的科学。
2) 尺度(Scale):某一现象或过程在空间、时间上所涉及到的范围和发生频率。
3) 生物圈(biosphere):地球上的全部生物和一切适合于生物栖息的场所。
包括岩石圈的上层、全部水圈和大气圈的下层。
4) 景观生态学(landscape ecology): 研究景观单元的类型组成,空间格局及其与生态学过程相互作用的科学。
(景观是由不同生态系统组成的异质性区域,生态系统在景观中形成斑块(patch))5) 全球生态学(global ecology): 研究全球性的环境问题与全球变化。
其主要理论为:地球表面温度和化学组成受地球所有生物总体的生命活动所主动调节,并保持动态平衡。
第一章生物与环境6) 环境(environment):某一特定生物体或生物群体以外的空间,以及直接或间接影响该生物体或生物群体生存的一切事物的总和。
7) 生境或栖息地(habitat): 指特定生物体或群体所处的物理环境。
8) 生态因子(ecological factor):环境中对生物的生长、发育、生殖、行为和分布有直接或间接影响的环境要素。
9) 相互作用或交互作用(interaction): 生物与生物之间的相互关系。
10) 反作用(counteraction):生物对环境的影响,一般称为反作用。
表现在生物的影响改变了环境因子的状况。
11) 利比希最小因子定律(Liebig’s law of the minimum): 植物的生长取决于处于最小量状况的营养物质的量。
即:每一种植物都需要一定种类和数量的营养物,如果其中有一种营养物完全缺失,植物就不能生存。
如果该种营养物数量极微,就会对植物的生长产生不良影响。
12) 限制因子(Limiting factor):在众多的环境因素中,任何接近或超过某种生物的耐受性极限而阻止其生存、生长、繁殖或扩散的因素,叫限制因子。
生态学名词解释30580
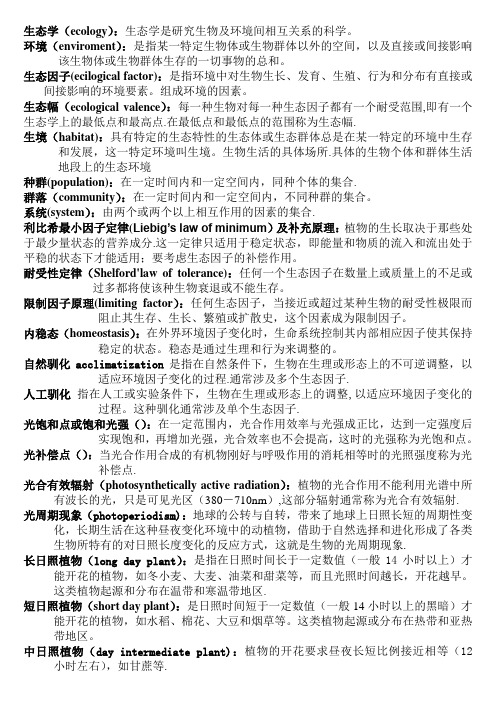
生态学(ecology):生态学是研究生物及环境间相互关系的科学。
环境(enviroment):是指某一特定生物体或生物群体以外的空间,以及直接或间接影响该生物体或生物群体生存的一切事物的总和。
生态因子(ecilogical factor):是指环境中对生物生长、发育、生殖、行为和分布有直接或间接影响的环境要素。
组成环境的因素。
生态幅(ecological valence):每一种生物对每一种生态因子都有一个耐受范围,即有一个生态学上的最低点和最高点.在最低点和最低点的范围称为生态幅.生境(habitat):具有特定的生态特性的生态体或生态群体总是在某一特定的环境中生存和发展,这一特定环境叫生境。
生物生活的具体场所.具体的生物个体和群体生活地段上的生态环境种群(population):在一定时间内和一定空间内,同种个体的集合.群落(community):在一定时间内和一定空间内,不同种群的集合。
系统(system):由两个或两个以上相互作用的因素的集合.利比希最小因子定律(Liebig’s law of minimum)及补充原理:植物的生长取决于那些处于最少量状态的营养成分.这一定律只适用于稳定状态,即能量和物质的流入和流出处于平稳的状态下才能适用;要考虑生态因子的补偿作用。
耐受性定律(Shelford'law of tolerance):任何一个生态因子在数量上或质量上的不足或过多都将使该种生物衰退或不能生存。
限制因子原理(limiting factor):任何生态因子,当接近或超过某种生物的耐受性极限而阻止其生存、生长、繁殖或扩散史,这个因素成为限制因子。
内稳态(homeostasis):在外界环境因子变化时,生命系统控制其内部相应因子使其保持稳定的状态。
稳态是通过生理和行为来调整的。
自然驯化acclimatization是指在自然条件下,生物在生理或形态上的不可逆调整,以适应环境因子变化的过程.通常涉及多个生态因子.人工驯化指在人工或实验条件下,生物在生理或形态上的调整,以适应环境因子变化的过程。
石油对人类的害处英语作文
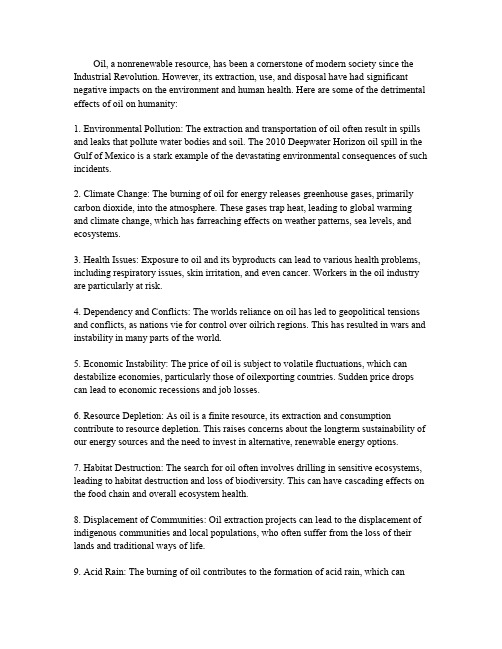
Oil, a nonrenewable resource, has been a cornerstone of modern society since the Industrial Revolution. However, its extraction, use, and disposal have had significant negative impacts on the environment and human health. Here are some of the detrimental effects of oil on humanity:1. Environmental Pollution: The extraction and transportation of oil often result in spills and leaks that pollute water bodies and soil. The 2010 Deepwater Horizon oil spill in the Gulf of Mexico is a stark example of the devastating environmental consequences of such incidents.2. Climate Change: The burning of oil for energy releases greenhouse gases, primarily carbon dioxide, into the atmosphere. These gases trap heat, leading to global warming and climate change, which has farreaching effects on weather patterns, sea levels, and ecosystems.3. Health Issues: Exposure to oil and its byproducts can lead to various health problems, including respiratory issues, skin irritation, and even cancer. Workers in the oil industry are particularly at risk.4. Dependency and Conflicts: The worlds reliance on oil has led to geopolitical tensions and conflicts, as nations vie for control over oilrich regions. This has resulted in wars and instability in many parts of the world.5. Economic Instability: The price of oil is subject to volatile fluctuations, which can destabilize economies, particularly those of oilexporting countries. Sudden price drops can lead to economic recessions and job losses.6. Resource Depletion: As oil is a finite resource, its extraction and consumption contribute to resource depletion. This raises concerns about the longterm sustainability of our energy sources and the need to invest in alternative, renewable energy options.7. Habitat Destruction: The search for oil often involves drilling in sensitive ecosystems, leading to habitat destruction and loss of biodiversity. This can have cascading effects on the food chain and overall ecosystem health.8. Displacement of Communities: Oil extraction projects can lead to the displacement of indigenous communities and local populations, who often suffer from the loss of their lands and traditional ways of life.9. Acid Rain: The burning of oil contributes to the formation of acid rain, which candamage forests, freshwater bodies, and even buildings and infrastructure.10. Ocean Acidification: The increased levels of carbon dioxide in the atmosphere also lead to ocean acidification, which threatens marine life and the balance of ocean ecosystems.In conclusion, while oil has been instrumental in driving industrialization and modernization, its harmful effects on the environment and human health cannot be ignored. It is crucial for societies to transition towards cleaner, more sustainable energy sources to mitigate these negative impacts and ensure a healthier future for all.。
Four-Laws-of-Ecology中英对照
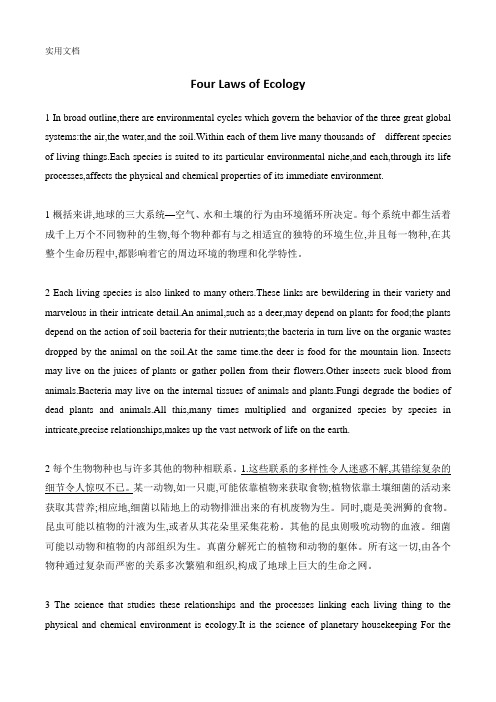
Four Laws of Ecology1 In broad outline,there are environmental cycles which govern the behavior of the three great global systems:the air,the water,and the soil.Within each of them live many thousands of different species of living things.Each species is suited to its particular environmental niche,and each,through its life processes,affects the physical and chemical properties of its immediate environment.1概括来讲,地球的三大系统—空气、水和土壤的行为由环境循环所决定。
每个系统中都生活着成千上万个不同物种的生物,每个物种都有与之相适宜的独特的环境生位,并且每一物种,在其整个生命历程中,都影响着它的周边环境的物理和化学特性。
2 Each living species is also linked to many others.These links are bewildering in their variety and marvelous in their intricate detail.An animal,such as a deer,may depend on plants for food;the plants depend on the action of soil bacteria for their nutrients;the bacteria in turn live on the organic wastes dropped by the animal on the soil.At the same time.the deer is food for the mountain lion. Insects may live on the juices of plants or gather pollen from their flowers.Other insects suck blood from animals.Bacteria may live on the internal tissues of animals and plants.Fungi degrade the bodies of dead plants and animals.All this,many times multiplied and organized species by species in intricate,precise relationships,makes up the vast network of life on the earth.2每个生物物种也与许多其他的物种相联系。
生态学专业术语英汉对照2
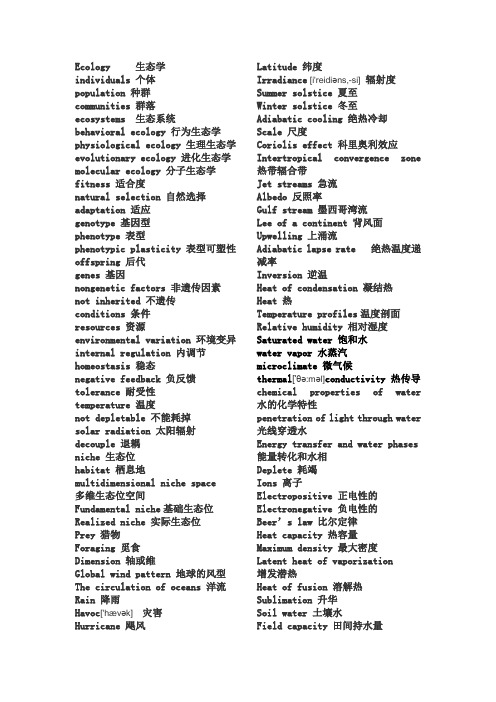
Ecology 生态学individuals 个体population 种群communities 群落ecosystems 生态系统behavioral ecology 行为生态学physiological ecology 生理生态学evolutionary ecology 进化生态学molecular ecology 分子生态学fitness 适合度natural selection 自然选择adaptation 适应genotype 基因型phenotype 表型phenotypic plasticity 表型可塑性offspring 后代genes 基因nongenetic factors 非遗传因素not inherited 不遗传conditions 条件resources 资源environmental variation 环境变异internal regulation 内调节homeostasis 稳态negative feedback 负反馈tolerance 耐受性temperature 温度not depletable 不能耗掉solar radiation 太阳辐射decouple 退耦niche 生态位habitat 栖息地multidimensional niche space 多维生态位空间Fundamental niche基础生态位Realized niche 实际生态位Prey 猎物Foraging 觅食Dimension 轴或维Global wind pattern 地球的风型The circulation of oceans 洋流Rain 降雨Havoc['hævək] 灾害Hurricane 飓风Latitude 纬度Irradiance [i'reidiəns,-si]辐射度Summer solstice 夏至Winter solstice 冬至Adiabatic cooling 绝热冷却Scale 尺度Coriolis effect 科里奥利效应Intertropical convergence zone热带辐合带Jet streams 急流Albedo 反照率Gulf stream 墨西哥湾流Lee of a continent 背风面Upwelling 上涌流Adiabatic lapse rate 绝热温度递减率Inversion 逆温Heat of condensation 凝结热Heat 热Temperature profiles温度剖面Relative humidity 相对湿度Saturated water 饱和水water vapor 水蒸汽microclimate 微气候thermal['θə:məl]conductivity 热传导chemical properties of water 水的化学特性penetration of light through water光线穿透水Energy transfer and water phases能量转化和水相Deplete 耗竭Ions 离子Electropositive 正电性的Electronegative 负电性的Beer’s law 比尔定律Heat capacity 热容量Maximum density 最大密度Latent heat of vaporization增发潜热Heat of fusion 溶解热Sublimation 升华Soil water 土壤水Field capacity 田间持水量The uptake of water by roots根对水的吸收Aquatic plants 水生植物Water availability 水的可利用性Plant productivity 植物生产力Permanent wilting point 永久萎焉点Potential evapotranspiration rate 潜在蒸发蒸腾速率Capillary pores 毛细管孔隙Resource