【2014】EPI,哺乳动物细胞的非CG位点的甲基化
表观遗传:组蛋白甲基化、磷酸化解决方案
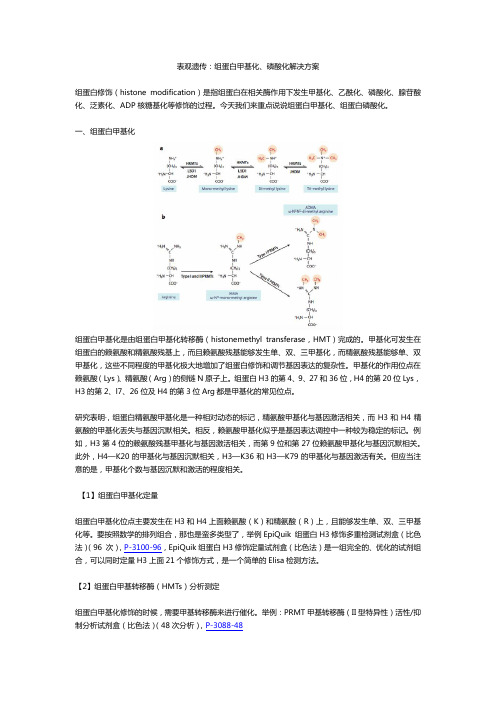
表观遗传:组蛋白甲基化、磷酸化解决方案组蛋白修饰(histone modification)是指组蛋白在相关酶作用下发生甲基化、乙酰化、磷酸化、腺苷酸化、泛素化、ADP核糖基化等修饰的过程。
今天我们来重点说说组蛋白甲基化、组蛋白磷酸化。
一、组蛋白甲基化组蛋白甲基化是由组蛋白甲基化转移酶(histonemethyl transferase,HMT)完成的。
甲基化可发生在组蛋白的赖氨酸和精氨酸残基上,而且赖氨酸残基能够发生单、双、三甲基化,而精氨酸残基能够单、双甲基化,这些不同程度的甲基化极大地增加了组蛋白修饰和调节基因表达的复杂性。
甲基化的作用位点在赖氨酸(Lys)、精氨酸(Arg)的侧链N原子上。
组蛋白H3的第4、9、27和36位,H4的第20位Lys,H3的第2、l7、26位及H4的第3位Arg都是甲基化的常见位点。
研究表明·,组蛋白精氨酸甲基化是一种相对动态的标记,精氨酸甲基化与基因激活相关,而H3和H4精氨酸的甲基化丢失与基因沉默相关。
相反,赖氨酸甲基化似乎是基因表达调控中一种较为稳定的标记。
例如,H3第4位的赖氨酸残基甲基化与基因激活相关,而第9位和第27位赖氨酸甲基化与基因沉默相关。
此外,H4—K20的甲基化与基因沉默相关,H3—K36和H3—K79的甲基化与基因激活有关。
但应当注意的是,甲基化个数与基因沉默和激活的程度相关。
【1】组蛋白甲基化定量组蛋白甲基化位点主要发生在H3和H4上面赖氨酸(K)和精氨酸(R)上,且能够发生单、双、三甲基化等。
要按照数学的排列组合,那也是蛮多类型了,举例EpiQuik 组蛋白H3修饰多重检测试剂盒(比色法)(96 次),P-3100-96,EpiQuik组蛋白H3修饰定量试剂盒(比色法)是一组完全的、优化的试剂组合,可以同时定量H3上面21个修饰方式,是一个简单的Elisa检测方法。
【2】组蛋白甲基转移酶(HMTs)分析测定组蛋白甲基化修饰的时候,需要甲基转移酶来进行催化。
表 观 遗 传 学
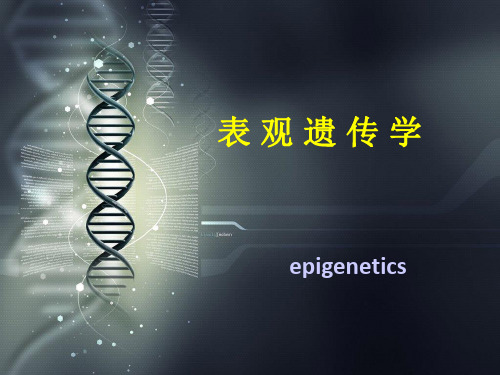
样本进行分析,可以开发历史上贮备的大量病理学资源。
组蛋白修饰
染色质蛋白并非只是一种包装蛋白,而是在DNA和细胞其他 组分之间构筑了一个动态的功能界面。
对植物研究发现miRNA 可诱导PHB基因甲基 化及染色质重塑。
DNA甲基化的生物学意义
调控基因表达, 在胚胎发育、细胞生长分化,衰老, 疾病等方面发挥重要作用。
维持染色体结构 X染色体失活 基因印记 肿瘤发生发展
DNA甲基化的检测方法
1.甲基化敏感的限制性内切酶法 2.基于重亚硫酸氢盐修饰的方法 3.基于甲基化DNA特异结合富集方法
相反,HDAC可移去乙酰基使组蛋白去乙酰化,稳定核 小体结构,诱导核小体聚集,形成更高级的染色体结构, 并抑制基本转录复合体组装,从而抑制转录。
组蛋白的甲基化
1. 主要发生在赖氨酸(K)或精氨酸(R)上;
2. Long-term;
3. HKMTs (histone lysine methyltransferases) vs. PRMTs (protein arginine methyltransferases)
MeCP2
Model for methylation-dependent gene silencing. The structural element of chromatin is the nucleosomal core, which consists of a 146-bp DNA sequence
5
4 SAM
DNMT
dCMP (~C~)
表观组学学习笔记-01表观遗传学简介
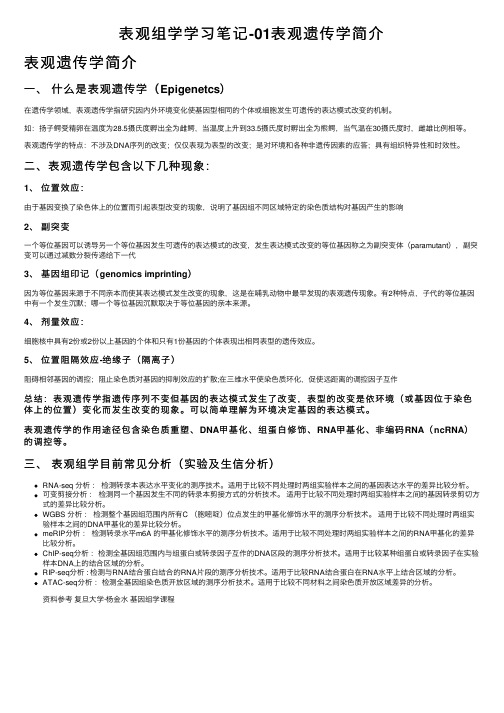
表观组学学习笔记-01表观遗传学简介表观遗传学简介⼀、什么是表观遗传学(Epigenetcs)在遗传学领域,表观遗传学指研究因内外环境变化使基因型相同的个体或细胞发⽣可遗传的表达模式改变的机制。
如:扬⼦鳄受精卵在温度为28.5摄⽒度孵出全为雌鳄,当温度上升到33.5摄⽒度时孵出全为熊鳄,当⽓温在30摄⽒度时,雌雄⽐例相等。
表观遗传学的特点:不涉及DNA序列的改变;仅仅表现为表型的改变;是对环境和各种⾮遗传因素的应答;具有组织特异性和时效性。
⼆、表观遗传学包含以下⼏种现象:1、位置效应:由于基因变换了染⾊体上的位置⽽引起表型改变的现象,说明了基因组不同区域特定的染⾊质结构对基因产⽣的影响2、副突变⼀个等位基因可以诱导另⼀个等位基因发⽣可遗传的表达模式的改变,发⽣表达模式改变的等位基因称之为副突变体(paramutant),副突变可以通过减数分裂传递给下⼀代3、基因组印记(genomics imprinting)因为等位基因来源于不同亲本⽽使其表达模式发⽣改变的现象,这是在哺乳动物中最早发现的表观遗传现象。
有2种特点,⼦代的等位基因中有⼀个发⽣沉默;哪⼀个等位基因沉默取决于等位基因的亲本来源。
4、剂量效应:细胞核中具有2份或2份以上基因的个体和只有1份基因的个体表现出相同表型的遗传效应。
5、位置阻隔效应-绝缘⼦(隔离⼦)阻碍相邻基因的调控;阻⽌染⾊质对基因的抑制效应的扩散;在三维⽔平使染⾊质环化,促使远距离的调控因⼦互作总结:表观遗传学指遗传序列不变但基因的表达模式发⽣了改变,表型的改变是依环境(或基因位于染⾊体上的位置)变化⽽发⽣改变的现象。
可以简单理解为环境决定基因的表达模式。
表观遗传学的作⽤途径包含染⾊质重塑、DNA甲基化、组蛋⽩修饰、RNA甲基化、⾮编码RNA(ncRNA)的调控等。
三、表观组学⽬前常见分析(实验及⽣信分析)RNA-seq 分析:检测转录本表达⽔平变化的测序技术。
适⽤于⽐较不同处理时两组实验样本之间的基因表达⽔平的差异⽐较分析。
DNA甲基化检测技术全攻略
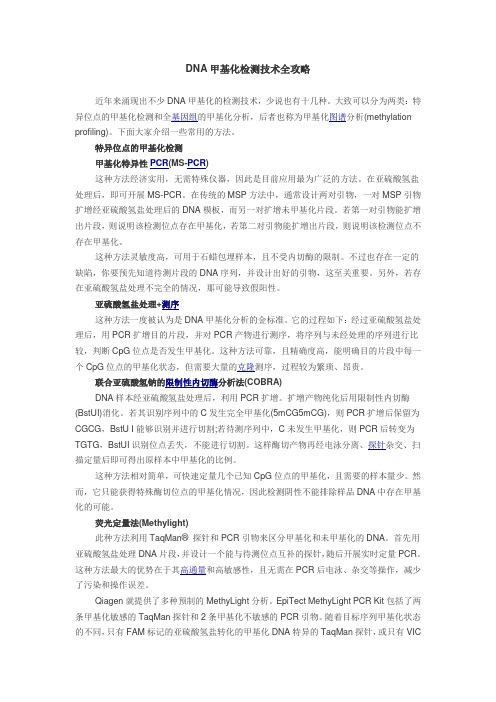
DNA甲基化检测技术全攻略近年来涌现出不少DNA甲基化的检测技术,少说也有十几种。
大致可以分为两类:特异位点的甲基化检测和全基因组的甲基化分析,后者也称为甲基化图谱分析(methylation profiling)。
下面大家介绍一些常用的方法。
特异位点的甲基化检测甲基化特异性PCR(MS-PCR)这种方法经济实用,无需特殊仪器,因此是目前应用最为广泛的方法。
在亚硫酸氢盐处理后,即可开展MS-PCR。
在传统的MSP方法中,通常设计两对引物,一对MSP引物扩增经亚硫酸氢盐处理后的DNA模板,而另一对扩增未甲基化片段。
若第一对引物能扩增出片段,则说明该检测位点存在甲基化,若第二对引物能扩增出片段,则说明该检测位点不存在甲基化。
这种方法灵敏度高,可用于石蜡包埋样本,且不受内切酶的限制。
不过也存在一定的缺陷,你要预先知道待测片段的DNA序列,并设计出好的引物,这至关重要。
另外,若存在亚硫酸氢盐处理不完全的情况,那可能导致假阳性。
亚硫酸氢盐处理+测序这种方法一度被认为是DNA甲基化分析的金标准。
它的过程如下:经过亚硫酸氢盐处理后,用PCR扩增目的片段,并对PCR产物进行测序,将序列与未经处理的序列进行比较,判断CpG位点是否发生甲基化。
这种方法可靠,且精确度高,能明确目的片段中每一个CpG位点的甲基化状态,但需要大量的克隆测序,过程较为繁琐、昂贵。
联合亚硫酸氢钠的限制性内切酶分析法(COBRA)DNA样本经亚硫酸氢盐处理后,利用PCR扩增。
扩增产物纯化后用限制性内切酶(BstUI)消化。
若其识别序列中的C发生完全甲基化(5mCG5mCG),则PCR扩增后保留为CGCG,BstU I能够识别并进行切割;若待测序列中,C未发生甲基化,则PCR后转变为TGTG,BstUI识别位点丢失,不能进行切割。
这样酶切产物再经电泳分离、探针杂交、扫描定量后即可得出原样本中甲基化的比例。
这种方法相对简单,可快速定量几个已知CpG位点的甲基化,且需要的样本量少。
《细胞生物学》学习题解答
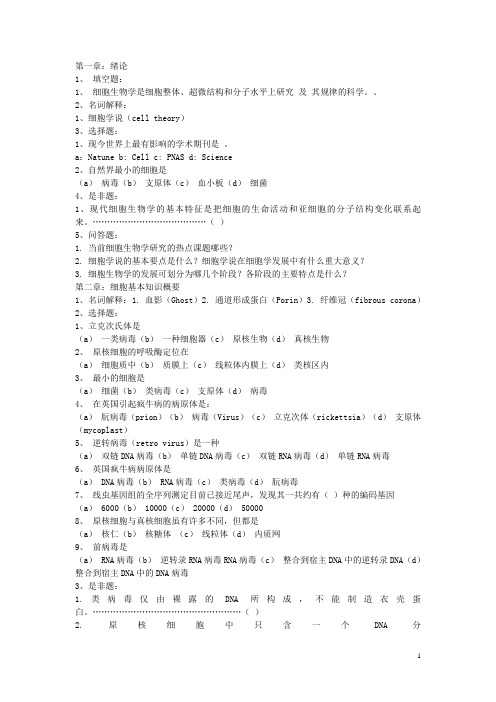
第一章:绪论1、填空题:1、细胞生物学是细胞整体、超微结构和分子水平上研究及其规律的科学。
、2、名词解释:1、细胞学说(cell theory)3、选择题:1、现今世界上最有影响的学术期刊是。
a:Natune b: Cell c: PNAS d: Science2、自然界最小的细胞是(a)病毒(b)支原体(c)血小板(d)细菌4、是非题:1、现代细胞生物学的基本特征是把细胞的生命活动和亚细胞的分子结构变化联系起来。
…………………………………()5、问答题:1. 当前细胞生物学研究的热点课题哪些?2. 细胞学说的基本要点是什么?细胞学说在细胞学发展中有什么重大意义?3. 细胞生物学的发展可划分为哪几个阶段?各阶段的主要特点是什么?第二章:细胞基本知识概要1、名词解释:1. 血影(Ghost)2. 通道形成蛋白(Porin)3. 纤维冠(fibrous corona)2、选择题:1、立克次氏体是(a)一类病毒(b)一种细胞器(c)原核生物(d)真核生物2、原核细胞的呼吸酶定位在(a)细胞质中(b)质膜上(c)线粒体内膜上(d)类核区内3、最小的细胞是(a)细菌(b)类病毒(c)支原体(d)病毒4、在英国引起疯牛病的病原体是:(a)朊病毒(prion)(b)病毒(Virus)(c)立克次体(rickettsia)(d)支原体(mycoplast)5、逆转病毒(retro virus)是一种(a)双链DNA病毒(b)单链DNA病毒(c)双链RNA病毒(d)单链RNA病毒6、英国疯牛病病原体是(a) DNA病毒(b) RNA病毒(c)类病毒(d)朊病毒7、线虫基因组的全序列测定目前已接近尾声,发现其一共约有()种的编码基因(a) 6000(b) 10000(c) 20000(d) 500008、原核细胞与真核细胞虽有许多不同,但都是(a)核仁(b)核糖体(c)线粒体(d)内质网9、前病毒是(a) RNA病毒(b)逆转录RNA病毒RNA病毒(c)整合到宿主DNA中的逆转录DNA(d)整合到宿主DNA中的DNA病毒3、是非题:1.类病毒仅由裸露的DNA所构成,不能制造衣壳蛋白。
表观遗传学&基因与基因组学

第十三章 表观遗传学第一节 概 述基因的表达相同的基因型不同的表型:一.表观遗传学(epigenetic)DNA的序列不发生变化、基因表达改变、并且这种改变可稳定遗传。
二.表观遗传学研究的内容:1.基因选择性转录、表达的调控。
2.基因转录后调控。
(表观遗传通常被定义为DNA的序列不发生变化但是基因表达却发生了可遗传的改变,也就是说基因型未变化而表型却发生了改变,这种变化是细胞内除了遗传信息以外的其他可遗传物质的改变,并且这种改变在发育和细胞增殖的过程中能稳定的传递下去。
表观遗传学研究内容具体来说主要包括DNA甲基化表观遗传、染色质表观遗传、表观遗传基因表达调控、表观遗传基因沉默、细菌的限制性基因修饰等。
从更加广泛的意义上来说,DNA甲基化、组蛋白甲基化和乙酰化、基因沉默、基因组印记、染色质重塑、RNA剪接、RNA编辑、RNA干扰、x染色体失活等等都可以归入表观遗传学的范畴,而其中任何一个过程的异常都将影响基因结构以及基因表达,导致某些复杂综合症、多因素疾病或癌症。
) 三.表观遗传修饰从多个水平上调控基因表达:1.RNA水平:非编码RNA可通过某些机制实现对基因转录以及转录后的调控,例如microRNA、RNA干扰等2.蛋白质水平:通过对蛋白质的修饰或改变其构象实现对基因表达的调控,例如组蛋白修饰3.染色质水平:通过染色质位置、结构的变化实现对基因表达的调控,例如染色质重塑以上几个水平之间相互关联,任何一方面的异常都将影响染色质结构和基因表达。
四.表观遗传学的研究意义:1.表观遗传学补充了“中心法则”所忽略的两个问题,即哪些因素决定了基因的正常转录和翻译以及核酸并不是存储遗传信息的唯一载体。
2.表观遗传信息可以通过控制基因的表达时间、空间和方式来调控各种生理反应。
所以许多用DNA序列不能解释的现象都能够找到答案。
3.与DNA序列的改变不同,许多表观遗传的改变是可逆的,这使表观遗传疾病的治愈成为可能。
表观遗传学:家畜遗传育种的新挑战
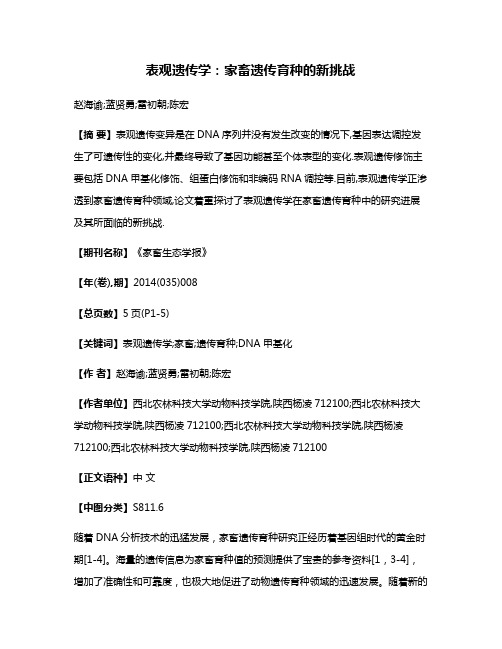
表观遗传学:家畜遗传育种的新挑战赵海谕;蓝贤勇;雷初朝;陈宏【摘要】表观遗传变异是在DNA序列并没有发生改变的情况下,基因表达调控发生了可遗传性的变化,并最终导致了基因功能甚至个体表型的变化.表观遗传修饰主要包括DNA甲基化修饰、组蛋白修饰和非编码RNA调控等.目前,表观遗传学正渗透到家畜遗传育种领域,论文着重探讨了表观遗传学在家畜遗传育种中的研究进展及其所面临的新挑战.【期刊名称】《家畜生态学报》【年(卷),期】2014(035)008【总页数】5页(P1-5)【关键词】表观遗传学;家畜;遗传育种;DNA甲基化【作者】赵海谕;蓝贤勇;雷初朝;陈宏【作者单位】西北农林科技大学动物科技学院,陕西杨凌712100;西北农林科技大学动物科技学院,陕西杨凌712100;西北农林科技大学动物科技学院,陕西杨凌712100;西北农林科技大学动物科技学院,陕西杨凌712100【正文语种】中文【中图分类】S811.6随着DNA分析技术的迅猛发展,家畜遗传育种研究正经历着基因组时代的黄金时期[1-4]。
海量的遗传信息为家畜育种值的预测提供了宝贵的参考资料[1,3-4],增加了准确性和可靠度,也极大地促进了动物遗传育种领域的迅速发展。
随着新的研究技术与方法层出不穷,家畜遗传育种学家们将主要精力聚焦在了重要家畜的全基因组测序分析上[5-7],如:猪、牛、绵羊、山羊、鸡、鸭等,以争取进一步提高基因组选择和QTL定位的准确性[3,8-9]。
然而到目前为止,仍然有一个崭新而且具有广阔前景的领域未能在家畜遗传育种中得到广泛的研究和有效的应用,即表观遗传学(Epigenetics)领域[10-13]。
为此,论文重点讨论了表观遗传学及其在家畜遗传育种中的研究进展以及所面临的新挑战。
表观遗传学是英文“Epigenetics”的中文翻译,在“Epigenetics”这个英文单词中,“Epi”源自于希腊语,表示“越过、超出、在…之外”之意[13-14]。
EpiMethy DNA 甲基化检测试剂 说明书
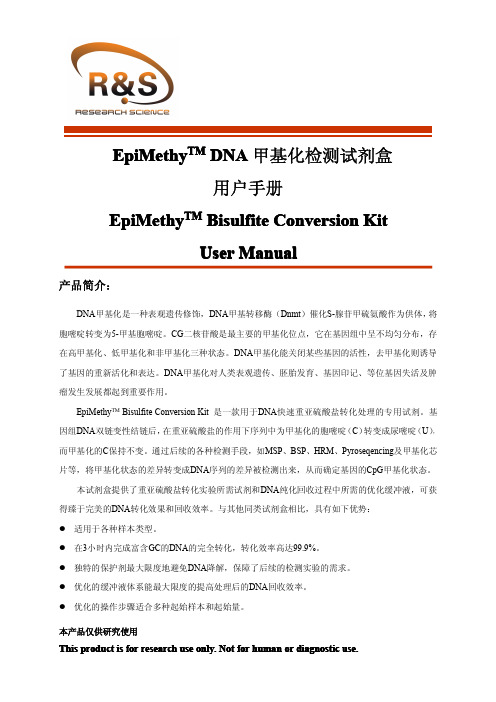
EpiMethy TM DNA甲基化检测试剂盒用户手册EpiMethy TM B isulfite Conversion KitUser Manual产品简介:DNA甲基化是一种表观遗传修饰,DNA甲基转移酶(Dnmt)催化S-腺苷甲硫氨酸作为供体,将胞嘧啶转变为5-甲基胞嘧啶。
CG二核苷酸是最主要的甲基化位点,它在基因组中呈不均匀分布,存在高甲基化、低甲基化和非甲基化三种状态。
