土壤重金属污染微生物形态
微生物对土壤中的重金属污染的修复
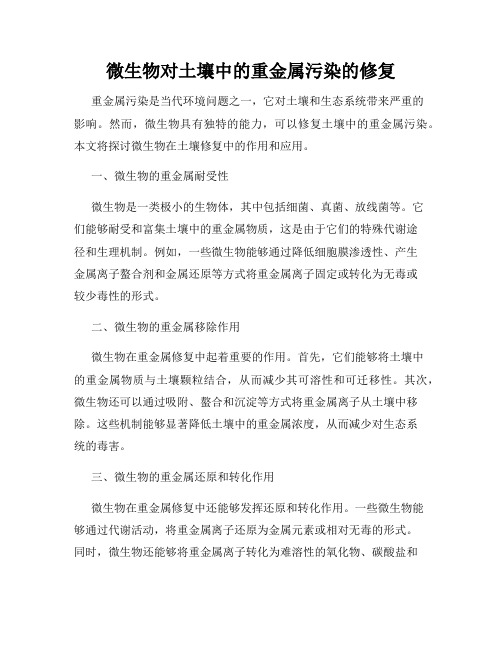
微生物对土壤中的重金属污染的修复重金属污染是当代环境问题之一,它对土壤和生态系统带来严重的影响。
然而,微生物具有独特的能力,可以修复土壤中的重金属污染。
本文将探讨微生物在土壤修复中的作用和应用。
一、微生物的重金属耐受性微生物是一类极小的生物体,其中包括细菌、真菌、放线菌等。
它们能够耐受和富集土壤中的重金属物质,这是由于它们的特殊代谢途径和生理机制。
例如,一些微生物能够通过降低细胞膜渗透性、产生金属离子螯合剂和金属还原等方式将重金属离子固定或转化为无毒或较少毒性的形式。
二、微生物的重金属移除作用微生物在重金属修复中起着重要的作用。
首先,它们能够将土壤中的重金属物质与土壤颗粒结合,从而减少其可溶性和可迁移性。
其次,微生物还可以通过吸附、螯合和沉淀等方式将重金属离子从土壤中移除。
这些机制能够显著降低土壤中的重金属浓度,从而减少对生态系统的毒害。
三、微生物的重金属还原和转化作用微生物在重金属修复中还能够发挥还原和转化作用。
一些微生物能够通过代谢活动,将重金属离子还原为金属元素或相对无毒的形式。
同时,微生物还能够将重金属离子转化为难溶性的氧化物、碳酸盐和磷酸盐等,从而减少其可迁移性。
这些转化过程有助于修复受重金属污染的土壤,并降低对环境的危害。
四、微生物修复的应用技术微生物修复技术是一种有效的重金属修复方法。
以下是几种常用的微生物修复技术:1. 微生物植物共生技术:利用植物根系与根际微生物的协同作用,修复受重金属污染的土壤。
植物通过根系分泌物质吸附和沉淀重金属离子,同时根际微生物通过降解重金属物质来修复土壤。
2. 微生物菌剂技术:选用具有重金属耐受性和转化能力的微生物制备菌剂,通过施加菌剂来修复受重金属污染的土壤。
这种方法具有操作简单、扩大应用范围的优点。
3. 微生物原位修复技术:将具有修复能力的微生物定向引入受重金属污染的土壤中,利用其对重金属的吸附、转化和还原等作用来修复土壤污染。
五、微生物修复技术的优势和挑战微生物修复技术具有许多优势,例如操作相对简单、环境友好、成本较低等。
微生物与土壤中重金属污染物的相互作用研究
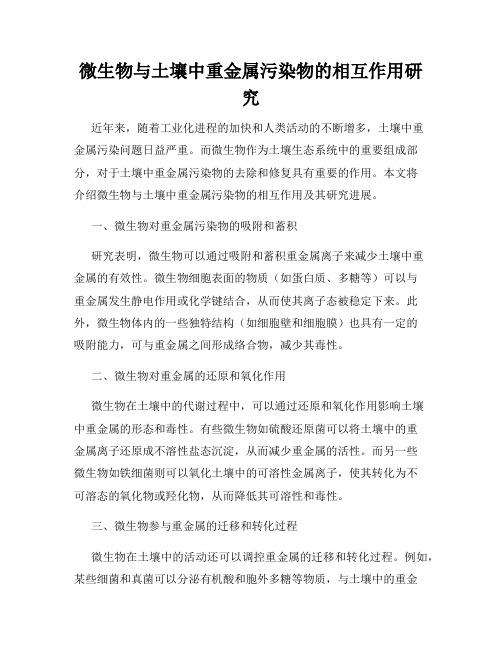
微生物与土壤中重金属污染物的相互作用研究近年来,随着工业化进程的加快和人类活动的不断增多,土壤中重金属污染问题日益严重。
而微生物作为土壤生态系统中的重要组成部分,对于土壤中重金属污染物的去除和修复具有重要的作用。
本文将介绍微生物与土壤中重金属污染物的相互作用及其研究进展。
一、微生物对重金属污染物的吸附和蓄积研究表明,微生物可以通过吸附和蓄积重金属离子来减少土壤中重金属的有效性。
微生物细胞表面的物质(如蛋白质、多糖等)可以与重金属发生静电作用或化学键结合,从而使其离子态被稳定下来。
此外,微生物体内的一些独特结构(如细胞壁和细胞膜)也具有一定的吸附能力,可与重金属之间形成络合物,减少其毒性。
二、微生物对重金属的还原和氧化作用微生物在土壤中的代谢过程中,可以通过还原和氧化作用影响土壤中重金属的形态和毒性。
有些微生物如硫酸还原菌可以将土壤中的重金属离子还原成不溶性盐态沉淀,从而减少重金属的活性。
而另一些微生物如铁细菌则可以氧化土壤中的可溶性金属离子,使其转化为不可溶态的氧化物或羟化物,从而降低其可溶性和毒性。
三、微生物参与重金属的迁移和转化过程微生物在土壤中的活动还可以调控重金属的迁移和转化过程。
例如,某些细菌和真菌可以分泌有机酸和胞外多糖等物质,与土壤中的重金属形成络合物,促进重金属的迁移。
同时,微生物的运动和代谢活动也可以改变土壤的物理和化学性质,间接影响重金属的迁移和转化。
四、微生物修复重金属污染土壤的应用研究基于微生物在重金属污染修复中的重要作用,研究者们开始利用和开发微生物技术来修复污染土壤。
目前,常见的微生物修复方法包括菌根菌修复、植物-微生物协同修复和基于基因工程的微生物修复等。
这些方法可以有效地减少土壤中重金属的含量,恢复土壤的生态功能,但仍存在着一些技术难题和风险,需要进一步的研究和实践。
综上所述,微生物与土壤中重金属污染物之间存在着复杂的相互作用关系。
微生物可以通过吸附和蓄积、还原和氧化、迁移和转化等方式影响土壤中重金属的形态和分布,从而影响其毒性和生物有效性。
土壤微生物对重金属污染的修复作用
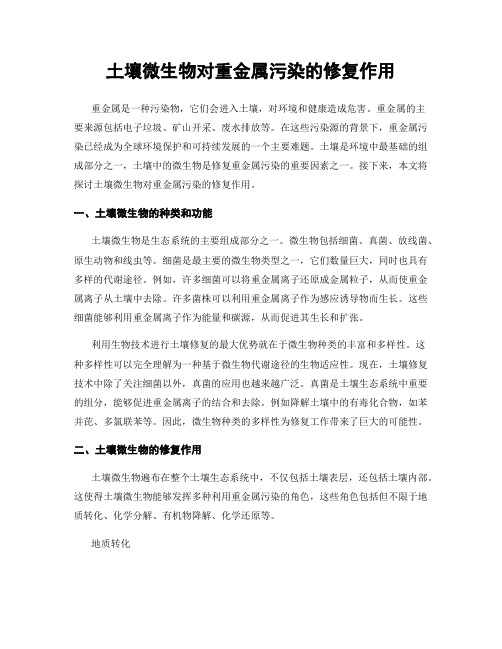
土壤微生物对重金属污染的修复作用重金属是一种污染物,它们会进入土壤,对环境和健康造成危害。
重金属的主要来源包括电子垃圾、矿山开采、废水排放等。
在这些污染源的背景下,重金属污染已经成为全球环境保护和可持续发展的一个主要难题。
土壤是环境中最基础的组成部分之一,土壤中的微生物是修复重金属污染的重要因素之一。
接下来,本文将探讨土壤微生物对重金属污染的修复作用。
一、土壤微生物的种类和功能土壤微生物是生态系统的主要组成部分之一。
微生物包括细菌、真菌、放线菌、原生动物和线虫等。
细菌是最主要的微生物类型之一,它们数量巨大,同时也具有多样的代谢途径。
例如,许多细菌可以将重金属离子还原成金属粒子,从而使重金属离子从土壤中去除。
许多菌株可以利用重金属离子作为感应诱导物而生长。
这些细菌能够利用重金属离子作为能量和碳源,从而促进其生长和扩张。
利用生物技术进行土壤修复的最大优势就在于微生物种类的丰富和多样性。
这种多样性可以完全理解为一种基于微生物代谢途径的生物适应性。
现在,土壤修复技术中除了关注细菌以外,真菌的应用也越来越广泛。
真菌是土壤生态系统中重要的组分,能够促进重金属离子的结合和去除。
例如降解土壤中的有毒化合物,如苯并芘、多氯联苯等。
因此,微生物种类的多样性为修复工作带来了巨大的可能性。
二、土壤微生物的修复作用土壤微生物遍布在整个土壤生态系统中,不仅包括土壤表层,还包括土壤内部。
这使得土壤微生物能够发挥多种利用重金属污染的角色,这些角色包括但不限于地质转化、化学分解、有机物降解、化学还原等。
地质转化一些微生物能够促进土壤物质循环,此外,这些微生物还能够降低土壤中重金属含量。
这些细菌能够代谢重金属离子,有机物降解产物和有机物。
土壤中的铁细菌可以帮助还原土壤中的二价铁和三价铁之间的络合物来加速污染物的去除。
微生物的一部分部分能够促进腐殖质的分解,从而提高土壤的吸附能力,以进一步降低土壤中重金属污染的危害。
细菌、真菌和酵母等也能通过降解土壤微生物中存在的有机物来减轻土壤中重金属的污染。
重金属在土壤中的迁移与转化研究
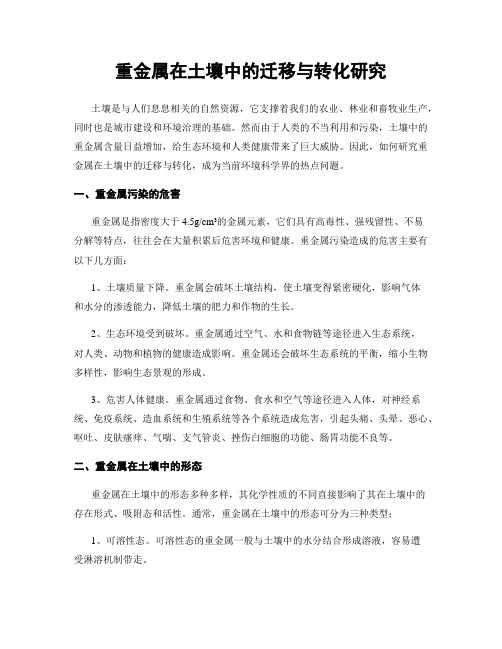
重金属在土壤中的迁移与转化研究土壤是与人们息息相关的自然资源,它支撑着我们的农业、林业和畜牧业生产,同时也是城市建设和环境治理的基础。
然而由于人类的不当利用和污染,土壤中的重金属含量日益增加,给生态环境和人类健康带来了巨大威胁。
因此,如何研究重金属在土壤中的迁移与转化,成为当前环境科学界的热点问题。
一、重金属污染的危害重金属是指密度大于4.5g/cm³的金属元素,它们具有高毒性、强残留性、不易分解等特点,往往会在大量积累后危害环境和健康。
重金属污染造成的危害主要有以下几方面:1、土壤质量下降。
重金属会破坏土壤结构,使土壤变得紧密硬化,影响气体和水分的渗透能力,降低土壤的肥力和作物的生长。
2、生态环境受到破坏。
重金属通过空气、水和食物链等途径进入生态系统,对人类、动物和植物的健康造成影响。
重金属还会破坏生态系统的平衡,缩小生物多样性,影响生态景观的形成。
3、危害人体健康。
重金属通过食物、食水和空气等途径进入人体,对神经系统、免疫系统、造血系统和生殖系统等各个系统造成危害,引起头痛、头晕、恶心、呕吐、皮肤瘙痒、气喘、支气管炎、挫伤白细胞的功能、肠胃功能不良等。
二、重金属在土壤中的形态重金属在土壤中的形态多种多样,其化学性质的不同直接影响了其在土壤中的存在形式、吸附态和活性。
通常,重金属在土壤中的形态可分为三种类型:1、可溶性态。
可溶性态的重金属一般与土壤中的水分结合形成溶液,容易遭受淋溶机制带走。
2、交换态。
交换态的重金属可与土壤中的颗粒物结合,形成为土壤中的不易迁移、不易淋溶的形态。
3、残渣态。
残渣态的重金属一般与土壤中的矿物质结合,成为土壤有机质的重要组成部分,几乎不参与活跃的物理、化学反应。
三、重金属在土壤中的迁移与转化重金属在土壤中的迁移与转化受到多种因素的影响,如土壤类型、土壤pH值、氧化还原电位、土壤有机质、微生物等等,下面分别进行讨论:1、土壤类型。
不同类型的土壤中,重金属的吸附能力和生物有效性会出现明显差异。
微生物对土壤中重金属污染物的影响研究
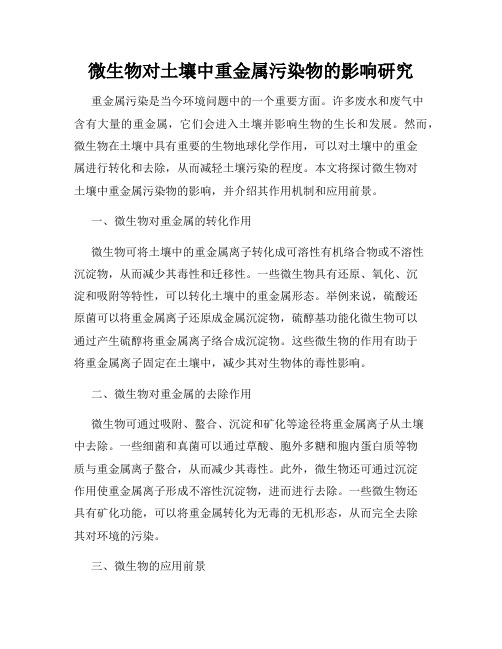
微生物对土壤中重金属污染物的影响研究重金属污染是当今环境问题中的一个重要方面。
许多废水和废气中含有大量的重金属,它们会进入土壤并影响生物的生长和发展。
然而,微生物在土壤中具有重要的生物地球化学作用,可以对土壤中的重金属进行转化和去除,从而减轻土壤污染的程度。
本文将探讨微生物对土壤中重金属污染物的影响,并介绍其作用机制和应用前景。
一、微生物对重金属的转化作用微生物可将土壤中的重金属离子转化成可溶性有机络合物或不溶性沉淀物,从而减少其毒性和迁移性。
一些微生物具有还原、氧化、沉淀和吸附等特性,可以转化土壤中的重金属形态。
举例来说,硫酸还原菌可以将重金属离子还原成金属沉淀物,硫醇基功能化微生物可以通过产生硫醇将重金属离子络合成沉淀物。
这些微生物的作用有助于将重金属离子固定在土壤中,减少其对生物体的毒性影响。
二、微生物对重金属的去除作用微生物可通过吸附、螯合、沉淀和矿化等途径将重金属离子从土壤中去除。
一些细菌和真菌可以通过草酸、胞外多糖和胞内蛋白质等物质与重金属离子螯合,从而减少其毒性。
此外,微生物还可通过沉淀作用使重金属离子形成不溶性沉淀物,进而进行去除。
一些微生物还具有矿化功能,可以将重金属转化为无毒的无机形态,从而完全去除其对环境的污染。
三、微生物的应用前景由于微生物在土壤中处理重金属污染中具有独特的优势,因此其应用前景广泛。
一方面,微生物修复可以在原地进行,不需要对土壤进行大规模开挖和运输,因此具有较低的成本和环境风险。
另一方面,微生物修复对土壤生态环境的破坏相对较小,能够保持土壤的水、肥结构,并且不会产生二次污染。
此外,微生物修复适用于不同类型的土壤和不同程度的污染,具有较高的适应性和灵活性。
然而,微生物修复技术在实际应用中还存在一些问题和挑战。
首先,不同微生物对不同重金属的转化和去除效果存在差异,因此需要针对具体的重金属污染物选择适宜的微生物种类。
其次,微生物修复过程需要一定的时间和环境条件,无法实现即时修复。
微生物在重金属污染土壤中的应用
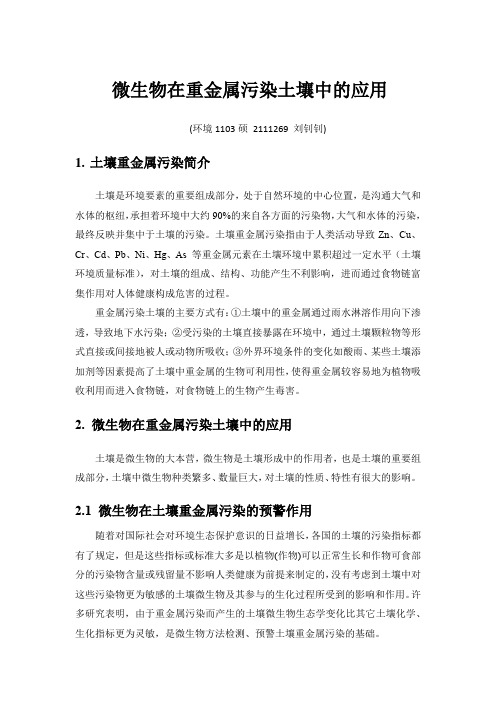
微生物在重金属污染土壤中的应用(环境1103硕2111269 刘钊钊)1.土壤重金属污染简介土壤是环境要素的重要组成部分,处于自然环境的中心位置,是沟通大气和水体的枢纽,承担着环境中大约90%的来自各方面的污染物,大气和水体的污染,最终反映并集中于土壤的污染。
土壤重金属污染指由于人类活动导致Zn、Cu、Cr、Cd、Pb、Ni、Hg、As 等重金属元素在土壤环境中累积超过一定水平(土壤环境质量标准),对土壤的组成、结构、功能产生不利影响,进而通过食物链富集作用对人体健康构成危害的过程。
重金属污染土壤的主要方式有:①土壤中的重金属通过雨水淋溶作用向下渗透,导致地下水污染;②受污染的土壤直接暴露在环境中,通过土壤颗粒物等形式直接或间接地被人或动物所吸收;③外界环境条件的变化如酸雨、某些土壤添加剂等因素提高了土壤中重金属的生物可利用性,使得重金属较容易地为植物吸收利用而进入食物链,对食物链上的生物产生毒害。
2. 微生物在重金属污染土壤中的应用土壤是微生物的大本营,微生物是土壤形成中的作用者,也是土壤的重要组成部分,土壤中微生物种类繁多、数量巨大,对土壤的性质、特性有很大的影响。
2.1 微生物在土壤重金属污染的预警作用随着对国际社会对环境生态保护意识的日益增长,各国的土壤的污染指标都有了规定,但是这些指标或标准大多是以植物(作物)可以正常生长和作物可食部分的污染物含量或残留量不影响人类健康为前提来制定的,没有考虑到土壤中对这些污染物更为敏感的土壤微生物及其参与的生化过程所受到的影响和作用。
许多研究表明,由于重金属污染而产生的土壤微生物生态学变化比其它土壤化学、生化指标更为灵敏,是微生物方法检测、预警土壤重金属污染的基础。
土壤微生物对各种污染物的胁迫响应较植物(作物)更为灵敏,如在欧共体规定的农田土壤重金属负荷标准之下,土壤微生物和微生物代谢过程已经发生了明显变化。
土壤微生物参与了80%~90%的土壤过程,几乎参与土壤中的一切生物及生物化学反应,在土壤功能及土壤过程中直接或间接地起重要作用,包括对动植物残体的分解、养分的储存转化及污染物的降解等,是土壤重金属污染的理想指标。
环境微生物课程论文-几种利用微生物修复土壤重金属方法的比较研究全解

几种利用微生物修复土壤重金属方法的比较研究摘要:总结了微生物修复土壤重金属污染方法的特点,对其原理进行了分析,比较了微生物刺激技术、微生物强化技术、植物-微生物联合修复三种方法,并对今后的微生物修复技术研究提出了建议。
关键词:重金属;微生物修复;菌根;根瘤菌0引言与常见的大气污染、水污染、工业固体废弃物污染相比,土壤重金属污染具有不可见性和隐蔽性.目前全国遭受不同程度污染的耕地面积已接近 2.0 ×107hm2,约占耕地面积的1/5,我国每年因重金属污染导致的粮食减产超过 1 ×107t,被重金属污染的粮食多达1.2 × 107t,合计经济损失至少200 亿元.土壤重金属污染日益严重导致土壤肥力退化、农作物产量降低和品质下降,严重影响环境质量和经济的可持续发展,威胁到人们的食品安全。
