Alpha谱仪实验
布鲁克红外alpha傅里叶红外光谱仪
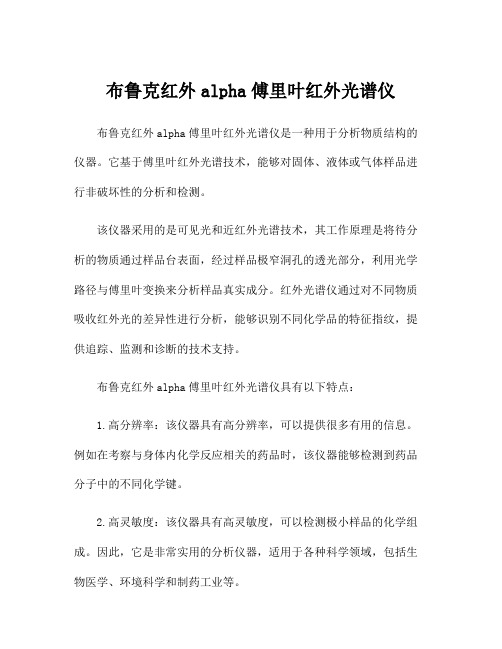
布鲁克红外alpha傅里叶红外光谱仪布鲁克红外alpha傅里叶红外光谱仪是一种用于分析物质结构的仪器。
它基于傅里叶红外光谱技术,能够对固体、液体或气体样品进行非破坏性的分析和检测。
该仪器采用的是可见光和近红外光谱技术,其工作原理是将待分析的物质通过样品台表面,经过样品极窄洞孔的透光部分,利用光学路径与傅里叶变换来分析样品真实成分。
红外光谱仪通过对不同物质吸收红外光的差异性进行分析,能够识别不同化学品的特征指纹,提供追踪、监测和诊断的技术支持。
布鲁克红外alpha傅里叶红外光谱仪具有以下特点:1.高分辨率:该仪器具有高分辨率,可以提供很多有用的信息。
例如在考察与身体内化学反应相关的药品时,该仪器能够检测到药品分子中的不同化学键。
2.高灵敏度:该仪器具有高灵敏度,可以检测极小样品的化学组成。
因此,它是非常实用的分析仪器,适用于各种科学领域,包括生物医学、环境科学和制药工业等。
3.容易使用:该仪器可以快速且易于使用。
相对于其他化学分析技术,如质谱法等,该仪器无需进行任何前处理的样品制备,具备便捷的操作和分析功效,可以快速地得出结果。
4.无需样品消耗:制备样品往往是科学研究的时间和金钱成本之一。
布鲁克红外alpha傅里叶红外光谱仪解决了这个问题。
这种技术完全不消耗样品,并且可以重复使用检测样品。
5.低维护:该仪器不需要频繁的校准、调整或维护。
这是非常优秀的功能之一,因为将科学家从固定的、繁琐和费时的校准工作中解放出来,从而可以集中精力进行实际的研究。
布鲁克红外alpha傅里叶红外光谱仪在许多不同领域都有应用价值。
例如,在生物体液、药品、食品、化妆品、化学工业品等领域,它被广泛使用。
在制药领域,该仪器被广泛应用于药品开发和制造过程中的质检。
检测药品中重要化合物和杂质的含量是制药过程的重要一环。
在环境科学领域,该仪器可以用来检测水和土壤中的污染物。
并且可以检测到大气颗粒物的化学成分,以确定空气污染的来源和可能的健康风险。
本科实验室实验指导书-阿尔法能谱测量
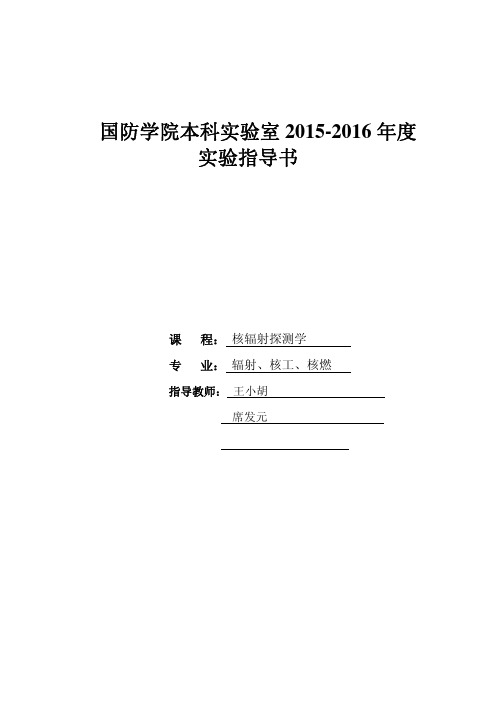
国防学院本科实验室2015-2016年度实验指导书课程:核辐射探测学专业:辐射、核工、核燃指导教师:王小胡席发元实验一: α射线能谱测量一、实验安排次数/实验:2次/实验 学时/次:2学时/次人数/次:10~20人/次二、实验目的及要求1.目的了解PN结半导体α谱仪的工作原理、特性。
掌握使用α谱仪测量α粒子能谱的方法。
掌握能量分辨率的概念,测量真空度对α粒子能量分辨率的影响。
在相同真空度条件下,测定α粒子能量分辨率、计数率随源-探距的变化。
2.要求预习PN结半导体α谱仪的工作原理;预习能量分辨率的基本概念,PN结半导体α谱仪能量分辨率的影响因素。
查询Am-241,Pu-239两种核素的α粒子能量。
三、实验仪器和试剂1.主要仪器:实验采用美国ORTEC公司生产的8路α谱仪。
仪器特点:每一路都有自己的探测器,真空室,真空计、探头偏压调节、前放、主放、脉冲产生器及漏电流检测器。
样品直径最大可达51mm。
探测器与被测样品之间有10档距离可选,相邻两档之间的距离差为4mm,最大距离可达44mm。
真空计:范围10mTorr到30Torr,具有真空/偏压锁定控制功能。
每路谱仪的探测器偏压、漏电流及真空度均可在软件相关界面上以数字和图形显示出来。
探测器偏压:范围0±100V,大小和正负极性可调节。
真空泵:每路谱仪的真空室采用高性能O圈密封,通过一个三位阀可以单独手动控制抽真空/保持/放气三个状态,互不影响;整个谱仪则合用一台真空泵,采用国际标准的NW25ISO-KF卡箍与真空泵相连。
2.放射源:采用Am-Pu混合源。
2π发射率分别为每秒282.2和每秒390.7。
四、实验内容1、调整α谱仪,测定谱仪能量分辨率。
利用Am-Pu混合源,对谱仪进行能量刻度。
2、测量真空度对α谱仪能量分辨率的影响。
3、测量源-探距对α粒子能量分辨率、探测器计数率的影响。
五、实验步骤1、调整α谱仪①打开PC机、8路α谱仪和真空泵;②点击PC桌面上“MAESTRO for Windows”;③选择探测器通道;④放置样品(Am-Pu源)⑤抽真空⑥设置探测器高压⑦点击Acquire-》Start 开始测量2、测量真空度对α谱仪能量分辨率的影响。
alpha傅里叶红外光谱使用说明书
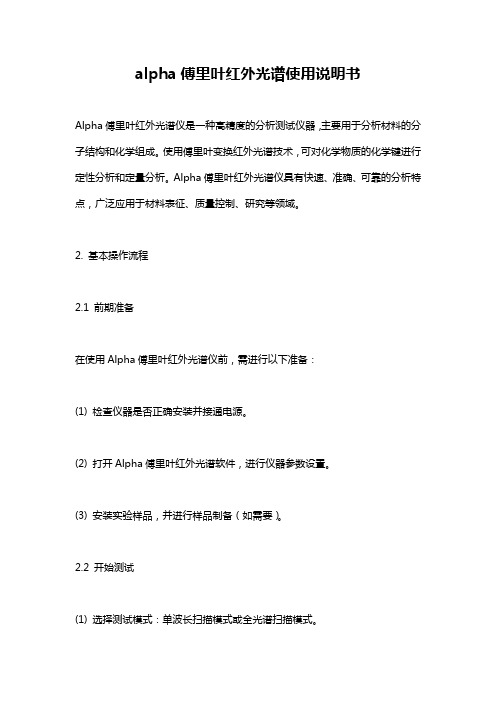
alpha傅里叶红外光谱使用说明书Alpha傅里叶红外光谱仪是一种高精度的分析测试仪器,主要用于分析材料的分子结构和化学组成。
使用傅里叶变换红外光谱技术,可对化学物质的化学键进行定性分析和定量分析。
Alpha傅里叶红外光谱仪具有快速、准确、可靠的分析特点,广泛应用于材料表征、质量控制、研究等领域。
2. 基本操作流程2.1 前期准备在使用Alpha傅里叶红外光谱仪前,需进行以下准备:(1) 检查仪器是否正确安装并接通电源。
(2) 打开Alpha傅里叶红外光谱软件,进行仪器参数设置。
(3) 安装实验样品,并进行样品制备(如需要)。
2.2 开始测试(1) 选择测试模式:单波长扫描模式或全光谱扫描模式。
(2) 设置光谱扫描参数,如扫描速度、波数范围等。
(3) 点击“开始扫描”按钮,进行光谱扫描。
(4) 完成扫描后,保存光谱数据。
2.3 分析光谱数据(1) 在Alpha傅里叶红外光谱软件中打开光谱数据文件。
(2) 对光谱数据进行处理和分析,如峰位测定、峰形分析、谱图比较等。
(3) 根据分析结果,对样品进行定性或定量分析。
3. 注意事项(1) 操作前请仔细阅读Alpha傅里叶红外光谱仪使用说明书,确保正确操作。
(2) 在使用Alpha傅里叶红外光谱仪进行样品测试前,需进行样品制备,保证样品质量。
(3) 在进行测试时,需注意调节仪器参数,如光谱扫描速度、波数范围、光谱分辨率等,确保测试结果的准确性和可靠性。
(4) 在保存光谱数据时,需注意选择适当的文件格式,如JCAMP-DX、CSV等。
(5) 使用结束后,需对仪器进行清洁和保养,保证仪器的正常运行。
傅里叶红外光谱仪alpha
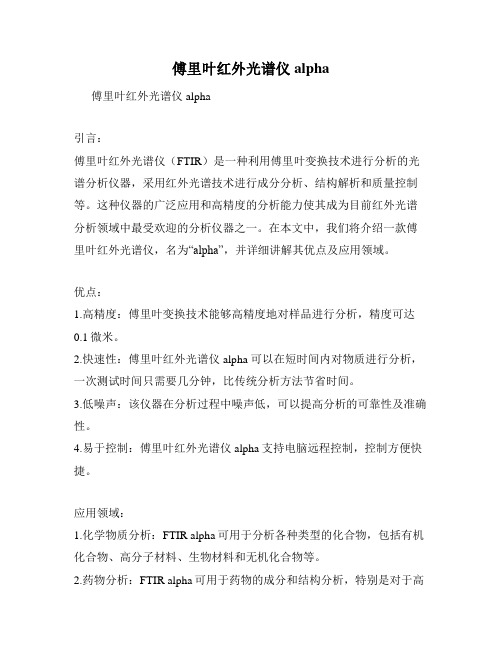
傅里叶红外光谱仪alpha傅里叶红外光谱仪 alpha引言:傅里叶红外光谱仪(FTIR)是一种利用傅里叶变换技术进行分析的光谱分析仪器,采用红外光谱技术进行成分分析、结构解析和质量控制等。
这种仪器的广泛应用和高精度的分析能力使其成为目前红外光谱分析领域中最受欢迎的分析仪器之一。
在本文中,我们将介绍一款傅里叶红外光谱仪,名为“alpha”,并详细讲解其优点及应用领域。
优点:1.高精度:傅里叶变换技术能够高精度地对样品进行分析,精度可达0.1微米。
2.快速性:傅里叶红外光谱仪 alpha 可以在短时间内对物质进行分析,一次测试时间只需要几分钟,比传统分析方法节省时间。
3.低噪声:该仪器在分析过程中噪声低,可以提高分析的可靠性及准确性。
4.易于控制:傅里叶红外光谱仪 alpha支持电脑远程控制,控制方便快捷。
应用领域:1.化学物质分析:FTIR alpha可用于分析各种类型的化合物,包括有机化合物、高分子材料、生物材料和无机化合物等。
2.药物分析:FTIR alpha可用于药物的成分和结构分析,特别是对于高价值药物的毒性和副作用分析具有重要意义。
3.环境分析:FTIR alpha可用于大气、水体和土壤污染物的检测和分析。
4.材料分析:FTIR alpha可用于材料的成分、结构及表面特性分析,可以广泛应用于化工、电子、能源等领域。
结论:傅里叶红外光谱仪 alpha 具有高精度、快速、低噪声及易于控制等优点。
其广泛应用于化学、生物、医药、环保等领域,成为目前最受欢迎的分析仪器之一。
在以后的科学研究和工业生产中,傅里叶红外光谱仪alpha 的重要性将得到进一步提高。
alpha傅里叶变换红外光谱仪
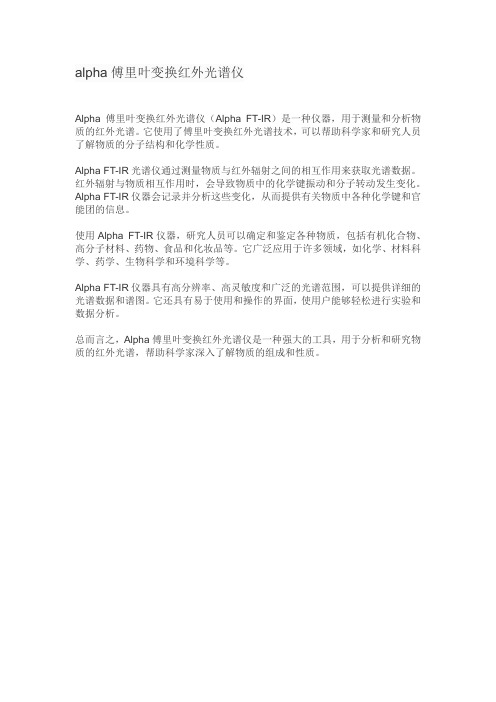
alpha傅里叶变换红外光谱仪
Alpha傅里叶变换红外光谱仪(Alpha FT-IR)是一种仪器,用于测量和分析物质的红外光谱。
它使用了傅里叶变换红外光谱技术,可以帮助科学家和研究人员了解物质的分子结构和化学性质。
Alpha FT-IR光谱仪通过测量物质与红外辐射之间的相互作用来获取光谱数据。
红外辐射与物质相互作用时,会导致物质中的化学键振动和分子转动发生变化。
Alpha FT-IR仪器会记录并分析这些变化,从而提供有关物质中各种化学键和官能团的信息。
使用Alpha FT-IR仪器,研究人员可以确定和鉴定各种物质,包括有机化合物、高分子材料、药物、食品和化妆品等。
它广泛应用于许多领域,如化学、材料科学、药学、生物科学和环境科学等。
Alpha FT-IR仪器具有高分辨率、高灵敏度和广泛的光谱范围,可以提供详细的光谱数据和谱图。
它还具有易于使用和操作的界面,使用户能够轻松进行实验和数据分析。
总而言之,Alpha傅里叶变换红外光谱仪是一种强大的工具,用于分析和研究物质的红外光谱,帮助科学家深入了解物质的组成和性质。
布鲁克红外alpha傅里叶红外光谱仪
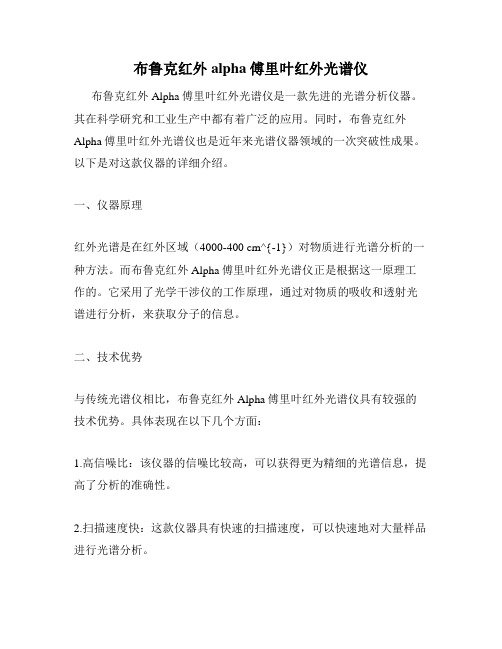
布鲁克红外alpha傅里叶红外光谱仪布鲁克红外Alpha傅里叶红外光谱仪是一款先进的光谱分析仪器。
其在科学研究和工业生产中都有着广泛的应用。
同时,布鲁克红外Alpha傅里叶红外光谱仪也是近年来光谱仪器领域的一次突破性成果。
以下是对这款仪器的详细介绍。
一、仪器原理红外光谱是在红外区域(4000-400 cm^{-1})对物质进行光谱分析的一种方法。
而布鲁克红外Alpha傅里叶红外光谱仪正是根据这一原理工作的。
它采用了光学干涉仪的工作原理,通过对物质的吸收和透射光谱进行分析,来获取分子的信息。
二、技术优势与传统光谱仪相比,布鲁克红外Alpha傅里叶红外光谱仪具有较强的技术优势。
具体表现在以下几个方面:1.高信噪比:该仪器的信噪比较高,可以获得更为精细的光谱信息,提高了分析的准确性。
2.扫描速度快:这款仪器具有快速的扫描速度,可以快速地对大量样品进行光谱分析。
3.高灵敏度:布鲁克红外Alpha傅里叶红外光谱仪的灵敏度较高,可以对微量物质进行分析,如痕量金属和有机物等。
4.非接触式测量:该仪器是一种非接触式测量仪器,在测试时无需对样品进行额外的处理,减小了对样品的影响。
三、应用领域由于其技术优势,布鲁克红外Alpha傅里叶红外光谱仪在科学研究和工业生产中都有着广泛的应用。
1.化学领域:可用于化学品的成分分析、结构确认和反应动力学研究等方面。
2.制药领域:可用于药品中有毒成分的检测以及不同药品疗效成分的分析。
3.食品行业:可用于食品成分的分析、添加剂的检测以及食品加工过程的监控。
4.环保领域:可用于环境水质的分析、大气污染物检测和土壤成分分析等。
四、结论总的来说,布鲁克红外Alpha傅里叶红外光谱仪是一款具有高性价比的光谱分析仪器。
它是当今科学研究和工业生产中不可或缺的一种测试工具。
它的出现,具有较大的推动作用,可以帮助人们更快速、准确地实现光谱分析这一过程。
红外光谱仪标准操作规程
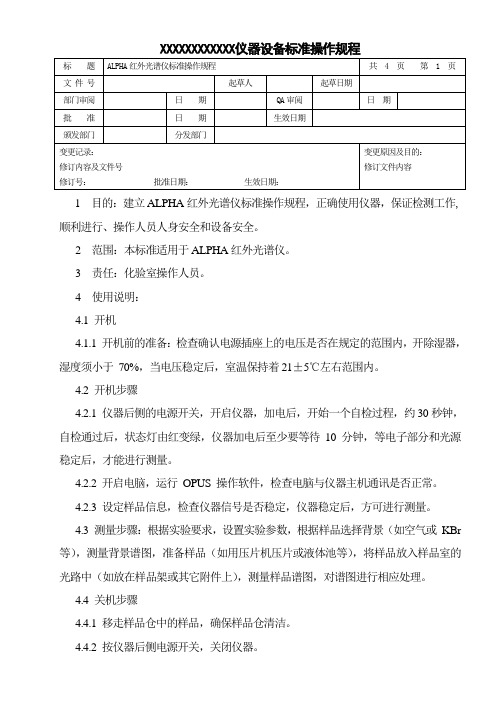
XXXXXXXXXXXX仪器设备标准操作规程1目的:建立ALPHA红外光谱仪标准操作规程,正确使用仪器,保证检测工作,顺利进行、操作人员人身安全和设备安全。
2 范围:本标准适用于ALPHA红外光谱仪。
3 责任:化验室操作人员。
4 使用说明:4.1 开机4.1.1 开机前的准备:检查确认电源插座上的电压是否在规定的范围内,开除湿器,湿度须小于70%,当电压稳定后,室温保持着21±5℃左右范围内。
4.2 开机步骤4.2.1 仪器后侧的电源开关,开启仪器,加电后,开始一个自检过程,约30秒钟,自检通过后,状态灯由红变绿,仪器加电后至少要等待10 分钟,等电子部分和光源稳定后,才能进行测量。
4.2.2 开启电脑,运行OPUS 操作软件,检查电脑与仪器主机通讯是否正常。
4.2.3 设定样品信息,检查仪器信号是否稳定,仪器稳定后,方可进行测量。
4.3 测量步骤:根据实验要求,设置实验参数,根据样品选择背景(如空气或KBr 等),测量背景谱图,准备样品(如用压片机压片或液体池等),将样品放入样品室的光路中(如放在样品架或其它附件上),测量样品谱图,对谱图进行相应处理。
4.4 关机步骤4.4.1 移走样品仓中的样品,确保样品仓清洁。
4.4.2 按仪器后侧电源开关,关闭仪器。
4.4.3 关闭电脑。
4.4.4 若有必要,还需要从电源插座上拔下电源线。
4.4.5 在记录本记录使用情况。
5 仪器的维护:5.1.1取出并再生干燥剂:封在筒中可更换的干燥剂(分子筛)能够保持干涉仪和探测器腔体中的空气干燥;尽管密封在腔体中,还是有必要再生干燥剂;如果频繁更换探测器的话,对探测器腔体更有必要再生干燥剂。
5.1.2大约每六个月或者至少当仪器上面的电子湿度指示等表明应该更换干燥剂时,再生或换干燥剂。
5.2更换干燥剂:干燥器筒在探测器和干涉仪腔体中,打开探测器腔体,取下探测器腔体左侧螺钉的塞子,转大约半圈,松开螺钉(6mm),移开盖子,拔出干燥器筒;5.2.1打开干涉仪腔体(TENSOR 27),按下光源腔体盖,打开盖子,会在面向干涉仪腔体的壁上看到一个螺钉,转大约半圈,松开螺钉(6 mm),取下干涉仪腔体盖子,拔出干燥器筒;5.2.2打开干涉仪腔体(TENSOR 37),转动仪器后面的把手,打开干涉仪腔体盖,取下干涉仪腔体盖,取出干燥剂管。
thermo scientific k-alpha 测量成分
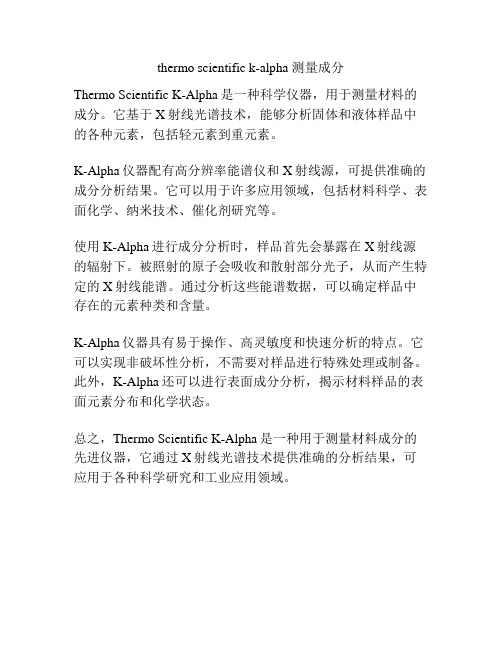
thermo scientific k-alpha 测量成分
Thermo Scientific K-Alpha是一种科学仪器,用于测量材料的成分。
它基于X射线光谱技术,能够分析固体和液体样品中的各种元素,包括轻元素到重元素。
K-Alpha仪器配有高分辨率能谱仪和X射线源,可提供准确的成分分析结果。
它可以用于许多应用领域,包括材料科学、表面化学、纳米技术、催化剂研究等。
使用K-Alpha进行成分分析时,样品首先会暴露在X射线源的辐射下。
被照射的原子会吸收和散射部分光子,从而产生特定的X射线能谱。
通过分析这些能谱数据,可以确定样品中存在的元素种类和含量。
K-Alpha仪器具有易于操作、高灵敏度和快速分析的特点。
它可以实现非破坏性分析,不需要对样品进行特殊处理或制备。
此外,K-Alpha还可以进行表面成分分析,揭示材料样品的表面元素分布和化学状态。
总之,Thermo Scientific K-Alpha是一种用于测量材料成分的先进仪器,它通过X射线光谱技术提供准确的分析结果,可应用于各种科学研究和工业应用领域。
α谱仪实验数据记录及分析
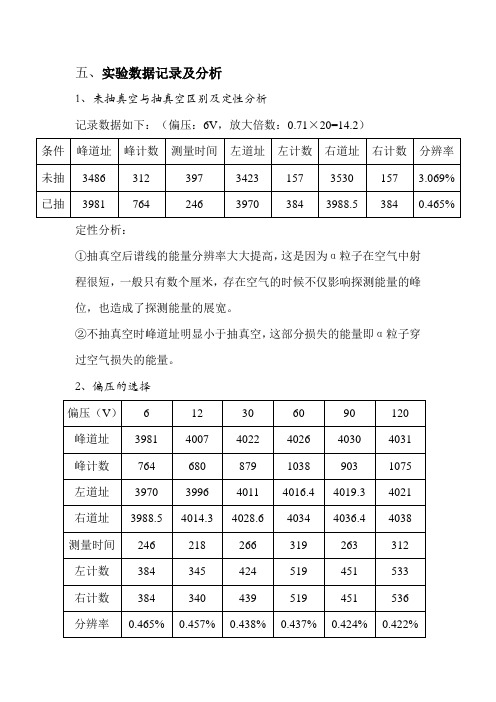
五、实验数据记录及分析1、未抽真空与抽真空区别及定性分析记录数据如下:(偏压:6V,放大倍数:0.71×20=14.2)条件峰道址峰计数测量时间左道址左计数右道址右计数分辨率未抽3486 312 397 3423 157 3530 157 3.069% 已抽3981 764 246 3970 384 3988.5 384 0.465% 定性分析:①抽真空后谱线的能量分辨率大大提高,这是因为α粒子在空气中射程很短,一般只有数个厘米,存在空气的时候不仅影响探测能量的峰位,也造成了探测能量的展宽。
②不抽真空时峰道址明显小于抽真空,这部分损失的能量即α粒子穿过空气损失的能量。
2、偏压的选择偏压(V) 6 12 30 60 90 120峰道址3981 4007 4022 4026 4030 4031峰计数764 680 879 1038 903 1075左道址3970 3996 4011 4016.4 4019.3 4021右道址3988.5 4014.3 4028.6 4034 4036.4 4038测量时间246 218 266 319 263 312左计数384 345 424 519 451 533右计数384 340 439 519 451 536分辨率0.465% 0.457% 0.438% 0.437% 0.424% 0.422%选择能量分辨率最低时对应的偏压即120V 为工作偏压。
