nature上柔性衬底核壳结构的量子点敏化和PN异质结结构电池的制备
高质量InAlGaN-GaN异质结材料及其器件研究
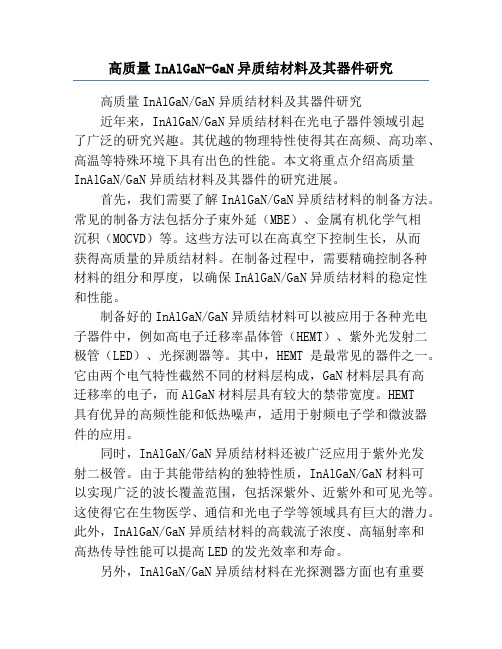
高质量InAlGaN-GaN异质结材料及其器件研究高质量InAlGaN/GaN异质结材料及其器件研究近年来,InAlGaN/GaN异质结材料在光电子器件领域引起了广泛的研究兴趣。
其优越的物理特性使得其在高频、高功率、高温等特殊环境下具有出色的性能。
本文将重点介绍高质量InAlGaN/GaN异质结材料及其器件的研究进展。
首先,我们需要了解InAlGaN/GaN异质结材料的制备方法。
常见的制备方法包括分子束外延(MBE)、金属有机化学气相沉积(MOCVD)等。
这些方法可以在高真空下控制生长,从而获得高质量的异质结材料。
在制备过程中,需要精确控制各种材料的组分和厚度,以确保InAlGaN/GaN异质结材料的稳定性和性能。
制备好的InAlGaN/GaN异质结材料可以被应用于各种光电子器件中,例如高电子迁移率晶体管(HEMT)、紫外光发射二极管(LED)、光探测器等。
其中,HEMT是最常见的器件之一。
它由两个电气特性截然不同的材料层构成,GaN材料层具有高迁移率的电子,而AlGaN材料层具有较大的禁带宽度。
HEMT具有优异的高频性能和低热噪声,适用于射频电子学和微波器件的应用。
同时,InAlGaN/GaN异质结材料还被广泛应用于紫外光发射二极管。
由于其能带结构的独特性质,InAlGaN/GaN材料可以实现广泛的波长覆盖范围,包括深紫外、近紫外和可见光等。
这使得它在生物医学、通信和光电子学等领域具有巨大的潜力。
此外,InAlGaN/GaN异质结材料的高载流子浓度、高辐射率和高热传导性能可以提高LED的发光效率和寿命。
另外,InAlGaN/GaN异质结材料在光探测器方面也有重要应用。
该材料具有宽波长范围的响应能力,可用于红外传感器和太阳能电池等光电子设备。
同时,高迁移率和低暗电流特性使其在低噪声条件下具有高灵敏度和快速响应速度。
然而,尽管InAlGaN/GaN异质结材料在光电子器件中具有广泛应用前景,但其研究仍面临一些挑战。
柔性衬底微晶硅太阳电池量子效率的研究

第39卷第5期 人 工 晶 体 学 报V o.l 39 N o .5 2010年10月J OURNAL O F S YNTHET IC CRY STA LSO c tober ,2010柔性衬底微晶硅太阳电池量子效率的研究刘 成,周丽华,叶晓军,钱子勍,陈鸣波(上海空间电源研究所,上海200233)摘要:通过对微晶硅太阳电池量子效率的测量,结合微区拉曼光谱和电学特性测试,讨论了本征层的硅烷浓度和等离子体辉光功率对太阳电池量子效率的影响。
发现本征层硅烷浓度增加时,电池的长波响应变差,材料结构由微晶相演变成非晶相;等离子体辉光功率的增加造成了电池短波响应的变化。
同时发现测量微晶硅太阳电池时使用掩膜板所得短路电流密度与量子效率积分获得的短路电流密度相差不大。
将优化后的沉积参数应用于不锈钢柔性衬底的非晶硅/微晶硅叠层太阳电池,获得了9.28%(AM 0,1353W /m 2)和11.26%(AM 1.5,1000W /m 2)的光电转换效率。
关键词:太阳电池;量子效率;柔性衬底;微晶硅;非晶硅/微晶硅中图分类号:O484;TK 514文献标识码:A文章编号:1000 985X (2010)05 1161 05Study on Quantu m Effici enci es ofM icrocrystalli ne SiliconSolar Cells on Flexi ble SubstratesLI U Cheng,Z HOU L i hua,Y E X iao j u n,QI AN Z i qing,C HEN M i n g bo(Shanghai Institute of Space Po w er sou rces ,Shanghai200233,Ch i na)(R eceive d 22M arc h 2010,acce p t ed 21Jul y 2010)Abstract :W it h the m easure m ent o f quant u m efficienc ies ,Ra m an spectra and e lectrical characteristics ,theeffects of silane concentrations and p las m a d i s charge po w ers on quantum efficiencies of m i c rocrysta lline silicon solar ce lls had been discussed .It is found that the long w avelength responses o f so l a r cells decrease w hen silane concentrations i n crease ,and the shortw aveleng t h responses o f solar cells changesw hen plas m a discharge powers i n crease .It is also found that the short circu it current density are al m ost the sa m e bet w een m easured by ill u m i n ated J V w ith m asks and by quant u m effic iency .W ith the opti m ized deposition para m eters ,a m or phous silicon /m icr ocrystalli n e silicon tande m so lar ce lls on sta i n less steel flex i b le substratesw ith conversi o n efficiency of 9.28%(AM 0,1353W /m 2)and 11.26%(AM 1.5,1000W /m 2)w ere obta i n ed .K ey w ords :so lar cells ;quantu m efficienc ies ;flex i b le substrates ;m icr ocr ystalli n e silicon ;a morphoussilicon /m icrocr ystalli n e silicon收稿日期:2010 03 22;修订日期:2010 07 21基金项目:上海市博士后科研资助计划项目(08R21420200);上海市引进技术的吸收与创新计划项目(07X I 2 016) 作者简介:刘 成(1980 ),男,湖南省人,博士后。
异质结发展现状及原理
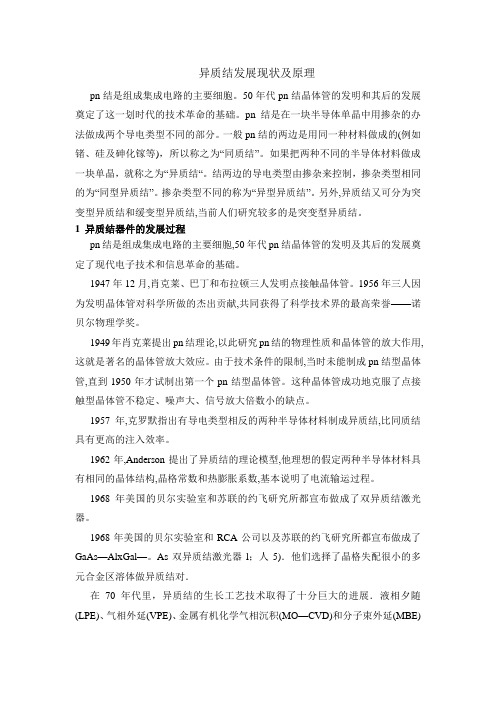
异质结发展现状及原理pn结是组成集成电路的主要细胞。
50年代pn结晶体管的发明和其后的发展奠定了这一划时代的技术革命的基础。
pn结是在一块半导体单晶中用掺杂的办法做成两个导电类型不同的部分。
一般pn结的两边是用同一种材料做成的(例如锗、硅及砷化镓等),所以称之为“同质结”。
如果把两种不同的半导体材料做成一块单晶,就称之为“异质结“。
结两边的导电类型由掺杂来控制,掺杂类型相同的为“同型异质结”。
掺杂类型不同的称为“异型异质结”。
另外,异质结又可分为突变型异质结和缓变型异质结,当前人们研究较多的是突变型异质结。
1 异质结器件的发展过程pn结是组成集成电路的主要细胞,50年代pn结晶体管的发明及其后的发展奠定了现代电子技术和信息革命的基础。
1947年12月,肖克莱、巴丁和布拉顿三人发明点接触晶体管。
1956年三人因为发明晶体管对科学所做的杰出贡献,共同获得了科学技术界的最高荣誉——诺贝尔物理学奖。
1949年肖克莱提出pn结理论,以此研究pn结的物理性质和晶体管的放大作用,这就是著名的晶体管放大效应。
由于技术条件的限制,当时未能制成pn结型晶体管,直到1950年才试制出第一个pn结型晶体管。
这种晶体管成功地克服了点接触型晶体管不稳定、噪声大、信号放大倍数小的缺点。
1957年,克罗默指出有导电类型相反的两种半导体材料制成异质结,比同质结具有更高的注入效率。
1962年,Anderson提出了异质结的理论模型,他理想的假定两种半导体材料具有相同的晶体结构,晶格常数和热膨胀系数,基本说明了电流输运过程。
1968年美国的贝尔实验室和苏联的约飞研究所都宣布做成了双异质结激光器。
1968年美国的贝尔实验室和RCA公司以及苏联的约飞研究所都宣布做成了GaAs—AlxGal—。
As双异质结激光器l;人5).他们选择了晶格失配很小的多元合金区溶体做异质结对.在70年代里,异质结的生长工艺技术取得了十分巨大的进展.液相夕随(LPE)、气相外延(VPE)、金属有机化学气相沉积(MO—CVD)和分子束外延(MBE)等先进的材料生长方法相继出现,因而使异质结的生长日趋完善。
《2024年CuInS2基量子点敏化太阳电池的掺杂特性、核壳结构及其吸附技术研究》范文
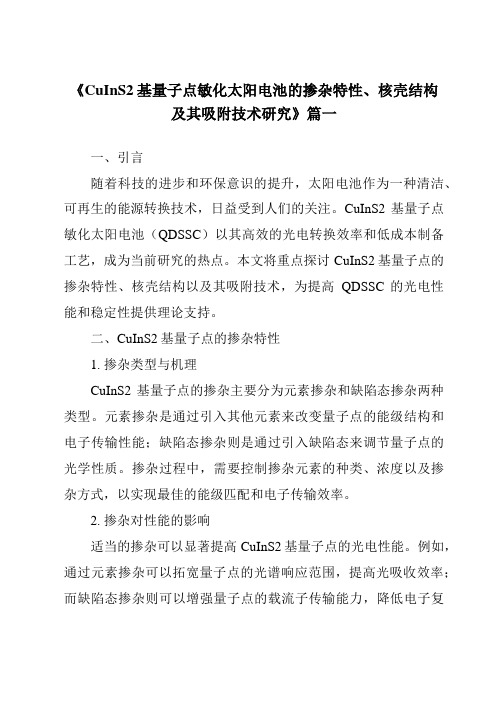
《CuInS2基量子点敏化太阳电池的掺杂特性、核壳结构及其吸附技术研究》篇一一、引言随着科技的进步和环保意识的提升,太阳电池作为一种清洁、可再生的能源转换技术,日益受到人们的关注。
CuInS2基量子点敏化太阳电池(QDSSC)以其高效的光电转换效率和低成本制备工艺,成为当前研究的热点。
本文将重点探讨CuInS2基量子点的掺杂特性、核壳结构以及其吸附技术,为提高QDSSC的光电性能和稳定性提供理论支持。
二、CuInS2基量子点的掺杂特性1. 掺杂类型与机理CuInS2基量子点的掺杂主要分为元素掺杂和缺陷态掺杂两种类型。
元素掺杂是通过引入其他元素来改变量子点的能级结构和电子传输性能;缺陷态掺杂则是通过引入缺陷态来调节量子点的光学性质。
掺杂过程中,需要控制掺杂元素的种类、浓度以及掺杂方式,以实现最佳的能级匹配和电子传输效率。
2. 掺杂对性能的影响适当的掺杂可以显著提高CuInS2基量子点的光电性能。
例如,通过元素掺杂可以拓宽量子点的光谱响应范围,提高光吸收效率;而缺陷态掺杂则可以增强量子点的载流子传输能力,降低电子复合率。
此外,掺杂还可以改善量子点的稳定性,延长QDSSC的使用寿命。
三、核壳结构的构建及其优势1. 核壳结构的设计与制备核壳结构是指在CuInS2基量子点外层包裹一层或多层其他材料,以提高量子点的稳定性和光电性能。
常用的外壳材料包括硫化锌(ZnS)、硫化镉(CdS)等。
制备过程中,需要控制核壳材料的厚度和均匀性,以实现最佳的电子传输和光吸收性能。
2. 核壳结构对性能的提升核壳结构能够有效地保护CuInS2基量子点免受外部环境的影响,提高其稳定性。
同时,核壳结构还能调节量子点的能级结构,优化电子传输路径,提高光电转换效率。
此外,核壳结构还能增强量子点的光谱响应范围和光吸收能力,进一步提高QDSSC的性能。
四、吸附技术研究1. 吸附剂的种类与选择吸附技术是QDSSC中关键的一环,通过在量子点表面吸附适当的物质来提高其光电性能和稳定性。
量子点敏化太阳能电池研究进展_卫会云
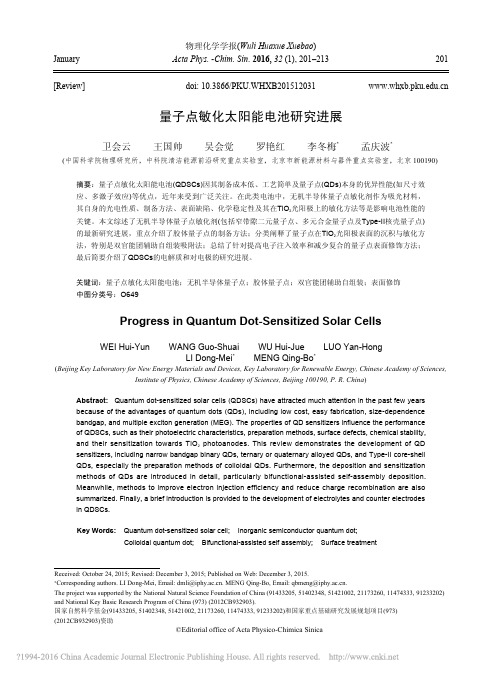
量子点敏化太阳能电池研究进展卫会云 王国帅 吴会觉 罗艳红 李冬梅* 孟庆波*(中国科学院物理研究所,中科院清洁能源前沿研究重点实验室,北京市新能源材料与器件重点实验室,北京 100190)摘要:量子点敏化太阳能电池(QDSCs)因其制备成本低、工艺简单及量子点(QDs)本身的优异性能(如尺寸效应、多激子效应)等优点,近年来受到广泛关注。
在此类电池中,无机半导体量子点敏化剂作为吸光材料,其自身的光电性质、制备方法、表面缺陷、化学稳定性及其在TiO 2光阳极上的敏化方法等是影响电池性能的关键。
本文综述了无机半导体量子点敏化剂(包括窄带隙二元量子点、多元合金量子点及Type-II 核壳量子点)的最新研究进展,重点介绍了胶体量子点的制备方法;分类阐释了量子点在TiO 2光阳极表面的沉积与敏化方法,特别是双官能团辅助自组装吸附法;总结了针对提高电子注入效率和减少复合的量子点表面修饰方法;最后简要介绍了QDSCs 的电解质和对电极的研究进展。