重金属对人类具有巨大的危害,能引起头痛、头晕、失眠、健忘、神经错乱、关节疼痛、结石、癌症,如肝癌、胃癌、肠癌、膀胱癌、乳腺癌、前列腺癌、乌脚病、畸形儿等[1]。
随着社会经济的发展,我国重金属污染已越来越严重,重金属污染事故频发。
近年来,随着土壤重金属防治方面研究增多,开发了越来越多的方法。
但是这些方法成本昂贵,可操作性差,且大部分是重金属稳定化技术,目前大都处在实验室研究阶段。
在此背景下,一些学者提出了利用微生物来修复土壤重金属污染方法,这对于传统的物理化学法是一种延伸。
1微生物修复土壤重金属污染的特点目前,用于土壤重金属污染治理的方法包括物理修复、化学修复和生物修复.物理修复、化学修复虽能达到一定的效果,但是能耗大、二次污染等问题也限制了其应用,尤其对于大面积有害的低浓度重金属污染,更是难以处理[2]。
微生物修复法具有处理费用低,对环境影响小、效率高等优点,加之微生物自身具有种类繁多,数量庞大,比表面积大等特点,所以利用一些真菌、细菌、放线菌等来修复重金属污染土壤具有很大的潜力,并且对重金属污染的耐性通常为真菌>细菌>放线菌。
重金属与土壤微生物的相互作用及污染土壤修复
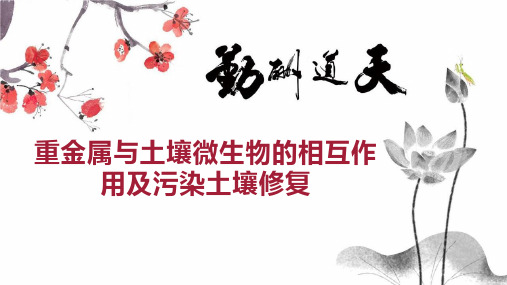
重金属对土壤生态系统的影响
重金属对土壤生态系统的影响
重金属进入土壤后,会对土壤微生物的多样性、群落结构和功能产生影响。 首先,重金属会降低土壤微生物的多样性,导致土壤生态系统的稳定性下降。其 次,重金属会改变土壤微生物的群落结构,导致某些微生物种群数量增加,而另 一些微生物种群数量减少。此外,重金属还会影响土壤微生物的功能,如降低土 壤微生物的酶活性,从而影响土壤生态系统的物质循环和能量流动。
重金属与土壤微生物的相互作用
其次,重金属会影响土壤微生物的基因表达。研究发现,重金属可诱导某些 微生物基因的表达,这些基因与重金属的抗性、富集和解毒有关。然而,过量的 重金属也会导致某些基因的表达受抑制,从而影响微生物的正常生长和代谢。
重金属与土壤微生物的相互作用
再者,重金属对土壤微生物的代谢功能也有一定的影响。例如,重金属可改 变土壤微生物的碳源利用和氮源利用能力,从而影响其代谢功能。此外,重金属 还会影响土壤微生物对有机物的降解和转化,进而影响土壤生态系统的物质循环。
内容摘要
在过去的几十年里,微生物修复技术得到了广泛。尤其是近年来,随着基因 组学、生物信息学和生物工程学的快速发展,微生物修复技术取得了显著进展。 其中,基因工程、生物传感器、植物修复和微生物联合应用等方面是研究的前沿 和热点。然而,这些技术在应用过程中仍存在一定的问题和局限性。例如,基因 工程菌的稳定性、生物传感器的灵敏度、植物修复的效率以及微生物联合应用的 效果等都需要进一步优化和提升。
结论
研究利用土壤微生物促进污染物降解和转化的新方法和新技术;(3)结合现 代生物技术手段(如基因组学、代谢组学和蛋白质组学等),解析土壤微生物在 污染土壤修复中的关键作用和调控机制;(4)加强研发具有高效、环保和可持 续性的污染土壤修复技术。通过这些研究工作,我们有望为解决重金属污染问题 提供更科学、有效的策略和技术手段。
微生物对重金属污染土壤的修复机制
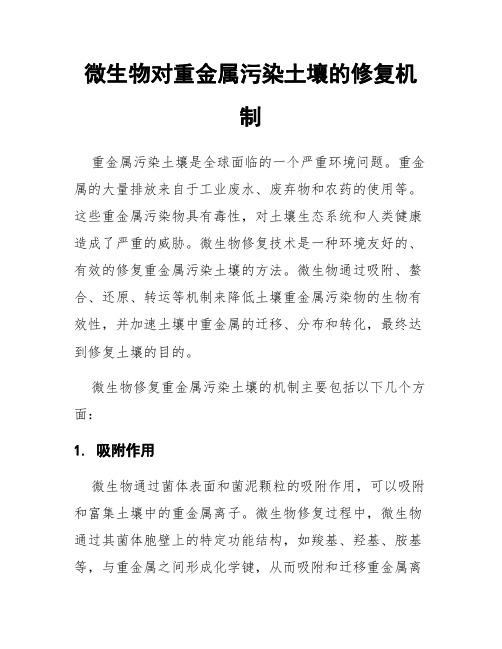
微生物对重金属污染土壤的修复机制重金属污染土壤是全球面临的一个严重环境问题。
重金属的大量排放来自于工业废水、废弃物和农药的使用等。
这些重金属污染物具有毒性,对土壤生态系统和人类健康造成了严重的威胁。
微生物修复技术是一种环境友好的、有效的修复重金属污染土壤的方法。
微生物通过吸附、螯合、还原、转运等机制来降低土壤重金属污染物的生物有效性,并加速土壤中重金属的迁移、分布和转化,最终达到修复土壤的目的。
微生物修复重金属污染土壤的机制主要包括以下几个方面:1. 吸附作用微生物通过菌体表面和菌泥颗粒的吸附作用,可以吸附和富集土壤中的重金属离子。
微生物修复过程中,微生物通过其菌体胞壁上的特定功能结构,如羧基、羟基、胺基等,与重金属之间形成化学键,从而吸附和迁移重金属离子。
此外,菌体表面带有一定的负电荷,可以吸附带正电荷的重金属离子。
2. 螯合作用微生物分泌的胞外多糖、有机酸和蛋白质等物质具有很强的螯合能力,能与重金属离子结合形成络合物。
这些络合物可以减少重金属的生物有效性,降低其对土壤生态系统的毒性。
3. 还原作用一些特定的微生物具有还原重金属的能力,能够将重金属离子转化为较为稳定的金属或其他形态。
例如,一些硫酸还原菌可以利用有机物作为电子供体,将重金属离子还原为金属或硫化物。
这样一来,重金属离子在土壤中的毒性得以降低,同时也减少了其生物有效性。
4. 转运作用微生物在修复过程中还起到了重要的转运作用。
它们通过活跃的代谢活动和膜传递系统,促进重金属在土壤中的迁移和分布。
微生物根际和菌根系统可以增强土壤团聚体结构,改善土壤物理性质,促进重金属的分散和迁移。
除了以上几个主要机制外,微生物修复重金属污染土壤还有一些次要的机制。
例如,一些细菌和真菌可以产生金属螯合物,与重金属形成络合物,从而减少重金属的生物有效性。
另外,微生物也可以分解土壤中的有机物和重金属络合物,从而降低其对土壤的毒性。
在微生物修复技术中,合理选择适当的微生物菌种非常重要。
微生物对重金属污染的生物修复
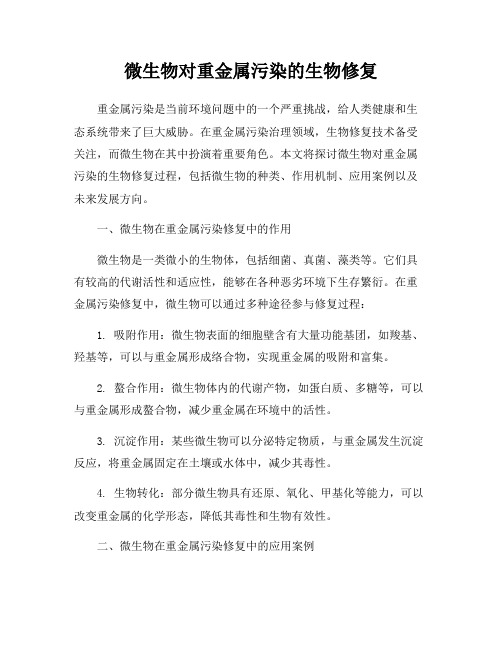
微生物对重金属污染的生物修复重金属污染是当前环境问题中的一个严重挑战,给人类健康和生态系统带来了巨大威胁。
在重金属污染治理领域,生物修复技术备受关注,而微生物在其中扮演着重要角色。
本文将探讨微生物对重金属污染的生物修复过程,包括微生物的种类、作用机制、应用案例以及未来发展方向。
一、微生物在重金属污染修复中的作用微生物是一类微小的生物体,包括细菌、真菌、藻类等。
它们具有较高的代谢活性和适应性,能够在各种恶劣环境下生存繁衍。
在重金属污染修复中,微生物可以通过多种途径参与修复过程:1. 吸附作用:微生物表面的细胞壁含有大量功能基团,如羧基、羟基等,可以与重金属形成络合物,实现重金属的吸附和富集。
2. 螯合作用:微生物体内的代谢产物,如蛋白质、多糖等,可以与重金属形成螯合物,减少重金属在环境中的活性。
3. 沉淀作用:某些微生物可以分泌特定物质,与重金属发生沉淀反应,将重金属固定在土壤或水体中,减少其毒性。
4. 生物转化:部分微生物具有还原、氧化、甲基化等能力,可以改变重金属的化学形态,降低其毒性和生物有效性。
二、微生物在重金属污染修复中的应用案例1. 铜污染修复:研究表明,一些铜耐受菌株如假单胞菌、硫酸亚铁细菌等,能够有效吸附和还原土壤中的铜离子,降低土壤铜浓度。
2. 镉污染修复:利用镉还原菌如硫酸还原菌、亚硝酸还原菌等,可以将土壤中的镉离子还原为不活跃的金属形态,减少其毒性。
3. 铅污染修复:一些铅耐受真菌如拟青霉、枯草芽孢杆菌等,通过吸附和螯合作用,可以有效清除土壤中的铅污染。
4. 镍污染修复:利用镍还原菌如硫酸还原菌、亚硝酸还原菌等,可以将土壤中的镍离子还原为不活跃的金属形态,降低土壤镍浓度。
三、微生物在重金属污染修复中的未来发展方向1. 多样性利用:未来可以进一步挖掘微生物资源,发现更多具有重金属耐受和修复能力的微生物种类,提高修复效率。
2. 基因工程改良:通过基因工程技术,可以改良微生物的代谢途径,增强其对重金属的吸附、转化和降解能力,提高修复效果。
典型土壤有机污染物赋存形态及影响因素

典型土壤有机污染物赋存形态及影响因素一、本文概述土壤是人类生存和发展的重要资源,然而,随着工业化和城市化的快速发展,大量有机污染物通过各种途径进入土壤环境,对土壤质量和生态环境造成了严重威胁。
这些有机污染物不仅影响土壤的理化性质,还会通过食物链进入人体,对人类的健康造成潜在风险。
因此,研究典型土壤有机污染物的赋存形态及其影响因素,对于制定有效的土壤污染防控和修复措施,保护土壤质量和生态环境具有重要意义。
本文旨在全面分析典型土壤有机污染物的赋存形态及其影响因素。
通过文献综述和实地调查,明确土壤中有机污染物的种类、分布和来源。
通过实验室研究,分析有机污染物在土壤中的赋存形态,包括吸附、溶解、转化等过程。
探讨土壤理化性质、微生物活动、环境因素等对有机污染物赋存形态的影响。
结合国内外相关研究成果,提出有效的土壤有机污染防控和修复建议,为土壤保护和生态环境建设提供科学依据。
通过本文的研究,可以深入了解典型土壤有机污染物的赋存形态及其影响因素,为土壤污染防控和修复提供理论支持和实践指导。
也为推动土壤科学和环境科学领域的发展,促进生态文明建设和可持续发展提供有益参考。
二、典型土壤有机污染物概述土壤有机污染物是指在土壤中存在的,来源于自然或人为活动产生的,具有潜在生态风险和健康风险的有机化合物。
这些污染物可以以多种形态存在于土壤中,如吸附态、溶解态、生物降解态等。
它们可能通过食物链进入生物体,对人体健康产生直接或间接影响。
典型的土壤有机污染物包括多环芳烃(PAHs)、多氯联苯(PCBs)、有机氯农药、石油烃、有机磷农药等。
这些污染物在土壤中的赋存形态和迁移转化行为受到多种因素的影响。
多环芳烃(PAHs)是一类由两个或多个苯环以线性、角状或簇状方式连接而成的化合物,主要来源于化石燃料的燃烧。
它们在土壤中的赋存形态受到土壤类型、有机质含量、pH值等因素的影响。
一般来说,多环芳烃在有机质含量高的土壤中更易吸附和积累。
土壤中重金属有效态
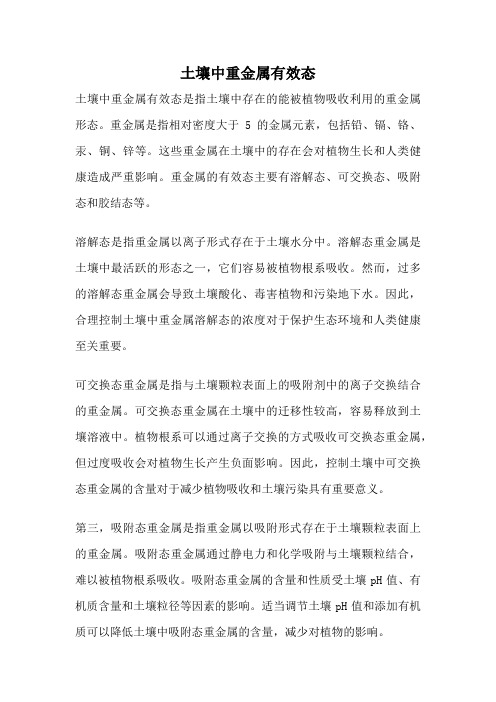
土壤中重金属有效态土壤中重金属有效态是指土壤中存在的能被植物吸收利用的重金属形态。
重金属是指相对密度大于5的金属元素,包括铅、镉、铬、汞、铜、锌等。
这些重金属在土壤中的存在会对植物生长和人类健康造成严重影响。
重金属的有效态主要有溶解态、可交换态、吸附态和胶结态等。
溶解态是指重金属以离子形式存在于土壤水分中。
溶解态重金属是土壤中最活跃的形态之一,它们容易被植物根系吸收。
然而,过多的溶解态重金属会导致土壤酸化、毒害植物和污染地下水。
因此,合理控制土壤中重金属溶解态的浓度对于保护生态环境和人类健康至关重要。
可交换态重金属是指与土壤颗粒表面上的吸附剂中的离子交换结合的重金属。
可交换态重金属在土壤中的迁移性较高,容易释放到土壤溶液中。
植物根系可以通过离子交换的方式吸收可交换态重金属,但过度吸收会对植物生长产生负面影响。
因此,控制土壤中可交换态重金属的含量对于减少植物吸收和土壤污染具有重要意义。
第三,吸附态重金属是指重金属以吸附形式存在于土壤颗粒表面上的重金属。
吸附态重金属通过静电力和化学吸附与土壤颗粒结合,难以被植物根系吸收。
吸附态重金属的含量和性质受土壤pH值、有机质含量和土壤粒径等因素的影响。
适当调节土壤pH值和添加有机质可以降低土壤中吸附态重金属的含量,减少对植物的影响。
胶结态重金属是指重金属与土壤胶结物质结合形成的复合物。
胶结态重金属对植物的吸收能力较低,但它们对土壤质地和结构的稳定性有着重要影响。
土壤中的胶结态重金属主要通过土壤微生物、根系分泌物和土壤有机质等因素进行转化。
因此,保护土壤微生物群落和增加土壤有机质含量是降低胶结态重金属含量的有效措施。
土壤中的重金属有效态包括溶解态、可交换态、吸附态和胶结态等形式。
了解不同重金属有效态的特点和影响因素,可以采取相应的措施来减少土壤中重金属的含量,保护生态环境和人类健康。
这一问题的解决需要从源头控制、土壤修复和科学耕作等方面综合考虑,以实现可持续发展和人与自然和谐共生。
重金属对土壤微生物群落结构的影响
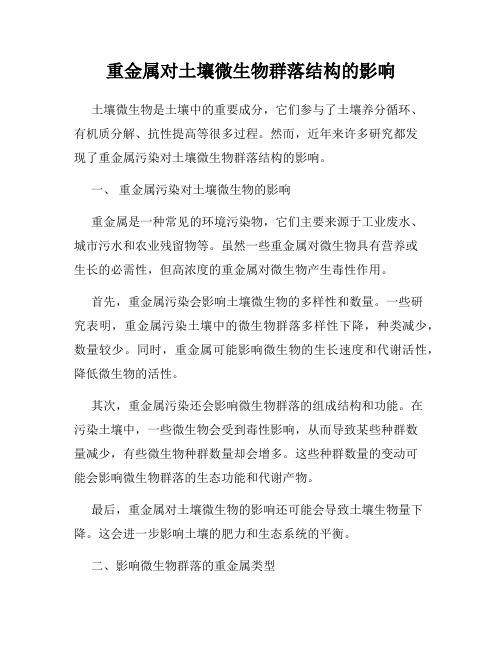
重金属对土壤微生物群落结构的影响土壤微生物是土壤中的重要成分,它们参与了土壤养分循环、有机质分解、抗性提高等很多过程。
然而,近年来许多研究都发现了重金属污染对土壤微生物群落结构的影响。
一、重金属污染对土壤微生物的影响重金属是一种常见的环境污染物,它们主要来源于工业废水、城市污水和农业残留物等。
虽然一些重金属对微生物具有营养或生长的必需性,但高浓度的重金属对微生物产生毒性作用。
首先,重金属污染会影响土壤微生物的多样性和数量。
一些研究表明,重金属污染土壤中的微生物群落多样性下降,种类减少,数量较少。
同时,重金属可能影响微生物的生长速度和代谢活性,降低微生物的活性。
其次,重金属污染还会影响微生物群落的组成结构和功能。
在污染土壤中,一些微生物会受到毒性影响,从而导致某些种群数量减少,有些微生物种群数量却会增多。
这些种群数量的变动可能会影响微生物群落的生态功能和代谢产物。
最后,重金属对土壤微生物的影响还可能会导致土壤生物量下降。
这会进一步影响土壤的肥力和生态系统的平衡。
二、影响微生物群落的重金属类型不同类型的重金属对微生物具有不同的毒性和生物学效应。
根据现有文献,汞、铅、镉、铬、镍和铜等重金属对土壤微生物的影响比较明显。
其中,汞和铅对微生物的毒性较高,汞在很低浓度下就能杀死大多数微生物,而铅对土壤微生物的影响是由于其对蛋白质酶的抑制作用。
镉、铬和镍则在高浓度下对微生物群落结构和数量产生影响。
铜在生物体内物质循环和电子传递等方面具有重要作用,但高浓度铜对土壤微生物的影响仍然相当大。
实验发现,高浓度铜会使微生物的生长受到抑制,群落结构发生变化,甚至造成一些微生物死亡。
三、微生物对重金属污染的响应机制尽管重金属对土壤微生物的影响非常大,但有些微生物可以通过多种方式适应和抵御重金属污染。
第一种适应机制是确保天然解毒剂的合成。
微生物可以通过生成金属结合蛋白、胞内螯合剂、某些络合物等天然解毒剂来降低对重金属的毒性反应。
微生物对土壤中重金属的修复与去除
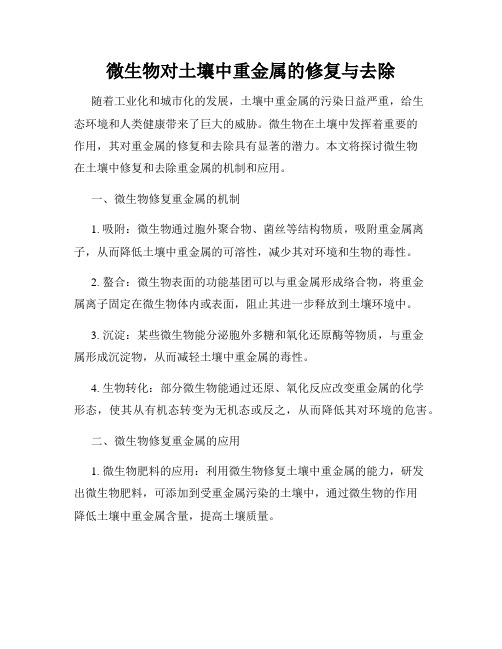
微生物对土壤中重金属的修复与去除随着工业化和城市化的发展,土壤中重金属的污染日益严重,给生态环境和人类健康带来了巨大的威胁。
