Thermo-mechanical and Experimental Analysis of Double Pass Line Heating
形状记忆聚合物记忆过程热力学性能分析

形状记忆聚合物记忆过程热力学性能分析黄天麟;孙慧玉【摘要】Assuming shape memory polymers to be isotropic, with consideration of temperature-dependent creep residual strain,a three-dimensional thermo-mechanical constitutive equation of shape memory polymers( SMPs) is derived from one-dimensional con-stitutive relationship based on viscoelastic theory. A user material subroutine program of the CAE software ABAQUS is compiled to simulate the memory process. Strain/temperature rates impact on the thermo-mechanical and shape memory properties of SMPs is analyzed and it shows a good agreement with the experimental data.%考虑蠕变残余应变的温度相关性,假设形状记忆聚合物为各向同性材料,将一维热力学本构方程扩展到三维。
基于该增量形式的本构,在有限元软件ABAQUS中开发了适用于模拟形状记忆聚合物形状记忆过程的材料接口子程序,对形状记忆聚合物记忆过程进行有限元模拟。
分析了加载速率和变温速率对形状记忆聚合物的热力学性能和记忆性能的影响,数值模拟的结果与实验吻合良好。
【期刊名称】《机械制造与自动化》【年(卷),期】2015(000)004【总页数】4页(P91-94)【关键词】形状记忆聚合物;三维本构;率相关;有限元方法【作者】黄天麟;孙慧玉【作者单位】南京航空航天大学,江苏南京210016;南京航空航天大学,江苏南京210016【正文语种】中文【中图分类】TB381形状记忆聚合物(SMPs)具有变形量大、易赋形、不锈蚀、质轻价廉、响应温度便于调整等优点,受到了广泛关注[1-3]。
Answers to Lesson 7 Part II 形容词和副词
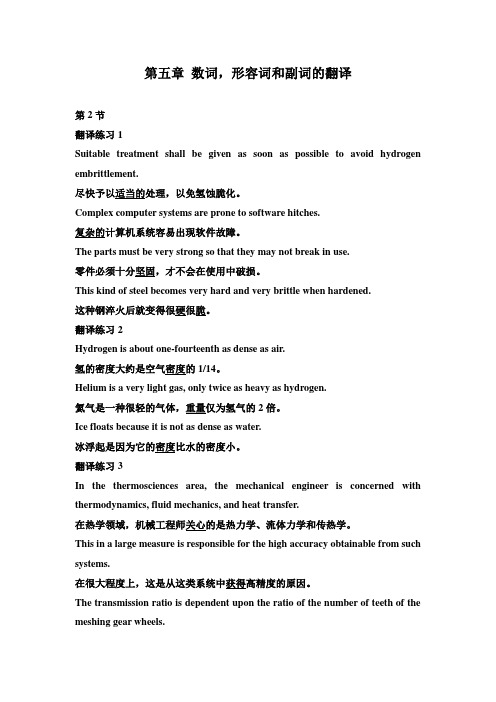
第五章数词,形容词和副词的翻译第2节翻译练习1Suitable treatment shall be given as soon as possible to avoid hydrogen embrittlement.尽快予以适当的处理,以免氢蚀脆化。
Complex computer systems are prone to software hitches.复杂的计算机系统容易出现软件故障。
The parts must be very strong so that they may not break in use.零件必须十分坚固,才不会在使用中破损。
This kind of steel becomes very hard and very brittle when hardened.这种钢淬火后就变得很硬很脆。
翻译练习2Hydrogen is about one-fourteenth as dense as air.氢的密度大约是空气密度的1/14。
Helium is a very light gas, only twice as heavy as hydrogen.氦气是一种很轻的气体,重量仅为氢气的2倍。
Ice floats because it is not as dense as water.冰浮起是因为它的密度比水的密度小。
翻译练习3In the thermosciences area, the mechanical engineer is concerned with thermodynamics, fluid mechanics, and heat transfer.在热学领域,机械工程师关心的是热力学、流体力学和传热学。
This in a large measure is responsible for the high accuracy obtainable from such systems.在很大程度上,这是从这类系统中获得高精度的原因。
乙酸蒸汽催化重整制氢的研究进展
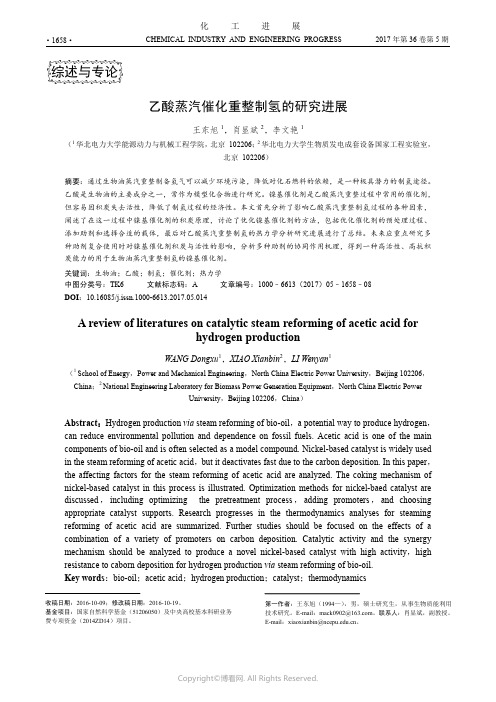
CHEMICAL INDUSTRY AND ENGINEERING PROGRESS 2017年第36卷第5期·1658·化 工 进展乙酸蒸汽催化重整制氢的研究进展王东旭1,肖显斌2,李文艳1(1华北电力大学能源动力与机械工程学院,北京 102206;2华北电力大学生物质发电成套设备国家工程实验室,北京 102206)摘要:通过生物油蒸汽重整制备氢气可以减少环境污染,降低对化石燃料的依赖,是一种极具潜力的制氢途径。
乙酸是生物油的主要成分之一,常作为模型化合物进行研究。
镍基催化剂是乙酸蒸汽重整过程中常用的催化剂,但容易因积炭失去活性,降低了制氢过程的经济性。
本文首先分析了影响乙酸蒸汽重整制氢过程的各种因素,阐述了在这一过程中镍基催化剂的积炭原理,讨论了优化镍基催化剂的方法,包括优化催化剂的预处理过程、添加助剂和选择合适的载体,最后对乙酸蒸汽重整制氢的热力学分析研究进展进行了总结。
未来应重点研究多种助剂复合使用时对镍基催化剂积炭与活性的影响,分析多种助剂的协同作用机理,得到一种高活性、高抗积炭能力的用于生物油蒸汽重整制氢的镍基催化剂。
关键词:生物油;乙酸;制氢;催化剂;热力学中图分类号:TK6 文献标志码:A 文章编号:1000–6613(2017)05–1658–08 DOI :10.16085/j.issn.1000-6613.2017.05.014A review of literatures on catalytic steam reforming of acetic acid forhydrogen productionWANG Dongxu 1,XIAO Xianbin 2,LI Wenyan 1(1 School of Energy ,Power and Mechanical Engineering ,North China Electric Power University ,Beijing 102206,China ;2 National Engineering Laboratory for Biomass Power Generation Equipment ,North China Electric PowerUniversity ,Beijing 102206,China )Abstract :Hydrogen production via steam reforming of bio-oil ,a potential way to produce hydrogen , can reduce environmental pollution and dependence on fossil fuels. Acetic acid is one of the main components of bio-oil and is often selected as a model compound. Nickel-based catalyst is widely used in the steam reforming of acetic acid ,but it deactivates fast due to the carbon deposition. In this paper ,the affecting factors for the steam reforming of acetic acid are analyzed. The coking mechanism of nickel-based catalyst in this process is illustrated. Optimization methods for nickel-baed catalyst are discussed ,including optimizing the pretreatment process ,adding promoters ,and choosing appropriate catalyst supports. Research progresses in the thermodynamics analyses for steaming reforming of acetic acid are summarized. Further studies should be focused on the effects of a combination of a variety of promoters on carbon deposition. Catalytic activity and the synergy mechanism should be analyzed to produce a novel nickel-based catalyst with high activity ,high resistance to caborn deposition for hydrogen production via steam reforming of bio-oil. Key words :bio-oil ;acetic acid ;hydrogen production ;catalyst ;thermodynamics第一作者:王东旭(1994—),男,硕士研究生,从事生物质能利用技术研究。
一种预测高低周复合疲劳寿命的新方法

Journal of Mechanical Strength2023,45(5):1229-1232DOI :10.16579/j.issn.1001.9669.2023.05.029∗20220105收到初稿,20220405收到修改稿㊂国家自然科学基金项目(51305348),陕西能源职业技术学院低碳清洁能源与智能制造科研创新团队(2021KYTD06)资助㊂∗∗石红梅,女,1992年生,陕西宝鸡人,汉族,陕西能源职业技术学院讲师,硕士,主要研究方向为高温设备材料的蠕变疲劳损伤㊂∗∗∗崔㊀璐,女,1979年生,陕西西安人,汉族,西安石油大学副教授,博士,主要研究方向为特殊环境下机械设备环境疲劳理论与工程应用㊂一种预测高低周复合疲劳寿命的新方法∗NEW METHOD TO PREDICT THE LIFE OF HIGH AND LOW CYCLECOMPOSITE FATIGUE LIFE石红梅∗∗1㊀崔㊀璐∗∗∗2㊀侯㊀伟3㊀王㊀澎4(1.陕西能源职业技术学院智能制造与信息工程学院,咸阳712000)(2.西安石油大学机械工程学院,西安710065)(3.咸阳市特种设备检验所,咸阳712000)(4.蜂巢动力系统(江苏)有限公司,镇江212214)SHI HongMei 1㊀CUI Lu 2㊀HOU Wei 3㊀WANG Peng 4(1.School of Intelligent Manufacturing and Information Engineering ,Shaanxi Energy Institute ,Xianyang 712000,China )(2.School of Mechanical Engineering ,Xiᶄan Shiyou University ,Xiᶄan 710065,China )(3.Xianyang Special Equipment Laboratory ,Xianyang 712000,China )(4.HYCET Engine System (Jiangsu )Co.,Ltd.,Zhenjiang 212214,China )摘要㊀发动机㊁内燃机㊁汽轮机转子等高温设备在工作中常受到高低周复合疲劳载荷的作用㊂在现有寿命模型基础上,提出了一种通过应变比-寿命比曲线预测高低周复合疲劳寿命的新方法㊂对三类不同材料的实验数据进行分析,表明了此方法具有一定的通用性㊂对寿命估算值与实验结果进行对比分析,估算值均在误差允许范围内,可以较好地预测高温设备材料的高低周复合疲劳寿命㊂关键词㊀高温设备材料㊀高低周复合疲劳载荷㊀寿命预测新方法中图分类号㊀TB301㊀㊀Abstract ㊀Engine,internal combustion engine,steam turbine rotors and other high-temperature components were oftensubjected to high and low cycle composite fatigue loads during operation.A new method by the curves of strain ratio (εHCF /εLCF )-life ratio (N LCF /HCF /N LCF )to predict the life of high and low cycle composite fatigue was advanced.The analysis of experimental data of three different materials showed that this method had certain versatility.The estimated values of life were within the error tolerance range by comparing the life estimation value with the experimental results,indicating that this methodcan better predict the life of high and low cycle composite fatigue for high temperature equipment materials.Key words㊀High temperature equipment materials ;High and low cycle composite fatigue loads ;New method topredict the lifeCorresponding author :SHI HongMei ,E-mail :919749277@The project supported by the National Natural Science Foundation of China (No.51305348),and the Research andinnovation team of low -carbon clean energy and intelligent manufacturing in Shanxi Energy Institute(No.2021KYTD06).Manuscript received 20220105,in revised form 20220405.0㊀引言㊀㊀许多高温设备在运行时都承受着复杂循环载荷的作用,如高周疲劳(High Cyde Fatigue,HCF)与低周疲劳(Low Cycle Fatigue,LCF)或高周疲劳与热机疲劳(Thermo-MechanicalFatigue,TMF )的复合作用[1]194-202[2]58-66[3]468-470[4]483-484[5]792-801[6]319-321㊂然而,传统的疲劳设计中并没有考虑到二者的交互作用,只是㊀1230㊀机㊀㊀械㊀㊀强㊀㊀度2023年㊀单独研究了两种疲劳失效形式㊂随着高温设备运行工况越来越复杂,进一步研究表明很多高温设备的疲劳寿命和高周疲劳载荷的附加有很大关系;随着高周疲劳载荷应变幅的增大,材料的疲劳寿命飞速降低[7-8]㊂因此,研究高温设备材料在高低周复合疲劳载荷下的寿命模型,成为了该领域的研究重点㊂对于高低周复合疲劳寿命模型的研究,较成熟的是裂纹扩展模型和累积损伤模型[9]629-632㊂基于裂纹扩展的机制模型认为叠加的高周疲劳载荷会增加裂纹扩展速率,减少构件的疲劳寿命,经实验验证可以较好地预测材料的疲劳寿命㊂赵振华等[9]629-632提出了线性和非线性疲劳损伤累积模型,其中非线性损伤累积模型研究了高低周复合循环比和应力(应变)幅比的关系,故而估算精度较好㊂这两种模型虽然得到了广泛的应用,但都只是将高低周疲劳载荷对材料寿命的影响进行了纯粹地叠加,而没有考虑二者的交互作用㊂因此,本文分析了高低周疲劳载荷对几种高温设备材料寿命的影响,提出一种预测高低周复合疲劳寿命的新方法㊂1㊀分析方法1.1㊀基础模型㊀㊀SCHWEIZER C等[1]194-202为了描述高低周复合载荷下疲劳裂纹的扩展行为,提出了以裂纹增长速率(d a/d N)和循环裂纹尖端位移(ΔCTOD)的相关性而展开的机制制型:d ad N block=d ad N total+ðblock d a d N HCF式中,d a/d N|block为高低周复合载荷下的裂纹增长速率;d a/d N|total为低周载荷下的裂纹增长速率;ðblock (d a/d N|HCF)为高周载荷下的裂纹增长速率㊂文中以汽轮机转子10%Cr钢为实验材料,分别进行了低周载荷和高低周复合载荷下的疲劳实验,得到了裂纹长度和循环周期的曲线,如图1所示㊂如此,已知裂纹长度,就可预测试件在该载荷下的循环周期(寿命)㊂而且此模型的预测结果和实验结果吻合度较好,可以很好地描述高低周复合载荷下疲劳裂纹的扩展行为㊂1.2㊀演化方法㊀㊀由图1可知,载荷加载初期,裂纹扩展很慢;当循环周期达到1500后,裂纹扩展速率加快,表现为裂纹长度迅速增大,而且高低周复合载荷下裂纹的扩展速率远大于纯低周疲劳载荷;裂纹长度增大到一定值时不再变化,循环周期趋于固定值,说明此时试件已经断图1㊀10%Cr钢在不同载荷下裂纹长度与循环周期的关系Fig.1㊀Relationship between crack length and life cycle of10%Cr steel under different loads裂,相对应的循环周期可以表示为试件的实验寿命㊂㊀㊀因为高周疲劳载荷的附加会大大降低材料的复合疲劳寿命,所以可推算得材料的高低周复合疲劳寿命与纯低周疲劳寿命之比(N LCF/HCF/N LCF)小于1,且大于0㊂受上述基础模型的启发,以10%Cr钢实验数据为例,做出高周应变-寿命比(εHCF-N LCF/HCF/N LCF)和高低周应变比-寿命比(εHCF/εLCF-N LCF/HCF/N LCF)两种曲线[10],如图2所示㊂图2㊀10%Cr钢的应变-寿命比和应变比-寿命比曲线Fig.2㊀Fitting curve that the strain-life ratio and the ratioby strain-life of10%Cr steel经曲线拟合,发现应变比-寿命比曲线的变化趋势更明显,拟合度较高㊂因此,提出可通过应变比-寿命比曲线来预测高温设备材料的复合疲劳寿命㊂此方法计算简单,其是否具有较高的准确度和通用性,需要大量的数据验证㊂㊀第45卷第5期石红梅等:一种预测高低周复合疲劳寿命的新方法1231㊀㊀2㊀方法验证2.1㊀实验数据分析㊀㊀铸造铝合金一般用于汽车发动机的汽缸盖㊁机体和活塞等部件,而这些部件往往遭受着复杂的热疲劳与机械疲劳载荷[3]468-470[4]483-484[5]792-801㊂钴基合金材料一般用于航空发动机或燃气涡轮机的燃烧室,而燃烧室在工作时既承受着由温度变化引起的热机疲劳载荷,也承受着由机械振动引起的高周疲劳载荷[6]319-321㊂球墨铸铁材料常用于内燃机的高温设备,尤其是汽缸盖部件[2]58-66㊂这些高温设备工作时不但遭受着由频繁启停机引起的低周疲劳载荷,而且承受着由点火压力和机械惯性振动引起的高周疲劳载荷㊂本文摘取以上三类材料在复合疲劳载荷下的实验数据,并对这三类不同材料在不同实验条件下的实验数据进行了分析,得到了其应变比-寿命比曲线,如图3所示㊂图3㊀不同材料的应变比-寿命比曲线Fig.3㊀Fitting curve that the ratio by strain-lifeof different materials由图3可知,所有数据的变化趋势一致,都是幂函数关系,且与10%Cr钢材料的应变比-寿命比曲线变化趋势一致㊂2.2㊀论证分析㊀㊀由以上不同材料的数据分析,可以得到如下结论: 1)虽然以上几种材料在组成成分上差别较大,但其大多都用在燃气涡轮机和航空发动机的燃烧室或燃烧器及汽车发动机的汽缸盖㊁机体和活塞等部件上,而这些高温部件在工作时不仅承受着由温度变化引起的热机疲劳载荷或者低周疲劳载荷,还承受着由机械振动及燃烧压力引起的高周疲劳载荷㊂这与10%Cr钢材料工作时的受力情况基本相同,故这几种材料具有可比性和代表性㊂2)同种材料,实验工况(温度㊁频率等)不同,其应变比-寿命比曲线计算值几乎在同一条曲线上,曲线变化趋势一致;不同材料,实验条件各不相同,但其应变比(εHCF/εLCF)-寿命比(N LCF/HCF/N LCF)曲线的变化趋势也相同㊂所有曲线都和10%Cr钢材料的曲线变化趋势一致,材料的应变比-寿命比呈幂函数变化关系㊂3)从数据分析的角度看,应变比-寿命比曲线反映的其实还是应变幅与疲劳寿命之间的关系,只不过将此关系用应变比-寿命比的数学关系反映出来了,更适合表达高周复合疲劳载荷下高低周应变福与疲劳寿命之间的关系㊂在疲劳实验中,当实验温度确定,在同样的应变幅下,同材料的疲劳寿命基本稳定在一定范围数值下,而应变比-寿命比曲线反应的含义与此实验现象是一致的,即应变幅值是决定疲劳寿命的最主要因素㊂因此,通过应变比(εHCF/εLCF)-寿命比(N LCF/HCF/ N LCF)曲线预测高温设备材料的高低周复合疲劳寿命时,具有一定的适用性㊂3㊀寿命估算㊀㊀10%Cr钢的应变比-寿命比计算值和对应的拟合曲线如图2(b)所示㊂图中黑色实心方块是根据实验数据计算的结果,平滑曲线是根据计算值在Origin中非线性拟合得到的曲线㊂在双对数坐标下,应变比与寿命比之间呈幂函数关系,拟合相似度为0.9777㊂得到这条拟合曲线后,已知实验条件(高低周载荷应变幅)和材料在纯低周载荷下的疲劳寿命,就可估算出材料在高低周复合载荷下的疲劳寿命㊂图4所示为通过拟合曲线估算的高低周复合疲劳寿命和实验结果的误差分析㊂由图4可知,此方法的计算精度在两倍误差范围内,且误差分布均匀㊂因此,该方法可以较好地估算高低周复合载荷下高温设备材料的疲劳寿命㊂图4㊀疲劳寿命估算值与实验值的比较Fig.4㊀Comparison of estimated and experimental values of fatigue life 4㊀结论与展望㊀㊀发动机㊁内燃机㊁汽轮机转子等高温部件在工作中常受到高低周复合疲劳载荷的作用,本文在现有寿命模型基础上,提出了一种预测高低周复合疲劳寿命的新方法㊂此方法通过应变比和寿命比反映了高低周疲劳载荷的交互作用,两者在双对数坐标下呈幂函数关系,三类不同材料的应变比-寿命比曲线表明此关系成立㊂文中还通过此关系估算了10%Cr钢的复合疲劳㊀1232㊀机㊀㊀械㊀㊀强㊀㊀度2023年㊀寿命,并与实验结果进行了对比,均在误差允许范围内,进而表明此方法可以估算高温设备材料的复合疲劳寿命㊂高低周复合疲劳参数复杂,影响因素众多,其中,温度和应变幅是最主要的影响因素㊂本文中得到的应变比-寿命比曲线是暂时忽略了温度的影响而得到的,通过实验数据的分析,不同温度下应变比-寿命比曲线的趋势基本是一致的㊂由此说明,此关系具有一定适用性,可以利用此关系估算高低周复合疲劳寿命㊂但为了更精确地预测疲劳寿命,可在后期通过实验及资料收集,得到更多实验数据,进行温度修正,使此关系能更精准地预测高温设备材料的复合疲劳寿命㊂参考文献(References)[1]㊀SCHWEIZER C,SEIFERT T,NIEWEG B,et al.Mechanisms andmodelling of fatigue crack growth under combined low and high cyclefatigue loading[J].International Journal of Fatigue,2011(33):194-202.[2]㊀METZGER M,NIEWEG B,SCHWEIZER C,et al.Lifetimeprediction of cast iron materials under combined thermomechanicalfatigue and high cycle fatigue loading using a mechanism-basedmodel[J].International Journal of Fatigue,2013(53):58-66. [3]㊀BECK T,LOEHE D,LUFT J,et al.Damage mechanisms of cast Al-Si-Mg alloys under superimposed thermal-mechanical fatigue andhigh-cycle fatigue loading[J].Materials Science and Engineering:A,2007,468/469/470:184-192.[4]㊀BECK T,HENNE I,LOEHE D.Lifetime of cast AlSi6Cu4undersuperimposed thermal mechanical fatigue and high-cycle fatigueloading[J].Materials Science and Engineering:A,2008,483/484:382-386.[5]㊀ZHENG X,ENGLER-PINTO C C,SU X,et al.Modeling of fatiguedamage under superimposed high-cycle and low-cycle fatigue loadingfor a cast aluminum alloy[J].Materials Science&Engineering A,2013(560):792-801.[6]㊀MOALLA M,LANG K-H,LOEHE D.Effect of superimposed highcycle fatigue loadings on the out-of-phase thermal-mechanical fatiguebehaviour of CoCr22Ni22W14[J].Materials Science andEngineering:A,2001:319/320/321:647-651.[7]㊀崔㊀璐,康文泉,吴㊀鹏,等.高低周复合疲劳工况下汽轮机转子钢寿命模型[J].科学技术与工程,2021,21(9):3612-3617.CUI Lu,KANG WenQuan,WU Peng,et al.Life model of steamturbine rotor steel under combined high and low cycle fatigueconditions[J].Science Technology and Engineering,2021,21(9):3612-3617(In Chinese).[8]㊀崔㊀璐,石红梅,李㊀臻,等.先进汽轮机转子材料蠕变疲劳损伤研究新进展[J].机械强度,2018,40(2):449-454.CUI Lu,SHI HongMei,LI Zhen,et al.Research on hightemperature and low cycle fatigue performance of ultra-supercriticalsteam turbine high and intermediate pressure rotor steel[A].Journalof Mechanical Strength,2013(7):51-52(In Chinese). [9]㊀赵振华,陈㊀伟,吴铁鹰.高低周复合载荷下的钛合金疲劳寿命估算[J].机械强度,2011,33(4):629-632.ZHAO ZhenHua,CHEN Wei,WU TieYing.Fatigue life estimationof titanium alloys under high and low cycle compound loads[J].Journal of Mechanical Strength,2011,33(4):629-632(InChinese).[10]㊀石红梅.复杂蠕变疲劳载荷对超超临界汽轮机转子钢寿命的影响[D].西安:西安石油大学,2017:42-46.SHI HongMei.The influence of complex creep fatigue loading on lifeof ultra supercritical steam turbine rotor steel[D].Xiᶄan:XiᶄanShiyou University,2017:42-46(In Chinese).。
热管理用快速凝固Al
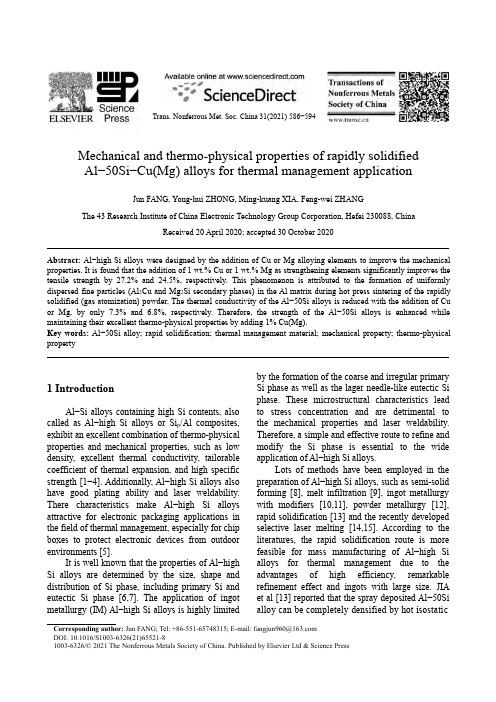