关键词:量子点敏化太阳能电池;无机半导体量子点;胶体量子点;双官能团辅助自组装;表面修饰中图分类号:O649Progress in Quantum Dot-Sensitized Solar CellsWEI Hui-Yun WANG Guo-Shuai WU Hui-Jue LUO Yan-HongLI Dong-Mei * MENG Qing-Bo *(Beijing Key Laboratory for New Energy Materials and Devices, Key Laboratory for Renewable Energy, Chinese Academy of Sciences,Institute of Physics, Chinese Academy of Sciences, Beijing 100190, P. R. China )Abstract: Quantum dot-sensitized solar cells (QDSCs) have attracted much attention in the past few years because of the advantages of quantum dots (QDs), including low cost, easy fabrication, size-dependence bandgap, and multiple exciton generation (MEG). The properties of QD sensitizers influence the performance of QDSCs, such as their photoelectric characteristics, preparation methods, surface defects, chemical stability,and their sensitization towards TiO 2 photoanodes. This review demonstrates the development of QD sensitizers, including narrow bandgap binary QDs, ternary or quaternary alloyed QDs, and Type-II core-shell QDs, especially the preparation methods of colloidal QDs. Furthermore, the deposition and sensitization methods of QDs are introduced in detail, particularly bifunctional-assisted self-assembly deposition.Meanwhile, methods to improve electron injection efficiency and reduce charge recombination are also summarized. Finally, a brief introduction is provided to the development of electrolytes and counter electrodes in QDSCs.Key Words: Quantum dot-sensitized solar cell; Inorganic semiconductor quantum dot;Colloidal quantum dot; Bifunctional-assisted self assembly; Surface treatment物理化学学报(Wuli Huaxue Xuebao )January Acta Phys. -Chim. Sin . 2016, 32 (1), 201–213201[Review]doi: 10.3866/PKU.WHXB201512031Received: October 24, 2015; Revised: December 3, 2015; Published on Web: December 3, 2015.*Corresponding authors. LI Dong-Mei, Email: dmli@. MENG Qing-Bo, Email: qbmeng@.The project was supported by the National Natural Science Foundation of China (91433205, 51402348, 51421002, 21173260, 11474333, 91233202)and National Key Basic Research Program of China (973) (2012CB932903).国家自然科学基金(91433205, 51402348, 51421002, 21173260, 11474333, 91233202)和国家重点基础研究发展规划项目(973)(2012CB932903)资助©Editorial office of Acta Physico-Chimica Sinica1 引言太阳能电池是一种利用光伏效应或光化学效应将太阳能转化为电能的能量转换形式。
量子点敏化太阳能电池
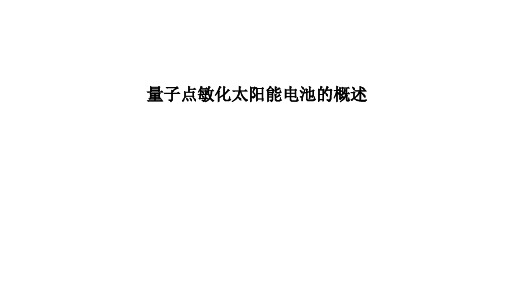
3. 量子点敏化太阳能电池的工作原理
理解后可重点讲一讲这个过程
光照下,量子点吸收光子后被激发,产生电子空穴对并发生分离,电子快速注入到TiO2导带并 经TiO2被外电路收集,量子点的空穴被电解质还 原回到基态,电解质在对电极处接收外电路流入 的电子完成再生,从而完成一个循环。光电转换 主要通过三个界面完成:(1) 量子点与金属氧化物 半导体界面;(2) 量子点和电解质界面和(3)电解 质与对电极界面。
第2代是无机薄膜太阳能电池(以CdS, GaAs或 CuInGaSn等为活性层)。与单晶硅太阳能电池相 比,这种电池的产品制造成本低、生产效率高、 可柔性制备,在市场中也占有一定份额。但它 们的电池效率和稳定性都较差,同时含有很多 稀有金属和有毒元素,因此限制了商业上的广 泛应用
第3代是新型太阳能电池(包括染料敏化、有机及钙 钛矿太阳能电池)。这类电池制备流程简单、成本较 低,且具有一定柔性,此外其光电转化效率高,有 很大的商业应用前景。特别地,量子点敏化太阳能 电池由于能量转换效率赢得了众多科研探索人员的 青睐,单个P-N结太阳能电池的理论能量转换效率 可达44%。
(3)优化作为量子点沉积基底的TiO2薄膜的比表面积,增多量子点的可沉积位点, 提高光阳极整体的敏化性能
5. 量子点敏化太阳能电池的独特优势
在光伏电池领域,量子点最具有吸引力的特点之一就是通 过调控粒径尺寸实现量子点的能带可调节性,进而实现对 光谱吸收范围的可调控性。量子点敏化太阳能电池直接受 益与此现象是通过粒径调控,实现从可见到近红外光区的 光谱吸收。由于太阳光的光谱辐射有一半以上是在红外区, 因此对红外光子的捕获对提高太阳能电池的光电转换效率 具有重要意义
p–GaN
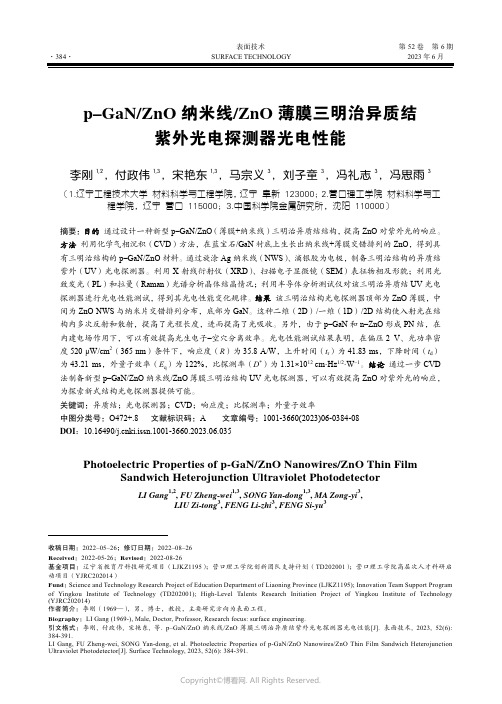
表面技术第52卷第6期p–GaN/ZnO纳米线/ZnO薄膜三明治异质结紫外光电探测器光电性能李刚1,2,付政伟1,3,宋艳东1,3,马宗义3,刘子童3,冯礼志3,冯思雨3(1.辽宁工程技术大学 材料科学与工程学院,辽宁 阜新 123000;2.营口理工学院 材料科学与工程学院,辽宁 营口 115000;3.中国科学院金属研究所,沈阳 110000)摘要:目的通过设计一种新型p–GaN/ZnO(薄膜+纳米线)三明治异质结结构,提高ZnO对紫外光的响应。
方法利用化学气相沉积(CVD)方法,在蓝宝石/GaN衬底上生长出纳米线+薄膜交错排列的ZnO,得到具有三明治结构的p–GaN/ZnO材料。
通过旋涂Ag纳米线(NWS)、滴银胶为电极,制备三明治结构的异质结紫外(UV)光电探测器。
利用X射线衍射仪(XRD)、扫描电子显微镜(SEM)表征物相及形貌;利用光致发光(PL)和拉曼(Raman)光谱分析晶体结晶情况;利用半导体分析测试仪对该三明治异质结UV光电探测器进行光电性能测试,得到其光电性能变化规律。
结果该三明治结构光电探测器顶部为ZnO薄膜,中间为ZnO NWS与纳米片交错排列分布,底部为GaN。
这种二维(2D)/一维(1D)/2D结构使入射光在结构内多次反射和散射,提高了光程长度,进而提高了光吸收。
另外,由于p–GaN和n–ZnO形成PN结,在内建电场作用下,可以有效提高光生电子–空穴分离效率。
光电性能测试结果表明,在偏压2 V、光功率密度520 μW/cm2(365 nm)条件下,响应度(R)为35.8 A/W,上升时间(t r)为41.83 ms,下降时间(t d)为43.21 ms,外量子效率(E q)为122%,比探测率(D*)为1.31×1012 cm·Hz1/2·W−1。
结论通过一步CVD 法制备新型p–GaN/ZnO纳米线/ZnO薄膜三明治结构UV光电探测器,可以有效提高ZnO对紫外光的响应,为探索新式结构光电探测器提供可能。
PLD法硫化物半导体敏化TiO_(2)纳米棒阵列薄膜的研究进展
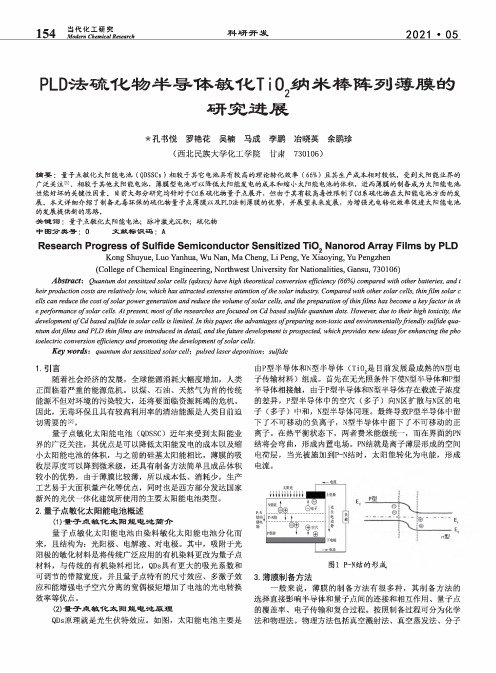
当代化工研究Modem Chemical Research154科研开发2021・05PLD法硫化物半导体敏化T i(J?纳米棒阵列薄膜的研究进展*孔书悦罗艳花吴楠马成李鹏冶晓英余鹏珍(西北民族大学化工学院甘肃730106)摘要:量子点敏化太阳能电池(QDSSCs)相较于其它电池具有较高的理论转化效率(66%)且其生产成本相对较低,受到太阳能业界的广泛关注切。
相较于其他太阳能电池,薄膜型电池可以降低太阳能发电的成本和缩小太阳能电池的体积,进而薄膜的制备成为太阳能电池性能好坏的关键性因素.目前大部分研究均针对于Cd系硫化物量子点展开,但由于其有较高毒性限制了Cd系硫化物在太阳能电池方面的发展。
本文详细介绍了制备无毒环保的硫化物量子点薄膜以及PLD法制薄膜的优势,并展望未来发展,为增强光电转化效率促进太阳能电池的发展提供新的思路。
关键词:量子点敏化太阳能电池;脉冲激光沉积;硫化物中图分类号:0文献标识码:AResearch Progress of Sulfide Semiconductor Sensitized TiO2Nanorod Array Films by PLD Kong Shuyue,Luo Yanhua,Wu Nan,Ma Cheng,Li Peng,Ye Xiaoying,Yu Pengzhen(College of Chemical Engineering,Northwest University for Nationalities,Gansu,730106) Abstract t Quantum dot sensitized solar cells(qdsscs)have high theoretical conversion efficiency(66%)compared with other batteries,and t heir p roduction costs are relatively loyv t which has attracted extensive attention of t he solar pared with other solar cells,thin f ilm solar c ells can reduce the cost ofsolar p ower generation and reduce the volume of s olar cells,and the p reparation of t hin f ilms has become a key f actor in th e performance of s olar cells.At p resent,most of t he researches are f ocused on Cd based sulfide quantum dots.Hoyvever,due to their high toxicity,the development of C d based sulfide in solar cells is limited.In this p aper,the advantages of p reparing non-toxic and environmentally f riendly sulfide quantum dot f ilms and PLD thin f ilms are introduced in detail,and the f uture development is prospected,which provides new ideas f or enhancing the p ho toelectric conversion efficiency and p romoting the development of s olar cells.Key words z quantum dot sensitized solar cell;pulsed laser deposition^sulfide1.引言随着社会经济的发展,全球能源消耗大幅度增加,人类正面临着严重的能源危机。