DNA甲基化能关闭某些基因的活性,去甲基化则诱导了基因的重新活化和表达。
DNA甲基化对人类表观遗传、胚胎发育、基因印记、等位基因失活及肿瘤发生发展都起到重要作用。
EpiMethy TM Bisulfite Conversion Kit是一款用于DNA快速重亚硫酸盐转化处理的专用试剂。
基因组DNA双链变性结链后,在重亚硫酸盐的作用下序列中为甲基化的胞嘧啶(C)转变成尿嘧啶(U),而甲基化的C保持不变。
通过后续的各种检测手段,如MSP、BSP、HRM、Pyroseqencing及甲基化芯片等,将甲基化状态的差异转变成DNA序列的差异被检测出来,从而确定基因的CpG甲基化状态。
本试剂盒提供了重亚硫酸盐转化实验所需试剂和DNA纯化回收过程中所需的优化缓冲液,可获得臻于完美的DNA转化效果和回收效率。
与其他同类试剂盒相比,具有如下优势:�适用于各种样本类型。
�在3小时内完成富含GC的DNA的完全转化,转化效率高达99.9%。
�独特的保护剂最大限度地避免DNA降解,保障了后续的检测实验的需求。
�优化的缓冲液体系能最大限度的提高处理后的DNA回收效率。
�优化的操作步骤适合多种起始样本和起始量。
本产品仅供研究使用产品包装:EpiMethy TM B isulfite Conversion Kit(Kit Size)MD2301-1(10次)MD2301-5(50次)M-Conversion Reagent1Tube5TubesM-Dilution Buffer300ul 1.5mlM-Dissolving Buffer100ul500ulM-Binding Buffer6ml30mlM-Wash Buffer6ml30mlM-Desulphonation Buffer2ml10mlM-Elution Buffer400ul2mlF-Spin Columns10ct.50ct.Collection Tubes10ct.50ct.Instruction Manual11贮藏条件:室温保存。
分子遗传学第10章
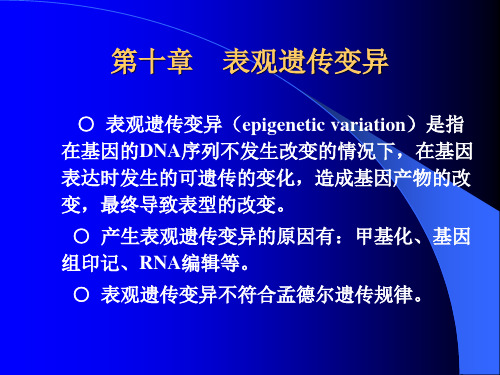
(二)原核细胞的DNA甲基化
○ 限制修饰系统
细菌能编码一种内切核酸酶,切断外源的DNA,建 立起一种自身保护机制。细菌又通过使内切核酸酶识别 位点中的特定碱基甲基化,来保护自身DNA免受内切核 酸酶的酶解。因而,每种DNA内切酶都有一种相关甲基 化酶。由此构成一个限制修饰系统。
○ Dam 甲基化
DNA腺嘌呤甲基化酶(Dam)GATC序列中A的甲基 化,使细胞识别DNA复制后母链和尚未甲基化的子链, 子链的非甲基化将指导修复酶从子链上切除错误的核苷 酸而不是母链上正确的核苷酸。
三 X染色体失活
(一)剂量补偿效应——转录速率的调节
○ 剂量补偿效应是指在XY型性别决定的生物中,性 连锁基因在XX和XY两种性别有相等或近乎相等的遗传效 应。就是说,在雌性和雄性个体中,由X染色体上基因编 码的酶(蛋白质)在数量上是相等或近乎相等的。 ○ 剂量补偿效应的机制之一——X染色体转录速率的 调节。 雌果蝇两条X染色体都有活性,但转录速率只是雄果 蝇X染色体的50%。主要是通过Sxl蛋白阻止雌性X染色体 过度表达来实现的。
○ 印记就是DNA的甲基化。目前在人和小鼠 身上已发现20多种印记基因。
图10-1 互交中亲本印记的效应
基因组印迹的机制是在配子形成过程中被打 上标记,通常是DNA甲基化。 图10-2 两个成熟的雌雄个体带有一个来自 母方的印迹基因和一个来自父方的正常基因。 1 胚胎和成熟体细胞中基因甲基化永久维持; 2 种系细胞中基因去甲基化; 3 雌性种系细胞中基因被重新甲基化; 4 精子带正常基因,卵子带甲基化印迹基因。
高考生物二轮复习微专题4 基因表达的调控

类型一
[解析]基因的甲基化不会改变基因的碱基排列顺序,A错误; 由柱形图可知,DNA甲基化后,基因转录产物mRNA减少,因此甲基化影响转录过程, 间接影响翻译过程,B错误; 由图可知,相比于广灵大尾羊,湖羊组第33和63位点上的甲基化程度较高,其余位点 甲基化程度相同,因此第33和63位点上的甲基化差异是影响AGPAT2基因表达量的 关键因素,C正确; 相比于广灵大尾羊,湖羊AGPAT2基因甲基化程度较高,转录受阻,该基因表达量较 小,由此可知,两种羊中AGPAT2基因的甲基化程度与其在脂肪组织中的表达量呈 负相关,D错误。
类型一
例2. [2023·河北石家庄一模] 某植物5号染色体上产生物碱基因B对不产生物碱基
因b为显性;7号染色体上从头甲基化酶基因D对无从头甲基化酶基因d为显性;甲基化 维持酶基因E对无甲基化维持酶基因e为显性,但所在染色体未知。从头甲基化酶可 将基因一条链带上甲基化,称为半甲基化;甲基化维持酶可将半甲基化状态下对应的 另一条链带上甲基化,称为全甲基化。具体过程如图Ⅰ所示。已知全甲基化的基因 无法表达,且基因甲基化会在减数分裂时消失。为研究甲基化相关酶对B/b基因甲基 化和基因表达的影响,科学家做了相关实验,如图Ⅱ所示(不考虑基因突变和染色体互 换)。回答下列问题:
于一对同源染色体上,根据题意,D和e连锁,d和E连锁,根据基因的自由组合定律,则
F1产生的配子种类及比例应为BdE∶BDe∶bDe∶bdE=1∶1∶1∶1。
类型一
(3)若F2表型及比例为产生物碱植株∶不产生物碱植株=1∶1。让F1自交,其后
代中含B基因但不产生物碱的植株占比为 3/8 。
[解析] (3)若F2表型及比例为产生物碱植株∶不产生物碱植株=1∶1,则F1的配子及 比例为Bde∶BDe∶bDE∶bdE=1∶1∶1∶1,即B与e连锁,b与E连锁,让F1自交,其后代 中含B基因但不产生物碱的植株基因型及比例为BbDDEe+BbDdEe=2/16+4/16=3/8。
hrm甲基化位点检测的敏捷性[常识]
![hrm甲基化位点检测的敏捷性[常识]](https://img.taocdn.com/s3/m/748c46226d175f0e7cd184254b35eefdc8d31564.png)
hrm甲基化位点检测的敏捷性[常识] HRM甲基化位点检测的灵敏性点击次数:142 发布日期:2010-10-12 来源:本站仅供参考,谢绝转载,否则责任自负利用LightScanner上的高分辨熔解曲线进行DNA甲基化位点检测的灵敏性引言DNA甲基化是一种重要的表观遗传CpG二核苷酸修饰形式,异常的DNA甲基化模式发生在许多基因启动子的CpG岛,这会增加患癌症的风险。
CpG岛高甲基化常导致基因沉默,与癌症、老化、基因印记、X-染色体失活密切相关,此外,全基因组CpG高甲基化中也证实了发生在肿瘤形成的早期。
传统的检测甲基化位点的方法是通过重亚硫酸盐处理,处理后原甲基化的胞嘧啶被保留,而非甲基化的胞嘧啶转变为胸腺嘧啶,然后进行直接测序。
甲基化的胞嘧啶没有发生改变,因此通过测序很容易识别。
本研究中我们系统的评价了通过LightScanner的高分辨溶解曲线检测甲基化的可靠性。
如果这种方法在检测甲基化中是可靠且灵敏度高,就可以减少测序的繁琐过程。
未处理的和经过SssI处理的pBluescript II SK(+)质粒用Qiagen EpiTect试剂盒进行重亚硫酸盐。
通过对有代表性的区域进行测序,确保样品完全甲基化以及亚硫酸盐处理。
引物设计在扩增片段大小在79-216bp的含有1-8个CpG位点的7个不同的片段。
100%甲基化的和未甲基化的样品按50%, 40%, 30%, 20%, 15%, 10%, 5%, 2%的比例混合,100%甲基化的和未甲基化的样品可以明显的区分开。
当两个样品按以上比例混合后,熔解曲线表现出重复的剂量放映关系,当50%混合时与未甲基化的标准品偏性最大。
高分辨溶解曲线的灵敏度足够检测甲基化位点,对小片段的灵敏度可达到2%,大片段的可以10%。
重要的DNA甲基化位点显示在B淋巴细胞增生性疾病,如慢性淋巴细胞白血病(CLL)中。
瘤抑制基因的外部沉默可以通过DNA甲基化抑制剂处理而发生转变。
DNA甲基化和癌症培训课件
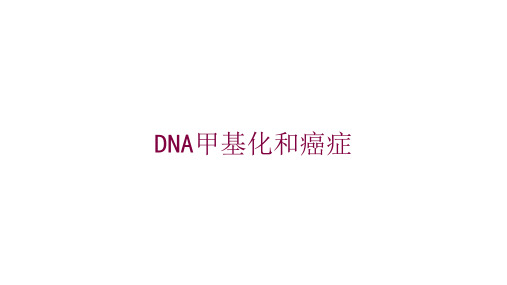
后者——怎么建、何时建、在哪建的附加信息
基因组不仅仅是序列包含遗传信息,而且其修饰也可以
记载遗传信息。
DNA甲基化和癌症
14
表观遗传的特点:广泛,多样, 复杂
DNA sequence
coding
Epigenetic programming
DNA甲基化和癌症
15
表观遗传学目前的主要研究发现
DNA甲基化 染色质重塑 RNA调控(RNA干扰,
他们往往在长大成人后在性格、健康方面往往
存在很大差异。
DNA甲基化和癌症
6
现象3: 肿瘤抑制基因被过量地甲基化而导致失去活性,而基因 的DNA序列并不发生变化。
DNA甲基化和癌症
7
现象4: 橘生淮南则为橘,生于淮北则为枳,叶徒相似, 其实味不同。同一种水果,在不同产地生长,其味 道,大小,外观可能相差很远。
DNA 甲基化
凋亡
DNA甲基化和癌症
基因表达的调节
分化
26
DNA甲基化的生物学意义
DNA甲基化直接影响基因的活化状态 DNA甲基化的生物学意义在于: A、 基因表达的时空调控 B、保护基因组 的稳定性
DNA甲基化和癌症
➢ 表观遗传学与DNA甲基化 ➢ DNA甲基化的检测手段 ➢ DNA甲基化与癌症
DNA甲基化和癌症
2
一、表观遗传学与DNA甲基化
DNA甲基化和癌症
3
DNA甲基化和癌症
4
现象1: 一个生物体的不同组织基因表达模式截然不同
表达模式迥异
基因型相同
DNA甲基化和癌症
5
现象2: 同卵双生的孪生子具有完全相同的基因组。但
基因选 择表达
细胞命运
ABO_正反定型不符的白血病患者血型鉴定与临床分析

㊃临床研究㊃D O I:10.3969/j.i s s n.1672-9455.2024.05.025A B O正反定型不符的白血病患者血型鉴定与临床分析*李茜,张智慧,高力立,彭沫溱,陈璐,赵梓昕,寸伟,罗臻ә,苏品璨云南昆明血液中心输血研究室,云南昆明650106摘要:目的对1例白血病患者A B O血型鉴定异常结果进行鉴定与回顾性分析,旨在为同类患者血型相容性检测提供参考㊂方法采用血清学检测进行A B O血型鉴定,采用聚合酶链反应-序列特异性引物进行A B O血型基因分型;采用亚硫酸氢盐测序检测A B O启动子甲基化状态㊂结果患者A B O血型正定型结果为O型,反定型结果为A型,正反定型结果不一致;试管法重复试验,4ħ时可见A1c凝集;吸收放散试验结果显示红细胞表面存在A抗原,提示正定型结果为A型;不规则抗体筛查阴性,直接抗人球蛋白试验阴性;基因分型检测基因型为A O1,表型为A型;甲基化检测发现患者A B O基因启动子区甲基化率为66.7%,明显高于随机选取的5例正常对照组标本平均甲基化率㊂结论白血病患者A B O抗原减弱可能导致血型误判,疾病状态下患者A B O基因启动子甲基化程度增高可能与其A B O抗原减弱密切相关,须结合分子生物学基因分型和血型基因甲基化检测方法进一步判断患者血型鉴定异常的原因,保障临床用血安全㊂关键词:白血病; A B O血型鉴定;血清学检测;聚合酶链反应-序列特异性引物;甲基化中图法分类号:R733.7;R457.1+1文献标志码:A文章编号:1672-9455(2024)05-0695-05输血作为一种临床救治患者的常用治疗手段,既可提高患者携氧功能,又能改善其凝血功能障碍,因此,输血前的A B O血型鉴定对保障临床输血安全尤为重要㊂A B O血型是由A B O基因编码决定的,一般情况下人体A B O血型不会发生改变㊂白血病是起源于造血干细胞或祖细胞的恶性克隆性疾病[1-2],白血病患者处于疾病状态可能引起自身体内血细胞或血浆成分发生改变,导致A B O抗原减弱或A B O抗体效价降低,出现A B O血型鉴定正反定型不相符的情况,极易引起血型误判,直接关系临床输血安全[3-4]㊂多种因素均可导致患者A B O抗原减弱,近年来,有研究发现,A B O基因启动子区甲基化程度与A B O抗原表达异常有关[5-8],但相关文献报道较少见㊂本研究通过血清学㊁聚合酶链反应-序列特异性引物(P C R-S S P)检测和A B O基因启动子区甲基化状态检测等检测手段对1例白血病患者的A B O血型鉴定检测异常结果㊁原因及处理方法进行了分析,初步探讨了白血病中A B O基因甲基化状态异常导致A B O抗原表达减弱在输血相容性检测中的临床意义,以期为临床诊疗提供参考依据,现报道如下㊂1资料与方法1.1一般资料血液标本来源于云南省肿瘤医院,患者,女,24岁,患恶性血液病,有2次输血史,患者知情同意本研究并签署知情同意书㊂患者于2022年2月3日初次诊断为白血病,目前患者白细胞计数(W B C)为206ˑ109/L,血红蛋白(H b)为51g/L,血小板计数(P L T)为38ˑ109/L,同时伴有脾脏肿大㊂患者严重贫血,急需输血,因其A B O血型鉴定正/反定型不一致(正定型结果为O型,反定型结果为A 型),医院将患者血液标本送检至血液中心进行A B O 血型鉴定和疑难交叉配血实验㊂1.2仪器 A B O血型定型检测卡配套孵育器和离心机(长春博迅生物技术有限责任公司),K A2200型血型血清学专用离心机(日本久保田公司),电热恒温水浴箱(上海博迅实业有限公司),冰箱(海尔集团海尔电冰箱有限公司),M a g C o r e H F16自动核酸提取仪(中国台湾R B C B i o s c i e n c e公司),T100P C R反应扩增仪(美国B i o-R a d L a b o r a t o r i e s公司),P o w e r P a c T M基础电泳仪(美国B i o-R a d L a b o r a t o r i e s公司), C h e m i D o c X R S+凝胶成像系统(美国B i o-R a d L a b o-r a t o r i e s公司),3730测序列分析仪(美国A B I公司), S W-C J-1D洁净工作台(江苏苏洁净化设备厂),D K-8D型电热恒温水槽(上海森信实验仪器有限公司), D Y Y-8型稳压稳流电泳仪(上海琪特分析仪器有限公司),Y X J-2离心机(湘仪离心机仪器有限公司),H6-1微型电泳槽(上海精益有机玻璃制品仪器厂),U-3010紫外-可见分光光度计(H i t a c h i公司),移液器(范围100~1000μL,20~200μL,0.5~10μL,德国艾本德E P P E N D O R F公司)㊂1.3试剂抗-A㊁抗-B血型定型试剂-单克隆抗体(上海血液生物医药有限责任公司,批号20220101), A B O血型卡正/反定型和R h D血型检测卡-微柱凝胶法(D i a M e d G m b H/B i o-R a d低离子抗人球蛋白卡,批号50093.11.28),抗-A1凝集素(上海血液生物医药㊃596㊃检验医学与临床2024年3月第21卷第5期 L a b M e d C l i n,M a r c h2024,V o l.21,N o.5*基金项目:云南省临床医学中心分中心开放项目(2020L C Z X K F-X Y19)㊂ә通信作者,E-m a i l:k m b l o o d l z@g m a i l.c o m㊂有限责任公司,批号20221019),抗-H单克隆抗体(上海血液生物医药有限责任公司,批号20221110),抗-A B抗体(D I A G A S T,批号464000),抗人球蛋白[抗免疫球蛋白G(抗-I g G),抗-C3d]检测试剂盒(上海血液生物医药有限责任公司,批号20225001),抗-I g G 检测试剂盒(上海血液生物医药有限责任公司,批号20215102),抗C3d检测试剂盒(上海血液生物医药有限责任公司,批号20225201),人A B O血型反定型用红细胞试剂盒(上海血液生物医药有限责任公司,批号20235313),低离子抗人球蛋白卡-微柱凝胶法(D i-a M e d G m b H/B i o-R a d,批号50531.76.10),不规则抗体检测试剂(人血红细胞,长春博迅生物技术有限责任公司,批号20221208),红细胞血型抗体筛选细胞(微柱凝胶法)S e r a s c a n D i a n a3(D i a g n o s t i c G r i f o l s, S.A.