Rate-Based Transport Control for Mobile Ad Hoc Networks
外文文献文献列表
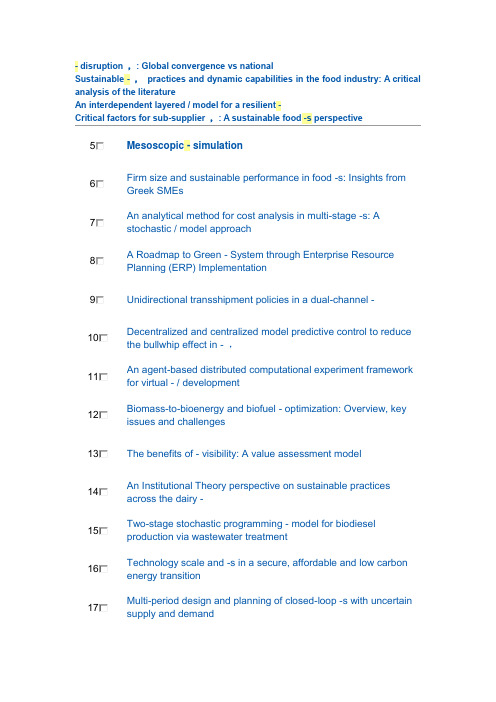
- disruption ,: Global convergence vs nationalSustainable - ,practices and dynamic capabilities in the food industry: A critical analysis of the literature5 Mesoscopic - simulation6 Firm size and sustainable performance in food -s: Insights from Greek SMEs7 An analytical method for cost analysis in multi-stage -s: A stochastic / model approach8 A Roadmap to Green - System through Enterprise Resource Planning (ERP) Implementation9 Unidirectional transshipment policies in a dual-channel -10 Decentralized and centralized model predictive control to reduce the bullwhip effect in - ,11 An agent-based distributed computational experiment framework for virtual - / development12 Biomass-to-bioenergy and biofuel - optimization: Overview, key issues and challenges13 The benefits of - visibility: A value assessment model14 An Institutional Theory perspective on sustainable practices across the dairy -15 Two-stage stochastic programming - model for biodiesel production via wastewater treatment16 Technology scale and -s in a secure, affordable and low carbon energy transition17 Multi-period design and planning of closed-loop -s with uncertain supply and demand18 Quality control in food - ,: An analytical model and case study of the adulterated milk incident in China19 - information capabilities and performance outcomes: An empirical study of Korean steel suppliers20 A game-based approach towards facilitating decision making for perishable products: An example of blood -21 - design under quality disruptions and tainted materials delivery22 A two-level replenishment frequency model for TOC - replenishment systems under capacity constraint23 - dynamics and the ―cross-border effect‖: The U.S.–Mexican border’s case24 Designing a new - for competition against an existing -25 Universal supplier selection via multi-dimensional auction mechanisms for two-way competition in oligopoly market of -26 Using TODIM to evaluate green - practices under uncertainty27 - downsizing under bankruptcy: A robust optimization approach28 Coordination mechanism for a deteriorating item in a two-level - system29 An accelerated Benders decomposition algorithm for sustainable - / design under uncertainty: A case study of medical needle and syringe -30 Bullwhip Effect Study in a Constrained -31 Two-echelon multiple-vehicle location–routing problem with time windows for optimization of sustainable - / of perishable food32 Research on pricing and coordination strategy of green - under hybrid production mode33 Agent-system co-development in - research: Propositions and demonstrative findings34 Tactical ,for coordinated -s35 Photovoltaic - coordination with strategic consumers in China36 Coordinating supplier׳s reorder point: A coordination mechanism for -s with long supplier lead time37 Assessment and optimization of forest biomass -s from economic, social and environmental perspectives – A review of literature38 The effects of a trust mechanism on a dynamic - /39 Economic and environmental assessment of reusable plastic containers: A food catering - case study40 Competitive pricing and ordering decisions in a multiple-channel -41 Pricing in a - for auction bidding under information asymmetry42 Dynamic analysis of feasibility in ethanol - for biofuel production in Mexico43 The impact of partial information sharing in a two-echelon -44 Choice of - governance: Self-managing or outsourcing?45 Joint production and delivery lot sizing for a make-to-order producer–buyer - with transportation cost46 Hybrid algorithm for a vendor managed inventory system in a two-echelon -47 Traceability in a food -: Safety and quality perspectives48 Transferring and sharing exchange-rate risk in a risk-averse - of a multinational firm49 Analyzing the impacts of carbon regulatory mechanisms on supplier and mode selection decisions: An application to a biofuel -50 Product quality and return policy in a - under risk aversion of a supplier51 Mining logistics data to assure the quality in a sustainable food -: A case in the red wine industry52 Biomass - optimisation for Organosolv-based biorefineries53 Exact solutions to the - equations for arbitrary, time-dependent demands54 Designing a sustainable closed-loop - / based on triple bottom line approach: A comparison of metaheuristics hybridization techniques55 A study of the LCA based biofuel - multi-objective optimization model with multi-conversion paths in China56 A hybrid two-stock inventory control model for a reverse -57 Dynamics of judicial service -s58 Optimizing an integrated vendor-managed inventory system for a single-vendor two-buyer - with determining weighting factor for vendor׳s ordering59 Measuring - Resilience Using a Deterministic Modeling Approach60 A LCA Based Biofuel - Analysis Framework61 A neo-institutional perspective of -s and energy security: Bioenergy in the UK62 Modified penalty function method for optimal social welfare of electric power - with transmission constraints63 Optimization of blood - with shortened shelf lives and ABO compatibility64 Diversified firms on dynamical - cope with financial crisis better65 Securitization of energy -s in China66 Optimal design of the auto parts - for JIT operations: Sequential bifurcation factor screening and multi-response surface methodology67 Achieving sustainable -s through energy justice68 - agility: Securing performance for Chinese manufacturers69 Energy price risk and the sustainability of demand side -s70 Strategic and tactical mathematical programming models within the crude oil - context - A review71 An analysis of the structural complexity of - /s72 Business process re-design methodology to support - integration73 Could - technology improve food operators’ innovativeness? A developing country’s perspective74 RFID-enabled process reengineering of closed-loop -s in the healthcare industry of Singapore75 Order-Up-To policies in Information Exchange -s76 Robust design and operations of hydrocarbon biofuel - integrating with existing petroleum refineries considering unit cost objective77 Trade-offs in - transparency: the case of Nudie Jeans78 Healthcare - operations: Why are doctors reluctant to consolidate?79 Impact on the optimal design of bioethanol -s by a new European Commission proposal80 Managerial research on the pharmaceutical - – A critical review and some insights for future directions81 - performance evaluation with data envelopment analysis and balanced scorecard approach82 Integrated - design for commodity chemicals production via woody biomass fast pyrolysis and upgrading83 Governance of sustainable -s in the fast fashion industry84 Temperature ,for the quality assurance of a perishable food -85 Modeling of biomass-to-energy - operations: Applications, challenges and research directions86 Assessing Risk Factors in Collaborative - with the Analytic Hierarchy Process (AHP)87 Random / models and sensitivity algorithms for the analysis of ordering time and inventory state in multi-stage -s88 Information sharing and collaborative behaviors in enabling - performance: A social exchange perspective89 The coordinating contracts for a fuzzy - with effort and price dependent demand90 Criticality analysis and the -: Leveraging representational assurance91 Economic model predictive control for inventory ,in -s92 - ,ontology from an ontology engineering perspective93 Surplus division and investment incentives in -s: A biform-game analysis94 Biofuels for road transport: Analysing evolving -s in Sweden from an energy security perspective95 - ,executives in corporate upper echelons Original Research Article96 Sustainable - ,in the fast fashion industry: An analysis of corporate reports97 An improved method for managing catastrophic - disruptions98 The equilibrium of closed-loop - super/ with time-dependent parameters99 A bi-objective stochastic programming model for a centralized green - with deteriorating products100 Simultaneous control of vehicle routing and inventory for dynamic inbound -101 Environmental impacts of roundwood - options in Michigan: life-cycle assessment of harvest and transport stages102 A recovery mechanism for a two echelon - system under supply disruption103 Challenges and Competitiveness Indicators for the Sustainable Development of the - in Food Industry104 Is doing more doing better? The relationship between responsible - ,and corporate reputation105 Connecting product design, process and - decisions to strengthen global - capabilities106 A computational study for common / design in multi-commodity -s107 Optimal production and procurement decisions in a - with an option contract and partial backordering under uncertainties108 Methods to optimise the design and ,of biomass-for-bioenergy -s: A review109 Reverse - coordination by revenue sharing contract: A case for the personal computers industry110 SCOlog: A logic-based approach to analysing - operation dynamics111 Removing the blinders: A literature review on the potential of nanoscale technologies for the ,of -s112 Transition inertia due to competition in -s with remanufacturing and recycling: A systems dynamics mode113 Optimal design of advanced drop-in hydrocarbon biofuel - integrating with existing petroleum refineries under uncertainty114 Revenue-sharing contracts across an extended -115 An integrated revenue sharing and quantity discounts contract for coordinating a - dealing with short life-cycle products116 Total JIT (T-JIT) and its impact on - competency and organizational performance117 Logistical - design for bioeconomy applications118 A note on ―Quality investment and inspection policy in a supplier-manufacturer -‖119 Developing a Resilient -120 Cyber - risk ,: Revolutionizing the strategic control of critical IT systems121 Defining value chain architectures: Linking strategic value creation to operational - design122 Aligning the sustainable - to green marketing needs: A case study123 Decision support and intelligent systems in the textile and apparel -: An academic review of research articles124 - ,capability of small and medium sized family businesses in India: A multiple case study approach125 - collaboration: Impact of success in long-term partnerships126 Collaboration capacity for sustainable - ,: small and medium-sized enterprises in Mexico127 Advanced traceability system in aquaculture -128 - information systems strategy: Impacts on - performance and firm performance129 Performance of - collaboration – A simulation study130 Coordinating a three-level - with delay in payments and a discounted interest rate131 An integrated framework for agent basedinventory–production–transportation modeling and distributed simulation of -s132 Optimal - design and ,over a multi-period horizon under demand uncertainty. Part I: MINLP and MILP models133 The impact of knowledge transfer and complexity on - flexibility: A knowledge-based view134 An innovative - performance measurement system incorporating Research and Development (R&D) and marketing policy135 Robust decision making for hybrid process - systems via model predictive control136 Combined pricing and - operations under price-dependent stochastic demand137 Balancing - competitiveness and robustness through ―virtual dual sourcing‖: Lessons from the Great East Japan Earthquake138 Solving a tri-objective - problem with modified NSGA-II algorithm 139 Sustaining long-term - partnerships using price-only contracts 140 On the impact of advertising initiatives in -s141 A typology of the situations of cooperation in -s142 A structured analysis of operations and - ,research in healthcare (1982–2011143 - practice and information quality: A - strategy study144 Manufacturer's pricing strategy in a two-level - with competing retailers and advertising cost dependent demand145 Closed-loop - / design under a fuzzy environment146 Timing and eco(nomic) efficiency of climate-friendly investments in -s147 Post-seismic - risk ,: A system dynamics disruption analysis approach for inventory and logistics planning148 The relationship between legitimacy, reputation, sustainability and branding for companies and their -s149 Linking - configuration to - perfrmance: A discrete event simulation model150 An integrated multi-objective model for allocating the limited sources in a multiple multi-stage lean -151 Price and leadtime competition, and coordination for make-to-order -s152 A model of resilient - / design: A two-stage programming with fuzzy shortest path153 Lead time variation control using reliable shipment equipment: An incentive scheme for - coordination154 Interpreting - dynamics: A quasi-chaos perspective155 A production-inventory model for a two-echelon - when demand is dependent on sales teams׳ initiatives156 Coordinating a dual-channel - with risk-averse under a two-way revenue sharing contract157 Energy supply planning and - optimization under uncertainty158 A hierarchical model of the impact of RFID practices on retail - performance159 An optimal solution to a three echelon - / with multi-product and multi-period160 A multi-echelon - model for municipal solid waste ,system 161 A multi-objective approach to - visibility and risk162 An integrated - model with errors in quality inspection and learning in production163 A fuzzy AHP-TOPSIS framework for ranking the solutions of Knowledge ,adoption in - to overcome its barriers164 A relational study of - agility, competitiveness and business performance in the oil and gas industry165 Cyber - security practices DNA – Filling in the puzzle using a diverse set of disciplines166 A three layer - model with multiple suppliers, manufacturers and retailers for multiple items167 Innovations in low input and organic dairy -s—What is acceptable in Europe168 Risk Variables in Wind Power -169 An analysis of - strategies in the regenerative medicine industry—Implications for future development170 A note on - coordination for joint determination of order quantity and reorder point using a credit option171 Implementation of a responsive - strategy in global complexity: The case of manufacturing firms172 - scheduling at the manufacturer to minimize inventory holding and delivery costs173 GBOM-oriented ,of production disruption risk and optimization of - construction175 Alliance or no alliance—Bargaining power in competing reverse -s174 Climate change risks and adaptation options across Australian seafood -s – A preliminary assessment176 Designing contracts for a closed-loop - under information asymmetry 177 Chemical - modeling for analysis of homeland security178 Chain liability in multitier -s? Responsibility attributions for unsustainable supplier behavior179 Quantifying the efficiency of price-only contracts in push -s over demand distributions of known supports180 Closed-loop - / design: A financial approach181 An integrated - / design problem for bidirectional flows182 Integrating multimodal transport into cellulosic biofuel - design under feedstock seasonality with a case study based on California183 - dynamic configuration as a result of new product development184 A genetic algorithm for optimizing defective goods - costs using JIT logistics and each-cycle lengths185 A - / design model for biomass co-firing in coal-fired power plants 186 Finance sourcing in a -187 Data quality for data science, predictive analytics, and big data in - ,: An introduction to the problem and suggestions for research and applications188 Consumer returns in a decentralized -189 Cost-based pricing model with value-added tax and corporate income tax for a - /190 A hard nut to crack! Implementing - sustainability in an emerging economy191 Optimal location of spelling yards for the northern Australian beef -192 Coordination of a socially responsible - using revenue sharing contract193 Multi-criteria decision making based on trust and reputation in -194 Hydrogen - architecture for bottom-up energy systems models. Part 1: Developing pathways195 Financialization across the Pacific: Manufacturing cost ratios, -s and power196 Integrating deterioration and lifetime constraints in production and - planning: A survey197 Joint economic lot sizing problem for a three—Layer - with stochastic demand198 Mean-risk analysis of radio frequency identification technology in - with inventory misplacement: Risk-sharing and coordination199 Dynamic impact on global -s performance of disruptions propagation produced by terrorist acts。
04 Coverage control for mobile sensing networks
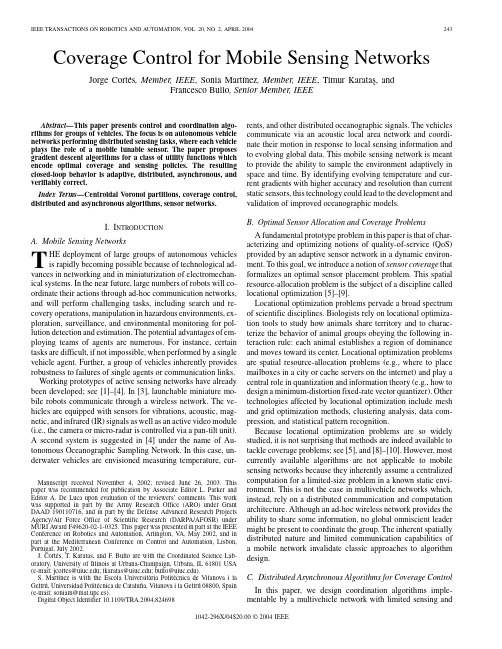
IEEE TRANSACTIONS ON ROBOTICS AND AUTOMATION, VOL. 20, NO. 2, APRIL 2004243Coverage Control for Mobile Sensing NetworksJorge Cortés, Member, IEEE, Sonia Martínez, Member, IEEE, Timur Karatas, and ¸ Francesco Bullo, Senior Member, IEEEAbstract—This paper presents control and coordination algorithms for groups of vehicles. The focus is on autonomous vehicle networks performing distributed sensing tasks, where each vehicle plays the role of a mobile tunable sensor. The paper proposes gradient descent algorithms for a class of utility functions which encode optimal coverage and sensing policies. The resulting closed-loop behavior is adaptive, distributed, asynchronous, and verifiably correct. Index Terms—Centroidal Voronoi partitions, coverage control, distributed and asynchronous algorithms, sensor networks.rents, and other distributed oceanographic signals. The vehicles communicate via an acoustic local area network and coordinate their motion in response to local sensing information and to evolving global data. This mobile sensing network is meant to provide the ability to sample the environment adaptively in space and time. By identifying evolving temperature and current gradients with higher accuracy and resolution than current static sensors, this technology could lead to the development and validation of improved oceanographic models. B. Optimal Sensor Allocation and Coverage Problems A fundamental prototype problem in this paper is that of characterizing and optimizing notions of quality-of-service (QoS) provided by an adaptive sensor network in a dynamic environment. To this goal, we introduce a notion of sensor coverage that formalizes an optimal sensor placement problem. This spatial resource-allocation problem is the subject of a discipline called locational optimization [5]–[9]. Locational optimization problems pervade a broad spectrum of scientific disciplines. Biologists rely on locational optimization tools to study how animals share territory and to characterize the behavior of animal groups obeying the following interaction rule: each animal establishes a region of dominance and moves toward its center. Locational optimization problems are spatial resource-allocation problems (e.g., where to place mailboxes in a city or cache servers on the internet) and play a central role in quantization and information theory (e.g., how to design a minimum-distortion fixed-rate vector quantizer). Other technologies affected by locational optimization include mesh and grid optimization methods, clustering analysis, data compression, and statistical pattern recognition. Because locational optimization problems are so widely studied, it is not surprising that methods are indeed available to tackle coverage problems; see [5], and [8]–[10]. However, most currently available algorithms are not applicable to mobile sensing networks because they inherently assume a centralized computation for a limited-size problem in a known static environment. This is not the case in multivehicle networks which, instead, rely on a distributed communication and computation architecture. Although an ad-hoc wireless network provides the ability to share some information, no global omniscient leader might be present to coordinate the group. The inherent spatially distributed nature and limited communication capabilities of a mobile network invalidate classic approaches to algorithm design. C. Distributed Asynchronous Algorithms for Coverage Control In this paper, we design coordination algorithms implementable by a multivehicle network with limited sensing andI. INTRODUCTION A. Mobile Sensing NetworksTHE deployment of large groups of autonomous vehicles is rapidly becoming possible because of technological advances in networking and in miniaturization of electromechanical systems. In the near future, large numbers of robots will coordinate their actions through ad-hoc communication networks, and will perform challenging tasks, including search and recovery operations, manipulation in hazardous environments, exploration, surveillance, and environmental monitoring for pollution detection and estimation. The potential advantages of employing teams of agents are numerous. For instance, certain tasks are difficult, if not impossible, when performed by a single vehicle agent. Further, a group of vehicles inherently provides robustness to failures of single agents or communication links. Working prototypes of active sensing networks have already been developed; see [1]–[4]. In [3], launchable miniature mobile robots communicate through a wireless network. The vehicles are equipped with sensors for vibrations, acoustic, magnetic, and infrared (IR) signals as well as an active video module (i.e., the camera or micro-radar is controlled via a pan-tilt unit). A second system is suggested in [4] under the name of Autonomous Oceanographic Sampling Network. In this case, underwater vehicles are envisioned measuring temperature, cur-Manuscript received November 4, 2002; revised June 26, 2003. This paper was recommended for publication by Associate Editor L. Parker and Editor A. De Luca upon evaluation of the reviewers’ comments. This work was supported in part by the Army Research Office (ARO) under Grant DAAD 190110716, and in part by the Defense Advanced Research Projects Agency/Air Force Office of Scientific Research (DARPA/AFOSR) under MURI Award F49620-02-1-0325. This paper was presented in part at the IEEE Conference on Robotics and Automation, Arlington, VA, May 2002, and in part at the Mediterranean Conference on Control and Automation, Lisbon, Portugal, July 2002. J. Cortés, T. Karatas, and F. Bullo are with the Coordinated Science Laboratory, University of Illinois at Urbana-Champaign, Urbana, IL 61801 USA (e-mail: jcortes@; tkaratas@; bullo@). S. Martínez is with the Escola Universitària Politècnica de Vilanova i la Geltrú, Universidad Politécnica de Cataluña, Vilanova i la Geltrú 08800, Spain (e-mail: soniam@mat.upc.es). Digital Object Identifier 10.1109/TRA.2004.8246981042-296X/04$20.00 © 2004 IEEE244IEEE TRANSACTIONS ON ROBOTICS AND AUTOMATION, VOL. 20, NO. 2, APRIL 2004communication capabilities. Our approach is related to the classic Lloyd algorithm from quantization theory; see [11] for a reprint of the original report and [12] for a historical overview. We present Lloyd descent algorithms that take into careful consideration all constraints on the mobile sensing network. In particular, we design coverage algorithms that are adaptive, distributed, asynchronous, and verifiably asymptotically correct. Adaptive: Our coverage algorithms provide the network with the ability to address changing environments, sensing task, and network topology (due to agents’ departures, arrivals, or failures). Distributed: Our coverage algorithms are distributed in the sense that the behavior of each vehicle depends only on the location of its neighbors. Also, our algorithms do not require a fixed-topology communication graph, i.e., the neighborhood relationships do change as the network evolves. The advantages of distributed algorithms are scalability and robustness. Asynchronous: Our coverage algorithms are amenable to asynchronous implementation. This means that the algorithms can be implemented in a network composed of agents evolving at different speeds, with different computation and communication capabilities. Furthermore, our algorithms do not require a global synchronization, and convergence properties are preserved, even if information about neighboring vehicles propagates with some delay. An advantage of asynchronism is a minimized communication overhead. Verifiable Asymptotically Correct: Our algorithms guarantee monotonic descent of the cost function encoding the sensing task. Asymptotically, the evolution of the mobile sensing network is guaranteed to converge to so-called centroidal Voronoi configurations (i.e., configurations where the location of each generator coincides with the centroid of the corresponding Voronoi cell) that are critical points of the optimal sensor-coverage problem. Let us describe the contributions of this paper in some detail. Section II reviews certain locational optimization problems and their solutions as centroidal Voronoi partitions. Section III provides a continuous-time version of the classic Lloyd algorithm from vector quantization and applies it to the setting of multivehicle networks. In discrete time, we propose a family of Lloyd algorithms. We carefully characterize convergence properties for both continuous and discrete-time versions (Appendix I collects some relevant facts on descent flows). We discuss a worst-case optimization problem, we investigate a simple uniform planar setting, and we present simulation results. Section IV presents two asynchronous distributed implementations of Lloyd algorithm for ad-hoc networks with communication and sensing capabilities. Our treatment carefully accounts for the constraints imposed by the distributed nature of the vehicle network. We present two asynchronous implementations, one based on classic results on distributed gradient flows, the other based on the structure of the coverage problem. (Appendix II briefly reviews some known results on asynchronous gradient algorithms.)Section V-A considers vehicle models with more realistic dynamics. We present two formal results on passive vehicle dynamics and on vehicles equipped with individual local controllers. We present numerical simulations of passive vehicle models and of unicycle mobile vehicles. Next, Section V-B describes density functions that lead the multivehicle network to predetermined geometric patterns. We present our conclusions and directions for future research in Section VI. D. Review of Distributed Algorithms for Cooperative Control Recent years have witnessed a large research effort focused on motion planning and coordination problems for multivehicle systems. Issues include geometric patterns [13]–[16], formation control [17], [18], gradient climbing [19], and conflict avoidance [20]. It is only recently, however, that truly distributed coordination laws for dynamic networks are being proposed; e.g., see [21]–[23]. Heuristic approaches to the design of interaction rules and emerging behaviors have been throughly investigated within the literature on behavior-based robotics; see [17], and [24]–[28]. An example of coverage control is discussed in [29]. Along this line of research, algorithms have been designed for sophisticated cooperative tasks. However, no formal results are currently available on how to design reactive control laws, ensure their correctness, and guarantee their optimality with respect to an aggregate objective. The study of distributed algorithms is concerned with providing mathematical models, devising precise specifications for their behavior, and formally proving their correctness and complexity. Via an automata-theoretic approach, the references [30] and [31] treat distributed consensus, resource allocation, communication, and data consistency problems. From a numerical optimization viewpoint, the works in [32] and [33] discuss distributed asynchronous algorithms as networking algorithms, rate and flow control, and gradient descent flows. Typically, both these sets of references consider networks with fixed topology, and do not address algorithms over ad-hoc dynamically changing networks. Another common assumption is that any time an agent communicates its location, it broadcasts it to every other agent in the network. In our setting, this would require a nondistributed communication setup. Finally, we note that the terminology “coverage” is also used in [34] and [35] and references therein to refer to a different problem called the coverage path-planning problem, where a single robot equipped with a limited footprint sensor needs to visit all points in its environment. II. FROM LOCATION OPTIMIZATION TO CENTROIDAL VORONOI PARTITIONS A. Locational Optimization In this section, we describe a collection of known facts about a meaningful optimization problem. References include the theory and applications of centroidal Voronoi partitions, see [10], and the discipline of facility location, see [6]. In the paper, we interchangeably refer to the elements of the network be the set as sensors, agents, vehicles, or robots. We letCORTÉS et al.: COVERAGE CONTROL FOR MOBILE SENSING NETWORKS245the position of the sensors and the partition of the space. This problem is referred to as a facility location problem and, in particular, as a continuous -median problem in [6]. Remark 2.2: Note that if we interchange the positions of any two agents, along with their associated regions of dominance, is not afthe value of the locational optimization function denotes the discrete group of perfected. Equivalently, if mutations of elements, then for all . To eliminate this discrete redundancy, one could take natural action on , and consider as the configuration space of for the position of the vehicles. B. Voronoi PartitionsFig. 