SDAS对A357合金时效过程的影响
合金时效的作用
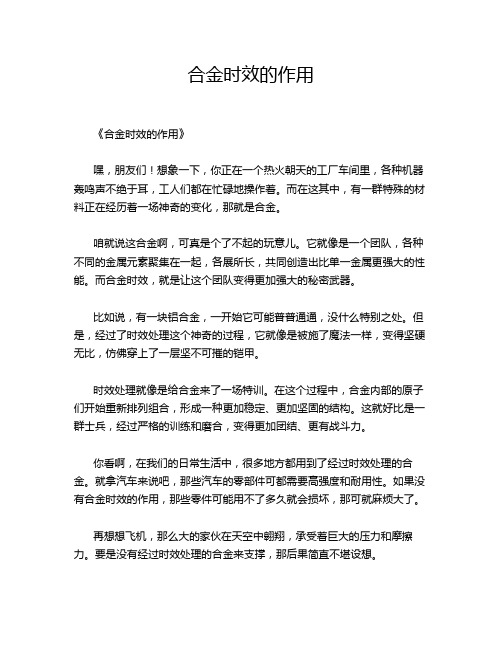
合金时效的作用《合金时效的作用》嘿,朋友们!想象一下,你正在一个热火朝天的工厂车间里,各种机器轰鸣声不绝于耳,工人们都在忙碌地操作着。
而在这其中,有一群特殊的材料正在经历着一场神奇的变化,那就是合金。
咱就说这合金啊,可真是个了不起的玩意儿。
它就像是一个团队,各种不同的金属元素聚集在一起,各展所长,共同创造出比单一金属更强大的性能。
而合金时效,就是让这个团队变得更加强大的秘密武器。
比如说,有一块铝合金,一开始它可能普普通通,没什么特别之处。
但是,经过了时效处理这个神奇的过程,它就像是被施了魔法一样,变得坚硬无比,仿佛穿上了一层坚不可摧的铠甲。
时效处理就像是给合金来了一场特训。
在这个过程中,合金内部的原子们开始重新排列组合,形成一种更加稳定、更加坚固的结构。
这就好比是一群士兵,经过严格的训练和磨合,变得更加团结、更有战斗力。
你看啊,在我们的日常生活中,很多地方都用到了经过时效处理的合金。
就拿汽车来说吧,那些汽车的零部件可都需要高强度和耐用性。
如果没有合金时效的作用,那些零件可能用不了多久就会损坏,那可就麻烦大了。
再想想飞机,那么大的家伙在天空中翱翔,承受着巨大的压力和摩擦力。
要是没有经过时效处理的合金来支撑,那后果简直不堪设想。
合金时效的作用可不仅仅是让材料变得更硬更强哦,它还能改善合金的其他性能呢。
就像一个全能选手,不仅力量强大,速度、耐力等方面也都很出色。
而且啊,时效处理的过程也挺有趣的。
就好像是一场精心策划的化学反应,各种元素在特定的条件下相互作用,最终产生出令人惊叹的效果。
有人可能会问了,那合金时效是不是很难呢?其实也没那么复杂啦。
科学家们和工程师们就像是一群聪明的魔法师,他们知道怎么去控制这个过程,让合金发挥出最大的潜力。
所以啊,可别小看了这合金时效。
它就像是一个默默无闻的英雄,在我们看不见的地方发挥着巨大的作用,让我们的生活变得更加安全、更加便捷。
总之,合金时效就是这么神奇,这么重要。
它让合金变得更加出色,为我们的现代生活提供了坚实的材料基础。
《2024年高压对Al-Mg-Si合金时效行为的影响》范文

《高压对Al-Mg-Si合金时效行为的影响》篇一一、引言铝基合金由于其在航空、汽车和电子工程等多个领域的广泛应用,其性能优化与处理工艺的深入研究具有重要意义。
其中,Al-Mg-Si合金作为一种典型的铝合金,具有优异的力学性能、加工性能以及耐腐蚀性能,是工程材料领域的常见选择。
本文主要关注的是高压环境下Al-Mg-Si合金的时效行为。
高压的引入对于该合金的性能有重要影响,能够加速原子运动、影响材料的结构及物理性质,进而影响其时效行为。
本文将从实验设计、结果分析等方面,深入探讨高压对Al-Mg-Si合金时效行为的影响。
二、实验设计本实验主要研究高压对Al-Mg-Si合金时效行为的影响。
首先,选取了不同成分的Al-Mg-Si合金作为研究对象,然后通过不同的高压处理条件(如压力大小、处理时间等)进行实验。
在实验过程中,我们采用了先进的X射线衍射仪、光学显微镜和扫描电镜等设备进行观察和分析。
通过时效处理和热处理后的力学性能测试,对比分析了不同高压条件下的时效行为变化。
三、实验结果与讨论(一)高压对Al-Mg-Si合金结构的影响实验结果表明,在高压环境下,Al-Mg-Si合金的晶格结构发生了明显的变化。
随着压力的增加,晶格参数和晶粒尺寸均有所减小,这表明高压能够促进原子间的紧密排列,提高合金的致密度。
此外,高压还可能引起合金中相的转变或相的细化,从而影响其力学性能和物理性质。
(二)高压对Al-Mg-Si合金时效行为的影响在时效过程中,高压对Al-Mg-Si合金的析出行为有显著影响。
在高压条件下,合金的析出速度加快,析出相的形态和分布也发生了明显的变化。
这些变化有利于提高合金的硬度和强度等力学性能。
同时,我们还发现,在一定的高压范围内,压力越大,合金的时效效果越好。
然而,当压力超过一定范围时,过高的压力可能会破坏合金的微观结构,导致时效效果下降。
(三)影响因素分析在高压条件下,合金元素的扩散速度加快,有利于合金元素的均匀分布和固溶度的提高。
用SAXS研究1420合金时效-回归-再时效析出δ'相
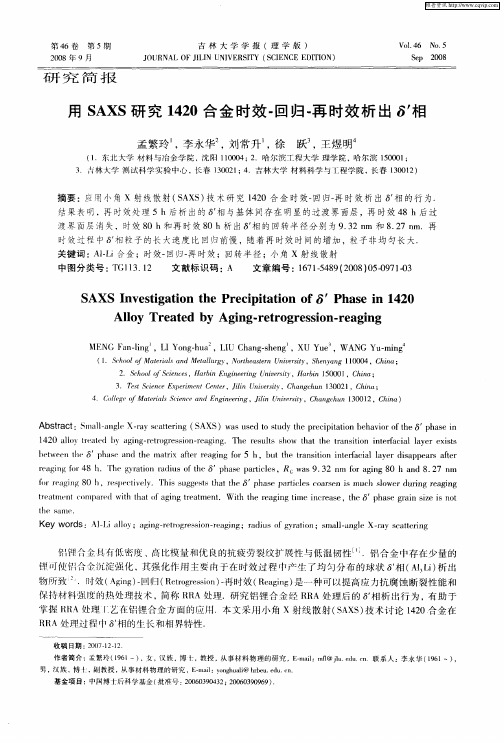
bewe n t e6 h s n h ti fe e g n o h.b tt e ta st n i tra ill y rd s p e r fe t e h p a e a d t e marx at rr a i g fr5 u h r n ii n er ca a e ia p a s at r o
M EN( Fa ln ,LIYo g h a ) n—i g n — u ,LI Ch ng s e g U a — h n ,XU Yue W ANG 。 Yu— i m ng
,
( .S h O o Ma r l a dMe l r) N r e s r n e i , h n a g 10 0 ,C ia 1 c O l / t i s n t l g ot at n U i r t S e y n 1 0 4 h ; ’ ea au , h e v sy n
1 2 l } r ae s g n —e r ge so —e g n . Th e u t h w h tt e ta sto it ra il ly r e it 4 0 a hy te td b ’a i g r to r s in r a i g e r s ls s o t a h r n iin n e f ca a e x ss
维普资讯
第4 6卷
第 5期
吉 林 大 学 学 报 (理 学 版 )
J U N L O I I N V R I Y ( C E C D T O O R A FJL N U I E ST S I N E E II N)
A357合金熔体结构变化的DSC分析

F OU N DRY T ECH NO LO GY ) 终止温 度 升温 587. 3 590 596 606. 3 587. 3 590 587. 3 590 降温 569. 6 570. 6 567. 4 565. 1 569. 6 570. 6 569. 6 570. 6 表3 原子键型 离解能 / ( kJ/ min)
表1 元素 含量 试验用铝硅合金 A357 的化学成分 Si 6. 88 Mg 0. 55 Mn < 0. 01 Fe 0. 12 w(%) Al balance T ab. 1 Chemical composition of A 357 allo y
。因