3、能量标定及相对强度测定 实验通过测量241Am 中峰能量为 5.486MeV 和239Pu 中峰能量为5.157MeV 所对应的峰道址进行能量标定,并求得239Pu α谱中未知能量峰的峰能量。
做出定标曲线,并进行拟合如下图由图像得能量道数关系为E=-0.01547+0.00137×C 求得未知峰能量为5.51248MeV 。
放射源峰道址 峰计数 左道址 右道址 左计数 右计数 测量时间 分辨率 已知源 241Am 4030 1129 4021.3 4038.5 564 564 338 0.427% 239Pu 3789 263 3773.6 3796.8 131 131 509 0.612% 未知源239Pu40357640224040.537385090.458%同时对241Am进行强度测量:峰位计数道址能谱总面积各峰的面积所占比例2148366.256% 一号峰(主峰)1129403032424二号峰3054001710721.919%。
α射线能谱测量

*************************************************************************** *西南科技大学《α射线能谱测量》报告设计名称α射线能谱测量学院班级学生姓名学号设计日期 2014年12月2014年10月制目录1实验目的 (1)2实验内容 (1)3实验原理 (1)α能谱 (1)α放射源 (2)α放谱仪 (3)探测器测量α射线能谱相关原理 (4)α谱仪的能量刻度和能量分辨率 (4)4实验仪器、器材 (5)5实验步骤 (5)6实验数据记录、处理 (6)7实验结论 (8)1实验目的α衰变中发射的α粒子能量及辐射几率的测量,对于核结构研究具有重要意义。
这些核数据的测量通常是用α磁谱仪或半导体α谱仪。
而本实验主要从以下几个方面进行:1、了解α谱仪工作原理与特性2、掌握α能谱测量原理及测量方法3、测量获取表中各种放射源在不同探源距下α能谱的数据与图像记录并进行刻度2实验内容测定α谱仪在不同源距下α能谱的数据,并通过计算获得相关能量分辨率。
同时,进行能量刻度。
3实验原理α能谱α粒子通过物质时,主要是与物质的原子的壳层电子相互作用发生电离损失,使物质产生正负离子对,对于一定物质,α在其内部产生一对离子所需的平均能量是一定的(即平均电能w),所以在物质中产生的正负离子对数与α粒子损失的能量成正比,即:EN=W公式中N为α粒子在物质中产生的正负离子对数目,E是在物质中损失的α粒子能量。
如果α粒子将其全部能量损失在物质内,E就是α粒子的能量。
由于α粒子在空气中的射程很短(在T=15℃,P=1大气压时,天然放射性核素衰变产生的α粒子,射程最大为Thc’(212Po) 为,能量最小232Th为),所以测量室应采用真空室,如上图1所示,采用真空泵将测量室抽成真空,这样与探测器接触的α粒子的能量才近似等于放射性核素经过α粒子放出的α粒子的初始能量(近似是因为不可能将测量室抽成绝对真空)。
布克尔ALPHA FT-IR光谱仪说明书

ALPHAThe very compact and smart FT-IR spectrometerAbout the foot-print of a laboratory notebook, ALPHA is a very compact FT-IR spectrometer perfectly suited for the daily routine. Plug & play set-up, easy-to-use software, combined with QuickSnap™ sampling modules assure the powerful and reliable FT-IR analysisyou expect from Bruker.Low-cost, small footprint FT-IR spectrometerQuality components with long lifetimeIntuitive instrument and software design for maximum ease of useFlexibility: Easily exchangeable QuickSnap™ sampling modules for every analytical questionIntelligence: Electronic coding of sampling modules and spectrometer componentsRockSolid TM: Robust, high performance results with Bruker´s well-proven interferometer designALPHA FT-IR SpectrometerMore than just ‘small’The ALPHA is more than just a compact FT-IR spectrometer: Its modular design always provides the adequate configuration for your analytical question. The ALPHA unites Bruker’s years of experience in infrared spectroscopy in a compact, rugged and intuitive design. Quality components and state-of-the-arttechnology ensure accurate and precise results within short measurement time. The ALPHA delivers excellent sensitivity, x-axis reproducibility and stability.Spectrometer components are continually monitored to ensure operation within their specification.Analysis where neededThe ALPHA is insensitive to vibrations, and can be placed almost anywhere; it can be moved easily and beimmediately operational. Its exclusive RockSolid TM interferometer design, delivers the performance andreproducible results people expect from a Bruker FT-IR spectrometer.A complete set of mobility options make the ALPHA an independent analyzerthat can be used just where it is needed most: close to the sample to save you time and cost per sample. The ALPHA can be transported easily from lab to lab, can be used in mobile laboratories, can directly fit into the fume hood or a glove box.ALPHA mobility options:Wireless communication Battery operation (>8h) Car battery operation Touch screen operationTransport caseanalyzeverifyquantifyidentifyQuickSnap™ sampling modulesThe QuickSnap TM sampling modules for the ALPHA offer full sampling flexibil-ity. They allow the analysis of almost any kind of sample (e.g. solids, liquids or gases) each with perfectly matched instrument configuration.With the push of a button the exchange of the sampling modules is performed quickly and easily. The sampling modules and even ATR plates are electronically coded. Therefore appropriate measurement parameters can be provided automatically for the current configuration.To match the requirements of different analytical questions Bruker offersvarious FT-IR sampling accessories for transmission, attenuated total reflection (ATR), external and diffuse reflection.QuickSnap™ module exchangeExtend Your Sampling CapabilitiesQuickSnapUniversal sampling moduleThe universal sampling module enables you to analyze all kinds of samples: solids,liquids and gases. This transmission sample compartment with its 2x3“ standardsample holder can house a variety of gas cells and liquid cells. Solid samples canbe investigated in a standard pellet holder or using a magnetic film holder. Sampleswith a reflective surface are analyzed with a dedicated accessory for reflectionmeasurements.Single reflection ATR: Platinum and Eco modulesAttenuated Total Reflection (ATR) is an easy-to-use FT-IR sampling method that is idealfor both solids and liquids.The Platinum-ATR is a single reflection ATR module with outstanding chemical andmechanical robustness. Its diamond crystal is brazed into tungsten carbide hard metal.This assembly allows the application of very high pressure so that even very hard samplescan be measured. A variety of sampling options for temperature controlled measure-ments and liquid flow through analysis complete the versatility of the Platinum-ATR.The Eco-ATR is a very cost-effective single reflection ATR module. It is optionally equippedwith either a ZnSe or Ge ATR-crystal.Multi reflection ATR moduleThe Multi reflection ATR module with horizontal ZnSe ATR crystal is very suitable forthe analysis of pastes, gels and liquids. Six internal reflections and an exceptionallyhigh light throughput provide highest ATR measurement sensitivity for the analysiseven of low concentrated sample components.DRIFT moduleThe DRIFT module is a very suitable option for the analysis of a broad variety of solidsamples: powders, inorganic material, gem stones, papers, textiles and many others.The DRIFT module is designed for easy sampling and high light throughput. Thisresults in an unmatched time-per-analysis for FT-IR diffuse reflection measurements.Module for external reflectionDedicated reflection modules allow contactless and non-destructive FT-IR analysis oflarge samples like coated metal, paper or textilefabrics. For the measurement the samples are placed in front or on top of the instru-ment, depending on the chosen module. Large or immobile samples such as surfacesof cars, airplanes, mural paintings or artwork can easily be analyzed as the ALPHA isplaced in front of the material/object. Optionally, an integrated video camera providesview of the sampling area.ALPHA validation manual supports the full instrument qualification process IQ, QQ, PQ.Fourier Transform Infrared (FT-IR) spectroscopy is a technique that has replaced many other expensive andtime-consuming methods over the years. The ALPHA is designed to be used for many years with low running costs. Its housing is made from robust metal.The ALPHA utilizes a modern diode laser with a lifetime of over 10 years. Its infrared source has been engineered for a lifetime of greater than 