石墨烯聚乳酸复合材料
聚乳酸纳米复合材料的研究进展
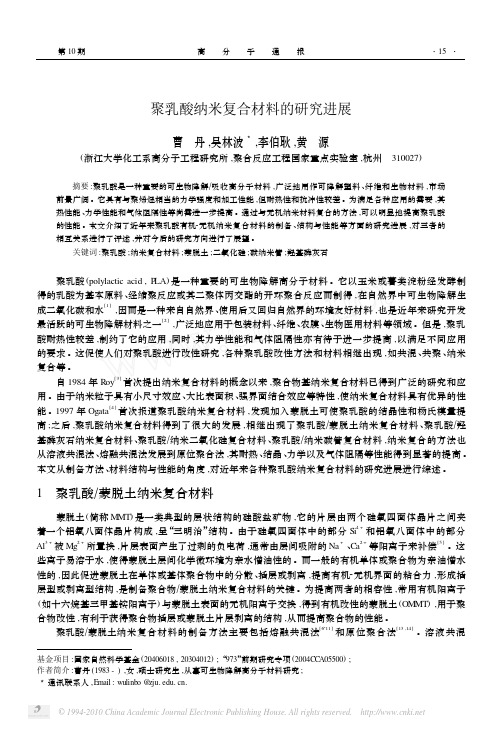
聚乳酸纳米复合材料的研究进展曹 丹,吴林波3,李伯耿,黄 源(浙江大学化工系高分子工程研究所,聚合反应工程国家重点实验室,杭州 310027) 摘要:聚乳酸是一种重要的可生物降解Π吸收高分子材料,广泛地用作可降解塑料、纤维和生物材料,市场前景广阔。
它具有与聚烯烃相当的力学强度和加工性能,但耐热性和抗冲性较差。
为满足各种应用的需要,其热性能、力学性能和气体阻隔性等尚需进一步提高。
通过与无机纳米材料复合的方法,可以明显地提高聚乳酸的性能。
本文介绍了近年来聚乳酸有机2无机纳米复合材料的制备、结构与性能等方面的研究进展,对三者的相互关系进行了评述,并对今后的研究方向进行了展望。
关键词:聚乳酸;纳米复合材料;蒙脱土;二氧化硅;碳纳米管;羟基磷灰石聚乳酸(polylactic acid,P LA)是一种重要的可生物降解高分子材料。
它以玉米或薯类淀粉经发酵制得的乳酸为基本原料、经缩聚反应或其二聚体丙交酯的开环聚合反应而制得,在自然界中可生物降解生成二氧化碳和水[1],因而是一种来自自然界、使用后又回归自然界的环境友好材料,也是近年来研究开发最活跃的可生物降解材料之一[2],广泛地应用于包装材料、纤维、农膜、生物医用材料等领域。
但是,聚乳酸耐热性较差,制约了它的应用,同时,其力学性能和气体阻隔性亦有待于进一步提高,以满足不同应用的要求。
这促使人们对聚乳酸进行改性研究,各种聚乳酸改性方法和材料相继出现,如共混、共聚、纳米复合等。
自1984年R oy[3]首次提出纳米复合材料的概念以来,聚合物基纳米复合材料已得到广泛的研究和应用。
由于纳米粒子具有小尺寸效应、大比表面积、强界面结合效应等特性,使纳米复合材料具有优异的性能。
1997年Ogata[4]首次报道聚乳酸纳米复合材料,发现加入蒙脱土可使聚乳酸的结晶性和杨氏模量提高;之后,聚乳酸纳米复合材料得到了很大的发展,相继出现了聚乳酸Π蒙脱土纳米复合材料、聚乳酸Π羟基磷灰石纳米复合材料、聚乳酸Π纳米二氧化硅复合材料、聚乳酸Π纳米碳管复合材料,纳米复合的方法也从溶液共混法、熔融共混法发展到原位聚合法,其耐热、结晶、力学以及气体阻隔等性能得到显著的提高。
石墨烯基复合材料的制备及性能研究
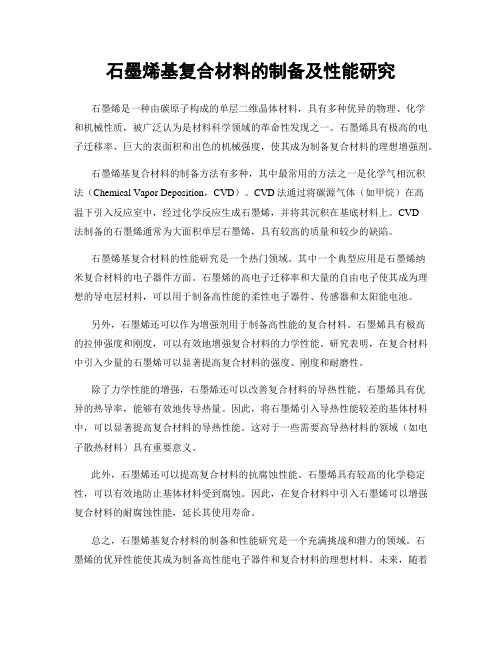
石墨烯基复合材料的制备及性能研究石墨烯是一种由碳原子构成的单层二维晶体材料,具有多种优异的物理、化学和机械性质,被广泛认为是材料科学领域的革命性发现之一。
石墨烯具有极高的电子迁移率、巨大的表面积和出色的机械强度,使其成为制备复合材料的理想增强剂。
石墨烯基复合材料的制备方法有多种,其中最常用的方法之一是化学气相沉积法(Chemical Vapor Deposition,CVD)。
CVD法通过将碳源气体(如甲烷)在高温下引入反应室中,经过化学反应生成石墨烯,并将其沉积在基底材料上。
CVD法制备的石墨烯通常为大面积单层石墨烯,具有较高的质量和较少的缺陷。
石墨烯基复合材料的性能研究是一个热门领域。
其中一个典型应用是石墨烯纳米复合材料的电子器件方面。
石墨烯的高电子迁移率和大量的自由电子使其成为理想的导电层材料,可以用于制备高性能的柔性电子器件、传感器和太阳能电池。
另外,石墨烯还可以作为增强剂用于制备高性能的复合材料。
石墨烯具有极高的拉伸强度和刚度,可以有效地增强复合材料的力学性能。
研究表明,在复合材料中引入少量的石墨烯可以显著提高复合材料的强度、刚度和耐磨性。
除了力学性能的增强,石墨烯还可以改善复合材料的导热性能。
石墨烯具有优异的热导率,能够有效地传导热量。
因此,将石墨烯引入导热性能较差的基体材料中,可以显著提高复合材料的导热性能。
这对于一些需要高导热材料的领域(如电子散热材料)具有重要意义。
此外,石墨烯还可以提高复合材料的抗腐蚀性能。
石墨烯具有较高的化学稳定性,可以有效地防止基体材料受到腐蚀。
因此,在复合材料中引入石墨烯可以增强复合材料的耐腐蚀性能,延长其使用寿命。
总之,石墨烯基复合材料的制备和性能研究是一个充满挑战和潜力的领域。
石墨烯的优异性能使其成为制备高性能电子器件和复合材料的理想材料。
未来,随着对石墨烯制备技术和性能研究的不断深入,相信石墨烯基复合材料将在各个领域展现出更多的应用前景。
新型功能性高分子材料的研究进展
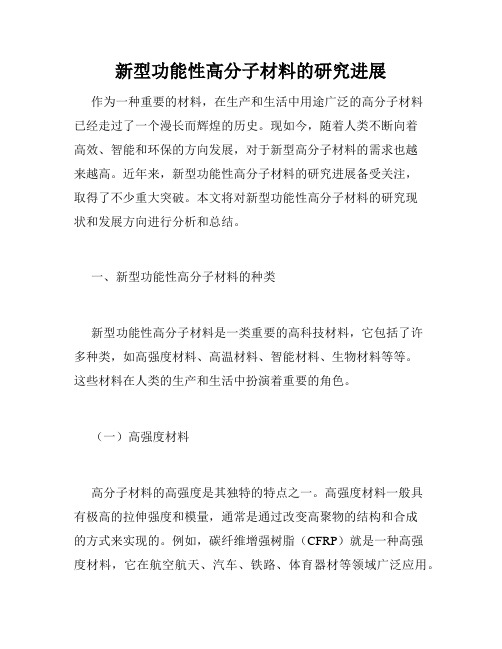
新型功能性高分子材料的研究进展作为一种重要的材料,在生产和生活中用途广泛的高分子材料已经走过了一个漫长而辉煌的历史。
现如今,随着人类不断向着高效、智能和环保的方向发展,对于新型高分子材料的需求也越来越高。
近年来,新型功能性高分子材料的研究进展备受关注,取得了不少重大突破。
本文将对新型功能性高分子材料的研究现状和发展方向进行分析和总结。
一、新型功能性高分子材料的种类新型功能性高分子材料是一类重要的高科技材料,它包括了许多种类,如高强度材料、高温材料、智能材料、生物材料等等。
这些材料在人类的生产和生活中扮演着重要的角色。
(一)高强度材料高分子材料的高强度是其独特的特点之一。
高强度材料一般具有极高的拉伸强度和模量,通常是通过改变高聚物的结构和合成的方式来实现的。
例如,碳纤维增强树脂(CFRP)就是一种高强度材料,它在航空航天、汽车、铁路、体育器材等领域广泛应用。
(二)高温材料高温材料也是一种重要的高分子材料。
高温材料的耐高温性能在一定程度上影响其应用范围和使用寿命。
目前,高温材料主要包括热塑性和热固性两类。
例如,聚苯硫脲(PPSU)是一种热塑性高温材料,其热稳定性、耐磨性和耐化学性能良好。
(三)智能材料智能材料是一种具有特定物理性质,可在外界刺激下自主感知和响应的材料。
目前,智能材料主要包括形状记忆材料、电致变材料、引热变形材料、光敏材料等等。
这些材料在人类的生产和生活中用途广泛,例如,形状记忆合金(SMA)可以广泛应用于机器人、医药等领域。
(四)生物材料生物材料也是一种新型的功能性高分子材料,它具有优良的生物相容性和生物活性。
生物材料主要包括生物可降解材料、生物惰性材料和生物活性材料三类。
如聚乳酸(PLA)是一种生物可降解材料,它已被广泛应用于医学领域。
二、随着人类对高效、智能、环保的需求不断增加,新型功能性高分子材料在不断推陈出新。
目前,新型功能性高分子材料的研究主要集中在以下几个方向:(一)高强度材料的研究高强度材料的研究是近年来新型功能性高分子材料的热点之一。
聚乳酸纳米复合材料的制备及性能
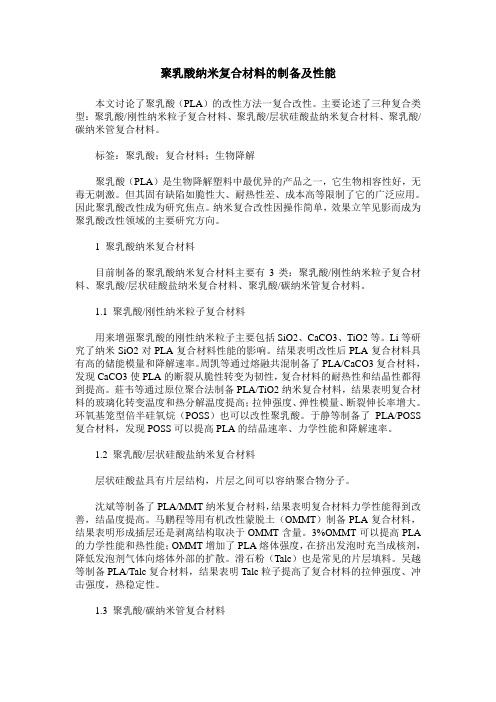
聚乳酸纳米复合材料的制备及性能本文讨论了聚乳酸(PLA)的改性方法一复合改性。
主要论述了三种复合类型:聚乳酸/刚性纳米粒子复合材料、聚乳酸/层状硅酸盐纳米复合材料、聚乳酸/碳纳米管复合材料。
标签:聚乳酸;复合材料;生物降解聚乳酸(PLA)是生物降解塑料中最优异的产品之一,它生物相容性好,无毒无刺激。
但其固有缺陷如脆性大、耐热性差、成本高等限制了它的广泛应用。
因此聚乳酸改性成为研究焦点。
纳米复合改性因操作简单,效果立竿见影而成为聚乳酸改性领域的主要研究方向。
1 聚乳酸纳米复合材料目前制备的聚乳酸纳米复合材料主要有3类:聚乳酸/刚性纳米粒子复合材料、聚乳酸/层状硅酸盐纳米复合材料、聚乳酸/碳纳米管复合材料。
1.1 聚乳酸/刚性纳米粒子复合材料用来增强聚乳酸的刚性纳米粒子主要包括SiO2、CaCO3、TiO2等。
Li等研究了纳米SiO2对PLA复合材料性能的影响。
结果表明改性后PLA复合材料具有高的储能模量和降解速率。
周凯等通过熔融共混制备了PLA/CaCO3复合材料,发现CaCO3使PLA的断裂从脆性转变为韧性,复合材料的耐热性和结晶性都得到提高。
莊韦等通过原位聚合法制备PLA/TiO2纳米复合材料,结果表明复合材料的玻璃化转变温度和热分解温度提高;拉伸强度、弹性模量、断裂伸长率增大。
环氧基笼型倍半硅氧烷(POSS)也可以改性聚乳酸。
于静等制备了PLA/POSS 复合材料,发现POSS可以提高PLA的结晶速率、力学性能和降解速率。
1.2 聚乳酸/层状硅酸盐纳米复合材料层状硅酸盐具有片层结构,片层之间可以容纳聚合物分子。
沈斌等制备了PLA/MMT纳米复合材料,结果表明复合材料力学性能得到改善,结晶度提高。
马鹏程等用有机改性蒙脱土(OMMT)制备PLA复合材料,结果表明形成插层还是剥离结构取决于OMMT含量。
3%OMMT可以提高PLA 的力学性能和热性能;OMMT增加了PLA熔体强度,在挤出发泡时充当成核剂,降低发泡剂气体向熔体外部的扩散。
石墨烯复合材料研究进展

石墨烯复合材料研究进展摘要:近年来石墨烯因其优良的力学、电学、热学和光学等特性, 且添加到基体材料中可以提高复合材料的性能,拓展其功能,因此石墨烯复合材料的制备成为研究热点之一。
本文介绍了国内外对石墨烯复合材料的研究,对石墨烯复合材料的研究进展及现状进行了详细的介绍,并对石墨烯复合材料的发展趋势进行了展望。
关键词:石墨烯;复合材料;研究进展一、引言石墨烯因其优异的物理性能和可修饰性, 受到国内外学者的广泛关注。
石墨烯的杨氏模量高达1TPa、断裂强度高达130GPa,是目前已知的强度性能最高的材料,同时是目前发现电阻率最小的材料, 只有约10-8Ω·m;拥有很高的电子迁移率,且具有较高的导热系数。
氧化石墨烯作为石墨烯的重要派生物,氧化石墨烯薄片在剪切力作用下很容易平行排列于复合材料中, 从而提高复合材料的性能。
本文总结介绍了几种常见的石墨烯复合材料。
二、石墨烯复合材料(1)石墨烯及氧化石墨烯复合材料膜聚乙烯醇(PVA)结构中有非常多的羟基,因此其能与水相互溶解,溶解效果很好。
GO和PVA都可以在溶液中形成均匀、稳定的分散体系。
干燥成型后,GO在PVA中的分散可以达到分子水平,GO表面丰富的含氧官能团可以与PVA的羟基形成氢键,因此添加少量的GO可以显著提高复合材料的力学性能。
樊志敏[1]等制备出了氧化石墨烯纳米带/TPU复合膜。
通过机械测试显示,当加入氧化石墨烯纳米带的量为2%时,复合薄膜的弹性模量和抗拉强度与不加氧化石墨烯纳米带的纯TPU薄膜相比都得到了非常大的提高,分别提高了160%和123%。
马国富[2]等人发现,在聚乙烯醇(PVA)和氧化石墨烯(GO)复合制备的得复合薄膜中,GO均匀的分散在PVA溶液中,PVA的羟基与GO表面的含氧基团发生相互作用复合而不分相。
加入GO之后,大大提高了复合膜的热稳定性,当加入的GO量为3%时,纳米复合膜力学性能测试出现最大值,此时断裂伸长率也出现了最大值,这表明在此GO含量时复合膜有最佳性能;与不加GO的纯PVA膜相比,当加入的GO量为3%时,耐水性也大大地提高。
新材料的突破与应用创新
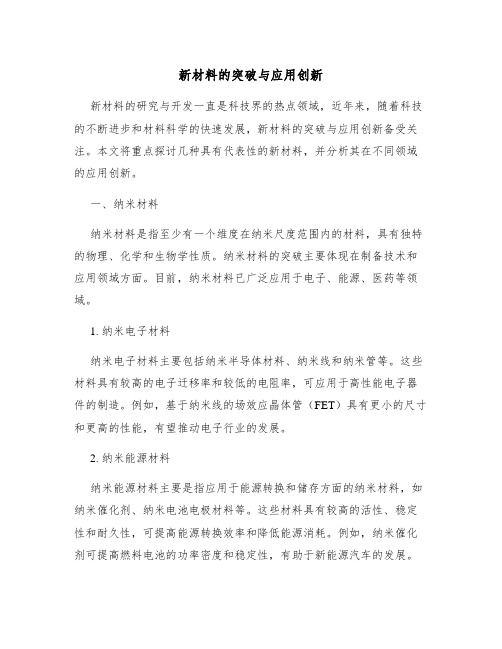
新材料的突破与应用创新新材料的研究与开发一直是科技界的热点领域,近年来,随着科技的不断进步和材料科学的快速发展,新材料的突破与应用创新备受关注。
本文将重点探讨几种具有代表性的新材料,并分析其在不同领域的应用创新。
一、纳米材料纳米材料是指至少有一个维度在纳米尺度范围内的材料,具有独特的物理、化学和生物学性质。
纳米材料的突破主要体现在制备技术和应用领域方面。
目前,纳米材料已广泛应用于电子、能源、医药等领域。
1. 纳米电子材料纳米电子材料主要包括纳米半导体材料、纳米线和纳米管等。