西班牙基立福公司,批号23007.01);核酸提取试剂盒(中国台湾R B C B i o s c i e n c e公司,批号P4101-17230);人类红细胞A B O血型基因分型试剂盒P C R-S S P法(天津秀鹏生物技术开发有限公司,批号202004001),M e t h y l E d g e T M B i s u l f i t e C o n v e r s i o n S y s-t e m(P r o m e g a,批号0000470334),焦碳酸二乙酯处理水(碧云天B e y o t i m e,批号111122230306),T a K a R a E p i T a q T M H S(T a K a R a,批号AM20984A),加样缓冲液(T a k a r a,批号A L G988A),B i g D y e测序反应试剂盒(A B I公司,批号91235489),P O P7测序胶(A B I公司,批号2211711),10ˑ电泳缓冲液(A B I公司,批号2208378)㊂1.4检测方法1.4.1血型血清学检测采集患者肘部静脉血进行检测,A B O血型正/反定型鉴定㊁间接抗人球蛋白试验㊁不规则抗体筛查采用微柱凝胶卡法和试管法;直接抗人球蛋白试验(D A T)采用试管法;将抗-A㊁抗-B 分别与经盐水洗涤3次的受检者浓缩红细胞等量混合进行吸收放散试验㊂所有试验操作均按照标准操作规范[9-10]和试剂盒说明书进行㊂1.4.2 P C R-S S P检测取患者乙二胺四乙酸外周抗凝全血400μL,使用自动核酸提取仪提取基因组D N A,采用微量紫外分光光度计检测D N A浓度和纯度㊂按照人类红细胞A B O血型基因分型试剂盒说明书进行P C R-S S P法基因分型P C R扩增试验㊂配制2.0%的琼脂糖凝胶,将5μL扩增P C R产物进行电泳检测,于120V恒定电压条件下电泳40m i n后使用凝胶成像系统成像,记录试验结果并根据试剂盒说明书结果分型表判定基因型㊂1.4.3 A B O基因启动子区甲基化检测采用亚硫酸氢盐测序法(B S P)检测标本A B O基因启动子区C p G岛甲基化状态㊂根据M e t h y l E d g e T M B i s u l f i t eC o n v e r s i o n S y s t e m试剂盒说明书,对患者基因组D N A进行亚硫酸氢盐修饰,使检测标本基因组D N A 中未发生甲基化的胞嘧啶通过亚硫酸氢盐处理后转化为尿嘧啶,甲基化的C p G胞嘧啶则不变㊂在N C B I 数据库下载A B O基因序列,应用M e t h y l p r i m e r e x-p r e s s s o f t w a r e v1.0和M e t h P r i m e r软件选取A B O 基因启动子区富含C p G位点的区域,并设计扩增引物,检测片段分别为175㊁160b p,共包含13个C p G 位点,分别编号为1~13,扩增引物由生工生物工程(上海)股份有限公司合成,上游引物序列1:A T G T T-G T T T A G G T T G G A G T G T A G T G,下游引物序列1: A C C A A C A T A A T A A A T C C T C C A T C T C;上游引物序列2:T T T T A A G T A G T T G G G G T T A T A G A T,下游引物序列2:A C C T A C A A T C C T A A C A C T T T C A A㊂以亚硫酸氢盐修饰后的患者基因组D N A为模板,根据T a K a R aE p i T a q T M H S说明书要求扩增目标序列㊂P C R反应体系为50μL,包括亚硫酸氢盐修饰后D N A模板2μL,T A K A R A E p i T a q T M H S (5U/μL)0.25μL,10ˑE p i T a q P C R B u f f e r(M g2+ f r e e)5μL,25mM M g C l25μL,2.5mM d N T P M i x-t u r e6μL,上下游引物各2μL,其余用无菌水补足㊂P C R条件为94ħ,1m i n;98ħ,10s;57ħ,30s;72ħ,30s;40个循环;72ħ终延伸10m i n;终止在4ħ㊂将P C R产物进行D N A琼脂糖凝胶电泳后对目的条带进行切胶,使用S a n P r e p柱式D N A胶回收试剂盒进行纯化回收;使用H i e f f C l o n e T M Z e r o T O P O-T A C l o n i n g K i t试剂盒对目的片段进行目的片段T A 克隆;使用生工质粒提取试剂盒S K8191U N I Q-10柱式质粒小量抽提试剂盒提取质粒D N A;将质粒使用P s tⅠ单酶切,琼脂糖凝胶电泳检查片段大小,确定是否含有目的片段,鉴定阳性克隆子;将阳性克隆子送生工生物工程(上海)股份有限公司进行序列分析㊂1.5统计学处理采用C h r o m a s(V e r s i o n2.3)软件分析测序峰图,使用甲基化分析软件B I Q A n a l y z e r分析目标序列C p G位点甲基化情况,并将B S P测序结果以圆圈图输出㊂目标序列中有13个C p G岛位点,计算C p G岛甲基化位点数占总C p G岛位点的百分比,计算甲基化率㊂以P<0.05为差异有统计学意义㊂2结果2.1 A B O血型鉴定患者A B O血型正定型结果为O型,反定型结果为A型,正反定型结果不一致,4ħ时试管法可见A1c凝集㊂见表1㊂不规则抗体筛查试验结果显示,患者血浆与3种抗体筛查细胞均无凝集㊂见表2㊂D A T结果显示,抗-I g G+C3d㊁抗-I g G㊁抗-C3d均为阴性㊂吸收放散试验结果显示,患者红细胞表面存在A抗原㊂见表3㊂2.2 P C R-S S P法检测 A B O基因分型检测出患者P C R-S S P基因分型格局1㊁2㊁4㊁6㊁8㊁9㊁11孔为阳性,3㊁5㊁7㊁10孔为阴性㊂对照人类红细胞A B O血型基因分型试剂盒(天津秀鹏生物技术开发有限公司)结果分型表判定患者基因型为A O1,表型为A型㊂见图1㊂㊃696㊃检验医学与临床2024年3月第21卷第5期 L a b M e d C l i n,M a r c h2024,V o l.21,N o.5表1 A B O 血型鉴定正反定型结果方法正定型抗-A 抗-B 抗-A B 抗-H 抗-A 1反定型A l cB cO c A 2c 自身c 微柱凝胶法(G e l )00///04+0/0试管法(R T -I S)0004+002+S000试管法(4ħ-I S)0004+02+3+000试管法(37ħ-I S)04+01+S注:0表示阴性;1+~4+表示阳性,强度以1~4数值表示;S表示凝集加强;/表示未检测㊂表2 不规则抗体筛查试验结果抗筛试剂红细胞R h -h rDCEceCWK e l lKkK p aJ saD u f f yF y aF y bK i d dJ k aJ k bⅠ++00+00+0n t 0++0Ⅱ+0++000+0n t ++++Ⅲ0++0++0n t++抗筛试剂红细胞L e w i sL e aL e bPP 1MN SMNSsL u t h .L u aC o l t .C o bX gX g a微柱凝胶法Ⅰ+0+0+0+00+0Ⅱ0+0+0+++0+0Ⅲ++++++注:0表示阴性;+表示阳性㊂表3 受检者吸收放散试验结果标本来源放散试验A 1cB c O c 患者2+00阴性对照注:0表示阴性;+表示阳性㊂2.3 A B O 基因启动子区甲基化检测 经B S P 检测A B O 基因启动子区部分序列的甲基化水平,待检测标本D N A 经亚硫酸氢盐试剂盒转化处理后甲基化的胞嘧啶不发生改变,经P C R 扩增和T A 克隆测序检测到该C G 位点仍为C G ;而未甲基化修饰的胞嘧啶则转化为尿嘧啶,经P C R 扩增和T A 克隆测序检测到该C G 位点转化为T G ㊂检测标本的甲基化率指检测区域中甲基化的C p G 数量与该区域C pG 总数量的比值㊂将A B O 基因启动子富含C p G 岛区域检测片段大小为175㊁160b p 序列进行TA 克隆测序㊂见图2~4㊂甲基化状态分析结果显示,患者标本AB O 基因启动子区甲基化率为66.7%,高于随机选取的5例正常对照组标本平均甲基化率(<25%),差异有统计学意义(P <0.05)㊂见图5㊂ 注:片段大小从下至上依次为100㊁250㊁500㊁750㊁1000b p 和2000b p;检测目的条带均同时检测内参质控带,内参为人类生长激素基因的保守片段,大小约为1000b p㊂图1 P C R -S S P基因分型注:箭头表示该C G 位点的C 为甲基化的碱基㊂图2 C pG 岛甲基化C G 位点㊃796㊃检验医学与临床2024年3月第21卷第5期 L a b M e d C l i n ,M a r c h 2024,V o l .21,N o .5注:箭头表示C G 位点的C 未被甲基化,C G 转化为T G ㊂图3 C pG 岛非甲基化C G位点 注:D Z 1㊁D Z 2㊁D Z 3㊁D Z 4㊁D Z 5为对照组标本,S C 为受检白血病患者标本;ʻ为非甲基化位点,Ә为甲基化位点;图中分别为患者标本和正常对照组标本A B O 基因启动子175b p 和160b p 区域甲基化状态分析情况㊂图4 患者A B O基因启动子区甲基化状态注:与正常对照组比较,aP <0.001㊂图5 患者标本与正常对照组标本甲基化情况比较3 讨 论白血病是一类造血干细胞异常的克隆性恶性疾病,由于发生病变的白细胞失去分化成熟的能力,停滞于其发育的不同阶段,进而引起患者骨髓和其他造血组织中病变细胞大量增殖积聚并浸润其他器官和组织,导致患者造血功能异常,出现病态造血而无法产生足量的正常血细胞,临床表现为贫血㊁出血㊁感染及各器官浸润症状,输血是白血病非常重要的辅助治疗手段之一[1-2]㊂在临床输血治疗过程中A B O 血型的正确鉴定尤为重要㊂人类A B O 血型抗原由A B O基因编码,A B O 基因位于人类第9号染色体的长臂,属于常染色体显性遗传,一般情况下不会发生变化[11]㊂有研究表明,急性髓系白血病患者处于其病情发作期可能出现红细胞血型抗原减弱㊁消失或变异,其原本的A B O 血型抗原无法正常检出,导致A B O 正/反定型不一致,极易引起血型误判,影响输血安全[12]㊂近年来,分子生物学基因分型技术㊁血型基因甲基化检测技术在A B O 疑难血型鉴定中的广泛应用,其将新技术与传统血型血清学鉴定技术相结合,可快速㊁准确地鉴定疑难A B O 血型,并且进一步合理解释疾病状态下抗原减弱的原因,为更加安全㊁合理地解决临床疑难血型鉴定问题提供了新思路㊂本例患者使用微柱凝胶法进行A B O 血型鉴定试验结果显示,正定型结果为O 型,反定型结果为A型,正反定型结果不一致㊂排除微柱凝胶卡㊁反定型试剂㊁离心设备运行及其他人为因素的干扰后采用试管法分别于室温㊁4ħ和37ħ条件下重复A B O 正反定型试验,结果显示,3个温度下正定型均为O 型,而反定型在4ħ时试管法可见A 1c 凝集,推测患者产生不规则抗-A 1抗体㊂微柱凝胶法进行不规则抗体筛查和试管法进行直接抗人球蛋白试验结果均为阴性,排除是自身抗体造成的A B O 正反定型不符[13]㊂结合患者处于白血病发作期,其A B O 血型鉴定正反定型不符可能是红细胞本身所致,经吸收放散试验后检出患者红细胞表面存在弱A 抗原㊂基于血液免疫学检测试验,由白血病疾病状态下导致受试者A 抗原减弱与A B O 亚型引起的红细胞抗原数量减少的试验检测结果较为相似,难以鉴别,需进一步结合其他检测技术加以鉴别㊂本研究使用分子生物学血型基因分型P C R -S S P 法进行检测,本例患者A B O 基因分型结果为A O 1,表型为A 型,即可排除A B O 亚型的可能,而其正定型结果为弱A 表型,有研究在髓系恶性肿瘤[4]㊁骨髓增生异常综合征[14-15]㊁急性髓系白血病[15-16]㊁慢性粒细胞白血病[17]㊁膀胱癌[18]和口腔癌[8,19]等患者中均检测到A B O 抗原减弱的现象,与本研究结果一致㊂目前,对于血型抗原减弱的确切原因尚未明确,且患者出现A B O 抗原减弱的现象不是持续的,在疾㊃896㊃检验医学与临床2024年3月第21卷第5期 L a b M e d C l i n ,M a r c h 2024,V o l .21,N o .5病缓解后还有可能恢复血型抗原表达[20]㊂推测为机体在白血病状态下的A B O基因表达调控机制发生了改变,而这种调控机制目前尚未明确㊂近年来,甲基化已成为肿瘤研究的热点,甲基化是一种表观遗传修饰方式,参与机体疾病状态下的基因表达调控㊂有研究发现,白血病患者A B O抗原减弱与D N A甲基化导致A B O基因沉默的关系更为密切[18]㊂基于此,推测患者A B O基因启动子区C p G岛甲基化状态的异常可能与其A抗原表达减弱有关㊂本研究对患者标本A B O基因启动子区175㊁160b p2个区域甲基化状态进行检测,结果显示,检测区域患者标本A B O基因启动子区甲基化率为66.7%,明显高于随机选取的5例正常对照组标本平均甲基化率(<25%),差异有统计学意义(P<0.05)㊂有研究表明,A B O基因启动子C p G岛甲基化程度与A B O抗原表达呈负相关,甲基化程度越高A B O基因表达越不活跃,从而导致A㊁B抗原表达均减少[5,8,18];且白血病患者存在不同程度A㊁B抗原减弱[21],与本研究结果一致㊂提示患者白血病状态下A B O基因启动子区甲基化程度增高可能与A B O抗原减弱之间存在较为密切的相关性,因机体抗原减弱的调控机制是多因素共同作用的结果,而A B O启动子甲基化导致的基因表达下调仅可能只是A B O抗原减弱的其中一个因素,其机制还需进一步探索㊂本研究结果提示,本例白血病患者在疾病发作期,其A B O基因启动子区甲基化状态异常可作为导致A B O抗原减弱的重要因素之一,从而干扰A B O正反定型鉴定㊂在临床输血实践中开展血清学疑难血型鉴定的同时进行分子生物学基因分型和血型基因甲基化检测可作为血清学方法的有效辅助手段,有效缩短了输血相容性试验检测时间,保障临床用血安全㊁合理㊁有效,为实现患者个体化血液管理㊁做到精准输血提供了新思路㊂参考文献[1]I MA I Y,MA R U Y,T A N A K A J.A c t i o n m e c h a n i s m s o fh i s t o n e d e a c e t y l a s e i n h i b i t o r s i n t h e t r e a t m e n t o f h e m a t o-l o g i c a l m a l i g n a n c i e s[J].C a n c e r S c i,2016,107(11):1543-1549.