A simplified wind power generation model for reliability evaluation
风电场机组功率曲线验证技术规程

英文回答:The technical protocol for the verification of wind power curves for wind power generation units is designed to regulate the relationship between wind power output and wind speed, and covers the identification of wind power curves, validation methods and processes, measuring equipment and requirements for wind power units。
The wind power curve isan important basis for the operation and management of wind farms and is important for their proper operation。
Validationof wind power curves is a key element in ensuring the proper operation of wind farms and is one of the technical preparations for wind power units to access the grid。
Validation of the wind field power curve is important and must be strictly implemented in accordance with technical specifications。
风电场机组功率曲线验证技术规程是为了规范风电机组输出功率与风速之间的关系曲线而制定的,其内容涵盖对风电机组功率曲线的确定、验证方法和流程、测量设备及要求等方面。
风力发电英文作文
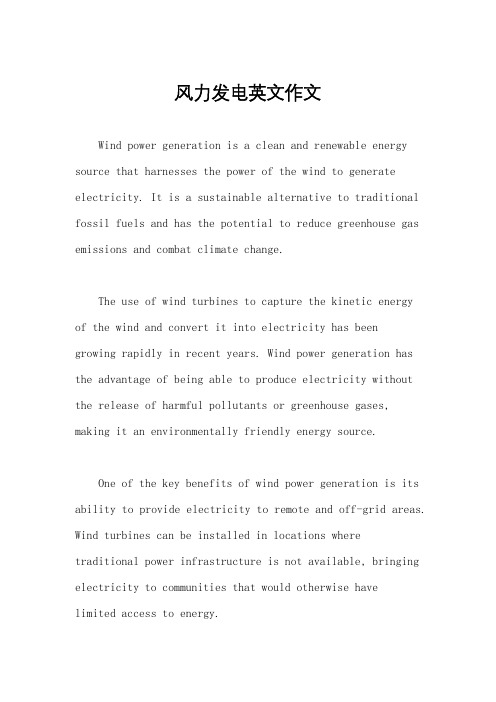
风力发电英文作文Wind power generation is a clean and renewable energy source that harnesses the power of the wind to generate electricity. It is a sustainable alternative to traditional fossil fuels and has the potential to reduce greenhouse gas emissions and combat climate change.The use of wind turbines to capture the kinetic energyof the wind and convert it into electricity has beengrowing rapidly in recent years. Wind power generation has the advantage of being able to produce electricity without the release of harmful pollutants or greenhouse gases, making it an environmentally friendly energy source.One of the key benefits of wind power generation is its ability to provide electricity to remote and off-grid areas. Wind turbines can be installed in locations wheretraditional power infrastructure is not available, bringing electricity to communities that would otherwise havelimited access to energy.In addition to its environmental benefits, wind power generation also has the potential to create jobs and stimulate economic growth. The development, installation, and maintenance of wind turbines require skilled labor, creating employment opportunities in the renewable energy sector.Despite its many advantages, wind power generation also faces challenges. One of the main challenges is the intermittent nature of wind energy, as the wind does not blow consistently. This variability in wind speed can make it difficult to rely solely on wind power generation for electricity supply.In conclusion, wind power generation is a promising and sustainable energy source that has the potential to play a significant role in the transition to a low-carbon economy. With ongoing technological advancements and investment in wind energy infrastructure, it is likely to become an increasingly important part of the global energy mix.。
平潭风力发电作文英语范文

平潭风力发电作文英语范文Chapter 1: IntroductionWind power is a rapidly growing source of renewable energy globally, with numerous advantages such as being a clean and sustainable option to replace fossil fuels. Among the many regions developing wind power projects, Pingtan Island (also known as Pingtan or Pintan), located in Fujian Province of China, stands out as a key site for wind power generation. Pingtan is an island with abundant wind resources, making it an ideal location for wind power development.Chapter 2: Wind Power Potential in PingtanPingtan has a unique geographic advantage, positioned at the northernmost tip of the Taiwan Strait. This location exposes the island to strong and consistent winds, especially during the winter months. These winds are primarily the result of the interaction between the Siberian high-pressure system and the Philippine low-pressure system. The average wind speed in Pingtan is estimated to be around 7 meters per second. With its favorable wind conditions, Pingtan has great potential for wind power generation.Chapter 3: Wind Farm Development in PingtanThe development of wind power in Pingtan started in 2011 with the construction of the Pingtan Offshore Wind Farm. This wind farm, located 15 kilometers southeast of Pingtan Island, consists of 59 wind turbines with a total installed capacity of 99.3 megawatts (MW). Since its completion, the Pingtan Offshore Wind Farm hasbeen successfully generating clean electricity to meet the energy needs of local residents.To further tap into Pingtan's wind power potential, the local government has plans to expand the wind farm. An additional 100 wind turbines are set to be installed, with a projected installed capacity of 250 MW. This expansion will bring the total capacity of the Pingtan Offshore Wind Farm to 349.3 MW, providing sustainable energy for both Pingtan Island and the surrounding areas.Chapter 4: Economic and Environmental BenefitsThe development of wind power in Pingtan has brought numerous economic and environmental benefits to the region. Economically, the construction and operation of wind farms have created job opportunities and stimulated local economic growth. Additionally, wind power has reduced the region's reliance on imported fossil fuels, leading to increased energy security and reduced energy costs.From an environmental perspective, wind power is a clean and sustainable energy source. By harnessing wind, Pingtan can reduce its carbon dioxide emissions and mitigate the effects of climate change. Furthermore, wind power generation does not produce harmful pollutants or waste, making it an environmentally friendly alternative to traditional energy sources.Conclusion:With its favorable wind resources, Pingtan Island has emerged as a key player in wind power development. The Pingtan Offshore Wind Farm and its planned expansion demonstrate the island's commitment to clean and sustainable energy generation. The economic and environmental benefits of wind power in Pingtan are evident, making it a model for other regions seeking to transition to renewable energy sources.Chapter 5: Challenges and SolutionsWhile the development of wind power in Pingtan has been promising, it has not come without its challenges. One major obstacle is the need for substantial investment in wind farm infrastructure. The construction and maintenance of wind turbines, as well as the installation of offshore transmission lines, require significant funding. To overcome this challenge, the local government has sought partnerships with private investors and international organizations to attract the necessary capital.Another challenge is the potential impact on the local ecosystem and wildlife. The offshore location of the wind farm can disrupt marine habitats and interfere with migratory patterns of birds and marine life. To mitigate these concerns, thorough environmental impact assessments have been conducted prior to the construction of the wind farm. Measures such as monitoring animal behavior and implementing noise reduction technologies have been implemented to minimize the impact on the environment. Chapter 6: Future OutlookThe future of wind power in Pingtan looks promising. With the planned expansion of the Pingtan Offshore Wind Farm, the islandis set to increase its wind power capacity significantly. This expansion will bring more clean and renewable energy to the region, further reducing its carbon footprint and dependence on fossil fuels.Furthermore, Pingtan's strategic location at the northernmost tip of the Taiwan Strait positions it as a potential hub for offshore wind power development. The island's strong, consistent winds and proximity to other coastal regions with high energy demand make it an ideal location for offshore wind farms. This presents an opportunity for Pingtan to become a renewable energy leader in the region and attract more investment in wind power projects.Chapter 7: Lessons for Other RegionsThe success of wind power development in Pingtan provides valuable lessons for other regions looking to harness their wind resources. First and foremost, conducting thorough feasibility studies and environmental impact assessments are crucial for the sustainable development of wind farms. Understanding wind patterns, potential environmental impacts, and the economic feasibility of projects ensures long-term success.Additionally, collaboration between the government, private sector, and international organizations is essential to overcome challenges such as funding and technical expertise. Developing partnerships and attracting investment can accelerate wind power development and ensure its long-term viability.Finally, Pingtan's experience highlights the importance of policysupport and a clear regulatory framework. Favorable policies and supportive regulations create a conducive environment for wind power development. This includes incentives for renewable energy projects, streamlined permitting processes, and transparent guidelines for environmental impact assessments.Conclusion:The development of wind power in Pingtan Island is a testament to the island's commitment to clean and sustainable energy generation. Overcoming challenges such as infrastructure investment, environmental concerns, and policy support, Pingtan has successfully harnessed its wind resources to generate clean electricity. The planned expansion of the Pingtan Offshore Wind Farm and its potential as a regional hub for offshore wind power development bode well for the future of renewable energy in Pingtan. The lessons learned from Pingtan's experience can serve as a blueprint for other regions seeking to tap into their wind power potential and transition to a more sustainable energy future.。
惠普彩色激光打印机 Pro M454 和惠普彩色激光多功能一体机 Pro M479 维修手册说明书
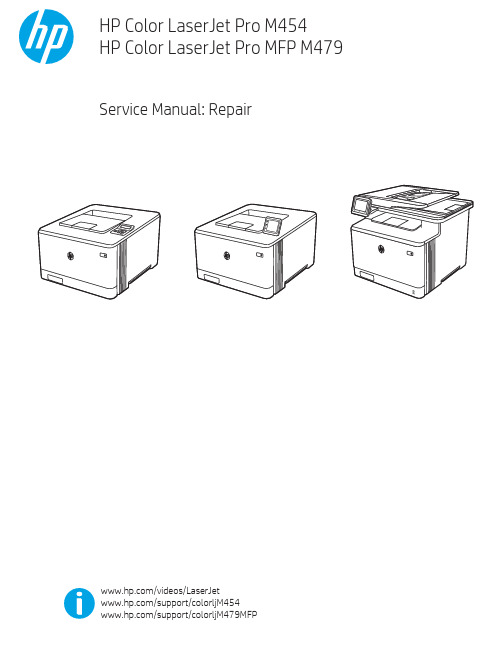
Table -1 Revision history Revision number 1
Revision date 6/2019
Revision notes HP LaserJet Pro M454 HP LaserJet Pro MFP M479 Repair manual initial release
Additional service and support for HP internal personnel HP internal personnel, go to one of the following Web-based Interactive Search Engine (WISE) sites: Americas (AMS) – https:///wise/home/ams-enWISE - English – https:///wise/home/ams-esWISE - Spanish – https:///wise/home/ams-ptWISE - Portuguese – https:///wise/home/ams-frWISE - French Asia Pacific / Japan (APJ) ○ https:///wise/home/apj-enWISE - English ○ https:///wise/home/apj-jaWISE - Japanese ○ https:///wise/home/apj-koWISE - Korean ○ https:///wise/home/apj-zh-HansWISE - Chinese (simplified)
Find information about the following topics ● Service manuals ● Service advisories ● Up-to-date control panel message (CPMD) troubleshooting ● Install and configure ● Printer specifications ● Solutions for printer issues and emerging issues ● Remove and replace part instructions and videos ● Warranty and regulatory information
风力发电外文文献翻译中英文
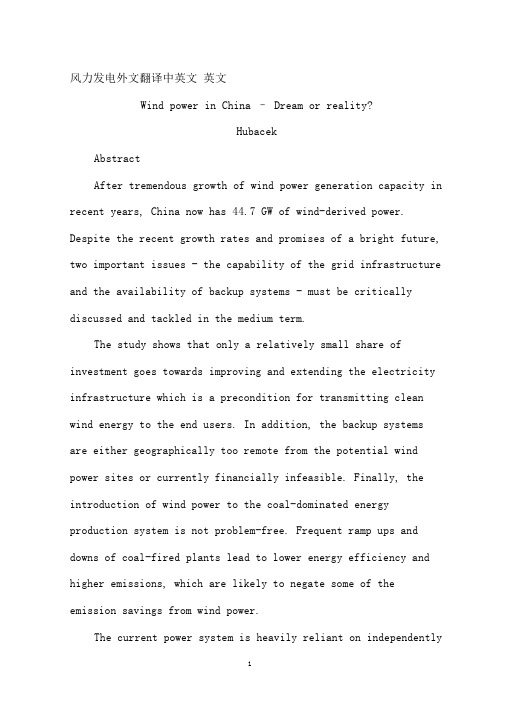
风力发电外文翻译中英文英文Wind power in China – Dream or reality?HubacekAbstractAfter tremendous growth of wind power generation capacity in recent years, China now has 44.7 GW of wind-derived power. Despite the recent growth rates and promises of a bright future, two important issues - the capability of the grid infrastructure and the availability of backup systems - must be critically discussed and tackled in the medium term.The study shows that only a relatively small share of investment goes towards improving and extending the electricity infrastructure which is a precondition for transmitting clean wind energy to the end users. In addition, the backup systems are either geographically too remote from the potential wind power sites or currently financially infeasible. Finally, the introduction of wind power to the coal-dominated energy production system is not problem-free. Frequent ramp ups and downs of coal-fired plants lead to lower energy efficiency and higher emissions, which are likely to negate some of the emission savings from wind power.The current power system is heavily reliant on independentlyacting but state-owned energy companies optimizing their part of the system, and this is partly incompatible with building a robust system supporting renewable energy technologies. Hence, strategic, top-down co-ordination and incentives to improve the overall electricity infrastructure is recommended.Keywords: Wind power, China, Power grids, Back-up systems1. IntroductionChina 'wsi nd energy industry has experienced a rapid growth over the last decade. Since the promulgation of the first Renewable Energy Law in 2006, the cumulative installed capacity of wind energy amounted to 44.7 GW by the end of 2010 [1]. The newly installed capacity in 2010 reached 18.9 GW which accounted for about 49.5% of new windmills globally. The wind energy potential in China is considerable, though with differing estimates from different sources. According to He et al. [2], the exploitable wind energy potential is 600–1000 GW onshore and 100–200 GW offshore. Without considering the limitations of wind energy such as variable power outputs and seasonal variations, McElroy et al. [3] concluded that if the Chinese government commits to an aggressive low carbon energy future, wind energy is capable of generating 6.96 million GWh of electricity by 2030, which is sufficient to satisfy China ' selectricity demand in 2030.The existing literature of wind energy development in China focuses on several discussion themes. The majority of the studies emphasize the importance of government policy on the promotion of wind energy industry in China [4], [5], [6], [7]. For instance, Lema and Ruby [8] compared the growth of wind generation capacity between 1986 and 2006, and addressed the importance of a coordinated government policy and corresponding incentives. Several studies assessed other issues such as the current status of wind energy development in China [9]; the potential of wind power [10]; the significance of wind turbine manufacturing [11]; wind resource assessment [5]; theapplication of small-scale wind power in rural areas [12]; clean development mechanism in the promotion of wind energy in China [4], social, economic and technical performance of wind turbines [13] etc.There are few studies which assess the challenge of grid infrastructure in the integration of wind power. For instance, Wang [14] studied grid investment, grid security, long-distance transmission and the difficulties of wind power integration at present. Liao et al. [15] criticised the inadequacy of transmission lines in the wind energy development. However, webelieve that there is a need to further investigate these issues since they are critical to the development of wind power in China. Furthermore, wind power