2 实验结果及分析 2. 1 综合热分析( DSC) 的实验结果 图 1 为铸造铝合 金 A357 在不 同的升温速率下, 由室温加热到 1 100 , 保温 10 m in, 再以同样的速率 降温到 200 的热流 - 温度曲线。 DSC 曲线 表明 : ! 合 金的 液相 线 温度 为 606. 3 ; ∀ 在液相以下, 加热过程出现了 3 个吸热峰; # 不 同加热速率的 DSC 曲线上 , 在液相线以上都不同程度 的出现了热效应峰。以图 1d 的升温过程为例, 在 821 ~ 925 处有明 显的放热峰, 或 者在 821~ 925 和 925~ 1 049 之间出现吸热反应; ∃加热和冷却时 2 条曲线并不重合 , 说明升温过程中发生的某些热反应 在降温过程中并没有完全恢复 ; % 加热速率越低, 曲线 上的波动越严重 , 当加热速率为 20 / m in 时, 曲线变
近年来大量涌现的实验事实表明
[ 1~ 4]
: 合金熔体
是一个由多种成分和多种尺度结构单元组成的呈熔融 状态的多相系统 , 其结构单元包括从单个原子到不同 尺度的原子团簇或原子集团 , 熔体中的这些原子集团 一方面在无序热运动中迁移碰撞, 不断地分裂解体 ; 另 一方面又有大量的原子或集团随热运动的涨落起伏, 并近程相互作用 , 不断地组成新的原子集团。这两种 运动过程并存, 是熔体结构显现出长程无序和在集团 区域内又短程有序的基本特征。 处于不同状态的合金熔体, 有不同特性的原子团 簇及其分布, 并直接影响到随后的凝固过程
《高压对Al-Mg-Si合金时效行为的影响》范文
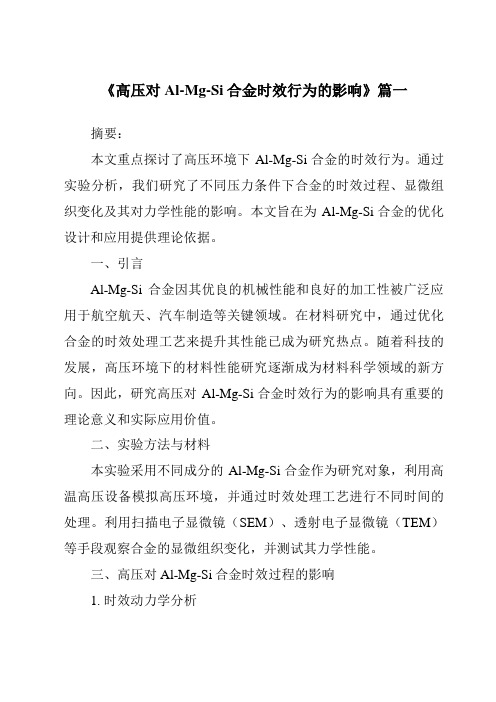
《高压对Al-Mg-Si合金时效行为的影响》篇一摘要:本文重点探讨了高压环境下Al-Mg-Si合金的时效行为。
通过实验分析,我们研究了不同压力条件下合金的时效过程、显微组织变化及其对力学性能的影响。
本文旨在为Al-Mg-Si合金的优化设计和应用提供理论依据。
一、引言Al-Mg-Si合金因其优良的机械性能和良好的加工性被广泛应用于航空航天、汽车制造等关键领域。
在材料研究中,通过优化合金的时效处理工艺来提升其性能已成为研究热点。
随着科技的发展,高压环境下的材料性能研究逐渐成为材料科学领域的新方向。
因此,研究高压对Al-Mg-Si合金时效行为的影响具有重要的理论意义和实际应用价值。
二、实验方法与材料本实验采用不同成分的Al-Mg-Si合金作为研究对象,利用高温高压设备模拟高压环境,并通过时效处理工艺进行不同时间的处理。
利用扫描电子显微镜(SEM)、透射电子显微镜(TEM)等手段观察合金的显微组织变化,并测试其力学性能。
三、高压对Al-Mg-Si合金时效过程的影响1. 时效动力学分析在高压环境下,Al-Mg-Si合金的时效过程呈现出加速现象。
随着压力的增加,合金中第二相析出速度加快,达到峰值时效状态的时间明显缩短。
这主要归因于高压环境下原子扩散速率的提高和相变驱动力增强。
2. 显微组织变化高压环境下,Al-Mg-Si合金的显微组织发生变化。
主要表现为第二相析出更加均匀、细小,基体中固溶体的晶格畸变程度增加,这有利于提高合金的力学性能。
四、高压对Al-Mg-Si合金力学性能的影响1. 硬度与强度随着压力的增加,Al-Mg-Si合金的硬度和强度均有所提高。
这主要归因于第二相的均匀析出和基体晶格畸变的增加,使得合金在受到外力作用时具有更好的抵抗变形能力。
2. 韧性及延展性虽然高压环境下合金的硬度和强度有所提高,但韧性及延展性并未受到明显影响,甚至在某些情况下有所改善。
这表明高压处理能够在提高Al-Mg-Si合金硬度的同时保持良好的韧性及延展性。
铝合金铸件凝固过程二次枝晶臂间距模拟计算
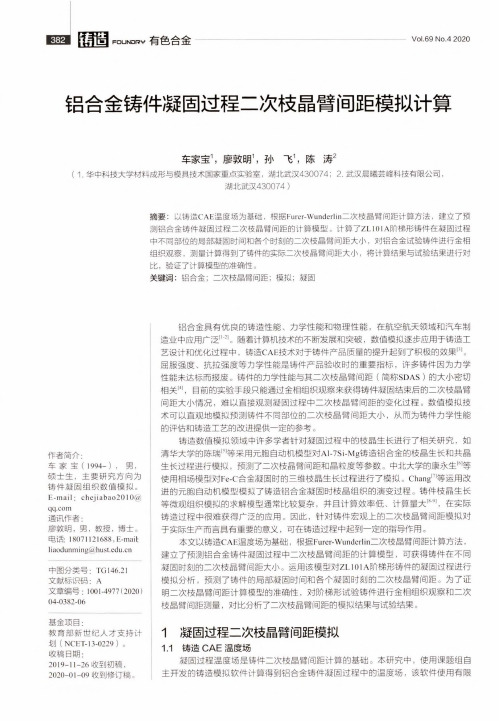
FOUNDRY有色合金Vol.69No.42020铝合金铸件凝固过程二次枝晶臂间距模拟计算车家宝S廖敦明’,孙飞",陈涛2(1.华中科技大学材料成形与模具技术国家重点实验室,湖北武汉430074;2.武汉晨曦芸峰科技有限公司,湖北武汉430074)摘要:以铸造CAE温度场为基础,根据Furer-Wunderlin二次枝晶臂间距计算方法,建立了预测铝合金铸件凝固过程二次枝晶臂间距的计算模型。
计算了ZL101A阶梯形铸件在凝固过程中不同部位的局部凝固时间和各个时刻的二次枝晶臂间距大小,对铝合金试验铸件进行金相组织观察,测量计算得到了铸件的实际二次枝晶臂间距大小,将计算结果与试验结果进行对比,验证了计算模型的准确性。
关键词:铝合金;二次枝晶臂间距;模拟;凝固作者简介:车家宝(1994-),男,硕士生,主要硏究方向为铸件凝固组织数值模拟。
E-mail:chejiabao2010@ 通讯作者:廖敦明,男,教授,博士。
电话:180****1688,E-mail:********************.cn中图分类号:TG146.21文献标识码:A文章编号:1001-4977(2020)04-0382-06基金项目:教育部新世纪人才支持计划(NCET-13-0229)。
收稿日期:2019-11-26收到初稿,2020-01-09收到修订稿。
铝合金具有优良的铸造性能、力学性能和物理性能,在航空航天领域和汽车制造业中应用广泛21。
随着计算机技术的不断发展和突破,数值模拟逐步应用于铸造工艺设计和优化过程中,铸造CAE技术对于铸件产品质量的提升起到了积极的效果'讥屈服强度、抗拉强度等力学性能是铸件产品验收时的重要指标,许多铸件因为力学性能未达标而报废。
铸件的力学性能与其二次枝晶臂间距(简称SDAS)的大小密切相关⑷,目前的实验手段只能通过金相组织观察来获得铸件凝固结束后的二次枝晶臂间距大小情况,难以直接观测凝固过程中二次枝晶臂间距的变化过程。
A357铸造铝合金特性研究的开题报告

A357铸造铝合金特性研究的开题报告
一、选题依据
随着航空航天、高速列车、汽车等高技术领域的迅猛发展,对材料
性能的要求越来越高,铸造铝合金作为轻质、高强度、优良的热膨胀系数、良好的导电性等特性,被广泛应用于航空、汽车、电子通讯等领域。
传统A356、A380等铸造铝合金有着熔点低、铸造性好、强度高等
优点,但受成分限制,无法满足更高的材料性能要求。
近年来,A357成
为了一种研究热点,其添加元素的特殊配比和制备工艺使其具备高强度、优异低温成形性和耐腐蚀性,成为广泛研究的对象。
二、研究内容
本研究将深入探究A357铸造铝合金的特性,重点包括以下内容:
1. A357铸造铝合金的化学成分及相结构分析;
2. A357铸造铝合金的热力学性能分析;
3. A357铸造铝合金的机械性能、热膨胀系数及耐腐蚀性能测试;
4. A357铸造铝合金的微观组织和取向分析。
三、研究意义
本项研究旨在深入探究A357铸造铝合金的特性,尤其是在机械性能、热膨胀系数及耐腐蚀性能方面的表现,在理论和实践方面都具有重要意义。
研究结果将为A357铸造铝合金的应用提供一定的理论基础和技术支持,为航空、汽车等领域的发展做出一定的贡献。
a357铝合金的半固态触变成形性能分析

由于液相的存在ꎬ会及时补充这部分体积ꎬ在变形
考虑到液压机操作空间的限制ꎬ模具加热装置采用
的最 终 阶 段 消 除 裂 纹ꎬ 得 到 表 面 质 量 较 好 的 模
电阻丝加热.在操作过程中ꎬ为了保护电热元件ꎬ使
锻件.
