GOCAD(外文资料) (18)
GOCAD(外文资料) (20)
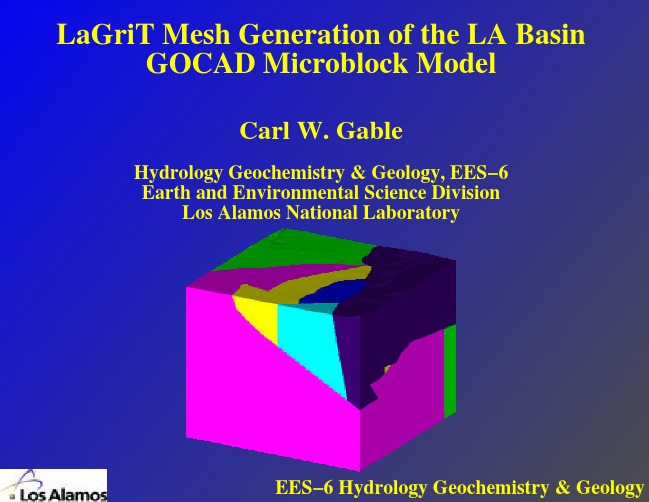
Tetrahedral Volume Mesh
− concatenate T−surf point distribution w/ octree point distribution − Apply 3D Delaunay tetrahedralization algorithm − Break edges that cross material boundary by point addition at location where edge intersects boundary
EES−6 Hydrology Geochemistry & Geology
Exploded View of Five Blocks
EES−6 Hydrology Geochemistry & Geology
GOCAD block model and close−up of triangulation
EES−6 Hydrology Geochemistry & Geology
Uniform finite difference grid to test geometry definition.
51**3 nodes
11**3 nodes
101**3 nodes
EES−6 Hydrology Geochemistry & Geology
EES−6 Hydrology Geochemistry & GeologyS1来自S4¡¡
¡
¡
¡
S2
¡
¡
¡
¡
¡
¡
¡
¡
¡
¡
R1
¡ ¡ ¡ ¡ ¡ ¡
EES−6 Hydrology Geochemistry & Geology
GOCAD软件总体介绍
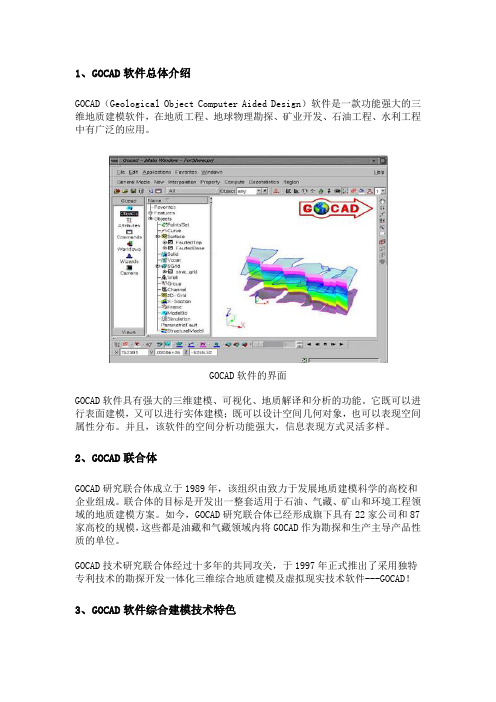
1、GOCAD软件总体介绍GOCAD(Geological Object Computer Aided Design)软件是一款功能强大的三维地质建模软件,在地质工程、地球物理勘探、矿业开发、石油工程、水利工程中有广泛的应用。
GOCAD软件的界面GOCAD软件具有强大的三维建模、可视化、地质解译和分析的功能。
它既可以进行表面建模,又可以进行实体建模;既可以设计空间几何对象,也可以表现空间属性分布。
并且,该软件的空间分析功能强大,信息表现方式灵活多样。
2、GOCAD联合体GOCAD研究联合体成立于1989年,该组织由致力于发展地质建模科学的高校和企业组成。
联合体的目标是开发出一整套适用于石油、气藏、矿山和环境工程领域的地质建模方案。
如今,GOCAD研究联合体已经形成旗下具有22家公司和87家高校的规模,这些都是油藏和气藏领域内将GOCAD作为勘探和生产主导产品性质的单位。
GOCAD技术研究联合体经过十多年的共同攻关,于1997年正式推出了采用独特专利技术的勘探开发一体化三维综合地质建模及虚拟现实技术软件---GOCAD!3、GOCAD软件综合建模技术特色要建模的地质目标,千姿百态,既要描述其几何形态,也要描述其所包含的地质属性特征。
但是无论多么复杂的地质体,归纳起来都可用点、线、面、体等四种类型的数据来描述。
基于这种观点,GOCAD中描述地质目标的数据定义有:•点集:描述离散数据;•线集:描述断层线、钻孔轨迹、测井曲线和河道等线状数据;•面集:描述层面、断面等面状数据;•体集:地震数据、遥感数据、地层网格、盐丘、封闭体等数据体。
3.1 GOCAD软件的对象GOCAD中的对象包括PointSet、Curve、Surface、Solid、Voxet、SGrid、Well、Group、Channel、2D-Grid、X-Section、Frame、Model3d等类型。
3.2 GOCAD软件中对象的属性GOCAD中不同类型对象包含的属性不同。
GOCAD 软件三维地质建模方法
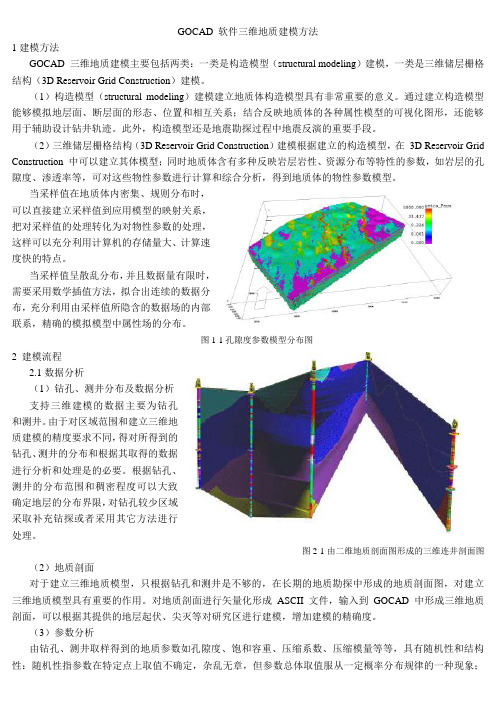
GOCAD 软件三维地质建模方法1建模方法GOCAD 三维地质建模主要包括两类:一类是构造模型(structural modeling)建模,一类是三维储层栅格结构(3D Reservoir Grid Construction)建模。
(1)构造模型(structural modeling)建模建立地质体构造模型具有非常重要的意义。
通过建立构造模型能够模拟地层面、断层面的形态、位置和相互关系;结合反映地质体的各种属性模型的可视化图形,还能够用于辅助设计钻井轨迹。
此外,构造模型还是地震勘探过程中地震反演的重要手段。
(2)三维储层栅格结构(3D Reservoir Grid Construction)建模根据建立的构造模型,在3D Reservoir Grid Construction 中可以建立其体模型;同时地质体含有多种反映岩层岩性、资源分布等特性的参数,如岩层的孔隙度、渗透率等,可对这些物性参数进行计算和综合分析,得到地质体的物性参数模型。
当采样值在地质体内密集、规则分布时,可以直接建立采样值到应用模型的映射关系,把对采样值的处理转化为对物性参数的处理,这样可以充分利用计算机的存储量大、计算速度快的特点。
当采样值呈散乱分布,并且数据量有限时,需要采用数学插值方法,拟合出连续的数据分布,充分利用由采样值所隐含的数据场的内部联系,精确的模拟模型中属性场的分布。
图1-1孔隙度参数模型分布图2 建模流程2.1数据分析(1)钻孔、测井分布及数据分析支持三维建模的数据主要为钻孔和测井。
由于对区域范围和建立三维地质建模的精度要求不同,得对所得到的钻孔、测井的分布和根据其取得的数据进行分析和处理是的必要。
根据钻孔、测井的分布范围和稠密程度可以大致确定地层的分布界限,对钻孔较少区域采取补充钻探或者采用其它方法进行处理。
图2-1由二维地质剖面图形成的三维连井剖面图(2)地质剖面对于建立三维地质模型,只根据钻孔和测井是不够的,在长期的地质勘探中形成的地质剖面图,对建立三维地质模型具有重要的作用。
gocad
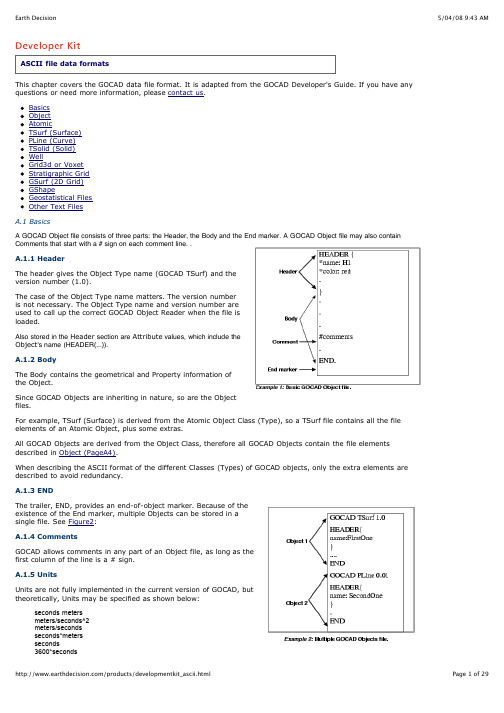
A GOCAD Object file consists of three parts: the Header, the Body and the End marker. A GOCAD Object file may also containused to call up the correct GOCAD Object Reader when the file isvalues, which include the0.44704*meters/seconds0.3048*meters/seconds0.3048*seconds*metersRestrictions: at most one scalar in front, at most one division.A.2 ObjectThe Object file elements define the Object style and the Object coordinate space and Units. Style AttributesThe HEADER block, delimited by {and}, contains the Style definition. Each line defines an Attribute of an Object. Attributes are strictly optional, except for the name of the Object. An Object must have a name.If preferred, an Attribute can be given on a separate line outside of the HEADER block; in that case the line should begin with the HDR keyword as shown in Example 3 (page 4).An example of Object Style element is:A.2.1 Coordinate SystemThe Object Coordinate System paragraph is optional for allobjects. If the paragraph is not present, the object is loaded inthe default coordinate system in the current session.The coordinate system description must be given after theHEADER block and before any other keyword giving the geometryof the object such as VRTX, PVRTX, ORIGIN, AXIS ....The Coordinate System must be given as follows:GOCAD_ORIGINAL_COORDINATE_SYSTEMNAME nameAXIS_NAME name_u name_v name_wAXIS_UNIT unit_u unit_v unit_wZPOSITIVE keywordEND_ORIGINAL_COORDINATE_SYSTEMIt is imperative that the Coordinate System definition is enclosed between the keywordsGOCAD_ORIGINAL_COORIDNATE_SYSTEM and END_ORIGINAL_COORDINATE_SYSTEM.The NAME and AXIS_NAME values are information string which gives the name of the local object coordinate system and its axes. The values must be specified by strings of characters enclosed in double-quotes, i.e. AXIS_NAME "X" "Y" "Z" or AXIS_NAME "X" "Y" "T"The AXIS_UNIT specifies the unit for each axes. The axis unit for the U and V axes must be identical, the axis unit for the W axis can be different from the unit of the U and V. It is possible to define units as AXIS_UNIT "m" "m" "m" or AXIS_UNIT "m" "m" "ft" but a definition such as AXIS_UNIT "m" "ft" 'km" is invalid. The AXIS_UNIT values must be specified by strings of characters enclosed by double-quotes.The ZPOSITIVE value indicates the direction of the Z axis. The values allowed for the keyword after ZPOSITIVE are either Depth (Z is increasing downwards) or Elevation (Z is increasing upwards)The coordinates are read in double precision converted to simple precision after coordinate system conversion. When an object is loaded into Gocad, its local coordinate system is compared with the current global coordinate system: if needed, the object geometrical coordinates are converted internally. When the coordinates are displayed, for example, for a picking in the 3D camera, the coordinates displayed are in the session coordinate system. When the object is saved back to a stand alone file, its geometrical coordinates are restored in its original coordinates system.A.3 AtomicThe Atomic data format defines the points, locations and Property values, of an Atomic (currently, this includes PointsSet, Curve, Surface, Solid and GShape). The Atomic inherits all of the Object file format elements, plus Atomic data.A.3.1 AtomicThis section describes the recommended Atomic file format.Since Property values are optional, a basic Atomic data line consists of three parts: Type, ID number, and data: VRTX ID X Y Z [CN]where the XYZ describes the location of that point. Additional information maybe attached at the end of each VRTX line to specify the Interpolation Restriction on that Atom (Control Node information).For example:A.3.1.1 Properties definitionIf there are Properties, they must bedeclared after the HEADER block andbefore the first point data line as:PROPERTIES Pname1 Pname2...PROPERTY_CLASSES class_name1class_name2 (optional, but if youdeclare one, you must declare all)UNITS unit1 unit2...(optional, but if youdeclare one, you must declare all)NO_DATA_VALUES v1 v2...(optional,but if you declare one, you mustdeclare all)ESIZES esize_1 esize_2... (optional, but if you declare one, you must declare all)The PROPERTIES line list all the properties that will be attached to the point. The ordering of the properties on the line correspond to the ordering of the properties values associated to the point definition.The PROPERTY_CLASSES line lists the property class names for each property defined in the PROPERTIES list. If one property class must be listed all property classes must be listed.The NO_DATA_VALUES line lists the no-data-values for each of the property defined in the property list. For vectorial properties, there is only one no-data-value and each property vector element should be equal to this value if it is to be treated as a no-data-value vectorial property.The UNITS line lists the units for each of the property defined in the property list (See Units for format specification of Units).The ESIZES line lists the dimension of each property defined in the property list. By default the dimension of a property is 1, but for example a property representing a 3D vector will be of dimension 3. For example. if you have a set of points with a 1 dimensional property (called "porosity") and a 3D vectorial property called "throw", the definition of the properties and one point will look like:PROPERTIES porosity throw ESIZES 1 3 PVRTX 1 X Y Z porosity_value throw_x throw_y throw_zwhere throw_x, throw_y and throw_z are the three components of the throw vector at the specified location.A.3.1.2 Point location and properties definitionThe data line which defines a point location and its properties look likes:PVRTX ID X Y Z PV1 PV2...where PV1, PV2... are Property values. For example.A.3.2 Parts (Subset)An Atomic Object can have its VRTXgrouped into subsets. In a VSet, the keywordis SUBVSET; in PLine, it is ILINE; in TSurf, itis TFACE; and in TSOLID, it is TVOLUME.A.3.3 Atomic-Old from C-GOCADThis section describes an older Atomicformat, mainly made to be compatiblewith C-GOCAD file format.The format consists of two sections, thelocation section and the Propertysection. The Property section isoptional.The location section is identical to thenew format:VRTX ID X Y ZThe Property value section contains a header and the list of Property values associated to a point defined in the previous section. The header defines the names of the DataPack Fields (Properties) associated with the Atomic. The following example Example 5 (page 8)declares three properties.FIELDS Pname1 Pname2(or PROPERTIES Pname1 Pname2...)PROPERTY_CLASSES class_name1 class_name2 (optional, but if you declare one, you must declare all)UNITS unit1 unit2...(optional, but if you declare one, you must declare all)NO_DATA_VALUES v1 v2...(optional, but if you declare one, you must declare all)ESIZES esize_1 esize_2... (optional, but if you declare one, you must declare all)The Property section define, for each point defined in the location section, the Property values associated with the Atomic's DataPackFields.REC ID1 ID2 PV1 PV2...Associated with a REC definition are two identifiers needed to be specified for compatibility reasons with earlier version. The two identifiers must be identical.An example of a Object file identical to the one given in Example 5 (page 8), but in this old data format is given below:.A.4 TSurf (Surface)The data file of a TSurf includes the inherited file elements of a Object and of an Atomic. The Atoms (Vertices) of the TSurf must be defined before the triangles.A.4.1 Geologic InformationBefore defining vertices and triangles, geologic information can be given.Geological information consists of GEOLIGICAL_TYPE, GEOLOGICAL_FEATURE and STRATIGRAPHIC_POSITION.The geological type of a TSurf can be specified using the following format:GEOLOGICAL_TYPE geological_type_namewhere geological_type_name can be one the following: top, intraformational, fault, unconformity, intrusive, topography, boundary, and ghost. The information is used during layer construction.The stratigraphic position is specified as follow:STRATIGRAPHIC_POSITION age timewhere age is the name of a stratigraphic unit. time is the numerical value of age (35000, etc.). This information is used during layer computation.The geological feature is specified as follow:GEOLOGICAL_FEATURE geologic_feature_namewhere geologic_feature_name is the name of the geologic feature that the surface represents. This information is used to associate surface to well markers (the geologic feature should have the same name than the well marker). A.4.2 TrianglesTriangles definition must occur after vertices definitions. Each Triangle is then defined by its three Vertices (Atoms) in the following format:TRGL id1 id2 id3where ids are the IDs of the already-defined Atoms (Vertices). For example, the ascii file corresponding to a TSurf named SQUARE, containing four vertices, and two triangles is shown in Figure1.A.4.3 Special Points: VRTX vs. ATOMIn addition to the VRTX or PVRTX point/vertex descriptor find in the Atomic format definition, on can find the additional line inside a TSurf ascii file:ATOM id1 id2 (where id1 > id2)where id1 is the index of the new ATOM and id2 is the index of the already existing VRTX node which XTZ this Atom shares.The goal of the ATOM keyword is to create a new ATOM but which shares an already existing vertex. An Atom node has its one Property values but it is spatially linked to the existing VRTX node. In other word, an ATOM and its referenced VRTX are collocated, but not connected. Triangles construction will use the vertex id or the atom id.An example of the use of such Atom record is given below:The input Tsurf file on the left will create a Surface shown at the center. The Surface has two TFaces, because two Triangles need to share an edge to be considered connected. When the Surface is saved, GOCAD will output the file on the right. It recognizes that the two Triangles both have one vertex at an identical location, but topologically, they can not be the same point. So, GOCAD creates a new point, ATOM 6, that shares the identical location of VRTX 3, but is an independent point in the sense that it has its own Property values (not in this case). However, had you have two more triangles in your input file, TRGL 2 4 3 and TRGL 6 5 1, the ATOM line would not have been created because the four triangles are then connected through direct or indirect edge-sharing.A.4.4 Parts/TFaceThe TFACE keyword is proposed to ensure identical indexing of the VRTX every time you save an Surface whose mesh you have not modified.In Figure2 the file on the right (the one output by GOCAD) not only has the extra ATOM line, it also two TFACE lines to separate VRTX (and ATOM) and TRGL from different TFaces. They arecurrently commented out for their full implementation is still to be determined by the GOCAD Committee.GOCAD will accept the simpler format shown on the right of Figure2 (where all the triangles and VRTX definition of all parts are all merged into one block) but will output the file shown on the right of Figure2.A.4.5 BordersAn edge of a TSurf can be a single piece or can be divided into multiple pieces. Each piece is called a Border, and it is separated from its neighboring Border by a Border Extremity. A Border Extremity is a designated node (VRTX, ATOM) on an edge of a TSurf that separates two Borders.Borders definition should appear at the end of the TSurf file format, in the format shown below:BSTONE atom_vrtx_idBORDER border_id bstone_id_1 bstone_id_2The BSTONE line defines an ATOM/VRTX as a Border Extremity.The BORDER line defines the border, border_id, as starting at vrtx_id1, which must have been declared as a Border Extremity in proceeding BSTONE lines, and continuing in the direction of vrtx_id2, which must be adjacent to vrtx_id1 (this border ends when it runs into another Border Extremity).The Border Extremities are always included in the list of points along the border.A.5 PLine (Curve)The Ascii PLine format inherits from the Object and from the Atomic formats. As for the TSurf, geologic information can be specified (See Geologic Information (PageA10)). For PLine geologic feature is used to relate multiple PLine to the same horizon.The Atoms of the Lines must be defined first. Each Segment in the PLine is then defined by its 2 apices in the following format:SEG id1 id2where ids are the IDs of the already-defined Atoms.For example, the ascii file corresponding to he PLine represented in Figure3 may look like:If no segments information is given, the atoms are assumed to form an open line, from the first atom to the last atom in the file order.If the PLine is composed of multiple parts, the parts can be combined or can be separated into ILines (or Isolated Lines). Each ILine is separated by a ILINE keyword and each ILine specify its own points and segments. In the same manner as for the TSurf, an ILINE keyword is proposed to ensure identical indexing of the VRTX every time you save a line which topology has not modified.A.6 TSolid (Solid)The Ascii TSolid format inherits from the Object and from the Atomic formats. The Atoms of the Atomic must be defined first. Each Tetrahedron in the Solid is then defined by its 4 apices in the following format.: TETRA id1 id2 id3 id4where ids are the IDs of the already-defined Atoms.For example, the ascii file corresponding to he Solid represented in Figure4 may look like:If the TSolid is composed of multiple parts, the parts can be combined or can be separated into TVolumes (or Tetrahedralize volumes). Each TVolume is separated by a TVOLUME keyword and each TVolume specify its own points and tetrahedras.