5 years and can be exchanged easily by the user after its end. The Platinum Diamond ATR module has a minimum lifetime of 10 years. These quality features are completed by the RockSolid TM interferometer with more than 10 years time of operation. Adding the low energy consumption these outstanding characteristics result in significant cost savings.10 years warranty on interferometer, laser and diamond ATR module5 years warranty on IR sourceValidationToday’s regulated laboratories must comply with extensive regulatory requirements. Bruker Optics offers a comprehensive system validation that provides the documentation and procedures neededfor an effective compliance. The ALPHAis prepared to fully support your validation needs; from the design qualification (DQ)to daily performance qualification (PQ). Bruker’s comprehensive system validation manual provides all related documentation and guides you through all the necessary steps of the validation procedures. Validation, instrument installation and annual certification are offered by Bruker’s factory trained, certified service engineers thereby further reducing the cost of compliance. The ALPHA incorporates a certified reference standard for fully-automated instrument test routines.With OPUS/Mentor your daily quality controlroutine is realized by a few mouse clicks. Theuser is guided through the complete analysis. OPUS/MentorOPUS/Mentor softwareThe OPUS/Mentor software with itseasy and intuitive user interface guidesthe operator through all the steps of ananalysis. The user interface can easily becustomized for dedicated applications orexperiments.Quality control made easyQuality control is one of the most importantapplications of the ALPHA. The predominantquestion in quality control is to verify thatthe sample meets its specifications.Consequently OPUS/Mentor provides theQuickCompare evaluation function asappropriate verification tool. The set-up ofQuickCompare methods is effortless andthe evaluation result is clear and safe.Furthermore, the identification of unknownsamples is of importance, e.g. of acontamination that caused a product failure.Identification results from an automatedsearch of an analogue reference spectrumin spectral libraries.Low Cost Of OwnershipThe Leader in FT-IR SpectroscopyTechnologies used are protected by one or more of the following patents:DE 102004025448; DE 19940981; US 5923422; DE 19704598The ALPHA is the culmination of what Bruker Opticshave pioneered and developed for infrared spectroscopy inover 30 years. Today, we offer the industry’s broadest and most advanced FT-IR product line.Our success stems from our commitment and dedication to provide you the proper analytical tool you require to solve a demanding research problem or run a daily quality controlroutine.Bruker Opticsis ISO 9001 ser class 1/opticsBruker Optics Inc.Billerica, MA · USAPhone +1 (978) 439-9899Fax +1 (978) 663-9177*********************Bruker Optik GmbH Ettlingen · GermanyPhone +49 (7243) 504-2000 Fax +49 (7243) 504-2050********************Bruker Hong Kong Ltd.Hong KongPhone +852 2796-6100Fax +852 2796-6109*******************.hkBruker Optics is continually improving its products and reserves the right to change specifications without notice. © 2014 Bruker Optics BOPT-4000054-05Mobile-IRPortable FT-IR spectrometer for the identification of unknowns anywhere! With its IP65 rated case, rugged design, it is ready for the field use.LUMOSLUMOS is a stand-alone FT-IR microscope with fullautomation. It is designed to combine best performance for visible inspection and infrared spectral analysis with highest user comfort.TENSOR SeriesEntry level research FT-IR spectrometer that has an exit port for external accessories such as FT-IR microscopy,TGA interface, PM-IRRAS or the high-throughput screening accessories.VERTEX SeriesResearch level FT-IR spectrometer that is fullycustomizable to meet your demanding requirements. Its vacuum option can eliminate atmospheric moisture absorptions for ultimate sensitivity and stability.TANGOAn ergonomic and easy-to-use FT-NIR spectrometer which can be applied for qualitative and quantitative analysis in laboratory as well as production environments.。
witec alpha 300m+激光共聚焦显微拉曼光谱仪使用与维护初探

232研究与探索Research and Exploration ·探讨与创新中国设备工程 2019.12(上)1928年,印度物理学家C. V. Raman 在用汞灯的单色光照射ccl 4 液体时,发现当光与ccl 4分子相互作用后,在液体的散射光中观测到了频率低于入射光频率的新谱线,通过对这些新谱线的研究可以得到ccl 4 分子结构的信息,因此这种效应被命名为Raman 效应,这种新谱线则被命名为拉曼光谱。
近年来,随着计算机技术、激光技术和高灵敏度探测器等的不断发展,拉曼光谱技术已经成为分析测试领域不可缺少的一部分。
一幅拉曼光谱图可包括单个或者多个拉曼散射峰,每个拉曼散射峰可反映所测物质的拉曼散射峰的峰强、峰位和峰宽等信息,与光照射物质时其某种分子的分子键振动和转动能级差一一对应,类似于人类指纹识别的效果,因而也称之为“指纹峰”,通过分析拉曼散射峰可得到所测物质材料的化学组成、晶体的结晶度、材料厚度以及形态等信息。
同时与AFM,SEM,TCSPC 联用,被广泛应用于高分子材料鉴定、生物分子鉴定、稀有矿物质鉴定等领域,在当今材料表征方法中占据重要地位。
1 基本工作原理Witec alpha 300M+激光共聚焦显微拉曼光谱仪的工作原理如图1所示,一束激光经过单模光纤入射到分光器,被分光器反射后进入显微物镜,经过显微物镜聚焦入射到已经聚焦好的样品表面处某微小区域内,该微区内的样品被入射激光照射后激发出拉曼信号,该信号和入射激光一起被样品反射回来后经过滤光片后被多模光纤接收,多模光纤接收的信号经过光谱仪探测分析,最终得到拉曼光谱图,其中白光的作用是对样品表面进行聚焦时的照明光源,“z-控制台”的作用是调节显微物镜沿z 轴方向的上下,“压电扫描台”的作用是调节样品x-y轴方向的位置。
图1 Witec alpha 300M+激光共聚焦显微拉曼光谱仪光路图Witec alpha 300M+激光共聚焦显微拉曼光谱仪使用与维护初探张秀英(南京工业大学先进材料研究院,江苏 南京 211816)摘要:激光共聚焦显微拉曼光谱仪是材料表征的一种重要工具,是从事拉曼光谱研究相关工作者的必备仪器。
核电工程外文翻译----国际空间站(空间站)上进行的阿尔法磁谱仪(ams02)实验

翻译原文Alpha Magnetic Spectrometer(AMS02)experiment on the International Space Station (ISS)Abstract The Alpha Magnetic Spectrometer experiment is realized in two phases. A precursor flight (STS-91) with a reduced experimental configuration (AMS01) has successfully flown on space shuttle Discovery in June 1998.The final version (AMS02) will be installed on the International Space Station (ISS) as an independent module inearly 2006 for an operational period of three years. The main scientific objectives of AMS02 include the searches for the antimatter and dark matter in cosmic rays. In this work we will discuss the experimental details as well as the improved physics capabilities of AMS02 on ISS.Keywords Alpha Magnetic Spectrometer, International Space Station, Antimatter, Dark matter IntroductionA possible existence of cosmologically large domains of antimatter or astronomical "anti objects" and the nature of dark matter in the universe are fundamental questions of the modern astroparticle physics and cosmology.The AMS02 experiment thanks to its large acceptance (~0.65 m2) and its particle identification capability, will study these fundamental aspects with unprecedented sensitivity. This requires the measurement of the physical quantities such as particle momentum, charge and velocity with highest possible degree of confidence.An unambiguous proof of existence of cosmicantimatter would be observation of antinuclei (Z>2)in cosmic rays. An observation even of a single antihelium or heavier nuclei would demonstrate that primordial antimatter indeed exists and it is not too far from us. The AMS02 will be able to distinguish a single antihelium nuclei among~10^9 estimated background particles over 3 years. The project is realized in two phases. In June 1998, a baseline configuration of the experiment has down on the space