这些材料具有较高的电子迁移率和较低的电阻率,可应用于高性能电子器件的制造。
例如,基于纳米线的场效应晶体管(FET)具有更小的尺寸和更高的性能,有望推动电子行业的发展。
2. 纳米能源材料纳米能源材料主要是指应用于能源转换和储存方面的纳米材料,如纳米催化剂、纳米电池电极材料等。
这些材料具有较高的活性、稳定性和耐久性,可提高能源转换效率和降低能源消耗。
例如,纳米催化剂可提高燃料电池的功率密度和稳定性,有助于新能源汽车的发展。
3. 纳米医药材料纳米医药材料是指应用于医药领域的纳米材料,如纳米药物载体、纳米生物传感器等。
这些材料具有较高的生物相容性和特定的生物活性,可实现药物的靶向输送和生物分子的灵敏检测。
例如,纳米药物载体可将药物有效递送到肿瘤细胞,提高治疗效果并减少副作用。
二、智能材料智能材料是指具有自适应、自修复、智能响应等特性的材料,可在外界刺激下产生相应的结构和性能变化。
智能材料的突破主要体现在材料设计、制备技术和应用领域方面。
目前,智能材料已广泛应用于航空航天、生物医学、建筑等领域。
1. 航空航天领域智能材料在航空航天领域的应用主要包括飞机机翼的变形、飞行器的自适应变形等。
通过智能材料实现机翼的变形,可提高飞行器的机动性和稳定性。
此外,智能材料还可应用于飞行器的损伤自修复,提高飞行器的安全性和可靠性。
2. 生物医学领域智能材料在生物医学领域的应用主要包括生物传感器、药物释放系统等。
石墨烯聚合物复合材料的研究现状及前景论文1 推荐
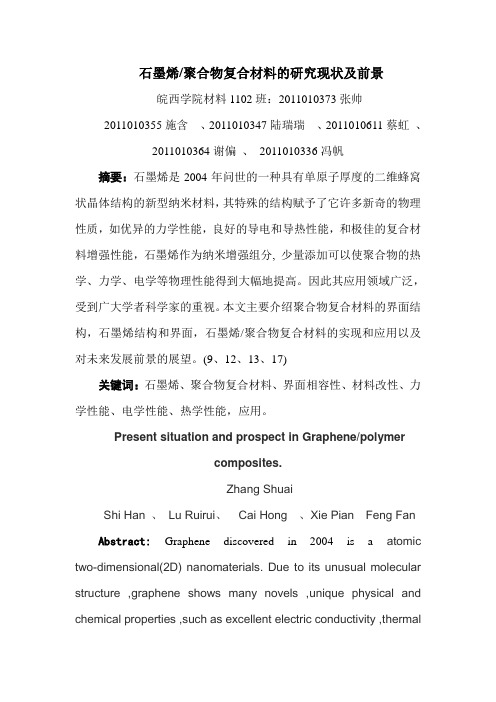
石墨烯/聚合物复合材料的研究现状及前景皖西学院材料1102班:2011010373张帅2011010355施含、2011010347陆瑞瑞、2011010611蔡虹、2011010364谢偏、2011010336冯帆摘要:石墨烯是2004年问世的一种具有单原子厚度的二维蜂窝状晶体结构的新型纳米材料,其特殊的结构赋予了它许多新奇的物理性质,如优异的力学性能,良好的导电和导热性能,和极佳的复合材料增强性能,石墨烯作为纳米增强组分, 少量添加可以使聚合物的热学、力学、电学等物理性能得到大幅地提高。
因此其应用领域广泛,受到广大学者科学家的重视。
本文主要介绍聚合物复合材料的界面结构,石墨烯结构和界面,石墨烯/聚合物复合材料的实现和应用以及对未来发展前景的展望。
(9、12、13、17)关键词:石墨烯、聚合物复合材料、界面相容性、材料改性、力学性能、电学性能、热学性能,应用。
Present situation and prospect in Graphene/polymercomposites.Zhang ShuaiShi Han 、Lu Ruirui、Cai Hong 、Xie Pian Feng Fan Abstract:Graphene discovered in 2004 is a atomic two-dimensional(2D)nanomaterials. Due to its unusual molecular structure ,graphene shows many novels ,unique physical and chemical properties ,such as excellent electric conductivity ,thermalconductivity ,thermal stability.Graphene as nano enhanced components, a small amount of added can make polymer thermal, mechanical, electrical and other physical properties are improved significantly.So its application field widely, have drawn the attention of the many scholars scientists.This paper mainly introduces the interface structure of polymer composite materials, graphene structure and interface, implementation and application of graphene/polymer composites as well as on the outlook for the future development prospect.Key words: Graphene,Polymer composite materials Material modification、Mechanical properties、Electrical performance、Thermal properties、application.一:石墨烯/聚合物的研究现状自年石墨烯发现以来,石墨烯的研究成果层出不穷,其中包括,生活领域,医用领域,电化学领域等。
聚乳酸复合材料
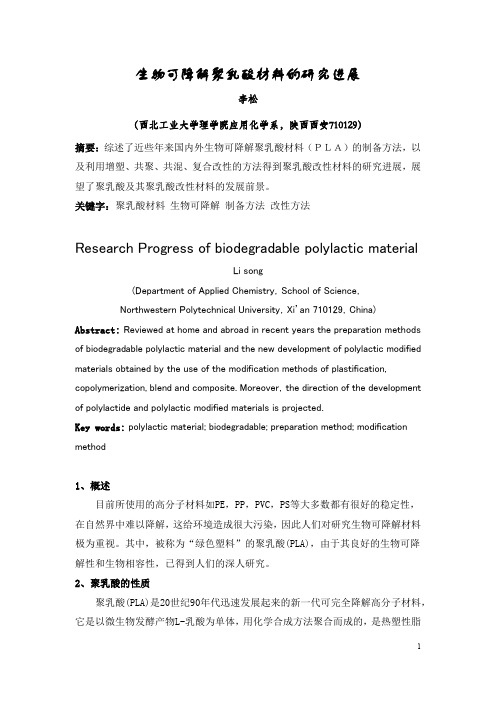
生物可降解聚乳酸材料的研究进展李松(西北工业大学理学院应用化学系,陕西西安710129)摘要:综述了近些年来国内外生物可降解聚乳酸材料(PLA)的制备方法,以及利用增塑、共聚、共混、复合改性的方法得到聚乳酸改性材料的研究进展,展望了聚乳酸及其聚乳酸改性材料的发展前景。
关键字:聚乳酸材料生物可降解制备方法改性方法Research Progress of biodegradable polylactic materialLi song(Department of Applied Chemistry,School of Science,Northwestern Polytechnical University,Xi’an 710129,China)Abstract:Reviewed at home and abroad in recent years the preparation methods of biodegradable polylactic material and the new development of polylactic modified materials obtained by the use of the modification methods of plastification, copolymerization, blend and composite. Moreover,the direction of the development of polylactide and polylactic modified materials is projected.Key words: polylactic material; biodegradable; preparation method; modification method1、概述目前所使用的高分子材料如PE,PP,PVC,PS等大多数都有很好的稳定性,在自然界中难以降解,这给环境造成很大污染,因此人们对研究生物可降解材料极为重视。
石墨烯材料在各个领域应用的进展

石墨烯材料在各个领域应用的进展1复合材料石墨烯由于具有极高的力学性能和电学性能,在作为聚合物基体的加强功能化添加剂方面被认为据有广泛的讨论前景。
2023年美国西北大学的Stankovich和RuofjF等人在Nature上报道了薄层石墨烯.聚苯乙烯纳米复合材料。
该讨论小组首先使用苯基异氰酸酯对完全氧化的石墨烯进行化学亲油改性,使之剥离和分散在有机溶剂中。
剥离的石墨烯均匀分散在聚苯乙烯溶液中,加入少量还原剂即可恢复石墨片层的导电性。
在还原过程中,聚苯乙烯的存在有效地阻拦了石墨纳米片层的聚集,这是该方法成功的关键。
该复合材料具有较低的渗阀值,在0.1%的体积分数下即可以导电,1%体积分数下导电率可达0.1Sm—1,可广泛应用于电子材料。
氧化态石墨烯只有在还原情况下才能发挥其优异的电学和力学行能,为了解决氧化石墨烯原位还原制备复合材料过程团聚现象的发生,加添石墨烯在各种聚合物单体中的浸润性,Stankovich利用苯乙烯磺酸钠包覆氧化态石墨烯,降低了石墨烯之间的接触面积,从而阻拦其在还原过程中不可逆自聚。
Haddon所领导的小组制备了石墨烯.环氧树脂纳米材料。
首先制备石墨烯的丙酮分散液,与环氧树脂均匀混合固化后得到复合材料。
热导率测试表明厚度小于2nm的石墨烯片特别适合作为环氧树脂的填料,在添加量达到25%时,热导率可以提升3000%,达6.44WmoKl。
复合材料杰出的热导性能重要由石墨烯的二维单原子层结构,高的纵横比,硬度和低的热界面阻力。
但该方法使用了溶剂,使得在所得复合材料中有显现微纳孔洞的可能。
石墨烯的添加不仅有利于聚合物基体电性能,热传导性能的改善,对于提高玻璃化变化温度,复合材料力学性能也具有重点意义。
Ruoff和Aksay等人在聚丙烯腈及聚甲基丙烯酸甲酯中加入仅1%及0.05%的石墨烯纳米片后,发觉他们的玻璃化变化温度提升30℃,此外包括杨氏模量,拉伸强度,热稳定性等一系列力学及热学性质得到提高。
石墨烯橡胶复合材料的性能
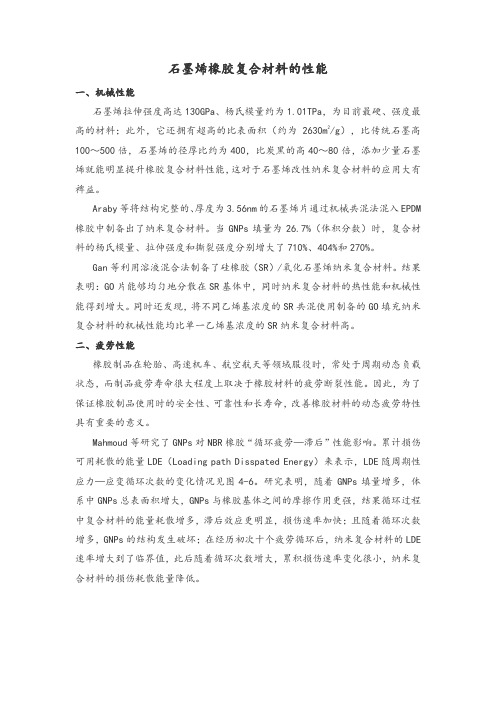
石墨烯橡胶复合材料的性能一、机械性能石墨烯拉伸强度高达130GPa、杨氏模量约为1.01TPa,为目前最硬、强度最高的材料;此外,它还拥有超高的比表面积(约为2630m2/g),比传统石墨高100~500倍,石墨烯的径厚比约为400,比炭黑的高40~80倍,添加少量石墨烯就能明显提升橡胶复合材料性能,这对于石墨烯改性纳米复合材料的应用大有裨益。
Araby等将结构完整的、厚度为3.56nm的石墨烯片通过机械共混法混入EPDM 橡胶中制备出了纳米复合材料。
当GNPs填量为26.7%(体积分数)时,复合材料的杨氏模量、拉伸强度和撕裂强度分别增大了710%、404%和270%。
Gan等利用溶液混合法制备了硅橡胶(SR)/氧化石墨烯纳米复合材料。
结果表明:GO片能够均匀地分散在SR基体中,同时纳米复合材料的热性能和机械性能得到增大。
同时还发现,将不同乙烯基浓度的SR共混使用制备的GO填充纳米复合材料的机械性能均比单一乙烯基浓度的SR纳米复合材料高。
二、疲劳性能橡胶制品在轮胎、高速机车、航空航天等领域服役时,常处于周期动态负载状态,而制品疲劳寿命很大程度上取决于橡胶材料的疲劳断裂性能。
因此,为了保证橡胶制品使用时的安全性、可靠性和长寿命,改善橡胶材料的动态疲劳特性具有重要的意义。
Mahmoud等研究了GNPs对NBR橡胶“循环疲劳—滞后”性能影响。
累计损伤可用耗散的能量LDE(Loading path Disspated Energy)来表示,LDE随周期性应力—应变循环次数的变化情况见图4-6。
研究表明,随着GNPs填量增多,体系中GNPs总表面积增大,GNPs与橡胶基体之间的摩擦作用更强,结果循环过程中复合材料的能量耗散增多,滞后效应更明显,损伤速率加快;且随着循环次数增多,GNPs的结构发生破坏;在经历初次十个疲劳循环后,纳米复合材料的LDE 速率增大到了临界值,此后随着循环次数增大,累积损伤速率变化很小,纳米复合材料的损伤耗散能量降低。
石墨烯_聚合物基复合材料3D打印成型研究进展