微生物在土壤中发挥着重要的作用,其对重金属的修复和去除具有显著的潜力。
本文将探讨微生物在土壤中修复和去除重金属的机制和应用。
一、微生物修复重金属的机制1. 吸附:微生物通过胞外聚合物、菌丝等结构物质,吸附重金属离子,从而降低土壤中重金属的可溶性,减少其对环境和生物的毒性。
2. 螯合:微生物表面的功能基团可以与重金属形成络合物,将重金属离子固定在微生物体内或表面,阻止其进一步释放到土壤环境中。
3. 沉淀:某些微生物能分泌胞外多糖和氧化还原酶等物质,与重金属形成沉淀物,从而减轻土壤中重金属的毒性。
4. 生物转化:部分微生物能通过还原、氧化反应改变重金属的化学形态,使其从有机态转变为无机态或反之,从而降低其对环境的危害。
二、微生物修复重金属的应用1. 微生物肥料的应用:利用微生物修复土壤中重金属的能力,研发出微生物肥料,可添加到受重金属污染的土壤中,通过微生物的作用降低土壤中重金属含量,提高土壤质量。
2. 微生物菌剂的使用:某些菌株能够耐受高浓度的重金属离子,通过菌剂的喷洒或施加到种植宿主上,将其引入受重金属污染的土壤中,进行修复。
3. 合成微生物群的应用:通过筛选和组合具有不同功能的微生物,形成合成微生物群,利用其协同作用,提高土壤中重金属的修复效果。
4. 基因工程应用:通过基因工程技术,改造微生物的基因,使其具有更高的重金属修复能力,提高修复效率和效果。
三、微生物去除重金属的机制1. 吸附:微生物通过复杂的细胞壁和胞外物质结构,吸附重金属离子,并将其转移至微生物体内。
2. 沉淀:某些微生物能分泌特定物质,与重金属形成沉淀物,沉降到底泥中,从而将重金属从土壤中去除。
3. 离子交换:微生物体内的离子通道和离子交换物质能与土壤中的重金属发生离子交换,实现重金属的去除。
4. 同化代谢:部分微生物可以通过同化代谢将重金属离子转化为无毒或低毒的物质,从而实现去除。
土壤中重金属污染元素的形态分布及其生物有效性_刘恩玲

土壤中重金属污染元素的形态分布及其生物有效性刘恩玲,王亮 (浙江省温州市农业科学院生态环境研究所,浙江温州325006)摘要 不同形态的重金属有不同的生物有效性,对环境的危害程度也不一样。
概述了土壤中重金属的形态划分、形态分布与转化及其生物有效性的研究进展。
关键词 土壤重金属;形态划分;形态转化;生物有效性中图分类号 S151.9 文献标识码 A 文章编号 0517-6611(2006)03-0547-02Distri bution of Different Hea vy Metal Form s in Soil and Their Bio-availa bilityLIU En-ling et al (Institute of Ecology and Environ ment,Wenz hou Acad emy of Agriculture Science,Wenz hou,Zhejian g325006)A bstract In addition to the different bio-availablities,the different forms of heavy metals d id the different d egrees of harm to environm en t.In the arti-cle,a s ystematic s um mary of the progress in the following aspect:t he forms of heavy metal,their d istribution,tran sform an d bio-availablities are m ad e. Key w ords Heavy m etal in soil;Di vision of form;Trans formation;Bio-availabilit y 随着工业生产特别是乡镇企业的发展,农村环境迅速恶化,污水灌溉、工厂排放的废气飘尘、汽车尾气中的铅镉及污泥和城市垃圾农用等原因造成农业生态领域的重金属污染日益严重[1]。
微生物与环境中的重金属污染
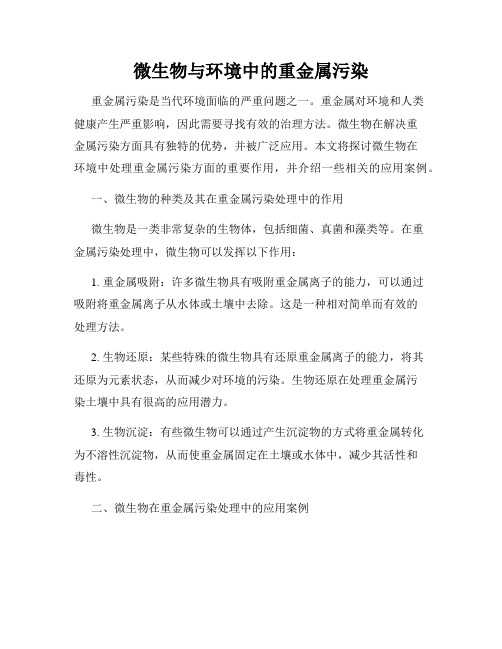
微生物与环境中的重金属污染重金属污染是当代环境面临的严重问题之一。
重金属对环境和人类健康产生严重影响,因此需要寻找有效的治理方法。
微生物在解决重金属污染方面具有独特的优势,并被广泛应用。
本文将探讨微生物在环境中处理重金属污染方面的重要作用,并介绍一些相关的应用案例。
一、微生物的种类及其在重金属污染处理中的作用微生物是一类非常复杂的生物体,包括细菌、真菌和藻类等。
在重金属污染处理中,微生物可以发挥以下作用:1. 重金属吸附:许多微生物具有吸附重金属离子的能力,可以通过吸附将重金属离子从水体或土壤中去除。
这是一种相对简单而有效的处理方法。
2. 生物还原:某些特殊的微生物具有还原重金属离子的能力,将其还原为元素状态,从而减少对环境的污染。
生物还原在处理重金属污染土壤中具有很高的应用潜力。
3. 生物沉淀:有些微生物可以通过产生沉淀物的方式将重金属转化为不溶性沉淀物,从而使重金属固定在土壤或水体中,减少其活性和毒性。
二、微生物在重金属污染处理中的应用案例1. 铜污染处理:某研究团队利用微生物的吸附能力,成功将废水中的铜离子降低到合理的安全标准。
通过添加具有吸附铜离子的微生物到废水中,可以快速、高效地去除铜离子。
2. 镉污染治理:一些微生物具有生物还原镉离子的能力。
通过培养这些微生物并将其添加到受镉污染的土壤中,可以将镉离子还原为不活性状态,有效治理镉污染。
3. 铅污染修复:使用一种特殊的微生物,可以实现生物沉淀铅污染土壤,从而减少铅污染的影响。
这种方法成本低廉且环境友好。
三、微生物在重金属污染治理中的优势和挑战微生物在重金属污染治理中具有多种优势,包括资源丰富、操作简便、成本较低等。
此外,微生物的应用还可以减少二次污染的风险,对环境和生态系统造成的影响比传统方法更小。
然而,微生物在重金属污染治理中也面临着一些挑战。
首先,不同种类的微生物对于不同的重金属有不同的处理效果,选择合适的微生物对治理效果至关重要。
微生物对土壤重金属污染的生物修复研究
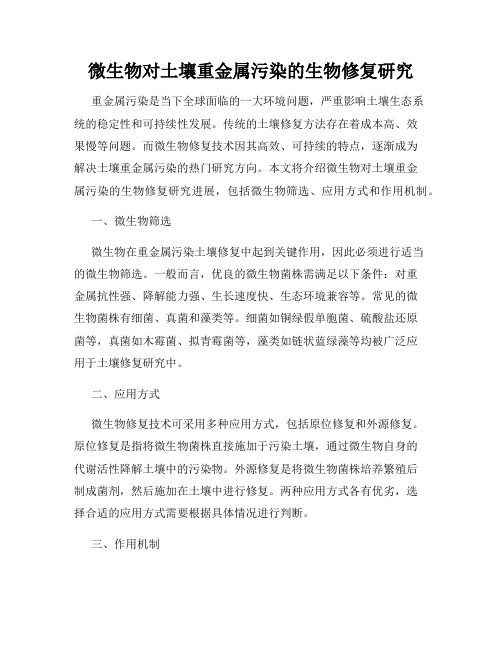
微生物对土壤重金属污染的生物修复研究重金属污染是当下全球面临的一大环境问题,严重影响土壤生态系统的稳定性和可持续性发展。
传统的土壤修复方法存在着成本高、效果慢等问题。
而微生物修复技术因其高效、可持续的特点,逐渐成为解决土壤重金属污染的热门研究方向。
本文将介绍微生物对土壤重金属污染的生物修复研究进展,包括微生物筛选、应用方式和作用机制。
一、微生物筛选微生物在重金属污染土壤修复中起到关键作用,因此必须进行适当的微生物筛选。
一般而言,优良的微生物菌株需满足以下条件:对重金属抗性强、降解能力强、生长速度快、生态环境兼容等。
常见的微生物菌株有细菌、真菌和藻类等。
细菌如铜绿假单胞菌、硫酸盐还原菌等,真菌如木霉菌、拟青霉菌等,藻类如链状蓝绿藻等均被广泛应用于土壤修复研究中。
二、应用方式微生物修复技术可采用多种应用方式,包括原位修复和外源修复。
原位修复是指将微生物菌株直接施加于污染土壤,通过微生物自身的代谢活性降解土壤中的污染物。
外源修复是将微生物菌株培养繁殖后制成菌剂,然后施加在土壤中进行修复。
两种应用方式各有优劣,选择合适的应用方式需要根据具体情况进行判断。
三、作用机制微生物修复土壤重金属污染的作用机制主要包括化学转化、生物吸附和生物浸提等。
微生物通过酵素的产生和分泌,能够将重金属离子转化为络合物、沉淀物或相对稳定的化合物,从而减少其对土壤环境和生物体的毒性。
微生物菌株表面的菌体、胞外多聚物等物质具有吸附重金属的能力,通过吸附作用,降低土壤中重金属的有效性。
此外,微生物菌株能够通过根系吸收和迁移重金属,达到了生物浸提的效果。
以铜绿假单胞菌为例,它具有铜离子还原酶和铜螯合酶,能够将土壤中的污染物铜离子还原成氢氧化铜或铜螯合物,进而沉淀或吸附在土壤中。
此外,该菌株菌体表面的胞外多聚物具有显著的吸附能力,能够吸附土壤中的重金属离子,使其形成不溶性沉淀物。
通过这些作用机制,铜绿假单胞菌能够有效修复铜污染的土壤。
综上所述,微生物对土壤重金属污染的生物修复研究已取得了显著的进展。
重金属污染土壤的微生物生态效应及其修复研究进展
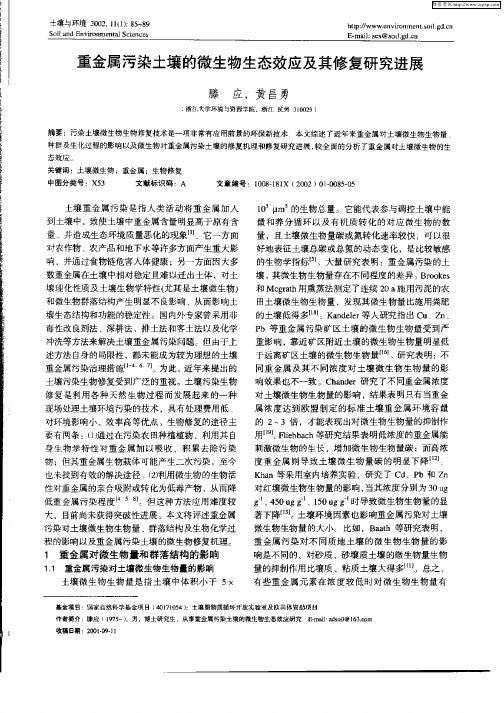
污染对土壤微生物生物量 、 群落结构及生物化学过 程的影响以及重金属污染土壤的微生物修复机理。
1 重 金 属对 微 生 物 量和 群落 结 构 的影 响
11 重金属污 染对 土壤微 生物 生物 量的影 响 土壤微 生 物 生 物 量是 指 土 壤 中体 积 小 于 5X
基金项 目 ・国家 自然科 学基 金项 目 { 0 71 4) 壤周 物质循 环开 放实 验室及 欧共 体资 助项 目、 4 115 :土 1 作者 简介 :哮应 【 95 .男 .博 士研 究生 .从事 重金 属柯 染土壤 的微 生物 生态 敢应研 究 t7 一)
态 效 应
关键 词 :土壤 微生 物 ;重金属 ;生物修 复
中图分类号 :X 3 5 文献标识码 :A 文章编号 :10 —8 X ( 0 2)0 —0 50 0 81 1 2 0 1 8 —5 0
土壤 重金 属 污 染 是 指 人 类 活 动将 重 金 属 加 人
到土壤中. 致使土壤中重金属含量明显高于原有含 量 、并造成 生态环 境质 量恶 化 的现象 。它一 方 面 】 对农作物 、 农产品和地下水等许多方面产生重大影 响, 并通过食物链危害人体健康 ; 另一方面因大多 数 重金属 在土壤 中相对 稳定且 难 以迁 出土体 ,对 土 壤理化性质及土壤生物学特性( 尤其是土壤微生物) 和微生物群落结构产生明显不 良影响, 从而影响土 壤生态结构和功能的稳定性 。国内外专家曾采用非 毒性改 良 剂法 、 深耕法 、 排土法和客土法以及化学
Ka h n等采用室内培养实验 ,研究 了 C 、 b和 _ d P , n 对红壤微生物生物量 的影响 , 当其浓度分别为 3 Og u
g‘ 5 g -、10u -时 导致 微 生物生 物量 的显 。 4 0u 5 gg g
土壤中重金属存在形态
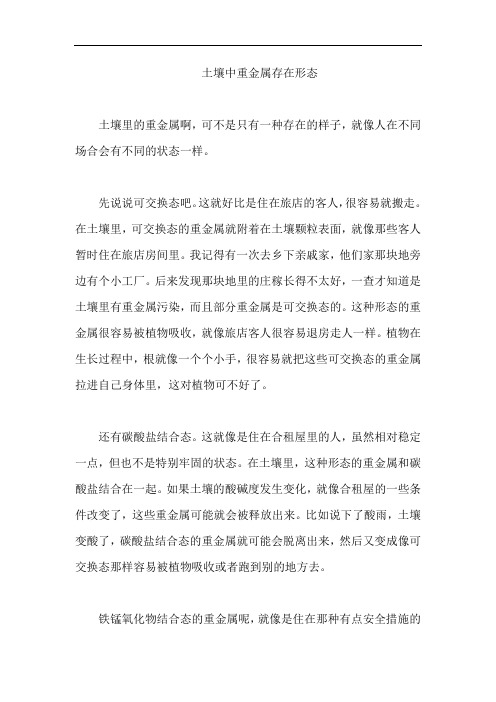
土壤中重金属存在形态土壤里的重金属啊,可不是只有一种存在的样子,就像人在不同场合会有不同的状态一样。
先说说可交换态吧。
这就好比是住在旅店的客人,很容易就搬走。
在土壤里,可交换态的重金属就附着在土壤颗粒表面,就像那些客人暂时住在旅店房间里。
我记得有一次去乡下亲戚家,他们家那块地旁边有个小工厂。
后来发现那块地里的庄稼长得不太好,一查才知道是土壤里有重金属污染,而且部分重金属是可交换态的。
这种形态的重金属很容易被植物吸收,就像旅店客人很容易退房走人一样。
植物在生长过程中,根就像一个个小手,很容易就把这些可交换态的重金属拉进自己身体里,这对植物可不好了。
还有碳酸盐结合态。
这就像是住在合租屋里的人,虽然相对稳定一点,但也不是特别牢固的状态。
在土壤里,这种形态的重金属和碳酸盐结合在一起。
如果土壤的酸碱度发生变化,就像合租屋的一些条件改变了,这些重金属可能就会被释放出来。
比如说下了酸雨,土壤变酸了,碳酸盐结合态的重金属就可能会脱离出来,然后又变成像可交换态那样容易被植物吸收或者跑到别的地方去。
铁锰氧化物结合态的重金属呢,就像是住在那种有点安全措施的房子里的人。
这种形态的重金属和土壤里的铁锰氧化物结合得比较紧密。
就像房子有了门锁一样,相对比较难出来。
不过要是土壤环境发生比较大的变化,比如说土壤里的氧气含量或者氧化还原电位改变了,这就像有人把房子的锁给弄坏了,这些重金属也会跑出来捣乱。
有机结合态的重金属就像是住在豪华公寓里的人,和土壤里的有机物结合得很紧密。
这就好比豪华公寓的设施齐全,住的人就很稳定。
一般情况下,这种形态的重金属不太容易出来活动。
但是如果土壤里的微生物大量繁殖或者死亡,就像公寓周围突然来了很多人或者发生了什么大事,微生物会分解有机物,这样有机结合态的重金属也可能会被释放出来。
残渣态的重金属就像是住在城堡里的人,是最稳定的。
这种重金属被包裹在土壤的矿物残渣里,就像城堡的城墙很坚固一样。
正常情况下,它们几乎不会出来,对环境和植物的影响也最小。
微生物修复重金属污染土壤的生态效益评估

微生物修复重金属污染土壤的生态效益评估第一章介绍随着工业化和城市化的加速发展,土壤污染已经成为了一个全球性的问题。
重金属污染是其中最常见的一种污染类型,而微生物修复是一种非常有效的治理方法。
本篇文章将探讨微生物修复重金属污染土壤的生态效益评估。
第二章微生物修复的机理微生物修复是一种利用微生物的生物学性质,在重金属污染土壤中减少或清除污染物的治理方法。
微生物修复主要是通过以下几个方面发挥作用:(1)生物吸附:微生物表面带有许多阴离子官能团,可以与阳离子如重金属结合。
(2)生物转化:微生物可以将重金属离子转化为难以吸收的纳米颗粒或微量元素,从而减少其毒性。
(3)生物浸出:微生物可以分泌有机酸和胞外多糖等物质,从而溶解土壤中的重金属离子,使其更容易被植物吸收。
第三章微生物修复的生态效益微生物修复的生态效益主要表现在以下三个方面:(1)降低土壤中重金属的浓度微生物利用其生物学特性,将重金属污染土壤中的重金属离子减少或清除,从而使土壤中的重金属浓度得到了降低。
这有助于减少对植物的毒性,促进植物的生长,从而增加土地的生产力。
(2)改善生态环境重金属的污染严重破坏了生态环境,微生物修复能够降低土壤中重金属的含量,使土壤中的生物和生境得到改善。
此外,微生物在修复过程中分泌有机物和胞外多糖等物质,有助于改善土壤质量。
(3)保护人体健康重金属污染对人体健康会造成一定的危害。
