上海800kv特高压直流输电系统设计
±800kV奉贤换流站的结构与功能特点
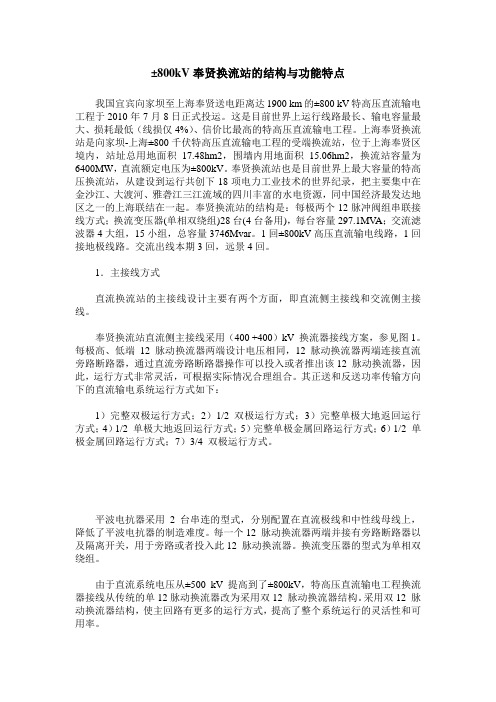
±800kV奉贤换流站的结构与功能特点我国宜宾向家坝至上海奉贤送电距离达1900 km的±800 kV特高压直流输电工程于2010年7月8日正式投运。
这是目前世界上运行线路最长、输电容量最大、损耗最低(线损仅4%)、信价比最高的特高压直流输电工程。
上海奉贤换流站是向家坝-上海±800千伏特高压直流输电工程的受端换流站,位于上海奉贤区境内,站址总用地面积17.48hm2,围墙内用地面积15.06hm2,换流站容量为6400MW,直流额定电压为±800kV。
奉贤换流站也是目前世界上最大容量的特高压换流站,从建设到运行共创下18项电力工业技术的世界纪录,把主要集中在金沙江、大渡河、雅砻江三江流域的四川丰富的水电资源,同中国经济最发达地区之一的上海联结在一起。
奉贤换流站的结构是:每极两个12脉冲阀组串联接线方式;换流变压器(单相双绕组)28台(4台备用),每台容量297.1MV A;交流滤波器4大组,15小组,总容量3746Mvar。
1回±800kV高压直流输电线路,1回接地极线路。
交流出线本期3回,远景4回。
1.主接线方式直流换流站的主接线设计主要有两个方面,即直流侧主接线和交流侧主接线。
奉贤换流站直流侧主接线采用(400 +400)kV 换流器接线方案,参见图1。
每极高、低端12 脉动换流器两端设计电压相同,12 脉动换流器两端连接直流旁路断路器,通过直流旁路断路器操作可以投入或者推出该12 脉动换流器,因此,运行方式非常灵活,可根据实际情况合理组合。
其正送和反送功率传输方向下的直流输电系统运行方式如下:1)完整双极运行方式;2)1/2 双极运行方式;3)完整单极大地返回运行方式;4)1/2 单极大地返回运行方式;5)完整单极金属回路运行方式;6)1/2 单极金属回路运行方式;7)3/4 双极运行方式。
平波电抗器采用 2 台串连的型式,分别配置在直流极线和中性线母线上,降低了平波电抗器的制造难度。
向家坝_上海_800kV特高压直流示范工程的低频谐振分析

第32卷 第10期 电 网 技 术 V ol. 32 No. 10 2008年5月Power System Technology May 2008文章编号:1000-3673(2008)10-0001-04 中图分类号:TM74 文献标识码:A 学科代码:470·4054向家坝—上海±800kV特高压直流示范工程的低频谐振分析郭贤珊1,马为民2(1. 国家电网公司特高压建设部,北京市西城区100031;2. 国网直流工程建设有限公司,北京市东城区 100005)Analysis on Low Frequency Resonance in ±800 kV UHVDC DemonstrationProject From Xiangjiaba to ShanghaiGUO Xian-shan1,MA Wei-min2(1.UHV Construction Department of SGCC,Xicheng District,Beijing 100031,China;2.State Grid DC Project Construction Company Limited,Dongcheng District,Beijing 100005,China)ABSTRACT: The harmonic impedance characteristic of DC system in ±800 kV UHVDC demonstration project from Xiangjiaba to Shanghai and the voltage and current levels of principle components in UHVDC system under various operation conditions are analyzed by EMTDC. It is pointed out that the series resonance may occur at the frequency near 50Hz and 100Hz. According to former engineering experiences in HVDC projects and based on basic principle of electrical engineering, it is proposed that the low frequency resonance in HVDC system can be effectively suppressed by configurating shunt filters or serial filters in HVDC system, and the features of the two methods are analyzed and compared, finally a recommended scheme for suppressing low frequency resonance in HVDC system is formed.KEY WORDS: UHVDC power transmission;low frequency resonance;shunt filter;serial filter;harmonic suppression摘要:仿真计算了向家坝—上海±800 kV特高压直流示范工程直流系统谐波阻抗特性,以及各种工况下系统中主要元件的电压和电流水平,指出该工程在50 Hz和100 Hz附近可能发生串联谐振。
特高压直流输电系统成套设计标准
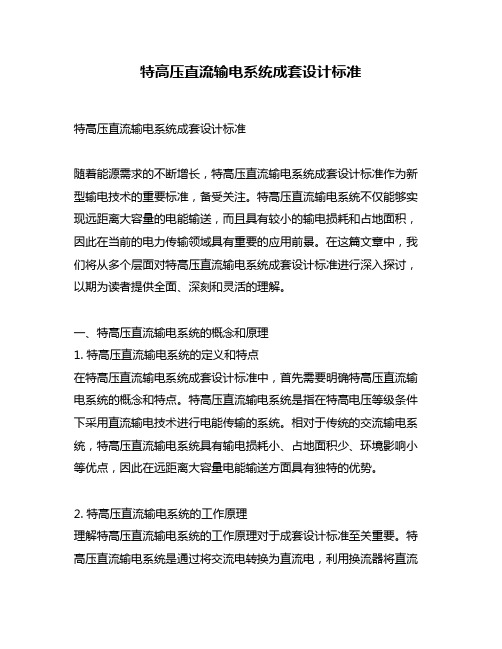
特高压直流输电系统成套设计标准特高压直流输电系统成套设计标准随着能源需求的不断增长,特高压直流输电系统成套设计标准作为新型输电技术的重要标准,备受关注。
特高压直流输电系统不仅能够实现远距离大容量的电能输送,而且具有较小的输电损耗和占地面积,因此在当前的电力传输领域具有重要的应用前景。
在这篇文章中,我们将从多个层面对特高压直流输电系统成套设计标准进行深入探讨,以期为读者提供全面、深刻和灵活的理解。
一、特高压直流输电系统的概念和原理1. 特高压直流输电系统的定义和特点在特高压直流输电系统成套设计标准中,首先需要明确特高压直流输电系统的概念和特点。
特高压直流输电系统是指在特高电压等级条件下采用直流输电技术进行电能传输的系统。
相对于传统的交流输电系统,特高压直流输电系统具有输电损耗小、占地面积少、环境影响小等优点,因此在远距离大容量电能输送方面具有独特的优势。
2. 特高压直流输电系统的工作原理理解特高压直流输电系统的工作原理对于成套设计标准至关重要。
特高压直流输电系统是通过将交流电转换为直流电,利用换流器将直流电输送到远距离,并在接收端将直流电转换为交流电进行供电。
特高压直流输电系统的核心设备是换流器,其性能和可靠性对整个系统的安全稳定运行具有重要影响。
二、特高压直流输电系统成套设计标准的总体要求1. 安全可靠特高压直流输电系统成套设计标准首先要求系统运行安全可靠。
在特高压直流输电系统中,设备的运行稳定性和可靠性对于保障电网的安全运行至关重要。
成套设计标准需要对设备的选用、安装、运行和维护等方面进行严格规定,以确保系统的安全可靠运行。
2. 高效节能特高压直流输电系统成套设计标准还要求系统具有高效节能的特点。
特高压直流输电系统的建设和运行成本较高,因此在设计标准中需要考虑如何提高系统的传输效率,降低能耗和成本。
这就需要对设备的技术指标、运行参数等方面进行合理规定,以实现系统的高效节能运行。
3. 灵活可控另外,特高压直流输电系统成套设计标准还需要考虑系统的灵活可控性。
向家坝—上海±800kv特高压直流输电线路1859#塔基护坡工程施工组织方案探讨

向家坝—上海±800kv特高压直流输电线路1859#塔基护坡工程施工组织方案探讨作者:蒋元林来源:《建筑工程技术与设计》2014年第27期[摘要] 本文笔者通过总结参与本塔基护坡项目施工的工程实践,对地质环境和工程施工条件复杂的边坡防护施工的特点、难点进行总结探讨,并提出相应的处治措施,并在施工组织方案中对各个施工环节的质量、安全等问题进行了探讨。
[关键词] 塔基护坡地质环境工程施工条件施工组织方案施工环节前言按向家坝-上海±800kv特高压直流输电线路工程湘Ⅰ标段1859#(以下简称“向上线1859#”)塔基护坡加固工程施工招标意见,根据中国电力工程顾问集团西北电力设计院提供的设计图纸要求,针对该塔基场地岩土工程特点及周围环境条件,结合以往类似工程的施工经验,编写本施工组织设计方案。
本施工组织方案设计作为向家坝-上海±800kv特高压直流输电线路工程1859#塔基护坡加固工程项目施工管理、安全生产、技术质量控制及施工措施方面的纲领性文件。
本人根据参与该工程项目前期准备、开工、施工及竣工验收各阶段的工程实践,充分考虑该项目的特点和施工难点,对关键点的施工组织方案进行探讨。
一、编制说明根据本工程的规模、特点及业主对工期的要求,结合我公司施工能力、经验及技术装备能力的基础上对目标工期、工程质量、项目管理机构设置、劳动组织、施工进度计划控制、机械设备及周转材料配备,主要分部分项工程施工方法,安全保证措施,文明施工环境保护措施、减低成本措施等尽可能充分考虑,突出科学性和可行性。
二、工程概况湖南省张家界市桑植县与慈利县交界的地段,有一座南北走向的大山,当地人称之为“厚山”。
1859#铁塔位于厚山西麓延伸地带的一座孤立山包的斜梁上,靠近峡谷内的公路,塔位坡度超过50°,相对公路路面的高差大于350m。
向上线1859#塔基为本工程路径中的最难点塔位之一。
本护坡工程项目设计单位按最小扰动原则和最低成本原则采用以下2种加固方法:1、塔腿区采用长锚杆喷射砼加固,其目的是加固塔腿和柱端基础地面。
向家坝-上海±800千伏特高压直流输电线路近日全线贯通

直驱发电机提高了约1%。 5
入 国际 先进 行列 的标 志性 工程。
此次 于 2 /6 9 2 区块 钻 获 的流 花 3 — 天 然 气发 现 是 中 42
海油有限公司与合作伙伴在南海东部海域珠江口盆地钻 获的第二个深水天然气发现。2 0 年8 0 4 月,中海油有限
公 司 的母 公司 中 国海 洋石 油 总公 司 与哈 斯基 能源 公 司就 珠 江 口盆 地 2 / 6 水 区块 签 订 石 油 合 同。 2 0 年 。这 92深 06
个 电站是国家西电东送的中线骨干电站, 电站能否安全
稳 定 运 行 关 系到 国家 整个 西 部 开发 的顺 利 进 行 ; 个 电 两
站机电设备安装的密度非常大, 是世界水电史上前所未
有的, 具有极 大 的 挑 战性 。 中, 其 向家 坝水 电站 为 国内 目 前单 机 容 量 最大 的水 轮 发 电 机 组 。
