On the calculation of protein-ligand binding constants
苏忠民教授简历
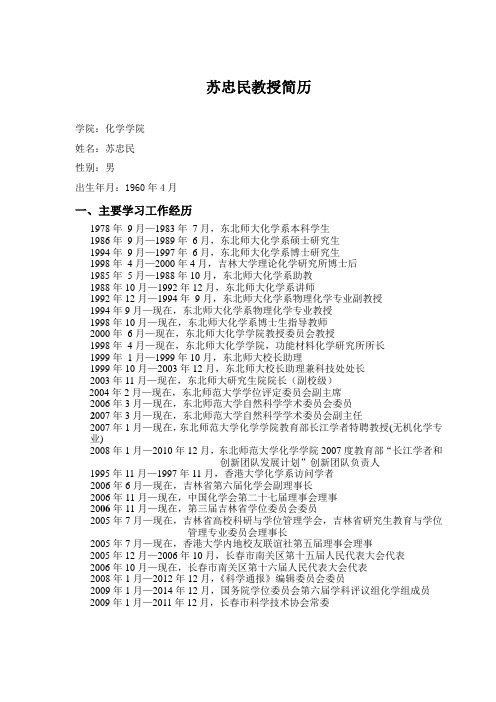
苏忠民教授简历学院:化学学院姓名:苏忠民性别:男出生年月:1960年4月一、主要学习工作经历1978年9月—1983年7月,东北师大化学系本科学生1986年9月—1989年6月,东北师大化学系硕士研究生1994年9月—1997年6月,东北师大化学系博士研究生1998年4月—2000年4月,吉林大学理论化学研究所博士后1985年5月—1988年10月,东北师大化学系助教1988年10月—1992年12月,东北师大化学系讲师1992年12月—1994年9月,东北师大化学系物理化学专业副教授1994年9月—现在,东北师大化学系物理化学专业教授1998年10月—现在,东北师大化学系博士生指导教师2000年6月—现在,东北师大化学学院教授委员会教授1998年4月—现在,东北师大化学学院,功能材料化学研究所所长1999年1月—1999年10月,东北师大校长助理1999年10月—2003年12月,东北师大校长助理兼科技处处长2003年11月—现在,东北师大研究生院院长(副校级)2004年2月—现在,东北师范大学学位评定委员会副主席2006年3月—现在,东北师范大学自然科学学术委员会委员2007年3月—现在,东北师范大学自然科学学术委员会副主任2007年1月—现在,东北师范大学化学学院教育部长江学者特聘教授(无机化学专业)2008年1月—2010年12月,东北师范大学化学学院2007度教育部“长江学者和创新团队发展计划”创新团队负责人1995年11月—1997年11月,香港大学化学系访问学者2006年6月—现在,吉林省第六届化学会副理事长2006年11月—现在,中国化学会第二十七届理事会理事2006年11月—现在,第三届吉林省学位委员会委员2005年7月—现在,吉林省高校科研与学位管理学会,吉林省研究生教育与学位管理专业委员会理事长2005年7月—现在,香港大学内地校友联谊社第五届理事会理事2005年12月—2006年10月,长春市南关区第十五届人民代表大会代表2006年10月—现在,长春市南关区第十六届人民代表大会代表2008年1月—2012年12月,《科学通报》编辑委员会委员2009年1月—2014年12月,国务院学位委员会第六届学科评议组化学组成员2009年1月—2011年12月,长春市科学技术协会常委二、主要研究方向或领域(一) 功能材料化学的理论和实验研究1.有机分子/聚合物的导电性质研究(1) 合成表征聚并苯半导体导电材料并进行改性研究,其可作为电极材料用于制作二次电池和双电层电容器,性能指标达到国际先进水平,并曾小批量投放市场。
含金属离子的蛋白靶点与小分子对接
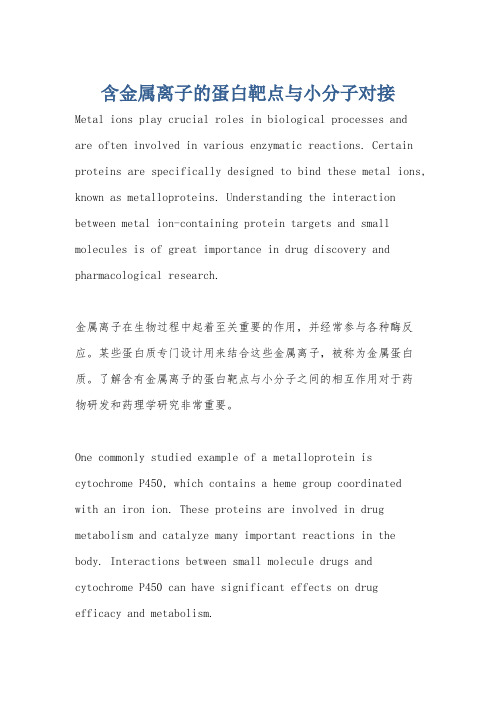
含金属离子的蛋白靶点与小分子对接Metal ions play crucial roles in biological processes and are often involved in various enzymatic reactions. Certain proteins are specifically designed to bind these metal ions, known as metalloproteins. Understanding the interaction between metal ion-containing protein targets and small molecules is of great importance in drug discovery and pharmacological research.金属离子在生物过程中起着至关重要的作用,并经常参与各种酶反应。
某些蛋白质专门设计用来结合这些金属离子,被称为金属蛋白质。
了解含有金属离子的蛋白靶点与小分子之间的相互作用对于药物研发和药理学研究非常重要。
One commonly studied example of a metalloprotein is cytochrome P450, which contains a heme group coordinatedwith an iron ion. These proteins are involved in drug metabolism and catalyze many important reactions in the body. Interactions between small molecule drugs and cytochrome P450 can have significant effects on drugefficacy and metabolism.一个常被研究的金属蛋白质的例子是细胞色素P450,其中包含一个与铁离子配位的血红素组分。
免疫学名词解释
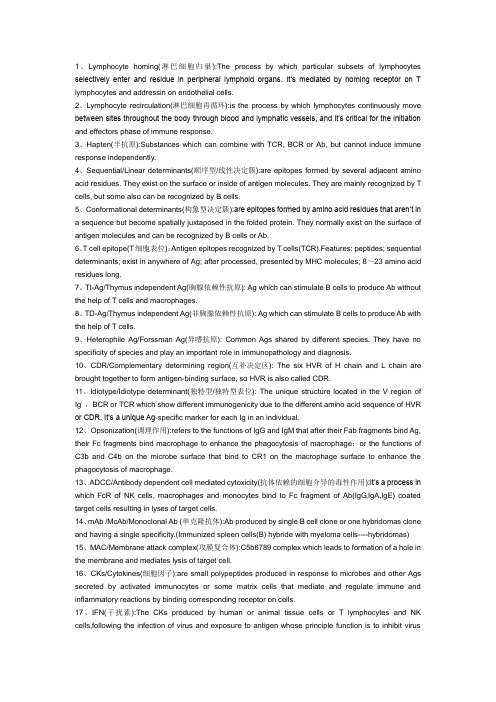
1、Lymphocyte homing(淋巴细胞归巢):The process by which particular subsets of lymphocytes selectively enter and residue in peripheral lymphoid organs. It’s mediated by homing receptor on T lymphocytes and addressin on endothelial cells.2、Lymphocyte recirculation(淋巴细胞再循环):is the process by which lymphocytes continuously move between sites throughout the body through blood and lymphatic vessels, and it’s critical for the initiation and effectors phase of immune response.3、Hapten(半抗原):Substances which can combine with TCR, BCR or Ab, but cannot induce immune response independently.4、Sequential/Linear determinants(顺序型/线性决定簇):are epitopes formed by several adjacent amino acid residues. They exist on the surface or inside of antigen molecules. They are mainly recognized by T cells, but some also can be recognized by B cells.5、Conformational determinants(构象型决定簇):are epitopes formed by amino acid residues that aren’t ina sequence but become spatially juxtaposed in the folded protein. They normally exist on the surface of antigen molecules and can be recognized by B cells or Ab.6、T cell epitope(T细胞表位):Antigen epitopes recognized by T cells(TCR).Features: peptides; sequential determinants; exist in anywhere of Ag; after processed, presented by MHC molecules; 8~23 amino acid residues long.7、TI-Ag/Thymus independent Ag(胸腺依赖性抗原): Ag which can stimulate B cells to produce Ab without the help of T cells and macrophages.8、TD-Ag/Thymus independent Ag(非胸腺依赖性抗原): Ag which can stimulate B cells to produce Ab with the help of T cells.9、Heterophile Ag/Forssman Ag(异嗜抗原): Common Ags shared by different species. They have no specificity of species and play an important role in immunopathology and diagnosis.10、CDR/Complementary determining region(互补决定区): The six HVR of H chain and L chain are brought together to form antigen-binding surface, so HVR is also called CDR.11、Idiotype/Idiotype determinant(独特型/独特型表位): The unique structure located in the V region of Ig ,BCR or TCR which show different immunogenicity due to the different amino acid sequence of HVR or CDR. It’s a unique Ag-specific marker for each Ig in an individual.12、Opsonization(调理作用):refers to the functions of IgG and IgM that after their Fab fragments bind Ag, their Fc fragments bind macrophage to enhance the phagocytosis of macrophage;or the functions of C3b and C4b on the microbe surface that bind to CR1 on the macrophage surface to enhance the phagocytosis of macrophage.13、ADCC/Antibody dependent cell mediated cytoxicity(抗体依赖的细胞介导的毒性作用):It’s a process in which FcR of NK cells, macrophages and monocytes bind to Fc fragment of Ab(IgG,IgA,IgE) coated target cells resulting in lyses of target cells.14、mAb /McAb/Monoclonal Ab (单克隆抗体):Ab produced by single B cell clone or one hybridomas clone and having a single specificity.(Immunized spleen cells(B) hybride with myeloma cells----hybridomas) 15、MAC/Membrane attack complex(攻膜复合体):C5b6789 complex which leads to formation of a hole in the membrane and mediates lysis of target cell.16、CKs/Cytokines(细胞因子):are small polypeptides produced in response to microbes and other Ags secreted by activated immunocytes or some matrix cells that mediate and regulate immune and inflammatory reactions by binding corresponding receptor on cells.17、IFN(干扰素):The CKs produced by human or animal tissue cells or T lymphocytes and NK cells,following the infection of virus and exposure to antigen whose principle function is to inhibit virusreplication or activate macrophage in both innate immunity and adaptive immunity.18、CAMs /Ams/cell adhesion molecules (黏附分子):The cell surface proteins involved in the interaction of cell-cell or cell-extracellular matrix. They play a crucial role in cell interaction, recognition, activation and migration by binding of receptor and ligand.19、CD/cluster of differentiation (分化簇):It is a group of cell surface molecules associated with the development and differentiation of immune cells.20、MHC/major histocompatibility complex(主要组织相容性复合体):A large cluster of linked genes located in some chromosomes of humanity or other mammals that encode major histocompatibility antigen and relate to allograft rejection, immune response and cell-cell recognition.21、HLA/Human leukocyte antigen(人类白细胞抗原):The major histocompatibility antigens for humanity which are associated with histocompatibility and immune response. They are alloantigens which are specific for each individual.22、HLA complex(HLA复合体):The MHC of humanity, a cluster of genes which encode for HLA and related to histocompatibility and immune response.23、MHC restriction(MHC 限制性):In interaction of T cell and APC or target cells, T cells not only recognize specific antigen but also recognize polymorphic residues of MHC molecules.24、PAMP/pathogen associated molecular pattern( 病原相关分子模式): The distinct structures or components that are common for many pathogens ,such as LPS, dsRNA of viruses etc.25、PRR/ pattern recognition receptor (模式识别受体): The receptors on macrophage that can recognize and bind PAMP on some pathogen, injured or apoptotic cells, including mannose receptor, scavenger receptor , toll like receptor etc.26、APC/Antigen presenting cells/Accessory cells/A cells(抗原递呈细胞): A group of cells which can uptake and process antigen and present antigen-MHC-Ⅰ/Ⅱcomplex to T cells, playing an important role in immune response.27、Cross-priming/Cross-presentation (交叉递呈): A mechanism by which a professional APC activates, a naïve CD8 CTL specific for the antigens of a third cell (e.g. a virus-infected or tumor cell)28、ITAM /immunoreceptor tyrosine-based activation motif(免疫受体酪氨酸活化基序): ITAM transduces activation signals from TCR, composing of tyrosine residues separated by around 18 aas. When TCR specially bind to antigen, the tyrosine becomes phosphorylated by the receptor associated tyrosine kinases to transduct active signals.29、TCR complex(TCR复合物): A group of membrane molecules on T cells that can specifically bind to antigen and pass an activation signal into the cell, consisting of TCR(αβ,γδ),CD3 (γε,δε)andδ-δ。
