Abstract tomography in medicine and process engineering
光学相干断层扫描血管成像术在糖尿病性视网膜病变中的应用
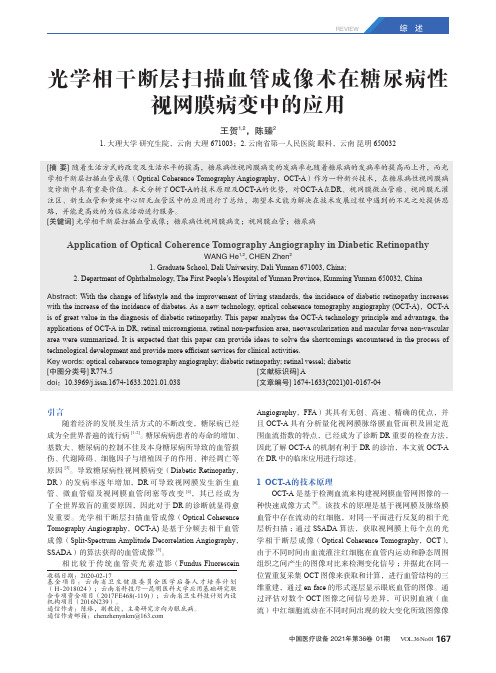
REVIEW引言随着经济的发展及生活方式的不断改变,糖尿病已经成为全世界普遍的流行病[1-2]。
糖尿病病患者的寿命的增加、基数大、糖尿病的控制不佳及本身糖尿病所导致的血管损伤、代谢障碍、细胞因子与增殖因子的作用、神经凋亡等原因[3]。
导致糖尿病性视网膜病变(Diabetic Retinopathy,DR)的发病率逐年增加,DR可导致视网膜发生新生血管、微血管瘤及视网膜血管闭塞等改变[4],其已经成为了全世界致盲的重要原因,因此对于DR的诊断就显得愈发重要。
光学相干断层扫描血管成像(Optical Coherence Tomography Angiography,OCT-A)是基于分频去相干血管成像(Split-Spectrum Amplitude Decorrelation Angiography,SSADA)的算法获得的血管成像[5]。
相比较于传统血管荧光素造影(Fundus Fluorescein Angiography,FFA)其具有无创、高速、精确的优点,并且OCT-A具有分析量化视网膜脉络膜血管面积及固定范围血流指数的特点,已经成为了诊断DR重要的检查方法,因此了解OCT-A的机制有利于DR的诊治,本文就OCT-A 在DR中的临床应用进行综述。
1 OCT-A的技术原理OCT-A是基于检测血流来构建视网膜血管网图像的一种快速成像方式[6]。
该技术的原理是基于视网膜及脉络膜血管中存在流动的红细胞,对同一平面进行反复的相干光层析扫描;通过SSADA算法,获取视网膜上每个点的光学相干断层成像(Optical Coherence Tomography,OCT),由于不同时间由血流灌注红细胞在血管内运动和静态周围组织之间产生的图像对比来检测变化信号;并据此在同一位置重复采集OCT图像来获取和计算,进行血管结构的三维重建,通过en face的形式逐层显示眼底血管的图像。
通过评估对数个OCT图像之间信号差异,可识别血液(血流)中红细胞流动在不同时间出现的较大变化所致图像像光学相干断层扫描血管成像术在糖尿病性视网膜病变中的应用王贺1,2,陈臻21. 大理大学研究生院,云南大理 671003;2. 云南省第一人民医院眼科,云南昆明 650032[摘 要]随着生活方式的改变及生活水平的提高,糖尿病性视网膜病变的发病率也随着糖尿病的发病率的提高而上升,而光学相干断层扫描血管成像(Optical Coherence Tomography Angiography,OCT-A)作为一种新兴技术,在糖尿病性视网膜病变诊断中具有重要价值。
中国迟发性运动障碍的药物治疗和研究现状及国内外差距

·学术天地·中国迟发性运动障碍的药物治疗和研究现状及国内外差距李睿,曾端,和申,沈一峰,李华芳摘要: 迟发性运动障碍(tardivedyskinesia,TD)是一种因长期使用抗精神病药而引发的严重药物不良反应,缺乏有效的治疗方法。
国内目前已尝试多种药物治疗TD,部分药物具有一定的疗效。
然而,相对国外已经批准新药且疗效确切的治疗现状,我国TD治疗与之有较大差距。
新型单胺类囊泡转运体2(vesicularmonoaminetransporter2,VMAT2)抑制剂的应用和相关研究是中国TD治疗的趋势和方向之一。
关键词: 迟发性运动障碍; 药物治疗; 戊苯那嗪; 氘代丁苯那嗪中图分类号: R749.053 文献标识码: A 文章编号: 1005 3220(2024)02 0166 03ThecurrentstatusofdrugtherapyandresearchontardymovementdisorderinChinaandthediffer encesathomeandabroad LIRui,ZENGDuan,HEShen,SHENYi feng,LIHua fang.DepartmentofPsychiatry,ShanghaiMentalHealthCenter,ShanghaiJiaoTongUniversitySchoolofMedicine,Shanghai200030,ChinaAbstract:Tardivedyskinesia(TD)isaseriousadversedrugreactioncausedbylong termuseofantipsy chotics,andtherehasbeenalackofeffectivetreatment.TDhasbeentreatedwithseveraldrugsinChina,andsomedrugshavecertainefficacy.However,TDtherapyinChinaisdifferentfromforeigncountrieswhichhaveapprovednewdrugsandhavedefinitetherapeuticeffects.TheapplicationandresearchofnovelVMAT2inhibi torsisoneofthetrendsanddirectionsofTDtherapyinChina.Keywords: tardivedyskinesia; drugtherapy; valbenazine; deutetrabenazine迟发性运动障碍(tardivedyskinesia,TD)临床表现以口 舌 颊三联征、四肢及躯干舞蹈样动作以及肌张力增高为主,严重影响患者的生活质量和社会功能。
医学影像学英文文献
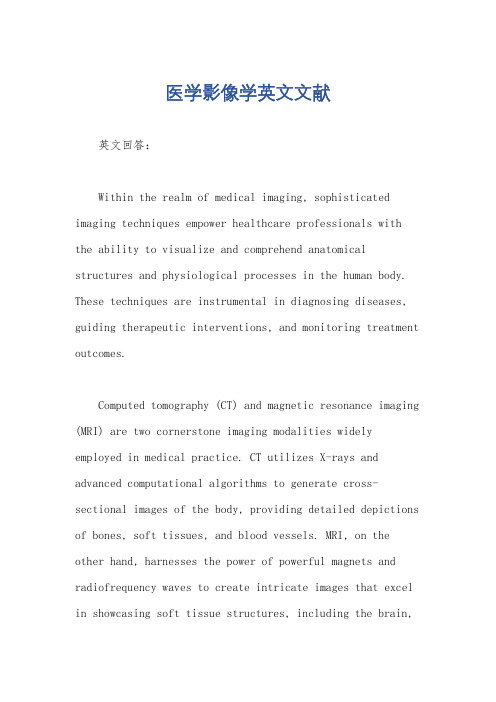
医学影像学英文文献英文回答:Within the realm of medical imaging, sophisticated imaging techniques empower healthcare professionals with the ability to visualize and comprehend anatomical structures and physiological processes in the human body. These techniques are instrumental in diagnosing diseases, guiding therapeutic interventions, and monitoring treatment outcomes.Computed tomography (CT) and magnetic resonance imaging (MRI) are two cornerstone imaging modalities widely employed in medical practice. CT utilizes X-rays and advanced computational algorithms to generate cross-sectional images of the body, providing detailed depictions of bones, soft tissues, and blood vessels. MRI, on the other hand, harnesses the power of powerful magnets and radiofrequency waves to create intricate images that excel in showcasing soft tissue structures, including the brain,spinal cord, and internal organs.Positron emission tomography (PET) and single-photon emission computed tomography (SPECT) are nuclear medicine imaging techniques that involve the administration of radioactive tracers into the body. These tracers accumulate in specific organs or tissues, enabling the visualization and assessment of metabolic processes and disease activity. PET is particularly valuable in oncology, as it can detect the presence and extent of cancerous lesions.Ultrasound, also known as sonography, utilizes high-frequency sound waves to produce images of internal structures. It is a versatile technique commonly employed in obstetrics, cardiology, and abdominal imaging. Ultrasound offers real-time visualization, making it ideal for guiding procedures such as biopsies and injections.Interventional radiology is a specialized field that combines imaging guidance with minimally invasive procedures. Interventional radiologists utilize imaging techniques to precisely navigate catheters and otherinstruments through the body, enabling the diagnosis and treatment of conditions without the need for open surgery. This approach offers reduced invasiveness and faster recovery times compared to traditional surgical interventions.Medical imaging has revolutionized healthcare by providing invaluable insights into the human body. The ability to visualize anatomical structures andphysiological processes in exquisite detail has transformed the practice of medicine, leading to more accurate diagnoses, targeted treatments, and improved patient outcomes.中文回答:医学影像学是现代医学不可或缺的一部分,它利用各种成像技术对人体的解剖结构和生理过程进行可视化和理解,在疾病诊断、治疗方案制定和治疗效果评估中发挥着至关重要的作用。
肠壁积气的影像学表现
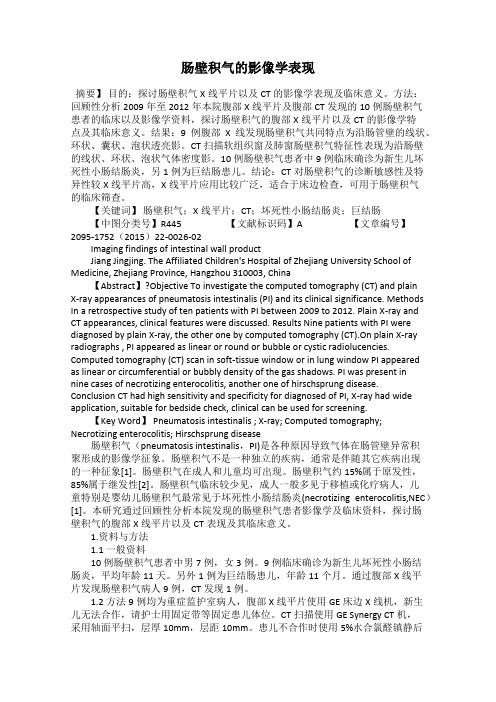
肠壁积气的影像学表现摘要】目的:探讨肠壁积气X线平片以及CT的影像学表现及临床意义。
方法:回顾性分析2009年至2012年本院腹部X线平片及腹部CT发现的10例肠壁积气患者的临床以及影像学资料,探讨肠壁积气的腹部X线平片以及CT的影像学特点及其临床意义。
结果:9例腹部X线发现肠壁积气共同特点为沿肠管壁的线状、环状、囊状、泡状透亮影。
CT扫描软组织窗及肺窗肠壁积气特征性表现为沿肠壁的线状、环状、泡状气体密度影。
10例肠壁积气患者中9例临床确诊为新生儿坏死性小肠结肠炎,另1例为巨结肠患儿。
结论:CT对肠壁积气的诊断敏感性及特异性较X线平片高,X线平片应用比较广泛,适合于床边检查,可用于肠壁积气的临床筛查。
【关键词】肠壁积气;X线平片;CT;坏死性小肠结肠炎;巨结肠【中图分类号】R445 【文献标识码】A 【文章编号】2095-1752(2015)22-0026-02Imaging findings of intestinal wall productJiang Jingjing. The Affiliated Children's Hospital of Zhejiang University School of Medicine, Zhejiang Province, Hangzhou 310003, China【Abstract】?Objective To investigate the computed tomography (CT) and plainX-ray appearances of pneumatosis intestinalis (PI) and its clinical significance. Methods In a retrospective study of ten patients with PI between 2009 to 2012. Plain X-ray and CT appearances, clinical features were discussed. Results Nine patients with PI were diagnosed by plain X-ray, the other one by computed tomography (CT).On plain X-ray radiographs , PI appeared as linear or round or bubble or cystic radiolucencies. Computed tomography (CT) scan in soft-tissue window or in lung window PI appeared as linear or circumferential or bubbly density of the gas shadows. PI was present innine cases of necrotizing enterocolitis, another one of hirschsprung disease. Conclusion CT had high sensitivity and specificity for diagnosed of PI, X-ray had wide application, suitable for bedside check, clinical can be used for screening.【Key Word】 Pneumatosis intestinalis ; X-ray; Computed tomography; Necrotizing enterocolitis; Hirschsprung disease肠壁积气(pneumatosis intestinalis,PI)是各种原因导致气体在肠管壁异常积聚形成的影像学征象。
a great deal of后面谓语动词

a great deal of后面谓语动词A Great Deal of Scientific Research in The Field of MedicineIntroductionScientific research plays a crucial role in the field of medicine by promoting innovation, advancing medical knowledge, and improving patient care. A great deal of research has been conducted in various domains, including disease prevention, diagnostic methods, therapeutic approaches, and public health interventions. In this article, we will explore some of the key areas in which a great deal of research has been undertaken, highlighting the impact it has had on healthcare practices worldwide.1. Disease Prevention and EpidemiologyDisease prevention is a fundamental aspect of healthcare, aiming to reduce the incidence and prevalence of various illnesses. A great deal of research has been focused on identifying risk factors associated with different diseases and developing effective preventive strategies. Epidemiological studies, in particular, have provided valuable insights into the distribution and determinants of diseases, aiding in the development of targeted interventions. For instance, extensive research in the field of cardiovascular diseases has led to the identification of risk factors such as smoking, high blood pressure, and obesity, which has subsequently influenced public health campaigns and policies.2. Diagnostic AdvancementsAdvancements in diagnostic methods have revolutionized medical practice, facilitating early detection and accurate diagnosis of diseases. A great deal of research has been dedicated to enhancing existing diagnostic techniques and developing novel approaches. For example, in the field of imaging, the development of magnetic resonance imaging (MRI) and positron emission tomography (PET) has significantly improved the visualization and characterization of diseases. Similarly, the application of molecular diagnostics, including polymerase chain reaction (PCR) and genetic testing, has allowed for precise identification of genetic disorders and targeted therapies.3. Therapeutic InnovationsTherapeutic research aims to find effective treatment options for various diseases, improving patient outcomes and quality of life. A great deal of research has been conducted to uncover novel therapeutic approaches, including drug development, immunotherapy, and regenerative medicine. The extensive research in the field of cancer has resulted in the discovery of targeted therapies, such as monoclonal antibodies and kinase inhibitors, which have revolutionized cancer treatment. Additionally, the advancements in stem cell research have paved the way for potential regenerative therapies, offering hope to patients with conditions like spinal cord injuries and neurodegenerative disorders.4. Public Health InterventionsPublic health research plays a vital role in identifying effective interventions to promote population health and prevent the spread of infectious diseases. A great deal of research has been undertaken tounderstand the social and environmental determinants of health and develop evidence-based public health policies. For instance, research on tobacco control has guided the implementation of smoking bans, taxation on tobacco products, and public awareness campaigns, resulting in significant reductions in tobacco-related diseases. Similarly, extensive studies on vaccination efficacy and immunization programs have led to the eradication or control of several infectious diseases globally.ConclusionIn conclusion, a great deal of scientific research has been conducted in the field of medicine, contributing to remarkable advancements in disease prevention, diagnostics, therapeutics, and public health interventions. The continuous efforts of researchers worldwide have not only expanded medical knowledge but also translated into improved patient care and population health outcomes. Moving forward, further research endeavors are essential to tackle emerging health challenges and ensure sustained progress in healthcare practices.。