is not a stand-alone energy source; it needs to be complemented by other energy sources when wind does not blow. Although the viability and feasibility of the combination of wind power with other power generation technologies have been discussed widely in other countries, none of the papers reviewed the situation in the Chinese context. In this paper, we discuss and clarify two major issues in light of the Chinese wind energy distribution process: 1) the capability of the grid infrastructure to absorb and transmit large amounts of wind powered electricity, especially when these wind farms are built in remote areas; 2) the choices and viability of the backup systems to cope with the fluctuations of wind electricity output.2. Is the existing power grid infrastructure sufficient?Wind power has to be generated at specific locations with sufficient wind speed and other favourable conditions. In China, most of the wind energy potential is located in remote areas with sparse populations and less developed economies. It means that less wind powered electricity would be consumed close to the source. A large amount of electricity has to be transmittedbetween supply and demand centres leading to several problems associated with the integration with the national power grid system, including grid investment, grid safety and grid interconnection.2.1.P ower grid investmentAlthough the two state grid companies-(SGCC) State Grid Corporation of China and (CSG) China Southern Grid - have invested heavily in grid construction, China 'pso wer grid is still insufficient to cope with increasing demand. For example, some coal-fired plants in Jiangsu, which is one of the largest electricity consumers in China, had to drop the load ratio to 60 percent against the international standard of 80 percent due to the limited transmission capacity [16]. This situation is a result of an imbalanced investment between power grid construction and power generation capacity. For example, during the Eighth Five-Year Plan, Ninth Five-Year Plan and Tenth Five-Year Plan,1 power grid investments accounted for 13.7%, 37.3% and 30% of total investment in the electricity sector, respectively. The ratio further increased from 31.1% in 2005 to 45.94% in 2008, the cumulative investment in the power grid is still significantly lower than the investments in power generation [17]. Fig. 1 gives a comparison of the ratios ofaccumulative investments in power grid and power generation in China, the US, Japan, the UK and France since 1978. In most of these countries, more than half of the electric power investment has been made on grid construction. By contrast, the ratio is less than 40% in China.According to the Articles 14 and 21 of the Chinese Renewable Energy Law, the power grid operators are responsible for thegrid connection of renewable energy projects. Subsidies are given subject to the length of the grid extension with standard rates. However, Mo [18] found that the subsidies were only sufficient to compensate for capital investment and corresponding interest but excluding operational and maintenance costs.Again, similar to grid connection, grid reinforcement requires significant amounts of capital investment. The Three Gorges power plant has provided an example of large-scale and long-distance electricity transmission in China. Similar to wind power, hydropower is usually situated in less developed areas. As a result, electricity transmission lines are necessaryt o deliver the electricity to the demand centres where the majority are located; these are the eastern coastal areas and the southern part of China. According to SGCC [19], the gridreinforcement investment of the Three Gorges power plants amounted to 34.4 billion yuan (about 5 billion US dollars). This could be a lot higher in the case of wind power due to a number of reasons. First, the total generating capacity of Three Gorges project is approximately 18.2 GW at this moment and will reach 22.4 GW when fully operating [20], whilst the total generating capacity of the massive wind farms amount to over 100 GW. Hence, more transmission capacities are absolutely necessary. Second, the Three Gorges hydropower plant is located in central China. A number of transmission paths are available, such as the 500 kV DC transmission lines to Shanghai (with a length of 1100 km), Guangzhou (located in Guangdong province, with a length of 1000 km) and Changzhou (located in Jiangsu province, with a length of 1000 km) with a transmission capacity of 3 GW each and the 500 kV AC transmission lines to central China with transmission capacity of 12 GW. By contrast, the majority of wind farm bases, which are located in the northern part of China, are far away from the load centres. For example, Jiuquan located in Gansu has a planned generation capacity of 20 GW. The distances from Jiuquan to the demand centres of the Central China grid and the Eastern China grid are 1500 km and 2500 km, respectively. For Xinjiang, the distances are even longer at 2500 km and 4000 km,respectively. As a result, longer transmission lines are required. Fig. 2 depicts the demand centres and wind farms in detail.2.2.Grid safetyThe second problem is related to grid safety. The large-scale penetration of wind electricity leads to voltage instability, flickers and voltage asymmetry which are likely to cause severe damage to the stability of the power grid [21]. For example, voltage stability is a key issue in the grid impact studies of wind power integration. During the continuous operation of wind turbines, a large amount of reactive power is absorbed, which lead to voltage stability deterioration [22]. Furthermore, the significant changes in power supply from wind might damage the power quality [23]. Hence, additional regulation capacity would be needed. However, in a power system with the majority of its power from base load provider, the requirements cannot be met easily [24]. In addition, the possible expansion of existing transmission lines would be necessary since integration of large-scale wind would cause congestion and other grid safety problems in the existing transmission system. For example, Holttinen [23] summarized the majorimpacts of wind power integration on the power grid at the temporal level (the impacts of power outputs at second, minute to year level on the power grid operation) and the spatial level (the impact on local, regional and national power grid). Besides the impacts mentioned above, the authors highlight other impacts such as distribution efficiency, voltage management and adequacy of power on the integration of wind power [23].One of the grid safety problems caused by wind power is reported by the (SERC) State Electricity Regulatory Commission [25]. In February and April of 2011, three large-scale wind power drop-off accidents in Gansu (twice) and Hebei caused power losses of 840.43 MW, 1006.223 MW and 854 MW, respectively, which accounted for 54.4%, 54.17% and 48.5% of the total wind powered outputs. The massive shutdown of wind turbines resulted in serious operational difficulties as frequency dropped to 49.854 Hz, 49.815 Hz and 49.95 Hz in the corresponding regional power grids.The Chinese Renewable Energy Law requires the power grid operators to coordinate the integration of windmills and accept all of the wind powered electricity. However, the power grid companies have been reluctant to do so due to the above mentioned problems as well as technical and economic reasons. For instance, more than one third of the wind turbines in China, amounting to 4 GW capacity, were not connected to the power grid by the end of 2008 [17]. Given that the national grid in China is exclusively controlled by the power companies – SGCC and CSG - the willingness ofthese companies to integrate wind energy into the electricity generation systems is critical.2.3.T he interconnection of provincial and regional power gridsThe interconnection of trans-regional power grids started at the end of 1980s. A (HVDC) high voltage direct current transmission line was established to link the Gezhouba2 dam with Shanghai which signifies the beginning of regional power grids interconnection. In 2001, two regional power grids, the North China Power Grid and Northeast China Power Grid were interconnected. This was followed by the interconnection of the Central China Power Grid and the North China Power Grid in 2003. In 2005, two other interconnection agreements were made between the South China Power Grid with North, Northeast and Central China Power Grid, and the Northwest China Power Grid and the Central China Power Grid. Finally, in 2009, the interconnection of Central China Power Grid and the East China Power Grid was made. In today ' s China, the Chinesepower transmission systems are composed of 330 kV and 500 kV transmission lines as the backbone and six interconnected regional power grids and one Tibet power grid [26].It seems that the interconnectivity of regional power grids would help the delivery of wind powered outputs from wind-rich regions todemand centres. However, administrative and technical barriers stillexist. First, the interconnectivity among regions is always considered as a backup to contingencies, and could not support the large-scale, long-distance electricity transmission [27]. In addition, the construction of transmission systems is far behind the expansion of wind power. The delivery of large amounts of wind power would be difficult due to limited transmission capacity. Furthermore, the quantity of inter-regional electricity transmission is fixed [27]. Additional wind power in theinter-regional transmission might have to go through complexadministrative procedures and may result in profit reductions of conventional power plants.3. Are the backup systems geographically available and technically feasible?Power system operators maintain the security of power supply by holding power reserve capacities in operation. Although terminologies used in the classification of power reserves vary among countries [28], power reserves are always used to keep the production and generation in balance under a range of circumstances, including power plant outages, uncertain variations in load and fluctuations in power generations (such as wind) [29]. As wind speed varies on all time scales (e.g. from seconds to minutes and from months to years), the integration of fluctuating wind power generation induces additional system balancing requirements on the operational timescale [29].A number of studies have examined the approaches to stabilize the electricity output from wind power plants. For example, Belanger and Gagnon [30] conducted a study on the compensation of wind power fluctuations by using hydropower in Canada. Nema et al. [31] discussed the application of wind combined solar PV power generation systems and concluded that the hybrid energy system was a viable alternative to current power supply systems in remote areas. In China, He et al. [2]investigated the choices of combined power generation systems. The combinations of wind-hydro, wind-diesel, wind-solar and wind-gas power were evaluated respectively. They found that, for instance, the wind-diesel hybrid systems were used at remote areas and isolated islands. This is because the wind-diesel hybrid systems have lower generation efficiency and higher generation costs compared to other generation systems. Currently, the wind-solar hybrid systems are not economically viable for large-scale application; thus, these systems have either been used at remote areas with limited electricity demand (e.g. Gansu Subei and Qinghai Tiansuo) or for lighting in some coastal cities [2]. Liu et al. [32] adopted the EnergyPLAN model to investigate the maximum wind power penetration level in the Chinese power system. The authors derived a conclusion that approximately 26% of national power demand could be supplied by wind power by the end of 2007. However, theauthors fail to explain the provision of power reserves at different time scales due to wind power integration.Because of the smoothing effects of dispersing wind turbines at different locations (as exemplified by Drake and Hubacek [33] for theU.K., Roques [34] for the E.U. and Kempton et al. [35] for the U.S.), the integration of wind power has a very small impact on the primary reserves which are available from seconds to minutes [36]. However, the increased reserve requirements are considerable on secondary reserves (available within 10 –15 min) which mainly consist of hydropower plants and gas turbine power plants [29]. Besides, the long-term reserves, which are used to restore secondary reserves after a major power deficit, will be in operation to keep power production and consumption in balance for a longer timescale (from several minutes to several hours). In the following subsection, we examine the availability of power plants providing secondary and long-term reserves and investigate the viability of energy storage system in China.中文中国的风力发电–梦想还是现实?胡巴切克摘要经过近几年风力发电能力的巨大增长,中国现在拥有 44.7 吉瓦的风力发电。
wind power generation作文及翻译风力发电
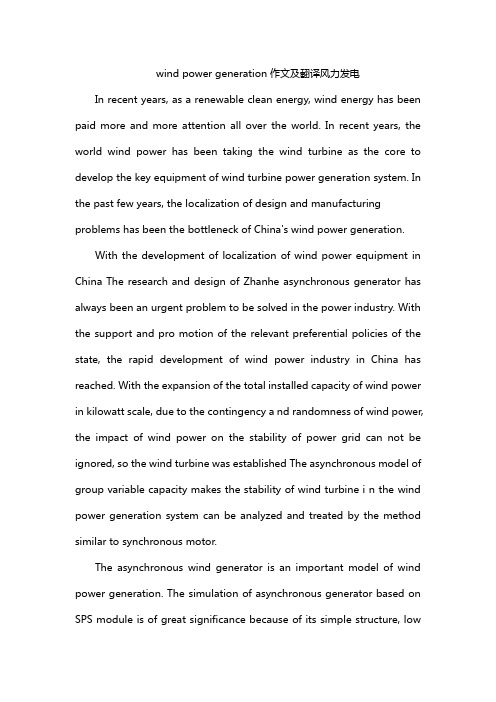
wind power generation作文及翻译风力发电In recent years, as a renewable clean energy, wind energy has been paid more and more attention all over the world. In recent years, the world wind power has been taking the wind turbine as the core to develop the key equipment of wind turbine power generation system. In the past few years, the localization of design and manufacturing problems has been the bottleneck of China's wind power generation.With the development of localization of wind power equipment in China The research and design of Zhanhe asynchronous generator has always been an urgent problem to be solved in the power industry. With the support and pro motion of the relevant preferential policies of the state, the rapid development of wind power industry in China has reached. With the expansion of the total installed capacity of wind power in kilowatt scale, due to the contingency a nd randomness of wind power, the impact of wind power on the stability of power grid can not be ignored, so the wind turbine was established The asynchronous model of group variable capacity makes the stability of wind turbine i n the wind power generation system can be analyzed and treated by the method similar to synchronous motor.The asynchronous wind generator is an important model of wind power generation. The simulation of asynchronous generator based on SPS module is of great significance because of its simple structure, lowprice and no strict control and control network equipment It is easier to connect with the power grid, but its speed can be changed to a certain extent, which will be able to absorb the transient process of wind energy. However, Xu Jizhu asynchronous generator grid is exciting and increases the demand for reactive power.Based on the requirements of asynchronous wind turbine control system, according to the actual operation model of wind turbine, and using Matlab/Simulink The simulation results are basically consistent with the actual operation of wind turbines.翻译:近年来,风能作为一种可再生的清洁能源日益受到世界各国的广泛关注,使得近几年世界风电一直以风电机组为核心发展风电机组发电系统的关键设备,在过去的几年里,设计和制造问题的本地化一直是中国风力发电的瓶颈,随着我国风力发电设备国产化工作的开展和异步发电机的研究与设计一直是电力行业迫切需要解决的问题,在国家相关优惠政策的支持和推动下,我国风电事业的快速发展已经达到了随着风电总装机容量千瓦规模的扩大,由于风电的偶然性和随机性,风电对电网稳定性的影响已不容忽视,建立了风电机组变容量异步模型,使风电机组在风力发电系统中的稳定性可以用类似于同步电机来分析处理它们的异步风力发电机是风力发电的一个重要模型,基于SPS模块的异步发电机仿真具有重要意义,因为其结构简单、价格低廉,而且不需要严格的控制和控制网络设备,可以更容易地与电网连接,但其转速可以在一定程度上改变,将能够吸收风能的暂态过程然而,许继柱异步发电机电网令人振奋,增加了电网对无功功率的需求,基于异步风电机组控制系统的要求开发了,根据建立的风力发电机组实际运行模型,并利用MATLAB/SIMULINK对其过程进行了仿真,仿真结果与实际运行的风电机组基本一致。