shuttle Discovery for 10 days mission on 51.70 orbit at altitudes between 320and 390km. From this mission (STS-91) we gathered precious information on detector performance in actual space conditions and on possible background sources.AMS01 has also measured, for the first time, with such an accuracy from space, cosmic ray fluxes in GeV region covering almost the whole Earth surface.The detector layout, performance and the physics resuits of AMSO1 during STS-91 flight (AMS-0l ) are described in detail elsewhere.In this work we will discuss the experimental configuration as well as the physics capabilities of AMS02 on the international Space Station.2.Details of the AMS02 experimentThe AMS02 is a large acceptance, high precision superconducting magnetic spectrometer designed to measure cosmic ray spectra of individual elements with Z<--25 up to TeV region. It can also measure the high energy gamma rays up to few hundreds GeV with very good y source pointing capability. Fig.l shows the details of AMS02 experiment.There are a total of 227,300 electronics channels each providing 16 bits of information with event rates up to 2 kH corresponding to a total raw data rate of over 1 Gbit/s. The DAQ electronics will reduce the event size, through proper filtering, to the allocated downlink data rate of 2 Mbit/s.All electronics and mechanical parts of AMS02 are tested for operation in vacuum. The effect of total ionization dose (up to 6 Gy/year) on all critical components is extensively tested.The detector has been designed to identify the cosmic rays but at the same time minimizing the multiple scattering and large angle nuclear scattering occurring inside the tracking part of the detector. Large acceptance for antihelium search, good particle rigidity and velocity resolutions as well as their redundant measurements and h/e rejection of >10^6 were other key parameters for its design.The AMS02 will weigh 6760 kg and will have a power consumption of 2 kW.In the following the AMS02 sub-detectors will be described from up to downstream. 2.1 Transition Radiation Detector (TRD)The Transition Radiation Detector is designed to separate a/p signals to distinguish e+ and p from relative backgrounds (p and e一respectively) with a rejection factor of 103一102 in the energy range from 10~300 GeV. This rejection factor combined with the Electromagnetic Calorimeter will provide an over-all e+/p rejection factor of 10^6 at 90% of e+ efficiency.The detector consists of 20 layers of 6 mm diameter straw tubes alternating with 20 mm layersof 10 hum polyethylene/polypropylene fiber radiator. The tubes are filled with a 80%~ 20% mixture of Xe÷CO2 at 1 bar from a recirculating gas system designed to operate in space for>3 years. The wall material of straw tubes is a 72 μm kapton foil. The upper and lower 4 layers run in the x direction (parallel to AMS02 magnetic field) while central layers run in the perpendicular y direction to provide bi-dimensional tracking and particle identification.2.2 Time of Flight (ToF) systemThe ToF system is designed to provide fast (first level) trigger to the experiment, measurementof time of flight of the particles traversing the detector with up/down separation better than 10-g, the measurement of the absolute charge of the particle (in addition to dE/dX measured from the Silicon Tracker) and the identification of electrons and positron from antiprotons and protons up to 1--2 GeV. The expected overall time resolution is≈ 140 ps for protons and better for heavier cosmic ray nuclei (see Fig.3 for intrinsic resolutions). It consists of four scintillator planes (see Fig.4) read by a total number of 144 Hamamatsu 85946 phototubes. The design of AMS02 ToF system is determined by the constraint of operation in 0.1~0.3 T stray magnetic field. The choice of PM and the shape of light guides are driven by this issue. Light guides are indeed tilted to reduce the angle between the magnetic field and PM axis.2.3 Superconducting Magnet (SCM)One of the challenging features of the AMS02 detector is its strong superconducting magnet.It is the first large superconducting magnet used in space and it has a bending power of B}LZ } 0.8 Tm^2 which will be essential to perform a sensitive search for antimatter(He)in the rigidity (p/Z) range from 0.1 GV to several TV.The magnet consists of 2}dipole coils together with 2 sets of smaller racetrack coils (see Fig.5) with a total cold mass of about 2300 kg. The racetrack coils is designed to increase the overall dipole field, to minimize the stray dipole field outside the magnet (max stray field at a radius of 3 m is 4 mT) in order to avoid an undesirable torque on the ISS caused by the interaction with the Earth magnetic field. All coils are wound from high purity aluminum-stabilized niobium-titanium conductor. The magnet will be operated at a temperature of 1.8 K and cooled by 2500 L of superfluid helium, which should be operational for three years without refilling (optimizedfor heat losses).2.4 Anticoincidence (AC)The AMS02 anticoincidence system (Fig.6) is designed to assure to trigger only on those particles passing through the aperture of the AMS02 superconducting magnet. It is placed inside the magnet free bore covering the inner surface of the superconducting magnet. AC system consists of thin scintillator slabs readout on both ends by PMs.2.5 Silicon Tracker (ST)The Silicon Tracker of AMS02 is designed to perform high precision measurements of the rigidity he sign of charge and the absolute charge of the particle traversing it.There are a total of 192 ladders with variable number of silicon ensors glued together and readout on one extremity y the front-end electronics. The lengths of the ladders vary from 36 cm to about with 60 cm (active part)corresponding to ≈6.4 m^2 of active double sided surface. Fig.8 shows a detailed design of a ladder and its main components.One of the key points in the assembling of long ladders is that it requires high precision in cutting of the sensors and during the ladder assembly. Fig.9 shows the differences between measured (through reference crosses on each sensor) and nominal (perfectly aligned) positions of the sensors of 73 ladders.The r.m.s. of distribution is about 4 μm. The readout electronics is based on low noise,low power (~0.7 mW/ch), high dynamic range(士70MIPs) V A_ HDR VLSI, preamplifier, shaper, sample and hold circuit connected to the silicon sensors through 700 pF decoupling capacitors.The performance of the AMS02 ladders has been tested with minimum ionizing particles and with heavy ions.Fig.10 shows the residuals of the reconstructed and expected positions of 400 GeV muons traversing the prototype ladders. The resulting spatial resolutions are on bending (non bending) directions for 400 GeV muons and 20 GeV/A helium particles respectively.The tracker cooling system bases on variable conductive heat-pipes in which the cooling fluid (C02) runs by the capillary forces. The heat is collected by 3 heat-pipe loops and then driven to