医学影像学英语

医学影像学英语Medical imaging refers to the use of various techniques to create visual representations of the interior of the human body for diagnostic and therapeutic purposes. There are several different modalities used in medical imaging, including:1. X-ray: X-ray imaging uses ionizing radiation to create images of bones and tissues. It is commonly used to diagnose fractures, infections, and lung conditions.2. Computed Tomography (CT): CT scans use a series of X-ray images taken from different angles to create cross-sectional images of the body. It provides detailed images of organs, blood vessels, and bones, and is particularly useful for imaging the brain, chest, and abdomen.3. Magnetic Resonance Imaging (MRI): MRI uses a strong magnetic field and radio waves to create detailed images of the body. It is particularly useful for imaging soft tissues, such as muscles, organs, and the brain.4. Ultrasound: Ultrasound imaging uses high-frequency sound waves to create images of the inside of the body. It is commonly used for imaging fetal development during pregnancy, as well as imaging the abdomen, pelvis, and blood vessels.5. Nuclear Medicine: Nuclear medicine imaging involves the use of small amounts of radioactive substances to create images of the body. It is primarily used to diagnose and treat diseases such as cancer, heart disease, and thyroid disorders.In addition to these modalities, there are also various specialized imaging techniques, such as positron emission tomography (PET), mammography, and fluoroscopy, that are used for specific purposes.Medical imaging plays a crucial role in diagnosing and monitoring various medical conditions. It allows healthcare professionals to visualize the internal structures of the body, identify abnormalities, and guide interventions and treatments. Medical imaging reports are often written in English in order to facilitate communication between healthcare professionals and ensure consistent terminology and standards.。
临床常用英文缩写
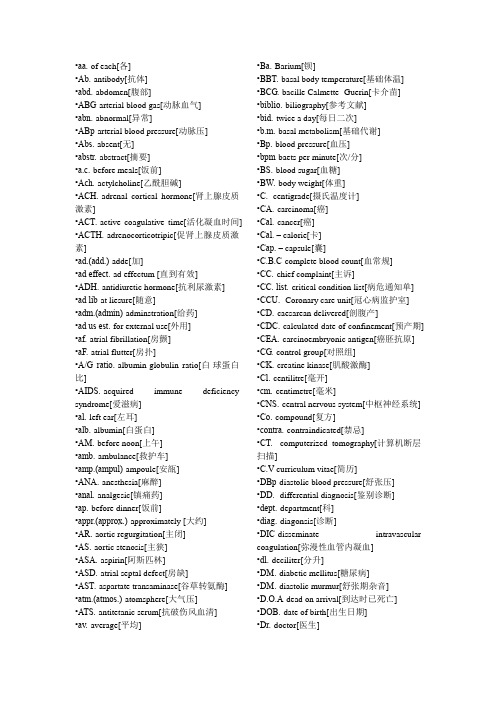
•aa.-of each[各]•Ab.-antibody[抗体]•abd.-abdomen[腹部]•ABG-arterial blood gas[动脉血气]•abn.-abnormal[异常]•ABp-arterial blood pressure[动脉压] •Abs.-absent[无]•abstr.-abstract[摘要]•a.c.-before meals[饭前]•Ach.-actylcholine[乙酰胆碱]•ACH.-adrenal cortical hormone[肾上腺皮质激素]•ACT.-active coagulative time[活化凝血时间] •ACTH.-adrenocorticotripic[促肾上腺皮质激素]•ad.(add.)-adde[加]•ad effect.-ad effectum [直到有效]•ADH.-antidiuretic hormone[抗利尿激素] •ad lib-at liesure[随意]•adm.(admin)-adminstration[给药]•ad us est.-for external use[外用]•af.-atrial fibrillation[房颤]•aF.-atrial flutter[房扑]•A/G ratio.-albumin-globulin ratio[白-球蛋白比]•AIDS.-acquired immune deficiency syndrome[爱滋病]•al.-left ear[左耳]•alb.-albumin[白蛋白]•AM.-before noon[上午]•amb.-ambulance[救护车]•amp.(ampul)-ampoule[安瓿]•ANA.-anesthesia[麻醉]•anal.-analgesic[镇痛药]•ap.-before dinner[饭前]•appr.(approx.)-approximately [大约] •AR.-aortic regurgitation[主闭]•AS.-aortic stenosis[主狭]•ASA.-aspirin[阿斯匹林]•ASD.-atrial septal defect[房缺]•AST.-aspartate transaminase[谷草转氨酶] •atm.(atmos.)-atomsphere[大气压]•ATS.-antitetanic serum[抗破伤风血清] •av.-average[平均] •Ba.-Barium[钡]•BBT.-basal body temperature[基础体温] •BCG.-bacille Calmette- Guerin[卡介苗] •biblio.-biliography[参考文献]•bid.-twice a day[每日二次]•b.m.-basal metabolism[基础代谢]•Bp.-blood pressure[血压]•bpm-baets per minute[次/分]•BS.-blood sugar[血糖]•BW.-body weight[体重]•C.- centigrade[摄氏温度计]•CA.-carcinoma[癌]•Cal.-cancer[癌]•Cal. – calorie[卡]•Cap. – capsule[囊]•C.B.C-complete blood count[血常规] •CC.-chief complaint[主诉]•CC. list.-critical condition list[病危通知单] •CCU.- Coronary care unit[冠心病监护室] •CD.-caesarean delivered[剖腹产]•CDC.-calculated date of confinement[预产期] •CEA.-carcinoembryonic antigen[癌胚抗原] •CG.-control group[对照组]•CK.-creatine kinase[肌酸激酶]•Cl.-centilitre[毫开]•cm.-centimetre[毫米]•CNS.-central nervous system[中枢神经系统] •Co.-compound[复方]•contra.-contraindicated[禁忌]•CT.- computerized tomography[计算机断层扫描]•C.V-curriculum vitae[简历]•DBp-diastolic blood pressure[舒张压] •DD.- differential diagnosis[鉴别诊断] •dept.-department[科]•diag.-diagonsis[诊断]•DIC-disseminate intravascular coagulation[弥漫性血管内凝血]•dl.-deciliter[分升]•DM.-diabetic mellitus[糖尿病]•DM.-diastolic murmur[舒张期杂音]•D.O.A-dead on arrival[到达时已死亡] •DOB.-date of birth[出生日期]•Dr.-doctor[医生]•DIW.-dextrose in water[葡萄糖液]•D-5-W,-5% dextrose in water[5%葡萄糖液] •DU-duodenal ulcer[十二指肠溃疡] •ECG.(EKG.)- electrocardiograph[心电图] •ECHO .-echogram[超声]•EDD.(EDC)-expected date of delivery (confinement)[预产期]•ENT. – ears, nose and throat[五官科] •EMG. – electromyogram[肌电图]•ER. – emergency room[急诊室]•et al.-and elsewhere[等等]•etc. – and so forth[等等]•F.(Fahr.)-Fahrenheit [华氏]•F- Female[女性]•F.B.S.- fasting blood sugar[空腹血糖] •FDP.-fibrinogen degradation products[纤维蛋白原降解产物]•FFA. – free fatty acid[游离脂肪酸] •FUO. – fever of unknown origin[不明原因发热]•FX. – fracture [骨折]•GH. – growth hormone[生长素]•GI.- gastrointestinal[消化]•GITS. – gastrointestinal therapy system[胃肠治疗系统]•gtt. – drops[滴]•GU.- gastric ulcer[胃溃疡]•Hb. – hemoglobin[血红蛋白]•HBp.-high blood pressure[高血压] •HCG. –human choroionic gonadotropic hormone[人绒毛膜促性腺激素]•HDL.- high density lipoprotein[高密度脂蛋白]•HR.-heart rate[心率]•ht.-height[身高]•HTN.-hypertension[高血压]•Hx.-history [病历]•Hypo.-hypodermic injection[皮下注射] •IABP.-intra – aortic balloon pacing[主动脉内囊反搏]•I/O.-intake and output [进出量]•ICU. – intensive care unit[重症监护病房] •ie. – that is [即]•Ig. – immunoglobulin[免疫球蛋白] •Im. – iutramuscular[肌内的]•INH.- inhalation[吸入]•INH.- isoniazid[异烟肼]•Inj.- injection[注射]•Int.- intern[实习生]•IP.- in-patient[住院病入]•Iu.- international unit[国防单位]•IV.-intravenously[静脉内]•J.- joule[焦耳]•K.U.B- Kidney,ureter and bladder[肾、输尿管和膀胱]•LBp.-low blood pressure [低血压]•LC. –laparoscopic cholecystectomy[腹腔镜胆囊切除术]•LDL.-Low density lipoprotein[低密度脂蛋白]•Liq. – liquid[液体]•LMP.- last menstrual period[未次月经] •LP. –lumbar puncture[腰穿]•M. –male[男性]•MCD.-mean corpuscular diameter[平均红细胞直径]•MCH.-mean corpuscular hemoglobin[平均红细胞血红蛋白量]•MCHC.-mean corpuscular hemoglobin concentration[平均红细胞血红蛋白浓度] •MCV.-mean corpuscular volume[平均红细胞体积]•MI.-myocardial infarction[心梗]•min.-minute[分]•mixt。
病灶定位技术的英语
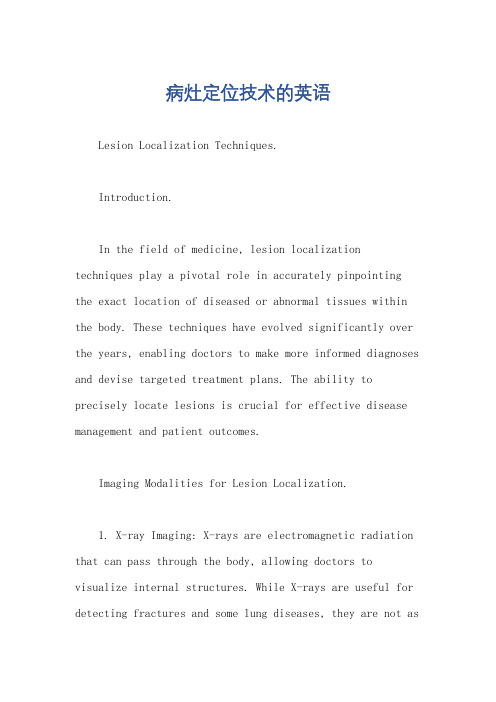
病灶定位技术的英语Lesion Localization Techniques.Introduction.In the field of medicine, lesion localization techniques play a pivotal role in accurately pinpointing the exact location of diseased or abnormal tissues within the body. These techniques have evolved significantly over the years, enabling doctors to make more informed diagnoses and devise targeted treatment plans. The ability to precisely locate lesions is crucial for effective disease management and patient outcomes.Imaging Modalities for Lesion Localization.1. X-ray Imaging: X-rays are electromagnetic radiation that can pass through the body, allowing doctors to visualize internal structures. While X-rays are useful for detecting fractures and some lung diseases, they are not assensitive as other imaging modalities for lesion localization.2. Ultrasound Imaging: Ultrasound uses sound waves to create images of internal organs. It is particularly useful for lesion localization in pregnant women, as it can safely visualize the fetus. It is also commonly used in the breast and thyroid.3. Computed Tomography (CT) Scans: CT scans combine multiple X-ray images taken from different angles to create cross-sectional views of the body. This technique is highly accurate for lesion localization and can detect even small abnormalities.4. Magnetic Resonance Imaging (MRI): MRI uses strong magnets and radio waves to generate detailed images of internal structures. It is excellent for soft tissue visualization and lesion localization, particularly in the brain and joints.5. Positron Emission Tomography (PET) Scans: PET scansdetect gamma rays emitted by radiolabeled substances injected into the patient. This technique is useful for lesion localization in cancer patients, as it can show tumor activity and spread.Advanced Lesion Localization Techniques.1. Fusion Imaging: This technique combines the data from multiple imaging modalities to create a single, comprehensive image. For example, PET-CT fusion imaging combines the functional information from a PET scan with the anatomical detail from a CT scan, improving lesion localization accuracy.2. Image-guided Interventions: These procedures use real-time imaging to guide surgical or therapeutic interventions. Examples include biopsy procedures, where a needle is guided to the lesion using ultrasound or CT imaging, or radiation therapy, where high-energy beams are precisely targeted to the lesion.3. Artificial Intelligence (AI) and Machine Learning:These technologies are revolutionizing lesion localization by enabling computers to analyze vast amounts of imaging data and detect patterns that may indicate disease. AI-powered algorithms can assist doctors in identifying lesions and even predicting their behavior.Conclusion.The evolution of lesion localization techniques has been transformative in medicine, enabling doctors to diagnose and treat patients more effectively. From traditional imaging modalities to advanced fusion imaging and AI-powered analysis, these techniques are constantly evolving to improve patient outcomes. As technology continues to advance, so will our ability to pinpoint and treat lesions with precision and accuracy.。
