石墨烯为基础的生物传感器的研究进展
石墨烯基纳米传感器

石墨烯基纳米传感器
石墨烯基纳米传感器是一种新型的高光敏度传感器,它利用石墨烯的优异性能制作而成。
这种传感器的关键在于使用了“滞留光线”的纳米结构,这种纳米结构能够比传统的传感器更长时间地捕获产生光线的电子微粒。
这就会导致产生一种更强的电信号,就像数码相机所拍摄的照片一样,它能够将这种电信号转变成图像。
石墨烯基纳米传感器在生物医学领域也有广泛的应用。
由于石墨烯呈现出极高的表面积和优异的电学性质,能够非常敏锐地检测DNA、蛋白质、细胞等生物分子的存在和数量变化,这对于疾病诊断和治疗具有重要意义。
例如,可以利用石墨烯基纳米传感器来检测生物分子和细胞的数量变化,从而判断疾病的发展情况。
此外,石墨烯基纳米传感器在环境监测领域也有广泛的应用。
由于石墨烯的电学性质会随着环境的变化而发生变化,因此可以利用石墨烯基纳米传感器来监测环境中的温度、湿度、气压等参数的变化情况。
总之,石墨烯基纳米传感器在多个领域都具有广泛的应用前景,它的优异性能和多功能性使其成为当前研究的热点之一。
石墨烯的应用现状及发展

石墨烯的应用现状及发展石墨烯是一种由碳原子形成的二维薄膜,具有单层结构、高比表面积、强的力学特性和电学特性等优良性质。
自2004年石墨烯被发现以来,人们已经发现了其在许多领域的广泛应用前景,包括电子学、能源、生物医学、化学催化和材料等领域。
本文将就石墨烯的现状及未来发展做一个概括性介绍。
1. 电子学应用石墨烯是电子迁移速度最快的材料之一,这使得石墨烯在电子学领域具有广阔的应用前景。
石墨烯的电学性质主要基于电荷移动和相互作用,它在高频电子器件、太阳能电池、柔性电子学和传感器等应用方面都有潜力。
2. 能源应用石墨烯的高电导性和低电子转移电阻使其成为能源存储材料的理想候选者。
石墨烯和其衍生物已在超级电容器、锂离子电池、燃料电池和太阳能电池等能源体系中被成功应用,同时还有石墨烯纳米线、石墨烯石墨烯氧化物等材料也正逐渐被广泛应用于新型能源系统中。
3. 生物医学应用石墨烯因其具有优异的生物相容性、生物功能化进一步拓展了它在生物医学领域的应用。
石墨烯在生物成像、控制释放和药物传递等方面发挥着重要作用。
石墨烯的电学和热学性质、强半导体特性使其成为一种重要的生物传感器,被用于在应用生物医学和生化传感领域的研究。
4. 化学催化石墨烯的高比表面积和化学稳定性赋予了它在催化领域的应用潜力。
石墨烯可以与不同的催化剂相结合形成多种复合材料,这些复合物在氧化还原催化、光催化和热催化等领域中拥有良好的应用前景,可以在催化剂的降低、催化过程的高选择性和催化剂重复利用等方面发挥重要作用。
5. 材料应用石墨烯的高比表面积和高电导率使得它成为一种理想的复合材料和增强材料,目前已经被广泛应用于汽车和航空领域等。
石墨烯纳米管等复合材料已经被用于制备纳米传感器,同时在消费电子、高性能运动器材等领域得到了广泛应用。
石墨烯的应用前景非常广泛,但是现有工艺、设备等硬件条件限制了大规模石墨烯材料的生产。
同时,石墨烯具有较高的价格,这也限制了其在一定程度上的应用。
石墨烯的应用现状及发展
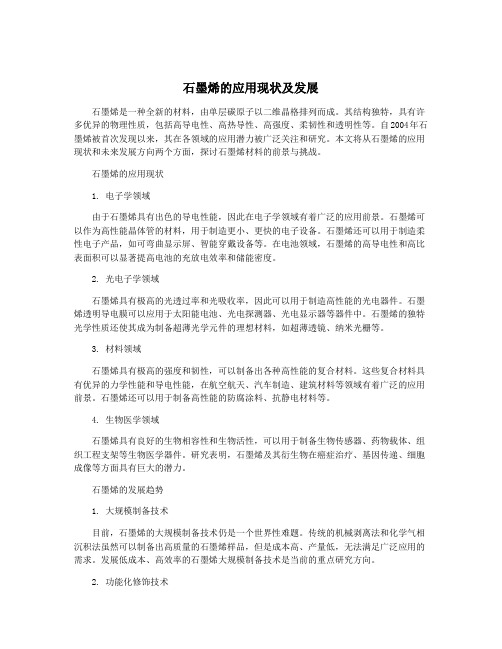
石墨烯的应用现状及发展石墨烯是一种全新的材料,由单层碳原子以二维晶格排列而成。
其结构独特,具有许多优异的物理性质,包括高导电性、高热导性、高强度、柔韧性和透明性等。
自2004年石墨烯被首次发现以来,其在各领域的应用潜力被广泛关注和研究。
本文将从石墨烯的应用现状和未来发展方向两个方面,探讨石墨烯材料的前景与挑战。
石墨烯的应用现状1. 电子学领域由于石墨烯具有出色的导电性能,因此在电子学领域有着广泛的应用前景。
石墨烯可以作为高性能晶体管的材料,用于制造更小、更快的电子设备。
石墨烯还可以用于制造柔性电子产品,如可弯曲显示屏、智能穿戴设备等。
在电池领域,石墨烯的高导电性和高比表面积可以显著提高电池的充放电效率和储能密度。
2. 光电子学领域石墨烯具有极高的光透过率和光吸收率,因此可以用于制造高性能的光电器件。
石墨烯透明导电膜可以应用于太阳能电池、光电探测器、光电显示器等器件中。
石墨烯的独特光学性质还使其成为制备超薄光学元件的理想材料,如超薄透镜、纳米光栅等。
3. 材料领域石墨烯具有极高的强度和韧性,可以制备出各种高性能的复合材料。
这些复合材料具有优异的力学性能和导电性能,在航空航天、汽车制造、建筑材料等领域有着广泛的应用前景。
石墨烯还可以用于制备高性能的防腐涂料、抗静电材料等。
4. 生物医学领域石墨烯具有良好的生物相容性和生物活性,可以用于制备生物传感器、药物载体、组织工程支架等生物医学器件。
研究表明,石墨烯及其衍生物在癌症治疗、基因传递、细胞成像等方面具有巨大的潜力。
石墨烯的发展趋势1. 大规模制备技术目前,石墨烯的大规模制备技术仍是一个世界性难题。
传统的机械剥离法和化学气相沉积法虽然可以制备出高质量的石墨烯样品,但是成本高、产量低,无法满足广泛应用的需求。
发展低成本、高效率的石墨烯大规模制备技术是当前的重点研究方向。
2. 功能化修饰技术石墨烯的很多优异性能是由其特殊的二维结构所决定的,但是这也使得石墨烯在某些方面表现出一定的局限性,比如化学稳定性差、易团聚等。
国家纳米科学中心石墨烯纳米生物传感器研究取得新进展
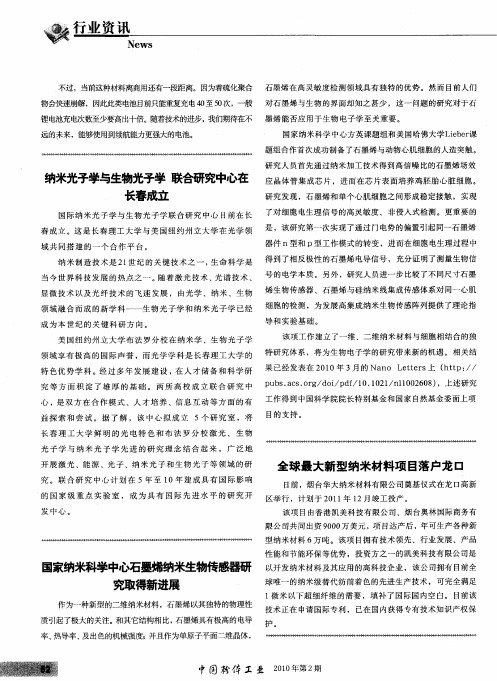
中 围糟 铸 工 韭 21年 期 0 第2 0
领 域融 合 而 成 的新 学 科一一 生 物光 子 学 和 纳米 光 子学 已经
成 为本 世 纪 的 关 键科 研 方 向。 美 国纽 约州 立 大学 布 法 罗 分校 在 纳 米学 、生 物 光子 学
细胞的检 测 ,为发展高 集成纳 米生物 传感 阵列 提供 了理 论指
导 和实验 基础 。
行业 资讯
Ne ws
不过 ,当前这种材料离商用还有一段距离。因为着硫化聚合 石墨烯 在高 灵敏度 检测领 域具 有独特 的优势 。然 而 目前 人们 物会快速崩解,因此此类电池目前只能重复充 电4 至 5 次 ,—般 对石 墨烯 与生物 的界面 却知之 甚少 ,这一 问题 的研究对 于石 J 0 D
球 唯一的 纳米级替 代纺前着 色 的先 进生产 技术 ,可 完全满足
1 米 以下超细 纤维 的需要 ,填 补 了国 际国 内空 白。 目前该 微
技术 正在 申请 国际专利 , 已在 国 内获得 专有技 术知识产 权保 质引起了极大的关注 。 和其它结构相 比, 石墨烯具有极高的电导 护 。
u s a s o g d i p f 0. 0 1 n l 0 6 8 ,上述研 究 究 等 方 面 积 淀 了 雄 厚 的 基 础 。 两 所 高 校 成 立 联 合 研 究 中 p b . c . r / o / d /l 1 2 / l 0 2 0 ) 心 ,是 双 方 在合 作 模 式 、人 才培 养 、信息 互 动 等 方 面 的 有 工作 得到 中国科学 院 院长特别基 金和 国家 自然基 金委面上项
该项 工作建 立了一 维 、二维纳 米材料 与细胞相 结合 的独
石墨烯场效应管生物传感器工作原理
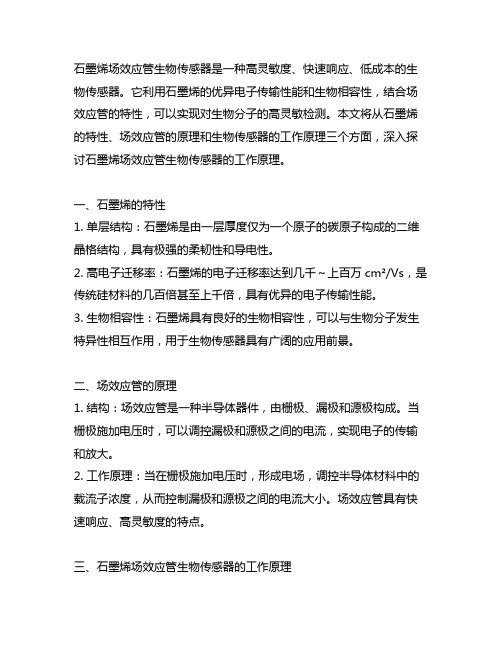
石墨烯场效应管生物传感器是一种高灵敏度、快速响应、低成本的生物传感器。
它利用石墨烯的优异电子传输性能和生物相容性,结合场效应管的特性,可以实现对生物分子的高灵敏检测。
本文将从石墨烯的特性、场效应管的原理和生物传感器的工作原理三个方面,深入探讨石墨烯场效应管生物传感器的工作原理。
一、石墨烯的特性1. 单层结构:石墨烯是由一层厚度仅为一个原子的碳原子构成的二维晶格结构,具有极强的柔韧性和导电性。
2. 高电子迁移率:石墨烯的电子迁移率达到几千~上百万cm²/Vs,是传统硅材料的几百倍甚至上千倍,具有优异的电子传输性能。
3. 生物相容性:石墨烯具有良好的生物相容性,可以与生物分子发生特异性相互作用,用于生物传感器具有广阔的应用前景。
二、场效应管的原理1. 结构:场效应管是一种半导体器件,由栅极、漏极和源极构成。
当栅极施加电压时,可以调控漏极和源极之间的电流,实现电子的传输和放大。
2. 工作原理:当在栅极施加电压时,形成电场,调控半导体材料中的载流子浓度,从而控制漏极和源极之间的电流大小。
场效应管具有快速响应、高灵敏度的特点。
三、石墨烯场效应管生物传感器的工作原理1. 生物识别:利用石墨烯的生物相容性,将生物分子(如蛋白质、DNA等)固定在石墨烯表面,实现对生物分子的高选择性识别。
2. 信号转换:当待检测的生物分子与固定在石墨烯表面的生物识别分子结合时,会影响石墨烯的电子结构,导致场效应管栅极的电压发生变化。
3. 电信号检测:通过检测场效应管栅极的电压变化,可以实现对生物分子的快速、高灵敏的检测。
由于石墨烯的优异电子传输性能,使得传感器具有极高的灵敏度和检测速度。
石墨烯场效应管生物传感器利用石墨烯的优异电子传输性能和生物相容性,结合场效应管的特性,可以实现对生物分子的高灵敏检测。
其工作原理简单清晰,具有广泛的应用前景,可用于生物医学领域、环境监测和食品安全等多个领域。
相信随着科学技术的不断进步,石墨烯场效应管生物传感器将在生物传感器领域发挥越来越重要的作用。
石墨烯在传感器中的性能与应用研究
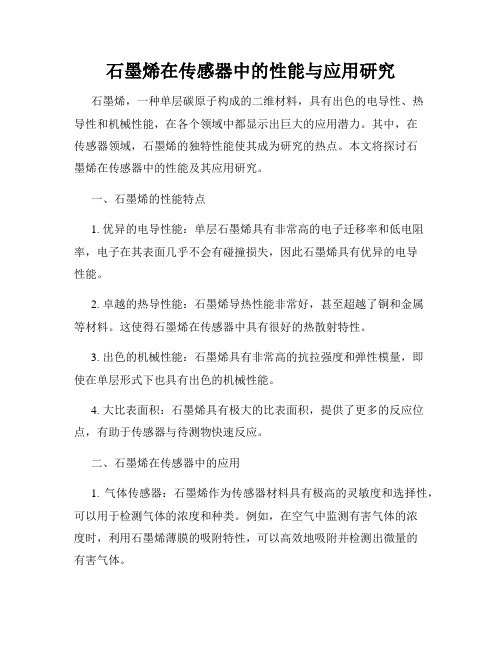
石墨烯在传感器中的性能与应用研究石墨烯,一种单层碳原子构成的二维材料,具有出色的电导性、热导性和机械性能,在各个领域中都显示出巨大的应用潜力。
其中,在传感器领域,石墨烯的独特性能使其成为研究的热点。
本文将探讨石墨烯在传感器中的性能及其应用研究。
一、石墨烯的性能特点1. 优异的电导性能:单层石墨烯具有非常高的电子迁移率和低电阻率,电子在其表面几乎不会有碰撞损失,因此石墨烯具有优异的电导性能。
2. 卓越的热导性能:石墨烯导热性能非常好,甚至超越了铜和金属等材料。
这使得石墨烯在传感器中具有很好的热散射特性。
3. 出色的机械性能:石墨烯具有非常高的抗拉强度和弹性模量,即使在单层形式下也具有出色的机械性能。
4. 大比表面积:石墨烯具有极大的比表面积,提供了更多的反应位点,有助于传感器与待测物快速反应。
二、石墨烯在传感器中的应用1. 气体传感器:石墨烯作为传感器材料具有极高的灵敏度和选择性,可以用于检测气体的浓度和种类。
例如,在空气中监测有害气体的浓度时,利用石墨烯薄膜的吸附特性,可以高效地吸附并检测出微量的有害气体。
2. 生物传感器:石墨烯可以作为生物传感器的载体,用于检测生物分子,如DNA、蛋白质等。
通过修饰石墨烯表面的生物分子,可以实现高灵敏度和快速的生物分子检测。
3. 应力传感器:由于石墨烯具有极好的机械性能,在应力传感器中也展现出很好的应用前景。
通过监测石墨烯薄膜的电阻变化,可以实时、非侵入性地测量物体的应变变化。
4. 光传感器:石墨烯对光的吸收和散射能力非常出色,因此在光传感器领域也有很大的潜力。
利用石墨烯的光学特性,可以实现高灵敏度、快速响应的光传感器。
5. 温度传感器:由于石墨烯的优异热导性能,可以用于制作高灵敏度的温度传感器。
石墨烯薄膜的电阻随温度的变化呈线性关系,因此可利用这一特性制作精确的温度传感器。
三、石墨烯传感器的优势与挑战1. 优势:a. 高灵敏度:石墨烯具有极高的灵敏度,可以检测极小浓度的待测物。
石墨烯气敏传感器的研究及其应用
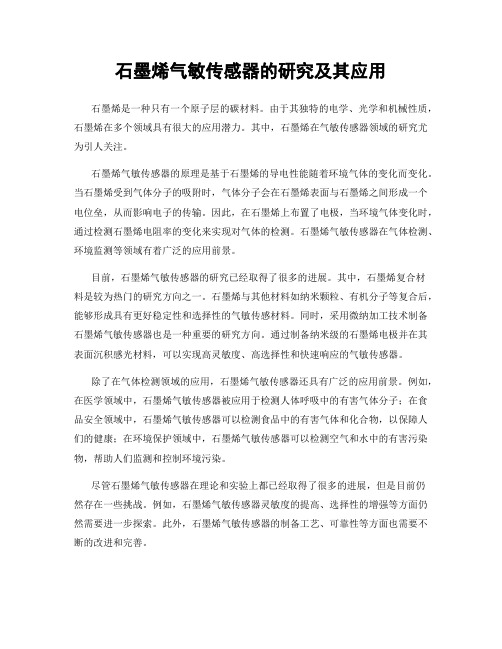
石墨烯气敏传感器的研究及其应用石墨烯是一种只有一个原子层的碳材料。
由于其独特的电学、光学和机械性质,石墨烯在多个领域具有很大的应用潜力。
其中,石墨烯在气敏传感器领域的研究尤为引人关注。
石墨烯气敏传感器的原理是基于石墨烯的导电性能随着环境气体的变化而变化。
当石墨烯受到气体分子的吸附时,气体分子会在石墨烯表面与石墨烯之间形成一个电位垒,从而影响电子的传输。
因此,在石墨烯上布置了电极,当环境气体变化时,通过检测石墨烯电阻率的变化来实现对气体的检测。
石墨烯气敏传感器在气体检测、环境监测等领域有着广泛的应用前景。
目前,石墨烯气敏传感器的研究已经取得了很多的进展。
其中,石墨烯复合材料是较为热门的研究方向之一。
石墨烯与其他材料如纳米颗粒、有机分子等复合后,能够形成具有更好稳定性和选择性的气敏传感材料。
同时,采用微纳加工技术制备石墨烯气敏传感器也是一种重要的研究方向。
通过制备纳米级的石墨烯电极并在其表面沉积感光材料,可以实现高灵敏度、高选择性和快速响应的气敏传感器。
除了在气体检测领域的应用,石墨烯气敏传感器还具有广泛的应用前景。
例如,在医学领域中,石墨烯气敏传感器被应用于检测人体呼吸中的有害气体分子;在食品安全领域中,石墨烯气敏传感器可以检测食品中的有害气体和化合物,以保障人们的健康;在环境保护领域中,石墨烯气敏传感器可以检测空气和水中的有害污染物,帮助人们监测和控制环境污染。
