题目基质金属蛋白酶在心衰中的作用(精)
基质金属蛋白酶

生命科学研究LIFE SCIENCE RESEARCH1999年 第3卷 第3期 Vol.3 No.3 1999基质金属蛋白酶吴二喜 王凤飞 Norman McKIE摘 要:基质金属蛋白酶是一类分解细胞外基质组分的锌蛋白酶。
它们在有机体生长发育中的细胞外基质逆转与重塑以及疾病中的病理损害起着极为重要的作用。
基质金属蛋白酶的表达和活性在不同细胞水平受到严密调控,如细胞因子、生长因子以及激素的调节。
基质金属蛋白酶以酶原形式分泌,随后被其它蛋白酶如胞浆素或非蛋白酶类化学物质如有机汞所激活。
所有基质金属蛋白酶都受到天然抑制剂金属蛋白酶组织抑制剂所抑制。
两者的不平衡导致许多疾病的发生,如肿瘤侵入及转移。
合成基质金属蛋白酶组织抑制剂所抑制,如Marimastat能控制肿瘤转移的发生及进一步扩散。
本文将对基质金属蛋白酶的特征、分子区域结构、底物特性、激活机制、调控方式等方面进行最新概述。
关键词:基质金属蛋白酶;金属蛋白酶组织抑制剂;胶原酶;明胶酶;基质酶;膜型基质金属蛋白酶中图分类号:Q51;Q55 文献标识码:AMatrix MetalloproteinasesWU Er-xi1*,WANG Feng-fei1*,Norman McKIE2(1.Department of Human Metabolism and Clinical Biochemistry,University of Sheffield Medical School,Beech Hill Road,Sheffield S10 2RX,UK; 2.Department of Rheumatology,University of Newcastle-upon-Tyne Medical School Framlington Place,Newcastle-upon-Tyne NE2 4HH,UK)Abstract:Matrix metalloproteinases (MMPs) are zinc proteinases that degrade compounds of the extracellular matrix (ECM). These enzymes play a pivotal role in turnover and remodelling of the ECM during organism growth and development and the pathological destruction of tissues in diseases. The activities of metalloproteinases are tightly controlled at several different cellular levels such as modulation by cytokines, growth factors and hormones. MMPs are secreted as zymogens which can be activated by other proteinases such as plasmin or non-proteolytic agents such as organomercurials. All MMPs are inhibited by their natural inhibitors,tissue inhibitors of matrix metalloproteinases(TIMPs). Imbalance between MMPs and TIMPs has been implicated in many diseases such as tumour invasion and metastasis. The synthetic MMP inhibitors such as Marimastat can prevent the growthand further spread of established metastases.Key words:MMPs;TIMPs;collagenases;gelatinases;stromelysins;membrane type MMPsT0 Introduction Matrix metalloproteinases (MMPs) are also called matrixins. Since the first MMP was discovered by Gross and Lapiere in 1962, numerous other MMPs have been described and characterized. To date at least 14 MMPs have been found (Table 1). According to their structural properties and substrate specificities, MMPs can be divided into sub-Table 1 The matrix metalloproteinase family*Enzymes MMP No.**EC No.Mr(kDa)latent/activeExtracellular matrixsubstratesCollagenases Interstitial collagenase MMP1EC 3.4.24.757/48Collagen Ⅰ,Ⅱ,Ⅲ,Ⅶ,Ⅹ;gelatin,entactin,tenascin,aggrecan,progelatinaseA,progelatinase BNeutrophil collagenase MMP8EC3.4.24.3485/65Collagen Ⅰ,Ⅱ,Ⅲ;aggrecanCollagenase 3MMP13 60/48Collagen Ⅰ,ⅡGelatinases Gelatinase A MMP2EC 3.4.24.2472/66Collagen Ⅰ,Ⅳ,Ⅴ,Ⅶ,Ⅹ;gelatin,fibronectin,laminin,aggrecan elastin,progelatinase BGelatinase B MMP9EC 3.4.24.3592/84Collagen Ⅳ,Ⅴ;gelatin,elastin,entactin,aggrecan,vitronectinStromelysins Stromelysin 1MMP3EC 3.4.24.1760/50Collagen Ⅱ,Ⅳ,Ⅸ,Ⅹ,Ⅺ;gelatin,laminin,fibronectin,elastin,tenascin,aggrecan,procollagenase,progelatinase B,neutrophil procollagenaseStromelysin 2MMP10EC 3.4.24.2253/47Collagen Ⅳ,laminin,fibronectin,elastin,aggrecan,procollagenaseStromelysin 3MMP11 65/45Serpins,α1-PI,α2-antiplasmin,insulin-like growth factor-bindingprotein-1Others Matrilysin MMP7EC 3.4.24.2328/21Collagen Ⅳ,gelatins,laminin,fibronectin,entactin,elastin,aggrecan,progelatinaseA,progelatinase B,procollagenaseMetalloelastase MMP12EC.3.4.24.6552/43ElastinMembrane type MMPs MT1-MMP MMP14 63/54Progelatinase A,procollagenase 3,collagen,proteoglycan,fibronectin,tenascinMT2-MMP MMP15 72/61Progelatinase A,procollagenase 3,collagen,proteoglycan,fibronectin,tenascinMT3-MMP MMP16 64/55Progelatinase A MT4-MMP MMP17 70/54Unknown*Compiled from sources including Sang and Douglas[2];Shingleton et al.[3];Nagase [4];Pei et al.[19];Mari et al.[44];Murphy et al.[96];Takino et al.[28,46]andCockett et al.[116]**Some MMPs have been omitted.MMP5 and MMP2 are the same enzyme[5],MMP4 and MMP6 have been described in only one laboratory and there are no sequence data to date [5].There are some novel MMPs which have been found recently,see text for details.groups: collagenases, gelatinases, stromelysins, membrane type MMPs (MT-MMP) andothers[1~4]. This review summarises the characteristics, domain structure, substratespecificity, activation mechanisms, regulation, functions of the MMPs.1 Main characteristics of the MMPs All MMPs have a number of common characteristics which are also helpful to identify new members. These properties[2, 5~7]are: 1)they share a common domain structure comprising signal, propeptide, catalytic, and C- terminal hemopexin-like domains (except MMP7) (Fig.1).Fig.1 The domain structure of the matrix metalloproteinases2)They are secreted as zymogens. 3)Their activation can be achieved by other proteinases or organomercurials. 4)Their proteinase activity is blocked by 1,10-phenanthroline and chelating agents. 5)Activation is accompanied by a loss of molecular weight. 6)The active site contains metal ion zinc. 7)Their activity is inhibited by tissue inhibitor of metalloproteinases (TIMPs). 8)The active enzymes cleave one or more components of the extracellular matrix. 9)The enzymes act in neutral pH and need calcium ions for stability.2 Domain structure of MMPs After comparing the primary amino acid sequences of the MMP members, it can be seen that these proteins are divided into several distinct domains that are conserved among family members[1]. The largest MMP member (MMP9) has 7 domains in order from N-terminal: signal, propeptide, catalytic, fibronectin-like,α2V collagen-like, hinge, and C-terminal hemopexin-like. The simplest member MMP7 has only signal, propeptide and catalytic domains. The newly discovered MT-MMPs have a transmembrane domain[8]. All MMPs produce a signal as a leader sequence which cells cleave prior to secretion. The propeptide is lost on activation. For example, the human fibroblast collagenase is synthesized as a preproenzyme of Mr 54 kDa (57 kDa in Table 1) with the signal peptide of19 amino acids[9]. The primary secretion products of human fibroblast collagenase consist ofa minor glycosylated form of Mr 57 kDa and a major unglycosylated polypeptide of Mr 52 kDa[9]. 81 amino acids are removed after proteolytic activation of human fibroblast collagenase[9]. The catalytic domain contains a conserved zinc binding site comprising the sequence HEXGHXXGXXH[2]. The zinc acts as an active site and is ligated by the three-histidine residues of the zinc binding consensus sequence. The glutamic acid residue in the conserved zinc binding site acts as the catalytic base and proton shuttle during proteolysis and is involved in the fixation of a zinc-bound water molecule[10]. The structural integrity of the zinc-binding active site is maintained by a conserved methionine that is called “Met-turn”[10]. The catalytic domain also contains a calcium-binding region where the calcium ion is believed to stabilize the enzyme[11]. With the exception of matrilysin (MMP7), the MMPs contain an additional feature, that is their hemopexin-like or vitronectin-like C-terminal domain[1]. This domain is thought to help determine substrate specificity[1,5]. Thiscould be true, since recently Gohlke et al.[12]have found that the topology and the side chain arrangements of gelatinase A (MMP2) and fibroblast collagenases are very similar, but there are significant differences in surface charge and contouring. They thought these differences may be a factor in allowing the MMP2 C-terminal domain to bind to TIMP2. Very recently, Brooks et al.[13,14]have found that MMP2 can bind αvβ3 through its hemopexin-like domain. Besides the prototype domain structure, the gelatinases contain three tandem domains with sequences similar to the collagen-binding domain of fibronectin. The fibronectin-like domain is thought to be involved in the binding of two gelatinases to their substrate[5]. The MT-MMPs contain a transmembrane domain and a furin recognition site which is also found in stromelysin 3[8,15](Fig.1).3 Substrate specificity of MMPs The collagenases mainly cleave interstitial collagens (type I, II and III), unlike other MMPs, their substrate specificity is well defined. Collagenase action on the α2-macroglobulin results in the cleavage of Gly-Leu Peptide bond[5].They cleave the Gly-Ile peptide bond of α1(I) chain of collagen and Gly-Leu peptide bond of α2(I) chain of collagen[5]. The substrate cleavage pattern for collagenases is that P1 "residue is invariably hydrophobic (Leu, Ile, Val) and that P1 is usually Gly or a hydrophobic residue[5,16]. The gelatinases cleave denatured collagens and type IV collagen. As shown in table 1, while gelatinase A (MMP2) can also cleave the fibronectin and laminin, major components of the basement membrane, gelatinase B (MMP9) can only cleave the basement membrane component entactin[2]. Stromelysin 1 and 2 have a broad pH optimum and more general activity and are able to degrade many ECM proteins including proteoglycans, gelatins, fibronectin, laminin, elastin, type IV collagen and type IX collagen[17]. Niedzwiecki et al.