Potential of Forest Wood Biomass in Bulgaria, Market and Possibilities for Its Utilization
森林碳汇的计算

CDM Capacity Development in Eastern and Southern AfricaCharlene WatsonLondon School of Economics and Political Sciencec.watson2@ DisclaimerThe views expressed in this publication are those of the author and do not necessarily represent those of the United Nations, UNDP, UNEP, UNEP Risoe Centre or their Member States.Forest Carbon Accounting: Overview & PrinciplesExecutive SummaryForests play an important role in the global carbon balance. As both carbon sources and sinks, they have the potential to form an important component in efforts to combat global climate change. Accounting for the carbon within forest ecosystems and changes in carbon stocks resulting from human activities is a necessary first step towards the better representation of forests in climate change policy at regional, national and global scales.The United Nations Development Programme (UNDP), as part of the UNDP-UNEP CDM Capacity Development Project for Eastern & Southern Africa, is seeking to promote carbon projects in sub-Saharan Africa, in the important bio-carbon sector and others. This report reinforces UNDP’s capacity building efforts by presenting the main principles, practices and challenges of carbon accounting in the forestry sector.Forest carbon accounting can be divided into three forms. Stock accounting assesses the magnitude of carbon stored in forest ecosystems at a single point in time. Emissions accounting assesses the net greenhouse gas emissions to the atmosphere resulting from forests. Emission reductions accounting assesses the decrease in emissions from project or policy activities, often so that they can be traded. Forest carbon accounting identifies the carbon-density of areas, providing information for low-carbon-impact land use planning. It prepares territories for accounting and reporting of emissions from the forestry sector. It allows comparison of the climate change impact of the forestry sector relative to other sectors, as well as allowing comparison between territories. Finally, it enables trade of project emission reductions on carbon markets and for emission reductions to be included in policy targets.Good practice in forest carbon accounting must be adhered to. In particular, transparency in methods and accuracy and precision in accounting are required for public and political acceptance of resultant estimates. A basic knowledge of the principles underlying forest carbon accounting is also beneficial. Understanding biomass dynamics and flows between carbon pools in forest ecosystems enables more effective accounting.The practice of forest carbon accounting requires clear identification of the accounting boundary in both space and time. Stratifying the forest into areas with similar carbon characteristics further improves the accuracy of carbon accounting. Data for accounting can be gathered from a variety of sources, including existing secondary data, remotely sensed data and primary data through field surveys. The amount of data from each source depends on the quality of the source as well as the trade-offs that must be made between accounting accuracy and costs of resources and time.All forest carbon accounting estimates contain uncertainty. Practitioners should identify, minimise where possible, and quantify this uncertainty through statistical analysis, published information and expert judgement. Uncertainty of model variables and components, once quantified, can be aggregated through simple propagation of errors or simulated through Monte Carlo analysis. The existence of substantial uncertainty can undermine efforts to reduce carbon emissions from forestry and can erode political support for the accounting process.Forest carbon accounting guidance from the Intergovernmental Panel on Climate Change (IPCC) has become the primary source of information for methods, accounting equations and parameters. However, IPCC guidance is vast and often difficult to navigate. In response, a number of tools for forest carbon accounting have emerged. These vary in terms of geographical coverage, forestry activities and the carbon pools accounted for, as well as the level of data input required. In light of such diversity, practitioners require an understanding of the forest carbon accounting process, irrespective of whether these tools are utilised.Despite substantial progress in the field of forest carbon accounting over the last decade, challenges still remain. Terminology relating to forests and managed lands is ambiguous and requires standardisation between stakeholders. More scientific research into forest biomass characteristics is also required to better incorporate the heterogeneity of forests, their growth dynamics and the fate of carbon in harvested wood products into forest carbon accounting methods.Forest carbon accounting is a multi-disciplinary task. Building capacity is essential. Investment is also necessary to improve and standardise carbon accounting methods. If future climate change policy and strategy are to adequately reflect the substantial role forests play in the global carbon balance, good forest carbon accounting is imperative.Table of ContentsExecutive Summary (2)List of Figures (5)1. Introduction (6)1.1. Report structure (6)1.2. What is forest carbon accounting? (6)2. Principles of forest carbon accounting (8)2.1. Accounting good practice (8)2.2. Biomass, carbon pools and stock accounting (9)2.3. Approaches to emission accounting (11)2.4. Accounting for emission reductions (12)2.4.1. Baselines (13)2.4.2. Additionality (13)2.4.3. Leakage (14)2.4.4. Permanence (14)3. Practice of forest carbon accounting (15)3.1. Establishing the accounting area (15)3.2.1. Collating existing forest data (17)3.2.2. Using remote sensing (17)3.2.3. Data from field sampling (19)3.3. Accounting for forest carbon stocks (19)3.3.1. Above-ground biomass (AGB) (19)3.3.2. Below-ground biomass (BGB) (20)3.3.3. Dead organic matter (wood) (21)3.3.4. Dead organic matter (litter) (21)3.3.5. Soil organic matter (SOM) (21)3.4. Accounting for forest carbon emissions (22)3.4.1. Accounting for carbon stock changes in carbon pools (22)3.4.2. Accounting for carbon stored in harvested wood products (HWPs) (22)3.4.3. Accounting for nitrous oxide and methane emissions from disturbances (24)3.5. Quantifying uncertainty in carbon accounting (25)4. Guidance and tools for forest carbon accounting (26)4.1. IPCC guidelines (26)4.2. Carbon accounting tools (26)4.3. Bilan Carbone (27)5. Challenges for forest carbon accounting (28)5.1. Clarifying terminology (28)5.1.1. Definition of ‘forest’ (28)5.1.2. Direct human-induced impacts (29)5.2. Forest Characteristics (30)5.2.1. Heterogeneity of forests (30)5.2.2. Forest growth and equilibrium (30)5.2.3. Accounting for harvested wood products (31)6. Conclusion (31)7. Appendices (33)7.1. Appendix I: References (33)7.2. Appendix II: Acronyms (38)7.3. Appendix III: Glossary (39)7.4. Appendix IV: Examples of default equations and data for forest carbon accounting (41)List of TablesTable 1. Good practice for forest carbon accounting (9)Table 2. Default forest biomass and annual biomass increment under tier 1 IPCC guidance (18)Table 3. Factors affecting forest carbon stocks (29)Table A4:1. Exemplary above-ground biomass regression equations for tropical trees (41)Table A4:2. Default mineral soil organic carbon stocks (41)List of FiguresFigure 1. Diagrammatic Representation of Carbon Pools (10)Figure 2. Generalised flow of carbon between pools (11)Figure 3. Outline of the practice of forest carbon accounting (16)1. Introduction1.1. Report structureThere has been considerable and growing interest in forest carbon and its role in international climate change policy. This interest stems from the substantial greenhouse gas (GHG) emissions that arise from the forestry sector and the potential for forests to deliver cheap-and-deep emission reductions. Forest Carbon Accounting: Overview & Principles presents the main principles, practices and challenges for carbon accounting in the forestry sector. In order to be accessible, the report is not overly technical and should not, therefore, be considered a stand-alone guide for forestry carbon accounting. It does, however, present guidance for good practice in accounting and indicates further sources of guidance. Section 1 outlines the historic, current and future needs for forest carbon accounting. Section 2 focuses on principles and good practice. The process of forest carbon accounting is outlined in Section 3. Section 4 highlights existing guidance and toolkits available for forestry carbon accounting and Section 5 presents the challenges and limitations to date. Section 6 concludes.1.2. What is forest carbon accounting?Carbon accounting is the practice of making scientifically robust and verifiable measurements of GHG emissions. Although characteristics of forests have been recorded for numerous historical purposes, accounting for carbon is a more recent addition to forest inventories. This follows the growing need to quantify the stocks, sources and sinks of carbon and other GHGs in the context of anthropogenic impacts on the global climate.Historically, forest inventories recorded stand structure, age, growth rate, biomass accumulation, and the wood densities of tree species. These have served both commercial purposes, such as determining merchantable timber volumes and use in the paper and pulp industry, as well as national or regional planning purposes, such as creating forest and land use inventories for land-use permits, land-use plans and agricultural expansion.In 1946, the Food and Agriculture Organisation (FAO) established the Forest Resource Assessment (FRA) which, published every five to ten years, compiles data gathered through national statistics and country-level reporting processes. Although criticised (see Grainger, 2008; Houghton, 2005), the FRA still provides the most comprehensive assessment of global forest cover, management and trends to date. In combination with the substantial body of forest science research literature, the FRA and similar forest inventories provide the background for carbon accounting.The forestry sector plays a vital role in the global balance of GHGs. Deforestation alone accounts for approximately 20% of anthropogenic emissions (FAO 2006; Stern, 2006) and the forestry sector represents upwards of 50% of global greenhouse gas mitigation potential (IPCC, 2007). As forests riseup the climate change agenda, three types of forest carbon accounting have developed: stock accounting, emissions accounting and project emission reductions accounting.∙Stock accountingForest carbon stock accounting often forms a starting point for emissions and project-level accounting. Establishing the terrestrial carbon stock of a territory and averagecarbon stocks for particular land uses, stock accounting allows carbon-dense areas to beprioritised in regional land use planning. An early form of forest carbon accounting,emissions and emission reductions accounting have evolved from the principlesestablished for stock accounting.∙Emissions accountingEmissions accounting is necessary to assess the scale of emissions from the forestrysector relative to other sectors. It also aids realistic goal-setting for GHG emissionstargets. Under the United Nations Framework Convention on Climate Change (UNFCCC)and the Kyoto Protocol, countries are mandated to undertake some land use, land usechange and forestry (LULUCF) carbon accounting (see Box 1). With a significant portionof developing country emissions arising from the LULUCF sector, the forestry sector islikely to play a prominent role in climate change strategies in these countries.∙Project emission reductions accountingCarbon accounting for forestry project emission reductions is required for both projectsundertaken under the flexible mechanisms of the Kyoto Protocol and the voluntarycarbon markets. Both necessitate good carbon accounting to ensure that emissionsreductions are real, permanent and verifiable. For projects to generate tradableemission reductions, accounting methods between countries, regions and projectsmust be standardised in both developed and developing countries.Past forest inventories and research outputs provide a substantial source of information on forest biomass characteristics. The challenge is to translate this information into carbon estimates, in particular increasing the coverage and/or scaling-up research that often focuses on ecological zones or specific territories. Ultimately, the quality of forest carbon estimates will be governed by a number of factors, not least time and financial resource constraints. Acknowledging that trade-offs between factors in the accounting process are inevitable, the carbon accounting process must adhere to good practice guidance if forestry is to be adopted more comprehensively in climate change policy.2. Principles of forest carbon accounting2.1. Accounting good practiceRegardless of the type of accounting – stock, emissions or project emission reductions – there are a number of principles for carbon accounting that should be followed (see Table 1). Adherence to good practice promotes better understanding, legitimacy and trust in the accounting system, which is critical for both political and public acceptance (Greenhalgh et al., 2006).Although publications commonly discuss ‘carbon’ accounting, completeness calls for the inclusion of other relevant GHGs in emissions and project emission reductions accounting. Thus, carbonaccounting often refers to accounting of carbon dioxide equivalent (CO2e), a metric which allows standardisation of the six major GHGs based on their global warming potential. In the forestry sector, management regimes influence the scale of methane (CH4) and nitrous oxide (N2O) emissions in addition to carbon