风力发电机作文英文
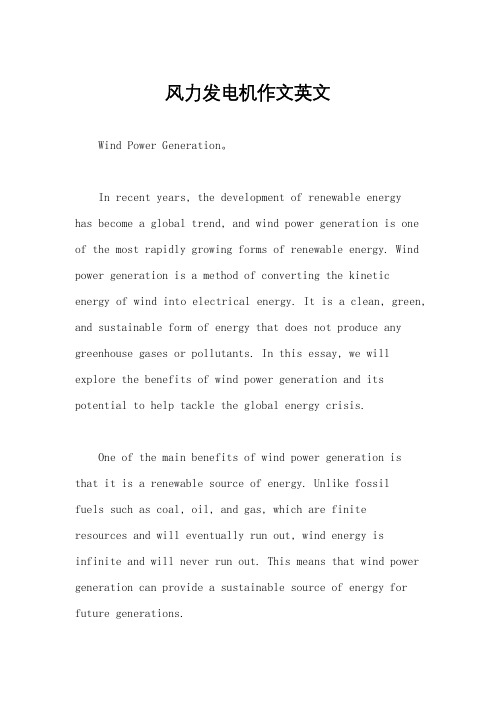
风力发电机作文英文Wind Power Generation。
In recent years, the development of renewable energyhas become a global trend, and wind power generation is one of the most rapidly growing forms of renewable energy. Wind power generation is a method of converting the kinetic energy of wind into electrical energy. It is a clean, green, and sustainable form of energy that does not produce any greenhouse gases or pollutants. In this essay, we will explore the benefits of wind power generation and its potential to help tackle the global energy crisis.One of the main benefits of wind power generation isthat it is a renewable source of energy. Unlike fossilfuels such as coal, oil, and gas, which are finiteresources and will eventually run out, wind energy isinfinite and will never run out. This means that wind power generation can provide a sustainable source of energy for future generations.Another benefit of wind power generation is that it is environmentally friendly. Wind turbines do not produce any greenhouse gases or pollutants, which means that they donot contribute to global warming or air pollution. This makes wind power generation a clean and green source of energy that can help reduce our carbon footprint andprotect the environment.In addition to being renewable and environmentally friendly, wind power generation also has economic benefits. Wind turbines can be installed in rural areas, which can provide jobs and stimulate economic growth in these regions. Wind power generation can also help to reduce our dependence on foreign oil, which can help to stabilize energy prices and improve energy security.Despite its many benefits, wind power generation is not without its challenges. One of the main challenges is that wind turbines can be expensive to install and maintain. However, as technology improves and economies of scale are achieved, the cost of wind power generation is expected tocontinue to decline.Another challenge is that wind power generation can be intermittent, meaning that it is not always available when it is needed. However, this can be mitigated by using energy storage systems, such as batteries, to store excess energy generated by wind turbines when it is available, and then release it when it is needed.In conclusion, wind power generation is a clean, green, and sustainable source of energy that has the potential to help tackle the global energy crisis. While it is not without its challenges, the benefits of wind power generation far outweigh the costs. As we continue to invest in renewable energy technologies, we can help to create a more sustainable and prosperous future for ourselves andfor generations to come.。
基于均值出力的风电调峰成本量化方法
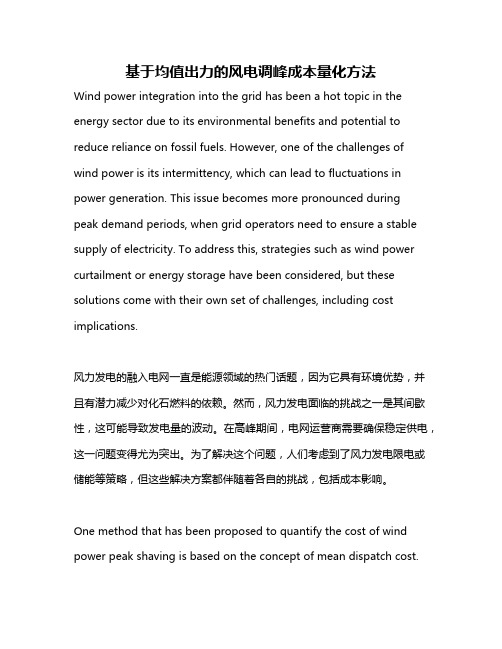
基于均值出力的风电调峰成本量化方法Wind power integration into the grid has been a hot topic in the energy sector due to its environmental benefits and potential to reduce reliance on fossil fuels. However, one of the challenges of wind power is its intermittency, which can lead to fluctuations in power generation. This issue becomes more pronounced during peak demand periods, when grid operators need to ensure a stable supply of electricity. To address this, strategies such as wind power curtailment or energy storage have been considered, but these solutions come with their own set of challenges, including cost implications.风力发电的融入电网一直是能源领域的热门话题,因为它具有环境优势,并且有潜力减少对化石燃料的依赖。
然而,风力发电面临的挑战之一是其间歇性,这可能导致发电量的波动。
在高峰期间,电网运营商需要确保稳定供电,这一问题变得尤为突出。
为了解决这个问题,人们考虑到了风力发电限电或储能等策略,但这些解决方案都伴随着各自的挑战,包括成本影响。
One method that has been proposed to quantify the cost of wind power peak shaving is based on the concept of mean dispatch cost.This approach involves calculating the difference between the average cost of dispatching electricity from wind power and the cost of dispatching electricity from other sources during peak periods. By comparing these costs, it is possible to estimate the financial implications of relying on wind power for peak shaving. However, this method may oversimplify the complexity of the electricity market and fail to capture all relevant factors that contribute to the cost of peak shaving.有一种提出的方法来量化风力调峰的成本,就是基于平均出力成本的概念。
电力英语词汇汇总
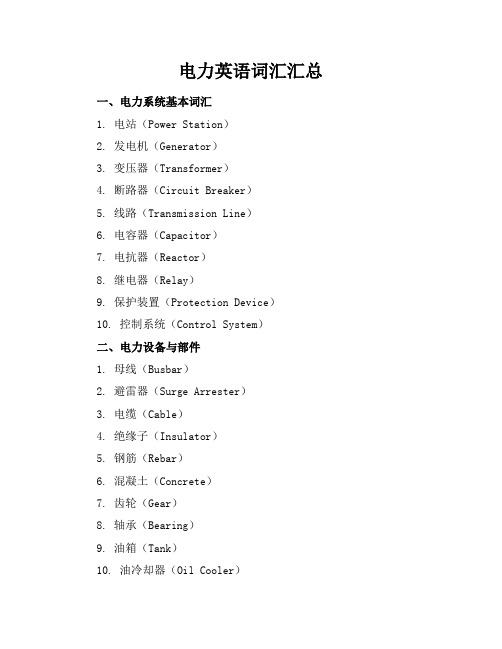
电力英语词汇汇总一、电力系统基本词汇1. 电站(Power Station)2. 发电机(Generator)3. 变压器(Transformer)4. 断路器(Circuit Breaker)5. 线路(Transmission Line)6. 电容器(Capacitor)7. 电抗器(Reactor)8. 继电器(Relay)9. 保护装置(Protection Device)10. 控制系统(Control System)二、电力设备与部件1. 母线(Busbar)2. 避雷器(Surge Arrester)3. 电缆(Cable)4. 绝缘子(Insulator)5. 钢筋(Rebar)6. 混凝土(Concrete)7. 齿轮(Gear)8. 轴承(Bearing)9. 油箱(Tank)10. 油冷却器(Oil Cooler)三、电力工程术语1. 电力工程(Electric Power Engineering)2. 设计规范(Design Specification)3. 施工图纸(Construction Drawing)4. 工程预算(Project Budget)5. 施工方案(Construction Scheme)6. 质量验收(Quality Acceptance)7. 安全生产(Safety Production)8. 环境保护(Environmental Protection)9. 节能减排(Energy Saving and Emission Reduction)10. 智能电网(Smart Grid)四、电力行业组织与机构1. 国家能源局(National Energy Administration)2. 电力公司(Electric Power Corporation)3. 电力设计院(Electric Power Design Institute)4. 电力科学研究院(Electric Power Research Institute)5. 电力行业协会(Electric Power Industry Association)6. 电力工会(Electric Power Trade Union)7. 电力市场(Electricity Market)8. 电力监管机构(Electric Power Regulatory Authority)9. 电力消费者协会(Electric Power Consumer Association)10. 国际电力组织(International Electric Power Organization)五、电力技术与发展1. 火力发电(Thermal Power Generation)2. 水力发电(Hydroelectric Power Generation)3. 核能发电(Nuclear Power Generation)4. 风能发电(Wind Power Generation)5. 太阳能发电(Solar Power Generation)6. 新能源(New Energy)7. 分布式发电(Distributed Generation)8. 电动汽车(Electric Vehicle)9. 能源互联网(Energy Internet)10. 电力系统自动化(Electric Power System Automation)六、电力运行与维护1. 电网调度(Power Grid Dispatching)2. 运行监控(Operation Monitoring)3. 设备巡检(Equipment Patrol Inspection)4. 预防性维修(Preventive Maintenance)5. 故障处理(Fault Handling)6. 状态检修(ConditionBased Maintenance)7. 安全操作(Safe Operation)8. 电力可靠性(Electric Power Reliability)9. 负荷预测(Load Forecasting)10. 电力质量(Power Quality)七、电力法律法规与政策1. 电力法(Electricity Law)2. 电力市场监管条例(Electricity Market Regulation)3. 电力设施保护条例(Regulations for the Protection of Electric Power Facilities)4. 电力供应与使用条例(Regulations on Electric Power Supply and Use)5. 电力价格政策(Electricity Pricing Policy)6. 电力体制改革(Electricity System Reform)7. 能源发展战略行动计划(Energy Development Strategy Action Plan)8. 环境保护法律法规(Environmental Protection Laws and Regulations)9. 节能减排政策(Energy Saving and Emission Reduction Policy)10. 电力行业发展规划(Electric Power Industry Development Plan)八、电力市场与交易1. 电力市场交易规则(Electricity Market Trading Rules)2. 电力中长期合同(Longterm Electricity Contract)3. 电力现货市场(Electricity Spot Market)4. 电价形成机制(Electricity Price Formation Mechanism)5. 售电公司(Electricity Sales Company)6. 用户侧响应(Customer Side Response)7. 跨区电力交易(Crossregional Electricity Trade)8. 电力市场分析(Electricity Market Analysis)9. 电力市场竞争(Electricity Market Competition)10. 电力市场风险管理与控制(Electricity Market Risk Management and Control)九、电力行业发展趋势1. 电力行业数字化转型(Digital Transformation of Electric Power Industry)2. 电力系统灵活性(Flexibility of Electric Power System)3. 电力储能技术(Electricity Storage Technology)4. 电力需求侧管理(Electricity Demand Side Management)5. 电力行业智能化(Intelligence of Electric Power Industry)6. 电力行业绿色低碳发展(Green and Lowcarbon Development of Electric Power Industry)7. 电力行业国际合作(International Cooperation inElectric Power Industry)8. 电力行业人才培养(Talent Training in Electric Power Industry)9. 电力行业科技创新(Technological Innovation in Electric Power Industry)10. 电力行业可持续发展(Sustainable Development of Electric Power Industry)十、电力行业热点问题1. 电力供需平衡(Electricity Supply and Demand Balance)2. 电力系统安全稳定(Safety and Stability of Electric Power System)3. 电力扶贫(Electricity Poverty Alleviation)4. 电动汽车充电基础设施建设(Electric Vehicle Charging Infrastructure Construction)5. 电力行业去产能(Capacity Reduction in Electric Power Industry)6. 电力行业环境保护(Environmental Protection in Electric Power Industry)7. 电力行业信用体系建设(Credit System Construction in Electric Power Industry)8. 电力行业反垄断(Antitrust in Electric Power Industry)9. 电力行业对外开放(Openingup of Electric Power Industry)10. 电力行业社会责任(Social Responsibility of Electric Power Industry)十一、电力技术创新与应用1. 智能电网技术(Smart Grid Technology)2. 分布式能源系统(Distributed Energy Systems)3. 微电网技术(Microgrid Technology)4. 能量管理系统(Energy Management System)5. 高压直流输电(High Voltage Direct Current Transmission)6. 超导技术(Superconductivity Technology)7. 电力电子技术(Power Electronics Technology)8. 量子计算在电力领域的应用(Application of Quantum Computing in Electric Power Field)9. 大数据与电力系统分析(Big Data and Electric Power System Analysis)10. 云计算在电力行业的应用(Application of Cloud Computing in Electric Power Industry)十二、电力工程项目管理1. 项目可行性研究(Project Feasibility Study)2. 项目立项(Project Approval)3. 项目招投标(Project Bidding)4. 项目合同管理(Project Contract Management)5. 项目进度控制(Project Schedule Control)6. 项目成本管理(Project Cost Management)7. 项目质量管理(Project Quality Management)8. 项目风险管理(Project Risk Management)9. 项目验收与移交(Project Acceptance and Handover)10. 项目后评价(Project Postevaluation)十三、电力行业职业素养与技能1. 电力工程师职业道德(Professional Ethics for Electrical Engineers)2. 电力行业职业技能培训(Vocational Skills Training in Electric Power Industry)3. 电力行业职称评定(Professional Title Evaluation in Electric Power Industry)4. 电力行业从业资格证书(Qualification Certificates in Electric Power Industry)5. 电力行业创新能力培养(Innovation Ability Training in Electric Power Industry)6. 电力行业团队协作(Team Collaboration in Electric Power Industry)7. 电力行业沟通与协调能力(Communication and Coordination Skills in Electric Power Industry)8. 电力行业应急处理能力(Emergency Handling Ability in Electric Power Industry)9. 电力行业法律法规知识(Legal Knowledge in Electric Power Industry)10. 电力行业国际视野(International Perspective in Electric Power Industry)十四、电力行业国际合作与交流1. 国际电力组织(International Electric Power Organizations)2. 国际电力展览会(International Electric Power Exhibitions)3. 国际电力技术交流(International Electric Power Technology Exchange)4. 国际电力项目合作(International Electric Power Project Cooperation)5. 国际电力市场分析(International Electric Power Market Analysis)6. 国际电力标准制定(International Electric Power Standards Development)7. 国际电力人才培养与合作(International Electric Power Talent Training and Cooperation)8. 国际电力政策研究(International Electric Power Policy Research)9. 国际电力环境保护合作(International Electric Power Environmental Protection Cooperation)10. 国际电力行业发展趋势探讨(Discussion on International Electric Power Industry Development Trends)。
风电专业术语大全英语

风能 /wind energy空气流动所具有的能量.风能资源 /wind energy resources大气沿地球表面流动而产生的动能资源.空气的标准状态 /standard atmospheric state空气的标准状态是指空气压力为101 325Pa,温度为15℃或绝对,空气密度m 3 时的空气状态.风速 /wind speed空间特定点的风速为该点空气在单位时间内所流过的距离.平均风速 /average wind speed给定时间内瞬时风速的平均值.年平均风速 /annual average wind speed时间间隔为一整年的瞬时风速的平均值.最大风速 /maximum wind speed10分钟平均风速的最大值.极大风速 /extreme wind speed瞬时风速的最大值.阵风 /gust超过平均风速的突然和短暂的风速变化.年际变化 /inter-annual variation以30年为基数发生的变化.风速年际变化是从第1年到第30年的年平均风速变化.风速或风功率密度年变化 /annual variation以年为基数发生的变化.风速或风功率变化年变化是从1月到12月的月平均风速或风功率密度变化.风速或风功率密度日变化 /diurnal variation以日为基数发生的变化.月或年的风速或风功率密度日变化是求出一个月或一年内,每日同一钟点风速或风功率密度的月平均值或年平均值,得到0点到23点的风速或风功率密度变化.风切变 /wind shear风速在垂直于风向平面内的变化.风切变指数 /wind shear exponent用于描述风速剖面线形状的幂定律指数.风速廓线 /wind speed profile, wind shear law又称“风切变律”,风速随离地面高度变化的数学表达式.湍流强度 /turbulence intensity标准风速偏差与平均风速的比率.用同一组测量数据和规定的周期计算.年风速频率分布 /annual wind speed frequency distribution在观测点一年时间内,相同的风速发生小时数之和占全年总小时数的百分比与对应风速的概率分布函数.威布尔分布 /Weibull distribution经常用于风速的概率分布函数,分布函数取决于两个参数,控制分布宽度的形状参数和控制平均风速分布的尺度参数.瑞利分布 /Rayleigh distribution控制分布宽度的形状参数值为2的威布尔分布,分布函数取决于一个调节参数——尺度参数,它控制平均风速的分布.风功率密度 /wind power density与风向垂直的单位面积中风所具有的功率.风能密度 /wind energy density在设定时段与风向垂直的单位面积中风所具有的能量. 风向 /wind direction风的流动方向在风速超过2m/s时测量.风向玫瑰图 /wind rose用极坐标表示不同风向相对频率的图解.风能玫瑰图 /wind energy rose用极坐标来表示不同方位风能相对大小的图解.主风向 /prevailing wind direction在风能玫瑰图中风能最大的方位.风能资源评估代表年 /representative year for wind energy resource assessment分析过去多年测风资料得到的一个典型年,其风能资源参数是未来风电场经营期内的预测平均值,作为估算风电机组年发电量的依据.测风塔 /wind measurement mast安装风速、风向等传感器以及风数据记录器,用于测量风能参数的高耸结构.风数据记录器 /wind data logger记录并初步处理测风数据的电子装置.风场 /wind site进行风能资源开发利用的场地、区域或范围.复杂地形带 /complex terrain风场的周围属地形显着变化的地带或有能引起气流畸变的障碍物地带.风力机/wind turbine将风的动能转换为另一种形式能的旋转机械.叶片/blade风力机的部件.叶片上的每个剖面设计成翼型,从叶根到叶尖,其厚度、扭角和弦长有一定分布规律,具有良好的空气动力外形.翼型/airfoil风轮叶片横截面的轮廓.叶片长度/length of blade叶片在展向上沿压力中心连线测得的最大长度.叶片叶根/root of blade叶片上与轮毂连接的部位.叶片叶尖/tip of blade叶片上在展向上与叶根距离最大的部位.叶片扭角/twist angle of blade叶片各剖面弦线和风轮旋转平面的夹角.叶片桨距角/pitch angle of blade叶尖剖面的弦线与风轮旋转平面的夹角.叶片安装角/setting angle of blade在叶片安装到轮毂上时设定的桨距角.顺桨/feather将桨距角变化到风轮叶片处于零升力或升力很小条件的状态.叶尖速度/tip speed风轮旋转时叶尖的线速度.叶尖速比/tip speed ratio叶尖速度与风速之比.风轮/rotor将风能转化为机械能的风力机部件,由叶片和轮毂组成.风轮直径/rotor diameter叶尖旋转圆的直径.风轮扫掠面积/swept area of rotor与风向垂直的平面上,风轮旋转时叶尖运动所生成圆的投影面积.风轮轴/rotor shaft风力机部件,与风轮连接传递机械能的旋转轴.轮毂/hub将叶片固定到旋转轴上的连接部件.轮毂高度/hub height从地面到风轮扫掠面中心的高度.水平轴风轮/horizontal axis rotor风轮轴基本上平行于水平面的风轮.垂直轴风轮/vertical axis rotor风轮轴线与水平面垂直的风轮.失速调节/stall regulated在风轮转速基本不变的条件下,利用叶片的气动力失速原理改变叶片升阻力,调节风电机组输出功率.桨距调节/pitch regulated利用叶片桨距角的变化改变叶片升阻力,调节风电机组输出功率.定桨距/fixed pitch叶片与轮毂固定连接,桨距角不可改变.变桨距/variable pitch叶片与轮毂通过轴承连接,桨距角可改变.定转速风轮/constant speed rotor风电机组运行时转速基本保持不变的风轮.变转速风轮/variable speed rotor风电机组运行时转速可以在一定范围内变化的风轮. 整流罩/nose cone装在轮毂上呈流线型的罩子.功率系数/power coefficient风轮所输出的功率与通过风轮扫掠面积从未扰动气流得到的功率之比.推力系数/thrust coefficient风作用在风轮上产生的轴向力与未扰动气流的动压及风轮扫掠面积的乘积之比.空气动力刹车/aerodynamic brake风轮旋转时增加叶片运动方向上的空气阻力,达到降低转速目的的制动装置.风力发电/wind power generation, wind power简称“风电”.将风所蕴涵的动能转换成电能的工程技术.风力发电机组/wind turbine generator system, WTGS简称“风电机组”.将风的动能转换为电能的系统.上风向式风电机组/upwind WTGS风先通过风轮再经过塔架的风电机组.下风向式风电机组/downwind WTGS风先通过塔架再经过风轮的风电机组.机舱/nacelle在水平轴风电机组顶部容纳发电机、传动系统和其他装置的部件.风电机组传动系统/drive train将风轮的机械能传递到发电机的系统.风电机组异步发电机/asynchronous generator, induction generator又称“感应发电机”.转速超过同步转速时,由定子绕组形成的旋转磁场与转子绕组中感应电流的磁场相互作用,输出电能的交流发电机.恒速恒频风电机组/constant speed-constant frequency WTGS并网运行后风轮转速不能随风速改变,由电网频率决定的风轮转速和电能频率在运行时基本保持不变的风电机组.变速恒频风电机组/variable speed-constant frequency WTGS风轮转速可以随风速在较宽的范围内改变,机组输出的电能频率与电网频率保持同步的风电机组.直驱式风电机组/direct drive WTGS, gearless WTGS又称“无齿轮箱式风电机组”.风轮直接驱动多极低速发电机的风电机组.半直驱式风电机组/multibrid technology WTGS风轮经过一级齿轮增速驱动多极中速发电机的风电机组.偏航机构/yawing mechanism使水平轴风电机组的风轮轴绕塔架垂直中心线旋转的机构.风电机组塔架/tower for WTGS支撑机舱和风轮的风电机组部件.桁架式塔架/lattice tower由钢管或角钢等组成的框架式结构的塔架.圆筒式塔架/tubular tower由钢板弯曲焊接成圆筒形结构的塔架.风电机组基础/foundation for WTGS与塔架连接支撑风电机组的构筑物.功率特性/power performance风电机组发电能力的表述. 净电功率输出/net electric power output风电机组输送给电网的电功率值.功率曲线/power curve描绘风电机组净电功率输出与风速的函数关系图和表.测量功率曲线/measured power curve描绘用正确方法测得并经修正或标准化处理的风力发电机组净电功率输出的图和表.它是测量风速的函数.风电机组额定功率/rated powerfor WTGS正常工作条件下,风力发电机组所能达到的设计最大连续输出电功率.风电机组最大功率/maximum powerfor WTGS正常工作条件下,风电机组输出的最高净电功率.切入风速/cut-in wind speed风电机组开始发电时,轮毂高度处的最低风速.额定风速/rated wind speed风电机组达到额定功率输出时轮毂高度处的设计风速.切出风速/cut-out wind speed风电机组保持额定功率输出时,轮毂高度处的最高风速.安全风速/survival wind speed风电机组结构所能承受的最大设计风速.年理论发电量/annual energy production利用功率曲线和轮毂高度代表年的风速频率分布,估算得到的一台风电机组一年时间内生产的全部电能.计算中假设可利用率为100%. 风电机组关机/shutdownfor WTGS从发电到静止或空转之间的风电机组过渡状态.风电机组正常关机/normal shutdownfor WTGS全过程都是在控制系统控制下进行的关机.风电机组紧急关机/emergency shutdownfor WTGS保护装置系统触发或人工干预下,使风电机组迅速关机.风电机组空转/idlingfor WTGS风电机组缓慢旋转但不发电的状态.风电机组可利用率/availabilityfor WTGS在评估期间内,风电机组处于能够运行发电、启动、停车或能够发电的待机状态的时数与这一期间内总时数的比值,用百分比表示. 风电机组控制系统/control systemfor WTGS接受风电机组信息和环境信息,调节风电机组,使其保持在工作要求范围内的系统.风电机组保护系统/protection systemfor WTGS确保风电机组运行在设计范围内的系统.风电场/wind power plant, wind farm由多台风力发电机组构成的发电厂.近海风电场/offshore wind farm距离海岸比较近,而且风电机组基础与海底连接的风电场.尾流效应损失/wake effect losses在风电场中,气流经过上游风电机组风轮后所形成的尾流对下游风电机组性能产生的影响和能量损失.风电机组电力汇集系统/power collection systemfor WTGS 汇集风电机组输出电能的电力连接系统. 包括风电机组电能输出端与电网连接点之间的所有电气设备.风电场电网连接点/grid connection point for wind farm 风电场电力汇集系统与电网输电线路的连接点.。