the radiators for dissipation (see Fig.13). The system will dissipate 192 W (1 W for each front-end hybrid pair) and fulfill the requirements of operating (survival) temperatures of-10℃to +25℃(-20℃to +40℃) and -10℃to +40℃(-20℃to +60℃) for silicon wafers and front-end hybrids respectively. The requirement for silicon wafer temperature stability per orbit is 3 K and for maximum accepted gradient between any silicon wafer fulfills 10 K. The total weight of ST is about 186 kg and the total power consumption is 734 W.The particle identification capabilities of the AMS-U2 proximity focused RICH detector will improve the confidence in the determination of the sign of the charge, will provide high level of redundancy required for high purity samples of positrons and antiprotons, will perform the identification of isotopes of mass A<~15-20, over a momentum range 1 GeV/c <p/A<-12 GeV/c and will identify the chemical composition of elements up to Fe (Z--26) to the upper rigidity limit of the spectrometer, p/Z<-1TV.The AMS02 RICH detector consists of a plane of radiator material, separated from the detector plane by a drift space in which Cherenkov rings can expand. A detector module includes a matrix of light guides coupled to a PM (Hamamatsu 87900-00-M16) connected to a socket and front-end electronics readout.The choice made by the Collaboration is to have an Aerogel radiator 3 cm thick with n=1.05 in order to cover the momentum interval w ith a velocity resolutionΔβ/β of about 1.5 * 10^3. In addition an 0.5 cm thick NaF placed at the central square, corresponding to the hole in the pixels plane, will improve the detection of particles traveling to the central hole direction.Since the Electromagnetic Calorimeter (ECAL) is located just below the RICH photomultipliers plane, the plane is designed with a central hole in order to avoid passive materials in front of ECAL. Fig.14 shows a 3-D design of RICH counter along with the performance test results obtained during the CERN SPS Test beam.2.7Electromagnetic Calorimeter (ECAL)In order to achieve good a/p separation (design rejection factor of一105) which is essential to perform accurate measurement of positron spectra (from few GeV up to一1 TeV), AMS02 comprises a fine grained sampling electromagnetic calorimeter (ECAL) capable of 3-D imaging of the shower development and of discrimination between hadronic and electromagnetic cascades.ECAL is a sampling device with a lead-scintillating fibers structure. It has a square parallelepiped shape with 65.8 cm side and 16.5 cm depth. It is segmented in 9 superlayers along its depth and each superlayer, of 18.5 mm total thickness, contains 11 grooved lead foils interleaved with 1 mm diameter scintillating fibers glued with an epoxy resin (average superlayer density of--6.8 1 0.3 g/cm3). The calorimeter has a radiation length of about l0mm, total thickness of almost 16 radiation lengths and it allows for 18 samplings in depth (10 in Y and 8 in X views).2.8 Star tracker (AMICA)The main purpose of Astro Mapper for Instrument Check of Attitude (AMICA) is to provideaccurate pointing direction for AMS02. AMICA will give a precise measurement of the AMS02 observing direction with a few arc-sec accuracy. The electronics unit is based on a VME bus which contains the processor (DSP21020) and housebeeping boards.3 AMS02 Physics3.1Antimatter searchOur region of the universe is certainly dominated by matter. Tiny amount of antiprotons and positrons present in cosmic rays can be explained as secondaries of ordinary matter (protons, electrons and nuclei consisting in protons and neutrons) and gamma rays interacting with interstellar material. At present day, from the direct observation we have Ny= 411.4/cm^3 and NB-> NB (at least in our neighborhood). According to Sakharov,}'4} to generate the baryon asymmetry three principles of karyogenesis should be fulfilled: non conservation of baryonic charge, breaking of C and CP invariance (symmetry breaking between particles and antiparticles) and the deviation from thermal equilibrium. There are several scenarios of karyogenesis which are based on the assumption of C and CP violation and give the cosmological baryon asymmetry with β=constant and no cosmological antimatter. However if charge symmetry is broken spontaneously, then in different CP domains the universe could be either baryonic or anti-baryonic. The size of these domains may be cosmologically large if after their formation the universe has passed through a period of inflation (exponential expansion). In principle, subsequent karyogenesis can lead to separate regions containing matter and antimatter galaxies. Recent reviews of (anti)-karyogenesis scenarios can be found in references.It has been shown that the ratio of extragalactic/galactic cosmic rays should increase with energy owing to the fact that the escape rate of the galactic cosmic rays from the galaxyincreases with energy. It is also argued that the galactic wind impedes the entrance of extragalactic cosmic rays and the cosmic rays may not propagate towards the Earth from tens of Mpc as required by some models. On the other hand it would not be accurate to estimate from how far the extragalactic nuclei can reach the Earth since we have very limited knowledge about the extragalactic field strength. It is however true stating that more sensitive test for extragalactic antimatter can be done at high rigidities (>hundreds of GV). Fig.18 shows expected AMS02 sensitivity to antihelium nuclei for 3 years of data taking period on ISS.Until recently, the search for antinuclei and antiproton has been carried out by stratospheric balloons,and on spacecrafts and no antinuclei was observed. The limits on antihelium/helium ratiopublished by various.Dark matterThe observation of stars in spiral galaxies enable rotational velocities of stars to calculate the mean density of the matter as a function of the distance from the galactic center. From the recent WMAP measurements of Cosmic Microwave Background (CMB) anisotropies, the total amount of matter is close to the critical density for a flat Universe with matter=0.27 1 0.04. The contribution of the luminous matter (stars, emitting clouds of gases) isΩ<0.01 and a precise determination of primeval abundance of deuterium provide strong limits on the value of baryondensityΩ=0.045+-0.005.