石墨烯/聚合物基复合材料3D打印成型研究进展发布时间:2021-01-18T06:38:09.593Z 来源:《中国科技人才》2020年第23期作者:熊金艳[导读] 自从石墨烯/聚合物基复合材料被发现以来,石墨烯材料因其新颖的特性而备受关注。
在电子学,光电子学和自旋电子学等各个领域都具有广泛的应用。
在3D打印成型研究的进展过程中,石墨烯/聚合物基复合材料更是发挥着重要的支撑作用。
三峡大学 443002摘要:自从石墨烯/聚合物基复合材料被发现以来,石墨烯材料因其新颖的特性而备受关注。
在电子学,光电子学和自旋电子学等各个领域都具有广泛的应用。
在3D打印成型研究的进展过程中,石墨烯/聚合物基复合材料更是发挥着重要的支撑作用。
实验和理论研究表明,石墨烯/聚合物基复合材料的整体结构具有更丰富的性能。
在各种报告的表述之中,由于石墨烯具有较高的比表面积,同时在室温下也有着较高的电子迁移率,因此可用于3D打印成型研究过程中。
本文从石墨烯/聚合物基复合材料的优势以及制备过程出发,分析石墨烯/聚合物基复合材料3D打印成型研究过程,最后对总结石墨烯/聚合物基复合材料3D打印成型技术的应用趋向。
关键词:3D打印成型研究;石墨烯/聚合物基复合材料;研究进展随着科学技术的飞速发展,材料领域也不断取得更多的成就,对于此前有所预测的材料而言,实验室也不断地合成更高性能的材料。
2004年,石墨烯在实验室中被成功制备,与此同时,石墨烯表现出更高的性能使其在各个领域的应用过程中都十分出彩,其研究价值与应用潜能得到了更多研究者们的关注。
一、石墨烯/聚合物基复合材料的优势以及制备过程石墨烯作为首个二维(2D)材料引起了科学家的广泛关注,石墨烯具有优良的化学和电子性质,其独特的二维晶体结构保障了石墨烯材料结构的稳定性。
除了其晶体结构稳定之外,石墨烯材料还具有其他重要的功能,例如:低电子噪声和高表面体积比等,备受关注的石墨烯材料制备的成本相对比较低,可以宏量制备。
《石墨烯-导电聚合物复合材料的制备及其电化学性能的研究》
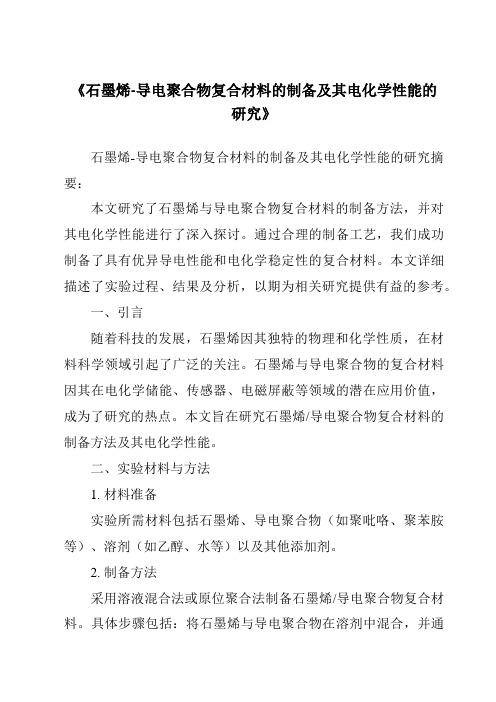
《石墨烯-导电聚合物复合材料的制备及其电化学性能的研究》石墨烯-导电聚合物复合材料的制备及其电化学性能的研究摘要:本文研究了石墨烯与导电聚合物复合材料的制备方法,并对其电化学性能进行了深入探讨。
通过合理的制备工艺,我们成功制备了具有优异导电性能和电化学稳定性的复合材料。
本文详细描述了实验过程、结果及分析,以期为相关研究提供有益的参考。
一、引言随着科技的发展,石墨烯因其独特的物理和化学性质,在材料科学领域引起了广泛的关注。
石墨烯与导电聚合物的复合材料因其在电化学储能、传感器、电磁屏蔽等领域的潜在应用价值,成为了研究的热点。
本文旨在研究石墨烯/导电聚合物复合材料的制备方法及其电化学性能。
二、实验材料与方法1. 材料准备实验所需材料包括石墨烯、导电聚合物(如聚吡咯、聚苯胺等)、溶剂(如乙醇、水等)以及其他添加剂。
2. 制备方法采用溶液混合法或原位聚合法制备石墨烯/导电聚合物复合材料。
具体步骤包括:将石墨烯与导电聚合物在溶剂中混合,并通过搅拌或超声处理使两者充分混合;然后进行聚合反应,得到复合材料。
三、电化学性能测试通过循环伏安法(CV)、恒流充放电测试、电化学阻抗谱(EIS)等方法,对制备的复合材料进行电化学性能测试。
四、结果与讨论1. 制备结果通过优化制备工艺,我们成功制备了具有良好分散性和导电性能的石墨烯/导电聚合物复合材料。
SEM和TEM结果表明,石墨烯与导电聚合物在纳米尺度上实现了良好的复合。
2. 电化学性能分析(1)循环伏安法(CV)测试:复合材料在充放电过程中表现出稳定的电化学行为,无明显极化现象。
(2)恒流充放电测试:复合材料具有较高的比电容和优异的循环稳定性。
在一定的电流密度下,其比电容随循环次数的增加而略有增加,表现出良好的充放电性能。
(3)电化学阻抗谱(EIS)分析:复合材料的内阻较小,电子传递速度快,表现出优异的电导率和良好的电荷传输能力。
通过分析不同因素(如石墨烯含量、聚合条件等)对电化学性能的影响,我们发现合理的复合比例和制备工艺是获得高性能复合材料的关键。
软骨再生领域的新材料研究

软骨再生领域的新材料研究随着科技的不断进步,医学领域也在不断发展壮大。
软骨再生这一领域也不例外,近年来不断有新的材料被用于软骨再生。
本篇文章将重点介绍软骨再生领域的新材料研究,包括目前最常用的三种材料以及新型材料的研究进展。
一、目前常用的软骨再生材料1. 胶原蛋白复合材料胶原蛋白复合材料是一种由胶原蛋白和其他材料混合而成的生物透析剂。
胶原蛋白具有良好的生物相容性,能够良好地与组织结合,同时具有一定的力学性能。
该材料被广泛应用于软骨修复和再生领域。
2. 玻璃酸钙玻璃酸钙是一种无机骨替代材料,具有良好的骨再生能力。
研究发现,玻璃酸钙也能促进软骨再生。
因此,玻璃酸钙被广泛应用于骨和软骨组织再生。
3. 聚乳酸聚乳酸是一种生物降解材料。
它具有很好的生物相容性和降解性能,对于软骨组织修复具有一定的作用。
同时,聚乳酸也具有适当的力学性能,可以满足软骨组织所需的一定的力学性能要求。
二、新型软骨再生材料的研究进展除了上述常用的材料,近年来也有不少新材料应用于软骨再生。
下文将重点介绍一下这些新材料。
1. 生物可降解高分子材料生物可降解高分子材料是一种新型的软骨再生材料,具有良好的生物相容性和可降解性能。
研究表明,生物可降解高分子材料可以促进软骨组织的再生和修复,同时也可以有效地缓解软骨退化的情况。
2. 石墨烯石墨烯是一种新型的材料,具有良好的物理性能和生物相容性。
最近的研究表明,石墨烯可以促进软骨细胞的增殖和生长,同时也可以促进软骨组织的再生。
因此,石墨烯被认为是一种有很大潜力的软骨再生材料。
3. 微胶束材料微胶束材料是一种新型的材料,它具有很小的粒径和较大的比表面积。
最新的研究表明,微胶束材料可以促进软骨细胞的生长和分化,有望成为一种有很大潜力的材料,用于软骨再生。
总之,软骨再生这一领域的发展严重依赖于新材料的研究和应用。
上述常用的材料以及新型材料的研究表明,这一领域取得了不错的进展。
随着科技的不断发展,相信在不久的将来,软骨再生领域将迎来更多更好的新材料,为软骨修复和再生提供更加完善的解决方案。
热喷涂中的喷涂材料的应用与开发新技术
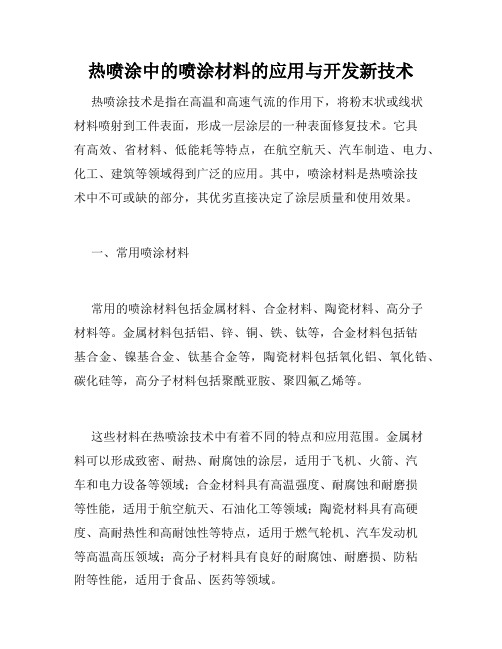
热喷涂中的喷涂材料的应用与开发新技术热喷涂技术是指在高温和高速气流的作用下,将粉末状或线状材料喷射到工件表面,形成一层涂层的一种表面修复技术。
它具有高效、省材料、低能耗等特点,在航空航天、汽车制造、电力、化工、建筑等领域得到广泛的应用。
其中,喷涂材料是热喷涂技术中不可或缺的部分,其优劣直接决定了涂层质量和使用效果。
一、常用喷涂材料常用的喷涂材料包括金属材料、合金材料、陶瓷材料、高分子材料等。
金属材料包括铝、锌、铜、铁、钛等,合金材料包括钴基合金、镍基合金、钛基合金等,陶瓷材料包括氧化铝、氧化锆、碳化硅等,高分子材料包括聚酰亚胺、聚四氟乙烯等。
这些材料在热喷涂技术中有着不同的特点和应用范围。
金属材料可以形成致密、耐热、耐腐蚀的涂层,适用于飞机、火箭、汽车和电力设备等领域;合金材料具有高温强度、耐腐蚀和耐磨损等性能,适用于航空航天、石油化工等领域;陶瓷材料具有高硬度、高耐热性和高耐蚀性等特点,适用于燃气轮机、汽车发动机等高温高压领域;高分子材料具有良好的耐腐蚀、耐磨损、防粘附等性能,适用于食品、医药等领域。
二、开发新型喷涂材料随着科技的不断进步,热喷涂技术对喷涂材料的要求越来越高。
特别是在军工、航天、新能源等高科技领域,需要更多性能更优异、适应更恶劣环境的涂层材料。
因此,开发新型喷涂材料已成为热喷涂技术领域的研究热点,具有重要的实际应用和经济价值。
1、纳米材料纳米材料指晶粒尺寸在1-100纳米之间的材料。
由于具有大比表面积、热稳定性好、力学强度大等特点,纳米材料在热喷涂技术中应用前景广阔。
例如,利用纳米氧化铝材料在钢结构表面喷涂处理,可显著提高钢材的抗腐蚀能力和耐热性。
此外,还有石墨烯、纳米碳管等纳米材料可以应用于喷涂材料的开发中。
2、复合材料复合材料是由两种或多种材料按一定比例混合而成的材料。
复合材料不仅可以结合多种材料的优点,而且还可以改善单纯材料的缺点。
在热喷涂技术中,复合材料喷涂材料具有热稳定性好、耐腐蚀性强、硬度高等特点。
石墨烯通用橡胶复合材料
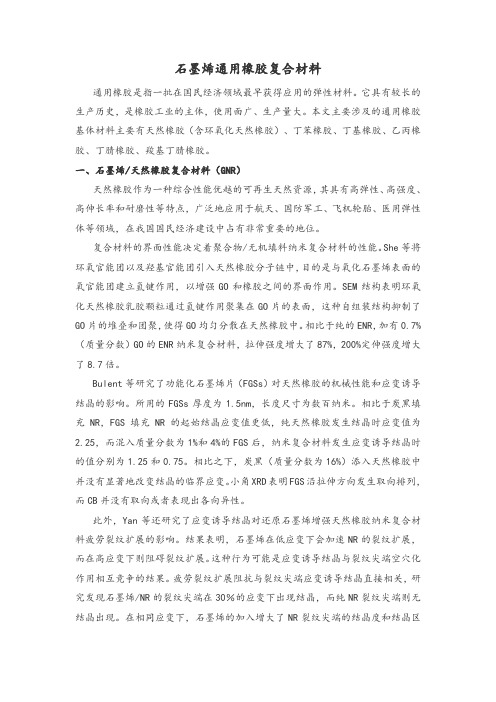
石墨烯通用橡胶复合材料通用橡胶是指一批在国民经济领域最早获得应用的弹性材料。
它具有较长的生产历史,是橡胶工业的主体,使用面广、生产量大。
本文主要涉及的通用橡胶基体材料主要有天然橡胶(含环氧化天然橡胶)、丁苯橡胶、丁基橡胶、乙丙橡胶、丁腈橡胶、羧基丁腈橡胶。
一、石墨烯/天然橡胶复合材料(GNR)天然橡胶作为一种综合性能优越的可再生天然资源,其具有高弹性、高强度、高伸长率和耐磨性等特点,广泛地应用于航天、国防军工、飞机轮胎、医用弹性体等领域,在我国国民经济建设中占有非常重要的地位。
复合材料的界面性能决定着聚合物/无机填料纳米复合材料的性能。
She等将环氧官能团以及羟基官能团引入天然橡胶分子链中,目的是与氧化石墨烯表面的氧官能团建立氢键作用,以增强GO和橡胶之间的界面作用。
SEM结构表明环氧化天然橡胶乳胶颗粒通过氢键作用聚集在GO片的表面,这种自组装结构抑制了GO片的堆叠和团聚,使得GO均匀分散在天然橡胶中。
相比于纯的ENR,加有0.7%(质量分数)GO的ENR纳米复合材料,拉伸强度增大了87%,200%定伸强度增大了8.7倍。
Bulent等研究了功能化石墨烯片(FGSs)对天然橡胶的机械性能和应变诱导结晶的影响。
所用的FGSs厚度为1.5nm,长度尺寸为数百纳米。
相比于炭黑填充NR,FGS填充NR的起始结晶应变值更低,纯天然橡胶发生结晶时应变值为2.25,而混入质量分数为1%和4%的FGS后,纳米复合材料发生应变诱导结晶时的值分别为1.25和0.75。
相比之下,炭黑(质量分数为16%)添入天然橡胶中并没有显著地改变结晶的临界应变。
小角XRD表明FGS沿拉伸方向发生取向排列,而CB并没有取向或者表现出各向异性。
此外,Yan等还研究了应变诱导结晶对还原石墨烯增强天然橡胶纳米复合材料疲劳裂纹扩展的影响。
结果表明,石墨烯在低应变下会加速NR的裂纹扩展,而在高应变下则阻碍裂纹扩展。
这种行为可能是应变诱导结晶与裂纹尖端空穴化作用相互竞争的结果。
- 1、下载文档前请自行甄别文档内容的完整性,平台不提供额外的编辑、内容补充、找答案等附加服务。
- 2、"仅部分预览"的文档,不可在线预览部分如存在完整性等问题,可反馈申请退款(可完整预览的文档不适用该条件!)。
- 3、如文档侵犯您的权益,请联系客服反馈,我们会尽快为您处理(人工客服工作时间:9:00-18:30)。