风力发电原理英语作文

风力发电原理英语作文Wind Power Generation Principle。
Wind power is one of the most promising renewable energy sources in the world today. It is a clean and sustainable source of energy that can be used to generate electricity. The principle of wind power generation is simple. The kinetic energy of the wind is converted into electrical energy through a process called wind power generation. The following is a detailed explanation of the wind power generation principle.Wind power generation is a process that involves the conversion of the kinetic energy of the wind intoelectrical energy. The process involves the use of wind turbines, which are designed to capture the kinetic energy of the wind and convert it into electrical energy. Wind turbines consist of three main components: the rotor, the generator, and the control system.The rotor is the part of the wind turbine that captures the kinetic energy of the wind. It consists of two or three blades that are attached to a hub. The blades are designedto be aerodynamic, which means that they are shaped in such a way that they can capture the maximum amount of wind energy. When the wind blows, it causes the blades to rotate.The generator is the part of the wind turbine that converts the kinetic energy of the wind into electrical energy. It consists of a rotor and a stator. The rotor is connected to the blades of the wind turbine and rotates when the wind blows. The stator is stationary and containsa series of coils that are connected to an electrical circuit. As the rotor rotates, it generates an electrical current in the coils of the stator.The control system is the part of the wind turbine that regulates the speed of the rotor. It consists of a set of sensors and a controller. The sensors measure the speed and direction of the wind, and the controller adjusts the position of the rotor to optimize the amount of energy that is captured.In summary, wind power generation is a process that involves the conversion of the kinetic energy of the wind into electrical energy. It is a clean and sustainable source of energy that can be used to generate electricity. Wind turbines consist of three main components: the rotor, the generator, and the control system. The rotor captures the kinetic energy of the wind, the generator converts it into electrical energy, and the control system regulates the speed of the rotor.。
主动配电网

1INTRODUCTIONAs the requirement of environmental protection is increasing day by day and one-time energy increasingly exhausted, develop clean pollution-free subsequent energy has become the top priority. Wind power as a kind of clean and renewable energy, is attached great importance to by all countries in the world. AC excitation variable-speed constant-frequency wind power generation system with doubly-fed induction generator (D FIG) is a new and efficient way to generate electricity, has significant advantages: not only can greatly improve the efficiency of energy conversion, reduce the prime mover of mechanical stress, but also can realize the decoupling control of active and reactive power, improve the adjusting ability and stability of power system.At present, there are some defects in AC excitation converter of doubly-fed induction generator with AC-AC frequency converter or double PWM converter. The former contains a large number of output voltage harmonic waves, the input side power factor is low, for the grid and generator are serious harmonic pollution; although the latter improves the input and output performance, needs to large volume of D C filter capacitor, and reduces the reliability of the system. The two-stage matrix converter (TSMC) is a new topological matrix converter, which has potential development in the last few years [1]. Without DC filter element, but with a simple topology, the power circuit is compact, easy to integration and modularization; Sinusoidal input and output current can be obtained, the energy can be two-way flow, and the system is reliable. Therefore, TSMC will be a kind of ideal excitation power This work is supported by National Nature Science Foundation under Grant 51467001, the national key project of university campus under Grant 2014XZZ03. supply for doubly-fed wind power generation system. Based on the characteristics of wind power generation, the simulation model of the stator flux oriented vector control system based on the TSMC is established. Since the input filter of the two-stage matrix converter reduces the input power factor in the network side, an adaptive control method is proposed to reduce the input power factor angle. In addition, the use of PR controller can realize adjusting the AC signal in the static coordinate system, don't need to coordinate rotation transformation, there is no coupling and feed forward compensation term, it is introduced into the rotor converter, the rotor PR control system were designed. Compared with the traditional double-loop PI control, the PR control reduces the difficulty of implementing control algorithms, and improves the robustness of the doubly-fed wind power generation control system.2THE TOPOLOGY STRUCTURE OF TSMC TSMC is similar to that of conventional AC - DC - AC converter topologies, which includes AC - DC (network side rectification stage) and DC - AC (rotor side inverter stage) two conversion circuits. D oubly-fed wind power generation system requires the rotor side energy bidirectional flow. Therefore, the 18 switch two-stage matrix converter is used as the excitation power source; the topological circuit is shown in figure 1. Under different constraints, the 18 switch topology circuit can be further simplified to give a variety of circuit topology, can be found in the literature 1.Control of Doubly-fed Wind Power Generation System Based onTwo-stage Matrix ConverterWang Junrui, Cao Xincheng, Zhang Jinning , Ji XuanweiSchool of Electrical and Information Engineering, the North University for Ethnics, Yinchuan 750021E-mail: jr09110111@Abstract: For the shortcomings of the traditional converter in variable-speed constant-frequency doubly-fed wind power generation system, this paper cites two-stage matrix converter (TSMC) as the excitation source of it. Based on the stator flux linkage oriented, the control system of the doubly-fed wind power generation is established. The TSMC input filter introduces a phase difference between the input current before and after the filter, which reduces input power factor of grid side, an adaptive input power factor control method is proposed to reduce the input power factor angle. With the principle of proportional resonant (PR) controller, PR control strategy used in rotor converter is proposed. In this method, the active and reactive current component in the vector control strategy is transformed in the stationary frame to adjust and to achieve decoupling the active and reactive power. The simulation results demonstrate the correctness and availability of the scheme presented in this paper.Key Words: two-stage matrix converter, doubly-fed wind power generation, input power factor, proportional resonantcontrol6177978-1-4673-9714-8/16/$31.00c 2016IEEEFig 1. 18 unidirectional switch composed of topology structurewith two - stage matrix converter3TSMC CONTROL STRATEGIESTSMC is a kind of AC/DC/AC converter, and the spacevector modulation strategy is divided into two parts:Rectifier level modulation and inverter level modulation.The purpose of the rectifier level modulation is to get theoutput polarity on the DC side is positive, with the inputcurrent and the input voltage changing according to thesine law, the input power factor is 1. Inverter levelmodulation is intended to be in the output frequency andamplitude can be adjusted by the three-phase outputvoltage. As shown in figure 1, the inverter level iscomposed of a single direction switch, which is the sameas the traditional voltage mode inverter. The controlstrategy adopts the traditional voltage space vectormodulation (SVPWM) [2-4]. As the control strategy is moretraditional, only the modulation strategy of the rectifierlevel is described in this paper [2], the modulation strategyof the inverter level is no longer described.3.1Rectification Level Space Vector ModulationIn order to simplify the analysis of the problem, thefollowing assumptions are made. Input three-phase powersupply voltage:°¯°®+==−====)32cos(cos)2cos(cos)cos(cosπωθπωθωθtUUutUUutUUuimcmscimbmsbimamsa(1)In the type,iωfor the input angular frequency, U m for theinput voltage amplitude value.The purpose of the rectifier level modulation is to the dcoutput voltage polarity is positive, and to obtain themaximum voltage utilization ratio, for which the inputphase voltage of the period is divided into 6 intervals, asshown in figure 2. The polarity of three-phase voltage ofeach interval is kept constant, the maximum amplitude ofwhich one phase voltage is positive or negative.The rectifier level space vector modulation is divided intotwo segments each switching period T s, by changing theswitch combination of these two segments and thecorresponding duty cycle to achieve the purpose ofmodulation. The following 1 interval as an example toillustrate.When the input voltage within the section of 1, u sa ispositive and the absolute value is maximum, u sb, u sc isnegative. To be as large as possible and output polarity DCFig 2. Division of the six sectionsvoltage with a positive, the a phase is always connected tothe DC positive electrode, the b and c phases are switchedto the DC negative electrode, the line voltage in the DCside of the rectifier level respectively u ab, u ac. So on, theinput voltage can be obtained in the other 5 intervals of theswitching period T s corresponding to the switchcombination and dc voltage.A d ab, and d ac, respectively for 1 interval DC voltage u ab, u accorresponding to the two time periods of duty ratio, thend ab + d ac =1.In order to ensure that the input current vector varies withthe input voltage vector and the input power factor is 1,then d ab, d ac should be proportional to the correspondinginput phase voltage amplitude:°°¯°°®−=−=−=−=sascacacsasbababuuduudθθθθcoscoscoscos(2)The local average DC voltage in a switching period T s:amacacababdcUududuθcos23=+= (3)In the same way, the duty cycle and the local average DCvoltage can be obtained by the switch in the T s of the otherswitching cycles:°¯°®==)coscoscosmax(coscos23cbaiimdcUuθθθθθˈˈ(4)3.2Rectifier Level Input Power Factor ControlAt present, the LC structure is used in the rectifier levelinput filter, as shown in figure 3. Usually in the modulationof the rectifier level, ignoring the input filter to the inputvoltage and current caused by the phase shift, and directthe input voltage u s and main circuit of input current i inbetween phase angle ij1 as the two-stage matrix converterinput power factor angle. In practice, however, the matrixconverter input power factor angle is not ij1,but thenetwork side input voltage u s and network side the anglebetween the input current i s are ij. Since the input filter isintroduced, the phase difference between the filter and theinput current is unavoidable. So even if the set with ij1=0,input voltage and current is not the same phase, the inputpower factor is not 1 [5-7].In wind power generation system, the general requirementof network side is running in the unit power factor, so theinput power factor of the rectifier level is controlled. 