1. Contour plot on a polygonal environment of the Gaussian density y ). function = exp( x0 0of nonnegative real numbers, be the set of positive natural numbers, and . be a convex polytope in , including its interior, Let denote the Euclidean distance function. We call and let a distribution density function if it reprea map sents a measure of information or probability that some event takes place over . In equivalent words, we can consider to be the bounded support of the function . Let be the location of sensors, each moving in the space . Because of noise and loss of resolution, the sensing performance at point taken from th sensor at the position degrades with the between and ; we describe this degradadistance . tion with a nondecreasing differentiable function Accordingly, provides a quantitative assessment of how poor the sensing performance is (see Fig. 1). mobile robots Remark 2.1: As an example, consider equipped with microphones attempting to detect, identify, and localize a sound source. How should we plan the robots’ motion in order to maximize the detection probability? Assuming the source emits a known signal, the optimal detection algorithm is a matched filter (i.e., convolve the known waveform with the received signal and threshold). The source is detected depending on the signal-to-noise-ratio (SNR), which is inversely proportional to the distance between the microphone and the source. Various electromagnetic and sound sensors have SNRs inversely proportional to distance. Within the context of this paper, a partition of is a collecwith disjoint interiors, tion of polytopes and are whose union is . We say that two partitions equal if and only differ by a set of -measure zero, for . all We consider the task of minimizing the locational optimization function (1)One can easily see that, at a fixed-sensors location, the optimal partition of is the Voronoi partition generated by the pointsWe refer to [9] for a comprehensive treatment on Voronoi diagrams, and briefly present some relevant concepts. The set is called the Voronoi diagram for the of regions . When the two Voronoi regions generators and are adjacent (i.e., they share an edge), is called a (and vice versa). The set of indexes (Voronoi) neighbor of of the Voronoi neighbors of is denoted by . Clearly, if and only if . We also define the face as . Voronoi diagrams can be defined with , and respect to various distance functions, e.g., the 1-, 2-, -norm over , see [36]. Some useful facts about the Euclidean setting are the following: if is a convex polytope in an -dimensional Euclidean space, the boundary of each is the union of -dimensional convex polytopes. In what follows, we shall writeNote that using the definition of the Voronoi partition, we have for all . Therefore(2) that is, the locational optimization function can be interpreted as an expected value composed with a “min” operation. This is the usual way in which the problem is presented in the facility location and operations research literature [6]. Remarkably, one can show [10] that(3) where we assume that the th sensor is responsible for measure. Note that the function ments over its “dominance region” is to be minimized with respect to both 1) the sensors location , and 2) the assignment of the dominance regions . The optimization is, therefore, to be performed with respect to with respect to the th sensor i.e., the partial derivative of only depends on its own position and the position of its Voronoi neighbors. Therefore, the computation of the derivative of with respect to the sensors’ location is decentralized in the sense246IEEE TRANSACTIONS ON ROBOTICS AND AUTOMATION, VOL. 20, NO. 2, APRIL 2004of Voronoi. Moreover, one can deduce some smoothness prop: since the Voronoi partition depends at least conerties of , for all , the function tinuously on is at least continuously differentiable on for some . C. Centroidal Voronoi Partitions Let us recall some basic quantities associated with a region and a mass density function . The (generalized) mass, centroid (or center of mass), and polar moment of inertia are defined asIII. CONTINUOUS AND DISCRETE-TIME LLOYD DESCENT FOR COVERAGE CONTROL In this section, we describe algorithms to compute the location of sensors that minimize the cost , both in continuous and in discrete time. In Section III-A, we propose a continuous-time version of the classic Lloyd algorithm. Here, both the positions and partitions evolve in continuous time, whereas the Lloyd algorithm for vector quantization is designed in discrete time. In Section III-B, we develop a family of variations of Lloyd algorithm in discrete time. In both settings, we prove that the proposed algorithms are gradient descent flows. A. A Continuous-Time Lloyd Algorithm Assume the sensors location obeys a first-order dynamical behavior described byAdditionally, by the parallel axis theorem, one can write (4) is defined as the polar moment of inertia of where . the region about its centroid Let us consider again the locational optimization problem (1), and suppose now we are strictly interested in the setting (5) . The parallel axis that is, we assume and theorem leads to simplifications for both the function its partial derivative Consider a cost function to be minimized and impose that follows a gradient descent. In equivalent conthe location a Lyapunov function, and trol theoretical terms, consider stabilize the multivehicle system to one of its local minima via dissipative control. Formally, we set (6) where is a positive gain, and where we assume that the is continuously updated. partition Proposition 3.1 (Continuous-Time Lloyd Descent): For the closed-loop system induced by (6), the sensors location con, i.e., the verges asymptotically to the set of critical points of set of centroidal Voronoi configurations on . Assuming this set is finite, the sensors location converges to a centroidal Voronoi configuration. Proof: Under the control law (6), we haveHere, the mass density function is define. It is convenient toTherefore, the (not necessarily unique) local minimum points are centroids of their for the location optimization function Voronoi cells, i.e., each location satisfies two properties simultaneously: it is the generator for the Voronoi cell , and it is its centroidAccordingly, the critical partitions and points for are called centroidal Voronoi partitions. We will refer to a sensors’ configuration as a centroidal Voronoi configuration if it gives rise to a centroidal Voronoi partition. Of course, centroidal Voronoi configurations depend on the specific distribution density function , and an arbitrary pair admits, in general, multiple centroidal Voronoi configurations. This discussion provides a proof alternative to the one given in [10] for the necessity of centroidal Voronoi partitions as solutions to the continuous -median location problem.By LaSalle’s principle, the sensors location converges to the , which is precisely the largest invariant set contained in set of centroidal Voronoi configurations. Since this set is clearly consists of invariant for (6), we get the stated result. If converges to one of them a finite collection of points, then (see Corollary 1.2). Remark 3.2: If is finite, and , then a sufficient condition that guarantees exponential convergence is be positive definite at . Establishing that the Hessian of this property is a known open problem, see [10]. Note that this gradient descent is not guaranteed to find the global minimum. For example, in the vector quantization and signal processing literature [12], it is known that for bimodal distribution density functions, the solution to the gradient flow reaches local minima where the number of generators allocated to the two region of maxima are not optimally partitioned.CORTÉS et al.: COVERAGE CONTROL FOR MOBILE SENSING NETWORKS247B. A Family of Discrete-Time Lloyd Algorithms Let us consider the following variations of Lloyd algorithm. verifying the Let be a continuous mapping following two properties: , 1) for all , where denotes the th component of ; is not centroidal, then there exists a such that 2) if . Property 1) guarantees that, if moved according to , the agents of the network do not increase their distance to its corresponding centroid. Property 2) ensures that at least one robot moves at each iteration and strictly approaches the centroid of its Voronoi region. Because of this property, the fixed points of are the set of centroidal Voronoi configurations. Proposition 3.3 (Discrete-Time Lloyd Descent): Let be a continuous mapping satisfying properties denote the initial sensors’ location. 1) and 2). Let converges to the set Then, the sequence of centroidal Voronoi configurations. If this set is finite, then converges to a centroidal the sequence Voronoi configuration. as an objective funcProof: Consider tion for the algorithm . Using the parallel axis theorem, , and therefore (7) for all , as long as with strict inequality if for any , . , with strict inequality if In particular, , where denotes the set of centroids of the partition . Moreover, since the Voronoi partition is the optimal one for fixed , we also have (8) with strict inequality if . Now, because of property 1) of , inequality (7) yieldsalgorithm can also be seen as a fixed-point iteration. Consider for the mappingsLet be defined by . is continuous (indeed, ), and corresponds to Clearly, , Lloyd algorithm. Now, for all . Moreover, if is not centroidal, then . Therefore, verifies the inequality is strict for all properties 1) and 2). C. Remarks 1) Note that different sensor performance functions in (1) correspond to different optimization problems. Provided one [cf. (1)], uses the Euclidean distance in the definition of the standard Voronoi partition computed with respect to the Euclidean metric remains the optimal partition. For arbitrary , it is not possible anymore to decompose into the sum and . Nevertheless, it is still of terms similar to possible to implement the gradient flow via the expression for the partial derivative (3). Proposition 3.5: Assume the sensors location obeys a first. Then, for the closed-loop order dynamical behavior, system induced by the gradient law (3), , the converges asymptotically to sensors location . Assuming this set is finite, the the set of critical points of sensors location converges to a critical point. 2) More generally, various distance notions can be used to define locational optimization functions. Different performance function gives rise to corresponding notions of “center of a region” (any notion of geometric center, mean, or average is an interesting candidate). These can then be adopted in designing coverage algorithms. We refer to [36] for a discussion on Voronoi partitions based on non-Euclidean distance functions, and to [5] and [8] for a discussion on the corresponding locational optimization problems. 3) Next, let us discuss an interesting variation of the original problem. In [6], minimizing the expected minimum distance in (2) is referred to as the continuous -median function problem. It is instructive to consider the worst-case minimum distance function, corresponding to the scenario where no information is available on the distribution density function. In other words, the network seeks to minimize the largest possible distance from any point in to any of the sensor locations, i.e., to minimize the functionand the inequality is strict if of . In additionis not centroidal by property 2)because of (8). Hence, , and the inequality is a is strict if is not centroidal. We then conclude that descent function for the algorithm . The result now follows from the global convergence Theorem 1.3 and Proposition 1.4 in Appendix I. Remark 3.4: Lloyd algorithm in quantization theory [11], [12] is usually presented as follows. Given the location of agents, : 1) construct the Voronoi partition corresponding to ; 2) compute the mass centroids of the Voronoi regions found in step 1). Set the new location of the agents to these centroids, and return to step 1). LloydThis optimization is referred to as the -center problem in [6] and [7]. One can design a strategy for the -center problem analog to the Lloyd algorithm for the -median problem. Each vehicle moves, in continuous or discrete time, toward the center of the minimum-radius sphere enclosing the polytope. We refer to [37] for a convergence analysis of the continuous-time algorithms. In what follows, we shall restrict our attention to the -median problem and to centroidal Voronoi partitions.248IEEE TRANSACTIONS ON ROBOTICS AND AUTOMATION, VOL. 20, NO. 2, APRIL 2004E. Numerical Simulations To illustrate the performance of the continuous-time Lloyd algorithm, we include some simulation results. The as a single cenalgorithm is implemented in setting, the code computes the tralized program. For the package bounded Voronoi diagram using the , and computes mass, centroid, and polar moment of inertia of polygons via the numerical . Careful attention was paid to integration routine numerical accuracy issues in the computation of the Voronoi diagram and in the integration. We illustrate the performance of the closed-loop system in Fig. 3. IV. ASYNCHRONOUS DISTRIBUTED IMPLEMENTATIONS In this section, we show how the Lloyd gradient algorithm can be implemented in an asynchronous distributed fashion. In Section IV-A, we describe our model for a network of robotic agents, and we introduce a precise notion of distributed evolution. Next, we provide two distributed algorithms for the local computation and maintenance of the Voronoi cells. Finally, in Section IV-C, we propose two distributed asynchronous implementations of Lloyd algorithm. The first one is based on the gradient optimization algorithms, as described in [32], and the second one relies on the special structure of the coverage problem. A. Modeling an Asynchronous Distributed Network of Mobile Robotic Agents We start by modeling a robotic agent that performs sensing, communication, computation, and control actions. We are interested in the behavior of the asynchronous network resulting from the interaction of finitely many robotic agents. A framework to formalize the following concepts is the theory of distributed algorithms; see [30]. Let us here introduce the notion of robotic agent with computation, communication, and control capabilities as the th element of a network. The th agent has a processor with the ability of allocating continuous and discrete states and performing operations on them. Each vehicle has access to its unique identifier . The th agent occupies a location and it is cafor any period of pable of moving in space, at any time time , according to a first-order dynamics of the form (11) The processor has access to the agent’s location and deter. The processor of the th agent mines the control pair has access to a local clock , and a scheduling sequence, such i.e., an increasing sequence of times and . The that processor of the th agent is capable of transmitting information to any other agent within a closed disk of radius . We to be a quantity controlassume the communication radius lable by the th processor and the corresponding communication bandwidth to be limited. We represent the information flow between the agents by means of “send” (within specified radius ) and “receive” commands with a finite number of arguments.Fig. 2. Notation conventions for a convex polygon.D. Computations Over Polygons With Uniform Density In this section, we investigate closed-form expression for the control laws introduced above. Assume the Voronoi region is a convex polygon (i.e., a polytope in ) with vertexes such as in Fig. 2. It is labeled convenient to define . Furthermore, we . By evaluating assume that the density function is the corresponding integrals, one can obtain the following closed-form expressions:(9) To present a simple formula for the polar moment of inertia, let and , for . Then, the polar moment of inertia of a polygon about its centroid becomesThe proof of these formulas is based on decomposing the polygon into the union of disjoint triangles. We refer to [38] for . analog expressions over Note also that the Voronoi polygon’s vertexes can be expressed as a function of the neighboring vehicles. The vertexes of the th Voronoi polygon that lie in the interior of are the circumcenters of the triangles formed by and any two neighbors adjacent to . The circumcenter of the triangle determined by , , and is(10) is the area of the triangle, and . where Equation (9) for a polygon’s centroid and (10) for the Voronoi cell’s vertexes lead to a closed-form algebraic expression for the control law in (6) as a function of the neighboring vehicles’ location.。
ECB (2000) Switch to varable rate tenders in main reˉnancing operations some technical det

The Rationale of Optical NetworkingCees de Laat1, Erik Radius2, Steven Wallace31Informatics Institute, University of Amsterdam, Kruislaan 403, 1098SJ, Amsterdam, The Netherlands2SURFnet Utrecht, The Netherlands3Advanced Network Management Lab, Indiana UniversityAbstractThe future of networking is to move to an entirely optical infrastructure. Several leading National Research Networking organizations are creating test-beds to pilot the new paradigm. This paper explores some thoughts about the different usage models of optical networks. Different classes of users are identified. The services, required by the Internet traffic from those different classes of users, are analyzed and a differentiated Internet architecture is proposed to minimize the cost per transported packet for the whole architecture.Key words: Lambda Networking, Optical Networking, switching, routing, DWDM, High performance, High throughput, Bandwidth on Demand.1 IntroductionIn various places in the research networking world test-beds are launched to study and deploy Lambda networking. In this contribution we discuss a differentiated architecture in which we can deliver different transport services for different classes of users. The current Lambda networking initiatives tend to only connect routers via SONET circuits. On the physical layer those circuits are mapped to colours of light on the fibre (sometimes 4 or more circuits are merged using Time Division Multiplexing (TDM) in one wavelength). While prices of those SONET circuits are rapidly dropping and the speeds are increasing, the main cost is going to be in the router infrastructure in which the circuits are terminated. Full Internet routers must be capable of finding the long prefix match for each routed packet in a next-hop database that is now over 100 megabytes in size. At 10 Gbit/s speeds an IP packet takes in the order of 100 ns to arrive, so the logic for doing Routing is becoming increasingly complex and, therefore, expensive. We will assume that a significant amount of the backbone traffic in fact does not need to be routed and, therefore, should stay at the optical or switching layer.2 Optical networking2.1 HistoryOptical transport technology has been around since the 1970s [4],[5], as it provided a reliable way of transporting high-bitrate signals over long distances. Its primary use was in telecom networks, for inter-city and international transport networks. An important milestone in the development of optical networking can be attributed to the erbium-doped fibre amplifier (EDFA) in the late 1980s. This EDFA provided signal amplification in a wide optical spectrum. The EDFA together with the development of the narrow line-width laser diode brought dense wavelength division multiplexing (DWDM) and terabits per second transport per fibre within reach. As mankind discovered the Internet, the hunger for bandwidth started to explode. As a result of this need for raw bandwidth, optical fibres were increasingly being deployed in regional and metro area networks, providing more bandwidth than the few megabits per second that traditional (copper-based) local loops in the access network can carry. While the cost of installing fibre into every household is still too high, it holds a clear promise for replacing the copper access line in the years to come. In the mean time, the abundance of dark fibres, hastily put into the ground since the early 90s in cities and regional business districts (but never lit since) provide a good opportunity for anyone with high bandwidth needs to get a cheap optical access loop into a carrier point of presence (POP). Transport networks are typically divided into different categories: Core, Regional and Access, each demanding diverse functions and optical networking elements. See Fig. 1 for a typical representation of this architecture as defined by Bonenfant et al [1].This optical network representation is typical for a next-generation telecom network, primarily SDH or SONET based, with some native Ethernet transport capabilities. The ‘true’ optical domain is in the core and regional network, shielded from the end-user in the access network by multi-service provisioning platforms (MSPPs).By contrast, the Internet community is increasingly using optical technology for short-haul purposes, i.g. for interconnecting switches and routers with Gigabit Ethernet interfaces. This technology is now very popular and due to the massive market relatively cheap compared to more traditional long haul telecom SONET equipment. So whenever it is feasible to get dark fibre on suitable distances it seems advantageous to apply gigabit Ethernet equipment for those connections.Gigabit Ethernet cannot easily be transported in traditional SONET networks (which uses OC-3 sized circuits of 155Mb/s each). It is successful in regional networks for dark fibre links up to approx. 100 kilometres.The recently finalized 10 Gb/s Ethernet (10GbE) WAN-phy standard specifies a SONET-framed physical interface type, becoming an alternative to 10Gb packet-over-SONET transport.Figure 1: Emerging optical transport network architecture.2.2 What’s in store: some trends in optical networksNow that EDFA and DWDM have boosted the usable fibre capacity into the Terabit/s realm, we observe optical element developments gearing towards optical switching techniques. Core switching techniques using lambda or fibre switching cross connects move the current static optical networks into a dynamical on the fly reconfigurable transport infrastructure. DWDM is nowadays used to provide cost-effective traffic grooming for the regional (metro) network, which may aggregate many disparate traffic types to a Core POP.On a line systems level, 10 Gb/s technology has matured and 40Gb/s is the next step. However, the current slowdown in the worldwide economy may stall the adoption of 40Gb/s systems for several years.For cost-effective and modest capacity increase in access rings, coarse WDM (CWDM) is gaining ground as a non-amplified, no-frills multiplexing technology.Further on the horizon, optical subsystems for optical packet switching may one day enable all-optical data networking and computing.With optical switching components inside the network, optical circuits may be setup and torn down dynamically and on demand. These dynamical features will only be used if adequate policy systems are developed and installed to control these new resources. One main reason of the failure of ATM 6 yearsback was the lack of this control, which inhibited the providers to offer Switched Virtual Circuits to Universities and end users.2.3 Related activities on Optical networkingThe research and education network community has expanded on the telecommunications-based connotation of “lambda networking” to include technologies and services that have one or more of the following attributes in common with this new optical technology:1)Transmission capacities of 2.4 and 10 gigabits per second. These capacities represent thetypical provisioned capacity of a wavelength in a DWDM system.2)The circuit nature of individual wavelength provisioned capacity. These individualwavelengths are provisioned as constant bit rate circuits. The term “light paths” has beencoined to describe end-to-end circuits.3)The lower cost of high capacity circuits in both long haul and metro systems.4)The ability to more directly interface high-speed local area network technologies (e.g. gigabitand 10 gigabit Ethernet) to telecommunications services.5)The ability to provision new services in a more automated fashion.Examples of “lambda networks” in this context include:•The Chicago Starlight facility. This facility is designed to provide interconnection services and co- location space for high-speed national and international research and educationnetworks. Interconnection will initially use one and ten gigabit Ethernet technology overlayer-two switching. Starlight's mantra, “bring us your lambdas”, implies connecting toStarlight with sufficient capacity and appropriate equipment to interconnect at gigabit speeds.•SURFnet's transoceanic 2.4Gb/s lambda between Amsterdam and Chicago, and its 2.4 Gb/s lambda between Amsterdam and Geneva. This connection is interfaced at both ends as anunprotected transparent OC48c, but time division multiplexing (TDM) equipment is installedat each end so that engineers may experiment with customer-provisioned circuits to supporttraffic engineering and quality of service. CA*net4’s current design document also describes a similar approach of using TDM based equipment as a functional model for future customer-provisioned lambda networking.•The Distributed Tera Scale Backbone. This network will consist of unprotected transparent OC192 SONET circuits terminating in Juniper M160 routers. One of its goals is to allow for a “virtual machine room”, where over provisioning of bandwidth in the wide area networkallows for transparent placement of devices across the multi-location machine room.In each of the above examples institutions are deploying and investigating various attributes of lambda network services, but there are no concrete plans to access the analogue lambdas themselves. Given the various overloaded meanings of “lambda networks”, the authors recommend the following extended nomenclature to more precisely identify particular networking techniques:R edefine a Lambda from the pure physics interpretation as being the wavelength of light to:“a Lambda is a pipe where you can inspect packets as they enter and when they exit, but principally not when in transit. In transit one only deals with the parameters of the pipe: number, colour, bandwidth”. This redefinition allows to study the concepts of optical networking using the current available technology and when true optical components become available, the older components can just be replaced.3 Factorizing the problem space3.1 MotivationOptical networking adds another dimension to the field of data-transport. The challenge is to use it as close as possible to the edge of the infrastructure because it promises sheer throughput capacity. The advantages seem numerous: high Quality of Service, protocol independent, cheap when calculated on throughput capacity. An estimation shows that at the moment of writing this article a 32 lambda optical switch costs about 80 k$, while a 32*10 Gb/s switch costs about 800 k$ and a full router with that capacity several millions. However, pure optical networks in its current form also have drawbacks such as: static point-to-point connection oriented, telephone system like, management overhead, etc. To understand where optical networking currently makes sense we need to decompose the problem space.We do that by first analyzing the current user constituency of the Internet, then defining scales and last determining what services are needed in which circumstances.3.2 User classificationWhen looking into the usage of the networks we can clearly classify 3 user groups. The first group (class A) are the typical home users. Those users can live with ADSL speeds (which is a moving target in time, currently order 1 Mb/s). They typically use that for WWW, mailing, streaming, messaging and peer-to-peer applications. They typically need full Internet routing and flows are generally very short lived.Fig 2: User classification according to the typical amount of bandwidth usage and type of connectivity used. Class A users are the low bandwidth full Internet access needing email and browser users, Class B are VPN’s, corporate networks, LAN’s which need Internet Uplink, Class C are the applications with the few big flows.A second group (class B) consists of the corporations, enterprises, Universities, virtual organisations and laboratories. Those operate obviously at LAN speeds, currently in the order of 1 Gb/s. Those need mostly layer 2 services, Virtual Private Networks and full Internet routing uplinks. For the business part they typically need many to many connectivity and collaborative support. Usually most of the traffic stays within the virtual organisation.The third type of users (class C) are the really high end applications, which need transport capacities far above 1 Gb/s. Examples in the science world are the radio astronomers who want to link radio telescopes around the world to correlate the data in real time to improve accuracy to pinpoint sources. Other examples are the data replication effort in the High Energy Physics field, data base correlation in Biology and earth observation data handling. Those applications tend to have long (>> minutes) lasting flows originating from a few places destined to a few places or just point to point. That traffic does not require routing, it always needs to take the same route from source to destination. If we estimate that the backbone load of the total sum of class A is of the same order of magnitude as the class B traffic being around a Gb/s in a country like the Netherlands, then the appearance of a 5 Gb/s class C user on the backbone of a provider is a disturbing event. Typically providers get nervous when their lines and interfaces get regularly populated with traffic loads of several 10’s of percents and they then start to discuss upgrading their costly infrastructure. But as seen above it does not seem to make sense to investin another round of full Internet routers if the major disturbing load on a backbone is coming from class C users, which do not need full Internet routing or even layer 2 switching.3.3 ScalesIt makes a big difference when discussing optical networking when one takes the scale of the environment in account. We also see three typical scales: the metro area, the national or regional scale and the transatlantic or worldwide scale. We discuss here the scale in round trip times in milliseconds (see fig 3). A one ms RTT equals light transport through 100 km fibre and back. The worldwide scale would then translate to 200 ms, since that gives 20.000 km or half the circumference of the earth. Taking orders of magnitude we get a scale of 20 ms or about half of the size of the USA or about the size of Western Europe. The two ms scale is around the size of Chicago or about half of the Netherlands.Fig 3: Scales of networking in round trip times on fibre. A RTT of 1 ms equals 100 km. Therefore, a 200 ms should be enough to reach the other side of the Earth.Given the current situation one can easily acquire dark fibre in the 2-millisecond scale. The number of Lambdas is then just limited by the DWDM equipment one deploys. Therefore, tens or hundreds of lambdas on that scale are within reach. On the 20 ms scale owning dark fibre may still be out of reach but owning a number of Lambdas on a fibre of a provider is very well possible. On the world scale of 200 ms owning a very few Lambdas is currently the limit. The estimation is that the number of Lambdas to work with is approximately:#lª200*e(t-2002)rttwhere t is the year and rtt is the distance in ms round trip time light speed through fibre.The usage of Lambdas and the way of multiplexing, therefore, depends on the scale at hand. Also the regional and world scales will usually involve more administrative domains that require Bandwidth on Demand and Authorization Authentication and Accounting architectures, which are the topic of another paper [3].4 ArchitectureGiven the classification of the users, the necessary services for their packets and the three different scales we can now discuss what architectures are optimal in the different situations. For that we make atable listing the scale versus the class of user (table 1). Given the different classes of users and the current state of the technology for the different 2, 20 and 200 ms scales we envision to build a provider backbone architecture in which we minimize the amount of routers. The routers are currently the most expensive parts. The traditional model in which at each university a POP with a router was placed which connects via one or few interfaces to the Router of the University does not scale to high speeds. The model is now to provide a DWDM device and to "transport" the A, B, C type connections from the University to either one of the more central core routers for A and B class traffic, switches for class B type traffic and/or directly to the destination if it consists of class C traffic. This means that at customer sites mostly optical equipment will be placed if the scale of the network makes it possible for the provider to own enough Lambda's or dark fibres and if the distances are suitable.2ms Metro 20 msNational/regional200WorldA SwitchingRoutingADSL/ATMRouting Router$B LANVPN’s(G)MPLS RoutingVPN’s(G)MPLSRoutingC Dark fibreOptical switchingEthernet Lambda switching Sub-Lambda’sSONET/SDHtable 1: Applicable technologies for different scales for different classes of users.At several strategic places in the core network switches and routers should be placed to act asVPN/VLAN or distributed Internet exchange islands, while routers can take care of the traffic needing full Internet routing. The proposed model architecture is shown in figure 4. This architecture seems suitable for the metro and most probably the national scale where the provider owns many Lambda's. On the edges connectivity needs to be established with the worldwide scale where the amount of Lambda's to destinations might be few or just single. The choices then are either Router-to-Router connection, switch to switch or a completely optical path. The disadvantage of these solutions is the discontinuity for the class C traffic. A fourth solution, which currently is being investigated in our test-bed (see section 5), is to terminate such a worldwide lambda in a TDM switch and to use the sub-channels as a kind of Lambdas. Some of the sub-channels can be dedicated for the