[2]B O Y I A D Z I S M,WH I T E S I D E T L.T h e e m e r g i n g r o l e so f t u m o r-d e r i v e d e x o s o m e s i n h e m a t o l o g i c a l m a l i g n a n c i e s [J].L e u k e m i a,2017,31(6):1259-1268.[3]张勇,刘剑荣,陈华良,等.1例疑难血型和疑难配血分析[J].实验与检验医学,2015,33(3):389-390.[4]B I A N C O T,F A R M E R B J,S A G E R E,e t a l.L o s s o f r e dc e l l A,B,a nd H a n t i ge n s i sf r e q u e n t i n m y e l o i d m a l ig n a n-c i e s[J].B l o o d,2001,97(11):3633-3639.[5]B I A N C O-M I O T T O T,HU S S E Y D J,D A Y T K,e t a l.D N A m e t h y l a t i o n o f t h e A B O p r o m o t e r u n d e r l i e s l o s s o fA B O a l l e l i c e x p r e s s i o n i n a s i g n i f i c a n t p r o p o r t i o n o f l e u-k e m i c p a t i e n t s[J].P L o S O n e,2009,4(3):e4788. [6]N I K P A Y M,R A V A T I S,M C P H E R S O N R.G e n o m e-w i d e s c r e e n i n g i d e n t i f i e s D N A m e t h y l a t i o n s i t e s t h a t r e g u l a t e t h e b l o o d p r o t e o m e[J].E p i g e n o m i c s,2022,14(13):10-15.[7]S H A O M,L Y U X P,T A N G P,e t a l.C o m p a r i s o n o f w e a kA B O a n t i g e n a n d n o r m a l A B O a n t i g e n i n p a t i e n t s w i t h a c u t e l e u k e m i a[J].J E x p H e m a t o l,2017,25(5):1307-1313.[8]P O K A L A A,P A R AMK U S AM G,T E J A S W I M L A,e ta l.H i s t o-b l o o d g r o u p a n t i g e n s i n o r a lc a n c e r a nd p o te n-t i a l l y m a l i g n a n t d i s o r d e r s[J].A s i a n P a c J C a n c e r P r e v, 2020,21(4):1163-1166.[9]尚红,王毓三,申子瑜.全国临床检测操作规程[M].4版.北京:人民卫生出版社,2015:118-143.[10]李勇,马学严.实用血液免疫学:血型理论和实验技术[M].北京:科学出版社,2006:588-701.[11]刘墅竹,姜琪,卢宝卫.急性白血病引起B型血型抗原减弱报告[J].实用医技杂志,2006,13(20):3717. [12]霍姿含,孙长杰,刘冰.22例A B O血型抗原减弱的血清学表现及原因分析[J].中国实验诊断学,2018,22(3): 510-511.[13]夏荣,张琦,陈勤奋.自身免疫性溶血性贫血患者输血前试验及临床输血专家共识[J].中国输血杂志,2017,30(7):663-665.[14]朱剑荧,胡利亚,兰炯采,等.骨髓增生异常综合征A B O血型鉴定观察[J].临床血液学杂志(输血与检验版), 2007,20(8):173-174.[15]O R A Z I A.H i s t o p a t h o l o g y i n t h e d i a g n o s i s a n d c l a s s i f i c a-t i o n o f a c u t e m y e l o i d l e u k e m i a,m y e l o d y s p l a s t i c s y n-d r o me s,a n d m y e l o d y s p l a s t i c/m y e l o p r o l if e r a t i v e d i s e a s e s[J].P a t h o b i o l o g y,2007,74(2):97-114.[16]S HA O M,L Y U X P,Y A N G Q K,e t a l.E f f e c t s o f D N Am e t h y l a t i o n o n A B O g e n e e x p r e s s i o n i n l e u k e m i a[J].Z h o n g h u a X u e Y e X u e Z a Z h i,2016,37(9):795-799.[17]S HA F I Q M,K A R I M F.R e d c e l l a n t i g e n l o s s i n a p a t i e n tw i t h c h r o n i c m y e l o i d l e u k e m i a:a c a s e o f A B O d i s c r e p a n-c y[J].T r a n s f u s A p h e r S c i,2015,52(1):103-104.[18]C H I HA R A Y,S U G A N O K,K O B A Y A S H I A,e t a l.L o s so f b l o o d g r o u p A a n t i g e n e x p r e s s i o n i n b l a d d e r c a n c e r c a u s e d b y a l l e l i c l o s s a n d/o r m e t h y l a t i o n o f t h e A B Og e n e[J].L a b I n v e s t,2005,85(7):895-907.[19]M O R T A Z A V I H,H A J I A N S,F A D A V I E,e t a l.A B O b l o o dg r o u p s i n o r a l c a n c e r:a f i r s t c a s e-c o n t r o l s t u d y i n a d e-f i n e d g r o u p o f I r a n i a n p a t i e n t s[J].A s i a n P a c J C a n c e rP r e v,2014,15(3):1415-1418.[20]侯瑞琴,杨洪燕,苗天红,等.422例A B O正反定型不符的原因分析及处理对策[J].中国输血杂志,2016,29(5): 509-511.[21]徐华,鲍国强,王宝燕,等.A B O基因启动子C p G岛甲基化与白血病的相关性[J].中国实验血液学杂志,2008,16(2):240-246.(收稿日期:2023-08-25修回日期:2023-12-20)㊃996㊃检验医学与临床2024年3月第21卷第5期 L a b M e d C l i n,M a r c h2024,V o l.21,N o.5。
- 1、下载文档前请自行甄别文档内容的完整性,平台不提供额外的编辑、内容补充、找答案等附加服务。
- 2、"仅部分预览"的文档,不可在线预览部分如存在完整性等问题,可反馈申请退款(可完整预览的文档不适用该条件!)。
- 3、如文档侵犯您的权益,请联系客服反馈,我们会尽快为您处理(人工客服工作时间:9:00-18:30)。
IntroductionDNA methylation involves the addition of a methyl group to the 5th carbon atom of cytosine to create 5-methylcytosine. In most mammalian cells, the majority of 5-methylcytosine is found immediately preceding (5′) guanine residues and is referred to as CpG methylation. The mammalian genome is interspersed with regions of high CpG density, known as CpG islands (CGIs), which overlap the promoter regions of ~70% of human genes.1DNA methylation has also been described in non-CpG con-texts, such as CpA, CpT, and CpC, which will be collectively referred to as non-CpG methylation throughout this review. First described in the plant genome,2 non-CpG methylation has since been found independent to, or coexisting with, CpG methyla-tion in various contexts within mammalian genomes. Non-CpG methylation occurs within various cell types and at specific stagesduring cell development; however, the functional significance of this in the mammalian genome is poorly understood.In this review, we will describe evidence for the existence of non-CpG methylation, specifically in mammalian genomes, and discuss the differences between CpG and non-CpG methylation, possible mechanisms of its establishment and maintenance, and the potential functions of this DNA modification.The Function of CpG Methylationin Mammalian CellsThe function of CpG methylation is context-dependent 3; how-ever, it is most clearly understood as a mechanism of controlling gene expression. Changes in CpG methylation, histone modifica-tions and nucleosome positioning can alter promoter DNA acces-sibility and regulate the recruitment of DNA binding proteins, such as transcription factors. CpG methylation can also repress transcription by directly blocking the binding of transcription activating proteins.4,5 Although CpG methylation at promoters is associated with transcriptional silencing, high levels of methyla-tion within the gene body are associated with highly expressed genes.6 The enrichment of CpG methylation within gene bod-ies, particularly at exonic regions, may