[18]tested the substrate specificity of the human stromelysin 1 and found the preferences at P3, P2, P1, P1 ", and P2 "are for the hydrophobic amino acids Pro, Leu, Ala, Nva, and Trp, respectively. The mature stromelysin 3 does not degrade any major ECM components. Up to now, the only known substrates for stromelysin 3 are α1-proteinase inhibitor, serine proteinase inhibitors, α2-antiplasmin, and insulin-like growth factor-binding protein-1 [19,20]. However, the substrate specificity overlaps among the MMP members. The gelatinase A (MMP2) can also cleave triple helical type I collagen generating the 3/4 and 1/4 length collagen fragments characteristic of interstitial collagenases[21]. The cleavage site is the same Gly-Ile/Leu bond as the interstitial collagenases[21]. In fact all of the MMPs cleave gelatin and fibronectin at some rate[1]. Almost every MMP degrades an octapeptide containing collagen sequence GPQGIAGQ[5].4 Activation mechanisms of MMPs The MMPs are secreted in a latent form that is subsequently converted into the mature enzymes. The inactive zymogen can be processed into active forms by numerous reagentsincluding proteinases such as trypsin and plasmin; conformational perturbants such as sodium dodecyl sulfate (SDS); heavy metals such as Au (I) compounds and organomercurials; oxidants such as NaSCN; disulfide reagents such as oxidized glutathione; and sulfhydryl alkylating agents such as N-ethylmaleimide[22]. The latent proform of MMPs contains the highly conserved PRCGVNPD sequence with an unpaired cysteine residue. The cysteine residue links to the active site zinc, which blocks the active site. A ‘cysteine switch’ activation mechanism has been proposed[22,23](Fig. 2). In other words, in the latent form of MMPs, the cysteine residue with the thiol group coordinates the catalytically essential zinc ion in the active site of enzyme. Some reagents such as SDS can dissociate the cysteine residue from the zinc ion[22]. From this hypothesis, each MMP should have an unpaired cysteine residue and a zinc-binding site. In fact, all MMPs contain the PRCGVNPD sequence with an unpaired cysteine residue in the propeptide domain and the HEXXHXXGXXH sequence in the catalytic domain. Very recently, it has been shown that he structure of human pro-MMP2 supports this hypothesis[24]. The loops within the propeptide domain act as bait for activating proteinases. The prodomain structure breaks down and its shielding of the catalytic cleft is withdrawn upon cleavage, which allow water to enter and hydrolyze the coordination of the cysteine residue to the zinc ion[24]. All MT-MMPs found to date and stromelysin 3 contain a consensus sequence RXR/KR, which has already been found to be essential in the activation of stromelysin 3 and MT1-MMP by furin [15,25,26]. MMPs also have the ability to activate one another[27]. MT1-MMP[8]and MT3-MMP[28]have been shown to activate MMP2. MMP7 can activate MMP1, MMP2, MMP3 and MMP9[29]. MMP3 activates MMP1[30], MMP8[31], MMP9[32]and MMP13[33]. MMP2 and MT1-MMP activate MMP13[34], while MMP10 activates MMP8[35]. It has also been well documented that MMPs and serine proteinases can act on the proforms of one another [36,37]. Plasmin can cleave the prodomains of MMPs such as collagenase and stromelysin and activate these enzymes[36,37]. MMP7 can catalyze the formation of low molecular weight pro-urokinase and urokinase[38].Fig.2 Cysteine switch mechanism for activation of MMPsProteinases such as trypsin and plasmin cleave the propeptide,ahead of the cysteine to generate intermediate forms.Alternatively,nonproteolytic agents such as aminiphenyl-mercuric acetate (APMA) and sodium dodecy1 sulfate (SDS) will modify the cysteine.In a second step,these intermediate forms can be autoproteolytically cleaved to remove the propeptide and confer permanent activity.5 Regulation of MMPs The activities of metalloproteinases are tightly controlled at several different cellular levels. There are five points: (i) modulation of gene expression by cytokines, growth factors and hormones; (ii) synthesis and secretion of proMMPs; (iii) selective expression of MMP genes in specific tissue/cell types; (iv) activation of proenzymes; and (v) inhibition of the active enzymes[7,36,39,40]. It is known that the MMPs are not constitutively expressed in most tissue types but their mRNAs can be induced by treatment with many kinds of agents such as cytokines, growth factors, hormones, tumour promoter and oncogene products. In some cases the induction of more than one MMP is coordinately regulated, for example, MMP1 and MMP3 are often coordinately expressed[40]. The synthesis of MMP1 and MMP3 can be upregulated by 12-O-tetradecanoyl-phorbol-13-acetate (TPA), interleukin 1 (IL1), tumor necrosis factor α(TNFα), epidermal growth factor (EGF), basic fibroblast growth factor (bFGF) and platelet derived growth factor (PDGF) and be downregulated by transforming growth factor β (TGF-β), interferon γ (IFN-γ) retinoic acid and dexamethasone[40], and MMP1 is down regulated by IL4[41]. The selective expression of MMP genes in specific tissue/cell types is one method of regulation of MMPs. Interstitial collagenase (MMP1) arises from connective tissue fibroblasts and macrophages. Neutrophil collagenase (MMP8) is only produced by cells of the neutrophil lineage. Stromelysin 1 (MMP3) is not widely expressed normally, but can be readily induced by growth factors, cytokines, tumour promoters and oncogene products in cultured mesenchymal cells such as chondrocytes and connective tissue fibroblasts. Stromelysin 2 (MMP10) is usually expressed in macrophages, keratinocytes and tumour cells[40,42]. Stromelysin 3 (MMP11) is often expressed in tumour stromal cells[37,40,43,44]. Gelatinase A (MMP2) is the most widespead of all MMPs and is frequently elevated in malignancies as well as occurring in connective tissue cells[36,40]. Gelatinase B (MMP9) is expressed in transformed and tumour-derived cells, neutrophil, corneal epithelial cells, cytotrophoblasts and keratinocytes[36]. Matrilysin is expressed in immature monocytes[36]. Metalloelastase (MMP12) is found in macrophages. MT1-MMP is expressed in tumour stroma cells[45]. MT2-MMP is produced by a human oral malignant melanoma and a human placenta[46]. MT3-MMP is expressed in normal tissues such as lung and kidney and cultured cells such as the squamous cell carcinoma cell line OSC-19 and human embryonal lung fibroblasts[28]. MT4-MMP is expressed in primary breast carcinomas and breast cancer cell lines examined[47]. All MMPs are inhibited by natural inhibitors called tissue inhibitors of metalloproteinases (TIMPs) of which four have been described (Table 2). TIMPs 1, 2 and 4 are secreted extracellularly in soluble form whereas TIMP3 binds to the ECM. All TIMPs share 35%~40% sequence identity and considerable higher structural similarity. The TIMPs bind with high affinity and 1∶1 molar ratio to active MMPs resulting in the loss of proteinase activity. All TIMPs contain 12 cysteine residues that have been shown to form disulfide bonds generating 6 loops. The mechanism of inhibition of MMPs by TIMPs is widely felt to be unclear. Murphy[39]. pointed out that the TIMP C-terminal domain has several different MMP binding sites that act to increase the rate of inhibition. Further studies such as site-directed mutagenesis and the crystal structure research of the complex of MMP and TIMP will facilitate understanding of the mechanism. TIMPs play a pivotal role in the regulation of ECM degradation/remodelling. Disruption of the balance between MMPs and TIMPs has been implicated in many diseases such as tumour invasion and metastasis. Besides their inhibitory activities, the TIMPs play a role in growth-promotion[48]. Some effective synthetic MMP inhibitors also can inhibit MMPs[49,50]. For example, the hydroxamate-based inhibitors such as BB94 (batimastat) and BB2516 (marimastat) can inhibit metastatic spread of tumoursand block the process of tumour neovasculariation[51~54]. The hydroxamate group in thesemolecules can combine with the zinc atom in the active site of MMPs[49,50].Table 2 Comparison of TIMP family members* TIMP1TIMP2TIMP3TIMP4 Molecular mass28kDa21kDa24kDa22kDa Glycosylation yes no no/yes no Binding properties proMMP9proMMP2ECM proMMP2Transcripts0.9kb 1.1 and 3.5kb 4.5,2.8,2.4and 1.2kb1.4,4.1,2.1,1.2,and 0.97kbModulation of gene expression by TGF-β1updown or noobvious effectup not determinedMajor sites ofexpressionOvary,bone Placenta Kidney,brain Heart Chromosome locationof human genexq1117q2522q12.1~13.22p25 *Compiled from sources including Stetler-Stevenson[117];Greene et al.