Router-to-Router connectivity to service the class A and some B traffic. Other sub-channels can be dedicated to extended vLAN support to provide protected networking environments for class B users or grid virtual organizations. The class C channels can use the remaining channels in a Bandwidth on Demand fashion, see [3] for a multi domain authorization and provisioning model.Fig 4: A differentiated architecture including optical switching, packet switching and routing. The dashed lines denote administrative domain boundaries.Several questions still need to be answered:1)If streams are very long lasting, why have a dynamical optical switching core instead of staticpatching?2)What is the cost model when including all interfaces on each layer?3)What is the cost model given a certain amount of over-provisioning of Lambda’s so that thereis something to switch?4)What optical switches can be used for which types of signals in different scales5)How to connect different scales infrastructures togetherIt is our intention to use our test-beds in collaboration with StarLight and others to investigate these novel new architectures, which are hopefully leading to an optical world.5 Testbed5.1 ArchitectureIn defining the next generation Dutch Lambda testbed we want to achieve the following goals:•Dark fibre infrastructure experiments with full optical components•Lambda based Internet Exchange prototype•International protected bandwidth connectivity for virtual organizations spanning multiple domains, scales and architecturesTo achieve these goals we construct a facility in Amsterdam, NetherLight, which serves as a proof of concept next generation Lambda based Internet Exchange. On a national scale we create a testbed where the DWDM and various optical components can find their place to test the models described in chapter 4. In the near future we will incorporate pure optical switching elements with millisecond setup times to study true lightpath provisioning.5.2 NetherLight testbed activitiesFor the near future we defined a test-bed to prototype the abovementioned architectural ideas. The Dutch testbed NetherLight is a next-generation Internet exchange facility with lambda switching capabilities in Amsterdam combined with a national differentiated transport infrastructure for class A, B and C type traffic. NetherLight focuses on the assessment of optical switching concepts and bandwidth provisioning for high-bitrate applications. In order to achieve these goals, NetherLight deploys TDM circuit switching and Ethernet VLAN switching today and lambda switching in the very near future. Connectivity to similar advanced testbeds is established by means of international lambdacircuits to StarLight in Chicago, IL, USA and the TeraGrid testbed, CERN, Geneva, Switzerland. Connections to other grid testbeds are in preparation. International collaborations include those with OMNInet in Chicago, TeraGrid, DataTAG at CERN, Canarie.Fig 5: NetherLight topology as being projected to be in place in beginning 2003.Figure 5 shows the NetherLight topology, end of 2002. It contains TDM (SONET) multiplexers in Amsterdam, Chicago and Geneva, for the termination of international OC48c circuits (to be upgraded to OC192c by late December, 2002). The TDM multiplexers (Cisco ONS15454 equipment) are used to map multiple GbE channels into the SONET circuits. In Chicago at StarLight, the ONS15454 connects at the LAN (GbE side) to the StarLight switch with 2xGbE ports, and to an ONS15454 from Canarie with 2xGbE for lightpath experiments. In Amsterdam at SARA, the ONS15454 LAN side connects to an Ethernet switch for layer 2 transport experiments by participating research groups. In CERN at Geneva, an ONS15454 multiplexer connects 2xGbE ports to an Ethernet switch for the DataTAG project at CERN.Connected to NetherLight are:•the University of Amsterdam,•the Dutch Institute for Astronomical Research ASTRON/JIVE,•StarLight, via the ONS15454 at Chicago,•Canarie, via a direct peering at StarLight.ASTRON/JIVE connected to the Amsterdam core of NetherLight via a DWDM line system carries several GbE lambda’s.6 Conclusions and Lessons LearnedWe think that optical networking techniques have the potential of delivering huge amounts of cheap tailored bandwidth to applications. However, we think that for cost and complexity reasons this should not be done with routed backbones but that a differentiated infrastructure delivers the best transport service for different classes of users. First tests with SURFnet’s for research only Lambda from Amsterdam to Chicago learned that unexpected traffic behaviours surface when real applications are put on the new infrastructure [2]. The properties of the layer one and two infrastructure have profound impact on the Layer 4 transport protocols. Multi domain path provisioning is still an open field [3].7 AcknowledgementsWe wish to thank the following persons for their stimulating discussions: Bill StArnaud, Kees Neggers, Tom DeFanti, Joe Mambretti, Erik-Jan Bos, Victor Reijs. This work is in large part funded by SURFnet and by the IST Program of the European Union (grant IST-2001-32459) via the DataTAG project.8 References[1] P. Bonenfant and A. Rodriguez Moral, "Regional optical transport networks," J. Opt. Netw. 1, 9-17 (2001), /abstract.cfm?URI=JON-1-1-9[2] Antony Antony, Johan Blom, Cees de Laat, Jason Lee, Wim Sjouw, “Microscopic Examination TCP flows over Trans-Atlantic Links”, IGRID2002 paper submitted to Future Generations Computer Systems, November 2002.[3] Leon Gommans, Cees de Laat, Bas van Oudenaarde, Arie Taal, “Authorization of a QoS Path based on Generic AAA", IGRID2002 paper submitted to Future Generations Computer Systems, November 2002.[4] “Optical Communication, Networks for the Next-Generation Internet”, Special Issue IEEE Network, November/December 2000, vol 14, No 6.[5] “IP-Optical Integration”, Special Issue IEEE Network, July/August 2001, vol 15, No 4. Authors:Cees de Laat is senior scientific staff member of the Informatics Institute at the University of Amsterdam. de Laat received a PhD in Physics from the University of Delft. Since then he has been active in data acquisition systems, heavy ion experiments and virtual laboratories. Over the past seven years he has been investigating applications for advanced research networks. His main focus is to achieve the "best" Internet Quality of Service matching for grid applications in both the computational sciences and the computer-based education domains, such as distance learning, virtual laboratories, remote experimenting and distributed computing.Currently Cees runs projects on the Dutch and European research networks. Topics include optical networking, lambda switching and provisioning, policy-based networking and Authorization, Authentication and Accounting architecture research. He participates in the European DataGrid project and the Dutch ASCII DAS project. He is responsible for the research on the Lambda switching facility ("NetherLight"), which is currently being built in Amsterdam as a peer to StarLight in Chicago. He implements research projects in the GigaPort Networks area in collaboration with SURFnet.He currently serves as Grid Forum Steering Group member, Area Director for the Peer to Peer area and GGF Liaison towards the IETF. He is co-chair of the IRTF Authentication, Authorization and Accounting Architecture Research group and member of the Internet Research Steering Group (IRSG). For recent work, publications and talks, please refer to his home page<http://www.science.uva.nl/~delaat>.Steven WallaceEmail: ssw@Steven Wallace brings to the Advanced Network Management Lab more than sixteen years of experience in data networking, computer programming and network management in both the public and private sectors. His most recent experience includes managing Internet2’s Abilene Project for Indiana University and serving as Manager and Senior Technical Advisor for Bloomington Data Services at Indiana University where he oversaw 35 employees and a $4 million budget. Wallace co-founded and served as vice president for a computer retail and system integration company with multiple locations, more than 20 employees, and annual sales exceeding $1 million. Other experience includes positions as Director of Networks, Manager of Network Operations and Principle Applications Programmer at Indiana University and several private Indiana firms.Over the past ten years, Wallace has focused on the design and operation of large high-speed data networks and the development of network-related management tools. His specific areas of interest and expertise include the optimal design of high-performance layer two networks (Ethernet broadcast domains), the creation of network management tools to support such environments, and research into end-to-end application performance.Outside of his work in the ANML, Wallace is an avid collector of early undersea telecommunications cables and a wide range of technical gadgets. He is a weather enthusiast and holds an amateur radio license. Wallace commutes daily by recumbent bicycle from his Bloomington, Indiana home.Erik Radius is a manager of Network Services at SURFnet, the national computer network for higher education and research in the Netherlands. He received a M.Sc. in Experimental Physics from the University of Amsterdam and since worked at the University of Twente and at KPN Research on optical network innovations for backbone, metro and access networks. After a brief stint in the Internet startup Bredband Benelux, during which he designed the optical part of a Fiber To The Home network infrastructure, he joined SURFnet to work on lambda networking and the closer integration of IP over optical networks. In that role, Erik is responsible for NetherLight, the experimental optical internet exchange in Amsterdam.<www.surfnetters.nl/radius/>。
国际贸易、运输、保险条款解释(R-S)
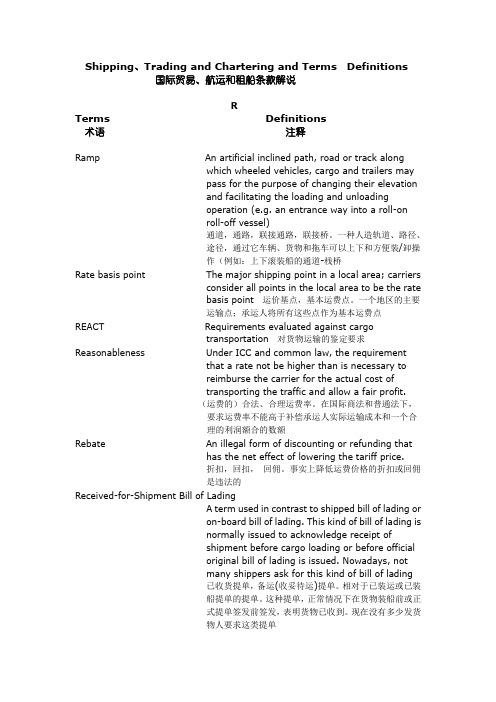
Shipping、Trading and Chartering and Terms Definitions国际贸易、航运和租船条款解说RTerms Definitions术语注释Ramp An artificial inclined path, road or track alongwhich wheeled vehicles, cargo and trailers maypass for the purpose of changing their elevationand facilitating the loading and unloadingoperation (e.g. an entrance way into a roll-onroll-off vessel)通道,通路,联接通路,联接桥。
一种人造轨道、路径、途径,通过它车辆、货物和拖车可以上下和方便装/卸操作(例如:上下滚装船的通道-栈桥Rate basis point The major shipping point in a local area; carriersconsider all points in the local area to be the ratebasis point 运价基点,基本运费点。
一个地区的主要运输点;承运人将所有这些点作为基本运费点REACT Requirements evaluated against cargotransportation 对货物运输的鉴定要求Reasonableness Under ICC and common law, the requirementthat a rate not be higher than is necessary toreimburse the carrier for the actual cost oftransporting the traffic and allow a fair profit.(运费的)合法、合理运费率。
BDCC...(IJCNIS-V6-N5-4)
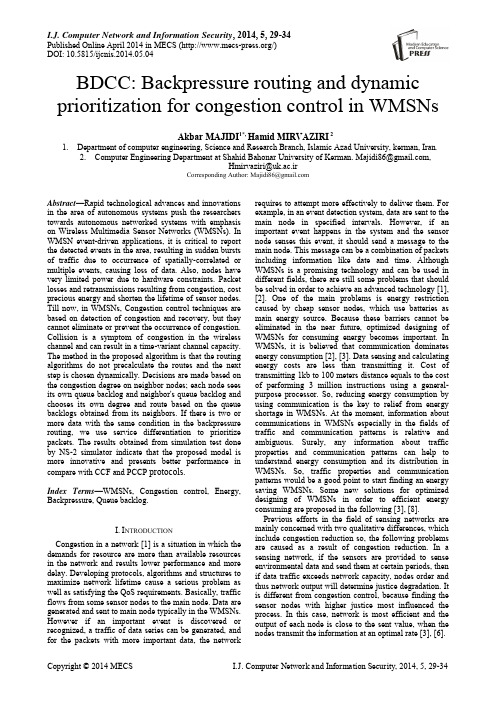
I.J. Computer Network and Information Security, 2014, 5, 29-34Published Online April 2014 in MECS (/)DOI: 10.5815/ijcnis.2014.05.04BDCC: Backpressure routing and dynamic prioritization for congestion control in WMSNsAkbar MAJIDI1*, Hamid MIRVAZIRI 21.Department of computer engineering, Science and Research Branch, Islamic Azad University, kerman, Iran.puter Engineering Department at Shahid Bahonar University of Kerman. Majidi86@,Hmirvaziri@uk.ac.irCorresponding Author: Majidi86@Abstract—Rapid technological advances and innovations in the area of autonomous systems push the researchers towards autonomous networked systems with emphasis on Wireless Multimedia Sensor Networks (WMSNs). In WMSN event-driven applications, it is critical to report the detected events in the area, resulting in sudden bursts of traffic due to occurrence of spatially-correlated or multiple events, causing loss of data. Also, nodes have very limited power due to hardware constraints. Packet losses and retransmissions resulting from congestion, cost precious energy and shorten the lifetime of sensor nodes. Till now, in WMSNs, Congestion control techniques are based on detection of congestion and recovery, but they cannot eliminate or prevent the occurrence of congestion. Collision is a symptom of congestion in the wireless channel and can result in a time-variant channel capacity. The method in the proposed algorithm is that the routing algorithms do not precalculate the routes and the next step is chosen dynamically. Decisions are made based on the congestion degree on neighbor nodes; each node sees its own queue backlog and neighbor's queue backlog and chooses its own degree and route based on the queue backlogs obtained from its neighbors. If there is two or more data with the same condition in the backpressure routing, we use service differentiation to prioritize packets. The results obtained from simulation test done by NS-2 simulator indicate that the proposed model is more innovative and presents better performance in compare with CCF and PCCP protocols.Index Terms—WMSNs, Congestion control, Energy, Backpressure, Queue backlog.I.I NTRODUCTIONCongestion in a network [1] is a situation in which the demands for resource are more than available resources in the network and results lower performance and more delay. Developing protocols, algorithms and structures to maximize network lifetime cause a serious problem as well as satisfying the QoS requirements. Basically, traffic flows from some sensor nodes to the main node. Data are generated and sent to main node typically in the WMSNs. However if an important event is discovered or recognized, a traffic of data series can be generated, and for the packets with more important data, the network requires to attempt more effectively to deliver them. For example, in an event detection system, data are sent to the main node in specified intervals. However, if an important event happens in the system and the sensor node senses this event, it should send a message to the main node. This message can be a combination of packets including information like date and time. Although WMSNs is a promising technology and can be used in different fields, there are still some problems that should be solved in order to achieve an advanced technology [1], [2]. One of the main problems is energy restriction caused by cheap sensor nodes, which use batteries as main energy source. Because these barriers cannot be eliminated in the near future, optimized designing of WMSNs for consuming energy becomes important. In WMSNs, it is believed that communication dominates energy consumption [2], [3]. Data sensing and calculating energy costs are less than transmitting it. Cost of transmitting 1kb to 100 meters distance equals to the cost of performing 3 million instructions using a general-purpose processor. So, reducing energy consumption by using communication is the key to relief from energy shortage in WMSNs. At the moment, information about communications in WMSNs especially in the fields of traffic and communication patterns is relative and ambiguous. Surely, any information about traffic properties and communication patterns can help to understand energy consumption and its distribution in WMSNs. So, traffic properties and communication patterns would be a good point to start finding an energy saving WMSNs. Some new solutions for optimized designing of WMSNs in order to efficient energy consuming are proposed in the following [3], [8]. Previous efforts in the field of sensing networks are mainly concerned with two qualitative differences, which include congestion reduction so, the following problems are caused as a result of congestion reduction. In a sensing network, if the sensors are provided to sense environmental data and send them at certain periods, then if data traffic exceeds network capacity, nodes order and thus network output will determine justice degradation. It is different from congestion control, because finding the sensor nodes with higher justice most influenced the process. In this case, network is most efficient and the output of each node is close to the sent value, when the nodes transmit the information at an optimal rate [3], [6].Two main operations in transmission layer of WMSNs are delivering reliable data and congestion control. To submit reliable data, this layer reviews and identifies missing data and recovers them. Data reliability is more important when the data is going to be used in critical operations. There are different types of congestion control such as data traffic, route discovery traffic, interface layer feedback and etc. This article is mainly concerned with data traffic and does not pay much attention to other kinds of traffic. It has been proved that if there is no control over the network congestion, bandwidth increase would not help solving congestion problem at all. Especially in the wireless networks, congestion wastes lots of energy and eliminates lots of data packets. So it is necessary to congestion control in order to improve network lifetime in WSNs. By using backpressure routing, connections are minimized; more important data are protected and delivered in the network, by using dynamic prioritization for service differentiation [5], [6].Many protocols have been set in the transmit layer for this purpose and we will review some of them in the following sections. There is an enormous diversity in the congestion scenarios, which are outside the scope of this article.This research has been formed as follows; section II includes a summary of related works and describes some of the WMSNs protocols. This Section is related to the issues encountered during designing different types of congestion and congestion control in WMSNs. BDCC is given in section III. Section IV gives us an evaluation of simulation results and the conclusion of the research is included in section VI.II.R ELATED W ORKSCongestion control is an important issue in transport protocols. Congestion is also a difficult problem in wireless sensor networks. It not only wastes the scarce energy due to a large number of retransmissions and packet drops, but also hampers the event detection reliability. Congestion in WSNs and WMSNs has a direct impact on energy efficiency and application QoS. Two types of congestion could occur in sensor networks [6]. The first type is node-level congestion that is caused by buffer overflow in the node and can result in packet loss, and increased queuing delay. Not only can packet loss degrade reliability and application QoS, but it can also waste the limited node energy and degrade link utilization. In each sensor node, when the packet-arrival rate exceeds the packet service rate, buffer overflow may occur. This is more likely to occur at sensor nodes close to the sink, as they usually carry more combined upstream traffic. The second type is link-level congestion that is related to the wireless channels which are shared by several nodes using protocols, such as CSMA/CD (carrier sense, multiple access with collision detection). In this case, collisions could occur when multiple active sensor nodes try to seize the channel at the same time.As was shown in [6], CCF is a non-work-conserving algorithm. To explain the non-work-conserving property of CCF, suppose that a root node is connected to two nodes A and B. The non-work-conserving property of CCF implies that the root node will wait until the required number of packets has been received and transmitted from node B before considering packets from node A. This also implies that CCF cannot effectively allocate the remaining capacity, resulting in a low throughput, especially, when some nodes do not have any packet to send. Further, as was shown in [6], CCF has another major problem. The rate adjustment in CCF relies only on packet service time which could lead to low utilization when some sensor nodes do not have enough traffic or there is a significant packet error rate.Priority based congestion control protocol (PCCP) was proposed in [6]. PCCP is an upstream congestion control protocol for WSNs which measures the congestion degree as the ratio of packet inter-arrival time to the packet service time. Based on the introduced congestion degree and node priority index, PCCP utilizes a cross-layer optimization and imposes a hop-by-hop approach to control congestion. It has also been shown that PCCP achieves efficient congestion control and flexible weighted fairness for both single-path and multipath routing. In wireless sensor networks data are normally generated and sent to the sink periodically. However, a burst of data traffic can also be suddenly generated when an important event is triggered or detected. So, in wireless sensor networks different data packets might have different importance. For packets containing information with higher importance, the network should make more effort in delivering them. This highlights a need for having service differentiation in sensor networks. Service differentiation in wireless sensor networks is a new research area and a few methods have been proposed [6], [7].Earlier works in the field of sensing networks are mainly concerned with two qualitative differences, which include congestion reduction [2]. So, the following problems are caused as a result of congestion reduction. In a sensing network, if the sensors are provided to sense environmental data, send them at certain periods, then if data traffic exceeds network capacity, nodes order and thus network output will determine justice degradation. It is different from congestion control, because finding the sensor nodes with higher justice has the highest impact on the process. In this case, the nodes transmit the information at an optimal rate, network is most efficient and the output of each node is close to the sent value. Adaptive Roll Control (ARC) indicates packet injecting to traffic flow like straight route traffic. Each node estimates the number of nodes in the neighborhood of Sink and the bandwidth is divided between straight route traffic and local traffic based on their priority. Division bandwidth of each node is an effort to achieve approximate justice. Reduction in the straight traffic transfer also affects upper nodes, which can reduce transmission rate [2], [6].A. Congestion and Congestion ControlBasically, congestion control is allocation of resources. Congestion control adjusts source transmission rate to avoid or reduce congestion. Congestion is an important problem in transmission protocols and network, especially wireless networks, due to lack of energy supplies. Congestion on the networks has a direct effect on energy efficiency and QoS appliance. There are two reasons that cause congestion in WMSNs: Node-level congestion, Link-level congestion [6].(Illustrated in Figure1) It has direct impact on energy efficiency and QoS.Fig. 1. Two types of congestions in WMSNs [6].B. Congestion Control SolutionThe main solution in congestion control is to predict congestion. But beside that, after congestion appeared, three main tasks should be done: Finding congestion, reporting congestion and adjusting transmission rate.In the old TCP protocol, congestion is found at the last node based on timeout. In WSNs, finding of corrupted packets and reporting is done in two ways: link-by-link& end-to-end. Medial nodes detect and report corrupted packets in the link-by-link method. End nodes detect and report corrupted packets in the end-to-end method. End-to-end method is not effective for WSNs. Because control messages use different Hops to detect corruption and it is very probable that the control messages became corrupt due to congestion or links malfunction. In the link-by-link method, detecting corruption and reporting is based on the neighbor nodes and they can resend the messages locally in case of corruption. This method is more efficient compared to end-to-end method [6], [7].III.D ETAILS OF THE P ROPOSED S CENARIOS (BDCC) Network tries to deliver more important packets of data, yet there is not a static value to show importance of any packet of data.So we use backpressure routing (also written "back-pressure routing" or "back pressure routing") algorithm to prioritize packets. In this algorithm, the routes are not selected beforehand, but the next step is chosen dynamically. These decisions are made based on congestion of neighbor nodes. Each node sees its own queue backlog and its neighbor's queue backlog and adjusts its own rate and select routes based on queue backlog of its neighbors [4], [5].A. Choosing the optimal commodity in Backpressure routing:An example shown in Figure 2, We assume that there are only three types of data in queue.Real time (Red), Not real time with high priority (Green) and Not real time with low priority (Blue). These are in correct numbered packets. Focusing on direct link (1, 2) differential queue backlog would be:Fig. 2. Differential Queue Backlog [5].So, optimum packet to send on links (1, 2) in the T slot would be not real time with high priority (Green). On the other hand, optimum packet to send on reversed links of (2, 1) in the T slot would be not real time with low priority (Blue). To make it simple, we assume that the topology has only 4 options, shown in Figure 3. The options in Figure 3 are provided in a matrix shown in Figure 4 [5].Fig. 3. Provided TopologyFig. 4. Matrix for Provided TopologyAs we can see, node number 6 cannot send or receive any data based on these possibilities. This may happen, because the node number 6 is out of transmission range at the moment. Total weighted scores for each possibility are:Choice (a):.Choice (b):.Choice (c):.Choice (d):.Since there are two nodes with total weight of 12, network controller in the backpressure routing can choose any or both of these options () ore choose one of the matrix [5]. Data priority in this paper is based on [6], [7]. That is, for the nodes with same weight, we use dynamic prioritization for service differentiation.B. Dynamic Prioritization for Service DifferentiationIf there are two or more data with same condition, we use dynamic prioritization for service differentiation. That is, network should do most efforts to deliver data packets with real time or high priority. Our BDCC method consists of three different traffic levels: Real time traffic level, not real time traffic level with high priority and not real time traffic level with low priority. Throughput and delay for BDCC is calculated from the equation below:Throu:HP = <Throu:NP = <Throu:LPDelay:HP< =Delay:NP< =Delay:LPEach node sees neighbor nodes and their queue backlog. Data is sent to nodes with less crowded buffer queues. If the buffer queues of all nodes are crowded, then data with real time priority are sent based on dynamic prioritization for service differentiation. After that, non-real time data with high priority and non-real time data with low priority are sent. If the buffer of any node is near to congestion threshold and real time data could not enter the queue, non-real time data with lower priority will be eliminated from queue until non-real time data with high priority move to this queue, new entrants data that real time data after real time queuing Placed. Note that the optimal decisions and good choices backpressure routing is true for dynamic priority algorithms to differentiate service. It means that, if the differential backlog queue does not match with optimal product selection decisions and the backpressure routings, dynamic priority algorithms do not apply to differentiate service for data and path.C: Congestion detection mechanism:Congestion detection mechanism uses two congestion indexes.First: Queuing delay is the primary congestion index. Second: Packet loss is the next congestion index. Congestion control strategies based on packet loss to keep high bandwidth is employed when delay based strategy act inefficiently. Proposed algorithm is applied to the network when these two indexes have been settled. In general case, proposed algorithm is not applied in normal case since computation is a time consumer manner. Proposed algorithm is applied to the nodes near the base station (which convey more traffic) after the congestion detection mechanism detected the congestion.IV.S IMULATION R ESULTSWe choose NS-2 simulator for researching based on investigation performed on simulator software including (OPNET, OMNET, MATLAB, NS-2, NS-3…) because of its flexibility and performance. Values assigned to the parameters for simulation are table 1.Table 1. Values of Parameters for simulationSimulator NS2 2.29-3 and 2.34Routing AODVSimulationduration(Sec)20, 40, 60, 80, 100, 120 Simulation area 1000m X 1000mNumber of nodes200Transmission range10 mMovement Chain topologyMAC Layer CCF, PCCPQueue Size200Packet rate 4 packets/secTraffic typeCBR (UDP)Data payload 512 bytes/packetAs it is shown in diagrams, we compared our BDCC with CCF and PCCP protocols. Five and six Figures approve and display average network delay and packet loss. This is the reason that the BDCC shows better performance .Fig. 5. Average network delayFig. 6. The Lost PacketsAs it has always been said, congestion control is directly related to energy consumption, figure seven expresses this fact that BDCC optimize the energy consumption and have a longer network lifetime in comparison with CCF and PCCP.Fig. 7. Power Consumption NetworkFigure eight and nine illustrated that, BDCC is better in terms of average throughput and packet delivery ratio.Fig. 8. Average throughputFig. 9. Packet delivery ratioFigure ten shows normalized routing load. Average efficiency of the BDCC is compared with listed protocols.V. C ONCLUSIONNew applications made possible by rapid improvements and miniaturization in hardware has motivated recent developments in wireless multimedia sensor networks (WMSNs). To provide the required quality of service for multimedia applications in WMSNs,congestion control is necessary. Each congestion control protocol should be able to detect congestion in advance, and allocate available rates to the sensor nodes accordingly.For some applications, there is a need to send real time traffic toward the sink node with low latency and high reliability so that immediate remedial and defensive actions can be taken, as appropriate. Further, when an important event occurs in the system, the sensor node that detected the event should send some alarm message to the sink. Usually this kind of high priority traffic is bursty. This means that high priority traffic is generated only for a short period of time while low priority traffic usually exists in the network and produce thousands of packets generated periodically. For such environments, service differentiation in wireless multimedia sensor networks becomes an important problem. To provide service differentiation in WMSNs, it is necessary to consider a different priority for each traffic source.In this paper we presented a new method for congestion control by using backpressure routings and service differentiation in WMSNs. We used backpressure routing in this article. This algorithm does not precalculate routes and next step is selected dynamically. Each node sees its own queue backlog and its neighbor's queue backlog and adjusts its own rate and selects routes based on queue backlog of its neighbors. We also used dynamic prioritization for service differentiation. If there are two or more data with same condition in backpressure routing, we use dynamic prioritization for service differentiation. That is, network should do most efforts to deliver data packets with instant or high priority.R EFERENCES[1] D. Chiu, R. Jain. Analysis of the increase and decreasealgorithms for congestion avoidance in computer networks. Computer Neworks and ISDN Systems 1989;17:1–14.[2]Akyildiz I, Melodia WT, Chowdhury KR. A survey onwireless multimedia sensor networks. Computer Networks 2007; 51:921–960.[3]Akyıldız IF, Su W, Sankarasubramaniam Y, Çayırcı E.Wireless Sensor Networks: Survey. Computer Networks 2002; 38:393–422.[4]Neely M. J and Urgaonkar R. Optimal BackpressureRouting in Wireless Networks with Multi-Receiver Diversity. Ad Hoc Networks 2009; 7: 862-881.[5]Wikipedia, The free encyclopedia en. BackpressureRouting.</wiki/Backpressure_routing>.[6]Yaghmaee MH, Adjerohb DA. Priority-based rate controlfor service differentiation and congestion control in wireless multimedia sensor networks. Computer Networks 2009; 53: 1798–1811.[7]LIN Q, Wang R, Jian. Novel congestion control approachin wireless multimedia sensor networks. Journal of China Universities of Posts and Telecommunications 2012; 18: 1–8.[8]Swastik Brahma, Mainak Chatterjee, Kevin Kwiat,Pramod K. Varshney. Traffic management in wireless sensor networks: Decoupling congestion control and fairness. Computer Communications 2012; 35:670-681 [9]Mattsson N. A DCCP module for ns-2. MSc, LuleåUniversity of Technology, Luleå, Sweden, 2004.[10]Harjot Bawa, Parminder Singh, Rakesh Kumar, “AnEfficient Novel Key Management Scheme for Enhancing User Authentication in A WSN", IJCNIS, vol.5, no.1, PP.56-64, DOI: 10.5815/ijcnis.2013.01.07.[11]Harjot Bawa, Parminder Singh, Rakesh Kumar,"AnEfficient Novel Key Management Scheme for Enhancing User Authentication in A WSN", IJCNIS, vol.5, no.1, pp.56-64,2013.DOI: 10.5815/ijcnis.2013.01.07.[12]Ozlem Durmaz Ince. A survey on multi-channelcommunication in wireless sensor networks. Computer Networks 2011; 55:3081–3099.[13]Yin X, Zhou X, Huang R, Fang Y, Li S. A fairness –aware congestion control scheme in wireless sensor networks. IEEE Transactions on Vehicular Technology 2009; 58:5225–5234.[14]Zawodniok M, Jagannathan S. Predictive congestioncontrol protocol for wireless sensor networks. IEEE Transactions on Wireless Communications 2007; 6:3955–3963.[15]Felemban E, Chang-Gun Lee, Ekici E. MMSPEED:multipath multi- SPEED protocol for QoS guarantee of reliability and timeliness in wireless sensor networks.IEEE Transactions on Mobile Computing 2006; 5:738–754.[16]Qinghua Wang. Traffic Analysis, Modeling and TheirApplications in Energy-Constrained Wireless-Sensor Networks - On Network Optimization and Anomaly Detection. Phd, Information Technology and Media Mid Sweden University, Sundsvall, Sweden, 2010.[17]Reza Fotohi, Shahram Jamali, FatemeSarkohaki,"Performance Evaluation of AODV, LHC-AODV, OLSR, UL-OLSR, DSDV Routing Protocols", IJITCS, vol.5, no.10, pp.21-29, 2013. DOI:10.5815/ijitcs.2013.10.03.[18]Reza Fotohi, Shahram Jamali, Fateme Sarkohaki,Shahram Behzad,"An Improvement over AODV Routing Protocol by Limiting Visited Hop Count", IJITCS, vol.5, no.9, pp.87-93, 2013. DOI: 10.5815/ijitcs.2013.09.09.Akbar MAJIDI: was born on january1986 in Ardabil-Germi, Iran. He receivedhis B.Sc. degree in Software Engineering,and M.Sc. degree in Architecture ofComputer Systems from Iran Universityof Science and Research Branch, Kerman,Iran, in 2013. His research interests arein Wireless Sensor Networks (WSNs),traffic and congestion control.Hamid MIRVAZIRI: has a B.ScfromShahidBahonar University of Kerman,M.Sc from Guilan University and Ph.Dfrom National University of Malaysia.His research area is in the field of signalprocessing and computer and networksecurity. Currently he is AssistantProfessor of Computer EngineeringDepartment Shahid Bahonar University of Kerman, Iran.。