Preparation of Polylactide/Graphene Composites From Liquid-Phase Exfoliated Graphite SheetsXianye Li,1Yinghong Xiao,2Anne Bergeret,3Marc Longerey,3Jianfei Che11Key Laboratory of Soft Chemistry and Functional Materials,Nanjing University of Science and Technology, Nanjing210094,China2Jiangsu Collaborative Innovation Center of Biomedical Functional Materials,Jiangsu Key Laboratory of Biomedical Materials,College of Chemistry and Materials Science,Nanjing Normal University,Nanjing210046,China3Materials Center,Ales School of Mines,30319Ales Cedex,FrancePolylactide(PLA)/graphene nanocomposites were pre-pared by a facile and low-cost method of solution-blending of PLA with liquid-phase exfoliated graphene using chloroform as a mutual solvent.Transmission electron microscopy(TEM)was used to observe the structure and morphology of the exfoliated graphene. The dispersion of graphene in PLA matrix was exam-ined by scanning electron microscope,X-ray diffrac-tion,and TEM.FTIR spectrum and the relatively low I D/I G ratio in Raman spectroscopy indicate that the structure of graphene sheets(GSs)is intact and can act as good reinforcement fillers in PLA matrix.Ther-mogravimetric analysis and dynamic mechanical analy-sis reveal that the addition of GSs greatly improves the thermal stability of PLA/GSs nanocomposites.More-over,tensile strength of PLA/GSs nanocomposites is much higher than that of PLA homopolymer,increasing from36.64(pure PLA)up to51.14MPa(PLA/GSs-1.0). POS.,35:396–403,2014.V C2013Society of Plastics EngineersINTRODUCTIONPolylactide(PLA),a renewable,sustainable,biode-gradable,and eco-friendly thermoplastic polyester,has balanced properties of mechanical strength[1],thermal plasticity[2],and compostibility for short-term commod-ity applications[3,4].It is currently considered as a promising polymer for various end-use applications for disposable and degradable plastic products[5–8].Never-theless,improvement in thermal and mechanical proper-ties of PLA is still needed to pursue commercial success. To achieve high performance of PLA,many studies on PLA-based nanocomposites have been performed by incorporating nanoparticles,such as clays[9,10],carbon nanotubes[11–13],and hydroxyapatite[14].However, research on PLA-based nanocomposites containing gra-phene sheets(GSs)or graphite nanoplatelets has just started[15–17].GSs exhibit unique structural features and physical properties.It has been known that GSs have excellent mechanical strength(Young’s modulus of1,060 GPa)[18],electrical conductivity of104S/cm[19],high specific surface area of2,630m2/g[20],and thermal sta-bility[21].Polymer nanocomposites based on graphene show substantial property enhancement at much lower fil-ler loadings than polymer composites with conventional micron-scale fillers,such as glass[22]or carbon fibers [23],which ultimately results in lower filler ratio and simple processing.Moreover,the multifunctional property enhancement of nanocomposites may create new applica-tions of polymers.However,the incorporation of graphene into PLA matrix is restricted by cost and yield.Although the weak interactions that hold GSs together in graphite allow them to slide readily over each other,the numerous weak bonds make it difficult to separate GSs homogeneously in sol-vents and polymer matrices[24].Many methods have been reported for exfoliation of graphite,such as interca-lation with alkali metals[25]or oxidation in strong acidic conditions[26–29].Recently,exfoliation of graphite in liquid-phase was found to be able to give oxide-free GSs with high quality and yield at relatively low cost[30–35].Correspondence to:Y.H.Xiao;e-mail:yhxiao@ or J.F.Che;e-mail:xiaoche@Contract grant sponsor:Specialized Research Fund for the DoctoralProgram of Higher Education of China;contract grant number:20123219110010;contract grant sponsor:Natural Science Foundation ofJiangsu Province of China;contract grant number:BK2012845;contractgrant sponsors:Priority Academic Program Development of JiangsuHigher Education Institutions(PAPD),contract grant sponsor:Financialsupport for short visit from Ales School of Mines,France.DOI10.1002/pc.22673Published online in Wiley Online Library().V C2013Society of Plastics EngineersPOLYMER COMPOSITES—2014This approach is direct,simple,and benign with no need of toxic chemicals and GSs can be dispersed at concentra-tions of up to17mg/mL in some high boiling point sol-vents[31],and0.5mg/mL in some low boiling point solvents[32].Here we report a new,facile,and low-cost method to prepare PLA/GSs nanocomposite materials with high ther-mal and mechanical performance.In particular,GSs were prepared via liquid-phase exfoliation in chloroform.After solution-blending with PLA,GSs dispersion can be read-ily turned into pared to traditional exfoliation media,such as dimethylformamide(DMF)or N-methyl-2-pyrrolidone(NMP),chloroform has obvious advantages of being eco-friendly due to its low toxicity and is easy to get rid of from PLA/GSs blend,thanks to its low boiling point.Graphene oxide(GO)with more oxide groups may be another candidate filler for