物 仅 为经 上 述 “ 勾兑 , 合 的 水。 , 混
据挪威专家介绍, 从理论上讲, 在任何淡水入海的地
方都 可 以建 造渗 透 能发 电 按 淡水 和海 水 之 间的总渗 透
能粗略计算, 这些发电厂每年的总发电量可达1o ̄ 1 o 6o U o太 7
瓦时, 相当于 欧盟地 区每年约 一半 电力需求。 这种 能源 既可 再生, 又不会产生 噪音或 排放 污染 物, 展前景 光明 。 发
_
_
_
_
区块的勘探再度取得重大突破。 继荔湾31 其合作伙伴 —后, 哈斯基石油中国有限公司日前再次钻获重要天然气发现。
哈斯 基石油 中国 有 限公 司是 加 拿 大上 市 的哈 斯 基能 源公
司的子公 司。
3.±800kV特高压直流输电工程换流站标准化设计文件之(三)-_接地极线路标准化设计指导书-V2.0_20150612
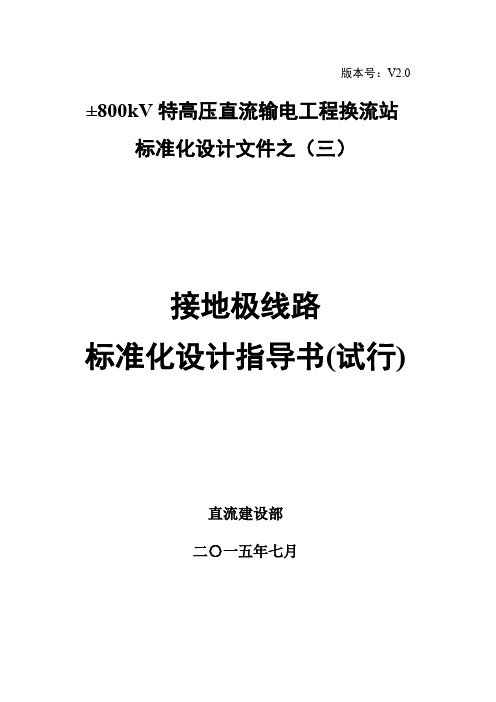
版本号:V2.0 ±800kV特高压直流输电工程换流站标准化设计文件之(三)接地极线路标准化设计指导书(试行)直流建设部二〇一五年七月±800kV特高压直流输电工程换流站标准化设计文件之(三)接地极线路标准化设计指导书(试行)批准:审核:郭贤珊黄勇宋胜利陈东编写:张宁刚王庆付颖王赞江岳魏鹏目录前言 (I)1 一般规定 (1)2 导地线选型 (2)2.1 导线选型 (2)2.1.1 导线选择主要原则 (2)2.1.2 导线载流量 (2)2.1.3 导线型号 (3)2.1.4 导线布置 (3)2.2 地线选型 (4)3 绝缘配合及防雷接地 (5)3.1绝缘配合 (5)3.1.1 绝缘子片数 (6)3.1.2 招弧角间隙 (6)3.1.3 空气间隙 (6)3.2防雷接地 (7)3.3地线绝缘设计 (8)4 导线对地和交叉跨越距离 (9)5 杆塔设计 (12)5.1杆塔结构设计原则 (12)5.1.1基本规定 (12)5.1.2杆塔优化设计原则 (13)5.2杆塔型式选择 (13)5.3杆塔荷载 (14)5.3.1杆塔荷载取值 (14)5.3.2杆塔荷载组合 (15)5.3.3其它规定 (18)5.4杆塔材料 (18)5.5杆塔防腐及绝缘设计 (19)5.5.1 基本规定 (19)5.5.2防腐要求 (19)6 基础设计 (20)6.1基础设计原则 (20)6.2基础选型 (20)6.2.1基本原则 (20)6.2.2常用的基础型式 (21)6.3基础材料 (21)6.4基础计算 (21)6.5基础防腐及绝缘设计 (21)6.5.1 基本规定 (21)6.5.2基础防腐设计 (22)6.6特殊地段基础处理 (22)7 单侧过负荷运行工况导线弧垂校核 (23)7.1接地极过负荷保护定值设计原则 (23)7.2接地极线路降功率工况运行时间 (23)7.3降功率工况的弧垂校核 (23)附录A 导线允许载流量计算方法 (25)附录B 灵州换流站接地极线路绝缘配置案例 (27)B.1 工作电压绝缘 (27)B.2 操作过电压绝缘 (28)C.1 导线型号 (33)C.2 额定电流状态下的导线温度 (34)C.3 一侧承受额定电流时的导线温度及弧垂 (35)C.4 过载时的对地及交叉跨越距离 (36)附录D 降功率工况的导线载流量分析 (38)D.1 一侧承受额定电流时的导线温度及弧垂 (38)D.2 过载时的对地及交叉跨越距离 (42)附录E 接地极线路设计标准指导书(试行)编写备忘录 (45)E.1 本设计指导书编写过程 (45)E.2 本设计指导书已解决的问题 (46)E.3 本设计指导书需解决的问题 (46)前言接地极线路是特高压换流站的配套工程。
_800kV特高压直流输电技术研究

第10期・・电力建设ElectricPowerConstruction第28卷第10期2007年10月Vol.28No.10Oct,20070引言金沙江水能资源十分丰富,是中国重要的能源基地之一。
干流全长2360km,多年平均径流量1550亿m3,落差3280m,可开发装机容量约9000万kW,年发电量约5000亿kW・h,占全国可开发水能资源的1/5。
适时开发金沙江意义深远。
金沙江下游乌东德、白鹤滩、溪洛渡、向家坝4个梯级的总装机容量达3800万kW,装机容量和年均发电量分别占金沙江的76%和63%。
其中溪洛渡、向家坝水电站总规模1860万kW,送电华东、华中,直流线路长度分别约2000km和1000km。
在历次论证中,通过对不同输电方式、不同输电电压等级以及各种组合输电方案的技术经济比较,都推荐采用纯直流输电方案。
基于国内外直流设备制造技术的进步,优化研究重点对采用±620kV直流5回、采用±800kV直流4回和3回的方案进行对比,研究结论认为溪洛渡、向家坝水电送出采用±800kV、6400MW的特高压直流输电方案在技术上是可行的,在经济上是合理的。
建设3回±800kV、4kA、6400MW的特高压直流输电工程,将电力输送到华东负荷中心和华中负荷中心,与建设5回±620kV,3720MW的超高压直流输电和4回±800kV,3kA,4800MW的特高压直流输电工程相比,考虑损耗后的综合投资分别节省约22亿元和105亿元,年费用低约5.7亿元和8.6亿元,而且可以大量节省走廊占地面积,具有重大的经济和社会意义。
2007年4月26日,国家发展和改革委正式核准建设第1回特高压直流输电工程———向家坝至上海±800kV特高压直流工程。
根据向家坝、溪洛渡水电站建设进度,工程应在2012年前建成投运。
为了确保工程按期、保质、高效地建成投产,保证向家坝、溪洛渡电站首批机组电力的送出,国家电网公司组织科研、设计和试验单位开展了一系列研究和设计工作,目前,第1回特高压直流工程的成套设计、初步设计已基本完成。
向家坝—上海800kV特高压直流工程中的关键技术方案

向家坝—上海±800kV特高压直流工程中的关键技术方案马为民,聂定珍,曹燕明(国网直流工程建设有限公司,北京市东城区100005)摘要:通过总结向家坝-上海±800kV特高压直流工程的咨询研究工作,阐述了该工程的技术方案,包括设计条件(特高压直流工程接入交流系统的条件、污秽条件和大件运输条件)、直流系统设计(电气主接线、额定值、动态响应、无功配置及可靠性可用率)、绝缘配合及外绝缘、换流站设计及主设备要求等关键技术问题的主要结论,为高压直流输电技术的发展和设备的研发及特高压直流工程的建设提供借鉴意见。
关键词:换流站;±800kV特高压直流;换流阀;绝缘配合;直流控制系统0 引言向家坝—上海±800kV特高压直流工程目前已获国家发展和改革委员会核准,特高压直流输电是一项崭新的技术,在建设过程中必然遇到新的技术问题。
受国家电网公司的委托,国网直流工程建设有限公司于2005年1月开始向家坝—上海±800kV特高压直流工程咨询研究。
本次咨询工作承担了国家电网公司科技部下达的8项直流特高压科研课题,并根据设备研发和工程建设的需要,开展了25项咨询专题研究。
其间系统组织召开了国际特高压工作组会议,成员包括ABB公司、SIEMENS公司及西安高压研究所,充分吸收采纳国内外先进技术及理念;与运行等单位联合召开技术研讨会,广泛征求运行人员的意见,总结运行经验;与设备制造厂进行技术研讨,优化技术参数,在此基础上提出了特高压直流系统的初步技术规范。
为验证上述技术规范的合理性,还开展了特高压直流系统的概念设计,最终确定了本工程的各项技术方案。
本文将对特高压直流工程的设计条件、直流系统设计、换流站设计以及主设备要求等关键技术方案进行深入探讨。
1 设计条件1.1 交流系统条件送端向家坝换流站通过3回4×400mm2的500kV线路接入系统。
受端上海换流站接入系统方案为:换流站本期通过3回4km500kV线路接入邻近的南汇变,其中2回出线导线截面为4×720mm2,1回导线截面为6×630mm2,另外预留1回备用。
特高压直流输电接地极研究

特⾼压直流输电接地极研究特⾼压直流输电接地极研究中⽂摘要:直流输电接地极设计⽬前基本依据以往的⼯程经验,由于其具有热⼒效应和电化效应,尚未形成统⼀的接地极设计标准。
从极址的选择、系统分析、跨步电压的验算以及新型材料的应⽤进⾏了阐述,为特⾼压直流输电接地极设计提供参考。
关键词:特⾼压直流输电;接地极;跨步电压;放热焊接。
0前⾔特⾼压直流输电是指800 kV及以上电压等级的直流输电及相关技术。
特⾼压直流输电的主要特点是输送容量⼤、电压⾼,可⽤于电⼒系统⾮同步联⽹。
⾃第⼀条500 kV 超⾼压直流输电⼯程葛洲坝上海直流输电⼯程建设⾄今,我国已建设⼗余条直流输电⼯程,接地极的设计取得较多的实际⼯程经验,但尚未出现统⼀的标准, 运⾏中也发现存在很多问题,本⽂以向家坝--上海800 kV 直流输电接地极设计为例论述设计过程。
1 特⾼压直流输电接地极的特点⽬前世界上已投运的直流接地极可以分为2类:陆地电极和海洋电极。
直流输电通常可采⽤3种接线⽅式,即单极线路、同极线路和双极线路。
根据⼯程实际,⼀般在送电投产后由单极线路过渡为双极线路。
极址条件不同,布置⽅式也不同。
从我国建设的直流⼯程接地极来看,⼤多数为陆地接地极。
强⼤的直流电流持续地、长时间地流过接地极时,接地极主要表现出电磁效应、热⼒效应和电化效应。
2 极址选择2 . 1 选址原则接地极的选址应遵循施⼯运⾏⽅便、易排除电极在运⾏中产⽣的⽓体和热量、电流分布⽐较均匀、造价⽐较低廉等原则。
同时, 极地应在⽆矿或矿产限制开采区,且当地⽓候为常温多⾬、⽔⼟保持功能较好。
2 . 2 地勘资料的准确性勘测数据应有较⾼的准确性,在实际⼯程勘查设计时,经常遇到的⼟壤模型是由不同电阻率的多层⼟壤地层构成。
⽬前较先进的⼟壤电阻率测试⽅法有⾼密电法,可以⽤2维图形⽅式直观地运算出深达50m的⼟壤电阻率分布图。
2 .3 向家坝—上海800kV直流输电极址概况向家坝—上海800kV特⾼压直流输电⼯程的送端换流站,额定电流为4kA,输电能⼒为6.4GW,直流额定电压为800kV;复龙换流站接地极⼯程即为该⼯程配套项⽬。
800kV6400MW特高压直流输电工程设计
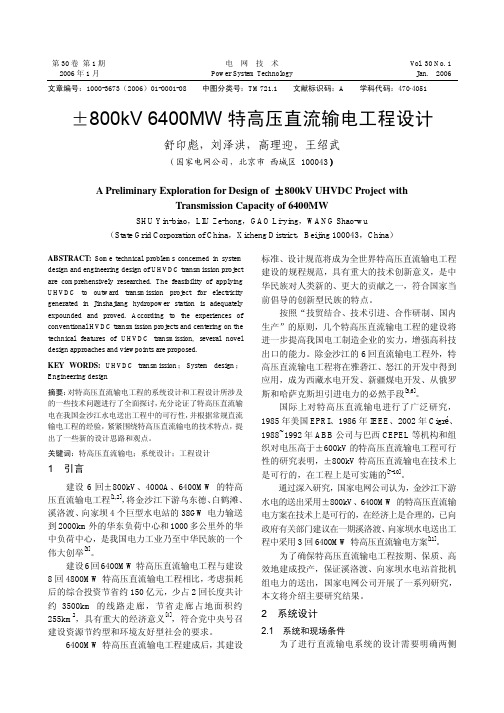