Trans.Nonferrous Met.Soc.China31(2021)586−594Mechanical and thermo-physical properties of rapidly solidifiedAl−50Si−Cu(Mg)alloys for thermal management applicationJun FANG,Yong-hui ZHONG,Ming-kuang XIA,Feng-wei ZHANGThe43Research Institute of China Electronic Technology Group Corporation,Hefei230088,ChinaReceived20April2020;accepted30October2020Abstract:Al−high Si alloys were designed by the addition of Cu or Mg alloying elements to improve the mechanical properties.It is found that the addition of1wt.%Cu or1wt.%Mg as strengthening elements significantly improves the tensile strength by27.2%and24.5%,respectively.This phenomenon is attributed to the formation of uniformly dispersed fine particles(Al2Cu and Mg2Si secondary phases)in the Al matrix during hot press sintering of the rapidly solidified(gas atomization)powder.The thermal conductivity of the Al−50Si alloys is reduced with the addition of Cu or Mg,by only7.3%and6.8%,respectively.Therefore,the strength of the Al−50Si alloys is enhanced while maintaining their excellent thermo-physical properties by adding1%Cu(Mg).Key words:Al−50Si alloy;rapid solidification;thermal management material;mechanical property;thermo-physical property1IntroductionAl−Si alloys containing high Si contents,also called as Al−high Si alloys or Si p/Al composites, exhibit an excellent combination of thermo-physical properties and mechanical properties,such as low density,excellent thermal conductivity,tailorable coefficient of thermal expansion,and high specific strength[1−4].Additionally,Al−high Si alloys also have good plating ability and laser weldability. There characteristics make Al−high Si alloys attractive for electronic packaging applications in the field of thermal management,especially for chip boxes to protect electronic devices from outdoor environments[5].It is well known that the properties of Al−high Si alloys are determined by the size,shape and distribution of Si phase,including primary Si and eutectic Si phase[6,7].The application of ingot metallurgy(IM)Al−high Si alloys is highly limited by the formation of the coarse and irregular primary Si phase as well as the lager needle-like eutectic Si phase.These microstructural characteristics lead to stress concentration and are detrimental to the mechanical properties and laser weldability. Therefore,a simple and effective route to refine and modify the Si phase is essential to the wide application of Al−high Si alloys.Lots of methods have been employed in the preparation of Al−high Si alloys,such as semi-solid forming[8],melt infiltration[9],ingot metallurgy with modifiers[10,11],powder metallurgy[12], rapid solidification[13]and the recently developed selective laser melting[14,15].According to the literatures,the rapid solidification route is more feasible for mass manufacturing of Al−high Si alloys for thermal management due to the advantages of high efficiency,remarkable refinement effect and ingots with large size.JIA et al[13]reported that the spray deposited Al−50Si alloy can be completely densified by hot isostaticCorresponding author:Jun FANG;Tel:+86-551-65748315;E-mail:******************DOI:10.1016/S1003-6326(21)65521-81003-6326/©2021The Nonferrous Metals Society of China.Published by Elsevier Ltd&Science PressJun FANG,et al/Trans.Nonferrous Met.Soc.China31(2021)586−594587 pressing(HIP)at570°C.Al alloys with Si contentof22%−50%were prepared by gas atomizationfollowed by hot pressing,and near fully densemicrostructure and excellent properties wereobtained[16].Al−30Si alloy prepared by spraydeposition can also be densified by hot pressing,and a continuous network of globular Si phase andan interpenetrating Al matrix were achieved[17].The Al−50Si alloy is widely used as electronicpackaging boxes,which has a high volume fractionof Si and approximately pure Al matrix.However,its strength should be improved in order to expandits application[5].The previous works of Al−highSi alloys for thermal management have beenfocused on the manufacturing technologies,parameters,and the subsequent properties.Generally,the properties of ingot metallurgyAl−high Si alloys can be modified through alloying,such as the A356,A380,and A390alloys[18].BEFFORT et al[19]reported that mechanicalproperties of the squeeze cast60vol.%SiC p/Alcomposites were also highly determined by the Zn,Cu and Mg elements in the Al matrix.However,less attention has been paid to the alloy compositionand the relationship between microstructuralevolution and properties of the Al−50Si alloy.Accordingly,in this work,Al−50Si,Al−50Si−1Cu and Al−50Si−1Mg alloys for electronicpackaging in thermal management weresuccessfully fabricated by rapid solidification(gasatomization)and powder metallurgy(hot pressing)route,and the microstructural characteristics,mechanical properties(tensile and bendingstrength)and thermo-physical properties wereparisons between the effect of Cu andMg addition on the Al−50Si alloys were analyzed based on the microstructural observations and macro-property tests.2ExperimentalPolycrystalline pure Si(99.9%,all the alloy compositions are in mass fraction unless otherwise mentioned)and pure Al(99.95%)were inductively melted at approximately1250°C.Then,Al−50Si pre-alloy powder was fabricated through a nitrogen gas atomization process,and the morphology of the powder particles is shown in Fig.1(a).After mechanical sieving,the Al−50Si pre-alloy powder with particle size less than74μm was mixed with Fig.1SEM morphologies of gas-atomized Al−50Si pre-alloy powder(a),electrolytic Cu powder(b)and inert gas-atomized Mg powder(c)with different shapes 1wt.%electrolytic Cu powder and1wt.%inertgas-atomized Mg powder,respectively.Mechanical mixing was applied for6h in the atmosphere of Ar with the mass ratio of ball to powder of4:1.The Cu and Mg powders having dendritic and spherical shapes are displayed in Figs.1(b)and1(c), respectively.The mixed powder was cold compacted at300MPa and hold for20s,and billets with relative density of approximately78%were obtained.Hot press sintering was employed on the cold compacted billets and held at560°C forJun FANG,et al/Trans.Nonferrous Met.Soc.China31(2021)586−594 58860min at45MPa.Finally,the samples with dimensions of d50mm×10mm were obtained. The hot-pressed alloys were solid solutionized at 500°C for4h and then aged at160°C for24h. Details of the fabrication process is reported in the previous work[16].Chemical compositions of the as-fabricated Al−50Si−X(X=0,Cu,and Mg)alloys were detected using an inductively coupled plasma optical emission spectrometer(IC-OES),and the results are illustrated in Table1.Morphologies of the Al−50Si pre-alloy powders,Cu powder and Mg powder were detected using a scanning electron microscope(SEM,Quanta−200).Hot-pressed samples for microstructural characterization were cut,ground,polished,and etched with Keller’s reagent.Field emission scanning electron microscope(FESEM,Sirion200)equipped with an energy dispersive spectroscopy(EDS)detector was used in the observation of microstructural details. The sizes of Si phase and secondary phases were measured using ImageJ software.The phases present in the Al−high Si alloys were further analyzed using X-ray diffraction(XRD)at a scanning angle of25°−80°.The room temperature tensile and three-point bending tests of samples were carried out on an electronic universal material testing machine (MTS850).The tensile specimens were made into a dumbbell shape according to the standard GB T228—2010with a gauge diameter of6mm. The dimensions of the three-point bending specimen are3mm×10mm×50mm.The tensile fractured surfaces of the specimens were observed using SEM.The Brinell hardness test of the alloy was performed at a load of7.35kN for30s on the polished samples.All the tensile and bending tests were repeated three times to obtain good reproducibility of data.Under the argon atmosphere,coefficient of thermal expansion of the Al−50Si−X alloys was measured in the temperature range of25−300°C using laser flash and calorimetric methods (NETZSCH LFA427/3/G).The sample has a size of 20mm×5mm×5mm and was required to be parallel and smooth at both ends.Thermal conductivity of the three kinds of alloys was performed on cylindrical slice specimens with dimensions of d10mm×3mm using NETZSCH DIL402C.Density of the alloys was measured by Archimedes method using a balance with the accuracy of0.1mg.3Results3.1Microstructural characteristicsTypical microstructures of the as-atomized Al−50Si pre-alloy powder and the hot-pressed Al−50Si−X alloys are shown in Fig.2.It can be seen from Fig.2(a)that the primary Si phase is highly refined to have a block-like morphology due to the large solidification rate and undercooling nature of gas atomization.The eutectic Si phase is also refined remarkably and its shape changes from needle-like with large aspect ratio in the as-cast alloy to bar-like with a low aspect ratio in the as-atomized powder.However,the primary Si seems to distribute mostly at the periphery of powder particles owing to the solidification sequence[20].After hot press,the gas-atomized Al−50Si pre-alloy powder is well densified and a pore-free microstructure is obtained,as shown in Figs.2(b−d). High density of defects,such as pores and cracks were observed in the Al−50Si alloy prepared by ingot metallurgy[21].Consequently,the measured density of the hot-pressed samples is near to the theoretical value.As the density of Cu(8.9g/cm3) is higher than that of Al(2.7g/cm3)while the density of Mg(1.7g/cm3)is lower than that of Al, the addition of Cu or Mg leads to a slight variation of density in the Al−50Si−X alloys.Table1Compositions of rapidly solidified(gas-atomized)and hot-pressed Al−50Si−X alloys measured by ICP-OES (wt.%)Material Si Mg Cu Zn Fe Mn Ti AlAl−50Si50.5<0.01<0.01<0.010.040.02<0.01Bal.Al−50Si−1Cu50.30.05 1.03<0.010.030.01<0.01Bal.Al−50Si−1Mg49.7 1.030.02<0.010.050.01<0.01Bal.Jun FANG,et al/Trans.Nonferrous Met.Soc.China31(2021)586−594589 Fig.2SEM morphologies of gas-atomized Al−50Si pre-alloy powder(a)and as-fabricated Al−50Si alloy(b),Al−50Si−1Cu alloy(c)and Al−50Si−1Mg alloy(d)having similar characteristics of Si phaseIt is seen that a semi-continuous networkstructure with smooth surface of the Si phase isformed in the Al matrix,as seen in Figs.2(b−d).The distribution of Si phase is quite homogeneousas compared with that of the as-atomized powder.Such characteristics of Si phase are highly differentfrom those of the as-cast Al−high Si alloys whichhave coarse and irregular(bar-like,plate-like,star-like,etc)primary Si with sharp corners as wellas needle-like eutectic Si with a large aspectratio[11,21].Furthermore,it is interesting to findthat the eutectic Si is completely absent in thehot-pressed samples due to the diffusion-controlled growth of Si phase and the Si−Si phase clustering in the solid-state sintering.There is no obvious change of the Si phase in the fabricated Al−50Si alloys with and without Cu(Mg)addition besides a little lower degree of the semi-continuous structure.X-ray diffractions were performed to detect the phases presented in the hot-pressed Al−50Si−X alloys,and the results are displayed in Fig.3.It is seen that the diffraction peaks ofα(Al)and Si phase are clearly observed in the samples.With the addition of Cu or Mg,small amounts of Al2Cu and Fig.3XRD patterns of as-fabricated Al−50Si−X alloys showing Al2Cu and Mg2Si secondary phases formed in Al−50Si−Cu/(Mg)alloys:(a)Al−50Si;(b)Al−50Si−1Cu;(c)Al−50Si−1MgMg2Si secondary phases are formed in the Al−50Si−Cu(Mg)alloys.It is noted that,different from the Al−50Si−1Cu alloy,no AlMg secondary phases are formed in the Al−50Si−1Mg alloy. However,as the content of Cu or Mg is only1%, the diffraction peaks of the Al2Cu and Mg2Si phases are not remarkable.Jun FANG,et al/Trans.Nonferrous Met.Soc.China 31(2021)586−594590To further investigate the secondary phases formed in the Al−50Si−Cu(Mg)alloys,magnified SEM observations were conducted and the results are shown in Fig.4.Other than the large Si particles,small needle-like Al 2Cu phase and bar-like Mg 2Si phase are present in the Al−50Si−Cu(Mg)alloys.This result is in consistent with the XRD patterns presented in Fig.3.Although the average sizes of the Al 2Cu and Mg 2Si secondary phases are less than 1μm,most of the Mg 2Si phase is larger than the Al 2Cu phase.Additionally,most of the Al 2Cu phases are dispersed in the center of the Al matrix.However,the Mg 2Si phase seems to distribute mostly near the surface of Si particles.This phenomenon can be attributed to the larger diffusion rate and supersaturation of Mg than those of Si in the Almatrix.Fig.4SEM morphologies and distribution of Al 2Cu (a)and Mg 2Si (b)secondary phases present in Al−50Si−Cu(Mg)alloys3.2Mechanical propertiesThe room temperature tensile tests were performed on the hot-pressed Al−50Si alloys with and without Cu(Mg)addition,and the tensile curves are depicted in Fig.5.The stress−strain response of the Al−50Si alloy is different from that containing Cu and Mg.A very slight plastic deformation of approximately 0.5%strain isobserved in the Al−50Si alloy.Remarkably enhanced ultimate tensile strength (UTS)is achieved in the Al−50Si−1Cu and Al−50Si−1Mg alloys.The plastic behavior is less evident,approximately 0.3%strain to fracture,with the addition of Cu or Mg.This phenomenon indicates that the addition of Cu(Mg)is beneficial to improving the strength of Al−50Si alloy but detrimental to the plasticity of the alloy.Additionally,the slope of the tensile stress−strain response of the Cu(Mg)-contained alloys becomes flatter and higher than that of the Al−50Si alloy,suggesting that the addition of Cu(Mg)also enhances the elastic modulus of thealloy.Fig.5Tensile stress−strain response of rapidly solidified Al−50Si−X alloys at room temperatureAverage values of the tensile strength,bending strength and hardness of the Al−50Si−X alloys were obtained from five parallel tests,and the results are shown in Fig.6.The strength of the Al−50Si alloy is significantly improved with the addition of Cu(Mg).Compared with the reference sample,the addition of 1%Cu raises the tensile and bending strength from 185.7and 288.6MPa to 236.2and 390.5MPa,with increments of 27.2%and 35.3%,respectively.Similarly,the addition of 1%Mg results in an enhancement of tensile and bending strength by 24.5%and 29.0%,respectively.At the same time,the addition of alloying elements also increases the hardness of the Al matrix.From Fig.6,it is also found that the strengthening effect of Cu is slightly higher than that of Mg.This phenomenon can be attributed to the fine and homogeneous distribution of the Al 2Cu secondary phase at the center of the Al matrix.Additionally,according to the image analysis from SEM results,the average size of Al 2Cu phase is a little smallerJun FANG,et al/Trans.Nonferrous Met.Soc.China31(2021)586−594591Fig.6Tensile strength,bending strength and hardness of rapidly solidified Al−50Si−X alloysthan that of the Mg2Si phase,which may also contribute to the higher strength of the Al−50Si−1Cu alloy.Tensile fractured morphologies of Al−50Si−X alloys are displayed in Fig.7.All samples show a clear brittle fracture feature.It is seen that the fracture planes of the alloys are vertical to the tensile direction and no visible macro-ductility fracture is observed.As seen from Fig.7(a),the crack source of the alloy with rather flat morphology is clearly observed.The crack progresses rapidly in a linear way through the sample when external pressure is applied.Figures 7(b−d)show that the Al matrix fractures by ductile rupture with tearing ridge while the Si phase fractures by cleavage surface.As there is a high volume fracture of Si phase(approximately53.7%) with semi-continuous structure,the Si particle dominated brittle fracture is the main mode of the Al−50Si alloys.The previous observation suggests that the crack tip moves through brittle fracture of the Si particles and finishes by ductile fracture of the Al matrix[22].Generally,metal matrix composites(MMCs)reinforced with high volume of reinforcement fracture in such particle dominatedFig.7Low magnification micrograph showing crack source of Al−50Si alloy(a)and high magnification micrographs of Al−50Si alloy(b),Al−50Si−1Cu alloy(c)and Al−50Si−1Mg alloy(d)Jun FANG,et al/Trans.Nonferrous Met.Soc.China 31(2021)586−594592mode [23,24].Additionally,dimples with small size are observed in the alloys due to the refined microstructure as a result of rapid solidification and solid-state sintering.However,three kinds of alloys show typical brittle fracture,and the difference among fractured morphologies is less visible.3.3Thermo-physical propertiesVariations of coefficient of thermal expansion (CTE)of the Al−50Si−X alloys as a function of temperature in the range of 25−300°C are shown in Fig.8.It is observed that the coefficient of thermal