An Approach to Modeling Adaptive Hypermedia for Children with Disabilities
2019年六月英语六级英语作文模板
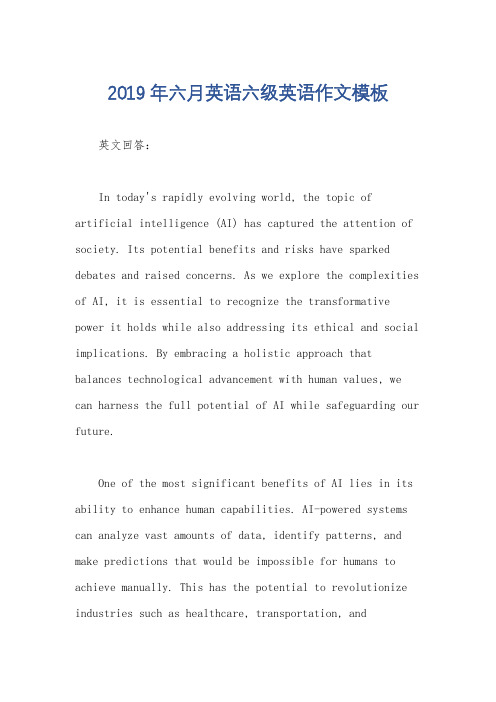
2019年六月英语六级英语作文模板英文回答:In today's rapidly evolving world, the topic ofartificial intelligence (AI) has captured the attention of society. Its potential benefits and risks have sparked debates and raised concerns. As we explore the complexities of AI, it is essential to recognize the transformative power it holds while also addressing its ethical and social implications. By embracing a holistic approach that balances technological advancement with human values, we can harness the full potential of AI while safeguarding our future.One of the most significant benefits of AI lies in its ability to enhance human capabilities. AI-powered systems can analyze vast amounts of data, identify patterns, and make predictions that would be impossible for humans to achieve manually. This has the potential to revolutionize industries such as healthcare, transportation, andmanufacturing, leading to improved efficiency, productivity, and innovation.Moreover, AI has the potential to address some of the world's most pressing challenges. By harnessing AI's computational power, we can develop novel solutions to environmental issues, design personalized learning experiences, and improve access to essential services. AI-driven systems can also assist in disaster relief efforts, providing real-time information and coordinating resourcesto save lives.Despite its immense promise, AI also presents potential risks that must be carefully considered. One concern is the potential for job displacement as AI-powered systems automate tasks that were previously performed by humans. It is crucial to address this challenge through robust education and re-skilling programs to ensure that workers can adapt to the changing job market.Another ethical concern is the use of AI insurveillance and data collection. While AI can enhancesecurity and improve public safety, it is essential to strike a balance between protecting individuals' privacy and utilizing AI for the common good. Clear regulations and transparent policies are needed to ensure responsible and ethical use of AI.Furthermore, the potential for bias in AI algorithms raises important ethical questions. If AI systems are trained on biased data, they may perpetuate and amplify these biases, leading to unfair or discriminatory outcomes. It is imperative to develop AI systems that are fair, unbiased, and inclusive, ensuring that they benefit all members of society.In addition to addressing ethical and social implications, it is crucial to foster collaboration and dialogue among stakeholders. Governments, industry leaders, researchers, and civil society organizations must work together to establish clear guidelines and regulations for the development and deployment of AI. This collaborative approach will help ensure that AI is used for the benefit of humanity and that its risks are effectively mitigated.By adopting a holistic approach that embraces both the benefits and risks of AI, we can create a future where AI empowers human potential and addresses societal challenges. Through responsible development, ethical considerations, and stakeholder collaboration, we can harness the transformative power of AI while safeguarding our human values.中文回答:人工智能的潜力和挑战。
免疫学No[1].9 - Hypersensitivity__ Reaction
![免疫学No[1].9 - Hypersensitivity__ Reaction](https://img.taocdn.com/s3/m/38b621d028ea81c758f5783d.png)
Type-I hypersensitivity
It is mediated by IgE which is normally found in very small amounts in the circulation and probably evolved to protect us against worm infestations. Allergic reaction(过敏反应)can occur to normally harmless antigens (such as pollen or foodstuffs) and microbial antigens (fungi, worms).
Types of hypersensitivity reactions
Type I: anaphylactic or immediate 速发型超敏反应 Type II: cytotoxic 细胞毒型超敏反应 Type III: Immune complex 免疫复合物型超敏反应 Type IV: cell mediated or delayed 迟发型超敏反应
1) Larger amounts of IgG would be produced than IgE and this excess IgG antibody would bind and remove the antigen before it could bind IgE on the mast cells or basophils and trigger degranulation; 2) IgG would also remove antigen before it could bind to and stimulate Th2 driven IgE producing B cells, thus decreasing the amount of antigen specific IgE produced.
自适应控制(Astrom著)Lecture1
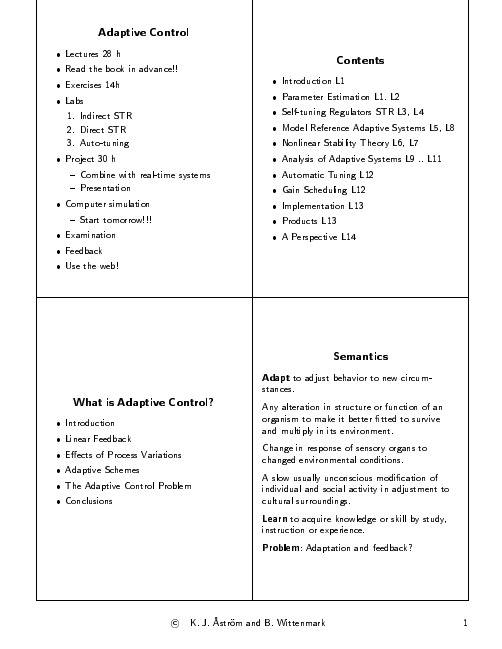
stances. Any alteration in structure or function of an organism to make it better tted to survive and multiply in its environment. Change in response of sensory organs to changed environmental conditions. A slow usually unconscious modi cation of individual and social activity in adjustment to cultural surroundings. Learn to acquire knowledge or skill by study, instruction or experience. Problem: Adaptation and feedback?
