Engineered bioretention for removal of nitrate from stormwater runoff
天津大学制药工程专业 考研《制药工艺学》中英文对译

天津⼤学《制药⼯艺学》中英⽂对译pharmaceutical technology制药⼯工艺学pharmaceutical pipeline制药链pharmacopoeia药典。
Roswell Park Memorial Instirute RPMI good manufacturing practices for drugs GMP制药⾏行行业medicines,drugs药品traditional Chinese medicines中药natural medicines天然药物chemical drugs化学药物biologics,biologic products⽣生物制品generics,generic drugs仿制药物me-too-drug仿制药biosimilars⽣生物类似药biotechnology⽣生物技术.Food and druge administration FDA biotechnology pharmaceutical,biopharmaceutical⽣生物制药nucleotide核苷酸nucleoside核⽢甘enzyme酶enzyme inhibitor酶抑制剂immunomodulator免疫调节剂penicillin⻘青霉素antibody engineering抗体⼯工程inducer诱导剂precursor前体prodrug前药transformation遗传转化.conversion⽣生物转化fermentation发酵.strain breeding菌种选育separation and purification分离纯化和提纯.cell growth phase/fermentationproduct synthesis phase/product secretion phase.Murashige&Skoog MScell autolysis phase/fermentation anaphase.generic通⽤用药物metabolism代谢.substrate培养基质primary/secondary metabolism初级/次级代谢.specific growth rate⽐比⽣生⻓长速率lag/log/decline/stationary/death phase延滞期/对数⽣生⻓长期/减数期/稳定期/死亡期coupling model⽣生⻓长与⽣生产偶联型.PEG聚⼄乙⼆二醇semi-coupling model⽣生⻓长与⽣生产半偶联型.starter culture培养物non-coupling model⽣生⻓长与⽣生产⾮非偶联型.storage保存protoplast fusion原⽣生质体融合.DMSO⼆二甲基亚砜master stock/cell bank MSB/MCB主菌种库.glycerol⽢甘油working stock/cell bank WSB/WCB⼯工作菌种库.Streptomyces链霉菌quality control QC质量量控制.cholramphenicol氯霉素China Center for Type Culture Collection CCTCC中国典型培养物保藏中⼼心China General Microbiological Culture Collection Center CGMCC中国普通微⽣生物保藏管理理中⼼心China Center of Industrial Culture Collection CICC中国⼯工业微⽣生物菌种保藏管理理中⼼心National Center for Medical Culture Collection(Bacteria)CMCC中国医学微⽣生物菌种保藏中⼼心America Type Culture Collection ATCCEuropean Collection of Cell Culture ECACCInstiture for Fermentation,Osaka IFONational Collection of Type Culture mNCTCmedium培养基.carbon source碳源nitrogen source氮源.mineral salt⽆无机盐macroelement⼤大量量元素.trace element microelement微量量元素growth factor⽣生⻓长因⼦子.precursor前体accelerant促进剂.fed medium补料料培养基agar琼脂粉.contaminated microbe杂菌contamination污染.phage噬菌体disinfection消毒.sterilization灭菌pathogen病原微⽣生物.filtration sterilization过滤灭菌filter过滤介质VVM空⽓气流量量(单位时间单位体积内通⼊入的标准状况下的空⽓气体积)primary culture原代培养.passage culture传代培养solid surface culture固体表⾯面培养.liquid submerged culture液体深层培养immobilized culture固定化培养.high cell density culture⾼高密度培养intermittent opration间歇式操作.discontinuous operation不不连续培养semi-continuous operation半连续培养.batch operation分批式操作fed batch operation补料料-分批式(流加)操作.chemostat恒化器器MPa罐压.dissolved oxygen DO溶解氧cell concentration菌体浓度.fermentation heat发酵热production heat产⽣生热.loss heat散失热biological heat⽣生物热.agitation heat搅拌热evaporation heat蒸发热.sensible heat显热radiant heat辐射热.oxygen supply供氧oxygen consumption耗氧.dissolved oxygen coefficient溶解氧系数oxygen transfer rate OTR氧传递速率.oxygen uptake rate OUR摄氧速率ventilation通⽓气.respiratory intensity呼吸强度oxygen saturation concentration氧饱和浓度.respiratory quotient RQ呼吸熵critical oxygen concentration临界氧浓度.fill补料料withdraw放料料.foam泡沫defoaming agent消沫剂.surfactant表⾯面活性剂dispersant分散剂.emulsifier乳化剂inertcarrier惰性载体.antibiotic抗⽣生素carbenicillin羧苄⻘青霉素/⻘青霉素G6-aminopenicillanic acid6-APA6-氨基⻘青霉烷酸.cephalosporin C CPC头孢菌素C erythromycin红霉素.amino acid氨基酸hybridomn杂交瘤.vitamin维⽣生素recombinant DNA technology重组DNA技术.recombinant DNA products rDNA制品plasmid质粒.replicon复制⼦子promoter启动⼦子.terminator终⽌止⼦子multiple cloning site MCS多克隆隆位点.transferability转移性incompatibility不不相容性.cloning vector克隆隆载体expression vector表达载体.shuttle vector穿梭载体intergration vector整合载体.inclusion body包涵体yeast酵⺟母.genetic engineering strain基因⼯工程菌yeast intergration plasmid YIP酵⺟母整合载体yeast episomal plasmid YEP酵⺟母附加载体yeast centromere plasmid YCP酵⺟母着丝粒载体centromere sequence CEN着丝粒序列列autonomously replicating sequences ARS⾃自主复制序列列yeast replicating plasmid YRP酵⺟母复制质粒polymerase chain reaction PCR聚合酶链式反应reverse transcription PCR RT-PCR反转录PCRcomplementary DNA cDNAavian myeloblastosis virus AMV禽源成髓细胞瘤病毒moloney murine leukemia virus MMLV⿏鼠源败⾎血病毒莫勒勒尼株diethyl pyrocarbonate DEPC焦碳酸⼆二⼄乙酯.denaturation变性annelling退⽕火.extension链延伸restriction endonuclease限制性核酸内切酶.ligase连接酶recombinant重组⼦子.interferon IFN⼲干扰素recombinant human interferon rhIFNtricarboxylic acid cycle TCA循环三羧酸循环pentose phosphate pathway PPP磷酸戊糖途径.glycosylation糖基化apoptosis凋亡.diploid cell⼆二倍体primary cell原代细胞.passage cell传代细胞immortal cell永久细胞系.Chinese hamster ovary CHO中国仓⿏鼠卵卵巢DHFR⼆二氢叶酸还原酶.methotrexate MTX甲氨蝶呤baby hamster kidney BHK幼仓⿏鼠肾脏dicistron双顺反⼦子long terminal repeat sequences LTRS逆转录病毒的⻓长末端重复序列列cytomegalovirus CMV⼈人巨噬病毒.ubiquitin泛素蛋⽩白bovine growth hormone.BGH⽜牛⽣生⻓长素.toppoisomerase拓拓扑异构酶internal ribosome entry site IRES核糖体进⼊入位点.serum⾎血清minimum essential medium MEM basal medium Eagle’s BME Dulbecco’s modified Eagle’s medium DMEMGlasgow’s modified Eagle’s medium GMEMJoklik’s Park Memorial Eagle’s medium JMEMRoswell Park Memorial Institute RPMIserum-free medium SEM⽆无⾎血清培养基.buffer solution缓冲液balance saline solution BSS平衡盐溶液monolayer anchorage-dependent culture单层贴壁培养.suspension culture悬浮培养microcarrier微载体.microencapsulation method微囊法phosphonate buffer solution PBS磷酸盐缓冲液.scale-down缩⼩小erythropoietin EPO红细胞⽣生成素.luria bertani LB recombinant human erythropoietin rhEPO重组⼈人红细胞⽣生成素synthon合成⼦子.synthetic equivalent合成等价物protocol solvent质⼦子性溶剂.micronization微晶化catalyst催化剂.phase transfer catalyst相转移催化剂TEBAC三⼄乙基苄基氯化铵Mokosza催化剂TO/CMAC三⾟辛基甲基氯化铵Starks催化剂.Brandstrom催化剂四丁基硫酸氢铵chirality⼿手性.enantiomers对应异构体configuration构型.chiral drug⼿手性药物enantiomeric excesses对映体过量量e.e.%.restrosynthesis追溯求源法resolution拆分.omeprazole奥美拉唑paclitaxel,Taxol紫杉醇.cephalosporin C CPC头孢菌素7-aminocephalosporanic acid7-ACA7-氨基头孢烷酸.cefalexin头孢氨苄tetrahydrofuran THF四氢呋喃.quality by design QbD质量量源于设计process analysis technology PAT过程分析技术quality target product profile QTPP⽬目标产品质量量概况critical material attribute CMA关键物料料属性critical process parameter CPP关键⼯工艺参数normal operation range NOR正常操作区间proven acceptable range PAR可接受的区间critical quality attribute CQA关键质量量属性bioreactor⽣生物反应器器key process parameter KPP重要⼯工艺参数.fermenter发酵罐complete stirred tank reactor CSTR全混流反应器器.yield得率piston fluid reactor PFR平推流反应器器.titer效价stirred tank reactor STR搅拌罐.scale-up放⼤大fixed bed reactor固定化床反应器器.draft tube导流筒packed bed reactor PBR填充床反应器器.bubble column⿎鼓泡塔fluidized bed reactor FBR流化床反应器器.air-lift reactor⽓气升式反应器器disk and turbine impeller涡流式搅拌桨.process validation⼯工艺验证marine style impeller推进式搅拌桨.process design⼯工艺设计process mass intensity PMI过程质量量强度.process qualification⼯工艺确认reaction mass efficiency RME反应质量量效率standard operation procedure SOP标准操作规程.continued process verification⼯工艺核实biochemical oxygen demand BOD⽣生化需氧量量.total nitrogen TN总氮chemical oxygen demand COD化学需氧量量.suspended subatance SS悬浮物mixed liquor suspended solids MLSS混合液悬浮固体total organic carbon TOC总有机碳.sludge volume SV污泥泥沉降⽐比sludge volume index SVI污泥泥指数piping&instrument diagram PID⼯工艺控制流程图。
EMEA-包括生物技术衍生制品在内的药品工艺变更可比性研究-质量事项(翻译)
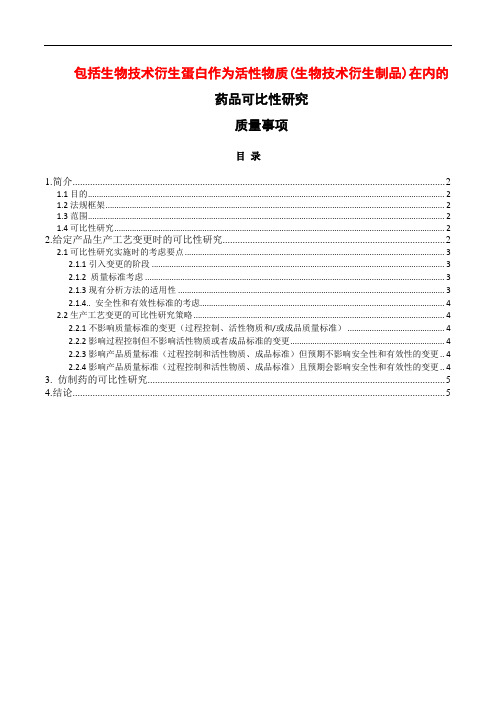
包括生物技术衍生蛋白作为活性物质(生物技术衍生制品)在内的药品可比性研究质量事项目录1.简介 (2)1.1目的 (2)1.2法规框架 (2)1.3范围 (2)1.4可比性研究 (2)2.给定产品生产工艺变更时的可比性研究 (2)2.1可比性研究实施时的考虑要点 (3)2.1.1引入变更的阶段 (3)2.1.2 质量标准考虑 (3)2.1.3现有分析方法的适用性 (3)2.1.4.. 安全性和有效性标准的考虑 (4)2.2生产工艺变更的可比性研究策略 (4)2.2.1不影响质量标准的变更(过程控制、活性物质和/或成品质量标准) (4)2.2.2影响过程控制但不影响活性物质或者成品标准的变更 (4)2.2.3影响产品质量标准(过程控制和活性物质、成品标准)但预期不影响安全性和有效性的变更 .. 42.2.4影响产品质量标准(过程控制和活性物质、成品标准)且预期会影响安全性和有效性的变更 .. 43. 仿制药的可比性研究 (5)4.结论 (5)1.简介1.1目的众所周知,生物技术药物、重组DNA和杂交瘤技术制备的蛋白药物都经常会发生工艺变更(活性成分和/或制剂)。
这些变更的主要原因为提高产品质量、增大产量和全球生产力,降低运营成本等。
这些变更可能发生在研发阶段或者上市之后。
无论这些变更发生在在什么阶段,都有必要比较变更前后的产品来确定变更的引入没有改变产品的理化性质和生物特性。
这些特性(可能体现在现有的过程控制或者放行标准)极为重要,是证明产品质量、安全性和有效性的基础。
这些特性的改变都可能导致产品安全性和有效性属性的改变。
因此,某个(a given product)药品工艺发生变更后,应该考虑做可比性研究。
该指南不涵盖在研发初期(即在做非临床研究和初期临床研究评价其初步安全性之前)引入的变更。
此外,当生产商进行生物仿制药的上市申请时,则应该考虑做可比性研究的必要性。
无论何种情况,做可比性研究的思路(逐步法)都很类似。
基于SWMM的低冲击开发雨洪控制过程模拟

基于SWMM的低冲击开发雨洪控制过程模拟李翠梅;罗贤达;黄锐【摘要】以苏州市某小区为例,采用SWMM模拟城市化后并采取LID措施(下凹式绿地、多孔路面等)时不同状况下的水文过程,评估不同LID措施的雨洪控制利用效果.结果表明,与现状模式情景相比,对于设计暴雨重现期为5a和10 a,采用多孔路面措施,洪峰削减率分别为60%、56.5%,平均径流系数分别减少27.2%、20.9%;采用下凹式绿地措施,峰现时刻分别推迟7、8 min;上述两种措施组合时,洪峰削减率分别为71.1%、64.5%,峰现时刻分别推迟11、9 min,平均径流系数分别减少37.5%、28%.【期刊名称】《兰州理工大学学报》【年(卷),期】2016(042)002【总页数】4页(P144-147)【关键词】低冲击开发;SWMM;雨洪控制及其利用;下凹式绿地【作者】李翠梅;罗贤达;黄锐【作者单位】苏州科技学院环境科学与工程学院,江苏苏州215009;苏州科技学院环境科学与工程学院,江苏苏州215009;佛山市水业集团有限公司,广东佛山528000;苏州科技学院环境科学与工程学院,江苏苏州215009【正文语种】中文【中图分类】TU992随着经济的快速发展及城镇化水平的不断提高,城市水资源危机、水环境污染、内涝灾害等问题日益突出.不透水面积的不断增加,改变了城市下垫面原有的水文状态.市政雨水管网的雨水排除压力增大,导致城市洪涝灾害频发.科学、合理地对雨洪进行控制利用显得格外重要.低冲击开发(low impact development,LID)是尽可能地维持或恢复开发前的水文机制,是一种分散的、小规模的源头控制机制和设计技术的雨水管理理念,能有效地缓解不透水面积增加造成的洪峰流量增加、径流系数增大、面源污染负荷加重的问题.近年来,欧美等发达国家在雨洪控制利用方面已形成了较为成熟的理论和技术体系.美国马里兰州的乔治王子郡首先提出低冲击开发理念,用来缓解城市化和不透水地表增加带来的负面影响[1].Liaw等[2]、Dietz等[3]和Bedan等[4]分析了LID理念与传统雨水处理的差别;Villarreal等[5]、Van Woert等[6]和Roseen等[7]对不同的LID措施进行模拟,分析在不同设计重现期下不同LID措施的雨洪控制作用;Kim等[8]、Dunnett等[9]及Myers等[10]利用现场试验研究不同LID措施的雨洪控制效果.国内对低冲击开发的应用尚处于起步阶段,但对低冲击开发的研究却不多.深圳光明新区率先在全国建立低冲击开发示范区,对光明新区的雨洪利用目标、技术手段、实施方法及相应政策进行探索与实践,为其他城市提供借鉴与指导作用[11].王建龙等[12]基于国内绿色建筑中雨洪控制作用的相关内容和现状,分析了低冲击开发在绿色建筑中的应用前景.晋存田团队[13]对透水砖和下凹式绿地在不同降雨频率下进行了雨洪控制效果的研究.目前低冲击开发的研究主要从微观尺度进行模拟与试验,尚未与城市的排水系统相结合.本文研究以苏州市某小区为例,运用暴雨管理模型模拟和评估不同低冲击开发措施的雨洪控制效果,为城市雨水控制利用技术的研究提供参照.美国环境保护署于1971年开发了一种综合性数学模型——暴雨管理模型(storm water management model, SWMM),主要用于模拟城市单一降水事件或长期(连续)的径流水量和水质模型.SWMM模型自1971年开发以来已被广泛应用于城市地区的暴雨洪水、合流式下水道、排污管道以及其它排水系统的规划、分析和设计[14].现在最新的版本为SWMM5.1,是一个动态的水文、水力和水质模拟模型.水文模拟增加了低冲击开发(low impact development, LID)模块,可以模拟生物滞留、渗渠、多孔路面、植草沟和雨水桶等5种分散的低冲击开发措施.通过对入渗、蒸发及滞蓄等水文过程的模拟,结合SWMM模型的水力和水质模块,实现对研究区域径流量、峰值流量等采取LID措施的模拟.其他的LID措施(如缓冲带、下凹式绿地等技术)都可以经过参数变换等相应处理后再进行模拟[15].LID在子汇水区域的布置方式主要有2种:1) 场地层面.在子流域上创建一个新的LID调控器,允许LID调控器被统一控制和接受来自上游子流域的出流作为该子流域的入流.此方式可以实现研究区域雨水处置过程的可视化,在小场地的LID水力模拟和水质控制效果模拟中比较适用.2) 子汇水区层面.向一个已经存在LID调控器的子流域加入一个或多个控制器,或向一个没有LID控制器子流域的区域直接添加LID.此方法可以将多个LID控制器混合置于一个子流域内,每一个调控器分别处理流域内没有设置调控器区域的水流部分,适用于较大区域的LID模拟.在任何时候只要LID引入到子流域中,那么其原有参数(如不透水率、特征宽度等)应重新确定.低冲击开发模式的原则为:尽量降低开发区域不透水面积的比例,尽量接近开发前的自然水文循环;充分利用地表的入渗能力延长雨水径流路径,增加雨水径流时间,减轻城市化进程对开发区域水文状态的冲击[16].低冲击开发的实施目标主要是控制雨水径流污染、减少径流总量、削减洪峰流量,满足雨水资源化、控制洪涝及改善水循环和水生态环境等多项需求.对于规划设计,首先应根据开发建设区域的自然环境和人文环境选择实施目标,例如南方地区偏重于城市暴雨洪涝灾害的控制,而北方地区则需要考虑地下水的补给[17].为研究LID措施的雨洪控制作用,遵循低冲击开发模式的原则,采用不同LID措施模拟分析其对雨洪控制的影响.渗透量大是下凹式绿地主要特点,因此把研究区域的现有绿化地块改造为下凹式绿地.控制调整好路面、绿地和雨水口3者的高程关系,使下凹式绿地的高程低于路面和雨水口的高程,则不透水区域上产生的径流先流入下凹式绿地,再流入下凹式绿地中的雨水口[18].本文研究中下凹式绿地的模拟面积为1.3 hm2,蓄水深度为100 mm.由于多孔路面可以使下垫面地表产生的径流尽快下渗到土壤的蓄水层中,因此将小区内的路面停车场等硬化面积改为多孔路面.多孔路面的模拟面积为0.695 hm2,面层厚度70 mm,糙率为0.014,空隙比为0.13;基层与垫层的厚度为250 mm,空隙比为0.43.将下凹式绿地和多孔路面组合模拟,低冲击开发措施组合模拟的参数设置及面积都和其单独模拟时相同,2种措施组合模拟时的总面积为1.995 hm2.3.1 研究区域概化苏州市某小区总面积6.5 hm2,其中不透水面积为3.94 hm2,占总面积的60%;透水面积为2.56 hm2,占总面积的40%.不透水面积由屋面、小区道路和停车场组成,透水面积为小区绿化.根据SWMM模型的应用要求,对小区的雨水管网进行概化,将整个小区的雨水管网概化成29个子汇水区,28条雨水管道,28个检查井节点,1个出水口,其概化图如图1所示.3.2 设计降雨模型计算苏州市暴雨强度公式:式中:q为设计暴雨强度,L/(s·hm2);t为降雨历时,min;P为设计降雨重现期,a.3.3 模型参数的确定研究区域结合SWMM模型应用.雨洪模拟参数主要参考模型用户手册中的典型值及相关研究[19-20].在子汇水区降雨产流过程中,选用Horton入渗模型进行雨水渗透模拟,最大入渗率为76 mm/h,最小入渗率为3.8 mm/h,衰减系数为3 h-1;地表汇流模拟采用非线性水库模型,其模拟过程采用动力波进行演算,模型参数参考SWMM模型用户手册的典型值;透水地表、不透水地表的洼蓄量和曼宁系数的典型值参照表1.为了评估低冲击开发措施在不同设计暴雨重现期下的雨洪控制效果,利用式(1)求出重现期P=5、10 a,降雨历时t=2 h的总降雨量分别为83、95 mm.设计雨型采用芝加哥降雨过程线模型,雨峰时刻的相对位置r=0.4,计算时间步长为1 min.运用SWMM模型模拟传统模式城市化及加入LID情景布设在设计暴雨重现期为P=5、10 a时各出口断面径流过程.各地表特性的洪峰流量、洪峰削减率、峰值流量时刻、平均径流系数及平均径流系数减小比例见表2.由表2可知,在设计暴雨重现期分别为5 a和10 a时,现状模式下的洪峰流量分别为0.45 m3/s和0.62 m3/s,峰现时刻分别为00:50和00:50,平均径流系数分别为0.696和0.737.相同设计暴雨重现期下,下凹式绿地洪峰流量分别为0.29m3/s和0.43 m3/s,峰现时刻分别为00:57和00:58,平均径流系数分别为0.579和0.624;与现状模式相比,下凹式绿地的洪峰流量削减率分别为35.6%、30.7%,峰现时刻分别推迟7、8 min,平均径流系数分别减少16.8%、15.3%.多孔路面洪峰流量分别为0.18 m3/s和0.27 m3/s,峰现时刻分别为00:56和00:57,平均径流系数分别为0.507和0.583;与现状模式相比,洪峰削减率分别为60.0%、56.5%,峰现时刻分别推迟6、5 min,平均径流系数分别减少27.2%、20.9%.上述2种LID措施组合模拟时的洪峰流量分别为0.13 m3/s和0.22 m3/s,峰现时刻分别为01:01和00:59,平均径流系数分别为0.435和0.531;与现状模式相比,洪峰削减率分别为71.1%、64.5%,峰现时刻分别推迟11、9 min,平均径流系数分别减少37.5%、28.0%.由模拟结果可以看出:1) LID措施单独模拟及组合模拟时,洪峰的削减率和地表平均径流系数减少幅度都随设计暴雨重现期的增加而减少,而且下凹式绿地对延长峰现时间的效果优于多孔路面,多孔路面对削减洪峰流量和减小平均径流系数优于下凹式绿地.2) 在添加LID措施模拟后,设计暴雨重现期为5 a时的雨洪控制效果优于10 a.这是因为设计重现期为10 a时地表的滞蓄量和入渗量达到饱和的时间要比5 a更快,当设计暴雨强度过大时,加入LID措施后的地表还未达到饱和,地表就产生地表径流.3) LID组合模拟时的雨洪控制利用效果优于各LID措施单独模拟.