depletion zone 资源枯竭区Halophytes 盐生植物Water balance in fish 鱼类的水平衡Amphibians 两栖类Water conservation by terrestrial animals 陆生动物的水保持Mammalian 哺乳动物Kidneys 肾脏Bladder 膀胱Beavers 河狸Osmoregulation 渗透调节Countercurrent exchange 逆流调节Hypertonic 高渗的Homeotherms 恒温动物Poikilotherms 变温动物Ectotherms 外温动物Endotherms 内温动物Temperature thresholds 温度阀Mechanisms 机理Enzyme 酶The thermoneutral zone 热中性区Dehydration 脱水Rates of development and growth发育和生长速度Acclimation and acclimatization 驯化和气候驯化Developmental threshold Temperature 发育温度阀Physiological time 生理时间Vernalization 春化Species distribution 物种分布Evolved response 进化反应Mean temperature 平均温度Isotherm 等温线Radiant energy 辐射能Photosynthesis 光合作用Efficiency of radiant energy conversion 辐射能的转换效率Changes in the intensity of radiation 辐射强度的变化Strategic and tactical response of plants to radiation 植物对辐射的战略和战术响应Compensation point 补偿点Photosynthetically active radiation (PAR)光和活性辐射Efficiency of Photosynthesis 光合作用效率Photosynthetic capacity 光合能力Diurnal and annual rhythms of solar radiation 太阳辐射日节律和年节律Resource depletion zone 资源耗竭带Strategic difference 战略差异Tactical response 战术响应Transpiration 蒸腾Net assimilation 净同化量Nutrient sources 营养物资源Nutrient budgets 营养预算Terrestrial communities 陆地群落Aquatic communities 水生群落Geochemistry 地球化学Global biogeochemical cycles 全球生物地球循环Mechanical weathering 机械风化Chemical weathering 化学腐蚀Wetfall 湿降落Dryfall 干降落Rainout component 雨水冲失成分Washout component 水冲失成分Streamflow 溪流Denitrification 脱氮Endorheic内陆湖泊Biogeochemistry 生物地球化学Hydrosphere carbon 水圈的碳Weathering 风化作用Nitrogen cycle 氮循环Phosphorus 氮Sediment 沉积型Lithospheric 岩石圈Sulfur 硫The fate of matter in the community 群落中物质的命运Producers 生产者Consumers 消费者Decomposers 分解者Autotrophs 自养生物Grazing mammals 草食哺乳动物Phytoplankton 浮游植物Zooplankton 浮游动物Bacteria 细菌Fungi 真菌Nonliving 无生命Food chains 食物链Primary and secondary production 初级和次级生产力Net Primary production 净初级生产力Aphotic zone 无光区Photic zone 透光区Primary consumers 初级消费者Secondary consumer 次级消费者Soil formation 土壤形成The soil profile 土壤剖面Primary classification:the great soil groups 主要分类:大土壤群Higher vegetation 高等植物Dynamic mixture 动态混合物Organic matter 有机质Cells 细胞Pedology 土壤学Subsoil 亚土壤Mineral soil 矿物质土壤Parent material 母质Soil series 土系Soil surveyor 土壤勘测员Succession 演替Ecosystem patterns 生态系统格局Soil horizons 土层Humic acids 腐植酸Great soil groups 土壤群Population size 种群大小Age and stage structure 年龄和时期结构Zygote 受精卵Unitary organism 单体生物Modular organism 构件生物Ramets 无性系分株Clone 无性系Genet 基株Evolutionary individuals 进化个体Immediate ecological impact 直接生态作用Stable age distribution 稳定年龄分布Age pyramid 年龄金字塔Stationary age distribution 固定的年龄分布Stage structure 时期结构Sizes classes 个体大小群Natality 出生率Mortality 死亡率Survivorship 存活率Life tables 生命表K-factor analysis k-因子分析The fecundity schedule 生殖力表Population growth 种群增长Density-independent Population growth 非密度制约性种群增长Density-dependent growth-the logistic equation 密度制约性种群增长:逻辑斯缔方程Life expectancy 生命期望Survivorship curve 存活曲线Cohort 同生群Age-specific survival rate 特定年龄存活率Key factors 关键因子Killing factor 致死因子Basic reproduction rate 基础繁殖率Carrying capacity 环境容纳量Estimating density 估计密度Mark release recapture 标记重捕法Density dependence密度制约Equilibrium population density平衡种群密度Relative density相对密度Allee effect阿利效应Exactly compensating准确补偿Undercompensating补偿不足Overcompensating过度补偿H4Population fluctuations 种群波动Chaos 混沌Expanding and contracting populations 增长种群和收缩种群Stable limit cycle 稳定极限环I1Competition 竞争Predation 捕食Parasitism 寄生Mutualism互利共生Intraspecific competition种内竞争Interspecific competition种间竞争Exploitation competition利用性竞争Interference competition干扰性竞争Cannibalism 自相残杀Altruism 利他主义Commensualism 偏利共生Amensualism偏害共生I2Dispersal扩散Territoriality领域性Niche shift生态位转移Allelopathy异株克生Competive asymmetry 竞争不对称Scramble competition争夺竞争Contest competition格斗竞争Zero net growth isocline零增长等斜线Self-thinning自疏Inbreeding近亲繁殖Reproductive value繁殖价值Leks 求偶场Competitive exclusion 竞争排斥Limiting similarity 极限相似性Competitive release 极限释放Character displacement 性状替换Apparent competition 表观竞争Enemy-free space 无敌空间Highly heterogeneous 高度异质性Gaps 断层Probability refuge 隐蔽机率J1Herbivores 食草动物Carnivores 食肉动物Omnivores 杂食动物Chemical defences 化学防御Behavioral strategies 行为对策Specialists 特化种Generalist 泛化种Monophagous单食者Oligophagous寡食者Polyphagous 多食者Parasites 寄生者J2Predator switching 捕食者转换Profitability of prey 猎物收益率Plant defence 植物防御The ideal free distribution 理想自由分布Functional response 功能反应Superpredation 超捕食K1Parasites 寄生物Modes of transmission 传播方式Social parasites 社会性寄生物Helminth worms 寄生蠕虫Insects 昆虫Necrotrophs 食尸动物Parasitoids 拟寄生物The cellular immune response 细胞免疫反应Vectors 媒介Optimal habitat use 最佳生境利用Brood parasitism 窝寄生Evolutionary constraint 进化约束Immunity 免疫Cevolution协同进化Gene for gene 基因对基因Mimics 模仿Herd immunity 群体免疫Antigenic stability 抗原稳定L1Pollination 传粉Symbiotic 共生性Obligate 专性Lichens 地衣Outcrossing 异型杂交Mitochondria 线粒体Chloroplasts 叶绿体M1Reproductive values 生殖价Hypothetical organism 假定生物Migration 迁移Senescence衰老Diapause 滞育Dormancy 休眠Longevity 寿命Enormous variation 巨大变异Energy allocation 能量分配Semelparity 单次生殖Iteroparity 多次生殖Carrying capacity 容纳量Current/future reproduction当前/未来繁殖Habitat disturbance 环境干扰The current/future reproductive output 当前/未来繁殖输出A high/low cost of reproduction 高/低繁殖付出Seed bank 种子库Torper蛰伏Hibernation 冬眠Cryptobiosis 隐生现象Aestivation 夏眠Migration 迁徙Morphological forms 形态学性状Generations世代Mechanistic level 机制水平Cooperation 合作Grouping-benefits 集群-好处Altruism 利他行为Group defens e 群防御Inclusive fitness 广义适合度Eusociality 真社会性Hymenoptera 膜翅目Haplodiploid 单倍二倍体Venomous sting毒刺N2Sex 性The costs of inbreeding 近交的代价Self-fertilization 自体受精Sexual versus asexual reproduction 有性和无性生殖Sex ratio 交配体制Monogyny 单配制Polygyny 一雄多雌制Polyandry 一雌多雄制Inbreeding depression 近交衰退Hermaphrodite 雌雄同体Recombine 重组Rare type advantage 稀少型有利Equal investment 相等投入Local mate competition局域交配竞争Epigamic 诱惑性Intrasexual selection 性内选择Intersexual selection 性间选择O1Alleles 等位基因Polymorphism 多型Genetic drift 遗传漂变Genetic bottleneck 遗传瓶颈Rare species 稀有物种Extinction 灭绝Chromosome染色体Genotype 遗传型Phenotype 表现型Gene pool 基因库Gel electrophoresis 凝胶电泳O2Gene flow 基因流Differentiation 分化Sibling species 姊妹种Genetic revolution 遗传演变Peripheral isolates 边缘隔离PTransfer efficiencies 转换效率(net)primary productivity (净)初级生产力Respiratory heat 呼吸热Grazer system 牧食者系统Food chains 食物链Pathways of nutrient flow营养物流Food webs 食物网QCommunity structure 群落结构Community boundaries 群落边界Guilds同资源种团Community organization 群落组织Species diversity 物种多样性Energy flow 能量流Superorganism 超有机体Species-poor/rich 物种贫乏/丰富Biomass stability 生物量稳定性Tundra 冻原Island biogeography 岛屿生物地理学Turnover rate 周转率Source of colonists 移植者源Relaxation松弛Edgespecies 边缘物种Interior species 内部物种Corridor 走廊Greenways 绿色通道Community assembly群落集合Grazers 食草动物Carnivores 食肉动物Keystone species 关键物种Dominance control 优势控制Habitat affinity生境亲和力Prey switching 猎物转换RSuccession 演替Climax Community 顶级群落Pioneer species 先锋物种Primary succession 原生演替Alluvial deposit 冲积层Secondary succession 次生演替Acidifying effect 酸化作用Opportunistic机会主义Cellulose 植物纤维素Lignin 木质素Resource ratio hypothesis 资源比假说Fluctuations 波动Cyclic succession 循环演替Disturbance 干扰Patch dynamics板块动态Mini-succession 微型演替Cambium 形成层Neotropical forest 新热带雨林Priority effect 优先效应SVegetation 植被Ecotones 群落交错区Climate map 气候图Biomes 生物群系Heat budget 热量预算Zonation 分带Grassland 草地Primary regions 基本区域Desertification 荒漠化Arctic tundra 北极冻原Alpine tundra 高山冻原Permafrost 永冻层Coniferous boreal forest北方针叶林Temperature forest 温带森林Tropical forest 热带森林Salinization 盐渍化Primary saltwater regions 基本盐水区域Opens oceans 开阔海洋Continental shelves 大陆架The intertidal zone 潮间带Salt marsh 盐沼Mudflats淤泥滩Mangroves 红树林Pelagic 浮游生物Photic zone 有光带Phyto plankton 浮游植物Nekton 自泳动物Benthic 底栖Rocky shore 岩岸Zonation 分带Streams 溪流Ponds 池塘Environmental concerns 环境关系Catchment area 集水区Temperature inversion 温度逆转Biomanipulation 生物处理TThe goals of harvesting 收获目标Quota limitation 配额限制Environmental fluctuation环境波动Maximum possible yiel最大可能产量Net recruitment 净补充量Surplus yield 过剩产量Age structure 年龄结构Population data 种群数据Stable equilibrium 稳定平衡Harvesting effort 收获努力Gun licences 猎枪执照Rod licences钓鱼许可证Upwelling of cold water冷水上升流Fisheries 渔业Ocean productivity 大洋生产力The tragedy of the common公共灾难Overexploitation 过捕Pollution 污染Global decline 全球性下降By-catch 附带收获Community perturbations 群落扰动Oil spills 原油泄漏Eutrophication 富营养化Algal blooms 水华Red tides 赤潮Biomagnification 生物放大作用UPest 有害生物Natural enemies 天敌Ruderal 杂草型Economic/aesthetic injury level 经济/美学损害水平Cultural 栽培Biological control 生物防治‘Silent spring’寂静的春天Chemical toxicity 化学毒性Evolution of resistance抗性进化Microbial insecticide微生物杀虫剂Inoculation接种Augmentation扩大Inundation 爆发VRare species 稀有种Genetic diversity 遗传多样性Extinction 灭绝Endemic species 特有种Habitat fragmentation 生境片段化Insularization 岛屿化Biodiversity 生物多样性Strategies for conservation保育对策Antarctic treaty 南极协议Ecotourism生态旅游WAir pollution空气污染Acid rain 酸雨Water pollutants 水体污染物Soil pollution 土壤污染Acid deposition 酸降Pathogens病源体Chemical oxygen demand 化学需氧量Anaerobes 厌氧菌The greenhouse effect 温室效应Carbon dioxide 二氧化碳Ozone 臭氧Photochemical smog 光化学烟雾XOverview 概述Soil erosion 土壤侵蚀Soil compaction 土壤硬结Contour ploughing等高耕作Cover crops 覆盖作物No-till farming 免耕农业。