}3}} The conclusion from these measurements is that most of the matter is non-luminous and non-baryonic. The weakly interacting massive particles (WIMPS), postulated in minimal supersymmetric standard model (MSSM) and in other R-parity conserving supersymmetric models, are particularly attractive to explain dark matter's nature. In this framework the lightest supersymmetric particle (LSP), stable neutralino, x, a neutral scalar boson being also its own antiparticle, is the most quoted candidate. Indirect signals may be produced by annihilation of neutralinos inside celestial bodies (Earth and Sun) where x`2s have been captured and accumulated.When x x annihilations take place in the galactic halo specific: signals would emerge.The stable cosmic ray species generated in neutralino annihilations include gamma rays, neutrinos,positrons, antiprotons and antideuterons and, in the same amounts, their counterparts with opposite lepton and baryon numbers.In the following we will discuss AMS02's capabilities, being the unique experiment to measure all neutralino annihilation products (except neutrinos) with the same apparatus.3.2.1 Gamma raysThe gamma rays are important tracers to probe the high energy processes in Universe. They travel over the entire Universe along straight lines without significant absorption and transport information about high/extreme energy interactions, objects or events from distant domains. Among proposed dark matter gamma ray sources, there are the Galactic center, the whole Milky Way halo, external galaxies and cosmological sources.About 20~30% of the energy released in WIMP annihilations goes into gamma rays. Most of them (-90%) are generated in the decay of neutral pions in fragmentation processes.A compilation of estimates of flux sensitivities is given in Fig.20 for space borne (upper,left part in the Figure) and Earth based (lower right) experiments showing which will be the progress innext decade arid what signal levels will be.Most of the high energy gamma ray data come from EGRET experiment on Gamma Ray Observatory (GRO). After the completion of GRO program (in June 2000) there is no experiment measuring high energy gamma rays in space. In upcoming decade there will be three experiments, AGILE (in 2004), AMS02 on ISS and GLAST (2007) which will be able to cover the energy spectrum region from 20 MeV up to about 300 GeV.Despite their low flux, high energy gamma rays bring valuable information complementary to those gathered from charged particles. Since they do not deflect on their path during the travel in the (inter) galactic magnetic field, it is possible to point to the galactic or extra galactic source directions. A good source pointing and capability to perform accurate spectral studies require good angular and energy resolutions respectively.3.2.2 AntiprotonsCalculation of secondary antiprotons, due to interactions of cosmic rays with Interstellar material,have greatly improved in recent years}4a,as1 and have shown that at low energy (below kinematic limit) the secondary spectrum is much flatter than previously believed and fits remarkably well the experimental data.}46} This makes the extraction of supersymmetric signal from the background more difficult. Although the measured antiproton flux gives rather stringent limits on MSSM models with the highest annihilation rates, the experimental upper limits may be used to bound from below the lifetime of hypothetical R-parity violating decaying neutralinos. In some other scenarios with a clumpy halo (which enhances the annihilation rate) there may be the possibility to detect heavy neutralinos through spectral features above several GeV.Fig.21 shows antiproton/proton ratio given by balloon experiments together with predictions by theoretical calculations. Solid curves are upper and lower limit assuming secondary production only. Dashed curve is a similar calculation by L. Bergstrom and P. Ullio.Instead, Fig.22 includes few thousands of antiprotons measured during last four decades by balloon borne experiments as well as AMSO1.The errors (both statistic and systematic) are larger at higher energies. The figure shows also the AMS02 capability to extend the energy range up to about 300 GeV with much higher statistics.3.2.3 Electrons and PositronsThere has been a measurement in a balloon-borne experiment (HEAT) with an excess ofpositrons around 7 GeV over that expected from ordinary sources. However, since there are many other possibilities to create positrons by astrophysical the interpretation is not yet conclusive. The measurement of positron indication for neutralinos spectra could give sources accurate a clear annihilation with rather precise determination of the neutralinos mass.3.2.4 AntideuteronsAntideuteron production from proton-proton collisions is a rare process and it may be less rare in neutralinos annihilation and recently has been pointed out that antideuterons in space could be more promising probe to look for the exotic sources than antiprotons.In particular considering the WIMP pair annihilation in the galactic halo, at energies below about 1 GeV per nucleon the primary antideuteron spectrum would be quite dominant over the secondary one (see Fig.24). The antideuterons measurements open up interesting perspectives for xx annihilation in space.The study of relative abundances of elements and isotopes will yield to a better understanding of origin, propagation, acceleration and confinement time of cosmic rays in our galaxy. The presence of Earth atmosphere restricts this kind of measurements to be carried out at high altitudes with as low as possible residual atmosphere or better, in space where the effect of Earth's atmosphere is negligible. Nowadays, the experiments aiming to perform elemental and isotopic measurements have suffered limited exposure time, residual atmosphere corrections and relative systematic errors and limited energy ranges.In galactic cosmic ray propagation models the diffusion coefficient as a function of momentum and the reacceleration are determined by the energy dependence of B/C ratio. The radioactive nuclei data are used, instead to derive a range for the height of the cosmic ray halo as well as to determine the residence time of the galactic cosmic rays in different propagation models. 4 Conclusions The AMS02 is scheduled for installation on the main external truss of the International Space Station in early 2006. Its three years exposure, large acceptance, state-of-art detectors and. superconducting magnet will allow accurate measurements of cosmic rays up to the unexplored TeV region.AMS02 will accurately measure the light element fluxes which are essential for better understanding of cosmic ray origin, propagation, and acceleration mechanisms .AMS02 will search for cosmological antimatter (antihelium and anamson) with unprecedented sensitivity. It is worth underlining that, among those planned for next decade, it will bethe unique detector capable of measuring simultaneously four different rare products of } annihilation. In addition the AMS02 will open up a window on exotic/other physics such as the study of strangelets.翻译中文国际空间站(空间站)上进行的阿尔法磁谱仪(ams02)实验摘要:阿尔法磁谱仪实验基于两种实验现象.简化实验配置(ams01)的首次航行(sts-91)已经在1996年6月在发现号航天飞机上成功进行.实验仪器配置的最终版本(ams02)将被安装在国际空间站(空间站)作为一个独立的模块,2006年初在经营期三年.ams02的主要科学目标包括寻找反物质和暗物质在宇宙射线.在这项工作中我们将讨论实验的细节比如说在在国际空间站上如何改进ams02的物理性能.关键词:阿尔法磁谱仪,国际空间站,反物质,暗物质1简介一个可能存在于宇宙学领域的反物质或天文“反”的对象和性质的暗物质在宇宙中是现代天体物理学和宇宙学的根本问题.该阿尔法磁谱仪实验由于其大接受范围(~0.65平方米)和较强的粒子的识别能力,将这些基本方面以前所未有的灵敏度研究.这要求测量的物理量如粒子的动量,电荷和速度与最高程度的信心.一个明确的证明存在宇宙反物质会观察反物质核在宇宙射线.一个观察一个单一反氦或较重的原子核,表明原始反物质确实存在,它是离我们不远的.该阿尔法磁谱仪02将能够在10^9个氦核区分一个反氦核之间的~估计背景颗粒超过3年.