组合模拟一方面可以增加地表透水面积,还可以达到削减洪峰流量、减少径流系数的目的,具有明显的雨洪控制效果.利用SWMM模拟城市化后的雨洪过程和下凹式路面、多孔路面及2种LID措施组合模拟情况下对城市雨洪控制作用的效果,结果表明:加入LID措施可以明显削减洪峰流量,延长峰值时刻、降低径流量和平均径流系数.下凹式绿地、多孔路面和2种LID措施组合模拟在设计重现期为5 a和10 a时对径流量、洪峰流量、径流系数、峰值时刻的雨洪控制效果不同,其中2种LID措施组合模拟的雨洪控制效果优于LID措施单独模拟,且设计降雨重现期为5 a时的雨洪控制效果要优于10 a.为了防止城市发生内涝的频率,在城镇化进程或者老城区改造中应积极推行低冲击开发理念,可采用下凹式绿地和多孔路面等多种措施组合的方式,根据实际情况和市政雨水管网的运行能力等,通过合理的方案确定低冲击开发措施的布设,可以实现经济效益、社会效益及环境效益的整体优化和雨洪控制利用的最大化.致谢:本文得到苏州科技学院研究生科研创新计划项目(SKCX13S_019,SKCX13S_023)的资助,在此表示感谢.【相关文献】[1] LEE J M,HYUN K H,CHOI J S,et al.Analysis of the impact of low impact development on runoff from a new district in korea [J].Water Science and Technology,2013,68(6):1315-1321.[2] LIAW C,CHENG M,TSAI Y.Low-impact development:An innovative alternative approach to stormwater management [J].Journal of Marine Science andTechnology,2000,8(1):41-49.[3] DIETZ M E,CLAUSEN J C.Stormwater runoff and export changes with development ina traditional and low impact subdivision [J].Journal of EnvironmentalManagement,2008,87(4):560-566.[4] BEDAN E S,CLAUSEN J C.Stormwater runoff quality and quantity from traditional and low impact development watersheds [J].Journal of the American Water Resources Association,2009,45(4):998-1008.[5] VILLARREAL E L,SEMADENI-DAVIES A,BENGTSSON L.Inner city stormwater control using a combination of best management practices [J].EcologicalEngineering,2004,22(4/5):279-298.[6] VANWOERT N D,ROWE D B,ANDRESEN J A,et al.Green roof stormwater retention:effects of roof surface,slope,and media depth [J].Journal of Environmental Quality,2005,34(3):1036-1044.[7] ROSEEN R M,BALLESTERO T P,HOULE J J,et al.Seasonal performance variations for storm-water management systems in cold climate conditions [J].Journal of Environmental Engineering,2009,135(3):128-137.[8] KIM H,SEAGREN E A,DAVIS A P.Engineered bioretention for removal of nitrate from stormwater runoff [J].Water Environment Research,2003,75(4):355-367.[9] DUNNETT N,NAGASE A,GRIME R B A P.Influence of vegetation composition on runoff in two simulated green roof experiments [J].Urban Ecosystems,2008,11(4):385-398. [10] MYERS B,BEECHAM S,VAN LEEUMWEN J A.Water quality with storage in permeable pavement basecourse [J].Water Management,2011,164(7):361-372.[11] 胡爱兵,任心欣,俞绍武,等.深圳市创建低影响开发雨水综合利用示范区 [J].中国给水排水,2010,26(20):69-72.[12] 王建龙,车伍,易红星.低影响开发与绿色建筑的雨水控制利用 [J].工业建筑,2009,39(3):123-125.[13] 晋存田,赵树旗,闫肖丽,等.透水砖和下凹式绿地对城市雨洪的影响 [J].中国给水排水,2010,26(1):40-42.[14] 赵冬泉,佟庆远,王浩正,等.SWMM模型在城市雨水排除系统分析中的应用 [J].给水排水,2009,35(5):198-201.[15] 王文亮,李俊奇,宫永伟,等.基于SWMM模型的低影响开发雨洪控制效果模拟 [J].中国给水排水,2012,28(21):42-44.[16] 王雯雯,赵智杰,秦华鹏.基于SWMM的低冲击开发模式水文效应模拟评估 [J].北京大学学报:自然科学版,2012,48(2):303-309.[17] 王琼珊,赵冬泉,王浩正.基于动态模拟的城市低影响开发规划流程探讨 [J].中国给水排水,2012,28(15):41-44.[18] 叶水根,刘红,孟光辉.设计暴雨条件下下凹式绿地的雨水蓄渗效果 [J].中国农业大学学报,2001,6(6):53-58.[19] 潘国庆.不同排水体制的污染负荷及控制措施研究 [D].北京:北京建筑工程学院,2008.[20] 刘俊,徐向阳.城市雨洪模型在天津市区排水分析计算中的应用 [J].海河水利,2001(1):9-11.。
生物分离工程的英语

生物分离工程的英语Biological Separation Engineering is a specialized field that focuses on the isolation and purification of biological products. It plays a crucial role in the pharmaceutical, food, and biotechnology industries, where the extraction ofbioactive compounds from natural sources is essential.The process typically begins with the selection of an appropriate feedstock, which could be anything from plant material to microorganisms. Once the feedstock is identified, it undergoes a series of steps to separate the desired components. These steps may include:1. Pre-treatment: This involves breaking down the complex structure of the feedstock to release the target molecules. Techniques such as mechanical disruption, enzymatic digestion, or chemical treatment may be used.2. Extraction: The target molecules are then extractedfrom the pre-treated material. This can be done using solvent extraction, where a solvent is used to dissolve the desired compounds, or by using methods like supercritical fluid extraction, which employs high-pressure gases to extract the compounds.3. Concentration: After extraction, the solution is often diluted and needs to be concentrated to increase the concentration of the target molecules. This can be achievedthrough evaporation, membrane filtration, or centrifugation.4. Purification: The concentrated solution may still contain impurities, so further purification is necessary. Chromatography is a common technique used at this stage, which separates molecules based on their affinity to the stationary phase.5. Polishing: The final step is to polish the purified product to ensure it meets the required specifications. This may involve additional rounds of purification or the use of specific techniques to remove any remaining impurities.Biological separation engineering is a complex process that requires a deep understanding of both the properties of the target molecules and the various separation techniques available. Advances in this field are continually improving the efficiency and selectivity of these processes, making it possible to produce high-quality biological products for a wide range of applications.。
制药工程专业英语课文翻译1 5 11 13 16单元原文加翻译
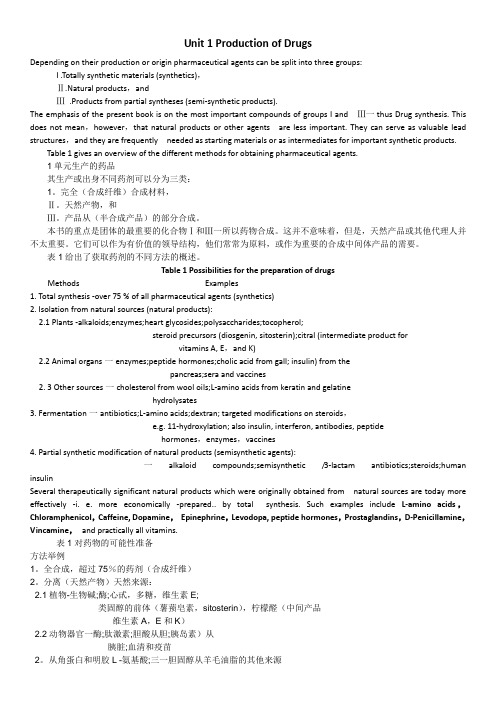
Unit 1 Production of DrugsDepending on their production or origin pharmaceutical agents can be split into three groups:I .Totally synthetic materials (synthetics),Ⅱ.Natural products,andⅢ.Products from partial syntheses (semi-synthetic products).The emphasis of the present book is on the most important compounds of groups I and Ⅲ一thus Drug synthesis. This does not mean,however,that natural products or other agents are less important. They can serve as valuable lead structures,and they are frequently needed as starting materials or as intermediates for important synthetic products.Table 1 gives an overview of the different methods for obtaining pharmaceutical agents.1单元生产的药品其生产或出身不同药剂可以分为三类:1。
完全(合成纤维)合成材料,Ⅱ。
天然产物,和Ⅲ。
产品从(半合成产品)的部分合成。
本书的重点是团体的最重要的化合物Ⅰ和Ⅲ一所以药物合成。
这并不意味着,但是,天然产品或其他代理人并不太重要。
它们可以作为有价值的领导结构,他们常常为原料,或作为重要的合成中间体产品的需要。
生物反应器处理醇醚有机废气工程应用
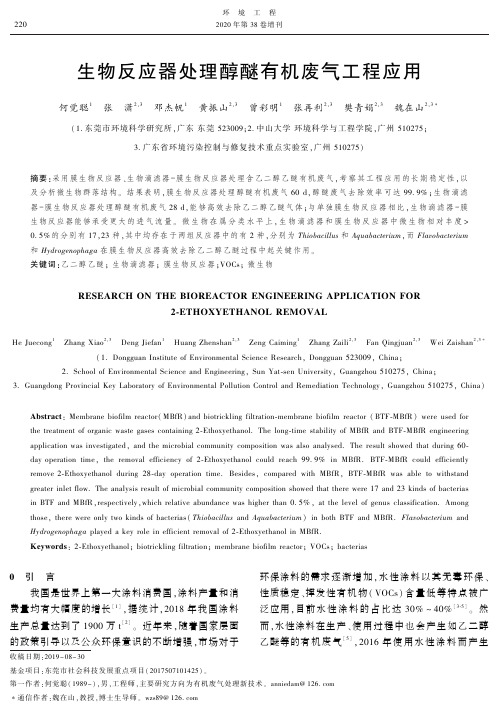
生物反应器处理醇醚有机废气工程应用何觉聪1㊀张㊀潇2,3㊀邓杰帆1㊀黄振山2,3㊀曾彩明1㊀张再利2,3㊀樊青娟2,3㊀魏在山2,3∗(1.东莞市环境科学研究所,广东东莞523009;2.中山大学环境科学与工程学院,广州510275;3.广东省环境污染控制与修复技术重点实验室,广州510275)摘要:采用膜生物反应器㊁生物滴滤器-膜生物反应器处理含乙二醇乙醚有机废气,考察其工程应用的长期稳定性,以及分析微生物群落结构㊂结果表明,膜生物反应器处理醇醚有机废气60d ,醇醚废气去除效率可达99.9%;生物滴滤器-膜生物反应器处理醇醚有机废气28d ,能够高效去除乙二醇乙醚气体;与单独膜生物反应器相比,生物滴滤器-膜生物反应器能够承受更大的进气流量㊂微生物在属分类水平上,生物滴滤器和膜生物反应器中微生物相对丰度>0.5%的分别有17,23种,其中均存在于两组反应器中的有2种,分别为Thiobacillus 和Aquabacterium,而Flavobacterium和Hydrogenophaga 在膜生物反应器高效去除乙二醇乙醚过程中起关键作用㊂关键词:乙二醇乙醚;生物滴滤器;膜生物反应器;VOCs ;微生物RESEARCH ON THE BIOREACTOR ENGINEERING APPLICATION FOR2-ETHOXYETHANOL REMOVALHe Juecong 1㊀Zhang Xiao 2,3㊀Deng Jiefan 1㊀Huang Zhenshan 2,3㊀Zeng Caiming 1㊀Zhang Zaili 2,3㊀Fan Qingjuan 2,3㊀Wei Zaishan 2,3∗(1.Dongguan Institute of Environmental Science Research,Dongguan 523009,China;2.School of Environmental Science and Engineering,Sun Yat-sen University,Guangzhou 510275,China;3.Guangdong Provincial Key Laboratory of Environmental Pollution Control and Remediation Technology,Guangzhou 510275,China)Abstract :Membrane biofilm reactor(MBfR)and biotrickling filtration-membrane biofilm reactor (BTF-MBfR)were used forthe treatment of organic waste gases containing 2-Ethoxyethanol.The long-time stability of MBfR and BTF-MBfR engineeringapplication was investigated,and the microbial community composition was also analysed.The result showed that during 60-day operation time,the removal efficiency of 2-Ethoxyethanol could reach 99.9%in MBfR.BTF-MBfR could efficientlyremove 2-Ethoxyethanol during 28-day operation time.Besides,compared with MBfR,BTF-MBfR was able to withstandgreater inlet flow.The analysis result of microbial community composition showed that there were 17and 23kinds of bacterias in BTF and MBfR,respectively,which relative abundance was higher than 0.5%,at the level of genus classification.Among those,there were only two kinds of bacterias(Thiobacillus and Aquabacterium )in both BTF and MBfR.Flavobacterium andHydrogenophaga played a key role in efficient removal of 2-Ethoxyethanol in MBfR.Keywords :2-Ethoxyethanol;biotrickling filtration;membrane biofilm reactor;VOCs;bacterias㊀㊀㊀㊀㊀㊀㊀㊀收稿日期:2019-08-30基金项目:东莞市社会科技发展重点项目(2017507101425)㊂第一作者:何觉聪(1989-),男,工程师,主要研究方向为有机废气处理新技术㊂anniedam@∗通信作者:魏在山,教授,博士生导师㊂wzs89@0㊀引㊀言我国是世界上第一大涂料消费国,涂料产量和消费量均有大幅度的增长[1],据统计,2018年我国涂料生产总量达到了1900万t [2]㊂近年来,随着国家层面的政策引导以及公众环保意识的不断增强,市场对于环保涂料的需求逐渐增加,水性涂料以其无毒环保㊁性质稳定㊁挥发性有机物(VOCs)含量低等特点被广泛应用,目前水性涂料的占比达30%~40%[3-5]㊂然而,水性涂料在生产㊁使用过程中也会产生如乙二醇乙醚等的有机废气[5],2016年使用水性涂料而产生的VOCs总量约为9.79万t[6],长期使用会对人体健康以及生态环境造成影响,因此针对水性涂料开展有机废气治理具有重要的意义㊂有机废气处理的常见方法主要有燃烧法㊁冷凝法㊁吸附法㊁吸收法和生物法等[7-16],其中生物法由于降解彻底㊁无二次污染㊁运行费用低㊁操作简单的优点而备受青睐[17,18]㊂生物滴滤器(biotrickling filtration, BTF)是使用较多的一种方法,在处理甲苯㊁苯乙烯及苯酚等有机废气已有大量研究[19-22],而膜生物反应器(membrane biofilm reactor,MBfR)是近些年来逐渐兴起的方法,因其具有较大的传质面积以及较好的VOCs去除效果[23],在VOCs治理领域越来越得到重视㊂目前膜生物反应器处理甲苯㊁二甲苯㊁乙酸乙酯等典型VOCs有机废气已经得到了较多的研究[24-27],但对于实际工程的应用研究却鲜有报道㊂本文针对水性涂料产生的乙二醇乙醚有机废气,采用BTF和MBfR进行工程应用,考察单独MBfR和BTF-MBfR 的长期运行稳定性,采用16S rRNA分析微生物群落结构,以期为水性喷涂有机废气生物处理的推广应用提供参考㊂1㊀材料与方法1.1㊀实验装置MBfR处理乙二醇乙醚工程应用如图1所示,实验装置包括气体混合系统㊁膜生物反应器和循环槽,采用膜组件参数为:膜丝外径0.7mm,内径0.58 mm,有效长度1m,根数24000根,外壳外径193mm,内径133mm,共6个,反应器有效体积0.038m3,总体积为3.9m3,总占地面积1.3m2㊂BTF-MBfR处理有机废气工程应用流程如图2所示,生物滴滤器内径为1.3m,装有高度为1.5m的空心球填料,占地面积1.4m2,孔隙率0.9,总体积为8.0m3㊂BTF-MBfR 中气体经过BTF后一部分进入到MBfR内,另一部分和MBfR净化后的气体混合后排放㊂在实验开始前,将前期研究获得的微生物菌种接种到MBfR装置内,并在循环液中加入营养液,随循环液的喷淋作用挂膜,时长30d㊂1.2㊀分析方法醇醚气体浓度通过挥发性有机物检测仪(RAE systems of PGM-7320,USA)测定;pH采用pH试纸测定;采用16S rRNA技术分析BTF和MBfR内的微生物群落结构㊂图1㊀MBfR工程应用示意图2㊀BTF-MBfR工程应用示意2㊀结果与分析2.1㊀MBfR降解乙二醇乙醚工程应用运行稳定性考察2018-11 2019-01,在东莞某公司对MBfR降解乙二醇乙醚气体进行了60d的运行稳定性考察㊂在乙二醇乙醚平均进气流量为2131.2m3/h㊁进气浓度50~400mg/m3㊁循环液喷淋量3.5m3/h㊁pH6~8的条件下,运行结果如图3所示㊂1~9d,乙二醇乙醚进气浓度为(60ʃ20)mg/m3,去除效率稳定在98%~ 99%;10~15d,受到生产车间工艺和生产周期的影响,装置运行期间受到冲击负荷的影响,乙二醇乙醚进气浓度在150~400mg/m3波动,平均去除效率为98.5%;16~17d,由于温度骤降,乙二醇乙醚去除效率明显下降,最低至96.5%;18~47d,随着温度的回升,乙二醇乙醚去除效率逐渐恢复并稳定在99.5%; 47~51d,由于循环液pH降低,去除效率发生波动,但依旧能够达到99%以上;52~60d,乙二醇乙醚去除效率稳定在99.5%㊂在整个运行期内,乙二醇乙醚去除效率均>95%,表明MBfR能够高效降解工厂产生的乙二醇乙醚气体㊂2.2㊀BTF-MBfR降解乙二醇乙醚稳定运行性分析2019-03 04,在平均进气流量为3974.4m3/h㊁进气浓度50~200mg/m3㊁BTF喷淋量为20m3/h, MBfR喷淋量为3.5m3/h㊁pH为6~8的条件下,对ʏ 去除效率; Җ 进气浓度㊂图3㊀MBfR 处理乙二醇乙醚工程应用稳定运行曲线BTF-MBfR 降解乙二醇乙醚气体进行28d 的运行稳定性考察,结果如图4所示㊂1~5d,在乙二醇乙醚进气浓度为(50ʃ20)mg /m 3,循环液pH 为6的条件下,去除效率较低为88%;第6天,调节反应器pH 至7后,乙二醇乙醚去除效率升高至96.9%;7~8d,在乙二醇乙醚进气浓度为(85ʃ5)mg /m 3,去除效率稳定在99%;9~13d,乙二醇乙醚进气浓度升高至(110ʃ10)mg /m 3,去除效率出现下降,最低为96.7%;14~22d,随运行时间的延长,乙二醇乙醚去除效率逐渐由96.5%升高至99.6%;23~28d,在乙二醇乙醚进气浓度为35~95mg /m 3时,去除效率稳定在99%,表明此时反应器能够适应低浓度的乙二醇乙醚废气㊂在运行期内,乙二醇乙醚平均去除效率>95%,表明BTF-MBfR 能够高效降解乙二醇乙醚气体㊂同单独的MBfR 相比,BTF-MBfR 能够承受更高的进气流量㊂ʏ去除效率; Ѳ进气浓度㊂图4㊀BTF-MBfR 处理乙二醇乙醚工程应用稳定运行曲线2.3㊀工程应用生物反应器内微生物群落结构研究采用16S rRNA 技术分析BTF 和MBfR 内的微生物群落结构㊂表1为微生物样品所含OUT 数及序列数统计㊂可知,BTF (Prac1)和MBfR (Prac2)样品测序深度较好㊂图5为Shannon 曲线,可知,随着测序深度的增加,两组样本曲线均趋于平坦,表明样本测序深度足够具有较强的可信度㊂2.3.1㊀门水平分析图6为两组样品在门水平上的物种相对丰度㊂㊀㊀表1㊀两组样品所含OTU 数及序列数统计样品名称有效序列数OUT 数BTF 61483400MBfR45073403Prac1; Prac2㊂图5㊀Shannon 曲线可知,BTF 中的优势菌门为Proteobacteria(65.63%)㊁Bacteroidetes (19.75%)㊁Firmicutes (5.41%)㊂MBfR 中优势菌门为Proteobacteria (38.09%)㊁Bacteroidetes (32.53%)㊁Armatimonadetes(6.18%)㊁Planctomycetes (4.07%)㊁Acidobacteria (3.62%)㊂Proteobacteria 和Bacteroidetes 能够将醇类物质氧化为羧酸类有机物[28,29],Planctomycetes 广泛应用于制药㊁市政工业废水处理厂,具有广谱降解能力[30]㊂图6㊀门水平物种相对丰度2.3.2㊀纲水平分析图7为两组样品在纲水平上的物种相对丰度㊂可知,BTF 中优势菌纲有Alphaproteobacteria (32.54%)㊁Gammaproteobacteria (31.69%)㊁Bacteroidia (19.20%)㊁Negativicutes (3.06%);MBfR 中优势菌纲有Bacteroidia (32.02%)㊁Alphaproteobacteria(24.24%)㊁Gammaproteobacteria(12.09%)㊁Planctomycetacia (4.05%)㊁Blastocatellia (2.40%)㊂Gammaproteobacteria㊁Bacteroidia 和Alphaproteobacteria 对多种有机物具有较强的降解作用[31-33]㊂2.3.3㊀属水平分析图8为两组样品在属水平上的物种相对丰度㊂可知,BTF 中相对丰度>0.5%的菌属有Chryseobacterium图7㊀纲水平物种相对丰度(16.18%)㊁Ottowia(16.07%)㊁Ancalomicrobium (10.23%)㊁Aquabacterium(6.41%)㊁Aeromonas (3.36%)㊁Azospirillum(2.78%)㊁Acidaminobacter (2.11%)㊁Thiobacillus(2.10%)㊁Anaerosporomusa (1.71%)㊁Telmatospirillum(1.53%)㊁Kribbia (1.10%)㊁Desulfovibrio(1.07%)㊁Sulfurospirillum (1.03%)㊁Acinetobacter(0.99%)㊁WCHB1-32 (0.85%)㊁Xanthobacter(0.76%)㊁Cloacibacterium (0.51%)㊂MBfR中相对丰度>0.5%的菌属有Rhodobacter(9.34%)㊁Novosphingobium(5.88%)㊁Flavobacterium(3.77%)㊁Algoriphagus(2.70%)㊁Sediminibacterium( 2.31%)㊁Hydrogenophaga (2.26%)㊁Reyranella(2.25%)㊁OLB12(2.11%)㊁Thiobacillus(1.37%)㊁Aquabacterium(1.33%)㊁Curvibacter(1.14%)㊁Sphingopyxis(1.12%)㊁Filimonas (1.00%)㊁Oligoflexus(0.95%)㊁Paludibaculum (0.91%)㊁Caulobacter(0.88%)㊁Planctopirus (0.75%)㊁Prosthecobacter(0.68%)㊁Limnohabitans (0.68%)㊁Cellvibrio(0.60%)㊁Emticicia(0.59%)㊁oc32(0.55%)㊁Runella(0.52%)㊁Hirschia(0.50%)㊂两组样品中相对丰度均>0.5%的有:Thiobacillus和Aquabacterium㊂两反应器的运行时长不同是导致二者内微生物种群存在较大差异的重要原因㊂BTF中的各菌属微生物对乙二醇乙醚的降解原理尚未得到研究,而通过宏基因组测序结果表明:MBfR中的Flavobacterium中含有能够代谢乙醇酸的基因glcD[34],Hydrogenophaga含有与醇醚降解相关的功能基因puuC,aldH;ACSS,acs;glcD㊂由此可推测, Flavobacterium和Hydrogenophaga能够快速降解乙二醇乙醚,在膜生物反应器高效去除乙二醇乙醚过程中起到关键作用㊂3㊀结㊀论1)MBfR处理醇醚有机废气工程应用的60d运行稳定性考察结果表明,在平均进气流量2131.2m3/图8㊀微生物在属水平上群落结构h㊁进气浓度50~400mg/m3㊁循环液喷淋量3.5m3/ h㊁pH6~8的条件下,醇醚废气去除效率可达99.9%㊂2)BTF-MBfR处理醇醚有机废气工程应用的28d运行稳定性考察结果表明,在平均进气流量为3974.4m3/h㊁进气浓度50~200mg/m3㊁BTF循环液喷淋量为20m3/h,MBfR循环液喷淋量3.5m3/h㊁pH 6.0~8.0的条件下,能够高效去除乙二醇乙醚气体㊂与单独膜生物反应器相比,能够承受更大的进气流量㊂3)在工程应用中,两组生物反应器相对丰度均> 0.5%的有:Thiobacillus和Aquabacterium㊂Flavobacterium 和Hydrogenophaga在膜生物反应器高效去除乙二醇乙醚过程中起关键作用㊂参考文献[1]㊀Lirong Hui,Xingang Liu,Qinwen Tan.VOC characteristics,sources and contributions to SOA formation during haze events inWuhan,Central China[J].Science of the Total Environment,2019,650:2624-2639.