生态学定律

生态学定律霍普金斯定律1918年霍普金斯提出的生物气候定律:在其它因素相同的条件下,北美温带地区,每向北移纬度1°向东移经度5°,或上升约122米,植物的阶段发育在春天和初夏将各延期四天;在晚夏和秋天则各提前四天等等。
葛洛格规则(Gioger's rule): 温血动物在温暖地区的个体黑色素增多,在干旱地区则红,黄,棕色为多,在寒冷地区色素逐渐减弱。
耐受性定律亦称为谢尔福德耐性定律(Shelford’s law of tolerance)是美国生态学家V.E. Shelford 于1913年提出的。
生物对其生存环境的适应有一个生态学最小量和最大量的界限,生物只有处于这两个限度范围之间生物才能生存,这个最小到最大的限度称为生物的耐受性范围。
生物对环境的适应存在耐性限度的法则称为耐受性定律。
具体可定义为:任何一种环境因子对每一种生物都有一个耐受性范围,范围有最大限度和最小限度,一种生物的机能在最适点或接近最适点时发生作用,趋向这两端时就减弱,然后被抑制。
这就是耐受性定律。
谢尔福德耐性定律在生物的生长和繁殖所需要的众多生态因子中,任何一个生态因子在数量上的过多过少或质量不足,都会成为限制因子。
即对具体生物来说,各种生态因子都存在着一个生物学的上限和下限(或称“阀值”),它们之间的幅度就是该种生物对某一生态因子的耐性范围(又称耐性限度)。
E. P. Odum (1973 )等对耐性定律作了如下补充:( 1 )同一种生物对各种生态因子的耐性范围不同,对一个因子耐性范围很广,而对另一因子的耐性范围可能很窄。
( 2 )不同种生物对同一生态因子的耐性范围不同。
对主要生态因子耐性范围广的生物种,其分布也广。
仅对个别生态因子耐性范围广的生物,可能受其它生态因子的制约,其分布不一定广。
( 3 )同一生物在不同的生长发育阶段对生态因子的耐性范围不同,通常在生殖生长期对生态条件的要求最严格,繁殖的个体、种子、卵、胚胎、种苗和幼体的耐性范围一般都要比非繁殖期的要窄。
国外对绿色发展效率的测度方法

国外对绿色发展效率的测度方法
国外对绿色发展效率的测度方法有很多种,以下是其中比较常见的几种:
碳足迹(Carbon Footprint)
碳足迹指的是人类活动所导致的温室气体排放量。
通过计算企业或国家的碳足迹,可以评估其在环保方面的表现和效率。
资源利用率(Resource Efficiency)
资源利用率是指在生产和消费过程中,采取的措施来节约和优化资源利用的程度。
这包括能源、原材料、水等各种资源的使用效率。
生命周期评价(Life Cycle Assessment)
生命周期评价是指对一个产品或服务从生产、使用到废弃全过程的环境影响进行评价。
这涉及到资源消耗、能源消耗、废弃物排放等方面,可以评估企业或国家的绿色效率。
可持续发展指数(Sustainable Development Index)
可持续发展指数是综合考虑经济、社会、环境等多个方面,评估一个国家或地区可持续发展水平的指标。
该指数可以反映出国家或地区在促进绿色发展方面的综合表现和效率。
以上是一些常见的国外对绿色发展效率的测度方法,除此之外还有很多其他的指标和方法。
对于目前的全球环境问题,各国在绿色发展方面都需要不断探索和创新,采取更加有效的措施来保护地球和人类生存环境。
霍普金斯生物气候定律

霍普金斯生物气候定律全文共四篇示例,供读者参考第一篇示例:霍普金斯生物气候定律是生态学领域里一个重要的理论,得名于盖尔·霍普金斯。
该理论表明了不同生物物种在环境中的分布规律,揭示了生物与环境之间的相互作用关系。
霍普金斯生物气候定律可以帮助我们更好地理解自然界的生态系统,为生态保护和生物多样性研究提供重要的理论基础。
霍普金斯生物气候定律主要包括两个基本原理,即物种多样性和生物地理分布。
在生态学中,物种多样性指的是一个生态系统中不同种群或种类的数量和种类的多寡。
霍普金斯生物气候定律指出,物种的多样性受到环境因素的影响,生物在不同的气候条件下会表现出不同的多样性。
在不同的气候区域,生物的种类和数量也会有所不同。
这一原理揭示了生物在环境中的适应性和分布规律,为生态系统的稳定性和多样性提供了重要的理论依据。
霍普金斯生物气候定律为我们认识自然界中生物与环境之间的相互作用提供了重要的理论支持。
通过研究这一定理,我们可以更好地理解生态系统的稳定性和多样性,为生物多样性保护和环境保护提供重要的理论依据。
希望通过不断深入的研究,我们能够更好地保护和管理自然界的生态系统,实现人类与自然的和谐共生。
【2000字】第二篇示例:霍普金斯生物气候定律是一种描述生物种群与环境之间相互作用关系的理论,由英国生态学家霍普金斯(Charles Sutherland Elton)提出。
霍普金斯生物气候定律认为,生物种群的种群数量与环境因素之间存在着一种稳定的关系,通过这种关系可以预测种群数量的变化趋势。
这一定律在生态学领域中有着重要的意义,对于研究生物种群与环境之间的相互关系和生态系统的稳定性具有深远的指导意义。
霍普金斯生物气候定律的提出源于霍普金斯对自然界观察和实验的总结与归纳。
他认为,生物种群数量与环境因子之间存在着一种动态平衡关系,即当环境因素发生变化时,生物种群数量也会随之发生变化,但最终会重新回到一种稳定状态。
这种稳定状态可以通过一定的数学模型来描述,即霍普金斯生物气候定律。
南方石质山地植被生态阈值

南方石质山地植被生态阈值英文回答:Ecological thresholds in vegetation of southern rocky mountains.Ecological thresholds refer to the critical points at which ecosystems undergo significant and often irreversible changes in response to environmental stressors. In the context of vegetation in southern rocky mountains, ecological thresholds play a crucial role in maintaining the health and functioning of these ecosystems.One important ecological threshold in southern rocky mountain vegetation is the fire threshold. Many plant species in these mountains have evolved to be fire-adapted, meaning they rely on periodic wildfires for their regeneration and growth. However, if the frequency or intensity of wildfires exceeds a certain threshold, it can lead to the loss of fire-adapted species and the dominanceof non-native or invasive species. This can result in a shift in vegetation composition and a decline in biodiversity.Another ecological threshold in southern rocky mountain vegetation is the water availability threshold. These mountains are often characterized by arid or semi-arid conditions, and water availability is a limiting factor for plant growth and survival. When water availability falls below a certain threshold, plants may experience water stress and become more susceptible to diseases and pests. This can lead to reduced plant productivity and even mortality.Furthermore, nutrient availability can also act as an ecological threshold in southern rocky mountain vegetation. Nutrients, such as nitrogen and phosphorus, are essential for plant growth. However, excessive nutrient inputs, such as from agricultural runoff or atmospheric deposition, can exceed the threshold at which plants can effectivelyutilize these nutrients. This can result in nutrient imbalances, nutrient leaching, and the proliferation ofnutrient-demanding invasive species.In addition to these ecological thresholds, human activities can also influence the vegetation dynamics in southern rocky mountains. For example, land use changes, such as deforestation or urbanization, can disrupt natural ecological processes and exceed the thresholds at which the vegetation can adapt. This can lead to habitat fragmentation, loss of biodiversity, and altered ecosystem functioning.In conclusion, ecological thresholds in southern rocky mountain vegetation are critical for maintaining the health and functioning of these ecosystems. Understanding and managing these thresholds is essential for ensuring the long-term sustainability of these ecosystems and the services they provide.中文回答:南方石质山地植被生态阈值。
南方石质山地植被生态阈值

南方石质山地植被生态阈值英文回答:The ecological threshold of vegetation in the southern rocky mountainous region refers to the point at which the vegetation community undergoes a significant change or collapse due to changes in environmental conditions. This threshold is influenced by various factors such as climate, soil conditions, and human activities.In terms of climate, factors such as temperature, rainfall, and sunlight play a crucial role in determining the ecological threshold. For example, if the average annual temperature exceeds a certain threshold, it may lead to the decline of certain plant species that are not adapted to high temperatures. Similarly, if there is a significant decrease in rainfall, it can result in water stress for vegetation, leading to a decline in plant growth and productivity.Soil conditions also play a significant role in determining the ecological threshold. The fertility of the soil, its water-holding capacity, and nutrient availability all influence the type and abundance of vegetation. If the soil becomes too degraded or nutrient-poor, it can limit the growth of plants and reduce their resilience to environmental stressors.Human activities, such as deforestation, overgrazing, and pollution, can also push the vegetation community beyond its ecological threshold. For example, excessive logging can lead to habitat loss and disrupt the natural regeneration of vegetation. Overgrazing by livestock can cause soil erosion and degradation, making it difficult for plants to grow. Pollution from industrial activities can introduce toxic substances into the environment, affecting the health and survival of vegetation.Understanding the ecological threshold of vegetation in the southern rocky mountainous region is important for conservation and management efforts. By identifying and monitoring these thresholds, we can take proactive measuresto prevent vegetation collapse and promote sustainable land use practices. For example, if we know that a certain plant species is sensitive to high temperatures, we can implement measures to mitigate the effects of climate change, such as planting shade trees or using irrigation techniques to cool the environment.In conclusion, the ecological threshold of vegetationin the southern rocky mountainous region is influenced by climate, soil conditions, and human activities. It is important to understand and monitor these thresholds to ensure the long-term health and resilience of the vegetation community.中文回答:南方石质山地植被的生态阈值指的是由于环境条件的变化,植被群落发生显著变化或崩溃的点。