2006年1月Power System Technology Jan. 2006 文章编号:1000-3673(2006)01-0001-08 中图分类号:TM721.1 文献标识码:A 学科代码:470·4051±800kV 6400MW特高压直流输电工程设计舒印彪,刘泽洪,高理迎,王绍武(国家电网公司,北京市西城区100043)A Preliminary Exploration for Design of ±800kV UHVDC Project withTransmission Capacity of 6400MWSHU Yin-biao,LIU Ze-hong,GAO Li-ying,WANG Shao-wu(State Grid Corporation of China,Xicheng District,Beijing 100043,China)ABSTRACT:Some technical problems concerned in system design and engineering design of UHVDC transmission project are comprehensively researched. The feasibility of applying UHVDC to outward transmission project for electricity generated in Jinshajiang hydropower station is adequately expounded and proved. According to the experiences of conventional HVDC transmission projects and centering on the technical features of UHVDC transmission, several novel design approaches and viewpoints are proposed.KEY WORDS:UHVDC transmission;System design;Engineering design摘要:对特高压直流输电工程的系统设计和工程设计所涉及的一些技术问题进行了全面探讨,充分论证了特高压直流输电在我国金沙江水电送出工程中的可行性,并根据常规直流输电工程的经验,紧紧围绕特高压直流输电的技术特点,提出了一些新的设计思路和观点。
800KV 特高压直流输电线路空气间隙设计
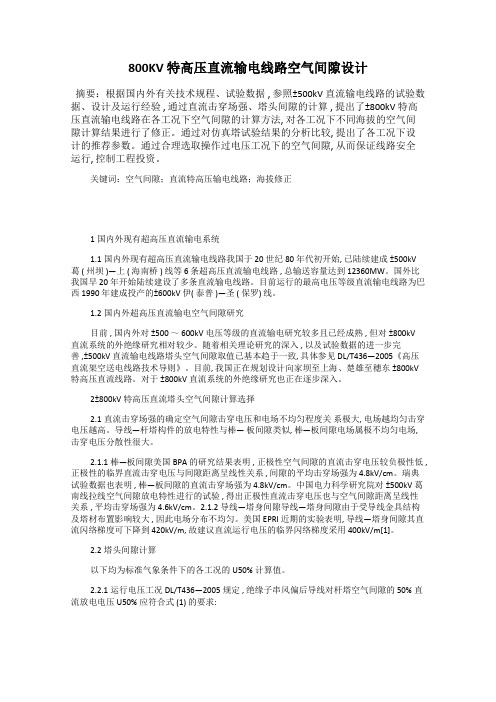
800KV 特高压直流输电线路空气间隙设计摘要:根据国内外有关技术规程、试验数据 , 参照±500kV 直流输电线路的试验数据、设计及运行经验 , 通过直流击穿场强、塔头间隙的计算 , 提出了±800kV 特高压直流输电线路在各工况下空气间隙的计算方法, 对各工况下不同海拔的空气间隙计算结果进行了修正。
通过对仿真塔试验结果的分析比较, 提出了各工况下设计的推荐参数。
通过合理选取操作过电压工况下的空气间隙, 从而保证线路安全运行, 控制工程投资。
关键词:空气间隙;直流特高压输电线路;海拔修正1国内外现有超高压直流输电系统1.1国内外现有超高压直流输电线路我国于20 世纪80 年代初开始, 已陆续建成 ±500kV葛 ( 州坝 )—上 ( 海南桥 ) 线等 6 条超高压直流输电线路 , 总输送容量达到 12360MW。
国外比我国早 20 年开始陆续建设了多条直流输电线路。
目前运行的最高电压等级直流输电线路为巴西 1990 年建成投产的±600kV 伊( 泰普 )—圣 ( 保罗) 线。
1.2国内外超高压直流输电空气间隙研究目前 , 国内外对 ±500 ~ 600kV 电压等级的直流输电研究较多且已经成熟 , 但对 ±800kV直流系统的外绝缘研究相对较少。
随着相关理论研究的深入 , 以及试验数据的进一步完善 ,±500kV 直流输电线路塔头空气间隙取值已基本趋于一致, 具体参见 DL/T436—2005《高压直流架空送电线路技术导则》。
目前, 我国正在规划设计向家坝至上海、楚雄至穗东 ±800kV 特高压直流线路。
对于 ±800kV 直流系统的外绝缘研究也正在逐步深入。
2±800kV 特高压直流塔头空气间隙计算选择2.1直流击穿场强的确定空气间隙击穿电压和电场不均匀程度关系极大, 电场越均匀击穿电压越高。
特高压直流输电工程一次系统设计概述
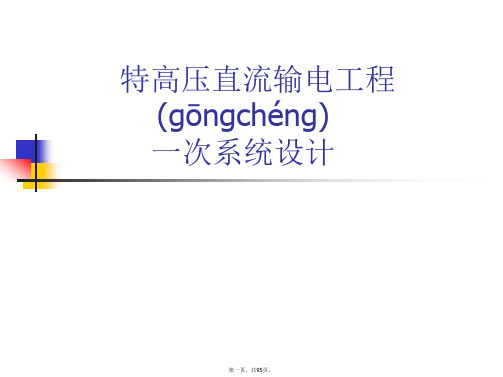
1954—2000年,世界上已投入运行的直流输电工程有63项,其中 架空线路17项,电缆(diànlǎn)线路8项,架空线和电缆(diànlǎn)混合 线路12项,背靠背直流工程26项。
(3)新型半导体换流设备的应用 20世纪90年代以后,新型氧化物半导体器件一绝缘栅双极晶体管 (IGBT)首先在工业驱动装置(zhuāngzhì)上得到广泛的应用。
第七页,共95页。
目前直流输电(shūdiàn)的应用主要在以下几个方面。 (1)远距离大容量输电(shūdiàn)直流输电(shūdiàn)线路的造价和运 行费用均交流输电(shūdiàn)低,而换流站的造价和运行用均比交流 变电所的高。
第八页,共95页。
第九页,共95页。
(2)电力系统(diàn lì xì tǒnɡ)联 网
第二页,共95页。
(2)晶闸管阀换流时期
1970年瑞典首先在哥特兰岛直流工程上扩建了直流电 压为50kv,功率为10MW,采用(cǎiyòng)晶闸管换流阀 的试验工程。
1972年世界上第一个采用(cǎiyòng)晶闸管换流的伊 尔河背靠背直流工程在加拿大投入运行。由于晶闸管换 流阀比汞弧阀有明显的优点,此后新建的直流工程均采 用(cǎiyòng)晶闸管换流阀。与此同时,原来采用(cǎiyòng) 汞弧阀的直流工程也逐步被晶闸管阀所替代。
第十三页,共95页。
3)直流电缆(diànlǎn)送电
直流电缆没有电容电流,输送容量不受距离(jùlí)的限制,而 交流电缆由于电容电流很大,其输送距离(jùlí)将受到限制。 交流电缆每相的电容电流可用下式表示
IC Un 2 f C0l
(1—1)
其临界距离(jùlí)可用下式表示
lcr
向家坝-上海±800kV特高压直流工程用并联电容器及装置的研制

—
{ 无 功 补偿 }
I -、p、nv ., , , , ,, ^ ,一 , ,
向家 坝一 上 海 ±80k 0 V特 高 压 直 流 工 程 用 并 联 电容 器 及 装 置 的研 制
刘水 平 苏 开 云 饶 , , 娣 杨 文 荣 ,
(. 1西安西电电力电容器有限责任公 司, 陕西 西安 708 ;. 1022西安高压 电器研究院有限责任公司, 陕西 西安 707 ) 13 7
LI S u ・ i g U h ip n , S Ka — u , RAO , YANG e r n U iy n Di W n-o g
( . ia D P w r a ai r C . Ld,X ’ n7 0 8 , hn ; 1 X ’nX o e p c os o, t. ia 10 2 C ia C t
to n h ac lto ft rd e di e e ta u r n r tc in s tig ft e i sa lto h w insa d t e c l u ain o he b g f r n ilc re tp o e t e t s o h n tlai n s o i f o n
ta tec pct s n s lt n ee p df H D r et sa tel d glvl nC ia h th a ai r a di t a o sdvl e o U V C po c i t h a i e i hn , o na i l o r j e n e
5.±800kV特高压直流输电工程换流站标准化设计文件之(五)- 换流站主建筑物标准化设计指导书-V2.0

版本号:V2.0 ±800kV特高压直流输电工程换流站标准化设计文件之(五)换流站主建筑物标准化设计指导书(试行)直流建设部二〇一五年七月±800kV特高压直流输电工程换流站标准化设计文件之(五)换流站主建筑物标准化设计指导书(试行)批准:审核:郭贤珊黄勇宋胜利胡劲松编写:王幼军王庆曹伟炜范新健饶冰目录1 换流站建筑物综述 (1)1.1主要建筑物火灾危险性类别、耐火等级 (1)1.2屋面防水等级 (2)1.3屋面排水 (2)1.4结构设计原则 (2)2 阀厅 (4)2.1阀厅设计总的要求 (4)2.2阀厅建筑尺寸 (7)2.3阀厅的结构型式 (8)2.4阀厅围护系统设计 (9)3 控制楼 (13)3.1控制楼设计原则 (13)3.2主控楼标准设计方案平面布置 (16)3.3辅控楼标准设计方案平面布置(方案一) (24)3.4辅控楼标准设计方案平面布置(方案二) (29)4 综合楼及其它建筑 (34)4.1综合楼 (34)4.2备品备件库 (35)4.3 GIS室 (35)4.4车库 (36)前言为规范±800kV特高压直流输电工程换流站项目的管理,在充分吸收了向家坝—上海、锦屏—苏南、哈密南—郑州、溪洛渡左岸—浙江金华、灵州—绍兴等特高压直流输电工程建设经验基础上,依托锡盟—泰州、上海庙—山东、晋北—南京±800kV特高压换流站工程设计优化成果,对±800kV换流站的主要建筑物的设计原则进行了统一,从而形成一套比较具有参考性的±800kV换流站主要建筑物标准设计方案,主要应用和指导后续±800kV换流站工程的主要建筑物设计工作,促进特高压直流工程建设质量与效率的提高,全面提升特高压直流工程建设水平。
1 换流站建筑物综述1.1主要建筑物火灾危险性类别、耐火等级±800kV换流站建筑物火灾危险性类别、耐火等级应符合表1.1规定。
±800kV直流架空输电线路运行规程
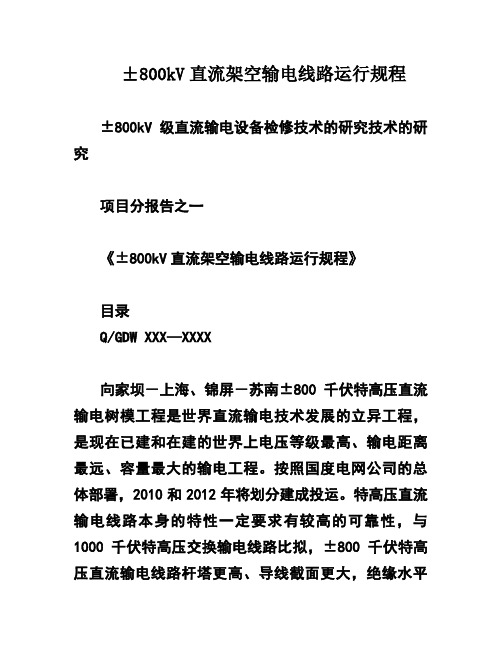
±800kV直流架空输电线路运行规程±800kV级直流输电设备检修技术的研究技术的研究项目分报告之一《±800kV直流架空输电线路运行规程》目录Q/GDW XXX—XXXX向家坝-上海、锦屏-苏南±800千伏特高压直流输电树模工程是世界直流输电技术发展的立异工程,是现在已建和在建的世界上电压等级最高、输电距离最远、容量最大的输电工程。
按照国度电网公司的总体部署,2010和2012年将划分建成投运。
特高压直流输电线路本身的特性一定要求有较高的可靠性,与1000千伏特高压交换输电线路比拟,±800千伏特高压直流输电线路杆塔更高、导线截面更大,绝缘水平更高,在线路的防污闪、防雷害方面提出了更高的要求。
为此在总结以往超高压、特高压交换线路运转经验的基础上,积极研讨特高压直流线路的运转标准和技术管理要求,是开展特高压直流线路运转维护准备事情确当务之急。
根据XXX《±800kV级直流输变电设备运行检修技术的研究》任务委托书,为确保±800kV级直流特高压输变电工程投运后安全经济运行,XXX承担了《±800kV直流架空输电线路运行规程》的研究工作,用来指导特高压直流线路运行维护工作,以提高特高压直流运行管理水平和运行可靠性。
2、依托工程±800kV向家坝-上海、锦屏-苏南直流树模工程。
3、研究内容1、研讨±800kV特高压直流线路运转维护管理基本要求;2、研讨±800kV特高压直流线路运转维护标准;3、研究±800kV特高压直流线路特殊区段的运行管理要求;1Q/GDW XXX—XXXX4、主要结论通过对±800kV特高压直流输电线路运行维护标准的研究,规定了±800kV直流架空送电线路运行工作的基本要求、技术标准,并对线路巡视检查、检测、在线监测、缺陷管理、维修、运行标准、技术管理等提出了具体要求。
