X-ray emission from active galactic nuclei with intermediate mass black holes
冲击波聚心的英文
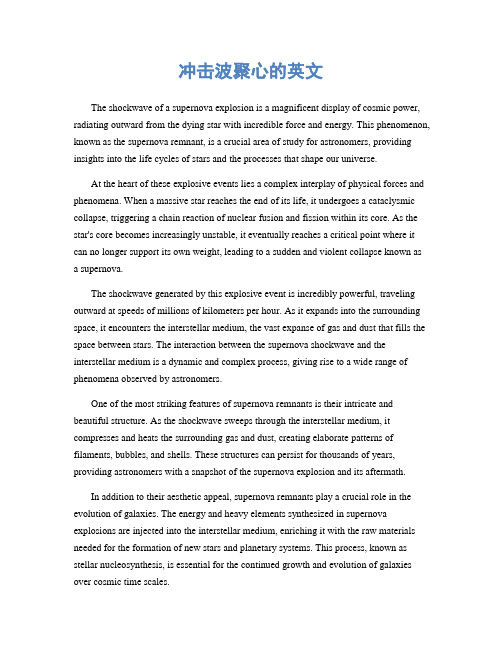
冲击波聚心的英文The shockwave of a supernova explosion is a magnificent display of cosmic power, radiating outward from the dying star with incredible force and energy. This phenomenon, known as the supernova remnant, is a crucial area of study for astronomers, providing insights into the life cycles of stars and the processes that shape our universe.At the heart of these explosive events lies a complex interplay of physical forces and phenomena. When a massive star reaches the end of its life, it undergoes a cataclysmic collapse, triggering a chain reaction of nuclear fusion and fission within its core. As the star's core becomes increasingly unstable, it eventually reaches a critical point where it can no longer support its own weight, leading to a sudden and violent collapse known as a supernova.The shockwave generated by this explosive event is incredibly powerful, traveling outward at speeds of millions of kilometers per hour. As it expands into the surrounding space, it encounters the interstellar medium, the vast expanse of gas and dust that fills the space between stars. The interaction between the supernova shockwave and the interstellar medium is a dynamic and complex process, giving rise to a wide range of phenomena observed by astronomers.One of the most striking features of supernova remnants is their intricate and beautiful structure. As the shockwave sweeps through the interstellar medium, it compresses and heats the surrounding gas and dust, creating elaborate patterns of filaments, bubbles, and shells. These structures can persist for thousands of years, providing astronomers with a snapshot of the supernova explosion and its aftermath.In addition to their aesthetic appeal, supernova remnants play a crucial role in the evolution of galaxies. The energy and heavy elements synthesized in supernova explosions are injected into the interstellar medium, enriching it with the raw materials needed for the formation of new stars and planetary systems. This process, known as stellar nucleosynthesis, is essential for the continued growth and evolution of galaxies over cosmic time scales.Furthermore, supernova remnants are also sites of intense physical processes, including the acceleration of charged particles to relativistic speeds. These high-energy particles can interact with magnetic fields and emit radiation across the entire electromagnetic spectrum, from radio waves to gamma rays. Studying the emission from supernova remnants allows astronomers to probe the underlying physics of particle acceleration and magnetic field dynamics in extreme environments.In recent years, advances in observational techniques have led to a deeper understanding of supernova remnants and their role in the universe. High-resolution imaging and spectroscopic observations have revealed the intricate details of shockwave dynamics and the distribution of heavy elements within these cosmic relics. Theoretical models and numerical simulations have provided valuable insights into the physical processes driving the evolution of supernova remnants and their impact on galactic ecosystems.Looking ahead, future observations with next-generation telescopes and space-based observatories promise to unveil even more secrets of supernova remnants. By studying these cosmic artifacts in greater detail, astronomers hope to unravel the mysteries of stellar death and the profound influence it has on the evolution of the cosmos. From the heart of a dying star to the farthest reaches of the universe, the shockwaves of supernova explosions continue to captivate our imagination and inspire scientific discovery.。
施勇 教育经历:!!!!!!!!!!!!!!!!!!!!!!!!!!!!!!!!!!!!!!!!!!!!
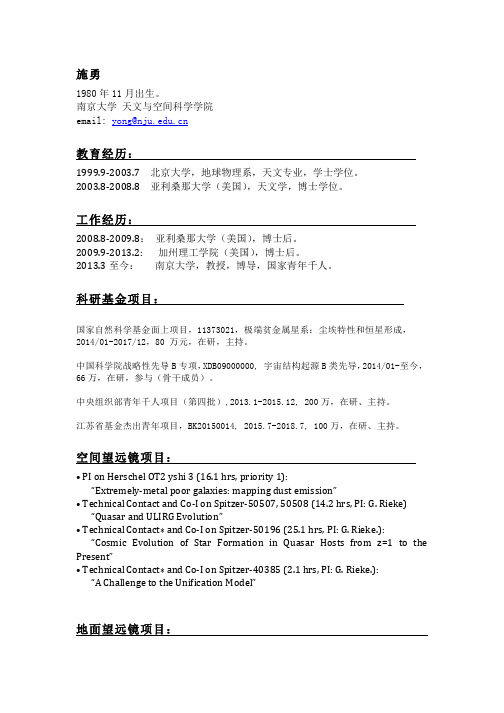
施勇1980年11月出生。
南京大学 天文与空间科学学院email: yong@教育经历: 1999.9-‐2003.7 北京大学,地球物理系,天文专业,学士学位。
2003.8-‐2008.8 亚利桑那大学(美国),天文学,博士学位。
工作经历: 2008.8-‐2009.8: 亚利桑那大学(美国),博士后。
2009.9-‐2013.2: 加州理工学院(美国),博士后。
2013.3至今: 南京大学,教授,博导,国家青年千人。
科研基金项目:国家自然科学基金面上项目,11373021,极端贫金属星系:尘埃特性和恒星形成,2014/01-2017/12,80 万元,在研,主持。
中国科学院战略性先导B专项,XDB09000000, 宇宙结构起源B类先导,2014/01-至今,66万,在研,参与(骨干成员)。
中央组织部青年千人项目(第四批),2013.1-2015.12, 200万,在研、主持。
江苏省基金杰出青年项目,BK20150014, 2015.7-2018.7, 100万,在研、主持。
空间望远镜项目: • P I o n H erschel O T2 y shi 3 (16.1 h rs, p riority 1):“Extremely-‐metal p oor g alaxies: m apping d ust e mission”• T echnical C ontact a nd C o-‐I o n S pitzer-‐50507, 50508 (14.2 h rs, P I: G. R ieke)“Quasar a nd U LIRG E volution”• T echnical C ontact∗ a nd C o-‐I o n S pitzer-‐50196 (25.1 h rs, P I: G. R ieke.):“Cosmic Evolution of Star Formation in Quasar Hosts from z=1 to the Present”• T echnical C ontact∗ a nd C o-‐I o n S pitzer-‐40385 (2.1 h rs, P I: G. R ieke.):“A C hallenge t o t he U nification M odel”地面望远镜项目:• K eck 10 m: D EIMOS• I RAM 30 m: 24 h rs (2014A), 59.5 h rs (2016A).• P alomar 200 i nch: D BSP; L FC; W IRC• C FHT: M egaCAM• B ok 2.3 m• A rizona R adio O bservatory N RAO-‐12m• A rizona R adio O bservatory S MT-‐10m学术服务:ApJ, A pJL, A&A, A J, S ciChina, R AA的审稿人Telescope A ccess P rogram 望远镜分配委员会委员论文发表情况汇总(共36篇)通讯作者 非通讯作者 总计Nature 1 0 1Nature子刊 0 1 117 18 35ApJ, ApJS, ApJL,MNRAS, A&A(全部为NatureIndex高影响力科学期刊)AJ 0 1 1总计 18 20 38第一或通讯作者论文: 18. Zhang, Z.; Shi, Y* et al. 2016, ApJL, 819, 27“Distributions of quasar hosts on the galaxy main-sequence plane”17. Zhou, L.; Shi, Y* et al. 2016, MNRAS, 458, 772“Spatially resolved dust emission of extremely metal poor galaxies”16. S hi, Y.*, W ang, J., Z hang, Z.-‐Y. e t a l. 2015, A pJL, 804, 11“The Weak Carbon Monoxide Emission in an Extremely Metal-‐poor Galaxy, Sextans A”15. S hi, Y.*, A rmus, L., H elou, G. e t a l. 2014, N ature, 514, 335–338“Inefficient s tar f ormation i n e xtremely m etal p oor g alaxies”14. Shi, Y.*, Rieke, G., Ogle, P. et al., 2014, ApJS, 214, 23 “Infrared spectra and photometry o f c omplete s amples o f P G a nd 2MASS q uasars”13. Shi, Y.*, Helou, G., Armus, L. 2013, ApJ, 777, 6 “A Joint Model Of X-‐ray And Infrared B ackgrounds. I I. C ompton-‐Thick A GN A bundance”12. Shi, Y.*, Helou, G., et al. 2013, ApJ, 764, 28 “A Joint Model of the X-‐Ray and Infrared E xtragalactic B ackgrounds. I. M odel C onstruc-‐ t ion a nd F irst R esults”11. Shi, Y.*, Helou, G., et al. 2011, ApJ, 733, 87 “Extended Schmidt Law: Roles Of Existing S tars I n C urrent S tar F ormation”10. Shi, Y.*, Rieke, G. H., et al. 2010, ApJ, 714, 115 “Unobscured Type 2 Active Galactic N uclei”9. Shi, Y.*, Rieke, G. H., et al. 2009, ApJ, 703, 1107 “Cosmic Evolution of Star Formation i n T ype-‐1 Q uasar H osts S ince z = 1”8. Shi, Y.*, Rieke, G. H., et al. 2009, ApJ, 697, 1764 “Role of Major Mergers In Cosmic S tar F ormation E volution”7. Shi, Y.*, Rieke, G. H. et al. 2008, ApJ, 688, 794 “BH Accretion in Low-‐Mass Galaxies S ince z∼1”6. Shi, Y.*, Ogle, P., Rieke, G. H. et al. 2007, ApJ, 669, 841 “Aromatic Features in AGN: S tar-‐Forming I nfrared L uminosity F unction o f A GN H ost G alaxies”5. Shi, Y.*, Rieke, G. H., Hines, D. C. et al. 2007, ApJ, 655, 781 “Thermal and Nonthermal I nfrared E mission f rom M87”4. Shi, Y.*, Rieke, G. H., Hines, D. C. et al. 2006, ApJ, 653, 127 “9.7 um Silicate Features i n A ctive G alactic N uclei: N ew I nsights i nto U nification M odels”3. Shi, Y.*, Rieke, G. H., Papovich, C. et al. 2006, ApJ, 645, 199 “Morphology of Spitzer 24 u m D etected G alaxies i n t he U DF: T he L inks b etween S tar F or-‐ m ation and G alaxy M orphology”2. Shi, Y.*, Rieke, G. H., Hines, D. C. et al. 2005, ApJ, 629, 88 “Far-‐Infrared Observations o f R adio Q uasars a nd F R I I R adio G alaxies”1.Shi, Y., & Xu, R. X.* 2003, ApJ, 596, 75 “Can the Age Discrepancies of NeutronStars B e C ircumvented b y a n A ccretion-‐assisted T orque?”其他作者论文:20. G uo R. e t a l. (Shi Y. 5th a uthor), 2016, A pJ a ccepted, a rXiv:1604.0712219. Chen, Y. et al. (Shi Y. 4th author), 2016, MNRAS accepted, “Boxy Hα EmissionProfiles i n S tar-‐Forming G alaxies”18. Bian, W. H. et al. (Shi Y. 4th author), 2016, MNRAS, 456, 4081, “Spectral principal component analysis of mid-infrared spectra of a sample of PG QSOs”17. Wang, J. et al. (Shi Y. 4th author), 2016, MNRAS, 455, 3986, “Dense-gas properties in Arp 220 revealed by isotopologue lines”16. Wang, J. et al. (Shi Y. 7th author), 2014, Nature Communication, 5, 5449 “SiO and C H3OH m ega-‐masers i n N GC 1068”15. Kirkpatrick, A. et al. (Shi Y. 10th author) , 2014, ApJ, 796, 135 “Early Science with the Large Millimeter Telescope: Exploring the Effect of AGN Activity on the Relationships b etween M olecular G as, D ust, a nd S tar F ormation”14. Wang, J. et al. et al. (Shi Y. 4th author) , 2014, ApJ, 796, 57 “Isotopologues o f Dense G as T racers i n N GC 1068”13. Jin, S. et al. (Shi Y. 4th author), 2014, ApJ, 787, 63 “Color-‐Magnitude Distribution o f F ace-‐on n earby G alaxies i n S loan D igital S ky S urvey D R7”12. D ale, D. e t a l. (Shi Y. 6th a uthor), 2014, A pJ, 784, 83 “A T wo-‐parameter M odel for the Infrared/Submillimeter/Radio Spectral Energy Distributions of Galaxies and A ctive G alactic N uclei”11. Wang, J. et al. (Shi Y. 3rd author), 2013, ApJL, 778, 39 “A SiO 2-‐1 Survey toward G as-‐rich A ctive G alaxies”10. Magdis, G. E. et al. (Shi Y. 22th author), 2013, A&A, 558, 136 “Mid-‐ to far infrared p roperties o f s tar-‐forming g alaxies a nd a ctive g alactic n uclei”9. Kim, Ji Hoon, et al. (Shi Y. 16th author), 2012, ApJ, 760, 120 “The 3.3 m Polycyclic A romatic H ydrocarbon E mission a s a S tar F ormation R ate I ndicator”8. Wang, J., et al. (Shi Y. 3rd author) 2011, MNRAS, 416, 21 “CS (5-‐4) survey towards n earby i nfrared b right g alaxies”7. T yler, K. D., R ieke, G. H. e t a l. (Shi Y. 9th a uthor) 2011, A pJ, 738, 56 “The N ature of S tar F ormation a t 24 m i n t he G roup E nvironment a t 0.3 < z < 0.55”6. Wu, Y., et al. (Shi Y. 2nd author) 2011, ApJ, 734, 40 “The Mid-‐infrared Luminosity Function at z < 0.3 from 5MUSES: Understanding the Star Formation/Active G alactic N ucleus B alance f rom a S pectroscopic V iew”5. W u, Y., e t a l. (Shi Y. 5th a uthor) 2010, A pJ, 723, 895 “Infrared L uminosities a nd Aromatic F eatures i n t he 24um F lux L imited S ample o f 5MUSES”4. Mason, R. E., et al. (Shi Y. 3nd author) 2009, ApJ, 693, 136 “The Origin of the Silicate E mission F eatures i n t he S eyfert 2 G alaxy N GC 2110”3. B allantyne, D. R., e t a l. (Shi Y. 2nd a uthor) 2006, A pJ, 653, 1070 “Does t he A GN Unified M odel E volve w ith R edshift? U sing t he X-‐Ray B ackground t o P re-‐ d ict t he Mid-‐Infrared E mission o f A GNs”2. J iang, L. e t a l. (Shi Y. 4th a uthor) 2006, A J, 132, 2127 “Probing t he E volution o f Infrared P roperties o f z ∼6 Q uasars: S pitzer O bservations”1. Wu, Y. et al. (Shi Y. 4th author) 2004, A&A, 426, 503 “A study of high velocity molecular o utflows w ith a n u p-‐to-‐date s ample”。
x射线吸收谱xas的英文
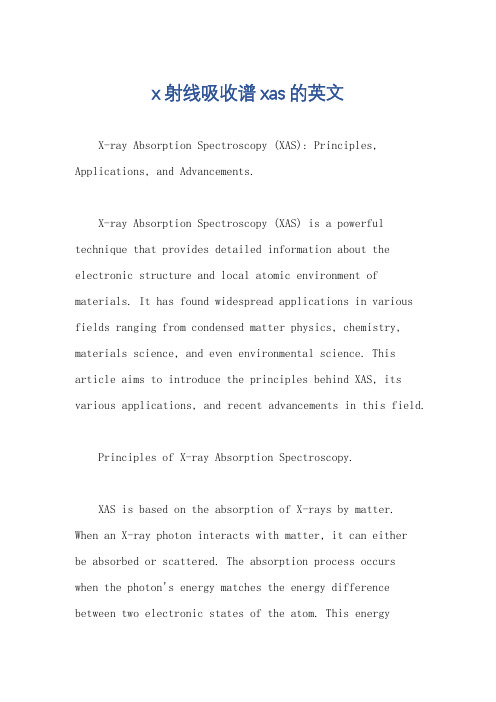
x射线吸收谱xas的英文X-ray Absorption Spectroscopy (XAS): Principles, Applications, and Advancements.X-ray Absorption Spectroscopy (XAS) is a powerful technique that provides detailed information about the electronic structure and local atomic environment of materials. It has found widespread applications in various fields ranging from condensed matter physics, chemistry, materials science, and even environmental science. This article aims to introduce the principles behind XAS, its various applications, and recent advancements in this field.Principles of X-ray Absorption Spectroscopy.XAS is based on the absorption of X-rays by matter. When an X-ray photon interacts with matter, it can eitherbe absorbed or scattered. The absorption process occurs when the photon's energy matches the energy difference between two electronic states of the atom. This energydifference is typically in the range of a few thousand to tens of thousands of electron volts, making X-rays an excellent probe for studying the inner electronic structure of atoms.In XAS experiments, a monochromatic X-ray beam is passed through a sample, and the intensity of the transmitted X-rays is measured as a function of energy. The absorption spectrum is then obtained by plotting the absorbed X-ray intensity against the incident X-ray energy. The shape and features of this spectrum are directlyrelated to the electronic structure and local atomic environment of the sample.There are two main types of XAS: X-ray Absorption Near-Edge Structure (XANES) and Extended X-ray Absorption Fine Structure (EXAFS). XANES probes the absorption near the absorption edge, providing information about the unoccupied states of the absorber atom. On the other hand, EXAFS focuses on the oscillatory structure that appears above the absorption edge and gives insights into the coordination geometry and bond lengths of the absorber atom.Applications of X-ray Absorption Spectroscopy.1. Materials Science: XAS is widely used in materials science to study the atomic-scale structure and electronic properties of various materials. It can provide information about the oxidation state, coordination geometry, and bond lengths of atoms in materials, which is crucial for understanding their physical and chemical properties.2. Chemistry: In the field of chemistry, XAS is often used to study the electronic structure and bonding in molecules and solids. It can reveal the oxidation states, ligand environments, and electronic configurations of atoms in complex molecules.3. Environmental Science: XAS has also found applications in environmental science, where it is used to study the speciation and transformation of elements in the environment. For example, it can be used to monitor the redox state of heavy metals in soils and waters, which is crucial for assessing their environmental risk.4. Energy Science: In the field of energy science, XAS is used to study materials for energy conversion and storage applications. For instance, it can provide insights into the electronic structure and atomic-scale structure of battery materials, which is essential for developing high-performance batteries.Advancements in X-ray Absorption Spectroscopy.With the continuous development of synchrotronradiation sources and advanced detector technologies, XAS has made significant advancements in recent years. Here are some of the key advancements in XAS:1. Higher Energy Resolution: Advancements in X-ray sources and detectors have led to improved energyresolution in XAS experiments. This higher resolution allows for more precise measurements of electronicstructure parameters and enhanced sensitivity to small changes in the local atomic environment.2. Faster Acquisition Times: With the advent of new detector technologies, XAS experiments can now be performed with much faster acquisition times. This reduction in measurement time not only improves experimental efficiency but also enables studies of dynamic processes and reactions in real time.3. Element-Specific Probing: Recent advancements in XAS have enabled element-specific probing, allowing researchers to study the electronic structure and local atomic environment of specific elements within a complex material. This capability is particularly useful in materials science and chemistry, where understanding the behavior of individual elements is crucial.4. In-Situ Studies: Another important advancement in XAS is the ability to perform in-situ studies, where the sample is exposed to various external stimuli (e.g., temperature, pressure, electric field) during the measurement. This approach allows researchers to study the evolution of materials' properties under realistic conditions, providing valuable insights into theirperformance and stability.In conclusion, X-ray Absorption Spectroscopy (XAS) is a powerful technique that provides detailed information about the electronic structure and local atomic environment of materials. Its applications span across various fields, and recent advancements in XAS have further expanded its capabilities and scope. With continued research and development, XAS is expected to play an even more critical role in understanding and developing advanced materials for various applications.。
天文学常用术语英汉对译及解释LaRivenbsp

天文学常用术语英汉对译及解释LaRive nbsp天文学常用术语英汉对译及解释0000术语解释aberration光行差由于地球的运动所导致的天体的视位置与真实位置之间的差异。
absolute magnitude绝对星等恒星的真正亮度。
定义为恒星在距离我们 10 秒差距 (32.6光年) 时的视星等。
absolute zero绝对零度理论上的最低温度,等于0开尔文(-459.67° F or -273.15° C)。
absorption lines吸收线光谱里的暗线。
来自天体的光,被原子或分子选择性的吸收,导致那部分的光从星光中被消去,留下一条条的暗线。
accretion disk吸积盘指白矮星、中子星或黑洞等致密天体周围,由于物质受到引力作用向中心天体落下所形成的盘状结构。
achromatic lens消色差透镜由两种不同材质的透镜组合而成,消色差透镜的用途是把两种不同颜色的光聚焦到同一点,或称为修正色像差。
active galactic nuclei活动星系核某些星系中的特别明亮的核,被认为是由于物质落向质量极大的黑洞而引起的。
adaptive optics自适应光学计算机控制的望远镜镜面,能做区域性变形,以补偿大气扰动所产生的散焦效应。
albedo反照率行星或卫星反射光能力的标示值,定义为所反射的光和入射光的比值。
反照率的值介于 0 (完美的黑体) 到 1 (完全反射)之间。
月球的反照率为 0.07,而金星为 0.6。
altazimuth mount地平装置一种望远镜支撑方式,使镜筒能在平行和垂直水平的方向自由移动。
altitude地平纬度、高度 1.在海平面以上的高度2..天体在天球上距离地平线的角度 anaglyph立体照片用两台相机拍摄出的一种照片。
将右边拍摄的影像(通常是红色)和左边拍摄的影像(通常是蓝色)叠加起来,通过特殊的色彩滤镜,就能看到三维的效果。