[118];Wick et al.[119];Chamber and Matrisian[120]and Olson et al.[121]6 MMPs in physiological processes and pathological destruction Normal expression of MMPs is associated with turnover and remodelling of the ECM during growth and development. Cawston[7]summarised the involvement of MMPs in the normal turnover of connective tissue matrix. The MMPs are involved in the normal physiological processes such as ovulation and embryo implantation, embryological development, angiogenesis, bone turnover, uterine resorption and cervical ripening[7]. The MMPs are associated with the pathological destruction of tissues in diseases[1,7]. They are implicated in the processes such as wound healing, corneal ulceration, tumour growth and metastasis, periodontal disease, rheumatoid arthritis, arteriosclerosis and aortic aneurism[1,7]. Considerable research has been directed toward understanding both the steps involved in tumour cell invasion and metastasis and the molecular mechanism of the process. MMPs are thought to be one of the main contributors to tumour invasion and metastasis since they can degrade all of the components of basement membranes which the tumour cellsmust traverse[55~58]. Now the gene-targeting experiments have facilitated the examinationsof the effects that their absence, mutation or overexpression, in various physiological and pathological processes[59]. In the following sections more detailed information is presented on several MMP family members.7 Collagenases I, II and III (MMP1, MMP8 and MMP13) The group of enzymes termed collagenases includes 3 members: MMP1 (interstitialcollagenase or fibroblast collagenase), MMP8 (neutrophil collagenase) and MMP13 (collagenase 3). Their sequences share around 50%[33]. They cleave all three α chains of native types I, II, III collagens at a single site resulting in fragments corresponding to three-quarters and one-quarter of their initial length by hydrolyzing the peptide bond Gly-[Ile orLeu][60~62]. They do not degrade collagen IV and V, which are cleaved by otherproteinases[63~66]. The collagenases can also cleave type X collagen[67]. The threecollagenases have their own preference in cleaving the collagens. The preferred substrate of collagenase 3 (MMP13) is collagen type II[33], fibroblast collagenase (MMP1) preferentially cleaves collagen type III[68]and neutrophil collagenase (MMP8) prefers to cleave type I collagen[69]. Also collagenase 3 has a much stronger gelatinolytic activity than its homologous counterparts MMP1 and MMP8[33]. To date MMP1 expression has not been found in rats or mice[33]. All collagenases are located in the human chromosome 11q22.3 cluster[70,71]and share a highly conserved gene structure[72,73]. The expression of MMP13 in cartilage and its preference to degrade type II collagen suggests that it plays a critical role in the arthritides[74]. X ray crystallographic analyses of MMP1 and MMP8 are available now. The structures show that their catalytic domains harbour two zinc ions and one or twocalcium ions[75~77]. The structure consists of a five-stranded β sheet and three α helices[75].8 Gelatinases A and B (MMP2 and MMP9) Gelatinases, also called type IV collagenases, contain two members: MMP2 and MMP9. They degrade denatured collagens, type IV, V, VII, X, and XII collagens,vitronectin, aggrecan, elastin, galectin 3 and laminin[78~83]. Recently, it has beendemonstrated that MMP2 degrades native interstitial collagens[21]. MMP2 is the most widely distributed MMP[1]. Overexpression of gelatinases has been demonstrated in many tumorsystems and has been linked to tumour invasion[84~87]. While the proMMP2 is often foundcomplexed with the TIMP2 and is activated by MT1-MMP[88,89], the proMMP9 is associated with the TIMP1[78,90]. The regulation of MMP2 and MMP9 gene expression is different[91]. The promoter of MMP9 gene has a TATA-like sequence and a TPA response element, while MMP2 gene has no TATA motif-like sequence in the vicinity of the start site for transcription and TPA response element in its promoter[91]. There is a structural difference between MMP2 and MMP9 that comprises an extended 54 amino acid hinge region sequence which shares some homology with the α2 chain of type V collagen[78]. Brooks et al.[13,14]have found that MMP2 can directly bind integrin αvβ3. They further demonstrated that MMP2 binds αvβ3 through its hemopexin-like domain. It is likely to be distinct from other αvβ3-directed ligands as MMP2 has no RGD sequence[13,14]. Brooks et al.[14]have also found that PEX, a fragment of MMP2, which contains the C-terminal hemopexin-like domain, prevents MMP2 binding to αvβ3 and blocks cells surfacecollagenolytic activity. PEX is likely to be a natural breakdown product of MMP2 since the active form (62 kDa) of MMP2 can be further processed to a smaller species (43 kDa) resulting from autocatalytic removal of the 29 kDa hemopexin-like domain without the presence of TIMP2[14,92]. Both MMP2 and MMP9 are located on human chromosome 16[93].9 Stromelysins 1, 2 and 3 (MMP3, MMP10 and MMP11) MMP3 (stromelysin 1), MMP10 (stromelysin 2) and MMP11 (stromelysin 3) belong to this group. Stromelysins have wide substrate specificity. Stromelysin 1 degrades aggrecan, fibronectin, gelatin, laminin, type II, IV, IX, X, XI collagens and elastin[2,94]. It also participates in activation of other proMMPs such as proMMP1, proMMP8 and proMMP9[31,32,80]. MMP3 is not readily expressed in tissue but can be induced by cytokines such as IL1 and TNFα, growth factors and tumour promoters such as PMA[1,5,95]. Stromelysin 2 cleaves aggrecan, laminin, fibronectin, elastin and type IV[2]. It is transcriptionally active in normal human cells such as keratinocytes and it encodes the secreted Stromelysin 2[42]. Stromelysin 3 is a newly characterized MMP. It can degrade serpin, α1-proteinase inhibitor (α1-PI), α2-antiplasmin, and insulin-like growth factor-binding protein-1[19,20]; the truncated stromelysin 3 can also degrade fibronectin, laminin, aggrecan and type IV[96]. Stromelysin 3 is often found expressed in stromal cells surrounding primary and metastatic carcinomas[43]and may be involved in promoting local tumor development. Recently it has been suggested that the tumor-specific processing of stromelysin 3 to the 35 kDa protein is likely to be an important regulatory mechanism, since the generation of 35 kDa stromelysin in tumour/stroma coculture requires basic fibroblast growth factor (bFGF) and an MMP-like enzyme[44]. Unlike other MMPs, prodomain of stromelysin 3 contains a furin cleavage site, and therefore stromelysin 3 can be processed directly to its 45 kDa active form by furin within the constitutive secretory pathway[15]. MMP3 and MMP10 are located in the human chromosome cluster 11q22.3[70,72], while MMP11 is mapped to the Q11.2 region of chromosome 22[97].10 Membrane type-matrix metalloproteinases (MMP15, MMP16, MMP17 and MMP18) Membrane type matrix metalloproteinases (MT-MMPs), comprising MT1-MMP, MT2-MMP2, MT3-MMP and MT4-MMP (MMP14, MMP15, MMP16 and MMP17, respectively) are a novel subgroup of MMPs which contain a transmembrane domain and a short cytoplasmic domain in addition to the signal, pro-, catalytic, hemopexin-like and C-terminal domains which are common to other MMPs. To date, four MT-MMPs have been described [8,28,47,98]. From the alignment of amino acid sequences for MT-MMPs[47], it can be seen that they have at least 30% sequence homology to each other. Like other MMPs, they contain cysteine switch sequence, zinc binding site and Met-turn. However, they have an insertion between the propeptide and the catalytic domain in addition to the above mentionedmembrane-binding domain and short cytoplasmic domain. This insertion as in stromelysin 3 contains a potential cleavage site of furin or furin like convertase[8,15]. It has already been demonstrated that furin can cleave this sequence in pro-stromelysin 3 and proMT1-MMP [15,25,26]. However, the proMT1-MMP is proteolytically activated by human plasmin[99]. Okumura et al.