普通生态学名词解释

死态教:死态教是钻研死物及环境间相互闭系的科教之阳早格格创做环境:是指某一特定死物体或者死物集体以中的空间,以及间接或者间接效用该死物体或者死物集体存正在的十足真物的总战.死态果子:是指环境中对付死物死少、收育、死殖、止为战分集有间接或者间接效用的环境果素.死态幅ecological amplitude:每一种死物对付每一种死态果子皆有一个耐受范畴,即有一个耐受范畴,既有一个死态上的最矮面战最下面.正在最矮面战最下面之间的范畴称为死态幅或者死态价ecological valence.大环境macroenvironment:指天区环境,天球环境战宇宙环境.小环境microenvironment:指对付死物有间接效用的毗邻环境,即指小范畴内的特定栖息天.大气候macroclimate:指离大天1.5m以上的气候,是有大范畴果素所决断的.小气候 microclimate:小环境中的气候.存正在果子:正在死态果子中通常是有肌体死计战收育所不可缺少的中界环境果素.死态环境:钻研的死物体或者死物集体以中的空间中,间接或者间接效用该死物体或者死物集体存正在战死少的十足果素的总战.死境habitat:具备特定的死态本性的死态体或者死态集体经常正在某一特定的环境中存正在战死少,那一特定环境喊死境.稀度约束果子density dependent factor:对付动物种群数量效用的强度随其种群稀度而变更,进而安排种群数量的果子,如食物天敌等死物果子.非稀度约束果子 density independent factor:效用强度不随种群稀度而变更的果子如温度降火等气候果子.节制果子limiting factor:所有死态果子,但是靠近或者超出某种死物的耐受极限而构制其存正在死少繁殖或者扩集时,那个果素称为节制果子.利比希最小果子定律:动物的死少与决于那些处于最少量状态的营养身分.耐受性定律:所有一个死态果子正在数量上或者品量上的缺乏或者过多皆将使该种死物出落或者不克不迭存正在.节制果子本理:一个死物或者一群死物的存正在战繁枯与决于概括的环境条件情景,所有靠近或者超出耐性节制的情景皆可道是节制情景或者节制果子.似昼夜节律:动物正在自然界所表示出去的昼夜节律除了由中界果素的昼夜周期所决断的以中,正在里里也有自收性战自运性的内源决断,果为那种离启中部天下的内源节律不是24小时,而是靠近 24小时,那种变更程序喊似昼夜节律.多型局里:种群内的个体正在形态、死殖力、体沉及其余死理死态习性上爆收好别,而出现种群内分歧死物型. 那种分歧不但表示正在♀♂相同,共性个体也有分歧.如飞虱少短翅; 社会性昆虫等阿朔妇程序:对付于夜出性动物处于恒乌的条件下,它们的昼夜周期支缩,对付于夜出性动物处于恒光的条件下,它们的昼夜周期延少,而且那种延少的巩固,那种延少越明隐.对付于日出性动物处于恒乌的条件下,它们的昼夜周期延少,对付于日出性动物处于恒光的条件下,它们的昼夜周期支缩,而且那种支缩随着光强的巩固,那种支缩越明隐.死物钟:是动物自己具备的定时体制.临界温度:死物矮于或者下于一定的温度时便会受到伤害,那一温度称为临界温度.热害:喜温死物正在0℃以上的温度条件下受到的伤害.冻害:死物正在冰面以下受到的伤害喊冻害.霜害:正在0℃受到的伤害喊霜害.超热:杂火正在整下40℃以去启初结冰,那种局里喊超热.符合性矮体温:它是一种受安排的矮体温局里,此时体温被安排很矮,靠近于环境温度的火仄,心律代开率及其余死理功能均相映的降矮,正在所有时间皆可自收的或者通过人为诱导,回复到本去的仄常状态.贝格曼程序:内温动物,正在比较热的气候区,身体体积比较大,正在比较温的气候区,身体体积比较小.阿伦程序:内温动物身体的凸出部分正在热热的天区有变小的趋势.乔丹程序:鱼类的脊椎数目正在矮温火域中比正在温温火域中多.死物教整度:死物死少收育的起面温度.灵验积温:死物完毕某个收育阶段所需的总热量.露面温度:气氛中火汽达到鼓战时的温度喊露面温度.相对付干度:大气中的本量火汽压与最大火汽压之好.鼓战好:最大火汽压与本量火汽压之好.x需火量:死产单位沉量搞物量所需的火量.t土壤量天:板滞身分的拉拢分歧百分比环境容纳量:对付于一个种群去道,设念有一个环境条件所允许的最大种群值以k表示,当种群达到k值时,将不再删少,此时k值为环境容纳量.死命表:用去形貌种群存正在与牺牲的统计工具.动背死命表:根据瞅察一群共一时间出死的死物牺牲或者存活的动背历程而或者得数据体例得死命表.固态死命表:根据某一特定时间对付种群做一个年龄结构考察,并根据截止而体例的死命表内禀删少率:正在不所有环境果素(食物、收天战其余死物)节制的条件下,又种群内正在果素决断的宁静的最大删殖速度称为种群的内禀删少率(intrinsic growth rate),记做 rm.驯化:是指正在真验条件下诱收的死理补偿体制,那种死理符合短时间即可完毕meta种群:一个大的兴旺的种群果环境传染,栖息天益害或者其余搞扰而破碎成许多孤坐的小种群时,那些小种群的共同体或者总体便称为联种群. (联种群又称集中种群)协共进化:一个物种的进化必定会改变效用于其余死物的采用压力,引起其余死物也爆收变更,那些变更反过去引起相闭物种的进一步变更.自然采用:种群内个体死殖乐成率的好别早早会改变种群的遗传本性.宁静化采用:种群的所有表型按某种尺度排列成一个序列,那么当自然采用有好处序列中位的表型并淘汰序列二端的表型.定背采用:自然采用更有好处其中一端的表型,而不利于另一端.团结采用:自然采用可共时对付序列二端的表型皆有利,虽然有利的程度大概分歧.最小可存正在种群持绝产量: 如果支获量不妨脆持少久宁静而不使种群数量低沉,该支获量被称为持绝产量.最大持绝产量:对付某一特定种群,持绝产量不是一个牢固稳定的值,依分歧的种群火仄易分歧管制技能可有很多值.若博得比持绝产量更大的产量制成种群数量的低沉,该持绝产量称为最大持绝产量.有害死物:有益于人类经济便宜或者有害于人类健壮的死物.区系:一定天区内所有动物的总体,区系有天理身分.植被:一定天区中动物群降的总体,可根据多种分歧的准则举止分类.死物群系(biome):是根据植被本性相似性对付死物群降战死态系统举止分类的体系.(为什么不是动物)群系(formation):植被分类的中级单位.植被区划:正在一定天段上依据植被典型及其天理分集的本性等区分出下、中、矮各级植被拉拢单位.(植被天区、植被天戴、植被区、植被小区)功能反应: 捕食者与猎物种群相互闭系模型掀穿出捕食者对付猎物稀度的变更可做出分歧典型反应.随着猎物稀度的减少,每个捕食者可捕获更多猎物或者可较快天捕获猎物,此局里称捕食者的功能反应比赛:死计正在共一天区的二个物种,由于利用相共的资材,引导每一个物种的数量低沉,即二种群相互爆收有害效用.戚眠:死物抵御姑且不利环境条件的一种非常灵验的死理体制.正在戚眠期,死物对付环境条件的耐受范畴便会比仄常活动时宽的多种群仄稳:指种群较万古间的保护正在险些共一火仄上,那一局里喊种群仄稳.种群大迸收:某种死物种群的数量正在短时间内慢遽降下,往往制成不利效用.死态侵犯:指由于人类蓄意识或者奇尔识把某种死物戴进相宜栖息战繁衍天区,种群不竭夸大,分集区逐步稳步的扩展,那个局里喊死态侵犯基果型:每一个体的基果拉拢.等位基果:决断一本性状的二个或者二个以上的基果拉拢.基果库:正在一个种群中,局部个体的基果拉拢.基果频次:正在一个基果库中,分歧基果所占的比率喊基果频次.基果型频次:正在一个基果库中,分歧基果型所占的比率喊基果型频次.哈-温定律:正在无限大的种群中,每一个体与种群内其余个体的接配机会均等,而且不其余搞扰果素(突变、漂移、自然采用等)各代的基果频次稳定,无论其基果型频次战基果频次怎么样,只经历一代,即达到遗传仄稳.遗传漂变:普遍爆收正在较小的种群中,果为正在一个很大的种群里,如果不爆收突变,根据哈-温定律,分歧的基果型频次将脆持仄稳状态,但是正在较小的种群中,既使无符合的变同爆收,种群内基果频次也会爆收变更,也便是由于断绝,不克不迭充分的随机接配,种群内基果不克不迭达到真足自由分散战拉拢时爆收的缺面所引起的,那样那些中性的或者不利性状正在种群中继承保存下去渐变群:采用压力正在天理空间上的连绝变更,引导基果频次或者表示型的渐变,产死一个具备变同梯度的集体.趋共符合:分歧种类的死物当死计正在相共或者相似的环境条件下,通过变同采用产死相共或者相似的形态或者死理本性以及相共或者相似的符合办法或者道路,那种局里喊趋共符合.趋同符合:共种类的死物当死计正在相共或者相似的环境条件下,通过变同采用产死分歧的形态或者死理本性以及分歧的符合办法或者道路,那种局里喊趋同符合.符合度:是指个体死产能存活后代、并能对付已去世代有孝敬的本收的指标死计史对付策:百般死物正在进化历程中产死百般特有的死计史,那种死计史是死物正在存正在历程中赢得存正在的对付策.K对付策:死物种群数量达到或者靠近环境容纳量的火仄,那种典型称做k对付策.毗邻效力:当种群稀度减少时,正在毗邻的个体之间所出现的相互效用.-3/2自疏规则:如果某培动物的支获稀度超出一定值时,种内对付资材的比赛不但是效用到植株死少收育的速度,而且效用动物的存活率,那一局里喊自疏局里范畴:指由个体、家庭或者其余社群单位所吞噬的并主动捍卫不让共种其余成员侵进的空间.范畴止为:死物以威胁或者间接打击驱赶侵犯者的止为.收域性:死物具备范畴止为的本性喊范畴性.集群:动物汇集正在所有喊集群阿里程序:动物种群有一个最适的种群稀度,果而种群过剩战种群过矮或者过稀或者过疏皆是不利的,皆大概对付种群爆收压制性的效用.社会等第:一群共种的动物中,每个个体的职位有一定程序性或者序位,其前提是支配-从属闭系,那种程序性喊社会等第.比赛排斥本理:正在环境资材上需要靠近的二个种类是不克不迭正在共一天区死计的.如果正在共一天区死计,往往正在栖息天、食性、活动时间等圆里有种分歧.若二个物种死态位真足沉叠,必定是一个物种牺牲,若使二个物种共时存正在,则要使死态位有好别,使死态位瓦解寄死:一种死物从另一种死物体液、构制或者已消化的物量获与营养,并制成对付宿主的妨害,那种局里喊寄死群降:正在一定时间内战一定空间内,分歧种群的集中种群:共一物种正在一定空间战一定时间的个体的集中体. 是具备潜正在互配本收的个体. 种.种群的稀度:每个单位空间内个体的数量或者死物量.稀度是种群最基础的参数, 也是种群要害的参数之一种群的分集型: 种群正在一个天区的分集办法,既个体怎么样正在空间摆设的.或者种群正在一定空间的个体扩集分集的一定形式.空间同量性:指死态教历程战格局正在空间分集上的不匀称性及其搀杂性.边沿效力:指缀块边沿部分由于受中围效用而表示出与缀块核心部分分歧的死态教本性的局里.死物百般性:死命有肌体及其好以存正在的死态概括体的百般化战变同性前提死态位:物种所吞噬的表里上的最大空间喊前提死态位.本量死态位:物种本量吞噬的死态位喊本量死态位.死态位:正在死态果子变更范畴内,不妨被死态元本量战潜正在吞噬、利用或者符合的部分,称做死态元的死态位死态位宽度:正在现有的资材谱中,一个死态元所能利用的百般资材总战的幅度.死态位沉叠:指分歧死态元的死态位之间相沉合的程度.死计型:分歧种类的动物之间或者动物之间由于趋共符合而正在形态、死理及符合办法等圆里表示出相似的典型群降最小里积:指起码要有那样大的里积及相映的空间,才搞包罗组成群降的大普遍死物种类.劣势种:对付群降的结媾战群降环境的产死有明隐统制效用的动物种称劣势种.修群种:群降中存留于主要条理中的劣势种.亚劣势种:个体数量与效用皆次于劣势种,但是正在决断群降本量战统制群降环境圆里仍起着一定效用的动物种.陪死种:为群降罕睹种类,它与劣势种相陪存留,但是不起主要效用.奇睹种或者罕睹种:正在群降中出现频次很矮的种类.多度:物种间个体数量对付比的估测指标.相对付稀度:某物种的个体数与局部物种个体数的比值.投影盖度:指动物天上部分笔曲投影里积占样大天积的百分比.基盖度:动物基部的覆盖里积.频度:某物种正在考察范畴内出现的频次.相对付沉量:单位里积或者容积内某一物种的沉量占局部物种总沉量的百分比死物百般性:死物中的百般化战变同性以及物种死境的死态搀杂性.死计型谱:群降内每类死计型的种数占总种数的百分比排列成一个系列.死态等值种:正在分歧天理位子但是环境相共或者相似的天区由于趋共进化而具备相共死计型的动物称为死态等值种.层间动物:群降除了自养、独力支撑的动物所产死的条理以中,另有一些如藤本动物、寄死、腐死动物,它们本去不独力产死条理,而是分别依附各条理中曲坐的动物体上共资材种团:以共一办法利用共共资材的物种集团本死演替:从本死裸天启初的演替.次死演替:从次死裸天启初的演替.演替系列:从死物定居启初曲到产死宁静的群降为止,那样的系列历程称为演替系列.顶级群降:一个群降演替达到宁静老练的群降.排序:把一个天区内所考察的群降样天依照相似度去排定百般天的位序,进而分解百般天之间及其与死境之间的相互闭系.群降演替:正在一定天区内,死物群降随时间而变更,由一种群降典型转形成另一种典型的历程.间接排序:根据一个或者多个已知的环境梯度举止排序的要收.间接排序:根据群降自己的属性比圆种的相闭性、群降相似性等导出抽象轴或者群降变更目标的排序.植被型:指正在植被型组内,把修群种死计型相共或者相似共时对付火热条件的死态闭系普遍的动物群降共同为植被型.植被型组:通常修群种死计型相似而且群降中貌相似的动物群降共同为植被型组.群系:通常是修群种或者共修种相共的动物群降共同为群系.群丛:通常是层片结构相共各层片的劣势种或者共劣种相共的动物群降系统:由二个或者二个以上相互效用的果素的集中.食物网:分歧的食物链间相互接叉而产死网状结构.营养级:食物链上每个位子上所有死物的总战.死态系统:是指一定时间战空间内,由死物身分战非死物身分相互效用而组成的具备一定结媾战功能的有机统一体死态仄稳:一个天区的死物与环境通过少久的相互效用,正在死物与死物、死物与环境之间修坐了相对付宁静的结构以及相映功能,此种状态即宁固态背反馈:大普遍死物的稳态体制以大概一般的办法起着效用;如果一个果子的里里火仄太下,该体制将缩小它;若火仄太矮,便普及它.那一历程称为背反馈.格中之一定律(能量利用的百分之十定律):食物链结构中,营养级之间的能量变化效用大概为格中之一,其余格中之九由于消耗者采食时的采用性浪费,以及呼吸战排鼓等而被消耗掉,那便是所谓的“格中之一定律”,也喊能量利用的百分之十定律.死物量:单位空间内,积蓄的有机物量的量.现存量:正在考察的时间内,单位空间中存留的活着的死物量初级死产力:单位时间、单位空间内,死产者汇集有机物量的量.总初级死产力:正在单位时间、空间内,包罗死产者呼吸消耗掉的有机物量正在内的所汇集有机物量的量.洁初级死产力:正在单位时间战空间内,去掉呼吸所消耗的有机物量之后死产者汇集有机物量的量共化效用:指被动物吸支的日光能中被光合效用所牢固的能量比率,或者主动物摄食的能量中被共化了的能量比率流利率:物量正在单位时间、单位里积或者单位体积内的移动量死态系统死物循环(死物小循环,系统里里循环):物量/元素正在死态系统各组成部分之间的变化或者循环.死物天球化教循环(*大循环):物量/元素正在分歧的系统间变化,并最后正在形式上完毕返回z动物次死化合物又称动物次死性代开产品,是相对付于基础代开产品而止的,是动物新陈代开的中间产品或者副产品, 对付动物的死少收育战保护肌体的基础死命历程无间接效用, 如萜类、酚类、死物碱等。
每日单词:threshold

每日单词:thresholdthreshold /ˈθreʃhəʊld/CET6丨IELTS丨TOEFL基本释义The threshold of a building or room is the floor in the doorway, or the doorway itself. A threshold is an amount, level, or limit on a scale. When the threshold is reached, something else happens or changes.n. 门槛,界限,临界点外刊例句Global emissions from fossil fuels and cement production in 2019 were 16% higher than in 2009. It will be even harder to limit climate change to less than 2°C above the pre-industrial level, the global threshold from which America’s target for 2050 comes.2019年全球化石燃料和水泥生产行业的总碳排放量较2009年上升了16%,而美国制定的2050年碳中和目标是将全球气候变化的温升限制在不超过工业化前水平2摄氏度,这将异常困难。