尽管石墨烯气敏传感器在理论和实验上都已经取得了很多的进展,但是目前仍然存在一些挑战。
例如,石墨烯气敏传感器灵敏度的提高、选择性的增强等方面仍然需要进一步探索。
此外,石墨烯气敏传感器的制备工艺、可靠性等方面也需要不断的改进和完善。
总之,石墨烯气敏传感器在气体检测、环境监测等领域具有很大的应用潜力。
石墨烯气敏传感器的研究不仅有助于提高人们的生活质量,还能够为环境保护、医学等领域的科学研究提供帮助。
随着石墨烯技术的不断发展和完善,相信石墨烯气敏传感器一定会有更为广泛的应用和更好的发展。
石墨烯在传感器技术中的应用前景
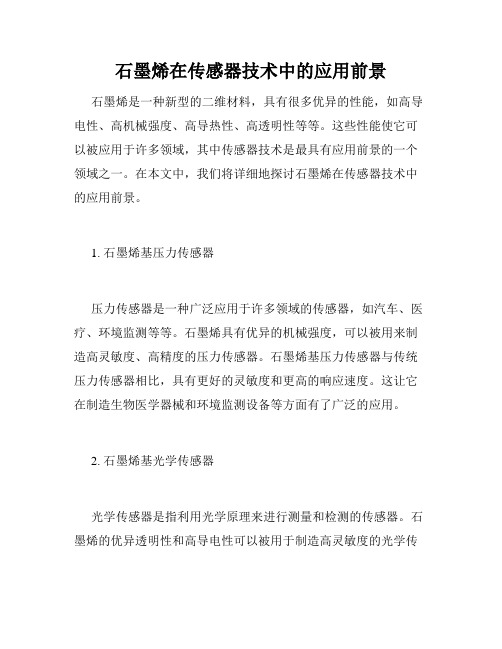
石墨烯在传感器技术中的应用前景石墨烯是一种新型的二维材料,具有很多优异的性能,如高导电性、高机械强度、高导热性、高透明性等等。
这些性能使它可以被应用于许多领域,其中传感器技术是最具有应用前景的一个领域之一。
在本文中,我们将详细地探讨石墨烯在传感器技术中的应用前景。
1. 石墨烯基压力传感器压力传感器是一种广泛应用于许多领域的传感器,如汽车、医疗、环境监测等等。
石墨烯具有优异的机械强度,可以被用来制造高灵敏度、高精度的压力传感器。
石墨烯基压力传感器与传统压力传感器相比,具有更好的灵敏度和更高的响应速度。
这让它在制造生物医学器械和环境监测设备等方面有了广泛的应用。
2. 石墨烯基光学传感器光学传感器是指利用光学原理来进行测量和检测的传感器。
石墨烯的优异透明性和高导电性可以被用于制造高灵敏度的光学传感器。
石墨烯基光学传感器在检测微生物、甲醛等有害气体、污染物质等方面有了广泛的应用。
3. 石墨烯基化学传感器化学传感器是用于检测和测量化学物质浓度、气体浓度等的传感器。
石墨烯具有高度的化学稳定性和电子传导性,可以被用来制造高灵敏度、高精度的化学传感器。
石墨烯基化学传感器在检测汽车尾气、空气污染物、药品成分、食品添加剂、病毒等方面有了广泛的应用。
4. 石墨烯基生物传感器生物传感器是指利用生物分子之间的相互作用和反应来进行测量和检测的传感器。
石墨烯具有优异的导电性和高度的生物相容性,可以被用来制造高灵敏度、高精度的生物传感器。
石墨烯基生物传感器在制造生物医学器械、检测生物标志物等方面有了广泛的应用。
5. 石墨烯基热传感器热传感器是一种测量温度变化的传感器,被广泛应用于许多领域,如电子、航空、化工等。
石墨烯具有优异的导热性,可以被用来制造高灵敏度、高精度的热传感器。
石墨烯基热传感器在制造电子元器件、汽车发动机温度检测等方面有了广泛的应用。
6. 石墨烯基气体传感器气体传感器是一种测量气体浓度的传感器,被广泛应用于环境监测、工业生产和生物医疗等领域。
石墨烯在生物传感器中的应用

石墨烯是一种具有独特结构和性质的二维碳纳米材料,具有优异的电、热、光学性能,在生物传感器领域具有广泛的应用前景。
本文将介绍石墨烯在生物传感器中的应用,包括石墨烯的结构特性、石墨烯在生物传感器中的应用原理以及石墨烯在生物传感器中的应用研究进展。
二、石墨烯的结构特性石墨烯是一种二维碳纳米材料,由一层厚度仅为0.34nm的碳原子构成,具有独特的结构特性。
石墨烯具有高强度、高导电性、高热导率、高抗拉强度、高抗折强度、高抗热稳定性等特性,可以有效抑制生物分子的氧化反应,并具有良好的生物相容性。
三、石墨烯在生物传感器中的应用原理石墨烯在生物传感器中的应用原理主要是利用其优异的电、热、光学性能,将生物分子与石墨烯结合,形成生物传感器,从而实现对生物分子的检测和分析。
石墨烯的优异性能可以有效抑制生物分子的氧化反应,并具有良好的生物相容性,可以有效提高生物传感器的灵敏度和稳定性。
四、石墨烯在生物传感器中的应用研究进展近年来,石墨烯在生物传感器领域的应用研究取得了显著进展。
研究人员利用石墨烯的优异性能,开发出了多种石墨烯生物传感器,用于检测和分析多种生物分子,如蛋白质、DNA、糖类、抗原等。
例如,研究人员利用石墨烯的优异性能,开发出了一种新型的石墨烯生物传感器,用于检测和分析蛋白质,可以有效提高检测灵敏度和稳定性。
此外,研究人员还利用石墨烯的优异性能,开发出了一种新型的石墨烯生物传感器,用于检测和分析DNA,可以有效提高检测灵敏度和稳五、结论石墨烯是一种具有独特结构和性质的二维碳纳米材料,具有优异的电、热、光学性能,在生物传感器领域具有广泛的应用前景。
近年来,石墨烯在生物传感器领域的应用研究取得了显著进展,可以有效提高生物传感器的灵敏度和稳定性。
未来,石墨烯在生物传感器领域的应用将会得到进一步发展,为生物传感器的研究提供新的思路和方法。
石墨烯在传感器领域中的应用

石墨烯在传感器领域中的应用石墨烯是一种由碳原子构成的单层薄膜材料,具有高导电性、高透明度和超强机械强度等优异特性,因此被广泛应用于多个领域,如电子、光学、能源和材料科学等。
在传感器领域中,石墨烯也被认为是一种具有巨大潜力的新型材料,因为其极高的灵敏度、快速的响应速度和良好的可重复性能够在诸多应用中发挥出色的作用。
1. 石墨烯在气体传感器方面的应用石墨烯气体传感器是一种基于石墨烯的传感器,其工作原理是通过检测气体分子与石墨烯表面之间相互作用引起的电性变化。
由于石墨烯具有大量可利用的表面积,它们能够高效地吸附气体分子,从而实现高灵敏度的检测。
另外,石墨烯还能够很快地响应气体的变化,并且具有很好的选择性,能够有效地区分不同种类的气体。
因此,石墨烯在气体传感器方面的应用具有广泛的前景,可以应用于空气污染监测、生化检测和气体检测等领域。
2. 石墨烯在生物传感器方面的应用生物传感器是一种能够检测生物分子的传感器,如蛋白质、DNA和细胞等。
由于石墨烯具有良好的生物相容性、高灵敏度和极低的检测限度,它们能够被广泛应用于医药和生物医学领域。
例如,基于石墨烯的蛋白质传感器在癌症诊断中能够识别一些癌症特异性蛋白质,从而帮助医生早早发现并治疗癌症。
另外,基于石墨烯的DNA传感器也能够检测基因的变异并对其进行分类,为疾病的诊断和治疗提供帮助。
3. 石墨烯在应力传感器方面的应用应力传感器是一种用于测量物体形变或受力的传感器,例如测量桥梁或建筑物的变形。
基于石墨烯的应力传感器由于具有可靠性高和灵敏度高的特点,大大拓展了应力传感器的应用领域。
基于石墨烯的微型应力传感器可以嵌入到纤维中,用于测量材料的应力分布,从而更好地了解材料的力学性能。
此外,基于石墨烯的智能应力传感器可以在机器人、汽车等领域,通过测量机器的变形来完成精准控制,提高机器的效率和安全性。
4. 石墨烯在环境传感器方面的应用环境传感器用于检测大气、水和土壤中的污染物质,例如二氧化碳、甲醛和重金属等。
生物传感器技术的发展现状和应用前景
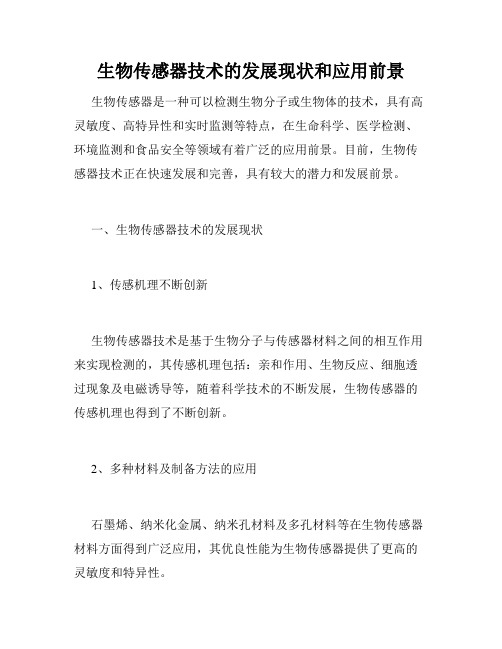
生物传感器技术的发展现状和应用前景生物传感器是一种可以检测生物分子或生物体的技术,具有高灵敏度、高特异性和实时监测等特点,在生命科学、医学检测、环境监测和食品安全等领域有着广泛的应用前景。
目前,生物传感器技术正在快速发展和完善,具有较大的潜力和发展前景。
一、生物传感器技术的发展现状1、传感机理不断创新生物传感器技术是基于生物分子与传感器材料之间的相互作用来实现检测的,其传感机理包括:亲和作用、生物反应、细胞透过现象及电磁诱导等,随着科学技术的不断发展,生物传感器的传感机理也得到了不断创新。
2、多种材料及制备方法的应用石墨烯、纳米化金属、纳米孔材料及多孔材料等在生物传感器材料方面得到广泛应用,其优良性能为生物传感器提供了更高的灵敏度和特异性。
制备方法上,喷墨、微影、光阻、热敏印刷、纳米印刷等制备技术也被广泛应用于生物传感器的制备。
3、先进检测技术的应用生物传感器的检测精度和灵敏度直接关系到生物传感器技术的发展水平,近年来,多种先进的检测技术被运用于生物传感器中,例如:表面增强拉曼光谱技术(SERS)、等离子体共振技术(PRS)、维克基光谱等技术,这些技术为生物传感器的检测提供了更加精确和全面的方法。
二、生物传感器技术的应用前景1、在生命科学研究中的应用生物传感器在生命科学研究中的应用较为广泛,例如:基因检测、蛋白质检测、信号转导等领域,可以用于癌症检测、药物研究等各个方面,大大方便了科研人员的实验操作。
2、在医疗检测中的应用传统的医学检测方法如采血、取样等方法有时会对患者造成二次伤害,而生物传感器技术可以对体内的分子进行实时监测,有机会在医学领域实现个性化医疗检测。
3、在环境监测中的应用生物传感器技术可以高灵敏度地监测环境中的有机物、无机物、微生物和毒物等,可用于空气、水质和土壤等方面的监测,为环境保护、公共安全等提供了新的手段和思路。
4、在食品安全中的应用在食品安全监测中,生物传感器技术可以对食品中的致病微生物,毒素及化学成分等进行检测,使食品检测更加安全、快捷和精准。
石墨烯吸附材料的制备与应用研究进展

石墨烯吸附材料的制备与应用研究进展一、本文概述石墨烯,作为一种独特的二维碳纳米材料,自2004年被科学家首次成功剥离以来,便以其优异的物理和化学性质引起了全球范围内的广泛关注。
其超大的比表面积、出色的电导性能、良好的热稳定性和极高的化学稳定性,使得石墨烯在吸附材料领域具有巨大的应用潜力。
本文旨在全面综述石墨烯吸附材料的制备方法、性能优化以及在不同领域的应用研究进展,以期为相关领域的科研工作者和工程师提供有价值的参考。
文章将概述石墨烯吸附材料的基本特性,包括其结构特点、吸附性能以及吸附机理等。
随后,我们将详细介绍石墨烯吸附材料的制备方法,包括化学气相沉积、氧化还原法、剥离法等多种方法,并分析各种方法的优缺点。
在此基础上,文章还将探讨如何通过对石墨烯进行改性或复合,进一步优化其吸附性能。
文章还将重点关注石墨烯吸附材料在环境保护、能源储存与转换、生物医学等领域的应用研究进展。
例如,在环境保护领域,石墨烯吸附材料可用于水处理、空气净化以及土壤修复等方面;在能源储存与转换领域,石墨烯吸附材料可用于锂离子电池、超级电容器等电化学器件中;在生物医学领域,石墨烯吸附材料则可用于药物递送、生物传感等方面。
文章将总结石墨烯吸附材料目前的研究现状和未来发展趋势,以期为相关领域的研究提供有益的参考和指导。
二、石墨烯吸附材料的制备方法石墨烯吸附材料的制备方法多种多样,主要包括化学气相沉积法、氧化还原法、剥离法以及模板法等。
化学气相沉积法(CVD):这是一种在气态环境中,通过化学反应在固体表面生成固态物质的过程。
在制备石墨烯时,通常使用含碳有机气体(如甲烷)在高温条件下分解,然后在催化剂(如铜或镍箔)表面生成石墨烯。
这种方法制备的石墨烯具有大面积、高质量的优点,适用于大规模生产。
氧化还原法:这种方法通常使用石墨作为原料,首先通过强氧化剂(如浓硫酸和硝酸)将石墨氧化成石墨氧化物,然后使用还原剂(如氢碘酸)将石墨氧化物还原成石墨烯。
石墨烯研究现状及应用前景-范本模板
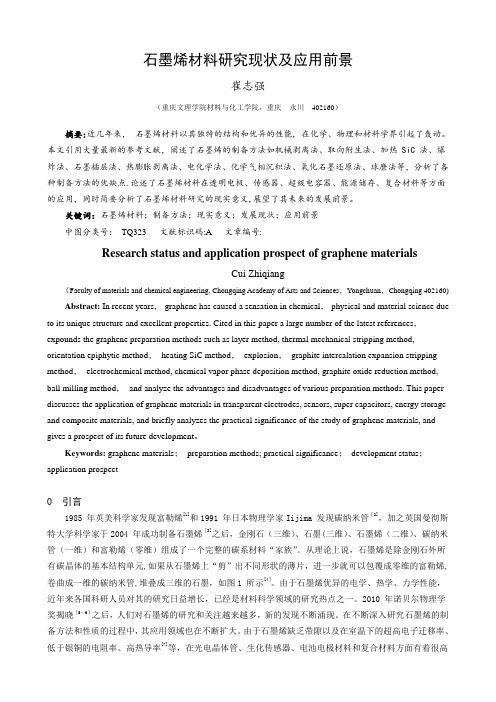
石墨烯材料研究现状及应用前景崔志强(重庆文理学院材料与化工学院,重庆永川402160)摘要:近几年来,石墨烯材料以其独特的结构和优异的性能, 在化学、物理和材料学界引起了轰动。
本文引用大量最新的参考文献,阐述了石墨烯的制备方法如机械剥离法、取向附生法、加热 SiC 法、爆炸法、石墨插层法、热膨胀剥离法、电化学法、化学气相沉积法、氧化石墨还原法、球磨法等,分析了各种制备方法的优缺点.论述了石墨烯材料在透明电极、传感器、超级电容器、能源储存、复合材料等方面的应用,同时简要分析了石墨烯材料研究的现实意义,展望了其未来的发展前景。