c K. J. str m and B. Wittenmark
Dual Control
uc Nonlinear control law u Process y
The Adaptive Control Problem
Principles Certainty Equivalence Caution Dual Control Controller structure Linear Nonlinear State Model Input Output Model Control Design Method Parameter Adjustment Method Speci cations Situation dependent? Optimality
0
5
保温与冷冻保存水果和小果实的质量管理模型说明书

Modeling of Kinetics of Mass-Exchange Processes for Fruit and Berry Production PreservationBazarnova Yu.G., Kuznetsova T.A., Aronova E.B.Higher School of Biotechnology and Food TechnologyPeter the Great St. Petersburg Polytechnic University,St.Petersburg, RussiaAbstract—At preservation of fruits and berries mass-exchange processes take place in liquid mediums, which define quality of the canned production. The composition of covering syrup should guarantee preservation of turgor, taste and color of fruits and berries and prevent fermentation processes. We carried out construction and adequacy examination of kinetic model for moisture osmotic transfer for fruit-berry material to covering syrups at thermal and refrigerating preservation. We obtained empirical coefficients. It was established that moisture migration rate depends on berry type and syrup concentration. Moisture loss for more than 30% results in berries turgor loss, which makes its further storage impractical. Using the obtained equation and empirical constants, we have established that the duration of the refrigeration storage of cowberry canned in 30% sugar syrups for the stated conditions was about 130 days.Keywords—kinetics modeling, mass-exchange processes, osmotic moisture transport, berries, syrup, preservationI.I NTRODUCTIONThe most common cause of deterioration in the texture of food products during refrigeration processing and storage is a change in its moisture content, including loss of moisture, an increase in humidity, or migration of moisture. Moisture migration can occur in multicomponent products if individual components are characterized by different indicators of water activity [1, 2]. The organoleptic perception of the product is governed by a combination of many changing factors. However, the correlation of organoleptic, physico-chemical and biochemical parameters is often absent. Testing the product during storage allows one to determine the indicators which are most important for assessing its quality and safety [3, 4].The processes of diffusive transfer of soluble components and osmotic transfer of moisture limit the period of cold storage of canned fruits and berries in liquid media. The study of the kinetics of mass transfer processes leading to the redistribution of soluble substances in the “berries-syrup” system is relevant in the development of technologies based on fruit and berry raw materials preserved in liquid media.For implementation of defrosting end storage of wild fruits and berries in liquid mediums in unregulated conditions the composition of covering syrups should guarantee quality (turgor, taste, color) of the fruits and berries and prevent fermentation processes [5,7]. The intensity of the diffusion-osmotic processes depends on a number of factors, including temperature, viscosity and density of the medium, the presence of various electrolytes. Therefore, when defrosting fruits and berries in liquid media, it is necessary to take into account the rheological properties of these media, the viscosity and density of which have a significant effect on the rate of diffusion of soluble substances.With respect to berries cell juice the sugar-covering syrups are hypertonic solutions. In order to prevent plasmolysis, one should choose its concentrations in such a way as to ensure alignment of the osmotic pressures. From the other side, osmotic pressure should be enough for microorganism cell dewatering, its plasmolysis and total inactivation [8,9].To assess the intensity of changes occurring in products during storage, and to obtain information about the influence of various factors on the storage time, the principles of modeling are used [10–12].The development of kinetic models suitable for practical use has some difficulties. Most often, they are developed on the basis of empirical data obtained from model experiments. In this case, the necessary condition is to check the adequacy of the obtained models to prove that they are reliable not only in laboratory conditions, but also when describing real food systems [13].The aims of the present work are to construct kinetic model of moisture osmotic transport process from the fruit-berry material to the covering syrups; carry out adequacy examination of the obtained model; obtain kinetic constants for various types of fruits and berries.II.M ATERIALS AND METHODSIn order to examine the adequacy of the developed mathematical model we used fresh and frozen cranberries and cowberries and syrups with sucrose content of 30, 40 and 50%. Analysis of sucrose content in berries and syrups was carried out by refractometry technique, which is widely used in many research areas [14-16].III.R ESULTS AND DISCUSSIONA.Construction of physical-mathematical modelFor construction of appropriate physical-mathematical model we will consider the following terms:International Conference on Smart Solutions for Agriculture (Agro-SMART 2018)V is juice volume in a berry, m 3;ν is total content of solvable sugars in a berry, mole; τ is time of berry storage in syrup;C s is sucrose concentration in a syrup, mole/m 3.C 0 is total sugar concentration in a berry, mole/m 3, which might be presented as:C ν=0, (1)at this C 0 < C s .Due to appearance of osmotic pressure the water moleculesfrom berry will migrate to the syrup through the berry surface layer. This layer is a membrane, which is permeable for water molecules and non- permeable for larger particles, in particularly, sucrose molecules. This process will continue until the sugar concentrations in a berry juice С(τ) and syrup C s become equal.The osmotic water transport through the surface layer of a berry is a slow (and, consequently, laminar) flow of viscous liquid through small channels. So we may use the Poiseuille [17] formula for liquid volume dV, passing during the time d τ, i.e. the following expression (2):τμξd dS N p dV o ⋅⋅⋅⋅=-2,(2)where:p 0 is osmotic pressure, Pa;N is amount of channels in a berry surface layer; S is sectional area of one channel, m 2;d is channel length which is equal to a berry surface layer width, m;μ is dynamic water viscosity, kg/(m .s);ξ is dimensionless coefficient, which depends on the channel shape (for a round channel ξ = 1/8π).Osmotic pressure is described by expression (3):()RT C C p s )(0τ-=, (3)where:R is universal gas constant, J/(mole .K); T is absolute temperature, K.By substituting (3) in (2) taking into account the following expressions:2;CdCdV CV ⋅=-=νν. (4)Let us obtain the following expression:dT R S N C k d C C k C dCC s s s ⋅⋅⋅⋅⋅⋅⋅=-=μνξτ2222;)(. (5)The expression (5) is easy to integrate. Its solution, whichmeets the initial condition C(0)= C 0 is the following (6):τk C C C C C C C C C C lпs s s s =+-⎭⎬⎫⎩⎨⎧--000)()(. (6)Further we introduce dimensionless constants:sC C a 0=; 0V V w =,(7)where: V 0, V are initial and current moisture volume in a berry, m3;And the expression (6) can be represented in the following way (8):τk a w a w a =-+⎭⎬⎫⎩⎨⎧--11ln . (8)Fig.1 presents relations between dimensionless concentration C/C s and dimensionless time k τ.Fig. 1. Relation between dimensionless concentration of sugar in a berry C/C s and dimensionless time k τ for various numerical values of dimensionless initial concentration a = C 0/C s.Empirical values of kinetic coefficient k, obtained using the expression (8) are presented in Table 1.TABLE I. C ONSTANTS OF MOISTURE OSMOTIC TRANSPORT RATE , K , DAYS –1 AT REFRIGERATORY STORAGE IN SYRUPS FOR CANNED CRANBERRIESAND COWBERRIES ; (4±2) ︒CFig.2 presents research data on residual moisture content incanned (a) and unfrozen in syrups (b) cowberries depending on its storage duration at temperature of (4±2)˚ С.It was established that turgor conservation for berries canned in syrups is reached at usage of sucrose concentration not more than 30%. It allows one to reduce