6178201628th Chinese Control and Decision Conference(CCDC)The voltage phase of the main circuit is α=∠ s mU U , the input current of the main circuit is 1()αφ=∠− in m II , the input current phase of the network is β=∠ s xI I . According to figure 3 can be obtained ˖°¯°®−==−)(1in s i in si in s I I C j U I L j U U ωω (5) Finishing,si in s i I LC I U C j )1(2ωω+=+ (6) The amount of each phase was substituted,βωϕααω∠+=−∠+∠x i m m i I LC I CU j )1()(21 (7) Can be obtained,LCI U C CU I I i m m i m i m x 21221sin 2)()(ϕωω+−+=(8) 1221sin 2)()(cos arcsin2ϕωωϕπβαϕm m i m i m m I U C CU I I −++−=−= (9)Visible, the actual power factor angle ij and filtercapacitor, grid side voltage amplitude, main circuit side input current amplitude, input voltage, frequency and input power factor angle setting value ij1related.Fig 3. Input LC filter with two-stage matrix converterAccording to the above analysis, the influence of the input filter on the input power factor is not negligible. To solve this problem, this paper presents an adaptive control method of input power factor, as shown in figure 4. First detected by three-phase input voltage value and current value, calculate the reactive power Q of the network side. To make the network side input power factor is 1, the reactive power for a given value of 0. PI controller is used to make the PI output according to the change of reactive power, real-time adjustment input phase shift angle ij1 set value between the grid side voltage and main circuit side input current.In doubly-fed wind power generation system, the rotor side energy can flow bi-directional. In the sub synchronous and synchronous speed, the network side input power factor is 1, and under the super synchronous speed, the input power factor of the grid side is -1. Due to the introduction of input filter, the phase angle of grid side input current in actual ahead of grid side input voltage, calculated reactive power feedback value is negative. When the doubly-fed motor in++Fig 4. Adaptive control of input power factordifferent operation speeds, ij1 set value in a different direction. In the sub synchronous and synchronous speed, the ij1 set value to make the ij1 lagged; and in super synchronous speed, ij1 set value to make ij1 advanced. Based on the above analysis, in the super synchronous speed, to calculate the reactive power value whichever is the opposite.Since the inverter stage of the two-stage matrix converter belongs to the voltage type inverter, therefore, in order to prevent anti-parallel diode is shorted by the intermediate D C link inverter stage switching devices, the need to ensure that the intermediate D C link voltage is always positive. This is a constraint on the input side power factor adjustment. In the intermediate D C voltage is positive assurance under the premise of rectification stage adjustment range input power factor angle for [- ʌ /6, ʌ /6]. In the PI regulation, the input power factor angle is adjusted by the output limit of PI, so as to ensure that the intermediate DC voltage is positive.Using the proposed control method, the input power factor can be changed by adjusting the setting value of the input phase shift angle for different loads and different operating conditions. However, in the case of no-load and light load, the output and input current amplitude is small, and the input power factor can be significantly reduced when the filter parameters and the input supply voltage are constant. In control to ensure that the intermediate D C voltage is positive, set the power factor angle adjustment range, and in the no-load and light load, can only reduce the input power factor angle, and can't fully achieve the input unit power factor.4 THE ROTOR SIDE CONVERTER PRCONTROL4.1 Doubly-fed Motor Mathematical ModelAccording to the stator side of the generator, the rotor side of the motor practice, based on the stator flux orientation, can be deduced from the two-phase synchronous rotating d-q-0 coordinate system of the mathematical model of the doubly-fed motor is [8-12]0ψψψ=−=®=−=¯ds s ds m dr s qs s qs m qrl i l i l i l i (10)°¯°®=+−=+−=+−=qr r qr r qs m qr dr r s s m dr r ds m dr i l i l i l i l l l i l i l σψσψψ (11)201628th Chinese Control and Decision Conference (CCDC)6179¯®−=−=−=+=2/3)(32/32/)(3ds s qs ds ds qs sqs s qs qs ds ds s i U i u i u Q i U i u i u P (12) °¯°®Δ++=Δ++=qrqr r qr r qr dr dr r dr r dru i dt dl i r u u i dt d l i r u σσ (13) °¯°®+−=Δ−=Δdr s r s s sm qr qr s r dr i l l l u i l u ωσψωωσ (14)In the type: u , i , Ȍ only respectively for voltage, current and flux; r , l respectively for resistance, inductance; subscript d and q respectively d , q component; subscript s and r respectively stator and rotor components; subscript m said rotor between the amount of interactions. Ȧs to turn the differential angle frequency; U s , ȥs , respectively the amplitude of the stator voltage and the stator flux vector; P s and Q s respectively for the stator output active power and reactive power; ı is the total magnetic flux leakage coefficient, )/(12r s m l l l ⋅−=σ.By the formula (12), it is known that the active power and reactive power of the doubly-fed motor can reach the decoupling control, and the independent decoupling control of the stator current i qs and i ds are required. In the type (14) the compensation terms for eliminating the rotor voltage and current cross coupling are presented. 4.2 PR ControllerPR controller [13] is composed of a proportional link and a generalized integral link, and its transfer function is22)(ω++=s sK K s G ip PR (15)In the formula, the K p is the proportionality constant, K i is the integral time constant; Ȧ is the resonant frequency. Figure 5 is the bode diagram of the PR controller, including K p =1, Ȧ=100ʌ, K i were taken 100 , 500 , 1000 , 3000, respectively. Above is the amplitude frequency characteristic curve, below is a characteristic curve of phase frequency. As can be seen from figure 5, the angular frequency Ȧ quite a narrow frequency band around the corresponding amplitude gain is greater than that of other frequency amplitude gain. The width of the frequency band is determined by the integral time constant K i . The smaller the K i , the narrower the width of the frequency band, and the wider, the width of the frequency band of K i . When the angular frequency of a given AC signal for the Ȧ, the amplitude of G PR can be infinite, the non steady state error tracking of AC input signals can be realized.In the control of the rotor side converter of doubly-fed wind power generation system, the active current and reactive current components of the traditional vector control strategy can be converted to the two-phase stationary coordinate system, and the PR controller is used to carry out the steady-state error of the AC input signal. The coordinate transformation of vector control strategy is simplified. In the rotor side converter control, the rotorcurrent frequency is constantly changing, and the change is slip frequency angular frequency Ȧs . So in the control, the controller can be used to adjust the current of the rotor, if the resonant frequency of the PR controller is the Ȧ=Ȧs .M a g n i t u d e (d B )10101010P h a s e (d e g )Bode DiagramFrequency (rad/sec)Fig 5. Bode diagram of PR controller4.3 Rotor Side Converter PR Control System Figure 6 shows the control system of the rotor side converter based on the PR controller. Compared with the traditional double closed loop PI control, this method reduces the difficulty of the control algorithm. By the graph, the rotor current active component and reactive component is converted into the AC quantity in the stationary coordinate system, and the AC quantity is regulated by PR controller. In the process of adjustment, the need to real-time detection slip angular frequency Ȧs to adjust PR controller, so as to realize the rotor side current ++−+−Fig 6. PR control system for rotor side converter5 SIMULATIONS AND RESULT ANALYSISIn order to verify the advantage of the two-stage matrix converter in doubly-fed wind power generation system, input power factor angle adjustment and PR vector transformation control effectiveness of active and reactive power independent control of the generator, the control system of the stator flux oriented vector control system with two-stage matrix converter is simulated. The parameters used in the simulation of AC excitation power generation system are as follows: two-stage matrix converter input inductance L=2mH, capacitance C=10μF; The rated power of three-phase six pole winding induction motor is 10KW; Stator resistance and leakage inductance6180201628th Chinese Control and Decision Conference (CCDC)are 0.379ȍ, 1.1mH; Rotor resistance and leakage inductance are 0.314ȍ, 2.2mH; Mutual inductance is 42.7mH; The rotational inertia is 0.39kg·m2.i /A , u /V(a) Sub synchronous speedt /si /A , u /Vu a(b) Synchronous speedt/s(c) Super synchronous speedFig 7.Rectifier open-loop control grid side A phase voltage current waveformi /A , u /V(a) Sub synchronous speedi /A , u /V(b) Synchronous speedi /A , u /V(c) Super synchronous speedFig 8. Input power factor rectification stage under adaptive control grid side A phase voltage and current waveforms Figure 7and figure 8 gives respectively the rectifier level of open loop control and join the adaptive control of inputpower factor, doubly-fed motor in sub synchronous, synchronous, super synchronous speed under three working conditions of grid voltage and current waveform. Contrast waveform, rectifier under the open loop control due to the introduction of input filter, the input power factor is not unit power factor. When the input power factor is added to the rectifier level, even though the doubly - fed motor is in different operating conditions, the input power factor angle can be reduced, so that the inputpower factor is close to unity power factor.i /A(a) Į shaft reference current and feedback currente i /A(b) Įshaft axial current errori /A(c) ȕ shaft reference current and feedback current Fig 9. PR Control of rotor current dynamic responseFigure 9 is the dynamic response of the rotor current under the PR control. As can be seen from figure 9, the two-phase stationary coordinate system, with the change of current frequency, the rotor current feedback value can quickly track the changes of a given value, after reaching the steady state, the steady-state error is very small, nearly zero. PR controller realizes the smooth transition of rotor current.Figure 10 is the middle of the DC side voltage and current waveform. From the DC voltage waveform, the DC side voltage is variable, but the polarity of the DC voltage is positive. The following diagram is a system by the sub synchronization, synchronization to super synchronous transition in the process of the DC side current waveform, it can be seen that the DC side current is also variable, but its polarity changes with the operating speed of the doubly-fed motor. 1s before the doubly-fed motor in the sub synchronous, synchronous speed, the average value of the current is positive, which shows the power of the converter to the rotor output. After 1s doubly-fed motor under super synchronous speed, the average of the current negative, the rotor to the converter output power.201628th Chinese Control and Decision Conference (CCDC)6181u d c /Vi d c /AFig 10. DC side voltage and currenti /AFigure 11. Three-phase current waveform of rotorFigure 11 is a dynamic waveform of the phase current of the rotor during the transition of the sub synchronization, synchronization to super synchronous. From the diagram can be seen, the frequency of the rotor current, phase sequence as the change of speed of unit and corresponding change, thus ensuring constant stator voltage frequency.t /si /A , u /V0.45Figure 12. Stator phase voltage and phase currentFigure 12 is the unit power factor control system of the stator phase current and phase voltage waveform. Under the condition of the reactive component given is 0, the frequency of the phase voltage and phase current is constant, and the phase is opposite, power made from the stator side to the grid .6 CONCLUSIONSThe two-stage matrix converter is a matrix converter with new topology structure, it is able to provide sinusoidal PWM output voltage, input power factor control, energy transmission reversible, without big advantages of energy storage element. In this paper, a two - stage matrix converter is introduced as the excitation source of the doubly-fed wind power generation system. The two-stage matrix converter is added to the input filter, so that the current fundamental frequency component of the filter is poor, and the input power factor is reduced. In this paper, an adaptive control method input power factor is proposed, which reduces the input power factor angle, and the input power factor is close to unity power factor. Combinationwith the characteristics of PR controller, and PR control of rotor side converter is proposed. The decoupling control of active and reactive power of doubly-fed motor is realized by this method. The control algorithm is simplified and the robustness of control system is improved.REFERENCES[1] Kolar J W, Baumann M, Schafmeister F, Novel three-phaseAC-DC-AC sparse matrix converter[C], Proceedings of 17th IEEE Aplied Power Electronics Conference and Exposition, 2:777-791, 2002.[2] Deng Wenlang, Yang Xingrong, Zhu Jianlin, et al. Spacevector modulation strategy of two-stage matrix converter with 18 switches and it is simulation study [J]. Proceedings of the CSEE, 25(15): 84-90, 2005.[3] Wei Lixiang, Lipo T A, Chan H. Matrix convertertopologies with reduced number of switches [J]. IEEE Trans on Industrial Electronics, 45(1): 675-680, 2003.[4] Wei Lixiang, Lipo T A. A novel matrix converter topologywith simple commutation[C]. Proceeding of 36th IEEE Industry Applications Society Conference (IAS2001), Chicago, 3:1749-1754, 2001.[5] Huber L, Borojevic D. Space vector modulated three-phaseto three-phase matrix converter with input power factor correction [J]. IEEE Trans on Industry Application, 31(6): 1234-1246, 1995.[6] Kwak Sangshin. Indirect matrix converter drives for unitydisplacement factor and minimum switching losses [J]. Electric Power Systems Research, 77(5-6): 447-454, 2007. [7] Mei Yang, Sun Kai, Huang Lipei. Input power factor controlfor matrix converters [J]. J Tsinghua Univ, 49(4): 369-472, 2009.[8] Bian Songjiang. Research on the key technology of VSCFwind power generation [D]. Hangzhou: Zhejiang University, 2003.[9] Liu Qihui. VSCF wind power generation system and controlresearch [D]. Hangzhou: Zhejiang University, 2005.[10] Pena R, Clare J C, Asher G M. D oubly fed inductiongenerator using back-to-back PWM converters and its application to variable-speed wind energy generation [J]. IEE Proceedings Electric power Applications, 143(3): 587-591, 1996.[11] Guo Jindong, Zhao Dongli, Lin Zixu, et al. Research of themegawatt level variable speed constant frequency wind power unit control system [J]. Proceedings of the CSEE, 27(6): 1-6, 2007. [12] Yuan Guofeng, Chai Jianyun, Lin Yongdong. Study onexcitation converter of variable speed constant frequency wind generation system [J]. Proceedings of the CSEE, 25(8): 90-94, 2005.[13] Chen Cheng. The variable speed constant frequencywind power generation system of dual PWMconverter control [D]. Tianjin: Tianjin University, 2008.6182201628th Chinese Control and Decision Conference (CCDC)。
DS2208数字扫描器产品参考指南说明书
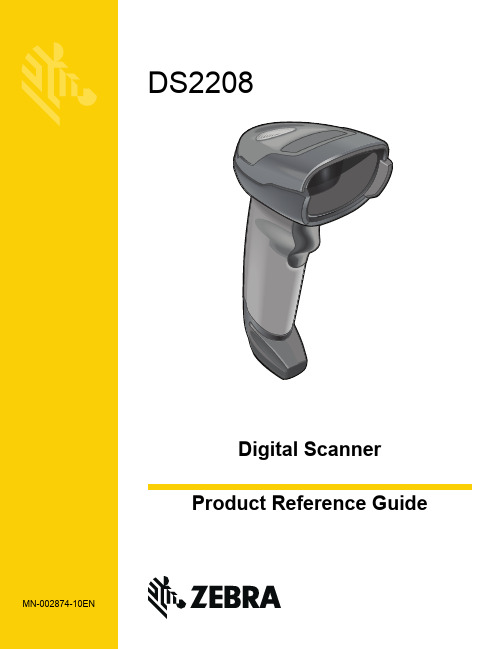
-05 Rev. A
6/2018
Rev. B Software Updates Added: - New Feedback email address. - Grid Matrix parameters - Febraban parameter - USB HID POS (formerly known as Microsoft UWP USB) - Product ID (PID) Type - Product ID (PID) Value - ECLevel
-06 Rev. A
10/2018 - Added Grid Matrix sample bar code. - Moved 123Scan chapter.