用英语介绍ct的工作原理700字作文

用英语介绍ct的工作原理700字作文全文共3篇示例,供读者参考篇1IntroductionComputed tomography (CT) is a valuable diagnostic tool in the field of medicine, providing detailed images of the body's internal structures. In this article, we will explore the working principle of CT scanners and how they produce high-quality images for medical diagnosis.Working Principle of CTCT scanners use a combination of X-rays and computer technology to create detailed cross-sectional images of the body. The patient lies on a table that slides through adoughnut-shaped machine called a gantry. Inside the gantry, an X-ray tube rotates around the patient, emitting thin beams ofX-rays. These X-rays pass through the body and are detected by sensors on the opposite side of the gantry.As the X-ray tube rotates, hundreds of images are taken from different angles. The data collected from these images is sent to a computer, which processes the information andreconstructs a three-dimensional image of the body's internal structures. This process is known as tomography, which means "slice imaging."Benefits of CTCT scans are widely used in medicine because they provide detailed images of soft tissues, organs, bones, and blood vessels. This allows doctors to diagnose a wide range of conditions such as cancer, heart disease, and traumatic injuries with great accuracy. CT scans are also used to guide surgical procedures, monitor treatment progress, and screen for certain diseases.ConclusionIn conclusion, CT scanners work by combining X-rays and computer technology to create detailed images of the body's internal structures. This diagnostic tool has revolutionized the field of medicine by providing doctors with valuable information for accurate diagnosis and treatment planning. As technology continues to advance, CT scanners will likely become even more sophisticated and versatile in the future.篇2Computed tomography (CT) scanning, also known as a CAT scan, is a powerful medical imaging technique that uses x-rays tocreate detailed cross-sectional images of the body. CT scans are commonly used to diagnose a wide range of conditions, including tumors, fractures, and internal injuries.The basic principle behind CT scanning is relatively simple. During a CT scan, the patient lies on a table that slides into a donut-shaped machine called a gantry. Inside the gantry, anx-ray tube rotates around the patient, emitting a series of thin x-ray beams. These beams pass through the body and are detected by a set of sensors located on the opposite side of the gantry.As the x-ray tube rotates around the patient, multiple images are captured from different angles. These images are then processed by a computer to create a series ofcross-sectional slices, or "tomograms," that show detailed views of the body's internal structures. The resulting images can be viewed as two-dimensional slices or combined to createthree-dimensional reconstructions.One of the key advantages of CT scanning is its ability to produce detailed images of soft tissues, bones, and blood vessels with a high level of clarity. This makes CT scans particularly useful for detecting abnormalities in the brain, lungs, abdomen, and other areas of the body. In addition, CT scans areoften used to guide minimally invasive procedures, such as biopsies and surgeries.Despite its many benefits, CT scanning does expose patients to a small amount of ionizing radiation, which can increase the risk of cancer over time. To minimize this risk, healthcare providers use the lowest possible dose of radiation required to obtain high-quality images. Patients who are pregnant or may be pregnant are typically advised to avoid CT scans, as the radiation can harm the developing fetus.In conclusion, CT scanning is a valuable tool for diagnosing a wide range of medical conditions. By combining x-rays with sophisticated computer technology, CT scans provide detailed images that help healthcare providers make accurate diagnoses and develop effective treatment plans. As technology continues to advance, CT scanning will likely play an increasingly important role in modern medicine.篇3CT (computed tomography) is a medical imaging technique that uses x-rays to create detailed cross-sectional images of the body. It is an essential tool in diagnosing and monitoring various medical conditions, including cancers, injuries, and infections. Inthis essay, we will explore the working principles of CT scanners and their importance in modern healthcare.A CT scanner consists of an x-ray tube that rotates around the patient while emitting a series of x-ray beams. These beams pass through the body and are picked up by detectors on the opposite side of the scanner. The detectors measure the amount of radiation that is absorbed by different tissues, producing a series of x-ray images.The x-ray images are then processed by a computer to create cross-sectional slices of the body. These slices provide detailed information about the internal structures of the body, allowing doctors to visualize organs, bones, and tissues in great detail. The images produced by a CT scanner are much clearer and more detailed than those produced by conventional x-ray machines, making it an invaluable tool in medical imaging.One of the key advantages of CT scanners is their ability to create three-dimensional images of the body. By combining multiple cross-sectional slices, doctors can reconstruct a 3D image of the patient’s anatomy, which can be rotated and viewed from different angles. This 3D imaging capability is particularly useful in planning surgical procedures and guidinginterventions, as it allows doctors to visualize the exact location and extent of abnormalities within the body.Another important feature of CT scanners is their ability to detect subtle differences in tissue density. Different tissues in the body absorb x-rays at different rates, which results in variations in the brightness of the images produced by a CT scanner. By analyzing these variations in tissue density, doctors can identify abnormalities such as tumors, blood clots, and infections that may be difficult to detect with other imaging techniques.In addition to their diagnostic capabilities, CT scanners are also used in monitoring the progress of certain medical treatments. For example, CT scans can be used to assess the response of tumors to chemotherapy or radiation therapy, allowing doctors to adjust treatment plans as needed. CT scans can also be used to guide minimally invasive procedures, such as biopsies and injections, by providing real-time imaging of the targeted area.Overall, CT scanners play a critical role in modern healthcare by providing detailed and accurate images of the body’s internal structures. Their ability to create 3D images, detect subtle tissue abnormalities, and monitor treatment responses makes them invaluable tools for diagnosing and managing awide range of medical conditions. As technology continues to advance, CT scanners are expected to become even more sophisticated, further enhancing their utility in clinical practice.。
探讨医学疗法的深度分析英文论文题目

探讨医学疗法的深度分析英文论文题目Abstract:This paper aims to delve into the intricacies of various medical therapies, examining their principles, effectiveness, and the evolving landscape of healthcare. By integrating diverse perspectives, we will explore traditional and modern treatments, comparing their benefits, limitations, and the role ofevidence-based medicine in guiding clinical decision-making.1. IntroductionThe field of medicine is constantly evolving, with novel therapies emerging alongside time-tested practices. This exploration begins by defining the scope of our inquiry, discussing the importance of understanding the complexities of medical therapies in improving patient outcomes.2. Traditional Medicine: Herbs, Acupuncture, and AyurvedaTraditional healing systems, such as herbal medicine, acupuncture, and Ayurveda, have deep historical roots. We analyze their holistic approaches, mechanisms of action, and the increasing interest in integrating them with Western medicine.3. Modern Medical Therapies: Pharmaceuticals and ProceduresFocusing on pharmaceuticals, we discuss the development of targeted therapies, pharmacogenomics, and the potential for personalized medicine. Procedures like chemotherapy, surgery, and radiation therapy will also be examined, emphasizing advancements and associated risks.4. Evidence-Based Medicine: The Foundation for Decision-MakingWe argue that evidence-based medicine (EBM) is crucial in evaluating the efficacy and safety of therapies. This section delves into how EBM promotes informed choices, challenges, and future directions for research.5. Complementary and Alternative Medicine (CAM)Exploring CAM's growing popularity, we analyze its role in integrative medicine, discussing the need for rigorous scientific evaluation and the potential for synergy with conventional treatments.6. Challenges and Ethical ConsiderationsThis section addresses the ethical dilemmas posed by rapidlyadvancing therapies, including access, affordability, and the potential for misuse or overuse of treatments.7. Future Directions: Emerging Technologies and ResearchA look at the future of medical therapies, including nanotechnology, gene editing, and telemedicine, highlights the opportunities and challenges these innovations present.8. ConclusionIn conclusion, this paper underscores the necessity of a comprehensive understanding of medical therapies to optimize patient care. By embracing an integrative approach, we can harness the strengths of both traditional and modern medicine, ultimately improving global health outcomes.中文摘要:本文旨在深入探讨医学疗法的深度分析,关注传统与现代疗法的交融。
医学影像学专业英语