一种PN型核电池及其制备方法[发明专利]
![一种PN型核电池及其制备方法[发明专利]](https://img.taocdn.com/s3/m/93aa2c726bec0975f565e2d5.png)
专利名称:一种PN型核电池及其制备方法专利类型:发明专利
发明人:陆敏
申请号:CN200910030431.1
申请日:20090410
公开号:CN101527176A
公开日:
20090909
专利内容由知识产权出版社提供
摘要:本发明公开了一种PN型核电池及其制备方法,通过两次MOCVD外延和一次HVPE外延的复合外延技术生长获得衬底-n型GaN掺杂层-p型GaN掺杂层结构的PN器件材料结构,再使用半导体微加工工艺溅射生成对应的接触电极,制备形成基本的电池器件,并将同位素耦合到p型接触电极上,封装制备完成核电池。
由于核电池的寿命取决于同位素的半衰期,故而本发明可以灵活使用防护简单的同位素种类(纯β同位素),大大提高了核电池的能量转换效率和能量密度(能量容积),延长了核电池的使用寿命,同时也为核废料变废为宝、合理利用创造了有效途径。
申请人:苏州纳米技术与纳米仿生研究所
地址:215125 江苏省苏州市工业园区独墅湖高教区若水路398号
国籍:CN
代理机构:南京苏科专利代理有限责任公司
代理人:陈忠辉
更多信息请下载全文后查看。
一种高效柔性染料敏化太阳能电池及其制备方法[发明专利]
![一种高效柔性染料敏化太阳能电池及其制备方法[发明专利]](https://img.taocdn.com/s3/m/dbabe102551810a6f424868b.png)
专利名称:一种高效柔性染料敏化太阳能电池及其制备方法专利类型:发明专利
发明人:黄素梅,张丁文,李晓冬,沈洁,尹希江
申请号:CN201210224477.9
申请日:20120702
公开号:CN103531362A
公开日:
20140122
专利内容由知识产权出版社提供
摘要:本发明公开了一种高效柔性染料敏化太阳能电池,包括光阳极、对电极、以及充满在两极间的电解质;其特征在于,所述光阳极包括导电基板、沉积在导电基板上的多孔金属氧化物薄膜、以及吸附在所述多孔金属氧化物薄膜上的染料;所述对电极包括导电基板、以及沉积在所述导电基板上的催化剂薄膜;其中,所述多孔金属氧化物薄膜中的金属氧化物颗粒之间通过搭桥形成颈部结构。
本发明还公开了高效柔性染料敏化太阳能电池的制备方法。
本发明增强了电池的光电流,提高电池效率,制备过程生产耗能减少,生产效率提高。
申请人:华东师范大学,新加坡理工学院
地址:200062 上海市普陀区中山北路3663号
国籍:CN
代理机构:上海麦其知识产权代理事务所(普通合伙)
代理人:董红曼
更多信息请下载全文后查看。
一种柔性钙钛矿太阳能电池及其制备方法[发明专利]
![一种柔性钙钛矿太阳能电池及其制备方法[发明专利]](https://img.taocdn.com/s3/m/beaaba6c842458fb770bf78a6529647d272834f1.png)
专利名称:一种柔性钙钛矿太阳能电池及其制备方法专利类型:发明专利
发明人:谭海仁,李禄东,王玉瑞
申请号:CN202111368243.7
申请日:20211118
公开号:CN114300618A
公开日:
20220408
专利内容由知识产权出版社提供
摘要:本发明公开了一种柔性钙钛矿太阳能电池及其制备方法,属于太阳能电池技术领域。
所述柔性钙钛矿太阳能电池为单结电池或叠层电池,在负载有底电极的柔性衬底上沉积有载流子传输层1、有机半导体分子桥接层和钙钛矿吸光层1,通过在氧化物载流子传输层1与钙钛矿吸光层1之间插入一层具有与氧化物相同导电类型的有机半导体分子桥接层,利用有机半导体分子桥接层的应力缓冲作用,即吸收并均化弯曲过程中氧化物传输层和钙钛矿吸光层1之间的弯曲应力,减少应力集中释放带来的器件破坏,从而显著提高柔性钙钛矿单结和叠层太阳能电池的弯曲耐折度。
申请人:南京大学
地址:210023 江苏省南京市栖霞区仙林大道163号
国籍:CN
代理机构:南京经纬专利商标代理有限公司
代理人:黄欣
更多信息请下载全文后查看。
一种超柔透明太阳能电池的制备方法[发明专利]
![一种超柔透明太阳能电池的制备方法[发明专利]](https://img.taocdn.com/s3/m/3b93f0074a35eefdc8d376eeaeaad1f346931135.png)
专利名称:一种超柔透明太阳能电池的制备方法专利类型:发明专利
发明人:柳佃义,姜倩晴,蒙蕤谦
申请号:CN202111232120.0
申请日:20211022
公开号:CN114171686A
公开日:
20220311
专利内容由知识产权出版社提供
摘要:本发明涉及太阳能电池技术领域,具体为一种超柔透明太阳能电池的制备方法,通过在硬质衬底表面制备可剥离高透明度超柔衬底,并在这类超柔衬底上用能有效利用紫外光或红外光的光电转换材料构建异质结,通过调节异质结材料的比例和膜层厚度,制备在可见光波段具有高透过率的透明单结太阳能电池或太阳能电池组件,最后分离超柔衬底和硬质衬底,实现超柔透明太阳能电池及其组件的制备。
申请人:西湖大学
地址:310024 浙江省杭州市石龙山街18号
国籍:CN
代理机构:北京轻创知识产权代理有限公司
代理人:朱广
更多信息请下载全文后查看。
TypeⅡ核壳异质结量子点敏化太阳能电池的开题报告

TypeⅡ核壳异质结量子点敏化太阳能电池的开题报告开题报告:TypeⅡ核壳异质结量子点敏化太阳能电池1. 研究背景面对能源短缺和全球气候变化,太阳能电池作为环保、可再生、可持续的清洁能源备受关注。
其中,量子点敏化太阳能电池成为了研究热点,其利用化学方法将纳米量子点吸附在半导体电极上,可以增强吸收光谱的范围,提高电池的光电转换效率。
而核壳异质结量子点是一种新型的量子点,具有优异的光电转换性能。
因此,采用核壳异质结量子点来敏化太阳能电池,可以进一步提高其性能。
2. 研究目的本论文旨在研究基于核壳异质结量子点的太阳能电池,探究其制备、性能表征以及电池效率的提高机制。
3. 研究内容及方法3.1 制备核壳异质结量子点采用微波辅助合成方法,通过控制反应温度、反应时间、配体种类和比例等因素,制备出适合敏化太阳能电池的核壳异质结量子点。
3.2 制备太阳能电池将制备好的核壳异质结量子点吸附在半导体电极上,构建太阳能电池,通过光电性能测试、X射线衍射、透射电子显微镜等手段对其性能进行表征。
3.3 提高电池效率的机理探究通过实验分析,研究核壳异质结量子点在光电转换中的作用机制,探究其对提高电池效率的贡献。
4. 预期结果通过制备核壳异质结量子点和构建太阳能电池,实现对该电池的性能表征。
同时,探究核壳异质结量子点在光电转换中的作用机制,为提高电池效率提供理论支撑。
最终,期望得到一种性能更优异的核壳异质结量子点敏化太阳能电池。
5. 研究意义研究核壳异质结量子点敏化太阳能电池,可以发掘新型量子点的光电性能,并将其应用于太阳能电池中,从而提高其性能和效率。
这将为可持续能源的发展提供新思路和技术支持,为应对全球能源和环境问题做出贡献。
InZnO 薄膜透明导电的制备及其柔性的表征
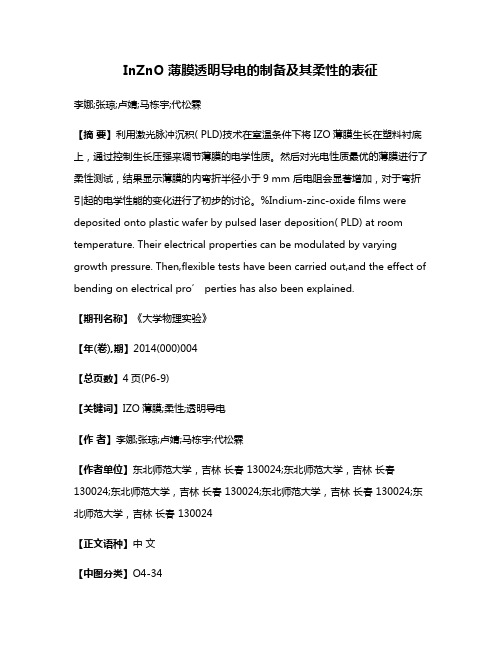
InZnO 薄膜透明导电的制备及其柔性的表征李娜;张琼;卢婧;马栋宇;代松霖【摘要】利用激光脉冲沉积( PLD)技术在室温条件下将IZO薄膜生长在塑料衬底上,通过控制生长压强来调节薄膜的电学性质。
然后对光电性质最优的薄膜进行了柔性测试,结果显示薄膜的内弯折半径小于9 mm 后电阻会显著增加,对于弯折引起的电学性能的变化进行了初步的讨论。
%Indium-zinc-oxide films were deposited onto plastic wafer by pulsed laser deposition( PLD) at room temperature. Their electrical properties can be modulated by varying growth pressure. Then,flexible tests have been carried out,and the effect of bending on electrical pro’ perties has also been explained.【期刊名称】《大学物理实验》【年(卷),期】2014(000)004【总页数】4页(P6-9)【关键词】IZO薄膜;柔性;透明导电【作者】李娜;张琼;卢婧;马栋宇;代松霖【作者单位】东北师范大学,吉林长春 130024;东北师范大学,吉林长春130024;东北师范大学,吉林长春 130024;东北师范大学,吉林长春 130024;东北师范大学,吉林长春 130024【正文语种】中文【中图分类】O4-34透明导电氧化物(TCO)薄膜兼具高导电性和高透明性而被广泛地应用到各种光电器件中,其中就包括太阳能电池[1-2],薄膜晶体管[3],平板显示器[4],和紫外发光二极管[5]。
目前对TCO 薄膜的研究越来越受到人们的关注,例如,多晶的In2O3∶Sn,ZnO∶Al,ZnO∶Ga 和非晶的InZnO(α-IZO),InGaZnO.虽然铟-基的氧化物是一种价格相对较高的材料,但是它们非晶态的薄膜具有独特的优势,如高的迁移率,好的柔性,大面积内的高均匀性,和低的制备温度。
PEN为衬底的柔性有机电致发光器件的光电性能
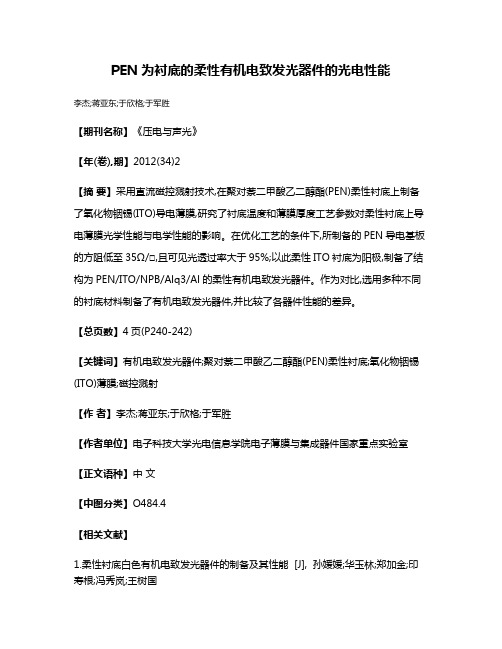
PEN为衬底的柔性有机电致发光器件的光电性能
李杰;蒋亚东;于欣格;于军胜
【期刊名称】《压电与声光》
【年(卷),期】2012(34)2
【摘要】采用直流磁控溅射技术,在聚对萘二甲酸乙二醇酯(PEN)柔性衬底上制备了氧化物铟锡(ITO)导电薄膜,研究了衬底温度和薄膜厚度工艺参数对柔性衬底上导电薄膜光学性能与电学性能的影响。
在优化工艺的条件下,所制备的PEN导电基板的方阻低至35Ω/□,且可见光透过率大于95%;以此柔性ITO衬底为阳极,制备了结构为PEN/ITO/NPB/Alq3/Al的柔性有机电致发光器件。
作为对比,选用多种不同的衬底材料制备了有机电致发光器件,并比较了各器件性能的差异。
【总页数】4页(P240-242)
【关键词】有机电致发光器件;聚对萘二甲酸乙二醇酯(PEN)柔性衬底;氧化物铟锡(ITO)薄膜;磁控溅射
【作者】李杰;蒋亚东;于欣格;于军胜
【作者单位】电子科技大学光电信息学院电子薄膜与集成器件国家重点实验室【正文语种】中文
【中图分类】O484.4
【相关文献】
1.柔性衬底白色有机电致发光器件的制备及其性能 [J], 孙媛媛;华玉林;郑加金;印寿根;冯秀岚;王树国
2.应用于柔性有机电致发光器件的导电衬底材料研究进展 [J], 黎晨;杨继萍;张都耀;李烨
3.柔性有机电致发光器件衬底阻透性能的测试方法 [J], 张树旺;王华林;苗壮;丁万昱;朱继国;柴卫平
4.柔性衬底黄光有机小分子电致发光器件 [J], 李传南;黄劲松;肖步文;侯晶莹;杨开霞;刘式墉
5.柔性有机电致发光器件制备及光电性能研究 [J], 孙媛媛;华玉林;徐峰;印寿根;郑加金;王树国;冯秀岚
因版权原因,仅展示原文概要,查看原文内容请购买。
- 1、下载文档前请自行甄别文档内容的完整性,平台不提供额外的编辑、内容补充、找答案等附加服务。
- 2、"仅部分预览"的文档,不可在线预览部分如存在完整性等问题,可反馈申请退款(可完整预览的文档不适用该条件!)。
- 3、如文档侵犯您的权益,请联系客服反馈,我们会尽快为您处理(人工客服工作时间:9:00-18:30)。