[99]proposed that this is an extracellular proteinase activator of proMT1-MMP whereas the intracellular mechanism of activation is mediated by furin or a furin-like enzyme. The second insertion is found in proteinase domain; the function of these 8 amino acids is not known. The third insertion which contains the hydrophobic transmembrane domain is located in the C-terminal. The transmembrane domain of MT-MMPs plays an essential role in the progelatinase A (proMMP2) activation function of MT-MMPs, although some portions of the truncated form remain on the cell surface after the removal of this domain[100]. In MT1-MMP, the activation complex is a trimer comprising MT1-MMP, progelatinase A and TIMP2[88,89]. A recent study has suggested that MT1-MMP is a TIMP2 receptor[101]. This is further confirmed by another recent study[73]that TIMP2 and MT1-MMP form a complex for activation of progelatinase A. TIMP2 and TIMP3 can efficiently inhibit MT1-MMP whereas TIMP1 is a poor inhibitor for MT1-MMP[102]. Will et al.[102] also demonstrated that TIMP2 and TIMP3 can bind more rapidly to the catalytic domain of MT1-MMP than to the catalytic domain of MMP2. At the same time, two groups showed that MMP14, MMP15 and MMP16 locate in chromosomes 14, 16 and 8, respectively [103,104]. The MT-MMP gene loci are dispersed whereas most MMPs are clustered on chromosome 11q22.3[70,71], suggesting that the MT-MMPs may be a genetically distinct subgroup of MMPs. It has been suggested that the MT-MMPs play a critical role in tumor cell invasion and in ECM degradation[8,57,102]. MT-MMPs also can degrade ECM components[25,105,106].11 Others This group includes MMPs such as matrilysin (MMP7) and metalloelastase (MMP12). Matrilysin, also called putative metalloproteinase 1 (pump 1), is the smallest member of the MMP family. Unlike other MMPs, it lacks a C-terminal hemopexin-like domain. MMP7 can degrade various ECM components. It also can activate other proMMPs such as proMMP1, 3, 2 and 9[29]. Metalloelastase (MMP12) is also called macrophage elastase (ME). The expression of human macrophage elastase (HME) is mainly restricted to tissue macrophages [107]. Besides the substrate elastin listed in Table 1, the purified recombinant HME can degrade fibronectin, laminin, entactin, type IV collagen, chondroinan sulfate, and heparan sulfate[108]. Chandler et al.[109]also demonstrated that HME can degrade myelin basic protein and processes a TNF α fusion protein. Therefore Gronski et al.[108]suggested that HME may be essential for macrophages to penetrate basement membranes and remodel injured tissue during inflammation. MMP7 and MMP12 are allocated in the human chromosome cluster 11q22.3[70,72,107].。
基质金属蛋白酶 识别位点
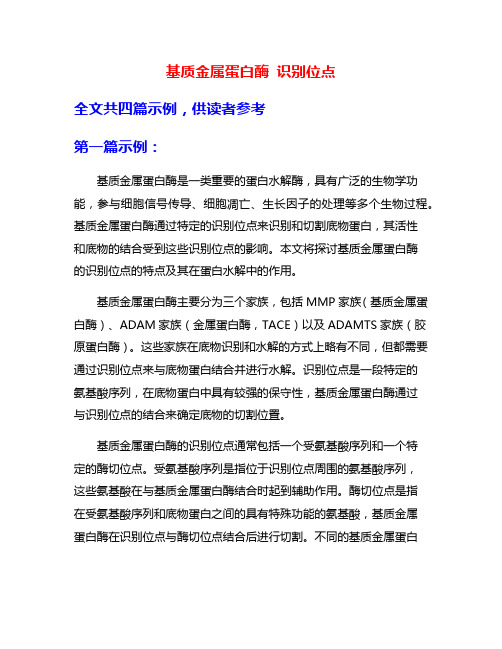
基质金属蛋白酶识别位点全文共四篇示例,供读者参考第一篇示例:基质金属蛋白酶是一类重要的蛋白水解酶,具有广泛的生物学功能,参与细胞信号传导、细胞凋亡、生长因子的处理等多个生物过程。
基质金属蛋白酶通过特定的识别位点来识别和切割底物蛋白,其活性和底物的结合受到这些识别位点的影响。
本文将探讨基质金属蛋白酶的识别位点的特点及其在蛋白水解中的作用。
基质金属蛋白酶主要分为三个家族,包括MMP家族(基质金属蛋白酶)、ADAM家族(金属蛋白酶,TACE)以及ADAMTS家族(胶原蛋白酶)。
这些家族在底物识别和水解的方式上略有不同,但都需要通过识别位点来与底物蛋白结合并进行水解。
识别位点是一段特定的氨基酸序列,在底物蛋白中具有较强的保守性,基质金属蛋白酶通过与识别位点的结合来确定底物的切割位置。
基质金属蛋白酶的识别位点通常包括一个受氨基酸序列和一个特定的酶切位点。
受氨基酸序列是指位于识别位点周围的氨基酸序列,这些氨基酸在与基质金属蛋白酶结合时起到辅助作用。
酶切位点是指在受氨基酸序列和底物蛋白之间的具有特殊功能的氨基酸,基质金属蛋白酶在识别位点与酶切位点结合后进行切割。
不同的基质金属蛋白酶具有不同的受氨基酸序列和酶切位点,这也决定了它们的底物特异性。
基质金属蛋白酶在识别位点与底物蛋白结合后,通过底物蛋白的构象变化来促进水解反应的进行。
一般来说,底物蛋白在与基质金属蛋白酶结合时会发生构象变化,使得酶切位点更容易被基质金属蛋白酶识别和切割。
这种构象变化是基质金属蛋白酶与底物蛋白结合的关键步骤,也是水解反应进行的前提。
第二篇示例:基质金属蛋白酶(Matrix Metalloproteinase,MMPs)是一类在许多生物学和病理学过程中起关键作用的蛋白酶。
它们参与细胞外基质的降解和重塑,促进组织的发育和修复,同时也在许多疾病的发生和发展中扮演重要角色。
识别基质金属蛋白酶的底物位点对于研究其功能和生物学效应至关重要。
本文将就基质金属蛋白酶的识别位点展开讨论,以帮助读者更好地理解这些重要的酶类的作用机制。
基质金属蛋白酶1
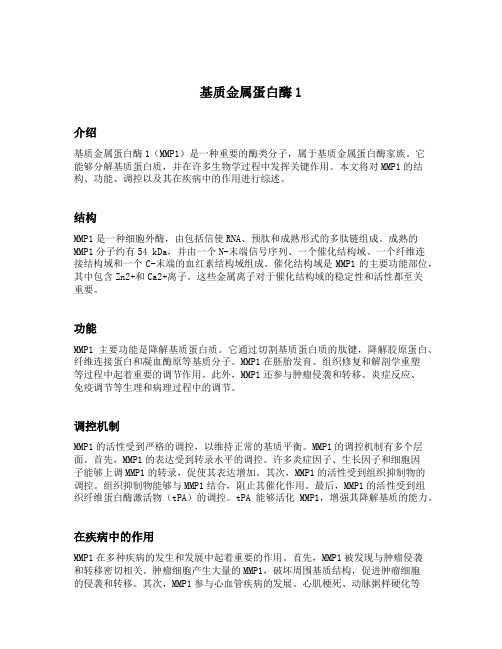
基质金属蛋白酶1介绍基质金属蛋白酶1(MMP1)是一种重要的酶类分子,属于基质金属蛋白酶家族。
它能够分解基质蛋白质,并在许多生物学过程中发挥关键作用。
本文将对MMP1的结构、功能、调控以及其在疾病中的作用进行综述。
结构MMP1是一种细胞外酶,由包括信使RNA、预肽和成熟形式的多肽链组成。
成熟的MMP1分子约有54 kDa,并由一个N-末端信号序列、一个催化结构域、一个纤维连接结构域和一个C-末端的血红素结构域组成。
催化结构域是MMP1的主要功能部位,其中包含Zn2+和Ca2+离子。
这些金属离子对于催化结构域的稳定性和活性都至关重要。
功能MMP1主要功能是降解基质蛋白质。
它通过切割基质蛋白质的肽键,降解胶原蛋白、纤维连接蛋白和凝血酶原等基质分子。
MMP1在胚胎发育、组织修复和解剖学重塑等过程中起着重要的调节作用。
此外,MMP1还参与肿瘤侵袭和转移、炎症反应、免疫调节等生理和病理过程中的调节。
调控机制MMP1的活性受到严格的调控,以维持正常的基质平衡。
MMP1的调控机制有多个层面。
首先,MMP1的表达受到转录水平的调控。
许多炎症因子、生长因子和细胞因子能够上调MMP1的转录,促使其表达增加。
其次,MMP1的活性受到组织抑制物的调控。
组织抑制物能够与MMP1结合,阻止其催化作用。
最后,MMP1的活性受到组织纤维蛋白酶激活物(tPA)的调控。
tPA能够活化MMP1,增强其降解基质的能力。
在疾病中的作用MMP1在多种疾病的发生和发展中起着重要的作用。
首先,MMP1被发现与肿瘤侵袭和转移密切相关。
肿瘤细胞产生大量的MMP1,破坏周围基质结构,促进肿瘤细胞的侵袭和转移。
其次,MMP1参与心血管疾病的发展。
心肌梗死、动脉粥样硬化等疾病中,MMP1介导的基质降解导致血管结构的破坏,加速病变的进展。
此外,MMP1在关节炎和炎症性肠病等炎症性疾病中也发挥重要作用。
应用前景由于MMP1在多种疾病中的重要作用,成为潜在的治疗靶点。
心力衰竭患者免疫功能的变化及其临床意义
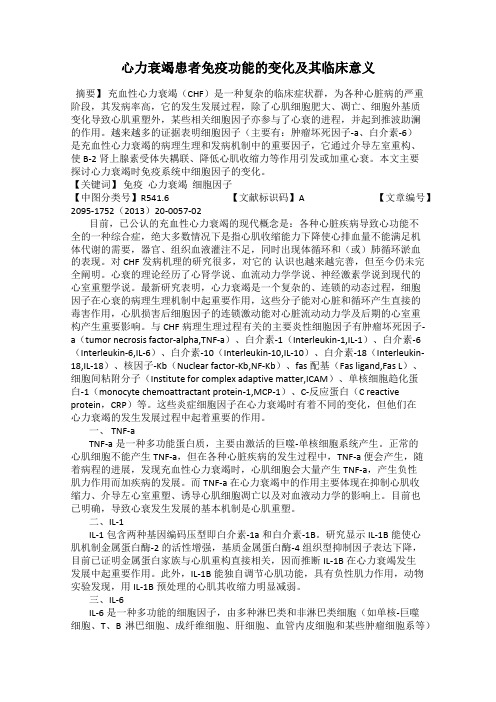
心力衰竭患者免疫功能的变化及其临床意义摘要】充血性心力衰竭(CHF)是一种复杂的临床症状群,为各种心脏病的严重阶段,其发病率高,它的发生发展过程,除了心肌细胞肥大、凋亡、细胞外基质变化导致心肌重塑外,某些相关细胞因子亦参与了心衰的进程,并起到推波助澜的作用。
越来越多的证据表明细胞因子(主要有:肿瘤坏死因子-a、白介素-6)是充血性心力衰竭的病理生理和发病机制中的重要因子,它通过介导左室重构、使 B-2肾上腺素受体失耦联、降低心肌收缩力等作用引发或加重心衰。
本文主要探讨心力衰竭时免疫系统中细胞因子的变化。
【关键词】免疫心力衰竭细胞因子【中图分类号】R541.6 【文献标识码】A 【文章编号】2095-1752(2013)20-0057-02目前,已公认的充血性心力衰竭的现代概念是:各种心脏疾病导致心功能不全的一种综合症,绝大多数情况下是指心肌收缩能力下降使心排血量不能满足机体代谢的需要,器官、组织血液灌注不足,同时出现体循环和(或)肺循环淤血的表现。
对CHF发病机理的研究很多,对它的认识也越来越完善,但至今仍未完全阐明。
心衰的理论经历了心肾学说、血流动力学学说、神经激素学说到现代的心室重塑学说。
最新研究表明,心力衰竭是一个复杂的、连锁的动态过程,细胞因子在心衰的病理生理机制中起重要作用,这些分子能对心脏和循环产生直接的毒害作用,心肌损害后细胞因子的连锁激动能对心脏流动动力学及后期的心室重构产生重要影响。
与CHF病理生理过程有关的主要炎性细胞因子有肿瘤坏死因子-a(tumor necrosis factor-alpha,TNF-a)、白介素-1(Interleukin-1,IL-1)、白介素-6(Interleukin-6,IL-6)、白介素-10(Interleukin-10,IL-10)、白介素-18(Interleukin-18,IL-18)、核因子-Kb(Nuclear factor-Kb,NF-Kb)、fas配基(Fas ligand,Fas L)、细胞间粘附分子(Institute for complex adaptive matter,ICAM)、单核细胞趋化蛋白-1(monocyte chemoattractant protein-1,MCP-1)、C-反应蛋白(C reactive protein,CRP)等。
心衰综述相关因子
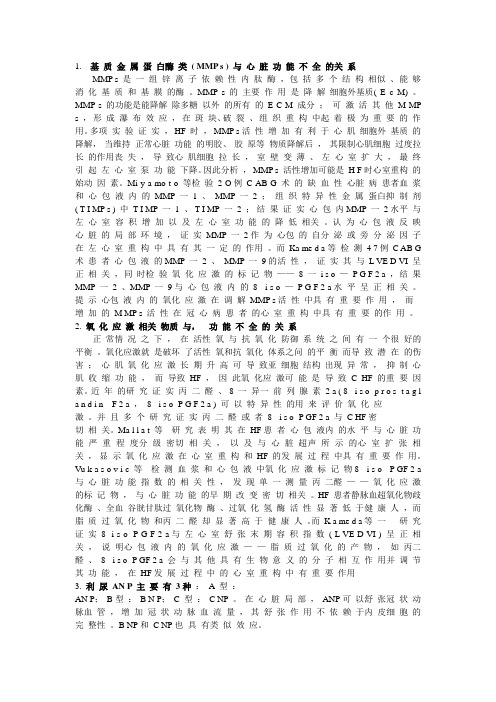
1. 基质金属蛋白酶类( MMP s ) 与心脏功能不全的关系MMP s 是一组锌离子依赖性内肽酶,包括多个结构相似、能够消化基质和基膜的酶。
MMP s 的主要作用是降解细胞外基质( E c M) 。
MMP s 的功能是能降解除多糖以外的所有的E C M 成分;可激活其他M MP s ,形成瀑布效应,在斑块、破裂、组织重构中起着极为重要的作用。
多项实验证实,HF 时,MMP s活性增加有利于心肌细胞外基质的降解,当维持正常心脏功能的明胶、胶原等物质降解后,其限制心肌细胞过度拉长的作用丧失,导致心肌细胞拉长,室壁变薄、左心室扩大,最终引起左心室泵功能下降。
因此分析,MMP s 活性增加可能是H F时心室重构的始动因素。
Mi y a mo t o 等检验2 O例C AB G 术的缺血性心脏病患者血浆和心包液内的MMP 一 1 、MMP 一 2 ;组织特异性金属蛋白抑制剂( T I MP s ) 中T I MP 一1 、T I MP 一2 ;结果证实心包内MMP 一2水平与左心室容积增加以及左心室功能的降低相关。
认为心包液反映心脏的局部环境,证实MMP 一2作为心包的自分泌或旁分泌因子在左心室重构中具有其一定的作用。
而Ka me d a等检测 4 7例C AB G 术患者心包液的MMP 一 2 、MMP 一9的活性,证实其与L VE D VI 呈正相关,同时检验氧化应激的标记物——8 一i s o —P G F 2 a ,结果MMP 一2 、MMP 一9与心包液内的8 - i s o —P G F 2 a水平呈正相关。
提示心包液内的氧化应激在调解MMP s活性中具有重要作用,而增加的M MP s 活性在冠心病患者的心室重构中具有重要的作用。
2. 氧化应激相关物质与,功能不全的关系正常情况之下,在活性氧与抗氧化防御系统之间有一个很好的平衡。
氧化应激就是破坏了活性氧和抗氧化体系之问的平衡而导致潜在的伤害;心肌氧化应激长期升高可导致亚细胞结构出现异常,抑制心肌收缩功能,而导致HF ,因此氧化应激可能是导致 C HF 的重要因素。
基质金属蛋白酶作用
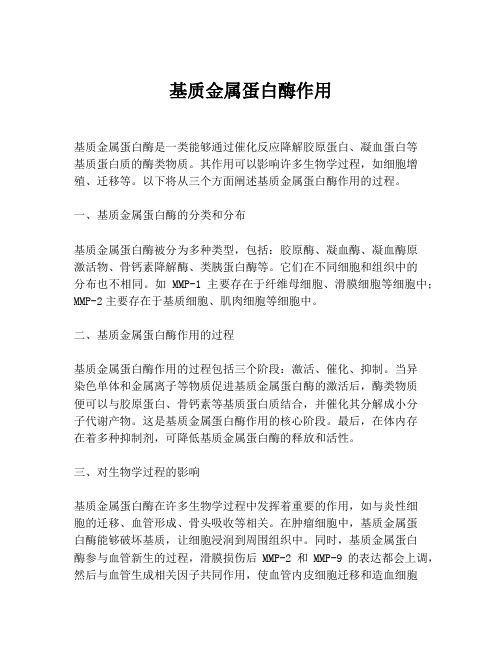
基质金属蛋白酶作用基质金属蛋白酶是一类能够通过催化反应降解胶原蛋白、凝血蛋白等基质蛋白质的酶类物质。
其作用可以影响许多生物学过程,如细胞增殖、迁移等。
以下将从三个方面阐述基质金属蛋白酶作用的过程。
一、基质金属蛋白酶的分类和分布基质金属蛋白酶被分为多种类型,包括:胶原酶、凝血酶、凝血酶原激活物、骨钙素降解酶、类胰蛋白酶等。
它们在不同细胞和组织中的分布也不相同。
如MMP-1主要存在于纤维母细胞、滑膜细胞等细胞中;MMP-2主要存在于基质细胞、肌肉细胞等细胞中。
二、基质金属蛋白酶作用的过程基质金属蛋白酶作用的过程包括三个阶段:激活、催化、抑制。
当异染色单体和金属离子等物质促进基质金属蛋白酶的激活后,酶类物质便可以与胶原蛋白、骨钙素等基质蛋白质结合,并催化其分解成小分子代谢产物。
这是基质金属蛋白酶作用的核心阶段。
最后,在体内存在着多种抑制剂,可降低基质金属蛋白酶的释放和活性。
三、对生物学过程的影响基质金属蛋白酶在许多生物学过程中发挥着重要的作用,如与炎性细胞的迁移、血管形成、骨头吸收等相关。
在肿瘤细胞中,基质金属蛋白酶能够破坏基质,让细胞浸润到周围组织中。
同时,基质金属蛋白酶参与血管新生的过程,滑膜损伤后MMP-2和MMP-9的表达都会上调,然后与血管生成相关因子共同作用,使血管内皮细胞迁移和造血细胞增殖,促进血管新生。
总之,基质金属蛋白酶是一类能够影响生物学过程的重要酶类物质。
它们的活性受多个因素影响,如细胞因子的浓度、蛋白酶抑制剂的存在等。
深入了解基质金属蛋白酶的基本结构和活性,有助于我们更好地理解生物过程并探索相关治疗方法。
心力衰竭患者同型半胱氨酸和基质金属蛋白-9的相关性研究
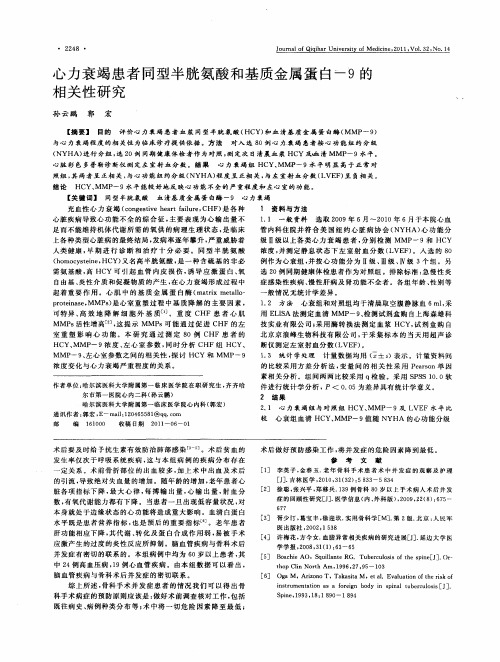
【 键 词 】 同型 半 胱 氨 酸 关
血 清基 质 金 属 蛋 白酶 一 9 心 力 衰竭
1 资 料 与 方 法
充 血 性 心 力 衰 竭 (o gsiehat alr, cn et er fi e CHF 是 各 种 v u ) 心脏 疾 病 导 致 心 功 能 不 全 的 综 合 征 , 要 表 现 为 心 输 出 量 不 主
北京京浪峰生物科技有 限公 司 , 于采集标 本的 当天用超声 诊
断 仪 测 定 左 室 射 血 分 数 ( VE ) L F
1 3 统计 学 处 理 .
计量 数 据 均 用 ( s 示 。计 量 资 料 问 z± )表
的 比较 采 用 方 差 分 析 法 , 量 间 的 相 关 性 采 用 P asn单 因 变 er o 素 相 关 分 析 。组 间两 两 比较 采 用 q 验 。采 用 S S 0 0软 检 P S 1.