通过微生物修复重金属污染土壤,可以有效地降低重金属对人体的毒性,保障人们的健康。
第四章生态效益的评估方法微生物修复重金属污染土壤的生态效益评估主要包含以下三个方面:(1)土壤重金属的浓度通过对修复前后土壤样品重金属浓度的检测,可以评估微生物修复对土壤中重金属含量的降低程度。
(2)植物生长情况通过对修复前后植物生长情况的观察和分析,可以评估微生物修复对植物生长的促进效果。
(3)土壤质量的改善通过对修复前后土壤质量的测定,包括土壤有机质含量、酸碱度、微生物数量等指标的检测,可以评估微生物修复对土壤质量的改善效果。
- 1、下载文档前请自行甄别文档内容的完整性,平台不提供额外的编辑、内容补充、找答案等附加服务。
- 2、"仅部分预览"的文档,不可在线预览部分如存在完整性等问题,可反馈申请退款(可完整预览的文档不适用该条件!)。
- 3、如文档侵犯您的权益,请联系客服反馈,我们会尽快为您处理(人工客服工作时间:9:00-18:30)。
ENVIRONMENTAL BIOTECHNOLOGYSilica fertilization and nano-MnO 2amendment on bacterial community composition in high arsenic paddy soilsJihai Shao 1&Yaxian He 1&Huiling Zhang 1&Anwei Chen 1&Ming Lei 1&Junfeng Chen 1&Liang Peng 1&Ji-Dong Gu 2,3Received:21September 2015/Revised:27October 2015/Accepted:29October 2015#Springer-Verlag Berlin Heidelberg 2015Abstract Silica fertilization and nano-MnO 2amendment are reported as useful approaches in lowering the accumulation of arsenic in rice grains,but the effects of silica fertilization or nano-MnO 2amendment on microbial community in the pad-dy soils containing high concentration of arsenic are still un-known.In order to elucidate this question,the structures and composition of microbial community in the paddy soils,in response to silica fertilization and nano-MnO 2amendment,were investigated using pyrosequencing technique.The re-sults indicated that Proteobacteria ,Chloroflexi ,and Acidobacteria were the main dominating phyla in these paddy soils.A decrease in the relative abundance of Chloroflexi and Cyanobacteria ,but an increase in the relative abundance of Acidobacteria was observed after silica fertilization and nano-MnO 2amendment.The changes of Acidobacteria ,Chloroflexi ,and Cyanobacteria were strongly correlated with pH and the concentration of bioavailable arsenic in the paddy soils.The α-diversity of bacteria in the paddy soils increased in response to silica fertilization at low amendment level,but decreased under silica or nano-MnO 2amendment at high amendment level.Results of β-diversity analysis indicated that the microbial communities in the control treatment sharedmore similarity with that of those received low level of nano-MnO 2amendment,and the two silica fertilization treatments also shared more similarity with each other.Keywords Arsenic .Paddy soil .Bacterial community .Silica .Pyrosequencing .Nano-MnO 2IntroductionArsenic pollution has been recognized as a major public health issue in some parts of the world,especially in Asia (Mukherjee et al.2006).Long-term arsenic exposure could damage internal organs and induce several cancers,e.g.,la-ryngeal cancer,bladder cancer,lung cancer,and skin cancer (McClintock et al.2012;Smith et al.2012).Rice is a very important staple food grain in Southeast Asian countries,in-cluding China,India,Bangladesh,and the Philippines.Arsenic in soils can be assimilated from root systems and then accumulated in rice,and arsenic in rice is regarded as a disas-ter for these countries (Azizur Rahman et al.2008;Zhu et al.2008).As for China and some other countries,another serious problem is the limitation of quality arable land without high level of pollutants considering the high population.For exam-ple,China has <9%of the world ’s cultivated land to feed 22%of the global population (Chen 2007).In addition,the limited quantities of arable lands continue to decrease due to urbanization and development (Tan et al.2005;Chen 2007).Food security and crisis could be aggravated if these countries give up arsenic-contaminated arable lands for food production completely.In order to safely utilize arsenic-contaminated ag-ricultural land for food production,various approaches were proposed to relieve the toxicity of arsenic in soils against rice and lower the accumulation of arsenic from soils into rice grains,e.g.,water regime (Xu et al.2008;Li et al.2009),silica*Ji-Dong Gujdgu@hku.hk1Collaborative Innovation Center of Grain and Oil Crops in South China,Hunan Agricultural University,Changsha 410128,People ’s Republic of China2Hunan Provincial Key Laboratory of Farmland Pollution Control and Agricultural Resources Use,Hunan Agricultural University,Changsha 410128,People ’s Republic of China3School of Biological Sciences,The University of Hong Kong,Pokfulam Road,Hong Kong SAR,People ’s Republic of ChinaAppl Microbiol BiotechnolDOI 10.1007/s00253-015-7131-yfertilization(Li et al.2009),and nano-MnO2amendment (Zhou et al.2015).Microorganisms are ubiquitous in soils and responsible for nutrient cycling.Microbial communities are enormously di-verse in paddy soils,and some of them can affect the growth of paddy rice seedlings or arsenic accumulation in rice grains (Pandey et al.2013;Wang et al.2009).The structure of mi-crobial community depends on biotic and abiotic factors tak-ing place in soils.Previous studies showed that arsenic con-tamination affected the composition of microbial community in paddy soils(Somenahally et al.2011).However,the effects of remedial approaches towards arsenic contamination and pollution on bacterial community remain unknown. Recently,silica fertilization(Li et al.2009)and nano-MnO2 amendment(Zhou et al.2015)are reported as effective ap-proaches to decrease the accumulation potential of arsenic into rice,particularly the grains.However,the effects of silica fer-tilization or nano-MnO2amendment on the structure of mi-crobial community in paddy soils are poorly understood.The majority of the soil microflora is difficult to culture using traditional isolation and culturing techniques(Amann et al.1995;Nocker et al.2007).It is estimated that only a very small fraction of the indigenous microbial community in soils (0.01–10%)could be cultured in artificial medium under lab-oratory conditions(Amann et al.1995;Richaume et al.1993). Thus,metagenomics and DNA sequencing-based methods are useful in the study of microbial ecology because they are culture-independent.PCR-based next-generation DNA-sequencing