x射线荧光光谱法 英文
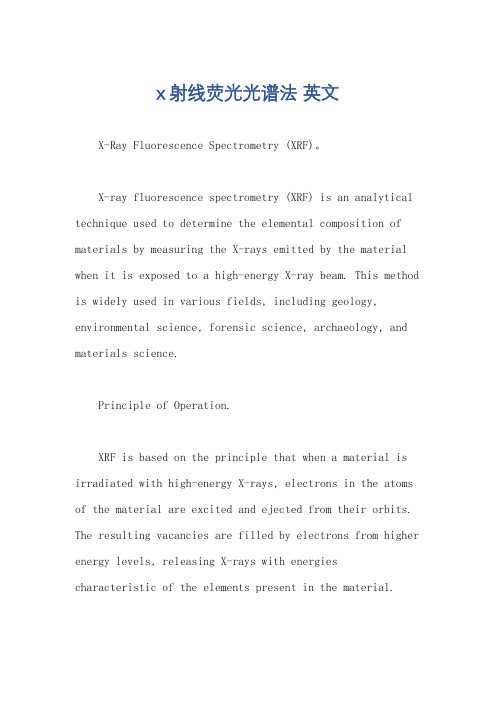
x射线荧光光谱法英文X-Ray Fluorescence Spectrometry (XRF)。
X-ray fluorescence spectrometry (XRF) is an analytical technique used to determine the elemental composition of materials by measuring the X-rays emitted by the material when it is exposed to a high-energy X-ray beam. This method is widely used in various fields, including geology, environmental science, forensic science, archaeology, and materials science.Principle of Operation.XRF is based on the principle that when a material is irradiated with high-energy X-rays, electrons in the atoms of the material are excited and ejected from their orbits. The resulting vacancies are filled by electrons from higher energy levels, releasing X-rays with energiescharacteristic of the elements present in the material.The energy of the emitted X-rays is specific to each element, and the intensity of the X-rays is proportional to the concentration of the element in the material. By measuring the energies and intensities of the emitted X-rays, it is possible to identify and quantify the elements present in the sample.Instrumentation.A typical XRF spectrometer consists of the following components:X-ray source: Generates high-energy X-rays that bombard the sample.Sample chamber: Holds the sample to be analyzed.Detector: Converts X-rays into electrical signals.Multichannel analyzer (MCA): Digitizes and analyzes the electrical signals from the detector.Types of XRF Spectrometers.There are several types of XRF spectrometers, each with its own advantages and limitations:Energy-dispersive XRF (EDXRF): Uses a solid-state detector to measure the energies of the emitted X-rays. EDXRF is relatively inexpensive and easy to operate, but it has lower energy resolution compared to other types of XRF spectrometers.Wavelength-dispersive XRF (WDXRF): Uses a crystal monochromator to separate the emitted X-rays by wavelength. WDXRF offers higher energy resolution than EDXRF, but it is more complex, expensive, and time-consuming to operate.Total reflection XRF (TXRF): Utilizes total reflection conditions to enhance the sensitivity for analyzing trace elements in liquids. TXRF is highly sensitive, but it requires sample preparation and is not suitable for solid samples.Applications of XRF.XRF is a versatile analytical technique with a wide range of applications:Geochemistry: Determining the elemental composition of rocks, minerals, and soils.Environmental science: Monitoring pollutants in air, water, and soil.Forensic science: Analyzing trace evidence, such as gunshot residue and paint chips.Archaeology: Studying the composition of artifacts and ancient materials.Materials science: Characterizing the elemental composition of metals, alloys, and other materials.Advantages of XRF.Nondestructive: Does not damage the sample being analyzed.Multi-elemental: Can identify and quantify multiple elements simultaneously.Rapid: Provides real-time analysis results.Sensitive: Can detect elements at trace levels.Versatile: Can be applied to various sample types, including solids, liquids, and powders.Limitations of XRF.Limited sensitivity: Cannot detect elements present in very low concentrations.Matrix effects: The presence of other elements in the sample can affect the accuracy of the analysis.Sample preparation: May require sample preparation,such as grinding or homogenization.Cost: XRF spectrometers can be expensive, especially WDXRF systems.Conclusion.X-Ray Fluorescence Spectrometry is a powerful analytical technique that provides valuable information about the elemental composition of materials. It is widely used in various fields and offers advantages such as non-destructiveness, multi-elemental analysis, and rapid results. However, it has limitations in sensitivity and potential matrix effects, which should be considered when selecting this technique for specific applications.。
Diffuse X-Ray Emission from the Quiescent Superbubble M17, the Omega Nebula
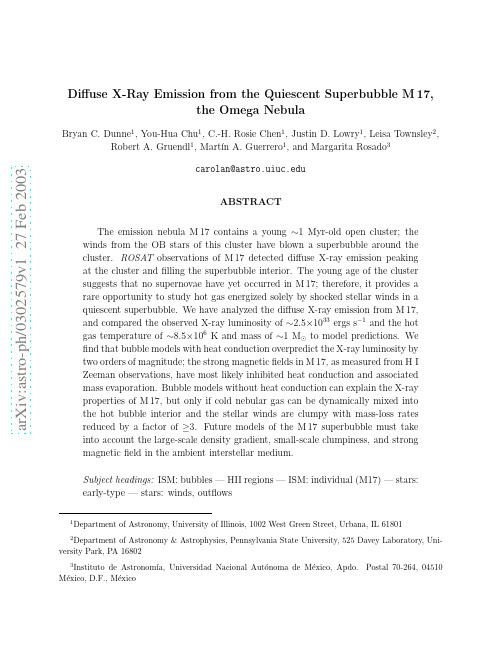
a rXiv:as tr o-ph/32579v127Fe b23Diffuse X-Ray Emission from the Quiescent Superbubble M 17,the Omega Nebula Bryan C.Dunne 1,You-Hua Chu 1,C.-H.Rosie Chen 1,Justin D.Lowry 1,Leisa Townsley 2,Robert A.Gruendl 1,Mart´ın A.Guerrero 1,and Margarita Rosado 3carolan@ ABSTRACT The emission nebula M 17contains a young ∼1Myr-old open cluster;the winds from the OB stars of this cluster have blown a superbubble around the cluster.ROSAT observations of M 17detected diffuse X-ray emission peaking at the cluster and filling the superbubble interior.The young age of the cluster suggests that no supernovae have yet occurred in M 17;therefore,it provides a rare opportunity to study hot gas energized solely by shocked stellar winds in a quiescent superbubble.We have analyzed the diffuse X-ray emission from M 17,and compared the observed X-ray luminosity of ∼2.5×1033ergs s −1and the hot gas temperature of ∼8.5×106K and mass of ∼1M ⊙to model predictions.We find that bubble models with heat conduction overpredict the X-ray luminosity by two orders of magnitude;the strong magnetic fields in M 17,as measured from H I Zeeman observations,have most likely inhibited heat conduction and associated mass evaporation.Bubble models without heat conduction can explain the X-ray properties of M 17,but only if cold nebular gas can be dynamically mixed into the hot bubble interior and the stellar winds are clumpy with mass-loss rates reduced by a factor of ≥3.Future models of the M 17superbubble must takeinto account the large-scale density gradient,small-scale clumpiness,and strong magnetic field in the ambient interstellar medium.Subject headings:ISM:bubbles —HII regions —ISM:individual (M17)—stars:early-type —stars:winds,outflows1.IntroductionMassive stars dynamically interact with the ambient interstellar medium(ISM)via their fast stellar winds and supernova ejecta.OB associations,with their large concentrations of massive stars,provide an excellent laboratory to study these interactions.The combined actions of the stellar winds and the supernovae from the massive stars in OB associations sweep up the ambient ISM to form expanding shells called superbubbles(Bruhweiler et al. 1980).The physical structure of a superbubble is very similar to that of a bubble blown by the stellar wind of an isolated massive star,as modeled by Castor,McCray,&Weaver (1975)and Weaver et al.(1977).Theoretically,an interstellar bubble consists of a shell of swept-up ISM with its interior filled by shocked fast wind at temperatures of106–108K.There are two basic types of models for wind-blown bubbles:energy-conserving and momentum-conserving.In the former,the shocked stellar wind is separated from the swept-up interstellar shell by a contact discontinu-ity,where heat conduction and mass evaporation may take place.The expansion of the shell is driven by the pressure of the hot interior gas(Dyson&de Vries1972;Castor et al.1975). In the momentum conserving bubbles,the fast stellar winds impinge on the swept-up shell directly,and the expansion is driven by the momentum of the fast stellar wind(Avedisova 1972;Steigman,Strittmatter,&Williams1975).One significant difference between a superbubble and a single star bubble is the possibil-ity that supernovae may occur inside a superbubble and introduce significant perturbations in the surface brightness and characteristic temperature of the X-ray emission,especially if a supernova explodes near the dense shell(Mac Low&McCray1988).This intermittent X-ray brightening has been observed in superbubbles in the Large Magellanic Cloud(LMC). Using Einstein and ROSAT observations,Chu&Mac Low(1990)and Dunne,Points,& Chu(2001)have reported diffuse X-ray emission from a large number of superbubbles in the LMC,and their X-ray luminosities all exceed the luminosities expected by Weaver et al.’s bubble model,indicating recent heating by supernovae.No LMC superbubbles in a quies-cent state,i.e.,without recent supernova heating,have been detected in X-rays by ROSAT (Chu et al.1995).It would be of great interest to detect diffuse X-ray emission from a qui-escent superbubble and compare it to model expectations,as this could provide a valuable diagnostic of bubble models.The emission nebula M17(from the catalog of Messier1850,α=18h21m,δ=−16◦10′(J2000.0),also known as the Omega Nebula,the Horseshoe Nebula,and NGC6618)is located on the eastern edge of a massive molecular cloud,M17SW(Lada,Dickinson,&Penfield 1974).M17exhibits a“blister-like”structure with an overall diameter of∼20′–25′,or∼10–12pc at an adopted distance of1.6kpc(Nielbock et al.2001).The nebula encompassesan open cluster with a stellar age of∼1Myr(Hanson,Howarth,&Conti1997).The open cluster is located on the western side of the nebula,which borders the molecular cloud. Arcuatefilaments extend eastward,creating a shell morphology,and suggesting that it is a young superbubble blown by the OB stars within.The young age of the cluster in M17 implies that no supernova explosions have occurred,thus M17provides an ideal setting to study the generation of hot gas solely by fast stellar winds inside a superbubble at a quiescent state.Recent Chandra observations of M17have revealed diffuse X-ray emission in the vicinity of the embedded open cluster(Townsley et al.2003).As a matter of fact,diffuse X-ray emis-sion from M17over a more extended area was previously detected in a ROSAT observation but was never reported.We have analyzed this diffuse X-ray emission from the interior of M17detected by ROSAT to determine the physical properties of the hot interior gas,and considered bubble models with and without heat conduction.Wefind that models with heat conduction produce results with the largest discrepancy from the observed X-ray luminos-ity and hot gas temperature and mass of the superbubble in M17.This paper reports our analysis of the ROSAT observations of M17and comparisons to a range of bubble models.2.Observations and Data Reduction2.1.ROSAT Archival DataWe have used an archival ROSAT Position Sensitive Proportional Counter(PSPC) observation to study the diffuse X-ray emission from M17and investigate the physical prop-erties of the hot,shocked gas interior to the superbubble.The PSPC is sensitive to X-rays in the energy range0.1–2.4keV and has an energy resolution of∼40%at1keV,with a field of view of∼2◦.Further information on the PSPC can be found in the ROSAT Mission Description(1991).The PSPC observation of M17(sequence number RP500311,PI:Aschenbach)was ob-tained on1993September12–13.It is centered on the nebula atα(J2000)=18h21m04.s78 andδ(J2000)=−16◦10′12.′′0,and has an exposure time of6.7ks.As M17has an angular size of∼20′–25′,the diffuse X-ray emission from the superbubble interior is well contained within the inner window support ring of the PSPC.The PSPC data were reduced using standard routines in the PROS4package under the IRAF5environment.2.2.Optical ImagingTo compare the spatial distribution of the X-ray-emitting gas with that of the cooler ionized gas in M17,we have obtained narrow-band Hαimages of this emission nebula.M17 was observed with the Mount Laguna1-m telescope on2002October28–31using a Tektronik 2K CCD.Afilter with peak transmission at6563˚A and a FWHM of20˚A was used to isolate the Hαline.Because the2K CCD has∼0.′′4pixels and afield-of-view of13.′6,the entire nebula could not be observed in a single exposure.Instead a total of twenty-four300s exposures were acquired at8positions to span the nebula.These exposures were combined to form a mosaic image of the nebula and to reject cosmic-ray events in the individual images using the methods outlined in Regan&Gruendl(1995).3.Analysis of the X-Ray EmissionSignificant X-ray emission is detected from M17in the PSPC observation,as can be seen in Figure1.To determine the nature and origin of this emission,we have analyzed its spatial distribution and spectral properties.We have examined the distribution of the X-ray emission and compared it to the optical morphology of the emission nebula.We have also extracted spectra from the PSPC data and modeled them to determine the physical conditions of the hot gas.3.1.Spatial Distribution of the X-ray EmissionTo study the spatial distribution of the X-ray emission from M17,the data were binned to5′′pixels and then smoothed with a Gaussian function ofσ=4pixels(see Figure1a). We have also taken our Hαmosaic and overlaid it with X-ray emission contours to study the extent of X-ray emission within the H II region(see Figure1b).Diffuse X-ray emission is observed to be well confined by the optical nebula.This diffuse emission shows no evidence of limb brightening,suggesting that the interior of M17is centrallyfilled with hot gas. The peak of the X-ray emission is coincident with the center of the open cluster in M17, where Chandra observations show a large number of point sources superposed on the diffuse emission(Townsley et al.2003).This spatial distribution suggests that the X-ray-emitting hot gas originates in the open cluster,as would be expected in a bubble blown by stellarwinds.As there is a massive molecular cloud to the west of M17,we expect the hot gas to expand more rapidly to the east;thisflow of hot gas to the east has then blown the blister-like bubble seen in optical images.Four additional point sources are detected in thefield around M17.The brightest of these point sources lies to the south of the X-ray peak and has been previously designated 1WGA J1820.6−1615in the WGA Catalog of ROSAT Point Sources(White,Giommi,& Angelini1994).This point source is coincident with OI352(Ogura&Ishida1976),an O8star on the southern edge of the open cluster in M17.The other three point sources lie on the northern edge of the emission nebula.These X-ray point sources,designated as1WGA J1820.8−1603,1WGA J1821.0−1600,and1WGA J1820.8−1556,are coincident with stars GSC606265−01977,SAO161369(a known O5star),and GSC06265−01808, respectively.These point sources are marked in Figure1a.3.2.X-Ray SpectraIn order to examine the spectra of the diffuse X-ray emission,wefirst excluded the point sources found in§3.1.Then,noting that M17is on the edge of a dense molecular cloud,we have sectioned the nebula into four regions to account for anticipated changes in the foreground absorption column density.These regions have been labeled A,B,C,and D and are displayed in Figure2.Additionally,we have selected a background annulus around the superbubble,as indicated in Figure2.The background-subtracted spectra were then extracted from the PSPC eventfiles.The observed X-ray spectra of the superbubble is a convolution of several factors:the intrinsic X-ray spectrum of the superbubble,the intervening interstellar absorption,and the PSPC response function.Because the interstellar absorption and the PSPC response function are dependent on photon energy,we must assume models of the intrinsic X-ray spectrum and the interstellar absorption to make the problem tractable.As the X-ray emission from the superbubble interior appears largely diffuse,we have used the Raymond &Smith(1977)thermal plasma emission model to describe the intrinsic X-ray spectra of the superbubble and the Morrison&McCammon(1983)effective absorption cross-section per hydrogen atom for the foreground absorption,assuming solar abundances for both the emitting and absorbing materials.We then simulated the observed spectrum,combining theassumed models for the intrinsic spectrum and the interstellar absorption with the responsefunction of the PSPC.The best-fit spectrum is found by varying parameters and comparingχ2for the simulated and observed spectra.We performed aχ2grid search of simulated spectralfits to determine the best-fit levelsfor the thermal plasma temperature,kT,and absorption column density,N H.Plots of thebestfits to the X-ray spectra are shown in Figure3,andχ2plots are presented in Figure4.Theχ2plots of regions A,B,and C indicate that the X-ray emission can befit by eitherhigher temperature plasma,∼0.7keV,with lower absorption column density,∼1020−21cm−2,or lower temperature plasma,∼0.2keV,with higher absorption column density,∼1022cm−2.This is a common problem for PSPC spectra with a limited number of counts because ofthe poor spectral resolution and soft energy coverage of the PSPC.The best modelfits favorthe higher temperature plasma and lower absorption column density solution.Indeed,thedetection of soft X-ray emission below0.5keV indicates that the solution with N H∼1022cm−2cannot be valid,as such a solution predicts no significant soft X-ray emission should bedetected.Furthermore,the high absorption column density solution predicts a foregroundabsorption column density for M17equal to the total Galactic H I column density along theline of sight(Dickey&Lockman1990).As M17is located in the plane of the Galaxy atl=15◦03′,b=−00◦40′and has a distance of1.6kpc,we do not expect the majority of theGalactic H I toward this direction to be located in front of the superbubble.From the modelfits,we calculated the unabsorbed X-rayflux,and therefore the X-rayluminosity,L X,of the diffuse X-ray emission from each source region.The normalizationfactor,A,of the thermal plasma model is equal to n e n p dV/4πD2,where n e and n p are the electron and proton number densities,V is the volume of the superbubble,and D is thedistance to the source.Assuming a He:H number ratio of1:10and that the X-ray emittinggas is completely ionized,wefind n e≃1.2n p,and that the volume emission measure can beexpressed as<n2e>fV,where f is the volumefilling factor.We have used the diameter ofthe superbubble,∼10–12pc,as the depth of the X-ray emitting gas in each source region.We determined the volume,V,of each source region by multiplying the surface area of theregion by the depth and a geometric correction factor of2/3(approximated by the volumeratio of a sphere to a cylinder).Taking the volumefilling factor to be f=0.5,we thencalculated rms n e in each region.The best-fit values of kT,A,N H,L X and rms n e are givenin Table1.Note that the modelfit to region D did not converge because it contains a largenumber of unresolved stellar sources as well as diffuse emission;only approximate values forthe X-ray luminosity and absorption column density are given.See Townsley et al.