[2]㊀王玲玲.2019年6月国内涂料市场行情走势分析[J].广州化工,2019,47(14):3-5.[3]㊀蒋亚辉,赵文增.绿色涂料的现状与发展趋势[J].信息化建设,2016,5:1008-1941[4]㊀齐祥安.工程机械对低VOC涂料的选择与应用[J].中国涂料,2015,30(4):15-21[5]㊀魏巍,王书肖,郝吉明.中国涂料应用过程挥发性有机物的排放计算及未来发展趋势预测[J].环境科学,2009,30(10):2809-2815[6]㊀高美平,邵霞,聂磊等.中国建筑涂料使用VOCs排放因子及排放清单的建立[J].环境科学,2019,40(3):1152-1162. [7]㊀黄青斌.挥发性有机物催化氧化技术及催化剂研究进展[J].化学试剂,2018.[8]㊀李纯爱.催化燃烧法处理喷漆有机废气的应用研究[J].环境与发展.2018,6:255-256.[9]㊀晋兵营,胡明江.MoS2/g-C3N4纳米纤维光催化降解柴油机苯系排放污染物[J].环境科学学报,2019,1:1-9.[10]㊀Haibao Huang,Haoxian Lu,Yujie Zhan.VUV photo-oxidation ofgaseous benzene combined with ozone-assisted catalytic oxidation:effect on transition metal catalyst[J].Applied Surface Science,2017,391:662-667.[11]㊀李婕,羌宁.挥发性有机物(VOCs)活性炭吸附回收技术综述[J].四川环境,2008,26(6):101-105[12]㊀徐孟孟,陈效,蒋轶锋,等.滤袋式膜生物反应器净化二甲苯[J].环境工程学报,2016,10(1):257-261.[13]㊀Yiqing Qi,Liming Shen,Jilei Zhang.Species and releasecharacteristics of VOCs in furniture coating process[J].Environmental Pollution,2019,245:810-819.[14]㊀Zhuangmin Zhong,Qingᶄe Sha,Junyu Zheng.Sector-based VOCsemission factors and source profiles for the surface coating industryin the Pearl River Delta region of China[J].Science of the TotalEnvironment,2017,583:19-28.[15]㊀吕晓宁.催化燃烧法处理污水处理场有机废气[J].环境与发展,2018,10:65-67[16]㊀耿凤华,张书武,宫磊.生物滴滤塔处理模拟印刷有机废气[J].化工环保,2018,2:217-221.[17]㊀苏俊朋,杜青平,李彦旭,等.鼠李糖脂强化生物滴滤器去除乙苯废气效能及菌群结构研究[J].环境科学学报,2018,38(9):3514-3521.[18]㊀陈波,张耀斌,吴丹,等.生物滴滤法去除低浓度苯乙烯[J].环境工程学报,2010,4(3):644-648[19]㊀席劲瑛,武俊良,胡洪营,等.工业VOCs气体处理技术应用状况调查分析[J].中国环境科学2012,32(11):1955-1960 [20]㊀何觉聪,黄倩茹,陈洲洋,等.生物滴滤塔处理苯酚气体研究[J].环境科学,2014,35(2):520-525[21]㊀任爱玲,赫环环,郭斌,等.生物滴滤塔净化含低浓度苯乙烯废气的研究[J].环境科学学报,2013,33(7):1840-1848 [22]㊀曾萍,宋永会,刘风华,等.不同表观气速对好氧颗粒污泥净化苯酚废气的影响[J].环境科学学报,2011,31(5):964-970 [23]㊀KUMARA,DEWLUF J,HERMAN V L,et al.Membrane-basedbiological waste gas treatment[J].Chemical Engineering Journal,2008,136(2/3):82-91.[24]㊀修光利,张晓峰,赵康,等.中空纤维膜生物反应器处理二甲苯废气[J].环境工程学报,2010,4(10):2287-2292. [25]㊀VOLCKAERT D,HEYNDERICKX P M,LANGENHOVE H V,etal.Performance of a composite membrane bioreactor for theremoval of ethyl acetate from waste air[J].BioresourceTechnology,2011,102(19):8893-8898.[26]㊀KUMAR A,DEWLUF J,VERCRUYSSEN A,et al.Performanceof a composite membrane bioreactor treating toluene vapors:inoculaselection,reactor performance and behavior under transientconditions[J].Bioresource Technology,2009,100(8):2381-2387.[27]㊀陈蓉,曹明福,王永忠,等.膜生物反应器处理甲苯废气的降解特性及传质过程强化[J].环境工程学报,2012,6(1):253-257.[28]㊀Chengmei Liao,Jiali Wu,Lean Zhou.Optimal set of electrodepotential enhances the toxicity response ofbiocathode toformaldehyde[J].Science of the Total Environment,2018,644:1485-1492.[29]㊀Xiaolong Yu,Fumitake Nishimura,Taira Hidaka.Effects ofmicrobial activity on perfluorinated carboxylic acids(PFCAs)generation during aerobic biotransformation of fluorotelomer alcoholsin activated sludge[J].Science of The Total EnvironmentVolumes,2018,7(610):776-785.[30]㊀Baisuo Zhao,Jie Liu,Craig Frear.Consolidated bioprocessing ofmicroalgalbiomass to carboxylates by a mixed culture of cow rumenbacteria using anaerobic sequencing batch reactor(ASBR)[J].Bioresource Technology,2016,222:517-522.[31]㊀Yu Jiang,Li Wei,Huining Zhang.Removal performance andmicrobial communities in a sequencing batch reactor treatinghypersaline phenol-laden wastewater[J].Bioresource Technology,2016,218:146-152.[32]㊀DOSTA J,NIETO J M,VILA J.Phenol removal from hypersalinewastewaters in a Membrane Biological Reactor(MBR):Operationand microbiological characterization[J].Bioresource Technology,2011,102:4013-4020.[33]㊀Jianguo Zhao,Yahe Li,Xiurong Chen.Effects of carbon sourceson sludge performance and microbial community for4-chlorophenolwastewater treatment in sequencing batch reactors[J].BioresourceTechnology,2018,255:22-28.[34]㊀PEREIRA B,LI Z J,MEY M D,et al.Efficient utilization ofpentoses for bioproduction of the renewable two-carbon compoundsethylene glycol and glycolate[J].Metabolic Engineering,2016,34:80-87.。
生物医学工程的英语
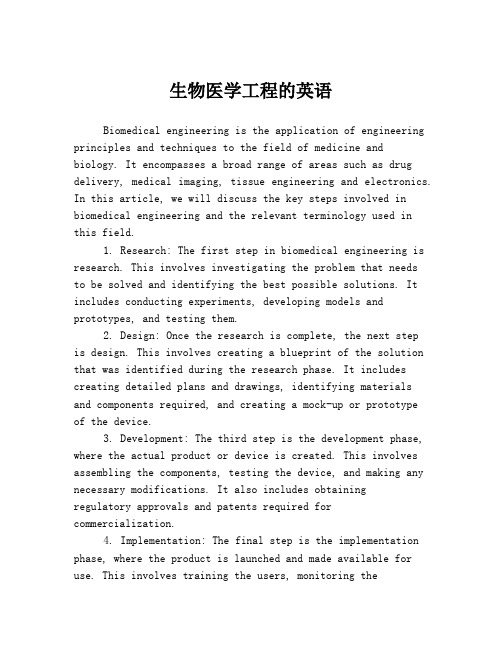
生物医学工程的英语Biomedical engineering is the application of engineering principles and techniques to the field of medicine and biology. It encompasses a broad range of areas such as drug delivery, medical imaging, tissue engineering and electronics. In this article, we will discuss the key steps involved in biomedical engineering and the relevant terminology used inthis field.1. Research: The first step in biomedical engineering is research. This involves investigating the problem that needsto be solved and identifying the best possible solutions. It includes conducting experiments, developing models and prototypes, and testing them.2. Design: Once the research is complete, the next stepis design. This involves creating a blueprint of the solution that was identified during the research phase. It includes creating detailed plans and drawings, identifying materials and components required, and creating a mock-up or prototypeof the device.3. Development: The third step is the development phase, where the actual product or device is created. This involves assembling the components, testing the device, and making any necessary modifications. It also includes obtainingregulatory approvals and patents required for commercialization.4. Implementation: The final step is the implementation phase, where the product is launched and made available for use. This involves training the users, monitoring theperformance of the device, and providing ongoing support and maintenance.Now let's look at some key terminology used inbiomedical engineering:1. Biomaterials: These are materials that are used to create medical devices or implants, which interact with biological systems. Examples include metals, polymers, ceramics, and composites.2. Biomechanics: This is the study of the mechanics of biological systems, such as bones, muscles, and tissues. It includes analyzing the structure and function of these systems, and developing models to predict their behaviorunder different conditions.3. Biomedical imaging: This involves the use ofdifferent imaging techniques to visualize the internal structures of the body. Examples include X-rays, CT scans, MRI, and ultrasound.4. Bioprocessing: This is the use of biological systemsor their components to produce drugs or other products. Examples include fermentation, chromatography, and cell culture.In conclusion, biomedical engineering is a rapidly growing field that combines the principles of engineering and medicine to improve healthcare outcomes. It involves research, design, development, and implementation of devices and technologies that can enhance diagnostics and treatment of diseases. Understanding the key steps and terminology used in this field is vital for anyone interested in this excitingarea of healthcare.。
CHEMICAL REMOVAL OF BIOMASS FROM WASTE AIR
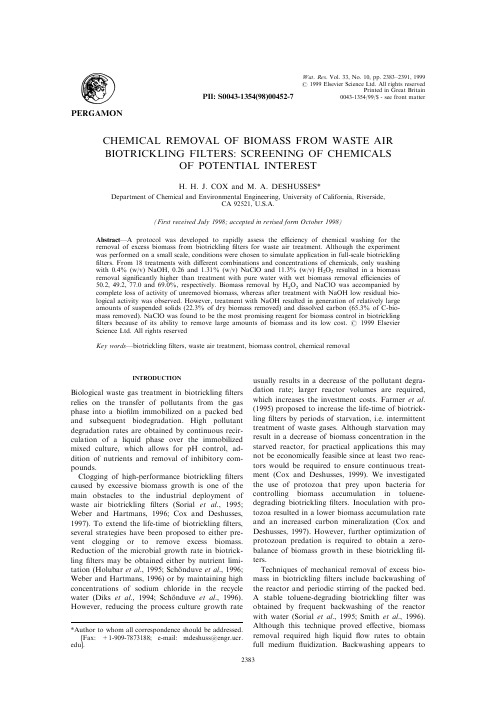
CHEMICAL REMOVAL OF BIOMASS FROM WASTE AIR BIOTRICKLING FILTERS:SCREENING OF CHEMICALSOF POTENTIAL INTERESTH.H.J.COX and M.A.DESHUSSES*Department of Chemical and Environmental Engineering,University of California,Riverside,CA 92521,U.S.A.(First received July 1998;accepted in revised form October 1998)Abstract ÐA protocol was developed to rapidly assess the e ciency of chemical washing for the removal of excess biomass from biotrickling ®lters for waste air treatment.Although the experiment was performed on a small scale,conditions were chosen to simulate application in full-scale biotrickling ®lters.From 18treatments with di erent combinations and concentrations of chemicals,only washing with 0.4%(w/v)NaOH,0.26and 1.31%(w/v)NaClO and 11.3%(w/v)H 2O 2resulted in a biomass removal signi®cantly higher than treatment with pure water with wet biomass removal e ciencies of 50.2,49.2,77.0and 69.0%,respectively.Biomass removal by H 2O 2and NaClO was accompanied by complete loss of activity of unremoved biomass,whereas after treatment with NaOH low residual bio-logical activity was observed.However,treatment with NaOH resulted in generation of relatively large amounts of suspended solids (22.3%of dry biomass removed)and dissolved carbon (65.3%of C-bio-mass removed).NaClO was found to be the most promising reagent for biomass control in biotrickling ®lters because of its ability to remove large amounts of biomass and its low cost.#1999Elsevier Science Ltd.All rights reservedKey words Ðbiotrickling ®lters,waste air treatment,biomass control,chemical removalINTRODUCTIONBiological waste gas treatment in biotrickling ®lters relies on the transfer of pollutants from the gas phase into a bio®lm immobilized on a packed bed and subsequent biodegradation.High pollutant degradation rates are obtained by continuous recir-culation of a liquid phase over the immobilized mixed culture,which allows for pH control,ad-dition of nutrients and removal of inhibitory com-pounds.Clogging of high-performance biotrickling ®lters caused by excessive biomass growth is one of the main obstacles to the industrial deployment of waste air biotrickling ®lters (Sorial et al.,1995;Weber and Hartmans,1996;Cox and Deshusses,1997).To extend the life-time of biotrickling ®lters,several strategies have been proposed to either pre-vent clogging or to remove excess biomass.Reduction of the microbial growth rate in biotrick-ling ®lters may be obtained either by nutrient limi-tation (Holubar et al.,1995;Scho nduve et al.,1996;Weber and Hartmans,1996)or by maintaining high concentrations of sodium chloride in the recycle water (Diks et al.,1994;Scho nduve et al.,1996).However,reducing the process culture growth rateusually results in a decrease of the pollutant degra-dation rate;larger reactor volumes are required,which increases the investment costs.Farmer et al.