-07 Rev. A
11/2019
Added: - SITA and ARINC parameters. - IBM-485 Specification Version.
No part of this publication may be reproduced or used in any form, or by any electrical or mechanical means, without permission in writing from Zebra. This includes electronic or mechanical means, such as photocopying, recording, or information storage and retrieval systems. The material in this manual is subject to change without notice.
风力机的尾流分析
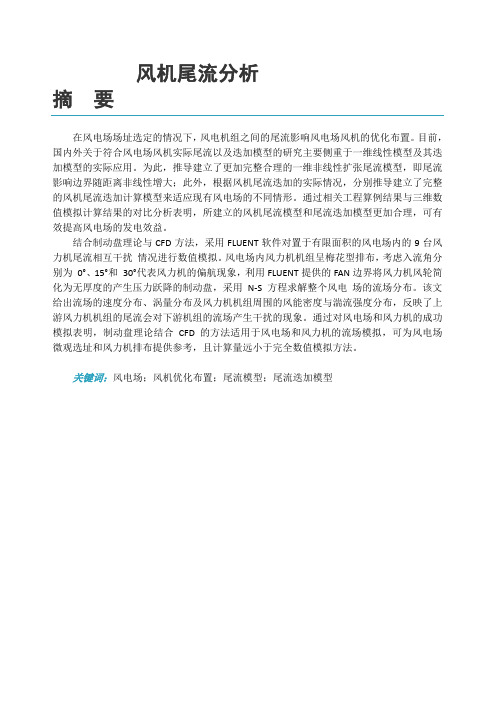
风机尾流分析摘要在风电场场址选定的情况下,风电机组之间的尾流影响风电场风机的优化布置。
目前,国内外关于符合风电场风机实际尾流以及迭加模型的研究主要侧重于一维线性模型及其迭加模型的实际应用。
为此,推导建立了更加完整合理的一维非线性扩张尾流模型,即尾流影响边界随距离非线性增大;此外,根据风机尾流迭加的实际情况,分别推导建立了完整的风机尾流迭加计算模型来适应现有风电场的不同情形。
通过相关工程算例结果与三维数值模拟计算结果的对比分析表明,所建立的风机尾流模型和尾流迭加模型更加合理,可有效提高风电场的发电效益。
结合制动盘理论与CFD方法,采用FLUENT软件对置于有限面积的风电场内的9台风力机尾流相互干扰情况进行数值模拟。
风电场内风力机机组呈梅花型排布,考虑入流角分别为0°、15°和30°代表风力机的偏航现象,利用FLUENT提供的FAN边界将风力机风轮简化为无厚度的产生压力跃降的制动盘,采用N-S 方程求解整个风电场的流场分布。
该文给出流场的速度分布、涡量分布及风力机机组周围的风能密度与湍流强度分布,反映了上游风力机机组的尾流会对下游机组的流场产生干扰的现象。
通过对风电场和风力机的成功模拟表明,制动盘理论结合CFD 的方法适用于风电场和风力机的流场模拟,可为风电场微观选址和风力机排布提供参考,且计算量远小于完全数值模拟方法。
关键词:风电场;风机优化布置;尾流模型;尾流迭加模型AbstractIn the case of wind farm site selection, layout optimization for wind turbine wake effects between wind turbine. At present, domestic and foreign about the practical application of the actual fan wake and overlay model of wind farm mainly focus on the one-dimensional linear model and its superposition model. Therefore, a more complete and reasonable derivation of one-dimensional nonlinear wake model, namely the slipstream boundary nonlinear increase with distance; in addition, according to the actual situation of WTG wake, respectively, are established by the fuller WTG flow superposition to adapt to different situation of existing wind farm model. Through the project example analysis results show that compared with thethree-dimensional numerical simulation of wind machine, the flow model and wake superposition model is more reasonable, can effectively improve the generation benefit of wind farm.Combined with the brake disc and CFD theory, using FLUENT software for wind farm in the limited area of the 9 sets of wind turbine wake interaction simulation. A wind farm wind turbine unit in the club arrangement, taking into account the yaw angle was 0 ° flow phenomenon, 15 ° and 30 ° representative wind machine, FAN boundary FLUENT provided by the use of the wind turbine is simplified to produce brake disc pressure jump down without thickness, by solving the N-S equation of the wind power field the flow field distribution. Wind energy density and turbulence intensity distribution is presented in this paper, the velocity distribution of flow field around the vorticity distribution and wind turbine generator, reflect the will of the flow field downstream units generate interference phenomenon of wind turbine generators upstream wake. The wind farm and wind turbine simulation shows that success, to simulate the brake disc theory combined with CFD method is applied to the wind farm and wind machine, can provide the micro-siting wind turbine arrangement and reference for wind farm, and the calculation method is far less than the amount of numerical simulation.Keywords: wind power; wind turbine layout optimization model; wake; wake superposition model引言随着风电技术的快速发展,以及风力发电在电力系统中比重的持续增加,大型风电场通常由几百台甚至上千台风电机组组成。
以wind power generation为关键词的作文及翻译
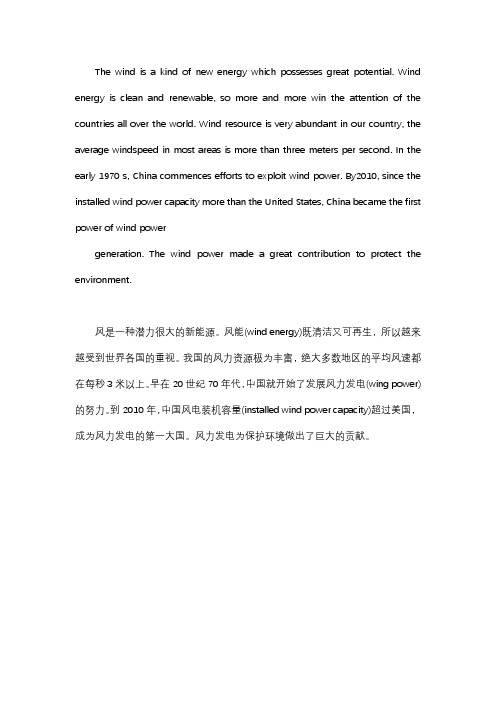
The wind is a kind of new energy which possesses great potential. Wind energy is clean and renewable, so more and more win the attention of the countries all over the world. Wind resource is very abundant in our country, the average windspeed in most areas is more than three meters per second. In the early 1970 s, China commences efforts to exploit wind power. By2010, since the installed wind power capacity more than the United States, China became the first power of wind powergeneration. The wind power made a great contribution to protect the environment.风是一种潜力很大的新能源。
风能(wind energy)既清洁又可再生,所以越来越受到世界各国的重视。
我国的风力资源极为丰富,绝大多数地区的平均风速都在每秒3米以上。
早在20世纪70年代,中国就开始了发展风力发电(wing power)的努力。
到2010年,中国风电装机容量(installed wind power capacity)超过美国,成为风力发电的第一大国。
风力发电为保护环境做出了巨大的贡献。
风能的利用英语作文
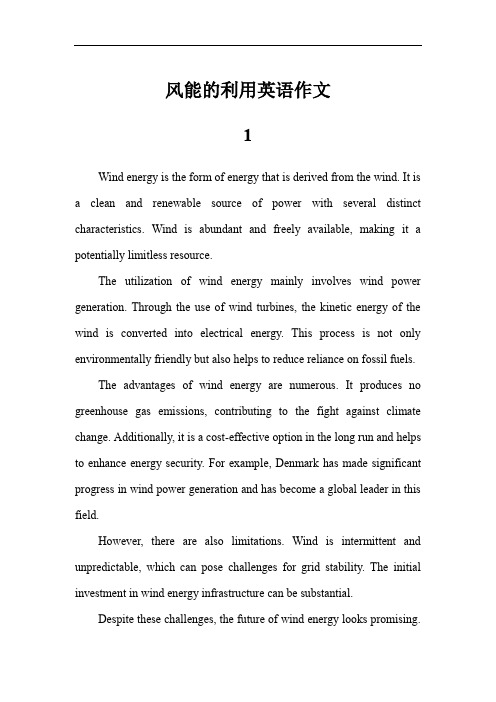
风能的利用英语作文1Wind energy is the form of energy that is derived from the wind. It is a clean and renewable source of power with several distinct characteristics. Wind is abundant and freely available, making it a potentially limitless resource.The utilization of wind energy mainly involves wind power generation. Through the use of wind turbines, the kinetic energy of the wind is converted into electrical energy. This process is not only environmentally friendly but also helps to reduce reliance on fossil fuels.The advantages of wind energy are numerous. It produces no greenhouse gas emissions, contributing to the fight against climate change. Additionally, it is a cost-effective option in the long run and helps to enhance energy security. For example, Denmark has made significant progress in wind power generation and has become a global leader in this field.However, there are also limitations. Wind is intermittent and unpredictable, which can pose challenges for grid stability. The initial investment in wind energy infrastructure can be substantial.Despite these challenges, the future of wind energy looks promising.Technological advancements are expected to improve the efficiency and reliability of wind turbines. More countries are likely to invest in wind energy to meet their growing energy demands and achieve sustainable development goals.In conclusion, wind energy holds great potential as a sustainable and clean energy source, but its full utilization requires continued innovation and strategic planning.2Wind energy is the form of energy that is derived from the wind. It is a clean and renewable source of power. Wind energy has several distinctive features. It is abundant and widely available, especially in areas with consistent wind patterns.The utilization of wind energy mainly includes wind power generation. In this process, large wind turbines are installed to convert the kinetic energy of the wind into electrical energy. This electricity can then be used to power homes, businesses, and industries.The advantages of wind energy are numerous. It is environmentally friendly, emitting no greenhouse gases during the generation process. It helps reduce reliance on fossil fuels and contributes to combating climate change. Additionally, it creates job opportunities in the manufacturing, installation, and maintenance of wind turbines. However, there are alsolimitations. Wind energy is intermittent, depending on the wind's availability. The initial investment for setting up wind farms can be high.For instance, in some Scandinavian countries, wind energy has made significant progress. They have successfully integrated a large proportion of wind power into their energy mix, reducing their carbon footprint and achieving greater energy independence.Looking to the future, the potential of wind energy is immense. With technological advancements, the efficiency of wind turbines will increase, and the costs will come down. More regions are expected to embrace wind energy as a crucial part of their energy strategies, leading to a cleaner and more sustainable world.3Wind energy has emerged as a significant and promising source of power in the modern world. It is a clean and renewable form of energy that holds great potential for meeting our ever-growing energy demands.One of the most common ways of harnessing wind energy is through wind turbines for electricity generation. These turbines convert the kinetic energy of the wind into electrical energy, which can then be distributed to power our homes and industries. Denmark, for instance, is a prime example of a country that has successfully utilized wind power. A significant portion of its electricity comes from wind turbines, making it aleader in this field.In addition to electricity generation, wind can also be used to drive mechanical devices. Windmills have been used for centuries to pump water or grind grains.The advantages of wind energy are numerous. It is a clean source, emitting no greenhouse gases or pollutants. It is also abundant and freely available. However, there are limitations. Wind is intermittent and its availability depends on weather conditions. Also, the initial setup cost of wind turbines can be high.Despite these challenges, the future of wind energy utilization looks bright. With technological advancements, we are likely to see more efficient and cost-effective ways of harnessing this energy. It is not only important for reducing our reliance on fossil fuels but also for creating a sustainable and environmentally friendly energy landscape.In conclusion, wind energy offers a sustainable and promising solution to our energy needs. Continued research and development in this area will undoubtedly lead to even greater benefits in the years to come.4Wind energy has emerged as a crucial and promising source of power in the modern world. Its significance lies in the need for sustainable and clean energy to address the global energy crisis andenvironmental challenges.The utilization of wind energy takes various forms. One of the most common is wind power generation. Large wind turbines are erected in windy areas to convert the kinetic energy of the wind into electrical energy. This has become a significant contributor to the power grid in many countries. For instance, Denmark has made remarkable progress in wind power, with a considerable portion of its electricity coming from wind turbines.In addition to power generation, wind energy is also finding applications in the transportation sector. Hybrid vehicles that incorporate wind-assisted propulsion systems are being developed to increase fuel efficiency and reduce emissions.The advantages of wind energy are numerous. It is a renewable resource, meaning it will never run out. It produces no greenhouse gas emissions, helping to combat climate change. Moreover, it is cost-effective in the long run and creates job opportunities in the manufacturing, installation, and maintenance of wind energy facilities.However, the utilization of wind energy also faces certain challenges. The intermittency of wind makes it less reliable compared to traditional energy sources. The initial investment for setting up wind farms can be substantial.In conclusion, the future of wind energy holds great potential. Withcontinuous technological advancements and increased investment, wind energy is likely to play an even more significant role in meeting our energy needs while protecting the environment. But to fully realize this potential, we need to overcome the existing challenges and make strategic and sustainable decisions.5Wind energy is the energy obtained from the wind. It is a clean and renewable source of power that has several distinct characteristics. The wind is abundant and freely available, making it a sustainable option for generating electricity.One of the primary ways wind energy is utilized is through wind power generation. Large wind turbines are installed in windy areas to convert the kinetic energy of the wind into electrical energy. This electricity can then be distributed to power homes, industries, and cities.The advantages of wind energy are numerous. It is a clean source of energy, producing no greenhouse gas emissions and thus helping to combat climate change. It is also a domestic source of energy, reducing reliance on imported fossil fuels and enhancing energy security. However, there are limitations as well. Wind is intermittent and its availability can vary, requiring backup power sources for consistent supply. The initial cost of setting up wind farms can be high, and there may be concernsabout the visual impact and noise pollution.Several countries and regions have successfully harnessed wind energy to address their energy needs. Denmark, for instance, has made significant progress in wind power integration into its energy grid. In the United States, states like Texas have large-scale wind farms contributing to the power supply.Looking ahead, the future of wind energy appears promising. Advances in technology are expected to make wind turbines more efficient and cost-effective. Research and development efforts are focused on improving energy storage solutions to overcome the intermittency challenge. With continued investment and innovation, wind energy is likely to play an even more significant role in the global energy mix, providing a sustainable and clean source of power for generations to come.。
Wind Power Generation
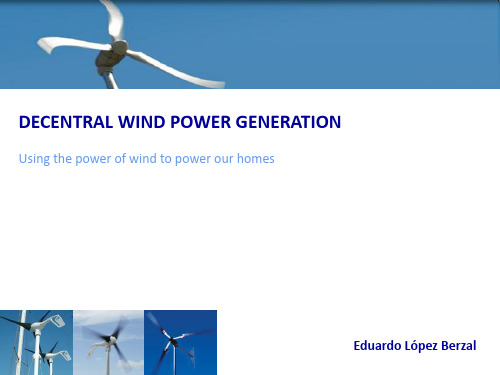
4.How to plan a stand-alone electronic systems 4.1 Assess your site
Assess your site (“go” – “no go” decision point)
1. Measure wind speed of your location (AEMED)
8750 € 10.500 €
Simple payback = 5,625 years
THANKS FOR YOUR ATTENTION
DECENTRAL WIND POWER GENERATION
Using the power of wind to power our homes
Eduardo López Berzal
INDEX
1. 2. 3. 4. 5. The power and potential of the wind. Non-grid connected systems. System components. How to plan a simple stand-alone electric system. Economics. How much does a system cost?