高速保护自动化数据传输说明书

Datasheet High Speed Guard Automated, high-performance data transfer for any enviromentKey Benefits›Sustains the industry’s fastest transfer rates of more than 9Gb/s on 10Gb networks with latencies as low as 1.3ms›Included in the U.S. NCDSMO Baseline for SABI and TSABI environments since 2001 and is designed to meet current Raise-The-Bar guidelines›Customer-maintainable for simplified configuration and management›High Speed Guard SP implementations support tactical and mobile forces and meet SWAP-C requirements›Enables real-time video streaming while providing unparalleled control and auditing›Supports multiple application protocols and adaptability for custom interfaces›Provides highly customizable data validation rules for maximum flexibility Rapid data transfer supporting all mission typesProtecting and streamlining how data is distributed between separated networks, in any environment, is essential to efficient and secure data sharing and collaboration. Customers’ most sensitive intelligence must often be sanitized and made accessible to various services, agencies, forces, and coalitions as quickly as possible. At the same time, data from a wide variety of sources must be transferred to protected enclaves from austere environments for processing and analysis. The sharing and movement of this data are essential to the rapid, accurate, and precise execution of our customers’ missions. The persistent threat of cyber attack, penetration, and data loss requires that only the most secure methods are used to maintain the highest standards of security.Forcepoint High Speed Guard & High Speed Guard SPForcepoint High Speed Guard is an integral part of many authorized systems enabling highly complex, bi-directional, automated data transfers between multiple domains (Figure 1). Forcepoint High Speed Guard and High Speed Guard SP for special-purpose implementations support both large enterprise environments and austere environments requiring specific size, weight, power, and cost (SWAP-C) considerations with comparatively low administration overhead. Forcepoint High Speed Guard has demonstrated the fastest bi-directional transfer rates of any guard technology. A typical Forcepoint High Speed Guard deployment is able to sustain transfer rates of more than 9 gigabits per second (Gb/s) on a commodity server. The operating system is derived from the Red Hat Enterprise Linux secure operating system with Security Enhanced Linux (SELinux) modules. Forcepoint High Speed Guard is included on the United States National Cross Domain Strategy Management Office (NCDSMO) Baseline list as an accredited transfer solution. Because it is an operationally accredited system, the Assessment and Authorization (A&A) process is streamlined for individual installations.Forcepoint High Speed Guard supports a wide variety ofdata transfer scenarios through the use of flexible transfer mechanisms and extensive data support. These include web services, real-time Moving Pictures Experts Group (MPEG2) video, transfer imagery of multiple formats, imagery metadata files, inter-system messaging, and a wide variety of proprietary data formats.A flexible approachHigh Speed Guard is highly flexible in its secure approachto multi-directional data movement through the inclusionof numerous transfer mechanisms and a wide array of inspection capabilities that, together, form robustsecurity policies.Security policy enforcementThe Forcepoint High Speed Guard provides built-in redundant filtering capabilities delivered by two filtering engines: Rule Engine and the Filtering Engine. Each engine provides consistent policy enforcement across all transfer mechanisms. Instead of pre-packaged point-and-click policies, the engines support full customization of inspection capabilities enabling the creation of complex security policies. This allows specific inspections and constraints for each deployment rather than generic controls based on file type. Almost any security policy can be expressed through the engines’ user-configurable interface languages. This allows new data formats to be added without product modification.Transfer mechanismsThe High Speed Guard transfer mechanisms provide a variety of fixed security protections and secure transfer methods. Forcepoint works with each customer to determine which mechanism(s) best supports their requirements. Many customers utilize multiple transfer mechanisms on a single system to reduce the size and cost of the solution (Figure 2). Any combination of transfer mechanisms can be used to provide multiple flows through a single system. Each flow is independently managed without affecting other operational flows. Providing separate security policies and configurations permits the broadest applicability possible.Streaming TransferWebServicesSIEMSWAP-CStreaming TransferFORCEPOINTHIGH SPEED GUARD/SPStructuredDataImages(Maps)StreamingVideoWeatherDataLargeData SetsNetwork CNetwork BNetwork ALowLatencyNetwork CNetwork BNetwork AFigure 1: Forcepoint High Speed Guard ArchitectureStreaming videoHigh Speed Guard provides unparalleled control and auditing of MPEG Transport Stream streaming video, supporting multiple formats like MPEG-2, MPEG-4, and H.264 encodings, along with STANAG 4609 (North Atlantic Treaty Organization (NATO) Standardization Agreement) compliant data. The built-in MPEG capability ensures that all data received is properly formatted and can process multiplexed streams individually. High Speed Guard extracts, audits, and validates the key length value (KLV) metadata within the MPEG stream; for example, classification and release caveats. Designed for flexibility, the streaming video transfer mechanism supports both unicast and multicast transfers and can send each input to multiple destinations across multiple networks.Service-Oriented Architecture web servicesUtilizing Hypertext Transfer Protocol (HTTP), with or without Secure Socket Layer (SSL), High Speed Guard has built-in support for web services. Ideally suited for SOAP over HTTP services, High Speed Guard supports complete inspectionof all HTTP headers and a full suite of parsing capabilities for the HTTP payload. This mechanism also provides extensive support for data sanitization and re-write, enabling the guard to deliver data that is different than what was transferred. The SOA web services transfer mechanism automatically parses and validates Multipurpose Internet Mail Extensions (MIME) segments and natively supports SOAP with Attachment (SWA) services for optimized data transmission.Adaptable lightweight messaging forUltra-High Data Rates (UDP & TCP)High Speed Guard supports almost any UPD- or TCP-based protocol with or without SSL. Many customers utilize this capability for the cross-domain transfer of custom protocols. Customers utilizing this protocol have demonstrated the transfer of broadcasts, as well as high-performance Java Messaging Services (JMS). Messaging latency can be as low as single- digit milliseconds or lower, providing exceptional support to low tolerance systems.High-Performance File StreamingThe Joint Architecture Study Data Transfer Protocol (JAS/ DTP), which is specifically designed and implemented forthe highest possible data transfer performance, is jointly defined by the National Geospatial-Intelligence Agency (NGA) and their mission partners to provide standardized high-performance data dissemination across a wide variety of networks and systems. High Speed Guard supports repeatable transfer rates of over 9Gb/s when using this protocol. This protocol provides exceptional support where a standardfile transfer protocol (FTP)-style data delivery would be appropriate but requires higher performance.HighBandwidthLowBandwidthFORCEPOINT HIGH SPEED GUARDMultiple SimultaneousTransfer Destinations Network A Figure 2: High-Performance file streaming/contactPublicly Available Information. This document does not contain information (e.g., technical data, technology) that is controlled under US International Traffic in Arms Regulations (ITAR) technical Cross-domain Simple Network Management Protocol (SNMP)Cross-domain SNMP provides the means to extend network management across domains. With this capability, enterprise network status can be received by a controlling domain.Automated Secure Transfer (AST)The AST mechanism provides a standard file “drop box” transfer capability that allows High Speed Guard to monitor external file servers for files to transfer. Using AST, High Speed Guard can monitor and re-create subdirectories, monitor multiple source directories, and transfer to multiple destinations across multiple domains. A unique feature of AST is the ability to send files that fail validation to a specific destination. For example, failed files could be automatically redirected to another guard such as Forcepoint Trusted Gateway System. High Speed Guard may also redirect failed files to a problem or trouble queue on the source system for further review. AST supports both Secure Copy (SCP) and FTP transfers.Administration and managementHigh Speed Guard architecture divides administrativetasks from critical data transfer tasks on separate hardware platforms. This separation permits the guard to be highly minimized and locked down, while administrators havecomplete access to the Administration Server for performing functions such as backup, restoration, configuration, logging, auditing, real-time alerting, and administrator accountmanagement. A single Administration Server supports 10 or more guards depending on the deployment. Consolidated logging and real-time alerting for the enterprise can bemanaged from a central area. The Administration Server itself can be accessed directly or remotely, depending on customer configuration requirements.Logging and auditingHigh Speed Guard is deployed with an audit configuration that meets standard requirements across the cross-domain community. Each deployment is enhanced with auditing specific to the data flows and security policies for that deployment. This unique auditing is driven by the Rule and Filter Engines, permitting the security policy to send any data deemed appropriate to the audit trail at any time. High Speed Guard supports local and remote log consolidation of the standard operating system syslog, binary auditing, and data transfer logging. All log and audit data is actively collected, parsed, and reduced for immediate administrator notification of security events and can be sent, in real time, to a collection server.System integrityHigh Speed Guard uses various mechanisms for file system integrity checking and local configuration monitoring. Integrity validation can occur at any interval as specified by customer policy, typically twice a day. Integrity failures result in a full server halt or service termination (i.e., transfer mechanisms are stopped), depending on customer policy.Configuration managementAdministration Server contains built-in configurationmanagement functionality. The configuration management system preserves a controlled baseline of all High Speed Guard configurations. System modifications are tracked through the configuration manager which runs in a dedicated area on the server. Use of configuration management enforces the maintenance of prior configuration versions and ensures strict adherence to two-person integrity controls.Assessment & Authorization (A&A)High Speed Guard is engineered to satisfy cross-domain security requirements for Top Secret/SCI and BelowInteroperability (TSABI) and Secret and Below Interoperability (SABI) A&A processes to include meeting current NSARaise-The-Bar guidelines. Forcepoint High Speed Guard is deployed worldwide and is part of systems authorized under Department of Defense (DoD) Risk Management Framework (RMF) IT, ICD 503, and National Institute of Standards & Technology 800-53 and 8500.2 security controls.ConclusionForcepoint’s cross-domain solutions have a proven track record of proactively preventing organizations from being compromised, while fostering the secure access andtransfer of information. This allows Forcepoint’s cross-domain solutions to strike the right balance betweeninformation protection and information sharing—a vital component to national security.。
轨道交通专业缩略词总结
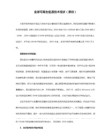
SSID 禁用服务集标志
SSPP 系统安全程序计划 System Safety Program Plan
STCP 系统测试和调试方案 System Testing & Commissioning Plan
TD-SCDMA 时分同步码分多址接入
FAT 工厂验收测试 Factory Acceptance Test
FLASH PROM 闪存型可编程序的只读存储器
GSM 全球移动通信系统, Global System for Mobile communication
I/O 输入/输出 Input/Output
ICDD 接口控制和定义文档 Interface Control & Defination Document
TID 追踪标识 Tracking Identity
TOR 轨道顶部 Top Of Rail
TSR 临时速度限制 Temporary Speed Restriction
TT 型式试验 Type Test
UDP 用户数据报协议 User Datagram Protocol
UPS 不间断电源 Uninterruptible Power Supply
RM 限制人工模式 Restricted Manual
RPR 弹性分组数据环 Resilient Packet Ring
SAP 服务接入点 Service Access Point
SBD 列车安全制动距离 Safety Braking Distance
SCADA (电力)监督控制和数据采集系统
ACS 计轴系统 Axile Counting System
chap12-Mobile IP and Wireless Application Protocol

Registration Process
Mobile node sends registration request to foreign agent requesting forwarding service Foreign agent relays request to home agent Home agent accepts or denies request and sends registration reply to foreign agent Foreign agent relays reply to mobile node
Open standard providing mobile users of wireless terminals access to telephony and information services
Wireless terminals include wireless phones, pagers and personal digital assistants (PDAs) Designed to work with all wireless network technologies such as GSM, CDMA, and TDMA Based on existing Internet standards such as IP, XML, HTML, and HTTP Includes security facilities
Mobile IP and Wireless Application Protocol
Chapter 12
Mobile IP Uses
Enable computers to maintain Internet connectivity while moving from one Internet attachment point to another Mobile – user's point of attachment changes dynamically and all connections are automatically maintained despite the change Nomadic - user's Internet connection is terminated each time the user moves and a new connection is initiated when the user dials back in
基于自适应快速终端滑模的车轮滑移率跟踪控制

DOI: 10.3785/j.issn.1008-973X.2021.01.020基于自适应快速终端滑模的车轮滑移率跟踪控制李静1,王晨1,张家旭1,2(1. 吉林大学 汽车仿真与控制国家重点实验室,吉林 长春 130011;2. 中国第一汽车集团有限公司智能网联研发院,吉林 长春 130011)摘 要:针对汽车对连续、快速和稳定的车轮滑移率跟踪控制的需求,提出基于自适应快速终端滑模的车轮滑移率跟踪控制策略. 基于Burckhardt 轮胎模型建立车轮滑移率跟踪控制模型,将模型简化过程中的不确定性考虑成复合干扰项,将轮胎侧向力对纵向力的影响考虑成未知参数. 利用双曲正切函数和终端吸引因子设计改进的跟踪微分器,平滑车轮滑移率跟踪误差和估计车轮滑移率跟踪误差的一阶导数. 以车轮滑移率跟踪控制模型和改进的跟踪微分器输出为基础,基于自适应快速终端滑模控制理论,设计对复合干扰项具有强鲁棒性的车轮滑移率跟踪控制律;基于投影算子理论设计自适应律来实时补偿未知参数,利用LaSalle 不变性原理证明了闭环系统的渐近稳定性. 利用车辆动力学软件仿真验证提出的控制律的可行性和有效性. 结果表明,提出的车轮滑移率跟踪控制策略具有精度高和鲁棒性强的优点.关键词: 汽车动力学;车轮滑移率跟踪控制;自适应控制;快速终端滑模控制中图分类号: U 461 文献标志码: A 文章编号: 1008−973X (2021)01−0169−08Wheel slip tracking control of vehicle based on adaptive fastterminal sliding mode control methodLI Jing 1, WANG Chen 1, ZHANG Jia-xu 1,2(1. State Key Laboratory of Automotive Simulation and Control , Jilin University , Changchun 130011, China ;2. Intelligent Network R&D Institute , China FAW Group Limited Company , Changchun 130011, China )Abstract: A novel wheel slip tracking control strategy was proposed based on adaptive fast terminal sliding modelcontrol method aiming at the requirement of the vehicle for the continuous, fast and stable wheel slip tracking control. The wheel slip tracking control model was established based on Burckhardt tire model. The uncertainty during the process of model simplification and the influence of the tire lateral force on the tire longitudinal force in the wheel slip tracking control model were considered as the lumped uncertainty and the unknown parameter,respectively. The modified tracking differentiator was deduced based on hyperbolic tangent function and terminal attraction factor in order to smooth the wheel slip tracking error and estimate the derivative of the wheel slip tracking error. The wheel slip rate tracking control law with strong robustness for the system uncertainty was proposed based on the adaptive fast terminal sliding mode control theory according to the wheel slip tracking control model and the outputs of the modified tracking differentiator. The adaptive law was proposed based on projection operator theory to compensate the unknown parameter. The asymptotic stability of the closed-loop system was proved using the LaSalle invariance principle. The feasibility and effectiveness of the proposed wheel slip tracking control strategy was verified based on vehicle dynamics simulation software. Results show that the proposed wheel slip tracking control strategy has high accuracy and strong robustness.Key words: vehicle dynamics; wheel slip tracking control; adaptive control; fast terminal sliding mode control收稿日期:2019−12−24. 网址:/eng/article/2021/1008-973X/202101020.shtml 基金项目:电动汽车智能辅助驾驶关键技术研究与产品开发项目(2016YFB0101000).作者简介:李静(1976—),男,教授,博导,从事汽车地面系统分析与控制的研究. /0000-0002-6126-1632. E-mail :****************通信联系人:张家旭,男,高级工程师. /0000-0001-6159-1965. E-mail :****************第 55 卷第 1 期 2021 年 1 月浙 江 大 学 学 报(工学版)Journal of Zhejiang University (Engineering Science)Vol.55 No.1Jan. 2021目前,安全、智能和环保汽车已经成为现阶段国内外汽车厂商的主要发展目标之一,连续、快速和稳定的车轮滑移率跟踪控制是汽车实现智能安全避障、制动能量回收等先进控制技术的重要基础,因此深入研究连续、快速和稳定的车轮滑移率跟踪控制方法具有重要的工程实用价值.车轮滑移率跟踪控制早期多应用于汽车的防抱死制动系统(anti-lock braking system,ABS)中,通过控制车轮滑移率使轮胎-地面附着系数最大,进而提高汽车制动稳定性和缩短汽车制动距离.由于车轮滑移率动态在汽车制动过程中呈现出复杂的非线性特点,早期多采用逻辑切换规则的控制方式实现车轮滑移率的强鲁棒跟踪控制[1-5]. Kuo等[1]利用车轮滑移率、角加速度和车轮制动力矩信息,设计ABS工作模式的逻辑切换控制规则,将轮胎-地面附着系数控制到最大值. Tanelli 等[2]将车轮制动力矩和车轮滑移率等信息作为切换控制信号,实现ABS工作模式的切换控制. 马付雷[4]基于车轮角加速度辅助车轮滑移率等信息设计逻辑切换规则,实现ABS工作模式的平滑切换控制. 上述基于逻辑切换规则的控制方式难以对任意目标滑移率进行高精度的稳定跟踪控制,无法满足安全、智能和环保汽车对车轮滑移率跟踪控制的需求.基于动态模型的控制方式更容易实现连续目标滑移率的跟踪控制,易满足安全、智能和环保汽车对车轮滑移率跟踪控制的需求. Pasillas-Lepine 等[6]采用Backstepping方法,设计车轮滑移率跟踪控制律. Shim等[7]基于滑模控制理论,设计车轮滑移率跟踪控制律. Yu等[8]采用最小化目标滑移率跟踪误差准则,设计车轮滑移率跟踪控制律. Mirzaei等[9]采用最优预测控制方法,设计车轮滑移率跟踪控制律. Amodeo等[10]采用二阶滑模控制方法,设计车轮滑移律跟踪控制律. Tanelli等[11]基于李雅普诺夫稳定性理论,推导出可智能区分车轮滑移率稳定和非稳定区域的车轮滑移率跟踪控制律. Johansen等[12]采用线性二次最优控制方法,设计具有实时调度特性的车轮滑移率跟踪控制律. Zhang等[13]设计干扰观测器,在线补偿车轮滑移率闭环系统的不确定性. Zhang等[14]基于耗散性理论,设计车轮滑移率跟踪控制律. He等[15]利用障碍李雅普诺夫函数,设计车轮滑移率跟踪控制律. He等[16]利用时变非对称障碍函数,设计车轮滑移率跟踪控制律. 上述基于动态模型的控制方式通常需要依靠简化的控制模型才能得到有效的控制方案,简化后的控制模型无法精确地表征车轮滑移率状态在汽车制动过程中呈现出的复杂非线性. 在基于动态模型的控制方式中,采用简化的控制模型设计控制方案之前需要解决简化的控制模型无法精确表征系统状态的问题.本文基于Burckhardt轮胎模型建立车轮滑移率跟踪控制模型,将模型简化过程中的不确定性考虑成系统的复合干扰项,同时将轮胎侧向力对纵向力的影响考虑成未知参数,从而解耦控制模型中的轮胎纵向力和侧向力. 利用双曲正切函数和终端吸引因子设计改进的跟踪微分器,平滑车轮滑移率跟踪误差和估计车轮滑移率跟踪误差的一阶导数,分别基于自适应快速终端滑模控制理论和投影算子理论设计车轮滑移率跟踪控制律和未知参数的自适应律. 仿真结果表明,所设计的车轮滑移率跟踪控制律具有控制精度高、鲁棒性强的优点,可以有效地抑制滑模控制固有的“抖振”现象.1 车轮滑移率跟踪控制模型如图1所示的车轮滑移率动态模型,基于Burckhardt轮胎模型,建立关于车轮角速度和汽车纵向速度的动力学方程:JωrF wl F wsT bT fm v xα式中:为车轮转动惯量,为车轮角速度,为车轮有效滚动半径,、分别为Burckhardt轮胎模型描述的轮胎纵向力和侧向力,为车轮制动力矩,为轮胎滚动阻力矩和机械摩擦阻力矩之和,为整车质量的1/4,为汽车纵向速度,为轮胎侧偏角.为了简化式(1)描述的关于车轮角速度和汽车纵向速度的动力学方程,将包含轮胎侧向力的因子与轮胎滚动阻力矩和机械摩擦阻力矩之和考虑成式(1)的扰动项,则式(1)修正为图 1 车轮滑移率动态模型Fig.1 Wheel slip dynamic model170浙江大学学报(工学版)第 55 卷d 1=−rF ws sin α−T f d 2=F ws sin α式中:,.Burckhardt 轮胎模型描述的轮胎纵向力可以表示为F z λl λres µres 式中:为车轮垂向载荷;为车轮纵向滑移率;为车轮合成滑移率;为轮胎-地面附着系数,可以表示为[17]ϑ1ϑ2ϑ3其中、和反映了轮胎的合成滑移率对轮胎-地面附着系数的影响.若用未知参数描述车轮侧向滑移率对轮胎纵向力的影响,则式(3)修正为cos α≈1假设,将式(5)代入式(2),可得Burckhardt 轮胎模型在制动工况下定义的车轮滑移率可以简化为对式(7)求二阶微分运算,可得对式(6)求一阶微分运算,可得x 1=λl −λd x 2=˙x1u =˙T b 由于车身惯量远大于车轮惯量,车轮滑移率的变化快于车身运动状态的变化. 将式(6)、(9)代入式(8),令,,,可得车轮滑移率跟踪控制模型为λd u 式中:为车轮目标滑移率;为控制输入;f (x )=v −1x {2F z µres (x 1+λd )(x 2+˙λd )/m −((1−x 1−λd )/m +r 2/J )F z ˙µres (x 1+λd )},x =[x 1,x 2]TG =r /(Jv x )d s =v x −1(d 2˙λl /m −˙d 2(1−λl )/m −r ˙d1/J )−¨λd |d s |⩽B d 其中为系统的状态向量;;d s 为系统的复合干扰项,,假设满足. 2 车轮滑移率跟踪控制器考虑式(10)描述的车轮滑移率跟踪控制模型,目标是设计改进的跟踪微分器、车轮滑移率跟踪控制律和未知参数自适应律,使得闭环系统可以高精度地跟踪车轮目标滑移率,对系统的复合干扰项具有较强的鲁棒性. 系统的总体架构如图2所示,改进的跟踪微分器用于平滑车轮滑移率跟踪误差,估计车轮滑移率跟踪误差的一阶导数. 车轮滑移率跟踪控制律基于改进的跟踪微分器的输出,计算车轮滑移率动态系统高精度的跟踪车轮目标滑移率所需要的车轮制动力矩变化率;利用未知参数自适应律实时估计和补偿车轮滑移率跟踪控制律中的未知参数,以提高车轮滑移率跟踪控制律对未建模动态的自适应能力. 车轮滑移率跟踪控制律的输出通过积分运算后作用于车轮滑移率动态系统,可以抑制系统的“抖振”现象.图 2 系统总体架构Fig.2 System overall architecture2.1 改进的跟踪微分器利用跟踪微分器可以准确地计算信号的一阶导数,为全状态反馈控制律设计奠定基础. 申帅等[18]利用跟踪微分器计算航空光电稳定平台的加速度信号,实现航空光电稳定平台的高增益加速度反馈控制. 谭诗利等[19]利用跟踪微分器计算高超声速飞行器反演控制律中的虚拟控制量,有效解决了高超声速飞行器反演控制律的微分膨胀问题. 为了提高跟踪微分器的收敛速度,利用双曲正切函数和终端吸引因子设计改进的跟踪微分器来平滑车轮滑移率跟踪误差,估计车轮滑移率跟踪误差的一阶导数,以避免利用传统的一阶惯性环节求解信号微分过程中产生的噪声放大效应. 基于双曲正切函数和终端吸引子,设计定理1描述的具有渐近稳定平衡原点的动态系统. 基于该动态系统,构建改进的跟踪微分器.(0,0)定理1 假设原点为如下系统的平衡点:第 1 期李静, 等:基于自适应快速终端滑模的车轮滑移率跟踪控制 [J]. 浙江大学学报:工学版,2021, 55(1): 169–176.171tanh(·)a 0>0a 1>0a 2>0b 0>00<p <1q 式中:为双曲正切函数. 若系统的匹配参数满足、、、、和为正奇数,则系统的平衡点是渐近稳定的.证明:定义Lyapunov 候选函数为z 1 0z 1tanh (b 0z 1)>0由双曲正切函数为奇函数,且时有可知,式(12)定义的Lyapunov 候选函数满足对式(12)求导,可得将式(11)代入式(13),可得定义集合z 2=0z 1=0N {z 1=0,z 2=0}由式(11)可知,当时,必有,因此集合的最大不变集为. 由LaSalle 不变性原理[20]可知,式(11)描述的系统平衡原点是渐近稳定的.根据文献[21]可知,基于式(11)构建改进的跟踪微分器为W >0ˆx 1ˆx 2x 1x 2W →∞ˆx 1→x1ˆx 2→x 2式中:W 为改进跟踪微分器的匹配参数,;和为车轮滑移率跟踪控制模型状态变量和的估计,且时,有和.2.2 车轮滑移率跟踪控制律基于改进跟踪微分器的输出,将式(10)描述的车轮滑移率跟踪控制模型转化为以式(18)为基础,设计车轮滑移率跟踪控制律,使得闭环系统可以高精度地跟踪车轮目标滑移率,且对系统的复合干扰项具有较强的鲁棒性.定义车轮滑移率跟踪误差滑模面为κ0κ0>0式中:为设计参数,.基于式(19),定义如下快速终端滑模面:κ1、κ2、、γκ1>0κ2>01.0<β<2.0γ>β式中:β为设计参数,,,,.由文献[22]可知,式(20)描述的快速终端滑模面可以在有限时间收敛到平衡点,收敛时间可以表示为F (·)式中:为高斯超几何函数,定义参见文献[23].定理2 考虑式(18)描述的车轮滑移率跟踪η1>0η2>0ˆθθ式中:η1、η2为设计参数,,;为未知参数的估计值,采用如下自适应律更新:θmin θmax κ3>0Proj θ(·)θ式中:和分别为未知参数的最小值和最大值;为待设计的自适应增益系数;为投影算子,可以保证未知参数的估计值满足如η1⩾B d 若设计参数,则车轮滑移率跟踪闭环系统的平衡原点是渐近稳定的.证明:定义Lyapunov 候选函数为˜θθ˜θ=θ−ˆθ式中:为未知参数的估计误差,定义为.对式(25)求导,可得将式(23)代入式(27),可得172浙 江 大 学 学 报(工学版)第 55 卷由投影算子的性质[24],可知η1⩾B d 若设计参数,则有将式(29)、(30)代入式(28),可得由式(30)可以定义如下不变集:˙s=0当时,根据式(19),可知˙s=0因此,当时,车轮滑移率跟踪闭环系统的状态轨迹将渐近收敛到平衡原点.σ=0˙s =0当时,根据式(20)可知,车轮滑移率跟踪闭环系统的状态轨迹将在有限时间内收敛到. 由式(33)可知,车轮滑移率跟踪闭环系统的状态轨迹将进一步渐近收敛到平衡原点.综上所述,由LaSalle 不变性原理[20]可知,闭环系统是渐近稳定的.˙s=0注1:在系统的复合干扰存在条件下,车轮滑移率跟踪闭环系统的状态轨迹可以渐近收敛到平衡原点,特别是在快速终端滑模面上的状态轨迹可以在有限时间内收敛到,说明由式(22)、(23)构成的车轮滑移率跟踪闭环系统具有较强的鲁棒性和良好的控制精度.注2:由式(22)可知,利用高阶滑模控制思想,将引起的“抖振”现象的不连续控制输入项包含于控制指令的一阶导数中;通过积分运算求解控制指令,从而有效抑制不连续控制输入项对系统性能的影响.3 仿真结果及分析a 0=10a 1=10a 2=10p =0.9q =3W =200κ0=3κ1=260κ2=150κ3=35γ=3β=1.5η1=2η2=12m =1416kg J =0.9kg ·m 2r=0.31m 基于车辆动力学仿真软件CarSim ,对设计的车轮滑移率跟踪控制律进行仿真验证,选择车辆前左轮和后右轮的仿真结果进行分析. 设计的改进跟踪微分器参数设置如下:,,,,,. 设计的车轮滑移率跟踪控制律参数设置如下:,,,,,,,. 仿真车辆参数设置如下:,,,车辆质心点到前轴和后轴的距离分别为1.016和1.562 m.3.1 斜坡信号工况在斜坡信号仿真工况中,车速和路面附着系数分别设置为33.34 m/s 和1,斜坡信号幅值和变化速率分别设置为0.1和0.03. 为了避免车速为零时控制律产生奇异性,仿真截止车速设置为5 m/s.仿真结果如图3、4所示. 图中,e fl 、e rr 分别为左前图 3 斜坡信号工况的跟踪误差及误差变化率Fig.3 Tracking error and its rate of ramp signal maneuver第 1 期李静, 等:基于自适应快速终端滑模的车轮滑移率跟踪控制 [J]. 浙江大学学报:工学版,2021, 55(1): 169–176.173前车轮和右后车轮滑移率跟踪误差变化率.从图3可知,设计的改进跟踪微分器可以较好地平滑车轮滑移率跟踪误差,且可以准确地估计车轮滑移率跟踪误差变化率. 从图4(a )~(c )、4(e )可知,车辆左前轮和右后轮轮速无明显波动,左前轮和右后轮的滑移率在系统存在复合干扰时可以快速、稳定地跟踪斜坡信号,且轮胎-地面附着系数可以跟随车轮滑移率平稳过渡到稳态阶段. 从图4(d )可知,在设计过程中利用积分运算求解车轮制动力矩,有效地抑制了滑模控制的“抖振”现象. 综上所述,所设计的车轮滑移率跟踪控制律可以在车辆垂向载荷动态变化过程中保证闭环系统具有良好的鲁棒性和控制精度.3.2 正弦信号工况在正弦信号仿真工况中,路面峰值附着系数设置为1,初始车速设置为33.34 m/s ,正弦信号幅值、偏移量和频率分别设置为0.045、0.055和9.42 rad/s.为了避免车速为零时控制律产生奇异性,仿真截止车速设置为5 m/s. 仿真结果如图5、6所示.从图5可知,所设计的改进跟踪微分器可以较好地平滑车轮滑移率跟踪误差,且可以无噪声放大效应地估计车轮滑移率跟踪误差变化率. 从图6(a )~(c )、(e )可知,车辆左前轮和右后轮轮速跟随正弦信号的周期性波动,左前轮和右后轮的滑移率在系统存在复合干扰时可以快速、稳定地跟踪正弦信号,无明显的相位滞后,且轮胎-地面附着系数可以跟随车轮滑移率周期性波动. 从图6(d )可知,利用积分运算求解车轮制动力矩可以有效地抑制滑模控制的“抖振”现象. 综上所述,所设计的车轮滑移率跟踪控制律可以在车辆垂向载荷动态变化过程中保证闭环系统具有良好的动态过程品质.4 结 论(1)基于Burckhardt 轮胎模型建立车轮滑移率跟踪控制模型,将模型简化过程中的不确定性考虑成系统复合干扰项,将轮胎侧向力对纵向力的影响考虑成未知参数,解耦控制模型中的轮胎纵向力和侧向力.(2)利用双曲正切函数和终端吸引因子设计改进的跟踪微分器,平滑车轮滑移率跟踪误差和估计车轮滑移率跟踪误差的一阶导数. 以车轮滑移率跟踪控制模型和改进的跟踪微分器输出为基础,基于自适应快速终端滑模控制理论和投影图 4 斜坡信号工况的车辆状态信息Fig.4 Vehicle state information of ramp signal maneuver174浙 江 大 学 学 报(工学版)第 55 卷算子理论推导出未知参数自适应律,对系统复合干扰项具有强鲁棒性的车轮滑移率跟踪控制律;利用LaSalle 不变性原理,证明了闭环系统的渐近稳定性.(3)利用车辆动力学软件仿真,验证所提出图 6 正弦信号工况的车辆状态信息Fig.6 Vehicle state information of sine signal maneuver图 5 正弦信号工况的跟踪误差及误差变化率Fig.5 Tracking error and its rate of sine signal maneuver第 1 期李静, 等:基于自适应快速终端滑模的车轮滑移率跟踪控制 [J]. 浙江大学学报:工学版,2021, 55(1): 169–176.175的控制律的可行性和有效性. 结果表明,设计的改进跟踪微分器可以较好地平滑车轮滑移率跟踪误差,且可以无噪声地估计车轮滑移率跟踪误差变化率;提出的车轮滑移率跟踪控制律具有良好的控制品质,可以有效地抑制滑模控制中固有的“抖振”现象.参考文献(References):KUO C Y, YEH E C. A four-phase control scheme of an anti-skid brake system for all road conditions [J]. Proceeding of the Institution of Mechanical Engineers, Part D: Journal of Automobile Engineering, 1992, 206(44): 275–283.[1]TANELLI M, OSORIO G, BERNARDO M D, et al. Existence, stability and robustness analysis of limit cycles in hybrid anti-lock braking systems [J]. International Journal of Control, 2009, 82(4): 659–678.[2]JING H H, LIU Z Y, CHEN H. A switched control strategy for antilock braking system with on/off valves [J]. IEEE Transactions on Vehicular Technology, 2011, 60(4): 1470–1484.[3]马付雷. 汽车ABS逻辑门限值控制算法研究与实现[D]. 重庆:重庆邮电大学, 2011.[4]王伟达, 丁能根, 张为, 等. ABS逻辑门限值自调整控制方法研究与试验验证[J].机械工程学报, 2010, 46(22): 90–104.WANG Wei-da, DING Neng-gen, ZHANG Wei, et al. Research and verification of the logic threshold self-adjusting control method for ABS [J]. Journal of Mechanical Engineering, 2010, 46(22): 90–104.[5]PASILLAS-LEPINE W, LORIA A, GERARD M. Design and experimental validation of a nonlinear wheel slip control algorithm [J]. Automatica, 2012, 48: 1852–1859.[6]SHIM T, CHANG S, LEE S. Investigation of sliding-surface design on the performance of sliding mode controller in antilock braking systems [J]. IEEE Transactions on Vehicular Technology, 2008, 57(2): 747–759.[7]YU H X, QI Z Q, DUAN J M, et al. Multiple model adaptive backstepping control for antilock braking system based on LuGre dynamic tyre model [J]. International Journal of Vehicle Design, 2015, 69(1-4): 168–184.[8]MIRZAEI M, MIRZAEINEJAD H. Optimal design of a non-linear controller for anti-lock braking system [J]. Transportation Research Part C, 2012, 24: 19–35.[9]AMODEO M, FERRARA A, TERZAGHI R, et al. Wheel slip control via second-order sliding-mode generation [J]. IEEE Transactions on Intelligent Transportation Systems, 2010, 11(1): 122–131.[10]TANELLI M, ASTOLFI A, SAVARESI S M. Robust nonlinear [11]output feedback control for brake by wire control systems [J].Automatica, 2008, 44: 1078–1083.JOHANSEN T A, PETERSEN I, KALKKUHL J, et al. Gain-scheduled wheel slip control in automotive brake systems [J].IEEE Transactions on Control Systems Technology, 2003, 11(6): 799–811.[12]ZHANG J X, LI J. Adaptive backstepping sliding mode control for wheel slip tracking of vehicle with uncertainty observer [J].Measurement and Control, 2018, 51(9/10): 396–405.[13]ZHANG J X, LI J. Robust backstepping sliding mode control with L2-gain performance for reference input wheel slip tracking of vehicle [J]. Information Technology and Control, 2019, 48(4): 660–672.[14]HE Y G, LU C D, SHEN J, et al. Design and analysis of output feedback constraint control for antilock braking system with time-varying slip ratio [J]. Mathematical Problems in Engineering, 2019, 2019: 1–11.[15]HE Y G, LU C D, SHEN J, et al. Design and analysis of output feedback constraint control for antilock braking system based on Burckhardt's model [J]. Assembly Automation, 2019, 39(4): 497–513.[16]KIENCKE U, NIELSEN L. Automotive control systems[M].Berlin: Springer, 2000.[17]申帅, 张葆, 李贤涛, 等. 基于跟踪微分器的加速度反馈控制[J]. 吉林大学学报: 工学版, 2017, 47(4): 1217–1224.SHEN Shuai, ZHANG Bao, LI Xian-tao, et al. Acceleration feedback control based on tracking differentiator [J]. Journal of Jilin University: Engineering and Technology Edition, 2017, 47(4): 1217–1224.[18]谭诗利, 雷虎民, 王鹏飞. 应用跟踪微分器的高超声速飞行器的反演控制[J]. 宇航学报, 2019, 40(6): 673–683.TAN Shi-li, LEI Hu-min, WANG Peng-fei. Backstepping control for hypersonic vehicle with a novel tracking differentiator [J].Journal of Astronautics, 2019, 40(6): 673–683.[19]姜长生, 吴庆宪, 费树岷, 等. 现代非线性系统鲁棒控制基础[M]. 哈尔滨: 哈尔滨工业大学出版社, 2012.[20]HAN J Q, WANG W. Nonlinear tracking differentiator [J].Journal of Systems Science and Mathematical Sciences, 1994, 14(2): 177–183.[21]YANG L, YANG J Y. Nonsingular fast terminal sliding-mode control for nonlinear dynamical systems [J]. International Journal of Robust and Nonlinear Control, 2011, 21: 1865–1879.[22]ABRAMOWITZ M, STEGUN I A. Handbook of mathematical functions: with formulas, graphs, and mathematical tables [M]. New York: Dover, 1972.[23]YAO B, TOMIZUKA M. Adaptive robust control of MIMO nonlinear systems in semi-strict feedback form [J]. Automatica, 2001, 37(9): 1305–1321.[24]176浙江大学学报(工学版)第 55 卷。
仪表自动化英语
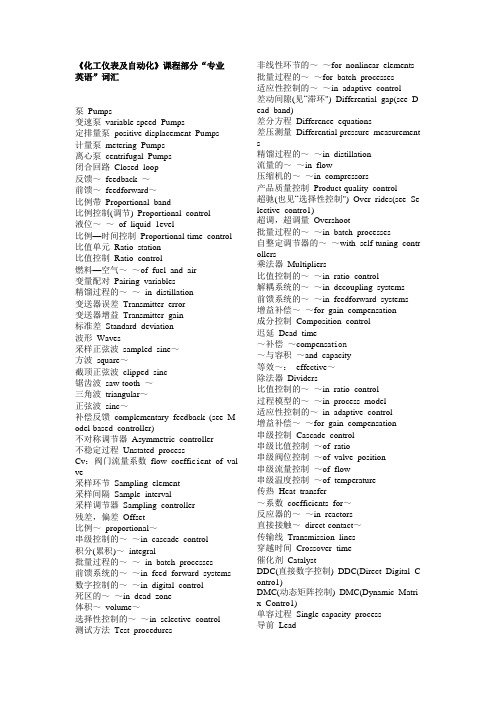