technologies,e.g.,454pyrosequencing and Illumina pyrosequencing,are proven as useful tools in reveal-ing the microbial ecology and are intensively used in the studying of microbial ecology in various niches from surface to subsurface(Wang et al.2014;Feng et al.2015;Yan et al. 2015;Yang et al.2014).In order to elucidate the effects of silica fertilization and nano-MnO2amendment on the struc-tures and composition of bacterial community in paddy soils, a field experiment was carried out,and Illumina pyrosequenc-ing method was used to investigate the effects of these reme-diation methods on the structures and composition of micro-bial community in paddy soils.Materials and methodsField experiment and sampling of non-rhizosphere paddy soilField experiment was carried out in2013at an arsenic-contaminated paddy field(N25°36′01.11″;E113°00′42.68″)in the country of Dengjiatang,Cunzhou District, Hunan Province,China.The total arsenic content in the paddy soil was72.71mg/kg on average.Slag based silica fertilizer (SiO2)and nano-MnO2were amended to the top surface of paddy soils at a level of1.8and18kg/m2,respectively,on May31,2013.Control was made without silica or nano-MnO2addition.Thus,there were five different treatments in this study:control,two silica fertilization treatments(1.8kg/ m2,Si-L;18kg/m2,Si-H),and two nano-MnO2amendment treatments(1.8kg/m2,Mn-L;18kg/m2,Mn-H).Each treat-ment contained three replicates.Surface paddy soils(0–20cm)were tilled after silica and nano-MnO2amendments and then flooded and incubated for7days for preparation of rice seedings.Rice seeding transplant was carried out on June6,2013.The fields were maintained flooded during the whole duration of the experiment.Non-rhizosphere paddy soil samples were taken on July29,2013at rice tillering stage. Paddy soils(0-10cm)were collected at three different loca-tions for each treatment using a4.5-cm-diameter soil sampling auger,put into sterile plastic bags,and transferred on ice back to the laboratory.They were stored at−80°C.Soil physicochemical analysisThe contents of total organic carbon(TOC),total phosphorus (TP),total nitrogen(TN),pH,Eh,and bioavailable arsenic of the paddy soil samples were determined.The contents of TOC in paddy soil samples were measured using the total organic carbon analyzer(TOC,Elementar vario TOC,Germany). Total nitrogen was analyzed with Kjeldahl method.Soil total phosphorus content was determined by alkaline digestion followed by molybdate colorimetric measurement(Murphy and Riley1962).Soil pH and Eh were determined using a pH-Eh meter(Leici,Shanghai,China).The bioavailable arse-nic content was assayed by extracting with0.1M of HCl according to the method described by Thomas(2006)and determining with atomic fluorescence spectrometry(AFS-920,Titan Instruments,Beijing,China).DNA extraction,PCR amplification,and pyrosequencing The soil samples from three replicates of the same treatment were mixed together and homogenized thoroughly.Microbial community DNA from paddy soil samples was extracted using SoilMaster DNA Extraction kit(Epicentre Biotechnologies,Madison,WI)according to manufacturer’s i n s t r u c t i o n s.T h e16S u n i v e r s a l p r i m e r s515F (G T G C C A G C M G C C G C G G)a n d907R (CCGTCAATTCMTTTRAGTTT)were used to amplify the V4-V5region of the16S ribosomal RNA(rRNA)gene(515–907).The oligonucleotide sequence barcode fused to the for-ward primer.The PCR reaction mixture(20μL volume) contained:4μL of fivefold FastPfu reaction buffer (TransGen Biotech,Beijing,China),2μL of dNTP mixture (2.5mM),0.4μL of each primer(5μmol/L),0.4μL of FastPfu polymerase,10ng of the template DNA,and ddH2O to make up the volume.The PCR thermal cyclingAppl Microbiol Biotechnolscheme was set as follows:initial denaturation at95°C for 5min,25cycles of denaturation at95°C for30s,annealing at 55°C for30s,and extension at72°C for30s followed by a final extension period at72°C for5min.PCR amplification was performed on an ABI GeneAmp PCR System9700 (Applied Biosystems,CA,USA).PCR products were exam-ined on a2%(w/v)agarose gel and further purified using AxyPrep DNA Gel Extraction Kit(Axygen Biosciences, Union City,CA,USA).Purified amplicons were quantified using QuantiFluor™-ST(Promega,USA)and paired-end se-quenced on an Illumina MiSeq platform at Majorbio Bio-Pharm Technology Co.,Ltd.(Shanghai,China)according to the standard protocols.Bioinformatics and statistical analysisRaw pyrosequencing data were demultiplexed and quality-filtered using Trimmomatic according to the method described by Lohse et al.(2012).Overlapping reads were merged into single long reads using FLASH(Magoc and Salzberg2011). The qualified sequences were clustered into operational taxo-nomic units(OTUs)with97%similarity cutoff using Usearch (version7.1/).The phylogenetic affiliation of each16S rDNA sequence was analyzed using RDP Classifier (version2.2/projects/rdp-classifier/) with a confidence threshold of0.7.The reference database was Silva(Release115http://www.arb-silva.de).Rarefaction curve and alpha diversity including Chao1estimator,ACE estimator,Shannon index,and Simpson index were analyzed using Mothur(version v.1.30.1/wiki/ Schloss_SOP#Alpha_diversity)(Schloss et al.2011).Venn diagram and heatmap figures were performed using R package(/).Principal component analysis(PCoA)and redundancy analysis(RDA)were per-formed using Canoco(version4.5,Microcomputer Power, Ithaca,NY,USA).The ANOV A analysis was performed using SPSS version13.0(SPSS Inc.,Chicago,USA). Sequencing data depositionThe raw data for this study were deposited in the SRA of NCBI(SRP059905).ResultsSoil physicochemical characteristicsThe effects of silica fertilization and nano-MnO2amendment on physicochemical characteristics of the paddy soil samples in this study are presented in Table1.Silica fertilization or nano-MnO2amendment had no significant effect on the con-tents of TN in the paddy