osmotic losses of the cell juice for canned and unfrozen in syrups berries.Fig. 2. Relation between residual moisture content for canned (a) and unfrozen in syrups (b) cowberries and storage duration. (4±2) ︒C. Empirical data is shown by dots, lines stand for calculation data.At berries preservation in isotonic syrups the osmotic concentration of berries cell juice is reaching the concentration of solvable dry syrup substances. So according to the expression (7)10→=sC a , the formula (8) can be rewritten as:τk w -=1. (9)IV. C ONCLUSIONSThe obtained expressions (6) and (8) might be used for development of composition of sugar-covering syrups and regulation of storage modes of preserved in covering syrups berries. They allow one to determine the residual moisture content in berries at any storage period [18].It was established that moisture migration rate depends on berry type and syrup concentration. Moisture loss for more than 30% results in berries turgor loss, which makes its further storage impractical.Using the expression (8) and empirical constants (Table 1), we have established that the duration of the refrigerator storage of cowberry canned in 30 % sugar syrups for the stated conditions was about 130 days. During this period cowberries preserve its taste and turgor.References[1] G. Balasubrahmanyam, A. K. Datta, “Prevention of moisture migration infondant coated biscuit”, J. of food engineering, vol. 21 (2), pp. 235-244, 1994.[2] T. P. Labusa, D. Riboh, “Theory and applications of Arrhenius kinetics tothe prediction of nutrient losses in food”, Food technology, vol. 36, pp. 56-61, 1982.[3] Yu.G. Bazarnova, “Study of content of some food supplements withantioxidant activity in wild herbs and berries”, Voprosy Pitaniia, vol. 76, №1, pp. 22-26, 2007.[4] Yu. G. Bazarnova, Food shelf life: calculation and testing (translated.from English, ed. R. Stele), St. Peterburg: Professiya, 2006, 460 p.[5] Yu. G. Bazarnova, T V. Shkotova, “The method for canning of wildberries” Pat. № 2286674 RF, MPK A23V 7/08, № 2004136398; of 10.11.06, 2006.[6] G. Skrede, R. E. Wrolstad, P. Lea and G. Enersen, “Color Stability ofStrawberry and Blackcurrant Syrups”, J. Food Sci., vol. 57, pp. 172–7, 1992.[7] M. J. Beker, J. E. Blumbergs, E. J. Ventina and A. I. Rapoport,“Characteristics of cellular membranes at rehydration of dehydrated yeast Saccharomyces cerevisiae”, Appl. Microbiol. Biotechnol., vol. 19, pp. 347–52, 1984.[8] M. J. Beker, A. J. Rapoport, P. B. Zikmanis and B.E. Damberga,“Dehydration as a yeast metabolism regulating factor”, Environmental Regulation of Microbial Metabolism: Proceedings of the Federation of European Microbiological Societies Symposium Held in Pushchino, USSR, pp 105–11, 1985.[9] N. M. Panagiotou, V. T. Karathanos and Z. B. Maroulis, “Effect ofosmotic agent on osmotic dehydration of fruits”, Dry. Technol., vol. 17, pp. 175–89, 1999.[10] J. Kristott, “Fats and oils” in The stability and shelf-life of food. D.Kilcast, P. Subramaniam, Eds. Boca Raton, FL: CRC Press, Woodhead Publishing, 2000, P. 279–309.[11] T. P. Labusa, M. K. Schmidt, “Accelerated shelf-life testing of food”,Food Technology, vol. 39, pp. 57–62, 1985.[12] Shelf-life of food — guide-lines for its determination and prediction.Institute of food science and technology (UK). London: IFST, 1993, 230 p.[13] Yu. G. Bazarnova, T. E. Burova, A. L. Ishevsky, V. M. Zyukanov,“Possibilities of applying the principles of chemical kinetics to assess the quality of food products”, Storage and processing of agricultural raw materials, vol.11, pp. 33–36, 2004.[14] E.S, Lur’e, A.I. Sharov Technological control of raw material at candyproduction. Moscow: Kolos, 2001.[15] A. V. Belashov, A. A. Zhikhoreva, V. G. Bespalov, V. I. Novik, N. T.Zhilinskaya, I. V. Semenova, O. S. Vasyutinskii, “Refractive indexdistributions in dehydrated cells of human oral cavity epithelium”, J. Opt.Soc. Am. B Opt. Phys., vol. 34, pp. 2538–43, 2017.[16]K. N. Semenov, G. O. Iurev, N. M. Ivanova, V. N. Postnov, V. V.Sharoyko, I. V. Prikhodko, I. V. Murin, “Physico-chemical properties of the C60-L-lysine water solutions” J. Mol. Liq., vol. 225, pp. 767–77, 2017. [17] S. P. Sutera and R. Skalak, “The History of Poiseuille’s Law”, Annu.Rev. Fluid Mech., vol. 25, pp. 1–20, 1993.[18] Yu. G. Bazarnova, V. E. Kucakova and S. V. Frolov, “Modeling ofosmotic processes at berries storage in a syrup”, Pishchevye tekhnologii-2006: Proceedings of the Second International Scientific Conference, p.14, 2006, October [17-19 October 2006, Odessa].。
积极行动铸传奇的英语作文
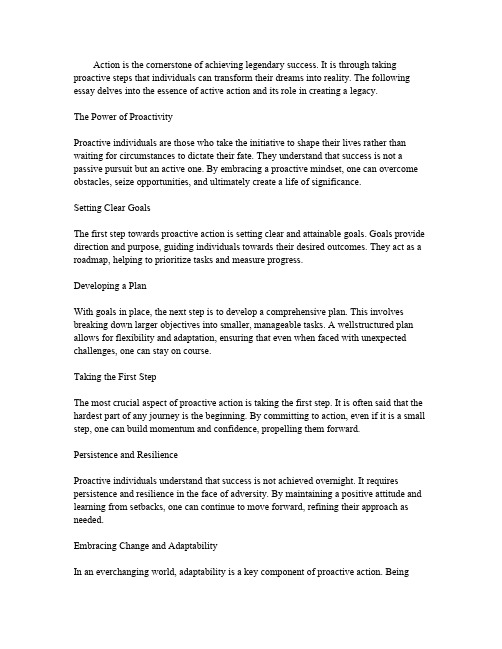
Action is the cornerstone of achieving legendary success.It is through taking proactive steps that individuals can transform their dreams into reality.The following essay delves into the essence of active action and its role in creating a legacy.The Power of ProactivityProactive individuals are those who take the initiative to shape their lives rather than waiting for circumstances to dictate their fate.They understand that success is not a passive pursuit but an active one.By embracing a proactive mindset,one can overcome obstacles,seize opportunities,and ultimately create a life of significance.Setting Clear GoalsThe first step towards proactive action is setting clear and attainable goals.Goals provide direction and purpose,guiding individuals towards their desired outcomes.They act as a roadmap,helping to prioritize tasks and measure progress.Developing a PlanWith goals in place,the next step is to develop a comprehensive plan.This involves breaking down larger objectives into smaller,manageable tasks.A wellstructured plan allows for flexibility and adaptation,ensuring that even when faced with unexpected challenges,one can stay on course.Taking the First StepThe most crucial aspect of proactive action is taking the first step.It is often said that the hardest part of any journey is the beginning.By committing to action,even if it is a small step,one can build momentum and confidence,propelling them forward.Persistence and ResilienceProactive individuals understand that success is not achieved overnight.It requires persistence and resilience in the face of adversity.By maintaining a positive attitude and learning from setbacks,one can continue to move forward,refining their approach as needed.Embracing Change and AdaptabilityIn an everchanging world,adaptability is a key component of proactive action.Beingopen to new ideas and willing to adjust ones plan in response to new information or circumstances demonstrates a commitment to growth and learning.The Role of Support NetworksWhile individual action is essential,the support of others can greatly enhance ones ability to take proactive steps.Building a network of mentors,peers,and collaborators can provide valuable insights,encouragement,and resources.Continuous Learning and ImprovementProactive individuals are lifelong learners.They continuously seek to improve their skills and knowledge,recognizing that personal development is a critical aspect of achieving and maintaining success.Leaving a LegacyThe ultimate goal of proactive action is not just personal success but also leaving a lasting legacy.This can take many forms,from creating a successful business to making a positive impact on ones community or contributing to a greater cause.In conclusion,proactive action is the driving force behind legendary achievements.It is the combination of clear goals,strategic planning,persistent effort,adaptability,and continuous learning that enables individuals to not only reach their own potential but also to inspire and influence others.By embracing a proactive approach to life,one can indeed forge a path to greatness and leave a lasting impact on the world.。
交通的未来:电动、自动和超越的英文作文