expansion increases linearly with the increase of testing temperature.The Al−high Si alloys can be regarded as Si particle reinforced Al matrix composites (Si p /Al)and the coefficient of thermal expansion of the alloy is mainly determined by the properties of the Al matrix and Si phase and the volume fraction of the Si phase according to the rule of mixture (ROM).As seen from Fig.2,there is little deviation of the volume fraction,size,and morphology of Si phase.Consequently,the coefficients of thermal expansion of the Al−50Si−X alloys are determined mainly by the properties of Al matrix.Owing to the presence of Al 2Cu and Mg 2Si secondary phase having lower coefficient of thermal expansion,the total thermal expansion of Al−50Si alloys is reduced.JIA et al [13]reported that no plastic deformation occurs in the Al matrix at low temperatures.The expansion of the alloys is caused by the combined expansion of the Al matrix and Si phase and results in the linearly increased coefficient of thermal expansion with increasingtemperature.Fig.8Coefficient of thermal expansion of rapidly solidified Al−50Si−X alloys in temperature range of 25−300°CThermal conductivity of the Al−50Si−X alloys is illustrated in Fig.9.Owing to the rapid solidification nature of gas atomization and the diffusion-controlled growth of Si phase during hot pressing,the Si phase has a semi-continuous structure with smooth surface,which contributes to the excellent thermal conductivity of the Al−50Si alloy,146.2W·m −1·K −1.At the same time,Si has low solid solubility in the Al matrix with equilibrium state,and a near pure Al matrix after hot pressing may also help for achieving high thermal conductivity of the alloy.However,the formation of the Al 2Cu and Mg 2Si secondary phases in the Al−50Si−Cu(Mg)alloys has a scattering effect on the free electron motion and hinders the thermal conduction [25].Consequently,the thermal conductivities of the Al−50Si alloy containing 1%Cu and 1%Mg are reduced by 7.3%and 6.8%,respectively.In comparison with the exceptionally improved strength of the Al−50Si alloy,this reduction of thermal conductivity is within the acceptable limit (≥120W·m −1·K −1).Fig.9Thermal conductivity of rapidly solidified and hot-pressed Al−50Si−X alloys at room temperature4Conclusions(1)Gas atomization endows the pre-alloyed Al−50Si alloy powder with highly refined primary and eutectic Si phase,and in combination with the subsequent solid-state hot-pressing,the Si phase with semi-continuous network structure is obtained.By adding 1%Cu or 1%Mg,Al 2Cu or Mg 2Si secondary phases are observed,respectively,but the influence on the Si phase characteristics is limited.(2)Tensile strength,bending strength and hardness of the Al−50Si alloys are significantlyJun FANG,et al/Trans.Nonferrous Met.Soc.China31(2021)586−594593improved with the addition of Cu or Mg, respectively,which is attributed to the strengthening effect of the fine secondary phases.The effect of Cu on mechanical properties is more remarkable compared with that of Mg.All the Al−50Si−X alloys show typical brittle fracture features having a clear cleavage surface.(3)The addition of Cu(Mg)is helpful for reducing the coefficient of thermal expansion of the Al−50Si−X alloys,but detrimental to the thermal conductivity.However,negligible difference in thermo-physical properties is observed in the Al−50Si−Cu(Mg)alloys.References[1]HOGG S C,LAMBOURNE A,OGILVY A,GRANT P S,Microstructural characterisation of spray formed Si−30Al for thermal management applications[J].Scripta Materialia, 2006,55(1):111−114.[2]KIMURA T,NAKAMOTO T,MIZUNO M,ARAKI H.Effect of silicon content on densification,mechanical and thermal properties of Al−x Si binary alloys fabricated using selective laser melting[J].Materials Science and Engineering A,2017,682:593−602.[3]ZHANG Wen-long,DING Dong-yan,GAO Ping.Highvolume fraction Si particle-reinforced aluminium matrix composites fabricated by a filtration squeeze casting route[J].Materials&Design,2016,90:834−838.[4]WANG Qian,ZHU Lin,CHEN Xiao-guang,YAN Jiu-chun,XIE Rui-shan,LI Pei-hao,WANG Zhi-hua,WANG Zhi-qi, LI Yun-tao,ZHOU Xiao-yu.Si particulate-reinforced ZnAl based composites joints of hypereutectic Al50Si alloys byultrasonic-assisted soldering[J].Materials&Design,2016, 107:41−46.[5]MAUDUIT D,DUSSERRE G,CUTARD T.Probabilisticrupture analysis of a brittle spray deposited Si−Al alloy under thermal gradient:Characterization and thermoelastic sizing guidelines[J].Materials&Design,2016,95:414−421.[6]CHEN Xu,ZHONG Yun-bo,ZHENG Tian-xiang,SHENZhe,WANG Jiang,FAN Li-jun,ZHAI Yong,PENG Ming-hu,ZHOU Bang-fei,REN Wei-li,LEI Zuo-sheng, REN Zhong-ming,HE Qiong.Refinement of primary Si in the bulk solidified Al−20wt.%Si alloy assisting by high static magnetic field and phosphorus addition[J].Journal of Alloysand Compounds,2017,714:39−46.[7]WANKHEDE D M,NARKHEDE B E,MAHAJAN S K,CHOUDHARI C M.Influence of pouring temperature and external chills on mechanical properties of aluminum silicon alloy castings[J].Materials Today:Proceedings,2018,5(9): 17627−17635.[8]WARD P J,ATKINSON H V,ANDERSON P R G,ELIAS LG,GARCIA B,KAHLEN L,RODRIGUEZ-IBABE J M.Semi-solid processing of novel MMCs based onhypereutectic aluminium-silicon alloys[J].Acta Materialia,1996,44(5):1717−1727.[9]CHEN Yu-yong,CHUNG D D L.Silicon-aluminiumnetwork composites fabricated by liquid metal infiltration[J].Journal of Materials Science,1994,29(23):6069−6075. [10]RAGHUKIRAN N,KUMAR R.Effect of scandium additionon the microstructure,mechanical and wear properties of the spray formed hypereutectic aluminum−silicon alloys[J].Materials Science and Engineering A,2015,641:138−147.[11]YU Wen-hui,ZHANG Yong,YAN Ting-liang,LIU Yue,JIANG Ao-lei,ZHENG Hong-liang,TIAN Xue-lei.Enhanced nucleation of primary silicon in Al−20wt.%Si alloy with Ni−Si inoculation[J].Journal of Alloys and Compounds,2017,693:303−307.[12]LIU Y Q,WEI S H,FAN J Z,MA Z L,ZUO T.Mechanicalproperties of a low thermal-expansion aluminum/siliconcomposite produced by powder metallurgy[J].Journal ofMaterials Science&Technology,2014,30(4):417−422. [13]JIA Yan-dong,CAO Fu-yang,SCUDINO S,MA Pan,LIHai-chao,YU Lei,ECKERT J,SUN Jian-fei.Microstructure and thermal expansion behavior of spray-deposited Al−50Si[J].Materials&Design,2014,57:585−591.[14]JIA Qing-bo,ROMETSCH P,KURNSTINER P,CHAO Qi,HUANG Ai-jun,WEYLAND M,BOURGEOIS L,WU Xin-hua.Selective laser melting of a high strengthAl−Mn−Sc alloy:Alloy design and strengtheningmechanisms[J].Acta Materialia,2019,171:108−118. [15]KANG Nan,CODDET P,LIAO Han-lin,CODDET C.Macrosegregation mechanism of primary silicon phase in selective laser melting hypereutectic Al−high Si alloy[J].Journal of Alloys and Compounds,2016,662:259−262. [16]CAI Zhi-yong,ZHANG Chun,WANG Ri-chu,PENGChao-qun,QIU Ke,FENG Yan.Preparation of Al−Si alloys by a rapid solidification and powder metallurgy route[J].Materials&Design,2015,87:996−1002.[17]WANG Feng,XIONG Bai-qing,ZHANG Yong-an,ZHUBao-hong,LIU Hong-wei,WEI Yan-guang.Microstructure, thermo-physical and mechanical properties of spray-deposited Si−30Al alloy for electronic packaging application [J].Materials Characterization,2018,59(10):1455−1457. [18]DAMAV ANDI E,NOUROUZI S,RABIEE S M,JAMAATIR.Effect of ECAP on microstructure and tensile properties of A390aluminum alloy[J].Transactions of NonferrousMetals Society of China,2019,29(5):931−940.[19]BEFFORT O,LONG Si-yuan,CAYRON C,KUEBLER J,BUFFAT P A.Alloying effects on microstructure and mechanical properties of high volume fraction SiC particle reinforced Al-MMCs made by squeeze casting infiltration[J].Composites Science and Technology,2007,67(3):737−745.[20]KALAY Y E,CHUMBLEY L S,ANDERSON I E,NAPOLITANO R E.Characterization of hypereutectic Al−Si powders solidified under far-from equilibrium conditions[J].Metallurgical and Materials Transactions A,2007,38(7): 1452−1457.[21]CAO Fu-yang,JIA Yan-dong,PRASHANTH K G,MA Pan,LIU Jing-shun,SCUDINO S,HUANG Feng,ECKERT J, SUN Jian-fei.Evolution of microstructure and mechanical properties of as-cast Al−50Si alloy due to heat treatment and P modifier content[J].Materials&Design,2015,74: 150−156.Jun FANG,et al/Trans.Nonferrous Met.Soc.China31(2021)586−594 594[22]CAI Zhi-yong,ZHANG Chun,WANG Ri-chu,PENGChao-qun,WU Xiang.Effect of copper content on microstructure and mechanical properties of Al/Si p composites consolidated by liquid phase hot pressing[J].Materials&Design,2016,110:10−17.[23]ZHANG Qi-guo,ZHANG Hong-xiang,GU Ming-yuan,JINYan-ping.Studies on the fracture and flexural strength of Al/Si p composite[J].Materials Letters,2004,58: 3545−3550.[24]BUFFIERE J Y,MAIRE E,VERDU C,CLOETENS P,PATEYRON M,PEIX G,BARUCHEL J.Damage assessment in an Al/SiC composite during monotonic tensile tests using synchrotron X-ray microtomography[J].Materials Science and Engineering A,1997,234−236: 633−635.[25]BLANK M,SCHNEIDER G,ORDONEZ-MIRANDA J,WEBER L.Role of the electron-phonon coupling on the thermal boundary conductance of metal/diamond interfaces with nanometric interlayers[J].Journal of Applied Physics, 2019,126:165302.热管理用快速凝固Al−50Si−Cu(Mg)合金的力学和热物理性能方军,钟永辉,夏明旷,张凤伟中国电子科技集团第四十三所,合肥230088摘要:通过加入Cu或Mg合金元素提高高硅铝合金的力学性能。
小尺度含水层热量运移试验研究及热弥散效应评估
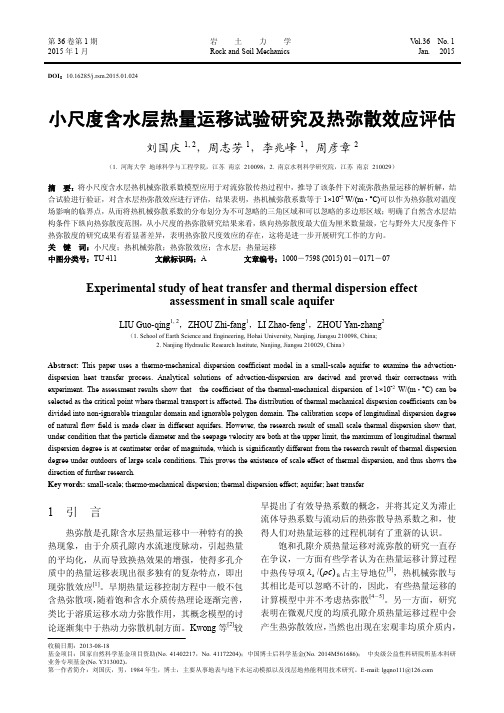
。另一方面,研究
表明在微观尺度的均质孔隙介质热量运移过程中会 产生热弥散效应, 当然也出现在宏观非均质介质内,
收稿日期:2013-08-18 基金项目:国家自然科学基金项目资助 (No. 41402217,No. 41172204);中国博士后科学基金(No. 2014M561686); 中央级公益性科研院所基本科研 业务专项基金 (No. Y313002)。 第一作者简介:刘国庆,男,1984 年生,博士,主要从事地表与地下水运动模拟以及浅层地热能利用技术研究。E-mail: lgqno111@
(1. School of Earth Science and Engineering, Hohai University, Nanjing, Jiangsu 210098, China; 2. Nanjing Hydraulic Research Institute, Nanjing, Jiangsu 210029, China)
(1. 河海大学 地球科学与工程学院,江苏 南京 210098;2. 南京水利科学研究院,江苏 南京 210029)
摘 要:将小尺度含水层热机械弥散系数模型应用于对流弥散传热过程中,推导了该条件下对流弥散热量运移的解析解,结 合试验进行验证,对含水层热弥散效应进行评估,结果表明,热机械弥散系数等于 1×10 2 W/(m·°C)可以作为热弥散对温度 场影响的临界点,从而将热机械弥散系数的分布划分为不可忽略的三角区域和可以忽略的多边形区域;明确了自然含水层结 构条件下纵向热弥散度范围,从小尺度的热弥散研究结果来看,纵向热弥散度最大值为厘米数量级,它与野外大尺度条件下 热弥散度的研究成果有着显著差异,表明热弥散尺度效应的存在,这将是进一步开展研究工作的方向。 关 键 词:小尺度;热机械弥散;热弥散效应;含水层;热量运移 中图分类号:TU 411 文献标识码:A 文章编号:1000-7598 (2015) 01-0171-07
硅烷偶联剂改性酚醛树脂的合成
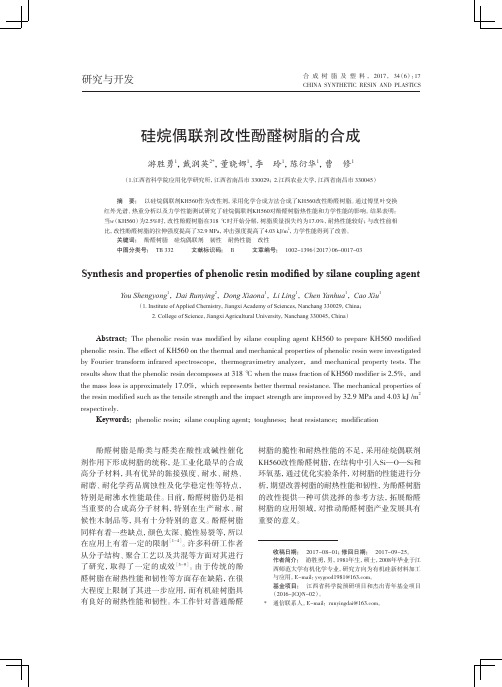
CHINA SYNTHETIC RESIN AND PLASTICS 研究与开发合 成 树 脂 及 塑 料 , 2017, 34(6): 17酚醛树脂是酚类与醛类在酸性或碱性催化剂作用下形成树脂的统称,是工业化最早的合成高分子材料,具有优异的黏接强度、耐水、耐热、耐磨、耐化学药品腐蚀性及化学稳定性等特点,特别是耐沸水性能最佳。
目前,酚醛树脂仍是相当重要的合成高分子材料,特别在生产耐水、耐候性木制品等,具有十分特别的意义。
酚醛树脂同样有着一些缺点,颜色太深、脆性易裂等,所以在应用上有着一定的限制[1-4]。
许多科研工作者从分子结构、聚合工艺以及共混等方面对其进行了研究,取得了一定的成效[5-9]。
由于传统的酚醛树脂在耐热性能和韧性等方面存在缺陷,在很大程度上限制了其进一步应用,而有机硅树脂具有良好的耐热性能和韧性。
本工作针对普通酚醛树脂的脆性和耐热性能的不足,采用硅烷偶联剂KH560改性酚醛树脂,在结构中引入Si—O—Si和环氧基,通过优化实验条件,对树脂的性能进行分析,期望改善树脂的耐热性能和韧性,为酚醛树脂的改性提供一种可供选择的参考方法,拓展酚醛树脂的应用领域,对推动酚醛树脂产业发展具有重要的意义。
硅烷偶联剂改性酚醛树脂的合成游胜勇1,戴润英2*,董晓娜1,李 玲1,陈衍华1,曹 修1(1.江西省科学院应用化学研究所,江西省南昌市 330029;2.江西农业大学,江西省南昌市 330045)摘要:以硅烷偶联剂KH560作为改性剂,采用化学合成方法合成了KH560改性酚醛树脂。
通过傅里叶变换红外光谱、热重分析以及力学性能测试研究了硅烷偶联剂KH560对酚醛树脂热性能和力学性能的影响。
结果表明:当w(KH560)为2.5%时,改性酚醛树脂在318 ℃时开始分解,树脂质量损失约为17.0%,耐热性能较好;与改性前相比,改性酚醛树脂的拉伸强度提高了32.9 MPa,冲击强度提高了4.03 kJ/m2,力学性能得到了改善。
A105_锻钢球阀激光深熔焊接工艺优化及组织性能研究
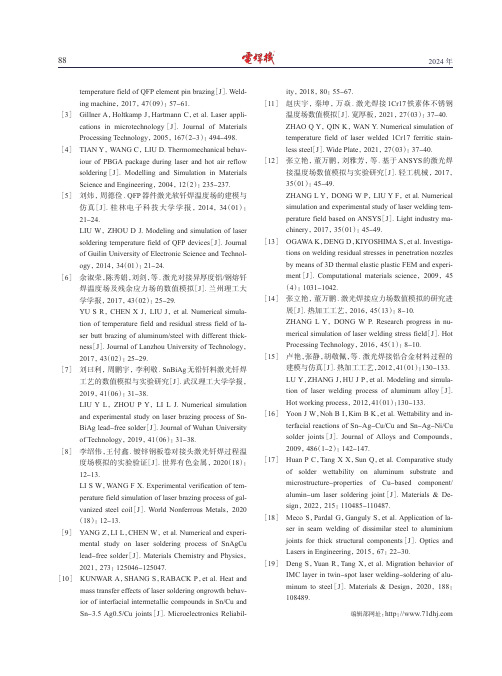
2024 年temperature field of QFP element pin brazing[J]. Weld‐ing machine, 2017, 47(09): 57-61.[3] Gillner A,Holtkamp J,Hartmann C,et al. Laser appli‐cations in microtechnology[J]. Journal of MaterialsProcessing Technology, 2005, 167(2-3): 494-498.[4] TIAN Y, WANG C, LIU D. Thermomechanical behav‐iour of PBGA package during laser and hot air reflowsoldering[J]. Modelling and Simulation in MaterialsScience and Engineering, 2004, 12(2): 235-237.[5] 刘炜,周德俭. QFP器件激光软钎焊温度场的建模与仿真[J]. 桂林电子科技大学学报, 2014, 34(01):21-24.LIU W, ZHOU D J. Modeling and simulation of lasersoldering temperature field of QFP devices[J]. Journalof Guilin University of Electronic Science and Technol‐ogy, 2014, 34(01): 21-24.[6] 余淑荣,陈秀娟,刘剑,等. 激光对接异厚度铝/钢熔钎焊温度场及残余应力场的数值模拟[J]. 兰州理工大学学报, 2017, 43(02): 25-29.YU S R, CHEN X J, LIU J, et al. Numerical simula‐tion of temperature field and residual stress field of la‐ser butt brazing of aluminum/steel with different thick‐ness[J]. Journal of Lanzhou University of Technology,2017, 43(02): 25-29.[7] 刘曰利,周鹏宇,李利敬. SnBiAg无铅钎料激光钎焊工艺的数值模拟与实验研究[J]. 武汉理工大学学报,2019, 41(06): 31-38.LIU Y L,ZHOU P Y,LI L J. Numerical simulationand experimental study on laser brazing process of Sn‐BiAg lead-free solder[J]. Journal of Wuhan Universityof Technology, 2019, 41(06): 31-38.[8] 李绍伟,王付鑫. 镀锌钢板卷对接头激光钎焊过程温度场模拟的实验验证[J]. 世界有色金属, 2020(18):12-13.LI S W,WANG F X. Experimental verification of tem‐perature field simulation of laser brazing process of gal‐vanized steel coil[J]. World Nonferrous Metals,2020(18): 12-13.[9] YANG Z,LI L,CHEN W, et al. Numerical and experi‐mental study on laser soldering process of SnAgCulead-free solder[J]. Materials Chemistry and Physics,2021, 273: 125046-125047.[10] KUNWAR A,SHANG S,RABACK P,et al. Heat and mass transfer effects of laser soldering ongrowth behav‐ior of interfacial intermetallic compounds in Sn/Cu andSn-3.5 Ag0.5/Cu joints[J]. Microelectronics Reliabil‐ity, 2018, 80: 55-67.[11] 赵庆宇,秦坤,万焱. 激光焊接1Cr17铁素体不锈钢温度场数值模拟[J]. 宽厚板, 2021, 27(03): 37-40.ZHAO Q Y, QIN K, WAN Y. Numerical simulation oftemperature field of laser welded 1Cr17 ferritic stain‐less steel[J]. Wide Plate, 2021, 27(03): 37-40.[12] 张立艳,董万鹏,刘雅芳,等. 基于ANSYS的激光焊接温度场数值模拟与实验研究[J]. 轻工机械, 2017,35(01): 45-49.ZHANG L Y, DONG W P, LIU Y F, et al. Numericalsimulation and experimental study of laser welding tem‐perature field based on ANSYS[J]. Light industry ma‐chinery, 2017, 35(01): 45-49.[13] OGAWA K,DENG D,KIYOSHIMA S,et al. Investiga‐tions on welding residual stresses in penetration nozzlesby means of 3D thermal elastic plastic FEM and experi‐ment[J]. Computational materials science,2009,45(4): 1031-1042.[14] 张立艳,董万鹏. 激光焊接应力场数值模拟的研究进展[J]. 热加工工艺, 2016, 45(13): 8-10.ZHANG L Y,DONG W P. Research progress in nu‐merical simulation of laser welding stress field[J]. HotProcessing Technology, 2016, 45(1): 8-10.[15] 卢艳,张静,胡敬佩,等. 激光焊接铝合金材料过程的建模与仿真[J]. 热加工工艺,2012,41(01):130-133.LU Y,ZHANG J,HU J P,et al. Modeling and simula‐tion of laser welding process of aluminum alloy[J].Hot working process, 2012,41(01):130-133.[16] Yoon J W,Noh B I,Kim B K,et al. Wettability and in‐terfacial reactions of Sn-Ag-Cu/Cu and Sn-Ag-Ni/Cusolder joints[J]. Journal of Alloys and Compounds,2009, 486(1-2): 142-147.[17] Huan P C,Tang X X,Sun Q,et al. Comparative study of solder wettability on aluminum substrate andmicrostructure-properties of Cu-based component/alumin-um laser soldering joint[J]. Materials & De‐sign, 2022, 215: 110485-110487.[18] Meco S,Pardal G,Ganguly S,et al. Application of la‐ser in seam welding of dissimilar steel to aluminiumjoints for thick structural components[J]. Optics andLasers in Engineering, 2015, 67: 22-30.[19] Deng S,Yuan R,Tang X,et al. Migration behavior of IMC layer in twin-spot laser welding-soldering of alu‐minum to steel[J]. Materials & Design,2020,188:108489.编辑部网址:http://88Electric Welding MachineVol.54 No.3Mar. 2024第 54 卷 第 3 期2024 年3 月A105锻钢球阀激光深熔焊接工艺优化及组织性能研究王申1, 王栋2, 冯涛3, 杨香1, 汪思鹏1, 魏志宏11.安徽理工大学 机械工程学院,安徽 淮南 2320012.安徽理工大学 人工智能学院,安徽 淮南 2320013.江苏诚功阀门科技有限公司,江苏 常州 213000摘 要:采用正交试验对5 mm 厚A105锻钢管件激光深熔焊接工艺参数进行优化,确定了各工艺参数对各响应量的影响程度及最优焊接工艺,对该最优工艺参数下焊接接头组织和力学特性进行研究。