追根溯源单词threshold来源古英语threscold,表示“门槛”,后来拼写受单词hold的影响,逐渐被写成threshold,含义也丰富起来。
基本的含义是指实体的“门槛,门口”,比如:She stopped at the threshold of the bedroom.她在卧室门口停了下来。
Just as he was crossing the threshold he heard voices behind him.正当他要跨进门槛,就听见背后有说话声。
备课素材:生态足迹及其分析法-高二上学期生物人教版选择性必修2
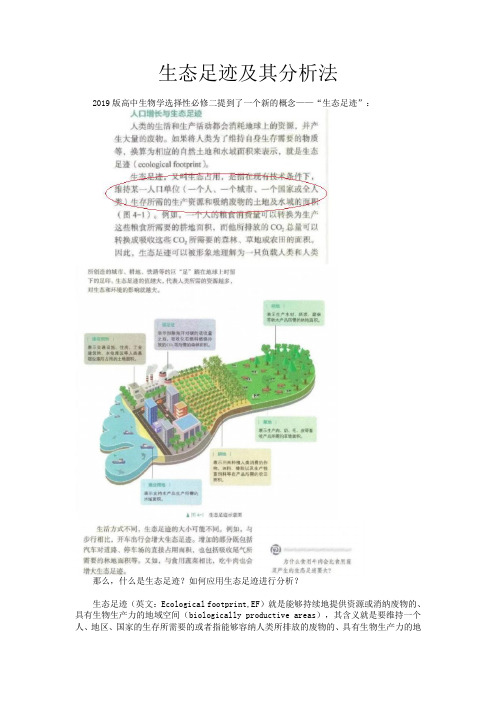
生态足迹及其分析法2019版高中生物学选择性必修二提到了一个新的概念——“生态足迹”:那么,什么是生态足迹?如何应用生态足迹进行分析?生态足迹(英文:Ecological footprint,EF)就是能够持续地提供资源或消纳废物的、具有生物生产力的地域空间(biologically productive areas),其含义就是要维持一个人、地区、国家的生存所需要的或者指能够容纳人类所排放的废物的、具有生物生产力的地域面积。
生态足迹估计要承载一定生活质量的人口,需要多大的可供人类使用的可再生资源或者能够消纳废物的生态系统,又称之为“适当的承载力”(appropriated carrying capacity)。
其应用意义是:通过生态足迹需求与自然生态系统的承载力(亦称生态足迹供给) 进行比较即可以定量的判断某一国家或地区可持续发展的状态,以便对未来人类生存和社会经济发展做出科学规划和建议。
在20世纪90年代初由加拿大大不列颠哥伦比亚大学规划与资源生态学教授里斯(Willian E.Rees)提出。
它显示在现有技术条件下,指定的人口单位内(一个人、一个城市、一个国家或全人类)需要多少具备生物生产力的土地(biological productive land)和水域,来生产所需资源和吸纳所衍生的废物。
生态足迹通过测定现今人类为了维持自身生存而利用自然的量来评估人类对生态系统的影响。
比如说一个人的粮食消费量可以转换为生产这些粮食的所需要的耕地面积,他所排放的二氧化碳总量可以转换成吸收这些CO2所需要的森林、草地或农田的面积。
因此它可以形象地被理解成一只负载着人类和人类所创造的城市、工厂、铁路、农田……的巨脚踏在地球上时留下的脚印大小。
它的值越高,人类对生态的破坏就越严重。
该指标的提出为核算某地区、国家和全球自然资本利用状况提供了简明框架,通过测量人类对自然生态服务的需求与自然所能提供的生态服务之间的差距,就可以知道人类对生态系统的利用状况,可以在地区、国家和全球尺度上比较人类对自然的消费量与自然资本的承载量。
基础生态学名词解释
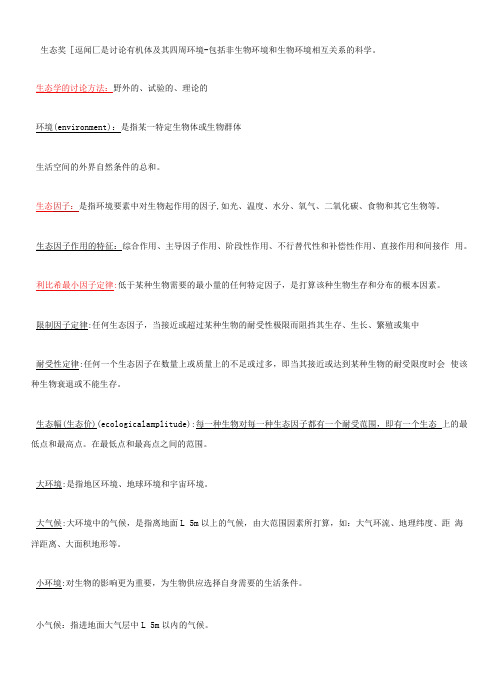
生态奖[逗闻匚是讨论有机体及其四周环境-包括非生物环境和生物环境相互关系的科学。
生态学的讨论方法:野外的、试验的、理论的环境(environment):是指某一特定生物体或生物群体生活空间的外界自然条件的总和。
生态因子:是指环境要素中对生物起作用的因子,如光、温度、水分、氧气、二氧化碳、食物和其它生物等。
生态因子作用的特征:综合作用、主导因子作用、阶段性作用、不行替代性和补偿性作用、直接作用和间接作用。
利比希最小因子定律:低于某种生物需要的最小量的任何特定因子,是打算该种生物生存和分布的根本因素。
限制因子定律:任何生态因子,当接近或超过某种生物的耐受性极限而阻挡其生存、生长、繁殖或集中耐受性定律:任何一个生态因子在数量上或质量上的不足或过多,即当其接近或达到某种生物的耐受限度时会使该种生物衰退或不能生存。
生态幅(生态价)(ecologicalamplitude):每一种生物对每一种生态因子都有一个耐受范围,即有一个生态上的最低点和最高点。
在最低点和最高点之间的范围。
大环境:是指地区环境、地球环境和宇宙环境。
大气候:大环境中的气候,是指离地面L 5m以上的气候,由大范围因素所打算,如:大气环流、地理纬度、距海洋距离、大面积地形等。
小环境:对生物的影响更为重要,为生物供应选择自身需要的生活条件。
小气候:指进地面大气层中L 5m以内的气候。
协同进化:一个物种的性状作为对另一物种性状的反应而进化,而后一物种的这一性状本身又是作为对前一物种性状的反应而进化的。
*自然界中捕食者对猎物种群大小的影响:任一捕食者的作用,只占猎物种总死亡率的很小一部分,因此去除捕食者对猎物种仅有微弱影响。
捕食者只是采用了对象种中超出环境所能支持的部分个体,所以对最终猎物种群大小无影响。
这时限制猎物种群的主要因素不是捕食者数量,而是其他因素。
*种内竞争的利与弊:从个体看,种内竞争可能是有害的,但是从整个种群看,淘汰了较弱个体,保存了较强个体,种内竞争有利于种群的进化与富强。
生态幅的名词解释

生态幅的名词解释
生态幅(ecological amplitude )又称生态价。
指某一生物对环境因子的耐受范围,即其生态上的最高点与最低点之间的范围。
主要由该物种的遗传特性决定。
生态幅由生物种适应范围较窄的环境因子(即限制因子)所限制,并由生物临界期的耐性决定。
例如,生物的繁殖期一般为临界期,这时某一生态因子的不足或过多,最易起限制作用,从而造成生物繁殖期的生态幅比营养期窄。
生态幅与环境相互作用决定了生物的分布区,地球上几乎没有任何不同的生物的分布区是完全重叠。
生态幅
生态幅(ecological amplitude)
1、每一种生物对每一种生态因子都有一个耐受范围,即有一个生态上的最低点和最高点。
在最低点和最高点(或称耐受性的上限和下限)之间的范围,称为生态幅。
例如,鲑鱼对温度这一生态因子的耐受范围是0~12℃,最适温为4℃。
2、一种生物对每一个环境因素都要求有适宜的量,过多或不足都会使其生命活动受到抑制,乃至死亡,这种生物对每一个因素的耐受范围,称为生态幅(ecological amplitude).
1。
- 1、下载文档前请自行甄别文档内容的完整性,平台不提供额外的编辑、内容补充、找答案等附加服务。
- 2、"仅部分预览"的文档,不可在线预览部分如存在完整性等问题,可反馈申请退款(可完整预览的文档不适用该条件!)。
- 3、如文档侵犯您的权益,请联系客服反馈,我们会尽快为您处理(人工客服工作时间:9:00-18:30)。
Derivation of ecotoxicitythresholds for uraniumSteve C.Sheppard a ,),Marsha I.Sheppard a ,Marie-Odile Gallerand b ,Barb Sanipelli aaECOMatters Inc.,Box 430,Pinawa,Manitoba,Canada R0E 1L0b Andra –Agence nationale pour la gestion des de ´chets radioactifs,Chaˆtenay-Malabry ce ´dex,France Received 11November 2003;received in revised form 21April 2004;accepted 29May 2004AbstractAssessment of the risk of impact from most radionuclides is based on the total radiological dose rate to the organism of concern.However,for uranium (U)there can be greater risk from chemical toxicity than radiological toxicity (depending on the isotopic composition).Chemical ecotoxicity of U is dependent on several environmental parameters.The most important are carbonate content,because of the formation of soluble carbonate complexes,and divalent cation content (Ca CC and Mg CC ),because of their competitive interaction with the uranyl ion (UO 2CC ).This study summarizes the literature available to set PNECs (predicted no-effect con-centrations)for chemical toxicity of U to non-human biota.The corresponding radiological doses were estimated,and as expected chemical toxicity proved to be the greater concern.There were limited data from some types of biota;however,PNECs for the types of biota of interest were as follows:terrestrial plants d 250mg U kg ÿ1dry soil;other soil biota d 100mg U kg ÿ1dry soil;freshwater plants d 0.005mg U L ÿ1water;freshwater invertebrates d 0.005mg U L ÿ1water; freshwater benthos d 100mg U kg ÿ1dry sediment;)Corresponding author.Tel.:C 12047532747;fax:C 12047538478.E-mail address:sheppards@ (S.C.Sheppard).0265-931X/$-see front matter Ó2004Elsevier Ltd.All rights reserved.doi:10.1016/j.jenvrad.2004.05.015Journal of Environmental Radioactivity 79(2005)55–83/locate/jenvrad56S.C.Sheppard et al./J.Environ.Radioactivity79(2005)55–83freshwaterfish at water hardnesses of:–!10mg CaCO3Lÿ1(very soft water)d0.4mg U Lÿ1water;–10–100mg CaCO3Lÿ1(soft water)d2.8mg U Lÿ1water;and–O100mg CaCO3Lÿ1(hard water)d23mg U Lÿ1water;or–as a function of hardness d0.26(hardness as mg CaCO3Lÿ1)mammals d0.1mg U kgÿ1body weight dÿ1.Ó2004Elsevier Ltd.All rights reserved.Keywords:Uranium;Ecotoxicity;Hardness;PNEC;Predicted no-effect concentration1.IntroductionAssessment of the risk of impact from most radionuclides is based on the total radiological dose rate to the organism of concern.However,for natural-isotopic-composition uranium(Nat U)and possibly iodine-129(129I)and technetium-99(99Tc), there is greater risk from chemical toxicity than radiological toxicity.Chemical toxicity exceeds radiological toxicity in importance when the decay half-life is very long(i.e.the radionuclide has very low specific activity),the element is not particularly bioaccumulative,and the radiological emissions have low relative biological impact.Uranium,a member of the actinide series,has an atomic number of92and ten radioactive isotopes,but238U(99.27%),235U(0.72%)and234U(0.0055%)are the three most abundant.The dominant isotope by mass,238U,has a physical half-life of 4.5!109a,giving it a very low specific activity(1.24!104Bq gÿ1U).In at least one detailed analysis of U radiotoxicity and chemical toxicity in humans(Kocher, 1989),the concentration of U in kidney exceeded the recommended limit for chemical toxicity(a value of0.1mg kgÿ1fresh weight kidney)while the correspond-ing annual effective dose equivalent remained below1mSv.Thus,in human risk assessments,the chemical toxicity of Nat U is the focus,and the same is expected for non-human biota.The objective of this work was to summarize the literature available to set PNECs (predicted no-effect concentrations)for chemical toxicity of U to non-human biota found in natural ecosystems of temperate biospheres.This paper summarizes the literature;more detailed discussions of the underlying papers are given in Sheppard (2003).2.Overview of uptake and behaviour of UThe environmental behaviour of U,Ra and other U and Th decay chain radio-nuclides has been extensively studied and the subject of previous reviews(Sheppard, 1980;Gascoyne,1992).A significant recent review of behaviour and toxicity wasundertaken by Environment Canada (PSL2,2002;Bird et al.,2002);some of the background information in this introductory section was drawn from that study.Although U has oxidation states of (C III)to (C VI),the two major oxidation states are U(C VI)and U(C IV).The chemical properties of U(C IV)are similar to Th(C IV)and Pu(C IV),but U(C IV)is readily oxidized to U(C VI)as UO 22C .The uranyl ion,UO 22C ,is the most stable U species in an oxidizing solution and the most prevalent form in the environment (Sheppard,1980).The oxidized U(C VI)(uranyl)ion complexes readily with carbonate,phosphate or sulfate ions.In these forms,U is soluble and readily transported.In contrast,under reducing conditions,such as those found in anoxic waters and sediment,U occurs in the tetravalent (U(C IV))state which has a strong tendency to bind to organic material (Koczy,1963)and to precipitate,and is therefore immobile (Gascoyne,1992).Metallic U and particles of insoluble U compounds are not very bioavailable (Durbin and Wrenn,1975).For detailed information,Ribera et al.