交通的未来:电动、自动和超越的英文作文The Future of Transportation: Electric, Autonomous, and BeyondAs we step into the future, the landscape of transportation is evolving at an unprecedented rate. No longer confined to traditional gasoline-powered vehicles, we are on the cusp of a revolution driven by electric, autonomous, and beyond-current-imagination technologies.The electric revolution has already begun. Electric vehicles (EVs) are no longer a novelty; they are becoming mainstream. With their zero-emission capabilities, EVs are not only environmentally friendly but also economically viable as battery technology improves and charging infrastructure expands. The future of EVs lies in longer ranges, faster charging, and more diverse models, including electric buses, trucks, and even aircraft.Autonomous vehicles are the next frontier. The promise of self-driving cars is not just about convenience but also about safety. With sensors, artificial intelligence, and vast amounts of data, autonomous vehicles have the potential to reduce accidents, traffic congestion, and energy consumption. While full autonomy may still be a few years away, we arealready seeing the integration of autonomous features in today's vehicles, such as adaptive cruise control and lane-keeping assist.Beyond autonomy, the future of transportation may involve entirely new modes of mobility. Flying cars, for instance, once the stuff of science fiction, are now being developed by several companies. These vehicles, combining the speed of air travel with the convenience of ground transportation, could revolutionize urban travel.Hyperloop, a proposed mode of transportation using vacuum tubes to transport pods at near-supersonic speeds, could revolutionize long-distance travel. While still in the early stages of development, the potential for faster, safer, and more environmentally friendly travel is immense.In conclusion, the future of transportation is bright and exciting. As we move towards an era of electric, autonomous, and innovative modes of travel, we are poised to experience a transformation in how we move from one place to another. The only limitation is our imagination and the will to pursue these revolutionary technologies.交通的未来:电动、自动和超越随着我们迈向未来,交通领域正以前所未有的速度发展。
Modeling hurricane waves and storm surge using integrally-coupled,
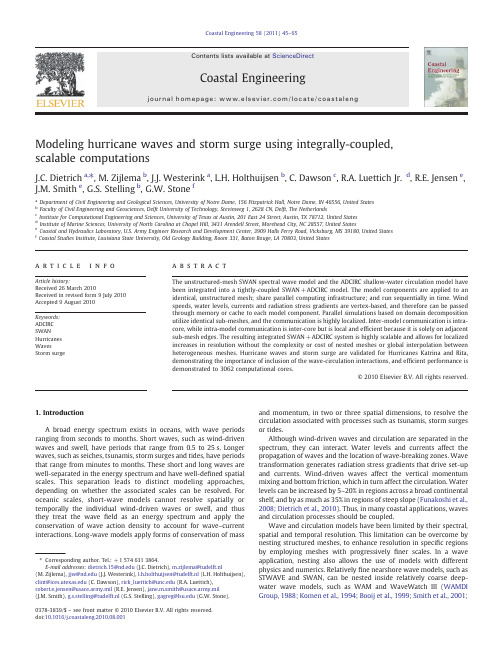
Modeling hurricane waves and storm surge using integrally-coupled,scalable computationsJ.C.Dietrich a ,⁎,M.Zijlema b ,J.J.Westerink a ,L.H.Holthuijsen b ,C.Dawson c ,R.A.Luettich Jr.d ,R.E.Jensen e ,J.M.Smith e ,G.S.Stelling b ,G.W.Stone faDepartment of Civil Engineering and Geological Sciences,University of Notre Dame,156Fitzpatrick Hall,Notre Dame,IN 46556,United States bFaculty of Civil Engineering and Geosciences,Delft University of Technology,Stevinweg 1,2628CN,Delft,The Netherlands cInstitute for Computational Engineering and Sciences,University of Texas at Austin,201East 24Street,Austin,TX 78712,United States dInstitute of Marine Sciences,University of North Carolina at Chapel Hill,3431Arendell Street,Morehead City,NC 28557,United States eCoastal and Hydraulics Laboratory,U.S.Army Engineer Research and Development Center,3909Halls Ferry Road,Vicksburg,MS 39180,United States fCoastal Studies Institute,Louisiana State University,Old Geology Building,Room 331,Baton Rouge,LA 70803,United Statesa b s t r a c ta r t i c l e i n f o Article history:Received 26March 2010Received in revised form 9July 2010Accepted 9August 2010Keywords:ADCIRC SWANHurricanes WavesStorm surgeThe unstructured-mesh SWAN spectral wave model and the ADCIRC shallow-water circulation model have been integrated into a tightly-coupled SWAN +ADCIRC model.The model components are applied to an identical,unstructured mesh;share parallel computing infrastructure;and run sequentially in time.Wind speeds,water levels,currents and radiation stress gradients are vertex-based,and therefore can be passed through memory or cache to each model component.Parallel simulations based on domain decomposition utilize identical sub-meshes,and the communication is highly localized.Inter-model communication is intra-core,while intra-model communication is inter-core but is local and ef ficient because it is solely on adjacent sub-mesh edges.The resulting integrated SWAN +ADCIRC system is highly scalable and allows for localized increases in resolution without the complexity or cost of nested meshes or global interpolation between heterogeneous meshes.Hurricane waves and storm surge are validated for Hurricanes Katrina and Rita,demonstrating the importance of inclusion of the wave-circulation interactions,and ef ficient performance is demonstrated to 3062computational cores.©2010Elsevier B.V.All rights reserved.1.IntroductionA broad energy spectrum exists in oceans,with wave periods ranging from seconds to months.Short waves,such as wind-driven waves and swell,have periods that range from 0.5to 25s.Longer waves,such as seiches,tsunamis,storm surges and tides,have periods that range from minutes to months.These short and long waves are well-separated in the energy spectrum and have well-de fined spatial scales.This separation leads to distinct modeling approaches,depending on whether the associated scales can be resolved.For oceanic scales,short-wave models cannot resolve spatially or temporally the individual wind-driven waves or swell,and thus they treat the wave field as an energy spectrum and apply the conservation of wave action density to account for wave –current interactions.Long-wave models apply forms of conservation of massand momentum,in two or three spatial dimensions,to resolve the circulation associated with processes such as tsunamis,storm surges or tides.Although wind-driven waves and circulation are separated in the spectrum,they can interact.Water levels and currents affect the propagation of waves and the location of wave-breaking zones.Wave transformation generates radiation stress gradients that drive set-up and currents.Wind-driven waves affect the vertical momentum mixing and bottom friction,which in turn affect the circulation.Water levels can be increased by 5–20%in regions across a broad continental shelf,and by as much as 35%in regions of steep slope (Funakoshi et al.,2008;Dietrich et al.,2010).Thus,in many coastal applications,waves and circulation processes should be coupled.Wave and circulation models have been limited by their spectral,spatial and temporal resolution.This limitation can be overcome by nesting structured meshes,to enhance resolution in speci fic regions by employing meshes with progressively finer scales.In a wave application,nesting also allows the use of models with different physics and numerics.Relatively fine nearshore wave models,such as STWAVE and SWAN,can be nested inside relatively coarse deep-water wave models,such as WAM and WaveWatch III (WAMDI Group,1988;Komen et al.,1994;Booij et al.,1999;Smith et al.,2001;Coastal Engineering 58(2011)45–65⁎Corresponding author.Tel.:+15746313864.E-mail addresses:dietrich.15@ (J.C.Dietrich),m.zijlema@tudelft.nl(M.Zijlema),jjw@ (J.J.Westerink),l.h.holthuijsen@tudelft.nl (L.H.Holthuijsen),clint@ (C.Dawson),rick_luettich@ (R.A.Luettich),robert.e.jensen@ (R.E.Jensen),jane.m.smith@ (J.M.Smith),g.s.stelling@tudelft.nl (G.S.Stelling),gagreg@ (G.W.Stone).0378-3839/$–see front matter ©2010Elsevier B.V.All rights reserved.doi:10.1016/j.coastaleng.2010.08.001Contents lists available at ScienceDirectCoastal Engineeringj o u r n a l h o m e p a g e :w ww.e l s ev i e r.c o m /l o c a t e /c o a s ta l e n gThompson et al.,2004;Gunther,2005;Tolman,2009).The nearshore wave models may not be ef ficient if applied to large domains,and the deep-water wave models may not contain the necessary physics or resolution for nearshore wave simulation.Until recently,wave models required nesting in order to vary resolution from basin to shelf to nearshore applications.These structured wave models can be coupled to structured circulation models that run on the same nested meshes (Kim et al.,2008).Unstructured circulation models have emerged to provide localized resolution of gradients in geometry,bathymetry/topogra-phy,and flow processes.Resolution varies over a range of scales within the same mesh from deep water to the continental shelf to the channels,marshes and floodplains near shore (Westerink et al.,2008).Unstructured meshes allow for localized resolution where solution gradients are large and correspondingly coarser resolution where solution gradients are small,thus minimizing the computa-tional cost relative to structured meshes with similar minimum mesh spacings.The coupling of