(2003)for a detailed analysis of the Chandra observations of region D.Combining our results from each of the source regions,wefind a total diffuse X-ray lu-minosity of∼2.5×1033ergs s−1in the ROSAT PSPC0.1–2.4keV band,a mean characteristic temperature of kT∼0.72keV or T∼8.5×106K,a mean electron density of∼0.09cm−3,and a total hot gas mass of∼1M⊙.We have calculated the total thermal energy in hot,shocked wind component of the superbubble to be E th∼1×1048ergs with a cooling timescale of t T∼40Myr.Given the stellar age of the open cluster,∼1Myr,we do not expect significant radiative cooling to have occurred.As a rough check,we multiply the current X-ray lumi-nosity by the age of the cluster andfind that the total energy radiated away by the X-ray emission is 10%of the total thermal energy.4.Discussionparisons with Model ExpectationsWe now compare the observed physical properties of M17determined above to theo-retical calculations from basic wind-blown bubble models.Although the M17superbubble is in an inhomogeneous ambient medium with a significant density gradient and the cluster is off-centered(a more complex scenario than is considered in basic bubble models),if the superbubble structure is indeed governed by the physical processes prescribed by these mod-els,we expect the properties of the diffuse X-ray emission to agree with predictions within similar orders of magnitude.Wefirst consider the wind-blown bubble model of Weaver et al.(1977)and will later consider a wind-blown bubble model without heat conduction.4.1.1.A Bubble with Heat ConductionIn the Weaver et al.model,heat conduction and mass evaporation act across the bound-ary between the hot interior gas and the nebular shell to lower the temperature and raise the density of the bubble interior.The temperature and electron density profiles of such a bubble have been calculated by Weaver et al.(1977),and the X-ray luminosities of such bubbles can be determined using two methods outlined by Chu et al.(1995).In thefirst method,we derive the expected X-ray luminosity from the observed physical properties of the gas in the104K ionized shell of swept-up ISM.In the second method,we use the spectral types of massive stars in M17to estimate the combined mechanical luminosity of the stellar winds and then derive the expected X-ray luminosity.These two methods use independent input parameters and thus allow us to check the consistency of the pressure-driven bubble model in addition to comparing the expected and observed X-ray luminosities.X-Ray Luminosity Method1:The Ionized ShellThe expected X-ray luminosity in the ROSAT band of0.1–2.4keV for the Weaver et al. (1977)wind-blown bubble model has been given by Chu et al.(1995),L X=(8.2×1027ergs s−1)ξI(τ)n10/70R17/7pcV16/7km/s,(1)whereξis the metallicity relative to the solar value and in this case we assume a value of unity,I(τ)is a dimensionless integral of value∼2,n0is the number density of the ambient medium in cm−3,R pc is the radius of the superbubble in pc,V km/s is the expansion velocity of the superbubble in units of km s−1.The ambient density n0cannot be measured directly, but assuming that the ram pressure of the expanding shell is equal to the thermal pressure of the ionized superbubble shell,the relation between the ambient density and the density of the ionized shell is given byn0=(9/7)n i kT i/(µa V2exp),(2) where n i is the electron number density in the ionized shell,T i∼104K is the electron temperature in the ionized shell,V exp is the expansion velocity of the bubble,andµa= (14/11)m H(Weaver et al.1977;Chu&Mac Low1990).Adopting a mean electron density of n i∼300cm−3(Felli,Churchwell,&Massi1984)and an observed V exp∼25km s−1(Clayton et al.1985),we calculated an ambient density of n0∼40cm−3.Given the superbubble radius of5–6pc,we have determined an expected X-ray luminosity of∼3×1035ergs s−1.X-Ray Luminosity Method2:Wind Luminosity from OB StarsWe can also calculate the expected X-ray luminosity in an energy-conserving,wind-blown bubble by the following equation from Chu et al.(1995),L X=(1.1×1035ergs s−1)ξI(τ)L33/3537n17/35t19/35Myr,(3)where L37is the mechanical luminosity of the stellar winds in units of1037ergs s−1,and t Myr is the age of the bubble in Myr.To remain independent of Method1,we do not use the value of n0determined for that method.Rather,we use the following relations between ambient density,radius,wind luminosity,bubble age,and expansion velocity,n0=(1.3×108cm−3)L37t3Myr R−5pc,(4)t Myr=(0.59Myr)R pc/V km/s,(5) (Weaver et al.1977;Chu et al.1995).We again take the expansion velocity to be∼25km s−1 (Clayton et al.1985)and the radius to be5–6pc and derive a bubble age of∼0.13Myr.To determine the wind luminosity of M17,we examined its massive stellar content. Hanson et al.(1997)identified nine O stars and four late-O/early-B stars in the open cluster. Using the spectral types of these massive stars,we have estimated their terminal stellar wind velocities,effective temperatures,and luminosities based on the stellar parameters given by Prinja,Barlow,&Howarth(1990)and Vacca,Garmany,&Shull(1996).We then calculated the mass-loss rates for the OB stars in M17by utilizing the empirically derived relationship between effective temperature,luminosity,and mass-loss rate of de Jager,Nieuwenhuijzen,& van der Hucht(1988).Table2lists the detected OB stars,their spectral types as determined from optical and K-band observations,their terminal wind velocities V∞,stellar effective temperatures T eff,stellar luminosities L,and their mass-loss rates˙M.We calculated the total mechanical luminosity of the stellar winds,L w=Σ(1/2)˙MV2∞,(6) from the OB stars to be∼1×1037ergs s−1.As noted by Felli et al.(1984),the identified OB stars can approximately account for the ionization of the emission nebula;we therefore expect our calculated wind mechanical luminosity to be reasonably complete as well.Assuming a relatively constant mechanical luminosity,wefind a total energy deposited by stellar winds of∼4×1049ergs over the life of the bubble.In addition,this value of the wind mechanical luminosity gives an ambient density of∼60cm−3and an expected X-ray luminosity of ∼5×1035ergs s−1.This X-ray luminosity value is consistent to within a factor of two with the value found by Method1.Although the two methods of determining the expected X-ray luminosity are consistent with each other,they do not agree with the X-ray luminosity derived from the PSPC obser-vation.The observed X-ray luminosity is∼100–200times lower than expected from Weaver et al.’s bubble model.It is possible that stellar winds are clumpy,as suggested by Moffat& Robert(1994),then the conventionally derived mass loss rates would be reduced by a factor of≥3.Even using the reduced mass loss rates,the expected X-ray luminosity is more than 40times too high.The observed temperature,density,and surface brightness of the hot gas in M17do not agree with the model expectations,either.The physical conditions of the shocked stellar winds in Weaver et al.’s model are heavily modified by heat conduction and the hot gas mass is dominated by the nebular mass evaporated across the interface.The predicted temperature is5.0–5.6×106K near the center and decreases outward,the predicted density is0.2–0.4cm−3near the center and increases outward,and the X-ray surface bright-ness is expected to show pared with observed properties,the expected temperature is too low,density is too high,and the X-ray morphology is inconsistent.The disagreements between observations and model expectations suggest that heat conduction may not play a dominant role in determining the physical conditions inside this superbubble.Heat conduction can be suppressed by the presence of magneticfields(Soker1994;Band &Liang1988).The magneticfield strength in M17has been measured via the H I Zeeman effect to be100–550µG,peaking near the interface between the H II region and the molecular cloud M17SW(Brogan et al.1999).Assuming a comparable magneticfield strength in the swept-up104K shell,wefind the Alfv´e n speed to be10–60km s−1which is comparable to or much greater than the isothermal sound velocity of the104K gas,10km s−1for H atoms. In addition,the magneticfield strength and isothermal sound velocity indicate a gyro-radius of 10km for protons in the swept-up shell.This suggests that the protons in the104K gas will be unable to escape the magneticfield and diffuse into the interior of the superbubble, inhibiting heat conduction and mass evaporation between the hot interior and the cool shell of the bubble.4.1.2.A Bubble without Heat ConductionWe now turn our consideration to a wind-blown bubble without heat conduction.The X-ray emission of a bubble interior depends on both the temperature and the amount of hot gas.We willfirst compare the plasma temperature expected from the shocked stellar winds to the observed hot gas ing the combined stellar winds mass-loss rate of 4.3×10−6M⊙yr−1(summed from Table2)and the integrated wind mechanical luminosity L w of1×1037ergs s−1as calculated in§4.1.1,we derive an rms terminal wind velocity of V∞∼2700km s−1.The post-shock temperature of the combined stellar winds is therefore expected to be∼8×107K.This temperature is an order of magnitude higher than that indicated by PSPC observations;to lower it to the observed temperature of∼8.6×106K requires the mixing in of cold nebular mass that is nearly10times the mass of the combined stellar winds.This mixing may be provided by turbulent instabilities at the interface between the shocked fast winds and the cold nebular shell(e.g.,Strickland&Stevens1998)or through the hydronamic ablation of clumps of cold nebular material distributed within the hot bubble interior(Pittard,Hartquist,&Dyson2001).Assuming that mixing has taken place,we next determine the hot gas mass expected as a result of mixing and compare it to the observed value(§3.2).Given the dynamical age of the superbubble,0.13Myr,a total stellar wind mass of∼0.56M⊙has been injected to the superbubble interior,and the expected total mass of the hot gas will be5–6M⊙.This is significantly greater than the observed value of∼1M⊙.This discrepancy can be reduced if we again consider the possibility of clumpy stellar winds(Moffat&Robert1994).With the mass-loss rates reduced by a factor of≥3,the expected hot gas mass is∼2M⊙,which would be in remarkable agreement with the observed value.We summarize the comparison between the observed X-ray emission and the various models in Table3,which lists the observed X-ray luminosity and hot gas temperature and mass as well as those expected from models with and without heat conduction for both homogeneous winds and clumpy winds.It is clear that bubble models with heat conduction have the largest discrepancies from the observations.The best agreement with observed properties is from bubble models without heat conduction but allowing dynamical mixing of cold nebular material with the hot gas.For models either with or without heat conduction, clumpy winds with reduced mass loss rates are needed to minimize the discrepancy between model expectations and observations.parisons with Other Wind-Blown BubblesDiffuse X-ray emission has been previously detected from other types of wind-blown bubbles,including planetary nebulae(PNe)and circumstellar bubbles blown by Wolf-Rayet (WR)stars.The X-ray emission from these circumstellar bubbles is qualitatively and quan-titatively different from that of M17.Chu,Gruendl,&Guerrero(2003)find that the X-ray emission from PNe and WR bubbles shows a limb-brightened spatial distribution,in sharp contrast to the centrally-filled spatial distribution in M17as described in§3.1.Further,Chu et al.(2003)note that PNe and WR bubbles exhibit hot gas temperatures of1–3×106K and electron densities of10–100cm−3,while the hot interior gas of M17exhibits a temperature of8.5×106K and a substantially lower electron density of∼0.09cm−3.The comparisons of morphology and temperature between the M17superbubble and small circumstellar bubbles show fundamental differences.The limb-brightened X-ray spa-tial distribution,low temperatures,and high electron densities of PNe and WR bubbles are qualitatively consistent with a hypothesis of heat conduction and mass evaporation occurring between the hot gas interior and the swept-up shell.However,the observed X-ray luminosi-ties for PNe and WR bubbles are both significantly lower(10–100times)than predicted by bubble models with heat conduction(Chu et al.2001;Wrigge,Wendker,&Wisotzki1994; Wrigge1999).It is possible that in these wind-blown bubbles,heat conduction has also been suppressed and that dynamical mixing,which allows a lower mass injection rate,occurs at the interface between the hot gas interior and the cool nebular shell.Exploring this question will require magneticfield measurements of PNe and WR bubbles.5.SummaryWe have presented analysis of a ROSAT observation of the emission nebula M17.The blister-like morphology seen in the optical images indicates that it is a superbubble blown by the winds of its OB stars in an inhomogeneous ISM.With a stellar age of∼1Myr,M17must be a young quiescent superbubble without any supernova heating.Diffuse X-ray emission is detected from M17and is confined within the optical shell.This suggests the presence of hot106–108K gas in the interior of M17,as is expected in a wind-blown bubble.Analysis of the diffuse X-ray emission indicates a characteristic gas temperature∼8.5×106K with a mean electron number density of0.09cm−3.We have considered bubble models with and without heat conduction and found that those with heat conduction overpredict the X-ray luminosity by two orders of magnitude. Furthermore,the magneticfield measured in M17is large enough to suppress heat conduc-tion and associated mass evaporation.Bubble models without heat conduction overestimate the hot gas temperature unless mixing with cold nebular gas has occurred.If nebular gas can be dynamically mixed into the hot bubble interior and if the stellar winds are clumpy with a lower mass-loss rate,the X-ray morphology and luminosity,and hot gas temperature and mass can be reasonably reproduced.M17provides us a rare opportunity to probe the physical conditions of hot gas energized solely by shocked stellar winds in a quiescent superbubble.While we have learned much from the current analysis,our model considerations were performed on a very basic level.More robust models are needed to accurately describe the evolution of a superbubble in a medium with a large-scale density gradient,small-scale clumpiness,and a strong magneticfield.We would like to thank the anonymous referee for the stimulating comments with have helped us to improve this paper.This research has made use of data obtained through the High Energy Astrophysics Science Archive Research Center Online Service,provided by the NASA/Goddard Space Flight Center.。
aaa1

How is this done?
• Read the textbook “Physics Concepts & Connections” • Come to the lectures • Participate in discussion section • Do the homework • Write the essay • Take the exams
What do you get?
• An understanding of the physical universe. • A grade – 15% HW – 15% essay -- 20% participation and presentation – 50% exam
(地球物体)
later.
向心的
(纬度)
埃拉托色尼(公元前三世纪的希腊 天文学家、数学家和地理学家)
(日食)
(类似过山车、极度尖叫游戏)
海 拔 高 度
(扭转光纤)
The Galactic Centre, Stellar dynamics in the innermost region, Radio and X-ray emission from Sgr A*, Near-infrared flares from the black hole
Goals of the course
• Learn a process for critical thinking, and apply it to evaluate physical theories • Use these techniques to understand the ideas underlying modern physics. • Implement the ideas in some basic problems. • Learn where physics is today,and where it is going.
