纳米给药系统相关文献
基于纳米技术的药物递送系统的研究
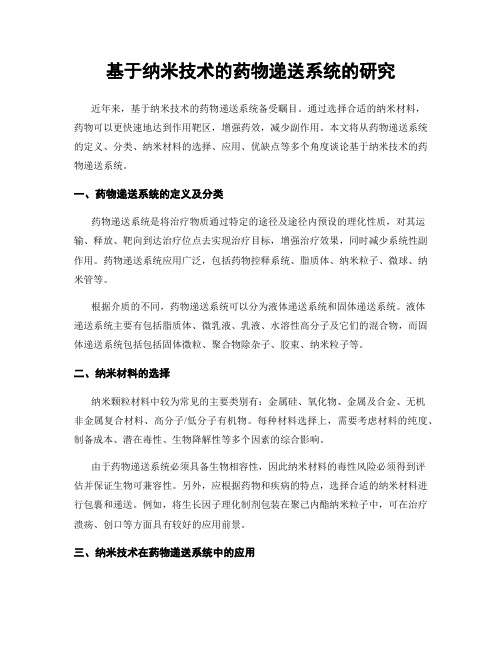
基于纳米技术的药物递送系统的研究近年来,基于纳米技术的药物递送系统备受瞩目。
通过选择合适的纳米材料,药物可以更快速地达到作用靶区,增强药效,减少副作用。
本文将从药物递送系统的定义、分类、纳米材料的选择、应用、优缺点等多个角度谈论基于纳米技术的药物递送系统。
一、药物递送系统的定义及分类药物递送系统是将治疗物质通过特定的途径及途径内预设的理化性质,对其运输、释放、靶向到达治疗位点去实现治疗目标,增强治疗效果,同时减少系统性副作用。
药物递送系统应用广泛,包括药物控释系统、脂质体、纳米粒子、微球、纳米管等。
根据介质的不同,药物递送系统可以分为液体递送系统和固体递送系统。
液体递送系统主要有包括脂质体、微乳液、乳液、水溶性高分子及它们的混合物,而固体递送系统包括包括固体微粒、聚合物除杂子、胶束、纳米粒子等。
二、纳米材料的选择纳米颗粒材料中较为常见的主要类别有:金属硅、氧化物、金属及合金、无机非金属复合材料、高分子/低分子有机物。
每种材料选择上,需要考虑材料的纯度、制备成本、潜在毒性、生物降解性等多个因素的综合影响。
由于药物递送系统必须具备生物相容性,因此纳米材料的毒性风险必须得到评估并保证生物可兼容性。
另外,应根据药物和疾病的特点,选择合适的纳米材料进行包裹和递送。
例如,将生长因子理化制剂包装在聚己内酯纳米粒子中,可在治疗溃疡、创口等方面具有较好的应用前景。
三、纳米技术在药物递送系统中的应用药物递送系统在药物运输、靶向治疗等方面应用广泛。
而在这些方面,基于纳米技术的药物递送系统表现出了比传统的递送系统更具有优势的特点。
纳米递送系统在药物释放的控制等方面可获得更高的精度;在递送过程中可以利用靶向分子实现特定组织及细胞的精准靶向;并且相比常规递送系统,纳米递送系统可运载的物质更广。
例如,通过适当修饰核蛋白,促使纳米粒子上的核酸与癌细胞相结合,并且通过细胞内压缩作用实现药物释放。
四、基于纳米技术的药物递送系统的优缺点优点:1. 减少副作用。
纳米技术在创新药物递送系统中的应用研究

汇报人:XXX
目录
纳米技术在药物递送系 统中的应用
纳米技术在创新药物研 发中的应用
纳米技术在药物递送 系统中的应用
纳米技术的优势
提高药物的溶解性和生物利用度 实现药物的定向和定位释放 增强药物的稳定性和长效性 降低药物的不良反应和副作用
纳米药物递送系统的分类
主动纳米药物递送 系统
被动纳米药物递送 系统
物理纳米药物递送 系统
化学纳米药物递送 系统
纳米技术纳米技术将药物直接送达病灶部位,提高药物疗效并降低副作用 案例2:设计具有生物降解性的纳米药物载体,实现药物的定向释放 案例3:利用纳米技术将传统中药进行现代化改造,提高其疗效和安全性 案例4:将纳米技术与基因治疗相结合,开发新型的基因治疗药物
药物副作用问题:纳米药物载体可以降低药物对正常细胞的损伤,减少药物副作用。
生产成本问题:纳米药物研发需要高昂的生产成本,可以通过技术创新和规模化生产等方 式降低成本。
THANK YOU
汇报人:XXX
纳米技术在创新药物 研发中的应用
纳米技术在创新药物研发中的优势
提高药物的生物 利用度
实现药物的定向 输送
增强药物的稳定 性
降低药物的不良 反应
纳米技术在创新药物研发中的技术难题及解决方法
药物递送效率问题:利用纳米技术可以提高药物在体内的递送效率,通过控制药物释放和 分布,实现精准治疗。
药物稳定性问题:纳米药物载体可以保护药物在体内免受降解和破坏,提高药物的稳定性 和药效。
纳米药物传递系统在癌症治疗中的应用研究
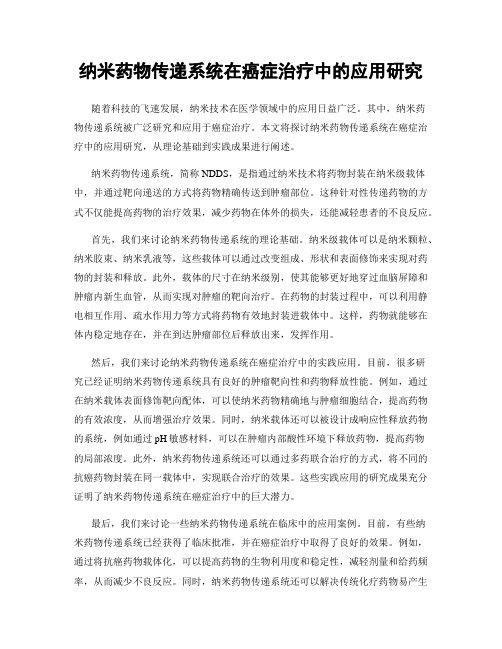
纳米药物传递系统在癌症治疗中的应用研究随着科技的飞速发展,纳米技术在医学领域中的应用日益广泛。
其中,纳米药物传递系统被广泛研究和应用于癌症治疗。
本文将探讨纳米药物传递系统在癌症治疗中的应用研究,从理论基础到实践成果进行阐述。
纳米药物传递系统,简称NDDS,是指通过纳米技术将药物封装在纳米级载体中,并通过靶向递送的方式将药物精确传送到肿瘤部位。
这种针对性传递药物的方式不仅能提高药物的治疗效果,减少药物在体外的损失,还能减轻患者的不良反应。
首先,我们来讨论纳米药物传递系统的理论基础。
纳米级载体可以是纳米颗粒、纳米胶束、纳米乳液等,这些载体可以通过改变组成、形状和表面修饰来实现对药物的封装和释放。
此外,载体的尺寸在纳米级别,使其能够更好地穿过血脑屏障和肿瘤内新生血管,从而实现对肿瘤的靶向治疗。
在药物的封装过程中,可以利用静电相互作用、疏水作用力等方式将药物有效地封装进载体中。
这样,药物就能够在体内稳定地存在,并在到达肿瘤部位后释放出来,发挥作用。
然后,我们来讨论纳米药物传递系统在癌症治疗中的实践应用。
目前,很多研究已经证明纳米药物传递系统具有良好的肿瘤靶向性和药物释放性能。
例如,通过在纳米载体表面修饰靶向配体,可以使纳米药物精确地与肿瘤细胞结合,提高药物的有效浓度,从而增强治疗效果。
同时,纳米载体还可以被设计成响应性释放药物的系统,例如通过pH敏感材料,可以在肿瘤内部酸性环境下释放药物,提高药物的局部浓度。
此外,纳米药物传递系统还可以通过多药联合治疗的方式,将不同的抗癌药物封装在同一载体中,实现联合治疗的效果。
这些实践应用的研究成果充分证明了纳米药物传递系统在癌症治疗中的巨大潜力。
最后,我们来讨论一些纳米药物传递系统在临床中的应用案例。
目前,有些纳米药物传递系统已经获得了临床批准,并在癌症治疗中取得了良好的效果。
例如,通过将抗癌药物载体化,可以提高药物的生物利用度和稳定性,减轻剂量和给药频率,从而减少不良反应。
药物递送系统中的纳米磁性技术研究进展
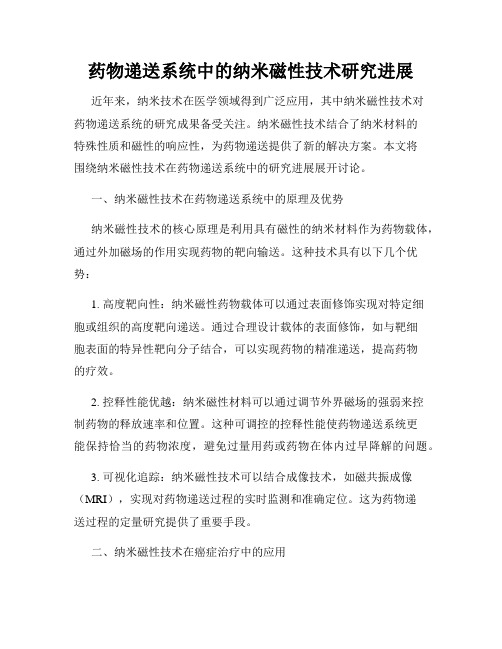
药物递送系统中的纳米磁性技术研究进展近年来,纳米技术在医学领域得到广泛应用,其中纳米磁性技术对药物递送系统的研究成果备受关注。
纳米磁性技术结合了纳米材料的特殊性质和磁性的响应性,为药物递送提供了新的解决方案。
本文将围绕纳米磁性技术在药物递送系统中的研究进展展开讨论。
一、纳米磁性技术在药物递送系统中的原理及优势纳米磁性技术的核心原理是利用具有磁性的纳米材料作为药物载体,通过外加磁场的作用实现药物的靶向输送。
这种技术具有以下几个优势:1. 高度靶向性:纳米磁性药物载体可以通过表面修饰实现对特定细胞或组织的高度靶向递送。
通过合理设计载体的表面修饰,如与靶细胞表面的特异性靶向分子结合,可以实现药物的精准递送,提高药物的疗效。
2. 控释性能优越:纳米磁性材料可以通过调节外界磁场的强弱来控制药物的释放速率和位置。
这种可调控的控释性能使药物递送系统更能保持恰当的药物浓度,避免过量用药或药物在体内过早降解的问题。
3. 可视化追踪:纳米磁性技术可以结合成像技术,如磁共振成像(MRI),实现对药物递送过程的实时监测和准确定位。
这为药物递送过程的定量研究提供了重要手段。
二、纳米磁性技术在癌症治疗中的应用癌症治疗是纳米磁性技术在药物递送系统中的一个重要应用领域。
目前,已有多种纳米磁性治疗药物递送系统在临床试验中展现出良好的疗效。
1. 磁性纳米粒子药物递送系统:磁性纳米颗粒作为药物载体,具有较大的比表面积和较强的磁响应性。
在磁场的作用下,药物可以被精确输送到靶细胞处,有效提高治疗效果。
2. 磁性纳米粒子联合光热疗法:将具有光热效应的纳米材料与磁性纳米粒子结合,可以实现联合光热疗法。
在外界磁场和激光的共同作用下,药物递送系统可以实现精确的热疗,杀灭癌细胞。
3. 磁性纳米粒子导引肿瘤靶向治疗:通过外加磁场的导引作用,磁性纳米粒子可以被定位于肿瘤部位。
这为高效药物递送、低剂量治疗提供了可能。
三、纳米磁性技术在神经系统疾病治疗中的应用除了癌症治疗,纳米磁性技术在神经系统疾病治疗中也显示出潜力。
医学研究的新兴技术利用纳米颗粒改善药物递送系统
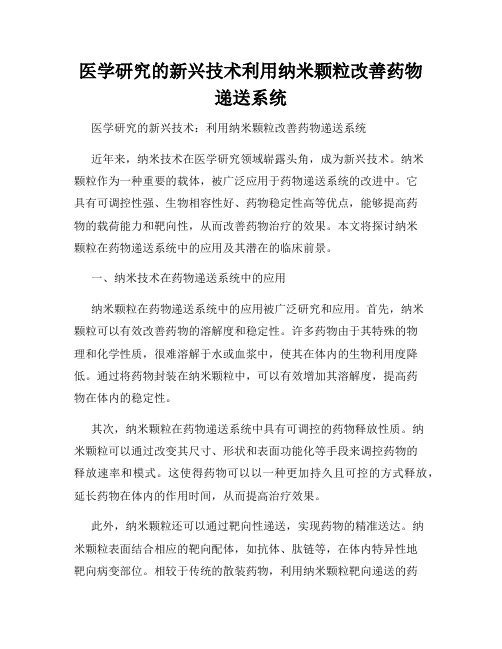
医学研究的新兴技术利用纳米颗粒改善药物递送系统医学研究的新兴技术:利用纳米颗粒改善药物递送系统近年来,纳米技术在医学研究领域崭露头角,成为新兴技术。
纳米颗粒作为一种重要的载体,被广泛应用于药物递送系统的改进中。
它具有可调控性强、生物相容性好、药物稳定性高等优点,能够提高药物的载荷能力和靶向性,从而改善药物治疗的效果。
本文将探讨纳米颗粒在药物递送系统中的应用及其潜在的临床前景。
一、纳米技术在药物递送系统中的应用纳米颗粒在药物递送系统中的应用被广泛研究和应用。
首先,纳米颗粒可以有效改善药物的溶解度和稳定性。
许多药物由于其特殊的物理和化学性质,很难溶解于水或血浆中,使其在体内的生物利用度降低。
通过将药物封装在纳米颗粒中,可以有效增加其溶解度,提高药物在体内的稳定性。
其次,纳米颗粒在药物递送系统中具有可调控的药物释放性质。
纳米颗粒可以通过改变其尺寸、形状和表面功能化等手段来调控药物的释放速率和模式。
这使得药物可以以一种更加持久且可控的方式释放,延长药物在体内的作用时间,从而提高治疗效果。
此外,纳米颗粒还可以通过靶向性递送,实现药物的精准送达。
纳米颗粒表面结合相应的靶向配体,如抗体、肽链等,在体内特异性地靶向病变部位。
相较于传统的散装药物,利用纳米颗粒靶向递送的药物可以更精准地作用于病变细胞,减少对正常细胞的影响,提高治疗效果。
二、纳米颗粒药物递送系统的临床前景纳米颗粒药物递送系统具有广阔的临床前景。
首先,它可以用于癌症治疗。
纳米颗粒具有靶向性和可调控的药物释放性质,可以实现对肿瘤组织的精准递送。
同时,纳米颗粒还可以携带多种药物,实现多重治疗,提高癌症治疗的效果。
其次,纳米颗粒药物递送系统对免疫系统的调控作用也备受关注。
透过控制纳米颗粒的粒径和表面性质,可以调节其在免疫系统中的相互作用,从而实现免疫调节、疫苗传递等多种临床应用。
此外,纳米颗粒还可以应用于神经系统疾病的治疗。
纳米颗粒具有较小的尺寸,可以穿过血脑屏障,将药物精确地送达至脑部病变区域,提高治疗效果。
新型纳米技术在药物递送系统中的应用研究
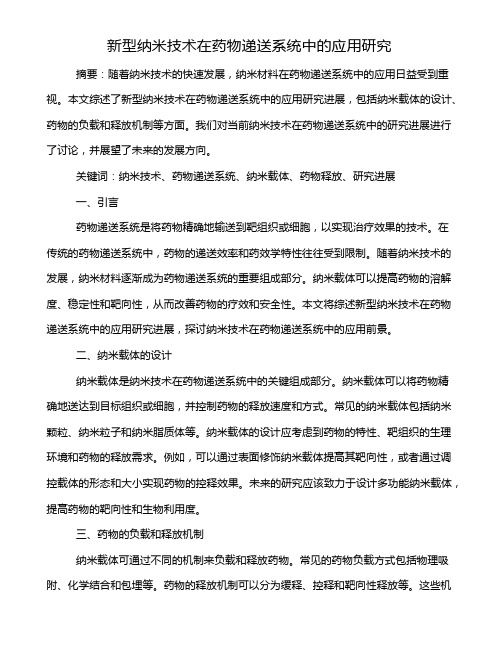
新型纳米技术在药物递送系统中的应用研究摘要:随着纳米技术的快速发展,纳米材料在药物递送系统中的应用日益受到重视。
本文综述了新型纳米技术在药物递送系统中的应用研究进展,包括纳米载体的设计、药物的负载和释放机制等方面。
我们对当前纳米技术在药物递送系统中的研究进展进行了讨论,并展望了未来的发展方向。
关键词:纳米技术、药物递送系统、纳米载体、药物释放、研究进展一、引言药物递送系统是将药物精确地输送到靶组织或细胞,以实现治疗效果的技术。
在传统的药物递送系统中,药物的递送效率和药效学特性往往受到限制。
随着纳米技术的发展,纳米材料逐渐成为药物递送系统的重要组成部分。
纳米载体可以提高药物的溶解度、稳定性和靶向性,从而改善药物的疗效和安全性。
本文将综述新型纳米技术在药物递送系统中的应用研究进展,探讨纳米技术在药物递送系统中的应用前景。
二、纳米载体的设计纳米载体是纳米技术在药物递送系统中的关键组成部分。
纳米载体可以将药物精确地送达到目标组织或细胞,并控制药物的释放速度和方式。
常见的纳米载体包括纳米颗粒、纳米粒子和纳米脂质体等。
纳米载体的设计应考虑到药物的特性、靶组织的生理环境和药物的释放需求。
例如,可以通过表面修饰纳米载体提高其靶向性,或者通过调控载体的形态和大小实现药物的控释效果。
未来的研究应该致力于设计多功能纳米载体,提高药物的靶向性和生物利用度。
三、药物的负载和释放机制纳米载体可通过不同的机制来负载和释放药物。
常见的药物负载方式包括物理吸附、化学结合和包埋等。
药物的释放机制可以分为缓释、控释和靶向性释放等。
这些机制可以通过调控载体的性质和结构来实现。
例如,可以通过改变载体的表面电荷、孔径大小和形态来调控药物的释放速度和方式。
未来的研究应该探索新的药物负载和释放机制,提高药物的生物利用度和抗药性。
四、研究进展目前,纳米技术在药物递送系统中已取得了一系列重要的研究进展。
例如,纳米载体可以提高抗肿瘤药物的靶向性,降低其毒副作用,进而提高治疗效果。
基于纳米技术的药物递送系统研究
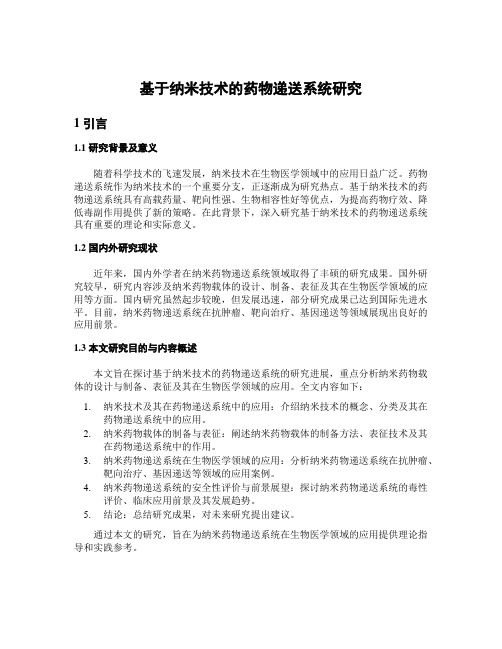
基于纳米技术的药物递送系统研究1 引言1.1 研究背景及意义随着科学技术的飞速发展,纳米技术在生物医学领域中的应用日益广泛。
药物递送系统作为纳米技术的一个重要分支,正逐渐成为研究热点。
基于纳米技术的药物递送系统具有高载药量、靶向性强、生物相容性好等优点,为提高药物疗效、降低毒副作用提供了新的策略。
在此背景下,深入研究基于纳米技术的药物递送系统具有重要的理论和实际意义。
1.2 国内外研究现状近年来,国内外学者在纳米药物递送系统领域取得了丰硕的研究成果。
国外研究较早,研究内容涉及纳米药物载体的设计、制备、表征及其在生物医学领域的应用等方面。
国内研究虽然起步较晚,但发展迅速,部分研究成果已达到国际先进水平。
目前,纳米药物递送系统在抗肿瘤、靶向治疗、基因递送等领域展现出良好的应用前景。
1.3 本文研究目的与内容概述本文旨在探讨基于纳米技术的药物递送系统的研究进展,重点分析纳米药物载体的设计与制备、表征及其在生物医学领域的应用。
全文内容如下:1.纳米技术及其在药物递送系统中的应用:介绍纳米技术的概念、分类及其在药物递送系统中的应用。
2.纳米药物载体的制备与表征:阐述纳米药物载体的制备方法、表征技术及其在药物递送系统中的作用。
3.纳米药物递送系统在生物医学领域的应用:分析纳米药物递送系统在抗肿瘤、靶向治疗、基因递送等领域的应用案例。
4.纳米药物递送系统的安全性评价与前景展望:探讨纳米药物递送系统的毒性评价、临床应用前景及其发展趋势。
5.结论:总结研究成果,对未来研究提出建议。
通过本文的研究,旨在为纳米药物递送系统在生物医学领域的应用提供理论指导和实践参考。
2 纳米技术及其在药物递送系统中的应用2.1 纳米技术概述2.1.1 纳米材料的定义与分类纳米材料是指至少在一个维度上尺寸在纳米级别的材料,通常为1至100纳米。
根据组成和结构,纳米材料可以分为以下几类:金属纳米材料、陶瓷纳米材料、有机纳米材料和复合纳米材料。
纳米药物递释系统的脑靶向研究进展
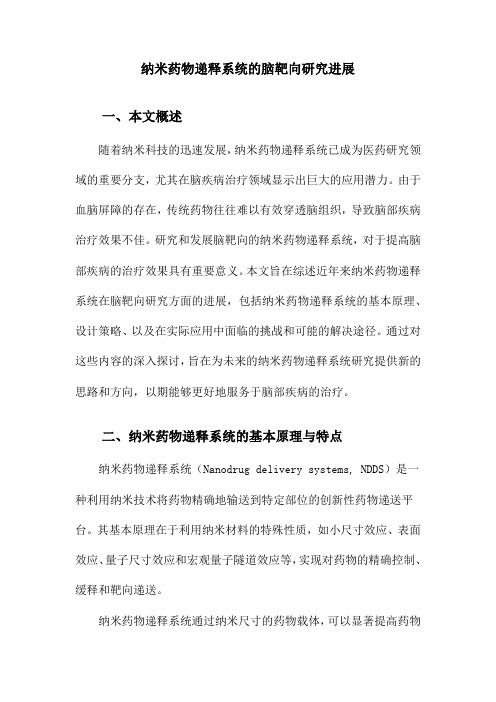
纳米药物递释系统的脑靶向研究进展一、本文概述随着纳米科技的迅速发展,纳米药物递释系统已成为医药研究领域的重要分支,尤其在脑疾病治疗领域显示出巨大的应用潜力。
由于血脑屏障的存在,传统药物往往难以有效穿透脑组织,导致脑部疾病治疗效果不佳。