[DIP azimuth dip][NORM X Y Z][MREF horizon_name][UNIT >rock_layer_name]Additional information (between [and]) can be attached to each Marker. This extra information includes the orientation (given as DIP or NORM) of the Marker, the Marker Reference Horizon (currently not utilized by GOCAD) and the Rock Unit (currently not utilized by GOCAD).The DIP information is given in Grads.What are Grads? It is an ancient French measurement of angles; there are 100 grads in a right angle (as opposed to 90 degrees in a right angle).The NORM information is always given in XYZ, representing the normal vector of the Marker. This is the preferred representation for Marker orientation (this is the way GOCAD will output Marker orientation information, instead of DIP).The optional information can be on the same line as the marker itself, or on lines that immediately follow the marker ascii code (MRKR).New WellMarker dip specificationsDIP angles are to be given in Grads, which is not a unit a lot of people are familiar with. GOCAD introduced a new dip specification DIPDEG where the two angles are given in degree.DIPDEG azimuth dipA.7.3 WellCurvesThe WellCurves section consists of two parts, the WellCurve Header section and the WellCurve Internal Data section. The header section defines the format of the Well Curves. The WellCurve data can be given in the same file, or in an external binary or ascii file.External Binary Data DeclarationIf the WellCurve data are stored in an external binary file, you must provide the name of the file, before any WellCurve Header information, using the following statement:BINARY_DATA_FILE filenameThe above statement gives the name of the binary file in which Well Curves are stored as a series of Z (measured depth) values and data values:P1Z1, P1Z2,...,P1Z20, P1V1, P1V2,...P1V20, P2Z1, P2Z2,...,P2Z12, P2V1, P2V2,..., P2V12, P3Z1,...etc.External ASCII Data DeclarationIf the data are stored in an external ASCII file, you must provide the following information before any WellCurve Header information:ASCII_DATA_FILE filenameDEPTH_COLUMN indexNCOLUMNS ncolNROWS nrowThe data are read in as a matrix of floating-point numbers and records are separated by blanks. The dimension of the matrix is defined by NCOLUMN and NROWs.Each column is a Property and NROWS specifies the number of data points per Property. The DEPTH_COLUMN specifies which column contains the measured depth data, which also means that in the external ASCII format, different Properties must all have data values at the same measured depth point.For example, the following file, named AsciiExample.wl.dat,P1V1 P2V1 P3V1 Z1 P4V1P1V2 P2V2 P3V2 Z2 P4V2P1V3 P2V3 P3V3 Z3 P4V3.....P1200 P2V200 P3V200 Z200 P4V200should be specified asASCII_DATA_FILE AsciiExampleDEPTH_COLUMN 4NCOLUMNS 5NROWS 200WellCurve HeaderEach WellCurve is defined inside a block beginning with WELL_CURVE and ending with END_CURVE. The format of the header is:NPTS nptsThis information will be used to read npts z values and npts data values from the binary file starting at Byte position seekpos. See Well with an External Binary Data File Example.A.7.4 Single Well File ExampleThe first example is a well file that includes the WellCurve data:A.7.5 Well with an External Binary Data File ExampleThe second example is a well with the WellCurve data stored in the external binary file described in External Binary Data Declaration:A.7.6 Well with an External ASCII Data File ExampleThe third example is a well with the WellCurve data stored in the external ASCII file described in External Binary Data Declaration:A.8 Grid3d or VoxetA Voxet is a rigid 3D Grid Object; it can carry multiple Properties. A Property in a Voxet is often referred to as a grid3d. It is derived from the Object Class.A common confusion comes from not realizing that UVW can mean the (i,j,k) indexing of the Voxet Nodes, but it can also mean the coordinate system in which the Voxet resides.In addition to its inherited Object file elements, a Voxet is further defined in two parts: the header section, which defines the geometry, and the grid3d sections which defines the Properties (grid3ds) and the Region section (which gives Region storage and Region information). Each Property in the Voxet has its own grid3d section that defines the Property and Property values.A.8.1 Geometry SectionUVW Coordinate System and Voxet DimensionAXIS_V, AXIS_W are required definitions. They define the coordinate system of the Voxet: represents the origin of the Voxet coordinate system, not the Voxet the origin.).For example, in Seismic AXIS_U represents typically the time or depth axis. An example will be: AXIS_U 0. 0.0004 (in A common confusion comes from not realizing that this set of vectors, AXES_OUVW, only defines the UVW coordinateThe upper two are the most common cases; i.e. the AXES UVW either define the entire volume dimension or they define a cell dimension. When GOCAD outputs a Voxet file, it uses the upper most format; i.e. the UVW axes define the entire volume.REGION region_name region_bit_marker_position_number (must be less than number_of_bits) An example of an Voxet ASCII file with Region information is given below:....AXIS_N 10 10 10FLAGS_ARRAY_LENGTH 1000FLAGS_BIT_LENGTH 10FLAGS_ESIZE 2FLAGS_OFFSET 0FLAGS_FILE v1__flags@@REGION RegionExample 6REGION HighPorosity 7REGION LowPerm 8REGION BadBuy 9ENDA.8.3 Grid3d/Property SectionThe grid3d section defines the property. Currently, Region information must be given before Property information.For each property there is a unique identifier, id, which is used to relate different Property statements to the same Property. The data can be in an external file in a binary format, or inside the file in ascii format.For each Property, the declaration must be the first line (PROPERTY id "property name") and the Property file name (PROP_FILE id filename) or data (DATA) must be the last line.PROPERTY id "property name"PROPERTY_CLASS id "property-class_name"PROP_UNIT id "unit"PROP_LEGAL_RANGE id min max (where min/max is a float or **none**PROP_SAMPLE_STATS id n x x2 min maxPROP_NO_DATA_VALUE id valuePROP_SAMPLE_STATS id n x x2 min maxPROP_ETYPE id data_type (either IEEE or IBM)PROP_FORMAT id file_format (either RAW or SEGY)PROP_ESIZE id element_size (either 1 or 4)PROP_FILE id filenamePROP_OFFSET id offsetDATAPROP_OFFSET indicates that the array of float value begins at the given offset (in bytes, = number of data points x PROP_ESIZE) in the PROP_FILE.PROP_ETYPE specifies the type of floating point value that is in the file. It can be IBM floating point value or IEEE floating point value.PROP_FORMAT specifies the format of the file. SEGY format implies that the data resides in a standard SEGY file. RAW specifies that the data is formatted as a C array where the fast axis is the Axis_U.The token DATA, if present, indicates that the Property data is to be read following that token. If this is the case, the floating point array of data must be in ascii RAW format.A.8.4 ExampleAn example of a Voxet file with an external Property file:GOCAD Voxet 0.01AXIS_O 2128403. -79200. 0.AXIS_U 0. 0. 55.AXIS_V 0. 220. 0.AXIS_W 220. 0. 0.AXIS_MIN 0.0 0.0 0.0AXIS_MAX 549. 227. 149.AXIS_N 550 228 150AXIS_D 1. 1. 1.AXIS_NAME "Z" "Y" "X"AXIS_UNIT "m" "m" "m"AXIS_TYPE even even evenPROPERTY 1 "Seismic"PROP_UNIT 1 " "PROP_ESIZE 1 4PROP_ETYPE 1 IEEEPROP_FILE 1 /tmp/seismic.nohdrA.9.1 Header SectionREGION_FLAGS_ARRAY_LENGTH length_of_the _array REGION_FLAGS_BIT_LENGTH number_of_bits (at least 1greater than the number of Regions) REGION_FLAGS_ESIZE number_of_bytes (should equal (number_of_bits+1)/8rounded up) REGION_FLAGS_OFFSET offset_inside_the_binary_file_for_the_first_data_point REGION_FLAGS_FILEbinary_file_name (values in the file increase first in U, then V, then W)An example of an SGrid file with Region information is given below:AXIS_N 41 21 31.....FLAGS_FILE MySGrid__flags@@REGION Reg_top_1 0REGION Reg_1_2 1REGION Reg_2_bot 2REGION Facies_Region_1 3REGION Facies_Region_2 4REGION Facies_Region_3 5REGION Facies_Region_4 6REGION Facies_Region_5 7REGION Facies_Region_6 8REGION BM_Inactive_Region 9REGION_FLAGS_ARRAY_LENGTH 26691REGION_FLAGS_BIT_LENGTH 10REGION_FLAGS_ESIZE 2REGION_FLAGS_OFFSET 0REGION_FLAGS_FILE MySGrid__region_flags@@PROPERTY 1 "Geol_Facies_1"PROPERTY_CLASS 1 "facgm".....A.9.3 Property SectionThe Property section defines the property at each node. Currently, Region information must be given before Property information. Each Property has its unique identifier, id, which is used to relate different Property statements to that Property. The data can be in an external file in a ascii or binary format.For each Property, the declaration must be the first line (PROPERTY id "property name") and theProperty file name (PROP_FILE id filename) or data (DATA) must be the last line.The definitions of the Property format is identical to Voxet. Please see Grid3d/Property Section (PageA31).PROPERTY id "property name"PROPERTY_CLASS id "property_class_name"PROP_UNIT id "unit"PROP_LEGAL_RANGE id min max (where min/max is a float or none)PROP_NO_DATA_VALUE id valuePROP_SAMPLE_STATS id n x x2 min maxPROP_ETYPE id data_type (must be IEEE, IBM, or SEGY)PROP_ESIZE id element_size (either 1 or 4)PROP_FILE id filenamePROP_NO_DATA_VALUE id no_data_valuePROP_OFFSET id offsetDATAThe fast axis is Axis_U. The data are specified as a C array.If the SGrid is cell-centered the size of the point array will be NX*NY*NZ but the size of the Property arrays will be (NX-1)*(NY-1)*(NZ-1).A.9.4 Ascii External FileThe geometry and the properties can both be read from an ASCII data file. First this file must be specified in the header as follows:ASCII_DATA_FILE filenameThe format of that file is:x y z p1 p2 p3... flag u v wwhere x, y, z specifies the location of that node; p1, p2, p3,... are the Property values at that node; flag specifies the connectivity flag of that node; and u, v, w specifies the index of the node.A.9.5 Split NodesIntroductionA node is a corner of a cell that is usually shared between 8 cells except when a fault affect this area (and on theborder where less cells are available).Faulting introduces split nodes.The ascii or binary file describing the geometry contains one x,y,z location. When a grid is faulted the nodes affected by the faulting have several x,y,z ; additional x,y,z information (the first one is in the ascii or binary file describing the geometry) is stored in the SGrid header file (usually defined with the extension ?s .sg ?t). The following set of examples are linked with the following SGrid depicted in Figure10FormatHere is an example of split node description :SPLIT 59 19 3 -225.76 177.902 1407.1 36 1 0 0 0 1 0 0 0 1This corresponds to the following information :SPLIT U V W X Y Z id Cell(u,v,w) Cell(u-1,v,w) Cell(u,v-1,w) Cell(u-1,v-1,w), Cell(u,v,w-1) Cell(u-1,v,w-1) Cell(u,v-1,w-1) Cell(u-1,v-1,w-1)This split node is attached to the grid regular node U V W. The first occurrence of a split node U V W means that the regular node is split. There can be up to 7 SPLIT lines per U V W.All the Cell(u,v,w) are boolean values (0 or 1).If Cell(u-1,v-1,w) is equal to 1, then this node (SPLIT) is a corner of the Cell(u_1,v-1,w).Remark : when a node is on the external border of the grid, it does not have 8 neighbor cells. For the missing cells the boolean value is set to 1Split FaceNodes are split along faces. When a face is split, split faces can be gathered in FaceSets. A FaceSet is described as: FACET_SET name number_of_faces cell_uvw_1 face_dir_1.... cell_uvw_2 face_dir_2where cell_uvw represents the cell number (U+V*NU+W*NU*NV) and face_dir is the face indicator (U=0, V=1, W=2).A.9.6 ExamplesA.9.6.1 External Binary filesAn example of an SGrid with one Property and external binary files (lines that start with an * are optional Attribute lines):GOCAD SGrid 0.01HEADER {name:s3}AXIS_N 32 32 32PROP_ALIGNMENT CELLSPOINTS_OFFSET 0POINTS_FILE s3__points@@FLAGS_OFFSET 0FLAGS_FILE s3__flags@@PROPERTY 1 "SGS_simulation_1"PROPERTY_CLASS 1 "porndx"PROP_UNIT 1 nonePROP_SAMPLE_STATS 1 62883 0.24624 0.000661522 0 0.415PROP_ESIZE 1 4PROP_ETYPE 1 IEEEPROP_ALIGNMENT 1 CELLSPROP_OFFSET 1 0PROP_FILE 1 s3_SGS_simulation_1@@ENDA.9.6.2 External Ascii FileBelow is the header file for the SGrid with one property.GOCAD SGrid 0.01 HEADER { name:s3 *painted:on } AXIS_N 32 32 32 PROP_ALIGNMENT CELLS ASCII_DATA_FILE s3__ascii@@ PROPERTY 1 "SGS_simulation_1" PROPERTY_CLASS 1 "porndx" PROP_UNIT 1 nonePROP_SAMPLE_STATS 1 29791 0.247145 0.000618586 0.154 0.414999 PROPERTY_CLASS_HEADER 1 "porndx" {*low_clip:0.183077 *high_clip:0.311214 *pclip:99 } ENDBelow are the first lines off an ASCII external file* * X Y Z SGS_simulation_1 Flag I J K * 145745 9416230 -3458.3147 0.229000002 1031 0 0 0 145995 9416230 -3459.58105 0.229790032 1039 1 0 0 146245 9416230 -3460.82251 0.229000002 1039 2 0 0 146495 9416230 -3462.18555 0.228938624 1039 3 0 0。
GOCAD 操作手册
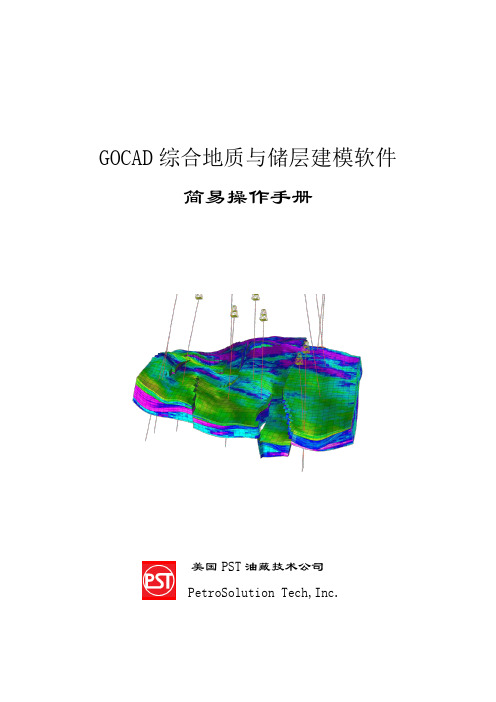
GOCAD综合地质与储层建模软件简易操作手册美国PST油藏技术公司PetroSolution Tech,Inc.目录第一节 GOCAD综合地质与储层建模软件简介┉┉┉┉┉┉┉┉┉┉┉┉┉┉1一、GOCAD特点┉┉┉┉┉┉┉┉┉┉┉┉┉┉┉┉┉┉┉┉┉┉┉┉┉1二、GOCAD主要模块┉┉┉┉┉┉┉┉┉┉┉┉┉┉┉┉┉┉┉┉┉┉┉1 第二节 GOCAD安装、启动操作┉┉┉┉┉┉┉┉┉┉┉┉┉┉┉┉┉┉┉┉2一、GOCAD的安装┉┉┉┉┉┉┉┉┉┉┉┉┉┉┉┉┉┉┉┉┉┉┉┉2二、GOCAD的启动┉┉┉┉┉┉┉┉┉┉┉┉┉┉┉┉┉┉┉┉┉┉┉┉3 第三节 GOCAD数据加载┉┉┉┉┉┉┉┉┉┉┉┉┉┉┉┉┉┉┉┉┉┉┉5一、井数据加载┉┉┉┉┉┉┉┉┉┉┉┉┉┉┉┉┉┉┉┉┉┉┉┉┉5二、层数据加载┉┉┉┉┉┉┉┉┉┉┉┉┉┉┉┉┉┉┉┉┉┉┉┉┉11三、断层数据加载┉┉┉┉┉┉┉┉┉┉┉┉┉┉┉┉┉┉┉┉┉┉┉┉11四、层面、断层面加载┉┉┉┉┉┉┉┉┉┉┉┉┉┉┉┉┉┉┉┉┉┉12五、地震数据加载┉┉┉┉┉┉┉┉┉┉┉┉┉┉┉┉┉┉┉┉┉┉┉┉12 第四节 GOCAD构造建模┉┉┉┉┉┉┉┉┉┉┉┉┉┉┉┉┉┉┉┉┉┉┉13一、准备工作┉┉┉┉┉┉┉┉┉┉┉┉┉┉┉┉┉┉┉┉┉┉┉┉┉┉13二、构造建模操作流程┉┉┉┉┉┉┉┉┉┉┉┉┉┉┉┉┉┉┉┉┉┉14三、构造建模流程总结┉┉┉┉┉┉┉┉┉┉┉┉┉┉┉┉┉┉┉┉┉┉40 第五节建立GOCAD三维地质模型网格┉┉┉┉┉┉┉┉┉┉┉┉┉┉┉┉41一、新建三维地质模型网格流程┉┉┉┉┉┉┉┉┉┉┉┉┉┉┉┉┉┉41二、三维地质模型网格流程┉┉┉┉┉┉┉┉┉┉┉┉┉┉┉┉┉┉┉┉41三、三维地质模型网格流程总结┉┉┉┉┉┉┉┉┉┉┉┉┉┉┉┉┉┉47 第六节 GOCAD储层属性建模┉┉┉┉┉┉┉┉┉┉┉┉┉┉┉┉┉┉┉┉┉48一、建立属性建模新流程┉┉┉┉┉┉┉┉┉┉┉┉┉┉┉┉┉┉┉┉┉48二、属性建模操作流程┉┉┉┉┉┉┉┉┉┉┉┉┉┉┉┉┉┉┉┉┉┉48三、属性建模后期处理┉┉┉┉┉┉┉┉┉┉┉┉┉┉┉┉┉┉┉┉┉┉66四、网格粗化┉┉┉┉┉┉┉┉┉┉┉┉┉┉┉┉┉┉┉┉┉┉┉┉┉┉74 第七节 GOCAD地质解释和分析┉┉┉┉┉┉┉┉┉┉┉┉┉┉┉┉┉┉┉┉78GOCAD综合地质与储层建模软件操作手册第一节GOCAD综合地质与储层建模软件简介Gocad是国际上公认的主流建模软件,在众多油公司和服务公司得到了广泛的应用。
GOCAD(外文资料) (30)

3D TGI Williston Basin – Gocad/Geocando projectNotes and IssuesIMPORTANT:In order for the project paths to function correctly (relative paths have not yet been implemented in Geocando) the zip file must be extracted to C:\The TGI Williston Basin 3D Geological model is based on a database of unit tops picked by geologists from selected drillholes penetrating the majority of the post-Precambrian stratigraphy and mapped unit edges derived from legacy mapping and drillhole intercepts. On average, five wells per township were selected from areas with sufficient drillhole density. The 3D surfaces were constructed using these picked tops from a total of 9012 wells, which includes 5046 wells from Saskatchewan, 2606 wells from Manitoba, and in order to reduce edge-effects, 771 wells from North Dakota and 589 from Montana. Of the 60 geological units in the TGI Williston Basin project, 42 were selected and modeled. The model was produced using Gocad earth modeling software.This model is entirely data driven with minimal human interpretation. Many fringe areas have a low data density, especially those areas close to unit edges. Because of this shortcoming, the expression of the unit edge (ie. escarpments) isn’t always accurately predicted.The TGI team was dedicated to maintaining accuracy and consistency in the picks and modeled information presented, however due to the size of the project, some errors may have been made. The TGI team is not liable for these errors. The user of this information accepts all responsibility for any work done on their part that uses all or part of this data. The data files included in this zip file have been exported from Gocad modeling software. These files can be opened using Gocad modeling software or the free Gocad viewer, Geocando. For ease of use, a Geocando project file has been included(Gocad_TGI_Model_Geocando.gtp). To use this file, open Geocando and navigate to File Æ Open Project…* Please see the ‘important issues’ section below for further information.This Geocando project includes:-42 modeled surfaces from Precambrian to rock surface-SRTM DEM ground surface-Original data pointsets used as the basis for model creationo Edge and tie points are not included-Cultural data in the form of borders and roadsGEOCANDO SOFTWARE FEATURESVertical ExaggerationWhen a project is first loaded into Geocando, the vertical exaggeration is set to 1x. In order to modify the vertical exaggeration, navigate to:1) View Æ Z-Scaling…2) Change the value to 75x for best resultsQuery toolSince the TGI Williston Basin 3D model is in real-world coordinates, you can query the model to extract coordinates from a point, and you can measure distances.Get Coordinates1) Info Æ Get Coordinates2) Click anywhere on the model. Coordinates will be displayed in the bottom left of the screenDistance tool1) Info Æ Measure Distances2) Click anywhere on the model to set the first measurement point. Click again in another location to set the second measurement point.* These tools can also be accessed using the shortcut buttons on the toolbar at the top of the screen.IMPORTANT ISSUESIn order for the project paths to function correctly (relative paths have not yet been implemented in Geocando) the zip file must be extracted to C:\If you wish to extract the file to another drive/directory, the Geocando project file (.gtp) must be modified to reflect the new path. Each surface has an associated path. The current path is: C:/3D_TGI_Williston_Basin.This issue should disappear as soon as Geocando supports relative paths.NOTE1)Many surfaces in the model are very close (vertically) to one another. Geocandorenders surfaces differently than Gocad, as a result of this difference, Geocandomay show the surfaces as interleaving. If you open the surfaces in Gocad you will not see this issue.2)Surfaces appear ‘rough’ in Geocando. Gocad’s interpolation engine renders thesurfaces with a much smoother appearance.。