之不被挤压模具、模座碰触到ꎬ在实验过程中ꎬ用瓷
585 ℃ ꎬ保温时间设定在 25 min.
2) 加热速度.
加热速度对坯料内的温度梯度和金属的消耗
够满足本实验的需要.其线圈的额定电压是 380 Vꎬ
第 27 卷 第 2 期
Vol.27 No 2
兰州工业学院学报
2020 年 4 月
Journal of Lanzhou Institute of Technology
Apr.2020
文章编号:1009-2269(202坯料的原始组织.首先用线切割
方式将坯料加工成 ϕ10 × 12 mm 的小圆柱体ꎬ在一
端部切割取样ꎬ经过粗磨、研磨和抛光之后ꎬ再用
15%的 NaOH 溶液进行浸蚀ꎬ在金相显微镜下观察
试样的微观组织.图 1 是初始态的显微组.
图 2 筒形件的挤压模具
图 1 半固态坯料的原始组织
表 1.
表 1 合金成分
Si
6.50 ~ 7.50
Fe
≤0.12
Cu
≤0.10
Mg
0.45 ~ 0.70
Mn
≤0.05
Zn
≤0.05
Ti
0.04 ~ 0.2
%
Al
余量
收稿日期: 2019 ̄12 ̄24
基金项目: 安徽省教育厅高校自然科学重点项目( KJ2019A0892) ꎻ安徽省高校优秀拔尖人才培育资助项目( gxgnfx2019071) ꎻ安徽三联
A357合金组织和性能的优化的开题报告
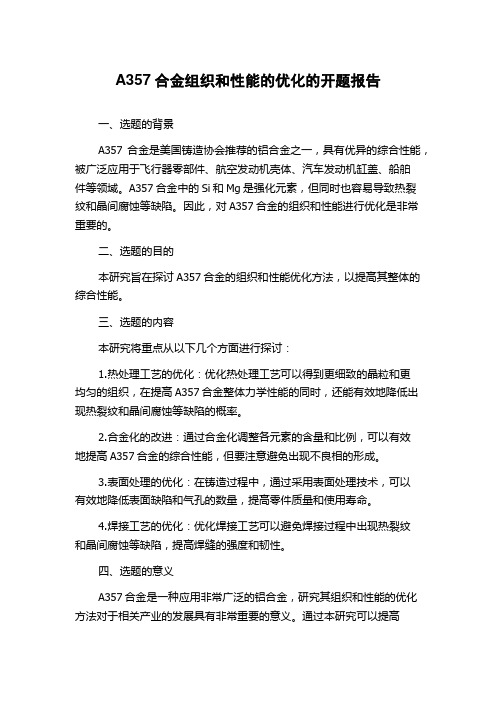
A357合金组织和性能的优化的开题报告
一、选题的背景
A357合金是美国铸造协会推荐的铝合金之一,具有优异的综合性能,被广泛应用于飞行器零部件、航空发动机壳体、汽车发动机缸盖、船舶
件等领域。
A357合金中的Si和Mg是强化元素,但同时也容易导致热裂纹和晶间腐蚀等缺陷。
因此,对A357合金的组织和性能进行优化是非常重要的。
二、选题的目的
本研究旨在探讨A357合金的组织和性能优化方法,以提高其整体的综合性能。
三、选题的内容
本研究将重点从以下几个方面进行探讨:
1.热处理工艺的优化:优化热处理工艺可以得到更细致的晶粒和更
均匀的组织,在提高A357合金整体力学性能的同时,还能有效地降低出现热裂纹和晶间腐蚀等缺陷的概率。
2.合金化的改进:通过合金化调整各元素的含量和比例,可以有效
地提高A357合金的综合性能,但要注意避免出现不良相的形成。
3.表面处理的优化:在铸造过程中,通过采用表面处理技术,可以
有效地降低表面缺陷和气孔的数量,提高零件质量和使用寿命。
4.焊接工艺的优化:优化焊接工艺可以避免焊接过程中出现热裂纹
和晶间腐蚀等缺陷,提高焊缝的强度和韧性。
四、选题的意义
A357合金是一种应用非常广泛的铝合金,研究其组织和性能的优化方法对于相关产业的发展具有非常重要的意义。
通过本研究可以提高
A357合金的整体综合性能,降低缺陷率,提高零件质量和使用寿命,促进相关产业的发展。
添加Cu对A357合金过时效行为及室温、高温拉伸和疲劳性能的影响

Trans. Nonferrous Met. Soc. China 30(2020) 2861−2878Effect of Cu addition on overaging behaviour, room and high temperature tensile and fatigue properties of A357 alloyLorella CESCHINI1, Simone MESSIERI2, Alessandro MORRI1,Salem SEIFEDDINE3, Stefania TOSCHI4, Mohammadreza ZAMANI31. Department of Industrial Engineering (DIN), Alma Mater Studiorum, University of Bologna,Viale Risorgimento 4, 40136 Bologna, Italy;2. Ducati Motor Holding, Via Cavalieri Ducati Antonio 3, 40132 Bologna, Italy;3. Department of Materials and Manufacturing, School of Engineering,Jönköping University, Gjuterigatan 5, 55111 Jönköping, Sweden;4. Department of Civil, Chemical, Environmental and Materials Engineering (DICAM), Alma Mater Studiorum,University of Bologna, Viale Risorgimento 4, Bologna, ItalyReceived 7 January 2020; accepted 16 July 2020Abstract: The aims of the present work are to evaluate the overaging behaviour of the investigated Cu-enriched alloy and to assess its mechanical behaviour, in terms of the tensile and fatigue strength, at room temperature and at 200 °C, and to correlate the mechanical performance with its microstructure, in particular with the secondary dendrite arm spacing (SDAS). The mechanical tests carried out on the overaged alloy at 200 °C indicate that the addition of about 1.3 wt.% Cu to the A357 alloy enables to maintain ultimate tensile strength and yield strength values close to 210 and 200 MPa, respectively, and fatigue strength at about 100 MPa. Compared to the quaternary (Al−Si−Cu−Mg) alloy C355, the A357−Cu alloy has greater mechanical properties at room temperature and comparable mechanical behaviour in the overaged condition at 200 °C. The microstructural analyses highlight that SDAS affects the mechanical behaviour of the peak-aged A357−Cu alloy at room temperature, while its influence is negligible on the tensile and fatigue properties of the overaged alloy at 200 °C.Key words: A357 alloy; C355 alloy; Al−Si−Cu−Mg casting alloy; tensile property; fatigue behaviour; high temperature; overaging1 IntroductionCast aluminium alloys are commonlyemployed to produce castings for automotiveapplications, in particular for engine parts, due totheir high specific strength, excellent castability,and good corrosion resistance. Among the mostimportant components, it is worth mentioning theengine block and the cylinder head. As reported byJA VIDANI and LAROUCHE [1], while in the past,such components were usually manufactured fromcast iron because of its mechanical strength andhigh-temperature resistance, nowadays, the currentincreasing demand to limit fuel consumption andreduce pollutant emissions gradually has leddesigners to prefer lighter materials. In this sense,aluminium alloys possess lower density and highspecific strength and are characterised by anexcellent thermal conductivity, which allows thecombustion heat to be extracted more rapidlycompared to cast iron.Among the most commonly employedalloys, ternary hypoeutectic alloys of Al−Si−Mg(e.g., A356 and A357 alloys) and Al−Si−Cu (e.g.,A319) types should be mentioned. Al−Si−Mg an dCorresponding author:AlessandroMORRI;E-mail:**************************DOI:10.1016/S1003-6326(20)65427-9Lorella CESCHINI, et al/Trans. Nonferrous Met. Soc. China 30(2020) 2861−2878 2862Al−Si−Cu alloys can be heat-treated to obtain elevated mechanical strength by precipitation hardening. According to JA VIDANI and LAROUCHE [1], peak-aged Al−Si−Mg alloys present good mechanical properties, elevated ductility and excellent castability; as a drawback, mechanical strength decreases with long-term exposure at high working temperatures (near or higher than 200 °C). Mg2Si precipitates easily undergo diffusion-controlled coarsening leading to a progressive loss of their effectiveness in hindering dislocation slip, which results in loss of mechanical strength of the α(Al) matrix under the overaged conditions. Conversely, owing to the high content of Cu (3−4 wt.%), Al−Si−Cu alloys generally exhibit a higher mechanical strength and a superior overaging resistance in comparison to Al−Si−Mg, but are characterised by the lower ductility and castability, with a higher tendency to interdendritic shrinkages and hot tearing [1−3].FARKOOSH and PEKGULERYUZ [3] pointed out that, in view of the specific conditions engine parts operate (which involve thermo- mechanical fatigue associated with the cyclic loading during engine heat-up and cool-down), a compromise between high tensile strength and ductility should be obtained to avoid undesired premature failure. Furthermore, the recent design trend has led to increase in the maximum engine working temperature and pressure, aiming to improve engine efficiency and to reduce fuel consumption and pollutant emissions by downsizing engines. As a result, the need for increased mechanical strength, superior thermal stability while maintaining good castability, led to the development of quaternary hypoeutectic Al−Si−Cu−Mg alloys, containing a limited content of Cu (≈1−2 wt.