研究和发展脑靶向的纳米药物递释系统,对于提高脑部疾病的治疗效果具有重要意义。
本文旨在综述近年来纳米药物递释系统在脑靶向研究方面的进展,包括纳米药物递释系统的基本原理、设计策略、以及在实际应用中面临的挑战和可能的解决途径。
通过对这些内容的深入探讨,旨在为未来的纳米药物递释系统研究提供新的思路和方向,以期能够更好地服务于脑部疾病的治疗。
二、纳米药物递释系统的基本原理与特点纳米药物递释系统(Nanodrug delivery systems, NDDS)是一种利用纳米技术将药物精确地输送到特定部位的创新性药物递送平台。
其基本原理在于利用纳米材料的特殊性质,如小尺寸效应、表面效应、量子尺寸效应和宏观量子隧道效应等,实现对药物的精确控制、缓释和靶向递送。
纳米药物递释系统通过纳米尺寸的药物载体,可以显著提高药物的溶解度和稳定性,从而改善药物的生物利用度。
纳米载体还可以通过改变药物的释放动力学,实现药物的缓释和持续释放,减少药物剂量和副作用,提高治疗效果。
纳米药物递释系统具有独特的靶向性。
通过修饰纳米载体的表面,可以使其主动或被动地靶向到特定的组织或细胞。
例如,利用脑毛细血管内皮细胞上的特殊受体,可以实现纳米药物递释系统对脑组织的靶向递送,从而提高药物在脑内的浓度,增强治疗效果。
纳米药物递释系统还具有良好的生物相容性和低毒性。
纳米载体通常采用生物相容性好的材料制备,如脂质体、聚合物纳米粒等,这些材料在体内可被降解吸收,不会造成长期毒性。
纳米载体还可以通过降低药物在体内的分布范围,减少药物对正常组织的损伤,进一步提高药物的安全性。
纳米药物递释系统通过其独特的纳米结构和性质,实现了药物的精确控制、缓释和靶向递送,为脑部疾病的治疗提供了新的思路和方法。
药物递送系统的纳米技术研究
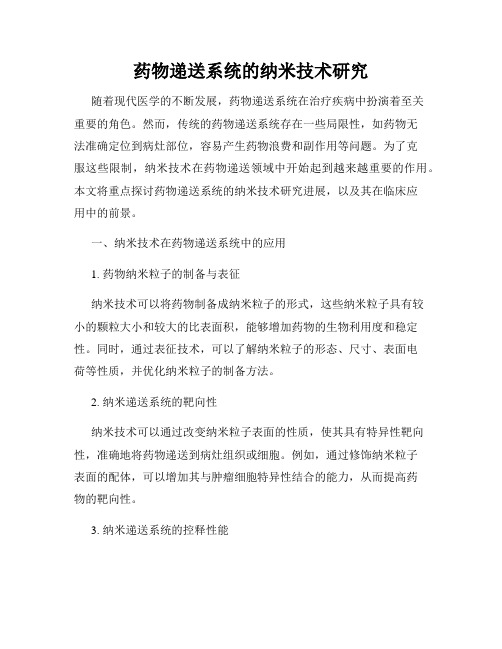
药物递送系统的纳米技术研究随着现代医学的不断发展,药物递送系统在治疗疾病中扮演着至关重要的角色。
然而,传统的药物递送系统存在一些局限性,如药物无法准确定位到病灶部位,容易产生药物浪费和副作用等问题。
为了克服这些限制,纳米技术在药物递送领域中开始起到越来越重要的作用。
本文将重点探讨药物递送系统的纳米技术研究进展,以及其在临床应用中的前景。
一、纳米技术在药物递送系统中的应用1. 药物纳米粒子的制备与表征纳米技术可以将药物制备成纳米粒子的形式,这些纳米粒子具有较小的颗粒大小和较大的比表面积,能够增加药物的生物利用度和稳定性。
同时,通过表征技术,可以了解纳米粒子的形态、尺寸、表面电荷等性质,并优化纳米粒子的制备方法。
2. 纳米递送系统的靶向性纳米技术可以通过改变纳米粒子表面的性质,使其具有特异性靶向性,准确地将药物递送到病灶组织或细胞。
例如,通过修饰纳米粒子表面的配体,可以增加其与肿瘤细胞特异性结合的能力,从而提高药物的靶向性。
3. 纳米递送系统的控释性能纳米技术可以制备控释性能较好的纳米递送系统,实现药物缓慢释放。
这种控释系统可以维持药物在有效浓度范围内,延长药物的作用时间,减少药物使用频率,从而降低药物的副作用。
二、药物递送系统的纳米技术研究进展1. 纳米递送系统的材料选择目前,常用的纳米药物递送系统材料包括聚合物、金属和无机材料等。
不同的材料具有不同的特性,适用于不同的药物递送需求。
例如,聚合物材料具有良好的生物相容性和可调控性,适用于制备控释性能较好的纳米递送系统。
2. 纳米递送系统的制备方法纳米递送系统的制备方法多种多样,包括溶剂沉淀法、乳化法、胶体共沉淀法等。
这些方法可以根据药物的特性和要求选择合适的制备方法,来制备具有优异性能的纳米递送系统。
3. 纳米递送系统的生物安全性评价在纳米递送系统的研究中,安全性评价是必不可少的环节。
科学家通过体内外实验评估纳米递送系统对机体的生物安全性,并优化纳米递送系统的结构和材料,以提高其生物相容性和稳定性。
纳米药物递送系统中药物的渗透性研究
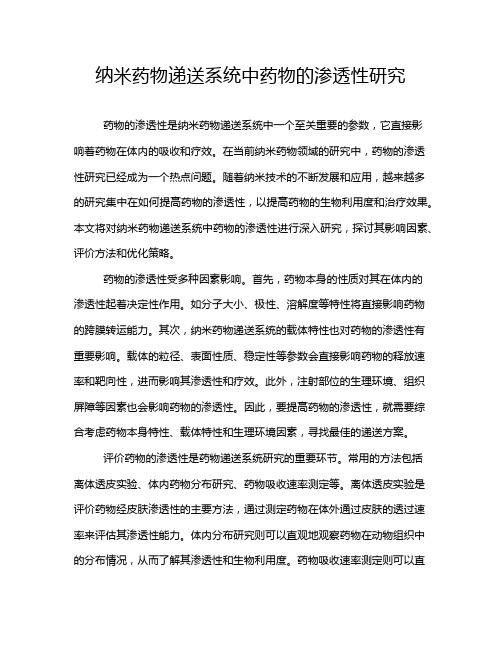
纳米药物递送系统中药物的渗透性研究药物的渗透性是纳米药物递送系统中一个至关重要的参数,它直接影响着药物在体内的吸收和疗效。
在当前纳米药物领域的研究中,药物的渗透性研究已经成为一个热点问题。
随着纳米技术的不断发展和应用,越来越多的研究集中在如何提高药物的渗透性,以提高药物的生物利用度和治疗效果。
本文将对纳米药物递送系统中药物的渗透性进行深入研究,探讨其影响因素、评价方法和优化策略。
药物的渗透性受多种因素影响。
首先,药物本身的性质对其在体内的渗透性起着决定性作用。
如分子大小、极性、溶解度等特性将直接影响药物的跨膜转运能力。
其次,纳米药物递送系统的载体特性也对药物的渗透性有重要影响。
载体的粒径、表面性质、稳定性等参数会直接影响药物的释放速率和靶向性,进而影响其渗透性和疗效。
此外,注射部位的生理环境、组织屏障等因素也会影响药物的渗透性。
因此,要提高药物的渗透性,就需要综合考虑药物本身特性、载体特性和生理环境因素,寻找最佳的递送方案。
评价药物的渗透性是药物递送系统研究的重要环节。
常用的方法包括离体透皮实验、体内药物分布研究、药物吸收速率测定等。
离体透皮实验是评价药物经皮肤渗透性的主要方法,通过测定药物在体外通过皮肤的透过速率来评估其渗透性能力。
体内分布研究则可以直观地观察药物在动物组织中的分布情况,从而了解其渗透性和生物利用度。
药物吸收速率测定则可以直接评估药物在体内的跨膜转运速率,为其渗透性提供定量数据。
这些方法的综合应用可以全面评价药物的渗透性,为下一步的优化设计提供依据。
针对提高药物的渗透性,在载体设计和制备方面有许多策略可供选择。
首先,可以优化纳米载体的结构和性质,如调控载体的粒径和表面性质,提高载体的稳定性和药物载荷量,增强其药物递送能力。
其次,可以利用靶向技术提高药物的渗透性。
通过修饰载体表面的靶向配体,实现对特定细胞或组织的选择性靶向释放,提高药物的局部渗透性和疗效。
此外,还可以利用生物膜渗透增强剂改善药物的渗透性。
纳米技术在口服给药系统中的应用综述
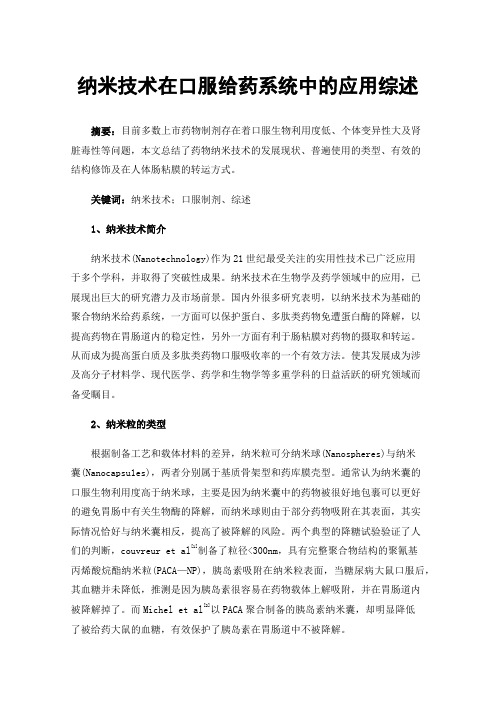
纳米技术在口服给药系统中的应用综述摘要:目前多数上市药物制剂存在着口服生物利用度低、个体变异性大及肾脏毒性等问题,本文总结了药物纳米技术的发展现状、普遍使用的类型、有效的结构修饰及在人体肠粘膜的转运方式。
关键词:纳米技术;口服制剂、综述1、纳米技术简介纳米技术(Nanotechnology)作为21世纪最受关注的实用性技术已广泛应用于多个学科,并取得了突破性成果。
纳米技术在生物学及药学领域中的应用,已展现出巨大的研究潜力及市场前景。
国内外很多研究表明,以纳米技术为基础的聚合物纳米给药系统,一方面可以保护蛋白、多肽类药物免遭蛋白酶的降解,以提高药物在胃肠道内的稳定性,另外一方面有利于肠粘膜对药物的摄取和转运。
从而成为提高蛋白质及多肽类药物口服吸收率的一个有效方法。
使其发展成为涉及高分子材料学、现代医学、药学和生物学等多重学科的日益活跃的研究领域而备受瞩目。
2、纳米粒的类型根据制备工艺和载体材料的差异,纳米粒可分纳米球(Nanospheres)与纳米囊(Nanocapsu1es),两者分别属于基质骨架型和药库膜壳型。
通常认为纳米囊的口服生物利用度高于纳米球,主要是因为纳米囊中的药物被很好地包裹可以更好的避免胃肠中有关生物酶的降解,而纳米球则由于部分药物吸附在其表面,其实际情况恰好与纳米囊相反,提高了被降解的风险。
两个典型的降糖试验验证了人们的判断,couvreur et al[1]制备了粒径<300nm,具有完整聚合物结构的聚氰基丙烯酸烷酯纳米粒(PACA—NP),胰岛素吸附在纳米粒表面,当糖尿病大鼠口服后,其血糖并未降低,推测是因为胰岛素很容易在药物载体上解吸附,并在胃肠道内被降解掉了。
而Michel et al[2]以PACA聚合制备的胰岛素纳米囊,却明显降低了被给药大鼠的血糖,有效保护了胰岛素在胃肠道中不被降解。
3、纳米粒的修饰如何提高纳米粒的生物利用度,进而提高药物的药用价值是目前研究的热点。
基于纳米技术的药物递送系统的设计与优化
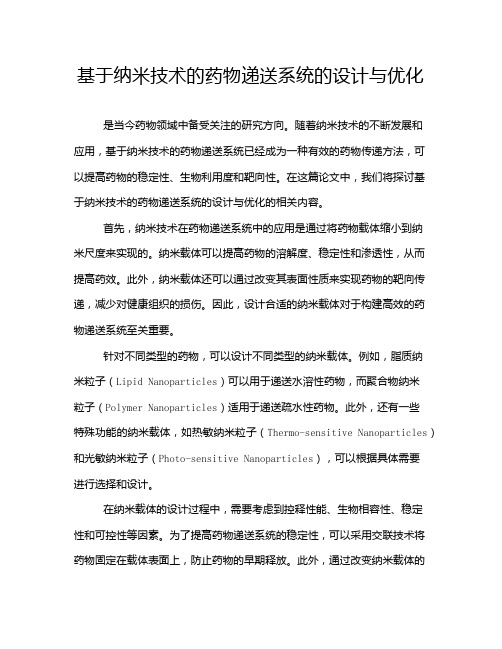
基于纳米技术的药物递送系统的设计与优化是当今药物领域中备受关注的研究方向。
随着纳米技术的不断发展和应用,基于纳米技术的药物递送系统已经成为一种有效的药物传递方法,可以提高药物的稳定性、生物利用度和靶向性。
在这篇论文中,我们将探讨基于纳米技术的药物递送系统的设计与优化的相关内容。
首先,纳米技术在药物递送系统中的应用是通过将药物载体缩小到纳米尺度来实现的。
纳米载体可以提高药物的溶解度、稳定性和渗透性,从而提高药效。
此外,纳米载体还可以通过改变其表面性质来实现药物的靶向传递,减少对健康组织的损伤。
因此,设计合适的纳米载体对于构建高效的药物递送系统至关重要。
针对不同类型的药物,可以设计不同类型的纳米载体。
例如,脂质纳米粒子(Lipid Nanoparticles)可以用于递送水溶性药物,而聚合物纳米粒子(Polymer Nanoparticles)适用于递送疏水性药物。
此外,还有一些特殊功能的纳米载体,如热敏纳米粒子(Thermo-sensitive Nanoparticles)和光敏纳米粒子(Photo-sensitive Nanoparticles),可以根据具体需要进行选择和设计。
在纳米载体的设计过程中,需要考虑到控释性能、生物相容性、稳定性和可控性等因素。
为了提高药物递送系统的稳定性,可以采用交联技术将药物固定在载体表面上,防止药物的早期释放。
此外,通过改变纳米载体的大小、形状和表面性质,还可以实现对药物的控制释放,提高药物的生物利用度。
除了纳米载体的设计外,优化药物递送系统的传输路径也是至关重要的。
传统的递送系统在经过消化道等过程时容易受到破坏,导致药物的生物利用度降低。
因此,可以通过口服纳米药物递送系统来提高药物的生物利用度。
纳米药物递送系统可以通过纳米载体的特殊结构和表面性质来避免药物在胃酸和胃酶中的分解,从而提高药物的吸收率和效果。
此外,优化纳米载体的表面修饰也是提高药物递送系统靶向性的有效手段。
药物递送系统的纳米颗粒制备研究
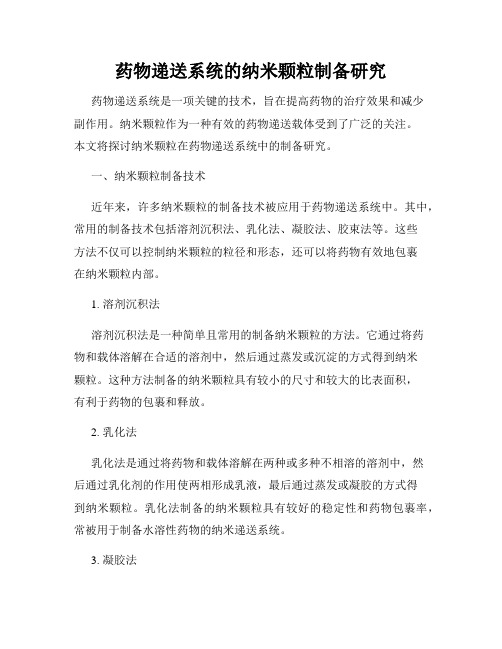
药物递送系统的纳米颗粒制备研究药物递送系统是一项关键的技术,旨在提高药物的治疗效果和减少副作用。
纳米颗粒作为一种有效的药物递送载体受到了广泛的关注。
本文将探讨纳米颗粒在药物递送系统中的制备研究。
一、纳米颗粒制备技术近年来,许多纳米颗粒的制备技术被应用于药物递送系统中。
其中,常用的制备技术包括溶剂沉积法、乳化法、凝胶法、胶束法等。
这些方法不仅可以控制纳米颗粒的粒径和形态,还可以将药物有效地包裹在纳米颗粒内部。
1. 溶剂沉积法溶剂沉积法是一种简单且常用的制备纳米颗粒的方法。
它通过将药物和载体溶解在合适的溶剂中,然后通过蒸发或沉淀的方式得到纳米颗粒。
这种方法制备的纳米颗粒具有较小的尺寸和较大的比表面积,有利于药物的包裹和释放。
2. 乳化法乳化法是通过将药物和载体溶解在两种或多种不相溶的溶剂中,然后通过乳化剂的作用使两相形成乳液,最后通过蒸发或凝胶的方式得到纳米颗粒。
乳化法制备的纳米颗粒具有较好的稳定性和药物包裹率,常被用于制备水溶性药物的纳米递送系统。
3. 凝胶法凝胶法是一种通过凝胶剂的作用使药物和载体形成凝胶状态,并通过后续处理得到纳米颗粒的方法。
凝胶法制备的纳米颗粒具有较大的孔隙结构和较好的药物包裹效果,适用于制备疏水性药物的纳米递送系统。
4. 胶束法胶束法是通过表面活性剂的作用将药物和载体形成胶束结构,并通过后续处理得到纳米颗粒的方法。
胶束法制备的纳米颗粒具有很高的药物包裹率和稳定性,常被应用于靶向递送系统的制备。
二、纳米颗粒在药物递送系统中的应用纳米颗粒在药物递送系统中具有广阔的应用前景。
首先,纳米颗粒可以通过改变表面性质来提高药物的溶解度和稳定性,从而增强药物的递送效果。
其次,纳米颗粒可以增加药物与靶标细胞之间的接触面积,提高药物的靶向性和疗效。
此外,纳米颗粒还可以通过调节纳米颗粒的粒径和形态来控制药物的释放速率和递送路径。
在纳米颗粒制备技术的基础上,科研人员和制药公司已经成功地开发了许多具有前景的纳米递送系统。
基于纳米技术的药物递送系统
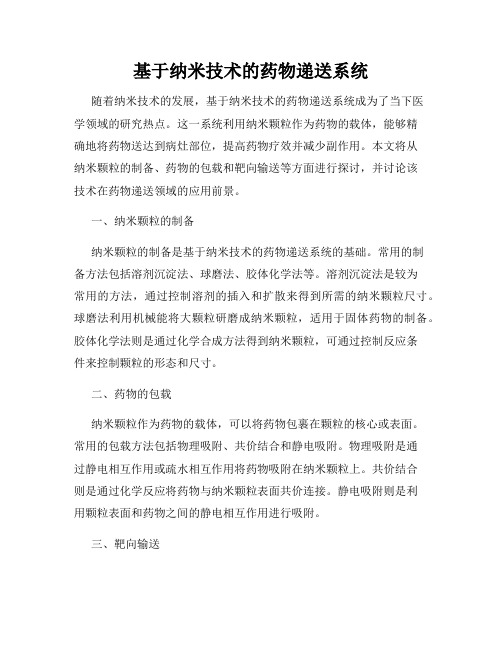
基于纳米技术的药物递送系统随着纳米技术的发展,基于纳米技术的药物递送系统成为了当下医学领域的研究热点。
这一系统利用纳米颗粒作为药物的载体,能够精确地将药物送达到病灶部位,提高药物疗效并减少副作用。
本文将从纳米颗粒的制备、药物的包载和靶向输送等方面进行探讨,并讨论该技术在药物递送领域的应用前景。
一、纳米颗粒的制备纳米颗粒的制备是基于纳米技术的药物递送系统的基础。
常用的制备方法包括溶剂沉淀法、球磨法、胶体化学法等。
溶剂沉淀法是较为常用的方法,通过控制溶剂的插入和扩散来得到所需的纳米颗粒尺寸。
球磨法利用机械能将大颗粒研磨成纳米颗粒,适用于固体药物的制备。
胶体化学法则是通过化学合成方法得到纳米颗粒,可通过控制反应条件来控制颗粒的形态和尺寸。
二、药物的包载纳米颗粒作为药物的载体,可以将药物包裹在颗粒的核心或表面。
常用的包载方法包括物理吸附、共价结合和静电吸附。
物理吸附是通过静电相互作用或疏水相互作用将药物吸附在纳米颗粒上。
共价结合则是通过化学反应将药物与纳米颗粒表面共价连接。
静电吸附则是利用颗粒表面和药物之间的静电相互作用进行吸附。
三、靶向输送纳米颗粒可以通过适当的功能化改性来实现药物的靶向输送。
功能化改性包括引入靶向配体、改变表面电荷和增加药物的稳定性等。
靶向配体可以与特定的受体结合,使纳米颗粒能够针对性地识别和富集在病变组织或细胞表面。
改变颗粒表面电荷可以影响颗粒在体内的分布和代谢,从而增加药物的靶向输送效果。
此外,要保证纳米颗粒在输送过程中的稳定性,避免药物的过早释放。
基于纳米技术的药物递送系统在药物疗效和安全性方面具有巨大的潜力。
首先,纳米颗粒载体能够提高药物的溶解度和稳定性,增加药物的生物利用度。
其次,通过纳米颗粒的靶向输送,药物可以准确地传送到病灶部位,并在目标组织或细胞内释放,降低对正常组织的损伤。
此外,纳米颗粒还能够提高药物的半衰期,减少用药次数,提高患者的依从性。
尽管基于纳米技术的药物递送系统在理论上具备良好的前景,但在实际应用中仍然面临一些挑战。
基于纳米技术的药物递送系统的体外评价研究
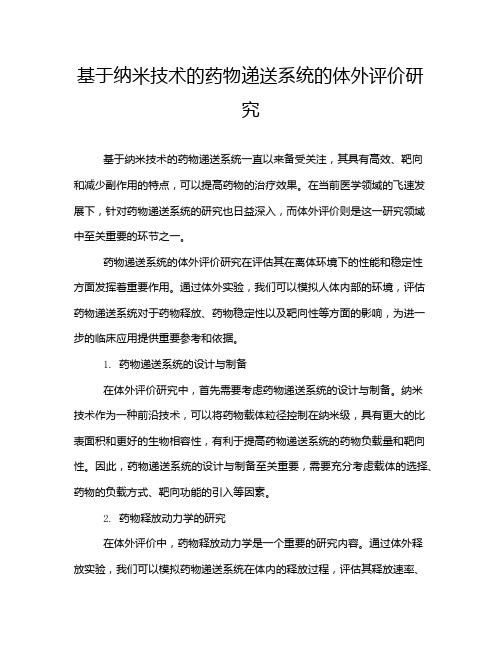