光谱法研究药物小分子与蛋白质大分子的相互作用的英文
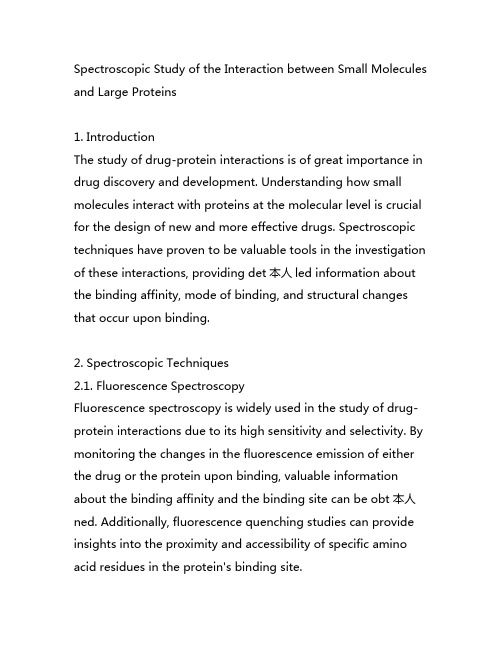
Spectroscopic Study of the Interaction between Small Molecules and Large Proteins1. IntroductionThe study of drug-protein interactions is of great importance in drug discovery and development. Understanding how small molecules interact with proteins at the molecular level is crucial for the design of new and more effective drugs. Spectroscopic techniques have proven to be valuable tools in the investigation of these interactions, providing det本人led information about the binding affinity, mode of binding, and structural changes that occur upon binding.2. Spectroscopic Techniques2.1. Fluorescence SpectroscopyFluorescence spectroscopy is widely used in the study of drug-protein interactions due to its high sensitivity and selectivity. By monitoring the changes in the fluorescence emission of either the drug or the protein upon binding, valuable information about the binding affinity and the binding site can be obt本人ned. Additionally, fluorescence quenching studies can provide insights into the proximity and accessibility of specific amino acid residues in the protein's binding site.2.2. UV-Visible SpectroscopyUV-Visible spectroscopy is another powerful tool for the investigation of drug-protein interactions. This technique can be used to monitor changes in the absorption spectra of either the drug or the protein upon binding, providing information about the binding affinity and the stoichiometry of the interaction. Moreover, UV-Visible spectroscopy can be used to study the conformational changes that occur in the protein upon binding to the drug.2.3. Circular Dichroism SpectroscopyCircular dichroism spectroscopy is widely used to investigate the secondary structure of proteins and to monitor conformational changes upon ligand binding. By analyzing the changes in the CD spectra of the protein in the presence of the drug, valuable information about the structural changes induced by the binding can be obt本人ned.2.4. Nuclear Magnetic Resonance SpectroscopyNMR spectroscopy is a powerful technique for the investigation of drug-protein interactions at the atomic level. By analyzing the chemical shifts and the NOE signals of the protein in thepresence of the drug, det本人led information about the binding site and the mode of binding can be obt本人ned. Additionally, NMR can provide insights into the dynamics of the protein upon binding to the drug.3. Applications3.1. Drug DiscoverySpectroscopic studies of drug-protein interactions play a crucial role in drug discovery, providing valuable information about the binding affinity, selectivity, and mode of action of potential drug candidates. By understanding how small molecules interact with their target proteins, researchers can design more potent and specific drugs with fewer side effects.3.2. Protein EngineeringSpectroscopic techniques can also be used to study the effects of mutations and modifications on the binding affinity and specificity of proteins. By analyzing the binding of small molecules to wild-type and mutant proteins, valuable insights into the structure-function relationship of proteins can be obt本人ned.3.3. Biophysical StudiesSpectroscopic studies of drug-protein interactions are also valuable for the characterization of protein-ligandplexes, providing insights into the thermodynamics and kinetics of the binding process. Additionally, these studies can be used to investigate the effects of environmental factors, such as pH, temperature, and ionic strength, on the stability and binding affinity of theplexes.4. Challenges and Future DirectionsWhile spectroscopic techniques have greatly contributed to our understanding of drug-protein interactions, there are still challenges that need to be addressed. For instance, the study of membrane proteins and protein-protein interactions using spectroscopic techniques rem本人ns challenging due to theplexity and heterogeneity of these systems. Additionally, the development of new spectroscopic methods and the integration of spectroscopy with other biophysical andputational approaches will further advance our understanding of drug-protein interactions.In conclusion, spectroscopic studies of drug-protein interactions have greatly contributed to our understanding of how small molecules interact with proteins at the molecular level. Byproviding det本人led information about the binding affinity, mode of binding, and structural changes that occur upon binding, spectroscopic techniques have be valuable tools in drug discovery, protein engineering, and biophysical studies. As technology continues to advance, spectroscopy will play an increasingly important role in the study of drug-protein interactions, leading to the development of more effective and targeted therapeutics.。
第九章 计算机辅助药物设计

第九章计算机辅助药物设计(CADD)9.1 靶向药物设计概述本世纪初曾有报道,国际上研制成功一种新药平均需要耗时10~12年,耗资2.0~3.5亿美元,这一费用在以每年20%的速度递增。
2018年有报道称,研制成功一种新结构药物耗资已达14亿美元。
目前药品发明专利保护期限为20年,如果药物上市前研究与开发(R&D)时间为10年,那么药品实际有效市场销售时间就仅有10年。
如能压缩R&D时间,不但能节约R&D的经费,而且能为市场赢得宝贵的时间,这将为产权拥有者带来巨大的经济效益和社会效益。
近年来,计算机辅助药物设计(Computer-aided Drug Design, CADD),成为国内外十分活跃的研究领域,成为新药研究的重要工具。
CADD是依据生物化学、酶学、分子生物学、遗传学等生命科学的研究成果,针对这些研究揭示的包括酶、受体、离子通道、核酸等潜在的药物作用靶点,并参考其他内源性配体以及天然药物的化学特征,合理设计药物分子的方法。
CADD方法能加速药物先导化合物的发现,因此已被许多国内外制药公司用于新药研究与开发,并且已经取得了极大的成功。
新药研究开发流程基本如下:近年来,“靶向给药”技术在临床中得到快速发展。
“靶向给药”亦称智能给药,是给患者用药的一种方式,其目标是使药物局部化、靶向作用和延长、保护药物与病变组织的相互作用。
传统给药方法,如:口服、血管注射等,药物是通过血液循环分布于整个身体各个组织器官,是通过跨生物膜吸收,对于大多数治疗药物仅有一小部分作用于用药部位器官。
靶向给药系统是有针对性的释放药物,主要针对作用的组织,而对其他组织的相对浓度较低,药物可以高浓度的到达活性位点。
靶向给药系统分为两类:(1)活性靶向给药,如:某些抗体药物;(2)被动靶向给药,例如:增强渗透性和滞留效应(EPR效应)。
药物作用生物靶点:与药物特异性结合的生物大分子统称为药物作用的生物靶点。
基于增强采样分子动力学模拟的蛋白质和小分子相互作用热力学和动力学研究
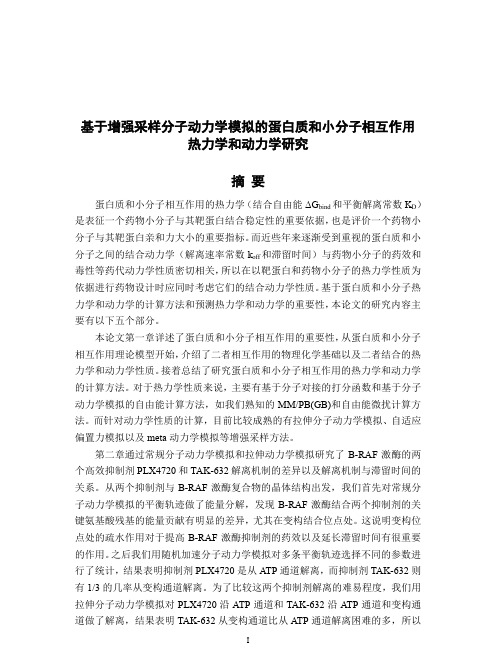
基于增强采样分子动力学模拟的蛋白质和小分子相互作用热力学和动力学研究摘要蛋白质和小分子相互作用的热力学(结合自由能ΔG bind和平衡解离常数K D)是表征一个药物小分子与其靶蛋白结合稳定性的重要依据,也是评价一个药物小分子与其靶蛋白亲和力大小的重要指标。
而近些年来逐渐受到重视的蛋白质和小分子之间的结合动力学(解离速率常数k off和滞留时间)与药物小分子的药效和毒性等药代动力学性质密切相关,所以在以靶蛋白和药物小分子的热力学性质为依据进行药物设计时应同时考虑它们的结合动力学性质。
基于蛋白质和小分子热力学和动力学的计算方法和预测热力学和动力学的重要性,本论文的研究内容主要有以下五个部分。
本论文第一章详述了蛋白质和小分子相互作用的重要性,从蛋白质和小分子相互作用理论模型开始,介绍了二者相互作用的物理化学基础以及二者结合的热力学和动力学性质。
接着总结了研究蛋白质和小分子相互作用的热力学和动力学的计算方法。
对于热力学性质来说,主要有基于分子对接的打分函数和基于分子动力学模拟的自由能计算方法,如我们熟知的MM/PB(GB)和自由能微扰计算方法。