CdT e Cu/AuSuperstrateCdS nanopillarElectron HoleFigure 1|CdS/CdTe SNOP cells.a ,Energy band diagram of a CdT e/CdS photovoltaic.b ,Cross-sectional schematic0.31 nm(112)(111)–abc2 µm500 nmFigure 2|SNOP cell at different stages of fabrication.a ,b ,SEM images of an as-made AAM with perfectly ordered pores (a )and a CdS nanopillar array after partial etching of the AAM (b ).c ,Transmission electron micrograph of the interface between a single-crystalline CdS nanopillar and a polycrystalline CdT e thin film.Inset:The corresponding diffraction pattern for which the periodically symmetric spots and multi-rings can be found.The symmetric spots are originated from the single-crystalline CdS nanopillar and the multi-rings are originated from the polycrystalline CdT e thin film.generation of carriers is significant in the entire device thickness and the 3D structure facilitates the efficient EHPs separation and collection.In addition,3D structures have been shown to enhance the optical absorption efficiency of the material 13,17,18.Specifically,photoelectrochemical studies of Cd(Se,Te)nanopillar arrays have shown that the nanopillar-array photoelectrodes exhibit enhanced collection of low-energy photons absorbed far below the surface,as compared with planar photoelectrodes 17.These results demonstrate the potential advantage of non-planar cell structures,especially for material systems where the bulk recombination rate of carriers is larger than the surface recombination rate.However,so far the conversion efficiency of the fabricated photovoltaics based on coaxial nanopillar arrays,grown by VLS,have been far from the simulation limits 16,with the highest reported efficiency of ∼0.5%(ref.11)arising from un-optimized nanopillar dimensions,poor nanopillar density and alignment,and/or low pn junction interface quality 12,13,although single-nanowire devices have demonstrated better efficiencies 5,14.Furthermore,controlled and cost-effective process schemes for the fabrication of large-scale solar modules that use highly dense and ordered arrays of single-crystalline nanopillar arrays have not been demonstrated.Here,some of the challenges summarized above are addressed through novel device structure engineering and fabrication process development.The fabrication process of our proposed 3D solar nanopillar (SNOP)cell uses highly periodic anodic alumina membranes (AAMs)as the template for the direct synthesis of single-crystalline nanostructures.This approach has been widely used for fabrica-tion of dense arrays of metallic,semiconductor and organic 1D nanostructures,owing to the ease of membrane fabrication and nanostructure geometric control 19–22.Highly regular AAMs with a thickness of ∼2µm and a pore diameter of ∼200nm were first formed on aluminium foil substrates (Fig.1c)by using previously reported processes (see Supplementary Fig.S1)23,24.Figure 2a shows a scanning electron microscopy (SEM)image of an AAM with long-range and near-perfect ordering after the anodization.A barrier-thinning process was applied to branch out the pore chan-nels and reduce the alumina barrier layer thickness at the bottom of the pores to a few nanometres 19,21.A ∼300-nm-thick Au layer was then electrochemically deposited at the bottom of the pore channels with an alternating current method (see the Methods section).The AAM with the electrodeposited Au catalytic layer was then placed in a thermal furnace to carry out the synthesis of the CdS nanopillar array by the VLS process (see the Methods section).To form the 3D nanopillar structures,the AAM was partially and controllably etched in 1N NaOH at room temperature.Notably,this etch solu-tion is highly selective and does not chemically react with the CdS nanopillars.Figure 2b shows a 3D nanopillar array with exposeddepth,H ∼500nm.The exposed depth was varied by tuning the etching time (see Supplementary Fig.S2)to enable a systematic study of the effect of the geometric configuration on the conversion efficiency.A p -type CdTe thin film with ∼1µm thickness (see Supplementary Fig.S3)was then deposited by chemical vapour de-position (see the Methods section)to serve as the photoabsorption layer owing to its near-ideal bandgap (E g =1.5eV)for solar energy absorption 15.Finally,the top electrical contact was fabricated by the thermal evaporation of Cu/Au (1nm/13nm),which enables low barrier contacts to the p -CdTe layer owing to the high workfunction of Au.It is worth noting that although the deposited Cu/Au bilayer was thin,the optical transmission spectrum (see Supplementary Fig.S4)shows that it has only ∼50%transparency,which results in a major cell performance loss because light is shone from the top during the measurements.Further top-contact optimization is required in the future,for instance,by exploring transparent conductive oxide contacts.The back electrical contact to the n -type CdS nanopillars was simply the aluminium support substrate,which greatly reduces the complexity of the fabrication.The entire device was then bonded from the top to a transparent glass support substrate with epoxy to encapsulate the structures.One of the primary merits of our fabrication strategy is the ability to produce high-density,single-crystalline nanopillar arrays on an amorphous substrate with fine geometric control,without relying on epitaxial growth from single-crystalline substrates.The single-crystalline nature of the grown CdS nanopillars is confirmed by transmission electron microscopy analysis with a near 1:1stoichiometric composition observed by energy-dispersive X-ray spectroscopy (see Supplementary Fig.S5).Notably,abrupt atomic interfaces with the polycrystalline CdTe layer are observed (Fig.2c).In addition,3D nanopillar or nanowire arrays,similar to the ones used in this work,have been demonstrated in the past to exhibit unique optical absorption properties 13,18.Similarly,we have observed reduced reflectivity from CdS nanopillar arrays especially when the inter-pillar distance is small (see Supplementary Fig.S6).This observation suggests that 3D nanopillar-based cell modules can potentially improve the light absorption while enhancing the carrier collection.An optical image of a fully fabricated SNOP cell is shown in Fig.3a with an active surface area of 5×8mm.The performance was characterized by using a solar simulator (LS1000,Solar Light)without a heat sink.Figure 3b demonstrates the I –V characteristics of a typical cell under different illumination intensities,P ,ranging from 17to 100mW cm −2(AM 1.5G).Specifically,an efficiency (η)of ∼6%is obtained with an open circuit voltage V oc ∼0.62V,short circuit current density J sc ∼21mA cm −2and fill factor FF ∼0.43under AM 1.5G illumination.The I –V curves cross over each otherJ (m A c m ¬2)Voltage (V)Intensity (mW cm ¬2)J s c (m A c m ¬2)Fill factorV oc (V)Intensity (mW cm ¬2)E f f i c i e n c y (%)GlassCu wireCdS/CdTe solar cellacbdFigure 3|Performance characterization of a representative SNOP cell.a ,An optical image of a fully fabricated SNOP cell bonded on a glass substrate.b ,I –V characteristics at different illumination intensities.c ,The short-circuit current density,J sc ,shows a near-linear dependence on the illuminationintensity,whereas the fill factor,FF ,slightly decreases with an increase of the intensity.d ,The open-circuit voltage,V oc ,slightly increases with the intensity and the solar energy conversion efficiency is nearly independent of the illumination intensity for P =17∼100mW cm −2.above V oc ,which can be attributed to the photoconductivity of CdS (ref.25).The dependency of the performance characteristics on the illumination intensity is shown in Fig.3c,d.As expected,J sc exhibits a near-linear dependency on the intensity because in this regime the photocurrent is proportional to the photon flux with a constant minority carrier lifetime.On the other hand,V oc increases only slightly from 0.55to 0.62V with a linear increase of J sc ,which we attribute to a slight thermal heating of the device (see Supplementary Fig.S7)because a cooling chuck was not used during the measurements 26.As the efficiency of a solar cell is expressed as η=V oc ×J sc ×FF /P and FF slightly decreases with light intensity,the extracted η∼6%shows minimal dependence on the illumination intensity as shown in Fig.3d.It should be noted that this modest efficiency is obtained without the use of an antireflective surface coating or concentrators.Although the conversion efficiency of our first-generation SNOP cells reported here is already higher than most of the previously reported photovoltaics based on nanostructured materials 11–13,further improvements are needed to meet the high-performance application requirements.Notably,the reported efficiency is higher than that of the planar CdS/CdTe cell with comparable CdTe film thickness 27,but lower than those with optimal CdTe film thicknesses.As confirmed by simulation (see Supplementary Figs S8and S9),we speculate that the efficiency can be readily enhanced in the future through further device and materials optimization,for instance,by using top contacts with higher optical transparency and lower parasitic resistances.Specifically,our top contacts result in a ∼50%efficiency loss owing to their lowtransparency level (see Supplementary Fig.S4),which can be readily improved in the future.To further examine the effect of the geometric configuration of the nanopillars on the overall conversion efficiency,devices with different embedded CdS nanopillar lengths,H ,(controlled by the etching time of the AAM,Supplementary Fig.S2)were fabricated and carefully characterized while maintaining the same overall CdTe thickness.As evident from Fig.4a,the conversion efficiency drastically and monotonically increases with H .Specifically,η=0.4%is obtained for H =0nm.In such a case,only the top surface of the CdS nanopillars is in contact with the CdTe film.As a result,only a small space charge region is obtained with low carrier collection efficiency.Most of the photogenerated carriers are lost by recombination in the CdTe film,especially through non-radiative recombination at the defect-rich grain boundaries.By increasing H ,the space charge region area is effectively increased with much improved carrier collection efficiency.In particular,the device conversion efficiency is increased by more than one order of magnitude when H is increased from 0to ∼640nm.To interpret the observed trend of the efficiency dependency on the geometric configuration,2D theoretical simulations were carried out by using a Sentaurus simulator (Fig.4b–d).The details of the simulation can be found in Supplementary Information.The simulated efficiency as a function of H ,shown in Fig.4b,is in qualitative agreement with the experimentally observed trend.Meanwhile,the recombination rate for H =0and 900nm is visualized and plotted in Fig.4c and d,respectively.It is clearly evident that the space charge and carrier collection region isE f f i c i e n c