医学影像学专业英语Title: Advancements in Medical Imaging TechnologyMedical imaging is a pivotal field within the broader domain of healthcare, providing critical insights into the diagnosis, treatment, and management of diseases. Over the years, this field has witnessed remarkable advancements, transforming the way medical professionals approach patient care. This essay delves into the evolution of medical imaging technology, highlighting its significance in modern medicine and discussing future prospects.The journey of medical imaging began with simple X-rays in the late 19th century, which allowed for the visualization of bones and other dense structures within the body. This groundbreaking discovery laid the foundation for more sophisticated imaging techniques that followed. The development of computed tomography (CT) scans in the 1970s marked a significant milestone, enabling detailed cross-sectional images of the body's internal structures. CT scans revolutionized the diagnosis and monitoring of various conditions, from traumatic injuries to cancerous growths.Magnetic Resonance Imaging (MRI) further advanced the capabilities of medical imaging by utilizing powerful magnetsand radio waves to produce high-resolution images of soft tissues. Unlike X-rays and CT scans, MRI does not involve ionizing radiation, making it a safer option for repeated use and particularly beneficial for imaging the brain, spinal cord, and joints. The introduction of functional MRI (fMRI) expanded the scope of MRI by allowing doctors to observe brain activity and understand how different regions of the brain communicate with each other.Ultrasound imaging, another non-invasive technique, employs high-frequency sound waves to create real-time images of organs and tissues. Its portability, safety, andcost-effectiveness make ultrasound an indispensable tool in prenatal care, cardiovascular assessments, and guiding invasive procedures.Positron Emission Tomography (PET) scans represent yet another leap forward in medical imaging. By measuring metabolic processes, glucose metabolism, and blood flow within the body, PET scans are invaluable for detecting cancerous tissues, assessing treatment efficacy, and studying brain disorders such as Alzheimer's disease.The integration of artificial intelligence (AI) into medical imaging represents the latest frontier in this field. AIalgorithms can analyze vast amounts of imaging data rapidly and with high accuracy, assisting radiologists in identifying abnormalities that might be overlooked by the human eye. Moreover, AI-powered tools are being developed to predict disease progression and personalize treatment plans based on individual patient data.Despite these advancements, challenges remain. Ensuring equitable access to advanced imaging technologies across different populations and geographies is a significant concern. Additionally, the interpretation of complex imaging data requires highly trained professionals, underscoring the need for continuous education and training in medical imaging.Looking ahead, the future of medical imaging holds immense promise. Innovations such as molecular imaging, which targets specific biological processes at the cellular level, and photoacoustic imaging, which combines laser excitation with ultrasound detection, are poised to further enhance our understanding of disease mechanisms. As these technologies continue to evolve, they will undoubtedly play a crucial role in advancing personalized medicine and improving patient outcomes.In conclusion, the field of medical imaging has come along way since its inception, driven by continuous innovation and technological advancements. These developments have not only transformed diagnostic capabilities but also paved the way for new therapeutic approaches. As we look to the future, the integration of emerging technologies like AI promises to usher in a new era of precision medicine, ultimately leading to better health outcomes for patients worldwide.。
医学影像相关的英语
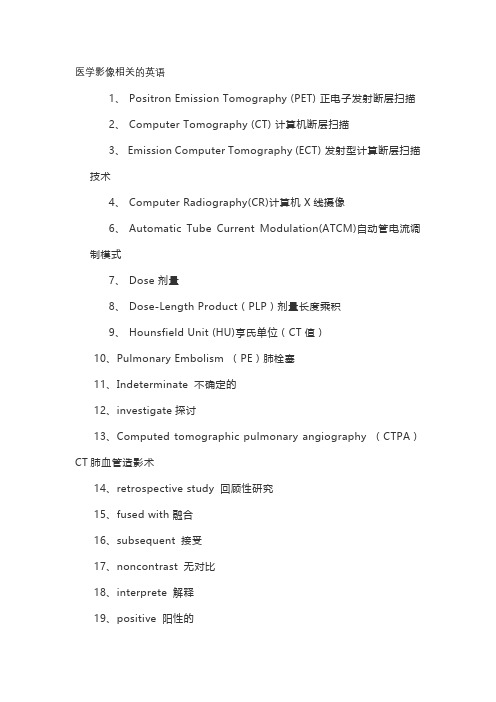
医学影像相关的英语1、Positron Emission Tomography (PET) 正电子发射断层扫描2、Computer Tomography (CT) 计算机断层扫描3、Emission Computer Tomography (ECT) 发射型计算断层扫描技术4、Computer Radiography(CR)计算机X线摄像6、Automatic Tube Current Modulation(ATCM)自动管电流调制模式7、Dose 剂量8、Dose-Length Product(PLP)剂量长度乘积9、Hounsfield Unit (HU)亨氏单位(CT值)10、Pulmonary Embolism (PE)肺栓塞11、Indeterminate 不确定的12、investigate探讨13、Computed tomographic pulmonary angiography (CTPA)CT肺血管造影术14、retrospective study 回顾性研究15、fused with融合16、subsequent 接受17、noncontrast 无对比18、interprete 解释19、positive 阳性的20、negative 阴性的21、false-negative 假阴性22、sensitivity 敏感的23、specificity 特异性24、predictive value 预测值25、clinical verdict 临床结论26、Hybrid Perfusion 混合灌注27、high attendant rates of morbidity and mortality 高发病率和死亡率28、fatality rates 病死率29、inherent 固有的30、dilemma 困境31、severity 严重度32、preventable 可预防的33、anticoagulant therapy 抗凝治疗34、priority 优先权35、visualization 显示36、multiple myeloma 多发性骨髓瘤37、allergy to intravenous contrast 静脉造影剂过敏38、saline flush 生理盐水冲洗39、trigger 触发40、ventricle 心室41、pitch factor 螺距系数42、in the supine position 仰卧位43、acquisition parameters 采集参数44、collimation 准直度45、gantry rotation speed 机架转速1、。
医学上的发明发现英语作文

医学上的发明发现英语作文英文回答:Medical Innovations and Discoveries.The field of medicine has witnessed a remarkable journey of scientific breakthroughs and technological advancements that have revolutionized healthcare. From the discovery of antibiotics to the advent of advanced imaging techniques, these innovations have profoundly impacted the way we prevent, diagnose, and treat diseases.Antibiotics.The discovery of antibiotics in the early 20th century marked a pivotal moment in medical history. Prior to their advent, bacterial infections were often fatal. Antibiotics, such as penicillin and streptomycin, have dramatically reduced the incidence and mortality rates associated with infections. Today, antibiotics are essential components ofmodern medicine, helping to combat a wide range ofbacterial diseases.Imaging Techniques.Advances in imaging technologies have revolutionizedthe diagnosis and monitoring of diseases. X-rays, computed tomography (CT), magnetic resonance imaging (MRI), and ultrasound provide clinicians with detailed cross-sectional images of the body's internal structures. These techniques enable accurate diagnoses and early detection of conditions, leading to more timely and effective treatments.Vaccines.The development of vaccines has played a crucial rolein preventing the spread of infectious diseases. Vaccines stimulate the body's immune system to produce antibodies against specific pathogens, thereby providing protection against future infections. Smallpox, polio, and measles are just a few examples of diseases that have been virtually eradicated thanks to the widespread use of vaccines.Surgical Techniques.Surgical techniques have also undergone significant advancements. Minimally invasive surgery, such as laparoscopy and robotic surgery, allows surgeons to perform complex procedures through small incisions. These techniques minimize pain, reduce scarring, and shorten recovery times for patients.Personalized Medicine.The rise of personalized medicine has empowered clinicians with the ability to tailor treatments to individual patients based on their genetic makeup and specific disease profiles. Genomic sequencing and biomarker analysis help identify patients who are likely to respond best to certain therapies, optimizing treatment outcomes.Artificial Intelligence (AI)。
医学影像诊断英语

医学影像诊断英语Diagnostic Imaging in MedicineThe field of diagnostic imaging has revolutionized the way healthcare professionals approach patient care. From the early days of X-rays to the cutting-edge technologies of modern imaging techniques, the evolution of this discipline has greatly enhanced our understanding of the human body and our ability to detect, diagnose, and monitor various medical conditions. In this essay, we will explore the significant role that diagnostic imaging plays in the field of medicine.One of the primary benefits of diagnostic imaging is its ability to provide healthcare professionals with a detailed and comprehensive view of the internal structures of the human body. Traditional physical examinations and laboratory tests can only provide limited information, but diagnostic imaging techniques, such as X-rays, computed tomography (CT) scans, magnetic resonance imaging (MRI), and ultrasound, allow healthcare providers to visualize organs, tissues, and even the smallest of anatomical details with remarkable clarity. This increased level of visual information has significantly improved the accuracy of diagnoses, enabling healthcareprofessionals to detect and identify a wide range of medical conditions at earlier stages, leading to more effective treatment and better patient outcomes.In the field of radiology, for instance, the use of X-rays has been a cornerstone of diagnostic imaging for over a century. X-rays, which are a form of electromagnetic radiation, are able to pass through the human body and create images that reveal the structure and density of internal organs and tissues. This technology has been instrumental in the diagnosis of fractures, lung diseases, and certain types of cancer, among other conditions. As technology has advanced, the quality and resolution of X-ray images have improved, allowing healthcare providers to make more informed decisions and provide more targeted treatments.Another important diagnostic imaging modality is the CT scan, which uses a series of X-ray images taken from different angles to create a detailed, three-dimensional representation of the body's internal structures. CT scans are particularly useful in the diagnosis of conditions that affect the brain, such as strokes, tumors, and head injuries, as well as in the evaluation of the abdominal and thoracic regions for a variety of medical issues.Magnetic resonance imaging (MRI), on the other hand, utilizes strong magnetic fields