X射线光电子能谱(XPS)

另外,原子中的电子既有轨道运动又有自旋运动。它们之间存在着耦合(电磁相
互)作用,使得能级发生分裂。对于ι >0的内壳层,这种分裂可以用内量子数j来
表示。其数值为:
j=
l + ms
=
l±
1 2
所以:对于ι =0,j=1/2。对于ι >0,则j= ι +½或者ι -½。也就是说,除了s能
级不发生分裂外,其他能级均分裂为两个能级:在XPS谱图中出现双峰。
3
电子能谱的基本原理
基本原理就是光电效应。
能量关系可表示:
hv = Eb + Ek + Er
电子结合能 电子动能
原子的反冲能量
Er
=
1(M
2
− m)υa*2
忽略 Er (<0.1eV)得
hv = Ek + Eb
4
对孤立原子或分子,Eb 就是把电子从所在轨道
移到真空需的能量,是以真空能级为能量零点的。
S能级的内量子数½通 常省略。如:C的1s 能级没有分裂,在 XPS谱图上只有一个 峰,表示为:C1s。
C1s
14
基本原理
4、电子结合能Eb: 一个自由原子或者离子的结合能,等于将此电子从所在的能级转移到无限远处所 需要的能量。对于气体样品,如果样品室和谱仪制作材料的影响可以忽略,那么 电子的结合能Eb可以从光子的入射能量hν以及测得的电子的动能Ek求出,即:
21
X射线光电子能谱分析的基本原理
5、XPS信息深度: 在XPS分析中,一般用能量较低的软X射线激发光电子(如:Al 和Mg的Kα线)。虽然软X射线的能量不高,但是仍然可以穿透 10nm厚的固体表层,并引起那里的原子轨道上的电子光电离。 产生的光电子在离开固体表面之前,要经历一系列的弹性(光 电子与原子核或者其他电子相互作用时不损失能量)和非弹性 散射(光电子损失能量)。弹性散射的光电子形成了XPS谱的 主峰;非弹性散射形成某些伴峰或者信号的背底。 一般认为:对于那些具有特征能量的光电子穿过固体表面时, 其强度衰减遵从指数规律。假设光电子的初始强度为I0,在固体 中经过dt距离,强度损失了dI,有:
X-rayemissionfrommassivestarswithmagneticfields
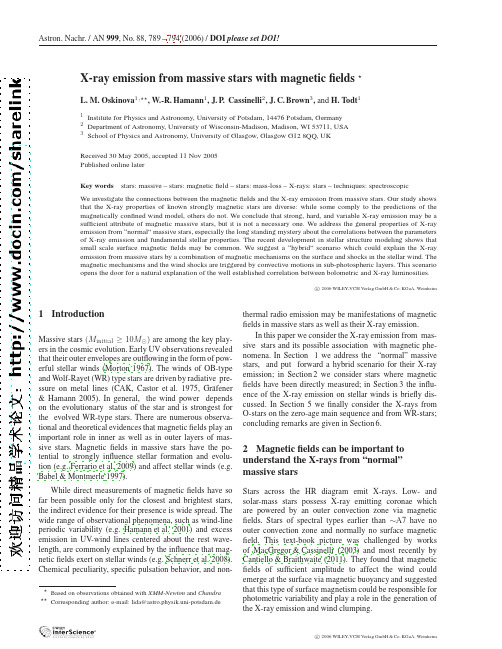
arX iv:111.5345v1[astro-ph.S R]24Oct211Astron.Nachr./AN 999,No.88,789–794(2006)/DOI please set DOI!⋆Based on observations obtained with XMM-Newton and Chandra⋆⋆Corresponding author:e-mail:*****************.uni-potsdam.de thermal radio emission may be manifestations of magnetic fields in massive stars as well as their X-ray emission.In this paper we consider the X-ray emission from mas-sive stars and its possible association with magnetic phe-nomena.In Section 1we address the “normal”massive stars,and put forward a hybrid scenario for their X-ray emission;in Section 2we consider stars where magnetic fields have been directly measured;in Section 3the influ-ence of the X-ray emission on stellar winds is briefly dis-cussed.In Section 5we finally consider the X-rays from O-stars on the zero-age main sequence and from WR-stars;concluding remarks are given in Section 6.2Magnetic fields can be important to understand the X-rays from “normal”massive stars Stars across the HR diagram emit X-rays.Low-and solar-mass stars possess X-ray emitting coronae which are powered by an outer convection zone via magnetic fields.Stars of spectral types earlier than ∼A7have no outer convection zone and normally no surface magnetic field.This text-book picture was challenged by works of MacGregor &Cassinelli (2003)and most recently by Cantiello &Braithwaite (2011).They found that magnetic fields of sufficient amplitude to affect the wind could emerge at the surface via magnetic buoyancy and suggested that this type of surface magnetism could be responsible for photometric variability and play a role in the generation ofthe X-ray emission and wind clumping.c 2006WILEY-VCH Verlag GmbH &Co.KGaA,Weinheim790L.M.Oskinova et al..:X-rays from massive stars with magneticfieldsM∗R−1∗,the velocity jump,i.e.the temperatures ofthe gas heated in the shock could be higher for more massivestars with higher T eff.This could explain the correlationsbetween T X and T effdiscovered by Walborn et al.(2009).3Magneticfields on massive stars are notnecessarily manifested via strong,hard andvariable X-raysFrom the small-scale magneticfields which we consideredin previous section,we now turn to the large-scale orga-nizedfields that may be fossil in origin.Babel&Montmerle(1997)studied the case of a star with a stellar wind and adipole magneticfield.They predicted that a collision be-tween the wind components from the two hemispheres inthe closed magnetosphere leads to a strong shock and char-acteristic X-ray emission.Based on this magnetically con-fined wind shock model(MCWS),the presence of a mag-neticfield on the O-type starθ1Ori C had been postulated.Direct confirmation of the magneticfield in this star byDonati et al.(2006a)proved that X-rays have large diagnos-tic potential in selecting massive stars with surface magneticfields.Using the parameters ofθ1Ori C,ud-Doula&Owocki(2002)and Gagn´e et al.(2005)performed MHD simula-c 2006WILEY-VCH Verlag GmbH&Co.KGaA,Weinheim Astron.Nachr./AN(2006)791792L.M.Oskinova et al..:X-rays from massive stars with magneticfieldsAstron.Nachr./AN(2006)793 Name Age Sp.Type log(L X/L bol)Presence of hard emission log(L X/L bol) Ref.Myr earliest O-type star earliest O-type star kT X>∼2keV ensemble of O stars(1)Hofner et al.(2002);(2)Rho et al.(2004);(3)Wolk et al.(2006);(4)Moffat et al.(2002);(5)L X is inferred using archival Chandra data;(6)Feigelson et al.(2002);(7)Cluster parameters from from Bonatto et al.(2006);other hand,some stars are weak X-ray sources,e.g.,Her36 (O7V)in the Hourglass and HD164492A(O7.5III)in the Trifid.Her36is of similar age and spectral type asθ1Ori C, yet the latter is significantly more X-ray luminous.The hardness of the X-ray spectrum is not an unambigu-ous indication of the presence of a magneticfield.X-ray temperatures of several keV are expected and have been ob-served in binary systems.The probability that a massive star in a young cluster is a binary is high,therefore it is not sur-prising that many stars listed in Table1display the presence of kT X>∼2keV plasma in their spectra.This is likely the case of the Kleimann star,a massive O4+O4binary ionizing the Omega Nebula cluster NGC6618.While its spectrum is rel-atively hard,the broad-band X-ray luminosity is consistent with the canonical L X≈10−7L bol relation which holds also for binaries(Oskinova2005).The X-ray observations of the Trifid Nebula(Rho et al. 2004)allowed to resolve the central ionizing source into discrete components and revealed that an O7.5III star has a soft(kT≈0.6keV)spectrum,while harder emission (kT≈6keV)is associated with a B-type star which is blen-ded with an unidentified source.Interestingly,X-rayflares were previously detected from B-type stars in two Orionis clusters:Trapezium andσOri(Sanz-Forcada et al.2004; Stelzer et al.2002).Often,young SFRs where massive stars are just forming are located close to more evolved massive star clusters,sug-gesting a possible causal connection between massive star feedback and star formation.An example of such SFR is ON2located close to the massive star cluster Berkeley87. Its X-ray properties were explored in Oskinova et al.(2010).The most evolved star in Berkeley87is a rare WO-type star,WR142.Stars of this spectral type are the ulti-mately latest evolutionary stage of a very massive stars(e.g. Sander et al.2011).There are no direct measurements of magneticfields on WR stars up to now(see Kholtygin et al., these proceed.).However,the X-ray properties of WR stars may provide indirect evidence that magnetism plays a role also in the latest stages of stellar evolution.Our XMM-Newton observation of the closest WO-type star WR142succeeded in detecting this object (Oskinova et al.2009).It was also detected by the Chandra observatory(Sokal et al.2010).Albeit the signal-to-noise of the observed spectrum was too poor for a detailed spec-tral analysis,from the hardness ratio Oskinova et al.(2009) concluded that the X-ray emission from this star is strongly absorbed in the wind,and is too hard to be explained by the wind-shock mechanism.We speculated that the hypo-thetical magnetic magneticfield can be responsible for this strong emission.Our analysis of the optical spectrum of this star yielded the fundamental stellar parameters:R∗=0.5R⊙,T eff= 160kK,M∗=5M⊙.Remarkedly,the optical emission line profiles in the spectrum of WR142have an unusual round shape.The formal rotational broadening models of these lines yield projected rotational velocity v sin i= 4000km s−1.Thus,the star may be rotating at break-up ve-locity.These stellar parameters compare well with those ex-pected for a SN orγ-ray burst progenitor(Paczy`n ski1998). 6Concluding remarksSurface magneticfields on massive stars are both predicted theoretically and confirmed observationally.All assive stars emit X-ray radiation,but the connection between X-rays and magnetism in massive stars is not yet fully understood. The MCWS model can explain the strong,hard,varable X-ray emission from some magnetic dipoles.However there is no quantitative explaination for the soft and the constant X-ray emission that is observed in the majority of magnetic massive stars.It was recently argued that all massive stars may have magneticfields on their surface(Cantiello&Braithwaite 2011).In this paper we propose a new scenario of X-ray emission from a massive star,where the plasma on the sur-face is heated by magnetic mechanisms while the plasma embedded in the stellar wind is heated by wind shocks.Both c 2006WILEY-VCH Verlag GmbH&Co.KGaA,Weinheim794L.M.Oskinova et al..:X-rays from massive stars with magneticfields。
Neutron Stars, Pulsars and Supernova Remnants concluding remarks
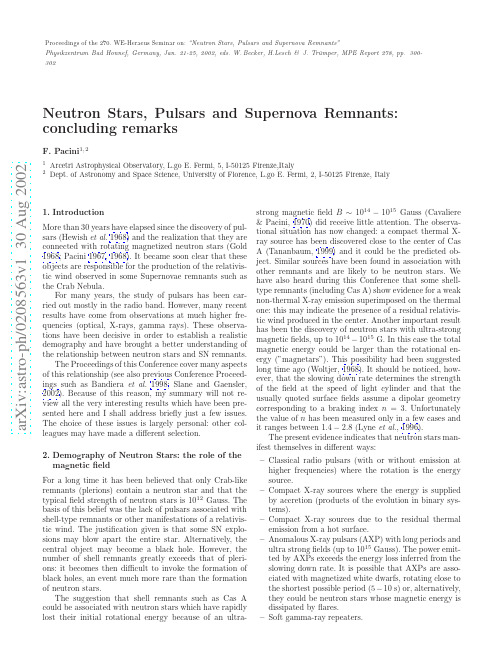
a r X i v :a s t r o -p h /0208563v 1 30 A u g 2002Proceedings of the 270.WE-Heraeus Seminar on:“Neutron Stars,Pulsars and Supernova Remnants”Physikzentrum Bad Honnef,Germany,Jan.21-25,2002,eds.W.Becker,H.Lesch &J.Tr¨u mper,MPE Report 278,pp.300-302Neutron Stars,Pulsars and Supernova Remnants:concluding remarksF.Pacini 1,21Arcetri Astrophysical Observatory,L.go E.Fermi,5,I-50125Firenze,Italy2Dept.of Astronomy and Space Science,University of Florence,L.go E.Fermi,2,I-50125Firenze,Italy1.IntroductionMore than 30years have elapsed since the discovery of pul-sars (Hewish et al.1968)and the realization that they are connected with rotating magnetized neutron stars (Gold 1968;Pacini 1967,1968).It became soon clear that these objects are responsible for the production of the relativis-tic wind observed in some Supernovae remnants such as the Crab Nebula.For many years,the study of pulsars has been car-ried out mostly in the radio band.However,many recent results have come from observations at much higher fre-quencies (optical,X-rays,gamma rays).These observa-tions have been decisive in order to establish a realistic demography and have brought a better understanding of the relationship between neutron stars and SN remnants.The Proceedings of this Conference cover many aspects of this relationship (see also previous Conference Proceed-ings such as Bandiera et al.1998;Slane and Gaensler,2002).Because of this reason,my summary will not re-view all the very interesting results which have been pre-sented here and I shall address briefly just a few issues.The choice of these issues is largely personal:other col-leagues may have made a different selection.2.Demography of Neutron Stars:the role of the magnetic field For a long time it has been believed that only Crab-like remnants (plerions)contain a neutron star and that the typical field strength of neutron stars is 1012Gauss.The basis of this belief was the lack of pulsars associated with shell-type remnants or other manifestations of a relativis-tic wind.The justification given is that some SN explo-sions may blow apart the entire star.Alternatively,the central object may become a black hole.However,the number of shell remnants greatly exceeds that of pleri-ons:it becomes then difficult to invoke the formation of black holes,an event much more rare than the formation of neutron stars.The suggestion that shell remnants such as Cas A could be associated with neutron stars which have rapidly lost their initial rotational energy because of an ultra-strong magnetic field B ∼1014−1015Gauss (Cavaliere &Pacini,1970)did receive little attention.The observa-tional situation has now changed:a compact thermal X-ray source has been discovered close to the center of Cas A (Tananbaum,1999)and it could be the predicted ob-ject.Similar sources have been found in association with other remnants and are likely to be neutron stars.We have also heard during this Conference that some shell-type remnants (including Cas A)show evidence for a weak non-thermal X-ray emission superimposed on the thermal one:this may indicate the presence of a residual relativis-tic wind produced in the center.Another important result has been the discovery of neutron stars with ultra-strong magnetic fields,up to 1014−1015G.In this case the total magnetic energy could be larger than the rotational en-ergy (”magnetars”).This possibility had been suggested long time ago (Woltjer,1968).It should be noticed,how-ever,that the slowing down rate determines the strength of the field at the speed of light cylinder and that the usually quoted surface fields assume a dipolar geometry corresponding to a braking index n =3.Unfortunately the value of n has been measured only in a few cases and it ranges between 1.4−2.8(Lyne et al.,1996).The present evidence indicates that neutron stars man-ifest themselves in different ways:–Classical radio pulsars (with or without emission at higher frequencies)where the rotation is the energy source.–Compact X-ray sources where the energy is supplied by accretion (products of the evolution in binary sys-tems).–Compact X-ray sources due to the residual thermal emission from a hot surface.–Anomalous X-ray pulsars (AXP)with long periods and ultra strong fields (up to 1015Gauss).The power emit-ted by AXPs exceeds the energy loss inferred from the slowing down rate.It is possible that AXPs are asso-ciated with magnetized white dwarfs,rotating close to the shortest possible period (5−10s)or,alternatively,they could be neutron stars whose magnetic energy is dissipated by flares.–Soft gamma-ray repeaters.2 F.Pacini:Neutron Stars,Pulsars and Supernova Remnants:concluding remarks In addition it is possible that some of the unidentifiedgamma ray sources are related to neutron stars.Thepresent picture solves some previous inconsistencies.Forinstance,the estimate for the rate of core-collapse Super-novae(roughly one every30-50years)was about a factorof two larger than the birth-rate of radio pulsars,suggest-ing already that a large fraction of neutron stars does not appear as radio pulsars.The observational evidence supports the notion of a large spread in the magnetic strength of neutron stars and the hypothesis that this spread is an important factor in determining the morphology of Supernova remnants.A very strongfield would lead to the release of the bulk of the rotational energy during a short initial period(say, days up to a few years):at later times the remnant would appear as a shell-type.A more moderatefield(say1012 Gauss or so)would entail a long lasting energy loss and produce a plerion.3.Where are the pulses emitted?Despite the great wealth of data available,there is no gen-eral consensus about the radiation mechanism for pulsars. The location of the region where the pulses are emitted is also controversial:it could be located close to the stellar surface or,alternatively,in the proximity of the speed of light cylinder.The radio emission is certainly due to a coherent pro-cess because of the very high brightness temperatures(T b up to and above1030K have been observed).A possible model invokes the motion of bunches of charges sliding along the curvedfield lines with a relativistic Lorentz fac-torγsuch that the critical frequencyνc∼c2π:Ψ∼10−2;B⊥∼104G;γ∼102−103.The model leads to the expectation of a very fast de-crease of the synchrotron intensity with period because of the combination of two factors:a)the reduced particles flux when the period increases;b)the reduced efficiency of synchrotron losses(which scale∝B2∝R−6L∝P−6)at the speed of light cylinder(Pacini,1971;Pacini&Salvati 1983,1987).The predictionfits the observed secular de-crease of the optical emission from the Crab Nebula and the magnitude of the Vela pulsar.A recent re-examination of all available optical data confirms that this model can account for the luminosity of the known optical pulsars (Shearer and Golden,2001).If so,the optical radiation supports strongly the notion that the emitting region is located close to the speed of light cylinder.4.A speculation:can the thermal radiation fromyoung neutron stars quench the relativisticwind?Myfinal remarks concern the possible effect of the ther-mal radiation coming from the neutron star surface upon the acceleration of particles.This problem has been inves-tigated for the near magnetosphere(Supper&Truemper, 2000)and it has been found that the Inverse Compton Scattering(ICS)against the thermal photons is impor-tant only in marginal cases.However,if we assume that the acceleration of the relativistic wind and the radiation of pulses occur close to the speed of light cylinder,the sit-uation becomes different and the ICS can dominate over synchrotron losses for a variety of parameters.The basic reason is that the importance of ICS at the speed of light distance R L scales like the energy density of the thermal photons uγ∝R−2L∝P−2;on the other hand, the synchrotron losses are proportional to the magnetic energy density in the same region u B∝R−6L∝P−6.Numerically,onefinds that ICS losses dominate over synchrotron losses ifT6>0.4B1/2121012G; P s is the pulsar period in seconds).The corresponding upper limit for the energy of the electrons,assuming that the acceleration takes place for a length of order of the speed of light distance and that the gains are equal to the losses is given by:E max≃1.2×103T6−4P s GeV.F.Pacini:Neutron Stars,Pulsars and Supernova Remnants:concluding remarks3Provided that the particles are accelerated and radi-ate in proximity of the speed of light cylinder distance,weconclude that the thermal photons can limit the acceler-ation of particles,especially in the case of young and hotneutron stars.It becomes tempting to speculate that thismay postpone the beginning of the pulsar activity untilthe temperature of the star is sufficiently low.The mainmanifestation of neutron stars in this phase would be aflux of high energy photons in the gamma-ray band,dueto the interaction of the quenched wind with the thermalphotons from the stellar surface.This model and its ob-servational consequences are currently under investigation(Amato,Blasi,Pacini,work in progress).ReferencesAloisio,R.,&Blasi,P.2002,Astrop.Phys.,Bandiera,R.,et al.1998,Proc.Workshop”The Relationshipbetween Neutron Stars and Supernova Remnants”,Mem.Societ Astronomica Italiana,vol.69,n.4Cavaliere,A.,&Pacini,F.1970,ApJ,159,170Gold,T.1968,Nature,217,731Hewish A.,et al.1968,Nature217,709Lyne,G.,et al.1996,Nature,381,497Pacini,F.1967,Nature,216,567Pacini,F.1968,Nature,219,145Pacini,F.1971,ApJ,163,L17Pacini,F.,&Salvati,M.1983,ApJ,274,369Pacini F.,&Salvati,M.1987,ApJ.,321,447Shearer,A.,and Golden,A.2001,ApJ,547,967Slane,P.,Gaensler,B.2002,Proc.Workshop”Neutron Starsin Supernova Remnants”ASP Conference Proceedings(inpress)Supper,R.,&Trumper,J.2000,A&A,357,301Tananbaum,B,et al.1999,IAU Circular7246Thompson,C.,Duncan,R.C.1996,ApJ,473,322Woltjer,L.1968,ApJ,152,179。