GOCAD 操作手册
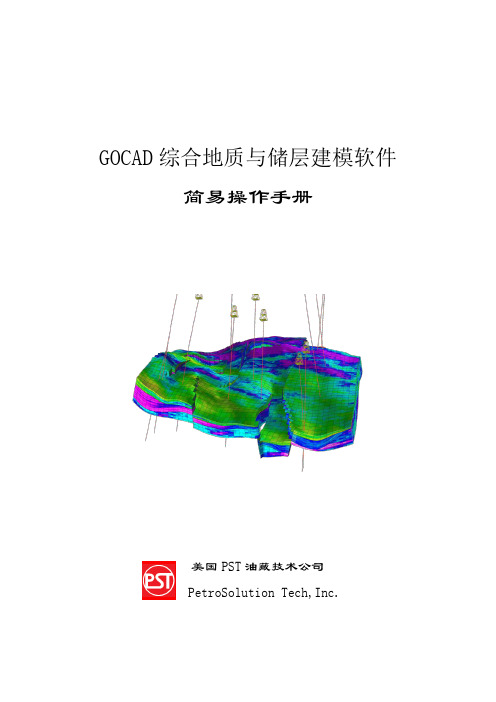
GOCAD综合地质与储层建模软件简易操作手册美国PST油藏技术公司PetroSolution Tech,Inc.目录第一节 GOCAD综合地质与储层建模软件简介┉┉┉┉┉┉┉┉┉┉┉┉┉┉1一、GOCAD特点┉┉┉┉┉┉┉┉┉┉┉┉┉┉┉┉┉┉┉┉┉┉┉┉┉1二、GOCAD主要模块┉┉┉┉┉┉┉┉┉┉┉┉┉┉┉┉┉┉┉┉┉┉┉1 第二节 GOCAD安装、启动操作┉┉┉┉┉┉┉┉┉┉┉┉┉┉┉┉┉┉┉┉2一、GOCAD的安装┉┉┉┉┉┉┉┉┉┉┉┉┉┉┉┉┉┉┉┉┉┉┉┉2二、GOCAD的启动┉┉┉┉┉┉┉┉┉┉┉┉┉┉┉┉┉┉┉┉┉┉┉┉3 第三节 GOCAD数据加载┉┉┉┉┉┉┉┉┉┉┉┉┉┉┉┉┉┉┉┉┉┉┉5一、井数据加载┉┉┉┉┉┉┉┉┉┉┉┉┉┉┉┉┉┉┉┉┉┉┉┉┉5二、层数据加载┉┉┉┉┉┉┉┉┉┉┉┉┉┉┉┉┉┉┉┉┉┉┉┉┉11三、断层数据加载┉┉┉┉┉┉┉┉┉┉┉┉┉┉┉┉┉┉┉┉┉┉┉┉11四、层面、断层面加载┉┉┉┉┉┉┉┉┉┉┉┉┉┉┉┉┉┉┉┉┉┉12五、地震数据加载┉┉┉┉┉┉┉┉┉┉┉┉┉┉┉┉┉┉┉┉┉┉┉┉12 第四节 GOCAD构造建模┉┉┉┉┉┉┉┉┉┉┉┉┉┉┉┉┉┉┉┉┉┉┉13一、准备工作┉┉┉┉┉┉┉┉┉┉┉┉┉┉┉┉┉┉┉┉┉┉┉┉┉┉13二、构造建模操作流程┉┉┉┉┉┉┉┉┉┉┉┉┉┉┉┉┉┉┉┉┉┉14三、构造建模流程总结┉┉┉┉┉┉┉┉┉┉┉┉┉┉┉┉┉┉┉┉┉┉40 第五节建立GOCAD三维地质模型网格┉┉┉┉┉┉┉┉┉┉┉┉┉┉┉┉41一、新建三维地质模型网格流程┉┉┉┉┉┉┉┉┉┉┉┉┉┉┉┉┉┉41二、三维地质模型网格流程┉┉┉┉┉┉┉┉┉┉┉┉┉┉┉┉┉┉┉┉41三、三维地质模型网格流程总结┉┉┉┉┉┉┉┉┉┉┉┉┉┉┉┉┉┉47 第六节 GOCAD储层属性建模┉┉┉┉┉┉┉┉┉┉┉┉┉┉┉┉┉┉┉┉┉48一、建立属性建模新流程┉┉┉┉┉┉┉┉┉┉┉┉┉┉┉┉┉┉┉┉┉48二、属性建模操作流程┉┉┉┉┉┉┉┉┉┉┉┉┉┉┉┉┉┉┉┉┉┉48三、属性建模后期处理┉┉┉┉┉┉┉┉┉┉┉┉┉┉┉┉┉┉┉┉┉┉66四、网格粗化┉┉┉┉┉┉┉┉┉┉┉┉┉┉┉┉┉┉┉┉┉┉┉┉┉┉74 第七节 GOCAD地质解释和分析┉┉┉┉┉┉┉┉┉┉┉┉┉┉┉┉┉┉┉┉78GOCAD综合地质与储层建模软件操作手册第一节GOCAD综合地质与储层建模软件简介Gocad是国际上公认的主流建模软件,在众多油公司和服务公司得到了广泛的应用。
GOCAD油藏三维综合地质建模技术
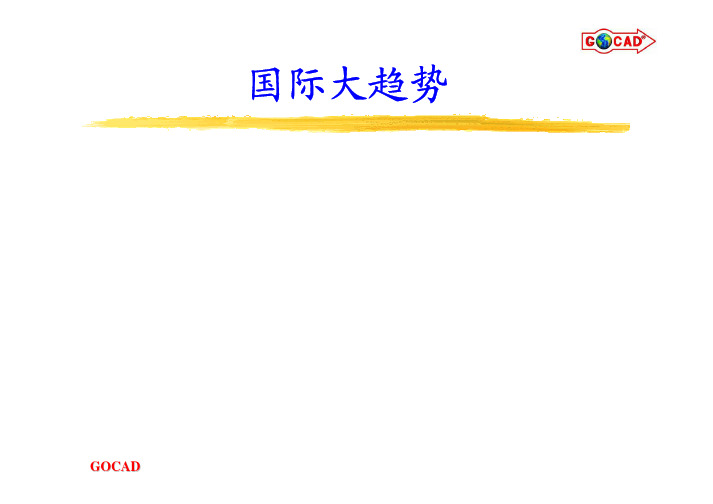
国际大趋势在勘探开发日益一体化的今天,国际上已相当普及从油气田规模到区块规模再到单个油气藏的三维定量综合地质建模。
对于中国普遍存在的复杂地质条件油气藏,三维综合地质建模更不可少,可以肯定地说,这也是国内勘探开发一体化发展的必然环节。
勘探开发一体化三维综合地质建模及虚拟现实技术您理想的综合地质建模解决方案主要内容国际石油工业界的建模共识 国际石油工业界的通力协作 GOCAD软件产品简介GOCAD综合建模技术特色GOCAD 综合建模技术简介国际石油工业界的建模共识现代油气藏科学管理两大支柱:静态描述+动态模拟•油气藏描述:目标―储层三维定量地质模型构造格架模型、储层结构模型、流动单元模型、参数分布模型•油气藏模拟:基础―储层三维定量地质模型三维油藏数值模拟成败的关键什么是储层三维定量地质模型呢?定量表征油气藏目前状态下,储层特征(储层结构及属性参数)在三维空间分布规律的地质模型。
储层地质模型分类一、按勘探开发阶段及模型精度分类(裘亦楠,1991)•概念模型(Conceptual model)•静态模型(Static model)•预测模型(Predictable model)储层概念模型:点坝砂体的半连通模式(据薛培华,1991)储层静态地质模型概念:描述某一具体油气田或区块的一个或一套储层的属性特征在三维空间上的变化和分布规律的地质模型。
目的:为编制开发方案及调整方案提供地质依据实现:小层平面图、油层剖面图、栅状图、三维分布图、切片图缺点:地震-信息覆盖面广但垂向分辨率低测井-井间参数内插与外推预测精度考虑较少,精度欠缺储层预测地质模型概念:对井间及其外围地区的储层参数进行高精度内插和外推预测的高质量地质模型。
目的:开发调整、井网加密或三次采油实现:地质随机建模技术、地震约束、井网控制精度:井间预测精度―数十米或数米级储层地质模型分类二、按储层表征内容或建模步骤分类•构造格架模型•储层结构模型•流动单元模型•参数分布模型储层结构地质模型储层相模型:相控建模技术应用的基础概念:描述储层几何形态以及三维空间分布的地质模型。
GOCAD(外文资料) (13)
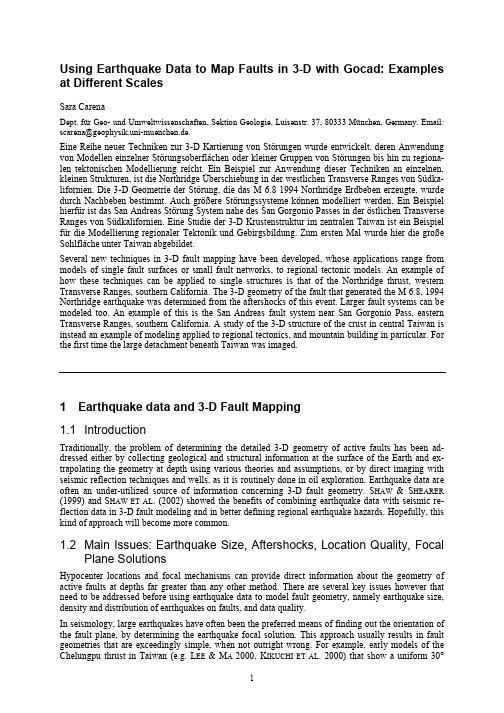
Using Earthquake Data to Map Faults in 3-D with Gocad: Examples at Different ScalesSara CarenaDept. für Geo- und Umweltwissenschaften, Sektion Geologie, Luisenstr. 37, 80333 München, Germany. Email: scarena@geophysik.uni-muenchen.de.Eine Reihe neuer Techniken zur 3-D Kartierung von Störungen wurde entwickelt, deren Anwendung von Modellen einzelner Störungsoberflächen oder kleiner Gruppen von Störungen bis hin zu regiona-len tektonischen Modellierung reicht. Ein Beispiel zur Anwendung dieser Techniken an einzelnen, kleinen Strukturen, ist die Northridge Überschiebung in der westlichen Transverse Ranges von Südka-lifornien. Die 3-D Geometrie der Störung, die das M 6.8 1994 Northridge Erdbeben erzeugte, wurde durch Nachbeben bestimmt. Auch größere Störungssysteme können modelliert werden. Ein Beispiel hierfür ist das San Andreas Störung System nahe des San Gorgonio Passes in der östlichen Transverse Ranges von Südkalifornien. Eine Studie der 3-D Krustenstruktur im zentralen Taiwan ist ein Beispiel für die Modellierung regionaler Tektonik und Gebirgsbildung. Zum ersten Mal wurde hier die große Sohlfläche unter Taiwan abgebildet.Several new techniques in 3-D fault mapping have been developed, whose applications range from models of single fault surfaces or small fault networks, to regional tectonic models. An example of how these techniques can be applied to single structures is that of the Northridge thrust, western Transverse Ranges, southern California. The 3-D geometry of the fault that generated the M 6.8, 1994 Northridge earthquake was determined from the aftershocks of this event. Larger fault systems can be modeled too. An example of this is the San Andreas fault system near San Gorgonio Pass, eastern Transverse Ranges, southern California. A study of the 3-D structure of the crust in central Taiwan is instead an example of modeling applied to regional tectonics, and mountain building in particular. For the first time the large detachment beneath Taiwan was imaged.1 Earthquake data and 3-D Fault Mapping1.1 IntroductionTraditionally, the problem of determining the detailed 3-D geometry of active faults has been ad-dressed either by collecting geological and structural information at the surface of the Earth and ex-trapolating the geometry at depth using various theories and assumptions, or by direct imaging with seismic reflection techniques and wells, as it is routinely done in oil exploration. Earthquake data are often an under-utilized source of information concerning 3-D fault geometry. S HAW & S HEARER (1999) and S HAW ET AL. (2002) showed the benefits of combining earthquake data with seismic re-flection data in 3-D fault modeling and in better defining regional earthquake hazards. Hopefully, this kind of approach will become more common.1.2 Main Issues: Earthquake Size, Aftershocks, Location Quality, FocalPlane SolutionsHypocenter locations and focal mechanisms can provide direct information about the geometry of active faults at depths far greater than any other method. There are several key issues however that need to be addressed before using earthquake data to model fault geometry, namely earthquake size, density and distribution of earthquakes on faults, and data quality.In seismology, large earthquakes have often been the preferred means of finding out the orientation of the fault plane, by determining the earthquake focal solution. This approach usually results in fault geometries that are exceedingly simple, when not outright wrong. For example, early models of the Chelungpu thrust in Taiwan (e.g. L EE & M A 2000, K IKUCHI ET AL. 2000) that show a uniform 30°58. Berg- und Hüttenmännischer Tagdipping plane to depths of 20 km are incorrect, because they assume that the fault keeps a constant strike and dip with increasing depth. In this case however the shallow main-shock focal mechanism is not representative of the entire geometrically complex fault, which flattens to a sub-horizontal de-tachment at 5-6 km depth (Y UE ET AL. 2005). In other instances, the slip direction in a large earth-quake does not coincide with the long-term slip direction (see the Northridge thrust, C ARENA & S UPPE 2002).Often most aftershocks are not located on the main fault that ruptured, but on nearby ones (L IU ET AL. 2003). Ambiguities could be avoided by imaging the entire fault network in the area using background seismicity and previous earthquake sequences. This approach can help us to recognize the principal plane by matching it with previous events with similar focal mechanisms. Microearthquakes (M≤3) are particularly useful for this purpose due to their abundance. Networks that can reliably record mi-croseismicity already exist in a few regions of the world, and more are being added.The most important issue to consider is the quality of the earthquake data. In recent years, several authors have developed new methods of improving earthquake hypocenter locations significantly (G OT ET AL. 1994, J ONES & S TEWART 1997, R UBIN ET AL. 1999, N ICHOLSON ET AL. 2000; R ICHARDS-D INGER & S HEARER 2000, W ALDHAUSER & E LLSWORTH 2002). In our work we gave preference to the clustering method of J ONES & S TEWART (1997) because it can be quickly applied to large earth-quake catalogs (hundreds of thousands of events), because the only information needed about the earthquakes are their location and location uncertainties, and because it does not result in the exclusion of some events. Of course, the more details we want to extract about the fault 3-D geometry, the more a need arises for combining different methods in order to get the best possible hypocenter locations. In fact, even when using the clustering method, the ideal approach is to start with an earthquake catalog that has already been relocated to minimize systematic errors as much as possible (J ONES & S TEWART 1997).Focal mechanisms are extremely useful in 3-D fault modeling because, besides providing confirmation of fault geometry when there is an abundance of hypocenter locations, in certain cases they provide enough additional information to image even faults with a small number of events on them. Unlike event locations, that only provide information about fault shape, focal mechanisms allow us to deter-mine fault type and direction of motion. Thus we fitted fault surfaces simultaneously to both earth-quake hypocenter locations and principal planes extracted from focal mechanisms whenever possible. The main problem with focal mechanisms is their reliability, which decreases with decreasing earth-quake size, as the event is recorded only at a few stations.1.3 Integration of Other Data TypesEarthquakes are not the only kind of data that can be successfully used in 3-D fault modeling, and earthquakes do not occur everywhere. Therefore other types of data should be integrated in the model whenever available to fill any gaps and to better constrain it. S HAW & S HEARER (1999) and S HAW ET AL. (2002), for instance, have shown how combining seismic reflection profiles with relocated earth-quake data can significantly improve results when imaging blind faults.Surface geological data, like strike and dip measurements, stratigraphy, and the detailed location of fault traces and earthquake surface breaks are also key data, because seldom large numbers of earth-quakes occur at depths shallower than a few kilometers. For example, the aftershocks of the Northridge earthquake occurred mostly below 3-5 km depth and did not provide any information about fault geometry, or even the presence of any faulting, at shallower depths (C ARENA & S UPPE 2002). In the case of the Chelungpu thrust in Taiwan, very few aftershocks of the 1999 Chi-Chi earthquake oc-curred on the thrust itself; most of them were deeper and occurred on nearby faults. Nevertheless, Y UE ET AL. (2005) were able to generate a detailed 3-D fault model on the basis of strike and dip data, inte-grating them with surface breaks and several seismic profiles, and then connecting their model with the deeper fault network imaged from seismicity.Digital elevation models are necessary to transform a map of fault traces or surface breaks into a 3-D trace that can be directly incorporated into the fault model. Fault depth measurements in wells are also a very good constraint when available, as they are yet another type of data that can give us information about fault geometry at shallow depths.Sara Carena Using Earthquake Data to Map Faults in 3-D with Gocad: Examples at Different Scales1.4 Some Techniques in 3-D Surface BuildingRealistic fault geometry is first of all a geometry that allows the fault to slip. Large jogs perpendicular to the long-term slip direction and large bumps are unrealistic, because not only they would impede slip itself, but also because slip on the fault would quickly destroy them. On the other hand, corruga-tions parallel to the slip direction can certainly exist and have been used by some authors to constrain the 3-D fault geometry when modeling faults. Based on faults whose geometry is well known due to direct observation of the fault surface or to numerous wells intersecting it,T HIBAUT ET AL. (1996) developed the concept of faults as “thread surfaces”. They improve the results of interpolating be-tween scattered points (a common characteristic of fault data) by assuming that faults behave in a way similar to a nut-and-bolt system, with the contact surface between the two blocks dented by grooves (threads) produced by slip on the fault. They obtain the orientation of the threads (which are lineations on the fault plane at any scale, from striae visible in the field, to corrugations of the fault surface with wavelengths up to 10 km or greater) from areas with denser data, and apply their thread criterion to the interpolation in areas of low data density, thus obtaining a consistent fault geometry everywhere. A similar approach is that of M ALLET ET AL. (1999) and M ALLET (2002), who show how fault corruga-tions imaged from actual data can be combined with the assumption that long-term slip must occur parallel to the corrugations to further improve a 3-D fault model, removing bumps on the surface that would impede slip and which are most likely the result of scattering or gaps in the data.2 A New Approach to 3-D Fault MappingOur approach requires handling up to several hundred thousands of earthquakes at once, and the abil-ity to integrate different data types into a single model. This is why Gocad was chosen as a modeling toolbox. While the main focus of Gocad is geomodeling, it was not designed specifically with han-dling earthquakes in mind, so we had to devise some procedures to be able to use it to manipulate earthquake data efficiently.We start from hypocenter locations that have been relocated if possible. The hypocenter locations are then clustered using the clustering method developed and described by J ONES & S TEWART (1997) and modified by N ICHOLSON ET AL. (2000). Clustering results in tighter earthquake distributions (a “sharper” image, see fig. 1), which makes the process of selecting subsets of earthquakes much easier.Fig. 1. Example of earthquake hypocenters (aftershocks of M 7.1, 1986, Loma Prieta, California, earth-quake) before (a) and after (b) application of the clustering algorithm. (c) and (d) show the mis-fit to the 3-D fault surface.58. Berg- und Hüttenmännischer TagFault geometry can be constrained by focal mechanisms as well. The comparison between earthquake hypocenter distribution and focal mechanisms in 3-D allows us to [1] distinguish between principal and auxiliary nodal planes, thus making it possible to select only the principal planes and vectors in the data set, [2] identify and map faults which have only a few events associated with them, [3] deter-mine the current slip direction on faults. Nodal planes and slip vectors are imported into Gocad as surfaces and lines. Once one of the two nodal planes has been identified as the principal plane, focal mechanisms can then be transformed into point sets and used directly in fault surface building.A third type of constraint that can be applied to fault geometry is surface traces, either in the form of known breaks caused by a specific earthquake (C ARENA & S UPPE 2002), or as mapped fault traces. Surface traces constrain the position of the top of the fault and may disclose a near-surface change in fault dip that could have gone undetected due to the general lack of earthquakes at shallow depths. Once earthquake hypocenter locations have been relocated and/or clustered, and all the other data types have been transformed into a suitable format, we separate clusters of earthquake hypocenters that illuminate different faults. This