而针对动力学性质的计算,目前比较成熟的有拉伸分子动力学模拟、自适应偏置力模拟以及meta动力学模拟等增强采样方法。
第二章通过常规分子动力学模拟和拉伸动力学模拟研究了B-RAF激酶的两个高效抑制剂PLX4720和TAK-632解离机制的差异以及解离机制与滞留时间的关系。
从两个抑制剂与B-RAF激酶复合物的晶体结构出发,我们首先对常规分子动力学模拟的平衡轨迹做了能量分解,发现B-RAF激酶结合两个抑制剂的关键氨基酸残基的能量贡献有明显的差异,尤其在变构结合位点处。
这说明变构位点处的疏水作用对于提高B-RAF激酶抑制剂的药效以及延长滞留时间有很重要的作用。
之后我们用随机加速分子动力学模拟对多条平衡轨迹选择不同的参数进行了统计,结果表明抑制剂PLX4720是从ATP通道解离,而抑制剂TAK-632则有1/3的几率从变构通道解离。
Protein-protein interaction 蛋白间的相互作用
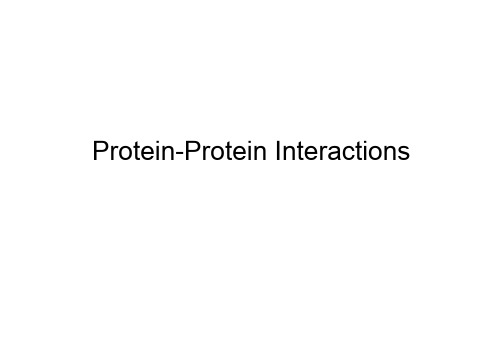
Protein-Protein InteractionsProtein Structure•The fundamental unit ofprotein structure is adomain.•This region of a polypeptidechain folds into a distinctstructure and mediates theprotein’s biologicalfunctionality.Abstract View of ProteinStructure•Proteins are representedas shaded areas and thedomains as geometricalfigures.•We assume that proteindomain (or domaincombination) interactionsare responsible forprotein interactions.Gen ome: 30.000 genesTranscript ome: 40-100.000 mRNAsProt eome:100-400.000 proteins >1.000.000 interactionsDimensions of InformationComplexityGenomics vs. Post-GenomicsHuman Genome Human Proteome TranscriptsProtein Interaction105106A proteomics circuit showing theinteraction of RNA splicing proteinsSource: Campbell/Heyer. Discovering genomics, proteomics, and bioinformaticsTerminologyProtein Complexes are groups of proteins that interact with each other at the same time and place, forming a single multi-molecular machine.E.g., anaphase-promoting complex, RNA-splicing andprotein export and transport complexes, etc. Functional modules consist of proteins that participate in a particular cellular process while binding each other at a different time and place (different conditions or phases of the cell cycle, in different cellular compartments, etc).E.g., yeast pheromone response pathway, MAPsignaling cascades, etc.How can we identify Protein-Protein Interactions ?1. In vitro interactions.2. In vivo interactions.3. How does this fit together?Identification of Protein-ProteinInteractions byCo-ImmunoprecipitationCo-Immunoprecipitation - PrincipleA variation ofCo-Immunoprecipitation:Reverse Co-Immunopreciptiation combined with cross-linking Spatial organisation of P-P-interactionsCross-linkerse.g. bifunctional sulfhydril-reactive crosslinkers:e.g. Maleimide-esterHomobifunctionalPeriodatecleavable!Identification of Protein-Protein Interactions by Pull-Down methodsprepare proteinThe GST fusion protein might have different origin: Bacterial and yeast expression systemsHigher eukaryotic expression systems (insect cells/ baculovirus)Various Affinity tags commonly used:GSTPoly-Histidine (IMAC)Calmoduline binding protein (CBP)………Multi-Tags e.g. TAP-tag:T andem Affinity Purification (TAP)Identification of Protein-Protein Interactions byProtein chipsCapture)Identification of Protein-Protein Interactions by Surface Plasmon Resonance(SRP)BackgroundTypical SRP dataGroups on Molecules Available for Immobilisation •Primary Amines •Carboxylic acids•Thiols•Sugars (aldehydes)•Lipids •Tags ԪBiotin ԪGST ԪFc ԪPoly-HIS2. In vivo interaction studiesIdentifying protein interactions in vivoProteomicsA more comprehensive yeast protein interaction networkDeletion phenotype:Red = lethalGreen = non-lethalOrange = slow growth Yellow = unknownSource: Jeong H et al (2004) Nature 411:41-42•An example of a scale-free network–Most nodes have fewconnections– A small number ofnodes (network hubs)are connected to alarge number of othernotesIdentification of Protein-Protein Interactions by Yeast 2-Hybrid SystemYeast two-hybrid system:a genetic assay for detecting protein-protein interactionsX YSuppose we have two proteins...One way of answering this question is to use the yeast two hybrid method.To understand the method we must firstconsider how gene expression is regulated in yeast......and the question,do these two protein bind each other?a genetic assay for detecting protein-protein interactionsRegulation of gene expression in yeastgeneUAS(upstream activation sequence)transcription activatorDNA binding domainactivation domaintranscription machinery and now back to the question...a genetic assay for detecting protein-protein interactionsXYDo these two protein bind?Regulation of gene expression in yeastgene UAS(upstream activation sequence)transcription activatorDNA binding domainactivation domaintranscription machinery reporter geneWe start with yeast possessing a reporter gene (a gene making a product that is easy to detect).And now we introduce genes coding for two hybrid proteins.....Use of the yeast two-hybrid systemYeast Two-Hybrid:Detecting protein-protein interactions in yeast---Interactions can be screened for strength。
蛋白质计算设计方法和应用展望
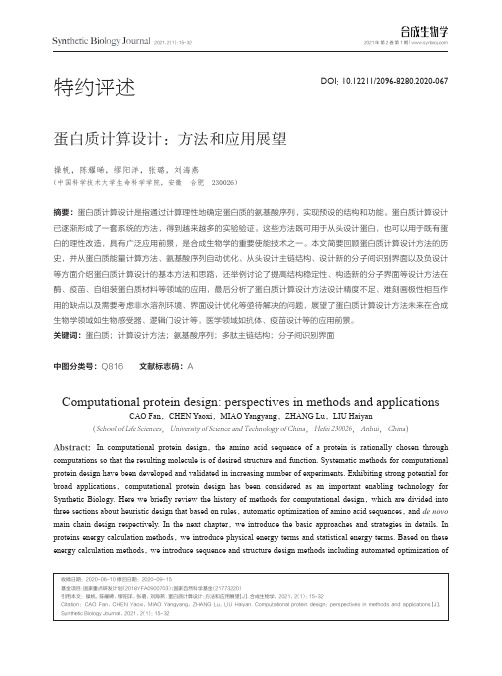
2021年第2卷第1期|Syn thetic Bio logy J ournal2021,2(1):15-32蛋白质计算设计:方法和应用展望操帆,陈耀晞,缪阳洋,张璐,刘海燕(中国科学技术大学生命科学学院,安徽合肥230026)摘要:蛋白质计算设计是指通过计算理性地确定蛋白质的氨基酸序列,实现预设的结构和功能。
蛋白质计算设计已逐渐形成了一套系统的方法,得到越来越多的实验验证。
这些方法既可用于从头设计蛋白,也可以用于既有蛋白的理性改造,具有广泛应用前景,是合成生物学的重要使能技术之一。
本文简要回顾蛋白质计算设计方法的历史,并从蛋白质能量计算方法、氨基酸序列自动优化、从头设计主链结构、设计新的分子间识别界面以及负设计等方面介绍蛋白质计算设计的基本方法和思路,还举例讨论了提高结构稳定性、构造新的分子界面等设计方法在酶、疫苗、自组装蛋白质材料等领域的应用,最后分析了蛋白质计算设计方法设计精度不足、难刻画极性相互作用的缺点以及需要考虑非水溶剂环境、界面设计优化等亟待解决的问题,展望了蛋白质计算设计方法未来在合成生物学领域如生物感受器、逻辑门设计等,医学领域如抗体、疫苗设计等的应用前景。
关键词:蛋白质;计算设计方法;氨基酸序列;多肽主链结构;分子间识别界面中图分类号:Q816文献标志码:AComputational protein design:perspectives in methods and applicationsCAO Fan ,CHEN Yaoxi ,MIAO Yangyang ,ZHANG Lu ,LIU Haiyan(School of Life Sciences ,University of Science and Technology of China ,Hefei 230026,Anhui ,China )Abstract:In computational protein design ,the amino acid sequence of a protein is rationally chosen through computations so that the resulting molecule is of desired structure and function.Systematic methods for computational protein design have been developed and validated in increasing number of experiments.Exhibiting strong potential for broad applications ,computational protein design has been considered as an important enabling technology for Synthetic Biology.Here we briefly review the history of methods for computational design ,which are divided into three sections about heuristic design that based on rules ,automatic optimization of amino acid sequences ,and de novo main chain design respectively.In the next chapter ,we introduce the basic approaches and strategies in details.In proteins energy calculation methods ,we introduce physical energy terms and statistical energy terms.Based on these energy calculation methods ,we introduce sequence and structure design methods including automated optimization of收稿日期:2020-06-10修回日期:2020-09-15基金项目:国家重点研发计划(2018YFA0900703);国家自然科学基金(21773220)引用本文:操帆,陈耀晞,缪阳洋,张璐,刘海燕.蛋白质计算设计:方法和应用展望[J ].合成生物学,2021,2(1):15-32Citation:CAO Fan,CHEN Yaoxi,MIAO Yangyang,ZHANG Lu,LIU putational protein design:perspectives in methods and applications [J ].Synthetic Biology Journal,2021,2(1):15-32DOI :10.12211/2096-8280.2020-067特约评述合成生物学第2卷amino acid sequences,de novo design of polypeptide backbones(with fragment assembling method or sequence independent backbone potentials),designing new interfaces for inter-molecule recognition such as protein-ligand interfaces and protein-protein interfaces,and the concept of negative design.Besides the history and detail of computational protein design methods that mentioned above,we also briefly discuss examples of using computational protein design to support application studies,including enhancing protein structural stability and redesign or de novo design of enzymes,vaccines and protein materials that related to interfaces design.These examples not only present current studies using the computational protein design methods,but also enlighten us on more broader applications in the future.Finally,we analyze some problems that need to be solved in the protein computational design method,such as inefficient in design accuracy,difficulty in characterizing polar interactions,and the need to consider the environment of non-aqueous solvents.We also discuss some aspects of possible application in synthetic biology like biological logic gates design and biosensor design,and application prospects in the medical field such as antibodies,vaccine design,etc.Keywords:protein;methods for computational design;amino acid sequences;polypeptide backbone structure; interface of intermolecular recognition蛋白质是执行生物功能的主要生物大分子,也是用于构筑合成生物系统的主要元件。