该项目的目的是实现两相.1998年六月,基线配置实验在发现号航天飞机任务10天51.70轨道在海拔320到390公里之间.从这个使命(sts-91)我们收集的珍贵信息探测器性能在实际空间的条件和可能的来源的背景.阿尔法磁谱仪一号也测量,为第一时间,准确性等空间,宇宙射线通量电子伏特地区几乎覆盖整个地球表面.该探测器布局,性能和物理结果阿尔法磁谱仪一号在sts-91飞行(阿尔法磁谱仪-01)详细描述的地方.在这项工作中我们将讨论实验配置以及物理能力阿尔法磁谱仪号2在国际空间站.2详细的阿尔法磁谱仪02实验是一个大型的阿尔法磁谱仪02验收,高精度超导磁光谱仪用于测量宇宙射线光谱个别要素——以<25为控制区.它也可以测量高能量伽马射线多达几百电子伏特非常好的源指向能力.图一显示的细节阿尔法磁谱仪02实验.总共有227300个电子渠道各提供16位的信息与事件率高达2的国家对应一个总的数据率超过1千兆位/秒数据采集电子将减少事件的大小,通过适当的过滤,以分配的下行数据速率为2兆位/秒.所有电子及机械配件阿尔法磁谱仪02测试在真空.影响电离总剂量(6戈瑞/年)的所有关键部件是广泛的测试.该探测器的目的是查明宇宙射线,同时尽量减少多重散射和大角度核散射发生在跟踪探测器的部分.大接受反氦搜索,良好的颗粒刚度和速度的决议以及冗余测量和/电子排斥>10^6其他关键参数的设计.该阿尔法磁谱仪02重6760公斤,将有一个功率消耗2千瓦.在下面的阿尔法磁谱仪02分将描述从上下游.2.1辐射探测器(公司)跃迁辐射探测器的目的是单独的一个磷信号区分正负和相对背景(磷和电子一分别)与排斥因素103一102的能量范围从10~300电子伏特.这种排斥因子结合电磁量能器将提供一个全面和+ /10^排斥因子6在90%的电子效率.探测器由20层6毫米直径的稻草管交替与20毫米的10层聚乙烯/聚丙烯纤维散热器的嗡嗡声.管子填充80%~20%混合氙÷二氧化碳在1酒吧从一个循环气系统设计运行在空间为>3年.墙体材料稻草管是一个72μ米复合箔.上下4层运行的方向(平行于阿尔法磁谱仪02磁场)而中央层在垂直方向提供二维跟踪,粒子鉴别.2.2时间飞行(飞行)系统飞行时间系统的目的是提供快速(第一级)触发的实验,测量飞行时间的粒子穿越探测器上/下分离比10克,测量的源电荷的粒子(除德/车测量从硅跟踪)和确定电子反质子与质子和正电子从高达1——2电子伏特.预计总时间分辨率≈140聚苯乙烯质子和重宇宙线核(见图3内在分辨率).它由四面(参见图4)宣读了总人数的144浜85946光电管.设计阿尔法磁谱仪02飞行系统是由约束操作0.1~0.3吨杂散磁场.选择时,光的形状是由这个问题指南.光导游确实是倾斜的角度减少之间的磁场和点轴.2.3超导磁体(供应链管理)一个具有挑战性的特征的阿尔法磁谱仪02探测器是其强大的超导磁铁.这是第一个大型超导磁体用的空间,它有一个弯曲的}楼主}0.8商标^2这将是至关重要的执行一个敏感的寻找反物质(他)的刚度(磷比值)范围从0.1货车的几个电视.磁偶极子线圈由2}连同2套小赛场线圈(参见图)与总冷量约2300公斤.赛马场线圈设计,增加整体偶极场,尽量减少杂散偶极场以外的磁铁(最大磁场在一个半径为3米是4机器翻译以避免不理想的扭矩对国际空间站的相互作用所造成的与地球磁场.所有线圈缠绕高纯度铝稳定铌钛铝导体.磁铁将维持在温度为1.8钾和2500升的超流氦冷却,而应运行三年无加气站(优化的热损失).2.4反符合 (交流)该阿尔法磁谱仪阿尔法磁谱仪二号电系统(图6)的目的是保证触发只对那些粒子通过光圈的阿尔法磁谱仪02超导磁铁.它是放置在磁铁免费孔内表面覆盖的超导磁体.交流系统由薄闪烁体板两端的读出.2.5硅跟踪器(ST)硅阿尔法磁谱仪02跟踪器设计进行高精度测量的刚度,他签署了电荷和电荷的源的粒子穿越它.总共有192个梯子可变数量的硅对待粘在一起,读出肢体上的前端电子学.长度的梯子从36厘米至60厘米(积极)相应的≈6.4米^2双面表面活性.图显示了一个详细设计的梯子,其主要成分.一个关键点,在组装长梯,是它需要高精度的传感器和切割组装的阶梯.图9显示之间的差异衡量(通过参考十字架,每个传感器)和名义(完全一致)的传感器的位置的73个梯子.有效值的分布是4μ米读出电子学是基于低噪声,低功耗(~0.7毫瓦),高动态范围超大规模集成电路,前置放大器,整形器,采样和保持电路连接到传感器通过700去耦电容器.性能的阿尔法磁谱仪02梯子已经过测试的最小电离粒子和重离子.fig.10表明残差的重建和预期的立场400电子伏特介子穿过原型梯子.由此产生的空间分辨率为8.5米7.1米(22μ米)弯曲(非弯曲)方向的400电子伏特的介子和20电子伏特/氦粒子分别.跟踪冷却系统变导电热管中的冷却液(二氧化碳)的毛细管力.热收集3热管循环再带动散热器耗散(参见图13).该系统将消耗192瓦特(1瓦特的每个前端混合对)和满足要求的操作(生存)的温度of-10℃+ 25℃(-20℃到+ 40℃)和- 10到+ 40℃(-20到+ 60℃)及晶片前端杂种分别.硅晶圆温度稳定性要求每轨道3钾和接受的最大梯度之间的任何硅晶片实现10的总重量是约186公斤,总耗电量为734W.粒子识别能力的阿尔法磁谱仪-u2近贴聚焦富探测器将提高信心的测定电荷符号,将提供高水平的冗余需要高纯度的样品的正电子和反质子,将执行鉴定同位素质量< ~15 - 20,在动量范围1电子伏特/ < / < - 12电子伏特/炭和将确定的化学组成元素铁的刚度限的光谱仪,磷/ < -1tv.丰富的阿尔法磁谱仪02探测器由一个平面散热器材料,分离检测平面由一个漂移的空间,可以扩大切伦科夫环.探测器模块包括一个光矩阵引导耦合到下午连接到插座和前端电子学读出. 本作的选择合作是有一个气凝胶散热器厚3厘米与1.05例以覆盖动量区间与速度分辨率Δβ/β约1.5* 10^3.另外一个0.5厘米厚的氟化钠放在中央广场,对应的孔在像素平面,将提高检测粒子的旅行到中央孔方向.由于电磁量能器(艾考尔)是位于下方的丰富的光电倍增管,平面,平面设计与中央孔,避免被动的材料在前面的.fig.14显示三维设计富有计数器随着性能试验获得的结果在欧洲核子研究中心的特殊试验束.2.7电磁量能器(ECAL)为了实现良好的磷分离(设计排斥因素一105),必须进行准确的测量正电子谱(从几个电子伏特到一1),阿尔法磁谱仪02包括细粒取样量能器(艾考尔)能够三维成像的发展和歧视之间的强子和电磁级联.艾考尔是一个取样装置与纤维结构.它有一个方形六面体形状的65.8厘米和16.5厘米的深度.它是分割9超级图层沿其深度和每个超级层的总厚度18.5毫米,包含11个槽铅箔交错的1毫米直径的光纤与环氧树脂胶.该热有一个辐射长度约l0mm,总厚度约16辐射长度,它允许18个采样深度.2.8 星象跟踪仪(公司)主要目的,天文仪器检查映射态度(公司)是提供准确的指向方向阿尔法磁谱仪02.这将使精确测量的阿尔法磁谱仪02观测方向几角秒的精度.该电子装置是基于总线包含处理器和家庭板. 3阿尔法号2物理结构3.1反搜索我们的宇宙中肯定是占主导地位的问题.少量的反质子和正电子在宇宙射线可以被解释为二级的普通物质(质子,电子和原子核由质子和中子)和伽玛射线相互作用与星际物质.目前,从直接观察我们纽约=411.4 /厘米3,^铌-铌(至少在我们附近).根据萨哈罗夫,产生重子不对称性三大原则:非核生成应履行保护重子荷,打破和处长不变性(对称破缺之间的粒子和反粒子)和偏离热平衡.有几种情况核生成所依据的假设和破坏,给宇宙重子不对称β=常数和宇宙反物质.但是如果电荷自发对称破了,然后在不同蛋白域宇宙可能是重子或反重子的.这些领域的规模可能是宇宙的大如果宇宙形成后经历了一个通货膨胀(指数膨胀原则,随后碎片可以导致不同的区域含有的物质和反物质星系.最近的评论(反)核生成情景中可以找到参考.它已经表明,比星系银河宇宙射线应增加–能源由於逃跑率从银河系银河系的宇宙射线增加能量.它还认为,银河风阻碍入口星系宇宙射线和宇宙射线可能不是向着地球数十所需要的一些预测模型.另一方面是不准确的估计从多远的星系核可以到达地球因为我们有非常有限的知识的河外星系的磁场强度.但这确实说明更敏感试验河外反物质可以在高硬度(>数以百计的货车).图18表明预期阿尔法磁谱仪02敏感性反氦核3年的数据,以期对国际空间站.反核子和反质子进行了平流层气球,和航天器和没有反核子观察.限制反氦氦比出版的各种实验以及模拟灵敏度阿尔法磁谱仪02显示在图19.暗物质弱相互作用重粒子(粒子),假定在最小超对称标准模型(mssm)和其他r-parity保护超对称模型,是特别有吸引力的解释暗物质的性质.在这一框架轻的超对称粒子(路径),稳定的中性,中性标量玻色子也被自己的反粒子,是最广为人知的候选人.间接信号可能产生的毁灭的中性伴子内天体(太阳与地球),已被抓获,并积累.当××湮灭发生在银河系晕具体:信号会出现.稳定的宇宙射线产生的中性湮灭包括伽马射线,中子,正电子,反质子和反氘核和,在同一数额,同行对轻子和重子数.我们在下面将讨论阿尔法磁谱仪2号的能力,是独特的实验测量中性产品(除湮没中微子)具有相同的仪器.3.2.1γ射线伽玛射线是重要的示踪探测高能过程中的宇宙.他们在整个宇宙线没有明显的吸收和运输信息,高能量的相互作用,物体或事件从遥远的领域.拟议中的暗物质的伽玛射线的来源,有银河系中心,整个银河系晕,外部星系和宇宙源.20~30%的能量释放在弱者湮灭到伽玛射线.他们中的大多数(90%)产生的衰减的中性π介子碎裂过程中.大多数高能量伽马射线数据来自白鹭实验伽玛射线天文台(夫人).完成后,公司计划(六月2000)是没有实验测量高能γ射线空间.在接下来的十年将有三个实验,阿尔法磁谱仪02国际空间尽管他们的低流量,高能量的伽马射线带来了宝贵的补充信息,这些收集到的带电粒子.因为他们不偏离其路径在旅行中(间)银河系磁场,它可能是指银河系或额外的银河源方向.一个很好的来源和能力进行准确的光谱研究需要良好的角和能量分辨率分别为站和转运体,将能够涵盖频谱能量区域从20到约300电子伏特.3.2.3反质子计算二次反质子相互作用,由于宇宙射线与星际物质,大大提高了在最近几年显示,在低能量(下运动极限)二级光谱较为扁平比以前认为适合的实验数据.这使得超对称信号提取从背景更加困难.虽然反质子通量测量作了较为严格的限制模型具有最高的灭绝率,实验上的限制可能被用来约束从下面的寿命违反假设衰减的中性伴子.在其他一些情况下与块状的光环(提高湮没率)可能有可能检测中性伴子通过光谱特征以上几个电子伏特图21表明质子/质子比由气球实验与预测的理论计算.固体曲线的上限和下限的假设二次生产.虚线曲线是一个类似的计算和ullio L.相反,图22包括数以千计的反质子测量在过去四年来的气球实验以及阿尔法磁谱仪o1.错误(包括统计和系统)是在更高的能量.图中显示的能力也阿尔法磁谱仪02延长能量高达300电子伏特的高得多的统计.3.2.4电子和正电子的有一个测量在气球实验(热)与过量的正电子在7电子伏特超过预期从普通的来源.然而,因为有许多其他的可能性创造正电子被天体物理解释尚未定论.测量正电子指示中性伴子谱可以给来源准确清晰湮没而精确测定的.中性伴子质量.反氘核生产从质子碰撞是一种罕见的过程中,它可能不那么罕见的中性伴子湮没和最近已经指出,反氘核空间可能更有前途探头寻找异国情调的来源比反质子.特别是考虑到懦夫对湮没在银河系晕,在能量低于约1电子伏特每核子小学反氘核谱是显性的中学之一(见fig.24).测。
阿尔法磁谱仪
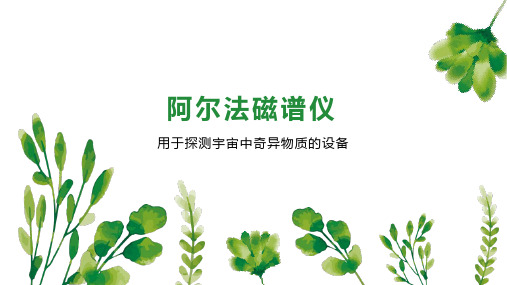
按照航天局计划,“奋进”号完成最后一次飞行后退役,“亚特兰蒂斯”号也完成“绝唱之旅”。美国航天 飞机全部退役,运行30多年的航天飞机项目宣告终结。
阿尔法磁谱仪升空后,在短时间内获得的数据就可使阿尔法磁谱仪正电子能谱的测量范围和精度超过正在空 中运行的PAMELA空间探测器(由意大利、俄罗斯联合研制),并有能力对空间电子能谱几个测量结果作出判断。 今后相当长的一段时期,阿尔法磁谱仪作为最主要的空间粒子探测器之一,对重大的科学前沿课题进行探索,是 意义重大的空间实验。
感谢观看
主结构的主体系外径为1.3米,内径为1.15米,高0.8米的空心高强度铝制圆柱体。永磁体呈条状插入主结构, 其磁场强度高达1400高斯。主结构要求高精度,在生产及装配过程中严格控制偏差,以使其与航天飞机对接时不 产生装配应力。
1996年4月第一台供地面试验的阿尔法磁谱仪主结构顺利完成生产,安装好永磁体后进行了振动试验及离心 试验,试验一次通过,技术状态良好。
项目合作
CERN暨欧洲核子中心的航空照片阿尔法磁谱仪重达6700千克,中国多家单位参加了研制,其中,中国科学院 高能物理研究所和中国运载火箭技术研究院与法国、意大利的两个单位合作,研制了阿尔法磁谱仪电磁量能器, 能够测量能量高达TeV的电子和光子,是寻找暗物质的关键子探测器。参加阿尔法磁谱仪国际合作的中国单位还 包括中国科学院电工研究所、上海交通大学、东南大学、山东大学、中山大学,以及中国台湾的“中央研究院” 物理研究所、“中央大学”、中山科学研究院等。
暗物质
AMS-一种粒子加速器,绰号为太空的大型强子对撞机。AMS的最初实验结果发现了可能是暗物质的痕迹。不 仅是AMS,很多地上甚至深埋地下的实验室也曾发现过暗物质的痕迹。
便携式傅立叶变换红外光谱仪ALPHA中文介绍

便携式傅立叶变换红外光谱仪ALPHA中文介绍便携式傅立叶变换红外光谱仪ALPHA是一种高精度、高效率的红外光谱仪。
它能够在更小的尺寸和便捷的操作下实现材料的快速分析和检测。
ALPHA搭载了先进的傅立叶变换红外(FTIR)技术,能够提供高分辨率和高灵敏度的光谱数据,广泛应用于材料科学、化学、医药、食品安全等领域。
ALPHA采用了紧凑的设计,外观小巧轻便,重量仅为1.9公斤。
这使得它非常适合移动分析与实地检测。
不论是在实验室、工场还是野外场景,ALPHA都能够提供高质量的红外光谱数据。
ALPHA具备广泛的波数范围选择,从550到7800 cm^-1,可以适应不同物质的分析需求。
通过傅立叶变换光谱技术,ALPHA能够捕捉并分析样品在红外光谱范围内的振动和伸缩信息,帮助用户快速准确地了解样品的结构和化学成分。
高性能的探测器是ALPHA的一大亮点。
它采用了湿式红外探测器,具有高灵敏度和快速响应的特点。
这意味着ALPHA在取样时间上十分高效,仅需几秒钟就能够获取准确可靠的光谱数据。
此外,高性能的探测器还能够提供窄带宽的光谱线,使得分析结果更加精确。
ALPHA支持多种采样方式,用户可以根据具体需求选择适合的采样方式。
例如,它可以通过直接固定在仪器顶部的透射采样盒进行透射光谱的测量。
此外,还可以使用反射采样配件来进行反射光谱分析。
这种灵活性使得ALPHA能够满足各种类型样品的分析需求。
除了高性能的硬件配置,ALPHA还配备了用户友好的软件界面。
软件提供了直观易用的操作界面,可以实时显示、保存和分析光谱数据。
用户可以通过该软件进行光谱数据处理、峰识别、峰拟合等操作。
软件还支持多种光谱库的导入和比对,方便用户对采集的光谱进行拟合和标识。
通过将ALPHA与云端服务器连接,用户可以轻松实现数据的分享和远程访问。
这为用户提供了更大的交流与合作空间。
此外,数据的云端存储和管理,也可以避免本地数据丢失的风险。
总之,便携式傅立叶变换红外光谱仪ALPHA以其高精度、高效率的特点成为材料分析、品质控制和环境监测等领域的理想选择。
阿尔法磁谱仪原理
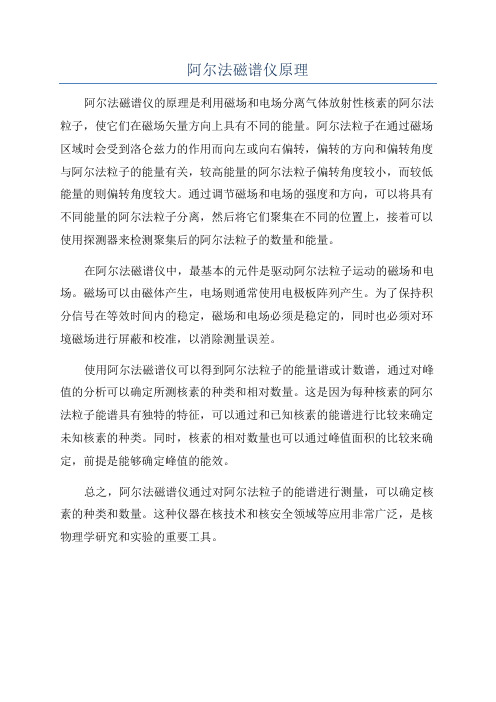
阿尔法磁谱仪原理
阿尔法磁谱仪的原理是利用磁场和电场分离气体放射性核素的阿尔法粒子,使它们在磁场矢量方向上具有不同的能量。
阿尔法粒子在通过磁场区域时会受到洛仑兹力的作用而向左或向右偏转,偏转的方向和偏转角度与阿尔法粒子的能量有关,较高能量的阿尔法粒子偏转角度较小,而较低能量的则偏转角度较大。
通过调节磁场和电场的强度和方向,可以将具有不同能量的阿尔法粒子分离,然后将它们聚集在不同的位置上,接着可以使用探测器来检测聚集后的阿尔法粒子的数量和能量。
在阿尔法磁谱仪中,最基本的元件是驱动阿尔法粒子运动的磁场和电场。
磁场可以由磁体产生,电场则通常使用电极板阵列产生。
为了保持积分信号在等效时间内的稳定,磁场和电场必须是稳定的,同时也必须对环境磁场进行屏蔽和校准,以消除测量误差。
使用阿尔法磁谱仪可以得到阿尔法粒子的能量谱或计数谱,通过对峰值的分析可以确定所测核素的种类和相对数量。
这是因为每种核素的阿尔法粒子能谱具有独特的特征,可以通过和已知核素的能谱进行比较来确定未知核素的种类。