J u n l fQiia ie st fM e ii , 0 1, 1 3 , .1 o r a qh rUnv riyo dcne 2 1 Vo. 2 No 4 o
心力 衰竭患者 同型半胱 氨酸和基质金属蛋 白一9的
相 关 性 研 究
孙 云鹏 郭 宏
评 价 心 力 衰 竭 患 者 血 浆 同型 半 胱 氨 酸 ( Y) 血 清 基 质 金 属 蛋 白 酶 ( HC 和 MMP 9 一 ) 【 要 】 目的 摘
需 氨 基 酸 , HC 可 引 起 血 管 内 皮 损 伤 , 导 应 激 蛋 白 、 高 Y 诱 氧 自由 基 、 性 介 质 和促 凝 物质 的 产 生 , 心 力 衰 竭形 成 过 程 中 炎 在 起 着 重 要 作 用 。 心 肌 中 的 基 质 金 属 蛋 白 酶 ( t xmea o mar tl - i l
基质金属蛋白酶的调节及其疾病的治疗
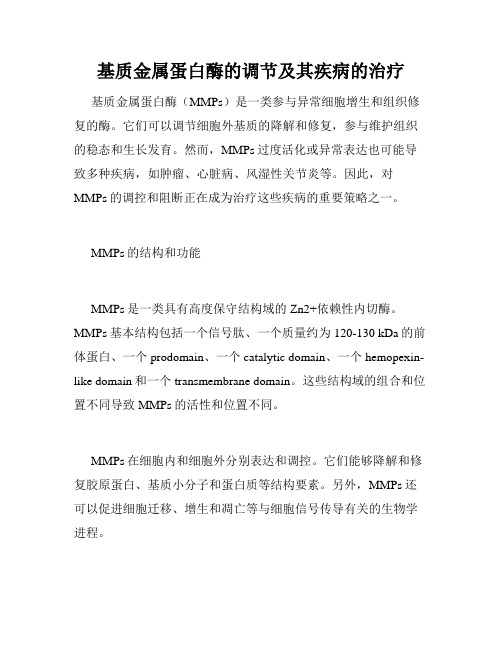
基质金属蛋白酶的调节及其疾病的治疗基质金属蛋白酶(MMPs)是一类参与异常细胞增生和组织修复的酶。
它们可以调节细胞外基质的降解和修复,参与维护组织的稳态和生长发育。
然而,MMPs过度活化或异常表达也可能导致多种疾病,如肿瘤、心脏病、风湿性关节炎等。
因此,对MMPs的调控和阻断正在成为治疗这些疾病的重要策略之一。
MMPs的结构和功能MMPs是一类具有高度保守结构域的Zn2+依赖性内切酶。
MMPs基本结构包括一个信号肽、一个质量约为120-130 kDa的前体蛋白、一个prodomain、一个catalytic domain、一个hemopexin-like domain和一个transmembrane domain。
这些结构域的组合和位置不同导致MMPs的活性和位置不同。
MMPs在细胞内和细胞外分别表达和调控。
它们能够降解和修复胶原蛋白、基质小分子和蛋白质等结构要素。
另外,MMPs还可以促进细胞迁移、增生和凋亡等与细胞信号传导有关的生物学进程。
MMPs的调控和疾病治疗MMPs的异常表达和活化在多种疾病中发挥作用。
例如,在肿瘤中,过度MMP-2活化可促进转移、侵袭和治疗难度;在心脏病中,MMP-9异常表达则能够促进心肌细胞凋亡和炎症反应。
因此,对MMPs的调控和阻断成为治疗这些疾病的重要策略之一。
目前,有多种方法可以调控和抑制MMPs的活性。
一些天然物质,如维生素D3和omega-3脂肪酸,已被证明可以调控MMPs的表达和活性。
此外,制备针对MMPs的抑制剂也成为治疗疾病的研究热点之一,这些抑制剂可以选择性抑制不同的MMPs亚型,并被广泛应用于肿瘤、心血管疾病的治疗中。
此外,其他一些方法也被应用于治疗相关疾病。
例如,在肿瘤治疗中,联合应用靶向药物和MMPs抑制剂能够提高治疗效果和减轻副作用。
在心脏病治疗中则使用心脏辅助装置来降低心脏负荷和减少MMPs的表达。
结论MMPs是一类重要的酶,在生物学进程中发挥重要作用。
心力衰竭患者血清MMP-9、LPO、cathepsin_S水平检测及其临床意义
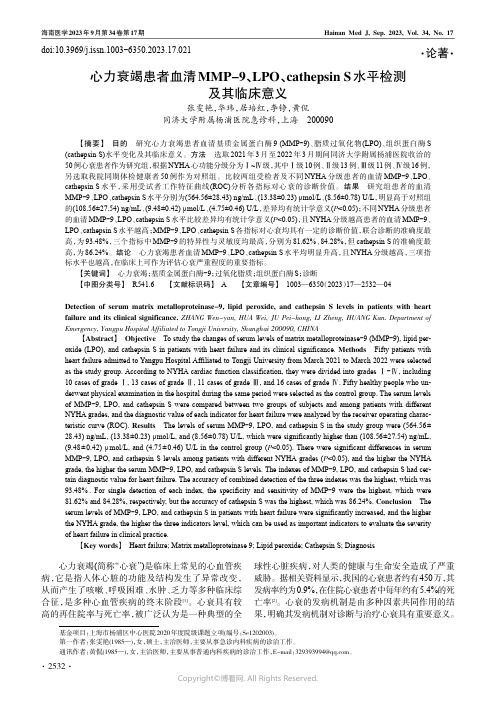
心力衰竭患者血清MMP-9、LPO 、cathepsin S 水平检测及其临床意义张雯艳,华玮,居培红,李铮,黄侃同济大学附属杨浦医院急诊科,上海200090【摘要】目的研究心力衰竭患者血清基质金属蛋白酶9(MMP -9)、脂质过氧化物(LPO)、组织蛋白酶S(cathepsin S)水平变化及其临床意义。
方法选取2021年3月至2022年3月期间同济大学附属杨浦医院收治的50例心衰患者作为研究组,根据NYHA 心功能分级分为Ⅰ~Ⅳ级,其中Ⅰ级10例、Ⅱ级13例、Ⅲ级11例、Ⅳ级16例,另选取我院同期体检健康者50例作为对照组。
比较两组受检者及不同NYHA 分级患者的血清MMP -9、LPO 、cathepsin S 水平,采用受试者工作特征曲线(ROC)分析各指标对心衰的诊断价值。
结果研究组患者的血清MMP -9、LPO 、cathepsin S 水平分别为(564.56±28.43)ng/mL 、(13.38±0.23)μmol/L 、(8.56±0.78)U/L ,明显高于对照组的(108.56±27.54)ng/mL 、(9.48±0.42)μmol/L 、(4.75±0.46)U/L ,差异均有统计学意义(P <0.05);不同NYHA 分级患者的血清MMP -9、LPO 、cathepsin S 水平比较差异均有统计学意义(P <0.05),且NYHA 分级越高患者的血清MMP -9、LPO 、cathepsin S 水平越高;MMP -9、LPO 、cathepsin S 各指标对心衰均具有一定的诊断价值,联合诊断的准确度最高,为93.48%,三个指标中MMP -9的特异性与灵敏度均最高,分别为81.62%、84.28%,但cathepsin S 的准确度最高,为86.24%。
结论心力衰竭患者血清MMP -9、LPO 、cathepsin S 水平均明显升高,且NYHA 分级越高,三项指标水平也越高,在临床上可作为评估心衰严重程度的重要指标。
基质金属蛋白酶测量意义
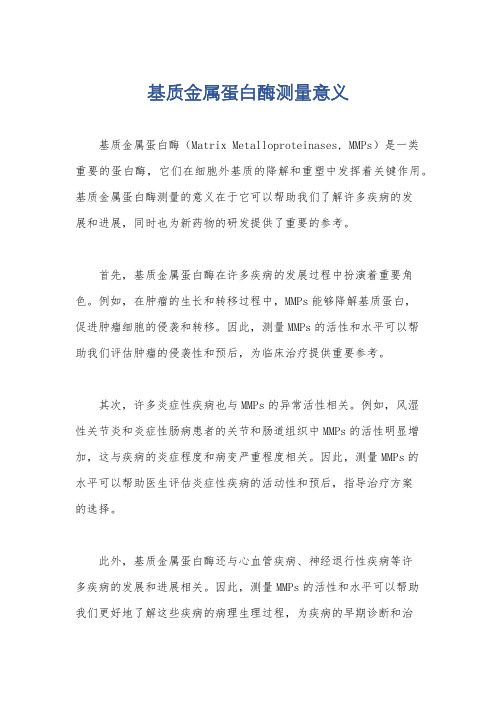
基质金属蛋白酶测量意义基质金属蛋白酶(Matrix Metalloproteinases, MMPs)是一类重要的蛋白酶,它们在细胞外基质的降解和重塑中发挥着关键作用。
基质金属蛋白酶测量的意义在于它可以帮助我们了解许多疾病的发展和进展,同时也为新药物的研发提供了重要的参考。
首先,基质金属蛋白酶在许多疾病的发展过程中扮演着重要角色。
例如,在肿瘤的生长和转移过程中,MMPs能够降解基质蛋白,促进肿瘤细胞的侵袭和转移。
因此,测量MMPs的活性和水平可以帮助我们评估肿瘤的侵袭性和预后,为临床治疗提供重要参考。
其次,许多炎症性疾病也与MMPs的异常活性相关。
例如,风湿性关节炎和炎症性肠病患者的关节和肠道组织中MMPs的活性明显增加,这与疾病的炎症程度和病变严重程度相关。
因此,测量MMPs的水平可以帮助医生评估炎症性疾病的活动性和预后,指导治疗方案的选择。
此外,基质金属蛋白酶还与心血管疾病、神经退行性疾病等许多疾病的发展和进展相关。
因此,测量MMPs的活性和水平可以帮助我们更好地了解这些疾病的病理生理过程,为疾病的早期诊断和治疗提供重要依据。
最后,基质金属蛋白酶作为潜在的药物靶点,其测量也为新药物的研发提供了重要的参考。
许多药物研发公司都在寻找能够调节MMPs活性的药物,以用于治疗肿瘤、炎症性疾病等。
因此,测量MMPs的活性和水平也为新药物的研发提供了重要的参考。
综上所述,基质金属蛋白酶测量具有重要的临床意义和研究价值,它可以帮助我们更好地了解疾病的发展和进展,为临床诊断和治疗提供重要参考,同时也为新药物的研发提供了重要的参考。
因此,基质金属蛋白酶测量在医学领域具有重要的意义。
冠心病心力衰竭患者血清基质金属蛋白酶水平及其意义
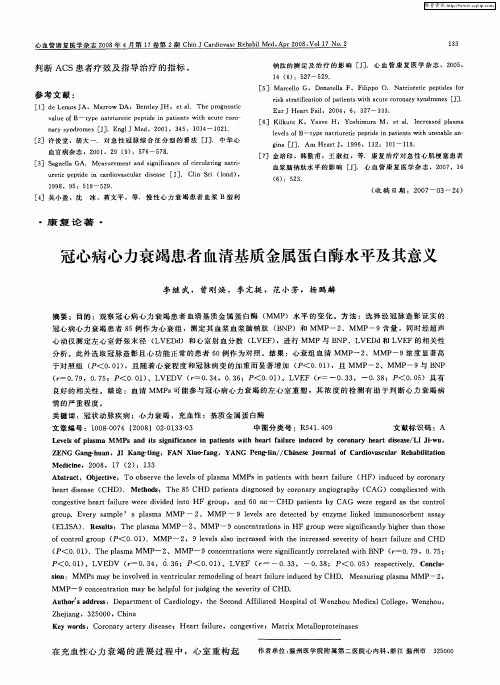
Eu a tFal 2 4, 6:3 — 3 3 rJ He r i, 00 27 3.