《化工仪表及自动化》课程部分“专业英语”词汇泵Pumps变速泵variable-speed Pumps定排量泵positive-displacement Pumps计量泵metering Pumps离心泵centrifugal Pumps闭合回路Closed loop反馈~feedback ~前馈~feedforward~比例带Proportional band比例控制(调节) Proportional control液位~~of liquid 1evel比例—时间控制Proportional-time control 比值单元Ratio station比值控制Ratio control燃料—空气~~of fuel and air变量配对Pairing variables精馏过程的~~in distillation变送器误差Transmitter error变送器增益Transmitter gain标准差Standard deviation波形Waves采样正弦波sampled sine~方波square~截顶正弦波clipped sinc锯齿波saw-tooth ~三角波triangular~正弦波sinc~补偿反馈complementary feedback(see M odel-based controller)不对称调节器Asymmetric controller不稳定过程Unstated processCv:阀门流量系数flow coeffi ci ent of val ve采样环节Sampling element采样间隔Sample interval采样调节器Sampling controller残差,偏差Offset比例~proportional~串级控制的~~in cascade control积分(累积)~integral批量过程的~~in batch processes前馈系统的~~in feed forward systems 数字控制的~~in digital control死区的~~in dead zone体积~volume~选择性控制的~~in selective control测试方法Test procedures 非线性环节的~~for nonlinear elements 批量过程的~~for batch processes适应性控制的~~in adaptive control差动间隙(见“滞环") Differential gap(see D ead band)差分方程Difference equations差压测量Differential-pressure measurement s精馏过程的~~in distillation流量的~~in flow压缩机的~~in compressors产品质量控制Product-quality control超驰(也见“选择性控制") Over rides(see Se lective contro1)超调,超调量Overshoot批量过程的~~in batch processes自整定调节器的~~with self-tuning contr ollers乘法器Multipliers比值控制的~~in ratio control解耦系统的~~in decoupling systems前馈系统的~~in feedforward systems 增益补偿~~for gain compensation成分控制Composition control迟延Dead time~补偿~compensat ion~与容积~and capacity等效~:effective~除法器Dividers比值控制的~~in ratio control过程模型的~~in process model适应性控制的~in adaptive control增益补偿~~for gain compensation串级控制Cascade control串级比值控制~of ratio串级阀位控制~of valve position串级流量控制~of flow串级温度控制~of temperature传热Heat transfer~系数coefficients for~反应器的~~in reactors直接接触~direct-contact~传输线Transmission lines穿越时间Cross o ver time催化剂CatalystDDC(直接数字控制) DDC(Direct Digital C ontro1)DMC(动态矩阵控制) DMC(Dynamic Matri x Contro1)单容过程Single-capacity process导前Lead导前—滞后补偿lead-lag compensation等百分比阀Equal-percentage valves电磁流量计Magnetic flow meter定时器Timer定位器,阀门~Positioners,Valve~动态补偿Dynamic compensation干燥器的~~for dryers锅炉燃烧的~~for boiler firing解耦的~~in decoupling精馏的~for distillation汽包水位控制的~~for drum-level contro l热交换器的~~for heat exchangers热流量计算的~~for heat-flow calculatio n优化器的~in optimizers蒸发器的~~for evaporators动态补偿器Dynamic compensators动态矩阵控制(DMC) Dynamic matrix cont rol动态增益Dynamic gain抖动Dither多变量过程Multivariable processes多变量调节器Multivariable controllers多变量系统的分解Decomposing multivaria te systems多容过程Multi-capacity processes多输出系统Multiple-output systems阀门Valves电磁~solenoid~电动~electric~气动~pneumatic~三通~three-way~调节阀control~阀门的可调范围Valve range ability阀门定位器Valve positioners阀门顺序动作Valve sequencing利用定位器实现~~using positioners利用选择器实现一~using selectors阀门特性Valve characteristics阀门响应Valve response阀门增益Valve gain阀位控制Valve-position control反馈Feedback串级系统的~~in cascade systems负~negative~干燥器控制系统的~~in dryer control sy stems关联系统的~~in interacting systems解耦系统的~~in decoupling systems 正~positive~反向响应Inverse response反应器Reactors反应速度Reaction rate非线性Nonlinearity变送器的~~in transmitters传热的~~in heat transfer阀门的~~in Valve非线性环节Nonlinear elements动态~dynamic~静态~steady-state~非线性调节器Nonlinear controllers非线性PID调节器PID~开关~on-off~三位~three-state~非自衡Non-self-regulation分布式控制Distributed control分析仪Analyzers采样~sampling~峰值位置Peak location浮动压力控制Floating pressure control负反馈Negative feedback符号Symbols负荷Load零~zero~负荷分布Load distribution负荷扰动(干扰) Load disturbances~的作用点point sofentry for~供应侧~supply-side需求侧~demand—side~负荷响应Load responsePI控制下的~~with PI controlPID控制下的~~with PID control副回路Secondary loop傅里叶序列Fourior series复式控制Dual-mode control干扰灵敏度Sensitivity tO disturbances周期性~periodic~干燥器Dryers功Work功率Power电~electric~热~thermal~水~hydraulic~关联,相互作用,相互干扰Interaction回路间的~~between loops回路间的局部~partial~between loops 控制作用之间的~~between control mod es惯性Inertia过程增益Process gain锅炉控制Boiler control~的干扰disturbances to~锅炉多变量控制multiple~回路增益Loop gain闭环~closed ~串级系统的~~in cascade systems非线性系统的~~in nonlinear systems开环~open~混合Mixing混合系统Blending systems火焰温度Flametem peratureIAE(绝对误差积分) IAE( Integrated absolu te error)IE(误差积分) Integrated ErrorISE(误差平方积分) Integral Square Error ITAE(时间绝对误差积分) Integral of Time and Absolute Error积分饱和Windup积分饱和防护,抗积分饱和Windup protec tion积分反馈Integral feedback积分过程Integrating process积分控制(调节) Integral control积分器Integrator积分调节器Integral controllers极限环Limit cycle热交换器heat exchangers基于矩阵的控制Matrix-based control基于模型的调节器Model—based controlle rs监控Supervisory control减温Attemperation间歇控制Batch control搅拌(见“混") Agitation(see Mixing)解耦Decoupling通过选择变量~~by choosing variables 适应性~adaptive~~的相对增益relative gain of ~绝对误差积分(1AE) Integrated Absolute Er ror均方根误差Root-mean-square(rms)error开方器Square-root extractor开关控制On-off control抗喘振控制Antis urge control抗积分饱和Anti windup可变结构Variable structuring可调范围Range ability变速泵的~~of variable-speed pumps阀门的~~of valves孔板流量计Orifice meter空气流量控制Air flow control控制的经济效益Economics of control 控制间隔Control interval控制难度Control difficulty控制算法Control algorithm冷凝器Condensers流量比Flow ratio~的调整manipulation of~~控制controlof~流量计Flow meter流量计的精度Accuracy of flow meter流量控制Flow control流阻Resistance to flow炉Furnace鲁棒性Robustness滤波器Filter非线性~nonlinear~数字~digital~马达Motor电动~:electric ~恒速电动~constant-speed ~变速电动~variable-speed气动~pneumatic~马力Horsepower模型Models动态~dynamic~能量平衡Energy balance前馈控制中的~~in feed forward control稳态~steady-state~能量转换Energy conversion耦合Coupling频率响应分析Frequency-response analysis平衡Equilibrium汽包锅炉假水位Shrink and swell in drum boilers气动调节器Pneumatic controllers前馈控制Feed forward control燃料—空气比控制Fuel-air ratio control 燃烧Combustion扰动,干扰Disturbances负荷~load~热力学Thermodynamics热流量计算Heat-flow calculation热流量控制Heat-flow control容积Capacity单容single~多容multiple~非自衡~non self-regulating~双容double~自衡~self-regulating~冗余仪表Redundant instrumentation三冲量水位控制Three-element level contr ol三位调节器Three-state controller设定值初始化Set-point initialization设定值响应Set-point response斜坡~ramp~湿度控制Moisture control时间常数Time constant等效~effective~时间迟后Time delay时间滞后Time lag时滞Lag传输~transport~多级~multiple~二阶~second-order~分布~distributed~负~negative~手动控制Manual control数字控制Digital control数字系统的分辨率Resolution in digital sy stems衰减Damping临界~critical~衰减比Decay ratio双容过程Two-capacity process速度控制Speed control速率限制Velocity limiting调节阀Control valves调节量Manipulated variable~的选择selection of~调节器Controllers调节器的饱和Saturation of controllers调节器的跟踪Tracking in controllers调节器的误差Error in controllers调节器动作Controller action调节器调整Controlleral adjustment调节器增益Controller gain调节作用,控制方式Control mode微分Derivative流量控制的~~in flow control微分方程Differential equations微分器Differentiators稳定性裕量Stability margin温度传感器Temperature sensor涡流流量计Vortex flow meter涡轮流量计Turbine meter限幅器Limiters相对干扰增益Relative disturbance gain相位裕量Phase margin斜坡补偿Ramp compensation斜坡扰动Ramp disturbances 信号选择器Signal selectors性能准则Performance crite rion压力补偿Pressure compensation压力控制Pressure control压缩机的喘振防护Surge protection of co mpressors烟道气体成分Flue-gas composition氧气分析仪Oxygen analyzer液面控制Level control液位控制Liquid-level control引风控制Draft control预热器Pre heaters增益补偿Gain compensation增益矩阵Gain matrix增益裕量Gain margin振荡Oscillation不衰减~undamped~等幅振荡Constant-amplitude~发散~expanding~衰减~damped ~振荡周期Period of oscillation整定Tuning蒸汽Steam过热~superheated~~加热heating with~~流量计~flow meter蒸汽压力Vapor pressure中值选择器Median selector自动—手动切换Auto/manual transfer自衡Self-regulation。
LTE-M终端设备技术规范(城市轨道交通车地综合通信系统(LTE-M)规范)
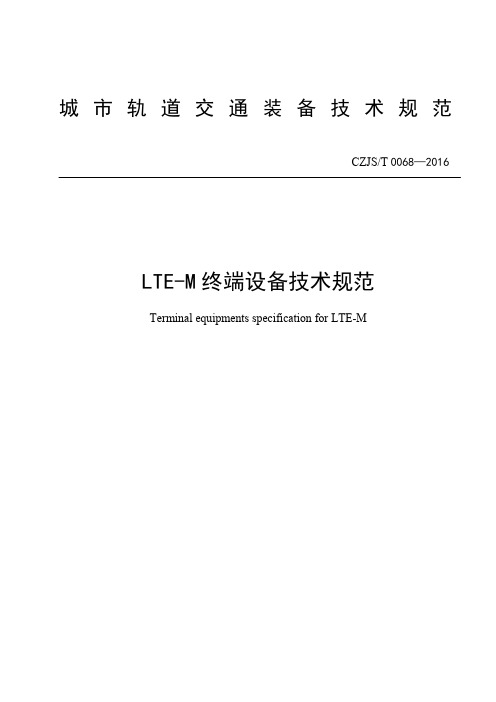
城市轨道交通装备技术规范CZJS/T 0068—2016LTE-M终端设备技术规范Terminal equipments specification for LTE-MCZJS/T 0068—2016目次前言 (III)1 范围 (1)2 规范性引用文件 (1)3 术语、定义和缩略语 (1)3.1 术语和定义 (1)3.2 缩略语 (2)4 LTE-M终端分类和功率等级 (3)4.1 车载台 (3)4.2 手持台 (4)4.3 固定台 (4)4.4 LTE-M终端功率等级 (4)5 LTE-M终端承载业务 (4)5.1 CBTC业务 (4)5.2 列车紧急文本下发业务 (4)5.3 列车运行状态监测业务 (4)5.4 列车视频监视业务 (4)5.5 PIS业务 (4)5.6 集群调度业务 (5)5.7 其它数据业务 (5)6 LTE-M终端物理层功能 (5)6.1 OFDMA/SC-FDMA参数 (5)6.2 帧结构 (5)6.3 物理信道 (5)6.4 多天线技术 (5)6.5 同步和小区搜索 (6)6.6 随机接入 (6)6.7 调制、编码和加扰 (6)6.8 参考信号 (6)6.9 资源分配 (7)6.10 功率控制、功率分配 (7)6.11 控制信道格式和控制信息 (7)6.12 CQI/PMI/RI反馈 (8)6.13 ACK/NACK反馈 (8)6.14 测量 (8)6.15 射频处理 (8)ICZJS/T 0068—20166.16 接口要求 (8)7 LTE-M终端层2功能 (8)7.1 信道类型 (8)7.2 MAC功能及过程 (9)7.3 RLC功能及过程 (10)7.4 PDCP功能及过程 (10)7.5 集群调度相关功能 (11)8 LTE-M终端层3及NAS层功能 (11)8.1 层3 RRC功能及过程 (11)8.2 NAS层功能及过程 (13)8.3 集群调度功能 (15)9 终端设备射频技术指标 (15)10 车载台 (15)10.1 LTE-M车载数据终端 (15)10.2 LTE-M车载集群终端 (17)11 手持台 (18)11.1 一般介绍 (19)11.2 基本功能需求 (19)11.3 集群调度业务需求 (19)11.4 无线功能需求 (19)11.5 环境和物理需求 (19)11.6 电源需求 (20)11.7 其它接口 (20)11.8 安全需求 (20)12 固定台 (20)附录A(资料性附录)不同业务QoS等级 (21)IICZJS/T 0068—2016LTE-M终端设备技术规范1 范围本规范规定了LTE-M终端设备的技术要求,规范定义了终端设备的业务要求、功能要求、性能要求和相关接口要求。
牵引车-飞机系统的路径跟踪控制(英文)
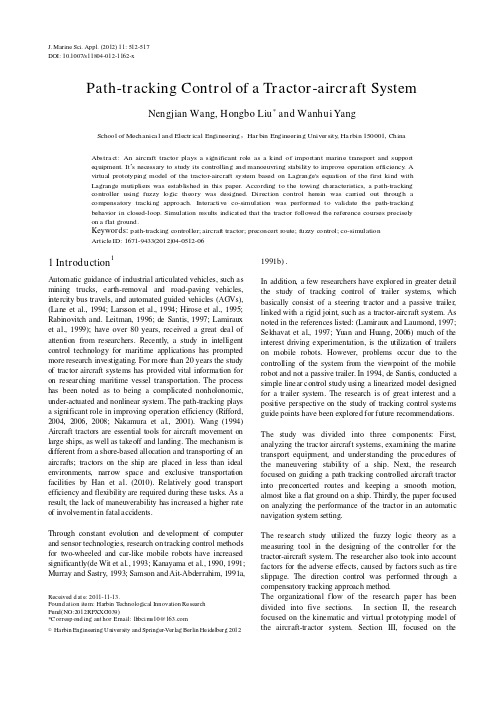
J.Marine Sci.Appl.(2012)11:512-517DOI:10.1007/s11804-012-1162-xPath-tracking Control of a Tractor-aircraft SystemNengjian Wang,Hongbo Liu*and Wanhui YangSchool of Mechanica l and Electr ical Engineering,Har bin Engineering Univer sity,Ha rbin150001,ChinaAbstra ct:An aircraft tractor plays a significant role as a kind of important marine transport and supportequipment.It’s necessary to study its controlling and manoeuvring stability to improve operation efficiency.Avirtual prototyping model of the tractor-aircraft system based on Lagrange's equation of the first kind withLagrange mutipliers was established in this paper.According to the towing characteristics,a path-trackingcontroller using fuzzy logic theory was designed.Direction control herein was carried out through acompensatory tracking approach.Interactive co-simulation was performed to validate the path-trackingbehavior in closed-loop.Simulation results indicated that the tractor followed the reference courses preciselyon a flat ground.Keywords:path-tracking controller;aircraft tractor;preconcert route;fuzzy control;co-simulationArticle ID:1671-9433(2012)04-0512-061Introduction1Automatic guidance of industrial articulated vehicles,such as mining trucks,earth-removal and road-paving vehicles, intercity bus travels,and automated guided vehicles(AGVs), (Lane et al.,1994;Larsson et al.,1994;Hirose et al.,1995; Rabinovitch and.Leitman,1996;de Santis,1997;Lamiraux et al.,1999);have over80years,received a great deal of attention from researchers.Recently,a study in intelligent control technology for maritime applications has prompted more research investigating.For more than20years the study of tractor aircraft systems has provided vital information for on researching maritime vessel transportation.The process has been noted as to being a complicated nonholonomic, under-actuated and nonlinear system.The path-tracking plays a significant role in improving operation efficiency(Rifford, 2004,2006,2008;Nakamura et al.,2001).Wang(1994) Aircraft tractors are essential tools for aircraft movement on large ships,as well as takeoff and landing.The mechanism is different from a shore-based allocation and transporting of an aircrafts;tractors on the ship are placed in less than ideal environments,narrow space and exclusive transportation facilities by Han et al.(2010).Relatively good transport efficiency and flexibility are required during these tasks.As a result,the lack of maneuverability has increased a higher rate of involvement in fatal accidents.Through constant evolution and development of computer and sensor technologies,research on tracking control methods for two-wheeled and car-like mobile robots have increased significantly(de Wit et al.,1993;Kanayama et al.,1990,1991; Murray and Sastry,1993;Samson and Ait-Abderrahim,1991a, Received d at e:2011-11-13.Found at ion item:Harbin Technological Innovation ResearchFund(NO:2012RFXXG039)*C orresp ond ing aut hor Email:lhbci************©Harbin Engineering Univers ity and Springer-Verlag Berlin Heidel berg20121991b).In addition,a few researchers have explored in greater detail the study of tracking control of trailer systems,which basically consist of a steering tractor and a passive trailer, linked with a rigid joint,such as a tractor-aircraft system.As noted in the references listed:(Lamiraux and Laumond,1997; Sekhavat et al.,1997;Yuan and Huang,2006)much of the interest driving experimentation,is the utilization of trailers on mobile robots.However,problems occur due to the controlling of the system from the viewpoint of the mobile robot and not a passive trailer.In1994,de Santis,conducted a simple linear control study using a linearized model designed for a trailer system.The research is of great interest and a positive perspective on the study of tracking control systems guide points have been explored for future recommendations.The study was divided into three components:First, analyzing the tractor aircraft systems,examining the marine transport equipment,and understanding the procedures of the maneuvering stability of a ship.Next,the research focused on guiding a path tracking controlled aircraft tractor into preconcerted routes and keeping a smooth motion, almost like a flat ground on a ship.Thirdly,the paper focused on analyzing the performance of the tractor in an automatic navigation system setting.The research study utilized the fuzzy logic theory as a measuring tool in the designing of the controller for the tractor-aircraft system.The researcher also took into account factors for the adverse ef fects,caused by factors such as tire slippage.The direction control was performed through a compensatory tracking approach method.The organizational flow of the research paper has been divided into five sections.In section II,the research focused on the kinematic and virtual prototyping model of the aircraft-tractor system.Section III,focused on theJournal of Marine S cience and Appl ication (2012)11:512-517513design of the fuzzy control system,while section IV contains simulation results.The paper concludes with remarks and recommendations in section V .2Model of a tr actor-aircraft system2.1Kinematic mod elThe model is based on a rigid multiply body that consists of a tractor,a drawbar,the undercarriage and fuselage,ignoring,for the moment,the flexibility of the tractor suspension and undercarriage buffer system.It is usually assumed that the wheels do not slip.The deformation of the tires is also ignored for the sake of simplicity.These assumptions are acceptable for tractor towing at low speeds:(1)Calculate the lateral component of constraint force onthe tractor-aircraft system junction.(2)The relative angles between the various parts are small,and the tractor front wheel steering angle is small.(3)Examine the wheels rolling resistance,back torque and air resistance.Primarily consider the lateral and the swaying motions of the tractor-aircraft system illustrated in Figure1.Fig.1Kinematic model of a tractor-aircraft system Dynamical equations of the tractor are shown as the following.11111121()cosy a y y m v u r F F F R (1)111122131cos z y y y a J r F d F d R d M (2)The drawbar and the nose landing gear dynamical equations are depicted as:2222323()y y y m v u r R R F (3)22243535z y y y J r R d R d F d (4)Dynamical equations of the fuselage and the rear landinggear are founded with the expression3333244()a y y m v u r F F R (5)3346247z y a y J r R d M F d (6)where i m represents the mass (the subscript i=1,2,3denotes the tractor,the drawbar and the aircraft respectively),i u is the marching velocity,i r is the sway rate and yi F is the cornering force on the tractor wheel,yi R is the lateral constraint reacting force on the articulation and the vertical moment of inertia is expressed with iz J ,ai F and ai M are the accessional lateral force and torque on the centroid,δis the tractor front wheel steering angles,12,d d are the distances from the tractor centroid to the front and rear axle,3d is the distance from the tractor centroid to the anterior to the drawbar,45,d d are the distances from the drawbar centroid to its foreside and rearward,67,d d are thedistances from the aircraft centroid to the front and rear axle.Cornering force on the tractor wheels yi F is defined as a function of the slip angle.When the lateral acceleration is less than 0.4g,the slip angle is generally no more than 4°-5°,the tire cornering properties are in the linear range.Cornering force is given by y i i i F k a ,where i k is thecornering stiffness,its value is negative,i a is the tire slipangle.The state equation of tractor-aircraft towing operation can be described by means of:K XL XM UN TS F(7)2.2Vir tual prototyping modelUsing the ADAMS/View program(Elliott,2000),a virtual prototyping model is created as shown in Figure 2.A centralized quality tractor model is established,which includes the body,suspension and steering system,tires and other components.The study shows evidence of a reduction in the drawbar to a cylindrical rod..The aircraft model is mainly composed of the fuselage,undercarriage and employs spring-dampers.As a result,nonlinear elastic damping effects in the spline curve takes place in the undercarriage buffer system.Nengjian W ang,et al.Path-tracking C ontrol of a T ract or-A ircraft System514Fig.2Virtual prototyping model of the tractor-aircraftsystemThe parameters of the tire and road can be set in the Fiala tyre model and mdi_2d_flat road model,such as:the vertical stiffness,vertical damping of the tire,the friction factor ,and graphics of the road.2.3Comparative analysis of kinetic model an d virtualprototype mod elA comparative analysis was conducted to set the tractor initial position on the ground coordinate system origin and zero degree for the initial direction.The simulation was carried out using a vertical speed of 5km/h.The step input was given to a steering wheel with the function:step (time,8,0,8.02,and 42d).The study compared the steady-state values of the kinetic model and the virtual prototype model,as shown in Table 1.It was established that the virtual prototype model is a good feature.Table 1Contrast of the Kinetics ParametersInvestigating variablesY aw rate of the tractor/((°)·s 1)Yaw rate of thedrawbar/((°)·s 1)Y aw rate of the aircraft/((°)·s 1)Lateral velocityof the tractor/(mm/s)Angle between the drawbar and the aircraft/(°)Angle betweenthe tractor and the drawbar/(°)Simulation value1.4361.4271.40445.904.1266.222Theoretic value 1.507 1.507 1.47846.60 4.395 6.549Absolute error 0.0740.0800.0740.7000.2690.327Relative error5.3%5.6%5.3%1.5%6.5%5.2%3Establishment of fuzzy control sysytemBased on the virtual prototype model of the tractor-aircraftsystem a Mamdani fuzzy control system is established (Shukla and Tiwari,2010).A block diagram of the fuzzy control system is visible in Figure 3.Distance deviation and angle deviation,which can be derived by drawing acomparison between the actual path,and the preconcerted routes are calculated as the input of the controller.The torque that controls the steering wheel angle sheers off betimes to eliminate the error is referred to as the output.Fig.3Block diagram of the controller3.1Path Reference fr ameUbiety between the tractor and the preconcerted route is shown in Figure4.The ground coordinate system OX YZ is used to describe the trajectory,whereas vehicle coordinated system oxyz is used to calculate the distance deviation Ed and angle deviation Ea.Path point P c (c=1,2,3,…n)connecting to the sequentially composed preconcerted path.The origin of the vehicle system of coordinates is (X 0,Z 0)on the ground coordinate and the relative angle between these two coordinated systems is .Fig.4Schematic diagram of the ubiety3.2Posit ion ControllerJournal of Marine S cience and Appl ication (2012)11:512-517515The functions of the fuzzification interface are to perform the following steps:measure the values among the input variables from the data acquisition interface,quantifying in order to transform the range of the observed values into the corresponding discourse of the language variables,and transforming the input data into proper linguistic values,that can be regarded as a form of fuzzy set.The subets of the in-out variables are decomposed into seven fuzzy partitions,denoted by PB (positive big),PM (positive medium),PS (positive small),Z (zero),NS (negative small),NM (negative medium),and NB (negative big),respectively.The domain of distance deviation,Ed is [–1000,1000],Unit:mm and of angle deviation Ea is [1.57,1.57],Unit:rad.Control axial torque on the steering wheel has a basic domain of [78400,78400]which unit is N ·mm.In-out variables in fuzzy set are on the fuzzy domain {6,4,2,0,2,4,6}.Analyzing the basic domain and the compartmentalization of the hierarchy,quantization factor of distance deviation Kd comes to a value of 0.006and that of angle deviation Ka is 0.267,while the control torque scale factor Kt is 13066.The membership function of in-out is shown in Figure5.Fig.5Membership functionThe rule table of fuzzy controller is shown in Table 2and the output surface of fuzzy control rules can be illustrated as shown in Figure6.There are four conditions of the tractor current position and preconcerted route determined by the distance and angle deviation:(a)0,0Ed Ea ;(b)0,0Ed Ea ;(c)0,0EdEa;(d)0,0EdEaTable 2Rule table of fuzzy controllerOutput Torque UEd NB NM NS Z PS PM PB Ed NB PB PB PB PM NS NS NS NM PB PB PM PS NS NS NS NS PB PM PM PS NS NS NS ZPM PM PM Z NM NM NM PS PS PS PS NS NM NM NB PM PS PS PS NS NM NB NB PBPSPSPSNMNBNBNBEd EaFig.6Output surface of fuzzy rules4Tracking behavior simulation analysisFor verifying the efficiency of the proposed controller,we realize this system on the virtual prototyping model created in section Ⅱ.Define the in-out adopting ADAMS/Controls and establish the control algorithms in Simulink Model.The study implemented control modules and designed software in the control system,and interactive simulation.The co-simulation model is shown in Figure7,which contains dynamic modules;path deviation calculation module,a fuzzy control module and a time limit module.The corresponding oscilloscope to record the distance and angle deviation and other important data were also established.Thus,the operations and some experimental results are presented in a series of pictures to demonstrate the efficiency of the proposedmethods.Fig.7Co-simulation mode l4.1Performance of the Virtu al Prototyping ModelGiven the tractor rear wheel,a axial torque with a step input:step (time,0,50000,180,1800000)and a drive function to the steering wheel with:step (time,0,0,1,168d),the simulation was carried out.The traction trajectory is shown in Figure 8.The simulations illustrated in Figs.7and 8,results indicate that the under-steer system increased the tractor turningNengjian W ang,et al.Path-tracking Control of a T ractor-A ircraft System 516radius and lateral velocity.t.The tractor's turning radius andlateral velocity are greater than those of the aircraft.Aforesaid analysis proves that the virtual prototyping modelhas good maneuveringstability.(a)Route of the Idle Load Tractor(b)Route of the Load-CarryingTractor(c)Route of the Passive AircraftFig.8TractionTrajectoryFig.9Turning Radius of theTractorFig.10Lateral Velocity Comparison4.2Tr acking Beh avior Und er th e Fuzzy Con trolTowing the aircraft at the speed of5.4km/h along route1(visible in Fig.11),simulations was carried out as follows:(a)Running with a step input:step(time,4,0,4.2,42d)(b)Control the system through co-simulation approachWe investigate the performance of the fuzzy control system.Figure12shows the tracking behavior under an operation ofclosed-loop input.The foundation of the fuzzy controllercould make up some adverse effects caused by tire slippage,etc,to a certain extent.Also the establishment plays animportant role in safe and efficient towingoperation.Fig.11PreconcertedRoutesFig.12Tracking TrajectoriesThe tractor drove in accordance with the intended route2asshown in Figure13,pulling the aircraft from point A todestination B at the speed of 5.4km/h.The trackingtrajectories also obtained the kinetics parameters during thetask from the co-simulation.Figure12shows the lateralvelocity and turn angle of the aircraft for wheel values.Themaximum kinetics parameters are also shown in Tab.4characterizing the towing performance.Therefore,using the designed controller to guide thetraction system tracking in an intended route under practicaltraction work conditions issafe.(a)L ateral Velocity of theTractor(b)Turn Angle of the Aircraft Fore-wheelFig.13Kinetics ParametersJournal of Marine S cience and Appl ication(2012)11:512-5175175ConclusionsFor the automatic guidance and stability control of the ship-based tractor-aircraft system,a fuzzy control system was designed.Firstly,taking into account lateral and the swaying motions,a nonlinear dynamic model is introduced.A virtual prototyping model,which has good maneuvering stability,is established.Furthermore,based on the fuzzy logic,the controller is derived based on the virtual prototyping model.The simulation results confirm the fuzzy control system effectively enables the traction system to track the preconcerted path well.Under the control of the designed controller,the tractor-aircraft system provided a good description of the dynamic behavior.ReferencesDe Santis RM(1994).Path-tracking for a tractor-trailer-like robot.Int J Robot Res,13(6),533-543.De Santis R(1997).Modeling and path-tracking for a load-haul-dump vehicle.J.Dynam.Sy st.Mea s.Contr.,119, 40-47.De Wit C,Khennouf H,Samson C,Sordalen OJ(1993).Nonlinear control design for mobile robots,recent trends in mobile robots.World Scientific Series in Robotics and A utomated Systems,11, 121-156.Elliott AS(2000).A highly efficient,general purpose approach for cosimulation with ADAMS.MDI North A mer,User Conf.,MI,. Han F,Yang BH,Wang HD,Bi YQ(2010).The optimizing research on aircraft handling workflow.Science Technologya nd Engineer ing,10(22),5602-04.Hirose S.Fukushima E,Tsukagoshi S(1995).Basic steering control methods for the articulated body mobile robot.IEEE Contr.Syst.Mag.,4,5-14.Kanayama Y,Kimura Y,Miyazaki F,Noguchi T(1990).A stable tracking control method for an autonomous mobile robot.IEEE Int Conf on Robotics and A utomation,Cincinnati,OH, 384-389.Kanayama Y,Kimura Y,Miyazaki F,Noguchi T(1991).A stable tracking control method for a non-holonomic mobile robot.Int Conf on Intelligent Robotics Systems,Osaka,Japan,1236-1241. Lamiraux F,Laumond JP(1997).A practical approach to feedback control for a mobile robot with trailer.IEEE Int Conf on Robotics a nd A utoma tion,leuv en,Belgium,3306-3311. Lamiraux F,Sekhavat S,Laumond J(1999).Motion planning and control for Hilare pulling a trailer.IEEE Tra ns.Robot.Automa t, 15,640-652.Lane J,King R(1994).Computer-assisted guidance of an underground mine truck.IEEE Int.Conf.Robotics a nd Automation,San Francisco,420-425.Larsson U,Zell C,Hyppa K,Wernesson A(1994).Navigating an articulated vehicle and reversing with a trailer.IEEE Int.Conf.Robotics a nd A utoma tion,San Francisco,2398-2404.Murray RM,Sastry S(1993).Nonholonomic motion planning: Steering using sinusoids.IEEE T r ans A utomat Contr,38(5), 700-716.Nakamura Y,Ezaki H,Tan Y,Chung W(2001).Design of steering mechanism and control of nonholonomic trailer systems.IEEE Transactions on Robotics and A utomation,17(3),367-374. Rabinovitch J,Leitman J(1996).Urban planning in Curitiba.Sci.A mer.,274(3),46-53.Rifford L(2008).Stabilization problem for nonholonomic control systems.Geometr ic Contr ol and N onsmooth A nalysis,Series on A dvances in Mathematics for A pplied Sciences,76,260-269.Rifford L(2006).The stabilization problem on surfaces.Control Theory a nd Stabilization II,64(1),55-61.Rifford L(2004).The stabilization problem:AGAS and SRS feedbacks.Optimal Control,Stabilization,and Nonsmooth Analysis.L ectures N otes in Control a nd Information Sciences, 301,173-184.Samson C,Ait-Abderrahim K(1991a).Feedback stabilization of a nonholonomic wheeled mobile Robot.Int Conf on Intelligent Robotics Systems,1242-1247.Samson C,Ait-Abderrahim K(1991b).Feedback control of a nonholonomic wheeled cart in cartesian space.IEEE Int Conf on Robotics and Automation,1136-1141.Sekhavat S,Lamiraux F,Laumond JP,Bauzil G,Ferrand A(1997).Motion planning and control for Hilare pulling a trailer.IEEE Int Conf on Robotics and A utomation,L euven,Belgium, 3306-3311.Shukla S,Tiwari M(2010).Fuzzy logic of speed and steering control system for three dimensional lines following of an autonomous vehicle.Inter national Journa l of Computer Science and Information Security,7(3),101-108.Wang Y(1994).Development of aircraft-towing tractor.Inter-national A viation,11(9),18-20.Yuan J,Huang YL(2006).Path following control for tractor-trailer mobile robots with two kinds of connection structures.IEEE/RSJ International Conference on Intelligent Robots and Systems,Beijing,China,2533-2538.Nengjian Wang was born in1962.He has been aprofessor at Harbi n Engineering University since2003.He has been a s upervisor for decades.Hisresearch covers a wide range of problems inmodern manufact uring systems theory,workshopand logistics scheduling and optimization,comput er-aided process planning and mechanicaldynamics.Hongbo Liu was born in1987.She is working ondoctoral degree at Harbin Engineering University.She mainly engages in computer simul ation,anal ysis of ai rcraft tracti on system dynamics andstabilit y control study.。
SIGCOMM 2015 TIMELY_RTT-based Congestion Control for the Datacenter
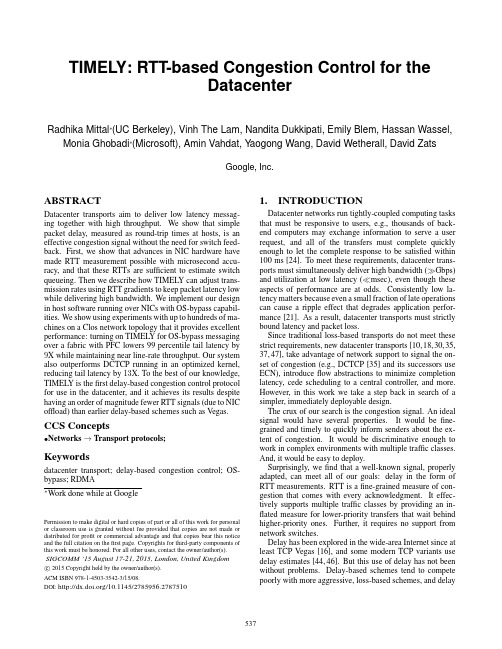