AND

T HE A STROPHYSICAL J OURNAL ,484:920È926,1997August 11997.The American Astronomical Society.All rights reserved.Printed in U.S.A.(STATISTICS OF FLUCTUATIONS IN THE SOLAR SOFT X-RAY EMISSIONS.AND S.U E N O 1M INESHIGEDepartment of Astronomy,Faculty of Science,Kyoto University,Sakyo-ku,Kyoto 606-01,JapanH.N EGOROInstitute of Space and Astronautical Science,Yoshinodai,Sagamihara,Kanagawa 226,JapanK.S HIBATASolar Physics Division,National Astronomical Observatory,Mitaka 181,JapanANDH.S.H UDSONSolar Physics Research Corporation,Institute of Space and Astronautical Science,Yoshinodai,Sagamihara,Kanagawa 226,JapanReceived 1996September 23;accepted 1997February 28ABSTRACTX-ray emission from the Sun Ñuctuates as a result of occasional Ñare events.We have calculated the power spectra of the solar soft X-ray variations using the photometric data of the GOES 6satellite.The data cover the period 1991September to 1994April,about 32months in total,and we have worked with 10minute averages.We Ðnd that the total power spectral densities (PSDs)have three distinct com-ponents;a Ñat or slightly decreasing low-frequency section,a 1/f -like moderate decline medium-frequency section,and steep decline high-frequency parts.The break frequencies separating three parts are Hz and 10~3.8Hz ,respectively.Such downward breaks are expected from the shot-f break^10~4.7noise (Ñarelike)character of solar X-ray emission,and we interpret the lower break frequency as indicat-ing an upper limit on Ñare timescales.These break frequencies do not vary appreciably with activity level.This suggests the existence of a universal mechanism for triggering Ñares in the solar corona.Moreover,the power-law index (where we assume PSD P f ~b ,f being frequency)of each part does not vary appreciably with the level of activity either;its average is b ^0.45,0.95,and 1.5,respectively.The overall shape of the PSD is quite similar to those of other astrophysical objects such as black hole can-didate stars and active galactic nuclei,albeit on a vastly di†erent scale.Subject headings:Sun:activity ÈSun:Ñares ÈSun:X-rays,gamma rays1.INTRODUCTIONIt is widely believed that solar Ñares are caused by a release of magnetic energy stored in the solar coronal Ðelds.If so,we may deÐne basic time and spatial scales,such as the Alfve n transit time over a pressure scale height.One might then expect that the pattern of Ñare occurrence would reÑect these typical scales.Interestingly,however,this is not the case:total energy outputs and peak intensities of Ñares are distributed rather smoothly.A power-law dependence of solar Ñares on peak intensity has been established through a number of observations (Drake 1971;Datlowe,Elcan,&Hudson et al.The 1974;Lin 1984;Dennis 1985).essence is that,although bigger Ñares occur less frequently than smaller Ñares,there is basically no preferential energy.We speculate that the occurrence of Ñares may not be the result of simple local physics but is somehow controlled globally.To investigate this possibility,thorough statistical studies of solar Ñares are indispensable.Many studies concerning the statistics of solar Ñares have been made so far,but most of them have treated solar Ñares as individual,independent events.To derive useful informa-tion,such as periodicities and noise properties,the tech-nique of power spectral densities (PSDs)is often used.In fact,there are some studies on long-term periodicities of solar Ñare occurrence et al.&Cliver (Rieger 1984;Kile Bai &Sturrock However,there have 1991;1991,1993).been few attempts to investigate high-frequency noise properties of these patterns.In the present study,we calcu-1ueno =kwasan.kyoto-u.ac.jp.late the PSD,using GOES 6data,and discuss the statistics of solar Ñares from viewpoints that di†er from previous ones.We shall describe the GOES 6data in The overall °2.light curves and the PSD will be presented in Solar °3.activity signiÐcantly decreased during this period.To see how the statistical nature changes with time,we divide the entire data set into 16subsets,each with a duration of 2months,and study the light curves and power spectra of each individual subset.The variation of each spectral parameter will also be displayed in In we focus on °3.°4the long-timescale periodicity of Ñare occurrence.The Ðnal section is devoted to summary and discussion on the sta-tistics of solar Ñares and its relation to X-ray Ñuctuation from other objects,such as black hole systems.2.DATAWe use the soft X-ray light curves from 1991September to 1994April obtained with GOES 6.The overall light curves are displayed in Clearly,the corona was Figure 1.rather active,producing large X-ray variations due to major Ñares only until the Ðrst half of 1993,and the number of large peaks decreased rapidly afterward.The GOES 6database contains soft X-ray Ñuxes (about 2È10keV)sampled at 3s intervals.Because of range changes in the logarithmic readout,the noise properties of a GOES light curve are complicated.In the concrete,the light curve with full time resolution shows steplike proÐles.To minimize this problem we have arbitrarily smoothed the data into 10minute bins,a total of 133,622samples in the 32month observation period.920FLUCTUATIONS IN SOLAR SOFT X-RAY EMISSION 921F IG .1a F IG .1bF IG .1.ÈAll of the soft X-ray light curves from the whole Sun obtained with GOES 6.The vertical axes are (a )on the linear scale and (b )on the logarithmic scale.3.POWER SPECTRUM OF SOLAR SOFT X -RAYVARIATIONS3.1.PSDs of Entire L ight CurvesWe calculate the PSD in the following way.At Ðrst,we transform the time of each data point,(j \1ÈJ ),into thet jcorresponding phase,for a given frequency,(i \1È/i ,j,f i N ).Next,we plot the normalized X-ray Ñux,(j \1ÈJ ),L (t j )against and Ðt these J data points bya straightx \sin (/i ,j )line:We also plot against y \cos L \a i x ]b i .L (t j )(/i ,j )and Ðt thedata again by a straight line:L \c i y ]d i .Finally,we sum the two squares of these declines,ai 2]c i 2,which corresponds to the PSD (except for a proportionalityconstant)at a frequency f i .The PSD of the entire time series is depicted in Figure 2.Here power spectra are averaged in frequency over equal intervals on the logarithmic scale;that is,we averaged over the intervals from to (i \1to N [1),where f i f i`1f i`1\fi (since 100.05^1.12).The peaks at lowfre-]100.05\1.12f i quencies reÑect structure in solar Ñare occurrence on theF IG .2.ÈPower spectral density of the whole time series of solar soft X-rays depicted in Power density is binned in the frequency direc-Fig.1.tion over the intervals from to where f i f i`1,f i`1\f i ]100.05\1.12f i.long timescale.At high frequencies,larger than D 10~5Hz,we can see a steepening of the spectrum to a 1/f -like decline.At Ðrst,we focus on the noise features at high frequencies.In order to increase the signal-to-noise ratio,we Ðrst calcu-lated PSDs for each of 16subsets with 2month durations (see for the deÐnition of subsets).We then averaged Table 1each PSD with frequency bins from to where f i f i`1,f i`1\fi]100.05,and Ðnally averaged the 16PSDs.shows Figure 3the averaged PSDs obtained in this way.Here the error bars show p /n 1@2,where p is the standard deviation of 16power spectra of each data subset and n is the number of data subsets,i.e.,n \16.We Ðnd that the solar PSDs appear to consist of two and possibly three distinct spectral domains.Break frequencies separating the three distinct parts with di†erent slopes are found at around f ^10~4.7Hz and f ^10~3.8Hz,respectively.These Ðxed frequencies were then used as limits of each domain for Ðts to power-law functions proportional to f ~b ,with b being a Ðtting parameter.The results of Ðtting are summarized in Table 2.Errors represent deviations of each PSD from the best-Ðt curve.It is interesting that these power-law indices are similar to those of a representative black hole candidate,Cyg X-1(cf.et al.see inset in and see Negoro 1995;Fig.3Table 2).What physical processes do these frequencies imply?Generally,if a single-Ñare light curve can be well represent-TABLE 1C ONTENTS OF S UBSETD ATASubset Start Time End Time Number (108s)(108s)Year and Month 1...... 3.9977280 4.05042181991Sep,Oct 2...... 4.0504320 4.10312741991Nov,Dec 3...... 4.1031360 5.15496801992Jan,Feb 4...... 4.1549760 4.20767101992Mar,Apr 5...... 4.2076800 4.26037441992May,Jun 6...... 4.2603840 4.31394181992Jul,Aug 7...... 4.3139520 4.36664701992Sep,Oct 8...... 4.3666560 4.41934981992Nov,Dec 9...... 4.4193600 4.47032901993Jan,Feb 10...... 4.4703360 4.52302981993Mar,Apr 11...... 4.5230400 4.57573701993May,Jun 12...... 4.5757440 4.62930501993Jul,Aug 13...... 4.6293120 4.68200901993Sep,Oct 14...... 4.6820160 4.73471391993Nov,Dec 15...... 4.7347200 4.78568701994Jan,Feb 16......4.78569604.83838981994Mar,Apr922UENO ET AL.Vol.484F IG.3.ÈPower spectral density for the32month data,which are averaged over time and frequency.The power spectrum of Cyg X-1obtained byNegorois also shown in the inset.(1992)ed by an exponential function of time[P exp([t/q),qbeing a constant],its power spectrum has aÑat-top proÐlewith a steep decline(P f~2)at high frequencies(LorentzianproÐle):S q (f)P(2nq f)21](2nq f)2.(1)In this case,the break frequency corresponds to f\(2nq)~1.To see the range of typical decay time q,we tried toÐt eachÑare light curve with an exponential func-tion for10bigÑares and20smallÑares present in the GOES data.Two such examples are displayed in One hasFigure4.TABLE2P OWER-L AW I NDEX OF T OTAL P OWER S PECTRUMFrequency RangeObject(Hz)Power-Law Index b Sun...........\10~4.70.45^0.0810~4.7to10~3.80.95^0.03[10~3.8 1.50^0.02 Cyg X-1......\10~1D0.010~1to100D0.9[100D1.5the longest decay time with s,whereas anotherqlongD8300has the shortest,s,among theÐtted data.Note,qshortD550however,that anyÑuctuations with even shorter duration (q\550s)have been suppressed byÐnite time resolution of our rebinning of the data(10minutes).The corresponding break frequencies are andflong^10~4.7fshort^10~3.5, respectively.These values agree well with the two break frequencies seen in The departure from the Lor-Figure3.entzian1/f2fallo†to high frequencies above10~4.7Hz could therefore be plausibly explained by a broad distribu-tion ofÑare decay times This means that we(Drake1971).may be able to reproduce by superposingFigure3Sq(f) with550\q\8300.In order to conÐrm this prediction,we performed PSD analyses of simulatedÑare light curves for the following two cases.In theÐrst case,we adopted20,000steps for the total observed time,and5and70steps for the shortest and longest durations ofÑares,respectively.In the second case, we again adopted20,000steps for the total time,but2and 20steps for the shortest and longest ones,respectively.In both simulations,the peak intensity distribution ofÑares was set to be N P P~1.8,which is consistent with previous observational studies.Time intervals between neighboring Ñares were simply assumed to be random,and the meanNo.2,1997FLUCTUATIONS IN SOLAR SOFT X-RAY EMISSION 923F IG .4.ÈSoft X-ray time proÐles of the solar Ñares (white squares )and the best-Ðt curve (solid line )for (a )the longest duration Ñare (1992May 3;22hr 48minutes)and (b )the shortest duration Ñare (1992May 28;13hr 26minutes)among the 32month data.interval equals 10and 9.1steps in the two cases,respec-tively.Moreover,Ñare durations were also taken to be random between the shortest and longest durations.We depict these two simulated light curves in and Figure 5smoothed power spectral densities in Apparently,Figure 6.the simulated light curves and PSDs are very similar to those observed.Quantitatively,we can predict that f short\10~1.50Hz and Hz in the Ðrst case,andf long\10~2.64Hz and Hz in the second f short \10~1.10f long \10~2.10case,by estimation from theshortest and the longest dura-tions,respectively.In fact,these values are found in simu-lated PSDs with good agreement.We thus (Fig.6),conÐrmed our interpretation of the shape of PSDs to be correct.The values of the upper break frequency,near 10~3.8Hz,are too close to the Nyquist frequency of our binning to beF IG .5.ÈSimulated light curves of Ñares.(a )Durations of Ñares are adopted as 5and 70steps,respectively,for the shortest and the longest ones.(b )Durations are set as 2and 20steps,respectively,for the shortest and the longest ones.These durations are taken to be at random between the shortest and longest durations.Time intervals between neighboring Ñares are also assumed to be random.On the other hand,the peak inten-sity distribution of Ñares was set to be N P P ~1.8.signiÐcant in this study.More quantitative discussion will be given in our next paper.Finally,we also examine periodicities in the occurrence of Ñares.In the PSDs we could Ðnd the apparent periodicities of 2.6,17.7,18.4,24.8,26.4,30.5,46.3,51.8,61.3,105,and 123days.&Sturrock also reported the period-Bai (1991)icities 25.5,51.0,76.5,102.0,127.5,and 153.0days before solar cycle 21.We have conÐrmed the periodicities of D 26,51,102,and 125days,but none with 76.5and 154days.Instead,we found the strong periodicity of 61.3days.These results are,however,very preliminary,and we need more detailed analysis to conÐrm our Ðndings in a future work.3.2.L ong -Term Changes of PSDsIn the previous subsection,we were concerned with the PSDs for the entire light curve.It is still open to question whether the power-law indices (or the break frequencies)of the PSDs remain the same all the time regardless of the di†erent magnitudes of Ñare activity.We thus next investi-gate the individual PSDs for each subset,Ðnding a variety of PSDs with di†erent shapes and magnitudes (see Table 3and Fig.5).To summarize,most PSDs show the three distinct com-ponents.If we denote the power-law indices of the com-ponents from lower to higher frequencies as and b 1,b 2,b 3,we usually Ðnd that is,the PSDis composed b 1\b 2\b 3;TABLE 3T IME V ARIATION OF P OWER -L AW I NDEXSubsetlog (f break )log (f break )Number b0b1(Hz)b2(Hz)b31.........0.031[4.550.66[3.70 1.52.........0.020[4.150.61[3.55 1.23.........0.17[4.600.92[3.80 1.34.........0.20[4.30 1.2[3.70 2.25.........0.11[4.65 1.1[3.90 3.06.........0.48[4.100.98[3.30 1.37.........0.46[4.55 1.8[3.750.908.........0.23[4.90 1.1[3.70 1.79.........0.036[4.500.73[3.85 1.410.........0.097[4.65 1.0[3.95 1.711.........0.18[4.300.87[3.60 2.112.........0.49[4.600.76[3.40 1.913...... 2.1[0.13[4.550.35[3.70 1.314.........0.38[4.15 1.1[3.45 1.715.........0.19[4.600.82[3.85 1.716......... 1.4[5.200.49[3.851.3924UENO ET AL.Vol.484F IG.6.ÈPower spectral density diagrams of simulated light curves ofÑares(a)PSD for the light curve with longer durations.(b)PSD for(Fig.5).the light curve with shorter durations.The di†erences of break frequenciescan be found.of aÑat part with a moderate decline part withb1D0,and a steep decline part with to f~2b2D f~1,b3D f~1.5from low to high frequencies,although these indices changefrom time to time There are not a few PSDs(Table3).having a1/f-like decline.Note again that the presence of thesteep decline part may be a†ected by theÐnite timeresolution.There are some exceptions:In the seventh subset,therelation among the power-law indices is Inb1\b3\b2.the16th subset,weÐnd In the13th subset,b2\b1^b3.the PSD appears to have four components.A steep declinealso appears at low frequencies(b1\b2\b3\b).On the other hand,the break frequencies did not changegreatly during the32months,although the meanÑuctua-F IG.7.ÈTime variations in the statistical properties of solarÑaresduring the32month data.