基于纳米技术的药物递送系统的体外评价研究基于纳米技术的药物递送系统一直以来备受关注,其具有高效、靶向和减少副作用的特点,可以提高药物的治疗效果。
在当前医学领域的飞速发展下,针对药物递送系统的研究也日益深入,而体外评价则是这一研究领域中至关重要的环节之一。
药物递送系统的体外评价研究在评估其在离体环境下的性能和稳定性方面发挥着重要作用。
通过体外实验,我们可以模拟人体内部的环境,评估药物递送系统对于药物释放、药物稳定性以及靶向性等方面的影响,为进一步的临床应用提供重要参考和依据。
1. 药物递送系统的设计与制备在体外评价研究中,首先需要考虑药物递送系统的设计与制备。
纳米技术作为一种前沿技术,可以将药物载体粒径控制在纳米级,具有更大的比表面积和更好的生物相容性,有利于提高药物递送系统的药物负载量和靶向性。
因此,药物递送系统的设计与制备至关重要,需要充分考虑载体的选择、药物的负载方式、靶向功能的引入等因素。
2. 药物释放动力学的研究在体外评价中,药物释放动力学是一个重要的研究内容。
通过体外释放实验,我们可以模拟药物递送系统在体内的释放过程,评估其释放速率、延时释放效果等参数。
通过分析药物的释放规律,可以为进一步优化药物递送系统提供重要的数据支持。
3. 药物稳定性的评估除了药物的释放动力学,药物的稳定性也是药物递送系统体外评价研究中需要关注的重点。
药物在递送系统中的稳定性直接影响着其药效和安全性,因此需要通过体外实验来评估药物在递送系统中的稳定性,包括药物的降解速率、分解产物的生成等方面。
4. 靶向性能的验证针对药物递送系统的体外评价研究还需要对其靶向性能进行验证。
在体外实验中,可以通过模拟靶向受体的存在,评估药物递送系统对特定受体的识别和结合能力,进而评估其靶向递送效果。
靶向性的验证是评价药物递送系统效果的重要指标之一。
5. 结论与展望通过以上对内容的探讨,我们可以看到,体外评价在药物递送系统的研究中起着至关重要的作用。
纳米材料在药物输送系统中的应用研究进展
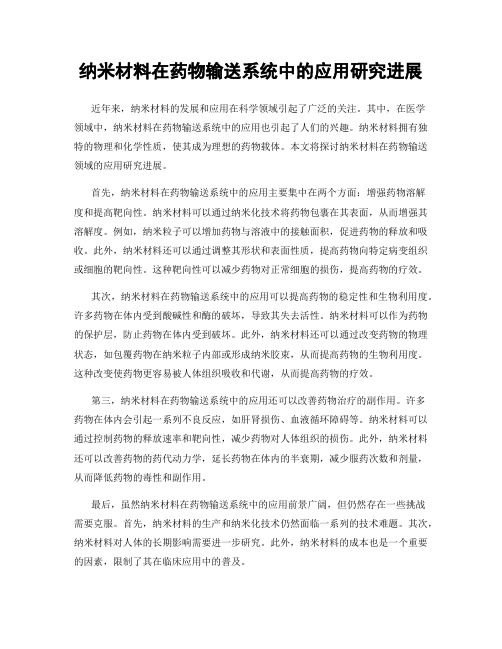
纳米材料在药物输送系统中的应用研究进展近年来,纳米材料的发展和应用在科学领域引起了广泛的关注。
其中,在医学领域中,纳米材料在药物输送系统中的应用也引起了人们的兴趣。
纳米材料拥有独特的物理和化学性质,使其成为理想的药物载体。
本文将探讨纳米材料在药物输送领域的应用研究进展。
首先,纳米材料在药物输送系统中的应用主要集中在两个方面:增强药物溶解度和提高靶向性。
纳米材料可以通过纳米化技术将药物包裹在其表面,从而增强其溶解度。
例如,纳米粒子可以增加药物与溶液中的接触面积,促进药物的释放和吸收。
此外,纳米材料还可以通过调整其形状和表面性质,提高药物向特定病变组织或细胞的靶向性。
这种靶向性可以减少药物对正常细胞的损伤,提高药物的疗效。
其次,纳米材料在药物输送系统中的应用可以提高药物的稳定性和生物利用度。
许多药物在体内受到酸碱性和酶的破坏,导致其失去活性。
纳米材料可以作为药物的保护层,防止药物在体内受到破坏。
此外,纳米材料还可以通过改变药物的物理状态,如包覆药物在纳米粒子内部或形成纳米胶束,从而提高药物的生物利用度。
这种改变使药物更容易被人体组织吸收和代谢,从而提高药物的疗效。
第三,纳米材料在药物输送系统中的应用还可以改善药物治疗的副作用。
许多药物在体内会引起一系列不良反应,如肝肾损伤、血液循环障碍等。
纳米材料可以通过控制药物的释放速率和靶向性,减少药物对人体组织的损伤。
此外,纳米材料还可以改善药物的药代动力学,延长药物在体内的半衰期,减少服药次数和剂量,从而降低药物的毒性和副作用。
最后,虽然纳米材料在药物输送系统中的应用前景广阔,但仍然存在一些挑战需要克服。
首先,纳米材料的生产和纳米化技术仍然面临一系列的技术难题。
其次,纳米材料对人体的长期影响需要进一步研究。
此外,纳米材料的成本也是一个重要的因素,限制了其在临床应用中的普及。
总之,纳米材料在药物输送系统中的应用具有重要的意义和潜力。
通过纳米化技术,药物的溶解度、靶向性、稳定性和生物利用度都可以得到提高。
纳米药物递送系统在疾病治疗中的应用研究
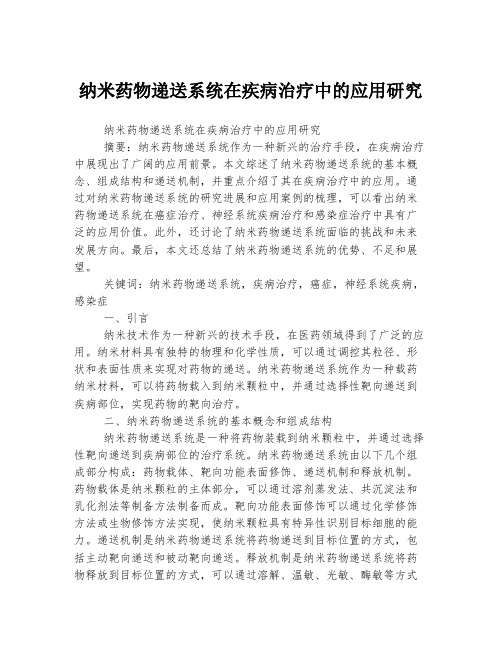
纳米药物递送系统在疾病治疗中的应用研究纳米药物递送系统在疾病治疗中的应用研究摘要:纳米药物递送系统作为一种新兴的治疗手段,在疾病治疗中展现出了广阔的应用前景。
本文综述了纳米药物递送系统的基本概念、组成结构和递送机制,并重点介绍了其在疾病治疗中的应用。
通过对纳米药物递送系统的研究进展和应用案例的梳理,可以看出纳米药物递送系统在癌症治疗、神经系统疾病治疗和感染症治疗中具有广泛的应用价值。
此外,还讨论了纳米药物递送系统面临的挑战和未来发展方向。
最后,本文还总结了纳米药物递送系统的优势、不足和展望。
关键词:纳米药物递送系统,疾病治疗,癌症,神经系统疾病,感染症一、引言纳米技术作为一种新兴的技术手段,在医药领域得到了广泛的应用。
纳米材料具有独特的物理和化学性质,可以通过调控其粒径、形状和表面性质来实现对药物的递送。
纳米药物递送系统作为一种载药纳米材料,可以将药物载入到纳米颗粒中,并通过选择性靶向递送到疾病部位,实现药物的靶向治疗。
二、纳米药物递送系统的基本概念和组成结构纳米药物递送系统是一种将药物装载到纳米颗粒中,并通过选择性靶向递送到疾病部位的治疗系统。
纳米药物递送系统由以下几个组成部分构成:药物载体、靶向功能表面修饰、递送机制和释放机制。
药物载体是纳米颗粒的主体部分,可以通过溶剂蒸发法、共沉淀法和乳化剂法等制备方法制备而成。
靶向功能表面修饰可以通过化学修饰方法或生物修饰方法实现,使纳米颗粒具有特异性识别目标细胞的能力。
递送机制是纳米药物递送系统将药物递送到目标位置的方式,包括主动靶向递送和被动靶向递送。
释放机制是纳米药物递送系统将药物释放到目标位置的方式,可以通过溶解、温敏、光敏、酶敏等方式实现。
三、纳米药物递送系统在癌症治疗中的应用研究纳米药物递送系统在癌症治疗中的应用研究已取得了重要进展。
通过调控纳米药物递送系统的大小、形状和表面性质,可以实现对肿瘤靶向治疗。
例如,通过改变纳米颗粒的粒径和表面性质,可以实现对肿瘤血管的靶向治疗。
药物纳米递送系统在治疗传染病中的应用研究
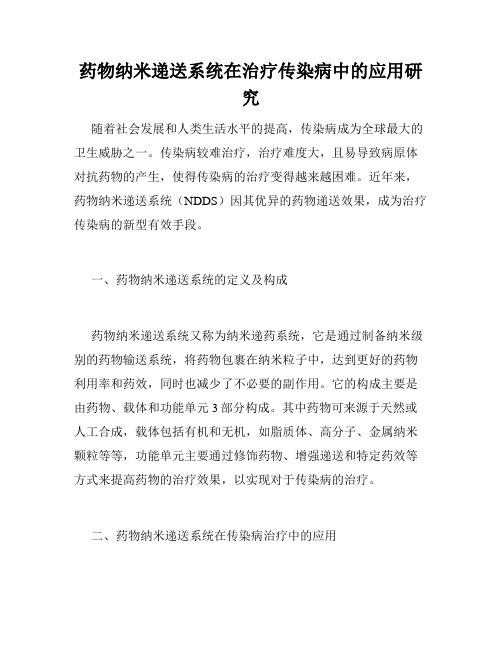
药物纳米递送系统在治疗传染病中的应用研究随着社会发展和人类生活水平的提高,传染病成为全球最大的卫生威胁之一。
传染病较难治疗,治疗难度大,且易导致病原体对抗药物的产生,使得传染病的治疗变得越来越困难。
近年来,药物纳米递送系统(NDDS)因其优异的药物递送效果,成为治疗传染病的新型有效手段。
一、药物纳米递送系统的定义及构成药物纳米递送系统又称为纳米递药系统,它是通过制备纳米级别的药物输送系统,将药物包裹在纳米粒子中,达到更好的药物利用率和药效,同时也减少了不必要的副作用。
它的构成主要是由药物、载体和功能单元3部分构成。
其中药物可来源于天然或人工合成,载体包括有机和无机,如脂质体、高分子、金属纳米颗粒等等,功能单元主要通过修饰药物、增强递送和特定药效等方式来提高药物的治疗效果,以实现对于传染病的治疗。
二、药物纳米递送系统在传染病治疗中的应用肝炎由肝病毒(HBV)、肝炎病毒 (HCV)、肝克罗恩氏病等病原体引发,属于一类常见的传染病。
肝炎的治疗过程常常需要长时间的药物治疗,且同时也存在很高的复发率,治疗难度极大。
因此,药物纳米递送系统在肝炎的治疗中被广泛研究和应用。
载体的构成和功能单元的修饰均影响累积和靶向性,经过研究得到的药物纳米递送系统将药物成功递送到靶目的地,使得减少了药物剂量和时间,同时也降低了副作用的产生。
2.结核病结核病由结核分枝菌(M.TB)引发,属于一类非常难以消灭的传染病,经常会引发肺部感染和其他身体部位的感染。
然而,传统抗结核药物常用于传染性早期,而对于慢性和复发性结核病的治疗则需要用到更为先进的药物,如异烟肼、利福平等。
在此背景下,药物纳米递送系统被认为是治疗结核病的突破口。
通过纳米级载体的制备和药物的修饰,将高效抗TB药物和靶向制备相结合到一起,实现了对M.TB的特异性干预,且IC50显著降低,疗效得到了明显提高。
艾滋病由艾滋病毒(HIV-1/HIV-2)感染引起,是一种世界上最致命的传染病之一。
白消安纳米给药系统研究进展
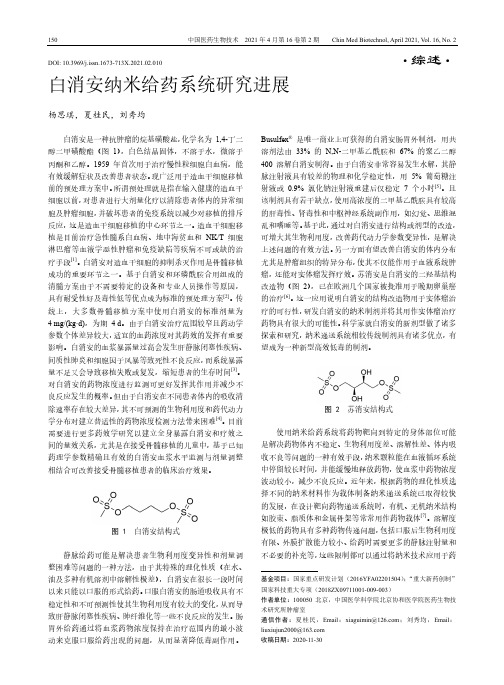
DOI: 10.3969/j.issn.1673-713X.2021.02.010·综述·白消安纳米给药系统研究进展杨思琪,夏桂民,刘秀均白消安是一种抗肿瘤的烷基磺酸盐,化学名为1,4-丁二醇二甲磺酸酯(图1),白色结晶固体,不溶于水,微溶于丙酮和乙醇。
1959 年首次用于治疗慢性粒细胞白血病,能有效缓解症状及改善患者状态。
现广泛用于造血干细胞移植前的预处理方案中。
所谓预处理就是指在输入健康的造血干细胞以前,对患者进行大剂量化疗以清除患者体内的异常细胞及肿瘤细胞,并破坏患者的免疫系统以减少对移植的排斥反应,这是造血干细胞移植的中心环节之一。
造血干细胞移植是目前治疗急性髓系白血病、地中海贫血和NK/T 细胞淋巴瘤等血液学恶性肿瘤和免疫缺陷等疾病不可或缺的治疗手段[1]。
白消安对造血干细胞的抑制杀灭作用是骨髓移植成功的重要环节之一。
基于白消安和环磷酰胺合用组成的清髓方案由于不需要特定的设备和专业人员操作等原因,具有耐受性好及毒性低等优点成为标准的预处理方案[2]。
传统上,大多数骨髓移植方案中使用白消安的标准剂量为4 mg/(kg·d),为期4 d。
由于白消安治疗范围较窄且药动学参数个体差异较大,适宜的血药浓度对其药效的发挥有重要影响。
白消安的血浆暴露量过高会发生肝静脉闭塞性疾病、间质性肺炎和细胞因子风暴等致死性不良反应,而系统暴露量不足又会导致移植失败或复发,缩短患者的生存时间[3]。
对白消安的药物浓度进行监测可更好发挥其作用并减少不良反应发生的概率。
但由于白消安在不同患者体内的吸收清除速率存在较大差异,其不可预测的生物利用度和药代动力学分布对建立普适性的药物浓度检测方法带来困难[4]。
目前需要进行更多药效学研究以建立全身暴露白消安和疗效之间的量效关系,尤其是在接受骨髓移植的儿童中,基于已知药理学参数精确且有效的白消安血浆水平监测与剂量调整相结合可改善接受骨髓移植患者的临床治疗效果。
纳米药物输送系统的研究
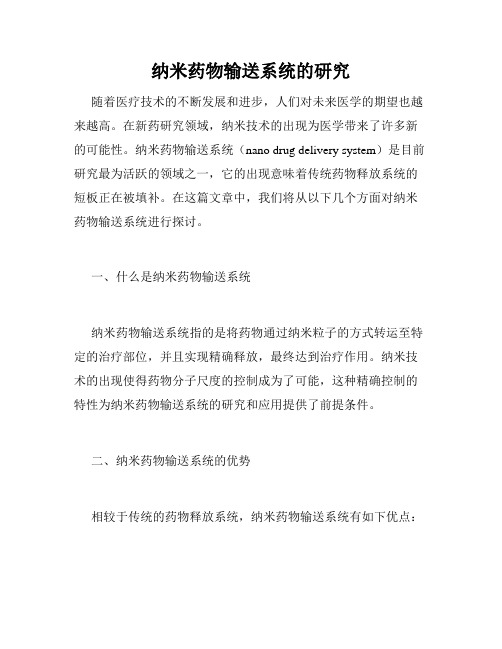
纳米药物输送系统的研究随着医疗技术的不断发展和进步,人们对未来医学的期望也越来越高。
在新药研究领域,纳米技术的出现为医学带来了许多新的可能性。
纳米药物输送系统(nano drug delivery system)是目前研究最为活跃的领域之一,它的出现意味着传统药物释放系统的短板正在被填补。
在这篇文章中,我们将从以下几个方面对纳米药物输送系统进行探讨。
一、什么是纳米药物输送系统纳米药物输送系统指的是将药物通过纳米粒子的方式转运至特定的治疗部位,并且实现精确释放,最终达到治疗作用。
纳米技术的出现使得药物分子尺度的控制成为了可能,这种精确控制的特性为纳米药物输送系统的研究和应用提供了前提条件。
二、纳米药物输送系统的优势相较于传统的药物释放系统,纳米药物输送系统有如下优点:1、精确性更高:药物可以精确地转运到治疗部位,并且精确释放,大大提高了疗效。
2、毒副作用更小:传统药物在体内会受到很多的影响,包括血管、组织等方面的障碍,而纳米粒子的小尺度对周围环境干扰更小,药物可以更加精准地作用于治疗部位,从而减少了毒副作用。
3、更好的药物稳定性:很多药物在体内的生物反应环境下极容易发生分解、降解等现象,而将药物包裹在纳米粒子之中可以有效保护药物,从而保证了药物的稳定性。
4、良好的可控制性:纳米颗粒的可控制性很强,从而可以对药物的释放过程进行精细控制,使其达到最佳治疗效果。
三、纳米药物输送系统的应用纳米药物输送系统的应用领域十分广泛,以下是一些典型的应用场景:1、治疗癌症:纳米药物输送系统可以将药物转运至肿瘤细胞的内部,从而达到治疗的目的。
在肿瘤治疗应用领域,目前已经有不少纳米药物输送系统的商业化产品上市了。
2、治疗炎症:纳米药物输送系统可以将药物转运至炎症部位,减轻病患的疼痛和不适。
3、改善药物传输途径:有些药物在体内的传输受限,而纳米药物输送系统可以将药物转运并使之更容易通过血脑屏障、肠屏障等障碍,从而达到更佳的治疗效果。
- 1、下载文档前请自行甄别文档内容的完整性,平台不提供额外的编辑、内容补充、找答案等附加服务。
- 2、"仅部分预览"的文档,不可在线预览部分如存在完整性等问题,可反馈申请退款(可完整预览的文档不适用该条件!)。
- 3、如文档侵犯您的权益,请联系客服反馈,我们会尽快为您处理(人工客服工作时间:9:00-18:30)。