(1995)proposed to increase the life-time of biotrick-ling ®lters by periods of starvation,i.e.intermittent treatment of waste gases.Although starvation may result in a decrease of biomass concentration in the starved reactor,for practical applications this may not be economically feasible since at least two reac-tors would be required to ensure continuous treat-ment (Cox and Deshusses,1999).We investigated the use of protozoa that prey upon bacteria for controlling biomass accumulation in toluene-degrading biotrickling ®lters.Inoculation with pro-tozoa resulted in a lower biomass accumulation rate and an increased carbon mineralization (Cox and Deshusses,1997).However,further optimization of protozoan predation is required to obtain a zero-balance of biomass growth in these biotrickling ®l-ters.Techniques of mechanical removal of excess bio-mass in biotrickling ®lters include backwashing of the reactor and periodic stirring of the packed bed.A stable toluene-degrading biotrickling ®lter was obtained by frequent backwashing of the reactor with water (Sorial et al.,1995;Smith et al.,1996).Although this technique proved e ective,biomass removal required high liquid ¯ow rates to obtain full medium ¯uidization.Backwashing appears toWat.Res.Vol.33,No.10,pp.2383±2391,1999#1999Elsevier Science Ltd.All rights reservedPrinted in Great Britain0043-1354/99/$-see front matterPII:S0043-1354(98)00452-7*Author to whom all correspondence should be addressed.[Fax:+1-909-7873188;e-mail:mdeshuss@].2383be limited to those biotrickling®lters containing support materials that are easily¯uidized and a 40%larger reactor volume is required to allow for bed expansion during¯uidization(Sorial et al., 1997).A proposed alternative to backwashing is to periodically stir the packed bed to shear o excess biomass(Wu bker et al.,1997).It is unclear how the latter proposal can be implemented at an industrial scale.Chemical removal of biomass is an attractive alternative for biomass control in biotrickling®lters but it remains a relatively unexplored area.So far, biomass removal from biotrickling®lters by chemi-cal washing has only been reported by Weber and Hartmans(1996),who used a sodium hydroxide solution.In the present contribution,we discuss results on the screening of various chemicals(oxi-dants,surfactants,bactericidal and hydrolyzing agents)to remove biomass from samples taken from a toluene-degrading biotrickling®lter. Experimental conditions were chosen in order to simulate future industrial treatment in biotrickling ®lters.As an inhibitory action on bacteria by the selected chemicals was expected,the biological ac-tivity of unremoved biomass was also measured.MATERIALS AND METHODSBiomass samplesBiomass was grown on the surface of2.5cm polypropy-lene Pall rings(Flexirings1,Koch Engineering,Wichita, KS)in a source biotrickling®lter(1.3m in height,0.152m in diameter)degrading toluene.The inoculum was a bac-terial species isolated from sludge using toluene as the sole carbon and energy pressed air with a metered amount of toluene resulting in an average inlet concen-tration of1.03g mÀ3was passed through the reactor from top to bottom at a volumetric load of64m3mÀ3hÀ1, resulting in a toluene load of65.9g mÀ3hÀ1.Liquid was recirculated concurrently with the air¯ow at a super®cial velocity of7.9m hÀ1and a mineral medium(Cox and Deshusses,1997)was continuously fed at a¯ow rate of 0.24l hÀ1.Pall rings with biomass were randomly taken from the upper part of the source biotrickling®lter after 79to147days of operation for the experiments described below.Experimental set-upRemoval of biomass was investigated in a small test bio-trickling®lter(0.3m in height,diameter0.152m)stacked with clean Pall rings.A schematic of the experimental set-up is shown in Fig.1and a picture of biomass attached on the Pall rings is shown in Fig.2.For each treatment,®ve rings with biomass were taken from the source bio-trickling®lter,weighed after10min of drying in air(wet biomass weight plus ring before treatment)and placed at regular intervals along the longitudinal axis of the test bio-trickling®lter.In most experiments,0.75l of liquid con-taining the tested chemical was recirculated for3h over the packed bed at a super®cial liquid velocity of7.9m hÀ1 using a centrifugal pump(model1P677A from Dayton Electric Mfg.Co.,Chicago,IL)and a needle valve and ¯owmeter combination.At the top of the test biotrickling ®lter,a liquid distribution system made of a container with equally spaced holes ensured homogenous distri-bution of the recycle liquid over the packed bed.All treat-ments lasted for3h(except for a treatment with hot water which lasted30min)and was ended by stopping the liquid recirculation.The liquid was then allowed to drain from the test biotrickling®lter for10min and collected for analysis of suspended solids and total dissolved carbon. The rings with unremoved biomass were carefully removed from the test biotrickling®lter,air-dried for10min and weighed(wet biomass weight plus ring after treatment). Unremoved biomass was separated from the Pall rings and immediately analyzed for biological activity in oxygen uptake rate(OUR)experiments.The Pall rings were cleaned,dried and weighed in order to calculate the amount of wet biomass before and after treatment.The e ciency of biomass removal was calculated as the percen-tage removal of wet biomass.In total,18di erent treatments were investigated.The principal action mechanism of each chemical is listed in Table1.The general procedure as outlined above was fol-lowed for the treatment with demineralized water(control experiment,H2O),0.04and0.4%(w/v)sodium hydroxide (NaOH),0.1and0.5%(w/v)sodium dodecylsulfate (SDS),0.01%(w/v)NaOH with0.1%(w/v)SDS,0.05Fig.1.Schematic of the experimental set-up.H.H.J.Cox and M.A.Deshusses2384and 0.2%(w/v)sodium azide (NaN 3),0.011,0.26and 1.31%(w/v)sodium hypochlorite (NaClO),1.1and 11.3%(w/v)hydrogen peroxide (H 2O 2),saturated iodine in 4%(v/v)ethanol [0.02%(w/v)I 2as determined by titration with Na 2S 2O 3],0.9%(w/v)ammonia (NH 3)and 0.9%(w/v)NH 3in combination with 0.22%(w/v)formaldehyde (HCO).For the treatment with demineralized water at el-evated temperature (H 2O,T =638C),a heating plate was used to maintain 1.2l of recycle liquid at 658C.This treat-ment lasted for only 30min and the average temperature inside the column was 638C.For the treatment with ozone,demineralized water was circulated over the column for 3h while ozone-containing air was passed counter-cur-rently at a ¯ow rate of 1.9l min À1.Ozone was prepared with an ozone generator model 100-O2from California Acrylic Industries (Pomona,CA).The ozone concen-tration in the gas phase was 0.5g m À3as determined by ti-tration with Na 2S 2O 3after trapping of ozone in 2%KI (APHA et al.,1985).Analytical proceduresThe dry matter content of the biomass was determined in quadruplicate by drying,at 958C overnight,known amounts of wet biomass,taken from the source biotrick-ling ®lter after 162days of operation.Analyses of the dried biomass for C,H and N content were done by Desert Analytics Laboratory (Tucson,AZ).Suspended solids in the liquid after treatment were determined bycentrifugation at 17,000g for 10min and by drying the pel-let at 958C overnight.Total dissolved carbon in the liquid after treatment was determined in triplicate by analyzing the supernatant using a model TOC-5050total carbon analyzer (Shimadzu,Kyoto,Japan).The activity of the biomass remaining on the rings after the treatment was determined in OUR experiments.Each of the 5rings was placed into 100ml of mineral medium and shaken vigor-ously to separate the biomass from the ring.OURs with and without 0.19mM toluene were determined in single experiments at room temperature in a custom made 57ml glass vial and by using an oxygen electrode and meter (Orion model 860,Boston,MA).OURs were related to the amount of unremoved,wet biomass and toluene-induced OURs were corrected for endogenous respiration.RESULTSCharacterization of biomass samplesBiomass sampling from the source biotrickling ®l-ter started after 79days of toluene supply,at which time the reactor contained 14.065kg wet biomass (approximately 60%of the reactor volume).The average amount of wet biomass on the Pall rings was 11.2g (average of 90rings,a range of 7.1to 16.5g with a standard deviation of 1.9g).Since the biomass content of the bioreactor was high,bio-mass was present both inside and on the outer sur-face of the rings.Assuming a bio®lm density of 1kg l À1and that Pall rings can be represented by empty cylinders,the maximum amount of wet bio-mass needed to ®ll the ring would be 12.3g.The average amount of biomass on the rings (11.2g)in-dicates that the amount of biomass on the outer surface was small compared to the amount ofbio-Fig.2.Picture of a clean Pall ring (left)and one covered with biomass (right)before cleaning.Since the biomass content was very high,a large portion of the inner volume of the ring was ®lled with biomass.Table 1.Principal action mechanisms of the chemicals tested toremove excess biomass in biotrickling ®lters CompoundMechanism H 2O none,control NaOHhydrolysis NaClO,H 2O 2,O 3,I 2oxidation SDS surfactant NaN 3toxic/reductionWater (638C),NH 3,NH 3+HCOotherRemoval of biomass from biotrickling ®lters 2385mass inside the ring.This was con®rmed by visual observation (Fig.2).The average dry matter con-tent of the biomass was 4.86%.Elemental analysis of the dried biomass showed a C:H:N ratio of 43.4:6.2:7.8%on a dry weight basis.Chemical removal of biomassThe control experiment with recirculation of demineralized water resulted in an average loss of wet biomass of 12.9%.Visual observation indicated that mainly biomass from the outer surface of the rings was removed,whereas biomass inside the rings was still present.Since no chemical reaction between water and biomass is expected,selective removal of biomass from the outer surface of the rings in this case was due to shear stresses caused by trickling of liquid on the rings in the column and/or to losses during handling of the rings.Table 2summarizes the wet biomass removal e -ciencies of the treatments investigated as well as pH measurements of the liquid before and after treat-ment.Most treatments had about the same removal e ciency as the control treatment with water.Only treatments with 0.4%(w/v)NaOH,0.26and 1.31%(w/v)NaClO and 11.3%(w/v)H 2O 2resulted in statistically signi®cant higher removal e ciencies (Student t -test,p >0.95).Biomass in the treatments using 0.26and 1.31%(w/v)NaClO and 11.3%(w/v)H 2O 2turned from an initial brown color to yel-lowish-white at the end of the treatment.This was not observed in treatments with 0.011%(w/v)NaClO nor with 1.1%(w/v)H 2O 2.In treatments using NaOH,only the 0.4%(w/v)NaOH appli-cation resulted in the remaining biomass becoming a highly viscous liquid.All treatments with SDS resulted in signi®cant parison of the pH during treatment and removal e ciencies at0.04and 0.4%NaOH (w/v)(Table 2)indicates that biomass removal induced by an alkaline environ-ment may be expected at a pH of about 12.4and higher.Analysis of the liquid after treatmentBiomass reduction may either be due to bio®lm detachment (cells and ¯ocs),to solubilization of biomass by chemical reaction or to oxidation of biomass to volatile compounds.In order to quan-tify these processes,the amounts of suspended solids and total dissolved carbon in the liquid after treatment were determined.It should be noted that larger ¯ocs tended to be captured on clean Pall rings below the test rings in the test biotrickling ®l-ter and thus determination of suspended solids in the liquid is an underestimation of bio®lm detach-ment.The liquid after treatment contained small amounts of suspended solids and dissolved carbon in cases of treatments with low biomass removal e ciencies,whereas the highest amounts were found in treatments with high biomass removal e -ciencies (Table 3).Since the total amounts of wet biomass on ®ve rings before and after treatment were known and biomass dry matter and carbon content were deter-mined,mass balances were calculated for the treat-ments with high removal e ciencies.As reported in Table 4,treatment with 0.4%(w/v)NaOH resulted in a slightly higher recovery of removed biomass as suspended solids,which may indicate that detach-ment of cells and bio®lm ¯ocs was a more import-ant process in this treatment as compared to the