%), combining benefits of both Mg and Cu additions [4−10]. The simultaneous presence of Cu and Mg provided through aging treatment and the formation of Q quaternary precipitates (Al5Cu2Mg8Si6) characterised by higher coarsening resistance than the binary Al2Cu or Mg2Si have been reported by FARKOOSH and PEKGULERYUZ [3].To design an alloy with a good compromise between high performance at the T6-state and overaging (related to the high Cu and Si levels), in which the hot tearing tendency induced by high Cu contents is minimised and good castability is maintained, is a challenge that needs to be addressed.The aim of the present work was therefore to assess the microstructure and mechanical properties of a modified quaternary Al−Si−Cu−Mg alloy developed to produce motorbike engine heads. The alloy is designed based on a typical A357 alloy, containing 7 wt.% Si and 0.6 wt.% Mg, but with the addition of about 1.3 wt.% Cu. Compared to A354 and C355 quaternary alloys [8−10], employed at the industrial scale for cast engine parts, the A357−Cu alloy presents medium values of Si (compared to 9 wt.% and 5 wt.% for A354 and C355, respectively) and Cu (1.5 wt.% and 1 wt.% for A354 and C355, respectively) and slightly high values of Mg (0.5 wt.% for both A354 and C355). In particular, this study aims to: (1) evaluate the solutioning and hot isostatic pressing (HIP) temperatures, in order to avoid incipient melting; (2) assess the effect of secondary dendrite arm spacing (SDAS) on the mechanical strength; (3) evaluate the overaging behaviour of the alloy, by assessing its overaging curves and (4) investigate tensile and fatigue behaviours at room temperature and at 200 °C, both at the T6 condition and after overaging. A comparison with the most widely used Al−Si−Mg alloys (A356 and A357) and the high-performance Al−Si−Cu−Mg alloy (C355) with different SDAS values was also carried out, in order to assess the performance of the new alloy characterised by solidification-induced microstructural features similar to those of real sand and die castings.2 ExperimentalCastings of A357−Cu alloy were produced in a laboratory furnace according to the following experimental procedure. The molten base AlSi7Mg alloy was kept at 750 °C for about 3 h. An appropriate amount of Al−Cu and Al−Sr master alloys were added to reach the target composition (≈ 1.3 wt.% Cu, 150×10−6 Sr). Mg content was then properly tuned by the addition of Al−Mg master alloy. The melt was then poured into a permanent Cu die, pre-heated at 250 °C and with a graphite coating to avoid interaction between molten metal and mould. The chemical composition of the cast alloy was measured with an optical emission spectrometer (OES), and the results are listed in Table 1.Lorella CESCHINI, et al/Trans. Nonferrous Met. Soc. China 30(2020) 2861−2878 2863Table 1Chemical compositions of A357−Cu casting and A356 and C355 alloys used for comparison, measured by OES (wt.%)Alloy Si Cu Mg Fe Mn Ti Sr Al A357−Cu 6.770 1.290 0.658 <0.03 <0.001 0.135 1.40×10−2Bal.C355 4.990 1.050 0.470 0.138 0.021 0.133 2.10×10−2Bal.A356 7.240 <0.001 0.420 0.138 0.007 0.120 1.50×10−2Bal.The solidified cylindrical bars were then processed to obtain the desired secondary dendrite arm spacing (SDAS), by remelting the bars through a Bridgman furnace allowing controlled cooling conditions as described by SEIFEDDINE [11]. The bars were remelted at 730 °C for 30 min under Ar atmosphere, and then were directionally solidified by fixed machine speeds and cooling media (Table 2) to obtain cast samples characterized by fine (FS) and coarse (CS) microstructures, with average SDAS values ranging between 20−25 µm and 50−70 µm, respectively. Samples were then subjected to hot isostatic pressing (HIP) at 490 °C for about 2 h, in order to eliminate internal porosity, and were then heat-treated by solutionizing at 530 °C for 20 h, quenching in the water at 60 °C and artificially aged at 190 °C for 5.5 h. Table 3 shows the processing conditions of the tested alloy and those of the C355 and A356 alloys used for comparison.Table 2Bridgman furnace processing parameters and target SDAS values for samples with fine and coarse microstructuresPulling velocity/(mm·s−1) Cooling Target SDAS/µm0.3 Water 20−25 (FS)0.03 Air 50−70 (CS)Table 3 Heat treatment conditions of studied alloys Alloy HIP Solution treatmentA357−Cu 490 °C, 2 h 530 °C, 20 hC355 490 °C, 2 h 530 °C, 24 hA356 490 °C, 2 h 535 °C, 4.5 hAlloy Quenching Aging at T6 conditionA357−Cu Water, 60 °C 190 °C, 5.5 hC355 Water, 60 °C 180 °C, 6 hA356 Water, 60 °C 160 °C, 4.5 hMicrostructural analyses were carried out on the as-cast and heat-treated samples, using optical microscopy (OM) and scanning electron microscopy equipped with energy-dispersive X-ray spectroscopy (SEM−EDS). Metallographic samples were cut from the as-cast and heat-treated samples, embedded in resin, then ground and polished by sandpapers and diamond suspensions up to 1 µm. Chemical etching with a 0.5% HF solution was then performed to highlighting microstructural features.The secondary dendrite arm spacing was obtained by image analysis of the optical micrographs obtained for the as-cast alloy, calculated as the average on 15 images at 50-times magnification. The area fraction of intermetallic compounds was evaluated by contrast image analysis on SEM micrographs. In order to normalize the investigated area to the coarseness of microstructure, 14 fields at 500-times and 16 fields at 1500-times were analysed for coarse and fine samples, respectively, as described by CESCHINI et al [9].Differential scanning calorimetry (DSC) analyses were carried out on as-cast and heat-treated samples, aiming to evaluate possible phase transformations occurring during heating. DSC was performed on 40 mg samples, under a purified argon atmosphere, employing a 10 °C/min scan rate in the temperature range of 25−680 °C.The overaging behaviour of A357−Cu alloy was evaluated by subjecting T6-treated samples to high-temperature soaking at 200, 245 and 290 °C for fixed time intervals up to 168 h, then evaluating the corresponding hardness variation. Brinell hardness tests were carried out with 2.5 mm ball and 62.5 kg load, according to the ASTM E 10−18 standard [12] hereafter referred to as HB. The average of at least six measurements for each overaging condition was reported in the alloy overaging curves.The tensile properties and fatigue behaviour of the A357−Cu alloy were evaluated in three different conditions: (1) at room temperature in the T6 condition (T6-RT), (2) at 200 °C in the T6 conditionLorella CESCHINI, et al/Trans. Nonferrous Met. Soc. China 30(2020) 2861−2878 2864(T6-200 °C) and (3) at 200 °C in the overaged condition induced by soaking at 210 °C for 41 h (OA-200 °C). The overaging temperature and time considered in the present study were chosen according to the