emissions. Methane emissions result from burning and decomposition of organic matter in oxygen-free environments, such as waterlogged soils. Nitrous oxide is emitted during burning, decomposition of organic matter, soil organic matter mineralisation and land fertilisation by nitrogen fertilisers. Although these gases tend to be produced in lower volumes than CO2 they have greater global warming potential. To adhere to good practice, CH4 and N2O emissions should be fully accounted for where significant. However, where minor, meaning less than 1% of the total (IPCC, 2003), such emissions can be omitted from accounting.Sources: Greenhalgh et al., 2006; Pearson et al., 2005; IPCC, 20002.2. Biomass, carbon pools and stock accountingForest biomass is organic matter resulting from primary production through photosynthesis minus consumption through respiration and harvest. Assessment of biomass provides information on the structure and functional attributes of a forest and is used to estimate the quantity of timber, fuel and fodder components (Brown, 1997). With approximately 50% of dry forest biomass comprised of carbon (Westlake, 1966), biomass assessments also illustrate the amount of carbon that may lost orsequestered under different forest management regimes. Carbon is lost to the atmosphere as CO2. To convert carbon in biomass to CO2, the tonnes of carbon are multiplied by the ratio of the molecular weight of carbon dioxide to the atomic weight of carbon (44/12). Estimating the biomass density of forest components is, therefore, the first step in forest carbon accounting.Carbon pools are components of the ecosystem that can either accumulate or release carbon and have classically been split into five main categories: living above-ground biomass (AGB), living below-ground biomass (BGB), dead organic matter (DOM) in wood, DOM in litter and soil organic matter (SOM) (see Figure 1). The classification of carbon pools is not strict and it is not the number of categories that is important but their completeness; pools must not be double-counted and significant pools should not be excluded (refer to Table 1, Section 2.1.). With harvested wood products (HWPs) increasingly recognised as an additional and potentially substantial carbon pool which exists outside of traditional forest boundaries (Lui & Han, 2009), many carbon pool classifications are being adapted to also include HWPs.Figure 1. Diagrammatic Representation of Carbon Pools(AGB above-ground biomass; BGB below-ground biomass; SOM soil organic matter;DOM dead organic matter; HWPs harvested wood products)A carbon source is a carbon pool from which more carbon flows out than flows in: forests can often represent a net source (rather than sink) of carbon due to the processes of decay, combustion and respiration. A carbon sink is a carbon pool from which more carbon flows in than out: forests can act as sink through the process of tree growth and resultant biological carbon sequestration (Brown, 2002). Forests can switch between being a source and a sink of carbon over time, with the stock of the forest referring to the absolute quantity of carbon held within a forest component at a specified time. The transfer of carbon between carbon pools is represented in Figure 2.Stock accounting sums carbon pools at a single point in time. Decisions on which carbon pools should be included are largely dependent on the availability of existing data, costs of measurement and the level of conservativeness required (MacDicken, 1997). Trees often represent the greatest fraction of total biomass of a forested area, with other carbon pools only a fraction of the total tree biomass. The understorey is estimated to be equivalent to 3% of above-ground tree biomass, dead wood 5-40%, and fine litter only 5% of that in the above-ground tree biomass. BGB is more variable, rangingbetween 4 - 230%, and can be more than two times greater than that in the above-ground tree biomass (Brown, 1997). AGB in trees also responds more rapidly and significantly as a result of land-use change than other carbon pools. As a consequence, the majority of carbon accounting efforts are focussed on tree AGB, for which there is a considerable forest science research base.Figure 2. Generalised flow of carbon between pools(source IPCC, 2006)2.3. Approaches to emission accountingAlthough many natural processes lead to emissions and removals of GHGs – for example, fires, insect attacks and local climate variability –anthropogenic activities such as slash and burn, fire management and harvesting have accelerated the release of GHGs from forests (Canadell et al., 2007). These forest management practices affect the balance of emissions into the atmosphere through biomass fluctuation, soil and litter disturbance (Sajwaj et al., 2008) and so have differing impacts on the various carbon pools.The purpose of emissions accounting is to quantify the exchange of GHGs between the atmosphere, terrestrial vegetation and soils through photosynthesis, respiration, decomposition and combustion. There are two main approaches to emissions accounting: the inventory approach and the activity-based approach, which are outlined below and mathematically represented in Box 2. Both approaches are supported under IPCC guidance (IPCC, 2003) and are based on the underlying assumption that the flows of GHGs to or from the atmosphere are equal to changes in carbon stocks in the biomass and soils.The inventory approach measures the difference in carbon stocks averaged between two points in time (Box 2, equation 1). Also called periodic accounting, or the stock-difference approach,measurement of stock change in this way can cover large areas and a variety of species and site conditions. The inventory-based system also captures non-linear changes in carbon stocks, for example biomass accumulation through growth. However, relying on the addition of carbon pools and assessments conducted in this way often leaves out smaller biomass components such as leaf biomass, ground vegetation and litter.In contrast, the activity-based approach estimates the net balance of additions to and removals from a carbon pool (Box 2, equation 2). The activity-based approach, also called the gain-loss or flux approach, estimates changes in carbon stocks by first establishing the rate of area change in land use and multiplying this by the response of carbon stocks under a particular land use. This biological response of a given land use is based indirectly on rates of carbon losses and gains by an area or it is directly measured with the aid of technology (for an example see Baldocchi, 2003). Where the gains and losses in carbon stock can be given as a standard rate of emissions per unit activity, an emissions factor replaces (C1– C2) in Equation 2 (Box 2). The activity-based approach is useful where individual carbon pools are difficult to measure and is less susceptible to short-term variation in carbon stocks. However, emission factors require non-linear carbon stock changes to be time-averaged and assumptions must be made explicit.In general, the accounting approach chosenmust reflect both purpose and acceptabilityto policy-makers, with decisions also likelyto rely on the availability and form ofexisting forest data within a territory. Asthe profile of the forestry sector rises upthe climate agenda, new accountingapproaches are being proposed (see Cowieet al., 2007). A consensus on whichaccounting approach to adopt into thefuture has yet to be reached and inventoryand activity-based accounting remain thedominant accounting approaches.2.4. Accounting for emission reductionsAccounting for emission reductions is most commonly required at the project level, but are also relevant when policy targets must be met. Where forestry carbon projects generate emission reductions, these can be traded as offsets either under the Kyoto Protocol or on the voluntary carbon markets.Accounting for emission reductions requires an understanding of a number of supplementary principles: the complexities of baseline establishment, demonstration of additionality, issues of leakage, and the permanence of emissions reductions. These principles have commonly been blamed for the limited demand and limited inclusion of the forestry sector in carbon trading mechanisms todate. These concepts are briefly outlined below and further guidance for project-specific forest carbon accounting is available from the World Resources Institute (Greenhalgh et al., 2006), World Bank and Winrock (Pearson et al., 2005) and the Voluntary Carbon Standard (VCS, 2008).2.4.1. BaselinesIn order to set emission reduction targets, a baseline scenario must be developed. Also called a counterfactual, this baseline scenario estimates what would have happened in the absence of a policy or project. It is required so that the mitigation impact of a project or policy can be quantified. In the forestry sector, the baseline is particularly important in attempts to reduce emissions from deforestation and degradation, and raises both technical and political considerations (Bond et al., 2009).Creation of a baseline scenario relies on a detailed understanding of the drivers of land use change. The drivers of deforestation, however, are complex and knowledge is still incomplete. A review by Giest and Lambin (2001) identify three aggregate proximate causes –agricultural expansion, wood extraction and expansion of infrastructure – and five broad categories of underlying driving forces: demographic, economic, technological, policy / institutional, and cultural / socio-political factors. They further identify a group of pre-disposing environmental factors, biophysical drivers and social trigger events that influence the rate of deforestation within a territory. The baseline must consider all of these complex and interlinked causes, forces and pre-disposing factors that vary greatly between countries, regions and over time. Substantial technical difficulties and uncertainties therefore arise when baselines are established.At the 9th COP of the UNFCCC, three baseline approaches for Reduced Emissions from Deforestation and Degradation (REDD) were proposed: extrapolating existing or historical rates of deforestation, also called business-as-usual; estimating changes in carbon stocks from land uses that represents economically attractive courses of action, taking into account barriers to investment; and estimating changes in carbon stocks from the most likely land use at the time the project starts (Bond et al., 2009). The choice of baseline methodology will impact country participation in climate change mitigation activities. For example, countries with historically high rates of deforestation will be rewarded more, and so will be more likely to participate, than those that have been active and successful in conserving forest areas. These latter countries are likely to prefer a perspective, or hybrid, approach to setting the baseline (for a more comprehensive review of baselines see Angelsen 2008 a,b).2.4.2. AdditionalityFor a project to be additional, it must be proven that emission reductions would not have occurred in the absence of a project. This is an important principle when emissions reductions at a project location are used to offset GHG emissions at another location. If there is no additionality, overall GHG emissions will increase as a result of the project activity.For afforestation and reforestation (AR) Clean Development Mechanism (CDM) projects of the Kyoto Protocol, Article 12 lays out additionality requirements that must be met for projects to be validated. The CDM AR Working Group, along with the CDM Executive Board, has developed a tool to demonstrate additionality, whereby project alternatives must first be identified. Additionality can then be proven through either a financial test (the CDM activity must be proven less economically orfinancially attractive than other alternatives) or a barriers test (investment, technological or prevailing practice barriers must be shown that they can only be overcome with CDM finance). A critical requirement of any emissions trading scheme, voluntary carbon market projects also require similar assessments of additionality.2.4.3. LeakageLeakage is a process by which emissions are reduced in one area but are also impacted outside of the area in question. Although positive leakage is a possibility, concern is directed to negative leakage, where emissions are merely shifted to another geographical area and fewer, or no, actual reductions are generated by the project activities (Sohngen & Brown, 2004). Leakage can be sub-divided into a number of categories including slippage, activity shifting, outsourcing, market effects and life-cycle emission shifting (for elaboration see Schwarze et al., 2002; IPCC, 2000; Moura-Costa et al., 2000; Schlamadinger & Marland, 2000; Brown et al., 1997) and can be one-time or recurrent.Adequate assessments of leakage are crucial and identification requires the main drivers of the project baseline to be properly addressed. A number of methods have been proposed to do this (see Aukland et al., 2002) and include socio-economic surveys, remote sensing and assessment of market effects. In general, the challenge of accounting for leakage should not be under-estimated in project development and project design should account for leakage through site selection policies, multi-component projects or leakage buffer and set-aside areas.2.4.4. PermanencePermanence refers to the persistence of emission reductions over time. Unlike other sectors, such as industry, energy, waste management and transport, there is a risk that forest carbon sinks, having delivered emissions reductions, may deteriorate or be depleted over the long term. This could be a result of natural disturbances including fire, pests and disease, or anthropogenic disturbances such as poor management and political instability leading to land-use change. Forestry emission reductions are, therefore, unlike those from other sectors in their certainty of delivery.This uncertainty is reflected in the UNFCCC 9th COP decision in 2003 to establish only temporary credit regimes for AR activities under the CDM of the Kyoto Protocol. Emission reductions generated from AR are effectively leased or rented in one of two forms and must be verified every five years, after which they are re-issued, renewed or replaced. Temporary Certified Emission Reductions (tCERs) expire at the end of the commitment period following the one in which they were issued. After verification, a tCER can either be re-issued (if the sequestered carbon remains intact) or the Annex I buyer must replace the expired tCER with a new tCER or a CER. Long-term Certified Emission Reductions (lCERs) expire at the end of the crediting period of the activity for which they were issued (and can, therefore, have a potential life of 60 years in the CDM AR context), but must be replaced in the interim if verification shows that sequestered carbon has decreased. At expiry, both tCERs and lCERs must be replaced with credits of their own kind (e.g. tCERs can be replaced by new tCERs but not by lCERs, and vice versa) or with permanent CERs.The added complications of temporary crediting have led to low prices and low market demand for tCERs and lCERs. However, outside of the Kyoto Protocol framework, alternative mechanisms to deal with the forestry project permanence risk have been developed. These include periodic, performance-。