soils.The content of TP was not influenced by silica fertilization,but was significantly lower than the control under nano-MnO2amendment at the level of 18kg/m2.The contents of TOC were lower than the control in the treatments of silica fertilization or nano-MnO2amend-ment.The pH of the soils that received high level of silica or nano-MnO2amendment was significantly lower than the con-trol treatment.Both silica fertilization and nano-MnO2amend-ment effectively lowered the bioavailable arsenic concentra-tion in these paddy soil samples,and the content of bioavail-able arsenic decreased with the increase of silica or nano-MnO2amendment levels.General characteristics of the pyrosequencing-derived dataFor the five paddy soil treatments,a total of194,594valid sequences were obtained after quality screening.The number of sequences per sample ranged from21,682to49,586with an average of38,919.The length of these valid sequences was mainly about300–400bp,accounting for98.82%of the total valid sequences.The average length of these valid sequences was396.43bp.Rarefaction analysis indicated that sequence obtained from the five soil samples reached near saturation at a genetic distance of3%(Fig.1).Bacterial richness and diversityThe valid sequences obtained for bacteria could be assigned to 5259OTUs using Usearch(version7.1/). These OTUs could be clustered into44phyla.The main phyla in these soil samples were Proteobacteria(36.95–38. 66%),Chloroflexi(8.64–15.34%),and Acidobacteria(10. 88–19.53%)(Fig.2).Other highly represented phyla included Nitrospirae(5.42–9.14%),Planctomycetes(6.37–7.66%),Cyanobacteria(1.09–7.06%),Bacteroidetes(3. 79–4.86%),candidate division WS3(2.80–3.98%), Actinobacteria(0.6–1.85%),and Gemmatimonadetes(1.37–1.52%)in decreasing order.Members of the phyla Spirochaetae,Firmicutes,Verrucomicrobia,and Elusimicrobia were also presented in most samples,but at low abundance.The effects of silica fertilization and nano-MnO2amend-ment on the bacterial richness and diversity in the paddy soil samples depend on the amendment materials and also the level applied.Proteobacteria was the highest presented phy-lum in all these paddy soil samples,and silica fertilization or nano-MnO2amendment showed no significantly influence on its relative abundance.Silica fertilization decreased the rela-tive abundance of Chloroflexi but increased the relative ratio of Acidobacteria in these paddy soil samples,and such effects were also observed on the treatment of high level nano-MnO2 amendment(Mn-H).This positive effect on Acidobacteria and negative effect against Chloroflexi led to the substitutionAppl Microbiol Biotechnolof Chloroflexi with Acidobacteria as the second highly pre-sented phylum in the two silica fertilization treatments and the Mn-H treatment.Cyanobacteria was another phylum signifi-cantly influenced by silica fertilizer and nano-MnO2amend-ments.The relative abundance of Cyanobacteria decreased drastically in response to silica fertilization or nano-MnO2 amendment,and the richness of Nitrospirae increased in re-sponse to two silicon fertilization treatments and the treatment of Mn-H.The alpha diversity of bacteria in paddy soil samples was also modified by silica fertilization and nano-MnO2amend-ments.The Chao1estimator,ACE estimator,Shannon index, and Simpson index were all presented in the same pattern to silica fertilization or nano-MnO2amendments(Fig.3).Theα-diversity of bacteria in the paddy soil samples increased to silica fertilization at an amendment level of1.8kg/m2but decreased at the amendment level of18kg/m2;nano-MnO2 amendment decreasedα-diversity at the amendment level of 18kg/m2.The number of OTUs shared by all these soil samples, according to Venn analysis,was1705,accounting for 32.42%of the total identified OTUs(Fig.4).In order to analyze the total number of shared OTUs between two sam-ples,the percentage of shared OTUs(accounted for the total observed OTUs of two target samples)were calculated based on Venn analysis.The results indicated that the shared OTUs between the control and the treatment of silica fertilization or nano-MnO2amendment were38.6%for low level of silica fertilization(Si-L),38.6%for high level of silica fertilization (Si-H),38.0%for low level of nano-MnO2amendment(Mn-L),and35.0%for Mn-H,respectively.A heatmap,based on the level of class,was generated using R packages pheatmap,which divided these five samples into two groups at first levels(Fig.5).One is composed of the samples Si-L and Si-H;the other of control,Mn-L and Mn-H.Bacterial community in the control treatment shared high similarity with the treatment of Mn-L,and they grouped into a branch apart from the treatment of Mn-H in the group.The results of PCoA analysis are shown in Fig.6.The first principal coordinate(PC1)and second principal coordinate (PC2)account for60.7and23.3%of the intersample vari-ances,respectively.The samples are located in three loci withTable1Effect of silica fertilization and nano-MnO2 amendment on the physicochemical characteristics of arsenic-contaminated paddy soilsTN(mg/kg)TP(mg/kg)Bioavailable As(mg/kg)TOC(g/kg)pH EhControl770±129a720±23a0.95±0.14a65.6±0.4a7.35±0.05a−15.0±1.41a Si-L660±102a640±3a0.66±0.05b62.5±0.3bc7.25±0.07ab−14.0±1.41a Si-H810±150a670±4a0.55±0.13bc63.0±0.2b7.26±0.01b−19.00±0.00b Mn-L710±91a620±6b0.55±0.01bc59.2±0.1c7.33±0.04ab−21.5±2.12b Mn-H890±7a650±62a0.36±0.02c58.4±0.4d7.25±0.01b−20.5±0.71b Data in table are means±SDDifferent letters after data mean significant difference(p<0.05)(ANOVA)Fig.1Rarefaction curves ofpyrosequencing based on V4-V5of16S rRNA gene at a geneticdistance of3%Appl Microbiol Biotechnolthe control and the treatment of Mn-L located in locus 1,the treatments of Si-L and Si-H in locus 2,and the treatment Mn-H in locus 3alone.DiscussionSilicon and arsenic are metalloids.They may share the same transport route during uptake by cell,and this has been