treatments with NaClO and H 2O 2.Marked di er-ences were found in the recovery of removed C-bio-mass as dissolved carbon,with values ranging from 18.5%for the treatment with 11.3%(w/v)H 2O 2toTable 2.Wet biomass removal e ciency from Pall rings during 3h treatment in a test biotrickling ®lterTreatment No.TreatmentWet biomass removal e ciency (%)a pH liquidstart end 1H 2O12.9(11.0)ND c 7.62H 2O,T =638C b 14.6(11.5)ND 8.030.4%(w/v)NaOH 50.2(22.4)12.812.440.04%(w/v)NaOH 10.3(9.8)12.09.550.5%(w/v)SDS 10.9(7.0) 6.78.060.1%(w/v)SDS10.4(12.0) 6.27.470.04%(w/v)NaOH +0.1%(w/v)SDS9.6(5.1)11.89.280.2%(w/v)NaN 320.4(8.0)7.87.990.05%(w/v)NaN 3 2.8(6.7)7.48.310 1.31%(w/v)NaClO 77.0(16.1)12.19.1110.26%(w/v)NaClO 49.2(13.2)11.49.0120.011%(w/v)NaClO 14.3(5.6)ND 8.01311.3%(w/v)H 2O 269.0(19.4) 4.3 6.514 1.1%(w/v)H 2O 228.2(16.0) 5.17.3150.02%(w/v)I 210.8(3.7) 5.47.4160.5g O 3m À3air 5.9(3.2)ND ND 170.9%(w/v)NH 36.5(6.4)11.59.3180.9%(w/w)NH 3+0.22%(w/v)HCO9.9(11.8)11.210.2aAverage of ®ve determinations,standard deviation (s n À1)in parentheses.b 30min treatment.c Not determined.H.H.J.Cox and M.A.Deshusses238665.3%for the treatment with0.4%(w/v)NaOH. No attempts were made to close dry matter and carbon balances,which would have required analy-sis of the liquid for dissolved solids and analysis of the gas phase for production of CO2and volatile organic compounds.However,assuming that the carbon content in suspended solids was the same as that in the biomass before treatment,the ratio of C-liquid produced to C-biomass removed in the treatments with0.4%(w/v)NaOH,0.26and1.31% (w/v)NaClO and11.3%(w/v)H2O2was88,60,63 and33%,respectively(Table4).These results may indicate that oxidation of biomass to gaseous or volatile compounds was signi®cant in the treatments using oxidants,such as NaClO and especially H2O2. Biological activity of unremoved biomass Endogenous and toluene-induced OURs of fresh biomass analyzed before chemical treatment were on average1.31and1.46m g O2gÀ1wet biomass-minÀ1,respectively.In the control experiment with water,unremoved biomass had approximately the same activity(Table5).This indicates that3h of trickling of a liquid on the test rings in the test bio-trickling®lter is in itself not a cause for loss of bio-logical activity.The data reported in Table5showsTable3.Removal of biomass from Pall rings as suspended solids and total dissolved carbon Treatment Suspended solids(SS)Dissolved carbon(C)total (mg)biomass recovered as SS(%)atotal(mg)biomass recovered as dissolved C(%)bH2O45 2.310 1.2H2O,T=638C128 6.240 4.50.4%(w/v)NaOH19411.224732.80.04%(w/v)NaOH81 3.311010.50.5%(w/v)SDS54 2.5132d14.30.1%(w/v)SDS70 3.30d00.04%(w/v)NaOH+0.1%(w/v)SDS31 1.5110d12.00.2%(w/v)NaN351 1.915 1.30.05%(w/v)NaN328 1.611 1.41.31%(w/v)NaClO24511.235337.30.26%(w/v)NaClO155 6.822422.60.011%(w/v)NaClO82 3.937 4.111.3%(w/v)H2O21879.910512.81.1%(w/v)H2O21727.739 4.00.02%(w/v)I277 4.1ND ND0.5g O3mÀ3air ND c ND ND ND0.9%(w/v)NH339 1.512511.10.9%(w/w)NH3+0.22%(w/v)HCO54 2.121d 1.9a Dry suspended solids in the liquid after treatment,as percentage of the total amount of dry biomass present on®ve Pall rings before treatment(dry biomass=0.0486Âwet biomass).b Total dissolved carbon in the liquid after treatment,as percentage of the total amount of C-biomass present on®ve Pall rings before treatment(C-biomass=0.4339Âdry biomass).c Not determined.d Values corrected for the presence of carbon containing reagents,assuming that the reagent concentration at the end of the treatment was the same as initially pre-sent.Table4.Mass balances for four treatments with the highest biomass removal e ciencyParameter Treatment0.4%(w/v) NaOH 0.26%(w/v)NaClO1.31%(w/v)NaClO11.3%(w/v)H2O2Dry biomassBiomass before treatment(g) 1.733 2.282 2.182 1.892 Biomass removed a(g)0.870 1.123 1.680 1.306 Recovered as suspended solids(g)0.1940.1550.2450.187 Recovery of removed biomass as suspended solids(%)22.313.814.614.3CarbonC-biomass before treatment(g)0.7520.9900.9470.821 C-biomass removed b(g)0.3780.4870.7290.567 Recovered as dissolved C(g)0.2470.2240.3530.105 Recovery of removed C-biomass as dissolved C(%)65.346.048.418.5 Total carbon recovery c(%)88606333a Assuming that the dry matter content in biomass before and after treatment is the same(0.0486g dry biomass gÀ1wet biomass).b Assuming that the carbon content of dry biomass before and after treatment is the same(0.4339g C gÀ1dry biomass).c(Suspended solidsÂ0.4339+dissolved carbon)/C-biomass removed.Removal of biomass from biotrickling®lters2387that chemical treatments caused various levels of inhibition of biological activity of the remaining biomass,depending on the chemical used.Interestingly,toluene oxidation generally appeared to be more inhibited than endogenous respiration,but this was not further investigated.From the four treatments with high biomass removal e ciencies,only unremoved biomass in the treatment with 0.4%(w/v)NaOH showed some level of biologicalactivity.In fact,toluene oxidation activity was found in only one sample out of the ®ve examined.Treatments with 0.26and 1.31%(w/v)NaClO and 11.3%(w/v)H 2O 2resulted in a complete loss of respiration activity of the unremoved biomass.In these cases it was found that residual H 2O 2and NaClO concentrations in suspensions for OUR ex-periments (i.e.after ten-fold dilution of unremoved biomass in mineral medium and assuming that the biomass was saturated with the reagent in a concen-tration as initially present at the start of the treat-ment)were su ciently high to cause complete inhibition of respiration activity of fresh and untreated biomass.DISCUSSIONThe e ciency of biomass removal of biomass from Pall rings was investigated in a laboratory test biotrickling ®lter at a super®cial liquid velocity commonly used in biotrickling ®lter operation.Experimental conditions were chosen to simulate chemical removal of biomass in full-scale biotrick-ling ®lters,with the exception being that the Pall rings with biomass in the test biotrickling ®lter were surrounded by clean Pall rings.The high ratio of re-agent to biomass may have facilitated reaction in the test biotrickling ®lter and,consequently,removal e ciencies as reported in Table 2may be higher than with actual treatment in biotrickling ®l-ters under otherwise identical conditions.Asindi-Fig.3.Correlation between toluene-induced oxygen uptake rate in the biomass remaining after treat-ment and wet biomass removal e ciency of the various treatments (see Table 2for identi®cation ofeach treatment).The inset shows target characteristics for various treatments.Table 5.Biological activity of unremoved biomass measured as the oxygen uptake rate (m g O 2g À1wet biomass min À1;average of ®ve determinations with standard deviation (s n À1)in parentheses)TreatmentEndogenous Toluene-induced H 2O1.21(0.27)1.92(0.54)H 2O,T =638C 00.4%(w/v)NaOH 0.76(0.52)0.12(0.26)0.04%(w/v)NaOH 1.27(0.22)0.06(0.11)0.5%(w/v)SDS 1.54(0.51)0.59(0.37)0.1%(w/v)SDS2.54(0.59)0.59(0.70)0.04%(w/v)NaOH +0.1%(w/v)SDS1.69(0.78)0.15(0.18)0.2%(w/v)NaN 30.55(0.11)0.27(0.21)0.05%(w/v)NaN 3 1.45(0.39)1.13(0.70)1.31%(w/v)NaClO 000.26%(w/v)NaClO 00.011%(w/v)NaClO 0.93(0.15)0.78(0.43)11.3%(w/v)H 2O 201.1%(w/v)H 2O 20.24(0.21)0.09(0.15)0.02%(w/v)I 2 1.63(0.41)0.64(0.32)0.5g O 3m À3air 1.21(0.58)0.82(0.38)0.9%(w/v)NH 30.67(0.33)0.05(0.09)0.9%(w/w)NH 3+0.22%(w/v)HCOH.H.J.Cox and M.A.Deshusses2388cated by the high standard deviation,removal e -ciencies per treatment sometimes varied consider-ably(Table2).Since the distribution of biomass on the ring was di erent for each sample,access of liquid to the biomass may have di ered as well. This may also have resulted in di erent ratios of inactive to active biomass in samples within each treatment,causing large¯uctuations in the biologi-cal activity of unremoved biomass as observed in OUR experiments(Table5).Chemical treatment of clogged biotrickling®lters should result in a high e ciency of biomass removal to reduce the frequency of cleaning. Ideally,chemical treatment should also have mini-mum adverse e ects on the activity of biomass remaining on the rings in order to reduce the time of recovery of the biotrickling®lter after treatment (Fig.3,Inset).In Fig.3,the toluene oxidation ac-tivity of the unremoved biomass after the treatment is plotted vs biomass removal e ciencies.Many treatments resulted in partial or complete inacti-vation of the biomass without signi®cant removal of biomass.These include the treatments with SDS (a surfactant commonly used with NaOH in an alkali lysis procedure to disrupt cell membranes; Sambrook et al.,1989),I2(an alternative for NaClO/Cl2in wastewater disinfection;White, 1972),NaN3(an inhibitor of the respiratory chain at concentrations of0.0065±0.065%;Heinen,1971) or water at638C.Biomass removal e ciencies could have been better for these treatments if higher reagent concentrations were tested.OUR exper-iments however show that these treatments should probably not be considered because of their toxicity at low concentration.The low biomass removal e -ciency in these and other treatments is most prob-ably due to the fact that cells in a bio®lm are embedded in a polymer matrix.This may have served as a means to increase cell detention but did not o er much protection against the di usion of aggressive chemicals.It further suggests that suc-cessful development of biomass washing strategies should consider chemicals that have a proven e ect on the components of the polymer matrix. Treatment with ozone did not result in biomass removal,although ozone is a stronger oxidant than NaClO(E0=1.24and0.90mV,respectively;Lide, 1996).Apparently the ozone concentration in the gas phase(0.5g mÀ3)and the corresponding con-centration in the liquid phase(0.1mg lÀ1,dimen-sionless Henry coe cient of0.2;White,1972)were too low to e ectively oxidize or remove biomass. This is further supported by Siddiqui et al.(1997) who observed that removal of biodegradable dis-solved organic carbon(BDOC)from drinking water sources was optimal at an applied ozone to DOC ratio of2:1(g gÀ1).Although ozone treatment of BDOC and immobilized biomass may not be directly comparable,the results of Siddiqui et al. (1997)indicate that the ozone to carbon ratio applied in our experiment was too low(0.25g gÀ1). The economical and ecological impacts of using a 2:1ratio to remove excess biomass in biotrickling ®lters is not yet known.No treatment was found that can combine a high biomass removal e ciency and high toluene oxi-dation activity of the biomass remaining after treat-ment(Fig.3).OUR experiments did not allow for distinction between loss of activity due to cell death during chemical treatment and permanent or tem-porary inhibition of still viable cells.Also,since unremoved biomass was not washed after treatment (to simulate industrial operation),residual reagents may have a ected OUR experiments.In control ex-periments with H2O2and NaClO,calculated re-sidual reagent concentrations were su ciently high to completely inhibit respiration of fresh biomass, even after a ten-fold dilution to suspend the bio-mass for OUR experiments.H2O2and NaClO are well-known disinfectants and concentrations used in this study were several orders of magnitude higher than that required for disinfection(White,1972). The present results indicate that rapid recovery of biotrickling®lters after treatment with NaClO or H2O2may be hindered by both cell death during treatment as well as the presence of residual reagent in unremoved biomass after treatment.This suggests that post treatments using a reducing agent in the case of a chemical wash with an oxidant or using an acid or pH bu er after an alkali treatment might be bene®cial.Such a post treatment would neutralize the active chemical and eliminate the e ect of any residual chemicals.This could poten-tially speed up the recovery of the process culture ®lter after chemical treatment.From the four treat-ments with signi®cantly higher biomass removal e ciencies,only biomass after treatment with0.4% (w/v)NaOH showed some biological activity in OUR experiments.This speaks in favor of the use of NaOH to control biomass accumulation in bio-trickling®lters.Weber and Hartmans(1996)indeed observed that the toluene elimination capacity of a biotrickling®lter was fully restored within one day after treatment with0.4%(w/v)NaOH.They also found that the NaOH washes resulted in an average removal of230g dry biomass from a71l reactor, i.e.3.2kg dry biomass mÀ3.The percentage biomass removal in the case of Weber and Hartmans(1996) could not be calculated and compared to the results of the present research,because the amount of bio-mass in their reactor was not determined.However, a removal of3.2kg dry biomass mÀ3reactor volume is insu cient for controlling biomass in high per-formance biotrickling®lters that are subject to rapid clogging.Our source-biotrickling®lter gained approximately0.37kg dry biomass mÀ3reactor-dayÀ1,which would require treatment with0.4% (w/v)NaOH once every8.7days to maintain a con-stant biomass concentration in the reactor.A more e ective reagent may be desired to obtain a higherRemoval of biomass from biotrickling®lters2389。