[11]温度对IGBT的影响
![[11]温度对IGBT的影响](https://img.taocdn.com/s3/m/685f7e5ec850ad02df804117.png)
Temperature Levels Effects on the Thermo-Mechanical Behaviour of Solder Attach During Thermal Cycling of Power Electronic ModulesM. Bouarroudj*, Z. Khatir*, S. Lefebvre*** INRETS-LTN, 2 av. du Général Malleret-Joinville, 94114 Arcueil, France** SATIE, ENS de Cachan, 61 Avenue du président Wilson, 94235 Cachan, FranceAbstract— The paper presents the effect of temperature amplitu e an wells level on the thermomechanical behaviour of power modules solder. Especially, we show the influence of these parameters on the crack initiation and propagation in the solder layer between Direct Bond Copper and base plate of high power IGBT mod ules. For this purpose, thermal cycling tests have been performed on IGBT power mod ules with three temperature profiles. For cycle#1 temperature vary between -40°C and 120°C, cycle#2 temperature vary from 40°C to 120°C and for cycle#3 temperature vary from -40°C to 40°C. These tests revealed that solder crack initiation and its propagation occur earlier for cycle#1 where temperature variation is higher. But the unexpected results concern those of cycle#2 and cycle#3. In spite of the fact that its have the same temperature variations, sol er crack initiations occur earlier an propagate faster in cycle#2 than in cycle#3 . These results show clearly that high and low temperature levels are important on sold er lifetime. In ord er to und erstand the experimental results, FE simulations have been performed (with ANSYS). The first obtained results show that stress variation d epend s on the low temperature level, lower is Tmin higher is stress variation. On the other hand, strain variation depends on high temperature level, higher is Tmax higher is strain variation. Finally, Complementary simulations with various high and low temperatures have been performed and revealed tow mechanical behaviour of the solder. When upper temperature is below a homologous temperature of 0.74Tm, shear strain variations remains in a relatively small range and shear stress variations have a linear d epend ence with the temperature variation. On the contrary, when Tmax is above 0.74 Tm, shear stresses variations reach a saturation value while inelastic shear strains increase significantly.Index Terms— IGBT, Power electronic mo d ules, Packaging, Thermal cycling tests, Finite Element Analysis (FEA)I.I NTRODUCTION THERMOMECHANICAL constraints in power modules are often caused by thermal cycling conditions [1,2]. Such constraints lead to crack initiation followed by crack propagation inside solder attach materials. In case of IGBT (Insulated Gate Bipolar Transistors) power modules, the weakest attach layers are the solders between DCB (Direct Copper Bonding) and base-plates due to the mismatch of coefficients of thermal expansion and the large areas of these solders [2-5]. So, lifetime assessment of a given power device packaging technology is an important stake. This can be achieved by using models that require both experimental and simulation results. In particular, accelerated tests may be used but it must be possible to extrapolate the lifetime results from accelerated conditions to normal operating conditions [6]. Generally, acceleration factor (AF) between two test conditions characterized by temperature variations 'T1 and 'T2, for solder lifetime in thermal cycling conditions are computed by using a simple Coffin-Manson relation [6,7] : AF =('T1/'T2)-m. This model of extrapolation is only related to the temperature variation and is probably too simple to be used in all operating conditions. Obviously, the highly non-linear behavior of solder joints makes the lifetime prognosis in real conditions difficult to estimate. So, it is important to understand the role played by each parameter of thermal cycle profiles in the solder joint lifetime. Some answers have been already reported concerning the effects of dwell time durations and temperature ramp rate in solder joint lifetime [8-11]. Pang et al. [12] have proposed scale factors for correlating flip chip solder joint fatigue life from an accelerated condition with a large 'T to smaller temperature range.In this paper, we show that large solder joints lifetime is not only dependent on temperature variation ('T) but also in other key parameters such as dwell temperature levels. In particular, we highlight how the upper and lower temperature levels (Tmax, Tmin) affect the shear stress and inelastic strain variations during thermal cycles and the role of these parameters in the acceleration factor. In a first step, thermal cycling tests were performed on integrated six-pack IGBT power modules (600V-200A). Several test conditions with two 'T and different upper and lower temperature levels have been done on which solder crack initiations have been detected and crack propagation rate have been measured. In a second step, these experimental tests are completed by finite element analyses (FEA) to show the mechanical behavior dependence of the solder behavior with temperature levels.II.T HERMAL C YCLING T ESTSWe have performed thermal cycling tests on IGBT power modules in order to investigate experimentally both the effect of several temperature variations ('T)with different high and low temperature levels (Tmax, Tmin) of thermal cycles. For this purpose, three thermal cycle profiles have been defined and applied to several power modules as shown in figure 1. Tested power modules are integrated inverter six-pack IGBT devices with three DBC alumina substrates attached to a copper base plate by solder alloys. Two modules (B8, E6) have undergone temperature cycling tests between -40°C and 120°C (cycle#1), four modules (A1, A2, E10, E11) were subjected to temperature cycling between 40°C and 120°C (cycle#2) and four other (C3, C8, E7, E9) to temperature cycling between -40°C and 40°C (cycle#3). Temperature rate variations are about 10°C/mn and dwelltime durations were approximately 15mn.Figure 1. Experimental thermal cyclesBefore thermal cycle tests, scan acoustic microscopy (SAM) images are realized in order to have the initial state of solder attach. Then, during thermal cycle tests, SAM images are regularly performed in order to detect crack solder initiations at each DCB corners. In a third step, SAM analyses are used to assess the crack propagation rates at each corner. Then, in order to take into account the disparity and inhomogeneous thickness of solder layers, a mean value of the propagation rate is calculated over all three DCBs of each power module.As a result, table I gives a summary of the experimental results. Crack initiation on cycle#1 occurred after only some tens cycles and propagates with a rate of about 20μm/cycle. For cycle#2 where the temperature amplitude was divided by 2 and with same upper temperature, crack initiation has been delayed. The first crack initiation appeared after about 200 to 300 cycles on some corners and propagates with quite same speed. Finally, for cycle#3, which has same temperature amplitude ('T) than cycle#2 but at different high and low temperature levels, crack initiation occurred after a mean value of about 900 cycles and propagates with lower rate of about 8 μm per cycle.Cycle#3 leads to higher number of cycles to first cracks and lower speed rate compared to cycle#1.Furthermore, cycle#3 leads also to significantly higher lifetime and propagation rate than for cycle#2 even with same temperature variation. These results clearly show that crack initiation and propagation in solder do not only depend on temperature amplitude but also on temperature levels (T max and T min ). For same temperature swings ('T),the higher temperature levels the shorter the solder lifetime.TABLE IExperimental results on thermal cyclingCycle type Modules 'T (°C)Number of cycle to crack initiationPropagation rate(μm/cycle)B820 23 Cycle#1(-40°C/120°C)E616050 19 A1300 17 A2300 15 E10300 20 Cycle#2(40°C/120°C)E1180150 20 C3500 5 C8900 8 E7900 8 Cycle#3(-40°C/40°C)E980900 8 Figure 2 shows some scan acoustic analyses (SAM) ofthe solder between DCB substrate and base plate. In order to show visible cracks, presented acoustic images in fig.2 are given long time after crack initiation.initial state after 250cycles(a)initial state after 450cycles(b)initial state after 1150cycles(c)Figure 2. Evolution of the solder crack under: (a) cycle#1, (b) cycle#2,(c) cycle#3Fig.2a presents solder at initial state and after 250 cycles of profile#1 where all corners are cracked and exhibits advanced fracture due to the relatively high propagation rate. Fig.2b gives the evolution after 450 cycles of profile#2. Although the presented picture is 150 cycles after first cracks, degradation remains very limited due to the low propagation rate. This is also the case for tests with profile#3 (Fig.2c) where after 1150 cycles, so near 600 cycles after first cracks, crack length remains very shorts, around 1mm from corners.III.F INITE E LEMENT S IMULATIONSA.Numerical s imulations of thermal cyclingIn order to understand the experimental test results, thermo-mechanical simulations have been carried out using finite element analysis (FEA). The objective is to highlight the dependence of shear stresses and plastic strains with high and low temperature levels during thermal cycling. For problem simplification, only one DCB substrate has been modelled in a 2D axi-symmetric structure with the solder layer and base plate assembly (fig.3).As the objectives of these simulations are only to show qualitatively the temperature level effects on mechanical behaviour for understanding purpose, the solder materialis assumed to be SnPb37 in the following simulations.Figure 3. Axisymetric FE model of the assembly Material data are given in table II, DCB ceramic is considered as linear, copper metallization is considered non-linear material (elasto-plastic) and solder is considered visco-plastic and modelled with the Anand model which parameters are given in table III [14]. In a first step, same temperature cycles than in experiment were applied (see fig.1). In a second step, complementary numerical analyses with more cycle profiles will be presented thereafter.TABLE IIMaterial physical parameters [13, 14]MaterialsCTE(10-6K-1)Young modulus(MPa)Yield stress(MPa)Tangent modulus(MPa)Cu17119×103 100 400 Al2O3 4.3370×103(elastic)SnPb3724.775842 -151.7 T(K) (Anand parameters)TABLE IIISolder Anand parameters of SnPb37 [14]s o(MPa)Q/R(K)A(S-1)ȟ mh0(MPa)S(MPa)n a12.419400 4.106 1.5 0.3031378.95 13.790.07 1.3Figures 4 and 5 give respectively the computed shearstresses and shear strains evolution on the solder attachfor simulated temperature profiles (cycle#1, #2 and #3),near the location of maximum stresses and strains (seepoint A in fig.3 or inserted diagram in figs.4 and 5).Table IV gives the result summary of these simulationswhere 'J in,'W,'W in are respectively cyclic variations ofinelastic shear strain, shear stress and inelastic workdensity. These variation values are given for the fifthsimulated thermal cycle for each case.TABLE IVSimulation results of thermal cycling.'T (°C) 'J in'W (MPa)'W in(J/cm3)Cycle#1(-40°C/120°C)160 0.061 44 1.24Cycle#2(40°C/120°C)80 0.049 19 0.68Cycle#3(-40°C/40°C)80 0.002 41 0.22Figure 4. Shear stress evolutionFigure 5. Shear strain evolutionWe can observe that for cycle#1, both shear stressesand shear strains values are significant. For cycle#2, the stress variation is nearly half that of the first profile but the strain variation is nearly the same. This is due to the fact that temperature variation occurred at high level (40°C<T<120°C), so the stresses are lower and the strains are similar. For cycle#3, the stress variation is quite similar with that of the first profile but the strain variation is very low. In this case, the same temperature variation than cycle#2 occurred at low temperature (-40°C<T<40°C) thus the stresses are quite similar than cycle#1 but strains are lower. From these results we can clearly conclude that stress values are monitored by the low temperature level and the strain by the high level one. Furthermore, we can observe that the magnitude of strain variations is relatively larger than for stress variations. For the same 'T reduction, from cycle#1 to cycle#2, stress variation is divided by a factor 2 whereas from cycle#1 to cycle#3, the strain variation has been divided by a factor 15. These numerical results are consistent with the experimental ones given in table III in the previous section.plementary numerical behavior of SnPb solderand effects of dwell temperature levelsIn order to make a large investigation of the influence of dwell temperature levels, complementary simulations have been done and presented in the following. All simulation cases done are given in fig.6. Each point of fig.6 represents a couple (Tmin, Tmax) investigated, all other parameters have been kept constant (15mn high and low dwell times, 10°C/mn temperature rates). In each simulation case, initial stresses, due to soldering process, have been taken into account with a cooling down phase from 183°C (eutectic temperature) to room temperature 20°C.Results obtained in the computed shear stress ('W) and plastic shear strain ('J p) variations during a cycle are given respectively in figs. 6 and 7 versus high temperature level (T max) for different low temperature levels (T min).From these results we can distinguish two regions separated by an upper dwell temperature level of about 65°C (grey area), corresponding to a homologous temperature of 0.74xT m where T m is the melting temperature (183°C). When maximum dwell temperature (Tmax) are lower than 65°C, 'J p remains in a relatively small range values below 0.5% and 'W has a linear dependence with the temperature variation. For our solder geometry and material: 'W (MPa) § 0.56 'T(K).Figure 6. Cyclic shear stress variations near the point A (fig.4) ofmaximum values.Figure 7. Cyclic plastic shear strain variations near the point A (fig.3) of maximum values vs. upper temperature levels.On the contrary, when Tmax is above 0.74xTm (65°C), which is almost always the case in practical use, for a given Tmin condition, shear stresses variations reach a saturation value while inelastic shear strains increase significantly. In other words, when Tmax is greater than 0.74xTm, increasing temperature cycling amplitude 'T leads to