contribute to exonic rec-ognition and splicing by promoting the pausing of the RNA polymerase II complex at exons and increasing the probability of co-transcriptional splicing.7,8 Other functions of CpG meth-ylation include the regulation of interactions between enhancers and promoters through the regulation of specific DNA-protein interactions. For example, nucleosome occlusion and hyper-methylation of the distal enhancers of the NANOG /OCT4 and glucocorticoid receptor promoters prevents them from activat-ing these genes.9,10 The expression of the imprinted IGF2 gene is regulated by methylation at sites within the IGF2-H19 imprinted locus, which prevents binding of the CTCF insulator and allows interaction of the IGF2 gene promoter with its enhancer.11 CpG*Correspondence to: Luke Hesson; Email: l.hesson@.au Submitted: 02/24/2014; Revised: 03/26/2014; Accepted: 04/02/2014;Published Online: 04/09/2014; /10.4161/epi.28741The evidence for functional non-CpG methylationin mammalian cellsvibha Patil, Robyn L ward, and Luke B Hesson*Adult Cancer Program; Lowy Cancer Research Centre and Prince of wales Clinical School; University of New South wales; Sydney, NSw AustraliaKeywords: DNA methylation, non-CpG methylation, non-CG methylation, epigeneticsAbbreviations: B29, Immunoglobin β-Chain; CDKN2A, cyclin-dependent kinase inhibitor 2A; CGI’s, CpG islands; CTCF, CCCTC-binding factor; DNMT, DNA methyltransferase; EBF, early B-cell factor; ESCs, embryonic stem cells; GSTP1, glutathione S-transferase pi 1; GVOs, germinal vesicle oocytes; H19, imprinted maternally expressed transcript; hESCs, human embryonic stem cells; IGF2, insulin-like growth factor 2; iPSCs, induced pluripotent stem cells; LUMA, luminometric-based assay; MSRE, methylation sensitive restriction endonuclease; PDK4, pyruvate dehydrogenase kinase, isozyme 4; OR, odourant receptor; PGC-1α, peroxisome proliferator-activated receptor gamma co-activator-1 α; SOX2, sex determining region Y-box 2; SYT11, synaptotagmin XI; TNF-α, tumor necrosis factor-α; TP53, tumor protein p53; T2DM, type 2 diabetes mellitus; WGBS, wholegenome bisulphite sequencingin mammalian genomes, the methylation of cytosine residues within CpG dinucleotides is crucial to normal devel-opment and cell differentiation. However, methylation of cytosines in the contexts of CpA, CpT, and CpC (non-CpG methylation) has been reported for decades, yet remains poorly understood. in recent years, whole genome bisulphite sequencing (wGBS) has confirmed significant levels of non-CpG methylation in specific tissues and cell types. N on-CpG methylation has several properties that distinguish it from CpG methylation. Here we review the literature describing non-CpG methylation in mammalian cells, describe the important characteristics that distinguish it from CpG methylation, and discuss its functional importance.©2014 L a n d e s B i o s c i e n c e . D o n o t d i s t r i b u t e .methylation is also important for genomic imprinting in other regions of the genome, as well as the transcriptional silencing of transposable elements, the control of tissue-specific gene expres-sion patterns,3 and X-chromosome inactivation.12Evidence for non-CpG Methylationin Mammalian CellsThough reported in the literature for many years,13 it was not until the development of WGBS that accurate base pair resolu-tion maps were generated and the extent of non-CpG methyla-tion in mammalian cells could be fully appreciated. Non-CpG methylation has now been described in stem cells and appears to be enriched in pluripotent cell types compared with most differentiated cell types. Studies comparing human embryonic stem cells (hESCs) and human fibroblast cell lines have revealed that non-CpG methylation, primarily at CpA sites, accounts for around 25% of all methylated cytosines in hESCs but is virtually absent in fibroblast cell lines.6,14,15 The relative abundance of non-CpG methylation in stem cells compared with differentiated cells was confirmed in a subsequent study comparing 76 genome-scale methylation maps across 32 distinct pluripotent cell lines and 10 differentiated cell types.16 However, recent reports show that non-CpG methylation is not restricted to pluripotent cells. For instance, non-CpG methylation is particularly abundant in mouse and human brain tissue.17,18 Analysis of human adult brain tissue (specifically the dorsolateral prefrontal cortex of healthy donors) showed that 666/2466 non-CpG dinucleotides tested across the genome were methylated across all 24 individuals tested.18 An interesting characteristic of non-CpG methylation is that it can occur in a variety of sequence contexts, but is most fre-quently found at CpA dinucleotides. In the human embryonic cell line HUES48, mean global levels of CpG, CpA, CpT, and CpC methylation were 67.85%, 6.68%, 1.48%, and 0.63% of all CpG, CpA, CpT, and CpC dinucleotides tested respectively.16 This trend in the frequency of methylation at each dinucleotide (CpG > CpA > CpT > CpC) has been described in all WGBS studies to date.6,14,17,19 Surrounding sequence context and cell type may also be important in determining non-CpG methylation. For example, in human and mouse brain, CpApC sites were most fre-quently methylated17,18 while in hESCs it was CpApG sites.6,16,18 In mouse germinal vesicle oocytes (GVOs), 65.5% of all methylcy-tosines occur in a non-CpG context, with TA m CA(G/C)C (where m indicates methylcytosine) representing more than 50% of all non-CpG methylation.19Methylation of CpG sites is usually symmetrical, meaning that a single CpG:GpC site is methylated at cytosine residues on both DNA strands. Due to sequence asymmetry, this is not the case with non-CpG methylation; for example, CpA dinucleotides are paired with complimentary TpG dinucleotides on the oppo-site strand (CpA:TpG). Consequently, non-CpG methylation is often only present on one DNA strand at any given site. WGBS from mouse GVOs has shown that 98% of methylated CpG sites were symmetrically methylated, whereas only 11% of methylated CpApG sites were methylated on both strands.19Non-CpG methylation has also been found in various path-ological contexts. Four independent studies analyzing breast cancer, prostate cancer, and brain lymphoma have reported methylation of non-CpG sites (mostly CpNpG sites, where N indicates A, C, T, or