2021届高考英语二轮复习题型突击专题05阅读理解之生态环保类含解析202103022221
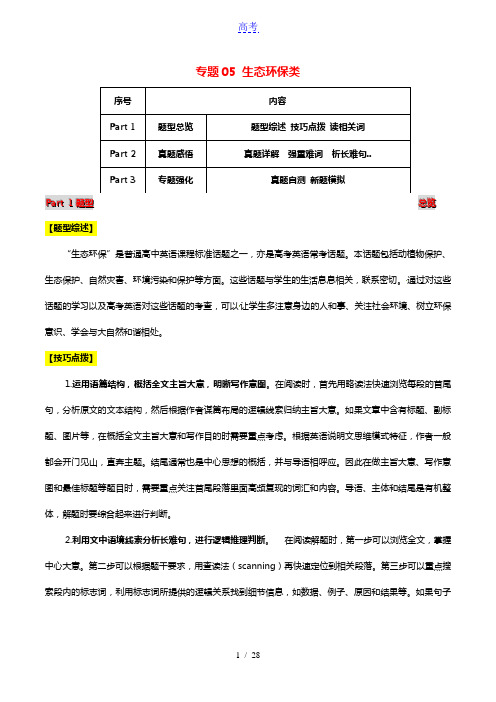
专题05 生态环保类序号内容Part 1 题型总览题型综述技巧点拨读相关词Part 2 真题感悟真题详解强重难词析长难句..Part 3 专题强化真题自测新题模拟P P a a r r t t11题题型型总总览览【题型综述】“生态环保”是普通高中英语课程标准话题之一,亦是高考英语常考话题。
本话题包括动植物保护、生态保护、自然灾害、环境污染和保护等方面。
这些话题与学生的生活息息相关,联系密切。
通过对这些话题的学习以及高考英语对这些话题的考查,可以让学生多注意身边的人和事、关注社会环境、树立环保意识、学会与大自然和谐相处。
【技巧点拨】1.运用语篇结构,概括全文主旨大意,明晰写作意图。
在阅读时,首先用略读法快速浏览每段的首尾句,分析原文的文本结构,然后根据作者谋篇布局的逻辑线索归纳主旨大意。
如果文章中含有标题、副标题、图片等,在概括全文主旨大意和写作目的时需要重点考虑。
根据英语说明文思维模式特征,作者一般都会开门见山,直奔主题。
结尾通常也是中心思想的概括,并与导语相呼应。
因此在做主旨大意、写作意图和最佳标题等题目时,需要重点关注首尾段落里面高频复现的词汇和内容。
导语、主体和结尾是有机整体,解题时要综合起来进行判断。
2.利用文中语境线索分析长难句,进行逻辑推理判断。
在阅读解题时,第一步可以浏览全文,掌握中心大意。
第二步可以根据题干要求,用查读法(scanning)再快速定位到相关段落。
第三步可以重点搜索段内的标志词,利用标志词所提供的逻辑关系找到细节信息,如数据、例子、原因和结果等。
如果句子成分复杂,有生词,也不要烦躁退缩,分析主句和从句或非谓语动词之间的关系,抓住行文逻辑的标志词,层层剖析,露出主干部分,就能明晰句意,弄懂作者的真实意图。
【读相关词】1.haze n. 雾霾2.conservation n. 保护3.recycle v.回收;循环利用4.ecological balance 生态平衡5.make proper use of 合理利用6.keep the balance of 保持……的平衡7.attach great importance to 十分重视8.bring ... under control 把……控制住9.be/bee aware of 意识到10.take the responsibility for/be responsible for 对……负责任11.mist n. 薄雾12.shortage n. 缺乏13.damage n.& vt. 毁坏,破坏14.destroy vt. 摧毁,破坏15.ruin vt. (使)毁坏;(使)毁灭n. (复)废墟;遗迹16.disappear vi. 消失17.threaten vt. 威胁18.be trapped in ruins 被困在废墟中19.rebuild one’s home 重建某人的家园20.extinct adj. 灭绝的21.preserve vt. 保护22.victim n. 牺牲品,受害者23.damage natural habitat 破坏自然栖息地24.endangered species濒危物种25.die out 灭绝26.natural reserve 自然保护区27.protect wildlife 保护野生动物28.cut down 砍倒29.raise the awareness of 唤醒……的意识30.live in harmony with 与……和谐相处31.pollute vt. 污染32.waste n. 废料33.rubbish n. 垃圾;废物34.litter n. 垃圾35.garbage n. 垃圾36.greenhouse effect 温室效应37.environmentallyfriendly adj. 环保的38.advocate vt. 拥护,支持,提倡39.preserve vt. 保护,保留,保存40.limited natural resources 有限的自然资源41.alternative energy 替代能源42.in harmony with nature 与自然和谐共处43.live a low-carbon life 过低碳生活44.prevent ...from ... 阻止……做……45.raise one’s environmental awareness提高某人的环保意识46.save and treasure our resources 节约并珍惜我们的资源47.take action/steps/measures to do sth. 采取措施做某事48.call on/appeal to sb. to do sth. 号召/呼吁某人做某事P P a a r r t t22真真题题感感悟悟【2020·全国新课标II】When you were trying to figure out what to buy for the environmentalist on your holiday list, fur probably didn’t cross your mind. But some ecologists and fashion (时装)enthusiasts are trying to bring back the market for fur made from nutria(海狸鼠).Unusual fashion shows in New Orleans and Brooklyn have(showcased)nutria fur made into clothes in different styles. “It sounds crazy to talk about guilt-free fur-unless you understand that the nutria are destroying vast wetlands every year”, says Cree McCree, project director of Righteous Fur.Scientists in Louisiana were so concerned that they decided to pay hunters $5 a tail. Some of the fur ends up in the fashion shows like the one in Brooklyn last month.Nutria were brought there from Argentina by fur farmers and let go into the wild. “The ecosystem down there can’t handle this non-native species(物种).It’s destroying the environment. It’s them or us.”says Michael Massimi, an expert in this field.The fur trade kept nutria check for decades,but when the market for nutria collapsed in the late 1980s,the cat-sized animals multiplied like crazy.Biologist Edmond Mouton runs the nutria control program for Louisiana. He says it’s not easy to convince people that nutria fur is green, but he has no doubt about it. Hunters bring in more than 300,000 nutria tails a year, so part of Mouton’s job these days is trying to promote fur.Then there’s Righteous Fur and its unusual fashion. Morgan says,”To give people a guilt-free option that they can wear without someone throwing paint on them-1 think that’s going to be a massive thing, at least here in New York.”Designer Jennifer Anderson admits it took her a while to e around to the opinion that using nutria fur for her creations is morally acceptable. She trying to e up with a label to attach to nutria fashions to show it is eco-friendly.28.What is the purpose of the fashion shows in New Orleans and Brooklyn?A.To promote guilt-free fur.B.To expand the fashion market.C.To introduce a new brand.D.To celebrate a winter holiday.29.Why are scientists concerned about nutria?A.Nutria damage the ecosystem seriously.B.Nutria are an endangered species.C.Nutria hurt local cat-sized animals.D.Nutria are illegally hunted.30.What does the underlined word “collapsed”in paragraph 5 probably mean? A.Boomed.B.Became mature.C.Remained stable.D.Crashed.31.What can we infer about wearing fur in New York according to Morgan?A.It’s formal.B.It’s risky.C.It’s harmful.D.It’s traditional.【答案】28.A29.A30.D31.B【解析】本文是说明文。
关于农林专业的英语作文
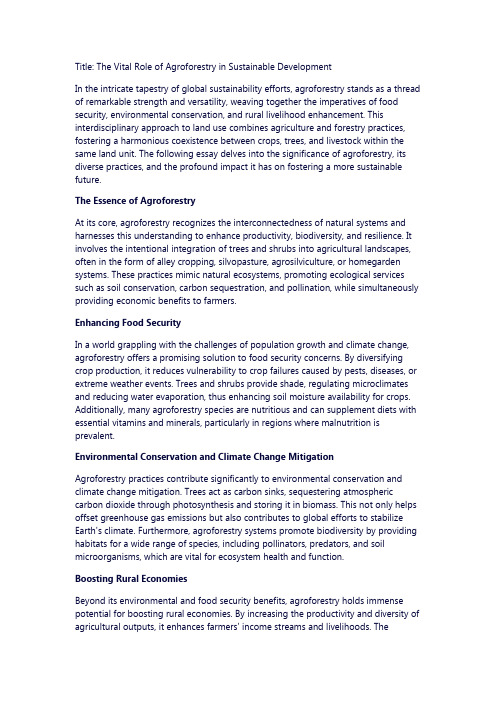
Title: The Vital Role of Agroforestry in Sustainable DevelopmentIn the intricate tapestry of global sustainability efforts, agroforestry stands as a thread of remarkable strength and versatility, weaving together the imperatives of food security, environmental conservation, and rural livelihood enhancement. This interdisciplinary approach to land use combines agriculture and forestry practices, fostering a harmonious coexistence between crops, trees, and livestock within the same land unit. The following essay delves into the significance of agroforestry, its diverse practices, and the profound impact it has on fostering a more sustainable future.The Essence of AgroforestryAt its core, agroforestry recognizes the interconnectedness of natural systems and harnesses this understanding to enhance productivity, biodiversity, and resilience. It involves the intentional integration of trees and shrubs into agricultural landscapes, often in the form of alley cropping, silvopasture, agrosilviculture, or homegarden systems. These practices mimic natural ecosystems, promoting ecological services such as soil conservation, carbon sequestration, and pollination, while simultaneously providing economic benefits to farmers.Enhancing Food SecurityIn a world grappling with the challenges of population growth and climate change, agroforestry offers a promising solution to food security concerns. By diversifying crop production, it reduces vulnerability to crop failures caused by pests, diseases, or extreme weather events. Trees and shrubs provide shade, regulating microclimates and reducing water evaporation, thus enhancing soil moisture availability for crops. Additionally, many agroforestry species are nutritious and can supplement diets with essential vitamins and minerals, particularly in regions where malnutrition is prevalent.Environmental Conservation and Climate Change MitigationAgroforestry practices contribute significantly to environmental conservation and climate change mitigation. Trees act as carbon sinks, sequestering atmospheric carbon dioxide through photosynthesis and storing it in biomass. This not only helps offset greenhouse gas emissions but also contributes to global efforts to stabilize Earth's climate. Furthermore, agroforestry systems promote biodiversity by providing habitats for a wide range of species, including pollinators, predators, and soil microorganisms, which are vital for ecosystem health and function.Boosting Rural EconomiesBeyond its environmental and food security benefits, agroforestry holds immense potential for boosting rural economies. By increasing the productivity and diversity of agricultural outputs, it enhances farmers' income streams and livelihoods. Theintegration of trees into farming systems also creates opportunities for value-added products, such as timber, fruits, nuts, and non-timber forest products, further diversifying farmers' markets and income sources. Moreover, agroforestry promotes sustainable land use practices, preserving natural resources for future generations and fostering resilience against economic shocks.ConclusionIn conclusion, agroforestry represents a powerful tool in the quest for sustainable development. By integrating agriculture and forestry practices, it addresses the intertwined challenges of food security, environmental conservation, and rural poverty. As the world grapples with the complex demands of the 21st century, embracing agroforestry becomes not just an option but a necessity. It offers a path towards a more resilient, equitable, and environmentally sustainable future, where humanity and nature can thrive in harmony.。
森林地上生物量遥感估算研究进展

森林地上生物量遥感估算研究进展一、本文概述Overview of this article随着全球生态环境问题的日益突出,对森林地上生物量的准确估算已成为生态学、林学和地球科学等领域的研究热点。
森林地上生物量是指森林生态系统中乔木层、灌木层、草本层等所有地上部分的生物量总和,其估算对于理解森林生态系统的碳循环、能量流动以及生物多样性保护等方面具有重要意义。
遥感技术以其高效、快速、无损的特点,在森林地上生物量估算中发挥了越来越重要的作用。
本文旨在对森林地上生物量遥感估算的研究进展进行全面梳理和评价,以期为未来的研究提供借鉴和参考。
With the increasingly prominent global ecological and environmental issues, accurate estimation of forest aboveground biomass has become a research hotspot in fields such as ecology, forestry, and earth science. Forest aboveground biomass refers to the total biomass of all aboveground parts of a forest ecosystem, including the tree layer, shrub layer, and herbaceous layer. Its estimation is ofgreat significance for understanding the carbon cycle, energy flow, and biodiversity conservation of forest ecosystems. Remote sensing technology has played an increasingly important role in estimating aboveground biomass in forests due to its efficient, fast, and non-destructive characteristics. This article aims to comprehensively review and evaluate the research progress of remote sensing estimation of forest aboveground biomass, in order to provide reference and inspiration for future research.文章首先回顾了遥感技术在森林地上生物量估算中的应用历程,分析了不同遥感数据源和方法在生物量估算中的优缺点。
CARBON SEQUESTRATION POTENTIAL OF INDIAN FORESTS

CARBON SEQUESTRATION POTENTIAL OF INDIAN FORESTSL and ROMA SINGHCentre for Atmospheric Sciences,Indian Institute of Technology,New Delhi-110016,IndiaE-mail:mlalecas.iitd.ernet.in(Received9June1997;accepted13November1998)Abstract.The forestry sector can not only sustain its carbon but also has the potential to absorb carbon from the atmosphere.India has maintained approximately64Mha of forest cover for the last decade.The rate of afforestation in India is one of the highest among the tropical countries,currently estimated to be2Mha per annum.The annual productivity has increased from0.7m3per hactare in 1985to1.37m3per hectare in1995.Increase in annual productivity directly indicates an increase in forest biomass and hence higher carbon sequestration potential.The carbon pool for the Indian forests is estimated to be2026.72Mt for the year1995.Estimates of annual carbon uptake increment suggest that our forests and plantations have been able to remove at least0.125Gt of CO2from the atmosphere in the year1995.Assuming that the present forest cover in India will sustain itself with a marginal annual increase by0.5Mha in area of plantations,we can expect our forests to continue to act as a net carbon sink in future.Keywords:anthropogenic emissions,carbon sequestration,carbon uptake,indian forests,productiv-ity1.IntroductionGreenhouse gases play a major role in maintaining the global energy balance;a small change in their concentration in the atmosphere can affect the climatic conditions on Earth.Carbon dioxide(CO2)is one of the main greenhouse gases. It is reported that the level of CO2is continuously rising in the atmosphere since preindustrial times.Observed increase in levels of atmospheric CO2(from∼280 ppmv in1800to∼315ppmv in1957to∼358ppmv in1996)has forced nations in recent years to assess their contributions to sources and sinks of CO2and to evaluate the processes that control CO2accumulation in the atmosphere.The major components of perturbations in the atmospheric carbon budget are anthropogenic emissions,ocean exchanges and terrestrial exchanges.Anthropogenic emissions of CO2are mostly due to fossil fuel combustion and deforestation.These emissions are likely to increase in the future as world’s population increases.The demand for fossil fuels and forest products is increasing continuously all over the globe.Deforestation and other changes in land use(including land management) cause significant exchanges of CO2between the land and the atmosphere.However, there is considerable uncertainty in estimated rates of deforestation and in estimates Environmental Monitoring and Assessment60:315–327,2000.