TIMEL Y:RTT-based Congestion Control for theDatacenterRadhika Mittal∗(UC Berkeley),Vinh The Lam,Nandita Dukkipati,Emily Blem,Hassan Wassel, Monia Ghobadi∗(Microsoft),Amin Vahdat,Y aogong Wang,David Wetherall,David ZatsGoogle,Inc.ABSTRACTDatacenter transports aim to deliver low latency messag-ing together with high throughput.We show that simple packet delay,measured as round-trip times at hosts,is an effective congestion signal without the need for switch feed-back.First,we show that advances in NIC hardware have made RTT measurement possible with microsecond accu-racy,and that these RTTs are sufficient to estimate switch queueing.Then we describe how TIMELY can adjust trans-mission rates using RTT gradients to keep packet latency low while delivering high bandwidth.We implement our design in host software running over NICs with OS-bypass capabil-ities.We show using experiments with up to hundreds of ma-chines on a Clos network topology that it provides excellent performance:turning on TIMELY for OS-bypass messaging over a fabric with PFC lowers99percentile tail latency by 9X while maintaining near line-rate throughput.Our system also outperforms DCTCP running in an optimized kernel, reducing tail latency by13X.To the best of our knowledge, TIMELY is thefirst delay-based congestion control protocol for use in the datacenter,and it achieves its results despite having an order of magnitude fewer RTT signals(due to NIC offload)than earlier delay-based schemes such as Vegas. CCS Concepts•Networks→Transport protocols;Keywordsdatacenter transport;delay-based congestion control;OS-bypass;RDMA∗Work done while at GooglePermission to make digital or hard copies of part or all of this work for personal or classroom use is granted without fee provided that copies are not made or distributed for profit or commercial advantage and that copies bear this notice and the full citation on thefirst page.Copyrights for third-party components of this work must be honored.For all other uses,contact the owner/author(s). SIGCOMM’15August17-21,2015,London,United Kingdomc 2015Copyright held by the owner/author(s).ACM ISBN978-1-4503-3542-3/15/08.DOI:/10.1145/2785956.27875101.INTRODUCTIONDatacenter networks run tightly-coupled computing tasks that must be responsive to users,e.g.,thousands of back-end computers may exchange information to serve a user request,and all of the transfers must complete quickly enough to let the complete response to be satisfied within 100ms[24].To meet these requirements,datacenter trans-ports must simultaneously deliver high bandwidth( Gbps) and utilization at low latency( msec),even though these aspects of performance are at odds.Consistently low la-tency matters because even a small fraction of late operations can cause a ripple effect that degrades application perfor-mance[21].As a result,datacenter transports must strictly bound latency and packet loss.Since traditional loss-based transports do not meet these strict requirements,new datacenter transports[10,18,30,35, 37,47],take advantage of network support to signal the on-set of congestion(e.g.,DCTCP[35]and its successors use ECN),introduceflow abstractions to minimize completion latency,cede scheduling to a central controller,and more. However,in this work we take a step back in search of a simpler,immediately deployable design.The crux of our search is the congestion signal.An ideal signal would have several properties.It would befine-grained and timely to quickly inform senders about the ex-tent of congestion.It would be discriminative enough to work in complex environments with multiple traffic classes. And,it would be easy to deploy.Surprisingly,wefind that a well-known signal,properly adapted,can meet all of our goals:delay in the form of RTT measurements.RTT is afine-grained measure of con-gestion that comes with every acknowledgment.It effec-tively supports multiple traffic classes by providing an in-flated measure for lower-priority transfers that wait behind higher-priority ones.Further,it requires no support from network switches.Delay has been explored in the wide-area Internet since at least TCP Vegas[16],and some modern TCP variants use delay estimates[44,46].But this use of delay has not been without problems.Delay-based schemes tend to compete poorly with more aggressive,loss-based schemes,and delay0.2 0.4 0.6 0.8 1 050100 150 200250C D FRTT (µs)Kernel TCP HW TimestampFigure 1:RTTs measured by hardware timestamps have a much smaller random variance than that by kernel TCP stack.estimates may be wildly inaccurate due to host and network issues,e.g.,delayed ACKs and different paths.For these reasons,delay is typically used in hybrid schemes with other indicators such as loss.Moreover,delay has not been used as a congestion sig-nal in the datacenter because datacenter RTTs are difficult to measure at microsecond granularity.This level of preci-sion is easily overwhelmed by host delays such as interrupt processing for acknowledgments.DCTCP eschews a delay-based scheme saying “the accurate measurement of such small increases in queueing delay is a daunting task.”[35]Our insight is that recent NIC advances do allow data-center RTTs to be measured with sufficient precision,while the wide-area pitfalls of using delay as a congestion signal do not apply.Recent NICs provide hardware support for high-quality timestamping of packet events [1,3,5,8,9],plus hardware-generated ACKs that remove unpredictable host response delays.Meanwhile,datacenter host software can be controlled to avoid competition with other transports,and multiple paths have similar,small propagation delays.In this paper,we show that delay-based congestion control provides excellent performance in the datacenter.Our key contributions include:1.We experimentally demonstrate how multi-bit RTT sig-nals measured with NIC hardware are strongly correlated with network queueing.2.We present Transport Informed by MEasurement of LatencY (TIMELY):an RTT-based congestion control scheme.TIMELY uses rate control and is designed to work with NIC offload of multi-packet segments for high performance.Unlike earlier schemes [16,46],we do not build the queue to a fixed RTT threshold.Instead,we use the rate of RTT variation,or the gradient ,to predict the onset of congestion and hence keep the delays low while delivering high throughput.3.We evaluate TIMELY with an OS-bypass messaging im-plementation using hundreds of machines on a Clos net-work topology.Turning on TIMELY for RDMA trans-fers on a fabric with PFC (Priority Flow Control)lowers 99percentile tail latency by 9X .This tail latency is 13X lower than that of DCTCP running in an optimized ker-nel.2.THE V ALUE OF RTT AS A CONGES-TION SIGNAL IN DATACENTERSExisting datacenter transports use signals from network switches to detect the onset of congestion and run with low levels of latency and loss [15,35,37].We argue that network0.2 0.4 0.6 0.8 1 0200400 600 8001000C D FRTT (µs)Queue Length Measured RTTFigure 2:RTTs measured at end-system track closely the queue occupancy at congested link.queueing delay derived from RTT measurements,without the need for any switch support,is a superior congestion sig-nal.RTT directly reflects latency.RTTs are valuable because they directly measure the quantity we care about:end-to-end latency inflated by network queueing.Signals derived from queue occupancy such as ECN fail to directly inform this metric.An ECN mark on a packet simply indicates that the queue measure corresponding to the packet exceeds a threshold.Rich use of QoS in the datacenter means it is not possible to convert this threshold into a single corresponding latency.Multiple queues with different priorities share the same output link,but the ECN mark only provides informa-tion about those with occupancy exceeding a threshold.Low priority traffic can experience large queueing delays with-out necessarily building up a large queue.In such circum-stances,queueing delay reflects the state of congestion in the network which is not reflected by queue occupancy of low priority traffic.Further,an ECN mark describes behavior at a single switch.In a highly utilized network,congestion oc-curs at multiple switches,and ECN signals cannot differen-tiate among them.The RTT accumulates information about the end-to-end path.It includes the NIC,which may also become congested but is ignored by most schemes.Finally,RTTs work even for the lossless network fabrics commonly used to support FCoE [2].In these fabrics,mere queue occu-pancy can fail to reflect congestion because of Priority Flow Control mechanisms used to ensure zero packet loss.RTT can be measured accurately in practice.A key prac-tical hurdle is whether RTTs can be measured accurately in datacenters where they are easily 1000X smaller than wide-area latencies.Many factors have precluded accurate mea-surement in the past:variability due to kernel scheduling;NIC performance techniques including offload (GSO/TSO,GRO/LRO);and protocol processing such as TCP delayed acknowledgements.This problem is severe in datacenters where each of these factors is large enough to overwhelm propagation and queuing delays.Fortunately,recent NICs provide hardware support to solve these problems [1,3,5,8,9]and can accurately record the time of packet transmission and reception without being affected by software-incurred delays.These methods must be used with care lest they overly tax the NIC.We describe our use of NIC support later in the paper.These NICs also provide hardware-based acknowledgements for some proto-cols.The combination of these features lets us take precise RTT measurements to accurately track end-to-end network0.2 0.4 0.6 0.8 1 0 50 100150 200 250 300 350E C NF r a c t i o nRTT (µs)0.2 0.4 0.6 0.8 1100-1500-2000-2500-3000-350E C N F r a c t i o nRTT Range (µs)Figure 3:Fraction of packets with ECN marks versus RTTs shown as scatter plot (top)and box plot (bottom).queues.The following experiment shows this behavior:we connect two hosts to the same network via 10Gbps links and send 16KB ping-pong messages without any cross traf-fic and on a quiescent network.Since there is no conges-tion,we expect the RTT measurements to be low and stable.Figure 1compares the CDF of RTTs measured using NIC hardware timestamps and RTTs measured via the OS TCP stack.The CDF for RTTs using NIC timestamps is nearly a straight line,indicating small variance.In contrast,the RTTs measured by kernel TCP are larger and much more variable.RTT is a rapid,multi-bit work queueing delay can be calculated by subtracting known propagation and se-rialization delays from an RTT.Surprisingly,we have found that this method provides richer and faster information about the state of network switches than explicit network switch signals such as ECN marks.As a binary quantity,ECN marks convey a single bit of information,while RTT mea-surements convey multiple bits and capture the full extent of end to end queueing delay aggregated across multiple switches rather than the presence of queueing exceeding a simple fixed threshold at any one of N switches.Since each packet may carry an ECN mark,it is plausible that the se-quence of marks can close this gap and convey multi-bit in-formation about the congestion level,as is done in DCTCP (which in practice is how ECN is used in datacenters).How-ever,modern practices such as 64KB segmentation offload undermine the independence of marks made closely in time because host sources are rge bursts at wire speed tend to see most packets either above or below the marking threshold.Assuming it is accurate,a single high RTT measurement immediately signals the extent of congestion.This RTT measurement works even with packet bursts for a flow sent along a single network path:the RTT of the last packet in the burst tracks the maximum RTT across packets since de-lays to earlier packets push out later packets.To show how well RTT tracks network queuing delay,we set up an incast experiment with 100flows on 10client machines simulta-neously transmitting to a single server.To incorporate NIC offload,we send 64KB messages and collect only a single RTT measurement per message on the client side.The bot-Figure 4:With ACK prioritization acknowledgements from the primary incast are not delayed by the data of the secondary incast.0.20.4 0.6 0.8 1C D FRTT (µs)Figure 5:In the presence of reverse congestion,RTT mea-surements with ACK prioritization are indistinguishable from RTTs that do not experience any reverse path congestion.tleneck link is a 10Gbps link to the server.We sample the switch queue each microsecond.Figure 2shows the CDF of RTTs as measured at the end system compared to the queue occupancy measured directly at the switch and shown in units of time computed for a 10Gbps link.The two CDFs match extremely well.In contrast,the ECN signal does not correlate well with RTT and hence with the amount of queuing.We set up a similar incast experiment,except now TCP senders per-form long transfers to a single receiver.The bottleneck is the 10Gbps link to the receiver,with an 80KB switch ECN marking threshold.We instrument the senders to measure the fraction of packets received with ECN marks in every round-trip time,using the advance of SND_UNA [41]to mark the end of an RTT round.We also measure the min-imum RTT sample in every round,as prior work has found it to be a robust descriptor untainted by delayed ACKs and send/receive offload schemes.Both the scatter and box plots 1[4]in Figure 3show only a weak correlation between the fraction of ECN marks and RTTs.Limitations of RTTs.While we have found the RTT sig-nal valuable,an effective design must use it carefully.RTT measurements lump queueing in both directions along the network path.This may confuse reverse path congestion experienced by ACKs with forward path congestion experi-enced by data packets.One simple fix is to send ACKs with higher priority,so that they do not incur significant queuing delay.This method works in the common case of flows that predominantly send data in one direction;we did not need more complicated methods.We conducted an experiment to verify the efficacy of ACK prioritization:we started two incasts (primary and sec-ondary )such that the ACKs of the primary incast share the same congested queue as the data segments of the secondary incast,as shown in Figure 4.Figure 5shows the CDF of 1Whiskers extend to the most distant point within 1.2X in-terquartile range.Points outside these limits are drawn indi-vidually.Figure6:TIMELY overview.RTTs from the primary incast for the following three cases: 1)no congestion in ACK path(no secondary incast);2)in the presence of congestion in ACK path;and3)ACKs from primary incast are prioritized to higher QoS queue in the presence of reverse congestion.Wefind that the reverse con-gestion creates noise in RTT measurements of the primary incast and elongates the tail latencies.Throughput of the primary incast is also impacted(and hence the smaller RTTs in the lower percentiles).With ACK prioritization,the RTTs measured in the primary incast are indistinguishable from those measured in the absence of reverse path congestion. In future work,it would be straightforward to calculate variations in one-way delay between two packets by embed-ding a timestamp in a packet(e.g.,TCP timestamps).The change in queuing delay is then the change in the arrival time minus send time of each packet.This method needs only clocks that run at the same rate,which is a much less stringent requirement than synchronized clocks.The other classic shortcoming of RTTs is that changing network paths have disparate delays.It is less of a problem in datacenters as all paths have small propagation delays. 3.TIMELY FRAMEWORKTIMELY provides a framework for rate control that is in-dependent of the transport protocol used for reliability.Fig-ure6shows its three components:1)RTT measurement to monitor the network for congestion;2)a computation en-gine that converts RTT signals into target sending rates;and 3)a control engine that inserts delays between segments to achieve the target rate.We implement TIMELY in host soft-ware with NIC hardware support,and run an independent instance for eachflow.3.1RTT Measurement EngineWe assume a traditional transport where the receiver ex-plicitly ACKs new data so that we may extract an RTT.We define the RTT in terms of Figure7,which shows the time-line of a message:a segment consisting of multiple packets is sent as a single burst and then ACKed as a unit by the receiver.A completion event is generated upon receiving an ACK for a segment of data and includes the ACK receive time.The time from when thefirst packet is sent(t send)until the ACK is received(t completion)is defined as the comple-tion time.Unlike TCP,there is one RTT for the set of packets rather than one RTT per1-2packets.There are several delay components:1)the serialization delay to transmit all packets in the segment,typically up to64KB;2)the round-trip wire delay for the segment and its ACK to propagate acrosstheFigure7:Finding RTT from completion time. datacenter;3)the turnaround time at the receiver to generate the ACK;and4)the queuing delay at switches experienced in both directions.We define the RTT to be the propagation and queueing de-lay components only.Thefirst component is a deterministic function of the segment size and the line rate of the NIC.We compute and subtract it from the total elapsed time so that the RTTs input to TIMELY’s rate computation engine are independent of segment size.The third component is suf-ficiently close to zero in our setting with NIC-based ACKs that we can ignore it.Of the remaining components,the second is the propagation delay including the packet-based store-and-forward behavior at switches.It is the minimum RTT andfixed for a givenflow.Only the last component–the queueing delay–causes variation in the RTT,and it is our focus for detecting congestion.In summary,TIMELY running on Host A(shown in Figure7)computes RTT as: RT T=t completion−t send−seg.sizeNIC line rateFor context,in a10Gbps network,serialization of64KB takes51µs,propagation may range from10-100µs,and 1500B of queuing takes1.2µs.We rely on two forms of NIC support to precisely measure segment RTTs,described next.ACK Timestamps.We require the NIC to supply the com-pletion timestamp,t completion.As shown in§2,OS times-tamps suffer from variations such as scheduling and inter-rupts that can easily obscure the congestion signal.t send is the NIC hardware time that’s read by host software just be-fore posting the segment to NIC.Prompt ACK generation.We require NIC-based ACK gen-eration so that we can ignore the turnaround time at the re-ceiver.An alternative would be to use timestamps to mea-sure the ACK turnaround delay due to host processing de-lay.We have avoided this option because it would require augmenting transport wire formats to include this difference explicitly.Fortunately,some modern NICs[1,3,5,8,9]provide one or both features,and our requirements are met naturally with a messaging implementation that timestamps segment ac-knowledgement.A specific implementation of TIMELY in the context of RDMA is described in§5.We believe our de-sign is more generally applicable to TCP with some care to work with the batching behavior of the NIC,correctly asso-ciate an ACK with the reception of new data,and compen-sate for ACK turnaround time.3.2Rate Computation EngineThis component implements our RTT-based congestion control algorithm as detailed in§4.The interface to the rate computation engine is simple.Upon each completion event, the RTT measurement engine provides the RTT in microsec-onds to the rate computation engine.While this is the only required input,additional timing information could also be useful,e.g.,the delay incurred in the NIC.There is no re-quirement for packet-level operation;in normal operation we expect a single completion event for a segment of size up to64KB due to NIC offload.The rate computation engine runs the congestion control algorithm upon each completion event,and outputs an updated target rate for theflow.3.3Rate Control EngineWhen a message is ready to be sent,the rate control en-gine breaks it into segments for transmission,and sends each segment to the scheduler in turn.For runtime efficiency,we implement a single scheduler that handles allflows.The scheduler uses the segment size,flow rate(provided by the rate computation engine),and time of last transmission to compute the send time for the current segment with the ap-propriate pacing delay.The segment is then placed in a pri-ority queue in the scheduler.Segments with send times in the past are serviced in round-robin fashion;segments with future send times are queued.After the pacing delay has elapsed,the rate control engine passes the segment to the NIC for immediate transmission as a burst of packets.Data isfirst batched into64KB segments,following which the scheduler computes the pacing delay to insert between two such batched segments.Note that64KB is the maximum batching size and is not a requirement,e.g.,the segment sizes for aflow that only has small messages to exchange at any given time will be smaller than64KB.We later present results for segment sizes smaller than64KB as well. TIMELY is rate-based rather than window-based because it gives better control over traffic bursts given the widespread use of NIC offload.The bandwidth-delay product is only a small number of packet bursts in datacenters,e.g.,51µs at 10Gbps is one64KB message.In this regime,windows do not providefine-grained control over packet transmissions. It is easier to directly control the gap between bursts by spec-ifying a target rate.As a safeguard,we limit the volume of outstanding data to a static worst-case limit.4.TIMELY CONGESTION CONTROL Our congestion control algorithm runs in the rate compu-tation engine.In this section,we describe our environment and key performance metrics,followed by our gradient-based approach and algorithm.4.1Metrics and SettingThe datacenter network environment is characterized by many bursty message workloads from tightly-coupled forms of computing over high bandwidth,low-latency paths.It is the opposite of the traditional wide-area Internet in many respects.Bandwidth is plentiful,and it isflow completion time(e.g.,for a Remote Procedure Call(RPC))that is the overriding concern.For short RPCs,the minimum comple-tion time is determined by the propagation and serialization delay.Hence,we attempt to minimize any queueing delay to keep RTTs low.The latency tail matters because appli-cation performance degrades when even a small fraction of the packets are late[21].Consistent low-latency implies low queueing delay and near zero packet loss,since recovery ac-tions may greatly increase message latency.Longer RPCs will have larger completion times because of the time it takes to transmit more data across a shared network.To keep this added time small,we must maintain high aggregate through-put to benefit allflows and maintain approximate fairness so that no oneflow is penalized.Our primary metrics for evaluation are tail(99th per-centile)RTT and aggregate throughput,as they determine how quickly we complete short and long RPCs(assuming some fairness).When there is a conflict between through-put and packet RTT,we prefer to keep RTT low at the cost of sacrificing a small amount of bandwidth.This is because bandwidth is plentiful to start with,and increased RTT di-rectly impacts the completion times of short transfers.In effect,we seek to ride the throughput/latency curve to the point where tail latency becomes unacceptable.Secondary metrics are fairness and loss.We report both as a check rather than study them in detail.Finally,we prefer a sta-ble design over higher average,but oscillating rates for the sake of predictable performance.4.2Delay Gradient ApproachDelay-based congestion control algorithms such as FAST TCP[46]and Compound TCP[44]are inspired by the sem-inal work of TCP Vegas[16].These interpret RTT increase above a baseline as indicative of congestion:they reduce the sending rate if delay is further increased to try and maintain buffer occupancy at the bottleneck queue around some pre-defined threshold.However,Kelly et al.[33]argue that it is not possible to control the queue size when it is shorter in time than the control loop delay.This is the case in datacen-ters where the control loop delay of a64KB message over a10Gbps link is at least51µs,and possibly significantly higher due to competing traffic,while one packet of queuing delay lasts1µs.The most any algorithm can do in these circumstances is to control the distribution of the queue oc-cupancy.Even if controlling the queue size were possible, choosing a threshold for a datacenter network in which mul-tiple queues can be a bottleneck is a notoriously hard tuning problem.TIMELY’s congestion controller achieves low latencies by reacting to the delay gradient or derivative of the queuing with respect to time,instead of trying to maintain a standing queue.This is possible because we can accurately measure differences in RTTs that indicate changes in queuing delay.A positive delay gradient due to increasing RTTs indicates a rising queue,while a negative gradient indicates a receding queue.By using the gradient,we can react to queue growth without waiting for a standing queue to form–a strategy that helps us achieve low latencies.Algorithm 1:TIMELY congestion control.Data :new_rttResult :Enforced ratenew_rtt_diff =new_rtt -prev_rtt ;prev_rtt =new_rtt ;rtt_diff =(1-α)·rtt_diff +α·new_rtt_diff ;α:EWMA weight parameternormalized_gradient =rtt_diff /minRTT ;if new_rtt <T low thenrate ←rate +δ;δ:additive increment stepreturn;if new_rtt >T high thenrate ←rate · 1-β· 1-T high new _rtt; β:multiplicative decrement factorreturn;if normalized_gradient ≤0thenrate ←rate +N ·δ;N =5if gradient <0for five completion events (HAI mode);otherwise N =1elserate ←rate ·(1-β·normalized_gradient)Delay gradient is a proxy for the rate mismatch at the bottleneck queue.We are inspired by RCP,XCP,PI,and QCN [10,28,32,39]that find explicit feedback on the rate mismatch has better stability and convergence properties than explicit feedback based only on queue sizes;the lat-ter can even cause the queue to be less accurately controlled.The key difference is that all of these prior controllers oper-ate at point queues in the network,while TIMELY achieves similar benefits by using the end-to-end delay gradient.The model we assume is N end hosts all sending data at a total rate y (t )into a bottleneck queue with drain rate C ,i.e.the outgoing rate is ≤C .We denote the queuing delay through the bottleneck queue by q (t ).If y (t )>C ,the rate at which the queue builds up is (y (t )−C ).Since queued data drains at a rate C ,the queuing delay gradient is givenby dq (t )dt=(y (t )−C )C .The gradient is dimensionless.It is positive for y (t )>C and signals how quickly the queue is building.The negative gradient when y (t )<C ,signals how quickly the queue is draining.Hence,the delay gradi-ent measured through RTT signals acts as an indicator for the rate mismatch at the bottleneck.This reasoning holds as long as there is some non-zero queue in the network.When there is zero queueing or queues are not changing in size,the measured gradient is also zero.TIMELY strives to match the aggregate incoming rate y (t )to the drain rate,C ,and so adapts its per-connection rate,R (t ),in proportion to themeasured error of (y (t )−C )C =dq (t )dt =d (RT T )dt.4.3The Main AlgorithmAlgorithm 1shows pseudo-code for our congestion con-trol algorithm.TIMELY maintains a single rate R (t )for each connection and updates it on every completion eventFigure 8:Gradient tracking zone with low and high RTT thresholds.using RTT samples.It employs gradient tracking,adjusting the rate using a smoothed delay gradient as the error signal to keep throughput close to the available bandwidth.Ad-ditionally,we employ thresholds to detect and respond to extreme cases of under utilization or overly high packet la-tencies.Figure 8shows the gradient zone along with the two thresholds.When the RTT is in the nominal operating range,the gradient tracking algorithm computes the delay gradient from RTT samples and uses it to adjust the sending puting the delay gradient.We rely on accurate RTT measurements using NIC timestamps (§3).To compute the delay gradient,TIMELY computes the difference between two consecutive RTT samples.We normalize this difference by dividing it by the minimum RTT,obtaining a dimension-less quantity.In practice,the exact value of the minimum RTT does not matter since we only need to determine if the queue is growing or receding.We therefore use a fixed value representing the wire propagation delay across the datacen-ter network,which is known ahead of time.Finally,we pass the result through an EWMA filter.This filter allows us to detect the overall trend in the rise and fall in the queue,while ignoring minor queue fluctuations that are not indicative of congestion.Computing the sending rate.Next,TIMELY uses the nor-malized gradient to update the target rate for the connec-tion.If the gradient is negative or equals zero,the network can keep up with the aggregate incoming rate,and therefore there is room for a higher rate.In this case,TIMELY probes for more bandwidth by performing an additive increment for the connection:R =R +δ,where δis the bandwidth ad-ditive increment constant.When the gradient is positive,the total sending rate is greater than network capacity.Hence,TIMELY performs a multiplicative rate decrement β,scaled by the gradient factor:R =R 1−βd (RT T (t ))dtThe delay gradient signal,which is based on the total incom-ing and outgoing rates,is common for all connections along the same congested path.The well-known AIMD property ensures that our algorithm achieves fairness across connec-tions [19].Connections sending at a higher rate observe a stronger decrease in their rate,while the increase in rate re-mains same for all connections.While the delay gradient is effective in normal operation,situations with significant under-utilization or high latency require a more aggressive response.Next we discuss how TIMELY detects and responds to these situations.Need for RTT low threshold T low .The ideal environment for our algorithm is one where packets are perfectly paced.。
优化相关counter介绍
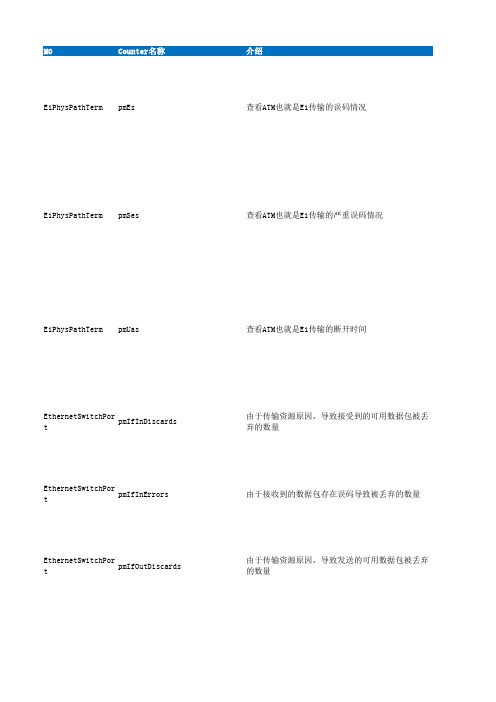
MO Counter名称介绍E1PhysPathTerm pmEs查看ATM也就是E1传输的误码情况E1PhysPathTerm pmSes查看ATM也就是E1传输的严重误码情况E1PhysPathTerm pmUas查看ATM也就是E1传输的断开时间EthernetSwitchPort pmIfInDiscards 由于传输资源原因,导致接受到的可用数据包被丢弃的数量EthernetSwitchPort pmIfInErrors由于接收到的数据包存在误码导致被丢弃的数量EthernetSwitchPort pmIfOutDiscards 由于传输资源原因,导致发送的可用数据包被丢弃的数量EthernetSwitchPort pmIfOutErrors由于发送的数据包存在误码导致被丢弃的数量IubDataStreams pmHsDataFramesLostSpi03HS数据帧丢失的次数IubDataStreams pmCapAllocIubHsLimitingRatioSpi0iub/iur传输带宽受限百分比IubDataStreams pmHsDataFrameDelayIubSpi03指示了传输时延的分布情况备注正常状态时每条传输都应该为0,如果有误码请根据实际情况及时进行处理。
该counter目前未发现有值,具体什么原因会导致尚不明。
一般情况都为0若传输断开1s则此counter就+1,代表传输存在闪断的情况,若此counter为3600,则代表改条传输已经断了,需要传输部门进行处理。
该counter目前未发现有值,具体什么原因会导致尚不明。
一般情况都为0可以用来查看IP传输存在的误码情况,正常情况下为0,不为0时建议根据实际情况进行处理。
该counter目前未发现有值,具体什么原因会导致尚不明。
一般情况都为0可以用来查看IP传输存在的误码情况,正常情况下为0,不为0时建议进行处理。
可以结合pmHsDataFramesReceivedSpi03进行计算,统计出丢帧率,如果丢帧率过高(长时间高于5%),则需要检查传输是否存在问题,例如:带宽不足、丢包、误码等问题。
机械制造及自动化专业外文翻译--掘进机在采矿和隧道业中的应用1
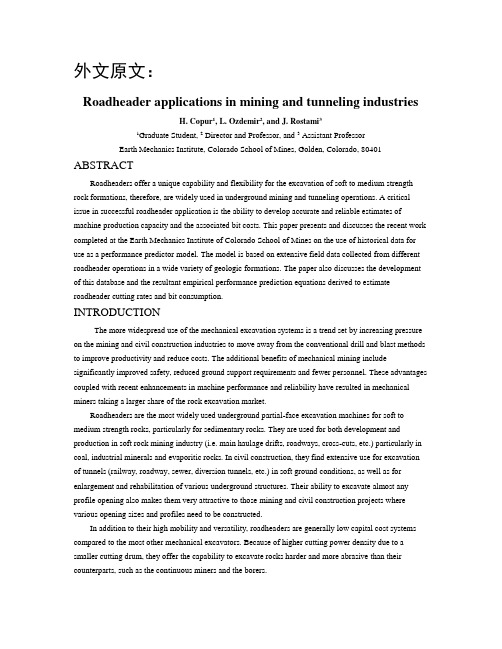