and radio waves to generate detailed imagesof the body's internal structures. Unlike X-rays and CT scans, MRI does not use ionizing radiation, making it a safer option for certain types of imaging, particularly in the diagnosis of neurological conditions and musculoskeletal injuries. MRI is also highly effective in the detection and monitoring of soft tissue diseases, such as multiple sclerosis, arthritis, and certain types of cancer.Ultrasound imaging, which uses high-frequency sound waves to create real-time images of the body's internal structures, is another important diagnostic tool. Ultrasound is particularly useful in the evaluation of the cardiovascular system, as it allows healthcare providers to visualize the heart and blood vessels, as well as in the assessment of pregnancy and fetal development. Ultrasound is also a valuable tool in the diagnosis of abdominal and pelvic conditions, such as gallbladder disease, kidney stones, and ovarian cysts.In addition to its diagnostic capabilities, diagnostic imaging also plays a crucial role in the planning and monitoring of medical treatments. For example, healthcare providers can use imaging techniques to guide the placement of surgical instruments, such as during minimally invasive procedures, or to monitor the progress of a patient's treatment, such as the response to cancer therapies. This integration of diagnostic imaging with treatment planning and monitoring has significantly improved the accuracy and effectiveness of medical interventions, leading to better patient outcomes andreduced healthcare costs.Moreover, the field of diagnostic imaging is continuously evolving, with the development of new and more advanced technologies. Innovations such as positron emission tomography (PET) scans, which can detect biochemical changes in the body, and hybrid imaging techniques, such as PET-CT and PET-MRI, which combine the strengths of multiple imaging modalities, have further enhanced the capabilities of diagnostic imaging in the detection and management of various medical conditions.As healthcare systems around the world strive to provide high-quality, cost-effective care, the importance of diagnostic imaging in modern medicine cannot be overstated. By providing healthcare professionals with detailed and accurate information about the state of a patient's health, diagnostic imaging has become an indispensable tool in the clinical decision-making process, leading to earlier diagnoses, more targeted treatments, and ultimately, better patient outcomes.In conclusion, the field of diagnostic imaging has played a pivotal role in the advancement of modern medicine. From the initial discovery of X-rays to the development of cutting-edge imaging technologies, the evolution of this discipline has transformed the way healthcare professionals approach patient care. By providingdetailed visual information about the internal structures of the human body, diagnostic imaging has become an essential component of comprehensive healthcare, enabling more accurate diagnoses, more effective treatments, and ultimately, improved patient outcomes.。
neuroscience 医学英语

neuroscience 医学英语Neuroscience, a field that delves into the intricate mysteries of the human brain and nervous system, has become an indispensable cornerstone in the realm of modern medicine. Its profound impact on our understanding of neurological disorders, cognitive functions, and the intricate pathways that govern our thoughts, emotions, and behaviors has revolutionized the way we approach healthcare. Through the lens of neuroscience, we have gained invaluable insights into the complexity of the human mind, unlocking new frontiers in diagnosis, treatment, and prevention of a myriad of neurological conditions.At the core of neuroscience lies the intricate study of the brain's anatomy, its intricate neural networks, and the intricate interplay between various neurotransmitters and receptors. By unraveling the intricacies of these microscopic processes, researchers have been able to shed light on the underlying mechanisms behind numerous neurological disorders, ranging from Alzheimer's disease and Parkinson's disease to epilepsy and stroke. This profound understanding has paved the way for groundbreaking advancements in therapeutic interventions, including targeted drug development, surgical procedures, and novel rehabilitation techniques.One of the most remarkable contributions of neuroscience to the field of medicine has been the development of cutting-edge neuroimaging techniques. Functional Magnetic Resonance Imaging (fMRI), Positron Emission Tomography (PET), and Electroencephalography (EEG) have provided unprecedented insights into the real-time functioning of the brain, enabling clinicians to visualize and analyze neural activity with unprecedented precision. These advanced imaging modalities have not only facilitated early diagnosis and monitoring of neurological conditions but have also opened up new avenues for understanding cognitive processes, such as memory, attention, and decision-making.Moreover, neuroscience has shed light on the intricate relationship between the brain and the body, revealing the profound impact of neurological processes on various physiological systems. This knowledge has led to groundbreaking discoveries in the treatment of chronic pain, sleep disorders, and even psychological conditions like depression and anxiety. By targeting specific neural pathways and modulating neurotransmitter levels, neuroscientists have developed novel therapeutic interventions that offer hope to countless individuals suffering from debilitating neurological and psychological conditions.Beyond its clinical applications, neuroscience has also played apivotal role in shaping our understanding of human behavior, cognition, and consciousness. Through the study of neural plasticity, researchers have uncovered the brain's remarkable ability to adapt and reorganize itself in response to environmental stimuli, injury, or learning experiences. This groundbreaking discovery has paved the way for innovative approaches in rehabilitation, cognitive enhancement, and educational strategies, empowering individuals to optimize their cognitive potential and overcome neurological challenges.As we venture deeper into the 21st century, the field of neuroscience continues to evolve at an unprecedented pace, driven by technological advancements and interdisciplinary collaborations. The integration of neuroscience with fields such as artificial intelligence, bioengineering, and nanotechnology has opened up exciting new frontiers, including the development of brain-computer interfaces, neural prosthetics, and targeted drug delivery systems. These cutting-edge innovations hold the promise of transforming the lives of individuals affected by neurological disorders, offering them newfound independence, enhanced cognitive abilities, and improved quality of life.Despite the remarkable progress made thus far, there remains an abundance of unanswered questions and unexplored territories within the realm of neuroscience. The quest to unravel the mysteriesof consciousness, the intricate mechanisms underlying neuroplasticity, and the intricate interplay between genetics and environmental factors in shaping brain development continues to captivate the minds of researchers worldwide. Additionally, the ethical and societal implications of neuroscientific discoveries, such as neuroenhancement and the potential for misuse of neurotechnology, pose complex challenges that demand careful consideration and responsible stewardship.In conclusion, neuroscience has emerged as a transformative force in the field of medicine, revolutionizing our understanding of the human brain and nervous system. Through its multidisciplinary approach and groundbreaking discoveries, it has paved the way for innovative diagnostic tools, therapeutic interventions, and preventive strategies, improving the lives of countless individuals afflicted by neurological disorders. As we continue to delve deeper into the intricate workings of the brain, neuroscience will undoubtedly unlock new frontiers in healthcare, empowering us to confront the challenges of the future with unprecedented knowledge, compassion, and resilience.。
医学影像学的英文

医学影像学的英文Medical Imaging in EnglishMedical imaging refers to the techniques and processes used to create images of the human body for clinical purposes. It plays a crucial role in the diagnosis and treatment of various medical conditions. In this article, we will explore the field of medical imaging and discuss its importance in the healthcare industry.One of the most common modalities used in medical imaging is X-ray imaging. X-rays are a form of electromagnetic radiation that can pass through the body, allowing healthcare professionals to visualize internal structures such as bones and organs. X-ray images are particularly useful in the diagnosis of fractures, lung conditions, and gastrointestinal issues.Another important modality in medical imaging is computed tomography (CT) scanning. CT scans use a combination of X-rays and computer technology to create cross-sectional images of the body. This allows for detailed examination of internal organs and tissues, making it an invaluable tool in the detection of tumors, infections, and other medical abnormalities.Magnetic resonance imaging (MRI) is another widely used modality in medical imaging. MRI uses magnetic fields and radio waves to generate detailed images of the body's internal structures. MRI is particularly useful in imaging soft tissues such as the brain, spinal cord, and muscles. It is often used to diagnose neurological disorders, joint injuries, and tumors.Ultrasound imaging, also known as sonography, uses sound waves to create images of the body's internal structures. Ultrasound is commonly used to visualize