increase significantly 'J p only, without any effect on shear stress variations ('W). Furthermore, as it can be seen in fig.7 for the highest Tmax values, the strain variation rises quasi-linearly with the upper temperature level (Tmax) with the same slope : G('J p)§ 1.10-3G(Tmax), whatever minimum temperature level (Tmin). For example, for Tmin=-40°C, and when Tmax increases from 100°C to 120°C, the increase in 'J p is around 2.2% in a other hand, for Tmin=80°C and for the same increase of Tmax from 100°C to 120°C, we have almost the same increase in 'J p, around 2%.Lifetime prognosis of the solder attach is sometimes related to shear strain variations as in Coffin-Manson [15] or Engelmaier [16] models and other times to inelastic work density as in Dravaux's [17]. For all the simulated cases, we have plotted the dependence of inelastic energy density per cycle ('W pl) with temperature swings ('T) atthe same point A (see fig.3), near the maximum value, infig.9.Figure 8. Plastic work density per cycle near the point A (fig.3).Obtained results clearly show that, when Tmax < 0.74xTm, inelastic energy density in the solder is due to both shear strains and stresses. Since in these conditions, shear strains are very limited and the magnitude of stress variations are in a ratio of only 4 (from 10 MPa for the lowest values to 40 MPa for the highest ones), the energy density remains relatively small, below 0.2 to 0.3 J/cm 3.On the contrary, when Tmax > 0.74xTm, cyclic plastic work density rises significantly with Tmax because shear strain variations exhibit large values (fig.8). It is clear that, in these conditions, the inelastic energy density is now driven essentially by inelastic shear strain. So we can conclude that when thermal cycles are performed in such condition that the high temperature level (Tmax) is greater than 0.74xTm, either strain based models or energy based models could be used for solder lifetime estimation. But, for Tmax < 0.74xTm, only energy based models should be used, strain based models are probably not suitable.As solder lifetime estimation is related to inelastic work density per cycle, from figure 10, it is clear that temperature variations ('T) is not the sole parameter which play a significant role in the cyclic energy density, thus in the solder lifetime. The dwell temperature levels have also a great influence on the lifetime of the solder attach. For example, for a same amplitude variation 'T = 100°C, cyclic plastic work is only 0.25 J/cm 3 when Tmin is -40°C but rises to 0.7 J/cm 3 for Tmin=0°C. This explain obtained results in experimental tests when for the same temperature amplitude, degradations occur first on the solders submitted at high temperature levels.Furthermore, results show clearly that for thermal cycling occurring at high ambient temperature (this is the case for Tmin=80°C), we have no large stresses inside the power assembly but very large strains and thus inelastic work densities in the solder, even for small 'T. All these results show the interest of solders with high Tm values for high temperature applications.C ONCLUSIONThis study has presented a detailed analysis of some key parameters and their influence during thermal cycling of large solder joints of power electronic assemblies. By both experimental and numerical approaches it has been shown that solder lifetime has not only a dependence with temperature variation ('T) but also with dwell temperature levels (Tmax and Tmin). For thermal cycle conditions whose maximum temperature level remains under a homologous temperature of about 0.74 Tm, whatever the temperature variation ('T), inelastic shear strains variations remains relatively low and shear stresses are linearly dependent with the temperature variation. In these conditions, inelastic energy density in the solder is due to both shear strains and stresses variations.In the contrary, for thermal cycling conditions whose Tmax is above a homologous temperature of about 0.74 Tm, whatever the temperature variation ('T), cyclic plastic work density rises significantly with Tmax, due only to plastic strain variations. So, either strain based models or energy based models could be used for solder lifetime estimation. But, for Tmax < 0.74 Tm, only energy based models should be used. Nevertheless, these results are based only on numerical simulations and must be verified by further experimental tests. Temperature swing ('T) is not the sole parameter which plays a significant role in the solder lifetime; dwell temperature levels have also a great influence.R EFERENCES[1]J.M. Thebaud et al., “Strategy for designing accelerated ageing tests to evaluate IGBT power modules lifetime in real operation mode”, IEEE Trans. Components and packaging technologies ,vol.26, n°2, pp.429-438, june 2003.[2]M. Ciappa, "Selected failure mechanisms of modern powermodules", Microelectronics Reliability , Vol.42, n°4-5, pp.653-667, April-May 2002.[3]K. Guth, P. Mahnke, “Improving the thermal reliability of largearea solder joints in IGBT power modules”, in Proc. CIPS ,Naples, 2006.[4]J. Yamada et al., "the latest High Performance and HighReliability IGBT Technology in New packages with Conventional Pin Layout", in Proc. PCIM conference , Nuremberg, 2003.[5]J.M. Thebaud et al., "Extensive Fatigue Investigation of SolderJoints in High Power Modules", in Proc.Electronic Components and Technology Conference , 2000[6]Finn Jensen, Electron c Component Rel ab l ty , John Wiley &Sons, 1995.[7]S.S. Manson, Thermal stress and low-cycle fatigue , Krieger, 1981 [8] C.J. Zhai, Sidarth and R. Blish, "Board Level Solder ReliabilityVersus Ramp Rate and Dwell Time During Temperature Cycling", IEEE Trans. devi ce and materi als reli abi li ty , vol.3, n°4, pp.207-212, Dec. 2003[9]S. Moreau, T. Lequeu and R. Jerisian, "Comparative study ofthermal cycling and thermal shocks tests on electronic components reliability",Mi croelectroni cs Reli abi li ty, vol.44, n°9-11 pp.1343-1347, Sept. 2004.[10]H.J. Shah and J.H. Kelly, "Effect of dwell time on thermal cyclingof the flip-chip joint", in Proc. Int. Microelectron. Symp. , pp.341-346, 1970.[11]V. Sarihan, "Energy Based Methodology for Damage and LifePrediction of solder joints under Thermal Cycling", IEEE Trans. on comp., packagi ng and manufac. technology , part-B, Vol.17, N°4, pp.626-631, Nov. 1994.[12]J.H.L. Pang, D.Y.R. Chong, and T.H. Low, "Thermal CyclingAnalysis of Flip-Chip Solder Joint Reliability", IEEE Trans. on comp. and packaging technologies, Vol.24, N°4, pp.705-712, Dec.2001[13]J.F. Shackelford and W. Alexander, “Materials science andengineering handbook”, CRC Press, 2001.[14] B. A. Zahn, "Comprehensive solder fatigue and thermalcharacterization of a silicon based multi-chip module package utilizing finite element analysis methodologies", Proceeding of the 52nd Electronic Components and Technology Conference, 2002. [15]S.S. Manson, "thermal stress and low-cycle fatigue", Krieger,1981[16]W. Engelmaier, “Fatigue Life of Leadless Chip Carrier solderjoints during power cycling”, IEEE Trans. on comp. hybrids and manuf. Technology, vol.6, n°3, sept. 1983.[17]R. Darveaux, “Effect of Simulation Methodology on Solder JointCrack Growth Correlation”, Proc. of the Electroni c Components and Technology Conference, 2000.。
Advanced Thermodynamics
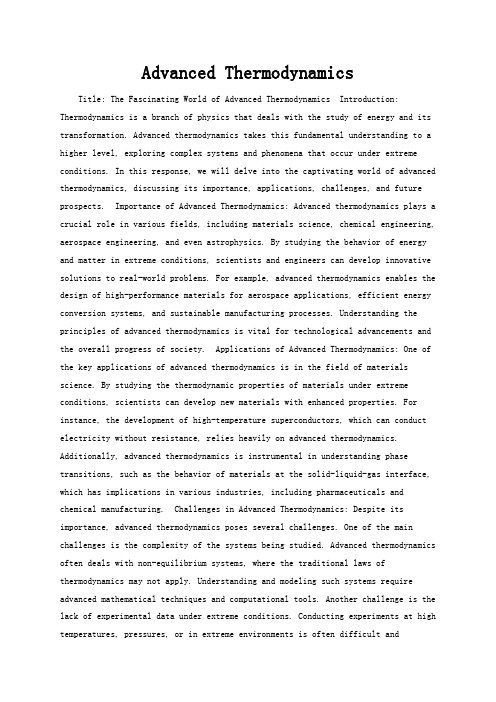
Advanced ThermodynamicsTitle: The Fascinating World of Advanced Thermodynamics Introduction: Thermodynamics is a branch of physics that deals with the study of energy and its transformation. Advanced thermodynamics takes this fundamental understanding to a higher level, exploring complex systems and phenomena that occur under extreme conditions. In this response, we will delve into the captivating world of advanced thermodynamics, discussing its importance, applications, challenges, and future prospects. Importance of Advanced Thermodynamics: Advanced thermodynamics plays a crucial role in various fields, including materials science, chemical engineering, aerospace engineering, and even astrophysics. By studying the behavior of energy and matter in extreme conditions, scientists and engineers can develop innovative solutions to real-world problems. For example, advanced thermodynamics enables the design of high-performance materials for aerospace applications, efficient energy conversion systems, and sustainable manufacturing processes. Understanding the principles of advanced thermodynamics is vital for technological advancements and the overall progress of society. Applications of Advanced Thermodynamics: One of the key applications of advanced thermodynamics is in the field of materials science. By studying the thermodynamic properties of materials under extreme conditions, scientists can develop new materials with enhanced properties. For instance, the development of high-temperature superconductors, which can conduct electricity without resistance, relies heavily on advanced thermodynamics. Additionally, advanced thermodynamics is instrumental in understanding phase transitions, such as the behavior of materials at the solid-liquid-gas interface, which has implications in various industries, including pharmaceuticals and chemical manufacturing. Challenges in Advanced Thermodynamics: Despite its importance, advanced thermodynamics poses several challenges. One of the main challenges is the complexity of the systems being studied. Advanced thermodynamics often deals with non-equilibrium systems, where the traditional laws of thermodynamics may not apply. Understanding and modeling such systems require advanced mathematical techniques and computational tools. Another challenge is the lack of experimental data under extreme conditions. Conducting experiments at high temperatures, pressures, or in extreme environments is often difficult andexpensive. Therefore, researchers heavily rely on theoretical models and simulations to gain insights into the behavior of complex systems. Future Prospects: The future of advanced thermodynamics looks promising, driven by advancements in computational power and experimental techniques. The development of sophisticated simulation tools, such as molecular dynamics and Monte Carlo methods, allows researchers to model complex systems more accurately. Furthermore, advancements in materials characterization techniques, such as high-resolution microscopy and spectroscopy, enable the direct observation and measurement of thermodynamic properties at the nanoscale. These advancements will not only deepen our understanding of advanced thermodynamics but also facilitate the design and development of novel materials and energy systems. Conclusion: In conclusion, advanced thermodynamics holds immense importance in various scientific and engineering disciplines. Its applications range from materials science to energy conversion systems, offering solutions to real-world challenges. However, the complexity of the systems being studied and the lack of experimental data pose significant challenges. Nevertheless, with the continuous advancements in computational power and experimental techniques, the future of advanced thermodynamics looks promising. By harnessing the principles of advanced thermodynamics, scientists and engineers can unlock new possibilities and drive innovation for a sustainable and technologically advanced future.。
部分造纸英文缩写及中文对照

部分造纸英文缩写及中文对照部分造纸英文缩写AAA = atomic absorption原子吸收ABS = acrylonitrile-buladrene styrene丙烯腈—丁;烯—苯乙烯ACAR = angular correlation of annihilation radiation消除辐射的角相关性AM = acrylamide丙烯酰胺AOX = adsorbable organic halides可吸附的有机卤化物AP = plkali pulp碱法纸浆APAM = anionic polyacrylamide阴离子型聚丙烯酰胺ASB = aerotion stabilization basin稳定曝气池AST = activated sludge treatment活性污泥处理BBCT = best convential pollutant cotrol technology最常用污染物控制技术BDMT = bone dry metric tons绝干公吨BME = bipolar membrane electro dialysis两极膜电透析BMP = best management practices最优管理实践BOD = biochemical oxygen demand生化耗氧量BP = boiling point沸点BPK = bleached papergrade kraft and soda(生产)白纸用硫酸盐和荷性纳法浆BPT = best practicable control technology最佳实用控制技术BTU = british thermal unit英热单位BW = basis weight定量CCAD = computer aided design计算机辅助设计CBLI = chemistry-based leak indicator化学(法)示漏器CC = consistency controller浓度调节器CFD = computational fluid dynamics计算流体动力学CI = colour index比色指数= cofidence interval置信区间CL = colored ledger彩色底板CLSM = confocal laser scanning microscopy共焦激光扫描显微镜CMC = carboxy methylated cellulose羧甲基纤维素COMS = compliance optimization modeling system寻优模型系统CP = chemical pulp化学浆= chemical pure化学纯CPPC = coordinated phosphate/pH chemistry controller配位磷酸盐/pH调节器CR = consistency regulator浓度调节器CRP = chloride removal process氯化物排出法CSD = condensate steam distillation column冷凝汽馏塔CTMP = chemical treatment in terms of sulphonation硫化期间的化学处理= chemithermomechanical pulp化学热磨机械浆CTU = centigrade thermal unit公制热量单位CV = coefficient variation偏离系数= crystal violet结晶紫DD = dioxide二氧化物DAF = dissolved air floatation(溶)气浮DCS = dissolved and colloidal substances溶解与胶态物= distributed control system集散控制系统DELS = Doppler electrophoretic light scattering多普勒电泳光扫描DIP = deinked pulp脱墨纸浆DKP = deinked kraft pulp脱墨牛皮纸浆DLK = double-line clippings双线限位DMS = dynamic mechanical spectroscopy动力谱学DMSO = dimethyl sulfoxide二甲亚砜DMT = dimethyl terephthalate对邻苯二甲酸二甲酯DO = dissolved oxygen溶解氧DP = degree of polymerization聚合度DSC = differential scanning calorimetry微分扫描量热法DVC = digital valve controller数字伐控制器EEC = embedded costs插入成本ECF = elemental chlorine free无元素氯(漂白)EDTA = ethylene eiamine tetraacetic acid乙二胺四乙酸EPC = experimental prismatic calcite实验棱镜方解石ERV = estimated replacement value预计取代值ESP = electrostatic precipitator静电滤尘器= emergency shutdown procedure事故停机程序EV A = ethylene vinyl acetate乙烯乙酸乙烯酯ESPRA = empire state paper research associates国立造纸研究会EVOH = ethylene-vinyl alcohol乙烯-乙烯醇FFAS = formamidine sulfinic acid甲脒亚磺酸FBB = folding box board折叠箱纸板FBK = fully bleached kraft全漂牛皮纸FC = flow controller流量控制器FID = free induction decays自由感应衰减FP = freezing point冰点;凝固点GGDP = gross domestic product本国生产总值GEMS = general energy and materials balance system通用能量和物料平衡系统GLC = gas-liquid chromatography气液色谱GPC = gel permeation chromatographic analysis凝胶渗透色谱分析GPM = gallons per minute加仑/分钟HHC = high consistency高浓HCR = high consistency refiner高浓磨浆机HD = high density高密度HPR = high production rate高生产率HPSEC = high-performance size-exclusion chromatography高性能粒度筛析色谱法HRT = hydraulic retention time水力停留时间HTH = high test hypochlorite高级漂粉HV = high voltage高压HW = hardwood硬木IIMPM = interactive multiplanar model相互作用的多面模型IPST = institute of paper science and technology造纸科技研究院IWC = international water consultants国际水质顾问团JJIT = just-in-time正好;准时KKP = kraft pulp牛皮浆;硫酸盐浆LLC = level controller液面控制器LCC = lignin-carbohydrate complexes木素-碳水化合物复合体LCL = lower control limits控制下限LCR = level cotroller and