关键词:石墨烯材料;制备方法;现实意义;发展现状;应用前景中图分类号:TQ323 文献标识码:A 文章编号:Research status and application prospect of graphene materialsCui Zhiqiang(Faculty of materials and chemical engineering, Chongqing Academy of Arts and Sciences,Yongchuan,Chongqing 402160) Abstract: In recent years,graphene has caused a sensation in chemical,physical and material science due to its unique structure and excellent properties. Cited in this paper a large number of the latest references,expounds the graphene preparation methods such as layer method, thermal mechanical stripping method, orientation epiphytic method,heating SiC method,explosion,graphite intercalation expansion stripping method,electrochemical method, chemical vapor phase deposition method, graphite oxide reduction method, ball milling method,and analyze the advantages and disadvantages of various preparation methods. This paper discusses the application of graphene materials in transparent electrodes, sensors, super capacitors, energy storage and composite materials, and briefly analyzes the practical significance of the study of graphene materials, and gives a prospect of its future development。
石墨烯的应用现状及发展
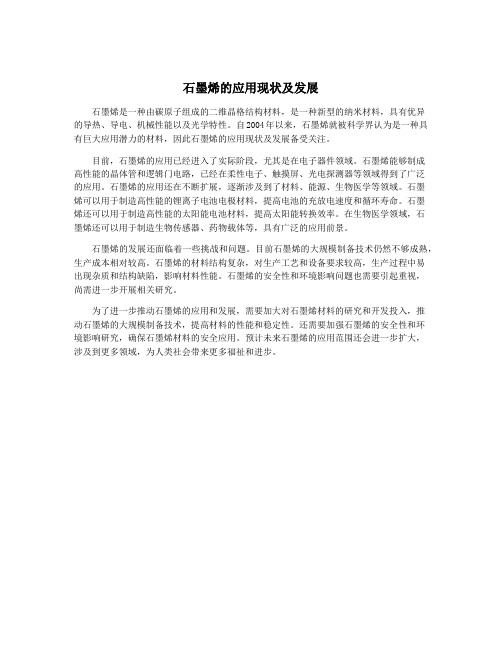
石墨烯的应用现状及发展
石墨烯是一种由碳原子组成的二维晶格结构材料,是一种新型的纳米材料,具有优异
的导热、导电、机械性能以及光学特性。
自2004年以来,石墨烯就被科学界认为是一种具有巨大应用潜力的材料,因此石墨烯的应用现状及发展备受关注。
目前,石墨烯的应用已经进入了实际阶段,尤其是在电子器件领域。
石墨烯能够制成
高性能的晶体管和逻辑门电路,已经在柔性电子、触摸屏、光电探测器等领域得到了广泛
的应用。
石墨烯的应用还在不断扩展,逐渐涉及到了材料、能源、生物医学等领域。
石墨
烯可以用于制造高性能的锂离子电池电极材料,提高电池的充放电速度和循环寿命。
石墨
烯还可以用于制造高性能的太阳能电池材料,提高太阳能转换效率。
在生物医学领域,石
墨烯还可以用于制造生物传感器、药物载体等,具有广泛的应用前景。
石墨烯的发展还面临着一些挑战和问题。
目前石墨烯的大规模制备技术仍然不够成熟,生产成本相对较高。
石墨烯的材料结构复杂,对生产工艺和设备要求较高,生产过程中易
出现杂质和结构缺陷,影响材料性能。
石墨烯的安全性和环境影响问题也需要引起重视,
尚需进一步开展相关研究。
为了进一步推动石墨烯的应用和发展,需要加大对石墨烯材料的研究和开发投入,推
动石墨烯的大规模制备技术,提高材料的性能和稳定性。
还需要加强石墨烯的安全性和环
境影响研究,确保石墨烯材料的安全应用。
预计未来石墨烯的应用范围还会进一步扩大,
涉及到更多领域,为人类社会带来更多福祉和进步。
石墨材料的生物医学应用研究进展
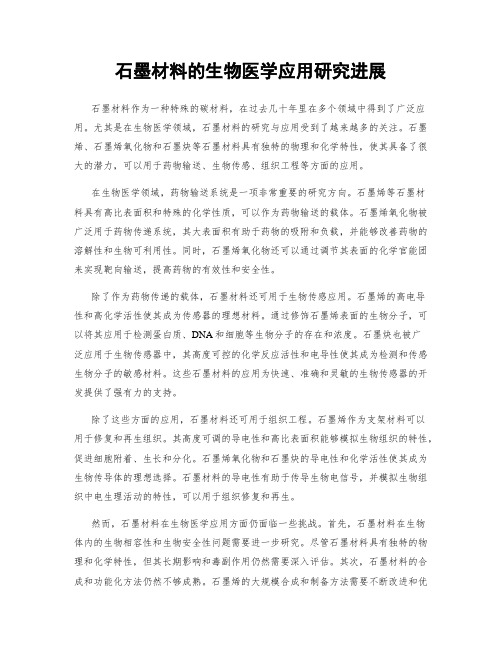
石墨材料的生物医学应用研究进展石墨材料作为一种特殊的碳材料,在过去几十年里在多个领域中得到了广泛应用。
尤其是在生物医学领域,石墨材料的研究与应用受到了越来越多的关注。
石墨烯、石墨烯氧化物和石墨炔等石墨材料具有独特的物理和化学特性,使其具备了很大的潜力,可以用于药物输送、生物传感、组织工程等方面的应用。
在生物医学领域,药物输送系统是一项非常重要的研究方向。
石墨烯等石墨材料具有高比表面积和特殊的化学性质,可以作为药物输送的载体。
石墨烯氧化物被广泛用于药物传递系统,其大表面积有助于药物的吸附和负载,并能够改善药物的溶解性和生物可利用性。
同时,石墨烯氧化物还可以通过调节其表面的化学官能团来实现靶向输送,提高药物的有效性和安全性。
除了作为药物传递的载体,石墨材料还可用于生物传感应用。
石墨烯的高电导性和高化学活性使其成为传感器的理想材料。
通过修饰石墨烯表面的生物分子,可以将其应用于检测蛋白质、DNA和细胞等生物分子的存在和浓度。
石墨炔也被广泛应用于生物传感器中,其高度可控的化学反应活性和电导性使其成为检测和传感生物分子的敏感材料。
这些石墨材料的应用为快速、准确和灵敏的生物传感器的开发提供了强有力的支持。
除了这些方面的应用,石墨材料还可用于组织工程。
石墨烯作为支架材料可以用于修复和再生组织。
其高度可调的导电性和高比表面积能够模拟生物组织的特性,促进细胞附着、生长和分化。
石墨烯氧化物和石墨炔的导电性和化学活性使其成为生物传导体的理想选择。
石墨材料的导电性有助于传导生物电信号,并模拟生物组织中电生理活动的特性,可以用于组织修复和再生。
然而,石墨材料在生物医学应用方面仍面临一些挑战。
首先,石墨材料在生物体内的生物相容性和生物安全性问题需要进一步研究。
尽管石墨材料具有独特的物理和化学特性,但其长期影响和毒副作用仍然需要深入评估。
其次,石墨材料的合成和功能化方法仍然不够成熟。
石墨烯的大规模合成和制备方法需要不断改进和优化,以满足实际应用的需求。
石墨烯在生物医学领域中的应用研究

石墨烯在生物医学领域中的应用研究石墨烯(Graphene)是由一层碳原子构成的二维材料,在它诞生的时候就引起了学术界的高度关注。
作为一种新型材料,石墨烯具有很高的导电性、导热性及机械强度,被认为是21世纪最具应用前景的材料之一。
近年来,石墨烯在生物医学领域的应用研究也引起了人们的极大兴趣。
一、石墨烯在生物医学领域的优势1.1高度可控性石墨烯至今已被广泛研究,并得到了大量的应用。
它有着出色的材料特性,具有独特的物理、化学和生物学特性。
相较于其他材料的特性,石墨烯具有更好的可控性和灵活性。
这意味着石墨烯可以被制作成各种形状、大小和结构,从而可以满足各种不同的生物医学应用需求。
1.2良好的生物相容性生物相容性是材料在生物体内的耐受性和生物不相容性的程度。
与碳纳米管等材料相比,石墨烯具有更好的生物相容性和生物稳定性。
这种材料对生物组织和细胞没有毒性和免疫原性,并且不会引发不良反应,这使得它成为一种理想的生物医学应用材料。
1.3优良的电化学特性石墨烯具有非常好的电化学特性,它的高导电性和高比表面积使得其成为一种理想的电化学传感器材料。
同时,通过功能化修饰可以使石墨烯的表面上具有不同的化学官能团,从而实现对不同种类物质的高灵敏度监测。
二、石墨烯在生物医学领域中的应用2.1药物传输石墨烯可以用作药物载体,在药物传输方面具有非常广阔的应用前景。
在药物传输的过程中,石墨烯纳米片可以被修饰,增加其与药物的相互作用,从而提高药物的载量、缩短药物释放时间和增加药物的稳定性。
与传统的药物传输材料相比,石墨烯纳米片具有保持药物活性不变和减少药物副作用的优势。
2.2生物传感器石墨烯可以用作生物传感器的制作材料,主要是因为它具有优秀的导电性和电化学特性。
石墨烯纳米片可以作为传感器的电极或传感元件,对微量生物分子、微生物和其他生物体内活性物质的检测具有极高的灵敏度和特异性。
同时,在制备生物传感器的过程中,利用石墨烯的高度可控性可以更好地控制传感器的灵敏度和特异性。
石墨烯材料在生物医学领域的应用
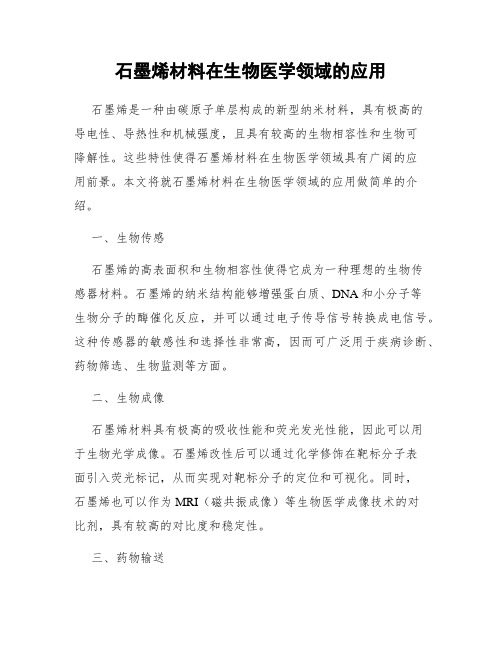
石墨烯材料在生物医学领域的应用石墨烯是一种由碳原子单层构成的新型纳米材料,具有极高的导电性、导热性和机械强度,且具有较高的生物相容性和生物可降解性。
这些特性使得石墨烯材料在生物医学领域具有广阔的应用前景。
本文将就石墨烯材料在生物医学领域的应用做简单的介绍。
一、生物传感石墨烯的高表面积和生物相容性使得它成为一种理想的生物传感器材料。
石墨烯的纳米结构能够增强蛋白质、DNA和小分子等生物分子的酶催化反应,并可以通过电子传导信号转换成电信号。
这种传感器的敏感性和选择性非常高,因而可广泛用于疾病诊断、药物筛选、生物监测等方面。
二、生物成像石墨烯材料具有极高的吸收性能和荧光发光性能,因此可以用于生物光学成像。
石墨烯改性后可以通过化学修饰在靶标分子表面引入荧光标记,从而实现对靶标分子的定位和可视化。
同时,石墨烯也可以作为MRI(磁共振成像)等生物医学成像技术的对比剂,具有较高的对比度和稳定性。
三、药物输送石墨烯材料的高表面积和生物相容性使它成为一种理想的药物输送系统。
石墨烯可以被用作药物包装材料作为药物传递的载体,同时还可以改善药物的生物利用度和稳定性。
石墨烯作为一种分子负载工具可以特异性地输送药物到癌细胞等病区域,减少对健康细胞的损伤,进一步提高治疗效果。
四、组织工程石墨烯材料可以和基质结合形成可注入的凝胶,使其可以在组织工程中作为一种生物支架。
石墨烯的生物可降解性和生物相容性可以避免免疫排斥和毒性反应。
石墨烯的高导电性和高导热性,可以促进组织细胞的生长和细胞分化,从而促进组织再生和修复。
结论石墨烯是一种独特的纳米材料,它的特性使得它成为生物医学领域的理想材料。
通过对石墨烯的合理改性和化学修饰,可以进一步提高其生物相容性和生物可降解性,从而广泛应用于生物传感、生物成像、药物输送、组织工程等领域,这为人们的健康和医学研究提供了极佳的帮助。
石墨烯纳米流体的制备及其在生物传感中的应用
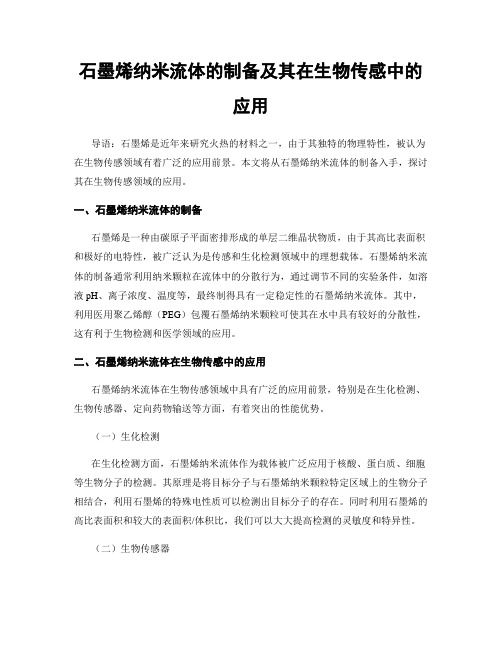
石墨烯纳米流体的制备及其在生物传感中的应用导语:石墨烯是近年来研究火热的材料之一,由于其独特的物理特性,被认为在生物传感领域有着广泛的应用前景。
本文将从石墨烯纳米流体的制备入手,探讨其在生物传感领域的应用。
一、石墨烯纳米流体的制备石墨烯是一种由碳原子平面密排形成的单层二维晶状物质,由于其高比表面积和极好的电特性,被广泛认为是传感和生化检测领域中的理想载体。
石墨烯纳米流体的制备通常利用纳米颗粒在流体中的分散行为,通过调节不同的实验条件,如溶液pH、离子浓度、温度等,最终制得具有一定稳定性的石墨烯纳米流体。
其中,利用医用聚乙烯醇(PEG)包覆石墨烯纳米颗粒可使其在水中具有较好的分散性,这有利于生物检测和医学领域的应用。
二、石墨烯纳米流体在生物传感中的应用石墨烯纳米流体在生物传感领域中具有广泛的应用前景,特别是在生化检测、生物传感器、定向药物输送等方面,有着突出的性能优势。
(一)生化检测在生化检测方面,石墨烯纳米流体作为载体被广泛应用于核酸、蛋白质、细胞等生物分子的检测。
其原理是将目标分子与石墨烯纳米颗粒特定区域上的生物分子相结合,利用石墨烯的特殊电性质可以检测出目标分子的存在。
同时利用石墨烯的高比表面积和较大的表面积/体积比,我们可以大大提高检测的灵敏度和特异性。
(二)生物传感器石墨烯纳米流体在生物传感器方面也有着广泛的应用前景,其原理主要利用石墨烯的特殊电性质,将其作为传感器的敏感元件,通过检测样品在石墨烯表面产生的特定电信号来达到检测的目的。
此外,利用石墨烯的导电特性,还可以制作出基于石墨烯的电化学生物传感器,实现对复杂环境中生物物质的快速检测。
(三)定向药物输送在医学方面,利用石墨烯的高比表面积和良好的生物相容性,可以将其制成具有定向性的药物输送载体,实现药物对特定位置的定向输送。
研究表明,将石墨烯纳米流体与药物包裹在一起,将其注射到肿瘤部位,可明显提高药物的效果,同时减少药物对正常细胞的损伤。
- 1、下载文档前请自行甄别文档内容的完整性,平台不提供额外的编辑、内容补充、找答案等附加服务。
- 2、"仅部分预览"的文档,不可在线预览部分如存在完整性等问题,可反馈申请退款(可完整预览的文档不适用该条件!)。
- 3、如文档侵犯您的权益,请联系客服反馈,我们会尽快为您处理(人工客服工作时间:9:00-18:30)。