范德华力和疏水作用力
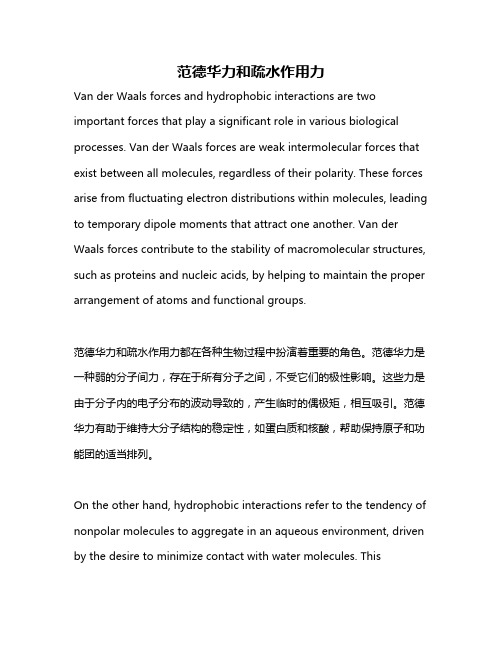
范德华力和疏水作用力Van der Waals forces and hydrophobic interactions are two important forces that play a significant role in various biological processes. Van der Waals forces are weak intermolecular forces that exist between all molecules, regardless of their polarity. These forces arise from fluctuating electron distributions within molecules, leading to temporary dipole moments that attract one another. Van der Waals forces contribute to the stability of macromolecular structures, such as proteins and nucleic acids, by helping to maintain the proper arrangement of atoms and functional groups.范德华力和疏水作用力都在各种生物过程中扮演着重要的角色。
范德华力是一种弱的分子间力,存在于所有分子之间,不受它们的极性影响。
这些力是由于分子内的电子分布的波动导致的,产生临时的偶极矩,相互吸引。
范德华力有助于维持大分子结构的稳定性,如蛋白质和核酸,帮助保持原子和功能团的适当排列。
On the other hand, hydrophobic interactions refer to the tendency of nonpolar molecules to aggregate in an aqueous environment, driven by the desire to minimize contact with water molecules. Thisphenomenon is crucial in the folding of proteins, as hydrophobic regions tend to bury themselves in the interior of the protein structure to avoid interaction with water. Hydrophobic interactions also play a role in the formation of lipid bilayers, where hydrophobic tails of phospholipids cluster together, creating a barrier that separates the interior of cells from the surrounding environment.另一方面,疏水作用是指非极性分子在水溶液环境中聚集的趋势,这是由于它们希望最小化与水分子的接触而驱动的。
药的副作用英文作文
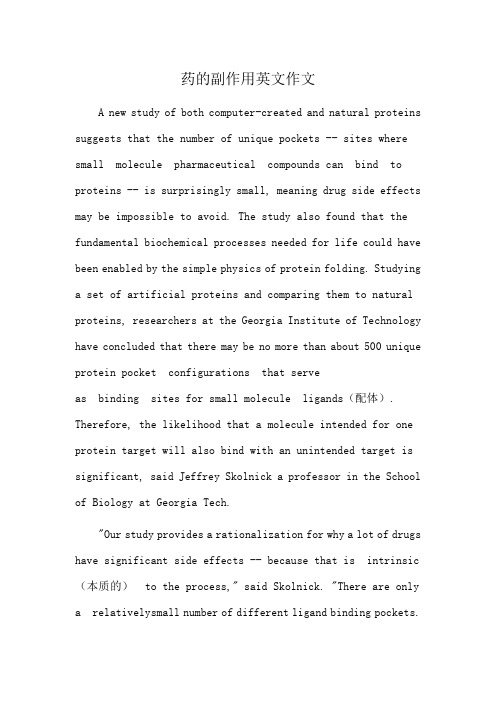
药的副作用英文作文A new study of both computer-created and natural proteins suggests that the number of unique pockets -- sites where small molecule pharmaceutical compounds can bind to proteins -- is surprisingly small, meaning drug side effects may be impossible to avoid. The study also found that the fundamental biochemical processes needed for life could have been enabled by the simple physics of protein folding. Studying a set of artificial proteins and comparing them to natural proteins, researchers at the Georgia Institute of Technology have concluded that there may be no more than about 500 unique protein pocket configurations that serveas binding sites for small molecule ligands(配体). Therefore, the likelihood that a molecule intended for one protein target will also bind with an unintended target is significant, said Jeffrey Skolnick a professor in the School of Biology at Georgia Tech."Our study provides a rationalization for why a lot of drugs have significant side effects -- because that is intrinsic (本质的)to the process," said Skolnick. "There are only a relativelysmall number of different ligand binding pockets.The likelihood of having geometry in an amino acid composition that will bind the same ligand turns out to be much higher than anyone would have anticipated. This means that the idea that a small molecule could have just one protein target can't be supported."Research on the binding pockets was scheduled to be published May 20 in the early online edition of thejournal Proceedings of the National Academy of Sciences. The research was supported by the National Institutes of Health (NIH).Skolnick and collaborator Mu Gao have been studying the effects of physics on the activity of protein binding and contrasting the original conditions created by the folding of amino acid residues against the role played by evolution in optimizing the process."The basic physics of the system providesthe mechanism for molecules to bind to proteins," said Skolnick, who is director of the Center for the Study of Systems Biology at Georgia Tech. "You don't need evolution to have a system that works on at least a low level. In other words, proteins are inherently capable of engaging in biochemicalfunction without evolution's selection. Beyond unintended drug effects, this has a lot of implications for thebiochemical component of the origins of life."Binding pockets on proteins are formed bythe underlying secondary structure of the amino acids, which is directed by hydrogen bonding in the chemistry. That allows formation of similar pockets on many different proteins, even those that are not directly related to one another."You could have the same or very similar pockets on the same protein, the same pockets on similar proteins, the same pockets on completely dissimilar proteins that haveno evolutionary relationship. In proteins that are related evolutionarily or that have similar structures, you could have very dissimilar pockets," said Skolnick, who is also a Georgia Research Alliance Eminent Scholar. "This helps explain why we see unintended effects of drugs, and opens up anew paradigm for how one has to think about discovering drugs."。
亲和蛋白a uhplc法

亲和蛋白a uhplc法英文回答:Affinity protein A is a commonly used method in thefield of biochemistry and biotechnology for thepurification and analysis of proteins. It is an effective technique for isolating and studying specific proteins from complex mixtures.The principle behind affinity protein A is the specific binding between the target protein and the protein A ligand. Protein A is a bacterial protein that has a high affinityfor the Fc region of immunoglobulins, particularly IgG. By immobilizing protein A on a solid support, such as a chromatography column or a magnetic bead, it is possible to selectively capture and purify IgG or other proteins that can bind to protein A.One of the major advantages of affinity protein A isits high selectivity and specificity. It allows for theisolation of a specific protein from a mixture containing a wide range of proteins. This is particularly useful in the purification of therapeutic antibodies, where high purityis essential for safety and efficacy.In addition to purification, affinity protein A can also be used for the analysis of protein-protein interactions. By immobilizing a target protein on a solid support and then incubating it with a protein mixture, itis possible to identify and study the proteins thatinteract with the target protein. This can provide valuable insights into the function and regulation of proteins in biological systems.中文回答:亲和蛋白A是生物化学和生物技术领域常用的一种蛋白质纯化和分析方法。
mdbthemetallopro...