(a)Variation of the power-law index at mediumfrequency.(b)Variation of the two break frequencies.The lower line(withlower frequencies)may show the longest durationÑare and the upper line(with higher frequencies)may show the shortest durationÑares.(c)Varia-tion of the solar activity.Values on the vertical axis show the soft X-rayÑux averaged over2months.tion amplitudes decreased appreciably.The variations inthe shapes of power spectra and the break frequencies arerather independent of the solar activity.In weFigure7depict the time variations of power-law indices,break fre-quencies,andÑare activity.The independence of the spec-tral parameters with time shows that the pattern ofÑareoccurrence does not depend on the level of activity.Thismay suggest the existence of a universal mechanism for trig-geringÑares in the solar corona.4.SUMMARY AND DISCUSSION4.1.Summary of Solar X-Ray FluctuationSoft X-rayÑuxes from the SunÑuctuate because large orsmallÑare events occur occasionally.By constructingpower spectra,we have found the following statistical fea-tures ofÑare occurrence.A1/f-likeÑuctuation appears at medium-frequencyregimes(f\10~4.7to10~3.8Hz)in the PSD.The breakfrequencies,which seem to correspond to inverses of themaximum and minimum duration ofÑares,are independentof the solar activity and do not change much with time.This1/f-like feature is quite reminiscent of X-ray variability fromother astronomical objects,such as Cyg X-1(black holecandidate)and the nuclei of active galaxies.We have alsofound that although the normalization of peak intensitieschanges,the overallÑuctuation properties(such as theNo.2,1997FLUCTUATIONS IN SOLAR SOFT X-RAY EMISSION925shape of the PSD)are more or less insensitive to the solar activity.From these features,we may conclude that the mecha-nism responsible for individual solarÑares is insensitive to the level of solar activity.Maybe the di†erent magnitudes of solar activity can be explained simply by the variation of the strength of helicity added to magneticÑux tubes in the solar convection zone.To connect this power-law distribution of solarÑares to the physics of individualÑares,&Hamilton andLu(1991) et al.propose that the solar coronal magneticÐeld Lu(1993)is in a self-organized critical state(SOC state),a critical state realized in a nonequilibrium open system Tang,(Bak,&Wiesenfeld The most outstanding feature of their 1988).model is that the system,following cellular automaton rules,spontaneously evolves to and steadily stays at an SOC state.In this state energy stored in magneticÐeld would be barely below its critical value,over which an energy-release event begins and leads to aÑare.The occurrence rate then shows a power-law dependence on size and lifetime of the event.We can expect1/f-likeÑuctuations on the energy dissipation in such a case,although the energy is injected randomly in time and space.If this is the case,solarÑares are like avalanches consisting of many small energy-release events,such as magnetic reconnec-tions.To summarize what we have learned through the present studies,the SOC model can naturally explain some of the observed statistical features,since the results are basically insensitive to the parameters of the physical system.If this model is usable,allÑarelike events,including nanoÑares and microÑares,can be understood in term of a common physical process.Although the present paper only presents the results of the PSD analyses,complementary information may be derived from the distributions of peak intensities of individ-ualÑares and intervals of adjacentÑares.It might be noted that the previous studies have shown that the distributions of peak intensities ofÑares can be well represented by power laws with indices c\1.8È1.9(cf.Yamashita,&Kakinuma,Enome et al.Dennis 1969;Drake1971;Datlowe1974;These distributions may contain 1985,1988;Shimizu1995).important physical information as follows:If this index is less than2.0,some additional heating mechanisms,such as nanoÑares are required to account for solar (Parker1988),coronal heating while otherwise coronal(Hudson1991),heating can be explained solely by various sizes ofÑares. Moreover,the distributions of intervals between adjacent peaks can be used to check whether the occurrence ofÑares is random or whether accumulation e†ects may exist. Detailed analyses using the GOES data will be presented in our next paper.4.2.Relationship to X-Ray Fluctuations fromBlack Hole ObjectsIt is also interesting to discuss the relationship between solar coronae and accretion disk coronae(cf.Galeev, Rosner,&Vaiana As mentioned1979;Takahara1979).already,1/f-likeÑuctuations are rather generally observed in X-ray radiation from stellar black hole candidates like Cyg X-1,neutron star X-ray binaries,and active galactic nuclei(see reviews by and&Makishima1988Pounds McHardy for more recent analysis see et al.1988;Negoroand references therein).The X-rayÈemitting regions of 1995these objects seem to be optically thin accretion disks domi-nated by magnetic pressure,where magneticÑares similar to solarÑares occasionally occur,giving rise to substantial Ñuctuations in X-ray emission(see Tajima,&Mat-Shibata,sumoto Kusunose,&Matsumoto 1990;Mineshige,1995, and references therein).It is thus of great importance to study the statistics of solar X-rayÑuctuations for consider-ing possible physical links between solar coronae and hot accretion disks.In particular,from PSDs the statistical features of solar and nonsolar X-rayÑuctuations are rather similar to each other.However,the absolute X-rayÑuxes from accretion disks are larger by many orders of magnitude than those from the solar corona,and the shapes of theÑare light curves are also rather di†erent from those of the X-ray shots in nonsolar sources.In the latter,mass accretion is another important factor in determining the shape of X-ray shot light curves.et al.in fact,have recently Manmoto(1996),succeeded in reproducing X-ray shot proÐles by time-dependent simulations of the accretion disk.When we consider these facts,it seems plausible that the mechanisms of creating solarÑares and X-rayÈemitting shots in accretion disks could be related(such as in an SOC model including magnetic reconnection;see,e.g.,Takeuchi, Mineshige,&Negoro In the solar interior,convec-1995).tion gives energy to the magneticÐeld.In accretion disks,in contrast,accreting gasÐrst acquires energy from gravita-tional potential well.The disk gas can then give energy to the magneticÐeld through ampliÐcation by the shearing motion of the gas and MHD instabilities associated with rotating disks.When the magnetic energy becomes compa-rable to internal energy of the disk gas,theÐeld will even-tually dissipate its energy via reconnection.The di†erence in shot characteristics,such as intensities,shot proÐles,and interval distributions,between the solar corona and an acc-retion disk source may arise from the di†erent physics involved.For accretion disks we mainly observe the accre-tion process of X-rayÈemitting blobs(powered by gravita-tional potential energy release due probably to magnetic reconnection),and such a source of energy is not available in the solar case.Furthermore,the solar soft X-ray radi-ation we analyze here does not represent the fundamental (direct)energy release in a solarÑare;it might be more appropriate to analyze the shot-noise properties of solar hard X-ray emission as a proxy for the fundamental energy-release mechanism.Finally,we need to o†er an explanation of the dimension-al constant we appear to have discovered,namely,the lower break frequency10~4.7Hz.It exceeds the Alfve n transit time of any basicÑare structure,but can be related to typical radiative cooling times forÑares limited in size scale to about1supergranule diameter(about50,000km).It is possible that this rough agreement suggests that the charac-teristic time is basically hydrodynamic in origin and does not reÑect the energy-release timescale itself.We are grateful to J.Kubota and T.Kato for useful comments and advice for analysis.This work was sup-ported in part by grants-in-aid from the Ministry of Educa-tion,Science,Sports,and Culture of Japan,06233101, 08640329(S.M.),and by NASA,NAS8-37334(H.S.H).926UENO ET AL.REFERENCEST.,&Sturrock,P.A.1991,Nature,350,Bai,1411993,ApJ,409,ÈÈÈ.476P.,Tang,C.,&Wiesenfeld,K.1988,Phys.Rev.A,38,Bak,364D.W.,Elcan,M.J.,&Hudson,H.S.1974,Sol.Phys.,39, Datlowe,155B.R.1985,Sol.Phys.,100,Dennis,465B.R.1988,Sol.Phys.,118,Dennis,49J.F.1971,Sol.Phys.,16,Drake,152A.A.,Rosner,R.,&Vaiana,G.S.1979,ApJ,229,Galeev,318H.S.1991,Sol.Phys.,133,Hudson,357T.,Yamashita,T.,&Enome,S.1969,Proc.Res.Inst.Atmo-Kakinuma,spherics Nagoya Univ.,16,127J.N.,&Cliver,E.W.1991,ApJ,370,Kile,442R.P.,Schwartz,R.A.,Kane,S.R.,Pelling,R.M.,&Hurley,K.C. Lin,1984,ApJ,283,421E.T.,&Hamilton,R.J.1991,ApJ,380,Lu,L89E.T.,Hamilton,R.J.,McTiernan,J.M.,&Bromund,K.R.1993,ApJ, Lu,412,841K.1988,in Physics of Neutron Stars and Black Holes,ed. Makishima,Y.Tanaka(Tokyo:Universal Academy),175T.,Takeuchi,M.,Mineshige,S.,Matsumoto,R.,&Negoro,H. Manmoto,1996,ApJ,464,L135S.,Kusunose,K.,&Matsumoto,R.1995,ApJ,445, Mineshige,l43H.1992,MasterÏs thesis,OsakaNegoro,Univ.H.,Kitamoto,S.,Takeuchi,M.,&Mineshige,S.1995,ApJ,452, Negoro,L49E.N.1988,ApJ,330,Parker,474K.A.,&McHardy,I.M.1988,in Physics of Neutron Stars and Pounds,Black Holes,ed.Y.Tanaka(Tokyo:Universal Academy),285E.,Share,G.H.,Forrest,D.J.,Kanbach,G.,Reppin,C.,&Chupp, Rieger,E.L.1984,Nature,312,623K.,Tajima,T.,&Matsumoto,R.1990,ApJ,350,Shibata,295T.1995,PASJ,47,Shimizu,251F.1979,Prog.Theor.Phys.,62,Takahara,629M.,Mineshige,S.,&Negoro,H.1995,PASJ,47,Takeuchi,617。
[指南]专业词汇(天体物理)
![[指南]专业词汇(天体物理)](https://img.taocdn.com/s3/m/28d39093bdeb19e8b8f67c1cfad6195f312be84a.png)
专业词汇(天体物理)1、Galactic background γ-ray radiation 银河γ背景辐射2、active galactic nuclei 活动星系核3、radio survey 射电巡天4、ultraviolet fluxes 紫外流量5、Roche-lobe overflow 洛希瓣超流6、abnormal redshift 反常红移7、absorption cross section 吸收截面8、acceleration mechanism 加速机制9、general electric synchrotron 广义电同步加速10、screening effect 屏蔽效应11、visible spectrum 可见光谱12、celestial matter 宇宙物质13、broad emission-line 宽发射线14、narrow emission-line 窄发射线15、planetary nebulae 行星状星云16、isotropic antenna 各向同性天线17、Seyfert galaxy 赛弗特星系18、ultraviolet continuum 紫外连续谱19、bolometric luminosity 光度20、variable time scale 可变时标21、continuum emission 连续辐射22、power law 幂率23、non-thermal 非热24、blazar spectra blazar光谱25、polarization 偏振26、doppler broadened 多普勒展宽27、碰撞激发 collisional excitation28、photoionization 光致电离29、horizon of the universe 宇宙视界30、Coulomb collision 库仑碰撞31、Coulomb coupling 库仑耦合32、Born approximation 玻恩近似33、absolute magnitude 绝对星等34、absolute parallax 绝对视差35、overmassive object 超大质量天体36、gravitational wave 引力波37、gravitational-wave astronomy 引力波天文学38、error distribution 误差分布39、chromatic aberration 色差40、characteristic function 特征函数41、CGRO 康普顿γ射线天文台(Compton Gamma-Ray Observatory)42、central lobe 中心瓣43、Rayleigh criterion 瑞利判据44、Rayleigh limit 瑞利极限45、quasi-stellar object 类星体(QSO)46、quiescent radiation 宁静辐射47、quiescent spectrum 宁静光谱48、radial-velocity survey 视向速度巡天49、absorption frequency 吸收频率50、background radiation 背景辐射51、light illumination 光照度52、radiant power 辐射功率53、anisotropic medium 各向异性介质54、plasma jet instability 各向异性等离子体喷流不稳定性55、cosmic rays 宇宙线56、Zeeman effect 塞曼效应57、annihilation radiation 湮灭辐射58、radiometric magnitude 辐射星等59、proton flux 质子流量60、Abell cluster 阿贝尔星系团61、autocorrelation coefficient 自相关系数62、dark matter 暗物质63、Maxwellion distribution 麦克斯韦分布64、black hole binary 黑洞双星65、blanketing factor 覆盖因子66、radio galaxy 射电星系67、Cauchy's dispersion formula 柯西色散公式68、intergalactic medium 星系际介质69、shock wave 激波70、background Compton scattering 背景康普顿散射71、dark energy 暗能量72、multiple galaxy 多重星系73、current density 流密度74、thermodynamic equilibrium 热动平衡75、thermal excitation 热激发76、synchrotron radiation 同步加速辐射77、synchro-cyclotron radiation 同步-回旋加速辐射78、center of curvature 曲率中心79、super-relativistic effect 极端相对论性效应80、active binary 活动双星81、compact binary 致密双星82、compact galaxy nucleus 致密星系核83、supernova explosion 超新星爆发84、supernova ejecta 超新星喷射物85、deflection angle 偏转角86、cosmic noise absorption 宇宙噪声吸收87、string theory 宇宙弦理论88、stripped plasma 全电离等离子体89、close binary star 密近双星90、stellar-mass black hole 恒星质量黑洞91、ultraviolet radiation 紫外辐射92、aberration 光行差93、spontaneous emission 自发发射94、curvature radiation 曲率辐射95、spontaneous transition 自发跃迁96、peculiar spectrum 特殊光谱97、particle horizon 粒子视界98、stimulated radiation 受激辐射99、stimulated emission 受激发射100、circular polarization 圆偏振101、damping radiation 阻尼辐射102、spherical harmonics 球谐函数103、parity nonconservation 宇称不守恒104、drifting zebras 漂移带105、parallax second 秒差距(parsec) (pc)106、geometric aberration 几何象差107、electric multipole radiation 电多极辐射108、Voigt effect 佛克特效应109、monotonic model 单调宇宙模型110、isochronous correspondence 等时对应111、gas nebula 气体星云112、cepheid parallax 造父视差113、diffusion equation 扩散方程114、advancing shock front 前进激波前115、advection dominated accretion 径移吸积流116、spherical potential 球对称势117、luminous emittance 发光度118、cosmic gusher 宇宙喷射源119、microwave background 微波背景120、herpolhode 空间极迹121、heat of desorption 退吸热122、termination shock 终端激波123、very hard binary 甚硬双星124、weak turbulence theory 弱湍流理论125、nova-like X-ray source 类新星 X 射线源126、longitudinal chromatic aberration 纵向色差127、statistical equilibrium 统计平衡128、Compton cross-section 康普顿截面129、irregular nebula 不规则星云130、stellar astrophysics 恒星天体物理131、background radiation intensity 背景辐射强度132、optical binary 光学双星133、astronomical coordinate system 天文坐标系统134、orbital inclination 轨道倾角135、critical mass 临界质量136、Kerr-Newman black hole 克尔-纽曼黑洞137、Kerr-Newman metric 克尔-纽曼度规138、chromatism 色差139、reflection at critical angle 临界角反射140、source brightness distribution 源亮度分布141、Laplace's nebular hypothesis 拉普拉斯星云假说142、photoionized plasma 光电离等离子体143、photoluminescence 光致发光144、relaxation time 弛豫时间145、cross-correlation function 互相关函数146、relaxed cluster 驰豫星团147、disk-like structure 盘状结构148、fast-spinning black hole 快自旋黑洞149、Fermi Gamma-ray Space Telescope 费米γ射线空间望远镜150、time reversal 时间反演。
x射线荧光光谱英文缩写

x射线荧光光谱英文缩写X-ray Fluorescence (XRF) Spectrometry: An Introduction and Applications.X-ray Fluorescence (XRF) spectrometry is a non-destructive analytical technique that utilizes the interaction of high-energy X-rays with matter to determine the elemental composition of materials. The technique is based on the fluorescence effect, where X-rays emitted from a source are absorbed by the sample, causing atoms within the sample to emit characteristic X-rays known as secondary or fluorescent X-rays. The emitted X-rays are then detected and measured to provide qualitative and quantitative information about the elements present in the sample.The fundamental principle of XRF spectrometry lies in the absorption and emission of X-rays by matter. When high-energy X-rays interact with a sample, they are absorbed by the atoms within the sample. This absorption process causes the inner-shell electrons of the atoms to be ejected,leaving vacancies in the electron shells. The outer-shell electrons then move down to fill these vacancies, releasing energy in the form of characteristic X-rays. The emitted X-rays have specific wavelengths or energies that are characteristic of the elements present in the sample.XRF spectrometers are typically divided into two categories based on the source of X-rays used: wavelength-dispersive XRF (WDXRF) and energy-dispersive XRF (EDXRF). In WDXRF spectrometers, a polychromatic X-ray source is used to excite the sample, and the emitted X-rays are then separated by a wavelength-dispersive monochromator into individual wavelengths. These wavelengths are then detected and measured using a suitable detector. In EDXRF spectrometers, a monochromatic X-ray source is used, and the emitted X-rays are detected and measured using an energy-dispersive detector that can resolve the X-rays based on their energy.XRF spectrometry has a wide range of applications in various industries and fields. In the metallurgical industry, XRF is commonly used for the determination ofelemental composition in metals, alloys, and ores. It is also used in the cement and glass industries to monitor the composition of raw materials and finished products. In environmental science, XRF spectrometry is employed for the analysis of soil, sediment, and water samples to assess pollution and contamination levels.In addition, XRF spectrometry plays a crucial role in the arts and antiquities industry. It can be used to authenticate artworks, such as paintings and sculptures, by analyzing the elemental composition of the pigments and materials used in their creation. Similarly, XRF can be used to identify and authenticate historical artifacts and jewelry by determining their elemental composition.The advantages of XRF spectrometry include its non-destructive nature, speed, and accuracy. Since the technique does not require any sample preparation or chemical reactions, it can be used on a wide variety of materials, including solids, liquids, and powders. Additionally, XRF spectrometers are relatively easy to operate and provide rapid results, making them suitable forboth laboratory and on-site applications.However, there are also some limitations to XRF spectrometry. One of the main limitations is that it is primarily suitable for the analysis of elements with atomic numbers greater than 11 (sodium). Lighter elements, such as hydrogen, helium, and lithium, cannot be detected using XRF spectrometry. Additionally, the technique is sensitive to surface conditions and can be affected by factors such as sample roughness, coating, and contamination.In conclusion, X-ray Fluorescence (XRF) spectrometry is a powerful analytical technique that provides rapid and accurate elemental composition analysis of materials. Its non-destructive nature, ease of operation, and wide range of applications make it a valuable tool in various industries and fields, including metallurgy, environmental science, arts and antiquities, and more. While there are some limitations to the technique, its overall advantages make it a preferred choice for elemental analysis in many scenarios.。