Biosensors and Bioelectronics 26 (2011) 4637–4648Contents lists available at ScienceDirectBiosensors andBioelectronicsj o u r n a l h o m e p a g e :w w w.e l s e v i e r.c o m /l o c a t e /b i osReviewRecent advances in graphene-based biosensorsTapas Kuila a ,Saswata Bose a ,Partha Khanra a ,Ananta Kumar Mishra b ,Nam Hoon Kim c ,Joong Hee Lee a ,b ,c ,∗aDepartment of BIN Fusion Technology,Chonbuk National University,Jeonju,Jeonbuk 561-756,Republic of KoreabBIN Fusion Research Center,Department of Polymer &Nano Engineering,Chonbuk National University,Jeonju,Jeonbuk 561-756,Republic of Korea cDepartment of Hydrogen and Fuel Cell Engineering,Chonbuk National University,Jeonju,Jeonbuk 561-756,Republic of Koreaa r t i c l ei n f oArticle history:Received 16February 2011Received in revised form 25May 2011Accepted 25May 2011Available online 2 June 2011Keywords:GrapheneBiocompatibility Enzyme biosensor Graphene electrode DNA sensor Gas sensora b s t r a c tA detailed overview towards the advancement of graphene based biosensors has been reviewed.The large surface area and excellent electrical conductivity of graphene allow it to act as an “electron wire”between the redox centers of an enzyme or protein and an electrode’s surface.Rapid electron transfer facilitates accurate and selective detection of biomolecules.This review discusses the application of graphene for the detection of glucose,Cyt-c,NADH,Hb,cholesterol,AA,UA,DA,and H 2O 2.GO and RGO have been used for the fabrication of heavy metal ion sensors,gas sensors,and DNA sensors.Graphene based FETs have also been discussed in details.In all these cases,the biosensors performed well with low working potentials,high sensitivities,low detection limits,and long-term stabilities.© 2011 Elsevier B.V. All rights reserved.Contents 1.Introduction ..........................................................................................................................................46381.1.Synthesis of graphene ........................................................................................................................46381.2.Electrochemical aspects of graphene ........................................................................................................46391.3.Biocompatibility of GO and graphene ........................................................................................................46401.4.Scope of this review ..........................................................................................................................46402.Graphene-based enzymatic electrodes ..............................................................................................................46402.1.Glucose oxidase biosensor ...................................................................................................................46402.2.Cytochrome c biosensor ......................................................................................................................46412.3.NADH biosensor ..............................................................................................................................46412.4.Hemoglobin biosensor .......................................................................................................................46412.5.HRP biosensor ................................................................................................................................46412.6.Cholesterol biosensor (4642)Abbreviations:A,adenine;AA,ascorbic acid;Ab,antibody;AuNP,gold nanoparticle;BDD,boron doped diamond;CdS,cadmium sulfide;CEA,carcinoembryonic antigen;CEEs,chloroethylethyl sulfide;-CD,-cyclodextrin;C,cytosine;CMG,chemically modified graphene;CNT,carbon nanotube;CNF,carbon nanofiber;CRG,chemically reduced graphene;CR-GO,chemically reduced graphene oxide;CS,chitosan;CVD,chemical vapor deposition;CV,cyclic voltammetry;Cyt-c,cytochrome c;DA,dopamine;DET,direct electron transfer;DMMP,dimethylmethylphosphonate;DNA,deoxyribonucleic acid;DNT,dinitrotoluene;D 2O,heavy water;DPV,differential pulse voltammetry;EDTA,ethylenediamine triacetic acid;EG,epitaxial graphene;EIS,electrochemical impedance spectroscopy;ET,electron transfer;Fe 3O 4,iron (II,III)oxide;FET,field effect transistor;G,guanine;GC,glass carbon;GO,graphene oxide;GOx,glucose oxidase;GR,graphene;Hb,hemoglobin;HCN,hydrogen cyanide;HOPG,highly oriented pyrrolytic graphite;HRP,horseradish peroxide;IL,ionic liquid;H 2O 2,hydrogen peroxide;IgE,immunoglobulin;ITO,indium tin oxide;LiTaO 3,lithium tantalite;MB,molecular beacons;Mb,methylene blue;MG,methylene green;MGNF,multilayer graphene nanoflake;MnO 2,manganese dioxide;NADH,nicotinamide adenine dinucleotide;NP,nanoparticle;PANIw,polyaniline nanowire;PB,Prussian blue;PBS,phosphate buffer solution;PE-CVD,plasma-enhanced chemical vapor deposition;PMMA,poly(methyl methacrylate);ppb,parts per billion;ppm,parts per million;PSS,polystyrene sulfonate;PSSA-g-PPY,poly(styrenesulfonic acid-g-pyrrole);PtNP,platinum nanoparticles;PPy,polypyrrole;RGO,reduced GO;rGSFs,reduced graphene sheet film (rGSF);RNA,ribo nucleic acid;RSD,relative standard deviation;SDBS,sodium dodecyl benzene sulfonate;Sic,silicon carbide;SRB,sulfate-reducing bacteria;ssDNA,single strand deoxyribonucleic acid;S/N,signal to noise background ratio;T,thymine;UA,uric acid.