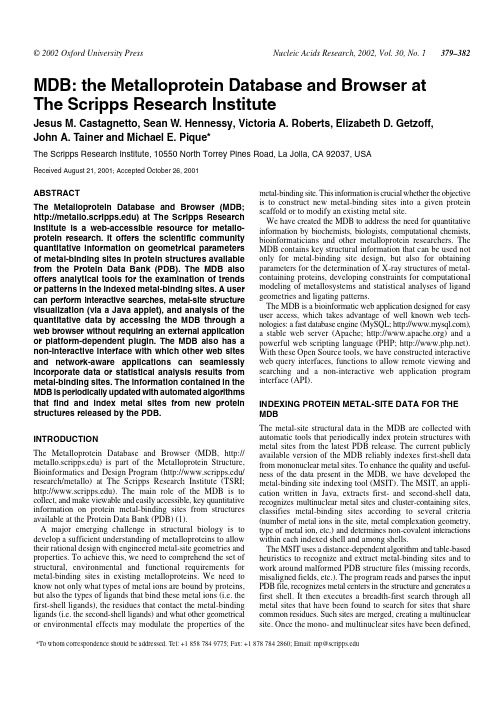
© 2002 Oxford University Press Nucleic Acids Research, 2002, Vol. 30, No. 1379–382 MDB: the Metalloprotein Database and Browser atThe Scripps Research InstituteJesus M. Castagnetto, Sean W. Hennessy, Victoria A. Roberts, Elizabeth D. Getzoff,John A. Tainer and Michael E. Pique*The Scripps Research Institute, 10550 North Torrey Pines Road, La Jolla, CA 92037, USAReceived August 21, 2001; Accepted October 26, 2001ABSTRACTThe Metalloprotein Database and Browser (MDB; ) at The Scripps Research Institute is a web-accessible resource for metallo-protein research. It offers the scientific community quantitative information on geometrical parameters of metal-binding sites in protein structures available from the Protein Data Bank (PDB). The MDB also offers analytical tools for the examination of trends or patterns in the indexed metal-binding sites. A user can perform interactive searches, metal-site structure visualization (via a Java applet), and analysis of the quantitative data by accessing the MDB through a web browser without requiring an external application or platform-dependent plugin. The MDB also has a non-interactive interface with which other web sites and network-aware applications can seamlessly incorporate data or statistical analysis results from metal-binding sites. The information contained in the MDB is periodically updated with automated algorithms that find and index metal sites from new protein structures released by the PDB.INTRODUCTIONThe Metalloprotein Database and Browser (MDB, http:// ) is part of the Metalloprotein Structure, Bioinformatics and Design Program (/ research/metallo) at The Scripps Research Institute (TSRI; ). The main role of the MDB is to collect, and make viewable and easily accessible, key quantitative information on protein metal-binding sites from structures available at the Protein Data Bank (PDB) (1).A major emerging challenge in structural biology is to develop a sufficient understanding of metalloproteins to allow their rational design with engineered metal-site geometries and properties. To achieve this, we need to comprehend the set of structural, environmental and functional requirements for metal-binding sites in existing metalloproteins. We need to know not only what types of metal ions are bound by proteins, but also the types of ligands that bind these metal ions (i.e. the first-shell ligands), the residues that contact the metal-binding ligands (i.e. the second-shell ligands) and what other geometrical or environmental effects may modulate the properties of the metal-binding site. This information is crucial whether the objective is to construct new metal-binding sites into a given protein scaffold or to modify an existing metal site.We have created the MDB to address the need for quantitative information by biochemists, biologists, computational chemists, bioinformaticians and other metalloprotein researchers. The MDB contains key structural information that can be used not only for metal-binding site design, but also for obtaining parameters for the determination of X-ray structures of metal-containing proteins, developing constraints for computational modeling of metallosystems and statistical analyses of ligand geometries and ligating patterns.The MDB is a bioinformatic web application designed for easy user access, which takes advantage of well known web tech-nologies: a fast database engine (MySQL; ), a stable web server (Apache; ) and a powerful web scripting language (PHP; ). With these Open Source tools, we have constructed interactive web query interfaces, functions to allow remote viewing and searching and a non-interactive web application program interface (API).INDEXING PROTEIN METAL-SITE DATA FOR THE MDBThe metal-site structural data in the MDB are collected with automatic tools that periodically index protein structures with metal sites from the latest PDB release. The current publicly available version of the MDB reliably indexes first-shell data from mononuclear metal sites. To enhance the quality and useful-ness of the data present in the MDB, we have developed the metal-binding site indexing tool (MSIT). The MSIT, an appli-cation written in Java, extracts first- and second-shell data, recognizes multinuclear metal sites and cluster-containing sites, classifies metal-binding sites according to several criteria (number of metal ions in the site, metal complexation geometry, type of metal ion, etc.) and determines non-covalent interactions within each indexed shell and among shells.The MSIT uses a distance-dependent algorithm and table-based heuristics to recognize and extract metal-binding sites and to work around malformed PDB structure files (missing records, misaligned fields, etc.). The program reads and parses the input PDB file, recognizes metal centers in the structure and generates a first shell. It then executes a breadth-first search through all metal sites that have been found to search for sites that share common residues. Such sites are merged, creating a multinuclear site. Once the mono- and multinuclear sites have been defined,*Towhomcorrespondenceshouldbeaddressed.Tel:+18587849775;Fax:+18787842860;Email:**************380Nucleic Acids Research, 2002, Vol. 30, No. 1second-shell residues and non-covalent interactions are identified. The geometrical data are written to structure files (in PDB, XML/CML and VRML formats) and the calculated data are inserted into the SQL database (Fig. 1).INTERACTIVE AND NON-INTERACTIVE ACCESS TO THE MDBInteractive interfacesThe MDB web site offers the researcher a series of query options, from the simple to the complex. Interactive interfaces are implemented either as simple HTML forms or as forms combined with an applet for real-time three-dimensional viewing of structures. In the MDB, you can search for metal-binding sites with HTML forms that allow you to:1.Specify PDB codes of proteins (e.g. 2sod or 1fer), resulting in alist of all metal-binding sites found within the indicated proteins.2.Select sites based on type of metal, number of ligands andother parameters. F or example, you can search for zinc-containing sites with four to six ligands found in protein structures with resolutions <2.0 Å. A more extensive HTML form allows even more restricted searches. For example, a specified number of ligands can be restricted to be of certain type (‘one must be water’) or a range of resolutions may be specified instead of an upper limit (‘resolution between 1.5 and 2.8 Å’).3.Perform a query using the SQL language, offering the usercomplete flexibility to examine any possible correlation among the data sets contained in the database. To assist the user, the database structure is documented (/ sql_docs/structure.html and / sql_docs/table_descriptions.html). A possible complex query would be to select all copper-binding sites that contain exactly one Asp or Glu, two His residues and a water molecule as the metal-ligating pattern and then to display the copper–water distance, the resolution and the date when the structure was released for each e an interface with a lightweight (70 kB in size) Javaapplet to view and manipulate the metal-binding sites found by the queries. This viewer allows the user to perform geometrical measurements, including atom to atom distances, valence angles and torsion angles. This applet also allows simple superpositions, stereo visualization, display of atoms within a given distance of the one selected, independent selection and movement of structures when several are being displayed, the ability to detach or reattach the viewer from/to the page (to enlarge the window), etc.Non-interactive interfacesThe non-interactive interfaces allow one to embed MDB data or visualization tools in any other web page or application. Currently there are three interfaces available.1.The remote query/viewer interface, which uses somesimple HTML code to allow transparent querying and visualization of metal-sites of interest (with the interactive Java viewer). Several sites are using this interface, including the IMB Jena Image Library of Biological Macromolecules (2), the PROMISE database (3) and the EF-Hand Calcium-Binding Proteins Data Library (http:// /cabp_database).2.An SQL query interface (/api)that allows application developers to call a particular URL on the MDB site, pass the appropriate parameters and obtain the results in program parsable format, such as comma delimited records (for spreadsheet analysis) or WDDX packets (/), or in a format ready to embed into a web page (HTML tables).3.An XML-RPC-based interface ()that accepts remote procedure calls from any application using the XML-RPC protocol, independent of the platform or programming language used by the application requesting the service. Added advantages are that the protocol supports introspection (i.e. an application can askthe server: ‘what procedures do you offer and how should I Figure 1. Algorithm used by the MSIT to identify and extract information about metal-binding sites in metalloproteins.Nucleic Acids Research, 2002, Vol. 30, No. 1381call them?’) and that there are XML-RPC libraries for most scripting and programming languages.This last interface (XML-RPC), which is under vigorous development, will comprise a whole set of callable methods (API) for the MDB. F or example, a program for metal-site design could use this interface to communicate directly with the MDB and request a list of observed ranges for a particular geometrical feature (distance, angle, etc.), and thus compare a proposed model value with those found in known metalloproteins. DATA ANALYSIS WITH THE MDBThe MDB has been used to analyze the distribution of geometrical parameters such as metal–ligand bond distances and side-chain torsion angles, and also to obtain the frequencies for ligating patterns in metal sites. These analyses have been used to assist in the determination of X-ray crystallographic structures, to validate and compare designed metal-site candidates, and to find ligating tendencies (such as differences between Cu–S distances when Cys and Met are metal ligands).These types of analyses have been very useful in our research, so we designed HTML forms to perform the analyses online. Using the forms, we can generate the distribution of the metal–ligand atom distances or the ligand patterns that are most common for a particular metal ion with a given coordination number. Figure 2 shows the result of analysis of the bond distance distribution for Zn-Nδ(His) (coordination number = 4 and fixed range of 1.5–3.0 Å). We find a normal distribution with an average Zn-Nδ(His) distance of 2.09 Å (SD 0.15) from 561indexed distances. Not shown in Figure 2 is a table listing each of the plotted bins, their corresponding counts and a button allowing the researcher to obtain (in another window) a list of the metal-binding sites in which a particular distance occurs. From that list, the scientist can choose to download the structure of the site (in PDB format) or view it using our Java viewer. Usually, this tool takes between 2 and 10 s to process a distribution (including plot, table, etc.) and behaves linearly with the number of matching distances. Improvements planned for this tool include discriminating distances from mono-nuclear, multinuclear or cluster sites and making the analysis on a dehomologized list of protein structures.A complementary analysis tool identifies ligand pattern tendencies of a particular metal ion in a specific coordination number. Using a simple form, we can choose what metal ion we are interested in and decide what coordination number it should present, and we will obtain a ranked list (by frequency count) of the ligand patterns that match those constraints. For example, if we were interested in designing a metal-binding site that matched the pattern CuL4, we would find that the three most frequent patterns are Cu(Cys)(Gly)(His)2, Cu(His)4 and Cu(His)3(H2O), and that the pattern Cu(Cys)4 does not appear in the data indexed in the MDB to date.RELATIONSHIP OF THE MDB WITH OTHER DATABASESThe MDB currently includes, in the lists generated fromqueries, links to the appropriate structure page at the PDB site (1) Figure 2. Distribution and statistics of the Zn-Nδ(His) distance (coordination number = 4, range 1.5–3.0) using the online histogram plotting tool.382Nucleic Acids Research, 2002, Vol. 30, No. 1and to the NIH Molecules R Us site (/ cgi-bin/pdb). The MDB is used by other databases to provide three-dimensional viewing of specific metal-binding sites through the use of our remote query/viewer tool. Sites using the MDB in this manner include the IMB Jena Image Library of Biological Macromolecules (2), the PROMISE database (3) and the E-F Hand Calcium-Binding Proteins Data Library (/cabp_database).We also make use of data from the PDB’s Het group dictionary (/het_dictionary.txt) and the lists of dehomologized structures from PDBSELECT (4) and from WHATIF SELECT (5). These lists have been manually converted into SQL tables so that they can be correlated with the other data being indexed in the MDB.AVAILABILITY AND ACCESS STATISTICSThe MDB is available at /, which includes interactive interfaces for querying and browsing the MDB, requiring just a common web browser. The non-interactiveinterfaces are described at /api (MDB’s web API) and /remote/ (remote query/viewer tool). New analytical tools and features are available for testing at /beta/. The use of the MDB by the research community has increased steadily since its inception back in early 1998. Table 1 summarizes some access statistics for the MDB from the third quarter of 1999 until the second quarter of 2001. In the last 2years the number of users has increased almost 3.5-fold and the number of documents viewed (counted as full page displays, not as individual hits in a document) has increased 3-fold. More importantly, the number of queries performed and the number of structures of metal-binding sites downloaded have increased by a factor of 4.5 and 2, respectively. ACKNOWLEDGEMENTSWe are grateful to all our users for their valuable and continuous feedback that drives improvements to the MDB. We would also like to acknowledge the work of Marij van Gorkom. Her enthusiasm helped us complete a prototype of the MSIT tool during her summer stay at TSRI. The MDB is part of the Metalloprotein Structure and Design Program Project at TSRI, funded by NIH grant P01-GM48495. The macromolecular Java viewer was developed as part of the Computational Center for Macromolecular Structure (/ CCMS), funded by NSF grant BIO/DBI 99-04559. REFERENCES1.Berman,H.M., Westbrook,J., Feng,Z., Gilliland,G., Bhat,T.N.,Weissig,H., Shindyalov,I.N. and Bourne,P.E. (2000) The Protein Data Bank. Nucleic Acids Res., 28, 235–242. Updated article in this issue:Nucleic Acids Res. (2002), 30, 245–248.2.Reichter,J., Jabs,A., Slickers,P. and Sühnel ,J. (2000) The IMB JenaImage Library of Biological Macromolecules. Nucleic Acids Res., 28, 246–249. Updated article in this issue: Nucleic Acids Res. (2002), 30,253–254.3.Degtyarenko,K.N., North,A.C.T. and Findlay,J.B.C (1999) PROMISE:a database of bioinorganic motifs. Nucleic Acids Res., 27, 233–236.4.Hobohm,U. and Sander,C. (1994) Enlarged representative set of proteinstructures. Protein Sci., 3, 522–524.5.Hooft,R.W.W., Sander,C. and Vriend,G. (1996) Verification of proteinstructures: side-chain planarity. J. Appl. Cryst., 29, 714–716.Table 1. Access statistics of the MDB web site from July 1999–June 2001 (outside users only)a Number of searches performed.b Number of structure files downloaded or viewed using the interactive Java viewer.c Number of pages viewed (complete documents).d Each host is counted only once per day.Year Quarter Queries a Structures b Pages c Hosts d 1999Jul–Sep14131327 8043 3431 Oct–Dec2983167912 2574762 2000Jan–Mar3711202514 9517522 Apr–Jun2522191413 0267733Jul–Sep5471157315 7727785Oct–Dec5414208018 6629292 2001Jan–Mar5716283219 76110 208 Apr–Jun6379228524 46811 987。
The chemistry of protein synthesis and folding
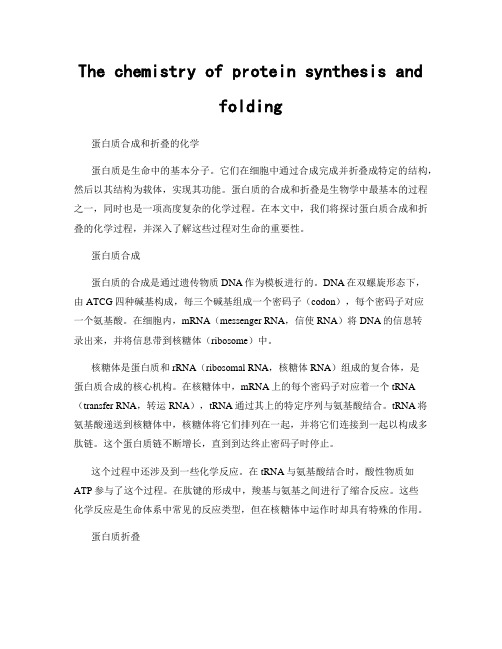
The chemistry of protein synthesis andfolding蛋白质合成和折叠的化学蛋白质是生命中的基本分子。
它们在细胞中通过合成完成并折叠成特定的结构,然后以其结构为载体,实现其功能。
蛋白质的合成和折叠是生物学中最基本的过程之一,同时也是一项高度复杂的化学过程。
在本文中,我们将探讨蛋白质合成和折叠的化学过程,并深入了解这些过程对生命的重要性。
蛋白质合成蛋白质的合成是通过遗传物质DNA作为模板进行的。
DNA在双螺旋形态下,由ATCG四种碱基构成,每三个碱基组成一个密码子(codon),每个密码子对应一个氨基酸。
在细胞内,mRNA(messenger RNA,信使RNA)将DNA的信息转录出来,并将信息带到核糖体(ribosome)中。
核糖体是蛋白质和rRNA(ribosomal RNA,核糖体RNA)组成的复合体,是蛋白质合成的核心机构。
在核糖体中,mRNA上的每个密码子对应着一个tRNA (transfer RNA,转运RNA),tRNA通过其上的特定序列与氨基酸结合。
tRNA将氨基酸递送到核糖体中,核糖体将它们排列在一起,并将它们连接到一起以构成多肽链。
这个蛋白质链不断增长,直到到达终止密码子时停止。
这个过程中还涉及到一些化学反应。
在tRNA与氨基酸结合时,酸性物质如ATP参与了这个过程。
在肽键的形成中,羧基与氨基之间进行了缩合反应。
这些化学反应是生命体系中常见的反应类型,但在核糖体中运作时却具有特殊的作用。
蛋白质折叠蛋白质链合成完成后,它们不断变形并定位到其所需的态势中,以完成其特定的功能。
这个过程称为折叠。
蛋白质折叠过程中涉及到的化学反应也非常困难。
这是因为蛋白质折叠需要快速且正确地进行,并且只有一个正确的结构才能起到特定的功能。
但是,这个过程中的化学反应不可预测性,使其成为生物学中一项具有挑战性的领域。
一些研究表明,蛋白质折叠需要水分子(water molecule)的参与,水分子的作用在于帮助蛋白质获得正确的结构,并帮助它们远离错误结构或聚积。
蛋白质-适配体相互作用预测的方法

蛋白质-适配体相互作用预测的方法蛋白质-适配体相互作用(protein-ligand interaction)是指蛋白质与其结合的小分子适配体(例如药物分子)之间的相互作用。
这种相互作用对于药物发现和蛋白质功能研究非常重要。
准确地预测蛋白质-适配体相互作用对于药物设计和疾病治疗具有重要意义。
在过去的几十年中,研究人员开发了许多方法来预测蛋白质-适配体相互作用,这些方法可以分为结构基于(structure-based)和序列基于(sequence-based)方法。
在结构基于的方法中,研究人员首先确定蛋白质的三维结构以及适配体的结构。
然后,利用计算方法预测蛋白质和适配体之间的相互作用能力。
这些方法包括基于分子力学的对接(docking)方法、分子动力学模拟(molecular dynamics simulation)方法和自由能计算(free energy calculation)方法。
对接方法通过搜索蛋白质和适配体之间的可能结合模式来预测相互作用。
动力学模拟方法则通过模拟蛋白质-适配体复合物的运动过程来获取相互作用的动力学信息。
而自由能计算方法通过计算结合自由能来评估蛋白质-适配体相互作用的稳定性。
相对于结构基于方法,序列基于的方法不需要蛋白质和适配体的具体结构信息。
这些方法基于蛋白质和适配体的氨基酸或化学特性来预测相互作用。
常见的序列基于方法包括序列比对(sequence alignment)、机器学习方法和结构模型方法。
序列比对方法通过比较蛋白质和适配体序列的相似性来预测相互作用。
机器学习方法则通过训练模型来预测相互作用,其中包括决策树、神经网络和支持向量机等方法。