[ 3 Ki ueK,Yav , Y s i r ,e 1 Icesd pama 6 l t k seH ohmuaM ta. nrae ls
l v l fB- t p a ru e i p p i e i a i n s wi n t b e a e e so - y e n t i r t e td n p te t t u s a l n— c h
n r y do s E3 n l d 0 1 4 : 11 — 1 2 . ays n rme J .E g Me ,2 0 ,3 5 0 4 0 1 J [] 许 俊 堂 ,胡 大一 . 对 急 性 冠 脉 综 合 征 分 型 的 看 法 E J 中 华 心 2 J.
血 官 病 杂 志 ,2 0 ,2 () 7 —5 8 0 1 9 9 :5 6 7 .
李 继武 ,曾刚焕 ,季 亢挺 ,范小芳 ,杨 鹏麟
摘 要 : 目的 :观 察 冠 心病 心 力 衰 竭 患 者 血清 基 质 金 属 蛋 白酶 ( MMP 水 平 的变 化 。 方 法 :选 择 经 冠 脉造 影 证 实 的 ) 冠 心 病 心 力 衰竭 患 者 8 5例 作 为 心 衰组 ,测 定 其 血 浆 血 浆 脑钠 肽 ( N ) 和 MMP一2 B P 、MMP 9含 量 ,同 时 经 超 声 一 心 动 仪 测 定 左 心 室 舒 张 末 径 ( VE ) 和心 室 射 血分 数 ( VE ) L Dd L F ,进 行 MMP与 B NP、L D VE d和 L F的相 关 性 VE
MMPs与心力衰竭
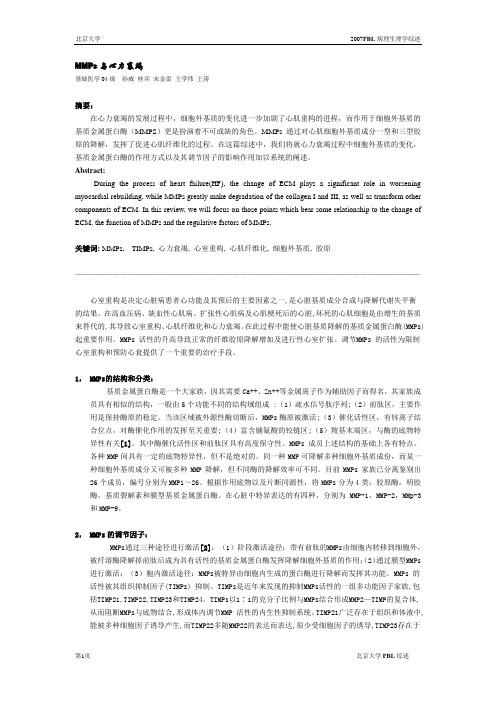
MMPs与心力衰竭基础医学04级孙威桂宾宋金雷王学伟王涛摘要:在心力衰竭的发展过程中,细胞外基质的变化进一步加剧了心肌重构的进程,而作用于细胞外基质的基质金属蛋白酶(MMPS)更是扮演着不可或缺的角色。
MMPs通过对心肌细胞外基质成分一型和三型胶原的降解,发挥了促进心肌纤维化的过程。
在这篇综述中,我们将就心力衰竭过程中细胞外基质的变化,基质金属蛋白酶的作用方式以及其调节因子的影响作用加以系统的阐述。
Abstract:During the process of heart failure(HF), the change of ECM plays a significant role in worsening myocardial rebuilding, while MMPs greatly make degradation of the collagen I and III, as well as transform other components of ECM. In this review, we will focus on those points which bear some relationship to the change of ECM, the function of MMPs and the regulative factors of MMPs.关键词: MMPs, TIMPs, 心力衰竭, 心室重构, 心肌纤维化, 细胞外基质, 胶原——————————————————————————————————————————————心室重构是决定心脏病患者心功能及其预后的主要因素之一,是心脏基质成分合成与降解代谢失平衡的结果。
在高血压病、缺血性心肌病、扩张性心肌病及心肌梗死后的心脏,坏死的心肌细胞是由增生的基质来替代的,其导致心室重构、心肌纤维化和心力衰竭。
在此过程中能使心脏基质降解的基质金属蛋白酶(MMPs) 起重要作用。
基质金属蛋白酶mmp3 正常值
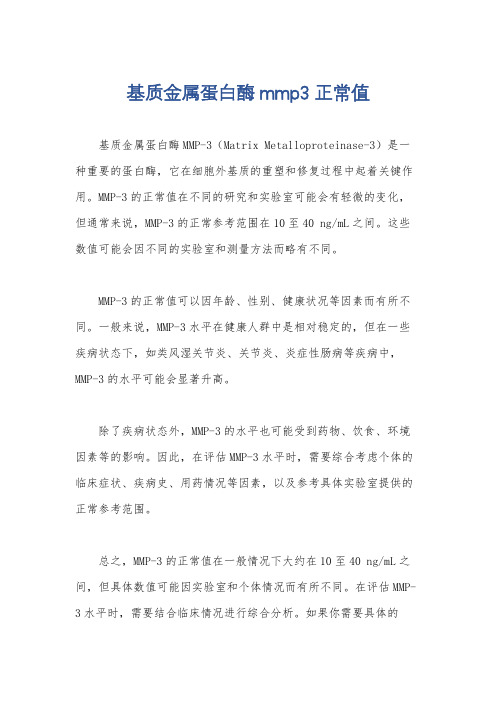
基质金属蛋白酶mmp3 正常值
基质金属蛋白酶MMP-3(Matrix Metalloproteinase-3)是一种重要的蛋白酶,它在细胞外基质的重塑和修复过程中起着关键作用。
MMP-3的正常值在不同的研究和实验室可能会有轻微的变化,但通常来说,MMP-3的正常参考范围在10至40 ng/mL之间。
这些数值可能会因不同的实验室和测量方法而略有不同。
MMP-3的正常值可以因年龄、性别、健康状况等因素而有所不同。
一般来说,MMP-3水平在健康人群中是相对稳定的,但在一些疾病状态下,如类风湿关节炎、关节炎、炎症性肠病等疾病中,MMP-3的水平可能会显著升高。
除了疾病状态外,MMP-3的水平也可能受到药物、饮食、环境因素等的影响。
因此,在评估MMP-3水平时,需要综合考虑个体的临床症状、疾病史、用药情况等因素,以及参考具体实验室提供的正常参考范围。
总之,MMP-3的正常值在一般情况下大约在10至40 ng/mL之间,但具体数值可能因实验室和个体情况而有所不同。
在评估MMP-3水平时,需要结合临床情况进行综合分析。
如果你需要具体的
MMP-3检测结果和解读,建议咨询专业医生或实验室进行详细咨询和解释。
基质金属蛋白酶(MMPs)种类及功能

基质金属蛋白酶(MMPs)种类及功能基质金属蛋白酶(MMPs),1962年首先被确定为一种胶原蛋白水解酶,在蝌蚪尾巴的吸收过程中导致ECM蛋白降解。
属于metzincins 超家族,是一类锌依赖性内肽酶,可降解ECM的各种蛋白质组分。
1、MMP家族结构特点在脊椎动物中,MMP家族由28个成员组成,至少23个在人体组织中表达,其中14个在脉管系统中表达。
基质金属蛋白酶通常根据其底物和其结构域的组织结构分为胶原酶(collagenases)、明胶酶(gelatinases)、溶血素(stromelysins)、基质溶素(matrilysins)、膜型MMPs(membrane-type (MT)-MMPs)和其他MMP。
MMP家族有一个共同的核心结构。
典型的MMPs由大约80个氨基酸的前肽、170个氨基酸的金属蛋白酶催化结构域、可变长度的连接肽或铰链区和约200个氨基酸的血红素蛋白结构域组成。
膜型MMPs(MT-MMPs)通常具有跨膜结构域和胞质结构域。
MMP-17和-25有一个糖基磷脂酰肌醇(GPI)锚。
MMP-23可通过其II型信号锚处于潜在的非活性形式,并且具有富含半胱氨酸和免疫球蛋白样脯氨酸的区域(图1)。
图1. MMP亚型及其结构2 、基质金属蛋白酶细胞来源及细胞学功能基质金属蛋白酶是由多种组织和细胞产生。
MMP由促炎细胞和子宫胎盘细胞分泌,包括成纤维细胞、成骨细胞、内皮细胞、血管平滑肌、巨噬细胞、中性粒细胞、淋巴细胞和细胞滋养细胞等。
真皮成纤维细胞和白细胞是MMP的主要来源,尤其是MMP-2。
血小板是MMP-1、MMP-2、MMP-3和MMP-14的重要来源。
它们存在于大多数结缔组织中。
MMP-1、MMP-2、MMP-3、MMP-7、MMP-8、MMP-9、MMP-12、MMP-13以及MT1-MMP 和MT3-MMP在各种血管组织和细胞中表达。
MMPs通常以非活性的proMMP形式分泌,它被包括其他MMP 在内的各种蛋白酶裂解为活性形式。
基质金属蛋白酶、金属蛋白酶组织抑制剂在心室重构中的作用和调节
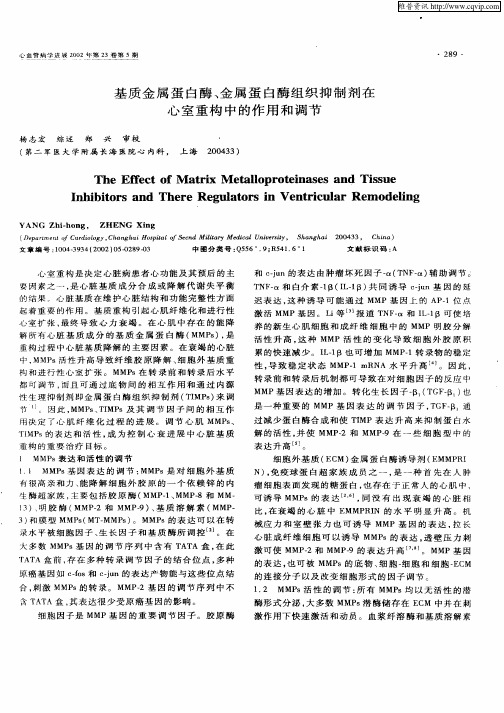
1 MMP s表 达 和 活 性 的调 节
细 胞 外 基 质 ( C 金 属 蛋 白酶 诱 导 剂 ( MMP I E M) E R N) 免 疫 球 蛋 白超 家 族 成 员 之 一 , 一 种 首 先 在 人 肿 , 是
1 1 MMP 基 因 表 达 的 调 节 : . s MMP s是 对 细 胞 外 基 质
和 cjn的 表 达 由 肿 瘤 坏 死 因 子 一 T F ) 助 调节 — u ( N — 辅 T F 和 白介 索 . N — 1 I 一p) 同诱 导 cj.基 因 的 延 ( L 1 共 — u
迟 表 达 , 种 诱 导 可 能 通 过 MMP 基 因 上 的 A 一 这 P 1位 点
维普资 9
基 质 金 属 蛋 白酶 、 属 蛋 白酶 组 织 抑 制 剂 在 金 心 室 重 构 中 的 作 用 和 调 节
杨 志 宏 综述 郑 兴 审校
( 二 军 医 大 学 附 属 长 海 医 院 心 内科 , 上 海 第 20 3 ) 0 4 3
激 活 MMP基 因 。 L 等 报 道 T — 和 I 一 可 使 培 i NF L1 养 的新 生 心 肌 细 胞 和 成 纤 维 细 胞 中 的 MMP明 胶 分 解 活 性 升 高 , 种 MMP活 性 的 变 化 导 致 细 胞 外 胶 原 积 这
心室 扩 张 , 终 导 致 心 力 衰 竭 。在 心 肌 中存 在 的 能 降 最 解 所 有 心脏 基 质 成 分 的 基 质 金 属 蛋 白 酶 ( MMP ), s 是
过 减 少 蛋 白酶 合 成 和使 T MP表 达 升 高 来 抑 制 蛋 白水 I
解 的活 性 , 使 MMP 2和 MMP 9在 一 些 细 胞 型 中 的 并 一 -
基质金属蛋白酶mmp介绍
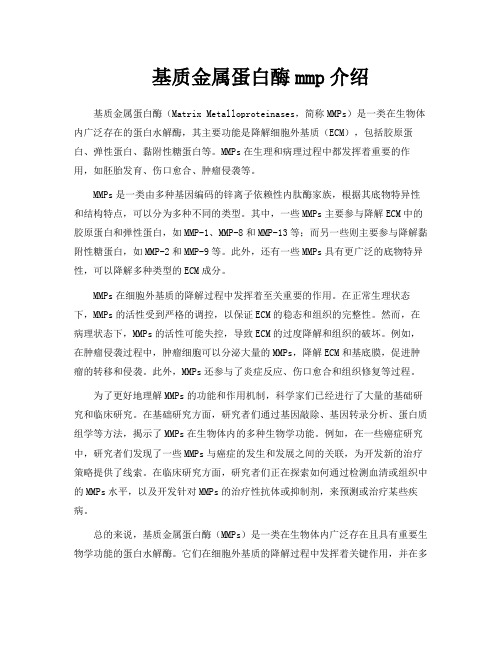
基质金属蛋白酶mmp介绍基质金属蛋白酶(Matrix Metalloproteinases,简称MMPs)是一类在生物体内广泛存在的蛋白水解酶,其主要功能是降解细胞外基质(ECM),包括胶原蛋白、弹性蛋白、黏附性糖蛋白等。
MMPs在生理和病理过程中都发挥着重要的作用,如胚胎发育、伤口愈合、肿瘤侵袭等。
MMPs是一类由多种基因编码的锌离子依赖性内肽酶家族,根据其底物特异性和结构特点,可以分为多种不同的类型。
其中,一些MMPs主要参与降解ECM中的胶原蛋白和弹性蛋白,如MMP-1、MMP-8和MMP-13等;而另一些则主要参与降解黏附性糖蛋白,如MMP-2和MMP-9等。
此外,还有一些MMPs具有更广泛的底物特异性,可以降解多种类型的ECM成分。