is the most subjective part of the procedure, as it has to be done manually, but in most cases the clusters are fairly obvious features when viewed in 3-D. Different operators performing the selection could include or exclude a few different hypocenters, but in the majority of cases where there is a recognizable cluster, these differences are limited to the outer edges of the cluster. Because the steps that follow the selection always include some form of averaging of the hypocenter locations, small differences in the initial selection of hypocenters will not have any appreciable influence on the final fault geometry.Occasionally, it is not possible to separate clusters adequately because they intersect forming X- or complex junctions. In such cases only better earthquake locations might solve the problem, or at least reduce uncertainties (for example, once earthquakes are relocated, an X-junction might turn out to have one through-going fault, and one slightly offset fault). At other times, faults come together in T- or Y- junctions. The earthquake clusters can usually be separated at such junctions, but there will be uncertainty as to where exactly the truncated fault stops. Also, at the junction earthquake density is often higher and there can be more scattering than far from it, thus when separating the clusters many earthquakes of the truncated fault might be included in the through-going fault cluster. Situations where T- and Y- junctions exist can of course be improved too by better relocation of the earthquakes. Another kind of useful information in separating these types of clusters is their timing: if the two faults are active in different periods of time, then the clusters can be separated by accounting for both posi-tion and timing of the events.As previously mentioned, any other data points relative to fault position should also be considered, for example the depth of a fault in oil wells, or seismic lines on which a fault has been identified (for an excellent example, see S HAW & S HEARER 1999, and S HAW ET AL. 2002). These additional data types are especially important: they are usually available for depths up to a few km below the surface, but this is precisely the depth range where often very few earthquakes occur, and allow us to image the shallow geometry of the fault.For each fault, all different types of data should be merged so that they can be used simultaneously to build a first approximation of the fault surface. In our case we transform all data into sets of points. We choose points as our basic data type because the majority of our data are earthquake hypocenter locations, which are already discrete points. We then build our surfaces directly from the set of points, setting constraints as needed and then smoothing the resulting surface with the Gocad Discrete Smooth Interpolator (DSI, M ALLET 2002). Several iterations of the DSI are usually necessary before all the irregularities in the surface that have a size below the minimum resolution of the data are smoothed out.2 Applications and ResultsWe have applied the techniques described above to several cases at different scales and in different tectonic settings, and three of these are briefly described below: [1] a thrust fault associated with a restraining bend (Northridge thrust, southern California), [2] a network of strike-slip and thrust faults (San Andreas fault and surrounding faults near San Gorgonio Pass, southern California), and [3] an entire orogen in a subduction margin setting (Taiwan).Sara Carena Using Earthquake Data to Map Faults in 3-D with Gocad: Examples at Different Scales2.1 Northridge Thrust, Southern CaliforniaThe aftershocks of the 1994 Northridge earthquake (M 6.8) illuminate the structure beneath the San Fernando Valley (northwestern Los Angeles) in 3-D. We combined aftershocks and geological data to build an image of the 3-D geometry of the north-vergent Northridge blind thrust to a depth of 21 km. The most striking feature of the imaged fault is mega-corrugations oriented parallel to the mean after-shock slip vector, with most of the 1994 slip confined to west of the largest corrugation (lateral ramp, fig. 2). We also imaged the partially overlying south-vergent San Fernando thrust, which broke to the surface in a complex rupture in 1971 (M 7.1). Both thrusts produce fault-related folding because of either fault propagation or fault bends (S UPPE 1983). This deeper folding however is masked by over-lying complex deformation in the cover, which is one reason why the Northridge thrust was not identi-fied until it ruptured in 1994. We used trishear fold modeling (E RSLEV 1991) based on our 3-D fault geometry to evaluate possible folding due to slip on the Northridge thrust as well as its interaction with the overlapping San Fernando thrust and with shallow structures in the cover. This example illus-trates the importance of earthquake data to structural geology and the value of its 3-D integration with surface and near-surface geological data.Fig. 2. 3-D model of the Northridge thrust. Coseismic slip distribution shown on the left, fault corruga-tions indicated on the right.2.2 San Andreas Fault Near San Gorgonio Pass, Southern California The 1200 km long San Andreas fault (SAF) loses its apparent continuity in southern California near San Gorgonio Pass (A LLEN 1957). This fact raises significant questions, given the dominant role of this fault in active California tectonics. What is the fundamental 3-D geometry and kinematics of the San Andreas fault system in this complex region? Is a through-going, San Andreas rupture from the Mojave desert to the Coachella valley possible? We explored the issue of 3-D continuity by mapping over 60 faults in this region to depths of 15-20 km from hypocenter locations and focal mechanisms. We were able to constrain the 3-D geometry of the SAF near San Gorgonio Pass from the 3-D geome-try of the fault network surrounding it, as the San Andreas itself appears to be aseismic here. The most likely configuration is for the San Andreas fault to merge into the shallow-dipping San Gorgonio Pass thrust northwest of Indio (fig. 3). We concluded that there is no direct continuity at present, but rather a network of faults, and the only kind of rupture possible for the SAF in this region is a complex rup-ture, involving both strike-slip and reverse faulting. GPS measurements also suggest that, despite the fact large motions must have occurred in the past, only minor ones are occurring today in this area (Y ULE & S IEH 2001, M EADE ET AL. 2002, Y ULE & S IEH 2003). Applying our findings about the fault geometry, we explored several simple earthquake scenarios, following K ING ET AL. (1994), to58. Berg- und Hüttenmännischer Tagdetermine the most favorable conditions for a through-going rupture of the San Andreas fault system from the Mojave desert to the Coachella valley (C ARENA ET AL. 2004).Fig. 3. Two different views of the detailed 3-D geometry of the San Andreas fault system between Cajon Pass and Indio. SGPT=San Gorgonio Pass thrust. F1, F2, F3 are tear faults. The gray surface below the faults represents the base of the seismogenic crust in this region.2.3 Taiwan OrogenActive deformation in the upper crust beneath central Taiwan is illuminated by 110,000 small (M=1 to M=4) earthquakes, including both background seismicity and aftershock swarms from larger events. When viewed in 3-D, it becomes clear that the seismicity is dominated by a major sub-horizontal band of events at about 10-15 km depth. The zone steepens below eastern Taiwan to 30°–90° and reaches depths of 30–60 km. We interpreted this feature as the Main Detachment of the mountain belt. Other planes of seismicity above and below abut against this detachment, indicating its through-going na-ture. Although the availability of focal mechanisms is limited because of the small size of most earth-quakes, the available mechanisms consistently show oblique slip with reverse component on the dip-ping part of the detachment. The imaged 3-D shape of the Main Detachment in relation to surface topography allows us a straightforward test of critical taper wedge mechanics and suggests that the first-order topography of Taiwan is controlled by the shape of the detachment. In fact, the reversal of topography at the crest of the mountain belt corresponds to the inflection of the Main Detachment under eastern Taiwan (fig. 4).Fig. 4. Relationship between topography and the Main Detachment.Sara Carena Using Earthquake Data to Map Faults in 3-D with Gocad: Examples at Different Scales This geometry is consistent with critical-taper wedge mechanics (D AVIS ET AL. 1983), and in particu-lar with homogeneous mechanical properties for the shallow brittle part of the wedge. The geometry of the detachment also indicates that it is very weak: the effective coefficient of friction on the detach-ment itself (µb*) that fits the data is 0.08 (C ARENA ET AL. 2002). Some of the most dangerous faults that break the surface, like the Chelungpu thrust, are connected to the Main Detachment at depth (Y UE ET AL. 2005).3 AcknowledgmentsI am grateful to Honn Kao and Robert Jones for their help with earthquake clustering. Many thanks also to Tony Dahlen for constructive discussion about critical taper wedge mechanics, and to John Suppe for the many insightful suggestions.The original earthquake catalog data used in this work are from the Southern California Earthquake Center (SCEC), and from the Taiwan Central Weather Bureau (CWB).4 ReferencesA LLEN, C.R. (1957): San Andreas fault zone in San Gorgonio Pass, southern California. –Geol. Soc. Am. Bull., 68: 315—350.C ARENA, S., & S UPPE, J. (2002): Three-dimensional imaging of active structures using earthquake aftershocks: the Northridge thrust, California. – J. Struct. Geol., 24: 887—904.C ARENA, S., S UPPE, J., AND K AO, H. (2002): Active detachment of Taiwan illuminated by small earthquakes and its control of first-order topography. – Geology, 30 (10): 935—938.C ARENA, S., S UPPE, J., AND K AO, H. (2004): Lack of continuity of the San Andreas fault in southern California: fault models and earthquake scenarios. – J. Geophys. Res.,109, B04313, doi:10.1029/2003JB002643.D AVIS, D., S UPPE, J., AND D AHLEN, F.A. (1983): Mechanics of fold-and-thrust belts and accretionary wedges. – J Geophys. Res., 88: 1153—1172.E RSLEV, E.A. (1991): Trishear fault-propagation folding. – Geology, 19 (6): 617—620.G OT, J.L., F RECHERT, J., K LEIN, F.W. (1994): Deep fault plane geometry inferred from multiplet relative reloca-tion beneath the south flank of Kilauea. – J Geophys. Res., 99: 15,375—15,386.J ONES, R.H., & S TEWART, R.C., 1997, A method for determining significant structures in a cloud of earthquakes. – J. Geophys. Res., 102: 8245—8254.K IKUCHI M.; Y AGI Y.; AND Y AMANAKA Y. (2000): Source Process of Chi-Chi, Taiwan Earthquake of September 21, 1999 Inferred from Teleseismic Body waves. – Bull. Earthq. Res. Inst. Univ. Tokyo, 75:1—13.K ING, G.C.P., S TEIN, R.S., AND L IN, J. (1994): Static stress changes and the triggering of earthquakes. – In: L ANGSTON, C.A. (Ed.): The 1992 Landers, California, earthquake sequence. – Bull. Seismol. Soc. Am., 84 (3): 935—953.L EE, S.-J., & M A, K.-F. (2000): Rupture process of the 1999 Chi-Chi, Taiwan, earthquake from the inversion of Teleseismic data. – Terrestrial, Atmospheric and Oceanic Sciences, 11 (3): 591— 608.L IU, J., S IEH, K., AND H AUKSSON, E. (2003), A structural interpretation of the aftershock "cloud" of the 1992 Mw7.3 Landers, California, earthquake. – Seism. Soc. Am. Bull., 93: 1333 — 1344.M ALLET, J.L. (2002): Geomodeling. New York, Oxford University Press: 624 p.M ALLET, J.L., M ASSOT, J., AND C OGNOT, R. (1999): Fault characterization. – Proceedings of the 19th Gocad Meeting, Nancy School of Geology, Nancy, France, June 14-17, 1999. ASGA (Association Scientifique pour la Géologie et ses Applications).M EADE, B., H AGER, B., AND K ING R. (2002): Block models of present day deformation in southern California constrained by geodetic measurements – Eos Trans. AGU, 83 (47), Fall Meet. Suppl., Abstract T62F-05.N ICHOLSON, T., S AMBRIDGE, M., AND G UDMUNDSSON, O. (2000): On entropy and clustering in earthquake hypocentre distributions. – Geophys. J. Int., 14: 37—51.R ICHARDS-D INGER, K., & S HEARER, P.M.. (2000): Earthquake Locations in Southern California Obtained Using Source Specific Station Terms. – J. Geophys. Res. 105 (5): 10939—10960.58. Berg- und Hüttenmännischer TagR UBIN, A.M., G ILLARD, D., AND G OT, J.L. (1999): Streaks of microearthquakes along creeping faults. – Nature, 400: 635—641.S HAW, J.H., P LESCH, A., D OLAN, J.F., P RATT, T.L., AND F IORE, F. (2002): Puente Hills blind-thrust system, Los Angeles, California. – Bull. Seismol. Soc. Am., 92 (8): 2946—2960.S HAW, J.H., S HEARER, P.M. (1999): An elusive blind-thrust fault beneath metropolitan Los Angeles. – Science, 283: 1516—1518.S UPPE, J. (1983): Geometry and kinematics of fault-bend folding. – Am. J. Sci., 283: 684—721.T HIBAUT, M., G RATIER, J.P., L EGER, M., M ORVAN, J.M.. (1996): An inverse method for determining three-dimensional fault geometry with thread criterion: application to strike-slip and thrust faults (Western Alps and California). – J. Struct. Geol., 18: 1127—1138.W ALDHAUSER, F., AND E LLSWORTH, W.L. (2002): Fault structure and mechanics of the Hayward Fault, Califor-nia, from double-difference earthquake locations. – J. Geophys. Res., 107: doi:10.1029/2000JB000084.Y UE, L.-F., S UPPE, J., AND H UNG, J.-H. (2005): Structural geology of a classic thrust belt earthquake: the 1999 Chi-Chi earthquake Taiwan (Mw = 7.6). – J. Struct. Geol., 27 (11): 2058—2083.Y ULE, D., & S IEH, K. (2001): The paleoseismic record at Burro Flats: evidence for a 300-year average recur-rence for large earthquakes on the San Andreas fault in San Gorgonio Pass, southern California. – GSA Cor-dilleran Section 97th Annual Meeting, and Pacific Section AAPG, Universal City, CA, 2001.Y ULE, D., & S IEH, K. (2003): Complexities of the San Andreas Fault near San Gorgonio Pass: implications for large earthquakes. – J. Geophys. Res., 108 (11): 2584, doi:10.1029/2001JB000451.。
GOCAD(外文资料) (4)
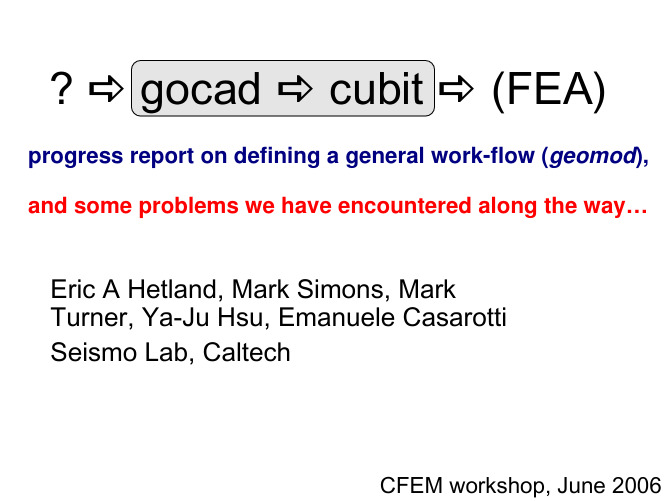
gocad
cubit callenges:
topology book-keeping in gocad is very different from bookkeeping in most FE meshers
each TSurf in gocad “owns” its own vertexes and borders, adjoining TSurfs do not reference common nodes
examples of lithospheric structure constraints:
active source seismology: wide-angle reflection/refraction lines (onshore&offshore), MCS lines (non-commercial products)
the CBM format (as I understood in 06/15/2006):
the CBM format (as I understood in 06/15/2006):
the CBM format (as I understood in 06/15/2006):
each volume is closed (and if parts were merged, each side of the volume shares vertexes along the edges), but adjoining volumes do not share a common surface, border surface is duplicated…
?
gocad
cubit
(FEA)
GOCAD(外文资料)(1)
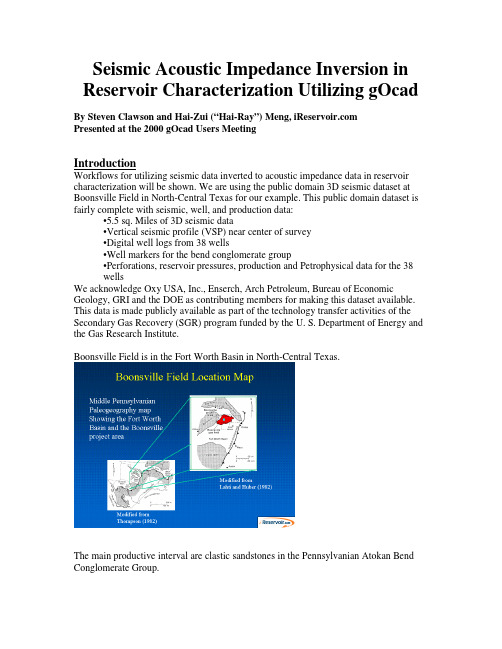