3.System components Wind turbines
3.System components Balance of System
INDEX
1. 2. 3. 4. 5. The power and potential of the wind. Non-grid connected systems. System components. How to plan a simple stand-alone electric system. Economics. How much does a system cost?
风力发电技术概述作文英语

风力发电技术概述作文英语Wind power generation technology is a sustainable and renewable energy source that harnesses the power of the wind to generate electricity. It involves the use of wind turbines, which are designed to capture the kinetic energy of the wind and convert it into electrical power.One of the key advantages of wind power generation is its environmental friendliness. Unlike fossil fuels, wind power does not produce harmful emissions or contribute to air pollution. This makes it a clean and green energy source that helps to reduce our reliance on non-renewable resources.Wind power generation technology has come a long way in recent years, with advancements in turbine design and efficiency leading to increased power output and lower costs. This has made wind power more competitive with traditional forms of energy generation, making it an attractive option for countries looking to reduce theircarbon footprint.In addition to its environmental benefits, wind power generation also has economic advantages. It creates jobs in the manufacturing, installation, and maintenance of wind turbines, and can provide a reliable source of income for landowners who lease their land for wind farm development.Overall, wind power generation technology offers a promising solution to the challenges of climate change and energy security. By harnessing the power of the wind, we can generate clean, renewable electricity and move towards a more sustainable future for our planet.。
WIND POWER GENERATOR, WIND POWER GENERATION SYSTEM

专利名称:WIND POWER GENERATOR, WIND POWERGENERATION SYSTEM, AND GENERATIONCONTROL METHOD OF WIND POWERGENERATOR发明人:HAYASHI, Yoshiyuki,SHIBATA, Masaaki申请号:EP08752729.7申请日:20080514公开号:EP2154362A1公开日:20100217专利内容由知识产权出版社提供专利附图:摘要:To provide a wind turbine generator, a wind turbine generator system, and apower-generation control method of a wind turbine generator that are capable of improving the power-generation capability and reducing the fatigue load on the windmill.A data accumulation unit 25 sequentially accumulates data sets of a generated output power P during operation of the wind turbine generator, an incoming wind speed Ws estimated on the basis of a wind speed measured at the anemometer, and a wind direction deviation, which is the difference between a wind direction θw measured at the anemoscope and the orientation of the nacelle; statistical analysis of the data accumulated is carried out by an analyzing unit 26; a distribution curve corresponding to the wind direction deviation of the generated output power at each incoming wind speed is determined; the wind direction deviation corresponding to the peak of the distribution curve is set as a correction value θd of the anemoscope; the correction value of the anemoscope for each incoming wind speed is stored in the wind-direction correction table 27; the wind direction Vw measured at the anemoscope is corrected with the correction value θd of the anemoscope for each incoming wind speed Ws; and power-generation control is carried out using the corrected wind direction as a control parameter.申请人:Mitsubishi Heavy Industries, Ltd.地址:16-5, Konan 2-chome Minato-ku Tokyo 108-8215 JP国籍:JP代理机构:Intes, Didier Gérard André更多信息请下载全文后查看。
风力发电场项目 英语
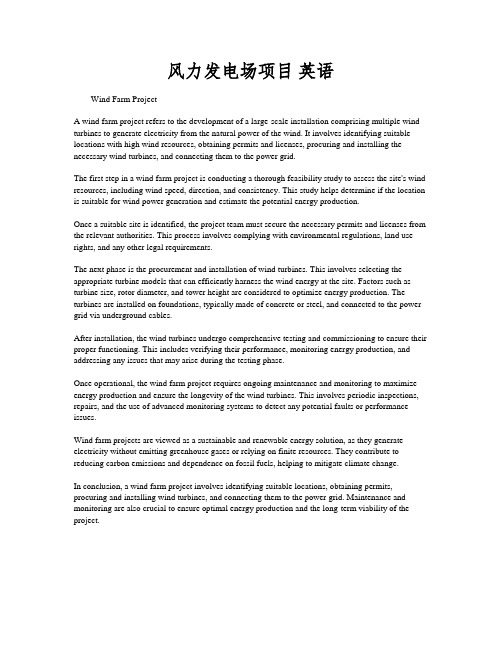
风力发电场项目英语Wind Farm ProjectA wind farm project refers to the development of a large-scale installation comprising multiple wind turbines to generate electricity from the natural power of the wind. It involves identifying suitable locations with high wind resources, obtaining permits and licenses, procuring and installing the necessary wind turbines, and connecting them to the power grid.The first step in a wind farm project is conducting a thorough feasibility study to assess the site's wind resources, including wind speed, direction, and consistency. This study helps determine if the location is suitable for wind power generation and estimate the potential energy production.Once a suitable site is identified, the project team must secure the necessary permits and licenses from the relevant authorities. This process involves complying with environmental regulations, land use rights, and any other legal requirements.The next phase is the procurement and installation of wind turbines. This involves selecting the appropriate turbine models that can efficiently harness the wind energy at the site. Factors such as turbine size, rotor diameter, and tower height are considered to optimize energy production. The turbines are installed on foundations, typically made of concrete or steel, and connected to the power grid via underground cables.After installation, the wind turbines undergo comprehensive testing and commissioning to ensure their proper functioning. This includes verifying their performance, monitoring energy production, and addressing any issues that may arise during the testing phase.Once operational, the wind farm project requires ongoing maintenance and monitoring to maximize energy production and ensure the longevity of the wind turbines. This involves periodic inspections, repairs, and the use of advanced monitoring systems to detect any potential faults or performance issues.Wind farm projects are viewed as a sustainable and renewable energy solution, as they generate electricity without emitting greenhouse gases or relying on finite resources. They contribute to reducing carbon emissions and dependence on fossil fuels, helping to mitigate climate change.In conclusion, a wind farm project involves identifying suitable locations, obtaining permits, procuring and installing wind turbines, and connecting them to the power grid. Maintenance and monitoring are also crucial to ensure optimal energy production and the long-term viability of the project.。
- 1、下载文档前请自行甄别文档内容的完整性,平台不提供额外的编辑、内容补充、找答案等附加服务。
- 2、"仅部分预览"的文档,不可在线预览部分如存在完整性等问题,可反馈申请退款(可完整预览的文档不适用该条件!)。
- 3、如文档侵犯您的权益,请联系客服反馈,我们会尽快为您处理(人工客服工作时间:9:00-18:30)。
A Simplified Wind Power Generation Modelfor Reliability EvaluationRajesh Karki,Senior Member,IEEE,Po Hu,Student Member,IEEE,and Roy Billinton,Life Fellow,IEEEAbstract—Renewable energy sources,especially wind turbine generators,are considered as important generation alternatives in electric power systems due to their nonexhausted nature and benign environmental effects.The fact that wind power penetra-tion continues to increase has motivated a need to develop more widely applicable methodologies for evaluating the actual benefits of adding wind turbines to conventional generating systems.Relia-bility evaluation of generating systems with wind energy sources is a complex process.It requires an accurate wind speed forecasting technique for the wind farm site.The method requires historical wind speed data collected over many years for the wind farm lo-cation to determine the necessary parameters of the wind speed models for the particular site.The evaluation process should also accurately model the intermittent nature of power output from the wind farm.A sequential Monte Carlo simulation or a multi-state wind farm representation approach is often used.This paper presents a simplified method for reliability evaluation of power systems with wind power.The development of a common wind speed model applicable to multiple wind farm locations is pre-sented and illustrated with an example.The method is further simplified by determining the minimum multistate representation for a wind farm generation model in reliability evaluation.The paper presents a six-step common wind speed model applicable to multiple geographic locations and adequate for reliability eval-uation of power systems containing significant wind penetration. Case studies on a test system are presented using wind data from Canadian geographic locations.Index Terms—Generation system,reliability evaluation,time-series model,wind power.I.I NTRODUCTIONW IND is one of the fastest growing energy sources,and is regarded as an important alternative to traditional power generating sources.Enhanced public awareness of the environ-ment has led to rapid wind power growth throughout the world to reduce greenhouse gas emissions associated with conventional energy generation.An energy policy known as the renewable portfolio standard(RPS)is being widely accepted around the world.Acceptance of the RPS is a commitment to produce a specified percentage of the total power generation from renew-able sources within a certain date.In the North America,many US states and Canadian provinces have agreed to generate be-tween5%and25%of electrical power from renewable energy resources by2010–2015.Most of this renewable energy willManuscript received September15,2005;revised September15,2005.Paper no.TEC-00177-2005.The authors are with the Power System Research Group,Department of Electrical Engineering,University of Saskatchewan,Saskatoon,SK S7N5A9Canada(e-mail:Rajesh.Karki@usask.ca,poh591@ask.ca, Roy Billinton@ask.ca).Digital Object Identifier10.1109/TEC.2006.874233come from wind as other renewable sources are not suitable for bulk power generation.The development of comprehensive reliability and cost-evaluation techniques becomes more important as wind power penetration levels in traditional power systems continue to in-crease in the near future.Both Monte Carlo simulation[1],[2] and analytical methods[3]–[5]have been utilized in adequacy assessment of generation systems containing wind power. Simulation methods can recognize the chronology of wind variation and its impact on a power system.Reference[6] presents an algorithm to simulate the hourly wind speed us-ing a time-series auto regressive and moving average(ARMA) model.The method requires actual hourly wind speed data col-lected over a long period of time for the particular geographic location to construct a wind speed simulation model for the specific site.This model can reflect the true probabilistic char-acteristics of wind speed for the wind site.The process for obtaining the proper ARMA model is quite complex,and is specific to the geographic location of the wind plex techniques are not readily applied in a practical world.It would be very useful from a practical application point of view if a common ARMA model could be developed that would work for multiple wind farm locations[7].It should also be noted that historical wind data are not available for all poten-tial wind farm locations.This paper presents the development of a common wind speed model that can generate wind speed prob-ability distributions for multiple wind farm sites if their annual mean wind speed and standard deviation are known.Histori-cal wind speed data from three Canadian sites(Swift Current, North Battleford,and Toronto,which have different geographic characteristics),are used in this paper to obtain the common wind speed model.The wind speed distribution obtained from the common wind speed model can then be used in an analytical method for reliability evaluation of power systems containing wind farms.A wind turbine generator(WTG)is modeled as a multistate unit in reliability evaluation using an analytical method[3]–[5]. The multistate model represents a WTG by a number of derated power output states using the wind speed model for the wind farm location and the power curve of the WTG.The model appropriately reflects thefluctuating characteristics of the site-specific wind.Reliability studies using the Roy Billinton Test System (RBTS)[8]are presented to determine the appropriate number of states in the multistate WTG model,and develop a simpli-fied model applicable to any wind farm connected to a power system.The development of a simplified6-step common wind speed model that can be applied to any wind farm in power0885-8969/$20.00©2006IEEEsystem reliability evaluation is presented.The validity of the developed method and its practicality is illustrated by evaluat-ing wind power penetration in the RBTS.II.S ITE-S PECIFIC W IND D A TA S IMULA TIONWind resource at a geographic location is highly variable. Power generated from WTG depends on the wind speed,which fluctuates randomly with time.Wind power studies,therefore, require accurate models to forecast wind speed variation for wind farm locations of interest.Wind speed distributions are often characterized by Weibull distributions,and methods using Weibull distributions[5]have been previously used to estimate wind speed data in wind power studies.Historical hourly data for the wind farm site collected over significant time are normally required to obtain the shaping parameters.An ARMA time-series model has been used by researchers [1],[2],[6]as an accurate method to forecast wind speed data at any particular location.The wind speed at any given time for a particular geographic location can be simulated using(1)SW t=µt+σt...y t(1) where SW t is the simulated wind speed for hour t from his-torical mean wind speedµt and standard deviationσt.Time-series values of y t can be obtained sequentially using(2),where ϕi(i=1,...,n)andθj(j=1,...,m)are the auto-regressive and moving average parameters of the model,respectively y t=φ1y t−1+φ2y t−2+...+φn y t−n+αt−αt−1θ1−αt−2θ2−...−αt−mθm(2) where{αt}is a normal white noise process with zero mean and a variance ofσ2a(i.e.,αt∈NID(0,σ2a),where NID denotes normally independently distributed.The order[n,m]and the values of the auto-regressive and moving average parameters shown in(2)can be obtained from many years of collected wind speed data using nonlinear least-square methods[9].A computer program was developed to estimate the parameters of ARMA(n,m)models for geographic wind farm sites using the algorithm provided in[9].Historical wind data was obtained for three different locations in Canada:Swift Current,North Battleford,and Toronto.Swift Current lies in the southern part of the Saskatchewan province and is home to one of the largest wind farms in Canada.North Battleford lies to the north in the same province,but does not have a good wind resource.Toronto lies at the great lakes and has a coastal wind regime.These three locations have diverse wind variation patterns and are therefore selected in the study. Historical data on hourly wind speeds for three years(from January1,2001to December31,2003),and the hourly mean and standard deviation of wind speeds from a15-year database (from January1,1989to December31,2003)were obtained from Environment Canada to determine the ARMA model for the Swift Current location.The parameters are shown in(3) y t=0.8782y t−1−0.0066y t−2+0.0265y t−3+αt−0.2162αt−1+0.0091αt−2(3)αt∈NID(0,0.557922).TABLE IM AIN S TA TISTICAL C HARACTERISTICS OF S IMULA TEDAND A CTUAL W IND S PEED D ATAEquations(4)and(5)show the time-series ARMA models obtained similarly for North Battleford and Toronto locations respectivelyy t=1.7901y t−1−0.9087y t−2+0.0948y t−3+αt−1.0929αt−1+0.2892αt−2(4)αt∈NID(0,0.4747622)y t=0.4709y t−1+0.5017y t−2−0.0822y t−3+αt+0.1876αt−1−0.2274αt−2(5)αt∈NID(0,0.55082).Hourly wind speeds were repeatedly simulated for a large number of yearly samples to obtain annual wind speed data for the three sites.Table I shows the mean value and standard de-viation of20years’average actual collected data and simulated wind speed data for a year for these sites.It can be seen that the simulated values are very close to those obtained from the actual data.The entire range of the simulated data is equally divided into N a intervals,and the SW ai(i=1,...,N a)is the midpoint of each interval.The probability P ai of a wind speed in an interval SW ai(i=1,...,N a)can be obtained using(6)by counting the number N ai(i=1,...,N a)of simulated wind speed data in the intervalP ai=N ai/(8760×N y)(6) where N y is the number of sample years simulated.The wind speeds SW ai at each of the N a intervals and their corresponding probabilities of occurrence P ai were calculated for the three sites.Fig.1shows the probability distributions of simulated wind speed data for these three sites.Wind speed probability distributions are often represented by Weibull distributions.Weibull distribution in general can have various shapes depending on the shaping parameters.The wind speed probability distributions obtained for the three diverse geographic locations,however,are close to normal distribution as shown in Fig.1.It can however be seen that the three curves are quite different,as the three selected sites have different types of wind regimes.Time-series ARMA model generate both positive and negative values of wind speeds as seen in Fig.1. Negative values have no physical meaning in this study,and are set to zeros as recommended in[7].KARKI et al.:A SIMPLIFIED WIND POWER GENERATION MODEL FOR RELIABILITY EV ALUATION535Fig.1.Probability distributions of simulated wind data.III.C OMMON W IND S PEED M ODEL FORM ULTIPLE W IND S ITESThe determination of a proper wind speed model for a wind farm location is a complicated process as described in Section II.The process also requires historical wind speed data collected over a significant period of time.It would be very useful for prac-tical applications,if a common wind speed model could be used for