MMPs在细胞外基质的降解过程中发挥着至关重要的作用。
在正常生理状态下,MMPs的活性受到严格的调控,以保证ECM的稳态和组织的完整性。
然而,在病理状态下,MMPs的活性可能失控,导致ECM的过度降解和组织的破坏。
例如,在肿瘤侵袭过程中,肿瘤细胞可以分泌大量的MMPs,降解ECM和基底膜,促进肿瘤的转移和侵袭。
此外,MMPs还参与了炎症反应、伤口愈合和组织修复等过程。
为了更好地理解MMPs的功能和作用机制,科学家们已经进行了大量的基础研究和临床研究。
在基础研究方面,研究者们通过基因敲除、基因转录分析、蛋白质组学等方法,揭示了MMPs在生物体内的多种生物学功能。
例如,在一些癌症研究中,研究者们发现了一些MMPs与癌症的发生和发展之间的关联,为开发新的治疗策略提供了线索。
在临床研究方面,研究者们正在探索如何通过检测血清或组织中的MMPs水平,以及开发针对MMPs的治疗性抗体或抑制剂,来预测或治疗某些疾病。
总的来说,基质金属蛋白酶(MMPs)是一类在生物体内广泛存在且具有重要生物学功能的蛋白水解酶。
它们在细胞外基质的降解过程中发挥着关键作用,并在多种生理和病理过程中发挥作用。
充血性心力衰竭患者血清基质金属蛋白酶-2浓度的变化及其临床意义
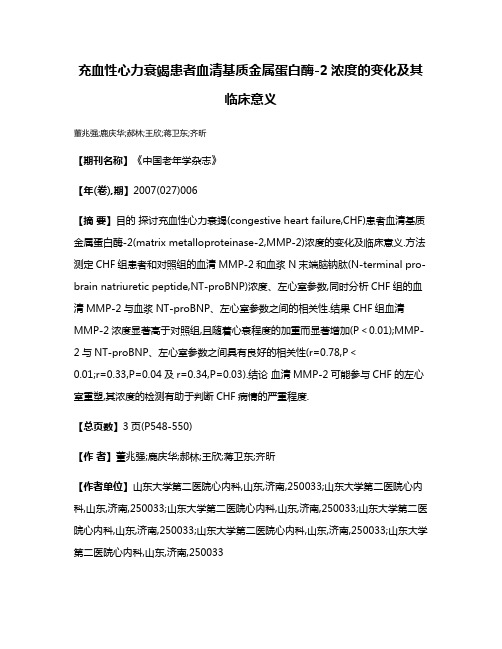
充血性心力衰竭患者血清基质金属蛋白酶-2浓度的变化及其临床意义董兆强;鹿庆华;郝林;王欣;蒋卫东;齐昕【期刊名称】《中国老年学杂志》【年(卷),期】2007(027)006【摘要】目的探讨充血性心力衰竭(congestive heart failure,CHF)患者血清基质金属蛋白酶-2(matrix metalloproteinase-2,MMP-2)浓度的变化及临床意义.方法测定CHF组患者和对照组的血清MMP-2和血浆N末端脑钠肽(N-terminal pro-brain natriuretic peptide,NT-proBNP)浓度、左心室参数,同时分析CHF组的血清MMP-2与血浆NT-proBNP、左心室参数之间的相关性.结果 CHF组血清MMP-2浓度显著高于对照组,且随着心衰程度的加重而显著增加(P<0.01);MMP-2与NT-proBNP、左心室参数之间具有良好的相关性(r=0.78,P<0.01;r=0.33,P=0.04及r=0.34,P=0.03).结论血清MMP-2可能参与CHF的左心室重塑,其浓度的检测有助于判断CHF病情的严重程度.【总页数】3页(P548-550)【作者】董兆强;鹿庆华;郝林;王欣;蒋卫东;齐昕【作者单位】山东大学第二医院心内科,山东,济南,250033;山东大学第二医院心内科,山东,济南,250033;山东大学第二医院心内科,山东,济南,250033;山东大学第二医院心内科,山东,济南,250033;山东大学第二医院心内科,山东,济南,250033;山东大学第二医院心内科,山东,济南,250033【正文语种】中文【中图分类】R541.6【相关文献】1.急性心肌梗死患者PCI术前后激活素A与血清基质金属蛋白酶浓度的变化及临床意义 [J], 赵晓辉;赵晓红;王慧;梅雪2.老年充血性心力衰竭患者血清基质金属蛋白酶的测定及其临床意义 [J], 赵忠普;杨艳秋;方卓;刘斌3.充血性心力衰竭患者激活素A、基质金属蛋白酶9及N端脑钠肽前体水平的变化及其临床意义 [J], 蒋健刚;陈金国;刘俊;王东平4.充血性心力衰竭患者血清基质金属蛋白酶-9的变化及临床意义 [J], 董兆强;郭静;鹿庆华;郝林;蒋卫东;齐昕5.充血性心力衰竭患者血清基质金属蛋白酶—3浓度的测定及其临床意义 [J], 鹿庆华;董兆强;齐昕;郝林;蒋卫东因版权原因,仅展示原文概要,查看原文内容请购买。
慢性充血性心力衰竭患者血清基质金属蛋白酶与肿瘤坏死因子-α变化的相关性研究
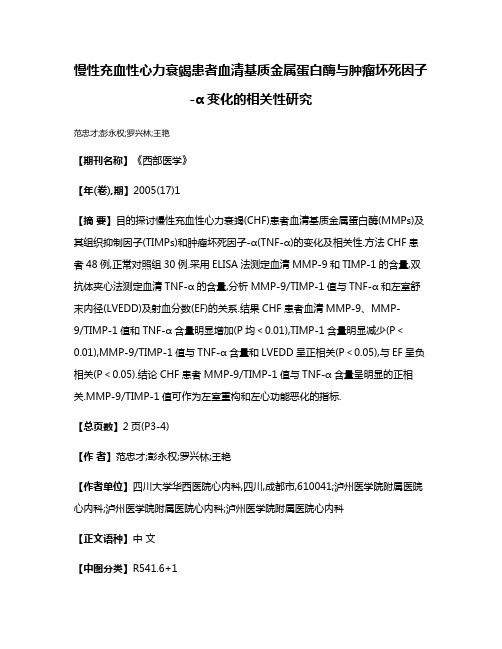
慢性充血性心力衰竭患者血清基质金属蛋白酶与肿瘤坏死因子-α变化的相关性研究范忠才;彭永权;罗兴林;王艳【期刊名称】《西部医学》【年(卷),期】2005(17)1【摘要】目的探讨慢性充血性心力衰竭(CHF)患者血清基质金属蛋白酶(MMPs)及其组织抑制因子(TIMPs)和肿瘤坏死因子-α(TNF-α)的变化及相关性.方法CHF患者48例,正常对照组30例.采用ELISA法测定血清MMP-9和TIMP-1的含量,双抗体夹心法测定血清TNF-α的含量,分析MMP-9/TIMP-1值与TNF-α和左室舒末内径(LVEDD)及射血分数(EF)的关系.结果CHF患者血清MMP-9、MMP-9/TIMP-1值和TNF-α含量明显增加(P均<0.01),TIMP-1含量明显减少(P<0.01),MMP-9/TIMP-1值与TNF-α含量和LVEDD呈正相关(P<0.05),与EF呈负相关(P<0.05).结论CHF患者MMP-9/TIMP-1值与TNF-α含量呈明显的正相关.MMP-9/TIMP-1值可作为左室重构和左心功能恶化的指标.【总页数】2页(P3-4)【作者】范忠才;彭永权;罗兴林;王艳【作者单位】四川大学华西医院心内科,四川,成都市,610041;泸州医学院附属医院心内科;泸州医学院附属医院心内科;泸州医学院附属医院心内科【正文语种】中文【中图分类】R541.6+1【相关文献】1.慢性充血性心力衰竭患者血清基质金属蛋白酶与骨桥蛋白变化的相关性研究 [J], 印芳颖;姜伟栋;孙景辉2.慢性充血性心力衰竭患者血清基质金属蛋白酶的液相芯片检测 [J], 邓元江;梁伟雄;陈云波;程淑意;王奇;陈海燕;赵益业;江俊珊;张卫3.充血性心力衰竭患者血清基质金属蛋白酶-2浓度的变化及其临床意义 [J], 董兆强;鹿庆华;郝林;王欣;蒋卫东;齐昕4.骨化三醇对慢性充血性心力衰竭患者血清脑钠肽、基质金属蛋白酶-9及左心室质量指数的影响——附39例检测报告 [J], 冯燕玲;李劲草;黄冰生;林桂雄;吴钰燕;卓裕丰;张鹏;解强;程颖5.促红细胞生成素对慢性充血性心力衰竭患者血清脑钠肽、基质金属蛋白酶-9及左心室质量指数的影响 [J], 李劲草;黄冰生;林桂雄;程颖因版权原因,仅展示原文概要,查看原文内容请购买。
基质金属蛋白酶底物

基质金属蛋白酶底物
基质金属蛋白酶底物是一类被基质金属蛋白酶(MMPs)酶解的蛋白质分子。
MMPs是一类重要的蛋白酶,它们参与了许多生理和病理过程,如细胞增殖、细胞迁移、组织修复和肿瘤转移等。
MMPs 的活性受到多种因素的调节,包括基质金属蛋白酶抑制剂(TIMPs)、细胞外基质(ECM)成分和细胞信号通路等。
基质金属蛋白酶底物是MMPs作用的靶标分子。
这些底物包括许多ECM成分,如胶原蛋白、纤维连接蛋白、弹性蛋白和透明质酸等。
此外,MMPs还可以酶解许多细胞表面受体和细胞因子,如TNF-α、IL-1β和VEGF等。
这些底物的酶解可以促进细胞迁移、血管生成和炎症反应等生理和病理过程。
MMPs的活性和底物特异性是由其结构和底物结构之间的相互作用决定的。
MMPs是一类锌离子依赖性蛋白酶,其结构包括一个N端信号肽、一个螺旋桨结构和一个C端催化结构。
底物结构中的特定氨基酸序列可以与MMPs的催化结构形成氢键和离子键,从而促进底物的酶解。
此外,底物的三维结构和ECM成分的组合也可以影响MMPs的活性和底物特异性。
基质金属蛋白酶底物在许多生理和病理过程中发挥着重要作用。
例如,在组织修复过程中,MMPs可以酶解ECM成分,促进细胞迁移和新生血管生成。
在肿瘤转移过程中,MMPs可以酶解ECM成分,促进肿瘤细胞的侵袭和转移。
因此,MMPs和其底物成为了治疗癌
症和其他疾病的潜在靶点。
基质金属蛋白酶底物是MMPs作用的靶标分子,其酶解可以促进许多生理和病理过程。
对MMPs和其底物的研究有助于深入了解这些过程的分子机制,并为治疗相关疾病提供新的靶点和策略。
- 1、下载文档前请自行甄别文档内容的完整性,平台不提供额外的编辑、内容补充、找答案等附加服务。
- 2、"仅部分预览"的文档,不可在线预览部分如存在完整性等问题,可反馈申请退款(可完整预览的文档不适用该条件!)。
- 3、如文档侵犯您的权益,请联系客服反馈,我们会尽快为您处理(人工客服工作时间:9:00-18:30)。
题目:基质金属蛋白酶在心衰中的作用——心力衰竭文献读书报告作者:04临床5班90401519 徐滢莹90401518 陈练90401520 高子清90401517 倪薇90401514 周阳90401516 张修举学科:病理生理学摘要:心肌重构是心力衰竭发生发展的重要机制,它不仅表现为心肌细胞的重塑,还表现为心肌基质纤维胶原的合成与降解之间动态平衡以及心肌基质胶原网的破坏、消失。
其中,基质金属蛋白酶(MMPs)是降解细胞外基质成分最主要的蛋白水解系统,在心肌重构中起重要作用。
而基质金属蛋白酶抑制物(TIMPs)则是MMPs的内源性特异性抑制剂。
MMPs和TIMPs之间的相互作用、动态平衡,在维持正常心脏几何形状和心功能中起重要作用。
关键词:基质金属蛋白酶,心肌重构,心力衰竭,基质金属蛋白酶抑制物心衰发生和发展过程中的一个重要环节——心肌重构,包括心肌细胞的重塑和非心肌细胞的变化,其中非心肌细胞的变化主要指心肌细胞外基质的变化。
由纤维胶原、基底膜蛋白、蛋白多糖和糖胺聚糖等组成的心肌细胞外基质(extracellular matrix, ECM)对于维持心脏形状及功能方面起重要作用。
心衰过程中,ECM成分合成和降解的失衡是心肌重构的主要因素。
MMPs是一族对ECM成分有特异降解作用的蛋白水解酶,它通过影响ECM的降解在心衰发生发展中起重要作用。
通过阅读文献,本文简单报告了我们关于“基质金属蛋白酶在心衰中的作用”的一些收获。
1、MMPs概述1.1MMPs的概念及分类MMPs 是一类锌、钙依赖性的蛋白水解酶家族,是细胞外基质降解的主要介质。