the fetus during pregnancy, as well as to assess the health of organs such as the heart, liver, and kidneys. Ultrasound is a safe and non-invasive imaging modality that is widely used in medical practice.Nuclear medicine is a specialized branch of medical imaging that uses radioactive substances to visualize and diagnose medical conditions. Techniques such as positron emission tomography (PET) and single-photon emission computed tomography (SPECT) are commonly used in nuclear medicine to detect diseases such as cancer, heart conditions, and neurological disorders.In addition to these modalities, medical imaging also encompasses a variety of other techniques such as fluoroscopy, mammography, and angiography. Each modality has its own strengths and limitations, and healthcare professionals must choose the most appropriate imaging technique based on the patient's condition and the clinical question at hand.The field of medical imaging is constantly evolving, with new technologies and techniques being developed to improve diagnostic accuracy and patient outcomes. Artificial intelligence (AI) and machine learning are increasingly being used in medical imaging to analyze images, detect abnormalities, and assist in diagnosis. These advances hold great promise for the future of medical imaging and have the potential to revolutionize healthcare delivery.In conclusion, medical imaging is an essential component of modern healthcare, providing healthcare professionals with valuable information todiagnose and treat medical conditions. From X-rays to MRI to nuclear medicine, the field of medical imaging offers a wide range of modalities to visualize the human body and provide insights into the underlying pathology. As technology continues to advance, the role of medical imaging in healthcare will only continue to grow in importance.。
影像 医学英语

医学影像与医学英语的融合之路In the field of healthcare, the integration of medical imaging and medical English plays a pivotal role in enhancing diagnostic accuracy, improving patient care, and facilitating international collaboration. As technology advances, the complexity of medical imaging techniques and terminologies increases, necessitating a strong foundation in medical English to keep up with the latest advancements. Medical imaging, encompassing modalities such as radiography, ultrasonography, computed tomography (CT), magnetic resonance imaging (MRI), and nuclear medicine, allows physicians to visualize the internal structures and functions of the human body non-invasively. Accurate interpretation of these images relies heavily on a clear understanding of anatomical terms, pathology, and imaging principles. Medical English, being the lingua franca of the medical community, facilitates the communication of these complex concepts across borders and disciplines.The importance of medical English in medical imaging is further emphasized by the globalization of healthcare. With the increasing number of international medical conferences,collaborations, and exchange programs, proficiency in medical English has become a prerequisite for professionals seeking to stay abreast of global trends and advancements. Additionally, as more patients seek medical care abroad, the ability to communicate effectively in English becomes crucial for ensuring smooth patient care and positive outcomes.Moreover, the integration of medical imaging and medical English is crucial for the education and training of future healthcare professionals. Medical students and residents must familiarize themselves with medical terminologies and imaging principles to diagnose and treat patients effectively. By incorporating medical English into their education, these future professionals will be better prepared to work in a globalized healthcare environment, collaborating with colleagues from diverse backgrounds and disciplines.In conclusion, the integration of medical imaging and medical English is not just a tool for communication but a necessity for enhanced diagnostic accuracy, improvedpatient care, and successful international collaboration.As healthcare continues to evolve, so must the proficiency of healthcare professionals in medical English to ensure the delivery of high-quality, patient-centered care.**医学影像与医学英语的融合之路**在医疗保健领域,医学影像与医学英语的融合对于提高诊断准确性、改善患者护理和促进国际合作至关重要。
骨转换标志物联合全身骨显像检测诊断早期骨转移的临床价值
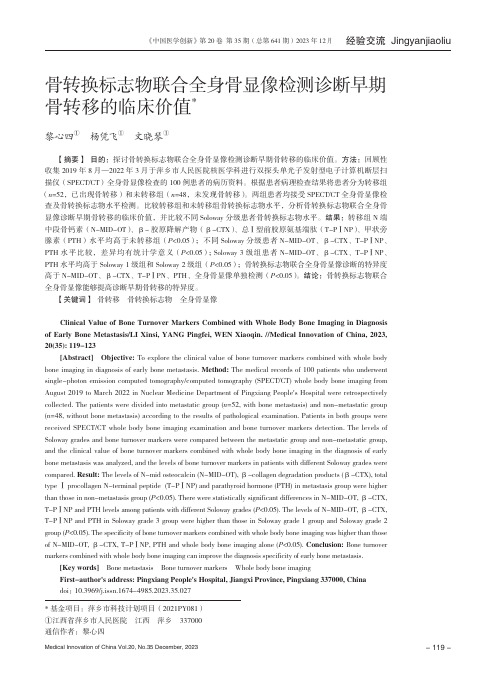
骨转换标志物联合全身骨显像检测诊断早期骨转移的临床价值*黎心四① 杨凭飞① 文晓琴① 【摘要】 目的:探讨骨转换标志物联合全身骨显像检测诊断早期骨转移的临床价值。
方法:回顾性收集2019年8月—2022年3月于萍乡市人民医院核医学科进行双探头单光子发射型电子计算机断层扫描仪(SPECT/CT)全身骨显像检查的100例患者的病历资料。
根据患者病理检查结果将患者分为转移组(n=52,已出现骨转移)和未转移组(n=48,未发现骨转移)。
两组患者均接受SPECT/CT全身骨显像检查及骨转换标志物水平检测。
比较转移组和未转移组骨转换标志物水平,分析骨转换标志物联合全身骨显像诊断早期骨转移的临床价值,并比较不同Soloway分级患者骨转换标志物水平。
结果:转移组N端中段骨钙素(N-MID-OT)、β-胶原降解产物(β-CTX)、总Ⅰ型前胶原氨基端肽(T-PⅠNP)、甲状旁腺素(PTH)水平均高于未转移组(P<0.05);不同Soloway分级患者N-MID-OT、β-CTX、T-PⅠNP、PTH水平比较,差异均有统计学意义(P<0.05);Soloway 3级组患者N-MID-OT、β-CTX、T-PⅠNP、PTH水平均高于Soloway 1级组和Soloway 2级组(P<0.05);骨转换标志物联合全身骨显像诊断的特异度高于N-MID-OT、β-CTX、T-PⅠPN、PTH、全身骨显像单独检测(P<0.05)。
结论:骨转换标志物联合全身骨显像能够提高诊断早期骨转移的特异度。
【关键词】 骨转移 骨转换标志物 全身骨显像 Clinical Value of Bone Turnover Markers Combined with Whole Body Bone Imaging in Diagnosisof Early Bone Metastasis/LI Xinsi, YANG Pingfei, WEN Xiaoqin. //Medical Innovation of China, 2023,20(35): 119-123 [Abstract] Objective: To explore the clinical value of bone turnover markers combined with whole bodybone imaging in diagnosis of early bone metastasis. Method: The medical records of 100 patients who underwentsingle-photon emission computed tomography/computed tomography (SPECT/CT) whole body bone imaging fromAugust 2019 to March 2022 in Nuclear Medicine Department of Pingxiang People's Hospital were retrospectivelycollected. The patients were divided into metastatic group (n=52, with bone metastasis) and non-metastatic group(n=48, without bone metastasis) according to the results of pathological examination. Patients in both groups werereceived SPECT/CT whole body bone imaging examination and bone turnover markers detection. The levels ofSoloway grades and bone turnover markers were compared between the metastatic group and non-metastatic group,and the clinical value of bone turnover markers combined with whole body bone imaging in the diagnosis of earlybone metastasis was analyzed, and the levels of bone turnover markers in patients with different Soloway grades werecompared. Result: The levels of N-mid osteocalcin (N-MID-OT), β-collagen degradation products (β-CTX), totaltype Ⅰ procollagen N-terminal peptide (T-PⅠNP) and parathyroid hormone (PTH) in metastasis group were higherthan those in non-metastasis group (P<0.05). There were statistically significant differences in N-MID-OT, β-CTX,T-PⅠNP and PTH levels among patients with different Soloway grades (P<0.05). The levels of N-MID-OT, β-CTX,T-PⅠNP and PTH in Soloway grade 3 group were higher than those in Soloway grade 1 group and Soloway grade 2group (P<0.05). The specificity of bone turnover markers combined with whole body bone imaging was higher than thoseof N-MID-OT, β-CTX, T-PⅠNP, PTH and whole body bone imaging alone (P<0.05). Conclusion: Bone turnovermarkers combined with whole body bone imaging can improve the diagnosis specificity of early bone metastasis. [Key words] Bone metastasis Bone turnover markers Whole body bone imaging First-author's address: Pingxiang People's Hospital, Jiangxi Province, Pingxiang 337000, China doi:10.3969/j.issn.1674-4985.2023.35.027*基金项目:萍乡市科技计划项目(2021PY081)①江西省萍乡市人民医院 江西 萍乡 337000通信作者:黎心四- 119 - 恶性肿瘤是目前对人类生命安全构成严重威胁的主要疾病之一。
生物医学工程专业英语
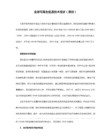
生物医学工程专业英语Unit 1 Biomedical Engineering (1)Lesson 1 A History of Biomedical Engineering (1)Lesson 2 What is a Biomedical Engineer? (7)Unit 2 Biomedical Instrumentation (15)Lesson 3 Basic Instrumentation Systems (15)Lesson 4 The Electrocardiogram (ECG) (26)Lesson 5 Measuring the Blood Pressure (31)Lesson 6 Heart Pacemaker (35)Unit 3 Medical Imaging (38)Lesson 7 An Introduction (38)Lesson 8 Basic Knowledge on X-rays in Medical Radiology (42)Part 1 X-RAYS (42)Part 2 The production of X-rays: X-ray spectra (46)Part 3 The interaction of X-rays with matters (52)Lesson 9 CT Scan (56)Lesson 10 Magnetic Resonance Imaging (60)Lesson 11 Ultrasonic Sensor (64)Lesson 12 Positron Emission Tomography (69)Unit 4 Hospital Management (76)Lesson 13 Hospital Information Systems (76)Lesson 14 Picture archiving and communication system (87)Unit 5 Biomaterial and Tissue Engineering (93)Lesson 15 Biomaterial (93)Lesson 16 Tissue Engineering (97)Unit 6 Rehabilitation Engineering and Biomechanics (110)Lesson 17 Rehabilitation (110)Lesson 18 Assistive Technology (114)Lesson 19 Biomechanics (122)Unit 1 Biomedical EngineeringLesson 1 A History of Biomedical EngineeringIn its broadest sense, biomedical engineering has been with us for centuries, perhaps even thousands of years. In 2000, German archeologists uncover a 3,000-year-old mummy from Thebes with a wooden prosthetic tied to its foot to serve as a big toe. Researchers said the wear on the bottom surface suggests that it could be the oldest known limb prosthesis. Egyptians also used hollow reeds to look and listen to the internal goings on of the human anatomy. In 1816, modesty prevented French physician Rene Laennec from placing his ear next to a young woman’s bare chest, so he rolled up a newspaper and listened through it, triggering the idea for his invention that led to today’s ubiquitous stethoscope.No matter what the date, biomedical engineering has provided advances in medical technology to improve human health. Biomedical engineering achievements range from early devices, such as crutches, platform shoes, wooden teeth, and the ever-changing cache of instruments in a doctor’s black bag, to more modern marvels, including pacemakers, the heart-lung machine, dialysis machines, diagnostic equipment, imaging technologies of every kind, and artificial