The effect of the linker on the hydrolysis rate ofdrug-linked ester bondsRonald G.Schoenmakers a,*,Petra van de Wetering a ,Donald L.Elbert b,1,Jeffrey A.Hubbell aaInstitute for Biomedical Engineering,ETH and University of Zurich,Moussonstrasse 18,CH-8044Zurich,SwitzerlandbDepartment of Biomedical Engineering,Washington University,Campus Box 1097,One Brookings Drive,St.Louis,MO 63130-4899,USAReceived 13August 2003;accepted 9December 2003AbstractTailoring the length of a sulfide containing linker adjusts the hydrolysis of a drug-linked ester bond to values appropriate for once-a-week administrations.A model drug of paclitaxel was coupled using a hydrolyzable linker to a poly(ethylene glycol)macromonomer,via a conjugate addition reaction between a thiol and an acrylamide.The macromonomers were synthesized in three steps with an average overall yield of 70%.By changing the length of the linker from 3-sulfanylpropionyl to 4-sulfanylbutyryl,the half-life time of the release of the drug could be increased from 4.2F 0.1to 14.0F 0.2days.Drug-containing hydrogels were prepared by radical photopolymerization of these macromonomers with either the 3-sulfanylpropionyl or the 4-sulfanylbutyryl linker.The release of the drug from these hydrogels followed similar trends as the release of the drug from the soluble polymer–drug conjugates.The synthetic methodology employed does not involve the use of coupling reagents in the final conjugation between the drug and the polymer,excluding the presence of potential toxic residuals.The conjugation method is relatively simple and is applicable to nearly any hydroxyl-containing drugs.D 2004Elsevier B.V .All rights reserved.Keywords:Prodrugs;Drug release;Conjugate addition;Hydrogels;Poly(ethylene glycol)1.IntroductionWater-soluble polymers can be conjugated to drugs to achieve a number of beneficial modifica-tions of the drug’s pharmacokinetics and pharmaco-distribution.With regard to macromolecular drugs,the clearance of protein drugs by the reticulo-endo-thelial system can be substantially reduced by con-jugation to water-soluble polymers; e.g.a 10-fold increase in the plasma circulation time of intrave-nously administered interferon-a 2b was observed for poly(ethylene glycol)(PEG)conjugated interferon-a 2b relative to its native form [1].For low molecular weight drugs,the fraction of drugs directed toward the hyper-permeable vasculature found in tumors relative to the less permeable vasculature found in most normal tissues can be increased by conjugation to water-soluble polymers; e.g.conjugation of the0168-3659/$-see front matter D 2004Elsevier B.V .All rights reserved.doi:10.1016/j.jconrel.2003.12.009*Corresponding author.Tel.:+41-1-6344556;fax:+41-1-632-1214.E-mail address:ronald.schoenmakers@mat.ethz.ch (R.G.Schoenmakers).1Fax:+/locate/jconrelJournal of Controlled Release 95(2004)291–300potent anticancer drug doxorubicin to N-(2-hydroxy-propyl)methacrylamide was observed to increase the fraction of drug targeting tumors,decrease systemic toxicity,and increase therapeutic efficacy[2].With hydrophobic low molecular weight drugs,conjuga-tion to water-soluble polymers can increase solubility and thus enable administration without the use of hydrophobic carrier vehicles like liposomes[3], emulsions[4],and poly(lactic-co-glycolic acid)-based nanoparticles[5];e.g.increased solubility and circu-lation times have been observed in water-soluble polymer-conjugates of doxorubicin[6],camptothecin [7],daunorubicin[8],podophyllotoxin[9].However, an important prerequisite of the resulting polymer–drug conjugates(polymeric prodrugs)is that the drug is regenerated in an acceptable time frame,and no toxic moieties are released or formed due to this in vivo transformation.It has been shown that an effectively permanent conjugation of PEG to pacli-taxel leads to higher circulation times,however,the PEG-conjugate is less active than the native com-pound[10].In addition to applications in polymeric prodrugs and polymer-linked drug conjugates with enhanced solubility,water-soluble polymers can also be used to prepare cross-linked drug delivery systems.The poly-meric carrier can be cross-linked to obtain a three-dimensional depot,from which the drug release pro-file is dependent on the hydrolysis rate of the linker between the drug and the polymer,thermodynamic partitioning of the drug within the cross-linked poly-mer,and the kinetics of diffusion of the drug from the pared to incorporation of drugs within a depot by physicochemical methods,covalent conju-gation provides an additional means by which to control the release rate,potentially decoupling the rate of release from the physicochemical character-istics of the drug and the depot matrix.In both soluble and cross-linked drug-conjugated polymers,of course the nature of the drug–polymer link plays a key role in the rate of drug release by generation of the native drug.Here,we focus on non-enzymatic hydrolytic release, e.g.not considering approaches developed for colonic release using aro-matic azo bonds that can be cleaved by microbial azoreductase activities[11]and intracellular release via lysosomally activated proteases[2,12].We further focus on hydrolytic mechanisms that are not triggered by biological changes in pH,as have been achieved using hydrazone linkages[13,14]and with selected orthoesters[15].Due to their relative sensitivity to hydrolysis,esters have generated substantial interest. However,the ester linkages that have been reported either hydrolyze too slowly for many extracellular applications at physiological pH[16]or too quickly [17–19].Here we present a novel ester linker,formed between a hydroxyl-containing drug and a mercap-toacid.We show that the number of methylene units that intervene between the ester link and the sulfide in the final product modulates the hydrolytic sensi-tivity of the ester in a rather sensitive manner,two yielding hydrolysis over a few days,and three yielding hydrolysis over a few weeks.The hydroly-sis of the ester that has a sulfide at the h or g position yields the native hydroxylated drug.The synthetic route utilized permits facile incorporation into cross-linked gel formulations,and we present an example using PEG-based cross-linked elastic gels. As a therapeutically interesting example,we select a model compound of the low aqueous-solubility anticancer drug paclitaxel,3-(S)-benzoylamino-2-(R)-hydroxy-3-phenyl-propionic acid methyl ester, employing its hydroxyl group in the side chain as the drug conjugation site.2.Materials and methodsAll reagents were obtained from commercial sour-ces and used as purchased.Dichloromethane(DCM) and N,N-dimethylformamide(DMF)were dried on molecular sieves(4A˚).Reactions were monitored by thin layer chromatography(TLC)on Fluka precoated silica gel60F-254(0.25mm)plates.Spots were visualized with UV light,ninhydrin or Cl2-TDM (N,N,N V,N V-tetramethyl-4,4V-diaminodiphenylmethane). Column chromatography was performed on Kieselgel 60(Machery-Nagel,CH).1H NMR spectra