《制药工程专业英语》Unit9,P96-98
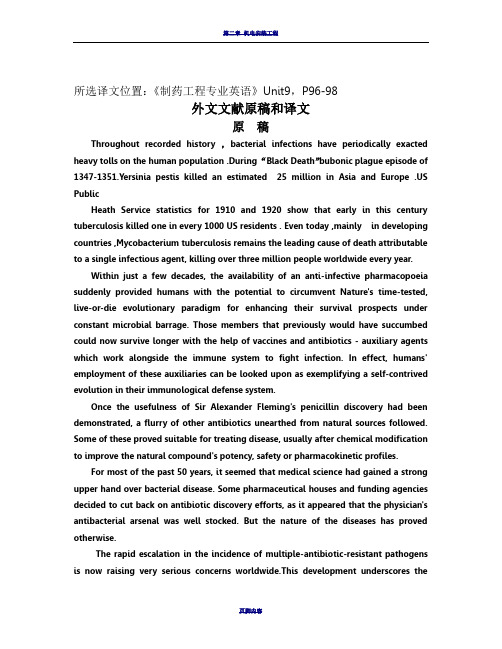
所选译文位置:《制药工程专业英语》Unit9,P96-98外文文献原稿和译文原稿Throughout recorded history ,bacterial infections have periodically exacted heavy tolls on the human population .During “Black Death”bubonic plague episode of 1347-1351.Yersinia pestis killed an estimated 25 million in Asia and Europe .US PublicHeath Service statistics for 1910 and 1920 show that early in this century tuberculosis killed one in every 1000 US residents . Even today ,mainly in developing countries ,Mycobacterium tuberculosis remains the leading cause of death attributable to a single infectious agent, killing over three million people worldwide every year.Within just a few decades, the availability of an anti-infective pharmacopoeia suddenly provided humans with the potential to circumvent Nature's time-tested, live-or-die evolutionary paradigm for enhancing their survival prospects under constant microbial barrage. Those members that previously would have succumbed could now survive longer with the help of vaccines and antibiotics - auxiliary agents which work alongside the immune system to fight infection. In effect, humans' employment of these auxiliaries can be looked upon as exemplifying a self-contrived evolution in their immunological defense system.Once the usefulness of Sir Alexander Fleming's penicillin discovery had been demonstrated, a flurry of other antibiotics unearthed from natural sources followed. Some of these proved suitable for treating disease, usually after chemical modification to improve the natural compound's potency, safety or pharmacokinetic profiles.For most of the past 50 years, it seemed that medical science had gained a strong upper hand over bacterial disease. Some pharmaceutical houses and funding agencies decided to cut back on antibiotic discovery efforts, as it appeared that the physician's antibacterial arsenal was well stocked. But the nature of the diseases has proved otherwise.The rapid escalation in the incidence of multiple-antibiotic-resistant pathogens is now raising very serious concerns worldwide.This development underscores thepowerful evolutionary capabilities of bacterial populations under the selective pressure imposed by antibiotic therapy .Antibiotic resistance Resistance problems are seen with both Gram-negative(for example Escherichia coli) and Gram-positive bacteria (such as Staphylococcus aureus), but most of the concerns are with the latter group of pathogens.Streptococcus is a respiratory Gram-positive pathogen responsible for 40,000 deaths a year in the US alone. A rapidly rising prevalence of penicillin-resistant S. pneumoniae infections is now problematic in many countries. One of the worst situations is in Hungary, where 70%of the S. from children tested in 1988-1989 were resistant to penicillin.Bacteria have evolved numerous ploys for defeatng antibiotic action——they inactivate the antibiotic by hydrolysis, acylation , phosphorylation or nucleotidylation reactions; aitre the antibiotic’s target site; or reduce the intracellular drug concentration by decreasing membrane permeability and/or actively pumping the drug out of the cell . With improved understanding of these mechanisms of resistance through molecular biology and biochemical techniques, medicinal chenmists have been provided with the targets for attempting to circumvent some of the resistance problems.A predominant resistance mechanism against the B-lactam drugs (such as Penicillin) involves enzymatic cleavage of the B-lactam ring. While the drug Methicillin was developed because it could withstand such action, strains of Methicillin-resistance S.ayreus (MRSA) emerged in 1961 , jusr two years after the drug first went into wide use . MRSA strains evolved so that they had an additional drug-target protein involved with cell wall biosynthesis, and this altered protein has a very low affinity for virtually all B-lactams. To make matters worse, mose MRSA strains are also resistant to many other classes of antibiotics,with the exception of the glycopeptide Vancomycin. Now seen around the globe, MRSA strains are very problematic in Japan ( where in some hospitials 60% of S. Aureus isolates are MRSA ), as well as in Spain , France , Italy and the US , each with a greater than 30% incidence.A particularly disturbing milestone was the 1988 emergence of Vancomycin-resistant enterococci (VRE). Some VRE now don’t respond to any available antibiotics. The enterococci have become the second mose frequently encountered hospital acquired pathogne in the US, where the incidence of VRE strains is now about15% of all clinical enterococcal isolates . Resistance to Vancomycin arises because a D-alanine-D-lactate residue ( which vancomycin binds to only poorly) has been substituted for the D-alanine-D-alanine residue normally found at the rerminus of a pentapeptide precursor involved in the bacteria’s cell wall biosynthesis.There is now a great concern that the genes conferring resistance in VRE to glycopeptides like Vancomycin will be naturally transferred to S.aureus, has been experimentally demonstrated feasible by William Noble at St Thomas' Hospital, London. As Vancomycin is the drug of last resort for treating MRSA infections, the anticipated natural acquisition of Vancomycin resistance in this virulent pathogen would result in the sobering return to pre-antibiotic era therapeutic failures, should no alternate effective therapy become available.ConclusionsMan's use of antibiotics has rapidly accelerated the dynamic evolutionary interplay between humans and bacteria. The recent rapid rise in multidrug-resistant Gram-positive bacterial infections worldwide has sounded a loud claxon for the need of new, effective therapies. The newer agents described here may provide physicians with a refurbished arsenal.The discovery of new bacterial drug targets through genomic research, as well as improvements in our understanding of bacterial resistant mechanisms, hold promise for the discovery of new means of treating multidrug-resistant bacterial infections. Given enough time, bacteria will eventually be able to develop resistance to any new antibacterial agent. Those drugs that can attack the pathogen through a novel mechanism may have reduced propensities to rapid resistance development.译文纵观历史记载,细菌感染的人口定期付出沉重的收费。
环境工程翻译译文
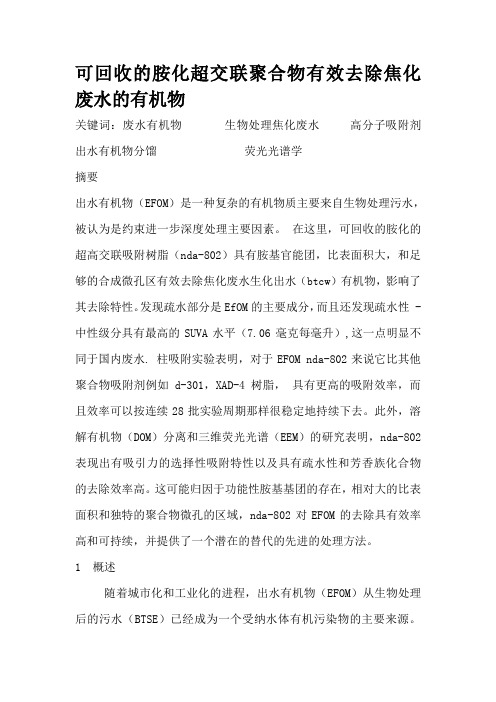
可回收的胺化超交联聚合物有效去除焦化废水的有机物关键词:废水有机物生物处理焦化废水高分子吸附剂出水有机物分馏荧光光谱学摘要出水有机物(EFOM)是一种复杂的有机物质主要来自生物处理污水,被认为是约束进一步深度处理主要因素。
在这里,可回收的胺化的超高交联吸附树脂(nda-802)具有胺基官能团,比表面积大,和足够的合成微孔区有效去除焦化废水生化出水(btcw)有机物,影响了其去除特性。
发现疏水部分是EfOM的主要成分,而且还发现疏水性 - 中性级分具有最高的SUVA水平(7.06毫克每毫升),这一点明显不同于国内废水. 柱吸附实验表明,对于EFOM nda-802来说它比其他聚合物吸附剂例如 d-301,XAD-4树脂,具有更高的吸附效率,而且效率可以按连续28批实验周期那样很稳定地持续下去。
此外,溶解有机物(DOM)分离和三维荧光光谱(EEM)的研究表明,nda-802表现出有吸引力的选择性吸附特性以及具有疏水性和芳香族化合物的去除效率高。
这可能归因于功能性胺基基团的存在,相对大的比表面积和独特的聚合物微孔的区域,nda-802对EFOM的去除具有效率高和可持续,并提供了一个潜在的替代的先进的处理方法。
1 概述随着城市化和工业化的进程,出水有机物(EFOM)从生物处理后的污水(BTSE)已经成为一个受纳水体有机污染物的主要来源。
EFOM在本质上是高度异质性(Quaranta等人。
,2012),天然有机物(NOM)主要是由来自地表水,可溶性微生物产物(SMP)的生物处理,有机化合物(SOC)的生产和使用有机化合物(Shon等人。
,2006b)。
一般来说,废水中COD大多数是由于EfOM,因此,有效去除EfOM成为主要的任务,但提高回收废水的质量或满足越来越严格的标准是有挑战性的任务。
大多数EfOM存在可溶性成分,而且以及构成了80%的COD (Shon等人。
,2006b),其有效去除仍然是一个具有挑战性的任务。
专业英语翻译

2. Of more resent introduction is mushroom cultivation which probably dates back many hundreds of years for Japanese shii-ta-ke cultivation and about 300 years for the Agaricus mushroom now widely cultivated throughout the temperate world.
译文:生物工程反应可以是分解代谢反应或合成代谢反应(也叫生物合成反应)。分解代谢反应中复杂的化合物被分解为简单物质(葡萄糖生成乙醇),而合成代谢反应或生物合成反应,是简单的分子合成为更复杂的物质(抗生素的合成)。
3.biotechnology includes fermentation process(ranging from beers and wines to bread, cheese, antibiotics and vaccines), water and waste treatment, parts of food technology, and an increasing range of novel applications(ranging from biomedical to metal recovery from low grade ores
译文: 生物工程是属于应用生物科学和技术的一个领域,它包含生物或其亚细胞组分在制造业、服务业和环境管理等方面的应用。
2.The reactions of biotechnological processes can be catabolic, in which complex compounds are broken down to simpler ones (glucose to ethanol ), or anabolic or biosynthetic, whereby simple molecules are built up into more complex ones(antibiotic synthesis).
生物工程专业英语
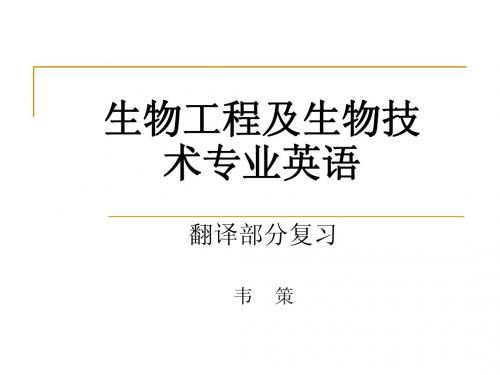
Cultivation of Escherichia coli XN-1 was carried out in the batch mode. A 5-L jar fermentor with a liquid working volume of 3 L was used for cultivation. The temperature was controlled at 32℃, and the pH was maintained at 7.0 by adding 28% ammonia water.
Unit 7 作业
At the end of cultivation (18 h), a cell density of 1.12 gDCW/l and succinic acid yields yield of 63–64% were obtained. 到发酵结束(18小时),干细胞量可达 到1.12g DCW/L,丁二酸的产率可达63– 64% 。 DCW——Dried Cell Weight
Unit 2 作业
The dissolved oxygen concentration was maintained at above 3.0 mg/L by controlling the agitation speed in the range 180 revolutions per minute (rpm). The aeration rate was maintained at 1.1L air volume per liter liquid volume per minute (vvm). The initial glucose concentration was 20 g/L, and the initial concentration of corn steep liquor was 15 g/L.
实验室污水有机物去除工艺流程
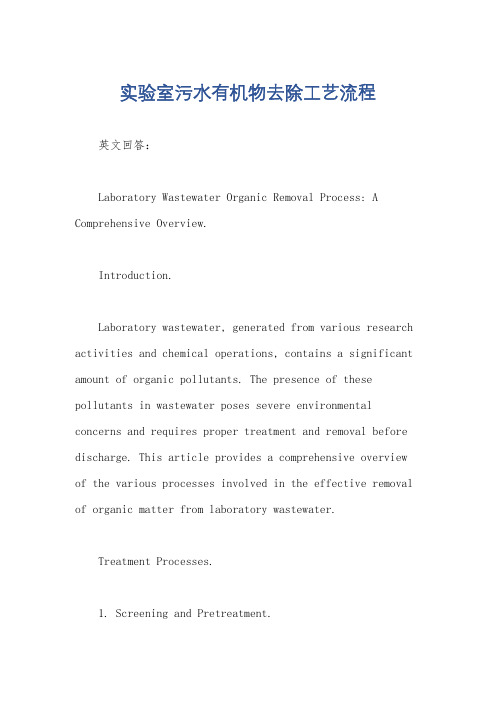
实验室污水有机物去除工艺流程英文回答:Laboratory Wastewater Organic Removal Process: A Comprehensive Overview.Introduction.Laboratory wastewater, generated from various research activities and chemical operations, contains a significant amount of organic pollutants. The presence of these pollutants in wastewater poses severe environmental concerns and requires proper treatment and removal before discharge. This article provides a comprehensive overview of the various processes involved in the effective removal of organic matter from laboratory wastewater.Treatment Processes.1. Screening and Pretreatment.The initial stage of wastewater treatment involves screening and pretreatment. This step removes large particles, debris, and solids that could interfere with downstream processes. Pretreatment also includes pH adjustment and the addition of coagulants to enhance the efficiency of subsequent treatment steps.2. Biological Treatment.Biological treatment is a widely adopted method for removing organic matter from wastewater. It utilizes microorganisms, such as bacteria and fungi, to biodegrade and assimilate organic pollutants. Activated sludge, trickling filters, and membrane bioreactors (MBRs) are common types of biological treatment systems.3. Advanced Oxidation Processes (AOPs)。
Biosurfactant-e nhanced bioremediation of aged polycyclic aromatic翻译..