typical average operating conditions of racing engine heads.Round dog-bone tensile specimens (L0=25 mm, gauge diameter d0=5 mm) were machined from the heat-treated and overaged (subjected to soaking at 210 °C for 41 h) bars. Tensile tests were carried out at room temperature and at 200 °C on a screw- testing machine according to the ISO 6892-1 and ISO 6892-2 [13,14]. Tensile data were obtained from the data acquisition system and calculated as the average of at least three samples for each investigated condition. Samples tested at 200 °C were maintained at that temperature for 90 min aiming to homogenize the temperature before running the test.Rotating bending fatigue tests were performed using the staircase method according to the UNI 3964 standard [15] to evaluate the 50% reliability endurance limit; fifteen samples for each investigated condition were tested. Fatigue samples were machined from the heat-treated and overaged (subjected to soaking at 210 °C for 41 h) bars; fatigue specimen sizes are shown in Fig. 1. Tests were carried out at 50 Hz of frequency, setting the run-out to 2×106 cycles. The A357−Cu alloy was tested both at room temperature and 200 °C, in the latter case allowing the temperature to homogenize before running the test for 60 min. The testing conditions are listed in Table 4.Fig. 1 Schematic diagram of fatigue sample (Unit: mm) Fractographic analyses were carried out on both tensile and fatigue fracture surfaces by means of a multi-focus microscope and SEM−EDS, aiming to assess the mechanisms of failure and the crack nucleation sites.A comparison of the overaging behaviour, the tensile and fatigue behaviour of the A357−Cu alloy with other (previously characterised [8,10,16]) ternary and quaternary Al-based alloys, namely the A356 (Al−Si−Mg) and C355 (Al−Si−Cu−Mg) alloys, was carried out.Table 4Tensile and rotating bending fatigue testing conditions (X=Performed, 0=Not performed)MicrostructuralclassHeattreatmentconditionTestingtemperatureTensiletestFatiguetest FineT6RoomtemperatureX XT6 200 °C X 0Overaged 200 °C X X CoarseT6RoomtemperatureX XT6 200 °C X 0Overaged 200 °C X X3 Results and discussion3.1 As-cast microstructureThe microstructure of the as-cast A357−Cu alloy consists of primary α(Al) dendrites surrounded by the eutectic structure (Fig. 2). Due to the Sr modification, eutectic Si particles appeared evenly distributed and generally characterised by a fine fibrous morphology. However, differently from fine samples, coarse samples presented some areas with partially modified eutectic Si (Fig. 2(b)). This can be ascribed to the effect of the cooling rate on the modification of eutectic silicon particles, in agreement with previous works on different Al−Si alloys [8,9]. Results of SDAS calculations are listed in Table 5, highlighting a good agreement between the expected SDAS values and the measured ones.3.2 Thermal analysesDifferential thermograms of as-cast and heat-treated A357−Cu samples are reported in Fig. 3. According to Refs. [17,18], Peaks 1−5 which were detected in the as-cast state of both FS and CS alloys, are consistent with the following reactions: Peak 1: Formation of precipitatesPeak 2: Melting of Al2Cu/Q-Al5Cu2Mg8Si6Peak 3: Melting of Al−Si eutecticPeak 4: Melting of α(Al)Calculated areas and onset temperature corresponding to P eaks 1−4 are listed in Table 6 and are in agreement with data reported in elsewhere [17]. During heating, the alloy firstlyLorella CESCHINI, et al/Trans. Nonferrous Met. Soc. China 30(2020) 2861−2878 2865Fig. 2 Optical micrographs of as-cast A357−Cu alloy with fine (a) and coarse (b) microstructuresTable 5 Expected and measured SDAS values calculated on optical micrographs of FS and CS samplesCondition SDAS/μm FS CS Target 20−25 50−70 Measured30±370±5experiences the precipitation of intermetallic phases, at 240 °C. According to the literatures [3,19], this exothermic peak corresponds to the formation of quaternary Q -Al 5Cu 2Mg 8Si 6 precipitates. By increasing the temperature, before melting of the Al −Si eutectic and α(Al) (Peaks 3 and 4, respectively), an endothermic reaction (Peak 2) is observed, corresponding to the melting of Cu- bearing intermetallics (Q -Al 5Cu 2Mg 8Si 6 and θ-Al 2Cu) that developed during alloy solidification [7,20]. It has been reported that polynary eutectic phases (Q -Al 5Cu 2Mg 8Si 6) with a low melting point areformed when the Cu content is more than 2 wt.%, and the onset melting temperature is around 507 °C, while the melting point of Al 2Cu is around 520 °C.Fig. 3 DSC thermograms during heating of FS and CS samplesTable 6 Peak areas and onset temperature calculated on DSC thermograms of as-cast alloysPeak FSCSHeat/ (J·g −1) Onset temperature/°C Heat/ (J·g −1) Onsettemperature/°C 1−13.07 235 −5.90 241 2 0.90 507 2.21 508 3 158.90 544 141.30 545 453.2261860.56618Such reaction is typical of quaternary alloys:the solution treatment temperature must be chosen according to this endothermic peak, to avoid the incipient melting and the generation of cavities within the casting. Solution treatment is therefore generally carried out: at a lower temperature, by optimisation of solution time, to fully homogenise and dissolve coarse solidification phases, or in multiple stages, the first at a temperature lower than that of the endothermic reaction (to dissolve the low melting compound) and the second at a higher temperature, to foster the alloying element ’s dissolution. In the latter case, if the alloy undergoes HIP, the HIP process replaces the first stage of the solution treatment; it is important, therefore, to carefully define the HIP temperature to dissolve coarse low-melting intermetallics and to avoid incipient melting.By comparing the thermograms of FS and CS samples in the as-cast condition, two mainLorella CESCHINI, et al/Trans. Nonferrous Met. Soc. China 30(2020) 2861−2878 2866differences should be observed, related to Peaks 1 and 2, respectively. Peak 1, associated with the precipitation of Q phase, is more relevant in the FS sample compared to the CS sample. This difference, resulting in different peak areas (Table 6), is ascribed to the higher level of supersaturation induced by the higher solidification rate employed for the production of FS sample. It is therefore inferred that FS sample, in the as-cast condition, contains a lower amount of strengthening Q phase than the CS sample [3]; the Q phase, however, precipitates from the supersaturated solution during heating increasing the Peak 2 area. On the other hand, a coarse microstructure presents a