recorder液面控制记录仪LDPE = low density poly ethylene低密度聚乙烯LDV = laser Doppler velocimetry激光多普勒测速法LIVG = low inlet velocity gasification process低入口速度气化工艺LPR = low production rate低生产率LRD = long rang dependence广范围相关LVDT = linear position transducer线性位移变送器LWC = lightweight coated低定量涂布的MMACT = maximum achievable control technology最大可达控制技术MAP = modified atmosphere packaging改良常压包装法MC = marginal cost边际成本= medium consistency中浓(度)MDI = methylendiphenyl diisocyanate亚甲苯二苯二异氰酸酯MeB = methylene blue亚甲基兰,四甲基兰MEK = methyl ethyl ketone甲(基)乙(基)酮MF = machine finished机械整饰的MG = machine glazed机械上光的= malachte green孔雀绿MISS = mixed liquor suspended solids (有机物与活性污泥)混合液中悬浮固体MOW = mixed office waste混合办公废纸MRP = matal removal process金属(离子)脱除过程MSW = municipal solid waste城市固体废物MVP = moisture vapor permeability水蒸汽渗透性MWL = milled wood lignin磨木木素NNC = nitrocellulose 硝化纤维素NF = nanofiltration超滤(毫微过滤)NMR = nuclear magnetic resonance核磁共振NSPS = new source performance standards新的资源性能标准NSSC = neutral sulfite semi-chemical pulp中性亚硫酸半化学浆OOCC = old corrugated container旧瓦楞纸箱OD = over dry绝干;烘干OEE = overall equipment efficiency总设备效率OIT = oxidative induction temperature氧化起始温度O&M = operating and maintenance 使用与维护ONP = old newspaper旧新闻纸OPP = oriented polypropylene取向聚丙烯OPR = oil penetration rates渗油率OWL = oxidized white liquor氧化白液PPAL = positron annihilation life time正电子湮没寿命PC = pressure controller压力调节器PCA = principal components analysis主成分分析PCC = precipitated calcium carbonate沉淀碳酸钙PCR = pressure controller and recorder压力调节记录仪PDSC = pressure differential scanning colorimetry压差扫描量热术PEMS = predictive emissions modeling system预测排放模型系统PEO = poly ethylene oxide聚氧化乙烯PGS = papergrade sulfite造纸用硫磺PGW = pressurized groundwood压力磨木浆PM = paper machine 造纸机;抄纸机PM/ECCM = preventive maintenance and essential care and condition monitoring预防维修/基本维修及状态监测PP = polypropylene聚丙烯PSES = pretreatment standards for existing sources现存资源预测标准PSM = process safety management(生产)过程安全管理PTFE = polytetrafluoroethylene聚四氟乙烯PTR = photothermal radiometry光热辐射分析法PVC = polyvinylchloride聚氯乙烯PVDC = polyvinyl dichloride聚二氯乙烯PVSK = polyvinylsulfate聚乙烯硫酸酯RRDH = rapid displacement heating快速置换加热法RH = relative humidity相对湿度RMP = refiner mechanical pulp木片磨木浆;盘磨机械浆RN = regular number纸板标准号RT = radiographic testing射线照相试验,X射线检验SSBK = solid bleached kraft(同质)漂白牛皮纸SBR = sequencing batch reactors程序化间歇反应器SC = super calendered超级压光的SDI = silt density index淤泥浓度指数SE = supplemental energy补充能量;辅助能SEC = size exclusion chromatographic粒度筛析色谱法SEM = scanning electron microscope扫描电子显微镜SEM-EDS = scanning electron microscope-energy dispersive spectrometry扫描电子显微镜—能量分散能谱测定法SGW = stone ground wood磨石磨木浆SIF = stress intensity factor应力强度系数;应力强化因子SOPs = standard operating procedures标准作业程序SP = sulphite pulp亚硫酸盐纸浆SPC = satislical process control过程控制SRT = solids retention time粒子留着时间SUB = solid unbleached board(同质)本色浆纸板SW = softwood软木;针叶树SWL = sulphite waste liguor亚硫酸盐废液TTAC = totally applied chlorine总用氯量TC = temperature controller温度调节器TCDF = tetrachlorodibenzofuran四氯二苯并呋喃TCF = totally chlorine-free全无氯(漂白)TCR = temperature controller and recorder温度调节记录仪TGA = thermal gravimetric analysis热重分析TLA = thin layer activation薄层活性化TMP = thermo mechanical pulp热磨机械浆TP = thermo-plastic热塑性的TQ = threshold quantity临界量(值)TRS = total reduced sulfur总还原硫TS = tensile strength抗张强度TSS = total suspended solids总悬浮固体量UUBB = unbeached board本色(浆)纸板UBK = unbeached kraft本色牛皮纸UCL = upper control limits控制上限UT = ultrasonic testing超声试验UV = ultraviolet紫外光VVOC = volatile organic compound挥发性有机化合物WW AS = waste-activated sludge废活性污泥WFMT = wet fluorescent magnetic particle test湿荧光磁粉试验WL = white ledger白色帐簿纸WLC = white-lined chipboard白浆衬里的粗纸板WP = wood pulp木浆WVTR = water vapor transmission rate水蒸汽传递速度YYI = yellow index返黄值;返黄指数YP = yield point屈服(软化)点。
基于热固耦合的双螺杆挤压机机筒有限元分析

第43卷 第7期 包 装 工 程2022年4月PACKAGING ENGINEERING ·218·收稿日期:2021-06-02基金项目:宁夏回族自治区重点研发计划(22019BFF02004)作者简介:张培建(1993—),男,天津科技大学硕士生,主攻多场耦合及优化设计。
通信作者:邢鸿雁(1969—),女,硕士,天津科技大学副教授,主要研究方向为CAD/CAE 。
基于热固耦合的双螺杆挤压机机筒有限元分析张培建1,2,邢鸿雁1,2,卫静怡1,2(1.天津市轻工与食品工程机械装备集成设计与在线监控重点实验室,天津 300222;2.天津科技大学 机械工程学院,天津 300222)摘要:目的 研究双螺杆挤压机在食品加工过程中出现卡顿、抱死的原因,为双螺杆挤压机设计及应用提供理论指导。
方法 应用ABAQUS 软件构建双螺杆挤压机仿真模型,通过传感器反馈的温度值设定温度载荷,同时以双螺杆挤压机运转时的低压工况、正常工况、极限工况分别设定压力载荷,运用热固耦合理论对机筒的温度分布、应力和变形进行仿真分析。
结果 在最大温度工况条件下,机筒轴向热变形量为2.356 mm ,总热变形量为2.358 mm ,x 方向上的热变形量为0.1324 mm ,y 方向上的热变形量为0.1592 mm ;在最大温度工况和极限压力耦合作用下,机筒总变形量为2.088 mm 。
温度引起的热膨胀是机筒变形的主要原因,机筒的轴向热变形量与总热变形量相当,并且远大于其他两方向的热变形量;与常温环境相比,在加热温度工况条件下机筒的变形量随着压力的增大而减小。
结论 要充分考虑温度对挤压机性能的影响,应用过程中要合理的设置温度参数。
关键词:双螺杆挤压机;机筒;热固耦合;有限元中图分类号:TB486;TH114+.7 文献标识码:A 文章编号:1001-3563(2022)07-0218-07DOI :10.19554/ki.1001-3563.2022.07.028 Finite Element Analysis of Twin-screw Extruder Cylinder Basedon Thermo-mechanical CouplingZHANG Pei-jian 1,2, XING Hong-yan 1,2, WEI Jing-yi 1,2(1.Tianjin Key Laboratory of Integrated Design and Online Monitoring of Light Industry and Food EngineeringMachinery and Equipment, Tianjin 300222, China; 2.School of Mechanical Engineering,Tianjin University of Science and Technology, Tianjin 300222, China) ABSTRACT: The work aims to study the causes of clatter and locking in the food extrusion process of twin-screw ex-truder, and to provide theoretical guidance for the design and application of twin-screw extruder. ABAQUS software was used to construct the simulation model of the twin-screw extruder. The temperature load was set according to the tem-perature feedback from the sensor. At the same time, the pressure load was set under the low pressure condition, normal condition and limit condition of the twin-screw extruder. The temperature distribution, stress and deformation of the cylinder were simulated and analyzed by the thermo-mechanical coupling theory. Under the maximum temperature condition, the axial thermal deformation of the cylinder was 2.356 mm, the total thermal deformation was 2.358 mm, the thermal deformation in the x direction was 0.1324 mm, and the thermal deformation in the y direction was 0.1592 mm. Under the coupling effect of maximum temperature condition and ultimate pressure, the total deformation of the barrel. All Rights Reserved.第43卷第7期张培建,等:基于热固耦合的双螺杆挤压机机筒有限元分析·219·was 2.088 mm. The thermal expansion caused by temperature was the main reason for the deformation of the cylinder, and the axial thermal deformation of the cylinder was equivalent to the total thermal deformation, and was much larger than that of the other two directions. Compared with the normal temperature environment, the deformation of the cylinder decreased with the increase of pressure under the condition of heating temperature. The effects of tem-perature on the performance of the extruder should be considered and reasonable temperature parameters should be set in application.KEY WORDS: twin screw extruder; cylinder; thermo-solid coupling; finite element随着生活水平的提高,人们对挤压膨化食品的营养和口感有更高的要求;膨化食品的生产通常是由双螺杆挤压机完成的。
纤维素纳米纤维增强聚乳酸
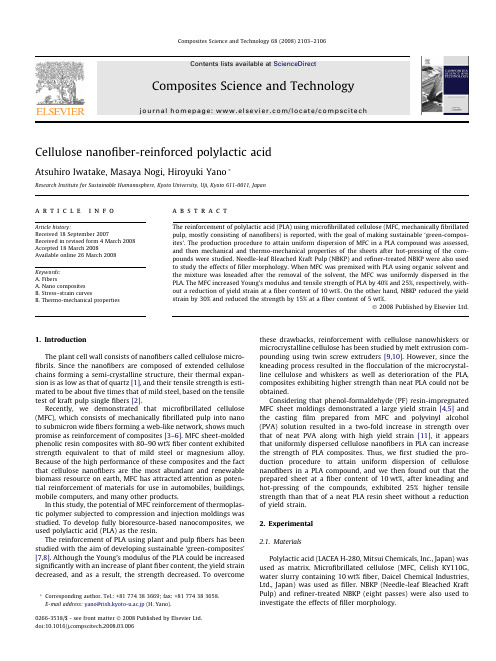
Cellulose nanofiber-reinforced polylactic acidAtsuhiro Iwatake,Masaya Nogi,Hiroyuki Yano *Research Institute for Sustainable Humanosphere,Kyoto University,Uji,Kyoto 611-0011,Japana r t i c l e i n f o Article history:Received 18September 2007Received in revised form 4March 2008Accepted 18March 2008Available online 26March 2008Keywords:A.FibersA.Nano compositesB.Stress–strain curvesB.Thermo-mechanical propertiesa b s t r a c tThe reinforcement of polylactic acid (PLA)using microfibrillated cellulose (MFC,mechanically fibrillated pulp,mostly consisting of nanofibers)is reported,with the goal of making sustainable ‘green-compos-ites’.The production procedure to attain uniform dispersion of MFC in a PLA compound was assessed,and then mechanical and thermo-mechanical properties of the sheets after hot-pressing of the com-pounds were studied.Needle-leaf Bleached Kraft Pulp (NBKP)and refiner-treated NBKP were also used to study the effects of filler morphology.When MFC was premixed with PLA using organic solvent and the mixture was kneaded after the removal of the solvent,the MFC was uniformly dispersed in the PLA.The MFC increased Young’s modulus and tensile strength of PLA by 40%and 25%,respectively,with-out a reduction of yield strain at a fiber content of 10wt%.On the other hand,NBKP reduced the yield strain by 30%and reduced the strength by 15%at a fiber content of 5wt%.Ó2008Published by Elsevier Ltd.1.IntroductionThe plant cell wall consists of nanofibers called cellulose micro-fibrils.Since the nanofibers are composed of extended cellulose chains forming a semi-crystalline structure,their thermal expan-sion is as low as that of quartz [1],and their tensile strength is esti-mated to be about five times that of mild steel,based on the tensile test of kraft pulp single fibers [2].Recently,we demonstrated that microfibrillated cellulose (MFC),which consists of mechanically fibrillated pulp into nano to submicron wide fibers forming a web-like network,shows much promise as reinforcement of composites [3–6].MFC sheet-molded phenolic resin composites with 80–90wt%fiber content exhibited strength equivalent to that of mild steel or magnesium alloy.Because of the high performance of these composites and the fact that cellulose nanofibers are the most abundant and renewable biomass resource on earth,MFC has attracted attention as poten-tial reinforcement of materials for use in automobiles,buildings,mobile computers,and many other products.In this study,the potential of MFC reinforcement of thermoplas-tic polymer subjected to compression and injection moldings was studied.To develop fully bioresource-based nanocomposites,we used polylactic acid (PLA)as the resin.The reinforcement of PLA using plant and pulp fibers has been studied with the aim of developing sustainable ‘green-composites’[7,8].Although the Young’s modulus of the PLA could be increased significantly with an increase of plant fiber content,the yield strain decreased,and as a result,the strength decreased.To overcomethese drawbacks,reinforcement with cellulose nanowhiskers or microcrystalline cellulose has been studied by melt extrusion com-pounding using twin screw extruders [9,10].However,since the kneading process resulted in the flocculation of the microcrystal-line cellulose and whiskers as well as deterioration of the PLA,composites exhibiting higher strength than neat PLA could not be obtained.Considering that phenol-formaldehyde (PF)resin-impregnated MFC sheet moldings demonstrated a large yield strain [4,5]and the casting film prepared from MFC and polyvinyl alcohol (PVA)solution resulted in a two-fold increase in strength over that of neat PVA along with high yield strain [11],it appears that uniformly dispersed cellulose nanofibers in PLA can increase the strength of PLA composites.Thus,we first studied the pro-duction procedure to attain uniform dispersion of cellulose nanofibers in a PLA compound,and we then found out that the prepared sheet at a fiber content of 10wt%,after kneading and hot-pressing of the compounds,exhibited 25%higher tensile strength than that of a neat PLA resin sheet without a reduction of yield strain.2.Experimental 2.1.MaterialsPolylactic acid (LACEA H-280,Mitsui Chemicals,Inc.,Japan)was used as matrix.Microfibrillated cellulose (MFC,Celish KY110G,water slurry containing 10wt%fiber,Daicel Chemical Industries,Ltd.,Japan)was used as filler.NBKP (Needle-leaf Bleached Kraft Pulp)and refiner-treated NBKP (eight passes)were also used to investigate the effects of filler morphology.0266-3538/$-see front matter Ó2008Published by Elsevier Ltd.doi:10.1016/pscitech.2008.03.006*Corresponding author.Tel.:+81774383669;fax:+81774383658.E-mail address:yano@rish.kyoto-u.ac.jp (H.Yano).Composites Science and Technology 68(2008)2103–2106Contents lists available at ScienceDirectComposites Science and Technologyj o ur na l h o me pa ge :w w w.e ls e v ie r.c o m/lo c a t e/c om p s c it e ch2.2.Preparation of PLA and MFC mixtureMFC slurry containing3gfiber was mixed with270g of acetone to make1wt%MFC suspension.When the MFC was thoroughly dispersed in the solvent by stirring,adequate amount of PLA was added gradually and stirring was kept for5h at ambient tempera-ture.The acetone and water of the mixture was evaporated under vacuum at70°C.The mixture from which water and acetone had been removed was kneaded by a twin rotary roller mixer(Labo Plastomill,Toyo Seiki Seisaku-sho,Ltd.,Japan).The compounding was carried out for12min at a rotary speed of40rpm at140°C. We hereafter call it the solvent method.As a comparison,MFC water slurry containing10wt%fiber was added directly to the melted PLA,and the mixture was kneaded at 140°C,which we hereafter refer as the direct mixing method.The compound was crushed into small pieces and compressed in a die at160°C and0.5MPa for5min followed by1MPa for another5min.The resulting sheet was0.3mm thick.Specimens 40mm long and5mm wide were prepared from the sheet.2.3.Mechanical testingTensile properties of the neat PLA and composite materials were measured using a universal mechanical testing machine(Instron 3365).The specimen gage length was20mm and the testing speed was set to1mm/min.Six composite specimens were tested for each set of samples.2.4.Dynamic mechanical thermal analysisTemperature dependency of dynamic viscoelastic properties of the composites were measured by the forced vibration method in tensile mode(RHEOVIBRON DDV-25FD,Orientec A&D Co.Ltd., Japan),with a chuck distance of20mm,preload of5g,frequency of1Hz,and heating rate of1.5°C/min.3.Results and discussion3.1.Premixing of MFC and PLA using acetoneThe tensile stress–strain curves of the sheets prepared from the compounds by the solvent method and direct mixing method are compared in Fig.1.MFC content in the sheets was5wt%.As can be seen from Fig.1,the Young’s modulus of the MFC/PLA compos-ite prepared by the solvent method is higher than that of a neat PLA sheet.In addition,contrary to the previous results of research on the microcrystalline cellulose-reinforced PLA composite[7],the MFC/PLA composites maintained the yield strain of neat PLA.As a result,the MFC/PLA composites exhibited higher tensile strength than that of neat PLA.Specifically,the average values of Young’s modulus and tensile strength of the PLA increased from3.4GPa and56.2MPa to4.3GPa and66.0MPa,respectively.Meanwhile, the MFC/PLA composites made by the direct mixing method did not show any improvement in Young’s modulus.In addition,the yield strain of the composites was smaller than that of neat PLA, resulting in the reduction of tensile strength by about10%.Fig.2shows the microscopic images of composites prepared by the solvent method and the direct mixing method.The image of the composite made by the solvent method shows that cellulose nanofibers were dispersed uniformly in the PLA,while many agglomerations were observed in the composite made by the direct mixing method.The reduction in yield strain of PLA in the latter case can be attributed to these agglomerates.The sheet prepared by the solvent method but without the kneading process exhibited a Young’s modulus of4.2GPa and a tensile strength of60.7MPa,indicating that the cellulose nano-fibers were more uniformly dispersed bykneading.Fig.1.Typical stress–strain curves comparing the solvent method,direct mixingmethod compounding,and neatPLA.Fig.2.Microscopy images of composites prepared by the solvent method(upper)and the direct mixing method(lower).2104 A.Iwatake et al./Composites Science and Technology68(2008)2103–21063.2.Effects offiller morphology on the mechanical properties of PLA compositesTo investigate the effects of microfibrillation of pulp on the mechanical properties of PLA composites,three types offiller,that is,NBKP,refiner-treated(eight passes)NBKP,and MFC were mixed with PLA by the solvent method.Thefiller content was5wt%.Fig.3shows typical morphology of thefillers.Pulp has a smooth surface with30–50l m in diameter,refiner-treated pulp has a fibrillated surface with a diameter similar to pulp and MFC is com-pletely disintegrated into nano to submicron widefibers forming a network.As shown in Fig.4,the addition of pulp slightly increased the Young’s modulus,but reduced the yield strain by30%and reduced the strength by15%.Thefibrillation of the pulp’s surface(refiner-treated)improved the Young’s modulus as well as the yield strain, resulting in strength increase by10%.Significant improvement was observed in the MFC-reinforced PLA.Since MFC attained the in-crease of Young’s modulus,as much as25%,without a reduction of the yield strain,the strength improved by20%compared to neat PLA.It should be emphasized that the reinforcement of refiner-treated pulp slightly increases the Young’s modulus of PLA, whereas MFC increases Young’s modulus of PLA by25%,as shown in Fig.4.Favier et al.