y (%)Nanopillar embeded height (nm)00.51.0 1.52.0 2.5X (µm)NW exposed length (nm)E f f i c i e n c y (%)12345600.51.0 1.52.02.532.52.01.51.00.5X (µm)0.51.0 1.52.02.5X (µm)Y (µm )2.01.51.00.5Y (µm )2.0E+216.0E+201.8E+205.5E+191.7E+195.0E+182.0E+216.0E+201.8E+205.5E+191.7E+195.0E+18SRHrecombination (cm ¬3 s ¬1)SRHrecombination (cm ¬3 s ¬1)abc dFigure 4|Effects of the nanopillar geometric configuration on the device performance.a ,Experimentally obtained efficiency of SNOP cells as a function of the embedded nanopillar height,H .NW:nanowire.The CdT e film thickness is maintained constant at ∼1µm.b ,Theoretical simulation of the SNOP cell efficiency as a function of H ,in qualitative agreement with the observed experimental trend shown in a .TF:thin film.Inset:Schematic diagram of the SNOP cell used for the simulation.c ,d ,Visualization of the Shockley–Read–Hall (SRH)recombination in SNOP cells with H =0(c )and 900nm (d ).The space charge and carrier collection region is quite small when H =0nm,resulting in a major carrier loss in the upper portion of the CdT e film throughrecombination,where there is a high EHP optical generation rate.However,the space charge and carrier collection region is significantly enlarged when H =900nm;thus,the total volumetric carrier recombination loss is greatly reduced.drastically enhanced for H =900nm,which reduces the total volumetric recombination of photogenerated carriers.In addition,further simulation confirms that the 3D configuration of SNOP cells enhances the performance as compared with conventional planar CdS/CdTe solar cells,especially for devices with short minority carrier diffusion lengths (see Supplementary Fig.S9).In these simulations,enhanced optical absorption (that is,reduced reflectance)due to the 3D geometric configuration of the nanopillars is ignored.However,the SNOP structure may be disadvantageous as compared with conventional planar-structured photovoltaics when interface recombination is the limiting factor for cell performance (for instance,when the bulk minority carrier lifetimes are long).Further exploration of minority carrier lifetimes in these structures is needed in the future.Mechanically flexible solar cells are of particular interest for a number of practical applications 3,28,29.In this regard,we fabricated bendable SNOP cells embedded in polydimethylsiloxane (PDMS;Fig.5a).Simply,a layer of PDMS (∼2mm thick)is cured on the top surface following the top-contact metallization process.The aluminium back substrate is then removed by a wet chemical etch,and a ∼200-nm-thick indium layer is deposited as the bottom contact to the n -CdS nanopillars.Finally,another layer of PDMS (∼2mm thick)is cured on the back side to finish the encapsulation process.Figure 5b shows an optical image of a fully fabricated,mechanically flexible cell.In such a device configuration,the nanopillars are placed in the neutral mechanical plane of the PDMS substrate,which minimizes the strain on the active elements.To examine the effect of strain,finite-element simulation (Comsol Multiphysics 3.3)was carried out (Fig.5c,d).From the simulation,when the substrate is mechanically bent with a curvature radius of 3cm,the 4-mm-thick PDMS substrate shows a maximum tensile and compressive strain of ∼8%at the top and bottom surfaces,respectively.However,because the active devices are only a few micrometres thick (nanopillar length ∼2µm)and are placed close to the centre of the PDMS substrate,the maximum observed strain in the nanopillars is only ∼0.01%,which suggests that the flexible photovoltaic devices can sustain large bending without structural degradation.The I –V characteristics and conversion efficiencies of a SNOP module under different bending conditions are shown in Fig.5e,f.It is clear that the bending of the devicesVoltage (V)J (m A c m ¬2)CdS nanopillarCdT eAAM In contactPDMSCu/Au contact(μm)εx εxm m164128(mm)-2-4-6-8-1002401234¬4¬3¬2¬101234 0.080.060.040.020¬0.02¬0.04¬0.06¬0.08¬2.0¬1.5¬1.0¬0.500.51.01.52.0Bending radius (cm)E f f i c i e n c y (%)34567(mm)(μm )acebdfαRDL(× 10¬4)Figure 5|Mechanically flexible SNOP cells.a ,b Schematic diagram (a )and optical image (b )of a bendable SNOP module embedded in PDMS.c ,d ,Theoretical simulation of the strain for a flexible SNOP cell (PDMS thickness ∼4mm),showing only ∼0.01%maximum strain in the nanopillars.e ,I –V characteristics of a flexible cell for various bending radii.f ,Performance characterization of a flexible SNOP cell,showing minimal change in V oc and ηon bending of the substrate.The inset shows a picture of the set-up for bending the flexible modules.affects the cell performance only marginally,and repetitive bending does not degrade the cell performance,as demonstrated in the Supplementary Video.Although the potency and the capabilities of the SNOP-cell module are demonstrated,further work is needed for performance optimization and low-cost process development.Specifically,although the 3D configuration of the proposed single-crystalline cells may potentially enable more efficient light absorption and carrier collection,further optimization of the contacts,in terms of both optical and electrical transparency,is needed to enable higher performances.The ability to directly grow single-crystalline structures on large aluminium sheets,as demonstrated in this work,is highly attractive for potentially lowering the materials processing