外文原文:Roadheader applications in mining and tunneling industriesH. Copur1, L. Ozdemir2, and J. Rostami31Graduate Student, 2 Director and Professor, and 3 Assistant ProfessorEarth Mechanics Institute, Colorado School of Mines, Golden, Colorado, 80401 ABSTRACTRoadheaders offer a unique capability and flexibility for the excavation of soft to medium strength rock formations, therefore, are widely used in underground mining and tunneling operations. A critical issue in successful roadheader application is the ability to develop accurate and reliable estimates of machine production capacity and the associated bit costs. This paper presents and discusses the recent work completed at the Earth Mechanics Institute of Colorado School of Mines on the use of historical data for use as a performance predictor model. The model is based on extensive field data collected from different roadheader operations in a wide variety of geologic formations. The paper also discusses the development of this database and the resultant empirical performance prediction equations derived to estimate roadheader cutting rates and bit consumption.INTRODUCTIONThe more widespread use of the mechanical excavation systems is a trend set by increasing pressure on the mining and civil construction industries to move away from the conventional drill and blast methods to improve productivity and reduce costs. The additional benefits of mechanical mining include significantly improved safety, reduced ground support requirements and fewer personnel. These advantages coupled with recent enhancements in machine performance and reliability have resulted in mechanical miners taking a larger share of the rock excavation market.Roadheaders are the most widely used underground partial-face excavation machines for soft to medium strength rocks, particularly for sedimentary rocks. They are used for both development and production in soft rock mining industry (i.e. main haulage drifts, roadways, cross-cuts, etc.) particularly in coal, industrial minerals and evaporitic rocks. In civil construction, they find extensive use for excavation of tunnels (railway, roadway, sewer, diversion tunnels, etc.) in soft ground conditions, as well as for enlargement and rehabilitation of various underground structures. Their ability to excavate almost any profile opening also makes them very attractive to those mining and civil construction projects where various opening sizes and profiles need to be constructed.In addition to their high mobility and versatility, roadheaders are generally low capital cost systems compared to the most other mechanical excavators. Because of higher cutting power density due to a smaller cutting drum, they offer the capability to excavate rocks harder and more abrasive than their counterparts, such as the continuous miners and the borers.ROADHEADERS IN LAST 50 YEARSRoadheaders were first developed for mechanical excavation of coal in the early 50s. Today, their application areas have expanded beyond coal mining as a result of continual performance increases brought about by new technological developments and design improvements. The major improvements achieved in the last 50 years consist of steadily increased machine weight, size and cutterhead power, improved design of boom, muck pick up and loading system, more efficient cutterhead design, metallurgical developments in cutting bits, advances in hydraulic and electrical systems, and more widespread use of automation and remote control features. All these have led to drastic enhancements in machine cutting capabilities, system availability and the service life.Machine weights have reached up to 120 tons providing more stable and stiffer (less vibration, less maintenance) platforms from which higher thrust forces can be generated for attacking harder rock formations. . The cutterhead power has increased significantly, approaching 500 kW to allow for higher torque capacities. Modern machines have the ability to cut cross-sections over 100m2 from a stationary point. Computer aided cutterhead lacing design has developed to a stage to enable the design of optimal bit layout to achieve the maximum efficiency in the rock and geologic conditions to be encountered. The cutting bits have evolved from simple chisel to robust conical bits. The muck collection and transport systems have also undergone major improvements, increasing attainable production rates. The loading apron can now be manufactured as an extendible piece providing for more mobility and flexibility. The machines can be equipped with rock bolting and automatic dust suppression equipment to enhance the safety of personnel working at the heading. They can also be fitted with laser-guided alignment control systems, computer profile controlling and remote control systems allowing for reduced operator sensitivity coupled with increased efficiency and productivity. Figure-1 shows a picture of a modern transverse type roadheader with telescopic boom and bolting system.Mobility, flexibility and the selective mining capability constitute some of the most important application advantages of roadheaders leading to cost effective operations. Mobility means easy relocation from one face to another to meet the daily development and production requirements of a mine. Flexibility allows for quick changes in operational conditions such asFigure-1: A Transverse Cutterhead Roadheader (Courtesy of Voest Alpine)different opening profiles (horse-shoe, rectangular, etc.), cross-sectional sizes, gradients (up to 20, sometimes 30 degrees), and the turning radius (can make an almost 90 degree turn). Selectivity refers to the ability to excavate different parts of a mixed face where the ore can be mined separately to reduce dilution and to minimize waste handling, both contributing to improved productivity. Since roadheaders are partial-face machines, the face is accessible, and therefore, cutters can be inspected and changed easily, and the roof support can be installed very close to the face. In addition to these, high production rates in favorable ground conditions, improved safety, reduced ground support and ventilation requirements, all resulting in reduced excavation costs are the other important advantages of roadheaders.The hard rock cutting ability of roadheaders is the most important limiting factor affecting their applications. This is mostly due to the high wear experienced by drag bits in hard, abrasive rocks. The present day, heavy-duty roadheaders can economically cut most rock formations up to 100 MPa (~14,500 psi) uniaxial compressive strength (UCS) and rocks up to 160 MPa (~23,000 psi) UCS if favorable jointing or bedding is present with low RQD numbers. Increasing frequency of joints or other rock weaknesses make the rock excavation easier as the machine simply pulls or rips out the blocks instead of cutting them. If the rock is very abrasive, or the pick consumption rate is more than 1-pick/m3, then roadheader excavation usually becomes uneconomical due to frequent bit changes coupled with increased machine vibrations and maintenance costs.A significant amount of effort has been placed over the years on increasing the ability of roadheaders to cut hard rock. Most of these efforts have focused on structural changes in the machines, such as increased weight, stiffer frames and more cutterhead power. Extensive field trials of these machines showed that the cutting tool is still the weakest point in hard rock excavation. Unless a drastic improvement is achieved in bit life, the true hard rock cutting is still beyond the realm of possibility with roadheaders. The Earth Mechanics Institute(EMI) of the Colorado School of Mines has been developing a new cutter technology, the Mini-Disc Cutter, to implement the hard rock cutting ability of disc cutters on roadheaders,as well as other types of mechanical excavators (Ozdemir et al, 1995). The full-scale laboratory tests with a standard transverse cutterhead showed that MiniDisc Cutters could increase the ability of the roadheaders for hard rock excavation while providing for lesser cutter change and maintenance stoppages. This new cutting technology holds great promise for application on roadheaders to extend their capability into economical excavation of hard rocks. In addition, using the mini-disc cutters, a drum miner concept has been developed by EMI for application to hard rock mine development. A picture of the drum miner during full-scale laboratory testing is shown in Figure-2.Figure-2: Drum Miner CutterheadFIELD PERFORMANCE DATABASEPerformance prediction is an important factor for successful roadheader application. This deals generally with machine selection, production rate and bit cost estimation. Successful application of roadheader technology to any mining operation dictates that accurate and reliable estimates are developed for attainable production rates and the accompanying bit costs. In addition, it is of crucial importance that the bit design and cutterhead layout is optimized for the rock conditions to be encountered during excavation.Performance prediction encompasses the assessment of instantaneous cutting rates, bit consumption rates and machine utilization for different geological units. The instantaneous cutting rate (ICR) is the production rate during actual cutting time, (tons or m3 / cutting hour). Pick consumption rate refers to the number of picks changed per unit volume or weight of rock excavated, (picks / m3 or ton). Machine utilization is the percentage of time used for excavation during the projectTable-I: Classification of the Information in the DatabaseINFORMATION GROUP DETAILSGeneral Information Type/purpose of excavation (roadway, railway, sewer, mining gallery, etc.), contractor, owner, consultant, location, starting andthe Istanbul Technical University has established an extensive database related to the field performance of roadheaders with the objective of developing empirical models for accurate and reliable performance predictions. The database contains field data from numerous mining and civil construction projects worldwide and includes a variety of roadheaders and different geotechnical conditions.The empirical performance prediction methods are principally based on the past experience and the statistical interpretation of the previously recorded case histories. To obtain the required field data in an usable and meaningful format, a data collection sheet was prepared and sent to major contractors, owners, consultants, and roadheader manufacturers. In addition, data was gathered from available literature on roadheader performance and through actual visits to job sites. This data collection effort is continuing.The database includes six categories of information, as shown in Table-I. The geological parameters in the database consist generally of rock mass and intact rock properties. The most important and pertinent rock mass properties contained in the database include Rock Quality Designation (RQD), bedding thickness, strike and dip of joint sets and hydrological conditions. The intact rock properties are uniaxial compressive strength, tensile strength, quartz content, texture and abrasivity. The rock formations are divided into separate zones to minimize the variations in the machine performance data to provide for moreaccurate analysis. This also simplifies the classification of the properties for each zone and the analysis of the field performance data.The major roadheader parameters included are the machine type (crawler mounted, shielded), machine weight, cutterhead type (axial, transverse), cutterhead power, cutterhead-lacing design, boom type (single, double, telescopic, articulated), and the ancillary equipment (i.e.grippers, automatic profiling, laser guidance, bit cooling and dust suppression by water jets, etc.).The operational parameters generally affect the performance of the excavator through machine utilization. The most important operational parameters include ground support, back up system (transportation, utility lines, power supply, surveying, etc.), ground treatment (water drainage, grouting, freezing, etc.), labor (availability and quality), and organization of the project (management, shift hours, material supply, etc.).CONCLUSIONSThe evaluation and analysis of the data compiled in the roadheader field performance database has successfully yielded a set of equations which can be used to predict the instantaneous cutting rate (ICR) and the bit consumption rate(BCR) for roadheaders. A good relationship was found to exist between these two parameters and the machine power (P), weight (W) and the rock compressive strength (UCS). Equations were developed for these parameters as a function of P, W and UCS. These equations were found mainly applicable to soft rocks of evaporatic origin. The current analysis is being extended to include harder rocks with or without joints to make the equations more universal. In jointed rock, the RQD value will be utilized as a measure of rock mass characteristics from a roadheader cuttability viewpoint. It is believed that these efforts will lead to the formulation of an accurate roadheader performance prediction model which can be used in different rock types where the roadheaders are economically applicable.中文译文:掘进机在采矿和隧道业中的应用摘要掘进机为方便的挖掘中硬岩石提供了一个独特的能力。
2003本系网络考试V1.0(答案)
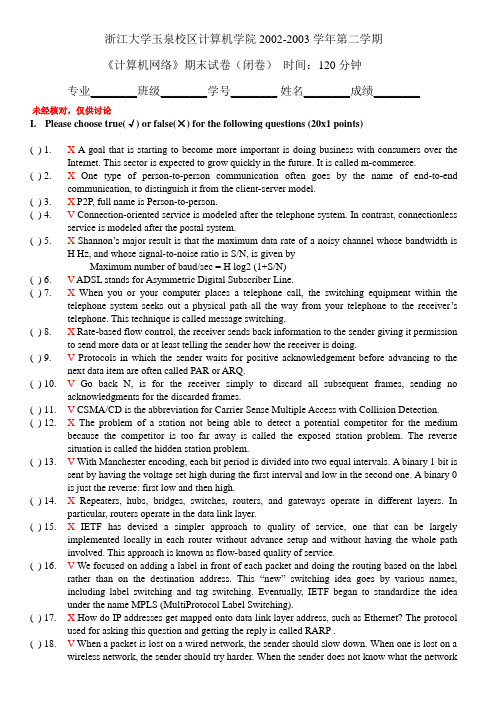
浙江大学玉泉校区计算机学院2002-2003学年第二学期《计算机网络》期末试卷(闭卷)时间:120分钟专业________班级________学号________ 姓名________成绩________未经核对,仅供讨论I.Please choose true(√) or false(×) for the following questions (20x1 points)( ) 1.X A goal that is starting to become more important is doing business with consumers over the Internet. This sector is expected to grow quickly in the future. It is called m-commerce.( ) 2.X One type of person-to-person communication often goes by the name of end-to-end communication, to distinguish it from the client-server model.( ) 3.X P2P, full name is Person-to-person.( ) 4.V Connection-oriented service is modeled after the telephone system. In contrast, connectionless service is modeled after the postal system.( ) 5.X Shannon’s major result is that the maximum data rate of a noisy channel whose bandwidth isH Hz, and whose signal-to-noise ratio is S/N, is given byMaximum number of baud/sec = H log2 (1+S/N)( ) 6.V ADSL stands for Asymmetric Digital Subscriber Line.( ) 7.X When you or your computer places a telephone call, the switching equipment within the telephone system seeks out a physical path all the way from your telephone to the receiver’s telephone. This technique is called message switching.( ) 8.X Rate-based flow control, the receiver sends back information to the sender giving it permission to send more data or at least telling the sender how the receiver is doing.( ) 9.V Protocols in which the sender waits for positive acknowledgement before advancing to the next data item are often called PAR or ARQ.( ) 10.V Go back N, is for the receiver simply to discard all subsequent frames, sending no acknowledgments for the discarded frames.( ) 11.V CSMA/CD is the abbreviation for Carrier Sense Multiple Access with Collision Detection. ( ) 12.X The problem of a station not being able to detect a potential competitor for the medium because the competitor is too far away is called the exposed station problem. The reverse situation is called the hidden station problem.( ) 13.V With Manchester encoding, each bit period is divided into two equal intervals. A binary 1 bit is sent by having the voltage set high during the first interval and low in the second one. A binary 0 is just the reverse: first low and then high.( ) 14.X Repeaters, hubs, bridges, switches, routers, and gateways operate in different layers. In particular, routers operate in the data link layer.( ) 15.X IETF has devised a simpler approach to quality of service, one that can be largely implemented locally in each router without advance setup and without having the whole path involved. This approach is known as flow-based quality of service.( ) 16.V We focused on adding a label in front of each packet and doing the routing based on the label rather than on the destination address. This “new”switching idea goes by various names, including label switching and tag switching. Eventually, IETF began to standardize the idea under the name MPLS (MultiProtocol Label Switching).( ) 17.X How do IP addresses get mapped onto data link layer address, such as Ethernet? The protocol used for asking this question and getting the reply is called RARP .( ) 18.V When a packet is lost on a wired network, the sender should slow down. When one is lost on a wireless network, the sender should try harder. When the sender does not know what the networkis, it is difficult to make the correct decision.( ) 19.X In RFC 822 header fields, Cc: meaning E-mail address of secondary recipient(s), Bcc: meaning E-mail address for carbon copies.( ) 20.V An organization that certifies public keys is now called a CA(Certification Authority).II.Please select one choice for every question. (30x1 points)1.If we look at the model in detail, we see that two process are involved, one on the clientmachine and one on the server machine. Communication takes the form of the client process sending a message over the network to the server process. The client process then waits for a reply message.When the server process gets the request, it performs the requested work or looks up the requested data and sends back a reply.(a) point-to-point (b) V client-server (c) end-to-end (d) peer-to-peer2.If a traveler plugs a notebook computer into the telephone jack in a hotel room, he has ________network.两个答案(a)V wired/fixed (b)V wired/mobile (c) wireless/fixed (d) wireless/mobile3.When a packet is sent from one router to another via one or more intermediate routers, the packet isreceived at each intermediate router in its entirety, stored there until the required output line is free, and then forwarded. A subnet organized according to this principle is called a ________ subnet.(a) circuit-switched (b) V store-and-forward (c) message-switched (d) soft-switched4.Nyquist proved that if an arbitrary signal has been run through a low-pass filter of bandwidth H, thefiltered signal can be completely reconstructed by making only ________ (exact) samples per second.(a) H (b) V 2H (c) 3H (d) 4H5.PCM, full name is ________ Code Modulation.(a) V Pulse (b) Packet (c) Point (d) Peer6. A PPP frame includes fields flag, address, control, ________, payload and checksum.(a) version (b) more (c) V protocol (d) flow7.The protocols used to determine who goes next on a multiaccess channel belong to a sublayer of thedata link layer called the ________.(a) LLC (b) V MAC (c) CSMA (d) SDLC8. A new MPLS header had to be added in front of the IP header. On a router-to-router line using PPP asthe framing protocol, the frame format, is as following sequence: ________.(a) MPLS, IP, PPP (b) PPP, IP, MPLS (c) V PPP, MPLS, IP (d) PPP,IP,TCP,MPLS9.IP addresses 191.1.2.3 is class ________ format.(a) A (b) V B (c) C (d) D10.The operation of the Internet is monitored closely by the routers. When something unexpected occurs,the event is reported by the ________.(a) ARP (b) RARP (c) V ICMP (d) BOOTP11.The original Internet interior gateway protocol was a distance vector protocol. That successor, calledOSPF, became a standard in 1990, is a ________ protocol.(a) V link state (b) broadcast (c) distance vector (d) Ad Hoc12.Port numbers below ________ are called well-known ports and are reserved for standard services.(a) 256 (b) V 1024 (c) 4096 (d) 100013.The Internet protocol suite supports a connectionless transport protocol, ________.(a) IP (b) TCP (c) V UDP (d) RTP14.URL(Uniform Resource Locator) have three parts: the ________, the DNS name of the machine onwhich the page is located, and a local name uniquely indicating the specific page.(a) V protocol (b) address (c) port number (d) name15.When a client requests a web page, the server can supply additional information along with therequested page. This information may include a ________, which is a small (at most 4KB) file (or string).(a) V cookie (b) water (c) car (d) book16.HTTP1.1, which supports ________ connections. With them, it is possible to establish a TCPconnection, send a request and get a response, and then additional requests and get additional response.(a) one time (b) V persistent (c) stop and wait (d) one-way17.Various schemes have been devised for digital signatures, using both symmetric-key and________-key algorithms.(a) V public (b) private (c) one time (d) one way18.Often, authentication is needed but secrecy is not, based on the idea of a one-way hash function thattakes an arbitrarily long piece of plaintext and from it computes a fixed-length bit string. This hash function, MD, often called a ________.(a) V message digest (b) packet-switched (c) message-detect (d) mini data19.________, which are overlay networks on top of public networks but with most of the properties ofprivate network..(a) PBX (b) V VPN (c) CDMA 1X (d) GPRS20.The positioning of SSL is between the application layer and the transport layer, accepting requestsfrom the browser and sending them down to TCP for transmission to the server. Once the secure connection has been established, SSL’s main job is handling compression and encryption. When HTTP is used over SSL, it is called ________.(a) HTTP (b) SSL (c) SHTTP (d) V HTTPS21.The range of frequencies transmitted without being strongly attenuated is called the____________________(a) baud (b) bps (c) V bandwidth (d)signal22.The two-wire connections between each subscriber’s telephone and the end office are known in thetrade as the ____________________(a) trunk (b) V local loop (c) link (d) switch23.___________________-based flow control, the protocol has a built-in mechanism that limits the rateat which senders may transmit data, without using feedback from the receiver.(a) ACK (b) V rate (c) feedback (d) NAK24.____________________, when it is used, a bad frame that is received is discarded, but the goodframes received after it are buffered.(a) V selective repeat (b) go back N (c) sliding window (d) stop and wait25.The problem of running out of IP addresses is not a theoretical problem that might occur at some pointin the distant future. Some people felt that a quick fix was needed for the short term. This quick fix came in the form of ____________________.(a) IP6 (b) DHCP (c) RARP (d) V NAT26.In the simplest form, web pages are ____________________, that is, are just files sitting on someserver waiting to be retrieved.(a) variable (b) active (c) dynamic (d) V static27.A large amount of content of page is ____________________ generated, using server-side scripts, aswell as client-side scripts.(a) variable (b) active (c) V dynamic (d) static28.Four primary parameters: reliability, delay, jitter, and bandwidth, together these determine the____________________.(a) performance of service (b) usage of service (c) V quality of service (d) price of service29.Public-key algorithms have the property that ____________________ keys are used for encryptionand decryption and that the decryption key cannot be derived from the encryption key. Theseproperties make it possible to publish the public key.(a) one time (b) random (c) same (d) V different30.The main public-key algorithm is ____________________ which derives its strength from the factthat it is very difficult to factor large numbers.(a)DES (b) AES (c) MD5 (d) V RSAIII.Please fill in the following blanks (28x1 points).1.Instant messaging _______ allows two people to type messages at each other in real time. A multipersonversion of this idea is the chat room, in which a group of people can type message for all to see.2.B2C, full name is Business-to-consumer.____________________.3.There are two types of transmission technology that are in widespread use. They are as following: 1._Broadcast link, 2. Point-to-point link.任意顺序4. A list of protocol used by a certain system, one protocol per layer, is called a protocol stack __5.Error ____________________ control is an important issue because physical communication circuitsare not perfect.6.An issue that occurs at every level is how to keep a fast sender from swamping a slow received withdata. This subject is called _ flow ___________________control7.These multiplexing schemes can be divided into two basic categories: FDM (Frequency DivisionMultiplexing) and TDM (Time ____________________ Division Multiplexing).8.We will look at four methods to make the start and end of each frame:(b)Character count(c)Flag bytes with byte stuffing(d)Starting and ending flags, with bit stuffing关键是指出面向位(e)Physical layer coding violations.9.The polynomial code is also known as a CRC 或cyclic redundancy check.____________________.10.The technique of temporarily delaying outgoing acknowledgements so that they can be hooked ontothe next outgoing data frame is known as piggybacking ____________________.11.The essence of all sliding _______ windows protocol is that at any instant of time, the sender maintainsa set of sequence numbers corresponding to frames it is permitted to send. These frames are said to fallwithin the sending ________ window. Similarly, the receiver also maintains a _ receiving ____________ window corresponding to the set of frames it is permitted to accept.12.HDLC protocol use the frame format included fields flag, address, control________________, dataand checksum13.MACA. The basic idea behind it is for the sender to stimulate the receiver into outputting a shortframe, so stations nearby can detect this transmission and avoid transmitting for the duration of the upcoming (large) data frame. Let us now consider how A sends a frame to B. A start by sending an _ RTS ___________________ frame to B. This short frame contains the length of the data frame than will eventually follow. Then B replies with a CTS frame. Upon receipt of the CTS frame, A begins transmission.14.Ethernet, the algorithm, called _ binary exponential backoff,_________, was chosen to dynamically adaptto the number of stations trying to send .ing virtual circuits requires a setup___ phase. Datagram subnet is not needed.(a) error control (b) flow control (c) setup (d) transmission16._ Distance vector routing___________________ algorithms operate by having each router maintain atable giving the best known distance to each destination and which line to use to get there. These tables are updated by exchanging information with the neighbors.17.The idea behind _ link state routing ___________________ is simple and can be stated as five parts.Each router must do the following:(f)Discover its neighbors and learn their network address.(g)Measure the delay or cost to each of its neighbors.(h)Construct a packet telling all it has just learned.(i)Send this packet to all other routers.(j)Compute the shortest path to every other router.18.Handing the general case of making two different networks interwork is exceedingly difficult.However, there is a common special case that is manageable. This case is where the source and destination hosts are on the same type of network, but there is a different network in between. The solution to this problem is a technique called __ tunneling __________________19.Connections are established in TCP by means of the _ three-way handshake ___________________.20.Naming in the Internet uses a hierarchical scheme called the domain name system 或DNS.21.Encryption methods have historically been divided into two categories: _substitution__________________ ciphers and transposition ____________________ ciphers任意顺序22.The fundamental job of a certificate is to bind a __ public key __________________ to the name of aprincipal (individual, company, etc.).23.Each _ packet filter ___________________ is a standard router equipped with some extra functionality.The extra functionality allows every incoming or outgoing packet to be inspected. Packets meeting some criterion are forwarded normally. Those that fail the test are dropped.24._Authentication___________________ is the technique by which a process verifies that itscommunication partner is who it is supposed to be and not an imposter.IV.Please answer the following questions.1.(10 points) Please discuss and compare the OSI reference model and the TCP/IP reference model. PH concerned with transmitting raw bits/deal with mechanical,electrical.timing interfaceDL to transform a raw transmission facility into a line that appears free of undetected transmission errors to the network layer/framing/flow controlN routing/internetworkT accept data from above, pass these to the network layer, and ensure that all arrive correctly at the other end./determines what type of service to provide to the users of the network./a true end-to-end layerS <EMPTY>P <EMPTY>A contains a variety of protocols that are commonly needed by usersCOMMONBased on the concept of a stack of independent protocolThe functionality of the layers is roughly similarDIFFERENCEOSI services,interface,protocolsIn the area of connectionless versus connection-oriented2.(12 points) Consider the graph below, each host has been assigned a IP address. Router A, B, C areused to link the hosts, and the subnet mask is 255.255.255.0.We use CISCO IOS command to configure the three router, the command syntax is:Configure IP address command:ip address <ip_address><subnet_mask>Configure IP static routing command:ip route <dest_network><subnet_mask> <router_address>RouterB HUBBridge SwitchHUBRouterC E0E0E1E0E1E110.10.10.10110.10.20.10110.10.30.10110.10.40.101RouterAPlease fill the following blank:1.Assign IP address to the Ethernet Interface of the three route A, B, C :Router A:Interface Ethernet0Ip address 10.10.10.X 255.255.255.0 Interface Ethernet1Ip address 10.10.20.X 255.255.255.0 Router B: Interface Ethernet0Ip address 10.10.20.X 255.255.255.0 Interface Ethernet1Ip address 10.10.30.X 255.255.255.0 Router C: Interface Ethernet0Ip address 10.10.20.X 255.255.255.0 Interface Ethernet1Ip address 10.10.40.X 255.255.255.02.Configure the static route of the three router :直连不管Router A:IP route 10.10.30.0 255.255.255.0 10.10.20.XIP route 10.10.40.0 255.255.255.0 10.10.20.XRouter B:IP route 10.10.10.0 255.255.255.0 10.10.20.X IP route 10.10.40.0 255.255.255.0 10.10.20.X Router C:IP route 10.10.10.0 255.255.255.0 10.10.20.XIP route 10.10.30.0 255.255.255.0 10.10.20.X。
摩克斯(Moxa)UC-8430系列微型无风扇工业嵌入式计算机说明书

UC-8430 SeriesOverviewThe UC-8430 embedded computer comes with 8 RS-232/422/485 serial ports, 3 Ethernet ports, dual displays, 4 digital input channels, 4 digital output channels, a CompactFlash socket, and 6 USB 2.0 hosts.The computer uses the Intel XScale IXP435 533 MHz RISC CPU. This powerful computing engine supports several useful communication functions, but will not generate too much heat. The built-in 32 MB NOR Flash ROM and 256 MB SDRAM give you enough memory to run your application software directly on the UC-8430, and the 32 MB NAND Flash can be used to provide additional data storage.Moreover, the 256 KB SRAM offers a better data retention mechanism for avoiding data loss. The UC-8430 computer comes with 8 RS-232/422/485 serial ports, digital I/O, and has 3 LAN ports, making it ideal as a communication platform for industrial applications thatrequire network redundancy. As an added convenience, the UC-8430 comes with dual VGA outputs; this is particularly helpful when establishing an industrial application at a remote field site.The UC-8430 comes with the Linux 2.6 or Windows CE 6.0 platform pre-installed to provide an open software operating system forsoftware program development. Software written for a desktop PC can be easily ported to the UC-8430 platform by using a common compiler, without needing to modify the code. This makes the UC-8430 an optimal solution for use with industrial applications, but with minimal cost and effort.A wide temperature model of the UC-8430, designed to operate reliably in temperatures ranging from -40 to 75°C, is also available.AppearanceFront ViewRear View(RS-232/422/485)LAN Port x 3, RJ45DO x 4Ready, Storage)Hardware SpecificationsComputerCPU: Intel XScale IXP435, 533 MHzOS (pre-installed): Linux, Window CE 6.0DRAM: 256 MB DDR2 SDRAM onboard (supports DDR2 up to 512 MB)SRAM: 256 KB, battery backupFlash:32 MB NOR Flash onboard to store OS32 MB NAND Flash onboard to store dataUSB: USB 2.0 host x 6StorageStorage Expansion: CompactFlash socketExpansion Bus: PCI/104Other PeripheralsAudio: SM502 chip with line-in/out interfaceDisplayGraphics Controller: SM502 chipDisplay Interface: 15-pin D-Sub connector x 2Resolution: CRT display mode with pixel resolution up to 1024 x 768 Ethernet InterfaceLAN: 3 auto-sensing 10/100 Mbps ports (RJ45)Magnetic Isolation Protection: 1.5 kV built-inSerial InterfaceSerial Standards: 8 RS-232/422/485 ports, software-selectable (8-pin RJ45)Console Port: RS-232 (TxD, RxD, GND), 4-pin pin header output (115200, n, 8, 1)Serial Communication ParametersData Bits: 5, 6, 7, 8Stop Bits: 1, 1.5, 2Parity: None, Even, Odd, Space, MarkFlow Control: RTS/CTS, XON/XOFF, ADDC® (automatic data direction control) for RS-485Baudrate: 50 bps to 921.6 kbps (supports non-standard baudrates; see user’s manual for details)Serial SignalsRS-232: TxD, RxD, DTR, DSR, RTS, CTS, DCD, GNDRS-422: TxD+, TxD-, RxD+, RxD-, GNDRS-485-4w: TxD+, TxD-, RxD+, RxD-, GNDRS-485-2w: Data+, Data-, GNDDigital InputInput Channels: 4, source typeInput Voltage: 0 to 30 VDCDigital Input Levels for Dry Contacts:• Logic level 0: Close to GND• Logic level 1: OpenDigital Input Levels for Wet Contacts:• Logic level 0: +3 V max.• Logic level 1: +10 V to +30 V (COM to DI)Connector Type: 10-pin screw terminal block (4 points, COM, GND) Isolation: 3 kV optical isolationDigital OutputOutput Channels: 4, sink typeOutput Current: Max. 200 mA per channelOn-state Voltage: 24 VDC nominal, open collector to 30 V Connector Type: 10-pin screw terminal block (4 points, GND) LEDsSystem: Power, Ready, Storage, Battery for SRAMLAN: 10M/Link x 3, 100M/Link x 3 (on connector)Serial: TxD x 8, RxD x 8Reset Button: Supports “Reset to Factory Default”Physical CharacteristicsHousing: SECC sheet metal (1 mm)Weight: 1 kgDimensions: 200 x 57 x 120 mm (7.87 x 2.24 x 4.72 in) Mounting: DIN-Rail, wallEnvironmental LimitsOperating Temperature:Standard Models: -10 to 60°C (14 to 140°F)Wide Temp. Models: -40 to 75°C (-40 to 167°F)Storage Temperature:Standard Models: -20 to 75°C (-4 to 167°F)Software SpecificationsOrdering InformationLinuxOS: Linux 2.6.23File System: JFFS2, NFS, Ext2, Ext3Internet Protocol Suite: TCP, UDP, IPv4, IPv6, SNMPv1, ICMP, ARP, HTTP, CHAP, PAP, DHCP, NTP, NFS, SMTP, Telnet, FTP, TFTP, PPP, PPPoEInternet Security: OpenVPN, iptables firewallWeb Server (Apache): Allows you to create and manage web sites; supports PHP and XMLTerminal Server (SSH): Provides secure encrypted communications between two un-trusted hosts over an insecure networkDial-up Networking: PPP Daemon for Linux that allows Unix machines to connect to the Internet through dialup lines, using the PPP protocol, as a PPP server or client. Works with ‘chat’, ‘dip’, and ‘diald’, among (many) others. Supports IP, TCP, UDP, and (for Linux) IPX (Novell).Watchdog: Features a hardware function to trigger system reset in a user specified time interval (Moxa API provided)Application Development Software:• Moxa API Library (Watchdog timer, Moxa serial I/O control, Moxa DI/DO API)• GNU C/C++ cross-compiler • GNU C library• GDB source-level debugging serverQT Embedded: Supports GUI developmentSoftware Protection: Encryption tool for user executable files (based on patented Moxa technology)Windows Embedded CE 6.0OS: Windows Embedded CE 6.0 R3File System: FATInternet Protocol Suite: TCP, UDP, IPv4, IPv6, SNMPv2, ICMP, IGMP, ARP, HTTP, CHAP, PAP, SSL, DHCP, SNTP, SMTP, Telnet, FTP, PPP Web Server (WinCE IIS): Supports ASP, ISAPI Secure Socket Layer (SSL 2/3) and Transport Layer Security (TLS/SSL 3.1) public key-based protocols, and Web Administration ISAPI Extensions Dial-up Networking: Supports RAS client API and PPP, Extensible Authentication Protocol (EAP), and RAS scriptingWatchdog: Features a hardware function to trigger system reset in a user specified time interval. (Moxa API provided)Application Development Software: • Moxa WinCE 6.0 SDK • Moxa API Library• C Libraries and Run-times• Component Services (COM and DCOM) • Microsoft® .NET Compact Framework 3.5• XML, including DOM, XQL, XPATH, XSLT, SAX, SAX2 • SOAP Toolkit Client • Winsock 2.2Available ModelsUC-8430-LX: RISC-based industrial embedded computer with 8 serial ports, 4 DIs, 4 DOs, 3 LANs, CompactFlash, Dual VGA, Audio, 6 USB, Linux OS, -10 to 60°C operating temperature UC-8430-CE: RISC-based industrial embedded computer with 8 serial ports, 4 DIs, 4 DOs, 3 LANs, CompactFlash, Dual VGA, Audio, 6 USB, Windows CE 6.0 OS, -10 to 60°C operating temperatureUC-8430-T-LX: RISC-based industrial embedded computer with 8 serial ports, 4 DIs, 4 DOs, 3 LANs, CompactFlash, Dual VGA, Audio, 6 USB, Linux OS, -40 to 75°C operating temperature UC-8430-T-CE: RISC-based industrial embedded computer with 8 serial ports, 4 DIs, 4 DOs, 3 LANs, CompactFlash, Dual VGA, Audio, 6 USB, Windows CE 6.0 OS, -40 to 75°C operating temperatureAmbient Relative Humidity: 5 to 95% (non-condensing)Anti-vibration: 2 g rms @ IEC-68-2-34, random wave, 5-500 Hz, 1 hr per axisAnti-shock: 20 g @ IEC-68-2-27, half sine wave, 30 msPower RequirementsInput Voltage: 12 to 48 VDC (3-pin terminal block)Power Consumption: 14 W • 270 mA @ 48 VDC • 533 mA @ 24 VDC • 1120 mA @ 12 VDCStandards and CertificationsSafety: UL 60950-1, EN 60950-1, CCC (GB9254, GB17625.1)EMC: EN55022 Class B, EN 55024-4-2, EN 55024-4-3, EN 55024-4-4, FCC Part 15 Subpart B Class BReliabilityAlert Tools: Built-in buzzer and RTC (real-time clock)Automatic Reboot Trigger: Built-in WDT (watchdog timer)MTBF (mean time between failures): 217,675 hrsWarrantyWarranty Period: 5 yearsDetails: See /warrantyNote: The Hardware Specifications apply to the embedded computer unit itself, but not to accessories. In particular, the wide temperature specification does not apply to accessories such as the power adaptor and cables.。