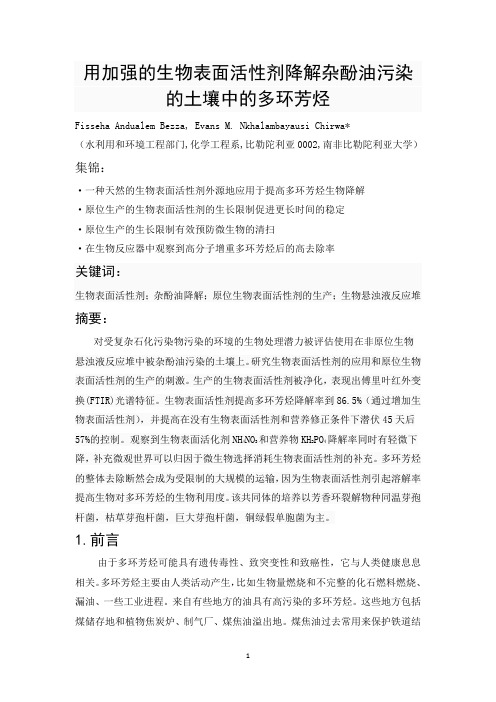
用加强的生物表面活性剂降解杂酚油污染的土壤中的多环芳烃Fisseha Andualem Bezza, Evans M. Nkhalambayausi Chirwa*(水利用和环境工程部门,化学工程系,比勒陀利亚0002,南非比勒陀利亚大学)集锦:·一种天然的生物表面活性剂外源地应用于提高多环芳烃生物降解·原位生产的生物表面活性剂的生长限制促进更长时间的稳定·原位生产的生长限制有效预防微生物的清扫·在生物反应器中观察到高分子增重多环芳烃后的高去除率关键词:生物表面活性剂;杂酚油降解;原位生物表面活性剂的生产;生物悬浊液反应堆摘要:对受复杂石化污染物污染的环境的生物处理潜力被评估使用在非原位生物悬浊液反应堆中被杂酚油污染的土壤上。
研究生物表面活性剂的应用和原位生物表面活性剂的生产的刺激。
生产的生物表面活性剂被净化,表现出傅里叶红外变换(FTIR)光谱特征。
生物表面活性剂提高多环芳烃降解率到86.5%(通过增加生物表面活性剂),并提高在没有生物表面活性剂和营养修正条件下潜伏45天后57%的控制。
观察到生物表面活化剂NH4NO3和营养物KH2PO4降解率同时有轻微下降,补充微观世界可以归因于微生物选择消耗生物表面活性剂的补充。
多环芳烃的整体去除断然会成为受限制的大规模的运输,因为生物表面活性剂引起溶解率提高生物对多环芳烃的生物利用度。
该共同体的培养以芳香环裂解物种同温芽孢杆菌,枯草芽孢杆菌,巨大芽孢杆菌,铜绿假单胞菌为主。
1.前言由于多环芳烃可能具有遗传毒性、致突变性和致癌性,它与人类健康息息相关。
多环芳烃主要由人类活动产生,比如生物量燃烧和不完整的化石燃料燃烧、漏油、一些工业进程。
来自有些地方的油具有高污染的多环芳烃。
这些地方包括煤储存地和植物焦炭炉、制气厂、煤焦油溢出地。
煤焦油过去常用来保护铁道结和电线杆,使它们防水。
它是油中产生多环芳烃污染物的主要原因。
木馏油是一种由200种化合物组成的复杂混合物,主要包括多环芳烃,以及酚醛和芳香氮、硫化合物的混合物。
生物工业发酵工程流程

生物工业发酵工程流程英文回答:Bioprocess engineering plays a crucial role in thefield of industrial biotechnology, particularly in the production of various valuable products through fermentation. Fermentation is a metabolic process carried out by microorganisms such as bacteria, yeast, or fungi, where they convert organic substances into useful products under controlled conditions.The bioprocess engineering flowchart typically consists of several key steps. Firstly, the selection and isolation of the desired microorganism is essential. This involves screening various microorganisms to identify the one with the desired characteristics, such as high productivity or specific metabolic capabilities. For example, in the production of ethanol, yeast strains with high ethanol tolerance and fermentation efficiency are selected.Once the microorganism is selected, the next step is to optimize the growth and production conditions. This includes determining the appropriate nutrient composition, pH, temperature, and oxygen levels for the fermentation process. The goal is to create an environment that maximizes the growth and productivity of the microorganism. For instance, in the production of citric acid, the fermentation process requires a pH of around 4-6 and a temperature of 30-35°C to achieve optimal yield.After optimizing the conditions, the actual fermentation process takes place. The selected microorganism is inoculated into a bioreactor, which provides a controlled environment for the fermentation process. The bioreactor allows for the monitoring and control of various parameters such as temperature, pH, agitation, and aeration. This ensures that the fermentation process proceeds efficiently and produces the desired product. For example, in the production of penicillin, the fermentation process is carried out in a stirred-tank bioreactor with controlled temperature and pH.During the fermentation process, the microorganism metabolizes the available nutrients and produces thedesired product. The progress of the fermentation is monitored through various analytical techniques, such as measuring the concentration of the desired product, biomass, and by-products. This allows for real-time adjustments tobe made if necessary to optimize the process and maximize product yield.Once the fermentation process is complete, the nextstep is the downstream processing. This involves separating and purifying the desired product from the fermentation broth. Techniques such as filtration, centrifugation, chromatography, and distillation are commonly employed to achieve this. For instance, in the production of recombinant proteins, chromatography is often used topurify the protein of interest from the complex mixture.Finally, the purified product is formulated and packaged for commercial use. This may involve additional processing steps, such as formulation with excipients, sterilization, and filling into appropriate containers. Thefinal product is then ready for distribution and sale.中文回答:生物工业发酵工程在工业生物技术领域中起着至关重要的作用,特别是在通过发酵生产各种有价值的产品方面。
生物工程专业英语
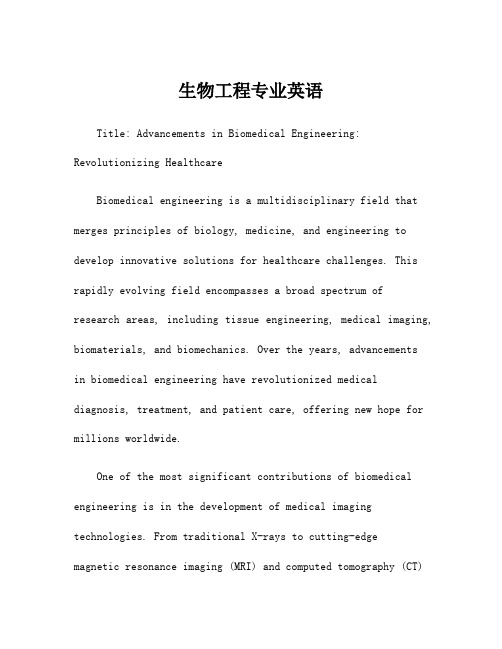
生物工程专业英语Title: Advancements in Biomedical Engineering: Revolutionizing HealthcareBiomedical engineering is a multidisciplinary field that merges principles of biology, medicine, and engineering to develop innovative solutions for healthcare challenges. This rapidly evolving field encompasses a broad spectrum of research areas, including tissue engineering, medical imaging, biomaterials, and biomechanics. Over the years, advancementsin biomedical engineering have revolutionized medical diagnosis, treatment, and patient care, offering new hope for millions worldwide.One of the most significant contributions of biomedical engineering is in the development of medical imaging technologies. From traditional X-rays to cutting-edgemagnetic resonance imaging (MRI) and computed tomography (CT)scans, these imaging modalities enable healthcareprofessionals to visualize internal structures of the human body with unprecedented clarity. Moreover, recent advancements in functional imaging techniques, such aspositron emission tomography (PET) and functional MRI (fMRI), have revolutionized our understanding of brain function and neurological disorders.In the realm of regenerative medicine, biomedical engineers are pioneering the development of tissueengineering techniques to repair or replace damaged tissues and organs. By combining scaffolds, cells, and growth factors, tissue engineers are creating artificial organs and tissues that mimic the structure and function of native tissues.These engineered tissues hold immense potential for treating conditions ranging from organ failure to severe burns,offering a promising alternative to traditionaltransplantation methods.Biomaterials play a crucial role in biomedical engineering by providing the foundation for medical devices, implants, and drug delivery systems. Advances in biomaterial science have led to the development of biocompatible materials that interact seamlessly with the human body, reducing the risk of rejection and complications. Nanotechnology has further expanded the capabilities of biomaterials, enabling precise drug targeting, enhanced imaging contrast, and controlled release of therapeutic agents.Biomechanics is another key area of biomedical engineering focused on studying the mechanical aspects of biological systems. By applying principles of physics and engineering, biomechanists analyze the movement, forces, and stresses within the human body to design orthopedic implants, prosthetic limbs, and assistive devices. These innovations improve mobility and quality of life for individuals withmusculoskeletal injuries or disabilities, enabling them to lead more active and independent lives.In addition to these areas, biomedical engineering encompasses a wide range of specialties, including medical robotics, bioinformatics, and personalized medicine.Robotics-assisted surgeries, powered exoskeletons, and wearable health monitoring devices are just a few examples of how technology is transforming healthcare delivery andpatient outcomes. Furthermore, advances in computational biology and bioinformatics are driving breakthroughs in genomics, proteomics, and drug discovery, paving the way for personalized therapies tailored to individual patients.Looking ahead, the future of biomedical engineering holds even greater promise, fueled by ongoing research and collaboration across disciplines. Emerging technologies such as 3D bioprinting, gene editing, and artificial intelligence are poised to revolutionize healthcare in ways we have yet toimagine. By harnessing the power of innovation and ingenuity, biomedical engineers will continue to push the boundaries of what is possible, ushering in a new era of personalized medicine and improved global health outcomes.In conclusion, biomedical engineering is at the forefront of transforming healthcare through innovation and technology. From medical imaging and tissue engineering to biomaterials and biomechanics, this interdisciplinary field is driving advancements that benefit patients worldwide. As we continue to unlock the mysteries of the human body and develop novel therapies, biomedical engineering will play a pivotal role in shaping the future of medicine and improving quality of life for generations to come.。
甾体雌激素在不同污水处理工艺中的去除规律
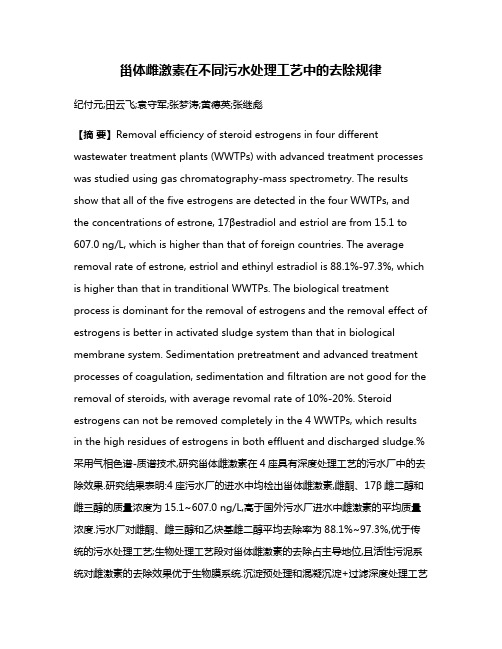
甾体雌激素在不同污水处理工艺中的去除规律纪付元;田云飞;袁守军;张梦涛;黄德英;张继彪【摘要】Removal efficiency of steroid estrogens in four different wastewater treatment plants (WWTPs) with advanced treatment processes was studied using gas chromatography-mass spectrometry. The results show that all of the five estrogens are detected in the four WWTPs, and the concentrations of estrone, 17βestradiol and estriol are from 15.1 to 607.0 ng/L, which is higher than that of foreign countries. The average removal rate of estrone, estriol and ethinyl estradiol is 88.1%-97.3%, which is higher than that in tranditional WWTPs. The biological treatment process is dominant for the removal of estrogens and the removal effect of estrogens is better in activated sludge system than that in biological membrane system. Sedimentation pretreatment and advanced treatment processes of coagulation, sedimentation and filtration are not good for the removal of steroids, with average revomal rate of 10%-20%. Steroid estrogens can not be removed completely in the 4 WWTPs, which results in the high residues of estrogens in both effluent and discharged sludge.%采用气相色谱-质谱技术,研究甾体雌激素在4座具有深度处理工艺的污水厂中的去除效果.研究结果表明:4座污水厂的进水中均检出甾体雌激素,雌酮、17β雌二醇和雌三醇的质量浓度为15.1~607.0 ng/L,高于国外污水厂进水中雌激素的平均质量浓度.污水厂对雌酮、雌三醇和乙炔基雌二醇平均去除率为88.1%~97.3%,优于传统的污水处理工艺;生物处理工艺段对甾体雌激素的去除占主导地位,且活性污泥系统对雌激素的去除效果优于生物膜系统.沉淀预处理和混凝沉淀+过滤深度处理工艺段对甾体雌激素的去除效果不佳,平均去除率为10%~20%.4座污水厂均不能彻底去除污水中的甾体雌激素,污水厂尾水及剩余污泥中残留较高质量浓度雌激素.【期刊名称】《中南大学学报(自然科学版)》【年(卷),期】2017(048)004【总页数】7页(P873-879)【关键词】甾体雌激素;污水处理工艺;去除作用;规律【作者】纪付元;田云飞;袁守军;张梦涛;黄德英;张继彪【作者单位】复旦大学环境科学与工程系,上海 200433;合肥工业大学土木与水利工程学院,安徽合肥,230009;中机国际工程设计研究院有限责任公司,湖南长沙,410007;复旦大学环境科学与工程系,上海 200433;合肥工业大学土木与水利工程学院,安徽合肥,230009;合肥工业大学土木与水利工程学院,安徽合肥,230009;复旦大学化学系,上海,200433;复旦大学环境科学与工程系,上海 200433【正文语种】中文【中图分类】X703.1甾体雌激素为四环脂烃类化合物,也称类固醇雌激素,是典型的内分泌干扰物(EDCs)。
生物技术专业外文翻译--优化微波萃取人类苯等离子体

中文译文:优化微波萃取人类苯等离子体P. Fernández & C. Vázquez & R. A. Lorenzo &A. M. Carro & I. Álvarez & P. Cabarcos摘要一个简单快速的微波萃取(MAE)方法,已经评估为固相萃取(SPE)的代替品,SPE对六地西泮的确定在欧洲很多国家被广泛规定在人体血浆。
对于MAE的优化,一个实验设计使用萃取时间、温度、溶剂体积作为影响参数。
一个期望函数被定义,为了同时优化MAE方法。
方差分析显示溶剂体积对所有的样本测试的优化有积极的影响,已经成为统筹全局的重要因素。
同时,萃取时间在四地西泮的萃取中的作用更为突出。
选择MAE的一种情况:温度89°C、时间13min、8mL的氯仿对应4mL的丙醇:89.8+0.3%到102.1+5.2%的回收率,因为苯二氮卓类运采用高效液相色谱法结合二极管阵列检测技术。
MAE和SPE的比较结果表明MPE方法更为实用,因为它处理样品简单高效。
苯二氮卓类用户就MAE的适用性在27种等离子种成功测试。
在短期内,苯二氮卓类(简称BZD)是非常有用的精神刺激药物,对紊乱症状很有帮助,比如焦虑、癫痫、失眠。
然而,严重的副作用源于长期经常使用治疗药物,自备配方或者使用过度剂量。
酒精和BZD所产生的效应相结合也许就是一种有助于过激行为的添加剂。
性犯罪和暴力行为可能和BZD的消费有联系,也会导致法医学的并发症。
依赖是可能的风险更大的强效,短效BZD就像阿普唑仑。
在开催眠和焦虑性的处方是,谨慎是必须的,因为长期使用就会产生极大的依赖性。
量化在等离子体也许有助于慢性定量优化,验证符合性和识别改变在药代动力学。
由于这些原因,可靠的优化,快速简单的方法,要控制BZD在等离子体要求节约时间和金钱,而且不丧失灵敏度和再现性分析方法。
液相色谱法已经成为常规测定的一个重要工具,由于它的特异性,速度和灵敏度。
生物工程生物技术专业英语翻译(二)
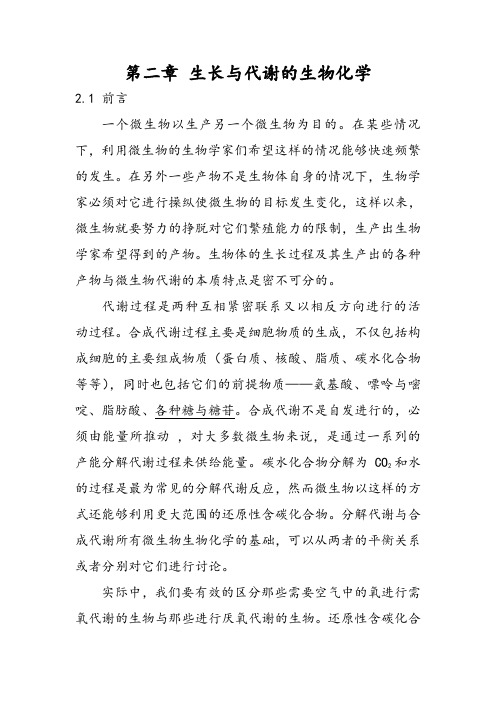
第二章生长与代谢的生物化学2.1 前言一个微生物以生产另一个微生物为目的。
在某些情况下,利用微生物的生物学家们希望这样的情况能够快速频繁的发生。
在另外一些产物不是生物体自身的情况下,生物学家必须对它进行操纵使微生物的目标发生变化,这样以来,微生物就要努力的挣脱对它们繁殖能力的限制,生产出生物学家希望得到的产物。
生物体的生长过程及其生产出的各种产物与微生物代谢的本质特点是密不可分的。
代谢过程是两种互相紧密联系又以相反方向进行的活动过程。
合成代谢过程主要是细胞物质的生成,不仅包括构成细胞的主要组成物质(蛋白质、核酸、脂质、碳水化合物等等),同时也包括它们的前提物质——氨基酸、嘌呤与嘧啶、脂肪酸、各种糖与糖苷。
合成代谢不是自发进行的,必须由能量所推动,对大多数微生物来说,是通过一系列的产能分解代谢过程来供给能量。
碳水化合物分解为CO2和水的过程是最为常见的分解代谢反应,然而微生物以这样的方式还能够利用更大范围的还原性含碳化合物。
分解代谢与合成代谢所有微生物生物化学的基础,可以从两者的平衡关系或者分别对它们进行讨论。
实际中,我们要有效的区分那些需要空气中的氧进行需氧代谢的生物与那些进行厌氧代谢的生物。
还原性含碳化合物与O2反应生成水和CO2,这是一个高效的放热反应过程。
因此,一个进行需氧代谢的生物要使用一小部分底物进行分解代谢以维持某一水平的合成代谢,即成长过程。
对于厌氧型生物,其底物的转化的过程基本上是一个不匀称的反应(氧化还原反应),产生很少的能量,因此,大部分底物都要被分解从而维持一定水平的合成代谢。
在生物体中这种差别能够明显的体现出来,比如酵母,它属于兼性厌氧生物,即它可在有氧条件下生长也可在无氧环境下生存。
需氧酵母使糖以同样的速度转化为CO2和水,相对产生高产量的新酵母。
而厌氧条件下,酵母菌生长缓慢,此时酵母被有效的转化为酒精和CO2。
2.2 代谢与能量分解代谢与合成代谢间的有效联系在于,各种分解代谢过程促进少量反应物的合成,而后又被用来促进全面的合成代谢反应。
- 1、下载文档前请自行甄别文档内容的完整性,平台不提供额外的编辑、内容补充、找答案等附加服务。
- 2、"仅部分预览"的文档,不可在线预览部分如存在完整性等问题,可反馈申请退款(可完整预览的文档不适用该条件!)。
- 3、如文档侵犯您的权益,请联系客服反馈,我们会尽快为您处理(人工客服工作时间:9:00-18:30)。
ENGINEERED BIORETENTION FOR REMOVALOF NITRATE FROM STORMWATER RUNOFFHunho Kim, Eric A. Seagren, and Allen P. DavisDepartment of Civil and Environmental EngineeringUniversity of MarylandCollege Park, MD 20742ABSTRACTControl of nitrate from urban stormwater runoff can have a significant impact on nitrate levels in local waters. One option for providing treatment to urban stormwater runoff is bioretention, a simple plant- and soil-based low impact treatment/infiltration facility. The goal of this study is to re-engineer the concept of bioretention to remove nitrate from urban runoff. Specifically, a modification to incorporate a continuously submerged anoxic zone with an overdrain is being evaluated for its capacity for nitrate removal via denitrification. Work to date has focused on selecting an electron donor and carbon source that will promote significant denitrification and be stable for extended periods of time in the subsurface. The electron donor and carbon source to be used in bioretention must be readily metabolizable, as well as low cost and readily available. Two sets of organic substrates for chemoorganotrophic denitrifying bacteria were evaluated: Set #1, alfalfa, newspaper, and leaf mulch compost; and Set #2, sawdust, wood chips, and wheat straw. An inorganic substrate for chemolithotrophic denitrifying bacteria, sulfur, was evaluated in experimental Set #3, in three configurations: large sulfur particles (2 to 2.36 mm) alone, large sulfur particles with limestone, and small sulfur particles (0.6 to 1.18 mm) with limestone. All materials were uniformly mixed with washed silica sand and transferred into 40 cm long by 6.4 cm inner diameter Plexiglas columns. A total of 4 columns were used for each experimental set, including a control column packed with washed silica sand only. The columns were seeded with the supernatant of settled secondary effluent and fed anoxic synthetic stormwater runoff. Based on the Set#1 and Set#2 experiments for the organic substrates, excellent nitrate removal was observed in columns containing alfalfa, newspaper, wheat straw, wood chips, and sawdust. However, based on total nitrogen removal and other water quality characteristics, newspaper and wood chips are the best candidates out of these sets. In Set#3, significantly better nitrate removal occurred in the column with the small sulfur particles/limestone compared to the large sulfur particles, probably as a result of the increased surface area of the sulfur available per unit volume of reactor. Further studies will be performed using the electron donors that gave the best nitrate removal efficiency and effluent quality in the experiments reported here: newspaper, wood chips, and small sulfur particles/limestone.KEYWORDSNitrate, Urban Runoff, Biological Treatment, DenitrificationINTRODUCTIONNitrogen, in particular nitrate, is a substance of critical concern with respect to water quality. Control of nitrate from urban stormwater runoff can have a significant impact on nitrate levels in local waters. Therefore, a low impact treatment facility to remove nitrate from stormwater runoff before it enters receiving waters would be extremely beneficial. One such approach is bioretention, a simple plant- and soil-based low impact treatment/infiltration facility for use in developed areas to provide treatment to stormwater runoff.Within the concept of bioretention for stormwater runoff treatment, two issues emerge with respect to nitrogen management. The first is the uptake of nitrogen compounds during the time scale of storm events. Based on previous research (Davis et al., 1998), ammonia is moderately removed from infiltrating stormwater due to sorptive interactions with the soil media. However, nitrate has near negligible affinity for soil components due to its anionic form, and consequently nearly no nitrate is removed. The second nitrogen issue in bioretention is on the long-term time scale. As organic and ammonia nitrogen are accumulated in the bioretention system, processes for their benign removal from the bioretention facility must be developed and optimized. The most desirable process is to promote the conversion of accumulated nitrogen species to nitrogen gas, with the off-gas release of nitrogen to the atmosphere. Work by Davis et al. (1998) indicates that this accumulated organic and ammonia nitrogen can be converted to nitrate during the days between storm events, presumably via the biologically-mediated processes of ammonification and nitrification, and that this nitrate is washed from the facility by succeeding rain events.The goal of this study is to systematically examine the removal of nitrate from urban runoff by re-engineering the concept of bioretention. Specifically, a modification to incorporate a continuously submerged anoxic zone with an overdrain is being evaluated for its capacity for nitrate removal via denitrification (See Figure 1 "Diagram of Modified Bioretention for Denitrification"). In this evaluation, conditions that optimize the denitrification reaction will be determined so that design parameters can be established for use in bioretention systems. The work to date, which is described in this paper, has focused on selecting an electron donor and carbon source