proven in rice (Ma et al.2008).The increase of silicon concentration could depress the uptake of arsenic and alleviate the toxicity of it.Nano-MnO 2and the complex of oxides and silica all couldeffectively adsorb arsenic and decrease its concentration in water (Con et al.2013;Bian et al.2012;Han et al.2011;Mahmood et al.2012).Thus,silica fertilizer and nano-MnO 2could lower the toxicity of arsenic through inhibiting the com-petitive uptake of arsenic by cells or decrease the concentra-tion of bioavailable arsenic in soils.According to the results of pyrosequencing and anal-ysis,Proteobacteria ,Chloroflexi ,andAcidobacteriaFig.2Relative abundance of the dominant bacterial phyla in arsenic-contaminated paddy soil samples.Si-L:silica fertilization at the level of 1.8kg/m 2;Si-H:silica fertilization at the level of 18kg/m 2;Mn-L:nano-MnO 2amendment at the level of 1.8kg/m 2;Mn-H:nano-MnO 2amendment at the level of 18kg/m2Fig.3Effect of silica fertilization and nano-MnO 2amendment on the α-diversity of bacteria in arsenic-contaminated paddy soil samples.a Chao1estimator.b ACE estimator.c Shannon index.d Simpson index.Differentlowercase letters within columns indicate statistically significant difference by one-way ANOV A (p<0.05)Fig.4Venn analysis of unique and shared OTUs of different treatments (3%distance level)Appl Microbiol Biotechnolwere the dominant phyla in all these paddy soil samples of this study (Fig.2).Such results are in agreement with previous studies (Xuan et al.2012;Feng et al.2015).The relative abundance and constituent of Proteobacteria was hardly influenced by silica fertiliza-tion or nano-MnO 2amendment (Figs.2and 5),and the main OTUs shared by all these soil samples in Fig.4are belonging to the phylum Proteobacteria .SomeFig.5Bacterial distribution of the top 100abundant classes among the five paddy soil samplesAppl Microbiol Biotechnolprevious studies indicated that Proteobacteria showed resistance to arsenic contamination (Lorenz et al.2006;Das et al.2013),and this may be a reason for its rel-atively high and constant abundance in these soil samples.Acidobacteria and Chloroflexi are the other two highly presented phyla in these paddy soils.Silica fertilization and nano-MnO 2amendment increased the relative ratio of Acidobacteria but decreased the relative abundance of Chloroflexi (Fig.2).Previous studies suggested that the abun-dance of Acidobacteria was strongly regulated by soil pH,and low pH was in favor of Acidobacteria (Jones et al.2009;Tripathi et al.2012).Results of RAD analysis in this study indicated that Acidobacteria showed a negative correlation with soil pH value,whereas Chloroflexi showed a positive correlation with soil pH value in this study (Fig.7).As shown in Table 1,the pH values of the soils that received silica or nano-MnO 2amendment were lower than the control.The de-crease of pH value in paddy soils may be a reason for the positive effect of silica and nano-MnO 2amendment on Acidobacteria .As indicated in Fig.7,the content of bioavail-able arsenic in soil is also negatively correlated with Acidobacteria but positively with Chloroflexi .The reduction of biotoxicity of arsenic by silica fertilization and nano-MnO 2amendment may be another reason for this phenomenon observed.Actinobacteria are widely distributed in soils and play an important role in organic matter turnover,carbon cycling,and suppression of some fungal pathogens (Jenkins et al.2010).Results of RAD analysis also suggested that the relative abun-dance of Actinobacteria was closely related with the contents of TOC in paddy soils (Fig.7).Some early studies indicated that Actinobacteria had high abundance (always more than 10%)in the soils (Nacke et al.2011;Davinic et al.2012).However,the relative abundance of Actinobacteria in this study ranged only from 0.6to 2.25%(Fig.2).Other studies reported that Actinobacteria had low relative abundance inarsenic-contaminated soils,whereas it had high relative abun-dance in the control soils (Sheik et al.2012;Lorenz et al.2006).Thus,arsenic contamination may be a plausible reason for the low relative abundance of Actinobacteria in soils of this study.The paddy soils in this study were under flooding during the whole experiment period,and the anaerobic condi-tion in paddy soils may be another reason for the lower rela-tive abundance of Actinobacteria in this study since most Actinobacteria are aerobic.Similar results were also presented in some paddy soils and activated sludge (Xuan et al.2012;Yang et al.2014).Cyanobacteria was highly presented in the control soil in this study and accounted for 7.06%of total reads in the con-trol (Fig.2).However,the relative abundance of Cyanobacteria decreased drastically to silica fertilization or nano-MnO 2amendment.Cyanobacteria ,such as Microcystis ,Nostoc ,and Synechocystis ,have various strate-gies to detoxify arsenic,including arsenite methylation,vola-tilization,arsenite reduction,and efflux (López-Maury et al.2003;Yin et al.2011;Pandey et al.2012).These strategies make Cyanobacteria resistant/tolerant to arsenic contamina-tion.This may be the reason for the high abundant ratio of Cyanobacteria in the control paddy soils.As indicated in Table 1,the content of bioavailable arsenic decreased under silica fertilization or nano-MnO 2amendment.The decrease of arsenic biotoxicity,in re-sponse to silica fertilization or nano-MnO 2amendment,may decrease the competition of Cyanobacteria ,which in turn decreased the relative abundance of this microor-ganism in soils.Cyanobacteria prefer the weak alkaline niches (Nayak and Prasanna 2007).The results of RAD analysis indicated that the richness of cyanobacteria was closely related to the pH of soil (Fig.7).The decrease of soil pH in response to silica or nano-MnO 2amendmentFig.6Principal component analysis on the similarity of bacterial communities in different paddy soil samples using