Seismic Acoustic Impedance Inversion in Reservoir Characterization Utilizing gOcad By Steven Clawson and Hai-Zui (“Hai-Ray”) Meng, Presented at the 2000 gOcad Users MeetingIntroductionWorkflows for utilizing seismic data inverted to acoustic impedance data in reservoir characterization will be shown. We are using the public domain 3D seismic dataset at Boonsville Field in North-Central Texas for our example. This public domain dataset is fairly complete with seismic, well, and production data:•5.5 sq. Miles of 3D seismic data•Vertical seismic profile (VSP) near center of survey•Digital well logs from 38 wells•Well markers for the bend conglomerate group•Perforations, reservoir pressures, production and Petrophysical data for the 38wellsWe acknowledge Oxy USA, Inc., Enserch, Arch Petroleum, Bureau of Economic Geology, GRI and the DOE as contributing members for making this dataset available. This data is made publicly available as part of the technology transfer activities of the Secondary Gas Recovery (SGR) program funded by the U. S. Department of Energy and the Gas Research Institute.Boonsville Field is in the Fort Worth Basin in North-Central Texas.The main productive interval are clastic sandstones in the Pennsylvanian Atokan Bend Conglomerate Group.A type log shows the interbedded sandstones and shales over about 1300 feet of section. The Bend Conglomerate is underlain by the Marble Falls Limestone, a platform carbonate. The Bend Conglomerates were sourced from the northwest on the Red River Arch as the Fort Worth Basin was forming during the Oachita orogeny. These Bend Conglomerate sandstones then pinchout to the southeast, outside of this project area as they become distal to the source, prograding into the Fort Worth Basin.Historical gas production has been from the lower most sequence in the Vineyard. Additional potential is expected in the middle sequences of the Runaway and Vineyardintervals.Conglomerate Group.This example seismic line shows the Bend Conglomerate Group structure. Most striking are the karst collapse features from dissolution of the underlying Ellenburger Limestone, some 2000 feet below the Atoka. These collapse features are seen to causecompartmentalization in the Bend Conglomerate sand bodies.Previous conclusions from the Bureau of Economic Geology’s GRI study are:1) Karsting from Ellenburger carbonates cause collapse features compartmentalizing thereservoir. Large range of compartment sizes exist.2) Need 3D seismic to image the collapse features.3) Seismic attributes can sometimes predict the reservoir faciesUpper Caddo: AmplitudeLower Caddo: Inst. FrequencyLower Bend Conglomerate sequences not definitive4) Reservoirs often exist as stacked compartments of genetic sequences.The utility of the seismic attributes derived from the amplitude data are limited and typically very dependant on the particular interval analyzed. In this project we integrate the well log data in with the seismic for a better defined reservoir model. This integration is accomplished by inverting the seismic amplitude data to acoustic impedance (AI) properties and depth converting the seismic so correlation with the well logs is possible. In this presentation I will only highlight the features of Structural Framework and Rock Property modeling in the overall Reservoir Modeling workflows:Structural Framework => Stratigraphic Gridding => Litholgy and Facies Mapping => Pressure Field => Rock Properties => Fracture Network and Stress Field =>Reservoir Fluids and Dynamic Response.Motivation for Reservoir Modeling include:1) Integration of all relevant and available data.2) Merge data of different scales:(Cores, Well logs, Seismic and Production).3) Dynamically update the model as new information becomes available.4) Measurement of errors and uncertainty as well as expected value.The specific workflows used are dependant on number and type of data available. In this case there is substantial well control and the seismic data is of high resolution (80Hz). Structural Framework WorkflowThe Structural Framework Workflow is shown below:Obtaining the Structural Framework from the seismic gives a much better description than from the well control alone. The karst features were not known until the 3D seismic data was acquired.Integration of the well marker tops and the seismic time horizons proceeds by 2 pathways:1) A reference horizon (the Caddo Limestone) was an excellent reflector that also tied the well tops. This is depth converted by a co-located co-Kriging method.2) Time horizons below this reference did not exactly tie the associated well markers due to tuning effects of the thin bedded Bend Conglomerate Group. For these horizons a velocity field was constructed from interpolating the sonic logs, calibrated to the seismic and checkshot survey. The depth was then created by the time and velocity relationship.3)The fault network will be incorporated in the future using a seismic continuity analysis. Depth conversion of the reference horizon is accomplished thru the strong correlation between the time and depth relationship at the well locations.Co-located co-Kriging of the seismic time and well marker depths produces a very accurate depth structure for the Caddo Limestone.Interpolating the sonic logs in the survey a interval velocity field is produced. Converting these interval velocities to average velocities (inverse Dix’s equation) provides the information on depth converting the intervening horizons.And here are the depth converted intervening seismic horizons.Rock Properties WorkflowThis rock property modeling workflow utilizes the seismic information obtained via inversion to acoustic impedance to better control the well log interpolation of rock properties. This is also accomplished with the accurate structural information that the seismic provides. This workflow is necessarily iterative due to the dependency of one data on another and the iteration between time (on the seismic data) and depth (for the log data) referencing.Seismic to Log Calibration is the first step in integrating the seismic amplitude data with the log properties. Starting off one may not know other than by qualitative correlation what the seismic wavelet is. In this case a reserse polarity wavelet is assumed. The synthetic is then tied to the seismic data, performing a constrained stretching and/or squeezing to fit major events. This stretching/squeezing is primarily due to dispersionbetween seismic velocities and sonic log velocities.A final seismic wavelet is then extracted. Always use more than a single seismic to log calibration tie. In this case 4 well ties were averaged for a consistent wavelet showing that the seismic wavelet is nearly –90degrees out of phase and slightly ringy. The ringing suggests that the deconvolution was not sufficient to collapse the source wavelet. Theseismic bandwidth is very good (20-80Hz).A background acoustic impedance model is needed to supply the low frequency component missing from the seismic trace data in the inversion. This first iteration uses asimple gridding of the 4 sonic logs in the survey.A model based inversion using Hampson-Russell Software’s Strata program shows the transform of the qualitative amplitude data into rock property information. The result is very dependant on the background model used and later we’ll see an improvedbackground model for a better result.Checking this inversion at our key well: B Yates 18D the seismic inverted acoustic impedance ties well qualitatively with well log acoustic impedance. Depth converting thisAI volume is also compared to the well log for quality control.Now that we ha ve seismically derived rock properties from the seismic in depth, let’s see how they correlate to the well logs. In general we see that:1) Low AI relates to shales from the gamma ray log.2) High AI relates to resisitve sandstones from the RT log.3) Correlation of AI to the porosity is more complicated since the shales measure a highporosity with low AI and the more porous sandstones are in an intermediate range of AI,while the tight sandstones are resistive and also high AI.properties show a rather low correlation coefficient.An observation of the relative scales of information is needed. The well logs of course are of higher resolution than the seismic data as shown in the lower variance of AI derived by the seismic data than that represented in the well log data. Smoothing the log curves is required to be able to statistically correlate the respective information. This correlation is also stongly influenced by the exact depth conversion of the seismic information to tie the wells. Due to the thin bedded nature of the Bend Conglomerate Group a mistie of only afew feet will severely effect the correlation.Cross-plotting the seismically derived AI to the smoothed well logs (20 feet averaging) increases the correlation, as now the data are on a more equal sample support resolution. These correlations are still low. These seismically derived AI values are also influencedby the simple background impedance model used in the inversion.in the survey.The well log acoustic impedance (AI) is highly correlated to the Log10(RT). The spatial variogram shows a fairly long range to the correlation in order to provide a goodbackground AI model for a 2nd iteration of inversion.First Kriging the Log10(RT) logs is performed. Next co_Kriging the 4 wells with acoustic impedance information is run. Spatially this new background impedance model is shown to provide spatial features not available with just the 4 wells with sonic logs. Areas near the well control have very high frequency information content. While away from well control the response is subdued towards an average from the Kriging system. Since the seismic is principally used for interpolating the interwell region this background impedance model is low pass filtered to 20Hz. This way the well control is only adding the very long wavelength trends to the inversion result. And the interwellregion should be justly controlled by the seismic data.rock properties.Qualitative correlation to the key well: B Yates 18D yields similar results as before.Now cross-plotting the seismically derived acoustic impedance and the log properties in depth shows a better correlation. These correlations are good enough to use in a co-located co-Kriging of the well log properties.Rock property models are now generated by co-located co-Kriging of the gamma ray logs for lithology discrimination and resistivity logs controlled by the seismically derived AIproperties.A reservoir model of sandstone porosity can be derived by the relationships of lithology to gamma ray and resistivity. Where these models of gamma ray and resistivity are related back to the seismically derived acoustic impedance.By segmenting the data into a sandstone region defined by where:Gamma ray is less than 90 andLog10(Resistivity) is greater than 0.8A sandstone porosity relationship is defined.Constructing the density model in the sandstone facies then is represented here.。
GOCAD(外文资料) (3)
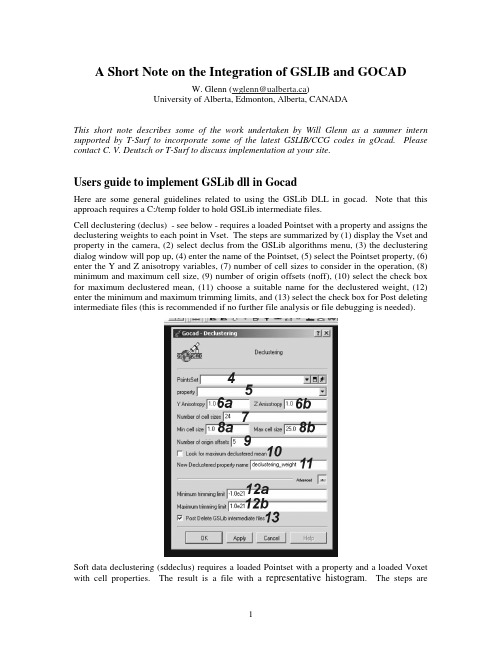
A Short Note on the Integration of GSLIB and GOCADW.Glenn(wglenn@ualberta.ca)University of Alberta,Edmonton,Alberta,CANADAThis short note describes some of the work undertaken by Will Glenn as a summer intern supported by T-Surf to incorporate some of the latest GSLIB/CCG codes in gOcad.Please contact C.V.Deutsch or T-Surf to discuss implementation at your site.Users guide to implement GSLib dll in GocadHere are some general guidelines related to using the GSLib DLL in gocad.Note that this approach requires a C:/temp folder to hold GSLib intermediate files.Cell declustering(declus)-see below-requires a loaded Pointset with a property and assigns the declustering weights to each point in Vset.The steps are summarized by(1)display the Vset and property in the camera,(2)select declus from the GSLib algorithms menu,(3)the declustering dialog window will pop up,(4)enter the name of the Pointset,(5)select the Pointset property,(6) enter the Y and Z anisotropy variables,(7)number of cell sizes to consider in the operation,(8) minimum and maximum cell size,(9)number of origin offsets(noff),(10)select the check box for maximum declustered mean,(11)choose a suitable name for the declustered weight,(12) enter the minimum and maximum trimming limits,and(13)select the check box for Post deleting intermediate files(this is recommended if no further file analysis or file debugging is needed).Soft data declustering(sddeclus)requires a loaded Pointset with a property and a loaded Voxet with cell properties.The result is a file with a representative histogram.The steps aresummarized by(1)display the Pointset with property and Voxet with cell property in the camera,(2)select sdeclus from the GSLib algorithms menu,(3)the soft declustering dialog window will pop up,(4)enter the name of the Voxet,(5)enter the name of the Vset,(6)enter the name of the Vset property,(7)enter the name of the Voxet property,(8) enter the number of secondary classes to consider,(9)GSLib sddeclus output file,(10) minimum and maximum trimming limits of data,and(11)post deleting intermediate files. This is recommended if no further file analysis or file debugging is needed.Stepwise conditional transformation(stepcon)requires a loaded Pointset with two or more properties.The result is a file with new colums for the transformed values and another file with the output transformation table.The steps are summarized by(1)display the Pointset with property and Voxet with cell property in the camera,(2)select stepcon from the GSLib..Algorithms menu,(3)the stepcon dialog window will pop up,(4)enter the name of the pointset,(5)enter the primary property,(6)enter the secondary property,(7)if there are other properties of the pointset that must be used,select the properties accordingly,(8)enter the number of secondary classes,(9)an optional transformation table file can be considered in the algorithm by indicating its location,(10)the GSLib output file containing the original data set with additional columns with the new conditioned data,(11)the GSLib output file containing the transformation table,(12)minimum and maximum data trimming limits,and(13)post deleting intermediate files.This is recommended if no further file analysis or file debugging is needed. The reverse of the stepwise conditional transformation(backstep)requires two data files and the transformation data.The result is the back transformed values.The steps are summarized by(1) select stepcon from the GSLib algorithms menu,(2)the backstepcon dialog window will popup, (3)enter the number of variables,(4)enter data file number1,(5)enter data file number2,(6) select check box for smoothed distribution,(7)enter the input transformation table,(8)enter theunivariate transformation table file for variable1,(9)enter the univariate transformation table file for variable2,and(10)select the output file.The sequential Gaussian simulation program with self healing(sgsim_sh)requires two pointsets and generates a new SGrid properties for each cell,a simulation output file,an MVF for simulation output file.The steps are summarized by(1)display the two Poinsets with property and SGrid in the camera,(2)select sgsim_sh from the GSLib algorithms menu,(3)the sgsim_sh dialog window will pop up,(4)enter the name of the primary Pointset,(5)enter the primary property,(6)a and b:select the weight checkbox if there is a weight property in the primary Pointset to be used in sgsim_sh and enter the property name,(7)a and b:select the secondary checkbox if there is a secondary property in the primary Pointset to be used in sgsim_sh and enter the property name,(8)enter the name of the secondary Poinset,(9)enter the secondary property to be used from the secondary pointset,(10)enter the Sgrid,(11)a and b:select the checkbox to consider the reference distribution file,(12)a and b:enter the minimum and maximum Z values, (13)a and b:enter the lower tail option and parameter,(14)a and b:Enter the Upper tail option and parameter,(15)enter the simulation output file,(16)enter the mvf file for simulation output, (17)enter the number of realizations,(18)a and b:Enter the minimum and maximum original data for simulation,(19)enter the number of simulation nodes,(20)select the checkbox to assign data to nodes,(21)select the checkbox to perform a multiple grid search,(22)enter the number of multiple searches,(23)enter the maximum data per octant(0=not used),(24)a,b,and c:-maximum search radii(hmax,hmin,vert),(25)a,b,and c:-angles for search ellipsoid,(26)a,b, and c:-size of covariance lookup table,(27)kriging type,(28)colocorr,(29)varred,(30)a,b,and c:a0v,a1v,acc,(31)select checkbox to transform secondary variable,(32)enter the nugget effect,(33)select the Variogram type,(34)enter the cc parameter,(35)a,b,and c:enter the angle parameters,and(36)a,b,and c:enter the a_hmax,a_hmin,and a_vert parameters.Note1A:the SGrid must be an translation of the original XYZ grid.Surface simulation(surfsim)requires a loaded Pointset generates four output files with the surface parameters.The steps are summarized by(1)display the poinset and Sgrid in the camera,(2)select surfsim from the GSLib..Algorithms menu,(3)the surfsim dialog window will pop up,(4)enter the name of the poinset,(5)select the Sgrid to use for the algorithm,(6)enter the Maximum number of surfaces,(7)enter the total thickness,(8) select the surface type–gauss-helmet-ellipse,(9)a,b,and c:Enter the L,M,and U height of surface,(10)a,b,and c:(simple)Enter the L length,(ellipse)M long axis,and(guass) Sigma X,(12)a,b,and c:(simple)Enter the L width,(ellipse)short axis,and(gauss) Sigma