©2000Kluwer Academic Publishers.Printed in the Netherlands.L AND ROMA SINGHof emission from changing land use in the drier,open canopy forests of the seasonal tropics(FAO,1993;Dixon et al.,1994).Several recent analysis suggest a sink of carbon in the regrowing Northern Hemisphere forests.A study by Goto and Yanagisava(1996)suggests that,during the period from1961to1991,the terrestrial ecosystem has removed a net amount of12.0Giga tonnes Carbon(GtC)from the atmosphere on a global mean basis, resulting from26.1GtC emission due to Land use changes and28.1GtC removal by fertilization effects.This suggests that the forestry sector can not only sustain its carbon but also has the potential to absorb carbon from the atmosphere.Un-fortunately,little has been done to improve our understanding of potential sinks in tropical forests.Available information on the initial biomass and rates of regrowth as a function of age for the tropical forests is incomplete.Improved observations for identifying terrestrial sinks are necessary for quantifying regional contributions to terrestrial sources and sinks.In developing countries like India,the demand of forest products is quite pro-nounced as,more than70%of the people in India living in rural areas,mostly rely on forests for their basic biomass needs,such as cattle feed,fuelwood,food and shelter.In spite of the increasing demand,India has been able to maintain approximately64Million hectare(Mha)of forest cover during the last decade.The rate of afforestation in India was1.67Mha yr−1during the last decade and currently it is2Mha per annum.Table I lists the progress of afforestation in India since1951. During the Ninth Five Year Plan period(1997–2002),about10Mha of land area is targeted for afforestation in India.This rate of afforestation is considered to be one of the highest among the tropical countries.The annual productivity of Indian forests is also reported to have increased from0.7m3per hectare in1985to1.37m3 per hectare in1995(FSI,1988;1996).An increase in annual productivity indicates an increase in the total standing biomass of Indian forests and its carbon uptake. In this paper,we report the current status of forest cover in India(including its growing stock and new plantations),annual productivity for the years1985,1990 and1995and its carbon sequestration potential.Assuming an increase of0.5Mha per annum in forest cover with3.2tonnes per hectare productivity,projections of net annual carbon uptake by the Indian forests are also estimated for the future.2.MethodologyForests play a vital role in carbon storage by the terrestrial biosphere to counter the anthropogenic emissions of CO2in the atmosphere.CO2is taken by plants during phospsynthesis,converted to organic compounds,and stored in the forest until it burns,decays or is removed in a harvest.CO2is returned to the atmosphere by respiration of the vegetation and decay of the organic matter in soils and litter. The gross CO2fluxes are large;roughly a seventh of the total atmospheric CO2 passes into vegetation each year and,in the absence of significant human inter-CARBON SEQUESTRATION POTENTIAL OF INDIAN FORESTS317TABLE IProgress of afforestation in IndiaPlan Period Area afforested Cumulative areain(Mha)plan period(Mha)First Plan(1951–56)0.050.05Second Plan(1956–61)0.310.36Third Plan(1961–66)0.580.94Post Third Plan(1866–69)0.45 1.39Fourth Plan(1969–74)0.71 2.10Fifth Plan(1974–79) 1.22 3.32Annual Plan(1979–80)0.22 3.54Sixth Plan(1980–85) 4.658.19Seventh Plan(1985–90)8.8817.07Eighth Plan(1992–97)7.8424.91Ninth Plan(1997–2002)10.0034.91(target)ference,this largeflux of CO2from the atmosphere to the terrestrial biosphere is balanced by the returned respirationfluxes.The estimates of biomass from grow-ing stock of forest stratum are crucial to assess the total carbon pool of forests. In this paper,we estimate the total biomass of Indian forests from the volume of growing stock recorded for the year1995(Table II).Biomass is expressed in tonnes of dry matter(t dm).For converting volume in m3to biomass in t dm,the given volume is multiplied to standard conversion and expansion factors available for different forest types.Expansion factors include small branches of vegetation, which remains undetected during volume estimation.Conversion and expansion factors(default value is0.95)used here are those prescribed in the IPCC guidelines for preparing national greenhouse gas inventories(IPCC,1995).The volume given for each reported forest stratum is multiplied by0.95to obatin the total biomass of that stratum.This biomass is then converted into carbon content by multiplying it with a factor0.45(as prescribed in the IPCC guidelines).The total carbon pool of Indian forests for the year1995is thus obtained.The total carbon pool does not provide an estimate of the annual carbonflux.To compute annual carbonflux we also need to know the annual forest productivity and annual extraction of wood from forests.The area occupied by various forest types in India(following the classification of Indian forest cover under fourteen forest types proposed by Champian and Seth,1968)has been assessed periodically since1983.The annual forest productivity and annual extraction of wood from forests is also being reported at regular ing these statistics,we haveL AND ROMA SINGHTABLE IIStanding biomass of Indian forests as reported for the year1995Forest stratum Total growing Total biomass Total carbonstock(103m3)(Mt)∗storage(Mt)∗∗Fir(Abies pindrow)153033145.3865.42 Spruce(Picea smithiana)95509.07 4.08 Fir-spruce3121529.6513.34 Blue-pine(Pinus wallichiana)8117577.1234.70 Deodar(Cedrus deodara)2747326.1011.75 Chir-pine(Pinus roxburghii)111960106.3647.86 Mixed conifers383932364.74164.13 Hardwood mixed with conifers4701844.6720.10 Upland hardwoods(Betula utilis etc.)111710106.1247.75 Teak(Tectona grandis)320546304.52137.03 Sal(Shorea robusta)515459489.69220.36 Bamboo(Dendrocalamus strictus)3637134.5515.55 Dipterocarpus(Dipterocarpus spp.)6830.650.29 Khasipine(Pinus khasya)7271 6.91 3.10 Khair(Acacia catechu)2406 2.29 1.03 Salai(Boswellia serrata)3107 2.95 1.33 Alpine pastures6190.590.27 (Rhododendron glaucum etc.)Western ghat evergreen4740345.0320.26 (Dysoxylun malabaricum etc.)Western ghat semi-evergreen3756035.6816.06 (Dalbergia latifolia etc.)Western ghat deciduous1835717.447.85 (Terminalia tomentosa etc.)Miscellaneous27940102654.311194.44TOTAL47408584503.822026.72∗For conversion and expansion of volume expressed as(m3)into biomass(tonnes of drymatter),a default value of0.95has been used.∗∗Carbon content of biomass is obtained by multiplying biomass with a factor0.45.attempted to compute here the annual carbonflux from Indian forests for the years 1985,1990and1995.Factors such as shifting cultivation,natural decay of wood and carbon release from soil are not considered in this comparative study.Carbon flux due to these factors is negligible as there is only marginal land use changes during the past decade relative to the period1951–80within which approximately 4.5Mha of the total forest area was reportedly diverted for non forestry purposes.CARBON SEQUESTRATION POTENTIAL OF INDIAN FORESTS319 Subsequent to the enactment of Forest Conservation Act1980,unabated division of forest land for non forestry purposes declined sharply.The present rate of division of forest land for non forest purposes is0.016Mha per year.In the recent past, the net forest area has only marginally declined as its diversion has been largely compensated by afforestation(FSI,1996).The forest Survey of India has listed the per hectare value of annual biomass increment for various natural forest types for the year1995(FSI,1996).We also take into account the annual national mean above-ground biomass productivity of forestry plantations to obtain total biomass increment for Indian forest.The forestry plantations under the national afforestation programme have a significantly higher productivity compared to natural forests and the afforestation area has been increas-ing consistently during the past decade.We have,therefore,included a fraction (1/4)of afforested area in our computations for estimating the annual carbonflux. The current extent of plantation forest cover used in this study is6.23Mha.The year wise data on biomass increment of different forest types are not available except for the year1995.In view of this,it has been assumed here that the annual biomass increment has remained unchanged during the period from1985to1995. This biomass increment is multiplied with the reported area of each of the forest types to get the total annual biomass increment for the year1985,1990and1995 (Table III).The total biomass increment thus obtained is different for each of these years as the area occupied by these forest types has changed over the period.The reported extraction from Indian forests is mainly for fuelwood and timber uses.Only40million cubic meters(M m3)of fuelwood and12M m3of timber have been extracted since1985(MOEF,1990;1996).These two categories of ex-tractions account for the primary losses of forest carbon pool.Wood used as timber does not release carbon immediately.It is,however,considered as carbon loss since part of the timber extracted during during previous years must have decayed in the years considered here.Fuelwood releases most of its carbon immediately while burning.The annual carbon loss(released back to atmosphere)from forests in India due to reported extraction is estimated to be22.23million tonnes(Mt)for the past decade.The net carbonflux for a specific year is calculated by substracting the annual carbon loss from the annual carbon uptake increment.The net annual carbon uptake is then multiplied by44/12to convert it into annual CO2removal from atmosphere.An estimate for the future annual carbon uptake/release through forestry can also be made based on the present state of Indian forests and an assumed sce-nario for afforestation rates,productivity and area available.The basic assumption considered here is that the natural forest cover will continue to sustain its present productivity and area.In addition to this,India has the potential to increase its forest plantation by at least25Mha in the next50years.The productivity of plantation forests are assumed to be3.2tonnes per hectare(t/ha)per year(Sudha,1997).The extraction of wood from the forest is assumed to double in the next25years and triple in50years.Based on these considerations,we have computed the net carbonL AND ROMA SINGHTABLE IIIForested area and total annual biomass increment for the years 1985,1990and 1995Forest type Annual Area occupied ∗(103ha)Totalbiomass Annual Biomass Incrementincrement (Kt)(t/ha)198519901995198519901995NATURAL FORESTTropical wet evergreen 1.203865.085289.303709.684638.106347.164451.62Tropical semi evergreen 1.181637.202575.701599.001931.903039.331886.82Tropical moist deciduous 0.8019421.7123054.7019379.8815537.3718443.7615503.90Littoral and swamp 1.07603.52383.40575.64645.77410.24615.94Tropical dry deciduous 0.6625103.7618083.3024432.7216568.4811934.9816125.60Tropical thorn 0.734468.601573.004285.323262.081140.293128.28Tropical dry evergreen 0.6283.47134.2063.9651.7583.2039.66Subtropical broad leaved hill forest 1.09256.82267.80255.84279.93291.90278.87Subtropical pine 1.323178.104567.503198.004195.096029.104221.36Subtropical dry evergreen 0.65173.351201.00127.92112.68780.6583.15Montane wet temperate 1.371373.972581.901279.201882.343537.201752.50Himalayan moist temperate 1.542317.762242.802174.643569.353453.913348.95Himalayan dry temperate 2.10173.3530.50127.92364.0464.05268.63Subalpine and alpine 1.511547.322027.7027502.802336.453061.834152.92PLANTATIONS 3.202051.504271.506231.506564.8013668.819940.80TOTAL 66255.5168284.5070191.5061940.1371405.5175799.04∗Source:FSI (1988;1992;1996)CARBON SEQUESTRATION POTENTIAL OF INDIAN FORESTS321flux from Indian forests for the years2020and2045(25and50years in the future with respect to the year1995).The majorfindings of this study are presented and discussed in the following section.3.Results and discussionThe natural forest cover for the years1985,1990and1995is reported to be64.20 Mha,64.01Mha and63.96Mha respectively by the Forest Survey of India(FSI, 1888;1992;1996).Even though there is a marginal decline in the reported area for natural forests over the period considered,we have afforested almost16Mha during the past decade.These afforesations are not always inside the recorded for-est area and hence,their extent is not appropriately recorded.The minimum size in the imagery that could be mapped in Indian national remote sensing periodic assessments is2mm×2mm,which corresponds to nearly25hectares on the ground.Moreover,there are cartographic constraints in delineation of forest cover in satellite based imagery.Since the resolution of the sensor is only36.25meters, the linear features such as road side,canal side and railway track side plantations of less than the resolution width neither can be recorded nor delineated.Similarly, young plantations where significant crown development has not yet taken place also are not recorded(FSI,1996).Due to the above-said constraints,new plantations with poor development of a significant crown cover were neglected in earlier studies as newly raised plantations could not be noticed in satellite imagery.New plantations have,however,signifi-cantly high productivity value as compared to natural forests and fully developed plantations.The biomass of25year old plantations is estimated to be twice that of 8year old plantations but the primary productivity values are the same(Bargali and Singh,1995).These plants absorb CO2more efficiently from the atmosphere.In this study,we have included new plantations in our computations for estimating the annual biomass increment.The statistics on forestry plantations under the national afforestation programme have been compiled based on available records of the Ministry of Environment and Forests,Government of India(MOEF,1990;1996). We have included only a fraction(1/4)of afforested area in our computations of annual biomass increment.A commonly adopted approach uses the areas under different ecosystem types and their average values of carbon storage to obtain terrestrial carboon pools. Ravindranath and Somashekar(1995)followed this approach to calculate total biomass and carbon pools for14forest types of India.According to their esti-mates,the total forest biomass and carbon storage in Indian forest was8357.9Mt and4178.95Mt,respectively,for the year1986.Dadhwal and Nayak(1993)fol-lowed both the area-based and volume-based approaches for estimating the pools of carbon in the biomass of Indian forests for the1980s.They reported that the area-based procedure may overestimate biomass carbon(almost four times)sinceL AND ROMA SINGHit does not consider the relative proportion of closed and open forests.Overesti-mation of forest biomass carbon by the area-based approach compared to the more accurate volume-based inventory has also been noted by Brown and Lugo(1984) and Houghton et al.