(输电)金沙江一期送电华中、华东±800kV直流输电工程 向家坝~上海±800kV直流输电工程(调整)
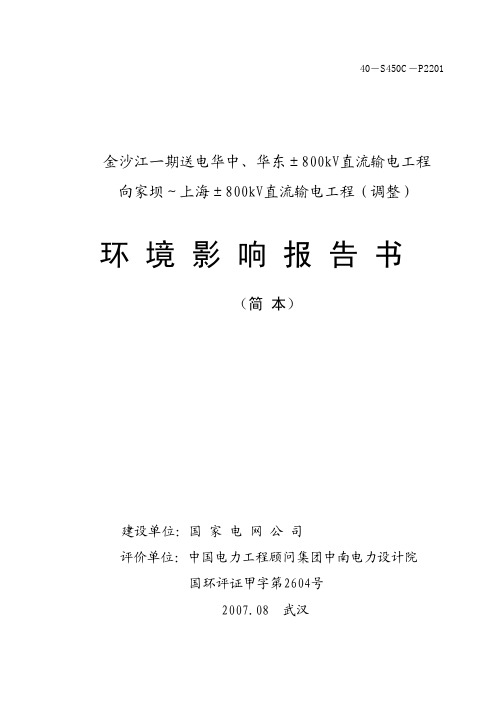
40-S450C-P2201金沙江一期送电华中、华东±800kV直流输电工程向家坝~上海±800kV直流输电工程(调整)环境影响报告书(简本)建设单位:国家电网公司评价单位:中国电力工程顾问集团中南电力设计院国环评证甲字第2604号2007.08武汉目录1前言 (1)1.1环境影响评价的背景 (1)1.2工程概况 (1)1.3设计工作过程 (3)1.4环评工作过程 (3)2编制依据 (4)2.1项目的名称及基本构成 (4)2.2编制依据 (5)2.2.1采用规范的名称及标准号 (5)2.2.2采用评价技术导则的名称及标准号 (5)2.2.3项目立项文件及相关文件 (6)2.2.4技术及工程资料 (6)2.2.5任务依据 (6)2.3评价范围、评价标准及评价因子 (6)2.3.1评价范围 (6)2.3.2评价标准 (7)2.3.3评价因子 (9)2.4评价工作重点 (10)3工程概况及工程分析 (11)3.1工程特性 (11)3.2换流站工程概况 (12)3.2.1送端换流站 (12)3.2.2受端换流站 (14)3.3线路工程概况 (15)3.3.1线路路径选择的原则 (15)3.3.2推荐线路概况 (16)3.3.3主要交叉跨越 (22)3.3.4线路地形情况 (23)3.3.5线路通过的行政区 (24)3.3.6导线 (24)3.3.7塔杆规划 (26)3.3.8导线对地距离 (29)3.3.9线路走廊及民房拆迁 (30)3.3.10电力设施规划控制区 (31)3.4计划工期 (31)3.5环境影响因子分析 (31)3.5.1环境影响因子识别 (31)3.5.2换流站环境影响因子 (32)3.5.3输电线路环境影响因子 (34)3.6工程环保特点及主要的环保问题 (34)3.7换流站和直流线路路径选择合理性分析 (35)3.7.1与法规相容性分析 (35)3.7.2与规划相容性分析 (35)4主要环境影响预测及评价结论 (37)4.1电磁环境影响预测及评价 (37)4.1.1输电线路电磁环境影响预测及评价 (37)4.1.2换流站电磁环境影响预测及评价 (37)4.1.3接地极及接地极线路环境影响预测和评价 (38)4.2声环境影响预测和评价 (38)4.2.1输电线路声环境影响预测 (38)4.2.2换流站声环境影响预测 (39)4.3水环境影响预测和评价 (39)4.4生态环境影响预测和评价 (39)5污染防治措施 (40)5.1运行期污染防治措施 (40)5.1.1输电线路运行期污染防治措施 (40)5.1.2换流站污染防治措施 (41)5.2施工期的污染防治措施 (42)5.2.1施工方法中采取的污染防治措施 (42)5.2.2施工过程中的生态保护措施 (44)5.3污染防治措施技术论证 (45)6结论 (47)1前言1.1环境影响评价的背景2006年6月20日,国家环境保护总局以环审[2006]277号《关于金沙江一期送电华中、华东±800千伏直流输电工程及锦屏一、二级送电华东±800千伏直流输电工程锦屏~苏南±800千伏直流输电工程环境影响报告书的批复》,对国家电网公司报送的《金沙江一期送电华中、华东±800千伏直流输电工程向家坝~上海±800kV直流输电工程环境影响报告书》进行了批复。
_800kV直流特高压输电线路的设计
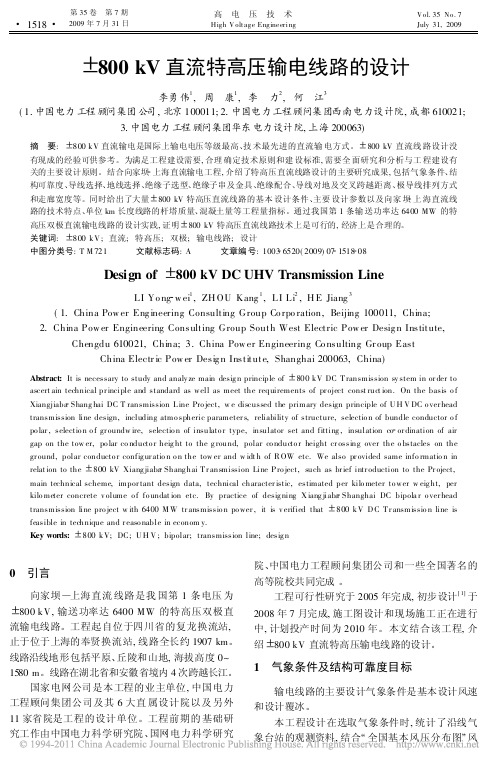
2
极导线选择
导线选择的基本目标是在满足各种技术性能要 求和环境限制条件下获得最为经济的导线束方案。 一般情况下导线选择应综合考虑输送容量、 功 率损耗、 导线允许发热、 机械特性、 工程造价和电磁 环境要求。就 800 kV 特高压线路而言 , 由直流电 晕引起的电磁环境问题更是极其重要的因素, 甚至 是导线选择和极导线布置的控制因素。直流电晕可 产生无线电干扰、 可听噪声、 电晕损耗、 地面合成电 场和地面离子电流等问题。工程设计中通过分析国 内外现有 500 kV 线路的运行经验 , 结合工程前期
重冰线路设计和运行经验表明由于冰凌荷载大导线承受拉应力很高如果此时再叠加较大的不平衡张力则将在线夹出口处出现导线断股现象铝绞线钢芯铝合金绞线等方案进行技术经济比较结合重冰区线路的运行经验最终在20mm重冰区采用与轻冰区相同的导线方案30mm重冰区则采用6aacsr72050钢芯铝合金绞线
# 1518 #
第 35 卷 第 7 期 2009 年 7 月 31 日
高
电
压
技
术
High V olt age Engineeri ng
V ol. 35 N o. 7 July 31, 2009
800 kV 直流特高压输电线路的设计
李勇伟 , 周 康 , 李 力 , 何 江 ( 1. 中国电力工程顾问集团公司 , 北京 100011; 2. 中国电力工程顾问集团西南电力设计院 , 成都 610021; 3. 中国电力工程顾问集团华东电力设计院, 上海 200063)
0
引言
向家坝 上海直流 线路是我 国第 1 条电压 为
院、 中国电力工程顾问集团公司和一些全国著名的 高等院校共同完成 。 工程可行性研究于 2005 年完成, 初步设计 [ 1] 于 2008 年 7 月完成, 施工图设计和现场施工正在进行 中 , 计划投产时间为 2010 年。本文结合该工程, 介 绍 800 kV 直流特高压输电线路的设计。
高压直流输电系统直流滤波器综合设计

* 收稿日期:2018-04-11 作者简介:徐亮亮(1989- ),男,江苏徐州市人,硕士,工程师,主要从事换流站电气二次设计。
100
2018年09月 增刊2
变电 高压直流输电系统直流滤波器综合设计
2 换流器及谐波成分
国 内 建 成 的 ±500 kV 换 流 站 和 在 建 的 ±800 kV 特高压换流站均采用 12 脉动换流器实 现整流和逆变,12 脉动换流器由 2 个 6 脉动换 流器串联而成,交流侧通过换流变并网。换流 变的阀侧绕组一个星接、一个角接,从而使 2 个 6 脉动换流器的交流侧得到相位差 30°的换 相电压。12 脉动换流器的 12 个换流阀在每个工 频周期内轮流导通,触发脉冲间隔 30°。12 脉 动换流器的原理接线见图 1。
另外由于触发角的不对称,换流变电感不平衡
和交流系统电压不对称,还会产生随机分布的
网侧
~
12脉动换流器
V1
V5 V9
U V7
V W
V11 V3
V2
V6
V10
U V8
V W
V12 V4
图1 12脉动换流器的原理接线图
利 用 MATLAB 对 直 流 输 电 系 统 进 行 仿 真,假定逆变侧的触发角 α=143°换相重叠角 μ=15°直流母线电压为 500 kV,仿真得到的稳 态结果见图 2,依次为直流母线电压波形和阀 V1 上的电压波形,对直流母线电压的谐波成分 进行分析,可得在直流侧含有 12 K 次特征谐波, 见图 3。
Integrated Design of DC Filter for HVDC Transmission System
XYU Liang-liang (East China Electric Power Design Institute Co., Ltd., Shanghai 200001, China) Abstract: DC filter project is an important part in the HVDC, it has a good good inhibitory effect against the harmonic and line output voltage. In order to systematically introduce the integrated design of DC filter, Firstly, the necessity of the DC filter is analyzed. Secondly, according to the impedance-frequency characteristic equation, the formula of the single-tuned and double-tuned filter is deduced. For the special method of DC filter protection, At the end, the configuration scheme of filter protection and the principle of CT configuration are given. Key words: characteristic harmonic; DTHP; equivalent disturbing current; DC filter protection.
向家坝_上海_800kV特高压直流输电线路的检测与运行维护

必要时派人看守。 4. 2 架空输电线路巡视的检查内容 检查内容
[ 5]
包括: ( 1) 沿线环境的线路安全;
( 2) 杆塔、 拉线和基础; ( 3) 导线和地线 ; ( 4) 绝缘 子、 连接器及金具 ; ( 5) 防雷设施和接地 ; ( 6) 附件 及其他设施。 800 kV 特高压直流输电工程, 高塔平均呼 高超过 60 m, 有的高达 130 m, 如此高塔, 要用肉 眼观察清楚相当困难 , 建议单位购置高倍望眼镜, 以能清楚观察输电杆塔上的异常现象。
( 1) 防雷保护以防绕击为主 雷电绕击导线的概率和保护角有很密切的关 系 , 避雷线保护角越小 , 绕击概率越低。减小保护
康宇 斌 , 等
向家坝
上海
800 kV 特高压直流输电线路的检测与运行维护
0115
角是降低
800 kV 特高压直流 输电线路雷电绕
缘子串覆冰闪络的布置形式 覆冰较重的区段也可采用大盘径空气动力型 绝缘子和传统钟罩形绝缘子交叉配置的方式 ( 插 花串 ) , 双联串应增大串间 距。在多次发 生舞动 的线路区段应加装防舞动装置, 减少脱冰舞动对 线路设备的影响
图 1 某线路红外色谱分析图
0116
2011, 39( 1)
通过红外成像色谱分析图片 , 见图 1 , 可以直 观地判断连接金具、 绝缘子等发热情况, 为线路的 状态评价提供可靠的科学依据。结合特高压输电 工程的特殊性, 在购置红外和紫外成像仪时, 建议 考虑度数大的镜头, 以方便检测塔高、 山区和不易 到达的地区。 目前高压、 超高压线路的各种在线监测方法 已经较多、 较成熟了, 为开展 800 kV 特高压直 流输电线路的状态检修奠定了良好的基础。从设 备寿命管理与预测技术、 设备可靠性分析技术、 设 备状态监测与故障诊断技术以及信息管理与决策 技术等方面 , 利用在线监测技术对容易发生微风 振动、 舞动、 风偏、 覆冰和绝缘子污秽闪络等线段 进行动态监控, 逐步实施特高压线路稳定的状态 检修工作。