more consistent Peak 2 in comparison to fine microstructure (2.214 vs 0.903 J/g), associated with the incipient melting of Cu-based phases. The lower solidification rate employed for CS sample, indeed, induced a higher level of segregation, thus a higher amount of low-melting compounds. After T6 treatment, it is observed that Peak 2 disappeared in both FS and CS samples. It is therefore inferred that Cu-based low melting compounds underwent complete dissolution, as a result of the solution treatment.3.3 Evolution of microstructure with solutiontreatmentThe evolution of microstructure with heat treatment was assessed by SEM analyses. In the as-cast state, Cu and Mg are entrapped in the coarse intermetallic particles formed during solidification. Their chemical composition, estimated by means of EDS analyses (Fig. 4), indicates that the stoichiometry of the observed particles is compatible with that of θ-Al2Cu (both in eutectic and blocky morphology), Mg2Si, π-Al8Mg3FeSi6, Q-Al5Mg8Cu2Si6[3,6,8−10]. Such intermetallics were observed both in FS and CS samples (Table 7), with a higher amount in the CS sample (about 1.60% area fraction) in comparison to the FS sample (about 1.40%). This result is in agreement with the previously reported DSC results (Fig. 2), showing higher energy associated with Peak 2 as a result of a higher segregation level induced by the lower solidification rate. Moreover, as previously observed in other quaternary alloys [8−10], the average size of intermetallic particles is strongly influenced by the solidification rate and, due to the higher time available for growth, the coarse microstructure is characterised by larger intermetallics in comparison to the fine one (Fig. 5). No β-Fe particles, were observed, either in fine or coarse microstructures, due to the low amount of Fe present in the alloy (~0.05 wt.%).The evolution of coarse intermetallic particles with heat treatment, in terms of response to solutioning, was then assessed. As expected, the total area fraction of intermetallic particles decreases after T6 heat treatment. It should be noticed that the residual amount of intermetallics (Table 7) is lower for fine alloy compared to coarse microstructure (about 0.80% and 0.90% area fraction for FS and CS samples, respectively). This should be ascribed to the effect of microstructural fineness on the response to solution treatment. As previously observed, intermetallic particles contained in the FS sample are characterised by smaller size than those present in CS sample; as a result, diffusion mechanisms and, therefore, the dissolution of particles are facilitated.With regard to the chemical composition of intermetallics after heat treatment, both in FS and CS samples, θ-Al2Cu particles were dissolved to an acceptable degree, independently from their morphology (blocky or eutectic). It is known that eutectic and blocky particles are subjected to different dissolution mechanisms [7,20,21]: while the dissolution of eutectic Al2Cu occurs by necking of the particle in several points, leading to subsequent fragmentation into small fragments and final dissolution, blocky phases undergo gradual dissolution of the entire particle, which generally requires longer time and higher temperatures. It is inferred that soaking the alloy at 530 °C for 20 h is sufficient to completely dissolve both the morphologies, regardless of size. Similarly, Mg2Si particles are completely dissolved after T6 treatment. This is somehow expected since these particles are easily brought into solution by heat treatment, as reported by SJÖLANDER and SEIFEDDINE [20]. Differently, from Al2Cu and Mg2Si, quaternary phases such as π-Al8Mg3FeSi6 and Q-Al5Mg8Cu2Si6were still observed in the solution treated condition, independently from the microstructural fineness. Q and π phases are known to be stable also after long-term exposure to high solution treatment temperatures. It is possible that partial fragmentation occurred, but no quantitative analyses were carried out to check this.Lorella CESCHINI, et al/Trans. Nonferrous Met. Soc. China 30(2020) 2861−2878 2867Fig. 4 SEM images and EDS analyses of intermetallics observed in FS (a −d) and CS (e −h) samples (The data in the brackets refer to elements present in the α(Al) matrix surrounding the analyzed phases)3.4 Mechanical behaviour3.4.1 Overaging effect on hardnessThe effect of high-temperature exposure on the hardness of the A357−Cu alloy is shown in Fig. 6,which includes the overaging curves at 200, 245, and 290 °C for time up to 168 h. The starting condition is the T6 state, corresponding to a peak hardness value equal to HB 126. It is possible toLorella CESCHINI, et al/Trans. Nonferrous Met. Soc. China 30(2020) 2861−2878 2868Table 7 Average intermetallic area fraction evaluated onFS and CS samples in as-cast state and after solutiontreatmentSampleAverage intermetallic area fraction/% As-cast state After solution treatmentFS 1.40 0.80 CS 1.60 0.90 observe that, in the first exposure time, increasing the overaging temperature from 200 to 290 °C leads to a more significant loss of hardness: after 4 h at 200 °C the hardness decrease is basically negligible (−2%), while at high temperatures of 245 and 290 °C loss of 21% and 45% with respect to the initial T6 condition is recorded. This behaviour can be ascribed to the diffusion-driven precipitatesFig. 5 SEM images of A357−Cu alloy in as-cast state for FS (a) and CS (b) samples and after T6 treatment for FS (c) and CS (d) samplesFig. 6 Overaging curves of T6 A357−Cu alloy at 200 °C, 245 °C and 290 °C (a) and corresponding focus on the first 24 h (b)Lorella CESCHINI, et al/Trans. Nonferrous Met. Soc. China 30(2020) 2861−2878 2869coarsening phenomena occurring at high temperatures. While after 4 h at 200 °C no effect is obtained, by soaking at 245 and 290 °C, diffusion mechanisms are fostered. As a result, the strengthening precipitates formed by aging are subjected to a rapid coarsening, leading to a loss of coherence with the surrounding α(Al) matrix and the consequent decrease of alloy strength. Increasing the overaging time leads to a further decrease in hardness, depending on the soaking temperature. At 200 °C, and soaking time longer than 16 h, a gradual hardness decrease is recorded, reaching the minimum value of HB 86 for the maximum investigated time (168 h), corresponding to a 32% decrease compared to the starting condition. At 245 and 290 °C, growth and coarsening kinetics are so fast that most of the total hardness loss, compared to the T6 condition, occurs in the