而结构模型方法则通过预测蛋白质和适配体的二级结构、三级结构或联系网络等结构信息来预测相互作用。
在蛋白质-适配体相互作用预测领域,还有一种新兴的方法是基于深度学习(deep learning)的方法。
这些方法通过构建深度神经网络来学习蛋白质和适配体之间的相互作用模式。
蛋白质结合 science

蛋白质结合 scienceProtein binding is a fundamental process in science that plays a crucial role in various biological functions. It refers to the interaction between proteins and other molecules, such as ligands, substrates, or other proteins. This binding process is essential for the proper functioning of cells, as it regulates cellular processes, signal transduction, and molecular recognition.One perspective to consider when discussing protein binding is the structural aspect. Proteins are composed of amino acids that fold into specific three-dimensional structures. The binding of molecules to proteins occurs through specific binding sites, which are often located in cavities or clefts on the protein surface. These binding sites possess complementary shapes and chemical properties to the molecules they bind. The binding interaction involves various non-covalent forces, such as hydrogen bonding, electrostatic interactions, hydrophobic interactions, and van der Waals forces. The precisearrangement of these forces determines the binding affinity and specificity between the protein and its ligand.From a functional perspective, protein binding iscrucial for many biological processes. Enzymes, for example, bind to their substrates to catalyze chemical reactions.The binding of a substrate to an enzyme's active site induces conformational changes that facilitate the conversion of the substrate into a product. Similarly, protein-protein interactions are vital for cellularsignaling pathways. Signaling proteins bind to each otherto transmit signals within cells, regulating processes such as cell growth, differentiation, and apoptosis. Protein binding also plays a role in gene regulation, astranscription factors bind to specific DNA sequences to control gene expression.Protein binding is not limited to small ligands orother proteins; it can also involve binding to larger structures. For instance, antibodies bind to antigens,which can be proteins, carbohydrates, or even small molecules. This binding is highly specific, allowingantibodies to recognize and neutralize foreign substancesin the body. Additionally, proteins can bind to cell membranes, such as receptors that interact with extracellular ligands. These interactions are crucial for cellular communication and signaling.Understanding protein binding has significant implications in drug discovery and development. Many drugs exert their therapeutic effects by binding to specific target proteins. By binding to these proteins, drugs can modulate their activity, block their function, or induce specific cellular responses. The study of protein-ligand interactions helps in the design and optimization of drugs, aiming to enhance their binding affinity and selectivity.In conclusion, protein binding is a fundamental process in science that occurs through specific binding sites on proteins. It is essential for various biological functions, including enzymatic catalysis, cellular signaling, and gene regulation. Protein binding involves specific structural and functional aspects, relying on complementary shapes and chemical properties between the protein and its ligand.Understanding protein binding is crucial in fields such as drug discovery, as it allows for the development of targeted therapies.。
The Chemistry and Biochemistry of Proteins

The Chemistry and Biochemistry ofProteinsProteins are one of the most important molecules in biology. They are responsible for carrying out many of the essential processes that take place in our bodies and are essential for life itself. Proteins are made up of chains of amino acids that are peeled together in a specific order. Through complex folding and chemical interactions, proteins can take on different shapes, which give them their distinct functions.Chemically, proteins are complex organic molecules that are composed of carbon, hydrogen, oxygen, nitrogen, and sometimes sulfur and phosphorus. They are made up of long chains of amino acids, linked together by peptide bonds. Amino acids are the building blocks of proteins. There are 20 different amino acids commonly found in proteins, each with its unique chemical structure and properties.The sequence of amino acids in a protein chain determines the protein’s structure and function. The order of amino acids in a protein is determined by the genetic code, which is a set of instructions encoded in the DNA of every organism. A gene is a section of DNA that codes for a specific protein. When a gene is activated, the information it contains is used to produce a specific protein.Once a protein is produced from a gene, it undergoes a process called folding. During folding, the long chain of amino acids twists and turns into a unique three-dimensional shape. The shape of the protein is critical to its function, as proteins interact with other molecules based on their shape. If a protein loses its shape, it may no longer be able to perform its intended function.There are several types of proteins with different functions. Enzymes, for example, are proteins that catalyze biochemical reactions in the body. Hormones are proteins that regulate various physiological processes. Antibodies are proteins that help the immune system defend against foreign substances. Structural proteins, like collagen and keratin, provide support and strength to tissues and organs.Biochemically, proteins are involved in many essential processes in the body. For example, proteins play a critical role in DNA replication, RNA transcription, and translation. Proteins are also involved in cellular signaling and communication, which are necessary for proper cell function.Proteins are also involved in metabolism, the set of biochemical reactions that occur in the body to produce energy and maintain bodily functions. One example of a metabolic process involving proteins is the breakdown of amino acids for energy. During times of energy deprivation, the body can break down proteins to release amino acids, which can then be metabolized for energy.Proteins are essential for life, and their importance is reflected in the numerous diseases that can result from protein-related defects. Many genetic diseases are caused by mutations in genes that affect protein production or function. For example, sickle cell anemia is caused by an abnormality in the protein hemoglobin, which carries oxygen in the blood.Overall, the chemistry and biochemistry of proteins are fascinating topics that are essential to understanding the complexities of life. Proteins are crucial for the proper functioning of the body, and their structure and function are tightly regulated to ensure optimal performance. The study of proteins is an ongoing and dynamic field with many new discoveries being made continuously.。
进修核磁共振工作总结
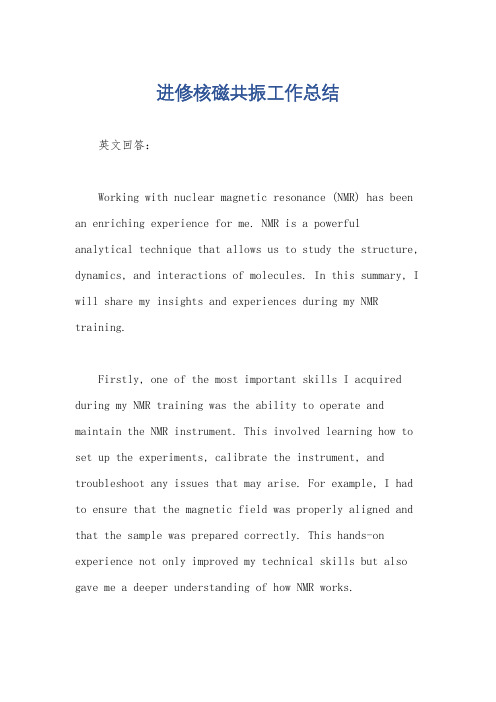
进修核磁共振工作总结英文回答:Working with nuclear magnetic resonance (NMR) has been an enriching experience for me. NMR is a powerfulanalytical technique that allows us to study the structure, dynamics, and interactions of molecules. In this summary, I will share my insights and experiences during my NMR training.Firstly, one of the most important skills I acquired during my NMR training was the ability to operate and maintain the NMR instrument. This involved learning how to set up the experiments, calibrate the instrument, and troubleshoot any issues that may arise. For example, I had to ensure that the magnetic field was properly aligned and that the sample was prepared correctly. This hands-on experience not only improved my technical skills but also gave me a deeper understanding of how NMR works.Another aspect of NMR that I found fascinating was the interpretation of spectra. NMR spectra provide valuable information about the chemical environment and connectivity of atoms in a molecule. However, interpreting these spectra can be challenging, especially when dealing with complex molecules. During my training, I learned how to identify different functional groups, determine the number of hydrogen atoms, and analyze coupling patterns. For instance, I remember analyzing a proton NMR spectrum of a drug molecule and identifying the presence of aromatic rings based on the chemical shifts and coupling constants.Furthermore, NMR experiments can be tailored to answer specific research questions. One of the techniques Ilearned was two-dimensional NMR, which provides detailed information about the correlations between atoms in a molecule. This technique allowed me to investigate the conformational changes of a protein upon binding to a ligand. By comparing the two-dimensional NMR spectra of the protein in the absence and presence of the ligand, I was able to identify the specific amino acid residues involvedin the binding interaction.In addition to the technical aspects, NMR training also emphasized the importance of data analysis and interpretation. I learned how to use software tools to process and analyze NMR data, such as peak picking, integration, and spectral fitting. These skills were essential for quantifying the concentration of compounds in a mixture or determining the purity of a sample. For example, I recall analyzing a mixture of organic compounds using NMR and successfully quantifying the concentrations of each component based on the integration values.Overall, my experience with NMR has been both challenging and rewarding. It has provided me with a solid foundation in analytical chemistry and enhanced my problem-solving skills. Working with NMR has also taught me the importance of attention to detail and patience, as even small errors in sample preparation or instrumentcalibration can affect the quality of the data. I am confident that the knowledge and skills I have gained through NMR training will be valuable in my future research endeavors.中文回答:在核磁共振(NMR)的工作中,我获得了很多宝贵的经验。