wave and circulation models has been imple-mented typically with heterogeneous meshes.A coupling application may have one unstructured circulation mesh and several structured wave meshes,and the models may pass information via external files (Funakoshi et al.,2008;Dietrich et al.,2010;Weaver and Slinn,2004;Ebersole et al.,2007;Chen et al.,2008;Pandoe and Edge,2008;Bunya et al.,2010).This ‘loose ’coupling is disadvantageous because it requires intra-model interpolation at the boundaries of the nested,structured wave meshes and inter-model interpolation between the wave and circulation meshes.This interpolation creates problems with respect to both accuracy and ef ficiency.Overlapping nested or adjacent wave meshes often have different solutions,and inter-mesh interpolation can smooth or enhance the integrated wave forcing.Furthermore,even if a component model is locally conservative,its interpolated solution will not necessarily be conservative.Finally,inter-model interpolation must be performed at all vertices of the meshes.This interpolation is problematic in a parallel computing environment,where the communication between sub-meshes is inter-model and semi-global.The sub-meshes must communicate on an area basis (i.e.,the information at all vertices on a sub-mesh mustbe shared).Global communication is costly and can prevent models from being scalable in high-performance computing environments.An emerging practice is to couple models through a generic framework,such as the Earth System Modeling Framework (ESMF)(Hill et al.,2004;Collins et al.,2005),the Open Modeling Interface (OpenMI)Environment (Moore and Tindall,2005;Gregersen et al.,2005)or the Modeling Coupling Toolkit (MCT)(Warner et al.,2008).These frameworks manage when and how the individual models are run,interpolate information between models if necessary,and make transparent the coupling to developers and users.However,these frameworks do not eliminate the fundamental problems of coupling when using heterogeneous meshes.Boundary conditions must be interpolated between nested,structured wave meshes,and water levels,currents and wave properties must be interpolatedbetweenFig.1.Schematic of parallel communication between models and cores.Dashed lines indicate communication for all vertices within a sub-mesh,and are inter-model and intra-core.Solid lines indicate communication for the edge-layer-based nodes between sub-meshes,and are intra-model andinter-core.Fig.2.ADCIRC SL15model domain with bathymetry (m).46J.C.Dietrich et al./Coastal Engineering 58(2011)45–65the unstructured circulation and structured wave meshes.This interpolation is costly,destroys the scalability of the coupled model,and thus limits the resolution that can be employed and the corresponding physics that can be simulated.The recent introduction of unstructured wave models makes nesting unnecessary.Resolution can be enhanced nearshore and relaxed in deep water,allowing the model to simulate ef ficiently the wave evolution.SWAN has been used extensively to simulate waves in shallow water (Booij et al.,1999;Ris et al.,1999;Gorman and Neilson,1999;Rogers et al.,2003),and it has been converted recently to run on unstructured meshes (Zijlema et al.,2010).This version of SWAN employs the unstructured-mesh analog to the solution technique from the structured version.It retains the physics and numerics of SWAN,but it runs on unstructured meshes,and it is both accurate and ef ficient in the nearshore and in deep water.In this paper,we describe a ‘tight ’coupling of the SWAN wave model and the ADCIRC circulation model.SWAN and ADCIRC are run on the same unstructured mesh.This identical,homogeneous mesh allows the physics of wave-circulation interactions to be resolved correctly in both models.The unstructured mesh can be applied on a large domain to follow seamlessly all energy from deep to shallow water.There is no nesting or overlapping of structured wave meshes,and there is no inter-model interpolation.Variables and forces reside at identical,vertex-based rmation can be passed without interpolation,thus reducing signi ficantly the communication costs.In parallel computing applications,identical sub-meshes and communication infrastructure are used for both SWAN and ADCIRC,which run as the same program on the same computational core.All inter-model communication on a sub-mesh is done through local memory or munication between sub-meshes is rmation is passed only to the edges of neighboring sub-meshes,and thus the coupled model does not require global communication over areas.Domain decomposition places neighbor-ing sub-meshes on neighboring cores,so communication costs are minimized.The coupled model is highly scalable andintegratesFig.3.ADCIRC SL15bathymetry and topography (m),relative to NAVD88(2004.65),for southernLouisiana.Fig.4.ADCIRC SL15mesh resolution (m)in southern Louisiana.47J.C.Dietrich et al./Coastal Engineering 58(2011)45–65seamlessly the physics and numerics from ocean to shelf tofloodplain. Large domains and high levels of local resolution can be employed for both models,allowing the accurate depiction of the generation, propagation and dissipation of waves and surge.The resulting SWAN +ADCIRC model is suited ideally to simulate waves and circulation and their propagation from deep water to complicated nearshore systems.In the sections that follow,the component SWAN and ADCIRC models are described,and the mechanics of their tight coupling is introduced.The coupled model is then validated through its application to hindcasts of Hurricanes Katrina and Rita.Finally,a benchmarking study shows SWAN+ADCIRC is highly scalable.2.Methods2.1.SWAN modelSWAN predicts the evolution in geographical space⇀x and time t of the wave action density spectrum N(→x,t,σ,θ),withσthe relative frequency andθthe wave direction,as governed by the action balance equation(Booij et al.,1999):∂N+∇⇀x⋅⇀c g+⇀UNh i+∂cθN+∂cσN=S tot:ð1ÞThe terms on the left-hand side represent,respectively,the change of wave action in time,the propagation of wave action in⇀x-space (with∇⇀x the gradient operator in geographic space,⇀c g the wave group velocity and⇀U the ambient current vector),depth-and current-induced refraction and approximate diffraction(with propagation velocity or turning rate cθ),and the shifting ofσdue to variations in mean current and depth(with propagation velocity or shifting rate cσ).The source term,S tot,represents wave growth by wind;action lost due to whitecapping,surf breaking and bottom friction;and action exchanged between spectral components in deep and shallow water due to nonlinear effects.The associated SWAN parameterizations are given by Booij et al.(1999),with all subsequent modificationsas Fig.5.Example of the METIS domain decomposition of the ADCIRC SL15mesh on1014computational cores.Colors indicate local sub-meshes and shared boundary layers.Table1Geographic location by type and number shown in Figs.6and7.Rivers and channels1Calcasieu Shipping Channel 2Atchafalaya River3Mississippi River4Southwest PassBays,lakes and sounds5Sabine Lake6Calcasieu Lake7White Lake8Vermilion Bay9Terrebonne Bay10Timbalier Bay11Lake Pontchartrain12Lake Borgne13Gulf of MexicoIslands14Grand Isle15Chandeleur IslandsPlaces16Galveston,TX17Tiger and Trinity Shoals 18New Orleans,LA Fig.6.Schematic of the Gulf of Mexico with locations of the12NDBC buoy stations used for the deep-water validation of SWAN during both Katrina and Rita.The hurricane tracks are also shown.48J.C.Dietrich et al./Coastal Engineering58(2011)45–65present in version40.72,including the phase-decoupled refraction–diffraction(Holthuijsen et al.,2003),although diffraction is not enabled in the present simulations.The unstructured-mesh version of SWAN implements an analog to the four-direction Gauss–Seidel iteration technique employed in the structured version,and it maintains SWAN's unconditional stability (Zijlema,2010).SWAN computes the wave action density spectrum N (⇀x,t,σ,θ)at the vertices of an unstructured triangular mesh,and it orders the mesh vertices so it can sweep through them and update the action density using information from neighboring vertices.It then sweeps through the mesh in opposite directions