∗Corresponding author at:Department of BIN Fusion Technology,Chonbuk National University,Jeonju,Jeonbuk 561-756,Republic of Korea.Tel.:+82632702342;fax:+82632702341.E-mail address:jhl@chonbuk.ac.kr (J.H.Lee).0956-5663/$–see front matter © 2011 Elsevier B.V. All rights reserved.doi:10.1016/j.bios.2011.05.0394638T.Kuila et al./Biosensors and Bioelectronics26 (2011) 4637–46482.7.Catechol biosensor (4642)3.Graphene-based non-enzymatic electrodes (4642)3.1.Hydrogen peroxide (4643)3.2.Ascorbic acid,uric acid and dopamine (4643)4.Graphene-based nano-electronic devices (4643)4.1.DNA sensors (4644)4.2.Heavy metal ion detection (4645)4.3.Gas sensors (4645)4.4.Field effect transistor (4646)5.Conclusions (4646)6.Scope of future work (4647)Acknowledgements (4647)References...........................................................................................................................................4647Fig.1.Schematic presentation of a biosensor.1.IntroductionThe historic development of enzyme electrodes by Leland C. Clark in1962opened a new area of research in medical science and technology(Clark and Lyons,1962).Research on enzyme electrodes in variousfields such as physics,chemistry,material science and biotechnology has resulted in more sophisticated and trustworthy biosensors.They are suitable for application in medicine,agricul-ture,biotechnology,as well as by the military and in bioterrorism detection and prevention(Wang,2008;Chaubey and Malhotra, 2002;Yogeswaran and Chen,2008).Recent work has examined ‘reagentless systems’,in which reagents are already immobilized and do not need to be added by the user(Schuhmann et al.,1993; Schmidt and Schuhmann,1995;Senillou et al.,1999).Biosensors comprise a selective interface in close proximity to or integrated with a transducer,which relays information about interactions between the surface of the electrode and the analyte either directly or through a mediator(Fig.1)(Yogeswaran and Chen,2008). Biosensors can be categorized depending on the transducing mech-anism:(i)resonant biosensors,(ii)optical-detection biosensors, (iii)thermal-detection biosensors,(iv)ion-sensitive FET biosen-sors,and(v)electrochemical biosensors(Chaubey and Malhotra, 2002).Electrochemical biosensors possess advantages over the oth-ers because their electrodes can sense materials present within the host without damaging the system(Chaubey and Malhotra,2002). However,the sluggish ET of the biomolecules limits electrochemi-cal efficiencies of the sensors(Kim et al.,2007;Huang et al.,2010a,b; Shao et al.,2010;Wang,2005).This is because of the structural features of the proteins and their unfavorable orientations at the surface of the electrodes.Generally electroactive prosthetic groups are embedded deep within the protein structure.To minimize the ET distance,nanomaterial“electron wires”,should be incorporated between the redox centers of the enzyme or protein and the elec-trode’s surface(Shao et al.,2010).Nanotechnology has allowed new applications of nanomaterials in electrochemical sensors and biosensors(Yogeswaran and Chen, 2008).Various nanomaterials,including metal NP,metal alloy NP, magnetic NP,nanowires,CNT,and CNF have been used as elec-trical connectors between the electrodes and the redox centers of the biomolecules(Shao et al.,2010;Kim et al.,2007;Huang et al., 2010a,b;Sun et al.,2001;Shi and Ma,2010;Haun et al.,2010;Yun et al.,2007).The use of metallic NP as biosensors is problematic because of their inconsistent signal amplification.The existence of CNT with metallic impurity is the main drawback while using in the modification of electrode(Pumera,2009,2010).Such metal-lic impurities are electrochemically active and can dominate the electrochemistry of CNT.Impurities present at50ppm can be tox-icological hazards as they can participate in redox reactions with the biomolecules(Pumera,2009).The discovery of graphene in2004,added a new dimension to electrochemical biosensor research(Novoselov et al.,2004).The use of graphene can avoid the problems associated with metal alloy NP and CNT.The unique properties of graphene(fast electron transportation,high thermal conductivity,excellent mechanical flexibility and good biocompatibility)give it potential applicability in electrochemical biosensors(Pumera,2009;Pumera et al.,2010; Allen et al.,2010;Brownson and Banks,2010).Table1shows the comparative study of biosensing efficiency between CNT-based and graphene-based biosensors.Proper conjugation between biolog-ical molecules such as enzymes,ssDNA,RNA,Ab,receptors,and aptamers needs to be developed for graphene based electrochem-ical sensing electrodes.Appropriate functionalization of graphene and the immobilization of biomaterials on it are important,as func-tional groups can create defects on graphene surfaces(Shao et al., 2010).1.1.Synthesis of grapheneThere are several reported methods for the syntheses of graphene(Choi et al.,2010;Dreyer et al.,2010;Kuila et al.,2010; Park and Ruoff,2009),including exfoliation and cleavage of natu-ral graphite,CVD,PE-CVD,electric arc discharge,micromechanical exfoliation of graphite,epitaxial growth on electrically insulat-ing surfaces,such as SiC,opening CNT and the solution-based reduction of GO(Choi et al.,2010;Kim et al.,2009;Kuila et al., 2010).Novoselov et al.(2004)discovered graphene sheets after the mechanical exfoliation of HOPG,a method now known as the scotch-tape method.Since their discovery,researchers haveT.Kuila et al./Biosensors and Bioelectronics26 (2011) 4637–46484639 Table1Comparative study of sensing efficiency between CNT and graphene-based biosensors/sensors.Sensor type Detected element Sensing materials RSD(%)a/response time b Detection limit ReferencesGlucose biosensor Glucose Graphene-PPy–3M±0.5M Alwarappan et al.(2010) Glucose CNT-PPy b15s0.2mM Wang and Musameh(2005) Glucose Graphene/Nafion a4.21 1.0×10−6M Chen et al.(2010)Glucose CNT/Nafion a2.8/b4s 3.0×10−9M Jeykumari andNarayanan(2008)CS–GR a5.3/0.02nM Kang et al.(2009) Glucose CS-CNT a5.00.05mM Zou et al.(2008)Glucose CS–GR-AuNP a6.00.6M Wu et al.