生物学英语——精选推荐

生物学英语生物学英语篇一:生物学基本名词详细解释(中英文对照)生物学基本名词详细解释(中英文对照)组织相容性Histocompatibility字面上讲是指不同组织共存的能力;严格地讲是指所有移植蛋白的一致性,这是阻止移植和器官排斥的需要。
组织相容性的分子基础是修饰几乎所有人类细胞表面的一套移植蛋白。
这些蛋白是由位于6号染色体上的一段称为主要组织相溶性复合体基因,MHC编码的。
这些蛋白高度多态。
例如,它们在不同的人中显示差异。
尽管很多人会有一些相同的MHC分子,极少数人有完全相同的MHC分子。
微小的差别导致这些蛋白质被移植受体的免疫系统识别为外来的而进行破坏。
对成功的移植来说这些蛋白质应该在供体和受体之间相匹配。
双胞胎相配的几率最高,接下来是兄弟姐妹。
在一般人群中只有10万分之一的比例是MHC匹配的,可以允许移植。
Literally, the ability of different tissues to “get along”; strictly, identity in all of the transplantation proteins, which is a requirement for the prevention of graft or organ rejection. Themolecular basis of histocompatibility is a set oftransplantation proteins that decorate the surface of nearly all human cells. These proteins are encoded by genes that are grouped on a part of chromosome 6 called the major histocompatibility complex, or MHC. These proteins are highly “polymorphic”i.e., they show variation in different individuals. Although many individuals may share some identical MHC molecules, a very low number share all the MHC molecules. The consequence of these minor differences is that these proteins are recognized by the transplant recipient’s immune system as being foreign, and so are targeted for destruction (since the immune system’s job is to eradicate any foreign proteins or cells that invade the body). For successful transplantation these proteins ideally should be matched between donor and recipient. Twins have the highest rate of match, followed by siblings. In the general population only 1 in 100,000 individuals is sufficiently “MHC matched”to another person to allow transplantation.X射线结晶学X-ray Crystallography阐述蛋白质、DNA或其它生物分子的原子水平的三维结构的技术。
- 1、下载文档前请自行甄别文档内容的完整性,平台不提供额外的编辑、内容补充、找答案等附加服务。
- 2、"仅部分预览"的文档,不可在线预览部分如存在完整性等问题,可反馈申请退款(可完整预览的文档不适用该条件!)。
- 3、如文档侵犯您的权益,请联系客服反馈,我们会尽快为您处理(人工客服工作时间:9:00-18:30)。
On the calculation of protein-ligand binding constantsAnatoly M.RuvinskyAlgodignB.Sadovaya,8,103379,Moscow,Russiaanatoly.ruvinsky@AbstractWe describe a general framework to calculate protein-ligand binding constants using the statistical-thermodynamic ap-proach and the harmonic well approximation.We found that the binding constant depends on the determinant of force constant matrix of the effective potential taking into account together with the protein-ligand interactions also nonpolar sol-vation energy.We show that for most cases binding entropy loss can not be separated into orientational and translational contributions due to the coupling of relative translational and rotational motions in the protein-ligand complex.1.IntroductionThe ability to predict reliable binding constants K b=exp(−(∆H−T∆S)/T)of small organic molecules to macro-molecules(e.g.proteins,DNA,RNA)is of tremendous pharmaceutical interest.Various methods[1-5]have been used to calculate binding changes of enthalpy∆H and entropy∆S.A free molecule,composed of N at atoms,has3translational and3rotational degrees of freedom associated with the free molecule motion,plus3N at−6vibrational degrees of freedom.During noncovalent binding the ligand becomes localized in a protein active site.Thus ligand degrees of freedom associated with the overall molecule motions are reduced into the vibrational degrees of freedom of the internal relative motions in the protein-ligand complex.The vibrational normal modes of free molecules do not contribute to the binding because vibrational spectra of protein and ligand are not changed in the noncovalent complex.The contribution of relative motions in the protein-ligand complex to the protein-ligand binding is considered in this paper.2.Calculations of the binding constantsThe protein-ligand complex is stabilized if the relative motion of molecules is localized in a well formed by electrostatic and Van der Waals forces.The oscillatory entropy contribution of the relative motion arises in the deep well,when the distance between oscillatory levels is much less then the depth of the well.For typical complexes the distance between oscillatory levels is≈10−3−10−2kcal/mol.So we can consider relative oscillating motions in the protein-ligand complex as a motion in the deep well and neglect the inharmonic effects.In classical approximation the binding constant of the protein-ligand complex formation is equal to[3]K b=N a V B8π2exp˜µp+˜µl+U p+U lT,(1)where N a is Avogadro number;V B=exp−U pl(r,θ,ϕ,ψ)+˜µpl(r,θ,ϕ,ψ)Td r sinθdθdϕdψ(2)is the binding volume;˜µp,l,pl accounts for the non-polar solvation effect and linearly depends on the corresponding solvent-accessible surfaces;U pl(r,θ,ϕ,ψ)is the energy of the complex in the solution in the implicit solvation model;U p,l are the energies of corresponding solutes;r is the vector of relative motions in the complex;(θ,ϕ,ψ)are Euler angels of relativeorientations.In Exp.(2)we use˜µp,l+U p,l=min{˜µ(i)p,l +U p,l}(i is a number of the molecular configuration)[3].In stable complexes relative motion of r isfinite and localized near r0.Relative orientational motions are free or restrained nearΩo=(θo,ϕo,ψo).Further we consider the case of relative orientational motions withα=1,2,3unrestrained degrees of freedom.Expanding the effective potential U eff(r,Ω)=U pl(r,Ω)+˜µpl(r,Ω)−T ln sinθnear the minimum(r0,Ωo)over the restrained coordinates up to the quadratic terms of deviations(x1,...,x6−α),we getU eff(r,Ω)=U eff(r o,Ωo)+6−αi,k=1G ik(r o,Ωo)x i x k(3)Here(x1,x2,x3)are deviations of r from the minimum,and(x4,...,x6−α)define orientational deviations of Euler angels.Inserting the expansion for U eff in Eq.(3)into Eq.(2)gives:K b=N a8αi=1V iT3−α/2π1−α/2det G(r o,Ωo)exp−U eff(r o,Ωo)−˜µp−˜µl−U p−U lT,(4)where Vθ=2and Vϕ=Vψ=2π.The number of unrestrained orientational degrees of freedom can be derived from the experimental temperature dependence of the binding constant.The pre-exponential factor in(4)describes the effect of conversion between ligand degrees of freedom and degrees of freedom of relative motions in the protein-ligand complex.The entropy loss of the binding process has the form of∆S=3−α/2+˜µp+˜µl−˜µpl(r o,Ωo)T−ln8N aαi=1V−1io oT3−α/2π1−α/2(5)Generally matrix G is not diagonal[4],and therefore only in special cases entropy loss∆S can be separated into angular and translational parts as were done,for example,in[1,2,3].Results of application of our method to the protein-ligand complexes using molecular mechanics and the knowledge-based approach will be presented.3.ConclusionsThis paper extends the method developed in[3]to calculate binding constants and the binding entropic contribution comes from changes in translational and rotational degrees of freedom during noncovalent molecular association.The analysis yields following conclusion regarding the entropy loss upon binding:Translational and rotational changes of the entropy can not be calculated separately except the very rare cases of the special effective potential,composed of the distant dependent term and the term depends on the relative orientations only.We show that the binding entropy loss depends on the determinant of the force constant matrix of the effective potential.References[1]A.Finkelstein,J.Janin,The price of lost freedom:entropy of bimolecular complex formatio.Prot Eng,3:1-3,1989.[2]L.M.Amzel,Loss of translational entropy in binding,folding,and catalysis.Proteins Str Func Genet,28:144-149,1997.[3]M.K.Gilson,J.A.Given,B.L.Bush,J.A.McCammon,The statistical-thermodynamic basis for computation of bindingaffinities:a critical review.Biophys J,72:1047-1069,1997.[4]I.G.Kaplan,Theory of molecular interactions.Elesevier,Amsterdam,1986.[5]S.M.Schwarzl,T.B.Tschopp,J.C.Smith,S.Fischer,Can the calculation of ligand binding free energies be improvedwith continuum solvent electrostatics and an ideal-gas entropy correction.J Comput Chem,23:1143-1149,2003.。