costs.In addition,the 3D configuration of the crystalline nanopillars can relax the materials requirements in terms of quality and purity,which can further lower the costs.Such materials cost reductions,however,are partially offset by thedevice fabrication costs,including the anodization steps and the top-contact formation.In the case of the last of these,exploration of various low-cost,conductive film deposition processes,such as ink jet printing may be a necessity in the future to further enhance the versatility of the proposed solar modules.MethodsAAM fabrication.Aluminium (Al)foil with a thickness of 0.25mm (99.99%Alfa Aesar)was cut into 1.2cm by 2.2cm pieces and cleaned in acetone and isopropyl alcohol.The substrates were electrochemically polished in a 1:3(v:v)mixture of perchloric acid and ethanol for 5min at 5◦C.As shown in Supplementary Fig.S1a,b,the cleaned Al substrates were imprinted twice with a straight-line diffraction grating (1,200lines mm −1,LightSmyth Technologies)with a pressure of ∼2.2×104N cm −2and 60◦rotation between the two imprints.The substrates were anodized in diluted H 3PO 4solution (1:600v /v in water)under a 195V d.c.bias for 1h at 1◦C.Supplementary Fig.S1c shows the SEM image of the substrate after the first anodization step.The first layer of AAM was etched away in a mixture of phosphoric acid (6wt%)and chromic acid (1.5wt%)at 63◦C in 1h.After etching,the second anodization step was carried out under the same condition for 64minto obtain∼2-µm-thick AAM,with a pore pitch of∼490nm and long-range perfect hexagonal ordering,as shown in Supplementary Fig.S1d.To carry out the subsequent Au electrodeposition,the barrier layer of the AAMs was thinned with a current ramping technique.Specifically,the AAMs were first etched in5wt%H3PO4at53◦Cfor4min to widen the pores to∼200nm. Then the substrates were anodized in0.2M H3PO4at1◦C with a starting voltage of∼160V and a current of∼1mA per substrate.The electrical current was then decreased by half every45min until the voltage reached36V.Then H3PO4was replaced by0.3M oxalic acid and the fourth anodization step was carried out with a starting voltage of∼38V and a current of∼1mA per substrate.Then the electrical current was decreased by half every10min until the voltage reached4.4V.After barrier thinning,the AAMs were briefly etched in5wt%H3PO4at53◦C for1min to further thin down the barrier layer.Then Au was electrochemically deposited into the pores with an alternating current method by using a Au electrodeposition solution(Technic gold25ES)and a potentiostat(SG300,Gamry Instruments).During the deposition,a60Hz sinusoidal voltage was applied for 10min,and the amplitude was adjusted from3.7to6V to maintain a peak current density of∼10mA cm−2at the negative deposition cycle.CdS nanopillar and CdTe thin-film growths.The nanopillar and thin-film growths were carried out in a1inch quartz tube furnace with two resistive heating zones.For the template-assisted,VLS growth of CdS nanopillars,CdS powder (∼1g,99.999%,Alfa Aesar)was used as the source and placed in the first heating zone.The AAM substrate(that is,the growth template)with the electroplated Au seeds was placed in the second heating zone.H2(50s.c.c.m.)was used as the transport gas with a chamber pressure of15torr.The source and sample heating zones were then heated to700and550◦C,respectively.After30min of growth, the furnace was turned off and cooled down naturally.The surface of the AAM with grown CdS nanopillars was cleaned by ion milling(1kV Ar+and∼80◦incident angle)for∼45min.The ion-mill-polished sample was then etched in1N NaOH at room temperature for50–60min to result in an exposed nanopillar length,H=400–600nm.CdTe thin film was deposited on the CdS nanopillar array in the same furnace. Before the deposition,CdS nanopillars were subjected to a5s HF(0.5wt%in deionized water)dip to remove the native oxide on the surface.CdTe powder (0.5g,99.999%,Alfa Aesar)was used as the source in the upper flow zone while the AAM sample was placed in the second zone.The base pressure was stabilized at19mtorr.Both the sample and the source zones were heated atthe same time to400◦C and650◦C,respectively.The growth lasted for50min followed by a cool down.Top-contact fabrication.The as-deposited CdTe film was ion milled(1kV Ar+ and80◦incident angle)for10min to obtain a flat surface for the ease of top-contact fabrication.It was then soaked in a CdCl2solution in methanol(12g l−1)at60◦C for20min,followed by a thermal annealing for5min at370◦C(ref.30).The annealing was carried out at760torr with200s.c.c.m.dry air co-flowing with 200s.c.c.m.N2.Next,the substrate was loaded into a thermal evaporator for the deposition of a1/13nm Cu/Au bilayer as the top contact electrode.Bonding of the modules on glass or PDMS.A thin copper wire was bondedto the top contact of the solar-cell device with silver paste.Then the substrate was attached to a glass slide with epoxy glue(Double Bubble,Hardman).For the mechanically flexible modules,instead of glass,PDMS was used for the encapsulation.To encapsulate the modules with PDMS,silicone elastomer(Sylgard 184,Dow Corning)was mixed with the curing agent(10:1weight ratio)at room temperature,then poured onto the module in a plastic dish to form a∼2mm layer, and cured at60◦C for6h.The Al substrate was then etched from the back side in a saturated HgCl2solution with high selectivity over the AAM,CdS nanopillar array, top contact and PDMS.The back side of the substrate was subjected to a brief ion-mill treatment(1kV neutralized Ar+,80◦incident angle with a water cooling chuck)for5∼10min.A∼200nm indium layer was then thermally evaporated on the back side of the substrate to electrically contact the CdS nanopillars.Finally,∼2-mm-thick PDMS was cured on the back side of the substrate to finish the encapsulation process.Received23February2009;accepted22May2009; published online5July2009References1.Bai,Y.et al.High-performance dye-sensitized solar cells based on solvent-freeelectrolytes produced from eutectic melts.Nature Mater.7,626–630(2008). 