until the wave energy has propagated sufficiently through geographical space in all direc-tions.It should be noted that,as a spectral model,SWAN does not attempt to represent physical processes at scales less than a wave length even in regions with veryfine-scale mesh resolution.Phase-resolving wave models should be employed at these scales if sub-wave length scaleflow features need to be resolved.However,this fine-scale mesh resolution may be necessary for other reasons,such as representing the complex bathymetry and topography of the region, or to improve the numerical properties of the computed solution.2.2.ADCIRC modelADCIRC is a continuous-Galerkin,finite-element,shallow-water model that solves for water levels and currents at a range of scales (Westerink et al.,2008;Luettich and Westerink,2004;Atkinson et al., 2004;Dawson et al.,2006).Water levels are obtained through solution of the Generalized Wave Continuity Equation(GWCE):∂2ζ∂t2+τ0∂ζ∂t+∂˜J x∂x+∂˜J y∂y−UH∂τ0∂x−VH∂τ0∂y=0;ð2Þwhere:˜J x =−Q x∂U∂x−Q y∂U∂y+fQ y−g∂ζ2∂x−gH∂∂xP s−αη+τsx;wind+τsx;waves−τbxρ0+M x−D xðÞ+U∂ζ∂t+τ0Q x−gH∂ζ∂x;ð3Þ˜J y =−Q x∂V−Q y∂V−fQ x−g∂ζ2−gH∂P s−αη+τsy;wind+τsy;waves−τbyρ0+M y−D y+V∂ζ+τ0Q y−gH∂ζ;ð4Þand the currents are obtained from the vertically-integrated momen-tum equations:∂U∂t+U ∂U∂x+V∂U∂y−fV=−g∂∂xζ+P sgρ0−αη+τsx;winds+τsx;waves−τbxρ0H+M x−D xH;ð5Þand:∂V∂t+U ∂V∂x+V∂V∂y+fU=−g∂∂yζ+P sgρ0−αη+τsy;winds+τsy;waves−τbyρ0H+M y−D yH;ð6Þwhere H=ζ+h is the total water depth;ζis the deviation of the water surface from the mean;h is the bathymetric depth;U and V are depth-integrated currents in the x-and y-directions,respectively;Q x=UH and Q y=VH arefluxes per unit width;f is the Coriolis parameter;g is the gravitational acceleration;P s is the atmospheric pressure at the surface;ρ0is the reference density of water;ηis the Newtonian equilibrium tidal potential andαis the effective earth elasticity factor;τs,winds andτs,waves are surface stresses due to winds and waves,respectively;τb is the bottom stress;M are lateral stress gradients;D are momentum dispersion terms;andτ0is a numerical parameter that optimizes the phase propagation properties(Atkinson et al.,2004;Kolar et al.,1994). ADCIRC computes water levelsζand currents U and V on an unstructured,triangular mesh by applying a linear Lagrange interpola-tion and solving for three degrees of freedom at every mesh vertex.2.3.Sharing informationSWAN is driven by wind speeds,water levels and currents computed at the vertices by ADCIRC.Marine winds can be input to ADCIRC in a variety of formats,and these winds are adjusted directionally to account for surface roughness(Bunya et al.,2010).ADCIRC interpolates spatially and temporally to project these winds to the computational vertices, and then it passes them to SWAN.The water levels and ambient currents are computed in ADCIRC before being passed to SWAN,where they are used to recalculate the water depth and all related wave processes (wave propagation,depth-induced breaking,etc.).The ADCIRC model is driven partly by radiation stress gradients that are computed using information from SWAN.These gradientsτs,waves are computed by:τsx;waves=−∂S xx−∂S xy;ð7Þand:τsy;waves=−∂S xy∂x−∂S yy∂y;ð8Þwhere S xx,S xy and S yy are the wave radiation stresses(Longuet–Higgins and Stewart,1964;Battjes,1972):S xx=ρ0g∬n cos2θ+n−12σNdσdθ;ð9ÞS xy=ρ0g∬n sinθcosθσNðÞdσdθ;ð10Þand:S yy=ρ0g∬n sin2θ+n−12σNdσdθ;ð11ÞFig.7.Schematic of southern Louisiana with numbered markers of the locations listed in Table1.Locations of the two CSI nearshore wave gauges and the hurricane tracks are also shown.49J.C.Dietrich et al./Coastal Engineering58(2011)45–65where n is the ratio of group velocity to phase velocity.The radiation stresses are computed at the mesh vertices using Eqs.(9)–(11).Then they are interpolated into the space of continuous,piecewise linear functions and differentiated to obtain the gradients in Eqs.(7)and (8),which are constant on each element.These element-based gradients are projected to the vertices by taking an area-weighted average of the gradients on the elements adjacent to each vertex.2.4.Coupling procedureADCIRC and SWAN run in series on the same local mesh and core.The two models “leap frog ”through time,each being forced with information from the other model.Because of the sweeping method used by SWAN to update the wave information at the computational vertices,it can takemuchFig.8.Hurricane Katrina signi ficant wave height contours (m)and wind speed vectors (m s −1)at 12-h intervals in the Gulf of Mexico.The six panels correspond to the following times:(a)2200UTC 26August 2005,(b)1000UTC 27August 2005,(c)2200UTC 27August 2005,(d)1000UTC 28August 2005,(e)2200UTC 28August 2005and (f)1000UTC 29August 2005.50J.C.Dietrich et al./Coastal Engineering 58(2011)45–65larger time steps than ADCIRC,which is diffusion-and also Courant-time-step limited due to its semi-explicit formulation and its wetting-and-drying algorithm.For that reason,the coupling interval is taken to be the same as the SWAN time step.On each coupling interval,ADCIRC is run first,because we assume that,in the nearshore and the coastal floodplain,wave properties are more dependent on circulation.At the beginning of a coupling interval,ADCIRC can access the radiation stress gradients computed by SWAN at times corresponding to the beginning and end of the previous interval.ADCIRC uses that information to extrapolate the gradients at all of its time steps in the current interval.These extrapolated gradients are used to force the ADCIRC solution as described previously.Once the ADCIRC stage is finished,SWAN is run for one time step,to bring it to the same moment in time as ADCIRC.SWAN can access the wind speeds,water levels and currents computed at the mesh vertices by ADCIRC,at times corresponding to the beginning and end of the current interval.SWAN applies the mean of those values to force its solution on its time step.In this way,the radiation stress gradients used by ADCIRC are always extrapolated forward in time,while the wind speeds,water levels and currents used by SWAN are always averaged over each of its time steps.2.5.Parallel coupling frameworkThe METIS domain-decomposition algorithm is applied to distribute the global mesh over a number of computational cores (Karypis and Kumar,1999).The decomposition minimizes inter-core communication by creating local sub-meshes with small ratios of the number of vertices within the domain to the number of shared vertices at sub-mesh interfaces.The decomposition also balances the computational load by creating local sub-meshes with a similar number of vertices;the local meshes decrease in geographical area as their average mesh size is decreased.A schematic of the communication is shown in Fig.1.Each local core has a sub-mesh that shares a layer of boundary elements with the sub-meshes on its neighbor cores.To update the information at these boundaries in either model,information is passed at the shared vertices on each sub-mesh.This communication is local between adjacent sub-meshes.Furthermore,only a small fraction of the vertices on any sub-mesh are shared.Thus the parallel,inter-core communication is localized and ef ficient.SWAN and ADCIRC utilize the same local rmation is stored at the vertices in both models,so it can be passed through local memory or cache,without the need for any network-based,inter-core communication.In contrast to loose coupling paradigms,in which the model components run on different sub-meshes and different cores,SWAN+ADCIRC does not destroy its scalability by interpolating semi-globally.The inter-model communication is intra-core.3.Hindcasts of Katrina and Rita 3.1.Parameters of hindcastsSWAN+ADCIRC will utilize the SL15mesh that has been validated for applications in southern Louisiana (Dietrich et al.,2010;BunyaFig.9.Hurricane Katrina winds and waves at 1000UTC 29August 2005in southeastern Louisiana.The panels are:(a)wind contours and vectors (m s −1),shown with a 10min averaging period and at 10m elevation;(b)signi ficant wave height contours (m)and wind vectors (m s −1);(c)mean wave period contours (s)and wind vectors (m s −1);and (d)radiation stress gradient contours (m2s −2)and