(2009a)Glucose CS-CNT-AuNP a4.95/b<6s 3.0×10−6M Wang et al.(2009b) Cholesterol oxidase Cholesterol PtNP-Graphene–0.5nM Dey and Raj(2010) Cholesterol PtNP-CNT a2.6–4.1/b<20s–Shi and Peng(2005) NADH Graphene 3.5–Tang et al.(2009) NADH GO b<1s0.1m Zhang et al.(2011a)NADH CNT a2.55×10−3M Musameh et al.,2002NADH CNT b4s2m Chakraborty and Raj(2007)HRP H2O2Graphene a4.48 1.05×10−7M Lu et al.(2010b) H2O2AuNP-Graphene a3.6 1.0×10−6M Zhou et al.(2010b)H2O2CNT b<1s 1.0×10−7M Xu et al.(2005)H2O2CNT-Mb a4.5 1.0m Xu et al.(2003)Hb H2O2Graphene– 5.1×10−7M Xu et al.(2010) H2O2CNT a9.35 2.41m Ding et al.(2010)H2O2CNT-sodium alginate a2.3416.41m Zhao et al.(2009)Nitrite Hb-CS-DMF/GR a2.1 1.8×10−7M Liu et al.(2011a,b)Electrochemical sensor AA,UA,DA MGNF a0.17M–Shang et al.(2008) DA-CD/GR b30s0.2M Tan et al.(2010) DA CMG–0.01M Hou et al.(2010) AA,UA,DA PSS-CNT a4,2.9,2.5 5.0×10−7M,1.5×10−7M,5.0×10−7M Manjunatha et al.(2010)AA,UA,DA PtNP-CNT– 1.9×10−5M,3.2×10−8M,2.7×10−8M,Dursun and Gelmez(2010)DNA sensor DNA EG–1M Lim et al.(2010) DNA GO–200nM Liu et al.(2010a,b)DNA GR/PANIw a1.15 3.25×10−13M Bo et al.(2011b)DNA CNT-Mb–0.252nM Li et al.(2005)Phenolic pollutants CNT-DNA a3 1.6×10−5M Zheng et al.(2009)DNA CNT a11/b3s 1.0×10−10M Cai et al.(2003)Gas sensors NO2Thermally reduced GO–2ppm Lu et al.(2009b,c) NO2and NH3,DNT Hydrazine reduced GO–<5ppm(NO2and NH3),28ppb DNT Fowler et al.(2009)NO2and NH3CNT b<600s2ppm Kong et al.(2000)NO2CNT b<600s44ppb Li et al.(2003)NH3CNT b∼100s10ppm Suehiro et al.(2003)NO2CNT b∼600s5–100ppb Valentini et al.(2004)NH3,CO,ethanol Mechanically exfoliated graphene–1ppb Schedin et al.(2007)HCN CNT–4000Robinson et al.(2008)CEES0.5DMMP0.1DNT0.1HCN Reduced GO–70Robinson et al.(2008)CEES0.5DMMP5DNT0.1sought cost effective methods of producing large-area,single lay-ers of graphene.CVD can produce large areas of single layer graphene(Reina et al.,2009).Li et al.(2009b)synthesized graphene by CVD using centimeter-scale copper substrates.Recently,Sun et al.(2010)synthesized graphene by CVD from solid PMMA deposited on a Cu substrate.Kim et al.(2009)synthesized graphene films on Ni substrates for stretchable transparent electrodes.Dong et al.(2009)prepared monolayers of graphene from naturalflake graphite using1,3,6,8-pyrenetetrasulfonic acid and D2O.Graphene can also be prepared on H2-etched surfaces of6H-SiC when heated to1250–1450◦C for a short time(1–20min)(Wu et al.,2009b). Another mass production method involves the chemical or thermal reduction of GO(Schniepp et al.,2006)and to date is the most com-mon and economical method.Graphene synthesized by chemical oxidation–reduction has been reported to possess many structural defects,which are advantageous for electrochemical applications (Shao et al.,2010).1.2.Electrochemical aspects of grapheneDET is the fundamental process in electrochemical reactions. Study of electrochemical responses of graphene towards different redox systems requires knowledge of the electrochemical aspects of graphene(Pumera,2009).It has been proposed that the pres-ence of oxygen containing groups on graphene surface can enhance the ET rate.Heterogeneous charge transfer for the oxidation of endiol groups occurs through a proton-coupled ET mechanism (Pumera,2009;Tang et al.,2009).Oxygen containing species take two protons from the endiol group and aid the oxidation reaction by reducing the overpotential voltage.Huang et al.(2011)mea-sured EIS of GC and carboxyl-functionalized graphene electrodes. Yang et al.(2009)prepared graphene-based electrodes using vase-line as binder.CV found a pair of well-defined redox waves at the electrodes when the graphene content in the vaseline solu-tion was10.0g mL−1.This indicates that the redox couple at such4640T.Kuila et al./Biosensors and Bioelectronics26 (2011) 4637–4648Table2Various types of enzymatic electrodes made from graphene and GO.In all the cases the mode of detection is change in electrical current.Type of biosensor Sensor materials RSD(%)Detection limit ReferencesGlucose Graphene-PPy–3M±0.5M Alwarappan et al.(2010) Metal decorated graphene–1M Baby et al.(2010)Graphene/Nafion 4.21 1.0×10−6M Chen et al.(2010)CVD grown graphene–0.1mM Huang et al.(2010a,b)CS–GR 5.30.02nM Kang et al.(2009)GO 5.8–Liu et al.(2010b)PVDF-protected graphene 3.22–14mM Shan et al.(2009)CS–GR-AuNP 4.7180M Shan et al.(2010a)GO––Wang et al.(2009c)Graphene-CdS 5.30.7mM Wang et al.(2011a)CS–GR-AuNP 6.00.6M Wu et al.(2009a)Graphene 2.510±2M Wu et al.(2010b)CMG–0.376mM Yang et al.(2010)Graphene–0.168mM Zeng et al.(2010a)CR-GO 4.3 2.0M Zhou et al.(2009)Graphene 3.25M Zhou et al.(2010a) NADH IL functionalized graphene 4.25M Shan et al.(2010b)Graphene 3.5–Tang et al.(2009)Hb Fe3O4–graphene 1.60.5M He et al.(2011)Graphene– 5.1×10−7M Xu et al.(2010)HRP Graphene 4.48 1.05×10−7M Lu et al.(2010b)SDBS–graphene– 1.0×10−7M Zeng et al.(2010b)AuNP–graphene 3.6 1.0×10−6M Zhou et al.(2010b)CS–GR/Fe3O4/HRP– 6.0×10−7M Zhou et al.