Canoco4.5Fig.7Cross analysis of bacterial community and soil physicochemical characteristics using redundancy analysis (Canoco 4.5)Appl Microbiol Biotechnolmay be another reason for the decrease of relative abun-dance of Cyanobacteria in paddy soils.Results of heatmap(Fig.5)and PCoA(Fig.6)anal-ysis all indicated that low level of nano-MnO2amend-ment had little effect on the structure of bacterial com-munity in arsenic-contaminated paddy soil,whereas high level nano-MnO2amendment and two silica fertilization treatments all significantly altered the structure of bac-terial community.Nano-MnO2was proposed to remedi-ate heavy metal contamination,while the toxicity of nano-MnO2had also been emphasized(Li et al.2014). Though the bioavailable arsenic in the treatment of Mn-L was lower than the control,the toxicity of nano-MnO2may partially offset the alleviation of toxicity from arsenic.We deduce that this is the reason for the little effect of low level nano-MnO2on the structure of bacterial community in arsenic-contaminated paddy soil. Theα-diversity analysis(Chao1estimator,ACE estima-tor,Shannon,and Simpson)indicated that silica fertili-zation increased soil microbial diversity at low amend-ment level but decreased it at high amendment level (Fig.3).The effects of silica fertilization on the struc-ture of bacterial community are still obscure.Ahn et al. (2012)reported that silica fertilizer decreased theα-diversity of bacteria in April in paddy soil but increased it in August.The bioavailable arsenic in two silica fer-tilization treatments was significantly lower than the control in this study,while two silica fertilization treat-ments have opposite effects on the bacterial diversity. We presently could not confirm the increase of bacterial diversity in the treatment of Si-L either it was induced by silica fertilizer itself or by the decrease of bioavail-able arsenic in paddy soils,but,we at least could de-duced that the decrease of bacterial diversity in the treatment of Si-H was induced by high-level silica amendment.As mentioned above,nano-MnO2may be toxic to bacteria;thus,we deduced that the decrease of bacterial diversity in the treatment of Mn-H was caused by the toxicity of nano-MnO2itself.Acknowledgments This work was supported by the Foundation of National Water Science and Technology Projects of China (2014ZX07206001-03),the Foundation of Furong Scholar Project of Hunan Province,and an honorary professorship.Funding This study was funded by National Water Science and Technology Projects of China(NO.2014ZX07206001-03),Furong Scholar Project of Hunan Province,and an honorary professorship (J-D Gu).Compliance with ethical standardsConflict of interest The authors declare that they have no competing interests.Ethical approval This article does not contain any studies with human participants or animals performed by any of the authors. ReferencesAhn JH,Song J,Kim BY,Kim MS,Joa JH,Weon HY(2012) Characterization of the bacterial and archaeal communities in rice field soils subjected to long-term fertilization practices.J Microbiol 50:754–765Amann RI,Ludwig W,Schleifer KH(1995)Phylogenetic identification and in situ detection of individual microbial cells without cultiva-tion.Microbiol Mol Biol Rev59:143–169Azizur Rahman M,Hasegawa H,Mahfuzur Rahman M,Mazid Miah MA,Tasmin A(2008)Arsenic accumulation in rice(Oryza sativa L.):human exposure through food chain.Ecotoxicol Environ Saf 69:317–324Bian ZQ,Xie L,Tian XH,Fan W,Zhang J(2012)Adsorption of As(Ш) and As(V)in water by nano-MnO2with three kinds of crystal struc-tures.J Environ Health29:645–648Chen J(2007)Rapid urbanization in China:a real challenge to soil pro-tection and food security.Catena69:1–15Con TH,Thao P,Dai TX,Loan DK(2013)Application of nano dimen-sional MnO2for high effective sorption of arsenic and fluoride in drinking water.Environ Sci2:69–77Das S,Jean JS,Kar S,Liu CC(2013)Changes in bacterial community structure and abundance in agricultural soils under varying levels of arsenic contamination.Geomicrobiol J30:635–644Davinic M,Fultz LM,Acosta-Martinez V,Calderón FJ,Cox SB,Dowd SE,Allen VG,Zak JC,Moore-Kucera J(2012)Pyrosequencing and mid-infrared spectroscopy reveal distinct aggregate stratification of soil bacterial communities and organic matter composition.Soil Biol Biochem46:63–72Feng Y,Yu Y,Tang H,Zu Q,Zhu J,Lin X(2015)The contrasting responses of soil microorganisms in two rice cultivars to elevated ground-level ozone.Environ Pollut197:195–202Han X,Li YL,Gu J-D(2011)Oxidation of As(III)by MnO2in the absence and presence of Fe(II)under acidic conditions.Geochim Cosmochim Acta75:368–379Jenkins S,Waite I,Blackburn A,Husband R,Rushton S,O’Donnell A (2010)Actinobacterial community dynamics in long term managed grasslands.19th World Congress of Soil Science,Brisbane Jones RT,Robeson MS,Lauber CL,Hamady M,Knight R,Fierer NA (2009)Comprehensive survey of soil acidobacterial diversity using pyrosequencing and clone library analyses.ISME J3:442–445Li RY,Stroud JL,Ma JF,McGrath SP,Zhao FJ(2009)Mitigation of arsenic accumulation in rice with water management and silicon fertilization.Environ Sci Technol43:3778–3783Li T,Shi T,Li X,Zeng S,Yin L,Pu Y(2014)Effects of nano-MnO2on dopaminergic neurons and the spatial learning capability of rats.Int J Environ Res Public Health11:7918–7930Lohse M,Bolger AM,Nagel A,Fernie AR,Lunn JE,Stitt M,Usadel B (2012)RobiNA:a user-friendly,integrated software solution for RNA-Seq-based transcriptomics.Nucleic Acids Res40:W622López-Maury L,Florencio J,Reye JC(2003)Arsenic sensing and resis-tance system in the cyanobacterium Synechocystis sp.strain PCC 6803.J Bacteriol18:5363–5371Lorenz N,Hintemann T,Kramarewa T,Katayama A,Yasuta T, Marschner P,Kandeler E(2006)Response of microbial activity and microbial community composition in soils to long-term arsenic and cadmium exposure.Soil Biol Biochem38:1430–1437Appl Microbiol Biotechnol。