first 24 h. In this condition, the alloy lost 37% and 54% of its initial hardness at 245 and 290 °C, respectively. At these temperatures, hardness curves present a plateau, representing the minimum hardness values of HB 61 and HB 51 at 245 and 290 °C, respectively, corresponding to total decrease of 52% and 60% with respect to the starting condition.A comparison of the overaging behaviour of the A357−Cu alloy with that of the A356 and A357 (Al−Si−Mg), and C355 (Al−Si−Cu−Mg) alloys is presented in Fig. 7 at temperatures of 200, 245 and 290 °C. The beneficial effect of adding Cu to A357 is clearly observable, leading to a significantly high residual hardness for the Cu-enriched alloy. Although the starting hardness is similar between the two alloys (HB 126 and HB 118 for A357−Cu and A357, respectively), the rate of hardness loss in the first 8 h is significantly higher for the A357 alloy. After about 48 h, a difference of about HB 20−30 is registered between the two alloys, remaining constant up to 168 h at all the tested overaging temperatures. Therefore, while the presence of Mg alone (generating Mg2Si by precipitation hardening) makes A357 suitable for the production of components that work at temperature lower than 200 °C, the addition of Cu (enabling the precipitation of Cu-bearing phases and in particular of Q′as reported also by FARKOOSH and PEKGULERYUZ [3]), makes it possible to maintain high hardness values at 200 °C also for long soaking time (168 h).The positive effect of Cu addition is even more evident at high temperatures. After 6 h of soaking at 245 °C and 290 °C, in fact, A357 alloy lost more than 50% of its initial hardness, while, at equal soaking time, the hardness losses of A357−Cu alloy are lower than 30% and 50% at 245 and 290 °C, respectively.The graphs, moreover, indicate that the overaging curves at 200 °C of the A356 and C355 alloys are superimposed to those of A357 and A357−Cu alloy, respectively, while at 245 and 290 °C, C355 and A357 alloys have slightly higher hardness compared to A357−Cu and A356, respectively.3.4.2 Tensile and fatigue testsResults of mechanical tests carried out on the A357−Cu alloy are summarised in Fig. 8, where data of fine (SDAS 30 µm) and coarse (SDAS 70 µm) microstructures are compared. The T6 heat-treated alloy was tested both at room temperature and high temperature (200 °C), while the overaged alloy (after soaking at 210 °C for 41 h) only at high temperature.The results of room temperature tensile characterisation confirmed the well-known beneficial effect of decreasing SDAS on tensile properties, as reported for other alloys [8,9]. The FS samples show higher yield strength (YS), ultimate tensile strength (UTS), elongation to failure (El), (≈5%, 8% and 40% respectively), compared to the CS ones. The intimate connection among UTS, El and SDAS has been documented in ternary Al−Si−Mg [22−25] and Al−Si−Cu systems [8,9]. The increase in YS, instead, is mainly related to the strengthening precipitates generated through precipitation hardening. The higher effectiveness of the solution treatment on smaller intermetallics present in FS, compared to larger intermetallics of the CS, may have lead to a higher degree of oversaturation [20,21] and consequently to the precipitation of a higher amount of strengthening precipitates during artificial aging. This is in agreement with microstructural observations, which shows a total area fraction of intermetallic particles after T6 heat treatment lower for the FS sample (≈0.8%) compared to the CS sample (≈0.9%). As previously observed, in fact, intermetallic particles contained in the CS alloy are characterised by a larger size than those present in FS samples. Their dissolution during solutioning, therefore, is more。
A357铸造铝合金疲劳裂纹偏折研究的开题报告

A357铸造铝合金疲劳裂纹偏折研究的开题报告该开题报告主要研究A357铸造铝合金疲劳裂纹偏折问题。
具体内容包括以下几个方面:1. 研究背景和意义:铝合金在航空、汽车、轨道交通等领域具有重要应用价值,而疲劳是铝合金材料失效的主要原因之一。
疲劳裂纹偏折是疲劳裂纹扩展过程中的重要现象,会导致裂纹扩展方向与力的作用方向有偏差,最终影响材料的使用寿命。
因此,研究A357铸造铝合金疲劳裂纹偏折问题,对于提高铝合金材料的疲劳性能,延长其使用寿命具有重要意义。
2. 研究现状分析:目前,关于铝合金材料疲劳裂纹偏折问题的研究已经取得了一定的进展。
主要采用数值模拟和实验研究相结合的方法,分析疲劳裂纹偏折的机理、影响因素以及对材料疲劳性能的影响。
但是,在A357铸造铝合金疲劳裂纹偏折问题的研究方面,尚缺乏系统性、深入的研究。
3. 研究内容:本研究通过建立A357铸造铝合金的疲劳裂纹偏折数值模型,采用数值模拟方法,研究不同裂纹形态、裂纹尺寸、载荷幅值等因素对疲劳裂纹偏折的影响,并与实验数据进行验证。
另外,本研究还将探究A357铸造铝合金疲劳裂纹偏折机制。
4. 研究方法和技术路线:(1)通过实验获取A357铸造铝合金的疲劳裂纹偏折数据。
(2)基于有限元方法,建立A357铸造铝合金疲劳裂纹偏折数值模型,分析裂纹形态、尺寸以及载荷等因素对疲劳裂纹偏折的影响。
(3)采用数值模拟和实验相结合的方法,验证模型的准确性。
(4)探究A357铸造铝合金疲劳裂纹偏折的机理和影响因素。
5. 预期结果:本研究将分析A357铸造铝合金疲劳裂纹偏折的机理和影响因素,建立疲劳裂纹偏折数值模型,并进行数值模拟和实验验证,为A357铸造铝合金材料的疲劳可靠性评估提供支持,为进一步提高铝合金材料的疲劳性能、延长使用寿命提供理论依据。
A357铸造铝合金疲劳裂纹扩展行为以及裂纹偏折

第28卷第10期 V ol.28 No.10 工 程 力 学 2011年 10 月 Oct. 2011 ENGINEERING MECHANICS197———————————————收稿日期:2010-02-23;修改日期:2010-07-30 基金项目:辽宁教育科学基金项目(20060659)作者简介:*徐 芳(1980―),女,辽宁大连人,助工,硕士,从事结构疲劳与损伤容限研究(E-mail: xuruiyao@);陈振中(1963―),男,辽宁沈阳人,教授,博士,沈阳航空航天大学航宇学院院长,主要从事结构疲劳断裂与可靠性分析研究文章编号:1000-4750(2011)10-0197-05A357铸造铝合金疲劳裂纹扩展行为以及裂纹偏折*徐 芳1,陈振中2(1. 中航工业沈飞民用飞机有限责任公司,辽宁,沈阳 110034;2. 沈阳航空航天大学航空宇航工程学院,辽宁,沈阳 110136)摘 要:该文研究了A357铸造铝合金在T5/T6两种热处理条件、应力比为0.05和0.7时的疲劳裂纹扩展行为,并用裂纹偏折模型对裂纹扩展速率曲线进行修正。
研究结果表明,裂纹沿着树状晶边界扩展导致显著的裂纹偏折。
在T5/T6两种热处理条件下,应力比r =0.05时的裂纹扩展速率均远小于应力比为0.7时的裂纹扩展速率,热处理方式对裂纹扩展速率没有影响。
应力比r =0.7时,偏折裂纹模型修正后的裂纹扩展速率没有明显变化;而应力比r =0.05时,偏折裂纹模型修正后的裂纹扩展速率较修正前有显著增加。
裂纹发生偏折时,采用同时考虑I 型和II 型裂纹的裂纹尖端有效应力强度因子描述裂纹扩展速率更合理也更准确。
关键词:A357铸造铝合金;疲劳裂纹扩展;裂纹偏折;断口;热处理 中图分类号:TU512.4 文献标志码:ATHE FATIGUE CRACK PROPAGATION BEHAVIOR AND CRACKDEFLECTION OF A357 CASTING ALUMINUM ALLOYS*XU Fang 1 , CHEN Zhen-zhong 2(1. Aviation Industry Corporation of China, Shenyang Aircraft Company Commercial Ltd., Shenyang, Liaoning 110034, China;2. School of Aerospace Engineering, Shenyang Aerospace University, Shenyang , Liaoning 110136, China)Abstract: The fatigue crack propagation behavior of A357 casting aluminum alloys at stress ratios of 0.05 and 0.7 under T5 and T6 heat treatments were studied and crack propagation curves were modified by a crack deflection model. The research results show that the fatigue crack grows along the boundary of dendrite cells to cause a remarkable crack deflection. The fatigue crack propagation rate at r =0.05 is smaller than that at r =0.7 for both T5 and T6 heat treatments. The effect of heat treatment on the fatigue crack propagation rates cannot be seen. The fatigue crack propagation rates modified by the crack deflection model predict no change at r =0.7, while a remarkable increase of the fatigue crack propagation rates can be seen at r =0.05. It is more reasonable and accurate to use the effective stress intensity factors for Mode I and II to describe the fatigue crack propagation rates when a crack deflection occurs.Key words: A357 casting aluminum alloy; fatigue crack propagation; crack deflection; fracture surface; heattreatment由于A356与A357铸造铝合金的强度高,铸造性优良、焊接性及耐蚀性良好,被广泛用于飞机、轮船和汽车上复杂铸件以及其它各种形状复杂、承受中等载荷的构件,如汽车汽缸盖及发动机滑块、汽车轮毂、活塞等[1―7]。