(1985).Using the growing stock data of each of the forest stratum(total volume is4740Million cu.m)reported for the year1995,the total biomass and carbon pool for the Indian forests are estimated to be4503.82Mt and 2026.72Mt respectively(Table II).Our carbon storage estimate(∼2027Mt)for the year1995is higher than that reported by Dadhwal and Nayak(1994Mt)for the year1985suggesting that the total biomass in the Indian forests has increased during the past decade.While estimating the carbon pool of forests,we must take into consideration the productivity of various types of forests and their individual contribution to annual carbonflux.Tropical rain forests are known to be most productive among various forest types.Rai and Pathak(1995)divided the total forest cover of India into seven main agro-ecological zones and estimated the potential productivity pattern for these zones of the country.According to their estimates,the lowest productivity is1.35m3per hectare per annum for the hot and cold desert area and the highest productivity is more than7m3per hectare per annum for the tropical rain forest. Of the14forest types considered in this study,tropical dry deciduous forests form the major portion of forest cover(accounts for38.2%of the total forest cover)in India.Tropical moist deciduous forests are another dominant type of forest in India and these cover almost30%of the total forest cover.The annual productivity strictly refers to the long term storage of carbon in the bole and branches of vegetation and does not include the carbonflow through litter fall.Live woody biomass(excluding leaves,reproductive parts and dead wood) is regarded as above ground standing biomass in our study.Only69%of the net primary productivity for any vegetation is considered as accumulating in the bole and large branches for long term storage(Ravindranath et al.,1997).Of the re-maining net primary productivity(31%)in a reference year,half is considered to be used as fuel wood(includes lumber)and the other half is left to decompose. The current consumption of the fuel wood component of the biomass has been estimated to be about296Mt and is projected to increase to about377Mt by the year2000.According to Ravindranath and Hall(1995),fuel wood harvesting may not be contributing to deforestation in any significant manner though it does con-tribute to forest degradation.Fuel wood harvest and consumption in a sustainable manner and decay of wood will not make any net CO2contribution assuming that the system remains in equilibrium(Singh et al.,1997).During the last decade the total forest cover in India has remained nearly stable but the annual productivity has shown a positive trend.Total annual carbon uptake increment is estimated to be27.8Mt,32.1Mt and34.1Mt for the years1985,1990 and1995respectively.This suggests that our forestry sector and plantations had the potential to remove about0.125Gt of CO2from the atmosphere in the year1995. However,the annual carbon loss(released back to atmosphere)of22.23millionCARBON SEQUESTRATION POTENTIAL OF INDIAN FORESTS323 tonnes(Mt)due to reported extraction from forests in India has to be subtracted from total annual carbon uptake increment to obtain net annual carbonflux.The net annual carbon uptake is thus obtained as5.64Mt,9.90Mt and11.88Mt for the years1985,1990and1995respectively.Figure1depicts the annual carbon uptake/release from forestry sector in India computed for these selected years.It is evident from Figure1that the net carbonflux is positive for each of the three years1985,1990and1995.The net CO2uptake for the years1985,1990and1995 as obtained by multiplying the net annual carbon uptake by the factor44/12is estimated to be0.02Gt,0.03Gt and0.04Gt respectively.We also estimate the annual carbonflux for the years2020and2045in this study.For this purpose,it is assumed that the extraction of wood from forests will double by the year2020and triple the current harvest in the year2045.The total natural forest cover is assumed to remain stable at about64Mha in the future and the plantation forest cover is assumed to increase in area by at least12.5Mha in the year2020and25Mha in the year2045(with a marginal increase by0.5 Mha per year in area of plantations).We assume here that the natural forest cover in India will sustain itself at an annual productivity of1.37m3per hectare while the plantations would have an annual productivity of3.2t/ha.This would lead to total annual carbon uptake of55.48Mt and73.48Mt for the years2020and2045 respectively.The annual carbon release to the atmosphere due to extraction of wood is estimated to be44.46Mt and66.69Mt for the respective years.The net annual carbon uptake would thus be11.02Mt and6.79Mt(0.04and0.02Gt of CO2equiv-alent)for the years2020and2045respectively.With the presently reported rate of productivity of Indian forests and plantations,the carbon sequestration potential of Indian forests are thus estimated to be4.1and9.8Gt and CO2by the years2020 and2045(cumulative CO2uptake from the atmosphere).Figure1includes the net annual carbon uptake computed for the years2020and2045based on the projected scenarios of natural and plantation forest covers.It is evident from this illustration that,even with modest efforts towards afforestation in India,we can expect the forests to continue to act as a net carbon sink in future.The productivity of any forest depends on the age of its vegetation.It is well established that forest plantations sequester carbon till maturity which would vary from25to75years depending upon the type of forests.At later stages,there is only marginal carbon sequestration.In natural forests,there is a net addition to standing biomass leading to carbon storage only until maturity.In mature forests all of the gross primary productivity is either used up in respiration or returned to soil as litter with no net addition to the standing biomass.These mature forest do not signifi-cantly contribute towards carbon uptake,through important for regeneration and thus in sustaining biodiversity.For harvesting purposes,planting forests are ideal because they have the highest annual productivity.However,according to Cannell (1995)replacing mature forests with new plantations may lead to a loss of carbon unless the plantations grow rapidly.Thus,new plantations on degraded and waste lands are the best options for carbon storage when these are planted/harvestedL AND ROMA SINGHFigure1.The annual carbonflux estimates from forestry sector in India for the selected years. periodically and used as a long term source of timber.In India,there is a large potential for expanding plantation forests.The net possible land categories avail-able for afforestation today stand at65.45Mha.The degraded forests cover almost 25%of the total available land(Figure2)and can be appropriately exploited for afforestation.CARBON SEQUESTRATION POTENTIAL OF INDIAN FORESTS325Figure2.The area under various land categories(percent of the total)available for afforestation in India.4.ConclusionForests are the major terrestrial sink for carbon in the world.They are also a po-tential source of biomass.This biomass can provide food,fuelwood,and wood for other domestic and industrial uses.With the increase in population,the demand of these products will also increase.To meet the ever growing demand,we not only need to sustain our forest biomass but should also aim at increasing it by implementing massive afforestation programmes in the coming years.The ongo-ing anthropogenic increases in CO2in the atmosphere can also be sequestered by increasing the rate of afforestation.The present rate of afforestation in the world is10.5Mha per annum.This is only marginal in comparison to the rate of forest degradation and deforestation.Thefindings reported here suggest that,even with modest efforts towards af-forestation,the Indian forests will continue to act as a net carbon sink in future.The study,however,does not include the changing rates of partitioning of photosyn-thates in the biomass,soil sequestration,litter composition etc.as a consequence of climate variability and change.Anthropogenic increases in atmospheric carbon dioxide is also likely to enhance the net primary productivity of forests(MooneyL AND ROMA SINGHet al.,1998).A net increase of about290Gt has been projected in global carbon stock due to CO2increases,climate change and vegetation redistribution(IGBP, 1998).India has a high population density and low per capita land share under forest cover.The replacement of forest land uses coupled with increasing need for forest products will not necessarily generate losses in forest volumes.Forest conservation and large afforestation efforts since in the1980s have stabilized the area under forest cover in India.The current rate of afforestation in India is one of the highest among the tropical countries.Through massive afforestation programmes launched in India,annual productivity of the forest biomass has shown a substantial increase during the past few years.A recent national study on vegetation status in Indian forests and tree plantations suggests that illegal tree cuttings are not so rampant either in primary forest or in plantations.Social forestry measures in recent years seem to have further arrested the degradation of forests.Effective control measures on the degradation of our natural forests and rehabilitation of major forest areas should continue to be adopted in future.AcknowledgmentsThis study was funded by the Indian Council of Forestry Research and Education, Dehradun under World Bank FREE Project.The authors acknowledge the support of Forest Survey of India for providing the data used in this study.ReferencesALGAS:1998,National Report on Asia Least-cost Greenhouse Gas Abatement Strategy,Ministry of Environment and Forests,Government of India,218pp.Bargali,S.S.and Singh,S.P.:1995,Dry matter dynamics,storage andflux of nutrients in an aged Eucalyptus plantation,Oecologia Montana4(12),9–14.Brown,S.and Lugo,A.E.:1984,Biomass of tropical forests–A new estimate based on forest volumes,Science223,1290.Cannell,M.:1995,Forest and the global carbon cycle in the past,present and future,European Forest Institute Report No.2,Finland,66pp.Champion,H.G.and Seth,S.K.:1968,A revised survey of forest types in India,Government of India Publication,Delhi,404pp.Dadhwal,V.K.and Nayak,S.R:1993,A preliminary estimate of biogeochemical cycle of carbon for India,Sci.and Cult.59(1and2),9–13.Dixon,R.K.,Brown,S.A.,Houghton,R.A.,Solomon,A.M.,Trexler,M.C.and Wisniewski,J.: 1994,Carbon pools andflux of global forest ecosystems,Science263,185–190.FAO(United Nations Food and Agriculture Organization):1993,Forest resources assessment1990, Tropical Countries,FAO Forestry Paper No.112,Rome Italy.FSI(Forest Survey of India):1988,The State of Forest Report1987,Ministry of Environment and Forests,Government of India.FSI(Forest Survey of India):1990,The State of Forest Report:1989,Ministry of Environment and Forests,Government of India.。
蜜谱市嫩翠学校高考英语一轮复习 Unit Environm
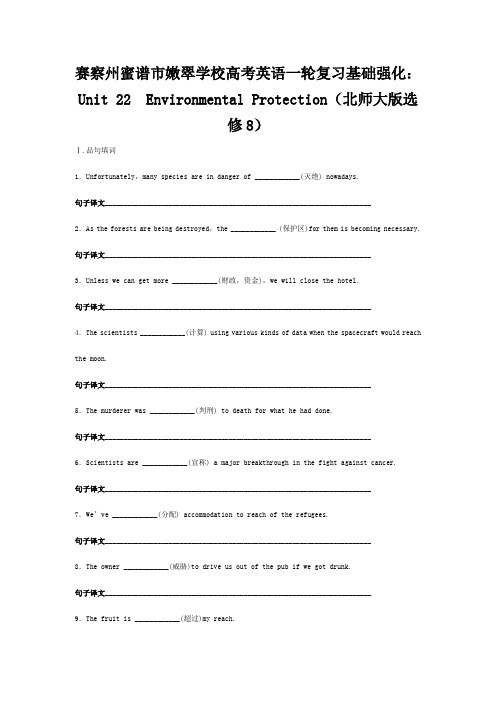
赛察州蜜谱市嫩翠学校高考英语一轮复习基础强化:Unit 22 Environmental Protection(北师大版选修8)Ⅰ.品句填词1.Unfortunately,many species are in danger of ____________(灭绝) nowadays.句子译文_______________________________________________________________________2.As the forests are being destroyed,the ____________ (保护区)for them is becoming necessary.句子译文_______________________________________________________________________3.Unless we can get more ____________(财政,资金),we will close the hotel.句子译文_______________________________________________________________________4.The scientists ____________(计算) using various kinds of data when the spacecraft would reach the moon.句子译文_______________________________________________________________________5.The murderer was ____________(判刑) to death for what he had done.句子译文_______________________________________________________________________6.Scientists are ____________(宣称) a major breakthrough in the fight against cancer.句子译文_______________________________________________________________________7.We’ve ____________(分配) accommodation to reach of the refugees.句子译文_______________________________________________________________________8.The owner ____________(威胁)to drive us out of the pub if we got drunk.句子译文_______________________________________________________________________9.The fruit is ____________(超过)my reach.句子译文_______________________________________________________________________10.We still don’t know exactly how the universe first came into ___________(存在).句子译文_______________________________________________________________________Ⅱ.短语识境refer to; trap; lead to; considerate; apart from;answer for; wrap up; carry off; all in all; on one’s behalf1.Even such a small mistake would perhaps ____________ disastrous consequences.句子译文_______________________________________________________________________2.____________,he is more learned than he was ten years ago.句子译文_______________________________________________________________________3.That just about____________it________for today.句子译文_______________________________________________________________________4.My colleagues are now on vocation,so I’m attending the meeting ____________.句子译文_______________________________________________________________________ 5.Whatever you do now,you must ____________ it one day.句子译文_______________________________________________________________________6.You might be more ____________ towards other people.句子译文_______________________________________________________________________7.She was ____________ in the burning house.句子译文_______________________________________________________________________8.Don’t ____________ this matter again,please.I don’t wa nt to talk about it any more.句子译文_______________________________________________________________________9.____________the cost,building a house also takes up a lot of time.句子译文_______________________________________________________________________10.She ___________ the first prize in the singing competition.句子译文_______________________________________________________________________Ⅲ.完成句子1.那位老师轮流帮助每一位学生,此事占用了他大量的时间。