Y,(13)a,b,and c:(simple)(not for ellipse&gauss)L,M,U width2,(14)select box for truncation,(15)select box for it variable,(16)a,b,and c:if trunction(14),Enter the L,M,and U of X0,(17)a,b,and c:if trunction(14),Enter the L,M,and U of Y0,(18) select box for itrans variable,(19)enter the nugget effect,(20)enter the cc variable,(21) a,b,and c:enter the L,M,and U Sigma of residual,(22)a,b,and c:enter angle_1, angle_2,and angle_3parameter,(23)a,b,and c:enter the a_hmax,a_hmin,and a_vert variables.Note2A:the SGrid must be a translation of the original XYZ grid.Create a Voxet from GSLIB takes a GSLIB data file and generates a file for GOCAD.The steps are summarized by(1)select Create_Vset_from_GSLib from the GSLib-tools menu,(2)enter an appropriate name for the Pointset,(3)select a GSLib data file from the file selector,(4)ct checkbox if GSLib data file contains a well prefix(ie.Is the first column in the GSLib data file a well number?),and(5)select checkbox if the GSLib data file contains a column for Z values(ie. Is the file in3-D or2-D format?).Load a Voxet.The steps are summarized by(1)select Load Voxet Property from the GSLib-tools menu,(2)choose the Voxet that will have the additional property,(3)give the new property a name,(4)choose the GSLib file A that contains the property,(5)select the checkbox if the GSLib file has a second property that is to be included in the Voxet,and (6)depending on step5,Enter the second property.Load a SGrid.The steps are summarized by(1)select the load_sgrid_property from the GSLib..tools menu,(2)choose the SGrid that will have the additional property,(3)enter a property name,(4)choose the GSLib file that contains the property,(5)select the checkbox if the GSLib file has a second property that is to be included in the SGrid,and (6).depending on step5,Enter the second property nameHow to integrate the GSLIB DLL in MSDEV C++:This is an example implementation of the IDECLUS function using the GSLIB.dll and GSLIB.lib.1download and install the VFRUN65AI.exe from:/fortran/kb/q1023.htmlthis exe will install the necessary dlls to run the DECLUS.dll or any other fortrandll created by visual fortran in C++code.2.Place the GSLIB.dll in the system path3.In the MSVisual Studio environment,update include the GSLIB.lib file in theProject->settings->Link(tab)->Object/library modules:linee the sample C++code to run the IDECLUS function inside theimplementation filesthe IDECLUS function requires a.dat and.par file inside a sample.cpp file:#include<string.h>…char instring[50];char outstring[50];strcpy(instring,"C:/temp/declus.par");strcpy(outstring,"C:/temp/data.out");/*Call Fortran routine-pass length of outstring explicitly*/int warnint=IDECLUS(instring,strlen(instring),outstring,50);//if warnint=0-Normal operation//if warnint=1-Missing parameter or input file//if warnint=2-Error in parameter file//if warnint=9-Extraneous Unknown error…inside a sample.h file:…extern"C"//must declare extern“C”to avoid C++name mangleing//IDECLUS being the name of the function{__declspec(dllimport)int__stdcall IDECLUS(char*STR_IN,int STR_IN_LEN,char*STR_OUT,int STR_OUT_LEN);}General Gocad Plugin informationFor future development of CCG Gocad plug-ins.The CCG Gocad plug-in was developed using the three stage implementation.Each plug-in element begins with the source stage. The source files are interfaced with the API stage.A single CLI command encapsulates the entire process of calling the API and source objects.Specific Libraries were tailored for the CCG Plug-in.the libraries are InputOutput,and Algortihms.The InputOutput library contained most of the file reading and writing.The Algortihms library contained all of the CCG_DLL.dll functionalities and GSLib parameter file creations.The source contains the GSLib function calls and extern”c”declarations.It also contains the file writing procedures for the GSLib intermediate files. The GAPI contains the calls to the source classes and performs error checks.It also prepares Gocad to receive properties and contains calls to update properties.The GAPI classes that the CCG has developed in summer2001have contained only public static functions(similar to Gocad api convention)that mainly return Boolean values as output. Inside the GAPI static functions the main source classes are called.In the CCG Gocad plug-in each library that was created,there was a corresponding GAPI file used to call the library’s code.The CLI and XCLI simplified the creation of the user interface windows.Inside the CLI commands the CCG used file selectors,Object selectors,property selectors,parameter entry fields,and Boolean variables to create a dialog to users.The CLI commands call the GAPI static functions.The menu-bars section defines the menu item names,CLI calls,and ordering of menu layout.The Gocad plugin takes care of the implementation of the menu-bar,so developers need only worry about the ordering of the menu items.Instructions on how to use each GSLib dll function in Gocad Using specific parameters and files.declus:Using this parameter file:START OF PARAMETERS:../data/cluster.dat \file with data1 2 0 3 \ columns for X, Y, Z, and variable-1.0e21 1.0e21 \ trimming limitsdeclus.sum \file for summary outputdeclus.out \file for output with data & weights1.0 1.0 \Y and Z cell anisotropy (Ysize=size*Yanis) 0 \0=look for minimum declustered mean(1=max)24 1.0 25.0 \number of cell sizes, min size, max size 5 \number of origin offsetsSteps:1.In GSLib..tools..Create VSet from GSLib,2.Enter the file cluster.dat as the GSLib file.3.make sure the check boxes are unchecked because cluster.dat does not have columns for well id orZ4.In GSLib..Algorithms..declus5.choose the Pointset previously entered and enter“Primary”property in the property boxe the default parameters(they are the same as the above)stepcon:Using this parameter file:START OF PARAMETERS:data.dat - file with data2 - number of variables to transform3 5 - columns for variable transformation-primary and Secon-1.0e21 1.0e21 - trimming limits10 - number of classes1 - input transformation table,yes=1,no=0scatsmth_k.trn - file with transformation table stepcon.out - file for outputstepcon.trn - file for output transformation tableSteps:1.In GSLib..tools..Create_Vset_from_GSLib,2.Enter the file cluster.dat as the GSLib file.3.Make sure the check boxes are unchecked because cluster.dat does not have columns for well id or Z4.In GSLib..Algorithms..stepcon5.choose the Pointset previously entered and enter“Primary”in the primary box and“Secondary”inthe secondary box.e the default parameters(they are similar to the ones above).7.Out put files should appear in the C:/temp folder.ssdeclus:Using this parameter file:START OF PARAMETERS:pairs.dat -file with paired calibration data1 2 - columns for secondary and primary-1.0 1.0e21 - trimming limitsseismic.dat -file with secondary data1 - column for secondary variable sddeclus.out -file for representative histogram50 -number of sec. classes to use (10-100)Steps:1.In GSLib..tools..Create_Vset_from_GSLib,2.Enter the file welldata.dat as the GSLib file.3.make sure the check boxes are checked because welldata.dat does have columns for well id and Z4.In Objects right click on Voxet..New..From_Objects_cage.5.give the Voxet a name,set Scale W dimention by1,set nu=81,nv=81,nw=787.Populate the Voxet nodes with properties from the file“seisimic.dat”.This file contains only onecolumn with the property“impedence”.The file data will expire before filling all Voxet nodes.8.go to Voxet..Attributes..select the Object and make the“volume”atoms visible.(this will slowthings down!)6.In GSLib..Algorithms..sddeclus7.Put in the above Voxet,Pointset,and“Porosity”property from Pointset in primary property and“impedance”in the secondary property.e the rest of the default parameters(similar to the above parameter file)uncheckPost_delete_par_file to view the intermediate GSLib files(ie.the pairs.dat file).backstep:Using this parameter file:START OF PARAMETERS:2 - number of variablessgsimscpor.out - data file number 1sgsimscper.out - data file number 21 - smoothed distribution, 1=yes, 0=noscatsmth_kfl.trn - file with input transformation table nspor.trn - univariate transformation table for variable 1 nsper.trn - univariate transformation table for variable 2 backfl.out - file for outputSteps:Not much to this Program,Just put in the files and Select where the output files go!sgsim_sh:Using this parameter file:START OF PARAMETERS:../data/cluster.dat -file with data1 2 0 3 0 4 - columns for X,Y,Z,vr,wt,sec.var.-1.0e21 1.0e21 - trimming limits1 -transform the data (0=no, 1=yes) sgsim.trn - file for output trans table0 - consider ref. dist (0=no, 1=yes) histsmth.out - file with ref. dist distribution1 2 - columns for vr and wt0.0 25.0 - zmin,zmax(tail extrapolation)1 0.0 - lower tail option, parameter1 25.0 - upper tail option, parameter1 -debugging level: 0,1,2,3GSLvm-SH.dbg -file for debugging outputGSLvm-SH.out -file for simulation outputGSLvm-SH-mvf.mvf -mvf file for simulation output mvffl 1 -number of realizations to generate25 0.5 2.0 -nx,xmn,xsiz25 0.5 2.0 -ny,ymn,ysiz1 0.5 1.0 -nz,zmn,zsiz123065 -random number seed0 8 -min and max original data for sim12 -number of simulated nodes to use1 -assign data to nodes (0=no, 1=yes)1 3 -multiple grid search (0=no, 1=yes),num 0 -maximum data per octant (0=not used) 15.0 15.0 10.0 -maximum search radii (hmax,hmin,vert)0.0 0.0 0.0 -angles for search ellipsoid31 31 1 -size of covariance lookup table2 0.774 1.0 -ktype: 0=SK,1=OK,2=LVM,3=EXDR,4=COLC1 0.1 1.0 0.02 -self healing (0=no, 1=yes)../data/ydata.dat - file with secondary variable(LVM,EXDR,COLC variable4 - column for secondary variable1 - transform secondary variable (0=no,1=yes)1 0.3 -nst, nugget effect1 0.7 0.0 0.0 0.0 -it,cc,ang1,ang2,ang312.0 12.0 12.0 -a_hmax, a_hmin, a_vertaSteps:1.In GSLib..tools..Create_Vset_from_GSLib,2.Enter the file cluster.dat as the GSLib file.3.make sure the check boxes are unchecked because cluster.dat does not have columns for well id or Z4.In GSLib..tools..Create_Vset_from_GSLib,5.Enter the file ydata.dat as the GSLib file.MAKE sure the name is not the same as above!!6.un-check check boxes because ydata.dat does not have columns for well id or Z7.create an SGrid..New..From_Step_Vectors.put orgin at0,0,-0.5to encapsulate the data,alsoset nu=51,nv=51,nw=2(these are the number of nodes)and leave the property_cell_centered8.Run the GSLib..Algorithms..sgsim_sh picking“Primary”as the pointset_vr_property,and“Secondary”as the sec_property.Also,pick the secondary pointset and its“Secondary”propertyand proper SGrid.9.DELETE the OLD properties if running again to avoid the Gocad database from rejecting theadditional properties with identical namessurfsim:Using this parameter file:START OF PARAMETERS:1 -number of realizationscond1.dat -input file of conditioning datacond1_.dat -output scaled conditioning dataW2000C1idx.out -output file of the indexW2000C1thk.out -output file of the thicknessW2000C1par.out -output of the parameters1500 0.5 1.0 -nx,xmn,xsiz20 0.5 1.0 -ny,ymn,ysiz100 0.5 1.0 -nz,zmn,zsiz100 -Maximum no. of surface200 -total thickness69069 -seed of random number3 -surface type (1-Helmet 2-Ellipse 3-gauss) 15.0, 35.0, 60.0 -L,M,U height of a surface100.0,150.,200.0 -L,M,U length (Simple), long axis (Ellipse), SigmaX (Gauss)20.0, 25., 30.0 -L,M,U width (Simple), short axis (Ellipse), SigmaY (Gauss)5.0, 7.5,10.0 -L,M,U width 2 (Simple) (not for Ellipse & Gauss)0.0,90.0,180.0 -L,M,U angle0 -truncation flag1000,1150,1300 -L,M,U of X0 if trunFlag = 010, 10, 10 -L,M,U of Y0 if trunFlag = 0-------------------------------------------0 -itrans0.00001, 0.00001, 0.00001 -L,M,U sigma of residual1 0.0001 -nst, nugget effect3 0.9999 0.0 0.0 0.0 -it,cc,ang1,ang2,ang330.0 30.0 1.0 -a_hmax, a_hmin, a_vertsteps:1.In GSLib..tools..Create_Vset_from_GSLib,2.Enter the file cond.dat as the GSLib file.3.make sure the check box for well id is unchecked because cond1.dat does not have a column forwell id4.create an SGrid from right clicking on SGrid..New..From_Step_ the SGrid andset the orgin to x=0.5,y=0.5,z=0.5,and set nu=1500,nv=20,and nw=1005.Run GSLib..Algorithms..surfsim using the default parameters。
GOCAD讲义
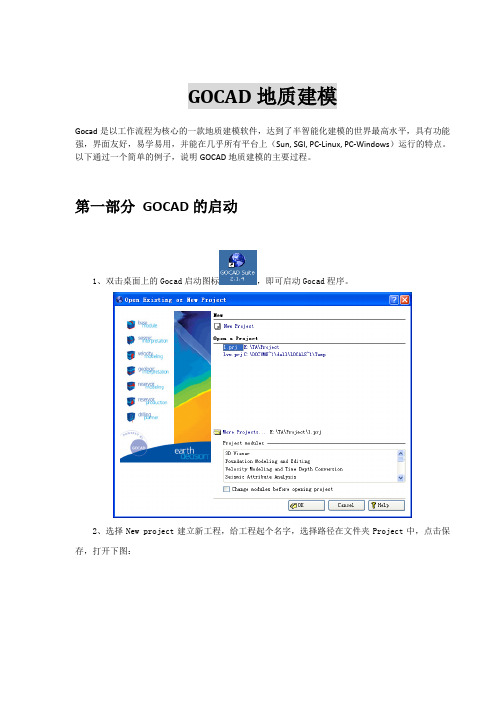
GOCAD地质建模Gocad是以工作流程为核心的一款地质建模软件,达到了半智能化建模的世界最高水平,具有功能强,界面友好,易学易用,并能在几乎所有平台上(Sun, SGI, PC-Linux, PC-Windows)运行的特点。
以下通过一个简单的例子,说明GOCAD地质建模的主要过程。
第一部分GOCAD的启动1、双击桌面上的Gocad启动图标,即可启动Gocad程序。
2、选择New project建立新工程,给工程起个名字,选择路径在文件夹Project中,点击保存,打开下图:3、选择Select All,点击OK即可。
4、弹出选择工程单位窗口如下。
此处选择平面上单位是英尺(Feet),深度单位也是英尺(Feet);时间选择毫秒;深度方向选择向上为增大;z值是时间域或深度域,选择深度域(最终如下图)。
选择完后点击OK。
5、打开如下Gocad主窗口。
第二部分GOCAD数据加载一、地震数据加载以SEGY格式为例,加载三维地震数据选择File > Import objects >Seismic Data > SEG-Y 3D as Voxet。
在Data文件夹下选择文件“tornado.sgy”,点击OK即可加载,如下图。
二、井数据加载1、井位数据加载不同的数据格式有不同的加载方法。
以普通的文本格式为例,包括井名、x坐标、y坐标、补心海拔、井深等。
(1)加载方式是:File > Import objects > Well data > (path)Locations from column-based File ,在Data\Wells文件夹中选择文件“WellPaths”,接受默认,按next两次,打开下图:(2)对问题What information do you have for the path? 选择X-Y-TVDSS-MD。
下来的两个问题各选Feet和Use a Column。
GOCAD(外文资料) (14)

Class Project LibraryIndependent Import & Export of GoCAD Data ObjectsGCAP provides an independent C++class library(Software Development Toolkit – SDK) to read/write GoCAD data for creating new data bridges. Defining principle data structures from GoCAD data models with methods to access GoCAD compatible files, the library has an efficient memory model with large file scalability. Full source code is available if required with the benefit that no other 3rd party software are required. A suite of example programs, including a VTK-based visualizer is available to simplify integrating GCAP into your applications. Currently Geological/Geophysical software is faced with unsolved problems of data management and visualisation of large multi-file and multi-entity geologic models.The aims of GCAP are to offer a C++class defining principle data structures from GoCAD models,with methods to read and write this data structure to GoCAD compatible files, using “out of core” scalable techniques.Motivation:With the development of GCAP, one such problem is solved that exists in the data management and visualisation of geologic models.Such models have grown significantly in storage and logical complexity in recent years. The field of software applications available to geologists and geophysicists has not kept pace with current practices and needs,especially as the minority of software applications that can address this complexity are in themselves very complex and difficult to use. While some applications exist, none offer an easy to use interface to other existing commercial or internal research applications.Existing Practices:GoCAD software and tools define a proprietary file transport standard for complex geologic models. This is supported by the vendor's native library API. This limits the scope of potential users to those organisations participating with GoCAD tools.Most 3rd party applications surveyed appear to only support a limited subset of GoCAD specifications, and have only written the portion of the data model support they were interested in. Many smaller groups have not been able to take up the full GoCAD system, creating an opportunity to provide an alternative with different design goals to the geological and geophysicalcommunity.Aims:GCAP seeks to achieve a significant advance in the data management and data visualisation of complex geologic 3D models, especially where models are defined using GoCAD format specifications. By integrating GCAP, full access to GoCAD hierarchical and multi-segment models can be manipulated at will from within your application, with the details of “out-of-core” scalability hidden from the developer.What is GCAP ?GCAP stands for G o C AD A ccess Class P roject. It is a library of C++ classes that can be used to read, store and write GoCAD data objects.The GCAP library consists of three main modules:1.GoCAD Data Object Module2.GoCAD File Reader Module3.GoCAD File Writer Module GCAP currently supports the following object types:1.Atomic or VSet2.TSurf3.TSolid4.Grid3D or Voxet5.GSurf6.SGridA full 50-page user manual and 200-page API Reference describes how to install and use GCAP in your application.Features:•Independent C++ Class Library•Scalability using "out-of-core" methods•Dictionary system allows application to pick and choose parts•Handles attributes and properties, not just raw geometry•Comprehensive professional documentation, hyperlinked help•Both Linux and Windows supported platformsContact InformationVisual Technology Services Ltd.The Courtyard, High Street, Ascot,Berkshire SL5 7HP, UKPhone: +44 (0) 7787 517529Email: info@[ Ver. 2, 02/11/2006 ]。