(1996)provide an excellent review of the behaviour,transfer and fate of U in both terrestrial and aquatic environments,including uptake by plants,animals,fish,aquatic invertebrates and plants and humans.3.Mechanisms of U toxicityAccidental exposures of workers and members of the public to releases of U hexafluoride (UF 6)in the mid-80s reinforced interest in the radiological and chemical toxicity of UF 6and its hydrolysis products.Radiological toxicity of U is primarily manifest in bone sarcomas,whereas chemical and combined chemical and radio-logical effects are reported as nephrotoxicity.Recently,interest in the chemical tox-icity of depleted U (DU)has increased following the Gulf War,where DU was used as strengthening material for various armor piercing munitions (Priest,2001).Also,a large industrial work force engaged in several types of U production operations,having intermittent exposures,has led to several toxicology studies of U to humans.Many of the studies using surrogates,such as rats or dogs,are relevant to this work.Depending on dose,route of administration and chemical form of administered U,exposure to U compounds can produce effects in a variety of mammalian organ systems,including kidney,liver,lung,cardiovascular and central nervous system (Hodge,1973cited by Diamond et al.,1989).The kidney is the most sensitive to uranyl U and is the critical target organ for humans after exposure to UF 6(Morrow et al.,1982).Ribera et al.(1996)state that chemical toxicity is particularly significant in compounds containing Nat U.Hence,UF 6and compounds,soluble in organic fluids,are extremely dangerous,while non-soluble compounds such as oxides (UO 2and U 3O 8),the form most commonly found in the environment,are much less dangerous.Further,Wrenn et al.(1985)stated that only the aqueous hexavalent ion UO 22C causes U chemical toxicity after oral intake.Renal injury observed in most mammalian toxicity studies is usually character-ized histologically by cellular injury and tubular necrosis (Diamond et al.,1989).However,often the repair of acute injury is rapid with complete restoration within 57S.C.Sheppard et al./J.Environ.Radioactivity 79(2005)55–8358S.C.Sheppard et al./J.Environ.Radioactivity79(2005)55–8335d after exposure,depending on the dose.Also associated with the injuries were abnormalities in renal function that are reversible making many of these toxicity studies difficult to assess(Diamond et al.,1989).Most international(USA and Australia)regulatory standards are set at3mg U kgÿ1kidney for injury in humans.The chemical toxicity to other organisms,such as earthworms,bivalves andfish, is usually judged by lack of response(no movement following physical prodding, losing the reflex of valve closure or death).For more detail on the cellular effects of U in animals,the reader is referred to Ribera et al.(1996)and Chassard-Bouchaud (1983,1987).4.Ecotoxicological approachIn general,the intent of ecotoxicology is to describe the potential impact of contaminants on ecosystem components so that good decisions can be made to protect the ecology of an environment.With most species,this means protecting the survival of a population.Thus,it is important to protect against effects on growth and reproduction,not just lethality.For endangered or highly valued species,the objective may be to protect individuals.Not all literature intended to convey ecotoxicity data is of sufficient quality to consider.Conversely,there are data not specifically intended for ecotoxicology that are useful from that perspective.It is recognized that in the absence of directly relevant and fully acceptable data,it is sometimes necessary to consider less-ideal data.Therefore,data acceptance criteria were developed.Data were considered potentially acceptable if they were from a peer-reviewed report or of similar calibre,the culture and experimental organisms and media were reported and were ecologically relevant,the results were consistent within the report (reproducible and monotonic with respect to U concentrations),and there was a credible statistical treatment and interpretation.Obviously,expert judgement was involved.Many papers will report multiple endpoints;indeed the preference in ecotoxicol-ogy is to apply a battery of bioassays because each contaminant will affect one bioassay more than another.In addition,it is common to report several endpoints for a given organism,such as for plants:emergence,growth,height and root elongation.The approach used in this study to select data for PNEC derivation was: -only the most sensitive,ecologically relevant endpoint for a given study and relevant organism is considered;-if different types of relevant organisms are used in a study,they are considered separately;-if a range of media characteristics,such as a series of soils,are used,and they had an important effect on the toxicity in a systematic way,they are considered separately.For the soils where results are considered the same,the effect concentration may be set as the geometric mean among those soils.Wherever possible,the results of studies in the literature were presented as EC25, the concentration of U where the performance of the endpoint was25%diminished compared to the control.This required some interpolation,but was considered preferable to summarizing results from a variety of effect levels as reported in the original papers.When this was not possible,the effect level reported by the researcher was used.5.Toxicity to terrestrial biota5.1.Toxicity to terrestrial plantsThe study showing the greatest sensitivity of plants to U in soil(Table1)is that of Aery and Jain(1998),who investigated the effect of U applied to a sandy-loam soil in 15-L pots.They measured a number of endpoints,many of which showed a toxic response to U.The most sensitive of these was for number of seeds per spike,with an Table1Toxicity information for terrestrial plants,with effect concentrations as mg U kgÿ1dry soilAuthors Biota Species Endpoint EffectconcentrationAery and Jain (1998)crop plant Triticum aestivum L.var.WH-147EC25for seed numberper spike0.5Shevchenko et al.(1987)crop plants corn,sorghum,cottonand sugar beetEC25for yield5Gulati et al. (1980)crop plants Triticum aestivum,Lycopersicon esculentumEC25for biomass yield6Meyer et al. (1998)native rangegrassAristida purpurea Nutt.(purple threeawn);Buchloedactyloides(Nutt.)Engelm.(buffalograss)andSchizachyrium scoparium(Michx.)Nash(littlebluestem)LOEC for biomassincrease50Zhukov andZudilkin(1971citedby Sheppard,1989)crop plant Probably Triticum aestivum EC11on yield50Sheppard et al.(1985)conifer Pinus sylvestris NOEC for growth O100Bufatin et al. (1986)crop plant rice(probably Oryza sativa)var.Krasnodarskiy424EC25for survival atharvest250Sheppard et al.(1992)crop plantsand coniferPhaseolus vulgaris,Zeamays,Lactuca sativa,Lycopersicon esculentum,Brassica rapa,Pinus strobusEC25for pod yield inbeans300Sheppard et al. (2004)grass Elymus lanceolatus NOEC for emergence,length and biomass100059 S.C.Sheppard et al./J.Environ.Radioactivity79(2005)55–8360S.C.Sheppard et al./J.Environ.Radioactivity79(2005)55–83EC25of0.5mg U kgÿ1dry soil(all soil concentrations in this paper are expressed on a soil dry weight basis).This study meets the data acceptance criteria established,but remains problematic because0.5mg U kgÿ1soil is below background concentrations in many settings.Four other studies(Table1),Shevchenko et al.