应用Sentinel-1影像纹理信息模型估测杉木林生物量
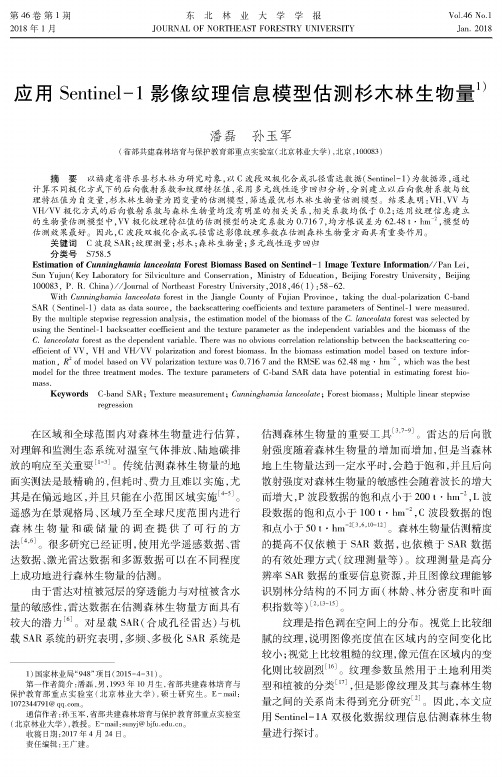
第46卷第1期2018年1月东北林业大学学报JOURNAL OF NORTHEAST FORESTRY UNIVERSIT^YVol.46 No.1Jan. 2018应用 S e n t i n e l-1 影像纹理信息模型估测杉木林生物量1潘磊孙玉军(省部共建森林培育与保护教育部重点实验室(北京林业大学),北京,100083)摘要以福建省将乐县杉木林为研究对象,以C波段双极化合成孔径雷达数据(Sentinel-1)为数据源,通过计算不同极化方式下的后向散射系数和纹理特征值,采用多元线性逐步回归分析,分别建立以后向散射系数与纹理特征值为自变量,杉木林生物量为因变量的估测模型,筛选最优杉木林生物量估测模型。
结果表明:VH、V V与VH/V V极化方式的后向散射系数与森林生物量均没有明显的相关关系,相关系数均低于0.2;运用纹理信息建立的生物量估测模型中,V V极化纹理特征值的估测模型的决定系数为0.7167,均方根误差为62.48 t•hnT2,模型的估测效果最好。
因此,C波段双极化合成孔径雷达影像纹理参数在估测森林生物量方面具有重要作用。
关键词 C波段SAR;纹理测量;杉木;森林生物量;多元线性逐步回归分类号 S758.5Estimation of Cunningharnm lanceolata Forest Biomass Based on Sentinel-1Image Texture Information//l^an Lei,Sun Yujun(Key Laboratory for Silviculture and Conservation,Ministry of Education,Beijing Forestry University,Beijing100083, P.R.China)//Journal of Northeast Forestry University,2018,46( 1):58-62.With Cunninghamia lanceolata f orest in the Jiangle County of Fujian Province,taking the dual-polarization C-band SAR (Sentinel-1)data as data source,the backscattering coefficients and texture parameters of Sentinel-1were measured.By the multiple stepwise regression analysis,the estimation model of the biomass of the geolata forest was selected byusing the Sentinel-1backscatter coefficient a nd the texture parameter as the independent variables and the biomass of thenceolata forest as the dependent variable.There was no obvious correlation relationship bet^veen the backscattering coefficient of V V,VH and VH/^VV polarization and forest biomass.In the biomass estimation model b mation,R2of model based on VV polarization texture was0.716 7 and the RMSE was62.48 mg•hm2,which was the bestmodel for the three treatment modes.The texture parameters of C-band SAR data have potential in estimating forest biomass.Keywords C-band SAR;Texture measurement;Cunninghamia lanceolate;Forest biomass;Multiple linear stepwise regression在区域和全球范围内对森林生物量进行估算,对理解和监测生态系统对温室气体排放、陆地碳排 放的响应至关重要[13。
英语作文forest potential
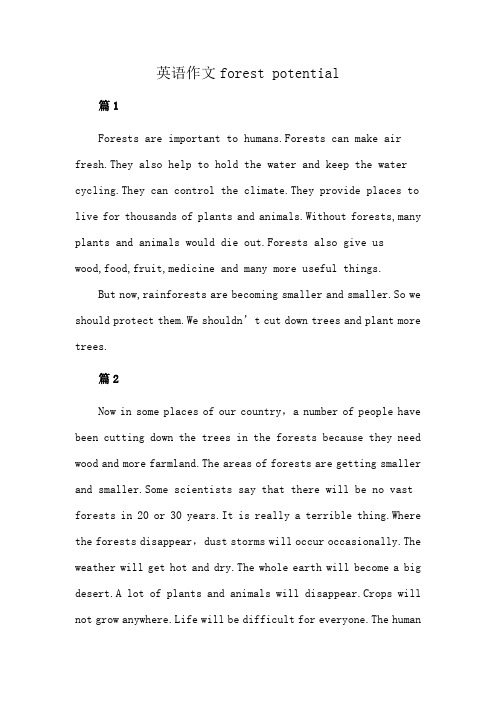
英语作文forest potential篇1Forests are important to humans.Forests can make air fresh.They also help to hold the water and keep the water cycling.They can control the climate.They provide places to live for thousands of plants and animals.Without forests,many plants and animals would die out.Forests also give us wood,food,fruit,medicine and many more useful things.But now,rainforests are becoming smaller and smaller.So we should protect them.We shouldn’t cut down trees and plant more trees.篇2Now in some places of our country,a number of people have been cutting down the trees in the forests because they need wood and more farmland.The areas of forests are getting smaller and smaller.Some scientists say that there will be no vast forests in 20 or 30 years.It is really a terrible thing.Where the forests disappear,dust storms will occur occasionally.The weather will get hot and dry.The whole earth will become a big desert.A lot of plants and animals will disappear.Crops will not grow anywhere.Life will be difficult for everyone.The humanbeings will be punished for their forest-destroying activities.Therefore,we should realize the importance of taking care of our forests.We should do our best to protect our living environment and keep our mountains green,the water clean,and the sky blue.篇3Now in some places of our country,a number of people have been cutting down the trees in the forests because they need wood and more farmland.The areas of forests are getting smaller and smaller.Some scientists say that there will be no vast forests in 20 or 30 years.It is really a terrible thing.Where the forests disappear,dust storms will occur occasionally.The weather will get hot and dry.The whole earth will become a big desert.A lot of plants and animals will disappear.Crops will not grow anywhere.Life will be difficult for everyone.The human beings will be punished for their forest-destroying activities.Therefore,we should realize the importance of taking care of our forests.We should do our best to protect our living environment and keep our mountains green,the water clean,and the sky blue.篇4One day,I eat breakfast,I suddenly heard the sound of a saw like lightning,Ill fly away,a look,turned out to be a lumberjack,I think:they must want to cut trees to do the house,I had an idea,the woodcutter said:"there are insects on the tree,I beg you to let the tree tree."He did not seem to hear,I flew to his shoulder,he pecked his head,he remained motionless for a while,environmental protection workers came and found him in the tree,picked him up.I call on people to protect the forest,or our homes will be destroyed,and the disaster will soon come to our human beings.篇5The weather became cold,and the bird flew back from the north.When he came to the forest to find his own home,it could not find the thick and easy tree of the past.Everywhere is a bald,sad tree stump,the bird is surprised,how is this,how can the family become so.It thought,"the logger must have destroyed its home."The bird was very sad to leave the place.We should protect the forest,not deforestation trees,more to care for the animal,they are humans good friends,they leavea quiet home.。
植树造林活动对地球的好处英语作文
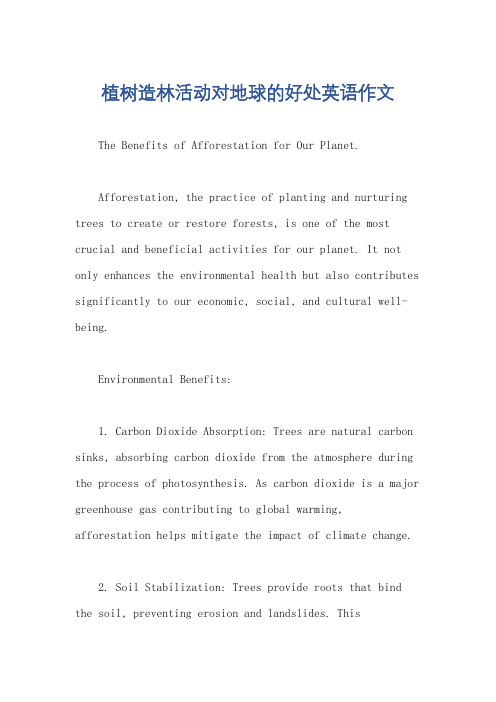
植树造林活动对地球的好处英语作文The Benefits of Afforestation for Our Planet.Afforestation, the practice of planting and nurturing trees to create or restore forests, is one of the most crucial and beneficial activities for our planet. It not only enhances the environmental health but also contributes significantly to our economic, social, and cultural well-being.Environmental Benefits:1. Carbon Dioxide Absorption: Trees are natural carbon sinks, absorbing carbon dioxide from the atmosphere during the process of photosynthesis. As carbon dioxide is a major greenhouse gas contributing to global warming,afforestation helps mitigate the impact of climate change.2. Soil Stabilization: Trees provide roots that bind the soil, preventing erosion and landslides. Thisstabilizes the soil, enhancing its fertility and reducing the risk of soil degradation.3. Water Conservation: Forests act as natural sponges, storing rainwater and releasing it gradually into streams and rivers. This helps maintain water levels during dry seasons, ensuring water supply for agriculture and wildlife.4. Biodiversity: Forests are habitats for a vast arrayof plant and animal species. Afforestation supports biodiversity, providing shelters and food sources for wildlife, thus maintaining ecological balance.5. Air Purification: Trees filter air, removing pollutants and dust particles. This improves air quality, making it healthier for humans and animals to breathe.Economic Benefits:1. Timber Resources: Forests provide a sustainable source of timber for construction, furniture, and other industrial uses. Managed afforestation ensures that theseresources are renewable and available for future generations.2. Agricultural Productivity: Trees provide shade and windbreaks, protecting crops from harsh weather conditions. This improves agricultural productivity and enhances food security.3. Ecotourism: Well-managed forests attract tourists, who come to enjoy nature, wildlife, and outdoor activities. This generates revenue for local communities and supports sustainable development.4. Medicinal Herbs: Many trees and plants in forests are sources of medicinal herbs. Afforestation preserves these resources, ensuring access to traditional medicines and providing opportunities for pharmaceutical research.Social and Cultural Benefits:1. Community Development: Afforestation activities often involve local communities, promoting social cohesionand unity. These activities create job opportunities, improve livelihoods, and enhance the overall well-being of community members.2. Education and Awareness: Afforestation programs often serve as platforms for environmental education, raising awareness about the importance of forests and conservation. This education fosters a culture of sustainability and respect for nature among young people.3. Cultural Values: Forests are oftenassociated with spiritual and cultural values. Afforestation preserves these values, providing spaces for religious practices, festivals, and other cultural events.4. Recreation and Leisure: Forests offer spaces for recreation and leisure activities such as hiking, camping, and bird watching. These activities promote physical and mental health, enhancing the quality of life for individuals and families.In conclusion, afforestation is a crucial activity forthe sustainable development of our planet. It benefits the environment, economy, society, and culture in numerous ways, ranging from carbon sequestration and soil stabilization to timber resources, community development, and cultural values. As we face the challenges of climate change and environmental degradation, it is imperative that weprioritize afforestation and other sustainable forestry practices to ensure a healthy and habitable planet forfuture generations.。
林木生长量的专业英语名词
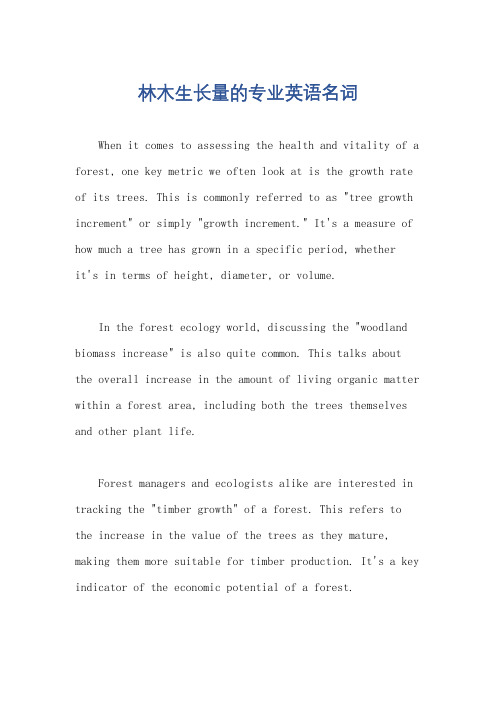
林木生长量的专业英语名词When it comes to assessing the health and vitality of a forest, one key metric we often look at is the growth rate of its trees. This is commonly referred to as "tree growth increment" or simply "growth increment." It's a measure of how much a tree has grown in a specific period, whetherit's in terms of height, diameter, or volume.In the forest ecology world, discussing the "woodland biomass increase" is also quite common. This talks about the overall increase in the amount of living organic matter within a forest area, including both the trees themselves and other plant life.Forest managers and ecologists alike are interested in tracking the "timber growth" of a forest. This refers to the increase in the value of the trees as they mature, making them more suitable for timber production. It's a key indicator of the economic potential of a forest.Another way to look at tree growth is through the lens of "vegetation productivity." This term encapsulates not just the quantity of growth but also the quality and efficiency of the trees' photosynthetic processes. It's a more holistic view of how well a forest is thriving.Finally, when discussing forest health and resilience, we might mention the "green biomass accumulation." This refers to the accumulation of carbon-rich organic matter, primarily from trees, that helps store carbon and mitigate climate change. It's a crucial aspect of sustainable forest management.。
砍树造成水土流失英语作文
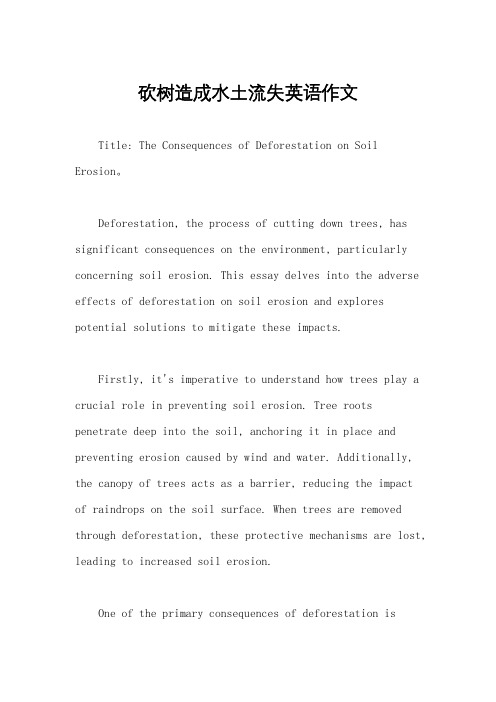
砍树造成水土流失英语作文Title: The Consequences of Deforestation on Soil Erosion。
Deforestation, the process of cutting down trees, has significant consequences on the environment, particularly concerning soil erosion. This essay delves into the adverse effects of deforestation on soil erosion and explores potential solutions to mitigate these impacts.Firstly, it's imperative to understand how trees play a crucial role in preventing soil erosion. Tree roots penetrate deep into the soil, anchoring it in place and preventing erosion caused by wind and water. Additionally, the canopy of trees acts as a barrier, reducing the impactof raindrops on the soil surface. When trees are removed through deforestation, these protective mechanisms are lost, leading to increased soil erosion.One of the primary consequences of deforestation isincreased surface runoff. Without trees to intercept rainfall and absorb water, rainwater flows over the bare ground, carrying away soil particles. This phenomenon exacerbates soil erosion, as the force of running water loosens and transports soil, leading to sedimentation in rivers, lakes, and other water bodies.Moreover, deforestation disrupts the delicate balance of ecosystems, leading to soil degradation. Trees contribute organic matter to the soil through leaf litter and root decomposition, which enriches the soil and enhances its ability to retain water and nutrients. In the absence of trees, soil fertility declines, making it more susceptible to erosion and degradation.Deforestation also contributes to habitat loss for numerous plant and animal species, further exacerbating soil erosion. The removal of trees disrupts the intricate web of life within forest ecosystems, leading to the loss of biodiversity. Without diverse vegetation to stabilize the soil and provide habitat for organisms, ecosystems become more vulnerable to erosion and degradation.Furthermore, deforestation exacerbates the effects of climate change, which in turn can amplify soil erosion. Trees act as carbon sinks, absorbing carbon dioxide from the atmosphere and storing it in their biomass. When trees are cut down and burned or left to decompose, carbon is released back into the atmosphere, contributing to the greenhouse effect and climate change. The alteration of precipitation patterns and increased frequency of extreme weather events associated with climate change can intensify soil erosion processes.To mitigate the adverse effects of deforestation onsoil erosion, concerted efforts are needed at local, national, and global levels. Reforestation andafforestation initiatives can help restore degraded landscapes and prevent further soil erosion. By planting native tree species and implementing sustainable land management practices, we can rebuild forest ecosystems and enhance their resilience to erosion.Additionally, land-use planning and regulation areessential to protect remaining forests from indiscriminate logging and deforestation. Implementing policies that promote sustainable forestry practices, such as selective logging and reforestation requirements, can help maintain forest cover and reduce soil erosion.Education and awareness-raising campaigns are also crucial in fostering a sense of stewardship for the environment and promoting responsible land management practices. By empowering local communities with knowledge about the importance of forests and the consequences of deforestation, we can mobilize grassroots efforts to protect and restore forest ecosystems.In conclusion, deforestation has significant consequences on soil erosion, leading to increased surface runoff, soil degradation, habitat loss, and exacerbating climate change. Addressing deforestation requires a multifaceted approach that includes reforestation, sustainable land management, policy interventions, and community engagement. By taking proactive measures to protect and restore forests, we can safeguard our soils andensure the health and resilience of ecosystems for future generations.。