GOCAD讲义
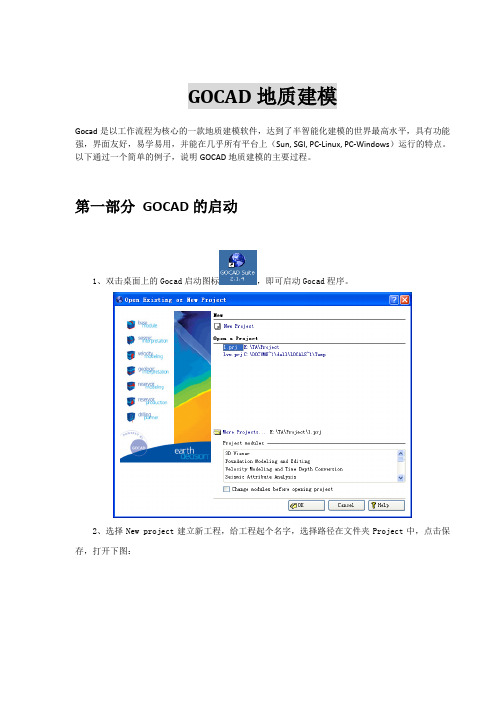
GOCAD地质建模Gocad是以工作流程为核心的一款地质建模软件,达到了半智能化建模的世界最高水平,具有功能强,界面友好,易学易用,并能在几乎所有平台上(Sun, SGI, PC-Linux, PC-Windows)运行的特点。
以下通过一个简单的例子,说明GOCAD地质建模的主要过程。
第一部分GOCAD的启动1、双击桌面上的Gocad启动图标,即可启动Gocad程序。
2、选择New project建立新工程,给工程起个名字,选择路径在文件夹Project中,点击保存,打开下图:3、选择Select All,点击OK即可。
4、弹出选择工程单位窗口如下。
此处选择平面上单位是英尺(Feet),深度单位也是英尺(Feet);时间选择毫秒;深度方向选择向上为增大;z值是时间域或深度域,选择深度域(最终如下图)。
选择完后点击OK。
5、打开如下Gocad主窗口。
第二部分GOCAD数据加载一、地震数据加载以SEGY格式为例,加载三维地震数据选择File > Import objects >Seismic Data > SEG-Y 3D as Voxet。
在Data文件夹下选择文件“tornado.sgy”,点击OK即可加载,如下图。
二、井数据加载1、井位数据加载不同的数据格式有不同的加载方法。
以普通的文本格式为例,包括井名、x坐标、y坐标、补心海拔、井深等。
(1)加载方式是:File > Import objects > Well data > (path)Locations from column-based File ,在Data\Wells文件夹中选择文件“WellPaths”,接受默认,按next两次,打开下图:(2)对问题What information do you have for the path? 选择X-Y-TVDSS-MD。
下来的两个问题各选Feet和Use a Column。
GOCAD(外文资料) (10)

Stanford Exploration Project,Report80,May15,2001,pages1–616Iterative velocity model building for3-D depth migration byintegrating GOCAD and A VSRobert G.Clapp and Biondo Biondi1ABSTRACTWe have developed a procedure for building a3-D velocity model starting from a2-D geological model in order to image3-D poststack data.The3-D model was built using GOCAD,and it was iteratively refined by interpreting the result of3-D poststack depth migrations using A VS.At the beginning of the iterative process,when only few reflectors are detectable in the migrated cube,we interpret only one surface.From the deformations measured on the selected reflector we compute a displacementfield for every point in the model.The application of the displacementfield to the GOCAD model used for migration leads to an improved model.The migration of the data with this improved model shows better focusing of some reflectors and allows a more complete interpretation of the structure.INTRODUCTIONOne of the most crucial problems in imaging3-D seismic data is the determination of the velocity model.With the ever increasing speed of parallel supercomputers new options for defining and refining the velocity model are becoming possible.These new options take ad-vantage of the speed of these super computers to integrate time consuming operations such as migration,into an interactive and iterative processingflow(Wyatt et al.,1992;Jones,1993). Sheppard and O’Brien(1993)took advantage of these new options by creating a3-D veloc-ity model by performing depth migration,interpreting the resulting image,and refining the geologic model.In addition,improved visualization software has made3-D model building, viewing,and manipulating an easier task.Della Malva and Williams(1992)used GOCAD to combine a series of2-D velocity models into a3-D model.We blended elements of both of these methods in an attempt to obtain a good3-D velocity model for3-D poststack migration. We started with a2-D geologic model,along with the prestack and poststack3-D data sets in which initially very few reflectors were identifiable.We migrated the data with2.5-D model created in GOCAD.We then viewed the3-D cube using A VS and picked a series of surfaces on various slices of the cube parallel to the direction of the initial2-D geologic model.From this series of surfaces we created a2-D displacement net for the cube.Choosing a single surface and then extrapolating its changes in depth to the entire geologic model does not accurately 1email:not available12Clapp and Biondi SEP-80 describe geologic sedimentation and the time variant nature of the stressfield accompanying it.The methodology is not a badfirst approximation,and proved effective in improving the migrated image and the accompanying geologic model.BUILDING THE3-D GEOLOGIC MODELThefirst challenge was to convert the2-D AIMS geologic model into a3-D GOCAD model. AIMS modelAIMS creates its model by constructing a series of surfaces defined by numerous line seg-ments.These line segments are defined in an ASCIIfile by a pair of coordinates representing the beginning and end of the line segment.In addition to the coordinates,each line contains the number of the corresponding surface.The surfaces are chosen so that only one velocity is represented below them and that velocity is defined in a horizon call.An example of a portion of an AIMS2-D modelfile is presented below:HORI40,,4600.0BEG40,2310.374,1108.975END40,2331.748,1058.843BEG40,2331.748,1058.843END40,2355.868,1000.688BEG40,2355.868,1000.688END40,2378.594,956.565BEG40,2378.594,956.565END40,2499.702,889.8913-D Model in GOCADAs described in Berlioux(?)a GOCAD3-D model is defined by a series of surfacesfiles and a partitionfile.Each surfacefile is divided into two parts.Thefirst part contains a series of points(vertices in the GOCAD nomenclature)upon which the surface lies.The second section is devoted to constructing a series of triangles from the defined points in order to give the surface continuity in two-dimensions.Figure1illustrates a simple model constructed from the surfacefile defined below:SEP-80Velocity model building3Figure1:A simple GOCAD model.bob-gocad1[NR]VRTX10.000.000.00VRTX2 2.000.000.00VRTX3 2.00 2.000.00VRTX40.00 2.000.00VRTX5 1.00 1.00 1.00TRGL125TRGL235TRGL345TRGL415The partitionfile defines a series of domains bounded by the various surfaces.The domains can then be assigned properties such as velocity or density.The ConversionRather than converting the AIMSfile manually into a series of GOCADfiles it was decided to develop a procedure that would accomplish this task automatically.The conversion program was written in standard SEP-like format to better allow it to be integrated into future processing flows.The input is simply the AIMSfile,parameters defining the extension in the cross-line direction,and an option to create a free surface.The output is a series of.tsfiles(GOCAD extension for a surfacefile)corresponding to the surfaces described by AIMS.Once the initial AIMSfile has been run through the conversion routine it is a relatively simple matter to create the GOCAD model.Figure2represents the GOCAD model corre-sponding to the input AIMS geologic model.In order to run the CM-5migration program it is necessary to convert the GOCAD model to SEP format.Figure3represents a2-D slice of the resulting velocity model using the program described in Berlioux(1993).4Clapp and Biondi SEP-80Figure2:GOCAD model corresponding to input AIMS model.bob-gocad2[NR] ../bob/./Figs/gocad3.pdfFigure3:Gridding output based on initial AIMS model.SEP-80Velocity model building5REFINING THE GEOLOGIC MODELOnce the initial geologic model had been built,the task was to better describe the3-D nature of the area.The velocity model was used as input to a depth migration program on the CM-5 developed at SEP(Biondi and Palacharla,1993).The resulting cube was then viewed using A VS.A VS pickerDave Nichols has created an A VS network,and written a module for that network which enables the user to pick a polyline on a2-D image and write that polyline in a GOCAD pline format.In order to create the displacementfield,we chose a2-D slice of the data cube parallel to the geologic model(see Figure4),then chose a surface that was possible to identify on all/most of the2-D images.We picked the surface on the image and saved it in afile corresponding to the slice number.Part of the recorded plinefile for Figure4can be seen below:Figure4:Slice324of3-D migrated cube.bob-avs3241[NR]VRTX132.00000058.0000000.000000VRTX242.00000054.0000000.000000VRTX344.00000053.0000000.000000VRTX448.00000055.0000000.0000006Clapp and Biondi SEP-80VRTX551.00000058.0000000.000000VRTX656.00000060.0000000.000000VRTX759.00000063.0000000.000000VRTX862.00000065.0000000.000000VRTX965.00000068.0000000.000000VRTX1067.00000070.0000000.000000VRTX1170.00000072.0000000.000000...SEG12SEG23SEG34SEG45SEG56SEG67SEG78SEG89SEG910SEG1011...We then attempted to pick the same pline on another in-line slice in order to approximately quantify the changes in depth along the cross-line direction of the geologic model.Figure5 shows a second slice in the in-line direction,note the change in the surface approximately80% of the way down the image in comparison to the same surface on Figure4.Creating GOCAD model from series of plinesCreating the3-D GOCAD model from the series of2-D plines was a two-step process.The first step was to create a2-D displacementfield.The displacementfield was created by:first, reading in the series of vertices making up the various plines,then resampling in the in-line direction using linear interpolation between the points,andfinally,subtracting the resampled values from a baseline corresponding to the original2-D geologic model.The corresponding displacement net is specified by a user defined density parameter in the in-line direction and by the number of slices of the3-D cube in which plines were chosen in the cross-line direction. It was not necessary to resample in the cross-line direction due to the combination of GOCAD and the gridding program doing the same linear interpolation as the program performs in the in-line direction.Once the2-D displacement net has been created,Figure6,the net is used to construct the same series,and in virtually the same manner,the.tsfiles constructed in the original2.5-D GOCAD model.Figure7represents the GOCAD model derived from the displacementfield shown in Figure6.Even though the initial data quality was poor,and the re-migrated image far fro m ideal (Figure8),the image does appear to be improved compared to the corresponding slice mi-grated with the initial2.5-D velocity model,Figure4.The improvement is especially notice-able in the middle of the image where an anticline has become better focused.SEP-80Velocity model building7Figure5:Slice220of3-D migrated cube.bob-avs220[NR]../bob/./Figs/displacement1.pdfFigure6:2-D displacement net.8Clapp and Biondi SEP-80Figure7:GOCAD model constructed from initial displacementfield calculations. bob-gocad4[NR]Figure8:Slice324of migrated cube with3-D velocity model.bob-avs3242[NR]SEP-80Velocity model building9CONCLUSIONSBy creating a2-D displacement net and applying it to the geologic model,the3-D poststack migration image did show some improvement.The velocity model could be further improved by re-choosing the surface on the series of2-D slices.The improved quality of the velocity function should enable a more consistent and improved picking,further enhancing the quality of the3-D model.Further,once the surfaces become more apparent in the migrated image,ex-tending the displacementfield into the third dimension by picking two or more surfaces might help improve the results and better approximate the true nature of sedimentation variation with time.In addition velocity inside the model could be better defined by intergrating geophysical data derived from such source as velocity analysis.ACKNOWLEDGEMENTSWe would like to thank Husky Oil for providing the dataset,and in particular Fred Kierulf with Husky Oil,for his enthusiastic support.At SEP,we are indebted with Arnaud Berlioux for the numerous hours spent explaining GOCAD and his gridding program,and Dave Nichols for explaining some of the intricacies of A VS and his network.REFERENCESBerlioux,A.,1993,3-D grid with GOCAD:SEP–79,301–318.Biondi,B.,and Palacharla,G.,1993,3-D wavefield depth extrapolation by rotated McClellan filters:SEP–77,27–36.Jones,I.F.,1993,3-D velocity model building via iterative one-pass depth migration:63rd Annual Internat.Mtg.,Soc.Expl.Geophys.,Expanded Abstracts,974–977.Malva,R.D.,and Williams,M.C.,1992,3-D model construction of overthrust geology for structural imaging of seismic by migration:62nd Annual Internat.Mtg.,Soc.Expl.Geo-phys.,Expanded Abstracts,1255–1256.Sheppard,F.,and O’Brien,M.,1993,Iterative3-D post stack depth migration:An interpretive seismic imaging tool:3-D Seismology:Integrated Comprehension of Large Data V olumes, SEG Summer Research Workshop,Technical Program and Abstracts,124–.Wyatt,K.D.,Towe,S.K.,Layton,J.E.,Wyatt,S.B.,von Seggern,D.H.,and Brockmeier, C.A.,1992,Ergonomics in3-D depth migration:62rd Annual Internat.Mtg.,Soc.Expl. Geophys.,Expanded Abstracts,944–947.。
GOCAD(外文资料) (28)

Open Spirit User Meeting1, Paris June 2004.Figure 1 OS 3D Viewer displaying data from OpenWorks, SeisWorks and Finder2.SummaryAbout 40 attended the EU Open Spirit (OS) user meeting held just after the annual meeting of the EAGE in Paris. Open Spirit Corp (OSC) appears to be gaining traction for its E&P middleware (see our section ‘What is Open Spirit’ below). This has been by dint of a constant marketing and technical effort over the last several years. The list of third party application vendors using the OS framework is getting longer. One independent vendor (SMT) has even made the bold step of offering an OS data sever. A recent OS innovation is the qualification program – so far only Hampson-Russell (Veritas) has signed up for this option3.OS is a developer’s tool above all else. End users should ideally be unaware of OS’s existence. Some larger oil companies develop their own software, for instance Shell’s 123DI and Total’s Sismage. For these tools, OS offers a convenient way to plug in-house developments into vendor data stores. Larger oils, especially those in the Schlumberger camp, may be using OS technology (such as CopySync) in their IT support and data management. Elsewhere, OS end users are found in third party software vendors such as EDS and SMT who use OS to link GoCad and the Kingdom Suite to vendor data stores.Schlumberger Information Solutions’ (SIS) position regarding OS is clear. They give strong support for OS – and promise integration within existing and new product lines. The only nuance here is that the support is stronger if the OS integration is performed and supported by SIS!Landmark’s presence (Murray Roth and Janet Hicks) was significant – after all, OS is a Schlumberger affiliate. Landmark is working on two pilots using OS – one an internal test of1 Visit the OpenSpirit website for more info and presentations.2 Image courtesy Open Spirit Corp.3 Announcements from SIS and EDS are expected ‘real soon now’.OS functions and performance, the other, a funded development project for a client. While Landmark’s evaluation of OS is still ‘work in progress’, the impression one got is of lukewarm support at best. Landmark offers enough encouragement to OS so as not to offend any key clients. Landmark was also significantly touting the merits of WITSML as an alternative, real-time focused, data sharing mechanism.As ever, there are many marketing decisions lurking behind the technology announcements. Is Schlumberger really moving to all .NET? Is Landmark moving to all Java? Will OS ever get beyond the position of a souped-up GeoFrame in the marketplace? What will likely determine OS’s role outside of Schlumberger is the attitude of the major oils. Will ChevronTexaco and Shell stick with OS? Will other majors come on board? Selling middleware is a tough call, but SAP and Tibco have both turned technology into a great business – with a little help from some of the same majors that seem to have cold feet when it comes to backing this E&P-specific middleware.HighlightsSchlumberger support strongLandmark’s position ‘explained’Shell and .NETSMT’s Open Spirit serverContentsOpenSpirit on the SIS Strategic Roadmap – Dominique Pajot, SIS (3)SIS Ocean/Coral/Seabed (3)OS role in Shell .NET migration – Winand Belmans, Shell (4)Landmark’s plans for OS – Janet Hicks, Landmark (5)Version 2.6 highlights – Clay Harter, Open Spirit Corp (6)An OS Server for SMT – Tom Smith, SMT (7)End user/developer presentations (7)Total’s use of Open Spirit (7)ChevronTexaco’s SeisVu seismic viewer (7)ChevronTexaco’s PWA reservoir model builder (7)Shell reports on growing use of Petrel (7)What is Open Spirit? (8)。
第3讲真三维GIS软件GOCAD介绍
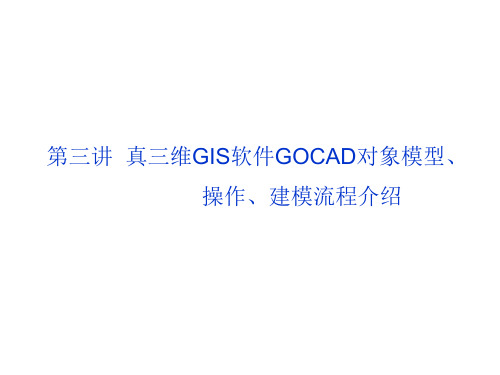
可以嵌入地质体.观看 Channelinstra.mx
Solid is a set of points connected by tetrahedron, a Solid is a 3-dimensional object. what is a tetrahedron? A three-sided pyramid, basically. A Solid is used to model volume, such as a velocity layer.