PLA. However,the preparation process of GO is more complex and the resulting GO is hard to disperse in organic sol-vents.Moreover,some dangerous reagents,such as sulfu-ric acid,may be used in GO preparation.Our approach presented here is direct,simple,and benign with no need of toxic chemicals.The liquid-phase exfoliated graphene is a promising candidate of nanocomposite fillers in prac-tical applications.Morphology,structures,and thermal and mechanical properties of PLA/GSs were systemati-cally studied and characterized.With GSs dispersing homogeneously in the matrix,the thermal stability of PLA is improved tremendously.E0of the resulting PLA/ GSs increases from6.87for pure PLA to212.15MPa for PLA/GSs-1.0at100 C,about30orders of magnitude higher compared to that of the unreinforced matrix. EXPERIMENTALMaterialsGraphite powder(1–44l m)was provided by Sino-pharm Chemical Reagent Co.,Ltd.PLA4032D(solid particles)was purchased from NatureWorks V R,USA, which can be converted into a biaxially oriented film with excellent optical properties,good machinability,and excellent twist and dead fold.Other reagents were pur-chased from commercial suppliers(Lingfeng Co.Shang-hai)and used without further purification.Preparation of GSsGraphite powder was dispersed in chloroform by soni-cation for30min(300W,2s on/2s off)with a tip soni-cation instrument(Scientz-IID,Ningbo Scientz Biotechnology Co.,Ltd.).The resulting dispersion was centrifuged at1000rpm for15min(TGL-16G,Shanghai Yuefeng Instruments&Meters Co.,Ltd).After centrifu-gation,the top80%of the supernatant of liquid-phase exfoliated GSs was decanted and collected for the follow-ing use.Preparation of PLA/GSs NanocompositesPLA/GSs nanocomposites were prepared through a solution-blending method,in which chloroform was used as a mutual solvent at different GSs loadings.PLA was com-pletely dissolved in chloroform at a concentration of100mg/ mL at45 C.The PLA solution was subsequently mixed with GSs dispersion at a certain ratio and stirred for4h at40 C to obtain the homogeneous PLA/GSs solution.After sonication for another10min,the mixed solution was poured into a Polytetrafluoroethylene(PTFE)mold and the solvent was evaporated at room temperature to obtain PLA/GSs nanocom-posite film with size of about8037030.50mm3.Finally, the film was dried at60 C under vacuum conditions for4 days to remove the residual solvent completely.For compari-son,PLA was mixed with GS of0.01,0.1,0.2,0.51.0,and 2.0wt%,respectively.The products are abbreviated as GSs-0.01,GSs-0.1,GSs-0.2,GSs-0.5,GSs-1.0,and GSs-2.0. CharacterizationIR spectrum was recorded using a Shimadzu FTIR-8400S spectrometer with pure KBr as the background. Raman spectra were taken on a Renishaw RM2000,using a3100objective lens with a514.5nm laser excitation. X-ray powder diffraction(XRD)analyses were carried out on a Bruker D8Advance diffractometer at40kV and40 mA with Cu Ka radiation(k50.15418nm),and the dif-fraction data were recorded for2h angles between16 and 19 .Scanning electron microscopy(SEM)observations were performed on a JEOL S-4800.The samples were coated with a thin layer of gold prior to SEM examination. Transmission electron microscopy(TEM)images of gra-phene and nanocomposite were obtained using a JEOL 2100,operated at200kV.TEM samples of composites were cryo-microtomed(Leica Ultracut)with a glass knife into 100nm thick slices and placed on400mesh Cu TEM grids.The composites were embedded in an epoxy matrix(TRA-BOND2115,Tra-Con)prior to the micro-tome.Bright field TEM micrographs were obtained with JEOL2100at an accelerating voltage of120kV.Ther-mogravimetric analysis(TGA)was performed using Shi-madzu DTG-60.Samples of neat PLA and PLA/GSs nanocomposites were heated to600 C from room tempera-ture at a rate of10 C/min in nitrogen atmosphere.The nitrogen flow rate was held at20mL/min.Dynamic mechanical analysis(DMA)was performed on a Metravib DMA-50dynamic mechanical analyzer(France).The aver-age sample size was253430.7mm3and the measure-ments were taken in tensile mode from0to140 C at a heating rate of3 C/min and frequency of1Hz.The tensile tests were carried out on an Instron1121machine at room temperature with a crosshead speed of50mm/min. RESULTS AND DISCUSSIONSA liquid-phase exfoliation method was applied to exfo-liate graphite powder in chloroform to obtain GSs.ByDOI10.1002/pc POLYMER COMPOSITES—2014397using this benign method,complicated oxidation–reduc-tion process and hazardous chemicals were avoided.After centrifugation and removal of the precipitation,a light-gray GSs dispersion with concentration as high as 0.4581mg/mL was obtained,which could remain stable for over a month.It is known that the typical color of GO disper-sion is brown-yellow.The distinct contrast between the colors suggests that there is nearly no oxidation in the process of exfoliation.TEM was applied to