(2011) Cholesterol PtNP–graphene–0.5nM Dey and Raj(2010)electrodes is diffusion-controlled.The anti fouling ability and elec-trocatalytic behavior towards electrochemical oxidations of NADH and H2O2at graphene-modified electrodes have also been evalu-ated(Lin et al.,2009).The proposed graphene-modified electrodes are expected to be useful in simple and effective electrochemical sensors and biofuel cells.1.3.Biocompatibility of GO and grapheneBiocompatibility of graphene or GO with microorganisms and living cells is very important.Extensive research efforts have been paid to study the biocompatibility of GO and graphene with the microorganisms(Akhavan and Ghaderi,2009).It has been found that GO decreases cell adhesion when enters into cytoplasm and nucleus(Wang et al.,2011b).It also causes lung inflammation and kidney failure.However,the blood circulation time in GO deposited lungs are much better than other carbon based nanofiller(Zhang et al.,2011c).The growth of Gram-positive,Gram-negative,and Escherichia coli bacteria is affected significantly by the sharp edges of the reduced graphene nanowalls(Akhavan and Ghaderi,2010; Hu et al.,2010).The ongoing research shows that the bacteria trapped within the aggregated graphene sheets are inactive with-out any chance for proliferation in a culture medium.But,after removing the aggregated graphene sheets from the surface of the bacteria by using sonication,they could be reactivated(Akhavan et al.,2011).Based on the cell culture experiments,Chen et al. (2008)showed that graphene paper may be biocompatible and therefore suitable for biomedical applications.Park et al.(2010)also proposed that graphene paper is noncytotoxic to three mammalian cell lines.Yang et al.(2011)showed that no obvious toxicity of pegy-lated graphene at the dose of20mg/kg to Balb/c female mice was observed in blood biochemistry,hematology,and histology analy-sis.However,the exact mechanism is still not clear.Therefore,to make it more biocompatible and to decrease or abolish the toxicity of GO is still a challengeable task for in vivo biomedical application.1.4.Scope of this reviewThis article reviews recent trends in the development of graphene-based electrochemical biosensors.Extensive research has been carried out on nanomaterial-based electrochemical biosensors;however,they suffer from limitations of sensitiv-ity,electrochemical stability,production cost,biocompatibility and detection time.This work contains three main sections that examine graphene-based enzymatic,non-enzymatic and nano-electronic devices.The electrochemical detection of glucose,Cyt-c, NADH,Hb,HRP and cholesterol has been considered in the dis-cussion of graphene-based enzyme electrodes.Non-enzymatic graphene-based electrodes for the detection of H2O2,AA,UA and DA are discussed in the second main section.Graphene-based nano-electronic devices for DNA sensing,heavy metal ion detection and gas sensing are reviewed in the last main section.2.Graphene-based enzymatic electrodesDirect electrochemistry of enzymes involves direct ET between the electrode and the active center of the enzymes without the participation of mediators or other reagents(Leger and Bertrand, 2008;Shao et al.,2010;Yao and Shiu,2008).New mediator-free (or reagentless)biosensors,enzymatic bioreactors,and biomedi-cal devices are sought that employ DET by immobilizing enzymes on conducting substrates.However,the redox centers of the biomolecules are usually embedded deep in their large three-dimensional structures.Recent research has shown that graphene can enhance DET between enzymes and electrodes.The use of metal NP with graphene has been reported to form exceptionally stable and cost-effective biosensors(Andreescu and Luck,2008;Xiao et al., 2003).Table2shows different types of enzymatic biosensor with their limit of detection and RSD.2.1.Glucose oxidase biosensorThe metabolic disorder of diabetes mellitus results in the defi-ciency of insulin and hyperglycemia and is reflected by blood glucose concentration higher or lower than the normal range of 80–120mg dL−1(4.4–6.6mM).The disease is a leading cause of death and disability(Wang,2008).Therefore,the diagnosis and management requires close monitoring of blood glucose levels. The application of graphene in highly sensitive and cost-effective biosensors can aid the aforementioned diseases(Wang et al.,T.Kuila et al./Biosensors and Bioelectronics26 (2011) 4637–464846412010b).Various groups have shown that graphene-based glucose biosensors exhibit good sensitivity,selectivity and reproducibility. The direct electrochemistry of GOx based on redox-active centers was confirmed by CV experiments in the potential range of0.8–0V to follow previously proposed reaction mechanisms(Yang et al., 2010):GO x(FAD)+2H++2e−↔GO x(FADH2)(In the absence of oxygen)(1) In the presence of oxygen,the reaction mechanism follows dif-ferent pathways:GO x(FADH2)+O2→GO x(FAD)+H2O2(2) GO x(FAD)+Glucose→GOx(FADH2)+Gluconolactone(3) Wang et al.(2009c)used electrochemically reduced single layers of graphene to detect glucose.The presence of CdS nanocrystal with graphene showed very low detection limit of0.7mM(Wang et al., 2011a).Shan et al.(2009)used a polyvinylpyrrolidone-protected graphene/polyethylenimine-functionalized IL/GOx electrochemi-cal biosensor to study the DET of GOx.CV measurements revealed characteristic reversible ET of the redox active center.Kang et al. (2009)fabricated CS–GR/GOx modified electrodes for direct elec-trochemical glucose sensing.The excellent performance of the biosensors was attributed to the large surface area to volume ratio and high conductivity of graphene and the good biocompatibility of chitosan with GOx.CS–GR/PtNP nanocompositefilm has also been used for the detection of glucose(Wu et al.,2009a,b).Introduc-tion of PtNP increased the sensor’s sensitivity,allowing a detection limit of0.6M glucose.The biosensor possessed good reproducibil-ity and long-term stability with negligible interference signals from AA and UA.Shan et al.