- 1、下载文档前请自行甄别文档内容的完整性,平台不提供额外的编辑、内容补充、找答案等附加服务。
- 2、"仅部分预览"的文档,不可在线预览部分如存在完整性等问题,可反馈申请退款(可完整预览的文档不适用该条件!)。
- 3、如文档侵犯您的权益,请联系客服反馈,我们会尽快为您处理(人工客服工作时间:9:00-18:30)。
Converter Valve Design and Valve Testing for Xiangjiaba – Shanghai ±800kV 6400MWUHVDC Power Transmission ProjectBaoliang Sheng,Jonatan Danielsson, Yanny Fu and Zehong LiuAbstract — Thyristor valve design merits are inherited and new developed high power rating thyristors are used in the valve design. The thyristor control unit (TCU) and snubber circuit are optimized for a high reliability and low loss of thyristor valves. Two 12-pulse converters are connected in series as one converter per pole. The converters use a double valve MVU structure.A careful designed rigorous type test program has been followed to verify the valve and converter design. A single valveis comprehensively dielectric type tested and operational type tested. High voltage valve hanging structure, low voltage valve hanging structure and MVU, plus the series connected valve simulator, are valve supporting structure dielectric tested and MVU dielectric tested. The test results indict that the valve voltage and converter design and protection are proper and thereis a substantial margin in operation since, for most of the operational tests, the test parameters were globally more severe than specified.Index Terms ⎯Xiangjiaba – Shanghai ±800kV UHVDC, HVDC converter, thyristor valve design, thyristor valve dielectric test, thyristor valve operational testI.I NTRODUCTIONBB was granted to design and manufacture the convertersused in the inverter station, Fengxia substation, for thefirst ±800kV 6400MW bulk power transmission project. Converter in this project comprises of two 12-pulse thyristor valves in series connection per pole. Those two 12-pulse thyristor valves are installed in a low voltage (0 – 400kV d.c.) valve hall and a high voltage (400kV – 800kV d.c.) valve hall respectively to build up the 800kV pole d.c. voltage.A double valve MVU structure is used to mount those valves in the valve halls. There are four MVUs in series connection per pole and phase with two of them located in the high voltage valve hall and the other two located in the low voltage valve hall. For a better transient over-voltage control on those MVUs surge arresters are connected from the top terminal of each 6-pulse bridge to ground, Figure 1.Baoliang Sheng and Jonatan Danielsson are with ABB AB, HVDC, 77180 Ludvika, Sweden (e-mails: baoliang.sheng@ and jonatan.danielsson@)Yanny Fu is with KEMA B.V., 6800 ET Arnhem, the Netherlands (e-mails: yanny.fu@)Zehong Liu is with SGCC, Beijing 100031, China (e-mails: zehong-liu@) The design of 800kV thyristor valves uses all the state-of-the-art technologies in HVDC thyristor valve design,including high power rating 8.5kV 6” electrically triggeredthyristors [1], thyristor module with compact design, andindividual thyristor local over-voltage protection.A comprehensive test program based on IEC 60700-1 [2]and client’s specification was followed in the valve designverification type test. A single valve exposed to the d.c.voltage test, a.c. voltage test, impulse voltage tests and non-periodic firing test in STRI’s high voltage laboratory. Thethyristor modules in this single valve were operational typetested afterwards in ABB’s high power test plant in a 6-pulseBack-to-Back based synthetic test circuit.Both high voltage (HV) MVU hanging structure and lowvoltage (LV) MVU were completely dielectric tested.Figure 1 Converter valves and valve over-voltage protection schemeThe MVU dielectric test focused on the verification ofvoltage withstand capability to surroundings and the partialdischarge levels being within specified limits. A pole potentialMVU, together with a series connected valve simulator, wasdielectric tested to verify the insulation of high potential 12-pulse converter in the HV valve hall and, the high potentialMVU in the LV valve hall was dielectric tested with the same Aprinciple to verify the insulation of low potential 12-pulse converter in the LV valve hall.II.T HYRISTOR V ALVE D ESIGNA. Thyristor valve requirementsThyristor valves in Fengxian substation of Xiangjiaba – Shanghai 800kV UHVDC are operated fundamentally as an inverter with the possibility to operate them as rectifier when needed. Thyristor valve requirements are concreted by the client specification and system design. Requirements on the thyristor valves are given in Table 1.Table 1 Thyristor valve requirementsMax. 