天文词汇中英文对照(推荐文档)
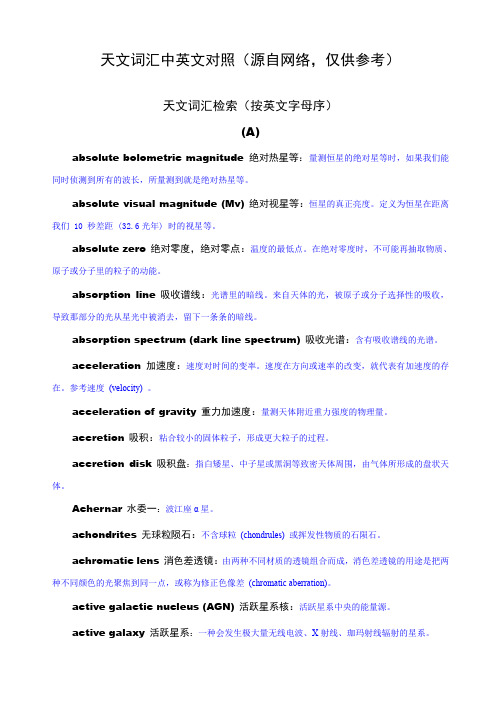
天文词汇中英文对照(源自网络,仅供参考)天文词汇检索(按英文字母序)(A)absolute bolometric magnitude绝对热星等:量测恒星的绝对星等时,如果我们能同时侦测到所有的波长,所量测到就是绝对热星等。
absolute visual magnitude (Mv)绝对视星等:恒星的真正亮度。
定义为恒星在距离我们 10 秒差距 (32.6光年) 时的视星等。
absolute zero绝对零度,绝对零点:温度的最低点。
在绝对零度时,不可能再抽取物质、原子或分子里的粒子的动能。
absorption line吸收谱线:光谱里的暗线。
来自天体的光,被原子或分子选择性的吸收,导致那部分的光从星光中被消去,留下一条条的暗线。
absorption spectrum (dark line spectrum)吸收光谱:含有吸收谱线的光谱。
acceleration 加速度:速度对时间的变率。
速度在方向或速率的改变,就代表有加速度的存在。
参考速度(velocity) 。
acceleration of gravity重力加速度:量测天体附近重力强度的物理量。
accretion吸积:粘合较小的固体粒子,形成更大粒子的过程。
accretion disk吸积盘:指白矮星、中子星或黑洞等致密天体周围,由气体所形成的盘状天体。
Achernar水委一:波江座α星。
achondrites无球粒陨石:不含球粒(chondrules) 或挥发性物质的石陨石。
achromatic lens消色差透镜:由两种不同材质的透镜组合而成,消色差透镜的用途是把两种不同颜色的光聚焦到同一点,或称为修正色像差(chromatic aberration)。
active galactic nucleus (AGN)活跃星系核:活跃星系中央的能量源。
active galaxy活跃星系:一种会发生极大量无线电波、X射线、珈玛射线辐射的星系。
active optics活动光学:由计算机控制,来改变光学组件的位置和形状,以补偿大气扰动所产生的散焦效应之光学系统。
窄线赛弗特I型星系中可能具有吸积盘热辐射起源的软X射线超

窄线赛弗特I型星系中可能具有吸积盘热辐射起源的软X射线超李晔【摘要】理论上,低质量高吸积率活动星系核的标准吸积盘黑体热辐射有可能形成软X射线波段的超出.然而,活动星系核的软X射线超温度为0.1 keV左右,显著高于标准吸积盘理论预言的最高有效温度.只有Yuan等人在2010年报道的RXJ1633+4718是一个例外,其软超温度为32.5±-8.06.0eV,显著小于0.1 keV,并与吸积盘理论预言的温度相符;此外,其光度也符合吸积盘的理论预期.因此该软X射线超很可能起源于吸积盘的热辐射.这一类源对于吸积盘理论和软X射线超的研究都有重要意义.文中使用ROSAT PSPC数据,在所有已知的窄线赛弗特I型星系(Narrow Line Seyfert I galaxies,NLSIs)中寻找类似于RXJ1633+4718的源,即软X射线超可能起源于吸积盘热辐射的源.分析了150个源的245条光谱,其中58个源的90条光谱具有显著的软X射线超.样本中软X射线超温度分布的平均值-Tsoft为0.10 keV,标准偏差为0.03 keV.除了RX J1633+4718外,只有3个源的软X射线超温度小于60 eV,而且温度接近吸积盘最高温度.这个结果表明,类似于RX J1633+4718的具有低温软X射线超的源很稀少.最后,在具有黑洞质量估计的26个源中,软超温度与黑洞质量MBH、爱丁顿比L/LEdd及预期吸积盘最高温度Tmax都没有明显的关联性,与前人的结果一致.%It is theoretically expected that the thermal radiation from accretion disks of Active Galactic Nuclei (AGNs) with low black hole mass would contribute to the soft X-ray band and result in an excess emission. However, the temperatures of the observed soft X-ray excess of AGNs are typically around 0.1 keV, significantly higher than those predicted by the standard disk model. There is only oneexception found so far, R.X J1633+4718, which has a significantly low soft excess temperature of 32.5l+8.0-6.0 eV, consistent with the maximum temperature as well as the luminosity of the predicted disk emission(Yuan et al. 2010).rnIn this paper, we search for RX J1633 like AGNs, whose soft X-ray excess temperature is close to the maximum temperature of accretion disk, in all known Narrow Line Seyfert I galaxies (NLSIs) observed with ROSAT PSPC in the archive. We analyzed the PSPC spectra of 150 NLSIs (245 observations) in total, 58 (90 observations) of which show significantly soft X-ray excess emission. In addition to RX J1633+4718, only 3 objects (4 observations) show soft X-ray excess temperatures less than 60 eV and close to the theoretical prediction of the disk emission. They form a sample of candidates for future follow-up studies. Our result indicates that RX J1633+4718 like objects are very rare. The correlations between the temperature of the soft X-ray excess and black hole mass as well as the Eddington ratio are also investigated, and no correlation is found. This confirms the previous results, and implies that the soft X-ray excess of most AGNs should originate from other mechanisms rather than the thermal emission of the accretion disk.【期刊名称】《天文学进展》【年(卷),期】2013(031)001【总页数】14页(P110-123)【关键词】活动星系核;吸积盘X射线热辐射;软X射线超【作者】李晔【作者单位】中国科学院国家天文台/云南天文台,昆明650011;中国科学院大学,北京100049;中国科学院国家天文台,北京100012【正文语种】中文【中图分类】P157.6活动星系核中心存在超大质量黑洞,物质落入中心黑洞的过程中形成吸积流,并产生辐射[1-3]。
The Power of Jets New Clues from Radio Circular Polarization and X-rays
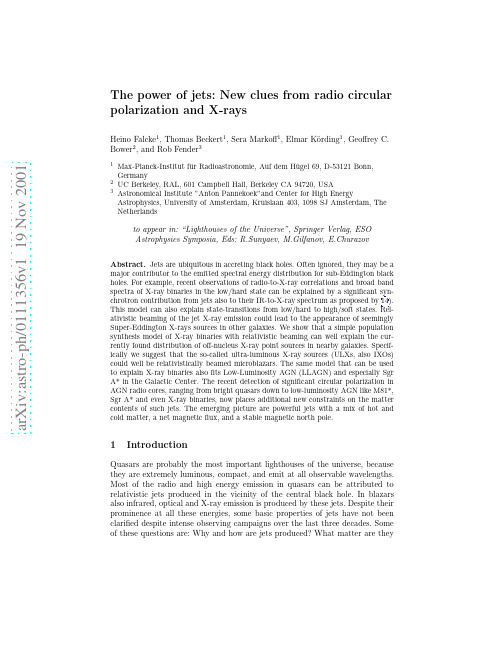
to appear in: “Lighthouses of the Universe”, Springer Verlag, ESO Astrophysics Symposia, Eds: R.Sunyaev, M.Gilfanov, E.Churazov
1 Max-Planck-Institut fu¨r Radioastronomie, Auf dem Hu¨gel 69, D-53121 Bonn, Germany
2 UC Berkeley, RAL, 601 Campbell Hall, Berkeley CA 94720, USA 3 Astronomical Institute ”Anton Pannekoek”and Center for High Energy
2
Falcke et al.
made of? How much energy is carried in the jet? Why are some jets more radioloud than others? What is the exact magnetic field configuration?
A characteristic feature of jets, also in X-ray binaries, is their flat-spectrum radio core which is best seen during phases of relative quiescence (e.g. as in GRS 1915+105, (5)). 10) found that the low/hard-state of the X-ray spectrum is correlated with the presence of a persistent flat-spectrum radio core. He also argued that this flat spectrum of the synchrotron emitting radio core extends up into the near-infrared and perhaps optical regime. This is, in fact, not surprising. The standard model for flat radio spectra (1; 11; 7) suggests that the emission arises from self-absorbed sections in a conical jet, where the smallest scales contribute at the highest frequencies (size ∝ ν−1). This is schematically shown in Fig. 1. The interesting point here is that the smallest scale in a system will be set by the size of the black hole. Hence stellar mass black holes be able to produce flat spectra up to a maximum frequency νssa,max that is much higher than the supermassive black holes in quasars – by a factor given by the ratio of the black hole masses which is of order 105−8. Therefore XRBs should be much more likely to exhibit direct synchrotron emission in NIR, UV and even X-rays than normal AGN.
类星体与活动星系核
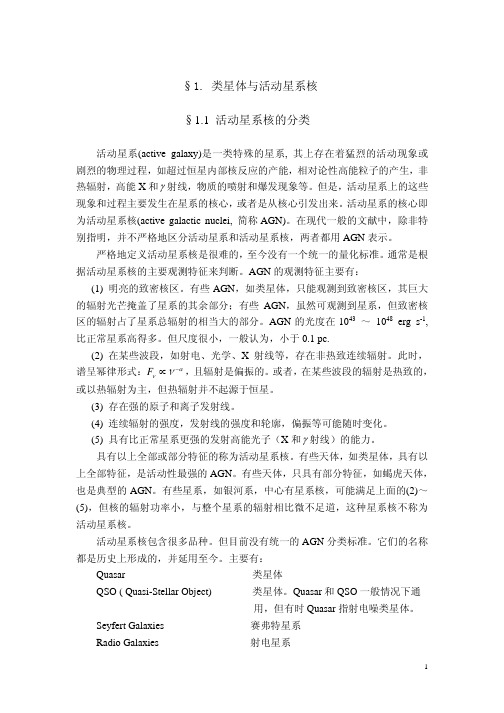
§1. 类星体与活动星系核§1.1 活动星系核的分类活动星系(active galaxy)是一类特殊的星系, 其上存在着猛烈的活动现象或剧烈的物理过程,如超过恒星内部核反应的产能,相对论性高能粒子的产生,非热辐射,高能X 和γ射线,物质的喷射和爆发现象等。
但是,活动星系上的这些现象和过程主要发生在星系的核心,或者是从核心引发出来。
活动星系的核心即为活动星系核(active galactic nuclei, 简称AGN)。
在现代一般的文献中,除非特别指明,并不严格地区分活动星系和活动星系核,两者都用AGN 表示。
严格地定义活动星系核是很难的,至今没有一个统一的量化标准。
通常是根据活动星系核的主要观测特征来判断。
AGN 的观测特征主要有:(1) 明亮的致密核区。
有些AGN ,如类星体,只能观测到致密核区,其巨大的辐射光芒掩盖了星系的其余部分;有些AGN ,虽然可观测到星系,但致密核区的辐射占了星系总辐射的相当大的部分。
AGN 的光度在1043 ~ 1048 erg s -1,比正常星系高得多。
但尺度很小,一般认为,小于0.1 pc.(2) 在某些波段,如射电、光学、X 射线等,存在非热致连续辐射。
此时,谱呈幂律形式:νF ∝αν−,且辐射是偏振的。
或者,在某些波段的辐射是热致的,或以热辐射为主,但热辐射并不起源于恒星。
(3) 存在强的原子和离子发射线。
(4) 连续辐射的强度,发射线的强度和轮廓,偏振等可能随时变化。
(5) 具有比正常星系更强的发射高能光子(X 和γ射线)的能力。
具有以上全部或部分特征的称为活动星系核。
有些天体,如类星体,具有以上全部特征,是活动性最强的AGN 。
有些天体,只具有部分特征,如蝎虎天体,也是典型的AGN 。
有些星系,如银河系,中心有星系核,可能满足上面的(2)~(5),但核的辐射功率小,与整个星系的辐射相比微不足道,这种星系核不称为活动星系核。
活动星系核包含很多品种。
- 1、下载文档前请自行甄别文档内容的完整性,平台不提供额外的编辑、内容补充、找答案等附加服务。
- 2、"仅部分预览"的文档,不可在线预览部分如存在完整性等问题,可反馈申请退款(可完整预览的文档不适用该条件!)。
- 3、如文档侵犯您的权益,请联系客服反馈,我们会尽快为您处理(人工客服工作时间:9:00-18:30)。
a r X i v :0801.3663v 1 [a s t r o -p h ] 23 J a n 2008Draft versionPreprint typeset using L A T E X style emulateapj v.10/09/06X-RAY EMISSION FROM ACTIVE GALACTIC NUCLEI WITH INTERMEDIATE MASS BLACK HOLESG.C.Dewangan 1,S.Mathur 2,R.E.Griffiths 1,&A.R.Rao 3Draft versionABSTRACTWe present a systematic X-ray study of eight active galactic nuclei (AGNs)with intermediate mass black holes (M BH ∼8−95×104M ⊙)based on 12XMM-Newton observations.The sample includes the two prototype AGNs in this class –NGC 4395and POX 52and six other AGNs discovered with the Sloan Digitized Sky Survey.These AGNs show some of the strongest X-ray variability with the normalized excess variances being the largest and the power density break time scales being the shortest observed among radio-quiet AGNs.The excess variance –luminosity correlation appears to depend on both the BH mass and the Eddington luminosity ratio.The break time scale –black hole mass relations for AGN with IMBHs are consistent with that observed for massive AGNs.We find that the FWHM of the H β/H αline is uncorrelated with the BH mass,but shows strong anticorrelation with the Eddington luminosity ratio.Four AGNs show clear evidence for soft X-ray excess emission (kT in ∼150−200eV).X-ray spectra of three other AGNs are consistent with the presence of the soft excess emission.NGC 4395with lowest L/L Edd lacks the soft excess emission.Evidently small black mass is not the primary driver of strong soft X-ray excess emission from AGNs.The X-ray spectral properties and optical-to-X-ray spectral energy distributions of these AGNs are similar to those of Seyfert 1galaxies.The observed X-ray/UV properties of AGNs with IMBHs are consistent with these AGNs being low mass extension of more massive AGNs;those with high Eddington luminosity ratio looking more like narrow-line Seyfert 1s while those with low L/L Edd looking more like broad-line Seyfert 1s.Subject headings:accretion,accretion disks —galaxies:active –galaxies:Seyfert —X-rays:galaxies1.INTRODUCTIONThe existence of astrophysical black holes in two mass ranges,stellar mass black holes with ∼10M ⊙in X-ray binaries and supermassive black holes (SMBHs)with masses in the range of ∼106−109M ⊙at the centers of active galaxies,is well supported by observations.Inter-mediate mass black holes (IMBHs),bridging the gap be-tween the stellar mass and supermassive black holes,re-main relatively unexplored.There was no observational evidence for such black holes for many years.Recent op-tical and X-ray observations have revived the possibility of existence of IMBHs.There are indirect evidence for IMBHs with masses of ∼104−106M ⊙at the center of some galaxies based on radiative signatures or dynamical measurements (Filippenko &Ho 2003;Barth et al.2004;Greene &Ho 2004,2007a).The AGNs with IMBHs form an important class for a number of reasons.First,the growth of SMBHs in Seyfert galaxies and quasars is still not understood.The nature of seed black hole (BH)is the major challenge to any cosmological BH growth model.Observations of IMBHs at the dynamical centers of nearby galaxies would provide significant constraints on the nature of seed black holes for cosmological growth models.The M BH −σ∗relation strongly suggests co-evolution of galaxies and BHs,but it is not known if this relation is established early in the evolution process.If this relation is established late in the galaxy evolution,1Department of Physics,Carnegie Mellon University,5000Forbes Avenue,Pittsburgh,PA 15213USA;email:gulabd@,griffith@2Astronomy Department,The Ohio State University,140West 18th Avenue,Columbus,OH 43210,USA 3Department of Astronomy and Astrophysics,Tata Institute of Fundamental Research,Mumbai 400005,Indiaas suggested by Di Matteo et al.(2003),then we should see deviations in the M BH −σ∗relation in a population of IMBHs that is not yet fully grown.In this growing phase,the accretion process and the disk-corona geome-try around the central IMBH could be markedly different than that around SMBHs in luminous AGNs.Therefore,it is crucial to study accretion onto the least massive cen-tral BHs in galaxies.AGNs with IMBHs also provide the missing link between the stellar mass and SMBHs and en-able us to test if the accretion physics is the same at all scales.They will also help to understand the accretion physics as it would be easier to isolate certain character-istics arising solely from an extreme physical parameter,in this case,low mass BH.AGNs with IMBHs may re-veal new accretion phenomena depending on the mode of accretion at low BH masses.It is also possible that they pass through an active phase of accretion and con-tribute to the observed background radiation at some level,particularly to the X-ray background.The merg-ers of intermediate mass black holes with masses 105M ⊙are expected to provide strong signals of gravitational waves for the Laser Interferometric Space Antenna (e.g.,Hughes 2002).The two prototypes examples of IMBH AGNs are the late-type spiral galaxy NGC 4395(Filippenko &Ho 2003)and the dwarf elliptical galaxy POX 52(Barth et al.2004).Greene &Ho (2004)discovered 19such AGNs using the Sloan Digital Sky Survey (SDSS).They have increased their sample by an order of magni-tude to 174using the fourth data release of the SDSS (Greene &Ho 2007a).NGC 4395has the emission properties of a type 1AGN,with broad optical and UV emission lines and a point-like hard X-ray source.Its X-ray emission is extremely rapidly variable (see2e.g.,Vaughan et al.2005).Filippenko&Ho(2003)ar-gued that the true mass of the black hole is likely to be∼104−105M⊙,while reverberation mapping of NGC4395has provided a BH mass of(3.6±1.1)×105M⊙(Peterson et al.2005).Another recently discovered AGN with an IMBH is POX52,a dwarf elliptical galaxy at z=0.021.It was discovered in an objective-prism search for emission line objects by Kunth et al.(1981)who also noted its star-like appearance in the Palomar Sky Survey images.Follow-up imaging and spectroscopic observa-tions by Kunth et al.(1987)revealed a dwarf galaxy with an AGN spectrum.The object was classified as a Seyfert 2galaxy by Kunth et al.(1981)based on theflux-ratios of narrow emission lines although a weak broad compo-nent of the Hβline was seen with a full width at half max-imum,FWHM∼840km s−1.The galaxy is indeed quite small with a diameter∼6.4kpc and its absolute magni-tude is only M V=−16.9.The luminosity of the nucleus is comparable to that of a mild Seyfert2nucleus but the ratio of nuclear to host-galaxy luminosity is1.1,a typical value for quasars(Kunth et al.1987).Barth et al.(2004) obtained a high quality optical spectra of POX52at the Keck that revealed an emission line spectrum very simi-lar to that of the dwarf Seyfert1galaxy NGC4395,with broad components to the permitted lines and reclassified POX52as a Seyfert1galaxy.This galaxy has a central velocity dispersion of36km s−1,which yields a mass of 1.4×105M⊙,again consistent with that derived from the Hβline width(Barth et al.2004).AGNs with IMBHs are rare compared to luminous AGNs.One explanation is that they are short-lived. Presumably,all AGNs grow fast in their early phase until their BH mass increases to106M⊙.During this fast-growth phase,the accretion rate may be close to Eddington or super-Eddington.The relative accretion rates of∼1for the SDSS AGNs with IMBH,derived by Greene&Ho(2004)from the optical data,favors the above scenario.However,all AGNs with IMBH are not accreting at high rates e.g.,NGC4395is under-lumnious for its BH mass with L/L Edd∼1.2×10−3 (Peterson et al.2005)or at most0.2(Vaughan et al. 2005)In fact,very little is known about the accretion process onto IMBHs in AGNs.The only AGN that has been well studied in X-rays is NGC4395.Iwasawa et al. (2000)presented the ASCA spectrum that showed a power-law continuum of photon indexΓ=1.7±0.3with a Fe K line marginally detected at∼6.4keV.The soft-X-ray emission below3keV is strongly attenuated by absorption.The X-ray spectrum in this absorption band showed a dramatic change in response to the variation in continuum luminosity.Iwasawa et al.(2000)concluded that the nuclear source of NGC4395is consistent with a scaled-down version of higher-luminosity Seyfert nu-clei.Vaughan et al.