were recorded on a Bruker(300.1MHz)spectrometer and chemical shifts are given in ppm(d)relative to TMS as internal standard.13C NMR spectra were recorded on the same spectrometer operating at75.5MHz. Mass spectrometry was carried out on an IonSpec HiResMALDI using2,5-dihydroxybenzoic acid as matrix.R.G.Schoenmakers et al./Journal of Controlled Release95(2004)291–300 2922.1.Syntheses2.1.1.Preparation of3-(S)-benzoylamino-2-(R)-hy-droxy-3-phenyl-propionic acid methyl ester(1) 3-(S)-Benzoylamino-2-(R)-hydroxy-3-phenyl-pro-pionic acid methyl ester(1)was prepared from3-(S)-benzoylamino-2-(R)-hydroxy-3-phenyl-propionic acid in methanol using thionyl chloride according to the method described by Brenner and Huber [20].1H-NMR(CDCl3)d3.38(s,1H,HCO H),3.83(s, 3H,OC H3),4.63(d,1H,C H OH),5.72and5.83(d d, 1H,C H Ph),7.01(d,1H,N H),7.25–7.53(m,23H, Ar),7.76(d t,2H,Ar).13C-NMR(CDCl3)d53.2(C HPh),54.8(H C OH), 73.2(O C H3),126.9–138.7(Ar),166.9(C OOMe), 173.4(C ONH).2.1.2.Preparation of3-(S)-benzoylamino-3-phenyl-2-(R)-(3-sulfanyl-propionyloxy)-propionic acid methyl ester(4a)2.1.2.1.3-Tritylsulfanylpropionic acid(2a).A solu-tion of0.87ml(10mmol)3-mercaptopropionic acid and2.86g(11mmol)triphenylmethanol in40ml DMF was stirred for30min at60j C.After the solution was cooled to ambient temperature,1.43ml (11.4mmol)boron trifluoride ethyl etherate was added and the reaction mixture was stirred at60j C for4h.The solution was concentrated in vacuo and the residue was dissolved in500ml5%NaHCO3.The aqueous solution was washed with250ml diethyl ether,acidified to pH3using1M KHSO4,and extracted twice with500ml ethyl acetate.The com-bined ethyl acetate fractions were washed with250ml brine and dried over Na2SO4.The product was obtained as a white solid in90%yield.1H-NMR(CDCl3)d2.23(t,2H,C H2S),2.46(t, 2H,C H2COOH),7.23–7.43(m,15H,Trt).2.1.2.2.3-(S)-benzoylamino-3-phenyl-2-(R)-(3-trityl-sulfanyl-propionyloxy)-propionic acid methyl ester (3a).A solution of120mg(0.4mmol)of1and 280mg(0.8mmol)3-tritylsulfanyl-propionic acid (2a)in4ml DCM and1ml DMF was cooled to0 j C.Then126A l(0.8mmol)diisopropylcarbodiimide (DIPC)and a few crystals of dimethylaminopyridine (DMAP)were added and the reaction was stirred at0j C for2h.After the reaction was complete based on TLC analysis of the reaction mixture,the solvents were removed in vacuo,and the crude product was dissolved in50ml DCM.The organic solution was washed with1M KHSO4,5%NaHCO3,and brine, consecutively,and dried over Na2SO4.After purifica-tion by column chromatography(silica,eluent:ethyl acetate/hexane1:2v/v),a white solid was obtained with a yield of74%.1H-NMR(CDCl3)d2.00–2.55(m,4H,C H2S andC H2COO),3.67and3.73(s,3H,OC H3),5.51and5.38(d,1H,C H O),5.72and5.83(d d,1H,C H Ph),6.35and6.96(d,1H,N H),7.15–7.59(m,23H,Ar and Trt),7.96and7.76(d t,2H,Ar).13C-NMR(CDCl3)d27.7and26.7(C H2S),35.7 and33.5(C H2COO),52.8and52.9(C HPh),53.3and 53.4(H C O),67.0and67.1(C(Ph)3Trt),74.8and74.5 (O C H3),126.6–144.7(Ar and Trt),165.4and166.9 (C OOMe),168.2(C OOCH),170.5(C ONH).2.1.2.3.3-(S)-benzoylamino-3-phenyl-2-(R)-(3-sul-fanyl-propionyloxy)-propionic acid methyl ester (4a).A solution of126mg of the trityl protected compound3a(0.2mmol)in DCM was added to10ml of a trifluoroacetic acid(TFA),triisopropylsilane (TIS),and water(95:2.5:2.5v/v/v)solution,and the reaction mixture was stirred at room temperature for1 h.The solvents were removed in vacuo,and the product was obtained after column chromatography (silica,eluent:ethyl acetate/hexane1:2v/v)in a quantitative yield.1H-NMR(CDCl3)d1.59–1.64(m,1H,S H),2.67–2.77(m,4H,C H2SH and C H2COO),3.76(s,3H, OC H3),5.50(d,1H,C H O),5.90(d d,1H,C H Ph), 7.04(d,1H,N H),7.26–7.80(m,8H,Ar),7.81(d t, 2H,Ar).13C-NMR(CDCl3)d19.6(C H2SH),38.2 (C H2COO),52.9(C HPh),53.4(C HOCO),74.6 (O C H3),126.6–137.4(Ar),166.9(C OOMe),168.3 (C OOCH),170.4(C ONH).MALDI-TOF MS:m/z=410.1(M+Na).2.1.3.Preparation of3-(S)-benzoylamino-3-phenyl-2-(R)-(4-sulfanyl-butyryloxy)-propionic acid methyl es-ter(4b)2.1.3.1.4-Tritylsulfanylbutyric acid(2b).A solution of0.48g(2mmol)4,4-dithiodibutyric acid in freshlyR.G.Schoenmakers et al./Journal of Controlled Release95(2004)291–300293distilled dioxane(20ml)was stirred under a nitrogen atmosphere.Then0.52ml(2.1mmol)tributylphos-phine and50A l water were added and the reaction mixture was stirred for4h at room temperature.After removing the solvents in vacuo the crude product was dissolved in25ml DMF together with1.15g(4.4 mmol)triphenylmethanol,and the solution was stirred for30min at60j C.After the solution was cooled to ambient temperature,580A l(4.6mmol)boron tri-fluoride ethyl etherate was added and the reaction mixture was stirred overnight at80j C.The solution was concentrated in vacuo and the product purified by column chromatography(silica,eluent:ethyl acetate/ hexane1:1v/v).After recrystallization from ethyl acetate/hexane the product was obtained as white needles with a yield of50%.1H-NMR(CDCl3)d1.67(m,2H,CH2C H2CH2), 2.27(m,4H,C H2S and C H2COOH),7.15–7.48(m, 15H,Trt),10.49(br s,1H,COO H).13C-NMR(CDCl3)d23.8(CH2C H2CH2),31.1 (C H2S),32.9(C H2COOH),66.7(C(Ph)3Trt), 126.6–144.8(Ar Trt),179.0(C OOH).2.1.3.2.3-(S)-benzoylamino-3-phenyl-2-(R)-(4-trityl-sulfanyl-butyryloxy)-propionic acid methyl ester (3b).This reaction of133mg(0.44mmol)of1 and177mg(0.49mmol)4-tritylsulfanylbutyric acid (2b)with76A l(0.49mmol)DIPC and a few crystals of DMAP was performed as described for compound 3a.The product was obtained as a white solid in77% yield after column chromatography(silica,eluent: ethyl acetate/hexane1:3to1:2v/v).1H-NMR(CDCl3)d1.60(m,2H,CH2C H2CH2), 2.20(m,2H,C H2COO),2.33(m,2H,C H2S),3.74(s, 3H,OC H3),5.40(d,1H,C H O),5.84(d d,1H,C H Ph),6.96(br d,1H,N H),7.16–7.54(m,23H,Ar and Trt),7.79(d t,2H,Ar).13C-NMR(CDCl3)d23.7(CH2C H2CH2),31.1 (C H2S),32.7(C H2COO),52.8(C HPh),53.4 (H C OCO),66.7(C(Ph)3Trt),74.3(O C H3),126.5–144.8(Ar and Trt),166.8(C OOMe),168.4(C OOCH), 171.7(C ONH).2.1.3.3.3-(S)-benzoylamino-3-phenyl-2-(R)-(4-sul-fanyl-butyryloxy)-propionic acid methyl ester (4b).The removal of the trityl group from the thiol was performed as described for compound4a.After column chromatography(silica,eluent:ethyl acetate/hexane1:3to1:2v/v)the product was obtained as a white solid in