[12]studied the effect of cellulose nanowhis-ker reinforcement of latex of poly(styrene-co-butyl acrylate)and found that the presence of percolated nanowhiskers,that is,the formation of a rigid network resulting from strong interactions between adjacent whiskers by hydrogen bonding,enabled effective reinforcement of the matrix and achieved a drastic increase of shear modulus at a whisker content of6wt%.Furthermore,Samir et al.[13]proved that the percolation effect is more pronounced when cellulose nanofiber is used as reinforcement due to the addi-tional entanglement effect of the elements.Thus,as can be specu-lated based on the comparison of SEM images(Fig.3),the fibrillation of pulp’s surface is not enough to create a strong network at a lowfiller content.On the other hand,microfibrillated cellulose creates afine network even at a lowfiller content such as 5wt%,restraining polymer deformation.3.3.Effects of MFC content on the mechanical and thermo-mechanical properties of PLA compositesThe effect offiller morphology indicated the importance of net-work formation in improving mechanical properties.Hence,the reinforcement effect of MFC on the mechanical and thermo-mechanical properties of PLA composites was studied as a function of MFC content.Fig.5shows the stress–strain curves of tensile test of MFC/PLA composites with differentfiber contents.As can be seen,the3wt% MFC/PLA composite showed a stress–strain curve similar to that of neat PLA.In other words,the effect of the addition of MFC could not be observed.However,5wt%MFC/PLA composites evidenced a reinforcing effect.Young’s modulus increased by25%compared to neat PLA while retaining the same yield strain of2.5%.Hence, the tensile strength of PLA increased from about50MPa to 70MPa.Similar results were observed in temperature dependency of storage modulus at1Hz(Fig.6).The storage modulus of3wt% MFC/PLA composites did not show a significant difference com-pared to PLA above100°C.On the other hand,5wt%MFC/PLA Fig.3.Filler morphology of Needle-leaf Bleached Kraft Pulp(NBKP),refiner-treated(eight passes)NBKP,and MFC.Scale bar:10lm.Fig.4.Effects of morphological changes offiller on stress–straincurves.Fig.5.Effects of MFC content(wt%)on stress–strain curves.A.Iwatake et al./Composites Science and Technology68(2008)2103–21062105composites showed a higher Young’s modulus than that of neat PLA.Additional improvements in Young’s modulus and strength were observed when the MFC content increased from 5wt%to 10wt%as shown in Fig.5.The average Young’s modulus and tensile strength of 10wt%MFC/PLA composites attained 4.7GPa and 75.0MPa,respectively,without a reduction of yield strain.It is worthy to note that the addition of 10wt%MFC could improve the Young’s modulus of PLA by 40%and the strength by 25%.However,a 15wt%or 20wt%addition of MFC made the compos-ites brittle and decreased their strength.Fracture initiating points such as flocculated nanofibers increased and dominated the mechanical properties of MFC/PLA composites.The improvement of the procedure for making high fiber content compounds is necessary to obtain further increments in strength as well as Young’s modulus of PLA.The constant storage modulus of 10wt%MFC/PLA composites above glass transition temperature (T g )of PLA,that is,from 70°C to 120°C should be emphasized (Fig.6).Similar phenomena were observed in cellulose nanowhisker reinforcement of latex of poly(styrene-co -butyl acrylate)[12]and MFC reinforcement of amylopectin-glycerol blend [14].The constant storage modulus suggests that the cellulose fiber network interconnected by hydro-gen bonds resists the applied stress independently of the softening of PLA.The result shows clearly the advantage of MFC over plant and pulp fibers in the reinforcement of thermoplastic polymer.4.ConclusionsThe reinforcement of PLA using microfibrillated cellulose (MFC)was studied to know the potential of reinforcement by a nanofibernetwork,with the goal of making sustainable ‘green-composites’.MFC was premixed with PLA using organic solvent and the mixture was kneaded to attain uniform dispersion of MFC in PLA.The uni-formly dispersed MFC reinforcement increased the Young’s modu-lus and tensile strength of PLA by 40%and 25%,respectively,without a reduction of yield strain at a fiber content of 10wt%.Fur-thermore,the storage modulus of the composites was kept con-stant above glass transition temperature of matrix polymer.MFC is a promising reinforcement of PLA composites.AcknowledgementsThe authors would like to thank Dr.A.N.Nakagaito,Research Institute for Sustainable Humanosphere,Kyoto University for valu-able suggestions.This research was supported by a Grant-in-Aid for Scientific Re-search (B)(No.1538012,2003.4–2007.3)from the Ministry of Edu-cation,Culture,Sports,Science,and Technology,Japan,and a Grant-in-Aid for Research and Development for regional innova-tion consortium (No.17S5018,2006.9–2007.3)from the Ministry of Economy,Trade and Industry,Japan.References[1]Nishino T,Matsuda I,Hirao K.All-cellulose composites.Macromolecules2004;37:7683–7.[2]Page DH,EL-Hosseiny F.The mechanical properties of single wood pulp fibres.JPulp Paper Sci 1983(September):99–100.[3]Yano H,Nakahara S.Bio-composites produced from plant microfiber bundleswith a nanometer unit web-like network.J Mater Sci 2004;39(5):1635–8.[4]Nakagaito AN,Yano H.Novel high-strength biocomposites based onmicrofibrillated cellulose having nano-order-unit web-like network structure.Appl Phys A 2005;80(1):155–9.[5]Nakagaito AN,Yano H.The effect of morphological changes from pulp fibertowards nano-scale fibrillated cellulose on the mechanical properties of high-strength plant fiber based composites.Appl Phys A 2004;78(4):547–52.[6]Nakagaito AN,Iwamoto S,Yano H.Bacterial cellulose:the ultimate nano-scalarcellulose morphology for the production of high-strength composites.Appl Phys A 2005;80(1):93–7.[7]Oksman K,Skrifvars M,Selin JF.Natural fibers as reinforcement in polylacticacid (PLA)p Sci Technol 2003;63(9):1317–24.[8]Huda MS,Drzal LT,Misra M,Mohanty AK,Williams K,Mielewski DF.A studyon biocomposites from recycled newspaper fiber and poly(lactic acid).Ind Eng Chem Res 2005;44(15):5593–601.[9]Mathew AP,Oksman K,Sain M.Mechanical properties of biodegradablecomposites from poly lactic acid (PLA)and microcrystalline cellulose (MCC).J Appl Polym Sci 2005;97(5):2014–25.[10]Oksman K,Mathew AP,Bondeson D,Kvien I.Manufacturing process ofcellulose whiskers/polylactic acid p Sci Technol 2006;66(15):2776–84.[11]Zimmermann T,Pohler E,Geiger T.Cellulose fibrils for polymer reinforcement.Adv Eng Mater 2004;6(9):754–61.[12]Favier V,Chanzy H,CavailléJY.Polymer nanocomposites reinforced bycellulose whiskers.Macromolecules 1995;28(18):6365–7.[13]Samir MASA,Alloin F,Paillet M,Dufresne A.Tangling effect in fibrillatedcellulose reinforced nanocomposites.Macromolecules 2004;37(11):4313–6.[14]Svagan AJ,Samir MASA,Berglund LA.Biomimetic polysaccharidenanocomposites of high cellulose content and high toughness.Biomacro-molecules2007;8(8):2556–63.Fig.6.Effects of MFC contents (wt%)on the temperature dependency of storage modulus.2106 A.Iwatake et al./Composites Science and Technology 68(2008)2103–2106。
基于热力耦合的金属切削过程分析及实验验证

基于热力耦合的金属切削过程分析及实验验证杨永刚,陈燕青(中国民航大学航空工程学院,天津300300)摘要院在传统切削仿真模型中袁工件或刀具模型设置为刚体袁不能有效反映切削过程中工件和刀具在热力耦合作用下的真实状态曰增大材料导热率不能准确反映切削液与材料表面的热交换过程遥针对传统模型存在的不足袁建立可反映材料加工特性的J-C 本构模型和动态失效模型袁通过添加对流换热来模拟冷却介质的冷却作用袁采用有限元软件ABAQUS 进行仿真遥分析了不同切削参数尧刀具形状和冷却介质对AISI-1045钢加工过程的影响袁并通过与实验数据对比验证仿真结果的正确性遥关键词院切削仿真曰切削力曰切削温度中图分类号院V250曰TP391.9文献标志码院A文章编号院1674原5590渊圆园18冤02原园园53原园6Analysis and experimental verification of metal cutting process based on thermo-mechanical couplingYANG Yonggang,CHEN Yanqing渊College of Aeronautical Engineering,CAUC,Tianjin 300300,China 冤Abstract:In traditional cutting simulation model,workpiece or tool model is set as rigid body,which can not effectivelyreflect the cutting process of workpiece and tool in thermodynamic coupling in real state;thermal conductivity increase of material can not accurately reflect the cutting fluid and material surface heat exchange process.J-C constitutive model and dynamic failure model are established to reflect the material processing characteristics.The cooling effect of cooling medium is simulated by adding convective heat transfer.Finite element software ABAQUS is used to simulate J-C constitutive model and dynamic failure model.The effects of different cuttingparameters,tool shapes and cooling mediums during the machining process of AISI-1045steel are analyzed,andthe correctness of simulation results is verified by comparison with experimental data.Key words:cutting simulation;cutting force;cutting temperature收稿日期院2017-09-14;修回日期院2017-11-17基金项目院中国航空工业集团公司资助项目(CXY2013MH35)作者简介院杨永刚(1978—),男,吉林四平人,副教授,博士,研究方向为机器人技术、飞行器制造技术.第36卷第2期圆园18年4月中国民航大学学报Vol.36No.2April 2018金属切削是一个复杂的工艺过程,涉及到弹性力学、断裂力学、热力学、摩擦学等多个学科。
METHOD AND DEVICE FOR THE THERMOMECHANICAL ANALYSI
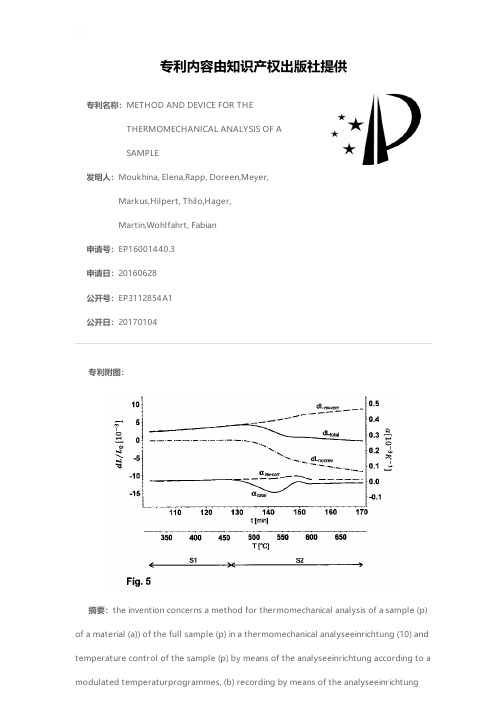
专利名称:METHOD AND DEVICE FOR THETHERMOMECHANICAL ANALYSIS OF ASAMPLE发明人:Moukhina, Elena,Rapp, Doreen,Meyer,Markus,Hilpert, Thilo,Hager,Martin,Wohlfahrt, Fabian申请号:EP16001440.3申请日:20160628公开号:EP3112854A1公开日:20170104专利内容由知识产权出版社提供专利附图:摘要:the invention concerns a method for thermomechanical analysis of a sample (p)of a material (a)) of the full sample (p) in a thermomechanical analyseeinrichtung (10) and temperature control of the sample (p) by means of the analyseeinrichtung according to a modulated temperaturprogrammes, (b) recording by means of the analyseeinrichtungderived datathe representative of an elongation (dl) of the sample (p) in the course of the temperature control units, (c) evaluating the data, a reversible component (dlrev) elongation (dl) and \/ or a reversible component (\u03b1rev) of the thermal coefficient of expansion (a) of the sample (p) to identify tteln,(d) to calculate a corrected reversible component (dlrev corr; \u03b1rev corr) elongation (dl) and \/ or the thermal coefficient of expansion (a) by means of a korrekturparameters (k)the using of the data identified a total elongation ratio (dltotal) and parameter (\u03b1total) and a reversible component (dlrev) elongation (dl) and parameter (\u03b1rev) iscalculated.erfindungsgem\u00e4\u00df has the temperaturprogramm a first segment (s1) with a first basisheizrate (\u03b21) and the korrekturparameter (k) on the basis of data from the first segment (s1) to estimate a temperature from a temperature dependent function of the relationship. n a field of the first segment (s1) calculatesin which the sample (p), a thermally induced transformation is, with the temperaturprogramm a second segment (s2) with a pared with the first basisheizrate (\u03b21) smaller second basisheizrate (\u03b22) owns and for calculation of the corrected reversible component (dlrev corr; \u03b1rev corr) elongation (dl) and \/ or the thermal coefficient of expansion (a) in the second segment (s2) on the basis of the d data from the first segment (s1) calculated korrekturparameter (k) is used.申请人:Netzsch Gerätebau GmbH地址:Wittelsbacherstrasse 42 95100 Selb DE国籍:DE更多信息请下载全文后查看。
ECCM18 - 18th European Conference on Composite Mat
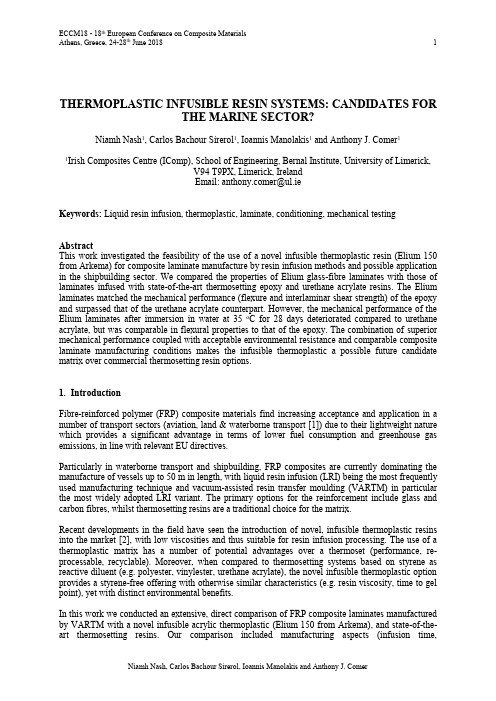