A357合金Sr变质

A357合金Sr变质工艺研究河南理工大学米国发 王 英 孔留安哈尔滨工业大学朱兆军 王宏伟 曾松岩中国科学院金属研究所张二林摘要研究了Al-10%Sr中间合金不同的加入量、加入温度、保温时间对A357合金变质效果的影响。
结果表明:Al-Sr合金具有较好的变质作用,加入量为0.02%Sr为宜,加入温度740℃以上;与Na变质相比,Sr 变质具有长效性。
关键词Al-Si合金Sr变质工艺1 引言亚共晶Al-Si(-Mg)合金中,共晶硅的形貌及尺寸均显著影响合金的力学性能。
共晶硅在未变质时呈针片状,严重割裂基体,导致合金力学性能的降低。
经过变质处理,共晶硅变为纤维状或层状,改善了合金的力学性能,尤其塑(韧)性得到明显提高。
因此对合金的变质处理研究一直倍受人们重视。
除Na以外,还发现K、Ca、Sr、Sb、Ba和RE等元素对共晶硅均有不同的变质作用,Na的变质效果最佳,可使共晶硅完全变为纤维状。
但在应用中存在有效时间短等问题,同时还发现经变质处理的亚共晶Al-Si(-Mg)合金经固溶和时效处理后力学性能并未得以提高。
经Sr 变质处理后,共晶硅相呈纤维状加少量层状组织。
Sr 变质处理具有如下优点[1,2]:Sr变质合金的力学性能略优于Na变质合金,Sr变质有效期为2~10h,且合金重熔后仍具有变质效果;Sr变质合金熔体的流动性和充填性优于Na变质合金,铸件表面光亮,外观好;Sr可以以Al-Sr中间合金加入,操作方便,不浸蚀炉衬和坩埚材料,无公害,不污染环境,且成本不很高;Sr变质不仅能够细化共晶硅,而且还具有细化共晶团的作用。
Sr作为一种理想的变质材料,已日益受到广泛的应用。
但过量添加Sr使熔体吸气倾向增大,易产生气孔缺陷,使合金密度降低。
为此,在熔体净化、晶粒细化的前提下[3~6],本文主要考察Al-10%Sr中间合金不同的加入量、加入温度、保温时间对合金变质效果的影响。
2 实验方法合金熔配在20kg电炉中进行,采用新型旋转转子除气机进行合金熔体的精炼处理;晶粒细化工艺为720加入4%的细化剂,保温5~10min,然后取样并浇注在尺寸为Φ20 mm×50mm、壁厚为1mm的石墨样杯中,观察合金的组织。
一种提高A357合金力学性能的方法[发明专利]
![一种提高A357合金力学性能的方法[发明专利]](https://img.taocdn.com/s3/m/19caf47d777f5acfa1c7aa00b52acfc788eb9f41.png)
(19)中华人民共和国国家知识产权局(12)发明专利申请(10)申请公布号 (43)申请公布日 (21)申请号 202010252497.1(22)申请日 2020.04.01(71)申请人 西北工业大学地址 710072 陕西省西安市友谊西路127号(72)发明人 陈忠伟 陈子雄 闫康 任聪聪 (74)专利代理机构 西北工业大学专利中心61204代理人 云燕春(51)Int.Cl.C22C 21/04(2006.01)C22C 1/03(2006.01)C22C 1/06(2006.01)(54)发明名称一种提高A357合金力学性能的方法(57)摘要本发明一种提高A357合金力学性能的方法,属于铝合金技术领域;使用一种钪(Sc)元素,通过熔炼添加到铝硅合金中,可以同时对铝合金起到晶粒细化作用和共晶Si的变质作用,对合金性能起到提升作用,即用一种元素同时充当了晶粒细化剂和变质剂,简化了生产工艺;并通过调整Sc元素的添加量,使得A357合金的抗拉强度和延伸率都得到提升,对常温下的力学性能有很大改善。
权利要求书1页 说明书4页 附图4页CN 111254328 A 2020.06.09C N 111254328A1.一种A357合金,其特征在于:所述A357合金成分的质量比为:Si为6.5-7.5wt.%,Mg 为0.45-0.7wt.%,Sc为0-1.0wt.%,余量为Al。
2.根据权利要求1所述A357合金,其特征在于:所述A357合金成分的质量比分别是Si为7%,Mg为0.6%,Sc为0.4-0.6%,余量为Al。
3.一种提高权利要求1所述A357合金力学性能的方法,其特征在于具体步骤如下:步骤一:以纯度为99.9%的铝和Al -2%Sc、Al -20%Si、Al -10%Mg三种成分的中间合金为原料,进行配料;通过所制备铝合金各成分质量比:Si为7%,Mg为0.6%,Sc为0-1.0%,余量为Al进行换算,得到Al -20%Si、Al -10%Mg、Al -2%Sc三种合金的质量比;步骤二:在电阻炉中进行合金熔炼:将实验原料按照熔点由低到高的顺序,依次添加到石墨坩埚中,顺序为铝、Al -20%Si、Al -10%Mg和Al -2%Sc,熔化后再加热到710℃,保证所有原料充分熔化;步骤三:用搅拌工具搅拌步骤二得到的熔体,使其成分混合均匀,随后加入除气剂和除渣剂,进行除气捞渣处理,以减少合金中缺陷的产生;步骤四:保温30min后进行合金浇铸;选用不锈钢铸型,铸造直径为60mm的圆柱锭,浇铸的六组铝合金铸锭中Sc元素含量均不相同,Sc元素的取值范围在0-1.0%之间;步骤五:对合金铸锭切割出的样品在扫描电镜SEM下进行观察,然后采用电子背散射衍射技术EBSD进行分析,统计合金晶粒的细化效果和共晶Si的变质效果;步骤六:按国家标准将铝合金铸锭加工成板状试样,在电子万能材料试验机上进行室温拉伸实验,检测合金的力学性能。
A357铝合金半固态成型工艺性能分析及其模拟的开题报告

A356/A357铝合金半固态成型工艺性能分析及其模拟的开题报告题目:A356/A357铝合金半固态成型工艺性能分析及其模拟一、研究背景随着汽车、飞机、船舶等各类应用领域对轻量化、高强度材料需求的不断增加,铝合金作为一种轻质、高强度的材料逐渐成为热门研究领域。
而半固态成型技术则是一种在模铸和锻造技术之间的新型材料成型技术。
A356/A357铝合金是常用的半固态成型材料之一,其在机械加工及航空、汽车等领域有广泛应用,因此研究A356/A357铝合金半固态成型工艺性能分析及其模拟有着重要的现实意义和研究价值。
二、研究目的和内容本文主要研究A356/A357铝合金半固态成型工艺性能分析及其模拟,探究其力学性能、金属流动及形成过程。
具体研究内容包括:1. 系统分析半固态成型工艺的功能适应性、操作性、工艺稳定性、成型效率等因素;2. 研究A356/A357铝合金半固态成型过程中的物理与化学变化,通过热力学分析掌握铝合金在不同成型温度下的相态转换规律;3.采用数值方法对半固态成型过程进行建模和仿真,运用有限元分析法探究成型温度、成型速率、应力应变等参数对成型性能的影响;4. 结合实验和模拟结果对半固态成型的成型能力和成型质量进行评估和验证。
三、研究方法1. 完成对该领域的文献资料收集和调研;2. 分析A356/A357铝合金半固态成型过程中的力学性能、材料变形、金属流动等因素,建立数值模型,运用有限元分析方法进行模拟,研究成型过程中的铝合金成型能力和成型质量;3. 根据实验结果和模拟分析结果进行比较和分析,评估研究成果,验证半固态成型过程的可行性和可靠性。
四、研究意义本文研究A356/A357铝合金半固态成型工艺性能分析及其模拟,可以为铝合金半固态成型技术的开发和工业化生产提供有力的理论支撑和技术指导。
同时,研究成果还可以拓展铝合金半固态成型工艺的研究领域,促进铝合金材料在轻量化、高强度领域的应用,也具有广泛的社会和经济意义。
7A55铝合金预拉伸板材的回归再时效处理

7A55铝合金预拉伸板材的回归再时效处理廖忠全;罗先甫;郑子樵;蔡彪;钟申【摘要】采用拉伸性能测试、慢应变速率拉伸测试、DSC分析、电导率测试及透射电镜观察,研究不同回归再时效(RRA)处理制度对7A55铝合金淬火预拉伸(W51)板材显微组织、拉伸性能和抗应力腐蚀(SCC)性能的影响.结果表明:采用120℃、24 h预时效,180℃、60 min回归处理和120℃、24 h终时效的RRA工艺,可使7A55合金获得与T6态相当的强度,而电导率大大提高,抗应力腐蚀性能接近T73态水平.7A55合金经适当的RRA处理后,晶内保持类似于T6状态的显微组织结构,为细小、弥散的η′相和极少量的η相;同时使晶界析出物的大小和分布特征与T73状态类似,为呈断续、孤立分布的粗化平衡相.【期刊名称】《中国有色金属学报》【年(卷),期】2012(000)009【总页数】7页(P2454-2460)【关键词】7A55铝合金;回归处理;再时效处理;应力腐蚀;微观组织【作者】廖忠全;罗先甫;郑子樵;蔡彪;钟申【作者单位】中南大学材料科学与工程学院,长沙 410083;中南大学材料科学与工程学院,长沙 410083;中南大学材料科学与工程学院,长沙 410083;中南大学材料科学与工程学院,长沙 410083;中南大学材料科学与工程学院,长沙 410083【正文语种】中文【中图分类】TG146.27000系高强高韧铝合金是航空、航天、兵器和交通运输等行业重要的结构材料之一[1−2]。
此系列合金虽然在峰时效(T6)状态下具有很高的强度,但抗应力腐蚀(SCC)性能较差。
采用双级过时效处理如T73、T74和T76,虽然可使合金得到较好的抗应力腐蚀性能,但强度相对于T6态,一般降低10%~15%[3]。
为了解决强度和抗应力腐蚀性能之间的矛盾,1974年以色列飞机公司的CINA[4]发明了回归再时效(RRA)工艺,采用这种热处理工艺能够在保持铝合金T6状态强度的基础上将抗应力腐蚀性能提高到接近T73水平,为此,各国开展了大量的研究工作[5−6]。
- 1、下载文档前请自行甄别文档内容的完整性,平台不提供额外的编辑、内容补充、找答案等附加服务。
- 2、"仅部分预览"的文档,不可在线预览部分如存在完整性等问题,可反馈申请退款(可完整预览的文档不适用该条件!)。
- 3、如文档侵犯您的权益,请联系客服反馈,我们会尽快为您处理(人工客服工作时间:9:00-18:30)。
o he i fu n e fS n t n e c s o DAS o gng k n tc fA3 7 a ly h s g e tsg i c n e t n r a e is p ro ma c s l n a i i e is o 5 lo a r a i n f a c o i c e s t e r n e .Th s i f i
a ay i wih o tc l mir s o e n l ss t p i a c o c p .Th r s ls h w h t e e u t s o t a ,un e he s me s l to c n ii n ,t e ha d e s fe d r t a o u in o d to s h r n s at r s l to te t n r d c s o u in r ame t e u e wih h S t t e DAS S n r a i ; t e g i g e k lg i c e sng h a en p a s a wi t e n r a i g f DAS t h i c e sn o S h .
p p r su i s t e i f e c s o D n a i g k n t s o 5 l y wi a d e s t se n tl g a hc a e t d e h n u n e f S AS o g n ie i f A3 7 A l t h r n s e tr a d me al r p i l c o h o
S S对 A 5 DA 3 7合金 时效过程 的影 响
王 友 彬 , 潘 杰花 , 胡 治 流 , 曾建 民
( 西 大 学 有 色 金 属材 料 及 其 加 工 新 技术 教 育部 重 点 实 验 室 , 宁 5 00 ) 广 南 3 04
摘 要 : 造 A— i Mg合 金 是 典型 的可 热 处理 强化 铝 合金 , 次枝 晶臂 间距 ( S对合 金 力 学性 铸 lS— 二 S DA ) 能有 非常显著 的影 响 , 究 S S对合 金 时效动 力学 的影响 有重要 意 义. 研 DA 采用硬 度计 进行 力 学性 能 测
S cn e d t am aig (D S h s ra if e c ntem ca ia poet s f l y T eeoe rsac eo dd n re r s cn S A ) a e tn u n eo eh ncl rp re l . h rf , erh i p g l h i o ao r e
Re r s i n a a y i a d d me so a n lssa e e ly d t sa l h a q ai n w o e r t n l y i e t e y g e s n l ss n i n in l a y i r mp o e o e t b i n e u t , h s ai ai st si d b o a s o o t i f
0 2月
有 色金 属科 学 与 工程
No f ro Me as c e c a d n e r us tl S i n e n En i e rn gn eig
Vo . , . 11 No 2
De 20 1 c. 0
文章编 号 :6 4 9 6 (0 0 0 — 0 0 0 17 — 6 9 2 1 ) 2 0 3 — 4
中 图分类号 :F 0 . T 8 51 文献标 识码 : A
Th n u n e fS eI f e c so DAS o i g Ki e iso 5 l y l n Ag n n t fA3 7 Al c o
W ANG u bn P Yo - i , AN i- u , J e h a HU i l , Zh- i ZENG in mi u Ja - n
试 、 学显 微镜 分析 金相 组 织 , 究 了 S AS对 A 5 光 研 D 3 7合金 时效 动 力学 的影响 . 究发现 , 相 同的 固 研 在
溶处 理条件 下 , 着 A 5 随 3 7合金 试 样二 次枝 晶臂 间距 的 增 大 , 固溶 处理 后 达到 的硬 度值 减 小 ; 同壁 不 厚 的合金 试样 的 时效峰 随 S S增 大而延 后 ; 时效 处理 实验 结 果进 行 回 归分析 和 量 纲分 析 , 到 DA 对 得 时效 力 学性 能 的数 学模 型 , 并证 明 了该数 学模 型的有 效性 . 关键 词 : 5 A3 7合金 ; 次枝 晶臂 间距 ; 二 时效 动力 学
Ab ta tT e A1 S - l y i a kn fc s n u n m l y ta a e s e  ̄h n d b e tt ame t. sr c : h - i Mg al s id o at g a miu al h tc n b t n e e y h a r t n s o i l o r e