wind vectors (m s −1).51J.C.Dietrich et al./Coastal Engineering 58(2011)45–65et al.,2010).The complex bathymetry/topography and mesh resolution are shown in Figs.2–4.This mesh incorporates local resolution down to 50m,but also extends to the Gulf of Mexico and the western North Atlantic Ocean.It includes a continental shelf that narrows near the protruding delta of the Mississippi River,suf ficient resolution of the wave-transformation zones near the delta and over the barrier islands,and intricate representation of the various natural and man-made geographic features that collect and focus storm surge in this region.The SL15mesh contains 2,409,635vertices and 4,721,496triangular elements.An example of the METIS domain decomposition of the SL15mesh on 1014cores is shown in Fig.5.Local sub-meshes are shown in separate colors,and the cores communicate via the layers of overlapping elements that connect these local meshes.Each parallel core utilizes the same unstructured local sub-mesh for both SWAN and ADCIRC.Notable geographic locations are summarized in Table 1and shown in Figs.6and 7.SWAN+ADCIRC has been validated via hindcasts of Katrina and Rita,which utilize optimized wind fields developed with an Interactive Objective Kinematic Analysis (IOKA)System (Cox et al.,1995;Cardone et al.,2007).The Katrina wind fields also have an inner core that is data-assimilated from NOAA's Hurricane Research Division Wind Analysis System (H*WIND)(Powell et al.,1996,1998).The wind speeds are referenced to 10m in height,peak 30min averaged “sustained ”wind speed,and marine exposure.They contain snapshots at 15min intervals on a regular 0.05°grid.The wind fields are read by ADCIRC,and then each local core interpolates onto its local sub-mesh.With the lone exception of the source of its radiation stress gradients,ADCIRC uses the same parameters as discussed in Bunya et al.(2010).The water levels are adjusted for the regional difference between LMSL and NAVD88(2004.65)and the seasonal fluctuation in sea level in the Gulf of Mexico.Bottom friction is parameterized using a Manning's n formulation,with spatially-variable values based on land classi fication.The Mississippi and Atchafalaya Rivers are forced with flow rates that are representative of the conditions during the storms.In addition,seven tidal constituents are forced on the open boundary in the Atlantic Ocean.ADCIRC applies a wind drag coef ficient due to Garratt (1977)with a cap of C d ≤0.0035.The SWAN time step and the coupling interval are 600s.The SWAN frequencies range from 0.031to 0.548Hz and are discretized into 30bins on a logarithmic scale (Δσ/σ≈0.1).The wave directions are discretized into 36sectors,each sector representing 10°.The present simulations use the SWAN default for wind input based on Snyder et al.(1981)and the modi fied whitecapping expression of Rogers et al.(2003),which yields less dissipation in lower frequency components and better prediction of the wave periods compared to the default formulation of Hasselmann (1974).Quadruplet nonlinear interactions are computed with the Discrete Interaction Approxima-tion (Hasselmann et al.,1985).For the shallow-water source terms,depth-induced breaking is computed with a spectral version oftheFig.10.Hurricane Katrina water levels and currents at 1000UTC 29August 2005in southeastern Louisiana.The panels are:(a)water level contours (m)and wind vectors (m s −1);(b)wave-driven set-up contours (m)and wind vectors (m s −1);(c)current contours (m s −1)and wind vectors (m s −1);and (d)wave-driven current contours (m s −1)and wind vectors (m s −1).52J.C.Dietrich et al./Coastal Engineering 58(2011)45–65model due to Battjes and Janssen (1978)with the breaking index γ=0.73,bottom friction is based on the JONSWAP formulation (Hasselmann et al.,1973)with friction coef ficient C b =0.067m 2s −3,and the triad nonlinear interactions are computed with the Lumped Triad Approximation of Eldeberky (1996).Although the resolution in the SL15mesh is well-suited to simulate waves and surge along the coastlines of Louisiana,Mississippi and Alabama,its relatively coarse resolution in the Caribbean Sea and Atlantic Ocean can create spurious wave refraction over one spatial element.Thus,wave refraction is enabled only in the computational sub-meshes in which the resolution of the bathymetry is suf ficient,speci fically in the northern Gulf of Mexico.SWAN applies a wind drag coef ficient due to Wu (1982)with a cap of C d ≤0.0035.In the validation sections that follow,the SWAN wave quantities will be compared to the measured data and also to the solution from a loose coupling to structured versions of WAM and STWAVE.WAM was run on a regular 0.05°mesh with coverage of the entire Gulf of Mexico,while STWAVE was run on four or five nested sub-meshes with resolution of 200m and coverage of southern Louisiana,Mississippi and Alabama.The details of this loose coupling can be found in Bunya et al.(2010)and Dietrich et al.(2010).For the validation herein,wave parameters from WAM and STWAVE were integrated to 0.41Hz,while parameters from SWAN were integrated to 0.55Hz.3.2.Hurricane KatrinaKatrina is a good validation case because of its size and scope.It was a large hurricane,with waves of 16.5m measured off the continental shelf and storm surge of 8.8m measured along the Mississippi coastline.But it also generated waves and storm surge over multiple scales and impacted the complex topography and levee protection system of southeastern Louisiana.To simulate the evolution of this hurricane,the coupled model must describe the system in rich detail and integrate seamlessly all of its components.3.2.1.Evolution of waves in deep waterBecause SWAN has not been used traditionally in deep water,we examine the behavior of its solution as Katrina moved through the Gulf of Mexico.Fig.8depicts the computed signi ficant wave heights at 12h intervals as Katrina enters the Gulf,generates waves throughout the majority of the basin,and then makes landfall in southern Louisiana.In its early stages,Katrina generated signi ficant wave heights of 6–9m in the eastern half of the Gulf.However,as the storm strengthened on 28August 2005,the signi ficant wave heights increased to a peak of about 22m at 2200UTC,and waves of at least 3m were generated throughout most of the Gulf.The impact of the hurricane on waves was widespread anddramatic.Fig.11.Signi ficant wave heights (m)during Hurricane Katrina at 12NDBC buoys.The measured data is shown with black dots,the modeled SWAN results are shown with black lines,and the modeled WAM results are shown with gray lines.53J.C.Dietrich et al./Coastal Engineering 58(2011)45–65。
欧拉方程求解静气动弹性问题
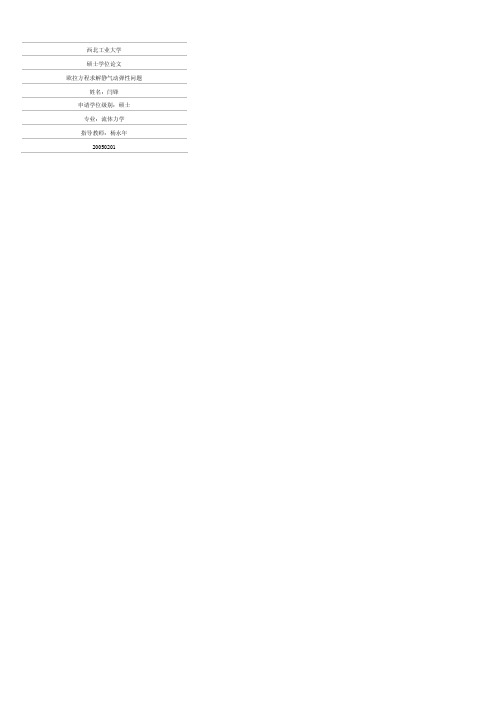
硕士学位论文
欧拉方程求解静气动弹性问题
姓名:***
申请学位级别:硕士
专业:流体力学
指导教师:***
20050201
两北工业人学硕士学位论文第三章飞行器气动弹性问题的研究
35.Guruswamy G P ENSAERO - A Multidisciplinary Program for Fluid/Structural Interaction Studies of Aerospace Vehicles 1990(2-4)
36.Bayyuk S A.Powell K G.vail Leer B Computation of flows with moving boundaries and fluid-structure interactions.[AIAA 97- 1771] 1997
43.Davis G A.Bendiksen O O Unsteady transonic two-dimensional Euler solutions using finite elements 1993
44.Batina J T Unsteady Euler airfoil Solutions using unstructured dynamic meshes.[AIAA 89-0115] 1989
7.MacCormack R W The Effect of Viscosity on Hypervelocity Impact Cratering.[AIAA paper 69-354]
x P D.Wendroff B System of Conservation Lows
9.郑诚行.熊小依机翼跨音速非线性静气动弹性计算 1991
- 1、下载文档前请自行甄别文档内容的完整性,平台不提供额外的编辑、内容补充、找答案等附加服务。
- 2、"仅部分预览"的文档,不可在线预览部分如存在完整性等问题,可反馈申请退款(可完整预览的文档不适用该条件!)。
- 3、如文档侵犯您的权益,请联系客服反馈,我们会尽快为您处理(人工客服工作时间:9:00-18:30)。