an81%yield.1H-NMR(CDCl3)d1.29(t,1H,S H),1.89(m,2H, CH2C H2CH2),2.53(m,4H,C H2SH and C H2COO),3.77(s,3H,OC H3),5.47(d,1H,C H O),5.89(d d,1H,C H Ph),6.99(d,1H,N H),7.28–7.57(m,8H,Ar),7.80 (m,2H,Ar).13C-NMR(CDCl3)d23.6(CH2C H2CH2),28.7 (C H2SH),32.1(C H2COO),52.9(C HPh),53.4 (C HOCO),74.4(O C H3),126.5–137.5(Ar),166.9 (C OOMe),168.4(C OOCH),171.7(C ONH).MALDI-TOF MS:m/z=424.1(M+Na).2.1.4.Conjugate addition and cross-linking reactions 2.1.4.1.Conjugate addition reaction between4a and PEG-3400diacrylamide.To a solution of10mg(25 A mol)4a and361mg(100A mol)PEG-3400diacry-lamide(5)in1ml dry methanol was added3.3A l(25 A mol)triethanolamine.PEG-3400diacrylamide was synthesized from PEG-3400diamine according to the procedure described by Elbert and Hubbell[21].The reaction mixture was stirred in the dark under an argon atmosphere at room temperature for4h.The solvent was removed by a nitrogen gas flow,and the residue was dissolved in1ml50mM aqueous acetic acid and purified using a PD10column(Pharmacia). The fractions containing PEG were collected and lyophilized.The product(6a)was obtained in a yield of91%(based on PEG-3400diacrylamide).2.1.4.2.Conjugate addition reaction between4b and PEG-3400diacrylamide.The conjugation of11mg (25A mol)4b with361mg(100A mol)PEG-3400 diacrylamide(5)was performed analogous to the conjugation reaction described as above resulting in macromonomer6b(90%yield).2.1.4.3.Formation of cross-linked PEG biomaterial containing covalently bound3-(S)-benzoylamino-2-(R)-hydroxy-3-phenyl-propionic acid methyl ester.To100A l of a10mg mixture of PEG-3400 diacrylamide(5)and PEG-3400a-monoacrylamide, N-mono(3-(S)-benzoylamino-2-(R)-hydroxy-3-phe-nyl-propionic acid methyl ester)(6)from above in a well of a96well plate was added N-vinyl pyrrolidone (0.35A l)and Eosin Y(1A l of a10mM solution in HEPES buffered saline,pH7.4).This solution wasR.G.Schoenmakers et al./Journal of Controlled Release95(2004)291–300 294exposed to visible light at about500nm(75mW/cm2) for1min,producing a cross-linked network.2.2.Release2.2.1.Release from PEG-linked conjugates in solutionA solution of50mg PEG-linked conjugate in1ml 10mM phosphate buffered saline(PBS),pH7.4was incubated at37j C.Periodically,samples of50A l were withdrawn to which1A l1M HCl was added to adjust the pH to4.The samples were stored atÀ30j C until analyzed.At the end of the release study100%release was determined by adjusting the pH to10.Because the methyl ester of the model drug was hydrolyzed during the release experiment,the formation of both 3-(S)-benzoylamino-2-(R)-hydroxy-3-phenyl-pro-pionic acid methyl ester and its acid were determined in order to obtain the total release of model drug from the polymer.The amount of the compounds was measured with HPLC using a reversed phase C18 column(Nova-Pak R C18, 3.9Â150mm,Waters Associates),a Waters2690Separation Module (Waters Associates)and a Waters996Photodiode Array Detector(Waters Associates).A linear gradient of0.1%TFA in water/acetonitrile75:25v/v to25:75 v/v in17.5min at a flow rate of1ml/min was used as elution system.The chromatograms were analyzed with Waters Millennium R32software(version 3.05.01).2.2.2.Release of conjugate from a photopolymerized hydrogelA10%w/v photopolymerized hydrogel from10 mg PEG-linked conjugate was incubated at37j C in1 ml PBS,pH7.4.Periodically,samples of50A l were withdrawn and replaced by fresh buffer.The amount of released compound was measured with HPLC as described above.2.3.Molecular modelingStructures were built using Chem3D7.0(Cam-bridgeSoft).The starting structures were optimized with a series of restricted Hartree-Fock calculations. The geometries of the structures were first optimized with the semi-empirical PM3method[22,23]using MOPAC[24].The obtained structures were then optimized using ab initio methods subsequently employing the small3-21G basis set[25]and the larger6-31G*basis set[26]using GAMESS[27].The atomic charges of the final conformations were cal-culated with the6-31G*basis set.2.4.CMC measurementsThe critical micelle concentrations(CMC)of the macromonomer solutions were determined using a pyrene fluorescence assay.Fluorescence measure-ments were performed on a Perkin Elmer LS50B fluorimeter.A stock solution of0.1M pyrene was made in THF.By diluting the stock solution to2Á10À6 M pyrene in PBS buffer(pH7.4),the pyrene working solution was obtained.Polymer solutions with varying concentrations were made in PBS.Just before the measurement,equal volumes of pyrene working so-lution and polymer solution were combined in a cuvette and stirred.For the measurement of pyrene emission spectra,pyrene was excited at339nm and emissions at373and383nm were taken as first and third vibronic peaks.3.Results and discussionThe conjugation of PEG,as an example of a water-soluble polymer that is synthetically convenient due to its well-defined,telechelic nature,to a model hydroxyl-containing drug,namely3-(S)-benzoyla-mino-2-(R)-hydroxy-3-phenyl-propionic acid methyl ester,is outlined in Fig.1.The first step in the conjugation was coupling of the linker,a protected mercaptoacid,to the hydroxyl moiety of the model drug.We employed the commercially available mer-captoacid3-mercaptopropionic acid and4-mercapto-butyric acid,which was obtained after reduction of its disulfide,4,4V-dithiodibutyric acid.Before coupling the linker to the model drug,the thiol group of the linker was protected with a trityl group using triphe-nylmethanol and boron trifluoride ethyl etherate.The protected linker was subsequently coupled to the hydroxyl group of the model drug via an esterifica-tion using DIPC in the presence of DMAP.Also,the trityl-protected mercaptoacetic acid was used,how-ever,the coupling product with the model drug could not be obtained.After removing the trityl group usingR.G.Schoenmakers et al./Journal of Controlled Release95(2004)291–300295TFA,TIS and water (95:2.5:2.5v/v/v)and purifica-tion,compound 4was attached to PEG-diacrylamide via a conjugate addition reaction between the thiol and acrylamide using triethanolamine as a base.The polymer–drug conjugate was obtained in 3steps with an overall yield of about 70%.One favorable feature of our synthetic scheme is that the drug is coupled to the