第三讲 真三维GIS软件GOCAD对象模型、 操作、建模流程介绍
GOCAD软件界面
2. WorkSpace
3. 3D Camera, where we view and work with our model objects.
4. Session History Area
5. Info Area,attribution operation,quickly button
复杂断层、地层面构建 地层面经常受断裂切割而不连续,在构建面时, 还要受不规则边界控制,相对于DEM表面的构 建,要复杂得多.
图9.3 TIN表面示意图
地层表面模拟
复杂表面的建立,要使用智能化向导工具WIZARD 生成.
演示复杂表面的生成.13FaultCurves.pl和 13toppart.vs
一个X-SECTION.
其他对象还有:MODEL3D,2D-G RID,FRAME,GROUP,用途不 多,先连接一下概念.以后可以应用.
关于构造建模.地层(储层)建模内容,下 次简单介绍,实习时候熟悉.
实体对象建立以后才可能进行建模,流程式 的操作,补角容易上手.
本周六下午两点半3S机房实习.
- 1、下载文档前请自行甄别文档内容的完整性,平台不提供额外的编辑、内容补充、找答案等附加服务。
- 2、"仅部分预览"的文档,不可在线预览部分如存在完整性等问题,可反馈申请退款(可完整预览的文档不适用该条件!)。
- 3、如文档侵犯您的权益,请联系客服反馈,我们会尽快为您处理(人工客服工作时间:9:00-18:30)。
Gocad Workflows for Expert-System Decision MakingJohn McGaughey, Gervais Perron, Sharon Parsons, and Tim Chalke Mira Geoscience Ltd May 2009AbstractWe present two examples of Gocad workflows that interrogate 3D earth models to assist critical geoscience business decision making. In both cases the general principle is to combine multiple model properties to determine spatial locations with desired statistical characteristics. The objective is to find (x, y, z) locations, otherwise difficult to discern, where certain special combinations of conditions exist. Targeting Workflow presents optimum targets to an explorationist seeking discovery. It takes as input multiple pre-computed properties and proximity relationships interpolated on a 3D grid (either voxet or sgrid) and outputs a classified and ranked list of targets. Typical input properties are lithology, formation, physical and geochemical rock properties, proximity relationships of structural and topological model objects, proximity to existing boreholes, and presence, proximity, and classification of geophysical anomalies. Geohazmap Workflow evaluates and monitors areas of potential geotechnical hazard. It is intended for use in underground or open pit mines as well as engineered rock structures for civil applications, such as tunnels and slopes. It takes as input a multitude of earth model objects such as rock quality models, stress, joints, faults, and excavation geometry. It also provides real-time monitoring of observations such as microseismic data, ground deformation, radar, and laser sighting. As with Targeting Workflow, it outputs a classified list of spatial locations, although in this case to be interpreted as zones of potential ground failure rather than as exploration targets. Geohazmap Workflow uses Gocad surfaces, rather than 3D grids, for supporting and computing the output properties, because geotechnical hazard is a natural property of surfaces rather than volumes. In both cases the workflows guide the user through the complex sequence of steps required for input data designation, characterization, quality control, normalization, and possible re-classification. Proven tools from the field of 2D-GIS spatial expert systems are deployed in 3D on either grids (Targeting Workflow) or surfaces (Geohazmap Workflow). These include the knowledge-driven techniques of Boolean Logic, Index Overlay, Multi-Class Index Overlay, and Fuzzy Logic, as well as the data-driven techniques of Weights-of-Evidence. Future work is contemplated to include other techniques such as Logistic Regression and possibly Neural Networks.IntroductionThe work described here is the development of commercial Gocad plug-ins for expert-system targeting of exploration drillholes and geotechnical hazards. In both cases a Gocad multi-disciplinary “common earth model” is created to support the various properties that serve as targeting criteria. The properties, held on either voxets or surfaces, are typically a mixture of interpreted rock properties such as lithology, alteration, and physical properties, and non-rock property target indicators such as depth, stress, proximity to faults, and geophysical anomalies. “Target” locations are identified, ranked, and classified by computing and analyzing a score at each location in the model. In the exploration case the score, called “mineral potential index”, is computed on a voxet. In the geotechnical hazard forecasting case the score, called “hazard”, is computed on a triangulated surface. A quantitative, probabilistic approach is taken by the workflows to computation of the score function by allowing the user to select amongst: a) a knowledge-driven framework in which expert knowledge is used to manually classify, score, and weight individual criteria; b) a data-driven framework in which training data of known valid targets in the model are used to classify and weight the input criteria; and c) classifications and scores from other models of similar settings are imported for application to a new model area. The workflows described here are inspired by a history of successful application of these methods in 2D GIS systems in mineral exploration going back to the 1980’s and 1990’s (see for example Bonham-Carter, 1994 and 1997), and more recent experimentation in 3D for exploration applications (Apel and Böhme,129th GOCAD-MEETING2006; Caumon et al., 2006). The Bayesian weights-of-evidence algorithm deployed at the core of Targeting Workflow is based on the work of Apel and Böhme (2006) with their “Predict” plug-in, presented at a previous Gocad Research Consortium meeting.1.Successful Targeting ProjectsWe have worked with many clients over the years in 3D earth modelling for both exploration and geotechnical hazard targeting, and learned what makes projects successful. The key to success is a process that is as simple in principle as it is too seldom followed with rigour in practice. Figure 1 shows the basic cyclical flow of project actions that lead to success. Variations on this theme work similarly well. The first five steps of the project can be completed, usually within a couple of days, by the project team. What we have found works well is an initial brainstorming session with the project team, facilitated by a neutral moderator, to quantitatively ascertain the targeting objective (for exploration this implies deposit size, grade, style, depth profile, and possibly other descriptions) and the conceptual model that establishes the geological context. There may be uncertainty in the conceptual model or possibly competing conceptual models advanced by the team. Typically agreement amongst the team on a quantitative objective and conceptual model is only arrived at after some meaningful discussion. Quantitative targeting criteria, required data, and overall model design (extents, depth, scale, resolution, etc.) follow fairly directly from a careful setup of objective and conceptual model. If there are competing conceptual models the model must be designed to support and test each of them. Although these first five project steps are fast and inexpensive to complete, we have often seen major projects start without them, proceeding directly to data compilation and model construction. Turning on the software should come only after careful planning.Figure 1 : Nominal steps of a successful targeting project. Data compilation is almost always the most expensive and time-consuming part of a targeting project, particularly when there is a lot of multi-disciplinary data and several custodians are involved. It is our experience that if projects falter, they typically falter at this stage, although the failure can usually be attributed to inadequate project planning and model design. Model construction simply requires an adept Gocad modeller and some time, typically a few weeks. If the project has been set up well, the resultingExpert-System WorkflowsMcGaughey et al.2/729th GOCAD-MEETINGmodel will give the expert-system workflows the best possible chance of success of identifying targets of business value.2.Targeting WorkflowTargeting Workflow is a Gocad workflow that takes as input a multi-variate voxet containing the set of targeting criteria and produces a target list as indicated by example in Figure 2. It takes the user through a sequence of pre-processing, prediction modelling, and post-processing steps. It provides a number of statistical investigations of input data and target validation procedures, in addition to offering both knowledge-driven and data-driven prediction modelling variants. At the highest level the workflow steps consist of: 1. Select modelling approach: a) Boolean Overlay, b) Weighted Boolean Overlay, c) Multi-class Index Overlay, d) Weights-of-evidence 2. Define model space and select evidential properties 3. Select training data 4. Re-classify evidential properties 5. Define thresholds based on expert opinion (knowledge-driven) or training data (data-driven) 6. Generate prediction model 7. Analysis and validation of prediction model 8. Target generation, classification, and ranking. Figure 3 shows a more detailed example, using the conceptually simple knowledge-driven, multi-class index overlay approach, of how various input data sets are classified into categories, each category assigned a score, and then each input variable weighted and combined into a simple mineral potential index.Figure 2: Idealized view of multi-variate targeting criteria as multiple voxet properties, output mineral potential index, and post-processed target list. This expert-system, 3D-GIS, approach to mineral exploration targeting has proven successful in discovering ore deposits in mature mining exploration camps that had otherwise not yielded new discovery in decades of exploration. This mode of analysis and interpretation of historical data, through Gocad’s superior 3D modelling technology, has been directly credited with discovery success (Martin et al., 2007).Expert-System WorkflowsMcGaughey et al.3/729th GOCAD-MEETINGFigure 3: Multi-class index overlay used with classifications, scores, and weights set by the expert team to interactively determine “mineral potential index”, the variable used to rank and classify targets.3.Geohazmap WorkflowGeohazmap Workflow is a Gocad workflow that accepts a range of real-time data to compute and display a user-defined hazard index on a mine or other geotechnical model. Its effectiveness rests on the power of Gocad, the underlying 3D modelling system. Geohazmap Workflow has been designed to be easily prototyped and rapidly customized through user workflow interaction. The ground failure whose risk is to be modelled by Geohazmap Workflow may take many forms, depending on user-defined site criteria. It is applicable to underground and open-pit mines, tunnelling, slope stability, and general seismic risk evaluation. It can be used to estimate risk of ground failure of any type, from slope failure to violent rockbursting. The Geohazmap Workflow approach is to define a list of input criteria that influence risk of ground failure (through the conceptual model), define how those criteria relate spatially to relative hazard through user-defined normalization functions, and then define the relative weighting of the criteria to the cumulative, general hazard index. The general hazard index is thus a spatially varying quantity which can be mapped on slope, stope, or development surfaces, in fact to any surface within an earth model. Many hazard components may be computed from the complex geometrical relationships of geology, stress, jointing, structure, rock mass properties, and engineered infrastructure. Thus it is critical that Geohazmap Workflow has access to a powerful underlying suite of raster and vector earth modelling and query tools, which is the rationale behind the development of Geohazmap Workflow as a Gocad workflow. Geohazmap Workflow has been designed to respond in real time to data updates. It presents a full visualization of hazard index with respect to all aspects of the mine geometry. Geohazmap Workflow has been prototyped at several sites, including both open-pit and underground coal mines and hard-rock mines. Figure 4 illustrates an intermediate computation from a case study in anExpert-System WorkflowsMcGaughey et al.4/729th GOCAD-MEETINGunderground gold mine in which Geohazmap Workflow was used to create a relative hazard index as a function of microseismicity, excavation geometry, stress, geological structure, and rock type.Figure 4. An intermediate computation made by Geohazmap Workflow in response to real-time microseismic data updates, showing distance to microseismic cluster-boundary edge on two vertical cross-sections and one plan elevation. The small spheres are microseismic events; the irregularly shaped “bubble” is a 3D isosurface contour at a threshold level of seismic event density (events per unit volume) over a user-defined time window. The lines are mine galleries.Expert-System WorkflowsMcGaughey et al.5/729th GOCAD-MEETINGFigure 5 shows the computation of a final hazard measure on infrastructure at an underground nickel mine. In this case study, the following hazard criteria were analyzed: 1. age of development 2. support type 3. mining method 4. stress state 5. proximity to structural faces, intersections, and boundaries 6. fault slip tendency 7. microseismic event density, local magnitude, and Es/Ep ratios 8. disking in core as an indirect stress indicator 9. rock quality 10. lithology 11. proximity to lithological contacts. The orange spheres shown in Figure 5 are the locations of historic falls of ground to fault-slip rockbursting. These locations served as the training data for a weights-of-evidence analysis. Posterior probability of groundfall is the property shown on the underground infrastructure. Because the system is designed to provide continuous monitoring of multiple inputs, and because it is able to consider variables and model states that are dynamic in time, Geohazmap Workflow serves as a convenient foundation for back-analysis in order to estimate or fine-tune the design of how the many individual hazard elements combine into a final estimation of hazard risk.Figure 5. Underground infrastructure painted with the posterior probability of groundfall due to faultslip rockbursting, based on a weights-of-evidence analysis using historical groundfall locations (depicted as orange spheres) as training data.Expert-System WorkflowsMcGaughey et al.6/729th GOCAD-MEETINGConclusionTargeting Workflow and Geohazmap Workflow are two new Gocad workflows that serve the same general purpose in two related business applications: discovering ore deposits and estimating geotechnical hazard. The common ground between them is expert-system identification of spatial locations of desired characteristics based on the statistical analysis of many “layers” of 3D input data. When used as part of projects that have thoughtfully considered quantitative objectives, geoscientifically sound conceptual models, and good project planning, they have already proven to deliver significant value in solving the difficult business problems of targeting exploration drilling and understanding geotechnical risk. The generality of the formulation means the same approach, even the same workflow software without modification, should find business value in other geoscientific applications where the objective is probabilistic identification of target locations based on multiple data layers. Examples from the mining industry include risk profiling in resource modelling, geometallurgical applications to understand and control mill feeds, and acid rock drainage minimization. Other examples of practical application assuredly exist in petroleum and environmental geoscience.ReferencesApel M., and Böhme, M., 2006. Predictive 3D mineral potential modelling. Proc. 26th Gocad Research Consortium Meeting, Nancy. Bonham-Carter, G.F., 1994. Geographic Information Systems for Geoscientists: Modelling with GIS. Computer Methods in the Geosciences. Pergamon Press, New York. 414 p. Bonham-Carter, G.F., 1997. GIS methods for integrating exploration data sets, in Gubins, A.G., ed., Proceedings of Exploration 97: Fourth Decennial Conference on Mineral Exploration, pp59-64. Caumon, G., Ortiz, J., and Rabeau, O., 2006. A comparative study of three mineral potential mapping techniques, Proc. IAMG 2006. Martin, L., Perron, G., and Masson, M., 2007. Discovery from 3D Visualization and Quantitative Modelling, in Milkereit, B., ed., Proceedings of Exploration 07: Fifth Decennial International Conference on Mineral Exploration, pp543-550.Expert-System WorkflowsMcGaughey et al.7/7。