that will promote significant denitrification and be stable for a long period of time in the subsurface. The electron donor and carbon source to be used in bioretention must be readily metabolizable, as well as low cost and readily available. Systems under evaluation include organic substrates for chemoorganotrophic denitrifying bacteria, and inorganic substrates for chemolithotrophic denitrification.METHODOLOGYBased on the selection criteria and past related research, one inorganic substrate--sulfur--and six organic substrates--alfalfa, leaf mulch compost, newspaper, sawdust, wheat straw and wood chips--were chosen as potential electron donors (e.g., Vogan, 1993; Blowes et al., 1994; Robertson and Cherry, 1995; Volokita et al., 1996; Schipper and Vojvodic-Vukovic, 1998; Shikora and Keeney, 1976; Zhang and Shan, 1999). The organic substrates were evaluated in two experimental sets: Set #1, alfalfa, newspaper, and leaf mulch compost; and Set #2, sawdust, wood chips, and wheat straw. The alfalfa, newspaper, and wheat straw were prepared by cuttingthe materials, and passing them through a 4-mm sieve; the sawdust and the leaf mulch compost were prepared by passing them through a 2-mm sieve. The wood chips were prepared by cutting them using a blender, and then passing the material through a 2-mm sieve. The inorganic substrate, sulfur, was evaluated in experimental Set #3. The sulfur (International Sulfur, Inc., Mt. Pleasant, TX) was sieved into two size fractions: “large” particles, ranging from 2 to 2.36 mm, and “small” particles, ranging from 0.6 to 1.18 mm. Three variations were compared in this set: large sulfur particles alone, large sulfur particles with limestone, and small sulfur particles with limestone. The limestone (Southdown, Inc., Easton, PA) was sieved to obtain a size range from 0.6 to 1.18 mm and was added to buffer the acid production during denitrification with sulfur as the electron donor.For each electron donor substrate, the total mass required for denitrification was calculated based on the nitrate loading for a 60-day experiment and using the appropriate reaction stoichiometry (McCarty, 1975). In the case of the organic electron donors, the total organic carbon (TOC) concentration used in the stoichiometric calculations was measured on a dry weight basis via a TOC analyzer (Shimadzu, Model 5000). In addition, the corresponding stoichiometric amount of limestone required for buffering in the sulfur experiments was also calculated (Zhang and Shan, 1999). The calculated material requirements were multiplied by a safety factor of 20 and the mass of material was uniformly mixed with silica sand that had been washed to minimize effects of residual organic carbon.The electron donor/sand mixtures were then transferred into 40 cm long by 6.4 cm inner diameter Plexiglas columns, with sampling ports that penetrated to the center installed every 10 cm along the column. A total of 4 columns were set up for each experiment including a control column, which was packed with washed silica sand only. The influent and effluent ports of the columns were separated from the packing material by two stainless steel screens. The influent screen was supported by a Plexiglas plate with 0.4 cm holes to promote even distribution of flow across the cross-sectional area of the column. The effluent screen was held in place by a rubber stopper. All four columns were operated at room temperature (22±2°C).The columns were seeded with the supernatant (settled at room temperature for 24 hours) of a secondary effluent sample from an activated sludge plant where denitrification was being performed. To inoculate the columns, the seed material was pumped into the column in an upflow mode and recycled through the column for 2 days.After the 2-day inoculation procedure, synthetic stormwater runoff was introduced into each column in an upflow mode at a flow rate of 4 cm/hr (2.2 mL/min). The synthetic stormwater runoff was made using tap water with addition of 2.0 mg/L nitrate as N, 120 mg/L CaCl2, 0.6 mg/L Na2HPO4 as P, and the pH adjusted to 7 (Davis et al., 1998). The tapwater was dechlorinated with NaHSO3 and continuously purged with N2 to remove O2, resulting in influent dissolved oxygen concentrations < 2 ppm. In all experiments, samples for analysis were taken daily for nitrate, and periodically for Total Kjeldahl Nitrogen (TKN), and turbidity. In addition, during the sulfur/limestone experiments, samples were also taken for sulfate, total alkalinity, and nitrite analysis. Nitrate and sulfate concentrations were quantified via ion chromatography (Dionex DX-100) using a AS4 column with a 1.3 mM Na2CO3/1.5 mM NaHCO3 eluent. Nitriteand TKN analysis were performed using Standard Methods 4500-NO2- B. Colorimetric Method and 4500-N org B. Macro-Kjeldahl Method, respectively (APHA et al., 1995). Turbidity levels were quantified using a HACH 2100N turbidimeter. Alkalinity was measured following Standard Method 2320 B. Titration Method (APHA et al., 1995).RESULTSThe experimental columns in each set were run for 35 to 40 days. This was sufficient time for the systems to reach a quasi-steady-state condition with respect to nitrate removal and the average percent nitrate removal during this period was calculated for each experimental set (See Figure 2 “Average Quasi-Steady-State Nitrate Removal (%)”). In Set#1, the nitrate removals observed in the alfalfa and newspaper columns were essentially 100%, while that for the leaf mulch compost column was about 60% (See Figure 2 “Average Quasi-Steady-State Nitrate Removal (%)”). The removal in the control column was only about 7%. However, treated water from the alfalfa column showed some odor, increased turbidity (≈ 27 NTU) and residual TKN (2-3 mg/L N), while that from the newspaper and mulch did not demonstrate any of these problems.The Set#2 organic electron donors, sawdust, wheat straw, and wood chips, all performed well, with >95% nitrate removal, compared to <10% for the control column (See Figure 2 “Average Quasi-Steady-State Nitrate Removal (%)”). However, the effluent quality based on other parameters was variable. In particular, the wheat straw column had somewhat high residual TKN (0.5-1.4 mg/L N), and high turbidity (up to about 16 NTU). The sawdust and wood chips had similar TKN levels in the effluent (roughly 0.3 mg/L N) during the quasi-steady-state period. The turbidity was somewhat higher in the wood chip effluent compared to the sawdust column (average 2.4 NTU compared to 0.8 NTU); however, the wood chip system provided consistently better nitrate removal than the sawdust system throughout the course of the experiment , and showed more rapid removal of nitrate along the length of the column.In Set#3, only the small sulfur particle/limestone combination performed well over the course of the experiment, with about 90% nitrate removal during the quasi-steady-state period (See Figure 2 “Average Quasi-Steady-State Nitrate Removal (%)”). The large sulfur only, and large sulfur/limestone columns produced only about 30% nitrate removal during the quasi-steady-state period, while the control had about 10% removal. Effluent sulfate concentrations in all three columns were approximately equal to the stoichiometric amount expected based on the nitrate removal. No clear trends were found in the alkalinity data; the buffering capacity seemed to be sufficient whether limestone was added to the columns or not, although the alkalinity levels in the sulfur-only column were generally somewhat lower. These results were expected based on the alkalinity levels in the simulated stormwater (roughly 30 mg/L as CaCO3), which should be sufficient to buffer the acid production at the tested nitrate concentration in the absence of added limestone. Interestingly, the small sulfur/limestone column effluent had relatively high nitrite levels during the quasi-steady-state period (about 0.5-0.6 mg/L N). Turbidity levels were low (<0.4 NTU) in all of the sulfur columns.DISCUSSIONBased on the Set#1 and Set#2 experiments for the organic substrates, alfalfa, newspaper, sawdust, wheat straw, and wood chips all appeared to be efficient electron donors and carbon sources for the removal of nitrate from stormwater runoff. However, it is also critical that the effluent water quality leaving the bioretention basin not be diminished compared to the influent and that the nitrate nitrogen removal from the system occurs via denitrification. Based on these criteria, the newspaper and wood chips are the best candidates, as they provide better effluent quality and total nitrogen removal than the other organic substrates. In the alfalfa and wheat straw systems, both of which had relatively substantial TKN in the column effluent, the nitrate was essentially completely removed from the pore water by the 20 cm height and there was qualitative evidence that sulfate reduction was occurring. One possible explanation for the high effluent TKN is that alfalfa and wheat straw have a lower C:N ratio than sawdust, wood chips, and newsprint (Rynk, 1992). Therefore, it is possible that more ammonification occurred in the alfalfa and wheat straw systems, resulting in increased TKN. It is also known that some microorganisms, including sulfate-reducing bacteria (Hansen, 1994), can reduce nitrate to ammonia in a dissimilative process, and it is possible that this is the source of the TKN in the effluent. This microbial process has been observed to be favored in anaerobic environments when carbon availability is high relative to nitrate availability (Tiedje et al., 1982), as was the case in these relatively short-term column systems. Dissimilatory reduction of nitrate to ammonia is an undesirable process in bioretention because nitrogen is conserved. This is a challenge in engineering bioretention basins for denitrification because of the relatively low nitrate levels, coupled with the need to provide suitable quantities of electron donor/carbon source to sustain the system for extended periods; however, this problem should decrease with time.The results in Set#3 with the sulfur systems are interesting. Although the same mass of sulfur was added to all three columns, and sufficient buffering capacity was present in all three cases, significantly better nitrate removal occurred in the column with the small sulfurparticles/limestone. This is probably a result of the increased number of sulfur particles and the increased surface area of the sulfur available per unit volume of reactor with the smaller sulfur particles. Thus, with the smaller particles, more surface area was available for contact with the nitrate-laden water, and for growth of the denitrifying microorganisms (Liu, 1992, referenced in Zhang and Shan, 1999). It is possible that with longer hydraulic retention times, the large sulfur particle systems would demonstrate improved nitrate removal efficiency (e.g., Koenig and Liu, 1996). Based on this evaluation, the small sulfur/limestone combination appears to be a good candidate system, although there is some concern at this point that relatively high levels of nitrogen may be leaving the system in the form of nitrite. This is being further investigated. Accumulation of nitrite is a characteristic of the chemolithotrophic denitrifying bacterium Thiobacillus denitificans (Baalsrud and Baalsrud, 1954). It may be that nitrite concentration levels can be decreased with longer residence times (e.g., Sikora and Keeney, 1976).One interesting finding from these experiments is that a suitable inoculum was provided in all cases by the settled supernatant of a secondary effluent sample. For example, in the case of theorganic substrates, which are all complex, cellulose-rich, carbon sources, no steps were taken to select for a cellulose-degrading inoculum. In addition, in the case of sulfur, a sufficient inoculum of chemolithotrophic denitrifying bacteria was provided in the secondary effluent. This is consistent with other research suggesting that these organisms are present in a variety of environments, including domestic wastewater (Zhang and Lampe, 1999; Zhang and Shan, 1999) CONCLUSIONSBased on the experiments performed, excellent nitrate removal from synthetic stormwater runoff was observed in anoxic sand-packed columns containing alfalfa, newspaper, wheat straw, wood chips, and sawdust. However, based on total nitrogen removal and other water quality characteristics, newspaper and wood chips are the best candidates out of these sets for providing the electron donor/carbon source in bioretention basins engineered for nitrate removal. These substrates are also attractive for other reasons as well. For example, they are both waste products that are readily available in many areas. Furthermore, these are both cellulose-rich substrates, and cellulose is an abundant renewable resource.The results with the sulfur and sulfur/limestone systems indicate that sulfur also holds promise as a electron donor for denitrification in engineered bioretention, in particular, with small sulfur particle sizes. Importantly, as discussed by others (Zhang and Shan, 1999), sulfur is also a relatively inexpensive resource ($0.018/kg; $16/ton).The next stage in this work includes the optimization of the anoxic zone (e.g., sizing, porous medium). This will be performed using the electron donors that gave the best nitrate removal efficiency and effluent quality in the experiments reported here: newspaper, wood chips, and small sulfur particles/limestone. In addition, the performance of the optimized system will be evaluated under conditions of intermittent loadings, such as are expected in the field. This is a unique challenge of bioretention that distinguishes it from many other engineered systems for biological denitrification.ACKNOWLEDGMENTSThis work was supported by a grant from the U.S. Geological Survey to the Maryland Water Resources Research Center.REFERENCESAPHA, AWWA and WPCF. (1995) Standard Methods for the Examination of Water and Wastewater, 19th Edition. Washington, D.C.Baalsrud, K., and Baalsrud, K.S. (1954) “Studies on Thiobacillus denitrificans.” Arch. Mikrobiol., Vol. 20, pp.34-62.Blowes, D.W., Robertson, W.D., Ptacek, C.J., and Merkley, C. (1994) “Removal of Agricultural Nitrate from Tile-Drainage Effluent Water using In-Line Bioreactors.” J. Contam. Hydrol., Vol. 15, No. 3, pp.207-221.Davis, A.P., Shokouhian, M., Sharma, H., Henderson, C., Winogradoff, D., and Coffman, L. (1998) "Bioretention for Treatment of Urban Stormwater Runoff: Laboratory and Field Results." WEFTEC '98, Surface Water Quality and Ecology I & II, Vol. 4, pp.421-434, Orlando FA, October 1998.Hansen, T.A. (1994) “Metabolism of Sulfate-Reducing Prokaryotes.” Antonie van Leeuwenhoek, Vol. 66, No. 1-3, pp.165-185.Koenig, A., and Liu, L.H. (1996) “Autotrophic Denitrification of Landfill Leachate using Elemental Sulphur.” Wat. Sci. Tech., Vol. 34, No. 5-6, pp.469-476.Liu, L.H. (1992) A Study on Nitrate Removal from Groundwater Served as Drinking Water by Autotrophic Denitrification. Ph.D. dissertation (in Chinese), Dept. of Environ. Eng., Tshinghua Univ., Beijing, China.McCarty, P.L. (1975) “Stoichiometry of Biological Reactions.” Prog. Water Technol., Vol. 7, pp.157-172.Robertson, W.D. and Cherry, J.A. (1995) “In Situ Denitrification of Septic-System Nitrate using Reactive Porous Media Barriers: Field Trials.” Ground water, Vol. 33, No. 1, pp.99-111.Rynk, R., M. (editor). (1992) On-Farm Composting Handbook. Northeast Regional Agricultural Engineering Service, Ithaca, NY.Schipper, L. and Vojvodic-Vukovic, M. (1998) “Nitrate Removal from Ground Water Using a Denitrification Wall Amended with Sawdust: Field Trial.” J. Environ. Qual., Vol. 27, pp.664-668.Sikora, L.J., and Keeney, D.R. (1976) “Evaluation of a Sulfur-Thiobacillus Denitrificans Nitrate Removal System.” J. Environ. Qual., Vol. 5, No. 3, pp.298-303.Tiedje, J.M., Sexstone, A.J., Myrold, D.D., and Robinson, J.A. (1982) “Denitrification: Ecological niches, competition, and survival.” Antonie van Leeuwenhoek, Vol. 48, No. 6,pp.569-583.Vogan, J.L. (1993) The use of Emplaced Denitrification Layers to Promote Nitrate Removal from Septic Effluent. M.S. Thesis, University of Waterloo, Ontario, Canada.Volokita, M., Belkin, S., Aberliovich, A. and Soares, M.I.M. (1996) “Biological denitrification of drinking water using newspaper.” Water Res., Vol. 30, No. 4, pp.965-971.Zhang, T.C., and Lampe, D.G. (1999) “Sulfur:Limestone autotrophic denitrification processes for treatment of nitrate-contaminated water: Batch experiments.” Wat. Res., Vol. 33, No. 3, pp.599-608.Zhang, T.C., and Shan, J. (1999) “In situ septic tank effluent denitrification using a sulfur-limestone process.” Water Environ. Res., Vol. 71, No.7, pp.1283-1291.Figure 1. Diagram of Modified Bioretention for Denitrification.RunoffTreated EffluentImpervious Layer020406080100120N i t r a t e R e m o v a l E f f i c i e n c y (%)020406080100120N i t r a t e R e m o v a l E f f i c i e n c y (%)Sulfur Sulfur/Limestone Sulfur/ Limestone020406080100120N i t r a t e R e m o v a l E f f i c i e n c y (%)WEFTEC 2000Figure 2. Average Quasi-Steady-State Nitrate Removal (%): (a) Set #1 Data, (b) Set #2 Data, and (c) Set #3 Data. Error Bars Represent ± One Standard Deviation.。