(1987),Gulati et al. (1980),Zhukov and Zudilkin(1971)and Bufatin et al.(1986),each have significant shortcomings.Methods,especially statistical treatment,are not well described.Sheppard et al.(1985)grew Scots pine in small pots with a range of U concentrations and included a mixed-contaminant soil with U.No effects relative to controls were observed at100mg added U kgÿ1dry soil.As a no-observed-effects-concentration (NOEC)value,this is not itself a definitive study but is supportive of other results.Sheppard et al.(1992)provided the next most sensitive study(Table1).Several different plant species,endpoints,soils and experiments were used,including large outdoor lysimeters and examination of second-generation effects in plants.Although the results are presented in a somewhat unorthodox manner,the study handily meets the acceptance criteria and has been cited by others for regulation development (Fleming et al.,2000;CCME,2002).One shortcoming is that the U concentration series used was an unbalanced design,with4replicates of the control,2–3replicates of treatments between10and100mg U kgÿ1soil,and no replicates at300and 1000mg U kgÿ1soil.The results clearly showed no effects below300mg U kgÿ1soil, but there was less precision to define exact effect levels above300mg U kgÿ1soil. Most of the data were not presented graphically,the EC25was between300and 1000mg U kgÿ1soil,and was500mg U kgÿ1soil for seedling emergence of several crop plants.The soils used by Sheppard et al.(1992)were stored moist,outdoors in covered, in-ground containers for over ten years,and were then used again for toxicity tests (Sheppard et al.,2004).The concentrations of U were re-measured and remained unchanged.In order to assure a toxic response,aliquots of the‘‘aged’’soil with 1000mg U kgÿ1soil were further spiked with U to achieve3000mg U kgÿ1.The plant bioassay,formally developed by Environment Canada,was a22-d bioassay with northern wheatgrass(Elymus lanceolatus),where emergence and shoot and root lengths and total plant biomass were measured.There was a statistically significant decrease in shoot and root length and in total plant biomass at3000mg U kgÿ1 compared to controls in a neutral pH loam,and a statistically significant decrease in root length at3000mg U kgÿ1compared to controls in afine sand with pH6.2. The solid/liquid partition coefficients,K d,for U in these soils were0.060and 0.29m3kgÿ1,respectively.The results,after aging the U in the soil for10years,show even less toxicity to plants than in the original study(Sheppard et al.,1992).The remaining studies of plant response to soil U(not shown in Table1,see Sheppard,2003),Meyer and McLendon(1997)and Meyer et al.(1998),showed EC25values above10000mg U kgÿ1soil,but are of marginal relevance because they used pulverised U metal from munitions that is relatively insoluble,or U as the mineral schoepite.They did note a hormesis-like response,with increased growth at 50mg U kgÿ1.Several other authors reported hormesis(e.g.,Gulati et al.,1980).In some cases,this could be because uranyl nitrate was used and the plant is responding to the nitrate(Gulati et al.,1980used uranyl acetate,which is less likely to cause aneffect as a result of the anion).There remains uncertainty in the ecotoxicology literature on how to interpret hormesis.In this analysis,it is assumed that positive response to U,regardless of mechanism,is not a regulatory concern and so only detrimental responses are considered.Because of the paucity of reliable studies on the toxicity of U in soil,it is relevant to consider the results of solution culture studies (Boileau et al.,1985;Cannon,1952;Murthy et al.,1984;Panda et al.,2001;Prister and Prister,1970;Weinberger and Shiva Shankara Murthy,1985).These must be interpreted with considerable caution as there is no proof that effects at a specific solution U concentration have any bearing on potential effects at the same U concentration in soil pore water.This is especially the case with U,where very marked accumulation of U in cell walls of the root epidermis is documented.In solution culture,it is possible that the U in solution is almost fully entrained on the root surface,resulting in a markedly different concentration at the uptake membranes compared to what was intended to be in the bulk solution.In general,solution culture results are expected to show toxicity at relatively low concentrations because of the absence of soil mediation.The studies listed above showed effect concentrations from 5to 200mg L ÿ1.The pH of the corresponding solutions was not consistently controlled (or reported),but in several it was about or above pH 7.Based on several reports of soil solid/liquid partition coefficients,a value of 4–30L kg ÿ1(geometric mean of 10L kg ÿ1)would correspond to the pH of the solution culture solutions.Thus,5–200mg L ÿ1in soil pore water might correspond to total soil concentrations of 50–2000mg U kg ÿ1dry soil.These are broadly consistent with the effect levels observed by Sheppard et al.(1992)in whole soils,and so support conclusions from that study.Solution culture data are not sufficiently relevant to indicate the possibility of ecologically relevant toxicity at concentrations lower than those observed in studies with soils,so the estimated effect at 50mg U kg ÿ1soil (based on an EC 25for tomato root growth)is considered only as guidance.Summarizing the studies above,the most reliable and relevant study (Sheppard et al.,1992)indicates an overall EC 25in the range of 300–500mg U kg ÿ1dry soil,and this is supported by the solution culture studies.Given that there are few studies,it is appropriate to apply a safety factor to these results.However,Sheppard et al.(1992)placed most emphasis on U concentrations in the range of 10–100mg U kg ÿ1,and on over 10soils and 6plant species there was no effect at these concentrations.Thus,any safety factor applied should not lower the PNEC to below 100mg U kg ÿ1.A value of 250mg U kg ÿ1dry soil is proposed as the PNEC to be protective of terrestrial plants.5.2.Toxicity to other soil biotaThis encompasses a large number of organisms,from microbes to animals,and is not fully independent of effects on plants.In an ecological setting,an effect on one soil-related organism may well affect several or all others,including plants.There have been few studies of effects of U on other soil biota,and the range of results (Table 2)reflects in part the range of types of biota.61S.C.Sheppard et al./J.Environ.Radioactivity 79(2005)55–83Cannon (1952)cited early work by Stoklasa and Penkava (1928)that showed effects on soil N-fixing microbes (Azotobacter )at 5mg U kg ÿ1dry soil.This work is difficult to confirm.In contrast,Sheppard et al.(1992)observed (qualitatively)no effect of 300mg U kg ÿ1on nodulation of legumes,another N-fixing process.Sheppard et al.(1992)also measured phosphatase enzyme activity in soils,following the logic that effects on nutrient cycling need to be examined and that the chemicalTable 2Toxicity information for soil biota other than plants,with effect concentrations as mg U kg ÿ1dry soil,except as notedAuthorsBiota Species Endpoint Effect concentration Cannon (1952)soil N-fixing microbes Azotobacter 5Sheppard et al.(2004)collembolan Onychiurus folsomi EC 20for survival and reproduction in fine sand,pH 6.292–190Sheppard et al.(1992)soil enzyme activity phosphatase enzyme activity EC 25for amount of p -nitrophenol released for phosphatase enzymes 100Meyer et al.(1998)soil microbes soil microbes EC 25for respiration,corresponding LOEC was 500mg/kg 160Sheppard andEvenden (1992)earth-worm Lumbricus sp.LOEC for implied survival 54d,soils !10%clay 300Sheppard et al.(2004)collembolan Folsomia candida EC 20for survival in fine sand,pH 6.2350Sheppard et al.