G) in the CDKN2A promoter.20-23 Non-CpG methylation has been reported at other important cancer-related genes, such as GSTP124 and TP5325 in endometrial andlung cancer specimens, respectively. Whether non-CpG meth-ylation is a contributing factor or a consequence of these diseasesis currently unclear.Measurement of Non-CpG MethylationNon-CpG methylation can be detected by either bisulphite sequencing or methylation sensitive restriction endonuclease (MSRE)-based methods. Bisulphite DNA conversion exploitsthe different sensitivities of cytosine and 5-methylcytosine to undergo deamination by bisulphite under acidic conditions.26This results in the conversion of cytosine to uracil, whereas5-methylcytosine remains non-reactive. This conversion cre-ates non-complimentary strands that must be amplified byPCR using separate pairs of primers (Fig. 1A). Alternatively,hairpin-bisulphite PCR can identify sites of 5-methylcytosineon complementary strands of restriction enzyme-fragmentedDNA by linking them with a hairpin oligonucleotide prior to bisulphite conversion.27 This method has successfully been usedto investigate the symmetry of CpG and non-CpG methyla-tion in mESCs.28 Detection of non-CpG methylation followingPCR of bisulphite converted DNA and sequencing is possible,but requires careful design of primer sequences. When design-ing PCR primers for bisulphite DNA it is usually assumed that cytosines within non-CpG dinucleotides are converted to ura-cil; however, this creates a bias for amplification of DNA thatis unmethylated at non-CpG sites (Fig. 1B). Also, non-CpG methylation confined to one DNA strand will not be detected if primers are specific for the unmethylated strand (Fig. 1C). Also,nested PCR amplification may cause an underestimation of non-CpG methylation29 and must be avoided. A final considerationis that bisulphite sequencing provides base pair resolution dataof DNA methylation; however, incomplete bisulphite conversionis a possible source of false positive methylation. The efficiencyof bisulphite conversion can be calculated after spiking sampleswith unmethylated lambda phage DNA.19 In addition to this control, consistent enrichment of non-CpG methylation at spe-cific sites between technical replicates, allele-specific methyla-tion and inducible methylation suggests the presence of genuinenon-CpG methylation.Methylcytosine capture assays utilizing proteins such asMBD2 and MeCP2, or the use of antibodies specific for5-methylcytidine, provide a means of enriching methylatedDNA for analysis. Although currently there is a lack of informa-tion regarding the affinity of MBD2 and MeCP2 for non-CpG methylation, a previous study has shown that non-CpG meth-ylation can be identified using antibodies specific for 5-meth-ylcytidine.30 While the majority of methylation detected using©214LandesBioscience.Donotdistribute.these affinity-based methods is likely to be within a CpG con-text, these methods may also detect non-CpG methylation.MSRE-based methods utilize enzymes that cannot digest DNA when specific cytosine residues within their recogni-tion sequence are methylated. For example, MspI is inhibited by methylation of the first cytosine in its recognition sequence (m CCGG). The combination of MSRE digestion of DNA with quantitative PCR using primers that flank the restriction site allows quantification of methylation by comparing the PCR sig-nals obtained before and after digestion.31 This relatively inex-pensive method only measures methylation at the recognition sites of the restriction enzyme used. The luminometric-based assay (LUMA), which also uses MSREs, was recently adapted to detect non-CpG methylation in human and rodent tissues.29 This technique simultaneously assesses methylation at particular restriction enzyme sites across the genome; however, it is unable to identify specific positions in the genome where methylation is located.Establishment and Maintenanceof Non-CpG MethylationIn mammalian cells DNA methylation is catalyzed by a fam-ily of DNA methyltransferases (DNMTs) known as DNMT1, DNMT3A, and DNMT3B.32 DNMT1 acts as the ‘maintenance’ methyltransferase, so-called due to its preference for hemimethyl-ated DNA, which is generated following DNA replication. This provides a mechanism by which CpG methylation is somatically heritable following cell division (Fig. 2A and B ). DNMT3A and DNMT3B, often referred to as “de novo” methyltransferases, are responsible for establishing patterns of DNA methylation in the early embryo.33 DNMT3L, which lacks methyltransferase activity, is essential for the establishment of genomic imprints inoocytes and for the silencing of retrotransposons within repeat sequences in male germ cells.32 In vitro substrate specificity of DNMT3A using oligonucleotides suggest this enzyme shows the substrate preference CpG > CpA > CpT,34,35 which matches the relative abundance of methylation at these sites in mammalian cells. The presence of non-CpG methylation is tightly linked to the expression of DNMT3A, DNMT3B, and DNMT3L, and it has been proposed that non-CpG methylation is a result of sus-tained expression of these proteins, particularly in non-dividing cells such as neurons and oocytes.17,18In vivo , studies using Dnmt1 knockout mouse ESCs have shown non-CpG methylation patterns can be maintained inde-pendently of Dnmt1.35 Loss of Dnmt3l in mice reduced CpA methylation in prospermatogonia from 15.2% to 2.8% of total methylation levels.36 Barres et al. found that DNMT3B deple-tion caused reduced non-CpG hypermethylation at the PGC-1α promoter in human primary muscle cells.30 Mouse GVOs lacking Dnmt3a or Dnmt3l show global reductions in both CpG and non-CpG methylation.19 Finally, loss of either Dnmt3l, Dnmt3a, Dnmt3b, or both Dnmt3a and Dnmt3b in ESCs revealed that non-CpG methylation was mediated by Dnmt3a and Dnmt3b, depends on the presence of Dnmt3L and is strongly correlated with the methylation of flanking CpG positions.28 Collectively, these studies show that non-CpG methylation is attributable to the de novo activity of the DNMT3A/B-DNMT3L complex. This, coupled with the fact that non-CpG methylation is asym-metrical, indicates that there is unlikely to be a mechanism of maintenance methylation at non-CpG sites. Therefore, in con-trast to CpG methylation, it is likely that non-CpG methylation