保护自然的原因和目的 英语作文
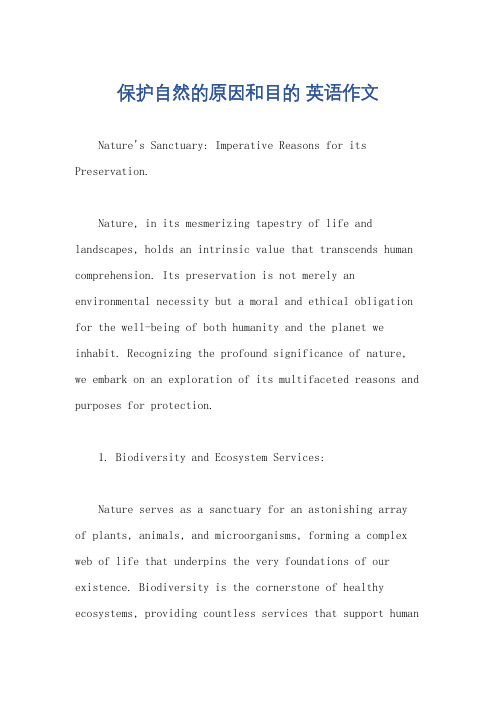
保护自然的原因和目的英语作文Nature's Sanctuary: Imperative Reasons for its Preservation.Nature, in its mesmerizing tapestry of life and landscapes, holds an intrinsic value that transcends human comprehension. Its preservation is not merely an environmental necessity but a moral and ethical obligation for the well-being of both humanity and the planet we inhabit. Recognizing the profound significance of nature, we embark on an exploration of its multifaceted reasons and purposes for protection.1. Biodiversity and Ecosystem Services:Nature serves as a sanctuary for an astonishing array of plants, animals, and microorganisms, forming a complex web of life that underpins the very foundations of our existence. Biodiversity is the cornerstone of healthy ecosystems, providing countless services that support humanendeavors.Forests, for instance, act as natural carbon sinks, absorbing greenhouse gases and mitigating climate change. Wetlands filter pollutants, purify water supplies, and provide habitats for diverse aquatic species. Pollinators, such as bees and butterflies, ensure the reproduction of numerous plants, including many food crops.The loss of biodiversity, driven by habitat destruction, climate change, and pollution, undermines the resilience of ecosystems and jeopardizes the services they provide. Preserving nature is thus essential for safeguarding the fabric of life and ensuring the stability of our planet.2. Climate Regulation:Nature plays a pivotal role in regulating the Earth's climate. Oceans, through their vast thermal mass, absorband release heat, influencing global temperatures. Forests and other vegetation sequester carbon dioxide, acting as carbon sinks that help mitigate climate change.The destruction of natural habitats, deforestation in particular, releases significant amounts of carbon dioxide into the atmosphere, contributing to the rise in greenhouse gas concentrations and exacerbating climate change. Preserving nature, on the other hand, helps maintain the balance of greenhouse gases and mitigate the severity of climate impacts.3. Health and Well-being:Nature offers a sanctuary for human health and well-being. Spending time in natural environments has been shown to reduce stress, improve mood, and boost cognitive function. Access to green spaces, parks, and open areas provides opportunities for physical activity, social interaction, and mental restoration.Moreover, contact with nature has therapeutic effectson mental health conditions, such as depression and anxiety. Studies have demonstrated that immersing oneself in natural settings can reduce symptoms, improve sleep, and enhanceoverall psychological well-being. Preserving nature is thus essential for promoting the health and happiness of our communities.4. Cultural and Spiritual Significance:Nature holds profound cultural and spiritual value for countless societies worldwide. From ancient to modern times, humans have drawn inspiration, sustenance, and spiritual connection from the natural world. Landscapes, wildlife,and natural phenomena have featured prominently in mythology, folklore, art, and literature across cultures.For indigenous communities in particular, nature is not merely a resource but a sacred entity, deeply intertwined with their cultural identity and spiritual practices. Preserving nature safeguards these invaluable cultural traditions and the well-being of the communities that rely on them.5. Future Generations:Protecting nature is a moral imperative for future generations. We have a responsibility to ensure that our planet remains a habitable and thriving place for generations to come. By preserving natural habitats, we safeguard the intricate web of life and the resources thatit provides.The choices we make today regarding nature conservation will shape the world that our children and grandchildren inherit. Preserving nature is an investment in their future, ensuring that they have access to clean air, water, healthy food, and the wonder and beauty of the natural world.Conclusion:The protection of nature is not a choice but anabsolute necessity for the present and future well-being of humanity and the planet. Its intrinsic value, its role in supporting life, regulating climate, enhancing health, fostering cultural traditions, and ensuring thesustainability of future generations demands our unwavering commitment to its preservation.It is through understanding the multifaceted reasons and purposes for protecting nature that we can develop comprehensive conservation strategies and foster a deep appreciation for the invaluable sanctuary it provides. By embracing a collaborative and holistic approach to nature conservation, we can create a legacy of stewardship that will preserve the splendor and vitality of the natural world for generations to come.。
预防人类砍树 英语作文
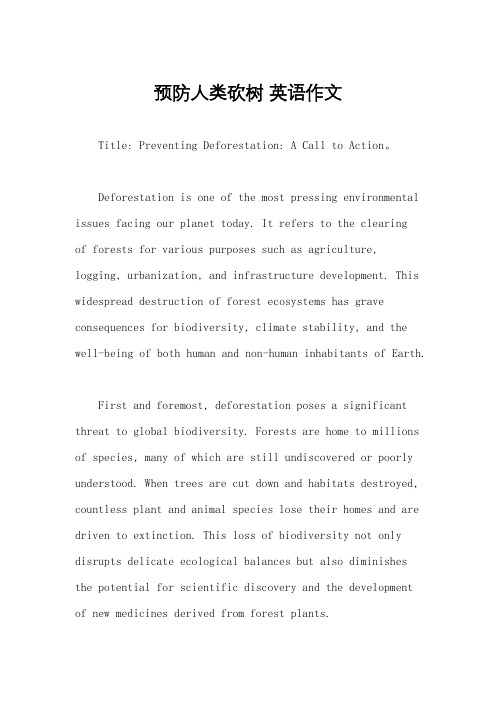
预防人类砍树英语作文Title: Preventing Deforestation: A Call to Action。
Deforestation is one of the most pressing environmental issues facing our planet today. It refers to the clearingof forests for various purposes such as agriculture, logging, urbanization, and infrastructure development. This widespread destruction of forest ecosystems has grave consequences for biodiversity, climate stability, and the well-being of both human and non-human inhabitants of Earth.First and foremost, deforestation poses a significant threat to global biodiversity. Forests are home to millions of species, many of which are still undiscovered or poorly understood. When trees are cut down and habitats destroyed, countless plant and animal species lose their homes and are driven to extinction. This loss of biodiversity not only disrupts delicate ecological balances but also diminishesthe potential for scientific discovery and the developmentof new medicines derived from forest plants.Moreover, deforestation exacerbates climate change by releasing large amounts of carbon dioxide into the atmosphere. Trees act as carbon sinks, absorbing carbon dioxide during photosynthesis and storing it in their biomass. When forests are cleared, this stored carbon is released back into the atmosphere, contributing to the greenhouse effect and global warming. In this way, deforestation not only accelerates climate change but also reduces the Earth's capacity to mitigate its effects.In addition to its environmental impacts, deforestation also has profound social and economic consequences. Forests provide essential ecosystem services such as clean water, air purification, and soil stabilization, which are vital for human health and well-being. Moreover, forests support millions of people around the world, particularly indigenous and local communities who depend on them for food, shelter, and livelihoods. When forests are destroyed, these communities lose not only their source of sustenance but also their cultural heritage and way of life.Given the gravity of the situation, urgent action is needed to prevent further deforestation and protect the world's remaining forests. One key strategy is to promote sustainable land management practices that balance the needs of people and the environment. This includes initiatives such as agroforestry, which integrates trees into agricultural systems to improve soil fertility, conserve water, and increase biodiversity. By adopting such practices, farmers can achieve higher yields while reducing pressure on natural forests.Furthermore, it is essential to strengthen laws and regulations aimed at protecting forests and enforcing them effectively. This may involve creating or expanding protected areas, establishing logging bans in sensitive ecosystems, and implementing mechanisms for monitoring and penalizing illegal deforestation activities. Additionally, governments and international organizations should provide incentives and support for forest conservation efforts, such as financial incentives for conservation, capacity-building initiatives, and technical assistance to help countries develop sustainable forest management plans.Education and awareness-raising are also crucial components of any strategy to prevent deforestation. By informing people about the importance of forests and the consequences of their destruction, we can mobilize public support for conservation efforts and empower individuals to take action in their own lives. This includes promoting sustainable consumption habits, supporting eco-friendly businesses, and participating in reforestation and restoration projects.In conclusion, deforestation is a complex and multifaceted issue that requires a coordinated and holistic approach to address. By implementing sustainable land management practices, strengthening forest protection laws, and raising awareness about the importance of forests, we can work together to prevent further deforestation and ensure a sustainable future for generations to come. The time to act is now. Let us join hands and protect our planet's precious forests before it is too late.。
树在生态系统中的作用的英语作文
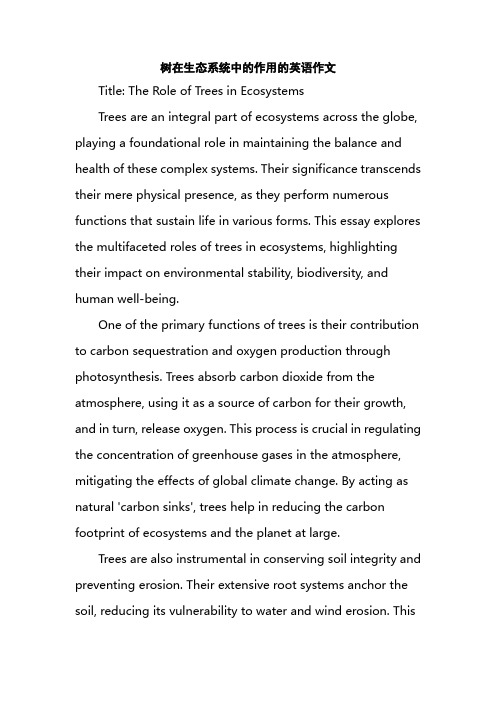
树在生态系统中的作用的英语作文Title: The Role of Trees in EcosystemsTrees are an integral part of ecosystems across the globe, playing a foundational role in maintaining the balance and health of these complex systems. Their significance transcends their mere physical presence, as they perform numerous functions that sustain life in various forms. This essay explores the multifaceted roles of trees in ecosystems, highlighting their impact on environmental stability, biodiversity, and human well-being.One of the primary functions of trees is their contribution to carbon sequestration and oxygen production through photosynthesis. Trees absorb carbon dioxide from the atmosphere, using it as a source of carbon for their growth, and in turn, release oxygen. This process is crucial in regulating the concentration of greenhouse gases in the atmosphere, mitigating the effects of global climate change. By acting as natural 'carbon sinks', trees help in reducing the carbon footprint of ecosystems and the planet at large.Trees are also instrumental in conserving soil integrity and preventing erosion. Their extensive root systems anchor the soil, reducing its vulnerability to water and wind erosion. Thisstabilization ensures that fertile topsoil remains in place, enabling the growth of other vegetation and maintaining the productivity of ecosystems. Furthermore, trees play a vital role in the cycling of nutrients, breaking down dead plant and animal matter into compost, which enriches the soil and supports the growth of various organisms.In addition to their ecological contributions, trees provide habitats for a myriad of species, thereby supporting biodiversity. Different layers of a forest, from the canopy to the understory, create diverse environments that cater to the specific needs of various creatures. These habitats offer food, shelter, and breeding grounds for insects, birds, mammals, and reptiles, among others. Thus, trees serve as keystone structures in ecosystems, around which the diversity of life revolves.Trees also have significant impacts on the hydrological cycle. They contribute to the regulation of water cycles by intercepting rainwater with their leaves, reducing runoff and increasing water infiltration into the soil. This process helps recharge groundwater supplies and maintains the availability of water resources for both ecosystems and human uses. Additionally, the shade provided by trees can moderate soiltemperatures and reduce evaporation rates, further preserving water content in the soil.Lastly, trees are of immense value to human society. They provide materials for construction, fuel, and medicinal purposes, and also offer aesthetic and recreational benefits. Forests and woodlands rich in tree diversity are often sites for ecotourism, allowing people to experience and appreciate the beauty and serenity of nature, which in turn fosters a conservation ethic.In conclusion, the role of trees in ecosystems is both profound and multifaceted. From influencing climate patterns and soil stability to promoting biodiversity and supporting human livelihoods, trees are truly the backbone of life on Earth. Their presence and health are indicators of the overallwell-being of ecosystems, making them invaluable assets that demand our utmost care and protection.。