MMPs 家族可被分成5 类:①胶原酶包括MMP21、8、13、18;②明胶酶包括MMP22、9;③基质酶包括MMP23、10、11;④间质酶包括MMP27、26;⑤其他类型包括MMP12、19、20、22、23、28等[1]。
它们不仅在基质降解中起作用,也调节胶原的合成。
正常的胶原蛋白可被升高的MMPs 降解并被缺乏连接结构的纤维间质取代,纤维化增加,MMPs 的正常表达是维持心肌结构和功能的重要因素。
1.2MMPs的调控MMPs的调控有三种途径:酶原合成调控、酶原活性调控和基质金属蛋白酶抑制物(TIMPs)内源性抑制作用的调控。
其中,包括转化生长因子β1 (TGF2β1 ) ,肿瘤坏死因子α(TNF2α) 和白介素1β( IL21β) ,细胞外基质金属蛋白酶诱导因子( EMMPRIN) 以及基质片段metrikines等调节因子,可诱导MMPs 基因的表达,使MMPs的活性升高。
TIMPs是近年来发现的抑制MMPs活性的一组多功能因子家族,包括TIMP21,TIMP22,TIMP23和TIMP24。
TIMPs以1∶1克分子比例与MMPs 结合形MMP2TIMP 的复合体,从而阻断MMPs 与底物结合,形成体内调节MMP 活性的内生性抑制系统。
TIMP21广泛存在于组织和体液中,被多种细胞因子诱导产生,而TIMP22多随MMP22的表达而表达,很少受细胞因子的诱导,TIMP23存在于细胞外基质中,TIMP24 仅在心肌中有高表达。
另外,压力负荷等物理刺激,如机械应力和室壁张力也对MMPs 具有调节作用,拉长心脏成纤维细胞可以诱导MMPs 的表达,透壁压力刺激引起室壁张力增加可使MMP22和MMP29的表达升高[2]。
由此可见,MMPs活性的变化与心衰的发展密切相关。
2、心衰中MMPs活性的变化心肌胶原基质可维持心肌细胞及心肌纤维的排列、限制心肌细胞过度拉长。
另外,心肌基质的改变还可造成心肌收缩和有效的射血之间失去协调性,并且对维持左室的几何形状有明显的支持作用,减轻泵功能受损。
左室泵功能下降早期伴随左室扩大、室壁变薄及心肌细胞拉长,其中一个重要的因素就是心肌胶原纤维的丧失。
研究显示,随着心衰程度的加重,MMP22、3、9表达加强。
MMP23又通过对胶原的降解,激活其他的MMPs而直接参与心肌重构,MMP23的表达增加导致MMP22、MMP29增加,使心肌胶原纤维降解增加,心肌胶原结构受到破坏,最终影响心肌的收缩力而影响心功能[3]。
另有研究也得出类似结论,即心力衰竭程度越重,MMP2、3、9表达增加。
MMP3通过对胶原的降解激活其它的MMPs而直接参与心肌重构,MMP3表达增加,势必导MMP2、MMP9增加,使心肌细胞外基质降解增加,心肌结构受到破坏,最终影响心肌的收缩力而影响心功能[4]。
前两项研究的结果看似矛盾——即在不同研究中表达加强的MMPs的种类不同。
但这其实并不矛盾,并且恰恰说明另一个结论——MMP在不同类型的CHF心肌中的表达水平不同。
如MMP2在扩张型心肌病标本中表达增加,而在冠心病心肌标本中表达并不改变。
MMP13在正常心肌中表达水平很低,而在终末期CHF心肌中则表达明显增加。
但是,不管其基本病因(冠脉病变及扩张型心肌病)如何,MMP9的活性均增加[5]。
在一次关于MMP9与急性心肌梗死后左室重构关系的研究中,也得出血浆MMP-9双相分布与急性心肌梗死后的左室重构和左室功能相关的结论[6],支持了以上的结论。
另外,文献中也提到,并不是心衰发展的每一期,MMPs的水平都升高。
在代偿型心肌肥大中,MMPs活性下调,而发展至失代偿性,即出现左室扩大时MMPs活性增高。
到终末期心衰心肌中MMPs表达种类则更加丰富,存在明显MMPs激活现象。
一些能降解许多胶原和基底膜成分的MMPs表达增加,同时伴随有纤维胶原结构的减少[5]。
以上,我们可以得出结论:MMPs活性的改变,在心肌重构中具有重要的意义,其改变的具体机制主要与心衰中MMPs和TIMPs的平衡失调有关。
3、心衰中MMPs和TIMPs的平衡失调在一项关于TIMP1的水平与左室收缩功能紊乱关系的研究中,研究者发现超声心动技术所测定的左室收缩功能和血浆中TIMP1的水平呈相反的关系。
观察结果证实TIMP1在各种心脏疾病的左室重塑中都扮演了重要的角色。
而抑制MMPs可以减少心衰动物的左室扩张。
说明左室重塑和由MMPs和TIMPs所介导的心脏细胞外基质积聚和降解的平衡紊乱有关[7]。
在另一项对照研究也表明,心衰组患者心功能越差,血浆MMP22、3、9水平逐渐递增,相反,血浆TIMP21水平逐渐降低,各心衰组心肌组织TIMP21蛋白表达明显低于正常心肌组织,且逐渐减少。
从研究中我们不难发现,。
在心衰发展中出现了内源性MMPs抑制性调控丧失,MMPs 表达增加和激活,从而导致MMP/TIMP平衡失调。
MMP/TIMP 的平衡失调受许多信号传导通路的影响,如局部浓度依赖性的生物活性分子的释放,即可使重构心肌内的MMPs和TIMPs 不平衡。
总之,心衰心肌中存在的MMP/TIMP 的不平衡有利于保持MMPs的持续性活性、ECM 的蛋白水解和相应的心肌重构,是心衰发展的重要机制。
4、来自反面的声音尽管以上大量研究证实,MMPs的激活是心肌重构的关键性特征,那么我们可以推测对MMP抑制可能会有利于影响心脏重塑。
然而,虽然实验模型研究结果是肯定的,但是最近进行的口服活性MMP抑制剂PG-116800的大型研究结果发现,其在心肌梗死后患者中未能防止左室重塑。
文献中显示,PG-116800治疗并不能显著改善LV舒张期容积,LV收缩期容积,LV射血分数和球度指数的变化以及死亡或再梗死发生率。
基质金属蛋白酶抑制剂PG-116800不能减轻LV重构或改善MI后临床预后[8]。
5、总结及展望综上所述,MMPs活性的增高与心肌的重构及心功能下降密切相关,在心衰发生发展中起到重要作用。
因此,调节MMPs的活性可能是心血管疾病治疗的一个新方向。
正面的研究让我们信心倍增,但是反面的声音给我们带来更多的是压力和挑战,如何更有效的将关于MMPs的基础研究有效的应用于临床,缩短基础进展与临床发展的差距,以早日解决心衰患者疾苦,必将是我们共同努力的目标。
参考文献:[1]刘军锋,贾克刚,刘运德基质金属蛋白酶与心脏重构及心肌病理损害的研究进展,中华老年心脑血管病杂志2007 年7 月第9 卷第7 期[2] 杨志宏心室重构及心衰时基质金属蛋白酶的活性变化,国外医学·生理、病理科学与临床分册第22 卷第5 期2005 年10 月[3] Jacob George, MD, Shuki Patal, MD, Dov Wexler, MD, Arie Roth, MD, David Sheps, MD, and Gad Keren, MD Circulating matrix metalloproteinase-2 but not matrix metalloproteinase-3, matrix metalloproteinase-9,or tissue inhibitor of metalloproteinase-1 predicts outcome in patients with congestive heart failure ,Tel Aviv, Israel ,American Heart Journal September 2005(484-487)[4] 杨永健,张鑫,杨大春p38MAPK及基质金属蛋白酶在心力衰竭病人心肌重构中的意义,中国病理生理杂志2007, 23(8):1631-1632、1641[5] 陈晓锋,顾振纶,唐礼江基质金属蛋白酶在心衰中作用的研究进展,中国药理学通报,2004 Feb;20(2):137~40[6] Kelly D./Cockerill G./Ng L.L. et al. Plasma matrix metalloproteinase-9 and left ventricular remodeling after acute myocardial infarction in man: A prospective cohort study ,EUR.HEART J. 2007,28/6(711-718)[7] Erdal Cavusoglu, MD,a,b Cyril Ruwende, MD, PhD,c Vineet Chopra, MD,b Sunitha Yanamadala, PhD,cCalvin Eng, MD,b Luther T. Clark, MD,a David J. Pinsky, MD,c and Jonathan D. Marmur, MDa Brooklyn andBronx, NY; and Ann Arbor,ML,Tissue inhibitor of metalloproteinase-1 (TIMP-1) is an independent predictor of all-cause mortality,cardiac mortality, and myocardial infarction,American Heart Journal 1108.e2 Cavusoglu et al May 2006[8] Hudson M.P./Armstrong P.W./Ruzyllo W. et al. Effects of Selective Matrix Metalloproteinase Inhibitor(PG-116800) to Prevent Ventricular Remodeling After Myocardial Infarction.Results of the PREMIER(Prevention of Myocardial Infarction Early Remodeling) Trial J.AM.COLL.CARDIOL.2006,48/1(15-20)。