(2005)have studied the exceptional X-ray variability of NGC4395.The variations observed are among the most violent seen in an AGN to date, with the fractional rms amplitude exceeding100%in the softest band.Greene&Ho(2007b)have performed snapshot(5ks exposure)Chandra ACIS X-ray observations of IMBH AGNs discovered with SDSS.They detected8of the 10AGNs with IMBH with a significance≥3σ.They measured the0.5−2keV photon indices in the range of 1−2.7,consistent with that for luminous AGNs,imply-ing that the BH mass is not the fundamental driver for the soft X-ray spectral shape.However,this result is not robust as some of the measurements are based on hard-ness ratios.Moreover,with5ks Chandra exposures,it was not possible to make detailed spectral and temporal analysis of any of the AGNs.In this paper,we present X-ray temporal and spectral study of a sample of8AGNs with IMBHs.These AGNs with M BH<106M⊙were selected based on the availabil-ity of XMM-Newton data.In Table1,we list these AGNs along with their general properties.The least massive AGN in our sample has only M BH∼83000M⊙.The accretion rates of these AGNs,estimated from optical observations,has a large range(L bol/L Edd∼10−3−3), suggesting a large diversity in the X-ray properties of these AGNs.We also present optical/UV emission ob-served with XMM-Newton optical monitor(OM).We de-scribe XMM-Newton observations and data reduction in Section2.In Section3,we present temporal analysis of the EPIC-pn data,followed by spectral analysis in Sec-tion4.We present the analysis of OM data and derive optical-to-X-ray spectral indices(αox)in Section5.We discuss the results in Section6,followed by a summary of our study in Section7.We assume the following cosmological parameters to calculate distances;H0=71km s−1Mpc−1,Ωm=0.27, andΩΛ=0.73.In the following,we abbreviate the names of the AGNs with IMBHs discovered with the SDSS e.g.,we mention SDSS J082912.67+500652.3as J0829+5006.2.OBSERVATION&DATA REDUCTIONAll the eight AGNs with IMBHs presented here were observed with XMM-Newton between2002May and2006 April.The European Photon Imaging Cameras(EPIC) pn(Str¨u der et al.2001)and MOS(MOS1and MOS2; Turner et al.2001)were operated in the imaging mode during all the observations.Table2lists the details of the X-ray observations.NGC4395,J0829+5006and J1357+6525were observed multiple times.The observa-tion datafiles were processed to produce calibrated event lists using the Science Analysis System(SAS v7.0.0).Ex-amination of the background rate above10keV showed that thefirst observation of NGC4395on2002June and thefirst,second and fourth observations of J0829+5006 were completely swamped out by theflaring particle background and therefore these observations were dis-carded from further study.The rest of observations were either clean or partly affected by the particle background and the intervals of high particle background were dis-carded from spectral analysis.We extracted the source spectra using the good EPIC-pn events in circular regions of radii in the25−40′′centered at the source position. We used event with pattern0−4(single and double pixel events)for all observations except for the long ob-servation of NGC4395.We used only the single pixel events for the long∼100ks observation of NGC4395. The background spectra were similarly extracted from nearby circular regions free of sources.Spectral response files were generated using the SAS tasks‘rmfgen’and ‘arfgen’.In Table1,we have listed the‘cleaned’expo-sure obtained from the EPIC-pn spectra.For temporal analysis we considered the full lengths of the observa-3TABLE1General properties of AGN with intermediate mass black holes observed with XMM-NewtonObject Position Redshift Galactic N H FWHM(km s−1)M BH(M⊙)L bolNGC4395122548.93+333247.80.0011.8515003.6×1051.2×10−3(1) POX52120256.90–205603.00.0213.857601.6×1050.5−1(2) SDSS J010712.03+140844.9010712.03+140844.90.07683.448307.2×1051.24(3) SDSS J024912.86-081525.6024912.86–081525.60.02953.677328.3×1040.71(3) SDSS J082912.67+500652.3082912.67+500652.30.04344.088705.2×1050.95(3) SDSS J114008.71+030711.4114008.71+030711.40.08111.915915.9×1052.98(3) SDSS J135724.52+652505.8135724.52+652505.80.1061.368729.5×1051.21(3) SDSS J143450.62+033842.5143450.71+033840.40.02842.4310892.0×1050.33(3)NGC43950112522001(1)2002-06-120112521901(2)2002-05-310.56±0.00712.50142830101(3)2003-11-301.13±0.00493.00112522701(4)2003-01-031.14±0.0145.9POX520302420101(1)2005-07-080.05±0.00181.1J0107+14080305920101(1)2005-07-220.27±0.00324.9J0249–08150303550101(1)2006-02-160.23±0.0084.0J0829+50060303550301(1)2006-03-270303550801(2)2006-03-280303550901(3)2006-04-260.95±0.022.30303551001(4)2006-04-26J1140+03070305920201(1)2005-12-030.66±0.00435.1J1357+65250305920301(1)2005-04-040.45±0.00519.20305920501(2)2005-05-160.55±0.011.90305920601(3)2005-06-230.42±0.00612.0J1434+03380305920401(1)2005-08-180.10±0.00222.0σ2NXS)(see Vaughan et al.2003and references therein).These quantities are listedin Table3for each lightcurve.NGC4395in the thirdobservation and POX52show the largest fractional vari-ability amplitudes of65.6±4.0%and73.6±9.3%,respec-tively.The fractional variability amplitude of NGC4395calculate here is similar within errors to that calculatedby Vaughan et al.(2005)who have performed a detailedanalysis of temporal characteristics of NGC4395us-ing the third XMM-Newton observation.Vaughan et al.(2005)also measured the the soft(0.2−0.7)band F varto be in excess of unity,making NGC4395the most vari-able AGN.In the case of POX52,some contribution tothe large F var may arise from incorrect background cor-rection as the long observation was partly affected withflaring background(see below).One of the objectives of this paper is to compare thevariability characteristics of AGNs with IMBHs withthat of NLS1s and broad-line Seyfert1galaxies(BLS1s).Leighly(1999a)presented a comprehensive X-ray vari-ability of a sample of NLS1s based on ASCA observa-tions.Nandra et al.(1997)presented a systematic timeseries analysis of Seyfert1galaxies consisting mostly ofBLS1s using ASCA data.It is preferable to use re-sults based on XMM-Newton observations.However,we did notfind systematic XMM-Newton study of sam-ples of NLS1s and BLS1s,similar to that performed4Fig.1.—XMM-Newton X-ray lightcurves of AGNs with IMBH derived from the EPIC-pn data with300s bins.TABLE3General properties of the EPIC-pn lightcurves of AGNs with IMBHObject Data Bin size Number mean rate rms Excess variance Normalized Excess F var(s)of bins(count s−1)(σ2XS )variance(σ2NXS)(%)5 Fig. 2.—Normalized excess variance and2−10keV X-rayluminosity for AGNs with IMBH,NLS1galaxies(Leighly1999a)and Syfert1galaxies(Nandra et al.1997).by Leighly(1999a)and Nandra et al.(1997).There-fore,we used results from Leighly(1999a)for NLS1andNandra et al.(1997)for BLS1s with the exclusion of twoNLS1s NGC4051and Mrk335.In Figure2,we haveplotted the normalized excess variance as a function of2−10keV luminosity for AGNs with IMBHs,NLS1sand BLS1s.As pointed out by Papadakis&Lawrence(1993)and Leighly(1999a),the normalized excess vari-ance depends on the length of observation.The nor-malized excess variance is the integration of the powerdensity spectrum over a certain frequency range impliedby the bin size and the length of the observation.Therednoise nature of the AGN variability means a strongdependance of excess variance on the length of the ob-servation.Therefore,care must be taken in interpret-ing Fig.2.Some of AGNs with IMBH were observedfor a short duration.To minimize the effect of observa-tion length,we have plotted only thosefive AGNs withIMBHs that were observed for at least30ks,thus makingthe length of observations similar to that for the NLS1sin Leighly(1999a)and BLS1s in Nandra et al.(1997).The prototype of AGNs with IMBH NGC4395lies atthe low luminosity end in Fig.2.The excess varianceof NGC4395is comparable to some of the most vari-able NLS1s.Vaughan et al.(2005)have noted that thevariations in the soft X-ray emission from NGC4395areamong the most violent seen in an AGN to date,with thefractional variability amplitude exceeding100%.Pre-viously,Nandra et al.(1997)and Leighly(1999a)havenoted that the excess variance is inversely correlatedwith the X-ray luminosity though with aflatter slope of∼−0.3for NLS1s compared with the slope of∼−1forBLS1s.Thefive AGNs with IMBHs follow the generaltrend of anticorrelation between sigma2and L2−10keVof Seyfert galaxies.While three of them follow the an-ticorrelation for BLS1,one(POX52)is clearly in theNLS1zone while NGC4395lies below the extrapolationof the BLS1trend.3.1.Power density spectraOnly two AGNs,NGC4395and POX52,were ob-served with long∼100ks exposures that allow us toprobe power density spectra(PDS)over a broad rangeof frequency.Vaughan et al.(2005)have already de-rived the PDS of NGC4395using the long observa-tion that showed a clear break from aflat spectrum(α∼1)to a steeper spectrum(α∼2)at a frequency0.5−3.0×10−3Hz.Here we present the PDS for POX52.To derive the PDS,we re-extracted the source andbackground lightcurves for POX52with time bins of500s.Figure1shows the background corrected EPIC-pn lightcurve of POX52.Fig.3also shows the EPIC-pnbackground level relative to the net source level.Thebackground rate varied in thefirst15ks of observationand then it was steady except for the aflare near theelapsed time of∼45000s.We calculated a power densityspectrum(PDS)using the background corrected EPIC-pn light curves sampled at500s.For this purpose,weexcluded thefirst15ks exposure to minimize the effectof varying background.We used the‘powspec’programin XRONOS.We rebinned the PDS in logarithmic spacewith a binsize of4and performed thefitting within theISIS(version1.4.8)spectralfitting environment.ThePDS is shown in Figure4(left panel).The power arisingfrom the Poisson errors has not been subtracted.ThePoisson errors dominate the PDS above a frequency of∼4×10−4Hz.A simple power-law(Power∝f−α)anda constant model resulted in a minimumχ2=15.6for23degrees of freedom(dof)withα=1.6±0.3.Theconstant power wasfixed to the value expected from thenoise.There is a weak excess of power at a frequncy of∼3×10−4Hz that is reminiscent of quasi-periodic os-cillations(QPO).However,niether the QPO-like featurenor a break in the PDS is statistically significant.Hencewe conclude that the PDS of POX52is consistent witha simple power law and the determination of a breakor the QPO-like feature will require future high signal-to-noise long X-ray observations.The non-detection ofa break may be due to the dominance of the Poissionnoise and the QPO-like feature above a frequency of∼2×10−4Hz below which the high frequency breakis likely to be present.J1140+0307is another AGN in our sample that hasgood signal-to-noise,∼40ks long X-ray lightcurve.Wehave derived the PDS of J1140+0307using the EPIC-pn data.First we extracted a lightcurve with7.34sbins.The source lightcurve was corrected for back-ground contribution though the background rate was sta-ble throughout the observation.As before we calculatedthe PDS and rebinned logarithmically into bins of sizes15.A simple power-law plus constant model provideda minimumχ2=213for177dof with power-law indexsteeper ing a broken power-law model inplace of the simple power-law improved thefit.However,all the parameters were not well constrained.We neededtofix the power-law indexα2after the break at its best-fit value to calculate the errors.This procedure resulted6Fig. 3.—The background subtracted EPIC-pn lightcurve of Pox52with500s bins and in the energy band of0.2−10keV. Also shown is the background level as dotted line in the same energy band.TABLE4Best-fit parameters for the power density spectrum ofPOX52&J1140+0307Model Parameter POX52J1140+0307Broken power-lawα1–1.0+0.8−0.9α2–3(fixed)×10−3f br(Hz)–0.7+1.2−0.5 Constant C–1.70+0.07−0.08χ2/dof–203.6/1767Fig.4.—Left:Power density spectrum of Pox52derived from the full band EPIC-pn lightcurve after excluding thefirst15ks exposure to minimized the effect of varying background.Right:Power density spectrum of J1140+0307and the best-fit models–a broken power law plus a constant(solid line)and a simple powerlaw plus a constant(dashed line).Fig.5.—Results of the spectral analysis of NGC4395.Upper left:EPIC-pn data obtained in2003November30,the absorbed power-law model and the deviations.Upper right:EPIC-pn data of2003November30,the best-fit model consisting of a power-law and an iron Kαline,modified by the Galactic absorption,intrinsic neutral and warm absorbers.Lower left:A comparison of unfolded EPIC-pn spectra of NGC4395obtained on2003November30and2002May31.Lower right:EPIC-pn data of2002May31,the best-fit model and the deviations.8TABLE5Parameters for NGC4395and POX52obtained from the best-fitting models to the EPIC-pn data.Component Parameter1NGC4395POX522003November2003January32002May312005July81‘(f)’indicates that the parameter value wasfixed.‘p’in the upper or lower error value indicates that the upper or lower confidence limit did not converge.2MCD normalization in units of n MCD=(R in/km)/(D/10kpc),where R in is the inner radius and D is the distance.9 dexΓ∼1.84.Evidently the multiple warm and neutralabsorbers strongly modify the spectrum below∼5keV.The parameters of the best-fit model are listed in Table5and the observed EPIC-pn spectrum of2003November30,the best-fit model and the deviations are shown inthe upper right panel of Fig.5.We have also analyzed the EPIC-pn spectrum ofNGC4395obtained on2002May31with a net exposureof12.5ks.The EPIC-pn data were grouped to a min-imum of20counts per spectral channel.Fig.5(lowerleft panel)shows a comparison of the unfolded EPIC-pn spectra of NGC4395obtained on2002May31and2003November30in a model independent way.UnlikeXSPEC,the unfolded spectrum in ISIS is derived in amodel-independent way as follows:[C(I)−B(I)]/∆tf unfold(I)=10Fig. 6.—Left:Observed EPIC-pn spectrum,absorbed power-law model and the deviations.Right:Observed EPIC-pn spectrum,the best-fit model consisting of a power law and an MCD modified by a multiple absorbing components–the Galactic,partially covering neutral absorption and a warm absorber.Also shown are the deviations of the observed data from the best-fit model.As afirst step,wefitted a simple power-law modifiedwith Galactic absorption to the individual spectra.Theresiduals of the observed data from the power-law modelshowed evidence for weak soft excess emission in the caseof J0249-0815,J1140+0307and J1357+6525.Additionof a multicolor disk blackbody component to the PLmodel improved thefit marginally for J0249-0815only ata level of97.4%(∆χ2=−8.5for two additional param-eters)based on the f-test.The best-fit parameters arekT in=169+43−50eV andΓ=1.72±0.30.For J1140+0307,the addition of the MCD component provided significantimprovement over the power-law model(∆χ2=−64.6for one additional parameter).Varying the absorptioncolumn did not improve thefit further.The best-fitparameters are kT in=181+3−7eV andΓ=2.4±0.1.J1357+6525has two observations.Both data show evi-dence for weak soft excess emission.The spectral shapesare similar withing errors for both the observations ex-cept for a narrow iron Kαline at6.65keV which hasbeen detected in thefirst observation at a level of99.3%based on the F-test.The best-fit parameters for theSDSS AGNs are listed in Table6.The simple power-law model provided statistically ac-ceptablefits to the pn data of J0107+1408,J0829+5006and J1434+0338with photon indicesΓ=2.4±0.1,2.65±0.08,2.05±0.07,respectively.The steep photonindices suggest possible presence of the soft X-ray ex-cess emission from these AGNs.However,addition of anMCD component did not improve any of thefits statisti-cally at95%level.To further investigate the presence ofthe soft excess component,wefitted the absorbed power-law model only to the hard2.5−10keV and found gen-erallyflatter indices.To estimate the strength of the softexcess emission from these AGNs,we added MCD com-ponents to the power-law model andfixed the power-lawindex to the best-fit values obtained for the2.5−10keVdata.Thefit qualities are similar to the simple power-lawfits.Thus the data are consistent with the pres-ence of the soft excess but do not require this componentstatistically.Therefore,we calculated90%upper-limitsto the strength of the soft excess emission.The upperlimits are listed in Table7.In Figure7,we show thestrength of the soft X-ray excess from the SDSS AGNswith IMBHs as the ratios of the observed data and thebest-fit2.5−10keV power-law model extrapolated tolower energies.For comparison,we have also plotted theratios for NGC4395and POX based on the best-fit ab-sorbed power law to the5−10keV data as the X-rayemission below∼5keV is strongly modified by the in-trinsic neutral and warm absorbes.5.UV EMISSION AND OPTICAL-TO-X-RAY SPECTRALINDEXAn additional advantage of XMM-Newton is the avail-ability of the OM that provides optical/UV data simul-taneously with the X-ray observations.All AGNs withIMBHs were observed with the OM in the imaging modewith one or more optical/UVfilters.The AGNs wereobserved in the UV for thefirst time,therefore we pro-vide here standard UV magnitudes andfluxes based onthe OM observations.The OM data were processed us-ing the SAS task omichain.In all cases,multiple ex-posures were taken in the samefilter and the standardmagnitudes andflux densities were derived based on theaverage count rates(Cohen2004).We list the averagecount rates,standard magnitude andflux densities foreach of the observations in Table8.We corrected theflux densities for the Galactic extinction by adopting theextinction law in Cardelli et al.(1989).We calculatedthe color excesses E(B-V)due to the Galactic reddeningfrom the Galactic hydrogen column density(N H)alongthe lines of sight to individual AGNs using the relationN H=5.8×1021×E(B−V)cm−2(2)(Bohlin et al.1978).The unreddenedflux densities arelisted in Table8.We have also calculated the optical-to-X-ray spectralindex(αox)defined asαox=−0.386log Lν(2500˚A)11Fig.7.—The ratios of EPIC-pn data and the best-fitting power-law model modified by the line of sight Galactic absorption.The power law wasfitted to2.5−10keV data for the IMBH AGNs discovered with the SDSS and extrapolated to lower energies to calculate the ratios.For NGC4395and POX52,the ratios were obtained byfitting the absorbed power law to the5−10keV band as the primary continua of the two AGNs are strongly modified below5keV by the multiple absorbing components.。