THERMOPLASTIC INFUSIBLE RESIN SYSTEMS: CANDIDATES FORTHE MARINE SECTOR?Niamh Nash1, Carlos Bachour Sirerol1, Ioannis Manolakis1 and Anthony J. Comer11Irish Composites Centre (IComp), School of Engineering, Bernal Institute, University of Limerick,V94 T9PX, Limerick, IrelandKeywords: Liquid resin infusion, thermoplastic, laminate, conditioning, mechanical testingAbstractThis work investigated the feasibility of the use of a novel infusible thermoplastic resin (Elium 150 from Arkema) for composite laminate manufacture by resin infusion methods and possible application in the shipbuilding sector. We compared the properties of Elium glass-fibre laminates with those of laminates infused with state-of-the-art thermosetting epoxy and urethane acrylate resins. The Elium laminates matched the mechanical performance (flexure and interlaminar shear strength) of the epoxy and surpassed that of the urethane acrylate counterpart. However, the mechanical performance of the Elium laminates after immersion in water at 35 o C for 28 days deteriorated compared to urethane acrylate, but was comparable in flexural properties to that of the epoxy. The combination of superior mechanical performance coupled with acceptable environmental resistance and comparable composite laminate manufacturing conditions makes the infusible thermoplastic a possible future candidate matrix over commercial thermosetting resin options.1. IntroductionFibre-reinforced polymer (FRP) composite materials find increasing acceptance and application in a number of transport sectors (aviation, land & waterborne transport [1]) due to their lightweight nature which provides a significant advantage in terms of lower fuel consumption and greenhouse gas emissions, in line with relevant EU directives.Particularly in waterborne transport and shipbuilding, FRP composites are currently dominating the manufacture of vessels up to 50 m in length, with liquid resin infusion (LRI) being the most frequently used manufacturing technique and vacuum-assisted resin transfer moulding (VARTM) in particular the most widely adopted LRI variant. The primary options for the reinforcement include glass and carbon fibres, whilst thermosetting resins are a traditional choice for the matrix.Recent developments in the field have seen the introduction of novel, infusible thermoplastic resins into the market [2], with low viscosities and thus suitable for resin infusion processing. The use of a thermoplastic matrix has a number of potential advantages over a thermoset (performance, re-processable, recyclable). Moreover, when compared to thermosetting systems based on styrene as reactive diluent (e.g. polyester, vinylester, urethane acrylate), the novel infusible thermoplastic option provides a styrene-free offering with otherwise similar characteristics (e.g. resin viscosity, time to gel point), yet with distinct environmental benefits.In this work we conducted an extensive, direct comparison of FRP composite laminates manufactured by VARTM with a novel infusible acrylic thermoplastic (Elium 150 from Arkema), and state-of-the-art thermosetting resins. Our comparison included manufacturing aspects (infusion time,recommended curing and post-curing schedule) as well as laminate quality, and mechanical and thermomechanical performance in both dry and wet (after immersion in water) conditions.2. Materials and Methods2.1. Manufacturing of Composite Laminates by VARTMComposite laminates (350 x 500 mm) were manufactured on glass or aluminium tools at room temperature. Unidirectional non-crimp glass fabric (Saertex UD NCF 996 gsm with PPG Hybon 2002 E-glass fibre) was the reinforcement of choice along with two thermosetting resins (urethane acrylate Crestapol 1210 from Scott Bader and epoxy Prime 27 from Gurit) and an infusible thermoplastic (Elium 150 from Arkema). The recommended ratios of hardener/catalyst/accelerator (as appropriate) from the supplier were used for each system. Four (4) plies of glass fabric were cut in the above mentioned dimensions and placed on the tool in a symmetric/unidirectional stacking sequence [0o]2S with a glass preform mass of approximately 650 g targeting a nominal laminate thickness of 3 mm. The infusion time was measured from the opening of the resin inlet to the closure of the outlet (outlet was closed on observing bubble-free resin in the outlet tube).2.2. Mechanical testing2.2.1. Interlaminar shear strength (ILSS)Interlaminar shear strength tests were conducted according to ISO 14130. Five (5) samples of 30 x 15 x 3 mm were used for each laminate to determine the apparent inter-laminar shear strength (ILSS). 2.2.2. 3-point bendingFlexure tests were conducted according to ISO 14125. Five (5) samples of 80 x 15 x 3 mm were used for each laminate to determine the flexural strength and flexural modulus for each case.2.3. Dynamic Mechanical AnalysisDynamic Mechanical Analysis was performed in a TA Instruments (USA) Q800 Dynamic Mechanical Analyser in 3-point bend testing mode, with a displacement amplitude of 10 μm and frequency of 1Hz. Laminate specimens with nominal dimensions of L x W x T equal to 50 x 12 x 3 mm were used. The specimens were heated from ambient temperature to an appropriate end temperature based on their expected T g at a rate of 5°C/min. Storage modulus (E’), loss modulus (E’’) and tanδ were recorded. 2.4. Scanning Electron MicroscopyCross-sections of the composite laminates were examined using a Hitachi SU-70 Analytical Field Emission SEM, at accelerating voltage of 10 kV and working distance of 10 mm. Specimens were examined as received, and after manual polishing (using P800 and P1000 SiC paper). The samples were sputter-coated with gold for 30 seconds using an Emitech K550 sputter coater before SEM observation.2.5. DensitySpecific gravity was calculated by the displacement method according to ASTM D792-08, using five (5) samples for each laminate with dimensions 25 x 12 x 3 mm.2.6. Fibre volume fraction (V f)Fibre volume fractions were determined by a resin burn-off test according to ISO 1172, using five (5) samples for each laminate.2.6. Water immersion studiesLaminate specimens for ILSS and flexure were immersed in distilled water in a water bath at 35 o C for 28 days, and tested after removal. Three samples were used for each laminate in each test (with the exception of Prime 27, for which two ILSS specimens were measured due to equipment issues).3. Results and DiscussionThe comparison of the composite laminates in this study was conducted across a number of aspects, namely manufacturability for resin infusion (resin/curing agent formulation, viscosity, infusion temperature and time, curing and post curing requirements), quality of produced laminates (achieved fibre volume fraction and T g), mechanical properties as-manufactured and effect of water immersion on mechanical properties.The manufacturing details for the three laminates are presented in Table 1. It is evident that the infusible thermoplastic is quite similar in terms of viscosity and infusion time at room temperature to its thermosetting counter parts. It also doesn’t require any post-curing according to the resin manufacturer; it can be left to cure at room temperature on the tool overnight, and is subsequently easily demoulded. This is a particularly attractive feature for complex shapes with curvature, which are quite abundant in shipbuilding.Table 1. VARTM manufacturing details for composite laminatesResin Details Resin:Curingagent(s)(w/w)Viscosity a(cP)ToolInfusiontime/T(min/o C)CuringschedulePost-curingscheduleUrethaneAcrylate CRESTAPOL1210 100:2:1:1b175 cP at25 °C(neat resin)Glass 11/21.1 1h at RT NonerequiredEpoxy PRIME 27100:28(Prime 27 slowhardener)285 cP at20 °C(mixture)Glass 15/18.81h at 45 °COvernightat RT7h at 65 o CAcrylic thermoplastic ELIUM 150100:2.5(benzoylperoxideLuperox A40FP-EZ9)100 cP at25 °C(neat resin)Glass 23/21.9 Overnightat RTNonerequireda: values from TDS; b: 2 parts by weight of Accelerator D (10% solution of dimethyaniline in styrene) : 1 part by weight of Accelerator G (1% cobalt solution in styrene) : 1 part by weight of peroxide catalyst (Trigonox 44B)All three laminates were shown to reach the expected T g levels when tested by DMA [3-5], indicating a complete cure cycle. Elium 150 is expected to show a heat deflection temperature of 109 o C, and indeed showed an onset transition in the storage modulus at 96 o C and a peak in the loss modulus at 107 o C (Figure 1).Mechanical properties of the as-manufactured composite laminates are summarized in Table 2. The achieved fibre volume fractions for all three resins were in the region of 56-58% (using the same UD glass fabric), indicating again that the infusible thermoplastic behaves in terms of manufacturing very similar to a thermosetting resin. Elium 150 clearly exceeded the urethane acrylate (Crestapol 1210) thermoset in both interlaminar shear and flexural strength values. Compared to the standard epoxy (Prime 27), Elium showed higher flexural and slightly lower interlaminar shear strength values. Overall, it is quite clear that Elium 150 is comparable in its performance to the epoxy laminate and outperforms the laminate made from a styrene-based resin in the dry condition.Figure 1. Dynamic mechanical analysis profile of the Elium 150/glass laminateTable 2. Mechanical (ILSS, flexure) and physical (V f, density) properties of composite laminates inthe Dry ConditionResin DetailsV f(%)Density(g/cm3)Apparent Inter-Laminar ShearStrength(MPa)*FlexuralStrength(MPa)*FlexuralModulus(GPa)*Urethane Acrylate CRESTAPOL 121057(±0.3%)2.017(±0.7%)42.09(±3.0%)790.61(±11.3%)34.52(±2.0%)Epoxy PRIME 2758(±0.9%)2.061(±0.5%)58.04(±2.4%)917.1(±2.4%)35.37(±2.8%)Acrylic thermoplastic ELIUM 15056(±1.0%)1.999(±0.4%)56.87(±3.6%)942.8(±3.8%)33.86(±1.6%)*Minimum required laminate property values in accordance with [6]: ILSS at least 15 MPa; Flexural Strength and Flexural Modulus for a laminate with equivalent fibre mass fraction (0.72) at least 367 MPa and 19.5 GPa, respectivelyScanning Electron Microscopy on cross-sections from the as-manufactured laminates provided information on the infusion quality/void content and the matrix/fibre interface. All laminates showed generally good fibre impregnation (a selection of SEM images in Figures 2a, 2b, 3a), as expected from their good mechanical properties (Table 2).a bc de f Figure 2. SEM images of the Elium 150/glass laminate cross-sections: as-manufactured (a, b; polished; Table 2) and after immersion in water for 28 days at 35 o C (c, d: polished; e, f: unpolished; Table 3). Longitudinal lines in the polished samples come from the polishing action/direction.The effect of prolonged water immersion on the mechanical properties of the three laminates is detailed in Table 3. Water absorption less than 70 mg (after 7 days of immersion), and a drop in mechanical properties no greater than 25% after 28 days are the main requirements for material qualification in shipbuilding [6].Figure 3. SEM images of the Prime 27/glass laminate polished cross-sections (a: as-manufactured; b: after immersion in water for 28 days at 35 o C). Longitudinal lines come from the polishing action/direction.Elium 150 appeared to absorb similar amounts of water to the epoxy. The urethane acrylate laminate recorded the lowest water uptake and the smallest drop in all mechanical properties. The Elium laminate showed the sharpest drop in ILSS value (37.5%) compared to the dry state value. In terms of flexural strength, Elium and Prime 27 were almost identical, with a drop of 17-18%. Flexural modulus was less affected for Elium. As in Table 1, Elium appears overall comparable with the state-of-the-art epoxy in terms of environmental resistance, with the exception of the ILSS values (yet with a high coefficient of variation); the latter may suggest a more affected matrix and/or Elium/glass interface due to water ingress (possibly also due to hydrolysis of the acrylic-based Elium matrix).Table 3. Comparsion of mechanical properties (Table 1) after immersion in water (35 o C, 28 days)Resin Details ApparentInter-LaminarShearStrength(MPa)Changecomparedto drystate(Table 1)(%)AveragemassuptakeILSSspecimens(mg)FlexuralStrength(MPa)Changecomparedto drystate(Table 1)(%)FlexuralModulus(GPa)Changecomparedto drystate(Table 1)(%)Averagemassuptakeflexurespecimens(mg)UrethaneAcrylate CRESTAPOL121041.90(±2.4%)-0.5 4.6(±30%)785.9(±1.5%)-0.6 35.34(±2.3%)+2.4 21.0(±16%)Epoxy PRIME 2748.45(±2.9%)-16.5 16.1(±7%)746.7(±5.0%)-18.6 33.80(±6.1%)-4.4 39.9(±2%)Acrylic thermoplastic ELIUM 15035.56(±14.5%)-37.5 9.7(±2%)779.8(±11.6%)-17.3 33.99(±1.0%)-0.4 34.7(±4%)For this reason, SEM was also conducted on laminate specimens after water immersion (Figures 2c, 2d, 2e, 2f, 3b) and compared to images obtained for the as-manufactured laminates. Crestapol 1210 (not shown here) showed very little difference in terms of SEM imaging in the dry and wet condition, in line with its minimal change in mechanical properties.In the case of Elium 150 (Figure 2), SEM suggested the presence of some localised resin-poor areas in the wet samples, potentially linked to possible hydrolysis of the acrylic matrix. Otherwise, there was again little visible difference overall by SEM between dry and wet samples, which can be partlya battributed to the overall low average mass uptake (Table 3) for all samples. Nevertheless, the effect on mechanical properties was significant for Prime 27 and Elium 150, as shown in Table 3.4. ConclusionsThis study highlighted the suitability of the novel infusible acrylic thermoplastic option for FRP laminate manufacture by liquid resin infusion in a shipbuilding environment. The laminates produced by the infusible thermoplastic were of high quality, and comfortably exceeded the minimum requirements set by classification societies [6] in ILSS and flexural properties (see note in Table 2). Elium 150 matched the mechanical performance of a state-of-the-art epoxy resin (Prime 27), and outperformed laminates produced with resins based on styrene reactive diluent technology (urethane acrylate Crestapol 1210 in this study).The drop observed in flexural properties for the thermoplastic laminates after immersion in water was comparable to that of the epoxy-based laminates and within the allowed 25% reduction compared to the dry state [6]. The reduction in ILSS values for Elium 150 (> 25%) observed in this study could be improved by e.g. employing the allowed post-curing schedule according to [6] (16 h at 40 o C), or selecting a glass fibre fabric with bespoke sizing for the Elium resin range.The new infusible acrylic thermoplastic combines good manufacturability in a liquid resin infusion context with epoxy-like laminate properties, and additionaly offers a re-processable, styrene-free resin option to the shipbuilding industry. Once a competitive price range could be established for the Elium range, it would be expected that its acceptance and adoption by the shipbuilding industry would increase accordingly.AcknowledgmentsThis work has been funded by the H2020 project FIBRESHIP (www.fibreship.eu) under grant agreement 723360.References[1] J. Summerscales. Marine applications of advanced fibre reinforced composites. WoodheadPublishing, Cambridge, 2016.[2] https:///en/media/news/news-details/Arkema-gains-ground-in-composites-andlaunches-a-revolutionary-range-of-Elium-liquid-resins/[3] Technical Data Sheet Crestapol 1210 (Scott Bader)[4] Technical Data Sheet Crestapol Prime 27 (Gurit)[5] Technical Data Sheet Elium 150 (Arkema)[6] Lloyds Register. Material and Qualification Procedures for Ships Book K Procedure 14-1 & 14-2.Revision 01 Dec 2013。
2012 高温屈曲 失效分析与防护

失效分析与预防
April,2012 Vol. 7,No. 2
热障涂层体系界面屈曲破坏实验测试研究
毛卫国1,2 ,苏 鹏2 ,张 瑜2 ,周 孟2 ,吴多锦2
( 1. 无损检测技术教育部重点实验室( 南昌航空大学) ,南昌 330063; 2. 湘潭大学 材料与光电物理学院,低维材料及其应用技术教育部重点实验室,湖南 湘潭 411105)
92
失效分析与预防
第7 卷
பைடு நூலகம்
Superalloy substrate
ZrO2 top-coat 100~400 μm Cooling air film
Bondcoat
Temperature
~100 μm
Thermal-barrier
coated
TGO
Turbine blade
Al2O3 1~10 μm
Loading
CCd monitoring Thermal couple
Indenter Sample
Cooling water Hot environment
( 1. Key Laboratory of Nondestrustive Testing( Nanchang Hangkong University) ,Nanchang 330063,China; 2. Key Laboratory of Low Dimensional Materials and Application Technology ( Ministry of Education) ,Faculty of Materials,
合理的解释,为进一步了解和研究热障涂层体系界面屈曲破坏问题起到重要的指导作用。