characterize the dispersion after centrifugation and it was found that almost all the sheets observed were flat and transparent (Fig.1A and B),indicating a mono-and few-layer struc-ture of GSs.The quality of graphene,mainly determined by the content of oxygen functional groups and structural defects,plays a crucial role in the final properties of GSs.From FTIR spectra shown in Fig.2A we see that unlike traditional GO,the as-made GSs show no absorbances for the C @O,A COOH,and C A O groups,of which charac-teristic features are often observed around 1,700and 1,000cm 21.Raman spectrum for GSs is shown in Fig.2B,with the spectra of graphite powder and chemically reduced graphenes (CRGs)as comparison.Three promi-nent bands can be seen:D band,G band,and 2D band appearing around 1,350,1,580,and 2,700cm 21,respec-tively.The band frequencies and intensity ratios of D and G bands are listed in Table 1.It is well known that the intensity ratio of D (I D )to G (I G )band can be used as an indicator of defect quantity.The fewer the defects,the lower the I D /I G value.As for graphene,these defects are generally attributed to basal plane defects and edge defects.After sonication,GSs are cut into smaller pieces and a number of new edges form,and thus I D /I G of the as-prepared GSs is higher than that of graphite powder.However,it is still much lower than that of CRGs,indi-cating that the structure of GSs is well preserved during liquid-phase exfoliation.To investigate the dispersibility of GSs in PLA matrix,SEM and TEM observations were performed.The SEM images of the fractured sections of PLA/GSs-0.1,PLA/GSs-1.0,and PLA/GSs-2.0and the TEM images of PLA/GSs-0.1are shown in Fig.3.SEM images show that GSs are dispersed homogeneously throughout the surfaces without any obvious large-size aggregates.The homoge-neous dispersing may be determined by two factors:(1)GS dispersion can maintain the stability during solution blending and (2)PLA macromolecules between GSs pre-vent the nano-sheets from aggregating and re-stacking during solvent evaporation.The cryofractured surfaces of composites with 0.1and 1.0wt%GSs (Fig.3A and B)are very dense and no GSs is observed to be pulled out from the surface,suggesting strong PLA–GSs interfacial adhesion.With increase of the GS content (2.0wt%,Fig.3C),there are more GSs “competing”for thematrix,FIG.1.TEM images of GSs deposited from GS dispersion.(A)An aggregate of GSs and (B)a single layer ofGS.FIG. 2.(A)FTIR spectra of GSs (a)and graphite powder (b).(B)Raman spectra for graphite powder,CRGs,and GSs.[Color figure can be viewed in the online issue,which is available at .]TABLE 1.Frequency of D,G and 2D bands and intensity ratio of D and G bands.Sample x D (cm 21)x G (cm 21)x 2D (cm 21)I D /I G Graphite 1357157927220.08CRGs 134515812683,2925 1.28GSs1352157627050.41398POLYMER COMPOSITES—2014DOI 10.1002/pcleading to less polymer cladding on the surface and weak-ened interfacial action,Consequently,the section rough-ness of the nanocomposites increases gradually.TEM image (Fig.3D)reveals that GSs maintain the inherent layered structure after being blended with PLA,and no obvious re-aggregation is observed.In order to confirm that the GSs in the polymer were not re-stacked,XRD measurements of pure PLA and PLA/GSs were carried out and the results are shown in Fig.4.The XRD pattern of PLA/GSs-0.1is almost the same as that of pure PLA,in which two characteristic dif-fraction peaks are shown at 16.3and 18.7 ,corresponding to (200)/(110)and (203)planes of crystalline PLA,respectively.The diffraction peak at 26 corresponding to graphite layer structure (002)is not observed in GSs due to the exfoliation.The scattering intensity profile does not reveal the presence of the diffraction peak of graphite,suggesting that the GSs do not aggregate in PLA matrix [36,37].As suggested by Ramanathan et al.,this result indicates that the dispersion of GSs in PLA matrix is close to single-sheet level [37].Recently,some researchers have demonstrated that the incorporation of graphenes could dramatically enhance the thermal stability of PLA at extremely low filling content [37,38].It is seen from Fig.5A that the incorporation of GSs into PLA makes the decomposition temperature of PLA moving to higher position compared with that ofPLA.With 0.01wt%GSs,the temperature at 5%weight loss of PLA increases about 20 C.When the content of GSs achieves 2wt%,the temperature at 5%weight loss of PLA increases by more than 150 C (Table 2).Differential thermal gravity (DTG)curves (Fig.5B)of pure PLA and PLA/GSs show that the weight loss rate ofPLA/GSsFIG.3.SEM images of PLA/GSs-0.1(A),PLA/GSs-1.0(B),and PLA/GSs-2.0(C).TEM image of PLA/GSs-0.1(D).FIG. 4.XRD spectra of pure PLA and PLA/GSs nanocomposites.