organs, implants and advanced prosthetics. The National Academy of Engineering estimates that there are currently about 32,000 bioengineers working in various areas of health technology.As an academic endeavor, the roots of biomedical engineering reach back to early developments in electrophysiology, which originated about 200 years ago. An early landmark in electrophysiology occurred in 1848 when DuBois Reymond published the widely recognized Ueber die tierische Elektrizitaet. Raymond’s contemporary, Hermann von Helmholtz, is credited with applying engineering principles to a problem in physiology and identifying the resistance of muscle and nervous tissues to direct current.In 1895, Wilhelm Roentgen accidentally discovered that a cathode-ray tube could make a sheet of paper coated with barium platinocyanide glow, even when the tube and the paper were in separate rooms. Roentgen decided the tube must be emitting some kind of penetrating rays, which he called “X”rays for unknown. This set off a flurry of research into the tissue-penetrating and tissue-destroying properties of X-rays, a line of research that ultimately produced the modern array of medical imaging technologies and virtually eliminated the need for exploratory surgery.Biomedical engineering’s unique mix of engineering, medicine and science emergedalongside biophysics and medical physics early this century. At the outset, the three were virtually indistinguishable and none had formal training programs.Between World War I and World War II a number of laboratories undertook research in biophysics and biomedical engineering. Only one offered formal training: the Oswalt Institute for Physics in Medicine, established in 1921 in Frankfurt, Germany, forerunner of the Max Planck Institute for Biophysics.The Institute’s founder, Friedrich Dessauer, pioneered research into the biological effects of ionizing radiation. The Oswalt Institute and the University in Frankfurt soon established formal ties that led to a Ph.D. program in biophysics by 1940. Research topics included the effects of X-rays on tissues and the electrical properties of tissues. The staff of 20 included university lecturers, research fellows, assistants and technicians.Following the Second World War, administrative committees began forming around the combined areas of engineering, medicine and biology. A biophysical society was formed in Germany in 1943. Five years later, the first conference of engineering in medicine and biology convened in the United States, under the auspices of the Institute of Radio Engineers (forerunner of the Institute of Electrical and Electronics Engineers), the American Institute for Electrical Engineering, and the Instrument Society of America. It was a small meeting. About 20 papers were delivered to an audience of fewer than 100. The first 10 annual conferences paid most of their attention to ionizing radiation and its implications. As conference topics broadened, so did attendance. The topic of the 1958 conference, Computers in Medicine and Biology, drew 70 papers and more than 300 attendees. By 1961, conference attendance swelled to nearly 3,000.The 1951 IRE convention generated enough interest in medical electronics that the IRE formed a Professional Group on Medical Electronics. An early action of this group was to collaborate on the Annual Conference on Electronic Instrumentation and Nucleonics in Medicine, which the AIEE[1]began about 1948. In 1954, the AIEE, the IRE and the ISA formed the Joint Executive Committee on Medicine and Biology, which began organizing the annual conferences.In 1963, the AIEE and the IRE merged to form the Institute of Electrical and Electronics Engineering. Contributing forces for the merger were the members of the AIEE and IRE technical committees for biomedical engineering. Most members favored it and had been collaborating with their counterparts in the other society for years.At the merger it was decided to carry over to the IRE system of Professional Groups. The IRE Professional Group on Medical Electronics became the IEEE Professional Group onBio-Medical Engineering (PGBME), the name change reflecting the fact that many members, particularly former AIEE members, were concerned with non-electronic topics.Also in the early 1960s the NIH[2]took three significant steps to support biomedical engineering. First, it created a program-project committee under the General Medical Sciences Institute to evaluate program-project applications, many of which served biophysics and biomedical engineering. Then it set up a biomedical engineering training study section to evaluate training-grant applications, and it established two biophysics study sections. A special “floating”study section processed applications in bioacoustics and biomedical engineering. Many applications did not make it to the biomedical engineering study section and ended up in radiology, physiology or other panels.The diversity of work in biomedical engineering and the diversity of background of the people contributing to this field made it difficult for a single organization to represent everyone[3]. In the 1960s there were efforts by some leaders of the PGBME, which became the IEEE Engineering in Medicine and Biology Society, to achieve greater autonomy within the IEEE in order to accommodate a more diverse membership. Because there were quite a few professional groups, several umbrella organizations were established to facilitate cooperation. In the late 1960s the Alliance for Engineering in Medicine and Biology was formed. In 1968, the Biomedical Engineering Society was formed to give "equal status to representatives of both biomedical and engineering interests and promote the increase of biomedical engineering knowledge and its utilization". Initially, the membership of the society consisted of 171 founding members and 89 charter members. Membership now numbers nearly 1,200 professional biomedical engineers, with another 1,600 student members.The society awarded the Alza Distinguished Lectureship from 1971 to 1993 to encourage the theory and practice of biomedical engineering. The BMES Distinguished Lectureship Award was founded in 1991 to recognize outstanding achievements in biomedical engineering. Other honors include a young investigator award, the BMES Distinguished Service Award, and the Presidential Award, established in 1999 to enable BMES presidents to recognize extraordinary leadership within the society.In addition to the professional societies, the field of biomedical engineering received a large ally when The Whitaker Foundation was created in 1975, upon the death of U.A. Whitaker. As an engineer and philanthropist, Whitaker recognized that major contributions to improving human health would come from the merging of medicine and engineering. Since its inception, the foundation has primarily supported interdisciplinary medical research andeducation, with the principal focus being on biomedical engineering. The foundation has become the nation’s largest private benefactor of biomedical engineering. By 2002, it had contributed more than $615 million to universities and medical schools to support faculty research, graduate students, program development, and construction of facilities.In 1990 the National Science Foundation and The Whitaker Foundation observed that in spite of the numerous academic programs calling themselves "bioengineering" or "biomedical engineering", there was no structure for this widely diversified field. Because many advances in biomedical engineering were generated through the collaboration of engineers and clinical scientists in a number of different fields, the evolution of biomedical engineering as a profession in the 1970s and 1980s was characterized by the emergence of separate professional societies with a focus on applications within their own field.As a step toward unification, the American Institute for Medical and Biological Engineering was created in 1992. AIMBE was born from the realization that an umbrella organization was needed to address the issues of public policy and public and professional education that comprise these engineering sciences. Ten societies saw the virtue of this approach and formed the original members of AIMBE. Today, its 17 society members work to "establish a clear and comprehensive identity for the field of medical and biological engineering, and improve intersociety relations and cooperation within the field of medical and biological engineering".The earliest academic programs began to take shape in the 1950s. Their establishment was aided by Sam Talbot of Johns Hopkins University, who petitioned the National Institutes of Health for funding to support a group discussion of approaches to teaching biomedical engineering. Ultimately three universities were represented in these discussions: The Johns Hopkins University, the University of Pennsylvania and the University of Rochester. These three institutions, along with Drexel University, were among the first to win important training grants for biomedical engineering from the National Institutes of Health.In 1973, discussions started about broadening the base of Pennsylvania’s graduate Department of Biomedical Electronic Engineering by including other activities and adopting and undergraduate curriculum. Its present graduate program is an extension of the earlier one.During the late 1960s and early 1970s, development at other institutions followed similar paths, but occurred more rapidly in most cases due to the growing opportunities of the field and in response to the important NIH initiative to support the development of the field. The earlier institutions were soon followed by a second generation of biomedical engineering programs and departments. These included: Boston University in 1966; Case WesternReserve University in 1968; Northwestern University in 