would need to be re-established de novo after each cell division in order to be maintained (Fig. 2C ). Consistent with this hypoth-esis, non-CpG methylation accumulates in non-dividing male mouse germ cells but is rapidly lost following the resumption of cell division.36Figure 1. Bisulphite PCR can cause underestimation of the levels of non-CpG methylation. (A ) A short region of genomic DNA with symmetrical CpG methylation (red spheres). Bisulphite converts cytosine to uracil (recognized as thymine during PCR), whereas 5-methylcytosine remains non-reactive. The sequence is then amplified (green arrow) using primers specific to the converted sequence. Primers are usually designed assuming that all cyto-sine in a non-CpG dinucleotide context are converted to uracil (and hence unmethylated). (B ) The same region of DNA with additional methylation at non-CpG sites. Methylation at non-CpG dinucleotides within the binding site of the primer will result in protection from bisulphite conversion and loss of primer complementarity. Under these circumstances all molecules containing non-CpG methylation fail to amplify (red cross) and are not detected. (C ) Non-CpG methylation, which is inherently asymmetrical, will remain undetected following PCR amplification (green arrow) using primers specific to the unmethylated strand.©2014 L a n d e s B i o s c i e n c e . D o n o t d i s t r i b u t e .The Function of Non-CpG MethylationReports describing the effects of non-CpG methylation on gene expression provide the most compelling evidence that it has a functional role in mammalian cells. Methylation of Cp m CpNpGpG sites within the promoter of the B29 gene has been shown to repress promoter activity in human B cells by directly blocking the binding of the early B cell factor (EBF) transcription factor.37 Non-CpG methylation of Sp transcription factor binding sites within the human SYT11 promoter region reduced the binding of Sp proteins and associated transcrip-tional activity.38 Other genes shown to be regulated by non-CpG methylation include PGC-1α and PDK4,30,39 which are involved in mitochondrial function and fuel utilization. A genome-scale promoter methylation analysis identified differential non-CpG methylation within the PGC-1α promoter in skeletal musclefrom type II diabetes mellitus (T2DM) patients.30 This meth-ylation negatively correlated with PGC-1α expression and was acutely inducible by exposure to systemic factors associated with insulin resistance such as tumor necrosis factor-α (TNF-α) or the free fatty acids palmitate and oleate.30 The direct effect of non-CpG methylation on promoter activity was confirmed using promoter reporter constructs containing a single methyl-ated CpC site located -112 bp from the PDK4 TSS. Methylation of this site alone resulted in ~20% reduced promoter activity.39 In the skeletal muscle of obese individuals, non-CpG methyla-tion levels within the PGC-1α gene promoter positively cor-related with markers of obesity including body mass index, C-reactive protein and leptin hormone levels, whereas non-CpG methylation at the PDK4 promoter negatively correlated with these parameters. Furthermore, non-CpG methylation in the PGC-1α and PDK4 promoters was restored to non-obese levels following gastric bypass surgery and weight loss.39 Also, acute bouts of exercise cause transient reductions in the levels of non-CpG methylation at the PGC-1α promoter, concomitant with increased PGC-1α expression.40 Interestingly, a study of mito-chondrial DNA methylation has also reported that ~50% of methylcytosine within the D-loop region, which contributes to the regulation of mitochondrial DNA replication and transcrip-tion, was within non-CpG dinucleotides.41 Collectively, these studies provide evidence that non-CpG methylation at gene pro-moters is associated with reduced gene expression. As discussed above, several lines of evidence implicate non-CpG methylation in the regulation of mitochondrial function. Finally, the obser-vation that non-CpG methylation can be regulated by TNF-α, free fatty acids or exercise suggest it may represent a mechanism of acutely regulating gene expression in response to environmen-tal changes.Because non-CpG methylation is relatively frequent in plu-ripotent stem cells, several studies have investigated how levels change when cells are reprogrammed to produce induced plu-ripotent stem cells (iPSCs).15,16 These studies demonstrated that non-CpG methylation reappears upon reprogramming. The role of non-CpG methylation in pluripotency has important impli-cations for regenerative medicine using stem cells. However, it is still unclear whether non-CpG methylation is a cause or a consequence of the pluripotent state. One study suggests non-CpG methylation patterns are independent from pluripo-tency-associated gene expression since depletion of the de novo methyltransferase DNMT3A in the hESC line HUES48 failed to cause significant changes to the expression of key pluripo-tency genes such as OCT4, NANOG , and SOX2.16 However, in this study CpA methylation levels were reduced by only 28% following DNMT3A depletion, and it was not determined whether DNMT3B functionally compensated for DNMT3A loss. Although studies have shown that cell reprogramming is associated with DNA methylation changes that reflect ESCs,42 Lister and colleagues have identified 22 separate megabase-scale differentially methylated regions (DMRs) that were hypometh-ylated in iPSCs compared with ESCs.15 These findings suggest there are hotspots of incomplete reprogramming of non-CpGmethylation in iPSCs, which may have important ramificationsFigure 2. Maintenance of CpG and non-CpG methylation. (A ) A short region of genomic DN A containing several sites of CpG methylation and non-CpG methylation (red spheres). (B ) During DNA replication the “maintenance methyltransferase” DN MT1 reinstates CpG methylation on the newly synthesized DN A strand (green) guided by CpG meth-ylation on the template DNA strand. (C ) Following DNA replication the “de novo methyltransferases” DN MT3A and DN MT3B, in complex with DNMT3L, re-establishes non-CpG methylation.