同时,核素的相对数量也可以通过峰值面积的比较来确定,前提是能够确定峰值的能效。
总之,阿尔法磁谱仪通过对阿尔法粒子的能谱进行测量,可以确定核素的种类和数量。
这种仪器在核技术和核安全领域等应用非常广泛,是核物理学研究和实验的重要工具。
布鲁克红外光谱仪阿尔法
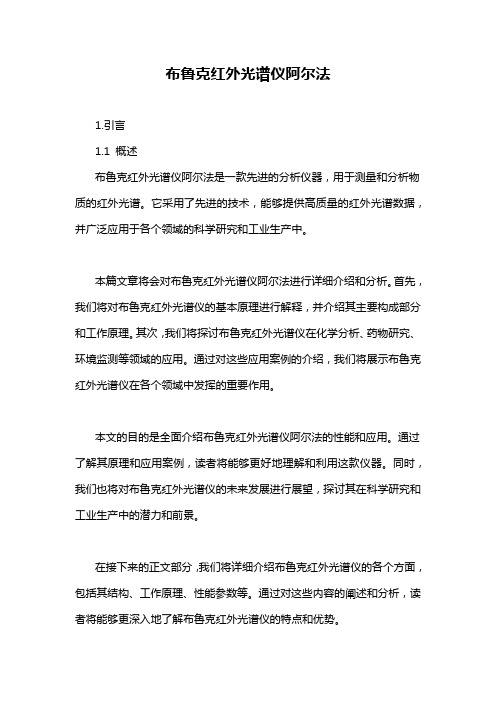
布鲁克红外光谱仪阿尔法1.引言1.1 概述布鲁克红外光谱仪阿尔法是一款先进的分析仪器,用于测量和分析物质的红外光谱。
它采用了先进的技术,能够提供高质量的红外光谱数据,并广泛应用于各个领域的科学研究和工业生产中。
本篇文章将会对布鲁克红外光谱仪阿尔法进行详细介绍和分析。
首先,我们将对布鲁克红外光谱仪的基本原理进行解释,并介绍其主要构成部分和工作原理。
其次,我们将探讨布鲁克红外光谱仪在化学分析、药物研究、环境监测等领域的应用。
通过对这些应用案例的介绍,我们将展示布鲁克红外光谱仪在各个领域中发挥的重要作用。
本文的目的是全面介绍布鲁克红外光谱仪阿尔法的性能和应用。
通过了解其原理和应用案例,读者将能够更好地理解和利用这款仪器。
同时,我们也将对布鲁克红外光谱仪的未来发展进行展望,探讨其在科学研究和工业生产中的潜力和前景。
在接下来的正文部分,我们将详细介绍布鲁克红外光谱仪的各个方面,包括其结构、工作原理、性能参数等。
通过对这些内容的阐述和分析,读者将能够更深入地了解布鲁克红外光谱仪的特点和优势。
最后,在结论部分,我们将对整篇文章进行总结,并对布鲁克红外光谱仪的未来发展进行展望。
我们相信,通过本文的介绍,读者将能够更加全面地了解和认识布鲁克红外光谱仪阿尔法,并对其在科学研究和工业生产中的应用有更深入的认识和理解。
文章结构部分主要介绍了本文的篇章组织和框架安排。
通过清晰的文章结构,读者可以更好地理解文章内容和思路,并能轻松地找到所需信息。
本篇长文主要分为引言、正文和结论三个部分。
1. 引言引言部分以简要介绍文章的背景和问题为开端,旨在吸引读者的兴趣并引出文章的主题。
在引言部分,我们将依次介绍概述、文章结构和目的三个方面。
1.1 概述文章概述部分将对布鲁克红外光谱仪阿尔法进行概括性的介绍,包括其基本特点和应用领域。
读者通过概述部分可以初步了解文章所要讨论的主要内容。
1.2 文章结构文章结构部分即本节所在的内容。
在这一部分,我们将详细介绍本文的篇章组织和框架安排,包括各个章节的主题和内容概要。
alpha谱仪应用
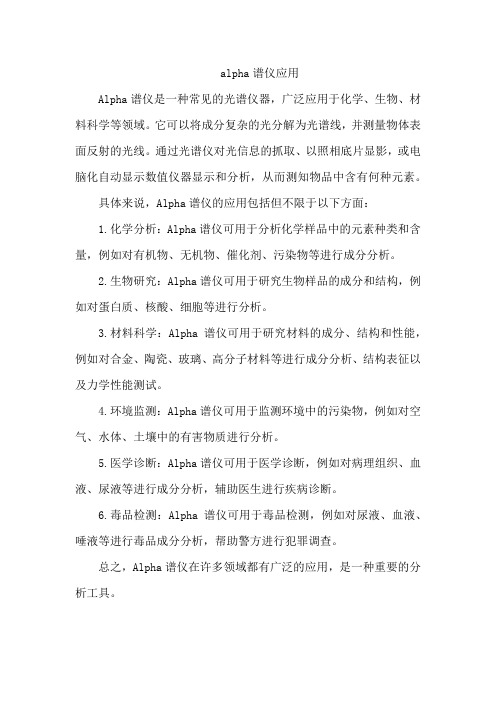
alpha谱仪应用
Alpha谱仪是一种常见的光谱仪器,广泛应用于化学、生物、材料科学等领域。
它可以将成分复杂的光分解为光谱线,并测量物体表面反射的光线。
通过光谱仪对光信息的抓取、以照相底片显影,或电脑化自动显示数值仪器显示和分析,从而测知物品中含有何种元素。
具体来说,Alpha谱仪的应用包括但不限于以下方面:
1.化学分析:Alpha谱仪可用于分析化学样品中的元素种类和含量,例如对有机物、无机物、催化剂、污染物等进行成分分析。
2.生物研究:Alpha谱仪可用于研究生物样品的成分和结构,例如对蛋白质、核酸、细胞等进行分析。
3.材料科学:Alpha谱仪可用于研究材料的成分、结构和性能,例如对合金、陶瓷、玻璃、高分子材料等进行成分分析、结构表征以及力学性能测试。
4.环境监测:Alpha谱仪可用于监测环境中的污染物,例如对空气、水体、土壤中的有害物质进行分析。
5.医学诊断:Alpha谱仪可用于医学诊断,例如对病理组织、血液、尿液等进行成分分析,辅助医生进行疾病诊断。
6.毒品检测:Alpha谱仪可用于毒品检测,例如对尿液、血液、唾液等进行毒品成分分析,帮助警方进行犯罪调查。
总之,Alpha谱仪在许多领域都有广泛的应用,是一种重要的分析工具。
阿尔法探测器 实验心得

阿尔法探测器实验心得通过该实验,对所学的知识有了进一步的了解。
在实验的过程中,出现了一些问题,不过最后都得以解决。
然而通过这些内容,使我对这些知识点更加印象深刻。
阿尔法(AMS)是在太空运行的最强大、最灵敏的粒子物理探测器,它不断探寻和推进物理学研究的最前沿。
作为一个磁谱仪,AMS的独特之处在于,它在空间中直接测量宇宙中的带电粒子和核子。
相对于以往的实验,AMS极大地提高了测量的准确度和精确度。
这归功于AMS运行时间长、接受度大、探测器系统冗余可靠、在CERN的加速器上进行的细致的束流测试,以及高度稳定、均匀的轨迹探测器温度控制。
这些特点保证了AMS的测量精度达到1%的水平,这要求物理及天文学家们创造出新的模型来解释这些精确的数据。
“宇宙中的辐射源(如恒星、大爆炸)产生的碳和氧的原子核,与恒星际物质发生碰撞,分裂成较轻的锂、铍和硼的原子核,到达我们地球大气层外时,既有碳、氧原子核,也有锂、铍和硼的原子核。
”聚焦在碳、氧的流强和硼-碳流强比测量数据分析的李样对其工作解释道:“硼与碳的流强比,提供关于宇宙线传播和传播过程中所穿越的恒星际物质的信息,可以用于对宇宙线传播模型进行限制。
通常人们以快速移动气体在磁化等离子体中的扩散过程来建立宇宙线传播的模型。
不同的磁化等离子体模型预言的硼碳流强比具有不同的特征。
硼碳流强比的测量对理解银河系宇宙线的传播机制有着重要的意义,可以有效限制宇宙线传播模型的各种参数。
”宇宙线传播模型的重要性还在于与新物理(如暗物质)有直接联系。
因为宇宙线在银河系传播过程中也会与恒星际物质作用,产生反质子、正电子等次级产物,而这些产物被认为是暗物质湮灭信号的本底。
暗物质湮灭信号的确定对宇宙线传播模型有较强的依赖关系,因此理解好宇宙线的传播才能更好地理解暗物质信号。
硼碳流强比一直是理论和实验测量最为理想的选择。
- 1、下载文档前请自行甄别文档内容的完整性,平台不提供额外的编辑、内容补充、找答案等附加服务。
- 2、"仅部分预览"的文档,不可在线预览部分如存在完整性等问题,可反馈申请退款(可完整预览的文档不适用该条件!)。
- 3、如文档侵犯您的权益,请联系客服反馈,我们会尽快为您处理(人工客服工作时间:9:00-18:30)。
α射线能谱测量实验报告核工1201 林勇 20120983一、实验目的:1、了解α谱仪的工作原理及其特性。
2、掌握应用谱仪测量α粒子能谱的方法。
3、测量获取表中各种放射源在不同真空度下的能谱图,为不同放射源、不同真空度、不同探源距下α能谱的解谱方法研究准备数据,同时为α能谱库的建立做一些探索性工作。
二、实验内容1、测定谱仪的能量分辨率,并进行能量刻度。
2、测量未知α源的能谱,并确定α粒子能量。
三、实验原理1、α放射源α放射源是以发射α粒子为基本特征的放射源。
α粒子能量一般为4-8MeV,在空气中的射程为2.5-7.5cm,在固体中的射程为10-20um。
由于α粒子穿透物质的能力弱,为此,设计制备α放射源时必须考虑源的自吸收。
目前工业用的α放射源主要有241Am、238Pu、239Pu、244Cm(锔)和210Po(钋)等,用量最大的是241Am源。
因为241Am容易生产,价格便宜,而且半衰期长。
常用α放射源核素数据2、α谱仪本次试验仪器拟采用西南科技大学国防重点试验室α能谱仪,该α谱仪为美国ORTEC公司生产的8通道α能谱仪,型号为:ALPHA-ENSEMBLE.ORTEC在α谱仪上采用超低本底和PIPS工艺(表面钝化、离子注入、可擦洗)硅探测器,同时真空舱室也为超低本底材料。
面积上提供300、450、490、600、900和1200平方毫米的选择,有效耗尽层100μm。
结构特性与性能指标:样品直径可从13mm至51mm。
探测器与被测样品之间有10档距离可选,相邻两档之间的距离差为4mm,最大距离可达44mm。
真空计:范围10mTorr到20Torr(1 Torr ≈ 133.322 Pa)。
探测器偏压:范围0±100V,大小和正负极性可调节。
漏电流检测器:范围0到10,000nA,显示分辨率3nA。
脉冲产生器;范围0到10MeV,稳定性<50ppm/ºC,脉冲的幅度可调。
数字化MCA(多道脉冲幅度分析仪):通过软件可设置系统转换增益(道数)为256、512、1024、2048或者4096道,细调增益为0.25到1;增益稳定性:≤150ppm/ºC;每个事件的转换时间(死时间):<2µs。
数字化稳谱、ADC的零点(ZERO)和下阈(LLD)均由计算机调节设置。
谱仪的探测器偏压、漏电流均可在软件相关界面上以数字和图形显示出来。
输入电源:120/240 V ac,50/60 Hz输入功率50W。
通讯:USB2.0接口。
每一个Alpha Ensemble最终提供一条电缆给PC。
应用软件:MAESTRO-32或AlphaVision工作条件:温度0ºC到50ºC,相对湿度≤ 95%。
分辨率与本底:基于使用450mm2 ULTRA-AS探测器和高质量的241Am点源,能量分辨率(FWHM):≤20KeV (探测器到源的距离等于探测器的直径),探测器效率:≥25% (探测器到源的距离小于10mm),本底:在3MeV以上,每小时计数≤1。
所有型号均可选择用于反冲抑制保护的样品盘选项。
主要特点:探测室、前放、主放和多道一体化,系统具有高度的可靠性;全部功能由计算机通过仿真软件控制;每一路都完全独立、互不干扰或影响;每一路谱仪可配不同规格型号探测器;容纳样品直径最大可达51mm,探测器面积最大可达1200mm2;系统可以扩展至8台共64路探测器。
3、α谱仪工作原理α粒子通过物质时,主要是与物质的原子的壳层电子相互作用发生电离损失,使物质产生正负离子对,对于一定物质,α在其内部产生一对离子所需的平均能量是一定的(即平均电能w),所以在物质中产生的正负离子对数与α粒子损失的能量成正比,即:EN=W公式中N为α粒子在物质中产生的正负离子对数目,E是在物质中损失的α粒子能量。
如果α粒子将其全部能量损失在物质内,E就是α粒子的能量。
半导体探测器是在六十年代发展起来的一种新型探测器,它以半导体为探测介质,其最大优点是能量分辨率高,脉冲上升时间短,体积小。
重原子核进行α衰变放出的α粒子是高速的氦原子核,质量数为4,带2个正电荷,其初速度约为1~2×109cm/s范围内。
由于α粒子在空气中的射程很短(在T=15℃,P=1大气压时,天然放射性核素衰变产生的α粒子,射程最大为Thc’(212Po) 为8.62cm,能量最小232Th为2.5cm),所以测量室应采用真空室,如上图1所示,采用真空泵将测量室抽成真空,这样与探测器接触的α粒子的能量才近似等于放射性核素经过α粒子放出的α粒子的初始能量(近似是因为不可能将测量室抽成绝对真空)。
α粒子在探测器中因电离、激发(由于α粒子的质量很大,所以与物质的散射作用很不明显。
α粒子在空气中的径迹是一条直线,这种直线很容易在威尔逊云室中看到。
)等效应而产生电流脉冲,其幅度与α粒子能量成正比。
电流信号经前置放大器、主放大器放大,出来的电信号通过多道分析器进行数据采集,最后通过计算机采集并显示其仪器谱(实验用α谱仪硬件连接及内部结构框图如图1所示)。
仪器谱以α粒子的能量(即脉冲幅度)为横坐标,某个能量段内α粒子数(或计数率)为纵坐标,即可计算样品中各单个核素发射的α粒子的能量与活度。
理论上,单能α粒子谱是线状谱,应是位于相应能量点处垂直于横坐标轴的单一直线,但由于α粒子入射方向、空气吸收、样品源自吸收的差异和低能粒子的叠加等原因,实际测得的是具有一定宽度的单个峰,其峰顶位置相应于α粒子的能量,谱线以下的面积为相应能量的α粒子的总计数率,峰的半高宽与峰顶能量比值的百分数则为α谱仪的能量分辨率。
图α谱仪硬件连接及内部结构框图但电信号经放大器放大之后,由于电路中寄生电容、电感的作用,电脉冲信号结束之后,输出端并不立即回到零点位。
电感性的寄生参量造成脉冲反冲,需要经过一段时间才能回到0电位,在更严重的情况下,甚至产生自激。
电容性的寄生参量同样使电路需要经过一段时间才能回到0电位。
虽然可以采用基线恢复或极零补偿等方式使输出端电位立即恢复,但是还达不到理想状态。
要是在恢复时间内又有第二个脉冲输入,其脉冲幅度将明显受到影响,在低能端形成拖尾。
如果电路中存在电容性的寄生参量,将高能端将产生拖尾。
在计数率较高时,若两个射线粒子(光子)几乎同时入射,探测器和电路的时间分辨能力不足以区分开而作为一个射线来记录,所得的脉冲能量为两个射线粒子(光子)的能量之和,从而在射线谱中得到一个能量很高的谱峰—和峰。
天然放射性物质进行α衰变放出的α粒子的能量在4~8MeV 之间(能量最大Thc’(212Po) 为8.785MeV,能量最小232Th为3.993MeV),核反应截面很小,产生卢瑟福散射的几率也很小,主要是与核外电子的相互作用。
α粒子与电子碰撞,将使原子电离、激发而损失能量。
α粒子的质量比电子大得多,当它通过吸收体时,需经过多次碰撞才损失较多的能量(因为在一次碰撞中,质量为M、能量为E的带电粒子,转移给电子(质量为m)的最大能量约为4EmM/(M+m)2)。
4、金硅面垒型探测器来测量α粒子能谱.金硅面垒探测器结构如图所示,是用一片N型硅,蒸上一层簿金层(100-2000A),接近金膜的那一层硅具有P型硅特性,这种方式形成的PN结靠近表面层,结区即为探测粒子的灵敏区。
探测器工作时加一反向偏压,带电粒子在空间电荷区(势垒区)内损失能量而产生电子—空穴对其数目同带电粒子的能量损失值E有关: N=E/W,式中N是产生的正、负离子对数,W是产生一对正、负离子对所需的平均能量, 正、负离子对,在一定的反向偏压作用下分别向探测器正、负极漂移,在输出电路产生电压脉冲,电压脉冲的幅度与核辐射在半导体探测器的灵敏区中的损失的能量成正比(若粒子在灵敏区损失其全部能量时);脉冲数与核辐射的强度成正比。
为了提高谱仪的能量分辨率,探测器放在真空室中;另外金硅面垒探测器一般具有光敏的特性,在使用过程中,应有光屏蔽措施。
5、半导体谱仪:半导体谱仪的组成如图1所示金硅面垒探测器是用一片N型硅,蒸上一薄层金(100-200),接近金膜的那一层硅具有P型硅的特性,这种方式形成的PN结靠近表面层,结区即为探测粒子的灵敏区。
探测器工作加反向偏压。
粒子在灵敏区内损失能量转变为与其能量成正比的电脉冲信号,经放大并由多道分析器测出幅度的分布,从而给出带电粒子的能谱。
偏置放大器的作用是当多道分析器的道数不够用时,利用它切割、展宽脉冲幅度,以利于脉冲幅度的精确分析。
为了提高谱仪的能量分辨率,探测器要放在真空室中。
另外金硅面垒探测器一般具有光敏的特性,在使用过程中,应有光屏蔽措施。
金硅面垒型半导体谱仪具有能量分辨率高、能量线性范围宽、脉冲上升时间快、体积小和价格便宜等优点,在粒子及其它重带电粒子能谱测量中有着广泛的应用。
带电粒子进入灵敏区,损失能量产生电子空穴对。
形成一对电子空穴所需的能量,与半导体材料有关,与入射粒子的类型和能量无关。
对于硅,在300K时,为3.62eV,77K 时为3.76eV。
对于锗,在77K时为2.96eV。
若灵敏区的厚度大于入射粒子在硅中的射程,则带电粒子的能量E全部损失在其中,产生的总电荷量Q等于。
为产生的电子空穴对数,e为电子电量。
由于外加偏压,灵敏区的电场强度很大,产生的电子空穴对全部被收集,最后在两极形成电荷脉冲。
通常在半导体探测器设备中使用电荷灵敏前置放大器。
它的输出信号与输入到放大器的电荷量成正比。
探测器的结电容是探测器偏压的函数,如果核辐射在探测器中产生电荷量为Q,那么探测器输出脉冲幅度是。
因此,由于探测器偏压的微小变化所造成的变化将影响输出脉冲的幅度。
事实上,电源电压的变化就可以产生偏压近种微小变化。
此外,根据被测粒子的射程调节探测器的灵敏区厚度时,也往往需要改变探测器的偏压。
要减少这些变化对输出脉冲幅度的影响,前级放大器对半导体探测器系统的性能越着重要的作用。
图2表示典型探测器的等效电路和前置放大器的第一级。
其中一K是放大器的开环增益,是反馈电容,是放大器的总输入电容,它等于是放大器插件电缆等寄生电容。
前置放大器的输入信号是,它的等到效输入电容近似等于,只要,那么前置放大器的输出电压为( 1 )这样一来,由于选用了电荷灵敏放大器作为前级放大器,它的输出信号与输入电荷Q 成正比,而与探测器的结电容无关。
确定半导体探测器偏压对N型硅,探测器灵敏区的厚度和结电容与探测器偏压的关系如下:( 2 )( 3 )其中为材料电阻率(。
因灵敏区的厚度和结电容的大小决定于外加偏压,所以偏压的选择首先要使入射粒子的能量全部损耗在灵敏区中和由它所产生的电荷完全被收集,电子空穴复合和陷落的影响可以忽略。
其次还需考虑到探测器的结电容对前置放大器来说还起着噪声的作用。