2.Kim,J.Y.et al.Efficient tandem polymer solar cells fabricated by all-solutionprocessing.Science317,222–225(2007).3.Yoon,J.et al.Ultrathin silicon solar microcells for semitransparent,mechanically flexible and microconcentrator module designs.Nature Mater.7, 907–915(2008).w,M.,Greene,L.E.,Johnson,J.C.,Saykally,R.&Yang,P.D.Nanowiredye-sensitized solar cells.Nature Mater.4,455–459(2005).5.Tian,B.Z.et al.Coaxial silicon nanowires as solar cells and nanoelectronicpower sources.Nature449,885–889(2007).6.Kempa,T.J.et al.Single and tandem axial p–i–n nanowire photovoltaic devices.Nano Lett.8,3456–3460(2008).7.Möller,H.J.Semiconductors for Solar Cells(Artech House,1993).8.Beaucarne,G.et al.Epitaxial thin-film Si solar cells.Thin Solid Films511,533–542(2006).9.Van Nieuwenhuysen,K.et al.Epitaxially grown emitters for thin film crystallinesilicon solar cells.Thin Solid Films517,383–384(2008).10.Schermer,J.J.et al.Thin-film GaAs epitaxial lift-off solar cells for spaceapplications.Prog.Photovoltaics13,587–596(2005).11.Garnett,E.C.&Yang,P.D.Silicon nanowire radial p–n junction solar cells.J.Am.Chem.Soc.130,9224–9225(2008).12.Czaban,J.A.,Thompson,D.A.&LaPierre,R.R.GaAs core–shell nanowiresfor photovoltaic applications.Nano Lett.9,148–154(2009).13.Tsakalakos,L.et al.Silicon nanowire solar cells.Appl.Phys.Lett.91,233117(2007).14.Kelzenberg,M.D.et al.Photovoltaic measurements in single-nanowire siliconsolar cells.Nano Lett.8,710–714(2008).15.Fahrenbruch,A.L.&Bube,R.H.Fundamentals of Solar Cells:PhotovoltaicSolar Energy Conversion(Academic,1983).16.Kayes,B.M.,Atwater,H.A.&Lewis,parison of the device physicsprinciples of planar and radial p–n junction nanorod solar cells.J.Appl.Phys.97,114302(2005).17.Spurgeon,J.M.,Atwater,H.A.&Lewis,N.S.A comparison between thebehavior of nanorod array and planar Cd(Se,Te)photoelectrodes.J.Phys.Chem.C112,6186–6193(2008).18.Hu,L.&Chen,G.Analysis of optical absorption in silicon nanowire arrays forphotovoltaic applications.Nano Lett.7,3249–3252(2007).19.Lee,W.,Scholz,R.,Niesch,K.&Gosele,U.A template-based electrochemicalmethod for the synthesis of multisegmented metallic nanotubes.Angew.Chem.Int.Ed.44,6050–6054(2005).20.Li,J.,Papadopoulos,C.&Xu,J.Nanoelectronics—growing Y-junction carbonnanotubes.Nature402,253–254(1999).21.Fan,Z.Y.et al.Electrical and photoconductive properties of vertical ZnOnanowires in high density arrays.Appl.Phys.Lett.89,213110(2006).22.Steinhart,M.et al.Polymer nanotubes by wetting of ordered porous templates.Science296,1997–1997(2002).23.Masuda,H.et al.Highly ordered nanochannel-array architecture in anodicalumina.Appl.Phys.Lett.71,2770–2772(1997).24.Mikulskas,I.,Juodkazis,S.,Tomasiunas,R.&Dumas,J.G.Aluminumoxide photonic crystals grown by a new hybrid method.Adv.Mater.13,1574–1577(2001).25.Corwine,C.R.,Pudov,A.O.,Gloeckler,M.,Demtsu,S.H.&Sites,J.R.Copper inclusion and migration from the back contact in CdTe solar cells.Sol.Energy Mater.Sol.Cells82,481–489(2004).26.Sze,S.M.Physics of Semiconductor Devices(Wiley–Interscience,1981).27.Marsillac,S.,Parikh,V.Y.&Compaan,A.D.Ultra-thin bifacial CdTe solar cell.Sol.Energy Mater.Sol.Cells91,1398–1402(2007).28.Fan,Z.&Javey,A.Solar cells on curtains.Nature Mater.7,835–836(2008).29.Lungenschmied,C.et al.Flexible,long-lived,large-area,organic solar cells.Sol.Energy Mater.Sol.Cells91,379–384(2007).30.Nakamura,K.,Fujihara,T.,Toyama,T.&Okamoto,H.Influence of CdCl2treatment on structural and electrical properties of highly efficient2-µm-thick CdS/CdTe thin film solar cells.Japan.J.Appl.Phys.141,4474–4480(2002). AcknowledgementsWe acknowledge G.F.Brown and J.Wu for help with simulations.This work was financially supported by Berkeley Sensor and Actuator Center.J.C.H.acknowledges an Intel Graduate Fellowship.All fabrication was carried out in the Berkeley Microfabrication Laboratory.The solar-cell experimental characterization was done at LBNL,and was supported by the Helios Solar Energy Research Center,which is supported by the Director,Office of Science,Office of Basic Energy Sciences of the US Department of Energy under Contract No.DE-AC02-05CH11231.Author contributionsZ.F.,H.R.,J.D.,A.M.,O.E.,Y.-L.C.and A.J.designed the experiments.Z.F.,H.R.,J.D., A.M.,O.E.,Y.-L.C.,J.C.H.,T.T.,L.A.R.,S.N.,K.Y.,M.W.,J.W.A.and A.J.carried out experiments.Z.F.,P.W.L.,J.W.A.and A.J.carried out simulations.Z.F.,H.R.,J.D.,A.M., O.E.,Y.-L.C.,J.C.H.,T.T.,L.A.R.,P.W.L.,S.N.,K.Y.,J.W.A.and A.J.contributed to analysing the data.Z.F.and A.J.wrote the paper and all authors provided feedback. Additional informationSupplementary information accompanies this paper on /naturematerials. Reprints and permissions information is available online at / reprintsandpermissions.Correspondence and requests for materials should be addressed to A.J.。