(2004)collembolan Onychiurus folsomi EC 20for survival and reproduction in 2loam soils,pH 7.5O 390Sheppard et al.(2004)collembolan Folsomia candida EC 20for survival and reproduction in 2loam soils,pH 7.5O 710Sheppard et al.(1992)earthworm Lumbricus sp.EC 25for earthworm survival 1000Sheppard andEvenden (1992)earthworm Lumbricus sp.NOEC for implied survival 54d,soils O 10%clay 1000Sheppard et al.(2004)earthworm Eisenia andrei NOEC for survival and reproduction,3soils 1000Norberg andMolin (1983)bacteria Zoogloea ramigera ,a common aerobic organism found in sewage treatmentplantsprolonged lag phase and decreased growth rate,solution culture5mg L ÿ1Labrot et al.(1996)earthworm Eisenia fetida EC 25for biomarkers,contact test1m g cm ÿ2Labrot et al.(1999)earthworm Eisenia fetida LC 5096h in contact test13m g cm ÿ2Ribera et al.(1996)earthworm Eisenia fetida andrei LC 50contact test 40m g cm ÿ262S.C.Sheppard et al./J.Environ.Radioactivity 79(2005)55–83analogy of U and P might result in some effects of U on P cycling in soil.The EC 25was as low as 100mg U kg ÿ1soil in an acidic sand and 400mg U kg ÿ1soil averaged across 10soils.Although consistent with effect levels observed for plants,there remains difficulty in the ecological interpretation of effects involving a soil enzyme system.Meyer et al.(1998)observed an LOEC for soil respiration at 500mg U kg ÿ1soil,with a corresponding EC 25of 160mg U kg ÿ1soil.This was at a specific time in their soil respiration study,at other times the EC 25was 1600mg U kg ÿ1soil.For other endpoints related to decomposition rate,the EC 25values were 1600–7000mg U kg ÿ1soil.These results suggest that the EC 25of 160mg U kg ÿ1soil may not be representative of the overall effect levels in the study:a higher value was more common.Ecological relevance is also less certain because of both the form of U,pulverised munitions metals,and the nature of the endpoints.The next most sensitive was a series of studies.The earliest examined survival of earthworms (Sheppard et al.,1992),and in a sandy soil they observed an EC 25of 1000mg U kg ÿ1soil.This soil was originally an acid forest soil,as fine sand,that was limed to pH 6.2to accommodate earthworm and plant bioassays.There was no effect of U in another neutral pH sandy-loam soil at U concentrations up to 1000mg U kg ÿ1soil.These were large,mature earthworms,and those surviving in the sandy-loam soil were actively processing soil,so that exposure to the soil U was assured.As a long-term exposure study,this is a credible NOEC value with direct ecological relevance.In a related study using 11different soils,Sheppard and Evenden (1992)reported on worm/soil U concentration ratios (CRs)for worms exposed to the soils for 54d.They did not report survival statistics,but concentrations in worms imply survival and the CR values indicate effects on the uptake process.In the absence of much ecotoxicology literature for U,this study is considered a surrogate.The results suggest survival (NOEC)at 1000mg U kg ÿ1soil if the soil clay content is above 10–20%,and a possible lowest observed effect concentration (LOEC)of 100–300mg U kg ÿ1soil if the clay content is less.The results for the organic soil are not useful.The effects on linearity of uptake were similar U concentrations,with an LOEC of 300–1000mg U kg ÿ1soil when the clay content is above 10%and an LOEC as low as 50–100mg U kg ÿ1soil for soils with lower clay contents.The limitations of these data are:the ecological relevance of the observations about linear uptake is not obvious,and the implied survival information is binary (yes/no)data rather than numerical scores.The soils used by Sheppard et al.(1992),including a loam soil not previously used in earthworm bioassays,were ‘‘aged’’moist and used again for toxicity tests (as described above for the plant bioassays and in Sheppard et al.,2004).Bioassays,formally developed by Environment Canada,for three different soil organisms were completed:an acute (14-d)survival and a chronic (56-d)reproduction bioassay with the earthworm Eisenia andrei ;a 28-d reproduction test with even-aged collembolan,Folsomia candida ,from a synchronous culture;and a 35-d reproduction test with mature adults of collembolan Onychiurus folsomi .There was no effect of 1000mg U kg ÿ1soil compared to controls on earthworm survival in any of the63S.C.Sheppard et al./J.Environ.Radioactivity 79(2005)55–8364S.C.Sheppard et al./J.Environ.Radioactivity79(2005)55–83soils,and no effect of1000mg U kgÿ1on reproduction in two of the soils.There was no reproduction in any treatment in the third soil.Clearly,E.andrei was not particularly sensitive to U.For F.candida,an EC20of350mg U kgÿ1soil for survival was observed in the limedfine sand with pH6.2,whereas reproduction was less sensitive in that soil and both survival and reproduction were less sensitive in the other soils(EC20from710to O3000mg U kgÿ1).Results for O.folsomi showed similar EC20in the neutral pH loam andfine sandy loam,from390to O1000mg U kgÿ1.Results for O.folsomi,a root-eating springtail,showed much greater sensitivity in the limedfine sand soil.In two different series of trials,survival and reproduction of this species in this soil was significantly affected.The EC20values were as low as 92mg U kgÿ1.There is no obvious explanation why this organism was especially sensitive.The soil was an acidic forest sand that had been limed10years earlier,and may not be typical habitat for O.folsomi.The remaining tests with earthworms are based on the OECD contact test,where worms are put into contact with moist,U-treatedfilter paper for a short interval and reported as m g U cmÿ2paper.Depending on the specific methods used,there is about 0.02cm3water cmÿ2paper.This test has some relevance for lipophilic organic compounds,especially pesticides,but is of severely limited value for inorganic con-taminants where effects are typically much slower and require some accumulation time in the tissues.The only reported sub-lethal effect level(Labrot et al.,1996)was for biomarkers at1m g cmÿ2,which assuming the above moisture content would (very crudely)coincide with50mg Lÿing a soil solid/liquid partition coefficient, K d,of10L kgÿ1,this would imply a soil concentration of500mg U kgÿ1soil.This is a highly speculative extrapolation,but somewhat supports the EC25from Sheppard et al.(1992).The study of Norberg and Molin(1983)is also somewhat removed from soil studies;they reported an EC25of5mg Lÿ1soil for Zoogloea ramigera,a common aerobic organism found in sewage treatment plants.The effect was a lag in growth in agar when U was present at pH7,and unfortunately did not change with U ing the5mg Lÿ1and the soil solid/liquid partition coefficient used earlier for this pH range(10L kgÿ1),a corresponding concentration in soil might be50mg U kgÿ1soil.This is lower than reported in the more relevant studies, and is not considered a definitive value.Summarizing the studies above,there is some indication from the phosphatase enzyme study of Sheppard et al.(1992),the collembolan test of Sheppard et al. (2004)and the soil respiration study of Meyer et al.(1998)that certain soil processes might be more sensitive to U than corresponding plant responses.Indeed,all3 studies included plants in their overall programs,and all reported some incidents of greater sensitivity in non-plant endpoints.However,the data are few,and the ecological relevance of the microbial endpoints is uncertain.The earthworm survival test of Sheppard et al.(1992)is more ecologically relevant and showed less sensitivity than corresponding plant bioassays.As a result,a PNEC of100mg U kgÿ1dry soil is proposed,to be lower than the PNEC for plants,but recognizing that there is a large diversity among the soil processes involved.。