1969; Carnegie Mellon, Duke University, Renssselaer and a joint program between Harvard and MIT[4] in 1970; Ohio State University and University of Texas, Austin, in 1971; Louisiana Tech, Texas A&M and the Milwaukee School of Engineering in 1972; and the University of Illinois, Chicago in 1973.The number of departments and programs continued to rise slowly but steadily in the 1980s and early 1990s. In 1992, The Whitaker Foundation initiated large grant programs designed to help institutions establish or develop biomedical engineering departments or programs. Since then, the numbers of departments and programs have risen to more than 90. Some of the largest and most prominent engineering institutions in the country, such as the Georgia Institute of Technology, have established programs and emerged as leaders in the field. Many other new and existing programs have benefited from the foundation’s support.A major development took place in late 2000 when President Clinton signed a bill creating the National Institute of Biomedical Imaging and Bioengineering at the NIH. According to NIBIB’s website, its mission is to "improve health by promoting fundamental discoveries, design and development, and translation and assessment of technological capabilities". The Institute coordinates with biomedical imaging and bioengineering programs of other agencies and NIH institutes to support imaging and engineering research with potential medical applications and facilitates the transfer of such technologies to medical applications.The newest of the NIH institutes, NIBIB spent much of 2001 building program and administrative staff, preparing a budget request, setting up office space, determining funding and grant identification codes and procedures, and identifying program (research, training, and communication) focus areas and opportunities. NIBIB assumed administration of the NIH's Bioengineering Consortium (BECON) in September 2001, and awarded its first research grant in April 2002.New Words and Expressionsmummy [ ] n. 木乃伊Thebes [ ] n. [史]底比斯(古希腊的主要城邦)ubiquitous [ ] adj. 到处存在的, (同时)普遍存在的prosthesis [ ] n. 弥补stethoscope [ ] n. 听诊器dialysis [ ] n.[化] 透析, 分离electrophysiology [ ] n. [物]电生理学barium [ ] n. 钡platinocyanide [ ] n. [化]铂氰化物,氰亚铂酸盐ionizing radiation 电离放射线cathode-ray 阴极射线instrumentation [ ] n. 使用仪器nucleonics [ ] n. [核]核子学, 原子核物理学bioacoustics [ ] n. [生]生物声学radiology [ ] n. X光线学, 放射线学, 放射医学, X光线科philanthropist [ ] n. 慈善家interdisciplinary [ ] adj. 各学科间的clinical [ ] adj. 临床的, 病房用的Notes[1]AIEE美国电机工程学会[2]NIH美国全国卫生研究所[3]生物医学工程领域工作的多样性以及工作在该领域人们的背景差异使得很难用单一的组织来代表每一个人。
医疗卫生领域英文译写规范

学习医疗卫生领域英文译写规范翻译者或者有过多次翻译行为的人,往往会比较谨慎,碰到一些名词都会考虑是否有固定译法。
对某些领域专业知识不了解,容易导致英语水平颇高的人犯“隔行如隔山”的翻译错误。
因此,对于译者而言,知识面广阔非常重要,同时也需要学习一些固定翻译的专业名词或术语。
公共服务领域英文译写规范有国标?很多领域都有相应的国际标准或国家标准,有些为人熟知,经常在广告宣传中有所耳闻,例如ISO9001。
但可能很少有人知道我国发布过GB/T 30240《公共服务领域英文译写规范》,这个国标于2017年5月22日发布,2017年12月1日实施,其中第7部分是关于医疗卫生领域的。
医疗卫生机构(health care and medical institution)是指具有医疗、预防、保健、医学教育和科研功能的单位或机构。
如何提高英文标志的准确度?现在各家医院的对外交流日益增多,很多医院的标志都是中英文双语的,但稍微看一下,很容易发现错误,有些甚至是比较低级的错误。
我国的医疗卫生机构若想提高标志文字的准确度,至少有三种方法:第一,在做标志之前认真学习这个规范,尽管它未必涵盖所有标志内容,但至少有比较大的参考价值;第二,出国研修的医务人员可以多看看英语国家医疗机构的标志是如何写的,拍照保存,再分类整理;第三,请英语国家的医疗机构工作人员审核英文译文。
附属医院和分院如何翻译?大学附属医院需要翻译出隶属关系时,“附属”译作affiliated, “附属医院”翻译方式有Affiliated Hospital of …或者Hospital Affiliated with/to;也可以省去不译,将大学名称置于医院名称之后,中间用“,”隔开。
医院的分院译作Branch Hospital,用of连接所隶属的总院名称,也可以采用“总院名称,专名+Branch”的译写方法。
专科医院名称如何翻译?专科医院一般都用最简单的单词表述。
- 1、下载文档前请自行甄别文档内容的完整性,平台不提供额外的编辑、内容补充、找答案等附加服务。
- 2、"仅部分预览"的文档,不可在线预览部分如存在完整性等问题,可反馈申请退款(可完整预览的文档不适用该条件!)。
- 3、如文档侵犯您的权益,请联系客服反馈,我们会尽快为您处理(人工客服工作时间:9:00-18:30)。
Knowledge-based modelling for dynamic electricaltomography in medicine and process engineeringRobert G Aykroyd1,Robert M West2,Sha Meng1and Richard A Williams31Department of Statistics,2Nuffield Institute for Health and3SPEME,University of Leeds.AbstractIn all applications of tomography,a section through an object is imaged using measurements taken from outside or on the boundary of the object.There are many applications of this tech-nique in areas such as medical imaging,industrial process monitoring and geophysics.Although many application only involve single snap-shots,more challenging examples involve sequences of multi-component data and allow the investigation of dynamically evolving processes.This paper will describe two examples:the mixing of liquids in a tank and the movement of heart and lungs in the human thorax.Different approaches to modelling,including choice of parameterisation and spatial and temporal prior modelling will be discussed.Results,ob-tained using MCMC techniques will be presented.In particular,theflexibility of the modelling approach,allowing a variety of prior knowledge to be incorporated and a variety of output summaries,will be highlighted.IntroductionIn tomography the aim is to reconstruct the inside of an object using measurements taken on the outside of the object.There are many applications of this approach in areas such as medical imaging,industrial process monitoring and geophysics.Here the focus is on electrical impedance tomography(EIT)where electrodes are attached to the boundary of the object and,whilst currents are applied between some electrodes,voltage measurements are recorded between other electrodes.The relationship between parameters (resistivities)and data(voltages)is nonlinear,and in a classical setting estimation requires regularization to overcome numerical instability.Calculation of voltages from a specified re-sistivity distribution requires the numerical solution of a PDE(see Vauhkonen et al.2001). The Bayesian approach provides a convenient setting in which to define,control and interpret regularization and has wide ranging applicability in many imaging problems.Although a pixel-based formulation is widely used and provides a generic approach,it is not always the most useful.In particular,problem-specific formulations,using information regarding known structure or physiological behaviour introduce reliable regularization through parameter reduction and allow important process quantities to be directly investigated without the need for post-processing of image reconstructions.For general background to Bayesian modelling and MCMC estimation see Besag et al.(1995)and Liu(2001).The various spatial and temporal prior models are combined with the likelihood distribution by Bayes’theorem and parameter estimation is then based on the posterior distribution using MCMC methods. Acknowledgement:The authors thank the EPSRC forfinancial support(GR/R22148/01).Example1:Mixing tankAn experiment was undertaken to investigate the mixing of two liquids in a laboratory tank(see West et al2004b).Initially the tank contains only tap water which is stirred.While the water is still revolving an aliquot of salty water is injected into theflow.The aliquot will gradually disperse and mix eventually leaving homogeneous,but slightly salty,tank contents.At different stages through the experiment our prior knowledge regarding the spatial and temporal smoothness changes.During thefirst few frames the tank contains a homogeneous solution(tap water)and the resistivity should be spatially and temporally very smooth.These frames have been used for calibration to estimate the contact impedances and the background resistivity.In the second stage an aliquot of low resistivity saline solution(tap water and potassium chloride)is injected into the tank and temporal smoothing breaks down completely. In the spatial distribution we expect a resistivity discontinuity at the aliquot boundary,with high smoothness elsewhere.As the aliquot begins to disperse there is moderate temporal smoothness and increasing spatial smoothness.At the end of this stage the solution should again be well mixed and so the endpoint will also be a homogeneous solution with resistivity between that of the initial contents and the saline solution.Example2:ThoraxA time sequence of resistivity distributions is considered which covers the whole heart–lung cycle.See West et al.(2003b)and reference therein for further details.Previous work has required post-processing of reconstructed images and the use of ad-hoc regions of interest to idenfity organ outline and hence estimate clinical parameters.Here explicit geometric models have been used which also incorporate temporal smoothness. The model states that the resistivity distribution is composed of a constant background and fixed spine,but with contracting and expanding regions for the heart and lungs.This provides very specific prior knowledge about the resistivity distribution–not just that it has disconti-nuities.Further,it is assumed that the location of the heart is known,but that its size and resistivity are to be estimated.Each of the lung is assumed to have a minimum size,corre-sponding to maximal expiration,but the actual shape,sizes and resistivities are unknown and will be estimated.As the process evolves the state is expected to change slowly and smoothly. This is modelled using temporal priors which are propagated within the frame sequence.Out-put includes clinically relevant quantities such as lung residual capacity,and heart ejection fractions.ReferencesBesag J,Green PJ,Higdon D,and Mengersen K(1995)Bayesian Computation and Stochastic Systems,Statistical Science,10,1–41.Liu,JS(2001)Monte Carlo Strategies in Scientific Computing,Springer-Verlag:Berlin,Hei-delberg.Vauhkonen M,Lionheart WRB,Heikkinen LM,Vauhkonen PJ,and Kaipio JP(2001)A MATLAB Package for the EIDORS Project to Reconstruct Two-dimensional EIT Images, Physiological Measurements,22,107–111.West RM,Aykroyd RG,Meng S,and Williams,RA(2004a)Markov chain Monte Carlo techniques and spatial-temporal modelling for medical EIT,Physiological Measurements. West RM,Meng S,Aykroyd RG,and Williams,RA(2004b)Spatial-Temporal Modelling for Electrical Impedance Imaging of a Mixing Process,Meas.Sci.and Tech.,in submission.。