[Color figure can be viewed in the online issue,which is available at .]DOI 10.1002/pcPOLYMER COMPOSITES—2014399nanocomposites gradually decrease with the increase of GSs,especially in the early stages of the decomposition process.The improvement of thermal stability may be due to the strong polymer–GSs interfacial action,which was confirmed by SEM and TEM in the preceding part.Due to the tremendous interfacial area between GSs and PLA,the resultant radicals from PLA thermal degradation would bind with the p conjugation system of GSs,and thus form inactive radicals to prevent further degradation,leading to lower initial decomposition rate.On the other hand,GSswith large specific surface area form a lamellar structure in PLA matrix,which plays the role of barrier and protective layers.GSs delay the penetration of oxygen and the escape of volatile degradation products,thus consequently boosts char formation.Figure 6A shows the spectra of dynamic storage mod-ulus (E 0)as a function of temperature for neat PLA and PLA/GSs.E 0is applied as a parameter of rigidity of materials.In the curve for pure PLA,two peaks of mod-ulus loss,at 31and 75 C,were observed.It is apparent that the first modulus loss is due to the minor changes of PLA (b changes),caused by the crank movement of side chain,end group,and bond angle of polymer chain.The second loss is due to the glass transition of PLA,which is in good agreement with the data released byNatureWorks VR .The addition of GSs increases the E 0of PLA/GSs nanocomposites within the test temperature range,especially within high temperature range.The E 0of PLA/GSs does not have any obvious decrease during PLA glass transition process and increases from6.87FIG.5.TGA curves of pure PLA and PLA/GSs nanocomposites (A).DTG curves of pure PLA and PLA/GSs nanocomposites (B).[Color fig-ure can be viewed in the online issue,which is available at .]TABLE 2.TGA data of pure PLA and PLA/GSs nanocomposites.Sample T 5%( C)T 50%( C)T 95%( C)Pure PLA 181.13354.08377.480.01%GSs 200.51355.08377.670.1%GSs 263.77354.46376.900.2%GSs 292.71355.32380.350.5%GSs 306.63355.79380.291%GSs 322.83362.59385.082%GSs332.11363.51385.19FIG. 6.Dynamic mechanical properties of PLA and PLA/GSs as a function of temperature.(A)Storage modulus log E 0and (B)damping factor tan d .[Color figure can be viewed in the online issue,which is available at .]400POLYMER COMPOSITES—2014DOI 10.1002/pc(pure PLA)to 212.15MPa (PLA/GSs-1.0)at 100 C,about 30orders of magnitude higher compared to that of pure PLA.The phenomenon can be explained as fol-lows.GSs,with a tremendous surface area,form a spe-cial spatial network structure in PLA matrix,leading to a strong interfacial reaction between matrix and fillers [39,40].Since GSs are stiffer material than PLA,the presence of GSs produces a more rigid interface in PLA matrix [41,42]and restricts the movement of polymer chains [43],resulting in greatly enhanced modulus of PLA matrix at high temperatures.Therefore,a GSs filled PLA composite with higher modulus at higher tempera-tures can be industrially manufactured.The temperature dependence of tan d for neat PLA and PLA/GSs is shown in Fig.6B.The peak glass transition temperature of PLA/GSs is not observed before the peak of pure PLA (75 C).Tensile strength of PLA/GSs composite films with different GSs contents were examined at room tempera-ture.Figure 7A shows the typical stress–strain curves of PLA/GSs and Fig.7B shows changes of tensile strength of PLA/GSs as a function of GS content.As shown inFig.7B,an increase in GSs loading from 0to 1.0wt%leads to a continuous increase of tensile strength.PLA/GSs-1.0has the improved tensile strength of 51.14MPa,compared to the unreinforced matrix (36.64MPa).The improvement ratio is as high as 39.57%.It is reasonable to conclude that the noticeable improvement of mechani-cal properties for PLA/GSs is attributed to the reinforce-ment effect of GSs dispersing homogeneously in PLA matrix.Further increment of GS loading to 2.0wt%results in a decrease in tensile strength instead.This can be explained by the reduced distance between GSs (refer to Fig.3C),which would cause highly intensified stress field between the closely spaced layers [44]and increased free volume [45].Figure 7C exhibits the varia-tion of the elongation at break with GS content in PLA/GSs.It can be seen that the elongation decreases with increasing GSs content,consistent with those reported in literatures [46,47].It can be explained by the fact that the strong interaction between GSs and PLA restricts the movement of the polymer chains.Figure 7D reveals the changes of PLA/GSs toughness as a function of GSs content.The toughness can be calculated from theareaFIG.7.Stress–strain curves (A),tensile strength (B),elongation at break (C),and toughness (D)of PLA/GSs as a function of GS content.