6-pulse bridge voltage 204kV d.c. Max. continuous current 4000A d.c.2 h overload current 4400A d.c. Peak over-current with subsequent blocking(one-loop fault current)40.8kAPeak over-current without subsequent blocking (three-loop fault current) 40.8kA, 42.2kA,42.2kAFiring delay angle at nominal power, α5° – 17.5° Extinction angle at nominal power, γ17° ± 1° Temporary a.c. system over-voltage loadrejection factor with de-blocked valves1.3Switching impulse voltage protection level ofvalve surge arrester386kVSteep-front impulse voltage protection level ofvalve surge arrester394kVB. Thyristor valve design6400MW bulk power transmission at ±800kV demands a high reliable converter. Thanks to the good performance records of thyristor converter valves in HVDC applications in the past decade many design merits [3] can be inherited directly in the design of 800kV thyristor valves. Successful development of high power rating 8.5kV 6” electrically triggered thyristors paved the way for a high current and voltage thyristor valve at a relative low quantity of thyristors in series connection.A single thyristor valve comprising 56 pieces of 8.5kV 6” thyristors and their associated circuits can fulfil the requirements of Table 1 well. 2 of 56 are redundant. Those 56 thyristor positions are group assembled in eight thyristor modules with seven thyristors in each module.Same as the valve design in Three-Gorges HVDC those thyristor modules are in series connection with reactor modules and layered up spirally as one complete single valve. The top end of this single valve terminates the bottom end of upper single valve. There are two single valves in series connection in each MVU.Thyristor control units (TCU) used in this project have been modified to match the gate driving requirement of 6” thyristors. Heat sinks have been enlarged and coolant flow rate was increased in order to effectively dissipate the losses from thyristors. Other robust valve design methods, such as thyristor series cooling in one thyristor module and TCU energization by a tapped voltage branch, are kept.C. Valve over-voltage protection designThyristor valve over-voltage protection is realized by valve surge arrester across each single valve and surge arresters installed on each bridge terminal to ground, Figure 1.The thyristor snubber circuit parameter has been carefully determined to ensure an even voltage distribution of the series connected thyristor levels, whereas the total losses of valve have been controlled to the minimum.The TCU provides an additional forward direction over-voltage protection and positive high voltage derivative protection by generating a firing signal to the gate of thyristor when the preset protective firing level or recovery protective firing level is reached.This additional over-voltage protection is a local protection on each individual thyristor level without involving the remote valve control unit. This protection function secures the thyristor when one or several fibre optic communications are broken or valve internal fault arises.III.D IELECTRIC T ESTA. Tests on a fully assembled single valveThese tests are performed on a complete valve equipped with eight thyrisitor modules. All valve components, except the valve surge arrester, in actual site installation are presented. The tests and test parameters in the single valve test are as follows:• d.c. voltage: ±320kV, 1 min. followed by ±260kV, 3 h (dry valve);• d.c. voltage: ±260kV, 1 min. followed by ±160kV, 5 min.(wet valve);• a.c. voltage: 273kV, 15 sec. followed by 187kV, 15 min.; •Switching impulse voltage: ±425kV, 5 shots each polarity (dry valve + wet valve);•Lightning impulse voltage: ±420kV, 5 shots each polarity (hot valve);•Seep-front impulse voltage: ±471kV, 5 shots each polarity (hot valve);•Non-periodic firing test: 5.3kA, 5 shots.Test demonstrated that the valve protective firing took place between +427kV and +428kV, immediately above the surge protection withstand level.Test results were quite a satisfactory and all tests were passed without any thyristor failure or component degradation.B. Tests on valve hanging structuresTwo valve hanging structures, differing mainly in length of insulators, are used in this project to suspend the low voltage (LV) MVUs (0 – 400kV d.c. voltage) and high voltage (HV) MVUs (400kV – 800kV d.c. voltage) respectively. The testprogram and test parameters adopted in the dielectric test of hanging structures are as follows:For HV MVU hanging structure • d.c. voltage: ±979·k atm kV, 1 min. followed by ±796kV,3 h;• Switching impulse voltage: ±1161·k atm kV, 5 shots eachpolarity;• Lightning impulse voltage: ±1270·k atm kV, 5 shots eachpolarity.For LV MVU hanging structure• d.c. voltage: ±326·k atm kV, 1 min. followed by ±265kV,3 h; • a.c. voltage: 310·k atm kV, 1 min. followed by 211kV, 30 min.;•• Switching impulse voltage: ±558·k atm kV, 5 shots eachpolarity;• Lightning impulse voltage: ±594·k atm kV, 5 shots eachpolarity; •Seep-front impulse voltage: ±454·k atm kV, 5 shots each polarity.C. Tests on MVUsThe immediate connection of two single valves and tower design of MVU in ABB’s valve structure physically waive the dielectric concern between two single valves as the dielectric strength between the two immediate connected layers of two single valves is lower than the one in single valve whenever in operation or in test. The MVU dielectric test therefore focused on the verification of external insulation of MVU, i.e, voltage withstanding capability of MVU to its surroundings and the verification of the partial discharge levels are within specified limits. To achieve this target the pole potential MVU was installed. For a correct voltage distribution representation in MVU test the other MVUs are substituted by valve simulators in test.Different valve simulators are used in different test duties in order to create the correct voltage on the low potential terminal of test MVU. A pure water cooled d.c. resistor was used in the MVU d.c. voltage test, a capacitor was used in the MVU switching impulse voltage test and a group of series connected valve reactors were used in MVU lightning impulse voltage test.Because the lightning impulse voltage isn’t decisive for the determination of air clearance in ultra high voltage no lightning impulse voltage test was done on the HV MVU. Considering the LV MVUs (0 – 400kV) and HV MVUs (400kV – 800kV) are installed in different valve halls and have different air clearances in the design, test on MVU was repeated under different test arrangements and test levels. LV MVU was lightning impulse voltage tested.The voltage levels used in the HV MVU and LV MVU dielectric test are listed below.For HV MVU • d.c. voltage: ±1306·k atm kV at high potential terminal and> ±980·k atm kV at low potential terminal, 1 min. followedby ±1061 kV at high potential terminal and > ±796 kV atlow potential terminal, 3 h;• Switching impulse voltage: ±1600·k atm kV at high voltageterminal and > ±1161·k atm kV at low voltage terminal,5 shots each polarity.For LV MVU• d.c. voltage: ±653·k atm kV at high potential terminal and> ±327·k atm kV at low potential terminal, 1 min. followed by ±530kV at high potential terminal and > ±265kV at low potential terminal, 3 h;Switching impulse voltage: ±788·k atm kV at high potential terminal and > ±558·k atm kV at low potential terminal, 5 shots each polarity;• Lightning impulse voltage: ±863·k atm kV at high potentialterminal and > ±594·k atm kV at low potential terminal,5 shots each polarity.Figure 2 A high voltage MVU installed in the high voltage laboratory fordielectric testIV. O PERATIONAL T ESTValve operational test was performed on the thyristor modules in a 6-pulse Back-to-Back based synthetic test circuit, Figure 3, [4] [5]. Eight modules in one single thyristor valve have been type tested in four test set-ups respectively. Most of the test duties were conducted on two thyristor modules in every set-up. Maximum operating duty (α=90ο) test, tests with transient forward voltage during the recovery period and valve fault currents tests were performed on one thyristor module each time.The project design specification, Table 1, is used to determine the test parameters in the synthetic operational test. The test results reported in Table 2 are the values applied on one thyristor module. All the test duties reported in Table 2started with a 10 minutes preheating with the heat-run test parameter after the specified test module(s) inlet cooling water is reached.GFigure 3 6-pulse back-to-back based synthetic test circuit1μs, 10μs and 100μs front time impulses are used in the tests of transient forward voltage during recovery period. Five impulses of each type were applied in the recovery protection (RP) zone of thyristor control unit (TCU) and three additional impulses were applied immediately after the RP zone.The objectives of the last test in Table 2, continuous protective firing, are to demonstrate the capability of continuous protective firing of TCU and the thermal capability of snubber circuit when fiber optic communication from ground potential valve control unit (VCU) to thyristor valve fails. This test is performed on one set-up with one pair of fiber optic disconnected to one thyristor position.Test oscillograms acquired in the heat-run test, transient forward voltage during recovery period and