(2010a)designed CS–GR/AuNP nanocompos-itefilms for glucose sensing,which exhibited good amperometric responses to glucose with a linear ranges of2–10mM(R=0.999)at −0.2V and2–14mM(R=0.999)at0.5V.This was attributed to the synergy effect of graphene and the AuNP.Zhou et al.(2010a)inves-tigated glucose sensitivity of Nafion-GR/AuNP biocompositefilms. This linear range of the sensor was15–5.8mM,with a detection limit of5mM(S/N=3).Zeng et al.(2010a)used organically modified graphene for enzyme-based glucose and maltose biosensing.The CV response of the modified electrode increased with increasing concentration of glucose in buffer solution.The detection limit and sensitivity were0.168mM(S/N=3)and0.261A mM−1cm−2,respectively. GO has also been used for the detection of glucose(Liu et al.,2010b; Song et al.,2010).Its biocompatibility with GOx led to a stable glu-cose biosensor with a sensitivity of8.045mA cm−2M−1.Yang et al. (2010)developed a glucose biosensor using CMG and IL.Chen et al. (2010)prepared Nafion-GR/GOxfilm-modified electrodes for the detection of glucose.The sensor showed good stability,with a RSD of4.21%(n=5).Huang et al.(2010a,b)used CVD-grown graphene to develop real-time glucose biosensors.Alwarappan et al.(2010) employed PPy-GR/GOx-based enzymatic biosensors for in vitro electrochemical glucose detection.Nafion-GR/PtNP or AuNP mod-ified electrodes exhibited best sensing performances,with a linear response up to30mM and an excellent detection limit of1M (Baby et al.,2010).Wang et al.(2010b)investigated the effect of nitrogen doping on the biosensing performances of graphene electrode.N-doped graphene exhibited excellent electrocatalytic activity for the reduction of H2O2,fast ET kinetics,high sensitivity and selectivity for glucose biosensing.2.2.Cytochrome c biosensorDET between Cyt-c and electrodes is receiving increasing research attention due to its biological functions(Xiang et al.,2008). However,bare GC does not exhibit a voltammetric response for Cyt-c detection due to slow ET kinetics at the electrode/electrolyte interface(Xiang et al.,2008).The development of carbon-based nanofiller has successfully overcome these problems.Wu et al.(2010a)tried to solve the same problem using CS–GR-modified electrodes to study the DET of Cyt-c.The formal potential of the CS–GR/Cyt-c modified GC electrode was estimated to be −0.28V.The ET rate constant of Cyt-c at the graphene electrode was estimated to be1.95s−1,which is much higher than that of the conventional electrodes.Therefore,Cyt-c on the surface of a CS–GR modified electrode maintains its bioactivity and shows an enzyme-like activity for the reduction of nitric oxide.2.3.NADH biosensorNADH is an important coenzyme that takes part in more than 300dehydrogenase enzymatic reactions(Liu et al.,2009).Elec-trocatalytic oxidation of NADH has been investigated as part of the development of dehydrogenase-based biodevices.However,its electrochemical oxidation at bare GC electrodes in neutral solu-tions occurs at a high overpotential(ca.0.5V)because of slow ET kinetics and electrode fouling(Raj and Ohsaka,2001).Therefore, the effective oxidation of NADH at low potentials would aid the development of NADH-based biodevices.Tang et al.(2009)studied the electrochemical behavior of NADH on rGSF.It showed increased ET kinetics and excellent electrocatalytic activity compared with bare GC electrodes.The peak potential of NADH oxidation shifted from0.75V on bare GC to0.42V on the rGSF/GC electrode.Liu et al.(2009)pre-pared a NADH sensor through noncovalent functionalization of graphene with water-soluble electroactive MG.The oxidation peak of NADH at a bare GC electrode occurs at+0.55V.However,this became+0.40V and+0.14V,respectively for electrodes of CRG and CRG functionalized with MG.Shan et al.(2010b)designed an IL-functionalized graphene biosensor for the electrochemical determination of NADH.The amperometric response at this elec-trode was more stable(95.4%and90%of initial activity remaining after10and30min,respectively,at1mM NADH solution)than that of the bare GC electrode(68%and46%).Moreover,the IL-CS–GR-modified electrode exhibited good linearity from0.25to2mM anda high sensitivity of37.43Am M−1cm−2.2.4.Hemoglobin biosensorHb is the most important part of blood and is responsible for transporting O2throughout the circulatory system.Change of Hb concentration in blood can cause several diseases and even death. Therefore,accurate determination of Hb content in blood is medi-cally very essential.Xu et al.(2010)used a CS–GR-modified electrode for the elec-troanalysis of Hb.The cyclic voltammogram of Hb at the CS–GR/GC electrode showed a well-resolved redox peak compared with a CS/GC electrode.The current response of Hb at the CS–GR/GC elec-trode increased linearly with scan rate from30to150mV s−1 (Fig.2),indicating a surface-controlled electrochemical process. He et al.(2011)studied the effect of magnetic NP on Hb detec-tion.The biosensor exhibited fast response time(<3s),broad linear response to H2O2in the range1.50–585M L−1and a rel-atively low detection limit of0.5M L−1(S/N=3).Wang et al. (2010a)designed CS–GR/Hb/GR/IL/GC electrode for the detection of nitromethane.The designed biosensor achieved a detection limit down to6.0×10−10M for quantitative nitromethane analysis.2.5.HRP biosensorAccurate determination of H2O2is useful in chemistry,biology, clinical control and environmental protection(Zhou et al.,2010b).。