[Color figure can be viewed in the online issue,which is available at .]DOI 10.1002/pcPOLYMER COMPOSITES—2014401under the stress–strain curve.The toughness of the com-posite,similar to its elongation at break,decreases with the addition of GSs.There is a trade-off between strengthening and toughening and a great challenge remains to realize simultaneous strength and toughness enhancement.CONCLUSIONIn summary,we reported a facile and low-cost route for the preparation of PLA-based nanocomposites con-taining GSs to improve properties for long-term applica-tions of degradable PLA.By this strategy,complicated oxidation–reduction process of conventional method for the fabrication of GSs can be avoided and the PLA/GSs nanocomposites can be obtained via a facile solvent blending of PLA with GS suspensions.The resultant GSs are highly exfoliated;thus the as-made dispersions of GSs in PLA are quite suitable for deposition of films. The addition of GSs in PLA greatly improves the ther-mal stability of the nanocomposites.The temperature at 5%weight loss increases by more than150 C when GSs content achieves2.0wt%.More important,the tensile strength of PLA/GSs nanocomposites is much higher than that of pure PLA,which increases from36.64for pure PLA up to51.14MPa for PLA/GSs-1.0 nanocomposite.REFERENCES1.Q.K.Meng,M.C.Heuzey,and P.J.Carreau,Int.Polym.Proc.,27,5(2012).2.Q.K.Meng,M.C.Heuzey,and P.J.Carreau,Polym.Degrad.Stab.,97,10(2012).3.I.H.Kim and Y.G.Jeong,J.Polym.Sci.Part B:Polym.Phys.,48,8(2010).4.E.E.M.Ahmad and A.S.Luyt,pos.,33,6(2012).5.D.K.Platt,Biodegradable Polymers Market report,SmithersRapra Limited,Shawbury,Shrewsbury,Shropshire,Limited, UK(2006).6.R.E.Drumright,P.R.Gruber,and D.E.Henton,Adv.Mater.,12,23(2000).7.P.H.Dubois and M.Murariu,J.Electroanal.Chem.,45,66(2008).8.E.T.H.Vink,K.R.R a bago,D.A.Glassner,and P.R.Gruber,Polym.Degrad.Stab.,80,3(2003).9.S.Boncel,M.Brzezinski,and J.Mrowiec-Bialon,Mater.Lett.,91,50(2013).10.M.Brzezinski,M.Boguslawska,and M.Ilcikova,Macro-molecules,45,21(2012).11.H.Quan,S.J.Zhang,and J.L.Qiao,Polymer,53,20(2012).12.H.H.Ge,F.Yang,and Y.P.Hao,J.Appl.Polym.Sci.,127,4(2013).13.G.Stoclet,R.Seguela,and J.M.Lefebvre,Macromolecules,43,3(2010).14.B.J.Jeon,Y.G.Jeonq,B.G.Min,W.S.Lyoo,and S.C.Lee,pos.,32,9(2011).15.M.P.Artur,C.Joana,A.P.T.David,M.M.Ad elio,and D.M.Fern~ao,Polym.Int.,62,1(2012).16.A.M.Pinto,M.Susana,I.C.Goncalves,F.M.Gama,A.M.Mendes,and F.D.Magalh~a es,Colloids Surf.B.,104,229 (2013).17.Y.W.Cao,J.C.Feng,and P.Y.Wu,Carbon,48,13(2010).18.C.G.Lee,X.D.Wei,J.W.Kysar,and J.Hone,Science,321,5887(2008).19.A.A.Balandin,S.Ghosh,W.Z.Bao,I.Calizo, D.Teweldebrhan,F.Miao,and u,Nano Lett.,8,3 (2008).20.H.K.Chae, D.Y.Siberio-P e rez,J.Kim,Y.Go,M.Eddaoudi, A.J.Matzger,M.O’keeffe,and O.M.Yaghi, Nature,427,6974(2004).21.D.H.Youn,Y.J.Yu,and H.K.Choi,S.H.Kim,S.Y.Choi,and C.G.Choi,Nanotechnology,24,7(2013).22.C.Courgneau,S.Domenek,R.Leboss e, A.Guinault,L.Av e rous,and V.Ducruet,Polym.Int.,61,2(2012).23.Y.X.Kong,J.Yuan,Z.M.Wang,and J Qiu,-pos.,33,9(2012).24.L.Dan and B.Richard,Kaner,Mater.Sci.,320,1170(2008).25.K.C.Kwon,K.S.Choi,B.J.Kim,J.L.Lee,and S.Y.Kim,J.Phys.Chem.C,116,50(2012).26.K.E.Carr,Carbon,8,155(1970).27.G.H.Chen,D.J.Wu,W.U.Weng,and C.L.Wu,Carbon,41,3(2003).28.W.G.Zheng,S.C.Wong,and H.J.Sue,Polymer,43,25(2002).29.Y.X.Pan,Z.Z.Yu,Y.C.Ou,and G.H.Hu,J.Polym.Sci.Part B:Polym.Phys.,38,12(2000).30.E.Y.Choi,W.S.Choi,Y.B.Lee,and Y.Y.Noh,Nanotech-nology,22,36(2011).31.U.Khan,H.Porwal,A.O’Neill,K.Nawaz,P.May,andJ.N.Coleman,Langmuir,27,15(2011).32.A.O.Neill,U.Khan,P.N.Nirmalraj,J.Boland,and J.N.Coleman,J.Phys.Chem.,115,13(2011).33.W.Liu and J.Wang,mun.,47,24(2011).34.L.Zhu,X.Zhao,Y.Li,X.Yu,C.Li,and Q.Zhang,Mater.Chem.Phys.,137,984(2013).35.K.H.Park,B.H.Kim,H.S.Song,J.Kwon,B.S.Kong,K.Kang,and S.Jeon,Nano Lett.,12,2871(2012).36.Y.K.Yang,C.E.He,R.G.Peng,A.Baji,X.S.Du,Y.L.Huang,X.L.Xie,and Y.W.Mai,J.Mater.Chem.,22,12(2012).37.T.Ramanathan,A.A.Abdala,S.Stankovich,D.A.Dikin,M.Herrera Alonso,R.D.Piner,D.H.Adamson,H.C.Schniepp,X.Chen,R.S.Ruoff,S.T.Nguyen,I.A.Aksay,R.K.Prud’Homme,and L.C.Brinson,Nat.Nanotechnol.,3,6(2008).38.S.Villar-Rodil,J.I.Paredes, A.Martinez-Alonso,andJ.M.D.Tascon,J.Mater.Chem.,19,22(2009).39.A.K.Rana,B.C.Mitra,and A.N.Banerjee,J.Appl.Polym.Sci.,71,4(1999).40.M.S.Huda,L.T.Drzala, A.K.Mohanty,and M.Misra,Compos.Sci.Technol.,66,11(2006).41.M.S.Huda,L.T.Drzala, A.K.Mohanty,and M.Misra,Compos.B,38,3(2007).402POLYMER COMPOSITES—2014DOI10.1002/pc42.S.N.Nazhat,M.Kelloma,P.Tormal,K.E.Tanner,and W.Bonfield,J.Biomed.Mater.Res.,58,4(2001).43.X.Zhao,Q.H.Zhang,D.J.Chen,and P.Lu,Macromole-cules,43,5(2010).44.D.Broek,Elementary Engineering Fracture Mechanics,KluwerAcademic Publishers,Dordrecht,The Netherlands(1986).45.L.An,Y.Z.Pan,X.W.Shen,H.B.Lu,and Y.L.Yang,J.Mater.Chem.,18,4928(2008).46.K.Nawaza,U.Khanb,N.Ul-Haqa,P.Mayb,A.O’Neillb,and J.N.Colemanb,Carbon,50,12(2012).47.C.F.Fabiana,R.S.B.Nara,P.G.Ana,S.A.Denise,and B.G.Griselda,J.Appl.Polym.Sci.,128,5(2012).DOI10.1002/pc POLYMER COMPOSITES—2014403。