©2014 L a n d e s B i o s c i e n c e . D o n o t d i s t r i b u t e .for the validity of using these cells in clinical applications, such as regenerative medicine.Non-CpG methylation may also play an important role in imprinting. Several studies have revealed that in mouse GVOs, non-CpG methylation is prevalent at maternally methylated imprint control regions.43,44 In mouse adult brain tissue, parent-of-origin-dependent non-CpG methylation was associated with transcriptionally repressed alleles at a further eight imprinted loci.17There has also been a report implicating non-CpG meth-ylation in regulating interchromosomal interactions between an enhancer element and odorant receptor (OR) genes within olfac-tory sensory neurons.45 The expression of a single OR gene within each olfactory sensory neuron is an essential feature in the organi-zation and function of the olfactory system.45 Using chromosome conformation capture (3C) techniques, Lomvardas et al. identi-fied a trans-acting enhancer region, known as the H element, on human chromosome 14 that was involved in the activation of only one OR allele in each neuron. In OR expressing cells CpA methylation was detected on one allele of the H enhancer whereas this methylation was absent in OR negative cells. The authors of this study speculated that non-CpG methylation may render one of the enhancer alleles non-functional, which would ensure the necessary expression of only one OR gene in each olfactory sensory neuron.45ConclusionsThere is now strong evidence for the existence of non-CpG methylation in specific tissues and cell types in mammals. Though the functional relevance of non-CpG methylation at most sites remains unknown, it appears to regulate the expression of at least some genes when present at promoters. However, the most intriguing characteristics of non-CpG methylation are poten-tially those that distinguish it from CpG methylation. Non-CpG methylation is prevalent only in specific tissues and cell types, or at particular regions of the genome. It is particularly prevalent in infrequently dividing cells such as neurons or skeletal muscle, or in cells with a long developmental hiatus such as gametes. Non-CpG methylation is inherently asymmetrical, which precludes it from the maintenance mechanisms that preserve CpG methyla-tion through cell division. Consequently, non-CpG methylation must be established de novo after each cell division. This feature of non-CpG methylation most likely explains its accumulation in non-dividing cells and scarcity in highly proliferative cells. Acute regulation of non-CpG methylation by acute bouts of exercise or specific growth factors and lipids suggests it may be a mecha-nism of regulating DNA function in response to environmental factors. Finally, the prevalence of non-CpG methylation in stem cells, as well as its reinstatement upon reprogramming suggests it could be important for pluripotency, but it is currently unclear whether it is a cause or consequence of the pluripotent state.The features of non-CpG methylation, as outlined above, have made this DNA modification difficult to study function-ally. Previous advances in our understanding of the function of CpG methylation were made by generating model systems lack-ing functional DNMT enzymes.46,47 However, the same enzymes are required for both CpG and non-CpG methylation, therefore, it is impossible to specifically target non-CpG methylation with-out also significantly altering CpG methylation. In addition, CpG and non-CpG methylation are often coincident and it is difficult to extrapolate the biological effects of one vs. the other. However, the growing number of reports describing non-CpG methylation in various contexts highlights the need to uncover its functional role in mammalian cells.Disclosure of Potential Conflicts of Interest No potential conflicts of interest were disclosed.AcknowledgmentsWe would like to thank Neil Youngson, Mathew Sloane, and Peter Zarzour for helpful discussions and careful reading of the manuscript. V.P. is supported by an Australian Postgraduate Award. R.L.W. is supported by funding from the Cancer Council NSW (.au) and Cancer Australia (.au). L.B.H. is supported by a Cancer Institute New South Wales Career Development Fellowship (Grant number: 09CDF226; . au).R eferences1. Saxonov S, Berg P, Brutlag DL. A genome-wide analy-sis of CpG dinucleotides in the human genome distin-guishes two distinct classes of promoters. Proc NatlAcad Sci U S A 2006; 103:1412-7; PMID:16432200;/10.1073/pnas.05103101032. Lindroth AM, Cao X, J ackson J P, ZilbermanD, McCallum CM, Henikoff S, J acobsen SE.Requirement of CHROMOMETHYLASE3 for maintenance of CpXpG methylation. Science 2001;292:2077-80; PMID:11349138; http://dx.doi.org/10.1126/science.10597453. J ones PA. Functions of DNA methylation: islands,start sites, gene bodies and beyond. Nat Rev Genet2012; 13:484-92; PMID:22641018; http://dx.doi.org/10.1038/nrg32304. Marx A, Kahan T, Simon I. Integrative analy-sis of methylome and transcriptome reveals theimportance of unmethylated CpGs in non-CpGisland gene activation. Biomed Res Int 2013;2013:785731; PMID:23936848; http://dx.doi.org/10.1155/2013/7857315. Thomson JP, Skene PJ, Selfridge J, Clouaire T, GuyJ, Webb S, Kerr AR, Deaton A, Andrews R, J amesKD, et al. CpG islands influence chromatin struc-ture via the CpG-binding protein Cfp1. Nature2010; 464:1082-6; PMID:20393567; http://dx.doi.org/10.1038/nature089246. Lister R, Pelizzola M, Dowen RH, Hawkins RD,Hon G, Tonti-Filippini J, Nery JR, Lee L, Ye Z, NgoQM, et al. Human DNA methylomes at base resolu-tion show widespread epigenomic differences. Nature2009; 462:315-22; PMID:19829295; http://dx.doi.org/10.1038/nature085147. Chodavarapu RK, Feng S, Bernatavichute YV, ChenPY, Stroud H, Yu Y, Hetzel JA, Kuo F, Kim J, CokusSJ, et al. Relationship between nucleosome position-ing and DNA methylation. Nature 2010; 466:388-92; PMID:20512117; /10.1038/nature091478. Shukla S, Kavak E, Gregory M, Imashimizu M,Shutinoski B, Kashlev M, Oberdoerffer P, SandbergR, Oberdoerffer S. CTCF-promoted RNA poly-merase II pausing links DNA methylation to splicing.Nature 2011; 479:74-9; PMID:21964334; http:///10.1038/nature104429. You J S, Kelly TK, De Carvalho DD, Taberlay PC,Liang G, Jones PA. OCT4 establishes and maintainsnucleosome-depleted regions that provide addi-tional layers of epigenetic regulation of its targetgenes. Proc Natl Acad Sci U S A 2011; 108:14497-502; PMID:21844352; /10.1073/pnas.1111309108©214LandesBioscience.Donotdistribute.。