linker first,allowing the linker-conjugated precursor to be purified by means available to small molecules.In the final step,the linker-conjugated drug is further conjugated to the polymer via a conjugated addition reaction,also known as Michael-type addition.This reaction be-tween polymer and derivatized drug does not require coupling reagents and generates no leaving group,residuals of either of which are often difficult to remove from the macromolecular product.Thus,in contrast to the methods in which the drug is subjected to several modification reactions before obtaining a polymer–drug conjugate,the presence of other reac-tants than used in the Michael-type addition can be excluded in our system.A further convenient feature of the synthetic route we chose may be seen in the generation of the cross-linked solid final form.By using an excess of PEG-diacrylamide in the conjugate addition to the linker-conjugated drug,a mixture of a heterobifunctional macromonomer 6with a homobi-functional PEG-diacrylamide (5)is obtained.Thus,the drug-linked macromonomer 6may be copolymer-ized with the PEG-diacrylamide that is also produced to obtain a final cross-linked PEG-based hydrogel.The aqueous solubilities of these polymer–drug conjugates 6a and 6b were similar to that of the PEG-diacrylamide employed.The release of the model drug in vitro under physiological conditions was first inves-tigated from the soluble,i.e.non-cross-linked,drug-conjugated polymer to allow measurement of release without concerns of the effect of the gel environment upon hydrolysis.The measured release profiles are shown in Fig.2and can be well described by first-order kinetics.Fig.2clearly shows the influence of the linker on the release rate,which is reflected in the exponential estimated half-lives for hydrolysis of 4.2F 0.1and 14.0F 0.2days for the 3-sulfanylpro-pionyl and 4-sulfanylbutyryl linkers,respectively.In order to get a better insight into the relation between structure and hydrolysis kinetics,molecular modeling experiments were performed.Besides the 3-sulfanylpropionyl and 4-sulfanylbutyryl linker,also the 2-sulfanylacetyl linker,which is expected to have a shorter half-life than the other two linkers,was included in the modeling experiments.To investigate the probability of a hydroxyl attack on the carbonyl group of the ester of interest,the atomic charge on the carbon atom of this carbonyl group was calculated.As can be seen in Fig.3,the atomic charge on the carbon atom decreases with an increasing number of methy-lene units between the ester bond and the sulfide.From these results it can be expected that the hydro-lysis rate of the ester decreases with an increasing linker length.This is in accordance with the observed difference in hydrolysis half-lives between the 3-sulfanylpropionyl and 4-sulfanylbutyryl linker.As described above,use of a slight excess of PEG-diacrylamide in the synthesis scheme results in a mixture of the macromonomer of the drug andPEG-Fig.1.Synthesis of the model drug-conjugated macromonomer,which can then be further polymerized to form a high polymer or cross-linked hydrogel (m =2:3-sulfanylpropionyl linker,m =3:4-sulfanylbutyryl linker).R.G.Schoenmakers et al./Journal of Controlled Release 95(2004)291–300296diacrylamide,which can then be polymerized into a cross-linked hydrogel drug delivery matrix by radical polymerization or a conjugate addition reaction with a compound containing multiple thiol groups.In this study,hydrogels were made from both3-(S)-ben-zoylamino-2-(R)-hydroxy-3-phenyl-propionic acid methyl ester-PEG conjugates6a and6b,by radical photopolymerization using the method described by Elbert and Hubbell[21].After preparation at a total solids content of10%w/v in water,the obtained hydrogels swelled an additional22F2%during the incubation in buffer at37j C to an equilibrium solids content of8%w/v.The release of the model drug conjugated into the hydrogels via both the3-sulfa-nylpropionyl or4-sulfanylbutyryl linker is presented in Fig.4.As observed for the PEG conjugates in solution,the release of the model drug is dependent on the linker length.However,in the case of the gels, the release profiles for both linkers could only be described by first-order kinetics until about45%of the total release.Above45%,the release gradually starts to deviate from a first order profile.This Fig.3.Optimized structures of the different linkers(A:2-sulfanylacetyl,B:3-sulfanylpropionyl,and C:4-sulfanylbutyryl)attached to the model drug3-(S)-benzoylamino-2-(R)-hydroxy-3-phenyl-propionic acid methyl ester,and the atomic charge on the carbon atom of the esterbond. Fig.2.Release of model drug from the PEG conjugates in solution(PBS,pH7.4,37j C,n=3,F S.D.n:3-sulfanylpropionyl linker;b:4-sulfanylbutyryl linker.R.G.Schoenmakers et al./Journal of Controlled Release95(2004)291–300297suggests a physicochemical effect that modulates release,in addition to hydrolysis from the linker,such as diffusion through the gel phase after hydro-lysis.Because both gels (with the 3-sulfanylpropionyl and with the 4-sulfanylbutyryl linker)have practically identical architecture,the release curves of the model drug from the gels approach and overlap each other in the course of time.It has been reported by several groups that PEG modified with hydrophobic end groups forms micelles [28–30].The drug-conjugated PEG macromonomer might also be able to form micelles consisting of a hydrophobic core in which the drug is concentrated,and a surrounding hydrophilic PEG layer.Due to the hydrophobic environment of the core the hydrolysis rate could be lower compared to the release rate in a hydrophilic environment like in a hydrogel.Whether the soluble polymer–drug conjugates were able to form micelles was checked with a pyrene assay.Indeed,the presence of hydrophobic nanodomains was observed,both in the case of the drug-conjugated macromonomer and the PEG-diacrylamide,with CMC values in the range 0.03–0.06mM,similar to values reported previously for PEGs monofunctional-ized with hydrophobic groups [28,29].However,comparing the release curves of the model drug fromthe polymer–drug conjugates in solution (Fig.2)and the release of the model drug from the hydrogels (Fig.4),it can be seen that release from the hydrogels is not significantly faster than release from the soluble polymer–drug conjugates for this effect to be consid-ered an important modulator of hydrolysis rate.This can be explained by the exchange of the polymers between the micelle and sol phases,as described by Yamamoto et al.