工业应用移动机器人术语标准说明书

目 次前言 ................................................................................ I II1 范围 (1)2 规范性引用文件 ...................................................................... 1 3 通用术语 . (1)4 导航方式术语 (2)5 转向方式术语 ........................................................................ 3 6 驱动方式术语 ........................................................................ 4 7 驱动结构术语 ........................................................................ 4 8 性能参数 ............................................................................ 5 9 运动方向术语 (6)10 机构与零部件 (6)11 辅助零部件术语 ..................................................................... 7 12 软件功能术语 .. (8)13 充电/供电方式 (9)14 安全术语 .......................................................................... 10 英文索引 .. (12)中文索引 (14)全国团体标准信息平台前 言本标准按照GB/T1.1给出的规则起草。
基于PLC控制的运料小车系统设计-毕业论文
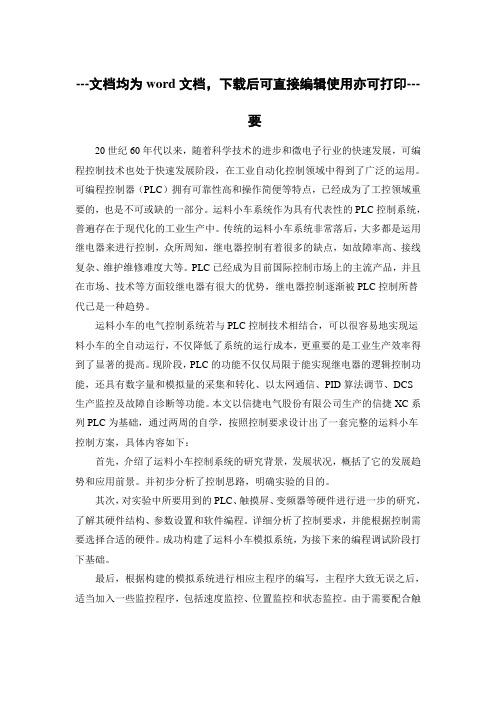
---文档均为word文档,下载后可直接编辑使用亦可打印---要20世纪60年代以来,随着科学技术的进步和微电子行业的快速发展,可编程控制技术也处于快速发展阶段,在工业自动化控制领域中得到了广泛的运用。
可编程控制器(PLC)拥有可靠性高和操作简便等特点,已经成为了工控领域重要的,也是不可或缺的一部分。
运料小车系统作为具有代表性的PLC控制系统,普遍存在于现代化的工业生产中。
传统的运料小车系统非常落后,大多都是运用继电器来进行控制,众所周知,继电器控制有着很多的缺点,如故障率高、接线复杂、维护维修难度大等。
PLC已经成为目前国际控制市场上的主流产品,并且在市场、技术等方面较继电器有很大的优势,继电器控制逐渐被PLC控制所替代已是一种趋势。
运料小车的电气控制系统若与PLC控制技术相结合,可以很容易地实现运料小车的全自动运行,不仅降低了系统的运行成本,更重要的是工业生产效率得到了显著的提高。
现阶段,PLC的功能不仅仅局限于能实现继电器的逻辑控制功能,还具有数字量和模拟量的采集和转化、以太网通信、PID算法调节、DCS生产监控及故障自诊断等功能。
本文以信捷电气股份有限公司生产的信捷XC系列PLC为基础,通过两周的自学,按照控制要求设计出了一套完整的运料小车控制方案,具体内容如下:首先,介绍了运料小车控制系统的研究背景,发展状况,概括了它的发展趋势和应用前景。
并初步分析了控制思路,明确实验的目的。
其次,对实验中所要用到的PLC、触摸屏、变频器等硬件进行进一步的研究,了解其硬件结构、参数设置和软件编程。
详细分析了控制要求,并能根据控制需要选择合适的硬件。
成功构建了运料小车模拟系统,为接下来的编程调试阶段打下基础。
最后,根据构建的模拟系统进行相应主程序的编写,主程序大致无误之后,适当加入一些监控程序,包括速度监控、位置监控和状态监控。
由于需要配合触摸屏的使用,一些中间标志位也是必不可少的。
考虑到实际工程项目中可能会出现的问题,对程序进行了进一步的完善,提高了系统稳定性和安全性。
- 1、下载文档前请自行甄别文档内容的完整性,平台不提供额外的编辑、内容补充、找答案等附加服务。
- 2、"仅部分预览"的文档,不可在线预览部分如存在完整性等问题,可反馈申请退款(可完整预览的文档不适用该条件!)。
- 3、如文档侵犯您的权益,请联系客服反馈,我们会尽快为您处理(人工客服工作时间:9:00-18:30)。
Rate-Based Transport Control for Mobile Ad HocNetworksHongqiang Zhai,Xiang Chen and Yuguang FangDepartment of Electrical&Computer EngineeringUniversity of Florida,Gainesville,Florida32611-6130Tel:(352)846-3043,Fax:(352)392-0044E-mail:zhai@ecel.ufl.edu,xchen@ecel.ufl.edu,and fang@ece.ufl.eduAbstract—The traditional congestion control mechanism,i.e., TCP,encounters a number of new challenges when applied in mobile ad hoc networks(MANET),such as wireless link error, medium contention,and frequent route failures.And very poor performance of TCP in MANET has been reported in many recent studies.In this paper,we focus on the problems resulting from the medium contention and propose a novel R ate B ased end-to-end C ongestion C ontrol scheme(RBCC).Wefirst illustrate that,under the impact of medium contention,window based congestion control algorithm is unstable and hence may not be appropriate for MANET because the optimum congestion window size is very small and may be even less than one,i.e., the source should send less than one packet in one round trip time(RTT).Based on the novel use of channel busyness ratio, which,we show,is an accurate sign of the network utilization and congestion status,a new rate control scheme has been proposed to efficiently and reliably support the transport service in MANET. In RBCC,a sublayer consisting of a leaky bucket is added under TCP to control the sending rate based on the network layer feedback at the bottleneck node.Extensive simulations show that our scheme significantly outperforms traditional TCP in terms of channel utilization,delay,and fairness.I.I NTRODUCTIONMobile ad hoc networks(MANETs)have found many ap-plications in battlefield,disaster rescue and conventions,where fixed communications infrastructures are not available and quick network configurations are needed.To provide reliable transport service over and hence fully exploit the potential of MANETs,efficient congestion control is of paramount importance.Unfortunately,traditional TCP congestion control mecha-nism performs very poorly,as shown in recent studies([1]–[7] and reference therein).TCP congestion control has an implicit assumption,which is that any packet loss is due to network congestion.However,this assumption is no longer valid in the MANET as packet losses may well be due to channel bit errors,medium contention,and route failures.To alleviate the impact of mobility,several schemes,such as those in[8]–[11],were proposed to distinguish between route failures from topology changes and network congestion through explicit route failure notifications.Other schemes,like those in[12],[13],do not use the network layer feedback but keep the TCP states unchanged when the sourcefirst detects the out-of-order packet and retransmission timeout.Several works have already noticed that greedy TCP can result in severe congestion in MANET and hence suffer per-formance degradation.Link-RED in[4]were proposed to mark or drop TCP packet according to observed packet collisions to notify the TCP source to reduce congestion window size before it becomes excessive large.[1]dynamically adjusted the congestion window limit according to path length of TCP flows.In[14],a neighborhood RED scheme was proposed to address TCP fairness problem resulted from medium con-tention.In this paper,we mainly focus on the problems arising from medium contention and show that a rate based congestion control protocol in stead of window based congestion control protocol should be more appropriate for MANET.In Section II,we illustrate that the optimum congestion window size of TCP may be less than one even in very simple topology, say chain topology,to maximize the end-to-end throughput and minimize the end-to-end delay.Therefore unstable per-formance is observed for TCP for large variation of sending rate.There is already one equation-based rate control scheme for Internet,i.e.,TFRC[23].However,the recent studies in[22] shows that TFRC is very conservative in MANETs due to inaccuracy of loss prediction.To conduct accurate end-to-end rate control,each node is in dire need of a robust and easy measured metric to control the feedback of each passing packet.For Internet and ATM network,lots of work have discussed the explicit and precise congestion feedback for end-to-end control,such as[15],[16]. These work are based upon the measure of packet loss,queue length,and link utilization,which are significantly different in MANETs.Severe medium contention is coupled with packet losses and large queue length when congestion happens in MANETs.And wireless link is shared by the neighboring nodes and is no longer a dedicated link between two nodes as in wired networks.Thus in Section II-B we propose to use channel busyness ratio as the timely and accurate sign of the network utilization as well as congestion.Then in Section III we propose a novel rate based con-gestion control protocol(RBCC)based upon the novel use of channel busyness ratio.Each forwarding node allocate the channel resource to the passingflows by monitoring andmod-Fig.1.Chain topology with9nodes.Small circles denote the transmission range,and the large circles denote the sensing rangeifying the feedbackfield of the data packets according to its measured channel busyness ratio.After receiving the feedback in the acknowledgement packets from the destination,the source accordingly adjust the sending rate.Thus,in RBCC, the sending rate of eachflow is determined by the channel utilization status at the bottleneck node.In Section IV,we evaluate the performance of the scheme through extensive simulations.Finally,conclusions and future work are given in Section V.II.M EDIUM C ONTENTION AND I TS I MPACTA.Optimal Congestion Window Size for TCP and Ideal Send-ing RateWe will show in this subsection that window based con-gestion control may not be an appropriate choice to support stable and reliable transport service in MANET because the optimal sending rate per RTT is very small and may be even less than one packet/RTT.Hence window based congestion control must results in instability.The authors of[19]have shown that,in chain topology like that of Fig.1,the maximum utilization of a chain of ad hoc nodes is1/4by scheduling the nodes four hops away to simultaneously transmit.Thus the optimal sending rate R o from the source should be just enough to make the above schedule feasible.Higher sending rate will result in packet collisions and losses,and hence long delay.At rate of R o, the packet will be delivered to the destination in shortest time without any medium collision and queueing dealy,and RTT will befixed and is denoted by RT T o here.Assuming there are N TCPflows from node1to node9,the optimal sending rate of each TCPflow isR oeachtcp=R o/N,(1)K eachtcp=RT T o×R o/N,(2) where K eachtcp is the number of packets sent by each TCP source per RT T o.We use simulation to illustrate R o when the802.11MAC is used.The channel bandwidth is2Mbps.A CBR/UDPflow with the same packet length as that of TCP DATA packets,i.e.,1000bytes,starts from node1and destines for node9.Another CBR/UDPflow with the same packet length of TCP ACK packets starts from node9and destines for node 1.Two UDPflows have the same sending rate.We gradually increase the sending rate until there are some packet losses for collisions in the300seconds simulation.The simulation results are summarized in Table I where the performance are also included when there are six TCPflows for comparison.TABLE IS IMULATION R ESULTS FOR TCP AND UDP F LOWSTraffic type UDP(node1to9)6TCPflows Aggregate throughput(Kbps)212205Average end-to-end delay(s)0.06870.627RTT(s)0.132∗ 1.120 Dropped packets/s0 1.276∗the sum of average end-to-end delay of the two UDPflowsThus the optimum aggregate sending rate is212Kbps≈25pkt/s given that there is no packet loss due to collisions. And RT T o=0.132s and K eachtcp=0.55pkt/RT T when N =6.The more TCPflows there are,the smaller K eachtcp is. In summary,to provide high throughput,short delay and stable performance with few packet collisions,window-based congestion control is not appropriate for MANET.And we will design an efficient rate-based congestion control algorithm in the following sections.B.Channel Busyness RatioWe propose to use an easily measured metric channel busyness ratio r b to track the current channel and network utilization at the location of each node.Notice that the IEEE 802.11is a CSMA-based MAC protocol,working on the physical and virtual carrier sensing mechanisms.The channel is determined busy when the measuring node is sending, receiving,or its network allocation vector(NA V)[17]indicates the channel is busy,and to be idle otherwise.In our previous work[24],[25],We have shown through both theoretical and simulation studies that the IEEE802.11 DCF protocol could achieve the maximal channel utilization when it is working at the optimal point corresponding to a certain amount of arriving traffic.If the arriving traffic is heavier than this threshold,the network enters into saturation, resulting in significant increase in delay and decrease in throughput due to severe collisions;on the other hand,if the arriving traffic is less than this threshold,there is almost no collisions and channel capacity is wasted.Further,if we denote by th b the channel utilization cor-responding to the optimal point,the available normalized throughput is proportional to th b−r b and r b is an accurate estimate of current channel utilization before the network achieves the maximal throughput.As shown in[24],th b isalmost the same for different number of active nodes andpacket size,and th b≈0.95(with RTS/CTS).In this paper we set th b to92%accordingly and leave3%space to avoidentering into saturation.III.R ATE-B ASED C ONGESTION C ONTROLOur proposed rate-based end-to-end control protocol(RBCC)is aimed to work around the optimal point in the sensethat the channel capacity is fully utilized while no congestion is caused.As mentioned earlier,in mobile ad hoc networks, the optimal window size for TCP is very small,typically less than5in packets and even less than1.Hence any change in congestion window may result in large throughput oscillation in each RTT,failing to stabilize the throughput.For this reason, RBCC,unlike TCP,employs rate based control,in order to stabilize the throughput of eachflow while making full use of the available bandwidth.A.Protocol OverviewRBCC controls the sending rate of eachflow by the explicit feedback carried in the ACKs.And each RBCC packet carries a congestion header as shown in Table II,which is used to communicate aflow’s state to the intermediate nodes and the feedback from the intermediate nodes further to the destination.Thefield r p is the sender’s current permit arriving rate,and thefield ci is the sender’s currently used control interval.They arefilled in by the sender and never modified in transit.The lastfield,fb,is initiated by the sender and all the intermediate nodes along the path may modify it to directly control the packet sending rate of the sources.The RBCC sender maintains an estimate of the round trip time rtt and accordingly calculates the control interval ci.It also adjusts the permit arriving rate r p according to the explicit feedback in the ACKs.Each time the sender transmits a packet, it attaches a congestion header to the packet with the latest r p and ci.All the nodes along theflow’s path,including the RBCC sender and receiver,keep monitoring the channel busyness ra-tio r b,and calculate the feedback accordingly.Then,according to the rules specified in5.3,the node will decide whether and how to modify the fbfield in the congestion header.The more congested node later in the path can overwrite the fbfield in the congestion header.Ultimately,the packet will contain the feedback from the bottleneck node along the path.When the feedback reaches the receiver,it is returned to the sender in an ACK packet,and the sender updates its permit arriving rate r p accordingly.The updated arriving rate r p is then used by the sender to control the permit arriving rate at the leaky bucket.TABLE IIC ONGESTION HEADERr p(sender’s permit arriving rate)ci(sender’s control interval)fb(feedback)A RBCC receiver is similar to a TCP receiver except that when acknowledging a packet,it copies the congestion header from the data packet to its ACK.B.Rate Control MechanismThe rate control mechanism of RBCC is illustrated in Fig.2.A leaky bucket is attached to the transport layer to control the sending rate of RBCC sender.The permit arriving rate of the leaky bucket is dynamically adjusted according to the explicitQueue of packetsof one per 1/rpsecFig.2.Rate control mechanismfeedback carried in the returned ACK.A RBCC sender always sets its congestion window cwnd to the receiver’s advertised window size in packets.Initially,when the RBCC sender sends out thefirst packet of aflow,r p=0,and ci is set to0to indicate to the intermediate nodes that the sender does not yet have a valid estimate of the round trip time rtt.The sender also initializes the fbfield to such that if bandwidth is available,this initialization allows the sender to reach the desired rate after one ci.When thefirst ACK returns,the sender calculates rtt and ci according to the method described next,sets r p=1/rtt,and sends out the second data packet.Thereafter,RBCC sends out a data packet only when the transmission window allows and a permit is available.The RBCC sender maintains an estimate of the smoothed round trip time srtt and calculate the control interval ci asci=max(srtt,5/r p).(3) When r p is large,i.e.,r p>5/srtt and ci=srtt,the sending rate will reach the path capacity after one srtt.Otherwise, this period equals5/r p.The value of the control interval thus ensures that,on average,there are at least5data packets being transmitted in this period.If the period is too long,the adjustment of the sending rate is sluggish to respond to the load change along the path.If the period is too short,the estimation of the feedback over short intervals at the nodes along the path leads to erroneous estimates,and sometimes there may be no feedback received in one control interval.The choice of5is the tradeoff between these two considerations. RBCC adjusts r p according to the fb in the ACK packet whenever a new ACK arrives,andrp=r p+fb.(4) The nodes along the path control the feedback such that the sending rate is allowed to reach the path capacity after one control interval ci.Retransmission timer RT O will expire when there is a packet loss.Notice that,in MANETs,queue overflow rarelyhappens for TCP flows.And packet losses mainly result from the failed transmission attempts at the MAC layer due to contention,collision,wireless channel error,or mobility-caused route failures.Subsequently,the link breakage will be reported to the routing protocol,which may further drop subsequent packets.Notice in this case,the original route is broken,thus the timeout signals not only the packet loss,but also the route breakage.To avoid long periods of pausing and hence waste of channel capacity,it is wise for RBCC to send out a probe message or just retransmit the lost packet in periodic intervals to detect whether a new route is established.Therefore,the response of RBCC to timeout is the fol-lowing.For the first timeout,the RBCC sender retransmits the corresponding packet,double the retransmission timer,and reset r p to 1/RT O .Note retransmitted packets have higher priority than normal packets.In other words,the retransmitted packets will be transmitted when the next permit arrives,no matter whether there are any other packets in the window.For the subsequent back-to-back timeouts before a new acknowledgements arrives,RBCC does not double its retransmission timer again,and nor does it reset r p .It also records the time when the first timeout in the window takes place to differentially treat the feedback information carried by the ACKs that arrive after the timeout and route repair.The feedback in those ACKs that acknowledge the packets that are sent prior to the timeout is simply ignored,since it is very likely the feedback was calculated before the route failure and hence becomes outdated.By contrast,the feedback in those ACKs that acknowledge the packets that are sent later than the timeout are used to adjust the permit arriving rate as normal.C.Feedback CalculationTo achieve both the efficiency and fairness,RBCC relies on the calculation of the explicit feedback in each average control interval denoted by ci .Specifically,in each ci ,each node first determines the aggregate feedback that is defined as the change in the total channel throughput at the MAC layer;then,the node will fairly allocate the change in the throughput to each flow traversing the node.Each node maintains a per-node estimation-control timer that is set to the most recent estimate of ci .It is calculated by using the information in the congestion header.Upon timeout the node updates its estimates and its control decisions.In each ci ,a node monitors all received and transmitted packets and accordingly update total input and output traffic S and the metrics S 1and S 2.And,S = jl j ,S 1= j1r pj =kci ×r pkr pk=ci ×K,S 2=j ci j r pj=cikci k ,K =S 1ci,ci new =S 2S 1,(5)where j is the index for each packet,l j is the length in bytes of the j th packet,k is the index for each flow,K is estimated total number of flows,ci ×r pk is the estimated total number ofFig.3.Chain topology with 5nodestransmitted packets of flow k in ci ,ci new is the new estimated value of ci .Notice that in all the summations in Equation 5,the node will update S ,S 1and S 2whenever it receives or transmits a packet because both receiving and transmission consumes the shared channel resource.For instance,at node 3in Fig.3,S =r 1+r 2+2×r 3where r i (1≤i ≤3)is the traffic of flow i ,and K =4which will be used in our fair allocation algorithm.To achieve efficiency,i.e.,maximizing the channel utiliza-tion while avoiding severe MAC layer contention,RBCC calculates the aggregate feedback ∆S in each ci as follows:∆S =th b −r br b×S,(6)After calculating ∆S ,the change in the total traffic or the aggregate feedback,RBCC needs to apportion it to individual flows in order to achieve fairness.We denote per packet feedback in bytes/s for packet j as pf j ,and∆S = j pf j ×ci,fb j =pf jl j (7)RBCC relies on an Additive-Increase Multiplicative-Decrease (AIMD)policy to converge to fairness:If ∆S >0,all flows increase the same amount of throughput.And if ∆S <0,each flow decreases the throughput proportionally to its current throughput.Thus,if ∆S >=0,the increasing amount of traffic rate for each flow C p ,and per packet feedback pf j will beC p =∆Sci ×K,pf j =C p r pj ×ci(8)Note that for those flows that either originate or terminate atthe node,the node counts each as one flow,whereas for those flows only pass the node,the node counts each as two flows,i.e.,one in and one out.This is because the flow passing the node contributes to both the input traffic and the output traffic.If ∆S <0,the per packet feedback is proportional to the throughput,i.e.,r pj ×l j ,and inversely proportional to the expected number of packets seen by the node in ci ,i.e.,r pj ×ci .Thus according to Equation (7),pf j =C nr pj ×l j r pj ×ci =C n ×l j ci ,C n =∆SS (9)IV.P ERFORMANCE E VALUATIONIn this section,we demonstrate through simulations that RBCC outperforms TCP in the MANET.In contrastto TCP,the new protocol dampens the oscillations of channel utiliza-tion,quickly converges to high utilization,short round trip time,small queue size,and fair bandwidth allocation.A.Simulation SettingsWe use network simulator ns-2to conduct theIn ns-2,the physical-layer propagation model is ground model.We adopt the default values in ns-2for transmission power and other physical parameters,which cate the transmission range is about 250m and the range is about 550m.The channel bandwidth is 2Mbps.payload size of each DATA packet is 1000bytes.The buffer size at each node is 50.In the simulation,a chain topology is used because it simple and allows us to clearly demonstrate the problems the advantages of RBCC over TCP.Under this topology,consider two routing schemes.The first one uses the computed shortest path,therefore has no routing which enables us to focus on the MAC and the transport and observe their interaction.The second one is AODV ,enables us to show how on-demand routing schemes affect performance of RBCC and TCP.B.Simulation ResultsThe chain topology consists of 9nodes as shown in 1.Nodes are separated by 200m.The pre-computed path routing is used.In this simulation,there are 6flows.The source is node 1and the destination is node 9.aggregate throughput,end-to-end delay,and packet rate is presented in Table III.TABLE IIIP ERFORMANCE OF RBCC AND TCP IN CHAIN TOPOLOGYPre-computed Path AODVTCP RBCC TCP RBCC Throughput(Kb/s)204.70230.84128.88202.68End-to-end delay(s)0.62650.069140.63300.1319Dropping(pkt/s)1.27590.03790.61380.082In Fig.4(a),the channel busyness ratio is presented.Each point in the curves is anaverage value during each second.It can be observed that RBCC quickly converges to high link utilization and remain stable,while TCP always oscillates in a large range.We also observe that different nodes see different channel busyness ratio.Since node 5is in the middle of the chain and thus encounters the most serious collisions,it’s channel busyness ratio is the largest.On the other hand,compared to node 1,node 9,as a destination,does not transmits any DATA packets,so it observes the smallest channel busyness ratio.Fig.4(b)demonstrates that RBCC has a much smaller round trip time,rtt ,than TCP.For RBCC,the average value of rtt is 0.1216s ,as opposed to 1.12s for TCP.Given that the measured throughput for each RBCC flow is 38.5Kbits/s,the transmission time for one packet is 0.22s ,which is larger than the average rtt .In other words,the window size for RBCC is less than 1.This is consistent with our previous analysis that the optimum window size may be less than one.For TCP,normally its window size is larger than one packet per rtt .Since TCP overestimates the window,it is no wonder that TCP incurs severe congestion and MAC collisions in MANETs,or the packet dropping rate due to MAC collisions is about 33times as high as that of RBCC traffic,as shown in Table III.In Fig.4(c),we see that as the sequence number of received packets is smoothly increasing,each RBCC flow has a stable throughput,and almost no packet needs to be retransmitted.Here the instantaneous throughput of a flow can be calculated by taking derivative of the sequence number with respect to time,i.e.,the slope of the curve in Fig.4(c).This further verifies that RBCC almost does not drop packets due to MAC collision or buffer overflow.On the contrary,TCP frequently retransmit lost packets.For clear demonstration,we only present the result from the start of the transmission,namely 10s ,to 50s ,although we observe the similar phenomenon for the rest of the simulation.As a result,the throughput of RBCC is 12.8%higher than that of TCP,as shown in Table III.Fig.4(d)shows that RBCC maintains a much smaller queue size at all the nodes than TCP.In fact,the average queue size of RBCC is always smaller than 1.This translates into a short end-to-end delay for RBCC,which is only 1/9of that of TCP,as shown in Table III.In addition,as pointed out earlier,a large queue size keeps node busy with contending the channel,which increases contention and causes packets to be dropped.Thus,a small queue size is desirable.This also explains whyTCP has a much larger packet dropping rate(in packets/s) than RBCC.We also simulate the same topology with the AODV routing algorithm.As seen in Table III,the aggregate TCP throughput drops37%compared with the case where the pre-computed shortest path is used.However,the aggregate RBCC through-put only drops12%.As a result,in this case,the aggregate throughput of RBCC is57%higher than that of TCP.V.C ONCLUSIONSCongestion control is critical to reliable transport service in MANETs.Traditional TCP suffers severe performance degradation and unfairness.Realizing the core cause is due to the poor interaction between traditional TCP and the802.11 MAC,we propose a systematic solution named Wireless Congestion Control Protocol(RBCC)to address this problem. The major contributions of this work is three-fold.First,we use simulation studies to show that window-based congestion control mechanism,say that of TCP,results in poor and unstable performance due to unique medium contention in MANET and hence argue that rate-based congestion control may be more appropriate for MANET.Second,we propose to use channel busyness ratio,which is a good sign of network congestion and available bandwidth at the MAC layer,to calculate explicit and precise feedback at the transport layer. Third,we propose an end-to-end congestion control protocol, which uses channel busyness ratio to adjust the sender’s rate so that the channel capacity can be fully utilized and fairness is improved.Simulation results show that our scheme significantly outperforms traditional TCP in terms of channel utilization,end-to-end delay,and fairness.A CKNOWLEDGEMENTThis work was supported in part by the U.S.Office of Naval Research under Young Investigator Award N000140210464 and under grant N000140210554.R EFERENCES[1]K.Chen,Y.Xue,and K.Nahrstedt.On setting TCP’s congestion windowlimit in mobile ad hoc networks.In Proc.IEEE ICC2003,May,2003.[2]X.Chen,H.Zhai,J.Wang and Y.Fang.TCP performance overmobile ad hoc networks.Canadian Journal of Electrical and Computer Engineering,V ol.29,No.1/2,p129-134,January/April2004.[3]Z.Fu,X.Meng,and S.Lu.How Bad TCP can Perform in Mobile Ad-Hoc Networks.In IEEE Symposium on Computers and Communications, July2002[4]Z.Fu,P.Zerfos,H.Luo,S.Lu,L.Zhang,M.Gerla.The Impact ofMultihop Wireless Channel on TCP Throughput and Loss.In proc.IEEE INFOCOM,March2003.[5]M.Gerla,R.Bagrodia,L.Zhang,K.Tang,and L.Wang.TCP overWireless Multihop Protocols:Simulation and Experiments.In Proc.IEEE ICC,June1999.[6]M.Gerla,K.Tang,and R.Bagrodia.TCP Performance in WirelessMultihop Networks.In Proc.IEEE WMCSA,Feb.1999.[7]S.Xu and T.Safadawi.Does the IEEE802.11MAC Protocol WorkWell in Multihop Wireless Ad Hoc Networks?IEEE Communications Magazine,pp.130-137,June2001.[8]K.Chandran,S.Raghunathan,S.Venkatesan,and R.Prakash.Afeedback-based scheme for improving TCP performance in ad hoc wireless networks.IEEE Personal communications,8(1):34-39,Feb.2001.[9]G.Holland and N.H.Vaidya.Analysis of TCP performance over mobilead hoc networks.In Proc.ACM MOBICOM,Aug.1999.[10]J.P.Monks,P.Sinha and V.Bharghavan.Limitations of TCP-ELFN forad hoc networks.In Proc.MOMUC,2000.[11]J.Liu and S.Singh.ATCP:TCP for mobile ad hoc networks.IEEEJSAC,V ol.19No.7,July2001.[12]T.D.Dyer and R.V.Boppana.A comparison of TCP performanceover three routing protocols for mobile ad hoc networks.In Proc.ACM Mobihoc,Oct.2001.[13] F.Wang and Y.Zhang.Improving TCP performance over mobile ad-hoc networks with out-of-order detection and response.In Proc.ACM MobiHoc,June2002.[14]K.Xu,M.Gerla,L.Qi,and Y.Shu.Enhancing TCP fairness in ad hocwireless networks using neighborhood RED.In Proc.ACM MobiCom, Sep.2003.[15]Y.Afek,Y.Mansour.Z.Ostfeld,Phantom:a simple and effectiveflowcontrol scheme.In Proc.ACM SIGCOMM,1996.[16] D.Katabi,M.Handley,and C.Rohrs.Congestion control for highbandwidth-delay product networks.In Proc.ACM SIGCOMM,2002.[17]IEEE standard for Wireless LAN Medium Access Control(MAC)andPhysical Layer(PHY)specifications,ISO/IEC8802-11:1999(E),Aug.1999[18]The network simulator ns-2./nsnam/ns.[19]J.Li,C.Blake,D.S.J.De Couto,H.I.Lee and R.Morris.Capacityof ad hoc wireless network.In Proc.ACM MobiCom,July2001. [20] C.E.Perkins,E.M.Royer,and S.R.Das.Ad Hoc On Demand DistanceVector(AODV)Routing.IETF RFC3561[21] C.E.Perkins,E.M.Royer,S.R.Das,and M.K.Marina.PerformanceComparison of Two On-demand Routing Protocols for Ad Hoc Net-works.IEEE Personal Communications,pp.16-28,Feb.2001. [22]K.Chen,and K.Nahrstedt.Limitations of Equation-based CongestionControl in Mobile Ad hoc Networks.In Proc.International Workshop on Wireless Ad Hoc Networking(WWAN2004)in conjuction with ICDCS-2004,March,2004[23]S.Floyd,M.Handley,J.Padhye,and J.Widmer.Equationbased con-gestion control for unicast applications.In Proc.ACM SIGCOMM,Aug.2000.[24]H.Zhai,X.Chen,and Y,Fang.How Well Can the IEEE802.11WirelessLAN Support Quality of Service?Accepted for publication in IEEE Transaction on Wireless Communications,2004.[25]H.Zhai,X.Chen,and Y.Fang.A Call Admission and Rate ControlScheme for Multimedia Support over IEEE802.11Wireless LANs.In Proc.First International Conference on Quality of Service in Heteroge-neous Wired/Wireless Networks(QShine’04),Oct.2004.。