可再生能源 英语作文

可再生能源英语作文The Importance of Renewable Energy in Our Future.In the face of a rapidly changing global landscape, the need for sustainable and renewable energy sources has become increasingly urgent. As the world moves towards decarbonization and environmental sustainability, renewable energy plays a pivotal role in this transition. It offers a clean, renewable, and environmentally friendly alternative to traditional fossil fuels, making it a critical component of our future energy mix.Renewable energy sources, such as solar, wind, hydroelectric, geothermal, and biomass, are derived from naturally replenishing resources. Unlike fossil fuels, which are finite and emit harmful greenhouse gases when burned, renewable energy does not contribute to climate change. It harnesses the power of nature to generate electricity and heat, without the need for combustion or the release of harmful emissions.Solar energy, for instance, converts sunlight into electricity using photovoltaic cells. It is a widely accessible resource, available in most parts of the world, and its potential is vast. With advances in technology, solar panels have become more efficient and cost-effective, making them a viable option for both residential and commercial use.Wind energy, on the other hand, harnesses the power of the wind to generate electricity. Wind turbines convert the kinetic energy of the wind into mechanical energy, which is then converted into electricity. Wind power is a popular renewable energy source, especially in areas with consistent wind patterns.Hydroelectric energy utilizes the force of moving water to generate electricity. It is a clean and renewable source of energy, but its availability is limited to areas with suitable water resources. Geothermal energy taps into the heat energy stored within the Earth's crust, while biomass energy comes from organic matter like wood, agriculturalwaste, and animal manure.The transition to renewable energy offers numerous benefits. Firstly, it helps to mitigate the impact of climate change by reducing greenhouse gas emissions. Burning fossil fuels is the primary source of carbon dioxide emissions, which trap heat in the Earth's atmosphere, leading to global warming. By shifting to renewable energy, we can significantly reduce these emissions and slow down the rate of climate change.Secondly, renewable energy is a sustainable long-term solution. Unlike fossil fuels, which will eventually run out, renewable energy sources are indefinitely available. By investing in renewable energy infrastructure, we can ensure a secure and sustainable energy supply for future generations.Moreover, renewable energy creates economic opportunities. The development and deployment of renewable energy projects require skilled labor, innovation, and investment. This creates jobs in the renewable energysector and drives economic growth. Additionally, renewable energy projects often involve community engagement and partnerships, promoting local development and social well-being.However, the transition to renewable energy is not without challenges. One of the main hurdles is the initial capital investment required to set up renewable energy projects. While the long-term benefits are significant, the upfront costs can be prohibitive for many. Additionally, the intermittency of some renewable energy sources, such as solar and wind, can pose challenges in terms of energy storage and grid stability.To overcome these challenges, governments and private sector entities need to collaborate and invest in renewable energy research and development. Innovations in battery storage, smart grids, and demand-side management can help to address the intermittency issue and ensure a reliable supply of renewable energy. Additionally, policies that encourage renewable energy deployment, such as subsidies, feed-in tariffs, and renewable energy targets, can help tolower the financial barriers and加速可再生能源的普及。
The secrets of the rainforest Biodiversity
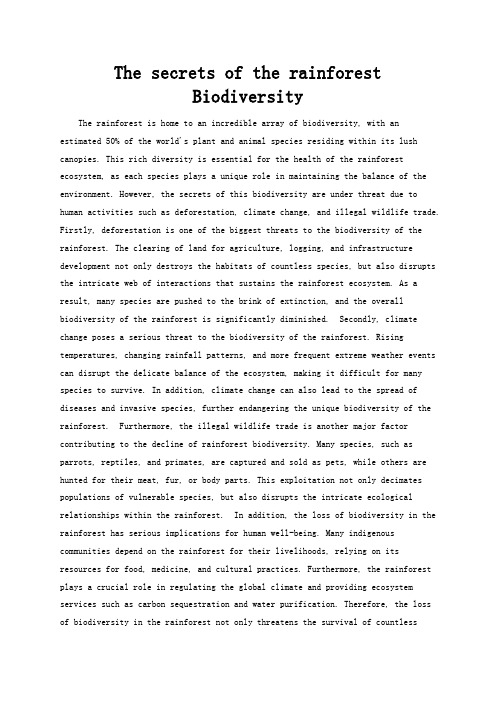
The secrets of the rainforestBiodiversityThe rainforest is home to an incredible array of biodiversity, with anestimated 50% of the world's plant and animal species residing within its lush canopies. This rich diversity is essential for the health of the rainforest ecosystem, as each species plays a unique role in maintaining the balance of the environment. However, the secrets of this biodiversity are under threat due to human activities such as deforestation, climate change, and illegal wildlife trade. Firstly, deforestation is one of the biggest threats to the biodiversity of the rainforest. The clearing of land for agriculture, logging, and infrastructure development not only destroys the habitats of countless species, but also disrupts the intricate web of interactions that sustains the rainforest ecosystem. As a result, many species are pushed to the brink of extinction, and the overall biodiversity of the rainforest is significantly diminished. Secondly, climate change poses a serious threat to the biodiversity of the rainforest. Rising temperatures, changing rainfall patterns, and more frequent extreme weather events can disrupt the delicate balance of the ecosystem, making it difficult for many species to survive. In addition, climate change can also lead to the spread of diseases and invasive species, further endangering the unique biodiversity of the rainforest. Furthermore, the illegal wildlife trade is another major factor contributing to the decline of rainforest biodiversity. Many species, such as parrots, reptiles, and primates, are captured and sold as pets, while others are hunted for their meat, fur, or body parts. This exploitation not only decimates populations of vulnerable species, but also disrupts the intricate ecological relationships within the rainforest. In addition, the loss of biodiversity in the rainforest has serious implications for human well-being. Many indigenous communities depend on the rainforest for their livelihoods, relying on itsresources for food, medicine, and cultural practices. Furthermore, the rainforest plays a crucial role in regulating the global climate and providing ecosystem services such as carbon sequestration and water purification. Therefore, the lossof biodiversity in the rainforest not only threatens the survival of countlessplant and animal species, but also jeopardizes the well-being of human societies around the world. In conclusion, the secrets of the rainforest biodiversity are under threat from various human activities, including deforestation, climate change, and illegal wildlife trade. The loss of biodiversity not only endangers the survival of countless plant and animal species, but also has far-reaching implications for the health of the rainforest ecosystem and human societies. It is crucial that concerted efforts are made to protect and preserve the biodiversity of the rainforest for the benefit of all life on Earth.。
树木高生长限制的几个假说
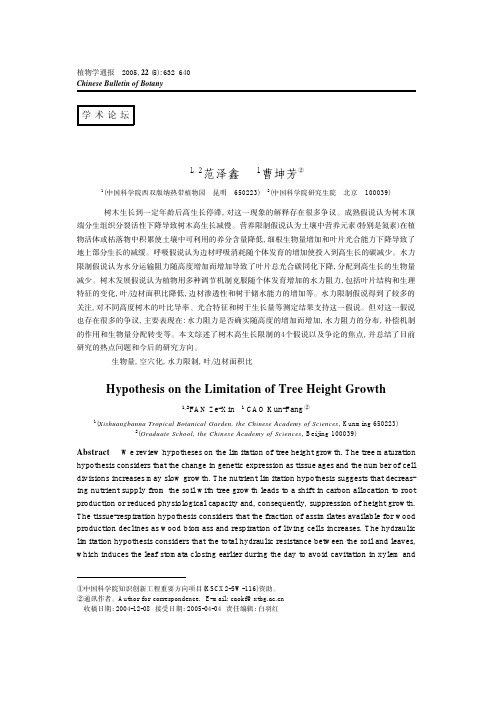
植物学通报 2005, 22 (5): 632 ̄640树木高生长限制的几个假说①1,2范泽鑫 1曹坤芳②1(中国科学院西双版纳热带植物园昆明 650223) 2(中国科学院研究生院北京 100039)摘要树木生长到一定年龄后高生长停滞, 对这一现象的解释存在很多争议。
成熟假说认为树木顶端分生组织分裂活性下降导致树木高生长减慢。
营养限制假说认为土壤中营养元素(特别是氮素)在植物活体或枯落物中积累使土壤中可利用的养分含量降低, 细根生物量增加和叶片光合能力下降导致了地上部分生长的减缓。
呼吸假说认为边材呼吸消耗随个体发育的增加使投入到高生长的碳减少。
水力限制假说认为水分运输阻力随高度增加而增加导致了叶片总光合碳同化下降, 分配到高生长的生物量减少。
树木发展假说认为植物用多种调节机制克服随个体发育增加的水力阻力, 包括叶片结构和生理特征的变化, 叶/边材面积比降低, 边材渗透性和树干储水能力的增加等。
水力限制假说得到了较多的关注, 对不同高度树木的叶比导率、光合特征和树干生长量等测定结果支持这一假说。
但对这一假说也存在很多的争议, 主要表现在: 水力阻力是否确实随高度的增加而增加, 水力阻力的分布, 补偿机制的作用和生物量分配转变等。
本文综述了树木高生长限制的4个假说以及争论的焦点, 并总结了目前研究的热点问题和今后的研究方向。
关键词生物量, 空穴化, 水力限制, 叶/边材面积比Hypothesis on the Limitation of Tree Height Growth1,2FAN Ze-Xin 1 CAO Kun-Fang②1(Xishuangbanna Tropical Botanical Garden, the Chinese Academy of Sciences, Kunming650223) 2(Graduate School, the Chinese Academy of Sciences, Beijing100039)Abstract We review hypotheses on the limitation of tree height growth. The tree maturation hypothesis considers that the change in genetic expression as tissue ages and the number of cell divisions increases may slow growth. The nutrient limitation hypothesis suggests that decreas-ing nutrient supply from the soil with tree growth leads to a shift in carbon allocation to root production or reduced physiological capacity and, consequently, suppression of height growth. The tissue-respiration hypothesis considers that the fraction of assimilates available for wood production declines as wood biomass and respiration of living cells increases. The hydraulic limitation hypothesis considers that the total hydraulic resistance between the soil and leaves, which induces the leaf stomata closing earlier during the day to avoid cavitation in xylem and①中国科学院知识创新工程重要方向项目(KSCX2-SW-116)资助。
植树造林英语作文

植树造林英语作文Title: The Importance of Afforestation and Reforestation。
Afforestation and reforestation are vital practicesthat contribute to the preservation of our environment and the sustainability of our planet. In this essay, we will delve into the significance of these activities, their benefits, and the role they play in combating environmental degradation and climate change.First and foremost, afforestation refers to the process of establishing forests in areas where there were previously no forests, while reforestation involves replanting trees in areas where forests have been depleted or destroyed. Both practices are essential for maintaining ecological balance and biodiversity.One of the primary benefits of afforestation and reforestation is their role in carbon sequestration. Treesact as natural carbon sinks, absorbing carbon dioxide from the atmosphere during photosynthesis and storing it intheir biomass. By increasing the number of trees through afforestation and reforestation efforts, we can effectively reduce the concentration of greenhouse gases in the atmosphere, mitigating the impacts of climate change.Moreover, forests play a crucial role in regulating local and regional climates. They help to moderate temperatures, regulate rainfall patterns, and prevent soil erosion. By planting trees strategically, we can create microclimates that are conducive to agricultural productivity and human well-being.Furthermore, forests are home to a diverse array of plant and animal species, many of which are endemic and endangered. Afforestation and reforestation efforts help to restore habitats and provide sanctuary for wildlife, thus promoting biodiversity conservation.In addition to their environmental benefits, afforestation and reforestation also have significantsocio-economic advantages. Forests provide valuable resources such as timber, fuelwood, and medicinal plants, which support livelihoods and economic development, particularly in rural communities. Furthermore, forests offer recreational opportunities such as hiking, camping, and eco-tourism, contributing to the tourism industry and local economies.However, despite the numerous benefits of afforestation and reforestation, these practices face several challenges and barriers. Deforestation, driven by agricultural expansion, logging, and urbanization, continues to threaten existing forests and hinder afforestation efforts. Additionally, inadequate policies, insufficient funding, and lack of community involvement often impedereforestation initiatives.To overcome these challenges and maximize the effectiveness of afforestation and reforestation efforts, comprehensive strategies and collaborative partnerships are necessary. Governments must enact and enforce robust environmental policies that promote sustainable land usepractices and protect forests from exploitation. International organizations, non-governmental organizations, and the private sector should invest in afforestation and reforestation projects and support local communities intheir conservation efforts.Furthermore, raising awareness about the importance of forests and engaging the public in tree-planting activities are crucial steps towards fostering a culture of environmental stewardship and sustainability. Educational campaigns, community workshops, and tree-planting eventscan inspire individuals to take action and contribute to forest restoration efforts.In conclusion, afforestation and reforestation are indispensable practices for safeguarding our environment, mitigating climate change, and promoting sustainable development. By investing in forest conservation and restoration, we can create a greener and more resilient planet for present and future generations. Let us joinhands in planting trees and nurturing forests for the well-being of all living beings.。
柳枝稷论文:黄土高原引种柳枝稷的生态适应性研究
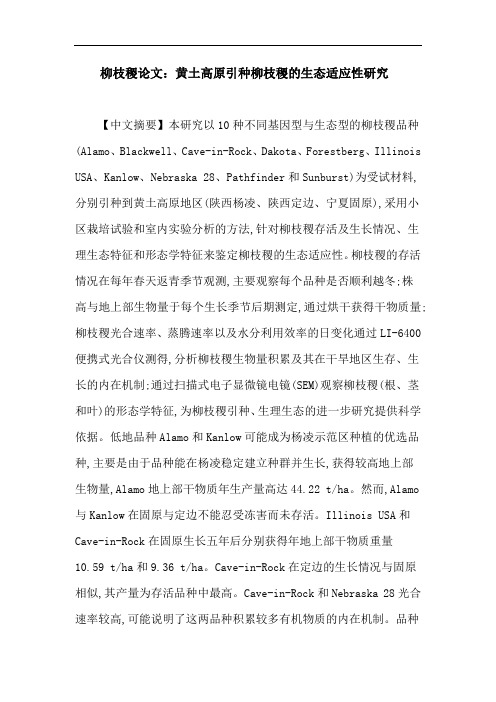
柳枝稷论文:黄土高原引种柳枝稷的生态适应性研究【中文摘要】本研究以10种不同基因型与生态型的柳枝稷品种(Alamo、Blackwell、Cave-in-Rock、Dakota、Forestberg、Illinois USA、Kanlow、Nebraska 28、Pathfinder和Sunburst)为受试材料,分别引种到黄土高原地区(陕西杨凌、陕西定边、宁夏固原),采用小区栽培试验和室内实验分析的方法,针对柳枝稷存活及生长情况、生理生态特征和形态学特征来鉴定柳枝稷的生态适应性。
柳枝稷的存活情况在每年春天返青季节观测,主要观察每个品种是否顺利越冬;株高与地上部生物量于每个生长季节后期测定,通过烘干获得干物质量;柳枝稷光合速率、蒸腾速率以及水分利用效率的日变化通过LI-6400便携式光合仪测得,分析柳枝稷生物量积累及其在干旱地区生存、生长的内在机制;通过扫描式电子显微镜电镜(SEM)观察柳枝稷(根、茎和叶)的形态学特征,为柳枝稷引种、生理生态的进一步研究提供科学依据。
低地品种Alamo和Kanlow可能成为杨凌示范区种植的优选品种,主要是由于品种能在杨凌稳定建立种群并生长,获得较高地上部生物量,Alamo地上部干物质年生产量高达44.22 t/ha。
然而,Alamo 与Kanlow在固原与定边不能忍受冻害而未存活。
Illinois USA和Cave-in-Rock在固原生长五年后分别获得年地上部干物质重量10.59 t/ha和9.36 t/ha。
Cave-in-Rock在定边的生长情况与固原相似,其产量为存活品种中最高。
Cave-in-Rock和Nebraska 28光合速率较高,可能说明了这两品种积累较多有机物质的内在机制。
品种间光合速率在一天当中的14:00点左右差异明显,大小依次为Cave-in-Rock > Blackwell > Sunburst > Forestberg > Pathfinder > Nebraska 28 > Dakota。