[31].Alternatively,the macromono-mer might be polymerized in the micellar phase.Both cases result in a similar exposure of the ester bond in the hydrogel and in the soluble polymer–drug conju-gate to the medium in the time frame of the release.4.ConclusionsEster linkages between drugs and polymeric carries have been utilized previously in polymeric prodrugs,however at physiological,extracellular pH,particular esters with desirable hydrolysis rates have not been described to enable formulations suitable for once-a-week pletely aliphatic esters of paclitaxel are hydrophobic and extremely stable [16].On the other hand,when the linker between drug and PEG is e.g.an amino acid (derivative)orsuccinicFig.4.Release of model drug from PEG-diacrylamide-cross-linked hydrogels formed by photopolymerization (PBS,pH 7.4,37j C,n =3,F S.D.n :3-sulfanylpropionyl linker;b:4-sulfanylbutyryl linker.R.G.Schoenmakers et al./Journal of Controlled Release 95(2004)291–300298acid,the half-lives are in the order of several hours to a few days,too short for many applications[17–19].Here,we present a conjugation methodology using a drug-linked ester bond with hydrolysis half-life times in the order of a few days to a few weeks. The number of methylene units between these two moieties could be employed to dial in a convenient hydrolysis rate.Although the details are under current investigation,it may be that the effect of an increasing number of methylene units on the ester half-life can be explained by a higher hydrophobicity in the vicinity of the drug-linked ester bond or an inducing effect of the sulfide on the ester bond.The synthetic methodology we report here to pre-pare sulfide containing ester linkages is relatively simple and is applicable to nearly any hydroxyl-con-taining drug;the drug must only be sufficiently stable to survive cleavage of the protecting group from the thiol prior to the conjugate addition reaction to form the final adduct.This versatile method readily formed PEG-based hydrogels after photopolymerization,and other routes to formation of gels or soluble polymer can be easily envisioned.AcknowledgementsThe authors would like to thank Rolf Ha¨fliger from the Laboratory of Organic Chemistry Mass Spectrom-etry Service,ETH Zu¨rich for recording the mass spectra.References[1]P.Glue,J.W.S.Fang,R.Rouzier-Panis,C.Raffanel,R.Sabo,S.K.Gupta,M.Salfi,S.Jacobs,The Hepatitus C Intervention Therapy Group,Pegylated interferon-a2b:pharmacokinetics, pharmacodynamics,safety,and preliminary efficacy data, Clin.Pharmacol.Ther.68(2000)556–567.[2]P.A.Vasey,S.B.Kaye,R.Morrison,C.Twelves,P.Wilson,R.Duncan,A.H.Thomson,L.S.Murray,T.E.Hilditch,T.Mur-ray,S.Burtles,D.Fraier,E.Frigerio,J.Cassidy,Phase I clinical and pharmacokinetic study of PK1[N-(2-hydroxypro-pyl)methacrylamide copolymer doxorubicin]:first member ofa new class of chemotherapeutic agents-drug–polymer con-jugates,Clin.Cancer Res.5(1999)83–94.[3] A.Sharma,R.M.Straubinger,Novel taxol formulations:prep-aration and characterization of taxol-containing liposomes, Pharm.Res.11(1994)889–896.[4] B.D.Tarr,T.G.Sambandan,S.H.Yalkowsky,A new paren-teral emulsion for the administration of taxol,Pharm.Res.4 (1987)162–165.[5] C.Fonseca,S.Simo˜es,R.Gaspa,Paclitaxel-loaded PLGAnanoparticles:preparation,physicochemical characterization and in vivo anti-tumoral activity,J.Control.Release83 (2002)273–286.[6]J.Kopecˇek,P.Rejmanova´,R.Duncan,J.B.Lloyd,Controlledrelease of drug model from N-[2-hydroxypropyl]methacryla-mide copolymers,Ann.N.Y.Acad.Sci.446(1985)93–104.[7]R.B.Greenwald,A.Pendri,C.D.Conover,C.Lee,Y.H.Choe,C.Gilbert,A.Martinez,J.Xia,D.Wu,M.M.Hsue,Campto-thecin20-PEG ester transport forms:the effect of spacer groups on antitumor activity,Bioorg.Med.Chem.6(1998) 551–562.[8] B.Rˇı´hova´,P.Kopecˇkova´,J.Strohalm,P.Rossmann,V.Vet-vicka,J.Kopecˇek,Antibody directed affinity therapy applied to the immune system:in vivo effectiveness and limited tox-icity of daunomycin conjugates to HPMA co-polymers and targeting antibody,Clin.Immunol.Immunopathol.46(1988) 100–114.[9]R.B.Greenwald,C.D.Conover,A.Pendri,Y.H.Choe,A.Martinez, D.Wu,S.Guan,Z.Yao,K.L.Shum,Drug delivery of anticancer agents:water soluble4-poly(ethylene glycol)derivatives of the lignan,podophyllotoxin,J.Control.Release61(1999)281–294.[10]R.B.Greenwald,A.Pendri,D.Bolikal,Highly water-solubletaxol derivatives:7-polyethylene glycol carbamates and car-bonates,.Chem.60(1995)331–336.[11]S.Sakuma,Z.R.Lu,B.Pecharova´,P.Kopecˇkova´,J.Kopecˇek,N-(2-Hydroxypropyl)-methacrylamide copolymer-9-amino-camptothecin conjugate:colon-specific drug delivery in rats, pat.Polym.17(2002)305–319.[12] D.A.Putnam,J.G.Shiah,J.Kopecˇek,Intracellularly biorecog-nizable derivatives of5-Fluorouracil.Implications for site-spe-cific delivery in the human condition,Biochem.Pharmacol.52(1996)957–962.[13]T.Etrych,M.Jelı´nkova´,B.Rˇı´hova´,K.Ulbrich,New HPMAcopolymers containing doxorubicin bound via pH-sensitive linkage:synthesis and preliminary in vitro and in vivo biolog-ical properties,J.Control.Release73(2000)89–102. [14]K.H.Bouhadir,G.M.Kruger,K.Y.Lee,D.J.Mooney,Sus-tained and controlled release of daunomycin from cross-linked poly(aldehyde guluronate)hydrogels,J.Pharm.Sci.89(2000) 910–919.[15]J.Heller,J.Barr,S.Y.Ng,K.Schwach-Abdellauoi,R.Gurny,Poly(ortho esters):synthesis,characterization,properties and uses,Adv.Drug Deliv.Rev.54(2002)1015–1039.[16]S.Ali,I.Ahmad,A.Peters,G.Masters,S.Minchey,A.Janoff,E.Mayhew,Hydrolyzable hydrophobic taxanes:synthesis andanti-cancer activities,Anti-Cancer Drugs12(2001)117–128.[17] A.Pendri,C.D.Conover,R.B.Greenwald,Antitumor activityof paclitaxel-2V-glycinate conjugated to poly(ethylene glycol):a water-soluble prodrug,Anti-Cancer Drug Des.13(1998)387–395.[18] A.E.Mathew,M.R.Mejillano,J.P.Nath,R.H.Himes,V.J.Stella,Synthesis and evaluation of some water-soluble pro-R.G.Schoenmakers et al./Journal of Controlled Release95(2004)291–300299。