benefit analysis of wind power sources located at different geographic sites.This approach will prove useful for prospec-tive wind farm locations lacking adequate historical data.The objective is to determine a general model that can be used to obtain power output from wind turbines located at different sites with reasonable accuracy.The probability distributions of wind speeds at the three se-lected geographic sites are shown in Fig.1in Section II.In this section,the probability distributions are plotted in terms of the annual mean wind speed valueµ,and the standard de-viationσfor the selected locations.The distribution consid-ers wind speeds up to10σto include extreme values despite their low probability of occurrences.The distribution is di-vided into N b number of interval steps,and each step has a length of10σ/N b,where the midpoint values of these steps are SW b i(i=1,...,N b),and one of the steps has a midpoint value ofµ.The steps SW b i are defined as in(7).For example,for a 100-step model,the midpoint values SW b i of the wind speed steps will be as follows:µ–4.9σ,µ–4.8σ,...,µ–0.1σ,µ,µ+ 0.1σ,µ+0.2σ,...,µ+5σ.The probability of each step P b i(i=1,...,N b)can be calculated by(8)SW b i=µ+(10σ/N b)×(i−0.5×N b)for even N b,and =µ+(10σ/N b)×(i−0.5×(N b+1))for odd N b(7) P b i=N b i/(8760×N y)(8) where N b i is the number of simulated wind speed data in the step SW b i(=1...,N b)bining wind speed models for different sites.The probability distributions plotted in terms ofµandσas discussed above are shown in Fig.2for the three locations.It can be seen that the three curves are very close.The three curves for the three different wind regimes can be combined to obtain a common wind speed model.The probability P c i(i=1,...,N b) for each step in the common wind speed model is obtained by taking the average value of P b i(i=1,...,N b)for these three wind sites.Fig.2also shows the curve for the common wind speed model.The common wind speed model can be used to obtain the wind speed probability distribution for any geographic location with wind speed characteristics close to the selected Canadian sites.The only data required are the annual mean wind speedµand the standard deviationσfor that site.Negative wind speed values are converted to zeroes in this process.It is shown in Section VI that the wind speeds and their probabilities obtained for a specific site using the common wind speed model can be used with reasonable accuracy in the reliability evaluation of power systems containing wind power.Fig.3shows a common wind speed model that can be used for multiple geographic locations.The application of the common wind speed model in Fig.3 is illustrated in this paper by applying it to a site in Regina, Saskatchewan.Theµandσvalues for Regina wind data are 19.53and10.06km/h,respectively.The common wind speed model is used to obtain the wind speeds and their corresponding probabilities for the example site.Table II shows the wind speeds in km/h and their corresponding probabilities.Only5out of the 100steps in the wind speed model are shown.It should be noted that the negative values are converted to zeroes in Table II.IV.C OMMON WTG P OWER G ENERA TION M ODEL FORR ELIABILITY E V ALUA TIONIt is important to accurately assess the electric power gen-erated by a WTG located at a particular geographic site in re-liability analysis.The generated power varies with the wind536IEEE TRANSACTIONS ON ENERGY CONVERSION,VOL.21,NO.2,JUNE2006mon wind speed model.TABLE IIW IND M ODEL FOR R EGINA S ASKA TCHEWANFig.4.Power curve of a WTG.speed at the wind farm site.The power output of a WTG canbe determined from its power curve,which is a plot of output power against wind speed.Fig.4shows a typical power curve of a WTG.A WTG is designed to start generating at the cut-in speed V ci (V c )and is shut down for safety reasons at the cut-out speed V co .Rated power P r is generated when the wind speed is between the rated speed V r and the cut-out speed V co .There is a nonlinear relationship between the power output and the wind speed when the wind speed lies within the cut-in speed V ci and the rated speed V r as shown in Fig.4.Fig.5.Development of a WTG model.Fig.5shows the development of the power generation model of a WTG located at a particular geographic site.The annual µand σwind speed data for the wind farm site is used in the common wind speed model shown in Fig.3to obtain the site-specific wind speed model.This site-specific wind speed model is then combined with the WTG power curve to obtain the WTG power generation model.Equation (9)is the mathematical expression for the power curve.The power generated P i (i =1,...,N b )corresponding to a given wind speed SW b i (i =1,...,N b )can therefore be obtained from (9)P i =0,0≤SW b i <V ci=P r (A +B ×SW b i +C ×SW 2b i ),V ci ≤SW b i <V r=P r ,V r ≤SW b i ≤V co =0,V co <SW b i(9)where the constants A,B ,and C are presented in [3].Wind speeds less than V ci and greater than V co produce zero output power from the WTG.The wind speed model steps cor-responding to these values can,therefore,be combined into a cumulative step,and the zero output probability P p0can be ob-tained from (10).Similarly,wind speed steps between V r and V co can be combined,and the probability of rated power output P pr can be obtained from (11)P p0=P ci for 0≤SW b i <V ci or V co <SW b i (10)P pr =P cifor V r ≤SW b i ≤V co(11)The application of the common WTG power generation model is illustrated in this paper by applying it to a WTG installed in Regina.WTG rated at 1.5MW,and with cut-in,rated,and cut-out wind speeds of 14.4,45,and 90km/h,re-spectively,are used in the studies.The site-specific wind speed model shown in Table II is combined with the power curve to obtain the WTG generation model.Table III shows the power output levels and their corresponding probabilities.The 100-step wind speed model in Table II is reduced to 33steps in the power generation model in Table III as different wind speeds producing the same power level are aggregated into single steps.KARKI et al.:A SIMPLIFIED WIND POWER GENERATION MODEL FOR RELIABILITY EV ALUATION 537TABLE IIIP OWER G ENERA TION M ODEL FOR A WTG IN REGINATABLE IVT RADITIONAL G ENERA TING U NIT R ELIABILITY D A TA(RBTS)A wind farm usually consists of many WTG units subjected to the same wind regime characterized by the geographic loca-tion.The total power generation model for the wind farm can be obtained by summing up the individual WTG power levels corresponding to each wind speed interval in the common wind speed model.V .V ALIDITY OF THE C OMMON W IND S PEED M ODEL FORR ELIABILITY S TUDIES The power generation model for a wind farm can be easily obtained from the common wind speed model shown in Fig.3using only the site-specific µand σdata and the WTG power curve.The validity of this common model for practical reliability applications is analyzed using a test system.Reliability studies using analytical techniques were conducted on the RBTS [8]to illustrate the practicality of a common wind speed model for different sites.The RBTS is a basic reliability test system that evolved from the research and teaching program conducted at the University of Saskatchewan.The RBTS consists of 11traditional generating units with a total capacity of 240MW.The generating unit ratings and reliability data are shown in Table IV.The system peak load is 185MW [8].A wind farm with 18WTG units was added to the RBTS.WTG rated at 1.5MW,and with cut-in,rated,and cut-out wind speeds of 14.4,45,and 90km/h,respectively,was used in the studies.The added wind capacity is 27MW,which is almost 10%of the total installed system capacity.The generating system adequacy of the test system was eval-uated for three different cases,in which the wind farm was considered at separate locations with North Battleford,Toronto,and Swift Current wind regimes.Table I shows the mean wind speed and the standard deviation for the three sites.Table V shows the loss of load expectation (LOLE)[10]for the RBTS before and after adding WTG at the different locations.The LOLE results were obtained by convolving theTABLE VLOLE C OMPARISON OF RBTS I NCLUDING WECS L OCA TEDA T D IFFERENT W IND SITESFig.6.LOLE comparison for North Battleford using its ARMA model and the common wind speed model.system generation model with the load model using an analytical technique.The conventional generating units were represented by a two-state model,in which the unit is either operating at rated power output or on forced outage.To account for the randomly varying power output from WTG units,they were represented by 100-state model in the studies.The annual load duration curve for the RBTS was used as the load model,and the data are provided in [8].The second row of Table V shows the indices using the site-specific ARMA models shown in (3)–(5)for the three locations.The third row shows the results obtained using the common wind speed model in Fig.3.It can be seen that the results are very close.It can also be seen from Table V that the adequacy of the RBTS is improved by adding the 27-MW wind farm.The improvement with the Swift Current site is more significant than that of the other two locations,as Swift Current has a higher mean wind speed,and therefore,provides a better wind resource.Table V shows that the common wind speed model produces accurate results for wind farm locations with different wind regimes.Power system reliability is very sensitive to changes in the system peak load.The accuracy of the common wind speed model is therefore analyzed for different peak load levels at the three geographic wind farm locations.Fig.6shows the system LOLE for different peak load levels with the wind farm located in North Battleford.The results obtained using the common wind speed model is compared with those obtained using the North Battleford ARMA model given in (4).Fig.6shows that the common wind speed model also produces very close results at different peak load levels.Fig.7shows similar results when the wind farm is considered to be in the Toronto location.The results show that the common wind speed model can be utilized in the reliability evaluation of power systems containing wind farms,538IEEE TRANSACTIONS ON ENERGY CONVERSION,VOL.21,NO.2,JUNE2006Fig.7.LOLE comparison for the Toronto Data using its ARMA model and the common wind speed model.and provide very close results compared to the site-specific ARMA models for the different sites.VI.S IMPLIFIED M ULTISTA TE WTG M ODELA conventional generating unit is usually represented by a two-state model in power system reliability evaluation.The two states are the unit-operating state with rated power output,and the unit-failure state with zero output.A WTG unit,however, cannot be represented by a two-state model since the power output can vary continuously and intermittently from zero to the rated value depending on the wind speed at the wind farm site. WTG units are therefore represented by multistate models in analytical methods used in power system reliability assessment. Accurate reliability assessment can be obtained by represent-ing a wind farm by a large number of discrete output states. This can be obtained from a wind speed model with a large number of discrete wind speed steps.The model can be simpli-fied by reducing the number of steps at the cost of accuracy.It is,therefore,important to determine the minimum number of steps that can be used in a simplified wind speed model to obtain reasonable accuracy for all practical purposes.Studies were done to evaluate the system LOLE with increas-ing peak load levels using different numbers of wind speed steps in the common wind speed model.The wind farm is assumed to be located in Swift Current.Fig.8shows the results of the study.It can be seen that using six or more steps in the wind speed model provides close results.The reliability contribution of a wind farm highly depends on the site wind regime.Studies similar to that shown in Fig.8were also conducted for a wind farm site with a different wind regime. Fig.9shows the system LOLE results for the case in which the wind farm is assumed to be located in Toronto.Fig.9also shows that a6-step wind speed model is adequate for reliability studies with reasonable accuracy.It should be noted that27MW of wind capacity is added to the RBTS in the above studies,which amounts to about a10%wind penetration.Since the reliability contribution of wind sources is highly dependent on the wind penetration level,it is important to determine the appropriate number of wind speed model steps at practical wind penetration levels.The existing wind penetration levels in most power systems are much lower than10%for Fig.8.Swift Current wind site with a10%wind power penetration level(18WTG).Fig.9.Toronto wind site with a10%wind power penetration level(18WTG). Fig.10.Swift Current wind site with a15%wind power penetration level(28WTG).which the above results are obtained.Studies conducted for wind penetration of1%showed that the number of steps had no impact on the system LOLE results.When the penetration level is insignificant,wind power has relatively little influence on the total system reliability performance.Reliability evaluation in power system planning should con-sider a long-term planning horizon.It is expected that wind power penetration will grow to10%–15%in the next10–15 years.Fig.10shows the results obtained when the wind farm added to the RBTS contains28WTG units,increasing the wind penetration to15%of the total installed system capacity.The results show that a six-step wind speed model is adequate for reliability studies with reasonable accuracy.KARKI et al.:A SIMPLIFIED WIND POWER GENERATION MODEL FOR RELIABILITY EV ALUATION539Fig.11.Six-step common wind speed model.TABLE VISix-S TEP W IND M ODEL FOR R EGINA SITETABLE VIIS IMPLIFIED P OWER G ENERA TION M ODEL FORTHE R EGINA W IND FARMThe 6-step common wind speed model that can be used for reliability studies of power systems with reasonable accuracy is shown in Fig.11.The model shows the six wind speed steps and the corresponding probabilities.The wind speeds for a ge-ographic site can be obtained from this model using (12),if µand σfor the site are known SW b i =µ+(i −3)×(5σ/3)for (i =1,...,6).(12)The application of the six-step common wind speed model is illustrated by applying it to a site in Regina.Table VI shows the wind speeds in km/h and their corresponding probabili-ties.It should be noted that the negative values are converted to zeroes.A simplified multistate power generation model for a WTG can be determined by combining the 6-step common wind speed model with the power curve equation (9).This method can be utilized to develop a simple but adequate multistate wind generation model.The only data required are the wind farm site annual µand σdata,and the power-curve data for the WTG units.For example,Table VII shows the simplified multistate power generation model for a wind farm consisting of 18WTG units in Regina.Fig.12shows the results of reliability assessment of the RBTS system with 10%wind penetration coming from the 27-MW Regina wind farm.The shaded bars show the LOLEresultsparison of different models for WTG power output by LOLEindex.using the simplified model in Table VII.The results obtained from the simplified model are compared with those obtained from a 100-step wind speed model derived from an accurate ARMA (4,3)model for Regina as shown in (13)y t =0.9336y t −1+0.4506y t −2−0.5545y t −3+0.1110y t −4+αt −0.2033αt −1−0.4684αt −2+0.2301αt −3(13)αt ∈NID(0,0.4094232)It can be seen from Fig.12that the simplified multistate WTG power generation model derived from the common 6-step wind speed model can provide very close results when compared with the 100-step model derived from the site-specific time-series ARMA wind speed model for Regina.The simplified model can therefore be used in the reliability evaluation of generating system including wind power with reasonable accuracy.VII.C ONCLUSIONIt becomes increasingly important to develop realistic reliabil-ity evaluation techniques that are practically useful for electric power industries that are expected to include a rapidly growing proportion of wind generation in the coming years.The bene-fits from wind sources are largely dictated by the wind regime at the wind farm site.It is,therefore,very important to obtain suitable wind speed simulation models and appropriate tech-niques to develop power generation model for WTG in reliability evaluation.It requires a significant amount of historical data and effort to develop a realistic wind speed model for a geographic site.Historical data from three Canadian sites with diverse wind regimes were used to obtain a common wind speed model in this paper.The common wind speed model can be applied to obtain a wind farm power generation model for any geographic location if the mean wind speed and standard deviation,and the WTG power curve parameters are known.Results from different analytical reliability studies are presented in this paper to show that a wind power generation model can be greatly simplified by using only 6steps in the common wind speed model.The technique to develop the simplified WTG multistate model for any geographic location is presented and illustrated with an example.The results show that the simplified method。