one-loop fault current with subsequent blocking voltage are illustrated in Figure 4, Figure and Figure 6. The upper red colored curve in those oscillograms is the test voltage across the test module and the lower blue colored curve is the test current.Figure 4 Periodic firing and extinction test / heat-run test (test set-up with twothyristor modules)V.T ESTS FOR V ALVE I NSENSITIVITY TO E LECTROMAGNETICD ISTURBANCEThose tests were combined in the dielectric test in section III.Figure 5 One-loop fault current with subsequent blocking voltage test[1][2][3]Baoliang Sheng, Hans-Ola. Bjarme and Hans Johansson, “ReliabilityEnhancement of HVDC Transmission by Standardization Thyristor Valves and Valve Testing”, in Proc. of the 6th International Conf. on Power T&D Technologies on Nov.10-12, 2007, Guangzhou, China[4]Baoliang Sheng, Hans-Ola. Bjarme, Pierre Riffon and Weimin Ma,“Operational Tests of the Three-Gorges ⎯ Changzhou HVDC Thyristor Valves by Using a Synthetic Test Circuit”, in Proc. of Cigré ICPS’2001 Conf. on Sep.03-05, 2001, Wuhan, China[5]Baoliang Sheng and Hans-Ola, “Proof of Performance ⎯ A synthetictest circuit for verifying HVDC thyristor valve design,” in ABB Review 03/2003, pp. 25 - 29VIII.B IOGRAPHIESBaoliang Sheng obtained his B.Sc degree from Xi’an Jiaotong University, China and his Ph.D. from Delft University of Technology, The Netherlands, in 1982 and 1995 respectively. From 1982 to 1992 he was a test and research engineer in XIHARI, China. He was a research engineer in KEMA, the Netherlands, and pursued his Ph.D. at Delft University of Technology from 1992 to 1996. He joined ABB Ludvika, Sweden in May 1996. He is Company Specialist in High Power Testing of Electrical Power Equipment and Senior Specialist in Design and Testing of HVDC Converter Valves and SVC Valves. He is Chairman of IEC TC115 Swedish subcommittee, convenor of IEC Strategic Group (SG2) on the standardization of ultra high voltage technologies, convenor of IEC SC22F WG15 on electrical testing of Voltage Sourced Converter (VSC) valves and member of several working groups in IEC SC22F and IEC TC33. He is Senior Member of IEEE.Jonatan Dainelssion obtained his Master degree in electrical engineering from Uppsala University, Sweden in 2007. He joined ABB Power Systems, HVDC, Ludvika, Sweden in September 2007 as thyristor valve design and test engineer.Yanny Fu graduated with her Master Degree from Xi’an Jiaotong University in 1985 in China and obtained her PhD degree from Technical University of Eindhoven in 1990 in The Netherlands. Since then, she has been working at KEMA and is a senior leading consultant at present. She is regular member Cigré SC B4 “HVDC and Power Electronics”, chair of Dutch national Cigré committee NSC B4, convennor of Cigré working group B4-51 “Study of Converter Voltage Transients Imposed on the HVDC converter transformers”, Dutch Member of IEC TC115 “HVDC systems above 100kV”. Dr. Fu received the Cigré technical committee award in 2007 for her “remarkable contribution to the work of Cigré Study Committee B4: HVDC and Power Electronics”.Zehong Liu obtained his Master degree in electrical engineering from China Electrical Power Research Institute (CEPRI), China, in 1984. He jointed State Grid Corporation of China (SGCC) after graduation. He is deputy secretary of IEC TC115, convenor of IEC TC115 WG1 on the design of ground electrodes for HVDC links and active member in several Cigré and IEC working groups dealing with ultra high voltage power transmission. He is deputy director general of Ultra High Voltage Construction Department of SGCC.Table 2 Operational test parameters per thyristor moduleTest Duty Duration I dc(A) I fault(kA)U p(kV)U f(kV)U r(kV)U imp(kV)Heat-run test 60 min. ≥ 4200 ≥ 22.1 ≥ 22.110 sec. ≥ 1400 ≥ 27.9 ≥ 27.9Max. temporary operating duty (α=90ο) test2 sec. ≥ 1470 ≥ 48.0Min. delay angle test 1 15 min. ≥ 4200 ≤ 4.9Min. delay angle test 2 1 min. ≥ 4200 ≤ 2.1Min. extinction angle test 1 15 min. ≥ 4200 ≤ 6.5Min. extinction angle test 2 1 min. ≥ 4200 ≤ 5.12 min. < 400 ≤ 4.9 Intermittent direct current tests10 sec. < 400 ≥ 33.3Tests with transient forward voltage during therecovery period≥ 4200 ≤ 6.5 ≥ 50.0One-loop fault current with re-applied forwardvoltage≥ 4200 ≥ 40.8 ≥ 28.2Three-loop fault current without re-appliedforward voltage≥ 4200 ≥ 41.7 ≥ 17.0 Continuous protective firing* 120 min. ≥ 4200 ≥ 22.1 ≥ 22.1 U p⎯ recovery voltage peak, including over-shoot U r⎯ power frequency recovery voltage peak in reverse directionU f⎯ forward voltage prior to the firing instant U imp⎯ forward transient voltage peak during recovery* test on one thyristor module only。