An Approach to Modeling Adaptive Hypermedia for Children with Disabilities JasminkaMezak NatasaHoic-BozicFaculty of Philosophy Faculty of PhilosophyDept. for informatics Dept. for informaticsjasminka@pefri.hr natasa.hoic@ri.tel.hrAbstractThis paper presents the outcome of the first stag e of the ongoing research that deals with the education of children with cerebral palsy usin the adaptive hypermedia courseware. Teachers can use software as an interactive multimedia desig ned unit of instruction to supplement traditional education teaching methods. This requirement acquires even greater significance in smaller non-English speakin countries where the need for educational software in their national lang uag es is more thoroug hly emphasized. If interactive multimedia is desig ned as an educational adaptive hypermedia system, it is even more helpful for persons with disabilities. We can enhance the learning process of such users by modeling adaptive presentations and adaptive navigation.1. In troductionIn the countries throughout the world all kind of new methods and means are used to improve education. To achieve improvement through the use of computer technology, it is necessary to educate teachers, introduce new learning model, and develop appropriate learning materials: educational software for learning-courseware. While in some countries these efforts are strategically planned, in others, such projects represent isolated initiatives and modest attempts which merely have a goal to build computer infrastructure and give schools and universities access to the Internet. In addition to our non-adequate hardware and network outfit, the problem is that in Croatian schools and universities computers are used rarely, except in subjects related to computer science.Children with cerebral palsy are treated as handicapped or disabled, but they are integrated into regular classrooms, regarding their motor impair. They also have extended special education for developing motor and psychophysics abilities what includes computers. Unfortunately, the use of technology within the special education classroom is often limited to rote practice using games or tasks such as word processing or drawing. In this paper we present our approach for developing adaptive hypermedia system with particular reference to student model. Students with the same abilities and condition may not respond in the same manner to the same software program. A program that is effective with one of them may not work well with the others.2. Adaptive hypermedia and assistivetechnologyAdaptive hypermedia (AH) is a relatively new area of research within the field of hypermedia. According to Brusilovsky [2], by adaptive hypermedia systems we mean all hypertext and hypermedia systems that reflect some features of the user in the user model and apply this model to adapt various visible aspects of the system to the user.An adaptive hypermedia system (AHS) adapts the presentation of content or links, based on the user model. We distinguish two major technologies in adaptive hypermedia: adaptive presentation and adaptive navigation support (ANS). Adaptive presentation adapts either the content of a document or the style of the text. ANS concentrates on changing the presentation of links.Adaptive hypermedia is particularly suitable for special education because all variety of presentation can be used in representing the knowledge in order to adapt to the user and to overcome his physical disabilities.3. The research3.1. Main objectivesThe idea of this project was to establish collaboration between Department of Informatics, Faculty of Philosophy in Rijeka and elementary school “Gornja Vezica” and to help the special educators to make the most appropriate decisions how to help handicapped children and how to evaluate their learning.The first step in this project is to coordinate sending and receiving materials for selecting the topics to be transfer into educational software and to make special educators familiar of using on-line communication and learning. The second step should consider that the specialeducators should be able to give the instruction onmethods concerning how to determine the appropriateness of hypermedia for scaffolding instruction during curricular modifications. The third step is development and evaluation of adaptive hypermedia courseware for disabled students from 1st to 4th grade of elementary school, which will include math lectures.3.2. BackgroundSince 1990 in the elementary school “Gornja Vezica” Rijeka is organized work of the Department for the extended expert procedure for children with cerebral paralysis. All children with impaired motor skills (i.e. physical invalidity or cerebral paralysis) who have psychophysical ability to attend regular or adoptable curriculum are involved into the work of this Department, beside their regular public education. The extended expert work is organized in the special needs classroom with didactical materials, computer classroom with 5 computers, speech pathologist cabinet and physiotherapy cabinet.3.3. MethodologyM any ways to structure knowledge in adaptive hypermedia have been proposed in the literature. However all of them have established two levels: structured contents and conceptual network with great variations how both levels are connected.Our approach is based on domain module (a module for knowledge representation), navigational module (a module with rules for conceptual network) and student module (a module with user classification depending on disabilities). Pedagogical knowledge is also included in the structure of the domain. It is the interrelationships between the knowledge of the content, the knowledge of the student's understanding of the content, and the knowledge of the strategies that will best facilitate learning according to these first two knowledges.Navigational module consists of rules that are responsible for generating adaptive hypermedia contents based on the relations between the domain concepts and the students' knowledge of these concepts.The most attention has been paid for the student module where both the user's knowledge and his abilities are included. In navigational module is also included the presentation module with certain rules which define what part of knowledge must be presented, in what order and which media will be used to represent that knowledge depending on users disability.4. Preliminary results and future plansThe specific learning needs of children in special education may often demand additional or adapted procedures. At this moment we are structuring the contents of domain module, defining navigational module and constructing the student module. The same knowledge has been presented in various ways with different kinds of media. The learning needs of the children will be defined in the student module as well as the interrelationships with the navigation (presentation) module. Among our future work plans we include the development of an adaptive hypermedia system that will allow content construction (domain module) independently from navigational and student module so schoolteachers and special education teachers can be ableto modify or adapt software described in the model.Such didactic courses should be also designed to be delivered using interactive technology for using in the context of distance education.5. Con clusionThis paper intends to describe an attempt in adapting hypermedia system to fulfil the learning needs of children with cerebral palsy. Developing the student module of such system is a delicate task that requires knowledge and collaboration of all: computer science teachers, topic lecturers and the special education teachers Currently, there is no requirement that the computer science teachersin Croatia have the basic background knowledge of special education and are required little in terms of technology for children with special needs in the classroom. Until the time when changes are made to require teachers to have the knowledge, children with special needs are going to be at a disadvantage in the regular classroom setting.6. Referen ces[1] J.W. Forgan, R.K. Weber and P.L. Schoon,"Special NeedsSoftware Evaluation: Choosing the Right One", Proceedingsof 11th International Conference of the Society for Information Technolog y and Teacher Education (SITE) 2000,San Diego CA: AACE, 2000, pp. 1968-1973[2] P. Brusilovsky, “M ethods and Techniques of AdaptiveHypermedia”, User Modeling and User-Adapted Interaction,6, pp. 87-129, 1996.[3] J.B. Pemberton,"Using Technology to Support SpecialEducation Certification Through Distance Education", Proceeding s of 8th International Conference of the Societyfor Information Technolog y and Teacher Education (SITE)1997,Orlando FL: AACE, 1997, pp. 1035-1036.。